- 1CAS Key Laboratory of Marine Ecology and Environmental Sciences, Institute of Oceanology, Chinese Academy of Sciences, Qingdao, China
- 2Laboratory for Marine Ecology and Environmental Science, Qingdao National Laboratory for Marine Science and Technology, Qingdao, China
- 3Center for Ocean Mega-Science, Chinese Academy of Sciences, Qingdao, China
- 4CAS Engineering Laboratory for Marine Ranching, Institute of Oceanology, Chinese Academy of Sciences, Qingdao, China
- 5College of Earth Sciences, University of Chinese Academy of Sciences, Beijing, China
Accelerating losses of seagrass meadows has led to efforts to restore these highly productive and beneficial ecosystems globally. Depth and light availability are critical determinants of seagrass restoration success. Eelgrass (Zostera marina L.) is the dominant seagrass species in the temperate northern hemisphere, but its global distribution has reduced dramatically. The main aims of this study were to determine: (1) the depth limit for Z. marina survival in Ailian Bay, north China, and (2) how light availability affects the growth and recruitment of Z. marina as a basis for identifying a suitable depth range for successful restoration. To achieve these aims, Z. marina shoots were transplanted from a nearby donor site, Swan Lake, to an experimental site, Ailian Bay, and the temporal responses of Z. marina shoots to light availability at water depths ranging from 1 to 8 m were investigated using in situ suspended cultures. Four suspended shoot transplantation experiments were conducted in 4 years. The results showed that the transplanted Z. marina shoots could survive and branch during an annual growth cycle, permanently underwater, at a depth ≤3 m. Due to the local turbidity of the waters in Ailian Bay, a depth of 4 m led to sufficient light deprivation (reduced to 6.48–10.08% of surface irradiance) to negatively affect seagrass shoot density and clonal reproduction. In addition, reproductive shoot density also tended to decline with water depth and light deprivation. Our results indicated that Z. marina population recruitment, through sexual and asexual (clonal growth) reproduction, were negatively affected by increasing water depth and light deprivation. These findings may provide a suitable depth range for the successful restoration of Z. marina in local coastal waters. They may also be applied to the management and restoration of Z. marina globally.
Introduction
Seagrass meadows are among the most productive plant communities, providing habitats, food, and nurseries for a variety of marine organisms (Costanza et al., 1997; Verweij et al., 2008; Barbier et al., 2011; Liu et al., 2013; Unsworth et al., 2018b), regulating nutrients (Barbier et al., 2011), and functioning as key sites for global carbon storage in the biosphere (Fourqurean et al., 2012). Unfortunately, due to the impact of multiple stressors (environmental, biological, and climatological) (Collier and Waycott, 2009; Unsworth et al., 2018a), seagrass meadows have been declining since 1990 at a rate of 7% per annum (Waycott et al., 2009). With the development of coastal construction, the turbidity of coastal waters has been increasing, thus the light availability has been reducing (Elsahragty and Kim, 2015). Reduced light availability due to increased anthropogenic nutrient loading and sedimentation has been identified as one of the primary causes of seagrass loss (Jennifer et al., 2003; Orth et al., 2006). Furthermore, light is often considered one of the most critical factors that controls the distribution and growth of eelgrass (Lee et al., 2007; Zhang et al., 2020).
The eelgrass Zostera marina L. is the dominant seagrass species throughout the Atlantic and Pacific coasts of the temperate northern hemisphere (Green and Short, 2003). In China, eelgrasses are distributed in the coastal waters of Shandong, Hebei, and Liaoning provinces (Zheng et al., 2013; Xu et al., 2020a,b). Historical distribution information (1950–2013) of eelgrass specimens (Biological Museum, Chinese Academy of Sciences, Qingdao, China) and previous literature (Yang and Wu, 1984; Editorial Board of China Bay Survey, 1991; Ye and Zhao, 2002; Guo et al., 2010; Zheng et al., 2013) have revealed that a large number of eelgrass meadows having contracted sharply or disappeared (more than 80%). This is due to increased anthropogenic nutrient loading and sedimentation, according to the National Seagrass Resource Survey (2015–2020). Similarly, previously abundant Z. marina in Ailian Bay, north China, has declined dramatically and is currently mainly distributed in the sea cucumber ponds, according to local fishermen.
Faced with an increasing rate of eelgrass decline, eelgrass restoration throughout its distribution area in temperate northern hemisphere has become an important management tool to mitigate seagrass losses and to enhance critical ecosystem functions (Marion and Orth, 2010; Zhou et al., 2014; van Katwijk et al., 2016; Liu et al., 2019). Unfortunately, only 37% of seagrass restoration efforts have been successful in recent years (van Katwijk et al., 2016). Insufficient light availability has been identified as the one of the primary causes of eelgrass transplantation failure (e.g., Moore et al., 1997; van Katwijk et al., 2016); therefore, understanding their ability to acclimate to a range of light availability may be the key to ensuring their survival in restoration efforts (Eriander, 2017; Zhang et al., 2020).
The light requirements of seagrass have been investigated in many studies, and comprehensively reviewed by Lee et al. (2007); it has been revealed that the minimum light requirements of seagrass vary with species (Lee et al., 2007; Statton et al., 2018) and also within species depending on site-specific conditions (Lee et al., 2007). Therefore, it is important to establish the local minimum light requirement for individual species in an area of interest (Bulmer et al., 2016). The maximum depth at which seagrass grows provides an insight into the local light environment and the minimum light requirements of that seagrass. This parameter is also described as the maximum depth limit or depth of colonization. Seagrass coverage declines with water depth, and a light availability gradient exists along this natural depth distribution (Duarte, 1995). Depth has been shown to be a critical determinant of seagrass restoration success, suggesting it is imperative for identifying the most resilient areas that are most suitable for conservation and restoration (Aoki et al., 2020). Therefore, the depth of colonization of eelgrass in Ailian Bay needs to be confirmed for future restoration efforts.
To date, many studies have been performed to determine the light requirements of seagrass (Abe et al., 2003; Beck et al., 2018). Previous seagrass light requirements were estimated in field investigations (Abal et al., 1994; Abe et al., 2003; Ochieng et al., 2010; Zhang et al., 2020) and laboratory experiments (Abal et al., 1994; Moore and Wetzel, 2000; Eriander, 2017). Laboratory experimental systems remove important contextual factors of natural ecosystems (Crain et al., 2008), such as changes in water levels (due to tides and waves), episodic turbidity, and epiphyte growth which all contribute to the reduction of light availability to seagrass (Abal et al., 1994). Field investigations have been conducted in natural seagrass beds, but not necessarily in severely degraded areas. Moreover, responses of seagrass to light reduction are also dependent on site-specific conditions (Lee et al., 2007).
In the present study, an in situ suspended culture experiment was conducted in an area of interest, to directly examine long-term responses of transplanted Z. marina shoots to a depth gradient. Four suspended shoot transplantation experiments were conducted in 4 years. The aims of this study were to determine: (1) the depth limit for the survival of eelgrass (Z. marina) in Ailian Bay, and (2) how light availability affects the growth and recruitment of Z. marina as a basis for identifying a suitable depth range for successful restoration. The results from this study could provide important implications for future management and restoration of eelgrass, to avert further loss and enhance the potential for recovery. Additionally, in situ suspended seagrass cultures are described that may serve as a useful methodology for future field simulation experimentation.
Materials and Methods
Study Sites
Donor Site
The donor site was located in Swan Lake, Rongcheng City, north China (Figure 1). Swan Lake is a 4.8-km2 marine lagoon that is connected to Rongcheng Bay by a narrow mouth, 86 m in width. The lagoon, a national nature reserve for the whooper swan, Cygnus cygnus, provides food resources and the largest wintering habitat for this bird in Asia (Wang et al., 2017). Zostera marina is distributed in the intertidal to subtidal zone, forming a meadow of ∼2.3 km2 (Zhou et al., 2015; Xu et al., 2019). The environmental conditions were shown in Table 1.
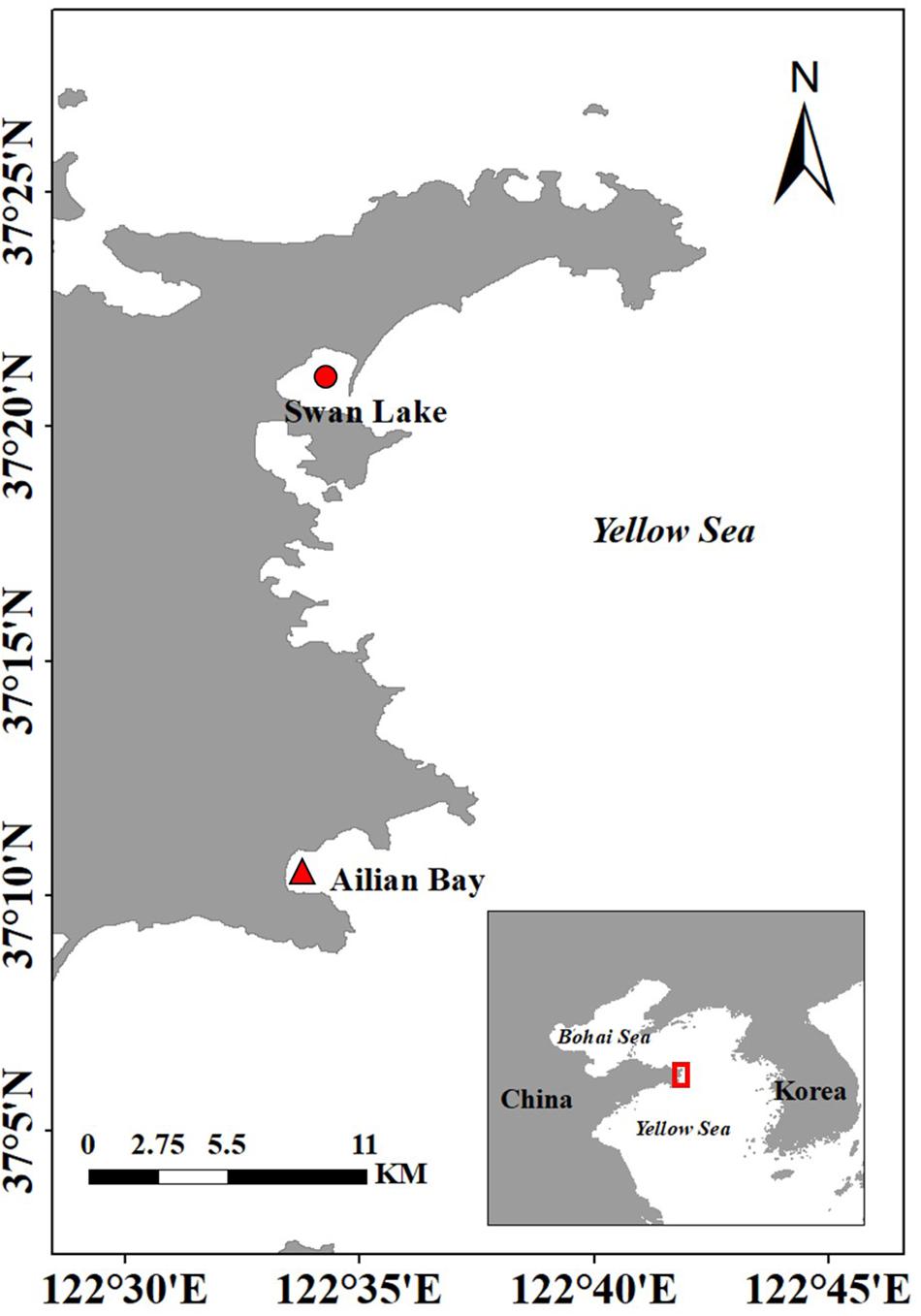
Figure 1. Location of the donor site in Swan Lake () and the experimental site in Ailian Bay (
), north China.
Experimental Site
The experimental site was located in Ailian Bay, Rongcheng City, ∼20 km from Swan Lake (Figure 1). It is a natural semi-enclosed bay. The bay is an important aquaculture area for north China (Yang et al., 2018), with more than 60% of it used for floating raft cultures of kelp or shellfish (scallops, oysters, and abalone). Z. marina is mainly distributed in sea cucumber ponds of this bay. The environmental conditions were shown in Table 1.
Collection of Adult Plants
Adult plants were collected from the intertidal area in Swan Lake at low tide. Z. marina materials were collected carefully with shovels, and adult shoots that had at least 1–2 cm of rhizome with roots were selected. Twenty centimeters of leaf blade and leaf sheath were retained, and the extra part was removed by scissors (Zhou et al., 2014; Liu et al., 2019). The transplantation period ran from April to September (Table 2).
Transplantations
Four suspended shoot transplantation experiments were performed in 4 years (2010, 2011, 2012, and 2014; see Table 2). Shoots were transplanted using a stone anchoring method (Zhou et al., 2014). This method involves anchoring a transplanting unit (PU) consisting of three to four shoots with rhizomes and roots to a small elongate stone 50–150 g in weight using biodegradable thread or thin rope (e.g., cotton thread or hemp string). The small stones were collected from the seashore in Swan Lake. PUs were buried in an experimental device (PE box or PVC pot; Table 2 and Figures 2B,C), which contained a bottom layer of sediment taken from the bank of Swan Lake. The rhizomes were placed at a depth of 2–4 cm in sediments and on the side of the stone. The initial shoot number per device for the four experiments ranged from 10 to 20 shoots (Table 2). The experimental devices planted with Z. marina materials were then transported to Ailian Bay and tied to the rafts with polyethylene ropes (Figure 2A). Four transplantation experiments were conducted along a depth gradient (Table 2). To balance the experimental devices in the water, a one 1-kg plumb ball was tied to the bottom of each device.
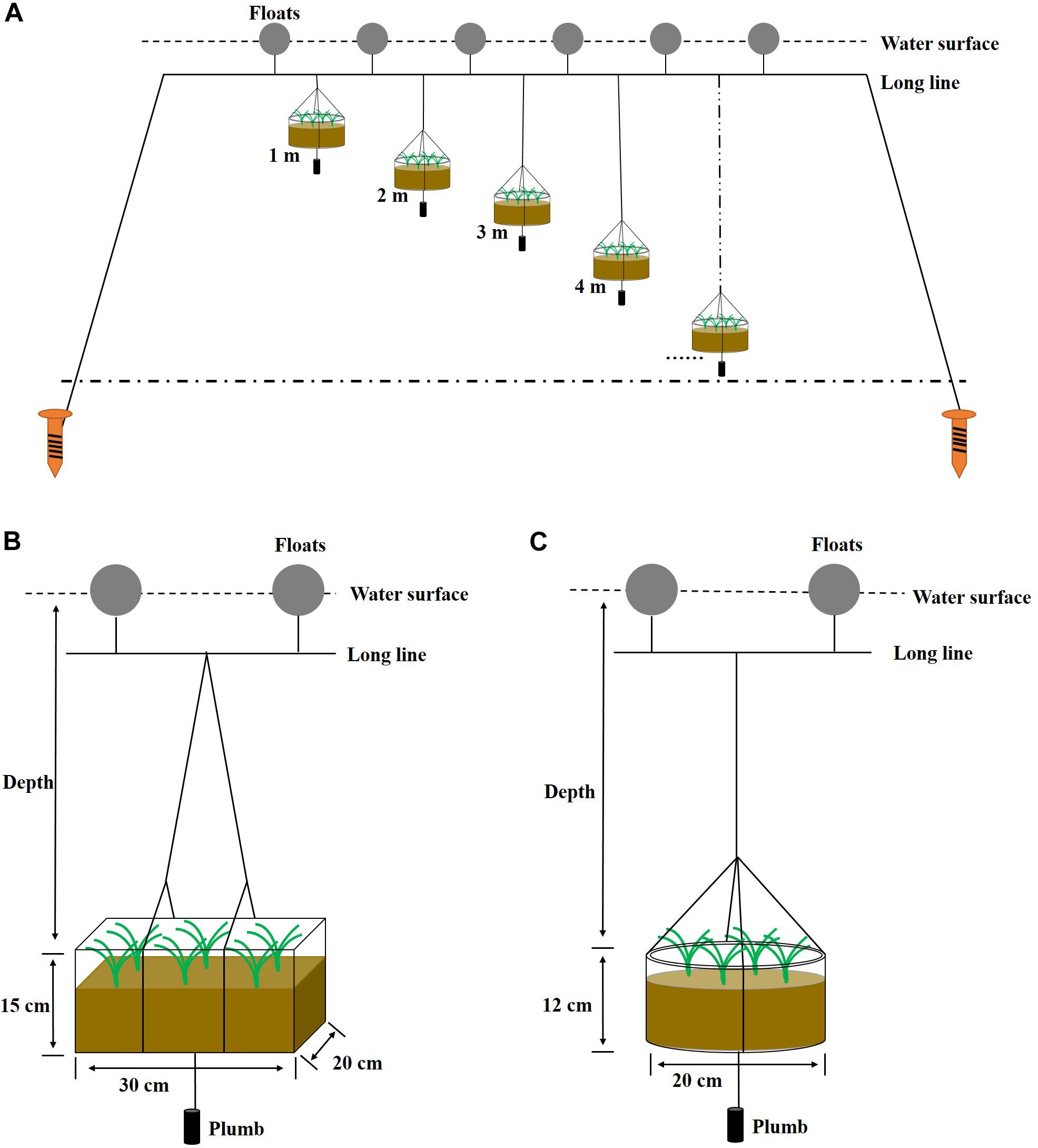
Figure 2. Floating raft (A), PE box (B), and PVC pot (C) used for suspended Z. marina transplantation experiments. PE boxes were used in 2010–2012, and PVC pots in 2014.
Monitoring Method
The main monitored parameters included shoot number and height in all four experiments, and flowering shoot number and shoot height were also monitored in 2014. Monitoring time and frequency were shown in Table 2.
Environmental Parameters
Water temperature (°C), salinity (ppt), dissolved oxygen content (DO, mg L–1), pH, chlorophyll content (μg L–1), and depth (m) were measured using a multi-parameter water quality sonde (YSI 6600, United States). The parameters were measured over a 2–15 min period at each depth. The measuring sonde was moved up and down the water column while several measurements were recorded at various depths (1, 2, 3, 4, and 6 m), all within an hour. Variations in water parameters with depth were measured five times in total during April, May, June, August, and November 2014.
Light availability (μmol photons m–2 s–1) was measured using an ECO-PARSB sensor (Sea-Bird Scientific, United States). The light sensor was moved up and down the water column with light availability measurements recorded at various depths (0, 1, 2, 3, 4, and 6 m), all within an hour. Light intensity was obtained at four different days/times in total, in May, August, and October 2014, and the light intensity was measured every minute.
Data Analyses
For the shoot transplantation experiment beginning in April 2014, differences among seagrass variables at the various depths were tested using a one-way ANOVA, and the specific treatment differences were identified using independent t-test. Simple linear regression was used to test the significance of the relationships between water depth and temperature. Before the analyses, the homogeneity of variance was tested using Levene’s test. Differences were considered significant at a probability level of p < 0.05. SPSS 20.0 was used for all data analyses. Simple linear regression analyses were considered significant at a probability level of p < 0.05.
Seagrass Responses to Transplantation Depth
To quantitatively analyze the responses of viable transplanted shoots to water depth and time from deployment in the 2014 experiment, the following linear model (see Zhang et al., 2020) was applied:
Where S(z) is shoot density, z is depth at each time point of each year, and a and b represent equation coefficients. Here, S(z) is the vegetative shoot density, not the total shoot density, because vegetative shoot density is more suitable for the evaluation of lateral shoot branching, and reproductive shoots disappear in August every year. Any depths at which the measured shoot density overlapped with zero were excluded from linear model fitting. All linear regressions were performed using R version 3.6.3.
Such analyses were not performed for reproductive density or shoot height because quantitative relationships, such as those expressed in equation (1), could not be identified from a visual inspection of the data.
Light Variation With Depth
To explore whether water clarity varied spatially, and thus determine if the depth limits obtained from the analysis were affected by any spatial variations in water clarity, the spatial correlation of water turbidity with depth was assessed (see Zhang et al., 2020). This was achieved using non-linear regression to fit each of the four distributions for the dependence of light intensity on depth to Beer’s Law (Kirk, 1985):
where, I represents the light availability at water depth z (m), I0 represents the surface light, and Kd∗ represents the light attenuation coefficient (m–1), which was not corrected for the sun’s location in the sky.
Improvement of the Experimental Device
Some PE boxes were overturned by marine currents during 2010–2011; therefore the design parameters of the experimental device were altered. In order to reduce the impact of currents on the experimental device, improvements to the stability of the device in seawater were attempted by reducing the drag force. The drag force is related to fluid density, frontal area of object, drag coefficient, and the velocity of fluid, and the shape of an object has a large effect on the amount of drag (Batchelor, 1967). This can be expressed as follows:
where, Fd is the drag force, ρ is the mass density of the fluid, μ is the flow speed of the object relative to the fluid, Cd is the drag coefficient, and A is the frontal area.
In order to change the shape (reducing drag coefficient) and reduce the frontal area of the experimental device, PVC pots were alternatively used in 2014. The drag coefficient ranges of the PE box (cuboid) and PVC pot (short cylinder) were 1.05–2.05 (Aaronaes et al., 2015) and <0.64 (Mohammed et al., 2016), respectively.
Results
Suspended Shoot Transplantation Experiment
In the shoot transplantation experiment beginning in June 2010, shoot densities at 2 m and 4 m increased by more than 100%, while there was no Z. marina remaining at 6 m and 8 m after approximately half a year of experimentation (Figure 3). After approximately a year of experimentation, the transplanted Z. marina at 2 m and 4 m successfully completed a 1-year life cycle, and reproductive shoots, with a maximum length of 102 cm, were found at 2 m. The shoot density at 2 m was four-fold greater than that at 4 m. There was also no Z. marina remaining at 6 m and 8 m after approximately a year of experimentation.
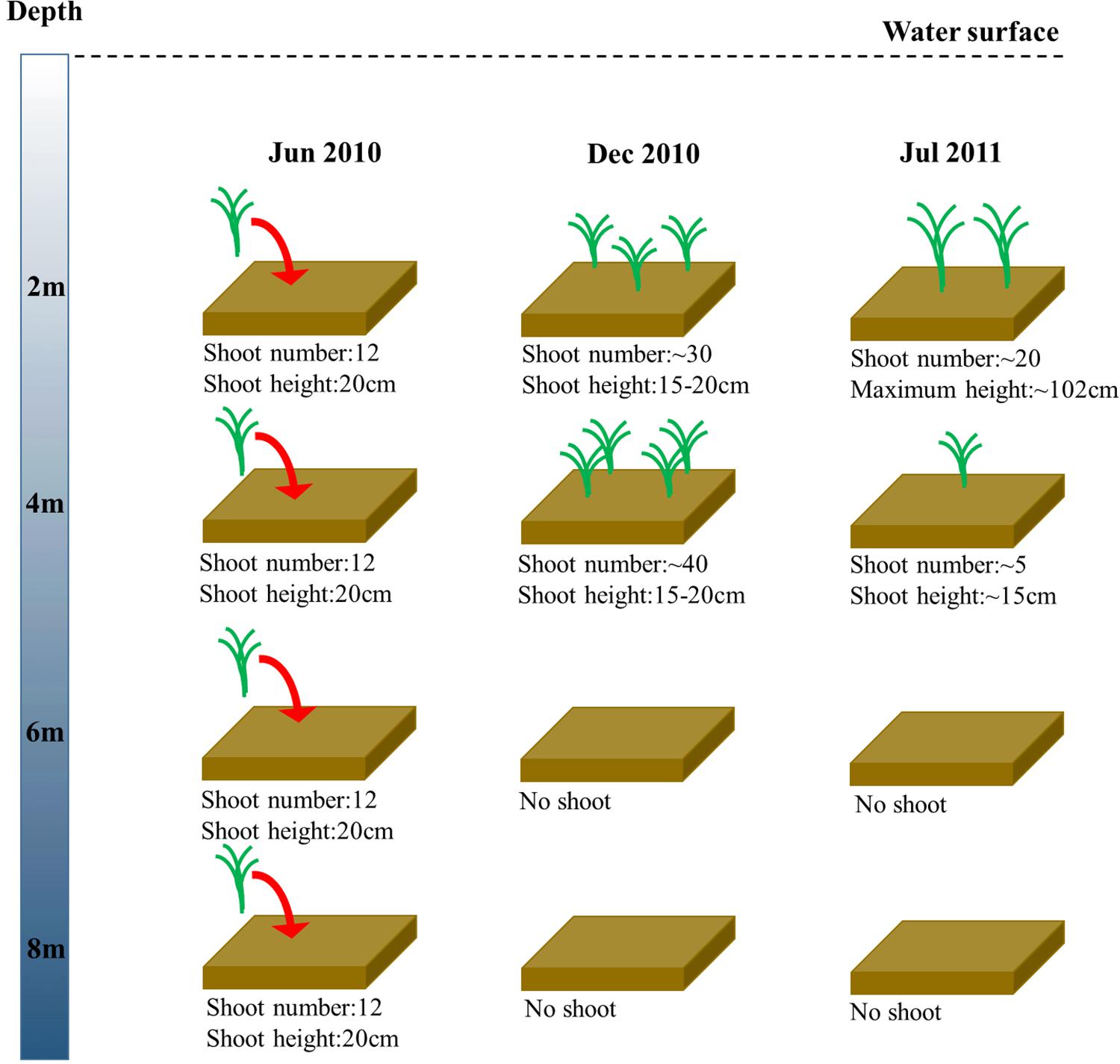
Figure 3. Changes in Z. marina shoot number and shoot height along a depth gradient in Ailian Bay in a shoot transplantation experiment beginning in June 2010.
In the shoot transplantation experiment beginning in April 2011, in order to test whether the depth between 4 and 6 m was suitable for eelgrass survival, Z. marina was transplanted at 5 m. After 3 months of experimentation, Z. marina survived only at 2 m and 4 m, and there was no Z. marina found at 5 m, 6 m, or 7 m (Figure 4).
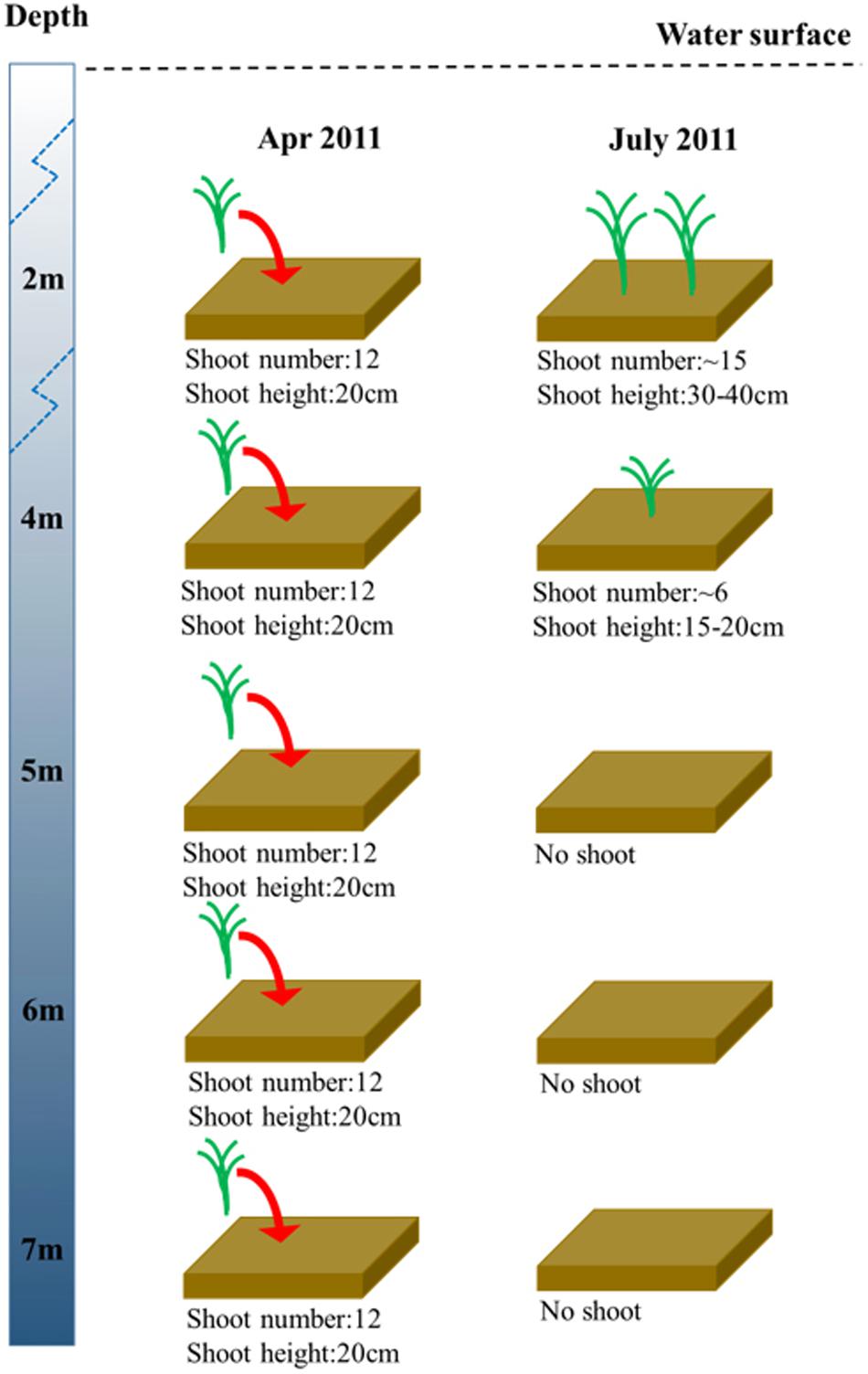
Figure 4. Changes in Z. marina shoot density and shoot height along a depth gradient in Ailian Bay in a shoot transplantation experiment beginning in April 2011.
In the shoot transplantation experiment beginning in September 2011, shoot densities decreased gradually in autumn and winter, increased slightly in March 2012, and then maintained at a low level (Figure 5A). From September to November 2012, shoot densities at 2 m and 3 m increased rapidly, and shoot densities at 2 m and 3 m increased approximately three- to four-fold, indicating that lateral shoots were germinating and growing rapidly during this period. In November, there was ∼90 shoots per box at 2 m and ∼30 shoots per box at 3 m. Shoot density at 4 m maintained at a low level, with approximately two shoots per box in November, which was significantly lower than that at 2 m and 3 m. In December 2011, there was no Z. marina remaining at 5 m and 6 m; therefore, 20 shoots per box were re-transplanted at 5 m and 6 m; however, Z. marina had completely disappeared again in August.
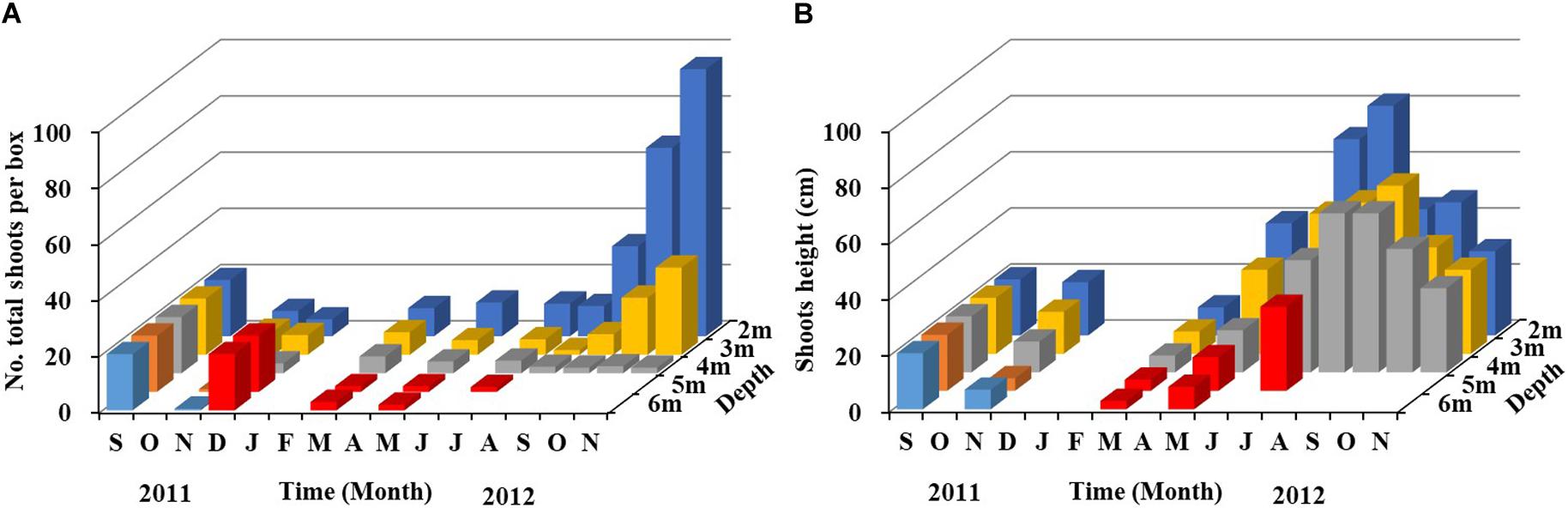
Figure 5. Temporal changes in Z. marina shoot density (A) and shoot height (B) along a depth gradient in Ailian Bay in a shoot transplantation experiment beginning in September 2011. Since Z. marina was not found at 5 m and 6 m in December 2011, 20 shoots per box were re-transplanted at 5 m and 6 m (red column). The values in the figure represent the means.
Shoot height at 2 m, 3 m, and 4 m decreased in autumn and winter, and increased significantly from spring to summer (Figure 5B). Shoot height decreased with increasing water depth. The maximum shoot height at 2 m was ∼80 cm in August 2012, and ∼60 cm at 3 m and 4 m in September. Plant height began to decrease after summer, and it was ∼30 cm at the above mentioned depths in November.
In the shoot transplantation experiment beginning in April 2014, total shoot densities at different depths were relatively stable in the first 3 months (Figure 6A). After the initial stable period, total shoot densities in shallow water (depths of 1 m and 2 m) increased rapidly, those at 3 m increased gradually, while those in deeper waters (depth >3 m) declined. The maximum total shoot densities at 1 m and 2 m were significantly higher than those at 3 m (p < 0.05), and approximately three-fold greater than those at 3 m. Very few shoots at 4 m depth survived until the end of the experiment, and shoots at 6 m depth had completely disappeared within 4 months. The temporal and spatial trends of vegetative shoots densities were consistent with those of the total shoots (Figure 6B). Flowering shoots were observed from May to August at 1 m, 2 m, 3 m, and 4 m, and were also sporadically observed at 6 m (Figures 6C,E).
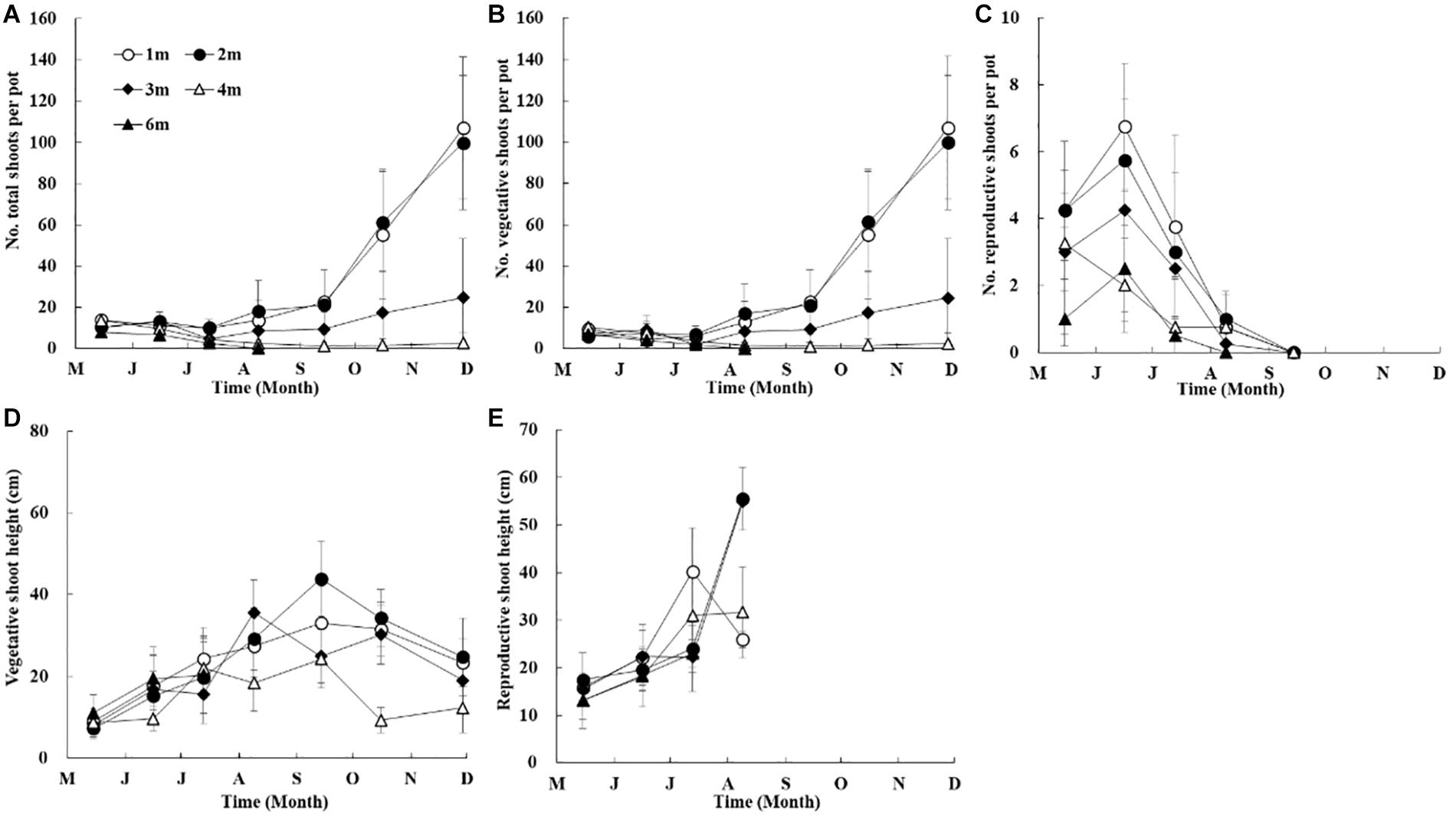
Figure 6. Temporal changes in Z. marina total shoot density (A), vegetative shoot density (B), reproductive shoot density (C), vegetative shoot height (D), and reproductive shoot height (E) along a depth gradient in Ailian Bay in a shoot transplantation experiment beginning in April 2014.
Shoot height at different depths increased gradually from May to August 2014 (Figure 6D). The maximum shoot height at 1 m and 2 m was ∼30 cm and ∼40 cm in September, respectively, and that at 3 m was ∼35 cm in August. Plant height began to decrease after summer, and it was ∼20 cm at the above-mentioned depths in November. However, the maximum shoot height at 4 m was ∼20 cm in September, and ∼10 cm in November (Figure 6D).
The linear regression of shoot density vs. depth revealed significant differences between depths in September and November for the 2014 experiment (p < 0.05, Figure 7).
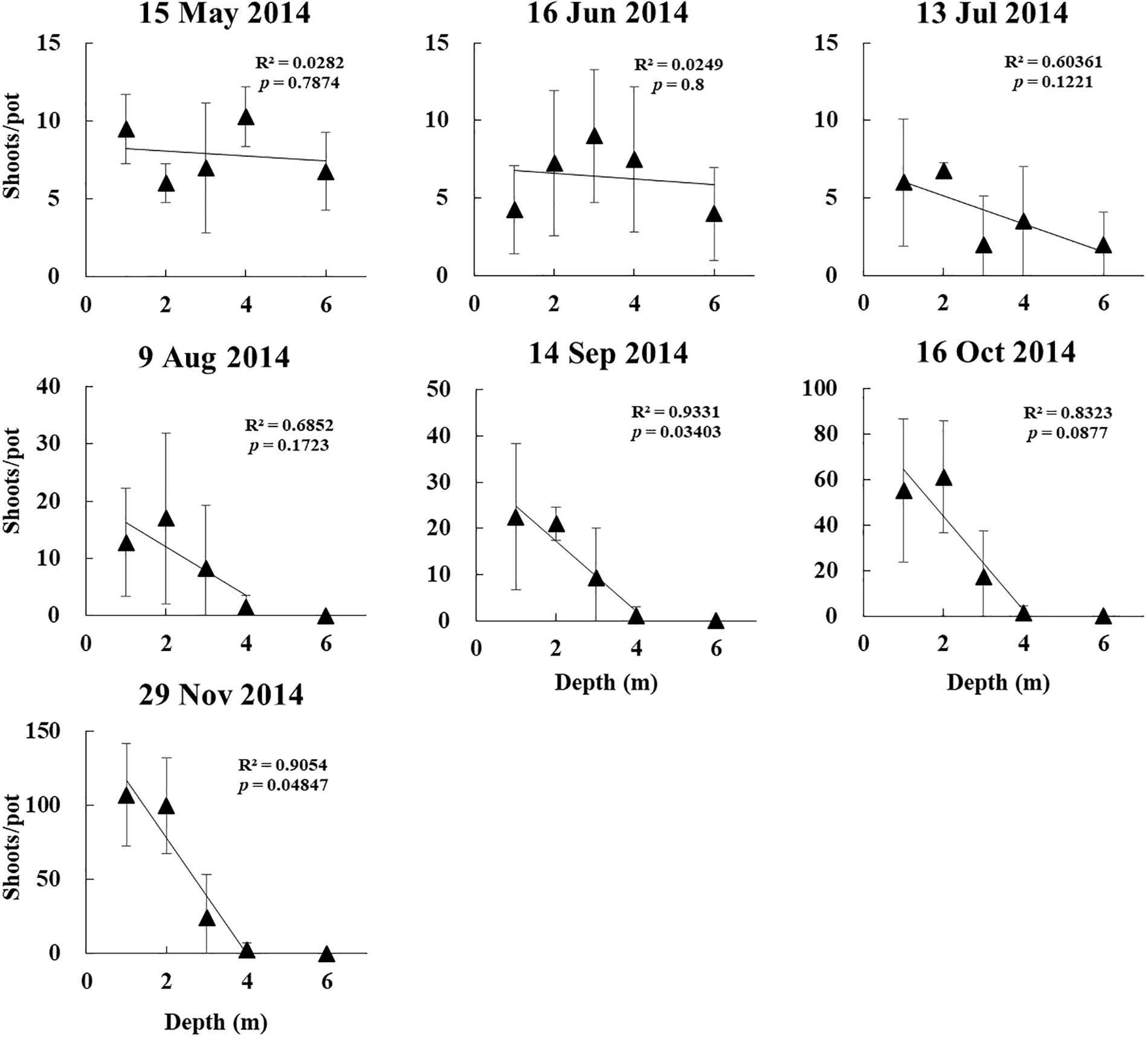
Figure 7. Linear regression of Z. marina vegetative shoot density vs. depth in a shoot transplantation experiments beginning in April 2014.
Correlations Between Water Parameters and Depth
Salinity, DO, and pH varied slightly with water depth. The chlorophyll content (Chl) changes with water depth were mostly slight. Temperature changes with water depth were relatively high in the warm season (April, May, June, and August 2014), ranging from 0.28 to 0.75°C/m (Figure 8). The regression of temperature vs. depth in May, June, August, and November were all significant (Figure 9; p < 0.05). Light intensity changed significantly as depth increased, and all four of the light vs. depth distributions adhered well to Beer’s Law (R2 ≥ 0.82; Figure 10). These results suggest that the water clarity in Ailian Bay did not substantially vary spatially with depth.
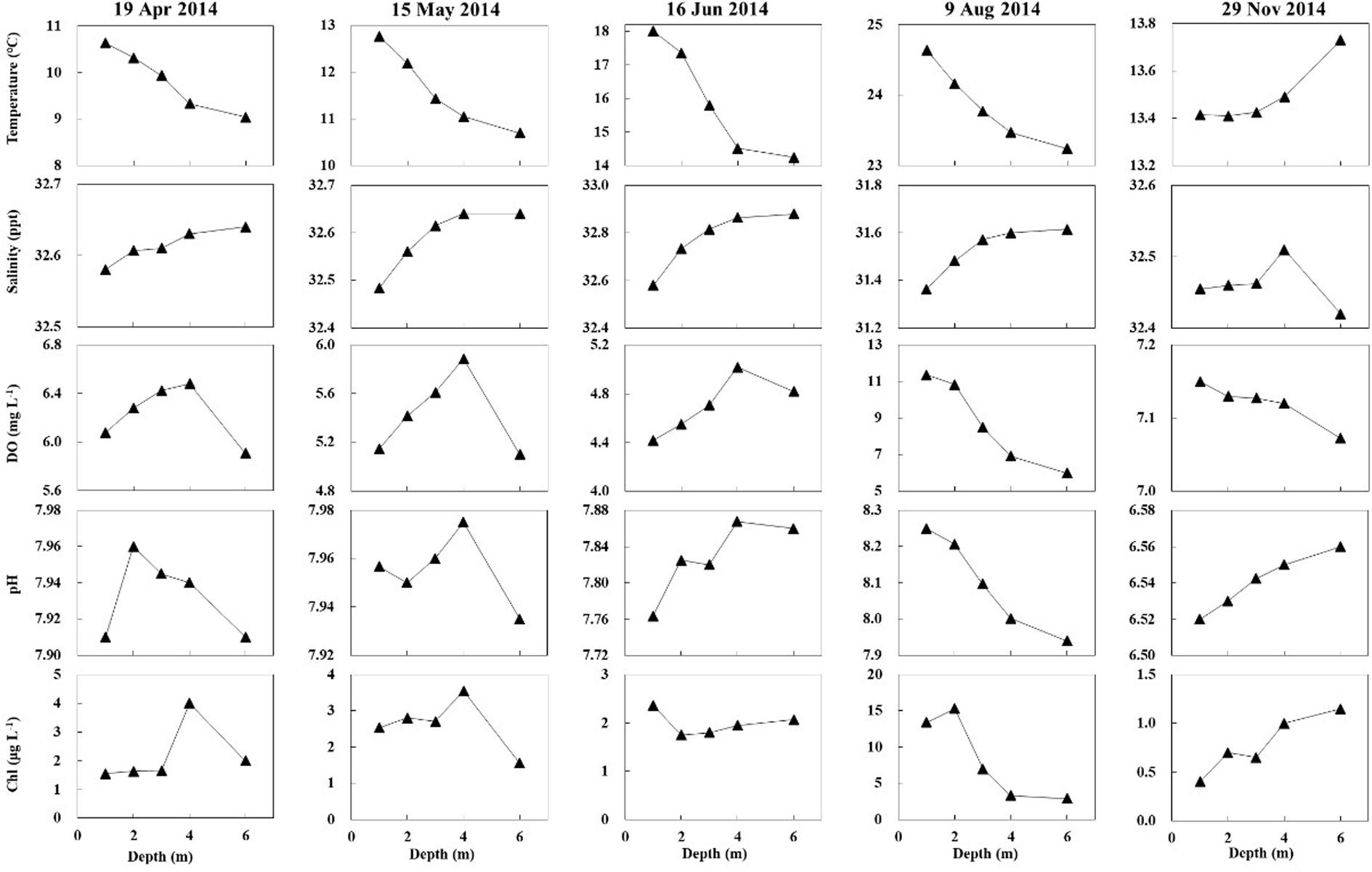
Figure 8. Changes in water temperature, salinity, dissolved oxygen (DO), pH, and chlorophyll content with depth in Ailian Bay in 2014.
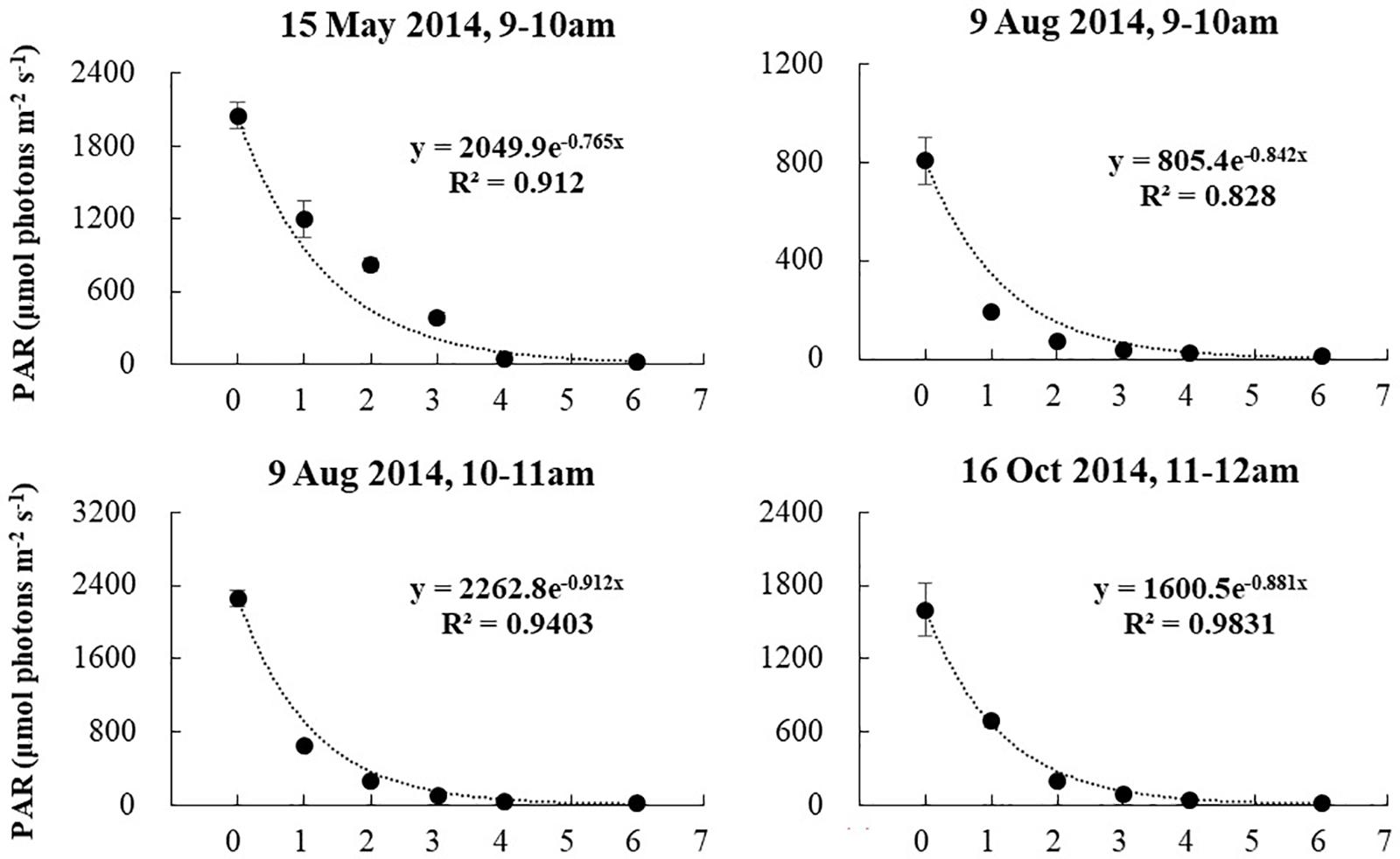
Figure 10. Variation in light availability with water depth in Ailian Bay at various dates and times during 2014. The fitted line is an exponent curve representing the fit of Beer’s law (equation 2) to the data.
Discussion
Zostera marina is a major habitat-forming species in north China. Its distribution may be threatened by changes in water clarity through increased sedimentation and nutrient input from agricultural and urban development (Thrush et al., 2004; Orth et al., 2006; Ralph et al., 2007). The present study defined the maximum depth limit for eelgrass (Z. marina) and provided a suitable depth range for successful restoration in local coastal waters, north China. More broadly, this study assessed the effect of light availability on the growth and recruitment of Z. marina conducted by in situ transplanted suspended cultures.
In situ Suspended Cultures and Improvement of the Experimental Device
Site selection for potential seagrass transplantation is crucial for restoration success, and environmental parameters limit suitable habitat availability for seagrass restoration (van Katwijk et al., 2016). One essential parameter determining seagrass restoration success is depth (Aoki et al., 2020). However, suggestions for site selection for seagrass restoration are mainly based on either in situ investigations or laboratory experiments. Laboratory experimental systems remove important contextual factors under natural conditions (e.g., waves, episodic turbidity, and epiphyte growth) (Abal et al., 1994; Crain et al., 2008), and the minimum light requirement for eelgrass in laboratory experiments has been shown to be lower than that in the field (Short, 1991). For field investigations, results might be difficult to generalize for other locations because seagrass responses to light reduction are dependent on site-specific conditions (Lee et al., 2007).
To overcome inherent weaknesses of the abovementioned experiments, an in situ suspended culture experiment was developed in this study to directly examine long-term responses of transplanted Z. marina shoots to a depth gradient, in order to determine the depth limit for eelgrass restoration. Moreover, in situ suspended cultures may prove to be a useful method for seagrass research, e.g., studying the effect of habitat type on eelgrass seed germination (unpublished data). Over the 4 years of in situ suspended experiments, the stability of the experimental device was improved by switching from PE boxes to PVC pots, based on device drag force comparisons. The drag force of the PE box was approximately double to six-fold that of the PVC pot; indicating a greater stability of the PVC pot, and consequently none of these were overturned by marine current. In addition, because it is much easier for the PE box to rotate around its vertical axis under the influence of current, the PVC pot appears to be a more stable device for suspended cultures of eelgrass. For marine areas with strong currents, a heavier plumb ball can be added at the bottom of the experimental device to improve the stability of the system.
Environmental Factors Contributing to the Depth Limit of Z. marina
Eelgrass shoot transplantation experiments provide evidence that eelgrass may have the ability to recolonize at an optimal depth of ≤3 m in Ailian Bay, where eelgrass has declined dramatically. The value of the Z. marina depth limit determined for Ailian Bay in this study was higher than that of the distribution depth (1–2 m; Xu et al., 2018, 2019) of natural populations at the donor site (Swan Lake). The depth limit in Ailian Bay is within the range reported previously, and Z. marina grows from the intertidal zone to depths of ∼10 m depending on water clarity (Jackson et al., 2013). However, even with sufficient light availability, seagrass can still decline if impacted by other environmental factors, such as increasing temperature (Marbà and Duarte, 2010) and high hydrogen sulfide content in sediment (Fraser and Kendrick, 2017). In China, land reclamation, clam harvesting, and mariculture have been suggested to be the main factors causing eelgrass habitat loss (Huang et al., 2006; Zheng et al., 2013).
Light availability, temperature, and inorganic nutrients are considered as major factors controlling seagrass growth (Lee et al., 2007). In the present study, light availability was identified as the most important factor determining the depth limit and growth of Z. marina, since there was little spatial variation vertically in other measured water parameters. In the present study, although Z. marina at 4 m depth persisted during the experimentation period, the shoot number, which was significantly lower than at shallow depths (≤3 m), appeared to show a downtrend. Z. marina at 4 m depth might disappear over a longer time scale with 1.75–2.88% of surface irradiance, since these values are lower than the minimum light requirement of 4–36% of surface irradiance for seagrass reported previously (Ralph et al., 2007). With 4.09–18.75% of surface irradiance, within the minimum light requirement of 4–36% (Ralph et al., 2007), the shoot number at 3 m depth presents seasonal variation with asexual reproduction in autumn. Therefore, Z. marina may be able to survival and recolonize at an optimal depth of ≤3 m in Ailian Bay over a longer time scale.
Sustained elevated temperatures can cause plant mortality (Hammer et al., 2018). Several studies have reported negative temperature effects on eelgrass morphology and survival over a range of 25–30°C (Orth and Moore, 1986; Touchette et al., 2003; Moore and Jarvis, 2008; Moore et al., 2014). The highest surface water temperature in Ailian Bay from August 2010 to September 2011 was 22.4°C, recorded by Liu (2012), indicating that eelgrass are not generally exposed to stressful temperatures in this area. Moreover, temperature has a small effect on eelgrass lateral branching (Eriander, 2017). For salinity, pH, and chlorophyll, the vertical variations in Ailian Bay were minimal, and thus likely have limited effects on seagrass productivity variation with depth (Lirman and Cropper, 2003). The DO concentrations at all depths were relatively high, except in June 2015 when phytoplankton abundance in the water column was high, leading to deeper areas experiencing anoxia (1–3 mg L–1) (Moore and Jarvis, 2008). Regarding nutrient concentrations, there are no significant differences between the surface water and bottom water (Li et al., 2018).
Correlating Z. marina Depth Limit to Minimum Light Requirements
As shown in Figure 9, all four of the light vs. depth distributions adhered well to Beer’s Law (R2 ≥ 0.82), indicating that the variation in turbidity with water depth was not substantial. Since long-term measurements of subsurface photosynthetically active radiation were not recorded for the duration of the experiment, it is difficult to convert the estimated depth threshold of 3 m to a similarly precise light threshold for Z. marina. However, combining all estimated values of Kd∗ shown in Figure 9, the light experienced at 3 m depth was between 6.48 and 10.08% of the surface irradiance. These values agree well with the minimum light requirement of 4–36% of surface irradiance for seagrass reported previously (Ralph et al., 2007).
Shoot Density as an Indicator of Seagrass Response to Light Reduction
In situ reductions of light are known to affect seagrasses in a variety of ways. Several studies have shown stunted growth, reduced biomass, and lower densities in response to reduced light (Abal et al., 1994; Collier et al., 2009, 2016). Shoot density is determined by lateral shoot production (Olesen and Sand-Jensen, 1994a), thus branching frequency is also reduced at low light availability. The production of new lateral branching shoots is essential for restoration success, and a high lateral branching frequency would aid in the establishment and expansion of transplants, thereby reducing the risk of failure due to stochastic events (Olesen and Sand-Jensen, 1994b). Furthermore, the present experiment conducted in autumn 2011 demonstrated shoot loss of 60–82.5% at ≤4 m depth over the winter of 2011, increasing the risk of complete transplant mortality over winter, and indicating that high lateral shoot production in autumn of 2012 may be essential for reducing the risk. Therefore, it would be more useful, for restoration purposes, to determine the minimum light requirement for high lateral branching rather than for general growth and maintenance (Eriander, 2017).
Implications for Restoration
Depth is a critical determinant of seagrass restoration success (Aoki et al., 2020). These results suggest that the greatest eelgrass survival may occur in areas ≤3 m depth, which are therefore the most suitable sites for restoration in Ailian Bay. Moreover, the current transplantation experiments have demonstrated greater survival and lateral branching frequency of shoots when restoration occurs in the spring (2014) than in the autumn (2011). Considering the similar conclusions reported by previous researchers, this indicates that restoration trials occurring in the spring and early summer will result in the greatest survival of shoots (Vichkovitten et al., 2007). This is because transplants have sufficient time to acclimatize, store carbohydrates, grow, and undergo lateral shoot branching during the first season (Eriander, 2017). In addition, the present findings suggest that the technique of suspending cultures of eelgrass, can be used to improve water quality, through regulating nutrient cycles, and attracting fish through the provision of food (e.g., zooplankton) and habitats.
Conclusion
This study presented a novel method (in situ suspended cultures) to directly examine long-term responses of transplanted Z. marina shoots to a depth gradient, in order to determine the depth limit (light requirement) for eelgrass restoration. The findings indicate that adult Z. marina transplants originating from a nearby matching donor site (Swan Lake) can successfully acclimatize and be used for restoration within degraded areas (Ailian Bay) requiring eelgrass restoration. These results provide a suitable depth range for successful restoration of eelgrass in Ailian Bay, north China. More broadly, this work may provide useful knowledge for the global management and restoration of seagrass.
Data Availability Statement
The raw data supporting the conclusions of this article will be made available by the authors, without undue reservation.
Author Contributions
SX: writing – original draft, conceptualization, methodology, investigation, and validation. PW, FW, PL, and BL: investigation, formal analysis, and writing – review and editing. XZ: data curation, formal analysis, investigation, and writing – review and editing. SY and YuZ: investigation and data curation. YiZ: funding acquisition, supervision, methodology, investigation, and writing – review and editing. All authors contributed to the article and approved the submitted version.
Funding
This research was supported by the National Key R&D Program of China (2019YFD0901300), the National Science and Technology Basic Work Program (2015FY110600), the Key Research Project of Frontier Sciences of CAS (QYZDB-SSW-DQC041-1), the International Partners Program of Chinese Academy of Sciences (133137KYSB20180069), and the Taishan Scholars Program (Distinguished Taishan Scholars).
Conflict of Interest
The authors declare that the research was conducted in the absence of any commercial or financial relationships that could be construed as a potential conflict of interest.
Acknowledgments
We would like to thank Yicheng Li, Hongrong Wang, and Jingtai Wang for their help in the field survey.
References
Aaronaes, A. S., Nilsson, H., and Neumann, N. (2015). Dynamic response of steel pipe rack structures subjected to explosion loads. Steel Constr. 8, 162–166. doi: 10.1002/stco.201510022
Abal, E., Loneragan, N. R., Bowen, P., Perry, C. J., Udy, J., and Dennison, W. C. (1994). Physiological and morphological responses of the seagrass Zostera capricorni aschers. to light intensity. J. Exp. Mar. Bio. Ecol. 178, 113–129. doi: 10.1016/0022-0981(94)90228-3
Abe, M., Hashimoto, N., Kurashima, A., and Maegawa, M. (2003). Estimation of light requirement for the growth of Zostera marina in central Japan. Fish. Sci. 69, 890–895. doi: 10.1046/j.1444-2906.2003.00704.x
Aoki, L. R., Mcglathery, K. J., Wiberg, P. L., and Al-Haj, A. (2020). Depth affects seagrass restoration success and resilience to marine heat wave disturbance. Estuar. Coast. 43, 316–328. doi: 10.1007/s12237-019-00685-0
Barbier, E. B., Hacker, S. D., Kennedy, C., Koch, E. W., Stier, A. C., and Silliman, B. R. (2011). The value of estuarine and coastal ecosystem services. Ecol. Monogr. 81, 169–193. doi: 10.1890/10-1510.1
Beck, M. W., Hagy, J. D., and Le, C. (2018). Quantifying seagrass light requirements using an algorithm to spatially resolve depth of colonization. Estuar. Coast. 41, 592–610. doi: 10.1007/s12237-017-0287-1
Bulmer, R., Kelly, S., and Jeffs, A. (2016). Light requirements of the seagrass, Zostera muelleri, determined by observations at the maximum depth limit in a temperate estuary, New Zealand. N. Zeal. J. Mar. Fresh. Res. 50, 183–194. doi: 10.1080/00288330.2015.1120759
Collier, C., and Waycott, M. (2009). Drivers of Change to Seagrass Distributions and Communities on the Great Barrier Reef: Literature Review of Gaps Analysis. Report to the Marine and Tropical Sciences Research Facility. Cairns: Cairns, Reef and Rainforest Research Centre Limited.
Collier, C. J., Adams, M. P., Langlois, L., Waycott, M., O’Brien, K. R., Maxwell, P. S., et al. (2016). Thresholds for morphological response to light reduction for four tropical seagrass species. Ecol. Indic. 67, 358–366. doi: 10.1016/j.ecolind.2016.02.050
Collier, C. J., Lavery, P. S., Ralph, P. J., and Masini, R. J. (2009). Shade-induced response and recovery of the seagrass Posidonia sinuosa. J. Exp. Mar. Biol. Ecol. 370, 89–103. doi: 10.1016/j.jembe.2008.12.003
Costanza, R., D’Arge, R., Groot, R. D., Farber, S., Grasso, M., Hannon, B., et al. (1997). The value of the world’s ecosystem services and natural capital. Nature 387, 253–260. doi: 10.1016/S0921-8009(98)00020-2
Crain, C. M., Kroeker, K., and Halpern, B. S. (2008). Interactive and cumulative effects of multiple human stressors in marine systems. Ecol. Lett. 11, 1304–1315. doi: 10.1111/j.1461-0248.2008.01253.x
Duarte, C. M. (1995). Submerged aquatic vegetation in relation to different nutrient regimes. Ophelia 41, 87–112. doi: 10.1080/00785236.1995.10422039
Editorial Board of China Bay Survey (1991). Survey of China Bays, Vol. 3. Beijing: China Ocean Press.
Elsahragty, M., and Kim, J. L. (2015). Assessment and strategies to reduce light pollution using geographic information systems. Proc. Eng. 118, 479–488. doi: 10.1016/j.proeng.2015.08.458
Eriander, L. (2017). Light requirements for successful restoration of eelgrass (Zostera marina L.) in a high latitude environment-acclimatization, growth and carbohydrate storage. J. Exp. Mar. Bio. Ecol. 496, 37–48. doi: 10.1016/j.jembe.2017.07.010
Fourqurean, J. W., Duarte, C. M., Kennedy, H., Marbà, N., Holmer, M., Mateo, M. A., et al. (2012). Global carbon stocks in seagrass ecosystems. Nat. Geosci. 5, 505–509. doi: 10.1038/ngeo1477
Fraser, M. W., and Kendrick, G. A. (2017). Belowground stressors and long-term seagrass declines in a historically degraded seagrass ecosystem after improved water quality. Sci. Rep. 7:14469. doi: 10.1038/s41598-017-14044-1
Green, E. P., and Short, F. T. (2003). World Atlas of Seagrasses. Berkeley, CA: University of California Press.
Guo, D., Zhang, P. D., Zhang, X. M., Li, W. T., Zhang, X. J., and Yuan, Y. D. (2010). Preliminary investigation and study on seagrass species of inshore areas in Shandong province. Trans. Oceanol. Limnol. 2, 17–21.
Hammer, K. J., Borum, J., Hasler-Sheetal, H., Shields, E. C., Sand-Jensen, K., and Moore, K. A. (2018). High temperatures cause reduced growth, plant death and metabolic changes in eelgrass Zostera marina. Mar. Ecol. Prog. Ser. 604, 121–132. doi: 10.3354/meps12740
Huang, X. P., Huang, L. M., Li, Y. H., Xu, Z. Z., Fong, J., Huang, D. J., et al. (2006). Main seagrass beds and threats to their habitats in the coastal sea of South China. Chin. Sci. Bull. 51, 136–142. doi: 10.1007/s11434-006-9136-5
Jackson, E. L., Griffiths, C. A., and Durkin, O. (2013). A Guide to Assessing and Managing Anthropogenic Impact on Marine Angiosperm Habitat - Part 1: Literature Review, Natural England Commissioned Report Natural England, UK, NECR111p. 1-128. Exeter: Natural England.
Jennifer, H., Just, C. N., and Ivan, V. (2003). Eelgrass Zostera marina loss in temperate estuaries: relationship to land-derived nitrogen loads and effect of light limitation imposed by algae. Mar. Ecol. Prog. Ser. 247, 59–73. doi: 10.3354/meps247059
Kirk, J. T. O. (1985). Effects of suspensoids (turbidity) on penetration of solar radiation in aquatic ecosystems. Hydrobiologia 125, 195–208. doi: 10.1007/BF00045935
Lee, K., Park, S. R., and Kim, Y. K. (2007). Effects of irradiance, temperature, and nutrients on growth dynamics of seagrasses: a review. J. Exp. Mar. Bio. Ecol. 350, 144–175. doi: 10.1016/j.jembe.2007.06.016
Li, H. M., Zhang, Y. Y., Liang, Y. T., Chen, J., Zhu, Y. C., Zhao, Y. T., et al. (2018). Impacts of maricultural activities on characteristics of dissolved organic carbon and nutrients in a typical raft-culture area of the Yellow Sea, North China. Mar. Pollut. Bull. 137, 456–464. doi: 10.1016/j.marpolbul.2018.10.048
Lirman, D., and Cropper, W. P. (2003). The influence of salinity on seagrass growth, survivorship, and distribution within Biscayne Bay, Florida: field, experimental, and modeling studies. Estuaries 26, 131–141. doi: 10.1007/BF02691700
Liu, B. J. (2012). Foundational Studies on Resource Investigation and Ecological Restoration of Eelgrass in Typical Bays in Shandong. Master’s thesis, The University of Chinese Academy of Sciences, Beijing.
Liu, X. J., Zhou, Y., Liu, B. J., Zhang, X. M., and Yang, H. S. (2019). Temporal dynamics of the natural and trimmed angiosperm Zostera marina L. (Potamogetonales: Zosteraceae), and an effective technique for transplantation of long shoots in a temperate tidal zone (northern China). Wetlands 39, 1043–1056. doi: 10.1007/s13157-019-01157-8
Liu, X. J., Zhou, Y., Yang, H. S., and Ru, S. G. (2013). Eelgrass detritus as a food source for the sea cucumber Apostichopus japonicus Selenka (Echinidermata: Holothuroidea) in coastal waters of North China: an experimental study in flow-through systems. PLoS One 8:e58293. doi: 10.1371/journal.pone.0058293
Marbà, N., and Duarte, C. M. (2010). Mediterranean warming triggers seagrass (Posidonia oceanica) shoot mortality. Glob. Chang. Biol. 16, 2366–2375. doi: 10.1111/j.1365-2486.2009.02130.x
Marion, S. R., and Orth, R. J. (2010). Innovative techniques for large-scale seagrass restoration using Zostera marina (eelgrass) seeds. Restor. Ecol. 18, 514–526. doi: 10.1111/j.1526-100X.2010.00692.x
Mohammed, M. G., Messerman, A., Mayhan, B. D., and Trauth, K. M. (2016). Theory and practice of the hydrodynamic redesign of artificial hellbender habitat. Herpetol. Rev. 47, 586–591.
Moore, K. A., and Jarvis, J. C. (2008). Environmental factors affecting recent summertime eelgrass diebacks in the lower Chesapeake Bay: implications for long-term persistence. J. Coast. Res. Spec. 55, 135–147. doi: 10.2112/SI55-014
Moore, K. A., Shields, E. C., and Parrish, D. B. (2014). Impacts of varying estuarine temperature and light conditions on Zostera marina (eelgrass) and its interactions with Ruppia maritima (widgeongrass). Estuar. Coast 37, 20–30. doi: 10.1007/s12237-013-9667-3
Moore, K. A., and Wetzel, R. L. (2000). Seasonal variations in eelgrass (Zostera marina L.) responses to nutrient enrichment and reduced light availability in experimental ecosystems. J. Exp. Mar. Biol. Ecol. 244, 1–28. doi: 10.1016/s0022-0981(99)00135-5
Moore, K. A., Wetzel, R. L., and Orth, R. J. (1997). Seasonal pulses of turbidity and their relations to eelgrass (Zostera marina L.) survival in an estuary. J. Exp. Mar. Biol. Ecol. 215, 115–134. doi: 10.1016/S0022-0981(96)02774-8
Ochieng, C. A., Short, F. T., and Walker, D. I. (2010). Photosynthetic and morphological responses of eelgrass (Zostera marina L.) to a gradient of light conditions. J. Exp. Mar. Biol. Ecol. 382, 117–124. doi: 10.1016/j.jembe.2009.11.007
Olesen, B., and Sand-Jensen, K. (1994a). Demography of shallow eelgrass (Zostera marina) populations-shoot dynamics and biomass development. J. Ecol. 82, 379–390. doi: 10.2307/2261305
Olesen, B., and Sand-Jensen, K. (1994b). Patch dynamics of eelgrass Zostera marina. Mar. Ecol. Prog. Ser. 106, 147–156. doi: 10.3354/meps106147
Orth, R. J., Carruthers, T. J. B., Dennison, W. C., Duarte, C. M., Fourqurean, J. W., Heck, K. L., et al. (2006). A global crisis for seagrass ecosystems. Bioscience 56, 987–996.
Orth, R. J., and Moore, K. A. (1986). Seasonal and year-to-year variations in the growth of Z. marina L. (eelgrass) in the Chesapeake Bay. Aquat. Bot. 24, 335–341. doi: 10.1016/0304-3770(86)90100-2
Ralph, P. J., Durako, M. J., Enríquez, S., Collier, C. J., and Doblin, M. A. (2007). Impact of light limitation on seagrasses. J. Exp. Mar. Biol. Ecol. 350, 176–193. doi: 10.1016/j.jembe.2007.06.017
Short, F. T. (1991). “Light limitation on seagrass growth,” in The Light Requirements of Seagrasses. Results and Recommendations of a Workshop., eds W. J. Kenworthy and D. E. Haunert (NOAA Tech. Memo. NMFS-SEFC-287. US Nat. Mar. Fish. Ser.), 85–94.
Statton, J., Mcmahon, K., Lavery, P. S., and Kendrick, G. A. (2018). Determining light stress responses for a tropical multi-species seagrass assemblage. Mar. Pollut. Bull. 128, 508–518. doi: 10.1016/j.marpolbul.2018.01.060
Thrush, S. F., Hewitt, J. E., Cummings, V. J., Ellis, J. I., Hatton, C., Lohrer, A., et al. (2004). Muddy waters: elevating sediment input to coastal and estuarine habitats. Front. Ecol. Environ. 2, 299–306. doi: 10.2307/3868405
Touchette, B. W., Burkholder, J. M., and Glasgow, H. B. (2003). Variations in eelgrass (Zostera marina L.) morphology and internal nutrient composition as influenced by increased temperature and water column nitrate. Estuaries 26, 142–155. doi: 10.1007/bf02691701
Unsworth, R. K. F., Ambo-Rappe, R., Jones, B. L., La Nafie, Y. A., Irawan, A., Hernawan, U. E., et al. (2018a). Indonesia’s globally significant seagrass meadows are under widespread threat. Sci. Total Environ. 634, 279–286. doi: 10.1016/j.scitotenv.2018.03.315
Unsworth, R. K. F., Nordlund, L. M., and Cullen-Unsworth, L. C. (2018b). Seagrass meadows support global fisheries production. Conserv. Lett. 12:e12566. doi: 10.1111/conl.12566
van Katwijk, M. M., Thorhaug, A., Marba, N., Orth, R. J., Duarte, C. M., Kendrick, G. A., et al. (2016). Global analysis of seagrass restoration: the importance of large-scale planting. J. Appl. Ecol. 53, 567–578. doi: 10.1111/1365-2664.12562
Verweij, M. C., Ivan, N., Ingmar, H., Ruseler, S. M., and Mason, P. R. D. (2008). Seagrass nurseries contribute to coral reef populations. Limnol. Oceanogr. 53, 1540–1547. doi: 10.4319/lo.2008.53.4.1540
Vichkovitten, T., Holmer, M., and Frederiksen, M. S. (2007). Spatial and temporal changes in non-structural carbohydrate reserve in eelgrass (Zostera marina L.) in Danish coastal waters. Bot. Mar. 50, 75–87. doi: 10.1515/BOT.2007.009
Wang, F., Xu, S. C., Zhou, Y., Wang, P. M., and Zhang, X. M. (2017). Trace element exposure of whooper swans (Cygnus cygnus) wintering in a marine lagoon (Swan Lake), northern China. Mar. Pollut. Bull. 119, 60–67. doi: 10.1016/j.marpolbul.2017.03.063
Waycott, M., Duarte, C. M., Carruthers, T. J. B., Orth, R. J., Dennison, W. C., Olyarnik, S., et al. (2009). Accelerating loss of seagrasses across the globe threatens coastal ecosystems. Proc. Natl. Acad. Sci. U.S.A. 106, 12377–12381. doi: 10.1073/pnas.0905620106
Xu, S., Xu, S. C., Zhou, Y., Zhao, P., Yue, S. D., Song, X. Y., et al. (2019). Single beam sonar reveals the distribution of the eelgrass Zostera marina L. and threats from the green tide algae Chaetomorpha linum K. in Swan-Lake lagoon (China). Mar. Pollut. Bull. 145, 611–623. doi: 10.1016/j.marpolbul.2019.06.022
Xu, S. C., Wang, P. M., Zhou, Y., Wang, F., Zhang, X. M., Gu, R. T., et al. (2020a). Sheaths of Zostera marina L. as ecological indicators of shoot length and the elemental stoichiometry of aboveground tissues. Mar. Pollut. Bull. 160:111545. doi: 10.1016/j.marpolbul.2020.111545
Xu, S. C., Xu, S., Zhou, Y., Yue, S. D., Qiao, Y. L., Liu, M. J., et al. (2020b). Sonar and in situ surveys of eelgrass distribution, reproductive effort, and sexual recruitment contribution in a eutrophic bay with intensive human activities: Implication for seagrass conservation. Mar. Pollut. Bull. 161:111706. doi: 10.1016/j.marpolbul.2020.111706
Xu, S. C., Wang, P. M., Zhou, Y., Zhang, X. M., Gu, R. T., Liu, X. J., et al. (2018). New insights into different reproductive effort and sexual recruitment contribution between two geographic Zostera marina L. populations in temperate China. Front. Plant Sci. 9:15. doi: 10.3389/fpls.2018.00015
Yang, X., Zhang, P., Li, W. T., Hu, C. Y., Zhang, X. M., and He, P. G. (2018). Evaluation of four seagrass species as early warning indicators for nitrogen overloading: implications for eutrophic evaluation and ecosystem management. Sci. Total Environ. 635, 1132–1143. doi: 10.1016/j.scitotenv.2018.04.227
Yang, Z. D., and Wu, B. L. (1984). Seagrass meadows and their epiphytes in Qingdao coastal area. J. Oceanogr. Huanghai Bohai Seas 2, 56–67.
Ye, C. J., and Zhao, K. F. (2002). Advances in the study on the marine higher plant eelgrass (Zostera marina L.) and its adaptation to submerged life in seawater. Chin. Bull. Bot. 19, 184–193.
Zhang, X. M., Zhou, Y., Adams, M. P., Wang, F., Xu, S. C., Wang, P. M., et al. (2020). Plant morphology and seed germination responses of seagrass (Zostera japonica) to water depth and light availability in Ailian Bay, northern China. Mar. Environ. Res. 162:105082. doi: 10.1016/j.marenvres.2020.105082
Zheng, F. Y., Qiu, G. L., Fan, H. Q., and Zhang, W. (2013). Diversity, distribution and conservation of Chinese seagrass species. Biodivers. Sci. 21, 517–526. doi: 10.3724/sp.j.1003.2013.10038
Zhou, Y., Liu, P., Liu, B. J., Liu, X. J., Zhang, X. M., Wang, F., et al. (2014). Restoring eelgrass (Zostera marina L.) habitats using a simple and effective transplanting technique. PLoS One 9:e92982. doi: 10.1371/journal.pone.0092982
Zhou, Y., Liu, X. J., Liu, B. J., Liu, P., Wang, F., Zhang, X. M., et al. (2015). Unusual pattern in characteristics of the eelgrass Zostera marina L. in a shallow lagoon (Swan Lake), north China: implications on the importance of seagrass conservation. Aquat. Bot. 120, 178–184. doi: 10.1016/j.aquabot.2014.05.014
Keywords: seagrass meadow, depth limit, light requirement, response, methodology, reproduction, restoration, Zostera marina
Citation: Xu SC, Wang PM, Wang F, Liu P, Liu BJ, Zhang XM, Yue SD, Zhang Y and Zhou Y (2020) In situ Responses of the Eelgrass Zostera marina L. to Water Depth and Light Availability in the Context of Increasing Coastal Water Turbidity: Implications for Conservation and Restoration. Front. Plant Sci. 11:582557. doi: 10.3389/fpls.2020.582557
Received: 12 July 2020; Accepted: 13 November 2020;
Published: 15 December 2020.
Edited by:
Richard K. F. Unsworth, Swansea University, United KingdomReviewed by:
Kathryn Margaret McMahon, Edith Cowan University, AustraliaChiara Mary Bertelli, Swansea University, United Kingdom
Copyright © 2020 Xu, Wang, Wang, Liu, Liu, Zhang, Yue, Zhang and Zhou. This is an open-access article distributed under the terms of the Creative Commons Attribution License (CC BY). The use, distribution or reproduction in other forums is permitted, provided the original author(s) and the copyright owner(s) are credited and that the original publication in this journal is cited, in accordance with accepted academic practice. No use, distribution or reproduction is permitted which does not comply with these terms.
*Correspondence: Yi Zhou, eWl6aG91QHFkaW8uYWMuY24=