- 1Laboratory of Applied Chemical Ecology, Federal Research Centre for Cultivated Plants, Institute for Plant Protection in Fruit Crops and Viticulture, Julius Kühn-Institut, Dossenheim, Germany
- 2Department of Plant Chemical Ecology, Technical University of Darmstadt, Darmstadt, Germany
Plant sap feeding insects like psyllids are known to be vectors of phloem dwelling bacteria (‘Candidatus Phytoplasma’ and ‘Ca. Liberibacter’), plant pathogens which cause severe diseases and economically important crop damage. Some univoltine psyllid species have a particular life cycle, within one generation they alternate two times between different host plant species. The plum psyllid Cacopsylla pruni, the vector of European Stone Fruit Yellows (ESFY), one of the most serious pests in European fruit production, migrates to stone fruit orchards (Prunus spp.) for mating and oviposition in early spring. The young adults of the new generation leave the Prunus trees in summer and emigrate to their overwintering hosts like spruce and other conifers. Very little is known about the factors responsible for the regulation of migration, reasons for host alternation, and the behavior of psyllids during their phase of life on conifers. Because insect feeding behavior and host acceptance is driven by different biotic factors, such as olfactory and gustatory cues as well as mechanical barriers, we carried out electrical penetration graph (EPG) recordings and survival bioassays with C. pruni on different conifer species as potential overwintering hosts and analyzed the chemical composition of the respective plant saps. We are the first to show that migrating psyllids do feed on overwintering hosts and that nymphs are able to ingest phloem and xylem sap of coniferous trees, but cannot develop on conifer diet. Analyses of plant saps reveal qualitative differences in the chemical composition between coniferous trees and Prunus as well as within conifer species. These differences are discussed with regard to nutritional needs of psyllid nymphs for proper development, overwintering needs of adults and restriction of ‘Ca. P. prunorum’ to Prunus phloem.
Introduction
Phloem and xylem tissue enables plants to allocate their resources from sources to sinks and distribute phytohormones to regulate physiological processes. Especially the phloem is rich in nutrients (Douglas, 2006), making it a suitable food source for sap-sucking insects. Although mechanical barriers like sclerenchymatous fibrous rings are able to hinder phloem-feeders from reaching the vascular bundles (George et al., 2017), the phloem is poorly chemically defended (Douglas, 2006). Since decades studies focused on the chemical composition of phloem sap and the nutrition of phloem-feeding insects. Most work was done in the field of crops, such as rice (Fukumorita and Chino, 1982), broad bean, clover, and peas (Sandström and Pettersson, 1994; Wilkinson and Douglas, 2003) and their pests (especially aphids), because of the economic importance and the role of aphids as model organisms. Information about the composition of phloem and xylem sap of coniferous plants is rare. Ziegler and Mittler (1959) extracted phloem sap from Picea abies by stylectomy and found sucrose as the only sugar in paper chromatography analysis. Later studies focused on induced defense mechanisms in bark phloem after bark beetle attack (Rohde et al., 1996), food quality of needles (Schopf et al., 1982; Fisher and Fisher, 1987) and impact of air pollution on nutrition of conifers (Zedler et al., 1986; Kainulainen et al., 1993). These studies give an impression of which metabolites could be found in plant sap of coniferous trees, but compounds were extracted from whole plant tissue (bark resp. needles). More explicit knowledge about plant sap composition is important for a better understanding of the biology of phloem-feeding insects that migrate between two different host plant species, e.g., psyllids (Hemiptera: Psyllidae).
Psyllids or jumping plant lice are plant sap feeding insects encompassing more than 3000 species. Most of them are oligophagous and use perennial dicotyledonous angiosperms as host plants for reproduction (Hodkinson, 2009; Mayer et al., 2009, 2011). In the genus Cacopsylla two different strategies can be observed: There are polyvoltine species reproducing and feeding exclusively on the same host plant and univoltine species with an obligate alternation of two host plants (Ossiannilsson, 1992; Hodkinson, 2009). The latter migrate between their reproduction host plants (respective fruit crops) and their overwintering host plants (conifers) (Mayer and Gross, 2007; Mayer et al., 2011). For identifying their particular host plants for feeding and reproduction, volatile signals are used in many species during migration (Soroker et al., 2004; Gross and Mekonen, 2005; Mayer et al., 2008a,b, 2009; Weintraub and Gross, 2013).
The plum psyllid, Cacopsylla pruni is the only known vector of one of the most serious pests in European fruit production, the cell wall lacking bacterium ‘Candidatus Phytoplasma prunorum’ (Carraro et al., 1998). The phloem dwelling bacterium induces the European Stone Fruit Yellows (ESFY) (Seemüller and Schneider, 2004). Because infected trees yield poorly and die quickly, this plant disease causes high economic losses in European fruit production every year. So far no curative approach was found against this disease. Unfortunately, it is not possible to cultivate this obligate cell parasite outside of the host plant or vector, which hampers research toward a cure. Therefore, the only measure to inhibit infection of stone fruit orchards is to prevent invasion of the vector insect, as C. pruni alternates between Prunus spp. and coniferous trees during its life cycle. After reproduction and development on Prunus spp., the young adults (emigrant stage) emigrate and spend the rest of the year on spruce and other conifers (Thébaud et al., 2009; Jarausch and Jarausch, 2016). In early spring they return to Prunus spp. for reproduction (remigrant stage). Very little is known about the reason for migration and feeding behavior of psyllids during their life on conifers (Thébaud et al., 2009). To date it remains unclear whether overwintering psyllids actually feed on conifers. Former experiments with the closely related hawthorn psyllid Cacopsylla melanoneura failed, although the maintenance of body condition and level of hydration suggested feeding (Jackson et al., 1990). Because it was shown that adult C. pruni did not survive the winter on one of their reproduction hosts Prunus spinosa (Carraro et al., 2002; Thébaud et al., 2009), and that some migrating species including C. pruni already start migration to their overwintering host during summer (Mayer and Gross, 2007; Mayer et al., 2009; Jarausch and Jarausch, 2016), we hypothesize that C. pruni needs to feed on overwintering host plants during this long period and therefore needs to leave deciduous Prunus trees to migrate to evergreen conifers, which show yearlong photosynthesis and phloem activity. On the other hand, reproduction on coniferous trees could be impossible for C. pruni, forcing them to migrate back to Prunus. A better knowledge of the vector biology is needed to develop new control strategies against vector insects and bacterial pathogens (Gross and Gündermann, 2016; Perilla-Henao and Casteel, 2016).
Here, we studied the feeding behavior of adults and nymphs on several conifer species as well as Prunus domestica, and conducted bioassays to unveil C. pruni’s ability to survive and develop on plant sap of overwintering hosts. Furthermore, we extracted the phloem/xylem sap of both Prunus spp. and conifers and analyzed sugars and organic acids including amino acids.
Materials and Methods
Insects
Cacopsylla pruni remigrants (overwintered adults) were caught by beating tray method from Prunus domestica trees located at the experimental field of the Julius Kühn-Institut in Dossenheim, Germany and at an experimental orchard of Dienstleistungszentrum Ländlicher Raum Rheinpfalz, Neustadt an der Weinstrasse, Germany in spring 2017. Psyllids were maintained on Prunus trees (cv. GF655/2 and Prunus spinosa) in cages housed in a climate chamber at 20°C during photophase and 16°C during scotophase (L16:D8). After mating and oviposition the field captured adults were transferred to cages with fresh plants. For survival experiments about 200 fifth instar nymphs were gently transferred to a new P. domestica (cv. Wavit) tree and emerged adults (emigrants) were collected daily.
Plants
Four conifer species, Abies alba (Silver fir), Larix decidua (European larch), Picea abies (Norway spruce), and Pinus sylvestris (Scots pine), and the P. domestica cultivar Wavit were used for experiments. Plants were grown under natural conditions in an insect safe environment. Hexythiazox (Ordoval, BASF, Ludwigshafen am Rhein, Germany) and Fenpyroximate (Kiron, Cheminova Deutschland GmbH & Co. KG, Stade, Germany) were applied once to P. domestica plants in April 2017 to prevent infestation with spider mites.
EPG-Recordings
To investigate whether C. pruni adults and nymphs feed on coniferous trees in general, the electrical penetration graph technique (EPG) was applied. EPGs were recorded using an 8 channel amplifier (model Giga-8d, EPG-Systems, Wageningen, Netherlands). Data acquisition and analysis was performed with Stylet+ software (EPG-Systems). To connect the psyllids to a copper electrode, a piece of fine gold wire (18 μm) was attached to the pronotum with a small droplet of water based silver glue (EPG-Systems). The electrode was attached to an EPG probe and the reference electrodes were placed in the soil of the test plants. Feeding behavior of C. pruni male and female emigrants (minimum age 6 weeks) was recorded in a climate chamber at 10°C with 60–65% RH for 16 h and of fifth instar nymphs (about 6 weeks old) at 20°C under the same conditions. Plants and insects were housed in a grounded self-constructed Faraday cage during recordings. Recordings were replicated 10 times for nymphs on each P. abies, A. alba, and P. domestica (cv. Wavit). Feeding behavior of emigrants was recorded on P. sylvestris (4 males and 6 females), P. abies (6 males and 4 females), A. alba (5 males and 5 females), and L. decudia (6 males and 4 females). To ensure that emigrants used for EPG recordings were not repelled by conifers (due to their developmental stage), C. pruni adults were caged with P. abies and A. alba twigs one day prior recordings and only emigrants which were found on conifer twigs were chosen for the experiment. Recordings were examined for occurrence of stylet penetration and waveforms indicating phloem and xylem uptake according to Bonani et al. (2010) and Civolani et al. (2011).
Bioassays
Survival
Survival of emigrants was studied on P. abies, A. alba, and P. domestica cv. Wavit plants. Transparent plastic cups (0.5 l capacity) were used as cages. The bottom of each cup was replaced by gauze for venting. A hole was punched into the lids to attach the cups on twigs of living plants. The lid was sealed with self-made modeling clay (composed of 42.6% water, 42.6% flour, 3.2% sunflower oil, 10.6% salt, and 1.1% citric acid) and five newly emerged emigrants (<24 h) were released in each cup. Living individuals were recorded daily over a period of 40 days. Additionally, the mortality of emigrants in the same type of cups, but without food supply (control), was observed. The experiment was replicated eight times for every plant species and five times without plants (control) under rearing conditions.
Development
For developmental experiments C. pruni nymphs of second and third instar were gently transferred with a fine brush from rearing plants to twigs with young flush of P. abies, A. alba, or P. domestica cv. Wavit, respectively. On each plant, five nymphs were caged in insect rearing sleeves (40 cm × 20 cm, 100 × 80 mesh/square inch, MegaView, Taiwan). The experiment was replicated seven times on each conifer species and five times on cv. Wavit. Experimental plants were housed under rearing conditions in a climate chamber. After 21 days cages were controlled consistently once a week for hatched C. pruni adults (emigrants). After 56 days all cages were opened and checked for living nymphs.
Xylem and Phloem Sap Sampling
Phloem and xylem saps were collected in June 2017 using modified centrifugation technique according to Hijaz and Killiny (2014). The twigs from young flush from P. domestica (cv. Wavit) and conifer species P. abies, A. alba, L. deciduas, and P. sylvestris were sliced into 2–3 cm pieces with a clean scalpel. The bottom of a 0.5 ml Eppendorf tube was removed with a razor blade and twig pieces were placed into the tube. The tube was immersed in a 1.5 ml tube. For collecting the phloem and xylem sap, the tubes were centrifuged at 12.000 rpm at 4°C for 10 min. The collected samples were stored at -80°C up to analysis. In the following, this collected mixture of phloem and xylem sap is referred as plant sap.
Plant Sap Derivatization
The sap samples were derivatized with methyl chloroformate (MCF) to focus the GC-MS analysis on amino and other organic acids (Smart et al., 2010). An aliquot of 20 μl plant sap was mixed with 180 μl sodium hydroxide (1 M) in a silanized glass vial. Then 167 μl methanol and 34 μl pyridine were added, followed by 20 μl MCF. The sample was vortexed for exactly 30 s, additionally 20 μl MCF were added and the sample was mixed again for 30 s. To extract the alkylated derivatives 150 μl chloroform were added to each sample and mixed for another 10 s. A 200 μl aliquot of sodium bicarbonate solution (50 mM) was added and mixed for 10 s again. After a double meniscus was formed, the aqueous phase was discarded and a few milligrams of anhydrous sodium sulfate were added to the organic layer to bind the remaining water. The supernatant was transferred to a GC-MS vial with a glass insert.
For the derivatization with trimethylsilyl (TMS) 5 μl aliquots of the sap samples were added to 60 μl of an internal standard solution (Ribitol in ultrapure water) and dried under nitrogen stream (Reacti-Vap, Thermo Fisher Scientific Inc., Waltham, MA, United States). Samples were derivatized by adding 70 μl methoxyamine hydrochloride solution (MOX) in pyridine (2%) and allow to incubate for 90 min at 37°C stirring at adjustment of 7 (Reacti-Therm, Thermo Fisher Scientific Inc.). 90 μl of N-methyl-N-(trimethylsilyl)trifluoroacetamide (MSTFA) were added and the silylation was allowed to react for 60 min at 37°C stirring at adjustment of 7 (Reacti-Therm, Thermo Fisher Scientific Inc.). The supernatant was transferred to a GC-MS vial with a glass insert.
Chemical Analysis
Derivatized samples were analyzed by gas chromatography coupled with mass spectrometry (GC-MS) using a PerkinElmer Clarus R 680 GC system coupled to a PerkinElmer quadrupole inert mass selective detector for molecular structure analysis. A non-polar Elite-5MS (Crossbond 5% diphenyl-95% dimethyl polysiloxane, PerkinElmer) capillary column (30 × 0.25 mm id × 0.25 μm film thickness) was used for GC separation. Carrier gas flow rate (Helium, Linde, Germany) was about 5 ml/min (column head pressure 150 kPa). Injection of 1 μl of the samples derivatized with MCF was done at 290°C injector temperature with a split flow of 1 ml/min. The initial oven temperature of 70°C was held for 3 min, followed by a temperature increase of 20°C/min up to 240°C held for 3.5 min and a further increase to 300°C at a rate of 20°C/min. The final temperature of 300°C was held for 2 min. The GC temperature program to analyze samples after silylation was as follows: the initial oven temperature of 80°C was held for 3 min, followed by an increase of 5°C/min up to 320°C. The final temperature of 320°C was held for 4 min. One microliter of each sample was injected at 220°C with a split flow of 5 ml/min. Transfer line and ion source temperatures were set to 250°C and 180°C, respectively. The quadrupole mass detector was operated in electron-impact (EI) mode at 70 eV. All data were obtained by collecting the full-scan mass spectra within the range of 35–550 m/z. Blank samples, reference standards and mixtures of alkanes (C8–C20 and C10–C40) were analyzed additionally according to both methods.
Identification and Quantification With AMDIS
GC-MS chromatograms were analyzed using “Automated Mass spectral Deconvolution and Identification System” (AMDIS, V. 2.71; National Institute of Standards and Technology NIST, Boulder, CO, United States). Detected compounds were identified by comparing characteristic ion fragmentation patterns, retention times and retention indices with standard compounds according to Weintraub and Gross (2013). For quantification, the peak areas were integrated after deconvolution with AMDIS. Identification criteria were applied as follows: match factor had to be ≥80% and the relative retention index deviation ≤5% from reference value. The settings for deconvolution were: component width: 32; adjacent peak subtraction: one; resolution: medium; sensitivity: medium; shape requirements: high; level: strong; maximum penalty: 20, and ‘no RI in library’: 20. Methionine, threonine, and serin were only found in traces (match < 80) and were therefore excluded from the analysis. Relative proportions of amino and organic acids were calculated by setting the sum of the selected compounds as 100%. Proportions of detected compounds after TMS derivatization were normalized to internal standard.
Chemicals and Standards
Alanine, aspartic acid, cysteine, glutamic acid, histidine, leucine, lysine, proline, threonine, tryptophan, valine, salicylic acid, pyridine, methanol, chloroform, methyl chloroformate (MCF), sodium bicarbonate, sodium sulfate, methoxyamine, ribitol, myo-inositol, xylose, pinitol, and iso-leucine were purchased from Sigma-Aldrich Chemie GmbH (Munich, Germany). Arginine and phenylalanine were purchased from SERVA Electrophoresis GmbH (Heidelberg, Germany). Glycine, methionine, serine, malic acid, caffeic acid, succinic acid, arabinose, and saccharose from Carl Roth GmbH & Co. KG (Karlsruhe, Germany). Asparagine, mannitol, glucose, and galactose from Merck KGaA (Darmstadt, Germany). Sorbitol and glutamine from AppliChem GmbH (Darmstadt, Germany). MSTFA from Macherey-Nagel GmbH & Co. KG (Düren, Germany). Citric acid was purchased from Acros Organics (Thermo Fisher Scientific, Geel, Belgium).
Statistical Analysis
Statistical analysis was done in R version 3.4.2 “Short Summer” (R Core Team, 2017). Visualizations were conducted with the ggplot2 package (Wickham, 2009). Death hazard from C. pruni emigrants on different host plants were compared by Cox’s proportional hazard regression through likelihood ratio test. Efron approximation was used for tie handling. The proportional hazards assumption for Cox regression model fit was confirmed using the cox.zph function of the survival package (Therneau, 2017). Non-metric multidimensional scaling (NMDS) plots were used to visualize Bray–Curtis dissimilarities of the chemical composition of xylem and phloem between plant species. NMDS was performed using the metaMDS function from vegan package (Oksanen et al., 2017). Scaling was standardized by Wisconsin double standardization. Significantly (p < 0.01, N = 10000) influential factors (chemical compounds) were plotted as arrows in NMDS plots. Dissimilarity matrix was calculated to test for discrimination of plant species by Permutational Multivariate Analysis of Variance (PERMANOVA). Additionally, the dispersion of groups was tested for multivariate homogeneity (PERMDISP).
Results
EPG-Recordings
To determine if C. pruni feeds on overwintering hosts (conifers), feeding behavior of emigrants was recorded on potential host plants. The recordings revealed that both male and female emigrants fed on plant saps of all four offered conifers: P. abies, A. alba, P. sylvestris, and L. decudia. Recordings from nymphs of C. pruni showed that they were also able to feed on P. abies and A. alba (Figure 1).
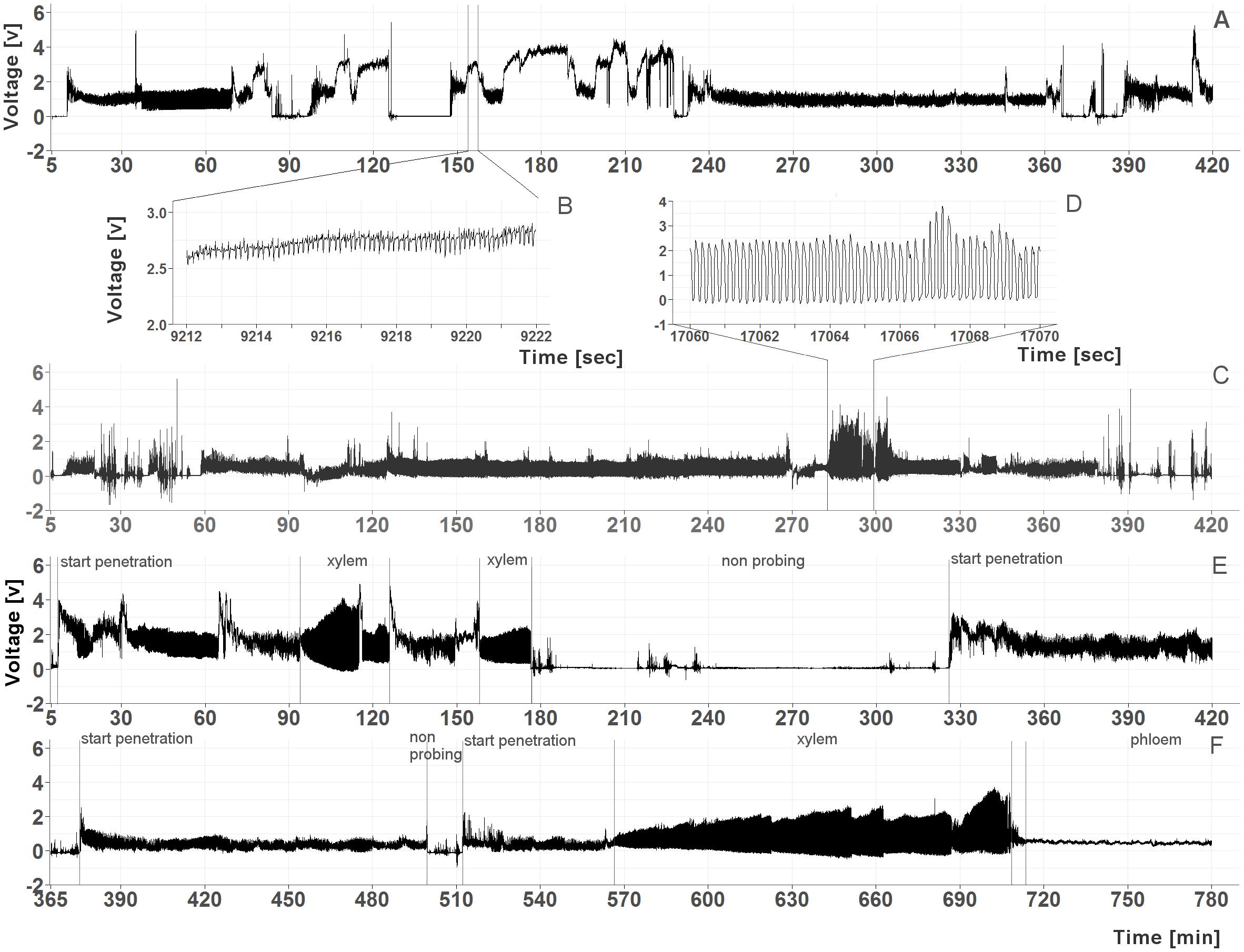
FIGURE 1. (A–D) Examples of EPG recordings from C. pruni nymphs (5th instar) on spruce (A) with a detailed magnification of phloem phase waveform (B) and on fir (C) with a detailed magnification of the waveform of xylem feeding (D). (E,F) Examples of recordings from a female C. pruni emigrant on larch (E) and a male emigrant on fir (F) with marked penetration and feeding phases.
Bioassays
Survival
Newly emerged C. pruni emigrants survived on P. abies and A. alba as long as on P. domestica cv. Wavit (Figure 2). The Cox regression model showed that death hazard differed significantly between host plants and controls without food supply (likelihood ratio = 81.76, df = 3, R2 = 0.431, p < 0.001). Death hazard for emigrants fed on P. domestica cv. Wavit did not differ from P. abies (likelihood ratio = 81.76, df = 3, R2 = 0.431, p = 0.803) and A. alba (likelihood ratio = 81.76, df = 3, R2 = 0.431, p = 0.846). Emigrants on all three potential host plant species had a significant lower death hazard than psyllids without food (control). The hazard ratio was reduced by 97, 97, and 96% if C. pruni was allowed to feed on P. abies, A. alba, or P. domestica cv. Wavit, respectively.
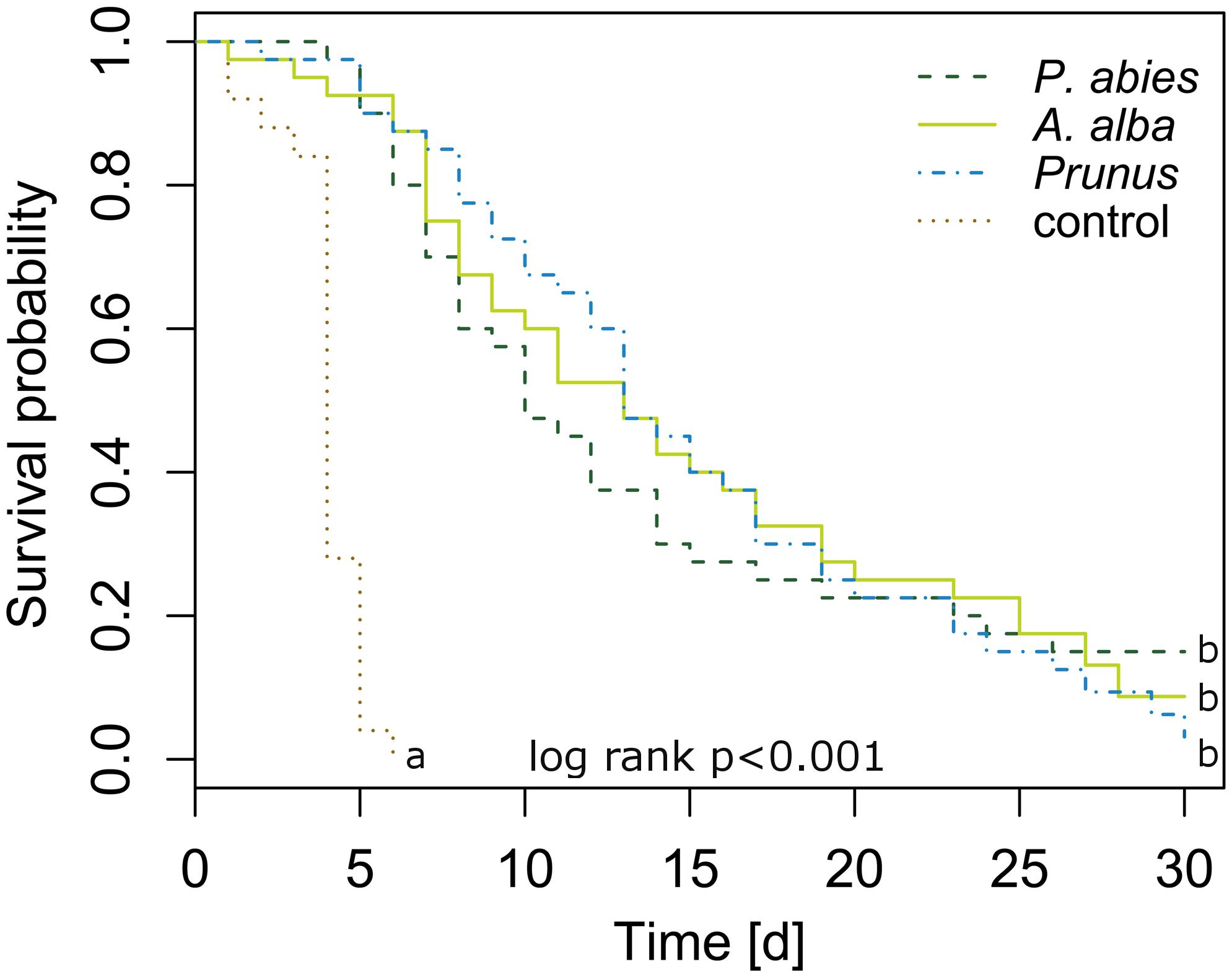
FIGURE 2. Kaplan–Meier curves visualizing the survival of newly emerged emigrants caged on P. abies (n = 40), A. alba (n = 40), P. domestica cv. Wavit (n = 40), or in cages without a plant (control, n = 25). Letters indicate significant differences between survival curves (likelihood ratio = 81.76, df = 3, R2 = 0.431, p < 0.001).
Development
After 56 days 92% of the C. pruni nymphs on P. domestica cv. Wavit emerged while none of the nymphs developed neither on P. abies nor A. alba (Figure 3). As no living nymphs could be found on the coniferous trees, we conclude that they all died in nymphal stage.
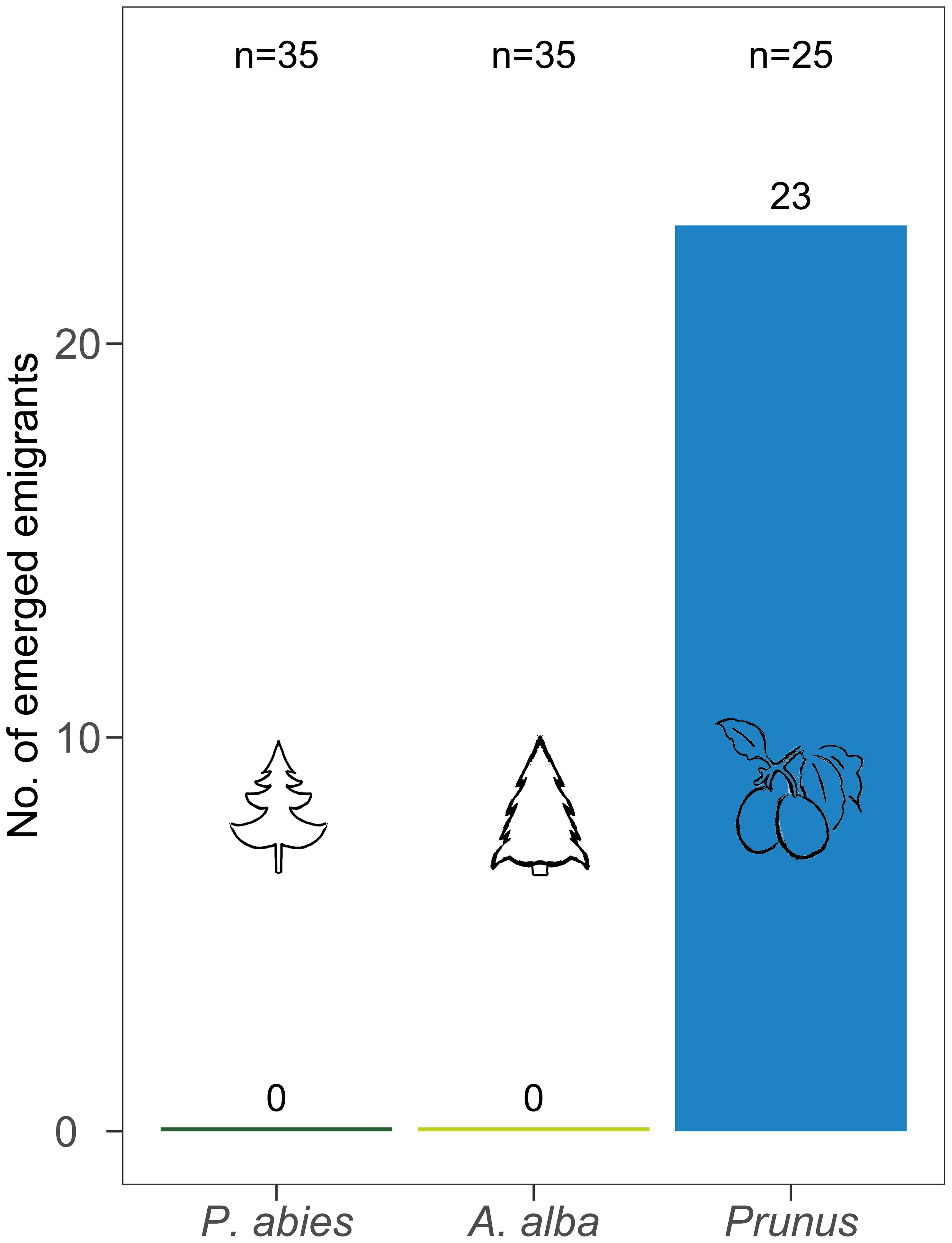
FIGURE 3. Number of emerged C. pruni emigrants from nymphs (2nd instar) on P. abies, A. alba, and P. domestica cv. Wavit.
Chemical Composition of Phloem and Xylem Content
Plant species differed significantly in the chemical composition of sugars and other compounds detected by GC-MS analysis after TMS derivatization of plant sap (PERMANOVA, df = 4, R2 = 60.83, N = 10000, P < 0.001). The dispersions differed not significantly between the groups (PERMDISP, df = 4, F = 0.42, N = 10000, P > 0.05), confirming that separation of species was due to their location. The NMDS plot illustrates the differences of chemical profiles (Figure 4).
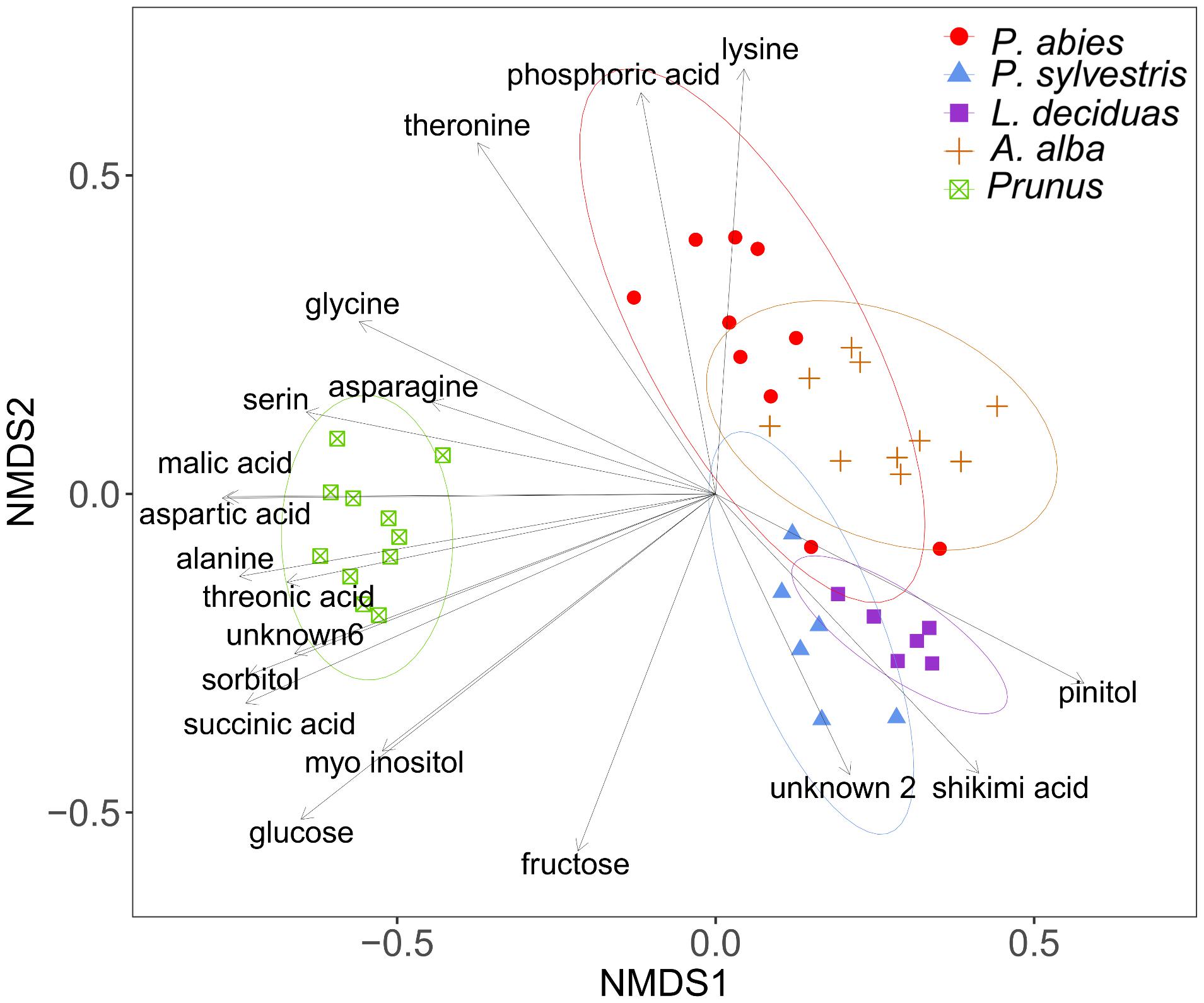
FIGURE 4. Visualization of Bray–Curtis dissimilarities with non-metric multidimensional scaling (NMDS) plots (stress = 0.14) of plant sap samples from spruce (n = 10), pine (n = 6), larch (n = 6), fir (n = 10), and P. domestica cv. Wavit (n = 11) after methoximation followed by trimethylsilylation.
Plant saps from P. domestica trees contained a high amount of sorbitol. This sugar alcohol constituted about 58% of the plant sap from P. domestica cv. Wavit but was not detected in samples from coniferous trees (Figure 5). In contrast, pinitol was exclusively found in plant sap from conifers. However, the most abundant component was quinic acid in all conifer samples (Figure 5). The relative abundance of quinic acid ranged from 30% in pine to 56% in spruce. Sap samples of P. domestica were composed of 80% sugars and sugar alcohols and 18% acids, whereas spruce, fir, pine, and larch samples consisted of 29, 41, 50, and 36% sugars and sugar alcohols and 69, 53, 43, and 61% acids, respectively.
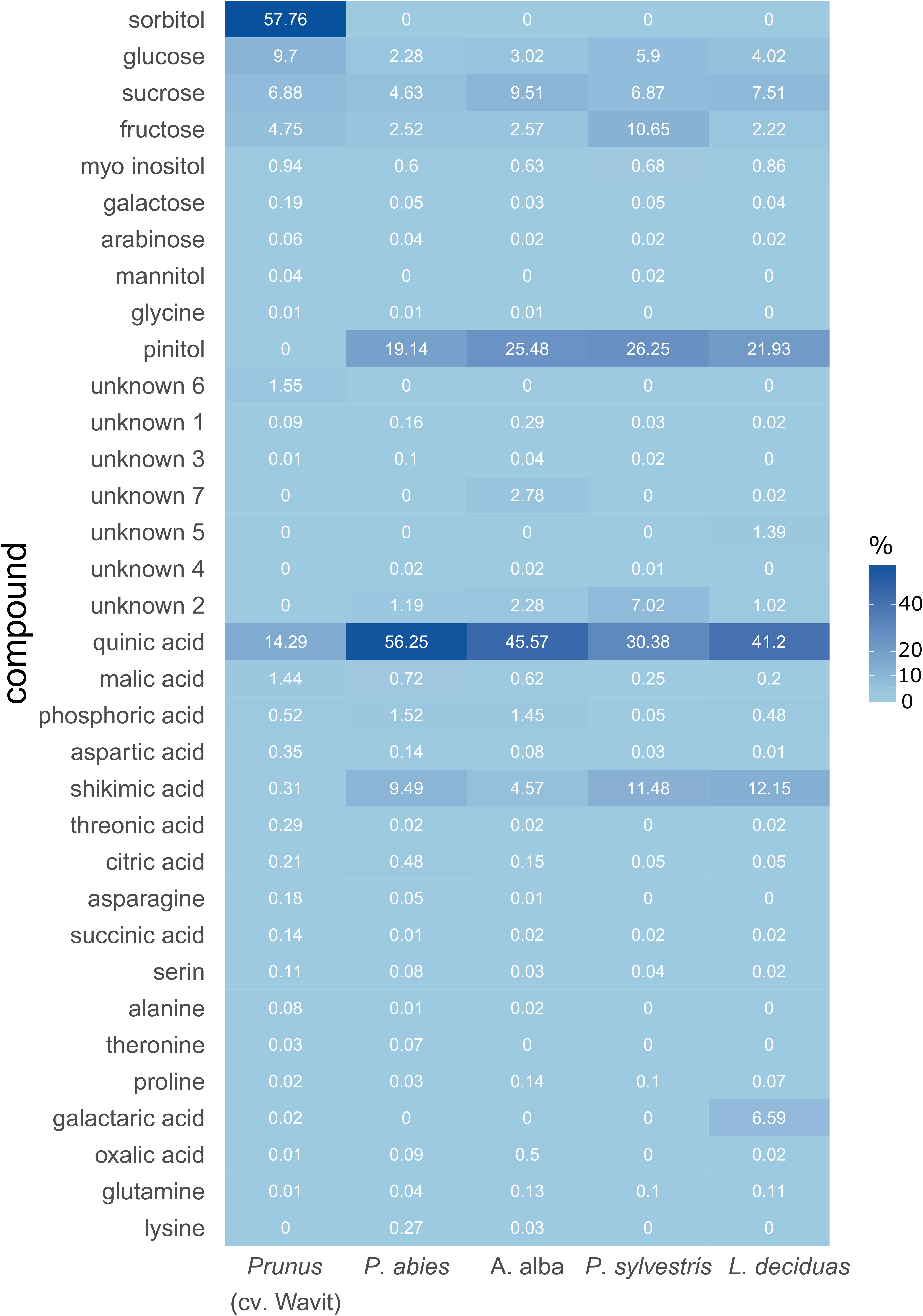
FIGURE 5. Composition of sugars and acids in vascular bundle content of P. domestica cv. Wavit (n = 11), spruce (n = 10), fir (n = 10), pine (n = 6), and larch (n = 6). Plant sap was collected by centrifugation and derivatized by trimethylsilylation after methoximation. Dark blue indicates a high relative abundance of the components, light blue a low abundance. Numbers are mean values of relative abundance.
The composition of amino acids and other organic acids differed significantly between the plant species (PERMANOVA, df = 4, R2 = 46.85, N = 10000, P < 0.001). The dispersions between the groups also differed significantly (PERMDISP, df = 4, F = 3.96, N = 10000, P < 0.01), indicating that the separation of the plant species could be effected by different variation within species (Figure 6). The NMDS plot shows caffeic acid and asparagine contributing to the separation of P. domestica cv. Wavit from coniferous trees (Figure 6). Caffeic acid was exclusively found in P. domestica cv. Wavit, while asparagine was more abundant in P. domestica cv. Wavit as in P. abies and A. alba (Figure 7).
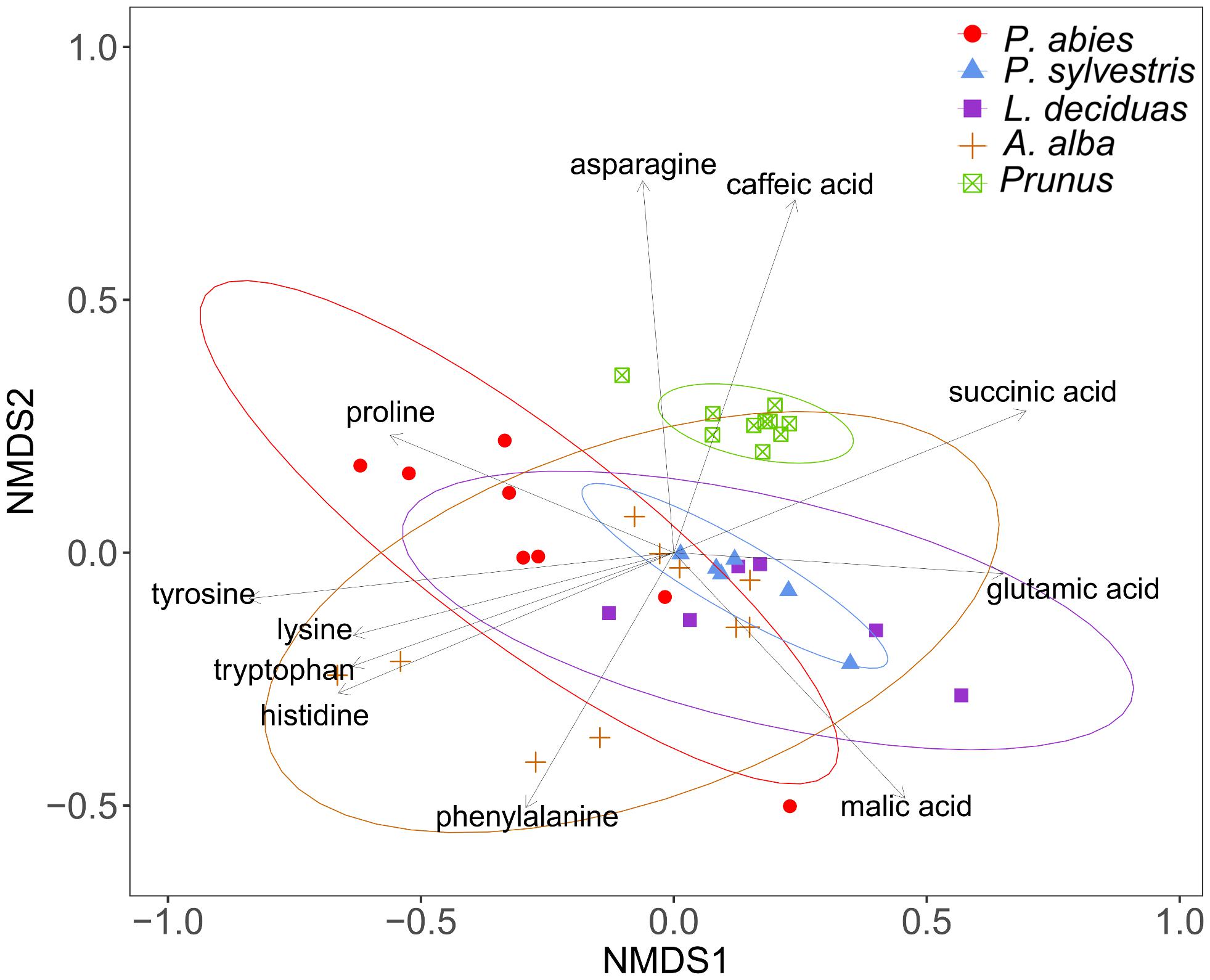
FIGURE 6. Visualization of Bray–Curtis dissimilarities with NMDS plots (Stress: 0.13) of plant sap samples from spruce (n = 8), pine (n = 6), larch (n = 6), fir (n = 10), and P. domestica cv. Wavit (n = 10) derivatization with methyl chloroformate.
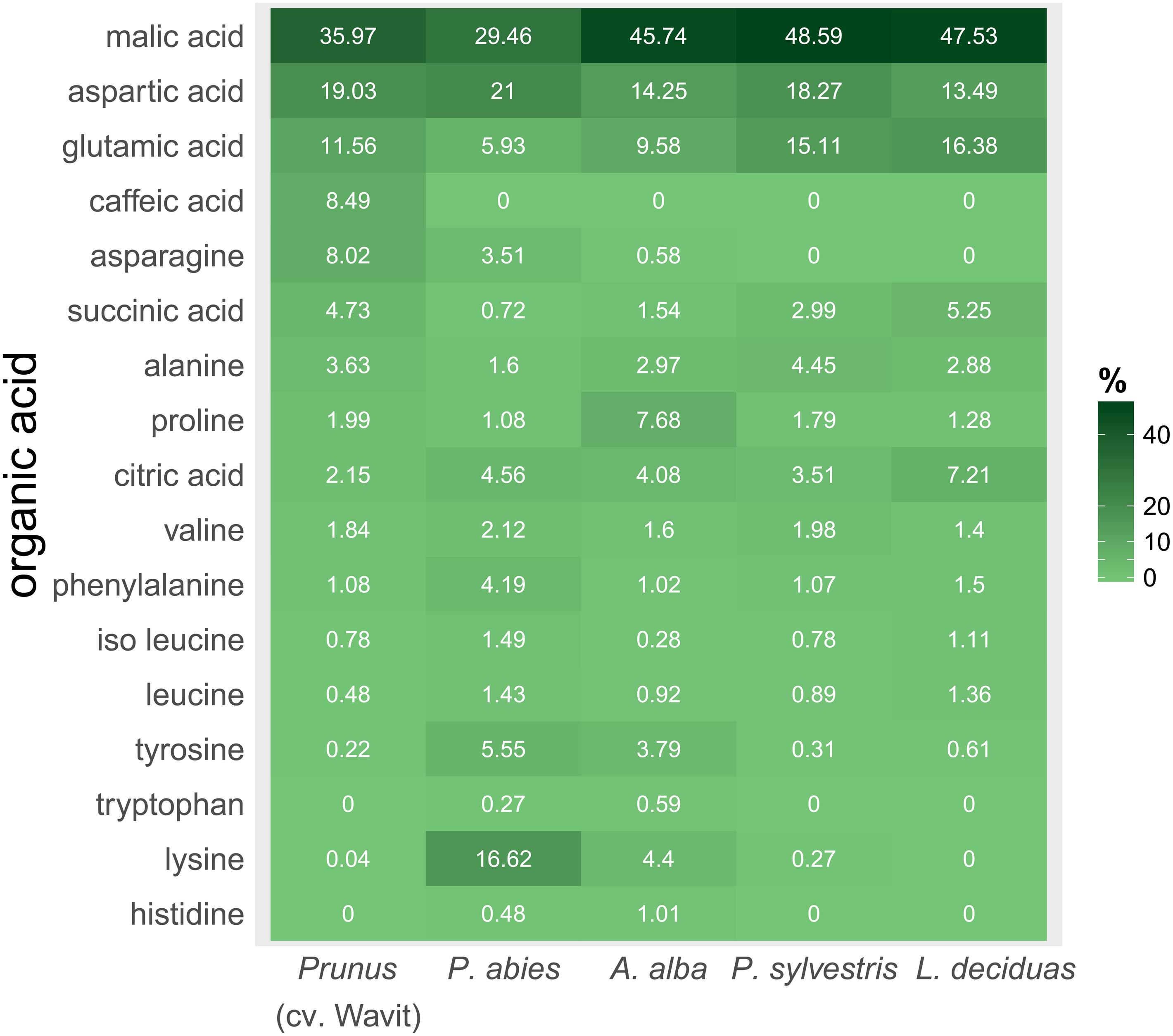
FIGURE 7. Composition of organic acids in vascular bundle content of P. domestica cv. Wavit (n = 10), spruce (n = 8), fir (n = 10), pine (n = 6), and larch (n = 6). Plant sap was collected by centrifugation and derivatization with methyl chloroformate. Dark green indicates a high relative abundance of a respective organic acid, light green a low abundance. Numbers are mean values of relative abundance.
The main organic acid component in the plant sap of all tested plant species was malic acid (29–48%). Aspartic acid was the second most abundant component in all plants, except in larch which contained more glutamic acid. Differences between the plant species were detected concerning the relative amounts of lysine in the plant sap composition. Lysine represented about 17% of the sap samples of spruce trees and was the third most abundant component in those trees, as glutamic acid was in fir (10%), pine (15%), and P. domestica cv. Wavit (12%) (Figure 7). Cysteine, methionine, and threonine were under detection limits in all samples. The NMDS plots indicate the responsibility of the essential amino acids tyrosine, tryptophan, lysine, and histidine on the separation of spruce and fir from P. domestica cv. Wavit (Figure 6).
Discussion
Electrical penetration graph recordings showed that C. pruni emigrants and nymphs are able to feed on the plant saps of spruce, pine, larch, and fir. EPGs recorded from 5th instar nymphs prove that nymphs are not repelled by metabolites of coniferous plants and able to reach the phloem and xylem tissue with their stylet. The question arises why C. pruni migrates to Prunus for reproduction when their progeny is able to ingest food from conifers. We suggest that there is no change in host acceptance of nymphs between different instars, but nutritional needs could change between nymphal development stages. Therefore we investigated the emergence of adults starting from the earliest possible instar (2nd). Because the impact of low food quality or inhibitory components may accumulate and negative influence raise over time, 5th instar nymphs may able to compensate a short period on a non-optimal diet while early instars would suffer more from low food quality than later ones. But it is of crucial importance, whether C. pruni is able to fully develop from egg to adult stage on coniferous trees. Bioassays revealed that adult psyllids survived on coniferous trees, while nymphs did not develop and died, although they were able to ingest plant sap from conifer needles. Thus, the chemical composition of the respective conifer saps influences the nymphal survival and development. Therefore the plant saps of overwintering hosts were subsequently analyzed and compared to sap content of their reproduction host plant (P. domestica).
The GC-MS analysis revealed enormous differences in the chemical compositions of plant sap of the Rosacea species P. domestica cv. Wavit and the four studied conifer species. Especially the lack of sorbitol in all four conifers as well as the high amount of quinic acid and pinitol (which was not detected in Prunus trees) could be challenging for phloem feeding insects, which alternate between Rosacea and conifers during their life cycle. Even though it was known that spruce needles contain quinic acid, shikimic acid, fructose, glucose, sucrose, and pinitol (Schopf et al., 1982), to date it was unclear, in which proportions they occur in the phloem and xylem sap of coniferous trees, and how their proportions differ between tree species.
Until today it was a widespread belief that conifers are used by migrating Cacopsylla species like C. pruni, C. picta, and C. melanoneura for shelter during winter time, exclusively (Burckhardt et al., 2014; Jarausch and Jarausch, 2016). In the presented study we were able to show for the first time, that conifers are not only shelter plants for migrating species belonging to the genus Cacopsylla, but also an important food resource enabling their overwintering. Thus, the term “shelter plant” should hereafter be replaced by “overwintering host” or just “alternate host” plant.
Due to the lack of knowledge that psyllids feed on conifers, the effect of coniferous phloem constituents like quinic acid, shikimic acid, and pinitol on psyllid feeding behavior and development was not studied before. Pinitol is a cyclic polyol, which serves as osmoprotectant and is involved in a broad spectrum of physiological processes in plants (Chiera et al., 2006; Kordan et al., 2011; Saxena et al., 2013). It is found in conifers, legumes (Fabaceae) and Caryophyllales such as Simmondsia chinensis (Angyal and Macdonald, 1952; Dittrich and Korak, 1984; Guo and Oosterhuis, 1995; Chiera et al., 2006). D-pinitol induces oviposition of the Grass Yellow Butterfly Eurema mandarina (Mukae et al., 2016). However, an influence of pinitol from the phloem of alfalfa on phloem-feeding pea aphid could not be found (Campbell and Binder, 1984).
There is evidence, that psyllid adults and nymphs are tolerant to high osmotic pressures of their diets (Hall et al., 2010; Russell and Pelz-Stelinski, 2015). Therefore, we hypothesize no negative influence of pinitol on C. pruni, even if it occurs in high amounts in overwintering hosts. Quite the contrary, pinitol could act as mechanism of protection against freezing stress, as shown for other polyols (Bale, 2002). The freezing temperature of the green spruce aphid is reduced in the presence of mannitol in aphid hemolymph (Parry, 1979). Whiteflies accumulate sorbitol for thermo- and osmoprotection (Hendrix and Salvucci, 1998). Sømme (1965) found an accumulation of sorbitol in overwintering eggs of European red mite (Panonychus ulmi).
We found that sorbitol is the most abundant component in sap samples of P. domestica cv. Wavit, which is in accordance with the fact that sorbitol is most often found in Rosacea (Loescher, 1987). Sorbitol is also known to be accumulated in the phloem of apple trees (Bieleski, 1969) and is the most abundant soluble sugar in the phloem of pear and apple fruits (Zhang et al., 2004, 2014). Nevertheless, adult C. pruni can tolerate high amounts of sorbitol or pinitol in their diet. EPG recordings suggest that C. pruni (both adults and nymphs) also ingest xylem content (unpublished results), which could be a regulatory reaction to reduce the phloem’s high osmotic pressure by dilution. Pompon et al. (2011) showed that aphids ingest more xylem sap after feeding on high concentrated sucrose diets to compensate osmotic unbalance. Moreover, for nymphal development the availability of amino acids (especially essential amino acids) could be of higher importance, as nitrogen content of food is an important limiting growth factor for phytophagous insects (Douglas, 2006). In accordance with Douglas (1993) we found asparagine besides aspartic acid and glutamic acid as one of the most abundant amino acids in young leaves of Prunus, while we found only low concentrations of glutamine in Prunus flush leaves. All plant species contained only low concentrations of the essential amino acids histidine, isoleucine, leucine, lysine, methionine, phenylalanine, threonine, tryptophan, and valine. To compensate for low quality of nitrogen in plant saps phloem feeders harbor microsymbionts (Douglas, 2006). Many psyllid species harbor the bacterial endosymbiont Carsonella ruddii, which provides its host with essential amino acids (Thao et al., 2000). Also representatives of the genus Wolbachia, Arsenophonus and other Enterobacteriaceae were found in psyllids (Baumann, 2005). Although the microsymbionts harbored by C. pruni are unidentified, differences in the symbiont community in adults and nymphs were not expected, because vertical transmission of endosymbionts was shown for many species. Furthermore, recent studies indicated the transovarial transmission of Arsenophonus in Cacopsylla pyricola (Cooper et al., 2017).
We suggest that the inability of C. pruni nymphs to develop on coniferous trees is due to differences in organic acid availability. The caffeic acid, which is exclusively found in cv. Wavit, could play a key role in host acceptance of C. pruni and maybe act as a phagostimulant. Caffeic acid was found in several stone fruits like peaches and plums, which are typical host plants of C. pruni (Carbonaro et al., 2002; Lombardi-Boccia et al., 2004). However, not all of the components responsible for the separation of cv. Wavit from the coniferous species need to be of biological relevance. To unravel which components are actually important for proper development or which ones may inhibit nymphal growth, feeding experiments with nymphs on artificial diets are crucial. The analysis of excreted honeydew could suggest important information on how psyllids process plant nutrients. This study also revealed differences between the plant saps of the investigated coniferous trees. Therefore, a detailed analysis of EPG recordings from nymphs on the different tree species could be needful to identify feeding stimulants or deterrents and will be investigated in future. This knowledge could be used for development of an artificial diet system for rearing of C. pruni and screening for potential toxins against psyllids (Jancovich et al., 1997; Hall et al., 2010). Interestingly, although some of the migrating psyllids like C. pruni harbor phloem-limited plant pathogenic bacteria (‘Ca. Phytoplasma’ or ‘Ca. Liberibacter’) and feed on conifers, the phytopathogens seem to be restricted to vector insects and their reproduction host plants (Gross, 2016). Because the genomes of Phytoplasma spp. lack metabolic genes but contain a lot of transporter systems, it is suggested that they depend strongly on the nutrition of their hosts (Oshima et al., 2004; Kube et al., 2008). Insight on the chemical composition of the phloem sap of host plants could support developing a culture media for phytoplasmas and may advance the research on phytoplasma diseases (Trivedi et al., 2016).
Conclusion
No mechanical nor chemical border prevents C. pruni adults and nymphs from feeding on conifers. Emigrants feed and survive on their overwintering hosts. Nymphs can feed on, but are not able to develop on spruce and fir. This is likely due to strong differences in the compositions of organic acids and sugars between plant saps of conifers and P. domestica. Furthermore, feeding experiments with nymphs on artificial diets should reveal which components are responsible for successful development of C. pruni. Additionally, more insight on phloem sap composition could open up new possibilities for phytoplasma cultivation and pathogen research.
Author Contributions
JGa and JGr designed the study, contributed to the interpretation of the data, approved the final version of the manuscript, and ensured the accuracy and integrity of the work. JGa conducted the experiments and analysis and wrote the first draft of the manuscript, which was revisited and edited by JGr. JGr supervised the project.
Funding
JGa was supported by a fund of the “Landwirtschaftliche Rentenbank” number 28RF4IP008.
Conflict of Interest Statement
The authors declare that the research was conducted in the absence of any commercial or financial relationships that could be construed as a potential conflict of interest.
Acknowledgments
We are grateful to Monika Bäuerle and Anja Frank for excellent experimental assistance and Felix Hergenhahn (JKI, Dossenheim, Germany) for cultivation of the plants. We thank Margit Rid (JKI, Dossenheim, Germany) for helpful advice on AMDIS. We are grateful to Eva Gross (Schriesheim, Germany) for language editing.
References
Angyal, S. J., and Macdonald, C. G. (1952). Cyclitols. Part I. isoPropylidene derivatives of inositols and quercitols. The structure of pinitol and quebrachitol. J. Chem. Soc. 686–695. doi: 10.1039/JR9520000686
Bale, J. S. (2002). Insects and low temperatures: from molecular biology to distributions and abundance. Philos. Trans. R. Soc. Lond. B 357, 849–862. doi: 10.1098/rstb.2002.1074
Baumann, P. (2005). Biology bacteriocyte-associated endosymbionts of plant sap-sucking insects. Annu. Rev. Microbiol. 59, 155–189. doi: 10.1146/annurev.micro.59.030804.121041
Bieleski, R. L. (1969). Accumulation and translocation of sorbitol in apple phloem. Aust. J. Biol. Sci. 22, 611–620. doi: 10.1071/BI9690611
Bonani, J. P., Fereres, A., Garzo, E., Miranda, M. P., Appezzato-Da-Gloria, B., and Lopes, J. R. S. (2010). Characterization of electrical penetration graphs of the Asian citrus psyllid, Diaphorina citri in sweet orange seedlings. Entomol. Exp. Appl. 134, 35–49. doi: 10.1111/j.1570-7458.2009.00937.x
Burckhardt, D., Ouvrard, D., Queiroz, D., and Percy, D. (2014). Psyllid host-plants (Hemiptera: Psylloidea): resolving a semantic problem. Fla. Entomol. 97, 242–246. doi: 10.1653/024.097.0132
Campbell, B. C., and Binder, R. G. (1984). Alfalfa cyclitols in the honeydew of an aphid. Phytochemistry 23, 1786–1787. doi: 10.1016/S0031-9422(00)83492-5
Carbonaro, M., Mattera, M., Nicoli, S., Bergamo, P., and Cappelloni, M. (2002). Modulation of antioxidant compounds in organic vs conventional fruit (Peach, Prunus persica L., and Pear, Pyrus communis L.). J. Agric. Food Chem. 50, 5458–5462. doi: 10.1021/jf0202584
Carraro, L., Ferrini, F., Ermacora, P., and Loi, N. (2002). Role of wild Prunus species in the epidemiology of European stone fruit yellows. Plant Pathol. 51, 513–517. doi: 10.1046/j.1365-3059.2002.00732.x
Carraro, L., Osler, R., Loi, N., Ermacora, P., and Refatti, E. (1998). Transmission of European stone fruit yelllows phytoplasna by Cacopsylla pruni. J. Plant Pathol. 80, 233–239. doi: 10.4454/jpp.v80i3.823
Chiera, J. M., Streeter, J. G., and Finer, J. J. (2006). Ononitol and pinitol production in transgenic soybean containing the inositol methyl transferase gene from Mesembryanthemum crystallinum. Plant Sci. 171, 647–654. doi: 10.1016/j.plantsci.2006.06.006
Civolani, S., Leis, M., Grandi, G., Garzo, E., Pasqualini, E., Musacchi, S., et al. (2011). Stylet penetration of Cacopsylla pyri; an electrical penetration graph (EPG) study. J. Insect Physiol. 57, 1407–1419. doi: 10.1016/j.jinsphys.2011.07.008
Cooper, W. R., Garczynski, S. F., Horton, D. R., Unruh, T. R., Beers, E. H., Peter, W. S., et al. (2017). Bacterial Endosymbionts of the Psyllid Cacopsylla pyricola (Hemiptera: Psyllidae) in the Pacific Northwestern United States. Environ. Entomol. 46, 393–402. doi: 10.1093/ee/nvx031
Dittrich, P., and Korak, A. (1984). Novel biosynthesis of D-pinitol in simmondsia chinensis. Phytochemistry 23, 65–66. doi: 10.1016/0031-9422(84)83079-4
Douglas, A. E. (1993). The nutritonal quality of phloem sap utilized by natural aphid populations. Ecol. Entomol. 18, 31–38. doi: 10.1111/j.1365-2311.1993.tb01076.x
Douglas, A. E. (2006). Phloem-sap feeding by animals: problems and solutions. J. Exp. Bot. 57, 747–754. doi: 10.1093/jxb/erj067
Fisher, M., and Fisher, M. (1987). The effect of previously infested spruce needles on the growth of the green spruce aphid, Elatobium abietinum, and the effect of the aphid on the amino acid balance of the host plant. Ann. Appl. Biol. 111, 33–41. doi: 10.1111/j.1744-7348.1987.tb01430.x
Fukumorita, T., and Chino, M. (1982). Sugar, amino acid and inorganic contents in rice phloem sap. Plant Cell Physiol. 23, 273–283. doi: 10.1093/oxfordjournals.pcp.a076347
George, J., Ammar, E.-D., Hall, D. G., and Lapointe, S. L. (2017). Sclerenchymatous ring as a barrier to phloem feeding by Asian citrus psyllid: evidence from electrical penetration graph and visualization of stylet pathways. PLoS One 12:e0173520. doi: 10.1371/journal.pone.0173520
Gross, J. (2016). Chemical communication between phytopathogens, their host plants and vector insects and eavesdropping by natural enemies. Front. Ecol. Evol. 4:104. doi: 10.3389/fevo.2016.00104
Gross, J., and Gündermann, G. (2016). “Principles of IPM in cultivated crops and implementation of innovative strategies for sustainable plant protection,” in Advances in Insect Control and Resistance Management, ed. A. R. Horowitz (Cham: Springer), 9–26.
Gross, J., and Mekonen, N. (2005). Plant odours influence the host finding behaviour of apple psyllids (Cacopsylla picta; C. melanoneura). IOBC WPRS Bull. 28, 351–355.
Guo, C., and Oosterhuis, D. M. (1995). Pinitol occurrence in soybean plants as affected by temperature and plant growth regulators. J. Exp. Bot. 46, 249–253. doi: 10.1093/jxb/46.2.249
Hall, D. G., Shatters, R. G., Carpenter, J. E., and Shapiro, J. P. (2010). Research toward an artificial diet for adult Asian citrus psyllid. Ann. Entomol. Soc. Am. 103, 611–617. doi: 10.1603/AN10004
Hendrix, D. L., and Salvucci, M. E. (1998). Polyol metabolism in homopterans at high temperatures: accumulation of mannitol in aphids (Aphididae: Homoptera) and sorbitol in whiteflies (Aleyrodidae: Homoptera). Comp. Biochem. Physiol. A Mol. Integr. Physiol. 120, 487–494. doi: 10.1016/S1095-6433(98)10058-2
Hijaz, F., and Killiny, N. (2014). Collection and chemical composition of phloem sap from Citrus sinensis L. Osbeck (sweet orange). PLoS One 9:e101830. doi: 10.1371/journal.pone.0101830
Hodkinson, I. D. (2009). Life cycle variation and adaptation in jumping plant lice (Insecta: Hemiptera: Psylloidea): a global synthesis. J. Nat. Hist. 43, 65–179. doi: 10.1080/00222930802354167
Jackson, C. S., Hodkinson, I. D., and Stanley, P. (1990). Cold-hardiness in the hawthorn psyllid Cacopsylla melanoneura (Förster)(Homoptera: Psylloidea). Entomologist 109, 224–230.
Jancovich, J. K., Davidson, E. W., Lavine, M., and Hendrix, D. L. (1997). Feeding chamber and diet for culture of nymphal Bemisia argentifolii (Homoptera: Aleyrodidae). J. Econ. Entomol. 90, 628–633. doi: 10.1093/jee/90.2.628
Jarausch, W., and Jarausch, B. (2016). A permanent rearing system for Cacopsylla pruni, the vector of ‘Candidatus Phytoplasma prunorum’. Entomol. Exp. Appl. 159, 112–116. doi: 10.1111/eea.12427
Kainulainen, P., Satka, H., Mustaniemi, A., Holopainen, J. K., and Oksanen, J. (1993). Conifer aphids in an air-polluted environment.: II. Host plant quality. Environ. Pollut. 80, 193–200. doi: 10.1016/0269-7491(93)90147-G
Kordan, B., Lahuta, L., Dancewicz, K., Sądej, W., and Gabryś, B. (2011). Effect of lupin cyclitols on pea aphid probing behaviour. J. Plant Prot. Res. 51, 171–178. doi: 10.2478/v10045-011-0030-z
Kube, M., Schneider, B., Kuhl, H., Dandekar, T., Heitmann, K., Migdoll, A. M., et al. (2008). The linear chromosome of the plant-pathogenic mycoplasma ‘Candidatus Phytoplasma mali’. BMC Genomics 9:306. doi: 10.1186/1471-2164-9-306
Loescher, W. H. (1987). Physiology and metabolism of sugar alcohols in higher plants. Physiol. Plant. 70, 553–557. doi: 10.1111/j.1399-3054.1987.tb02857.x
Lombardi-Boccia, G., Lucarini, M., Lanzi, S., Aguzzi, A., and Cappelloni, M. (2004). Nutrients and antioxidant molecules in yellow plums (Prunus domestica L.) from conventional and organic productions: a comparative study. J. Agric. Food Chem. 52, 90–94. doi: 10.1021/jf0344690
Mayer, C. J., and Gross, J. (2007). Different host plant odours influence migration behaviour of Cacopsylla melanoneura (Förster), an insect vector of the apple proliferation phytoplasma. IOBC WPRS Bull. 30, 177–184.
Mayer, C. J., Jarausch, B., Jarausch, W., Jelkmann, W., Vilcinskas, A., and Gross, J. (2009). Cacopsylla melanoneura has no relevance as vector of apple proliferation in Germany. Phytopathology 99, 729–738. doi: 10.1094/PHYTO-99-6-0729
Mayer, C. J., Vilcinskas, A., and Gross, J. (2008a). Pathogen-induced release of plant allomone manipulates vector insect behavior. J. Chem. Ecol. 34, 1518–1522. doi: 10.1007/s10886-008-9564-6
Mayer, C. J., Vilcinskas, A., and Gross, J. (2008b). Phytopathogen lures its insect vector by altering host plant odor. J. Chem. Ecol. 34, 1045–1049. doi: 10.1007/s10886-008-9516-1
Mayer, C. J., Vilcinskas, A., and Gross, J. (2011). Chemically mediated multitrophic interactions in a plant-insect vector-phytoplasma system compared with a partially nonvector species. Agric. For. Entomol. 13, 25–35. doi: 10.1111/j.1461-9563.2010.00495.x
Mukae, S.-Y., Ohashi, T., Matsumoto, Y., Ohta, S., and Ômura, H. (2016). D-pinitol in fabaceae: an oviposition stimulant for the common grass yellow butterfly, Eurema mandarina. J. Chem. Ecol. 42, 1122–1129. doi: 10.1007/s10886-016-0775-y
Oksanen, J., Blanchet, F. G., Friendly, M., Kindt, R., Legendre, P., McGlinn, D., et al. (2017). Vegan: Community Ecology Package. R Package Version 2.4-4. Available at: https://CRAN.R-project.org/package=vegan
Oshima, K., Kakizawa, S., Nishigawa, H., Jung, H.-Y., Wei, W., Suzuki, S., et al. (2004). Reductive evolution suggested from the complete genome sequence of a plant-pathogenic phytoplasma. Nat. Genet. 36, 27–29. doi: 10.1038/ng1277
Ossiannilsson, F. (1992). “The psylloidea (Homoptera) of Fennoscandia and Denmark,” in Fauna entomologica Scandinavica 26, ed. F. Ossiannilsson (Leiden, NY: Brill).
Parry, W. H. (1979). Acclimatisation in the green spruce aphid, Elatobium abietinum. Ann. Appl. Biol. 92, 299–306. doi: 10.1111/j.1744-7348.1979.tb03877.x
Perilla-Henao, L. M., and Casteel, C. L. (2016). Vector-borne bacterial plant pathogens: interactions with hemipteran insects and plants. Front. Plant Sci. 7:1163. doi: 10.3389/fpls.2016.01163
Pompon, J., Quiring, D., Goyer, C., Giordanengo, P., and Pelletier, Y. (2011). A phloem-sap feeder mixes phloem and xylem sap to regulate osmotic potential. J. Insect Physiol. 57, 1317–1322. doi: 10.1016/j.jinsphys.2011.06.007
R Core Team (2017). R: A Language and Environment for Statistical Computing. Vienna: R Foundation for Statistical Computing.
Rohde, M., Waldmann, R., and Lunderstädt, J. (1996). Induced defence reaction in the phloem of spruce (Picea abies) and larch (Larix decidua) after attack by Ips typographus and Ips cembrae. For. Ecol. Manage. 86, 51–59. doi: 10.1016/S0378-1127(96)03802-9
Russell, C. W., and Pelz-Stelinski, K. S. (2015). Development of an artificial diet and feeding system for juvenile stages of the Asian citrus psyllid, Diaphorina citri. Entomol. Exp. Appl. 154, 171–176. doi: 10.1111/eea.12268
Sandström, J., and Pettersson, J. (1994). Amino acid composition of phloem sap and the relation to intraspecific variation in pea aphid (Acyrthosiphon pisum) performance. J. Insect Physiol. 40, 947–955. doi: 10.1016/0022-1910(94)90133-3
Saxena, S. C., Kaur, H., Verma, P., Petla, B. P., Andugula, V. R., and Majee, M. (2013). ““Osmoprotectants: potential for crop improvement under adverse conditions”,” in Plant Acclimation to Environmental Stress, eds N. Tuteja and G. S. Singh (New York, NY: Springer), 197–232.
Schopf, R., Mignat, C., and Hedden, P. (1982). As to the food quality of spruce needles for forest damaging insects. J. Appl. Entomol. 93, 244–257. doi: 10.1111/j.1439-0418.1982.tb03595.x
Seemüller, E., and Schneider, B. (2004). ‘Candidatus Phytoplasma mali’, ‘Candidatus Phytoplasma pyri’ and ‘Candidatus Phytoplasma prunorum’, the causal agents of apple proliferation, pear decline and European stone fruit yellows, respectively. Int. J. Syst. Evol. Microbiol. 54, 1217–1226. doi: 10.1099/ijs.0.02823-0
Smart, K. F., Aggio, R. B. M., van Houtte, J. R., and Villas-Boas, S. G. (2010). Analytical platform for metabolome analysis of microbial cells using methyl chloroformate derivatization followed by gas chromatography-mass spectrometry. Nat. Protoc. 5, 1709–1729. doi: 10.1038/nprot.2010.108
Sømme, L. (1965). Changes in sorbitol content and supercooling points in overwintering eggs of the European red mite (Panonychus ulmi (Koch)). Can. J. Zool. 43, 881–884. doi: 10.1139/z65-089
Soroker, V., Talebaev, S., Harari, A. R., and Wesley, S. D. (2004). The role of chemical cues in host and mate location in the pear psylla Cacopsylla bidens (Homoptera: Psyllidae). J. Insect Behav. 17, 613–626. doi: 10.1023/B:JOIR.0000042544.35561.1c
Thao, M. L., Moran, N. A., Abbot, P., Brennan, E. B., Burckhardt, D. H., and Baumann, P. (2000). Cospeciation of psyllids and their primary prokaryotic endosymbionts. Appl. Environ. Microbiol. 66, 2898–2905. doi: 10.1128/AEM.66.7.2898-2905.2000
Thébaud, G., Yvon, M., Alary, R., Sauvion, N., and Labonne, G. (2009). Efficient transmission of ‘Candidatus phytoplasma prunorum’ Is delayed by eight months due to a long latency in its host-alternating vector. Phytopathology 99, 265–273. doi: 10.1094/PHYTO-99-3-0265
Therneau, T. M. (2017). A Package for Survival Analysis in S. R Package Version 2.41-3. Available at: https://github.com/therneau/survival
Trivedi, P., Trivedi, C., Grinyer, J., Anderson, I. C., and Singh, B. K. (2016). Harnessing host-vector microbiome for sustainable plant disease management of phloem-limited bacteria. Front. Plant Sci. 7:1423. doi: 10.3389/fpls.2016.01423
Weintraub, P., and Gross, J. (2013). “Capturing insect vectors of phytoplasmas,” in Phytoplasma: Methods in Molecular Biology Methods and Protocols, eds M. J. Dickinson, and J. Hodgetts (Totowa, NJ: Humana Press), 61–72.
Wickham, H. (2009). ggplot2: Elegant Graphics for Data Analysis. Use R. New York, NY: Springer. doi: 10.1007/978-0-387-98141-3
Wilkinson, T. L., and Douglas, A. E. (2003). Phloem amino acids and the host plant range of the polyphagous aphid, Aphis fabae. Entomol. Exp. Appl. 106, 103–113. doi: 10.1046/j.1570-7458.2003.00014.x
Zedler, B., Plarre, R., and Rothe, G. M. (1986). Impact of atmospheric pollution on the protein and amino acid metabolism of spruce Picea abies trees. Environ. Pollut. 40, 193–212. doi: 10.1016/0143-1471(86)90094-2
Zhang, H.-P., Wu, J.-Y., Tao, S.-T., Wu, T., Qi, K.-J., Zhang, S.-J., et al. (2014). Evidence for apoplasmic phloem unloading in pear fruit. Plant Mol. Biol. Rep. 32, 931–939. doi: 10.1007/s11105-013-0696-7
Zhang, L.-Y., Peng, Y.-B., Pelleschi-Travier, S., Fan, Y., Lu, Y.-F., Lu, Y.-M., et al. (2004). Evidence for apoplasmic phloem unloading in developing apple fruit. Plant Physiol. 135, 547–586. doi: 10.1104/pp.103.036632
Keywords: phloem, chemical composition, psyllid, development, overwintering, host alternation, migration, conifer
Citation: Gallinger J and Gross J (2018) Unraveling the Host Plant Alternation of Cacopsylla pruni – Adults but Not Nymphs Can Survive on Conifers Due to Phloem/Xylem Composition. Front. Plant Sci. 9:484. doi: 10.3389/fpls.2018.00484
Received: 11 January 2018; Accepted: 29 March 2018;
Published: 13 April 2018.
Edited by:
Mariana Alves Stanton, Universidade de São Paulo, BrazilReviewed by:
Sean Michael Prager, University of Saskatchewan, CanadaNabil Killiny, University of Florida, United States
Copyright © 2018 Gallinger and Gross. This is an open-access article distributed under the terms of the Creative Commons Attribution License (CC BY). The use, distribution or reproduction in other forums is permitted, provided the original author(s) and the copyright owner are credited and that the original publication in this journal is cited, in accordance with accepted academic practice. No use, distribution or reproduction is permitted which does not comply with these terms.
*Correspondence: Jürgen Gross, SnVlcmdlbi5Hcm9zc0BqdWxpdXMta3VlaG4uZGU=