- 1Key Laboratory of Resource Biology and Biotechnology in Western China (Ministry of Education), College of Life Sciences, Northwest University, Xi'an, China
- 2College of Biopharmaceutical and Food Engineering, Shangluo University, Shangluo, China
- 3Xi'an Botanical Garden of Shaanxi Provence, Institute of Botany of Shaanxi Provence, Xi'an, China
Gynostemma BL., belonging to the family Cucurbitaceae, is a genus containing 17 creeping herbaceous species mainly distributed in East Asia. It can be divided into two subgenera based on different fruit morphology. Herein, we report eight complete chloroplast genome sequences of the genus Gynostemma, which were obtained by Illumina paired-end sequencing, assembly, and annotation. The length of the eight complete cp genomes ranged from 157,576 bp (G. pentaphyllum) to 158,273 bp (G. laxiflorum). Each encoded 133 genes, including 87 protein-coding genes, 37 tRNA genes, eight rRNA genes, and one pseudogene. The four types of repeated sequences had been discovered and indicated that the repeated structure for species in the Subgen. Triostellum was greater than that for species in the Subgen. Gynostemma. The percentage of variation of the eight cp genomes in different regions were calculated, which demonstrated that the coding and inverted repeats regions were highly conserved. Phylogenetic analysis based on Bayesian inference and maximum likelihood methods strongly supported the phylogenetic position of the genus Gynostemma as a member of family Cucurbitaceae. The phylogenetic relationships among the eight species were clearly resolved using the complete cp genome sequences in this study. It will also provide potential molecular markers and candidate DNA barcodes for future studies and enrich the valuable complete cp genome resources of Cucurbitaceae.
Introduction
Gynostemma BL., which belongs to the family Cucurbitaceae, is a genus containing 17 creeping herbaceous species mainly grown in moist mountains, forests, thickets, and streamside (Chen et al., 2011). According to different fruit morphology, all species are divided into 2 subgenera: Subgen. Gynostemma with berries and Subgen. Triostellum with capsules. The species of the former have a wide distribution in East Asia, especially in subtropical China, Japan, Myanmar, and India, whereas the latter are endemic to southern China (Chen, 1995). The most widespread species (G. pentaphyllum) and seven microspecies were studied in our current study, four of these (G. pentaphyllum, G. longipes, G. pubescens, and G. burmanicum) belong to Subgen. Gynostemma, and the remaining four (G. cardiospermum, G. laxiflorum, G. caulopterum, and G. pentagynum) are Subgen. Triostellum species.
In the past, most known studies of the genus Gynostemma mainly focused on extracting bioactive components (Yin et al., 2004), chemistry, or pharmacology (Razmovski-Naumovski et al., 2005; Tsai et al., 2010). As a traditional Chinese medicinal herb, G. pentaphyllum has a high content of saponin and dissociative amino acids. It is useful in clinical and medical science because of its hypoglycemic activity, anticancer effects, and immunity enhancement (Xie et al., 2010). In fact, most of the species from the genus Gynostemma are valuable in the production of medicine, whereas the differences lie in the amount of active ingredients (Liu et al., 2006). Currently, with the development and utilization of medicinal plants, the use of natural medicine by people is growing. Therefore, identification of wild species is particularly important. However, it is difficult to define a classification of species within the genus Gynostemma because of hybridization, and more transitional taxa were found in the wild. Thus, it is necessary to develop more genomic resources for population genetics and DNA barcodes for species identification within the genus Gynostemma.
Additionally, the few genetic studies on this genus was restricted to the development of molecular markers (Zhou et al., 2010; Liao et al., 2011), the identification and relationships among a minority of species, and the population genetics of single species (Wang et al., 2008a; Jiang et al., 2009). However, these studies only used a few specific fragments of DNA that may lead to an incomplete conclusion. Therefore, we prefer to use the whole genome, especially the complete chloroplast genome, to resolve the problems discussed herein.
The chloroplast (cp) is an important organelle found in green plants where photosynthesis and energy transformation occurs. It is inherited in a maternal manner in the majority of plants. Compared with the nuclear genome and mitochondria genome, the cp genome is smaller in size and is a circular double-stranded DNA molecule. Matrilineal inheritance allows stable transgene expression without gene contamination. Moreover, the cp genome has a moderate rate of nucleotide evolution, but shows a large difference in the rate of divergence between coding (CDS) and non-coding (CNS) regions. This makes the cp genome suitable for phylogenetic studies at different taxonomic levels (Li et al., 2013).
An increasing number of cp genomes of higher plants have been obtained since the first complete cp genome of Nicotiana tabacum was determined (Shinozaki et al., 1986; Li et al., 2016). However, only a few complete cp genomes of Cucurbitaceae have been reported, and even fewer Gynostemma species. In angiosperms, most cp genomes are made up of four parts: a pair of inverted repeats (IRa and IRb), one large single-copy region (LSC) and one small single-copy region (SSC) (Palmer, 1985). Because of the expansion and contractions of the IR regions, the size of cp genomes have ranged from 120–160 kb (Wang et al., 2008b). The comparative analysis of the complete cp genomes provided information on genome structure. It also played an important role in understanding the cp genome evolution, species identification, and phylogenetic relationships (Yang et al., 2016).
In the present study, the comparative analysis of eight complete Gynostemma cp genomes was conducted to explore the features and structural differentiation of the sequences, as well as enrich the valuable complete cp genome resources of the family Cucurbitaceae. The simple sequence repeats (SSRs), which are also known as microsatellites, could serve as potential molecular polymorphic markers for genetic diversity and genetic structure of Gynostemma populations in the future. Highly variable regions would provide candidate DNA barcodes for coming studies. This study reconstructed the phylogenetic relationship and verified the morphologic phylogenetic position of the genus Gynostemma in Cucurbitaceae. Furthermore, our study will contribute to further studies on the phylogenetic analysis within the genus Gynostemma and enhanced our profound understanding of the systematic evolution of Cucurbitaceae.
Materials and Methods
Plant Materials and DNA Extraction
Fresh and healthy leaves were collected from adult plants for eight species (Table 1). Voucher specimens were deposited in the Key Laboratory of Resource Biology and Biotechnology (Shaanxi, China). The chloroplast genomic DNA of G. pentaphyllum was isolated from fresh leaves using the density gradient centrifugation method (Sandbrink et al., 1989) and the CTAB extraction method, and each of the total genomic DNA for the seven microspecies (G. longipes, G. pubescens, G. burmanicum, G. cardiospermum, G. laxiflorum, G. caulopterum, and G. pentagynum) were extracted from silica-dried leaf material with a simplified CTAB protocol (Doyle, 1987).
Illumina Sequencing, Assembly, and Annotation
Illumina raw reads were generated from an Illumina Hiseq 2500 platform. For G. pentaphyllum, the raw reads were assembled using program SPAdes (Bankevich et al., 2012) to obtain the original scaffold. Gapcloser (Luo et al., 2012) and GapFiller (Boetzer and Pirovano, 2012) were used to fill in the gaps and connect the different scaffolds according to the overlap regions. The gaps containing an ambiguous base “N” were validated with PCR amplification productions by designing pairs of primers (Table S1) using Primer3 version 4.0.0 (Untergasser et al., 2012). PrInSeS-G (Massouras et al., 2010) was used to correct the error of bases and indels during assembly. The cp genome was annotated using CpGAVAS (Liu et al., 2012). All the coding DNA sequence (CDS) regions were predicted using EMBOSS (Rice et al., 2000), and then the predicted genes were blasted in eight public databases (NR, CDD, KOG, PFAM, SWISS-PROT, TREMBL, GO, and KEGG) using BLAST with a cut-off e-value of e < 1.0 × 10−5, and the similarity degree was set to at least 30%.
Regarding the seven microspecies, the quality-trimmed raw reads were proceeded by CLC Genomics Workbench v7.5 (CLC Bio, Aarhus, Denmark) software with the default parameters set. Reference-guided assembly was performed twice to reconstruct the cp genomes with the program MITObim v1.7 (Hahn et al., 2013) using the obtained G. pentaphyllum and published Cucumis melo var. melo (GenBank accession number: JF412791) as references. A few gaps in the assembled cp genomes were corrected by the Sanger sequencing method (Table S1). The program DOGMA (Wyman et al., 2004) was used to annotate the complete cp genomes, and corrected by comparing with the complete cp genomes of the references mentioned above using GENEIOUS R8 (Biomatters Ltd., Auckland, New Zealand). The circular cp genome maps were drawn using the online program OGDRAW (http://ogdraw.mpimp-golm.mpg.de/).
Repeated Sequences Identification
We searched four types of repeated sequences in all eight species. Size and location of dispersed and palindromic repeats were detected using the program REPuter (Kurtz et al., 2001), in which the similarity percentage of two repeat copies was at least 90 % and the parameter of minimal repeat size was 30 bp. The online program Tandem Repeats Finder (http://tandem.bu.edu/trf/trf.html) was used to find the tandem repeat sequences, which was at least 10 bp in length. The alignment parameters for match, mismatch, and indels were set at 2, 7, and 7, respectively. Microsatellites (SSRs) were performed by MISA (Thiel et al., 2003) with thresholds of 10, 5, 4, 3, 3, and 3 for mono-, di-, tri-, tetra-, penta-, and hexa-nucleotide, respectively.
Sequence Divergence Analysis
The variable sites across the complete cp genomes, containing LSC, SSC, and IR regions of all species, were computed by DnaSP v5.0 (Librado and Rozas, 2009). MEGA 5.0 (Tamura et al., 2011) was used for statistics on nucleotide substitutions (NSs) and indels of the cp genome sequences to investigate the sequence divergence patterns. The percentage of variable characters for each coding and non-coding region was calculated based on the method of Zhang et al. (2011).
Whole Chloroplast Genomes Comparison
To discover the interspecific variation among the complete cp genome sequences of eight Gynostemma species, we used the program mVISTA (Frazer et al., 2004) to visualize the alignments with annotations using G. pentaphyllum as a reference. MAFFT version 7.017 (Katoh and Standley, 2013) was used for multiple alignments of seven microspecies cp genomes with G. pentaphyllum. The IR region borders and gene rearrangement among 10 species (eight Gynostemma species, the close relative C. mole var. melo, and the first reported complete cp genome N. tabacum) were ascertained by the plug-in program Mauve in GENEIOUS R8 to analyze the expansion variation in junction regions.
Phylogenetic Relationship
For the purpose of reconstructing the phylogenetic relationships and verifying the phylogenetic position of the genus Gynostemma in the family Cucurbitaceae, nine published complete cp genome sequences from the orders Cucurbitales and Fagales were also selected in our analyses (Table S2). Sequences were aligned using the program MAFFT version 7.017 (Katoh and Standley, 2013) and manually edited where necessary. Because of the differentiation of molecular evolutionary rates among the different cp genome regions, phylogenetic relationship analyses were performed using the following six datasets: (1) the complete cp genome sequences; (2) 75 common protein coding genes (CDS); (3) one inverted repeats region (IRb); (4) the large single copy region (LSC); (5) the small single copy region (SSC), and (6) the consensus sequences of 10 highly variable regions. The best-fitting model for each dataset based on the Akaike information criterion was determined by Modeltest 3.7 (Posada and Crandall, 1998). Bayesian inference (BI) was implemented with MrBayes 3.12 (Ronquist and Huelsenbeck, 2003) using the settings as following: Markov chain Monte Carlo simulations (MCMC) algorithm for 100,000 generations with four incrementally heated chains, starting from random trees, and sampling one out of every 100 generations. The first 25% of trees were discarded as burn-in (Meng et al., 2008; Ma et al., 2014). The maximum likelihood (ML) trees were reconstructed with RAxML v7.2.8 (Stamatakis, 2006) performed with 1,000 replicates. In all analyses, Castanea mollissima and Castanea pumila var. pumila (Fagaceae) were regarded as outgroups.
Results
Gene Prediction and Genome Features
For the cp genome of the widespread G. pentaphyllum, 586,299,000 bp reads were produced by Illumina sequencing, including 470,640,574 bp high-quality reads (>Q20), accounting for 80.77% of the total reads with an average length of 251.8 bp. The basic information of sequencing products is shown in Table S3. After preliminary assembly and gene prediction, we obtained a 157,761 bp sequence with four gaps of 176 bp unknown bases, and predicted 91 protein-coding genes, 30 tRNA, and 8 rRNA in total. The predicted protein-coding genes were blasted in the public databases of the known functional genes (Table S4, Figure S1), of which 97.8% could be found in NR databases for species distribution (Figure S2). The top-hit three species, C. melo var. melo, Cucumis sativus, and Corynocarpus laevigata, were identified as belonging to the order Cucurbitales. Moreover, we designed four pairs of primers that successfully amplified the ambiguous regions (Table S1). The initial automatic annotation information of the genome sequence was corrected by comparison with the cp genome of C. melo var. melo as a reference. Finally, the complete cp genome of G. pentaphyllum was fixed to 157,576 bp, which encoded 133 genes, including 87 protein-coding genes, 37 tRNA genes, eight rRNA genes, and one pseudogene (Tables 2, 3, Figure 1).
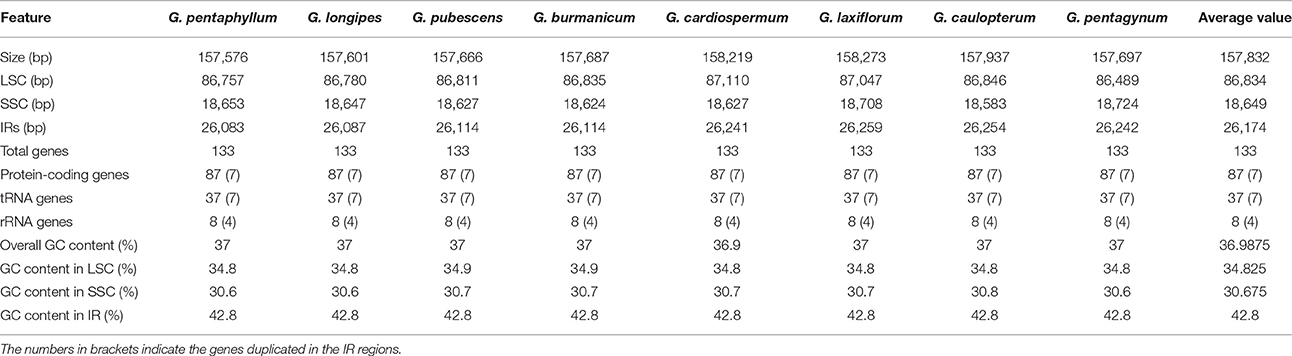
Table 2. Characters of Illumina sequencing and assembly of chloroplast genome of eight Gynostemma species.
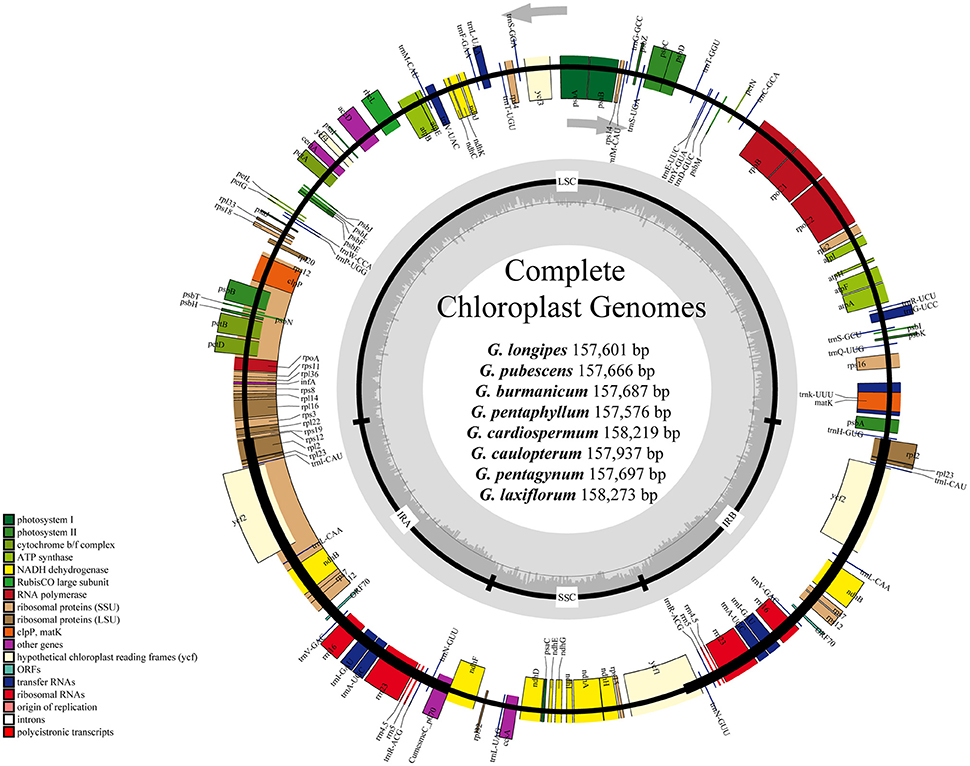
Figure 1. Gene maps of chloroplast genomes of Gynostemma BL. Genes on the inside of the large circle are transcribed clockwise and those on the outside are transcribed counterclockwise. The genes are color-coded based on their functions. Dashed area represents the GC composition of the chloroplast genome.
The consensus sequences of the seven cp genomes of the microspecies were assembled by mapping each Illumina raw read to the reference genome sequences. The seven microspecies cp genomes ranged from 157,601 bp (G. longipes) to 158,273 bp (G. laxiflorum), and the average length was 157,832 bp. All reads and coverage of the cp genomes are displayed in Table 1. The gene number and varieties were consistent with G. pentaphyllum (Table 2, Figure 1). The annotated circular cp genome sequences were submitted to the GenBank and accession numbers were obtained (Table 1).
All of the cp genomes displayed a typical quadripartite structure, two copies of inverted repeats (IRs, 26,174 bp average) segregated by two SC regions, namely a large single copy region (LSC, 86,834 bp average) and small single copy region (SSC, 18,649 bp average, Table 2). Comparison of cp genome sequences among eight Gynostemma species and seven other Cucurbitales plants (Table S5) showed no dramatic difference in compared features (Figure 2). The GC content percentage of G. cardiospermum (36.9%) was less than any other genome (37.0%), whereas the GC content of the IR region was clearly higher than that of any other region of each cp genome (Table 2). The cp genomes encoded an identical set of 133 functional genes, of which 18 were duplicated in the IR region, including seven protein-coding genes, seven transfer RNA (tRNA) genes, and four ribosomal RNA (rRNA) genes. Twenty-three genes had introns, four of them (two rps12, one clpP and one ycf3) with two introns. The gene infA, which was a translation-related gene, was identified as a pseudogene (Table 3).
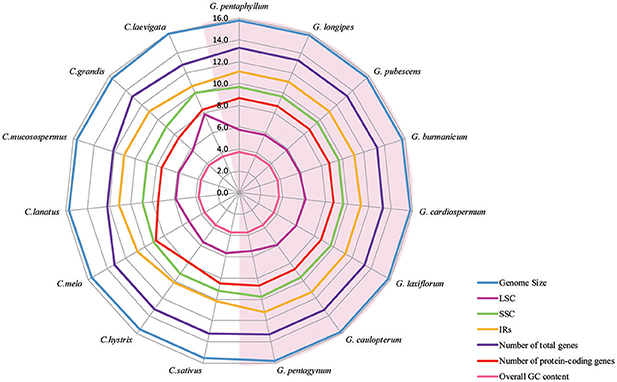
Figure 2. A radar-plot comparing features of the complete chloroplast genomes of 15 accessions of Cucurbitales species. Showing, from inside to out, overall GC content, LSC region size, number of protein-coding genes, SSC region size, IR region size, number of total genes and genome size.
Repeat Analysis and Simple Sequence Repeats (SSR)
The types and distribution of repeated sequences and the presence of SSRs were analyzed in the cp genomes of eight Gynostemma species. Sixteen dispersed repeats, 19 palindromic repeats, and seven tandem repeats were discovered in the G. pentaphyllum cp genome. In the G. cardiospermum cp genome, the numbers of these three types of repeated sequences were 27, 22, and 18, respectively. The repeat number of the other six microspecies are shown in Figure 3B. Repeated sequences of Subgen. Triostellum species were much greater than those of Subgen. Gynostemma species. Most repeats (73.32%) were 30–45 bp in length (Figure 3A). The repeated sequences were mostly distributed in the intergenic spacers (IGS) and intron regions (Figure 3D), but some repeats were also found in coding regions (CDS), such as ycf2, psaB, and trnS, among others (Tables S6, S7). Moreover, SSRs of the eight cp genomes were analyzed (Figure 3C). Among them, SSRs of G. pubescens and G. burmanicum (77) were the greatest and that of G. pentagynum (58) were the lowest. In total, 561 SSRs were discovered, of which 77.76% were distributed in the CNS and 125 SSRs were found in the CDS (Figure 3D, Table S8). For the four structural regions in cp genomes, 429 SSRs were found in LSC, whereas 20, 92, and 20 were in IRb, SSC, and IRa, respectively (Figure 3E). It appeared that the SSRs were distributed unevenly across the cp genomes. There were 304, 126, 44, 69, and 16 mono-, di-, tri-, tetra-, and penta-nucleotide repeats, respectively. It was noteworthy that G. pentagynum had two hexanucleotides SSRs (Figure 3C). Among these SSRs, mononucleotide repeats were common and accounted for 54.19% of the total, whereas dinucleotides accounted for 22.46%, and the other polynucleotide SSRs occurred with less frequency (Figure 3C).
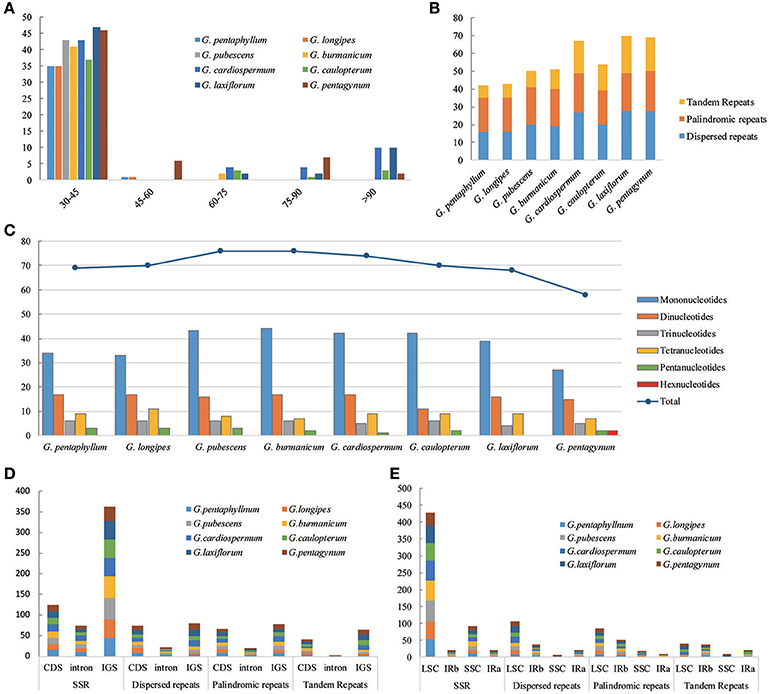
Figure 3. The distribution, type and presence of repeated sequences and simple sequence repeats (SSR) in the cp genome of eight Gynostemma species. (A) Number of repeat sequences by length; (B) Number of three repeat types in the eight chloroplast genomes; (C) Number of SSR types in the eight chloroplast genomes; (D,E) Location of the all repeats from eight species.
Sequence Divergence
The percentage of variation for eight Gynostemma cp genomes in the CNS ranged from 0 to 45.98%, with a total of 10.86%, which was much higher than that in the CDS (0–7.14, 2.11% in total, Table 4, Figure 4), indicating that the CDS were much more conservative than the CNS. Furthermore, the mean percentage of variation in IRs (2.59 and 0.51%) was lower than that of LSC (12.52 and 1.93%) or SSC (16.84 and 4.13%), which demonstrated that the IR region had fewer mutations and was highly conserved. The genes rps16, matK, rpl22, rps15, and ndhF were the top five genes exhibiting higher variability (variation percentage ≥4.21%, Figure 4A) than other genes. It was notable that gene ndhD in G. caulopterum was 144 bp shorter than others because of an indel of AT in the initiation region (Figure S3). We further analyzed the sequence divergence patterns in all of the cp genomes. Finally, 3,425 nucleotide substitution (NS) loci with 3,885 bp and 1,116 indel loci with 4,750 bp were found in the aligned sequence.
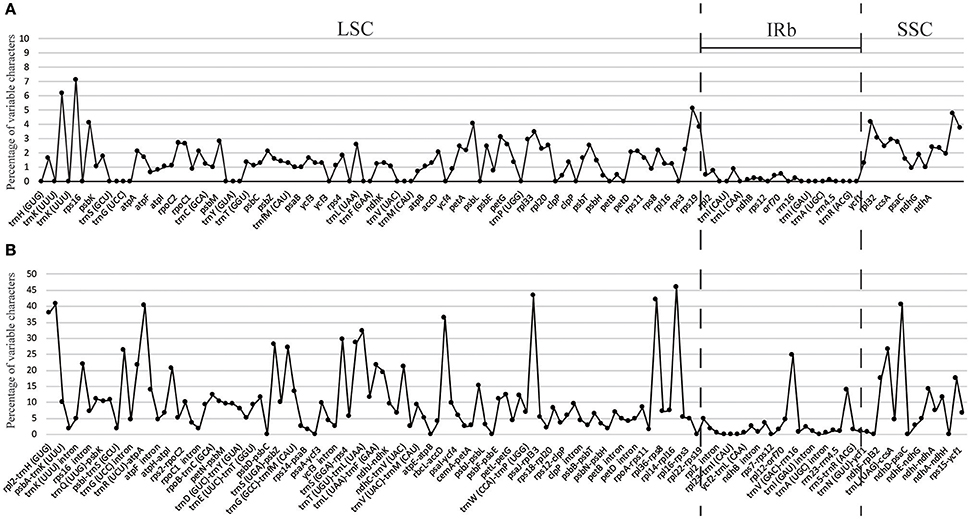
Figure 4. Percentage of variable characters in aligned eight Gynostemma chloroplast genomes. (A) Coding region (CDS) and (B) Noncoding region (CNS); These regions are oriented according to their locations in the chloroplast genome.
Comparison of Border Regions and Sequence Identity
The border regions of the eight Gynostemma cp genomes were compared with C. melo var. melo and N. tabacum to analyze the expansion and contraction variation in junction regions. The IR regions of 10 cp genomes ranged from 25,342 bp (N. tabacum) to 26,259 bp (G. laxiflorum) in size, of which rps19, ycf1, ndhF, rpl2, and trnH genes were present at the junctions of the LSC/IR and SSC/IR borders. Considerable variation was observed in the expansion and contraction of IR regions (Figure 5). For the LSC/IR borders, the gene rps19 in the LSC of Gynostemma species extended 2–20 bp into the IRb region, whereas N. tabacum contracted four bp instead. The gene trnH in the LSC region contracted 32–89 bp from the junction region of IRa/LSC. The gene rpl2 in the IRa region also contracted by a different number of bases (53–71 bp). In contrast, the SSC/IR boundary regions were relatively stable. The gene ycf1 in the IRb region and gene ndhF in the SSC region interlaced at the IRb/SSC border, and ycf1 in the SSC region was astride the border of SSC/IRa. Gene ndhF and ycf1 in the SSC region extended the same number of bases among the eight Gynostemma species (12 and 1,186 bp, respectively), whereas gene ycf1 in the IRb region exhibited an interesting expansion: Subgen. Gynostemma species extended 26 bp into the SSC region, whereas the Subgen. Triostellum species extended 32 bp instead. We also compared the cp genomes of 10 species mentioned above to identify genome rearrangement events for each species. It was shown that no rearrangement events occurred in the eight Gynostemma plants (Figure S4).
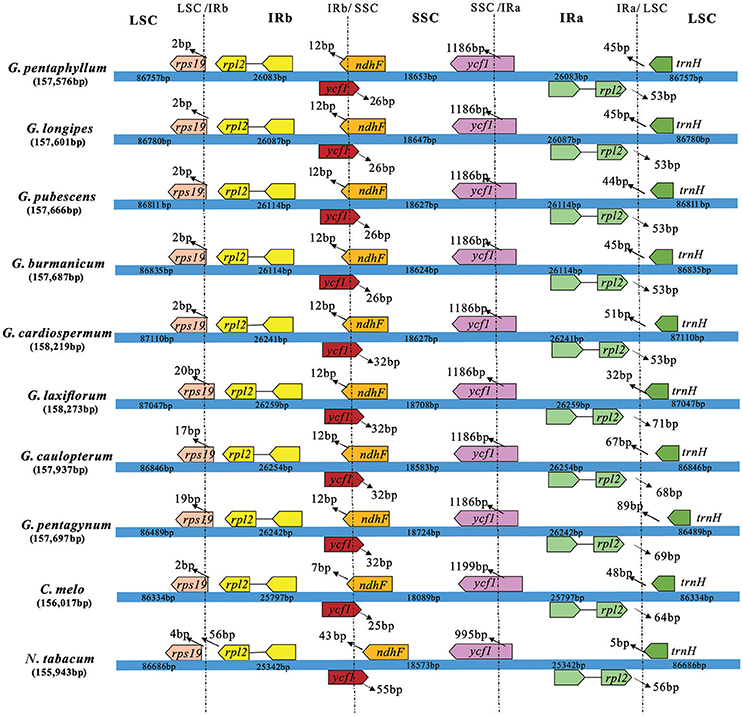
Figure 5. Comparison of the border positions of LSC, SSC, and IR regions in four chloroplast genomes. Boxes above or below the main line represent the genes at the IR/SC borders.
The overall sequence identity of eight Gynostemma cp genomes were performed with G. pentaphyllum as a reference (Figure 6). The alignment showed high sequence similarity. However, most of the significant divergence was found in the CNS. Among them, a high degree of divergence included ycf3-trnS (GGA), trnT (UGU)-trnL (UAA), rpl32-trnL (UAG), ccsA-ndhD, and petA-psbJ. The most similar sequences were in four rRNA genes, photosystem I genes, cytochrome b/f complexes, and ATP synthase genes. As previously mentioned, the IR regions exhibited lower sequence divergence than did the SC regions, suggesting that they were highly conserved.
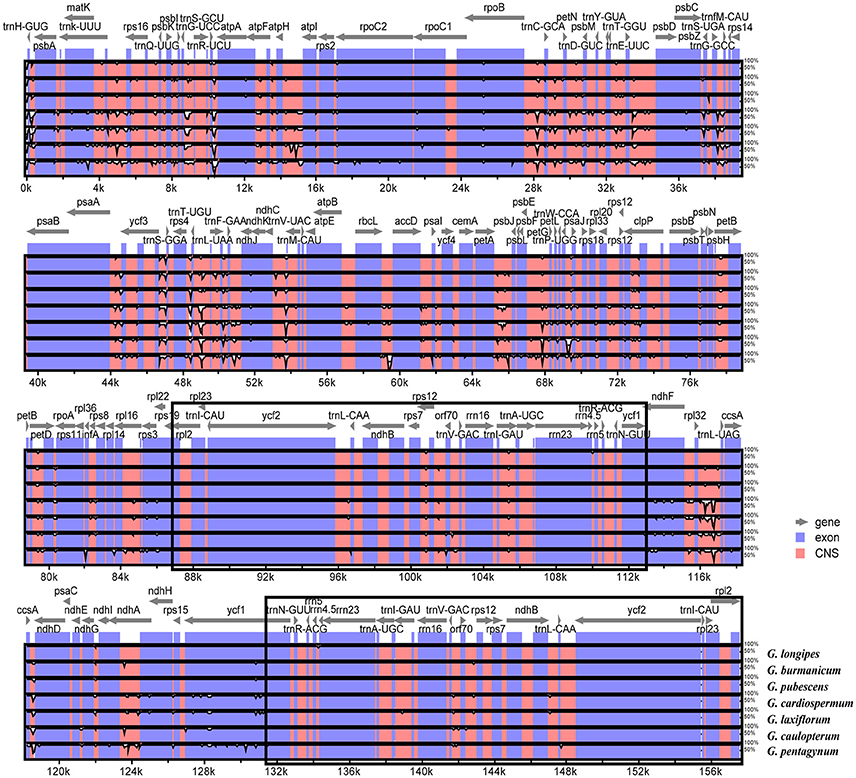
Figure 6. Sequence identity plots among eight sequenced chloroplast genomes, with G. pentaphyllum as a reference by using mVISTA. The vertical scale indicates the identity percentage ranging from 50 to 100%. The horizontal axis corresponds to the coordinates within the chloroplast genome. Coding and non-coding regions are marked in blue and pink, respectively. Annotated genes are displayed along the top. The black boxes show the two IR regions.
Phylogenetic Analysis
All the BI and ML trees reconstructed based on the six datasets were congruent in identification of the phylogenetic position of the genus Gynostemma in the family Cucurbitaceae. The best-fit models for each dataset used in BI and ML analysis are displayed in Table 5. The phylogeny produced from the analysis of 17 complete cp genome sequences was well-supported. All nodes of the phylogenetic trees were strongly supported by 1.00 Bayesian posterior probabilities in BI analysis and 51–100% bootstrap values in ML analysis (Figure 7). It was shown that C. laevigata was the earliest diverging lineage in this group, which was identified as a sister to other species. All species of the Subgen. Gynostemma (G. pentaphyllum, G. longipes, G. pubescens, and G. burmanicum) and Subgen. Triostellum (G. cardiospermum, G. laxiflorum, G. caulopterum, and G. pentagynum) formed a Gynostemma clade. Combined with the species of the genera Cucumis, Coccinia, and Citrullus, indicated the family Cucurbitaceae was monophyletic. The phylogenetic trees of other datasets also showed a similar and clear internal relationship of Cucurbitales plants (Figure S5).
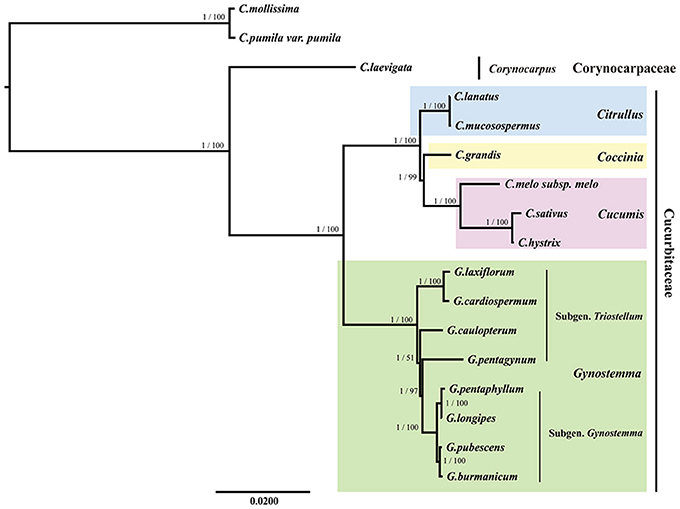
Figure 7. Phylogenetic relationship of the 17 species inferred from BI and ML analyses based on the complete cp genome sequences. The Bayesian posterior probabilities and bootstrap values of ML analyses are shown beside the clades. Castanea mollissima and Castanea pumila var. pumila were used as the outgroups.
Discussion
Chloroplast Sequence Variation and Evolution
We determined the complete nucleotide sequences of eight Gynostemma cp genomes using Illumina paired-end sequencing combined with the Sanger sequencing method. Because no perfect assembler program has been created, de novo assembly always generates misassembled contigs; thus, assembled contigs must be checked and corrected by read-mapping and further scanned for any gaps of lower coverage (Naito et al., 2013; Nashima et al., 2015). Despite the occurrence of gene loss-and-gain events and the rearrangements in several genomes of land plants (Palmer, 1987; Fu et al., 2016), the eight cp genomes we studied displayed the typical quadripartite structure with two IRs and two SCs. In terms of gene content, each of the cp genomes encoded 133 genes containing with 87 protein-coding genes, 37 tRNA genes, eight rRNA genes, and one pseudogene. The function, order, and GC-content of these genes were all highly conserved as noted for other angiosperms (Palmer, 1991). The cp genomes of all the Gynostemma species contained more AT and had a GC content of 36.9–37.0%, similar to previously published Olea and Diospyros genomes (Mariotti et al., 2010; Fu et al., 2016), and possibly caused by the high GC content of the rRNA gene sequences located in IR regions.
Pseudogenes are functionless relatives of genes that have lost their ability to code a protein (Vanin, 1985), and are generally regarded as the last stop for genomic material and are often thought to be “junk DNA” (Zheng et al., 2007). Nonetheless, recent research suggested that pseudogenes were evolutionary relics of functional components in the genome that provide important information about the history of the gene and genome evolution (Balakirev and Ayala, 2003; Zou et al., 2009). Although not protein-coding, the DNA of pseudogenes may be similar to other kinds of non-coding DNA, which may have a regulatory function (Poliseno et al., 2010). In this study, gene infA, which is located between genes rpl36 and rps8 in the LSC region was identified as a pseudogene because of the presence of several internal stop codons. The gene infA acts as a transcription anti-terminator and has RNA chaperone activity (Phadtare et al., 2007). It also exists as a pseudogene in many other cp genomes [e.g., Syzygium cumini and Ananas comosus (Asif et al., 2013; Nashima et al., 2015)], or is lost [Arabidopsis thaliana and Alstroemeria aurea (Sato et al., 1999; Do et al., 2013)]. However, many other pseudogenes, for instance, the ycf15 gene in Quercus spinose (Du et al., 2015), rpl22 and rps18 genes in the Paeoniaceae (Dong et al., 2013) were predicted to be lost or normal in our study.
Previous research supports that repeated sequences may play an important role in rearranging sequences and producing variation that cp genomes lost through slipped-strand mispairing and illegitimate recombination (Cavalier Smith, 2002). The presence of dispersed, palindromic, and tandem repeats in Gynostemma cp genomes were reported. The repeats in Subgen. Triostellum species were greater than those in Subgen. Gynostemma species. The majority were distributed in the IGS and intron regions, which were the highly variable regions in the cp genomes. The cp SSRs have been detected in Oryza sativa (Ishii and McCouch, 2000), Haloxylon ammodendron, and Haloxylon persicum (Dong et al., 2016). We also identified 561 SSRs within eight species, 77.72 % of them were distributed in the IGS and intron regions. Overall, the SSRs can be used to analyze the population genetics and evolutionary studies based on their polymorphism leading to sensitive genetic diversity, population structure, and phylogeographic studies at the inter- and intrapopulation levels (Pauwels et al., 2012; Naydenov et al., 2016). Thus, Gynostemma cp SSRs could contribute to evolutionary and molecular ecological knowledge, which warrants further research.
Although the extant studies point that the cp genome of herbaceous plants has been evolving rapidly (Zhong et al., 2009) and has several structural changes such as gene inversion (Doyle et al., 1992) and gene loss-and-gain events (Diekmann et al., 2009), no rearrangement events were found in all of our species after global alignment with the published cp genomes of C. melo var. melo, and N. tabacum. The cp genomes are highly conserved in terms of size and genomic structure, whereas usually it is different among species within a family (Sun et al., 2013). In addition, the IR regions are important for stabilizing cp genome structure (Maréchal and Brisson, 2010). IR regions are highly conserved, but our results indicated that some position changes occurred in the IR/SC border areas. They may have been caused by the contraction and expansion events of the IR region, which was mainly responsible for length mutations of cp genomes and is a common evolutionary phenomenon in plants (Kim and Lee, 2004). However, borders between two regions among the eight Gynostemma species showed high similarity, especially for gene ndhF located in the SSC region and ycf1 in the IRb region located in the LSC. It was interesting that the gene positions for Subgen. Gynostemma species were stable but for those of Subgen. Triostellum species were variable and differed from one another.
The sequence identity revealed low differentiation among species within a single subgenus, but great differences between the cp genomes of two subgenera. As expected, in conformity with most angiosperms, IRs and CDS were more conserved than SCs and CNS. This was consistent with the result of sequence divergence where the mean percentage of variations in IRs was lower than that of the SCs. Remarkably, the top five genes exhibiting higher variability and the high-divergence regions, which were always identified as hotspots, had been described. Therefore, further work on developing universal primers and candidate DNA barcodes for these regions would be necessary to judge whether it is conducive to the assessment of the phylogenetic relationships among Gynostemma species.
Phylogenetic Analysis
There has been an increasing number of studies using complete cp genome sequences for assessing phylogenetic relationships among angiosperms (Bock et al., 2014; Raman et al., 2016). Our phylogenetic trees indicated a very clear internal relationship of restricted Cucurbitales plants with high bootstrap values. The phylogenetic trees showed that the eight species of Gynostemma clustered into Cucurbitaceae and paralleled the sister taxa of Cucumis, Coccinia, and Citrullus species. This is consistent with the phylogenetic position of the genus Gynostemma in the morphological classification by Chen (Chen, 1995). However, our study was just a glimpse of phylogenetic relationships for the species of Gynostemma, we will estimate and discuss the interior phylogenetic relationships within the genus Gynostemma with more comprehensive and in-depth analyses, and enhance our profound understanding of the systematic evolution of Cucurbitaceae in the future.
Author Contributions
GZ and XZ conceived and designed the experiment. XZ and TZ performed the experiments and analyzed the data. YZ and GB collected the samples. XZ wrote the paper. TZ and NK helped to revise the paper. All authors read and approved the final manuscript.
Conflict of Interest Statement
The authors declare that the research was conducted in the absence of any commercial or financial relationships that could be construed as a potential conflict of interest.
Acknowledgments
This work was supported by the National Natural Science Foundation of China (grant number 31270364 and J1210063) and the Program for Changjiang Scholars and Innovative Research Team in University (PCSIRT) (grant number IRT1174). We are grateful to Yanci Yang, Shuyun Zeng, and Yiheng Hu from College of Life Sciences in Northwest University for their useful advice and helpful data analysis.
Supplementary Material
The Supplementary Material for this article can be found online at: http://journal.frontiersin.org/article/10.3389/fpls.2017.01583/full#supplementary-material
Table S1. Primers for low coverage regions of eight Gynostemma species.
Table S2. List of species and their accession numbers in GenBank included in the phylogenetic analyses of complete chloroplast genomes.
Table S3. Basic Information of Illumina Paired-end Sequencing Products of G. pentaphyllum.
Table S4. The result of comparison for predicted protein-coding genes and the public databases.
Table S5. Characteristics of chloroplast genomes of 15 different accessions of Cucurbitales species.
Table S6. List of tandem repeats in the chloroplast genome of eight Gynostemma species.
Table S7. List of dispersed repeats and palindromic repeats in the chloroplast genome of eight Gynostemma species.
Table S8. List of SSRs in the chloroplast genome of eight Gynostemma species.
Figure S1. Percentage of annotated genes in eight public databases.
Figure S2. Species distribution of predicted protein-coding genes of G. pentaphyllum in NR databases.
Figure S3. Alignment of gene ndhD in eight Gynostemma species. The purple box with a letter M presents initiation codons.
Figure S4. Genome rearrangement events of eight Gynostemma species, comparing with C. melo var. melo and N. tabacum.
Figure S5. Phylogenetic relationship of the 17 species inferred from BI and ML analyses based on five datasets (A. CDS; B. IR; C. LSC; D. SSC, and E. consensus sequences of 10 highly variable regions). The Bayesian posterior probabilities and bootstrap values of ML analyses are shown beside the clades. Castanea mollissima and Castanea pumila var. pumila were used as the outgroups.
References
Asif, H., Khan, A., Iqbal, A., Khan, I. A., Heinze, B., and Azim, M. K. (2013). The chloroplast genome sequence of Syzygium cumini (L.) and its relationship with other angiosperms. Tree Genet. Genomes 9, 867–877. doi: 10.1007/s11295-013-0604-1
Balakirev, E. S., and Ayala, F. J. (2003). Pseudogenes: are they junk or functional DNA? Annu. Rev. Genet. 37, 123–151. doi: 10.1146/annurev.genet.37.040103.103949
Bankevich, A., Nurk, S., Antipov, D., Gurevich, A. A., Dvorkin, M., Kulikov, A. S., et al. (2012). SPAdes: a new genome assembly algorithm and its applications to single-cell sequencing. J. Comput. Biol. 19, 455–477. doi: 10.1089/cmb.2012.0021
Bock, D. G., Kane, N. C., Ebert, D. P., and Rieseberg, L. H. (2014). Genome skimming reveals the origin of the Jerusalem Artichoke tuber crop species: neither from Jerusalem nor an artichoke. New Phytol. 201, 1021–1030. doi: 10.1111/nph.12560
Boetzer, M., and Pirovano, W. (2012). Toward almost closed genomes with GapFiller. Genome Biol. 13:R56. doi: 10.1186/gb-2012-13-6-r56
Cavalier Smith, T. (2002). Chloroplast evolution: secondary symbiogenesis and multiple losses. Curr. Biol. 12, R62–R64. doi: 10.1016/S0960-9822(01)00675-3
Chen, S. (1995). A classificatory system and geographical distribution of the genus Gynostemma, B. L. (Cucurbitaceae). Acta Phytotaxon. Sin. 33, 403–410.
Chen, S., Lu, A., and Charles, J. (2011). Flora of China, Vol. 19. Beijing: Missouri Botanical Garden Press.
Diekmann, K., Hodkinson, T. R., Wolfe, K. H., van den Bekerom, R., Dix, P. J., and Barth, S. (2009). Complete chloroplast genome sequence of a major allogamous forage species, perennial ryegrass (Lolium perenne L.). DNA Res. 16, 165–176. doi: 10.1093/dnares/dsp008
Do, H. D. K., Kim, J. S., and Kim, J. H. (2013). Comparative genomics of four Liliales families inferred from the complete chloroplast genome sequence of Veratrum patulum O. Loes. (Melanthiaceae). Gene 530, 229–235. doi: 10.1016/j.gene.2013.07.100
Dong, W., Xu, C., Cheng, T., and Zhou, S. (2013). Complete chloroplast genome of Sedum sarmentosum and Chloroplast genome evolution in saxifragales. PLoS ONE 8:e77965. doi: 10.1371/journal.pone.0077965
Dong, W., Xu, C., Li, D., Jin, X., Li, R., Lu, Q., et al. (2016). Comparative analysis of the complete chloroplast genome sequences in psammophytic Haloxylon species (Amaranthaceae). PeerJ 4:e2699. doi: 10.7717/peerj.2699
Doyle, J. J. (1987). A rapid DNA isolation procedure for small quantities of fresh leaf tissue. Phytochem. Bull. 19, 11–15.
Doyle, J. J., Davis, J. I., Soreng, R. J., Garvin, D., and Anderson, M. J. (1992). Chloroplast DNA inversions and the origin of the grass family (Poaceae). Proc. Natl. Acad. Sci. U.S.A. 89, 7722–7726. doi: 10.1073/pnas.89.16.7722
Du, F. K., Lang, T., Lu, S., Wang, Y., Li, J., and Yin, K. (2015). An improved method for chloroplast genome sequencing in non-model forest tree species. Tree Genet. Genomes 11:114. doi: 10.1007/s11295-015-0942-2
Frazer, K. A., Pachter, L., Poliakov, A., Rubin, E. M., and Dubchak, I. (2004). VISTA: computational tools for comparative genomics. Nucleic Acids Res. 32 (Suppl. 2), W273–W279. doi: 10.1093/nar/gkh458
Fu, J., Liu, H., Hu, J., Liang, Y., Liang, J., Wuyun, T., et al. (2016). Five complete chloroplast genome sequences from diospyros: genome organization and comparative analysis. PLoS ONE 11:e0159566. doi: 10.1371/journal.pone.0159566
Hahn, C., Bachmann, L., and Chevreux, B. (2013). Reconstructing mitochondrial genomes directly from genomic next-generation sequencing reads—a baiting and iterative mapping approach. Nucleic Acids Res. 41:e129. doi: 10.1093/nar/gkt371
Ishii, T., and McCouch, S. R. (2000). Microsatellites and microsynteny in the chloroplast genomes of Oryza and eight other Gramineae species. Theor. Appl. Genetics 100, 1257–1266. doi: 10.1007/s001220051432
Jiang, L., Guo, Z., Wang, C., and Zhao, G. (2009). ITS sequence analysis of Gynostemma pentaphyllum from different habitats in China. Chin. Tradit. Herb. Drugs 7:042.
Katoh, K., and Standley, D. M. (2013). MAFFT multiple sequence alignment software version 7: improvements in performance and usability. Mol. Biol. Evol. 30, 772–780. doi: 10.1093/molbev/mst010
Kim, K. J., and Lee, H. L. (2004). Complete chloroplast genome sequences from Korean Ginseng (Panax schinseng Nees) and comparative analysis of sequence evolution among 17 vascular plants. DNA Res. 11, 247–261. doi: 10.1093/dnares/11.4.247
Kurtz, S., Choudhuri, J. V., Ohlebusch, E., Schleiermacher, C., Stoye, J., and Giegerich, R. (2001). REPuter: the manifold applications of repeat analysis on a genomic scale. Nucleic Acids Res. 29, 4633–4642. doi: 10.1093/nar/29.22.4633
Li, J., Chen, C., and Wang, Z. (2016). The complete chloroplast genome of the Dendrobium strongylanthum (Orchidaceae: Epidendroideae). Mitochon. DNA Part A 27, 3048–3049. doi: 10.3109/19401736.2015.1063128
Li, X., Gao, H., Wang, Y., Song, J., Henry, R., Wu, H., et al. (2013). Complete chloroplast genome sequence of Magnolia grandiflora and comparative analysis with related species. Sci. China Life Sci. 56:189. doi: 10.1007/s11427-012-4430-8
Liao, H., Zhao, Y., Zhou, Y., Wang, Y., Wang, X., Lu, F., et al. (2011). Microsatellite markers in the traditional Chinese medicinal herb Gynostemma pentaphyllum (Cucurbitaceae). Am. J. Bot. 98, e61–e63. doi: 10.3732/ajb.1000456
Librado, P., and Rozas, J. (2009). DnaSP v5: a software for comprehensive analysis of DNA polymorphism data. Bioinformatics 25, 1451–1452. doi: 10.1093/bioinformatics/btp187
Liu, C., Shi, L., Zhu, Y., Chen, H., Zhang, J., Lin, X., et al. (2012). CpGAVAS, an integrated web server for the annotation, visualization, analysis, and GenBank submission of completely sequenced chloroplast genome sequences. BMC Genomics 13:715. doi: 10.1186/1471-2164-13-715
Liu, S., Lin, R., and Hu, Z. (2006). Comparison of stem and leaf structures and total gypenosides among 5 species of Gynostemma. J. Fu. Agric. Forest. Univ. 35, 495–499.
Luo, R., Liu, B., Xie, Y., Li, Z., Huang, W., Yuan, J., et al. (2012). SOAPdenovo2: an empirically improved memory-efficient short-read de novo assembler. Gigascience 1:18. doi: 10.1186/2047-217X-1-18
Ma, P., Zhang, Y., Zeng, C., Guo, Z., and Li, D. (2014). Chloroplast phylogenomic analyses resolve deep-level relationships of an intractable bamboo tribe arundinarieae (Poaceae). Syst. Biol. 63, 933–950. doi: 10.1093/sysbio/syu054
Maréchal, A., and Brisson, N. (2010). Recombination and the maintenance of plant organelle genome stability. New Phytol. 186, 299–317. doi: 10.1111/j.1469-8137.2010.03195.x
Mariotti, R., Cultrera, N. G., Díez, C. M., Baldoni, L., and Rubini, A. (2010). Identification of new polymorphic regions and differentiation of cultivated olives (Olea europaea L.) through plastome sequence comparison. BMC Plant Biol. 10:211. doi: 10.1186/1471-2229-10-211
Massouras, A., Hens, K., Gubelmann, C., Uplekar, S., Decouttere, F., Rougemont, J., et al. (2010). Primer-initiated sequence synthesis to detect and assemble structural variants. Nat. Methods 7, 485–486. doi: 10.1038/nmeth.f.308
Meng, Y., Wen, J., Nie, Z., Sun, H., and Yang, Y. (2008). Phylogeny and biogeographic diversification of Maianthemum (Ruscaceae: Polygonatae). Mol. Phylogenet. Evol. 4, 424–434. doi: 10.1016/j.ympev.2008.07.017
Naito, K., Kaga, A., Tomooka, N., and Kawase, M. (2013). De novo assembly of the complete organelle genome sequences of azuki bean (Vigna angularis) using next-generation sequencers. Breed. Sci. 63, 176–182. doi: 10.1270/jsbbs.63.176
Nashima, K., Terakami, S., Nishitani, C., Kunihisa, M., Shoda, M., Takeuchi, M., et al. (2015). Complete chloroplast genome sequence of pineapple (Ananas comosus). Tree Genet. Genomes 11:60. doi: 10.1007/s11295-015-0892-8
Naydenov, K. D., Naydenov, M. K., Alexandrov, A., Vasilevski, K., Gyuleva, V., Matevski, V., et al. (2016). Ancient split of major genetic lineages of European Black Pine: evidence from chloroplast DNA. Tree Genet. Genomes 12:68. doi: 10.1007/s11295-016-1022-y
Palmer, J. D. (1985). Comparative organization of chloroplast genomes. Annu. Rev. Genet. 19, 325–354. doi: 10.1146/annurev.ge.19.120185.001545
Palmer, J. D. (1987). Chloroplast DNA evolution and biosystematic uses of chloroplast DNA variation. Am. Nat. 130, S6–S29. doi: 10.1086/284689
Palmer, J. D. (1991). Plastid chromosomes: structure and evolution. Mol. Biol. Plastids 7, 5–53. doi: 10.1016/B978-0-12-715007-9.50009-8
Pauwels, M., Vekemans, X., Godé, C., Frérot, H., Castric, V., and Saumitou-Laprade, P. (2012). Nuclear and chloroplast DNA phylogeography reveals vicariance among European populations of the model species for the study of metal tolerance, Arabidopsis halleri (Brassicaceae). New Phytol. 193, 916–928. doi: 10.1111/j.1469-8137.2011.04003.x
Phadtare, S., Kazakov, T., Bubunenko, M., Pestova, T., and Severinov, K. (2007). Transcription antitermination by translation initiation factor IF1. J. Bacteriol. 189, 4087–4093. doi: 10.1128/JB.00188-07
Poliseno, L., Salmena, L., Zhang, J., Carver, B., Haveman, W. J., and Pandolfi, P. P. (2010). A coding-independent function of gene and pseudogene mRNAs regulates tumour biology. Nature 465, 1033–1038. doi: 10.1038/nature09144
Posada, D., and Crandall, K. A. (1998). Modeltest: testing the model of DNA substitution. Bioinformatics 14, 817–818. doi: 10.1093/bioinformatics/14.9.817
Raman, G., Choi, K. S., and Park, S. (2016). Phylogenetic Relationships of the Fern Cyrtomium falcatum (Dryopteridaceae) from Dokdo Island based on chloroplast genome sequencing. Genes 7:115. doi: 10.3390/genes7120115
Razmovski-Naumovski, V., Huang, T. H. -W., Tran, V. H., Li, G. Q., Duke, C. C., and Roufogalis, B. D. (2005). Chemistry and pharmacology of Gynostemma pentaphyllum. Phytochem. Rev. 4, 197–219. doi: 10.1007/s11101-005-3754-4
Rice, P., Longden, I., and Bleasby, A. (2000). EMBOSS: the European molecular biology open software suite. Trends Genet. 16, 276–277. doi: 10.1016/S0168-9525(00)02024-2
Ronquist, F., and Huelsenbeck, J. P. (2003). MrBayes 3: Bayesian phylogenetic inference under mixed models. Bioinformatics 19, 1572–1574. doi: 10.1093/bioinformatics/btg180
Sandbrink, J., Vellekoop, P., Van Ham, R., and Van Brederode, J. (1989). A method for evolutionary studies on RFLP of chloroplast DNA, applicable to a range of plant species. Biochem. Syst. Ecol. 17, 45–49. doi: 10.1016/0305-1978(89)90041-0
Sato, S., Nakamura, Y., Kaneko, T., Asamizu, E., and Tabata, S. (1999). Complete structure of the chloroplast genome of Arabidopsis thaliana. DNA Res. 6, 283–290. doi: 10.1093/dnares/6.5.283
Shinozaki, K., Ohme, M., Tanaka, M., Wakasugi, T., Hayashida, N., Matsubayashi, T., et al. (1986). The complete nucleotide sequence of the tobacco chloroplast genome: its gene organization and expression. EMBO J. 5:2043. doi: 10.1007/BF02669253
Stamatakis, A. (2006). RAxML-VI-HPC: maximum likelihood-based phylogenetic analyses with thousands of taxa and mixed models. Bioinformatics 22, 2688–2690. doi: 10.1093/bioinformatics/btl446
Sun, Y., Moore, M. J., Meng, A., Soltis, P. S., Soltis, D. E., Li, J., et al. (2013). Complete plastid genome sequencing of trochodendraceae reveals a significant expansion of the inverted repeat and suggests a paleogene divergence between the two extant species. PLoS ONE 8:e60429. doi: 10.1371/journal.pone.0060429
Tamura, K., Peterson, D., Peterson, N., Stecher, G., Nei, M., and Kumar, S. (2011). MEGA5: molecular evolutionary genetics analysis using maximum likelihood, evolutionary distance, and maximum parsimony methods. Mol. Biol. Evol. 28, 2731–2739. doi: 10.1093/molbev/msr121
Thiel, T., Michalek, W., Varshney, R., and Graner, A. (2003). Exploiting EST databases for the development and characterization of gene-derived SSR-markers in barley (Hordeum vulgare L.). Theor. Appl. Genet. 106, 411–422. doi: 10.1007/s00122-002-1031-0
Tsai, Y. C., Lin, C. L., and Chen, B. H. (2010). Preparative chromatography of flavonoids and saponins in Gynostemma pentaphyllum and their antiproliferation effect on hepatoma cell. Phytomedicine 18, 2–10. doi: 10.1016/j.phymed.2010.09.004
Untergasser, A., Cutcutache, I., Koressaar, T., Ye, J., Faircloth, B. C., Remm, M., et al. (2012). Primer3—new capabilities and interfaces. Nucleic Acids Res. 40:e115. doi: 10.1093/nar/gks596
Vanin, E. F. (1985). Processed pseudogenes: characteristics and evolution. Annu. Rev. Genet. 19, 253–272. doi: 10.1146/annurev.ge.19.120185.001345
Wang, C., Zhang, H., Qian, Z., and Zhao, G. (2008a). Genetic differentiation in endangered Gynostemma pentaphyllum (Thunb.) Makino based on ISSR polymorphism and its implications for conservation. Biochem. Syst. Ecol. 36, 699–705. doi: 10.1016/j.bse.2008.07.004
Wang, R., Cheng, C., Chang, C., Wu, C., Su, T., and Chaw, S. (2008b). Dynamics and evolution of the inverted repeat-large single copy junctions in the chloroplast genomes of monocots. BMC Evol. Biol. 8:36. doi: 10.1186/1471-2148-8-36
Wyman, S. K., Jansen, R. K., and Boore, J. L. (2004). Automatic annotation of organellar genomes with DOGMA. Bioinformatics 20, 3252–3255. doi: 10.1093/bioinformatics/bth352
Xie, Z., Liu, W., Huang, H., Slavin, M., Zhao, Y., Whent, M., et al. (2010). Chemical composition of five commercial Gynostemma pentaphyllum samples and their radical scavenging, antiproliferative, and anti-inflammatory properties. J. Agric. Food Chem. 58, 11243–11249. doi: 10.1021/jf1026372
Yang, Y., Zhou, T., Duan, D., Yang, J., Feng, L., and Zhao, G. (2016). Comparative analysis of the complete chloroplast genomes of five Quercus species. Front. Plant Sci. 7:959. doi: 10.3389/fpls.2016.00959
Yin, F., Hu, L., and Pan, R. (2004). Novel dammarane-type glycosides from Gynostemma pentaphyllum. Chem. Pharmaceut. Bullet. 52, 1440–1444. doi: 10.1248/cpb.52.1440
Zhang, Y., Ma, P., and Li, D. (2011). High-throughput sequencing of six bamboo chloroplast genomes: phylogenetic implications for temperate woody bamboos (Poaceae: Bambusoideae). PLoS ONE 6:e20596. doi: 10.1371/journal.pone.0020596
Zheng, D., Frankish, A., Baertsch, R., Kapranov, P., Reymond, A., Choo, S. W., et al. (2007). Pseudogenes in the ENCODE regions: consensus annotation, analysis of transcription, and evolution. Genome Res. 17, 839–851. doi: 10.1101/gr.5586307
Zhong, B., Yonezawa, T., Zhong, Y., and Hasegawa, M. (2009). Episodic evolution and adaptation of chloroplast genomes in ancestral grasses. PLoS ONE 4:e5297. doi: 10.1371/journal.pone.0005297
Zhou, J., Luo, Y., Wu, Y., Li, K., Jiang, J., and Xu, P. (2010). RAPD primers screening and genetic diversity analysis of eight germplasms of Gynostemma pentaphyllum from Guangxi. Genomics Appl. Biol. 29, 273–278.
Keywords: Gynostemma BL., chloroplast genome, characterization, comparison, repeats, phylogeny
Citation: Zhang X, Zhou T, Kanwal N, Zhao Y, Bai G and Zhao G (2017) Completion of Eight Gynostemma BL. (Cucurbitaceae) Chloroplast Genomes: Characterization, Comparative Analysis, and Phylogenetic Relationships. Front. Plant Sci. 8:1583. doi: 10.3389/fpls.2017.01583
Received: 05 June 2017; Accepted: 29 August 2017;
Published: 12 September 2017.
Edited by:
Alessio Mengoni, University of Florence, ItalyReviewed by:
Umesh K. Reddy, West Virginia State University, United StatesLuis Delaye, Center for Research and Advanced Studies of the National Polytechnic Institute (CINVESTAV), Mexico
Copyright © 2017 Zhang, Zhou, Kanwal, Zhao, Bai and Zhao. This is an open-access article distributed under the terms of the Creative Commons Attribution License (CC BY). The use, distribution or reproduction in other forums is permitted, provided the original author(s) or licensor are credited and that the original publication in this journal is cited, in accordance with accepted academic practice. No use, distribution or reproduction is permitted which does not comply with these terms.
*Correspondence: Guifang Zhao, Z2Z6aGFvQG53dS5lZHUuY24=