- 1Dipartimento di Biologia e Biotecnologie “Charles Darwin,” Sapienza Università di Roma, Rome, Italy
- 2Dipartimento di Medicina Clinica, Sanità Pubblica e Scienze della Vita e dell’Ambiente, Università degli Studi dell’Aquila, L’Aquila, Italy
Several oligosaccharide fragments derived from plant cell walls activate plant immunity and behave as typical damage-associated molecular patterns (DAMPs). Some of them also behave as negative regulators of growth and development, and due to their antithetic effect on immunity and growth, their concentrations, activity, time of formation, and localization is critical for the so-called “growth-defense trade-off.” Moreover, like in animals, over accumulation of DAMPs in plants provokes deleterious physiological effects and may cause hyper-immunity if the cellular mechanisms controlling their homeostasis fail. Recently, a mechanism has been discovered that controls the activity of two well-known plant DAMPs, oligogalacturonides (OGs), released upon hydrolysis of homogalacturonan (HG), and cellodextrins (CDs), products of cellulose breakdown. The potential homeostatic mechanism involves specific oxidases belonging to the family of berberine bridge enzyme-like (BBE-like) proteins. Oxidation of OGs and CDs not only inactivates their DAMP activity, but also makes them a significantly less desirable food source for microbial pathogens. The evidence that oxidation and inactivation of OGs and CDs may be a general strategy of plants for controlling the homeostasis of DAMPs is discussed. The possibility exists of discovering additional oxidative and/or inactivating enzymes targeting other DAMP molecules both in the plant and in animal kingdoms.
Introduction
The cell wall represents the interface between plants and the environment and acts as a physical barrier to protect plants against biotic and abiotic stresses. Main components of the cell wall are the polysaccharides cellulose, hemicellulose, and pectin, which interact among themselves and with lignin to form a complex structure that influences the shape, rigidity, growth, and differentiation of plant cells (Bacete and Hamann, 2020; Zhang and Zhang, 2020). All three types of polysaccharides are also a repository of extracellular damage-associated molecular patterns (DAMPs), potentially released during microbial infections or upon mechanical damage (De Lorenzo et al., 2018; Hou et al., 2019). DAMPs are likely to be released also upon cell wall remodeling that occurs during plant growth and development. Microlesions caused, for example, by cell expansion, lateral root formation, or organ abscission, may cause cell wall damage and affect the so-called cell wall integrity (CWI; Anderson and Kieber, 2020; Gigli-Bisceglia et al., 2020). In these cases, endogenous cell wall degrading enzymes (CWDEs) can release, at low levels, oligosaccharide fragments of the same nature as those accumulated in larger quantities during more destructive mechanical injuries or plant cell wall degradation caused by pathogen-encoded CWDEs (De Lorenzo et al., 2018, 2019). Some DAMPs are recognized by pattern-recognition receptors and are capable of inducing pattern-triggered immunity (PTI) even in the absence of infection (De Lorenzo et al., 2018).
Unlike mammals, plants do not have an adaptive immune system but, instead, entirely rely on the ability of individual cells to recognize pathogens and to activate defense responses (Gust et al., 2017). The plant cell must be able to discriminate between the possibilities that cell wall degradation is caused by a physiological or a pathological accumulation of DAMPs. The time of formation, the appropriate concentration, and the correct distribution of the active molecules may help distinguish a pathological from a physiological event. In the former case, plants need to respond quickly, intensively, and systemically to the danger, whereas in the latter case, they may only need to activate local immune responses, preventing a massive immune response that may hamper growth (Li et al., 2020). In general, plants cannot dissipate too much energy in defense vs. growth and need to maintain the correct growth-defense trade-off. To this purpose, they require continuous balancing of the defense pathways and signaling.
Hyper-immunity generated by DAMPs can cause deleterious effects in plants like in animals. Uncontrolled or prolonged production of DAMPs may promote hyperinflammation or chronic inflammation through the adaptive immune system in animals (Land, 2020), whereas in plants, it mainly causes hypersensitivity and death of individual cells and reduced growth of the entire organism. In this review, we discuss how several extracellular DAMPs released from the polysaccharides of the plant cell wall may be kept under homeostatic control by oxidizing/inactivating enzymes (Figure 1) and how a similar mechanism may have general relevance for other DAMPs.
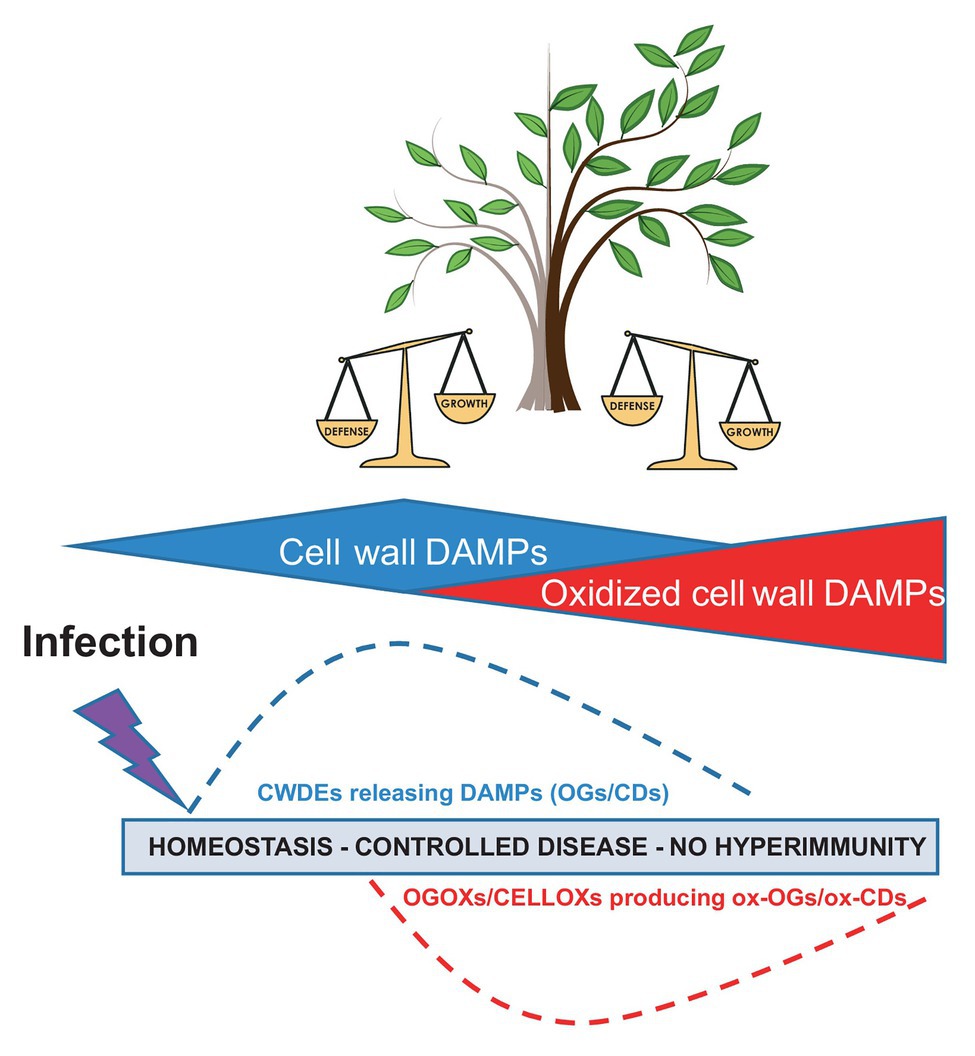
Figure 1. Damage-associated molecular patterns (DAMPs) and oxidized DAMPs in immunity, homeostasis of signals, and “growth-defense trade-off” (modified from Relja and Land, 2020). CWDEs, cell wall degrading enzymes; OGs, oligogalacturonides; CDs, cellulose fragments; ox-OGs, oxidized oligogalacturonides; and ox-CDs, oxidized cellulose fragments.
Dynamics of the Cell Wall and Release of Regulatory Fragments
In order to provide structural support during growth and protection against diseases, the composition and structure of the plant cell wall is continuously changing during development or upon biotic and abiotic stresses (Vaahtera et al., 2019). During both development and disease, CWI is often compromised and cell wall components undergo dynamic changes (Gigli-Bisceglia et al., 2020). These changes may cause a direct enzymatic release of fragments possessing growth-regulating/DAMP activity from each of the major wall components. The impairment of CWI itself could function as a mechano-sensory signal of damage that may also regulate cellular events, such as meristem patterning and the cell cycle (Bacete and Hamann, 2020). Notably, microtubule organization, orientation, and deposition of cellulose microfibrils are reorganized, and several CWDEs are activated in response to mechanical stimuli (Vaahtera et al., 2019).
Pectin, which is abundant in the middle lamella and in the primary walls, influences wall porosity and thickness and may play a role in the maintenance of CWI due to its marked sensitivity to mechanical deformation. A recent model indicates that cell shape and expansion as well as the turgor force exerted by the cell wall are driven by 15–30 nm nanofilaments of homogalacturonan (HG), the main component of pectin (Haas et al., 2020). These nanofilaments, located perpendicularly to the cotyledon surface, expand upon demethylation (Haas et al., 2020). Among the cell wall components, pectin appears to be more readily degraded upon tissue damage and microlesions that may arise during growth and development (Gigli-Bisceglia et al., 2020). Pectin also represents the first target of CWDEs produced by invading pathogens. The breakdown fragments of HG, the oligogalacturonides (OGs), act as regulators of plant growth and development and, if accumulated at high concentration, act as elicitors of plant defense (DAMPs). Interestingly, an alteration of the lignin content of cell walls has been reported to induce the expression of genes encoding CWDEs, such as pectate lyase, xyloglucan (XG) endo-transglycosylases, and ARABIDOPSIS DEHISCENCE ZONE POLYGALACTURONASE (ADPG1). Especially, ADPG1 may be responsible for the release of elicitor-active OGs, which could explain why a reduction in lignin content often enhances disease resistance rather than reducing it (Gallego-Giraldo et al., 2020).
Xyloglucans, hemicelluloses interacting with cellulose through hydrogen bonds, may control the rate of cell wall expansion and plant growth (York et al., 1985; Cosgrove, 2018). XG-derived fucose-containing oligosaccharides have been reported to display growth regulating activity (York et al., 1985; Zablackis et al., 1995). On the other hand, XG-derived fragments have been reported to act as DAMPs (Claverie et al., 2018).
Arabinoxylan (AX), another hemicellulose present in both the primary and secondary walls, consists of copolymers of two pentoses: arabinose and xylose. Very recently, by analyzing mutants in Arabidopsis Response Regulators (ARRs), which mediate cytokinin signaling and modulate the disease resistance (Bacete and Hamann, 2020), it was demonstrated that the AX-derived pentasaccharide 33-α-L-arabinofuranosyl-xylotetraose (XA3XX) triggers a strong immune response in Arabidopsis and enhances disease resistance of some crop plants (Mélida et al., 2020).
Cellulose crystalline microfibrils are present at high levels together with hemicelluloses and lignin in the secondary wall (Cosgrove, 2014). The inhibition of cellulose biosynthesis triggers NADPH oxidase-dependent production of hydrogen peroxide and lignin in the elongation zone of the root. Lignin deposition is a compensatory response to reinforce cell walls that occur upon cell wall modifications or degradation (Denness et al., 2011; Vaahtera et al., 2019). Arabidopsis mutants impaired in the perception of cell wall damage and reactive oxygen species (ROS) perception/signaling show decreased or absent lignin deposition in response to cell wall damage, suggesting that the damage causes the release of DAMPs such as OGs that activate lignification to reinforce the secondary wall (Gallego-Giraldo et al., 2018, 2020). Most of the genes involved in cell wall damage are also implicated in responses to biotic stresses (Denness et al., 2011). Cellulose breakdown products, i.e., cellodextrins (CDs), also act as elicitors of plant defense (Aziz et al., 2007; Souza et al., 2017; Johnson et al., 2018).
Recently, a non-branched 1,3-β-D-(Glc)-hexasaccharide has been identified as a major fungal microbe-associated molecular pattern (MAMP). Because linear β-1,3-glucans are present in plants and accumulate as callose papillae at the site of infection (Albersheim et al., 2011; Chowdhury et al., 2014), the possibility exists that this type of oligosaccharide is released during callose synthesis or degradation to act as a DAMP (Mélida et al., 2018).
Mannans are mainly considered as storage polysaccharides that provide energy for the growing seeds, and their presence and functional importance as structural components of both primary and secondary walls has been highlighted by several authors (Schröder et al., 2009; Marcus et al., 2010). Recently, mannan oligosaccharides (MOS) have been reported as novel cell wall DAMPs (Zang et al., 2019).
In conclusion, the plant cell wall is a dynamic structure that during growth and development can depolymerize its components by auto-degradation, recycle the released fragments, and rebuild the entire structure (Barnes and Anderson, 2018). Alterations of cellulose, xylan, glucuronoxylan, pectin, xyloglucans, and lignin often enhance disease resistance due to their recalcitrance to pathogen-mediated degradation, or, as reported in the case of altered lignin content, provoke the release of DAMPs that activate defense responses (Gallego-Giraldo et al., 2020). During growth, in the absence of pathogens, released fragments, like OGs, may play a physiological role as growth regulators and be recruited as DAMPs if a pathological event occurs (Figure 2).
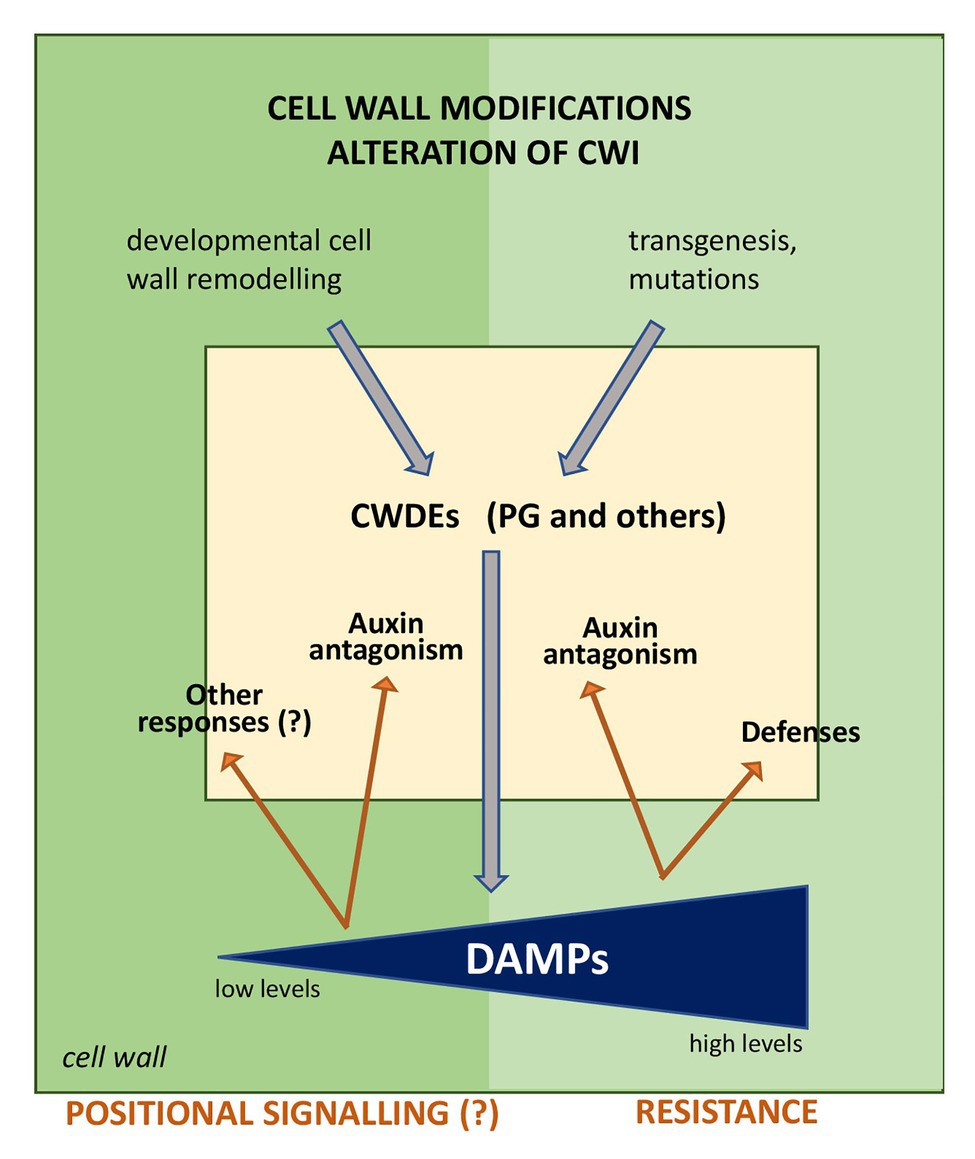
Figure 2. Possible outcomes of the release of DAMPs during alteration of cell wall integrity (CWI). Alterations of the cell wall composition due to transgenesis (for example, reduced lignin content) or mutations lead to a signaling cascade that induces the expression of endogenous cell wall degrading enzymes (CWDEs), among which a polygalacturonase (PG) may be responsible for the release of oligogalacturonides (OGs) that activate defense responses. In normal conditions, i.e., in the absence of pathogens, alterations of CWI during growth, and developmental events, such as cell expansion and division, wall remodeling, secondary root formation, abscission, etc. may be mediated by and/or induce the expression of CWDEs, including PGs. CWDEs release wall fragments that may act as signals for the regulation of growth in a short range cell-cell communication (positional signaling). The question mark indicates events to be elucidated.
The Mode of Action of Cell Wall Damps
Oligogalacturonides
The best described wall-associated DAMPs are the OGs, which derive from the partial degradation of HG (Cervone et al., 1989; Orozco-Cardenas and Ryan, 1999) and likely act as indicators of CWI under stress conditions (Rojo et al., 1999; Aziz et al., 2004; De Lorenzo et al., 2011; Ferrari et al., 2013; Savatin et al., 2014). OGs antagonize auxin (Branca et al., 1988; Bellincampi et al., 1995) by inhibiting the transcription of auxin-induced genes belonging to the IAA and SAUR families as well as the activity of the auxin-responsive promoter DR5 (Savatin et al., 2011). During microbial infections, the accumulation of OGs is facilitated by the interaction of microbial polygalacturonases (PGs) with specific PG-inhibiting proteins (PGIPs; Kalunke et al., 2015; Haeger et al., 2020). Notably, the transgenic expression of a PGIP-PG chimera referred to as the “OG-machine” is able to produce in vivo endogenous OGs, enhancing Arabidopsis resistance against Botrytis cinerea, Pectobacterium carotovorum, and Pseudomonas syringae (Benedetti et al., 2015). OGs bind the extracellular domain of the Arabidopsis wall-associated receptor kinase1 (WAK1; Brutus et al., 2010; Gramegna et al., 2016). Treatments with OGs trigger a wide range of defense responses, including calcium increase, release of ROS and nitric oxide, production of phytoalexins, glucanase, and chitinase, and deposition of callose in the cell wall (Davis et al., 1986; Davis and Hahlbrock, 1987; Broekaert and Pneumas, 1988; Bellincampi et al., 2000; Aziz et al., 2007; Galletti et al., 2008; Rasul et al., 2012). OGs activate the transcription factors WRKY40 and WRKY33, and several genes involved in defense, such as EDS5/SID1, SID2/ICS1, NPR1, SAG101, PAD4, ACS7, LOX3, and LOX4 as well as the indole glucosinolate biosynthetic genes CYP83B1, CYP79B2, CYP79B3, SUR1, and MYB51 (Denoux et al., 2008; Souza et al., 2017). OGs activate phosphorylation of the MAP kinases AtMPK3 and AtMPK6 (Galletti et al., 2011; Mattei et al., 2016) and upregulate phenylalanine ammonia lyase, stilbene synthase (De Lorenzo et al., 1987; Aziz et al., 2007), and serine-protease inhibitors (Ferrari et al., 2003). Treatments with OGs increase the Arabidopsis resistance to B. cinerea through the expression of PAD3, which is required for the basal resistance to this fungus (Aziz et al., 2004), and, if applied to roots, induce systemic resistance in tomato plants against B. cinerea (Gamir et al., 2020).
Cellodextrins
Cellodextrins are linear β-1,4 gluco-oligosaccharides with a degree of polymerization (DP) ranging from 3 to 9 derived from cellulose fragmentation. In grapevine, a CD with a DP of seven induces rapid ROS production and transient elevation of cytosolic calcium levels. Notably, grapevine cells pretreated with CD7 are refractory to a successive treatment with the same oligomer but respond to a subsequent application of OGs, suggesting that CDs and OGs activate different signaling pathways. CDs upregulate the expression of defense genes encoding phenylalanine ammonia lyase, stilbene synthase, chitinases, glucanases, and serine-protease inhibitors, and enhance the resistance of plants against B. cinerea (Aziz et al., 2007). In Arabidopsis, cellobiose (DP 2), cellotriose (DP 3), and cellotetraose (DP 4) cause an increase of intracellular calcium in a fast and transitory way, the early activation of MPK3 and MPK6, and the upregulation of some defense-related genes like those involved in the biosynthesis of glucosinolates. Cellotriose derived from the endophytic fungus Piriformospora indica also elicits plant defense responses (Johnson et al., 2018). Treatment with cellobiose has been reported to induce resistance to P. syringae pv tomato DC3000 (Souza et al., 2017). On the other hand, seedlings grown on high levels of cellobiose exhibit an increase in biomass and the induction of β-glucosidase that provides glucose as a useful carbon source for the growth of the plant itself, as well as for microbes. This is in contrast with the notion that the activation of defenses impairs growth and casts some doubts on the activity of cellobiose as a DAMP (Souza et al., 2017). Indeed, in a study, on the activity of a berberine bridge enzyme-like (BBE-like) oxidase (see below), only CDs with DP higher than two showed a significant elicitor activity (Locci et al., 2019).
Xyloglucans
Xyloglucan oligomers have been recently identified as DAMPs in both grapevine and Arabidopsis. In Arabidopsis, they induce phosphorylation of MPK3 and MPK6, PMR4-dependent callose deposition, enhanced expression of defense genes, including PR1, PAD3, PR2, and PLANT DEFENSIN 1.2 (PDF1.2) as well as an increased jasmonic acid (JA)-, salicylic acid (SA)-, and ethylene (ET)-dependent resistance against B. cinerea. In grapevine, XG oligomers induce accumulation of the phytoalexin resveratrol and resistance against B. cinerea (Claverie et al., 2018; Heloir et al., 2019).
Arabinoxylans
Arabinoxylan oligosaccharides have been recently identified as novel DAMPs (Mélida et al., 2020). This group of hemicelluloses consists of a main backbone of β-1,4-linked D-xylose residues decorated with single L-arabinose residues linked to the C2/C3 position of a D-xylose unit (Ebringerová and Heinze, 2000). The pentasaccharide XA3XX triggers an oxidative burst, a rapid calcium influx, the phosphorylation of MPK3 and MPK6, and the upregulation of genes involved in innate immunity, including several PTI marker genes (CYP81F2, WRKY53, PHI1, FRK1, and NHL10). Moreover, tomato plants treated with XA3XX are more resistant to P. syringae pv tomato DC3000, and XA3XX-treated pepper plants are more resistant to Sclerotinia sclerotiorum (Mélida et al., 2020).
β-1,3-Glucans
Arabidopsis plants treated with 1,3-β-d-(Glc)6 isolated from the pathogenic fungus Plectosphaerella cucumerina show the CERK1-mediated expression of several immunity-associated responses like elevation of cytoplasmic calcium concentration and MAPK cascades (Mélida et al., 2018). Because β-1,3-glucans are present in the callose of the plant papillae that are formed at the sites of infection, 1,3-β-d-(Glc)6 may be considered as both MAMP and DAMP.
Oligomannans
Oligomannans with DP 2–6 enhance defense responses and expression of genes related with NO and ROS accumulation as well as the expression of PR-1 and LOX in both Nicotiana benthamiana and rice. In rice, MOS trigger the upregulation of the expression of MAPK12 and MAPK6, and lead to the accumulation of phytoalexins. Treatments with MOS protect rice and tobacco against Xanthomonas oryzae and Phytophthora nicotianae, respectively (Zang et al., 2019).
Pathways and Signals Involved in the Growth-Defense Trade-Off
Due to their energy cost and consequent metabolic limitations, growth and defense are constantly regulated by a balanced trade-off of antithetic pathways, both of which are most likely affected by stimulatory and inhibitory signals (Huot et al., 2014; Vaahtera et al., 2019). As stated above, homeostatic control of the defense response is important to avoid deleterious effects due to a hyper-immune response that causes reduced growth and/or extensive cellular death. Under such circumstances, attenuation of the pathways leading to defense or a reduction in the levels of the signals triggering such pathways is expected to occur (Karasov et al., 2017; Li et al., 2020). The crosstalk between the pathways controlled by ET, JA, SA, and by other growth regulators may influence immune and developmental processes in opposite directions (Denancé et al., 2013; Guo et al., 2018). Over-expression of pathways that activate immunity often enhances pathogen resistance while negatively affecting plant growth and may cause anatomical and physiological responses, such as dwarfism, accelerated senescence, delayed flowering, sterility, or reduced seed production.
Many other signals, besides ET, JA, and SA, are known to influence the growth/defense trade-off. ROS in the apoplast are among these signals as their concentration and homeostasis is critical for maintaining the correct balance between growth and defense (Farvardin et al., 2020). A low level of H2O2 in the cell wall, for example, promotes growth and concomitantly suppresses the plant defense (Neuser et al., 2019). Other signals such as OGs antagonize auxin by inhibiting adventitious root formation, stem elongation, and pericycle cell differentiation. At the molecular level, OGs downregulate auxin-induced expression of the DR5 promoter as well as the early auxin-regulated genes IAA5, IAA19, IAA20, IAA22, SAUR16, SAUR AC1, and GH3.3. On the other hand, auxin antagonizes the protection exerted by OGs against B. cinerea (Branca et al., 1988; Bellincampi et al., 1993; Ferrari et al., 2008; Savatin et al., 2011). Furthermore, a high level of endogenous OGs strongly reduces growth while a systemic and prolonged accumulation of OGs causes a hypersensitive-like response characterized by extensive cell death (Benedetti et al., 2015). Interestingly, OGs-auxin antagonism is independent of the extracellular accumulation of H2O2 produced by NADPH oxidase (Bellincampi et al., 1996, 2000) and is also independent of ET, JA, and SA signaling. The antagonism takes place downstream in the auxin signaling pathway, most likely at the post-translational level (Savatin et al., 2011).
Oligogalacturonides and Cellodextrins are Enzymatically Oxidized by BBE-Like Proteins
Oxidized OGs were first identified in leaf diffusates of transgenic plants expressing the “OG-machine,” a chimeric protein generated by fusing a fungal endo-polygalacturonase with a plant PGIP (Benedetti et al., 2018). The OG-machine is capable of releasing elicitor-active OGs on command under the control of a chemically inducible promoter (Benedetti et al., 2015). The analysis of these transgenic plants revealed the presence of atypical oligomers displaying oxygen at the C1 position of the reducing end due to the conversion of a galacturonic acid into galactaric acid (Figure 3; Benedetti et al., 2018). A flavin adenine dinucleotide (FAD)-dependent and sulfite-sensitive enzyme, named oligogalacturonide oxidase (OGOX)1, capable of oxidizing OGs, was subsequently purified and found to belong to the family of BBE-like proteins, which consists of 28 members in Arabidopsis considering that one of the 27 genes, i.e., At4g20830, encodes for two OGOX1 isoforms, BBE19 and BBE20 (Benedetti et al., 2018; Table 1; Figure 4). OGOX1 specifically oxidizes OGs releasing H2O2 derived from the oxidization of the reduced FAD cofactor by O2 that, in turn, restores the activity of the enzyme (Figure 3). Three additional BBE-like proteins of the Arabidopsis BBE family, OGOX2, OGOX3, and OGOX4, oxidize OGs at a different pH (Benedetti et al., 2018). Another member of the gene family encodes an enzyme that catalyzes the conversion of indole cyanohydrin to indole-3-carbonyl nitrile, a metabolite with a role in defense (Boudsocq et al., 2010; Rajniak et al., 2015), whereas two other BBE proteins encode monolignol oxidases (Daniel et al., 2015). Finally, a BBE protein member named Cellodextrin Oxidase (CELLOX) was shown to oxidize and convert the glucose at the reducing end of CDs into gluconic acid (Locci et al., 2019). Another BBE protein, BBE23, is characterized by a 62.5% amino acid identity with CELLOX and is likely a potential paralog. CELLOX and BBE23 display the highest amino acid identity with Nectarin V, a glucose oxidase from ornamental tobacco (Nicotiana langsdorffii x Nicotiana sanderae; Carter and Thornburg, 2004; Table 1; Figure 4). Recently, BBE8 was hypothesized to oxidize some wall-derived oligosaccharides in guard cells in order to facilitate stomatal opening in response to infection by P. syringae and Salmonella enterica (Rodrigues Oblessuc et al., 2019). However, the activity of BBE8 as an oxidase of oligosaccharides was not proven. Similarly, the substrate specificity of BBE28 has not been identified yet despite the fact that the crystal structure of the enzyme has been solved (Daniel et al., 2016). So far, the substrates of 19 out of the 28 Arabidopsis BBE-like family members have not been identified (Table 1). The scenario of undetermined functions of these BBE-like enzymes becomes even more complex if plants with larger BBE-like families are considered such as, for example, the Western Poplar (Populus trichocarpa), which comprises 64 BBE-like members (Wallner et al., 2012).
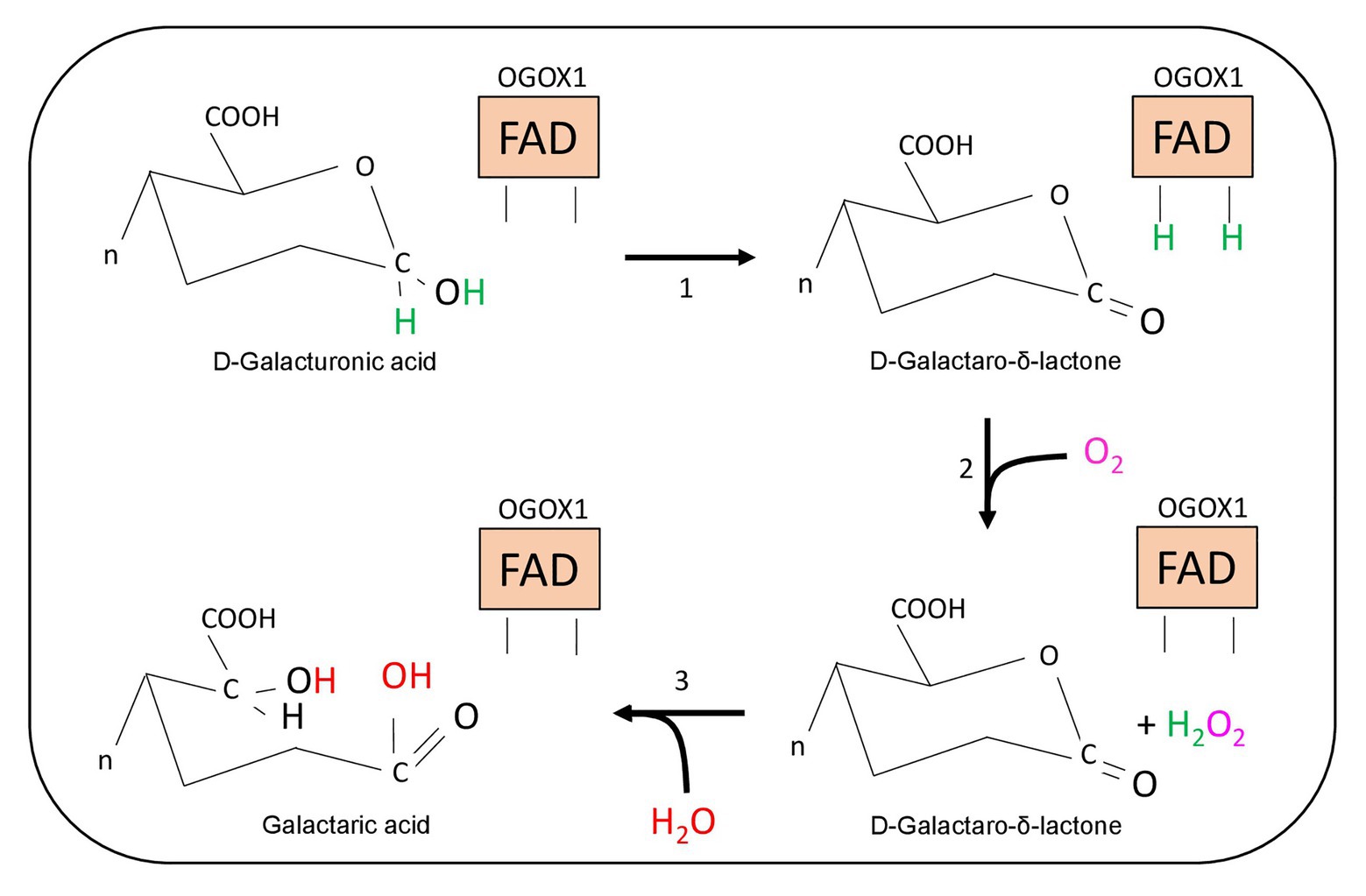
Figure 3. Schematic representation of the enzymatic reaction catalyzed by OGOX1. The oxidized FAD-cofactor of OGOX1 oxidizes the galacturonic acid to a lactone at the reducing-end of OGs (step 1). Molecular oxygen oxidizes the reduced FAD(H2)-cofactor and releases hydrogen peroxide (step 2). The final hydrolysis transforms the lactone into galactaric acid (step 3).
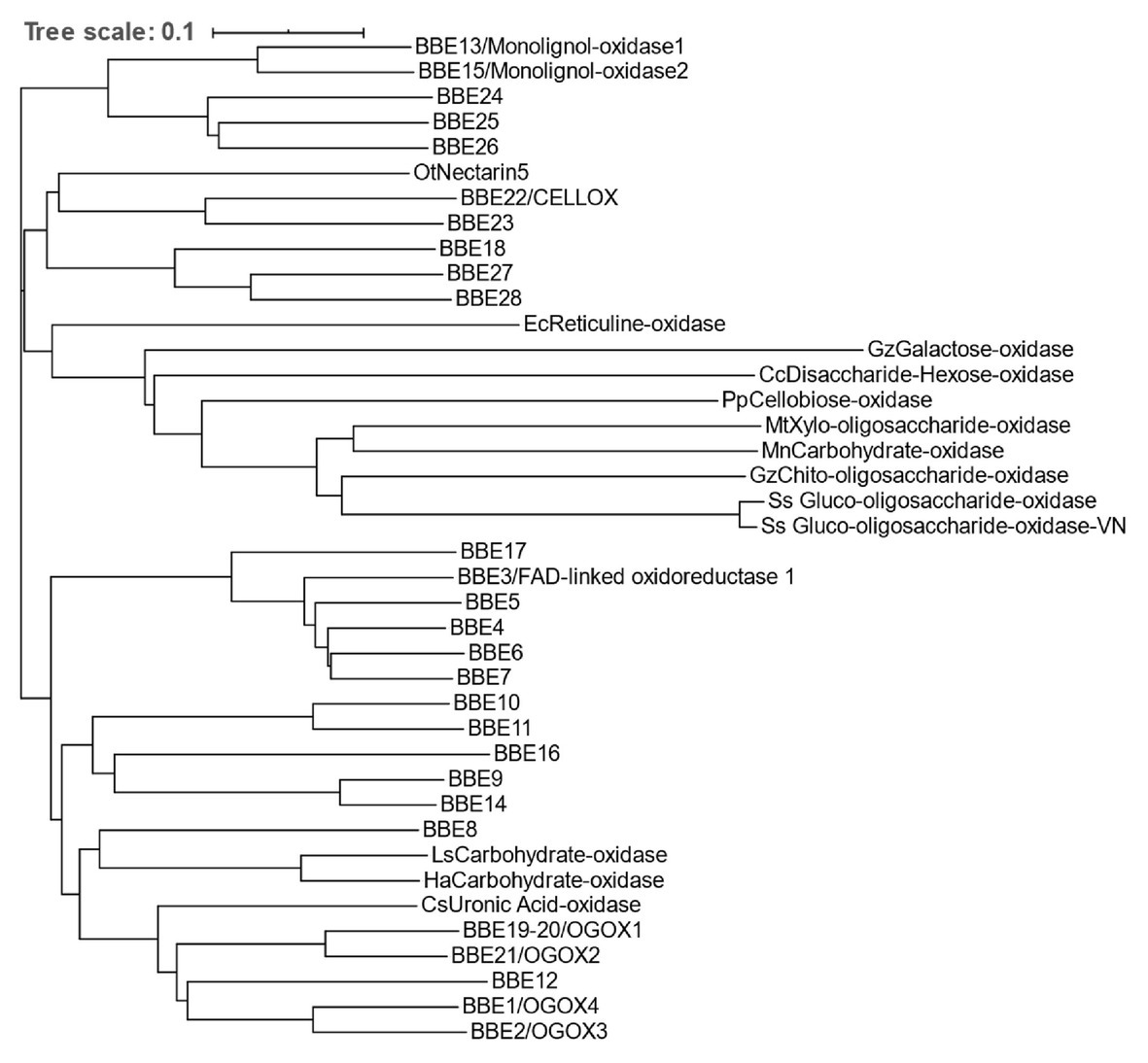
Figure 4. Homology tree of BBE-like proteins and FAD-linked oxidases. The BBE-like superfamily of A. thaliana (BBE1-28) and FAD-linked oxidases with mono- and oligo-saccharide oxidase activity from fungi, moss, and plants are indicated in the protein homology tree. Reticuline oxidase from Eschscholzia californica is also indicated. The identification code of each flavoprotein is reported in Table 1. (Cc, Chondrus crispus; Cs, Citrus sinensis; Ec, Eschscholzia californica; Gz, Gibberella zeae; Ha, Helianthus annuus; Ls, Lactuca sativa; Mn, Microdochium nivale; Mt, Myceliophthora thermophila; Ot, Ornamental tobacco – Nicotiana langsdorffii x N. sanderae; Pp, Physcomitrella patens; Ss, Sarocladium strictum; CELLOX, cellodextrin oxidase; and OGOX, oligogalacturonide oxidase).
Oxidases of Mono- and Oligosaccharides in Plants and Microbes
Oxidases of mono- and oligosaccharides are widespread among plants and microbes but only in a few cases their physiological role has been investigated. To date, OGs, CDs, XG, and AX fragments, 1,3-β-d-(Glc)6 and MOS are the oligosaccharides shown to display a DAMP activity (see above). However, FAD-dependent oxidases have only been found for two of these oligosaccharides (OGs and CDs). On the other hand, (oligo)saccharides that apparently do not have DAMP activity have been found to be substrates of enzymatic oxidation both in plants and in microbes, such as Gibberella zeae, Sarocladium strictum, and Myceliophthora thermophila, as well as in the moss Physcomitrella patens (Table 1; Figure 4; Toplak et al., 2018). Sarocladium strictum, for example, produces mono- and disaccharide oxidases as well as gluco- and xylo-oligosaccharide oxidases which, despite sharing a high sequence identity with each other, are characterized by different substrate specificities (Lee et al., 2005; Vuong et al., 2013; Table 1). An enzyme from G. zeae (Figure 4; Heuts et al., 2007) oxidizes chito-oligosaccharides derived from fungal cell walls that are known to be powerful elicitors of plant defense (Wan et al., 2008). However, it is not known whether the chito-oligomers lose their elicitor activity upon oxidation as in the case OGs and CDs. Finally, an oxidase from M. thermophila exhibits a strong substrate preference toward xylo-oligosaccharides (Ferrari et al., 2016).
A potential physiological role of microbial FAD-dependent oligosaccharide oxidases is to provide H2O2 to support the hydrolysis of substrates, such as crystalline cellulose (Villares et al., 2017), chitin, and xylan by enzymes referred to as lytic polysaccharide mono-oxygenases (LPMOs; Nakagawa et al., 2015; Couturier et al., 2018). The copper-containing active site of LPMOs must be reduced after each oxidative-cleavage reaction and electrons may be restored by the H2O2 produced by the FAD-depended enzymes (Filandr et al., 2020; Giovannoni et al., 2020).
In conclusion, oxidases of mono- and oligosaccharides are widely distributed among plants and microbes, but their role is difficult to decipher and may depend on the nature of the producing organism, the substrate specificity of the enzyme, and the metabolic role of the substrate. The plant-encoded oxidizing enzymes acting on DAMPs can attenuate the excessive activity of these elicitors of defense. The same kind of enzymes produced by pathogens may sustain the activity of microbial LPMOs in the degradation of resistant cell wall substrates such as crystalline cellulose. Pathogenic microorganisms may recruit the plant derived FAD-dependent oxidases to sustain the activity of LPMOs and cause a diversion (hijacking) of the host responses into an advantageous trait for the pathogens.
Final Considerations: The Role of Oxidases in the Maintenance of Signal Homeostasis and Growth-Defense Trade-Off
As a co-product of the enzymatic oxidation of OGs, CDs, and possibly of other DAMPs, H2O2 is expected to be present temporally and locally in limited zones of the tissues, where a breach in the wall is generated. This may occur upon local injury caused by biotic or abiotic stress or upon a localized loss of CWI during growth and development like, for example, during the formation of lateral roots (Peretto et al., 1992). It is known that H2O2 is involved not only in the strengthening and repairing of the plant tissues (Farvardin et al., 2020) but also in signaling during both immunity and development (Mhamdi and Van Breusegem, 2018; Huang et al., 2019; Sies and Jones, 2020). Spatial distribution of different ROS and their finely-tuned balance drive plant morphogenetic processes (Mhamdi and Van Breusegem, 2018). It can be argued that the enzymatic oxidation of wall polysaccharide fragments by the FAD-dependent oxidases is also a mechanism for a strictly localized production of limited amounts of H2O2. This production of H2O2 is expected to occur only where breaks are made by CWDEs in the wall, and therefore only in one or few cells, and may have a biological significance, for example, as a signal for a very short range cell-cell communication. In addition, the oxidation of the reducing end of the cell wall polysaccharides may hamper the trans-glycosylation that is critical for cell expansion (Franková and Fry, 2020), thereby contributing to the growth-defense trade-off.
Both OGs and CDs play a dual function as elicitors of plant immunity and as a carbon source sustaining the growth of phytopathogenic microbes. Oxidation of these oligosaccharides protects against hyper-immunity and blocks pathogen growth by making them more difficult to metabolize. Consequently, transgenic Arabidopsis plants overexpressing OGOX1 and CELLOX are more resistant to B. cinerea because the fungus does not grow well when fed with a mixture of oxidized oligosaccharides (Benedetti et al., 2018; Locci et al., 2019). It is relevant, in this context, that a marked accumulation of oxidized di-galacturonic acid has been detected as a final product of tissue degradation in Arabidopsis plants infected with B. cinerea (Voxeur et al., 2019). The recalcitrance to enzymatic hydrolysis of the oxidized oligosaccharides is also favored by the basification of the apoplastic pH normally occurring as a defense response to microbial attacks. OGOXs and CELLOX display a high optimum pH of activity while the microbial pectinases and cellulases display an optimal activity between pH 3 and 6.
The recognition of DAMPs by pattern recognition receptors is thought to occur ubiquitously across the tree of life (Heil and Land, 2014; De Lorenzo et al., 2018). In mammals and plants, DAMP-mediated immunity exhibits both common and divergent features (De Lorenzo et al., 2018). Elicitors such as DAMPs and MAMPs elicit what is referred to as PTI. Attenuation or suppression of PTI is expected to protect the organism from the deleterious effects of hyperactivated immunity that negatively affect growth (Li et al., 2020). Currently, attenuation of PTI, through phosphorylation and dephosphorylation of the MAPKs, protein degradation by the proteasome, or regulation of WRKY transcription factors is known to occur downstream of MAMP perception (Li et al., 2020). However, not much is known about the attenuation/clearance of DAMPs that must occur to avoid a continuous activation of defense once a corresponding danger has ceased to exist. Moreover, the homeostasis of endogenous molecules that normally have a physiological role and become DAMPs upon pathogen attack needs to be quickly restored, as in the case of OGs that are released in plants not only upon microbial attacks but also in the healthy plants possibly to regulate growth and development in concert with hormones (Pontiggia et al., 2015).
The complexity of the cell wall does not allow an easy in vivo investigation of the release, activity, or degradation of oligosaccharide fragments. To this purpose, an excellent tool by which the cause-effect of the biological activity of OGs has been clearly documented is the “OG-machine” expressed in Arabidopsis. Using these plants, it is possible to release elicitor-active OGs under the control of an inducible promoter. Released OGs in vivo activate immunity, confer protection against fungal and bacterial pathogens, and, if accumulated in excess, cause an increase of salicylic acid, reduction of growth, leaf discoloration, and chlorosis and, finally, cell death; i.e., a typical hypersensitive response (Figure 5; Benedetti et al., 2015). The first oxidase specifically acting on DAMPs was identified in these plants, where the enzyme is constitutively expressed (Benedetti et al., 2018). The same plants not only express high levels of OG-oxidases but also an oxidase acting on CDs (Locci et al., 2019). Notably, recent studies show that cellulose and pectin interact (Du et al., 2020; Palacio-Lopez et al., 2020) and that cellulose microfibers and HG nanofilaments form a single cohesive network (Haas et al., 2020; Zhang and Zhang, 2020).
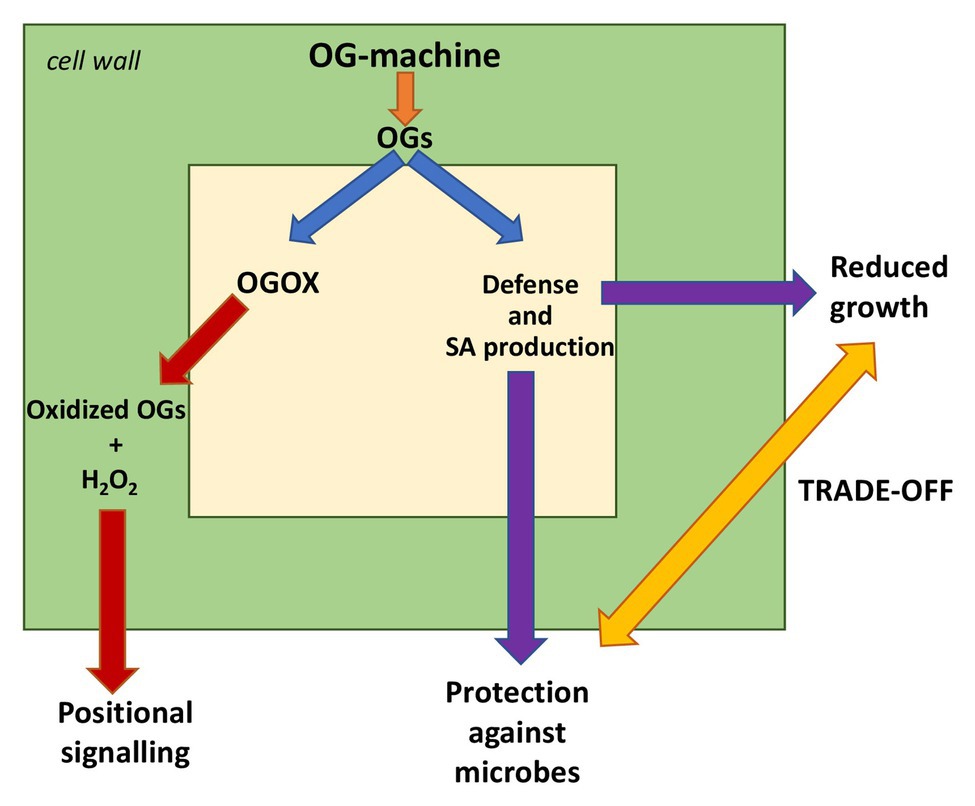
Figure 5. Cellular responses to the “OG-machine” action. The plants expressing the OG-machine under an inducible promoter produce OGs on command. These activate defenses and salicylic acid (SA) formation that, on one side, confer protection against pathogenic fungi and bacteria and, on the other side, reduce the plant growth (trade-off). Excess of accumulated OGs causes cell death (hyper-immunity). Homeostasis of OGs is maintained by an oxidase (OGOX) producing hydrogen peroxide that in turn may act as a transduction signal. Oxidized OGs cannot be utilized by some microbes as a carbon source; this contributes to plant resistance.
Oxidases can attenuate DAMP activity of OGs and CDs but a relevant question is why plants have evolved such a type of activity if they can potentially mitigate or abolish the DAMP signal by using the vast variety of endogenous degrading enzymes (pectinases and cellulases). One possible answer is found in the evidence that the final products of hydrolysis of pectin and cellulose are utilized as a carbon source by microbes, and therefore may contribute to plant susceptibility. The combination of hydrolases and oxidases, instead, produces oligosaccharide fragments that cannot be readily catabolized by microbes and, consequently, contributes to enhance the plant resistance (Locci et al., 2019).
In humans, inflammation is not only caused by a hyper-accumulation of inducible DAMPs but also by an imbalance of suppression/inhibition of DAMPs or by an insufficient generation of the so-called “SAMPs,” where “S” stands for “suppressing DAMPs” (Land, 2018; Relja and Land, 2020). SAMPs include molecules such as prostaglandin E2 (PGE2), annexin A1 (AnxA1), and specialized pro-resolving mediators (SPMs; Land, 2018). The attention on SAMPs is increasing because of their potential therapeutic applications in pathological processes of hyper-inflammation like those currently observed in patients affected by COVID-19, and which, in certain cases, may lead to severe autoimmune disorders (Gallo et al., 2015; Land, 2020). DAMP oxidases can be considered in the broadest sense as DAMP suppressors: i.e. SAMPs that maintain a balanced level of signals in plants. The identification of plant SAMPs may have significant biotechnological applications to overcome the limitations imposed by the growth/defense trade-off and by an excessive accumulation of DAMPs causing a deleterious hyper-immunity.
Author Contributions
DP, MB, and SC contributed to writing. FC and GL supervised the work and edited the final version of the paper. All authors contributed to the article and approved the submitted version.
Funding
This work was supported by the Italian Ministry of University and Research (MIUR-PRIN2017 Grant N° 2017ZBBYNC).
Conflict of Interest
The authors declare that the research was conducted in the absence of any commercial or financial relationships that could be construed as a potential conflict of interest.
References
Albersheim, P., Darvill, A., Roberts, K., Sederoff, R., and Staehelin, A. (2011). Plant cell walls: from chemistry to biology. New York: Garland Science, Taylor and Francis Group.
Anderson, C. T., and Kieber, J. J. (2020). Dynamic construction, perception, and remodeling of plant cell walls. Annu. Rev. Plant Biol. 71, 39–69. doi: 10.1146/annurev-arplant-081519-035846
Aziz, A., Gauthier, A., Bézier, A., Poinssot, B., Joubert, J. M., Pugin, A., et al. (2007). Elicitor and resistance-inducing activities of beta-1,4 cellodextrins in grapevine, comparison with beta-1,3 glucans and alpha-1,4 oligogalacturonides. J. Exp. Bot. 58, 1463–1472. doi: 10.1093/jxb/erm008
Aziz, A., Heyraud, A., and Lambert, B. (2004). Oligogalacturonide signal transduction, induction of defense-related responses and protection of grapevine against Botrytis cinerea. Planta 218, 767–774. doi: 10.1007/s00425-003-1153-x
Bacete, L., and Hamann, T. (2020). The role of mechanoperception in plant cell wall integrity maintenance. Plants 9:574. doi: 10.3390/plants9050574
Barnes, W. J., and Anderson, C. T. (2018). Release, recycle, rebuild: cell-wall remodeling, autodegradation, and sugar salvage for new wall biosynthesis during plant development. Mol. Plant 11, 31–46. doi: 10.1016/j.molp.2017.08.011
Bellincampi, D., Cardarelli, M., Zaghi, D., Serino, G., Salvi, G., Gatz, C., et al. (1996). Oligogalacturonides prevent rhizogenesis in rolB-transformed tobacco explants by inhibiting auxin-induced expression of the rolB gene. Plant Cell 8, 477–487. doi: 10.1105/tpc.8.3.477
Bellincampi, D., Dipierro, N., Salvi, G., Cervone, F., and De Lorenzo, G. (2000). Extracellular H2O2 induced by oligogalacturonides is not involved in the inhibition of the auxin-regulated rolB gene expression in tobacco leaf explants. Plant Physiol. 122, 1379–1385. doi: 10.1104/pp.122.4.1379
Bellincampi, D., Forrest, R., Nuss, L., Salvi, G., DeLorenzo, G., and Cervone, F. (1995). Extracellular accumulation of an auxin-regulated protein in Phaseolus vulgaris L. cells is inhibited by oligogalacturonides. J. Plant Physiol. 147, 367–370. doi: 10.1016/s0176-1617(11)82169-3
Bellincampi, D., Salvi, G., Delorenzo, G., Cervone, F., Marfa, V., Eberhard, S., et al. (1993). Oligogalacturonides inhibit the formation of roots on tobacco explants. Plant J. 4, 207–213. doi: 10.1046/j.1365-313X.1993.04010207.x
Benedetti, M., Pontiggia, D., Raggi, S., Cheng, Z., Scaloni, F., Ferrari, S., et al. (2015). Plant immunity triggered by engineered in vivo release of oligogalacturonides, damage-associated molecular patterns. Proc. Natl. Acad. Sci. U. S. A. 112, 5533–5538. doi: 10.1073/pnas.1504154112
Benedetti, M., Verrascina, I., Pontiggia, D., Locci, F., Mattei, B., De Lorenzo, G., et al. (2018). Four Arabidopsis berberine bridge enzyme-like proteins are specific oxidases that inactivate the elicitor-active oligogalacturonides. Plant J. 94, 260–273. doi: 10.1111/tpj.13852
Boudsocq, M., Willmann, M. R., McCormack, M., Lee, H., Shan, L., He, P., et al. (2010). Differential innate immune signalling via Ca2+ sensor protein kinases. Nature 464, 418–422. doi: 10.1038/nature08794
Branca, C., De Lorenzo, G., and Cervone, F. (1988). Competitive inhibition of the auxin-induced elongation by a-D-oligogalacturonides in pea stem segments. Physiol. Plant. 72, 499–504. doi: 10.1111/j.1399-3054.1988.tb09157.x
Broekaert, W. F., and Pneumas, W. J. (1988). Pectic polysaccharides elicit chitinase accumulation in tobacco. Physiol. Plant. 74, 740–744. doi: 10.1111/j.1399-3054.1988.tb02046.x
Brutus, A., Sicilia, F., Macone, A., Cervone, F., and De Lorenzo, G. (2010). A domain swap approach reveals a role of the plant wall-associated kinase 1 (WAK1) as a receptor of oligogalacturonides. Proc. Natl. Acad. Sci. U. S. A. 107, 9452–9457. doi: 10.1073/pnas.1000675107
Carter, C. J., and Thornburg, R. W. (2004). Tobacco nectarin V is a flavin-containing berberine bridge enzyme-like protein with glucose oxidase activity. Plant Physiol. 134, 460–469. doi: 10.1104/pp.103.027482
Cervone, F., De Lorenzo, G., Salvi, G., Bergmann, C., Hahn, M. G., Ito, Y., et al. (1989). “Release of phytoalexin elicitor-active oligogalacturonides by microbial pectic enzymes” in Signal molecules in plants and plant-microbe interactions. NATO ASI series. Vol. H36. ed. B. J. J. Lugtenberg (Heidelberg, FRG: Springer Verlag), 85–89.
Choosri, W., Paukner, R., Wührer, P., Haltrich, D., and Leitner, C. (2011). Enhanced production of recombinant galactose oxidase from Fusarium graminearum in E. coli. World J. Microbiol. Biotechnol. 27, 1349–1353. doi: 10.1007/s11274-010-0585-2
Chowdhury, J., Henderson, M., Schweizer, P., Burton, R. A., Fincher, G. B., and Little, A. (2014). Differential accumulation of callose, arabinoxylan and cellulose in nonpenetrated versus penetrated papillae on leaves of barley infected with Blumeria graminis f. sp. hordei. New Phytol. 204, 650–660. doi: 10.1111/nph.12974
Claverie, J., Balacey, S., Lemaitre-Guillier, C., Brule, D., Chiltz, A., Granet, L., et al. (2018). The cell wall-derived xyloglucan is a new DAMP triggering plant immunity in Vitis vinifera and Arabidopsis thaliana. Front. Plant Sci. 9:1725. doi: 10.3389/fpls.2018.01725
Cosgrove, D. J. (2014). Re-constructing our models of cellulose and primary cell wall assembly. Curr. Opin. Plant Biol. 22, 122–131. doi: 10.1016/j.pbi.2014.11.001
Cosgrove, D. J. (2018). Diffuse growth of plant cell walls. Plant Physiol. 176, 16–27. doi: 10.1104/pp.17.01541
Couturier, M., Ladevèze, S., Sulzenbacher, G., Ciano, L., Fanuel, M., Moreau, C., et al. (2018). Lytic xylan oxidases from wood-decay fungi unlock biomass degradation. Nat. Chem. Biol. 14, 306–310. doi: 10.1038/nchembio.2558
Custers, J. H., Harrison, S. J., Sela-Buurlage, M. B., van Deventer, E., Lageweg, W., Howe, P. W., et al. (2004). Isolation and characterisation of a class of carbohydrate oxidases from higher plants, with a role in active defence. Plant J. 39, 147–160. doi: 10.1111/j.1365-313X.2004.02117.x
Daniel, B., Pavkov-Keller, T., Steiner, B., Dordic, A., Gutmann, A., Nidetzky, B., et al. (2015). Oxidation of monolignols by members of the berberine-bridge enzyme family suggests a role in plant cell wall metabolism. J. Biol. Chem. 290, 18770–18781. doi: 10.1074/jbc.M115.659631
Daniel, B., Wallner, S., Steiner, B., Oberdorfer, G., Kumar, P., van der Graaff, E., et al. (2016). Structure of a berberine bridge enzyme-like enzyme with an active site specific to the plant family brassicaceae. PLoS One 11:e0156892. doi: 10.1371/journal.pone.0156892
Davis, K. R., Darvill, A. G., Albersheim, P., and Dell, A. (1986). Host-pathogen interactions. XXIX. Oligogalacturonides released from sodium polypectate by endopolygalacturonic acid lyase are elicitors of phytoalexins in soybean. Plant Physiol. 80, 568–577. doi: 10.1104/pp.80.2.568
Davis, K. R., and Hahlbrock, K. (1987). Induction of defense responses in cultured parsley cells by plant cell wall fragments. Plant Physiol. 85, 1286–1290. doi: 10.1104/pp.84.4.1286
De Lorenzo, G., Brutus, A., Savatin, D. V., Sicilia, F., and Cervone, F. (2011). Engineering plant resistance by constructing chimeric receptors that recognize damage-associated molecular patterns (DAMPs). FEBS Lett. 585, 1521–1528. doi: 10.1016/j.febslet.2011.04.043
De Lorenzo, G., Ferrari, S., Cervone, F., and Okun, E. (2018). Extracellular DAMPs in plants and mammals: immunity, tissue damage and repair. Trends Immunol. 39, 937–950. doi: 10.1016/j.it.2018.09.006
De Lorenzo, G., Ferrari, S., Giovannoni, M., Mattei, B., and Cervone, F. (2019). Cell wall traits that influence plant development, immunity, and bioconversion. Plant J. 97, 134–147. doi: 10.1111/tpj.14196
De Lorenzo, G., Ranucci, A., Bellincampi, D., Salvi, G., and Cervone, F. (1987). Elicitation of phenylalanine ammonia-lyase in Daucus carota by oligogalacturonides released from sodium polypectate by homogeneous polygalacturonase. Plant Sci. 51, 147–150. doi: 10.1016/0168-9452(87)90186-5
Denancé, N., Sánchez-Vallet, A., Goffner, D., and Molina, A. (2013). Disease resistance or growth: the role of plant hormones in balancing immune responses and fitness costs. Front. Plant Sci. 4:155. doi: 10.3389/fpls.2013.00155
Denness, L., McKenna, J. F., Segonzac, C., Wormit, A., Madhou, P., Bennett, M., et al. (2011). Cell wall damage-induced lignin biosynthesis is regulated by a reactive oxygen species- and jasmonic acid-dependent process in Arabidopsis. Plant Physiol. 156, 1364–1374. doi: 10.1104/pp.111.175737
Denoux, C., Galletti, R., Mammarella, N., Gopalan, S., Werck, D., De Lorenzo, G., et al. (2008). Activation of defense response pathways by OGs and Flg22 elicitors in Arabidopsis seedlings. Mol. Plant 1, 423–445. doi: 10.1093/mp/ssn019
Du, J., Kirui, A., Huang, S., Wang, L., Barnes, W. J., Kiemle, S., et al. (2020). Mutations in the pectin methyltransferase QUASIMODO2 influence cellulose biosynthesis and wall integrity in Arabidopsis thaliana. Plant Cell 32, 3576–3597. doi: 10.1105/tpc.20.00252
Ebringerová, A., and Heinze, T. (2000). Xylan and xylan derivatives—biopolymers with valuable properties, 1. Naturally occurring xylans structures, isolation procedures and properties. Macromol. Rapid Commun. 21, 542–556. doi: 10.1002/1521-3927(20000601)21:9<542::Aid-marc542>3.0.Co;2-7
Farvardin, A., Gonzalez-Hernandez, A. I., Llorens, E., Garcia-Agustin, P., Scalschi, L., and Vicedo, B. (2020). The apoplast: a key player in plant survival. Antioxidants 9:604. doi: 10.3390/antiox9070604
Ferrari, S., Galletti, R., Pontiggia, D., Manfredini, C., Lionetti, V., Bellincampi, D., et al. (2008). Transgenic expression of a fungal endo-polygalacturonase increases plant resistance to pathogens and reduces auxin sensitivity. Plant Physiol. 146, 669–681. doi: 10.1104/pp.107.109686
Ferrari, A. R., Rozeboom, H. J., Dobruchowska, J. M., van Leeuwen, S. S., Vugts, A. S., Koetsier, M. J., et al. (2016). Discovery of a xylooligosaccharide oxidase from Myceliophthora thermophila C1. J. Biol. Chem. 291, 23709–23718. doi: 10.1074/jbc.M116.741173
Ferrari, S., Savatin, D. V., Sicilia, F., Gramegna, G., Cervone, F., and Lorenzo, G. D. (2013). Oligogalacturonides: plant damage-associated molecular patterns and regulators of growth and development. Front. Plant Sci. 4:49. doi: 10.3389/fpls.2013.00049
Ferrari, S., Vairo, D., Ausubel, F. M., Cervone, F., and De Lorenzo, G. (2003). Tandemly duplicated arabidopsis genes that encode polygalacturonase-inhibiting proteins are regulated coordinately by different signal transduction pathways in response to fungal infection. Plant Cell 15, 93–106. doi: 10.1105/tpc.005165
Filandr, F., Man, P., Halada, P., Chang, H., Ludwig, R., and Kracher, D. (2020). The H2O2-dependent activity of a fungal lytic polysaccharide monooxygenase investigated with a turbidimetric assay. Biotechnol. Biofuels 13:37. doi: 10.1186/s13068-020-01673-4
Foumani, M., Vuong, T. V., and Master, E. R. (2011). Altered substrate specificity of the gluco-oligosaccharide oxidase from Acremonium strictum. Biotechnol. Bioeng. 108, 2261–2269. doi: 10.1002/bit.23149
Franková, L., and Fry, S. C. (2020). “Activity and action of cell-wall transglycanases” in The plant cell wall: Methods and protocols. ed. Z. A. Popper (New York, NY: Springer, New York), 165–192.
Gallego-Giraldo, L., Liu, C., Pose-Albacete, S., Pattathil, S., Peralta, A. G., Young, J., et al. (2020). Arabidopsis dehiscence zone polygalacturonase 1 (ADPG1) releases latent defense signals in stems with reduced lignin content. Proc. Natl. Acad. Sci. U. S. A. 117, 3281–3290. doi: 10.1073/pnas.1914422117
Gallego-Giraldo, L., Pose, S., Pattathil, S., Peralta, A. G., Hahn, M. G., Ayre, B. G., et al. (2018). Elicitors and defense gene induction in plants with altered lignin compositions. New Phytol. 219, 1235–1251. doi: 10.1111/nph.15258
Galletti, R., Denoux, C., Gambetta, S., Dewdney, J., Ausubel, F. M., De Lorenzo, G., et al. (2008). The AtrbohD-mediated oxidative burst elicited by oligogalacturonides in Arabidopsis is dispensable for the activation of defense responses effective against Botrytis cinerea. Plant Physiol. 148, 1695–1706. doi: 10.1104/pp.108.127845
Galletti, R., Ferrari, S., and De Lorenzo, G. (2011). Arabidopsis MPK3 and MPK6 play different roles in basal and oligogalacturonide- or flagellin-induced resistance against Botrytis cinerea. Plant Physiol. 157, 804–814. doi: 10.1104/pp.111.174003
Gallo, P. M., Rapsinski, G. J., Wilson, R. P., Oppong, G. O., Sriram, U., Goulian, M., et al. (2015). Amyloid-DNA composites of bacterial biofilms stimulate autoimmunity. Immunity 42, 1171–1184. doi: 10.1016/j.immuni.2015.06.002
Gamir, J., Minchev, Z., Berrio, E., Garcia, J. M., De Lorenzo, G., and Pozo, M. J. (2020). Roots drive oligogalacturonide-induced systemic immunity in tomato. Plant Cell Environ. doi: 10.1111/pce.13917 [Epub ahead of print]
Gigli-Bisceglia, N., Engelsdorf, T., and Hamann, T. (2020). Plant cell wall integrity maintenance in model plants and crop species-relevant cell wall components and underlying guiding principles. Cell. Mol. Life Sci. 77, 2049–2077. doi: 10.1007/s00018-019-03388-8
Giovannoni, M., Gramegna, G., Benedetti, M., and Mattei, B. (2020). Industrial use of cell wall degrading enzymes: the fine line between production strategy and economic feasibility. Front. Bioeng. Biotechnol. 8:356. doi: 10.3389/fbioe.2020.00356
Gramegna, G., Modesti, V., Savatin, D. V., Sicilia, F., Cervone, F., and De Lorenzo, G. (2016). GRP-3 and KAPP, encoding interactors of WAK1, negatively affect defense responses induced by oligogalacturonides and local response to wounding. J. Exp. Bot. 67, 1715–1729. doi: 10.1093/jxb/erv563
Groen, B. W., De Vries, S., and Duine, J. A. (1997). Characterization of hexose oxidase from the red seaweed Chondrus crispus. Eur. J. Biochem. 244, 858–861. doi: 10.1111/j.1432-1033.1997.00858.x
Guo, Q., Yoshida, Y., Major, I. T., Wang, K., Sugimoto, K., Kapali, G., et al. (2018). JAZ repressors of metabolic defense promote growth and reproductive fitness in Arabidopsis. Proc. Natl. Acad. Sci. U. S. A. 115, E10768–E10777. doi: 10.1073/pnas.1811828115
Gust, A. A., Pruitt, R., and Nürnberger, T. (2017). Sensing danger: key to activating plant immunity. Trends Plant Sci. 22, 779–791. doi: 10.1016/j.tplants.2017.07.005
Haas, K. T., Wightman, R., Meyerowitz, E. M., and Peaucelle, A. (2020). Pectin homogalacturonan nanofilament expansion drives morphogenesis in plant epidermal cells. Science 367, 1003–1007. doi: 10.1126/science.aaz5103
Haeger, W., Henning, J., Heckel, D. G., Pauchet, Y., and Kirsch, R. (2020). Direct evidence for a new mode of plant defense against insects via a novel polygalacturonase-inhibiting protein expression strategy. J. Biol. Chem. 295, 11833–11844. doi: 10.1074/jbc.RA120.014027
Heil, M., and Land, W. G. (2014). Danger signals—damaged-self recognition across the tree of life. Front. Plant Sci. 5:578. doi: 10.3389/fpls.2014.00578
Heloir, M. C., Adrian, M., Brule, D., Claverie, J., Cordelier, S., Daire, X., et al. (2019). Recognition of elicitors in grapevine: from MAMP and DAMP perception to induced resistance. Front. Plant Sci. 10:1117. doi: 10.3389/fpls.2019.01117
Heuts, D. P. H. M., Janssen, D. B., and Fraaije, M. W. (2007). Changing the substrate specificity of a chitooligosaccharide oxidase from Fusarium graminearum by model-inspired site-directed mutagenesis. FEBS Lett. 581, 4905–4909. doi: 10.1016/j.febslet.2007.09.019
Hou, S., Liu, Z., Shen, H., and Wu, D. (2019). Damage-associated molecular pattern-triggered immunity in plants. Front. Plant Sci. 10:646. doi: 10.3389/fpls.2019.00646
Huang, H., Ullah, F., Zhou, D. X., Yi, M., and Zhao, Y. (2019). Mechanisms of ROS regulation of plant development and stress responses. Front. Plant Sci. 10:800. doi: 10.3389/fpls.2019.00800
Huot, B., Yao, J., Montgomery, B. L., and He, S. Y. (2014). Growth-defense tradeoffs in plants: a balancing act to optimize fitness. Mol. Plant 7, 1267–1287. doi: 10.1093/mp/ssu049
Johnson, J. M., Thurich, J., Petutschnig, E. K., Altschmied, L., Meichsner, D., Sherameti, I., et al. (2018). A poly(a) ribonuclease controls the cellotriose-based interaction between Piriformospora indica and its host Arabidopsis. Plant Physiol. 176, 2496–2514. doi: 10.1104/pp.17.01423
Kalunke, R. M., Tundo, S., Benedetti, M., Cervone, F., De Lorenzo, G., and D’Ovidio, R. (2015). An update on polygalacturonase-inhibiting protein (PGIP), a leucine-rich repeat protein that protects crop plants against pathogens. Front. Plant Sci. 6:146. doi: 10.3389/fpls.2015.00146
Karasov, T. L., Chae, E., Herman, J. J., and Bergelson, J. (2017). Mechanisms to mitigate the trade-off between growth and defense. Plant Cell 29, 666–680. doi: 10.1105/tpc.16.00931
Kulys, J., Tetianec, L., and Schneider, P. (2001). Specificity and kinetic parameters of recombinant Microdochium nivale carbohydrate oxidase. J. Mol. Catal. B Enzym. 13, 95–101. doi: 10.1016/S1381-1177(00)00233-2
Land, W. G. (2018). Damage-associated molecular patterns in human diseases: Injury-induced innate immune responses. Vol. 1. Springer.
Land, W. G. (2020). Use of DAMPs and SAMPs as therapeutic targets or therapeutics: a note of caution. Mol. Diagn. Ther. 24, 251–262. doi: 10.1007/s40291-020-00460-z
Lee, M. -H., Lai, W. -L., Lin, S. -F., Hsu, C. -S., Liaw, S. -H., and Tsai, Y. -C. (2005). Structural characterization of glucooligosaccharide oxidase from Acremonium strictum. Appl. Environ. Microbiol. 71, 8881–8887. doi: 10.1128/aem.71.12.8881-8887.2005
Li, P., Lu, Y. -J., Chen, H., and Day, B. (2020). The lifecycle of the plant immune system. Crit. Rev. Plant Sci. 39, 1–29. doi: 10.1080/07352689.2020.1757829
Locci, F., Benedetti, M., Pontiggia, D., Citterico, M., Caprari, C., Mattei, B., et al. (2019). An Arabidopsis berberine bridge enzyme-like protein specifically oxidizes cellulose oligomers and plays a role in immunity. Plant J. 98, 540–554. doi: 10.1111/tpj.14237
Marcus, S. E., Blake, A. W., Benians, T. A., Lee, K. J., Poyser, C., Donaldson, L., et al. (2010). Restricted access of proteins to mannan polysaccharides in intact plant cell walls. Plant J. 64, 191–203. doi: 10.1111/j.1365-313X.2010.04319.x
Mattei, B., Spinelli, F., Pontiggia, D., and De Lorenzo, G. (2016). Comprehensive analysis of the membrane phosphoproteome regulated by oligogalacturonides in Arabidopsis thaliana. Front. Plant Sci. 7:1107. doi: 10.3389/fpls.2016.01107
Mélida, H., Bacete, L., Ruprecht, C., Rebaque, D., Del Hierro, I., Lopez, G., et al. (2020). Arabinoxylan-oligosaccharides act as damage associated molecular patterns in plants regulating disease resistance. Front. Plant Sci. 11:1210. doi: 10.3389/fpls.2020.01210
Mélida, H., Sopena-Torres, S., Bacete, L., Garrido-Arandia, M., Jorda, L., Lopez, G., et al. (2018). Non-branched beta-1,3-glucan oligosaccharides trigger immune responses in Arabidopsis. Plant J. 93, 34–49. doi: 10.1111/tpj.13755
Mhamdi, A., and Van Breusegem, F. (2018). Reactive oxygen species in plant development. Development 145:dev164376. doi: 10.1242/dev.164376
Nakagawa, Y. S., Kudo, M., Loose, J. S. M., Ishikawa, T., Totani, K., Eijsink, V. G. H., et al. (2015). A small lytic polysaccharide monooxygenase from Streptomyces griseus targeting α‐ and β‐chitin. FEBS J. 282, 1065–1079. doi: 10.1111/febs.13203
Neuser, J., Metzen, C. C., Dreyer, B. H., Feulner, C., van Dongen, J. T., Schmidt, R. R., et al. (2019). HBI1 mediates the trade-off between growth and immunity through its impact on apoplastic ROS homeostasis. Cell Rep. 28, 1670.e1673–1678.e1673. doi: 10.1016/j.celrep.2019.07.029
Orozco-Cardenas, M. L., and Ryan, C. A. (1999). Hydrogen peroxide is generated systemically in plant leaves by wounding and systemin via the octadecanoid pathway. Proc. Natl. Acad. Sci. U. S. A. 96, 6553–6655. doi: 10.1073/pnas.96.11.6553
Palacio-Lopez, K., Sun, L., Reed, R., Kang, E., Sørensen, I., Rose, J. K. C., et al. (2020). Experimental manipulation of pectin architecture in the cell wall of the unicellular charophyte, Penium margaritaceum. Front. Plant Sci. 11:1032. doi: 10.3389/fpls.2020.01032
Peretto, R., Favaron, F., Bettini, V., De Lorenzo, G., Marini, S., Alghisi, P., et al. (1992). Expression and localization of polygalacturonase during the outgrowth of lateral roots in Allium porrum L. Planta 188, 164–172. doi: 10.1007/BF00216810
Pontiggia, D., Ciarcianelli, J., Salvi, G., Cervone, F., De Lorenzo, G., and Mattei, B. (2015). Sensitive detection and measurement of oligogalacturonides in Arabidopsis. Front. Plant Sci. 6:258. doi: 10.3389/fpls.2015.00258
Rajniak, J., Barco, B., Clay, N. K., and Sattely, E. S. (2015). A new cyanogenic metabolite in Arabidopsis required for inducible pathogen defence. Nature 525, 376–379. doi: 10.1038/nature14907
Rasul, S., Dubreuil-Maurizi, C., Lamotte, O., Koen, E., Poinssot, B., Alcaraz, G., et al. (2012). Nitric oxide production mediates oligogalacturonide-triggered immunity and resistance to Botrytis cinerea in Arabidopsis thaliana. Plant Cell Environ. 35, 1483–1499. doi: 10.1111/j.1365-3040.2012.02505.x
Relja, B., and Land, W. G. (2020). Damage-associated molecular patterns in trauma. Eur. J. Trauma Emerg. Surg. 46, 751–775. doi: 10.1007/s00068-019-01235-w
Rodrigues Oblessuc, P., Vaz Bisneta, M., and Melotto, M. (2019). Common and unique Arabidopsis proteins involved in stomatal susceptibility to Salmonella enterica and Pseudomonas syringae. FEMS Microbiol. Lett. 366:fnz197. doi: 10.1093/femsle/fnz197
Rojo, E., Leon, J., and Sanchez-Serrano, J. J. (1999). Cross-talk between wound signalling pathways determines local versus systemic gene expression in Arabidopsis thaliana. Plant J. 20, 135–142. doi: 10.1046/j.1365-313x.1999.00570.x
Savatin, D. V., Ferrari, S., Sicilia, F., and De Lorenzo, G. (2011). Oligogalacturonide-auxin antagonism does not require posttranscriptional gene silencing or stabilization of auxin response repressors in Arabidopsis. Plant Physiol. 157, 1163–1174. doi: 10.1104/pp.111.184663
Savatin, D. V., Gramegna, G., Modesti, V., and Cervone, F. (2014). Wounding in the plant tissue: the defense of a dangerous passage. Front. Plant Sci. 5:470. doi: 10.3389/fpls.2014.00470
Schröder, R., Atkinson, R. G., and Redgwell, R. J. (2009). Re-interpreting the role of endo-beta-mannanases as mannan endotransglycosylase/hydrolases in the plant cell wall. Ann. Bot. 104, 197–204. doi: 10.1093/aob/mcp120
Sies, H., and Jones, D. P. (2020). Reactive oxygen species (ROS) as pleiotropic physiological signalling agents. Nat. Rev. Mol. Cell Biol. 21, 363–383. doi: 10.1038/s41580-020-0230-3
Souza, C. A., Li, S., Lin, A. Z., Boutrot, F., Grossmann, G., Zipfel, C., et al. (2017). Cellulose-derived oligomers act as damage-associated molecular patterns and trigger defense-like responses. Plant Physiol. 173, 2383–2398. doi: 10.1104/pp.16.01680
Toplak, M., Wiedemann, G., Ulicevic, J., Daniel, B., Hoernstein, S. N. W., Kothe, J., et al. (2018). The single berberine bridge enzyme homolog of Physcomitrella patens is a cellobiose oxidase. FEBS J. 285, 1923–1943. doi: 10.1111/febs.14458
Vaahtera, L., Schulz, J., and Hamann, T. (2019). Cell wall integrity maintenance during plant development and interaction with the environment. Nat. Plants 5, 924–932. doi: 10.1038/s41477-019-0502-0
Villares, A., Moreau, C., Bennati-Granier, C., Garajova, S., Foucat, L., Falourd, X., et al. (2017). Lytic polysaccharide monooxygenases disrupt the cellulose fibers structure. Sci. Rep. 7:40262. doi: 10.1038/srep40262
Voxeur, A., Habrylo, O., Guenin, S., Miart, F., Soulie, M. C., Rihouey, C., et al. (2019). Oligogalacturonide production upon Arabidopsis thaliana-Botrytis cinerea interaction. Proc. Natl. Acad. Sci. U. S. A. 116, 19743–19752. doi: 10.1073/pnas.1900317116
Vuong, T. V., Vesterinen, A. H., Foumani, M., Juvonen, M., Seppala, J., Tenkanen, M., et al. (2013). Xylo- and cello-oligosaccharide oxidation by gluco-oligosaccharide oxidase from Sarocladium strictum and variants with reduced substrate inhibition. Biotechnol. Biofuels 6:148. doi: 10.1186/1754-6834-6-148
Wallner, S., Dully, C., Daniel, B., and Macheroux, P. (2012). “Berberine bridge enzyme and the family of bicovalent flavoenzymes” in Flavoproteins, Vol. 1. Berlin: De Gruyter, 1–30.
Wan, J., Zhang, X. C., Neece, D., Ramonell, K. M., Clough, S., Kim, S. Y., et al. (2008). A LysM receptor-like kinase plays a critical role in chitin signaling and fungal resistance in Arabidopsis. Plant Cell 20, 471–481. doi: 10.1105/tpc.107.056754
Wei, Y., Tan, Y. L., Ang, E. L., and Zhao, H. (2020). Identification and characterization of citrus peel uronic acid oxidase. ChemBioChem 21, 797–800. doi: 10.1002/cbic.201900546
Winkler, A., Hartner, F., Kutchan, T. M., Glieder, A., and Macheroux, P. (2006). Biochemical evidence that berberine bridge enzyme belongs to a novel family of flavoproteins containing a bi-covalently attached FAD cofactor. J. Biol. Chem. 281, 21276–21285. doi: 10.1074/jbc.M603267200
York, W. S., Darvill, A. G., McNeil, M., Stevenson, T. T., and Albersheim, P. (1985). Isolation and characterization of plant cell walls and cell wall components. Methods Enzymol. 118, 3–40. doi: 10.1016/0076-6879(86)18062-1
Zablackis, E., Huang, J., Muller, B., Darvill, A. G., and Albersheim, P. (1995). Characterization of the cell-wall polysaccharides of Arabidopsis thaliana leaves. Plant Physiol. 107, 1129–1138. doi: 10.1104/pp.107.4.1129
Zang, H., Xie, S., Zhu, B., Yang, X., Gu, C., Hu, B., et al. (2019). Mannan oligosaccharides trigger multiple defence responses in rice and tobacco as a novel danger-associated molecular pattern. Mol. Plant Pathol. 20, 1067–1079. doi: 10.1111/mpp.12811
Keywords: growth-defense trade-off, Berberine bridge enzyme-like (BBE-like) proteins, cell wall DAMPs, oligo-saccharide oxidase, oxidized oligogalacturonides, oxidized cellodextrins
Citation: Pontiggia D, Benedetti M, Costantini S, De Lorenzo G and Cervone F (2020) Dampening the DAMPs: How Plants Maintain the Homeostasis of Cell Wall Molecular Patterns and Avoid Hyper-Immunity. Front. Plant Sci. 11:613259. doi: 10.3389/fpls.2020.613259
Edited by:
Massimo E. Maffei, University of Turin, ItalyReviewed by:
Antonio Molina, Polytechnic University of Madrid, SpainFrederick M. Ausubel, Harvard Medical School, United States
Copyright © 2020 Pontiggia, Benedetti, Costantini, De Lorenzo and Cervone. This is an open-access article distributed under the terms of the Creative Commons Attribution License (CC BY). The use, distribution or reproduction in other forums is permitted, provided the original author(s) and the copyright owner(s) are credited and that the original publication in this journal is cited, in accordance with accepted academic practice. No use, distribution or reproduction is permitted which does not comply with these terms.
*Correspondence: Giulia De Lorenzo, Z2l1bGlhLmRlbG9yZW56b0B1bmlyb21hMS5pdA==
†These authors have contributed equally to this work