- 1Key Laboratory of National Forestry and Grassland Administration, International Center for Bamboo and Rattan, Beijing, China
- 2National Location Observation and Research Station of the Bamboo Forest Ecosystem in Yixing, National Forestry and Grassland Administration, Beijing, China
Strip clearcutting can significantly reduce the harvesting costs of moso bamboo forests. Although bamboo is characterized by rapid accumulation of biomass, it is still a concern that this management method may reduce long-term productivity. Nutrient cycling has long been considered essential for forests to maintain high primary productivity. However, nutrient cycling of bamboo forests after strip cutting has not been previously reported. We conducted a strip clearcutting experiment and surveyed the litter dynamics for 1 year. We assessed changes in litter nutrients in response to the cutting and calculated the nutrient resorption efficiency and litter decomposition rate to evaluate the effect on nutrient use efficiency and nutrient return. Our results showed that strip cutting had no significant effect on litter production and nutrient return in the moso bamboo forest (p > 0.05). However, annual litter biomass and nutrient return in reserved belts (RB) were significantly higher than those in the control (CK) (p < 0.05). P and K resorption efficiencies in RB were significantly higher than in CK during certain periods of bamboo growth (p < 0.05). We also observed that the annual decay constant of CK was significantly higher than that of plots that were strip clearcut (SC) (p < 0.05). Our results suggest that strip cutting does not affect nutrient use efficiency or storage in the short term.
Introduction
Bamboo is an important forest type in China (Su et al., 2019a). More than 500 species of bamboo in 39 genera are spread across 13 provinces (Jiang, 2007). Moso bamboo (Phyllostachys edulis (Carrière) J. Houz.) covers 4.67 million ha (72.96%) of the total bamboo forest area in China (Jiang, 2007). Moso bamboo trees can be harvested after 4 years and have gradually become a substitute for wood (Su et al., 2019a), being widely adopted as construction material, furniture, biomass energy, and other related uses (Jiang, 2007). Bamboo shoots are also a tasty and nutritious food (Jiang, 2007). Therefore, moso bamboo plays an important role in the development of the forest economy in China (Zhao et al., 2018a).
Moso bamboo is characterized by clonal integration and is a typical forest comprising plants of different ages (Su et al., 2019a). In traditional harvesting, trees older than 4 years require their age to be identified by an experienced farmer (Zhao et al., 2018b). However, due to development of the social economy and population urbanization, the rising costs caused by labor shortages have reduced the enthusiasm of bamboo farmers. Fortunately, the demand to reduce the management costs of bamboo forests has been effectively supported. The strip-cutting model has been studied in a major region of moso bamboo forest as a possible solution to reduce the high cutting cost (Li et al., 2018; Zeng et al., 2019b; Zhan et al., 2020). Felled bamboo is taken out of the cutting site for sale. Previous studies have analyzed the natural restoration status of postharvest plots with different widths, and suggested that 8 m is the best width for strip cutting (Li et al., 2018; Xianli, 2019; Zhan, 2019). However, bamboo forest development mainly relies on the recycling of nutrients from soil organic matter to sustain fertility. Rotation is a common management practice. Therefore, there are some concerns that these practices may reduce long-term productivity (Turner and Lambert, 2013).
Litterfall is the main bridge between vegetation and mineral soil and plays a central role in the nutrient cycle of forest ecosystems (Huang et al., 2017; Johnson and Turner, 2019). By assessing differences in litterfall yield and chemical properties, we can gain important insights into the impact of logging on the ecological function of moso bamboo communities (Zhou et al., 2007). Litter, through nutrient deposition, has been reported to provide more than 70% of plant growth nutrients (Adolfo et al., 2016). Litter substrate (Lihua et al., 2014) and environmental conditions (Bothwell et al., 2014) determine the decomposition rate of organic matter return. Nutrient resorption refers to the transport of movable proteins, carbohydrates, and other nutrients from aging tissues and organs to other tissues to ensure that they remain in the plant and are available for physiological processes and future growth demands (Tang et al., 2013). The nutrient content of leaves is closely related to soil nutrition and bamboo yield in the following year (Chen et al., 2009). When the availability of soil nutrients decreases, the internal reabsorption level is higher, indicating that nutrient concentration in the litter also decreases (Johnson and Turner, 2019). Research on the dynamics of nutrient resorption in leaves will improve our understanding of nutrient retention, utilization, and adaptation to the development environment of plants (Proe and Millard, 1995). At the stand level, a change in nutrient resorption is reflected by the nutrient concentration and mass of litter (Turner and Lambert, 2015). This is of great interest, as it is directly related to the nutrient return of the stand.
We have studied the effects of strip cutting on bamboo restoration characteristics (Su et al., 2019b; Zeng et al., 2019a), undergrowth vegetation diversity (Zhan et al., 2020), and soil nutrient characteristics (Zeng et al., 2019b; Zhan, 2019). However, the effect of strip cutting on litterfall and the nutrient cycling of moso bamboo forests is unclear. This may limit our ability to predict the long-term productivity of bamboo forests. Hence, it is necessary to gain a wider understanding of how cutting affects litter conditions (e.g., productivity and related nutrients) to guide the restoration and realization of healthy and sustainable management of cut bamboo forests. In this study, we quantified litterfall and nutrient return, determined the nutrient resorption efficiency as well as the rates of litter decomposition of different treatments of moso bamboo to determine the patterns of nutrient release on the floor by thinning practices. We tested the hypotheses that (1) strip cutting has no significant effect on nutrient return; (2) cutting reduces the density of bamboo forests and provides a sufficient nutrient environment for the growth of stand development; and (3) cutting may affect the litter decomposition rate. This study was conducted on experimental bamboo plantations in subtropical China.
Materials and Methods
Study Site
Our study was performed in the Yixing Forest Farm (31°15′1″-31°15′12″ N, 119°44′2″-119°44′8″ E), located in southern Jiangsu Province, China. This region is dominated by low mountainous and hilly terrain, and the soil type is identified as yellow clay soil according to the classification and codes for Chinese soil (GB/T 17296-2009). The area is characterized by a maritime monsoon climate. The lowest and highest annual temperatures are −4.5°C and 38.8°C, respectively, with an annual average temperature of 16.5 °C (data from Yixing Forest meteorological station, located 1 km from the field station). There are 129 rainy days per year, with an average annual precipitation of 1229.9 mm. The growing period can reach approximately 250 days. The farm was established in March 1950 with 120 ha of monodominant moso bamboo forest. There are no pests, diseases, or fertilization treatments in the region. The average bamboo DBH and height is 8.13 cm and 13.37 m, respectively, and the average tree density is 3375 stems ha–1. The on-year and off-year can be clearly distinguished. The prominent understory species include Hedyotis chrysotricha, Carex breviculmis, Paederia cruddasiana, Oxalis corniculata, and Salvia prionitis.
Strip clearcutting involves cutting all trees in the plot and removing them from the experimental site. Two reserved belts were set between each cut belt. The function of the reserved plots was to provide nutrients to the strip-cut belts through physiological integration. And we need to know that physiological integration is one of the important characteristics of clonal plants. When the linked clonal ramet is located in different resource patches, the material absorbed from the resource-rich patch can be shared by other connected ramets outside the patch, that is, the ability to resist disturbance is improved (Su et al., 2019a). No management practices were carried out in the harvest plots and reserve plots during the restoration period. The test was performed at the same site quality. This study designed three different types of sites as treatments, including unharvested plots as a control (CK), plots that were strip clearcut (SC) in February 2019, and the reserved belt (RB). The cutting plots were 24 m × 20 m, including a strip-cutting belt 8 m wide and 20 m long, and two reserved plots of the same size. Each treatment included four replicates. Moreover, four trenches 50 cm wide and 50 cm deep were excavated around the plot to cut off rhizomes, eliminating the effect of long-distance nutrient transport. A control treatment with (8 m × 20 m) uncut plots (CK) was also included. The slope was approximately 6°. The location of the study area and field experiment design and sampling sites was shown in Figure 1.
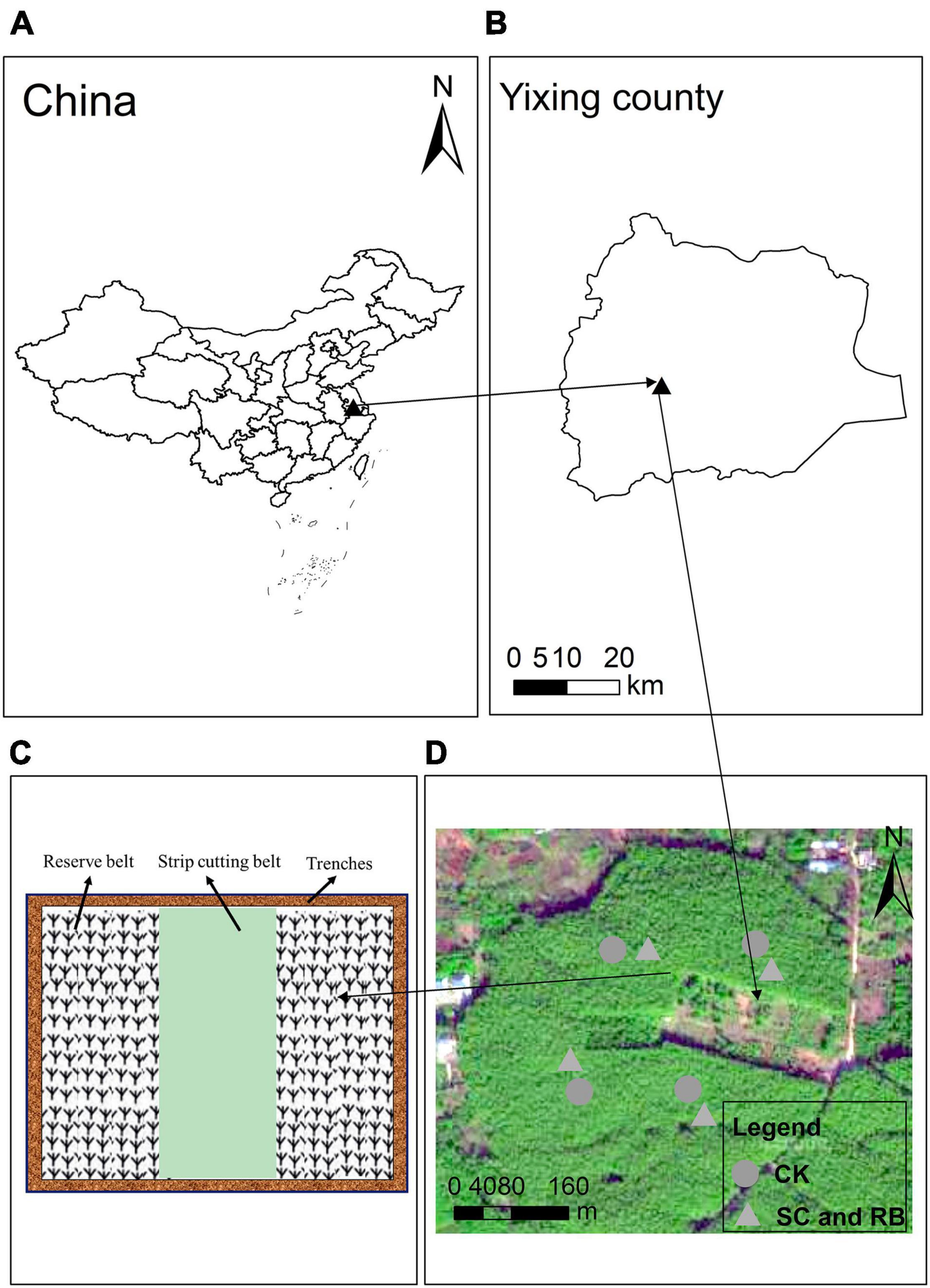
Figure 1. Location of the study area (A,B) and field experiment design (C,D) and sampling sites (SC, RB, and CK) Moso bamboo forests.
Litterfall Measurements
Litterfall was measured between October 2019 and September 2020. Three litter traps (1 m × 1 m) at a height of 1 m were randomly placed along the median line in the SC, RB, and CK plots. The litter was collected monthly and dried at 65°C to a constant weight. An electronic balance (0.01 g) was used to weigh the samples. The samples were then ground into a fine powder, passed through a sieve with a diameter of 0.15 mm, and stored in a sealing bag to analyze the nutrient content.
Mature Leaves Collection
Fresh leaves were collected from two stands in July 2019. Samples were taken according to the upper, middle, and lower layers along the tree heights. Ten bamboo trees were selected from each SC plot, and 20 bamboo trees were selected from four age grades in the CK plot. The leaves collected from the same plot were mixed into one composite sample. According to the physiological characteristics of moso bamboo, a 1-year-old bamboo was labeled as “du,” and thereafter marked as a sub-degree bamboo according to a 2-year vegetative cycle (Tang et al., 2016). For example, 2- to 3-year-old bamboos are labeled II “du,” and III “du” represented a 4- to 5-year-old bamboo forest. Therefore, we sampled new leaves in July 2020. The nutrient content of mature leaves from October 2019 to June 2020 was determined using the July 2019 survey. Mature leaves from July to September 2020 were represented by samples from July 2020. Freshly fallen litter and fresh leaves were dried at 65°C to a constant weight. The samples were then ground into a fine powder and passed through a sieve with a diameter of 0.15 mm, and the nutrient content was determined.
Litter Decomposition
Freshly fallen litter was collected on nylon mesh screens in all stands from July to September 2019. We then used this to create litter decomposition bags. Part of the litter was collected, ground into a fine powder, and passed through a sieve with a diameter of 0.15 mm. The chemical properties were measured as the initial values of the litter bags. Litter decomposition bags (20 cm × 30 cm in size) were made of 1 mm mesh nylon net. Next, 20.00 g of dried litter was placed in each bag to measure the initial weight. In August 2019, we randomly placed bags in each plot. The bag was attached to the soil and fixed using a PVC pipe. Three litter bags were collected from each plot at 3-month intervals for 1 year. The recycled bag was washed with clean water to remove excess soil, dried at 65 °C to a constant weight, and then weighed. The samples were then ground into a fine powder, passed through a sieve with a diameter of 0.15 mm, and stored in a sealing bag to analyze the nutrient content.
Soil Sampling
In each plot, sequential soil coring was used to extract 10 soil cores in October 2020. Sampling was conducted at a depth of 0–10 cm. Cores from the same layer were mixed into a composite sample. Coarse roots were removed from the mixed samples through a 2 cm sieve, and the soil chemical properties were determined after air-drying.
Chemical Analysis
The soil organic carbon (SOC) and total nitrogen (TN) content was determined using an elemental analyzer (ECS 4024 CHNSO; Costech, Picarro, Italy). Total phosphorus (TP) content was determined following the molybdenum-antimony resistance colorimetric method (concentrated H2SO4-HClO4) using an automatic chemical analyzer (Smartchem 300; AMS, Italy). Total potassium (TK) content was determined using a flame photometer (M410; Sherwood, United Kingdom).
Calculations and Statistics
Nutrient return was calculated as the product of litter mass and litter nutrient concentrations (Sayer et al., 2020). The nutrient resorption efficiency of plant leaves was calculated using the following formula (Guo et al., 2021):
The Olson litter decomposition index model was adopted to calculate the decomposition rate (Olson, 1963):
The element remaining (ER) in the litter bag for each period (Xi) was determined using the following formula (Ren et al., 2018):
where, Ri (%) denotes the resorption efficiency of nutrient i (N,P,K) in percent, C0 is the nutrient content of mature leaves, Ct is the nutrient content of litters at time t, M0 is the initial dry mass of litter, Mt is the remaining dry mass of litter decomposed at time t (in years), k is the annual decay constant, t0.5 is the time for 50% of mass loss, and t0.95 is the time for 95% of mass loss.
One-way analysis of variance (ANOVA) was used to test the differences between the three treatment plots. Means were separated by the least significant difference (LSD) test, and statistical significance was set at p < 0.05. Principal component analysis (PCA) was used to examine the associations between annual litter yield, nutrient return, and soil characteristics. All statistical analyses were performed in R (version 3.6.2), and the data calculated using Excel 2016. We examined the assumptions of normality and homogeneous variance using the Shapiro-Wilk test and Leven’s test, respectively. PCA was calculated using the FaceFactoMineR package. All graphs were drawn using the ggplot2 package.
Results
Litterfall and Nutrient Return
The trends of monthly litter in the three sites were the same, with two increase-decrease cycles. As shown in Table 1, the litter biomass from March and April 2020 in RB was significantly higher than in SC and CK (p < 0.05). From July to September 2020, the litter yield of SC was significantly lower than that of CK and RB (p < 0.05). We also found that the annual litter yield of RB was significantly higher than that of CK and SC (p < 0.05).

Table 1. Monthly and annual litter yield of strip clearcut (SC), reserve belt (RB), and unharvested (CK) plots.
The annual return amounts of N, P, and K in RB were significantly higher than those in SC and CK (Figure 2, p < 0.05). However, there was no significant difference in C return between the three plot types (Figure 2, p > 0.05).
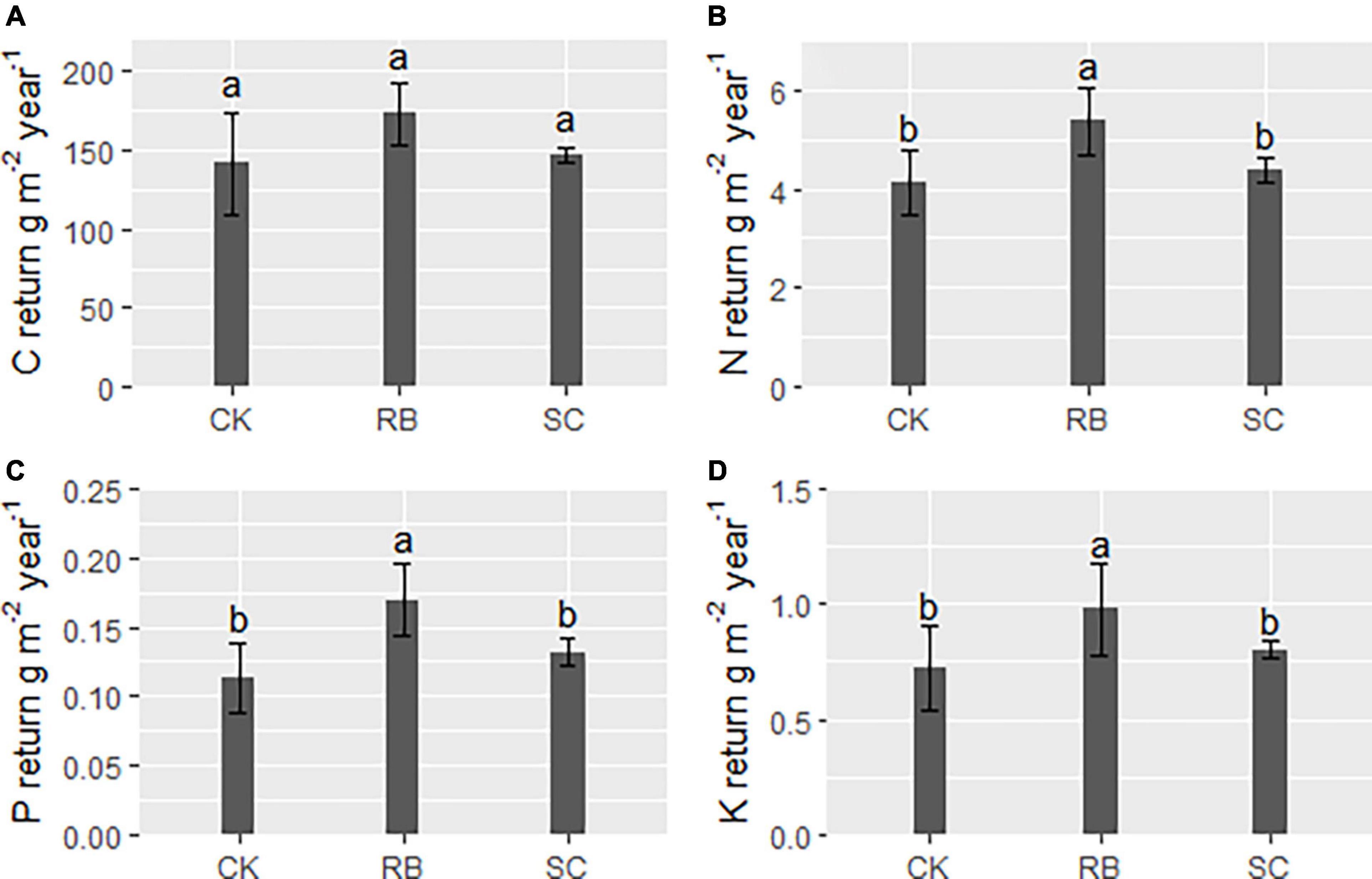
Figure 2. Return of elements (A, carbon; B, nitrogen; C, phosphorus; and D, Potassium) via litter to forest soil in strip clearcut (SC), reserve belt (RB), and unharvested (CK) plots within a year. Different lowercase letters indicate a significant difference in element return between the three type plots (ANOVA and LSD test, P < 0.05). Error bars indicate standard deviation (n = 4).
Nutrient Resorption Efficiency
From September 2019 to August 2020, the N, P, and K resorption efficiencies in the three types of plots showed two increase-decrease cycles. For all treatments, N resorption efficiency was the highest in May 2020. In May and August 2020, N resorption efficiency in SC was significantly lower than in the CK (Table 2). For all treatments, P resorption efficiency was the highest in April 2020, where there was no significant difference between the treatments. However, P resorption efficiency in RB was significantly higher than in CK in February and June 2020. For all treatments, K resorption efficiency was the highest in December 2019, where there was no difference between the three treatments. In January and April 2020, K resorption efficiency in SC was significantly lower than in CK. From July to September 2020, K resorption efficiency in SC was significantly higher than in the CK. In June and July 2020, the K reabsorption efficiency of RB was significantly higher than that of CK (Table 2).
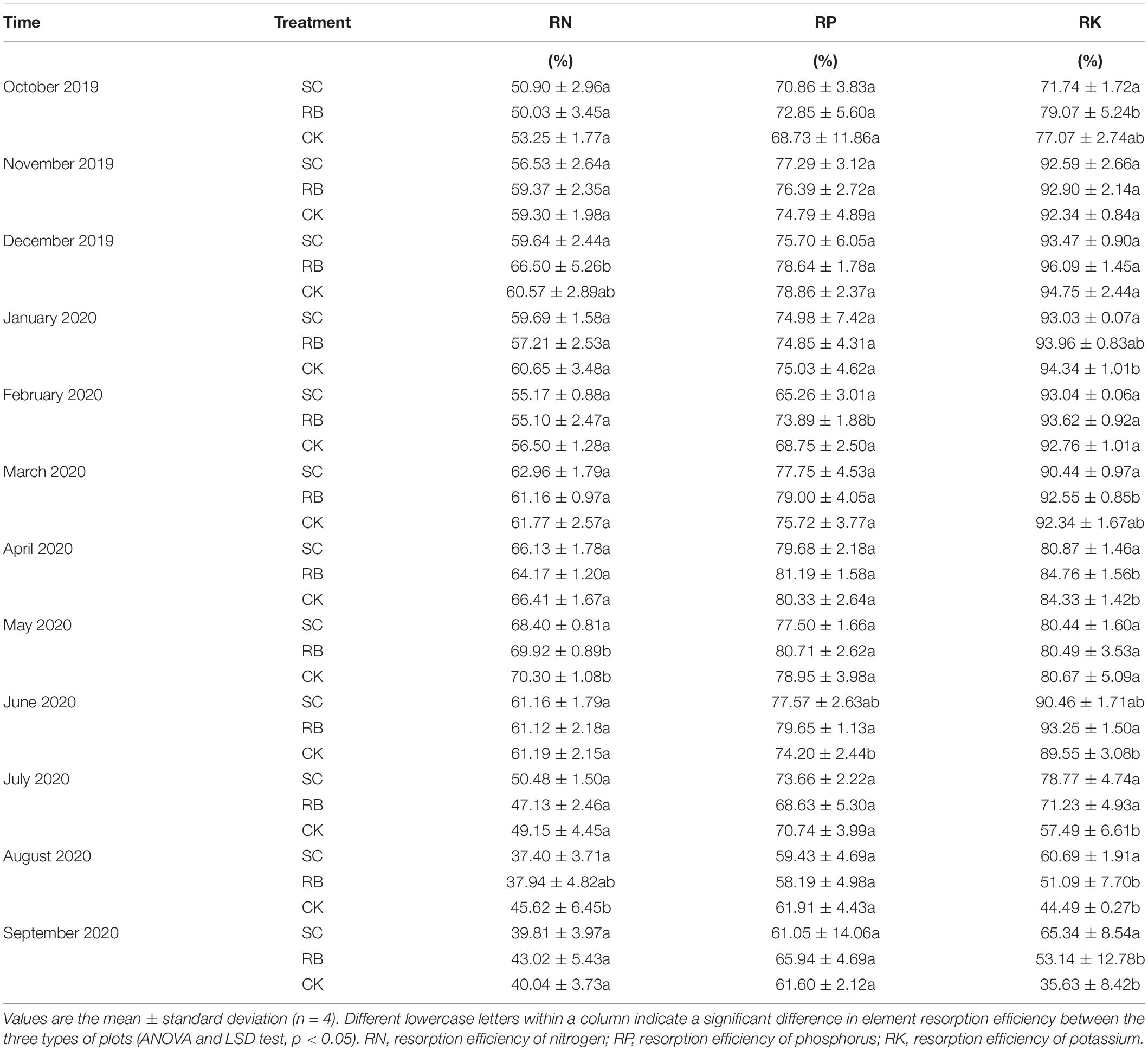
Table 2. Nutrient resorption efficiency of moso bamboo in strip clearcut (SC), reserve belt (RB), and unharvested (CK) plots.
Litter Decomposition
The k value of CK was significantly higher than that of the SC (Table 3, p < 0.05). After decomposing for 1 year, the residual rate of the dry mass of litter in CK, SC, and RB showed no significant difference (p > 0.05). The decomposition time of 50 and 95% mass loss of the litter in CK was significantly lower than that in SC (p < 0.05).

Table 3. Decomposition parameters of litter in strip clearcut (SC), reserve belt (RB), and unharvested (CK) plots.
Bioelement Dynamics
The concentration dynamics of the remaining four bioelements exhibited various patterns during the year-long decomposition process in the three treatment plots (Figure 3). The remaining concentration of C in CK exhibited two increase-decrease cycles, and the final concentration had increased (Figure 3A). C concentration decreased in the initial stage (decrease-increase-decrease) in SC, but increased in the initial stage (increase-decrease) in RB. The final C concentrations in both sites decreased. N concentration in the three types of plots decreased in the initial stage, but the concentrations fluctuated upward in CK and SC during the decomposition process (Figure 3B). In general, litter from all types of sample sites released a partial amount (>20%) of N during the decomposition process in 1 year. P concentration in CK and RB decreased in the initial stage (decrease-increase-decrease) (Figure 3C). In contrast, P concentration increased in the initial stage (increase-decrease-increase) in SC. The final P concentration decreased in all plots. K concentrations in SC, RB, and CK remained consistent with initial content (Figure 3D).
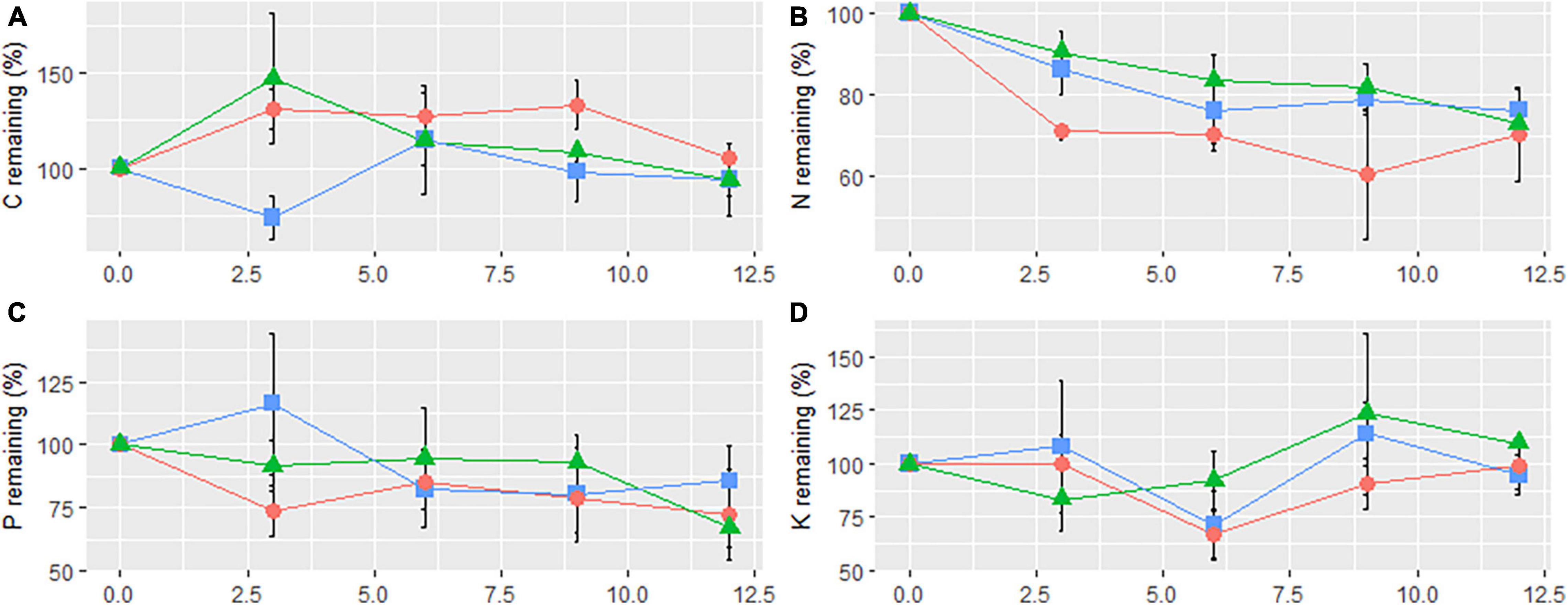
Figure 3. Elements remaining of carbon (A), nitrogen (B), phosphorus (C), and potassium (D) of litter bag on strip clearcut (SC, rectangle), reserve belt (RB, triangle), and unharvested (CK, circle) plots. The error bars indicate the standard deviation (n = 4).
Discussion
Effect of Strip Cutting on Litterfall and Nutrient Return
In subtropical bamboo ecosystems with limited nutrition, strip cutting can effectively reduce harvesting costs. Moso bamboo is a fast-growing grass, and the nutrients needed in the different stages of bamboo growth are mainly provided by the physiological integration of the trees in the RB (Su et al., 2019a), but the development of stands relying only on the soil nutrient cycle maintains fertility. The production and nutrient content of litter plays a key role in the soil nutrient pool (Lihua et al., 2014). Litterfall is used as an indicator of functional recovery to assess tropical cloud forest restoration (Guadalupe et al., 2021). Our study found that annual litterfall did not differ significantly between SC and CK, but increased significantly in RB. This result does not coincide with other types of forests, such as Cunninghamia lanceolata (Jian et al., 2021), poplars (Berthelot et al., 2000), and the Sari Bumi Kusuma forest (Prasetyo et al., 2015). There are numerous possible mechanisms involved in litterfall production. First, the litter yield was closely related to the density of bamboo, and the number of bamboo trees in SC was significantly reduced (Zeng et al., 2019a). Second, the age of the bamboo trees also affected litter yield. New trees change leaves after 1 year of growth, whereas trees older than 1 year change leaves every 2 years (Su, 2012). Moreover, the allocation of biomass in the cutting plots was also the main factor affecting litter return. Bamboo in strip cutting plots may increase photosynthesis by increasing leaf biomass, which can provide more nutrients for the development of the rhizome system. In addition, we cannot eliminate the possibility that litters from the RB fell into the strip cutting plot due to wind.
In this study, there was no difference in the return amount of different nutrient elements between SC and CK, which indicates that strip cutting does not decrease nutrient return. This finding is consistent with our hypothesis. However, the return amounts of N, P, and K in RB were significantly higher than those in CK and SC. This correlated with changes in litter yield (Figure 3). Conversely, there was no difference in C return between the three treatments. This difference may be due to the low nutrient content in the leaves of old bamboo. Guo (2014) tested nutrient content in the leaves of 1- to 6-year-old bamboo trees and found that C content in bamboo leaves decreased gradually with age, and that N and P content in bamboo leaves indicated a “W” shaped change with age.
Effect of Strip Cutting on Nutrient Resorption Efficiency
According to the theory of dynamic equilibrium in ecological stoichiometry, when the external environment fluctuates within a certain narrow range, a steady-state mechanism can be formed in the organism to maintain stable nutrient elements and achieve dynamic equilibrium (Zhang et al., 2003). Strip cutting removed all aboveground biomass from the plot and altered the forest microenvironment. Nutrient resorption provides nutrients to developing tissue instead of the soil (Johnson and Turner, 2019). Nutrients in the leaves can be resorbed in plants through resorption and redistribution to adapt to a low supply of nutrients in the soil (Regina et al., 2000). Su (2012) studied the different growth stages of moso bamboo and found that N consumption was higher in the mature stage and vigorous vegetative growth stage, whereas the demand for P and K was higher in the whip stage of pregnant bamboo shoots. Our results indicated a decrease in N, P, and K resorption efficiency from June to September in 2020, which may indicate lower retranslocation of this element from the leaves to other organs during leaf renewal.
Nitrogen is essential for achieving good yields in a moso bamboo forest, because the demand for and uptake of N is the highest in each growing period (Su, 2012). In the three treatment plots, the resorption efficiency of N tended to increase during the growth cycle (Table 2). This enhances the use efficiency of N, which might maintain balance in the internal supply of nutrients between the soil and the plant, with trees taking advantage of the most accessible routes (Miller et al., 1979; Turner and Lambert, 2015). The nutrient reabsorption capacity of N was strong, indicating that the availability of soil N was weak. Under N stress, moso bamboo can increase nutrient resorption efficiency and store more nutrients in the old leaves when the nutrient supply is sufficient. We found that N resorption efficiency in CK was significantly higher than in the SC in May and August 2020, which could reduce the limit of N, possibly owing to the high density of bamboo in CK. Phosphorus is an essential micronutrient for higher plants and is usually a highly mobile and frequently translocated element (White, 2012). Our study found that there was no difference in the reabsorption efficiency of P between SC and CK, but P resorption efficiency in RB was significantly higher than in CK in February and June 2020 (Table 2). Previous reports have also discussed different trends in seasonal variation, showing that P translocates from the leaves to other organs (Umemura and Takenaka, 2014). Potassium accumulates in meristems and young tissues, and is assimilated to the roots of higher plants (White, 2012). Umemura and Takenaka (2014) investigated K loss from the leaves and observed K resorption from mature leaves to growing organs. In our study, K showed the highest resorption efficiency (Table 2), due to the retranslocation of K. Additionally, the leaching of rainfall also has a great influence on K loss. Sakai and Tadaki (1997) studied the K+ concentration of forest rainfall in Phyllostachys pubescens and verified that K+ had leached from bamboo leaves.
Effect of Strip Cutting on Litter Decomposition and Bioelement Dynamics
Litter decomposition is a basic biogeochemical cycle in forest ecosystems (Lihua et al., 2014). There are a number of factors that affect the litter decomposition rate, including temperature (Bothwell et al., 2014), precipitation (Wieder et al., 2009), litter substrate quality (Astel et al., 2009), and soil nutrient availability (Keeler et al., 2009). In the present study, we found that litter decomposition rates were significantly higher in CK than in SC (Table 3). There was a slower release of nutrients from litter decomposition in SC, which contributed to the storage of soil nutrients. Moso bamboo is a fast-growing plant. It requires a high nutrient cycling rate in high-density stands. The decrease in nutrient deposition in the postharvest plots may be influenced by bamboo density. A previous study suggested that, in a higher fertility soil, plants tend to obtain nutrients from the soil more efficiently, rather than to accelerate litter decomposition and absorption (Wright and Westoby, 2010). We suggest that the reduction in bamboo density has created a more nutrient-rich environment in postharvest sites. In addition, the effect of the functional diversity of soil fauna on the decomposition rate over a short period of time should also be considered (Bohlen et al., 2004). Luan et al. (2020) found that the expansion of moso bamboo slowed down the decomposition rate of litter, but this negative effect was reversed when the role of macrofauna was excluded. Forest clearing significantly decreased the species richness of soil macrofauna and altered the composition (Mathieu et al., 2010). Therefore, strip cutting may change soil fauna diversity and composition, which results in a lower litter decomposition rate in SC.
After 1 year, the three treatment plots indicated a net release of N and P, whereas C and K release had an opposite trend (Figure 3). Parton et al. (2007) found that only the average C/N ratio of the litter was less than 40, indicating that net N release had occurred. A previous study, focused on nutrient dynamics in Pleioblastus amarus and Bambusa pervariabilis × Dendrocalamopsis daii stands, found that the trophic dynamic pattern and the remaining final quantities were mainly determined by their initial matrix quality (Lihua et al., 2014). This observation is consistent with our findings. The pattern of bioelement dynamics and the elements remaining in RB and CK changed consistently (Figure 3). However, in SC, the change differed. After decomposing for 3 months, nutrient release in SC indicated a net C release and net P enrichment. This may be due to the demands of decomposers and the availability of nutrients in the environment (Lihua et al., 2014).
Associations Between Annual Litter Yield and Nutrient Return and Soil Characteristics
The first two principal components, component 1 (Dim.1) and component 2 (Dim.2) explained 67.6% and 21.2% of the variation, respectively (Figure 4). PCA indicated that SOC, TN, TP, and TK were negatively correlated with k. The return of C, N, P, and K was positively correlated with litter yield. This change could be attributed to changes in the stand density. Moso bamboo is characterized by its rapid growth, and before harvesting, bamboo plantations with a relatively high biomass make full use of the space and resources in soil (light, temperature, and nutrition), and resource competition is intense. Cutting reduced the density of standing bamboo and the transport of nutrients between the ramets of the clonal plants through the bamboo rhizome. This practice created an abundant, nutrient-rich environment for new trees. Therefore, a reduction in the litter decomposition rate can decrease nutrient loss and increase nutrient storage.
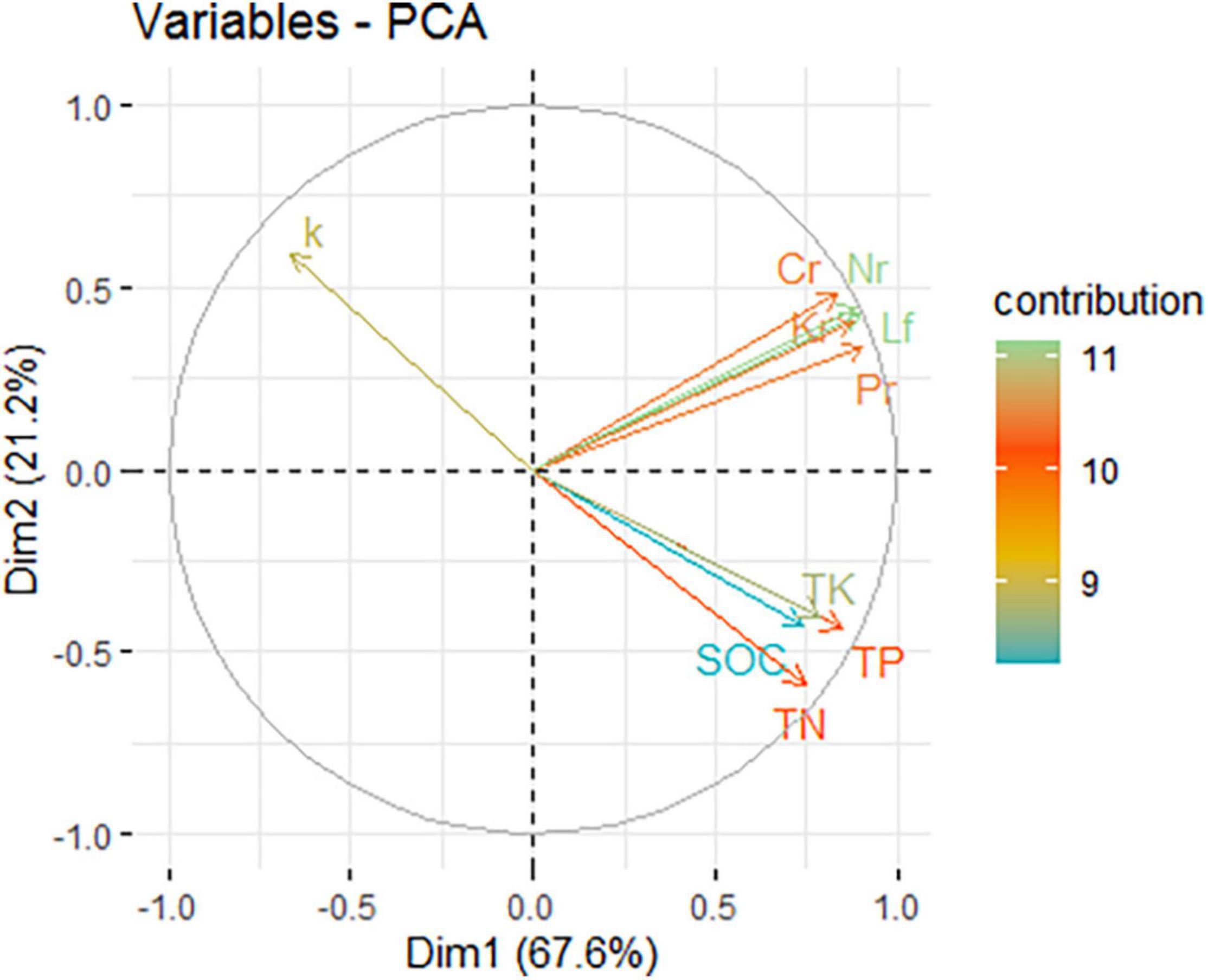
Figure 4. Principal component analysis of variables. The arrow line of the variables is plotted as the correlation coefficients between them and the first two principal components in the unit circle; colors indicate the contributions (%) of variables to the variance in a given principal component. SOC, soil organic carbon; TN, total nitrogen; TP, total phosphorous; TK, total potassium; Lf, annual litter yield; Cr, C return through litter in a year; Nr, N return through litter in a year; Pr, P return through litter in a year; Kr, K return through litter in a year; k, annual decay constant.
Conclusion
After cutting, only New bamboo grew in SC plot, while CK and RB plot had three kinds of bamboo of different ages. However, this study clearly indicated that strip cutting did not reduce annual litter yield or nutrient return. The seasonal variation trend of litter yield in the three treatment plots was consistent, but it was found that the annual litter yield and nutrient return of N, P, and K in RB increased significantly, and PCA indicated that there was a close correlation between litter yield and nutrient return. The reabsorption efficiency of N in SC was significantly lower than in CK at the leaf changing stage. In RB, the reabsorption efficiency of P was significantly higher than in CK during shoot pregnancy and leaf changes. The difference in the reabsorption efficiency of K mainly occurred after leaf change had completed, being significantly higher in SC than in CK. Moreover, litter decomposition tests revealed that the decomposition rate of SC was significantly lower than that of CK, and the litter decomposition rate was negatively correlated with soil nutrients after a short recovery time. Based on these findings, we propose that the reduction in bamboo forest density in the strip-cutting plots provided a sufficient nutrient environment for new trees. Therefore, it is necessary to conduct a long-term study to quantify nutrient flow to explore the response of nutrient cycling to strip cutting and guide the management of bamboo forests.
Data Availability Statement
The original contributions presented in the study are included in the article/supplementary material, further inquiries can be directed to the corresponding authors.
Author Contributions
SF and FG designed the study and improved the English language and grammatical editing. YZ wrote the first draft of manuscript and performed the data analysis. YZ and XY did the field works. LH gave guidance and methodological advice. All authors contributed to the discussion, revision and improvement of the manuscript.
Funding
This work was supported by the Fundamental Research Funds of the International Center for Bamboo and Rattan (1632021009).
Conflict of Interest
The authors declare that the research was conducted in the absence of any commercial or financial relationships that could be construed as a potential conflict of interest.
Publisher’s Note
All claims expressed in this article are solely those of the authors and do not necessarily represent those of their affiliated organizations, or those of the publisher, the editors and the reviewers. Any product that may be evaluated in this article, or claim that may be made by its manufacturer, is not guaranteed or endorsed by the publisher.
References
Adolfo, C. C., Lourdes, C. H., and Sandra, R. O. (2016). Mass, nutrient pool, and mineralization of litter and fine roots in a tropical mountain cloud forest. Sci. Total Environ. 575, 876–886. doi: 10.1016/j.scitotenv.2016.09.126
Astel, A., Parzych, A., and Trojanowski, J. (2009). Comparison of litterfall and nutrient return in a Vaccinio uliginosi – Betuletum pubescentis and an Empetro nigri – Pinetum forest ecosystem in northern Poland. For. Ecol. Manag. 257, 2331–2341. doi: 10.1016/j.foreco.2009.03.026
Berthelot, A., Ranger, J., and Gelhaye, D. (2000). Nutrient uptake and immobilization in a short-rotation coppice stand of hybrid poplars in north-west France. For. Ecol. Manag. 128, 167–179.
Bohlen, P. J., Scheu, S., Hale, C. M., Mclean, M. A., Migge, S., Groffman, P. M., et al. (2004). Non-native invasive earthworms as agents of change in northern temperate forests. Front. Ecol. Environ. 2, 427–435. doi: 10.2307/3868431
Bothwell, L., Selmants, P., Giardina, C., and Litton, C. (2014). Leaf litter decomposition rates increase with rising mean annual temperature in Hawaiian tropical montane wet forests. PeerJ 2:e685. doi: 10.7717/peerj.685
Chen, Z., Yao, X., and Tian, X. (2009). Establishment of leaf nutrition, soil fertility and output models in Phyllostachys pubescens. Nonwood For. Res. 27, 53–56. (In Chinese with English abstract),
Guadalupe, W.-L., Martha, B.-M., Fabiola, L.-B., and Javier, T. (2021). Litterfall, vegetation structure and tree composition as indicators of functional recovery in passive and active tropical cloud forest restoration. For. Ecol. Manag. 493:119260. doi: 10.1016/j.foreco.2021.119260
Guo, B. (2014). Ecological Stoichiometry Characteristics of Carbon, Nitrogen and Phosphorus in Phyllostachys pubescens Forest of Different Productivity Levels. Beijing: Chinese Academy of Forestry.
Guo, W., Qi, L., Lei, G., Hu, X., Zhang, J., Shu, Q., et al. (2021). Leaf stoichiometry and nutrient reabsorption efficiency of Phyllostachys edulis and its varieties. J. Nanjing Forest. Univ. (Nat. Sci. Edn.) 45, 79–85. (In Chinese with English abstract),
Huang, G., Zhao, H., and Li, Y. (2017). Litter decomposition in hyper-arid deserts: photodegradation is still important. Sci. Total Environ. 601-602, 784–792. doi: 10.1016/j.scitotenv.2017.05.213
Jian, Y., Wu, J., Sheng, W., Nie, G., Zheng, C., and Jiang, P. (2021). Effects of thinning and stand types on litter stock and soil water-holding capacity. J. Zhejiang A&F Univ. 38, 320–328. (In Chinese with English abstract),
Johnson, D. W., and Turner, J. (2019). Nutrient cycling in forests: a historical look and newer developments. For. Ecol. Manag. 444, 344–373. doi: 10.1016/j.foreco.2019.04.052
Keeler, B. L., Hobbie, S. E., and Kellogg, L. E. (2009). Effects of long-term nitrogen addition on microbial enzyme activity in eight forested and grassland sites: implications for litter and soil organic matter decomposition. Ecosystems 12, 1–15. doi: 10.1007/s10021-008-9199-z
Li, X., Lai, J., Yu, Z., Li, S., Chen, L., He, T., et al. (2018). Effect of different clear cutting band on physiological indexes of Phyllostachys pubescens. Mod. Agric. Sci. Technol. 18, 122–125.
Lihua, T., Hongling, H., Tingxing, H., Jian, Z., Xianwei, L., Li, L., et al. (2014). Litterfall, litter decomposition, and nutrient dynamics in two subtropical bamboo plantations of China. Pedosphere 24, 84–97. doi: 10.1016/s1002-0160(13)60083-1
Luan, J., Liu, S., Li, S., Whalen, J. K., Wang, Y., Wang, J., et al. (2020). Functional diversity of decomposers modulates litter decomposition affected by plant invasion along a climate gradient. J. Ecol. 109, 1236–1249. doi: 10.1111/1365-2745.13548
Mathieu, J., Rossi, J. P., Mora, P., Lavelle, P., Martins, P., and Grimaldi, C. R. (2010). Recovery of soil macrofauna communities after forest clearance in Eastern Amazonia, Brazil. Conserv. Biol. 19, 1598–1605. doi: 10.1111/j.1523-1739.2005.00200.x
Miller, H. G., Cooper, J. M., Miller, J. D., and Pauline, O. J. L. (1979). Nutrient cyles in pines and their adaptation to poor soils. Can. J. For. Res. 9, 19–26. doi: 10.1139/x79-004
Olson, J. S. (1963). Energy storage and the balance of producers and decomposers in ecological systems. Ecology 44, 322–331. doi: 10.2307/1932179
Parton, W., Silver, W. L., Burke, I. C., Grassens, L., Harmon, M. E., Currie, W. S., et al. (2007). Global-scale similarities in nitrogen release patterns during long-term decomposition. Science 315, 361–364. doi: 10.1126/science.1134853
Prasetyo, E., Hardiwinoto, S., Supriyo, H., and Widiyatno, W. (2015). Litter production of logged-over forest using Indonesia selective cutting system and strip planting (TPTJ) at PT. Sari Bumi Kusuma. Proc. Environ. Sci. 28, 676–682. doi: 10.1016/j.proenv.2015.07.079
Proe, M. F., and Millard, P. (1995). Effect of P supply upon seasonal growth and internal cycling of P in Sitka spruce (Picea sitchensis (Bong.)Carr.) seedlings. Plant Soil 168, 313–317. doi: 10.1007/978-94-011-0455-5_36
Regina, S. I., Leonardi, S., and Rapp, M. (2000). Foliar nutrient dynamics and nutrient-use efficiency in Castanea sativa coppice stands of southern Europe. Forestry 74, 1–10. doi: 10.1093/forestry/74.1.1
Ren, L., Liu, S., Wang, Y., Cai, C., Luan, J., and Chen, C. (2018). Decomposition characteristics of litter of Phyllostachys edulis and Dicranopteris pedata. For. Res. 31, 91–97.
Sakai, Y., and Tadaki, Y. (1997). Characteristics of stem flow and throughfall in a Madake and in a Mousouchiku stands. Bamboo J. 14, 28–35.
Sayer, E. J., Rodtassana, C., Sheldrake, M., Bréchet, L., and Tanner, E. (2020). Revisiting nutrient cycling by litterfall—Insights from 15 years of litter manipulation in old-growth lowland tropical forest. Adv. Ecol. Res. 62, 173–223. doi: 10.1016/bs.aecr.2020.01.002
Su, W. (2012). Fertilization Theory and Practice for Phyllostachys edulis Stand Based on Growth and Nutrient Accumulation Rules. Beijing: Chinese Academy of Forestry.
Su, W., Fan, S., Zhao, J., and Cai, C. (2019a). Effects of various fertilization placements on the fate of urea–15N in moso bamboo forests. For. Ecol. Manag. 453:117632.
Su, W., Zeng, X., Fan, S., and Ni, H. (2019b). Effects of strip clear-cutting on the allocation of non-structural carbohydrates and aboveground biomass of Phyllostachys edulis. Chin. J. Ecol. 37, 2934–2940. (In Chinese with English abstract),
Tang, L., Han, W., Chen, Y., and Fang, J. (2013). Editor’s choice: resorption proficiency and efficiency of leaf nutrients in woody plants in eastern China. J. Plant Ecol. 6, 408–417. doi: 10.1093/jpe/rtt013
Tang, X., Qi, L., Fan, S., Guan, F., and Zhang, H. (2016). Soil respiration and net ecosystem production in relation to intensive management in Moso bamboo forests. Catena 137, 219–228.
Turner, J., and Lambert, M. (2013). Analysing inter-rotational productivity and nutrition in a New South Wales radiata pine plantation. New For. 44, 785–798.
Turner, J., and Lambert, M. J. (2015). Analysis of nutrient use efficiency (NUE) in Eucalyptus pilularis forests. Aust. J. Bot. 62, 558. doi: 10.1071/bt14162
Umemura, M., and Takenaka, C. (2014). Retranslocation and localization of nutrient elements in various organs of moso bamboo (Phyllostachys pubescens). Sci. Total Environ. 493, 845–853. doi: 10.1016/j.scitotenv.2014.06.078
White, P. J. (2012). “Long-distance transport in the xylem and phloem,” in Marschner’s Mineral Nutrition of Higher Plants, ed. P. Marschner (Cambridge, MA: Academic Press), 49–70.
Wieder, W. R., Cleveland, C. C., and Townsend, A. R. (2009). Controls over leaf litter decomposition in wet tropical forests. Ecology 90, 3333–3341. doi: 10.1890/08-2294.1
Wright, I. J., and Westoby, M. (2010). Nutrient concentration, resorption and lifespan: leaf traits of Australian sclerophyll species. Funct. Ecol. 17, 10–19.
Xianli, Z. (2019). Recovery Characteristics and Influencing Factors of Moso Bamboo Forests under Different Strip Clearcutting in South Anhui Province. Ph.D. thesis. Beijing: Chinese Academy of Forestry.
Zeng, X., Su, W., Fan, S., Jing, X., and Chu, H. (2019b). Soil quality assessment in Moso bamboo forests under different strip clearcutting. Chin. J. Ecol. 38, 3015–3023.
Zeng, X., Su, W., Fan, S., and Jin, Y. (2019a). Qualitative recovery characteristics of moso bamboo forests under strip clearcutting. Acta Bot. Boreali Occident. Sin. 39, 917–924.
Zhan, M. (2019). Characteristic Research on Rapid Regeneration of Strip Harvesting Bamboo (Phyllostachys edulis) Forest in Jiangsu Province. Beijing: Chinese Academy of Forestry.
Zhan, M., Guan, F., Yan, Y., Zhang, M., and Zheng, Y. (2020). Effects of strip harvesting on species diversity of undergrowth in bamboo (Phyllostachys edulis) forest. Acta Ecol. Sin. 40, 4169–4179. (In Chinese with English abstract),
Zhang, L.-X., Bai, Y.-F., and Han, X.-G. (2003). Application of N: P stoichiometry to ecology studies. Acta Bot. Sin. 45, 1009–1018.
Zhao, J., Su, W., Fan, S., Cai, C., Su, H., and Zeng, X. (2018a). Ammonia volatilization and nitrogen runoff losses from moso bamboo forests after different fertilization practices. NRC Res. Press 49, 213–220.
Zhao, J., Wang, B., Li, Q., Yang, H., and Xu, K. (2018b). Analysis of soil degradation causes in Phyllostachys edulis forests with different mulching years. Forests 9:149.
Keywords: moso bamboo forest, strip clearcutting, litterfall, resorption efficiency, nutrient return
Citation: Zheng Y, Guan F, Fan S, Yan X and Huang L (2022) Dynamics of Leaf-Litter Biomass, Nutrient Resorption Efficiency and Decomposition in a Moso Bamboo Forest After Strip Clearcutting. Front. Plant Sci. 12:799424. doi: 10.3389/fpls.2021.799424
Received: 21 October 2021; Accepted: 20 December 2021;
Published: 27 January 2022.
Edited by:
Arun Jyoti Nath, Assam University, IndiaReviewed by:
Uttam Kumar Sahoo, Mizoram University, IndiaRajesh Kaushal, Indian Institute of Soil and Water Conservation (ICAR), India
Copyright © 2022 Zheng, Guan, Fan, Yan and Huang. This is an open-access article distributed under the terms of the Creative Commons Attribution License (CC BY). The use, distribution or reproduction in other forums is permitted, provided the original author(s) and the copyright owner(s) are credited and that the original publication in this journal is cited, in accordance with accepted academic practice. No use, distribution or reproduction is permitted which does not comply with these terms.
*Correspondence: Fengying Guan, Z3VhbmZ5QGljYnIuYWMuY24=; Shaohui Fan, ZmFuc2hAaWNici5hYy5jbg==