- 1University of Chinese Academy of Sciences, Beijing, China
- 2Key Laboratory of Biofuels, Chinese Academy of Sciences, Shandong Provincial Key Laboratory of Energy Genetics, Qingdao Institute of Bioenergy and Bioprocess Technology, Chinese Academy of Sciences, Qingdao, China
- 3College of Resources and Environment, Qingdao Agricultural University, Qingdao, China
Plant CCCH zinc finger proteins control growth, development, and stress responses mainly at the post-transcriptional level. Currently, limited reports are available about the roles of plant CCCH proteins in drought tolerance. In this study, we provided evidence showing that PdC3H17 from Populus deltoides × P. euramericana involves drought tolerance and response. Overexpression of PdC3H17 in poplar caused dwarf, resulted in higher stem water potential, and showed increased photosynthetic and ROS-scavenging abilities, thereby enhancing tolerance to drought stress, compared to controls. Accordingly, after drought treatment the stem elongation and thickening rates of these overexpression lines were higher than those of the controls. However, overexpression of the coding region excluding the CCCH domain of PdC3H17 roughly exhibited WT-like physiological and drought-resistant phenotypes, indicating the requirement of the CCCH domain for PdC3H17 controlling these processes. In addition, N-terminal sequence of PdC3H17 was found to possess transcriptional activity ability in yeast cells. Together, our results suggest that PdC3H17 may depend on its CCCH domain to control drought tolerance in Populus.
Introduction
Poplar (Populus spp.), a fast-growing tree species, is widely used for timber, pulp, and paper, and has potential as a source of bioenergy (Du and Groover, 2010). Poplars are drought-sensitive woody species and have evolved versatile mechanisms to mitigate drought stress, including reducing transpiration, scavenging reactive oxygen species (ROS), generating abscisic acid (ABA), and altering plant morphology (Harfouche et al., 2014). Accumulating evidence in Populus shows that a large amount of genes participate in controlling these processes under drought conditions. For example, PdEPF1, a member of the epidermal patterning factor (EPF) family in Populus nigra × (Populus deltoides × Populus nigra), regulates water use efficiency and drought tolerance by modulating stomatal density (Wang et al., 2016). PeCHYR1, an ubiquitin E3 ligase in Populus euphratica, enhances drought tolerance via ABA-induced stomatal closure by ROS production (He et al., 2018). The AREB1 transcription factor influences histone acetylation of PtrNAC006, PtrNAC007, and PtrNAC120, resulting in key physiological alterations conducive to drought tolerance and resilience and thereby changing drought responses in Populus trichocarpa (Li et al., 2019). Currently, it remains unclear that the regulatory mechanisms underlying drought response and tolerance in tree species.
The CCCH zinc finger family contains a typical C3H-type motif and members of this family had already been identified in organisms from yeast to human (Chai et al., 2015). Plant CCCH proteins play vital roles in a wide variety of growth, development, and stress responses, and may perform both transcriptional and posttranscriptional regulation (Bogamuwa and Jang, 2014). In Arabidopsis, the subfamily IX members of CCCH proteins are shown to mediate stress signaling based on qRT-PCR analysis (Wang et al., 2008). This is validated by subsequent functional analyses. AtTZF1/AtC3H23 functions as a regulator of ABA- and GA-mediated growth and drought response (Lin et al., 2011). Overexpression of AtTZF2/AtC3H20 or AtTZF3/AtC3H49, the homologs of AtTZF1, improves ABA hypersensitivity, reduces transpiration, and enhances drought tolerance (Lee et al., 2012). OsTZF1 is an ortholog of AtTZF1 in rice and confers tolerance to drought and high-salt stresses by regulating stress-related genes (Jan et al., 2013). OsC3H47 decreases ABA sensitivity and promotes drought and salt tolerance in rice seedlings (Wang et al., 2015). GhTZF1, an ortholog of AtTZF1 in cotton, regulates drought stress responses and delays leaf senescence by inhibiting ROS accumulation in transgenic Arabidopsis (Zhou et al., 2014). Recently, a non-tandem CCCH protein, IbC3H18, is shown to act as a nuclear transcriptional activator and enhances salt, drought, and oxidation stress tolerance in sweet potato (Zhang et al., 2019), suggesting that in addition to subfamily IX, the members of other CCCH subfamilies in Arabidopsis may participate in controlling drought tolerance.
Our previous study demonstrated that Populus contains 91 CCCH gene family members, 90% of which are physically distributed on the duplicated blocks (Chai et al., 2012). Thirty-four paralogous pairs are identified in these CCCHs, of which 22 pairs (65%) may be created by the whole genome segment duplication. Of them, PdC3H17 and PdC3H18, a pair of paralogs, are direct targets of PdMYB3 and PdMYB21, which are second-level master switches of the transcription network for wood formation (Ye and Zhong, 2015), and function as positive regulators of secondary xylem development in both Arabidopsis and poplar (Chai et al., 2014). PdC3H17 has two orthologs (AtC3H14 and AtC3H15, belonging to subfamily II in CCCHs) in Arabidopsis (Wang et al., 2008; Chai et al., 2014). AtC3H14 and AtC3H15 redundantly control stem elongation and secondary cell wall thickening as well as anther development (Kim et al., 2014; Chai et al., 2015). However, the roles of the CCCH proteins in response to environmental stresses in trees have not yet been elucidated.
In this study, we provided evidence showing that PdC3H17 controls drought response depending on its CCCH domain in a hybrid poplar. Overexpression of PdC3H17 in poplar resulted in stronger drought-tolerance phenotype, correlating with a significant increase in both leaf ROS-scavenging abilities and stem xylem vessel cell numbers, compared to control plants. However, transgenic poplars overexpressing the coding region excluding the CCCH domain of PdC3H17 roughly exhibited wild type-like phenotypes. Combined with other biochemical evidence, we suggest that PdC3H17 may be a novel regulator of drought tolerance in poplar.
Materials and Methods
Transcriptional Activation Assay in Yeast
The full-length coding region (PdC3H17) and the fragments including (PdC3H17ΔN) or excluding (PdC3H17ΔC) the CCCH domain of PdC3H17 (Figure 1A) were separately fused in frame with the GAL4 DNA-binding domain in pGBKT7 (Clontech) using the appropriate primers containing the EcoRI site. PdC3H17: (Forward, 5′-ATGGAGGCCGAATTCATGGAGAAAACAGAATCACCA-3′; Reverse, 5′-GATCCCCGGGAATTCTCAACGAGGACCCAGCAGTAACC-3′). PdC3H17ΔC: (Forward, 5′-ATGGAGGCCGAATTCATGGAGAAAACAGAATCACCA-3′; Reverse, 5′-GATCCCCGGGAATTCCGTGAAGGTTTTGGCGGGAA-3′). PdC3H17ΔN: (Forward, 5′-ATGGAGGCCGAATTCATGCAAGGGATGTGGAAGACAG-3′; Reverse, 5′-GATCCCCGGGAATTCGTCAGTGAGGGAGTGGCGAAA-3′). The recombinant vectors and the pGBKT7 empty vector were transformed into yeast strain AH109. The yeast liquid cultures were dropped on the synthetic dropout (SD)/Trp‐ and SD/Trp‐/His‐/Ade (adenine)‐agar media. The transcriptional activation activity of each protein was evaluated according to their growth status and the activity of α-galactosidase.
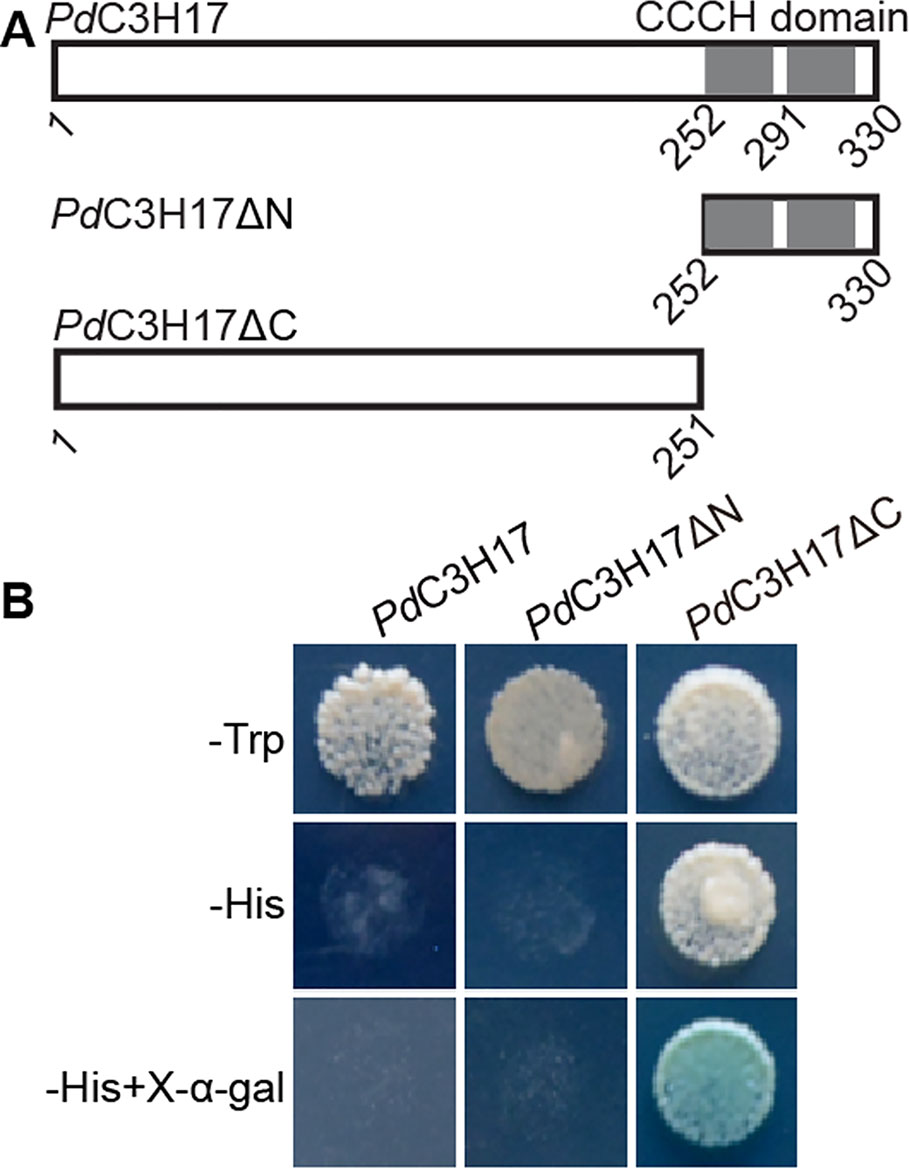
Figure 1 Transactivation analysis of different regions of PdC3H17 fused with the GAL4 DNA-binding domain in yeast. (A) Diagram of PdC3H17, PdC3H17ΔN, and PdC3H17ΔC proteins. (B) Only PdC3H17ΔC is able to activate the expression of the HIS3 reporter gene.
Vector Construction and Plant Transformation
Full-length PdC3H17 and PdC3H17ΔC were separately ligated downstream of the 35S promoter in pCAMBIA1300-GFP vector to generate the overexpression constructs. Primers for PdC3H17 were (Forward, 5′-CGGGGTACCATGGAGAAAACAGAATCACCA-3′, the KpnI site marked with underline) and (Reverse, 5′-CGGGATCCTCAACGAGGACCCAGCAGTAACC-3′, the BamHI site). Primers for PdC3H17ΔC were (Forward, 5′-CGGGGTACCATGGAGAAAACA GAATCACCA-3′, the KpnI site) and (Reverse, 5′-CGGGATCCCGTGAAGGTTTTGGCGGAA-3′, the BamHI site). The resulting construct was introduced into Populus deltoides × P. euramericana cv “nanlin895” via leaf disc method (Chai et al., 2014). The transgenic poplar plantlets were selected on a medium containing 5 mg L−1 hygromycin and identified through PCR at the DNA level and qRT‐PCR at the mRNA level. PCR primers: 35Spro: ATGACGCACAATCCCACTATCC; PdC3H17R: 5′-CAGTGAGGGAGTGGCGAAAG-3′; PdC3H17ΔCR: 5′-TCGTTTGCATTAAACACCTCCA-3′. qRT-PCR primers: PdC3H17 (Forward: 5′-CAAGTGGCAAGAGACAGGCA-3′; Reverse: 5′-CAGTGAGGGAGTGGCGAAAG-3′) and PdC3H17ΔC (Forward: 5′-ACTCAACTCGGTCTCGTGTG-3′; Reverse: 5′-TCGTTTGCATTAAACACCTCCA-3′). Regenerated plantlets were acclimatized in a mist chamber for 30 days, and transferred to a greenhouse with a 16/8 h light/dark photoperiod at 25°C to 28°C.
Quantitative Real-Time RT-PCR (qRT-PCR)
Total RNA isolation and first-strand cDNA synthesis were performed as described previously (Chai et al., 2014). The qRT-PCR assays were conducted on a LightCycler®480 Detection System (Roche) using a SYBR Premix Ex Taq II (TaKaRa) kit. The expression was normalized using reference gene PdUBQ (BU879229, Forward, 5′-GTTGATTTTTGCTGGGAAGC-3′; Reverse, 5′-GATCTTGGCCTTCACGTTGT-3′), and determined by the 2−ΔΔCT method (Livak and Schmittgen, 2001). Data represent the average of at least three biological replicates.
Microscopy
Cross section of the basal stems was produced from 3-month-old transgenic poplars as described previously (Chai et al., 2014). For each construct, at least six plants of two lines were examined. Briefly, 0.5-cm stem segments were submerged in 4% paraformaldehyde for 3 days, then dehydrated in a graded ethanol series, and finally incubated in pure paraplast. The paraplast-embedded stems were sectioned to a thickness of 7 μm using a Leica RM 2235 microtome (Leica) and adhered to Superfrost Plus microscope slides (Thermo Fisher). Stem sections were stained with Toluidine Blue-O (TBO, 1% w:v) and then observed using an Olympus DX51 microscope.
Drought Treatment
Three-month-old transgenic poplar lines encountered drought treatment, in which the soil RWC was reduced from 70% following described previously (Wang et al., 2016). Control plants were kept in the same conditions, except that the soil RWC was maintained at 70%. Control and transgenic plants were grown in suitably sized pots, and each pot had a tray. Photographs were taken after treatment for 20 days.
Measurement of Physiologic Parameters
At 20th day of drought treatment, net photosynthetic rate, stomatal conductance, transpiration, and chlorophyll a+b content were detected in the 5th to 7th leaves of control and transgenic lines using Li-6400 Photosynthesis System (Li-Cor Biosciences, Lincoln, NE). The 4th to 6th leaves from the top of the plants were sampled for measurement of the amounts of malondialdehyde (MDA), hydrogen peroxide (H2O2), four ROS-scavenging enzymes (superoxide dismutase, SOD; peroxidase, POD; ascorbate peroxidase, APX; and catalase, CAT) and three osmotic adjustments (proline, soluble protein, and soluble sugar). At least five biological replicates were performed for each genotype. The levels of H2O2, SOD, POD, APX, CAT, and soluble protein were measured according to the methods described by Shi et al. (2014).
The levels of MDA, proline, and soluble sugar were detected with the commercial kits following the instructions (Nanjing Jiancheng Bioengineering Institute, China). For determination of MDA content, 0.1 g of sample was extracted with 1 mL of 20% (w/v) trichloroacetic acid (TCA). The homogenate was centrifuged at 3500g for 20 min, 2 mL supernatant was added to 2 mL of 20% TCA containing 0.5% (w/v) triobarbituric acid (TBA). After heating in 95°C for 30 min and cooling in ice bath, the proline level was calculated based on absorbance at 532 and 600 nm. The value for non-specific absorption at 600 nm was subtracted from the value at 532 nm. For determination of proline content, 0.1 g of sample was ground and extracted in 3% (w/v) sulphosalicylic acid. After reacting with acid ninhydrin solution, the proline level was calculated based on absorbance at 520 nm. For the determination of soluble sugar content, 0.1 g of sample was extracted in 5 ml of 80% (v/v) ethanol at 80 C for 40 min and centrifuged at 12000 rpm for 10 min. The supernatants were dipigmented by litter activated charcoal at 80°C for 30 min. The mixture of 0.1 ml of the extracts and 3 ml of 0.15% (w/v) anthrone reagent (0.3 g anthrone was dissolved in 200 ml of 7.74 M H2SO4) was heated at 90°C for 20 min. The level of soluble sugar was examined at 620 nm of absorbance by making the specification curve with known concentration of glucose.
Water potential was measured under well-watered condition or with drought treatment for 20 days. For each genotype, at least six plants of two lines were selected. A TP-PW-II Water Potential System (TPYN SciTech, Hangzhou, China) was used for the measurement of stem water potential according to the manufacturer's instructions. Statistical analyses were performed based on data from two independent experiments.
Statistical Analysis
All data were presented as mean ± standard errors. The statistical significance of differences between data was evaluated using one-way analysis of variance (ANOVA) followed by Duncan's multiple range test (P < 0.05).
Results
Transcriptional Activation Analysis of PdC3H17
Our previous study showed that Populus PdC3H17 functions as positive regulators of secondary xylem development in both Arabidopsis and poplar (Chai et al., 2014). To elucidate the transcriptional properties of PdC3H17, multiple fusion proteins were constructed, in which the coding region and selected portions of PdC3H17 were fused to the GAL4 DNA-binding domain. All of the transformed yeast cells grew well on SD/Trp-medium. Transcription of the HIS reporter gene was significantly activated by GAL4-PdC3H17 N-terminal region that excludes the CCCH domain (ΔC), but not by the GAL4-full-length PdC3H17 protein (FL) and the GAL4-CCCH domain (ΔN) (Figure 1). These results indicated that the N-terminal sequence of PdC3H17 may possess transcriptional activation capacity.
Phenotypes of Transgenic Poplars Overexpressing PdC3H17 or PdC3H17ΔC
The structure and size of xylem vessel cells are key factors affecting water transport in plants and are important determinants of drought tolerance (Fisher et al., 2007; Li et al., 2019). To investigate whether PdC3H17 participates in controlling drought response, we generated transgenic poplar lines overexpressing the full length coding region (PdC3H17OE) or the fragment excluding the CCCH domain (PdC3H17ΔCOE) of PdC3H17 (Figure 1A). For each construct, at least 30 independent transgenic lines were generated and two lines with highest expression of the transgene were selected for further characterization (Figures 2A, B). Consistent with our previous phenotypic observation (Chai et al., 2014), PdC3H17OE lines exhibited dwarf compared with WT controls (Figure 2C). Considering that AtC3H14 and AtC3H15 redundantly control cell elongation in stems (Kim et al., 2014; Chai et al., 2015), we suggest that PdC3H17 may play a negative role in controlling stem elongation. Interestingly, we found that PdC3H17ΔCOE lines roughly showed wild type-like phenotypes (Figure 2), suggesting that overexpression of PdC3H17 inhibits stem elongation likely depending on its CCCH domain.
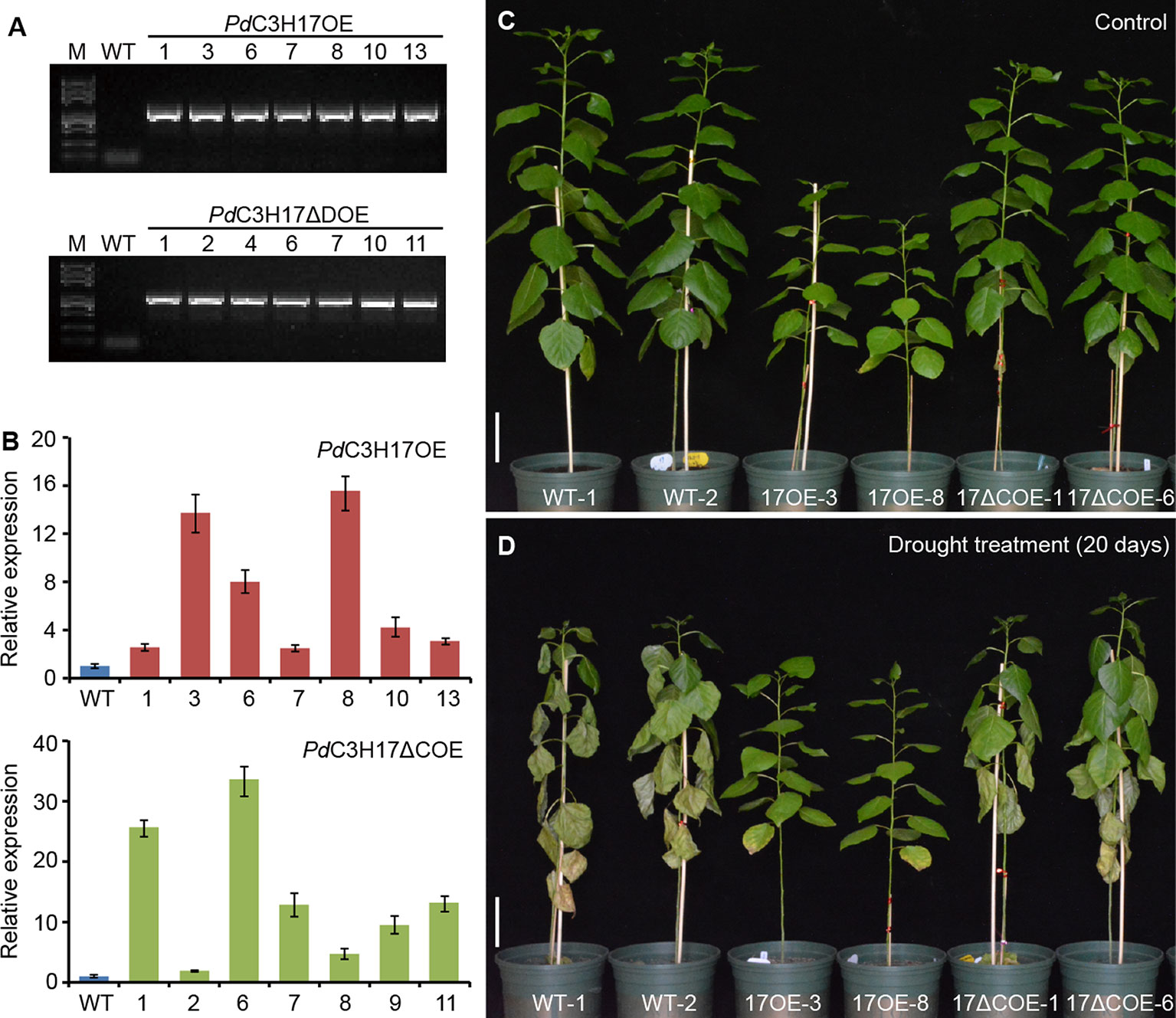
Figure 2 Morphological differences between wild-type and transgenic poplars overexpressing PdC3H17 or PdC3H17ΔC after well-watered or drought stress conditions for 20 days. (A) PCR identification of representative PdC3H17 and PdC3H17ΔC overexpression poplar lines using pro35S and gene-specific primers. Wild-type (WT) plants were used as the control. (B) qRT-PCR analysis of PdC3H17 expression in WT, PdC3H17OE, or PdC3H17ΔCOE poplar lines. (C, D) Drought response of 3-month-old representative WT, PdC3H17OE (17OE), and PdC3H17ΔCOE (17ΔCOE) poplar lines at the 20th day of well-watered (C) or drought (D) treatment. Bars = 10 cm.
Different Drought Responses of PdC3H17 and PdC3H17ΔC Overexpression Poplars Are Associated With the Changes of Their Stem Xylem Vessel Cell Number
To determine the biological role of PdC3H17 in drought stress, WT, PdC3H17OE, and PdC3H17ΔCOE plants grown in soil for 3 months were exposed to drought treatment by withholding water. Most leaves of the WT poplars were seriously wilted on day 20, whereas those of PaC3H17OE plants remained normal (Figure 2D). The drought response of PdC3H17ΔCOE plants was slightly stronger than that of WT plants but significantly weaker than that of PdC3H17OE plants. Under the normally watered condition, WT and PdC3H17ΔCOE plants remained drastically faster growth than PdC3H17OE plants (Figure 2C).
We further investigated the effects of PdC3H17 or PdC3H17ΔC overexpression on alterations in physiology that may contribute to drought response. It is known that higher stem xylem water potential can prevent drought-induced hydraulic failure and enhance drought resistance (Choat et al., 2012). Our results indicated that stem xylem water potential was the highest in PdC3H17OE plants, followed in PdC3H17ΔC OE plants, and the lowest in WT plants under drought stress (Figure 3A), which was consistent with their visible phenotypes (Figure 2D). By contrast, the three genotypes showed similar stem water potential under control condition (Figure 3A). We then analyzed the morphology of stem xylem cells. As indicated in Figures 3B, C the vessel number per unit of area was the greatest in PdC3H17OE plants, following in PdC3H17ΔCOE plants, and the smallest in WT plants. No significant difference for cell size of stem xylem vessels was observed between the three genotypes. It is possible that the increase of stem vessel cell number in PdC3H17 and PdC3H17ΔC overexpression poplars contributes to more effective water transport, thereby promoting drought tolerance.
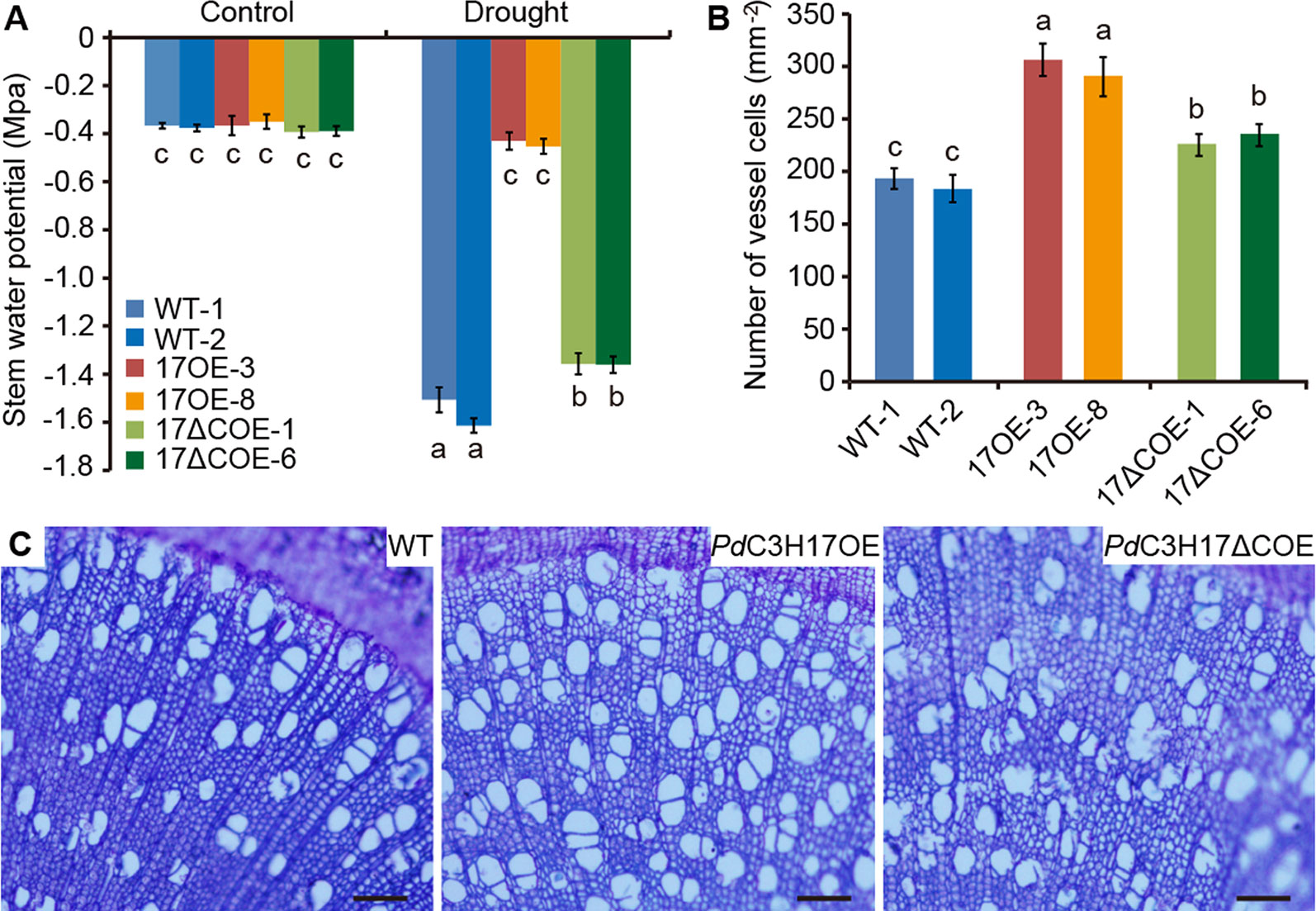
Figure 3 Stem water potential and xylem vessel cell numbers of WT, PdC3H17OE, and PdC3H17ΔCOE poplars. (A) Statistical analysis of stem water potential of 4-month-old WT, PdC3H17OE (17OE), and PdC3H17ΔCOE (17ΔCOE) poplars under well-watered and drought stress conditions for 20 days. (B) Statistical analysis of the numbers of stem xylem vessel cells in 3-month-old WT, 17OE, and 17ΔCOE poplars. At least six plants from two lines in each genotype were selected for measurement of stem water potential and xylem vessel cells. Data are presented as mean ± SD. Different letters above bars denote statistical significance between treatments of samples (P < 0.05). (C) Basal stem sections of 3-month-old representative WT, PdC3H17OE, and PdC3H17ΔCOE plants. Bars = 100 μm.
Photosynthetic Capacities Are Differentially Repressed in PdC3H17 and PdC3H17ΔC Overexpression Poplars After Drought Treatment
Four photosynthetic parameters (chlorophyll a+b content, stomatal conductance, net photosynthetic rate, and transpiration) were detected in WT, PdC3H17OE, and PdC3H17ΔCOE poplars (Figure 4). At 20th day of normal-watered condition, chlorophyll a+b content was roughly similar among three genotypes (Figure 4A). However, stomatal conductance, transpiration, and net photosynthetic rate in PdC3H17OE plants were markedly lower than those in WT and PdC3H17ΔCOE plants (Figures 4B–D). When treated by drought stress for 20 days, four photosynthetic parameters were decreased in all detected plants, but these decreases were more obvious in both WT and PdC3H17ΔCOE plants than in PdC3H17OE plants (Figure 4). These results suggest that overexpression of PdC3H17 increases drought tolerance likely depending on its CCCH domain.
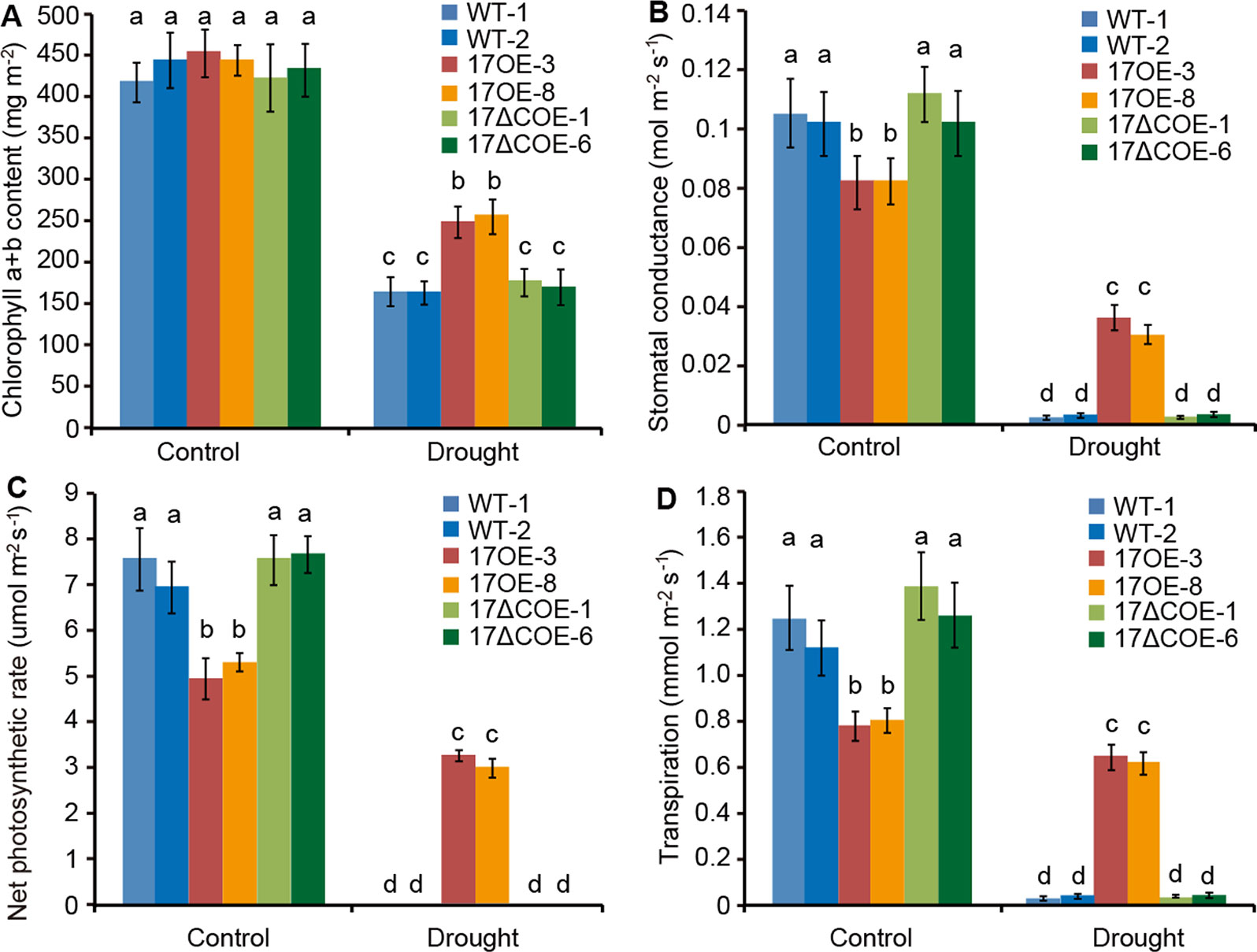
Figure 4 Variation in photosynthetic parameters of 3-month-old PdC3H17OE (17OE) and PdC3H17ΔCOE (17ΔCOE) plants relative to those of WT plants after well-watered or drought stress conditions for 20 days. (A) Chlorophyll a+b content. (B) Stomatal conductance. (C) Net photosynthetic rate. (D) Transpiration. At least six plants of two lines in each genotype were measured. Data are presented as mean ± SD. Different letters above bars denote statistical significance between treatments of leaves (P < 0.05).
The ROS-Scavenging Abilities Are Differentially Increased in PdC3H17 and PdC3H17ΔC Overexpression Poplars After Drought Treatment
ROS play a key role in the acclimation process of plants to drought stress (Choudhury et al., 2017). We thus examined whether overexpression of PdC3H17 or PdC3H17ΔC affects the accumulation of ROS in transgenic poplars. The levels of H2O2, a major ROS, were slightly higher in PdC3H17OE plants than in WT and PdC3H17ΔCOE plants under control condition. However, at 20 days of drought stress a lower accumulation of H2O2 was observed in PdC3H17OE plants relative to other two genotypes (Figure 5A). Further, the PdC3H17ΔCOE plants showed less increase of H2O2 level than WT plants. These results indicated that overexpression of PdC3H17 and PdC3H17ΔC in poplar differentially affected drought-induced ROS accumulation. Similarly, the increased levels of MDA, an indicator of cytomembrane oxidative damage (Shi et al., 2014), were the highest in WT plans and the lowest in PdC3H17OE plants after drought treatment (Figure 5B), suggesting that WT plants may undergo more serious membrane damage than these transgenic plants.
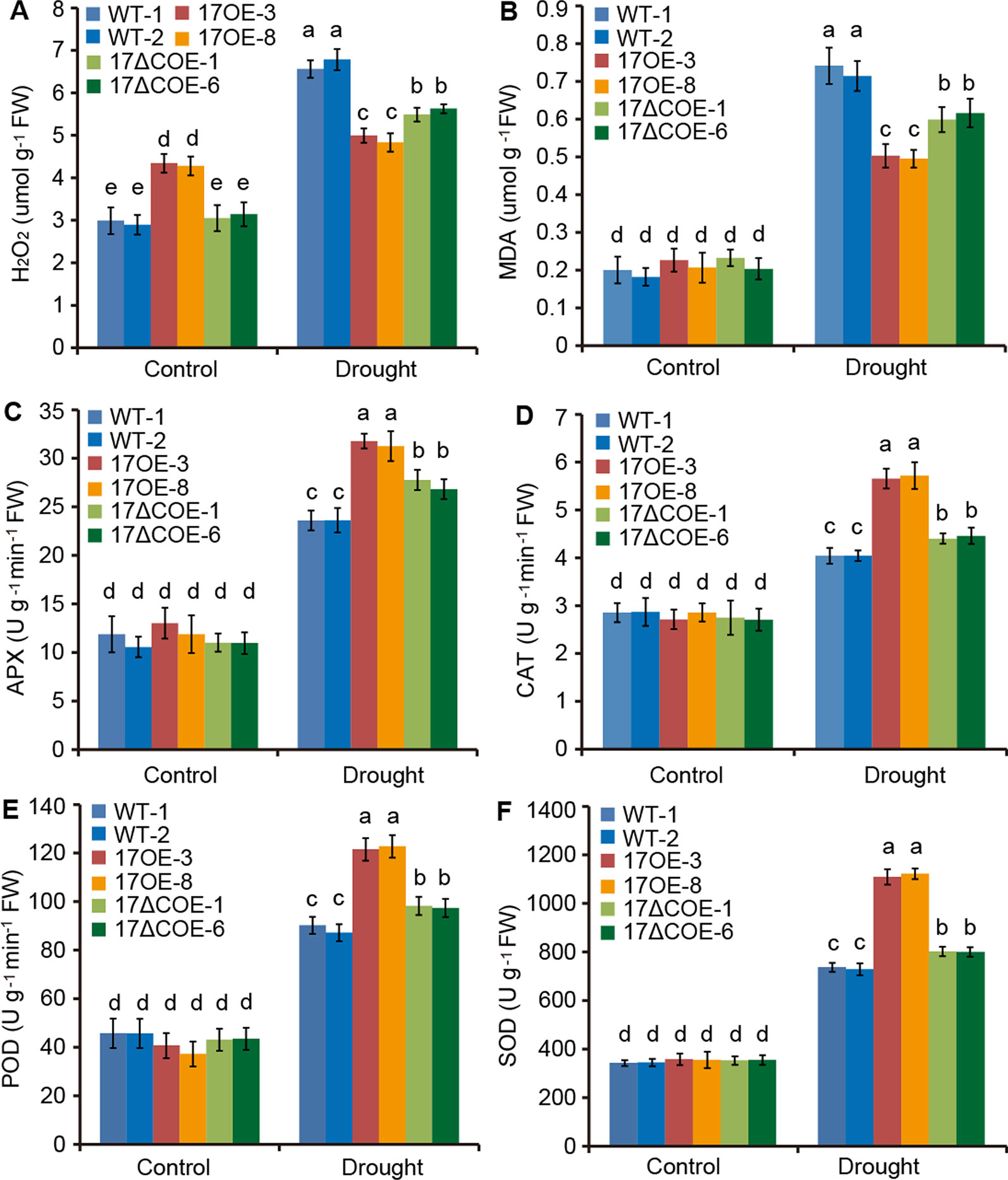
Figure 5 Quantitative analyses of H2O2 and four antioxidants in 3-month-old WT, PdC3H17OE (17OE), and PdC3H17ΔCOE (17ΔCOE) poplars after well-watered or drought stress conditions for 20 days. (A) H2O2, hydrogen peroxide. (B) MDA, malondialdehyde. (C) APX, ascorbate peroxidase. (D) CAT, catalase. (E) POD, peroxidase. (F) SOD, superoxide dismutase. At least six plants of two lines in each genotype were measured. Data are presented as mean ± SD. Different letters above bars denote statistical significance between treatments of leaves (P < 0.05).
6APX, CAT, POD, and SOD are crucial antioxidants that can scavenge ROS (Choudhury et al., 2017). We further detected the activities of these four enzymes in drought-treated and control WT and transgenic poplars. Corresponded with the H2O2 levels, the activities of APX, CAT, POD, and SOD were most obviously elevated in PdC3H17OE plants, following PdC3H17ΔCOE plants, and less significantly increased in WT plants when treated by drought (Figures 5C–F). Besides, free proline, soluble protein, and soluble sugar contents were measured in these poplars (Figures 6A–C). Under control condition, PdC3H17OE plants showed higher levels of soluble protein and soluble sugar than WT and PdC3H17ΔCOE plants (Figures 6B–C). After drought stress for 20 days, free proline, soluble protein, and soluble sugar were more obviously accumulated in PdC3H17OE plants than in other two genotypes (Figures 6A–C). These data indicated that PdC3H17 overexpression may enhance the ROS scavenging capacity and thereby confer plant resistance to drought stress.
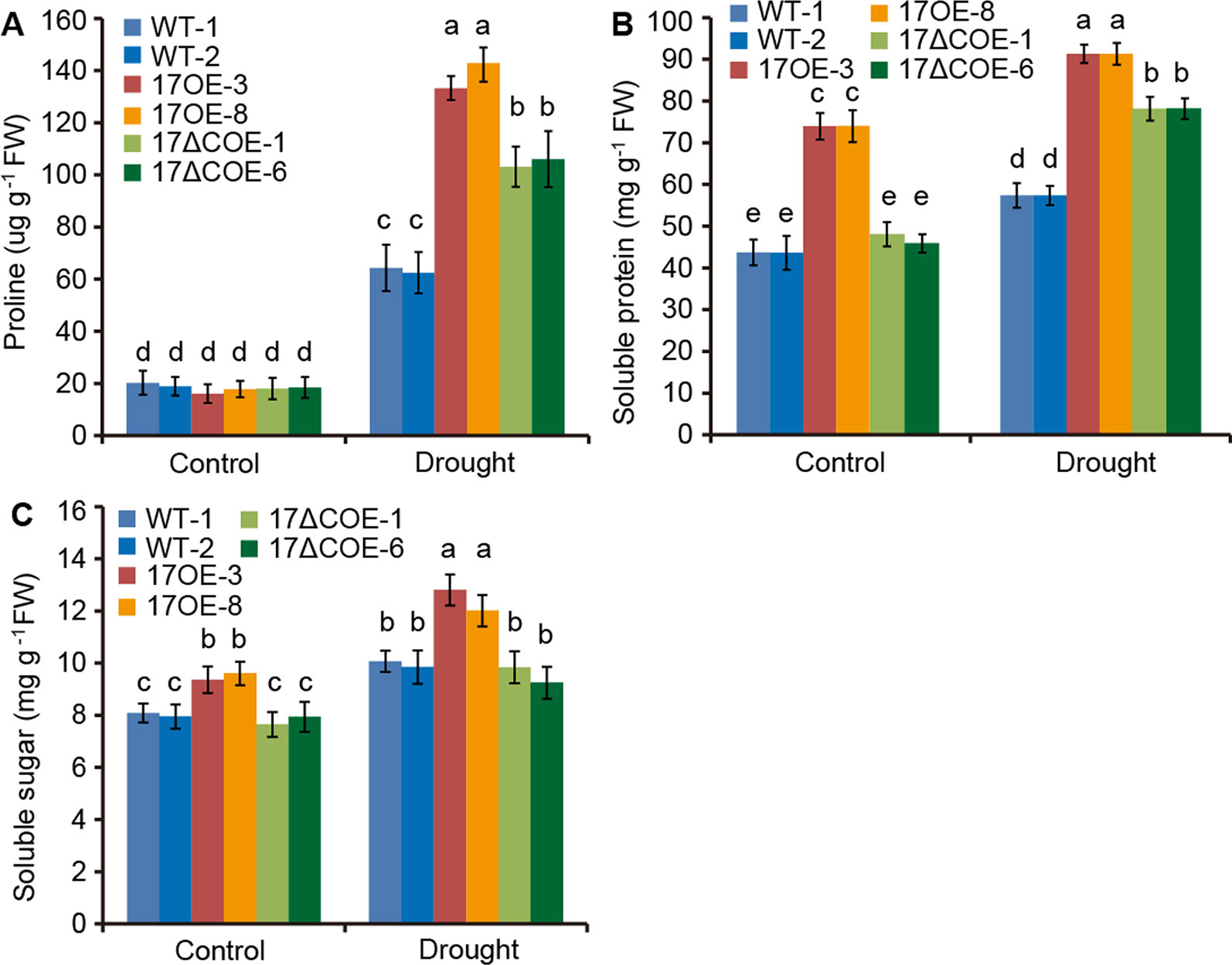
Figure 6 Quantitative analyses of osmotic adjustment substances in 3-month-old WT, PdC3H17OE (17OE), and PdC3H17ΔCOE (17ΔCOE) poplars after well-watered or drought stress conditions for 20 days. (A) Free proline. (B) Soluble protein. (C) Soluble sugar. At least six plants of two lines in each genotype were measured. Data are presented as mean ± SD. Different letters above bars denote statistical significance between treatments of leaves (P < 0.05). P < 0.05.
Weaker Growth Inhibition is Observed in PdC3H17 Overexpression Poplars During Drought Stress
We further investigate whether overexpression of PdC3H17 or PdC3H17ΔC in poplar affects stem growth in the absence of water stress. Statistical analyses revealed that drought treatment resulted in a significantly greater decrease of both stem elongation and thickening rates in WT and PdC3H17ΔCOE poplars than in PdC3H17OE poplars compared to control condition (Figure 7). Furthermore, these decreases were more obvious in WT plants than in PdC3H17ΔCOE plants. Therefore, PdC3H17OE poplars maintained higher growth than WT plants under long-term water deficit conditions.
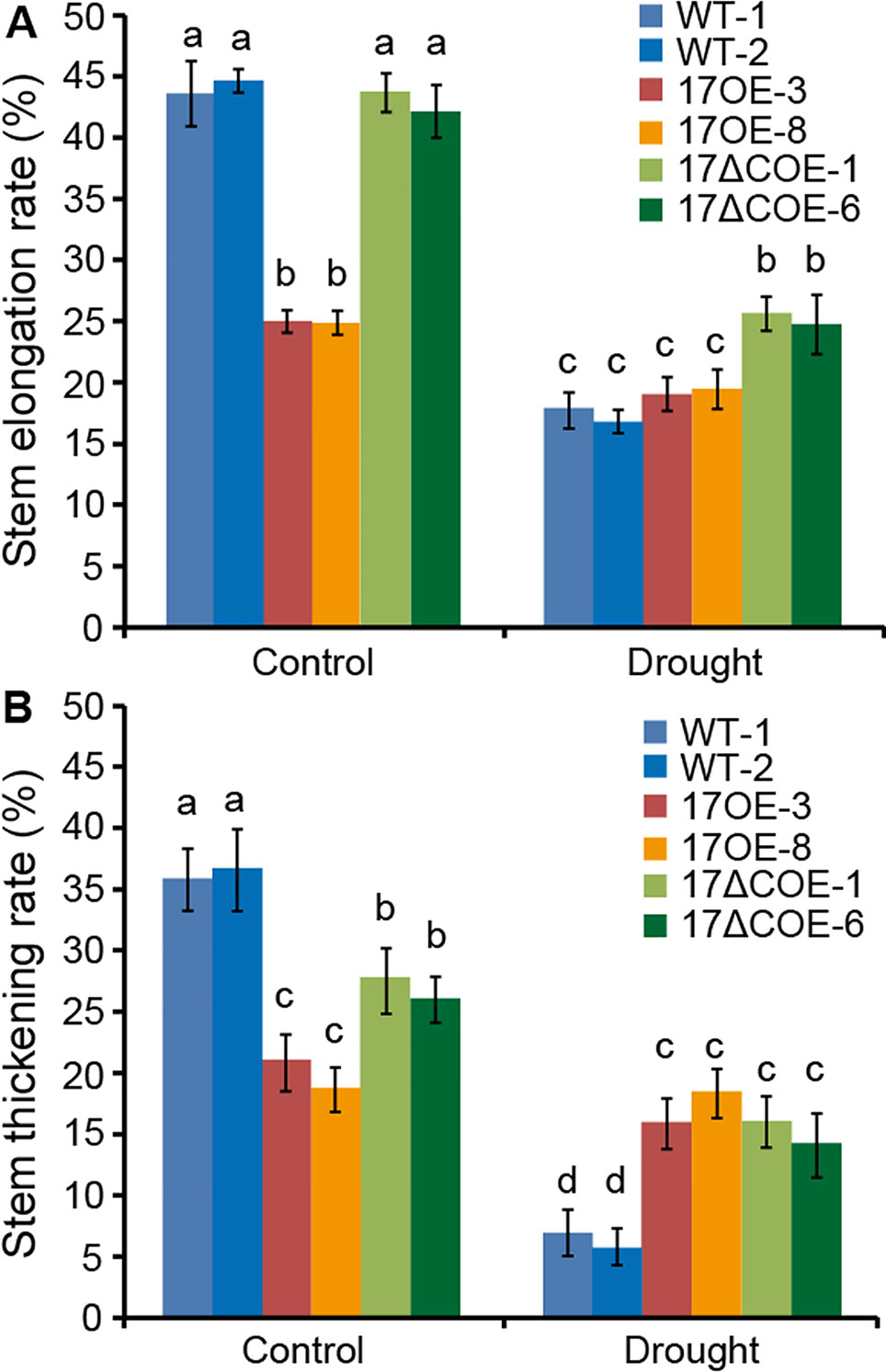
Figure 7 Stem elongation and thickening rates of 3-month-old WT, PdC3H17OE (17OE), and PdC3H17ΔCOE (17ΔCOE) poplars after well-watered or drought stress conditions for 20 days. (A) Stem elongation rate. (B) Stem thickening rate. At least six plants of two lines in each genotype were measured. Data are presented as mean ± Different letters above bars denote statistical significance between treatments of samples (P < 0.05).
Discussion
Drought is the primary abiotic stress responsible for inhibiting poplar growth (Yin et al., 2004; Monclus et al., 2006; Harfouche et al., 2014). Clarifying the regulatory mechanisms of drought tolerance in poplar is essential for ecological conservation and wood production. In this study, we provide evidence showing that PdC3H17 is a novel regulator of drought response and tolerance. Overexpression of PdC3H17 in a hybrid poplar resulted in a significant increase in drought tolerance in comparison with WT plants, correlating with visible alterations of their physiological parameters. As far as we know, this is the first report of CCCH-mediated drought response in woody species.
Wood is made up of xylem, the conductive tissue that transports water from soil to leaves and provides mechanical support for the entire plant (Evert, 2006). Hydraulic conductivity in xylem is highly related to cell size and number of stem xylem vessels (Fisher et al., 2007; Li et al., 2019). For instance, transgenic poplars overexpressing PtrNAC006, PtrNAC007, or PtrNAC120 have smaller vessel lumen area and more vessel cells than WT plants, resulting in stronger drought tolerance (Li et al., 2019). Here, our observations of stem sections revealed that the PdC3H17OE poplars had more xylem vessel cells than WT controls, correlating with higher stem water potential in these transgenic plants (Figure 3). These may result in stronger stem hydraulic conductance in PdC3H17OE plants than in WT plants. Stress-resistant plants often have a dwarf form (Hu et al., 2005; Yu et al., 2008). Because the dwarf is benefit for reducing water and energy consumption and facilitates energy redistribution. Our current results revealed that non-stressed PdC3H17OE poplars showed growth inhibition phenotypes with reduced plant height and decreased stomatal conductance, transpiration, and net photosynthetic rate (Figures 2 and 4). Further, PdC3H17OE lines showed higher stem elongation and thickening rates than WT plants under water‐deficit condition (Figure 7). Therefore, PdC3H17OE poplars enhance drought tolerance likely due to greater hydraulic conductance and dwarf phenotype.
H2O2 is one of the major ROS in plants, and its level is significantly increased under stress conditions (Bhattacharjee, 2012; Choudhury et al., 2017). To minimize oxidative damage, plants have developed sophisticated ROS-scavenging mechanisms. Here, we found that drought stress led to mass accumulation of H2O2 in all detected poplars (Figure 5A). However, the accumulation of H2O2 in WT plants was obviously higher than that in PdC3H17OE plants (Figure 5A), and meanwhile the stomatal conductance and photosynthesis in the WT lines almost reached zero (Figures 4B, C). Accordingly, the activities of four major ROS-scavenging enzymes (APX, CAT, POD, and SOD) were increased less significantly in WT plants than in PdC3H17OE plants after drought treatment (Figures 5C–F). Thus, another possible reason for strong drought tolerance of PdC3H17OE poplars may be more effective activation of the antioxidant system.
Tristetraprolin (hTTP) and AtC3H14 are the orthologs of PdC3H17 in human and Arabidopsis, respectively (Chai et al., 2012). The two proteins have the DNA/RNA binding abilities and may perform transcriptional regulation in the nucleus and RNA regulation in cytoplasm (Lai et al., 1999; Kim et al., 2014; Chai et al., 2015). The best-known CCCH protein is human hTTP, which binds to AU-rich elements (AREs; AUUUA) in the 3′-untranslated region (UTR) of target genes such as TNF-alpha, and performs post-transcriptional regulation (Lai et al., 1999). Further, the CCCH domain of hTTP is necessary for the deadenylation and degradation of target mRNAs in cytoplasmic foci (Lai et al., 1999; Michel et al., 2003). RNA-EMSA data showed that AtC3H14 from Arabidopsis also requires its CCCH domain for target RNA binding (Kim et al., 2014). Our current results revealed that PdC3H17OE poplars exhibited dwarf and drought-tolerance phenotypes whereas morphology and drought response of PdC3H17ΔCOE poplars are more similar to WT plants (Figure 2), suggesting that overexpression of PdC3H17 in a hybrid poplar may confer drought tolerance and inhibit stem elongation depending of its CCCH domain. Considering that PdC3H17, like hTTP and AtC3H14, shares typical CX8CX5CX3H motifs and is targeted to cytoplasmic foci (Chai et al., 2014), we speculate that PdC3H17 might function in drought tolerance and stem elongation through CCCH domain-dependent and post-transcriptional regulation in Populus. Further studies need to be conducted to resolve these ambiguities. Interestingly, we found that the N-terminal sequence of PdC3H17, but not full-length PdC3H17, had transcriptional activation ability in yeast cells (Figure 1). LIC, a CCCH protein in rice, is shown to function as a negative regulator of the Brassinosteroid (BR) signaling pathway through direct suppression of BZR1 targets (Zhang et al., 2012). Thus, it is possible that PdC3H17, like LIC, acts as a transcriptional repressor of stem development and stress resistance, in addition to functioning at the post-transcriptional level. This hypothesis needs to be further validated experimentally.
In conclusion, we developed transgenic PdC3H17- or PdC3H17ΔC-expressing poplar plants. PdC3H17OE poplar was slightly dwarf, had more stem xylem vessel cells, and enhanced ROS-scavenging abilities, thereby reinforcing plant tolerance to drought stress. In contrast, PdC3H17ΔCOE poplar showed WT-like phenotypes, indicating the requirement of its CCCH domain for drought response. Our previous study demonstrated that PdC3H17OE poplars had significantly wider xylem and thicker secondary cell walls than WT controls after growth of 4 months (Chai et al., 2014). Therefore, PdC3H17 may have potential for use in the genetic improvement of drought tolerance and wood production in Populus.
Data Availability Statement
The datasets generated for this study are available on request to the corresponding authors.
Author Contributions
GC designed the experiment, performed data processing, and drafted the manuscript. YZhu, CW, YZha, SC, DW, and QL prepared the materials and performed the experiments. GZ conceived the study and revised the manuscript. All authors read and approved the final version of the manuscript.
Funding
Financial support for this work was obtained from National Key Scientific Research Project of China (2016YFD0600104), National Key Program on Transgenic Research (2018ZX08020002), National Natural Science Foundation of China (31670606, 31570670, 31770315, and 31700526), Major Basic Research Project of Shandong Natural Science Foundation (ZR2018ZC0335), Shandong Provincial Natural Science Foundation (ZR2017BC096, ZR2017BC078, and ZR2019BC006), and Taishan Scholar Program of Shandong (to GZ).
Conflict of Interest
The authors declare that the research was conducted in the absence of any commercial or financial relationships that could be construed as a potential conflict of interest.
References
Bhattacharjee, S. (2012). The language of reactive oxygen species signaling in plants. J. Bot. 2012, 1–22. doi: 10.1155/2012/985298
Bogamuwa, S. P., Jang, J. C. (2014). Tandem CCCH zinc finger proteins in plant growth, development and stress response. Plant Cell Physiol. 55, 1367–1375. doi: 10.1093/pcp/pcu074
Chai, G., Hu, R., Zhang, D., Qi, G., Zuo, R., Cao, Y., et al. (2012). Comprehensive analysis of CCCH zinc finger family in poplar (Populus trichocarpa). BMC Genomics 13, 253. doi: 10.1186/1471-2164-13-253
Chai, G., Qi, G., Cao, Y., Wang, Z., Yu, L., Tang, X., et al. (2014). Poplar PdC3H17 and PdC3H18 are direct targets of PdMYB3 and PdMYB21, and positively regulate secondary wall formation in Arabidopsis and poplar. New Phytol. 203 (2), 520–534. doi: 10.1111/nph.12825
Chai, G., Kong, Y., Zhu, M., Yu, L., Qi, G., Tang, X., et al. (2015). Arabidopsis C3H14 and C3H15 have overlapping roles in the regulation of secondary wall thickening and anther development. J. Exp. Bot. 66, 2595–2609. doi: 10.1093/jxb/erv060
Choat, B., Jansen, S., Brodribb, T. J., Cochard, H., Delzon, S., Bhaskar, R., et al. (2012). Global convergence in the vulnerability of forests to drought. Nature 491, 752–755. doi: 10.1038/nature11688
Choudhury, F. K., Rivero, R. M., Blumwald, E., Mittler, R. (2017). Reactive oxygen species, abiotic stress and stress combination. Plant J. 90 (5), 856–867. doi: 10.1111/tpj.13299
Du, J., Groover, A. (2010). Transcriptional regulation of secondary growth and wood formation. J. Integer. Plant Biol. 52 (1), 17–27. doi: 10.1111/j.1744-7909.2010.00901.x
Evert, R. F. (2006). Esau’s plant anatomy: meristems, cells, and tissues of the plant body: their structure, function, and development (Hoboken, NJ: John Wiley & Sons).
Fisher, J. B., Goldstein, G., Jones, T. J., Cordell, S. (2007). Wood vessel diameter is related to elevation and genotype in the Hawaiian tree Metrosideros polymorpha (Myrtaceae). Am. J. Bot. 94 (5), 709–715. doi: 10.3732/ajb.94.5.709
Harfouche, A., Meilan, R., Altman, A. (2014). Molecular and physiological responses to abiotic stress in forest trees and their relevance to tree improvement. Tree Physiol. 34 (11), 1181–1198. doi: 10.1093/treephys/tpu012
He, F., Wang, H. L., Li, H. G., Su, Y., Li, S., Yang, Y., et al. (2018). PeCHYR1, a ubiquitin E3 ligase from Populus euphratica, enhances drought tolerance via ABA-induced stomatal closure by ROS production in Populus. Plant Biotechnol. J. 16 (8), 1514–1528. doi: 10.1111/pbi.12893
Hu, L., Lu, H., Liu, Q., Chen, X., Jiang, X. (2005). Overexpression of mtlD gene in transgenic Populus tomentosa improves salt tolerance through accumulation of mannitol. Tree Physiol. 25 (10), 1273–1281. doi: 10.1093/treephys/25.10.1273
Jan, A., Maruyama, K., Todaka, D., Kidokoro, S., Abo, M., Yoshimura, E., et al. (2013). OsTZF1, a CCCH-tandem zinc finger protein, confers delayed senescence and stress tolerance in rice by regulating stress-related genes. Plant Physiol. 161, 1202–1216. doi: 10.1104/pp.112.205385
Kim, W. C., Kim, J. Y., Ko, J. H., Kang, H., Kim, J., Han, K. H. (2014). AtC3H14, a plant-specific tandem CCCH zinc-finger protein, binds to its target mRNAs in a sequence-specific manner and affects cell elongation in Arabidopsis thaliana. Plant J. 80, 772–784. doi: 10.1111/tpj.12667
Lai, W. S., Carballo, E., Strum, J. R., Kennington, E. A., Phillips, R. S., Blackshear, P. J. (1999). Evidence that tristetraprolin binds to AU-rich elements and promotes the deadenylation and destabilization of tumor necrosis factor alpha mRNA. Mol. Cell. Biol. 19 (6), 4311–4323. doi: 10.1128/mcb.19.6.4311
Lee, S. J., Jung, H. J., Kang, H., Kim, S. Y. (2012). Arabidopsis zinc finger proteins AtC3H49/AtTZF3 and AtC3H20/AtTZF2 are involved in ABA and JA responses. Plant Cell Physiol. 53, 673–686. doi: 10.1093/pcp/pcs023
Li, S., Lin, Y. J., Wang, P., Zhang, B., Li, M., Chen, S., et al. (2019). The AREB1 transcription factor influences histone acetylation to regulate drought responses and tolerance in Populus trichocarpa. Plant Cell. 31 (3), 663–686. doi: 10.1105/tpc.18.00437
Lin, P. C., Pomeranz, M. C., Jikumaru, Y., Kang, S. G., Hah, C., Fujioka, S., et al. (2011). The Arabidopsis tandem zinc finger protein AtTZF1 affects ABA- and GA-mediated growth, stress and gene expression responses. Plant J. 65, 253–268. doi: 10.1111/j.1365-313X.2010.04419.x
Livak, K. J., Schmittgen, T. D. (2001). Analysis of relative gene expression data using real-time quantitative PCR and the 2(−Delta Delta C (T)) method. Methods 25, 402–408. doi: 10.1006/meth.2001.1262
Michel, S. L. J., Guerrerio, A. L., Berg, J. M. (2003). Selective RNA binding by a single CCCH zinc-binding domain from Nup475 (Tristetraprolin). Biochemistry 42 (16), 4626–4630. doi: 10.1021/bi034073h
Monclus, R., Dreyer, E., Villar, M., Delmotte, F. M., Delay, D., Petit, J. M., et al. (2006). Impact of drought on productivity and water use efficiency in genotypes of Populus deltoides x Populus nigra. New Phytol. 169 (4), 765–777. doi: 10.1111/j.1469-8137.2005.01630.x
Shi, H., Ye, T., Zhu, J. K., Chan, Z. (2014). Constitutive production of nitric oxide leads to enhanced drought stress resistance and extensive transcriptional reprogramming in Arabidopsis. J. Exp. Bot. 65 (15), 4119–4131. doi: 10.1093/jxb/eru184
Wang, D., Guo, Y. H., Wu, C. G., Yang, G. D., Li, Y. Y., Zheng, C. C. (2008). Genome-wide analysis of CCCH zinc finger family in Arabidopsis and rice. BMC Genomics 9, 44. doi: 10.1186/1471-2164-9-44
Wang, W., Liu, B., Xu, M., Jamil, M., Wang, G. (2015). ABA-induced CCCH tandem zinc finger protein OsC3H47 decreases ABA sensitivity and promotes drought tolerance in Oryza sativa. Biochem. Biophys. Res. Commun. 464, 33–37. doi: 10.1016/j.bbrc.2015.05.087
Wang, C., Liu, S., Dong, Y., Zhao, Y., Geng, A., Xia, X., et al. (2016). PdEPF1 regulates water-use efficiency and drought tolerance by modulating stomatal density in poplar. Plant Biotechnol. J. 14 (3), 849–860. doi: 10.1111/pbi.12434
Ye, Z. H., Zhong, R. (2015). Molecular control of wood formation in trees. J. Exp. Bot. 66, 4119–4131. doi: 10.1093/jxb/erv081
Yin, C. Y., Duan, B. L., Wang, X., Li, C. Y. (2004). Morphological and physiological responses of two contrasting poplar species to drought stress and exogenous abscisic acid application. Plant Sci. 167, 1091–1097. doi: 10.1016/j.plantsci.2004.06.005
Yu, H., Chen, X., Hong, Y., Wang, Y., Xu, P., Ke, S., et al. (2008). Activated expression of an Arabidopsis HD-START protein confers drought tolerance with improved root system and reduced stomatal density. Plant Cell. 20 (4), 1134–1151. doi: 10.1105/tpc.108.058263
Zhang, C., Xu, Y., Guo, S., Zhu, J., Huan, Q., Liu, H., et al. (2012). Dynamics of brassinosteroid response modulated by negative regulator LIC in rice. PloS Genet. 8, e1002686. doi: 10.1371/journal.pgen.1002686
Zhang, H., Gao, X., Zhi, Y., Li, X., Zhang, Q., Niu, J., et al. (2019). A non-tandem CCCH-type zinc-finger protein, IbC3H18, functions as a nuclear transcriptional activator and enhances abiotic stress tolerance in sweet potato. New Phytol. 223, 1918–1936. doi: 10.1111/nph.15925
Keywords: the CCCH domain, PdC3H17, drought tolerance, ROS scavenging activity, xylem vessel cell number, Populus
Citation: Zhuang Y, Wang C, Zhang Y, Chen S, Wang D, Liu Q, Zhou G and Chai G (2020) Overexpression of PdC3H17 Confers Tolerance to Drought Stress Depending on Its CCCH Domain in Populus. Front. Plant Sci. 10:1748. doi: 10.3389/fpls.2019.01748
Received: 02 August 2019; Accepted: 12 December 2019;
Published: 24 January 2020.
Edited by:
Eric Ruelland, UMR7618 Institut D’Écologie et des Sciences de L’Environnement de Paris (IEES), FranceReviewed by:
Severine Planchais, Université Pierre et Marie Curie, FranceJin Zhang, Oak Ridge National Laboratory (DOE), United States
Copyright © 2020 Zhuang, Wang, Zhang, Chen, Wang, Liu, Zhou and Chai. This is an open-access article distributed under the terms of the Creative Commons Attribution License (CC BY). The use, distribution or reproduction in other forums is permitted, provided the original author(s) and the copyright owner(s) are credited and that the original publication in this journal is cited, in accordance with accepted academic practice. No use, distribution or reproduction is permitted which does not comply with these terms.
*Correspondence: Guohua Chai, Y2hhaWdoQHFhdS5lZHUuY24=; Gongke Zhou, emhvdWdrQHFpYmVidC5hYy5jbg==