- Key Laboratory of South China Agricultural Plant Molecular Analysis and Genetic Improvement and Guangdong Provincial Key Laboratory of Applied Botany, South China Botanical Garden, Chinese Academy of Sciences, Guangzhou, China
The phytohormones gibberellic acid (GA) and abscisic acid (ABA) are widely recognized as essential endogenous regulators that mostly play antagonistic roles in plant developmental processes and environmental responses. A variety of both internal and external cues oppositely regulate GA and ABA biosynthesis and catabolism, which directly and indirectly affect their signaling pathways and subsequent responses. Recent discoveries have revealed direct molecular links between GA- and ABA-signaling components, which provide novel insights into their antagonistic regulation. In this review, we mainly focus on these recent reports and the growing understanding of GA and ABA antagonism in metabolic regulation and signaling interactions, and attempt to clarify the problems and challenges involved in exploring the complicated regulatory events associated with these two phytohormones.
Introduction
In higher plants, the classic phytohormones abscisic acid (ABA) and gibberellic acid (GA) antagonistically regulate various developmental stages, such as seed dormancy and germination, root growth, leaf development, flowering time, and responses to environmental cues, such as light, temperature, and abiotic stresses (Weiss and Ori, 2007; Vanstraelen and Benková, 2012; Golldack et al., 2013).
Gibberellic acid form a large class of diterpenoid compounds in plants and fungi, but only a handful of GAs are bioactive and essential for plant growth and development (Yamaguchi, 2008). In GA biosynthesis and catabolism, various non-bioactive GAs act as precursors and intermediates in the conversion to bioactive GAs, which are mainly catalyzed by GA 20-oxidases (GA20ox) and GA 3-oxidases (GA3ox). Otherwise, the existing bioactive GAs are deactivated to non-bioactive forms, which are catalyzed by GA 2-oxidases (GA2ox) or another P450 monooxygenase (e.g., elongated uppermost internode) in Arabidopsis or rice (Sun, 2011). Thus, GA20ox and GA3ox are key synthetic enzymes of bioactive GAs and positively regulate the GA metabolism, while GA2ox and ELONGATED UPPERMOST INTERNODE are crucial catabolic enzymes of bioactive GAs and negatively regulate GA metabolism.
The sesquiterpene metabolite ABA plays crucial roles in plant development as well as in adaptation to abiotic stresses (Nambara and Marion-Poll, 2005). Cellular ABA biosynthesis occurs mainly in chloroplasts and cytoplasm. First, the carotenoid zeaxanthin is catalyzed to the all-trans violaxanthin in the chloroplast by zeaxanthin epoxidase (ZEP). Second, the intermediate violaxanthin is catalyzed to xanthoxin by nine-cis epoxycarotenoid dioxygenase (NCED), which is transported from the chloroplast to the cytoplasm. Then, xanthoxin is converted to abscisic aldehyde and oxidized to bioactive ABA by short-chain dehydrogenase reductase and abscisic aldehyde oxidase in the cytoplasm (Seo and Koshiba, 2002). The bioactive ABA is catalyzed to hydroxy ABA (ABA deactivation) by cytochrome P450 monooxygenase (CYP707A) superfamily members (Nambara and Marion-Poll, 2005). Thus, the key ABA synthetic enzymes are ZEP, NCED, short-chain dehydrogenase reductase, and abscisic aldehyde oxidase, which positively regulate ABA metabolism, while CYP707As negatively regulate ABA metabolism by catalyzing the ABA deactivation reaction.
In the current model of GA action that has been well-identified in rice and Arabidopsis, DELLA proteins interact with key regulatory factors, transcriptionally regulating downstream genes to restrain plant growth and development, while GA promotes growth by releasing DELLA-inhibition to activate the GA response (Davière and Achard, 2013). Similarly, ABA mediates its signaling pathway through a double-negative regulatory system. PP2C represses the positive ABA regulator SnRK2 activity, while ABA recognizes PP2C via receptor to releases activated SnRK2 that phosphorylates downstream targets, such as ABA RESPONSIVE ELEMENT BINDING FACTOR proteins (ABFs), to activate the ABA response (Cutler et al., 2010).
The essential phytohormones GA and ABA play diverse and contrary roles in plant growth and development processes at the physiological, biochemical, and molecular levels. However, the molecular mechanisms behind the antagonistic actions of these two hormones are not fully understood and have only been investigated using elusive and complicated regulatory networks. Here, we review recent advances in two aspects of GA and ABA antagonistic interactions, metabolic regulation, and core molecular signaling.
The Antagonistic Regulation of GA and ABA Metabolic Genes in Plant Development and Stress Responses
The antagonistic regulation of metabolic genes is a main feature of the GA and ABA interactions, and these interactions have mostly negative correlations at different developmental stages, including light-mediated seed germination, seed maturation and dormancy, root growth, and in response to stresses, such as cold, high temperature, and high salt.
Studies of the photoreversible seed germination of Arabidopsis revealed the opposing regulatory mechanisms of GA and ABA metabolism. For instance, bioactive GA biosynthesis is up-regulated by the light-mediated phytochrome pathway, which mainly activates the transcription of GA biosynthetic genes GA3ox1 and GA3ox2 and represses the transcription of the GA deactivation gene GA2ox2 to promote active GA accumulation in the light-regulated seed germination process (Yamaguchi et al., 1998; Oh et al., 2007). At the same time, ABA biosynthesis and inactivation are also phytochrome-regulated by down-regulating the ABA biosynthesis gene NCED6 and activating the ABA deactivation gene CYP707A2 in a manner opposite to that of GA metabolism (Seo et al., 2006). More importantly, high GA levels in the ABA-deficient mutants are observed, indicating that ABA is involved in the suppression of GA metabolic genes (GA3ox1/2 and GA3ox1/2/3) in seed germination, while the inhibition of GA elevates ABA metabolism by promoting the ABA biosynthetic genes (ABA1, NCED6, and NCED9) and repressing the ABA catabolic gene (CYP707A2) in the GA-deficient mutant seeds (Seo et al., 2006; Oh et al., 2007). Many recent studies focused on the regulation of GA and ABA metabolism to illuminate the potential regulatory mechanism involved in light-mediated seed germination. A light-mediated basic helix-loop-helix leucine zipper domain containing transcription factor (TF) in Arabidopsis, PHYTOCHROME-INTERACTING FACTOR3-LIKE5 (PIL5), inhibits GA metabolism in seed germination by indirectly repressing the GA synthetic genes GA3ox1 and GA3ox2 and activating a GA catabolic gene GA2ox2, and it increases ABA levels by indirectly activating the ABA synthetic genes ABA1, NCED6, and NCED9 and repressing an ABA catabolic gene CYP707A2 (Oh et al., 2007). In addition, the zinc finger protein DOF AFFECTING GERMINATION1, functioning downstream of PIL5, directly down-regulates the GA biosynthetic gene GA3ox1 in light-dependent seed germination (Gabriele et al., 2010). Similarly, Arabidopsis SOMNUS/Tandem CCCH zinc finger 4 (SOM/TZF4) and its homologs TZF5 and TZF6 modulate the expression of ABA and GA metabolic genes and function in the light-, GA-, and ABA-mediated regulation of seed germination responses (Kim et al., 2008; Bogamuwa and Jang, 2013). The expression changes in ABA and GA metabolic genes in the som/tzf4 mutant lead to lower ABA levels and elevated GA levels. Meantime, SOM is directly downstream of PIL5, which regulates ABA and GA metabolic genes partly through SOM (Kim et al., 2008). Moreover, CHOTTO1, a double-AP2 domain-containing TF, represses the GA biosynthetic gene GA3ox1 and activates ZEP and NCED9 genes to regulate ABA and GA metabolism during Arabidopsis seed germination (Yano et al., 2009).
In addition to light-mediated seed germination, another study on temperature-controlled seed dormancy clarified the temperature-dependent expression patterns of GA and ABA metabolic genes in the seasonal seed dormancy of Arabidopsis (Footitt et al., 2011). Increased dormancy by low temperature in the winter is correlated with the up-regulated expression of ABA synthesis (NCED6) and GA catabolism (GA2ox2) genes. Conversely, decreased dormancy by high temperature in the spring and summer is accompanied with increased ABA catabolism (CYP707A2) and GA synthesis (GA3ox1) (Footitt et al., 2011). In a study of the regulatory mechanism, ABA-INSENSITIVE 4 (ABI4), an AP2 domain-containing TF, was reported as the potential mediator between ABA and GA metabolism to promote seed dormancy by directly repressing the ABA inactivation genes CYP707A1 and CYP707A2 and inhibiting GA metabolism genes in Arabidopsis (Shu et al., 2013). The MYB96 TF regulates seed dormancy through ABA and GA metabolism by directly activating the ABA biosynthetic genes NCED2 and NCED6 and also repressing GA biosynthetic genes GA3ox1 and GA20ox1 (Lee et al., 2015).
In seed development, seed morphogenesis (embryogenesis) and seed maturation are considered two major physiological processes. A high level of ABA and low level of GA have been observed during seed maturation processes in some published studies (Hays et al., 2001; Yamaguchi et al., 2007), however, the GA and ABA levels during embryogenesis in developing seeds have been less reported. Embryo development and seed maturation are controlled by the B3 domain containing the TFs, LEAFY COTYLEDON2 and FUSCA3 (FUS3) in Arabidopsis, which antagonistically modulate ABA and GA metabolism by directly repressing the GA synthetic gene GA3ox2 and promoting ABA synthesis through light-dependent DELLA stimulation and ABI3 activity (Curaba et al., 2004; Gazzarrini et al., 2004; Piskurewicz et al., 2009). Similarly, the B3 TF GERMINATION DEFECTIVE 1 directly or indirectly modulates GA homeostasis by suppressing rice (Oryza sativa) LEAFY COTYLEDON2/FUS3-LIKE 1 and then regulates GA metabolic gene (OsGA3ox, OsGA20ox, and OsGA2ox) expression involved in seed maturation, germination, and seedling development (Guo et al., 2013).
The GA–ABA antagonism not only dominates seed development, dormancy, and germination, but also contributes to other developmental processes, which are also mostly promoted or suppressed by an opposite and specific TF-regulated ABA/GA ratio. The GA- AND ABA-RESPONSIVE ZINC FINGER factor modulates ABA and GA metabolism in Arabidopsis root tissue-formation by regulating CYP707A1, GA20ox2/3/4/5, and GA3ox1/3/4 (Lee et al., 2016). ABI4, of which protein stability is oppositely regulated by GA and ABA, directly activates NCED6 and GA2ox7 transcription, suggesting the important role of ABI4 in ABA and GA antagonism during post-germination stages (Shu et al., 2016). In addition, OsAP2-39, may balance ABA and GA metabolism in multiple physiological processes, such as the size of root systems, flowering time, and pollen grain morphology, by directly regulating ABA and GA biosynthetic or inactivation genes (Yaish et al., 2010). Notably, both ABI4 and OsAP2-39 belong to the AP2 domain-containing TFs (ATFs), implying ATFs play key roles in the metabolism regulation of ABA and GA, which has been summarized recently (Shu et al., 2018).
Abiotic stresses (i.e., high temperature, cold, and high salt) trigger the relevant stress responses by affecting the balance of ABA and GA contents. High temperature increases the ABA level and decreases the GA level in Arabidopsis seeds (Toh et al., 2008). The increasing ABA level in imbibed seeds contributes to the up-regulation of the ABA synthetic enzyme genes ZEP, NCED2, NCED5, and NCED9 at high temperatures. However, the decrease in the GA level at high temperature is largely the result of the suppression of the GA synthetic enzyme genes GA20ox1, GA20ox2, GA20ox3, GA3ox1, and GA3ox2 (Toh et al., 2008). The Arabidopsis seed development master regulator FUS3 activates heat-related and ABA metabolic genes and inhibits the GA metabolic genes to delay germination at high temperatures (Chiu et al., 2012). Similarly, the DELLA proteins GAI or RGA, ABI3 and ABI5 directly activate SOM, which regulates ABA and GA metabolic genes during the high-temperature response in Arabidopsis (Lim et al., 2013). In addition, cold and high-salt stresses also result in changes in the ABA/GA ratio (Golldack et al., 2013). Low temperature- and ABA-induced C-REPEAT/DROUGHT-RESPONSIVE ELEMENT BINDING FACTOR 1 enhances the accumulation of RGA by inhibiting GA metabolism by promoting the expression of the GA catabolic gene GA2ox (Achard et al., 2008). The Arabidopsis AP2 domain-containing TF DWARF AND DELAYED FLOWERING 1 directly promotes the GA-inactivated gene GA2ox7 to repress GA metabolism during high-salinity stress in Arabidopsis (Magome et al., 2008).
Thus, we can reasonably infer that the antagonistic regulation of GA and ABA metabolism mainly occurs by activating and repressing the opposing metabolic genes (NCED/GA2ox or CYP707A/GA3ox family) to maintain a hormonal balance during plant growth and development and to respond to environmental cues. The pattern information of phytohormone distribution could be acquired by recent data and tools, which will help us to gain novel insights into ABA and GA action in a temporal and spatial view (Waadt et al., 2015).
The Antagonistic Interaction Between Core GA- and ABA-Signaling Components
Our knowledge regarding ABA- and GA-signaling pathways has substantially improved over the past two decades, although direct molecular interactions between core ABA- and GA-signaling components remains to be elucidated. Other phytohormone signals have been well-demonstrated and provide good references for the investigation of the direct interactions. For example, JASMONIC ACID ZIM proteins-DELLAs interaction for crosstalk between JA and GA (Hou et al., 2010; Yang et al., 2012), BRASSINAZOLE RESISTANT 1-DELLAs interaction for crosstalk between brassinosteroids (BR) and GA (Li et al., 2012), BRASSINOSTEROID INSENSITIVE 2-ABI5 interaction for crosstalk between BR and ABA (Hu and Yu, 2014), ARABIDOPSIS RESPONSE REGULATOR proteins-DELLAs for crosstalk between cytokinin and GA (Marín-de la Rosa et al., 2015), and DWARF 14-DELLAs for crosstalk between strigolactones and GA (Nakamura et al., 2013). Encouragingly, some novel interpretations may have revealed the direct molecular links between ABA and GA signaling.
The antagonistic GA–ABA signaling and downstream transcriptional regulations have been well-illustrated in barley aleurone cells. The GA- and ABA-mediated downstream genes encoding α-amylases are induced by GA but regulated by ABA in an opposite manner, and the key TF GAMYB is essential for activating α-amylase expression and is itself regulated positively by GA and negatively by ABA through core GA- and ABA-signaling components DELLA and PKABA1 (SnRK2 homolog), respectively (Gomez-Cadenas et al., 2001; Kaneko et al., 2004). However, the downstream targets of the antagonistic GA–ABA signaling are still not clear in the model plant Arabidopsis owing to the missing analogous α-amylase gene and relevant GAMYB TF.
In the core GA-mediated signaling, the DELLA repressors are mainly degraded through the ubiquitin–proteasome system. SLY1 recruits the Skp, Cullin, and F-box E3 ubiquitin ligase to the GA-GID1-DELLA complex and causes DELLA polyubiquitination and degradation in the 26S proteasome system (Steber et al., 1998; Murase et al., 2008). In addition to ubiquitination, the core signaling components are regulated by ubiquitin-like modifiers, such as small ubiquitin-related modifier (SUMO). Recently, the E3 SUMO ligase SIZ1 was found to negatively regulate ABA signaling through the sumoylation of ABI5, a core component of ABA signaling during seed germination in Arabidopsis (Miura et al., 2009). Interestingly, SIZ1 directly and positively regulates a GA-signaling component by sumoylating SLY1 (Kim et al., 2015), implying that SIZ1 functions antagonistically as a direct molecular link between SLY1 promotion and ABI5 inhibition in ABA and GA signaling.
ANAPHASE-PROMOTING COMPLEX/CYCLOSOME (APC/C) is another E3 ligase complex in the E3 family of enzymes and is necessary for many cellular events. Recently, the SnRK2-APC/C Tiller Enhancer (TE) regulatory module revealed a novel regulatory link underlying the antagonistic action of GA and ABA in rice. In this model, ABA inhibits APC/CTE activity by phosphorylating TE by activating the OsSnRK2s, which may break the interaction between TE and the OsPYLs and subsequently stabilize the ABA receptor. In contrast, GA inhibits the OsSnRK2s and may promote the APC/CTE-mediated degradation of the OsPYLs (Lin et al., 2015).
DELLAs form a central connection between GA and other signaling pathways, including ABA signaling. The ABA-promoted accumulation of DELLA proteins in roots depends on ABI1, a core ABA-signaling repressor, and the phenotype of a quadruple-DELLA mutant resembles that of the gain-of-function mutant abi1-1, being resistant to the growth-inhibitory effects of ABA (Achard et al., 2006; Guo et al., 2014). By contrast, ABI1 does not mediate DELLAs’ stability induced by salt stress (Duan et al., 2013), suggesting that the regulation of ABI on DELLA proteins occurs specifically upon exogenous ABA applications. Similarly, the tomato DELLA protein PROCERA promotes stomatal closure in guard cells in an ABA-dependent manner (Nir et al., 2017). In transcriptional regulation, DELLAs regulate a set of downstream genes by interacting with the TFs involved in ABA signaling. For example, the Arabidopsis GA-signaling repressors GAI and RGA function in response to heat stress by interacting with core ABA-signaling TFs ABI3 and ABI5, which directly activate SOM expression and integrate ABA and GA signaling (Lim et al., 2013). In addition, Arabidopsis NUCLEAR FACTOR-Y C homologs (NF-YC3, 4, and 9) also interact with the core GA signaling repressor RGA-LIKE2 (RGL2) to form NF-YC-RGL2 modules that target the key ABA-signaling TF gene ABI5 to regulate a set of GA- and ABA-responsive genes during seed germination (Liu et al., 2016). Interestingly, a recent study showed that NF-YC9 promotes ABA responses in early seedling growth and stomatal closure by binding ABI5 to increase ABA sensitivity (Bi et al., 2017), which further supports the hypothesis that the NF-YCs–DELLAs–ABFs/ABI5 module integrates the antagonistic GA and ABA signaling in seed germination and post-germination stages.
Conclusion and Perspectives
To summarize, the plant hormones ABA and GA antagonistically mediate multiple physiological processes, and their balance is critical to normal development and stress responses. The GA and ABA antagonistic crosstalk comprises two main layers (Figure 1): one is the metabolic homeostasis of ABA and GA, which are controlled by distinct TF regulators in response to specific endogenous and environmental signals, leading to opposite patterns of ABA and GA accumulation; and the other one is the direct molecular interaction between core ABA- and GA- signaling components, which orchestrates a rapid and efficient response to developmental changes and external challenges by quickly mediating the antagonistic interaction of ABA and GA.
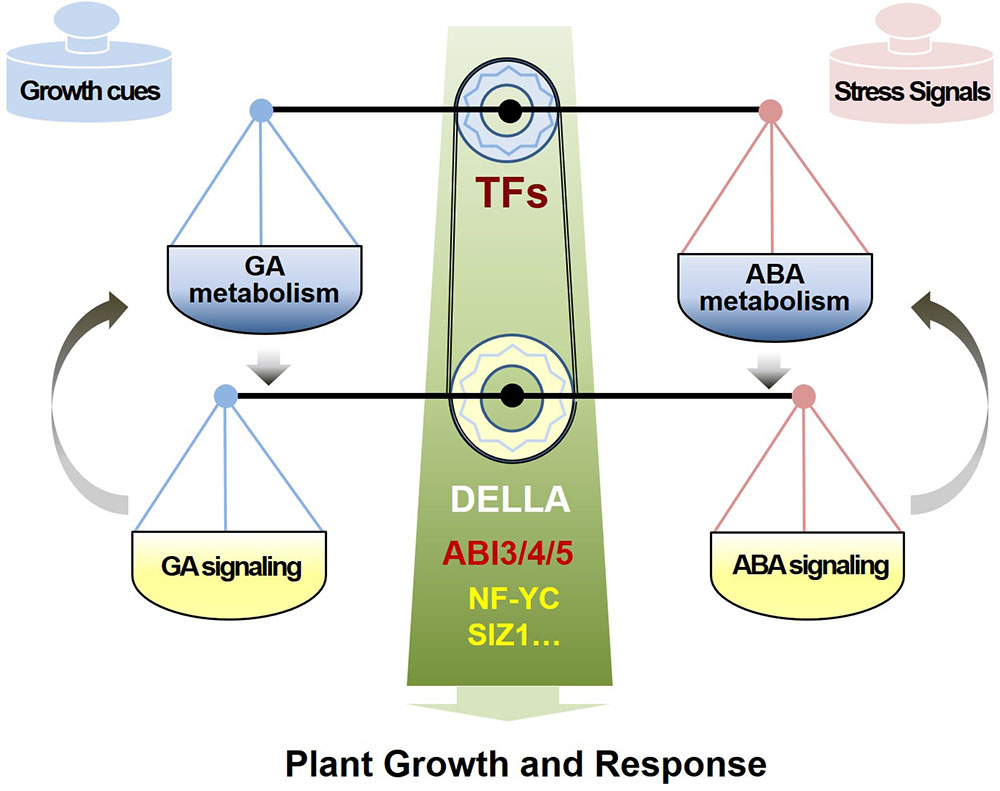
FIGURE 1. The model of antagonism of ABA and GA in metabolism regulation and signaling interaction. Phytohormones GA and ABA antagonistically mediate plant normal growth and response by two regulation layers, including the metabolic homeostasis of ABA and GA controlled by TF regulators in response to various environmental signals, and the direct molecular interaction between ABA and GA signaling components or other mediators, which orchestrate an efficient response to the cues of growth and stress.
In recent years, many studies have shed light on the antagonistic regulation of GA and ABA in plants; however, the intricate regulatory network involved has made it difficult to develop a clear picture of plant growth and development as mediated by crosstalk between GA and ABA. Several notable points are worthy of further investigations: first, phytohormones relay signals through signaling pathways, while signaling components have feedback effects on phytohormone metabolism, which contributes to a beneficial hormone homeostasis balance for plant normal growth. Thus, how does the antagonistic regulation of metabolic and signaling pathways between GA and ABA synergistically control plant growth and development? Second, as the essential growth regulators, GA and ABA perform separate roles in different cells and tissues during various developmental stages. How is the GA–ABA antagonism precisely modulated in an accurate temporal and spatial manner to process a specific development such as germination or dormancy? Third, several epigenetic regulators, such as BRAHMA, HDAs, and REF6, have been shown to be involved in GA or ABA response (Hou et al., 2014; Ryu et al., 2014; Peirats-Llobet et al., 2016). Significantly, the GA–ABA antagonistic mediator NF-YC has a functional interaction with the epigenetic factors HDA15 or REF6 (Hou et al., 2014; Tang et al., 2017), implying that NF-YC may mediate GA–ABA antagonism by epigenetic regulation. In addition, some studies reported that non-coding RNAs, which act the overlapping or distinct functions in various molecular events, especially transcription regulation, are also related to GA or ABA response (Yu et al., 2012; Yan et al., 2016; Huang et al., 2017; Qin et al., 2017). Thus, it is worthy to investigate whether and how epigenetic factors and non-coding RNAs play roles in the crosstalk between GA and ABA.
Author Contributions
XL and XH wrote the manuscript; XL and XH contributed to the discussion and approved the final manuscript.
Funding
This work was supported by grants from the National Natural Science Foundation of China (No. 31301055) and the Natural Science Foundation of Guangdong Province (No. 2017A030313211).
Conflict of Interest Statement
The authors declare that the research was conducted in the absence of any commercial or financial relationships that could be construed as a potential conflict of interest.
Acknowledgments
We apologize to those authors whose excellent work could not be cited due to space limitations.
References
Achard, P., Cheng, H., De Grauwe, L., Decat, J., Schoutteten, H., Moritz, T., et al. (2006). Integration of plant responses to environmentally activated phytohormonal signals. Science 311, 91–94. doi: 10.1126/science.1118642
Achard, P., Gong, F., Cheminant, S., Alioua, M., Hedden, P., and Genschik, P. (2008). The cold-inducible CBF1 factor-dependent signaling pathway modulates the accumulation of the growth-repressing DELLA proteins via its effect on gibberellin metabolism. Plant Cell 20, 2117–2129. doi: 10.1105/tpc.108.058941
Bi, C., Ma, Y., Wang, X. F., and Zhang, D. P. (2017). Overexpression of the transcription factor NF-YC9 confers abscisic acid hypersensitivity in Arabidopsis. Plant Mol. Biol. 95, 425–439. doi: 10.1007/s11103-017-0661-1
Bogamuwa, S., and Jang, J. C. (2013). The Arabidopsis tandem CCCH zinc finger proteins AtTZF4, 5 and 6 are involved in light-, abscisic acid- and gibberellic acid-mediated regulation of seed germination. Plant Cell Environ. 36, 1507–1519. doi: 10.1111/pce.12084
Chiu, R. S., Nahal, H., Provart, N. J., and Gazzarrini, S. (2012). The role of the Arabidopsis FUSCA3 transcription factor during inhibition of seed germination at high temperature. BMC Plant Biol. 12:15. doi: 10.1186/1471-2229-12-15
Curaba, J., Moritz, T., Blervaque, R., Parcy, F., Raz, V., Herzog, M., et al. (2004). AtGA3ox2, a key gene responsible for bioactive gibberellin biosynthesis, is regulated during embryogenesis by LEAFY COTYLEDON2 and FUSCA3 in Arabidopsis. Plant Physiol. 136, 3660–3669. doi: 10.1104/pp.104.047266
Cutler, S. R., Rodriguez, P. L., Finkelstein, R. R., and Abrams, S. R. (2010). Abscisic acid: emergence of a core signaling network. Annu. Rev. Plant Biol. 61, 651–660. doi: 10.1146/annurev-arplant-042809-112122
Davière, J. M., and Achard, P. (2013). Gibberellin signaling in plants. Development 140, 1147–1151. doi: 10.1242/dev.087650
Duan, L., Dietrich, D., Ng, C. H., Chan, P. M., Bhalerao, R., Bennett, M. J., et al. (2013). Endodermal ABA signaling promotes lateral root quiescence during salt stress in Arabidopsis seedlings. Plant Cell 25, 324–341. doi: 10.1105/tpc.112.107227
Footitt, S., Douterelo-Soler, I., Clay, H., and Finch-Savage, W. E. (2011). Dormancy cycling in Arabidopsis seeds is controlled by seasonally distinct hormone-signaling pathways. Proc. Natl. Acad. Sci. U.S.A. 108, 20236–20241. doi: 10.1073/pnas.1116325108
Gabriele, S., Rizza, A., Martone, J., Circelli, P., Costantino, P., and Vittorioso, P. (2010). The Dof protein DAG1 mediates PIL5 activity on seed germination by negatively regulating GA biosynthetic gene AtGA3ox1. Plant J. 61, 312–323. doi: 10.1111/j.1365-313X.2009.04055.x
Gazzarrini, S., Tsuchiya, Y., Lumba, S., Okamoto, M., and McCourt, P. (2004). The transcription factor FUSCA3 controls developmental timing in Arabidopsis through the hormones gibberellin and abscisic acid. Dev. Cell 7, 373–385. doi: 10.1016/j.devcel.2004.06.017
Golldack, D., Li, C., Mohan, H., and Probst, N. (2013). Gibberellins and abscisic acid signal crosstalk: living and developing under unfavorable conditions. Plant Cell Rep. 32, 1007–1016. doi: 10.1007/s00299-013-1409-2
Gomez-Cadenas, A., Zentella, R., Walker-Simmons, M. K., and Ho, T. H. (2001). Gibberellin/abscisic acid antagonism in barley aleurone cells: site of action of the protein kinase PKABA1 in relation to gibberellin signaling molecules. Plant Cell 13, 667–679. doi: 10.1105/tpc.13.3.667
Guo, W., Cong, Y., Hussain, N., Wang, Y., Liu, Z., Jiang, L., et al. (2014). The remodeling of seedling development in response to long-term magnesium toxicity and regulation by ABA-DELLA signaling in Arabidopsis. Plant Cell Physiol. 55, 1713–1726. doi: 10.1093/pcp/pcu102
Guo, X., Hou, X., Fang, J., Wei, P., Xu, B., Chen, M., et al. (2013). The rice GERMINATION DEFECTIVE 1, encoding a B3 domain transcriptional repressor, regulates seed germination and seedling development by integrating GA and carbohydrate metabolism. Plant J. 75, 403–416. doi: 10.1111/tpj.12209
Hays, B., Mandel, R., and Pharis, R. (2001). Hormonesin zygotic and microspore embryos of Brassica napus. Plant Growth Regul. 35, 47–58. doi: 10.1023/A:1013831116996
Hou, X., Lee, L. Y., Xia, K., Yan, Y., and Yu, H. (2010). DELLAs modulate jasmonate signaling via competitive binding to JAZs. Dev. Cell 19, 884–894. doi: 10.1016/j.devcel.2010.10.024
Hou, X., Zhou, J., Liu, C., Liu, L., Shen, L., and Yu, H. (2014). Nuclear factor Y-mediated H3K27me3 demethylation of the SOC1 locus orchestrates flowering responses of Arabidopsis. Nat. Commun. 5:4601. doi: 10.1038/ncomms5601
Hu, Y., and Yu, D. (2014). BRASSINOSTEROID INSENSITIVE2 interacts with ABSCISIC ACID INSENSITIVE5 to mediate the antagonism of brassinosteroids to abscisic acid during seed germination in Arabidopsis. Plant Cell 26, 4394–4408. doi: 10.1105/tpc.114.130849
Huang, W., Peng, S., Xian, Z., Lin, D., Hu, G., Yang, L., et al. (2017). Overexpression of a tomato miR171 target gene SlGRAS24 impacts multiple agronomical traits via regulating gibberellin and auxin homeostasis. Plant Biotechnol. J. 15, 472–488. doi: 10.1111/pbi.12646
Kaneko, M., Inukai, Y., Ueguchi-Tanaka, M., Itoh, H., Izawa, T., Kobayashi, Y., et al. (2004). Loss-of-function mutations of the rice GAMYB gene impair alpha-amylase expression in aleurone and flower development. Plant Cell 16, 33–44. doi: 10.1105/tpc.017327
Kim, D. H., Yamaguchi, S., Lim, S., Oh, E., Park, J., Hanada, A., et al. (2008). SOMNUS, a CCCH-type zinc finger protein in Arabidopsis, negatively regulates light-dependent seed germination downstream of PIL5. Plant Cell 20, 1260–1277. doi: 10.1105/tpc.108.058859
Kim, S. I., Park, B. S., Kim, D. Y., Yeu, S. Y., Song, S. I., Song, J. T., et al. (2015). E3 SUMO ligase AtSIZ1 positively regulates SLY1-mediated GA signalling and plant development. Biochem. J. 469, 299–314. doi: 10.1042/BJ20141302
Koornneef, M., and vanderVeen, J. H. (1980). Induction and analysis of gibberellin sensitive mutants in Arabidopsis thaliana (L.). Heynh. Theor. Appl. Genet. 58, 257–263. doi: 10.1007/BF00265176
Lee, H. G., Lee, K., and Seo, P. J. (2015). The Arabidopsis MYB96 transcription factor plays a role in seed dormancy. Plant Mol. Biol. 87, 371–381. doi: 10.1007/s11103-015-0283-4
Lee, S. A., Jang, S., Yoon, E. K., Heo, J. O., Chang, K. S., Choi, J. W., et al. (2016). Interplay between ABA and GA modulates the timing of asymmetric cell divisions in the Arabidopsis root ground tissue. Mol. Plant 9, 870–884. doi: 10.1016/j.molp.2016.02.009
Li, Q. F., Wang, C., Jiang, L., Li, S., Sun, S. S., and He, J. X. (2012). An interaction between BZR1 and DELLAs mediates direct signaling crosstalk between brassinosteroids and gibberellins in Arabidopsis. Sci. Signal. 5:ra72. doi: 10.1126/scisignal.2002908
Lim, S., Park, J., Lee, N., Jeong, J., Toh, S., Watanabe, A., et al. (2013). ABA-INSENSITIVE3, ABA-INSENSITIVE5, and DELLAs interact to activate the expression of SOMNUS and other high-temperature-inducible genes in imbibed seeds in Arabidopsis. Plant Cell 25, 4863–4878. doi: 10.1105/tpc.113.118604
Lin, Q., Wu, F., Sheng, P., Zhang, Z., Zhang, X., Guo, X., et al. (2015). The SnRK2-APC/CTE regulatory module mediates the antagonistic action of gibberellic acid and abscisic acid pathways. Nat. Commun. 6:7981. doi: 10.1038/ncomms8981
Liu, X., Hu, P., Huang, M., Tang, Y., Li, Y., Li, L., et al. (2016). The NF-YC-RGL2 module integrates GA and ABA signalling to regulate seed germination in Arabidopsis. Nat. Commun. 7:12768. doi: 10.1038/ncomms12768
Magome, H., Yamaguchi, S., Hanada, A., Kamiya, Y., and Oda, K. (2008). The DDF1 transcriptional activator upregulates expression of a gibberellin-deactivating gene, GA2ox7, under high-salinity stress in Arabidopsis. Plant J. 56, 613–626. doi: 10.1111/j.1365-313X.2008.03627.x
Marín-de la Rosa, N., Pfeiffer, A., Hill, K., Locascio, A., Bhalerao, R. P., Miskolczi, P., et al. (2015). Genome wide binding site analysis reveals transcriptional coactivation of cytokinin-responsive genes by DELLA proteins. PLoS Genet. 11:e1005337. doi: 10.1371/journal.pgen.1005337
Miura, K., Lee, J., Jin, J. B., Yoo, C. Y., Miura, T., and Hasegawa, P. M. (2009). Sumoylation of ABI5 by the Arabidopsis SUMO E3 ligase SIZ1 negatively regulates abscisic acid signaling. Proc. Nat. Acad. Sci. U.S.A. 106, 5418–5423. doi: 10.1073/pnas.0811088106
Murase, K., Hirano, Y., Sun, T. P., and Hakoshima, T. (2008). Gibberellin-induced DELLA recognition by the gibberellin receptor GID1. Nature 456, 459–463. doi: 10.1038/nature07519
Nakamura, H., Xue, Y. L., Miyakawa, T., Hou, F., Qin, H. M., Fukui, K., et al. (2013). Molecular mechanism of strigolactone perception by DWARF14. Nat. Commun. 4:2613. doi: 10.1038/ncomms3613
Nambara, E., and Marion-Poll, A. (2005). Abscisic acid biosynthesis and catabolism. Annu. Rev. Plant Biol. 56, 165–185. doi: 10.1146/annurev.arplant.56.032604.144046
Nir, I., Shohat, H., Panizel, I., Olszewski, N., Aharoni, A., and Weiss, D. (2017). The tomato DELLA protein PROCERA acts in guard cells to promote stomatal closure. Plant Cell 12, 3186–3197. doi: 10.1105/tpc.17.00542
Oh, E., Yamaguchi, S., Hu, J. H., Yusuke, J., Jung, B., Paik, I., et al. (2007). PIL5, a phytochrome-interacting bHLH protein, regulates gibberellin responsiveness by binding directly to the GAI and RGA promoters in Arabidopsis seeds. Plant Cell 19, 1192–1208. doi: 10.1105/tpc.107.050153
Peirats-Llobet, M., Han, S. K., Gonzalez-Guzman, M., Jeong, C. W., Rodriguez, L., Belda-Palazon, B., et al. (2016). A direct link between abscisic acid sensing and the chromatin-remodeling ATPase BRAHMA via core ABA signaling pathway components. Mol. Plant 9, 136–147. doi: 10.1016/j.molp.2015.10.003
Piskurewicz, U., Turecková, V., Lacombe, E., and Lopez-Molina, L. (2009). Far-red light inhibits germination through DELLA-dependent stimulation of ABA synthesis and ABI3 activity. EMBO J. 28, 2259–2271. doi: 10.1038/emboj.2009.170
Qin, T., Zhao, H., Cui, P., Albesher, N., and Xiong, L. (2017). A nucleus-localized long non-coding RNA enhances drought and salt stress tolerance. Plant Physiol. 175, 1321–1336. doi: 10.1104/pp.17.00574
Ryu, H., Cho, H., Bae, W., and Hwang, I. (2014). Control of early seedling development by BES1/TPL/HDA19-mediated epigenetic regulation of ABI3. Nat. Commun. 5:4138. doi: 10.1038/ncomms5138
Seo, M., Hanada, A., Kuwahara, A., Endo, A., Okamoto, M., Yamauchi, Y., et al. (2006). Regulation of hormone metabolism in Arabidopsis seeds: phytochrome regulation of abscisic acid metabolism and abscisic acid regulation of gibberellin metabolism. Plant J. 48, 354–366. doi: 10.1111/j.1365-313X.2006.02881.x
Seo, M., and Koshiba, T. (2002). Complex regulation of ABA biosynthesis in plants. Trends Plant Sci. 7, 41–48. doi: 10.1016/S1360-1385(01)02187-2
Shu, K., Chen, Q., Wu, Y., Liu, R., Zhang, H., Wang, P., et al. (2016). ABI4 mediates antagonistic effects of abscisic acid and gibberellins at transcript and protein levels. Plant J. 85, 348–361. doi: 10.1111/tpj.13109
Shu, K., Zhang, H., Wang, S., Chen, M., Wu, Y., Tang, S., et al. (2013). ABI4 regulates primary seed dormancy by regulating the biogenesis of abscisic acid and gibberellins in Arabidopsis. PLoS Genet. 9:e1003577. doi: 10.1371/journal.pgen.1003577
Shu, K., Zhou, W., and Yang, W. (2018). APETALA 2-domain-containing transcription factors: focusing on abscisic acid and gibberellins antagonism. New Phytol. 217, 977–983. doi: 10.1111/nph.14880
Steber, C. M., Cooney, S. E., and McCourt, P. (1998). Isolation of the GA-response mutant sly1 as a suppressor of ABI1-1 in Arabidopsis thaliana. Genetics 149, 509–521.
Sun, T. P. (2011). The molecular mechanism and evolution of the GA–GID1–DELLA signaling module in plants. Curr. Biol. 21, R338–R345. doi: 10.1016/j.cub.2011.02.036
Tang, Y., Liu, X., Liu, X., Li, Y., Wu, K., and Hou, X. (2017). Arabidopsis NF-YCs mediate the light-controlled hypocotyl elongation via modulating histone acetylation. Mol. Plant 10, 260–273. doi: 10.1016/j.molp.2016.11.007
Toh, S., Imamura, A., Watanabe, A., Nakabayashi, K., Okamoto, M., Jikumaru, Y., et al. (2008). High temperature-induced abscisic acid biosynthesis and its role in the inhibition of gibberellin action in Arabidopsis seeds. Plant Physiol. 146, 1368–1385. doi: 10.1104/pp.107.113738
Vanstraelen, M., and Benková, E. (2012). Hormonal interactions in the regulation of plant development. Annu. Rev. Cell Dev. Biol. 28, 463–487. doi: 10.1146/annurev-cellbio-101011-155741
Waadt, R., Hsu, P. K., and Schroeder, J. I. (2015). Abscisic acid and other plant hormones: methods to visualize distribution and signaling. Bioessays 37, 1338–1349. doi: 10.1002/bies.201500115
Weiss, D., and Ori, N. (2007). Mechanisms of cross talk between gibberellin and other hormones. Plant Physiol. 144, 1240–1246. doi: 10.1104/pp.107.100370
Yaish, M. W., El-kereamy, A., Zhu, T., Beatty, P. H., Good, A. G., Bi, Y. M., et al. (2010). The APETALA-2-Like transcription factor OsAP2-39 controls key interactions between abscisic acid and gibberellin in rice. PLoS Genet. 6:e1001098. doi: 10.1371/journal.pgen.1001098
Yamaguchi, S. (2008). Gibberellin metabolism and its regulation. Annu. Rev. Plant Biol. 59, 225–251. doi: 10.1146/annurev.arplant.59.032607.092804
Yamaguchi, S., Kamiya, Y., and Nambara, E. (2007). “Regulation of ABA and GA levels during seed development and germination in Arabidopsis,” in Annual Plant Reviews: Seed Development Dormancy and Germination, Vol. 27, eds K. F. Bradford and H. Nonogaki (Hoboken, NJ: John Wiley & Sons), 224–247.
Yamaguchi, S., Smith, M. W., Brown, R. G., Kamiya, Y., and Sun, T. (1998). Phytochrome regulation and differential expression of gibberellin 3beta-hydroxylase genes in germinating Arabidopsis seeds. Plant Cell 10, 2115–2126.
Yan, J., Zhao, C., Zhou, J., Yang, Y., Wang, P., Zhu, X., et al. (2016). The miR165/166 mediated regulatory module plays critical roles in ABA homeostasis and response in Arabidopsis thaliana. PLoS Genet. 12:e1006416. doi: 10.1371/journal.pgen.1006416
Yang, D. L., Yao, J., Mei, C. S., Tong, X. H., Zeng, L. J., Li, Q., et al. (2012). Plant hormone jasmonate prioritizes defense over growth by interfering with gibberellin signaling cascade. Proc. Natl. Acad. Sci. U.S.A. 109, E1192–E1200. doi: 10.1073/pnas.1201616109
Yano, R., Kanno, Y., Jikumaru, Y., Nakabayashi, K., Kamiya, Y., and Nambara, E. (2009). CHOTTO1, a putative double APETALA2 repeat transcription factor, is involved in abscisic acid-mediated repression of gibberellin biosynthesis during seed germination in Arabidopsis. Plant Physiol. 151, 641–654. doi: 10.1104/pp.109.142018
Keywords: gibberellic acid, abscisic acid, antagonistic interaction, metabolic pathway, signaling pathway
Citation: Liu X and Hou X (2018) Antagonistic Regulation of ABA and GA in Metabolism and Signaling Pathways. Front. Plant Sci. 9:251. doi: 10.3389/fpls.2018.00251
Received: 30 December 2017; Accepted: 12 February 2018;
Published: 26 February 2018.
Edited by:
Paola Vittorioso, Sapienza Università di Roma, ItalyReviewed by:
Kai Shu, Sichuan Agricultural University, ChinaLucio Conti, Università degli Studi di Milano, Italy
Copyright © 2018 Liu and Hou. This is an open-access article distributed under the terms of the Creative Commons Attribution License (CC BY). The use, distribution or reproduction in other forums is permitted, provided the original author(s) and the copyright owner are credited and that the original publication in this journal is cited, in accordance with accepted academic practice. No use, distribution or reproduction is permitted which does not comply with these terms.
*Correspondence: Xingliang Hou, aG91eGxAc2NpYi5hYy5jbg==