- Department of Bioresource Engineering, McGill University, Sainte-Anne-de-Bellevue, QC, Canada
Rapid technology development in controlled environment (CE) plant production has been applied to a large variety of plants. In recent years, strawberries have become a popular fruit for CE production because of their high economic and nutritional values. With the widespread use of light-emitting diode (LED) technology in the produce industry, growers can manipulate strawberry growth and development by providing specific light spectra. Manipulating light intensity and spectral composition can modify strawberry secondary metabolism and highly impact fruit quality and antioxidant properties. While the impact of visible light on secondary metabolite profiles for other greenhouse crops is well documented, more insight into the impact of different light spectra, from UV radiation to the visible light spectrum, on strawberry plants is required. This will allow growers to maximize yield and rapidly adapt to consumer preferences. In this review, a compilation of studies investigating the effect of light properties on strawberry fruit flavonoids is provided, and a comparative analysis of how light spectra influences strawberry’s photobiology and secondary metabolism is presented. The effects of pre-harvest and post-harvest light treatments with UV radiation and visible light are considered. Future studies and implications for LED lighting configurations in strawberry fruit production for researchers and growers are discussed.
Introduction
Strawberry (Fragaria × ananassa) is a valuable crop cultivated worldwide. All strawberry species belong to the genus Fragaria and are members of Rosaceae, a family that contains many economically significant crops, primarily fruits such as apple (Malus domestica), pear (Pyrus communis), and peach (Prunas persica). The most cultivated strawberry species produced in North America is F. × ananassa, arising from breeding between two species: F. × virginiana and F. × chiloensis (Stewart and Folta, 2010). Strawberry fruits provide a wide range of sensory elicitation and health benefits to the consumer, including high fiber, micronutrient, and ascorbic acid content (Giampieri et al., 2012; Afrin et al., 2016; Battino et al., 2016). Additionally, strawberry fruits are part of a growing trend that highlights plant-derived antioxidants for their proven health benefits (Nile and Park, 2014). These consumer-liking and health-promoting properties have consequently led to strawberry’s strong economic role in the fruit industry. In 2016, the global total production of strawberries was over US$17.7 billion, and this value has substantially increased over the last 10 years (FAO, 2016).
The production of strawberry fruit and their nutritional value are highly impacted by the surrounding environment; therefore, strawberries are often produced in controlled environments (CEs) where lighting and temperature are controlled (Samtani et al., 2019). The use of artificial lighting is a common approach for flower initiation and improved fruit yield (López-Aranda et al., 2011; Hidaka et al., 2014). The critical photoperiod for strawberry flower initiation varies depending upon cultivars and interactions with temperature (Bradford et al., 2010; López-Aranda et al., 2011; Heide et al., 2013). Inhibition of flower initiation at high temperature has been reported for strawberry plants with flowering habits under different photoperiods (Ito and Saito, 1962; Heide, 1977; Serçe and Hancock, 2005). As such, it is suggested that it is inadequate to classify strawberry cultivars solely based on their flowering habits (i.e., short-day, long-day, and day length-insensitive) without considering temperature effects (Durner et al., 1984). Artificial light properties, including wavelength and intensity, also play an important role in strawberry fruit production and quality (Nadalini et al., 2017; Zahedi and Sarikhani, 2017). For instance, sole blue light treatment enhances strawberry (F. × ananassa cv. Elsanta) fruit production, approximately 25% more than other light sources (Nadalini et al., 2017). End-of-day 735-nm radiation treatment results in a higher strawberry sucrose level (Zahedi and Sarikhani, 2017). These studies have proven that the use of artificial lighting systems allows growers to optimize fruit production and meet consumers’ sensory desires (Nadalini et al., 2017; Zahedi and Sarikhani, 2017).
Plant-derived antioxidants are produced through secondary metabolic pathways, and act as an essential protective barrier against both biotic and abiotic stressors, including light stress (Pocock, 2015; Nadalini et al., 2017). Secondary metabolites such as flavonoids and quinones protect plants from oxidation caused by free radical scavenging (Lü et al., 2010). The extent of secondary metabolite accumulation further influences plant and fruit features, such as specific coloration and antioxidant properties that consumers adore (Akula and Ravishankar, 2011). The high level of total antioxidant capacity contained in strawberry fruit enables the neutralization of free radicals and reduces oxidative stress in the human body (Afrin et al., 2016). The most prevalent secondary metabolites in strawberry fruits are flavonoids, including anthocyanins (Aaby et al., 2012), which are associated with antioxidative and anti-inflammatory properties. Flavonoids predominantly protect plants from UV radiation (Panche et al., 2016), and anthocyanins protect plants from blue and green light (Landi et al., 2020a). In recent years, known antioxidant properties of strawberry fruit have prompted the rise of its global consumption.
Considering the important role that strawberry plants play in the fruit industry, several reviews on strawberry production have been conducted, including flower initiation, development, handling, flavor profile, and health benefits (López-Aranda et al., 2011; Heide et al., 2013; Afrin et al., 2016; Baicu and Popa, 2018; Yan et al., 2018). However, there is limited information available on the impact of light properties on strawberry productivity and secondary metabolite accumulation. Secondary metabolite accumulation in strawberries is impacted by interactive effects between light wavelengths, developmental stages, and lighting strategies (i.e., pre-harvesting and post-harvesting; Erkan et al., 2008; Kadomura-Ishikawa et al., 2013). To this end, this review attempts to compile and compare available research on the impact of light properties within the wavelength ranges of UV radiation (<380 nm) to the visible light spectrum (380–730 nm) on strawberry fruit production, as well as the major group of secondary metabolites, flavonoid compounds. This may lead to improved strawberry fruit production and quality with additional health values in antioxidant activity, while possibly allowing for knowledge transfer to other berry plants grown in CEs.
Flavonoid Profile and Function in Strawberry Fruits
Plant secondary metabolites have several functions in light signaling and defending against abiotic stresses (Thirumurugan et al., 2018). The most prevalent class of secondary metabolites in strawberry fruits is phenolic compounds, which have at least one phenol unit (aromatic organic ring) in their chemical structures. Phenolic compounds are further divided into different sub-groups, including coumarins, flavonoids, phenolic acids, and tannins. Flavonoids are widely found in foods and beverages of plant origin (i.e., fruits and vegetables; Rozema et al., 2002; Delgado et al., 2019). Flavonoids are easily recognized as flower pigments – they are responsible for the color and aroma of flowers (Dewick, 2001). Flavonoids can be further sub-classified to different subgroups: anthocyanins, flavonols, and flavanols (Aaby et al., 2012; Alvarez, 2014). Over 10,000 flavonoids have been reported, representing the third largest group of naturally occurred secondary metabolites, after terpenoids and alkaloids (Martens et al., 2010). Most flavonoids absorb wavelengths between 315 and 400 nm; therefore, they play an important role in UV radiation screening and as antioxidants for plants (Kotilainen et al., 2009). Sunlight and UV radiation exposure directly impact the extent of flavonoid accumulation in plants (Downey et al., 2006).
Anthocyanins
Anthocyanins are the most prevalent phenolic compound found in the outer cell layers of various fruits, constituting up to 40% of total phenols in some strawberry cultivars (Aaby et al., 2012). In strawberry, the major anthocyanin is pelargonidin 3-glucoside, with reported anti-inflammatory effects (Da Silva et al., 2007; Amini et al., 2017). Although anthocyanin accumulation is implicated in UV–B protection (280–315 nm), it also occurs under stress conditions involving visible light and far-red radiation (Carvalho and Folta, 2016; Dou et al., 2017). Anthocyanins are the pigments responsible for coloration in flowers and fruits, often serving as visual signal for insect-mediated pollination and seed dispersers (Turturică et al., 2015). Anthocyanin stability largely depends on light, temperature, pH, and the co-pigmentation with other flavonoids (i.e., flavonols; Martens et al., 2010; Turturică et al., 2015). Anthocyanin color is pH-dependent because of its ionic nature; anthocyanin pigments appear red under acidic conditions and blue under alkaline conditions (Khoo et al., 2017). In strawberry plants, anthocyanin accumulates quickly in the late stages of ripening, beginning when fruits turn from white to red and increase more than 10-fold in red, ripe berries (Kadomura-Ishikawa et al., 2013). These phytochemicals largely contribute to antioxidant capacity, impacting the nutritional benefits of the fruit (Aaby et al., 2012; Kadomura-Ishikawa et al., 2013). About 70% of total antioxidant capacity comes from anthocyanins, highlighting its importance among plant secondary metabolites (Wang and Millner, 2009; Giampieri et al., 2012).
Flavonols
Flavonols are abundantly found in a variety of fruits and vegetables including apples, grapes, and berries, and are reportedly associated with antioxidant potential and reduced risk of vascular disease in humans (Panche et al., 2016). In cultivated strawberry, the major flavonols are quercetin and kaempferol (Labadie et al., 2020). Flavonols are often the main flavonoids at the beginning of the fruit development, but at the ripening stage the flavonoid pathway switches to anthocyanin production (Chassy et al., 2012). Compared to anthocyanins, flavonols contribute more to antioxidant protection against UV-B radiation (Ferreyra et al., 2012; Zoratti et al., 2014); however, they are more sensitive to light properties (Carbone et al., 2009). Studies have reported that flavonol accumulation is highly reduced under shadow treatment in grape (Vitis vinifera) skins and is influenced by light levels in grape berry (Vitis berlandieri × V. vinifera; Pereira et al., 2006; Matus et al., 2009). Apart from functioning as a tissue-protector against UV radiation, flavonols act as flower pigments that attract and defend against insects (Gronquist et al., 2001). Flavonols influence plants’ responses to gravity, but these effects were observed in mutants only (Owens et al., 2008).
Flavanols
Flavanols, also called flavan-3-ols, are the most common dietary flavonoids. They are used as functional ingredients in food processing to control microbial levels and provide oxidative stability (Aron and Kennedy, 2008). Flavanols consist of monomeric units (i.e., catechins and epicatechin), in addition to oligomeric and polymeric compounds (proanthocyanidins, also called condensed tannins; Al-Dashti et al., 2018). Like anthocyanins and flavonols, flavanol accumulation is developmental stage-dependent (Zhang et al., 2013). For instance, supplemental UV radiation increases flavanol content during development but not in mature grape berries (V. vinifera cv. Cabernet Sauvignon). Flavanols help plants protect against harmful pathogens, such as microbes and fungi, as well as insects and herbivorous animals (Aron and Kennedy, 2008). As for flavanols’ dietary effects, they may improve vascular function and nitric oxide availability, as well as modulate metabolism and respiration (Al-Dashti et al., 2018). Being flavanol polymers, it has been reported that proanthocyanidins possess antioxidative and cardio-preventive properties (Monagas et al., 2010).
Plant Photomorphogenetic Responses and Flavonoid Biosynthesis Under UV Radiation
Biologically active radiation extends from 300 to 800 nm. UV radiation lies in the wavelength range below 380 nm, followed by the visible spectrum between 380 and 720 nm. Outdoors or in an environment lit without supplemental light, approximate 6% of solar radiation is UV radiation, comprising 95% UV-A radiation (315–380 nm) and 5% of UV-B radiation (280–315 nm). UV-C radiation (<280 nm) does not penetrate to Earth’s surface because of the ozone layer. Currently, the major focus of UV radiation-plant investigations is on the UV-B wavelength range, and the number of studies targeting UV-A radiation is relatively small (Verdaguer et al., 2017).
Secondary metabolite formation, including phenolic compounds and antioxidants, is a plant’s response to UV-A and UV-B radiation (Caldwell and Britz, 2006), and most flavonoids absorb light in the UV-A radiation range (Cerovic et al., 2002). High levels of UV radiation can cause damage to plants at different levels, including DNA and lipids, leading to impaired gene transcription and photosynthesis (Kunz et al., 2006; Khudyakova et al., 2017). Plant responses to UV-A and UV-B radiation are summarized in Figure 1A. UV radiation alters plant morphology and biomass accumulation during both vegetative and reproductive stages (Müller-Xing et al., 2014; Bernal et al., 2015), and UV-A radiation is perceived by several photoreceptors, including cryptochromes and phytochromes (Figure 1B; Mockler et al., 2003; Folta and Carvalho, 2015). Cryptochromes (cry1 and cry2) are flavin-type blue light photoreceptors (320–500 nm) that have been implicated in numerous developmental and circadian signaling pathways (Banerjee and Batschauer, 2005; Jones, 2018). Phytochromes (phyA to phyE) are light-sensitive proteins with photo-reversible conformers: Pr and Pfr (Folta and Carvalho, 2015). Phytochrome Pr, the inactive form of phytochrome, has a primary absorption peak at 660 nm and a secondary absorption peak located at 380 nm. Absorption peaks of the active form shift approximately 20–70 nm, toward longer wavelengths (408 and 730 nm; Stutte et al., 2009).
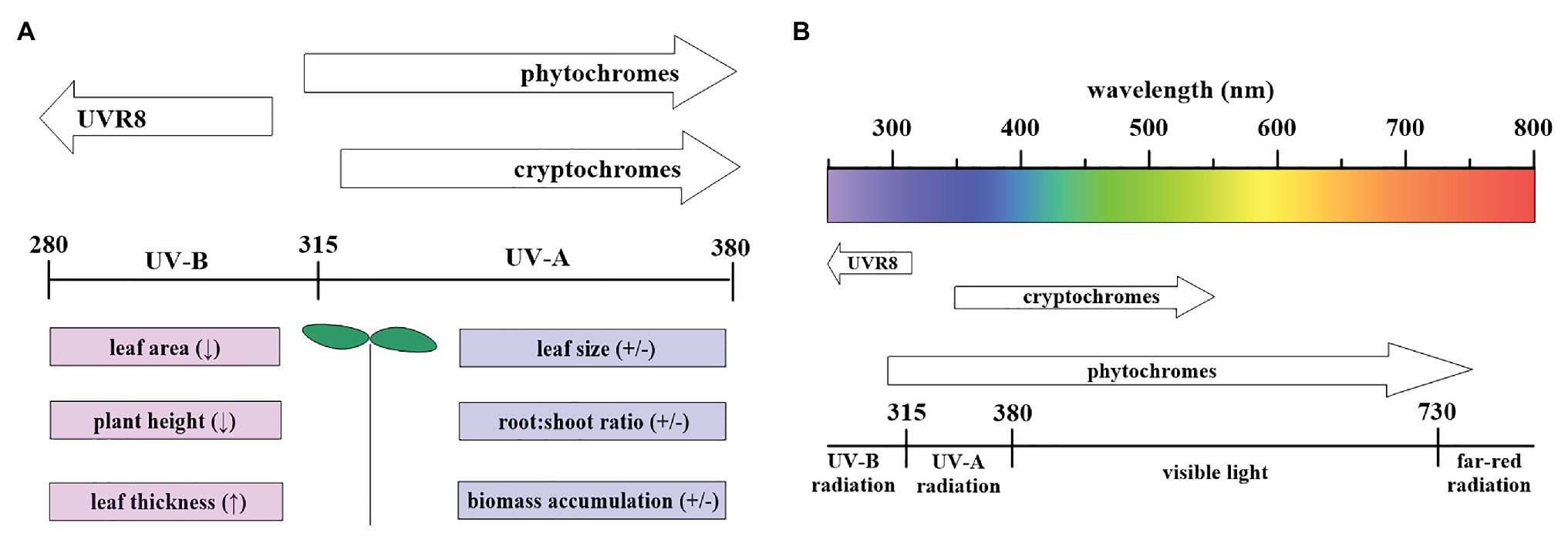
Figure 1. (A) Plant responses to UV-A and UV-B radiation, with both positive and negative effects induced by UV-A radiation. (B) Plant photoreceptors involved in flower initiation with their corresponding spectral regions, adapted from Folta and Carvalho (2015) and Jones (2018).
The mechanisms underlying this process are poorly investigated in plants, and varied plant responses to UV-A radiation regarding leaf size, morphology and biomass accumulation are reported (Biswas and Jansen, 2012; Kataria and Guruprasad, 2012; Verdaguer et al., 2017). Leaf size is one of the most important determinants of light capture and productivity. Increased rosette size was observed under different accessions of Arabidopsis thaliana grown indoors under 1.59 W m−2 UV-A radiation and 30 μmol m−2 s−1 of white light (4,000 K; Biswas and Jansen, 2012). The use of UV-blocking films further revealed that UV-A radiation increases total leaf area in soybean (Glycine max) when grown in a greenhouse (Zhang et al., 2014). To the contrary, the solar spectrum, without UV-A and UV-B radiation, induced a larger leaf size when compared to UV-A and UV-B radiation in different varieties of sorghum (Sorghum bicolor; Kataria and Guruprasad, 2012). Published data shows no clear link between the impact of UV-A radiation and biomass accumulation, as inconsistent responses have been reported for UV-A-mediated biomass responses (Kataria and Guruprasad, 2012; Zhang et al., 2014). Some studies demonstrated stimulatory effects on biomass accumulation under UV-A radiation (Bernal et al., 2013; Zhang et al., 2014), while others reported inhibitory effects (Kataria et al., 2013). One study concluded that the genotype determines UV-A-mediated responses in plants; however, the study was solely conducted using A. thaliana ecotypes (Cooley et al., 2001).
Such contradictory findings may be due to changes in morphology and photosynthetic activity, as well as accumulation of secondary metabolites (Verdaguer et al., 2017). Apart from the impact of plant physiological properties on UV-A radiation, different UV-A radiation conditions might contribute to these conflicting findings. Most UV-A-mediated response studies were conducted with UV-blocking films and the solar spectrum as radiation sources (Zhang et al., 2014; Khudyakova et al., 2017). The use of UV-blocking films only enables the reflection of a certain percentage of UV radiation from solar radiation, and their cut-off wavelengths vary depending on the manufacturer (Katsoulas et al., 2020). In this scenario, although all studies reported the same radiation treatments (i.e., UV-A radiation), radiation spectra might differ. Distinct UV spectra and radiation properties could potentially lead to different plant responses. Furthermore, users cannot select for specific wavelengths passing through the UV-blocking films, as is possible with bandpass optical filters (i.e., blocking UV-A radiation only). As such, UV-A + UV-B treatments are often used as a baseline to compare and discuss the impact of UV-A radiation. Although potential interactive effects between UV-A and UV-B radiation have not yet been reported, they should still be considered and determined with future research.
The most frequently reported UV-B-induced morphological changes are a decrease in leaf area and/or an increase in leaf thickness (Figure 1A; Klem et al., 2012; Robson and Aphalo, 2012; Doupis et al., 2016). UV-B radiation results in leaf changes (i.e., chlorosis, necrosis, and desiccation), and declines in plant height and shoot growth. These observations may serve as a protective mechanism since UV-B can damage photosystems (Gupta et al., 2017). Additionally, secondary metabolite responses differ under UV-B radiation (Schreiner et al., 2012; Hectors et al., 2014). For instance, polyamine and tocopherol levels upregulate quickly (within less than 24 h) in A. thaliana (Hectors et al., 2014), whereas flavonoids accumulate at a lower rate, with steady states usually reached after several days (Kusano et al., 2011). Furthermore, dose-dependent responses by flavonoids have been reported; a moderate UV-B dose (ambient radiation) induces flavonoid rutin production, which decreases under both reduced and enhanced UV-B dosage. Ambient UV-B dosage stimulates rutin accumulation, and accumulated rutin is more evident in buckwheat (Fagopyrum esculentum) leaves than in flowers (Kreft et al., 2002; Jansen et al., 2008). This suggests that different protectants respond differently based on UV radiation dosage, and that the relative abundance of different flavonoid species differs after UV radiation treatment, implying that distinct dose-response curves for each individual flavonoid compounds exist (Reifenrath and Müller, 2007).
UV-B radiation is perceived by the UV-B photoreceptor UV resistance locus 8 (UVR8), which promotes pest resistance and increases flavonoid accumulation. Under a low dose of UV-B radiation, UVR8 photoreceptor signaling is mediated through the RING-finger type ubiquitin E3 ligase CONSTITUTIVE PHOTOMORPHOGENIC1 (COP1; Figure 2; Lau and Deng, 2012; Peng et al., 2013). COP1 promotes the expression of ELONGATED HYPOCOTYL5 (HY5) in A.thaliana (Lau and Deng, 2012) and apple (Malus × domestica; Peng et al., 2013). Specifically, the presence of low UV-B radiation results in UVR8 monomerization, and UVR8 monomers interact with COP1 to initiate the UV-B signaling pathway. The UVR8-COP1 complex then activates HY5 binding to the promoter region of different R2R3 MYBs, and this leads to flavonoid accumulation in the nucleus (Peng et al., 2013; Jenkins, 2017). Under a high dose of UV-B radiation, UV-B signaling may occur independently of UVR8, possibly via mitogen-activated protein kinase (MAPK) signaling (Besteiro et al., 2011).
The negative effects of UV-C radiation on plant development are well established (Urban et al., 2016). Overexposure of UV-C radiation can lead to shortened shelf-life for fresh produce and a reduction in photosynthetic efficiency (De Oliveira et al., 2016; Li et al., 2019). UV-C radiation inflicts considerable damage on lipids and DNA; hence, it is often credited with the most bactericidal activity within the UV wavelength range (Santos et al., 2013). In the context of plant secondary metabolism, it is important to note that UV-C radiation induces the accumulation of phenolics and flavonoids (Nigro et al., 2000; Erkan et al., 2008). However, because of higher energy contained in each photon, the focus on UV-C plant applications for secondary metabolites is largely placed on low doses and on pre‐ and post-harvesting treatments (Urban et al., 2016).
The Impact of UV Radiation on Strawberry Fruit Flavonoids
Two approaches are often used to manipulate wavelength in CE production: UV-blocking films (i.e., pure polyethylene) and light-emitting diodes (LEDs; Singh et al., 2015; Katsoulas et al., 2020). Both technologies can manipulate wavelengths, yet they have different constraints and effective spectrum ranges. Earlier studies have reported the impact of UV dosage (combined UV-A and UV-B radiation) on strawberry fruit flavonoid levels, predominantly using UV-blocking films (Josuttis et al., 2010; Tsormpatsidis et al., 2011). Strawberry (cvs. Everest, Elsanta) fruits grown under films with high UV transparency (UV-A + UV-B) have higher anthocyanin and phenolic content (cyanidin 3-glucoside, quercetin 3-glucuronide, and kaempferol 3-glucoside) than the strawberry fruits grown under UV-blocking film (Josuttis et al., 2010; Tsormpatsidis et al., 2011). Moreover, UV radiation affects strawberry fruit firmness and color, in which fruit ripened with UV radiation was smaller, firmer, and darker compared to fruit grown under UV-blocking film (Tsormpatsidis et al., 2010; Ordidge et al., 2012). These earlier studies provide insightful information on the impact of UV radiation on strawberry fruit quality and flavonoid contents. However, reported effects on the impact of UV radiation include both UV-A and UV-B radiation. Specific wavelength or radiation treatments within the UV wavelength range cannot be achieved by using solar UV radiation and UV-blocking films. It is unknown if interactive effects within the UV range exist for strawberry flavonoid accumulation.
Unlike UV-blocking films, LEDs offer higher controllability of light properties, such as specific wavelength(s), photoperiod adjustment, and a wide range of intensities (Zoratti et al., 2014; Alrifai et al., 2019; Wu et al., 2019). Many recent studies show the potential of manipulating plant growth and regulating plant secondary metabolite profiles with LED lighting on numerous greenhouse crops within the visible spectrum (Stewart and Folta, 2010; Cocetta et al., 2017; Landi et al., 2020b). In recent years, steady progress has been made regarding wall-plug efficiency and the life-span of UV-LEDs (Kneissl, 2016). UV-LEDs may be superior to UV-blocking films when investigating UV radiation. However, the majority of strawberry studies using UV-LEDs is for enhanced strawberry fruit production, not insect and disease control (Kanto et al., 2009; Suthaparan et al., 2016). To our knowledge, only one study using UV-LED on strawberry (cvs. Maehyang and Seolhyang) flavonoid level has been reported to date, demonstrating that anthocyanin content increased in the Seolhyang cultivar when irradiated with combined 254, 306, and 352-nm LED radiation (Kim et al., 2011). Insufficient data suggest there is a clear lack of studies in this regard, and further study is required to elucidate the impact of narrow UV-A and UV-B spectra on strawberry flavonoids and other secondary metabolite accumulation.
Studies on strawberry secondary metabolites and UV-C radiation have concentrated on pre‐ and post-harvest treatment (Erkan et al., 2008; Xu et al., 2017a, 2019). This might be because of the availability of UV-C radiation sources (i.e., UV discharge lamps with a major peak at 255 nm) and the adverse effect of UV-C radiation on plant development (Kim et al., 2011). Low dose UV-C radiation at the post-harvesting stage has been applied to many valuable crops to reduce postharvest losses due to fungal growth and fruit decay (Marquenie et al., 2002). This UV-C radiation treatment is recognized as an effective method to enhance secondary metabolite concentrations in strawberry fruit within the food industry (Xu et al., 2017a; Saini and Keum, 2018; Li et al., 2019). UV-C radiation improves the antioxidant capacity and reduces softening of fresh-cut strawberry fruit (Erkan et al., 2008; Pombo et al., 2009; Li et al., 2019). Erkan et al. (2008) first observed that low dose UV-C radiation (0.43–4.30 kJ m−2) promoted the antioxidant capacity and phenolic content in strawberry fruit storage at 10°C (post-harvest), yet few effects were observed for anthocyanin accumulation. A recent study reported that both total phenolic compounds and anthocyanin in strawberry fruit significantly increased (>20%) with post-harvest treatment using UV-C radiation at 4°C (Li et al., 2019). Low dose UV-C radiation (4.1 kJ m−2) slowed down strawberry fruit softening and degradation (Pombo et al., 2009).
Unlike post-harvest treatment, pre-harvest treatment with UV-C radiation is a relatively new approach to improving fruit quality that shows promise (Xie et al., 2015; Severo et al., 2017; Xu et al., 2017a). With UV-C radiation, strawberry fruit exhibit an increase in sucrose, ascorbic acid, and phytochemical profiles (ellagic acid and kaempferol-3-glucuronide). It is possible that UV-C radiation might affect fruit quality via the action of plant hormones, as it may be involved in abscisic acid signaling. A recent study showed that pre-harvest UV-C radiation of strawberries had a dose-dependent effect on secondary metabolite levels. Upon harvest, strawberry fruit that underwent UV-C radiation had a higher overall level of anthocyanins and flavonols at 15 kJ m−2, and this level dropped to that of the control under the highest UV-C dose (29.4 kJ m−2; Xu et al., 2017b). However, pre-harvest UV-C radiation also induced a decrease in volatile compounds responsible for aroma, impacting its flavor profile (Severo et al., 2017; Table 1).
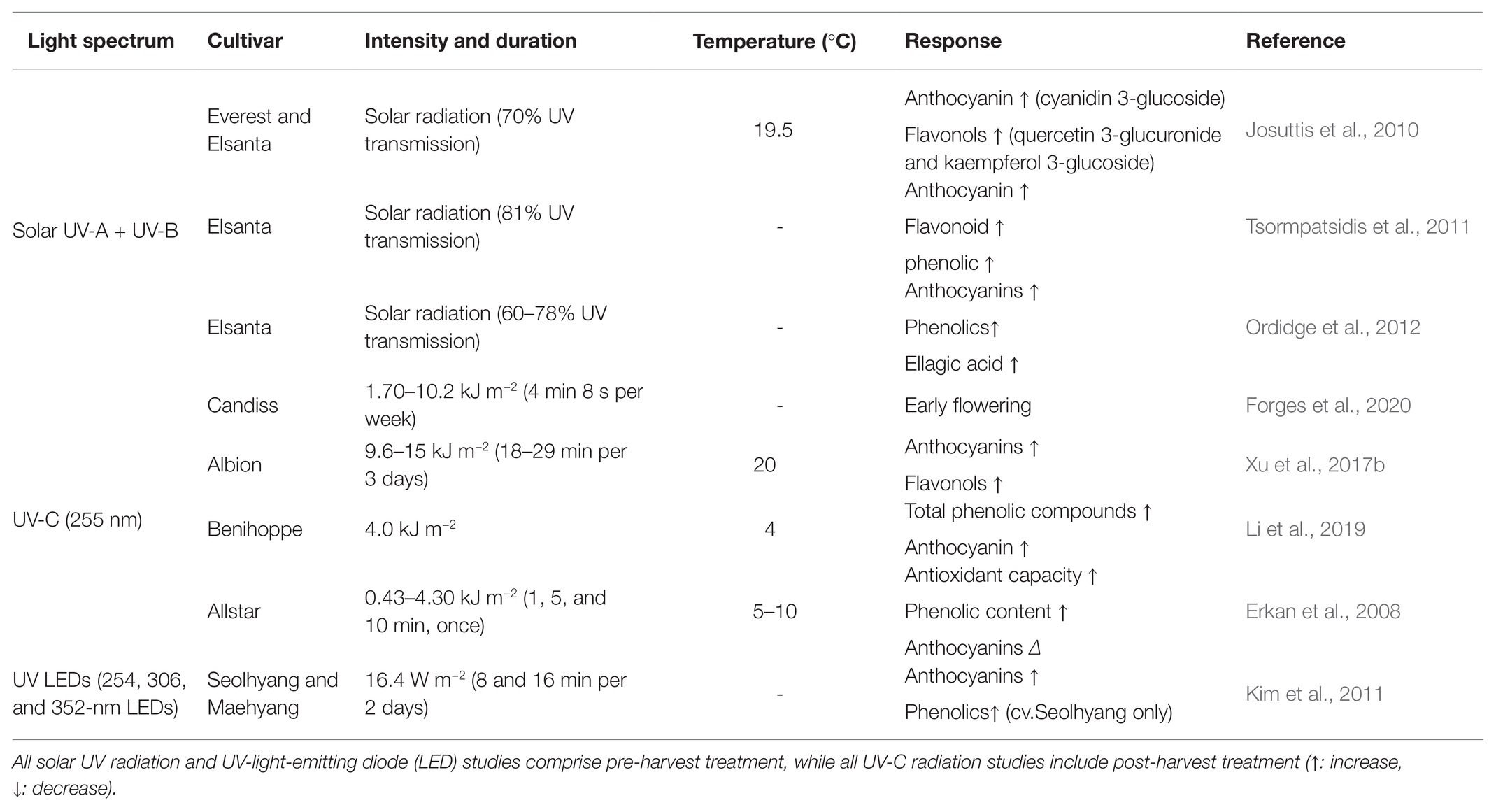
Table 1. Strawberry anthocyanin, phenolic, and plant responses under UV radiation compared to control lighting (solar spectrum without UV radiation).
The Impact of Visible Light and Far-Red Radiation on Strawberry Development and Flavonoid Accumulation
Key transcription factors for flavonoid biosynthesis have been identified in many fruit crops, such as apple, grapevine, and woodland strawberry (Jaillon et al., 2007; Velasco et al., 2010; Shulaev et al., 2011). These studies indicate that R2R3 MYB transcription factors are the primary regulators of fruit flavonoid biosynthesis in response to changing light conditions within the visible light spectrum. Contrary to its positive regulator role under UV-radiation, COP1 acts as a repressor of flavonoid biosynthesis under visible light (Shulaev et al., 2011; Zoratti et al., 2014). Specifically, COP1 is exported from the nucleus to the cytoplasm, allowing nuclear-localized transcription factors to accumulate and induce expression of genes that are directly regulated by R2R3 MYB transcription factors (Lau and Deng, 2012; Zoratti et al., 2014). Within the visible spectrum, blue light most prominently affects fruit flavonoid accumulation, particularly anthocyanins in unripe strawberries (Zoratti et al., 2014). The relevance of blue light photoreceptors and anthocyanin accumulation was demonstrated at the molecular level, and it was observed that the elevated expression of phototropin 2 FaPHOT2 corresponded to an increase in anthocyanin content (Kadomura-Ishikawa et al., 2013), In addition, blue light leads to overexpression of cryptochrome, resulting in anthocyanin accumulation (Giliberto et al., 2005). Blue light is perceived by cryptochromes that influence different plant developmental steps, including flowering induction and fruit secondary metabolite production (Fantini and Facella, 2020). Increases in flavonoid/anthocyanin biosynthesis with blue light have been reported in tomato (Solanum lycopersicum cv. Moneymaker; Lopez et al., 2012) and grape (cv. Malbec; Gonzalez et al., 2015). The impact of blue light and supplemental red light on anthocyanin accumulation has also been investigated in many greenhouse crops (Liu et al., 2018).
Attention on strawberry secondary metabolites is mainly placed on anthocyanin accumulation in CE agriculture, and investigations are mainly conducted with two approaches: (i) sole LED lighting as a growing light with post-harvest treatment; and (ii) different lighting strategies that result in different strawberry phenolic compound/anthocyanin biosynthesis responses (Kadomura-Ishikawa et al., 2013; Miao et al., 2016; Nadalini et al., 2017). Table 2 summarizes current research on the modulation of strawberry flavonoid and fruit productivity under the visible spectrum. Opposed to other greenhouse crops, conflicting data for blue-light-mediated responses and strawberry fruit flavonoid accumulation exist. It appears that blue light is not the most effective wavelength for anthocyanin production in strawberry fruit (Besteiro et al., 2011; Piovene et al., 2015; Nadalini et al., 2017). Blue LED light (e.g., 436 and 470-nm light) induces a higher number of flower clusters and increases final yield for strawberry plants (Nadalini et al., 2017; Magar et al., 2018). A reduction in anthocyanin (pelargonidin-3-glucoside) and phenolic concentrations was observed in strawberry fruit grown under sole 436-nm LED light when compared to the fluorescent light spectrum, with peaks at 430, 450, 540, and 620 nm (Nadalini et al., 2017). Different ratios of 630 and 450 nm (0.7–5.5) LED light has no impact on flavonoid concentrations for strawberry fruit when compared to fluorescent light (Piovene et al., 2015). In the same study, lower flavonoid concentrations were reported in basil leaves (Ocimum basilicum) grown under red and blue LED light when compared to basil grown under fluorescent light. The authors concluded that the impact of blue light on flavonoid biosynthesis is species-dependent (Nadalini et al., 2017). Light bandwidth might also attribute to varied responses under blue light. Contrary to other strawberry studies, Miao et al. (2016) reported increased anthocyanins (cyanidin 3-glucoside) in the strawberry cultivar “Yueli” grown under blue plastic film (Miao et al., 2016). Although details of the blue light spectrum used in this study are not known, it is possible that the blue light produced with blue plastic film has a broad spectrum that is similar to the one from UV-blocking films. A broader spectrum of blue light (>25 nm LED bandwidth) could lead to overexpression of both phototropin and cryptochrome, two main blue light photoreceptors. Further investigation into the impact of blue light bandwidths, as well as how blue light influences flavonoid accumulation in different strawberry plant tissues, may answer this question.
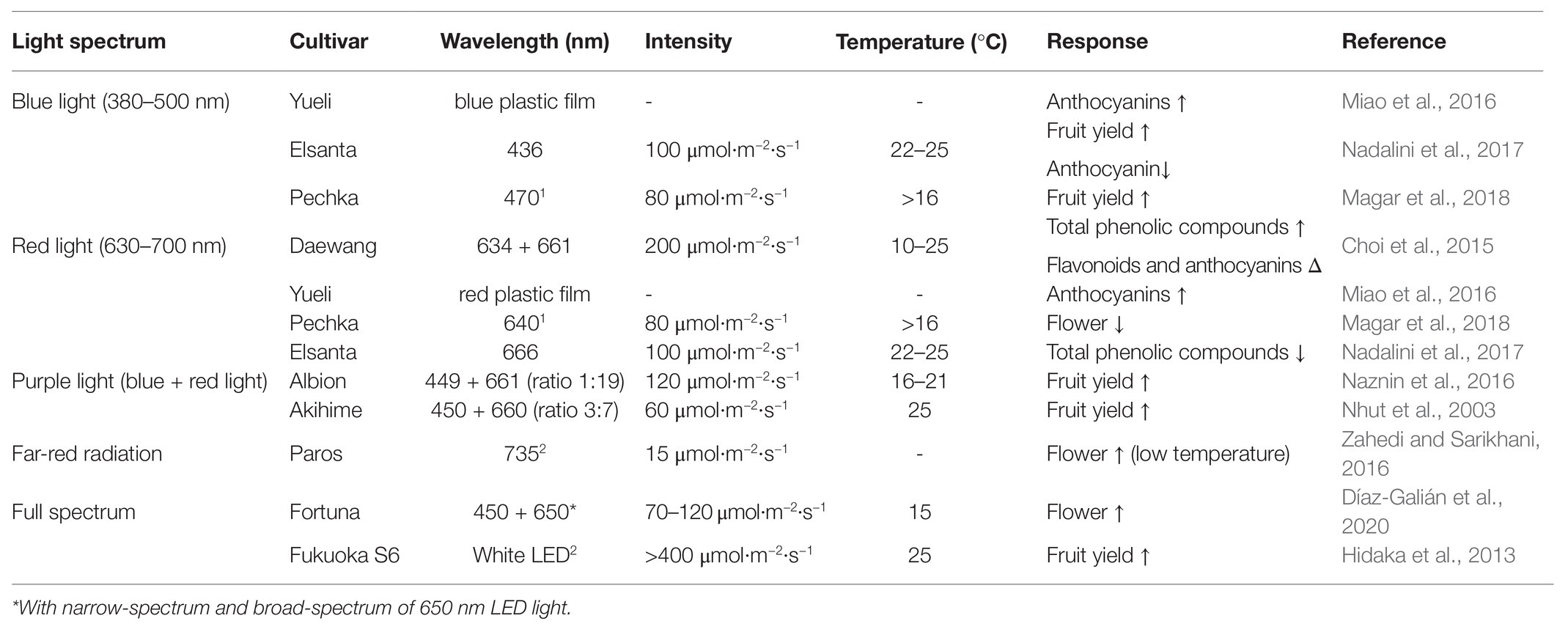
Table 2. Strawberry anthocyanin, phenolic, and plant responses under the assigned light exposition compared to control (white light produced by fluorescent lamps; 1: post-harvesting treatment, 2: supplemental lighting, ↑: increase, Δ: same as control, ↓: decrease).
In the presence of red light and far-red radiation, activated photoreceptors (phytochromes) repress COP1 function and allow its export from the nucleus, thus inducing flavonoid gene expression (Lau and Deng, 2012; Tossi et al., 2019). The impact of red light on strawberry secondary metabolites and development was reported by Choi et al. (2015), who compared the flavonoid and phenolic compounds of strawberry (cv. Daewang) when grown under 448, 634, and 661-nm LED light in a growth chamber, and with supplementary light in a plastic greenhouse. Upon fruit maturation and testing, higher levels of total phenolic compounds were observed under red light (634 + 661 nm) treatment in the growth chamber, yet there was no significant difference in the amount of anthocyanin and flavonoid between any of the treatments. This was confirmed in a later study with 666-nm LED and strawberry (cv. Elsanta; Nadalini et al., 2017). Similar to the conflicting data obtained with the blue film, opposite anthocyanin responses to “red light” produced with red film and red LED light were reported (Miao et al., 2016). Strawberry (cv. Yueli) grown under red film had a significant impact on total anthocyanin concentration and individual anthocyanins (pelargonidin 3-glucoside and pelargonidin 3-malonyglucoside; Miao et al., 2016). Although the detailed light spectrum under these plastic films was not presented (Miao et al., 2016), differences in secondary metabolite responses to light spectrum imply that specific wavelengths can differentially affect secondary metabolite accumulation in strawberry plants.
Unlike LED light in the visible spectrum, sole far-red radiation has less impact on secondary metabolite accumulation. Yet, it plays an import role in flowering and has proven an effective method for improved flowering with short duration at the end of the day (Zahedi and Sarikhani, 2016, 2017). The authors tested the end-of-day approach with 735-nm LED radiation using different exposure durations and temperatures on various developmental stages for strawberry (cv. Paros). They concluded that flowering can be induced in 12-week old and older strawberry plants through 32 daily cycles of 735-nm LED radiation at a cooler temperature (Zahedi and Sarikhani, 2016). A similar conclusion on flowering initiation was made when end-of-day lighting was combined with mixed red light and far-red radiation (Rantanen et al., 2014).
Although beyond the scope of this review, it is noteworthy to mention that purple light, with different ratios of red and blue LED light as well as intensity, results in increased stolon production, higher photosynthetic activity, and fruit productivity in strawberry plant (Nhut et al., 2003; Wu et al., 2009; Piovene et al., 2015; Naznin et al., 2016). An increase in strawberry flowering and fruit yield occurs with full-spectrum and white LED light (Hidaka et al., 2013; Díaz-Galián et al., 2020). In addition to the purple light spectrum, intensity is critical for influencing strawberry growth (Zhou et al., 2005; Choi et al., 2015). This response appears cultivar-dependent (Smeets, 1976, 1980). An increased daily light integral result in higher dry matter accumulation, propagation efficiency, and quality of strawberry runner plants (Miyazawa et al., 2009; Zheng et al., 2019). In summary, the importance of wavelength and its bandwidth should be emphasized and light spectra with different emitting wavelengths and lighting strategies clearly affect flavonoid content and fruit productivity in strawberry plants. The shift from broad-spectrum to narrow spectrum artificial lighting system is gaining momentum, but there is a paucity of studies comparing broad-spectrum to narrow-spectrum light. This is compounded by conflicting findings for the blue-light-mediated response in strawberry fruit. More CE research could further elucidate the impact of different wavelengths and underlying mechanisms, while determining interactions with temperature-dependent processes, to improve fruit properties, including yield, quality, and nutritional value.
UV Radiation and Photobiological Safety
UV radiation triggers the accumulation of flavonoids and other secondary metabolites (Caldwell and Britz, 2006). However, widespread use remains elusive, mainly caused by a limited selection of UV radiation sources and the photobiological hazard it presents to humans (Voke, 1999). Several types of UV radiation sources (i.e., gas-discharge lamps, fluorescent bulbs, and LEDs) are not often used in plant photobiology studies. UV gas-discharge lamps radiate a sharp 255-nm spectrum, and are accompanied by many disadvantages, including low radiation output and limited effective radiation area (Sarigiannis et al., 2012). These disadvantages limit further investigation into the impact of UV radiation on plants. Unlike conventional UV radiation sources, UV-LEDs available on the market have a wide range of wavelength selection. By adding aluminum nitride (AlN) to the GaN diodes, emitting UV wavelengths ranges cover from 220 to 380 nm. Unlike earlier UV devices with less than a 100-h lifespan (L50, 50% of light bulbs fail at 100 h), current UV-LEDs emitting at 280–310 nm now boast a lifespan of at least 3,000 h (Würtele et al., 2011; Fujioka et al., 2014; Kneissl, 2016), with some exceeding more than 10,000 h (Glaab et al., 2015). Although these UV-LEDs still have lower reliability and longevity than LEDs in visible spectrum, they have become an emerging radiation source for research involving UV germicidal irradiation, such as water treatment and microbial inactivation (Song et al., 2016; Kebbi et al., 2020).
A major concern when applying UV radiation in plant production facilities is UV photobiology safety. While employing any UV radiation source, there is a potential risk of being exposed to hazardous ocular and skin UV radiation (Ichihashi et al., 2003; Laube et al., 2004), and UV photobiology safety precautions for users are necessary (Lau, 2013). Controls to prevent skin and eye injuries should be placed, and protective housing that restricts or reduces UV exposure is an effective approach. If personnel requires to entering UV-radiation environment, personal protective equipment is highly recommended. Many international bodies have published guidelines that assess and evaluate photobiological eye safety based on wavelength and exposure (European Union, 2006; International Electrotechnical Commission, 2006). For radiation between 180 and 400 nm, the exposure limit is 30 J cm−2 within 8-h per day. Note that when users assess the UV radiation environment, spectral weighting functions need to be applied. Sunglasses provide adequate, as most sunglasses are able to greatly reduce the amount of UV radiation (Tuchinda et al., 2006; Wu and Lefsrud, 2018).
Concluding Remarks and Future Outlook
Here, we review aspects of photobiology and flavonoid accumulation that are relevant to strawberry plant production. Studies showing our interest in enhancing strawberry plant growth, development, metabolites, and crop status span nearly 100 years. Information on photobiology research can be utilized to tailor artificial light spectra, which can target the development of flavonoid content in strawberry fruits. More specifically that different wavelengths will elicit varied responses in the growth and quality of fruit production. Practically applied, optimized light recipes reduce the necessary electrical inputs, while increasing the crop yields and quality. Pre-harvesting UV treatment combined the UV-A to UV-C wavelengths is a powerful tool for stimulating flavonoid biosynthesis in strawberry fruits; however, UV-C radiation alone impacts flavor profile. In post-harvesting treatments, UV-C radiation shows promising results on enhancing secondary metabolites. We expect that UV LEDs will be increasingly used to stimulate desirable fruit metabolites, while requiring only short doses (minutes per day) to elicit a response. Evidence indicates that blue LED light can enhance flavonoid accumulation in greenhouse crops, but this is not explicitly seen with strawberry plants. Blue-light-mediated responses in strawberry fruit flavonoid accumulation are bandwidth-dependent, rather than wavelength-dependent. Based on literature reported, a spectrum with board blue light spectrum or with multiple peaks in the blue wavelength range targeting both phototropin and cryptochrome is optimal and recommended for enhancing flavonoid accumulation. Understanding the action spectrum (or spectral dose-response curve) of flavonoid biosynthesis in different tissues is important to improve the precision of flavonoid production and the antioxidant capacity in strawberry CE production, making these “super foods” more super. Continued investigation of this variation in flavonoid response to light spectrum will provide important knowledge on light signaling machinery, such as COP1-mediated pathways, in strawberry, and possibly other fruit producing species.
Based on research reviewed, we believe there is value in pursuing further research on the implication of light spectra on strawberry secondary metabolites to improve crop quality for human health. The following areas should be considered for further study to fill knowledge gaps: (1) the impact of pre-harvest UV-A on secondary metabolite accumulation. Flavonoids absorb majorly in the UV-A spectrum, yet there is minimal research available on the direct impact of UV-A on flavonoid accumulation in strawberries. (2) Further investigations into the impact of pre-harvest UV-C radiation, considering post-harvest research is very promising. UV-C LED sources with different wavelengths are highly available, and the accessibility to both researchers and producers make results more accessible. (3) Determination of the impact of specific visible light wavelengths across both cultivars and temperature, especially with white LED light. (4) Investigations into blue: red light ratios in purple lighting during vegetative growth and flowering to determine impact on secondary metabolite profile in strawberries. (5) Degradation monitoring of flavonoids during post-harvest storage and transport, to determine timeframe of benefits imposed by augmented light spectrum.
Author Contributions
RW and B-SW led the writing of this paper. B-SW and SM were the major editors. SM and ML contributed over 40% of the writing for the paper. ML is the correspondence point person. All authors contributed to the article and approved the submitted version.
Conflict of Interest
The authors declare that this study was funded in part by Gardyn Inc. The funder was not involved in study design, collection, data analysis, the drafting of this manuscript, or the decision to submit it for refereed publication.
Acknowledgments
We would like to thank Mitacs (grant number IT16220) and Gardyn Inc. for their continuous support and funding of this project.
References
Aaby, K., Mazur, S., Nes, A., and Skrede, G. (2012). Phenolic compounds in strawberry (Fragaria x ananassa Duch.) fruits: composition in 27 cultivars and changes during ripening. Food Chem. 132, 86–97. doi: 10.1016/j.foodchem.2011.10.037
Afrin, S., Gasparrini, M., Forbes-Hernandez, T. Y., Reboredo-Rodriguez, P., Mezzetti, B., Varela-LóPez, A., et al. (2016). Promising health benefits of the strawberry: a focus on clinical studies. J. Agric. Food Chem. 64, 4435–4449. doi: 10.1021/acs.jafc.6b00857
Akula, R., and Ravishankar, G. A. (2011). Influence of abiotic stress signals on secondary metabolites in plants. Plant Signal. Behav. 6, 1720–1731. doi: 10.4161/psb.6.11.17613
Al-Dashti, Y. A., Holt, R. R., Stebbins, C. L., Keen, C. L., and Hackman, R. M. (2018). Dietary flavanols: a review of select effects on vascular function, blood pressure, and exercise performance. J. Am. Coll. Nutr. 37, 553–567. doi: 10.1080/07315724.2018.1451788
Alrifai, O., Hao, X., Marcone, M. F., and Tsao, R. (2019). Current review of the modulatory effects of LED lights on photosynthesis of secondary metabolites and future perspectives of microgreen vegetables. J. Agric. Food Chem. 67, 6075–6090. doi: 10.1021/acs.jafc.9b00819
Alvarez, M. A. (ed.) (2014). “Plant secondary metabolism” in Plant biotechnology for health. Springer, 15–31.
Amini, A. M., Muzs, K., Spencer, J. P., and Yaqoob, P. (2017). Pelargonidin-3-O-glucoside and its metabolites have modest anti-inflammatory effects in human whole blood cultures. Nutr. Res. 46, 88–95. doi: 10.1016/j.nutres.2017.09.006
Aron, P. M., and Kennedy, J. A. (2008). Flavan-3-ols: nature, occurrence and biological activity. Mol. Nutr. Food Res. 52, 79–104. doi: 10.1002/mnfr.200700137
Baicu, A. A., and Popa, M. E. (2018). Trends in prolonging the post-harvest life of strawberries–a review. The Annals of the University Dunarea de Jos of Galati Fascicle VI-Food Technology 42, 9–16.
Banerjee, R., and Batschauer, A. (2005). Plant blue-light receptors. Planta 220, 498–502. doi: 10.1007/s00425-004-1418-z
Battino, M., Forbes-Hernandez, T., Gasparrini, M., Afrin, S., Mezzetti, B., and Giampieri, F. (2016). The effects of strawberry bioactive compounds on human health. Acta Hortic. 1156, 355–362. doi: 10.17660/ActaHortic.2017.1156.54
Bernal, M., Llorens, L., Badosa, J., and Verdaguer, D. (2013). Interactive effects of UV radiation and water availability on seedlings of six woody Mediterranean species. Physiol. Plant. 147, 234–247. doi: 10.1111/j.1399-3054.2012.01660.x
Bernal, M., Verdaguer, D., Badosa, J., Abadía, A., Llusià, J., Peñuelas, J., et al. (2015). Effects of enhanced UV radiation and water availability on performance, biomass production and photoprotective mechanisms of Laurus nobilis seedlings. Environ. Exp. Bot. 109, 264–275. doi: 10.1016/j.envexpbot.2014.06.016
Besteiro, M. A. G., Bartels, S., Albert, A., and Ulm, R. (2011). Arabidopsis MAP kinase phosphatase 1 and its target MAP kinases 3 and 6 antagonistically determine UV-B stress tolerance, independent of the UVR8 photoreceptor pathway. Plant J. 68, 727–737. doi: 10.1111/j.1365-313X.2011.04725.x
Biswas, D. K., and Jansen, M. A. (2012). Natural variation in UV-B protection amongst Arabidopsis thaliana accessions. Emir. J. Food Agric. 24, 621–631. doi: 10.9755/ejfa.v24i6.621631
Bradford, E., Hancock, J. F., and Warner, R. M. (2010). Interactions of temperature and photoperiod determine expression of repeat flowering in strawberry. J. Am. Soc. Hortic. Sci. 135, 102–107. doi: 10.21273/JASHS.135.2.102
Caldwell, C. R., and Britz, S. J. (2006). Effect of supplemental ultraviolet radiation on the carotenoid and chlorophyll composition of green house-grown leaf lettuce (Lactuca sativa L.) cultivars. J. Food Compos. Anal. 19, 637–644. doi: 10.1016/j.jfca.2005.12.016
Carbone, F., Preuss, A., De Vos, R. C., D’amico, E., Perrotta, G., Bovy, A. G., et al. (2009). Developmental, genetic and environmental factors affect the expression of flavonoid genes, enzymes and metabolites in strawberry fruits. Plant Cell Environ. 32, 1117–1131. doi: 10.1111/j.1365-3040.2009.01994.x
Carvalho, S., and Folta, K. (2016). Green light control of anthocyanin production in microgreens. Acta Hortic. 1134, 13–18. doi: 10.17660/ActaHortic.2016.1134.2
Cerovic, Z., Ounis, A., Cartelat, A., Latouche, G., Goulas, Y., Meyer, S., et al. (2002). The use of chlorophyll fluorescence excitation spectra for the non-destructive in situ assessment of UV-absorbing compounds in leaves. Plant Cell Environ. 25, 1663–1676. doi: 10.1046/j.1365-3040.2002.00942.x
Chassy, A. W., Adams, D. O., Laurie, V. F., and Waterhouse, A. L. (2012). Tracing phenolic biosynthesis in Vitis vinifera via in situ C-13 labeling and liquid chromatography–diode-array detector–mass spectrometer/mass spectrometer detection. Anal. Chim. Acta 747, 51–57. doi: 10.1016/j.aca.2012.08.012
Choi, H. G., Moon, B. Y., and Kang, N. J. (2015). Effects of LED light on the production of strawberry during cultivation in a plastic greenhouse and in a growth chamber. Sci. Hortic. 189, 22–31. doi: 10.1016/j.scienta.2015.03.022
Cocetta, G., Casciani, D., Bulgari, R., Musante, F., Kołton, A., Rossi, M., et al. (2017). Light use efficiency for vegetables production in protected and indoor environments. Eur. Phys. J. Plus 132:43. doi: 10.1140/epjp/i2017-11298-x
Cooley, N. M., Higgins, J., Holmes, M., and Attridge, T. (2001). Ecotypic differences in responses of Arabidopsis thaliana L. to elevated polychromatic UV-A and UV-B+ A radiation in the natural environment: a positive correlation between UV-B+ A inhibition and growth rate. J. Photochem. Photobiol. B 60, 143–150. doi: 10.1016/S1011-1344(01)00140-3
Da Silva, F. L., Escribano-Bailón, M. T., Alonso, J. J. P., Rivas-Gonzalo, J. C., and Santos-Buelga, C. (2007). Anthocyanin pigments in strawberry. LWT-Food Sci. Technol. 40, 374–382. doi: 10.1016/j.lwt.2005.09.018
De Oliveira, I. R., Crizel, G. R., Severo, J., Renard, C. M., Chaves, F. C., and Rombaldi, C. V. (2016). Preharvest UV-C radiation influences physiological, biochemical, and transcriptional changes in strawberry cv. Camarosa. Plant Physiol. Biochem. 108, 391–399. doi: 10.1016/j.plaphy.2016.08.012
Delgado, A. M., Issaoui, M., and Chammem, N. (2019). Analysis of main and healthy phenolic compounds in foods. J. AOAC Int. 102, 1356–1364. doi: 10.5740/jaoacint.19-0128
Dewick, P. M. (ed.) (2001). “The shikimate pathway: aromatic amino acids and phenylpropanoids” in Medicinal natural products: A biosynthetic approach. 2nd Edn. (Chichester, UK: John Wiley & Sons, Ltd), 137–186.
Díaz-Galián, M. V., Torres, M., Sanchez-Pagán, J. D., Navarro, P. J., Weiss, J., and Egea-Cortines, M. (2020). Enhancement of strawberry production and fruit quality by blue and red LED lights in research and commercial greenhouses. S. Afr. J. Bot. doi: 10.1016/j.sajb.2020.05.004 (in press).
Dou, H., Niu, G., Gu, M., and Masabni, J. G. (2017). Effects of light quality on growth and phytonutrient accumulation of herbs under controlled environments. Horticulturae 3:36. doi: 10.3390/horticulturae3020036
Doupis, G., Bosabalidis, A., and Patakas, A. (2016). Comparative effects of water deficit and enhanced UV-B radiation on photosynthetic capacity and leaf anatomy traits of two grapevine (Vitis vinifera L.) cultivars. Theor. Exp. Plant Physiol. 28, 131–141. doi: 10.1007/s40626-016-0055-9
Downey, M. O., Dokoozlian, N. K., and Krstic, M. P. (2006). Cultural practice and environmental impacts on the flavonoid composition of grapes and wine: a review of recent research. Am. J. Enol. Vitic. 57, 257–268.
Durner, E. F., Barden, J., Himelrick, D., and Poling, E. (1984). Photoperiod and temperature effects on flower and runner development in day-neutral, junebearing, and everbearing strawberries. J. Am. Soc. Hortic. Sci. 109, 396–400.
Erkan, M., Wang, S. Y., and Wang, C. Y. (2008). Effect of UV treatment on antioxidant capacity, antioxidant enzyme activity and decay in strawberry fruit. Postharvest Biol. Technol. 48, 163–171. doi: 10.1016/j.postharvbio.2007.09.028
European Union (2006). Directive 2006/25/EC of the European Parliament and of the Council of 5 April 2006 on the minimum health and safety requirements regarding the exposure of workers to risks arising from physical agents (artificial optical radiation). Official Journal of the European Union 114, 38–59.
Fantini, E., and Facella, P. (2020). Cryptochromes in the field: how blue light influences crop development. Physiol. Plant. 169, 336–346. doi: 10.1111/ppl.13088
FAO (2016). FAOSTAT online database [Online]. Available: http://www.fao.org/faostat/en/#data/QV (Accessed June 30 2020).
Ferreyra, M. L. F., Rius, S., and Casati, P. (2012). Flavonoids: biosynthesis, biological functions, and biotechnological applications. Front. Plant Sci. 3:222. doi: 10.3389/fpls.2012.00222
Folta, K. M., and Carvalho, S. D. (2015). Photoreceptors and control of horticultural plant traits. HortScience 50, 1274–1280. doi: 10.21273/HORTSCI.50.9.1274
Forges, M., Bardin, M., Urban, L., Aarrouf, J., and Charles, F. (2020). Impact of UV-C radiation applied during plant growth on pre-and post-harvest disease sensitivity and fruit quality of strawberry. Plant Dis. 104, 3239–3247. doi: 10.1094/PDIS-02-20-0306-RE
Fujioka, A., Asada, K., Yamada, H., Ohtsuka, T., Ogawa, T., Kosugi, T., et al. (2014). High-output-power 255/280/310 nm deep ultraviolet light-emitting diodes and their lifetime characteristics. Semicond. Sci. Technol. 29:084005. doi: 10.1088/0268-1242/29/8/084005
Giampieri, F., Tulipani, S., Alvarez-Suarez, J. M., Quiles, J. L., Mezzetti, B., and Battino, M. (2012). The strawberry: composition, nutritional quality, and impact on human health. Nutrition 28, 9–19. doi: 10.1016/j.nut.2011.08.009
Giliberto, L., Perrotta, G., Pallara, P., Weller, J. L., Fraser, P. D., Bramley, P. M., et al. (2005). Manipulation of the blue light photoreceptor cryptochrome 2 in tomato affects vegetative development, flowering time, and fruit antioxidant content. Plant Physiol. 137, 199–208. doi: 10.1104/pp.104.051987
Glaab, J., Ploch, C., Kelz, R., Stölmacker, C., Lapeyrade, M., Ploch, N. L., et al. (2015). Temperature induced degradation of InAlGaN multiple-quantum well UV-B LEDs. Mater. Res. Soc. Symp. Proc. 1792:mrss 15–2102646. doi: 10.1557/opl.2015.446
Gonzalez, C. V., Fanzone, M. L., Cortés, L. E., Bottini, R., Lijavetzky, D. C., Ballare, C. L., et al. (2015). Fruit-localized photoreceptors increase phenolic compounds in berry skins of field-grown Vitis vinifera L. cv. Malbec. Phytochemistry 110, 46–57. doi: 10.1016/j.phytochem.2014.11.018
Gronquist, M., Bezzerides, A., Attygalle, A., Meinwald, J., Eisner, M., and Eisner, T. (2001). Attractive and defensive functions of the ultraviolet pigments of a flower (Hypericum calycinum). Proc. Natl. Acad. Sci. U. S. A. 98, 13745–13750. doi: 10.1073/pnas.231471698
Gupta, S. K., Sharma, M., Deeba, F., and Pandey, V. (2017). “Plant response: UV-B avoidance mechanisms” in UV-B radiation: From environmental stressor to regulator of plant growth. eds. V. P. Singh, S. M. Prasad, and P. Parihar (West Sussex, UK: Wiley-Blackwell), 217–258.
Hectors, K., Van Oevelen, S., Geuns, J., Guisez, Y., Jansen, M. A., and Prinsen, E. (2014). Dynamic changes in plant secondary metabolites during UV acclimation in Arabidopsis thaliana. Physiol. Plant. 152, 219–230. doi: 10.1111/ppl.12168
Heide, O. M. (1977). Photoperiod and temperature interactions in growth and flowering of strawberry. Physiol. Plant. 40, 21–26. doi: 10.1111/j.1399-3054.1977.tb01486.x
Heide, O., Stavang, J., and Sønsteby, A. (2013). Physiology and genetics of flowering in cultivated and wild strawberries–a review. J. Hortic. Sci. Biotechnol. 88, 1–18. doi: 10.1080/14620316.2013.11512930
Hidaka, K., Dan, K., Imamura, H., Miyoshi, Y., Takayama, T., Sameshima, K., et al. (2013). Effect of supplemental lighting from different light sources on growth and yield of strawberry. Environ. Control Biol. 51, 41–47. doi: 10.2525/ecb.51.41
Hidaka, K., Okamoto, A., Araki, T., Miyoshi, Y., Dan, K., Imamura, H., et al. (2014). Effect of photoperiod of supplemental lighting with light-emitting diodes on growth and yield of strawberry. Environ. Control Biol. 52, 63–71. doi: 10.2525/ecb.52.63
Ichihashi, M., Ueda, M., Budiyanto, A., Bito, T., Oka, M., Fukunaga, M., et al. (2003). UV-induced skin damage. Toxicology 189, 21–39. doi: 10.1016/S0300-483X(03)00150-1
International Electrotechnical Commission (2006). “Photobiological safety of lamps and lamp systems (IEC 62471).” Geneva: IEC.
Ito, H., and Saito, T. (1962). Studies on the flower formation in the strawberry plants. Tohoku J. Agr. Res. 13, 191–203.
Jaillon, O., Aury, J. -M., Noel, B., Policriti, A., Clepet, C., Casagrande, A., et al. (2007). The grapevine genome sequence suggests ancestral hexaploidization in major angiosperm phyla. Nature 449, 463–467. doi: 10.1038/nature06148
Jansen, M. A., Hectors, K., O’brien, N. M., Guisez, Y., and Potters, G. (2008). Plant stress and human health: do human consumers benefit from UV-B acclimated crops? Plant Sci. 175, 449–458. doi: 10.1016/j.plantsci.2008.04.010
Jenkins, G. I. (2017). Photomorphogenic responses to ultraviolet-B light. Plant Cell Environ. 40, 2544–2557. doi: 10.1111/pce.12934
Jones, M. A. (2018). Using light to improve commercial value. Hortic. Res. 5:47. doi: 10.1038/s41438-018-0049-7
Josuttis, M., Dietrich, H., Treutter, D., Will, F., LinnemannstöNs, L., and KrüGer, E. (2010). Solar UVB response of bioactives in strawberry (Fragaria× ananassa Duch. L.): a comparison of protected and open-field cultivation. J. Agric. Food Chem. 58, 12692–12702. doi: 10.1021/jf102937e
Kadomura-Ishikawa, Y., Miyawaki, K., Noji, S., and Takahashi, A. (2013). Phototropin 2 is involved in blue light-induced anthocyanin accumulation in Fragaria x ananassa fruits. J. Plant Res. 126, 847–857. doi: 10.1007/s10265-013-0582-2
Kanto, T., Matsuura, K., Yamada, M., Usami, T., and Amemiya, Y. (2009). UV-B radiation for control of strawberry powdery mildew. Acta Hortic. 842, 359–362. doi: 10.17660/ActaHortic.2009.842.68
Kataria, S., and Guruprasad, K. (2012). Intraspecific variations in growth, yield and photosynthesis of sorghum varieties to ambient UV (280–400 nm) radiation. Plant Sci. 196, 85–92. doi: 10.1016/j.plantsci.2012.07.011
Kataria, S., Guruprasad, K., Ahuja, S., and Singh, B. (2013). Enhancement of growth, photosynthetic performance and yield by exclusion of ambient UV components in C3 and C4 plants. J. Photochem. Photobiol. B 127, 140–152. doi: 10.1016/j.jphotobiol.2013.08.013
Katsoulas, N., Bari, A., and Papaioannou, C. (2020). Plant responses to UV blocking greenhouse covering materials: a review. Agronomy 10:1021. doi: 10.3390/agronomy10071021
Kebbi, Y., Muhammad, A. I., Sant’ana, A. S., Do Prado-Silva, L., Liu, D., and Ding, T. (2020). Recent advances on the application of UV-LED technology for microbial inactivation: progress and mechanism. Compr. Rev. Food Sci. Food Saf. 19, 3501–3527. doi: 10.1111/1541-4337.12645
Khoo, H. E., Azlan, A., Tang, S. T., and Lim, S. M. (2017). Anthocyanidins and anthocyanins: colored pigments as food, pharmaceutical ingredients, and the potential health benefits. Food Nutr. Res. 61:1361779. doi: 10.1080/16546628.2017.1361779
Khudyakova, A. Y., Kreslavski, V. D., Shirshikova, G. N., Zharmukhamedov, S. K., Kosobryukhov, A. A., and Allakhverdiev, S. (2017). Resistance of Arabidopsis thaliana L. photosynthetic apparatus to UV-B is reduced by deficit of phytochromes B and A. J. Photochem. Photobiol. B 169, 41–46. doi: 10.1016/j.jphotobiol.2017.02.024
Kim, S. -K., Bae, R. -N., and Chun, C. -H. (2011). Changes in bioactive compounds contents of ‘Maehyang’ and ‘Seolhyang’ strawberry fruits by UV light illumination. Korean J. Hortic. Sci. Technol. 29, 172–180.
Klem, K., Ač, A., Holub, P., Kováč, D., Špunda, V., Robson, T. M., et al. (2012). Interactive effects of PAR and UV radiation on the physiology, morphology and leaf optical properties of two barley varieties. Environ. Exp. Bot. 75, 52–64. doi: 10.1016/j.envexpbot.2011.08.008
Kneissl, M. (2016). “A brief review of III-nitride UV emitter technologies and their applications” in III-nitride ultraviolet emitters. eds. M. Kneissl and J. Rass (Heidelberg: Springer), 1–25.
Kotilainen, T., Venäläinen, T., Tegelberg, R., Lindfors, A., Julkunen-Tiitto, R., Sutinen, S., et al. (2009). Assessment of UV biological spectral weighting functions for phenolic metabolites and growth responses in silver birch seedlings. Photochem. Photobiol. 85, 1346–1355. doi: 10.1111/j.1751-1097.2009.00597.x
Kreft, S., Štrukelj, B., Gaberščik, A., and Kreft, I. (2002). Rutin in buckwheat herbs grown at different UV-B radiation levels: comparison of two UV spectrophotometric and an HPLC method. J. Exp. Bot. 53, 1801–1804. doi: 10.1093/jxb/erf032
Kunz, B. A., Cahill, D. M., Mohr, P. G., Osmond, M. J., and Vonarx, E. J. (2006). Plant responses to UV radiation and links to pathogen resistance. Int. Rev. Cytol. 255, 1–40. doi: 10.1016/S0074-7696(06)55001-6
Kusano, M., Tohge, T., Fukushima, A., Kobayashi, M., Hayashi, N., Otsuki, H., et al. (2011). Metabolomics reveals comprehensive reprogramming involving two independent metabolic responses of Arabidopsis to UV-B light. Plant J. 67, 354–369. doi: 10.1111/j.1365-313X.2011.04599.x
Labadie, M., Vallin, G., Petit, A., Ring, L., Hoffmann, T., Gaston, A., et al. (2020). Metabolite quantitative trait loci for flavonoids provide new insights into the genetic architecture of strawberry (Fragaria x ananassa) fruit quality. J. Agric. Food Chem. 68, 6927–6939. doi: 10.1021/acs.jafc.0c01855
Landi, M., Agati, G., Fini, A., Guidi, L., Sebastiani, F., and Tattini, M. (2020a). Unveiling the shade nature of cyanic leaves: a view from the “blue absorbing side” of anthocyanins. Plant Cell Environ. 1–11. doi: 10.1111/pce.13818 [Epub ahead of print]
Landi, M., Zivcak, M., Sytar, O., Brestic, M., and Allakhverdiev, S. I. (2020b). Plasticity of photosynthetic processes and the accumulation of secondary metabolites in plants in response to monochromatic light environments: a review. Biochim. Biophys. Acta Bioenerg. 1861:148131. doi: 10.1016/j.bbabio.2019.148131
Lau, E. K. (2013). “Understanding radiation safety of high-intensity light-emitting diodes” in 2013 IEEE Symposium on Product Compliance Engineering (ISPCE); October 7–9, 2013; Austin, TX, USA, 1–3.
Lau, O. S., and Deng, X. W. (2012). The photomorphogenic repressors COP1 and DET1: 20 years later. Trends Plant Sci. 17, 584–593. doi: 10.1016/j.tplants.2012.05.004
Laube, T., Apel, H., and Koch, H. -R. (2004). Ultraviolet radiation absorption of intraocular lenses. Ophthalmology 111, 880–885. doi: 10.1016/j.ophtha.2003.08.031
Li, M., Li, X., Han, C., Ji, N., Jin, P., and Zheng, Y. (2019). UV-C treatment maintains quality and enhances antioxidant capacity of fresh-cut strawberries. Postharvest Biol. Technol. 156:110945. doi: 10.1016/j.postharvbio.2019.110945
Liu, Y., Tikunov, Y., Schouten, R. E., Marcelis, L. F., Visser, R. G., and Bovy, A. (2018). Anthocyanin biosynthesis and degradation mechanisms in Solanaceous vegetables: a review. Front. Chem. 6:52. doi: 10.3389/fchem.2018.00052
Lopez, L., Carbone, F., Bianco, L., Giuliano, G., Facella, P., and Perrotta, G. (2012). Tomato plants overexpressing cryptochrome 2 reveal altered expression of energy and stress-related gene products in response to diurnal cues. Plant Cell Environ. 35, 994–1012. doi: 10.1111/j.1365-3040.2011.02467.x
López-Aranda, J. M., Soria, C., Santos, B. M., Miranda, L., Domínguez, P., and Medina-Mínguez, J. J. (2011). Strawberry production in mild climates of the world: a review of current cultivar use. Int. J. Fruit Sci. 11, 232–244. doi: 10.1080/15538362.2011.608294
Lü, J. M., Lin, P. H., Yao, Q., and Chen, C. (2010). Chemical and molecular mechanisms of antioxidants: experimental approaches and model systems. J. Cell. Mol. Med. 14, 840–860. doi: 10.1111/j.1582-4934.2009.00897.x
Magar, Y., Ohyama, K., Noguchi, A., Amaki, W., and Furufuji, S. (2018). Effects of light quality during supplemental lighting on the flowering in an everbearing strawberry. Acta Hortic. 1206, 279–284. doi: 10.17660/ActaHortic.2018.1206.39
Marquenie, D., Lammertyn, J., Geeraerd, A., Soontjens, C., Van Impe, J., Nicolaı, B., et al. (2002). Inactivation of conidia of Botrytis cinerea and Monilinia fructigena using UV-C and heat treatment. Int. J. Food Microbiol. 74, 27–35. doi: 10.1016/S0168-1605(01)00719-X
Martens, S., Preuß, A., and Matern, U. (2010). Multifunctional flavonoid dioxygenases: flavonol and anthocyanin biosynthesis in Arabidopsis thaliana L. Phytochemistry 71, 1040–1049. doi: 10.1016/j.phytochem.2010.04.016
Matus, J. T., Loyola, R., Vega, A., Peña-Neira, A., Bordeu, E., Arce-Johnson, P., et al. (2009). Post-veraison sunlight exposure induces MYB-mediated transcriptional regulation of anthocyanin and flavonol synthesis in berry skins of Vitis vinifera. J. Exp. Bot. 60, 853–867. doi: 10.1093/jxb/ern336
Miao, L., Zhang, Y., Yang, X., Xiao, J., Zhang, H., Zhang, Z., et al. (2016). Colored light-quality selective plastic films affect anthocyanin content, enzyme activities, and the expression of flavonoid genes in strawberry (Fragaria× ananassa) fruit. Food Chem. 207, 93–100. doi: 10.1016/j.foodchem.2016.02.077
Miyazawa, Y., Hikosaka, S., Goto, E., and Aoki, T. (2009). Effects of light conditions and air temperature on the growth of everbearing strawberry during the vegetative stage. Acta Hortic. 842, 817–820. doi: 10.17660/ActaHortic.2009.842.180
Mockler, T., Yang, H., Yu, X., Parikh, D., Cheng, Y. -C., Dolan, S., et al. (2003). Regulation of photoperiodic flowering by Arabidopsis photoreceptors. Proc. Natl. Acad. Sci. U. S. A. 100, 2140–2145. doi: 10.1073/pnas.0437826100
Monagas, M., Urpi-Sarda, M., Sánchez-Patán, F., Llorach, R., Garrido, I., Gómez-Cordovés, C., et al. (2010). Insights into the metabolism and microbial biotransformation of dietary flavan-3-ols and the bioactivity of their metabolites. Food Funct. 1, 233–253. doi: 10.1039/c0fo00132e
Müller-Xing, R., Xing, Q., and Goodrich, J. (2014). Footprints of the sun: memory of UV and light stress in plants. Front. Plant Sci. 5:474. doi: 10.3389/fpls.2014.00474
Nadalini, S., Zucchi, P., and Andreotti, C. (2017). Effects of blue and red LED lights on soilless cultivated strawberry growth performances and fruit quality. Eur. J. Hortic. Sci. 82, 12–20. doi: 10.17660/eJHS.2017/82.1.2
Naznin, M., Lefsrud, M., Gravel, V., and Hao, X. (2016). Using different ratios of red and blue LEDs to improve the growth of strawberry plants. Acta Hortic. 1134, 125–130. doi: 10.17660/ActaHortic.2016.1134.17
Nhut, D. T., Takamura, T., Watanabe, H., Okamoto, K., and Tanaka, M. (2003). Responses of strawberry plantlets cultured in vitro under superbright red and blue light-emitting diodes (LEDs). Plant Cell Tissue Organ Cult. 73, 43–52. doi: 10.1023/A:1022638508007
Nigro, F., Ippolito, A., and Lattanzio, V., Di Venere, D., and Salerno, M. (2000). Effect of ultraviolet-C light on postharvest decay of strawberry. J. Plant Pathol. 82, 29–37.
Nile, S. H., and Park, S. W. (2014). Edible berries: bioactive components and their effect on human health. Nutrition 30, 134–144. doi: 10.1016/j.nut.2013.04.007
Ordidge, M., García-Macías, P., Battey, N. H., Gordon, M. H., John, P., Lovegrove, J. A., et al. (2012). Development of colour and firmness in strawberry crops is UV light sensitive, but colour is not a good predictor of several quality parameters. J. Sci. Food Agric. 92, 1597–1604. doi: 10.1002/jsfa.4744
Owens, D. K., Alerding, A. B., Crosby, K. C., Bandara, A. B., Westwood, J. H., and Winkel, B. S. (2008). Functional analysis of a predicted flavonol synthase gene family in Arabidopsis. Plant Physiol. 147, 1046–1061. doi: 10.1104/pp.108.117457
Panche, A., Diwan, A., and Chandra, S. (2016). Flavonoids: an overview. J. Nutr. Sci. 5:e47. doi: 10.1017/jns.2016.41
Peng, T., Saito, T., Honda, C., Ban, Y., Kondo, S., Liu, J. H., et al. (2013). Screening of UV-B-induced genes from apple peels by SSH: possible involvement of MdCOP1-mediated signaling cascade genes in anthocyanin accumulation. Physiol. Plant. 148, 432–444. doi: 10.1111/ppl.12002
Pereira, G. E., Gaudillere, J. -P., Pieri, P., Hilbert, G., Maucourt, M., Deborde, C., et al. (2006). Microclimate influence on mineral and metabolic profiles of grape berries. J. Agric. Food Chem. 54, 6765–6775. doi: 10.1021/jf061013k
Piovene, C., Orsini, F., Bosi, S., Sanoubar, R., Bregola, V., Dinelli, G., et al. (2015). Optimal red: blue ratio in led lighting for nutraceutical indoor horticulture. Sci. Hortic. 193, 202–208. doi: 10.1016/j.scienta.2015.07.015
Pocock, T. (2015). Light-emitting diodes and the modulation of specialty crops: light sensing and signaling networks in plants. HortScience 50, 1281–1284. doi: 10.21273/HORTSCI.50.9.1281
Pombo, M. A., Dotto, M. C., Martínez, G. A., and Civello, P. M. (2009). UV-C irradiation delays strawberry fruit softening and modifies the expression of genes involved in cell wall degradation. Postharvest Biol. Technol. 51, 141–148. doi: 10.1016/j.postharvbio.2008.07.007
Rantanen, M., Kurokura, T., Mouhu, K., Pinho, P., Tetri, E., Halonen, L., et al. (2014). Light quality regulates flowering in FvFT1/FvTFL1 dependent manner in the woodland strawberry Fragaria vesca. Front. Plant Sci. 5:271. doi: 10.3389/fpls.2014.00271
Reifenrath, K., and Müller, C. (2007). Species-specific and leaf-age dependent effects of ultraviolet radiation on two Brassicaceae. Phytochemistry 68, 875–885. doi: 10.1016/j.phytochem.2006.12.008
Robson, T. M., and Aphalo, P. J. (2012). Species-specific effect of UV-B radiation on the temporal pattern of leaf growth. Physiol. Plant. 144, 146–160. doi: 10.1111/j.1399-3054.2011.01546.x
Rozema, J., Björn, L. O., Bornman, J., Gaberščik, A., Häder, D. -P., Trošt, T., et al. (2002). The role of UV-B radiation in aquatic and terrestrial ecosystems—an experimental and functional analysis of the evolution of UV-absorbing compounds. J. Photochem. Photobiol. B 66, 2–12. doi: 10.1016/S1011-1344(01)00269-X
Saini, R. K., and Keum, Y. -S. (2018). Significance of genetic, environmental, and pre-and postharvest factors affecting carotenoid contents in crops: a review. J. Agric. Food Chem. 66, 5310–5324. doi: 10.1021/acs.jafc.8b01613
Samtani, J. B., Rom, C. R., Friedrich, H., Fennimore, S. A., Finn, C. E., Petran, A., et al. (2019). The status and future of the strawberry industry in the United States. HortTechnology 29, 11–24. doi: 10.21273/HORTTECH04135-18
Santos, A. L., Oliveira, V., Baptista, I., Henriques, I., Gomes, N. C., Almeida, A., et al. (2013). Wavelength dependence of biological damage induced by UV radiation on bacteria. Arch. Microbiol. 195, 63–74. doi: 10.1007/s00203-012-0847-5
Sarigiannis, D., Karakitsios, S., Antonakopoulou, M., and Gotti, A. (2012). Exposure analysis of accidental release of mercury from compact fluorescent lamps (CFLs). Sci. Total Environ. 435, 306–315. doi: 10.1016/j.scitotenv.2012.07.026
Schreiner, M., Mewis, I., Huyskens-Keil, S., Jansen, M., Zrenner, R., Winkler, J., et al. (2012). UV-B-induced secondary plant metabolites-potential benefits for plant and human health. Crit. Rev. Plant Sci. 31, 229–240. doi: 10.1080/07352689.2012.664979
Serçe, S., and Hancock, J. F. (2005). Inheritance of day-neutrality in octoploid species of Fragaria. J. Am. Soc. Hortic. Sci. 130, 580–584. doi: 10.21273/JASHS.130.4.580
Severo, J., De Oliveira, I. R., Bott, R., Le Bourvellec, C., Renard, C. M., Page, D., et al. (2017). Preharvest UV-C radiation impacts strawberry metabolite content and volatile organic compound production. LWT-Food Sci. Technol. 85, 390–393. doi: 10.1016/j.lwt.2016.10.032
Shulaev, V., Sargent, D. J., Crowhurst, R. N., Mockler, T. C., Folkerts, O., Delcher, A. L., et al. (2011). The genome of woodland strawberry (Fragaria vesca). Nat. Genet. 43, 109–116. doi: 10.1038/ng.740
Singh, D., Basu, C., Meinhardt-Wollweber, M., and Roth, B. (2015). LEDs for energy efficient greenhouse lighting. Renew. Sust. Energ. Rev. 49, 139–147. doi: 10.1016/j.rser.2015.04.117
Smeets, L. (1976). Effect of light intensity on stamen development in the strawberry cultivar ‘Glasa’. Sci. Hortic. 4, 255–260. doi: 10.1016/0304-4238(76)90048-0
Smeets, L. (1980). Effect of the light intensity during flowering on stamen development in the strawberry cultivars ‘Karina’and ‘Sivetta’. Sci. Hortic. 12, 343–346. doi: 10.1016/0304-4238(80)90049-7
Song, K., Mohseni, M., and Taghipour, F. (2016). Application of ultraviolet light-emitting diodes (UV-LEDs) for water disinfection: a review. Water Res. 94, 341–349. doi: 10.1016/j.watres.2016.03.003
Stewart, P. J., and Folta, K. M. (2010). A review of photoperiodic flowering research in strawberry (Fragaria spp.). Crit. Rev. Plant Sci. 29, 1–13. doi: 10.1080/07352680903436259
Stutte, G. W., Edney, S., and Skerritt, T. (2009). Photoregulation of bioprotectant content of red leaf lettuce with light-emitting diodes. HortScience 44, 79–82. doi: 10.21273/HORTSCI.44.1.79
Suthaparan, A., Solhaug, K., Bjugstad, N., Gislerød, H., Gadoury, D., and Stensvand, A. (2016). Suppression of powdery mildews by UV-B: application frequency and timing, dose, reflectance, and automation. Plant Dis. 100, 1643–1650. doi: 10.1094/PDIS-12-15-1440-RE
Thirumurugan, D., Cholarajan, A., Raja, S. S., and Vijayakumar, R. (2018). “An introductory chapter: secondary metabolites” in Secondary metabolites-sources and applications. ed. R. Vijayakumar (London, UK: IntechOpen), 3–21.
Tossi, V. E., Regalado, J. J., Iannicelli, J., Laino, L. E., Burrieza, H. P., Escandón, A. S., et al. (2019). Beyond Arabidopsis: differential UV-B response mediated by UVR8 in diverse species. Front. Plant Sci. 10:780. doi: 10.3389/fpls.2019.00780
Tsormpatsidis, E., Henbest, R., Battey, N. H., and Hadley, P. (2010). The influence of ultraviolet radiation on growth, photosynthesis and phenolic levels of green and red lettuce: potential for exploiting effects of ultraviolet radiation in a production system. Ann. Appl. Biol. 156, 357–366. doi: 10.1111/j.1744-7348.2010.00393.x
Tsormpatsidis, E., Ordidge, M., Henbest, R. G., Wagstaffe, A., Battey, N. H., and Hadley, P. (2011). Harvesting fruit of equivalent chronological age and fruit position shows individual effects of UV radiation on aspects of the strawberry ripening process. Environ. Exp. Bot. 74, 178–185. doi: 10.1016/j.envexpbot.2011.05.017
Tuchinda, C., Srivannaboon, S., and Lim, H. W. (2006). Photoprotection by window glass, automobile glass, and sunglasses. J. Am. Acad. Dermatol. 54, 845–854. doi: 10.1016/j.jaad.2005.11.1082
Turturică, M., Oancea, A. M., Râpeanu, G., and Bahrim, G. (2015). Anthocyanins: naturally occuring fruit pigments with functional properties. The Annals of the University Dunarea de Jos of Galati Fascicle VI-Food Technology 39, 9–24.
Urban, L., Charles, F., De Miranda, M. R. A., and Aarrouf, J. (2016). Understanding the physiological effects of UV-C light and exploiting its agronomic potential before and after harvest. Plant Physiol. Biochem. 105, 1–11. doi: 10.1016/j.plaphy.2016.04.004
Velasco, R., Zharkikh, A., Affourtit, J., Dhingra, A., Cestaro, A., Kalyanaraman, A., et al. (2010). The genome of the domesticated apple (Malus× domestica Borkh.). Nat. Genet. 42, 833–839. doi: 10.1038/ng.654
Verdaguer, D., Jansen, M. A., Llorens, L., Morales, L. O., and Neugart, S. (2017). UV-A radiation effects on higher plants: exploring the known unknown. Plant Sci. 255, 72–81. doi: 10.1016/j.plantsci.2016.11.014
Voke, J. (1999). Radiation effects on the eye. Part 3b-ocular effects of ultraviolet radiation. OT 8, 37–40.
Wang, S. Y., and Millner, P. (2009). Effect of different cultural systems on antioxidant capacity, phenolic content, and fruit quality of strawberries (fragaria× aranassa duch.). J. Agric. Food Chem. 57, 9651–9657. doi: 10.1021/jf9020575
Wu, B. -S., Hitti, Y., Macpherson, S., Orsat, V., and Lefsrud, M. G. (2019). Comparison and perspective of conventional and LED lighting for photobiology and industry applications. Environ. Exp. Bot. 171:103953. doi: 10.1016/j.envexpbot.2019.103953
Wu, C., Hsu, S., Chang, M., and Fang, W. (2009). Effect of light environment on runner plant propagation of strawberry. Acta Hortic. 907, 297–302. doi: 10.17660/ActaHortic.2011.907.48
Wu, B. -S., and Lefsrud, M. G. (2018). Photobiology eye safety for horticultural LED lighting: transmittance performance of eyewear protection using high-irradiant monochromatic LEDs. J. Occup. Environ. Hyg. 15, 133–142. doi: 10.1080/15459624.2017.1395959
Würtele, M., Kolbe, T., Lipsz, M., Külberg, A., Weyers, M., Kneissl, M., et al. (2011). Application of GaN-based ultraviolet-C light emitting diodes–UV LEDs–for water disinfection. Water Res. 45, 1481–1489. doi: 10.1016/j.watres.2010.11.015
Xie, Z., Charles, M. T., Fan, J., Charlebois, D., Khanizadeh, S., Rolland, D., et al. (2015). Effects of preharvest ultraviolet-C irradiation on fruit phytochemical profiles and antioxidant capacity in three strawberry (Fragaria× ananassa Duch.) cultivars. J. Sci. Food Agric. 95, 2996–3002. doi: 10.1002/jsfa.7064
Xu, Y., Charles, M. T., Luo, Z., Mimee, B., Tong, Z., Roussel, D., et al. (2019). Preharvest UV-C treatment affected postharvest senescence and phytochemicals alternation of strawberry fruit with the possible involvement of abscisic acid regulation. Food Chem. 299:125138. doi: 10.1016/j.foodchem.2019.125138
Xu, Y., Charles, M. T. R. S., Luo, Z., Mimee, B., Veronneau, P. -Y., Rolland, D., et al. (2017b). Preharvest ultraviolet C irradiation increased the level of polyphenol accumulation and flavonoid pathway gene expression in strawberry fruit. J. Agric. Food Chem. 65, 9970–9979. doi: 10.1021/acs.jafc.7b04252
Xu, Y., Charles, M. T., Luo, Z., Roussel, D., and Rolland, D. (2017a). Potential link between fruit yield, quality parameters and phytohormonal changes in preharvest UV-C treated strawberry. Plant Physiol. Biochem. 116, 80–90. doi: 10.1016/j.plaphy.2017.05.010
Yan, J. W., Ban, Z. J., Lu, H. Y., Li, D., Poverenov, E., Luo, Z. S., et al. (2018). The aroma volatile repertoire in strawberry fruit: a review. J. Sci. Food Agric. 98, 4395–4402. doi: 10.1002/jsfa.9039
Zahedi, S. M., and Sarikhani, H. (2016). Effect of far-red light, temperature, and plant age on morphological changes and induction of flowering of a ‘June-bearing’strawberry. Hortic. Environ. Biotechnol. 57, 340–347. doi: 10.1007/s13580-016-0018-8
Zahedi, S., and Sarikhani, H. (2017). The effect of end of day far-red light on regulating flowering of short-day strawberry (Fragaria× ananassa Duch. сv. Paros) in a long-day situation. Russ. J. Plant Physiol. 64, 83–90. doi: 10.1134/S1021443717010198
Zhang, L., Allen, L. H. Jr., Vaughan, M. M., Hauser, B. A., and Boote, K. (2014). Solar ultraviolet radiation exclusion increases soybean internode lengths and plant height. Agric. For. Meteorol. 184, 170–178. doi: 10.1016/j.agrformet.2013.09.011
Zhang, Z. -Z., Che, X. -N., Pan, Q. -H., Li, X. -X., and Duan, C. -Q. (2013). Transcriptional activation of flavan-3-ols biosynthesis in grape berries by UV irradiation depending on developmental stage. Plant Sci. 208, 64–74. doi: 10.1016/j.plantsci.2013.03.013
Zheng, J., He, D., and Ji, F. (2019). Effects of light intensity and photoperiod on runner plant propagation of hydroponic strawberry transplants under LED lighting. Int. J. Agric. Biol. Engin. 12, 26–31. doi: 10.25165/j.ijabe.20191205.5265
Zhou, Y. -H., Guo, D. -P., Zhu, Z. -J., and Qian, Q. -Q. (2005). Effects of in vitro rooting environments and irradiance on growth and photosynthesis of strawberry plantlets during acclimatization. Plant Cell Tissue Organ Cult. 81, 105–108. doi: 10.1007/s11240-004-2775-9
Keywords: LED, light spectrum, secondary metabolite, UV, visible light
Citation: Warner R, Wu B-S, MacPherson S and Lefsrud M (2021) A Review of Strawberry Photobiology and Fruit Flavonoids in Controlled Environments. Front. Plant Sci. 12:611893. doi: 10.3389/fpls.2021.611893
Edited by:
Marie-Theres Hauser, University of Natural Resources and Life Sciences Vienna, AustriaReviewed by:
Vance Whitaker, University of Florida, United StatesGiovanni Agati, Nello Carrara Institute of Applied Physics (IFAC), Italy
Copyright © 2021 Warner, Wu, MacPherson and Lefsrud. This is an open-access article distributed under the terms of the Creative Commons Attribution License (CC BY). The use, distribution or reproduction in other forums is permitted, provided the original author(s) and the copyright owner(s) are credited and that the original publication in this journal is cited, in accordance with accepted academic practice. No use, distribution or reproduction is permitted which does not comply with these terms.
*Correspondence: Mark Lefsrud, bWFyay5sZWZzcnVkQG1jZ2lsbC5jYQ==