- 1Institute of Water Saving Agriculture in Arid Areas of China, Northwest A&F University, Yangling, China
- 2Institute of Soil and Water Conservation, Northwest A&F University, Yangling, China
- 3College of Water Resources and Architectural Engineering, Northwest A&F University, Yangling, China
- 4Language Culture College, Northwest A&F University, Yangling, China
The vertical root distribution and rooting depth are the main belowground plant functional traits used to indicate drought resistance in arid and semiarid regions. The effects of the slope aspect on the aboveground traits are visible but not the belowground deep root traits. We aimed to investigate the fine root traits of the locust tree (Robinia pseudoacacia L.) planted on southerly and northerly aspects, and the variations in the rooting depth in regions with different rainfall, as well as assessing how deep rooting, might affect the response to drought in a loess region. We selected three study sites with different rainfall amounts, with six sampling plots at each site (three each with southerly and northerly aspects). Soil core samples were collected down to the depth where no roots were present. The locust trees tended to develop deeper fine roots rather than greater heights. The tree height and diameter were greater for locust trees on northerly aspects, whereas trees on southerly aspects had significantly deeper rooting depths. Fine root traits (root length, root area, and root dry weight density) were higher in the southerly aspect for both Changwu and Ansai, but lower in Suide. The ratio of the root front depth tree height ranged from 1.04 to 3.17, which was higher on southerly than northerly aspects, and it increased as the rainfall decreased. Locust tree growth traits (belowground fine root and aboveground tree height) were positively correlated with the mean annual rainfall. The soil moisture content of the topsoil decreased as the rainfall decreased, but the pattern varied in the deep layer. Our results suggest that the variations in the belowground rooting depth under different slope aspects may be related to plant survival strategies. The vertical extension of the rooting depth and tree height may be key functional traits that determine plant growth in drought-prone regions.
Introduction
Determining plant functional traits might help us to understand the responses of plants to environmental variations (Reich et al., 2003). Solar radiation, temperature, and soil moisture are the main environmental factors that contribute to plant growth. The slope aspect is an important topographical factor because it can change the intensity and duration of solar radiation, thereby affecting the temperature and soil moisture in local areas (Cantón et al., 2004). The contrasting effects of northerly and southerly slopes can produce different climates, where the greater solar radiation on southerly slopes can increase the temperature and evapotranspiration to yield more arid conditions in the Northern Hemisphere (Finkel et al., 2001; Gutiérrez-Jurado et al., 2013). These climatic differences can cause plants to develop different functional traits, especially in terms of the tree height, canopy size, leaf structure, and branch density (Niinemets, 2006; Rozendaal et al., 2006). Finkel et al. (2001) and Nevo (2012) showed that the aboveground biomass was lower in a plantation on a southerly slope, whereas Kou et al. (2013) and Fan et al. (2012) obtained the opposite results. These differences of slope aspect and soil moisture in environmental factors could affect the aboveground morphological traits of plants (Vitória et al., 2019) and they might reflect the growth strategies employed by plants to resist drought (Liu and Ma, 2015). However, little information is available regarding the deep root traits of plants.
Fine roots (diameter < 2mm) play important roles in the absorption of water and nutrients (Chahine, 1992), especially the deep fine roots, which can absorb deep water in the soil to maintain plant transpiration during the dry season (Nepstad et al., 1994; Liu and Diamond, 2005; Oliveira et al., 2005). The ability of plants to access water reflects its adaptation to drought (Vadez, 2014). The fine root distribution and the rooting depth are the main belowground functional traits that might determine the volume of soil water acquired, thereby characterizing the growth and survival strategies of plants in water-limited ecosystems (Collins and Bras, 2007). Akkermans et al. (2012) showed that the rooting depth was a major variable that controls evapotranspiration during the dry season in African forests according to soil–vegetation–atmosphere transfer models, and it might be a direct indicator of a plant’s adaptability to drought. However, few studies have quantified the fine root distribution relative to rooting depth due to the difficulties of root sampling (Wang et al., 2009; Maeght et al., 2013; Freycon et al., 2014). In addition, studies have rarely investigated the deep fine root distributions under contrasting slope aspects (Kurze et al., 2017). Previous studies mainly concentrated on the differentiation of ecotypes on a large-scale aridity gradient (Wang and Gao, 2003; Volis, 2007; Kurze et al., 2017). According to the IPCC et al. (2013), the aridity will intensify in many regions throughout the world in the present century and the annual precipitation will decrease. Thus, deep fine root traits in regions with different rainfall amounts are valuable data for assessing hydrobiological processes and the adaptability of plants to environmental variations.
The Loess Plateau region of China is an ideal area for studying the root distributions in a deep and homogeneous soil layer because the deep loess layer is not affected by any interference due to differences in the groundwater level. The locust tree (Robinia pseudoacacia L.) is an exotic species that has been planted widely in China to conserve soil and water since the 1950s due to its drought tolerance, rapid growth, and simple propagation (Wang, 2017). The area planted with locust trees since the 1950s exceeds 70,000ha in the arid and semiarid Loess Plateau region (Guo et al., 1998). The locust tree is characterized by high water consumption and it can readily exhaust the available soil water. However, it is highly resistant to drought when the minimum survival requirement is maintained. Zhang et al. (2018) found that the fine root area density in locusts tree exhibited a decreasing trend from a wetter site to a drier site, and the fine roots exhibited similar rooting patterns along a precipitation gradient throughout the whole 3m soil profile. Nicolescu et al. (2018) showed that the locust tree was fast growing with the maximum tree height being reached in the first 5years and the peak diameter in the first 10years. The locust tree has a high capacity for adaptability and it can be utilized in projects that aim to convert farmland into forestland, it can severely desiccate the deep soil layer (Jia et al., 2017), thereby resulting in environmental deterioration with low productivity and efficiency.
In the present study, we comprehensively analyzed the vertical extent comprising the tree height and the rooting depth for the locust tree. We hypothesized that the rooting depth would increase as the rainfall decreased and it was deeper in northerly than southerly aspect according to the theory of optimal resource partition which indicated that plant root could extend deeper layer to absorb deeper soil water to resist drought in drier areas. The main aims of this study were as: (1) to investigate the deep fine root distribution for locust trees under different slope aspects; (2) to analyze the trends in the fine roots in regions with different rainfall amounts; and (3) to assess the vertical extents of the rooting depth and tree height as key functional traits for determining plant growth. The results obtained in this study provide new insights into the vertical extent of the deep roots and the ratio of the belowground rooting depth relative to the aboveground tree height in areas with different rainfall amounts, thereby helping us to understand plant growth and survival strategies.
Materials and Methods
Study Sites
This study was conducted during summer between July and August 2018 in the Loess Plateau region of China, where the deep loess layer and ground water depth are more than 50m (Ma et al., 2013; Zhu et al., 2018). Thus, this region is an excellent area for studying deep roots. In our study area, we investigated three different sites in Changwu, Ansai, and Suide counties across a distance of 337km. In this region, the climate is cold with four distinct seasons characterized by dry winters and warm summers in Changwu and Ansai (Dwb), and the arid conditions characterized by steppe and cold (Bsk) in Suide according to the Köppen-Geiger climate type (Peel et al., 2007). The highest sunlight, heat, and rainfall levels mostly occur in July, August, and September, and more than 50% of the total annual rainfall occur in these 3months (Table 1). The locations of the sites are shown in Figure 1. We sampled the northerly and southerly aspects at all sites with three samples from each.
At each site, we selected six sampling plots on the upper slopes (10×10m, with three on southerly aspects and three on northerly aspects) to quantitatively investigate the characteristics of the locust tree plantations. The sites were chosen carefully to minimize confounding environmental factors, where the altitude, inclination, and tree growth stage were similar on both aspects (three replicates). All sites were located in well-vegetated areas where the depth of the surface litter was about 1cm in the locust tree stands. The sampling point was located on the same side of the tree along the contour line. The sampling point and two adjacent locust trees (2m between the trees and 4m between the tree rows) at a similar growth stage in the same row formed an isosceles triangle with a side length of 1.2m from the tree trunk. We transformed the slope into flat ground with a shovel and fixed sampling shelves with two wooden baseboards (1.2m in length and 0.3m in width), where a plumb was used to determine the level. All weeds and the litter layer were removed every 10days at 30, 20, and 10days before sampling period within the sampling area to eliminate any interference from other plant roots.
The maximum rainfall infiltration depth was 2m (Liu et al., 2010). We defined the deep soil as the soil layer below 2m. Liu et al. (2014) showed that the deep soil moisture content (below 2m) remained fairly stable with almost no seasonal variation based on long-term observations in the study area. Thus, we assumed that the deep soil moisture was not affected by the rainfall in the sampling period and it could represent the soil moisture regime consumed by plants over a long period. According to previous studies, the sampling period during the summer is characterized by the peak abundance of fine roots (Burke and Raynal, 1994), minimum soil water storage (Duan et al., 2017), and the most severe drought throughout the year. Therefore, the data collected represented the responses of the fine roots to the soil water regime and other environmental factors in the corresponding sampling period.
Stand Characteristics
The locust tree stands at the three sites had similar densities (5,000 trees ha−1), with 2m between the trees and 4m between the tree rows, and the canopy was closed with coverage of more than 85%, except on the southerly aspect in Ansai where the coverage was 70%. The stands were all artificial forests, which were in middle age in Ansai (18years) and mature age in Changwu and Suide (37 and 35years) (Table 2); the trees were all at the growth period based on the regulations for the main tree species (LY/T 2908–2017, China), which define the age of locust trees as young at ≤15years, middle at 16–25years, and mature at 31–40years. The tree height and mean diameter at breast height were determined. We measured the tree height with an optical height meter (CGQ-1, Harbin Optical Instrument Factory Co. Ltd., China) and the mean diameter with a measuring tape. First, we measured the horizontal distance between the trees and the optical height meter with a measuring tape. We then targeted the top of the tree through an observation hole and determined the sight point carefully by aligning the pointer in the plumb position. We pulled the trigger and the pointer was locked. Hence, tree height was determined by the pointer scale values. The coverage represents the percentage of the vertical projection area of the plant aboveground relative to the sampling plot area, and it was calculated using the formula for the elliptical projected area of the canopy: , where X and Y denote the major and minor axis lengths, respectively (Phillips and Macmahon, 1981).
Fine Root Sampling and Analysis
Fine roots were sampled using the soil coring method (with a specialized soil auger made in China called a “Luoyang shovel” internal diameter=16cm), as described previously by Ma et al. (2013). In fact, a massive effort was done to achieve such work through very deep soil excavation. Luoyang shovel with larger internal diameter could greatly improve the sampling efficiency, it took about 2.5h for two persons to drill down to 8m depth, 4h for two persons to 12m depth, and 8h for three persons to 18m depth. Soil samples were collected at 20cm intervals throughout the whole soil layer until no roots were found in two consecutive layers. We set the “two consecutive layers” criterion for root front depth which meant that root front extended downward to the depth where no root occurred below this depth in two consecutive layers. Deep roots were defined as roots found below 2m. The vertical tree extension was defined as root front depth plus tree height.
Roots were separated from soil particles by washing through a 0.2mm sieve under running tap water. The root samples were placed in plastic bags, tagged with identifiers, and stored at 4°C until they were processed. Tree roots were distinguished from grass roots based on their morphology and color (Sun et al., 2015). Dead roots were identified based on their color and flexibility, and then discarded (live roots were yellowish brown, whereas dead roots were dark). We found a few dead roots in the top 1.2m soil depth and almost no decay roots occurred in deeper soil layer. Fine roots (diameter < 2mm) were collected and placed on transparent rectangular plastic plates filled with water, which allowed the roots to spread and float on the surface, before scanning at a resolution of 300 dpi. The area, length, and diameter were measured for the fine roots with WinRHIZO (Regent Instruments Inc., Canada). The fine root length density (RLD, m m−3) was defined as the root length per unit soil volume. D50 and D95 were used to denote the soil depths above which 50% or 95% of the total RLD were located, respectively. The fine root area density (RAD, m2 m−3) was defined as the root area per unit soil volume. The fine root dry weight density (RDWD, g m−3) was defined as the dry weight of roots per unit soil volume, which was determined after washing and cleaning, and drying for 24h at 65°C. Nothing was present in the deep layer except for soil and roots. We found only a few roots above 1cm in diameter below 3m. The eolian soil was homogeneous among the six sampling points at each site in the loess region. At present, the soil coring method is a feasible approach for identifying deep roots by actual field sampling.
Soil Moisture Analyses
Soil bulk density, soil field capacity, and soil wilting point were measured in 0–1m layer in the laboratory. Soil bulk density and soil filed capacity were determined by the cutting ring method proposed by Zhong and Zhang (2014). The wilting point was measured following the method described by Wei et al. (2004), who defined the wilting point as the soil water content when the soil water suction was equal to 1.47MPa.
After separating the roots, each soil core sample was dried to a constant weight at 105°C and the gravimetric soil moisture content was determined using the following formula.
Soil organic carbon, soil total nitrogen, and soil total phosphorus concentration were measured on the southerly and northerly aspect in the whole sampling soil depth in Changwu (unpublished data); we found that soil nutrients were concentrated on surface layer (0–40cm) and varied little with soil depth. Soil organic carbon content was larger on northerly than southerly aspect, while the total nitrogen and phosphorus content kept fairly consistent on different slope aspect. Soil organic carbon, total nitrogen, and phosphorus content were largest in Changwu, while they remained roughly similar values in Ansai and Suide (Zhang et al., 2018).
Statistical Analysis
A general linear model and multi-way analysis of variance were used to test the effects of the soil depth, slope aspect, site, and the interactions between the slope aspect × site, slope aspect × soil depth, site × soil depth, and slope aspect × soil depth × site on the fine root diameter, RLD, RAD, and RDWD. The slope aspect and site were treated as fixed effects, and the soil depth as a continuous variable and covariate. F-tests were conducted to assess the significance of the site, slope aspect, and their interaction. We log-transformed the root biomass values to satisfy the requirements for homoscedasticity and normality. All analyses were conducted with SPSS 11.0 (SPSS Inc., Chicago, Illinois, United States). Duncan’s test was used to determine significant differences (p < 0.05).
Results
Fine Root Traits
The fine roots of the locust trees exhibited similar trends, where RLD, RAD, and RDWD all decreased sharply in the top 2.6m of the soil, varied slightly from 2.6–5m, and then remained stable below 5m (Figure 2). The maximum RLD and RAD occurred in the top 0.2m on the northerly aspect in Ansai, and the maximum RDWD on the southerly aspect in Changwu. More than 50% of the total RLD was concentrated in the upper 2.2m (except for 3.4m on the northerly aspect in Changwu) and less than 5% below 8.6m (Figure 3).
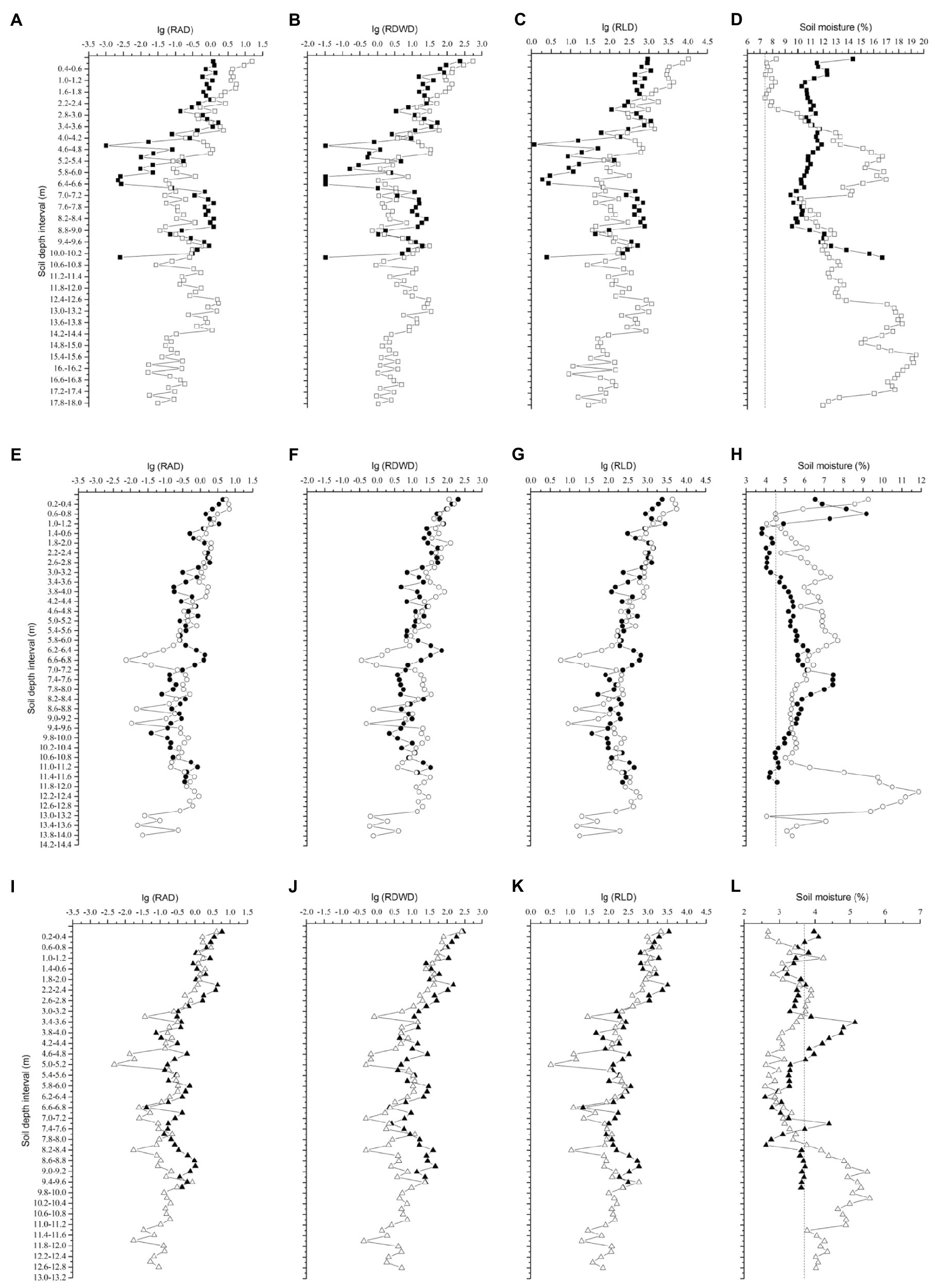
Figure 2. Changes in RAD, RDWD, RLD, and soil moisture down to the root front with soil depth. RAD is the root area density (m2 m−3), RDWD is the root dry weight density (gm−3), and RLD is the root length density (mm−3). Dashed lines denote the wilting point at each site. Solid and empty rectangles, circles, and triangles represent northerly and southerly aspects at the sites Changwu (A,B,C,D), Ansai (E,F,G,H), and Suide (I,J,K,L), respectively.
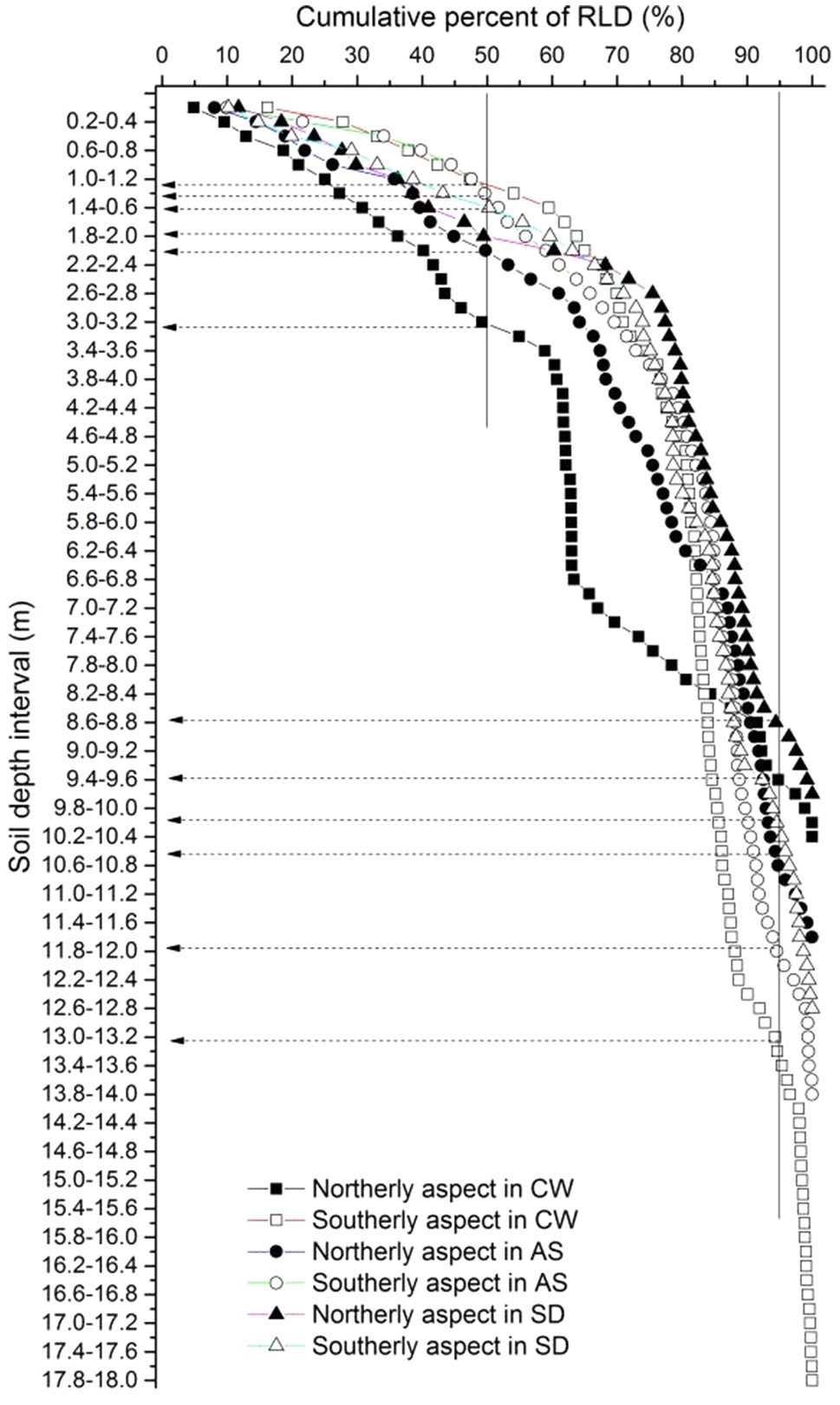
Figure 3. Cumulative percentages of fine root length density down to the root front with soil depth. Solid lines denote the 50 and 95% total root length densities. Dashed lines are the corresponding soil depths above which 50 and 95% of the total root length densities are located. Solid and empty rectangles, circles, and triangles represent northerly and southerly aspects at the sites Changwu, Ansai, and Suide, respectively.
We found that trees on southerly aspects had higher RLD, RAD, and RDWD values, except for Suide (Table 3). The total RLD, RAD, and RDWD values decreased as the rainfall decreased on southerly aspects. However, the total RAD and RDWD increased on northerly aspects, while the total RLD values were similar in Ansai and Suide, and greater than those in Changwu. Though fine root traits (RLD, RAD, and RDWD) decreased with the rainfall amount, there were no significant differences among the three sites (Table 4). The effects of the soil depth on RLD, RAD, and RDWD were highly significant (p < 0.01). The slope aspect had significantly different effects on RLD (p < 0.01) and RAD (p < 0.05) but not on RDWD. The mean root diameter varied little and it tended to be uniform throughout the whole soil depth (Table 3).
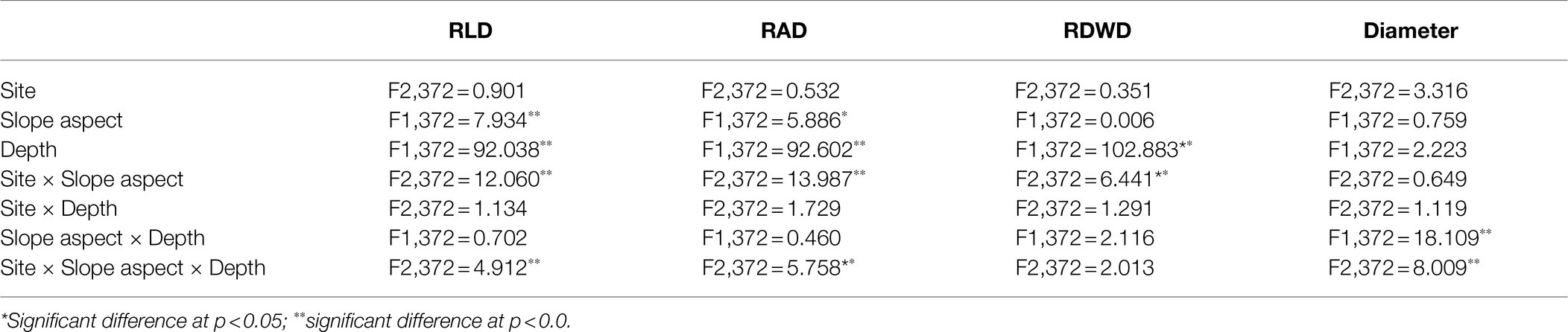
Table 4. F-values for the effects of site, slope aspect, and depth, and the interactions between site × slope aspect, site × depth, slope aspect × depth, and site × slope aspect × depth based on fine root length density (RLD, m m−3), fine root area density (RAD, m2 m−3), fine root dry weight density (RDWD. g m−3), and fine root diameter (mm).
Vertical Tree Extension
Our results showed that the vertical extents of the trees varied among sites and slope aspect (Figure 4; Table 2). The tree height and diameter at breast height decreased as the rainfall amount decreased, and they were also higher on northerly than southerly aspects (Table 2). The mean root front depth also decreased as the rainfall amount decreased, but it was much deeper on southerly than northerly aspects in the same site, where the difference was significant. Root front depth ranged from 9.6m on the northerly aspect in Suide to 18m on the southerly aspect in Changwu. The heights of the locust trees tended to be shorter than the depths of the root front. The ratio between the belowground root front depth and aboveground tree height ranged from 1.04 to 3.17, which was higher on southerly than northerly aspects, and it increased as the rainfall amount decreased. The vertical tree extension values were also larger on southerly than northerly aspects but they decreased as the rainfall amount decreased (Table 2).
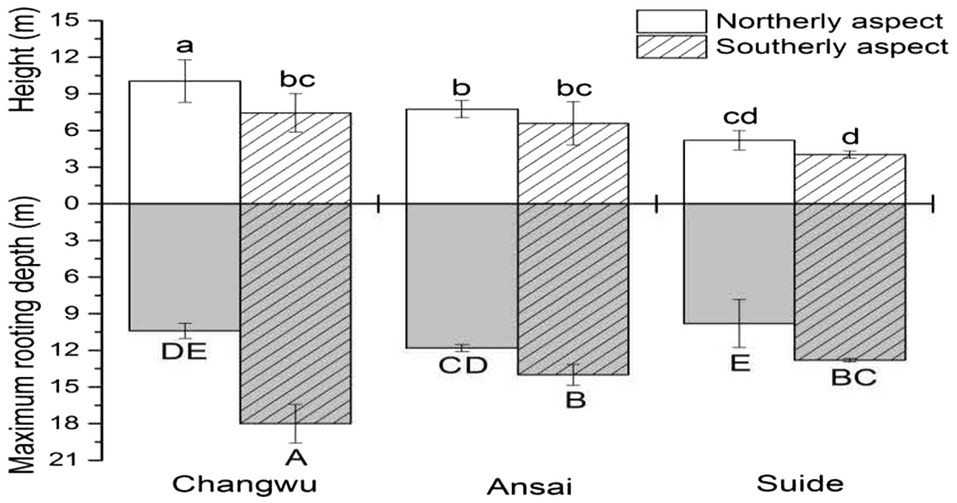
Figure 4. Mean stand heights and the depth of the root front at Changwu, Ansai, and Suide. Vertical bars indicate standard deviations. Lowercase letters indicate significant differences in stand height and uppercase letters indicate significant differences in the depth of the root front (n=3, p < 0.05).
Soil Moisture
The mean soil moisture contents in the 0–2m layer were higher on northerly than southerly aspects at each site, which were 11.55 and 7.8% in Changwu, 5.93 and 5.75% in Ansai, and 3.6 and 3.15% in Suide (lower than the wilting point of 3.7%), respectively. However, the tendency of mean soil moisture contents from 2m to the root front depth was opposite, which were 11.32 and 14.1% in Changwu, 5.34 and 6.65% in Ansai, and 3.57 and 3.83% in Suide, respectively. We found that the mean soil moisture contents of the top soil layer were higher on northerly than southerly aspects at each site and they decreased as the rainfall decreased. The soil moisture contents varied below 2m (Figures 2D,H,L).
Discussion
Deep Root Traits
Our results indicated that the mean RLD, RAD, and RDWD decreased as the rainfall amount decreased. Locust trees on southerly aspects had higher root biomasses, but the opposite was found in Suide, possibly because the lower root diameter and weaker growth led to smaller biomasses (Table 3) due to severe soil moisture depletion lower than wilting point. We found that the total RLD on southerly aspects was approximately three times higher in Changwu compared with that in Suide (Table 3; 62967.3mm−3 vs. 21161.9m m−3). A previous study also showed that the root biomass was four times higher in mesic populations (Kurze et al., 2017). Volis et al. (2002) and Mendola et al. (2015) determined a similar trend to our results for an annual grass. There may be intensified plant–plant competition for belowground water and nutrient resources in mesic environments (Metz and Tielbőrger, 2016). If the competition supersedes the effect of regional climate on the availability of belowground resource, i.e., less resources are available per individual in mesic sits, then the allocation of resources to the roots will be higher in a mesic environment compared with an arid environment (Kurze et al., 2017). However, these results were opposite to those predicted by optimal resource partitioning, where the investment in roots was expected to be higher under lower soil water conditions (Bloom et al., 1985). Aronson et al. (1992) also found that the allocation to roots was higher in an arid population during the early life stage. While this trend gradually reversed with age, thereby indicating that the maturing plant allocated more biomass to seed production rather than roots which might reflect a strategy for maximizing reproductive production at the expense of vegetative growth.
Pinheiro et al. (2016) found that the fine root densities decreased in the topsoil but remained roughly similar at depths of 0.25 and 6m. We also found that RLD, RDWD, and RAD decreased sharply in the top soil, but they remained stable below 5m (Figure 2). Schenk and Jackson (2002a) showed that D50 and D95 were located in the upper 0.3m and 2m, respectively. However, we found that D50 and D95 moved down to greater depths, especially D95, according to the experimental field measurements. The cumulative percentage RLD did not differ greatly in the shallow layers between northerly and southerly aspects, where the difference was mainly due to roots extending deeper on southerly aspects (Figure 3). Christina et al. (2011) and Laclau et al. (2013) showed that less than 5% of the fine root biomass of Eucalyptus grandis seedlings was present below a depth of 8m. We obtained similar results where less than 5% of the fine RLD was located below 8.6m (Figure 3). Christina (2015) reported that very low densities of the fine roots of E. grandis below a depth of 10m could meet most of the water requirements during dry periods after canopy closure. Indeed, an extremely low deep fine root density might contribute to improving tree survival in extremely arid regions. We speculated that variability in the rooting depth might be related to the survival of trees on different slope aspects in arid regions.
Soil Moisture
Plants can maintain a balance between the supply and demand of water by regulating the proportions of the aboveground and belowground plant parts (Tian et al., 2014). Tian et al. (2014) reported that the root: shoot ratio increased when Haloxylon seedlings suffered from severe water stress and a new water balance mechanism formed, which facilitated survival under soil moisture contents below 1.5%. We found that root front depth and root biomass (RLD, RAD, and RDWD) decreased as rainfall amount decreased and rainfall amount was positively related to soil moisture in the whole soil layer in three sampling sites. Severe soil moisture depletion up to the wilting point of 3.7% in Suide led to slow and stagnant growth, where the locust trees tended to be smaller and older, probably because of the rapid growth and high consumption of soil water in the early stage, and soil moisture could not meet the water requirements and environmental deterioration occurred accompanied by soil dry layer and degraded forest.
The infiltration of rainfall is quite complex and it is primarily influenced by factors, such as the soil texture, soil pore space, soil surface feature, slope position, and slope gradient. Ribolzi et al. (2011) found that the infiltration depth increased as the slope gradient increased. However, Jiang and Huang (1984) showed that the infiltration of rainfall decreased as the slope gradient increased due to the gradual decrease in the infiltration rate and increased runoff. Thus, the soil moisture content was higher on gentle slopes than steep slopes. In our study, we selected similar slope positions and soil surface feature to avoid the influence of rainfall infiltration. The soil moisture is an important factor for root growth (Zhou and Shangguan, 2007). We found that the mean soil moisture content in the 0–2m depth was higher on northerly than southerly aspects at each site (Figures 2D,H,L), possibly due to the greater photosynthetically active radiation and higher evapotranspiration on southerly compared with northerly aspects. Our results are consistent with those reported by Deng and Zhang (2010), whereas Sun et al. (2007) obtained the opposite results in the 1m surface layer. Wang (2017) found that the soil moisture content was higher in the topsoil and the tree height was lower on a shady slope compared with a sunny slope, where the main factor related to tree growth was soil aeration rather than the water content. We concluded that the soil moisture in the topsoil might have been affected by environmental factors, such as rainfall and soil transpiration, but the soil moisture content in the deep soil may have reflected differences of long-term rainfall in the soil and deep root characteristics, where an alternating pattern was detected at different sites (Figures 2D,H,L).
Effects of Slope Aspect on Tree Height and Rooting Depth
In contrast to our hypothesis, we found that the effect of the slope aspect was greater on the belowground plant parts than the aboveground parts (Figure 4). The locust trees had greater rooting depths and smaller tree heights on southerly aspects than northerly aspects, possibly because the southerly aspects could receive more solar radiation, thereby leading to greater transpiration and lower relative humidity, and the available soil moisture by locust trees in surface soil layer was less, thus exhibited smaller tree height. As we all know, the aboveground growth depended on the water taken up by the roots (Salmerón-Miranda et al., 2007), we found that root extended deeper in southerly than northerly aspect, which showed that root could absorb deeper water from deeper soil layer to sustain plant growth, while it did not indicate a lower water moisture content because the mean deep soil moisture content was calculated from 2m to the root front depth., The reduced root in northerly aspect may reflect the strategy of belowground part to maximize reproductive allocation (Kurze et al., 2017). Thus, the locust trees appear to have adapted to the different water conditions caused by the different slope aspects, where they exhibited diverse survival strategies (vertical tree extension, i.e., tree height plus rooting depth) to cope with drought. Petrů et al. (2006) obtained similar results and found that the height of Biscutella was lower in arid populations. May et al. (2013) and Šímová et al. (2015) also found that the rooting depth differed greatly between northerly and southerly aspects, where the tree height varied along a regional rainfall gradient. However, Christina et al. (2011) showed that the vertical tree extents above and below ground were almost the same in Eucalyptus plantations in Brazil. Kurze et al. (2017) suggested that the relative investment in the root: shoot ratio was higher in mesic populations. However, we found that the ratio of the rooting depth relative to the tree height increased as the rainfall amount decreased, thereby indicating that the locust trees allocated more biomass to the belowground parts to cope with drought conditions.
Larson and Funk (2016) suggested that the rooting depth may be the key factor for differentiating the performance of plants under drought conditions. However, few previous studies have determined the correlation between the rooting depth and the mean annual rainfall. Bhattachan et al. (2012) reported that there was no obvious relationship between the rooting depth and mean annual precipitation in water-limited ecosystem. Zhou et al. (2020) found that the potential rooting depth was negatively correlated with the mean annual rainfall across Southern African savannas, and they suggested that the root strategies were highly diverse in more arid sites. They estimated that the rooting depth was 4.58m for drought-resistant Birrea based on an exponential decay function. However, we determined deeper rooting depths by conducting full vertical excavations and based on actual measurements. Contrary to our hypothesis, we found that the rooting depth and vertical tree extension all decreased as the rainfall amount decreased (Figure 4). Zou (1986) showed that annual rainfall of 550mm was the boundary for the vegetation type distribution from forest steppe to forest vegetation. Our experimental areas had annual rainfall amounts ranging from 443 to 580mm (Table 1). Thus, the difference in the annual rainfall combined with the evapotranspiration produced by the slope aspect could have affected plant growth, thereby reflecting the plant survival strategy. The locust trees were relatively vigorous in terms of both their aboveground and belowground parts in the areas with more rainfall, but not in arid areas because the low rainfall and poor water supply led to weaker growth. We considered that the rooting depth could be used as a single indicator to represent a plant’s survival strategy, and the tree height combined with the rooting depth as two criteria to understand the strategy employed by a plant to adapt to drought stress.
The changes in the rooting pattern with stand age might vary according to the root adaptation strategies relative to the availability of water and nutrients. Zhang et al. (2018) obtained a curve with a single peak for the roots as the trees aged, and the coefficient of the rooting distribution to stand age (β) peaked in 18-year-old locust trees (β=0.9912) with a greater rooting depth but then decreased sharply in 35-year-old stands (β=0.9847) with a shallower rooting depth. Chang et al. (2012) found that the rooting patterns were similar for 8- and 30-year-old locust stands in the Loess Plateau region. However, we could only investigate 18-year-old locust trees in the area sampled in Ansai. In addition, we found that the 18-year-old locust trees in Ansai were in a vigorous growth stage, whereas the trees aged over 35years were in a stage of decline in Suide and Changwu (Table 2). According to a study by Zhang et al. (2018), the rooting depth might have become shallower with age in Ansai, and it would be greater in Changwu than Ansai at the same stand age. The difference between Ansai and Suide means that it is also necessary to compare tree samples with similar ages.
The rooting depth and biomass allocation are important belowground functional traits (Liu and Ma, 2015). Our deep sampling procedure was laborious and time consuming, so we only sampled three trees on each slope aspect per site. The sample size was limited but given the valuable quantitative data obtained, we consider that sampling three trees may have been representative of the actual root distributions. We found only a few roots with lengths of about 1cm below 2m in the soil cores, and our previous study also showed that that deep root biomass varied little with the age of the trees (Ma et al. IPCC et al., 2013). We concluded that a few deep root observed in our study might play an important role in improving locust tree resistance to drought and sustaining tree growth. Our sampling was an integration of annual growth that encompassed the water availability of the year given the limited infiltration of the periodic rainfall events. As described above, rainfall infiltration depth was no more than 2m (Liu et al., 2010), deep soil moisture (below 2m) would not affected by the periodic rainfall, i.e., the wettest part of the year versus both wet and dry period, it could reflect the effect of long-term meteorological factors on local area, rather than the short-term ones. Ma et al. (2012) also showed that the root density decreased with the horizontal distance from the tree trunk. Thus, the coring points selected in our study in the same position relative to the tree trunk might have avoided differences in the horizontal root distribution. The specific sampling positions might have influenced the shallow root distribution more than that of the deep roots. Therefore, comparing the deep root distribution at the same horizontal sampling position in different sites was a suitable approach.
The rooting depth is recognized as a key trait that underlies the resistance or resilience of trees under drought events (Nardini and Valentino, 2016). Our findings suggest that the vertical extension of the rooting depth and tree height might be key functional traits that determine plant growth in drought-affected areas. We found that the rooting depth of locust trees was positively correlated with the mean annual rainfall, but further studies of the shifts in the belowground root traits (e.g., root xylem size) and aboveground functional traits (e.g., leaf area) are needed to advance our understanding of the roles of the roots in the response to drought (Choat et al., 2018). Nevo (2012) suggested that differences in the slope aspect may be suitable for assessing the mechanisms that allow plants to adapt to different environments under climate change. Considering both the aboveground and belowground effects will be critical for predicting future changes in forest ecosystem function (Collins et al., 2020). Our results provide useful insights into how environmental factors might control the belowground and aboveground functional traits of plants.
Data Availability Statement
The original contributions presented in the study are included in the article/supplementary material, and further inquiries can be directed to the corresponding author.
Author Contributions
ML and LX were responsible for manuscript drafting and editing. WY was contributed to experimental design and analysis. YJ was contributed to field sampling and root cleaning. CJ was contributed to Interprate the data of work, and revise our manuscript critically. All authors contributed to the article and approved the submitted version.
Funding
This study was supported by the National Science Foundation of China (project number 41671510) and the Key Program of Research and Development in Shaanxi Province (project number 2020NY-155).
Conflict of Interest
The authors declare that the research was conducted in the absence of any commercial or financial relationships that could be construed as a potential conflict of interest.
Publisher’s Note
All claims expressed in this article are solely those of the authors and do not necessarily represent those of their affiliated organizations, or those of the publisher, the editors and the reviewers. Any product that may be evaluated in this article, or claim that may be made by its manufacturer, is not guaranteed or endorsed by the publisher.
Acknowledgments
We would like to thank the instrument shared platform of Food Science and Engineering College of NWAFU for assistance with the freeze dryer analysis. We are grateful to Duncan Jackson for correcting the English language.
References
Akkermans, T., Lauwaet, D., Demuzere, M., Vogel, G., Nouvellon, Y., Ardö, J., et al. (2012). Validation and comparison of two soil-vegetation-atmosphere transfer models for tropical Africa. J. Geophys. Res. Biogeosci. 117, 1–15. doi: 10.1029/2011JG001802
Aronson, J., Kigel, J., Shmida, A., and Klein, J. (1992). Adaptive phenology of desert and Mediterranean populations of annual plants grown with and without water stress. Oecologia 89, 17–26. doi: 10.1007/BF00319010
Bhattachan, A., Tatlhego, M., Dintwe, K., O’Donnell, F., Caylor, K. K., et al. (2012). Evaluating ecohydrological theories of woody root distribution in the Kalahari. PLoS One 7:e33996. doi: 10.1371/journal.pone.0033996
Bloom, A. J., Chapin, F. S. C., and Mooney, H. A. (1985). Resource limitation in plants - an economic analogy. Annu. Rev. Ecol. Syst. 16, 363–392. doi: 10.1146/annurev.es.16.110185.002051
Burke, M. K., and Raynal, D. J. (1994). Fine root growth phenology, production, and turnover in a northern hardwood forest ecosystem. Plant Soil 162, 135–146. doi: 10.1007/BF01416099
Cantón, Y., Del Barrio, G., Solé-Benet, A., and Lázaro, R. (2004). Topographic controls on the spatial distribution of ground cover in the Tabernas badlands of SE Spain. Catena 55, 341–365. doi: 10.1016/S0341-8162(03)00108-5
Chahine, M. T. (1992). The hydrological cycle and its influence of climate. Nature 359, 373–380. doi: 10.1038/359373a0
Chang, R. Y., Fu, B. J., Liu, G. H., Yao, X. L., and Wang, S. (2012). Effects of soil physicochemical properties and stand age on fine root biomass and vertical distribution of plantation forests in the loess Platear of China. Ecol. Res. 27, 827–836. doi: 10.1007/s11284-012-0958-0
Choat, B., Brodribb, T. J., Brodersen, C. R., Duursma, R. A., Lopez, R., and Medlyn, B. E. (2018). Triggers of tree mortality under drought. Nature 558, 531–539. doi: 10.1038/s41586-018-0240-x
Christina, M. (2015). Modeling the Water-Carbon Cycles at Tree Scale in Eucalyptus Plantations under Contrasting Water and Nutrient Constraints. France: SupAgro Montpellier.
Christina, M., Laclau, J. P., Gonçalves, J. L. M., Jourdan, C., Nouvellon, Y., and Bouillet, J. P. (2011). Almost symmetrical vertical growth rates above and below ground in one of the world's most productive forests. Ecosphere 2, 1–10. doi: 10.1890/ES10-00158.1
Collins, D. B. G., and Bras, R. L. (2007). Plant rooting strategies in water-limited ecosystems. Water Resour. Res. 43:W06407. doi: 10.1029/2006WR005541
Collins, C. G., Spasojevic, M. J., Alados, C. L., Aronson, E. L., Benavides, J. C., Cannone, N., et al. (2020). Belowground impacts of alpine woody encroachment are determined by plant traits, local climate and soil conditions. Glob. Chang. Biol. 26, 7112–7127. doi: 10.1111/gcb.15340
Deng, L., and Zhang, W. H. (2010). Natural development pattern of Robinia pseudoacacia plantations in loess hilly region. Sci. Silvae Sin. 46, 15–22.
Duan, L., Huang, M., Li, Z., Zhang, Z., and Zhang, L. (2017). Estimation of spatial mean soil water storage using temporal stability at the hillslope scale in black locust (Robinia pseudoacacia) stands. Catena 156, 51–61. doi: 10.1016/j.catena.2017.03.023
Fan, Y. Q., Zhou, G. M., Shi, Y. J., Dong, D. J., and Zhou, Y. F. (2012). Relationship of slope aspect and positon on biomass and carbon storage in a Phyllostachys edulis stand. J. Zhejiang. Agric. Univ. 29, 321–327.
Finkel, M., Fragman, O., and Nevo, E. (2001). Biodiversity and interslope divergence of vascular plants caused by sharp microclimatic differences at “evolution canyon II”, lower Nahal Keziv, upper Galilee. Israel. Isr. J Plant Sci 49, 285–296.
Freycon, V., Wonkam, C., Fayolle, A., Laclau, J.-P., Lucot, E., Jourdan, C., et al. (2014). Tree roots can penetrate deeply in African semi-deciduous rain forests: evidence from two common soil types. J. Trop. Ecol. 31, 13–23.
Guo, X. P., Zhu, J. Z., Yu, X. X., and Luo, J. (1998). The preliminary discussion of reforming the low-yield locust in loess plateau. Res. Soil. Water. Conserv. 5, 77–82.
Gutiérrez-Jurado, H. A., Vivoni, E. R., Cikoski, C., Harrison, J. B. J., Bras, R. L., and Istanbulluoglu, E. (2013). On the observed ecohydrologic dynamics of a semiarid basin with aspect-delimited ecosystems. Water Resour. Res. 49, 8263–8284. doi: 10.1002/2013WR014364
IPCC Stocker, T., Qin, D., Plattner, G. K., Tignor, M., Allen, S. K., et al. (2013). The physical science basis. Contrib. Work. Group I. Fifth. Assess. Rep. Intergovernmental. Panel. Clim. Change 996.
Jia, X. X., Shao, M. A., Zhu, Y. J., and Luo, Y. (2017). Soil moisture decline due to afforestation across the loess plateau, China. J. Hydrol. 546, 113–122. doi: 10.1016/j.jhydrol.2017.01.011
Jiang, D. S., and Huang, G. J. (1984). Simulation test of the influence of slope gradient on rainfall infiltration. Bull. Soil. Water. Conserv. 4, 10–13.
Kou, M., Jiao, J. Y., Du, H. D., Wang, Z. J., Wang, Q. L., and Su, Y. (2013). Species diversity and biomass of herbosa at different site conditions in the hilly-gullied loess plateau. J. Northwest. Univ. 28, 12–18.
Kurze, S., Bareither, N., and Metz, J. (2017). Phenology, roots and reproductive allocation, but not the LHS scheme, shape ecotypes along an aridity gradient. Perspect. Plant. Ecol. Evol. Syst. 29, 20–29. doi: 10.1016/j.ppees.2017.09.004
Laclau, J. P., da Silva, E. A., Rodrigues Lambais, G., Bernoux, M., le Maire, G., Stape, J. L., et al. (2013). Dynamics of soil exploration by fine roots down to a depth of 10 m throughout the entire rotation in Eucalyptus grandis plantations. Front. Plant Sci. 4:243. doi: 10.3389/fpls.2013.00243
Larson, J. E., and Funk, J. L. (2016). Seedling root responses to soil moisture and the identification of a belowground trait spectrum across three growth forms. New Phytol. 210, 827–838. doi: 10.1111/nph.13829
Liu, J., and Diamond, J. (2005). China’s environment in a globalizing world. Nature 435:1179. doi: 10.1038/4351179a
Liu, X. J., and Ma, K. P. (2015). Plant functional traits-concepts, applications and future directions. Scientia. Sinica. Vitae. 45, 325–339. doi: 10.1360/N052014-00244
Liu, X. L., Ma, L. H., Yang, R. H., Wu, P. T., and Wang, Y. K. (2014). Deep soil water depletion characteristic of jujube plantation in loess semiarid region. Trans. Chin. Soc. Agric. Mach. 45, 139–145.
Liu, W., Zhang, X. C., Dang, T., Ouyang, Z., Li, Z., Wang, J., et al. (2010). Soil water dynamics and deep soil recharge in a record wet year in the southern loess plateau of China. Agric. Water Manag. 97, 1133–1138. doi: 10.1016/j.agwat.2010.01.001
Ma, L. H., Liu, X. L., Wang, Y. K., and Wu, P. T. (2013). Effects of drip irrigation on deep root distribution, rooting depth, and soil water profile of jujube in a semiarid region. Plant Soil 373, 995–1006. doi: 10.1007/s11104-013-1880-0
Ma, L. H., Wu, P. T., and Wang, Y. K. (2012). Spatial distribution of roots in a dense jujube plantation in the semiarid hilly region of the Chinese loess plateau. Plant Soil 354, 57–68. doi: 10.1007/s11104-011-1041-2
Maeght, J. L., Rewald, B., and Pierret, A. (2013). How to study deep roots—and why it matters. Front. Plant Sci. 4:299. doi: 10.3389/fpls.2013.00299
May, F., Giladi, I., Ristow, M., Ziv, Y., and Jeltsch, F. (2013). Plant functional traits and community assembly along interacting gradients of productivity and fragmentation. Perspect. Plant. Ecol. Evol. Syst. 15, 304–318. doi: 10.1016/j.ppees.2013.08.002
Mendola, M. L., Baer, S. G., Johnson, L. C., and Maricle, B. R. (2015). The role of ecotypic variation and the environment on biomass and nitrogen in a dominant prairie grass. Ecology 96, 2433–2445. doi: 10.1890/14-1492.1
Metz, J., and Tielbőrger, K. (2016). Spatial and temporal aridity gradients provide poor proxies for plant-plant interactions under climate change: a large-scale experiment. Funct. Ecol. 30, 20–29.
Nardini, A., Valentino, C., Borgo, A. D., Savi, T., Stenni, B., Bertoncin, P., et al. (2016). Rooting depth, water relations and non-structural carbohydrate dynamics in three woody angiosperms differentially affected by an extreme summer drought. Plant. Cell. Environ. 39, 618–627. doi: 10.1111/pce.12646
Nepstad, D. C., de Carvalho, C. R., Davidson, E. A., Jipp, P. H., Lefebvre, P. A., Negreiros, G. H., et al. (1994). The role of deep roots in the hydrological and carbon cycles of Amazonian forests and pastures. Nature 372, 666–669. doi: 10.1038/372666a0
Nevo, E. (2012). “evolution canyon,” a potential microscale monitor of global warming across life. Proc. Natl. Acad. Sci. U. S. A. 109, 2960–2965. doi: 10.1073/pnas.1120633109
Nicolescu, V. N., Hernea, C., Bakti, B., Keser, Z., Antal, B., and Rédei, K. (2018). Black locust (Robinia pseudoacacia L.) as a multi-purpose tree species in Hungary and Romania: a review. J. For. Res. 29, 1449–1463. doi: 10.1007/s11676-018-0626-5
Niinemets, U. (2006). The controversy over traits conferring shade-tolerance in trees: ontogenetic changes revisited. J. Ecol. 94, 464–470. doi: 10.1111/j.1365-2745.2006.01093.x
Oliveira, R. S., Bezerra, L., Davidson, E. A., Pinto, F., Klink, C. A., Nepstad, D. C., et al. (2005). Deep root function in soil water dynamics in cerrado savannas of Central Brazil. Funct. Ecol. 19, 574–581. doi: 10.1111/j.1365-2435.2005.01003.x
Peel, M. C., Filayson, B. L., and McMahon, T. A. (2007). Updated world map of the Köppen-Geiger climate classification. Hydrol. Earth Syst. Sci. 11, 1633–1644. doi: 10.5194/hess-11-1633-2007
Petrů, M., Tielbörger, K., Belkin, R., Sternberg, M., and Jeltsch, F. (2006). Life historyvariation in an annual plant under two opposing environmental constraints along an aridity gradient. Ecography, 66–74. doi: 10.1111/j.2005.0906-7590.04310.x
Phillips, D. L., and Macmahon, J. A. (1981). Competition and spacing patterns in desert shrubs. J. Ecol. 69, 97–115. doi: 10.2307/2259818
Pinheiro, R. C., De Deus, J. C., Nouvellon, Y., Campoe, O. C., Stape, J. L., Aló, L. L., et al. (2016). A fast exploration of very deep soil layers by eucalyptus seedlings and clones in Brazil. For. Ecol. Manag. 366, 143–152. doi: 10.1016/j.foreco.2016.02.012
Reich, P. B., Wright, I. J., Cavender-Bares, J., Craine, M., Oleksyn, J., Westoby, M., et al. (2003). The evolution of plant functional variation: traits, spectra, and strategies. Int. J. Plant Sci. 164, S143–S164. doi: 10.1086/374368
Ribolzi, O., Patin, J., Bresson, L. M., Latsachack, K. O., Mouche, E., Sengtaheuanghoung, O., et al. (2011). Impact of slope gradient on soil surface features and infiltration on steep slopes in northern Laos. Geomorphology 127, 53–63. doi: 10.1016/j.geomorph.2010.12.004
Rozendaal, D. M. A., Hurtado, V. H., and Poorter, L. (2006). Plasticity in leaf traits of 38 tropical tree species in response to light; relationships with light demand and adult stature. Funct. Ecol. 20, 207–216. doi: 10.1111/j.1365-2435.2006.01105.x
Salmerón-Miranda, F., Båth, H., Eckersten, H., Forkman, J., and Wivstad, M. (2007). Aboveground nitrogen in relation to estimated total plant uptake in maize and bean. Nutr. Cycl. Agroecosyst. 79, 125–139. doi: 10.1007/s10705-007-9102-x
Schenk, H. J., and Jackson, R. B. (2002a). The global biogeography of roots. Ecol. Monogr. 72, 311–328.
Šímová, I., Violle, C., Kraft, N. J. B., Storch, D., Svenning, J.-C., Boyle, B., et al. (2015). Shifts in trait means and variances in north American tree assemblages: species richness patterns are loosely related to the functional space. Ecography 38, 649–658. doi: 10.1111/ecog.00867
Sun, T., Dong, L. L., Mao, Z. J., and Li, Y. Y. (2015). Fine root dynamics of trees and understorey vegetation in a chronosequence of Betula platyphylla stands. For. Ecol. Manag. 346, 1–9. doi: 10.1016/j.foreco.2015.02.035
Sun, Y. L., Wang, H. Y., and Liu, X. P. (2007). Soil water of artifical Robinia pseudoacacia L. on loess plateau. J. Qingdao. Univ. (Nat. Sci. Ed) 24, 123–126.
Tian, Y., Tashpolat, T., Li, Y., Tang, L. S., and Fan, L. L. (2014). The survival and above/below ground growth of Haloxylon ammodendron seedling. Acta Ecol. Sin. 34, 2012–2019.
Vadez, V. (2014). Root hydraulics: the forgotten side of roots in drought adaptation. Field. Crops. Res. 165, 15–24. doi: 10.1016/j.fcr.2014.03.017
Vitória, A. P., Alves, L. F., and Santiago, L. S. (2019). Atlantic forest and leaf traits: an overview. Trees 33, 1535–1547. doi: 10.1007/s00468-019-01864-z
Volis, S. (2007). Correlated patterns of variation in phenology and seed production in populations of two annual grasses along an aridity gradient. Evol. Ecol. 21, 381–393. doi: 10.1007/s10682-006-9108-x
Volis, S., Mendlinger, S., and Ward, D. (2002). Differentiation in populations of Hordeum spontaneum Koch along a gradient of environmental productivity and predictability: plasticity in response to water and nutrient stress. Biol. J. Linn. Soc. 75, 301–312. doi: 10.1111/j.1095-8312.2002.tb02071.x
Wang, Q. H. (2017). Effects of aspect and soil texture on growth of Robinia pseudoacacia. Prot. Sci. Technol., 28–30.
Wang, R., and Gao, Q. (2003). Climate-driven changes in shoot density and shoot biomass in Leymus chinensis (Poaceae) on the north-East China transect (NECT). Glob. Ecol. Biogeogr. 12, 249–259. doi: 10.1046/j.1466-822X.2003.00027.x
Wang, Z. Q., Liu, B. Y., Liu, G., and Zhang, Y. X. (2009). Soil water depletion depth by planted vegetation on the loess plateau. Sci. China. Ser D 52, 835–842.
Wei, Y. C., Liu, Z. X., and Kang, L. L. (2004). Derivation and verification of soil hydrodynamic parameters in cinnamon soil. J. Hydraul. Eng. 3, 81–85.
Zhang, Z. D., Huang, M. B., and Zhang, Y. K. (2018). Vertical distribution of fine-root area in relation to stand age and environmental factors in black locust (Robinia pseudoacacia) forests of the Chinese loess plateau. Can. J. For. Res. 48, 1148–1158. doi: 10.1139/cjfr-2018-0149
Zhong, C., and Zhang, J. B. (2014). Comparative analysis on measurement methods of field water capacity. Agr. Tech. 34, 29–30.
Zhou, Z. C., and Shangguan, Z. P. (2007). Vertical distribution of fine roots in relation to soil factors in Pinus tabulaeformis Carr. Forest of the loess plateau of China. Plant Soil 291, 119–129. doi: 10.1007/s11104-006-9179-z
Zhou, Y., Wigley, B. J., Case, M. F., Coetsee, C., and Staver, A. C. (2020). Rooting depth as a key woody functional trait in savannas. New Phytol. 227, 1350–1361. doi: 10.1111/nph.16613
Zhu, Y. J., Jia, X., and Shao, M. (2018). Loess thickness variations Across the loess plateau of China. Surv. Geophys. 39, 715–727. doi: 10.1007/s10712-018-9462-6
Keywords: fine root, functional trait, root distribution, slope aspect, vertical tree extension
Citation: Lihui M, Xiaoli L, Jie C, Youke W and Jingui Y (2021) Effects of Slope Aspect and Rainfall on Belowground Deep Fine Root Traits and Aboveground Tree Height. Front. Plant Sci. 12:684468. doi: 10.3389/fpls.2021.684468
Edited by:
Boris Rewald, University of Natural Resources and Life Sciences Vienna, AustriaReviewed by:
Emily Palm, University of Florence, ItalyChristophe Jourdan, Institut National de la Recherche Agronomique (INRA), France
Copyright © 2021 Lihui, Xiaoli, Jie, Youke and Jingui. This is an open-access article distributed under the terms of the Creative Commons Attribution License (CC BY). The use, distribution or reproduction in other forums is permitted, provided the original author(s) and the copyright owner(s) are credited and that the original publication in this journal is cited, in accordance with accepted academic practice. No use, distribution or reproduction is permitted which does not comply with these terms.
*Correspondence: Liu Xiaoli, NDA0MjcwMjM0QHFxLmNvbQ==