- 1Dipartimento di Agraria, University of Naples Federico II, Portici, Italy
- 2School of Agricultural, Forestry, Food and Environmental Science, University of Basilicata, Potenza, Italy
Biochar is nowadays largely used as a soil amendment and is commercialized worldwide. However, in temperate agro-ecosystems the beneficial effect of biochar on crop productivity is limited, with several studies reporting negative crop responses. In this work, we studied the effect of 10 biochar and 9 not pyrogenic organic amendments (NPOA), using pure and in all possible combinations on lettuce growth (Lactuca sativa). Organic materials were characterized by 13C-CPMAS NMR spectroscopy and elemental analysis (pH, EC, C, N, C/N and H/C ratios). Pure biochars and NPOAs have variable effects, ranging from inhibition to strong stimulation on lettuce growth. For NPOAs, major inhibitory effects were found with N poor materials characterized by high C/N and H/C ratio. Among pure biochars, instead, those having a low H/C ratio seem to be the best for promoting plant growth. When biochars and organic amendments were mixed, non-additive interactions, either synergistic or antagonistic, were prevalent. However, the mixture effect on plant growth was mainly dependent on the chemical quality of NPOAs, while biochar chemistry played a secondary role. Synergisms were prevalent when N rich and lignin poor materials were mixed with biochar. On the contrary, antagonistic interactions occurred when leaf litter or woody materials were mixed with biochar. Further research is needed to identify the mechanisms behind the observed non-additive effects and to develop biochar-organic amendment combinations that maximize plant productivity in different agricultural systems.
Introduction
Biochar is a carbon-rich product defined as “a solid material obtained from the thermo-chemical conversion of biomass in an oxygen limited environment” (International Biochar Initiative [IBI], 2012). Biochar is generated through pyrolysis, i.e., a thermal process carried-out at temperatures that range from 250°C to >900°C and under limited oxygen availability. Many positive interplays with soils are related to the biochar including the liming effect (Van Zwieten et al., 2010), the increase of water retention capacity (Novak et al., 2012) and the capability to adsorb phytotoxic organic molecules (Oleszczuk et al., 2012), stimulate the activity of beneficial microbes (Warnock et al., 2007) and suppress soilborne pathogens (Bonanomi et al., 2015). The beneficial effects of biochar on crops production have been known since ancient times. In Amazon basin, pre-Columbian populations developed the “terra preta” soils, also known as “Amazonian dark earth,” by repeating cycles of vegetation burning combined with the application of organic amendments including leaf litter, nutrient-rich kitchen wastes, and fecal materials (Kammann et al., 2016). However, scientific evidence in support of such ancient agricultural practice has taken place only in the last two decades (Lehmann and Joseph, 2015). For these reasons, biochar is nowadays largely used as a soil amendment and commercialized worldwide (Jirka and Tomlinson, 2015).
The meta-analyses of Jeffery et al. (2011) and Biederman and Harpole (2013), however, highlight that the beneficial effect of biochar on crop productivity is context dependent, with several studies reporting negative crop responses (Wisnubroto et al., 2010; Calderón et al., 2015; Knox et al., 2015). In this regards, the combined use of biochar with other, non-pyrolyzed organic amendments (i.e., compost, manure, plant litter, etc.) has been proposed, but, to date, only incomplete results are available on this topic. On the other hand, a flourishing number formulations, in which biochar is combined with different types of organic matter to obtain the so called “terra preta”-like planting substrates, have been commercialized (e.g., Wolf and Wedig, 2007). The recent review by Kammann et al. (2016) acknowledges that very few studies that directly combined organic amendments with biochars are available. Furthermore, most of the research concerns co-composting, e.g., the technique where biochar is added to compost at the beginning of the process. Most of these studies reported that “activated” biochar by co-composting consistently promotes plant growth, with better performances than those observed when composts and biochars are used individually (Joseph et al., 2013; Schulz et al., 2013). As recently demonstrated, plant growth improvement by co-composted biochar is largely mediated by nutrients capture, especially nitrate, and their subsequent slow release (Kammann et al., 2015).
This body of evidence suggests that mixing biochar with fresh organic matter is a powerful factor to explicate the potential positive effects on higher plants by the application of such organic amendments. However, because of the limited and fragmented knowledge, reliable guidelines about the types and amount of organic materials that should be mixed with biochars to maximize plant growth are lacking. The chemical diversity of biochars, related to the quality of the initial organic feedstock and pyrolysis conditions, together with the large variety of organic matter types used in agriculture (e.g., composts, crop residues, humic substances, peat and organic wastes from agro-industry) define a great number of possible biochar-organic matter combinations. In this context, the general aim of this work was to study the effects of biochars and organic matters alone and in mixture on crop performances. For this purpose, we analyzed the effect of 10 biochars and 9 not pyrogenic organic amendment types (thereafter indicate as NPOA), alone or in all possible mixtures (i.e., 90 combinations), on lettuce growth (Lactuca sativa L.). We selected different organic matter types, ranging from compost to leaf litter, in order to cover materials with a wide spectrum of organic carbon chemistry and total nitrogen content. All organic materials were characterized by standard chemical analyses and by 13C-cross-polarization magic angle spinning (CPMAS) nuclear magnetic resonance (NMR) spectroscopy (Kögel-Knabner, 2002) to investigate the link between organic matter chemistry and crop responses. Specific aims of the study were to (i) assess the effect of 10 biochars, 9 NPOA types, and their 90 combinations on growth of lettuce; (ii) explore the relationships between organic feedstocks and biochar chemistry, as defined by 13C-CPMAS NMR spectroscopy and elemental analysis (pH, EC, C, N, C/N, and H/C ratios), and growth of lettuce; (iii) identify, starting from the initial chemical composition, the biochar/organic matter combinations that maximize plant growth.
Materials and Methods
Organic Amendment Collection
Nine NPOAs were selected because represent materials with a wide range of organic carbon chemistry and total nitrogen (N) content: F.O.R.S.U., is the organic fraction of municipal solid waste (Naples municipality, Southern Italy), wood chips from sawmill, Medicago sativa L. hay, Zea mays L. stalks, a compost from cattle manure, and four freshly fallen leaf litter types (Quercus ilex L., Fagus sylvatica L., Robinia pseudoacacia L., and Populus alba L.). In the case of leaf litter, for each species freshly abscised leaves were collected from randomly selected plants (N > 10), air dried for 25 days until reaching constant weight, and then was stored at room temperature.
Biochar Production
Ten biochar types were used in this study: two were purchased and eight produced in our laboratory. The eight biochar types were made from four feedstock (F.O.R.S.U., Medicago hay, Zea stalks, and wood chips) that were subject to pyrolysis at two temperatures (300 and 550°C) for 5 h in a muffle furnace. Activated carbon (AC) and a biochar from Fagus wood were purchased from Sigma–Aldrich Co., and a local market, respectively. All NPOAs and biochars were powdered in a blender to obtain <2 mm particles and then stored in air-tight containers.
Chemical Analyses and 13C-CPMAS NMR Characterization
Electrical conductivity (EC) and pH of the NPOAs and biochars were measured by using a pH-meter (Basic 20 CRISON) and conductivity meter (CRISON) in suspensions of 1:2.5 and 1:5 organic material:water, respectively. NPOAs and biochars were characterized for total H, C, and N content by flash combustion of micro samples (5 mg) using an Elemental Analyser NA 1500 (Fison 1108 Elemental Analyzer, Thermo Fisher Scientific).
All materials were characterized by 13C-CPMAS NMR obtained in the solid state under the same conditions to allow a quantitative comparison of spectra. A Bruker AV-300 spectrometer equipped with a 4 mm wide-bore MAS probe was used. NMR spectra were obtained with MAS of 13000 Hz of rotor spin, 1 s of recycle time, 1 ms of contact time, 20 ms of acquisition time, 2000 scans. Samples were packed in 4 mm zirconium rotors with Kel-F caps. The pulse sequence was applied with a 1H ramp to account for non-homogeneity of the Hartmann-Hahn condition at high spin rotor rates. The 13C-CPMAS NMR spectrum was automatically integrated to calculate the area of the peaks which appeared in the chosen region. Seven spectral regions were selected as identified by previous studies (Kögel-Knabner, 2002; Bonanomi et al., 2016a): 0–45 ppm = alkyl C; 46–60 ppm = methoxyl and N-alkyl C; 61–90 ppm = O-alkyl C; 91–110 ppm = di-O-alkyl C; 111–140 ppm = H- and C- substituted aromatic C; 141–160 ppm O-substituted aromatic C (phenolic and O-aryl C); 161–190 ppm carboxyl C.
Plant Bioassay
A plant bioassay was carried-out to determine the effect of NPOAs and biochars on the growth of lettuce (Lactuca sativa L.), a vegetable cultivated worldwide. Seeds of lettuce were purchased at a local market.
The bioassay was carried-out with 9 NPOAs and 10 biochars used pure plus the untreated control. In addition, all possible NPOA-biochar mixtures were made, resulting in 90 organic matter combinations, replicated 10 times with a total of 1,100 experimental units. Each organic amendment was applied in a pot alone at 2% (10 g-1 per pot of dry matter), and carefully mixed with the soil; the unamended soil was also mixed in the same manner. The pots of the two-organic matter mixtures (90 combinations) were amended with 2% of dry organic amendment (10 g-1 per pot) having a loading ratio of 50:50. The unamended soil was set as the control.
For this bioassay, about 800 kg of a previously characterized fertile soil were collected from the top 20 cm layer in an agricultural field. Soil was collected in a very productive area of about 5,000 ha cultivated under greenhouses located in the Salerno area (Southern Italy). The study site is characterized by a Mediterranean climate with a mean annual temperature of 15.9°C and monthly temperatures ranging from 23.6°C in August to 9.0°C in January. Mean annual rainfall does not exceed 988 mm with a relatively dry summer (84 mm). Low-technology, unheated polyethylene-covered greenhouses (height ∼4 m) are the main crop protection structures used in this area (Bonanomi et al., 2016b). According to Soil Survey Staff classification (Soil Survey Staff, 1998), the soil was Lithic Haplustolls (Supplemenatry Table S1). The soil was sieved in the laboratory (mesh size < 2 mm). Pots (14 cm diameter and 16 cm height) were filled with 500 g of air-dried soil and planted with 1 pre-germinated 7-day-old seedlings. Then, pots were placed in a greenhouse (20 ± 5°C during the day and 14 ± 5°C at night) following a completely random design with regular rotation every 10 days. Pots were wetted with distilled water every 3 days until water holding capacity was reached. After 90 growing days, above-ground plant part were harvested, dried (80°C in a ventilated oven until constant weight was reached), and dry weighted.
Data Analysis
Not Pyrogenic Organic Amendment and Biochar Chemistry
One-way ANOVA was used to assess differences in litter chemistry as evaluated by chemical analyses and 13C CPMAS NMR. Spectral data from 13C NMR analysis were also explored with a multivariate cluster analysis (CA) in order to provide a synthetic representation of the variability of NPOA and biochar chemistry.
Not Pyrogenic Organic Amendment and Biochar Used Pure
Concerning the bioassay results, the species response data were expressed as a percentage of the respective control, then subjected to one-way ANOVA to assess the effect of NPOAs and biochars used pure on the growth of target plant species. Pairwise differences were tested using Duncan post hoc test, and statistical significance was set at P < 0.05. To analyze the relationship between the target species in the bioassays and NPOA and biochar biochemistry, correlation was extensively calculated between plant growth and 13C NMR data, as well as basic chemical parameters (i.e., pH, EC, C and N content, C/N and H/C ratios) for the same test material. Plant growth was also tested for correlation with 13C-CPMAS NMR spectral regions (N = 7) selected from reference literature (Kögel-Knabner, 2002; Bonanomi et al., 2016a). Finally, 13C NMR spectral data were submitted to multivariate principal component analysis (PCA), in order to assess the multiple relationships among NPOM and biochar chemistry with plant growth. Following the approach indicated by Legendre and Legendre (1998) for supplementary variables, bioassay growth was also plotted as a loading vector in the bi-dimensional PCA space even if it was not used to compute the eigenvalues of the same ordination space. Analyses were performed with Statistica 10 (Statsoft, Inc., Tulsa, OK, United States).
Not Pyrogenic Organic Amendment and Biochar Used in Mixture
Mixed NPOMs and biochars were analyzed by a traditional method used to specifically evaluate synergism and antagonism of non-additive effects. In this case, lettuce growth observed in the presence of different mixtures have been compared with the values expected from the component NPOA and biochar used pure. This approach resembles that used to address the effect of litter mixing on mass loss during decomposition (Wardle et al., 1997; Bonanomi et al., 2010). For all mixtures, the expected lettuce growth was calculated as described by Salamanca et al. (1998) as follow:
where ELG is the expected mass loss (%), OLGi is the observed lettuce growth in case of amendment with NPOM or biochar alone, and PLGi is the proportion between NPOM and biochar in the mixture. For each NPOA-biochar mixture, paired t-test was used to assess significant differences between observed and expected values (Salamanca et al., 1998; Wardle et al., 2006). Classification of NPOA-biochar interactions about lettuce growth in the mixtures followed that used by Gartner and Cardon (2004): additive (no significant differences between observed and expected values), non-additive synergistic (observed higher than expected), non-additive antagonistic (observed lower than expected).
Finally, with the aim of identifying the chemical parameters of the NPOAs and biochars that maximize plant growth when the materials were mixed, a linear regression was performed using the ‘lm’ function in R version 3.3.0 (R Core Team, 2016). With this approach, we identified the significance of a set of descriptors of NPOA and biochar chemistry (pH, EC, C and N content, C/N and H/C ratios, spectral regions from 13C CPMAS NMR) used as predictors on a response variable (i.e., observed-expected ratio of plant growth). In addition, the quantification of the relative contribution (expressed as percent of explained variance) of each predictor for the response variable was assessed by using the natural decomposition method (lmg, Lindeman et al., 1980) as well as bootstrap estimates for the confidence intervals of the metrics, with the package ‘relaimpo’ (Grömping, 2006).
Results
Biochar and Not Pyrogenic Organic Amendment Chemistry
Initial nutrient concentrations and organic C chemical fractions significantly varied between biochars and NPOAs (Table 1). The highest N concentration was found in leaf litter from N fixer species (i.e., Medicago and Robinia), in compost, F.O.R.S.U. and biochars derived from N rich feedstocks (Table 1). C/N ratio showed large variations, ranging from the 639 value of wood biochar made at 300°C to the 6.41 value of Medicago hay (Table 1). EC was high for compost, F.O.R.S.U., Medicago hay and biochars derived from these feedstocks (Table 1). For NPOAs the pH showed small variations, ranging from 5.35 to 7.10 values of Fagus and Medicago litter, respectively. Biochar, instead, showed a much larger variation of pH, ranging from 5.67 of AC to 11.60 of Medicago biochar made at 550°C (Table 1). The H/C ratio of NPOAs was in all case >1.71 with the only exception of compost (1.32). In contrast, pyrolyzation drastically reduced the H/C ratio giving values ranging from 0.21 of AC to 1.11 of Medicago biochar made at 550°C (Table 1).
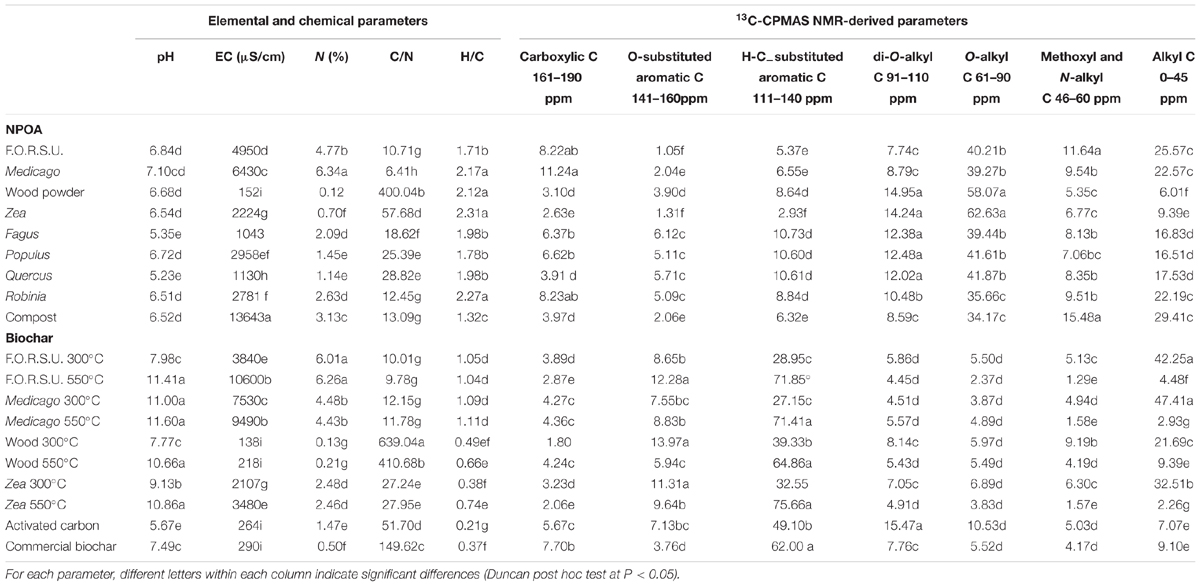
TABLE 1. Main characteristics of not pyrogenic organic amendment (NPOA) and biochar assessed by elemental and 13C cross-polarization magic angle spinning CPMAS nuclear magnetic resonance (NMR) analyses.
The 13C-CPMAS NMR data of NPOAs and biochars, with regard to C molecular types corresponding to reference spectral regions, revealed consistent changes of the material organic chemistry (Table 1). Among NPOAs, 13C NMR spectra showed consistent differences of C molecular types, with major chemical changes observed for the alkyl-C fraction (0–45 ppm), significantly lower for Zea and wood, and the methoxyl and N-alkyl C fraction (46–60 ppm) that was higher in F.O.R.S.U., compost, Medicago, and Robinia litter (Table 1). The relative abundance of the O-alkyl-C fraction (61–90 ppm) was higher for wood and Zea, and quite low for compost (Table 1). The carboxylic C fraction (161–190 ppm) ranged from 11.24 to 2.63% of Medicago hay and Zea residues, respectively. The H-C-substituted aromatic C region (111–140 ppm) was very high for lignin rich plant litter (i.e., Fagus, Populus, and Quercus) (Table 1). Interestingly, the chemistry of biochars was dramatically different from that of NPOAs. In particular, di-O-alkyl-C, methoxyl and N-alkyl C, and carboxylic C fractions were very low compared to those of NPOAs (Table 1). The O-alkyl-C region was abundant only in biochars made at 300°C, but disappeared in all biochars made at higher temperatures (Table 1). In contrast with the other spectral regions, the aromatic C fractions (141–160 ppm, but especially that at 111–140 ppm) showed a sharp increase in biochars compared to NPOAs, with the highest values recorded for biochar made at temperatures >300°C (Table 1).
Cluster analysis of NPOA and biochar spectra showed a remarkable difference between the two groups (Figure 1). NPOAs were mostly grouped together at low distance values, with only wood and Zea that had major distances, indicating some species-specific chemical differences (Figure 1). The dendrogram of biochars based on 13C NMR spectra showed two main clusters segregating at high distances and including materials made at either low (300°C) and high-intermediate (550°C, AC and commercial biochar) temperatures (Figure 1). Moreover, within the low-temperature cluster, most samples belonging to the same feedstock were consistently aggregated at the lowest distance levels, while within the high-temperature cluster sample aggregation was independent of the feedstock of origin (Figure 1).
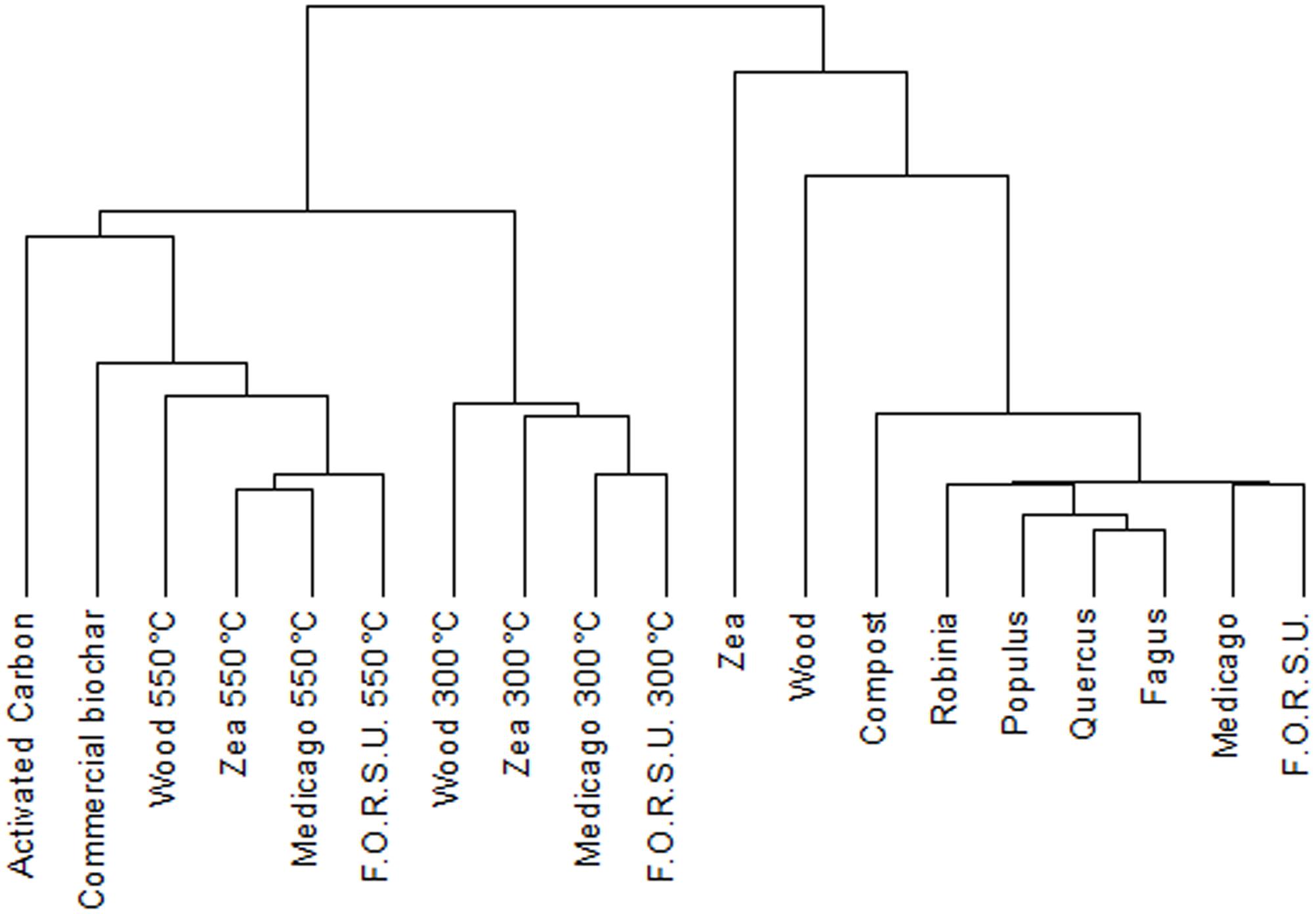
FIGURE 1. Dendrogram from cluster analysis of the 19 organic materials (10 biochar and 9 not pyrogenic organic amendment).
Effect of Biochar and Not Pyrogenic Organic Amendment on Plant Growth
The effect of NPOAs and biochars used alone on plant growth was largely affected by the type material (Figures 2A,B). With NPOAs lettuce showed various responses, being inhibited by Fagus, Quercus, and Zea, not affected by wood powder and promoted by the remaining materials, especially Medicago hay and F.O.R.S.U. (Figure 2A). Among biochars, five materials stimulated lettuce growth compared to untreated control, three had no significant effects and only two showed a moderate inhibition (i.e., F.O.R.S.U. and Zea biochar made at 300°C) (Figure 2B).
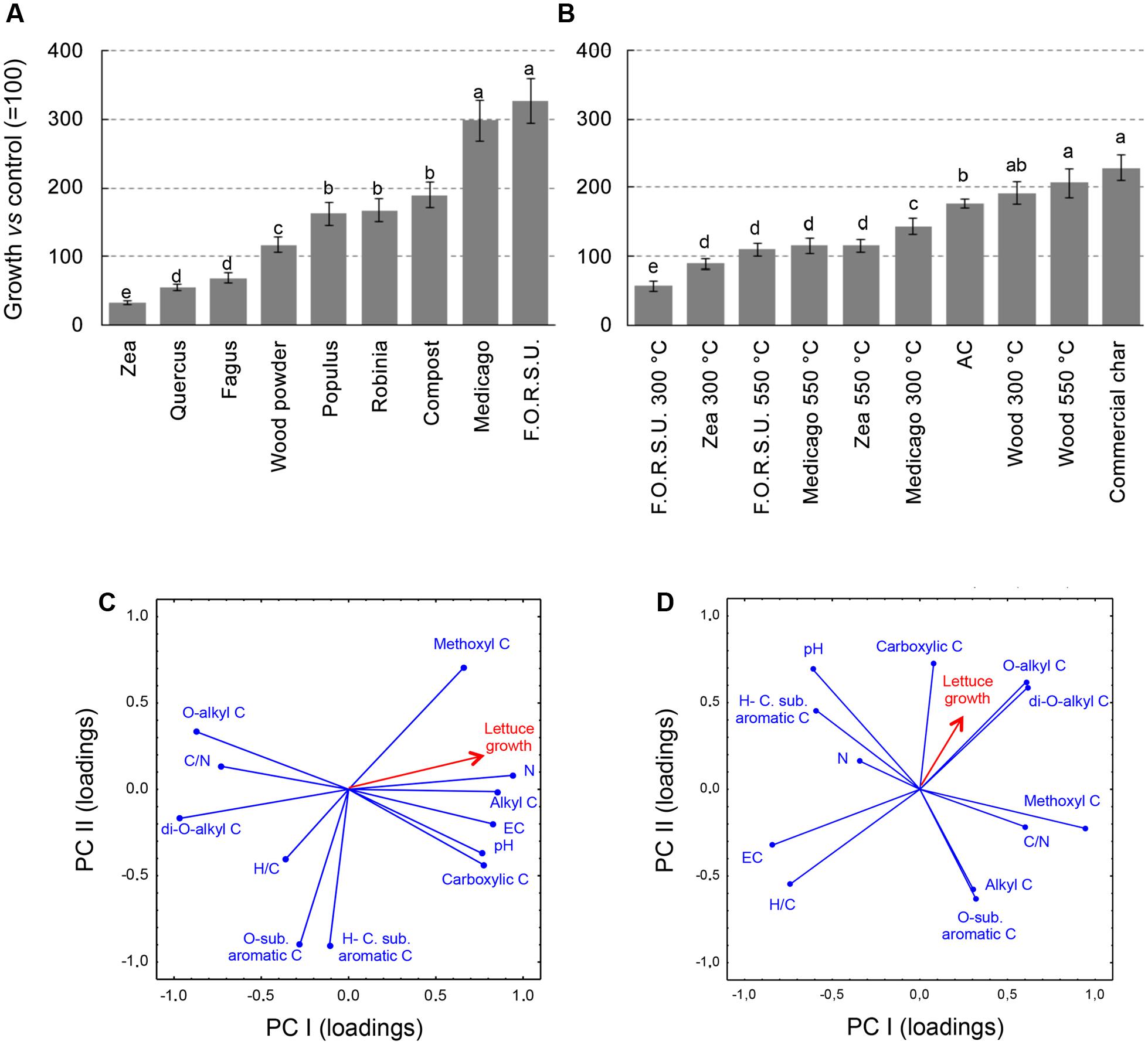
FIGURE 2. Growth of lettuce (Lactuca sativa) plants in pot amended with not pyrogenic organic amendment (A) and biochar (B) at 2% (w/w). Values are expressed as percentage of untreated controls (=100%). Different letters indicate significant difference with comparison within each organic amendment type (Duncan test at P < 0.05). Principal component analysis (PCA) between not pyrogenic organic amendment (C) and biochar (D), based on basic chemical parameters and seven 13C NMR spectral regions with lettuce growth. Panel also shows target lettuce growth as supplementary variables following Legendre and Legendre (1998).
The PCA results provided a synthetic picture of the chemistry-dependent effects on the target plant responses caused by NPOAs and biochars (Figures 2C,D). PCA provided a satisfactory ordination of the NPOA and biochar chemical parameters in relation to the sensitivity of the tested plant, with the eigenvalues of the first two components accounting for 76.53% (53.13 and 23.40%) and 62.47% (35.82 and 26.65%) of the total variance for NPOAs and biochars, respectively. Figures 2C,D reports the loading vectors that represent the organic matter chemistry parameters plotted in the bi-dimensional space. For NPOAs, the first and second PCA components highlight the importance of pH, N content, EC, C/N ratio, and several NMR regions to affecting plant growth. Lettuce growth was largely explained by the negative correlations with C/N ratio as well as O-alkyl C and di-O-alkyl C regions, and positive correlations with EC, N content, and the carboxylic C, methoxyl C, and alkyl C NMR regions (Figure 2C). For biochars, instead, lettuce growth was explained to its negative correlations with EC and H/C ratio and positive correlations with O-alkyl C, di-O-alkyl, and carboxylic C NMR regions (Figure 2D).
Synergism, Antagonism, and Additive Effects in NPOA-Biochar Mixtures
Non-additive and additive effects on plant growth were found in 87.78 and 12.22% of the tested mixtures, respectively (Figure 3). Antagonistic responses were more common than synergistic interactions, with 59 and 20 cases, respectively. The magnitude of synergistic and antagonistic interactions ranged from -87.99% to +156.51% of the expected plant growth (Figure 3).
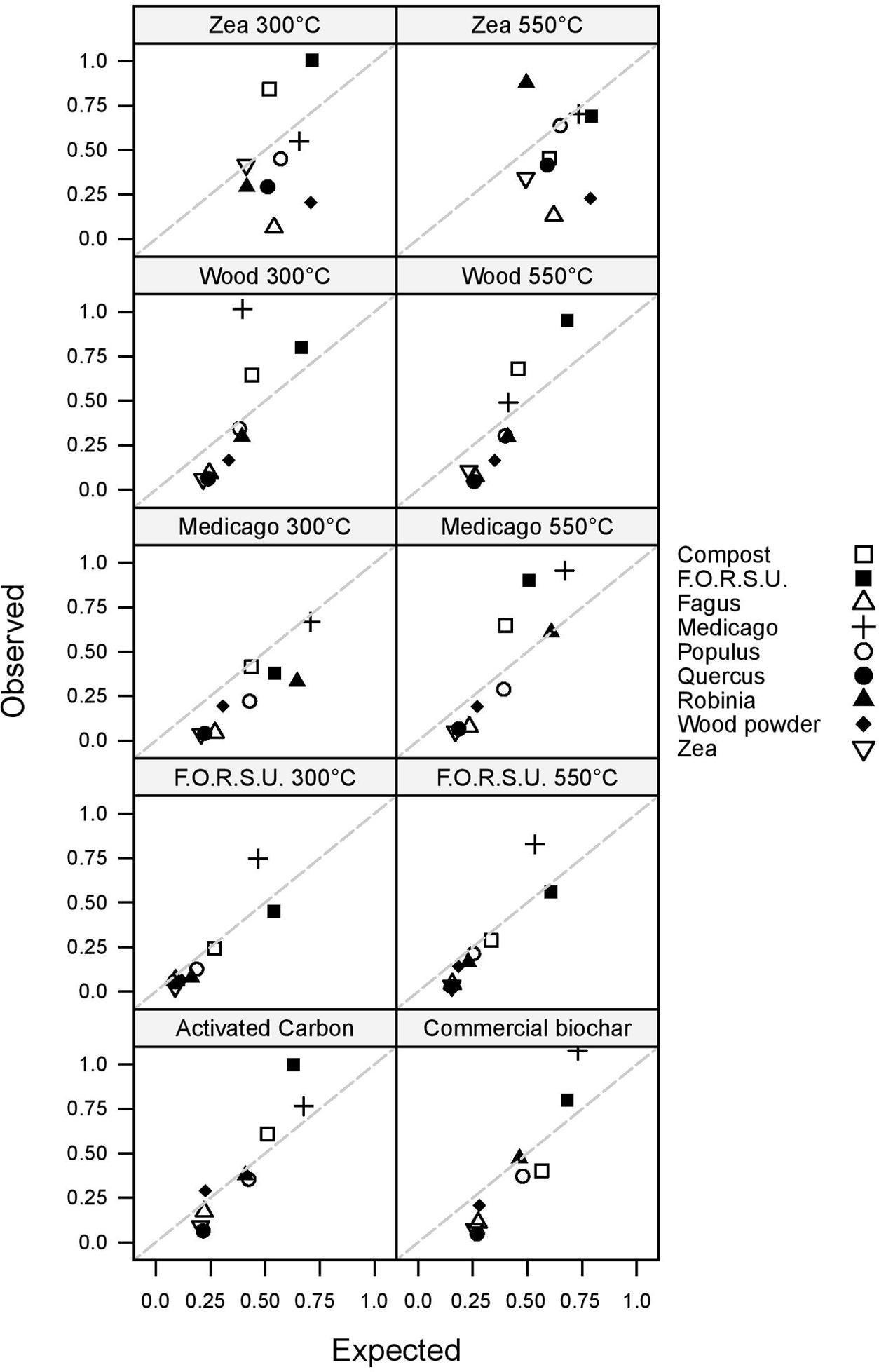
FIGURE 3. Observed vs. expected values of lettuce growth in mixtures composed of not pyrogenic organic amendment and biochar. Values above and below the 1:1 dashed line indicate synergistic and antagonistic interactions, respectively.
Biochar and NPOA types in the mixture affected the outcome of non-additive interactions. For instance, when Medicago hay and F.O.R.S.U. were used as NPOA, synergistic interactions with biochars were recorded in 7 and 6 out of 10 cases, respectively (Figure 3). The deviation between observed and expected growth among all biochar types was +36.74%, +18.72%, and +15.74%, for Medicago, F.O.R.S.U. and compost, respectively (Figure 4). Mixtures with Populus and Robinia litter showed synergistic, non-additive, and antagonistic interactions, with the latter being more common than the other two (Figure 3). In contrast, the mixtures containing Quercus litter as NPOA gave an antagonistic interaction in 90% of the cases, with no synergistic responses (Figure 3). Similar results were found for Fagus and Zea litter as well as with wood which showed 80% of antagonistic interactions and 20% of non-additive responses (Figure 3). The deviation between observed and expected growth with all biochar types was negative for Robinia and wood powder (Figure 4). For Quercus, Fagus, and Zea litter, the deviation between observed and expected growth was -64.05%, -63.15%, and -58.42%, respectively (Figure 4).
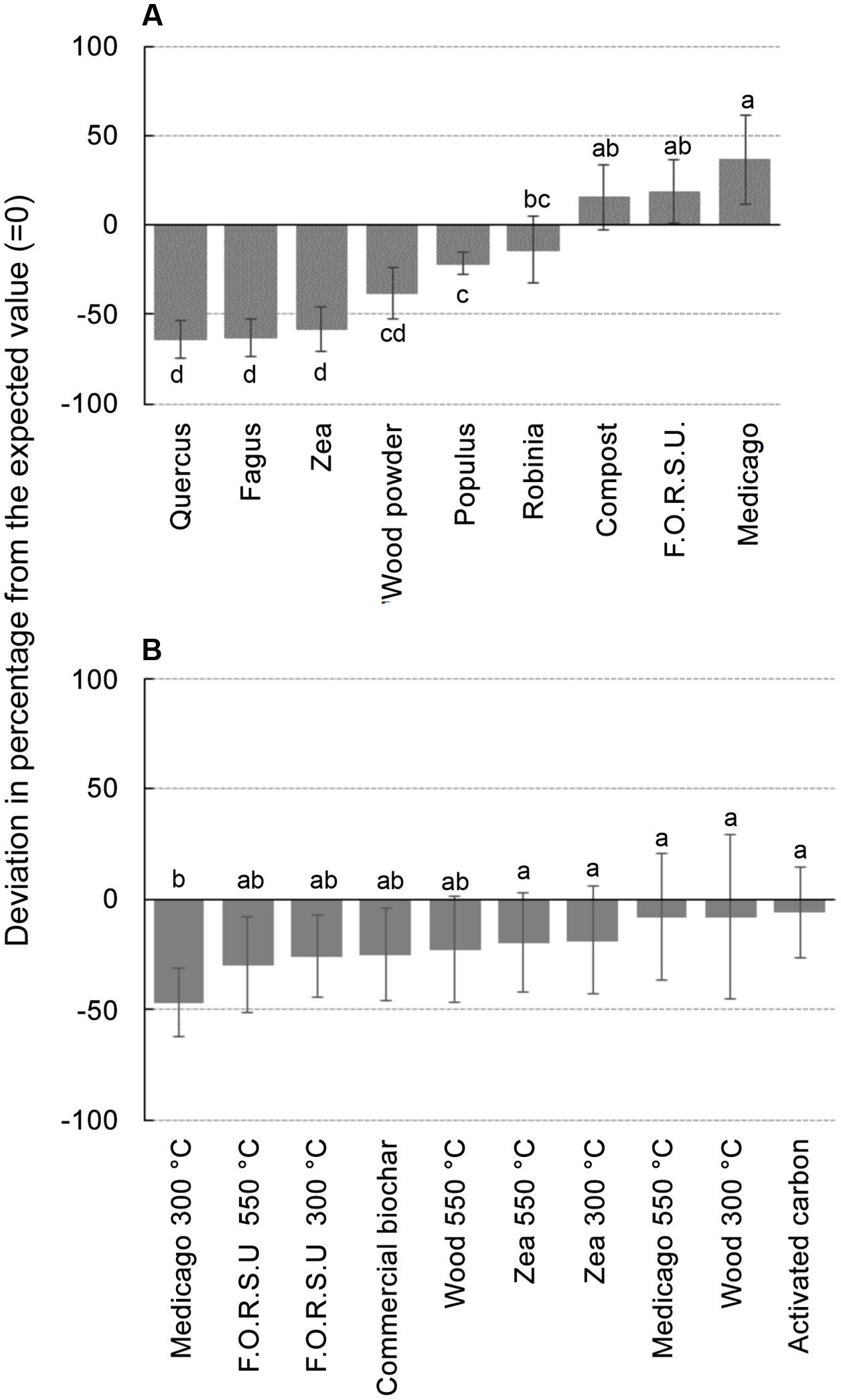
FIGURE 4. Deviation between observed growths of lettuce in different mixtures compared with the calculated values expected from the growth observed with not pyrogenic organic amendment (NPOA) and biochar used in isolation. In (A) vales are the average of 10 biochar combined with each NPOA type, in (B) values are the average of 9 NPOA combined with each biochar type. Different letters indicate significant differences (Duncan test at P < 0.05).
With biochars, the interactions were additive, i.e., the deviation from expected values was not significant, for AC, Zea, and wood biochars made at 300 and 550°C and for Medicago char made at 550°C. For the remaining biochars (commercial, Medicago 300°C, and F.O.R.S.U. 300 and 550°C), the deviation from expected values was negative with all NPOA types, meaning that antagonist interactions were more important (Figure 4).
Predicting Non-additive Effects from Not Pyrogenic Organic Amendment and Biochar Chemistry
Deviation from expected values are adequately described by the linear model [F(9,80) = 8.92, P < 0.001, Figure 5]; half of the total variance in observed-expected ratio of plant growth is explained by the chemistry of the mixtures. In particular, the significance of individual coefficients indicated a positive influence of carboxylic C (161–190 ppm), C/N ratio, and N content (for P < 0.001, P < 0.01, and P < 0.05, respectively) and a negative effect of H/C ratio (for P < 0.01) on the observed-expected ratio of growth. On the other hand, EC and the other C-types corresponding to NMR spectral regions did not reveal statistical significance. Moreover, the relative explained variance vary drastically among chemical parameters, i.e., between 3.5 and 20.3% (Figure 5). In this regard, it is noteworthy that the significant variables that contributed the most (20.3% of the relative explained variance) were the carboxylic C (161–190 ppm) followed by the H/C ratio (15.0%), N content (14.8%), and C/N ratio (7.2%). The residual portion of the relative variance is attributed to the non-significant variables.
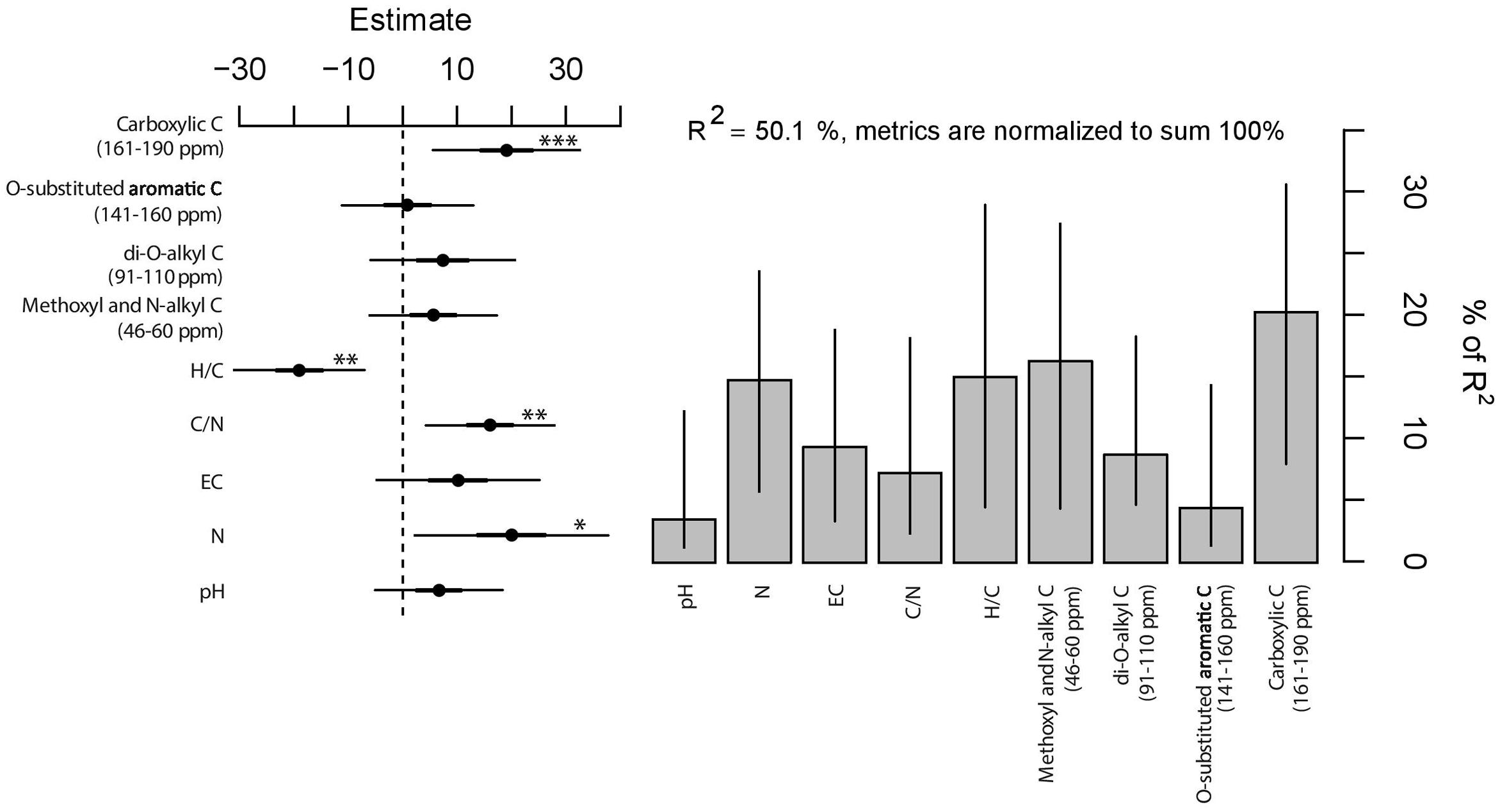
FIGURE 5. Estimates (filled circles) and 95% confidence interval (bars) of linear regression (left panel). ∗∗∗, ∗∗, and ∗ indicate significance for P < 0.001, P < 0.01, and P < 0.05, respectively. Right panel shows the relative importance (y-axis, percentage of variance explained) of predictors with the bootstrapped 95% confidence interval (bars).
Discussion
Pure Biochar and Not Pyrogenic Organic Amendment
Biochars and NPOAs used pure have contrasting effects on the growth of lettuce, showing clear relationships with their chemical characteristics. Effects of NPOAs on lettuce growth range from intense inhibition (-67% by Zea residues) to strong stimulation (+226% by F.O.R.S.U.). The major inhibitory effect was found with N poor materials characterized by high C/N and H/C ratios. Our finding of a severe plant root inhibition by undecomposed litter of Fagus, Quercus, and Zea is consistent with previous studies carried-out in natural ecosystems (Dorrepaal et al., 2007; Lopez-Iglesias et al., 2014; Meiners, 2014). Lettuce growth inhibition by undecomposed leaf litter can partially be attributed to microbial N immobilization (Hodge et al., 2000) and/or to the phytotoxic activity of putative allelopathic compounds (Bonanomi et al., 2011). The N immobilization hypothesis sustains that when organic matter with high C/N ratio is incorporated in soil reduce N availability, thus impairing root system development. The main mechanism is related to the behavior of saprophytic microorganisms in soil. In fact, to efficiently exploit soil organic matter microbes require both organic C and N in a relatively constant stoichiometric ratio. Organic C or N limit microbial growth, and when C/N ratio of organic matter is above the threshold value of ∼30–35 mineral N is taken from the soil, incorporated in microbial biomass and temporarily immobilized (Hodge et al., 2000). Such a hypothesis is indirectly supported by the present work, because plant growth is inversely related to substrate C/N ratio that, in turn, is directly correlated to N immobilization (Palm et al., 2001). However, C/N ratio can only partially explain the observed inhibitory effect because the wood, characterized by the highest C/N ratio, had a neutral effect on plant growth. This discrepancy could be explained by the quality of organic C of the NPOA. We found, in fact, that plant growth was inversely related to the relative abundance of the O-alkyl and di-O-alkyl NMR regions that are mainly associated with sugar and polysaccharides (Kögel-Knabner, 2002). This result suggests that organic amendments rich in labile C, e.g., sugars and relatively simply polysaccharides, may induce a faster N immobilization with a more negative impact on crop growth than materials rich in lignin (e.g., powdered wood). An alternative hypothesis is that plant litter can release, either directly or mediated by microbial activity, allelopathic compounds with harmful effects on root growth (Bonanomi et al., 2011). The identification of the ultimate causes of litter inhibitory is out of the aim of this work, but our approach by using 13C NMR indicates that lettuce growth is negatively affected by O-alkyl and di-O-alkyl C, but positively associated with signals related to plant tissue lignification. On the other hand, we found that lettuce growth was promoted by NPOAs with a relatively high content of methoxyl C and carboxyl C NMR regions, as well as a high N content. These results confirm the well-known positive effect of mineral and organic N sources on plant development (Marschner, 2012).
Concerning biochars, the effect on lettuce growth was less variable than that observed with NPOAs. Lettuce response ranged from moderate inhibition with F.O.R.S.U. biochar made at 300°C, to a highly significant stimulation observed with AC and the three biochars derived from wood. 13C NMR spectra showed that during pyrolysis all materials are subjected to a decrease of labile C molecular compounds, especially at high temperatures, and a relative increase of aromatic fractions. Noteworthy, all biochars pyrolyzed at 300°C showed a peak in the alkyl fraction, mainly related to aliphatic compounds, including the so-called bio-oils (Amonette and Joseph, 2009). However, the alkyl C region was absent in biochar made at 550°C or higher temperatures (e.g., AC and commercial wood biochar). Biochars made at high temperature or derived from wood sources showed a quite low H/C atomic ratio. This index, which has been proposed to define biochar quality in the context of bioremediation (Xiao et al., 2016), seems to be the best parameter to identify the biochar with the ability to promote plant growth. Finally, it is notable that total N content of biochars (ranging from 0.13 to 6.26% of wood 300°C and F.O.R.S.U. 550°C, respectively) had a limited power to explain its effect on lettuce growth. Probably, during pyrolysis most of the organic N was gradually transformed into pyridine-like compounds, with a consequent drastic reduction of its bio-availability for plants (Bagreev et al., 2001; Chan and Xu, 2009).
Not Pyrogenic Organic Amendment-Biochar Mixtures
In our experiments non-additive interactions, either synergistic or antagonistic, were prevalent. However, the majority of these interactions showed antagonistic effects, i.e., plant growth was lower than that expected by using each organic amendment alone. When biochars were mixed with N rich materials (i.e., Medicago hay, compost or F.O.R.S.U.), synergistic interactions were prevalent.
According to the recent review of Kammann et al. (2016), co-composted biochars have a remarkable plant growth-promoting effect compared to biochars used pure, but no-systematic studies were done to understand the interactive effects of biochars with NPOAs. In our experiment, additive effects were rare considering the expected vs. observed pooled data (11 cases out of 90 combinations). Contrarily to the general trends reported in the literature (Kammann et al., 2016), antagonistic interactions were more common than synergistic ones. However, some important methodological differences between the present and previous studies should be considered. In this study, the organic substrates mixed with biochars span an ample range of N content and C chemistry, while previous studies used only compost (Joseph et al., 2013; Schulz et al., 2013), or selected nutrient-rich materials such as urine (Schmidt et al., 2015).
Our results, being based on a wide range of NPOA types, provided a complete picture to understand the complex relationships between biochar and organic amendments. First, our data highlight that NPOA identity is more important than biochar types as a determinant of plant growth in the mixture. In fact, the deviation from expected values ranged from -29 to -5% for biochar, while for NPOAs the deviation spans from -64 to +36%. This means that most biochars appear suitable as a component of the mixture, with the exception of biochar made from F.O.R.S.U. and Medicago at 300°C. The same is not true for NPOAs, because only some materials are able to synergize when are used in combination with biochars. Synergistic interactions were observed with N rich, lignin poor substrates (i.e., compost, F.O.R.S.U., and Medicago hay). This result is in agreement with previous findings concerning biochar co-composting, where the best synergism was achieved blending C rich biochars with N rich organic materials (Kammann et al., 2016). The mechanisms proposed to explain the synergism between biochars and compost is based on the improvement of soil structure and water availability (Novak et al., 2012), the promotion of beneficial microbes (Warnock et al., 2007), and the enhanced plant-available nutrients (Liu et al., 2012). A recent study (Kammann et al., 2015) showed that co-composted biochars became highly enriched with nutrients (i.e., nitrate, ammonium, and phosphate) and dissolved organic carbon that is slowly released in soil. In addition to nutrients, low- and high-molecular weight organic C compounds can accumulate on biochar surfaces and in pores, having the potential to affect plant growth. In fact, many low-molecular weight organic C compounds are known to be phytotoxic at high concentrations, but have hormonal effects at low concentrations (Joseph et al., 2013). In this regards, Medicago sativa hay and F.O.R.S.U. could be phytotoxic if applied at high rate (Chefetz et al., 1996; Chung et al., 2000), but in presence of biochars such a negative effect could be reversed. Our study, however, was not designed to identify the mechanisms behind antagonistic and synergistic interactions between NPOAs and biochars. In the soil-biochar-NPOA mixtures, all the aforementioned cited mechanisms could be operative, but further studies are needed to clarify their relative contribution.
This study is the first reporting antagonist interactions between NPOAs and biochars. The occurrences of antagonistic interactions are well predictable based on the chemistry of the original components. Antagonisms were observed with wood powder and all leaf litter types, materials which are characterized by low N content combined with a high lignin content and high H/C ratio. Noteworthy, the combination among biochars with Robinia leaf litter showed an intermediate behavior, with several additive interactions and some moderate antagonistic interactions. This leaf litter is the only material characterized by a high N concentration and a relatively high lignin content. These results clearly indicate that the combination of biochars with low quality litter types (i.e., high lignin, high H/C ratio, and low N content) would enhance the probability of antagonistic interactions on plant growth. The mechanisms underlying such antagonist interactions are unknown, although a short-term, intense N immobilization could be hypothesized.
Conclusion
This is the first study that systematically explored the interaction between biochars and organic amendments mixed in the soil and demonstrates that non-additive interactions, either synergistic or antagonistic, were prevalent. The results provided evidence that, at least in the short-term, chemical quality of NPOAs is more important than biochar characteristics in affecting plant responses. In addition, we found that the final effect of the mixtures can be predictable based on the chemical characteristic of their components, with synergistic interactions being prevalent when N rich, lignin poor materials are mixed with biochars. On the contrary, antagonistic interactions occurred when leaf litter or woody materials were mixed with biochars. We acknowledge the limitations of this study which was carried-out by using only one soil type, one target species, and one single loading ratio. Although the selected NPOAs represent a wide range of organic matter chemistry and nitrogen content, several common organic amendments like peat, fresh manure, and humus-like compounds were not included. Therefore, understanding the interactions between biochar and the above-mentioned organic amendment should not be overlooked, with special attention to humic substances that promote root proliferation and growth (García et al., 2016).
Mixing NPOAs with biochar have great potential for plant growth promotion, but their variable performances may be a limiting factor for their extensive use in agriculture. It appears that the positive properties of specific NPOA-biochar combinations far outweigh the growth promotion observed when their component were used separately. However, as long as the impact of NPOA-biochar blending on plant growth remains unpredictable, farmers may be justified in ignoring it as a profitable agronomic technique. In this regard, among other challenges, (i) developing practice guidelines that to ensure reliable biochar mixture performance on plant productivity in different agricultural systems and, (ii) get insight in the chemical and microbiological nature at the base of the synergistic as well the antagonistic interactions among biochar, not-pyrogenic organic matters and soil are strong efforts that researchers will face forward.
Author Contributions
GB conceived the project. FI, GC, BN, and NL collected litter samples, produced the biochar, performed the experiments, and make chemical analyses. AR, GB, and AS analyzed the data. The manuscript was written by GB, FS, and AS with some contributions from all the other authors. All authors read and approved the final version of the manuscript.
Conflict of Interest Statement
The authors declare that the research was conducted in the absence of any commercial or financial relationships that could be construed as a potential conflict of interest.
Acknowledgments
The 13C-CPMAS NMR measurements were performed at the CERMANU-Interdepartmental Research Centre, University of Napoli Federico II.
Supplementary Material
The Supplementary Material for this article can be found online at: http://journal.frontiersin.org/article/10.3389/fpls.2017.01570/full#supplementary-material
References
Amonette, J. E., and Joseph, S. (2009). “Characteristics of biochar: microchemical properties,” in Biochar for Environmental Management: Science and Technology, eds J. Lehmann and S. Joseph (London, UK: Earthscan),k33–52.
Bagreev, A., Bandosz, T. J., and Locke, D. C. (2001). Pore structure and surface chemistry of adsorbents obtained by pyrolysis of sewage sludge-derived fertilizer. Carbon 39, 1971–1979. doi: 10.1016/S0008-6223(01)00026-4
Biederman, L. A., and Harpole, W. S. (2013). Biochar and its effects on plant productivity and nutrient cycling: a meta-analysis. GCB Bioenergy 5, 202–214. doi: 10.1111/gcbb.12037
Bonanomi, G., De Filippis, F., Cesarano, G., La Storia, A., Ercolini, D., and Scala, F. (2016b). Organic farming induces changes in soil microbiota that affect agro-ecosystem functions. Soil Biol. Biochem. 103, 327–336. doi: 10.1016/j.soilbio.2016.09.005
Bonanomi, G., Incerti, G., Antignani, V., Capodilupo, M., and Mazzoleni, S. (2010). Decomposition and nutrient dynamics in mixed litter of Mediterranean species. Plant Soil 331, 481–496. doi: 10.1007/s11104-009-0269-6
Bonanomi, G., Incerti, G., Barile, E., Capodilupo, M., Antignani, V., Mingo, A., et al. (2011). Phytotoxicity, not nitrogen immobilization, explains plant litter inhibitory effects: evidence from solid-state 13C NMR spectroscopy. New Phytol. 191, 1018–1030. doi: 10.1111/j.1469-8137.2011.03765.x
Bonanomi, G., Ippolito, F., and Scala, F. (2015). A “black” future for plant pathology? Biochar as a new soil amendment for controlling plant diseases. J. Plant Pathol. 97, 223–234.
Bonanomi, G., Ippolito, F., Senatore, M., Cesarano, G., Incerti, G., Saracino, A., et al. (2016a). Water extracts of charred litter cause opposite effects on growth of plants and fungi. Soil Biol. Biochem. 92, 133–141. doi: 10.1016/j.soilbio.2015.10.003
Calderón, F. J., Benjamin, J., and Vigil, M. F. (2015). A comparison of corn (Zea mays L.) residue and its biochar on soil C and plant growth. PLOS ONE 10:e0121006. doi: 10.1371/journal.pone.0121006
Chan, K. Y., and Xu, Z. (2009). “Biochar: nutrient properties and their enhancement,” in Biochar for Environmental Management: Science and Technology, eds J. Lehmann and S. Joseph (London, UK: Earthscan),k67–84.
Chefetz, B., Hatcher, P. G., Hadar, Y., and Chen, Y. (1996). Chemical and biological characterization of organic matter during composting of municipal solid waste. J. Environ. Qual. 25, 776–785. doi: 10.2134/jeq1996.00472425002500040018x
Chung, I.-M., Seigler, D., Miller, D. A., and Kyung, S.-H. (2000). Autotoxic compounds from fresh alfalfa leaf extracts: identification and biological activity. J. Chem. Ecol. 26, 315–327. doi: 10.1023/A:1005466200919
Dorrepaal, E., Cornelissen, J. H., and Aerts, R. (2007). Changing leaf litter feedbacks on plant production across contrasting sub-arctic peatland species and growth forms. Oecologia 151, 251–261. doi: 10.1007/s00442-006-0580-3
García, A. C., de Souza, L. G. A., Pereira, M. G., Castro, R. N., García-Mina, J. M., Zonta, E., et al. (2016). Structure-property-function relationship in humic substances to explain the biological activity in plants. Sci. Rep. 6:20798. doi: 10.1038/srep20798
Gartner, T. B., and Cardon, Z. G. (2004). Decomposition dynamics in mixed-species leaf litter. Oikos 104, 230–246. doi: 10.1111/j.0030-1299.2004.12738.x
Grömping, U. (2006). Relative importance for linear regression in R: the package relaimpo. J. Stat. Softw. 17, 1–27. doi: 10.18637/jss.v017.i01
Hodge, A., Robinson, D., and Fitter, A. (2000). Are microorganisms more effective than plants at competing for nitrogen? Trends Plant Sci. 5, 304–308. doi: 10.1016/S1360-1385(00)01656-3
International Biochar Initiative [IBI] (2012). Standardized Product Definition and Product Testing Guidelines for Biochar that is Used in Soil. Ibi Biochar Standards. Available at: http://www.biochar-international.org/sites/default/files/Guidelines_for_Biochar_That_Is_Used_in_Soil_Final.pdf [accessed August 7, 2017].
Jeffery, S., Verheijen, F. G. A., van der Velde, M., and Bastos, A. C. (2011). A quantitative review of the effects of biochar application to soils on crop productivity using meta-analysis. Agric. Ecosyst. Environ. 144, 175–187. doi: 10.1016/j.agee.2011.08.015
Jirka, S., and Tomlinson, T. (2015). State of the Biochar Industry 2014: A Survey of Commercial Activity in The Biochar Sector. International Biochar Initiative. Available at: http://www.biochar-international.org/sites/default/files/State_of_the_Biochar_Industry_2013
Joseph, S., Graber, E. R., Chia, C., Munroe, P., Donne, S., Thomas, T., et al. (2013). Shifting paradigms: development of high-efficiency biochar fertilizers based on nano-structures and soluble components. Carbon Manag. 4, 323–343. doi: 10.4155/cmt.13.23
Kammann, C., Glaser, B., and Schmidt, H. P. (2016). “Combining biochar and organic amendments,” in Biochar in European Soils and Agriculture: Science and Practice, eds S. Shackley, G. Ruysschaert, K. Zwart, and B. Glaser (New York: Routledge), 136–164.
Kammann, C. I., Schmidt, H. P., Messerschmidt, N., Linsel, S., Steffens, D., Müller, C., et al. (2015). Plant growth improvement mediated by nitrate capture in co-composted biochar. Sci. Rep. 5:11080. doi: 10.1038/srep11080
Knox, O. G. G., Oghoro, C. O., Burnett, F. J., and Fountaine, J. M. (2015). Biochar increases soil pH, but is as ineffective as liming at controlling clubroot. J. Plant Pathol. 97, 149–152.
Kögel-Knabner, I. (2002). The macromolecular organic composition of plant and microbial residues as inputs to soil organic matter. Soil Biol. Biochem. 34, 139–162. doi: 10.1016/S0038-0717(01)00158-4
Lehmann, J., and Joseph, S. (2015). Biochar for Environmental Management: Science, Technology and Implementation, 2nd Edn. Oxon, UK: Routledge.
Lindeman, R. H., Merenda, P. F., and Gold, R. Z. (1980). Introduction to Bivariate and Multivariate Analysis. Glenview, IL: Scott Foresman.
Liu, J., Schulz, H., Brandl, S., Miehtke, H., Huwe, B., and Glaser, B. (2012). Short-term effect of biochar and compost on soil fertility and water status of a Dystric Cambisol in NE Germany under field conditions. J. Plant Nutr. Soil Sci. 175, 698–707. doi: 10.1002/jpln.201100172
Lopez-Iglesias, B., Olmo, M., Gallardo, A., and Villar, R. (2014). Short-term effects of litter from 21 woody species on plant growth and root development. Plant Soil 381, 177–191. doi: 10.1007/s11104-014-2109-6
Marschner, H. (2012). Marschner’s Mineral Nutrition of Higher Plants, 3rd Edn. London: Academic Press.
Meiners, S. J. (2014). Functional correlates of allelopathic potential in a successional plant community. Plant Ecol. 215, 661–672. doi: 10.1007/s11258-014-0331-1
Novak, J. M., Busscher, W. J., Watts, D. W., Amonette, J. E., Ippolito, J. A., Lima, I. M., et al. (2012). Biochars impact on soil-moisture storage in an ultisol and two aridisols. Soil Sci. 177, 310–320. doi: 10.1097/SS.0b013e31824e5593
Oleszczuk, P., Rycaj, M., Lehmann, J., and Cornelissen, G. (2012). Influence of activated carbon and biochar on phytotoxicity of air-dried sewage sludges to Lepidium sativum. Ecotoxicol. Environ. Saf. 80, 321–326. doi: 10.1016/j.ecoenv.2012.03.015
Palm, C. A., Gachengo, C. N., Delve, R. J., Cadisch, G., and Giller, K. E. (2001). Organic inputs for soil fertility management in tropical agroecosystems: application of an organic resource database. Agric. Ecosyst. Environ. 83, 27–42. doi: 10.1016/S0167-8809(00)00267-X
R Core Team (2016). R: A Language and Environment for Statistical Computing. Vienna: R Foundation for Statistical Computing.
Salamanca, E. F., Kaneko, N., and Katagiri, S. (1998). Effects of leaf litter mixtures on the decomposition of Quercus serrata and Pinus densiflora using field and laboratory microcosm methods. Ecol. Eng. 10, 53–73. doi: 10.1016/S0925-8574(97)10020-9
Schmidt, H. P., Pandit, B. H., Martinsen, V., Cornelissen, G., Conte, P., and Kammann, C. I. (2015). Fourfold increase in pumpkin yield in response to low-dosage root zone application of urine-enhanced biochar to a fertile tropical soil. Agriculture 5, 723–741. doi: 10.3390/agriculture5030723
Schulz, H., Dunst, G., and Glaser, B. (2013). Positive effects of composted biochar on plant growth and soil fertility. Agron. Sustain. Dev. 33, 817–827. doi: 10.1007/s13593-013-0150-0
Soil Survey Staff (1998). Keys to Soil Taxonomy. Washington, DC: USDA-Natural Resources Conservation Service.
Van Zwieten, L., Kimber, S., Morris, S., Chan, K. Y., Downie, A., Rust, J., et al. (2010). Effects of biochar from slow pyrolysis of papermill waste on agronomic performance and soil fertility. Plant Soil 327, 235–246. doi: 10.1007/s11104-009-0050-x
Wardle, D. A., Bonner, K. I., and Nicholson, K. S. (1997). Biodiversity and plant litter: experimental evidence which does not support the view that enhanced species richness improves ecosystem function. Oikos 79, 247–258. doi: 10.2307/3546010
Wardle, D. A., Yeates, G. W., Barker, G. M., and Bonner, K. I. (2006). The influence of plant litter diversity on decomposer abundance and diversity. Soil Biol. Biochem. 38, 1052–1062. doi: 10.1016/j.soilbio.2005.09.003
Warnock, D. D., Lehmann, J., Kuyper, T. W., and Rillig, M. C. (2007). Mycorrhizal responses to biochar in soil and concepts and mechanisms. Plant Soil 300, 9–20. doi: 10.1007/s11104-007-9391-5
Wisnubroto, E. I., Hedley, M., Hina, K., and Camps-Arbestain, M. (2010). The Use of Biochar from Biosolids on Waitarere Sandy Soils: Effect on the Growth of Rye Grass. Palmerston: Massey University.
Wolf, R., and Wedig, H. (2007). Process for Manufacturing a Soil Conditioner. U.S. Patent No WO 2010055139 A1.
Keywords: organic amendment, crop productivity, C/N ratio, N content, organic matter quality, “terra preta”, 13C CPMAS NMR
Citation: Bonanomi G, Ippolito F, Cesarano G, Nanni B, Lombardi N, Rita A, Saracino A and Scala F (2017) Biochar As Plant Growth Promoter: Better Off Alone or Mixed with Organic Amendments? Front. Plant Sci. 8:1570. doi: 10.3389/fpls.2017.01570
Received: 08 May 2017; Accepted: 28 August 2017;
Published: 15 September 2017.
Edited by:
Puneet Singh Chauhan, National Botanical Research Institute (CSIR), IndiaReviewed by:
Jose M. Garcia-Mina, University of Navarra, SpainAnton Hartmann, Helmholtz Zentrum München (HZ), Germany
Copyright © 2017 Bonanomi, Ippolito, Cesarano, Nanni, Lombardi, Rita, Saracino and Scala. This is an open-access article distributed under the terms of the Creative Commons Attribution License (CC BY). The use, distribution or reproduction in other forums is permitted, provided the original author(s) or licensor are credited and that the original publication in this journal is cited, in accordance with accepted academic practice. No use, distribution or reproduction is permitted which does not comply with these terms.
*Correspondence: Giuliano Bonanomi, Z2l1bGlhbm8uYm9uYW5vbWlAdW5pbmEuaXQ=