- Department of Biotechnology, School of Chemical and Life Sciences, Jamia Hamdard, New Delhi, India
Genus Ocimum of Labiatae is well known in all traditional medicinal systems like Ayurveda, Unani, Siddha, and Homeopathy. The pharmaceutical activities of different species of Ocimum attributed to all plant parts. Roots are the most significant vital organ of the plant, as they absorb water and nutrients from soil and transport to aerial parts of the plants. Roots of Ocimum were found helpful with free-radical scavenging activity to improve physical and mental strength as well as to treat diabetes, malaria, and liver problems. Antibacterial activity of Ocimum roots and its main component, rosmarinic acid, is very beneficial to protect against several human pathogens, including bacteria and viruses. Being so important in every way, roots of Ocimum need healthy rhizosphere. Bacteria, fungi, nematodes, types of soil, fungicide, pesticides, salt, radioactive elements, as well as heavy metal contaminations, affect roots and overall growth of Ocimum in positive or negative ways. Each component of rhizosphere (natural, treatment or contamination) affects the roots, which highlights current ecological scenario to discover biosafe and more productive approaches. For such prestigious organ of Ocimum, development of in vitro root cultures and hairy root cultures assists to reduce the efforts and timing of the traditional cultivation process along with elimination of negative factors in rhizosphere. Different strains of Agrobacterium rhizogenes, various media compositions, as well as discrete treatments, like elicitors, on nonidentical species or cultivars of Ocimum boost the root induction, biomass, and accumulation of phytoceuticals differently. Hairy roots and in vitro roots of Ocimum accumulate higher quantity of therapeutic metabolites. These metabolites include several phenolics (like rosmarinic acid, 3-hydroxybenzoic acid, m-coumaric acid, p-coumaric acid, caffeic acid, ferulic acid, vanillic acid, chicoric acid, and lithospermic acid), triterpenes (such as betulinic acid, 3-epimaslinic acid, alphitolic acid, euscaphic acids, oleanolic acid, and ursolic acid) as well as flavonoids (flavones, flavonols, and dihydroflavonols). This review highlights pharmaceutical applications of Ocimum roots, a great deal of rhizosphere components and in vitro culturing techniques to enhance biomass as well as chief phytoceuticals.
Introduction
Genus Ocimum (Family Labiatae) is among the most important plants with therapeutic potential due to its worldwide cultivation, including, Australia, Malaysia, Africa (east, north, and west), India, China, Taiwan, and several Arab countries (Mondal et al., 2009; Baliga et al., 2013; Kulkarni and Adavirao, 2018). O. sanctum L. (or Ocimum tenuiflorum), O. basilicum, O. canum, O. gratissimum, O. americanum, O. camphora, O. kilimandscharicum, and O. micranthum are explored medicinally important species of Ocimum (Pattanayak et al., 2010; Rahman et al., 2011; Kulkarni and Adavirao, 2018; Das et al., 2020; Singh et al., 2020).
All parts (root, stem, leaves, flower, and seeds), as well as whole the plant of Ocimum, have been recommended in traditional medicinal systems. Potential therapeutic properties of Ocimum include adaptogenic, anabolic, analgesic, antiaging, antiallergic, antiarthritic, anticancer, anticataract, anticoagulant, anticonvulsant, antidiabetic, antidiuretic, antiemetic, antifertility, antihistaminic, anti-inflammatory, antilipidemic, antimicrobial, antioxidant, antipyretic, antispasmodic, antistress, antiulcer, cardioprotective, chemopreventive, diaphoretic, hepatoprotective, hypolipidemic, immunomodulatory, immune stimulant, larvicidal, mosquito repellent, neuroprotective, radioprotective, and other activities (Gupta et al., 2002; Prakash and Gupta, 2005; Singh et al., 2007; Rahman et al., 2011; Baliga et al., 2013; Jamshidi and Cohen, 2017; Wakchaure et al., 2017; Kulkarni and Adavirao, 2018; Gautam et al., 2020; Kurepa and Smalle, 2021).
Mostly, aerial parts of Ocimum have been reported with active biological components, including flavonoids, saponins, tannins, and triterpenoids in stems and leaves (Pattanayak et al., 2010), as well as aldehydes, phenol, phenylpropanoids, and terpenes in seeds (Mondal et al., 2009). A wide range of metabolites has been identified from Ocimum, including apigenin, apigenin-7-O-glucuronide, α-terpineol, β-bisabolene, bornyl acetate, camphene, campesterol, carnosic acid, carvacrol, caryophyllene, circimaritin, cirsilineol, α-elemene, eugenol, euginal, isothymusin, isothymonin, linalool, limatrol, luteolin, luteolin-7-O glucuronide, methyl chavicol, molludistin, myretenal, α- and β-pinenes, oleanlic acid, orientin, rosmarinic acid, sitosterol, stigmasterol, ursolic acid, and vicenin (Gupta et al., 2002; Pattanayak et al., 2010; Shilpa et al., 2010; Baliga et al., 2013; Vyas and Mukhopadhyay, 2014; Wakchaure et al., 2017; Chaudhary et al., 2020; Singh et al., 2020).
Ocimum sanctum, which belongs to Ayurvedic and Unani medicinal system, is the most studied indigenous plant of the Indian subcontinent. The plant is also called as Holy Tulsi and known to have two varieties, Krishna or Hari Tulsi and Ram or Shri Tulsi, with similar biochemical and therapeutic properties. The importance of tulsi made it the “queen of herbs,” “elixir of life,” “matchless one,” “incomparable one,” “a herb for all reasons,” or “mother medicine of nature” (Wakchaure et al., 2017; Kulkarni and Adavirao, 2018; Gautam et al., 2020; Nadar et al., 2020; Singh et al., 2020; Kurepa and Smalle, 2021). O. sanctum is an erect, branched, annual herb with a square stem, which grows up to 18 inches (75 cm) into low bushes and has specific aroma (Gupta et al., 2002; Prakash and Gupta, 2005; Mondal et al., 2009; Pattanayak et al., 2010; Shilpa et al., 2010; Rahman et al., 2011; Kulkarni and Adavirao, 2018). Therapeutic metabolic of O. sanctum is responsible for the defense mechanism of the plant (Vyas and Mukhopadhyay, 2014). Antimicrobial properties of Tulsi have been utilized and available in the form of hand sanitizer, mouthwash, preservatives, and water purifier (Cohen, 2014). O. basilicum, which is also called sweet basil, is another utmost studied Ocimum species known for its pharmaceutical significance and the responsible metabolites (Marzouk, 2009).
Researchers have mentioned all parts of the Ocimum with pharmaceutical significance. Most studies focused on therapeutic meaning of aerial parts of the plants, especially related to essential oil, eugenol, and rosmarinic acid. Roots of the plant also possess same medicinal importance as aerial parts are enriched with considered amount and variety of phytoceuticals.
This review highlights the importance of Ocimum roots by bringing available research (Figure 1). Because of emphasized therapeutical benefits of Ocimum roots, a systematic learning of its root and rhizosphere is a prerequisite. Due to the significance of rhizosphere and increasing contamination of soil and water, a summarized effect of soil type, microbes, nematodes, agricultural treatments, and contaminants (from industries) in rhizosphere on roots and growth of the whole plant of Ocimum is discussed in detail. Variable rhizosphere of Ocimum roots under in vivo conditions affects nutritional uptake, plant growth, and its medicinal properties, which can be overcome by in vitro cultures. In order to establish productive root culture, optimization of basal media, plant growth hormone, Agrobacterium rhizogenes strain, and elicitor treatment is vital; thus, it is briefly discussed in this review.
Pharmacological Roots
Like other parts of plant, roots of Ocimum sp. conquer the therapeutic potential due to metabolic content of roots. Most studies used different types of root extracts to investigate curative activity of roots of O. sanctum, O. basilicum, O. canum, and O. campechianum. Detailed information on species-specific analysis for particular disease or pathogen is summarized in Table 1.
Hepatoprotective Activity
Induced hepatotoxicity (using carbon tetrachloride, CCl4) in female albino rats resulted in enhanced lipid peroxidation, liver transaminases, and malondialdehyde (MDA), which ultimately leads to cell necrosis. Marzouk (2009) analyzed comparative hepatotoxic-inhibitory effect of extracts from aerial parts, normal roots, hairy roots, as well as purified six-triterpenes of Ocimum basilicum. Purified compounds, betulinic acid, and euscaphic aid (ursane derivative), and extract of hairy roots proved to be the best with hepatoprotective activity. Effect of CCl4 to induce hepatotoxicity and the counter-effect of hairy root extract has been presented in Figure 2A.
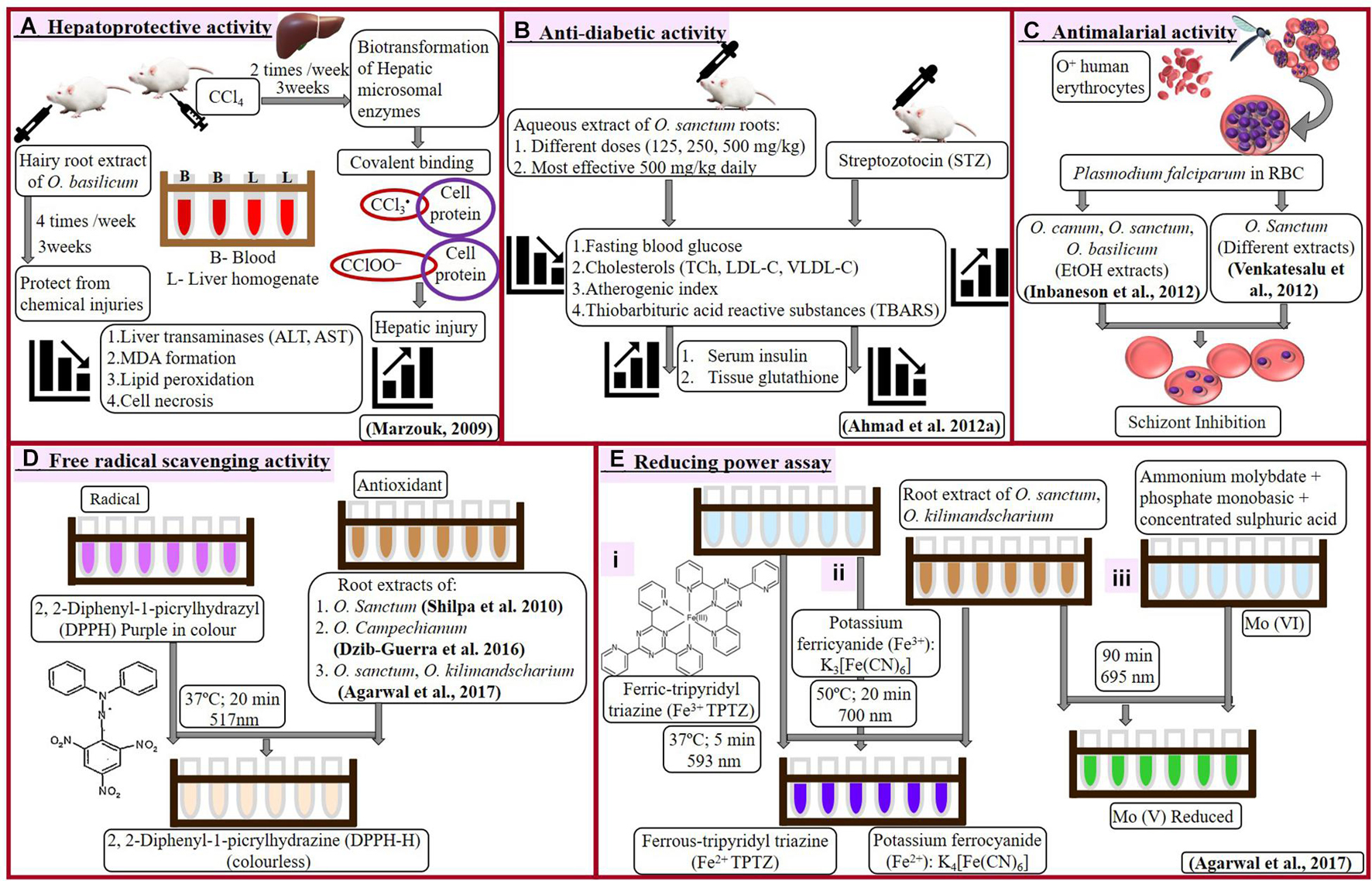
Figure 2. Hepatoprotective (A), antidiabetic (B), antimalarial (C), and antioxidant (D-free radical scavenging and E-reducing power assay) activities of Ocimum sp.
Antidiabetic Activity
Ahmad et al. (2012a) examined blood glucose (fasting) and lipid profile in streptozotocin (STZ)-induced diabetic rats under influence of aqueous root extract of O. sanctum (Figure 2B). Even single dose of streptozotocin significantly elevated blood glucose, total cholesterol, low-density lipoprotein, very low-density lipoprotein along with reduction in levels of insulin and tissue glutathione (GSH). Administration of root extracts reduced elevated metabolites and recovered a level of insulin and GSH, which was more effective at a higher dose of 500 mg/kg. This biochemical profiling reveals the antidiabetic character of O. sanctum root extract.
Antimalarial Activity
Antimalarial activity of Ocimum roots extracts against Plasmodium falciparum was analyzed by Inbaneson et al. (2012) and Venkatesalu et al. (2012). P. falciparum cultivated on O+ human erythrocytes was treated with different extracts of Ocimum. Inbaneson et al. (2012) studied ethanolic extracts of roots, stems, and leaves of O. canum, O. sanctum, O. basilicum; however, Venkatesalu et al. (2012) examined hexane, chloroform, ethyl acetate, acetone, and methanol extracts of O. sanctum roots along with different parts of six other plants (roots of Adhatoda vasica, pulp of Carica papaya, leaves of Caesalpinia pulcherrima, and Erythroxylum monogynum, as well as whole plants of Lantana camara and Phyllanthus niruri). Inhibition in parasitic growth was calculated as reduction in parasitemia by counting schizonts against 200 counts of an asexual parasite and expressed by inhibitory concentration (IC50) value.
Maximum extract yield (16.79%) and antiplasmodial activity were associated with leaves of O. sanctum (IC50 35.58 μg/ml). Among Ocimum species, roots were remarked with lowest percentage yield of extracts (2.42–2.9%) and antimalarial activity (IC50 > 100 to 78.69 μg/ml), followed by flowers, stems, and leaves. However, roots of O. basilicum were most suitable to inhibit P. falciparum (IC50 78.69 μg/ml) as compared with roots of other Ocimum species (Inbaneson et al., 2012). When compared with other plants, chloroform and methanolic root extract of O. sanctum (similar to methanolic extract of Caesalpinia pulcherrima and Adhatoda vasica) showed better antiplasmodial activity with minimum IC50 value of 11.47 and 14.04 μg/ml (Venkatesalu et al., 2012).
Treatment of Ocimum root extract prevented the erythrocytes injury and reduced spread of P. falciparum into fresh RBCs (Figure 2C). Such influence of Ocimum root extract can be very helpful in treating malaria, especially caused by drug-resistant Plasmodium.
Antioxidant Activity
Shilpa et al. (2010) investigated DPPH (2, 2-Diphenyl-1-picrylhydrazyl) radical scavenging activity in ethyl acetate extract of leaves from field-grown as well as in vitro roots and callus of O. sanctum. Reaction to reduce purple-colored DPPH radical into colorless DPPH-H has been shown in Figure 2D. Increasing concentration (50–100 μg/ml) of in vitro (adventitious) root extract demonstrated increase in free radical scavenging activity from 73 to 83%. Callus was observed with minimum activity (38.6%), followed by leaves with 77% scavenging activity. In vitro roots reduced DPPH radical with 6% more efficiency as compared to in vivo leaves. This summarizes that extract of in vitro roots possesses maximum antioxidant activity as compared to in vitro-grown callus, leaves, or in vivo leaves.
Dzib-Guerra et al. (2016) studied many plants from Yucatecan traditional medicine system, including roots, leaves, and stems of Ocimum campechianum (Figure 2D). Aqueous traditional preparations, as well as different extracts of these plants, were analyzed for anti-advanced glycation end (AGE) product inhibition and DPPH radical scavenging activity. There was no significant anti-AGE activity observed with O. campechianum (IC50 > 1), which was maximum in Cassia fistula (IC50 0.2–0.5). However, traditional preparation of O. campechianum exhibited better radical scavenging activity (EC50 150) as compared to leaf, stem, and root extracts of the plant (EC50 235 to > 300).
Agarwal et al. (2017) analyzed antioxidant potential of root extracts of O. sanctum and O. kilimandsacharicum, using different experiments (Figures 2D,E). Butanol, chloroform, ethyl acetate (EtAc), and hexane fractions of methanolic extract were used to test inhibition of DPPH activity and reduction of ferric to ferrous (using ferric tripyridyl triazine, Fe3+ TPTZ complex, as well as potassium ferricyanide). The ferric-reducing antioxidant power (FRAP) was calculated by comparing each Ocimum extract with the quantity of ferrous [ferrous sulfate equivalent required for the same change in optical density using FeSO4 as standard following the protocol of Luqman et al. (2012)]. Ethyl acetate fraction of Ocimum root extract holds maximum antioxidant potential followed by butanol, methanol, chloroform, aqueous, and hexane fraction. Root extract (EtAc fraction) of O. kilimandsacharicum possesses better DPPH scavenging potential (85 ± 2%) and ferric reduction (80.98 ± 3.50 ferrous sulfate equivalents, FSE) as compared with O. sanctum (80 ± 2%; 77.05 ± 3 FSE). Another test of iron reduction from potassium ferricyanide to potassium ferrocyanide expressed higher activity with EtAc fraction of O. kilimandsacharicum (1.64 ± 0.23) as compared with O. sanctum (2.14 ± 0.36) at 700 nm. Total antioxidant capacity was assessed as Molybdenum reduction from Mo (VI) to Mo (V) as ascorbic acid equivalent (AsE). Similarly, EtAc fraction of O. kilimandsacharicum and O. sanctum were detected with higher (7.56 ± 0.47 to 11.31 ± 1.02 μg AsE/mg, respectively) Mo reduction. Therefore, ethyl acetate extracts are best to prefer for antioxidant formulations using roots of O. kilimandsacharicum as compared with roots of O. sanctum. This finding suggests that root extract of O. sanctum is preferred, showing higher antioxidant potential over O. kilimandsacharicum.
Effect of Ocimum on Physical Ability
Maity et al. (2000, 2003) analyzed effect of methanolic root extract from field-grown O. sanctum with swimming performance of adult male albino mice and pentobarbitone sodium-induced hypnosis in Swiss albino mice (Figures 3A,B). Dose-dependent decrease in mean swimming time of mice has been analyzed (Maity et al., 2000) with root extract treatment, along with dose-dependent decrease in number of errors and sleeping time (Maity et al., 2003). Effect of O. sanctum root extract on central nervous system was similar to antidepressant remedy and confirms adaptogenic activity of O. sanctum root.
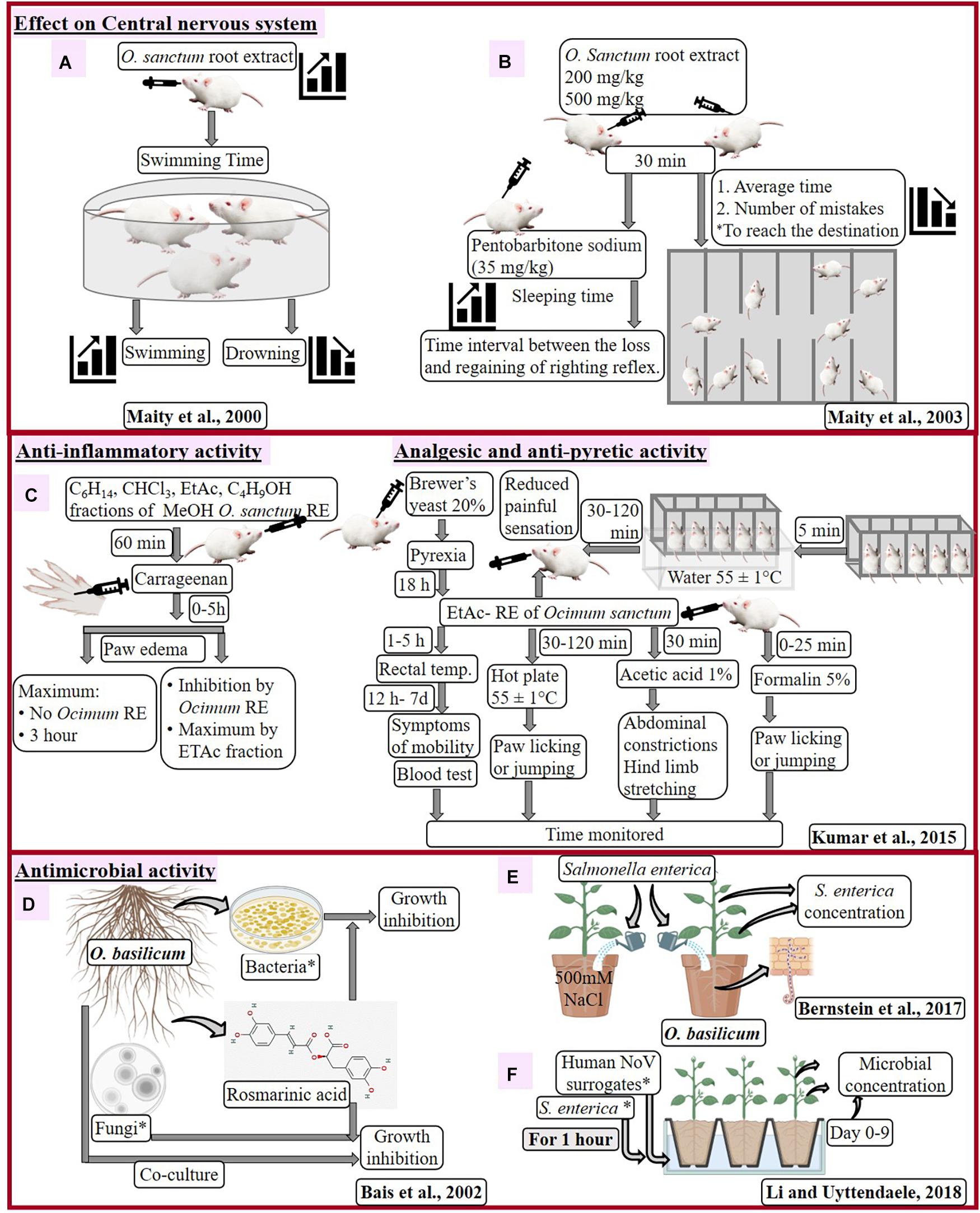
Figure 3. Effect of Ocimum sanctum root extract on central nervous system of mouse (A,B), pharmacological activities of O. sanctum RE in the mouse model (C) and antimicrobial activity of Ocimum basilicum (D–F). *C6H14, hexane; CHCl3, chloroform; EtAc, ethyl acetate; C4H9OH, butanol; RE, root extract; Bacteria: Xanthomonas campestris, Pseudomonas fluorescens, P. aeruginosa (strains PAO1 and PA14), Erwinia carotovora; Fungal isolates: Alternaria brassicae, A. solani, Phytophthora megasperma, P. parasitica, P. drechsleri, P. ultimum, A. niger, Rhizoctonia solani, Fusarium oxysporum, Pythium aphanidermatum, Verticillium dahliae; serovar Typhimurium SL 1344, serovar Thompson RM1987 and serovar Thompson FMFP 899; S. enterica: 3-strains serovar Typhimurium SL 1344, serovar Thompson RM1987 and serovar Thompson FMFP 899; human noroviruses (NoV) surrogates: Murine norovirus-1, i.e., MNV-1 and Tulane virus, i.e., TV.
Kumar et al. (2015) inspected effect of different fractions (hexane, chloroform, ethyl acetate, and butanol) of root methanolic extract of O. sanctum on Swiss albino mice for different disorders (Figure 3C). Treatment with root extracts (especially ethyl acetate, at 30 to 300 mg/kg) inhibited the paw edema (13–60%), showing anti-inflammatory activity, heat-induced painful sensation (31–51%), chemical-induced pain (22–31%), paw licking (22–51%), and number of writhing (24–53%), showing analgesic activity as well as reduction of temperature (29–38%), showing anti-pyretic activity in dose-dependent manner. Such response of O. sanctum root extract could defy several after effects observed due to nonsteroidal anti-inflammatory drugs, like diclofenac sodium, therefore healthier as anti-inflammatory, antipyretic, and analgesic agents.
Effect of Roots of O. basilicum and Rosmarinic Acid on Microbes
Inhibition in different types of bacterial and fungal growth recommends rosmarinic acid (RA) with constitutive antimicrobial activity. Bais et al. (2002) enhanced accumulation of RA in hairy root cultures of O. basilicum, which positively correlated with treatment of fungal cell wall elicitors (CWE). However, in the testing compound, RA has negative effect on growth of microbes, as evidenced by the zone of inhibition (Figure 3D). Out of tested bacteria of rhizosphere, Xanthomonas campestris, Pseudomonas fluorescens, P. aeruginosa (strains PAO1 and PA14), and Erwinia carotovora displayed concentration-dependent increase in the zone of inhibition. Among these bacteria, P. aeruginosa (human pathogen) was highly sensitive to RA treatment. Increased inhibition was observed with higher titers and higher amount of RA in case of Pythium ultimum and P. aphanidermatum, respectively, while delayed inhibition was observed in Aspergillus niger and Fusarium oxysporum on increasing the concentration of RA. Microscopic examination reveals morphogenic alteration in fungi due to RA treatment like clumping of hyphae (P. drechsleri), rounded spores (in place of dumbbell shaped), damaged cell surface, damaged hyphae, broken intersepta (A. niger), prolific cell division, and damaged nucleoid along with condensed genetic material (P. aeruginosa). The antifungal mechanism of O. basilicum was briefly studied by co-culturing hairy roots with fungal hyphae. Root tips overcame fungal growth having droplets (exudates) with RA at the region of fungal contacts.
These findings suggest that metabolites like RA might get released in rhizosphere to combat microbial infection. The antimicrobial nature of O. basilicum roots and RA challenges infection from soil-born microbes.
In situ Effect of O. basilicum on Human Pathogen
Contaminated soil and water with human pathogens help them to penetrate and contaminate plant tissues. Interaction of plants and pathogens influences morphological, molecular, and physiological changes in plants, as well as pathogens. Plant and pathogen interaction becomes more interesting and significant when it comes to widely used traditional medicinal plants. Bernstein et al. (2017) studied effects of Salmonella enterica serovar Newport (strain 96E01153C-TX) on O. basilicum (Figure 3E) as colony forming unit (CFU). Li and Uyttendaele (2018) examined the pathogenicity of S. enterica serovar Typhimurium (strain SL 1344) and S. enterica serovar Thompson (strains RM1987 and FMFP 899), along with two types of human noroviruses (NoV) surrogates, Murine norovirus-1 (MNV-1), and Tulane virus (TV) on O. basilicum (Figure 3F) as CFU and plague-forming unit (PFU). Effects of O. basilicum on these pathogens are discussed below:
Bernstein et al. (2017) inoculated soil, leaf (petiole), and leaf sap of 60-day-old O. basilicum (with and without 0–500-mM NaCl treatment) with 106 CFU g/soil, 109 CFU ml/l and 103 CFU ml/l suspension of S. enterica, respectively. Salmonella concentration was determined in potting mixture, shoots, leaves, and leaf sap. Root penetration of S. enterica as well as its concentration in soil, leaves, and leaf sap was not affected by salt treatment. Salmonella was undetected in aerial parts of the plant when inoculated in soil. S. enterica counts in leaves reduced by 2.9 × 103 CFU/g/day within 6 h and becomes undetected after 16–24 h. However, sigmoidal increase in growth of S. enterica was observed in leaf sap of plants. Concentration of S. enterica and total aerobic bacteria were decreased (1–1.5 log CFU/g) from 24 h to 5 days (Bernstein et al., 2017). Although salt stress induces lots of changes (root branching, root mass, stomatal conductance, and transpiration rate) in plants, it did not affect interaction of pathogen with Ocimum. Salinity neither affected survival of S. enterica nor its integration into roots of O. basilicum. Metabolic composition, as well as active innate immunity of intact leaves, attributed to the growth inhibition of S. enterica, as compared with leaf sap.
Li and Uyttendaele (2018) prepared 8.46 log-PFU/ml and 8.60 log-CFU/ml solutions of NoVs and S. enterica strains, respectively, to infect leaves of 4-week-old and roots and 6-week-old O. basilicum. Pathogens were quantified using titration by plaque assay for virus as well as pre- and post-enrichment quantification for bacteria. Consistent reduction of >5.5-log, >3.3-log, and 3 to 4-log was observed with MNV-1, TV, and S. enterica strains, respectively, in leaves after 3 days of inoculation through leaves. S. enterica Thompson FMFP 899 can be identified as most infectious due to better survival with 5.5 to a 6.7-log CFU/sample leaf from 6 to 9 days of leaf infection. Internalization of pathogens via roots toward leaves was tested for MNV-1 and S. enterica serovar Thompson FMFP 899 after 1 h, followed by 1, 3, 6, and 9 days. MNV-1 was reduced from 540, 580, 400 PFU/g tissues (at Day 1) to 280, 80, < 4 PFU/g tissues (at Day 3); after that, virus was unable to detect. Only one in three colonies of S. enterica serovar Thompson FMFP 899 was detected after 3–6 days with S. enterica serovar Thompson FMFP 899.
Human noroviruses and S. enterica are most infectious pathogens of food industry with maximum transmission and internalization into plant tissues due to soil and water contamination. Time-dependent reduction of these pathogens was observed after infection via leaves and roots due to antimicrobial nature of the plant. These pathogens negatively affect the food crops, including lettuce, green onion, kale, mustard, and spinach. These pathogens spread faster due to highly contaminating nature; however, antimicrobial nature of Ocimum prevents their growth.
Effect of Rhizosphere on Ocimum Plants
Rhizosphere affects growth and health of overall plants. Therefore, biotic factors like bacteria and nematodes, as well as abiotic ones, such as salt, greatly influence the overall significance of the medicinal plants (Table 2).
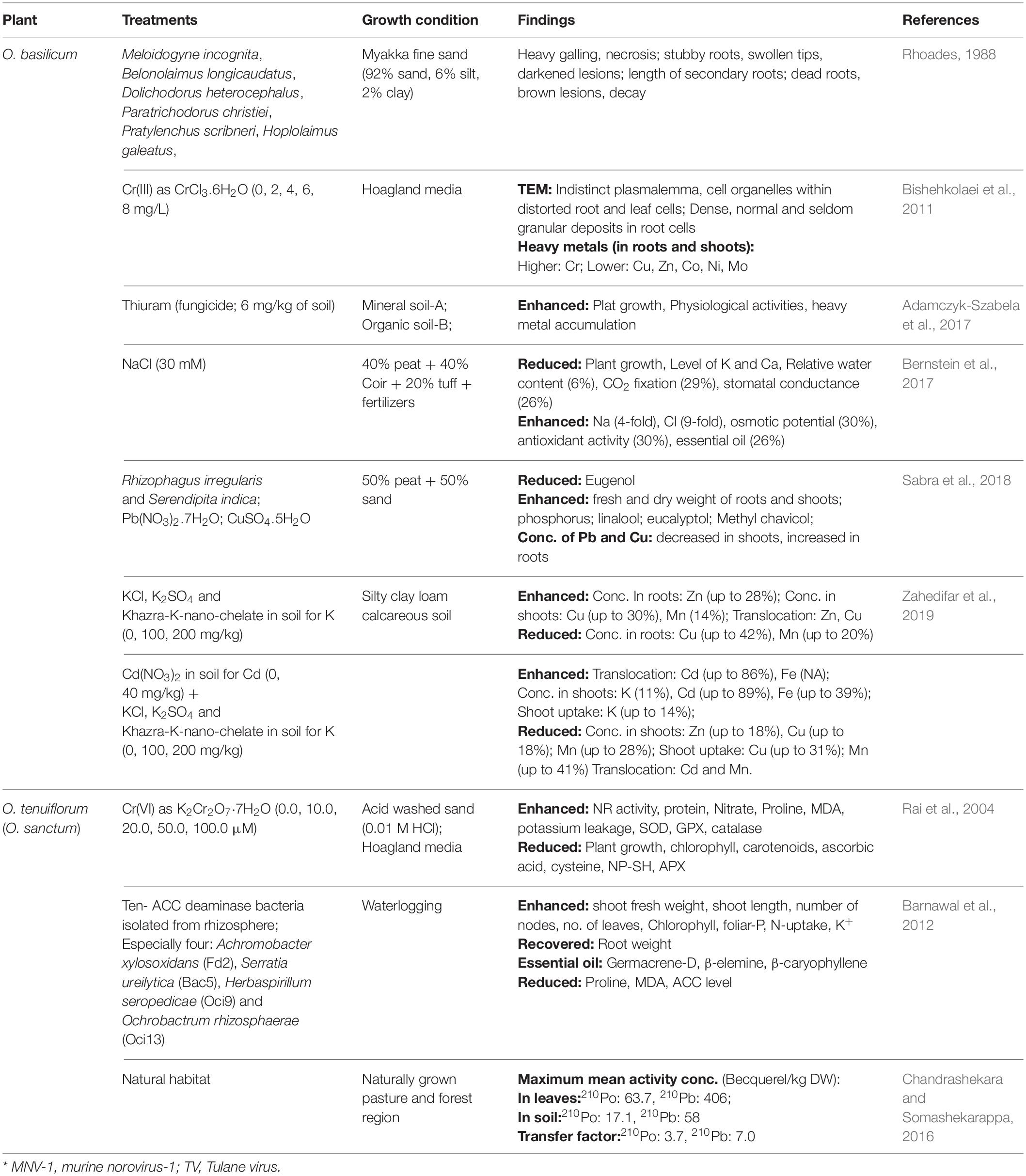
Table 2. Effect of microorganisms (nematode, deaminase bacteria, and symbiotic fungi) and different abiotic stresses (salt heavy metal and radioactive metal) on roots and the whole plant of Ocimum sp.
Growth-Promoting Bacteria in Rhizosphere of O. sanctum
Rhizosphere of Ocimum sanctum was analyzed by Barnawal et al. (2012) under waterlogged condition to isolate bacteria containing 1-aminocyclopropane-1-carboxylic acid (ACC) deaminase. Among isolated 10 bacteria, four strains were verified for growth-promoting activity for waterlogged plants and identified as Achromobacter xylosoxidans (Fd2), Serratia ureilytica (Bac5), Herbaspirillum seropedicae (Oci9), and Ochrobactrum rhizosphaerae (Oci13) through rDNA sequencing. Barnawal et al. (2012) studied O. sanctum plants (10 weeks old) after treating with isolated 10-bacterial strains for 4 weeks and flooded them for the next 15 days.
Strain Fd2 was observed with maximum growth-promoting effect under waterlogging, with maximum increase in shoot FW (46.5%), shoot length (76.3%), number of nodes (72%), number of leaves (41.9%), and maximum recovered root weight (37%). Non-waterlogged O. sanctum plants exhibited maximum shoot FW (59.8%) and minimum reduction in root weight (16%) with Oci13 strain, while maximum shoot length (22%), number of leaves (26.8%), and number of nodes (39.4%) with Bac5 strain. Germacrene-D, β-elemine, and β-caryophyllene were observed as major components of essential oil, with no significant changes due to waterlogging or ACC-deaminase-containing rhizobacteria. Enhanced chlorophyll content in O. sanctum was caused by waterlogging reduction and in the presence of rhizobacteria. Inoculation with Fd2 resulted in maximum chlorophyll content (49.5%) in waterlogging. Inoculation with ACC-deaminase bacteria reverted the effects of waterlogging by maximizing reduction in elevation of proline (18%), MDA [22%, by strain Fd2 and the ACC level (62%, by Bac5 strain)]. However, strains Oci13, Oci9, and Fd2 were observed with maximized foliar-P (22.5%), N-uptake (13.6%), and K+ content, respectively (Barnawal et al., 2012).
Study of Barnawal et al. (2012) emphasized significance of microbes like ACC-deaminase-containing rhizobacteria in growth of O. sanctum and also to overcome the effects of stresses like waterlogging.
Effect of Different Nematodes on Growth of O. basilicum
Rhoades (1988) analyzed soil and root of unhealthy O. basilicum. Roots of these plants were damaged due to the presence of few nematodes, Meloidogyne incognita, Belonolaimus longicaudatus, Dolichodorus heterocephalus, Pratylenchus scribneri, Hoplolaimus galeatus, and Paratrichodorus christiei. To confirm pathogenicity of these nematodes, Rhoades (1988) poured suspension of eggs, juveniles, and adults of different nematodes around rooted cutting (10–12-m tall) of the plant. Eggs of M. incognita (5,000 or 15,000 per pot), as well as juveniles and adults of Belonolaimus longicaudatus, Dolichodorus heterocephalus, Pratylenchus scribneri (l,000 or 2,000 per pot), Hoplolaimus galeatus (7,500 or 15,000 per pot), and Paratrichodorus christiei (150 per pot) were used to make suspension. Rhoades (1988) evaluated soil, plant growth, and yield, as well as root injury till 10 months. Plant growth and yield were unaffected till 5 months of all nematode inoculation. D. heterocephalus and H. galeatus had no significant effect on growth of O. basilicum, even after 5 months; however, other nematodes significantly reduced growth and yield from 5 to 10 months. Different nematodes injured roots in different ways. M. incognita caused heavy galling with necrosis, B. longicaudatus produced stubby roots with swollen tips and darkened lesions, P. christiei reduced length of secondary roots, while P. scribneri almost killed roots of O. basilicum.
Several nematodes can be pathogenic to Ocimum and compromise the production. Therefore, prior to cultivation, soil must be examined for pathogenic organisms. Abd-Elgawad (2021) and Renčo et al. (2021) confirmed the wide availability and pathogenic effect of these nematodes along with other species of nematodes.
Salt Stress to O. basilicum
Bernstein et al. (2017) briefly studied the effect of saline treatment (30 mM of NaCl) on growth, physiology, as well as essential oil and metal accumulation in seedlings of O. basilicum (30 days old), for 30 days. Although salinity reduced shoots, roots, along with areas of individual leaves, shoots were observed with utmost reduction. Exposure of NaCl-enhanced Na (4-fold) and Cl (9-fold) with reduction in the levels of K and Ca. Salinity-induced reduction in relative water content (6%), CO2 fixation (29%), and stomatal conductance (26%) was observed along with increase in osmotic potential (30%), antioxidant activity (30%), and essential oil (26%) content.
Bernstein et al. (2017) highlighted the negative impact of increased salinity in soil on O. basilicum, which significantly reduced the growth, physiology, and metabolic contents of the plant.
Metal Uptake With Different Soil Compositions and Fungicide Treatments
Roots are primarily considered as the transport system to provide water and nutrients (present in soil) to aerial parts of plants. This way, it becomes essential to understand the performance of the root system in the current scenario of soil pollution for translocation of different metals. Adamczyk-Szabela et al. (2017) examined growth and physiological activities of O. basilicum (1 month old) germinated on two different soil types with and without thiuram (fungicide) treatment for 14, 28, and 42 days (Table 2). Soil-A from uncultivated farmland (for 2 years) and soil-B from organic rich commercial land were confirmed with acidic nature and no heavy metal contamination. Time-dependent increase in all physiological activities was observed in plants of both soil-A and soil-B, which further enhanced after fungicide treatment, except for intracellular CO2.
Roots of Ocimum basilicum (grown in both soils) were observed with higher content of Co, as well as lower content of Cd and Zn at 14th day, which alters till 42nd day of fungicide treatment. The accumulation patterns of Pb, Ni, and Mn for soil-A and Cu for soil-B were similar to Co. Similarly, Cu and Pb showed a similar pattern of Cd, with soil-A and soil-B, respectively. Mn content in shoots of O. basilicum gradually increased in soil-A, while, in soil B, increased at 42nd day after gradual decrease till 28th day of thiuram treatment. Thiuram treatment had no effect on Mn translocation factor (TF, metal distribution), which was highest with both soils, as well as Zn and Cd uptake (Transfer coefficients, TC), which was maximum with soil-A and soil-B, respectively. However, TCs for other metals vary with treatment. With plants of soil-A, TF for Mn and Zn was increased at 14th day, and then gradually decreased. Plants grown in soil-B were observed with higher TF value for Mn, Zn, Pb, and Ni on 42nd day of thiuram treatment.
Thiuram has low water solubility and rather adsorbs to soil particles. It degrades easily in organic soil (acidic). Thiuram enhanced metal uptake in soil-A (for Co, Pb, Ni, and Mn) as compared with soil-B (Co and Cu). Degradation of thiuram due to existing humic acids and lesser accessibility of metals due to stable metal complexes in soil B is the reason behind lower heavy-metal uptake in soil-B. Therefore, it is important to consider the nature of soil and plantation of Ocimum and requirement of fungicide.
Metal Uptake With Different Forms of Chromium Contamination
Heavy utilization of chromium is well-known in catalyst application, electroplating, leather tanning, pigment manufacturing, metal finishing, and steel production. Gigantic consumption of carcinogenic chromium becomes the cause of soil and water pollution. Due to therapeutic nature of Ocimum, Cr exposure to O. tenuiflorum as Cr(VI) using K2Cr2O7⋅7H2O (0 to 100 μM), as well as to O. basilicum as Cr (III) using CrCl3.6H2O (0–8 mg/l), was examined by Rai et al. (2004) and Bishehkolaei et al. (2011), respectively (Table 2).
Chromium concentration increased in plant tissues with increase in Cr concentration and duration of exposure. Roots of O. tenuiflorum accumulated more Cr as compared to leaves of same. Highest accumulation of Cr (419.50 μg g–1 dry weight, DW) was observed in roots of O. tenuiflorum at 72 h of 100-μM Cr treatment. Chromium uptake reduced plant growth, biomass, total chlorophyll, chlorophyll a, chlorophyll b, and carotenoids in dose- and time-dependent manner. Similar to plant growth, maximum reduction in nitrate reductase activity (64%), protein content (77.45%), and accumulation of nitrate (3.59-fold) was recorded with 100-μM Cr exposure for 72 h in leaves. Same conditions inhibited ascorbic acid (52.83%), cysteine (77.33%), nonprotein thiol (NP-SH, 60.87%), and activity of APX (76.22%). However, proline content (574.94 μmol g/FW), MDA content (5-fold), potassium leakage (which leads to cell membrane disruption), as well as activities of SOD (3.29-fold), GPX (5.56-fold), and catalase (1.63-fold) were enhanced with increasing dose and time. TLC of leaf tissues confirmed enhanced eugenol accumulation due to Cr exposure. However, maximum increase in eugenol content (24.61%) was observed with treatment of 20-μM Cr for 72 h. Based on physiological and metabolic changes, O. tenuiflorum can tolerate chromium-induced stress (Rai et al., 2004).
Root cortical and leaf mesophyll cells were analyzed through TEM, which reveals indistinct plasmalemma and cell organelles within distorted cells when treated with higher concentrations (4–8 mg/l) of Cr (III). Root cortical cells of Cr (III)-treated plants exhibited dense, normal, and seldom granular deposits along the periplasmic zone of cell wall, the innermost layer of cell wall, and cytoplasm, respectively. Granular deposits of root cortical cells consist of Cr as the dominant element along with Cu, Zn, Co, Ni, and Mo. Increasing concentration of (≥4 mg/l) Cr (III) treatment resulted in decreased amount of other heavy metals in roots as well as shoots. This could be due to either Cr-inhibited activity of H+- ATPase associated with plasma membrane, or competitive interaction with plasma membrane. However, accumulation of Cr was higher in roots (up to 1130.9 ± 51.5-mg/g DW) as compared to shoots (up to 57.2 ± 2.7-mg/g DW) of O. basilicum, with maximum concentration of Cr (Bishehkolaei et al., 2011).
Ocimum tenuiflorum exhibited reduced growth and chlorophyll with enhanced eugenol and antioxidant enzymes as protective measures to stress. Both, O. tenuiflorum and O. basilicum accumulated lesser Cr in shoots as compared to roots. Cr (IV) gets reduced to Cr(III), which later confiscated in the vacuole of root cells in order to prevent Cr translocation in aerial parts of the plant. This is the natural defense mechanism to prevent Cr toxicity in Ocimum plants and is the reason behind the adaptive behavior of O. tenuiflorum and O. basilicum during chromium exposure (Rai et al., 2004; Bishehkolaei et al., 2011).
Metal Uptake With Natural Radioactive Elements
Chandrashekara and Somashekarappa (2016) analyzed activities of natural radionuclides 210Po and 210Pb in leaves of O. sanctum (natural habitat) and associated soil samples, along with other 11 medicinal plants and 11 soil samples (Table 2). Mean activity concentration of radionuclides varied from 11.2 to 42.9 Becquerel/kg DW in soil and 3.3 to 63.7 Becquerel/kg DW in tested plants for 210Po, as well as 36.1 to 124 Becquerel/kg DW in soil and 12 to 406 Becquerel/kg DW in tested plants for 210Pb. Leaves of O. sanctum were observed with maximum mean activity concentration of both radioactive elements 210Po (63.7 Becquerel/kg DW) and 210Pb (406 Becquerel/kg DW) among 12 selected medicinal plants. However, soil samples related to O. sanctum were observed with medium range mean activity concentration 210Po (17.1 Becquerel/kg DW) and 210Pb (58 Becquerel/kg DW). Higher concentration of elements in leaves suggested more translocation from roots, which was proved with observed maximum transfer factor (TF) of O. sanctum for 210Po (3.7) and 210Pb (7.0) as compared with other 11 medicinal plants. Chandrashekara and Somashekarappa (2016) calculated these natural radionuclides to understand the safety of medicinal plants based on their radiological behavior. Chandrashekara and Somashekarappa (2016) assumed the role of leave morphology, surface area, shedding time, age of leaf, along with height of the plant in the uptake of 210Po and 210Pb. The documentation of radionuclides uptake and accumulation in medicinal plants like Ocimum are necessary steps before consuming them in the drug and food industry.
Metal Uptake With Pb and Cu Contamination Affected by Endophytic Fungi
Sabra et al. (2018) treated potting mixture of O. basilicum (4 weeks old) with Rhizophagus irregularis (AM) and/or Serendipita indica (beneficial endophyte) for 5 weeks, as well as with 400 or 200 mg/l of Pb [as Pb(NO3)2.7H2O] and/or Cu (as CuSO4.5H2O) for 10 days. Maximum mycorrhization of R. irregularis (63%) in roots of O. basilicum when inoculated with both fungi, however, reduced to 46% in the presence of both heavy metals. Highest increase in fresh and dry weight of roots and shoots of plants was testified with R. irregularis alone, followed by treatment of S. indica and both fungi. Treatment of S. indica positively influenced shoot and root dry weight with and without contaminating Pb and Cu, respectively. P uptake in shoots by plants was highly promoted by R. irregularis with Pb and/or Cu contamination; however, S. indica promoted P uptake with and without Pb treatment only. Maximum reduction in shoot Pb content (48%) was observed with treatment of both fungi, followed by R. irregularis and S. indica in Pb contamination. However, Cu content in shoots decreased with treatment of S. indica and both fungi on Cu- and/or Pb-contaminated soil. Pb and Cu contents in roots were enhanced in roots grown on soil treated with both fungi and metal treatment. Single-metal treatment had no significant effect on root metal accumulation. Effect of fungal and metal treatments on metabolites in shoots of O. basilicum was also analyzed by them. R. irregularis enhanced the level of two phenylpropenes, linalool (1–7-mg/g DW) and eucalyptol (from 0.1-to-0.8-mg/g DW) even in uncontaminated or single-metal (Pb or Cu)-contaminated condition. Eugenol (terpene) content was reduced from 2.2 to 0.5-mg/g DW, only with S. indica colonization along with Pb treatment. Methyl chavicol (terpene) was enhanced from 5- to 7.2-μg/g DW by mycorrhization of roots in only uncontaminated soil.
Sabra et al. (2018) discussed the involvement of beneficial fungi to promote growth and metabolic composition of O. basilicum and overcome aftereffects of heavy metal stress (Table 2). Variations in the accumulation pattern of phenylpropenes and terpenes were observed due to unrelated biosynthetic pathways. Such growth-promoting microbes become noteworthy to endorse plant growth in tough environmental circumstances.
Metal Uptake Affected by Different K-Fertilizers and Cd Treatments
Zahedifar et al. (2019) germinated seeds of O. basilicum in soil treated with Cd as Cd(NO3)2 (0- and 40-mg/kg soil), along with KCl, K2SO4, and Khazra-K-nano-chelate ( 0-, 100-, 200-mg/kg soil), for 10 weeks. Cd treatment increased K concentration in shoots of O. basilicum by about 11% in all K-treatments, while the shoot uptake was decreased by 12, 14, and 8% with KCl, K2SO4, and K-nano-chelate. The use of K in the form of K-nano-chelate reduced Cd accumulation. The potassium supplement (without Cd) enhanced Zn (up to 28%) in roots, Cu (up to 30%), and Mn (14%) in shoots as well as reduced Cu (up to 42%) and Mn (up to 20%) in roots of O. basilicum. Lesser stability of CdCl2 (as compared with CdSO4) enhanced the phyto-availability of Cd, hence accumulation of Cd in shoots with 40-mg Cd and 100-mg KCl. Enhanced translocation (86, 82, and 76%) of Cd from roots to shoots, as well as Cd concentration (89, 86, and 81%) in shoots, was observed with 40 mg of Cd along with different K-treatments. Cd treatment (with the K supplement) reduced shoot concentrations of Zn (up to 18%), Cu (up to 18%), Mn (up to 28%), shoot uptake of Cu (up to 31%), and Mn (up to 41%) of O. basilicum. Increasing concentration of Cd possesses inhibitory effect on translocation of Cd and Mn, which was also reduced due to K supplementation. Treatment of Cd enhanced shoot Fe (up to 39%) and Fe transfer from roots to shoots, which was further accompanied by the K supplement. Translocation of Zn and Cu seemed to be unaffected by Cd treatment and increased by the K supplement. Transfer of Cd from roots to shoots was observed maximum with K in the form of nano-chelate. Table 2 explains the effect of different K-fertilizers on heavy metal uptake in roots and shoots of O. basilicum in Cd-contaminated soil. The result signifies K-nano-chelate as a preferable source of potassium fertilizer for the growth of O. basilicum.
Root Uptake of Nanoparticles and Their Effect on the Whole Plant
Study and involvement of nanoparticles are increasing every day. Nanoparticles are being used in several industries, including medicine, paint, plastic, and agriculture. These nanoparticles can affect plants through direct use in agriculture or indirect as a waste or a contaminant. The effect of nanoparticles in O. basilicum has been extensively studied by (Tan et al., 2017, 2018a, b) using nanoparticles of Ti and Cu.
TiO2 Nanoparticles as Pollutants
Tan et al. (2017, 2018a) studied plants germinated on soil treated with 0–750 mg/kg of three different types of nano-TiO2 (unmodified, hydrophobic, and hydrophilic) for first (Tan et al., 2017), second, or both cultivation cycles (Tan et al., 2018a). Subsequently, growth parameters, physiological activities, antioxidant enzyme activities, and nutrient/metal distribution were examined. Details of experiments have been mentioned in Figure 4A.
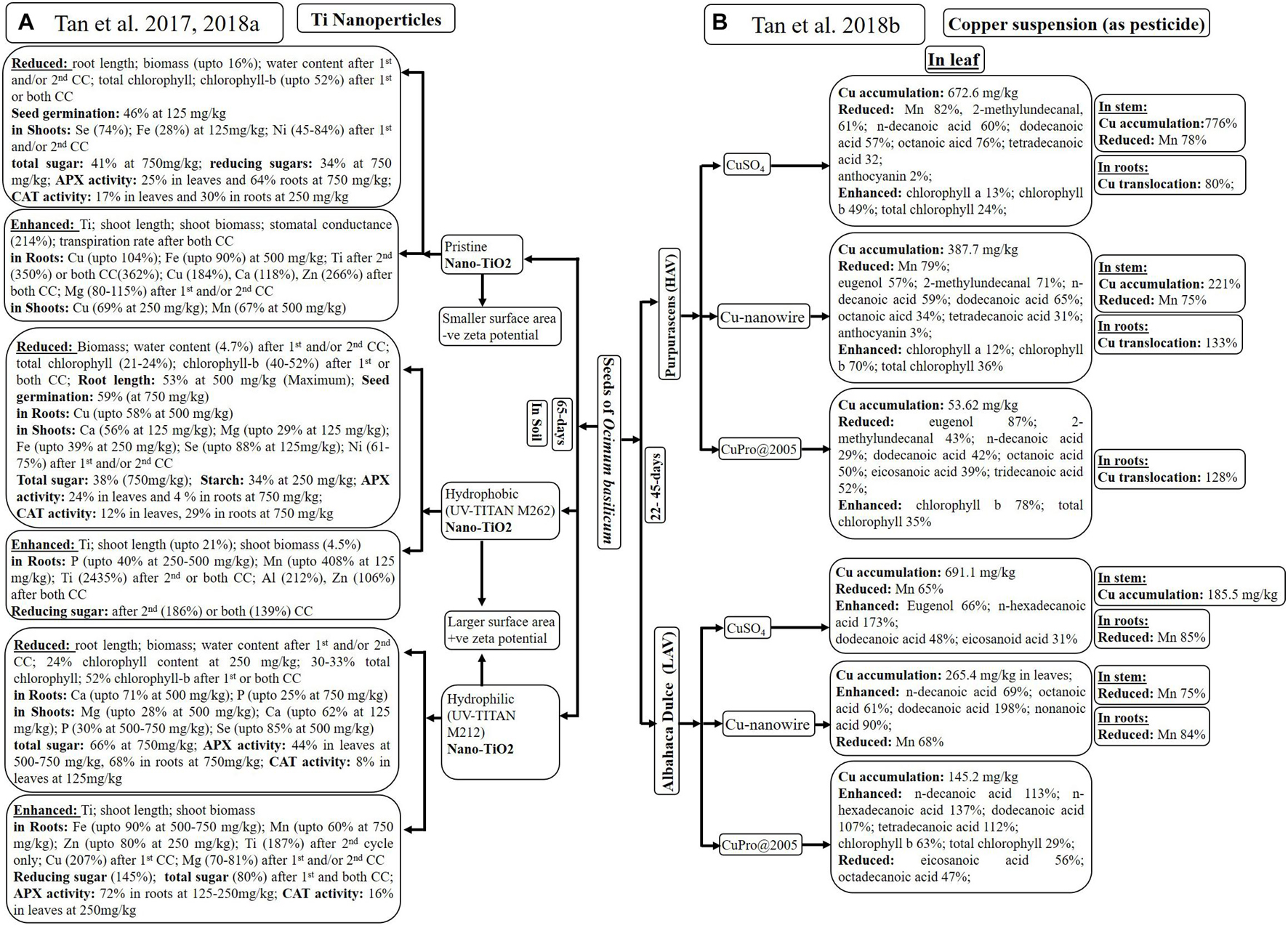
Figure 4. Analysis of plant growth as well as accumulation of elements and metabolites after introduction of: Ti-nanoparticles (A) and Cu-suspensions (B) through root system of Ocimum basilicum. *CC: cultivation cycle; HAV: High anthocyanin variety; LAV: Low anthocyanin variety.
Tan et al. (2017, 2018a) observed maximum Ti accumulation with hydrophobic nanoparticles, although all nanoparticles enhanced Ti content in a dose-dependent manner. There was no significant difference in Ti translocation from roots to shoots using any of nano-TiO2 (Tan et al., 2017). Tan et al. (2017, 2018a) experienced variability in macro- and micronutrient content with different types of nano-TiO2 at different concentrations. Variability among few nutrients in leaves and shoots has been given in Figure 4A.
Only hydrophobic and unmodified nano-TiO2 inhibited seed germination (up to 59%), root length (up to 53%), biomass (up to 16%), and water content (up to 16%) of O. basilicum (Tan et al., 2017). However, higher concentration of nanoparticles appeared to have no effect on the germination rate, root length, the photosynthetic rate, and chlorophyll-a in any cultivation cycle (Tan et al., 2018a). Tan et al. (2018a) observed maximum increase in shoot length (up to 21%) and shoot biomass (4.5%) after first and/or second cycle of treatment with hydrophobic nanoparticles, followed by uncoated and hydrophilic nano-TiO2. Nanoparticles treatment during first and/or both cycles reduced water content (up to 4.7%) total chlorophyll (up to 33%) and chlorophyll-b (up to 52%). Tan et al. (2017, 2018a) mostly experienced reduction in total sugar, reducing sugars and starch after nanoparticles treatment. Only treatment with hydrophobic nanoparticles for second (186%) or both cycles (139%) enhanced reducing sugar in O. basilicum. All nano-TiO2 significantly reduced APX activity in leaves (up to 44%) and roots (up to 29%) of O. basilicum at 500–750 mg/kg of nano-TiO2. However, only hydrophilic nanoparticles first enhanced APX activity (72%) in roots at lower concentration (125–250 mg/kg) due to interference of the -OH group.
Being used in several industries, nano-TiO2 gets released in water and soil, which ultimately affects the plants. Tan et al. (2017, 2018a) presumed that, by changing the properties of nano-TiO2, their uptake and effect on plants can be reduced. All three types of nanoparticles affected the plant in different ways, which was continued for the next cultivation cycle. Pristine nano-TiO2 enhanced gas exchange; hydrophobic nano-TiO2 increased shoot length and biomass, reducing sugar with reduced chlorophyll contents in one or both cultivation cycles. Hydrophilic nano-TiO2 reduced total sugar in first cultivation cycle, which was improved in the next cycle. Therefore, selection of nanoparticles totally depends on the desired morphological or physiological character.
Cu-Nanowire- as Pesticide
Tan et al. (2018b) studied effect of Cu suspensions [CuSO4, Cu(OH)2 nanowires and CuPro@2005, as pesticides] on high and low anthocyanin varieties of O. basilicum (22 days old) through spray every 3rd day (till 4.8-mg Cu/pot). The differences in physiological activities, metal as well as metabolic accumulation of dark opal, “Purpurascens” (high-anthocyanin variety, HAV) and dulce, “Albahaca Dulce” (low anthocyanin variety, LAV) varieties (45 days old), are mentioned in Figure 4B.
Ionization of Cu2+ with CuSO4 suspension results in higher accumulation of Cu in leaves (691.1 mg/kg) and stems (185.5 mg/kg) of LAV, followed by leaves (672.6 mg/kg) and stems (776%) of HAV plants. Only HAV variety demonstrated higher Cu translocation from shoots to roots, using CuSO4 (80%), Cu(OH)2 nanowires (133%), and CuPro (128%) due to more dissolution of Cu in acidic nature of HAV leaves. Smaller size of Cu(OH)2 nanowires promoted accumulation by facilitating stomatal entry of nanoparticles as compared to CuPro@2005. Only Mn content reduced in leaves (65–82%), stems (up to 78%), and roots (up to 84%) of both varieties after exposure of CuSO4 and Cu(OH)2 nanowires. Cu-induced alteration in Mn has been reported in several other plants.
Tan et al. (2018b) observed elevated levels of most of the important metabolites, including eugenol (66%), in LAV variety. Variety HAV exhibited reduction in 2-methylundecanal (71, 43, and 61%) and eugenol (57 and 87%) when treated with Cu(OH)2 nanowires, CuPro@2005, and CuSO4, respectively. Handling of CuSO4, Cu(OH)2 nanowires, and CuPro@2005 with HAV variety dropped the concentration of n-decanoic acid (60, 59, and 29%), dodecanoic acid (57, 65, and 42%), and octanoic acid (76, 34, and 50%), respectively. However, the same Cu suspensions enhanced dodecanoic acid (48, 198, and 107%), n-decanoic acid (0, 69, and 113%), octanoic acid (20, 61, and 5%), and nonanoic acid (55, 90, and 10%) in LAV variety. Saturated fatty acids like eicosanoic acid (56%) and octadecanoic acid (47%) were decreased with CuPro@2005 treatment due to its negative effect on LAV variety. Enhanced chlorophyll content was observed with only CuPro@2005 in LAV and with all three treatments in HAV.
Anthocyanin content influenced Cu uptake along with physiological and metabolic changes in presence of Cu suspensions. Acidic nature of HAV, as well as higher accumulation of Cu, promoted more chloroplast development and reduction of essential oil as well as fatty acids, as compared to LAV. Application of Cu as nanowire has least Cu accumulation and translocation along with enhanced fatty and essential oil in LAV as compared to HAV. Therefore, Cu nanowire can be used as potential pesticide for O. basilicum.
Adventitious Root Culture
The most vital part of the plant – roots – exists underground and helps in healthy growth and stability of the plant. Economic benefits increase the significance and the demand of Ocimum roots, which get affected by rhizosphere. Therefore, in vitro studies on roots of medicinal plants like O. sanctum seek attention of researchers.
Till date, only Shilpa et al. (2010) mentioned the development of adventitious in vitro root cultures (Figure 5). Sterilized leaves were inoculated on MS media supplemented with different concentrations of NAA, in combination with BA, kinetin or 2, 4-dichlorophenoxyacetic acid. Root proliferation was achieved in obtained rhizogenic calli on MS media containing auxins only. MS liquid supplemented with different concentrations and combinations of NAA and BA was used for the further subculture of roots. Maximum callus induction was observed during the 1st week of culture, caused by NAA (2 mg/l) in combination with Kn (0.2 mg/l) or BA (1.3 mg/l), as well as NAA (0.5 mg/l) with 2,4-D (1.5 mg/l). However, only NAA with Kn showed root induction during the 2nd week of culture. Further root growth was achieved when induced roots were transferred to MS media containing NAA (0.5 mg/l) with 2,4-D (1.5 mg/l). No roots formation was observed with MS media with or without 2,4-D in the absence of NAA. Maximum root biomass of 1,460 ± 0.8 mg/l (dry weight) was accomplished in the liquid MS medium supplemented with 4 mg/l of NAA and 1.3 mg/l of BA. Root induction reduced with the reducing concentration of NAA, and callus induction increased with increasing concentration of BA. Therefore, the medium with the high auxin to cytokinin ratio is best for in vitro root culture.
In vitro root cultures present a better way to accumulate secondary metabolites with medicinal significance. In case of O. sanctum, NAA, in combination with BA, is required for rapid growth of adventitious roots from leaf explants and achieving maximum biomass.
Hairy Root Culture: Agrobacterium rhizogenes-Mediated Transformation
Similar to in vitro root culture, hairy roots are the best mode to enhance biomass and accumulate metabolites specific to root tissues using bacterial infection. Gram negative bacteria Agrobacterium rhizogenes induce the hairy root production with the help of their rol-genes, which integrate into plant DNA after infection. These hairy roots can be cultured in vitro at a large scale using bioreactors. Valuable phytochemicals from several plants have been in production using hairy root culture technology (Pedreño and Almagro, 2020; Rat et al., 2021; Roy, 2021). Hence, several researchers have put their efforts in development of hairy root cultures of plants with significance, using various strains of wild as well as modified Agrobacterium rhizogenes. Different methods of transformation of O. sanctum and O. basilicum using different media, antibiotics, and elicitors treatments have been described in Figure 5. Produced hairy roots were analyzed for different aspects like biomass, genetic transformation, and phytochemical and medicinal behavior, as described below:
Bacterial Strain Used for Transformation
Strain ATCC 15834 (Tada et al., 1996; Bais et al., 2002; Vyas and Mukhopadhyay, 2014) and strain LBA 9402 (Skorupinska-Tudek et al., 2007; Marzouk, 2009) of A. rhizogenes were preferred during most studies to infect O. sanctum and O. basilicum. Other strains of A. rhizogenes used were MAFF 03-01724 (Tada et al., 1996), A4, Arqua1-pTSC5, 8196, and 11325 (Srivastava et al., 2016b, c).
Studies suggest that strains A-4, ATCC 15834, and LBA 9402 of A. rhizogenes were preferred to induce hairy roots as strains Arqua1-pTSC5, 8196, and 11325 were least effective to induce hairy roots from Ocimum.
Media and Explant Used for Transformation
Mostly, MS media with or without agar was used for hairy root culture of O. basilicum and O. sanctum. However, G5 and WP mediums were also tried to culture hairy roots by Tada et al. (1996) and Skorupinska-Tudek et al. (2007). The use of claforan and cefotaxime is recommended, as these successfully cleared bacterial infection after co-cultivation (in the dark for 2 days). Only Marzouk (2009) used ampicillin for the same.
Mostly, young leaves of in vitro-germinated seedlings were used to infect with A. rhizogenes. Studies also reported the use of stems (Bais et al., 2002; Marzouk, 2009), as well as hypocotyl and cotyledon (Vyas and Mukhopadhyay, 2014; Srivastava et al., 2016b), for transformation purposes. Vyas and Mukhopadhyay (2014) studied green and red varieties of O. sanctum, and (Srivastava et al., 2016b, c) analyzed three cultivars of O. basilicum (Subja, Holy Green and Red Rubin) for development of hairy root cultures.
Therefore, leaves from green variety of O. sanctum and Subja cultivar of O. basilicum are better to achieve more clones and higher biomass on MS media after treatment of cefotaxime or claforan.
Transformation Frequency of Hairy Roots
Leaves of green variety of Ocimum sanctum have been reported with maximum transformation frequency of 70%, followed by excised shoots of green form and red form (58 and 43%, respectively). Leaves of red form showed transformation frequency of 42%. Lowest transformation frequency of 26 and 21% was observed with hypocotyl of both red and green forms, respectively (Vyas and Mukhopadhyay, 2014).
Earliest root induction was observed with A. rhizogenes strain A4 in Subja cultivar (7 days), followed by Holy Green (10 days) and Red Rubin (13 days) cultivar. Late root induction (15 days) with a low survival rate in all cultivars was observed with A. rhizogenes strains ARqua1-pTSC5 and 11325, while strain 8196 was unable to induce root in any tissue. Maximum transformation efficiency of 70% was observed with leaves of Subja cultivar of O. basilicum (Srivastava et al., 2016b).
Morphology of Hairy Roots
Insertion, copy number, and position of left and right fragments of T-DNA influence morphological assortment among hairy roots of different varieties, which determine phytochemical characteristic of hairy roots. Hairy roots from two varieties (red and green) of O. sanctum were mostly unbranched and densely covered with root hairs. Leaves of green variety produced higher number of hairy roots due to more phenylpropenes (signaling phenolics) as compared to red variety with more anthocyanins (Vyas and Mukhopadhyay, 2014).
Hairy roots of all the cultivars were observed with different characteristics. Subja cultivar was observed with maximum thickness (0.45 mm), Holy Green was observed with maximum number of root tips (1,317), and Red Rubin with maximum root length (1,112 cm) after 60 days of culture (Srivastava et al., 2016b). Microscopic analysis revealed no significant difference in root morphology of O. basilicum, even after 90 days of co-culture with R. irregularis (Srivastava et al., 2016c). All co-cultured hairy roots, as well as roots of seedlings, were observed with hyphae (intra- and extra-radical), arbuscules (active and collapsed), vesicles, and extra-radical spores. Maximum hyphal spread (dense) was observed with hairy roots of Red Rubin cultivar during the pre-symbiotic phase (20 days). During the symbiotic phase (after 40 days), hairy roots of Subja cultivar were observed with highest extraradical sporulation, as well as root length colonization percentage (32%) of R. irregularis. Therefore, co-cultivation with R. irregularis enhanced the overall characteristics and biomass of hairy roots in all cultivars of O. basilicum.
Enhanced Biomass and Root Induction
Tada et al. (1996) and Bais et al. (2002) recorded maximum biomass when hairy roots of O. basilicum were infected with strain ATCC 15834 of A. rhizogenes. Tada et al. (1996) observed rapid proliferation in hairy roots of O. basilicum in WP and MS mediums after 3 weeks. Highest calculated biomass was 14.06 % DW (inoculum weight: 0.2-g FW) after 6 weeks (Tada et al., 1996) and 65 ± 5-g/l FW (inoculum weight: 100-mg FW) after 4 weeks (Bais et al., 2002) in MS medium.
Hairy root cultures of O. basilicum were subjected to treatments of salicylic acid (SA), jasmonic acid (JA), chitosan, and fungal cell wall elicitors (CWE) from Phytophthora cinnamon and Phytophthora drechsleri (Bais et al., 2002). Higher biomass of 65 ± 5-g/l FW (6.51-fold) of hairy roots (from 100-mg FW) could be further enhanced by 1.28-fold (up to 105.65 ± 7.9-g/l FW from 100-mg FW) using elicitors treatment (CWE). Although reduced biomass (10-g/l FW) was observed with other elicitors like SA, JA, and chitosan (Bais et al., 2002).
Similarly, when started with 3–4 hairy root tips to compare biomass among three cultivars (Subja, Holy Green, and Red Rubin) of O. basilicum, time-dependent increase was observed from 20 days (4–27-mg DW) to 60 days (59–112-mg DW) of culture. Subja and Holy Green cultivars accounted for highest biomass of 114- and 116-mg DW, respectively, as compared with 59-mg DW of Red Rubin (Srivastava et al., 2016b).
Lately, hairy root culture has been major focus to explore many plants. Hairy roots can be the best source of the desired metabolite and do not require growth regulators for culture. These studies demonstrated highly productive nature of hairy root cultures of pharmaceutically vital plant like Ocimum to enhance biomass, which ultimately resulted in heightened phytoceuticals for the therapeutic industry.
Phytochemical Investigation
Accumulated metabolites directly reflect the commercial importance of all plant parts, including roots, of medicinal plants. A number of studies mentioned phytochemical profile of leaves and seeds of Ocimum sp., but revelation of metabolic composition of roots remained understated. Ahmad et al. (2012a; 2012b) and Kumar et al. (2015) analyzed natural root tissues of field-grown O. sanctum, while hairy roots of O. sanctum (Skorupinska-Tudek et al., 2007) and O. basilicum (Tada et al., 1996; Bais et al., 2002; Marzouk, 2009) were mostly studied from methanolic root extracts for phytochemicals due to their commercial application. Solvent used for extraction of diverse explants of varied culture conditions to isolate and identify different metabolites has been mentioned in Table 3.
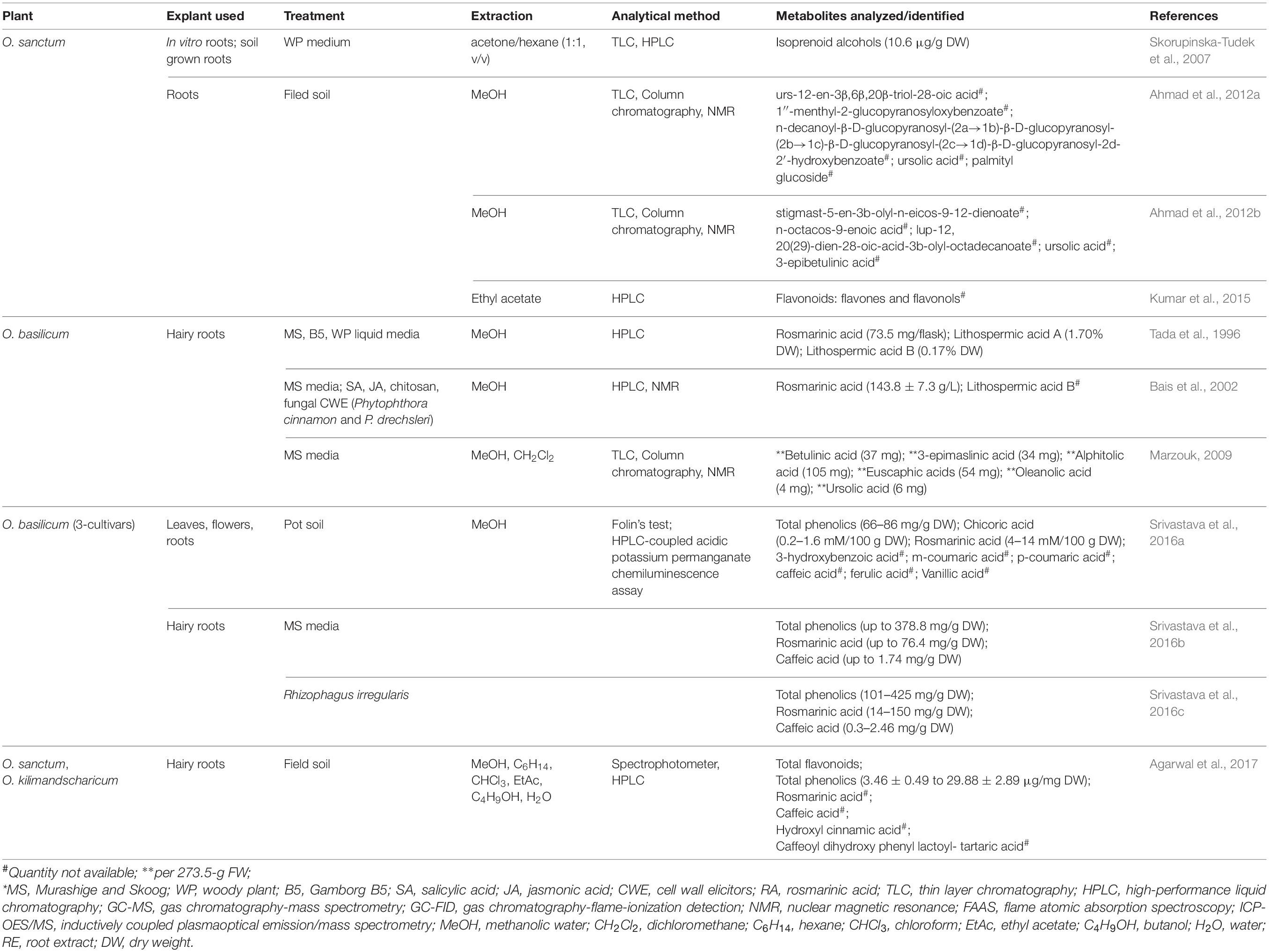
Table 3. Identification of phytochemicals from soil-grown, in vitro, and hairy roots of Ocimum basilicum and Ocimum sanctum.
Rosmarinic Acid and Phenolics: Due to Different Bacterial Strains and Media Composition
Roots of Ocimum accumulate rosmarinic acid (RA) as the principal antioxidant phenolic compound, as compared to other tissues of the plants (Bais et al., 2002). Secondary metabolite content, which varies in hairy roots based on different culture conditions (like Agrobacterium strain, media composition, and elicitors treatment), is discussed below:
Hairy roots of O. basilicum obtained after infection of A. rhizogenes (strains ATCC 15834 and MAFF 03-01724) were cultured on three different types of liquid media (MS, B5, and WP). Methanolic extract of lyophilized hairy roots was analyzed through HPLC for amount of rosmarinic acid (RA) and related phenolics (lithospermic acids A and B). Higher concentration of RA was obtained from hairy root culture obtained from infection of MAFF 03-01724 in MS media (14.1%), as well as from infection of ATCC 15834 strain on B5 media (14%). Hairy roots cultured on MS media after infection of A. rhizogenes strain MAFF 03-01724 accumulated up to 73.5 mg/flask of RA (maximum). Lithospermic acid A was observed maximum (1.7%) in hairy root clones with MAFF 03-01724 infection, cultured in MS media. Accumulation of lithospermic acid B (0.17%) was observed in B5 and WP media (Tada et al., 1996).
Hairy roots of O. basilicum obtained using strain MAFF 03-01724 produced a higher level of rosmarinic acid and lithospermic acid A in the presence of MS media. However, when the media was replaced with B5 and WP, a greater number of phenolics were extracted. Optimization of macronutrients has benefits in root growth (Arab et al., 2018) and Agrobacterium-mediated transformation (Hata et al., 2021). Therefore, selection of effective media can be very advantageous to attain the desired trait in Ocimum.
Difference in Rosmarinic Acid Due to Elicitors Treatments
Hairy roots of Ocimum basilicum obtained from infection of A. rhizogenes (ATCC 15834) were analyzed for RA with and without elicitor(s) treatment. Chitosan SA, JA, and fungal CWE (P. cinnamon and P. drechsleri) were used to treat hairy root cultures. HPLC revealed maximum accumulation of RA in hairy roots as compared to leaves, shoots, and roots of in vitro-grown plants. Hairy roots accumulated 2.98%-g-FW-higher RA, as compared to non-transformed roots. Elicitation due to CWE further enhanced RA content by 8.1 ± 0.59%-g FW on 28th day of culture. Specifically, CWE of P. cinnamon and P. drechsleri increased concentration of RA (in dose-dependent manner) on 12th day by 1.54-fold (125.8 ± 9.4 g/l) and 1.64-fold (143.8 ± 7.3 g/l), respectively (Bais et al., 2002).
Thus, results indicate that accumulation of RA in root tissue of O. basilicum can be increased by synergistic effect of transformation and elicitors.
Isoprenoids and Triterpenes in Hairy Roots
Extracts (lipid fractions) of hairy roots, roots, stems, and leaves of O. sanctum were analyzed for polyisoprenoid by Skorupinska-Tudek et al. (2007) and compared with extracts of few more plants (TLC and HPLC). Total isoprenoid content was lowest in root tissues (2–6-μg/g DW) of O. sanctum followed by stem (3–9-μg/g DW) and leaves (77–100-μg/g DW). This is because the photosynthetic tissues accumulate higher amounts of polyprenols in the presence of sunlight. Hairy roots of O. sanctum grown in WP media showed significant quantity of isoprenoids (up to 15-μg/g DW). Therefore, even the metabolite with lower concentration in normal roots of O. sanctum can be extracted in higher amount using hairy root culture.
Marzouk (2009) presented similar a TLC-screening pattern of triterpenes in normal and hairy roots (273.5-g FW); however, 3-fold more extractives were collected from a hairy root of O. basilicum (4 weeks old). NMR analysis of these purified extracts has led to isolation and identification of six major triterpenes. These triterpenes were betulinic acid (37 mg), 3-epimaslinic acid (34 mg), alphitolic acid (105 mg), euscaphic acids (54 mg), oleanolic acid (4 mg), and ursolic acid (6 mg). Among these oleanolic acid, 3-epimaslinic acid and euscaphic acids were reported for the first time from O. basilicum. All the identified terpenoids proved to be hepatoprotective in nature.
Phytochemical Difference Among Cultivars
Three cultivars (Subja, Holy Green, and Red Rubin) of O. basilicum were studied for difference in polyphenolics (3-hydroxybenzoic, m-coumaric, p-coumaric, caffeic, ferulic, vanillic, chicoric, and rosmarinic acids) by Srivastava et al. (2016a, b, c). Ethanolic extract of different plant parts of 90-day-old plants (Srivastava et al., 2016a), hairy roots (Srivastava et al., 2016b), and co-cultured hairy roots with 75-day-old spores of Rhizophagus irregularis were quantified using HPLC (Srivastava et al., 2016c).
Highest accumulation of rosmarinic acid and chicoric acid was reported in leaves and roots of Holy Green. Flowers of Subja gave higher and the Red Rubin showed undetected levels of chicoric acid (Srivastava et al., 2016a). Maximum accumulation of RA experienced in hairy roots at 40, 50, and 60 days in Subja (71.03 ± 12.67-mg/g DW), Holy green (69.49 ± 1.79-mg/g DW), and Red Rubin (76.41 ± 3.71-mg/g DW) cultivars, respectively. Subja cultivar showed 5-fold-higher accumulation of RA in hairy roots as compared to non-transformed roots. Among all cultivars, hairy roots of Holy green were detected with maximum CA accumulation (1.74–0.17-mg/g DW) at 50 days. Although Subja cultivars accumulated highest amount of RA till 40 days, Holy green cultivar can be better to maximize accumulation of both RA and CA till 50 days of culture (Srivastava et al., 2016b). Colonization with R. irregularis influenced concentration of RA and CA in hairy roots (Srivastava et al., 2016c). Colonized hairy roots of Subja, Holy Green, and Red Rubin cultivars observed with maximum 1.9-fold (60 days), 2.1-fold (30 days), and 1.6-fold (30 days) increase in RA content, respectively. Co-culture with R. irregularis improved accumulation of CA in 30-day-old hairy roots of Subja (6.7-fold), followed by Holy Green (3.3-fold) and Red Rubin (1.3-fold).
The leaves of all three cultivars were detected with similar (66.99 to 85.53-mg/g DW) total phenolic content. Subja cultivar was observed with significantly higher total phenolic content in flowers (68.20 ± 5.54 mg/g DW), roots (80.69 ± 4.58-mg/g DW) and the whole plant (219.22 ± 4.50-mg/g DW) when compared with other cultivars of O. basilicum (Srivastava et al., 2016a). Hairy roots of Holy green cultivars were calculated for maximum amount (139.1–378.8-mg/g DW) of total phenolics from 20 to 60 days of culture, followed by hairy roots of Subja cultivar (127.7–354.9-mg/g DW). Total phenolics in cultivar Red Rubin increased slowly till 40 days, which enhanced significantly from 161.6 to 374.9-mg/g DW from 40 to 60 days of culture (Srivastava et al., 2016b). Total phenolic content of Subja and Red Rubin hairy roots was enhanced by 44–50-mg/g DW at 60 days of co-culture with R. irregularis (Srivastava et al., 2016c). More endogenous IAA (up to 0.039 μg/mg) was quantified using HPLC in hairy roots of all cultivar of O. basilicum, although content of IAA varies among different clones of same cultivars (Srivastava et al., 2016b).
Srivastava et al. (2016a) experienced highest antioxidant potential in roots of Subja and leaves of other cultivars. However, flowers of Subja were observed with greater antioxidant potential as compared to flowers of Holy Green and Red Rubin. Srivastava et al. (2016b) stated time-dependent increase (2-fold) in total antioxidant potential with no significant difference among hairy roots of three cultivars. Srivastava et al. (2016c) boosted antioxidant potential up to 4-, 2-, and 5-fold for total phenolics as well as 5-, 4-, and 12-fold for RA in hairy roots of Subja, Holy Green, and Red Rubin cultivars after colonization with R. irregularis.
Thus, it can be concluded that rosmarinic acid is major antioxidant in all cultivars of O. basilicum. Caffeic acid (CA) was also spotted as a minor metabolite in hairy roots of O. basilicum. Time-dependent increased accumulation of these metabolites and total phenolics was achieved through hairy root culture in all three cultivars. Increase in antioxidant potential from normal roots to transformed hairy roots and elicited hairy roots (with R. irregularis) represent enhanced metabolic composition of culture. Although roots and leaves of Holy green cultivar accumulated maximum phenolics, including RA, hairy roots of Subja cultivar could be considered best for extraction due to its morphology with enhanced biomass, antioxidant potential, total phenolics, and RA (40 days old).
Metabolites in Roots of Field-Grown Plants
Ahmad et al. (2012a) analyzed methanolic roots extract of O. sanctum (2.5 kg) for isolation of three new phytoconstituents. These compounds were identified as stigmast-5-en-3b-olyl-n-eicos-9-12-dienoate, n-octacos-9-enoic acid, and lup-12, 20(29)-dien-28-oic-acid-3b-olyl-octadecanoate, along with known compounds of ursolic acid and 3-epibetulinic acid.
Another study of methanolic root extracts of field-grown O. sanctum by Ahmad et al. (2012b) identified five phytochemicals using nuclear magnetic resonance (NMR), fast atom bombardment (FAB) mass, 1H-1H correlation spectroscopy (COSY), and heteronuclear multiple-bond correlation (HMBC) spectral techniques. Two compounds, ursolic acid and palmityl glucoside, have already been reported. Three unknown identified compounds were called as urs-12-en-3β,6β,20β-triol-28-oic acid, 1″-menthyl-2-glucopyranosyloxybenzoate, and n-decanoyl-β-D-glucopyranosyl-(2a→1b)-β-D-glucopyranosyl-(2b→1c)-β-D-glucopyranosyl-(2c→1d)-β-D-glucopyranosyl-2d-2′-hydroxybenzoate.
Kumar et al. (2015) analyzed different fractions (hexane, chloroform, ethyl acetate, and butanol) of root methanolic extracts from field-grown O. sanctum. These extracts were monitored using high-performance liquid chromatography (HPLC) fingerprints to categorize flavonoids (flavones, flavonols, and dihydroflavonols), including glycone and aglycones. Kumar et al. (2015) provided preliminary phytochemical screening as chromophoric groups and did not quantify the metabolites.
Improvement in metabolic awareness of Ocimum roots generates more possibilities to explore and utilize roots as starting material. In vitro-established root cultures are best to harvest desired product using the best possible combination for maximum accumulation of phytoceuticals present.
Conclusion
Promising therapeutic activities, like hepatoprotective, antimicrobial, antimalarial, and antidiabetic, are associated with Ocimum roots. Although antimicrobial nature of Ocimum roots protects from several pathogens, conversely, microbes of rhizosphere also affect the roots in both ways. Chemical nature of soil, soil treatments (fungicide and pesticides), as well as microbes and contaminations (radioactive, salt, and heavy metal), define the suitability of soil in negative or positive manner. Hence, rhizosphere is very crucial as it furnishes supplements to signals via roots to the whole plant. Effect of different rhizospheric elements on roots, as well as whole plants, provides the better understanding of required eco-friendly and biosafe procedure for industries, including agriculture and bioprocess industries.
In vitro techniques stand best among such procedures, which include adventitious and hairy root cultures as promising candidates to support the phytochemical industry by reducing manpower and duration of field cultivation. Ocimum roots being very useful due to significant amount and variety of phytoceuticals were thoroughly studied. Improved biomass and quantity of phytoceuticals were achieved through adventitious or hairy root cultures of different species as well as cultivars of Ocimum. Phytochemical profile of roots with different treatments simplifies higher production of phytoceuticals.
Author Contributions
VP and RS has drafted the review under the guidance of AN and written the review article. AN has further critically read the article. All authors contributed to the article and approved the submitted version.
Conflict of Interest
The authors declare that the research was conducted in the absence of any commercial or financial relationships that could be construed as a potential conflict of interest.
Publisher’s Note
All claims expressed in this article are solely those of the authors and do not necessarily represent those of their affiliated organizations, or those of the publisher, the editors and the reviewers. Any product that may be evaluated in this article, or claim that may be made by its manufacturer, is not guaranteed or endorsed by the publisher.
Abbreviations
MS, Murashige and Skoog; WP, woody plant; B5, Gamborg B5; FW, fresh weight; DW, dry weight; NAA, α-naphthaleneacetic acid; Kn, Kinetin; 2, 4-D, 2, 4-dichlorophenoxyacetic acid; BAP, 6-benzylaminopurine; PGR, plant growth regulator; DPPH, 2, 2-Diphenyl-1-picrylhydrazyl; NRA, nitrate reductase activity; NP-SH, nonprotein thiol; MDA, malondialdehyde; CAT, catalase; APX, ascorbate peroxidase; GPX, guaiacol peroxidase; SOD, superoxide dismutase; TLC, thin layer chromatography; HPLC, high-performance liquid chromatography; GC-MS, gas chromatography-mass spectrometry; GC-FID, gas chromatography-flame-ionization detection; NMR, nuclear magnetic resonance; FAAS, flame atomic absorption spectroscopy; ICP-OES/MS, inductively coupled plasmaoptical emission spectrometry/mass spectrometry; SA, salicylic acid; JA, jasmonic acid; CWE, cell wall elicitors; RA, rosmarinic acid; K, potassium; CFU, colony-forming unit; PFU, plaque-forming unit.
References
Abd-Elgawad, M. M. M. (2021). Biological control of nematodes infecting eggplant in Egypt. Bull. Natl. Res. Cent. 45:6. doi: 10.1186/s42269-020-00463-0
Adamczyk-Szabela, D., Romanowska-Duda, Z., Lisowska, K., and Wolf, W. M. (2017). Heavy metal uptake by herbs. V. Metal accumulation and physiological effects induced by Thiuram in Ocimum basilicum L. Water Air Soil Pollut. 228:334. doi: 10.1007/s11270-017-3508-0
Agarwal, K., Singh, D. K., Jyotshna, J., Ahmad, A., Shanker, K., Tandon, S., et al. (2017). Antioxidative potential of two chemically characterized Ocimum (Tulsi) species extracts. Biomed. Res. Therapy 4:1574. doi: 10.15419/bmrat.v4i9.366
Ahmad, M. Z., Ali, M., and Mir, S. R. (2012a). Anti-diabetic activity of Ocimum sanctum L. roots and isolation of new phytoconstituents using two-dimensional nuclear magnetic resonance spectroscopy. J. Pharmacogn. Phytother. 4, 75–85. doi: 10.5897/JPP12.008
Ahmad, M. Z., Ali, M., and Mir, S. R. (2012b). New phytoconstituents from the roots of Ocimum sanctum L. J. Pharma. Res. 5, 548–550.
Arab, M. M., Yadollahi, A., Eftekhari, M., Ahmadi, H., Akbari, M., and Khorami, S. S. (2018). Modeling and optimizing a new culture medium for in vitro rooting of G×N15 Prunus Rootstock using artificial neural network-genetic algorithm. Sci. Rep. 8:9977. doi: 10.1038/s41598-018-27858-4
Bais, H. P., Walker, T. S., Schweizer, H. P., and Vivanco, J. M. (2002). Root specific elicitation and antimicrobial activity of rosmarinic acid in hairy root cultures of Ocimum basilicum. Plant Physiol. Biochem. 40, 983–995. doi: 10.1016/S0981-9428(02)01460-2
Baliga, M. S., Jimmy, R., Thilakchand, K. R., Sunitha, V., Bhat, N. R., Saldanha, E., et al. (2013). Ocimum sanctum L. (Holy Basil or Tulsi) and its phytochemicals in the prevention and treatment of cancer. Nutr. Cancer 65, 26–35. doi: 10.1080/01635581.2013.785010
Barnawal, D., Bharti, N., Maji, D., Chanotiya, C. S., and Kalra, A. (2012). 1-Aminocyclopropane-1-carboxylic acid (ACC) deaminase-containing rhizobacteria protect Ocimum sanctum plants during waterlogging stress via reduced ethylene generation. Plant Physiol. Biochem. 58, 227–235. doi: 10.1016/j.plaphy.2012.07.008
Bernstein, N., Sela Saldinger, S., Dudai, N., and Gorbatsevich, E. (2017). Salinity stress does not affect root uptake, dissemination and persistence of Salmonella in sweet-basil (Ocimum basilicum). Front. Plant. Sci. 8:675. doi: 10.3389/fpls.2017.00675
Bishehkolaei, R., Fahimi, H., Saadatmand, S., Nejadsattari, T., Lahouti, M., and Yazdi, F. T. (2011). Ultrastructural localisation of chromium in Ocimum basilicum. Turkish J. Bot. 35, 261–268.
Chaudhary, A., Sharma, S., Mittal, A., Gupta, S., and Dua, A. (2020). Phytochemical and antioxidant profiling of Ocimum sanctum. J. Food Sci. Technol. 57, 3852–3863. doi: 10.1007/s13197-020-04417-2
Chandrashekara, K., and Somashekarappa, H. M. (2016). 210Po and 210Pb in medicinal plants in the region of Karnataka, Southern India. J. Environ. Radioact. 160, 87–92. doi: 10.1016/j.jenvrad.2016.04.036
Cohen, M. M. (2014). Tulsi-Ocimum sanctum: a herb for all reasons. J. Ayurveda Integr. Med. 5, 251–259. doi: 10.4103/0975-9476.146554
Das, S., Barman, S., Teron, R., Bhattacharya, S. S., and Kim, K. H. (2020). Secondary metabolites and anti-microbial/anti-oxidant profiles in Ocimum spp. Role of soil physico-chemical characteristics as eliciting factors. Environ. Res. 188:109749. doi: 10.1016/j.envres.2020.109749
Dzib-Guerra, W. D., Escalante-Erosa, F., García-Sosa, K., Derbré, S., Blanchard, P., Richomme, P., et al. (2016). Anti-advanced glycation end-product and free radical scavenging activity of plants from the Yucatecan flora. Pharmacognosy Res. 8, 276–280. doi: 10.4103/0974-8490.188883
Gautam, S., Gautam, A., Chhetri, S., and Bhattarai, U. (2020). Immunity against COVID-19: potential role of Ayush Kwath. J. Ayurveda Integr. Med. doi: 10.1016/j.jaim.2020.08.003 [Epub ahead of print].
Gupta, S. K., Prakash, J., and Srivastava, S. (2002). Validation of traditional claim of Tulsi, Ocimum sanctum Linn as a medicinal plant. Indian J. Exp. Biol. 40, 765–773.
Hata, T., Mukae, K., Satoh, S., Matsuo, M., and Obokata, J. (2021). Preculture in an enriched nutrient medium greatly enhances the Agrobacterium-mediated transformation efficiency in Arabidopsis T87 cultured cells. Plant Biotechnol. (Tokyo) 38, 179–182. doi: 10.5511/plantbiotechnology.20.1211b
Inbaneson, S. J., Sundaram, R., and Suganthi, P. (2012). In vitro antiplasmodial effect of ethanolic extracts of traditional medicinal plant Ocimum species against Plasmodium falciparum. Asian Pac. J. Trop. Med. 5, 103–106. doi: 10.1016/S1995-7645(12)60004-2
Jamshidi, N., and Cohen, M. M. (2017). The clinical efficacy and safety of Tulsi in humans: a systematic review of the literature. Evid. Based Complement. Altern. Med. 2017:9217567. doi: 10.1155/2017/9217567
Kulkarni, K. V., and Adavirao, B. V. (2018). A review on: indian traditional shrub Tulsi (Ocimum sanctum): the unique medicinal plant. J. Med. Plants Stud. 6, 106–110.
Kumar, A., Agarwal, K., Maurya, A. K., Shanker, K., Bushra, U., Tandon, S., et al. (2015). Pharmacological and phytochemical evaluation of Ocimum sanctum root extracts for its antiinflammatory, analgesic and antipyretic activities. Pharmacogn. Mag. 11, S217–S224. doi: 10.4103/0973-1296.157743
Kurepa, J., and Smalle, J. A. (2021). Composition of the metabolomic bio-coronas isolated from Ocimum sanctum and Rubia tinctorum. BMC Res. Notes 14:6. doi: 10.1186/s13104-020-05420-8
Li, D., and Uyttendaele, M. (2018). Potential of human norovirus surrogates and Salmonella enterica contamination of pre-harvest Basil (Ocimum basilicum) via leaf surface and plant substrate. Front. Microbiol. 9:1728. doi: 10.3389/fmicb.2018.01728
Luqman, S., Srivastava, S., Kumar, R., Maurya, A. K., and Chanda, D. (2012). Experimental assessment of Moringa oleifera leaf and fruit for its antistress, antioxidant, and scavenging potential using in vitro and in vivo assays. Evid Based Complement Alternat. Med. 2012:519084. doi: 10.1155/2012/519084
Maity, T. K., Dinda, S. C., and Pal, M. (2003). Studies on some psychopharmacological activities of Ocimum sanctum root extract. Indian J. Traditional Knowl. 2, 284–288.
Maity, T. K., Mandal, S. C., Saha, B. P., and Pal, M. (2000). Effect of Ocimum sanctum roots extract on swimming performance in mice. Phytother. Res. 14, 120–121. doi: 10.1002/(SICI)1099-1573(200003)14:2<120::AID-PTR557>3.0.CO;2-0
Marzouk, A. M. (2009). Hepatoprotective triterpenes from hairy root cultures of Ocimum basilicum L. Z. Naturforsch. C. 64, 201–209. doi: 10.1515/znc-2009-3-409
Mondal, S., Mirdha, B. R., and Mahapatra, S. C. (2009). The science behind sacredness of Tulsi (Ocimum sanctum Linn.). Indian J. Physiol. Pharmacol. 53, 291–306.
Nadar, B. G., Usha, G. V., and Lakshminarayan, N. (2020). Comparative evaluation of efficacy of 4% tulsi extract (Ocimum sanctum), fluoridated and placebo dentifrices against gingivitis and plaque among 14-15 years school children in Davangere City, India – A triple blinded randomized clinical trial. Contemp. Clin. Dent. 11, 67–75. doi: 10.4103/ccd.ccd_109_19
Pattanayak, P., Behera, P., Das, D., and Panda, S. K. (2010). Ocimum sanctum Linn. A reservoir plant for therapeutic applications: an overview. Pharmacogn. Rev. 4, 95–105. doi: 10.4103/0973-7847.65323
Pedreño, M. A., and Almagro, L. (2020). Carrot hairy roots: factories for secondary metabolite production. J. Exp. Bot. 71, 6861–6864. doi: 10.1093/jxb/eraa435
Prakash, P., and Gupta, N. (2005). Therapeutic uses of Ocimum sanctum Linn (Tulsi) with a note on eugenol and its pharmacological actions: a short review. Indian J. Physiol. Pharmacol. 49, 125–131.
Rahman, S., Islam, R., Kamruzzaman, M., Khasrul, A., and Jamal, H. M. A. (2011). Ocimum sanctum L: review of phytochemical and pharmacological profile. Am. J. Drug Discov. Devel. 2011, 1–15.
Rai, V., Vajpayee, P., Singh, S. N., and Mehrotra, S. (2004). Effect of chromium accumulation on photosynthetic pigments, oxidative stress defence system, nitrate reduction, proline level and eugenol content of Ocimum tenuiflorum L. Plant Sci. 167, 1159–1169. doi: 10.1016/j.plantsci.2004.06.016
Rat, A., Naranjo, H. D., Krigas, N., Grigoriadou, K., Maloupa, E., Alonso, A. V., et al. (2021). Endophytic bacteria from the roots of the medicinal plant Alkanna tinctoria Tausch (Boraginaceae): exploration of plant growth promoting properties and potential role in the production of plant secondary metabolites. Front. Microbiol. 12:633488. doi: 10.3389/fmicb.2021.633488
Renčo, M., Čerevková, A., and Homolová, Z. (2021). Nematode communities indicate the negative impact of Reynoutria japonica invasion on soil fauna in ruderal habitats of Tatra national park in Slovakia. Glob. Ecol. Conserv. 26:e01470. doi: 10.1016/j.gecco.2021.e01470
Rhoades, H. L. (1988). Effects of several phytoparasitic nematodes on the growth of Basil, Ocimum basilicum. J. Nematol. 20, 22–24.
Roy, A. (2021). Hairy root culture an alternative for bioactive compound production from Medicinal Plants. Curr. Pharm. Biotechnol. 22, 136–149. doi: 10.2174/1389201021666201229110625
Sabra, M., Aboulnasr, A., Franken, P., Perreca, E., Wright, L. P., and Camehl, I. (2018). Beneficial root endophytic fungi increase growth and quality parameters of Sweet Basil in heavy metal contaminated soil. Front. Plant Sci. 9:1726. doi: 10.3389/fpls.2018.01726
Shilpa, K., Selvakkumar, C., Senthil, A. K., and Lakshmi, B. S. (2010). In vitro root culture of Ocimum sanctum L. and evaluation of its free radical scavenging activity. Plant Cell Tissue Organ Cult. 101, 105–109. doi: 10.1007/s11240-009-9661-4
Singh, S., Lal, R. K., and Singh, B. (2020). “Genetic variability in Ocimum L. germplasm: medicinal and economic potential for value addition and product development,” in Botanical Leads for Drug Discovery, ed. B. Singh (Singapore: Springer). doi: 10.1007/978-981-15-5917-4_11
Singh, S., Taneja, M., and Majumdar, D. K. (2007). Biological activities of Ocimum sanctum L. Fixed oil- an overview. Indian J. Exp. Biol. 45, 403–412.
Skorupinska-Tudek, K., Pytelewska, A., Zelman-Femiak, M., Mikoszewski, J., Olszowska, O., Gajdzis-Kuls, D., et al. (2007). In vitro plant tissue cultures accumulate polyisoprenoid alcohols. Acta Biochim. Pol. 54, 847–852. doi: 10.18388/abp.2007_3184
Srivastava, S., Adholeya, A., Conlan, X. A., and Cahill, D. M. (2016a). Acidic potassium permanganate chemiluminescence for the determination of antioxidant potential in three cultivars of Ocimum basilicum. Plant Foods Hum. Nutr. 71, 72–80. doi: 10.1007/s11130-016-0527-8
Srivastava, S., Conlan, X. A., Adholeya, A., and Cahill, D. M. (2016b). Elite hairy roots of Ocimum basilicum as a new source of rosmarinic acid and antioxidants. Plant Cell Tiss. Organ Cult. 126, 19–32. doi: 10.1007/s11240-016-0973-x
Srivastava, S., Conlan, X. A., Cahill, D. M., and Adholeya, A. (2016c). Rhizophagus irregularis as an elicitor of rosmarinic acid and antioxidant production by transformed roots of Ocimum basilicum in an in vitro co-culture system. Mycorrhiza 26, 919–930. doi: 10.1007/s00572-016-0721-4
Tada, H., Murakami, Y., Omoto, T., Shimomura, K., and Ishimaru, K. (1996). Rosmarinic acid and related phenolics in hairy root cultures of Ocimum basilicum. Phytochemistry 42, 431–434. doi: 10.1016/0031-9422(96)00005-2
Tan, W., Du, W., Barrios, A. C., Armendariz, R. Jr., Zuverza-Mena, N., Ji, Z., et al. (2017). Surface coating changes the physiological and biochemical impacts of nano-TiO2 in basil (Ocimum basilicum) plants. Environ. Pollut. 222, 64–72. doi: 10.1016/j.envpol.2017.01.002
Tan, W., Du, W., Darrouzet-Nardi, A. J., Hernandez-Viezcas, J. A., Ye, Y., Peralta-Videa, J. R., et al. (2018a). Effects of the exposure of TiO2 nanoparticles on basil (Ocimum basilicum) for two generations. Sci. Total Environ. 636, 240–248. doi: 10.1016/j.scitotenv.2018.04.263
Tan, W., Gao, Q., Deng, C., Wang, Y., Lee, W. Y., Hernandez-Viezcas, J. A., et al. (2018b). Foliar exposure of Cu(OH)2 nanopesticide to basil (Ocimum basilicum): variety-dependent copper translocation and biochemical responses. J. Agric. Food Chem. 66, 3358–3366. doi: 10.1021/acs.jafc.8b00339
Venkatesalu, V., Gopalan, N., Pillai, C. R., Singh, V., Chandrasekaran, M., Senthilkumar, A., et al. (2012). In vitro anti-plasmodial activity of some traditionally used medicinal plants against Plasmodium falciparum. Parasitol. Res. 111, 497–501. doi: 10.1007/s00436-012-2834-9
Vyas, P., and Mukhopadhyay, K. (2014). Development of a rapid and high frequency Agrobacterium rhizogenes mediated transformation protocol for Ocimum tenuiflorum. Biologia 69, 765–770. doi: 10.2478/s11756-014-0375-7
Wakchaure, R., Ganguly, S., and Praveen, P. K. (2017). “Ocimum sanctum (Tulsi), the queen of herbs: a review,” in Biochemistry and Therapeutic Uses Of Medicinal Plants, eds A. M. Abbas, Y. K. Sharma, M. Abid, and M. M. A. A. Khan (New Delhi: Discovery Publishing House Pvt. Ltd), 166–173.
Keywords: root, hairy roots, rhizosphere, medicinal, metabolites, Agrobacterium rhizogenes, Ocimum
Citation: Pandey V, Swami RK and Narula A (2021) Harnessing the Potential of Roots of Traditional Power Plant: Ocimum. Front. Plant Sci. 12:765024. doi: 10.3389/fpls.2021.765024
Received: 27 August 2021; Accepted: 30 September 2021;
Published: 01 November 2021.
Edited by:
Luigi Lucini, Catholic University of the Sacred Heart, ItalyReviewed by:
Mehdi Mohebodini, University of Mohaghegh Ardabili, IranAleksandra Krolicka, University of Gdańsk, Poland
Copyright © 2021 Pandey, Swami and Narula. This is an open-access article distributed under the terms of the Creative Commons Attribution License (CC BY). The use, distribution or reproduction in other forums is permitted, provided the original author(s) and the copyright owner(s) are credited and that the original publication in this journal is cited, in accordance with accepted academic practice. No use, distribution or reproduction is permitted which does not comply with these terms.
*Correspondence: Alka Narula, YWxrYS5uYXJ1bGFAcmVkaWZmbWFpbC5jb20=