- 1Laboratoire Reproduction et Deìveloppement des Plantes, École Normale Supérieure de Lyon, Université Claude Bernard Lyon 1, CNRS, INRA, Université de Lyon, Lyon, France
- 2Équipe ARBOREAL, “Agriculture Biodiversité et Valorisation”, Institut Agronomique Néo-Calédonien (IAC), Païta, New Caledonia
The ovules and seeds of most angiosperm groups are enclosed by two integuments, whose evolutionary origins are considerably separated in time, as the inner integument arose over 300 million years ago (MYA) in an ancestor of all living seed plants, while the outer integument arose, perhaps as recently as 164 MYA, in an ancestor of all living angiosperms. Studies of the model angiosperm Arabidopsis thaliana indicate that the mechanisms of development of the inner and outer integuments depend on largely different sets of molecular players. However, it was not known, in most cases, whether these differences were already present in early flowering plants, or arose later in the Arabidopsis lineage. Here, we analyze the expression patterns of integument regulators in Amborella trichopoda, the likely sister to all other living angiosperms. The data obtained indicate that regulators of the YABBY, KANADI, and homeodomain-leucine zipper class III transcription factor families have largely conserved their integument-specific expression profiles in the Amborella and Arabidopsis lineages since the most recent common ancestor (MRCA) of living angiosperms. We identified only one case, involving the paralogous genes ETTIN and AUXIN RESPONSE FACTOR4, in which integument-specific expression patterns had clearly diverged between Amborella and Arabidopsis. We use the data obtained to partially reconstruct molecular mechanisms of integument development in the MRCA of living angiosperms and discuss our findings in the context of alternative hypotheses for the origin of the angiosperm outer integument.
Introduction
The ovules and seeds of most seed plants are covered by one or two integuments. These maternal tissues function to (1) protect the internal tissues of the ovule and later the seed, (2) define a route, via the micropyle, for pollen or pollen-tube entry, and (3) contribute in many cases to the regulation of seed hydration and dormancy (Linkies et al., 2010). Gymnosperms possess a single integument, whereas the majority of angiosperm groups, including the most basally diverging of these, possess two integuments, of which the inner integument is considered homologous to the single integument of gymnosperms. The origin of the inner integument therefore dates from before the separation of the living angiosperm and gymnosperm lineages, believed to have occurred over 300 million years ago (MYA), while the outer integument must have arisen somewhere along the angiosperm stem lineage, perhaps as recently as ∼164 MYA: a reasonable estimated date for the most recent common ancestor (MRCA) of living angiosperms (Moore et al., 2010). Within angiosperms, several groups have undergone a secondary reduction to a single integument (Endress, 2011), notably including the asterids, which contain over 80,000 species.
Much of what is known of the molecular mechanisms of integument development comes from the study of the model angiosperm Arabidopsis thaliana (hereafter referred to as Arabidopsis), which possesses anatropous, bitegmic ovules. Ovule initiation in Arabidopsis begins with the expression of the homeobox transcription factor WUSCHEL (WUS) (see Mathews and Kramer, 2012), which is known to promote cell proliferation in meristematic tissues. The ovule thus shares characteristics of meristems, such as the stem apical meristem and floral meristem, both of which produce lateral organs on their flanks. In the case of the bitegmic ovule, the lateral organs produced in this way are the inner and outer integuments, which arise from the chalazal tissue in the central region of the elongating ovule primordium. The inner integument arises first, followed closely by the outer integument (Endress, 2011).
Early expression of WUS at the apex of the Arabidopsis ovule primordium defines the presumptive nucellar tissue. The outgrowth of the integuments from the chalazal region, immediately below the nucellus, depends on the expression of three homeodomain-leucine zipper class III (HD-ZIP III) transcription factors, PHABULOSA (PHB), PHAVOLUTA (PHV), and CORONA (CNA) (Kelley et al., 2009), which act redundantly, and together with other factors, to limit the basal spread of WUS expression (Yamada et al., 2016). Expression of these HD-ZIP III factors defines the adaxial (towards the growth axis) zone of the presumptive inner integument. The inner and outer integument primordia are associated with the formation of auxin maxima, and it has been proposed that ETTIN/AUXIN RESPONSE FACTOR3 (ETT/ARF3) and ABERRANT TESTA SHAPE/KANADI4 (ATS/KAN4), both of which are expressed in the abaxial (away from the growth axis) domain of the inner integument, act to remove auxin from the zone between the two integument primordia (Kelley et al., 2012). Interestingly, Lora et al. (2015) found that in certain species of Prunus whose ovules contain only one integument, expression of the ETT ortholog was absent from the chalaza and inner integument. These authors accordingly proposed the loss of ETT expression to represent a potential evolutionary mechanism for the reduction from two integuments to one in these species.
HD-ZIP III and KANADI family factors are believed to control abaxial–adaxial tissue polarity in lateral organs through the regulation of a common set of direct target genes, many of which are related to auxin signaling or dynamics (Reinhart et al., 2013; Huang et al., 2014). HD-ZIP III factors regulate these targets positively to promote adaxial tissue identity, while KANADI factors regulate the same genes negatively to promote abaxial tissue identity. In Arabidopsis, there is a clear division of labor between the inner and outer integuments, as ATS is the principal KANADI family member expressed in the inner integument (McAbee et al., 2006), while this role is played redundantly by KAN1 and KAN2 in the outer integument (Eshed et al., 2004; McAbee et al., 2006). In the HD-ZIP III family, PHB, PHV, and CNA are expressed specifically in the inner integument, while REV is expressed in both integuments (Sieber et al., 2004b; Kelley et al., 2009).
Tissue outgrowth in angiosperm lateral organs, including for example leaves and carpels, is typically associated with the expression of YABBY transcription factors. These factors are expressed in the abaxial tissue domain, and can act either positively or negatively on the transcription of downstream targets, apparently through protein–protein interactions with distinct sets of co-factors (Bonaccorso et al., 2012; Simon et al., 2017). Despite their abaxial-specific expression profiles, YABBYs are believed not to define abaxial tissue identity per se, but rather to facilitate lateral organ outgrowth by promoting the expression of WUS-related transcription factors in marginal meristems (Nakata et al., 2012). The YABBY gene INNER NO OUTER (INO), is expressed specifically in the abaxial zone of the Arabidopsis outer integument, and an orthologue of this gene shows a largely similar expression pattern in the basally diverging angiosperm Nymphaea (Nymphaeales, Nymphaeaceae) (Yamada et al., 2003, 2011), suggesting INO’s tissue-specific role to have been conserved from early stages of angiosperm evolution.
With very few exceptions (such as that of INO, mentioned above), the mechanisms of integument development have been investigated to date uniquely in Arabidopsis, and it is therefore not known, in most cases, which of these were already present in early flowering plants and which evolved later in the Arabidopsis lineage. In the present work, we investigate the conservation of mechanisms of integument development from very early stages of angiosperm evolution by analyzing the expression patterns of the orthologs of Arabidopsis integument regulators in the basally diverging angiosperm Amborella trichopoda (hereafter referred to as Amborella). Most recent molecular phylogenetic studies place Amborella, the only known representative of Amborellales, as sister to all other living angiosperms (Stevens, 2001 onwards). According to these studies, Amborellales is the first of the three earliest diverging “ANA-grade” angiosperm orders, the remaining two being Nymphaeales and Austrobaileyales. Amborella is a dioecious scrambling shrub, endemic to the subtropical rainforests of the southern pacific island of New Caledonia. Its female flowers produce 5–6 unfused carpels, each containing a single, pendant, bitegmic ovule. These ovules are of more-or-less orthotropous symmetry, though show a distinct longitudinal curvature, which may be due to developmental constraints within the ovary, or may represent the residual effect of an ancestral anatropous symmetry (Endress and Igersheim, 2000).
Here, we focus on integument regulators of the YABBY, KANADI, HD-ZIP III, and ARF transcription factor families. We use gene expression patterns to identify molecular mechanisms of integument development that have probably been conserved since the MRCA of living flowering plants, and others that appear to have undergone changes in either the Amborella or Arabidopsis lineages. We discuss the data obtained in the context of alternative hypotheses for the origin of the outer integument in a distant common ancestor of living angiosperms.
Materials and Methods
Phylogenetic Reconstruction
Phylogenetic reconstructions in the KANADI and HD-ZIP III families were performed using a wide taxonomic sampling of angiosperms. Sequences for inclusion in phylogenetic analyses were selected by TBLASTN searching (Altschul et al., 1997) of the NCBI/NIH non-redundant nucleotide database1 and the Amborella genome database2 using Arabidopsis integument development proteins. Protein sequences were aligned using MUSCLE in the SeaView phylogeny package (Gouy et al., 2010). Sites were selected using G-BLOCKS, using all three options provided to minimize the stringency of selection. Phylogenies were generated from amino acid alignments in PhyML (Guindon et al., 2009) using the following parameters: model: LG; branch support: aLRT; amino acid equilibrium frequencies: model-given; invariable sites: optimized; across-site rate variation: optimized; tree searching operations: best of NNI and SPR; and starting tree: BioNJ.
Gene Expression Studies
Full-length nucleotide sequences corresponding to Amborella orthologs or pro-orthologs of Arabidopsis integument regulators were PCR-amplified from an Amborella female flower cDNA library (Fourquin et al., 2005) using the primers shown in Supplementary Table S1. Digoxigenin-labeled riboprobes were generated from these and used in non-radioisotopic in situ hybridization to tissue sections of Amborella flower buds at approximately Stage 7 (Buzgo et al., 2004), and of female flowers at anthesis, using the protocol given by Vialette-Guiraud et al. (2011). Gene expression patterns were observed and photographed under bright field illumination using a Leica Axio Imager M2 inverted microscope fitted with a Leica AxioCam MRc digital camera.
Anatomical Observations
Amborella ovule anatomy was revealed in sections of fixed female flowers, prepared and photographed as for in situ hybridization. These sections were stained with 0.05% (w/v) toluidine Blue-0 in 0.1 M sodium phosphate buffer (pH 6.8).
Results
Clear Orthologs of Several Arabidopsis Integument Regulators Can Be Identified in Amborella
Several previous studies have focused on the molecular phylogeny of gene families involved in the regulation of integument development. Accordingly, the Amborella ortholog of the Arabidopsis YABBY-family integument regulator INO has previously been reported (Finet et al., 2016), as have those of the paralogous and partially redundant auxin response family regulators ETT and AUXIN RESPONSE FACTOR4 (ARF4) (Finet et al., 2010). However, published phylogenies of the KANADI and HD-ZIP III families have not to our knowledge previously included sequences from Amborella.
Phylogenetic reconstructions of the HD-ZIP III family in the present study (Supplementary Figure S1), generated from the protein alignment shown in Supplementary Figure S2, succeeded in identifying clear Amborella orthologs of the Arabidopsis genes REV and CNA, and a clear pro-ortholog of the Arabidopsis genes PHB and PHV, which accordingly appear to be derived from a duplication within the crown group of living angiosperms. In all these cases, the Amborella genes identified as orthologs or pro-orthologs of Arabidopsis integument regulators occupied basal positions within their respective clades, in agreement with the likely phylogenetic placement of Amborella.
Phylogenetic reconstruction of the KANADI family (Supplementary Figure S3), generated from the protein alignment shown in Supplementary Figure S4, succeeded in identifying a clear Amborella ortholog of the Arabidopsis inner integument regulator ATS (KAN4), which grouped in a small basal clade of ATS-like sequences. Relationships of gene orthology between Amborella sequences and the Arabidopsis outer integument regulators KAN1 and KAN2 were less clear in phylogenetic reconstructions.
The results obtained in these reconstructions, combined with those of previously published work, permitted detailed analyses in the present study of the expression in Amborella female flower tissues of the genes: Atr.INO (XM_006840616), Atr.ATS, (XM_006856161.3), AtrPHB/PHV (XM_006845952.3), Atr.REV (XM_006846714.3), Atr.ARF4 (XM_020671056), and Atr.ETT (XM_011627988.2). The longer alternative splicing variant of Atr.ARF4 (Finet et al., 2010) was used for in situ hybridization studies.
The Amborella Orthologue of the YABBY Gene INO Is Narrowly Expressed in the Abaxial Domain of the Outer Integument, As Is INO in Arabidopsis
Atr.INO proved to be specifically expressed in the most abaxial cell layer of the outer integument. Its expression was first noted in the outer integument, towards the chalazal-end of the ovary (Figure 1A), spreading towards the micropyle-end with the growth of the outer integument (Figure 1B). This expression pattern closely resembles that of INO in Arabidopsis. Atr.INO expression was stronger in early stages of Amborella ovule development, becoming absent in the mature ovule (Figure 1C). These data reinforce observations in Nymphaea (Yamada et al., 2003) that already suggested Arabidopsis INO to have conserved its function in outer integument development from early stages of angiosperm evolution. Expression patterns of INO orthologs in Arabidopsis and Amborella ovules, together with those of the other factors studied (below), are summarized in Figure 2.
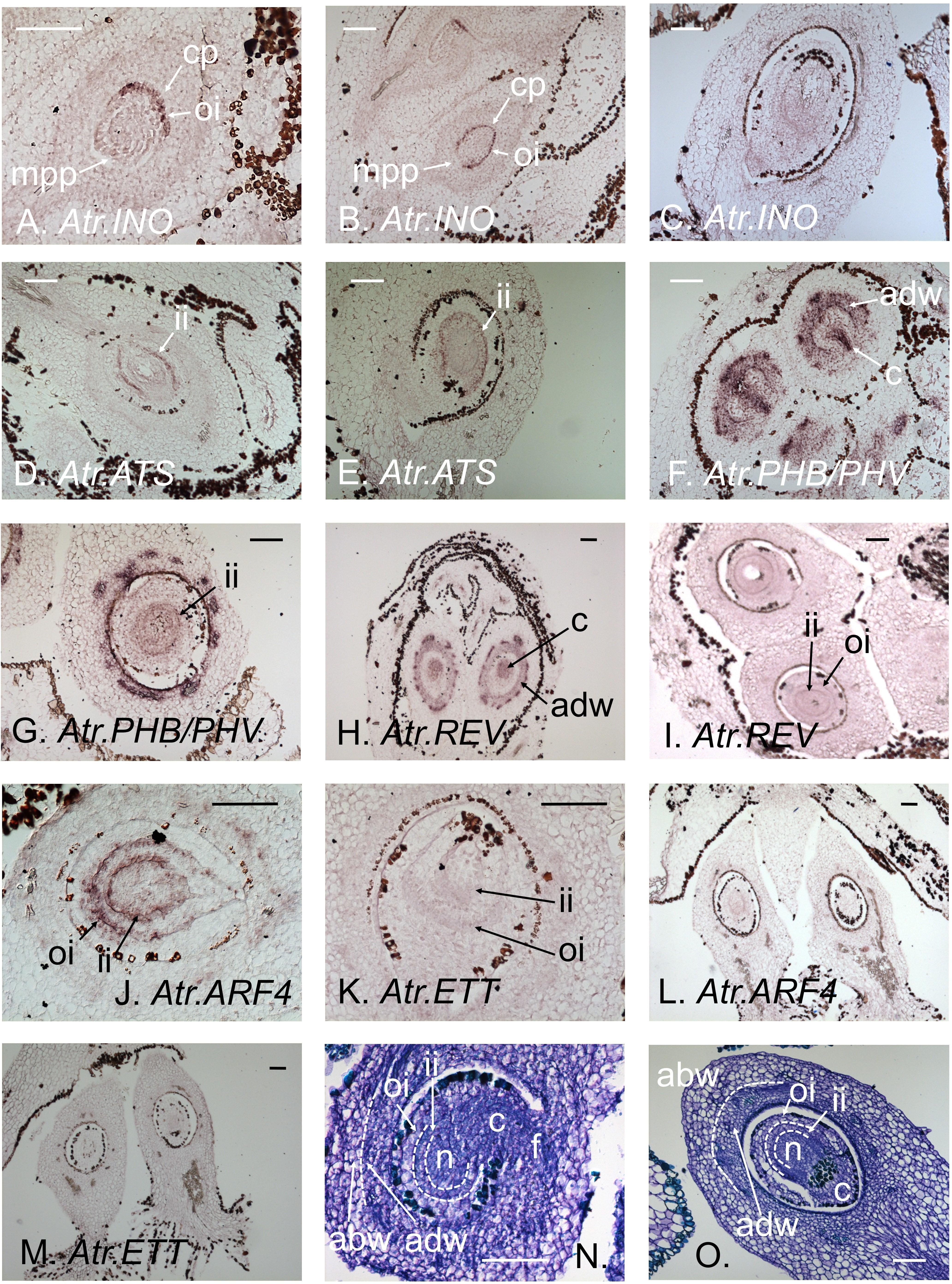
FIGURE 1. In situ hybridizations of integument regulators to female Amborella flower sections. (A) Atr.INO expression in the growing outer integument at early Stage 7. (B) Atr.INO expression in the near fully grown outer integument at late Stage 7. (C) Atr.INO expression is no longer apparent in the outer integument (LS) in mature flowers. (D) Atr.ATS expression in the inner integument at floral bud Stage 7. (E) Atr.ATS expression in the inner integument persists in the mature flower. (F) Narrow Atr.PHB/PHV expression in the chalaza and strong expression in the adaxial domain of the ovary wall at early Stage 7. (G) Weak Atr.PHB/PHV expression persists in the abaxial domain of the inner integument at late Stage 7. (H) Atr.REV is extensively expressed in the chalaza, and strongly expressed in the adaxial domain of the ovary wall at early Stage 7. (I) Weak Atr.REV expression persists in the adaxial domains of both integuments at late Stage 7. (J) Atr.ARF4 expression in the abaxial domain of both integuments at early Stage 7. (K) Very weak Atr.ETT expression in the abaxial domain of both integuments at early Stage 7. (L) Atr.ARF4 expression is no longer apparent in the integuments in the mature flower. (M) Atr.ETT expression is no longer apparent in the integuments in the mature flower. (N,O) Toluidine-blue stained sections of Stage 7 and mature ovules, respectively, showing anatomical details. Tissues zones in panels (N,O) have been delimited with dotted white lines for clarity. Developmental stages are as defined by Buzgo et al. (2004). abw, abaxial domain of the ovary wall; adw, adaxial domain of the ovary wall; c, chalaza; cp, chalazal pole; f, funiculus; ii, inner integument; mpp, micropyle pole; n, nucellus; and oi, outer integument. Scale bars = 100 μm.
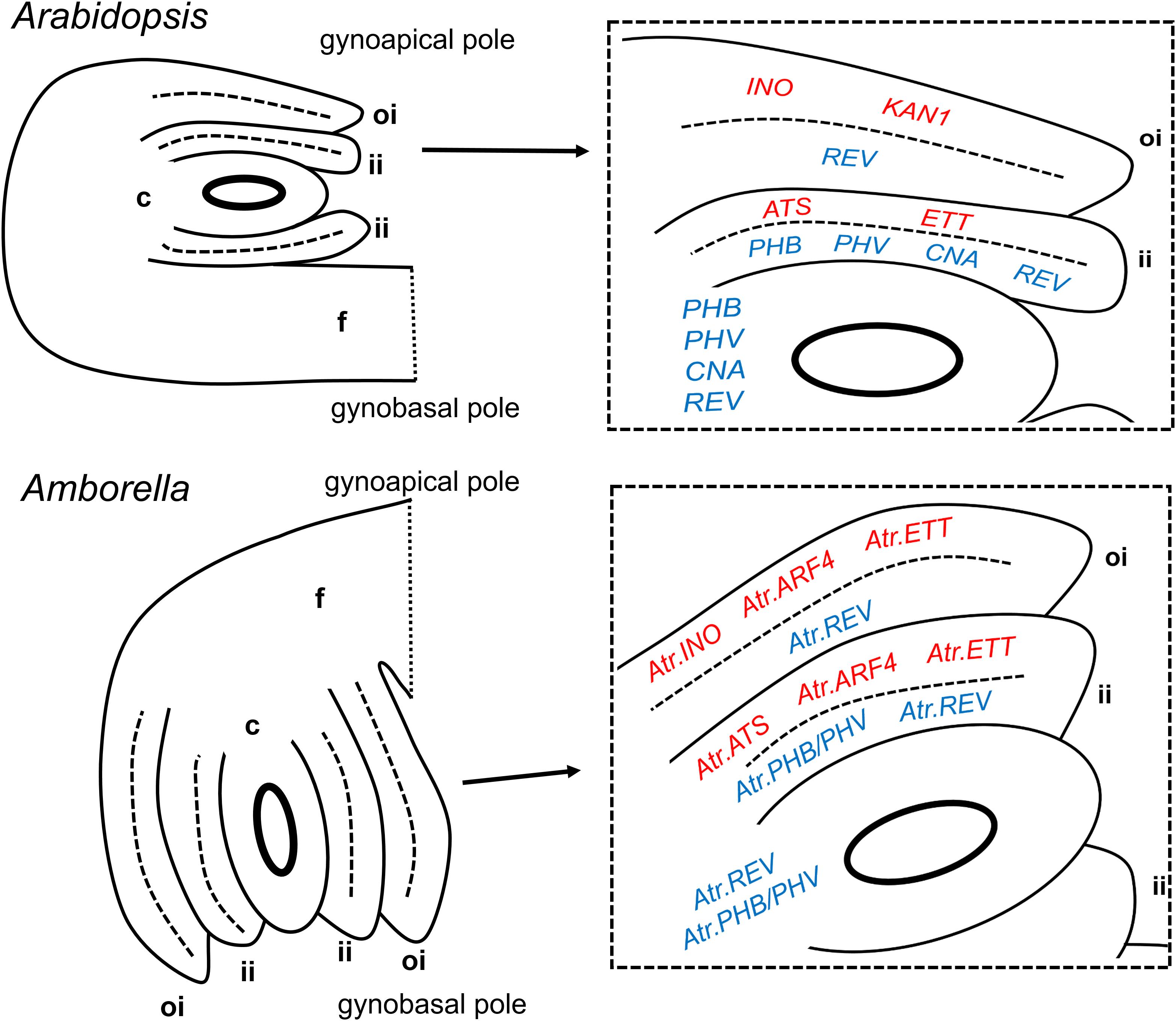
FIGURE 2. Summary of expression patterns of ovule integument regulators in Arabidopsis thaliana and Amborella trichopoda. Abaxial-promoting genes are shown in red in enlarged diagrams, adaxial-promoting genes are shown in blue. Mature ovules are shown, though the genes indicated may be maximally expressed in the tissues shown at earlier stages. Entire ovules (on the left) are shown in their natural orientations relative to the longitudinal axis of the gynoecium/carpel. c, chalaza; f, funiculus; ii, inner integument; and oi = outer integument.
The Amborella Orthologue of the KANADI Family Gene ATS (KAN4) Is Expressed Abaxially in the Inner Integument, As Is ATS in Arabidopsis
Atr.ATS was found to be expressed specifically in the abaxial domain of the inner integument (Figure 1D), as is ATS in Arabidopsis. This expression persisted into the final stages of ovule development in almost mature flowers (Figure 1E). No expression of Atr.ATS was apparent in any female reproductive tissue other than the inner integument. These data strongly suggest ATS orthologs to have conserved their function in inner integument development in both the Amborella and Arabidopsis lineages since the MRCA of living flowering plants.
Amborella HD-ZIP III Genes Show Integument-Specific Profiles Similar to Those of Their Arabidopsis Orthologs
We found Atr.PHB/PHV, the Amborella pro-ortholog of Arabidopsis PHB and PHV, to be strongly expressed at early stages of development in the chalazal region of the ovule, which gives rise to the integuments, and very strongly expressed in the adaxial tissues of the ovary wall (Figure 1F). At later developmental stages, Atr.PHB/PHV appeared weakly expressed in the adaxial zone of the inner integument (Figure 1G). At early stages of ovule development, Atr.REV showed a similar expression pattern to Atr.PHB/PHV in the chalaza and ovary wall, though arguably with a slightly wider expression in the chalaza (Figure 1H). At later stages of ovule development, reduced levels of Atr.REV expression were visible in the adaxial regions of both integuments (Figure 1I). The association of Atr.PHB/PHV expression with the inner integument, and that of Atr.REV with both integuments (Figures 1G,I), suggests the paralog-specific conservation of function between the Amborella and Arabidopsis lineages since their separation at the base of the living flowering plant clade. Atr.PHB/PHV and Atr.REV expression patterns are nonetheless very similar, and these genes are clearly expressed together in parts of the chalaza, the inner integument and the abaxial region of the ovary wall.
The Amborella Orthologs of the Paralogous Auxin Response Factors ETT and ARF4 Are Expressed Abaxially in Both Integuments, Showing Partial Conservation With Expression Patterns in Arabidopsis
ETT and ARF4 act redundantly in the definition of abaxial tissue identity in the Arabidopsis leaf (Pekker et al., 2005), though ETT appears to play the major role in this process in both the carpel wall and inner integument. An earlier study of the orthologs of these factors in Amborella (Finet et al., 2010) suggested that Atr.ARF4 may show a higher level of expression than Atr.ETT in female reproductive tissues and perhaps therefore play the major developmental role. In the present study, both Atr.ARF4 and Atr.ETT were found to be expressed at early stages in the abaxial domain of both integuments (Figures 1J,K), with Atr.ARF4 giving the stronger hybridization signal. Expression levels of both genes diminished in mature integuments (Figures 1L,M). A switch may therefore have occurred in the major paralog active in the inner integument, with ETT playing the principal role in Arabidopsis, while Atr.ARF4 is more highly expressed in Amborella. In addition, whereas the role of ETT is limited to the inner integument in Arabidopsis, Atr.ARF4 and Atr.ETT are expressed in the abaxial domains of both integuments in Amborella.
It is interesting to note that Arabidopsis ETT has been found to interact physically with ATS, both of which are expressed specifically in the inner integument (Kelley et al., 2012). Atr.ETT and Atr.ARF4 may similarly interact with Atr.ATS in the Amborella inner integument, but this interaction could not occur in the outer integument as Atr.ATS is not apparently expressed in that tissue (Figures 1D,E). Of course, Atr.ETT and Atr.ARF4 might interact in the outer integument with other Amborella KANADI proteins (Supplementary Figure S4) whose expression patterns were not investigated in the present study.
Toluidine-blue stained sections of the carpel wall and ovule in a Stage 7 (Figure 1N) and a mature (Figure 1O) female flower are provided to help interpret anatomical details of in situ hybridization images shown in Figures 1A–M.
Discussion
Reconstructed Gene Expression Patterns Suggest That Largely Distinct Sets of Regulators Controlled Inner and Outer Integument Development in Early Flowering Plants, As In Present-Day Arabidopsis
The data presented here strongly suggest the extensive conservation of integument-specific developmental roles of genes of the YABBY, KANADI, and HD-ZIP III families in both the Amborella and Arabidopsis lineages since the MRCA of living flowering plants. It was previously known that inner and outer integument development in Arabidopsis depended on substantially distinct sets of molecular players. The current study provides evidence that most of these differences in molecular controls are ancient, and already existed in early flowering plants. The only clear exception noted in the present study, in which molecular mechanisms seem to have diverged in one or both of the Amborella and Arabidopsis lineages, concerns the paralogous ARF family members ETT and ARF4. ETT contributes to abaxial tissue identity uniquely in the inner integument of Arabidopsis, whereas orthologs of both ETT and ARF4 are expressed in the abaxial domain of both integuments in Amborella. The inner integument expression of these factors may, as for that of ETT in Arabidopsis, act to prevent the coalescence of the two integument primordia. However, these factors may play a further role in the establishment and/or maintenance of abaxial/adaxial polarity in the Amborella outer integument, compared to their orthologs in Arabidopsis. Analysis of gene expression patterns in further ANA-grade taxa could be used to determine whether these ARF genes evolved more restricted inner integument-specific roles in the Arabidopsis lineage, or whether, conversely, their expression patterns became more generalized to both integuments, specifically in the Amborella lineage.
It is interesting to note that ETT expression is limited to the abaxial domain of the inner integument in bitegmic species of Prunus (rosid I clade) (Lora et al., 2015), as it is in Arabidopsis. If ETT/ARF4 expression was lost from the outer integument in the Arabidopsis lineage, rather than gained in the Amborella lineage, it would therefore seem likely that this change happened before the divergence of the rosid I and II clades, which is estimated to have occurred some 105 MYA (Moore et al., 2010).
We have used the data presented here, together with information from published studies, to construct a partial molecular model for the control of integument development in the MRCA of living angiosperms (Figure 3). Predicted intermolecular interactions shown in this model are based on functional data from Arabidopsis alone and the conservation of tissue-colocalization of the molecules concerned in Amborella.
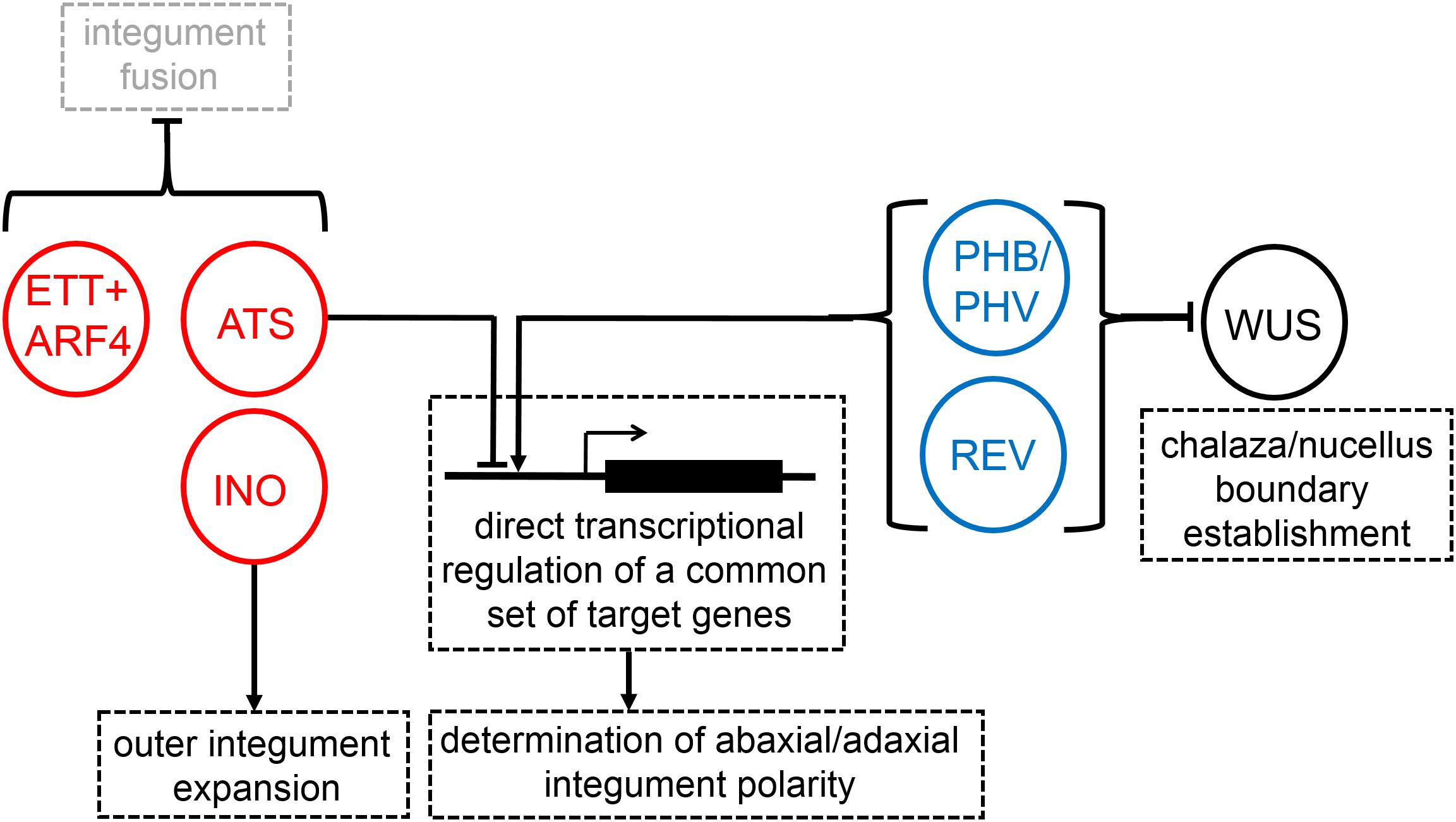
FIGURE 3. A partial reconstruction of the molecular mechanisms of integument development in the most recent common ancestor of living flowering plants. Pointed and barred arrows represent cases of positive and negative regulation, respectively. Abaxial-promoting factors are shown in red, adaxial-promoting factors are shown in blue.
Implications of Reconstructed Integument Development Mechanisms for the Origin of the Outer Integument and Flowering Plants
Like the carpel, the outer integument is a pleisiomorphic feature of angiosperms. Two contrasting hypotheses have been proposed to account for the origin of this structure, prior to the radiation of living angiosperms. One of these hypotheses (reviewed by Doyle, 2008), based mainly on paleobotanical data, proposes that the angiosperms evolved from cupulate seed–ferns, possibly resembling the known fossil groups Caytoniales or Glossopteridales. In most known members of these taxa, several ovules occur within laminar cupules that are either borne on the margins of a female rachis (in Caytoniales), or emerge from the axil or midrib of a megasporophyll (in Glossopteridales). According to this “cupule hypothesis”, the bitegmic angiosperm ovule would have evolved from a unitegmic gymnosperm ovule by a reduction in the number of ovules-per-cupule to one, the cupule thereby becoming the outer integument. The Caytoniales-type cupule may be a particularly strong candidate as a precursor to the angiosperm outer integument as the orientation of the ovule within this type of cupule could have led directly to the anatropous ovule arrangement (Doyle, 2008), which may have been pleisiomorphic in angiosperms.
The main alternative hypothesis for the origin of the outer integument, as discussed by Mathews and Kramer (2012), is that this structure simply arose through a modular reiteration of the single integument already present in species along the angiosperm stem lineage. Indeed, the development of the outer and inner integuments proceeds in a remarkably similar manner in most angiosperms, and the overexpression of WUS leads, in transgenic Arabidopsis, to the production of supernumerary integuments (Gross-Hardt et al., 2002; Sieber et al., 2004a), demonstrating that extra integuments can be generated from pre-existing developmental mechanisms by a simple molecular change in an upstream regulator. Not all strong candidates for ancestors, or close stem-lineage relatives of the angiosperms, possessed cupules. Notably, Bennettitales, which possessed many angiosperm-like characteristics, including a perianth, a bisexual axis (in some taxa), non-saccate pollen, net-veined leaves, and oleananes (highly resistant terpenoid compounds, also present in angiosperms), possessed no structure resembling an ovule-enclosing cupule. A mechanism based on the modular reiteration of a pre-existing single integument might, therefore, explain the origin of the angiosperm outer integument in a potential bennettialian ancestor.
The data presented here strongly suggest that the precise mechanisms of integument development were already substantially distinct in the inner and outer integuments some 164 MYA in the MRCA of living flowering plants. This conclusion clearly favors the hypothesis of a distant or indirect homology between the inner and outer integuments, such as might be explained by the more direct origin of the outer integument from an ovule-containing seed–fern cupule. It does not provide support for the possible origin of the outer integument by a modular reiteration of the inner integument, as this mechanism would be expected to yield two integuments whose development initially depended, in early flowering plants, on near-identical sets of molecular regulators.
Author Contributions
GA performed the experiments and phylogenetic analyses. AV supervised the experimental work and phylogenetic analyses. AA-R supervised the experimental work. BF and GG provided the plant material and contributed to writing the paper. CS planned and supervised the research and wrote the paper.
Funding
This research was supported by ANR grant “ORANGe” 2013-18 to CS. GA was supported by a normalien undergraduate scholarship, and AV was employed as an agrégée préparatrice (teacher/researcher) of the ENS-Lyon.
Conflict of Interest Statement
The authors declare that the research was conducted in the absence of any commercial or financial relationships that could be construed as a potential conflict of interest.
Acknowledgments
We are grateful to the regional authorities of the province Nord and province Sud of New Caledonia for permission to collect living Amborella material.
Supplementary Material
The Supplementary Material for this article can be found online at: https://www.frontiersin.org/articles/10.3389/fpls.2018.01352/full#supplementary-material
FIGURE S1 | Maximum likelihood phylogeny of angiosperm HD-ZIP III family proteins. aLRT branch-support values are shown at nodes. Amborella and Arabidopsis sequences mentioned in the text are highlighted. Key to species names: Ara tha: Arabidopsis thaliana; AmTr: Amborella trichopoda; Bra dis: Brachypodium distachyon; Bra nap: Brassica napus; Bra rapa: Brassica rapa; Cam sat: Camelina sativa; Cit sin: Citrus sinensis; Fra ves: Fragaria vesca; Gly max: Glycine max; Med tru: Medicago truncatula; Mus acu: Musa acuminata; Nel nuc: Nelumbo nucifera; Ory bra: Oryza brachyantha; Ory sat: Oryza sativa; Pop tri: Populus trichocarpa; Pru per: Prunus persica; Raph sat: Raphanus sativus; Ric com: Ricinus communis; Sol lyc: Solanum lycopersicum; Sol tub: Solanum tuberosum; The cac: Theobroma cacao; Vig ang: Vigna angularis; Vig rad: Vigna radiate; Vit vin: Vitis vinifera; and Zea may: Zea mays.
FIGURE S2 | Alignment of angiosperm HD-ZIP III family proteins used to produce the phylogeny shown in Supplementary Figure 1. Sites used in phylogenetic analysis are underlined.
FIGURE S3 | Maximum likelihood phylogeny of angiosperm KANADI family proteins. aLRT branch-support values are shown at nodes. Amborella and Arabidopsis sequences mentioned in the text are highlighted. Key to species names: Ara tha: Arabidopsis thaliana; AmTr: Amborella trichopoda; Bra dis: Brachypodium distachyon; Bra nap: Brassica napus; Bra rapa: Brassica rapa; Cam sat: Camelina sativa; Cit sin: Citrus sinensis; Fra ves: Fragaria vesca; Gly max: Glycine max; Med tru: Medicago truncatula; Mus acu: Musa acuminate; Nel nuc: Nelumbo nucifera; Ory bra: Oryza brachyantha; Ory sat: Oryza sativa; Pop tri: Populus trichocarpa; Pru per: Prunus persica; Raph sat: Raphanus sativus; Ric com: Ricinus communis; Sol lyc: Solanum lycopersicum; Sol tub: Solanum tuberosum; The cac: Theobroma cacao; Vig ang: Vigna angularis; Vig rad: Vigna radiate; Vit vin: Vitis vinifera; and Zea may: Zea mays.
FIGURE S4 | Alignment of angiosperm KANADI family proteins used to produce the phylogeny shown in Supplementary Figure 3. Sites used in phylogenetic analysis are underlined.
TABLE S1 | Oligonucleotide primers used to amplify Amborella integument regulators.
Footnotes
References
Altschul, S. F., Madden, T. L., Schaffer, A. A., Zhang, J. H., Zhang, Z., Miller, W., et al. (1997). Gapped BLAST and PSI-BLAST: a new generation of protein database search programs. Nucleic Acids Res. 25, 3389–3402. doi: 10.1093/nar/25.17.3389
Bonaccorso, O., Lee, J. E., Puah, L., Scutt, C. P., and Golz, J. F. (2012). FILAMENTOUS FLOWER controls lateral organ development by acting as both an activator and a repressor. BMC Plant Biol. 12:176. doi: 10.1186/1471-2229-12-176
Buzgo, M., Soltis, P. S., and Soltis, D. E. (2004). Floral developmental morphology of Amborella trichopoda (Amborellaceae). Int. J. Plant Sci. 165, 925–947. doi: 10.1086/424024
Doyle, J. A. (2008). Integrating molecular phylogenetic and paleobotanical evidence on origin of the flower. Int. J. Plant Sci. 169, 816–843. doi: 10.1086/589887
Endress, P. K. (2011). Angiosperm ovules: diversity, development, evolution. Ann. Bot. 107, 1465–1489. doi: 10.1093/aob/mcr120
Endress, P. K., and Igersheim, A. (2000). Reproductive structures of the basal angiosperm Amborella trichopoda (Amborellaceae). Int. J. Plant Sci. 161, S237–S248. doi: 10.1086/317571
Eshed, Y., Izhaki, A., Baum, S. F., Floyd, S. K., and Bowman, J. L. (2004). Asymmetric leaf development and blade expansion in Arabidopsis are mediated by KANADI and YABBY activities. Development 131, 2997–3006. doi: 10.1242/dev.01186
Finet, C., Floyd, S. K., Conway, S. J., Zhong, B., Scutt, C. P., and Bowman, J. L. (2016). Evolution of the YABBY gene family in seed plants. Evol. Dev. 18, 116–126. doi: 10.1111/ede.12173
Finet, C., Fourquin, C., Vinauger, M., Berne-Dedieu, A., Chambrier, P., Paindavoine, S., et al. (2010). Parallel structural evolution of auxin response factors in the angiosperms. Plant J. 63, 952–959. doi: 10.1111/j.1365-313X.2010.04292.x
Fourquin, C., Vinauger-Douard, M., Fogliani, B., Dumas, C., and Scutt, C. P. (2005). Evidence that CRABS CLAW and TOUSLED have conserved their roles in carpel development since the ancestor of the extant angiosperms. Proc. Natl. Acad. Sci. U.S.A. 102, 4649–4654. doi: 10.1073/pnas.0409577102
Gouy, M., Guindon, S., and Gascuel, O. (2010). SeaView version 4: a multiplatform graphical user interface for sequence alignment and phylogenetic tree building. Mol. Biol. Evol. 27, 221–224. doi: 10.1093/molbev/msp259
Gross-Hardt, R., Lenhard, M., and Laux, T. (2002). WUSCHEL signaling functions in interregional communication during Arabidopsis ovule development. Genes Dev. 16, 1129–1138. doi: 10.1101/gad.225202
Guindon, S., Dufayard, J.-F., Hordijk, W., Lefort, V., and Gascuel, O. (2009). PhyML: fast and accurate phylogeny reconstruction by maximum likelihood. Infect. Genet. Evol. 9, 384–385.
Huang, T., Harrar, Y., Lin, C., Reinhart, B., Newell, N. R., Talavera-Rauh, F., et al. (2014). Arabidopsis KANADI1 acts as a transcriptional repressor by interacting with a specific cis-element and regulates auxin biosynthesis, transport, and signaling in opposition to HD-ZIPIII factors. Plant Cell 26, 246–262. doi: 10.1105/tpc.113.111526
Kelley, D. R., Arreola, A., Gallagher, T. L., and Gasser, C. S. (2012). ETTIN (ARF3) physically interacts with KANADI proteins to form a functional complex essential for integument development and polarity determination in Arabidopsis. Development 139, 1105–1109. doi: 10.1242/dev.067918
Kelley, D. R., Skinner, D. J., and Gasser, C. S. (2009). Roles of polarity determinants in ovule development. Plant J. 57, 1054–1064. doi: 10.1111/j.1365-313X.2008.03752.x
Linkies, A., Graeber, K., Knight, C., and Leubner-Metzger, G. (2010). The evolution of seeds. New Phytol. 186, 817–831. doi: 10.1111/j.1469-8137.2010.03249.x
Lora, J., Hormaza, J. I., and Herrero, M. (2015). Transition from two to one integument in Prunus species: expression pattern of INNER NO OUTER (INO), ABERRANT TESTA SHAPE (ATS) and ETTIN (ETT). New Phytol. 208, 584–595. doi: 10.1111/nph.13460
Mathews, S., and Kramer, E. M. (2012). The evolution of reproductive structures in seed plants: a re-examination based on insights from developmental genetics. New Phytol. 194, 910–923. doi: 10.1111/j.1469-8137.2012.04091.x
McAbee, J. M., Hill, T. A., Skinner, D. J., Izhaki, A., Hauser, B. A., Meister, R. J., et al. (2006). ABERRANT TESTA SHAPE encodes a KANADI family member, linking polarity determination to separation and growth of Arabidopsis ovule integuments. Plant J. 46, 522–531. doi: 10.1111/j.1365-313X.2006.02717.x
Moore, M. J., Soltis, P. S., Bell, C. D., Burleigh, J. G., and Soltis, D. E. (2010). Phylogenetic analysis of 83 plastid genes further resolves the early diversification of eudicots. Proc. Natl. Acad. Sci. U.S.A. 107, 4623–4628. doi: 10.1073/pnas.0907801107
Nakata, M., Matsumoto, N., Tsugeki, R., Rikirsch, E., Laux, T., and Okada, K. (2012). Roles of the middle domain-specific WUSCHEL-RELATED HOMEOBOX genes in early development of leaves in Arabidopsis. Plant Cell 24, 519–535. doi: 10.1105/tpc.111.092858
Pekker, I., Alvarez, J. P., and Eshed, Y. (2005). Auxin response factors mediate Arabidopsis organ asymmetry via modulation of KANADI activity. Plant Cell 17, 2899–2910. doi: 10.1105/tpc.105.034876
Reinhart, B. J., Liu, T., Newell, N. R., Magnani, E., Huang, T., Kerstetter, R., et al. (2013). Establishing a framework for the Ad/Abaxial regulatory network of Arabidopsis: ascertaining targets of class III HOMEODOMAIN LEUCINE ZIPPER and KANADI regulation. Plant Cell 25, 3228–3249. doi: 10.1105/tpc.113.111518
Sieber, P., Gheyselinck, J., Gross-Hardt, R., Laux, T., Grossniklaus, U., and Schneitz, K. (2004a). Pattern formation during early ovule development in Arabidopsis thaliana. Dev. Biol. 273, 321–334. doi: 10.1016/j.ydbio.2004.05.037
Sieber, P., Petrascheck, M., Barberis, A., and Schneitz, K. (2004b). Organ polarity in Arabidopsis. NOZZLE physically interacts with members of the YABBY family. Plant Physiol. 135, 2172–2185. doi: 10.1104/pp.104.040154
Simon, M. K., Skinner, D. J., Gallagher, T. L., and Gasser, C. S. (2017). Integument development in Arabidopsis depends on interaction of YABBY protein INNER NO OUTER with coactivators and corepressors. Genetics 207, 1489–1500. doi: 10.1534/genetics.117.300140
Stevens, P. F. (2001). Angiosperm Phylogeny Website. Version 14. Available at: http://www.mobot.org/MOBOT/research/APweb/
Vialette-Guiraud, A. C., Alaux, M., Legeai, F., Finet, C., Chambrier, P., Brown, S. C., et al. (2011). Cabomba as a model for studies of early angiosperm evolution. Ann. Bot. 108, 589–598. doi: 10.1093/aob/mcr088
Yamada, T., Ito, M., and Kato, M. (2003). Expression pattern of INNER NOOUTER homologue in Nymphaea (water lily family, Nymphaeaceae). Dev. Genes Evol. 213, 510–513. doi: 10.1007/s00427-003-0350-8
Yamada, T., Sasaki, Y., Hashimoto, K., Nakajima, K., and Gasser, C. S. (2016). CORONA, PHABULOSA and PHAVOLUTA collaborate with BELL1 to confine WUSCHEL expression to the nucellus in Arabidopsis ovules. Development 143, 422–426. doi: 10.1242/dev.129833
Keywords: integument, Amborella trichopoda, ovule, angiosperms, HD-ZIP III, YABBY, KANADI, AUXIN RESPONSE FACTOR
Citation: Arnault G, Vialette ACM, Andres-Robin A, Fogliani B, Gâteblé G and Scutt CP (2018) Evidence for the Extensive Conservation of Mechanisms of Ovule Integument Development Since the Most Recent Common Ancestor of Living Angiosperms. Front. Plant Sci. 9:1352. doi: 10.3389/fpls.2018.01352
Received: 23 June 2018; Accepted: 28 August 2018;
Published: 19 September 2018.
Edited by:
Annette Becker, Justus-Liebig-Universität Gießen, GermanyReviewed by:
Charles Gasser, University of California, Davis, United StatesMasaru Ohme-Takagi, Saitama University, Japan
Copyright © 2018 Arnault, Vialette, Andres-Robin, Fogliani, Gâteblé and Scutt. This is an open-access article distributed under the terms of the Creative Commons Attribution License (CC BY). The use, distribution or reproduction in other forums is permitted, provided the original author(s) and the copyright owner(s) are credited and that the original publication in this journal is cited, in accordance with accepted academic practice. No use, distribution or reproduction is permitted which does not comply with these terms.
*Correspondence: Charles P. Scutt, Y2hhcmxpZS5zY3V0dEBlbnMtbHlvbi5mcg==