- School of Biological Sciences, Faculty of Biology, Medicine and Health, University of Manchester, Manchester, United Kingdom
TARGET OF RAPAMYCIN (TOR) kinase has been recognised as a key developmental regulator in both plants and animals. Despite their distinct developmental programmes, all eukaryotes studied possess a functional TOR kinase, which integrates environmental and nutrient signals to direct growth and development. This is particularly important in plants, as they are sessile and must sense and respond to external signals to coordinate multicellular growth appropriately. Thus, the investigation of TOR is essential for plant developmental studies in the context of the resources available for growth. Recently, links have been shown between TOR and plant development from embryogenesis through to senescence, however more investigation is crucial to fully elucidate TOR function in each developmental process.
TOR Is a Key Plant Developmental Regulator
Mounting evidence suggests that integrated signaling and metabolic networks play an instructive role in developmental programs and responses to environmental changes and stresses (Li and Sheen, 2016; Krejci and Tennessen, 2017). Remarkably, the TARGET OF RAPAMYCIN (TOR) protein kinase has been identified as a “master regulator” of such networks in all eukaryotes, from single-celled yeast and algae, to complex multicellular organisms such as plants, animals and humans (Dobrenel et al., 2016). Nutrients and growth factors activate TOR, whilst energy deprivation, starvation and stresses are responsible for its inactivation (Dobrenel et al., 2016).
Since the discovery of the TOR inhibitor rapamycin from the soil bacterium Streptomyces hygroscopicus (Sehgal et al., 1975), and its use to identify and isolate TOR in yeast (Heitman et al., 1991; Kunz et al., 1993), mammals (Sabatini et al., 1994) and plants (Menand et al., 2002), our knowledge and understanding of TOR signaling mechanisms and function has progressed immensely. Nevertheless, the study of plant TOR has been largely limited to the model plant Arabidopsis thaliana (and select few other plant species, see: Nanjareddy et al., 2016; De Vleesschauwer et al., 2018) and further investigation is crucial if we are to fully elucidate TOR function in diverse developmental processes across the plant kingdom.
The Plant TOR Kinase Complex
A single large TOR gene exists in Arabidopsis, Chlamydomonas reinhardtii, most animals and humans (Xiong and Sheen, 2014). TOR encodes a highly conserved Ser/Thr kinase (Menand et al., 2002; Zoncu et al., 2011), belonging to the phosphatidylinositol 3-kinase-related kinase family (Heitman et al., 1991). In plants, TOR functions as a complex [TARGET OF RAPAMYCYIN COMPLEX1 (TORC1)] with the core components REGULATORY-ASSOCIATED PROTEIN OF TOR (RAPTOR) and LETHAL WITH SEC THIRTEEN 8 (LST8) (Dobrenel et al., 2016). Whether other plant specific components exist remains to be determined. All sequenced plant species possess orthologs of the RAPTOR and LST8 genes (Anderson et al., 2005; Deprost et al., 2005; Duan et al., 2006; Mahfouz et al., 2006; Diaz-Troya et al., 2008; Moreau et al., 2012).
Two homologs of the mammalian RAPTOR gene exist in Arabidopsis, RAPTOR1 (or RAPTOR1B, AT3G08850) and RAPTOR2 (or RAPTOR1A, AT5G01770) (Anderson et al., 2005; Deprost et al., 2005). In silico analyses reveal that RAPTOR1 is highly expressed throughout development, whereas RAPTOR2 expression is markedly lower. As there is only one RAPTOR gene in algae (Diaz-Troya et al., 2008), it has been suggested that RAPTOR2 arose by a duplication of the ancestral RAPTOR gene in the land plant lineage and is a redundant copy (Deprost et al., 2005), however more detailed RAPTOR phylogenies are needed to test when this occurred. In some reports raptor2 mutants display no obvious phenotypic defects (Deprost et al., 2005), further supporting redundancy, however a slight increase in autophagy was detected in Arabidopsis seedlings and protoplasts (Pu et al., 2017). The protein structure of RAPTOR is conserved in plants; RAPTOR1 has three HEAT motifs followed by seven WD-40 repeats, which are important for protein interactions (Deprost et al., 2005). The conservation of the TOR and RAPTOR1 interaction via the TOR HEAT motifs has been confirmed by coimmunoprecipitation experiments in tobacco leaves (Mahfouz et al., 2006), however higher resolution imaging of the complex would be useful to compare to recent electron microscopy studies of TORC1 and TORC2 in mammals and yeast to study structural conservation (Adami et al., 2007; Aylett et al., 2016; Karuppasamy et al., 2017). Future work into plant-specific RAPTOR interactions in different tissues would also prove informative for elucidating any direct interactions with plant development pathways.
All of the plant genomes checked contain an LST8 gene, however two LST8 genes (LST8-1 and LST8-2) have been found in Arabidopsis thaliana and A. lyrata as a result of a gene duplication event in their common ancestor (Moreau et al., 2012). As in other eukaryotes, the Arabidopsis LST8-1 (AtLST8-1) protein contains seven WD-40 repeats. GUS reporter expression analyses reveal that LST8-1 is expressed throughout plant development, particularly in the aerial tissues. Yeast and Arabidopsis LST8 proteins share 51% sequence identity, and yeast expressing the AtLST8-1 coding sequence were able to grow normally, demonstrating that AtLST8-1 is a homolog of yeast LST8 with conserved function. As with RAPTOR, the interaction of LST8 with plant-specific components in different tissues will reveal potential pathways by which developmental phenotypes arise.
TOR Function During Plant Development
Phylogenetic studies show that TOR, RAPTOR and LST8 gene trees are congruent with the land plant species tree (Deprost et al., 2005; Moreau et al., 2012; Sapre et al., 2018), suggesting that this protein complex is highly conserved and therefore likely to be very important across the plant kingdom. However, how TORC was recruited during the evolution of multicellularity and plant specific processes is unclear. As well as controlling photosynthesis, autophagy and senescence (Deprost et al., 2007; Liu and Bassham, 2010; Moreau et al., 2012; Ren et al., 2012; Xiong et al., 2013; Li et al., 2015), TOR is critical for promoting different aspects of plant development under favourable conditions throughout a plant's lifespan.
Embryogenesis
In flowering plants, seed formation is characterized by double fertilization of the female gametophyte, giving rise to two distinct tissues: the zygote and the endosperm (Dumas and Rogowsky, 2008). The endosperm grows as a syncytium until it reaches around 200 nuclei, before cellularization. The Arabidopsis loss of function KO tor mutant endosperm reaches approximately 48 (± 13) nuclei and cellularization does not occur (Menand et al., 2002). Embryos of Arabidopsis null tor mutants arrest early at the dermatogen stage, with cells in metaphase still present. While cell division itself is thus not inhibited by the disruption of AtTOR in the embryo, cell growth is supressed (Menand et al., 2002). This is consistent with wide-scale downregulation of translation machinery and cell wall modifying enzymes such as CELLULASE SYNTHASE 6 (CESA6) and EXPANSIN B1 (EXPB1) after AtTOR inhibition (Xiong et al., 2013). On the other hand, the role of RAPTOR1 in embryogenesis is unclear. raptor1 T-DNA insertion lines had viable embryos, suggesting that AtTOR function in embryogenesis is independent of RAPTOR1 (Anderson et al., 2005). However, further work found the same line (SALK_078159) to be embryo lethal (Deprost et al., 2005), therefore varying light and temperature growth conditions could affect the phenotypic severity.
Germination
AtTOR has been implicated as a key mediator of environmental signals with seed germination (Xiong et al., 2013). To drive the transition from heterotrophic to photoautotrophic growth in Arabidopsis seedlings, glucose‐AtTOR signaling activates broad gene sets involved in the cell cycle and anabolic processes, and suppresses gene sets controlling catabolic processes (Xiong et al., 2013). This in turn activates root growth via glycolysis-mitochondria-ETC (electron transport chain) relays (Xiong et al., 2013). Furthermore, photosynthesis-derived sugars are necessary for hormones (auxin, brassinosteroid, cytokinin, and gibberellin) to promote rapid root elongation and reactivate the quiescent root during this transition to photoautotrophy (Xiong et al., 2013).
Two independent raptor1 mutants (SALK_101990 and SALK_022096) had seeds with delayed germination and reduced stress resistance, resulting in reduced viability (Salem et al., 2017). Furthermore, seed-coat pigmentation and mucilage production was reduced, accompanied by changes in metabolic content, such as increased free amino acids, and decreased protective secondary metabolites and storage proteins. This is consistent with the transcriptional reprogramming of gene sets involved in central and secondary metabolism in response to glucose-AtTOR signaling in seedlings (Xiong et al., 2013). There were also increases in abscisic acid, auxin and jasmonic acid, all known to inhibit germination.
Seedling Development
The transition from dark-grown (etiolated) to light-grown (de-etiolated) seedlings is accompanied by several morphological changes; elongation rate is reduced, the apical hook opens, true leaves undergo expansion and mature chloroplasts develop. The inhibition of AtTOR in seedlings, via either asTORis (active site ATP-competitive TOR inhibitors) or genetic suppression, reduces cotyledon greening, chloroplast development and seedling growth (Deprost et al., 2007; Dong et al., 2015; Li et al., 2015; Xiong et al., 2017). 40S ribosomal protein S6 KINASE (S6K) is a phosphorylation target of TOR, and promotes chloroplast development and seedling growth via the regulation of BR INSENSITIVE 2 (BIN2) (See review: Shi et al., 2018).
An exciting link has been made between light and the activation of AtTOR-RPS6 (RIBOSOMAL PROTEIN S6) in de-etiolating seedlings. Light is first perceived by photoreceptors such as phytochrome A and cryptochromes, leading to the inactivation of the negative regulator CONSTITUTIVE PHOTOMORPHOGENESIS 1 (COP1), which triggers the activation of the auxin pathway and thus AtTOR-dependent phosphorylation of RPS6 (Figure 1). Accordingly, mutant seedlings lacking functional AtTOR, RPS6A or RPS6B displayed delayed cotyledon opening (Chen et al., 2018). It has also been shown that auxin can activate TOR via Rho-like small GTPase 2 (ROP2) (Schepetilnikov et al., 2013; Schepetilnikov et al., 2017). TOR may therefore coordinate light and auxin levels to ensure a timely switch in the development of de-etiolating seedlings.
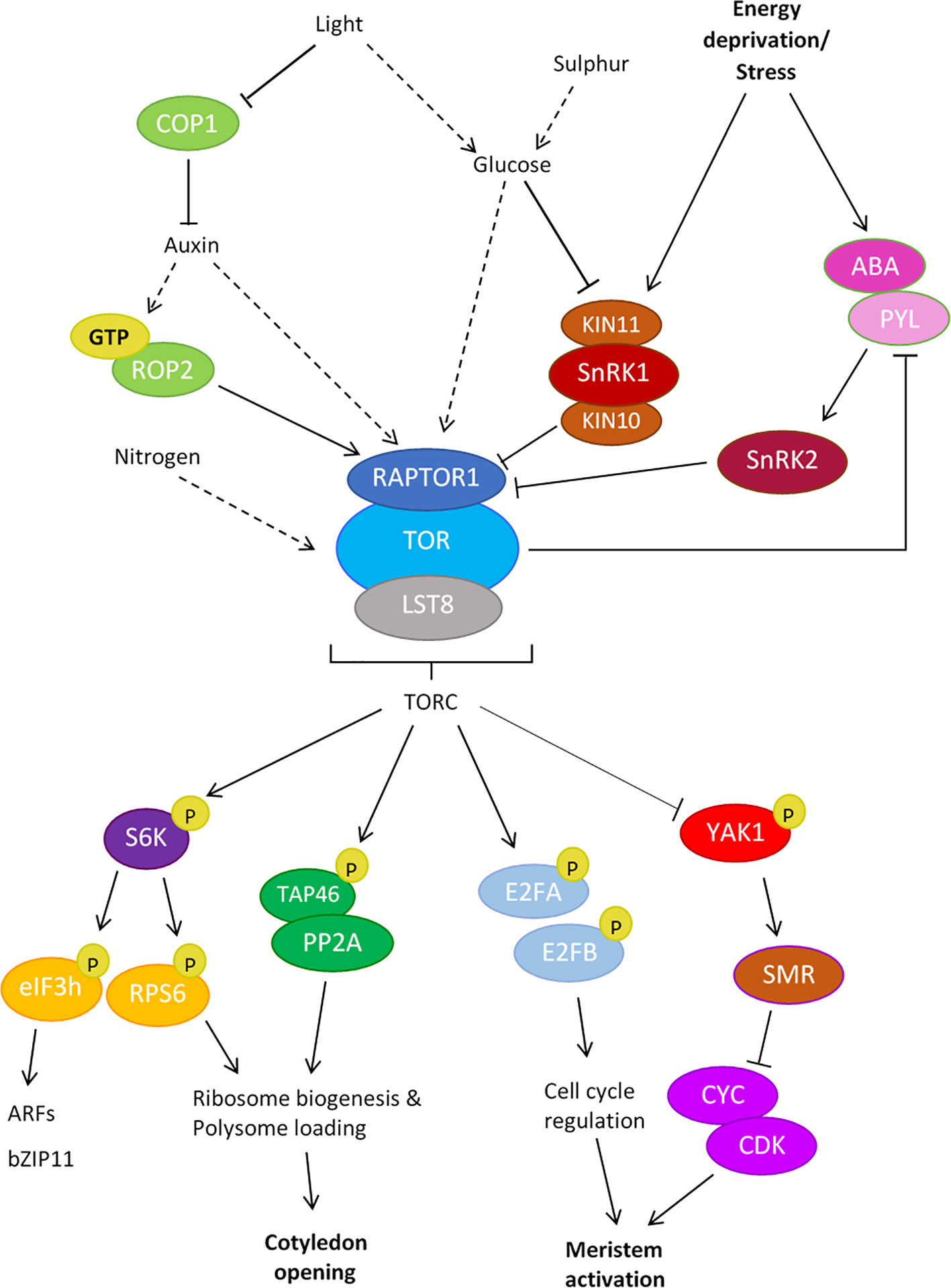
Figure 1 Upstream and downstream targets of the TOR Complex (TORC) in plants. Upstream regulators of plant TORC1: Light, glucose and nutrients are known activators of the TOR pathway. Light activates the TOR pathway via the inactivation of the negative regulator COP1, triggering the activation of the auxin pathway, leading to TOR activation during seedling de-etiolation. Light is also known to trigger GTP-ROP2/auxin activation of TOR signaling in the shoot apex. Light and glucose coordinate to inactivate the TOR antagonist SnRK1, leading to the indirect activation of TORC. The TORC kinase also senses sulfur availability indirectly through glucose signaling; sulfur deficiency induces low glucose levels, leading to the negative regulation of TOR signaling. Stress signals trigger ABA binding of PYL receptors, which activate SnRK2s. SnRK2s directly phosphorylate RAPTOR1, inhibiting TORC signaling to promote stress responses. Downstream targets of plant TORC1: Direct phosphorylation targets of TORC1 include PP2A (via the subunit TAP46), E2FA/B and S6K, leading to the activation of cellular processes throughout development. elF3H and RPS6 are direct phosphorylation targets of S6K-P. The YAK1 kinase is inhibited by active TOR, relieving the inhibition of CYC/CDKs by SMRs to allow cell proliferation in the meristem. Solid arrows indicate direct interaction, dashed arrows indicate indirect interaction.
Meristem Development
The Shoot Apical Meristem (SAM) and the Root Apical Meristem (RAM) maintain undifferentiated stem cells responsible for the formation of the above- and below-ground organs, and AtTOR is known to be expressed in these tissues (Menand et al., 2002). Consistently, delayed shoot growth has been observed in tor knockdown and raptor1 mutant lines (Menand et al., 2002; Xiong et al., 2013; Pfeiffer et al., 2016). Recent data showed that in 4-5 day old Arabidopsis seedlings, AtTOR-E2FA phosphorylation activates the RAM by activating S phase, whilst AtTOR, activated by light-Auxin-ROP2 signaling, phosphorylates both E2FA and E2FB to activate S phase in the SAM (Figure 1; Xiong et al., 2013; Li et al., 2017). AtTOR is thus a likely candidate for the integrator of environmental signals from distant organs to direct meristem activity in both roots and shoots.
YET ANOTHER KINASE 1 (YAK1) has recently been reported as a downstream target of the AtTOR pathway and a major regulator of RAM activity (Barrada et al., 2019). YAK1 was discovered through a pharmaco-genetic screen; yak1 loss-of-function mutants are resistant to the asTORis AZD-8055, whilst Arabidopsis overexpressing YAK1 are hypersensitive. Treatment of WT plants with AZD-8055 reduces the number of dividing cells in the meristematic zone (MZ) inducing early differentiation. In yak1 mutants MZ size was not reduced in the presence of the inhibitor. Furthermore, when treated with pINDY, an ATP-competitive inhibitor of the animal AtYAK1 homolog DYRK1A (DUAL SPECIFICITY TYROSINE PHOSPHORYLATION REGULATED KINASE 1A), MZ size was restored in WT plants treated with AZD-8055. These results indicate that YAK1 controls cell proliferation in the MZ in a TOR-dependent manner.
Cell proliferation is controlled by CYCLIN DEPENDENT KINASES (CDKs), which are regulated by the periodic activation of cyclins (CYC). CDK-CYC activity is regulated by plant-specific CDK inhibitors such as SIAMESE (SIM) and SIAMESE-RELATED (SMR) (See review: Inagaki and Umeda, 2011). Various promoter-driven GUS reporter lines tracking the expression of SIM, SMR, and CYC genes revealed that, in the absence of AtTOR activity, YAK1 induces the expression of SMR genes, which in turn repress CDKs to promote differentiation (Barrada et al., 2019). Contrarily, YAK1 inhibition by AtTOR kinase promotes growth, by lifting the repression of CDKs and CYC, to maintain proliferation (Figure 1). Furthermore, physical interaction between AtYAK1 and RAPTOR1 has been confirmed by both yeast two-hybrid assay and biomolecular fluorescence complementation (BiFC) assays in plant cells (Forzani et al., 2019). A phosphoproteomics analysis in growth-induced Arabidopsis cell culture further demonstrated TOR-dependent phosphorylation of two conserved Ser residues of AtYAK1 (Van Leene et al., 2019). It has thus been suggested that TORC1 binds to AtYAK1 through the component RAPTOR and inactivates it by phosphorylation (Forzani et al., 2019), perhaps revealing how the TOR-YAK1-SMR-CYC/CDK interaction functions to regulate RAM activity and maintenance.
Patterning of the SAM by WUSCHEL (WUS) and CLAVATA (CLV) genes has also been connected to AtTOR activity (See review: Somssich et al., 2016). Interestingly, when three day old Arabidopsis seedlings were grown in AZD-8055, the activity of the WUS promoter in pWUS::3xVENUS-NLS lines decreased (Pfeiffer et al., 2016). AtTOR may therefore activate WUS expression, promoting meristem activity in favourable conditions. However, these seedlings were grown for three days in AZD-8055 liquid culture, so it is unclear to what extent long term metabolic changes are controlling WUS expression. Conversely, no expression changes of the root meristem patterning gene, WUSCHEL RELATED HOMEOBOX 5 (WOX5) were observed when treated with asTORis, suggesting that AtTOR may not regulate meristem patterning in the RAM (Xiong et al., 2013). Further determining the exact role of AtTOR in the SAM and RAM will prove critical for understanding environment dependent meristem activity.
Plant and Leaf Size
AtTOR is also involved in size regulation. ß-estradiol inducible and ethanol inducible AtTOR silencing plants show a reduction in plant biomass, including reduced cell size and ultimately reduced leaf size (Deprost et al., 2007; Xiong and Sheen, 2012), consistent with a T-DNA raptor1 SALK line (Anderson et al., 2005). Accordingly, lst8-1 mutants have reduced size, as well as increased shoot branching (Moreau et al., 2012). Ser-Thr PROTEIN PHOSPHATASE 2A (PP2A) contains a conserved regulatory subunit TAP46 (TYPE 2A-PHOSPHATASE-ASSOCIATED PROTEIN 46KD) (TAP42 in yeast), which is directly phosphorylated by AtTOR (Figure 1; Ahn et al., 2011). Disruption of TAP46 expression results in global translation defects, decreased polysome accumulation and methionine incorporation, and in turn smaller plants as above (Ahn et al., 2015). Furthermore, a recent study has confirmed that TOR inhibition with asTORis prevents leaf primordia initiation in 10 day old Arabidopsis plants, causing a reduction in leaf number (Mohammed et al., 2018).
Conversely, overexpression of both TAP46 and AtTOR results in larger seeds and plants (Deprost et al., 2007; Ahn et al., 2015), with bigger leaves due to larger epidermal cells and longer petioles. AtTOR domain overexpression lines possess twisted leaves and siliques (Deprost et al., 2007; Ahn et al., 2011). Together these studies clearly indicate the involvement of AtTOR in leaf development, however it is unclear whether AtTOR only directly controls global cell cycle regulators and cell growth machinery (Xiong et al., 2013; Li et al., 2017), or affects leaf development genes such as the OVATE FAMILY PROTEINS (OFPs) (Wang et al., 2011) to target specific leaf development pathways.
Nutrients such as nitrogen (N), phosphate (Pi), and sulfur (S) play crucial roles in the promotion of plant growth and recent studies suggest that AtTOR functions in these processes. For example, S availability coordinates glucose signaling to activate AtTOR (Figure 1; Dong et al., 2015). Furthermore, nitrate, a major N source, behaves as a nutrient signal to promote system-wide shoot and root growth in Arabidopsis (Liu et al., 2017). Notably, Arabidopsis seedlings modified to overexpress AtTOR show hypersensitivity to high nitrate inhibition of roots (Deprost et al., 2007). By sensing the nutrient content in the cell, AtTOR kinase is able to initiate growth at a time when sufficient resources are available for healthy plant development.
Abscisic acid (ABA) signaling has been implicated as a critical player in the inhibition of plant growth under stress and recent studies propose TOR is the key mediator of this process (Wang et al., 2018). Upon stress induction, ABA binds PYR1/PYL/RCAR (PYL) receptors, triggering the activation of SnRK2s (SUC NON-FERMENTING 1-RELATED KINASE 2). SnRK2s phosphorylate RAPTOR, thereby inhibiting TORC signaling and promoting stress-induced growth inhibition. When favourable conditions return, TOR phosphorylates PYL receptors, preventing ABA binding, and, critically, inhibiting the activity of ABA-independent PYLs. This interaction between ABA core signaling components and TORC represents a conserved regulatory mechanism to maximise fitness under stress and promote growth recovery in its absence.
Other regulators of plant growth via TOR include SnRK1, a conserved glucose/energy sensor protein kinase. Glucose can activate AtTOR indirectly via the inactivation of SnRK1 (Figure 1; Baena-Gonzalez and Sheen, 2008). Arabidopsis SnRK1 (AtSnRK1) forms a heterotrimeric complex with the catalytic subunits KIN10 and KIN11 (Baena-Gonzalez and Sheen, 2008), and KIN10 has been shown to directly interact with and phosphorylate RAPTOR (Nukarinen et al., 2016). Thus, TORC1 and AtSnRK1 dominate a complex network, acting antagonistically to direct plant growth.
Flowering
Alongside altering organ size and initiation, AtTOR disruption delays flowering time (Deprost et al., 2007), which is also evident in raptor1 and lst8-1 mutants (Anderson et al., 2005; Moreau et al., 2012). The transition to flowering time is controlled by myriad external and internal factors, such as plant age, sugar availability, photoperiod and temperature (See review: Cho et al., 2017). These signals converge on factors such as LEAFY to convert the SAM into an Inflorescence Meristem (IFM) (Blazquez et al., 1997). Future work is necessary to determine whether AtTOR interacts with these pathways directly or indirectly, linking AtTOR delayed flowering phenotypes and sensitivity to day length with flowering time control.
Following the establishment of the IFM, flower primordia are initiated at its flanks, producing a Floral Meristem (FM) and flanking floral organ primordia. Mutation of lst8-1 produces flowers with smaller floral organs, but no changes in organ patterning or number have been reported (Moreau et al., 2012), suggesting that LST8 may be independent from the ABC patterning genes (See review: Irish, 2017). Abnormal flower phenotypes have also been recorded in raptor1 SALK lines, but not described fully (Anderson et al., 2005). Furthermore, tor knockdown flowers have yet to be investigated, and are necessary to determine whether flower development is under the control of AtTOR as well as LST8 and RAPTOR1. It is unclear whether the phenotypes are due to direct changes to cell cycle/growth genes, and/or interactions with genes specific to floral development, such as AUXIN RESPONSE FACTOR 8 (ARF8) and BIGPETALp (BPEp) (Szecsi et al., 2006; Varaud et al., 2011).
Interestingly, ectopic expression of Lily S6K (LIS6K) in A. thaliana produces flowers with shortened petals and stamens, due to reduced cell expansion and normal cell division (Tzeng et al., 2009). S6K is a conserved target of TOR; it was shown that S6K binds to RAPTOR for phosphorylation by TOR in plants (Mahfouz et al., 2006). S6K1 in turn phosphorylates the subunit h of eukaryotic Initiation Factor 3 (eIF3h), which promotes loading of mRNAs that carry upstream open reading frames (uORFs) within their 5' untranslated regions (5'UTRs) into the ribosome for translation re-initiation (Figure 1) (Schepetilnikov et al., 2013). Plant specific genes such as AUXIN RESPONSE FACTORS (ARFs) and BASIC LEUCINE ZIPPER 11 (bZIP11) are encoded by uORF-mRNAs and therefore their translation reinititation may be under the control of TOR via S6K (Schepetilnikov et al., 2013). It would be interesting to investigate if ARFs with important roles in development such as MONOPTEROS (ARF5) and ARF2 (Schruff et al., 2006; Chapman and Estelle, 2009) are activated by S6K in different tissue types such as the leaves and flower, and whether this S6K activation is dependent on TOR activity under different environmental conditions.
Concluding Remarks and Future Perspectives
The TOR signaling pathway is vital to integrate information about the nutrient and energy status of cells and tissues to direct the appropriate developmental and physiological response (Dobrenel et al., 2016). Our understanding of plant TOR has boomed over the past years, with studies beginning to expand beyond the model plant Arabidopsis. Evidence is clearly emerging that TOR has a conserved regulatory role in photosynthetic organisms, acting in conjunction with the antagonist SnRK1 to adapt growth and metabolism according to nutrient and hormone signals. Developmental pathways are highly interconnected and it will be interesting to determine how these interact with TOR signaling in a tissue specific manner, particularly at later developmental stages and in novel plant species. The synthesis of such processes will require bioinformatic pathway analysis to build networks at the RNA expression, protein expression, and protein modification levels, for a complete understanding of TOR activity in each tissue. Crucially, these signaling pathways may be even more critical in plants than in animals and yeast, since plant immobility prevents their escape from hostile environments or nutrient scarcity, placing increased importance on their developmental plasticity in response to the environment.
Author Contributions
KM drafted the manuscript and all authors revised it.
Conflict of Interest
The authors declare that the research was conducted in the absence of any commercial or financial relationships that could be construed as a potential conflict of interest.
Acknowledgments
This work was supported by a Biotechnology and Biological Sciences Research Council research grant (BBSRC no. BB/I012982/1 to MK) and a BBSRC DTP studentship (BB/J014478/1 to VS).
References
Adami, A., Garcia-Alvarez, B., Arias-Palomo, E., Barford, D., Llorca, O. (2007). Structure of TOR and its complex with KOG1. Mol. Cell 27, 509–516. doi: 10.1016/j.molcel.2007.05.040
Ahn, C. S., Han, J. A., Lee, H. S., Lee, S., Pai, H. S. (2011). The PP2A regulatory subunit Tap46, a component of the TOR signaling pathway, modulates growth and metabolism in plants. Plant Cell 23, 185–209. doi: 10.1105/tpc.110.074005
Ahn, C. S., Ahn, H. K., Pai, H. S. (2015). Overexpression of the PP2A regulatory subunit Tap46 leads to enhanced plant growth through stimulation of the TOR signalling pathway. J. Exp. Bot. 66, 827–840. doi: 10.1093/jxb/eru438
Anderson, G. H., Veit, B., Hanson, M. R. (2005). The Arabidopsis AtRaptor genes are essential for post-embryonic plant growth. BioMed. Cent. Biol. 3, 12. doi: 10.1186/1741-7007-3-12
Aylett, C. H., Sauer, E., Imseng, S., Boehringer, D., Hall, M. N., Ban, N., et al. (2016). Architecture of human mTOR complex 1. Science 351, 48–52. doi: 10.1126/science.aaa3870
Baena-Gonzalez, E., Sheen, J. (2008). Convergent energy and stress signaling. Trends In Plant Sci. 13, 474–482. doi: 10.1016/j.tplants.2008.06.006
Barrada, A., Djendli, M., Desnos, T., Mercier, R., Robaglia, C., Montane, M. H., et al. (2019). A TOR-YAK1 signaling axis controls cell cycle, meristem activity and plant growth in Arabidopsis. Development 146, dev.171298. doi: 10.1242/dev.171298
Blazquez, M. A., Soowal, L. N., Lee, I., Weigel, D. (1997). LEAFY expression and flower initiation in Arabidopsis. Development 124, 3835–3844.
Chapman, E. J., Estelle, M. (2009). Mechanism of auxin-regulated gene expression in plants. Annu. Rev. Genet. 43, 265–285. doi: 10.1146/annurev-genet-102108-134148
Chen, G. H., Liu, M. J., Xiong, Y., Sheen, J., Wu, S. H. (2018). TOR and RPS6 transmit light signals to enhance protein translation in deetiolating Arabidopsis seedlings. Proc. Natl. Acad. Sci. U. S. A. 115, 12823–12828. doi: 10.1073/pnas.1809526115
Cho, L. H., Yoon, J., An, G. (2017). The control of flowering time by environmental factors. Plant J. 90, 708–719. doi: 10.1111/tpj.13461
De Vleesschauwer, D., Filipe, O., Hoffman, G., Seifi, H. S., Haeck, A., Canlas, P., et al. (2018). Target of rapamycin signaling orchestrates growth-defense trade-offs in plants. New Phytol. 217, 305–319. doi: 10.1111/nph.14785
Deprost, D., Truong, H. N., Robaglia, C., Meyer, C. (2005). An Arabidopsis homolog of RAPTOR/KOG1 is essential for early embryo development. Biochem. Biophys. Res. Commun. 326, 844–850. doi: 10.1016/j.bbrc.2004.11.117
Deprost, D., Yao, L., Sormani, R., Moreau, M., Leterreux, G., Nicolai, M., et al. (2007). The Arabidopsis TOR kinase links plant growth, yield, stress resistance and mRNA translation. EMBO Rep. 8, 864–870. doi: 10.1038/sj.embor.7401043
Diaz-Troya, S., Florencio, F. J., Crespo, J. L. (2008). Target of rapamycin and LST8 proteins associate with membranes from the endoplasmic reticulum in the unicellular green alga Chlamydomonas reinhardtii. Eukaryotic Cell 7, 212–222. doi: 10.1128/EC.00361-07
Dobrenel, T., Caldana, C., Hanson, J., Robaglia, C., Vincentz, M., Veit, B., et al. (2016). TOR signaling and nutrient sensing. Annu. Rev. Plant Biol. 67 (1), 261–285. doi: 10.1146/annurev-arplant-043014-114648
Dong, P., Xiong, F. J., Que, Y. M., Wang, K., Yu, L. H., Li, Z. G., et al. (2015). Expression profiling and functional analysis reveals that TOR is a key player in regulating photosynthesis and phytohormone signaling pathways in Arabidopsis. Front. Plant Sci. 6, 677. doi: 10.3389/fpls.2015.00677
Duan, H. Y., Li, F. G., Wu, X. D., Ma, D. M., Wang, M., Hou, Y. X. (2006). The cloning and sequencing of a cDNA encoding a WD repeat protein in cotton (Gossypium hirsutum L.). DNA Sequence 17, 49–55. doi: 10.1080/10425170500476418
Dumas, C., Rogowsky, P. (2008). Fertilization and early seed formation. C. R. Biol. 331, 715–725. doi: 10.1016/j.crvi.2008.07.013
Forzani, C., Duarte, G. T., Van Leene, J., Clément, G., Huguet, S., Paysant-Le-Roux, C., et al. (2019). Mutations of the AtYAK1 Kinase Suppress TOR Deficiency in Arabidopsis. Cell Rep. 27, 3696–3708.e3695. doi: 10.1016/j.celrep.2019.05.074
Heitman, J., Movva, N. R., Hall, M. N. (1991). Targets for cell-cycle arrest by the immunosuppressant rapamycin in yeast. Science 253, 905–909. doi: 10.1126/science.1715094
Inagaki, S., Umeda, M. (2011). “Cell-Cycle Control and Plant Development,” in International Review of Cell and Molecular Biology. Ed. Jeon, K. W. (Academic Press), 227–261. doi: 10.1016/B978-0-12-386035-4.00007-0
Irish, V. (2017). The ABC model of floral development. Curr. Biol. 27, R887–R890. doi: 10.1016/j.cub.2017.03.045
Karuppasamy, M., Kusmider, B., Oliveira, T. M., Gaubitz, C., Prouteau, M., Loewith, R., et al. (2017). “Cryo-EM structure of Saccharomyces cerevisiae target of rapamycin complex 2,” Nat. Commun. 8, 1729. doi: 10.1038/s41467-017-01862-0
Krejci, A., Tennessen, J. M. (2017). Metabolism in time and space - exploring the frontier of developmental biology. Development 144, 3193–3198. doi: 10.1242/dev.150573
Kunz, J., Henriquez, R., Schneider, U., Deuterreinhard, M., Movva, N. R., Hall, M. N. (1993). Target of Rapamycin in Yeast, TOR2, is an essential Phosphatidylinositol kinase homolog required for G(1) progression. Cell 73, 585–596. doi: 10.1016/0092-8674(93)90144-F
Li, L., Sheen, J. (2016). Dynamic and diverse sugar signaling. Curr. Opin. In Plant Biol. 33, 116–125. doi: 10.1016/j.pbi.2016.06.018
Li, L. X., Song, Y., Wang, K., Dong, P., Zhang, X. Y., Li, F. G., et al. (2015). TOR-inhibitor insensitive-1 (TRIN1) regulates cotyledons greening in Arabidopsis. Front. In Plant Sci. 6, 861. doi: 10.3389/fpls.2015.00861
Li, X. J., Cai, W. G., Liu, Y. L., Li, H., Fu, L. W., Liu, Z. Y., et al. (2017). Differential TOR activation and cell proliferation in Arabidopsis root and shoot apexes. Proc. Natl. Acad. Sci. U. S. A 114, 2765–2770. doi: 10.1073/pnas.1618782114
Liu, Y. M., Bassham, D. C. (2010). TOR is a negative regulator of autophagy in Arabidopsis thaliana. PloS ONE 5, e11883. doi: 10.1371/journal.pone.0011883
Liu, K. H., Niu, Y. J., Konishi, M., Wu, Y., Du, H., Chung, H. S., et al. (2017). Discovery of nitrate-CPK-NLP signalling in central nutrient-growth networks. Nature 545, 311–31+. doi: 10.1038/nature22077
Mahfouz, M. M., Kim, S., Delauney, A. J., Verma, D. P. S. (2006). Arabidopsis TARGET OF RAPAMYCIN interacts with RAPTOR, which regulates the activity of S6 kinase in response to osmotic stress signals. Plant Cell 18, 477–490. doi: 10.1105/tpc.105.035931
Menand, B., Desnos, T., Nussaume, L., Berger, F., Bouchez, D., Meyer, C., et al. (2002). Expression and disruption of the Arabidopsis TOR (target of rapamycin) gene. Proc. Natl. Acad. Sci. U. S. A 99, 6422–6427. doi: 10.1073/pnas.092141899
Mohammed, B., Bilooei, S. F., Doczi, R., Grove, E., Railo, S., Palme, K., et al. (2018). Converging light, energy and hormonal signaling control meristem activity, leaf initiation, and growth. Plant Physiol. 176, 1365–1381. doi: 10.1104/pp.17.01730
Moreau, M., Azzopardi, M., Clement, G., Dobrenel, T., Marchive, C., Renne, C., et al. (2012). Mutations in the Arabidopsis Homolog of LST8/G beta L, a partner of the target of rapamycin kinase, impair plant growth, flowering, and metabolic adaptation to long days. Plant Cell 24, 463–481. doi: 10.1105/tpc.111.091306
Nanjareddy, K., Blanco, L., Arthikala, M. K., Alvarado-Affantranger, X., Quinto, C., Sanchez, F., et al. (2016). A Legume TOR protein kinase regulates rhizobium symbiosis and is essential for infection and nodule development. Plant Physiol. 172, 2002–2020. doi: 10.1104/pp.16.00844
Nukarinen, E., Nagele, T., Pedrotti, L., Wurzinger, B., Mair, A., Landgraf, R., et al. (2016). Quantitative phosphoproteomics reveals the role of the AMPK plant ortholog SnRK1 as a metabolic master regulator under energy deprivation. Sci. Rep. 6, 31697. doi: 10.1038/srep31697
Pfeiffer, A., Janocha, D., Dong, Y. H., Medzihradszky, A., Schone, S., Daum, G., et al. (2016). Integration of light and metabolic signals for stem cell activation at the shoot apical meristem. Elife 5, e17023. doi: 10.7554/eLife.17023
Pu, Y., Luo, X., Bassham, D. C. (2017). TOR-Dependent and -Independent Pathways Regulate Autophagy in Arabidopsis thaliana. Front. Plant Sci. 8, 1204. doi: 10.3389/fpls.2017.01204
Ren, M. Z., Venglat, P., Qiu, S. Q., Feng, L., Cao, Y. G., Wang, E., et al. (2012). Target of Rapamycin Signaling Regulates Metabolism, Growth, and Life Span in Arabidopsis. Plant Cell 24, 4850–4874. doi: 10.1105/tpc.112.107144
Sabatini, D. M., Erdjumentbromage, H., Lui, M., Tempst, P., Snyder, S. H. (1994). RAFT1 - A Mammalian Protein That Binds to FKBP12 in a Rapamycin-Dependent Fashion and is Homologous to Yeast TORS. Cell 78, 35–43. doi: 10.1016/0092-8674(94)90570-3
Salem, M. A., Li, Y., Wiszniewski, A., Giavalisco, P. (2017). Regulatory-associated protein of TOR (RAPTOR) alters the hormonal and metabolic composition of Arabidopsis seeds, controlling seed morphology, viability and germination potential. Plant J. 92, 525–545. doi: 10.1111/tpj.13667
Sapre, S., Tiwari, S., Thakur, V. V. (2018). Phylogenetic analysis of target of rapamycin (TOR) kinase gene of some selected plants species. Biosci. Biotechnol. Res. Commun. 11, 476–480. doi: 10.21786/bbrc/11.3/17
Schepetilnikov, M., Dimitrova, M., Mancera-Martinez, E., Geldreich, A., Keller, M., Ryabova, L. A. (2013). TOR and S6K1 promote translation reinitiation of uORF-containing mRNAs via phosphorylation of eIF3h. EMBO J. 32, 1087–1102. doi: 10.1038/emboj.2013.61
Schepetilnikov, M., Makarian, J., Srour, O., Geldreich, A., Yang, Z. B., Chicher, J., et al. (2017). GTPase ROP2 binds and promotes activation of target of rapamycin, TOR, in response to auxin. EMBO J. 36, 886–903. doi: 10.15252/embj.201694816
Schruff, M. C., Spielman, M., Tiwari, S., Adams, S., Fenby, N., Scott, R. J. (2006). The AUXIN RESPONSE FACTOR 2 gene of Arabidopsis links auxin signalling, cell division, and the size of seeds and other organs. Development 133, 251–261. doi: 10.1242/dev.02194
Sehgal, S. N., Baker, H., Vezina, C. (1975). Rapamycin (AY-22,989), a new antifungal antibiotic. II. Fermentation, isolation and characterization. J. antibiot. 28, 727–732. doi: 10.7164/antibiotics.28.727
Shi, L., Wu, Y., Sheen, J. (2018). TOR signaling in plants: conservation and innovation. Development 145, dev160887. doi: 10.1242/dev.160887
Somssich, M., Je, B. I., Simon, R., Jackson, D. (2016). CLAVATA-WUSCHEL signaling in the shoot meristem. Development 143, 3238–3248. doi: 10.1242/dev.133645
Szecsi, J., Joly, C., Bordji, K., Varaud, E., Cock, J. M., Dumas, C., et al. (2006). BIGPETALp, a bHLH transcription factor is involved in the control of Arabidopsis petal size. EMBO J. 25, 3912–3920. doi: 10.1038/sj.emboj.7601270
Tzeng, T. Y., Kong, L. R., Chen, C. H., Shaw, C. C., Yang, C. H. (2009). Overexpression of the Lily p70(s6k) gene in arabidopsis affects elongation of flower organs and indicates TOR-dependent regulation of AP3, PI and SUP translation. Plant Cell Physiol. 50, 1695–1709. doi: 10.1093/pcp/pcp114
Van Leene, J., Han, C., Gadeyne, A., Eeckhout, D., Matthijs, C., Cannoot, B., et al. (2019). Capturing the phosphorylation and protein interaction landscape of the plant TOR kinase. Nat. Plants 5, 316–327. doi: 10.1038/s41477-019-0378-z
Varaud, E., Brioudes, F., Szecsi, J., Leroux, J., Brown, S., Perrot-Rechenmann, C., et al. (2011). AUXIN RESPONSE FACTOR8 regulates arabidopsis petal growth by interacting with the bHLH transcription factor BIGPETALp. Plant Cell 23, 973–983. doi: 10.1105/tpc.110.081653
Wang, S. C., Chang, Y., Guo, J. J., Zeng, Q. N., Ellis, B. E., Chen, J. G. (2011). Arabidopsis ovate family proteins, a novel transcriptional repressor family, control multiple aspects of plant growth and development. PloS ONE 6, e23896. doi: 10.1371/journal.pone.0023896
Wang, P., Zhao, Y., Li, Z., Hsu, C.-C., Liu, X., Fu, L., et al. (2018). Reciprocal regulation of the TOR kinase and ABA receptor balances plant growth and stress response. Mol. Cell 69, 100–112. e106. doi: 10.1016/j.molcel.2017.12.002
Xiong, Y., Sheen, J. (2012). Rapamycin and Glucose-Target of Rapamycin (TOR) protein signaling in plants. J. Biol. Chem. 287, 2836–2842. doi: 10.1074/jbc.M111.300749
Xiong, Y., Sheen, J. (2014). The role of target of rapamycin signaling networks in plant growth and metabolism. Plant Physiol. 164, 499–512. doi: 10.1104/pp.113.229948
Xiong, Y., McCormack, M., Li, L., Hall, Q., Xiang, C. B., Sheen, J. (2013). Glucose-TOR signalling reprograms the transcriptome and activates meristems. Nature 496, 181–18+. doi: 10.1038/nature12030
Xiong, F. J., Zhang, R., Meng, Z. G., Deng, K. X., Que, Y. M., Zhuo, F. P., et al. (2017). Brassinosteriod insensitive 2 (BIN2) acts as a downstream effector of the Target of Rapamycin (TOR) signaling pathway to regulate photoautotrophic growth in Arabidopsis. New Phytol. 213, 233–249. doi: 10.1111/nph.14118
Keywords: TARGET OF RAPAMYCIN (TOR), plant development, nutrient sensing, meristem patterning, leaf development, flower induction
Citation: McCready K, Spencer V and Kim M (2020) The Importance of TOR Kinase in Plant Development. Front. Plant Sci. 11:16. doi: 10.3389/fpls.2020.00016
Received: 22 October 2019; Accepted: 09 January 2020;
Published: 05 February 2020.
Edited by:
Mary Byrne, University of Sydney, AustraliaReviewed by:
Benoit Menand, Institut de biosciences et biotechnologies (BIAM), FranceLyuba A. Ryabova, UPR2357 Institut de biologie moléculaire des plantes (IBMP), France
Copyright © 2020 McCready, Spencer and Kim. This is an open-access article distributed under the terms of the Creative Commons Attribution License (CC BY). The use, distribution or reproduction in other forums is permitted, provided the original author(s) and the copyright owner(s) are credited and that the original publication in this journal is cited, in accordance with accepted academic practice. No use, distribution or reproduction is permitted which does not comply with these terms.
*Correspondence: Minsung Kim, bWluc3VuZy5raW1AbWFuY2hlc3Rlci5hYy51aw==