- 1College of Agronomy, Henan Agricultural University, Zhengzhou, China
- 2State Key Laboratory of Wheat and Maize Crop Science, Henan Agricultural University, Zhengzhou, China
- 3National Engineering Research Centre for Wheat, Henan Agricultural University, Zhengzhou, China
Unreasonably high irrigation levels and excessive nitrogen (N) supplementation are common occurrences in the North China Plain that affect winter wheat production. Therefore, a 6-yr-long stationary field experiment was conducted to investigate the effects of irrigation and N regimes on root development and their relationship with soil water and N use in different soil layers. Compared to the non-irrigated treatment (W0), a single irrigation at jointing (W1) significantly increased yield by 3.6–45.6%. With increases in water (W2, a second irrigation at flowering), grain yield was significantly improved by 14.1–45.3% compared to the W1 treatments during the drier growing seasons (2010–2011, 2012–2013, and 2015–2016). However, under sufficient pre-sowing soil moisture conditions, grain yield was not increased, and water use efficiency (WUE) decreased significantly in the W2 treatments during normal precipitation seasons (2011–2012, 2013–2014, and 2014–2015). Irrigating the soil twice inhibited root growth into the deeper soil depth profiles and thus weakened the utilization of soil water and NO3-N from the deep soil layers. N applications increased yield by 19.1–64.5%, with a corresponding increase in WUE of 66.9–83.9% compared to the no-N treatment (N0). However, there was no further increase in grain yield and the WUE response when N rates exceeded 240 and 180 kg N ha−1, respectively. A N application rate of 240 kg ha−1 facilitated root growth in the deep soil layers, which was conducive to utilization of soil water and NO3-N and also in reducing the residual NO3-N. Correlation analysis indicated that the grain yield was significantly positively correlated with soil water storage (SWS) and nitrate nitrogen accumulation (SNA) prior to sowing. Therefore, N rates of 180–240 kg ha−1 with two irrigations can reduce the risk of yield loss that occurs due to reduced precipitation during the wheat growing seasons, while under better soil moisture conditions, a single irrigation at jointing was effective and more economical.
Introduction
The North China Plain covers an area of 3.2 × 105 km2. Due to climatic conditions, a highly intensive winter wheat and summer maize double-cropping system is the dominant, high-yielding agricultural production system (Zhao et al., 2007), supplying more than 50% of the winter wheat (Triticum aestivum L.) and 33% of the summer maize (Zea mays L.) produced in China (National Bureau of Statistics, 2013). The crop rotation system consumes 800–850 mm of water annually (about 450 mm for winter wheat and 360 mm for summer maize). However, annual precipitation in the region ranges from 500 to 600 mm, with an average of 200–220 mm falling during the wheat growing season (Liu et al., 2001; Yang et al., 2015). Water is the main limiting factor in the North China Plain, and crop production relies mainly on groundwater irrigation (Hu et al., 2010; Zhang et al., 2010; Li et al., 2015). As a result, groundwater tables are dropping ~1.5 m per year, which is threatening the development of sustainable agriculture (Fang et al., 2010; Li et al., 2015). In general, there are 4–5 irrigations supplied by some farmers during the wheat growing seasons in this region, which leads to a lower water use efficiency (WUE; Sun et al., 2010). Appropriate irrigation regimes are therefore fundamental to increase WUE so as to develop sustainable wheat production in the North China Plain (Li et al., 2015). Current evidence suggests that with deficit irrigation, nearly 22% of irrigation water might be saved without any significant reduction in wheat yield (Hamid et al., 2010; Shaughnessy et al., 2012; Li et al., 2015). Therefore, it is important to obtain a balance between high yield and reduced water supply in winter wheat by increasing its WUE in this region (Zhong and Shangguan, 2014).
Nitrogen (N) is fundamental plant nutrient that affects productivity and WUE in wheat (Zhou et al., 2011; Devadas et al., 2014; Wang et al., 2015) and improves soil fertility (Hai et al., 2010). The increasing use of N fertilizer has been shown to significantly increase grain yields by 50–60% in the dry-land of the Loess Plateau (Fan et al., 2005) and by 20–80% in a rainfed Mediterranean environment (Bassoa et al., 2010). Zhong and Shangguan (2014) reported that the wheat grain yield and WUE increased as the N rate increased from 0 to 270 kg N ha−1, while excessive N application had no positive effect on either parameter. However, a large range of N application rates exists in the North China Plain, some of which exceed 300 kg N ha−1. In fact, excessive N application not only causes a waste of resources and economic losses, but also has an adverse effect on wheat yield and the environment (Godfray et al., 2010; Hvistendahl, 2010). In the North China Plain, 221–620 kg N ha−1 of residual nitrate was detected in the 0–1.0 m soil profile at N application rates of 200–300 kg N ha−1 after two wheat-maize rotations (Fang et al., 2006). Therefore, it is highly important to use water and N fertilizer in a reasonable manner in order to reduce the cost of resources in this region.
Root distribution is often utilized in the analysis of soil-root-shoot-atmosphere interactions (Smit et al., 1994; Asseng et al., 1997; Dai et al., 2014). The temporal and spatial distribution of the wheat root system affects potential water and nutrient absorption (Hund et al., 2009; Dai et al., 2014; Xu et al., 2016). Irrigation and nitrogen regimes affect the root distribution in wheat, which strongly influences soil water consumption (Zhang et al., 2009; Wang et al., 2014) and soil NO3-N content (Xu et al., 2016). In general, slight water shortages and nitrogen deficiency during vegetative growth of wheat cause an increase in vertical root penetration, decreasing the root length density (RLD) in the top soil layer and increasing the RLD in deeper layers (Li et al., 2010; Wang et al., 2014; Xu et al., 2016). Roots in the deep soil layers (>0.8 m) are essential for nutrient and moisture absorption and, therefore, growth and final yield in wheat (Dai et al., 2014; Xu et al., 2016).
Although some studies have been conducted on root systems, water-N use, and yield under different fertilization and crop management schemes (Zhou et al., 2011; Shen et al., 2012; Chu et al., 2016), fixed–position experiments on irrigation and nitrogen regimes in wheat-maize fields have been relatively poorly studied in the North China Plain, especially under high yielding conditions. In addition, the relationships between root growth and the use of soil water and N in different soil layers remain to be further investigated. Moreover, the yield and WUE in wheat is also influenced by meteorological factors and seasonal variation (Kang et al., 2002). Therefore, a 6-yr-long stationary field experiment was initiated in 2010 in the western part of the North China Plain. The objectives of the present investigation were as follows: (1) to examine the impacts of irrigation regimes and N application rates on wheat grain yield; (2) to establish relationships between root development and soil water reduction amount and NO3-N content at different soil depths, and (3) to determine the optimal combination of irrigation and nitrogen application rate for winter wheat in the North China Plain.
Materials and Methods
Experimental Site
The field experiments were performed in six winter wheat-summer maize rotations from 2010 to 2016 at Henan Agricultural University Experimental Station, located in the west of the North China Plain (113°38′39″ E, 34°47′51″ N). The soil is an alluvial soil (Gong et al., 2007), belonging to the Ochri-Aquic Cambosols (32.3% sand, 57.7% silt, 10.0% clay). In the cultivated layer (0.2 m), the pH was 7.82 with a field capacity of 23.9% volumetric soil water and a bulk density of 1.28 g m−3. The amounts of organic matter, total N, available phosphorus and available potassium were 17.47 g kg−1, 0.84 g kg−1, 18.83 mg kg−1, and 252.56 mg kg−1, respectively. The experimental site is characterized by a north temperate continental monsoon climate zone with an annual mean temperature of 14.3°C and annual sunshine duration of 2300 h. The average (1951–2006) annual rainfall is 617.1 mm, with the majority (60–75%) occurring in the summer months (June to September), and an average of 201.2 mm falling during the wheat growing season. Precipitation during the experimental seasons for wheat and maize is shown in Figure 1. Relatively dry weather prevailed during the wheat growing seasons of 2010–2011, 2012–2013, and 2015–2016; rainfall was 84.8, 183.9, and 194.3 mm, respectively, compared to the long-term average of 201.2 mm (1951–2006). However, the rainfall pattern showed monthly variability. During the 2012–2013 and 2015–2016 wheat growing seasons, there was only 13.1 and 7.9 mm of rainfall from jointing to flowering (March 10 to April 15), which was a major limiting factor to wheat production (Xue et al., 2006; Karam et al., 2009).
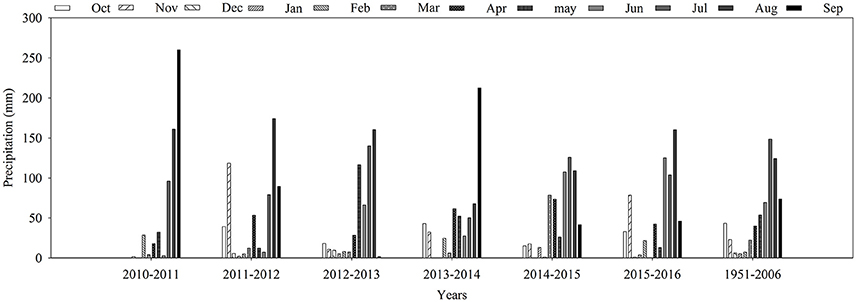
Figure 1. Annual precipitation by month (October to September) during the six wheat-maize growing seasons from 2010–2011 to 2015–2016 and the corresponding monthly averages from 1951 to 2006 at the experimental site.
Experimental Design and Crop Management
The experiment, a typical winter wheat-summer maize rotation, started with the winter wheat season of October 2010, and ended after the winter wheat harvest in June 2016, covering 11 successive seasonal crops in the same experimental plot. Each experimental plot was 7 m in length and 2.9 m in width. The plots were arranged in a split-plot design with four replicates. The main plots were assigned to three irrigation regimes: no irrigation water applied after sowing (W0), a single irrigation at jointing (W1), and two irrigations, one each at jointing and flowering (W2) during the wheat growing seasons. During the maize growing seasons, in all treatments irrigation was provided based on critical growth stages, such as the V12 stage, tasseling stage, and elongation stage during the 2012, 2013, and 2014 maize growing seasons, respectively (Table 1). Irrigation was applied by a movable sprinkler system, and 75 mm was applied each time as measured by a water meter. The treatment details are given in Table 1. Subplots were treated with five nitrogen rates: 0, 90, 180, 240, and 300 kg N ha−1 during wheat growing season, and 0, 112.5, 225, 300, and 375 kg N ha−1 during the maize growing season; these are referred to as N0, N1, N2, N3, and N4, respectively.
Wheat (cv. “Aikang 58” until 2012, then “Yumai 49–198”) was sown on 12 October each year at a sowing rate of 225 kg ha−1 with 0.2 m row spacing. The wheat was harvested in late May-early June of the following year. Phosphorus was applied as triple superphosphate (P2O5; 46%) at a rate of 150 kg P ha−1, and potash was applied as potassium chloride (K2O; 46%) at a rate of 100 kg K ha−1. Half the amount of nitrogen (urea; 46% N), and all phosphorus and phosphate was spread uniformly over the soil surface and then drilled into the upper 0.15 m at sowing. The other half of the nitrogen was applied at the jointing stage for each treatment. Maize (cv. “Zhengdan 958”) was planted by hand immediately after the wheat harvest in the same plots. P2O5 was applied at 90 kg ha−1, K2O at 120 kg ha−1, and 30% of the nitrogen for each treatment was applied at the elongation stage of maize, with the remaining 70% applied at the V12 stage.
Additional protective measures were taken to ensure healthy growth such as the spray application of insecticides, fungicides, and herbicides. Accordingly, no significant incidence of pests, diseases, or weeds was observed in any treatment during any of the growing seasons.
Soil Samples and Analysis
Soil cores were collected every 0.2 m to a final depth of 1.6 m using a soil auger (0.05 m diameter) before sowing and after harvest in all experimental plots. Two cores were collected from each plot. Samples were then analyzed for soil NO3-N content (SNC; mg kg−1). Briefly, samples of fresh soil were extracted by shaking with a 2 M KCl solution (soil: solution ratio: 1:5) for 1 h. After filtering, the extract solution was analyzed using a continuous flow analyzer. Soil NO3-N accumulation (SNA) was then calculated using the following formula:
Where, ρb (g cm−3) is the soil bulk density, and h (cm) is the soil thickness. The gravimetric water content (θ, g g−1) of the samples was determined by drying them to a constant weight in an oven at 105°C (Bao, 2000). Soil water storage (SWS, mm) was calculated as:
In addition, soil water storage reduction amount (SWR) was calculated as the SWS at sowing minus the SWS at maturity for the different soil layers.
The evapotranspiration (ET) of the wheat was calculated by the following formula:
where ET (mm) is evapotranspiration, I (mm) is the amount of irrigation water that was measured using the water meters, P (mm) is the precipitation that was measured with the weather station at the site shown in Figure 1, ΔS (mm) is the SWS in the soil layer from 0 to 1.6 m at sowing minus the SWS at harvest, R (mm) is surface runoff (there was no surface runoff, so this variable was ignored). U is the upward capillary flow into the root zone (mm), and D is the downward drainage out from the root zone (mm). Because the groundwater table was ~10.2–11.2 m below the soil surface during the 6-year period, capillary flow is negligible (Liu and Wei, 1989). Drainage from the root zone was estimated using the following equation:
Where K (θ) is the unsaturated water conductivity when soil moisture is θ (cm day−1), ΔH is the soil water potential between the top-soil and sub-soil (cm), ΔZ is the soil thickness (cm), and Δt is the period of time (in days). SWC was measured in the 1.6 m soil profile in this study, since more than 90% of the root system of wheat plants occupies only the first 1.0 m soil layer in the North China Plain, as was shown by several previous studies (Zhang et al., 2009; Wang et al., 2014; Xu et al., 2016). Therefore, drainage out from the root zone can be ignored in the North China Plain, including at our experimental site (Lv et al., 2011). Water use efficiency (WUE; kg ha−1 mm−1) in wheat is defined as grain yield divided by ET over the whole wheat growing season.
Root Sampling
Roots were sampled at maturity by removing soil blocks during the 2013–2014 wheat growing season. Briefly, blocks were dug at 0.2 m intervals to a vertical depth of 1.6 m in six separate layers. Each sampling area was 0.4 m in length (perpendicular to the rows, providing access to plants in two rows) and 0.4 m in width (parallel to the rows). The roots with soil were then transferred to a 100–mesh nylon bag and submerged in water for 1 h. Any remaining soil was then washed from the roots using a low-pressure garden hose, and the clean roots were transferred to a sieve (0.25 mm2 mesh) suspended in a trough partially filled with water. The cleaned roots were imaged using a scanner for the gray-scale scanning (Epson perfection V700 photo). The files were then analyzed using WinRHIZO 2008 in order to measure root length. Root length density (RLD; mm cm−3), the unit volume of soil root length, was determined using the following formula:
where L is the total root length (mm) and V is the volume of the soil sample (cm3).
Statistical Analysis
Statistical analyses were performed using SPSS 17.0 software. Irrigation regime, nitrogen rates, and their interactions were taken as fixed factors, while the growing season was considered to be a random factor due to unpredictable weather conditions. Analysis of variance (ANOVA) was conducted for grain yield, SWS and SNA, ET and WUE, and for SWS, SNC, and RLD at each soil layer. The means of each treatment were compared with Duncan's multiple range test at the 0.05 probability level (at p ≤ 0.05). Pearson correlations were used to analyze the relationship between RLD and SWC (n = 72) and SNC (n = 36), and between yield and water-nitrogen use parameters (n = 144). Figures were prepared using Sigmaplot 12.3.
Results
Wheat Yield
Wheat yields averaged from 2055 to 8690 kg ha−1 in all treatments during the six growing seasons (Figure 2). Compared with the non-irrigated treatment (W0), irrigation at jointing (W1) significantly increased yield by 25.7, 3.6, 31.8, 13.6, 5.6, and 45.6% in the growing seasons from 2010–2011 to 2015–2016, respectively (Figure 2, Table 2). With increased irrigation (i.e., W2), wheat yield was significantly increased by 14.1, 45.3, and 29.3% compared to W1 in the 2010–2011, 2012–2013, and 2013–2016 growing seasons, respectively, in which the rainfall was lower or unevenly distributed during the wheat growing seasons (Figure 1). Correlation analysis (Table 5) revealed a strong positive correlation between rainfall and wheat yield (r = 0.601**). However, no significant differences were found between W1 and W2 in the 2011–2012, 2013–2014, and 2014–2015 growing seasons (Figure 2, Table 2). This indicated that overfull irrigation did not result in higher yields in normal precipitation growing seasons. Wheat yields in irrigated treatments varied less over time compared to non-irrigated treatments, indicating that irrigation not only increased but also stabilized wheat yield. The CV for wheat grain yield in W0 was higher than in the irrigated treatments (W1 and W2), indicating that the precipitation and its distribution may be other important factors affecting wheat yield (Figure 1).
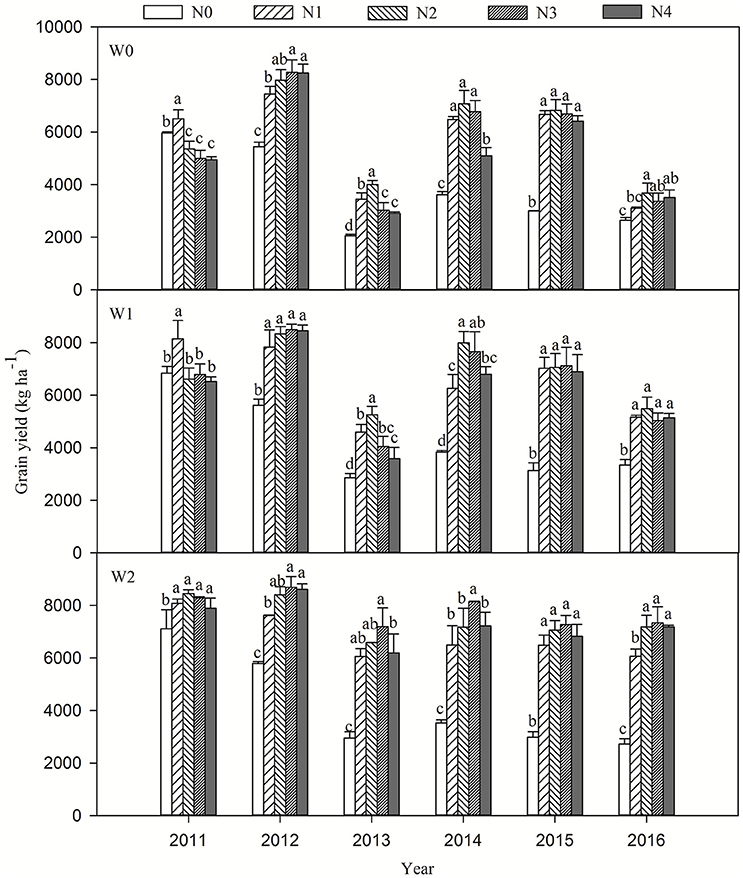
Figure 2. Effects of irrigation and nitrogen application regimes on grain yield in winter wheat from the 2010–2011 to 2015–2016 growing seasons. Different letters above the bars indicate significant differences between wheat yields in the different N treatments at the 5% level under the same irrigation conditions. N0, no applied N; N1, 90 kg ha−1; N2, 180 kg ha−1; N3, 240 kg ha−1; N4, 300 kg ha−1.
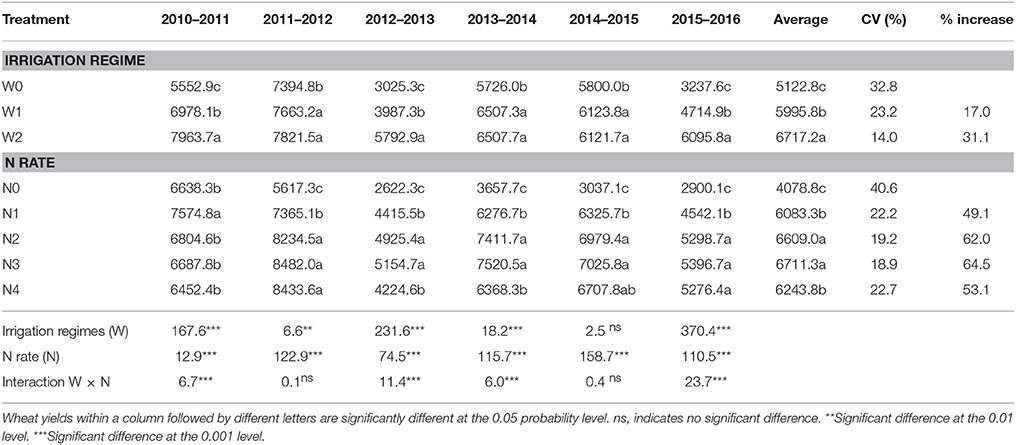
Table 2. Mean wheat grain yields for irrigation treatments across N rates and for N rates across irrigation treatments (kg ha−1) and F-values from analysis of variance for the 2010–2011 to 2015–2016 growing seasons.
N application significantly improved wheat yield, but excessive N rates did not have a positive effect on yield (Figure 2). In the growing seasons from 2010–2011 to 2015–2016, the wheat yield (averaged across irrigation and N treatments) was increased by 3.6, 44.7, 78.5, 88.5, 122.6, and 76.8% in the N treatments compared to the N0 control treatment, respectively (Figure 2, Table 2), suggesting that the effect of N fertilizer on wheat yield became greater as the experimental seasons progressed. Averaged across the six growing seasons, the highest yield of 6711.3 kg ha−1 was in the N3 treatment, but there was no significant difference between N2 and N3. Statistical analysis showed that the interaction between irrigation regimes and N rates on yield was significant (Table 2). The W2N3 treatment produced the maximum yield, and the W0N0 treatment gave the lowest yield (Figure 2).
Soil Water Storage (SWS)
Maximum SWS (averaged across irrigation and N treatments) before sowing of wheat (388.9 mm) was recorded in the 2011–2012 growing season, whereas the minimum SWS of wheat (274.9 mm) occurred in the 2012–2013 growing season (Table 3), which resulted in the highest and the lowest grain yields in the corresponding seasons (Table 2). No difference in SWS before sowing was observed among the three irrigation regimes in the 2013–2014 and 2014–2015 growing seasons, which resulted in reduced yield increases in the irrigated treatments (Table 2). SWS before sowing in N0 was higher than in the N treatments, and irrigation therefore did not increase yield under no-N conditions. N3 had the lowest SWS before sowing, while W2 gave the highest yield (Figure 2). This suggests that irrigation can increase yield under N application conditions, but that it could not balance the adverse effect of N stress on grain yield under conditions of N deficiency.
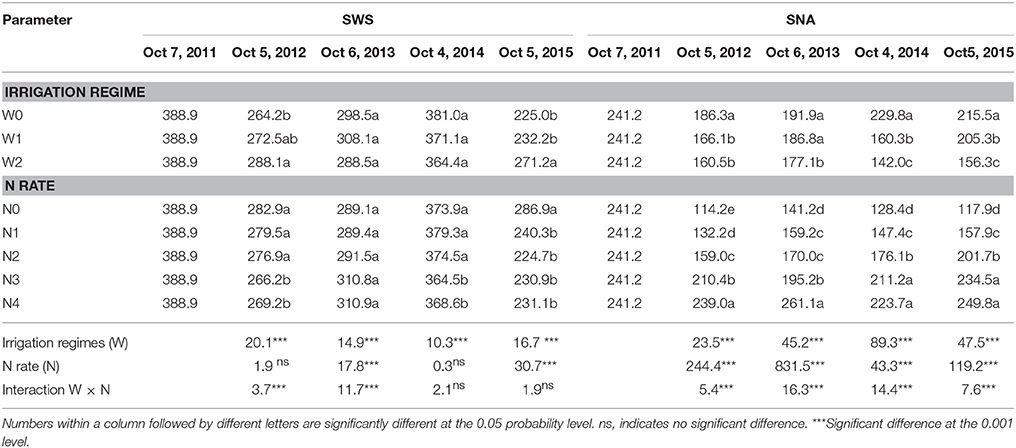
Table 3. The soil water storage (SWS; mm) and soil NO3-N accumulation (SNA; kg ha−1) in the 0–1.6 m soil profile prior to sowing winter wheat.
Plots of the soil water reduction amount (SWR) in the different soil layers are shown in Figure 3. Water was mainly consumed in the 0.4–1.2 m deep layers, with the highest SWR in the 0.6–1.0 m layer. The proportion of SWR in the 1.0–1.6 m layer was higher in the drier growing season 2012–2013 (32.0%) than in the normal precipitation growing season 2013–2014 (22.4%). Similarly, SWR in W0 was higher than in W1 and W2 at all soil layers (Figure 3). N rates had a significant effect on SWR, since N application increased SWR in all soil layers. The N3 treatment gave the highest SWR (Figure 3). Moderate water applied (W1) and appropriate N rates facilitated longer root growth, which resulted in more water being consumed in the deeper soil layers.
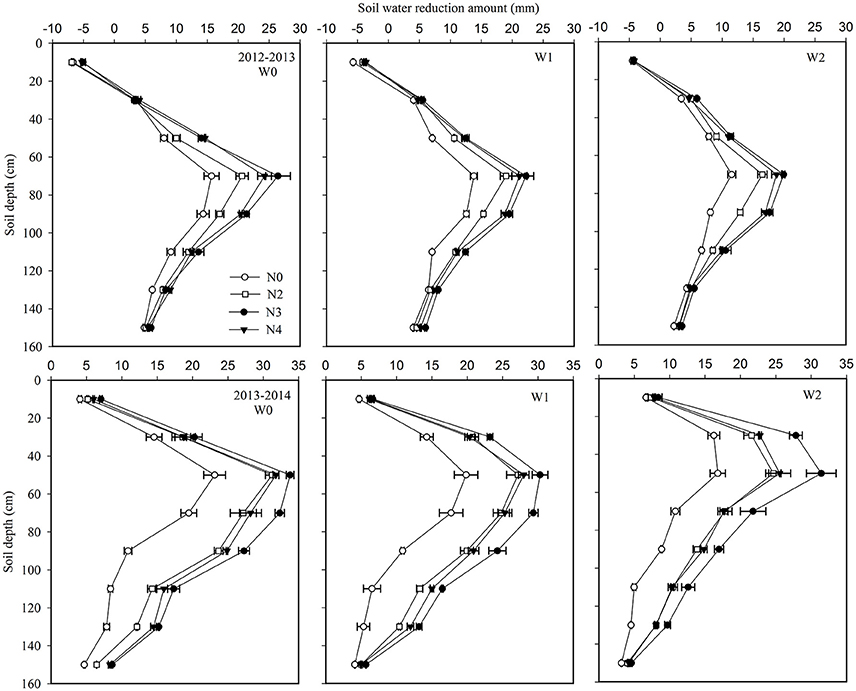
Figure 3. Effects of irrigation and nitrogen regimes on soil water reduction amount at 0.2 m soil depth increments to 1.6 m in the 2012–2013 and 2013–2014 growing seasons. Horizontal bars represent the standard errors.
ET and WUE
Irrigation caused a marked increase in evapotranspiration (ET). Compared to the un-irrigated treatments, ET in the W2 treatment increased by 45.2% during the drier growing seasons (averaged across 2012–2013 and 2015–2016), but only increased by 18.6% during growing seasons with normal precipitation (averaged across 2013–2014 and 2014–2015; Table 3). The W2 treatment showed a significant reduction in WUE in the 2013–2014 and 2014–2015 growing seasons, but gave the maximum WUE values during the 2012–2013 and 2015–2016 growing seasons (Table 4), which might be attributed to less rainfall and uneven distribution (Figure 1). N fertilizer caused an increase in ET that averaged 32.5, 38.2, and 34.5 mm for the N180, N240, and N300 treatments compared to N0, respectively (Table 3). Compared to the N0 treatment, N treatment significantly increased WUE by 76.4, 69.0, 96.1, and 70.9% (averaged across irrigation and N treatments) for the 2012–2013 to 2015–2016 growing seasons, respectively (Table 4). However, there was no further increase in WUE when the nitrogen application rate exceeded 180 kg N ha−1.
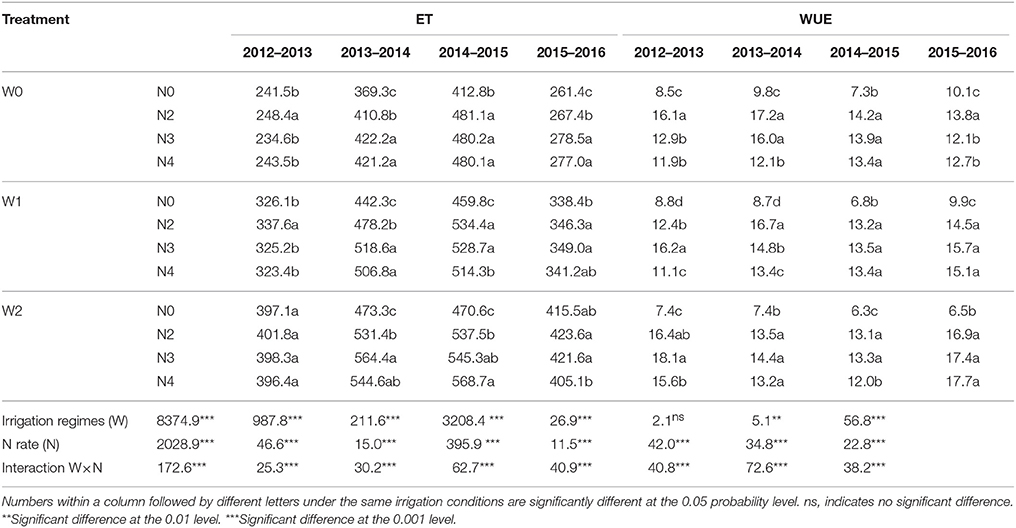
Table 4. Effects of irrigation and nitrogen regimes on evapotranspiration (ET; mm) and water use efficiency (WUE; kg ha−1 mm−1) of winter wheat for the 2012–2013 to 2015–2016 growing seasons.
Soil NO3-N Content (SNC)
W2 gave the lowest soil NO3-N content (SNC), while W0 had the highest SNC in the top 0.4 m soil layer. However, from 0.8 m downwards, SNC in the irrigated treatments was greater than in the W0 control (Figure 4). This suggests that irrigation increased the levels of residual NO3-N in the deep soil layers. Compared to N0, all N treatments significantly increased SNC in the 0–1.6 m soil layer at both flowering and maturity (Table 3, Figure 4). SNC in the N3 treatment was higher than in N2 in the 0.6–1.4 m soil layers at flowering, while no difference was found between N2 and N3 at maturity, indicating that N3 improved absorption of NO3-N in the 0.6–1.4 m soil layers during the later stages of growth. This might be attributed to plants in the N3 treatment having the most extensive root systems in the deep soil layers. SNC in the 0.8–1.6 m soil layers in the N4 treatment was 37.7 and 86.9% higher than in N3 at flowering and maturity, respectively (Figure 2), implying that there was more residual NO3-N in the deep soil layer in N4, which exceeded the ability of wheat to absorb it. Table 3 also shows that the soil nitrogen supply in N0 gradually declined as the experimental seasons progressed, which was in agreement with the slight decrease observed in grain yield in the N0 treatment (Figure 2).
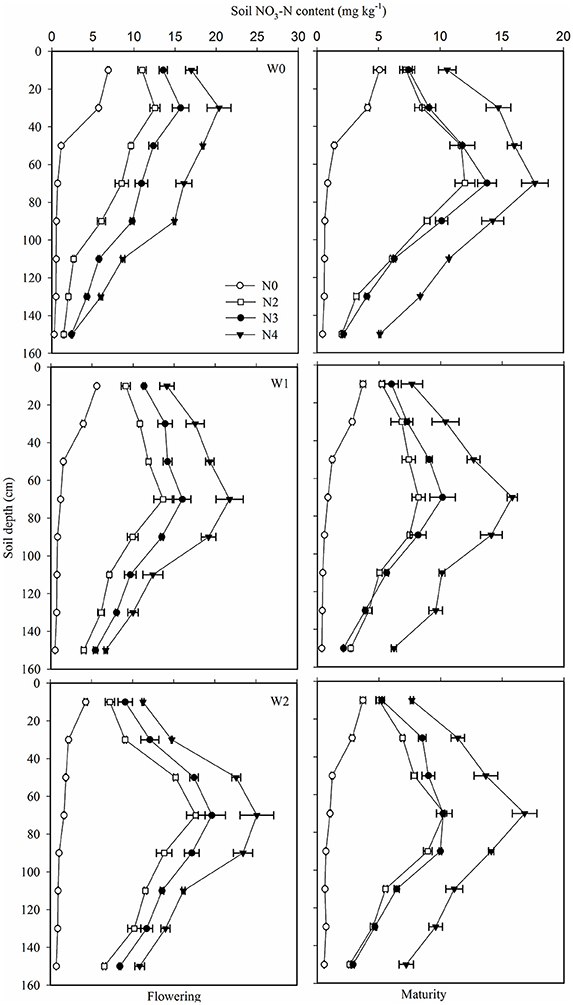
Figure 4. Effect of irrigation and nitrogen regimes on NO3-N content at flowering (left panels) and maturity (right panels) at 0.2 m increments to a depth of 1.6 m in the 2013–2014 growing season. Horizontal bars represent the standard errors.
Root Length Density (RLD)
Root length density (RLD) declined with increasing soil depth. Averaged across the irrigation and N treatments and both growing seasons, the highest RLD was 21.0 mm cm−3 in the 0–0.2 m soil layer, gradually decreasing to 10.1, 6.0, 4.9, 3.7, 3.0, 2.5, and 2.2 mm cm−3 at 0.2 m intervals through the soil column to a depth of 1.6 m. The W2 treatment had the highest RLD, while W0 plants had the smallest root system in the top 0.4 m soil layer (Figure 5). The RLD in the W0 and W1 treatments was greater than in W2 from 0.8 m downwards. The N application rates of 180 and 240 kg ha−1 (N2 and N3) gave higher RLD values at depths from 0.4 to 1.6 m, while a higher RLD was only found in the 0–0.4 m soil layer in the N4 treatment. The relationships between RLD distribution and SWR and SNC for the different soil profiles were analyzed at 0.4-m intervals (Table 6). Positive relationships between RLD and SWR, and RLD and SNC were found in each soil layer for the non-irrigated and no-N treatments. SWC and SNC at soil depths of 0–0.4 and 0.8–1.6 m exhibited significant positive correlations with RLD for the W1 and N2 treatments, while a significant correlation was only found in the upper soil profile (0–0.4 m) for the W2 and N4 treatments. This suggests that elevated water and N use in deep soil layers under optimum irrigation and N regimes can be attributed to a deeper root system.
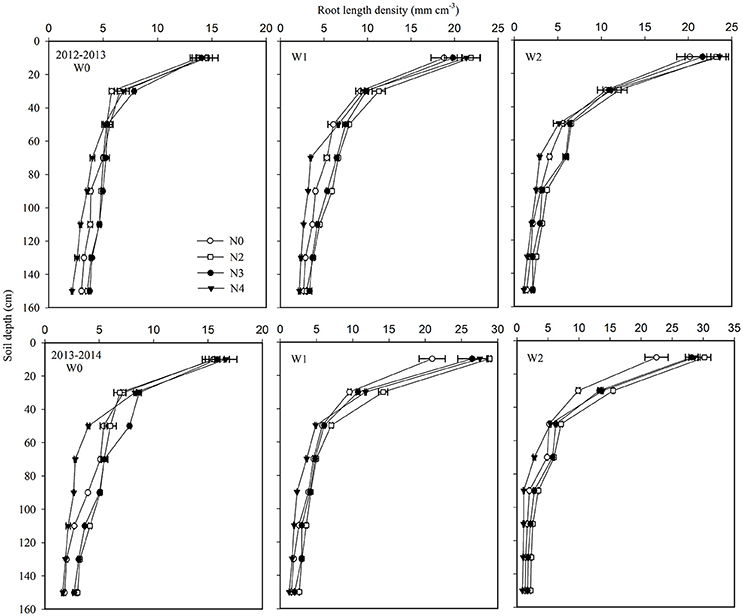
Figure 5. Effects of irrigation and nitrogen regimes on root distribution at 0.2 m depth increments to 1.6 m at maturity in the 2012–2013 and 2013–2014 growing seasons. Horizontal bars represent the standard errors.
Discussion
When the crop growing season was dry, irrigation was necessary in order to offset a water deficit. It has been previously reported that supplemental irrigation and limited or deficit irrigation greatly affects both crop yield and WUE (Zhang et al., 1999; Wang et al., 2001; Sun et al., 2010). Compared to rainfed conditions, grain yield with supplemental irrigation was significantly increased by 8.0% in the North China Plain (Li et al., 2015), and by 25–35% in the Lake Chad Basin of Africa (Karam et al., 2009). Optimal irrigation levels and mild soil water stress conditions (65–70% of field capacity) significantly increased yield and WUE in wheat (Zhang et al., 2003; Liu et al., 2016). However, some studies have indicated that deficit irrigation significantly decreased wheat yield (Adelian et al., 2012; Chen et al., 2015). In the present study, compared to the W0 control treatment, grain yield increased by 3.6–45.6% in W1 and by 5.8–91.5% in W2. No significant difference was found between the W1 and W2 treatments during normal precipitation growing seasons, in which the SWS before sowing was relatively high. Correlation analysis showed a significant positive correlation between SWS before sowing and grain yield (Table 5), which might be attributed to the precipitation and irrigation during the maize growing seasons. In the high SWS growing seasons of 2011 and 2014, the September rainfall was 260.0 and 212.3 mm, respectively (Figure 1). In 2013, although there was less rainfall in September, irrigation was applied during the late growing stage of maize (Table 1). This suggested that, for wheat production, it is critical to make use of additional water from summer maize. In the experimental area, precipitation was sufficient to meet the needs of maize production, and irrigation usually had no beneficial effect on maize yield (data not shown). Correlation analysis showed no significant correlation between precipitation and maize yield, but wheat yield was significantly influenced by precipitation (r = 0.601**). During the drier growing seasons, the relative increase in wheat yield was higher (54.4%) than that during normal precipitation growing seasons (8.0%). High soil moisture content throughout the seasons resulted in high levels of water consumption but did not lead to higher grain yields (Kang et al., 2002; Chen et al., 2015). Qiu et al. (2008) suggested that high grain yield in wheat can be obtained by no- or one-time irrigation in the North China Plain. Our results showed that irrigation at the wheat jointing stage can produce high grain yields with a sufficient pre-sowing soil moisture level. With increases in water (i.e., W2), ET increased, but grain yield did not increase. WUE decreased significantly during normal precipitation growing seasons. But during the drier growing seasons, grain yield, ET, and WUE increased simultaneously. Growers can make decisions for the second irrigation (from booting to flowering) based on precipitation, soil water content and wheat growth. These informations can be obtained from local service centers of agricultural technology in China.
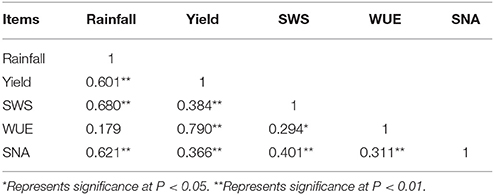
Table 5. Pearson correlations of soil water storage (SWS) before sowing and soil NO3-N accumulation (SNA) before sowing with rainfall, yield, and water use efficiency (WUE) in winter wheat.
N fertilizer is the basis of high yield (Wang et al., 2011; Sinclair and Rufty, 2012) and WUE (Shangguan et al., 2000; Brueck and Senbayram, 2009). Grain yield and WUE both increased at N application rates of 200–300 kg N ha−1 under sufficient soil water conditions (Sepaskhah and Tafteh, 2012). Deng et al. (2006) found that N fertilizer increased wheat yield by 71.3% and WUE by 20.1% in north and northwest China. In the present study, compared to N0, the N treatments increased grain yield by 49.1–64.5% and WUE by 66.9–83.9%, respectively. However, there was no further increase in grain yield or WUE when N rates exceeded 240 and 180 kg N ha−1, respectively. Excessive N application and irrigation resulted in high residual soil NO3-N after the wheat harvest (Wang et al., 2010, 2015), and 172 kg N ha−1 at a soil depth of 0.9 m was previously observed under high N fertilizer conditions in the North China Plain (Cui et al., 2010). After 6 years of N application, there was about twice as much SNA at sowing in the soil profile in the N4 treatment as in the N0 control, but there was ~27.5% less in the W2 treatment than in W0 control (Table 3). The distribution of soil NO3-N was also determined by the movement of soil water, and followed with water infiltration (Perego et al., 2012; Sharma et al., 2012). This indicated that NO3-N leached out of the 1.0 m soil layer in the wheat growing seasons with each irrigation amount of 75 mm (Liu et al., 2005), and leached out of the 2.0 m soil layer when each irrigation amount was 100 mm (Gu et al., 2015). In the present study, SWR declined from 0.8 m downwards due to high SWC below 0.8 m soil depth at harvest, and the peaks of SNA occurred in the 0.6–0.8 m soil layer after 3 years.
Root distribution is essential for nutrient and moisture absorption, and therefore, growth and final yield in crops (Zhang et al., 2009; Li et al., 2010). Roots in the deep soil profile (>0.8 m) play an important role in the utilization of soil water after flowering in wheat (Li et al., 2010; Xu et al., 2016) and, therefore, increasing WUE (Zhang et al., 2004). In the present study, a significant positive relationship was found between RLD and both SWR and SNC in the single irrigation treatment at jointing combined with N application rates of 180–240 kg N ha−1 in both upper and deep soil layers, which confirmed that wheat not only utilizes soil water and N from the upper soil layers but also from the deep layers. With late irrigation and N application (after the flowering stage), less irrigation water and N are used by wheat (Kang et al., 2000; Li et al., 2010). Under the sufficient water (W2) and excessive N supplementation (N4) treatments, water and N were not limiting factors, reducing the need for roots to absorb water and N from deeper soil layers (Table 6). These findings indicate that appropriate N application (180–240 kg ha−1) with a single irrigation at jointing can improve the ability of wheat to absorb soil water and N in the 0.6–1.4 m soil layer during the growing season, which might be attributed to the more extensive root system in the deeper soil layers.
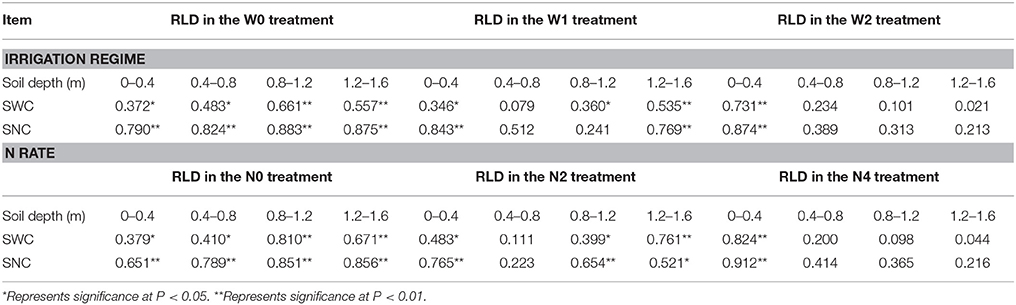
Table 6. Correlation coefficients between root length density (RLD) and soil water consumption (SWC) and soil NO3-N content (SNC) at different soil depths.
The F-value gives an indication of statistical significance, and the size of the effect of the treatments can be obtained from the variables measured (Wang et al., 2010). Our results show that N fertilizer rates had higher F-values than irrigation regimes during normal precipitation growing seasons, but irrigation regimes had higher F-values than did N rates during the drier growing seasons. This might be attributed to the relatively high SNA before sowing in the 1.6 m soil profile (from 148.6 to 243.0 kg N ha−1). Correlation analyses also showed that grain yield and WUE were significantly influenced by SNA before sowing. Abad et al. (2004) found that if the starting SNA in the 0–0.9 m soil profile was < 157 kg N ha−1, the yield would increase clearly with N applications up to 100 kg N ha−1 and high initial SNA reduced the response of yield to N rates.
Adequate water supply increases the availability of N fertilizer, while an adequate N fertilizer supply is a benefit to utilizing additional water from summer maize rainfall (Tilling et al., 2007; Di Paolo and Rinaldi, 2008; Sadras et al., 2012; Wang et al., 2015). Guo et al. (2012) concluded that the optimum N application rates in dry, normal, and wet years were 45, 135, and 180 kg ha−1, respectively, in a rainfed wheat cropping system in the semiarid Loess Plateau of China. Under well-water conditions, yield and WUE are enhanced under adequate N application rates of 200–300 kg N ha−1 (Sinclair and Rufty, 2012). Under current Huang-Huai climate conditions, application of N at rates of 180 and 240 kg N ha−1 produced the maximum grain yield under non-irrigated and irrigated conditions, respectively. The appropriate irrigation (a single irrigation at jointing) and N application rates (180–240 kg ha−1) favorably promoted the use of soil water and NO3-N mainly by increasing the number of roots in the deep soil layers, which is conducive to achieving high WUE with relatively high grain yield.
Conclusions
Our experiments revealed that N is the predominant factor that limits grain yield during normal precipitation growing seasons. During the drier growing seasons, irrigation had a relatively larger effect on wheat yield. Irrigation at the jointing stage gave higher yields and WUE during normal precipitation growing seasons. However, an additional irrigation at flowering (W2) was necessary in the drier growing seasons. Appropriate N application rates facilitated root development, which not only resulted in higher yields and WUE, but also reduced residual NO3-N. When irrigation was combined with N rates of 180–240 kg N ha−1, it favorably enhanced the use of soil water and NO3-N in both the upper and deeper soil layers by regulating root growth, which is conducive to attaining high grain yields and WUE.
Author Contributions
CW: conceived of and designed the study; WL and GM: analyzed the data and wrote the manuscript; WL, SL, and JW: carried out the field measurements and root analysis; HL, WF, and YX: critically reviewed the manuscript; DM and GK: assisted with manuscript writing and editing. All authors approved the final version of the manuscript.
Conflict of Interest Statement
The authors declare that the research was conducted in the absence of any commercial or financial relationships that could be construed as a potential conflict of interest.
Acknowledgments
This work was supported by the National Key Research and Development Program of China [grant numbers 2016YFD0300100, 2016YFD0300404] and the Twelfth Five-Year National Food Production Technology Project [grant numbers 2015BAD26B01, 2013BAD07B07].
References
Abad, A., Lloveras, J., and Michelena, A. (2004). Nitrogen fertilization and foliar urea effects on durum wheat yield and quality and on residual soil nitrate in irrigated mediterranean conditions. Field Crops Res. 87, 257–269. doi: 10.1016/j.fcr.2003.11.007
Adelian, D., Haghighi, B. J., Alizadeh, O., and Aseyfi, Z. (2012). Study and comparison of figures wheat yield on deficit irrigation. Int. J. Agron. Plant Prod. 3, 527–534.
Asseng, S., Richter, C., and Wessolek, G. (1997). Modelling root growth of wheat as the linkage between crop and soil. Plant Soil 190, 267–277. doi: 10.1023/A:1004228201299
Bassoa, B., Cammarano, D., Troccoli, A., Chen, D., and Ritchie, J. T. (2010). Long-term wheat response to nitrogen in a rainfed Mediterranean environment: field data and simulation analysis. Eur. J. Agron. 33, 132–138. doi: 10.1016/j.eja.2010.04.004
Brueck, H., and Senbayram, M. (2009). Low nitrogen supply decreases water-use efficiency of oriental tobacco. J. Plant Nutr. Soil Sci. 172, 216–223. doi: 10.1002/jpln.200800097
Chen, R., Cheng, W., Cui, J., Liao, J., Fan, H., Zheng, Z., et al. (2015). Lateral spacing in drip-irrigated wheat: the effects on soil moisture, yield, and water use efficiency. Field Crops Res. 179, 52–62. doi: 10.1016/j.fcr.2015.03.021
Chu, P., Zhang, Y., Yu, Z., Guo, Z., and Shi, Y. (2016). Winter wheat grain yield, water use, biomass accumulation and remobilisation under tillage in the North China plain. Field Crops Res. 193, 43–53. doi: 10.1016/j.fcr.2016.03.005
Cui, Z., Chen, X., and Zhang, F. (2010). Current nitrogen management status and measures to improve the intensive wheat–maize system in China. J. Hum. Environ. 39, 376–384. doi: 10.1007/s13280-010-0076-6
Dai, X., Xiao, L., Jia, D., Kong, H., Wang, Y., Li, C., et al. (2014). Increased plant density of winter wheat can enhance nitrogen–uptake from deep soil. Plant Soil 384, 141–152. doi: 10.1007/s11104-014-2190-x
Deng, X., Shan, L., Zhang, H., and Turner, N. C. (2006). Improving agricultural water use efficiency in arid and semiarid areas of China. Agric. Water manage. 80, 23–40. doi: 10.1016/j.agwat.2005.07.021
Devadas, R., Simpfendorfer, S., Backhouse, D., and Lamb, D. W. (2014). Effect of stripe rust on the yield response of wheat to nitrogen. Crop J. 2, 201–206. doi: 10.1016/j.cj.2014.05.002
Di Paolo, E., and Rinaldi, M. (2008). Yield response of corn to irrigation and nitrogen fertilization in a Mediterranean environment. Field Crops Res. 105, 202–210. doi: 10.1016/j.fcr.2007.10.004
Fan, T., Stewart, B. A., Yong, W., Junjie, L., and Guangye, Z. (2005). Long-term fertilization effects on grain yield, water-use efficiency and soil fertility in the dryland of Loess Plateau in China. Agric. Ecosyst. Environ. 106, 313–329. doi: 10.1016/j.agee.2004.09.003
Fang, Q., Ma, L., Green, T. R., Yu, Q., Wang, T., and Ahuja, L. R. (2010). Water resources and water use efficiency in the North China Plain: current status and agronomic management options. Agric. Water Manage. 97, 1102–1116. doi: 10.1016/j.agwat.2010.01.008
Fang, Q., Yu, Q., Wang, E., Chen, Y., Zhang, G., Wang, J., et al. (2006). Soil nitrate accumulation, leaching and crop nitrogen use as influenced by fertilization and irrigation in an intensive wheat-maize double cropping system in the North China Plain. Plant Soil 284, 335–350. doi: 10.1007/s11104-006-0055-7
Godfray, H. C. J., Beddington, J. R., Crute, I. R., Haddad, L., Lawrence, D., Muir, J. F., et al. (2010). Food security: the challenge of feeding 9 billion people. Science 327, 812–818. doi: 10.1126/science.1185383
Gong, Z., Zhang, G., and Chen, Z. (2007). Pedogenesis and Soil Taxonomy. Chapter 16: Fundamental Properties of Soil Orders and Chapter 21: Reference of Chinese Genetic Soil Classification With Chinese Soil Taxonomy. Beijing: Science Press.
Gu, L., Liu, T., Zhao, J., Dong, S., Liu, P., Zhang, J., et al. (2015). Nitrate leaching of winter wheat grown in lysimeters as affected by fertilizers and irrigation on the north china plain. J. Integr. Agric. 14, 374–388. doi: 10.1016/S2095-3119(14)60747-4
Guo, S., Zhu, H., Dang, T., Wu, J., Liu, W., Hao, M., et al. (2012). Winter wheat grain yield associated with precipitation distribution under long-term nitrogen fertilization in the semiarid Loess Plateau in China. Geoderma 189, 442–450. doi: 10.1016/j.geoderma.2012.06.012
Hai, L., Li, X., Li, F., Suo, D., and Guggenberger, G. (2010). Long-term fertilization and manuring effects on physically-separated soil organic matter pools under a wheat-wheat-maize cropping system in an arid region of China. Soil Biol. Biochem. 42, 253–259. doi: 10.1016/j.soilbio.2009.10.023
Hamid, D. J., Karim, N. N., and Mohsen, A. (2010). Effect of deficit irrigation regimes on yield, yield components and some quality traits of three bread wheat cultivars (Triticum aestivum L.). Int. J. Agric. Crop Sci. 4, 234–237.
Hu, Y., Moiwo, J. P., Yang, Y., Han, S., and Yang, Y. (2010). Agricultural water saving and sustainable groundwater management in Shijiazhuang Irrigation District, North China Plain. J. Hydrol. 393, 219–232. doi: 10.1016/j.jhydrol.2010.08.017
Hund, A., Ruta, N., and Liedgens, M. (2009). Rooting depth and water use efficiency of tropical maize inbred lines, differing in drought tolerance. Plant Soil 318, 311–325. doi: 10.1007/s11104-008-9843-6
Hvistendahl, M. (2010). China's push to add by subtracting fertilizer. Science 327, 801–801. doi: 10.1126/science.327.5967.801
Kang, S., Shi, W., and Zhang, J. (2000). An improved water-use efficiency for maize grown under regulated deficit irrigation. Field Crops Res. 67, 207–214. doi: 10.1016/S0378-4290(00)00095-2
Kang, S., Zhang, L., Liang, Y., Hu, X., Cai, H., and Gu, B. (2002). Effects of limited irrigation on yield and water use efficiency of winter wheat in the Loess Plateau of China. Agric. Water Manage. 55, 203–216. doi: 10.1016/S0378-3774(01)00180-9
Karam, F., Kabalan, R., Breidi, J., Rouphael, Y., and Oweis, T. (2009). Yield and water-production functions of two durum wheat cultivars grown under different irrigation and nitrogen regimes. Agric. Water Manage. 96, 603–615. doi: 10.1016/j.agwat.2008.09.018
Li, Q., Bian, C., Liu, X., Ma, C., and Liu, Q. (2015). Winter wheat grain yield and water use efficiency in wide-precision planting pattern under deficit irrigation in North China Plain. Agric. Water Manage. 153, 71–76. doi: 10.1016/j.agwat.2015.02.004
Li, Q., Dong, B., Qiao, Y., Liu, M., and Zhang, J. (2010). Root growth, available soil water, and water-use efficiency of winter wheat under different irrigation regimes applied at different growth stages in North China. Agric. Water Manage. 97, 1676–1682. doi: 10.1016/j.agwat.2010.05.025
Liu, C., and Wei, Z. (1989). Agricultural Hydrology and Water Resources in the North China Plain. Beijing: Chinese Scientific Press.
Liu, C., Yu, J., and Kendy, E. (2001). Ground water exploitation and its impact on the environment in the North China Plain. Water Int. 26, 265–272. doi: 10.1080/02508060108686913
Liu, E., Mei, X., Yan, C., Gong, D., and Zhang, Y. (2016). Effects of water stress on photosynthetic characteristics, dry matter translocation and WUE in two winter wheat genotypes. Agric. Water Manage. 167, 75–85. doi: 10.1016/j.agwat.2015.12.026
Liu, G., Wu, W., and Zhang, J. (2005). Regional differentiation of non-point source pollution of agriculture-derived nitrate nitrogen in groundwater in Northern China. Agric. Ecosyst. Environ. 107, 211–220. doi: 10.1016/j.agee.2004.11.010
Lv, L., Wang, H., Jia, X., and Wang, Z. (2011). Analysis on water requirement and water-saving amount of wheat and corn in typical regions of the North China Plain. Front. Agric. China 5, 556–562. doi: 10.1007/s11703-011-1149-4
Perego, A., Basile, A., Bonfante, A., Mascellis, R. D., Terribile, F., Brenna, S., et al. (2012). Nitrate leaching under maize cropping systems in po valley (italy). Agric. Ecosyst. Environ. 147, 57–65. doi: 10.1016/j.agee.2011.06.014
Qiu, G., Wang, L., He, X., Zhang, X., Chen, S., Chen, J., et al. (2008). Water use efficiency and evapotranspiration of winter wheat and its response to irrigation regime in the North China Plain. Agric. Forest Meteorol. 148, 1848–1859. doi: 10.1016/j.agrformet.2008.06.010
Sadras, V. O., Lawson, C., Hooper, P., and McDonald, G. K. (2012). Contribution of summer rainfall and nitrogen to the yield and water use efficiency of wheat in mediterranean-type environments of south australia. Eur. J. Agron. 36, 41–54. doi: 10.1016/j.eja.2011.09.001
Sepaskhah, A. R., and Tafteh, A. (2012). Yield and nitrogen leaching in rapeseed field under different nitrogen rates and water saving irrigation. Agric. Water Manage. 112, 55–62. doi: 10.1016/j.agwat.2012.06.005
Shangguan, Z., Shao, M., and Dyckmans, J. (2000). Nitrogen nutrition and water stress effects on leaf photosynthetic gas exchange and water use efficiency in winter wheat. Environ. Exp. Bot. 44, 141–149. doi: 10.1016/S0098-8472(00)00064-2
Sharma, P., Shukla, M. K., Sammis, T. W., Steiner, R. L., and Mexal, J. G. (2012). Nitrate-nitrogen leaching from three specialty crops of new mexico under furrow irrigation system. Agric. Water Manage. 109, 71–80. doi: 10.1016/j.agwat.2012.02.008
Shaughnessy, S. A. O., Evett, S. R., Colaizzi, P. D., and Howell, T. A. (2012). Grain sorghum response to irrigation scheduling with the time-temperature threshold method and deficit irrigation levels. Trans. ASABE 55, 451–461. doi: 10.13031/2013.41395
Shen, J., Zhao, D., Han, H., Zhou, X., and Li, Q. (2012). Effects of straw mulching on water consumption characteristics and yield of different types of summer maize plants. Plant Soil Environ. 58, 161–166.
Sinclair, T. R., and Rufty, T. W. (2012). Nitrogen and water resources commonly limit crop yield increases, not necessarily plant genetics. Global Food Secur. 1, 94–98. doi: 10.1016/j.gfs.2012.07.001
Smit, A. L., Groenwold, J., and Vos, J. (1994). The wageningen rhizolaba facility to study soil-root-shoot-atmosphere interactions in crops. Plant Soil 161, 275–287. doi: 10.1007/BF00046400
Sun, H., Shen, Y., Yu, Q., Flerchinger, G. N., Zhang, Y., Liu, C., et al. (2010). Effect of precipitation change on water balance and WUE of the winter wheat–summer maize rotation in the North China Plain. Agric. Water Manage. 97, 1139–1145. doi: 10.1016/j.agwat.2009.06.004
Tilling, A. K., O'Leary, G. J., Ferwerda, J. G., Jones, S. D., Fitzgerald, G. J., Rodriguez, D., et al. (2007). Remote sensing of nitrogen and water stress in wheat. Field Crops Res. 104, 77–85. doi: 10.1016/j.fcr.2007.03.023
Wang, C., Liu, W., Li, Q., Ma, D., Lu, H., Feng, W., et al. (2014). Effects of different irrigation and nitrogen regimes on root growth and its correlation with above-ground plant parts in high-yielding wheat under field conditions. Field Crops Res. 165, 138–149. doi: 10.1016/j.fcr.2014.04.011
Wang, H., Zhang, L., Dawes, W., and Liu, C. (2001). Improving water use efficiency of irrigated crops in the North China Plain-Measurements and modeling. Agric. Water Manage. 48, 151–167. doi: 10.1016/S0378-3774(00)00118-9
Wang, J., Liu, W., and Dang, T. (2011). Responses of soil water balance and precipitation storage efficiency to increased fertilizer application in winter wheat. Plant Soil 347, 41–51. doi: 10.1007/s11104-011-0764-4
Wang, Q., Li, F., Zhao, L., Zhang, E., Shi, S., Zhao, W., et al. (2010). Effects of irrigation and nitrogen application rates on nitrate nitrogen distribution and fertilizer nitrogen loss, wheat yield and nitrogen uptake on a recently reclaimed sandy farmland. Plant Soil 337, 325–339. doi: 10.1007/s11104-010-0530-z
Wang, X., Shi, Y., Guo, Z., Zhang, Y., and Yu, Z. (2015). Water use and soil nitrate nitrogen changes under supplemental irrigation with nitrogen application rate in wheat field. Field Crops Res. 183, 117–125. doi: 10.1016/j.fcr.2015.07.021
Xu, C., Tao, H., Tian, B., Gao, Y., Ren, J., and Wang, P. (2016). Limited-irrigation improves water use efficiency and soil reservoir capacity through regulating root and canopy growth of winter wheat. Field Crops Res. 196, 268–275. doi: 10.1016/j.fcr.2016.07.009
Xue, Q., Zhu, Z., Musick, J. T., Stewart, B. A., and Dusek, D. A. (2006). Physiological mechanisms contributing to the increased water-use efficiency in winter wheat under deficit irrigation. J. Plant Physiol. 163, 154–164. doi: 10.1016/j.jplph.2005.04.026
Yang, X., Chen, Y., Pacenka, S., Gao, W., Ma, L., Wang, G., et al. (2015). Effect of diversified crop rotations on groundwater levels and crop water productivity in the North China Plain. J. Hydrol. 522, 428–438. doi: 10.1016/j.jhydrol.2015.01.010
Zhang, H., Wang, X., You, M., and Liu, C. (1999). Water-yield relations and water use efficiency of winter wheat in the North China Plain. Irrigat. Sci. 19, 37–45. doi: 10.1007/s002710050069
Zhang, J., Mauzerall, D. L., Zhu, T., Liang, S., Ezzati, M., and Remais, J. V. (2010). Environmental health in China: progress towards clean air and safe water. Lancet 375, 1110–1119. doi: 10.1016/S0140-6736(10)60062-1
Zhang, X., Chen, S., Sun, H., Wang, Y., and Shao, L. (2009). Root size, distribution and soil water depletion as affected by cultivars and environmental factors. Field Crops Res. 114, 75–83. doi: 10.1016/j.fcr.2009.07.006
Zhang, X., Pei, D., and Chen, S. (2004). Root growth and soil water utilization of winter wheat in the North China Plain. Hydrol. Process. 18, 2275–2287. doi: 10.1002/hyp.5533
Zhang, X., Pei, D., and Hu, C. (2003). Conserving groundwater for irrigation in the North China Plain. Irrig. Sci. 21, 159–166. doi: 10.1007/s00271-002-0059-x
Zhao, R., Chen, X., Zhang, F., Zhang, H., Schardor, J., and Roemheld, V. (2007). Fertilization and nitrogen balance in a wheat-maize rotation system in Northern China Plain. Agron. J. 98, 938–945. doi: 10.2134/agronj2005.0157
Zhong, Y., and Shangguan, Z. (2014). Water consumption characteristics and water use efficiency of winter wheat under long-term nitrogen fertilization regimes in Northwest China. PLoS ONE 9:e98850. doi: 10.1371/journal.pone.0098850
Keywords: irrigation regime, nitrogen regime, root length density, grain yield, water use, soil NO3-N content
Citation: Liu W, Ma G, Wang C, Wang J, Lu H, Li S, Feng W, Xie Y, Ma D and Kang G (2018) Irrigation and Nitrogen Regimes Promote the Use of Soil Water and Nitrate Nitrogen from Deep Soil Layers by Regulating Root Growth in Wheat. Front. Plant Sci. 9:32. doi: 10.3389/fpls.2018.00032
Received: 11 August 2017; Accepted: 09 January 2018;
Published: 01 February 2018.
Edited by:
Puneet Singh Chauhan, National Botanical Research Institute (CSIR), IndiaReviewed by:
Sotirios Archontoulis, Iowa State University, United StatesRobert John French, Department of Agriculture and Food, Australia
Copyright © 2018 Liu, Ma, Wang, Wang, Lu, Li, Feng, Xie, Ma and Kang. This is an open-access article distributed under the terms of the Creative Commons Attribution License (CC BY). The use, distribution or reproduction in other forums is permitted, provided the original author(s) and the copyright owner are credited and that the original publication in this journal is cited, in accordance with accepted academic practice. No use, distribution or reproduction is permitted which does not comply with these terms.
*Correspondence: Chenyang Wang, xmzxwang@163.com
†These authors have contributed equally to this work.