- 1Triticeae Research Institute, Sichuan Agricultural University, Chengdu, China
- 2College of Resources, Sichuan Agricultural University, Chengdu, China
- 3Joint International Research Laboratory of Crop Resources and Genetic Improvement, Sichuan Agricultural University, Chengdu, China
Tetraploid Thinopyrum elongatum, which has superior abiotic stress tolerance characteristics, and exhibits resistance to stripe rust, powdery mildew, and Fusarium head blight, is a wild relative of wheat and a promising source of novel genes for wheat improvement. Currently, a high-resolution Fluorescence in situ hybridization (FISH) karyotype of tetraploid Th. elongatum is not available. To develop chromosome-specific FISH-based markers, the hexaploid Trititrigia 8801 and two accessions of tetraploid Th. elongatum were characterized by different repetitive sequences probes. We found that all E-genome chromosomes could be unambiguously identified using a combination of pSc119.2, pTa535, pTa71, and pTa713 repeats, and the E-genome chromosomes of the wild accessions and the partial amphiploid failed to exhibit any significant variation in the probe hybridization patterns. To verify the validation of these markers, the chromosome constitution of eight wheat- Th. elongatum hybrid derivatives were analyzed. We revealed that these probes could quickly detect wheat and tetraploid Th. elongatum chromosomes in hybrid lines. K16-712-1-2 was a 1E (1D) chromosome substitution line, K16-681-4 was a 2E disomic chromosome addition line, K16-562-3 was a 3E, 4E (3D, 4D) chromosome substitution line, K15-1033-8-2 contained one 4E, two 5E, and one 4ES⋅1DL Robertsonian translocation chromosome, and four other lines carried monosomic 4E, 5E, 6E, and 7E chromosome, respectively. Furthermore, the E-genome specific molecular markers analysis corresponded perfectly with the FISH results. The developed FISH markers will facilitate rapid identification of tetraploid Th. elongatum chromosomes in wheat improvement programs and allow appropriate alien chromosome transfer.
Introduction
As one of the most important cultivated cereals, the wheat (Triticum aestivum L., 2n = 6x = 42, AABBDD) grain production was forecast at 754.8 million tonnes in 2017, and ranked second only to maize in the world (FAO, 2017). However, the progress in wheat breeding is hampered by a relatively narrow range of genetic diversity (Dubcovsky and Dvorak, 2007). To solve this problem, it is good to develop cultivars with superior tolerance to biotic and abiotic stress. Wild relatives have a rich reservoir of useful alien genes that can be introduced into common wheat by distant hybridization to enrich the genetic diversity of wheat (Friebe et al., 1996; Mujeeb-Kazi et al., 2013; Ceoloni et al., 2017).
As a tertiary gene pool species for common wheat, Thinopyrum elongatum (syn. Agropyron elongatum, Lophopyrum elongatum) has always been a focus of wheat breeders. The taxon comprises a complex of polyploidy series based on the E-genome: diploid (2n = 2x = 14, EE), tetraploid (2n = 4x = 28, EEEE) and decaploid (2n = 10x = 70, EEEEEEStStStSt). It is commonly believed that the E-genome of the diploid is one of the basic genomes of Th. elongatum polyploids (Dvořák and Chen, 1984). Tetraploid Th. elongatum harbors genes that protect against many pathogens and adverse conditions including stripe rust, powdery mildew, FHB, smut, cold, drought, and high salinity (Dvořák et al., 1988; Jauhar and Peterson, 1996; Fedak, 1999). It also serves as an important wild gene pool to increase the genetic diversity of common wheat (Guo et al., 2015). To date, most introgressions from Th. elongatum into wheat have been produced using diploid and decaploid Th. elongatum (Sepsi et al., 2008; Ceoloni et al., 2017; Lou et al., 2017). Few attempts have been made to transfer the tetraploid Th. elongatum genes into wheat (Chen et al., 2013; Li et al., 2016; Dai et al., 2017a,b).
Fluorescence in situ hybridization (FISH) is a widely used cytogenetic tool in genomic studies of chromosome alterations (Alkhimova et al., 1999). The utility of FISH depends on the availability of suitable probes that provide chromosome-specific labeling patterns. FISH has become a new powerful tool for differentiating wheat chromosomes from those of its relatives (Sepsi et al., 2008; Zhuang et al., 2015). Afa family, pAs1, pSc119.2, pTa535, pTa713, pTa794, and pTa71 repetitive sequences are usually used as probes in FISH analysis to distinguish wheat A-, B-, and D-genome chromosomes and wheat wild species chromosomes (Rayburn and Gill, 1986; Fedak and Han, 2005; Molnár-Láng et al., 2010; Tang et al., 2014; Du et al., 2017; Kang et al., 2017). Linc et al. (2012) set up a detailed FISH karyotype of the E-genome of diploid Th. elongatum using probes pSc119.2, Afa family, and pTa71 probes, and revealed that significant intraspecific chromosome polymorphism in different accessions of diploid Th. elongatum with diverse geographical origin. Georgieva et al. (2011) detected significant signals on most chromosomes of Th. intermedium (2n = 6x = 42, EEJJStSt) with pSc119.2, Afa family, and pTa71 probes; only three pairs were not detected because they did not hybridize with any of the probes used. To date, knowledge of tetraploid Th. elongatum FISH patterns remains poor. A lack of standard karyotypes of tetraploid Th. elongatum has hampered its use for chromosome-mediated gene transfer into wheat.
The hexaploid Trititrigia 8801 (2n = 6x = 42, AABBEE), a genetically stable partial amphidiploid line, was produced by hybridization of T. durum (2n = 4x = 28, AABB) with tetraploid Th. elongatum (Guo et al., 2015). Trititrigia 8801 is commonly used as a bridge parent to transfer desirable genes from wild wheat species into cultivated wheat (Dai et al., 2017b). Currently, a standard FISH karyotype of tetraploid Th. elongatum has not been established. In addition, we cannot depend solely on the FISH karyotype of diploid Th. elongatum to identify the E chromosomes of tetraploid Th. elongatum due to polymorphism of the E-genome chromosomes from the two ploidy levels of Th. elongatum. Therefore, development of a FISH map for tetraploid Th. elongatum could help in alien chromosome identification in wheat- Trititrigia 8801 hybrid derivatives. Tetraploid Th. elongatum is an important genetic resource for wheat improvement and many wheat–tetraploid Th. elongatum alien chromosome introgression lines have been developed. The objectives of the present research were mainly to: (i) set up a FISH karyotype of the E-genome chromosomes of tetraploid Th. elongatum using different repetitive sequence probes; and (ii) accurately identify wheat–tetraploid Th. elongatum alien chromosome introgression lines and reveal genetic diversity among wheat cultivars by FISH-based markers.
Materials and Methods
Plant Materials
Materials employed in the current study are listed in Table 1. The hexaploid Trititrigia 8801 (2n = 6x = 42, AABBEE), which has the characteristics of cold, drought, and salt tolerance, and superior resistance to Fusarium head blight, rust, and powdery mildew, was kindly supplied by Dr. George Fedak (Eastern Cereal and Oilseed Research Center, Ottawa, Canada). Two accessions of tetraploid Th. elongatum (2n = 4x = 28, EEEE) with different origin were used for studying chromosome polymorphism: PI 531749 (Italy) and PI531750 (Greece), from the American National Plant Germplasm System (Pullman, WA, United States). The native wheat cultivars Shumai482 (SM482), Shumai921 (SM921), Shumai51 (SM51), Chuanmai104 (CM104), and Chuannong16 (CN16) are ideal recurrent parents for wheat breeding programs in southwestern China because they possess a comprehensive array of good agronomic characters, but most are susceptible to stripe rust pathogens prevalent in China. Eight derivative lines K16-712-1-2, K16-681-4, K16-562-3, K17-1089-1, K17-1084-3, K17-1065-5, K16-2521-1-2, and K15-1033-8-2 were obtained by hybridization of native wheat cultivars with Trititrigia 8801. For GISH analysis, the wheat cultivar ‘J-11’ (2n = 6x = 42, AABBDD) genome was used as blocking DNA, and the entire genomic DNA of tetraploid Th. elongatum PI531750 was used as a probe. Chinese Spring (CS, T. aestivum L., 2n = 6x = 42, AABBDD) was used as a control in molecular marker analysis.
GISH and FISH Analysis
Germinating seed root tips were collected and treated with nitrous oxide for 2 h and 90% acetic acid for 10 min, and the digested with pectinase and cellulose (Yakult Pharmaceutical Industry Co., Ltd., Tokyo, Japan), using the procedure of Komuro et al. (2013). Slides were prepared for GISH as previously described by Han et al. (2006). Total genomic DNA of tetraploid Th. elongatum PI531750 and J-11 was isolated using the cetyltrimethylammonium bromide (CTAB) method (Allen et al., 2006). The tetraploid Th. elongatum genomic DNA was labeled, as a probe, with fluorescein-12-dUTP using the nick translation mix (Thermo Fisher Scientific, Eugene, OR, United States). J-11 DNA was used for blocking, and as probe DNA and blocking agent DNA in the proportion of 1:150. GISH hybridization and detection were performed following the method of Han et al. (2009). A total volume of 10 μL of hybridization mixture containing 10 ng/μL labeled probe DNA, blocking DNA and buffer (2 × SSC and 1 × TE), was loaded per slide, denatured by heating at 85°C for 5 min, incubated for 8 h at 37°C, and washed in 2 × SSC. Chromosomes were counterstained with DAPI (4-6-diamino-2-phenylindole) solution (Vector Laboratories, Inc., Burlingame, CA, United States). Photomicrographs of GISH chromosomes were taken with an Olympus BX-51 (Olympus Corporation, Tokyo, Japan) microscope equipped with a DP-70 CCD camera and all images were processed with Photoshop CS 5.0 (Adobe Systems Incorporated, San Jose, CA, United States).
After the GISH analysis, the slides were washed with 2 × SSC and used for multicolor FISH. Synthetic oligonucleotide probes, including Oligo-pSc119.2, Oligo-pTa535, Oligo-pTa71, pAs1 (Tang et al., 2014), Oligo-pTa713 (Komuro et al., 2013), Oligo-pTa794 (Gerlach and Dyer, 1980), (ACT)5, (CTT)5, and Afa family (TAM 5′-GTTGAAACTTGGCATGGTATCATAATTTCACCCACATAGCATGT GCTAAAAAGTTGAGAGGGTTAC), were designed according to Nagaki et al. (1995). All the probes were synthesized by TSINGKE (Chengdu, China). The FISH procedure was also performed according to Han et al. (2006), with slight modifications. The probe mixture (0.35 μL of each probe in 2 × SSC and 1 × TE buffer, pH 7.0, total volume = 10 μL) was dropped on a slide, covered with a coverslip, stored in a moist box at 37°C for 2 h and washed in 2 × SSC at room temperature. The procedures for detection and visualization of FISH signals were the same as in the aforementioned GISH protocol.
Molecular Marker Analysis
Seven Th. elongatum 1-7E specific markers were used to characterize the chromatin in the eight wheat-Th. elongatum introgression lines (all primer sequences are shown in Table 2). Trititrigia 8801 and tetraploid Th. elongatum PI531750 were used as positive controls, while CS, SM482, SM921, SM51, CM104, and CN16 were used as negative controls. The total volume of the amplification reaction system was 25 μL, including 1.0 μL of template DNA (100 ng/μL), 12.5 μL of 2 × Taq Master Mix for PAGE (Dye plus), 1.0 μL of 10 μM of each of the two primers, and 9.5 μL ddH2O. The PCR reaction procedure involved pre-denaturing at 94°C for 5 min; 35 cycles of 94°C for 45 s, 55-60°C for 1 min, and 72°C for 30 s; a final extension of 10 min at 72°C; and holding at 4°C. The PCR amplification products were detected by 3% agarose gel electrophoresis and visualized with a gel imaging system (Bio-Rad Laboratories, lnc., United States).
Results
Characterizing Karyotype of Tetraploid Th. elongatum E-Genome Chromosomes
The GISH results showed that Trititrigia 8801 carried 14 E-genome chromosomes (Figure 1A). The GISH signals were then washed away and the same slide was used for FISH. After screening and combining FISH probes including (ACT)5, (CTT)5, pSc119.2, pAs1, pTa535, Afa family, pTa71, pTa713, and pTa794, the (ACT)5 and (CTT)5 microsatellites failed to show any signals on any E-genome chromosomes (Figures 1B,C). The seven pairs of E-genome chromosomes of tetraploid Th. elongatum could be accurately identified in Trititrigia 8801 using the repeat combination pSc119.2, pTa535, pTa71, and pTa713; additionally, the probe pTa535 could also be replaced by pAs1 or Afa family (Table 3 and Figure 2). Two accessions of tetraploid Th. elongatum failed to show significant intraspecific chromosome polymorphism after FISH using repetitive clones (Figure 3). The probe hybridization patterns of the E-genome chromosomes were similar between the wild accessions and Trititrigia 8801, except for 7E chromosomes using pTa713 probe (Figures 2, 3).
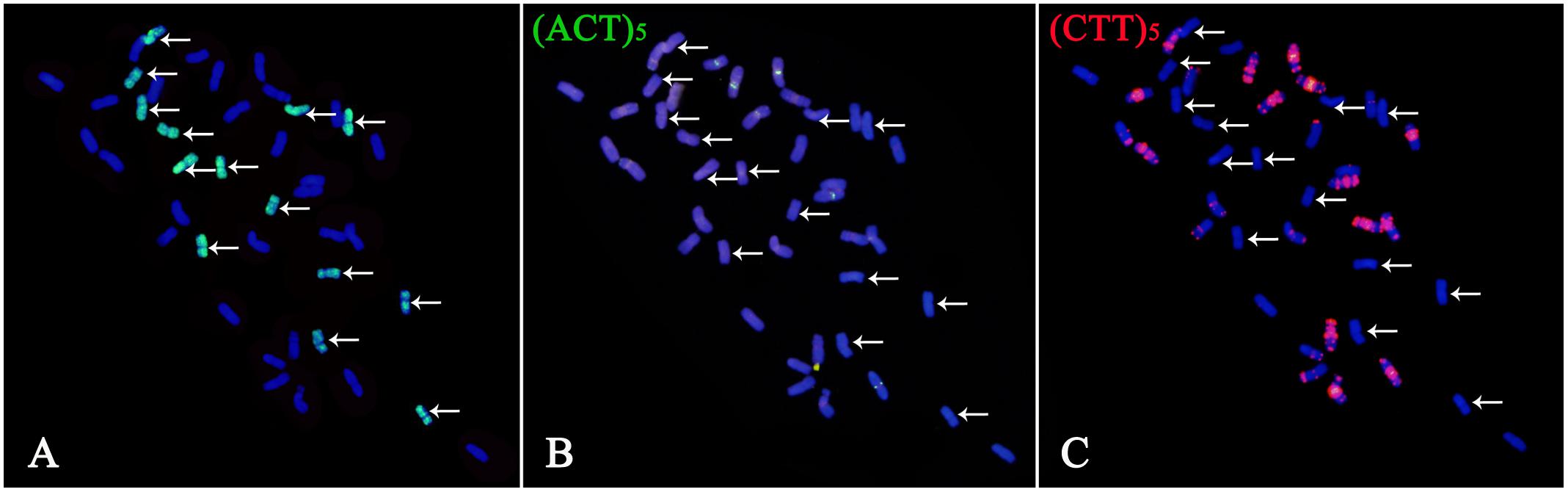
FIGURE 1. Karyotyping of mitotic metaphase chromosomes of Trititrigia 8801 by sequential GISH-FISH. Arrows indicate E-genome chromosomes. (A) Tetraploid Thinopyrum elongatum genomic DNA was used as a probe for GISH (green). (B,C) (ACT)5 and (CTT)5 probe signals were pseudo-colored as green and red in the FISH patterns, respectively. The probes (ACT)5 and (CTT)5 failed to show any signals on the E-genome chromosomes.
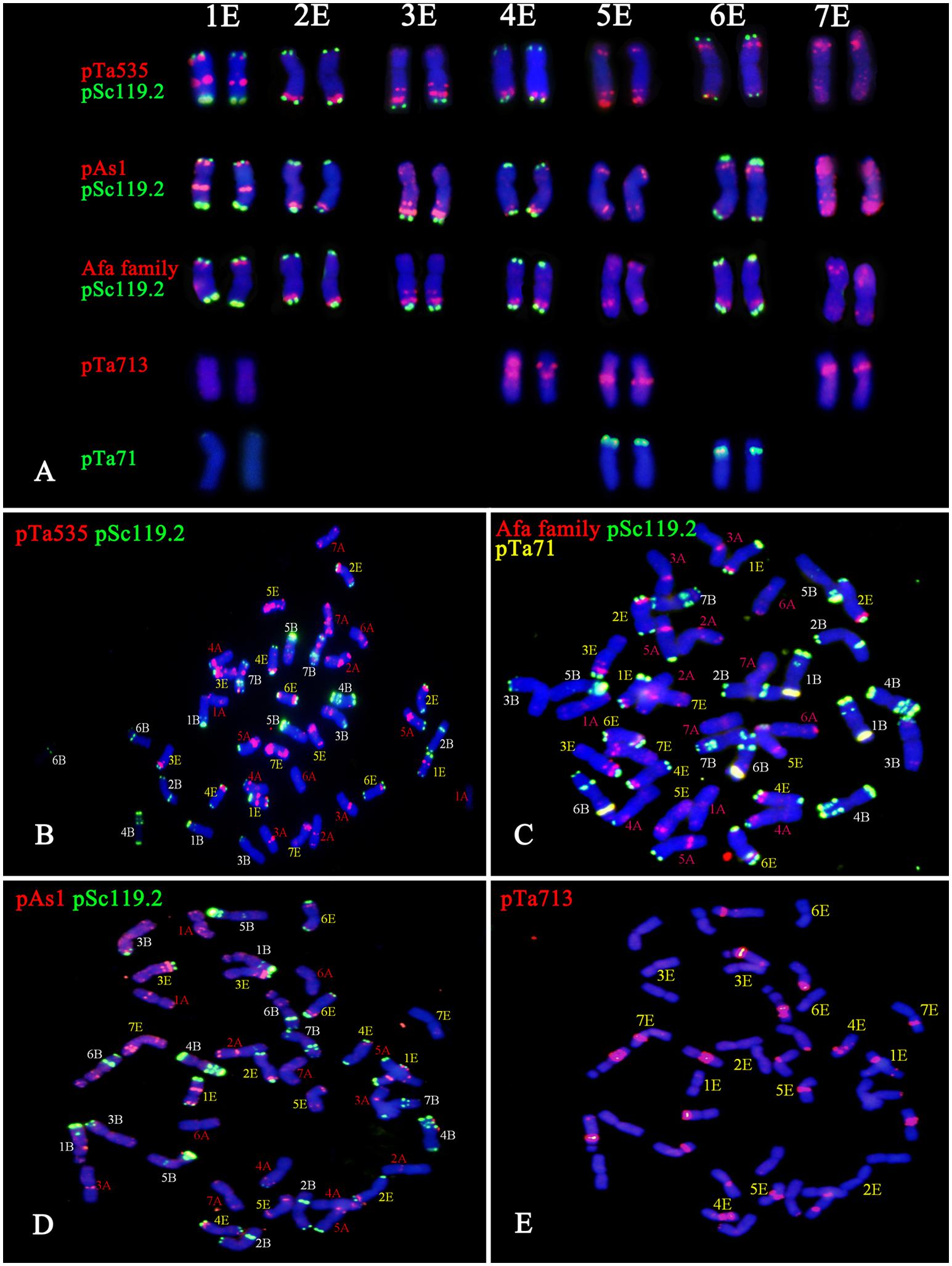
FIGURE 2. FISH patterns of oligo probes in Trititrigia 8801. (A) FISH patterns of the 1E-7E chromosomes using pSc119.2 (green), pTa535 (red), Afa family (red), pAs1 (red), pTa713 (red), and pTa71 (green). (B) FISH identification of 8801 using pTa535 (red) and pSc119.2 (green). (C) FISH identification of 8801 using Afa family (red), pSc119.2 (green), and pTa71 (yellow). (D) FISH identification of 8801 using pAs1 (red) and pSc119.2 (green). (E) FISH identification of 8801 using pTa713 (red).
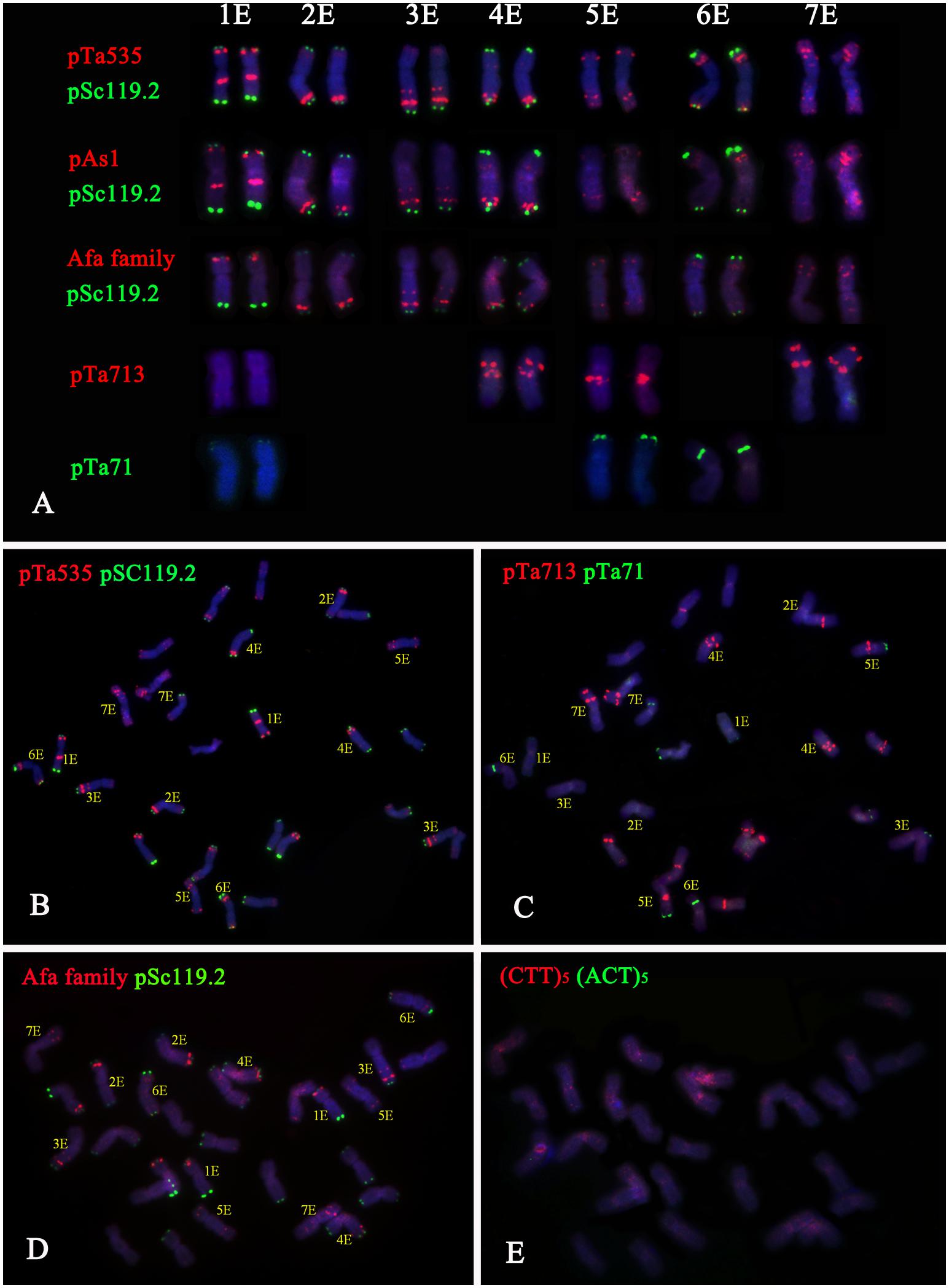
FIGURE 3. FISH patterns of the tetraploid Th. elongatum. (A) FISH patterns of the 1E-7E chromosomes using pSc119.2 (green), pTa535 (red), Afa family (red), pAs1 (red), pTa713 (red), and pTa71 (green). (B) FISH identification of PI531750 using pTa535 (red) and pSc119.2 (green). (C) FISH identification of PI531750 using pTa713 (red) and pTa71 (green). (D) FISH identification of PI531749 using Afa family (red) and pSc119.2 (green). (E) FISH identification of PI531749 using (ACT)5 and (CTT)5. The probes (ACT)5 and (CTT)5 failed to show any signals on the E-genome chromosomes.
Chromosome 1E carried strong terminal pSc119.2 signals on both arms, and strong pTa535 signals were found on the subterminal region of the short arm and near the centromere region of the long arm. The pAs1 signals on chromosome 1E were similar to those of pTa535, but differed from those of the Afa family probe, which had subterminal signals on the short arm. Faint pTa71 and pTa713 hybridization sites were detected on the short arm and centromere region of 1E chromosome, respectively. Chromosome 2E was characterized by strong terminal signals of pSc119.2 on both arms and subterminal pTa535 signals on the long arm. Clear terminal pSc119.2 signals and two subterminal pTa535 signals were detected on the long arm of chromosome 3E, and the short arm produced faint telomeric pTa535 signals. Chromosome 4E had similar pSc119.2 and pTa535 signals compared with chromosome 2E, but showed faint pTa535 signals subterminally on the short arm. However, strong pTa713 signals were detected near the centromere region of chromosome 4E, which distinguished it from chromosome 2E. We failed to detect any pSc119.2 signals on chromosome 5E, but pTa535 signals were found on the terminal region of the short arm and the terminal-subterminal region of the long arm. The probe pTa713 gave strong signals near the centromere on the long arm of chromosome 5E. Chromosome 6E was identified by strong terminal signals of pSc119.2 and subterminal signals of pTa535 on both arms. Notably, chromosomes 1E, 5E, and 6E carried different 45S rDNA signals. Chromosome 7E lacked any specific pSc119.2 signals, while strong pTa535 signals were located in terminal-subterminal positions and strong pTa713 signals were found near the centromere on the short arm. In addition, the pAs1 and Afa family signals were similar to those of pTa535 on the E chromosomes of groups 2, 3, 4, 5, 6, and 7 (Figure 2).
Alien Chromosome Identification of Hybrid Progenies Using Efficient Oligo Probes
GISH analysis was used to detect E-genome chromosomes in eight wheat-Trititrigia 8801 hybrid derivatives. Then, according to the FISH pattern developed for the E-genome chromosomes of tetraploid Th. elongatum, the chromosome constitutions of these lines were accurately identified (Table 4 and Figures 4, 5). The FISH analysis showed that the line K16-712-1-2 was a 1E (1D) chromosome substitution line, containing 14 A-genome (1A-7A), 14 B-genome (1B-7B), 12 D-genome (2D-7D), and one pair of 1E chromosomes (Figures 4A,B). The probe signals indicated that the line K16-681-4 was a disomic chromosome addition line with a pair of 2E chromosomes (2n = 44) (Figures 4C–E). Line K16-562-3 was a 3E, 4E (3D, 4D) chromosome substitution line that contained 14 A-genome, 14 B-genome, 10 D-genome, and two pairs of E-genome chromosomes (Figures 4F–H). Line K17-1089-1 (2n = 43) consisted of one 4E chromosome and complete sets of A-, B-, and D-genome chromosomes (Figures 4I–K). There were 40 wheat chromosomes and one 5E chromosome in K17-1084-3 (Figures 5A–C). Line K17-1065-5 (2n = 42) carried all A-genome and B-genome (plus one 4B) chromosomes, and 12 D-genome (lacking two 6D) plus one 6E chromosomes (Figures 5D–F). Line K16-2521-1-2 was a monosomic addition line that contained the complete sets of A-, B-, and D-genome chromosomes and one 7E chromosomes (Figures 5G–I). Additionally, line K15-1033-8-2 contained 41 chromosomes of common wheat, three E-genome (one 4E and two 5E) chromosomes, and one wheat-Th. elongatum Robertsonian translocation chromosome (4ES⋅1DL) (Figures 5J–L).
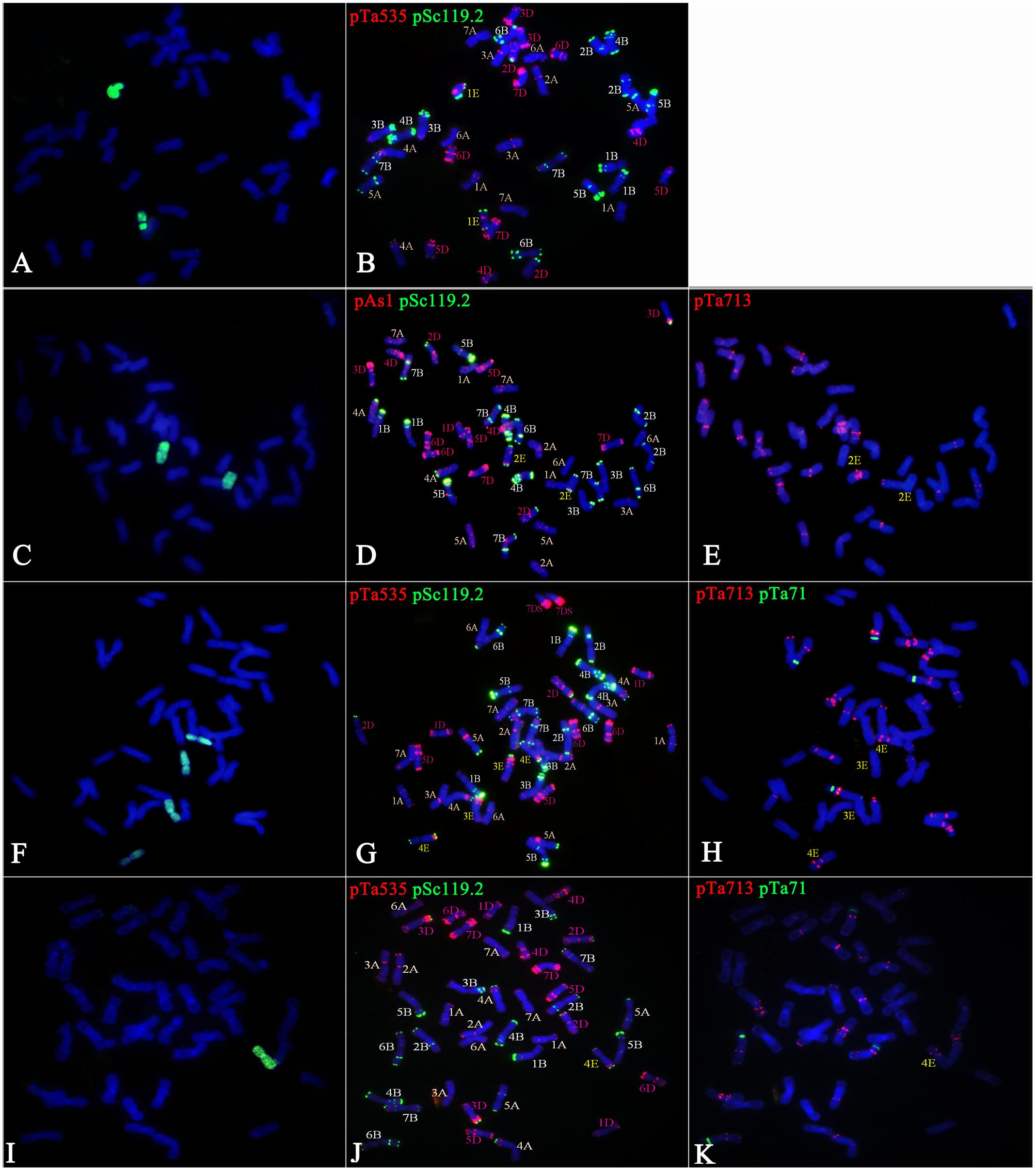
FIGURE 4. Chromosome identification of hybrid progenies. Images on the right of each panel show FISH results using pSc119.2 (green), pTa535 (red), pAs1 (red), pTa713 (red), and pTa71 (green), and those on the left show corresponding GISH patterns. Chromosomes in blue and green are wheat and Th. elongatum chromosomes in the GISH patterns, respectively. (A,B) Line K16-712-1-2 had a complete set of 28 A/B chromosomes, 12 D (2D-6D) chromosomes, and a pair of 1E chromosomes. (C–E) Line K16-681-4 had 13 A (lacking one 3A), 16 B (four 7B), 13 D (lacking one 1D), and one pair of 2E chromosomes. (F–H) Line K16-562-3 had all 28 A/B chromosomes, 10 D (1D, 2D, 5D, 6D, and 7D) chromosomes, and a pair each of 3E and 4E chromosomes. (I–K) Line K17-1089-1 had a complete set of 42 A/B/D chromosomes and one 4E chromosomes.
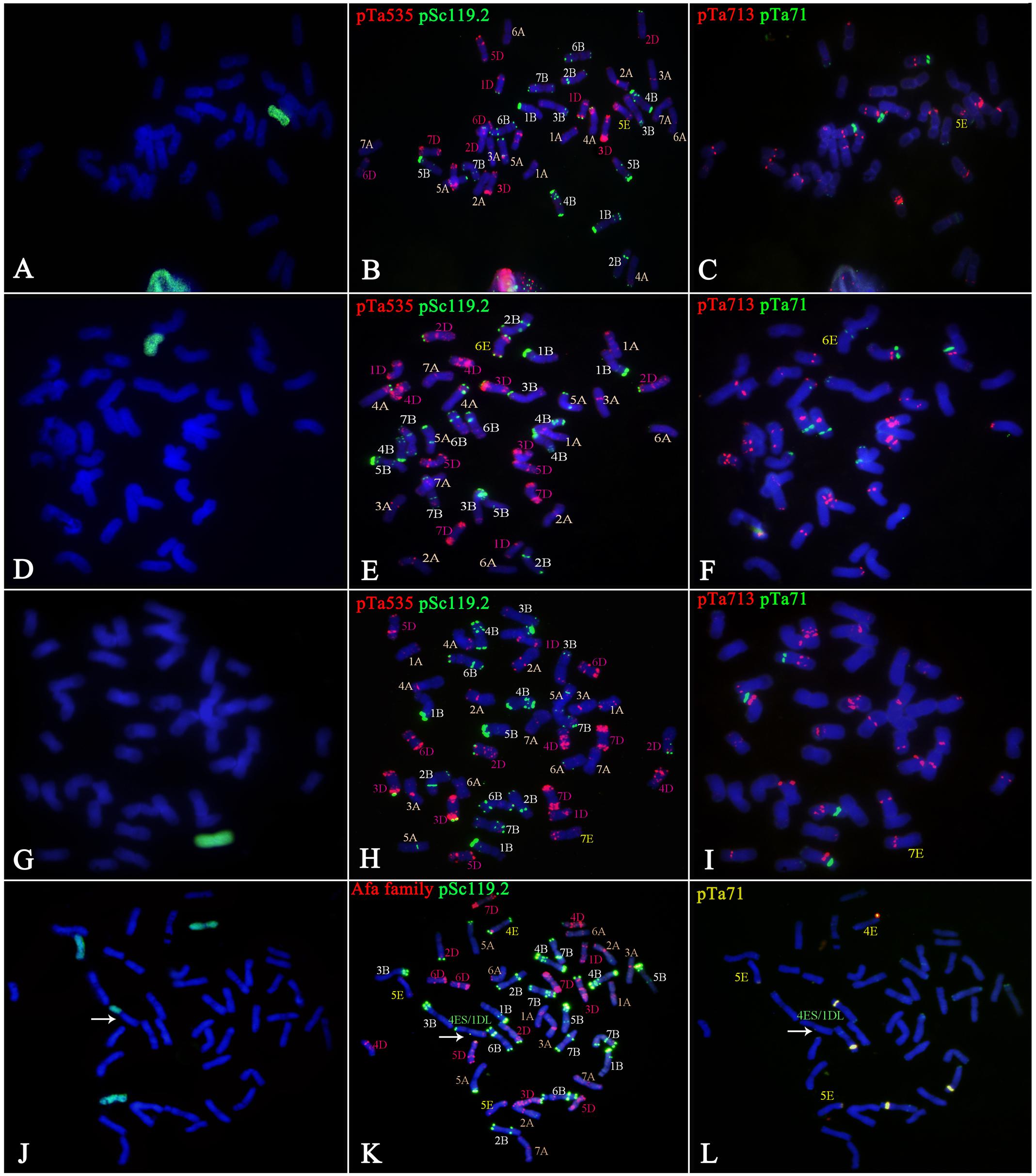
FIGURE 5. Chromosome identification of the hybrid progenies. Images on the right of each panel show FISH results using pSc119.2 (green), pTa535 (red), pAs1 (red), pTa713 (red), and pTa71 (green), and those on the left show corresponding GISH patterns. Chromosomes in blue and green are wheat and Th. elongatum chromosomes in the GISH patterns, respectively. (A–C) Line K17-1084-3 had a complete set of 28 A/B chromosomes, 12 D (lacking one 4D and 5D) chromosomes, and one 5E chromosomes. (D–F) Line K17-1065-5 had 14 A, 15 B (plus one 4B), 12 D (1D-5D, 7D), and one 6E chromosomes. (G–I) Line K16-2521-1-2 had a complete set of wheat chromosomes and one 7E chromosomes. (J–L) K15-1033-8-2 had 12A (1A-3A, 5A-7A), 16 B (four 7B), 13 D (lacking one 1D), 3 E (one 4E and two 5E), and one 4ES1DL translocation chromosomes (arrow).
Molecular Marker Analysis
The chromosome constitutions of the eight hybrid progenies were verified using E-genome specific molecular markers. The PCR results are shown in Figure 6. As expected, amplification products with the 1E, 2E, 4E, 5E, 6E, and 7E specific markers were observed in lines K16-712-1-2, K16-681-4, K17-1089-1, K17-1084-3, K17-1065-5, K16-2521-1-2, respectively, and the parents Trititrigia 8801 and tetraploid Th. elongatum PI531750. Similarly, line K16-562-3 showed bands of 3E and 4E chromosomes, and 4E and 5E chromosomes were detected in line K15-1033-8-2. Thus, the PCR results for the chromosome constitutions of the hybrid progenies were consistent with the FISH analysis.
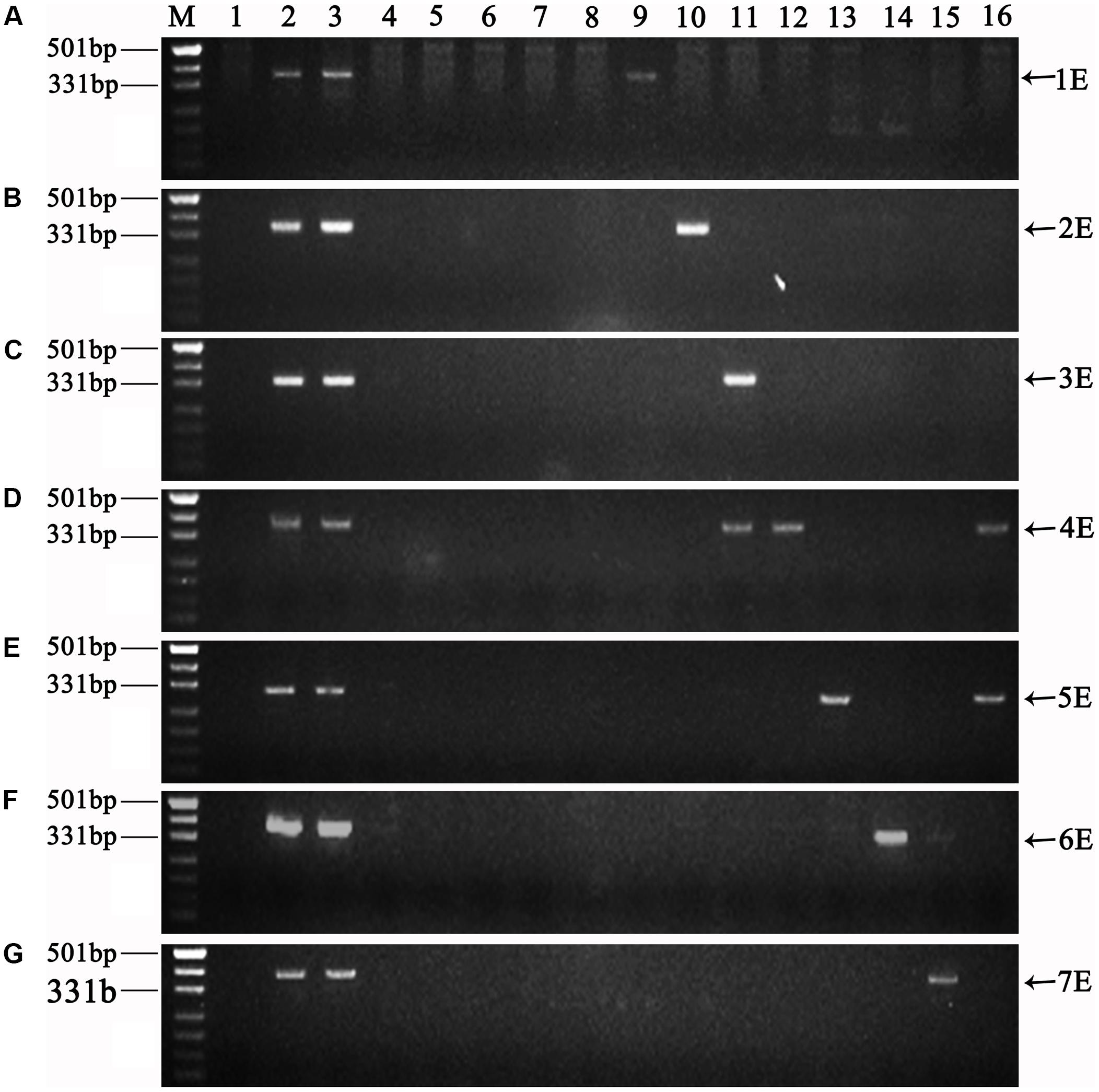
FIGURE 6. Amplification patterns of E-genome specific molecular markers. (A-G) Specific molecular markers of chromosomes 1E to 7E. Lanes: M Marker (DL500); 1 CS; 2 Tetraploid Th. elongatum PI531750; 3 8801; 4 SM482; 5 SM921; 6 SM51; 7 CM104; 8 CN16; 9 K16-712-1-2; 10 K16-681-4; 11 K16-562-3; 12 K17-1089-1; 13 K17-1084-3; 14 K17-1065-5; 15 K16-2521-1-2; 16 K15-1033-8-2. Arrows show the diagnostic amplification products of tetraploid Th. elongatum 1E-7E chromosomes.
Discussion
The ultimate objective of this study was to set up an E-genome specific FISH karyotype of tetraploid Th. elongatum using different probes, and determine the chromosome constitutions of wheat- tetraploid Th. elongatum progenies. A FISH pattern for tetraploid Th. elongatum was produced using the probes pSc119.2, pTa535, pTa713, and pTa71, enabling the exact FISH characterization of the E-genome chromosomes in wheat-tetraploid Th. elongatum hybrids.
Many studies have shown that repetitive DNA sequences are major components of plant genomes. For example, they can account up to 80% of the genome size in common wheat and barley (Flavell et al., 1974; Mascher et al., 2017; Wang et al., 2017). Using repetitive sequences as probes, FISH has proved to be a valuable tool for accurate identification of individual chromosomes within a species from the distribution of hybridization sites (Rayburn and Gill, 1986) or illustrating the phylogenetics of polyploidy species by analyzing the intraspecific and interspecific divergence (Taketa et al., 2000; Arzani et al., 2005; Zhao et al., 2018). The microsatellites (ACG)n, (ACT)n, (GAA)n, and complementary repeat (CTT)n have been used to identify the chromosomes of common wheat, rye, and Aegilops species (U-, M-, S-, and D-genomes) (Molnár et al., 2011; Schneider and Molnár-Láng, 2012; Badaeva et al., 2015; Zhao et al., 2016). However, the sequences (ACT)5 and (CTT)5 failed to show any signals on the E-genome chromosomes in the present study, which is consistent with previous studies (Sepsi et al., 2008; Linc et al., 2012). The probe pTa535 from wheat repetitive sequences enables the discrimination of A- and D-genome chromosomes (Komuro et al., 2013). In this study, pTa535 produced characteristic patterns on all chromosomes of tetraploid Th. elongatum, and facilitated the detection of the E-genome chromosomes in the wheat background. The pSc119.2 probes from rye repetitive sequences was used to identify the B-genome and 4A, 5A, 2D, 3D, and 4D chromosomes of wheat and R-genome chromosomes of rye (Cuadrado et al., 1997; Tang et al., 2014). pSc119.2 has also been successfully used to detect some Thinopyrum chromosomes, including those of Th. intermedium, Th. ponticum, Th. bessarabicum, and diploid Th. elongatum (Lapitan et al., 1987; Charette and Andre, 2003; Georgieva et al., 2011; Linc et al., 2012; Du et al., 2017). Previous studies on Triticeae have shown that, in most species, combinations of pSc119.2 and other FISH probes do not permit the complete identification of all chromosomes. In our results, five chromosomes (1E, 2E, 3E, 4E, and 6E) hybridized with pSc119.2, which made them distinguishable from the pattern of the well-known CS chromosomes, but there was no difference between chromosomes 2E and 4E. However, using the probe pTa713, clear signals were visible in the pericentromeric regions of the 1E, 4E, 5E, and 7E chromosomes. Therefore, we concluded that FISH with pSc119.2 and pTa713 enabled the identification of all tetraploid Th. elongatum chromosomes. pTa794 is a 420 bp-long sequence of wheat encoding the 5S rRNA gene (Gerlach and Dyer, 1980). The pTa71 sequence is a clone from common wheat with a size of 9 kb and encodes 18S, 5.8S, and 26S rRNA genes (Gerlach and Bedbrook, 1979). The 45S rDNA sites preferentially occur in the chromosomes of homoeologous groups 1, 5, and 6 (Badaeva et al., 1996). The activity of the 45S rDNA genes is usually linked with NORs and secondary constrictions in the Triticeae tribe. Previous results using molecular markers indicated that the chromosome 1ES of diploid Th. elongatum carried 5S rDNA sites, and the 5E and 6E chromosomes were characterized by two clear 45S rDNA sites (Dvořák et al., 1989; Fominaya et al., 1997). In Linc (2012), pTa71 failed to show any signals on the diploid Th. elongatum 6ES chromosome by FISH, but showed distinct subterminal 45S rDNA sites on chromosome 5ES. However, the present results showed that 5S rDNA sites were located on the 1ES chromosome of tetraploid Th. elongatum and 45S rDNA sites were simultaneously located on the short arms of the 1E, 5E, and 6E chromosomes. Polyploid speciation usually and rapidly cause genomic changes (Adams and Wendel, 2005). The 1E, 5E, and 6E chromosomes in this study showed different 45S rDNA sites compared with the diploid Th. elongatum, which can probably be attributed to evolutionary and environmental causes. Hence, using specific probes, the FISH karyotype of tetraploid Th. elongatum and the identities of eight wheat–tetraploid Th. elongatum alien chromosome introgression lines were characterized in a simple, fast, and accurate way. Our results demonstrate that FISH karyotyping can facilitate the use of tetraploid Th. elongatum in alien gene introgression into wheat.
Fluorescence in situ hybridization karyotype studies have been reported for various species in the Triticeae, such as T. aestivum, Secale cereale, Ae. variabilis, and Agropyron cristatum (Badaeva et al., 2004; Tang et al., 2014; Zhao et al., 2016; Linc et al., 2017). In the genus Thinopyrum, karyotypes for diploid Th. elongatum (Linc et al., 2012), Th. bessarabicum (Du et al., 2017), and Th. intermedium (Georgieva et al., 2011) have been completed and used to identify alien chromosomes in wheat introgression lines. In our study, we discriminated the chromosome constitutions of eight wheat-tetraploid Th. elongatum hybrid progenies by combining FISH karyotyping, GISH, and E-genome specific molecular markers. These progenies included a 1E (1D) substitution line, a 2E disomic addition line, a 3E, 4E (3D, 4D) disomic substitution line, and four lines with monosomic 4E, 5E, 6E, and 7E chromosomes, respectively. Furthermore, one line, K15-1033-8-2, contained a Robertsonian translocation chromosome, which was successfully distinguished. The results suggested that E-genome chromosomes of tetraploid Th. elongatum can be quickly and accurately identified by FISH karyotyping in the background of common wheat. Because some lines with monosomic alien chromosomes are cytogenetically unstable, the stable disomic substitution lines and translocation lines will be obtained by the selfing of the monosomic addition lines. These wheat-tetraploid Th. elongatum progeny lines will extend the genetic resources available for wheat breeding, and enrich the genetic diversity and improve the yield and quality of wheat. Further work is needed to evaluate the agronomic performance and disease resistance of these addition, substitution and translocation lines.
Author Contributions
HK conceived and designed the research. DL, TL, YlW, and XZ conducted the experiments. WZ, YW, JZ, LX, XF, and LS participated in the preparation of the reagents and materials. HZ, YZ, and HK analyzed the data. DL and HK wrote the manuscript. All authors read and approved the manuscript.
Funding
This work was funded by the National Key Research and Development Program of China (2016YFD0102000 and 2017YFD0100905), the National Natural Science Foundation of China (Nos. 31501311 and 31771781), and the Science and Technology Bureau of Sichuan Province (No. 2016HH0048).
Conflict of Interest Statement
The authors declare that the research was conducted in the absence of any commercial or financial relationships that could be construed as a potential conflict of interest.
Acknowledgments
We thank Dr. George Fedak, Eastern Cereal and Oilseed Research Center, Ottawa, Canada, for kindly supplying the Trititrigia 8801 material used in this study. We also thank the reviewers of the manuscript for their useful comments.
References
Adams, K. L., and Wendel, J. F. (2005). Polyploidy and genome evolution in plants. Curr. Opin. Plant Biol. 8, 135–141. doi: 10.1016/j.pbi.2005.01.001
Alkhimova, A. G., Heslop-Harrison, J. S., Shchapova, A. I., and Vershinin, A. V. (1999). Rye chromosome variability in wheat-rye addition and substitution lines. Chromosome Res. 7, 205–212. doi: 10.1023/A:1009299300018
Allen, G. C., Flores-Vergara, M. A., Krasynanski, S., Kumar, S., and Thompson, W. F. (2006). A modified protocol for rapid DNA isolation from plant tissues using cetyltrimethylammonium bromide. Nat. Protoc. 1, 2320–2325. doi: 10.1038/nprot.2006.384
Arzani, A., Peng, J. H., and Nlv, L. (2005). DNA and morphological markers for a Russian wheat aphid resistance gene. Euphytica 139, 167–172. doi: 10.1007/s10681-004-2898-4
Badaeva, E. D., Amosova, A. V., Goncharov, N. P., Macas, J., Ruban, A. S., Grechishnikova, I. V., et al. (2015). A set of cytogenetic markers allows the precise identification of all A-genome chromosomes in diploid and polyploid wheat. Cytogenet. Genome Res. 146, 71–79. doi: 10.1159/000433458
Badaeva, E. D., Amosova, A. V., Samatadze, T. E., Zoshchuk, S. A., Shostak, N. G., Chikida, N. N., et al. (2004). Genome differentiation in aegilops. 4. evolution of the U-genome cluster. Plant Syst. Evol. 246, 45–76. doi: 10.1007/s00606-003-0072-4
Badaeva, E. D., Friebe, B., and Gill, B. S. (1996). Genome differentiation in Aegilops. 2. physical mapping of 5s and 18s-26s ribosomal RNA gene families in diploid species. Genome 39, 1150–1158. doi: 10.1139/g96-145
Ceoloni, C., Forte, P., Kuzmanović, L., Tundo, S., Moscetti, I., De Vita, P., et al. (2017). Cytogenetic mapping of a major locus for resistance to Fusarium head blight and crown rot of wheat on Thinopyrum elongatum 7EL and its pyramiding with valuable genes from a Th. ponticum homoeologous arm onto bread wheat 7DL. Theor. Appl. Genet. 130, 2005–2024. doi: 10.1007/s00122-017-2939-8
Charette, A. B., and Andre, B. (2003). Chromosome characterization in Thinopyrum ponticum (Triticeae, Poaceae) using in situ hybridization with different DNA sequences. Genet. Mol. Biol. 26, 505–510. doi: 10.1590/S1415-47572003000400014
Chen, S., Gao, Y., Zhu, X., Zhang, C., Cao, W., Fedak, G., et al. (2015). Development of E-chromosome specific molecular markers for in a wheat background. Crop Sci. 55, 2777–2785. doi: 10.2135/cropsci2014.08.0539
Chen, S., Huang, Z., Dai, Y., Qin, S., Gao, Y., Zhang, L., et al. (2013). The development of 7E chromosome-specific molecular markers for Thinopyrum elongatum based on SLAF-seq technology. PLoS One 8:e65122. doi: 10.1371/journal.pone.0065122
Cuadrado, A., Vitellozzi, F., Jouve, N., and Ceoloni, C. (1997). Fluorescence in situ hybridization with multiple repeated DNA probes applied to the analysis of wheat-rye chromosome pairing. Theor. Appl. Genet. 94, 347–355. doi: 10.1007/s001220050422
Dai, Y., Duan, Y., Chi, D., Liu, H., Huang, S., Cao, W., et al. (2017a). Chromosome identification by new molecular markers and genomic in situ hybridization in the triticum-secale-thinopyrum trigeneric hybrids. Genome 60, 687–694. doi: 10.1139/gen-2017-0025
Dai, Y., Duan, Y., Liu, H., Chi, D., Cao, W., Xue, A., et al. (2017b). Molecular cytogenetic characterization of two Triticum–Secale–Thinopyrum trigeneric hybrids exhibiting superior resistance to Fusarium head blight, leaf rust, and stem rust race Ug99. Front. Plant Sci. 8:797. doi: 10.3389/fpls.2017.00797
Du, P., Zhuang, L., Wang, Y., Yuan, L., Wang, Q., Wang, D., et al. (2017). Development of oligonucleotides and multiplex probes for quick and accurate identification of wheat and Thinopyrum bessarabicum chromosomes. Genome 60, 93–103. doi: 10.1139/gen-2016-0095
Dubcovsky, J., and Dvorak, J. (2007). Genome plasticity a key factor in the success of polyploid wheat under domestication. Science 316, 1862–1866. doi: 10.1126/science.1143986
Dvořák, J., and Chen, K. C. (1984). Phylogenetic relationships between chromosomes of wheat and chromosome. Genome 26, 128–132.
Dvořák, J., Edge, M., and Ross, K. (1988). On the evolution of the adaptation of Lophopyrum elongatum to growth in saline environments. Proc. Natl. Acad. Sci. U.S.A. 85, 3805–3809. doi: 10.1073/pnas.85.11.3805
Dvořák, J., Zhang, H. B., Kota, R. S., and Lassner, M. (1989). Organization and evolution of the 5s ribosomal RNA gene family in wheat and related species. Génome 32, 1003–1016. doi: 10.1139/g89-545
Fedak, G. (1999). Molecular aids for integration of alien chromatin through wide crosses. Genome 42, 584–591. doi: 10.1139/g99-046
Fedak, G., and Han, F. (2005). Characterization of derivatives from wheat-Thinopyrum wide crosses. Cytogenet. Genome Res. 109, 360–367. doi: 10.1159/000082420
Flavell, R. B., Bennett, M. D., Smith, J. B., and Smith, D. B. (1974). Genome size and the proportion of repeated nucleotide sequence DNA in plants. Biochem. Genet. 12, 257–269. doi: 10.1007/BF00485947
Fominaya, A., Molnar, S., Fedak, G., Armstrong, K. C., Kim, N. S., and Chen, Q. (1997). Characterization of Thinopyrum distichum chromosomes using double fluorescence in situ hybridization, RFLP analysis of 5s and 26s rRNA, and c-banding of parents and addition lines. Genome 40, 689–696. doi: 10.1139/g97-791
Friebe, B., Jiang, J., Raupp, W. J., Mcintosh, R. A., and Gill, B. S. (1996). Characterization of wheat-alien translocations conferring resistance to diseases and pests: current status. Euphytica 91, 59–87. doi: 10.1159/000082420
Georgieva, M., Sepsi, A., Molnar-Lang, M., and Tyankova, N. (2011). Molecular cytogenetic analysis of Triticum aestivum and Thinopyrum intermedium using the FISH technique. Compt. Rend. Acad. Bulg. Sci. 64, 1713–1718.
Gerlach, W. L., and Bedbrook, J. R. (1979). Cloning and characterization of ribosomal RNA genes from wheat and barley. Nucleic Acids Res. 7, 1869–1885. doi: 10.1093/nar/7.7.1869
Gerlach, W. L., and Dyer, T. A. (1980). Sequence organization of the repeating units in the nucleus of wheat which contain 5s-RNA genes. Nucleic Acids Res. 8, 4851–4865. doi: 10.1093/nar/8.21.4851
Guo, X., Shi, Q., Jing, W., Hou, Y., Wang, Y., and Han, F. (2015). Characterization and genome changes of new amphiploids from wheat wide hybridization. J. Genet. Genomics 42, 459–461. doi: 10.1016/j.jgg.2015.06.006
Han, F., Gao, Z., and Birchler, J. A. (2009). Reactivation of an inactive centromere reveals epigenetic and structural components for centromere specification in maize. Plant Cell 21, 1929–1939. doi: 10.1105/tpc.109.066662
Han, F., Lamb, J., and Birchler, J. (2006). High frequency of centromere inactivation resulting in stable dicentric chromosomes of maize. Proc. Natl. Acad. Sci. U.S.A 103, 3238–3243. doi: 10.1073/pnas.0509650103
Jauhar, P. P., and Peterson, T. S. (1996). Thinopyrum and Lophopyrum as sources of genes for wheat improvement. Cereal Res. Commun. 24, 15–21.
Kang, H., Wang, Y., Diao, C., Li, D., Wang, Y., Zeng, J., et al. (2017). A hexaploid triticale 4D (4B) substitution line confers superior stripe rust resistance. Mol. Breed. 37:36. doi: 10.1007/s11032-017-0642-8
Komuro, S., Endo, R., Shikata, K., and Kato, A. (2013). Genomic and chromosomal distribution patterns of various repeated DNA sequences in wheat revealed by a fluorescence in situ hybridization procedure. Genome 56, 131–137. doi: 10.1139/gen-2013-0003
Lapitan, N. L. V., Gill, B. S., and Sears, R. G. (1987). Genomic and phylogenetic relationships among rye and perennial species in the triticeae1. Crop Sci. 27, 682–687. doi: 10.1139/g10-121
Li, H., Liu, H., Dai, Y., Huang, S., Zhang, J., Gao, Y., et al. (2016). Transmitting characters of individual E chromosomes of Thinopyrum elongatum in Triticum turgidum background. Hereditas 38, 1020–1029.
Linc, G., Gaál, E., Molnár, I., Icsó, D., Badaeva, E., and Molnár-Láng, M. (2017). Molecular cytogenetic (FISH) and genome analysis of diploid wheatgrasses and their phylogenetic relationship. PLoS One 12:e0173623. doi: 10.1371/journal.pone.0173623
Linc, G., Sepsi, A., and Molnárláng, M. (2012). A FISH karyotype to study chromosome polymorphisms for the Elytrigia elongata E genome. Cytogenet. Genome Res. 136, 138–144. doi: 10.1159/000334835
Lou, H., Dong, L., Zhang, K., Wang, D. W., Zhao, M., Li, Y., et al. (2017). High throughput mining of E-genome specific SNPs for characterizing Thinopyrum elongatum introgressions in common wheat. Mol. Ecol. Res. 17, 1318–1329. doi: 10.1111/1755-0998.12659
Mascher, M., Gundlach, H., Himmelbach, A., Beier, S., Twardziok, S. O., Wicker, T., et al. (2017). A chromosome conformation capture ordered sequence of the barley genome. Nature 544, 427–433. doi: 10.1038/nature22043
Molnár, I., Cifuentes, M., Schneider, A., Benavente, E., and Molnárláng, M. (2011). Association between simple sequence repeat-rich chromosome regions and intergenomic translocation breakpoints in natural populations of allopolyploid wild wheats. Ann. Bot. 107, 65–76. doi: 10.1093/aob/mcq215
Molnár-Láng, M., Cseh, A., Szakács, E., and Molnár, I. (2010). Development of a wheat genotype combining the recessive crossability alleles kr1kr1kr2kr2 and the 1BL.1RS translocation, for the rapid enrichment of 1RS with new allelic variation. Theor. Appl. Genet. 120, 1535–1545. doi: 10.1007/s00122-010-1274-0
Mujeeb-Kazi, A., Kazi, A. G., Dundas, I., Rasheed, A., Ogbonnaya, F., Kishii, M., et al. (2013). Chapter four – genetic diversity for wheat improvement as a conduit to food security. Adv. Agron. 122, 179–257. doi: 10.1016/B978-0-12-417187-9.00004-8
Nagaki, K., Tsujimoto, H., Isono, K., and Sasakuma, T. (1995). Molecular characterization of a tandem repeat, Afa family, and its distribution among Triticeae. Genome 38, 479–486. doi: 10.1139/g95-063
Rayburn, A. L., and Gill, B. S. (1986). Isolation of a D-genome specific repeated DNA sequence from Aegilops squarrosa. Plant Mol. Biol. Rep. 4, 102–109. doi: 10.1007/BF02732107
Schneider, A., and Molnár-Láng, M. (2012). Detection of various U and M chromosomes in wheat–Aegilops biuncialis hybrids and derivatives using fluorescence in situ hybridisation and molecular markers. Czech J. Genet. Plant Breed. 48, 169–177. doi: 10.17221/45/2012-CJGPB
Sepsi, A., Molnár, I., Szalay, D., and Molnárláng, M. (2008). Characterization of a leaf rust-resistant wheat–Thinopyrum ponticum partial amphiploid BE-1, using sequential multicolor GISH and FISH. Theor. Appl. Genet. 116, 825–834. doi: 10.1007/s00122-008-0716-4
Taketa, S., Ando, H., Takeda, K., Harrison, G. E., and Heslopharrison, J. S. (2000). The distribution, organization and evolution of two abundant and widespread repetitive DNA sequences in the genus Hordeum. Theor. Appl. Genet. 100, 169–176. doi: 10.1007/s001220050023
Tang, Z., Yang, Z., and Fu, S. (2014). Oligonucleotides replacing the roles of repetitive sequences pAs1, pSc119.2, pTa-535, pTa71, CCS1, and pAWRC.1 for FISH analysis. J. Appl. Genet. 55, 313–318. doi: 10.1007/s13353-014-0215-z
Wang, L., Shi, Q., Su, H., Wang, Y., Sha, L., Fan, X., et al. (2017). St2-80: a new FISH marker for St genome and genome analysis in Triticeae. Genome 60, 553–563. doi: 10.1139/gen-2016-0228
Zhao, L., Ning, S., Yi, Y., Zhang, L., Yuan, Z., Wang, J., et al. (2018). Fluorescence in situ hybridization karyotyping reveals the presence of two distinct genomes in the taxon Aegilops tauschii. BMC Genomics 19:3. doi: 10.1186/s12864-017-4384-0
Zhao, L., Ning, S., Yu, J., Hao, M., Zhang, L., Yuan, Z., et al. (2016). Cytological identification of an Aegilops variabilis chromosome carrying stripe rust resistance in wheat. Breed. Sci. 66, 522–529. doi: 10.1270/jsbbs.16011
Keywords: Fluorescence in situ hybridization karyotype, tetraploid Thinopyrum elongatum, repetitive sequences, molecular marker, hybrid derivatives
Citation: Li D, Li T, Wu Y, Zhang X, Zhu W, Wang Y, Zeng J, Xu L, Fan X, Sha L, Zhang H, Zhou Y and Kang H (2018) FISH-Based Markers Enable Identification of Chromosomes Derived From Tetraploid Thinopyrum elongatum in Hybrid Lines. Front. Plant Sci. 9:526. doi: 10.3389/fpls.2018.00526
Received: 29 January 2018; Accepted: 04 April 2018;
Published: 01 May 2018.
Edited by:
Petr Smýkal, Palacký University, Olomouc, CzechiaReviewed by:
István Molnár, Centre for Agricultural Research (MTA), HungaryXiyin Wang, North China University of Science and Technology, China
Copyright © 2018 Li, Li, Wu, Zhang, Zhu, Wang, Zeng, Xu, Fan, Sha, Zhang, Zhou and Kang. This is an open-access article distributed under the terms of the Creative Commons Attribution License (CC BY). The use, distribution or reproduction in other forums is permitted, provided the original author(s) and the copyright owner are credited and that the original publication in this journal is cited, in accordance with accepted academic practice. No use, distribution or reproduction is permitted which does not comply with these terms.
*Correspondence: Houyang Kang, aG91eWFuZy5rYW5nQHNpY2F1LmVkdS5jbg==
†These authors have contributed equally to this work.