- 1College of Agronomy and Biotechnology, Hebei Normal University of Science and Technology, Qinhuangdao, China
- 2The State Key Laboratory for Biology of Plant Diseases and Insect Pests, Institute of Plant Protection, Chinese Academy of Agricultural Sciences, Beijing, China
Elevated atmospheric CO2 (eCO2) and increased nitrogen (N) fertilization significantly change the nutritional quality of plants and influence the growth and development of insects. However, little is known about plant metabolism and plant-insect interactions under eCO2 and increased N fertilization, especially C4 plants. Thus, the combined effects of eCO2 and increased N fertilization on maize-Ostrinia furnacalis interactions were tested in this study. Our data demonstrated that both eCO2 and increased N fertilization increased starch content, while increased N fertilization promoted the N content in maize. The combined effects of eCO2 and increased N fertilization did not influence the total non-structural carbohydrates (TNC):N ratio in maize. The jasmonic acid level of maize was enhanced by increased N fertilization and O. furnacalis infestation. The total phenolics content and defensive enzyme activities of maize increased under eCO2, increased N fertilization and O. furnacalis infestation. Protective enzyme activities were enhanced, while digestive enzyme activities, mean relative growth rate, body mass and efficiency of conversion of ingested food decreased for O. furnacalis feeding on maize grown under eCO2 and increased N fertilization. Therefore, eCO2 and increased N fertilization increased starch and N accumulation, and did not influence the TNC:N ratio, however, eCO2 and N promoted the resistance-related secondary metabolites (with or without O. furnacalis induced) of maize, which ultimately decreased the fitness of O. furnacalis to the host. These results will help to better understand the metabolic mechanisms of plants and the plant-insect interaction under eCO2 and increased N fertilization in the context of future climate change scenarios.
Introduction
Industrialization has increased atmospheric CO2 concentration from 280 ppm at the beginning of the Industrial Revolution to 400 ppm today and the value is expected to double by the end of the century (IPCC, 2014). Generally, carbohydrate content in plants increases with elevated atmospheric CO2 (eCO2), due to higher photosynthetic rates (Long et al., 2006; Reddy et al., 2010; Kimball, 2016). Plants grown in eCO2 are observed to have lower nitrogen (N) concentration compared to plants grown in ambient CO2. However, the interpretations for lower N concentration are inconsistent across the literatures. There exist several reasons for this: the dilution effect due to enhanced production of carbohydrates (Novriyanti et al., 2012), the decrease in the specific uptake rates of N by roots under eCO2 (Taub and Wang, 2008), or that eCO2 directly inhibits plant nitrogen metabolism (Bloom et al., 2012). Therefore, more sophisticated approaches to nitrogen fertilization should be employed to enhance N concentration and improve plant quality under eCO2 (Bloom et al., 2014).
Present and future eCO2 is predicted to have a profound effect on plant-insect interactions due to changes in the C:N ratio and chemical compositions based on primary and secondary metabolites (Zavala et al., 2017). This change impacts the food quality of the host plant which subsequently influences insect growth and development. For example, eCO2 increases the total non-structural carbohydrates (TNC) content in maize plants and decreases the N content, causing insects to consume more plant tissue to obtain enough N-based nutrients, and extends their development time (Yin et al., 2010; Xie et al., 2015). At the same time, eCO2 also influences plant secondary metabolites that affect insect growth and development (Reddy et al., 2004; Chen et al., 2005b). For example, under eCO2, the contents of tannin and gossypol are increased and Bt protein synthesis is decreased in cotton (Chen et al., 2005b), the content of total phenolics is increased in rape seed (Reddy et al., 2004), and the defensive enzyme activities against aphids are decreased in Medicago truncatula (Guo et al., 2014).
Increased N fertilization is expected to enhance the N content in plants grown under eCO2. Previous studies have shown that increased N fertilization of wheat plants increases nitrate reductase activity, whereas eCO2 reduces it, and the combined effects result in additional protein content (Pal et al., 2005; Xu et al., 2011). However, Sudderth et al. (2005) indicated that higher N availability in the presence of eCO2 increased foliar N content in Amaranthus viridis (C4 plant), but had no effect on Solanum dulcamara (C3 plant). Therefore, we reason that the responses of C3 and C4 plants to increased N fertilization under eCO2 are entirely different.
Several studies have indicated that the availability of N fertilization determines plant allocation of defensive compounds in response to eCO2, which subsequently alters insect performance (Kinney et al., 1997; Hättenschwiler and Schafellner, 1999; Henn and Schopf, 2001; Saxon et al., 2004). However, changes in nutritional and defensive metabolisms of plants grown under eCO2 and increased N fertilization, as well as insect fitness remain unclear.
Plants have evolved a series of defense systems against insects, one of which is the jasmonic acid (JA) signaling pathway (Kawazu et al., 2012). Several studies have examined the insect-induced plant defense response via JA under eCO2, however, these responses have varied for different plant and insect species (Casteel et al., 2008; Zavala et al., 2008; Lu et al., 2018). In view of the above-mentioned facts, even if N fertilization increases the protein content in plant tissue under eCO2, it is not clear if this will be able to influence JA-mediated secondary metabolites.
Asian corn borer, Ostrinia furnacalis (order Lepidopteran), consumes C4 plant-maize. One to seven generations of this insect occur per year in northern, cool temperate regions to southern tropical areas (Zhou et al., 1995). They cause a 10–30% yield loss in most maize production areas in China (Wang et al., 2000). Our previous study showed that the fitness of O. furnacalis in maize was adversely affected by an eCO2-mediated decrease in maize nutritional quality (higher TNC:N ratio) during a field experiment (Xie et al., 2015). Based on this result and the evidence for different responses of C3 and C4 plants to increased N fertilization under eCO2, as mentioned above, the interactions between maize and O. furnacalis may change due to the combined effects of eCO2 and increased N fertilization.
The specific objectives of this study were to (1) quantify the effects of eCO2 and increased N fertilization in maize on C and N primary metabolites and O. furnacalis induced defense-related secondary metabolites, (2) determine the successive performance of O. furnacalis to the host. Results from our study help to understand the metabolic mechanism of the plant and the plant-insect interactions under eCO2 and increased N fertilization, which will subsequently aid in adjusting pest control strategies under the context of future climate change.
Materials and Methods
Plant Preparation and Treatments With CO2 and Nitrogen
The experiment was carried out in six environmental chambers with three environmental chambers maintained at ambient CO2 concentrations (∼380 ppm) and three environmental chambers maintained at elevated CO2 concentrations (∼750 ± 15 ppm). The ambient CO2 was the surrounding air entering the environmental chamber facilities, whereas the elevated CO2 was supplied from direct gas tanks. Details of the automatic control system for CO2 levels were described by Chen et al. (2005a). The environmental chambers were maintained at 28 ± 1°C and 60–70% RH, with a photoperiod of 16:8 h (L:D) and illumination (90 μmol m−2 s−1) provided by fluorescent lamp. Maize seeds were planted in trays (26.5 cm × 18.5 cm × 7.5 cm) filled with a sand, peat and vermiculite mixture (1:1:1). Three N fertilization levels were established, low N (100 mg N/kg soil mixture); middle N (200 mg N/kg soil mixture), and high N (300 mg N/kg soil mixture) under each CO2 level (i.e., in each environmental chamber). In preliminary experiments, low N produced adequate growth of maize plants, while high N significantly enhanced growth. Each tray was planted with 20 seedlings, three trays were used in each N fertilization level. Of these three trays, maize plants from two trays were used for O. furnacalis rearing experiment, and from the remaining tray was used for metabolic analysis. After collecting tissue for metabolic analysis, the tray with maize plants was removed from the environmental chamber to avoid the wounding signals transmission among the plants. All trays were provided the same amount of water (100 mL) each day. To account for possible spatial variability from micro environmental factors, the environmental chambers were arranged in three blocks of two adjacent chambers per block.
Insect Rearing
The O. furnacalis neonates used in this study were obtained from a laboratory colony that originated from a field population and had been maintained on a regular artificial diet (Zhou et al., 1995) for 5–6 generations in the lab. The centrifuge tubes (50 mL) were used as O. furnacalis larval rearing containers in the environment chamber as above description. Centrifugal tube orifice was covered with three layers of gauze. A piece of maize leaf (1 cm × 4 cm) from the same environment chamber was placed in a centrifuge tube and fed by a neonate larva (∼12 h). When larva developed to the 4th instar, a short stem (5 cm long) was supplied to it instead of leaf tissue (Zhou et al., 1995). Twenty larvae were reared in each treatment, with three replications per treatment (total 60 larvae per treatment). The leaf or stem issues were replaced with fresh leaves or stems every other day until larvae pupated. Meanwhile the unconsumed leaf- or stem-tissues and frass were collected and weighed after oven drying at 80°C for 72 h. The water content was calculated by daily drying of the fresh leaf or stem to obtain the dry weight of larval food consumption. The neonate (∼12 h) and mature larvae (before pupation) fresh weight were measured and the duration (in days) of larval and pupal stages were also recorded. The conventional, ratio-based nutritional indices, including mean relative growth rate (MRGR), efficiency of conversion of ingested food (ECI) and of digested diet (ECD), and approximate digestibility (AD) were determined gravimetrically following the methods of Rayapuram and Baldwin (2006) and Chen et al. (2007). The amount of food consumption, frass produced, larval body weight, and weight gain were all calculated as dry weights. Formulas for calculation of the indices measured are shown in Table 1.
The O. furnacalis feeding maize plant from each treatment were used to test the activity of digestive and protective enzymes. The digestive enzymes included trypsin, total protease, lipase and amylase, the protective enzymes included catalase (CAT), peroxidase (POD), and superoxide dismutase (SOD). 10 larvae (4–5th instar) from each treatment were selected and were grinded in physiological saline (0.9%). Homogenates were centrifuged at 10,000 × g for 10 min, and the supernatants were subjected to enzyme activity analysis according to the kit instructions (MM-126401 for Trypsin, MM-3439701 for total protease, MM-3442501 for lipase, MM-3439801 for amylase, MM-3440701 for CAT, MM-3440801 for POD, MM-3439601 for SOD, Jiangsu Kete Biological Technology Co., Ltd., China).
Primary and Secondary Metabolites Analysis of Maize
For primary metabolic analysis, one leaf was collected at random from each maize plant on the 20th day after sowing, a total of 5 leaves were collected in each tray and mixed for leaf samples. The TNC (primarily soluble sugars and starch) were analyzed using the method of Tissue and Wright (1995). The nitrogen content was determined according to the Official Methods of Analysis of AOAC International (2009), using a Kjeltec N analyzer (Model KDY-9830; Foss automated Kjeltec instruments, Beijing, China).
For analysis of secondary defense metabolism, 2nd to 3rd instar larvae of O. furnacalis were placed on each maize leave. Damaged areas from approximately 1 cm around the feeding sites were taken from the leaves using a knife. Leaf samples were collected 2 h after infestation (Guo et al., 2018). One leaf was collected at random from each maize plant on the 20th day after sowing, a total of 5 leaves were harvested in each tray and mixed for leaf samples. After harvesting, leaf samples were immediately frozen in liquid nitrogen and then stored at −80°C until use. Uninfested leaves were used as controls and treated as described above. For JA analysis, frozen leaves (0.1 g) were ground in 1:10 [leaf: extracting solution (w/v) ratio]. The extracting solution contained methyl alcohol, formic acid, and pure water (15/1/4, v/v/v). Homogenates were centrifuged at 8,000 × g for 20 min. The supernatants were subjected to JA content analysis according to the kit instructions (MM-0887001, Keteshengwu, Nanjing, China). For the remaining analysis, frozen leaves (0.1 g) were ground in buffer (1:10 w/v ratio; pH 7.0–7.4). Homogenates were centrifuged at 10,000 × g for 10 min. The supernatants were then analyzed for total phenolics content, the activities of polyphenol oxidase (PPO), POD, phenylalanine ammonia-lyase (PAL), CAT, and proteinase inhibitors (PIs) according to the kit instructions (MM-123301 for total phenolics, MM-3269901 for PPO, MM-3596001 for POD, MM-089901 for PAL, MM-079901 for CAT, MM-063301 for PIs, Jiangsu Kete Biological Technology Co., Ltd., China).
Statistical Analyses
The main effects of CO2, N, infestation and block (pairs of adjacent environmental chambers) on plant secondary defense metabolism (JA and total phenolics content; and PPO, POD, CAT, PAL, and PIs activities) were tested by four-factor ANOVA according to a model (Sudderth et al., 2005). A split-split plot design with CO2 and block was used as the main effects, N was used as the subplot effect, and O. furnacalis presence or absence was used as the sub-subplot effect (SAS Institute, 2006). The effects of CO2 N and block on plant primary metabolism (starch, soluble sugar, and N content and TNC:N ratio) and O. furnacalis performance (trypsin, total protease, amylase, lipase, catalase, POD, and SOD activities; and larvae, pupal stage duration, food consumption, MRGR, ECI, ECD, AD metrics) were tested by three-factor ANOVA using a split-plot design with CO2 and block as the main effects and N as the subplot effect.
Results
C and N Primary Metabolism in Maize
The C and N primary metabolism of maize was influenced by eCO2 and increased N fertilization (Table 2 and Figures 1A–D). Relative to the ambient CO2 treatment, the starch content of maize was significantly increased by 4.69% in plants grown under eCO2 (Table 2 and Figure 1A). Relative to the low N fertilization treatment, plants grown with middle and high N fertilizations showed a significant increase in the starch content (9.38 and 12.41%, respectively; Table 2 and Figure 1A), and N content (9.38 and 12.42%, respectively; Table 2 and Figure 1C). However, eCO2 and increased N fertilization did not influence the soluble sugar content and TNC:N ratio of maize, while the interaction between them was significant on N content and TNC:N ratio (Table 2 and Figures 1B,D).
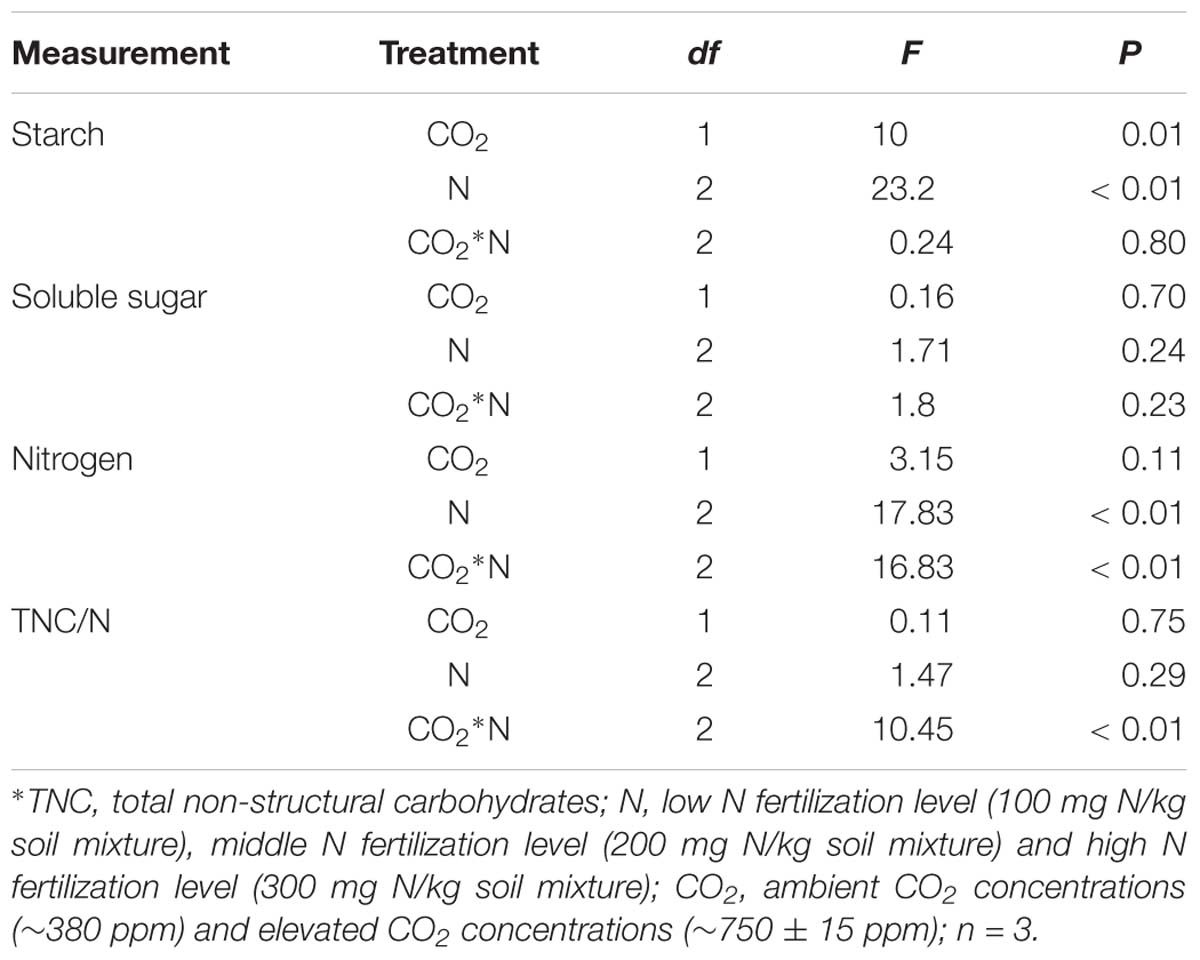
Table 2. Summary of ANOVA results for effects of elevated CO2 and increased nitrogen fertilization on chemical components of maize.
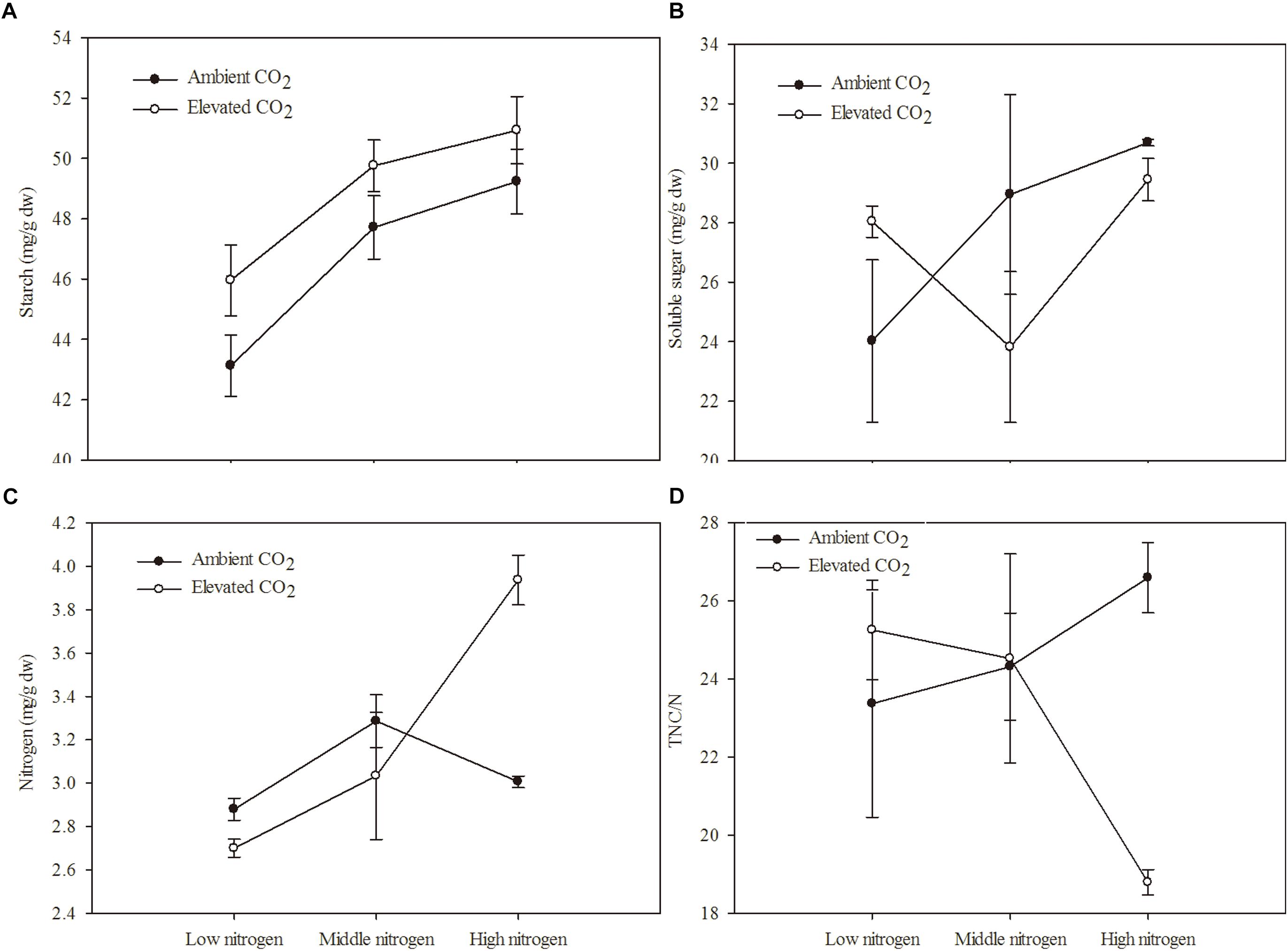
Figure 1. Chemical components of maize grown under different levels of CO2 and nitrogen fertilization conditions (mean ± SE, n = 3). (A) Starch, (B) soluble sugar, (C) nitrogen (N) and (D) total non-structural carbohydrates (TNC)/N.
O. furnacalis Induced Defense-Related Secondary Metabolites in Maize
The chemical defense of maize was significantly influenced by eCO2, increased N fertilization and O. furnacalis infestation (Table 3 and Figures 2A,B). Relative to ambient CO2, the total phenolics content of maize was increased by 5.13% under eCO2 (Table 3 and Figure 2B). Relative to low N fertilization, the JA content of maize increased by 11.64 and 34.99% in middle and high N fertilization treatments, while the total phenolics content increased by 11.75% in the high N fertilization (Table 3 and Figure 2A). O. furnacalis infestation increased the JA and total phenolics content of maize by 15.06 and 7.69%, respectively, compared with uninfested tissues (Table 3 and Figures 2A,B). Furthermore, the interactions between CO2 and infestation and between N and infestation on total phenolics content were significant (Table 3 and Figure 2B).
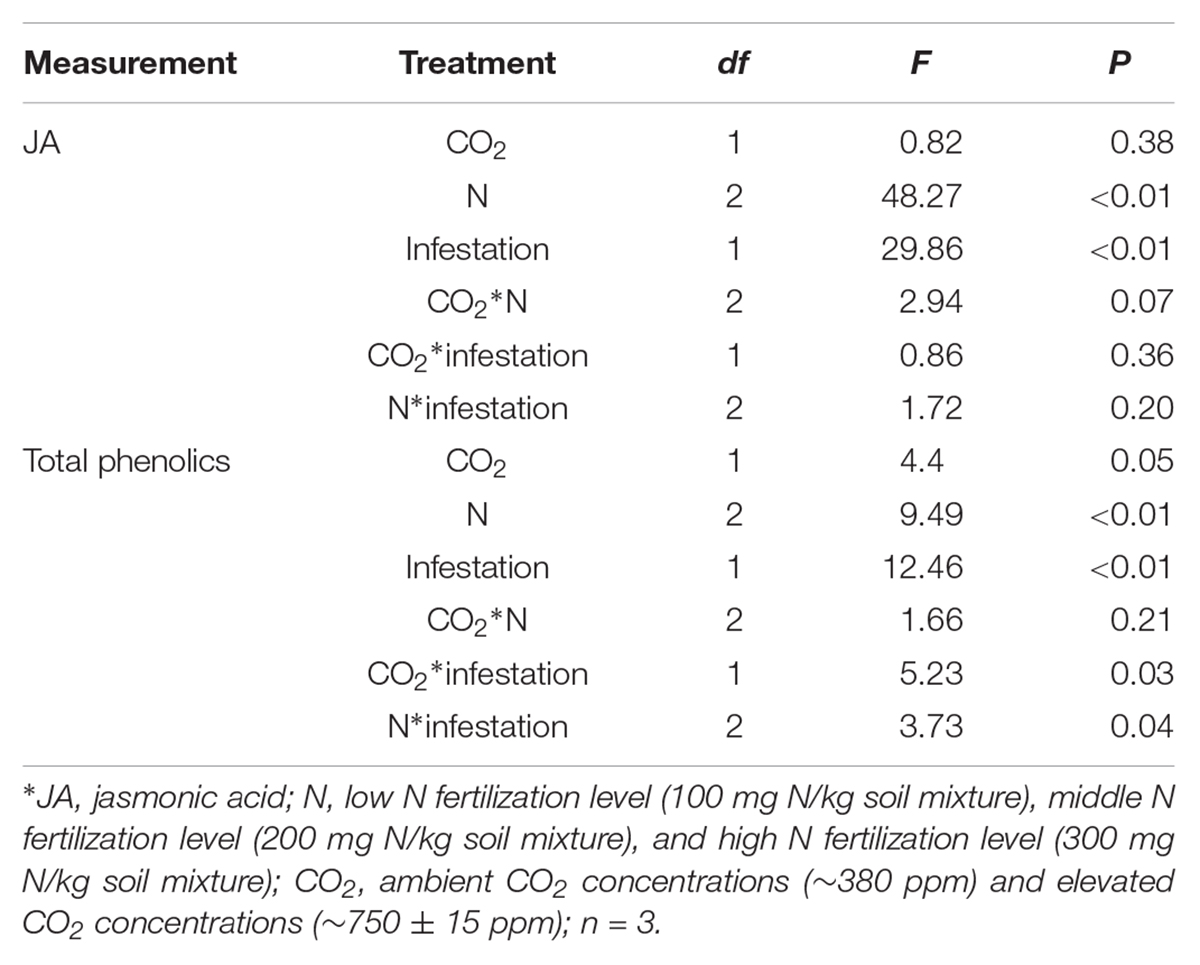
Table 3. Summary of ANOVA results for effects of elevated CO2 and increased nitrogen fertilization on chemical defenses of maize.
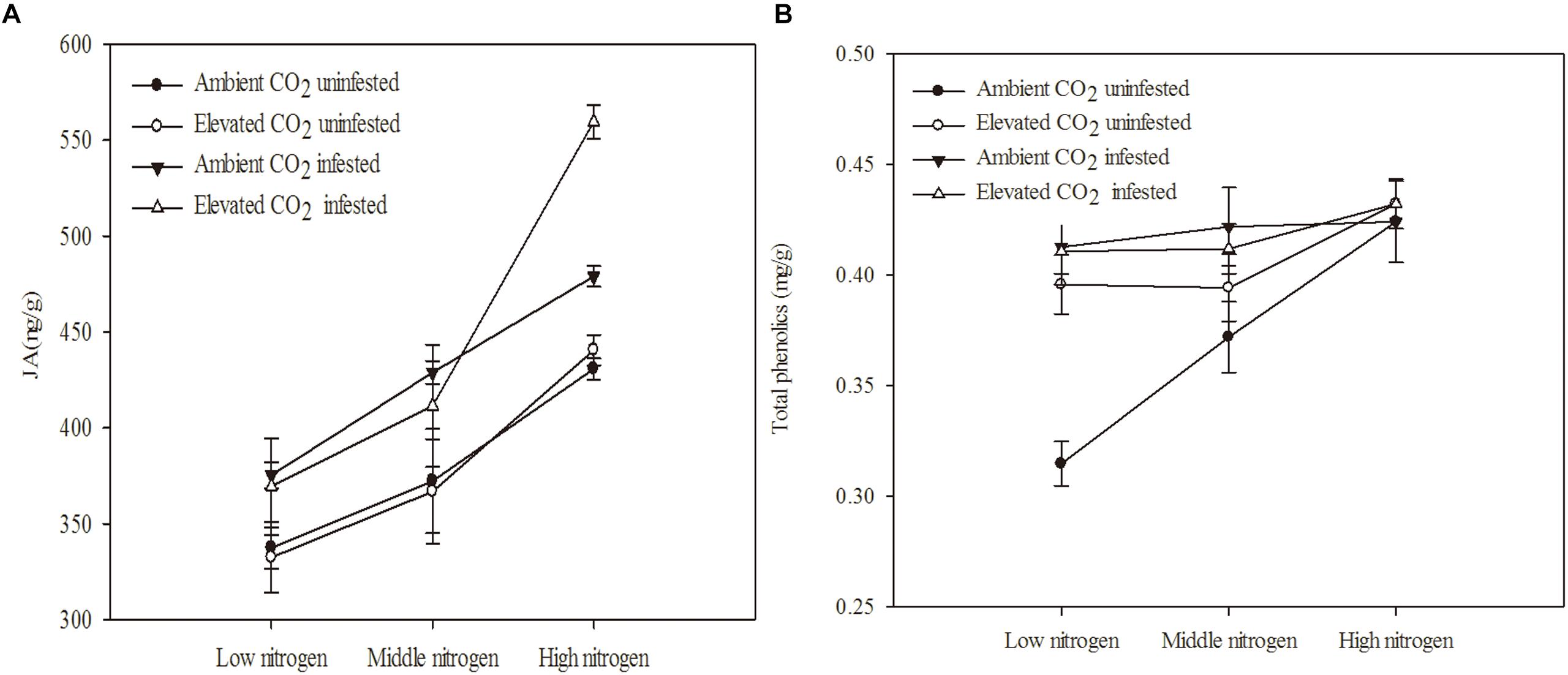
Figure 2. Defensive chemical components of maize grown under different levels of CO2 and nitrogen fertilization conditions (mean ± SE, n = 3). (A) Jasmonic acid (JA) and (B) total phenolics.
The defensive enzyme activities of maize were significantly influenced by eCO2, increased N fertilization and O. furnacalis infestation (Table 4 and Figures 3A–E). Relative to ambient CO2, the POD, PPO and PAL activities of the maize were increased by eCO2 (Table 4 and Figures 3A,B,D). Relative to low N fertilization, the POD, PAL, CAT, and PIs activities were increased in the middle and high N fertilization treatments, whereas the PPO activities were increased in only the high N fertilization (Table 4 and Figures 3A–E). O. furnacalis infestation increased the POD, PPO, PAL, and PIs activities of maize, compared with the uninfested tissues (Table 4 and Figures 3A–C,E). The interactions between CO2 and infestation and between N and infestation on CAT activity, and between N and infestation on PIs were significant (Table 4 and Figures 3C,E).
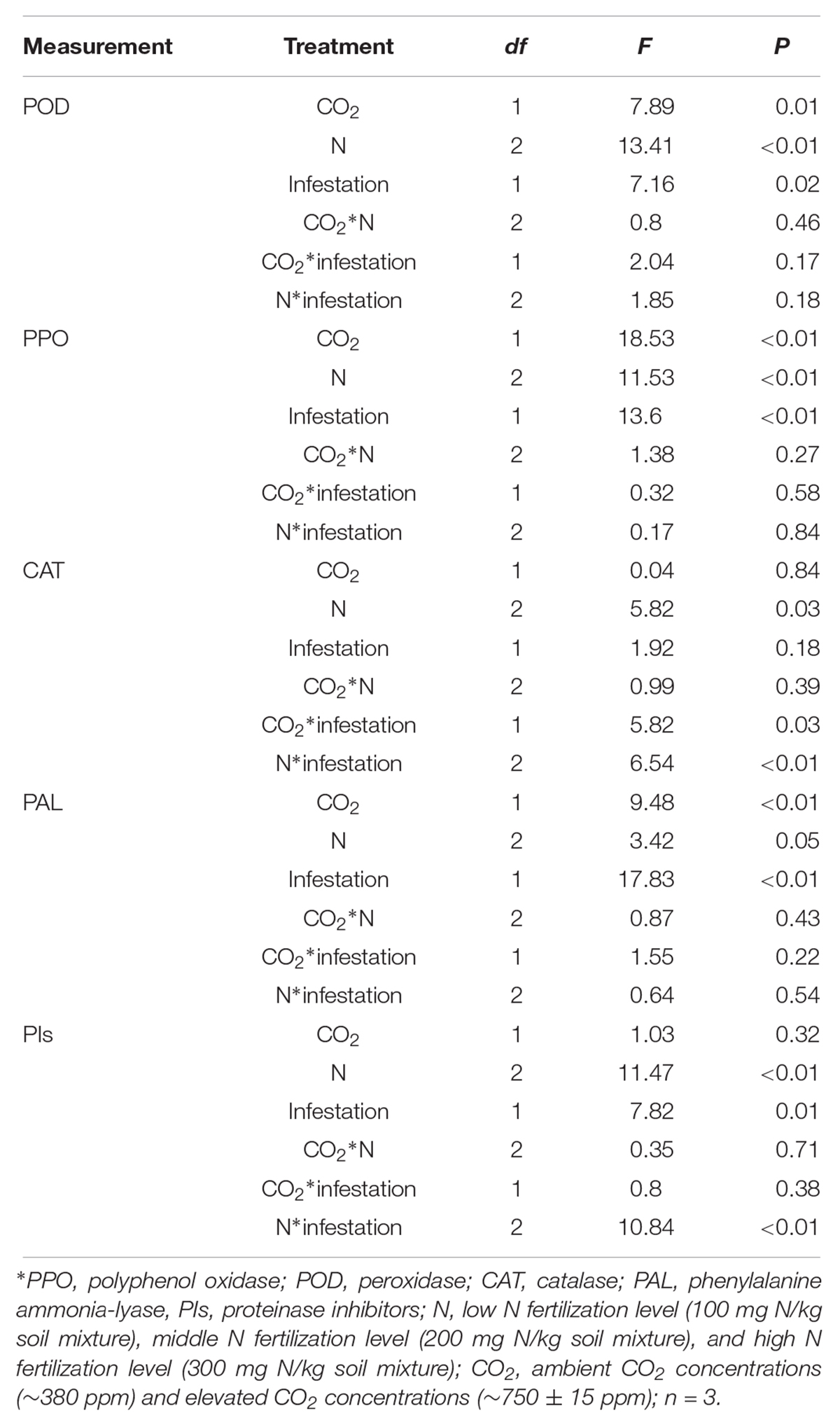
Table 4. Summary of ANOVA results for effects of elevated CO2 and increased nitrogen fertilization on defensive response of maize.
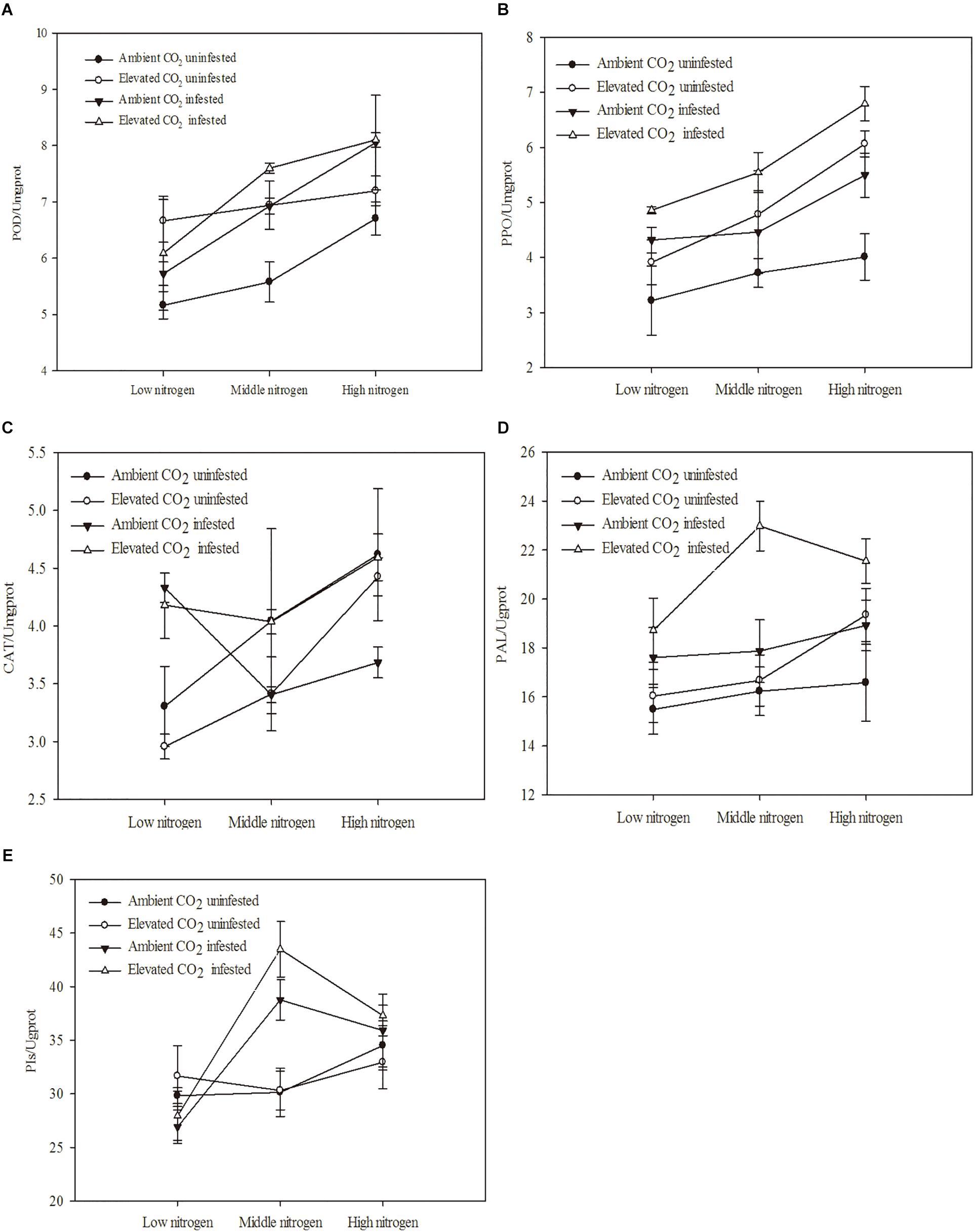
Figure 3. Defensive response of mazie grown under different levels of CO2 and nitrogen fertilization conditions (mean ± SE, n = 3). (A) Peroxidase (POD) activity, (B) polyphenol oxidase (PPO) activity, (C) catalase (CAT) activity, (D) phenylalanine ammonia-lyase (PAL) activity, and (E) proteinase inhibitors (PIs).
The Growth and Development of O. furnacalis
The digestive and protective system enzyme activities varied for O. furnacalis feeding on maize grown under different levels of CO2 and N fertilization (Table 5 and Figures 4A–G). Compared with ambient CO2, amylase activity decreased and SOD activity increased for O. furnacalis feeding on maize grown under eCO2 (Table 5 and Figures 4C,G). Compared with low N fertilization, the trypsin, total protease and amylase activities decreased for O. furnacalis feeding on maize grown in the middle and high N fertilization treatments, whereas the CAT and SOD activities increased for O. furnacalis feeding on maize grown at high N fertilization and two increasing N fertilization treatments, respectively (Table 5 and Figures 5A–C,E).
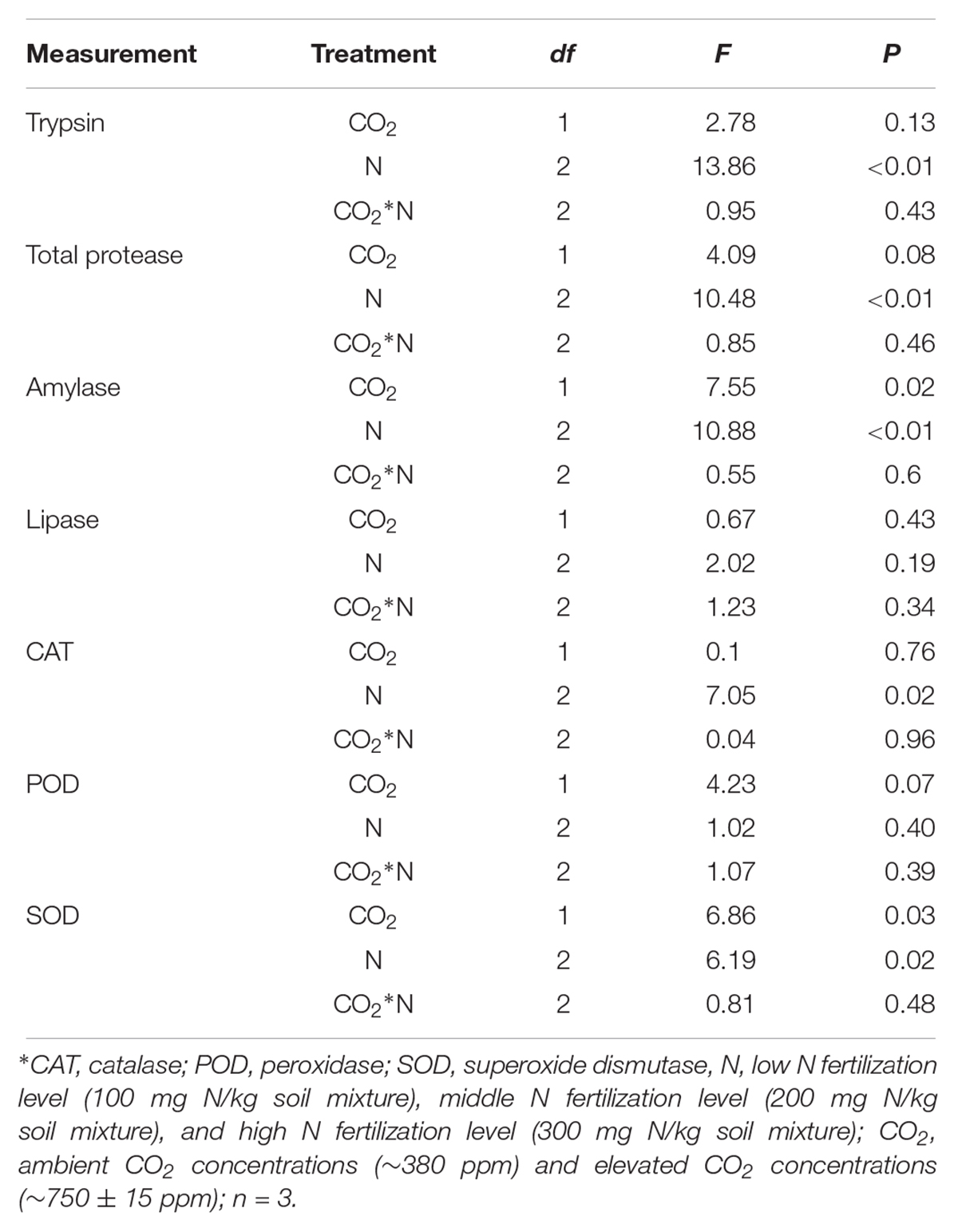
Table 5. Summary of ANOVA results for effects of elevated CO2 and increased nitrogen fertilization on digestive and protective system enzymes of Ostrinia furnacalis.
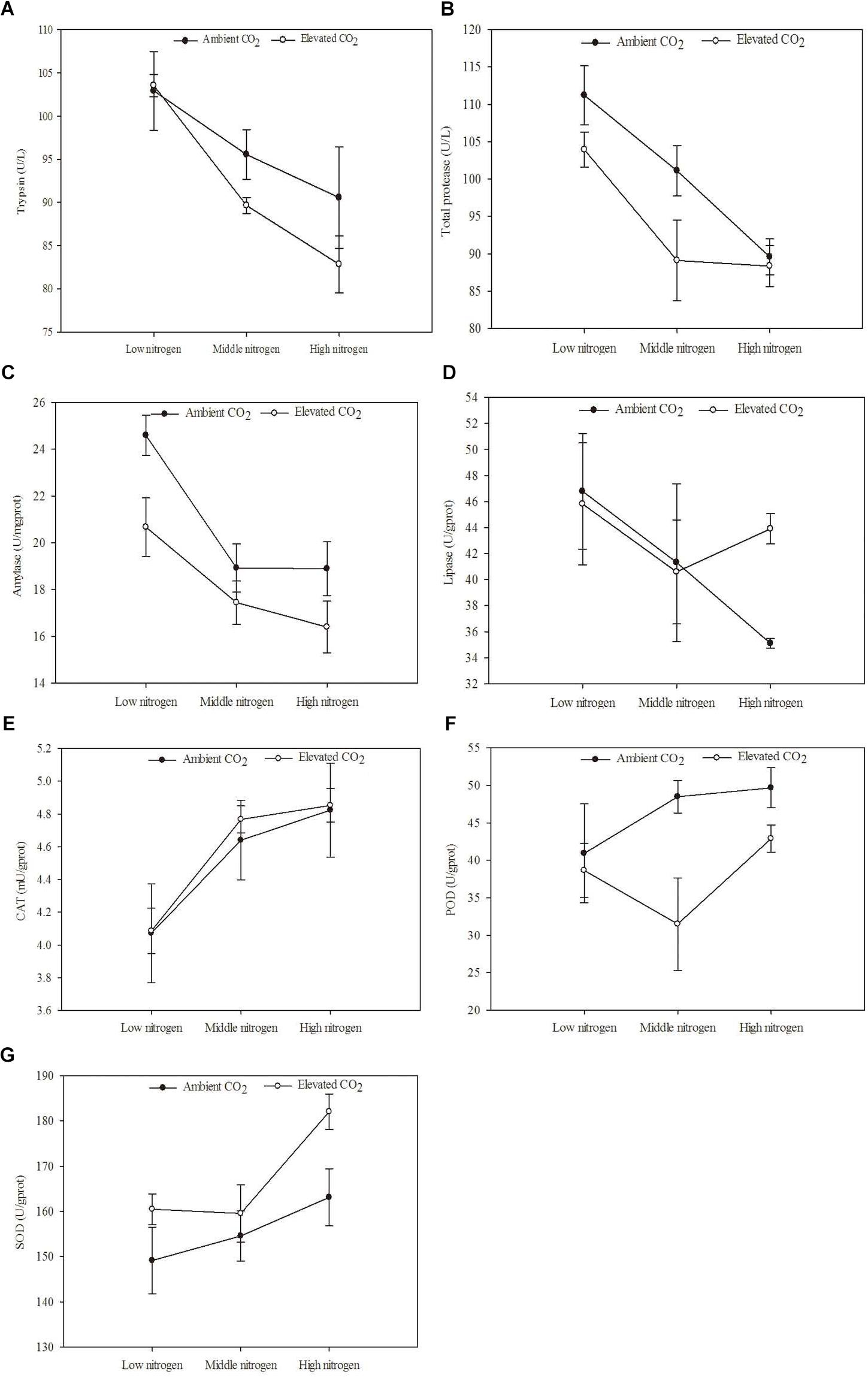
Figure 4. Defense chemical components of Ostrinia furnacalis feeding on maize grown under different levels of CO2 and nitrogen fertilization conditions (mean ± SE, n = 3). (A) Trypsin activity, (B) total protease activity, (C) amylase activity, (D) lipase activity, (E) catalase (CAT) activity, (F) peroxidase (POD) activity, and (G) superoxide dismutase (SOD) activity.
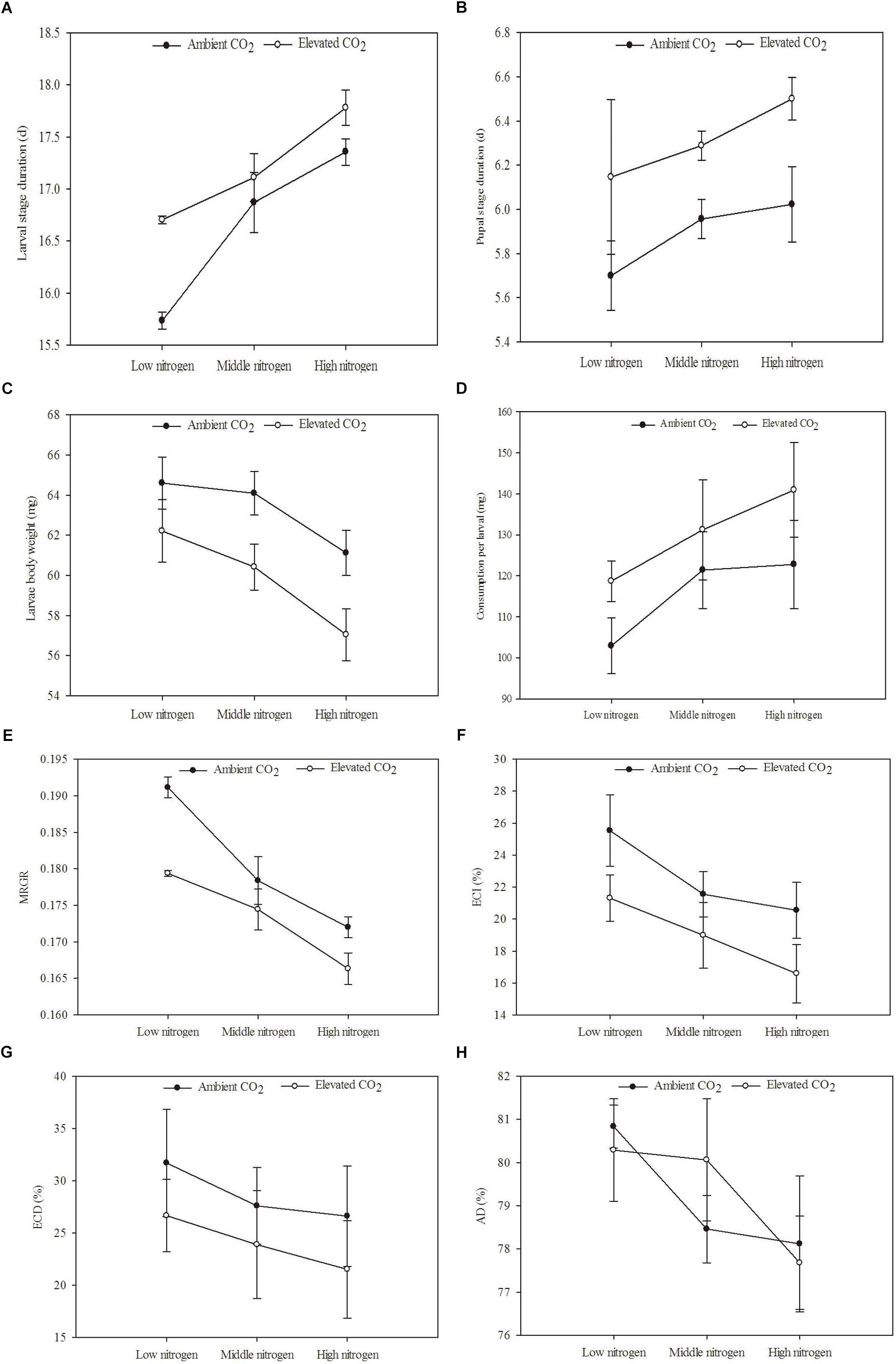
Figure 5. Development and digestibility of O. furnacalis feeding on mazie grown under different levels of CO2 and nitrogen fertilization conditions (mean ± SE, n = 3). (A) Larvae stage duration, (B) pupal stage duration, (C) larvae body mass, (D) food consumption, (E) mean relative growth rate (MRGR), (F) efficiency of conversion of ingested food (ECI), (G) efficiency of conversion of digested food (ECD), and (H) approximate digestibility (AD).
The development and digestibility of O. furnacalis were changed after feeding on maize grown under different levels of CO2 and N fertilization (Table 6 and Figures 5A–H). Relative to ambient CO2, eCO2 extended the duration of the larval and pupal stage by 3.99 and 7.13%, respectively; reduced larval body mass by 5.64%; and decreased MRGR, ECI, and ECD by 4.15, 18.89, and 19.23%, respectively (Table 6 and Figures 5A–C,E,F). Relative to low N fertilization, the middle and high N fertilization treatments extended the larval stage duration by 4.75 and 8.32%, and decreased MRGR by 4.32 and 9.52%, respectively; in the high N fertilization, the larval body mass and ECI were decreased by 7.31 and 26.12%, respectively (Table 6 and Figures 5A,C,E,F).
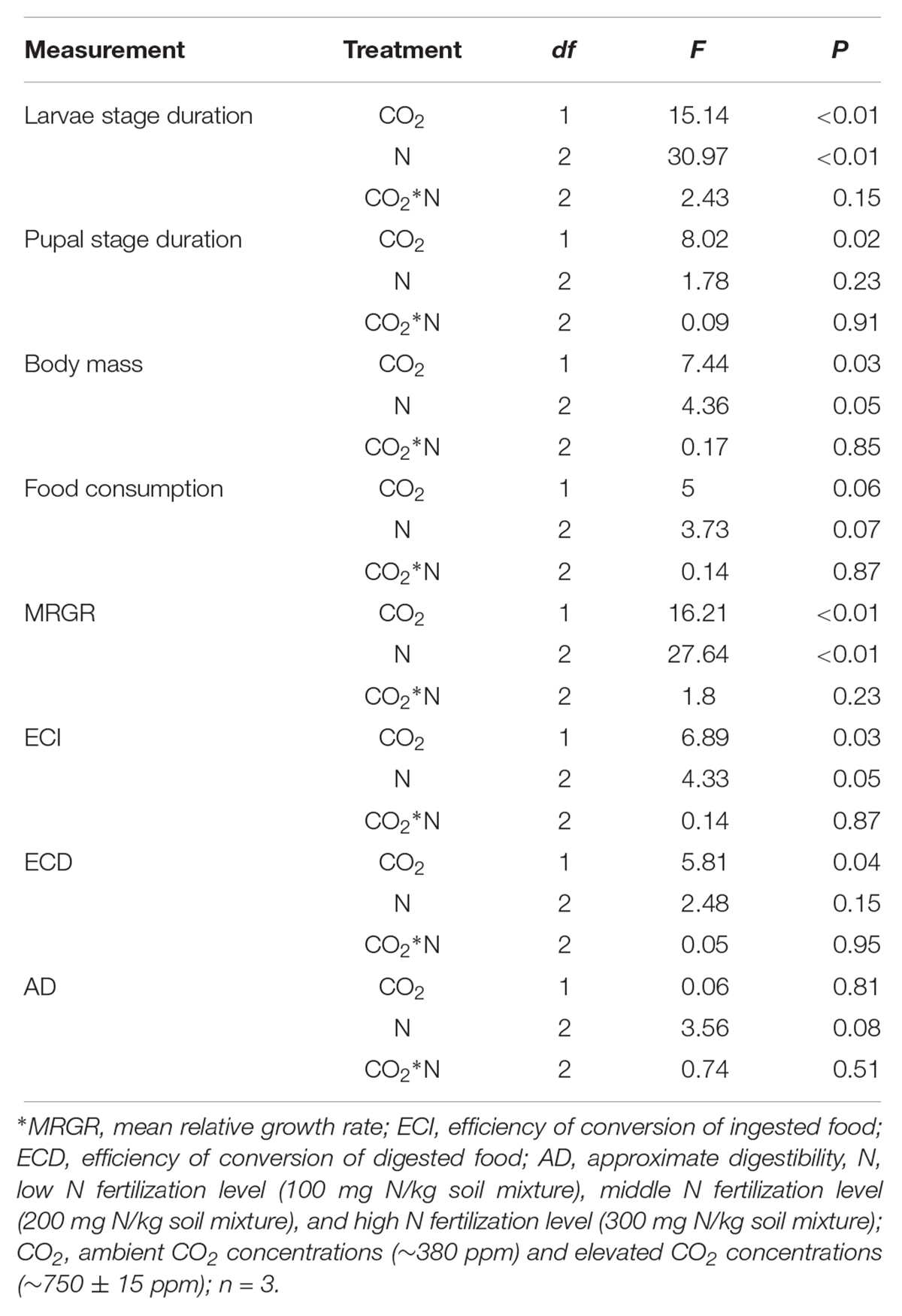
Table 6. Summary of ANOVA results for effects of elevated CO2 and increased nitrogen fertilization on development and digestibility of O. furnacalis.
Discussion
Both eCO2 and increased N fertilization increased the starch content of the maize in this experiment. A previous study found that the C4 photosynthesis in maize was enhanced by increasing N nutrition under eCO2 (Cousins and Bloom, 2003), thus the accumulation of photosynthetic products (carbohydrate) might increase in C4 plants. The eCO2 did not reduce the N content. However, increased N fertilization enhanced the N content in this experiment. Thus, eCO2 and increased N fertilization increased C and N primary metabolites, although the combined effects of eCO2 and increased N fertilization did not influence the TNC:N ratio. However, our previous results from a different study indicate that eCO2 decreases the N content and increases the TNC:N ratio in maize. In this experiment, the altered response of N content to eCO2 may be due to the close interaction between CO2 and N fertilization (P < 0.05, Pal et al., 2005). In other words, increased N fertilization could offset the decreasing N content of maize grown under eCO2.
Numerous experiments have shown that defensive metabolisms of plants are influenced by eCO2, increased N fertilization, or insect infestation (Lou and Baldwin, 2004; Guo et al., 2012; Zavala et al., 2013; Guo et al., 2018; Lu et al., 2018). However, relatively little is known about their combined effects on the defensive metabolism of plants. In this study, defensive metabolisms of maize were enhanced by eCO2, increased N fertilization and insect infestation. We observed that the eCO2, increased N fertilization or insect infestation effects were additive, but there were few interactions between factors. The accumulation of JA level and activation of antioxidative enzymes (POD, PPO, and CAT) and PIs play an important role in regulating chewing-induced resistance of plants to insects (Scott et al., 2010; Nahar et al., 2011; Kawazu et al., 2012; Kerchev et al., 2012; Wang and Wu, 2013; War et al., 2015). In this study, both eCO2 and increased N fertilization improved defensive enzymes (POD, PPO, and PAL) activities, and increased N fertilization also improved JA level, CAT, and PIs activities. Similarly, a previous study found that the defensive enzyme activities in maize were increased under eCO2 or increased N fertilization (Zhang et al., 2007; Zhao et al., 2008). Lou and Baldwin (2004) indicated that JA levels decreased in plants grown with low N fertilization, which was due to the decreased expression levels of JA-related genes in plants grown in low N fertilization. Our observations may be explained by sufficient CO2 and N driving the maize metabolites, which enahnced the JA level and defensive enzymes activities at the same time (Nunes-Nesi et al., 2010). Like other chewing insects, O. furnacalis infestation can also induce the defensive metabolism of maize (Guo et al., 2018). In the present study, O. furnacalis infestation increased JA level, total phenolic content and defensive enzyme (POD, PPO, PAL, and PIs) activities even under eCO2 and increased N fertilization conditions, i.e., the maize defensive response still exist under eCO2 and increased N fertilization. The activity of PAL, which is a principle enzyme involved in a rate-limiting step in phenolic biosynthesis, could be induced by some enviromental factors (Hartley et al., 2000; Zhao et al., 2009). In this study, PAL activity was enhanced by increased N fertilization and O. furnacalis infestation, which subsequently raised the total phenolic content in these treatments. At the same time, there is a positive interaction between O. furnacalis infestation and CO2 or N fertilization on the total phenolics content in maize plants. We conclude that the defensive metabolism of maize plants grown under elevated CO2 and higher N fertilization could be enhanced, especially during O. furnacalis infestation.
Plant proteinase inhibitors (PIs) are able to reduce the feeding fitness of chewing insects by suppressing insect gut proteases (Govind et al., 2010). The results in this experiment also indicated that increased N fertilization increased PIs activity in maize, and accordingly, increased N fertilization decreased the activities of O. furnacalis digestive enzymes (trypsin, total protease, and amylase). The eCO2 also decreased amylase activity, while both eCO2 and high N fertilization increased protective enzyme activities. Thus, O. furnacalis digestive ability was decreased and the defensive response was enhanced during feeding on maize plants grown under eCO2 and increased N fertilization.
Both eCO2 and increased N fertilization extended the larvae development time; and decreased the MRGR, body mass and ECI of O. furnacalis. These observations could be explained by the results above, due to the fact that TNC:N ratio did not changed, but the defensive metabolism of maize plants was increased by eCO2 and increased N fertilization. Thus, the increased defensive metabolism subsequently may increase O. furnacalis defensive response, slowed growth and decreased food digestibility and utilization (Xie et al., 2013; Guo et al., 2018; Lu et al., 2018). Henn and Schopf (2001) demonstrated increased consumption and decreased ECI for Lymantria dispar fed on trees grown under ambient CO2 and low N fertilization. Considering our results, the different effects of eCO2 and increased N fertilization on ECI may be due to the N availability and the level of defensive metabolism for host species.
Conclusion
Our data demonstrate that eCO2 and increased N fertilization increased C and N primary metabolites. The combined effects of eCO2 and increased N fertilization did not influence the TNC:N ratio, because increased N fertilization could offset the decreasing N content of maize grown under eCO2. The resistance-related secondary metabolites (with or without O. furnacalis induced) in maize were enhanced by eCO2 and increased N fertilization, which increased the O. furnacalis defensive response, slowed its growth; and decreased its food digestibility and utilization. Thus, increased N fertilization will increase starch and N accumulation, do not influence the TNC:N ratio. But increased N fertilization promote resistance of maize to O. furnacalis feeding, which may decrease the fitness of O. furnacalis to its host in future eCO2 scenarios.
Furthermore, the metabolic mechanisms of plants and insects may vary with plant and insect species, the insect fitness over multiple generations to host plant and insect enemy behavior may also change under eCO2 and increased N fertilization. The optimal N application rates should be employed to improve plant growth and insect population control under eCO2. Thus, more research will be needed to elucidate the effects of eCO2 and increased N fertilization on interaction of plant-insect, which will help to predict the plant damage in agroecosystem.
Author Contributions
HuX wrote the manuscript. HaX designed the experiments. HaX and SW performed the experiments. ZW and KH provided the insect, reagents, and materials.
Funding
This research was supported by the National Key Research and Development Program (2017YFD0201800), the Natural Science Foundation of Hebei Province (C2017407008), and China Agriculture Research System (CRAS-02).
Conflict of Interest Statement
The authors declare that the research was conducted in the absence of any commercial or financial relationships that could be construed as a potential conflict of interest.
References
AOAC International (2009). “AOAC Official Method 2001.11 Protein (crude) in animal feed, forage (plant tissue), grain and oilseeds,” in Official Methods of Analysis of AOAC International, eds J. W. Horwitz and L. George (Gaithersburg, MD: AOAC International).
Bloom, A. J., Asensio, J. S. R., Randall, L., Rachmilevitch, S., Cousins, A. B., and Carlisle, E. A. (2012). CO2 enrichment inhibits shoot nitrate assimilation in C3 but not C4 plants and slows growth under nitrate in C3 plants. Ecology 93, 355–367. doi: 10.1890/11-0485.1
Bloom, A. J., Burger, M., Kimball, B. A., and Pinter, P. J. Jr. (2014). Nitrate assimilation is inhibited by elevated CO2 in field-grown wheat. Nat. Clim. Change 4, 477–480. doi: 10.1038/nclimate2183
Casteel, C. L., O’Neill, B. F., Zavala, J. A., Bilgin, D. D., Berenbaum, M. R., and DeLucia, E. H. (2008). Transcriptional profiling reveals elevated CO2 and elevated O3 alter resistance of soybean (Glycine max) to Japanese beetles (Popillia japonica). Plant Cell Environ. 31, 419–434. doi: 10.1111/j.1365-3040.2008.01782.x
Chen, F. J., Ge, F., and Parajulee, M. N. (2005a). Impact of elevated CO2 on tri-trophic interaction of Gossypium hirsutum, Aphis gossypii, and Leis axyridis. Environ. Entomol. 34, 37–46. doi: 10.1603/0046-225X-34.1.37
Chen, F. J., Wu, G., Ge, F., Parajulee, M. N., and Shrestha, R. B. (2005b). Effects of elevated CO2 and transgenic Bt cotton on plant chemistry, performance, and feeding of an insect herbivore, the cotton bollworm. Entomol. Exp. Appl. 115, 341–350. doi: 10.1111/j.1570-7458.2005.00258.x
Chen, F. J., Wu, G., Parajulee, M. N., and Ge, F. (2007). Long-term impacts of elevated carbon dioxide and transgenic Bt cotton on performance and feeding of three generations of cotton bollworm. Entomol. Exp. Appl. 124, 27–35. doi: 10.1111/j.1570-7458.2007.00563.x
Cousins, A. B., and Bloom, A. J. (2003). Influence of elevated CO2 and nitrogen nutrition on photosynthesis and nitrate photo-assimilation in maize (Zea mays L.). Plant Cell Evriron. 26, 1525–1530. doi: 10.1046/j.1365-3040.2003.01075.x
Govind, G., Mittapalli, O., Griebel, T., Allmann, S., Bocker, S., and Baldwin, I. T. (2010). Unbiased transcriptional comparisons of generalist and specialist herbivores feeding on progressively defenseless Nicotiana attenuata plants. PLoS One 5:e8735. doi: 10.1371/journal.pone.0008735
Guo, H. J., Sun, Y. C., Li, Y. F., Liu, X. H., Zhang, W. H., and Ge, F. (2014). Elevated CO2 decreases the response of the ethylene signaling pathway in Medicago truncatula and increases the abundance of the pea aphid. New Phytol. 201, 279–291. doi: 10.1111/nph.12484
Guo, H. J., Sun, Y. C., Ren, Q., Zhu-Salzman, K., Kang, L., Wang, C. Z., et al. (2012). Elevated CO2 reduces the resistance and tolerance of tomato plants to Helicoverpa armigera by suppressing the JA signaling pathway. PLoS One 7:e41426. doi: 10.1371/journal.pone.0041426
Guo, J. F., Qi, J. F., He, K. L., Wu, J. Q., Bai, S. X., Zhang, T. T., et al. (2018). The Asian corn borer Ostrinia furnacalis feeding increases the direct and indirect defence of mid-whorl stage commercial maize in the field. Plant Biotechnol. J. 17, 88–102. doi: 10.1111/pbi.12949
Hartley, S. E., Jones, C. G., Couper, G. C., and Jones, T. H. (2000). Biosynthesis of plant phenolic compounds in elevated atmospheric CO2. Global Chang. Biol. 6, 497–506. doi: 10.1046/j.1365-2486.2000.00333.x
Hättenschwiler, S., and Schafellner, C. (1999). Opposing effects of elevated CO2 and N deposition on Lymantria monacha larvae feeding on spruce trees. Oecologia 118, 210–217. doi: 10.1007/s004420050720
Henn, M. W., and Schopf, R. (2001). Response of beech (Fagus sylvatica) to elevated CO2 and N: influence on larval performance of the gypsy moth Lymantria dispar (Lep., Lymantriidae). J. Appl. Entomol. 125, 9–10. doi: 10.1046/j.1439-0418.2001.00592.x
IPCC (2014). “Impacts, adaptation and vulnerability,” in Working Group II Contribution to the Fifth Assessment Report of the Intergovernmental Panel on climate Change, 1132, eds C. B. Field, V. R. Barros, D. J. Dokken, K. J. Mach, M. D. Mastrandrea, T. E. Bilir, et al. (Cambridge: Cambridge University Press).
Kawazu, K., Mochizuki, A., Sato, Y., Sugeno, W., Murata, M., Seo, S., et al. (2012). Different expression profiles of jasmonic acid and salicylic acid inducible genes in the tomato plant against herbivores with various feeding modes. Arthropod Plant Inte. 6, 221–230. doi: 10.1007/s11829-015-9412-x
Kerchev, P. I., Fenton, B., Foyer, C. H., and Hancock, R. D. (2012). Plant responses to insect herbivory: interactions between photosynthesis, reactive oxygen species and hormonal signalling pathways. Plant Cell Environ. 35, 441–453. doi: 10.1111/j.1365-3040.2011.02399.x
Kimball, B. A. (2016). Crop responses to elevated CO2 and interactions with H2O, N, and temperature. Curr. Opin. Plant Biol. 31, 36–43. doi: 10.1016/j.pbi.2016.03.006
Kinney, K. K., Lindroth, R. L., Jung, S. M., and Nordheim, E. V. (1997). Effects of CO2 and NO3- availability on deciduous trees: phytochemistry and insect performance. Ecology 78, 215–230. doi: 10.1890/0012-9658
Long, S. P., Ainsworth, E. A., Leakey, A. D. B., Nosberger, J., and Ort, D. R. (2006). Food for thought: lower-than-expected crop yield stimulation with rising CO2 concentrations. Science 312, 1918–1921. doi: 10.1126/science.1114722
Lou, Y. G., and Baldwin, I. T. (2004). Nitrogen supply influences herbivore-induced direct and indirect defenses and transcriptional responses in Nicotiana attenuata. Plant Physiol. 135, 496–506. doi: 10.1104/pp.104.040360
Lu, C. K., Qi, J. F., Hettenhausen, C., Lei, Y. T., Zhang, J. X., Zhang, M., et al. (2018). Elevated CO2 differentially affects tobacco and rice defense against lepidopteran larvae via the jasmonic acid signaling pathway. J. Intergr. Plant Biol. 60, 412–431. doi: 10.1111/jipb.12633
Nahar, K., Kyndt, T., De Vleesschauwer, D. D., Hofte, M. M., and Gheysen, G. (2011). The Jasmonate pathway is a key player in systemically induced defense against root knot nematodes in rice. Plant Physiol. 157, 305–316. doi: 10.1104/pp.111.177576
Novriyanti, E., Watanabe, M., Kitao, M., Utsugi, H., Uemura, A., and Koike, T. (2012). High nitrogen and elevated [CO2] effects on the growth, defense and photosynthetic performance of two eucalypt species. Environ. Pollut. 170, 124–130. doi: 10.1016/j.envpol.2012.06.011
Nunes-Nesi, A., Fernie, A. R., and Stitt, M. (2010). Metabolic and signaling aspects underpinning the regulation of plant carbon nitrogen interactions. Mol. Plant 3, 973–996. doi: 10.1093/mp/ssq049
Pal, M., Rao, L. S., Jain, V., Srivastava, A. C., Pandey, R., Raj, A., et al. (2005). Effects of elevated CO2 and nitrogen on wheat growth and photosynthesis. Biol. Plant. 49, 467–470. doi: 10.1007/s10535-005-0031-8
Rayapuram, C., and Baldwin, I. T. (2006). Using nutritional indices to study LOX3-dependent insect resistance. Plant Cell Environ. 29, 1585–1594. doi: 10.1111/j.1365-3040.2006.01534.x
Reddy, A. R., Rasineni, G. K., and Raghavendra, A. S. (2010). The impact of global elevated CO2 concentration on photosynthesis and plant productivity. Curr. Sci. 99, 46–57. doi: 10.1371/journal.pone.0011405
Reddy, G. V. P., Tossavainen, P., Anne-Marja, N., and Holopainen, J. K. (2004). Elevated atmospheric CO2 affects the chemical quality of Brassica plants and the growth rate of the specialist, Plutella xylostella, but not the generalist, Spodoptera littoralis. J. Agr. Food Chem. 52, 4185–4191. doi: 10.1021/jf049358v
Saxon, M. E., Davis, M. A., Pritchard, S. G., Runion, G. B., Prior, S. A., Stelzer, H. E., et al. (2004). Influence of elevated CO2, nitrogen, and Pinus elliottii genotypes on performance of the redheaded pine sawfly, Neodiprion lecontei. Can. J. For. Res. 34, 1007–1017. doi: 10.1139/x03-273
Scott, M. I., Thaler, S. J., and Scott, G. F. (2010). Response of a generalist herbivore Trichoplusiani to jasmonate-mediated induced defense in tomato. J. Chem. Ecol. 36, 490–499. doi: 10.1007/s10886-010-9780-8
Sudderth, E. A., Stinson, K. A., and Bazzaz, F. A. (2005). Host-specific aphid population responses to elevated CO2 and increased N availability. Global Chang. Biol. 11, 1997–2008. doi: 10.1111/j.1365-2486.2005.01006.x
Taub, D. R., and Wang, X. (2008). Why are nitrogen concentrations in plant tissues lower under elevated CO2? A critical examination of the hypotheses. J. Integr. Plant Biol. 50, 1365–1374. doi: 10.1111/j.1744-7909.2008.00754.x
Tissue, D. T., and Wright, S. J. (1995). Effects of seasonal water availability on phenology and the annual shoot carbohydrate cycle of tropical forest shrubs. Funct. Ecol. 9, 518–527. doi: 10.2307/2390018
Wang, L., and Wu, J. (2013). The essential role of jasmonic acid in plant-herbivore interactions-using the wild tobacco Nicotiana attenuata as a model. J. Genet. Genomics 40, 597–606. doi: 10.1016/j.jgg.2013.10.001
Wang, Z. Y., Lu, X., He, K. L., and Zhou, D. R. (2000). Review of history, present situation and prospect of the Asian maize borer research in China. J. Shenyang Agric. Uni. 31, 402–412.
War, A. R., Paulraj, M. G., Ignacimuthu, S., and Sharm, H. C. (2015). Induced resistance to Helicoverpa armigera through exogenous application of jasmonic acid and salicylic acid in groundnut, Arachis hypogaea. Pest Manag. Sci. 71, 72–82. doi: 10.1002/ps.3764
Xie, H. C., Cai, W. Z., Wang, Z. Y., and He, K. L. (2013). Effects of elevated atmospheric CO2 on plant, herbivorous insect, and its natural enemy: a review. Chi. J. Appl. Ecol. 24, 3595–3602.
Xie, H. C., Liu, K. Q., Sun, D. D., Wang, Z. Y., Lu, X., and He, K. L. (2015). A field experiment with elevated CO2-mediated changes to C4 crop-herbivore interactions. Sci. Rep. U.K. 5:13923. doi: 10.1603/EC13302
Xu, Y. B., Shen, Y. F., and Li, S. Q. (2011). Effect of elevated CO2 concentration and nitrogen application on translocation of dry matter and nitrogen restored before anthesis in winter wheat. Acta Agron. Sin. 37, 1465–1474.
Yin, J., Sun, Y. C., Wu, G., and Ge, F. (2010). Effects of elevated CO2 associated with maize on multiple generations of the cotton bollworm, Helicoverpa armigera. Entomol. Exp. Appl. 136, 12–20. doi: 10.1111/j.1570-7458.2010.00998.x
Zavala, J. A., Casteel, C. L., DeLucia, E. H., and Berenbaum, M. R. (2008). Anthropogenic increase in carbon dioxide compromises plant defense against invasive insects. Proc. Natl. Acad. Sci. U.S.A. 105, 5129–5133. doi: 10.1073/pnas.0800568105
Zavala, J. A., Gog, L., and Giacometti, R. (2017). Anthropogenic increase in carbon dioxide modifies plant-insect interactions. Ann. Appl. Biol. 170, 68–77. doi: 10.1111/aab.12319
Zavala, J. A., Nabity, P. D., and DeLucia, E. H. (2013). An emerging understanding of mechanisms governing insect herbivory under elevated CO2. Annu. Rev. Entomol. 58, 79–97. doi: 10.1146/annurev-ento-120811-153544
Zhang, L. X., Li, S. X., Zhang, H., and Liang, Z. S. (2007). Nitrogen rates and water stress effects on production, lipid peroxidation and antioxidative enzyme activities in two maize (Zea mays L.) genotypes. J. Agron. Crop Sci. 193, 387–397. doi: 10.1111/j.1439-037X.2007.00276.x
Zhao, L. Y., Chen, J. L., Cheng, D. F., Sun, J. R., Liu, Y., and Tian, Z. (2009). Biochemical and molecular characterizations of Sitobion avenae-induced wheat defense responses. Crop Prot. 28, 435–442. doi: 10.1016/j.cropro.2009.01.005
Zhao, T. H., Sun, J. W., Zhao, Y. X., Fu, Y., Wang, Y., and Shi, Y. (2008). Effects of elevated CO2 and O3 concentration and combined on ROS metabolism and anti-oxidative enzymes activities of maize (Zea mays L). Acta Ecol. Sin. 28, 3644–3653. doi: 10.3321/j.issn:1000-0933.2008.08.017
Keywords: climate change, elevated CO2, N fertilization, plant-insect interaction, metabolites
Citation: Xu H, Xie H, Wu S, Wang Z and He K (2019) Effects of Elevated CO2 and Increased N Fertilization on Plant Secondary Metabolites and Chewing Insect Fitness. Front. Plant Sci. 10:739. doi: 10.3389/fpls.2019.00739
Received: 27 January 2019; Accepted: 17 May 2019;
Published: 04 June 2019.
Edited by:
Scott Alan Heckathorn, The University of Toledo, United StatesCopyright © 2019 Xu, Xie, Wu, Wang and He. This is an open-access article distributed under the terms of the Creative Commons Attribution License (CC BY). The use, distribution or reproduction in other forums is permitted, provided the original author(s) and the copyright owner(s) are credited and that the original publication in this journal is cited, in accordance with accepted academic practice. No use, distribution or reproduction is permitted which does not comply with these terms.
*Correspondence: Haicui Xie, aGN4aWUyMDA4QDEyNi5jb20=