- 1Department of Biology, Colorado State University, Fort Collins, CO, United States
- 2Department of Biology, Laramie County Community College, Cheyenne, WY, United States
- 3Department of Sciences and Mathematics, Texas A&M University-San Antonio, San Antonio, TX, United States
- 4Advanced Light Source, Lawrence Berkeley National Laboratory, Berkeley, CA, United States
Endophytes can enhance plant stress tolerance by promoting growth and affecting elemental accumulation, which may be useful in phytoremediation. In earlier studies, up to 35% elemental selenium (Se0) was found in Se hyperaccumulator Astragalus bisulcatus. Since Se0 can be produced by microbes, the plant Se0 was hypothesized to be microbe-derived. Here we characterize a fungal endophyte of A. bisulcatus named A2. It is common in seeds from natural seleniferous habitat containing 1,000–10,000 mg kg-1 Se. We identified A2 as Alternaria tenuissima via 18S rRNA sequence analysis and morphological characterization. X-ray microprobe analysis of A. bisulcatus seeds that did or did not harbor Alternaria, showed that both contained >90% organic seleno-compounds with C-Se-C configuration, likely methylselenocysteine and glutamyl-methylselenocysteine. The seed Se was concentrated in the embryo, not the seed coat. X-ray microprobe analysis of A2 in pure culture showed the fungus produced Se0 when supplied with selenite, but accumulated mainly organic C-Se-C compounds when supplied with selenate. A2 was completely resistant to selenate up to 300 mg L-1, moderately resistant to selenite (50% inhibition at ∼50 mg Se L-1), but relatively sensitive to methylselenocysteine and to Se extracted from A. bisulcatus (50% inhibition at 25 mg Se L-1). Four-week old A. bisulcatus seedlings derived from surface-sterilized seeds containing endophytic Alternaria were up to threefold larger than seeds obtained from seeds not showing evidence of fungal colonization. When supplied with Se, the Alternaria-colonized seedlings had lower shoot Se and sulfur levels than seedlings from uncolonized seeds. In conclusion, A. tenuissima may contribute to the Se0 observed earlier in A. bisulcatus, and affect host growth and Se accumulation. A2 is sensitive to the Se levels found in its host’s tissues, but may avoid Se toxicity by occupying low-Se areas (seed coat, apoplast) and converting plant Se to non-toxic Se0. These findings illustrate the potential for hyperaccumulator endophytes to affect plant properties relevant for phytoremediation. Facultative endophytes may also be applicable in bioremediation and biofortification, owing to their capacity to turn toxic inorganic forms of Se into non-toxic or even beneficial, organic forms with anticarcinogenic properties.
Introduction
Selenium is not only toxic at elevated concentrations but also an essential micronutrient for many organisms including humans. The gap between Se deficiency and toxicity is narrow, and both are problems worldwide. Selenium is toxic due to its similarity to sulfur (S). Selenium readily replaces S in proteins, interfering with their function (Stadtman, 1990). In the Western United States, where many soils have elevated Se concentrations, chronic ingestion of high-Se plants by livestock has been reported to result in large livestock losses (Rosenfeld and Beath, 1964; Wilber, 1980).
Selenium serves no known essential function in plants, nor in fungi (Zhang and Gladyshev, 2009). In some microbes and fungi, Se is potentially used as a weak electron acceptor under anaerobic conditions (Heider and Böck, 1993). Selenium can also be beneficial to plants: it has been reported to increase growth and antioxidant activity (Hartikainen, 2005). At higher levels, Se offers plants protection against a wide variety of herbivores (Hanson et al., 2003; Freeman et al., 2006a).
Plants readily take up and assimilate Se into organic compounds, due to the similarities of Se and S (Schiavon and Pilon-Smits, 2017b). Hyperaccumulators can accumulate and tolerate up to 15,000 mg Se kg-1, and are also unique in that they preferentially take up Se over S and allocate Se to the reproductive tissues, i.e., flowers and seeds (Quinn et al., 2011a; Valdez Barillas et al., 2012; El Mehdawi et al., 2018). Selenium accumulation in plants can be used for phytoremediation as well as biofortification (Schiavon and Pilon-Smits, 2017a).
Several hypotheses have been proposed for why plants hyperaccumulate toxic elements like Se: inadvertent uptake, drought tolerance, elemental tolerance, allelopathy, and elemental defense against herbivores and pathogens (Boyd and Martens, 1992). For Se hyperaccumulators, the evidence for the elemental defense hypothesis is well supported. Selenium has been shown to protect plants from a variety of generalist, Se-sensitive herbivores, for a review see El Mehdawi and Pilon-Smits (2012). There is also evidence that hyperaccumulators may deposit Se in the surrounding soil as a form of elemental allelopathy against Se-sensitive neighboring plants (El Mehdawi et al., 2011a).
While Se-sensitive ecological partners suffer in their interactions with Se hyperaccumulators, Se-resistant partners may exploit the high-Se niche offered by hyperaccumulator plants. Se-resistant herbivores have been found to feed on hyperaccumulator seeds and leaves. In some of these herbivores resistance is based on tolerance and in others it is based on exclusion (Freeman et al., 2006a, 2010; Valdez Barillas et al., 2012). Furthermore, Se-tolerant neighboring plants of hyperaccumulators in the field were shown to benefit from their proximity to hyperaccumulators: they exhibited enhanced Se levels, which made them less susceptible to herbivory (El Mehdawi et al., 2011b). Selenium tolerance in these ecological partners was often associated with the accumulation of organic Se (e.g., methylselenocysteine, MeSeCys) in their tissues. Selenium hyperaccumulators also contain mostly MeSeCys, which may explain their extreme Se tolerance. MeSeCys cannot be incorporated into protein, and thus Se toxicity is avoided (Terry et al., 2000).
Relatively little is known about how Se affects the plant–microbe interactions of hyperaccumulators. Depending on whether the associated microbe lives in the rhizoplane (surface of roots), phyllosphere (surface of leaves), or as endophyte (inside plant tissues), it may experience different Se levels, and with that, Se toxicity (Valdez Barillas et al., 2011, 2012). The microbe’s relationship with the plant may involve pathogenicity, mutualism, and commensalism. Some microbes may perform beneficial functions for the hyperaccumulator: stimulating growth, aiding in nutrient and water acquisition, or fighting off pathogens. In hyperaccumulators, microbes may also affect the acquisition, speciation, and accumulation of the hyperaccumulated element (de Souza et al., 1999; Di Gregorio et al., 2006; Alford et al., 2010).
There is evidence that Se can protect plants from Se-sensitive microbial pathogens. In a study with non-hyperaccumulator Brassica juncea, Se was shown to protect plants from two Se-sensitive fungal pathogens, Alternaria brassicicola and a Fusarium oxysporum (Hanson et al., 2003). There is also evidence for the presence of Se-resistant microbes that live in association with hyperaccumulators (Wangeline et al., 2011). A litter decomposition experiment on seleniferous soil revealed that there were more culturable microbes (colony forming units per gram) on high-Se leaf litter from hyperaccumulators than on low-Se litter from related species collected from the same site (Quinn et al., 2011b). This finding may suggest that specialist Se-resistant decomposing microbes are present at seleniferous sites. Furthermore, a Se-resistant Rhizobacterium apparently lives in association with the hyperaccumulator Astragalus bisulcatus (Fabaceae), since this species produces high-Se nodules (Valdez Barillas et al., 2012). This bacterium may affect plant Se speciation, since the nodules accumulated a high fraction of elemental Se (Se0) (Valdez Barillas et al., 2012; Alford et al., 2014). Other endophytic bacteria were found to colonize this and other hyperaccumulators, which were also found to produce elemental Se (Staicu et al., 2015; Sura-de Jong et al., 2015).
Interestingly, roots of Se hyperaccumulators collected from the field contained high fractions of Se0 (up to 35%) while greenhouse-grown counterparts contained exclusively organic selenocompounds with a C-Se-C configuration (Se attached to two organic groups, Lindblom et al., 2013a). Based on these findings it was hypothesized that microbes are responsible for the production of Se0 observed in hyperaccumulators in their natural habitat. To test this hypothesis, hyperaccumulator plants were grown from surface-sterilized seeds and inoculated with several root-associated fungi shown earlier to be able to produce Se0. However, no significant effect on plant Se speciation was observed (Lindblom et al., 2013b, 2014).
In this study we test another hyperaccumulator-associated fungus that appears to be a seed-transmitted endophyte that asymptomatically colonizes stems and leaves of A. bisulcatus in their natural habitat. It was found to emerge regularly from surface-sterilized seeds of A. bisulcatus, and small spored Alternaria species could readily be cultured from surface-sterilized stem and leaf tissue (Valdez Barillas et al., 2012). This endophytic fungus clearly has a close association with the hyperaccumulator and thus maximal opportunity to impact plant Se speciation. In this work we identify this fungal endophyte using a combination of molecular and morphological characters, characterize the Se-related properties of the pure isolate (Se tolerance, Se metabolic properties), and test its impact on plant Se speciation, Se accumulation, and growth.
Materials and Methods
Biological Material
Astragalus bisulcatus seeds were collected in Pineridge Natural Area, Fort Collins, CO, United States during 2008–2011. The seeds were stored in coin envelopes inside a silica gel desiccator at 4°C until use. For endophyte isolation, the seeds were first surface-scarified using a scalpel blade and surface-sterilized with 50% bleach for 5 min, then rinsed three times with sterile water. Seeds were transferred to petri dishes with half strength water agar and were allowed to germinate at room temperature. Fungal mycelia growing from the seed were then transferred to half-strength malt extract agar (0.5 MEA, Difco, Detroit, MI, United States) via hyphal tipping. Hyphal tipping was repeated at least two times to ensure the fungal was a pure culture. The isolate was designated as A2.
Sample Preparation for X-Ray Microprobe Analyses
Astragalus bisulcatus seeds were surface-sterilized as described above and germinated on 0.5 strength Murashige and Skoog (1962) basal salts agar medium containing 30 mg L-1 Na2SeO4. Two seeds were selected for X-ray microprobe analyses: one that showed the presence of A2 fungal mycelium and one that did not. These seeds were frozen at -80°C until analysis.
Agar plugs (0.5 cm × 0.5 cm) of A2 fungal mycelia were transferred to liquid malt extract medium containing 30 mg L-1 Na2SeO4 or 30 mg L-1 Na2SeO3. Sections of approximately 3 mm3 of A2 fungus mycelia were washed briefly in 1 mM sulfate to removed adsorbed Se. Each section was immediately placed inside a separate 0.5 ml centrifuge plastic tube, frozen in liquid nitrogen, and stored at -80°C.
Determination of Fungal Se Tolerance
For the analysis of A2 fungal tolerance to different seleno-compounds, the fungus was cultivated under continuous fluorescent light at 22°C in sealed Petri dishes containing 0.5 strength MEA supplemented with Na2SeO4 or Na2SeO3 at 0, 10, 30, or 300 mg L-1. Fungal tolerance was also tested on different concentrations of MeSeCys (0, 10, 30, 60, 150 mg L-1) in 0.5 MEA as well as on extract made from the flowers of A. bisulcatus added at these same Se concentrations to 0.5 MEA.
Fungal Identification
The A2 fungus was grown on V-8 juice agar with continuous light in unsealed plates. Potato carrot agar was used for slide culture conditions and comparison colony conditions with a 8 h light - 16 h dark cycle in unsealed plates. Morphological characterization was carried out as described below in the results section.
For molecular identification of the A2 fungus, DNA extraction, Polymerase chain reaction (PCR) and sequencing were done using the ITS 1 and 4 primers (White et al., 1990), following the protocol by Vincelli and Tisserat (2008).
Analysis of Plant Growth and Se Accumulation as Influenced by Endophytic Alternaria
Seeds of A. bisulcatus were first scarified for 10 min with concentrated sulfuric acid, and then further surface-sterilized by rinsing for 20 min in 20% bleach, followed by five 10-min rinses in sterile water. Seeds were then germinated on sterile filter paper under continuous light at 23°C in a plant growth cabinet. Upon germination, seedlings were separated into those that naturally contained the endophyte and two that did not, and transferred to culture tubes containing autoclaved potting soil. Half of the seedlings in each group, (A2-associated and seeds without A2), were watered with 80 μM selenate in liquid 0.5 MS medium while the other half were given medium without Se. The culture tubes were sealed with breathable tape and opened only to add fresh medium. There were ten replicates per treatment (40 total). The experiment was terminated after 4 weeks. At that point half of the replicates in the control group had died (i.e., the group without the fungus and without Se added).
Elemental Analysis
At harvest the plant roots were washed and then dried for 48 h at 45°C. Samples were digested in nitric acid as described by Zarcinas et al. (1987). Inductively coupled plasma atomic emission spectrometry (ICP-AES) was used to determine Se and S concentrations in the acid digest (Fassel, 1978).
X-Ray Microprobe Analyses
Elemental distribution and chemical speciation in the tissues were determined using μ X-ray fluorescence mapping (XRF) and μ X-ray absorption near-edge structure (XANES) spectroscopy, respectively, at the Advanced Light Source beamline 10.3.2 of the Lawrence Berkeley National Lab (Marcus et al., 2004). Frozen samples were transferred onto a Peltier stage kept at -25°C to reduce potential beam radiation damage. μXRF elemental maps were recorded at 13 keV, using a 15 μm (H) × 6 μm (V) beam, 15 μm × 15 μm pixel size, 50 ms dwell time per pixel. The chemical forms of Se in particular areas of interest were further investigated using Se K-edge XANES, at the tissue locations indicated in Figures 1, 4. XANES provides information about the oxidation state and, when compared to well-characterized Se standard compounds, information about its chemical speciation (Pickering et al., 1999). XRF maps and XANES spectra were recorded with a seven element Ge solid state detector (Canberra, ON, Canada). Spectra were deadtime corrected, pre-edge background subtracted, and post-edge normalized using standard procedures (Kelly et al., 2008). Red amorphous elemental selenium (white line position set at 12660 eV) was used to calibrate the spectra. Least square linear combination (LSQ) fitting of Se XANES spectra was performed in the 12630–12850 eV range, using a library of standard seleno-compounds. As Se standards a library of 52 compounds was used (Fakra et al., 2018). All data processing and analyses were performed with a suite of custom LabVIEW (National Instruments, Austin, TX, United States) programs available at the beamline. Se valence-state scatter plots of the sample and standard compounds data were also obtained using MATLAB, following methods described in details elsewhere (Fakra et al., 2018).
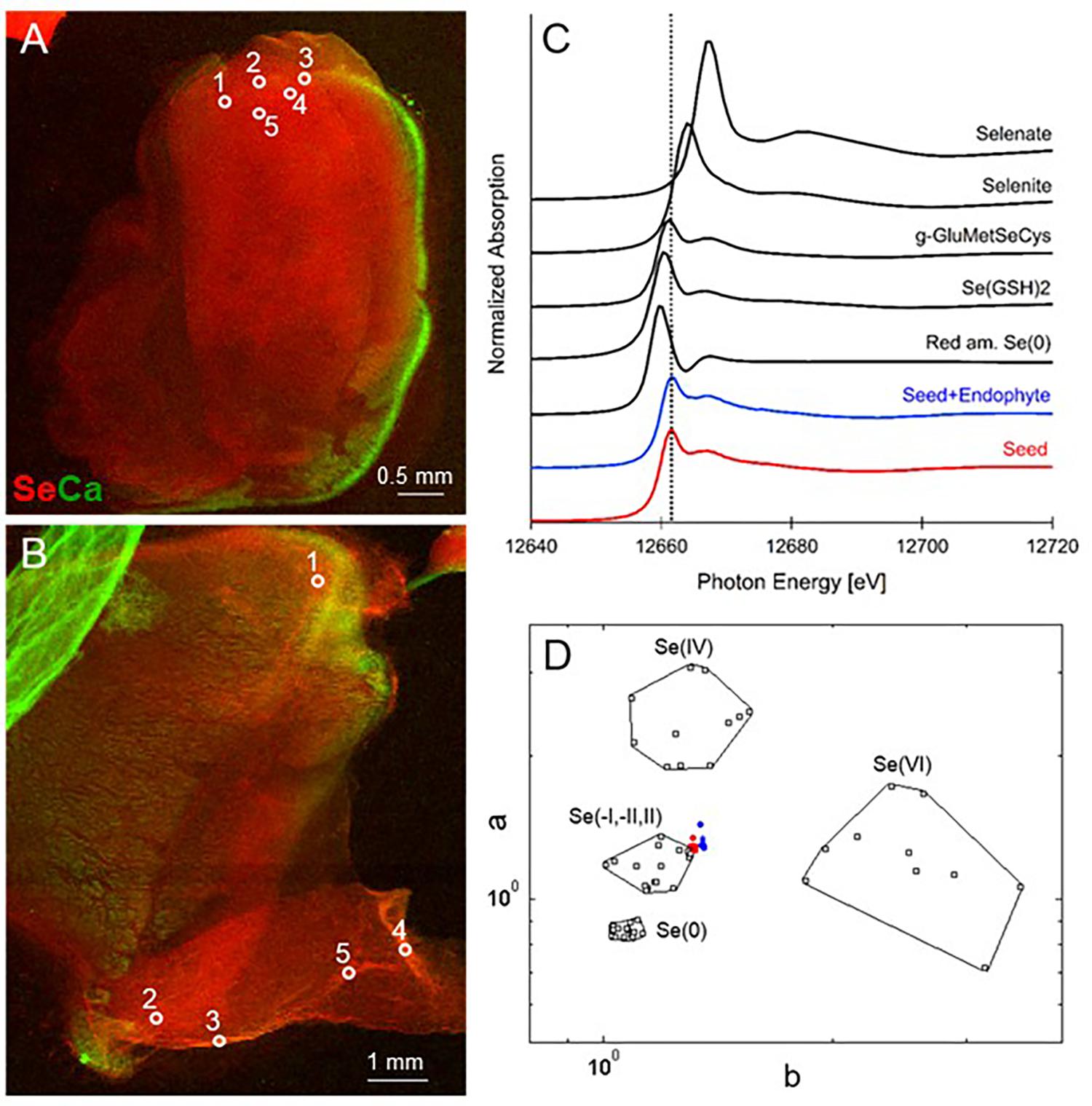
FIGURE 1. Selenium distribution and speciation in germinating A. bisulcatus seeds. (A,B) are bicolor-coded XRF maps showing the distribution of Se (in red) and Ca (in green). (A) Seed not containing fungal endophyte A2, (B) seed containing A2. Locations where XANES spectra were collected are indicated by white circles. The seedlings are oriented with their radicle at the bottom, pointing left (A) or right (B) and the cotyledons toward the top. Selenium is clearly localized in the seed embryo; the seed coat contains Ca. (C) Average XANES spectrum obtained from five locations for each sample, compared to Se-bearing standards. (D) Se valence-state scatter plot obtained from XANES spectra of the seed (red dots), seed+endophyte (blue dots) compared to Se standards (black empty squares). The hexagonal datapoints correspond to the average spectrum for each sample.
Statistical Analysis
The software JMP-IN (3.2.6, SAS Institute, Cary, NC) was used for statistical data analysis. Analysis of variance followed by a post hoc Tukey Kramer test was used when comparing averages of Se content and averages of plant biomass among selenium treated and untreated A. bisulcatus replicates. A student t-test was used for pairwise comparisons between two means (using an alpha error = 0.5). It was verified that the assumptions underlying these tests (normal distribution, equal variance) were met.
Results
X-Ray Microprobe Analysis of Seeds
When seeds of A. bisulcatus were surface-sterilized and germinated on sterile filter paper, about half of them contained an endophytic fungus, which was designated A2. Germination trials typically have shown around 50% infestation by Alternaria in A. bisulcatus germinated seeds based on visual estimation. To characterize the distribution and chemical speciation of Se in A. bisulcatus seeds, XRF and XANES analysis were performed on non-colonized vs. Alternaria-colonized seeds. Also, a valence plot was made, for a quick comparison of the fungal Se data with Se standards of known valence. Regardless of the presence or absence of fungus, Se was found in the embryo but not detected in the seed coat (Figure 1). There was no clear difference in Se speciation between Alternaria-colonized and uncolonized seeds (Table 1). Both contained predominantly (86–90%) organic Se with C-Se-C configuration, that fitted best with the Se standard γ-glutamyl-methylSeCys but may also include other C-Se-C compounds like SeMet or methyl-SeCys. In both seeds, there were small fractions of other selenocompounds that correspond with Se(IV) and Se(VI) oxidation states (forms of selenite and selenate, respectively) that fitted best with various metal selenate standards (Zn, Fe, and Cu selenate, particularly). The micro-XRF spectra (MCA) that were collected on each Se XANES spot indeed detected Ca, Fe, Zn, and Cu; at the energy we were exciting the sample with (13 keV), we were not very sensitive to elements below Ca (such as K, Cl, and S).
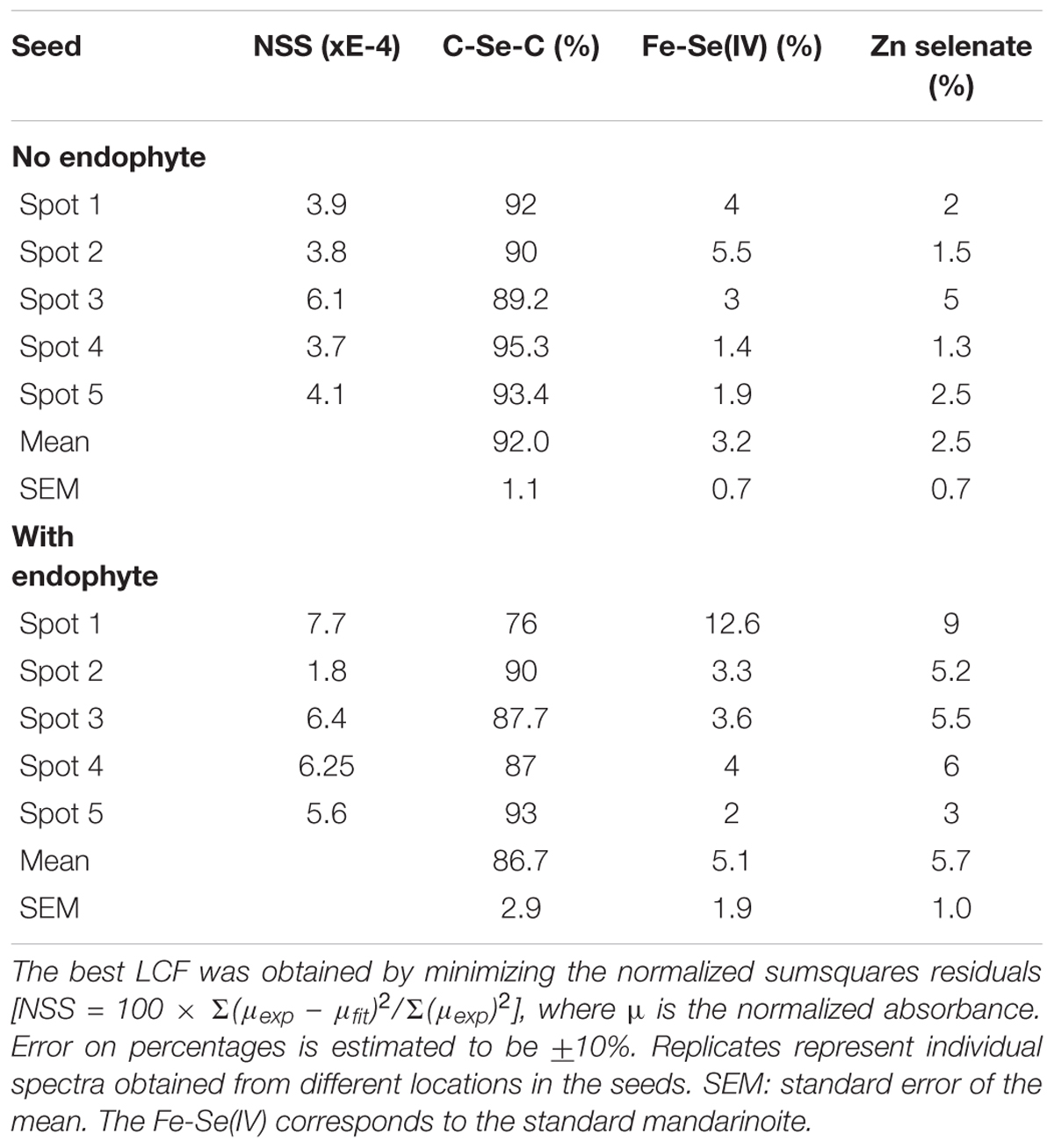
TABLE 1. Astragalus bisulcatus seed Se speciation results obtained from least squares linear combination fitting (LCF) of the XANES spectra collected at the spots shown (as white circles) in Figure 1, using 52 standard seleno-compounds.
The identity of A2 was initially investigated by DNA sequencing of the internal transcribed spacer region of ribosomal genes (ITS 1 and 4) of the small ribosomal subunit. As shown in Figure 2A, the sequence from A2 showed 100% sequence similarity with the known plant pathogen Alternaria tenuissima and 99.6% similarity with Alternaria astragali, a rhizosphere fungus associated with A. bisulcatus (Wangeline and Reeves, 2007). Small-spored Alternaria, particularly A. alternata and A. tenuissima, are difficult to distinguish using solely molecular techniques (Andrew et al., 2009), so morphological characteristics were included for identification (Simmons, 2007). The references were updated accordingly (Simmons, 2007). To date A. tenuissima is grouped among other small-spored Alternaria that show no association between host, geographic origin and phylogenetic lineage, and is considered by some as an unresolved group associated with Alternaria alternata (Andrew et al., 2009).
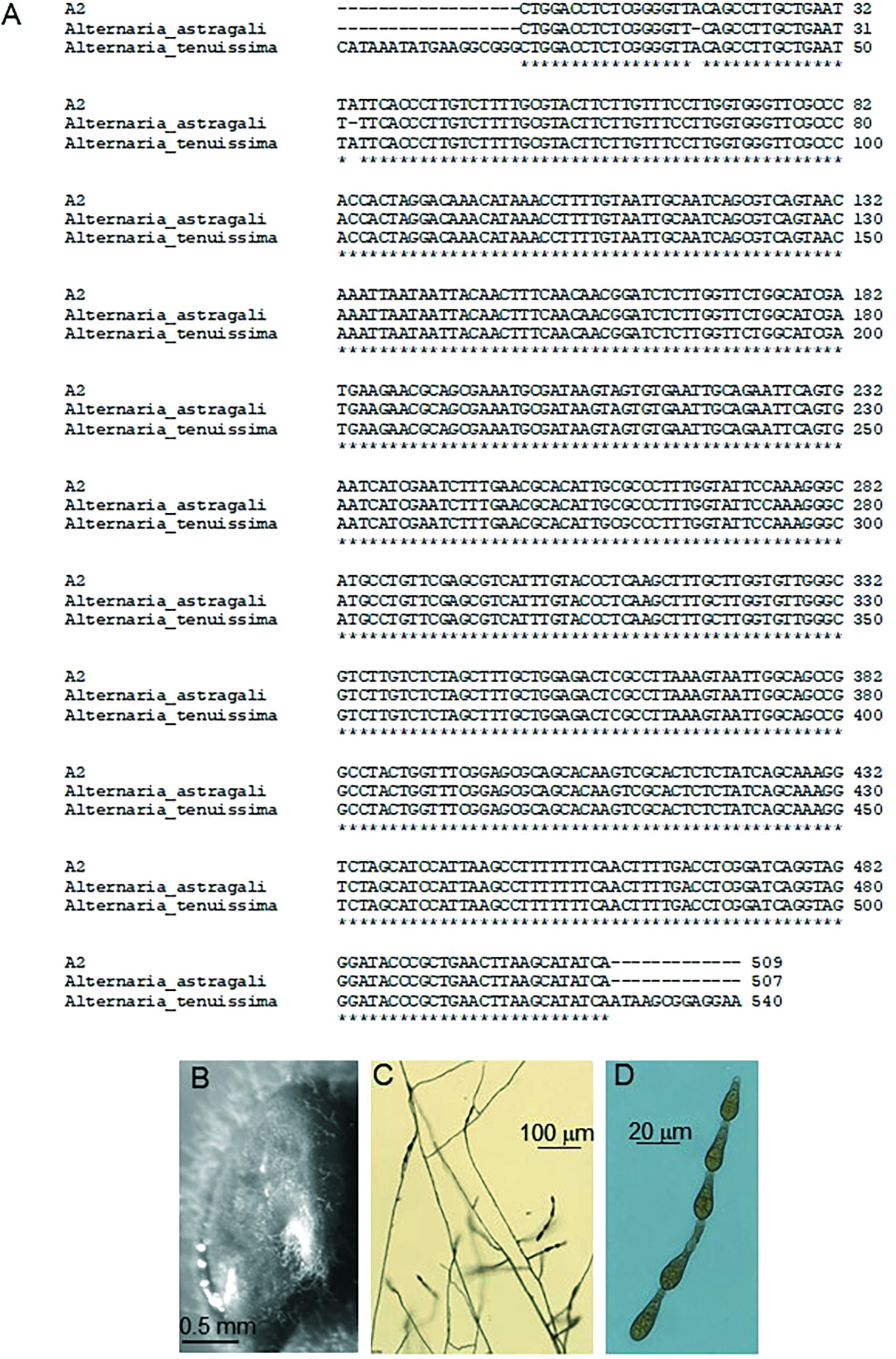
FIGURE 2. Information used to identify A2 fungus. (A) ITS sequence alignment with Alternaria astragali (99%) and Alternaria tenuissima (100%). (B) Surface-sterilized A. bisulcatus seed with A2 endophyte mycelia emerging from the seed coat. (C) Conidiophores and conidia of A2, and (D) magnified conidia.
Fungal Isolate A2 Taxonomic Description
The A2 fungal strain was identified as A. tenuissima (Nees and T. Nees: Fr.) Wiltshire, anamorph (no known teleomorph), with the following specific morphology.
Colony at 7–8 days, PCA and V-8: colony 5–6 cm diameter. On PCA is a surface network of interwoven radial hyphae, gray to buff with little sporulation and no discernible rings. Also present is a dense mat of reduced aerial hyphae. The reverse is dark green to black and uniform. The colony on V-8 is similar to PCA. Mycelium cottony, with an advancing colony edge of approximately 10 mm. Colony has dark mycelial rings alternating with dense aerial rings when infrequently present. Mycelia gray, buff, and medium to dark brown. Mycelia layers upward with increasing age. The reverse is dark green to black.
Conidiophores (40×) produced on V-8 range on average between 130–155 μm in length (Figures 2C,D). Juvenile conidia are ovoid and have no definable beak, while most mature into a body narrowly ellipsoid with a long beak. The conidiophores are mostly simple but may have low amounts of branching (1–2), sometimes proliferating at 1–2 conidiogenous sites. Conidia chains of 2–6 (8) are produced (most common 4). Older areas of mycelial growth can have a distinctive rope-like formation, commonly up to or less commonly surpassing 11 μm wide.
Conidium bodies (100×) are ovoid or long ellipsoid; commonly with a long beak or less commonly with no distinctive beak. Conidium length, with beak, ranging from 14 to 39 μm with 2–5 transverse septa, width ranging from 6 to 11 μm with 0–3 longitudinal septa, and beak length from 1.5 to 17 μm. Noteworthy is that mature conidia have beaks >10 μm, and continue to divide, becoming septate in both the beak (2–3 cells) and body gaining additional longitudinal septa. Also noteworthy that roughly 50% of conidia have zero longitudinal septa. Few conidium walls are rough or thickened.
Selenium Tolerance of the A. tenuissima A2 Strain
The Se tolerance of the A2 fungus to different forms and levels of Se when grown on MEA is shown in Figure 3. The fungus was most tolerant to selenate: its growth was still 90% of the control when supplied with 300 mg Se L-1 (Figure 3A). The fungus was also fairly tolerant to selenite: it showed 50% inhibition around 100 mg Se L-1 (Figure 3B). To test A2 growth as a function of plant-derived Se, flower material was extracted in water and added to the growth medium at different dilutions. The Se concentration in the extract was determined using ICP-AES. The fungus was significantly inhibited by the plant extract, showing 50% inhibition around 25 mg Se L-1 (Figure 3C). To test whether this was likely due to the Se, particularly C-Se-C, or (also) to other growth inhibiting compounds in the flowers, fungal growth was also determined as a function of MeSeCys concentration. When pure MeSeCys was added to the medium, the growth of A2 was 50% inhibited around 20 mg Se L-1 (Figure 3D), i.e., A2 growth was similarly inhibited by pure MeSeCys and by the Se extracted from A. bisulcatus.
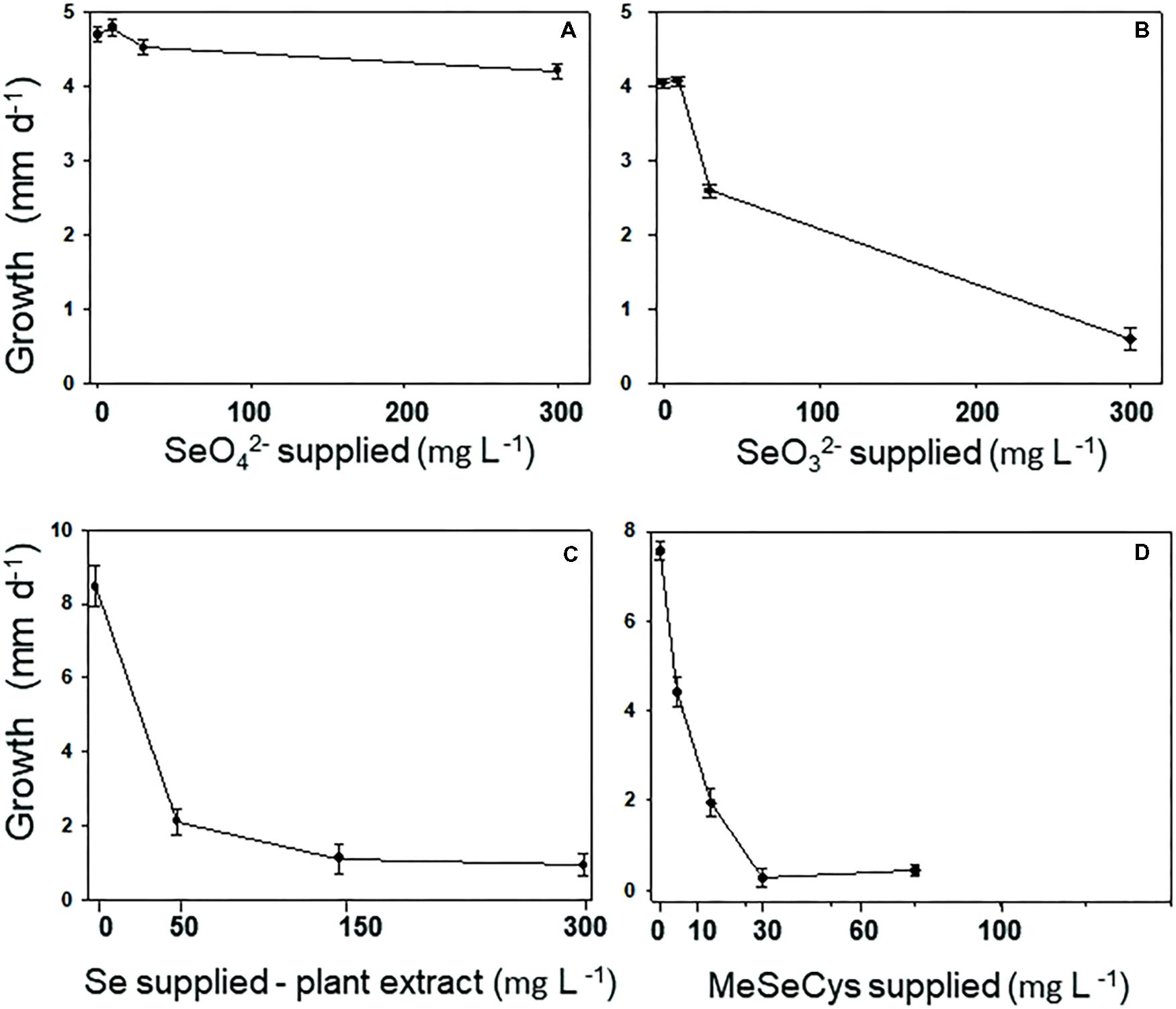
FIGURE 3. Fungus A2 is resistant to selenate, moderately resistant to selenite, but relatively sensitive to organic MeSeCys and hyperaccumulator-derived Se (= MeSeCys). A2 resistance was measured as growth of the colony per day on varying concentrations of (A) selenate, (B) selenite, (C) extract from the flowers of Astragalus bisulcatus, and (D) methyl-selenocysteine.
X-Ray Microprobe Analysis of A2 Mycelium Grown on Medium With Selenate or Selenite
X-ray microprobe analysis was carried out on mycelia of A2 that was grown on fungal growth media spiked with selenate or selenite. XRF maps and XANES spectra are shown in Figure 4, as well as a valence plot for a quick comparison of the fungal Se data with Se standards of known valence. The detailed Se speciation results are listed in Table 2. When the fungus was supplied with selenite (SeO32-), 83% of Se in the mycelia was present as Se0; the remainder was best matching the seleno-diglutathione (Se-GSH2) standard. When supplied with selenate (SeO42-) the fungus accumulated a large fraction (67%) of Se as C-Se-C, as well as a substantial fraction of selenate (27%), and a minor fraction of Se-GSH2 (6%).
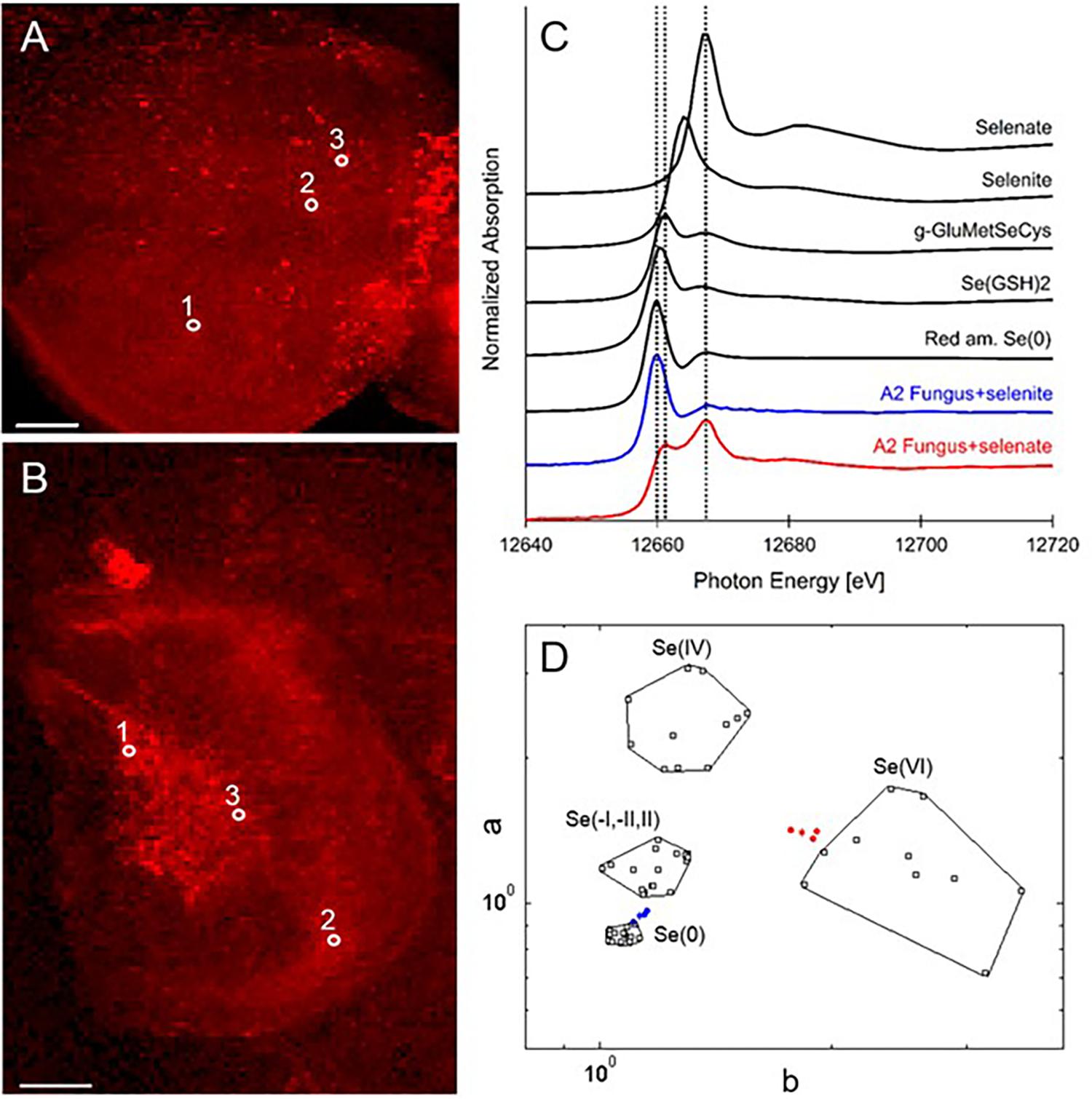
FIGURE 4. Selenium distribution and speciation in A2 fungal material (mycelium) collected after growth on MEA medium spiked with selenate or selenite. (A) XRF Se map of A2 supplied with selenate. (B) XRF Se map of A2 supplied with selenite. Scale bars are 500 microns. (C) Average XANES spectrum obtained from three locations for each sample (white circles in A,B), compared to Se standards. (D) Se valence-state scatter plot obtained from XANES spectra of A2 supplied with selenate (in red), A2 supplied with selenite (in blue), compared to Se standards (in black). The hexagonal datapoints correspond to the average spectrum for each sample.
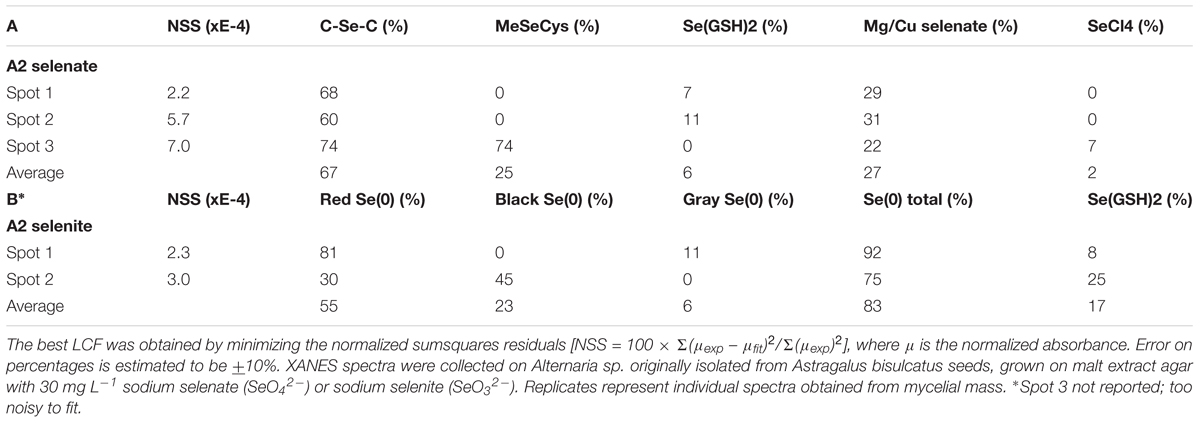
TABLE 2. Selenium speciation results obtained from least squares LCF of experimental XANES spectra collected from the locations shown in Figure 4 with standard seleno-compounds.
Effect of A. tenuissima (A2) on A. bisulcatus Growth and Se and S Accumulation
Seeds with hyphae emerging during germination were separated from those that did not show hyphae, and the two groups were cultivated on sterile peat moss for 4 weeks with or without selenate, the main form of bioavailable Se found in soil. The A2-containing seedlings had reached a twofold to threefold greater dry weight compared to uncolonized seedlings (Figures 5A,B). The addition of Se did not significantly affect A. bisulcatus growth for either group (Figures 5A,B). The presence of the A2 fungus was also associated with reduced shoot Se and S levels (Figures 5C,D).
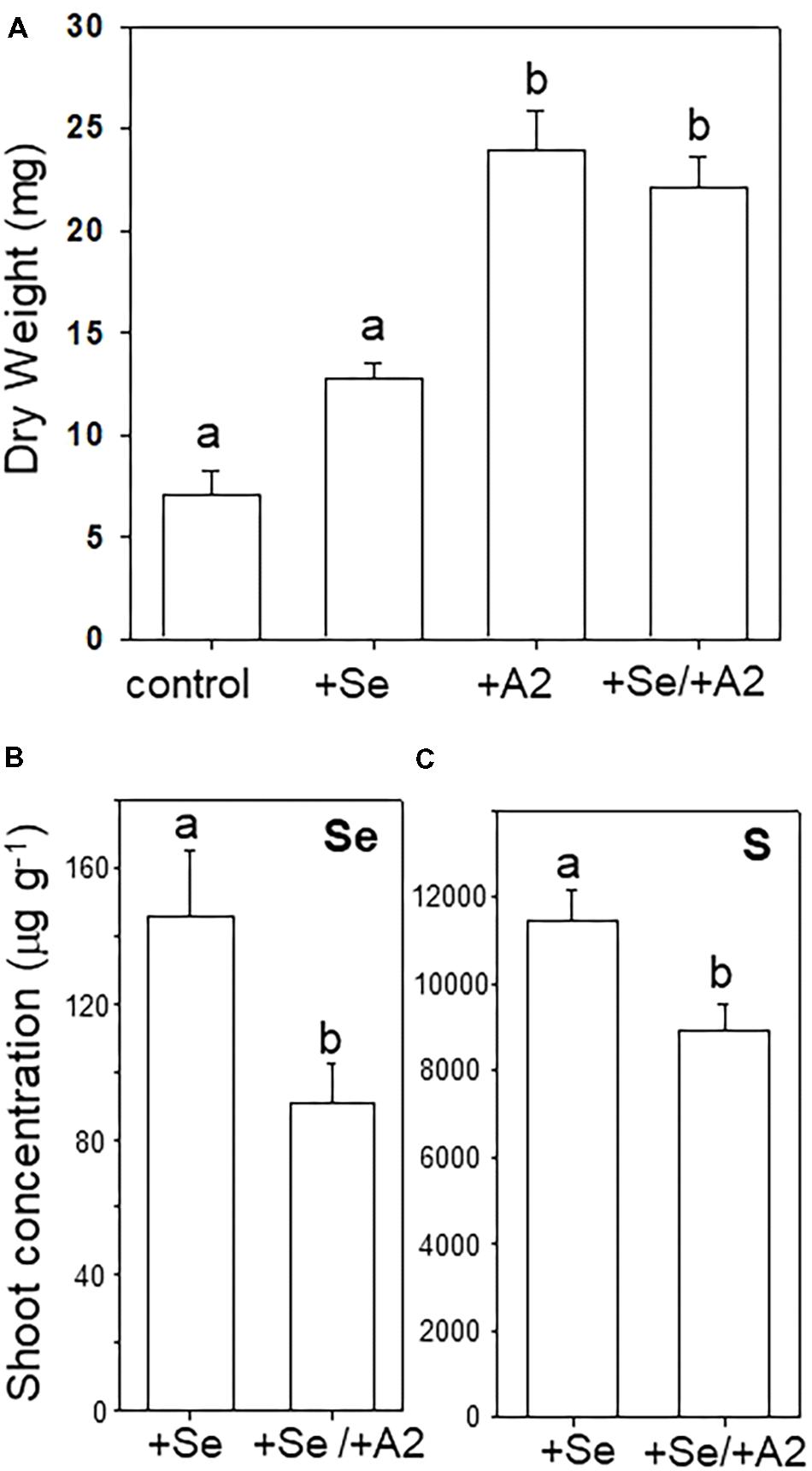
FIGURE 5. Effect of the presence of Alternaria endophyte on growth and Se and S accumulation in its host, Se hyperaccumulator A. bisulcatus. (A) Plant biomass. (B) Shoot Se concentration, (C) shoot sulfur concentration. Different letters above bars indicate significant differences (p < 0.05) between treatment means (n = 10).
Discussion
Hyperaccumulators of toxic elements are an interesting potential resource for discovery of microbes with properties useful in phytoremediation or bioremediation. Like all plants, Se hyperaccumulators harbor a variety of endophytic and rhizosphere bacteria and fungi (Wangeline et al., 2011; Sura-de Jong et al., 2015). While Se hyperaccumulator A. bisulcatus accumulates Se mainly in organic C-Se-C forms (methyl-SeCys, especially), up to 30% elemental Se0 was reported in the roots and stem of mature field-collected plants, as well as in seedlings germinated from field-collected seeds (Valdez Barillas et al., 2012; Lindblom et al., 2013a). The A2 fungal strain, identified here as A. tenuissima, may contribute to this fraction of Se0 in A. bisulcatus. It could be cultured from about 50% of field-collected, surface-sterilized A. bisulcatus seeds. In addition, small-spored Alternaria species, of which A. tenuissima is a member, could be cultured from surface-sterilized roots and stems of A. bisulcatus. In pure culture supplied with selenite, the A2 fungus was shown here to be capable of producing Se0. While A. tenuissima is known as a potential plant pathogen with a wide host range (Mishra and Parakash, 1975), there is no evidence from this study that it acts as a pathogen on A. bisulcatus. Alternaria-containing seedlings grew better than seedlings not containing this endophyte, so it actually may be growth-promoting. However, it is possible that the relationship between A. bisulcatus and A2 depends on the conditions, particularly the Se level, the overall nutrient supply and the health status of the plant.
The Se speciation in germinating Alternaria-colonized or uncolonized A. bisulcatus seeds was similar: 86–90% C-Se-C. This was likely MeSeCys and glutamyl-MeSeCys, as has been previously reported for seeds (Nigam and McConnell, 1969); MeSeCys has also been found to be the main form of Se in leaves (Freeman et al., 2006b) and flowers of this species (Valdez Barillas et al., 2012). In contrast, an earlier study by Valdez Barillas et al. (2012) found a seed in a late-stage Alternaria infestation to contain 22% Se0, both in the seed and the mycelium growing from the seed. These results suggest that this Alternaria can convert the C-Se-C in the seed to Se0, perhaps as a tolerance mechanism. Grown in pure culture, A2 produced Se0 when supplied with selenite. Conversion of more toxic forms of Se to insoluble, inert Se0 is known to be a tolerance mechanism for many microbes (Gharieb et al., 1994 and citations therein; Gadd, 1993; Lovely, 1993). A2 appears to have a different Se resistance mechanism for selenate, since it contained a variety of organic selenocompounds (C-Se-C) when supplied with this form of Se. The tolerance of A2 to selenate was much higher than for selenite. Surprisingly, although A2 grew very well on seeds that contained upward of 1,000 mg Se kg-1 (Galeas et al., 2007; Quinn et al., 2011a), it was already 50% inhibited by 25 mg kg-1 Se extracted from A. bisulcatus flowers, as well as by 20 mg kg-1 MeSeCys. A possible explanation for the ability of A2 to successfully grow on these high-Se plants could be that A2 occupies areas of the plant where there is relatively less Se, such as the interface between the seed coat and the seed embryo, and in the apoplast. As shown here, the seed coat contains very little Se, and in earlier studies energy dispersive X-ray analysis of hyperaccumulator leaves revealed that Se is generally stored in the vacuole in Se hyperaccumulators, and not in the apoplast (Freeman et al., 2006c, 2010). Thus, A2 may not encounter toxic Se levels in the living plant, like it does when grown on pure selenocompounds or homogenized plant extract.
The ITS sequence alignment identification of the fungus revealed an interesting similarity to another fungal-symbiont of A. bisulcatus, A. astragali (A3), which was originally isolated from the rhizoplane of surface-sterilized roots (Wangeline and Reeves, 2007). An additional Alternaria species, A. seleniiphila (A1) was isolated from the rhizoplane of hyperaccumulator Stanleya pinnata (Wangeline and Reeves, 2007). Both A1 and A3 were characterized for Se tolerance and speciation by Lindblom et al. (2013a). The Se-related characteristics of A2 are somewhat similar to A1 and A3. All are capable of reducing selenite to Se0 and all are fairly tolerant to selenate. All three also stimulated the growth of their hyperaccumulator host.
Perhaps related to its effect on Se speciation toward more insoluble Se0 in roots, the A2-containing A. bisulcatus seedlings showed significantly lower Se and S levels in their shoots. In previous studies where hyperaccumulators were inoculated with the related Alternaria species A1 and A3, there was a reduction in root-to-shoot translocation (Lindblom et al., 2013b, 2014). The same may be the case for A2; the root biomass was too small to determine root elemental concentrations. The possible mechanism for reduced translocation could be the production of Se0 in the rhizosphere or inside the root apoplast, trapping Se in a non-soluble and therefore non-translocatable form. In this context it is interesting to note that pure A2 cultures produced mainly C-Se-C compounds from selenate (the form provided in the seedling study), and elemental Se from selenite. Thus, if the A2 endophyte produced elemental Se in the root, the plant may have reduced the selenate to selenite first.
This study helps us understand the ecology of Alternaria fungi in relation to various hosts. This fungus is best known for its capacity to colonize different hosts including many domesticated crop species, where it may or may not act as a pathogen. Zou et al. (2018), however, report an Alternaria sp. fungal endophyte that acted as a plant growth promoting fungus. The increases in shoot and root biomass observed in that study were attributed to a plant metabolic upregulation induced by the fungal endophyte. In this non-domesticated Se hyperaccumulator A. tenuissima behaves asymptomatically similarly to other vertically transmitted fungal endophytes, perhaps due to the host’s elemental defense (the high levels of this toxic element may negatively affect pathogens) and limited access to nutrients by the host’s apoplast. However, it is apparently capable of colonizing the hyperaccumulator’s tissues and perpetuating its genetic line by keeping its host alive and colonizing the seeds. This is another example of Alternaria’s phenotypic plasticity, showing its ability to colonize a diverse range of hosts via different mechanisms and under different types of ecological interactions.
Author Contributions
SL performed the experiments and wrote the manuscript. AW and BD helped with the fungal identification and advised concerning fungal cultivation. SL, SF, and JVB helped with X-ray microprobe analysis. EP-S oversaw the project and helped with manuscript preparation.
Funding
Funding for these studies was provided by National Science Foundation Grant # IOS-0817748 to EP-S and NIH Grant # P20 RR016474 from the INBRE Program of the National Center for Research Resources to AW. Its contents are solely the responsibility of the authors and do not necessarily represent the official views of NSF or NIH. The Advanced Light Source is supported by the Office of Science, Basic Energy Sciences, and Division of Materials Science of the U.S. Department of Energy (DE-AC02-05CH11231).
Conflict of Interest Statement
The authors declare that the research was conducted in the absence of any commercial or financial relationships that could be construed as a potential conflict of interest.
Acknowledgments
We thank Ned Tisserat for helping with the molecular identification of the A2 fungus.
References
Alford, E. A., Pilon-Smits, E. A., and Paschke, M. (2010). Metallophytes – a view from the rhizosphere. Plant Soil 337, 33–50. doi: 10.1007/s11104-010-0482-3
Alford, E. R., Lindlbom, S. D., Pittarello, M., Freeman, J. L., Fakra, S. C., Marcus, M. A., et al. (2014). Roles of rhizobial symbionts in selenium hyperaccumulation in Astrgalus (Fabaceae). Am. J. Bot. 101, 1895–1905. doi: 10.3732/ajb.1400223
Andrew, M., Peever, T. L., and Pryor, B. M. (2009). An expanded multilocus phylogeny does not resolve morphological species within the small-spored Alternaria species complex. Mycologia 101, 95–109. doi: 10.3852/08-135
Boyd, R. S., and Martens, S. N. (1992). “The raison d’être for metal for metal hyperaccumulation by plants,” in The Vegetation of Ultramafic (Serpentine) Soils, eds A. J. M. Baker, J. Proctor, and R. D. Reeves (Andover: Intercept), 279–289.
de Souza, M. P., Chu, D., May, Z., Zayed, A. M., Ruzin, S. E., Schichnes, D., et al. (1999). Rhizosphere bacteria enhance selenium accumulation and volatilization by Indian mustard. Plant Physiol. 199, 565–573. doi: 10.1104/pp.119.2.565
Di Gregorio, S., Lampis, S., Malorgio, F., Petuzzelli, G., Pezzarossa, B., and Vallini, G. (2006). Brassica juncea can improve selenite and selenate abatement in selenium contaminated soils through the aid of its rhizospheric bacterial population. Plant Soil 285, 233–244. doi: 10.1007/s11104-006-9010-x
El Mehdawi, A. F., Jiang, Y., Guignardi, Z. S., Esmat, A., Pilon, M., Pilon-Smits, E. A., et al. (2018). Influence of sulfate supply on selenium uptake dynamics and expression of sulfate/selenate transporters in selenium hyperaccumulator and non-hyperaccumulator Brassicaceae. New Phytol. 217, 194–205. doi: 10.1111/nph.14838
El Mehdawi, A. F., and Pilon-Smits, E. A. (2012). Ecological aspects of plant selenium hyperaccumulation. Plant Biol. 14, 1–10. doi: 10.1111/j.1438-8677.2011.00535.x
El Mehdawi, A. F., Quinn, C. F., El-Mehdawi, A. F., Quinn, C. F., and Pilon-Smits, E. A. (2011a). Effects of selenium hyperaccumulation on plant–plant interactions: evidence for elemental allelopathy. New Phytol. 191, 120–131. doi: 10.1111/j.1469-8137.2011.03670.x
El Mehdawi, A. F., Quinn, C. F., and Pilon-Smits, E. A. (2011b). Selenium hyperaccumulators facilitate selenium-tolerant neighbors via phytoenrichment and reduced herbivory. Curr. Biol. 21, 1440–1449. doi: 10.1016/j.cub.2011.07.033
Fakra, S. C., Luef, B., Castelle, C. J., Mullin, S. W., Williams, K. H., Marcus, M. A., et al. (2018). Correlative cryogenic spectromicroscopy to investigate selenium bioreduction products. Environ. Sci. Technol. 52, 503–512. doi: 10.1021/acs.est.7b01409
Fassel, V. A. (1978). Quantitative elemental analysis by plasma emission spectroscopy. Science 202, 183–191. doi: 10.1126/science.202.4364.183
Freeman, J. L., Quinn, C. F., Marcus, M. A., Fakra, S., and Pilon-Smits, E. A. (2006a). Selenium tolerant diamondback moth disarms hyperaccumulator plant defense. Curr. Biol. 16, 2181–2192.
Freeman, J. L., Zhang, L. H., Marcus, M. A., Fakra, S., and Pilon-Smits, E. A. (2006b). Spatial imaging, speciation and quantification of selenium in the hyperaccumulator plants Astragalus bisulcatus and Stanleya pinnata. Plant Physiol. 142, 124–134.
Freeman, J. L., Zhang, L. H., Marcus, M. A., Fakra, S., McGrath, S. P., and Pilon-Smits, E. A. (2006c). Spatial imaging, speciation and quantification of selenium in the hyperaccumulator plants Astragalus bisulcatus and Stanleya pinnata. Plant Physiol. 142, 124–134.
Freeman, J. L., Tamaoki, M., Stushnoff, C., Quinn, C. F., Cappa, J. J., Devonshire, J., et al. (2010). Molecular mechanisms of selenium tolerance and hyperaccumulation in Stanleya pinnata. Plant Physiol. 153, 1630–1652. doi: 10.1104/pp.110.156570
Gadd, G. M. (1993). Interactions of fungi with toxic metals. New Phytol. 124, 25–60. doi: 10.1111/j.1469-8137.1993.tb03796.x
Galeas, M. L., Zhang, L. H., Freeman, J. L., Wegner, M., and Pilon-Smits, E. A. H. (2007). Seasonal fluctuations of selenium and sulfur accumulation in selenium hyperaccumulators and related non-accumulators. New Phytol. 173, 517–525. doi: 10.1111/j.1469-8137.2006.01943.x
Gharieb, M. M., Wilkinson, S. C., and Gadd, G. M. (1994). Reduction of selenium oxyanions by unicellular, polymorphic and filamentous fungi: cellular location of reduced selenium and implications for tolerance. J. Ind. Microbiol. 14, 300–311. doi: 10.1007/BF01569943
Hanson, B., Garifullina, G. F., Lindblom, S. D., Wangeline, A., Ackley, A., Kramer, K., et al. (2003). Selenium accumulation protects Brassica juncea from invertebrate herbivory and fungal infection. New Phytol. 159, 461–469. doi: 10.1046/j.1469-8137.2003.00786.x
Hartikainen, H. (2005). Biogeochemistry of selenium and its impact on food chain quality and human health. J. Trace Elem. Med. Biol. 18, 309–318. doi: 10.1016/j.jtemb.2005.02.009
Heider, J., and Böck, A. (1993). Selenium metabolism in microorganisms. Adv. Microb. Physiol. 35, 74–107. doi: 10.1016/S0065-2911(08)60097-1
Kelly, S. D., Hesterberg, D., and Ravel, B. (2008). “Analysis of soils and minerals using X-ray absorption spectroscopy,” in Methods Soil Analysis, eds A. L. Ulery and L. R. Drees (Madison: Soil Science Society-America). 367–464. doi: 10.2136/sssabookser5.5.c14
Lindblom, S. D., Fakra, S. C., Landon, J., Schulz, P., Tracy, B., and Pilon-Smits, E. A. (2014). Inoculation of selenium hyperaccumulator Stanleya pinnata and related non-accumulator Stanleya elata with hyperaccumulator rhizosphere fungi - Effects on Se accumulation and speciation. Physiol. Plant 150, 107–118. doi: 10.1111/ppl.12094
Lindblom, S. D., Fakra, S. C., Landon, J., Schulz, P., Tracy, B., and Pilon-Smits, E. A. (2013a). Co-cultivation of Astragalus racemosus and Astragalus convallarius with selenium-hyperaccumulator rhizosphere fungi: Effects on plant growth and accumulation of selenium and other elements. Planta 237, 717–729. doi: 10.1007/s00425-012-1789-5
Lindblom, S. D., Valdez-Barillas, J. R., Fakra, S. C., Marcus, M. A., Wangeline, A. L., and Pilon-Smits, E. A. (2013b). Influence of microbial associations on selenium localization and speciation in roots of Astragalus and Stanleya hyperaccumulators. Exp. Environ. Bot. 88, 33–42. doi: 10.1016/j.envexpbot.2011.12.011
Lovely, D. R. (1993). Dissimilatory metal reduction. Annu. Rev. Microbiol. 47, 263–290. doi: 10.1146/annurev.mi.47.100193.001403
Marcus, M. A., MacDowell, A. A., Celestre, R., Manceau, A., Miller, T., Padmore, H. A., et al. (2004). Beamline 10.3.2 at ALS: a hard X-ray microprobe for environmental and materials sciences. J. Synchrotron. Radiat. 11, 239–247. doi: 10.1107/S0909049504005837
Mishra, B., and Parakash, O. (1975). Alternaria [tenuissima] leaf spot of soybean from India. Indian J. Mycol. Plant Pathol. 5:95.
Murashige, T., and Skoog, F. (1962). A revised medium for rapid growth and bioassays with tobacco tissue culture. Physiol. Plant 15, 437–497. doi: 10.1111/j.1399-3054.1962.tb08052.x
Nigam, S. N., and McConnell, W. B. (1969). Seleno amino compounds from Astragalus bisulcatus isolation and identification of γ-L-glutamyl-Se-methyl-seleno-L-cysteine and Se-methylseleno-L-cysteine. Biochim. Biophys. Acta Gen. Subj. 192, 185–190. doi: 10.1016/0304-4165(69)90354-7
Pickering, I. J., George, G. N., Van Fleet Stalder, V., Chasteen, T. G., and Prince, R. C. (1999). X ray absorption spectroscopy of selenium-containing amino acids. J. Biol. Inorg. Chem. Soc. 6, 791–794. doi: 10.1007/s007750050352
Quinn, C. F., Prins, C. N., Freeman, J. L., Gross, A. M., Hantzis, L., Reynolds, R. J., et al. (2011a). selenium accumulation in flowers and its effects on pollination. New Phytol. 192, 727–737. doi: 10.1111/j.1469-8137.2011.03832.x
Quinn, C. F., Wyant, K. A., Wangeline, A. L., Shulman, J., Galeas, M. L., Valdez, J. R., et al. (2011b). Enhanced decomposition of selenium hyperaccumulator litter in a seleniferous habitat- evidence for specialist decomposers? Plant Soil 341, 51–61. doi: 10.1007/s11104-010-0446-7
Rosenfeld, I., and Beath, O. A. (1964). Selenium, Geobotany, Biochemistry, Toxicity, and Nutrition. New York, NY: Acad Press.
Schiavon, M., and Pilon-Smits, E. A. (2017a). Selenium biofortification and phytoremediation phytotechnologies – A review. J. Environ. Qual. 46, 10–19. doi: 10.2134/jeq2016.09.0342
Schiavon, M., and Pilon-Smits, E. A. (2017b). Tansley review: the fascinating facets of plant selenium accumulation – biochemistry, physiology, evolution and ecology. New Phytol. 213, 1582–1596. doi: 10.1111/nph.14378
Simmons, E. G. (2007). Alternaria: an Identification Manual. Utrecht: CBS Fungal Biovidersity Centre.
Stadtman, T. C. (1990). Selenium biochemistry. Annu. Rev. Biochem. 59, 111–127. doi: 10.1146/annurev.bi.59.070190.000551
Staicu, L. C., Ackerson, C. J., Hunter, W. J., Cornelis, P., Ye, L., Berendsen, R. L., et al. (2015). Pseudomonas moraviensis subsp. stanleyae: a bacterial endophyte capable of efficient selenite reduction to elemental selenium under aerobic conditions. J. Appl. Microbiol. 119, 400–410. doi: 10.1111/jam.12842
Sura-de Jong, M., Reynolds, R. J., Richterova, K., Musilova, L., Hrochova, I., Frantik, T., et al. (2015). Selenium hyperaccumulators harbor a diverse endophytic bacterial community characterized by extreme selenium tolerance and plant growth promoting properties. Front. Plant Sci. 6:113. doi: 10.3389/fpls.2015.00113
Terry, N., Zayed, A. M., de Souza, M. P., and Tarun, A. S. (2000). Selenium in higher plants. Annu. Rev. Plant Physiol. Plant Mol. Biol. 51, 401–432. doi: 10.1146/annurev.arplant.51.1.401
Valdez Barillas, J. R., Quinn, C. F., Freeman, J. L., Lindblom, S. D., Marcus, M. S., Fakra, S. C., et al. (2012). Selenium distribution and speciation in hyperaccumulator Astragalus bisulcatus and associated ecological partners. Plant Physiol. 159, 1834–1844. doi: 10.1104/pp.112.199307
Valdez Barillas, J. R., Quinn, C. F., and Pilon-Smits, E. A. (2011). Selenium Accumulation in plants—phytotechnological applications and ecological implications. Int. J. Phytorem. 13(Suppl 1), 166–178. doi: 10.1080/15226514.2011.568542
Vincelli, P., and Tisserat, N. (2008). Nucleic acid-based detection of plant pathogens in applied plant pathology. Plant Dis. 92, 660–669. doi: 10.1094/PDIS-92-5-0660
Wangeline, A. L., and Reeves, F. B. (2007). Two new alternaria species from selenium-rich habitats in the rocky mountain front range. Mycotaxon 99, 83–89.
Wangeline, A. L., Valdez, J. R., Lindblom, S. D., Bowling, K. L., Reeves, F. B., and Pilon-Smits, E. A. (2011). Selenium tolerance in rhizosphere fungi from Se hyperaccumulator and non-hyperaccumulator plants. Amer. J. Bot. 98, 1139–1147. doi: 10.3732/ajb.1000369
White, T. J., Bruns, T., Lee, S., and Taylor, J. (1990). “Amplification and direct sequencing of fungal ribosomal RNA genes for phylogenetics,” in PCR Protocols: a Guide to Methods and Applications, eds M. A. Innis, D. H. Gelfand, J. J. Sninsky, and T. J. White (New York,NY: Academic Press), 315–322.
Wilber, C. G. (1980). Toxicology of selenium: a review. Clin. Toxicol. 17, 171–230. doi: 10.3109/15563658008985076
Zarcinas, B. A., Cartwright, B., and Spouncer, L. R. (1987). Nitric acid digestion and multi element analysis of plant material by inductively coupled plasmaspectrometry. Commun. Soil Sci. Plan Anal. 18, 131–146. doi: 10.1080/00103628709367806
Zhang, Y., and Gladyshev, V. N. (2009). Comparative genomics of trace elements: emerging dynamic view of trace element utilization and function. Chem. Rev. 109, 4828–4861. doi: 10.1021/cr800557s
Keywords: hyperaccumulation, selenium, endophyte, Alternaria, Astragalus, x-ray analysis
Citation: Lindblom SD, Wangeline AL, Valdez Barillas JR, Devilbiss B, Fakra SC and Pilon-Smits EAH (2018) Fungal Endophyte Alternaria tenuissima Can Affect Growth and Selenium Accumulation in Its Hyperaccumulator Host Astragalus bisulcatus. Front. Plant Sci. 9:1213. doi: 10.3389/fpls.2018.01213
Received: 14 May 2018; Accepted: 27 July 2018;
Published: 20 August 2018.
Edited by:
Nuria Ferrol, Consejo Superior de Investigaciones Científicas (CSIC), SpainReviewed by:
Luisa Lanfranco, Università degli Studi di Torino, ItalyYu-Feng Li, Institute of High Energy Physics (CAS), China
Copyright © 2018 Lindblom, Wangeline, Valdez Barillas, Devilbiss, Fakra and Pilon-Smits. This is an open-access article distributed under the terms of the Creative Commons Attribution License (CC BY). The use, distribution or reproduction in other forums is permitted, provided the original author(s) and the copyright owner(s) are credited and that the original publication in this journal is cited, in accordance with accepted academic practice. No use, distribution or reproduction is permitted which does not comply with these terms.
*Correspondence: Elizabeth A. H. Pilon-Smits, ZXBzbWl0c0Bjb2xvc3RhdGUuZWR1