- 1School of Resources and Environmental Science, Northeast Agricultural University, Harbin, China
- 2Institute of Natural Resources and Ecology, Heilongjiang Academy of Sciences, Harbin, China
- 3College of Life Sciences, Heilongjiang University, Harbin, China
- 4College of Life Science, Northeast Forestry University, Harbin, China
In this study, the effects of inoculating arbuscular mycorrhizal fungi (Glomus mosseae) on the growth, chlorophyll content, photosynthetic gas exchange parameters, and chlorophyll fluorescence characteristics of Lolium perenne L. in cadmium (Cd) contaminated soil were investigated. The results showed that the root vigor of L. perenne declined, while the chlorophyll content significantly decreased with the increase of Cd content, especially the chlorophyll a content in leaves. The photosynthetic carbon assimilation capacity and PSII activity of L. perenne leaves were also significantly inhibited by Cd stress, especially the electron transfer at the receptor side of PSII, which was more sensitive to Cd stress. The infection level of G. mosseae on L. perenne roots was relatively high and inoculation with G. mosseae increased the mycorrhizal infection rate of L. perenne roots up to 50–70%. Due to the impact of the mycorrhizal infection, the Cd content in L. perenne roots was significantly increased compared to non-inoculated treatment; however, the Cd content in the aboveground part of L. perenne was not significantly different compared to the non-inoculated treatment. After inoculation with G. mosseae, the root vigor of L. perenne increased to some extent, alleviating the chlorophyll degradation in L. perenne leaves under Cd contaminated soil. Infection with G. mosseae can improve the stoma limitation of L. perenne leaves in Cd contaminated soil and increase the non-stomatal factors including the tolerance of its photosynthetic apparatus to Cd, to improve photosynthetic capacity. G. mosseae infection can improve the photosynthetic electron transport capacity of PSII in L. perenne leaves under Cd stress and promotes the activity of the oxygen-evolving complex to different degrees at the donor side of PSII and the electron transport capacity from QA to QB on the receptor side of PSII. Thus, this guarantees that L. perenne leaves inoculated with G. mosseae in Cd contaminated soil have relatively higher PSII activity. Therefore, inoculation with G. mosseae can improve the capacity of Cd tolerance of L. perenne with regard to various aspects, such as morphological characteristics and photosynthetic functions, and reduce the toxicity of Cd on L. perenne.
Introduction
Over the past 50 years, global cadmium (Cd) emission in the environment reached 2.2 × 104 t (Singh et al., 2003). Cd is an unnecessary and toxic element for living organisms, and when humans intake excess Cd, functional impairment of the kidneys and osteoporosis will be induced (Alfvén et al., 2000). In addition, Cd has carcinogenic, teratogenic, and mutagenic effects on human beings (Qian et al., 2009). Cd influences the physiological and biochemical processes of plants, such as inhibiting seed germination and plant growth (Peralta et al., 2001; Rizwan et al., 2017), affecting the uptake of mineral elements (Murtaza et al., 2017; Qaswar et al., 2017), resulting in leaf necrosis (Ciecko et al., 2001; López-Millán et al., 2009), restraining nutrient absorption (Hall, 2002; Dong et al., 2006), changing the structure and function of mitochondria, blocking the synthesis of carbohydrates and proteins (Kieffer et al., 2008), causing a disorder of the hormonal metabolism (Masood et al., 2012), increasing reactive oxygen species (Zhang et al., 2007), and causing leakage of cellular electrolytes (Rodrıguez-Serrano et al., 2006; Gill et al., 2015). Cd stress influences plant photosynthetic capacity (Kola and Wilkinson, 2005). Studies have indicated that Cd stress has resulted in the destruction of the chlorophyll structure in Populus deltoides × P. nigra leaves, and the number of chloroplasts declined, the chloroplast membrane swelled, the membrane structure blurred and even disappeared, and the thylakoids became disordered (Zhang et al., 2014). Cd also leads to the blockage of plant solar energy utilization (Krantev et al., 2008) and affects the carbon assimilation process (Xue and Gao, 2017). However, previous studies have found that low concentrations of Cd stress result in the increase of the large and small subunits of Rubisco, as well as increase Rubisco activity in Typha angustifolia leaves (Bah et al., 2010) and the activities of relevant photosynthetic carbon assimilation enzymes of Arabidopsis thaliana (Semane et al., 2010) and Glycine max L. (Hossain et al., 2012). However, relatively high concentrations of Cd could combine with the functional groups of some enzymes to inhibit their activities (Tukaj et al., 2007). For example, the content and activity of Rubisco in Populus tremula L. leaves obviously declined under Cd stress (Marmiroli et al., 2013).
Arbuscular mycorrhizal fungi (AMF), which obtain nutrients through infecting host plant root systems and are the most widely distributed symbiont, can infect the root systems of over 80% of vascular plants (Li, 1998). The hypha of AMF can serve as an important channel for soil nutrients entering plants, hence it can also promote the absorption of nutrients by host plants (Karagiannidis et al., 2002), promote plant growth, yield, and quality (Bowles et al., 2016; Rozpądek et al., 2016), improve plant photosynthetic capacity and PSII function (Chen et al., 2017a; Mathur et al., 2018), as well as accelerate plant growth and enhance plant stress resistance through impacting root exudation (Chen et al., 2017b). Some studies also found that AMF could improve plant tolerance to heavy metal pollution (Del et al., 1999a,b). Under heavy metal stress, through chelation and hyphae immobilization (Gonzalez-Chavez et al., 2002; Rufyikiri et al., 2004) or the complexes formed by cysteine in the mycorrhiza combined with heavy metals, AMF can retain heavy metals within roots (Dehn and Schüepp, 1990), inhibiting heavy metal transport from roots to the aboveground parts of the plant, effectively reducing heavy metal toxicity and promoting plant growth (Tullio et al., 2003; Christie et al., 2004). Pallara et al. (2013) selected Populus alba as the experiment material and found that inoculation with Glomus mosseae was beneficial for inducing the expression of genes encoding chelate synthetase in leaves and controlling cellular antioxidant levels, thus improving host plant tolerance to heavy metals. In addition, AMF hyphae had a relatively higher cation exchange capacity and heavy metal adsorption capacity, which helped plants infected by AMF to achieve improved heavy metal tolerance (Joner et al., 2000).
Lolium perenne L. is an annual graminaceous monocot plant that is easily grown and has a relatively large biomass, strong regeneration capacity, and pest resistance; it is an important cultivated forage plant and green manure plant with a high economic and ecological value (Hebeisen et al., 2010; Chang et al., 2017). Moreover, L. perenne has been shown to have very strong resistance to heavy metals and can concentrate heavy metals (Lial and Huang, 2002). Therefore, L. perenne is commonly used as pasture and grass for golf courses and is also used for the phytoremediation of areas with heavy metal contamination. Many relevant studies have shown that L. perenne could promote plant resistance to heavy metals. For example, the inoculation of AMF can improve the plant capacity for nutrient adsorption including in Astragalus sinicus L. and Zea mays L (Chen and Zhao, 2009; Zhang et al., 2010). It can also increase the retention of heavy metals in plant roots and decrease the distribution of heavy metals in the aboveground part of host plants (Lins et al., 2006; Słomka et al., 2011), or decrease the heavy metal content in host plants through improving host plant growth, while increasing biomass (Andrade et al., 2009). Previous studies have found that inoculation with AMF could improve plant absorption of heavy metals (Aloui et al., 2012). However, few studies have been conducted on the effects of AMF on the photosynthetic characteristics of L. perenne in Cd contaminated soil. Thus, this research intended to study the effects of AMF (G. mosseae) inoculation on the growth, chlorophyll content, photosynthetic gas exchange parameters, and chlorophyll fluorescence parameters of L. perenne in soil contaminated by different Cd concentrations from the perspective of plant photosynthetic mechanisms, with the purpose of providing fundamental data for revealing the mechanism of AMF improving L. perenne resistance to Cd stress.
Materials and Methods
Experimental Materials
The experiments were conducted in the Laboratory of Soil Science, Northeast Agricultural University (Harbin, Heilongjiang Province, China) between March and June, 2016. The culture medium utilized well-mixed turfy soil and cleaned river sand at a ratio of 1:1 (v/v), which was sterilized in a high-pressure sterilizer at 121°C for 2 h to kill native mycorrhiza and other microbes in the soil. The mycorrhizal fungi used in this study were G. mosseae, and the inoculum was purchased from the Chinese Arbuscular Mycorrhizal Fungi Germplasm Resources Information System, labeled as “BGCAH01.” After propagation for four months using Trifolium repens L. as host plant, the soil containing fungal spores, hyphae, and the infected root section of the host plant was used as inoculum. The plant species used was L. perenne, which was provided by the Heilongjiang Academy of Agricultural Sciences. Before use, the seeds were immersed in an H2O2 solution with volume percent fraction of 10% for 10 min to kill infectious microbes on the surface of the seeds. The seeds were then washed with sterilized water and processed to accelerate germination in a sterile culture dish in a manual climatic box with conditions as follows: temperature of 25/23°C (light/dark), light intensity of 400 μmol⋅m-2⋅s-1, photoperiod of 12/12 h (light/dark), and relative humidity of approximately 75%.
Different Cd levels were tested including 0, 30, 90, and 180 mg⋅kg-1. Under each Cd concentration, two treatments were conducted: one treatment inoculated with G. mosseae (+AMF) and the other without AMF inoculation (CK). In the +AMF treatment, 50 g of soil containing G. mosseae were added into every 1 kg of culture matrix; while in the CK treatment, the same amount of sterile soil was added into the matrix. After the sterilized soils with/without fungi were mixed well with the respective treated matrixes, they were used to fill in the cultivation pots (completely intermixed). The seeds processed with accelerated germination were planted evenly in culture pots with a diameter of 12 cm and a height of 15 cm. About 50 seeds were sown in each pot, and 0.5 cm of sterile soil covered the soil surface. Each treatment consisted of 5 pot replicates, with a total of 40 pots. The culture pots were placed in a manual climatic box set to 25°C, with a light intensity of 400 μmol⋅m-2⋅s-1, photoperiod of 12/12h (light/dark), and relative humidity of 75%. The plants were irrigated and seedling management was conducted regularly. The relative water content was maintained at approximately 80% in the soil in the pots containing different treatments. When the seedling height reached about 20–30 cm, and after significant differences in plant phenotype appeared between various treatments, relevant data was measured.
Parameter Measurements and Methods
Measurement of Growth Parameters
After the plant height was measured, the plants were removed from the culture matrix. The root length of each plant was measured after the culture matrix on the root surface was removed. Any remaining water on the root surface was wiped with absorbent paper, the aboveground and underground parts were placed into an aluminum box respectively, at 105°C for 30 min, and then dried at 60°C for 30 h until a constant weight was reached; then, the biomass was weighed. The aboveground biomass, underground biomass, and root to shoot ratio of each plant were calculated, wherein the root to shoot ratio = underground biomass/aboveground biomass.
Measurement of Mycorrhizal Infection Rate, Chlorophyll Content, and Root Vigor
The Trypan blue staining method was used to measure the mycorrhizal infection and after dyeing, the ratio of infected roots (i.e., mycorrhizal infection rate) was measured under a microscope using the modified cross-bonded method. Mycorrhizal infection rate (%) = the root with arbuscules/measured root amount × 100. The acetone-ethanol solvent extraction was applied for the determination of chlorophyll content. The fully expanded top leaves of L. perenne in each treatment were selected. The collected L. perenne leaves were soaked in a 1:1 mixture of acetone and ethanol in darkness and the samples were shaken periodically until the green completely faded from the leaves. The absorbance values at 663, 646, and 470 nm were determined, and the calculation of chlorophyll content was conducted according to the method described by Porra (2002). Root vigor was measured following the TTC method (Shao et al., 2009). The dry samples in the aboveground and underground parts of L. perenne were digested using a Microwave Digestion System (MARS6, American CEM) with nitric acid digestive solution until the samples were a clear liquor. Then, samples were diluted to a constant volume, and an atomic absorption spectrophotometer (AA7700, SHIMADZU, Japan) was applied to determine the Cd content.
Measurement of Photosynthetic Gas Exchange Parameters
The measurement of photosynthetic parameters was carried out between 9:00 a.m. and 11:00 a.m. The CIRAS-2 portable photosynthesis system (PPsystem company, United Kingdom) was used to measure photosynthetic parameters such as net photosynthetic rate (Pn), stomatal conductivity (Gs), transpiration rate (Tr), and intercellular CO2 concentration (Ci) of fully expanded leaves located at the top of each treated plant. The leaves were tiled to fill the whole leaf chamber during determination. During the measurements, the CIRAS-2 self-made light source and built-in CO2 cylinder were used, the light intensity was set to 800 μmol⋅m-2⋅s-1, and the CO2 concentration within the fixation system was 400 μL⋅L-1.
Measurement of Chlorophyll Fluorescence Parameters
Fully expanded leaves at the top of each treated plant were selected and the leaves were processed with 30-min dark adaptation using the dark adaptation clips. Then, the Handy-PEA multi-function plant efficiency analyzer (Hansatech Company, United States) was used to measure the OJIP curves in each leaf after dark adaptation, and this measurement was replicated five times. The OJIP curves were induced by 3,000 μmol⋅m-2⋅s-1 pulsing red light. Then, the relative fluorescence intensity between 10 μs and 1 s was measured and points O, J, I, and P on the OJIP curve corresponded to the time of 0, 2, 30, and 1,000 ms. The relative fluorescence intensity at point O was defined as 0, and that at point P was defined as 1. The OJIP curve was standardized based on the formula VO-P = (Ft - Fo)/(Fm - Fo); then, the relative variable fluorescence at point K (0.3 ms), point J (2 ms), VK and VJ could be obtained. The difference between the standardized OJIP curve of L. perenne leaves under different Cd concentrations and in the treatment with no Cd was taken and presented as ΔVO-P. Then, the JIP-test was conducted on the OJIP curves and the chlorophyll fluorescence parameter was obtained, including maximal photochemical efficiency of PSII (Fv/Fm), photosynthetic performance index based on absorption of light energy (PIABS), light energy absorbed by per unit of reaction center (ABS/RC), the energy for electron transport derived from the light energy absorbed per unit reaction center (ETo/RC), energy dissipated by per unit reaction center (DIo/RC), light energy absorbed per unit area (ABS/CSm), the energy for electron transport derived from the light energy absorbed by per unit area (ETo/CSm), and energy dissipated per unit area (DIo/CSm). The calculation methods for the abovementioned parameters were based on research conducted by Strasser et al. (1995).
Data Processing Methods
Excel and SPSS software were used to conduct statistical analyses on the measured data. The data in the figure shows mean ± SD. A one-way analysis of variance (ANOVA) and least significant difference (LSD) test were adopted to compare the differences between treatments.
Results and Analysis
The Effects of Inoculating L. perenne With G. mosseae on Plant Growth Characteristics in Cadmium Contaminated Soil
Table 1 shows that the effect of AMF inoculation on root/shoot ratio of L. perenne was insignificant; however, the effect on belowground biomass (P < 0.01) and other growth parameters was significant (P < 0.001). Soil Cd significantly affected the growth parameters of L. perenne (P < 0.001) but showed no significant interaction with AMF inoculation.
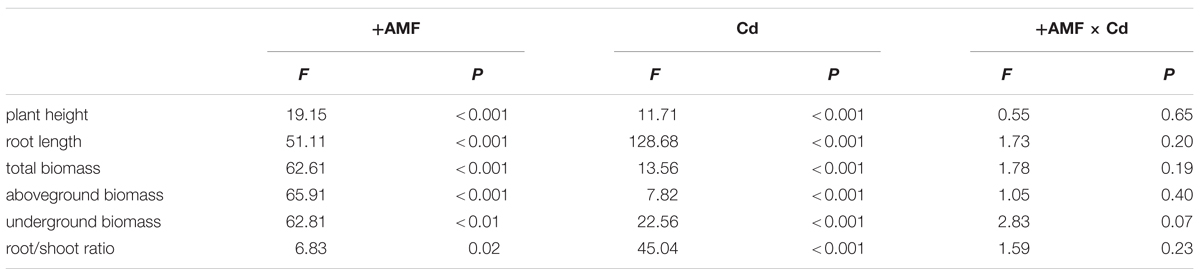
TABLE 1. Two-way ANOVAs examining the effects of AMF inoculation (+AMF), soil Cd (Cd), and their interaction (+AMF × Cd) on plant height, root length, total biomass, aboveground biomass, underground biomass, and root/shoot ratio.
With the increase of Cd content in the soil, the plant height, root length, and biomass of L. perenne presented significantly decreasing trends (Figures 1A,B). However, when Cd content was 30 mg⋅kg-1, compared to the treatment without Cd stress, L. perenne only had a decreased root length, but the plant height and biomass of the different plant parts did not change, and the root to shoot ratio even showed an increasing trend. Under different soil Cd content, the plant height, root length, and biomass of L. perenne when inoculated with G. mosseae (treatment +AMF) all increased to some extent compared to the CK treatment. When the Cd content in the soil was 180 mg⋅kg-1, the plant height, root length, total biomass, and aboveground and underground biomass of L. perenne in the treatment +AMF were higher than those in the CK by 16.46% (P > 0.05), 18.60% (P < 0.05), 23.33% (P < 0.05), 22.86% (P < 0.05), and 24.00% (P < 0.05), respectively (Figures 1A–E), but the root shoot ratio of the treatment +AMF had no significant difference compared to the CK (Figure 1F).
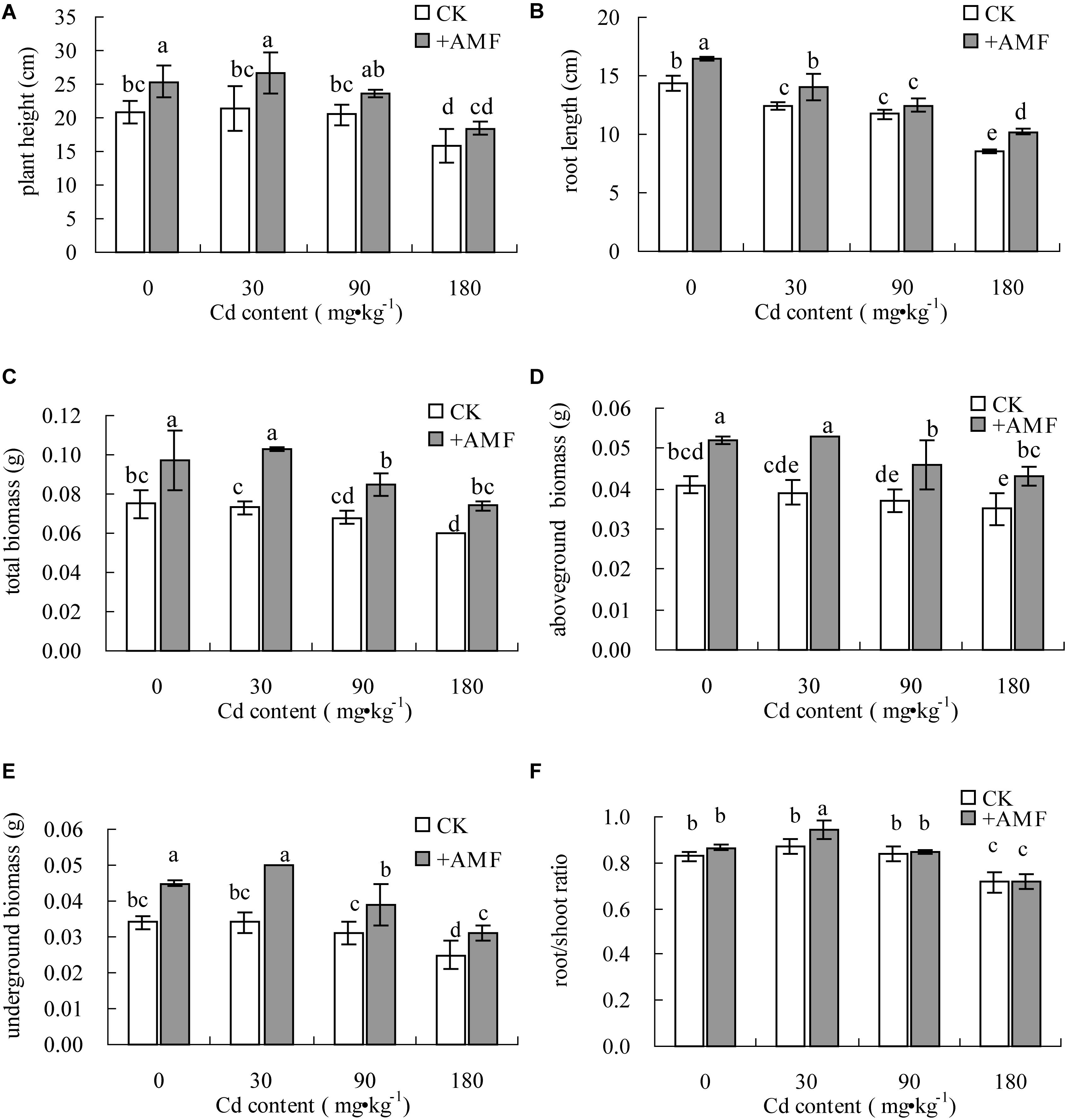
FIGURE 1. The effects of inoculating Lolium perenne with G. mosseae on the plant height (A), toot length (B), total biomass (C), aboveground biomass (D), underground biomass (E), and root/shoot ratio (F) of the plant in cadmium contaminated soil. Different lowercase letters indicate a significant difference (P < 0.05) between the treatment and control.
Effects of G. mosseae Inoculation on the Cd Content in the Underground and Aboveground Parts of L. perenne in Cd Contaminated Soil
As shown in Table 2, AMF inoculation significantly affected the belowground Cd content of L. perenne (P < 0.001) but it had no significant effect on aboveground Cd content (P > 0.05). Soil Cd showed significant effect on both belowground and aboveground Cd content (P < 0.001). The interaction between AMF inoculation and soil Cd had a significant effect on belowground Cd content, but not on aboveground Cd content.
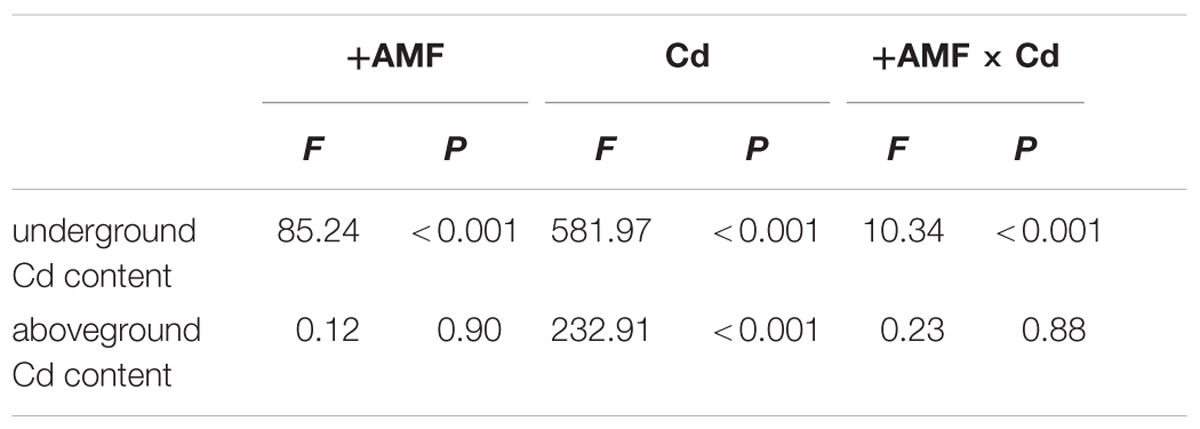
TABLE 2. Two-way ANOVAs examining the effects of AMF inoculation (+AMF), soil Cd (Cd), and their interaction (+AMF × Cd) on Cd content in the underground and aboveground.
Figures 2A,B show that both the Cd contents in the underground and aboveground parts of L. perenne represented significant increasing tendencies with the increasing Cd content in soil, and the Cd content in the underground part of L. perenne was higher than in the aboveground plant parts. Inoculation with G. mosseae significantly improved the absorption of Cd in L. perenne roots. The Cd content in the underground plant parts in the +AMF treatment were significantly higher than the plants not inoculated, except in the treatments without Cd, as there was no significant difference found in the inoculated and non-inoculated treatments. Although G. mosseae inoculation improved the absorption of Cd in L. perenne, the Cd content in the aboveground part of L. perenne in the +AMF treatment was not significantly different from the Cd content in the aboveground part of the non-inoculated L. perenne.
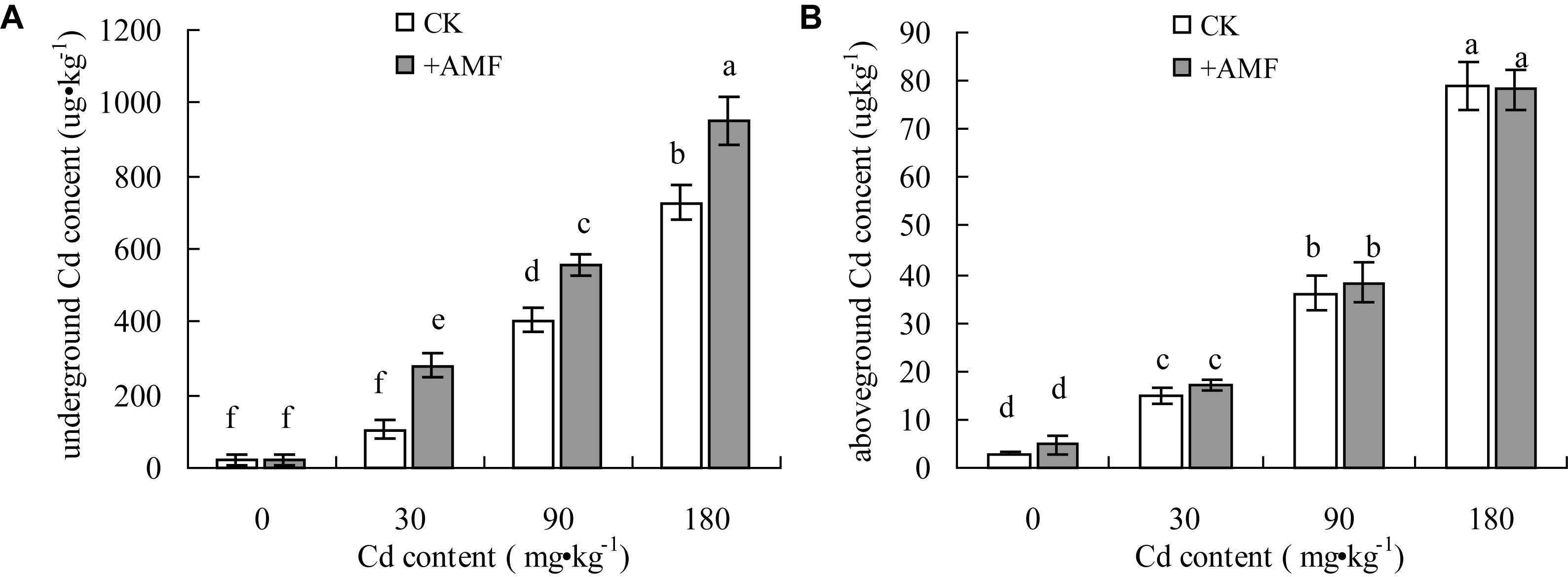
FIGURE 2. The effects of inoculation with G. mosseae on the Cd content in the underground (A) and aboveground (B) parts of L. perenne grown in Cd contaminated soil. Different lowercase letters indicate a significant difference (P < 0.05) between the treatment and control.
Effects of L. perenne Inoculation With G. mosseae on Mycorrhizal Infection Rate and Root Vigor in Cadmium Contaminated Soil
The effect of AMF inoculation and soil Cd on mycorrhizal infection rate and root vigor of L. perenne was significant (Table 3). Moreover, there was a significant interaction effect between these two factors on mycorrhizal infection rate, but not on root vigor (P > 0.05).

TABLE 3. Two-way ANOVAs examining the effects of AMF inoculation (+AMF), soil Cd (Cd), and their interaction (AMF × Cd) on mycorrhizal infection rate and root vigor.
The mycorrhizal infection rate of L. perenne root systems in the treatment +AMF increased by approximately 50–70%, which presented an obvious decreasing trend with the increase of Cd content in the soil (Figure 3A). When the soil Cd content reached 180 mg⋅kg-1, the infection rate of the root system in the treatment +AMF declined by 41.31% (P < 0.05) compared to the treatment without Cd. The root vigor of L. perenne also showed a decreasing trend with the increase of Cd content in the soil (Figure 3B). Due to the impact of the G. mosseae infection, the root vigor of L. perenne in the +AMF treatments under different soil Cd content was higher than that in the treatment without Cd.
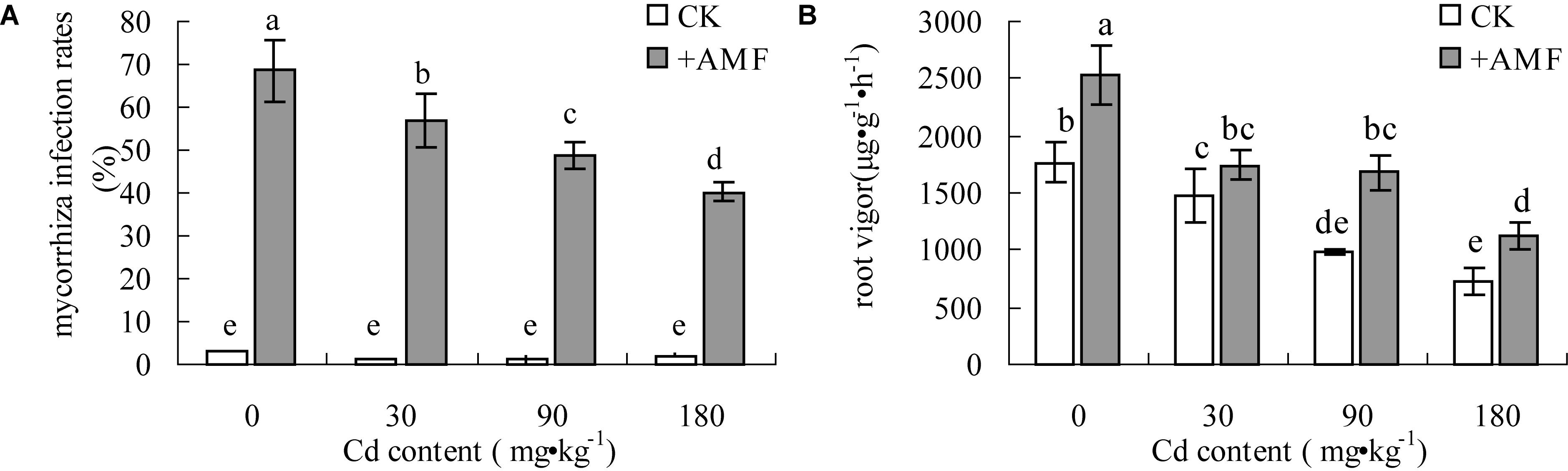
FIGURE 3. The effects of inoculating L. perenne with G. mosseae on mycorrhizal infection rate (A) and root vigor (B) in cadmium contaminated soil. Different lowercase letters indicate a significant difference (P < 0.05) between the treatment and control.
Effects of L. perenne Inoculation With G. mosseae on the Chlorophyll Content of Leaves From Plants Grown in Cadmium Contaminated Soil
The effects on various chlorophyll contents are shown in Table 4. The effect of AMF inoculation on contents of chlorophyll a, total chlorophyll, and chlorophyll a/b of L. perenne was significant (P < 0.01), but for the chlorophyll b content, it was not significant (P > 0.05). Soil Cd had a significant effect on contents of chlorophyll a, chlorophyll b, total chlorophyll, and chlorophyll a/b (P < 0.001). However, there was no significant interaction effect between these two factors on chlorophyll contents and ratio of L. perenne.

TABLE 4. Two-way ANOVAs examining the effects of AMF inoculation (+AMF), soil Cd (Cd), and their interaction (+AMF × Cd) on chlorophyll a content, chlorophyll b content, total chlorophyll a content, and chlorophyll a/b.
With the increase of soil Cd content, the chlorophyll a, chlorophyll b, and total chlorophyll content of L. perenne leaves all declined (Figures 4A–C). Moreover, the degree of decrease of the chlorophyll a content was higher than that of chlorophyll b; thus, the chlorophyll a/b value also decreased with the increasing soil Cd content (Figure 4D). In the treatments with different soil Cd content, inoculation with G. mosseae could increase the chlorophyll content of L. perenne leaves; however, the difference in the chlorophyll b content of L. perenne leaves between the treatment +AMF and the CK was not significant while both the differences in chlorophyll a and total chlorophyll content reached significant levels. Inoculation with G. mosseae could increase the chlorophyll a/b value of L. perenne leaves under Cd stress.
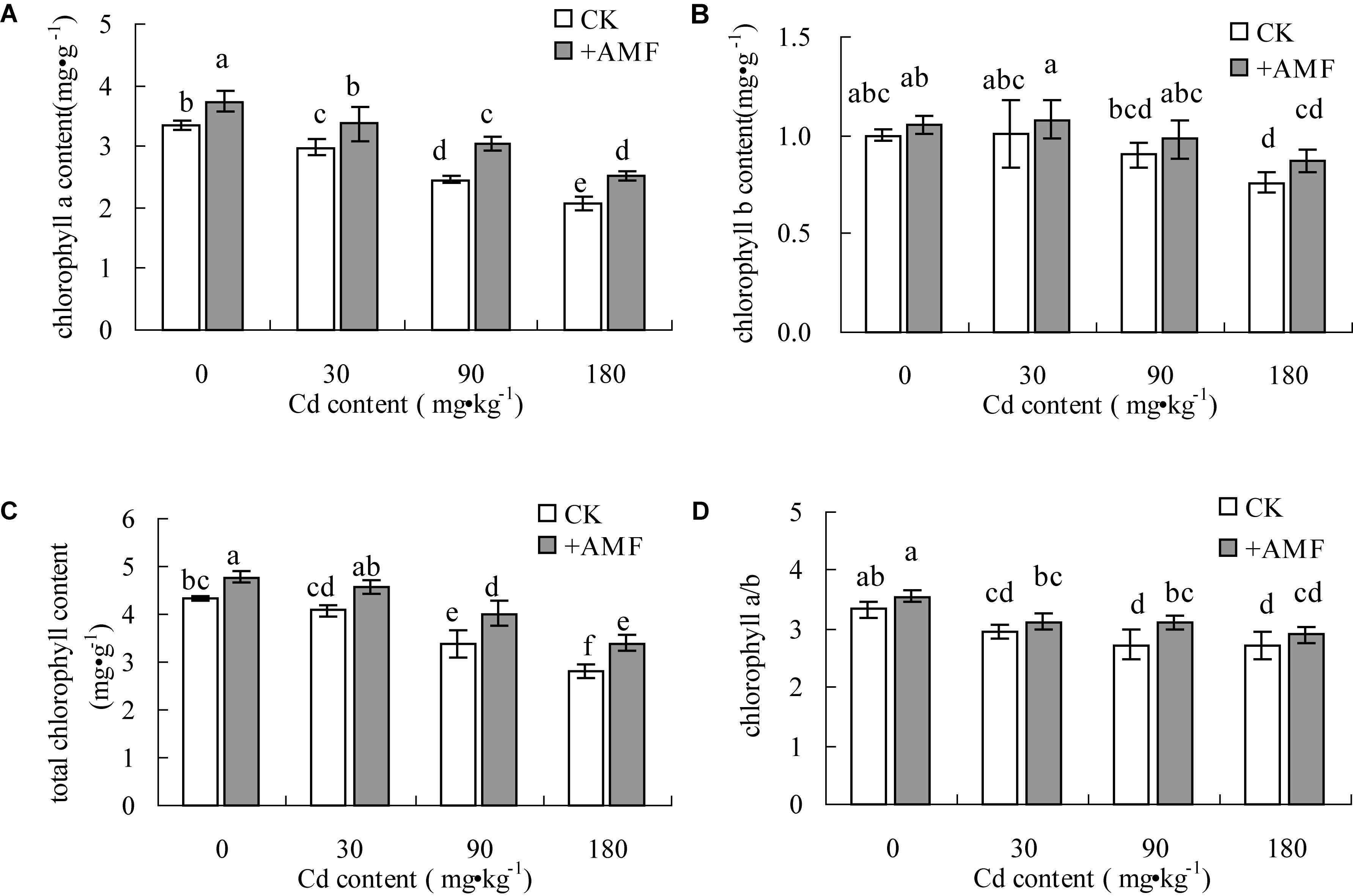
FIGURE 4. The effects of inoculation with G. mosseae on the chlorophyll a content (A), chlorophyll b content (B), total chlorophyll a content (C), and chlorophyll a/b (D) of L. perenne leaves in cadmium contaminated soil. Different lowercase letters indicate a significant difference (P < 0.05) between the treatment and control.
Effects of G. mosseae Inoculation on the Photosynthetic Characteristics of L. perenne Leaves in Cadmium Contaminated Soil
The effect of AMF inoculation on net photosynthetic rate, stomatal conductance, and transpiration rate of L. perenne was significant (P < 0.01, Table 5). Soil Cd also had a significant effect on these parameters (P < 0.001). However, these two factors had no significant effect on intercellular CO2 concentration of L. perenne (P > 0.05) and there was no significant interaction effect between these two factors on the photosynthetic gas exchange parameters.

TABLE 5. Two-way ANOVAs examining the effects of AMF inoculation (+AMF), soil Cd (Cd), and their interaction (AMF × Cd) on net photosynthetic rate, stomatal conductance, transpiration rate, and intercellular CO2 concentration.
The photosynthetic gas exchange parameters of L. perenne leaves were obviously affected by soil Cd content (Figure 5). With the increase of the soil Cd content, the Pn, Gs, and Tr all presented declining tendencies (Figures 5A–C). When the soil Cd content increased to 180 mg⋅kg-1, the Ci of L. perenne leaves in the CK increased by 8.98% (P > 0.05) compared to the Ci of the 90 mg⋅kg-1 Cd treatment (Figure 5D). Inoculation with G. mosseae could evidently improve the Gs of L. perenne leaves, increasing both Tr and Pn. When the soil Cd content increased to 180 mg⋅kg-1, the Pn, Gs, and Tr of L. perenne leaves in the treatment +AMF increased by 33.20% (P < 0.05), 37.79% (P < 0.05), and 10.06% (P > 0.05), respectively, compared to CK.
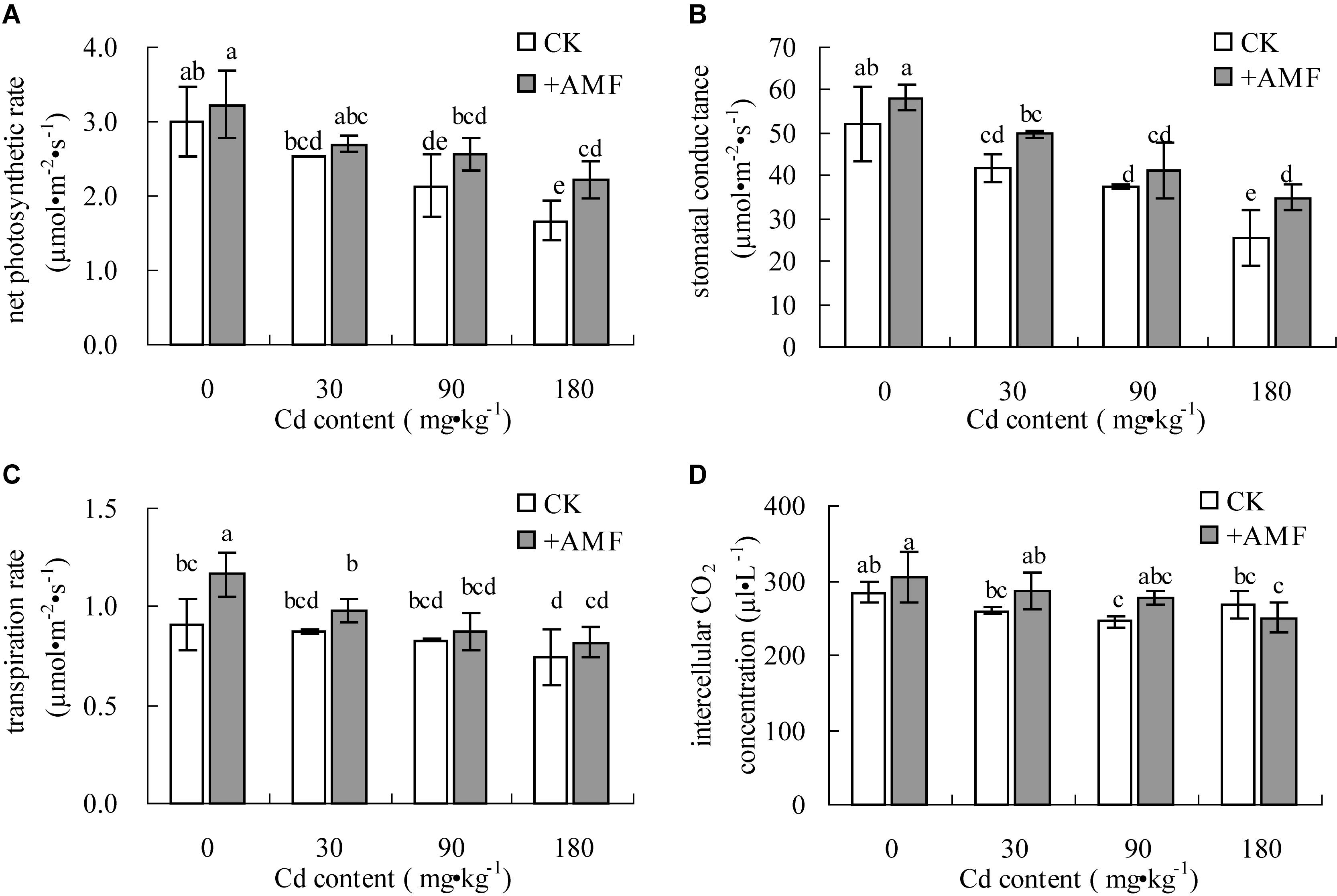
FIGURE 5. The effects of inoculation with G. mosseae on the net photosynthetic rate (A), stomatal conductance (B), transpiration rate (C), and intercellular CO2 concentration (D) of L. perenne leaves in cadmium contaminated soil. Different lowercase letters indicate a significant difference (P < 0.05) between the treatment and control.
Effects of G. mosseae Inoculation on the OJIP Curves of L. perenne Leaves in Cadmium Contaminated Soil
Soil Cd content could evidently influence the shape of OJIP curves in L. perenne leaves (Figures 6A,B). With the increase of soil Cd content, the relative fluorescence intensity at point O increased, while that at point, P presented an obvious decreasing tendency; the reduction of Fm was the most significant, and the OJIP curve became smoother with increasing soil Cd content. AMF inoculation had a significant effect on Fo of L. perenne (P < 0.001, Table 6), but not on Fm (P > 0.05). Soil Cd exerted a significant effect on Fo and Fm (P < 0.001). However, there was no significant interaction effect between these two factors on Fo and Fm. In the treatment +AMF, the variation of the OJIP curve in L. perenne leaves was obviously smaller in the CK. Quantitative analysis of the relative fluorescence intensity at point O and P (Fo and Fm) showed that when soil Cd treatments were 0 and 30 mg⋅kg-1, the difference of Fo in L. perenne leaves between the treatment +AMF and CK was not significant. However, when the Cd content was 90 and 180 mg⋅kg-1, the Fo of L. perenne leaves in the treatment +AMF was significantly lower than in the CK. Although under the Cd content treatments, the Fo of L. perenne leaves in the treatment +AMF were all higher than in the CK, but the difference was not significant (Figures 6C,D).
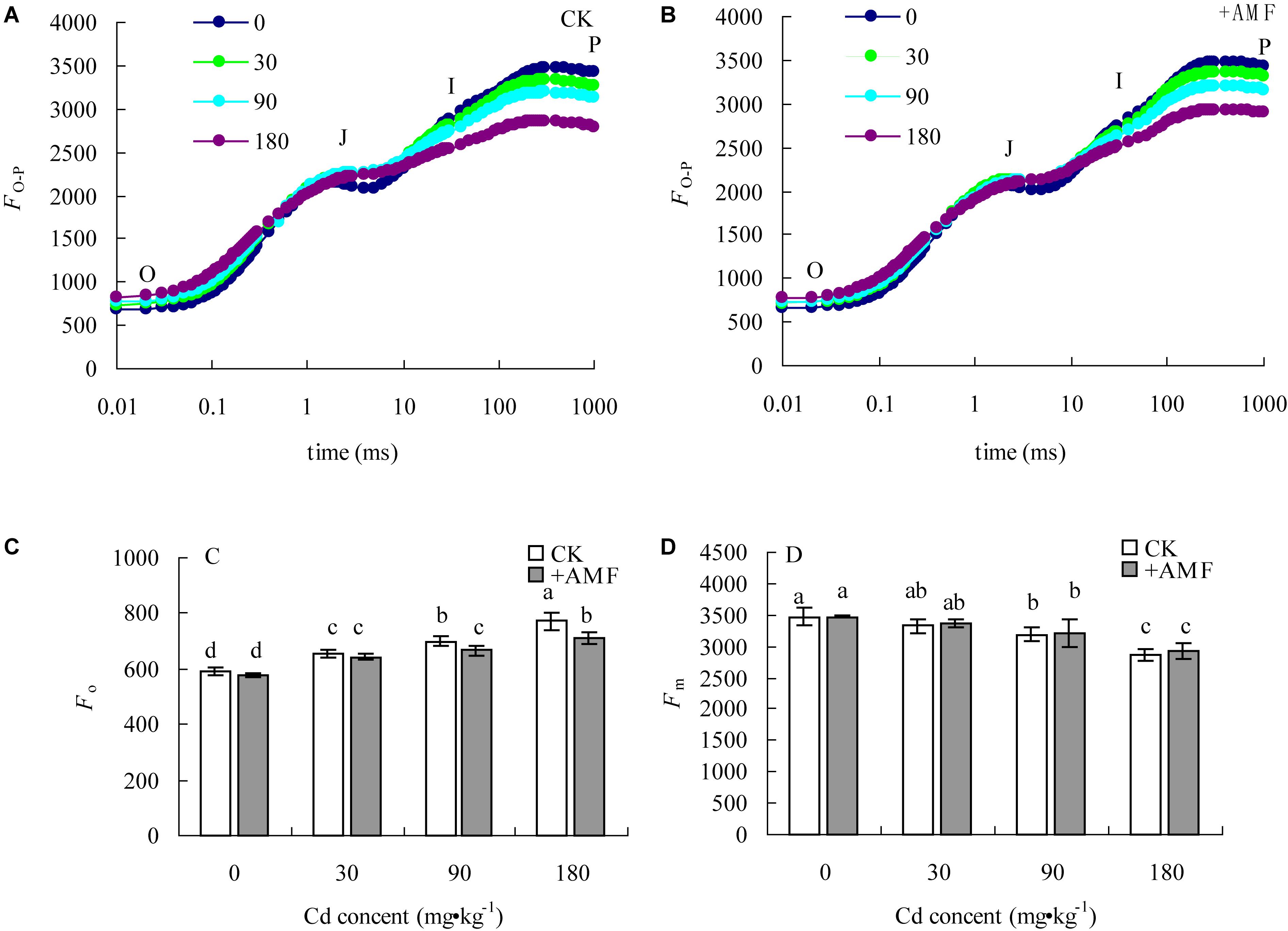
FIGURE 6. The effects of inoculation with G. mosseae on the OJIP curve (A,B), Fo (C), and Fm (D) of L. perenne leaves in cadmium contaminated soil. Different lowercase letters indicate a significant difference (P < 0.05) between the treatment and control. (A,B) data is the average of five repetitions.
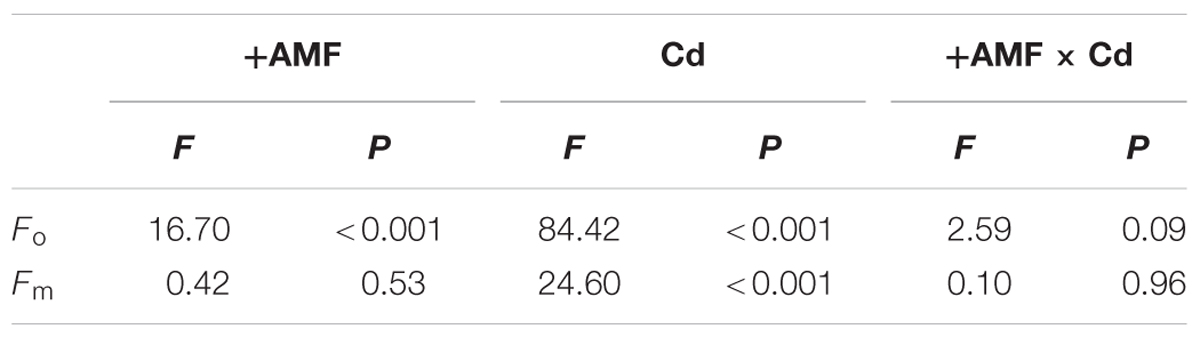
TABLE 6. Two-way ANOVAs examining the effects of AMF inoculation (+AMF), soil Cd (Cd), and their interaction (AMF × Cd) on Fo and Fm.
Effects of G. mosseae Inoculation on Electron Transport at the Donor and Receptor Sides of PSII of L. perenne Leaves in Cadmium Contaminated Soil
The relative fluorescence intensity at point O was defined as 0 and that at point P was defined as 1. After the OJIP curves of L. perenne leaves under different treatments were standardized (Figures 7A–D), the data showed that with the increase of soil Cd content, VJ increased, and the relative variable fluorescence at point J (2 ms) had the most obvious increase. However, the increase of VJ in the treatment +AMF was significantly lower than that in the CK. The standardized OJIP curves under different soil Cd content treatments compared with that in the treatment where the Cd content was 0 showed that in addition to the significantly increased relative variable fluorescence at point J, there was also an evident K point (at 0.3 ms). According to the quantitative analysis of VJ and VK variations, with an increase of soil Cd content, VJ and VK significantly increased and the increase of VJ was significantly greater than that of VK.
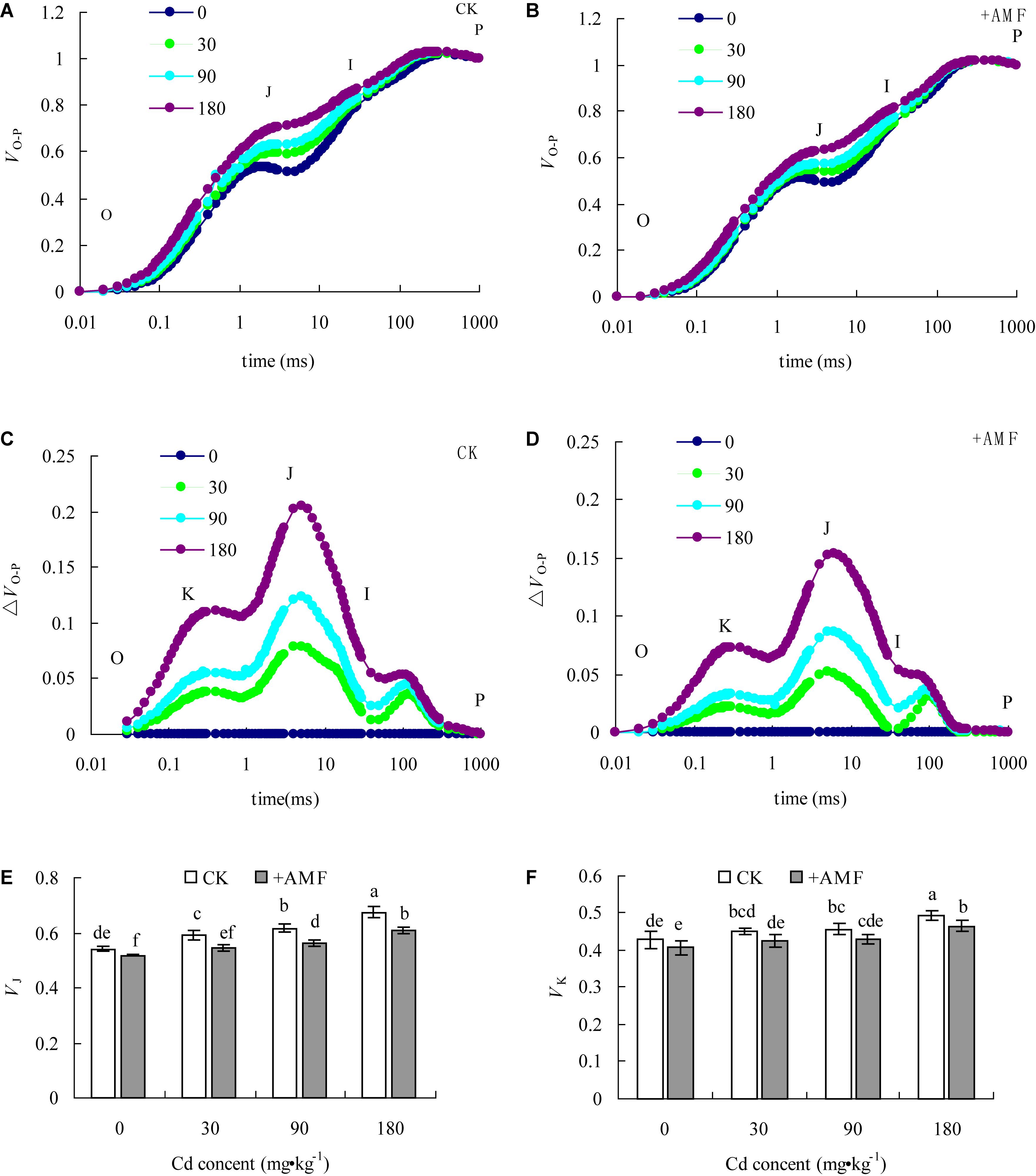
FIGURE 7. The effects of inoculation with G. mosseae on the VO-P (A,B), ΔVO-P (C,D), VJ (E), and VK (F) of L. perenne leaves in cadmium contaminated soil. Different lowercase letters indicate a significant difference (P < 0.05) between the treatment and control. (A,D) data is the average of five repetitions.
Table 7 shows that the effect of AMF inoculation and soil Cd on VJ and VK of L. perenne was significant (P < 0.001), but their interaction effect on VJ and VK was not significant. In different soil Cd content treatments, VJ and VK of in the treatment +AMF were obviously lower than those in the CK. In addition, in different soil Cd content treatments, the VJ in the treatment +AMF was significantly lower than in the CK, while the difference in VK between the two treatments only reached a significant level when Cd content was 180 mg⋅kg-1 in Cd contaminated soil (Figures 7E,F).
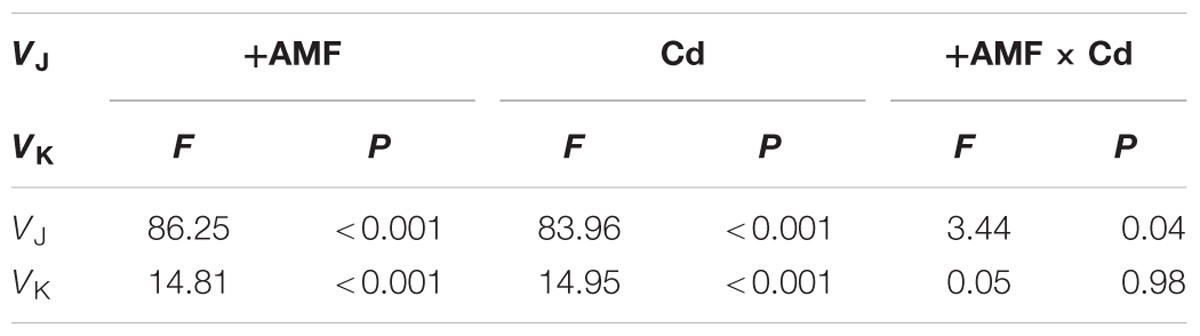
TABLE 7. Two-way ANOVAs examining the effects of AMF inoculation (+AMF), soil Cd (Cd), and their interaction (AMF × Cd) on VJ and VK.
Effects of G. mosseae Inoculation on Photochemical Activities of PSII of L. perenne Leaves in Cadmium Contaminated Soil
The AMF inoculation and soil Cd had significant effect on Fv/Fm and PIABS of L. perenne (P < 0.001), which is shown in Table 8, but they had no significant interaction effect on these parameters.
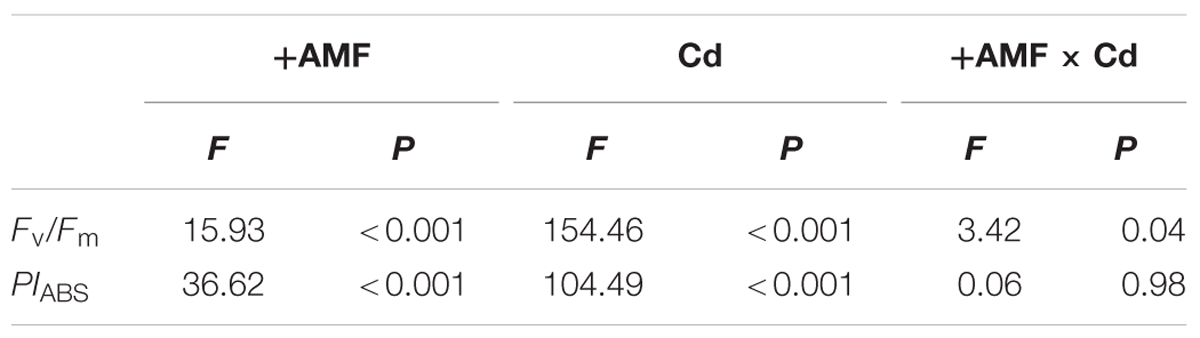
TABLE 8. Two-way ANOVAs examining the effects of AMF inoculation (+AMF), soil Cd (Cd), and their interaction (AMF × Cd) on Fv/Fm and PIABS.
In soil without Cd contamination, there was no significant difference between Fv/Fm in the L. perenne leaves between the treatment +AMF, and the CK (Figure 8A); however, the PIABS of the treatment +AMF exceeded that of the CK by 17.08% (P < 0.05), which was significant (Figure 8B). With the increase of soil Cd content, the Fv/Fm and PIABS of the L. perenne leaves both presented obvious declining trends; the decrease of PIABS was the greatest. When the soil Cd content was between 0 and 90 mg⋅kg-1, the Fv/Fm in the leaves from L. perenne in the +AMF treatment were not significantly different from that in the CK treatment. When the soil Cd content was 180 mg⋅kg-1, the Fv/Fm in the leaves from L. perenne in the +AMF treatment increased by 4.82% (P < 0.05) compared to the CK, while PIABS in the +AMF treatment under different soil Cd contents were all significant higher than the CK.
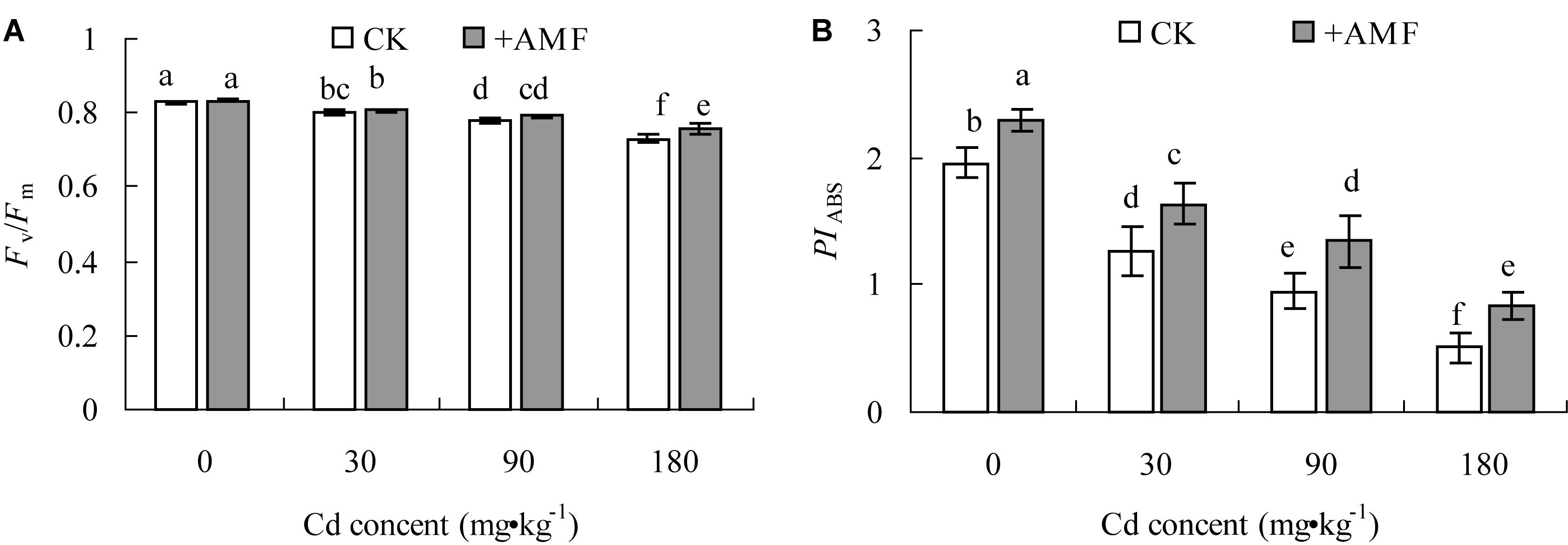
FIGURE 8. The effects of inoculation with G. mosseae on the Fv/Fm (A) and PIABS (B) of L. perenne leaves in cadmium contaminated soil. Different lowercase letters indicate a significant difference (P < 0.05) between the treatment and control.
Effects of G. mosseae Inoculation on Light Energy Absorption and Utilization Parameters of L. perenne Leaves in Cadmium Contaminated Soil
The AMF inoculation showed significant effect on ABS/RC, ETo/RC, DIo/RC, ETo/CSm, and DIo/CSm (P < 0.001, Table 9), but an insignificant effect on ABS/CSm (P > 0.05). Soil Cd also had a significant effect on these parameters (P < 0.001). However, the interaction effect between AMF inoculation and soil Cd on these parameters was not significant.
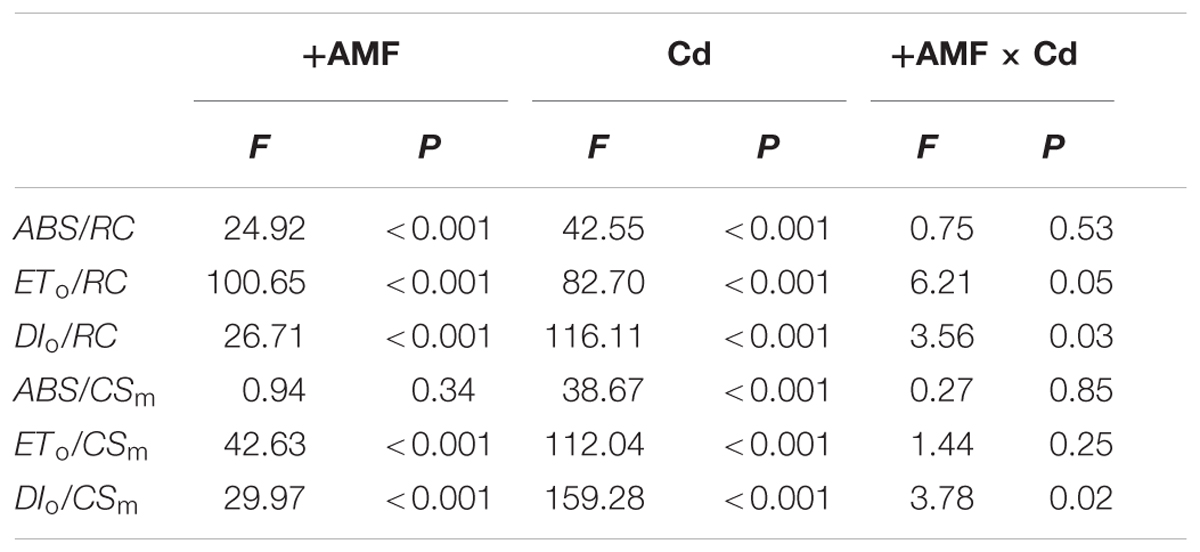
TABLE 9. Two-way ANOVAs examining the effects of AMF inoculation (+AMF), soil Cd (Cd), and their interaction (AMF × Cd) on ABS/RC, ETo/RC, DIo/RC, ABS/CSm, ETo/CSm, and DIo/CSm.
Cadmium (Cd) stress significantly changed the light energy absorption and utilization parameters per unit reaction center and per unit area of L. perenne leaves (Figures 9A,B). With the increase of Cd content, the ABS/RC increased evidently, while the ABS/CSm presented an obvious decreasing trend. Cd stress also resulted in a significant decrease of ETo/RC and ETo/CSm as well as the increase of DIo/RC and DIo/CSm; the decrease of ETo/CSm and increase of DIo/RC were much greater. However, inoculating L. perenne with G. mosseae obviously mitigated the variation of each of the energy allocation parameters in the Cd treatments.
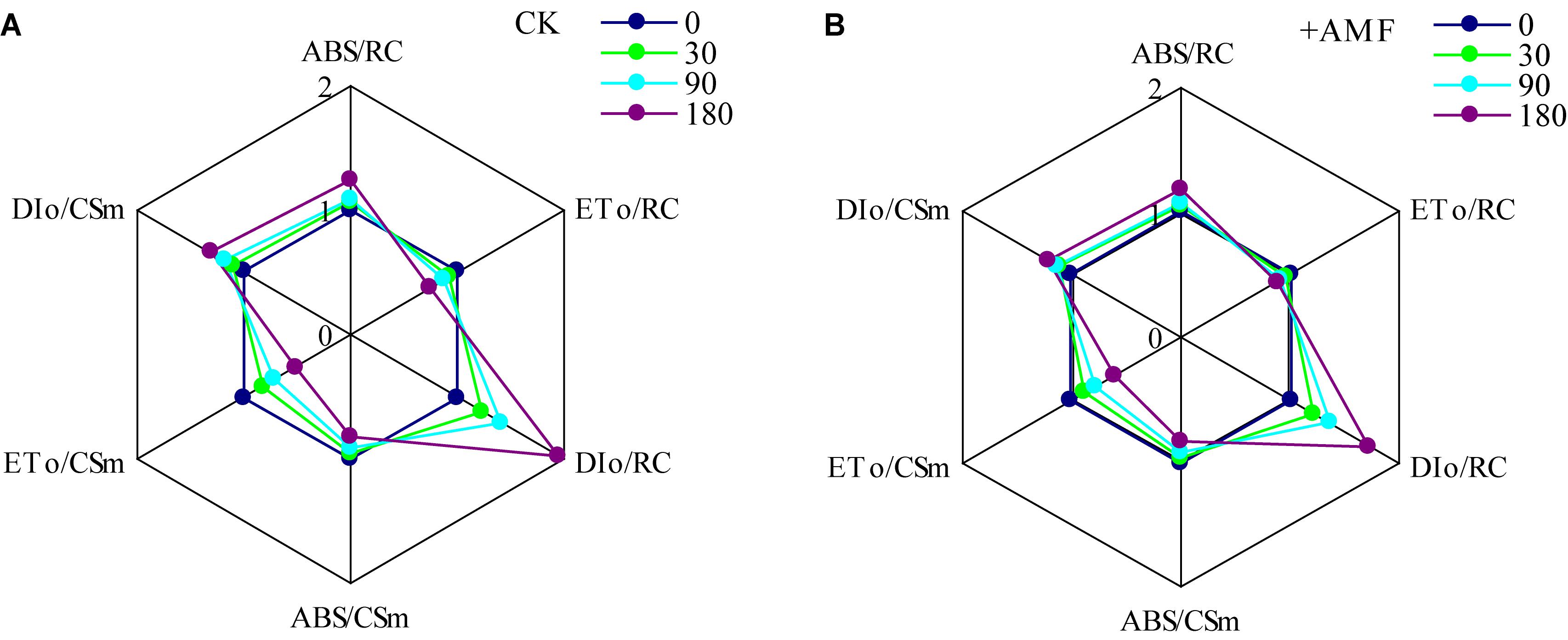
FIGURE 9. The effects of inoculating L. perenne with G. mosseae on light energy absorption and utilization parameters (A,B) in plants grown in cadmium contaminated soil. (A,B) data is the average of five repetitions.
Discussion
Cadmium (Cd) can result in the suppression of plant growth (Liang et al., 2005). Plants must adapt to adverse environmental conditions both morphologically and physically to maintain normal growth and ensure the normal accumulation of dry mass, thus maintaining normal physical processes such as water absorption by roots and photosynthesis of the aboveground parts (Zhang H.H. et al., 2012). In this experiment, the growth of L. perenne in Cd contaminated soil was obviously inhibited; plant height, root length, and biomass accumulation obviously declined with the increase of soil Cd content. However, when soil Cd content was 30 mg⋅kg-1, the root to shoot ratio increased to some extent compared to the treatment without Cd, which might be an adaptation to Cd stress. However, with the increase of soil Cd content, the root to shoot ratio gradually decreased, indicating that Cd in the soil would first result in the blockage of L. perenne root growth and development. It is possible that L. perenne roots would accumulate Cd and alleviate the effects of Cd on aboveground plant growth. The infection level of L. perenne roots by G. mosseae was relatively high, and due to this infection, the root vigor of L. perenne in the treatment +AMF was significantly higher than that in the CK under different Cd contamination levels, hence increasing the root length and root biomass of L. perenne grown under Cd stress. Inoculation with G. mosseae increased the Cd content in L. perenne roots but had no significant effect on the Cd content in aboveground plant parts. This might be related to the Cd immobilization by the AMF or the increased biomass in the aboveground parts of L. perenne, which diluted the Cd concentration.
In addition to the toxicity to plant roots, the effects of Cd on plant growth also included the inhibition of plant photosynthesis under relatively high Cd concentrations, which was one of the important reasons for the suppression of plant growth. Excessive Cd absorption in Phaseolusvul garis destroyed the structure of the chloroplasts, influenced chlorophyll synthesis or accelerated its degradation rate (Siedlecka and Krupa, 1996), and destroyed mesophyll cells in leaves of rye (Secale cereale L. cv. Pastar), increasing the resistance of CO2 transport in mesophyll cells, which resulted in the decrease or loss of key enzyme activity for photosynthesis (Krupa et al., 1999). Simultaneously, Cd stress also resulted in the decrease of Rubsico affinity to CO2 and the stability of the photosynthetic apparatus as well as the blocking of electron transport along the electron transport chain, inducing free radical accumulation and leading to oxidative damage of cells (Shah et al., 2001; Olmos et al., 2003). In this study, with the increase of soil Cd content, the chlorophyll content of L. perenne leaves declined and the chlorophyll a/b value also presented a decreasing tendency, indicating that in L. perenne leaves, chlorophyll a was more sensitive to Cd than chlorophyll b. Chlorophyll a served as a light energy receptor and the reaction center for photoreaction in plants, which was responsible for converting light energy to electrical energy and conducting electron transport on the electron transport chain. Therefore, Cd stress resulted in the decrease of the light energy capturing capacity of L. perenne leaves and led to a declining light energy utilization capacity. Although the +AMF treatment increased chlorophyll a and b content compared to the CK treatment when grown in soil contaminated with Cd, the chlorophyll b content between the treatments was not significantly different, but the chlorophyll a content was significantly different. This indicates that inoculation with G. mosseae improved the light energy acquisition in leaves of L. perenne when grown on Cd contaminated soil by increasing the chlorophyll a.
Studies have also shown that Cd can result in disordered stacking of grana, the disappearance of stroma lamella and the decrease of chloroplast function leading to the suppression of the capacity for photosynthetic carbon assimilation in plants (Zhang et al., 2005; Deng et al., 2014). The Cd content in the aboveground parts of the +AMF treatment grown on soil with different Cd contents was not significantly different compared to the CK, but the photosynthetic gas exchange parameters between the two treatments were significantly different. In this study, with the increase of soil Cd content, Pn, Gs, and Tr presented obvious declining tendencies. Inoculating L. perenne with G. mosseae evidently improved the stomatal limitation of leaves, hence increasing its Tr and Pn, which was favorable for the accumulation of assimilates. In the +AMF treatment, the stomatal aperture in L. perenne leaves might be related to the change of endogenous hormones, including plant cytokinins, induced by inoculation with AMF (Cosme and Wurst, 2013). When soil Cd content increased to 180 mg⋅kg-1, the Ci of L. perenne leaves in the treatment +AMF showed a continuous decreasing trend, while that of the CK increased to some extent compared to plants grown in 90 mg⋅kg-1 of Cd. This indicated that soil Cd content of 180 mg⋅kg-1 had an inhibiting effect on the photosynthesis of L. perenne, which was also related to the destruction of the photosynthetic apparatus in leaves by Cd as well as reducing its CO2 utilization ability, and Ci increased even when stomatal conductance decreased. However, Ci in the treatment +AMF was lower than that in the CK when Cd content reached 180 mg⋅kg-1, indicating that inoculation with G. mosseae could protect the physical functions of the photosynthetic apparatus in the host and increase CO2 assimilation capacity. Inoculation with G. mosseae could improve the photosynthetic capacity of L. perenne leaves by increasing the stomatal aperture when plants are grown in soil with a low level of Cd contamination. However, when the soil Cd content was 180 mg⋅kg-1 and the non-stomatal factors impacted photosynthesis, inoculation with G. mosseae could also improve the photosynthetic capacity through non-stomatal factors including increasing the CO2 fixation capability of L. perenne leaves. This might be related to the effects of AMF on the activities of some enzymes such RuBP carboxylase/oxygenase (RuBisCO), D-fructose-1,6-bisphosphatase (FBPase), D-fructose-6-phosphatase (F6P), and ribulose-5-phosphatekinase (Ru5PK) (Chen et al., 2017c). Previous studies have found that heavy metal ions could react with nutrients, such as phosphate radicals (HPO42-, H2PO4-), resulting in restrictions in absorption of mineral nutrition elements such as phosphorus (Liu et al., 2017). However, inoculation with AMF could improve the plant’s ability to absorb minerals, especially phosphorus (Li et al., 1991; Ahmed et al., 2006; Dong et al., 2008; Xu et al., 2008), which has been shown to play an important role in normal photosynthetic carbon assimilation processes. Therefore, inoculation with G. mosseae could improve the photosynthetic carbon assimilation capability of L. perenne leaves possibly due to the improvement of the absorption of phosphorus by roots; however, this requires further research.
The chlorophyll fluorescence technique is an important method to study the plant photosynthetic apparatus, especially the function of PSII (Misra et al., 2012; Kalaji et al., 2016, 2017). Heavy metal stress could significantly decrease the PSII activity in leaves and inhibit the electron transfer process (Mathur et al., 2016). In this study, with the increase of soil Cd content, the Fo on the OJIP curve of L. perenne leaves increased, while the Fm presented a decreasing trend; both Fv/Fm and PIABS, the parameters reflecting PSII photochemical activity of L. perenne leaves, evidently declined. PIABS showed a stronger decrease than Fv/Fm, reflecting the sensitivity of this PSII parameter (Kalaji et al., 2014). However, the decrease of Fv/Fm and PIABS in the +AMF treatment was evidently lower than those in the CK, which indicated that inoculation with G. mosseae could mitigate the decrease of PSII photochemical activity in L. perenne leaves. To further analyze the reason why inoculation with G. mosseae could promote PSII photochemical activity in L. perenne leaves under Cd stress, the OJIP curves of the different treatments were standardized. The results showed that, compared to the treatment with a soil Cd content of 0, both the relative variable fluorescence VJ and VK at point J (2 ms) and point K (0.3 ms) increased evidently; VJ increased in particular. Some studies found that in adverse environmental conditions, the blocking of photosynthetic electron transport usually occurred at the electron receptor side and the electron donor side of the PSII reaction center. At the electron receptor side, transport from QA (primary electron receptor of the PSII electron transport chain) to QB (secondary electron receptor of the PSII electron transport chain) was the main inhibited site, while at the electron donor side, the activity of the oxygen-evolving complex (OEC) was one of the main parts sensitive to adverse environmental conditions (Abbasi and Komatsu, 2004; Park et al., 2004; Zhang et al., 2018). The relative variable fluorescence VJ at point J (2 ms) on the OJIP curve could reflect the amount of accumulation of QA-. Specifically, the enhancement of VJ indicated the blockage of electron transport from QA to QB on the electron receptor side of PSII (Haldimann and Strasser, 1999; Zhang et al., 2016) and the increase of VK was considered the specific tag of damaged OEC activity on the electron donor side of PSII (Zhang Z. et al., 2012). Therefore, the decline of PSII photochemical activity in L. perenne leaves under Cd stress was mainly related to the inhibition of electron transport from QA to QB on the PSII electron receptor side caused by Cd stress and the reduction of OEC activity in the PSII electron donor side. The PSII receptor side was especially sensitive to Cd stress. However, under different soil Cd treatments, the increase of the VJ and VK in L. perenne leaves in the treatment +AMF were significantly lower than in the CK, which showed that inoculation with G. mosseae could promote PSII activity through stabilizing electron transport at the PSII receptor and donor sides. Inoculation with G. mosseae could improve the PSII function in L. perenne leaves by improving the absorption of nutrients, reducing the distribution of heavy metals in the aboveground part of the host plants (Lins et al., 2006; Chen and Zhao, 2009; Słomka et al., 2011; Zhang H.H. et al., 2012), which might also be related to the effects of AMF on the activities of some enzymes and hormones in the host plants or the induction on the expressions of related resistance genes which further initiate the anti-oxidation system. For example, inoculation with G. mosseae could significantly improve the activities of secondary metabolism-related enzymes including polyphenol oxidase, peroxidase, and phenylalanine ammonia-lyase in Cabernet sauvignon roots, alleviating injuries to plant cell membranes caused by Cd stress (Qu et al., 2009). Infection with AMF could induce a significant increase in the content of osmotic adjustment substrates, such as proline, in Coffea arabica leaves (Andrade et al., 2010). Inoculation with Glomus intraradices and G. mosseae could induce the expression of heavy metal stress-related genes in Populus alba plants grown in soil contaminated with heavy metals and increase the polyamine level (Cicatelli et al., 2010). In addition, inoculation with G. mosseae increased the biomass of L. perenneplants (Figure 1) and decreased the Cd concentration in L. perenne leaves, which might also play an important role in reducing the photoinhibition caused by Cd stress. However, the absorption and distribution mechanism of Cd in L. perenne plants through inoculation with G. mosseae requires further studies.
Under stress, plants generally improve their adaptability through adjusting energy distribution (Misra et al., 2001). Soil Cd stress evidently changed light energy absorption and utilization parameters per unit reaction center and per unit area of L. perenne leaves. With the increase of soil Cd content, although the light energy absorbed per unit area, ABS/CSm, presented an obvious declining trend, while the light energy absorbed per unit of the reaction center, ABS/RC, increased. This indicates that with the increase of the Cd content, the amount of active reaction centers per unit area of L. perenne leaves declined to some extent, forcing the enhancement of the remaining active reaction centers. Cd stress might result in the inactivation of partial reaction centers in L. perenne leaves, which was also a type of adaptation mechanism for PSII reaction centers adapting to soil Cd stress (Mlinariæ et al., 2017; Zhang et al., 2017). Nevertheless, Cd stress resulted in the significant decrease on the proportion of light energy absorbed by L. perenne leaves used for photochemical reactions, while the proportion of waste heat dissipation increased greatly. However, inoculation with G. mosseae significantly mitigated each energy allocation parameter of L. perenne leaves under Cd stress. This showed that inoculation with G. mosseae could promote PSII photochemical activity and helped the host to adapt to Cd stress through optimizing light energy allocation and utilization in L. perenne.
Conclusion
Soil Cd pollution will first influence L. perenne roots, resulting in the decline of root vigor and the decrease of underground biomass accumulation as well as the decline of chlorophyll content and photosynthetic capacity of leaves. Inoculation with G. mosseae slightly increased the Cd content in L. perenne roots but did not aid in the transport and distribution of Cd to the aboveground parts of L. perenne. Inoculation with G. mosseae can significantly increase the root vigor and chlorophyll content of L. perenne in Cd contaminated soil. In addition, the photosynthetic carbon assimilation capacity of L. perenne leaves and PSII photochemical activity also increased to different degrees. Therefore, inoculating L. perenne with G. mosseae can promote the host’s tolerance to Cd via morphological characteristics and photosynthetic functions as well as reduce the toxicity of Cd to L. perenne.
Author Contributions
NX, HaZ, and HuZ conceived and designed the experiments. All the authors performed the experiments and analyzed the data. NX and HuZ wrote the manuscript and prepared the figures and/or tables. NX, HuZ, HaZ, and SG reviewed drafts of the paper.
Funding
This research was supported by The National Natural Science Fund (Grant Nos. 31500323, 31370426, and 31500410).
Conflict of Interest Statement
The authors declare that the research was conducted in the absence of any commercial or financial relationships that could be construed as a potential conflict of interest.
References
Abbasi, F. M., and Komatsu, S. (2004). A proteomic approach to analyze salt-responsive proteins in rice leaf sheath. Proteomics 4, 2072–2081. doi: 10.1002/pmic.200300741
Ahmed, F. R. S., Killham, K., and Alexander, I. (2006). Influences of arbuscular mycorrhizal fungus Glomus mosseae on growth and nutrition of lentil irrigated with arsenic contaminated water. Plant Soil. 283, 33–41. doi: 10.1007/s11104-005-0415-8
Alfvén, T., Elinder, C. G., Carlsson, M. D., Grubb, A., Hellstrom, L., Persson, B., et al. (2000). Low level cadmium exposure and osteoporosis. J. Bone Miner. Res. 15, 1579–1586. doi: 10.1359/jbmr.2000.15.8.1579
Aloui, A., Dumas-Gaudot, E., Daher, Z., Tuinen, D., Aschi-Smit, S., and Morandi, D. (2012). Influence of arbuscular mycorrhizal colonisation on cadmium induced Medicago truncatula root isoflavonoid accumulation. Plant Physiol. Biochem. 60, 233–239. doi: 10.1016/j.plaphy.2012.08.014
Andrade, S. A., Gratão, P. L., Schiavinato, M. A., Silveira, A. P. D., Azevedo, R. A., and Mazzafera, P. (2009). Zn uptake, physiological response and stress attenuation in mycorrhizal jack bean growing in soil with increasing Zn concentrations. Chemosphere 75, 1363–1370. doi: 10.1016/j.chemosphere.2009.02.008
Andrade, S. A., Silveira, A. P., and Mazzafera, P. (2010). Arbuscular mycorrhiza alters metal uptake and the physiological response of Coffea arabica seedlings to increasing Zn and Cu concentrations in soil. Sci. Total Environ. 408, 5381–5391. doi: 10.1016/j.scitotenv.2010.07.064
Bah, M. A., Sun, H. Y., Chen, F., Zhou, J., Dai, H. X., Zhang, G. P., et al. (2010). Comparative proteomic analysis of Typha angustifolia leaf under chromium, cadmium and lead stress. J. Hazard. Mater. 184, 191–203. doi: 10.1016/j.jhazmat.2010.08.023
Bowles, T. M., Barrios-Masias, F. H., Carlisle, E. A., Cavagnaro, T. R., and Jackson, L. E. (2016). Effects of arbuscular mycorrhizae on tomato yield, nutrient uptake, water relations, and soil carbon dynamics under deficit irrigation in field conditions. Sci Total Environ. 56, 1223–1234. doi: 10.1016/j.scitotenv.2016.05.178
Chang, Z., Sun, B., and Li, D. (2017). Water withholding contributes to winter hardiness in perennial ryegrass (Lolium perenne L.). Eur. J. Hortic. Sci. 82, 31–37. doi: 10.17660/eJHS.2017/82.1.4
Chen, M., Yang, G., Sheng, Y., Li, P. Y., Qiu, H. Y., Zhou, X. T., et al. (2017a). Glomus mosseae inoculation improves the toot system architecture, photosynthetic efficiency and flavonoids accumulation of liquorice under nutrient stress. Front Plant Sci. 8:931. doi: 10.3389/fpls.2017.00931
Chen, M., Yang, G., Liu, D. H., Li, M. H., Qiu, H. Y., Guo, L. P., et al. (2017b). Inoculation with Glomus mosseae improves the growth and salvianolic acid b accumulation of continuously cropped Salvia miltiorrhiza. Appl. Sci. 7:692. doi: 10.3390/app7070692
Chen, S., Zhao, H., Zou, C., Li, Y. S., Chen, Y. F., Wang, Z. H., et al. (2017c). Combined inoculation with multiple arbuscular mycorrhizal fungi improves growth, nutrient uptake and photosynthesis in cucumber seedlings. Front. Microbiol. 8:2516. doi: 10.3389/fmicb.2017.02516
Chen, X. H., and Zhao, B. (2009). Arbuscular mycorrhizal fungi mediated uptake of nutrient elements by Chinese milk vetch (Astragalus sinicus L.) grown in lanthanum spiked soil. Biol. Fertil. Soils 45, 675–678. doi: 10.1007/s00374-009-0379-6
Christie, P., Li, X., and Chen, B. (2004). Arbuscular mycorrhiza can depress translocation of zinc to shoots of host plants in soils moderately polluted with zinc. Plant Soil 261, 209–217. doi: 10.1023/B:PLSO.0000035542.79345.1b
Cicatelli, A., Lingua, G., Todeschini, V., Biondi, S., Torrigiani, P., and Castiglione, S. (2010). Arbuscular mycorrhizal fungi restore normal growth in a white poplar clone grown on heavy metal-contaminated soil, and this is associated with upregulation of foliar metallothionein and polyamine biosynthetic gene expression. Ann. Bot. 106, 791–802. doi: 10.1093/aob/mcq170
Ciecko, Z., Wyszkowski, M., Krajewski, W., and Zabielska, J. (2001). Effect of organic matter and liming on the reduction of cadmium uptake from soil by triticale and spring oilseed rape. Sci. Total Environ. 281, 37–45. doi: 10.1016/S0048-9697(01)00800-2
Cosme, M., and Wurst, S. (2013). Interactions between arbuscular mycorrhizal fungi, rhizobacteria, soil phosphorus and plant cytokinin deficiency change the root morphology, yield and quality of tobacco. Soil Biol. Biochem. 57, 436–443. doi: 10.1016/j.soilbio.2012.09.024
Dehn, B., and Schüepp, H. (1990). Influence of VA mycorrhizae on the uptake and distribution of heavy metals in plants. Agric. Ecosyst. Environ. 29, 79–83. doi: 10.1016/0167-8809(90)90258-F
Del, V. C., Barea, J. M., and Azcon-Aguilar, C. (1999a). Assessing the tolerance to heavy metals of arbuscular mycorrhizal fungi isolated from sewage sludge-contaminated soils. Appl. Soil Ecol. 11, 261–269. doi: 10.1016/S0929-1393(98)00153-X
Del, V. C., Barea, J. M., and Azcon-Aguilar, C. (1999b). Diversity of arbuscularmycorrhizal fungus populations in heavy-metal-contaminated soils. Appl. Environ. Microbiol. 65, 718–723.
Deng, G., Li, M., Li, H., Yin, L., and Li, W. (2014). Exposure to cadmium causes declines in growth and photosynthesisin the endangered aquatic fern (Ceratopteris pteridoides). Aquat. Bot. 112, 23–32. doi: 10.1016/j.aquabot.2013.07.003
Dong, J., Wu, F. B., and Zhang, G. P. (2006). Influence of cadmium on antioxidant capacity and four microelement concentrations in tomato seedlings (Lycopersicon esculentum). Chemosphere 64, 1659–1666. doi: 10.1016/j.chemosphere.2006.01.030
Dong, Y., Zhu, Y. G., Smith, F. A., Wang, Y., and Chen, B. (2008). Arbuscular mycorrhiza enhanced arsenic resistance of both white clover (Trifolium repens Linn.) and ryegrass (Lolium perenne L.) plants in an arsenic-contaminated soil. Environ. Pollut. 155, 174–181. doi: 10.1016/j.envpol.2007.10.023
Gill, R. A., Ali, B., Islam, F., Farooq, M. A., Gill, M. B., Mwamba, T. M., et al. (2015). Physiological and molecular analyses of black and yellow seeded Brassica napus regulated by 5-aminolivulinic acid under chromium stress. Plant Physiol. Biochem. 94, 130–143. doi: 10.1016/j.plaphy.2015.06.001
Gonzalez-Chavez, C., Harris, P. J., Dodd, J., and MeHarg, A. A. (2002). Arbuscular mycorrhizal fungi confer enhanced arsenate resistance on Holcus lanatus. New Phytol. 155, 163–171. doi: 10.1046/j.1469-8137.2002.00430.x
Haldimann, P., and Strasser, R. J. (1999). Effects of anaerobiosis as probed by the polyphasic chlorophyll a fluorescence rise kinetic in pea (Pisum sativum L.). Photosynth. Res. 62, 67–83. doi: 10.1023/A:1006321126009
Hall, J. L. (2002). Cellular mechanisms for heavy metal detoxification and tolerance. J. Exp. Bot. 53, 1–11. doi: 10.1093/jexbot/53.366.1
Hebeisen, T., Lüscher, A., Zanetti, S., Fischer, B. U., Hartwig, U., Frehner, M., et al. (2010). Growth response of Trifolium repens L. and Lolium perenne L. as monocultures and bi-species mixture to free air CO2 enrichment and management. Glob. Chang. Biol. 3, 149–160. doi: 10.1046/j.1365-2486.1997.00073.x
Hossain, Z., Hajika, M., and Komatsu, S. (2012). Comparative proteome analysis of high and low cadmium accumulating soybeans under cadmium stress. Amino Acids. 43, 2393–2416. doi: 10.1007/s00726-012-1319-6
Joner, E. J., Briones, R., and Leyval, C. (2000). Metal-binding capacity of arbuscular mycorrhizal mycelium. Plant Soil 226, 227–234. doi: 10.1023/A:1026565701391
Kalaji, H. M., Jajoo, A., Oukarroum, A., Brestic, M., Zivcak, M., Samborska, I. A., et al. (2016). Chlorophyll a, fluorescence as a tool to monitor physiological status of plants under abiotic stress conditions. Acta Physiol. Plant. 38:102. doi: 10.1007/s11738-016-2113-y
Kalaji, H. M., Schansker, G., Brestic, M., Bussotti, F., Calatayud, A., Ferroni, L., et al. (2017). Erratum to: frequently asked questions about chlorophyll fluorescence, the sequel. Photosynth. Res. 132, 13–66. doi: 10.1007/s11120-016-0318-y
Kalaji, H. M., Schansker, G., Ladle, R. J., Goltsev Video, Bosa, K., Allakhverdiev, S. I., Brestic, M., et al. (2014). Frequently asked questions about in vivo chlorophyll fluorescence: practical issues. Photosynth. Res. 122, 121–158. doi: 10.1007/s11120-014-0024-6
Karagiannidis, N., Bletsos, F., and Stavropoulos, N. (2002). Effect of Verticillium, wilt (Verticillium dahliae, Kleb.) and mycorrhiza (Glomus mosseae) on root colonization, growth and nutrient uptake in tomato and eggplant seedlings. Sci. Hortic. 94, 145–156. doi: 10.1016/S0304-4238(01)00336-3
Kieffer, P., Dommes, J., Hoffmann, L., Hausman, J. F., and Renaut, J. (2008). Quantitative changes in protein expression of cadmium-exposed poplar plants. Proteomics 8, 2514–2530. doi: 10.1002/pmic.200701110
Kola, H., and Wilkinson, K. J. (2005). Cadmium uptake by a green alga can be predicted by equilibrium modelling. Environ. Sci. Technol. 39, 3040–3047. doi: 10.1021/es048655d
Krantev, A., Yordanova, R., Janda, T., Szalai, G., and Popova, L. (2008). Treatment with salicylic acid decreases the effect of cadmium on photosynthesis in maize plants. J. Plant Physiol. 165, 920–931. doi: 10.1016/j.jplph.2006.11.014
Krupa, Z., Siedlecka, A., and Kleczkowski, L. A. (1999). Cadmium affected level of inorganic phosphate in rye leaves influences Rubisco subunits. Acta Physiol. Plant. 21, 257–261. doi: 10.1007/s11738-999-0040-x
Li, S. (1998). Different arbuscular mycorrhizal fungal species are potential determinants of plant community structure. Ecology 79, 2082–2091. doi: 10.1890/0012-9658(1998)079[2082:DAMFSA]2.0.CO;2
Li, X. L., George, E., and Marschner, H. (1991). Extension of the phosphorus depletion zone in VA-mycorrhizal white clover in a calcareous soil. Plant Soil 136, 41–48. doi: 10.1007/BF02465218
Lial, M., and Huang, C. Y. (2002). Effects of organic acids on the toxicity of cadmium during rye grass growth. Chin. J. Appl. Ecol. 13, 109–112.
Liang, Y., Wong, J. W., and Wei, L. (2005). Silicon-mediated enhancement of cadmium tolerance in maize (Zea mays L.) grown in cadmium contaminated soil. Chemosphere 58, 475–483. doi: 10.1016/j.chemosphere.2004.09.034
Lins, C. E. L., Cavalcante, U. M. T., Sampaio, E. V. S. B., Messias, A. S., and Maia, L. C. (2006). Growth of mycorrhized seedlings of Leucaena leucocephala (Lam.) de Wit. in a copper contaminated soil. Appl. Soil Ecol. 31, 181–185. doi: 10.1016/j.apsoil.2005.06.004
Liu, M., Sun, J., Li, Y., and Xiao, Y. (2017). Nitrogen fertilizer enhances growth and nutrient uptake of Medicago sativa inoculated with Glomus tortuosum grown in Cd-contaminated acidic soil. Chemosphere 167, 204–211. doi: 10.1016/j.chemosphere.2016.09.145
López-Millán, A. F., Sagardoy, R., Solanas, M., Abadía, A., and Abadía, J. (2009). Cadmium toxicity in tomato (Lycopersicon esculentum) plants grown in hydroponics. Environ. Exp. Bot. 65, 376–385. doi: 10.1016/j.envexpbot.2008.11.010
Marmiroli, M., Imperiale, D., Maestri, E., and Marmiroli, N. (2013). The response of Populus spp. to cadmium stress: chemical, morphological and proteomics study. Chemosphere 93, 1333–1344. doi: 10.1016/j.chemosphere.2013.07.065
Masood, A., Iqbal, N., and Khan, N. A. (2012). Role of ethylene in alleviation of cadmium-induced photosynthetic capacity inhibition by sulphur in mustard. Plant Cell Environ. 35, 524–533. doi: 10.1111/j.1365-3040.2011.02432.x
Mathur, S., Kalaji, H. M., and Jajoo, A. (2016). Investigation of deleterious effects of chromium phytotoxicity and photosynthesis in wheat plant. Photosynthetica 54, 185–192. doi: 10.1007/s11099-016-0198-6
Mathur, S., Sharma, M. P., and Jajoo, A. (2018). Improved photosynthetic efficacy of maize (Zea mays) plants with arbuscular mycorrhizal fungi (AMF) under high temperature stress. J Photochem. Photobiol. B Biol. 180, 149–154. doi: 10.1016/j.jphotobiol.2018.02.002
Misra, A. N., Misra, M., and Singh, R. (2012). “Chlorophyll fluorescence in plant biology,” in Biophysics, Chap. 7, ed. A. N. Misra (London: IntechOpen), 171–192
Misra, A. N., Srivastava, A., and Strasser, R. J. (2001). Utilization of fast chlorophyll a, fluorescence technique in assessing the salt/ion sensitivity of mung bean and Brassica seedlings. J. Plant Physiol. 158, 1173–1181. doi: 10.1078/S0176-1617(04)70144-3
Mlinariæ, S., Antunoviæ, D. J., Skendroviæ, B. M., Cesar, V., and Lepedus, H. (2017). Differential accumulation of photosynthetic proteins regulates diurnal photochemical adjustments of PSII in common fig (Ficus carica L.) leaves. J. Plant Physiol. 209, 1–10. doi: 10.1016/j.jplph.2016.12.002
Murtaza, G., Javed, W., Hussain, A., Qadir, M., and Aslam, M. (2017). Soil applied zinc and copper suppress cadmium uptake and improve the performance of cereals and legumes. Int. J. Phytoremediat. 19, 199–206. doi: 10.1080/15226514.2016.1207605
Olmos, E., Martínez-Solano, J. R., Piqueras, A., and Hellín, E. (2003). Early steps in the oxidative burst induced by cadmium in cultured tobacco cells (BY-2 line). J. Exp. Bot. 54, 291–301. doi: 10.1093/jxb/erg028
Pallara, G., Todeschini, V., Lingua, G., Camussi, A., and Racchi, M. L. (2013). Transcript analysis of stress defence genes in a white poplar clone inoculated with the arbuscular mycorrhizal fungus Glomus mosseae, and grown on a polluted soil. Plant Physiol. Biochem. 63, 131–139. doi: 10.1016/j.plaphy.2012.11.016
Park, C. J., Kim, K. J., Shin, R., Park, J. M., Shin, Y. C., and Paek, K. H. (2004). Pathogenesis-related protein 10 isolated from hot pepper functions as a ribonuclease in an antiviral pathway. Plant J. Cell Mol. Biol. 37, 186–198. doi: 10.1046/j.1365-313X.2003.01951.x
Peralta, J. R., Gardea Torresdey, J. L., Tiemann, K. J., Gomez, E., Arteage, S., Rascon, E., et al. (2001). Up take and effects of five heavy metals on seed germination and plant growth in alfalfa (Medicago sativa L.). Environ Contam. Toxicol. 66, 727–734.
Porra, R. J. (2002). The chequered history of the development and use of simultaneous equations for the accurate determination of chlorophylls a and b. Photosynth. Res. 73, 149–156. doi: 10.1023/A:1020470224740
Qaswar, M., Hussain, S., and Rengel, Z. (2017). Zinc fertilisation increases grain zinc and reduces grain lead and cadmium concentrations more in zinc-biofortified than standard wheat cultivar. Sci Total Environ. 605, 454–460. doi: 10.1016/j.scitotenv.2017.06.242
Qian, H. F., Li, J. J., Sun, L. W., Wei, C., Sheng, G. D., Liu, W. P., et al. (2009). Combined effect of copper and cadmium on Chlorella vulgaris growth and photosynthesis-related gene transcription. Aquat. Toxicol. 94, 56–61. doi: 10.1016/j.aquatox.2009.05.014
Qu, Y. P., Fang, Y. L., Liu, Y. L., Song, S. R., Zhang, A., and Zhou, G. R. (2009). Effects of AM fungal on the secondary metabolites of grape under cadmium stress. J. Northwest For. Univ. 24, 101–105.
Rizwan, M., Ali, S., Hussain, A., and Asma, M. (2017). Effect of zinc-lysine on growth, yield and cadmium uptake in wheat (Triticum aestivum L.) and health risk assessment. Chemosphere 187, 35–42. doi: 10.1016/j.chemosphere.2017.08.071
Rodrıguez-Serrano, M., Romero-Puertas, M. C., Zabalza, A., Corpas, F. J., Gomez, M., Rio, L. A., et al. (2006). Cadmium effect on oxidative metabolism of pea (Pisum sativum L.) roots. Imaging of reactive oxygen species and nitric oxide accumulation in vivo. Plant Cell Environ. 29, 1532–1544. doi: 10.1111/j.1365-3040.2006.01531.x
Rozpądek, P., Rąpałakozik, M., Wêżowicz, K., Grandin, A., Karlsson, S., and Wazny, R. (2016). Arbuscular mycorrhiza improves yield and nutritional properties of onion (Allium cepa). Plant Physiol Biochem. 107, 264–272. doi: 10.1016/j.plaphy.2016.06.006
Rufyikiri, G., Huysmans, L., Wannijn, J., Hees, M. V., Leyval, C., and Jakobsen, I. (2004). Arbuscular mycorrhizal fungi can decrease the uptake of uranium by subterranean clover grown at high levels of uranium in soil. Environ. Pollut. 130, 427–436. doi: 10.1016/j.envpol.2003.12.021
Semane, B., Dupae, J., Cuypers, A., Noben, J. P., Tuomainen, M., Tervahauta, A. I., et al. (2010). Leaf proteome responses of Arabidopsis thaliana exposed to mild cadmium stress. J. Plant Physiol. 167, 247–254. doi: 10.1016/j.jplph.2009.09.015
Shah, K., Kumar, R. G., Verma, A., and Hellan, E. (2001). Effect of cadmium on lipid peroxidation, superoxide anion generation and activitivies of antioxidant enzymes in growing rice seedlings. Plant Sci. 161, 1135–1144. doi: 10.1016/S0168-9452(01)00517-9
Shao, X. J., Yang, H. Q., Qiao, H. T., Zhang, L., and Shu, Z. Y. (2009). Effects of CdCl2 on grape root mitochondrial characteristics and root activity. Chin. J. Appl. Ecol. 20, 1390–1394.
Siedlecka, A., and Krupa, Z. (1996). Interaction between cadmium and iron and its effects on photosynthetic capacity of primary leaves of Phaseolusvul garis. Plant Physiol. Biochem. 34, 833–841.
Singh, O. V., Labana, S., Pandey, G., Budhiraja, R., and Jain, R. X. (2003). Phytoremediation: an overview of metallic ion decontamination from soil. Appl. Microbiol. Biotechnol. 61, 405–412. doi: 10.1007/s00253-003-1244-4
Słomka, A., Kuta, E., Szarek-Łukaszewska, G., Godzik, B., Kapusta, P., Tylko, G., et al. (2011). Violets of the section Melanium, their colonization by arbuscular mycorrhizal fungi and their occurrence on heavy metal heaps. J. Plant Physiol. 168, 1191–1199. doi: 10.1016/j.jplph.2011.01.033
Strasser, R. J., Srivastava, A., and Govindjee. (1995). Polyphasic chlorophyll a fluorescence transient in plants and cyanobacteria. Photochem. Photobiol. 61, 32–42. doi: 10.1111/j.1751-1097.1995.tb09240.x
Tukaj, Z., Bascik-Remisiewicz, A., Skowronski, T., and Tukaj, C. (2007). Cadmium effect on the growth, photosynthesis, ultrastructure and phytochelatin content of green microalga Scenedesmus armatus: a study at low and elevated CO2 concentration. Environ. Exp. Bot. 60, 291–299. doi: 10.1016/j.envexpbot.2006.12.002
Tullio, M., Pierandrei, F., Salerno, A., and Rea, E. (2003). Tolerance to cadmium of vesicular arbuscular mycorrhizae spores isolated from a cadmium-polluted and unpolluted soil. Biol Fertil. Soils 37, 211–214.
Xu, P. L., Christie, P., Liu, Y., Zhang, J. L., and Li, X. L. (2008). The arbuscular mycorrhizal fungus Glomus mosseae can enhance arsenic tolerance in Medicago truncatula by increasing plant phosphorus status and restricting arsenate uptake. Environ. Pollut. 156, 215–220. doi: 10.1016/j.envpol.2008.01.003
Xue, Z. C., and Gao, H. Y. (2017). The difference of photosynthetic responses to the cadmium stress between a wild soybean (Glycine soja Sieb. et Zucc.) and a cultivated soybean. Bull. Environ. Contam. Toxicol. 99, 405–410. doi: 10.1007/s00128-017-2143-1
Zhang, F., Wan, X. Q., and Zhai, J. (2014). Effects of nitrogen supplement on chlorophyll synthesis and chloroplast ultrastructure of poplar plants under cadmium stress. J. Nuclear Agric. Sci. 28, 485–491.
Zhang, F. Q., Wang, Y. S., Luo, Z. P., and Dong, J. D. (2007). Effect of heavy metal stress on antioxidative enzymes and lipid peroxidation in leaves and roots of two mangrove plant seedlings (Kandelia candel and Bruguiera gymnorrhiza). Chemosphere 67, 44–50. doi: 10.1016/j.chemosphere.2006.10.007
Zhang, H., Feng, P., Yang, W., Sui, X., Li, X., Li, W., et al. (2018). Effects of flooding stress on the photosynthetic apparatus of leaves of two Physocarpus cultivars. J. For. Res. 29, 1049–1059. doi: 10.1007/s11676-017-0496-2
Zhang, H. H., Tang, M., Chen, H., Zheng, C. L., and Niu, Z. C. (2010). Effect of inoculation with AM fungi on lead uptake, translocation and stress alleviation of Zea mays L. seedlings planting in soil with increasing lead concentrations. Eur. J. Soil Biol. 46, 306–311. doi: 10.1016/j.ejsobi.2010.05.006
Zhang, H.-h., Xu, N., Li, X., Jin, W.-w., Tian, Q., Gu, S.-y., et al. (2017). Overexpression of 2-Cys Prx increased salt tolerance of photosystem II in tobacco. Int. J. Agric. Biol. 19, 735–745. doi: 10.17957/IJAB/15.0348
Zhang, H. H., Zhang, X. L., Li, X., Ding, J. N., Zhu, W. X., Qi, F., et al. (2012). Effects of NaCl and Na2CO3 stresses on the growth and photosynthesis characteristics of Morus alba seedlings. Chin. J. Appl. Ecol. 23, 625–631.
Zhang, H. H., Zhong, H. X., Wang, J. F., Sui, X., and Xu, N. (2016). Adaptive changes in chlorophyll content and photosynthetic features to low light in Physocarpus amurensis Maxim and Physocarpus opulifolius “Diabolo”. Peer J. 4:e2125. doi: 10.7717/peerj.2125
Zhang, J., Liang, Y. C., Lou, Y. S., and Hua, H. (2005). Effect of cadmium on photosynthesis parameters, leaf soluble sugar and plant growth in two rice cultivars. Plant Nutr. Fertil. Sci. 11, 774–780.
Keywords: Lolium perenne L., cadmium (Cd), arbuscular mycorrhizal fungi, photosynthetic characteristics, PSII
Citation: Zhang H, Xu N, Li X, Long J, Sui X, Wu Y, Li J, Wang J, Zhong H and Sun GY (2018) Arbuscular Mycorrhizal Fungi (Glomus mosseae) Improves Growth, Photosynthesis and Protects Photosystem II in Leaves of Lolium perenne L. in Cadmium Contaminated Soil. Front. Plant Sci. 9:1156. doi: 10.3389/fpls.2018.01156
Received: 08 February 2018; Accepted: 20 July 2018;
Published: 13 August 2018.
Edited by:
Hazem M. Kalaji, Warsaw University of Life Sciences, PolandReviewed by:
Giacomo Cocetta, Università degli Studi di Milano, ItalyAnjana Jajoo, Devi Ahilya Vishwavidyalaya, India
Amarendra Narayan Misra, Khallikote University, India
Garcia-Sanchez Francisco, Centro de Edafología y Biología Aplicada del Segura (CEBAS), Spain
Copyright © 2018 Zhang, Xu, Li, Long, Sui, Wu, Li, Wang, Zhong and Sun. This is an open-access article distributed under the terms of the Creative Commons Attribution License (CC BY). The use, distribution or reproduction in other forums is permitted, provided the original author(s) and the copyright owner(s) are credited and that the original publication in this journal is cited, in accordance with accepted academic practice. No use, distribution or reproduction is permitted which does not comply with these terms.
*Correspondence: Nan Xu, eHVuYW4wNDUxQDEyNi5jb20= Haixiu Zhong, NjE1NzI5NDc4QHFxLmNvbQ==; cmQwMDE3QHFxLmNvbQ== Guang Y. Sun, c3VuZ3lAdmlwLnNpbmEuY29t
†These authors have contributed equally to this work