- 1Postdoctoral Research Station of Crop Science, College of Agronomy, Shenyang Agricultural University, Shenyang, China
- 2College of Agronomy, Shenyang Agricultural University, Shenyang, China
- 3National Field Observation and Research Station of Shenyang Agro-Ecosystems, Shenyang, China
Enhanced ultraviolet-B (UV-B) radiation and elevated tropospheric ozone alone may inhibit the growth of agricultural crops. However, research regarding their combined effects on growth and biochemical properties of roots is still scarce. Using open top chambers, we monitored the response of growth, secondary metabolites, endogenous hormones and enzyme activities of soybean roots to elevated O3 and enhanced UV-B individually and in combination at stages of branching, flowering and podding. Our results indicated that the root biomass decreased by 23.6, 25.2, and 27.7%, and root oxidative capacity declined by11.2, 39.9, and 55.7% exposed to elevated O3, enhanced UV-B, and O3 + UV-B, respectively, compared to the control treatment. Concentrations of quercetin and ABA were significantly increased, while concentrations of total polyphenol and P-coumaric acid responded insignificantly to elevated O3, enhanced UV-B, and O3 + UV-B during the whole period of soybean growth. Elevated O3, enhanced UV-B and O3 + UV-B showed significant negative effects on superoxide dismutase (EC 1.15.1.1) activity at flowering stage, on activities of peroxidase (EC 1.11.1.7) and catalase (EC 1.11.1.6) at podding stage, on ascorbate peroxidase activity during the whole period of soybean growth. Moreover, compared to hormones and enzyme activity, secondary metabolisms showed stronger correlation with root growth exposed to elevated O3 and enhanced UV-B individually and in combination. Our study concluded that combined effects of O3 and UV-B radiation significantly exacerbated the decline of soybean root growth, and for annual legumes, the inhibited root growth exposed to O3 and/or UV-B radiation was mostly associated with secondary metabolisms (especially flavonoids).
Introduction
Tropospheric O3 has increased worldwide as a result of industrialization and anthropogenic activities, and its concentration has already exceeded the threshold levels for the protection of vegetation (Vingarzan, 2004). The increase of tropospheric O3 concentration can reduce plant growth and crop yields not only by its direct injury on leaves and alteration of photosynthetic systems, but also indirectly through its impacts on resource allocation (Goumenaki et al., 2010). Moreover, global changes in the chemical composition of the atmosphere with a substantial reduction of the stratospheric O3 layer have led to a notable increase in the solar UV-B reaching the earth surface (Ballaré et al., 2011). Although stratospheric O3 has been slowly recovering, no statistically significant decreases in UV-B radiation attributable to the recovery of stratospheric O3 have yet been detected (Bais et al., 2015). Numerous studies have shown that enhanced UV-B radiation can affect physiological and biochemical processes of many plant species (Jansen et al., 1998; Newsham and Robinson, 2009; Bussell et al., 2012; Li X. et al., 2012; Mazza et al., 2013). Under natural field conditions, plants are usually affected by elevated O3 and enhanced UV-B radiation simultaneously. However, most of our knowledge today depends on the individual effects of O3 and UV-B radiation on the productivity and quality of some important agricultural crops and plants, and studies regarding their combined effect on crop yields and plant growth are still scarce (Ambasht and Agrawal, 2003; Tripathi et al., 2011; Rinnan et al., 2013; Tripathi and Agrawal, 2013).
Several studies regarding combined effect of enhanced UV-B and elevated O3 on physiological characters and yield components in different plant species have found a wide variety of responses. For example, Miller et al. (1994) did not find any effect of enhanced UV-B (3.02, 6.24 and 8.98 kJ m-2) on soybean yield in the presence or absence of O3 (24, 49 and 83 nL L-1). Ambasht and Agrawal (2003) noticed negative effects of combination of elevated O3 concentration (0.07 μmol mol-1) and enhanced UV-B radiation (7.1 kJ m-2) on biomass, yield, photosynthetic rate, chlorophyll, carotenoid and ascorbic acid contents and CAT activity, whereas positive effects on total phenol content and POX activity. However, most of these studies focused on the above-ground system. Compared to above-ground responses, below-ground responses to O3 may be larger in magnitude and may have larger, long-term consequences for ecosystem productivity and carbon, nutrient and water cycling (Andersen, 2003; Laurence and Andersen, 2003). Similarly, previous study showed that the growth of root was also affected by enhanced UV-B radiation (Xu et al., 2013; Yokawa et al., 2013; Yokawa and Baluška, 2015). However, little is known about the root responses to the combined effects of elevated ozone and enhanced UV-B radiation.
It is well known that plants generate antioxidant enzymes (Mittler, 2002; Blokhina et al., 2003), such as SOD, APX, POD, glutathione reductase (GR), guaiacol peroxidase (GPX), PAL, and CAT, and protective secondary metabolites (Paul and Gwynn-Jones, 2003; Mewis et al., 2012; Escobar-Bravo et al., 2017; Wang et al., 2017), such as flavonoids and phenolic acids, in response to environmental changes. Phenolic acids (such as ferulic acid and P-coumaric acid) are mainly responsible for providing plant pest and disease resistance and protection from UV radiation. Flavonoids (such as quercetin and rutin) in root exudates are inducers of genes responsible for the nodulation process of rhizobium in specific legumes and are responsible for triggering action and signaling cascades (Walker et al., 2003; Peer et al., 2004). Previous studies have showed that UV-B exposure might initiate signaling through UV RESISTANCE LOCUS 8 (UVR8) and then induce the changes of metabolites and antioxidant enzymes involved in protection against enhanced UV-B radiation (Binder et al., 2009; Hao et al., 2009; Huang et al., 2016; Liu et al., 2016). Several studies suggest that elevated O3 concentration induced oxidative damage in plant cells leading to changes in concentrations of secondary metabolites and activities of enzymes (Rai and Agrawal, 2008; Tripathi et al., 2011). Furthermore, plant hormones play important roles in regulating developmental processes and signaling networks involved in plant responses to environmental change. When plants are subject to environmental change, the concentrations of some plant endogenous hormones might be different, and the differences of endogenous hormones not only influence the adaptive response to environmental changes but also affect the normal growth and development (Dodd, 2005; Albacete et al., 2008; Li et al., 2011). Unfortunately, the changes of concentrations of secondary metabolites, hormones and activities of enzymes during the growth of roots of plants under coupling condition of elevated O3 concentration and enhanced UV-B radiation remain largely unknown.
Soybean (Glycine max) ranks among the most O3-sensitive agricultural crops (Mills et al., 2007). The effects of enhanced O3 concentrations and enhanced UV-B radiation on aboveground growth, morphology and yield of soybean have been studied widely (Li et al., 2002; Kakani et al., 2003; Feng and Kobayashi, 2009; VanLoocke et al., 2012; Kataria et al., 2017). For instance, Ashmore (2005) found that an increase of O3 concentration from 30 to 60 ppb for 7 h day-1 could decrease soybean yield by 16%. Liu et al. (2013) found that enhanced UV-B radiation (13 kJ m-2 d-1) decreased plant height, dry weight of individual stem and yield per plant of three soybean cultivars on average by 15.5, 16.9, and 43.7%, respectively. However, the effects of elevated O3 concentrations and/or enhanced UV-B radiation on root growth of soybean have not been investigated. Furthermore, a soybean developmental stage classification based on significant changes in external morphological characteristics has been in place since 1971 and plays important roles in soybean cultivation (Fehr et al., 1971). The root growth at different developmental stages (such as branching stage, flowering stage, and podding stage) is critical in agriculture and ecology, while little is known about the effects of elevated O3 concentrations and/or enhanced UV-B radiation on root growth of soybean at different developmental stages.
The objective of this study was to examine how elevated O3 concentrations (110 ± 10 nmol mol-1 for 8 h per day) and/or enhanced UV-B radiation (9.50 kJ d-1 m-2 for 8 h per day) affect secondary metabolites, endogenous hormones and enzyme activities of soybean roots at stages of branching, flowering and podding (from June 20 to August 12), using the open top chambers (OTC). We hypothesized that: (1) elevated O3 and enhanced UV-B individually or in combination inhibited root growth via changes of secondary metabolites, endogenous hormone and enzyme activities, and (2) the effect of O3 + UV-B is synergistic on root growth.
Materials and Methods
Experimental Site and Design
The experimental site is located in the Shenyang Experimental Station of Ecology, Chinese Academy of Sciences (41°310′N, 123°220′E). This region has a continental monsoon climate with a mean annual temperature of 7.0–8.0°C, annual precipitation of 650–700 mm, and an annual non-frost period of 147–164 days. The soil at the study site is classified as an aquic brown soil (silty loam Hapli-Udic Cambosols in Chinese Soil Taxonomy), with 11.28 g kg-1 organic C, 1.20 g kg-1 total N, 0.41 g kg-1 total P, pH (H2O) 6.7 at 0–15 cm depth.
Experimental Design
The study was conducted on soybean (G. max) plants grown in open-top chambers (OTCs), which were established in 2008. The OTCs were 1.15 m in diameter and 2.4 m in height with a 45° sloping frustum and the minimum distance between any two chambers was 4 m. Each chamber was constructed from an iron framework, clad with standard horticultural glass, with a plenum incorporated just below the mouth of the chamber (at 2.4 m from ground-level) to reduce incursion of ambient air. From June to September, Mean temperature of the day was about 25.6°C and mean relative air humidity throughout the day was 50.6% in the OTCs. The mean value of UV-B radiation was about 8.64 kJ d-1 m-2 during clear sky conditions from June to September in the OTCs. The mean value of O3 concentration was about 45 ± 5 nmol mol-1 and the average value of AOT40 was 3.5 ppm h during clear sky conditions from June to September in the OTCs.
The experimental design was based on completely randomized plots including four treatments belonging to 12 OTCs: (1) control (hereinafter referred to as CK, O3 concentration, 45 ± 5 nmol mol-1 + UV-B radiation, 8.64 kJ d-1 m-2); (2) elevated O3 concentration (110 ± 10 nmol mol-1 + UV-B radiation, 8.64 kJ d-1 m-2); (3) enhanced UV-B radiation (9.50 kJ d-1 m-2 + O3 concentration, 45 ± 5 nmol mol-1); (4) O3 + UV-B (elevated O3 concentration, 110 ± 10 nmol mol-1 + enhanced UV-B radiation, 9.50 kJ d-1 m-2). There were no air filtered chambers in all OTCs. Each treatment had three replicated OTCs and each OTC was divided into three compartments in order to avoid variability within the same chamber, so there were three replications for each treatment.
For the elevated O3 treatment and the combined treatment, O3 was generated from pure oxygen by an electrical discharge O3 generator (GP-5J, China) and then mixed with ambient air in the OTCs to achieve the target O3 concentration. The O3 concentrations were continuously monitored by O3 analyzers (S-900 Aeroqual, New Zealand), and were controlled by computers using a professional program for O3 dispensing and monitoring (Zhao et al., 2010). Meanwhile, during O3 fumigation from June to September in the OTCs, the mean of the daily 8 h O3 concentration (M8) in elevated O3 treatment averaged 114.4 nmol mol-1 with a maximum of 125.1 nmol mol-1 and in combined treatment averaged 115.7 nmol mol-1 with a maximum of 124.0 nmol mol-1. In addition, the average value of AOT40 in elevated O3 treatment and combined treatment during the whole fumigation season was 37.3 and 37.7 ppm h, respectively.
UV-B was artificially supplied with UV-B radiation by 40 W fluorescent tubes (F40T8/30; length was 120 cm; Beijing Lighting Research Institute, peak value was 305 nm; Figure 1) held in mobile and adjustable frames over each pot row. In control treatment (CK) and elevated O3 treatment, UV-B tubes were covered with 0.13 mm polyester filters to absorb radiation below 320 nm (UV-B + UV-C). In treatments of enhanced UV-B radiation and combined treatment, UV-B tubes were covered with 0.08 mm cellulose diacetate filters to absorb radiation below 280 nm (UV-C). The UV-B radiation of two tubes with cellulose diacetate filters on the sample surface was 9.50 kJ d-1 m-2, equivalent to those about 10% enhanced in average of UV-B radiation on the sample surface in Shenyang during clear sky conditions in summer. The polyester filters and cellulose diacetate filters were changed at week intervals. The distance between the top canopies of the plants and the lamps was maintained at 40 ± 2 cm by the mobile frames. UV radiation was monitored by UV radiometer (UV 340B, China). Plants were exposed to elevated O3 or/and UV-B radiations for 8 h (09:00–17:00) per day in the middle of the photoperiod from June 20 to August 12.
Soil at 0–15 cm layer was collected at the study site and then was mixed thoroughly after removing roots and organic residues. After sieving (2 mm), the soils were used in the pots of soybean cultivar. The potted soybean cultivar was Tiefeng 29, which was seeded in each pot (26 cm long × 36 cm wide × 45 cm deep) on May 20 in 2016. Five plants in three leaves stage were established in each pot. There were nine pots in total in each OTC [three collected periods (branching stage, flowering stage and podding stage) × 3 compartments]. The pots were periodically watered to prevent water deficit. Each whole pot of soil (26 cm long × 36 cm wide × 45 cm deep), which contained the whole roots of five plants, was collected at branching stage (June 30, 2016), flowering stage (July 24, 2016), and podding stage (August 12, 2016). Each whole pot of soil was immersed in water for about 60 min and then roots and root nodules were carefully collected in a 0.5 mm sieve with the aid of running water. Debris, weeds, and dead roots were removed by hand. The samples of root and root nodules were then combined so that there was one root sample for each pot. The roots and root nodules were blotted dry with tissue paper and biomass of roots and root nodules was measured. Then each root sample was divided into two sub-samples. One (fresh sample) was stored at 4°C to analyze root activity and the relative electrical conductivity and the other (frozen sample) was immediately frozen in liquid nitrogen and stored at -70°C until further analysis.
Biochemical Analyses
Lipid peroxidation in soybean root was determined by measuring MDA formation, using the thiobarbituric acid (TBA) method as previously described by Buege and Aust (1978). Frozen roots (500 mg) were ground into a fine powder and then were homogenized in trichloroacetic acid (TCA). After centrifugation, the supernatants were mixed with 0.5% TBA (prepared in 20% TCA). The mixture was incubated at 95°C for 30 min and the reaction was stopped by placing the mixture on ice for 5 min. After centrifugation, the absorbance of the supernatant was measured at 532 and 600 nm. After subtracting the non-specific absorbance (A600 nm), the MDA concentration was determined by its extinction coefficient of 155 mM-1 cm-1 and expressed as nmol g-1 of fresh weight.
Fresh roots (200 mg) were washed with no ion water and were cut into tubes with 20 mL of ionized water. After shaking 30 min, DDS-11A Type conductivity meter was used to determine the conductivity as E1. And then the solutions were incubated at boiling water bath for 10 min. After cooling, the solutions were used to determine the total conductivity as E2. No ion water conductivity was considered as E0. The relative electrical conductivity (R) is calculated according to the method of Zhao et al. (1992) as: R = [(E1 - E0)/(E2 - E0)] × 100%.
Root activity was analyzed by a modified α-naphthylamine oxidation method (Sakai and Yoshida, 1957; Ando et al., 1983). Fresh roots (1 g) were immersed in 50 μg mL-1 of α-naphthylamine solution for 2 h at 25°C. 2 mL of α-naphthylamine solution was pipetted out and reacted with 10 mL of 0.1% sulfanilic acid and 2 mL of NaNO2. The absorbance of the colored solution was determined at 530 nm.
Total flavonoids concentration was determined by modified method of Geissman (1955). Frozen samples (500 mg) were extracted with ethanol for 8 h and then the sample solution was distilled. After washed by ether, the sample solution was measured by spectrophotometry at 510 nm. Total polyphenol concentration was determined by the Folin-Ciocalteu method (Waterman and Mole, 1994). In brief, frozen sample (500 mg) with folin reagent and Na2CO3 was incubated at 75°C in a water bath for 10 min after extracted by 60% methanol for 2 h. Then the sample was measured by spectrophotometry at 760 nm. An Agilent (Waldbronn, Germany) 1100 HPLC series, which consists of a degasser, binary pump, auto-sampler, thermostat, and photodiode array detector, with a C18-column (Hypersil ODS, 250 mm × 4.6 mm) was used to determine the concentration of rutin, quercetin, ferulic acid and P-coumaric acid (Montedoro et al., 1992; Huang et al., 2016). Pure compound of rutin, quercetin dehydrate, ferulic acid and P-coumaric acid (Sigma, China) were used as external standards.
Extraction, purification, and determination of endogenous levels of IAA, ABA, and ZR by an indirect ELISA technique were performed as described by Teng et al. (2006). The frozen samples (1 g) were ground under liquid nitrogen, extracted with ice-cold 80% methanol (v/v) containing 1 mmol L-1 butylated hydroxytoluene to prevent oxidation, and then stored overnight at 4°C for 16 h in the dark. After centrifugation at 4°C, the supernatants were passed through a C18 Sep-Pak cartridge (Waters, Milford, MA, United States). The efflux was collected and dried in N2, and dissolved in 0.01 mol L-1 phosphate buffer solution (pH 7.4) and concentrations of IAA, ZR, and ABA were determined in an enzyme-linked immunosorbent assay (ELISA) following methods described in previous publications (Yang et al., 2001; Zhu et al., 2005).
Activities of SOD (EC 1.15.1.1) were assessed by the method of Beauchamp and Fridovich (1971). The frozen samples (500 mg) were immersed in EDTA–phosphate buffer (pH 7.8). After filter and centrifugation, the supernatant was used to determine SOD activity by inhibition of the photochemical reduction of nitro-blue tetrazolium (NBT) at 560 nm. POD (EC 1.11.1.7) was extracted by homogenizing 500 mg frozen roots with 5 ml 0.1 mol L-1 Tris-HCl buffer (pH 8.5) at 0°C. After filter and centrifugation, the supernatant with 0.2 mol L-1 phosphate buffer (pH 6.0), 0.028 mL H2O2 and 0.019 mL guaiacol was used to determine POD activity at 470 nm (Kochba et al., 1977). APX activity was determined by the method of Nakano and Asada (1981). 500 mg frozen roots were homogenized in 50 mmol L-1 phosphate buffer (pH 7.6), 0.1 mmol L-1 EDTA, 0.5 mmol L-1 ascorbate and 0.1 mmol L-1 H2O2. After centrifugation, the supernatant was used to determine APX activity at 290 nm.
Catalase (CAT; EC 1.11.1.6) activity was extracted by homogenizing 500 mg frozen roots at 4°C in 100 mol L-1 cold phosphate buffer (pH 7.2) containing 0.5% Triton-X. Activity of CAT was assessed in 100 μL phosphate buffer, 400 μL 200 mmol L-1 H2O2 and 100 μL enzyme extract. A decrease in H2O2 was measured at 240 nm, and activity of CAT was measured according to the method of Aebi (1984). Phenylalanine ammonia-lyase (PAL, EC 4.3.1.5) activity was determined by the method of Havir and Hanson (1968) and Bradford (1976). The calculation was based on the extinction coefficient (9500 M-1 cm-1) for trans-cinnamic acid. One unit of activity for PAL was defined as the amount of enzyme which caused the formation of 1 μmol trans-cinnamic acid per hour. Lipoxygenase (LOX) was determined by the method of Buranasompob et al. (2007). 500 mg frozen roots were used for the extraction of LOX in phosphate buffer (pH 7.5). After added solid ammonium sulfate and centrifugation, the supernatant was used to determine APX activity.
Statistical Analysis
The differences of MDA concentration, relative electrical conductivity, root biomass, root nodule biomass and root activity in the four treatments of CK, elevated O3, enhanced UV-B radiation and O3 + UV-B were evaluated by one-way analysis of variance (ANOVA) (SPSS 16.0). Multiple comparisons among means of MDA concentration, relative electrical conductivity, root biomass, root nodule biomass and root activity under the four treatments were performed with the Tukey’s multiple comparisons test. Three-way ANOVA was used to evaluate the effects of the elevated O3 concentration, enhanced UV-B radiation and growth stage (branching, flowering and podding stages) on root growth, MDA concentration, relative electrical conductivity, secondary metabolisms, endogenous hormones, and enzyme activities. Biometric and biochemical properties of soybean roots not meeting assumptions of normality and homogeneity of variance were log-transformed before statistical analysis. We used paired T test to analyze the differences between control treatment and the treatments of elevated O3, enhanced UV-B and O3 + UV-B in secondary metabolisms, endogenous hormones and enzyme activities of soybean roots at stage of branching, flowering and podding. Multivariate analyses were analyzed using Canoco 5.0. Six secondary metabolisms, six enzymes and six hormones of soybean at four treatments were log-transformed (using the “standardize species” option in Canoco 5.0) before unconstrained principal component analysis (PCA). Furthermore, to better highlight the effects of biochemical properties (secondary metabolisms, endogenous hormones, and enzyme activities) of soybean roots on root growth (root biomass and root nodule biomass) and root activities, constrained redundancy analyses (RDA) were used. Significance was based on permutation test using 999 permutations and using a split-plot design (Lepš and Šmilauer, 2003; Li et al., 2015).
Results
Root Growth and Activity
Biomass of root and nodule as well as root activity were significantly lower in the treatments of elevated O3, enhanced UV-B radiation and O3 + UV-B than CK treatments during the whole period of soybean growth (Figure 2). There were no significant differences in root biomass between elevated O3 treatment and enhanced UV-B treatment at stages of flowering and podding (Figure 2A). Root biomass, nodule biomass and root activity were significantly lower in O3 + UV-B treatment than those in the other three treatments during the whole period of soybean growth (Figure 2).
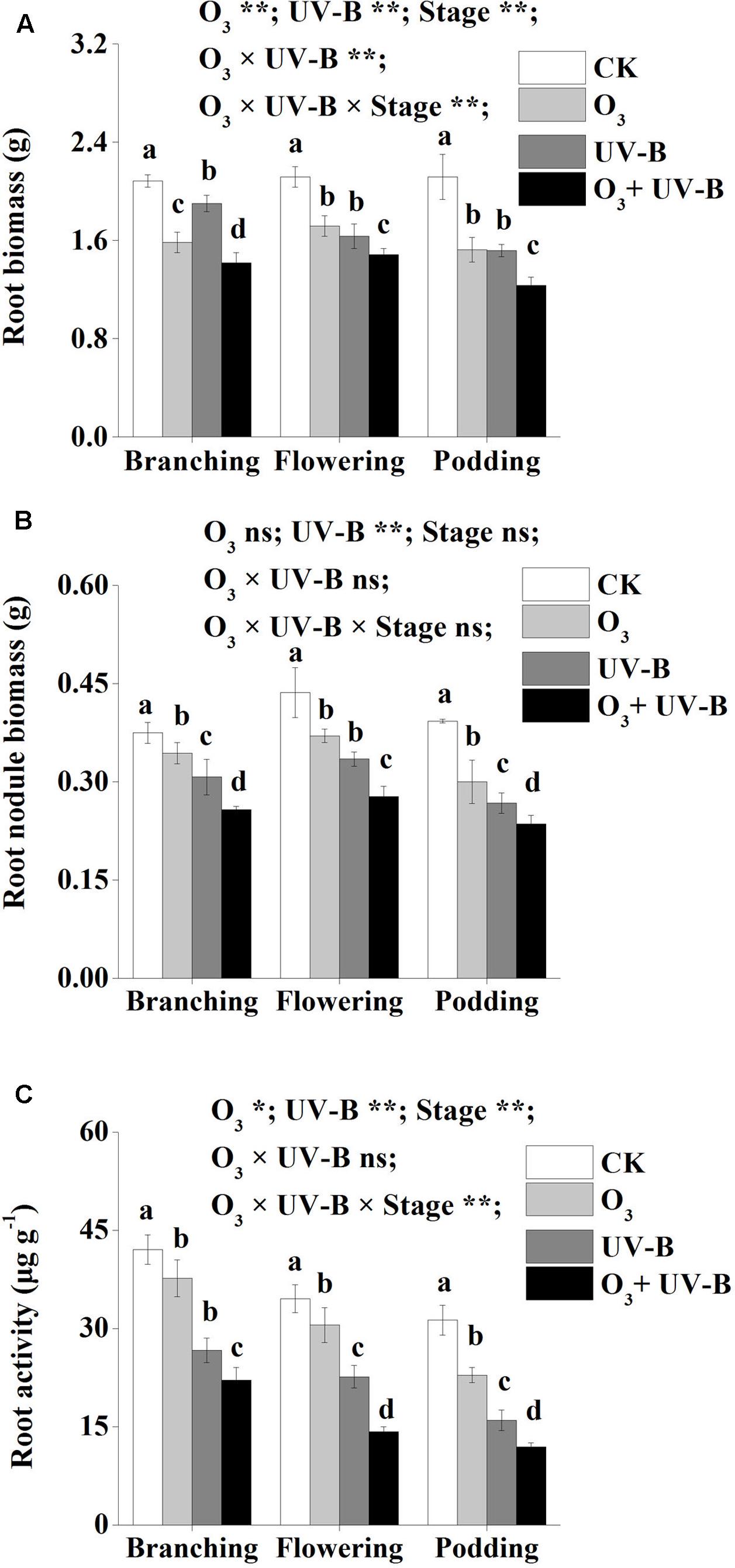
FIGURE 2. Root biomass (A), root nodule biomass (B) and root activity (C) of soybean in response to control treatment (CK), elevated O3 treatment (O3), enhanced UV-B radiation treatment (UV-B) and O3 + UV-B treatment (O3 + UV-B) at stages of branching, flowering and podding. Different letters above the bars represent significant differences from Tukey’s multiple comparisons among four treatments (P < 0.05). For each parameter, results of three-way ANOVA are reported, with asterisks showing the significance of factors [elevated O3 treatment (O3), enhanced UV-B treatment (UV-B) and growth stage of soybean (Stage)] and their interaction for: ∗∗P < 0.001, ∗P < 0.05, ns: insignificant.
Furthermore, the interaction of elevated O3 concentration and enhanced UV-B radiation was found to be significant for root biomass (O3 × UV-B term at P < 0.001; Figure 2). The interactive effects was found to be insignificant for the parameters of root nodule biomass and root activity (O3 × UV-B term at P = 0.074, P = 0.492, respectively).
MDA and Relative Electrical Conductivity of Soybean Roots
Compared to CK treatment, elevated O3, enhanced UV-B and elevated O3 + enhanced UV-B increased MDA concentration and relative electrical conductivity of soybean roots during the whole period of soybean growth (Figure 3). There was no significant difference in MDA concentration and relative electrical conductivity exposed to elevated O3 and elevated O3 + enhanced UV-B during the whole period of soybean growth. Furthermore, the interaction of elevated O3 and enhanced UV-B was found to be significant for MDA concentration and relative electrical conductivity of soybean root (O3 × UV-B term all at P < 0.05).
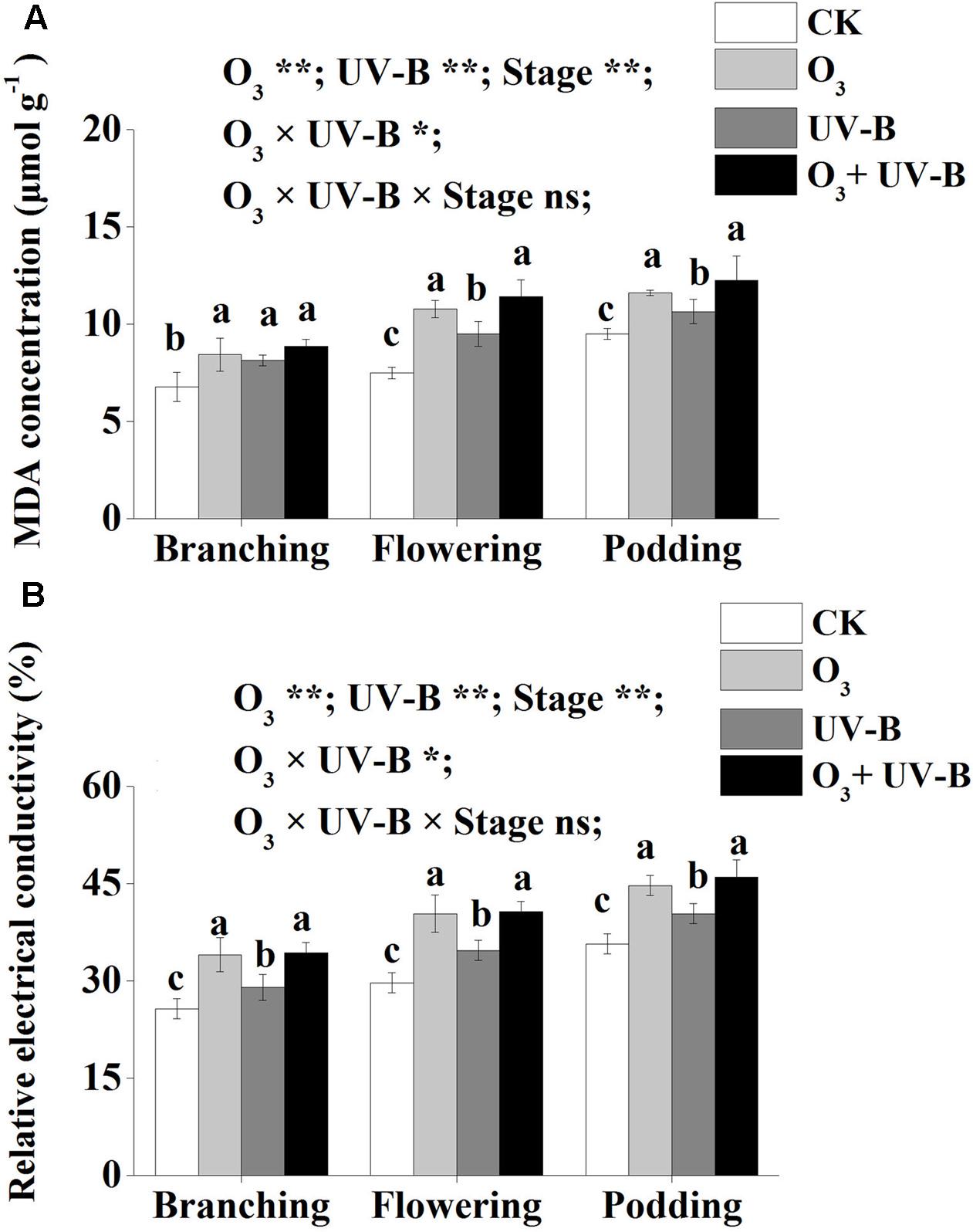
FIGURE 3. Malondialdehyde concentration (μmol g-1) (A) and relative electrical conductivity (%) (B) of soybean roots in response to control treatment (CK), elevated O3 treatment (O3), enhanced UV-B and O3 + UV-B treatment (O3 + UV-B) at stages of branching, flowering and podding. Different letters above the bars represent significant differences from Tukey’s multiple comparisons among four treatments (P < 0.05). For each parameter, results of three-way ANOVA are reported, with asterisks showing the significance of factors (elevated O3 treatment (O3), enhanced UV-B and growth stage of soybean (Stage)] and their interaction for: ∗∗P < 0.001, ∗P < 0.05; ns, insignificant.
Biochemical Properties of Soybean Roots
Flavonoids concentration of soybean roots was significantly increased by exposure to O3 + UV-B at flowering stage and podding stage (Table 1). Three treatments of elevated O3, enhanced UV-B radiation and O3 + UV-B significantly increased quercetin concentration during the whole period of soybean growth. Enhanced UV-B radiation and O3 + UV-B showed significant positive effects on rutin concentration of soybean root at stages of flowering and podding. There were no significant differences in polyphenol and P-coumaric acid concentrations of soybean root among the four treatments. Ferulic acid concentration of soybean root was significantly increased by exposure to elevated O3, enhanced UV-B radiation and O3 + UV-B at podding stage.
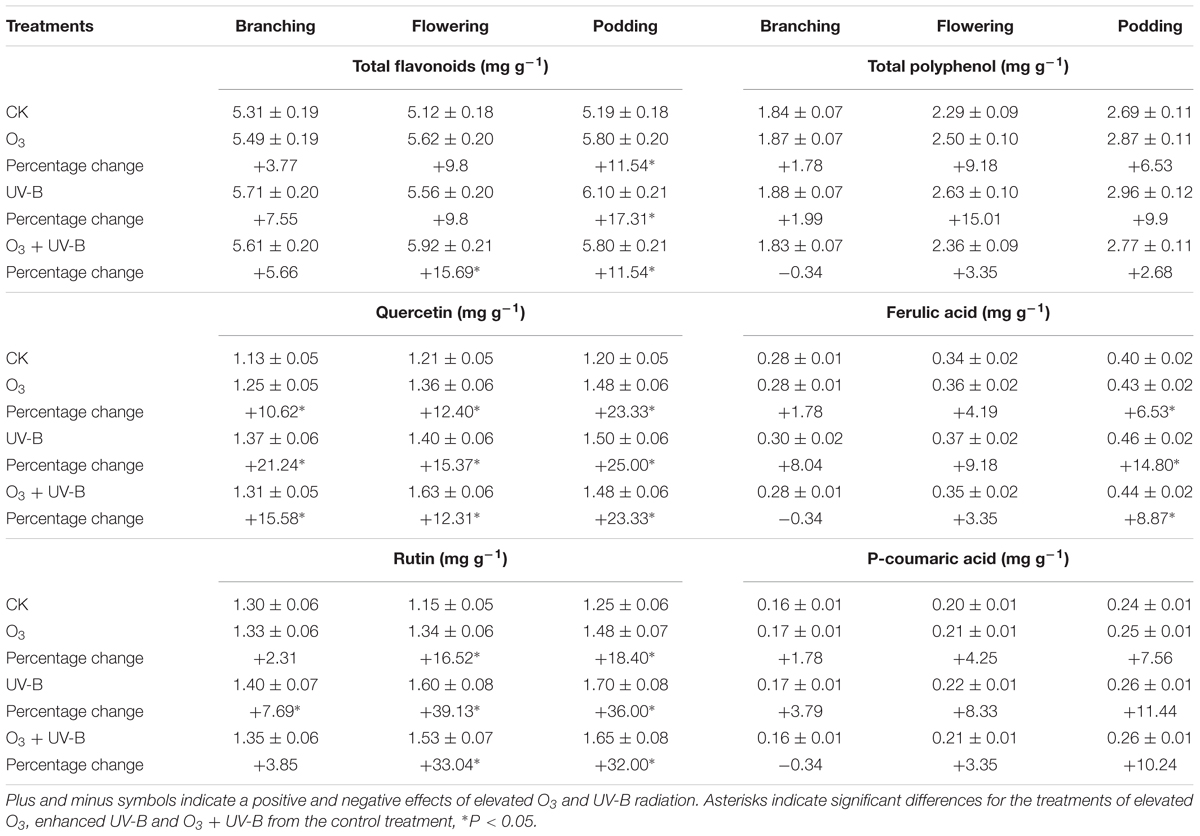
TABLE 1. Differences in secondary metabolisms of soybean roots in response to control treatment (CK), elevated O3 treatment (O3), enhanced UV-B treatment (UV-B) and O3 + UV-B treatment (O3 + UV-B) at stages of branching, flowering, and podding (mean ± SD, n = 3).
Elevated O3, UV-B radiation and O3 + UV-B showed significant positive effects on ABA concentration during the whole period of soybean growth (Table 2). ZR concentration was significantly increased exposed to elevated O3, enhanced UV-B radiation and O3 + UV-B at stages of branching and podding. ZR concentration was significantly decreased exposed to enhanced UV-B radiation and O3 + UV-B at flowering stage. Elevated O3, enhanced UV-B radiation and O3 + UV-B showed significant negative effects on IAA concentration at podding stage, and on ZR/ABA ratio at flowering stage, and on ratios of IAA/ABA and (IAA+ZR)/ABA at stages of branching, flowering and podding.
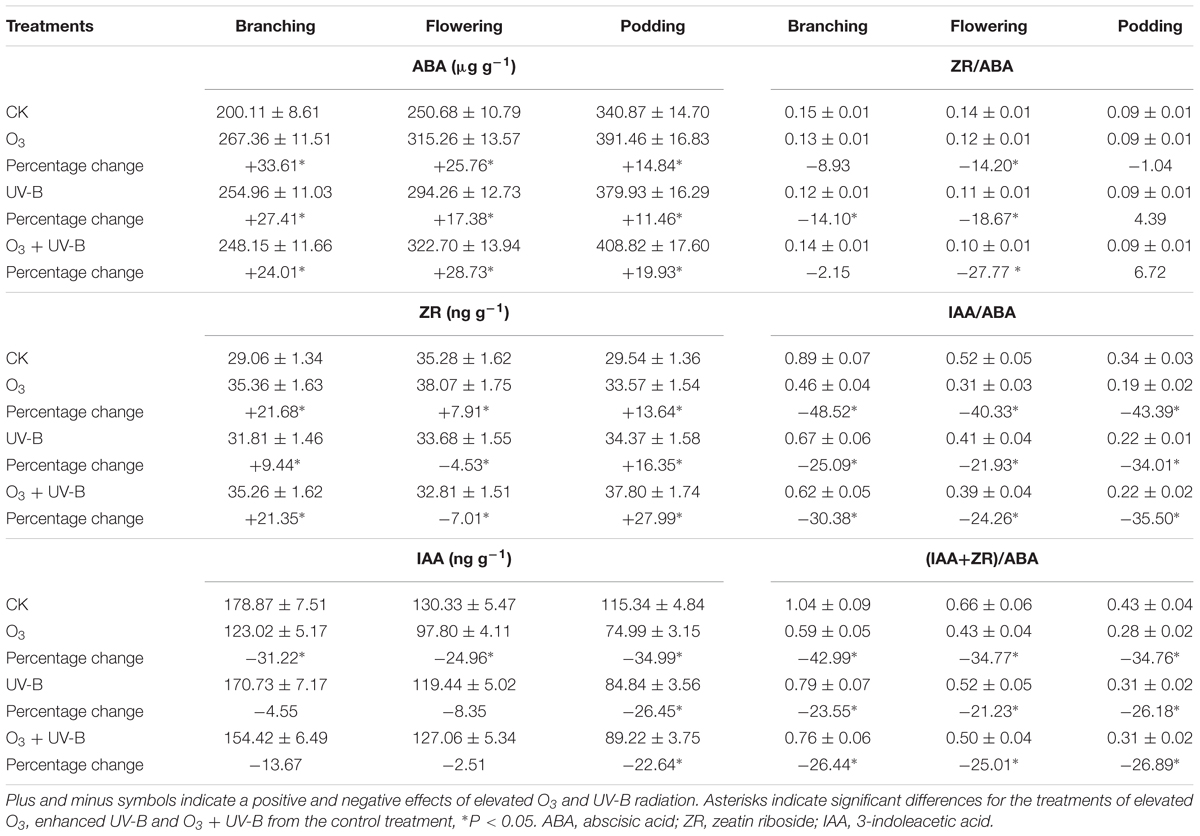
TABLE 2. Differences in endogenous hormones of soybean roots in response to control treatment (CK), elevated O3 treatment (O3), enhanced UV-B treatment (UV-B) and O3 + UV-B treatment (O3 + UV-B) at stages of branching, flowering, and podding (mean ± SD, n = 3).
Elevated O3, enhanced UV-B radiation and O3 + UV-B significantly inhibited SOD activity at flowering stage, and POD activity at podding stage, and APX activity during the whole period of soybean growth, and CAT activity at podding stage (Table 3). Concerning PAL activity, elevated O3 showed significant negative effects, and enhanced UV-B radiation showed significant positive effects during the whole period of soybean growth. Elevated O3, enhanced UV-B radiation and O3 + UV-B showed significant positive effects on LOX activity during the whole period of soybean growth.
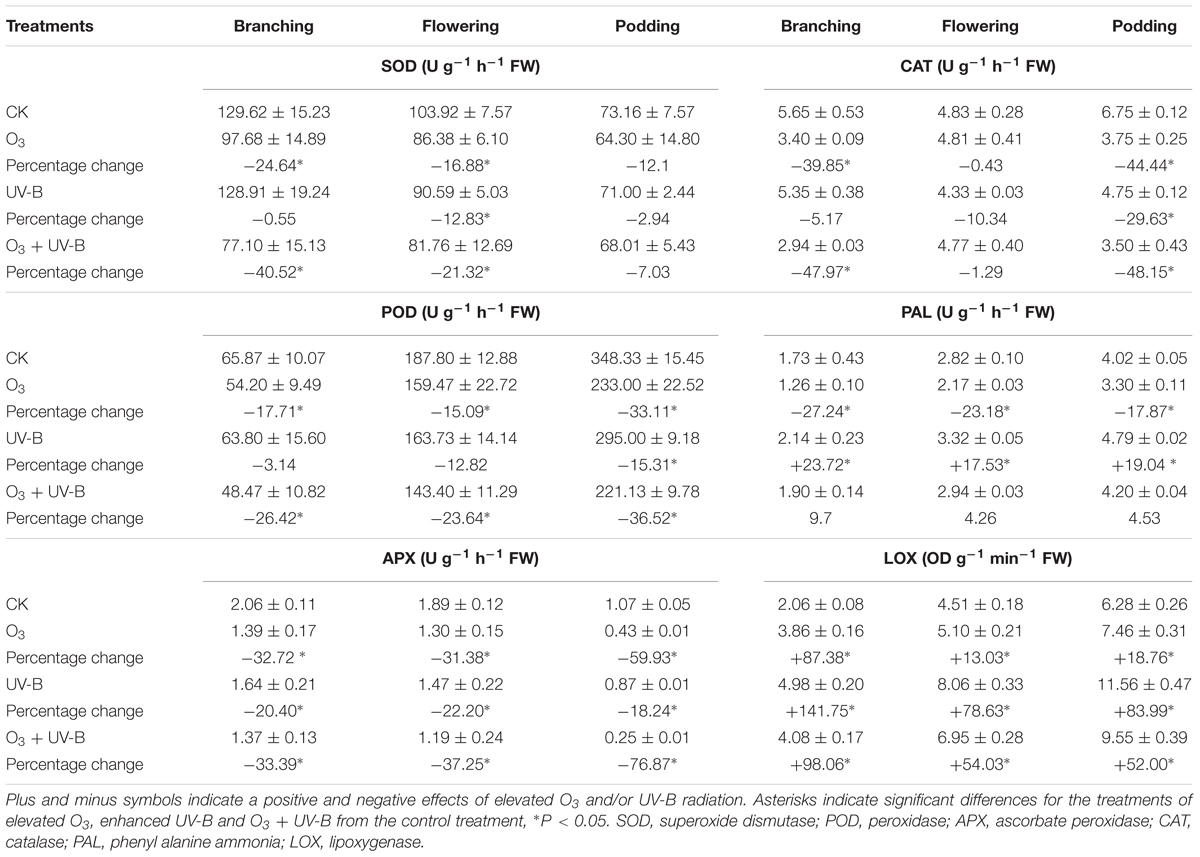
TABLE 3. Differences in enzyme activities of soybean roots in response to control treatment (CK), elevated O3 treatment (O3), enhanced UV-B radiation treatment (UV-B) and O3 + UV-B treatment (O3 + UV-B) at stages of branching, flowering, and podding (mean ± SD, n = 3).
According to PCA analysis, the effects of elevated O3, UV-B radiation and O3 + UV-B on the concentrations of secondary metabolisms, endogenous hormones and enzyme activities were differed from each other (Figure 4). In the PCA analysis of all secondary metabolisms, CK treatment and elevated O3 treatment were separated on the first axis, and the UV-B radiation treatment and O3 + UV-B treatment were separated on the second axis. As to endogenous hormones, the CK treatment was separated on the first axis, and the elevated O3 treatment, UV-B radiation treatment and O3 + UV-B treatment were separated on the second axis. Furthermore, the first axis discriminated CK treatment and O3 + UV-B treatment, and the elevated O3 treatment and UV-B radiation treatment were separated on the second axis in the PCA analysis of all enzyme activities.
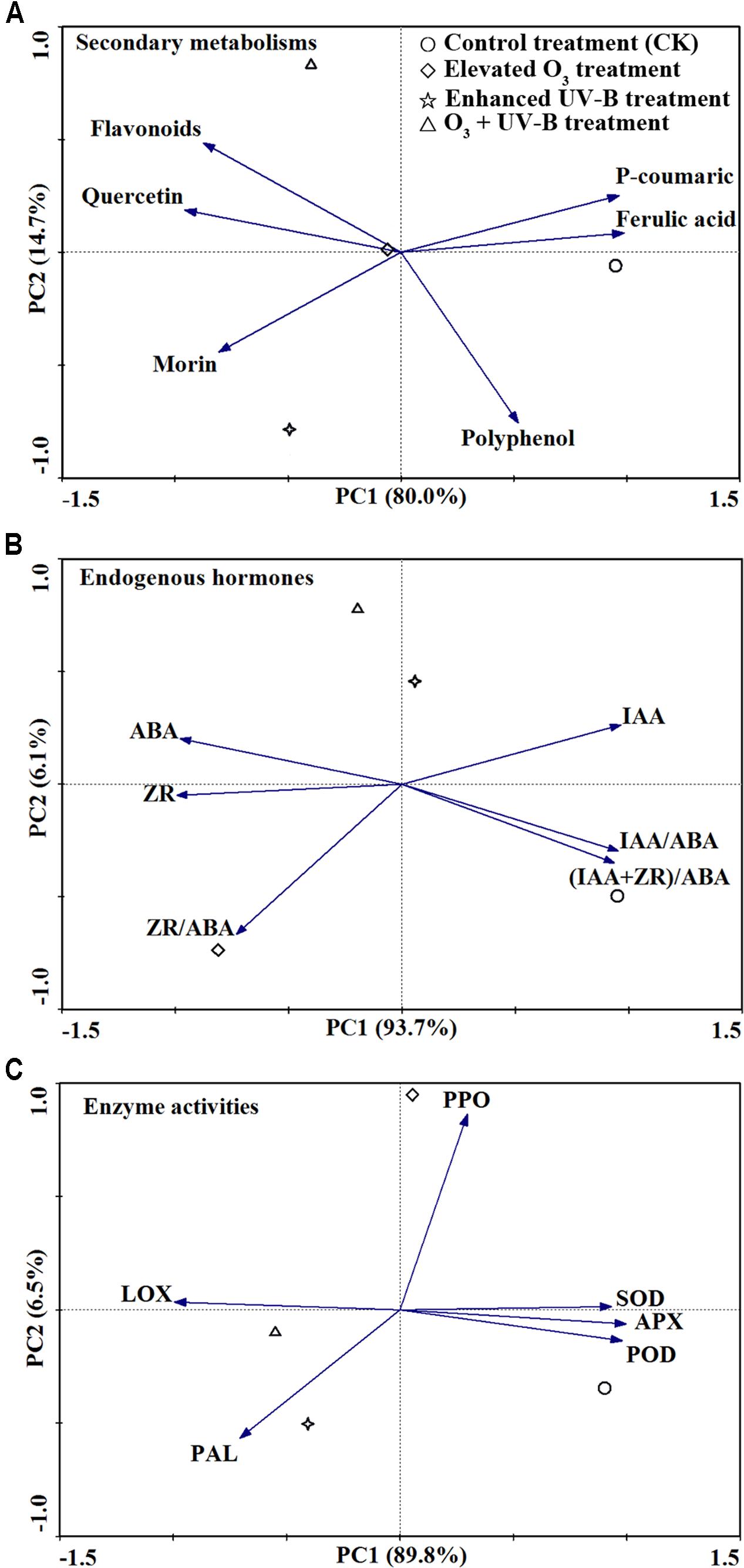
FIGURE 4. Principal component analysis analysis of secondary metabolisms (A), endogenous hormones (B) and enzyme activities (C) in response to control treatment (CK), elevated O3 treatment, enhanced UV-B radiation treatment and O3 + UV-B treatment at stages of branching, flowering, and podding.
Multivariate analysis of secondary metabolisms and endogenous hormones showed a significant interactive effect of elevated O3 and enhanced UV-B on all parameters (O3 × UV-B term all at P < 0.05; Table 4). There were significant interactive effects of elevated O3 concentration and enhanced UV-B radiation on the activities of APX and LOX (O3 × UV-B term at P = 0.044, P < 0.001, respectively).
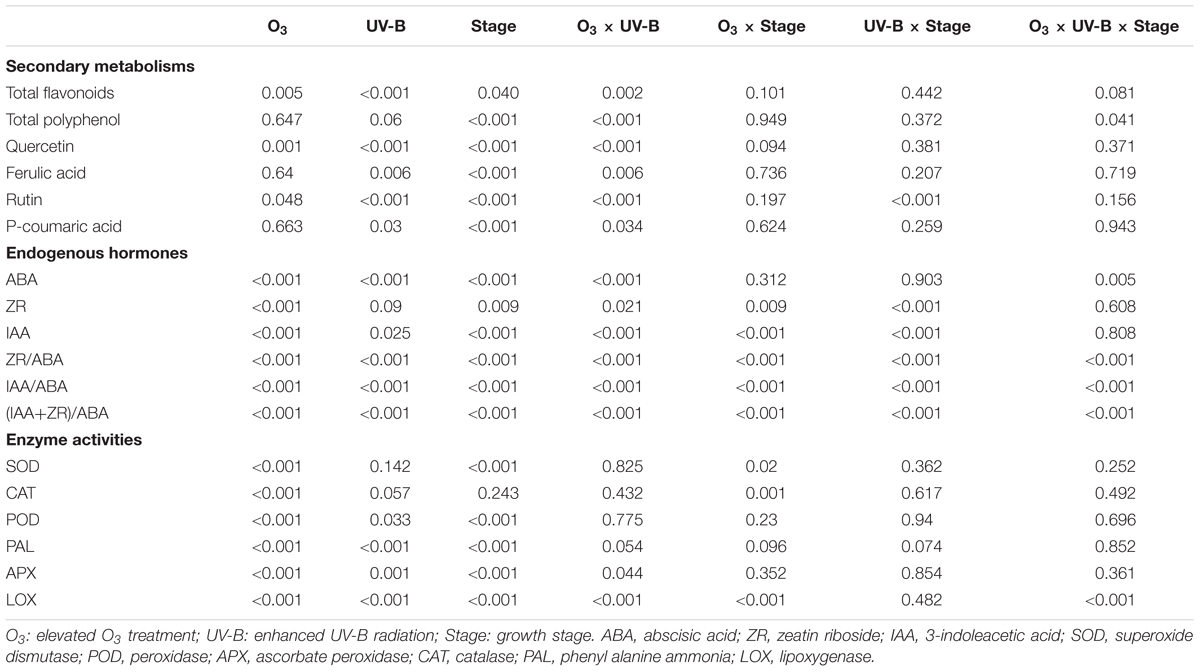
TABLE 4. P-values of 3-way ANOVA results showing the effect of the elevated O3 concentration, the enhanced UV-B radiation and the growth stage on secondary metabolisms, endogenous hormones, and enzyme activities of soybean roots.
Modulation of Biochemical Properties on Root Growth and Activity
Redundancy analyses analyses showed that the explained variation in secondary metabolisms and enzyme activities by effects of elevated O3 and UV-B radiation on root biomass was significant (P = 0.024, P = 0.002, P = 0.044, P = 0.026, respectively; Table 5). The explained variation in secondary metabolisms and endogenous hormones by effects of O3 + UV-B on root biomass was significant (all at P = 0.002). Concerning nodule biomass, explained variations in secondary metabolisms by UV-B radiation effects and in endogenous hormones by O3 + UV-B effect were significant (P = 0.038 and P = 0.002, respectively).
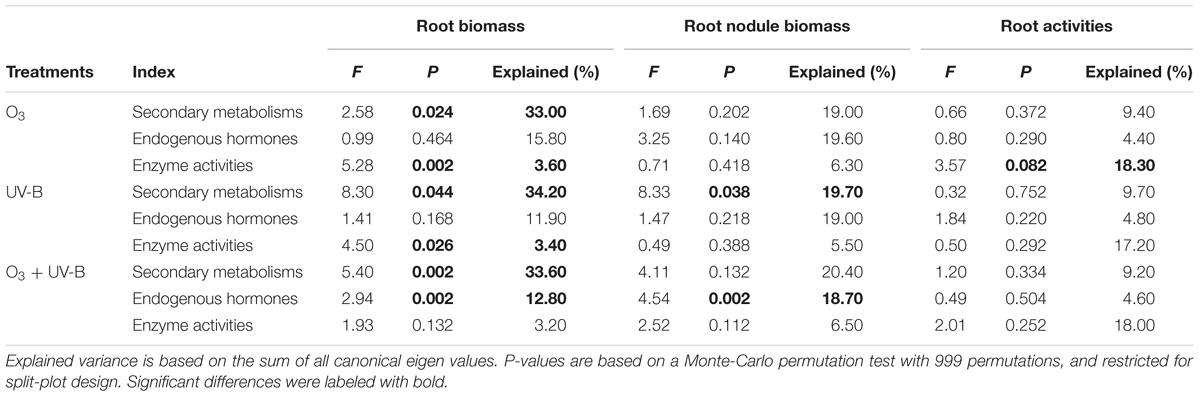
TABLE 5. Results of redundancy analysis (RDA) of the modulation of secondary metabolisms, endogenous hormones and enzyme activities in response to elevated O3 treatment (O3), enhanced UV-B radiation treatment (UV-B) and O3 + UV-B treatment (O3 + UV-B) on root biomass, root nodule biomass and root activities.
Discussion
Our study found that elevated O3 concentration and enhanced UV-B radiation individually decreased root biomass at each developmental stages (branching stage, flowering stage, and podding stage). Root growth is highly dependent on carbohydrate availability (Muller et al., 1998; Walter et al., 2009). The balance between carbohydrate assimilation, storage, and growth and the allocation between shoot and root are crucial for root growth (Schurr et al., 2006). Elevated O3 is known to produce a decline in the availability of carbon in leaves by impairing stomatal function and influencing Rubisco carboxylation capacity, which can alter carbon balance of plants, decreasing the allocation of carbon to roots and shoots and leading to an altered biomass of shoots and roots (Andersen, 2003; Fiscus et al., 2005; Matyssek et al., 2008). Meanwhile, enhanced UV radiation also could alter biomass of shoots and roots in many plant species, because exceeding ambient UV radiation intensity could directly affect photosynthesis systems and phyto-hormones and indirectly impact stomatal closure (Kakani et al., 2003; Yadav et al., 2017). Thus, to better understanding the mechanism of root growth in response to elevated O3 and enhanced UV-B radiation, plant biomass allocation (root biomass: shoot biomass and root nodule biomass: shoot biomass) needs to be further studied. Furthermore, the significant effects of O3 × UV-B were shown in the present study indicating that the combined effects of elevated O3 concentration and enhanced UV-B radiation were synergistic on root biomass of soybean. Thus, combined effects of elevated O3 and enhanced UV-B significantly exacerbated the decreased biomass of soybean roots.
Moreover, in accordance with our hypothesis, biomass of root nodule significant decreased in the presence of both treatments of elevated O3 and enhanced UV-B radiation individually or in combination. Decreased biomass of root nodule might decrease N uptake of roots, and therefore might exacerbate root senescence or death. Meanwhile, the effects of O3 × UV-B on nodule biomass were insignificant in the present study indicating that the combined effects of elevated O3 and enhanced UV-B on nodule biomass were additive.
Root activity is a physiological index reflecting the ability of roots to absorb water and nutrients, to synthesize certain compounds, and to either oxidize or reduce elements in the surrounding rhizosphere. Previous studies showed that enhanced UV-B radiation and elevated O3 significantly decreased root activity of rice (Dai et al., 1992). Similarly, in present study, elevated O3 and enhanced UV-B radiation individually as well as in combination inhibited the root activity at each developmental stages (branching stage, flowering stage, and podding stage). Meanwhile, the inhibition effect of coupling elevated O3 and enhanced UV-B radiation on root activity was more significant (55.7%) than individual treatment (11.2 and 39.9%, respectively). However, the effects of O3 × UV-B on root activity were insignificant in the present study indicating that the combined effects of elevated O3 and enhanced UV-B on root activity were simply additive. Moreover, elevated O3 and combination effects of O3 and UV-B radiation significantly decreased POD concentration of soybean roots during the whole incubation in present study. Previous studies showed that hydrogen peroxide (H2O2) could be oxidized by α-naphthylamine in the presence of iron oxidase (mainly POD) (Schopfer and Liszkay, 2006), thus, there was a positive correlation between POD activity and oxidation intensity of α-naphthylamine.
O3 is known to affect the plasma function by disorganizing the membrane structure and altering membrane permeability through lipid peroxidation and electrolyte leakage (Calatayud et al., 2003). In the present study, MDA concentration and relative electrical conductivity of soybean roots were drastically enhanced by elevated O3 at three developmental stages, indicating that O3 intensified accumulation of ROS (reactive oxygen species) induced by oxidative stress and degree of lipid peroxidation of root issue membrane throughout the whole growing period. Similarly, MDA concentrations and relative electrical conductivity of soybean roots during the whole period of soybean growth were also enhanced by enhanced UV-B, indicating that enhanced UV-B radiation also induced oxidative stress. Furthermore, O3 + UV-B radiation generally displayed higher MDA concentration and relative electrical conductivity than enhanced UV-B radiation during the whole growing period of soybean roots, and according to multivariate analysis, the effects of O3 × UV-B on MDA concentration and relative electrical conductivity were significant in the present study. Thus supplemental O3 aggravated the oxidative effects of UV-B radiation.
Phenols are mainly responsible for providing plant pest and disease resistance and protection. In present study, there generally was no significant change in phenols concentration at three developmental stages. Differently, Ambasht and Agrawal (2003) and Tripathi et al. (2011) reported increases in phenols of crop leaves under treatment of UV-B radiation and/or O3. Moreover, the present study demonstrated a considerable difference in the flavonoids concentration in response to elevated O3 and/or enhanced UV-B radiation. Li B. et al. (2012) also found that enhanced UV-B radiation increased flavonoids concentration of roots. Some studies found that flavonoids (such as kaempferol) were an inhibitor of auxin transport (Peer et al., 2004; Taylor and Grotewold, 2005), and auxin was a key player on organizing node for environmental/hormonal modulation of root hair growth (Lee and Cho, 2013). In the present study, three treatments of elevated O3, enhanced UV-B radiation and elevated O3 + enhanced UV-B radiation significantly increased IAA concentration at podding stage. The accumulation of flavonoids presumably resulted in the reduction of roots development through inhibiting auxin transport in the root (Brown et al., 2001). Quercetin and rutin (sometime called vitamin P) was known to have strong antioxidant activity which could alleviate the damage exposed to UV-B radiation (Edreva, 2005). In the present study, concentrations of quercetin and rutin of roots were significantly increased at stages of flowering and podding. Similarly, Huang et al. (2016) showed that contents of quercetin and rutin of fine roots were increased when exposed to enhanced UV-B radiation.
Plants have several enzymatic antioxidants (such as SOD, CAT, POD, and APX) to defend themselves against oxidative stress (Gill and Tuteja, 2010). Previous studies have found various trends in enzyme activity of leaves in response to elevated O3 concentration and/or enhanced UV-B radiation mainly based on the above-ground system. Kumari et al. (2009) showed that a reduction in SOD activity of leaves of Abelmoschus esculentus L. after 10 days and then subsequent increment after 20 days exposed to enhanced UV-B radiation (10.4 kJ m-2). Rai and Agrawal (2008) found a significant increase in SOD activity in rice leaves under elevated O3. Tripathi et al. (2011) found an increment in activity of SOD, CAT, and APX of leaves of Linum usitatissimum L. in response to O3 (+10 ppb) and UV-B radiation (+7.2 kJ m-2 d-1) individually as well as in combination. Rao et al. (1996) and Baumbusch et al. (1998) also reported increased CAT and POD activity in UV-B radiation, O3, and UV-B + O3 in leaves, while Rao et al. (1996) did not find any major change in CAT activity after O3 treatment (200 ppb). In the present study, activities of SOD, CAT, POD, and APX of roots were all significantly decreased in response to O3 and UV-B radiation individually as well as in combination, while, there were insignificant effects of O3 × UV-B on the activity of SOD, CAT, and POD. Thus, the combined effects of elevated O3 and enhanced UV-B on the activity of SOD, CAT and POD were simply additive. SOD is the primary enzyme responsible for the dismutation of superoxide anions and hence decreasing the risk of hydroxyl radicals from superoxide anions (Arora et al., 2002). And for detoxification of H2O2, other enzymes (such as POD, APX, and CAT) were found inside the cell. Production rate of superoxide anions and hydroxyl radicals increased significantly with the high dose of enhanced UV and/or elevated O3, which might result in enzyme activity exceeding its threshold. Thus, activities of SOD, CAT, POD, and APX were all significantly decreased in the present study.
According to redundancy analysis, secondary metabolisms showed stronger relationship with root growth in response to elevated O3, enhanced UV-B radiation and elevated O3 + enhanced UV-B radiation compared to hormones and enzyme activity, probably because the secondary metabolites are more sensitive to elevated O3 and enhanced UV-B radiation compared to hormones and enzyme activity, especially for annual legumes. Secondary metabolites (such as flavonoids, phenol) are considered as a first line to limit the defense of environmental change (Bartwal et al., 2013). UV-B radiation and O3 lead to not only ROS generation and oxidative stress, but also changes of hormones and activity of antioxidant enzyme. However, previous studies showed that the exact set of enzymes activated is dependent on the plant species and no consensus has been reached as to the most important antioxidant enzyme system in defense of environmental changed (Soheila, 2000; Caldwell et al., 2007). Thus, for annual legumes in present study, the variation of root growth exposed to O3 and/or UV-B radiation was mostly associated with flavonoids.
In the present study, the effects of O3 × UV-B on root biomass, flavonoids, and hormones were significant indicating that the changes of root biomass, flavonoids, and hormones in response to coupling UV radiation and O3 were always greater than that in response to individual effects. Thus, supplemental elevated O3 might exacerbate the damage of enhanced UV-B on soybean root growth, contrary to some previous studies mainly based on the above-ground system (Zeuthen et al., 1997; Baumbusch et al., 1998; Tripathi et al., 2011). For example, Zeuthen et al. (1997) found that UV-B and O3 in combination enhanced the negative effects on photosynthesis of beech compared with UV-B and O3 alone. Baumbusch et al. (1998) reported that, generation of ROS of pine leaf was counteracted by combination effects. Tripathi et al. (2011) showed that generation of ROS of linseed in response to combined effects of UV-B and O3 was lower than that to individual effects.
Conclusion
The major objective of our study was to assess the effects of elevated O3 and enhanced UV-B radiation individually as well as in combination on root growth and activity of soybean, and we found that elevated O3 and enhanced UV-B radiation individually inhibited root growth and activity of soybean. Elevated O3 and enhanced UV-B individually as well as in combination induced oxidative damage of soybean roots leading to changes in secondary metabolites (especially flavonoids), endogenous hormone and enzyme activities of soybean roots. We found that, compared to endogenous hormones and enzyme activity, secondary metabolisms (especially flavonoids) showed stronger relationship with root growth in response to elevated O3, enhanced UV-B radiation and their combination. Consequently, the inhibited root growth was mostly associated with flavonoids. Moreover, the effect of UV-B + O3 was generally greater than those of their individual stresses on root biomass, flavonoids, and hormones.
Author Contributions
BM and R-RT detected the changes of secondary metabolites of soybean roots. YW detected the changes of endogenous hormone of soybean roots. WW and J-SY completed the experiment of the effect of elevated ozone and enhanced UV-B on root growth of soybean. T-HZ guided the whole research as the corresponding author.
Conflict of Interest Statement
The authors declare that the research was conducted in the absence of any commercial or financial relationships that could be construed as a potential conflict of interest.
Acknowledgments
This work was supported by the National Natural Science Foundation of China (30970448; 31570404) and China Postdoctoral Science Foundation (2016M601342). We thank editor, two referees, professor De-Hui Zeng and Dr. Qiong Zhao for his valuable comments and suggestions that greatly improved the manuscript.
Abbreviations
ABA, abscisic acid; APX, ascorbate peroxidase; CAT, catalase; IAA, 3-indoleacetic acid; LOX, lipoxygenase; MDA, malondialdehyde; PAL, phenylalanine ammonia; POD, peroxidase; SOD, superoxide dismutase; ZR, zeatin riboside.
References
Aebi, H. (1984). Catalase in vitro. Method Enzymol. 105, 121–126. doi: 10.1016/S0076-6879(84)05016-3
Albacete, A., Ghanem, M. E., Martinez-Andujar, C., Acosta, M., Sanchez- Bravo, J., Martinez, V., et al. (2008). Hormonal changes in relation to biomass partitioning and shoot growth impairment in salinized tomato (Solanum lycopersicum L.) plants. J. Exp. Bot. 59, 4119–4131. doi: 10.1093/jxb/ern251
Ambasht, N. K., and Agrawal, M. (2003). Effects of enhanced UV-B radiation and tropospheric ozone on physiological and biochemical characteristics of field grown wheat. Biol. Plant. 47, 625–628. doi: 10.1023/B:BIOP.0000041076.95209.c3
Andersen, C. P. (2003). Source-sink balance and carbon allocation below ground in plants exposed to ozone. New Phytol. 157, 213–228. doi: 10.1046/j.1469-8137.2003.00674.x
Ando, T., Yoshida, S., and Nishiyama, I. (1983). Nature of oxidizing power of rice roots. Plant Soil 72, 57–71. doi: 10.1007/BF02185094
Arora, A., Sairam, R. K., and Srivastava, G. C. (2002). Oxidative stress and antioxidative system in plants. Curr. Sci. 82, 1227–1238.
Ashmore, M. R. (2005). Assessing the future global impacts of ozone on vegetation. Plant Cell Environ. 28, 949–964. doi: 10.1111/j.1365-3040.2005.01341.x
Bais, A. F., McKenzie, R. L., Bernhard, G., Aucamp, P. J., Ilyas, M., Madronich, S., et al. (2015). Ozone depletion and climate change: impacts on UV radiation. Photochem. Photobiol. Sci. 14, 19–52. doi: 10.1039/C4PP90032D
Ballaré, C. L., Caldwell, M. M., Flint, S. D., Robinson, A., and Bornman, J. F. (2011). Effects of solar ultraviolet radiation on terrestrial ecosystems. Patterns, mechanisms and interactions with climate change. Photochem. Photobiol. Sci. 10, 226–241. doi: 10.1039/C0PP90035D
Bartwal, A., Mall, R., Lohani, P., Guru, S. K., and Arora, S. (2013). Role of secondary metabolites and brassinosteroids in plant defense against environmental stresses. J. Plant Growth Regul. 32, 216–232. doi: 10.1007/s00344-012-9272-x
Baumbusch, L. O., Eiblmeier, M., Schnitzler, J. P., Heller, W., Sandermann, H Jr, and Polle, A. (1998). Interactive effect of ozone and low UV-B radiation on antioxidants in spruce (Picea abies) and pine (Pinus sylvestris) needles. Physiol. Plant. 104, 248–254. doi: 10.1034/j.1399-3054.1998.1040213.x
Beauchamp, C. O., and Fridovich, I. (1971). Superoxide dismutase: improved assay and an assay applicable to acrylamide gels. Anal. Biochem. 44, 276–287. doi: 10.1016/0003-2697(71)90370-8
Binder, B. Y. K., Peebles, C. A. M., Shanks, J. V., and San, K. Y. (2009). The effects of UV-B stress on the production of terpenoid indole alkaloids in Catharanthus roseus hairy roots. Biotechnol. Prog. 25, 861–865. doi: 10.1002/btpr.97
Blokhina, O., Virolainen, E., and Fagerstedt, K. V. (2003). Antioxidants, oxidative damage and oxygen deprivation stress: a review. Ann. Bot. 91, 179–194. doi: 10.1093/aob/mcf118
Bradford, M. M. (1976). A rapid and sensitive method for the quantitation of microgram quantities of protein utilizing the principle of protein-dye binding. Anal. Biochem. 72, 248–254. doi: 10.1016/0003-2697(76)90527-3
Brown, D. E., Rashotte, A. M., Murphy, A. S., Normanly, J., Tague, B. W., Peer, W. A., et al. (2001). Flavonoids act as negative regulators of auxin transport in vivo in Arabidopsis. Plant Physiol. 126, 524–535. doi: 10.1104/pp.126.2.524
Buege, J. A., and Aust, S. D. (1978). Microsomal lipid peroxidation. Methods Enzymol. 52, 302–310. doi: 10.1016/S0076-6879(78)52032-6
Buranasompob, A., Tang, J., Powers, J. R., Reyes, J., Clark, S., and Swanson, B. G. (2007). Lipoxygenase activity in walnuts and almonds. LWT Food Sci. Technol. 40, 893–899. doi: 10.1016/j.lwt.2006.05.003
Bussell, J. S., Gwynn-Jones, D., Griffith, G. W., and Scullion, J. (2012). Above- and below-ground responses of Calamagrostis purpurea to UV-B radiation and elevated CO2 under phosphorus limitation. Physiol. Plant. 145, 619–628. doi: 10.1111/j.1399-3054.2012.01595.x
Calatayud, A., Iglesias, D. J., Talon, M., and Barreno, E. (2003). Effects of 2-month ozone exposure in spinach leaves on photosynthesis, antioxidant systems and lipid peroxidation. Plant Physiol. Biochem. 41, 839–845. doi: 10.1016/S0981-9428(03)00123-2
Caldwell, M. M., Bornman, J. F., Ballaré, C. L., Flint, S. D., and Kulandaivelu, G. (2007). Terrestrial ecosystems, increased solar ultraviolet radiation, and combined effects with other climate change factors. Photochem. Photobiol. Sci. 6, 252–266. doi: 10.1039/B700019G
Dai, Q., Coronel, V. P., Vergara, B. S., Barnes, P. W., and Quintos, A. T. (1992). Ultraviolet-B radiation effects on growth and physiology of four rice cultivars. Crop Sci. 32, 1269–1274. doi: 10.2135/cropsci1992.0011183X003200050041x
Dodd, I. C. (2005). “Root-to-shoot signalling: assessing the roles of ‘up’ in the up and down world of long-distance signalling in planta,” in Root Physiology: From Gene to Function, eds H. Lambers and T. D. Colmer (Dordrecht: Springer), 251–270.
Edreva, A. (2005). The importance of non-photosynthetic pigments and cinnamic acid derivatives in photoprotection. Agric. Ecosyst. Environ. 106, 135–146. doi: 10.1016/j.agee.2004.10.002
Escobar-Bravo, R., Klinkhamer, P. G., and Leiss, K. A. (2017). Interactive effects of UV-B light with abiotic factors on plant growth and chemistry, and their consequences for defense against arthropod herbivores. Front. Plant Sci. 8:278. doi: 10.3389/fpls.2017.00278
Fehr, W. R., Caviness, C. F., Burmood, D. T., and Pennington, J. S. (1971). Stage of development descriptions for soybeans. Crop Sci. 11, 929–931. doi: 10.2135/cropsci1971.0011183X001100060051x
Feng, Z. Z., and Kobayashi, K. (2009). Assessing the impacts of current and future concentrations of surface ozone on crop yield with meta-analysis. Atmos. Environ. 43, 1510–1519. doi: 10.1016/j.atmosenv.2008.11.033
Fiscus, E. L., Booker, F. L., and Burkey, K. O. (2005). Crop responses to ozone: uptake, modes of action, carbon assimilation and partitioning. Plant Cell Environ. 28, 997–1011. doi: 10.1111/j.1365-3040.2005.01349.x
Geissman, T. A. (1955). “Anthocyanins, chalcones, aurones, flavones and related water-soluble plant pigments,” in Moderne Methoden der Pflanzenanalyse/Modern Methods of Plant Analysis, eds K. Paech and M. V. Tracey (Berlin: Springer), 450–498.
Gill, S. S., and Tuteja, N. (2010). Reactive oxygen species and antioxidant machinery in abiotic stress tolerance in crop plants. Plant Physiol. Biochem. 48, 909–930. doi: 10.1016/j.plaphy.2010.08.016
Goumenaki, E., Taybi, T., Borland, A., and Barnes, J. (2010). Mechanisms underlying the impacts of ozone on photosynthetic performance. Environ. Exp. Bot. 69, 259–266. doi: 10.1016/j.envexpbot.2010.04.011
Hao, G., Du, X., Zhao, F., Shi, R., and Wang, J. (2009). Role of nitrucoxide in UV-B-induced activation of PAL and stimulation of flavonoid biosynthesis in Ginkgo biloba callus. Plant Cell Tissue Organ Cult. 97, 175–185. doi: 10.1007/s11240-009-9513-2
Havir, E. A., and Hanson, K. R. (1968). L-Phenylalanine ammonia-lyase. I. Purification and molecular size of the enzyme from potato tubers. Biochemistry 7, 1896–1903. doi: 10.1021/bi00845a038
Huang, X., Yao, J., Zhao, Y., Xie, D., Jiang, X., and Xu, Z. (2016). Efficient rutin and quercetin biosynthesis through flavonoids-related gene expression in Fagopyrum tataricum Gaertn. hairy root cultures with UV-B irradiation. Front. Plant Sci. 7:63. doi: 10.3389/fpls.2016.00063
Jansen, M. A. K., Gaba, V., and Greenberg, B. M. (1998). Higher plants and UV-B radiation: balancing damage, repair and acclimation. Trends Plant Sci. 3, 131–135. doi: 10.1016/S1360-1385(98)01215-1
Kakani, V. G., Reddy, K. R., Zhao, D., and Sailaja, K. (2003). Field crop responses to ultraviolet-B radiation: a review. Agric. For. Meteorol. 120, 191–218. doi: 10.1016/S1360-1385(98)01215-1
Kataria, S., Baghel, L., and Guruprasad, K. N. (2017). Alleviation of adverse effects of ambient UV stress on growth and some potential physiological attributes in soybean (Glycine max) by seed pre-treatment with static magnetic field. J. Plant Growth Regul. 36, 550. doi: 10.1007/s00344-016-9657-3
Kochba, J., Lavee, S., and Spiegel-Roy, P. (1977). Differences in peroxidase activity and isoenzymes in embryogenic ane non-embryogenic ‘Shamouti’ orange ovular callus lines. Plant Cell Physiol. 18, 463–467. doi: 10.1093/oxfordjournals.pcp.a075455
Kumari, R., Singh, S., and Agrawal, S. B. (2009). Combined effects of Psoralens and ultraviolet-B on growth, pigmentation and biochemical parameters of Abelmoschus esculentus L. Ecotoxicol. Environ. Saf. 72, 1129–1136. doi: 10.1016/j.ecoenv.2008.12.009
Laurence, J. A., and Andersen, C. P. (2003). Ozone and natural systems: understanding exposure, response and risk. Environ. Int. 29, 155–160. doi: 10.1016/S0160-4120(02)00158-7
Lee, R. D., and Cho, H. T. (2013). Auxin, the organizer of the hormonal/environmental signals for root hair growth. Front. Plant Sci. 4:448. doi: 10.3389/fpls.2013.00448
Lepš, J., and Šmilauer, P. (2003). Multivariate Analysis of Ecological Data Using CANOCO. Cambridge: Cambridge University Press.
Li, B., Krumbein, A., Neugart, S., Li, L., and Schreiner, M. (2012). Mixed cropping with maize combined with moderate UV-B radiations lead to enhanced flavonoid production and root growth in faba bean. J. Plant Interact. 7, 333–340. doi: 10.1080/17429145.2012.714407
Li, Q., Yang, Y., Bao, X., Liu, F., Liang, W., Zhu, J., et al. (2015). Legacy effects of elevated ozone on soil biota and plant growth. Soil Biol. Biochem. 91, 50–57. doi: 10.1016/j.soilbio.2015.08.029
Li, X., Zhang, L., Li, Y., Ma, L., Bu, N., and Ma, C. (2012). Changes in photosynthesis, antioxidant enzymes and lipid peroxidation in soybean seedlings exposed to UV-B radiation and/or Cd. Plant Soil 352, 377–387. doi: 10.1007/s11104-011-1003-8
Li, X., Zhang, L., Li, Y., Ma, L., Chen, Q., Wang, L. L., et al. (2011). Effects of elevated carbon dioxide and/or ozone on endogenous plant hormones in the leaves of Ginkgo biloba. Acta Physiol. Plant. 33, 129–136. doi: 10.1007/s11738-010-0528-4
Li, Y., Zu, Y. Q., Chen, J. J., and Chen, H. Y. (2002). Intraspecific responses in crop growth and yield of 20 soybean cultivars to enhanced ultraviolet-B radiation under field conditions. Field Crops Res. 78, 1–8. doi: 10.1016/S0378-4290(02)00084-9
Liu, B., Liu, X. B., Li, Y. S., and Herbert, S. J. (2013). Effects of enhanced UV-B radiation on seed growth characteristics and yield components in soybean. Field Crops Res. 154, 158–163. doi: 10.1016/j.fcr.2013.08.006
Liu, J., Tie, H., Chen, H., and Han, R. (2016). The distribution of profilin in root-tip cells of wheat seedlings exposed to enhanced UV-B radiation. Front. Life Sci. 9, 44–51. doi: 10.1080/21553769.2015.1075434
Matyssek, R., Sandermann, H., Wieser, G., Booker, F., Cieslik, S., Musselman, R., et al. (2008). The challenge of making ozone risk assessment for forest trees more mechanistic. Environ. Pollut. 156, 567–582. doi: 10.1016/j.envpol.2008.04.017
Mazza, C. A., Giménez, P. I., Kantolic, A. G., and Ballaré, C. L. (2013). Beneficial effects of solar UV-B radiation on soybean yield mediated by reduced insect herbivory under field conditions. Physiol. Plant. 147, 307–315. doi: 10.1111/j.1399-3054.2012.01661.x
Mewis, I., Schreiner, M., Nguyen, C. N., Krumbein, A., Ulrichs, C., Lohse, M., et al. (2012). UV-B irradiation changes specifically the secondary metabolite profile in broccoli sprouts: induced signaling overlaps with defense response to biotic stressors. Plant Cell Physiol. 53, 1546–1560. doi: 10.1093/pcp/pcs096
Miller, J. E., Booker, F. L., Fiscus, E. L., Heagle, A. S., Pursley, W. A., Vozzo, S. F., et al. (1994). Ultraviolet-B radiation and ozone effects on growth, yield, and photosynthesis of soybean. J. Environ. Qual. 23, 83–91. doi: 10.2134/jeq1994.00472425002300010012x
Mills, G., Buse, A., Gimeno, B., Bermejo, V., Holland, M., Emberson, L., et al. (2007). A synthesis of AOT40-based response functions and critical levels of ozone for agricultural and horticultural crops. Atmos. Environ. 41, 2630–2643. doi: 10.1016/j.atmosenv.2006.11.016
Mittler, R. (2002). Oxidative stress, antioxidants and stress tolerance. Trends Plant Sci. 7, 405–410. doi: 10.1016/S1360-1385(02)02312-9
Montedoro, G., Servili, M., Baldioli, M., and Miniati, E. (1992). Simple and hydrolyzable phenolic compounds in virgin olive oil. 1. Their extraction, separation, and quantitative and semiquantitative evaluation by HPLC. J. Agric. Food Chem. 40, 1571–1576. doi: 10.1021/jf00021a019
Muller, B., Stosser, M., and Tardieu, F. (1998). Spatial distributions of tissue expansion and cell division rates are related to irradiance and to sugar content in the growing zone of maize roots. Plant Cell Environ. 21, 149–158. doi: 10.1046/j.1365-3040.1998.00263.x
Nakano, Y., and Asada, K. (1981). Hydrogen peroxide is scavenged by ascorbate-specific peroxidase in spinach chloroplasts. Plant Cell Physiol. 22, 867–880. doi: 10.1093/oxfordjournals.pcp.a076232
Newsham, K. K., and Robinson, S. A. (2009). Responses of plants in polar regions to UVB exposure: a meta-analysis. Glob. Change Biol. 15, 2574–2589. doi: 10.1111/j.1365-2486.2009.01944.x
Paul, N. D., and Gwynn-Jones, D. (2003). Ecological roles of solar UV radiation: towards an integrated approach. Trends Ecol. Evol. 18, 48–55. doi: 10.1016/S0169-5347(02)00014-9
Peer, W. A., Bandyopadhyay, A., Blakeslee, J. J., Makam, S. I., Chen, R. J., Masson, P. H., et al. (2004). Variation in expression and protein localization of the PIN family of auxin efflux facilitator proteins in flavonoid mutants with altered auxin transport in Arabidopsis thaliana. Plant Cell 16, 1898–1911. doi: 10.1105/tpc.021501
Rai, R., and Agrawal, M. (2008). Evaluation of physiological and biochemical responses of two rice (Oryza sativa L.) cultivars to ambient air pollution using open top chambers at a rural site in India. Sci. Total Environ. 407, 679–691. doi: 10.1016/j.scitotenv.2008.09.010
Rao, M. V., Paliyath, G., and Ormrod, D. P. (1996). Ultraviolet-B and ozone-induced biochemical changes in antioxidant enzymes of Arabidopsis thaliana. Plant Physiol. 110, 125–136. doi: 10.1104/pp.110.1.125
Rinnan, R., Saarnio, S., Hapala, J. K., Mörsky, S. K., Martikainen, P. J., Silvola, J., et al. (2013). Boreal peatland ecosystems under enhanced UV-B radiation and elevated tropospheric ozone concentration. Environ. Exp. Bot. 90, 43–52. doi: 10.1016/j.envexpbot.2012.10.009
Sakai, Y., and Yoshida, T. (1957). Studies on the Conditions Affecting the Outbreak of Physical Damping of Rice Seedlings, So-called “Murenae” in Frame Nursery. Part 1. Observation on the Oxidative Activity of Roots by Means of α-Naphthylamine Oxidation. Hokkaido: Japan National Agricultural Experiment Station.
Schopfer, P., and Liszkay, A. (2006). Plasma membrane-generated reactive oxygen intermediates and their role in cell growth of plants. Biofactors 28, 73–81. doi: 10.1002/biof.5520280202
Schurr, U., Walter, A., and Rascher, U. (2006). Functional dynamics of plant growth and photosynthesis—from steady-state to dynamics—from homogeneity to heterogeneity. Plant Cell Environ. 29, 340–352. doi: 10.1111/j.1365-3040.2005.01490.x
Soheila, A. H. (2000). Plant responses to ultraviolet-B (UV-B: 280–320 nm) stress: what are the key regulators? Plant Growth Regul. 32, 27–39. doi: 10.1023/A:1006314001430
Taylor, L. P., and Grotewold, E. (2005). Flavonoids as developmental regulators. Curr. Opin. Plant Biol. 8, 317–323. doi: 10.1016/j.pbi.2005.03.005
Teng, N., Wang, J., Chen, T., Wu, X., Wang, Y., and Lin, J. (2006). Elevated CO2 induces physiological, biochemical and structural changes in leaves of Arabidopsis thaliana. New Phytol. 172, 92–103. doi: 10.1111/j.1469-8137.2006.01818.x
Tripathi, R., and Agrawal, S. B. (2013). Interactive effect of supplemental ultraviolet B and elevated ozone on seed yield and oil quality of two cultivars of linseed (Linum usitatissimum L.) carried out in open top chambers. J. Sci. Food Agric. 93, 1016–1025. doi: 10.1002/jsfa.5838
Tripathi, R., Sarkar, A., Rai, P. S., and Agrawal, S. B. (2011). Supplemental ultraviolet-B and ozone: impact on antioxidants, proteome and genome of linseed (Linum usitatissimum L. cv. Padmini). Plant Biol. 13, 93–104. doi: 10.1111/j.1438-8677.2010.00323.x
VanLoocke, A., Betzelberger, A. M., Ainsworth, E. A., and Bernacchi, C. J. (2012). Rising ozone concentrations decrease soybean evapotranspiration and water use efficiency whilst increasing canopy temperature. New Phytol. 195, 164–171. doi: 10.1111/j.1469-8137.2012.04152.x
Vingarzan, R. (2004). A review of surface ozone background levels and trends. Atmos. Environ. 38, 3431–3442. doi: 10.1016/j.atmosenv.2004.03.030
Walker, T. S., Bais, H. P., Grotewold, E., and Vivanco, J. M. (2003). Root exudation and rhizosphere biology. Plant Physiol. 132, 44–51. doi: 10.1104/pp.102.019661
Walter, A., Silk, W. K., and Schurr, U. (2009). Environmental effects on spatial and temporal patterns of leaf and root growth. Annu. Rev. Plant Biol. 60, 279–304. doi: 10.1146/annurev.arplant.59.032607.092819
Wang, H., Gui, M., Tian, X., Xin, X., and Wang, T. (2017). Effects of UV-B on vitamin C, phenolics, flavonoids and their related enzyme activities in mung bean sprouts (Vigna radiata). In. J. Food Sci. Technol. 52, 827–833. doi: 10.1111/ijfs.13345
Waterman, P. G., and Mole, S. (1994). Analysis of Phenolic Plant Metabolites: The Methods in Ecology Series. Oxford: Blackwell Scientific Publications, 238.
Xu, W., Ding, G., Yokawa, K., Baluška, F., Li, Q. F., Liu, Y., et al. (2013). An improved agar-plate method for studying root growth and response of Arabidopsis thaliana. Sci. Rep. 3:1273. doi: 10.1038/srep01273
Yadav, S., Shrivastava, A. K., Agrawal, C., Sen, S., Chatterjee, A., Rai, S., et al. (2017). “Impact of UV-B exposure on phytochrome and photosynthetic machinery: from cyanobacteria to plants,” in UV-B Radiation: From Environmental Stressor to Regulator of Plant Growth, eds V. P. Singh, S. Singh, S. M. Prasad, and P. Parihar (Hoboken, NJ: John Wiley & Sons).
Yang, J., Zhang, J., Wang, Z., Zhu, Q., and Wang, W. (2001). Hormonal changes in the grains of rice subjected to water stress during grain filling. Plant Physiol. 127, 315–323. doi: 10.1104/pp.127.1.315
Yokawa, K., and Baluška, F. (2015). Pectins, ROS homeostasis and UV-B responses in plant roots. Phytochemistry 112, 80–83. doi: 10.1016/j.phytochem.2014.08.016
Yokawa, K., Kagenishi, T., and Baluška, F. (2013). Root photomorphogenesis in laboratory-maintained Arabidopsis seedlings. Trends Plant Sci. 18, 117–119. doi: 10.1016/j.tplants.2013.01.002
Zeuthen, J., Mikkelsen, T. N., Paludan-Müller, G., and Ro-Poulsen, H. (1997). Effects of increased UV-B radiation and elevated levels of tropospheric ozone on physiological processes in European beech (Fagus sylvatica). Physiol. Plant. 100, 281–290. doi: 10.1111/j.1399-3054.1997.tb04784.x
Zhao, T. H., Wang, J. L., Wang, Y., Sun, J. W., and Cao, Y. (2010). Effects of reactive oxygen species metabolic system on soybean (Glycine max) under exogenous chitosan to ozone stress. Bull. Environ. Contam. Toxicol. 85, 59–63. doi: 10.1007/s00128-010-0039-4
Zhao, Y., Aspinall, D., and Paleg, L. G. (1992). Protection of membrane integrity in Medicago saliva L. by glycinebetaine against the effects of freezing. J. Plant Physiol. 140, 541–543. doi: 10.1016/S0176-1617(11)80785-6
Keywords: flavonoids, endogenous hormones, enzyme activities, soybean roots, open-top chambers
Citation: Mao B, Wang Y, Zhao T-H, Tian R-R, Wang W and Ye J-S (2017) Combined Effects of Elevated O3 Concentrations and Enhanced UV-B Radiation of the Biometric and Biochemical Properties of Soybean Roots. Front. Plant Sci. 8:1568. doi: 10.3389/fpls.2017.01568
Received: 22 May 2017; Accepted: 28 August 2017;
Published: 11 September 2017.
Edited by:
Angeles Calatayud, Instituto Valenciano de Investigaciones Agrarias, SpainCopyright © 2017 Mao, Wang, Zhao, Tian, Wang and Ye. This is an open-access article distributed under the terms of the Creative Commons Attribution License (CC BY). The use, distribution or reproduction in other forums is permitted, provided the original author(s) or licensor are credited and that the original publication in this journal is cited, in accordance with accepted academic practice. No use, distribution or reproduction is permitted which does not comply with these terms.
*Correspondence: Tian-Hong Zhao, enRoMTk5OUAxNjMuY29t