- Plant Sciences and Plant Pathology Department, Montana State University, Bozeman, MT, United States
The wheat curl mite (WCM, Aceria tosichella, Keifer) is an eriophyid mite species complex that causes damage to cereal crops in the Northern Great Plains by feeding damage and through the transmission of plant viruses, such as wheat streak mosaic virus. Insecticide treatments were evaluated in the greenhouse and field for efficacy at managing the WCM complex on wheat. Treatments tested were carbamates, organophosphates, pyrethroids, a neonicotinoid seed treatment, mite growth inhibitors, and Organic Materials Review Institute–approved biocontrols, soaps, and oils. Treatment with carbamates, organophosphates, and pyrethroids decreased WCM in greenhouse trials compared with untreated controls 14 days after infestation. The seed treatment, mite growth inhibitors, and organic pesticides did not reduce WCM populations effectively and consistently. The timing of application was tested using a sulfur solution as the experimental treatment. Treating plants with sulfur seven days after mite infestation reduced mites compared with the untreated control. In contrast, prophylactically applied sulfur and sulfur applied 14 days after mite infestation were not effective. When tested under field conditions with plots infested with viruliferous mites, there was no yield difference detected between untreated control plots and plots sprayed with insecticides. Select carbamates, organophosphates, and pyrethroids have a potential for use in greenhouse mite management when appropriate.
Introduction
The wheat curl mite (WCM, Aceria tosichella, Keifer) is an eriophyid mite species complex that causes feeding damage to cereal crops (Harvey et al., 2000) and vectors a number of viral pathogens, such as wheat streak mosaic virus (WSMV), triticum mosaic virus, High Plains wheat mosaic virus, and Brome streak mosaic virus (Slykhuis, 1955; Seifers et al., 1997, 2009; Stephan et al., 2008). Economical losses to these cereal viruses have been reported in epidemics since the 1950s in Montana (Brey et al., 1998) and other states in the Northern Great Plains region of the United States (Burrows et al., 2015). Infection with WSMV alone can be highly damaging to cereal crops, and research indicates that viruses in tandem can compound damage (Tatineni et al., 2010; Byamukama et al., 2014). WSMV is a member of the Potyviridae family (Stenger et al., 1998), and symptoms include a yellow mosaic on leaves, plant stunting, decreased grain yield, and plant death (Staples and Allington, 1956). Management recommendations include removing the “green bridge” plants that can allow mites to overwinter and oversummer, such as grassy weeds and volunteer wheat (Thomas and Hein, 2003; Jiang et al., 2005), altering planting date to avoid the mite vector (Hunger et al., 1992), and sources of plant tolerance or resistance to the mite or viruses (Martin et al., 1984; Price et al., 2014).
The difficulty of managing the WCM species complex can be partially attributed to the variability in genetic lineages within the complex that spans a large plant host range (Skoracka et al., 2013), with 90 host species recorded (Skoracka et al., 2010). The WCM species complex can therefore survive on multiple grass species and move by wind currents to re-infest in subsequent cropping seasons by surviving on weeds, alternative hosts, and winter wheat germinating in the fall (Coutts et al., 2008; Ito et al., 2012; Ranabhat et al., 2018).
Insecticides and acaricides are often used to curtail eriophyid mites on high value cash crops, such as citrus, coconuts, olives, and others (Kalaisekar et al., 2000; Pushpa and Nandihalli, 2008). Using chemicals to treat the WCM, and by proxy the viruses it vectors, is less feasible for cereal crops because of the low input and low return nature of production. An option that has been used for plant pest management since the early days of agriculture is sulfur (Tweedy, 1981), and researchers have used it to dust plants or in a foliar solution for greenhouse WCM management (Jeppson et al., 1975; Conner et al., 1991; Li et al., 2007).
A number of synthetic insecticides have also been tested for management of the WCM complex, such as carbamate carbofuran (Harvey et al., 1979) and organophosphate terbufos (Fritts et al., 1999), which are no longer labeled for cereals because of off-target effects. Similar broad-spectrum insecticides run the risk of reducing natural mite predators in the field (Stilwell, 2009) and beneficial organisms like pollinators (Moore et al., 1998; Nash et al., 2008). There are also concerns for human health, causing some insecticides to be put under regulatory review. A relevant example is chlorpyrifos (Racke, 1993; Ventura et al., 2019). The goal of this study was to evaluate the efficacy of insecticide treatments on the WCM complex in the greenhouse under controlled conditions and in field studies, with varying modes of action tested. Included were synthetic products and Organic Materials Review Institute (OMRI)-approved products for organic cereal growers.
Materials and Methods
Wheat Curl Mite Population
Mites were sourced from a colony established from WCM collected from the Arthur H. Post Agronomy Research Farm, Bozeman, MT, as described by Ito et al. (2012). Other mite populations collected from this region clustered with North American “Type 1” WCM; however, the mite biotype used in this research was not evaluated. The colony was maintained in a plant growth chamber at 18–24°C. Mite populations were raised on spring wheat cultivar Choteau, in MSU mix soil [1:1:1 Canadian sphagnum peat moss, mineral soil mix, and Aquagro 2000G (Aquatrols, Paulsboro, NJ, United States)].
Greenhouse Insecticide Efficacy Trials
Twenty treatments (Table 1) were analyzed in the greenhouse for efficacy against WCM-infested spring wheat plants (Triticum aestivum L. cv. Choteau). Experiments were conducted in the Plant Growth Center at Montana State University. Due to the large number of treatments tested and the time-consuming nature of mite counting, treatments were grouped by mode of action and tested in four separate experiments. Each experiment was performed three times and included a mite-infested control without insecticide. Experiments were organized as a completely randomized design with eight replications per treatment. Wheat seeds were planted in MSU mix (described above) in 12.7 × 9 cm pots at conditions of 24 ± 4°C (16 h day) and 18 ± 4°C (8 h night), watered daily or as necessary, and thinned to one plant, the experimental unit. Wheat seeds were coated with thiamethoxam prior to planting, courtesy of Syngenta®.
Wheat curl mites were transferred to experimental plants from the growth chamber colony at growth stage DC 13–14 (Zadoks et al., 1974) by attaching infested leaves (6 cm in length with 30 WCMs) using paper clips to the ligule end and adaxial surface of the youngest expanded leaf to allow mites to move from the leaf piece to the experimental plant. After 2 days, leaf pieces and paper clips were removed from plants, and the number of mites remaining on the leaf piece was counted to estimate the approximate number of mites out of the 30 WCMs applied that transferred to the experimental plant. At the time of WCM transfer, plants were enclosed in a plastic cover [14 cm height, 8.5 cm diameter, Pro-Kal containers (Fabri-Kal, Kalamazoo, MI, United States)] with three cutouts (5 × 2 cm) sealed with a fine nylon lab pak mesh (25 μm; Sefar AG, Heiden, Switzerland) to prevent mite spread between plants. Pots were positioned eight per tray into black plastic 1,020 flats with no holes, for ease of watering due to plant covers.
Foliar treatments were applied 14 days after infestation (DAI) using a spray booth (Generation III Research Sprayer, DeVries Manufacturing, Hollandale, MN, United States) calibrated to run at 3.78 kph and spray a length of 1.8 m and at a pressure of 275 kPa with nozzle height 35 cm above the top of the plant. An 8002vs nozzle was used for application, manufactured by TeeJet® (Springfield, IL), and pesticides were prepared and mixed with water to make 500 ml of solution. Active ingredient concentrations were the highest labeled rates acceptable for wheat. The granular aldicarb treatment was applied to the soil and watered as per label directions. Three to four days after treatment, whole plant mite number was calculated by counting mites under a dissecting microscope at 10× magnification. Mites were determined to be living based on movement, opacity, and white color.
To account for the fact that plants did not all have the same number of mites transferred initially, a population growth rate was calculated to determine how populations responded to treatments. This calculation used the Malthusian population growth model P(t) = P0ert given that P0 is the number of mites initially transferred to experimental plants, P(t) is the total plant number of mites at experiment end, “r” is the population growth rate, and “t” is time (in days) that mites were on plants (Yaninek et al., 1989). For ease of understanding differences in treatments, as population growth rate can be difficult to comprehend, population growth rate was then used to calculate adjusted total WCM, with the equation P(t) = 30er14 for the eight replicates, from which an average was calculated. This equation gives an estimated total mite number if all pots had exactly 30 mites transferred to start with, held constant at 14 days.
Sulfur was used in a treatment timing experiment, repeated twice. Foliar sulfur treatments were applied using the spray booth, calibrated as above. Treatments were spraying sulfur prophylactically 1 day prior to mite application, 7 DAI, and 14 DAI.
Statistical Analysis of Greenhouse Trials
Data from all three trials were not combined because of contamination with a morphologically similar mite, the cereal rust mite (Abacarus hystrix), in the final trial repetition. A linear model with treatment as a fixed effect and total WCM number as the response variable was run using R software version 3.4.3. A Tukey test was conducted using the “multcomp” package (Hothorn et al., 2008), and mean comparison letters were generated. The assumption of normality was checked using Q–Q plots and the assumption of homogeneity of variances with standardized residual plots.
Effects of Insecticides on Viruliferous Mite Inoculated Field Plots
Field trials were conducted on spring wheat (Tritcum aestivum cv. Choteau) in 2013 and winter wheat (cv. Genou) in 2014 at the Lutz Farm in Bozeman, Montana (45°48′38.5″N, 111°03′03.1″W, elevation 1,409 m). The soil at the farm is black dog silt loam, with an approximate pH of 7.3. The selected field design was a randomized complete block with seven blocks, with a total of 70 plots (2.75 × 2.45 m) of eight rows with 60 wheat seeds/m2, with buffer regions of 2 m between plots. Buffer strips of wheat were grown on the edge of field plots to check for natural WCM presence. Buffer zones were checked for natural WCM presence and mite movement from experimental plots twice in the growing season by sampling 20 leaves, selected randomly. WCMs were not detected in buffer zones in both years.
Field plots were inoculated in spring with WCM viruliferous for WSMV at growth stage DC 21 (Zadoks et al., 1974). WCMs were grown on spring wheat cv. Choteau that had been mechanically inoculated with an isolate of WSMV designated “Conrad-I” as described in Ito et al. (2012). Winter wheat plots were not inoculated in the fall. Plots were infested with 150 mites each from the source population used in greenhouse trials (described above), by attaching leaf pieces to one centrally located plant per plot.
Treatments were applied using a CO2-charged backpack sprayer with 4, 8,002 VS nozzles by TeeJet® (Springfield, IL) spaced one foot apart 18 days after mite infestation, at DC 39 (Zadoks et al., 1974). Ten treatments, including an inoculated but untreated control (sprayed with water), were randomized in seven blocks. Treatments selected were dimethoate, chlorpyrifos, zeta-cypermethrin, lambda-cyhalothrin, hexythiazox, neem oil with azadirachtin, Isaria fumosorosea Apopka, potassium salts, and sulfur using the same active ingredient amount as described for greenhouse trials (Table 1). In 2014, eight treatments were the same, while methomyl and etoxazole replaced I. fumosorosea Apopka and sulfur treatments because of the difficulty in the application of these products with the spray boom in the previous year. Sampling for WCM and WSMV was conducted 14 days posttreatment. Thirty fully expanded leaves were collected per plot in a systematic manner from the inner 1.75 m and inner six rows and stored on ice in 10.2 × 15.2 cm plastic sample bags (Fisher Scientific, Pittsburgh, PA, United States). Leaves were checked for mite presence under a dissecting microscope at a magnification of 10×. The leaves were then frozen at −20°C until virus detection with ELISA, conducted using the methodology described in Ito et al. (2012). At wheat maturity plots were harvested using a research combine (Wintersteiger, Salt LakeCity, UT, United States), and samples were returned to Montana State University to be dried and cleaned. Whole plot yield was calculated from grain weight using plot lengths taken on the day of harvest. Samples were also analyzed for moisture, test weight, and protein content at the MSU cereal quality laboratory.
Statistical Analysis of Field Trials
Yield was the selected response variable using a mixed-effects model with the “lme” function in the “nlme” package in R version 4.0.2 (Pinheiro et al., 2021). The fixed effects included in the model were WCM per plot, virus presence, and insecticide treatment. Block was included in the model as a random effect. WSMV infection at the plot scale was calculated as a proportion of sampled leaves testing positive for WSMV, and logit transformed to meet model assumptions, with untransformed data displayed. Residuals for normality of the model were checked using Q–Q plots, and the final model was selected in a sequential removal of components from a full model that checked interaction terms between factors. No interactions were found between factors, so interaction terms were excluded from the final statistical model.
Results
In greenhouse trials, the carbamates, organophosphates, and pyrethroids tested reduced the total WCM number on plants compared to untreated control plants (p < 0.001) (Table 2). Active ingredients aldicarb, chlorpyrifos, and methomyl were more effective at reducing WCM than dimethoate.
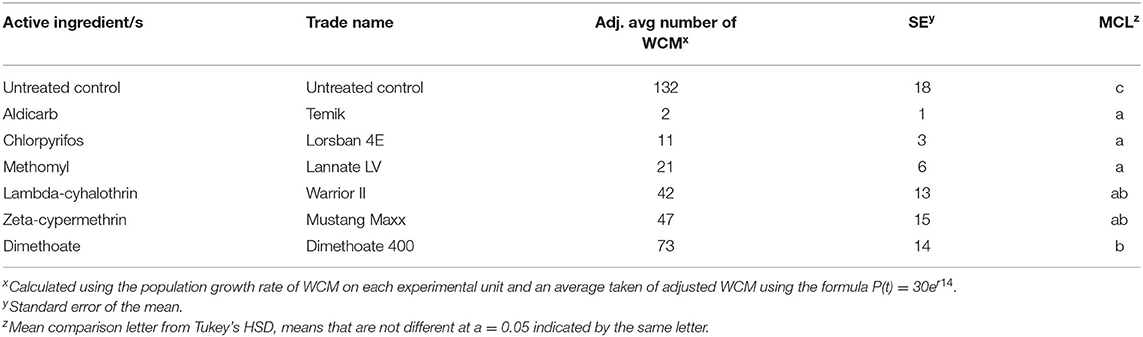
Table 2. Effect of carbamate, organophosphate, and pyrethroid treatments on average WCM total per plant.
The mite growth inhibitors, organic biocontrol, and organic pesticides did not reduce wheat curl mite numbers when applied 14 DAI under greenhouse conditions (Table 3).
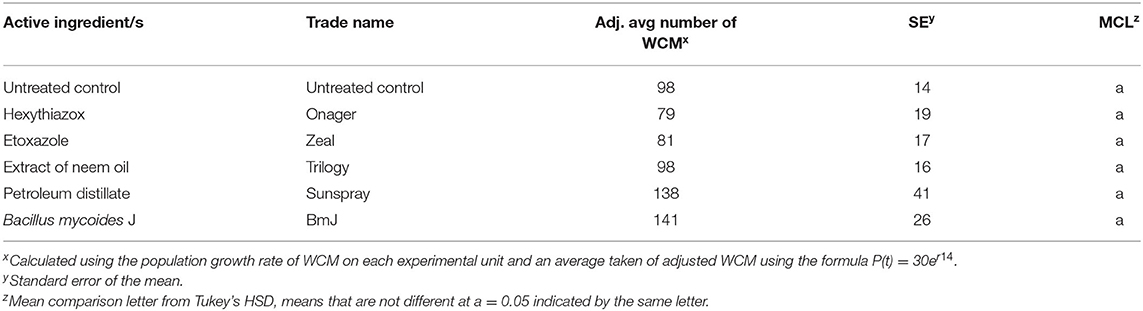
Table 3. Effect of mite growth inhibitor, organic biocontrol, and organic pesticide treatments on average WCM total per plant.
Additional organic pesticides tested did not reduce the total WCM number on plants compared with untreated controls (Table 4).
The seed treatment tested did not reduce mites compared with controls (Table 5).
The timing of sulfur treatment had an impact on the efficacy of treatment (Table 6). There was a difference between temporal treatments with sulfur applied seven DAI reducing WCM numbers and no difference between the prophylactic treatment, the 14 DAI treatment, and the untreated control (p = 0.028).
In the 2013 spring wheat field trial, there was no evidence of treatment improving yield compared to untreated controls (p = 0.912). Mite numbers detected per leaf were relatively low on sampled leaves (data not shown), while the presence of WSMV was easily discerned (Table 7). The presence of virus in plots did indicate an impact on yield (p = 0.015). In the 2014 winter wheat plots, no impact of treatment was detected on yield (p = 0.218), and the presence of mite and virus was much lower on the winter wheat than the year prior on spring wheat (Table 7).
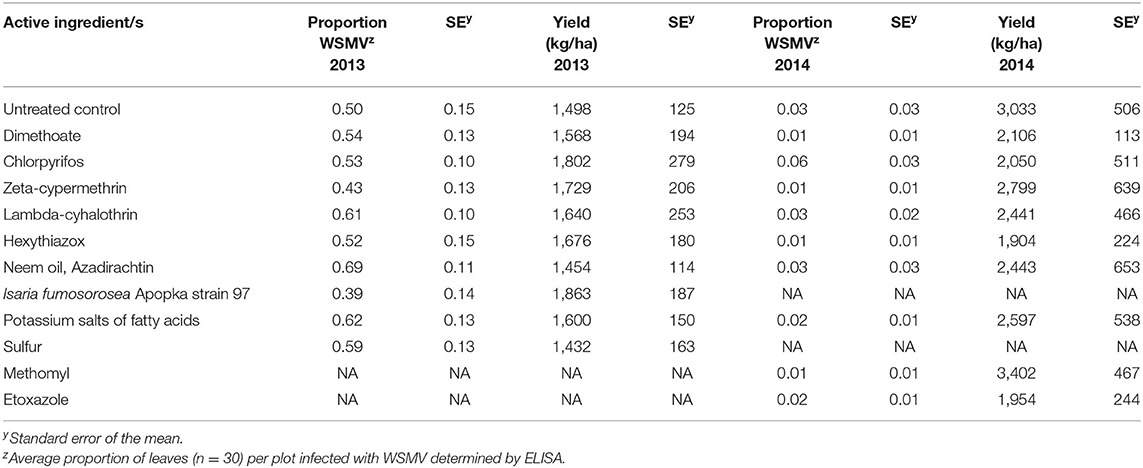
Table 7. Wheat streak mosaic virus presence and grain yield for spring wheat and winter wheat field plots.
Discussion
The aim of this study was to evaluate the efficacy of insecticides on WCM populations in controlled greenhouse conditions and in the field. Insecticides provide an additional tool in the management of other eriophyid mite species and have the potential for use in conjunction with other integrated pest management strategies. In a controlled environment, treatments effective at reducing WCM numbers on spring wheat were the organophosphate, carbamate, and pyrethroid insecticides. Active ingredients chlorpyrifos, methomyl, zeta-cypermethrin, lambda-cyhalothrin, and dimethoate were useful in managing greenhouse mite populations. These treatments are registered for use in the United States for cereals. Active ingredient aldicarb consistently reduced WCM; however, it is no longer registered for use on cereal crops in the United Sates. Chlorpyrifos, although available, is not recommended for management. This product is banned in the European Union, and its use is contested and controversial in the United Sates because of concerns with human safety.
Non-synthetics tested in this study did not reduce WCM populations at the selected rates and 14 DAI application timing. In other systems, non-synthetic options have been useful for eriophyid mite management. Neem oil is a prevalent treatment for the eriophyid coconut mite Aceria guerreronis on immature nuts (Pushpa and Nandihalli, 2008; Bagde et al., 2014). Demeton-S-methyl application, an organic product, was shown to reduce damage to coconuts from A. guerreronis in India (Muthiah and Bhaskaran, 1999). Garlic essential oil had acaricidal activity on eriophyid olive mites (Mossa et al., 2018). Compared with other eriophyid mites, management of the WCM on wheat is hindered when mite populations are high and wheat leaves curl inward under pressure, protecting WCM from the elements (Somsen and Sill, 1970). Contact foliar treatments may not be appropriate for this reason.
Under field conditions, spraying insecticides to control WCM as a method to help prevent WSMV damage and therefore prevent yield loss was not beneficial in the years these trials were run. On spring wheat plots, virus presence was relatively high and took a toll on grain yield, while mites were not easily detected in plots. In winter wheat, virus presence was too low to detect any impact of treatment for vector management and may require the addition of a fall inoculation for higher disease pressure. A previous study on Montana wheat fields indicated that spring inoculation led to higher virus presence on winter wheat than fall inoculation, with wheat cultivars differing in mite and virus tolerance (Miller et al., 2014). WCMs are dispersed by wind, and mite movement is often difficult to track, which can make scouting for the mite challenging and leads to variability in WCM presence each year. Natural WCM presence at the field site was not detected in buffer strips of wheat; however, it is possible that spring wheat plots were impacted by naturally occurring mites. WCMs have been found in the county in years prior and are spread by wind.
Timing of inoculation and timing of insecticide treatment are important factors for these studies. In the greenhouse, manipulating the timing of sulfur application revealed that treating plants infested with viruliferous mites at 7 DAI was more successful than treating at 14 DAI. Spraying earlier when mite numbers are potentially lower, and leaves are uncurled, requires regular scouting to detect the mite or virus, which may not be realistic for producers in large-scale cereal production.
Using insecticides for vector management in cereal production raises additional factors to consider. Concerns include environmental impacts of synthetic treatments, cost of treatment for producers compared with returns, target organism resistance to treatments, impact on beneficial organisms and naturally occurring mite predators (such as thrips) (Stilwell, 2009), and impacts to human health. Resistance becomes a concern when multiple sprays are needed in a season and pressure is high on target organisms. For the WCM complex, it has a high rate of reproduction and fast generational turnover that a few mites surviving after foliar spray can lead to a new generation in 10 days (Somsen and Sill, 1970). Multiple sprays may be required to see a yield benefit from treatment, which is likely not cost-effective in a cereal system, and extra treatments increase the risk for vector resistance. In addition, a taxonomic study conducted on the wheat curl mite has shown that it is likely a cryptic species complex (Skoracka et al., 2012, Skoracka et al., 2017). Two distinct WCM types exist in North America, Type 1 and Type 2, with tested Montana populations identified as Type 1 (Hein et al., 2012). It is unknown if WCM biotypes will respond differently to chemical treatments. Resistance to treatments could also differ across biotypes within the WCM complex with persistent and prolonged exposure to insecticides. In summary, greenhouse populations of WCM were reduced with select carbamates, organophosphates, and pyrethroids. Greenhouse and research facilities often deal with unwanted pests, and the WCM can be problematic in facilities where cereals are regularly propagated. This study demonstrated that select insecticides were effective at reducing WCM populations and may be useful in protecting such plants. Although efficacy was seen in greenhouse conditions, an effect of treatment was not seen in inoculated field trials. Using insecticides for WCM management in the field may not be feasible, and elucidating application timing, treatment rates, and cost analysis is required before it is considered appropriate. Therefore, it is important to consider the holistic system and evaluate the risk–reward ratio to insecticide application and other management options before incorporating an insecticide treatment into integrated mite management for management of viral pathogens.
Data Availability Statement
The raw data supporting the conclusions of this article will be made available by the authors, without undue reservation.
Author Contributions
MB conceived experiments, acquired funding, assisted with experimental design, and provided manuscript edits. CM conducted experiments, ran analyses, and wrote the manuscript. Both authors contributed to the article and approved the submitted version.
Funding
This project was funded by the United States Department of Agriculture (USDA) Pest Management award number 2012-34381-20120.
Conflict of Interest
The authors declare that the research was conducted in the absence of any commercial or financial relationships that could be construed as a potential conflict of interest.
Acknowledgments
The authors would like to thank Monica Brelsford, Matt Moffet, and David Baumbauer.
References
Bagde, A. S., Patil, P. D., and Pashte, V. V. (2014). Studies on efficacy of neem bio-pesticides against eriophyid mite (Aceria guerreronis Keifer.). Bioscan 9, 341–346.
Brey, C. W., Johnson, G. D., and Blodgett, S. L. (1998). Survey of Montana grasses for wheat curl mite (Acari: Eriophyidae), the vector of Wheat streak mosaic virus. J. Agric. Entomol. 15, 173–181.
Burrows, M. E., Thomas, C. S., Mcroberts, N., Bostock, R. M., Coop, L., and Stack, J. P. (2015). Coordination of diagnostic efforts in the Great Plains: wheat virus survey and modelling of disease onset. Plant Dis. 100, 1037–1045. doi: 10.1094/PDIS-04-15-0467-FE
Byamukama, E., Wegulo, S., Tatineni, S., Hein, G., Graybosch, R., Baenziger, P. S., et al. (2014). Quantification of yield loss caused by Triticum mosaic virus and Wheat streak mosaic virus in winter wheat under field conditions. Plant Dis. 98, 127–133. doi: 10.1094/PDIS-04-13-0419-RE
Conner, R. L., Thomas, J. B., and Whelan, E. D. P. (1991). Comparison of mite resistance for control of wheat streak mosaic. Crop Sci. 31, 315–318. doi: 10.2135/cropsci1991.0011183X003100020018x
Coutts, B., Strickland, G., Kehoe, M., Severtson, D., and Jones, R. (2008). The epidemiology of Wheat streak mosaic virus in Australia: case histories, gradients, mite vectors, and alternative hosts. Crop Pasture Sci. 59, 844–853. doi: 10.1071/AR07475
Fritts, D., Michels Jr, G., and Rush, C. (1999). The effects of planting date and insecticide treatments on the incidence of high plains disease in corn. Plant Dis. 83, 1125–1128. doi: 10.1094/PDIS.1999.83.12.1125
Harvey, T. L., Martin, T. J., and Seifers, D. L. (2000). Effect of nonviruliferous wheat curl mites on yield of winter wheat. J. Agric. Urban Entomol. 17, 9–13.
Harvey, T. L., Martin, T. J., and Thompson, C. A. (1979). Controlling wheat curl mite and Wheat streak mosaic virus with systemic insecticide. J. Econ. Entomol. 72, 854–855. doi: 10.1093/jee/72.6.854
Hein, G. L., French, R., Siriwetwiwat, B., and Amrine, J. W. (2012). Genetic characterization of North American populations of the wheat curl mite and dry bulb mite. J. Econ. Entomol. 105, 1801–1808. doi: 10.1603/EC11428
Hothorn, T., Bretz, F., and Westfall, P. (2008). Simultaneous inference in general parametric models. Biometric. J. 50, 346–363. doi: 10.1002/bimj.200810425
Hunger, R., Sherwood, J., Evans, C., and Montana, J. (1992). Effects of planting date and inoculation date on severity of wheat streak mosaic in hard red winter wheat cultivars. Plant Dis. 76, 1056–1060. doi: 10.1094/PD-76-1056
Ito, D., Miller, Z., Menalled, F., Moffet, M., and Burrows, M. (2012). Relative susceptibility among alternative host species prevalent in the Great Plains to Wheat streak mosaic virus. Plant Dis. 96, 1185–1192. doi: 10.1094/PDIS-09-11-0746-RE
Jeppson, L. R., Keifer, H. H., and Baker, E. W. (1975). Mites Injurious to Economic Plants. Oakland, CA: University of California Press.
Jiang, W., Garrett, K. A., Peterson, D. E., Harvey, T. L., Bowden, R. L., and Fang, L. (2005). The window of risk for emigration of Wheat streak mosaic virus varies with host eradication method. Plant Dis. 89, 853–858. doi: 10.1094/PD-89-0853
Kalaisekar, A., Naidu, V. G., and Rao, N. V. (2000). Citrus rust mite, Phyllocoptruta oleivora (Ashmead) (Eriophyidae: Acarina): effect of its damage on fruit quality and its chemical control. Ind. J. Plant Protect. 28, 132–134.
Li, H., Conner, R., Liu, Z., Li, Y., Chen, Y., Zhou, Y., et al. (2007). Characterization of wheat-triticale lines resistant to powdery mildew, stem rust, stripe rust, wheat curl mite, and limitation on spread of WSMV. Plant Dis. 91, 368–374. doi: 10.1094/PDIS-91-4-0368
Martin, T. J., Harvey, T. L., Bender, C. G., and Seifers, D. L. (1984). Control of Wheat streak mosaic virus with vector resistance in wheat. Phytopathology 74, 963–964. doi: 10.1094/Phyto-74-963
Miller, Z., Menalled, F., Ito, D., Moffet, M., and Burrows, M. (2014). Impacts of crop variety and time of inoculation on the susceptibility and tolerance of winter wheat to Wheat streak mosaic virus. Plant Dis. 98, 1060–1065. doi: 10.1094/PDIS-12-13-1210-RE
Moore, M., Huggett, D., Gillespie, W. Jr, Rodgers Jr, J., and Cooper, C. (1998). Comparative toxicity of chlordane, chlorpyrifos, and aldicarb to four aquatic testing organisms. Arch. Environ. Contam. Toxicol. 34, 152–157. doi: 10.1007/s002449900299
Mossa, A.-T. H., Afia, S. I., Mohafrash, S. M., and Abou-Awad, B. A. (2018). Formulation and characterization of garlic (Allium sativum L.) essential oil nanoemulsion and its acaricidal activity on eriophyid olive mites (Acari: Eriophyidae). Environ. Sci. Pollut. Res. 25, 10526–10537. doi: 10.1007/s11356-017-0752-1
Muthiah, C., and Bhaskaran, R. (1999). Screening of coconut genotypes and management of eriophyid mite Aceria guerreronis (Eriophyidae: Acari) in Tamil Nadu. Indian Coconut J. 30, 10–11.
Nash, M. A., Thomson, L. J., and Hoffmann, A. A. (2008). Effect of remnant vegetation, pesticides, and farm management on abundance of the beneficial predator Notonomus gravis (Chaudoir)(Coleoptera: Carabidae). Biol. Control 46, 83–93. doi: 10.1016/j.biocontrol.2008.03.018
Pinheiro, J., Bates, D., DebRoy, S., Sarkar, D., and R Core Team. (2021). nlme: Linear and Nonlinear Mixed Effects Models. R package version 3.1-152. Available online at: https://CRAN.R-project.org/package=nlme
Price, J. A., Simmons, A. R., Rashed, A., Workneh, F., and Rush, C. M. (2014). Winter wheat cultivars with temperature-sensitive resistance to Wheat streak mosaic virus do not recover from early-season infections. Plant Dis. 98, 525–531. doi: 10.1094/PDIS-04-13-0455-RE
Pushpa, V., and Nandihalli, B. (2008). Bioefficacy of botanicals in the management of coconut eriophyid mite, Aceria guerreronis Keifer. J. Plant Prot. Environ. 5, 90–94.
Racke, K. D. (1993). Environmental fate of chlorpyrifos. Rev. Environ. Contam. Toxicol. 131, 1–150. doi: 10.1007/978-1-4612-4362-5_1
Ranabhat, N. B., Seipel, T., Lehnhoff, E. A., Miller, Z. J., Owen, K. E., Menalled, F. D., et al. (2018). Temperature and alternative hosts influence Aceria tosichella infestation and Wheat streak mosaic virus infection. Plant Dis. 102, 546–551. doi: 10.1094/PDIS-06-17-0782-RE
Seifers, D. L., Harvey, T. L., Martin, T., and Jensen, S. G. (1997). Identification of the wheat curl mite as the vector of the High Plains virus of corn and wheat. Plant Dis. 81, 1161–1166. doi: 10.1094/PDIS.1997.81.10.1161
Seifers, D. L., Martin, T. J., Harvey, T. L., Fellers, J. P., and Michaud, J. P. (2009). Identification of the wheat curl mite as the vector of Triticum mosaic virus. Plant Dis. 93, 25–29. doi: 10.1094/PDIS-93-1-0025
Skoracka, A., Kuczyński, L., De Mendonça, R. S., Dabert, M., Szydło, W., Knihinicki, D., et al. (2012). Cryptic species within the wheat curl mite Aceria tosichella (Keifer)(Acari: Eriophyoidea), revealed by mitochondrial, nuclear and morphometric data. Invertebr Syst. 26, 417–433. doi: 10.1071/IS11037
Skoracka, A., Kuczynski, L., Szydlo, W., and Rector, B. (2013). The wheat curl mite Aceria tosichella (Acari: Eriophyoidea) is a complex of cryptic lineages with divergent host ranges: evidence from molecular and plant bioassay data. Biol. J. Linnean Soc. 109, 165–180. doi: 10.1111/bij.12024
Skoracka, A., Lewandowski, M., Rector, B. G., Szydło, W., and Kuczyński, L. (2017). Spatial and host-related variation in prevalence and population density of wheat curl mite (Aceria tosichella) cryptic genotypes in agricultural landscapes. PLoS ONE 12, 1–17. doi: 10.1371/journal.pone.0169874
Skoracka, A., Smith, L., Oldfield, G., Cristofaro, M., and Amrine, J. W. (2010). Host-plant specificity and specialization in eriophyoid mites and their importance for the use of eriophyoid mites as biocontrol agents of weeds. Exp. Appl. Acarol. 51, 93–113. doi: 10.1007/s10493-009-9323-6
Slykhuis, J. T. (1955). Aceria tulipae Keifer (Acarina: Eriophyidae) in relation to the spread of wheat streak mosaic. Phytopathology 45, 116–128.
Somsen, H. W., and Sill, W. H. Jr. (1970). The Wheat Curl Mite, Aceria tulipae Keifer, in Relation to Epidemiology and Control of Wheat Streak Mosaic. Manhattan, NY: Kansas State University Agricultural Experiment Station. 1–24.
Staples, R., and Allington, W. B. (1956). Streak Mosaic of Wheat in Nebraska and Its Control. Lincoln, OR: University of Nebraska College of Agriculture Experiment Station.
Stenger, D. C., Hall, J. S., Choi, I.-R., and French, R. (1998). Phylogenetic relationships within the family Potyviridae: Wheat streak mosaic virus and Brome streak mosaic virus are not members of the genus Rymovirus. Phytopathology 88, 782–787. doi: 10.1094/PHYTO.1998.88.8.782
Stephan, D., Moeller, I., Skoracka, A., Ehrig, F., and Maiss, E. (2008). Eriophyid mite transmission and host range of a Brome streak mosaic virus isolate derived from a full-length cDNA clone. Arch. Virol. 153, 181–185. doi: 10.1007/s00705-007-1065-3
Stilwell, A. R. (2009). Remote Sensing to Detect the Movement of Wheat Curl Mites Through the Spatial Spread of Virus Symptoms, and Identification of Thrips as Predators of Wheat Curl Mites. Lincoln, OR: Ph.D, University of Nebraska-Lincoln.
Tatineni, S., Graybosch, R. A., Hein, G. L., Wegulo, S. N., and French, R. (2010). Wheat cultivar-specific disease synergism and alteration of virus accumulation during co-infection with Wheat streak mosaic virus and Triticum mosaic virus. Phytopathology 100, 230–238. doi: 10.1094/PHYTO-100-3-0230
Thomas, J. A., and Hein, G. L. (2003). Influence of volunteer wheat plant condition on movement of the wheat curl mite, Aceria tosichella, in winter wheat. Exp. Appl. Acarol. 31, 253–268. doi: 10.1023/B:APPA.0000010384.12678.46
Tweedy, B. G. (1981). Inorganic sulfur as a fungicide. Residue Rev. 78, 43–68. doi: 10.1007/978-1-4612-5910-7_3
Ventura, C., Zappia, C., Lasagna, M., Pavicic, W., Richard, S., Bolzan, A., et al. (2019). Effects of the pesticide chlorpyrifos on breast cancer disease. Implication of epigenetic mechanisms. J. Steroid Biochem. Mol. Biol. 186, 96–104. doi: 10.1016/j.jsbmb.2018.09.021
Yaninek, J., Gutierrez, A., and Herren, H. (1989). Dynamics of Mononychellus tanajoa (Acari: Tetranychidae) in Africa: experimental evidence of temperature and host plant effects on population growth rates. Environ Entomol. 18, 633–640. doi: 10.1093/ee/18.4.633
Keywords: eriophyid, mite, wheat, cereal, insecticide
Citation: Murphy CY and Burrows ME (2021) Management of the Wheat Curl Mite and Wheat Streak Mosaic Virus With Insecticides on Spring and Winter Wheat. Front. Plant Sci. 12:682631. doi: 10.3389/fpls.2021.682631
Received: 18 March 2021; Accepted: 10 May 2021;
Published: 08 June 2021.
Edited by:
Christina Cowger, United States Department of Agriculture, United StatesReviewed by:
Wiktoria Szydło, Adam Mickiewicz University, PolandRobert Wright, University of Nebraska-Lincoln, United States
Copyright © 2021 Murphy and Burrows. This is an open-access article distributed under the terms of the Creative Commons Attribution License (CC BY). The use, distribution or reproduction in other forums is permitted, provided the original author(s) and the copyright owner(s) are credited and that the original publication in this journal is cited, in accordance with accepted academic practice. No use, distribution or reproduction is permitted which does not comply with these terms.
*Correspondence: Mary E. Burrows, bWJ1cnJvd3NAbW9udGFuYS5lZHU=