- Departamento de Biologia Vegetal, Instituto de Biologia, Universidade Estadual de Campinas, São Paulo, Brazil
The number of integuments found in angiosperm ovules is variable. In orchids, most species show bitegmic ovules, except for some mycoheterotrophic species that show ovules with only one integument. Analysis of ovules and the development of the seed coat provide important information regarding functional aspects such as dispersal and seed germination. This study aimed to analyze the origin and development of the seed coat of the mycoheterotrophic orchid Pogoniopsis schenckii and to compare this development with that of other photosynthetic species of the family. Flowers and fruits at different stages of development were collected, and the usual methodology for performing anatomical studies, scanning microscopy, and transmission microscopy following established protocols. P. schenckii have ategmic ovules, while the other species are bitegmic. No evidence of integument formation at any stage of development was found through anatomical studies. The reduction of integuments found in the ovules could facilitate fertilization in this species. The seeds of P. schenckii, Vanilla planifolia, and V. palmarum have hard seed coats, while the other species have seed coats formed by the testa alone, making them thin and transparent. P. schenckii, in contrast to the other species analyzed, has a seed coat that originates from the nucellar epidermis, while in other species, the seed coat originates from the outer integument.
Introduction
Flowers are highly variable structures, resulting in a great morphological diversity and a variety of adaptive processes in angiosperms (Endress, 1994; Friis et al., 2011). Variations in flower size and number of whorls, besides the presence or absence of fused floral parts, are caused by differences that occur during the development of floral organs. Plants exhibit open organization, which means that their organs are generally exposed, and that they do not have any organ or parts of organs internalized, with the exception of carpels (Endress, 2015). In turn, carpels can be free or united, becoming curved during their initial development, with edges getting closed or sealed when they are fully developed (Endress, 2006). While most floral organs are exposed, mainly due to the action of pollinating agents (e.g., animals, wind, and water), ovules are completely enclosed in the carpel a condition known as angiospermy (Endress, 2006).
Ovules are female reproductive structures that develop in the seeds (Bouman, 1984; Endress, 2011). Despite their relatively stable basic structure, ovules have a wide diversity of form, varying in terms of their position in the ovary, size, curvature, number and thickness of integument, funiculus length, and degree of vascularization (Endress, 2011). For angiosperms, there are records of bitegmic, unitegmic, and ategmic species (Bouman, 1984; Endress, 2011). Although most angiosperms are bitegmic, variation in the number and thickness of integuments can be observed at different taxonomic levels, such as in families and genera. For example, in Olacaceae, there are described bitegmic, unitegmic, and ategmic species (Brown et al., 2010). In Melastomataceae, ovules are bitegmic; however, in species of the same genus, the number of the outer integument layers can vary from two to many (Caetano et al., 2018).
Previous studies have described the main function of integuments as the delimitation of the micropyle, and protection to the embryo sac and embryo (Herrero, 2001); however, they may also have other functions in species of different families. For example, the inner epidermis of the inner integument can function as a secretory tissue, playing a role in the nutrition of the embryonic sac. This layer of cells is known as integumentary tapetum (Kapil and Tiwari, 1978). Another hypothesis is that the number of integument layers could be related to the fruit type and seed dispersal mode. A study performed with several species of Melastomataceae tried to confirm if there was a relationship between ovules with multiseriate outer integument and fleshy fruits (Caetano et al., 2018). The data obtained did not confirm this relation; however, ancestral state reconstruction shows a tendency for ovules with multiseriate outer integument to occur in fleshy fruit clades. Recent studies conducted with Arabidopsis show that the number of ovule integument layers is related to gene and hormone expression (Bencivenga et al., 2012; Gomez et al., 2016; Coen and Magnani, 2018) and may be responsible for the seed coat diversity observed in angiosperms. After fertilization, the integument layers go through different pathways to establish a protective barrier for the embryo (Windsor et al., 2000). There is an immense diversity in seed structure, such as size, color, texture, and shape; this diversity is related to dispersal and germination strategies (Boesewinkel and Bouman, 1984), and may have been initially determined by the arrangement and number of ovule integuments.
In most angiosperms, the formation of ovules is complete when anthesis starts. In Orchidaceae, however, a different pattern is observed, where in the development of ovules and their respective placental proliferation are conditioned to the pollination event (Swamy, 1949a). In general, orchids have low reproductive success because of low pollination rates (Cozzolino and Widmer, 2005). Thus, ovules will be produced only if there is guaranteed seed formation, in order to prevent unnecessary energy expenditure (Arditti, 1992). The formation of the integuments in orchids occurs simultaneously with the formation of the embryonic sac. To date, in most species studied, the embryonic sac is bitegmic (Swamy, 1949a; Yeung and Law, 1997; Mayer et al., 2011). However, four unitegmic species, which are all mycoheterotrophic, have been described in previous studies (Abe, 1976; Arekal and Karanth, 1981; Krawczyk et al., 2016; Li et al., 2016).
Mycoheterotrophic plants are aclorophyllated and are completely dependent on carbon available through their association with fungi throughout their life cycle (Leake, 1994). Recent phylogeny using plastid and mitochondrial genomes in Orchidaceae show that mycoheterotrophic species evolved several times independently (Li et al., 2019). In the family, 235 species with this condition are described (Merckx et al., 2013), and little is known about the reproductive process of these species. Owing to the importance of the seed coat in the life cycle of plants, and because it is considered a stable characteristic, understanding its structure and development can reveal information relevant to its functional aspects, such as dispersal and seed germination (Bouman, 1984; Windsor et al., 2000; Endress, 2011). Thus, the objective of this work was to analyze the origin and development of the seed coat of the mycoheterotrophic orchid Pogoniopsis schenckii Cogn, and to compare this development with that of other species in the family that have chlorophyll and present different mechanisms of seed dispersal, Polystachya estrellensis Rchb.f., Elleanthus brasiliensis Rchb.f., Isochilus linearis (Jacq) Barb.Rodr., and Cleistes libonii (Rchb. f.) Schltr.—species that exhibit anemochory, and Vanilla planifolia Jacks. ex Andrews and Vanilla palmarum (Salzm ex. Lindl.) Lindl.—species showing evidence of zoochory (Cribb, 1999). Pogoniopsis schenckii is an endemic mycoheterotrophic species found in the Brazilian Atlantic Forest. Prior studies indicate a tendency of reduction in the number of integuments in species of mycoheterotrophic plants, including orchids (Abe, 1976; Arekal and Karanth, 1981; Maas and Ruyters, 1986; Bouman et al., 2002; Endress, 2011; Krawczyk et al., 2016; Li et al., 2016). Thus, our hypothesis is that P. schenckii also exhibits reduction in the number of integuments, leading to a greater exposure of the ovule and simplification of the seed coat involving the embryo, which may facilitate the penetration of fungal hyphae. In this context, structural information on the reproductive organs of mycoheterotrophic species, especially P. schenckii, can contribute to the elucidation of processes related to the symbiosis between fungi and mycoheterotrophic species. In addition, since the mode of seed dispersal of P. schenckii is not known, characterization of the stages of development of its seeds can contribute to the understanding of the ecological interactions involved in the dispersal and colonization of new habitats.
Material and Methods
Species Studied and Literature Review
Pogoniopsis schenckii Cogn. -Epidendroideae- is aclorophyllated and remains underground for almost its entire life cycle. During its reproductive phase, a floral stem appears above ground level; afterwards, flowers and fruits develop. Polystachya estrellensis Rchb. f., Elleanthus brasiliensis Rchb. f., Isochilus linearis (Jacq) Barb. Rodr., belonging to the subfamily Epidendroideae, and Cleistes libonii (Rchb. f.) Schltr., Vanilla planifolia Jacks. ex Andrews, and Vanilla palmarum (Salzm ex. Lindl.) Lindl., belonging to the subfamily Vanilloideae, are photosynthetic species that are found in all Brazilian regions, and in different phytogeographical domains, such as the Cerrado, Atlantic Forest, and Amazon. Voucher specimens were deposited at the Herbarium of the University of Campinas (HUEC), Campinas, São Paulo, Brazil, and the registration numbers are: 196921, 205027, 161354, 197343, 205047, 205028, and 20502.
A literature review was carried out to verify the number of species that developed bitegmic ovules, and the number of species that developed unitegmic ovules. The following keywords were used to search publication databases: embryo development in Orchidaceae, embryology in Orchidaceae, and integuments in Orchidaceae.
Anatomic Analyses
To analyze the integument development freshly opened flowers off all species were collected. For E. brasilliensis and V. palmarum fruits from natural pollinations at different developmental stages were collected. For P. schenckii freshly opened flowers were marked and monitored. For the other species we carried out experimental self-pollination in flowers during the first day of anthesis. The flowers were then monitored and fruits at different stages of development were collected with 15, 20, 25, 30, 60, and 90 opening flower/days after pollination. All material was fixed in Karnovsky (Karnovsky, 1965), dehydrated in serial dilutions of ethanol, and were infiltrated with hydroxyethylmethacrylate (Gerrits and Smid, 1983). The samples were sectioned at 4 µm thickness using a Leica RM2245 rotary microtome, stained with Toluidine Blue 0.05% in phosphate buffer, pH 4.5 (Sakai, 1973), and mounted using Entellan® synthetic resin (Merck®). The slides were analyzed under an Olympus BX51 optical microscope and photographed with an Olympus DP71 digital camera.
Scanning Electron Microscopy
Botanical material was fixed in Karnovsky’s solution (Karnovsky, 1965), dehydrated using a serial dilution of ethanol and critical point dried under carbon dioxide (CO2) in a Balzers model CPD 030 Critical Point Dryer. The material was then mounted on metal supports and coated with colloidal gold for 220 s on the Bal-Tec model SCD 050 equipment. Analysis and electron micrograph recordings were performed using a LEO VP 435 scanning electron microscope at 20 kV, at the Institute of Biology/UNICAMP.
Transmission Electron Microscopy
To analyze the changes occurring during the development of P. schenckii seed coat, ovules, and seeds at different stages of development were fixed using 2.5% glutaraldehyde in 0.2 M sodium cacodylate buffer, pH 7.25, for 24 h (Mc Dowel and Trump, 1976). Post-fixation was performed with 1.0% osmium tetroxide (OsO4) in sodium cacodylate buffer for 12 h in the dark (Gabriel, 1982). The material was dehydrated in a series of increasing concentrations of acetone solution and soaked in LR White® resin according to the manufacturer’s instructions. The ultrafine sections were prepared with Leica ultramicrotome using diamond knife. The ultrafine sections were contrasted with uranyl acetate (Bozzola and Russel, 1998) and lead citrate (Hanaich et al., 1986), examined under a Philips EM 100 transmission electron microscope at 80 kV, and documented with Eastman Kodak 5302 (35 mm) film.
Results
Literature review showed that 97 species of orchids of different subfamilies, 2 species of Vanilloideae, 8 species of Cypripedioideae, 31 species of Orchidoideae, and 56 species of Epidendroideae, had been evaluated till date. Regarding these species, 93 presented bitegmic ovules, and 4 presented unitegmic ovules (Table 1 and Supplementary Table 1). With the 6 new species included in this study, a total of 103 species have been evaluated in terms of the type of ovule integuments.
All species analyzed in this study are teninucellate and bitegmic, except for P. schenckii (Figures 1A–J), which has an ategmic ovule. P. schenckii is the first species within Orchidaceae possessing this characteristic. There is no growth of integuments at any stage of development (Figures 1A, B, I, J). The embryonic sac is only covered by the nucellar epidermis, which exhibit cells with high metabolism, thin walls, and evident nucleus (Figures 1A, B, E, F). Owing to the absence of integuments, there is no micropyle formation in the ovule of the species (Figures 1B, I, J, K).
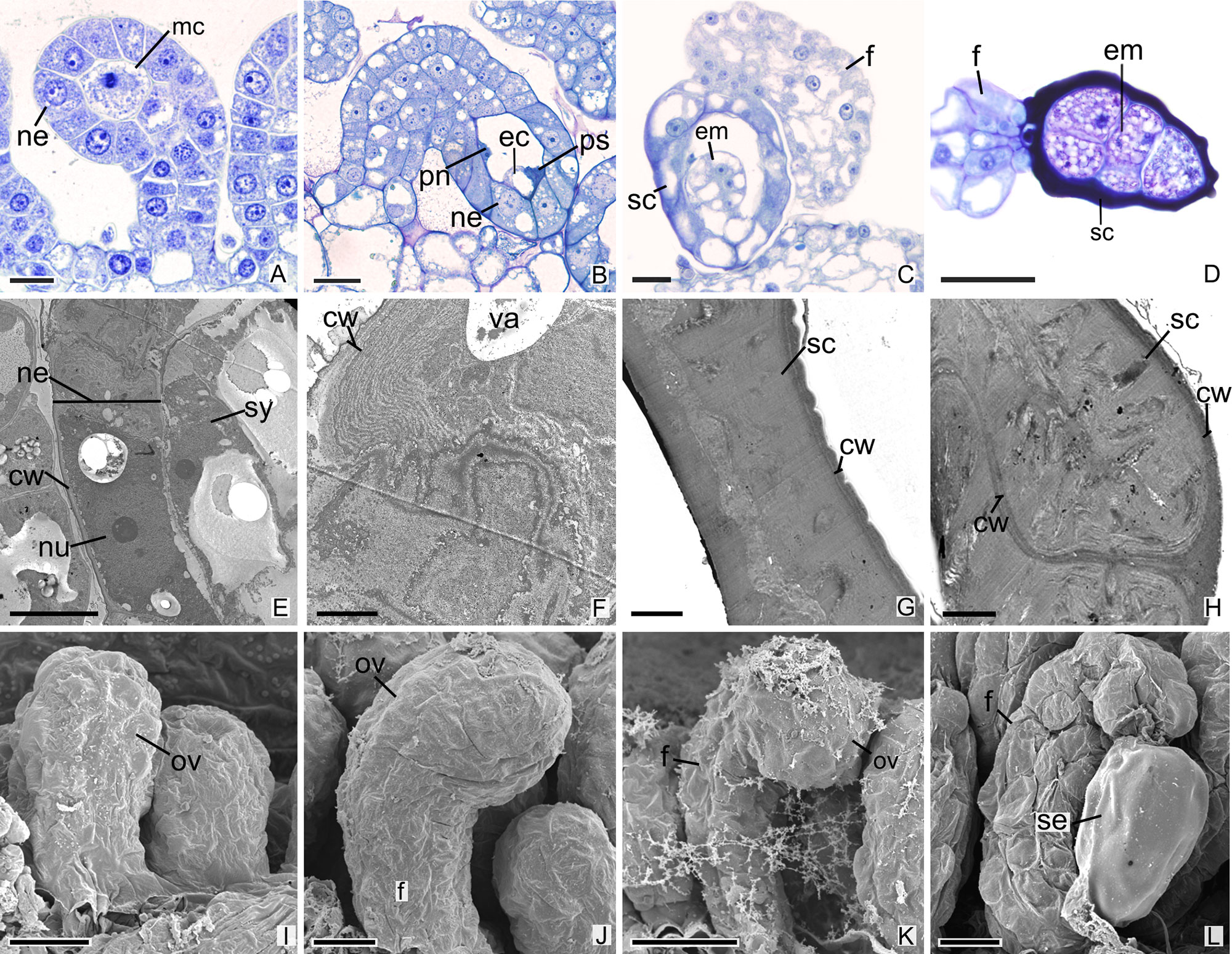
Figure 1 Longitudinal sections of ovules and seeds of Pogoniopsis schenckii. (A) Megaspore mother cell. Note that there is no formation of integuments. (B) Penetrated synergid, egg cell, and polar nuclei. (C) Young seed. (D) Embryo with five cells. (E–H) Transmission microscopy electromyography. (E) Embryo sac and nucellar epidermis. (F) Details of the nucellar epidermis which presents cells with thin walls and evident nucleus. (G) Seed coat that originates from the nucellar epidermis. (H) Details of the hard seed coat. Note their accumulation of substances that confers the cytoplasm a dense aspect. (I–L) Scanning microscopy electromyography. (I–K) Ovule in development. Note that there is no formation of integuments. (L) Seed. cw, cell wall; ec, egg cell; em, embryo; f, funiculus; mc, megaspore mother cell; ov, ovule; ne, nucellar epidermis; nu, nuclei; pn, polar nuclei; ps, penetrated synergid; sc, seed coat; se, seed; sy, synergid; va, vacuolo. Scale bars A, C, I = 20 µm; B, D, K, L = 50 µm; E, J = 10 µm; F–H = 2 µm.
In other species, the development of integuments occurs from periclinal divisions in the epidermal cells located at the base of the ovules, which is seen in both the subfamily Epidendroideae (Figures 2A–P) and in the subfamily Vanilloideae (Figures 3A–Q). During ovule development, the inner integument grows and recovers the nucellar epidermis. In P. estrellensis, E. brasiliensis, I. linearis, and C. libonii, the outer integument grows and covers the inner integument (Figures 2A–D, G–L, M–O and 3A–E). In P. estrellensis, the outer integument has three layers (Figure 2C). The initial development of the integuments in V. planifolia and V. palmarum show that the outer integument also has three layers (Figure 3P). In other species, the outer integument has two layers, and in all the species analyzed, the inner integument has two layers (Figures 2M and 3B).
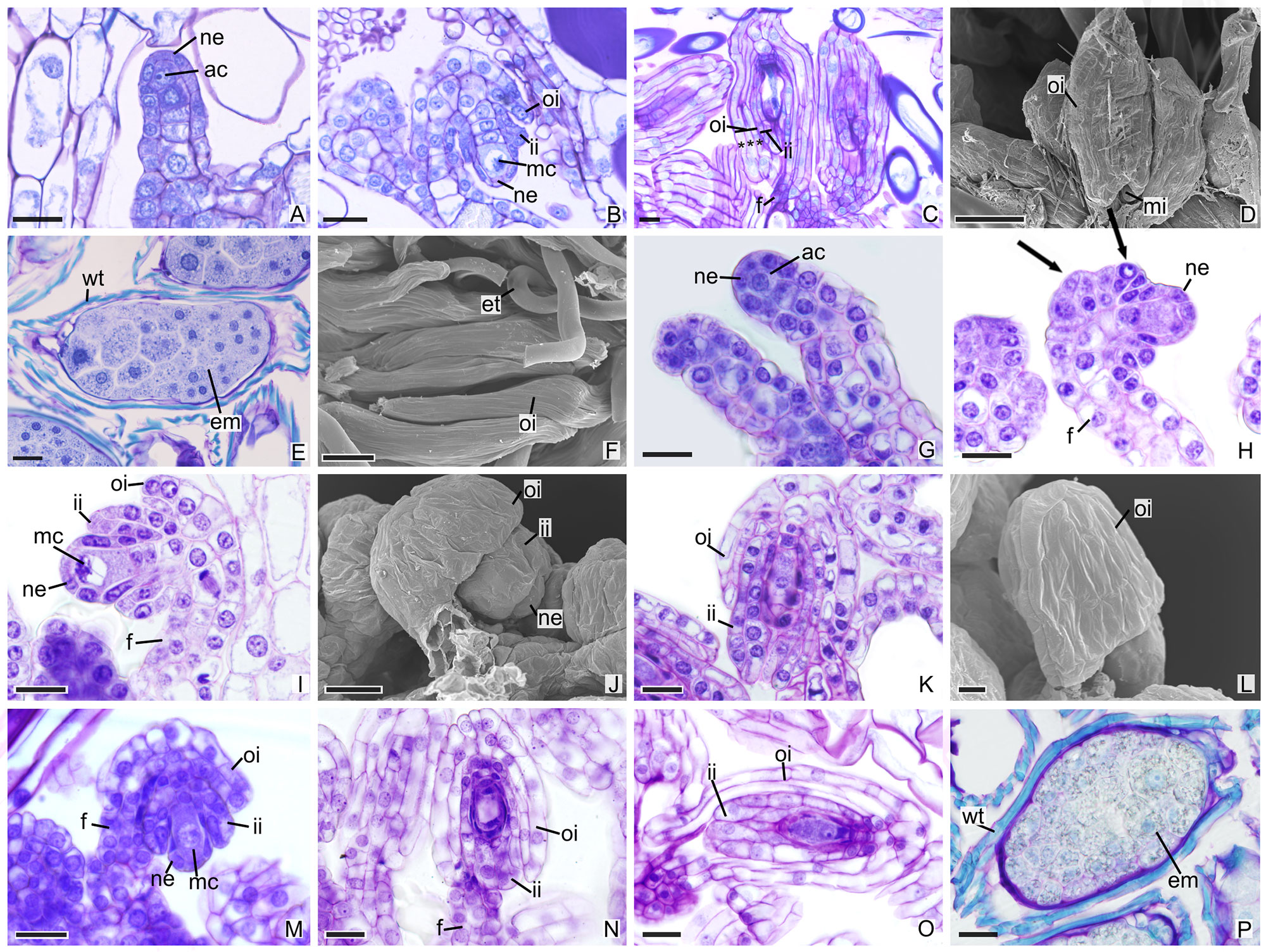
Figure 2 Longitudinal sections of ovules and seeds of Epidendroideae species. (A–F) Ovules and seeds of Polystachya estrellensis. (D, F) Scanning microscopy electromyography. (A) Differentiation of the initial archesporial cell. (B) Megaspore mother cell and formation of integuments. (C) Embryo sac. *indicates the outer integument with three layers. (D) Embryo sac. (E, F) Seed. (G–L) Ovules of Elleanthus brasiliensis. (J, L) Scanning microscopy electromyography. (G) Differentiation of the initial archesporial cell. (H) Formation of integuments indicated by arrows. (I) Megaspore mother cell. (J–K) Formation of integuments. (L) Embryo sac with the outer integument developed. (M–P) Ovules and seeds of Isochilus linearis. (M) Megaspore mother cell and formation of integuments. (N, O) Embryo sac with the integuments developed. P. Seed. ac, initial archesporial cell; em, embryo; f, funiculus; ii, inner integument; mc, megaspore mother cell; mi, micropyle; ne, nucellar epidermis; oi, outer integument; wt, wall thickening. Scale bars A–C; E; G-K; M-P = 20 µm; D, F = 50 µm; L = 10 µm.
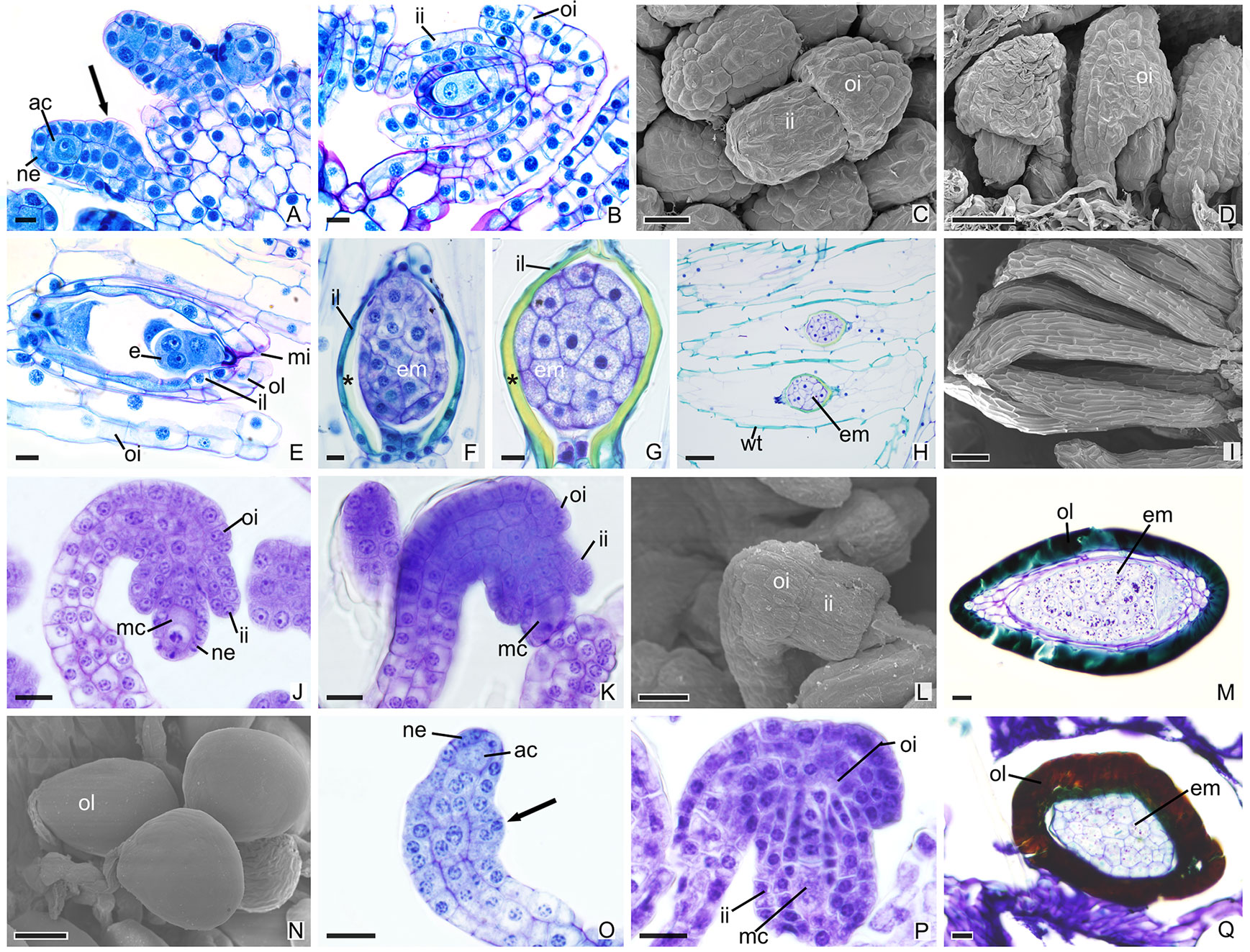
Figure 3 Longitudinal sections of ovules and seeds of Vanilloideae species. (A–I) Ovules and seeds of Cleistes libonii. (C, D, I) Scanning microscopy electromyography. (A) Differentiation of the initial archesporial cell. Arrows indicate the initial formation of integuments. (B) Megaspore mother cell and formation of integuments. (C, D) Development of integuments. (E) Initial development of embryo. (F–G) Embryo. *indicate the extracellular exudate. (H–I) Seeds. (J–N) Ovules and seeds of Vanilla planifolia. (L, N) Scanning microscopy electromyography. (J–K) Megaspore mother cell and formation of integuments. (L) Development of integuments. (M, N) Seeds with hard dark-colored coat. (O–Q) Ovules and seed of Vanilla palmarum. (O) Differentiation of the initial archesporial cell. Arrows indicate the initial formation of integuments.(P) Megaspore mother cell and formation of integuments. Note the outer integument with three layers. (Q) Seed with hard dark-colored coat. ac, initial archesporial cell; em, embryo; f, funiculus; ii, inner integument; il, inner layer of the inner integument; mc, megaspore mother cell; mi, micropyle; ne, nucellar epidermis; oi, outer integuments; ol, out layer of the inner integument; wt, wall thickening. Scale bars A, B, E–G, J–M, O–Q = 20 µm; C = 50 µm; D, H, N = 100 µm; I = 200 µm.
After fertilization, changes are observed in the integument of all species. In P. schenckii (i.e., about 25 days after the floral opening), the seed coat becomes hard and forms from the nucellar epidermis itself (Figures 1C, D, G, H, L). It is possible to observe that during development of the seed coat there is an accumulation of substances that confers the cytoplasm a dense aspect (Figures 1G, H). When mature, the seed presents a brown-colored integument (Figure 4A). In P. estrellensis, E. brasiliensis, and I. linearis, the inner integument is fully absorbed, and the outer integument undergoes elongation. In the outer integument, the inner layer is absorbed, and the outer layer gives rise to the seed testa, which, when mature, is impregnated with lignin and surrounds the embryo (Figures 2D–F, P and 4B–D). In C. libonii, at the beginning of seed development (i.e., about 40 days after fertilization), the inner layer of the inner integument begins to possess a dense cytoplasm and an evident nucleus (Figures 3E, F). Sixty days after fertilization, it is possible to observe the presence of a natural yellow-colored secretion surrounding the embryo (Figures 3G, H and 4E). In the mature seed, only the outer tegument and this secreted layer that covers the embryo remain as coat, whereas the inner layer of the internal integument is reabsorbed (Figures 2E, P and 4E). This substance is probably secreted by the cells of the inner layer of the inner integument. In V. planifolia and V. palmarum, the mature seed has a hard dark-colored coat (Figures 3M, Q and 4F, G).
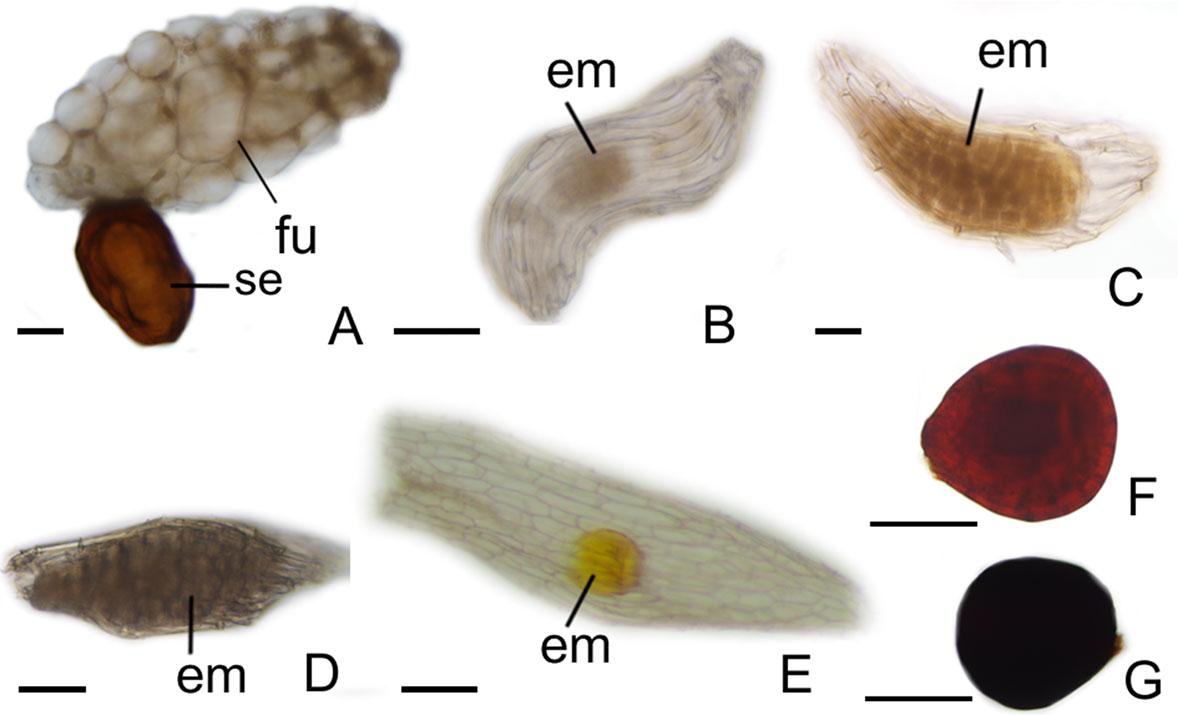
Figure 4 Aspects of seeds. (A) Pogoniopsis schenckii. (B) Polystachya estrellensis. (C) Elleanthus brasiliensis. (D) Isochilus linearis. (E) Cleistes libonii. (F) Vanilla planifolia. (G) Vanilla palmarum. em, embryo; fu, funiculus; se, seed . Scale bars A, B, C, D, E = 150 µm; F, G = 75 µm.
Discussion
For the first time the presence of ategmic ovules, as observed in P. schenckii, are described in Orchidaceae. Bitegmic ovules are commonly in orchids (Swamy, 1949a), but a reduction in ovule integuments are commonly observed in mycoheterotrophic species, which are unitegmic (Tohda, 1967; Abe, 1976; Arekal and Karanth, 1981; Krawczyk et al., 2016; Li et al., 2016). Anatomical analyses show that the ovules of P. schenckii develop normally, and that there is no evidence of development of integument in the ovules of the species at all time points. In this way, the nucellar epidermis is responsible for surrounding the embryo sac, and in the mature seed, for surrounding the embryo. This result differs from the pattern found in other species analyzed in this study, which had seed coats originating from the outer integument.
Reduction of integuments occurs independently in different groups. They have been described in mycoheterotrophic species of Gentianales (Gentianaceae), parasite species of Santalales (Balanophoraceae, Loranthaceae, Olacaeae, and Santalaceae), and in a photosynthetic species of Aquifoliales (Cardiopteridaceae) (Maas and Ruyters, 1986; Bouman et al., 2002; Brown et al., 2010; Polli et al., 2016; Sato and Maria Gonzalez, 2016; Suaza-Gaviria et al., 2016; Tobe, 2016; Gonzalez et al., 2019). Molecular studies show that in ategmic ovules of Santalales, the genes associated with the expression of the integument are expressed in the periphery of the ovary, and that the reduction found in these species is the result of the fusion between the integument and the nucellus (Brown et al., 2010). In P. schenckii, the reduction of integuments leads to a total loss of the micropyle. However, this structural reduction does not seem to compromise reproduction, since the synergids continue to secrete substances for pollen tube attraction. The absence of integuments could facilitate the penetration of the synergids (Figure 1B) and subsequent fertilization. Mycoheterotrophic orchids have ovules with simpler structures, and the absence of a distinct micropyle is common in unitegmic species (Tohda, 1967; Abe, 1976; Arekal and Karanth, 1981; Li et al., 2016). The micropyle is responsible for directing the pollen tube; moreover, both the micropyle and secretions released by the synergid that promotes pollen tube attraction facilitate fertilization (Cheung and Wu, 2001; Okuda et al., 2009; Chen and Fang, 2016).
The differentiation of ovules in the species studied occurred after the stimulation of pollination, and the development of the integuments in P. estrellensis, I. linearis, E. brasiliensis, C. libonii, V. planifolia, and V. palmarum occurs simultaneously with the events of the megasporogenesis, as observed in other species of the family (Swamy, 1949a; Sood, 1985; Sood, 1986; Sood and Rao, 1986; Mayer et al., 2011; Li et al., 2016; Duarte et al., 2019). In most orchids, the outer integument has two layers of cells (Swamy 1949a; Wirth and Withner, 1959). However, in P. estrellensis, the outer integument was observed to have three layers, and the Vanilla species presented ovules with outer integuments that had three to four layers of cells in V. imperiallis, and four to six layers of cells in V. planifolia (Swamy, 1947; Nishimura and Yukawa, 2010; Kodahl et al., 2015). It is believed that the outer multiseriate integument in Vanilla would be related to the larger size of the seed found in the species of the genus (Kodahl et al., 2015).
Of all species analyzed, P. schenckii, V. planifolia, and V. palmarum have seeds with hard coat. Preliminary results show that in P. schenckii, dispersal in the species is very restricted (personal data). It was found to not be related to anemochory; moreover, dispersal by animals was not observed. Based on this, it seems that the hard coat has other unknown functions. Population genetics studies have been conducted, seeking to understand how this restricted dispersal can affect the dynamics of the populations of the species (Alves, unpublished data). Besides the hard coat P. schenckii presents seed with a large funiculus, differing from the other analyzed species. Preliminary analyzes show that the funiculus assists in the penetration of fungal hyphae after dispersion (personal data). Seeds with hard coat have also been described for other mycoheterotrophic orchids. For Cyrtosia japonica, seeds with coats originating from the outer integument and inner integument (Yang and Lee, 2014) are registered. In C. japonica, the seed presents an outer integument with four layers, and the outermost layer later becomes sclerified (Yang and Lee, 2014). It is suggested that the observed lignification protects the embryo when it passes through the alimentary tract of its dispersers (Rodolphe et al., 2011; Yang and Lee, 2014). In Yonia japonica, the seed also presents a lignified coat; however, the fruits and seeds of the species are dispersed by insects (Suetsugu, 2018). Similar to Cyrtosia, it is believed that the lignified seed coat in Y. japonica is an adaptation that protects the seed during digestion (Suetsugu, 2018).
It is assumed that V. planifolia and V. palmarum undergo endozoochory dispersal (Cribb, 1999; Kodahl et al., 2015). In these species, as in others belonging to Vanilloideae, seeds with hard coats exist as a strategy for the dispersal of the genus (Kodahl et al., 2015); in addition, hard coats would protect seeds that can be dispersed over long distances. In other species, seeds were observed to have a thin and transparent coat, which is seemingly a common feature in Orchidaceae. Seeds from Orchidaceae have small sizes, and are called “dust seeds” (Swamy, 1949b; Arditti and Ghani, 2000). The rather small size observed in orchid seeds was traditionally thought to be an adaptation to long-distance wind dispersal events (Arditti and Ghani, 2000). However, recent molecular studies have shown discordant patterns that show the orchid seeds ability to reach long distances (Cozzolino et al., 2003; Trapnell and Hamrick, 2004). Many other species present dispersal patterns limited to a few meters (Chung et al., 2004; Chung et al., 2005; Ren et al., 2017).
Dark-colored hard seed coats, as observed in P. schenckii, V. planifolia, and V. palmarum, have already been described for Apostasia (Swamy, 1947). Occurrence of phytomelanin deposition in Asparagales seeds is described in the literature (Dahlgren et al., 1985). Phytomelanin is a dark and insoluble pigment that is found in different parts of plants and exhibit distinct transport load and structural stability (Nicolaus et al., 1964; Cordero and Casadevall, 2017). Phytomelanin’s main function is to confer protection to different conditions, such as environmental variations, harmful radiation, extreme temperatures, and chemical and mechanical stress (Roulin, 2014; Cordero and Casadevall, 2017). The dark-colored integument in the species studied may result from phytomelanin deposition. However, studies are still needed to clarify this issue.
The results obtained show novelties in the development of the seed coat in Orchidaceae. P. schenckii has an ategmic ovule and has a hard seed coat that originates from the nucellar epidermis. Mycoheterotrophic plants have numerous modifications in their morphology, reproductive biology, and physiology (Leake, 1994; Bidartondo, 2005), the most prominent among loss of photosynthetic function and severe ruptures in the plastid genome (Graham et al., 2016). The genomic losses observed may be related not only to photosynthetic processes, but also to the absence of genes that present other functions, such as genes related to reproductive functions. Anatomical analyses show that there is no evidence of integument development in the ovules of P. schenckii. Thus, the reduction of the integuments found in the species may be due to the absence of gene expression, or even the absence of genes linked to the development of the integument; however, molecular studies are necessary to elucidate this issue.
Data Availability Statement
The datasets generated for this study are available on request to the corresponding author.
Author Contributions
MA carried the anatomical analysis, analysis of scanning and transmission microscopy, and writing the manuscript. FP supervised the work and writing the manuscript. MN carried anatomical analyses in Cleistes libonii and JM was responsible for collecting the material, carried anatomical analyses, writing the manuscript, and supervised the work.
Funding
This study was financed in part by the Coordenação de Aperfeiçoamento de Pessoal de Nível Superior - Brasil (CAPES) - Finance Code 001. Juliana Lischka Sampaio Mayer thank FAPESP (2015/26479-6), FAEPEX 0944/14, CNPq (447453/2014-9), and CNPQ (310184/2016-9) for funding support.
Conflict of Interest
The authors declare that the research was conducted in the absence of any commercial or financial relationships that could be construed as a potential conflict of interest.
Acknowledgments
We thank The Espaço da Escrita - Pró-Reitoria de Pesquisa - UNICAMP - for the language services provided and the access to equipment and assistance provided by the Electron Microscope Laboratory (LME/UNICAMP). We thank the Instituto Florestal (Parque Estadual da Serra do Mar, Núcleo Santa Virginia and Núcleo Picinguaba) for the development of the study on protected public land and Carlos Eduardo Pereira Nunes for his help in field activities.
Supplementary Material
The Supplementary Material for this article can be found online at: https://www.frontiersin.org/articles/10.3389/fpls.2019.01447/full#supplementary-material
Supplementary Table 1 | List of the type of integuments in species of Orchidaceae. Ateg = ategmic ovules; biteg = bitegmic ovules; uniteg = unitegmic ovules.
References
Abe, K. (1972). Contributions to the embryology of the Family Orchidaceae. VI. Development of the embryo sac in 15 species of Orchids. Sei Rep. Tohoku Univ. Ser. IV (Biol) 36, 135–178.
Abe, K. (1976). A reinvestigation of the development of the Embryo Sac in Gastrodia elata Blume (Orchidaceae). Ann. Bot. 40, 99–102. doi: 10.1093/oxfordjournals.aob.a085119
Abe, K. (1977). Development of the Embryo Sac in Amitostigma kinoshitae (Makino) Schltr. (Orchidaceae). Ann. Bot. 41, 897–899. doi: 10.1093/oxfordjournals.aob.a085367
Arditti, J. (1992). Fundamentals of orchid biology. Arditti, J, editor. New York: John Wiley & Sons Inc.
Arditti, J., Ghani, A. K. A. (2000). Tansley Review No. 110.: Numerical and physical properties of orchid seeds and their biological implications. New Phytol. 145, 367–421. doi: 10.1046/j.1469-8137.2000.00587.x
Arekal, G. D., Karanth, K. A. (1981). The embryology of Epipogium roseum (Orchidaceae). P1. Syst. Evol. 138, 1–7. doi: 10.1007/bf00984604
Bencivenga, S., Simonini, S., Benkova, E., Colombo, L. (2012). The transcription factors BEL1 and SPL are required for cytokinin and auxin signaling during ovule development in Arabidopsis. Plant Cell 24, 2886–2897. doi: 10.1105/tpc.112.100164
Bidartondo, M. I. (2005). The evolutionary ecology of mycoheterotrophy: Tansley review. New Phytol. 167, 335–352. doi: 10.1111/j.1469-8137.2005.01429.x
Boesewinkel, F. D., Bouman, F. (1984). “Embryology of angiosperms,” in The seed structure. Ed. Johri, B. M. (Verlag: Springer), 597–610.
Bouman, F. (1984). “Embryology of Angiosperms,” in The ovule. Ed. Johri, B. M. (Verlag: Springer), 123–157.
Bouman, F., Cobb, L., Devente, N., Goethals, V., Maas, P. J. M., Smets, E. (2002). “Gentianaceae systematics and natural history,” in The seeds of Gentianaceae. Eds. L. Struwe, L., Albert, V. A. (Cambridge: Cambridge University Press), 498–572.
Bozzola, J. J., Russel, L. D. (1998). Electron microscopy. principles and techniques for biologists. Boston: Jones e Bartlett Publishers.
Brown, R. H., Nickrent, D. L., Gasser, C. S. (2010). Expression of ovule and integument associated genes in reduced ovules of Santalales: ovule gene expression in Santalales. Evol. Dev. 12, 231–240. doi: 10.1111/j.1525-142X.2010.00407.x
Caetano, A. P. S., Basso-Alves, J. P., Cortez, P. A., Brito, V. L. G. D., Michelangeli, F. A., Reginato, M., et al. (2018). Evolution of the outer ovule integument and its systematic significance in Melastomataceae. Bot. J. Linn. Soc 186, 224–246. doi: 10.1093/botlinnean/box093
Chen, J. C., Fang, S. C. (2016). The long pollen tube journey and in vitro pollen germination of Phalaenopsis orchids. Plant Reprod. 29, 179–188. doi: 10.1007/s00497-016-0280-z
Cheung, A. Y., Wu, H. (2001). Pollen tube guidance — right on target. Science 293, 1441–1442. doi: 10.1126/science.1065051
Chung, M. Y., Nason, J. D., Chung, M. G. (2004). Spatial genetic structure in populations of the terrestrial orchid Cephalanthera longibracteata (Orchidaceae). Am. J. Bot. 91, 52–57. doi: 10.3732/ajb.91.1.52
Chung, M. Y., Nason, J. D., Chung, M. G. (2005). Spatial genetic structure in populations of the terrestrial orchid Orchis cyclochila (Orchidaceae). Plant Syst. Evol. 254, 209–219. doi: 10.1007/s00606-005-0341-5
Coen, O., Magnani, E. (2018). Seed coat thickness in the evolution of angiosperms. Cell. Mol. Life Sci. 75, 2509–2518. doi: 10.1007/s00018-018-2816-x
Cordero, R. J. B., Casadevall, A. (2017). Functions of fungal melanin beyond virulence. Fungal Biol. Rev. 31, 99–112. doi: 10.1016/j.fbr.2016.12.003
Cozzolino, S., Cofasso, D., Pellegrino, G., Masachhio, A., Widmer, A. (2003). Fine-scale phylogeographical analysis of Mediterranean Anacamptis palustris (Orchidaeae) populations based on chloroplast minisatellite and microsatellite variation. Mol. Ecol. 12, 2783–2792. doi: 10.1046/j.1365-294x.2003.01958.x
Cozzolino, S., Widmer, A. (2005). Orchid diversity: an evolutionary consequence of deception? Trends Ecol. Evol. 20, 487–494. doi: 10.1016/j.tree.2005.06.004
Cribb, P. J. (1999). “Genera Orchidacearum: Volume 1: General Introduction, Apostasioideae, Cypripedioideae,” in Morfology. Eds. Pridgeon, A. M., Cribb, P. J., Chase, M. W., Rasmussen, F. N. (Oxford: Oxford University Press Inc.), 13–23.
Dahlgren, R. M. T., Clifford, H. T., Yeo, P. F. (1985). The Families of the Monocotyledons. Heidelberg: Springer-Verlag.
Dong-mei, L., Xiulin, Y., Chengye, L., Xiaoying, H. (2006). Growth paths of pollen tubes in ovary of Phaius tankervilliae (Aiton) Bl. J. Trop. Subtrop. Bot. 14, 130–133. doi: 10.3969/j.issn.1005-3395.2006.2.007
Duarte, M. O., Oliveira, D. M. T., Borba, E. L. (2019). Ontogenesis of ovary and fruit of Acianthera johannensis (Pleurothallidinae, Orchidaceae) reveals a particular female embryology. Flora 259, 151462. doi: 10.1016/j.flora.2019.151462
Endress, P. K. (1994). Diversity and evolutionary biology of tropical flowers. Cambridge: Cambridge University Press.
Endress, P. K. (2006). Angiosperm floral evolution: morphological developmental framework. Adv. Bot. Res. 44, 1–61. doi: 10.1016/S0065-2296(06)44001-5
Endress, P. K. (2011). Angiosperm ovules: diversity, development, evolution. Ann. Bot. 107, 1465–1489. doi: 10.1093/aob/mcr120
Endress, P. K. (2015). Patterns of angiospermy development before carpel sealing across living angiosperms: diversity, and morphological and systematic aspects: Angiospermy Development Across Living Angiosperms. Bot. J. Linn. Soc. 178, 556–591. doi: 10.1111/boj.12294
Fredrikson, M., Carlsson, K., Franksson, O. (1988). Confocal scanning laser microscopy, a new technique used in an embryological study of Dactylorhiza maculata (Orchidaceae). Nord. J. Bot. 8, 369–374. doi: 10.1111/j.1756-1051.1988.tb00513.x
Fredrikson, M. (1990). Embryological study of Herminium monorchis (Orchidaceae) using confocal scanning laser microscopy. Am. J. Bot. 77, 123–127. doi: 10.1002/j.1537-2197.1990.tb13535.x
Fredrikson, M. (1992). The development of the female gametophyte of Epipactis (Orchidaceae) and its inference for reproductive ecology. Am. J. Bot. 79, 63–68. doi: 10.1002/j.1537-2197.1992.tb12624.x
Friis, E. M., Crane, P. R., Pedersen, K. R. (2011). Early flowers and angiosperm evolution. Cambridge; New York: Cambridge University Press.
Gerrits, P. O., Smid, L. (1983). A new less toxic polymerization system for the embedding of soft tissues in glycol methacrylate and subsequent preparing of serial sections. J. Microsc. 132, 81–, 85. doi: 10.1111/j.1365-2818.1983.tb04711.x
Gomez, M. D., Ventimilla, D., Sacristan, R., Perez-Amador, M. A. (2016). Gibberellins regulate ovule integument development by interfering with the transcription factor ATS. Plant Physiol. 172, 2403–2415. doi: 10.1104/pp.16.01231
Gonzalez, A., Sato, H., Marazzi, B. (2019). Embryology in Helosis cayennensis (Balanophoraceae): structure of female flowers, fruit, endosperm and embryo. Plants 8, 74. doi: 10.3390/plants8030074
Gurudeva, M. R. (2011). Development of embryo sac in Zeuxine gracilis (Breda) bl. (orchidaceae). J. Indian Bot. Soc 90, 191–194.
Graham, S. W., Lam, V. K. Y., Merckx, V. S. F. T. (2016). Plastomes on the edge: the evolutionary breakdown of mycoheterotroph plastid genomes. New Phytol. 214, 48–55. doi: 10.1111/nph.14398
Hanaich, T., Sato, T., Iwamoto, T., Malavasi, Y. J., Hoshiro, M., Mizuno, N. A. (1986). Stable lead by modification of Sato method. J. Electron. Microscrosc. 35, 304–306. doi: 10.1093/oxfordjournals.jmicro.a050582
Herrero, M. (2001). Ovary signals for directional pollen tube growth. Sex Plant Reprod. 14, 3–7. doi: 10.1007/s004970100082
Kapil, R. N., Tiwari, S. C. (1978). The integumentary tapetum. Bot. Rev. 44, 457–490. doi: 10.1007/BF02860847
Karnovsky, M. J. (1965). A formaldehyde-glutaraldehyde fixative o high osmolarity of use in eletron microscopy. J. Cell Biol. 27, 137–138.
Kodahl, N., Johansen, B. B., Rasmussen, F. N. (2015). The embryo sac of Vanilla imperialis (Orchidaceae) is six-nucleate, and double fertilization and formation of endosperm are not observed: embryo sac of Vanilla imperialis. Bot. J. Linn. Soc 177, 202–213. doi: 10.1111/boj.12237
Krawczyk, E., Rojek, J., Kowalkowska, A. K., Kapusta, M., Znaniecka, J., Minasiewicz, J. (2016). Evidence for mixed sexual and asexual reproduction in the rare European mycoheterotrophic orchid Epipogium aphyllum, Orchidaceae (ghost orchid). Ann. Bot. 118, 159–172. doi: 10.1093/aob/mcw084
Law, S. K., Yeung, E. C. (1989). Embryology of Calypso bulbosa. I. Ovule development. Am. J. Bot. 76, 1668–1674. doi: 10.1002/j.1537-2197.1989.tb15151.x
Leake, J. R. (1994). The biology of myco-heterotrophic (‘saprophytic’) plants. New Phytol. 127, 171–216. doi: 10.1111/j.1469-8137.1994.tb04272.x
Lee, Y. I., Lee, N., Yeung, E. C., Chung, M. C. (2005). Embryo development of Cypripedium formosanum in relation to seed germination in vitro. J. Am. Soc Hortic. Sci. 130, 747–753. doi: 10.21273/JASHS.130.5.747
Lee, Y. I., Yeung, E. C., Lee, N., Chung, M. C. (2008). Embryology of Phalaenopsis amabilis var. formosa: embryo development. Bot. Stud. 49, 139–146.
Lee, Y. I., Yeung, E. C. (2012). Embryology of the lady’s slipper orchid, Paphiopedilum delenatii: ovule development. Bot. Stud. 53, 8.
Li, Y. Y., Chen, X.-M., Guo, S. X., Lee, Y. I. (2016). Embryology of two mycoheterotrophic orchid species, Gastrodia elata and Gastrodia nantoensis: ovule and embryo development. Bot. Stud. 57, 18. doi: 10.1186/s40529-016-0137-7
Li, Y. X., Li, Z. H., Schuitman, A., Chase, M. W., Li, J. W., Huang, W. C., et al. (2019). Phylogenomics of Orchidaceae based on plastid and mitochondrial genomes. Mol. Phylogenet. Evol. 139, 1–11. doi: 10.1016/j.ympev.2019.106540
Liu, F., Tian, M., Wang, C. X., Maojiang, G., Quanjan, L. (2012). Studies on fruit growth and embryo development of the endangered species Cypripedium japonicum. J. Plant Resour. Environ. 21, 28–35.
Lu-Han, W., Jyh-Shyan, T., Hai-Shan, C. (2016). Embryological studies on Spiranthes sinensis (Pers.) Ames. Flora Morphol. Distrib. Funct. Ecol. Plants 224, 191–202. doi: 10.1016/j.flora.2016.07.019
Maas, P. J. M., Ruyters, P. (1986). Voyria and Voyriella (Saprophytic Gentianaceae) [Monograph 41]. Flora 40/42, 1–93. www.jstor.org/stable/4393786.
Maheshwari, P., Naraynaswami, S. (1951). Embryological studies on Spiranthes australis Lind1. Bot. J. Linn. Soc 53, 474–756. doi: 10.1111/j.1095-8339.1952.tb01558.x
Mayer, J. L. S., Carmello-Guerreiro, S. M., Appezzato-da-Glória, B. (2011). Anatomical development of the pericarp and seed of Oncidium flexuosum Sims (ORCHIDACEAE). Flora Morphol. Distrib. Funct. Ecol. Plants 206, 601–609. doi: 10.1016/j.flora.2011.01.009
Mc Dowel, E. M., Trump, B. (1976). Histological fixatives for diagnostic light and electron microscopy. Arch. Pathol. Lab. Med. 100, 517–527.
Merckx, V. F. S. T., Freudenstein, J. V., Kissling, J., Christenhusz, M. J. M., Stotler, R. E., Crandall-Stotler, B., et al. (2013). “Mycoheterotrophy,” in Taxonomy and classification. Ed. Merckx, V. F. S. T. (New York: Springer), 19–102.
Mohana Rao, P. R., Sood, S. K. (1979). Embryology of Habenaria densa (Orchidaceae). Bot. Notiser 132, 145–148.
Nicolaus, R. A., Piatelli, M., Fattorusso, E. (1964). The structure of melanins and melanogenesis: IV. On some natural melanins. Tetrahedron 20, 1163–1172. doi: 10.1016/s0040-4020(01)98983-5
Nishimura, G., Yukawa, T. (2010). Dark material accumulation and sclerotization during seed coat formation in Vanilla planifolia Jacks. ex Andrews (Orchidaceae). Bull. Natl. Mus. Nat. Sci. 36, 33–37.
Okuda, S., Tsutsui, H., Shiina, K., Sprunck, S., Takeuchi, H., Yui, R., et al. (2009). Defensin-like polypeptide LUREs are pollen tube attractants secreted from synergid cells. Nature 458, 357–361. doi: 10.1038/nature07882
Polli, A., Souza, L. A., Almeida, O. J. G. (2016). Structural development of the fruits and seeds in three mistletoe species of Phoradendron (Visceae: Santalaceae). Rodriguésia 67, 649–659. doi: 10.1590/2175-7860201667309
Ren, M.-X., Cafasso, D., Cozzolino, S., Pinheiro, F. (2017). Extensive genetic differentiation at a small geographical scale: reduced seed dispersal in a narrow endemic marsh orchid. Anacamptis Robusta Bot. J. Linn. Soc 183, 429–438. doi: 10.1093/botlinnean/bow017
Rodolphe, G., Severine, B., Michel, G., Pascale, B. (2011). “The dynamical processes of biodiversity - case studies of evolution and spatial distribution,” in Biodiversity and evolution in the vanilla genus. Ed. Grillo, O. (Croatia: InTech). doi: 10.5772/24567
Roulin, A. (2014). Melanin-based colour polymorphism responding to climate change. Glob. Change Biol. 20, 3344–3350. doi: 10.1111/gcb.12594
Sato, H. A., Maria Gonzalez, A. (2016). Floral development and anatomy of pistillate flowers of Lophophytum (Balanophoraceae), with special reference to the embryo sac inversion. Flora 219, 35–47. doi: 10.1016/j.flora.2016.01.002
Sakai, W. S. (1973). Simple method for differential staining of paraffin embedded plant material using toluidine blue O. Stain Technol. 48, 247–249. doi: 10.3109/10520297309116632
Sood, S. K. (1985). Gametophytes, integuments initiation and embryogeny in Microstylis cylindrostachya (Orchidaceae, Epidendreae). Proc. Indian Acad. Sci. 6, 379–387. doi: 10.1007/bf03053676
Sood, S. K. (1986). Gametogenesis, integuments initiation and embryogeny in three species of Habenaria (Orchidaceae, Orchideae). Proc. Indian Acad. Sci. 6, 487–494. doi: 10.1007/bf03053543
Sood, S. K., Mohana Rao, P. R. (1986). Gametophytes, embryogeny and pericarp of Microstylis wallichii lindl. (Orchidaceae). Bot. Mag. Tokyo 99, 351–359. doi: 10.1007/BF02488715
Sood, S. K. (1988). Development of gametophytes, embryogeny and pericarp in Goodyera repens (Orchidaceae, Neottieae). Proc. lndian Acad. Sci. 2, 149–156. doi: 10.1007/bf03053400
Sood, S. K., Mohana Rao, P. R. (1988). Studies in the embryology of the diandrous orchid Cypripedium cordigerum (Cypripedieae, Orchidaceae). Plant Syst. Evol. 160, 159–168. doi: 10.1007/BF00936043
Sood, S. K. (1989). Embryology and systematic position of Liparis (Orchidaceae). Plant Syst. Evol. 166, 1–9. doi: 10.1007/BF00937871
Sood, S. K. (1992). Embryology of Malaxis saprophyta, with comments on the systematic position of Malaxis (Orchidaceae). P1. Syst. Evol. 179, 95–105. doi: 10.1007/bf00938022
Suaza-Gaviria, V., Pabón-Mora, N., González, F. (2016). Development and Morphology of Flowers in Loranthaceae. Int. J. Plant Sci. 177, 559–578. doi: 10.1086/687280
Suetsugu, K. (2018). Seed dispersal in the mycoheterotrophic orchid Yoania japonica: further evidence for endozoochory by camel crickets. Plant Biol. 20, 707–712. doi: 10.1111/plb.12731
Swamy, B. G. L. (1945). Embryo sac and fertilization in Cypripedium spectabile. Bot. Gaz. 107, 291–295.
Swamy, B. G. L. (1946a). The embryology of Zeuxine sulcata Lindl. New Phytol. 45, 132–136. doi: 10.1111/j.1469-8137.1946.tb05050.x
Swamy, B. G. L. (1947). On the life-history of Vanilla planifolia. Bot. Gaz. 108, 449–456. doi: 10.1086/335429
Swamy, B. G. L. (1949a). Embryological Studies in the Orchidaceae. I. Gametophytes. Am. Midl. Nat. 41, 184. doi: 10.2307/2422025
Swamy, B. G. L. (1949b). Embryological studies in the orchidaceae. II. Embryogeny. Am. Midl. Nat. 41, 202. doi: 10.2307/2422026
Tobe, H. (2016). Embryology of Cardiopteris (Cardiopteridaceae, Aquifoliales), with emphasis on unusual ovule and seed development. J. Plant Res. 129, 883–897. doi: 10.1007/s10265-016-0845-9
Tohda, H. (1967). An embryological study of Hetaeria skikokiana, a saprophytic orchid in Japan. Sei Rep. Tohoku Univ. Ser. IV (Biol) 33, 83–95.
Trapnell, D. W., Hamrick, J. L. (2004). Partitioning nuclear and chloroplast variation at multiple spatial scales in the neotropical epiphytic orchid Laelia rubescens. Mol. Ecol. 13, 2655–2666. doi: 10.1111/j.1365-294X.2004.02281.x4
Windsor, J. B., Symonds, V. V., Mendenhall, J., Lloyd, A. M. (2000). Arabidopsis seed coat development: morphological differentiation of the outer integument. Plant J. 22, 483–493. doi: 10.1046/j.1365-313x.2000.00756.x
Wirth, M., Withner, C. L. (1959). “The orchids: a scientific survey,” in Embryology and development in the Orchidaceae. Ed. Withner, C. L. (New York: Ronald Press), 155–188.
Yang, C. K., Lee, Y. I. (2014). The seed development of a mycoheterotrophic orchid, Cyrtosia javanica Blume. Bot. Stud. 55, 44. doi: 10.1186/s40529-014-0044-8
Yeung, E. (1996). Embryology of Cymbidium sinense: embryo development. Ann. Bot. 78, 105–110. doi: 10.1006/anbo.1996.0101
Yeung, E. C., Law, S. K. (1989). Embryology of Epidendrum ibaguense. I. Ovule development. Can. J. Bot. 67, 2219–2226. doi: 10.1139/b89-283
Yeung, E. C., Law, S. K., (1997). “Orchid biology reviews and perspectives, VII,” in Ovule and megagametophyte development in orchids. Eds. Arditti, J., Pridgeon, A. M. (New York: Springer Science), 31–68.
Zhang, X. S., O’Neill, S. D. (1993). Ovary and gametophyte development are coordinately regulated by auxin and ethylene following pollination. Plant Cell 5, 403–418. doi: 10.1105/tpc.5.4.403
Keywords: anatomy, integument, Epidendroideae, saprophytic, Vanilloideae
Citation: Alves MF, Pinheiro F, Niedzwiedzki MP and Mayer JLS (2019) First Record of Ategmic Ovules in Orchidaceae Offers New Insights Into Mycoheterotrophic Plants. Front. Plant Sci. 10:1447. doi: 10.3389/fpls.2019.01447
Received: 05 July 2019; Accepted: 17 October 2019;
Published: 29 November 2019.
Edited by:
Jen-Tsung Chen, National University of Kaohsiung, TaiwanReviewed by:
David Smyth, Monash University, AustraliaDennis William Stevenson, New York Botanical Garden, United States
Copyright © 2019 Alves, Pinheiro, Niedzwiedzki and Mayer. This is an open-access article distributed under the terms of the Creative Commons Attribution License (CC BY). The use, distribution or reproduction in other forums is permitted, provided the original author(s) and the copyright owner(s) are credited and that the original publication in this journal is cited, in accordance with accepted academic practice. No use, distribution or reproduction is permitted which does not comply with these terms.
*Correspondence: Mariana Ferreira Alves, bWFyaWFuYWZlYWx2ZXNAZ21haWwuY29t; Juliana Lischka Sampaio Mayer, amltYXllckB5YWhvby5jb20uYnI=