- 1Key Laboratory of Agro-environment and Climate Change of Agriculture Ministry, Institute of Environment and Sustainable Development in Agriculture, Chinese Academy of Agricultural Sciences, Beijing, China
- 2Jiangsu Key Laboratory for Bioresources of Saline Soils, Jiangsu Provincial Key Laboratory of Coastal Bio-agriculture, Jiangsu Provincial Key Laboratory of Coastal Wetland Bioresources and Environmental Protection, Jiangsu Synthetic Innovation Center for Coastal Bio-agriculture, School of Wetlands, Yancheng Teachers University, Yancheng, China
- 3Key Laboratory of Tea Quality and Safety Control, Ministry of Agriculture, Tea Research Institute, Chinese Academy of Agricultural Sciences, Hangzhou, China
- 4College of Agronomy, Heilongjiang Bayi Agricultural University, Daqing, China
Recently, several reports have suggested that the growth and grain yield of wheat are significantly influenced by high atmospheric carbon dioxide concentration (CO2) because of it photosynthesis enhancing effects. Moreover, it has been proposed that plants with large carbon sink size will benefit more from CO2 enrichment than those with small carbon sink size. However, this hypothesis is yet to be test in winter wheat plant. Therefore, the aim of this study was to examine the effect of elevated CO2 (eCO2) conditions on the quantum efficiency of photosystem II (PSII) photochemistry in large ear-type (cv. Shanhan 8675; greater ear C sink strength) and small multiple ear-type (cv. Early premium; greater vegetative C source strength) winter wheat varieties. The experiment was conducted in a free air CO2 enrichment (FACE) facility, and three de-excitation pathways of the primary reaction of PSII of flag leaf at the anthesis stage were evaluated under two CO2 concentrations (ambient [CO2], ∼415 μmol⋅mol–1, elevated [CO2], ∼550 μmol⋅mol–1) using a non-destructive technique of modulated chlorophyll fluorescence. Additionally, the grain yield of the two varieties was determined at maturity. Although elevated CO2 increased the quantum efficiency of PSII photochemistry (ΦPSII) of Shanhan 8675 (SH8675) flag leaves at the anthesis stage, the grain number per ear and 1,000-kernel weight were not significantly affected. In contrast, the ΦPSII of early premium (ZYM) flag leaves was significantly lower than that of SH8675 flag leaves at the anthesis stage, which was caused by an increase in the regulatory non-photochemical energy dissipation quantum (ΦNPQ) of PSII, suggesting that light energy absorbed by PSII in ZYM flag leaf was largely dissipated as thermal energy. The findings of our study showed that although SH8675 flag leaves exhibited higher C sink strength and quantum efficiency of PSII photochemistry at the anthesis stage, these factors alone do not ensure improved grain yield under eCO2 conditions.
Introduction
According to the IPCC (The Intergovernmental Panel on Climate Change) report, atmospheric CO2 concentration has been on an increase since the industrial revolution and is predicted to increase to 550 μmol⋅mol–1 in 2,050 and 1,020 μmol⋅mol–1 (RCP8.5) by the end of the century (Stocker et al., 2013; Dier et al., 2019). Atmospheric CO2 is an essential environmental factor necessary for photosynthesis, and it is commonly believed that photosynthesis is stimulated by elevated CO2 (eCO2) in C3 crops, because the ribulose-1,5-bisphosphate carboxylase-oxygenase (RuBisCO) is not substrate-saturated under the current ambient CO2 (aCO2) concentrations (Long et al., 2006; Aranjuelo et al., 2013). As one of the most important C3 food crop, wheat (Triticum aestivum L.) has been demonstrated to be highly sensitive to climatic and environmental variations (Misra and Chen, 2015; Pandey et al., 2018; Urban et al., 2018). Several studies have examined the effects of eCO2 on wheat photosynthesis; however, most of the studies focus on the dark phase of photosynthesis. Moreover, the effect of eCO2 on the primary reaction of photosystem II (PSII) in wheat is not fully understood. Primary reactions of photosystems mainly involve converting light energy into a primary form of chemical energy (Mathis and Rutherford, 1987). Effective photosynthesis involves optimum light absorption by the photosystem and the use of absorbed light quanta in subsequent oxygen-evolving reactions (Barber, 2016). Therefore, there is a need to examine the primary reaction of PSII in wheat photosynthetic organs under future eCO2 environments for sustainable wheat production.
Earlier studies on crop responses to elevated CO2 suggested significant genotypic variability in growth and yield (Ziska et al., 2012; Tausz et al., 2013; Tausz-Posch et al., 2015; Erice et al., 2019). The differences in light energy dissipative mechanisms between varieties in response to eCO2 might offer opportunities for the selection and breeding of high grain yield varieties for future production conditions. In cereals, it has been suggested that the source-sink relationship is a key factor for photosynthetic efficiency response to elevated CO2 (Uddling et al., 2008; Tausz et al., 2013). It has been proposed that plants are capable of avoiding photosynthetic downregulation because of their ability to increase C sink strength (Aranjuelo et al., 2009). It is of great interest to know how elevated CO2 will influence photosynthetic CO2 fixation, photoassimilates metabolism, and source-sink relationships in different varieties.
In wheat plants, photoassimilates accumulate mainly in the form of starch in the steams and in the form of sucrose in the flag-leaf before heading. After heading, the stored sugar is remobilized and transported to the ears, the new sink organs. The contribution of carbohydrate assimilated before anthesis to grain yield is in the range of 20∼40% of grain yield (Cock and Yoshida, 1972). However, little information has been reported on the carbon metabolism and allocation of photoassimilates in wheat varieties with different ear types and sizes under elevated CO2. Identifying wheat varieties that can permit full utilization of photosynthetic capacity is crucial for breeding high-photosynthesis potential varieties that are suitable for growth under elevated CO2 environments. Hence, the main objective of this study was to analyze the responses of large ear type and small multiple ear-type winter wheat varieties to elevated CO2 concentrations using modulated chlorophyll fluorescence detection technology.
The modulated chlorophyll fluorescence detection technology can rapidly capture fluorescence signals originating only from the plants and highly sensitive physiological responses to plant physiological status, particularly the responses of PSII activity to environmental changes (Feng et al., 2015; Goltsev et al., 2016; Banks, 2018; Osipova et al., 2019). Moreover, it can also identify the physiological conditions of plants at larger spatial and temporal scales (Zarco-Tejada et al., 2002). Additionally, chlorophyll fluorescence detection can explain the stepwise flow of energy through PSII from light absorption, dissipation, and electron transport for photochemical reactions (Kalaji et al., 2014). Therefore, in the present study, we adopted chlorophyll fluorescence detection technology to explore the effects of eCO2 on the quantum efficiency of PSII photochemistry in large ear and small multiple ear-type wheat varieties. The objectives of the study were: (i) to analyze the effect of eCO2 on chlorophyll fluorescence, photochemistry, and thermal dissipation in large ear and small multiple ear-type wheat varieties; (ii) to determine whether large ear type winter wheat variety with greater ear C sink strength (var. Shanhan 8675) possess higher quantum efficiency of PSII than that does the small multiple ear-type variety (cv. Early premium) under eCO2 environment; and (iii) to analyze the correlation between yield parameters and photosynthetic parameters, and to explore their responses to eCO2. The main hypothesis of this study is that the PSII primary photochemistry reaction of large-ear wheat variety responds positively (higher quantum efficiency of PSII photochemistry and lower non-photochemical energy dissipation quantum) to elevated CO2.
Materials and Methods
Experimental Site and Mini-FACE System
The experiment was conducted in a wheat-maize rotation mini-free air carbon dioxide enrichment system of Chinese Academy of Agricultural Sciences (CAAS-FACE system) in Changping (40°10′N, 116°14′E), Beijing, China, from 2016 to 2017. The soil (0–0.20 m) used for the study was a clay loam with pH (soil:water ratio of 1:5) of 8.4, organic C content of 14.10 g⋅kg–1, total N of 0.82 g⋅kg–1, available phosphorus of 19.97 mg⋅kg–1, and ammonium acetate extractable potassium of 79.77 mg⋅kg–1. The mean rainfall and temperature during the wheat growth period were 203 mm and 8.0°C, respectively (Figure 1). The Mini-FACE system consisted of 12 experimental plots, including six eCO2 rings (550 ± 17 μmol⋅mol–1) and six ambient CO2 (aCO2) rings (415 ± 16 μmol⋅mol–1), each with a diameter of 4 m. The experimental plots were at least 14 m apart to minimize cross-contamination of CO2 between the experimental treatments (Han et al., 2015). The CO2 enrichment treatment was accomplished using eight steel release pipes arranged like octagon corners, which released CO2 gas (Figure 2). In the case of eCO2 treatment, CO2 enrichment commenced 1 week after sowing and terminated at maturity. The CO2 concentration was maintained at 550 ± 17 μmol⋅mol–1 throughout the study period.
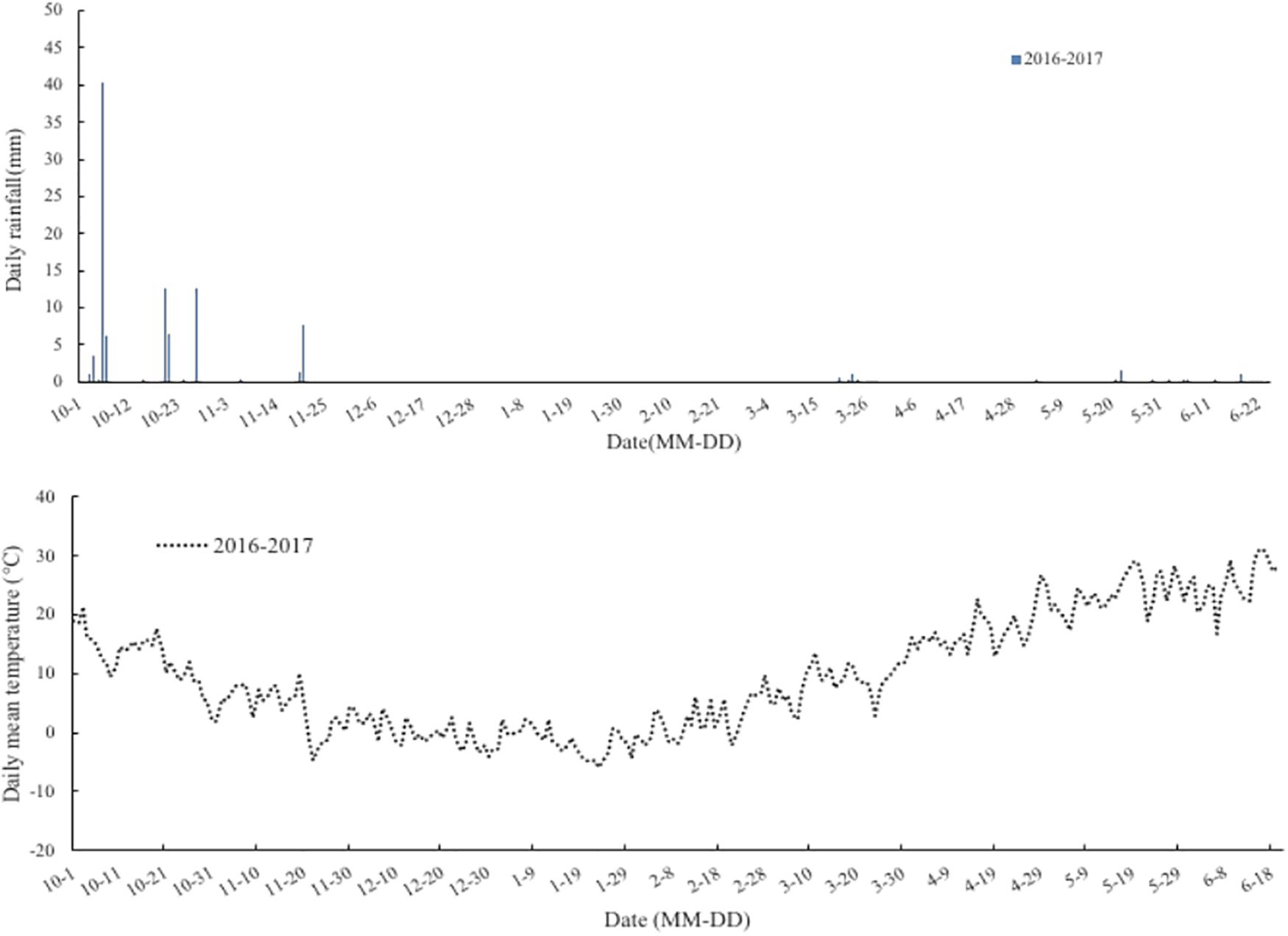
Figure 1. Rainfall (mm) and daily temperature (°C) at the wheat-maize rotation CAAS-FACE system in Changping, Beijing, China, from sowing of winter wheat until maturity during 2016–2017 experiment years.
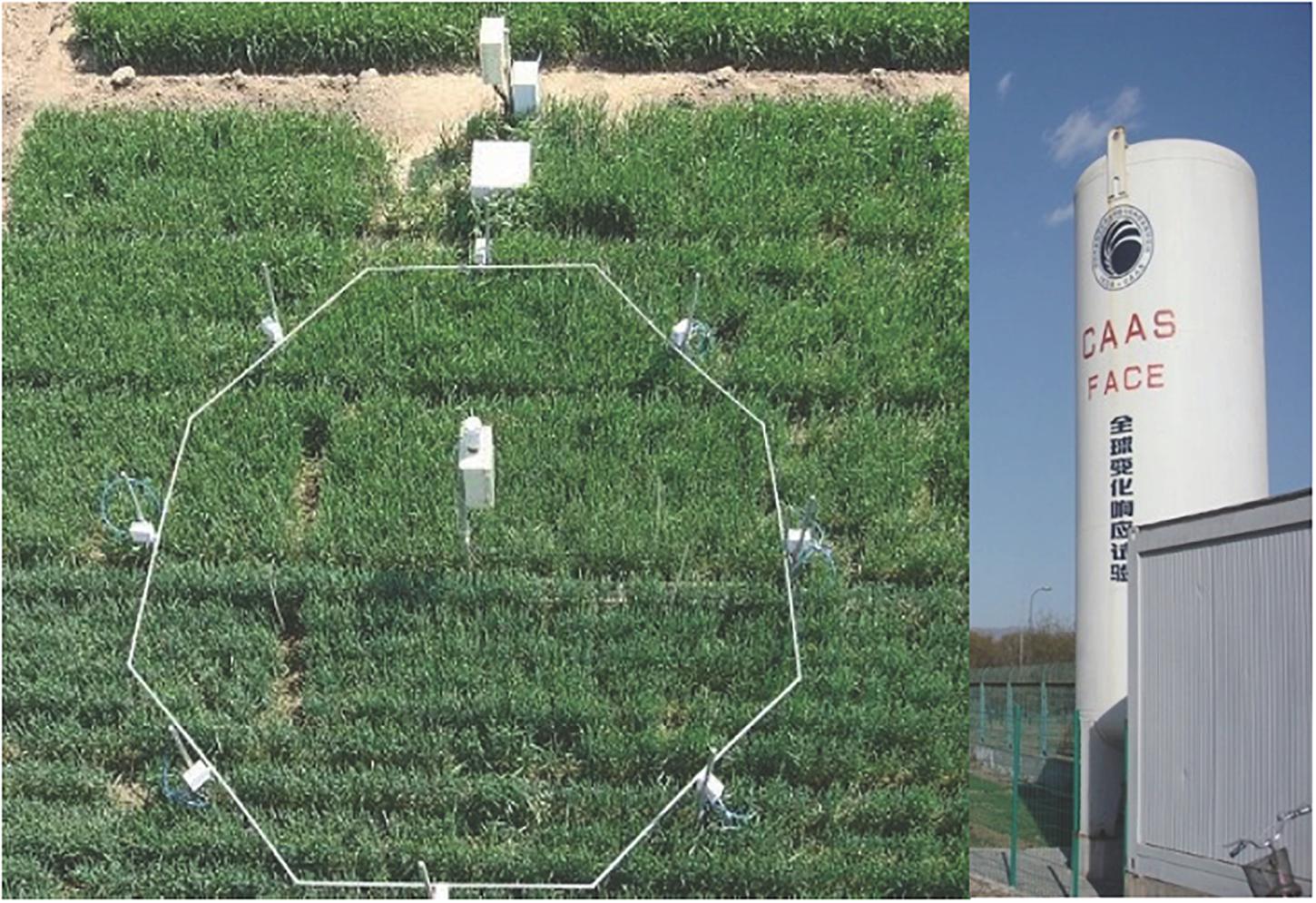
Figure 2. Mini-free air carbon dioxide enrichment system of Chinese Academy of Agricultural Sciences (CAAS-FACE system) in Changping, Beijing, China.
Plant Material and Fertilization
Two winter wheat (Triticum aestivum L.) varieties, SH8675 and ZYM, were selected for this study. According to the ear traits and harvest index (HI), SH8675 is regarded as a large-ear variety, while ZYM is regarded as a small-ear variety (Table 1). The winter wheat varieties were sown in each of the CO2 treatment plots at the same time, with a plot area of 3.75 m2. The planting density of SH8675 and ZYM wheat was 333 plants per square meter and rows interval was 20 cm in elevated CO2 rings (∼550 μmol⋅mol–1) and ambient CO2 rings (∼415 μmol⋅mol–1), with three replicates per treatment. The varieties were planted randomly in each plot to minimize the effects of soil variation. Granular urea (N, 46%), diammonium phosphate (N:P2O5 13:44%), and potassium chloride (K2O, 60%) were applied as basal fertilizers at the rates of 100 kg⋅hm–2, 165 kg⋅hm–2, and 90 kg⋅hm–2, respectively. At the jointing stage, granular urea was applied as side dressing at a rate of 100 kg⋅hm–2 on April 28, 2017. Irrigation was applied twice during the entire growing season of the winter wheat: the wintering irrigation at a rate of 750 m3⋅hm–2 on November 23, 2016, and spring irrigation at a rate of 750 m3⋅hm–2 was applied at the jointing stage after side dressing fertilization.
Chlorophyll Fluorescence Measurements
Chlorophyll fluorescence parameters were measured using a pulse amplitude modulation fluorometer (MINI-PAM, Heinz Walz, Germany). Chlorophyll fluorescence measurements were performed using intact flag leaves (three plants from each CO2 treatment) at 9:00–11:30 at half-way anthesis stage (DC 65) (Zadoks et al., 1974). Generally, SH8675 reaches anthesis (213 d) earlier than ZYM (216 d). However, in the present study, both varieties reached anthesis on the same day under CO2 and eCO2 conditions. The leaves were dark-adapted for 20 min with leaf clips to determine the ambient temperature fluorescence of dark-adapted leaf when all reaction centers are open and closed (Fo and Fm, respectively). Fo was measured under a weakly modulated measuring light (< 1μmol photons m–2s–1), and the leaves were immediately illuminated with an intense saturating pulse light (8,000 μmol photons m–2s–1, pulse time, 1s) to obtain Fm. The leaves were then light-adapted for 20 min, then turn on the actinic irradiation until the fluorescence reaches a steady state, the steady-state chlorophyll fluorescence (Fs) was measured, and Fm′ in the light-adapted state was estimated under saturated pulse light. According to previous studies, other parameters were calculated using the formulae given in Table 2.
NSC Measurement and Calculation
Non-structural carbohydrates (NSC) were extracted from plants at the anthesis stage. Leaf samples were placed in paper bags, deactivated at 150°C, and then dried at 80°C to a constant weight. The samples were ground and sieved through a 0.5 mm sieve. Sucrose and starch contents were measured using a resorcinol reagent and 3,5-dinitrosalicylic acid colorimetry reagent according to the procedures described by Wang et al. (2019). The sucrose and starch contents of the samples were determined spectrophotometrically using a multimode microplate reader (Infinite 200 PRO Nano Quant, Tecan, Switzerland). In this study, sugar and starch concentrations were estimated for NSC (Pan et al., 2011).
Statistical Analysis
Statistical analysis of the data generated in this study was performed using SPSS 18.0 and EXCEL 2016. The experiment was arranged in a split-plot design with the plots arranged in randomized complete blocks; and the CO2 concentrations (ambient or elevated CO2) were the whole-plot treatment and the winter wheat varieties with different ear-types were the split-plot treatment. A general linear model was used to estimate the main effects of CO2 and variety, as well as their interactions. Analysis of variance (ANOVA) was used to test for statistical significance to determine the differences between treatment means. Mean values were compared using the least significant difference (LSD) test and the means were considered significant at p < 0.05.
Results
Chlorophyll Fluorescence Yield and Attenuation
There was a 24.7% decrease (p < 0.05) in the Fm′ of ZYM and a 14.0% decrease (p < 0.05) in the Fs of SH8675 under eCO2 condition (Table 3). However, there were no significant differences in the Fm′ and Fs of the two varieties under eCO2 condition (Table 3). There was a 14.8 and 15.4% decrease (p < 0.05) in the ΔFv and ΔFv/Fo ratio of ZYM, respectively, under eCO2 condition; moreover, the ΔFv/Fo ratio of ZYM was significantly lower (p < 0.05) than that of SH8675 under eCO2 condition (Table 3). There was a 10.9% increase (p < 0.05) in the Rfd of SH8675 under eCO2 condition (Table 3); however, there was no significant difference in the Rfd of the two varieties under eCO2 condition (Table 3).
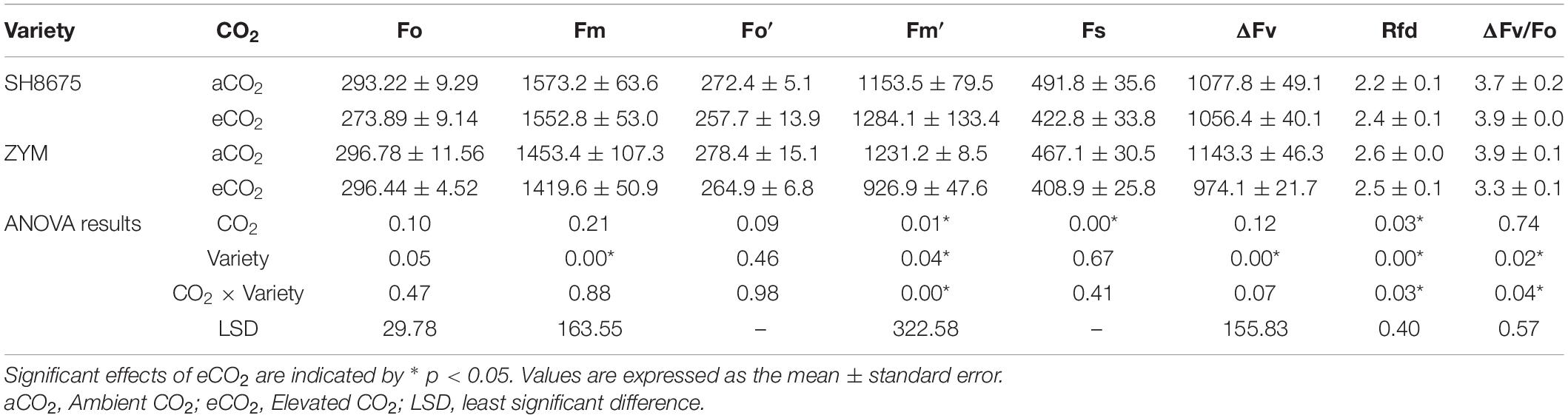
Table 3. Effects of elevated CO2 on chlorophyll fluorescence emission and attenuation of two winter wheat varieties.
Chlorophyll Fluorescence Quenching Coefficients
Elevated CO2 had no significant effect on the qP of the two winter wheat varieties; moreover, the effect of the varieties on qP was not significant (Figure 3A). However, eCO2 increased (p < 0.05) the qN of ZYM and SH8675 by 130.3 and 64.8%, respectively. Moreover, the qN of ZYM was significantly higher (p < 0.05) than that of SH8675 (Figures 3B,C), indicating that eCO2 significantly increased the thermal dissipation potential (more light energy absorbed by PSII was dissipated thermally) of ZYM compared with that of SH8675.
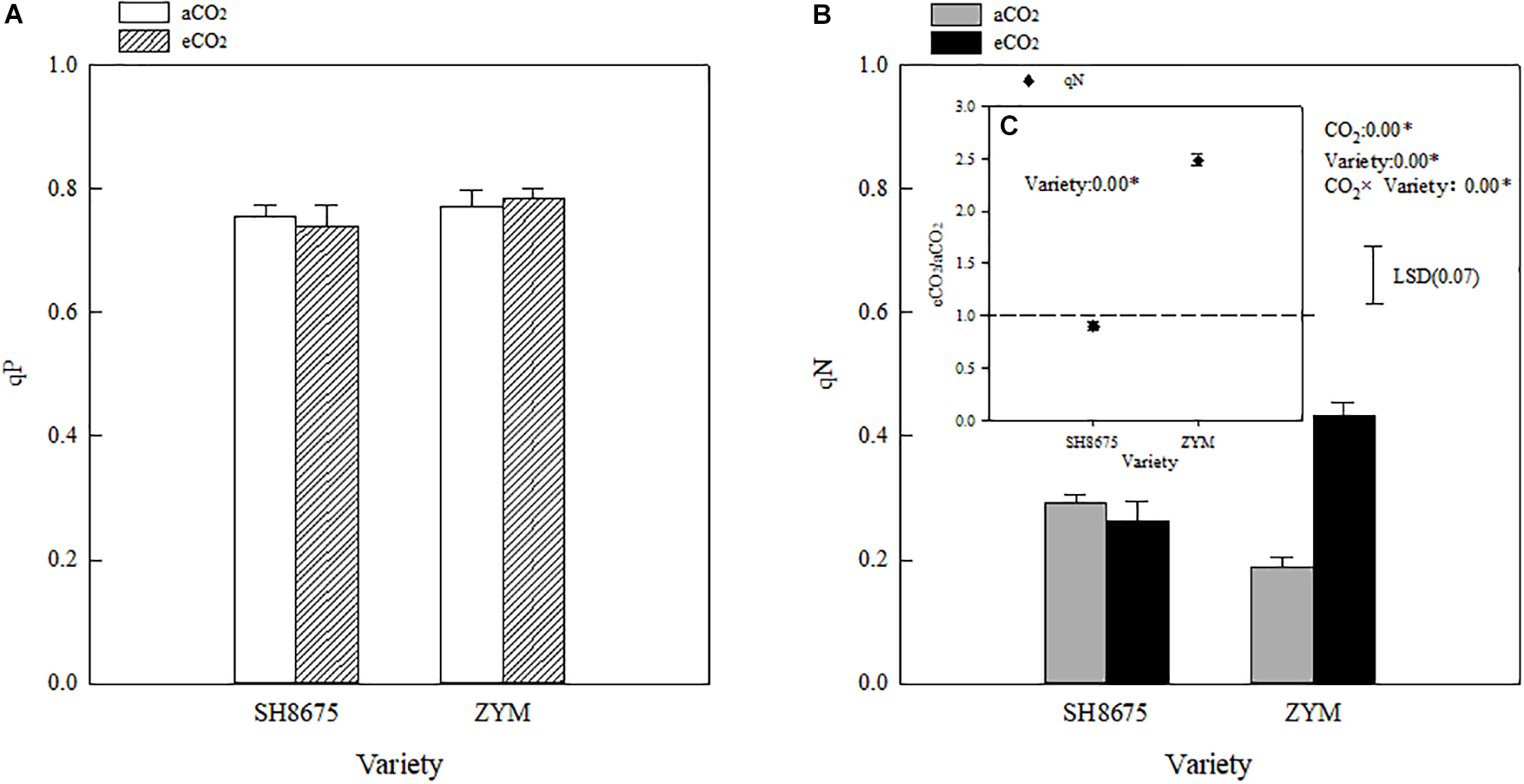
Figure 3. Effects of eCO2 on the qP and qN of two winter wheat varieties (A–C). Measurements were carried out on intact flag leaves. Data represent the mean of three plants from each plot ± SD (standard error) bars. ANOVA results with * indicate significance at p < 0.05. Vertical bar in Figure 3B indicates LSD (p < 0.05) for qN. Differences in the qN of the varieties in response to eCO2 is showed in Figure 3C.
Non-photochemical Excitation Energy Dissipation
ΦNPQ and ΦNO are positively related to light energy utilization in photochemical reactions. Elevated CO2 increased (p < 0.05) the ΦNPQ of ZYM and SH8675 by 106.4 and 50.9%, respectively (Figure 4A). However, the ΦNPQ of ZYM was significantly higher than that of SH8675 (Figure 4C), indicating that eCO2 significantly increased the thermal dissipation of ZYM, which resulted in lower quantum efficiency of PSII photochemistry. While elevated CO2 had no significant effect on the ΦNO of ZYM and SH8675 (Figures 4B,D).
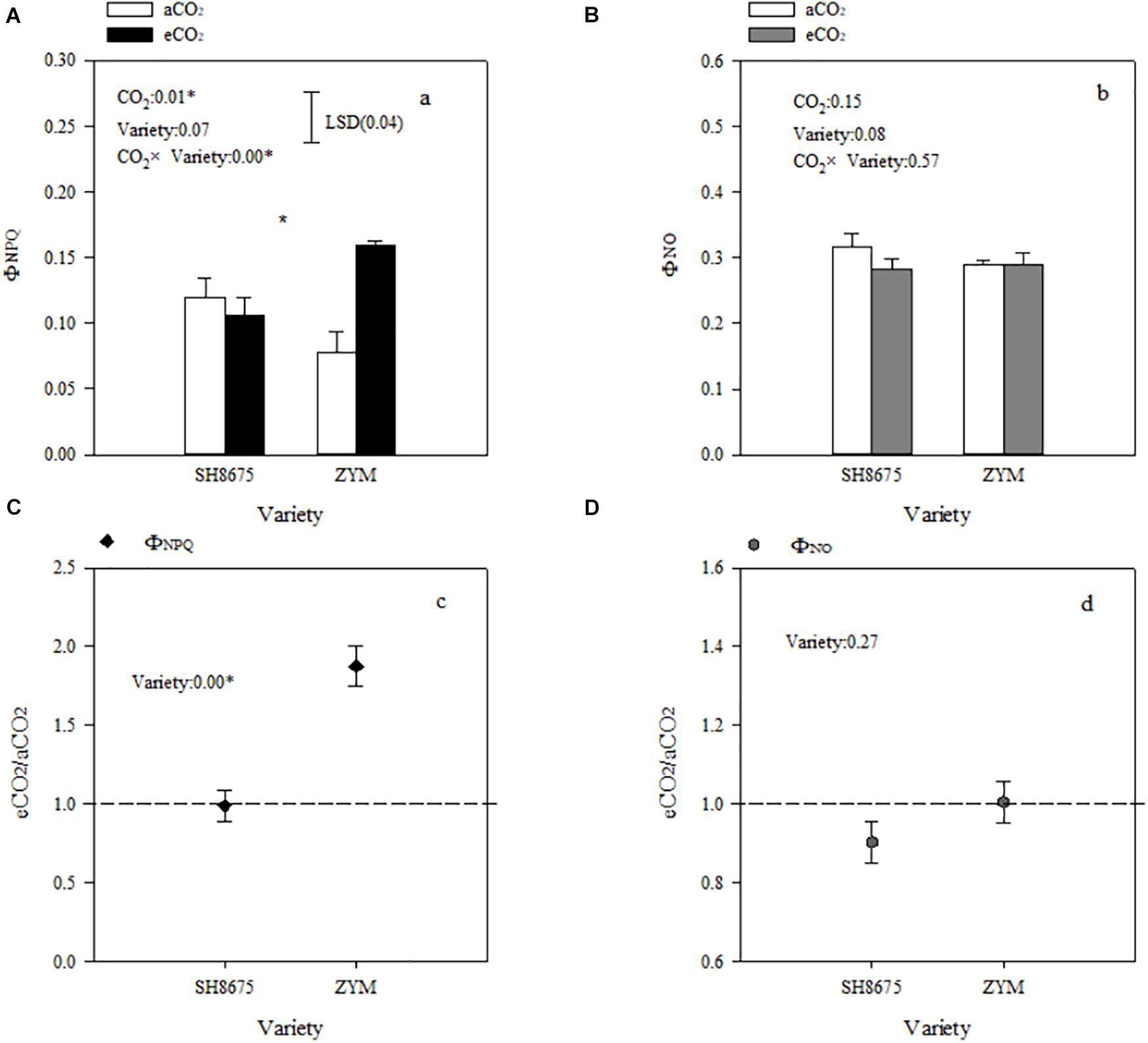
Figure 4. Effects of elevated CO2 on non-photochemical dissipation of two winter wheat varieties. Measurements were carried out on intact flag leaves. Data represent the mean of three plants from each variety plot ± SD (standard error) bars. ANOVA results with * indicate significance at p < 0.05. Vertical bars in (A,B) indicate LSD (p < 0.05) for ΦNPQ and ΦNO, respectively. Differences of ΦNPQ and ΦNO between varieties in responses to eCO2 are shown in (C,D), respectively.
Photosynthetic Activity of PSII
Elevated CO2 did not significantly affect the Fv/Fm ratio of the two varieties (Figure 5A). However, elevated CO2 increased the ΦPSII of SH8675 by 16.3% (p < 0.05), but decreased that of ZYM by 9.9%.
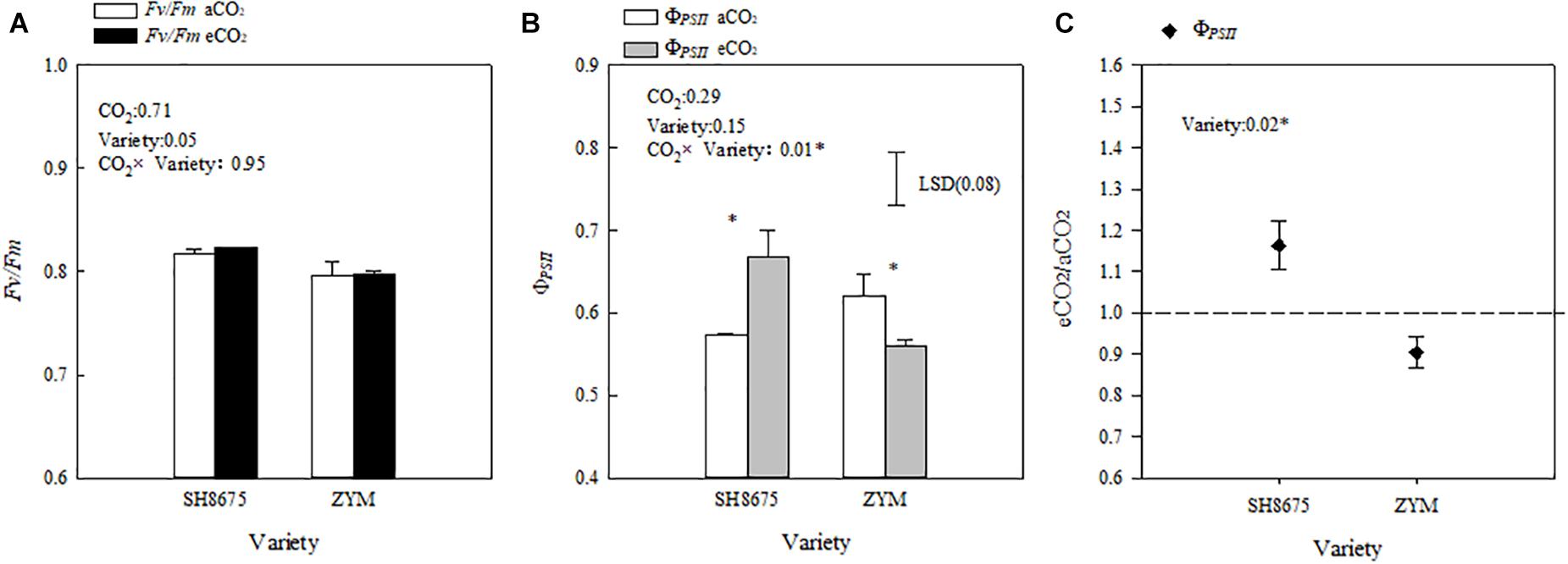
Figure 5. Effects of elevated CO2 on the ΦPSII and Fv/Fm ratios of two winter wheat varieties (A–C). Measurements were carried out on intact flag leaves. Data represent the mean of three plants from each plot ± SD (standard error) bars. ANOVA results with * indicate significance at p < 0.05. Vertical bars in (B,C) indicate LSD (P < 0.05) for ΦPSII and Fv/Fo ratio, respectively. Differences of ΦPSII between varieties in responses to eCO2 are shown in (C).
Carbohydrate Contents of Flag Leaves and Biomass at Anthesis Stage
For the large-ear variety, the levels of sucrose increased by 15.9% and the levels of starch in the flag leaves declined by 18.6% under elevated CO2 for SH8675. Contrast with the small multiple ear variety-ZYM, a larger amount of starch accumulated in the high-CO2 grown leaves than in the controls, while the sucrose contents were decreased by CO2 enrichment (Table 4). Additionally, there was a significant increase in the ear weight per unit area of the two varieties under eCO2 condition. However, the ear weight per unit area of SH8675 was 30.2% higher (p < 0.05) than that of ZYM (Table 4).
Grain Number per Ear and 1,000-Kernel Weight
There were no significant differences in the grain number per ear and 1,000-kernel weight between the varieties under eCO2 condition (Figure 6A). However, the grain number per ear of SH8675 was 31.1% higher (p < 0.05) than that of ZYM (increased by 16.3%) under eCO2 condition (Figure 6A). Although, aCO2 did not significantly affect grain numbers per ear and 1,000-kernel weight of the two varieties, SH8675 had a greater 1,000-kernel weight than that did ZYM under the two CO2 treatments (Figure 6B).
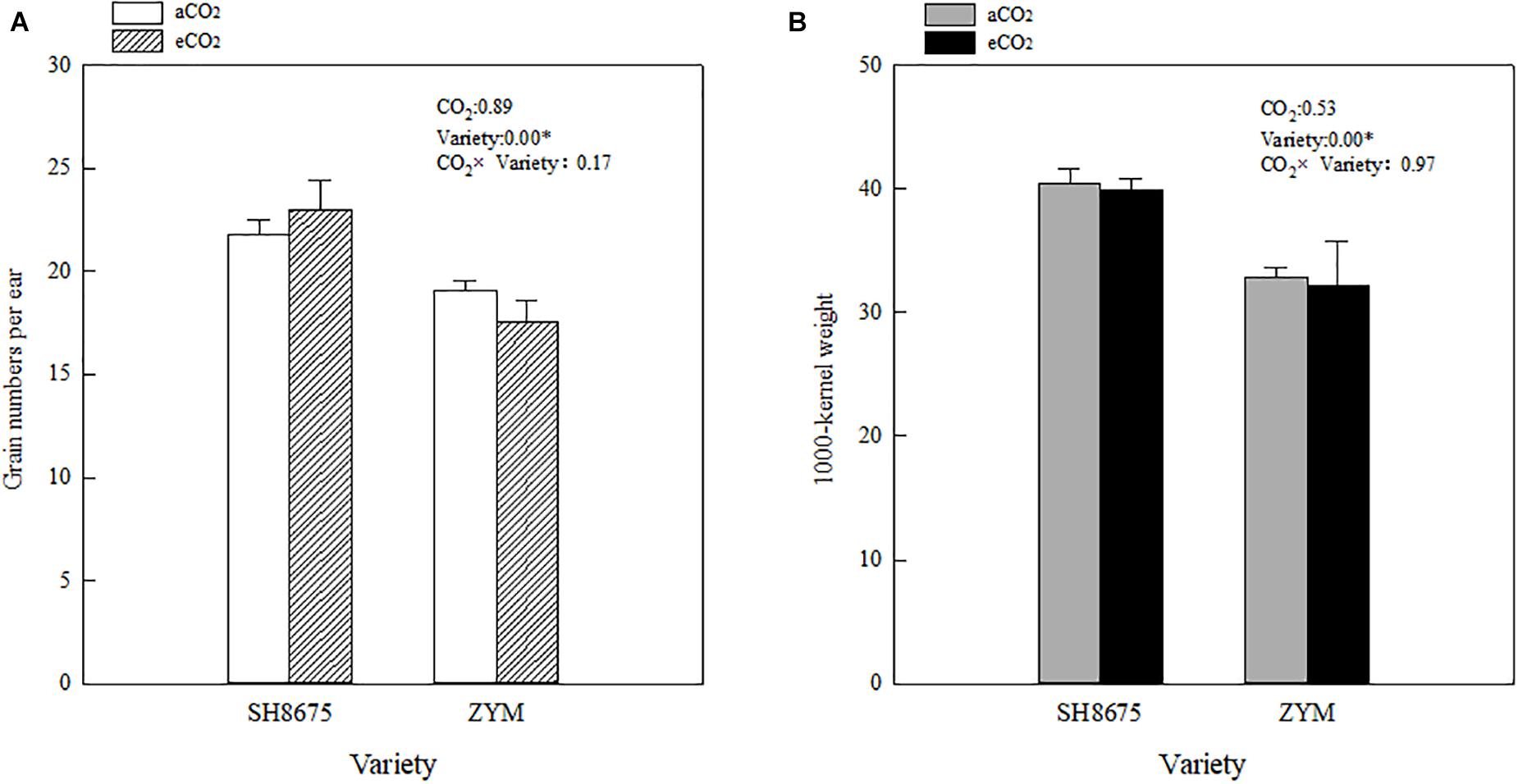
Figure 6. Effects of elevated CO2 on grain number per ear and 1,000-kernel weight of two winter wheat varieties (A,B). Measurements were carried out at maturity stage. Data represent the mean of three plants from each plot ± SD (standard error) bars. ANOVA results with * indicate significance at p < 0.05.
Discussion
In the present study, we examined the effect of elevated CO2 on the primary reaction of PSII and carbon allocation in two winter wheat varieties with different ear C sink strengths. The results of the study showed that the greater ear C sink strength of SH8675 was beneficial for improved quantum efficiency of PSII photochemistry (ΦPSII) and the carbon allocation of the flag leaf under eCO2 at the anthesis stage. The carbohydrate content response to elevated CO2 varied in different ear type wheat varieties. For the high CO2 grown leaves, the starch content of SH8675 was significantly lower than that of the control, while that of ZYM was opposite. These results suggest that the high-CO2 grown leaves may function as stronger sinks for small multiple ear variety than the control leaves. It seems that excess carbohydrates produced by ZYM exposed to elevated CO2 and originally destined for storage in the stems and ears might be accumulated in the flag leaves, which are normally weak sinks.
However, the grain number per ear and 1,000-kernel weight of the wheat plants were not significantly affected by CO2 concentrations or varieties (Figure 6). In contrast, a lower ΦPSII was observed in the flag leaf of ZYM at the anthesis stage, which was caused by an increase in the ΦNPQ of PSII, suggesting that light energy absorbed by PSII in ZYM flag leaf was largely dissipated as thermal energy compared to that utilized for photochemical reaction. Furthermore, the results of correlation analysis showed that although eCO2 induced significant changes in the quantum efficiency of PSII photochemistry, these changes were not significantly correlated with grain number per ear and 1,000-kernel weight at the maturity stage in both wheat varieties (Figure 7). This result indicates that large ear type with high ear C sink strength alone does not necessary ensure effective utilization of eCO2 for grain yield. However, at the anthesis stage, ear C sink strength improved the quantum efficiency of PSII photochemistry of flag leaf in response to eCO2 condition (Figures 7, 8).
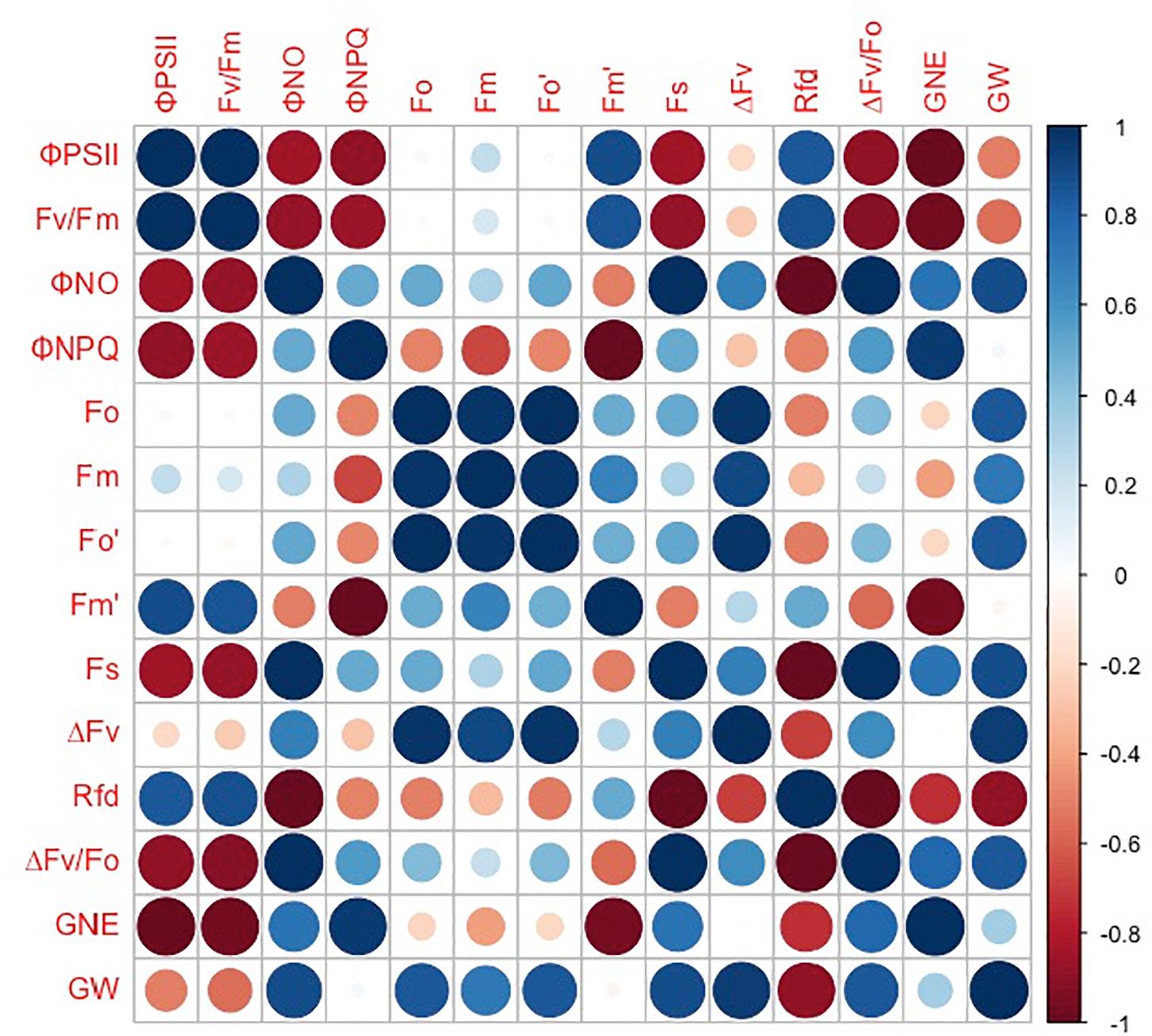
Figure 7. Correlations between grain number per ear (GNE), 1,000-kernel weight (GW), ΦPSII, Fv/Fm, ΦNO, ΦNPQ, Fo, Fm, Fo′, Fm′, Fs, ΔFv, Rfd, and ΔFv/Fo in SH8675. Spearman’s rank correlation coefficient-based correlograms of the measured parameters on plants grown under elevated CO2. The color of each square indicates the value of the correlation coefficient for each pair of traits following the color scale of the vertical color bar. The red and blue circles indicate negative or positive correlations between parameters, respectively.
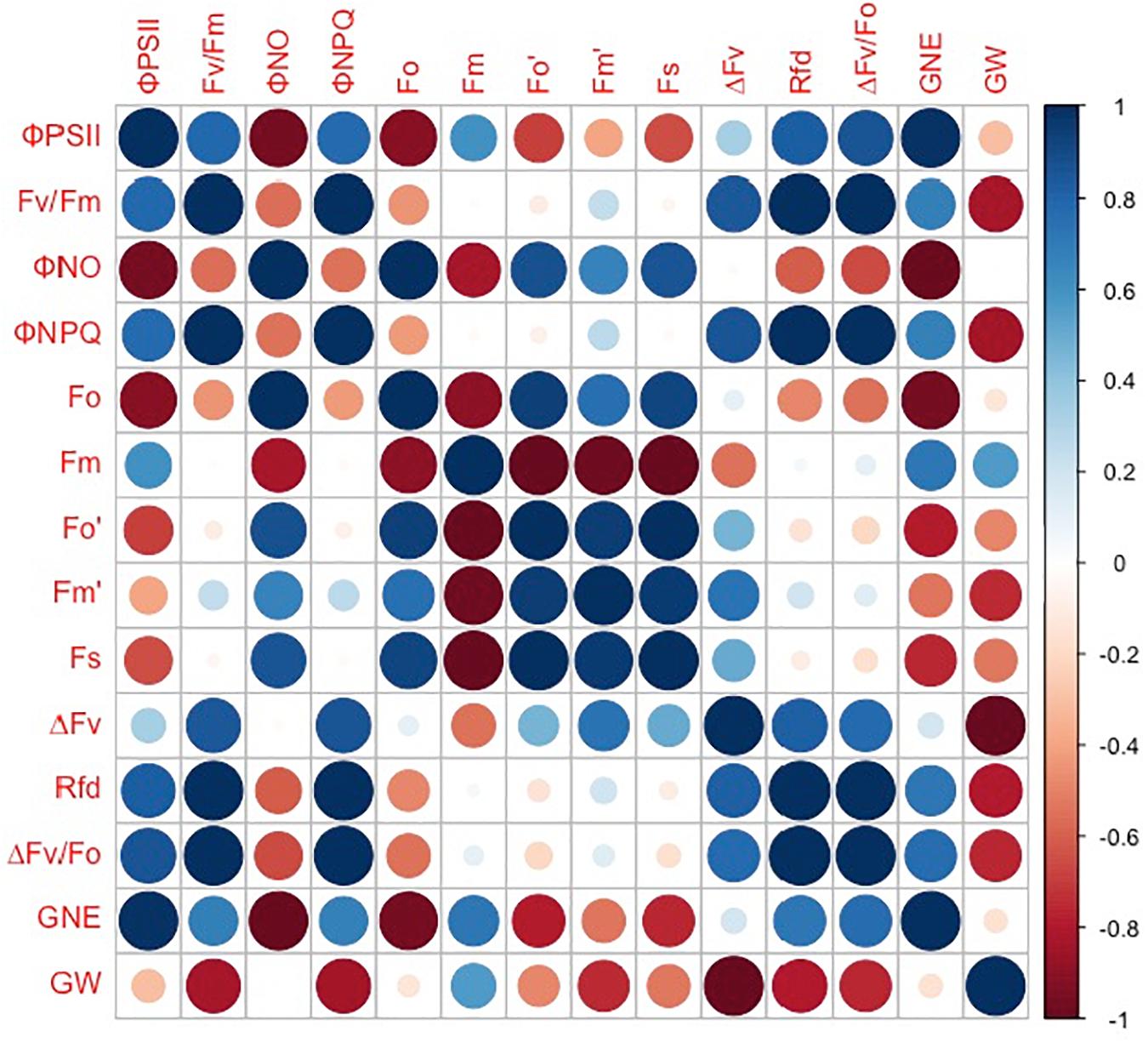
Figure 8. Correlations between grain number per ear (GNE), 1,000-kernel weight (GW), ΦPSII, Fv/Fm, ΦNO, ΦNPQ, Fo, Fm, Fo′, Fm′, Fs, ΔFv, Rfd, and ΔFv/Fo in ZYM. Spearman’s rank correlation coefficient-based correlograms of the measured parameters on plants grown under elevated CO2. The color of each square indicates the value of the correlation coefficient for each pair of traits following the color scale of the vertical color bar. The red and blue circles indicate negative or positive correlations between parameters, respectively.
Light absorption by PSII is converted into energy, and most of the excitation energy is used for photosynthesis, a portion of the excitation energy is dissipated as heat, and a small percentage is emitted in the form of fluorescence (Maxwell and Johnson, 2000). Previous research has reported that elevated CO2 significantly increases PSII photochemical activity in cereal crops (Wang et al., 2015). Besides that, eCO2 led to decreases in both photorespiration rates and oxidative pressure was reported frequently (Leakey et al., 2009; Marçal et al., 2021). In the present study, the PSII photochemical activities of the two varieties in response to elevated CO2 were different (Figure 4C). There was a significant decrease in the ΦPSII of ZYM under eCO2 condition (Figure 5B), which was caused by an increase in the qN of ZYM (Figure 3B), as indicated by the high ΦNPQ of the flag leaves of ZYM (Figure 4A). Increased thermal dissipation in light-harvesting complexes competes with photochemistry for absorbed excitation energy, resulting in a decreased ΦPSII (Yamamoto, 2016; Chen et al., 2018; Li et al., 2019). Therefore, it can be speculated that a large proportion of absorbed excitation energy of PSII in ZYM was dissipated as thermal energy, with lesser amount of energy used in photochemical processes. In contrast, eCO2 caused a 11.9 and 10.3% decrease in ΦNPQ and ΦNO of SH8675, respectively (Figures 4A,B); the ΦPSII of SH8675 increased with increase in CO2 concentration from 415 μmol⋅mol–1 to 550 μmol⋅mol–1 (Figure 5B). Additionally, the Rfd value of SH8675 was significantly increased by eCO2, indicating that the potential photosynthetic activity of SH8675 was higher under eCO2 than that under CO2. Rfd is a vital indicator of the photosynthetic activity of plant leaf (Tuba et al., 1994), with a higher Rfd value indicating a higher photosynthetic rate (Lichtenthaler et al., 2005). Previous research has shown that plants increase non-photochemical quenching, with a down-regulation of PSII activity that causes a decrease in the photosynthetic carbon metabolism (Aljazairi et al., 2014; Mathobo et al., 2017). However, in this study, although eCO2 reduced the ΦPSII of the flag leaf of ZYM at the anthesis stage, the grain number per ear and 1,000-kernel weight did not change significantly. Similarly, although eCO2 increased the ΦPSII of the flag leaf of SH8675 at anthesis stage, the number per ear and 1,000-kernel weight were not significantly affected. These results lead us to ask if the responses of three de-excitation pathways to elevated CO2 differ due to different ear types in winter wheat varieties. The quantum efficiency of PSII photochemistry (ΦPSII) can be used to estimate the photosynthetic performance of the two varieties under both CO2 concentrations. In the present study, the wheat varieties were sensitive to eCO2. The ΦPSII of SH8675 and ZYM were positively and negatively affected by elevated CO2, respectively. Hence, when CO2 increased to 550 μmol⋅mol–1, the PSII of SH8675 had a higher energy conversion efficiency than that did ZYM (Figures 5B,C). By analyzing the agronomic characteristics of these two winter wheat varieties, we found that the ear and leaf weights of SH8675 had the same response trend to elevated CO2 as that of ΦPSII (Table 4). Additionally, eCO2 increased the sucrose ratio of the NSC of SH8675 flag leaf, but reduced the starch ratio (Table 4). Sucrose is the primary product of the source and substrate sink, and plays an important role in NSC metabolism and transfer into the ear (Griffiths et al., 2016; Weichert et al., 2017). Therefore, the above results indicated that under elevated CO2 condition, the flag leaf of the large-ear variety exhibited enhanced capacity for light energy utilization and an efficient translation of carbohydrates into the ear at the anthesis stage. Thus, efficient carbohydrate transport is important for the efficient utilization of light energy by winter wheat flag leaves, which is necessary for sustainable wheat farming under future climate change scenario. This is confirmed by the results of previous studies, which showed that sink-source imbalance can cause an accumulation of total non-structural carbohydrates (soluble sugar and starch) in source leaves, leading to a decrease in the photosynthetic capacity of leaves (Kasai, 2008; Daisuke et al., 2019). However, for the small multiple-ear variety, ZYM, a large quantity of energy absorbed by the flag leaves was largely dissipated as thermal energy, with limited amount being utilized for photochemical reaction under eCO2 condition. This could also be explained by the carbohydrate transfer theory, in which we analyzed NSC data and found that eCO2 increased the starch ratio of the NSC content of ZYM flag leaf, which can cause a decrease in the translocation of carbohydrates and subsequently, a decrease in ear weight (Table 4).
Furthermore, the effect of eCO2 on the ear weight of the two varieties at anthesis did not reflect in the grain number per ear and 1,000-kernel weight of the varieties at maturity stage. The reasons for this will be subject to further research. The methods need to be improved to explore the enzymatic activities of carbon metabolism and metabolites produced in photorespiration pathway, this is the limitations of the approach used in this study.
Conclusion
In summary, the findings of the present study suggest that the high ear C sink strength of SH8675 improved the quantum efficiency of PSII photochemistry of the flag leaf in response to elevated CO2 and the translation of carbohydrates into the ear at the anthesis stage. In contrast, light energy absorbed by PSII in the ZYM flag leaf was largely dissipated as thermal energy, with relatively lesser amount being utilized for photochemical reaction; this resulted in a decrease in the translocation of carbohydrate to the ear and consequently a decrease in ear weight at the anthesis stage. However, the improvement in the quantum efficiency of PSII photochemistry of SH8675 flag leaf was not significantly correlated with grain number per ear and 1,000-kernel weight at maturity stage. Overall, the findings of our study indicate that high light utilization and high C sink strength alone does not necessarily ensure increased grain yield in wheat under eCO2 conditions.
Data Availability Statement
The raw data supporting the conclusions of this article will be made available by the authors, without undue reservation.
Author Contributions
YTL designed the study, performed the research and statistical analysis, and wrote the manuscript. SZ and YJL performed the experimental studies, data acquisition, and data analysis. XH conceived the idea and approved the final version of the manuscript. XL and EL contributed to conception and design of the study. YF provided intellectual content for this manuscript. All authors contributed to manuscript revision, read, and approved the submitted version.
Funding
This work was supported by the National Key Research and Development Project (2016YFD0300401 and 2019YFA0607403).
Conflict of Interest
The authors declare that the research was conducted in the absence of any commercial or financial relationships that could be construed as a potential conflict of interest.
Acknowledgments
We would like to thank Dr. Golam Jalal Ahammed for his review and constructive suggestions.
References
Aljazairi, S., Arias, C., Sanchez, E., Lino, G., and Nogues, S. (2014). Effects of pre-industrial, current and future CO2 in traditional and modern wheat genotypes. J. Plant Physiol. 171, 1654–1663. doi: 10.1016/j.jplph.2014.07.019
Aranjuelo, I., Pardo, A., Biel, C., Savé, R., Azcón-bieto, J., and Nogués, S. (2009). Leaf carbon management in slow-growing plants exposed to elevated CO2. Glob. Chang. Biol. 15, 97–109. doi: 10.1111/j.1365-2486.2008.01829.x
Aranjuelo, I., Sanz-Saez, A., Jauregui, I., Irigoyen, J. J., Araus, J. L., Sanchez-Diaz, M., et al. (2013). Harvest index, a parameter conditioning responsiveness of wheat plants to elevated CO2. J. Exp. Bot. 64, 1879–1892. doi: 10.1093/jxb/ert081
Baker, N. R., and Rosenqvist, E. (2004). Applications of chlorophyll fluorescence can improve crop production strategies: an examination of future possibilities. J. Exp. Bot. 55, 1607–1621. doi: 10.1093/jxb/erh196
Banks, J. M. (2018). Chlorophyll fluorescence as a tool to identify drought stress in Acer genotypes. Environ. Exp. Bot. 155, 118–127. doi: 10.1016/j.envexpbot.2018.06.022
Barber, J. (2016). Photosystem II: the water splitting enzyme of photosynthesis and the origin of oxygen in our atmosphere- Corrigendum. Q. Rev. Biophys. 49:e16.
Chen, J., Burke, J. J., and Xin, Z. (2018). Chlorophyll fluorescence analysis revealed essential roles of FtsH11 protease in regulation of the adaptive responses of photosynthetic systems to high temperature. BMC Plant Biol. 18:11. doi: 10.1186/s12870-018-1228-2
Cock, J. H., and Yoshida, S. (1972). Accumulation of 14C-labelled carbohydrate before flowering and its subsequent redistribution and respiration in the rice plants. Proc. Crop Sci. Soc. Jpn. 41, 226–234. doi: 10.1626/jcs.41.226
Daisuke, S., Eriko, B., and Ichiro, T. (2019). Interspecific differences in how sink-source imbalance causes photosynthetic downregulation among three legume species. Ann. Bot. 123, 715–726. doi: 10.1093/aob/mcy204
Dier, M., Sickora, J., Erbs, M., Weigel, H. J., Zorb, C., and Manderscheid, R. (2019). Positive effects of free air CO2 enrichment on N remobilization and post-anthesis N uptake in winter wheat. Field Crops Res. 234, 107–118. doi: 10.1016/j.fcr.2019.02.013
Erice, G., Sanz-Saez, A., Gonzalez-Torralba, J., Mendez-Espinoza, A. M., Urretavizcaya, I., Nieto, M. T., et al. (2019). Impact of elevated CO2 and drought on yield and quality traits of a historical (Blanqueta) and a modern (Sula) durum wheat. J. Cereal Sci. 87, 194–201. doi: 10.1016/j.jcs.2019.03.012
Feng, W., He, L., Zhang, H.-Y., Guo, B.-B., Zhu, Y.-J., Wang, C.-Y., et al. (2015). Assessment of plant nitrogen status using chlorophyll fluorescence parameters of the upper leaves in winter wheat. Eur. J. Agron. 64, 78–87. doi: 10.1016/j.eja.2014.12.013
Goltsev, V. N., Kalaji, H. M., Paunov, M., Baba, W., Horaczek, T., Mojski, J., et al. (2016). Variable chlorophyll fluorescence and its use for assessing physiological condition of plant photosynthetic apparatus. Russ. J. Plant Physiol. 63, 869–893. doi: 10.1134/s1021443716050058
Griffiths, C. A., Paul, M. J., and Foyer, C. H. (2016). Metabolite transport and associated sugar signalling systems underpinning source/sink interactions. Biochim. Biophys. Acta Bioenerg. 1857, 1715–1725. doi: 10.1016/j.bbabio.2016.07.007
Han, X., Hao, X. Y., Lam, S. K., Wang, H. R., Li, Y. C., Wheeler, T., et al. (2015). Yield and nitrogen accumulation and partitioning in winter wheat under elevated CO2: A 3-year free-air CO2 enrichment experiment. Agr. Ecosyst. Environ. 209, 132–137. doi: 10.1016/j.agee.2015.04.007
Kalaji, H. M., Gert, S., Ladle, R. J., Vasilij, G., Karolina, B., Allakhverdiev, S. I., et al. (2014). Frequently asked questions about in vivo chlorophyll fluorescence: practical issues. Photosynth. Res. 122, 121–158.
Kasai, M. (2008). Regulation of leaf photosynthetic rate correlating with leaf carbohydrate status and activation state of Rubisco under a variety of photosynthetic source/sink balances. Physiol. Plant. 134, 216–226. doi: 10.1111/j.1399-3054.2008.01105.x
Klughammer, C., and Schreiber, U. (2008). Complementary PS II quantum yields calculated from simple fluorescence parameters measured by PAM fluorometry and the Saturation Pulse method. PAM Appl. Notes 1, 27–35.
Kramer, D. M., Johnson, G., Kiirats, O., and Edwards, G. E. (2004). New fluorescence parameters for the determination of Q(A) redox state and excitation energy fluxes. Photosynth. Res. 79, 209–218. doi: 10.1023/b:pres.0000015391.99477.0d
Leakey, A. D. B., Ainsworth, E. A., Bernacchi, C. J., Rogers, A., Long, S. P., and Ort, D. R. (2009). Elevated CO2 effects on plant carbon, nitrogen, and water relations: six important lessons from FACE. J. Exp. Bot. 60, 2859–2876. doi: 10.1093/jxb/erp096
Li, P., Li, B., Seneweera, S., Zong, Y., Li, F. Y., Han, Y., et al. (2019). Photosynthesis and yield response to elevated CO2, C4 plant foxtail millet behaves similarly to C3 species. Plant Sci. 285, 239–247. doi: 10.1016/j.plantsci.2019.05.006
Lichtenthaler, H. K., Buschmann, C., and Knapp, M. (2005). How to correctly determine the different chlorophyll fluorescence parameters and the chlorophyll fluorescence decrease ratio R-Fd of leaves with the PAM fluorometer. Photosynthetica 43, 379–393. doi: 10.1007/s11099-005-0062-6
Long, S. P., Ainsworth, E. A., Leakey, A. D., Nosberger, J., and Ort, D. R. (2006). Food for thought: lower-than-expected crop yield stimulation with rising CO2 concentrations. Science 312, 1918–1921. doi: 10.1126/science.1114722
Marçal, D. M. S., Avila, R. T., Quiroga-Rojas, L. F., Souza, R. P. B. D., and Damatta, F. M. (2021). Elevated [CO2] benefits coffee growth and photosynthetic performance regardless of light availability. Plant Physiol. Bioch. 158, 524–535. doi: 10.1016/j.plaphy.2020.11.042
Mathis, P., and Rutherford, A. W. (1987). Chapter 4 The primary reactions of photosystems I and II of algae and higher plants. New Compr. Biochem. 15, 63–96. doi: 10.1016/s0167-7306(08)60135-0
Mathobo, R., Marais, D., and Steyn, J. M. (2017). The effect of drought stress on yield, leaf gaseous exchange and chlorophyll fluorescence of dry beans (Phaseolus vulgaris L.). Agr. Water Manage. 180, 118–125. doi: 10.1016/j.agwat.2016.11.005
Maxwell, K., and Johnson, G. (2000). Chlorophyll fluorescence—a practical guide. J. Exp. Bot. 51, 659–668. doi: 10.1093/jxb/51.345.659
Misra, B. B., and Chen, S. X. (2015). Advances in understanding CO2 responsive plant metabolomes in the era of climate change. Metabolomics 11, 1478–1491. doi: 10.1007/s11306-015-0825-4
Osipova, S. V., Permyakov, A. V., Permyakova, M. D., Rudikovskaya, E. G., Verchoturov, V. V., and Rudikovsky, A. V. (2019). Tolerance of the photosynthetic apparatus in recombinant lines of wheat adapting to water stress of varying intensity. Photosynthetica 57, 160–169. doi: 10.32615/ps.2019.007
Pan, J., Cui, K., Wei, D., Huang, J., Xiang, J., and Nie, L. (2011). Relationships of non-structural carbohydrates accumulation and translocation with yield formation in rice recombinant inbred lines under two nitrogen levels. Physiol. Plant. 141, 321–331. doi: 10.1111/j.1399-3054.2010.01441.x
Pandey, A. K., Ghosh, A., Agrawal, M., and Agrawal, S. B. (2018). Effect of elevated ozone and varying levels of soil nitrogen in two wheat (Triticum aestivum L.) cultivars: growth, gas-exchange, antioxidant status, grain yield and quality. Eecotox. Environ. Safe 158, 59–68. doi: 10.1016/j.ecoenv.2018.04.014
Stocker, T. F., Talley, L. D., Wallace, J. M., Qin, D., Manning, M., Chen, Z., et al. (2013). Change 2007: the Physical Science Basis. Contribution of Working Group I to the Fourth Assessment Report of the Intergovernmental Panel on Climate Change [Solomon, S]. Comput. Geo. 18, 95–123.
Tausz, M., Tausz-Posch, S., Norton, R. M., Fitzgerald, G. J., Nicolas, M. E., and Seneweera, S. (2013). Understanding crop physiology to select breeding targets and improve crop management under increasing atmospheric CO2 concentrations. Environ. Exp. Bot. 88, 71–80. doi: 10.1016/j.envexpbot.2011.12.005
Tausz-Posch, S., Dempsey, R. W., Seneweera, S., Norton, R. M., Fitzgerald, G., and Tausz, M. (2015). Does a freely tillering wheat cultivar benefit more from elevated CO2 than a restricted tillering cultivar in a water-limited environment? Eur. J. Agron. 64, 21–28. doi: 10.1016/j.eja.2014.12.009
Tuba, Z., Lichtenthaler, H., Csintalan, Z., Nagy, Z., and Szente, K. (1994). Reconstitution of chlorophylls and photosynthetic CO2 assimilation upon rehydration of the desiccated poikilochlorophyllous plant Xerophyta scabrida (Pax) Th. Dur. et Schinz. Planta 192, 414–420.
Uddling, J., Gelang-Alfredsson, J., Karlsson, P. E., Selldén, G., and Pleijel, H. (2008). Source–sink balance of wheat determines responsiveness of grain production to increased CO2 and water supply. Agr. Ecosyst. Environ. 127, 215–222. doi: 10.1016/j.agee.2008.04.003
Urban, O., Hlavacova, M., Klem, K., Novotna, K., Rapantova, B., Smutna, P., et al. (2018). Combined effects of drought and high temperature on photosynthetic characteristics in four winter wheat genotypes. Field Crops Res. 223, 137–149. doi: 10.1016/j.fcr.2018.02.029
Wang, M. J., Xie, B. Z., Fu, Y. M., Dong, C., Hui, L., and Liu, G. H. (2015). Effects of different elevated CO2 concentrations on chlorophyll contents, gas exchange, water use efficiency and PSII activity on C3 and C4 cereal crops in a closed artificial ecosystem. Photosynth. Res. 126, 351–362. doi: 10.1007/s11120-015-0134-9
Wang, W., Li, Q., Tian, F., Deng, Y., Wang, W., Wu, Y., et al. (2019). Wheat NILs contrasting in grain size show different expansin expression, carbohydrate and nitrogen metabolism that are correlated with grain yield. Field Crops Res. 241:107564. doi: 10.1016/j.fcr.2019.107564
Weichert, H., Hoegy, P., Mora-Ramirez, I., Fuchs, J., Eggert, K., Koehler, P., et al. (2017). Grain yield and quality responses of wheat expressing a barley sucrose transporter to combined climate change factors. J. Exp. Bot. 68, 5511–5525. doi: 10.1093/jxb/erx366
Yamamoto, Y. (2016). Quality Control of Photosystem II: the Mechanisms for Avoidance and Tolerance of Light and Heat Stresses are Closely Linked to Membrane Fluidity of the Thylakoids. Front. Plant Sci. 7:1136. doi: 10.3389/fpls.2016.01136
Zadoks, J. C., Chang, T. T., and Konzak, C. F. (1974). A decimal code for the growth stages of cereals. Weed Res. 14, 415–421. doi: 10.1111/j.1365-3180.1974.tb01084.x
Zarco-Tejada, P. J., Miller, J. R., Mohammed, G. H., Noland, T. L., and Sampson, P. H. (2002). Vegetation stress detection through chlorophyll a+b estimation and fluorescence effects on hyperspectral imagery. J. Environ. Qual. 31, 1433–1441. doi: 10.2134/jeq2002.1433
Ziska, L. H., Bunce, J. A., Shimono, H., Gealy, D. R., Baker, J. T., Newton, P. C., et al. (2012). Food security and climate change: on the potential to adapt global crop production by active selection to rising atmospheric carbon dioxide. Proc. Biol. Sci. 279, 4097–4105. doi: 10.1098/rspb.2012.1005
Keywords: elevated CO2, chlorophyll fluorescence, quantum efficiency, PSII photochemistry, winter wheat variety
Citation: Li Y, Li X, Li Y, Zhuang S, Feng Y, Lin E and Han X (2021) Does a Large Ear Type Wheat Variety Benefit More From Elevated CO2 Than That From Small Multiple Ear-Type in the Quantum Efficiency of PSII Photochemistry? Front. Plant Sci. 12:697823. doi: 10.3389/fpls.2021.697823
Received: 20 April 2021; Accepted: 22 June 2021;
Published: 20 July 2021.
Edited by:
Maxwell Ware, Colorado State University, United StatesReviewed by:
Catello Di Martino, University of Molise, ItalyTamara Golovko, Institute of Physiology, Komi Scientific Center (RAS), Russia
Copyright © 2021 Li, Li, Li, Zhuang, Feng, Lin and Han. This is an open-access article distributed under the terms of the Creative Commons Attribution License (CC BY). The use, distribution or reproduction in other forums is permitted, provided the original author(s) and the copyright owner(s) are credited and that the original publication in this journal is cited, in accordance with accepted academic practice. No use, distribution or reproduction is permitted which does not comply with these terms.
*Correspondence: Xue Han, aGFueHVlQGNhYXMuY24=