- 1State Key Laboratory of Crop Gene Exploration and Utilization in Southwest China, Sichuan Agricultural University, Chengdu, China
- 2Rice Research Institute, Sichuan Agricultural University, Chengdu, China
Photoperiod is acknowledged as a crucial environmental factor for plant flowering. According to different responses to photoperiod, plants were divided into short-day plants (SDPs), long-day plants (LDPs), and day-neutral plants (DNPs). The day length measurement system of SDPs is different from LDPs. Many SDPs, such as rice, have a critical threshold for day length (CDL) and can even detect changes of 15 minutes for flowering decisions. Over the last 20 years, molecular mechanisms of flowering time in SDP rice and LDP Arabidopsis have gradually clarified, which offers a chance to elucidate the differences in day length measurement between the two types of plants. In Arabidopsis, CO is a pivotal hub in integrating numerous internal and external signals for inducing photoperiodic flowering. By contrast, Hd1 in rice, the homolog of CO, promotes and prevents flowering under SD and LD, respectively. Subsequently, numerous dual function regulators, such as phytochromes, Ghd7, DHT8, OsPRR37, OsGI, OsLHY, and OsELF3, were gradually identified. This review assesses the relationship among these regulators and a proposed regulatory framework for the reversible mechanism, which will deepen our understanding of the CDL regulation mechanism and the negative response to photoperiod between SDPs and LDPs.
Introduction
The earth’s rotation around its tilt axis is the primary principle of external forces in the world, which repeatedly manifests in diurnal cycles and seasonal fluctuations. Plants have relied on exact and regular variations in day length to trigger the right flowering time (Imaizumi and Kay, 2006; Amasino, 2010; Andres and Coupland, 2012; Anwer and Davis, 2013; Sun et al., 2021). Garner and Allard, in the 1920s, discovered that many plants flowered at the proper time independent of their sowing dates, which was first described as photoperiodic phenomenon (Garner and Allard, 1920; Garner and Allard, 1923). Then, photoperiod is acknowledged as a crucial pathway for flowering time (Song et al., 2015). According to different responses to photoperiod, plants were divided into short-day plants (SDPs), long-day plants (LDPs), and day-neutral plants (DNPs) (Thomas and Vince-Prue, 1996; Izawa, 2007). While LDPs prefer longer days for flowering and DNPs flower regardless of day length, SDPs flower earlier on shorter days. According to physiological investigations, SDPs have a different day length measurement system from LDPs (Thomas, 1998). However, what causes the difference in their photoperiodic response remains unclear.
In photoperiodic flowering, the critical threshold for day length (CDL) refers to the photoperiod that distinguishes between flowering and vegetative growth. LDP Arabidopsis does not detect any CDL and flowers steadily earlier as the day length gets longer (Izawa, 2007; Wang et al., 2021b). However, SDP rice has a 13.5 h CDL and can even detect changes of 15 minutes daily to decide when to flower (Thomas and Vince-Prue, 1996; Nishida et al., 2002; Itoh et al., 2010; Sun et al., 2021) (Figure 1A). Furthermore, SDPs observed the dark period’s length rather than the day’s length. This conclusion was reached after observing a night-break response phenomenon (NB). Many SDPs, but not LDPs, are significantly inhibited from flowering during the night by a light pulse (Hamner and Bonner, 1938) (Figure 1B). Therefore, the CDL and NB are two important indicators for detecting differences in day length response between SDPs and LDPs.
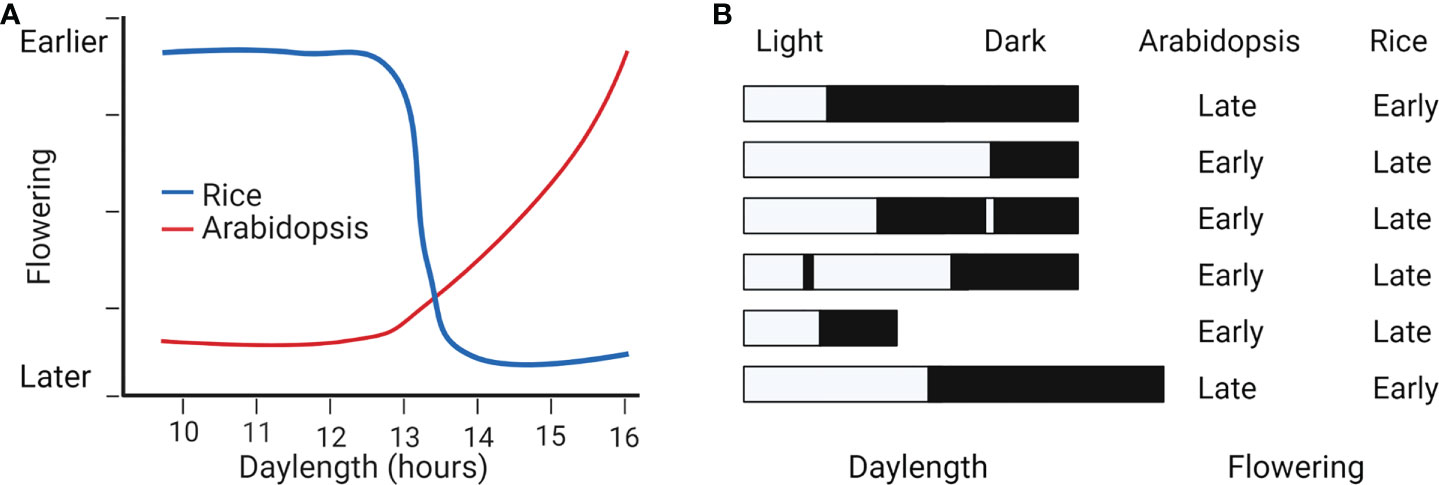
Figure 1 Different photoperiod and NB responses between SDP rice and LDP Arabidopsis. (A) Opposite responses to photoperiod in rice and Arabidopsis. Flowering in Arabidopsis gradually becomes earlier with increasing day length. However, rice has a ≈13.5 h CDL and detects changes of 15 minutes for flowering transition. (B) Effect of dark length to flowering in rice and Arabidopsis. Rice flowering is early in the long dark. Arabidopsis flowering is early in long day or short dark. NB treatment induces flowering in Arabidopsis but suppresses flowering in rice (Thomas and Vince-Prue, 1996).
Two main photoperiodic measurement models have been developed due to decades of physiological study into how plants detect day length. According to the external coincidence model, a photo-inducible phase of the circadian cycle and the illuminated portion of the light signal must coincide for photoperiodic responses to be elicited. In this model, light has two distinct effects, training the circadian system and causing photoperiodic responses during the photo-inducible phase of the cycle. According to the internal coincidence model, the internal consistency of circadian rhythms is the only way to induce the photoperiodic response (Bunning, 1936).
In the past 20 years, the flowering molecular mechanisms in rice and Arabidopsis have gradually clarified, which offers a chance to ascertain the molecular difference in day length measurement between the two types of plants (Izawa, 2007). Numerous studies suggest that functional differences in CONSTANS (CO) and Heading date 1 (Hd1) play essential roles. Hd1 is the first bi-functional flowering gene that was found in rice. Subsequently, numerous bi-functional regulators, such as phytochromes, Grain Number, Plant Height and Heading Date 7 (Ghd7), Days to Heading 8 (DTH8), Oryza sativa Pseudo-Response Regulator 37 OsPRR37), Oryza sativa GIGANTEA (OsGI), Oryza>sativa LATE ELONGATED HYPOCOTYLN (OsLHY), and Oryza sativa EARLY FLOWERING 3 (OsELF3), were identified. Bi-functional regulator here is defined as the effectors that could promote and inhibit flowering in different day length conditions (such as Hd1), as well as the modifiers (such as Ghd7, DTH8) that could modify the effectors and affect their dual functions (Table 1). However, no bi-functional flowering genes were found in Arabidopsis. It was proposed that the reversal mechanism is crucial to investigating the CDL regulation mechanism and will fill in our understanding of the negative response to photoperiod between SDPs and LDPs (Yano et al., 2001; Izawa, 2007). This review assesses the relationships between these dual function regulators and proposes a putative regulatory framework for the reversible mechanism in rice.
Photoperiodic flowering pathways in arabidopsis and rice
CO is a hub in photoperiodic flowering in Arabidopsis
The external coincidence hypothesis could partly explain the photoperiodic flowering in Arabidopsis (Imaizumi and Kay, 2006). The key flowering regulator CO is hypothesized to be a hub in integrating numerous internal and external signals into photoperiodic flowering (Shim et al., 2017) (Figure 2A). CO produces a B-box-type zinc-finger transcriptional activator that stimulates the florigen gene FLOWERING LOCUS T (FT) in a light-dependent manner (Yanovsky and Kay, 2002). GIGANTEA (GI) serves as a bridge between CO and the circadian clock by positively regulating the expression of CO. This GI-CO-FT pathway works as a key mechanism for daylength dependent flowering promotion pathway in Arabidopsis (Shim et al., 2017).
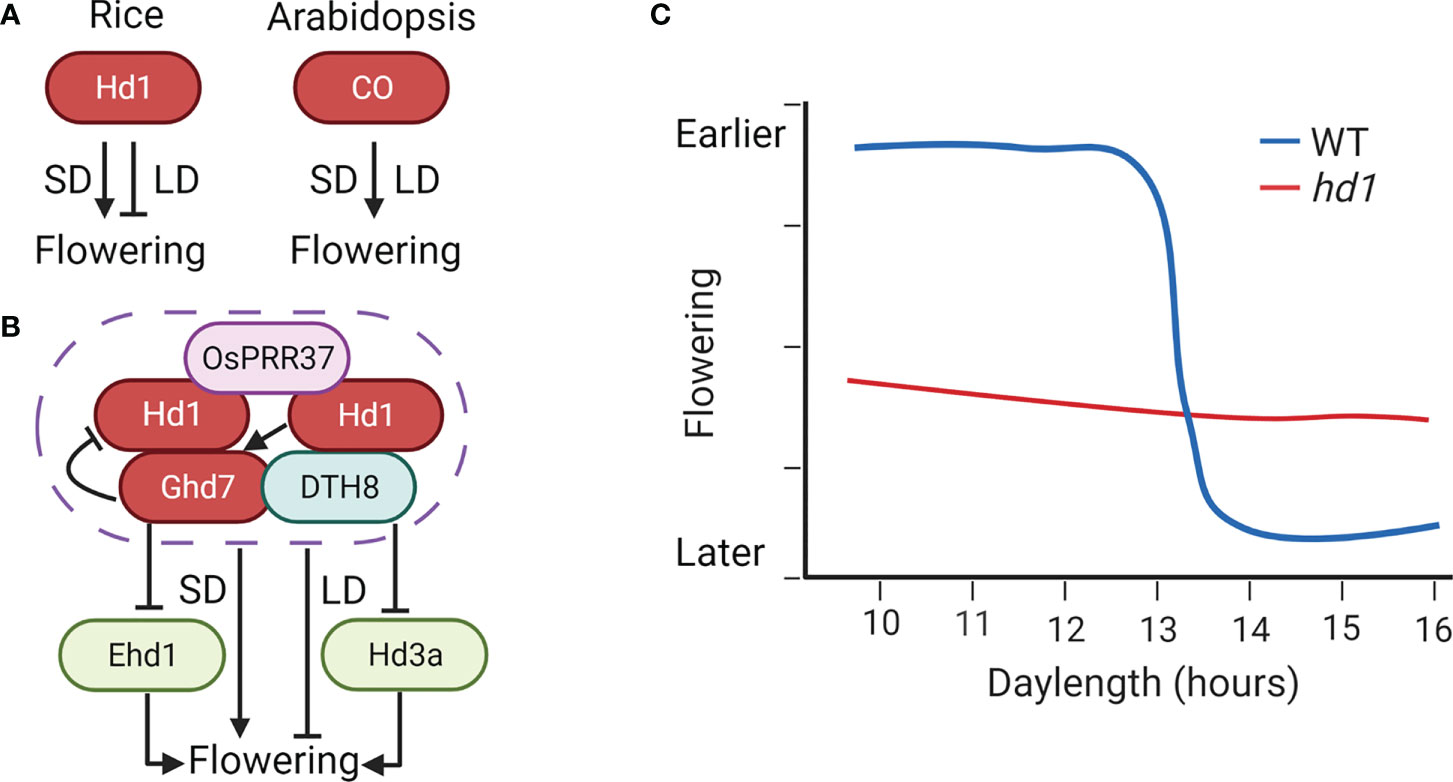
Figure 2 Bifunctional Hd1 and its enhancers Ghd7, DTH8, and OsPRR37 in rice. (A) The opposite function between Hd1 and CO under LD. (B) Three enhancers of bifunctional Hd1. Ghd7, DTH8, and OsPRR37 could improve the dual function of Hd1, thus enhancing the photosensitivity of flowering in rice. (C) Hd1 predominantly regulates photoperiodic response in rice. Arrows represent positive regulation, inhibitors represent negative regulation, and graphical overlap represents protein physical interaction.
Furthermore, the internal circadian clock system sets the CO expression gate, and the light directly influences the stability and activity of CO protein (Yanovsky and Kay, 2003; Hayama and Coupland, 2004). Therefore, flowering time is controlled by the coincidence of light with a specific circadian phase defined by strong CO expression. Then, increased quantities of FT mRNA are created by this exogenous coincidence, which eventually encourages the transition from vegetative to reproductive growth (Yanovsky and Kay, 2002; Bouche et al., 2016).
Unique photoperiodic flowering pathways in rice
In rice, a sophisticated network with more than 80 flowering genes has been investigated, and the molecular mechanism of day length recognition gradually surfaced (Cao et al., 2021; Zhou et al., 2021). Rice has two florigen genes, Heading date 3a (Hd3a) and RICE FLOWERING LOCUS T 1 (RFT1) (Komiya et al., 2008). There are primarily two pathways for photoperiodic flowering, a unique LD flowering suppression route, Ghd7-Early Heading Date 1 (Ehd1)-Hd3a/RFT1, and an OsGI-Hd1-Hd3a/RFT1 flowering pathway, which is conserved to the GI-CO-FT pathway of Arabidopsis (Sun et al., 2014; Chen et al., 2022). Among them, both Ehd1 and Ghd7 are unique flowering regulators in rice. Ehd1 encodes a B-type response regulator and forms homo-dimers to operate as a transcription factor (Doi et al., 2004; Cho et al., 2016). Ehd1 promotes heading by upregulating the expression of Hd3a and RFT1 in both long day (LD) and short day (SD), forming an Ehd1-Hd3a/RFT1 module in rice (Chen et al., 2022). Ghd7 encodes a CCT-domain transcription factor, prefers to express under LD and confers flowering repression (Xue et al., 2008; Itoh et al., 2010).
CDL gates florigen gene Hd3a expression in rice
Different from FT in Arabidopsis, rice florigen gene Hd3a expression is gated by CDL, which is consistent with physiological research (Thomas and Vince-Prue, 1996; Nishida et al., 2002; Itoh et al., 2010; Sun et al., 2021). Hd3a expression is at fairly high levels in less than 13 hours (h) of day length conditions, but it drops off at day lengths of 13.5 h and becomes undetectable at day lengths of more than 14 h. Two unique mechanisms regulated by Ehd1 and Ghd7 are thought to control the CDL of Hd3a. When blue light corresponds with the morning phase defined by OsGI-dependent circadian clocks, Hd3a production is stimulated by Ehd1 expression. On the other hand, Ghd7 is acutely induced when phytochrome signals and photosensitive phases coincide, and this induction suppresses Ehd1 expression the following morning (Itoh et al., 2010).
Bifunctional Hd1 and its enhancers in rice
Hd1, a homolog of Arabidopsis CO, is a significant quantitative trait locus (QTL) that predominantly regulates photoperiodic response in rice (Yano et al., 2000). Interestingly, Hd1 serves in stimulating heading under SD and in repressing heading under LD, in contrast to the active role of CO (Yano et al., 2000) (Figure 2A). In certain genetic backgrounds of rice, hd1 mutant almost loses the photosensitivity of flowering (Figure 2C). By contrast, this bifunctional phenomenon was not found in flowering regulators in Arabidopsis characterized so far (Bouche et al., 2016). Furthermore, the amount of Hd1 mRNA was not significantly influenced by photoperiod changes, proving that the function of Hd1 is certainly not at the transcription level. Therefore, post-transcriptional regulation may thus be the key to controlling the Hd1 function (Yano et al., 2000).
Many investigations revealed that Ghd7, DTH8, and OsPRR37 could improve the dual function of Hd1, thus enhancing the photosensitivity of rice. Ghd7, DTH8, and OsPRR37 are initially identified as flowering inhibitors and pleiotropic genes controlling plant height, heading date, and yield (Xue et al., 2008; Wei et al., 2010; Yan et al., 2011; Dai et al., 2012; Fujino et al., 2013; Koo et al., 2013; Liu et al., 2013; Yan et al., 2013; Gao et al., 2014; Du et al., 2017). Generally, Ghd7 is crucial in converting Hd1, and Ghd7 alone can determine the bi-function of Hd1. The protein complex generated by Ghd7-Hd1 proteins specifically binds to the cis-regulatory region of Ehd1 (Nemoto et al., 2016). This protein-protein interaction between Ghd7 and Hd1 is also likely to suppress or impede the active ability of Hd1 (Zhang et al., 2017). DTH8 is a putative HAP3 subunit and directly interacts with Hd1 for the transcriptional repression of Hd3a (Wei et al., 2010; Du et al., 2017). But DTH8 alone does not appear to determine the dual function of Hd1. Furthermore, by binding to the promoter region of Ghd7, DTH8 could form a protein complex with Hd1 to stimulate the transcription of Ghd7 (Wang et al., 2019). Additionally, there is also a physical interaction between Ghd7 and DTH8, suggesting that they could control rice flowering synergistically (Li et al., 2015b) (Figure 2B). For OsPRR37, we will discuss it separately in the circadian clock section. Moreover, different haplotype combinations of Hd1, Ghd7, DTH8, and OsPRR37 are important for photoperiodic adaptation in rice. In the Minghui63 background, Ghd7 or OsPRR37 reverse the Hd1 function collectively rather than separately (Zhang et al., 2017). The greatest digenic interaction in the functional Hd1 backgrounds was Ghd7 by DTH8 under LD, whereas Ghd7 by OsPRR37 under SD (Zhang et al., 2019; Zhou et al., 2021; Chen et al., 2022).
Phytochromes
The nature of phytochromes
The transmission of the initial photoperiodic signals to the final flower-forming element through three steps, light perceived, circadian clock, and signal output (Song et al., 2015). In the first step, light is perceived by various photoreceptors, such as phytochromes (red/far-red light receptor) and cryptochromes (blue light receptor) (Bae and Choi, 2008; Shim et al., 2017). Phytochromes are created as chromoproteins, where the apoprotein is joined to a billin chromophore. Exposure to red light (R) could change phytochrome conformations from inactive Pr to active Pfr. The active Pfr can then be transformed back to inactive Pr by either a gradual reaction caused by darkness or a rapid response caused by exposure to far-red light (FR) (Mancinelli, 1994; Quail, 1997; Fankhauser, 2001). Therefore, phytochromes can act as the developmental switch (Li et al., 2011). The inactive Pr form is initially localized in the cytoplasm and enters the nucleus after converting to the active Pfr form (Van Buskirk et al., 2012; Klose et al., 2015). In the nucleus, phytochromes interact with several regulatory components (such as PILs or PIFs) to control the expression of downstream genes and mediate light responses (Xu et al., 2015; Pham et al., 2018) (Figure 3).
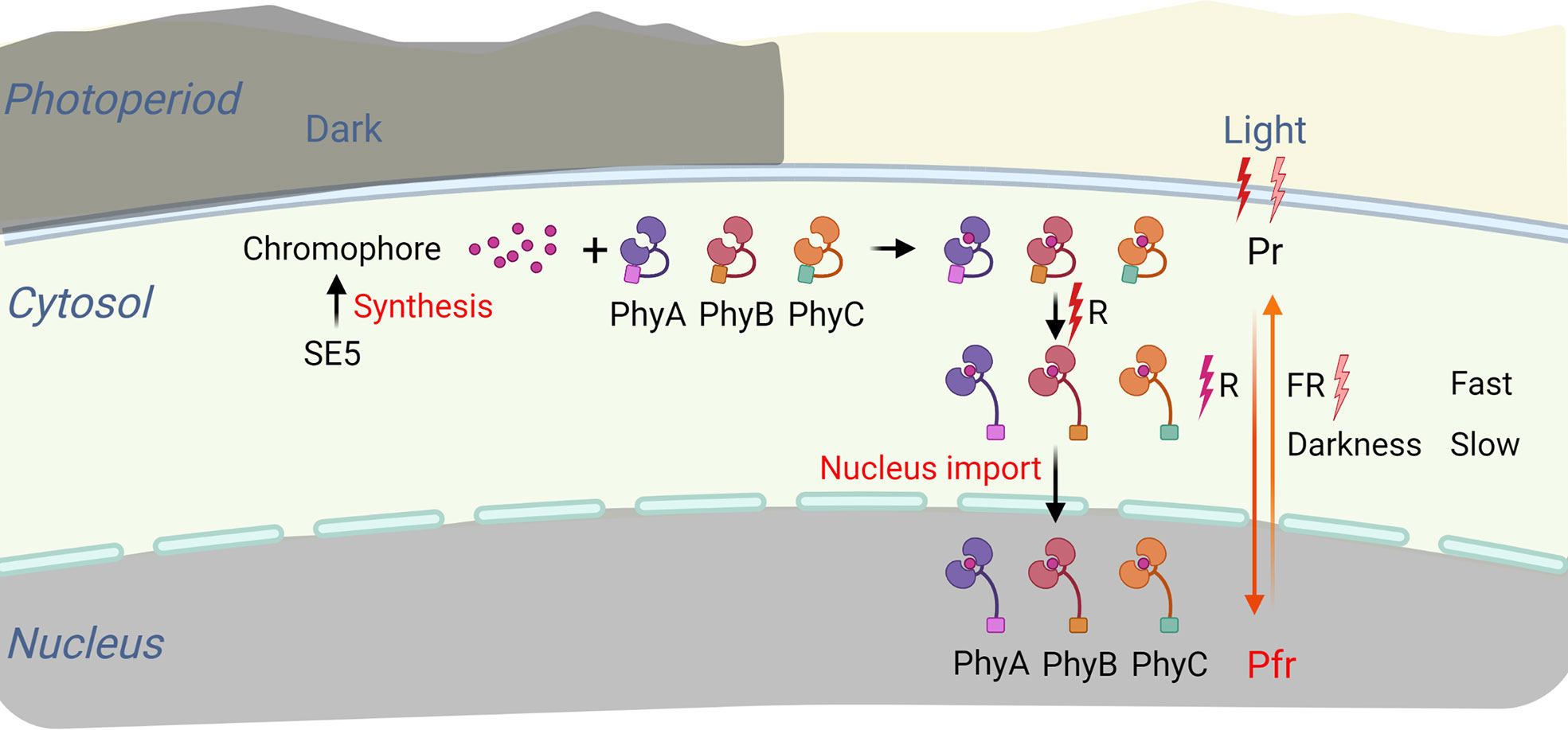
Figure 3 Phytochromes import to the nucleus after converting from inactive Pr form to active Pfr form. Phytochromes could change from inactive Pr to active Pfr under Red light (R). The active Pfr can be reverted to the inactive Pr by either a slow reaction caused by darkness or a quick response caused by exposure to far-red light (FR). When the inactive Pr form transforms into the active Pfr form, phytochromes leave their initial location (cytoplasm) and enter the nucleus.
In higher plants, phytochrome genes are encoded by a small family. The phytochrome in Arabidopsis consists of five members, PhyA to PhyE (Sharrock and Quail, 1989). However, only three phytochromes, PhyA, PhyB, and PhyC, exist in rice (Mathews and Sharrock, 1997) (Figure 3). The distinct phytochrome signal route between Arabidopsis and rice may be due to the presence or absence of PhyD/E (Pham et al., 2018). Phytochromes are also classified based on their stability in light and darkness. The PhyB to PhyE are all type II (light steady), whereas PhyA is a type I (light labile) (Li et al., 2015a). When exposed to R or white (W) light, PhyA levels rapidly decrease, but they are most plentiful in seedlings developed in the dark. PhyB is the most abundant phytochrome in plants cultivated in the light, while PhyC to PhyE are less abundant (Strasser et al., 2010; Hu et al., 2013; Cheng et al., 2021).
Phytochromes and flowering
Takano (2005) did excellent work examining the function of all possible combinations of PhyA to PhyC single and double mutants in rice flowering. Depending on the day length, these mutants display various heading phenotypes (Takano et al., 2005). Under nature long day (nLD), phyB and phyC are engaged in decreasing flowering time, while phyA does not change the heading date. Double mutant phyB phyC flowers as early as phyB or phyC single mutant, demonstrating that the two phytochromes have a similar influence. Although phyA single mutant cannot alter the flowering, the double mutants phyA phyB and phyAphyC flower much earlier than phyB or phyC. These findings suggest that phyA mutation alone does not significantly affect flowering time. However, in the background of phyB or phyC mutants, phyA mutation significantly influences the flowering time under nLD (Takano et al., 2005).
Under SD, the phyC mutant displayed almost the same heading as the wild type, and the phyA mutant showed a minor later flowering, while the phyB mutant flowered earlier. phyA phyC and phyB phyC double mutants flowered simultaneously with those of the phyA or phyB single mutants, indicating that PhyC has no appreciable influence on the floral induction under SD. Interestingly, in contrast to earlier flowering in LD, phyA phyB double mutant flowered later in SD and even later than in LD, making it a simulated LDP (Takano et al., 2005). These findings suggest a close association between phytochromes and Hd1 in the inversion mechanism of flowering regulation.
Hd1 and phytochromes
All phytochromes have the same chromophore. The chromophore is synthesized by the phytochromobilin-synthetic pathway, which is seriously damaged in an Arabidopsis mutant hy1. Therefore, the hy1 mutant significantly reduces the function of the phytochromes (Cheng et al., 2021). PHOTOPERIODIC SENSITIVITY 5 (SE5), a homolog of HY1, was the first flowering gene cloned in rice (Izawa et al., 2000). The mutant se5 has less than 1% chromophore concentration, significantly hindering phytochrome production. As a result, se5 has a very limited ability to send light signals for day length measurement and exhibits a very early flowering phenotype with no photoperiodic response, deservedly accompanied by the loss of CDL (Izawa et al., 2000) (Figure 4A, B).
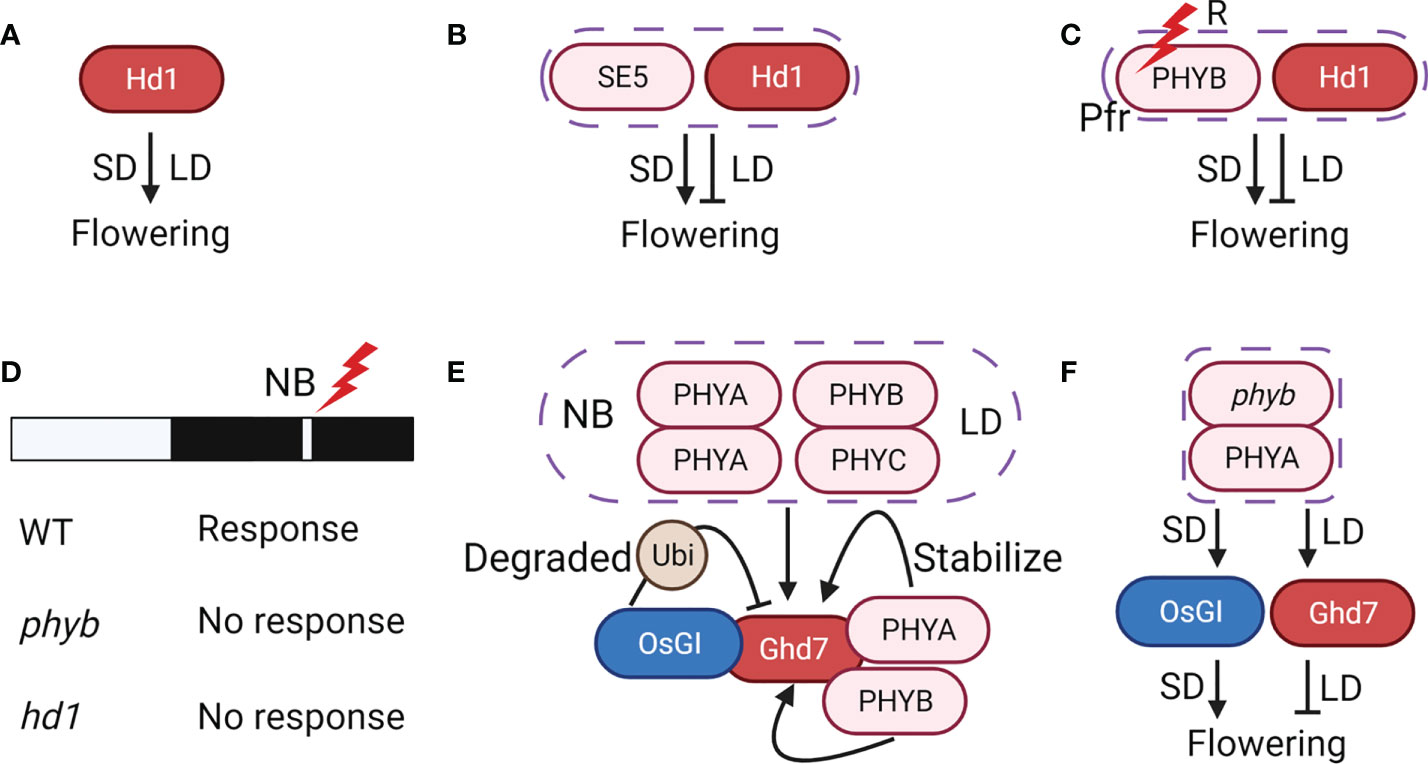
Figure 4 A strong connection exists between the reversal mechanism and phytochromes. (A) Hd1 alone is a flowering activator. (B) Hd1 changes to be a flowering suppressor with SE5 in LD. (C) Hd1 changes to be a flowering suppressor with PhyB in LD. (D) PhyB and Hd1 are critical to NB response. (E) Phytochromes, NB and LD regulate Ghd7 positively. OsGI degrades Ghd7 through the ubiquitination mechanism. (F) PhyA promotes the expression of OsGI under SD and Ghd7 under LD in phyb background. PhyA induces flowering under SD but represses it under LD without PhyB. Arrows represent positive regulation, inhibitors represent negative regulation, and graphical overlap represents protein physical interaction.
Interestingly, Hd1 constantly promoted flowering in se5 under SD and LD, indicating that the functional conversion of Hd1 depends on phytochromes (Thomas and Vince-Prue, 1996; Izawa et al., 2002; Andres et al., 2009) (Figure 4A, C). Further study showed that the dual function of Hd1 that phytochromes switch may be involved in determining the CDL as the threshold for rice flowering (Izawa et al., 2000). Therefore, Hd1 and phytochromes are both critical in sensing CDL, suggesting a strong link between them in regulating CDL.
Furthermore, experiments with non-24-hour light/dark cycles showed that Hd1 serves as a circadian clock output. However, the circadian phase setting of Hd1 expression is not affected in se5 under SD and LD (Izawa et al., 2002). Therefore, the function convertible Hd1 may be posttranscriptionally regulated by coincidence with different forms of phytochromes (Izawa et al., 2002). In the dark, Pfr could slowly transform into Pr (Elich and Chory, 1997; Eichenberg et al., 2000). According to End-Of-Day FR (EOD-FR) treatment observations, the Pfr form of PhyB could persist through the night and contribute to flowering inhibition under SD (Takano et al., 2005). Therefore, Hd1 serves as a flowering repressor by interacting with the Pfr form. In contrast, Hd1 activates flowering without this interaction. The overlap between Hd1 and Pfr depends greatly on the photoperiod since the Pfr diurnal pattern differs from the Hd1 expression rhythm. Hd1 should have a larger overlap period under LD than under SD, thus making it a flowering gene with two distinct functions (Izawa et al., 2002) (Figure 4C). However, it has not been reported whether phytochromes could directly interact with Hd1. However, phytochromes could regulate the modifiers of Hd1 by protein interaction, such as Ghd7 and OsGI, thus affecting the function of Hd1 (Osugi et al., 2011; Zheng et al., 2019).
It seems that NB and CDL share a set of molecular mechanisms. Short exposure to light during the night (NB) imitates SD to LD and delays flowering in SDPs. However, different spectra of light have different NB effects. Red and blue light NB downregulated Hd3a expression, but far-red light NB did not. The impact of red light NB on Hd3a could be reversed by subsequent far-red light treatment. Interestingly, phyB mutants do not respond to NB, and their flowering time is not affected by NB, suggesting that PhyB is essential to NB (Ishikawa et al., 2009) (Figure 4D). Furthermore, PhyB-mediated flowering suppression by NB is Hd1 dependent, which is also a primary cause of flowering suppression under LD (Figure 4D). However, rather than the change of Hd1 expression controlled by the circadian clock, flowering is predominantly controlled by the direct action of light. As the Hd1 protein level is unaffected by light, protein activity but not the stability of Hd1 is the actual cause of photoperiodic flowering (Ishikawa et al., 2011).
Ghd7 and phytochromes
Ghd7 mRNA levels increase as the day length becomes longer in the WT, but there are no appreciable changes in the se5 mutant. Additionally, the expression of Ghd7 was also strictly controlled by NB. Consequently, phytochromes might act as a mediator for light signals to induce Ghd7 expression (Itoh et al., 2010). Since Ghd7 expression could be induced in phyB phyC but not in phyA phyC or phyA phyB double mutants, PhyA alone is adequate to promote Ghd7 expression (Osugi et al., 2011) (Figure 4E). However, Ghd7 expression is inhibited in the phyA phyC or phyA phyB double mutants, proving that PhyB and PhyC could work collectively to generate Ghd7 expression under phyA mutant background. Therefore, Ghd7 expression can be induced by light signals sent by the PhyA/PhyA homodimer or the PhyB/PhyC heterodimer (Figure 4E). Despite the phytochrome action of entraining the circadian clock in rice, phytochromes do not set the Ghd7 expression gate (Osugi et al., 2011).
Circadian clock is critical to the inversion mechanism
OsGI
GI is identified as a flowering promoter in Arabidopsis. While, in rice, several studies of OsGI are not completely consistent. Early flowering under LD and late flowering under SD, similar to the hd1 mutant, were caused by decreased OsGI expression, according to the first study using OsGI RNAi in the Norin 8 background (Hayama et al., 2003). A null mutant of OsGI by CRISPR/Cas9 method (cv. Nipponbare) flowered about 25 days earlier and confirmed this conclusion only in LD (Wang et al., 2021a). Therefore, OsGI might act upstream and control flowering in the same direction as Hd1 (Figure 5A). However, hd1 osgi-1 double mutants flowered intermediate between hd1 and osgi-1, indicating that OsGI functions somewhat differently from Hd1 (Izawa et al., 2011). In contrast, the osgi-1 mutant (cv. Norin 8) prolonged flowering under SD, but did not show appreciable changes under LDs (Izawa et al., 2011). A T-DNA insertion mutant (cv. Dongjin) displayed an entirely distinct phenotype with null OsGI expression. It flowered 36 days later under SD (12 hours of light) and 9 days later under LD (14.5 hours of light) (Lee and An, 2015).
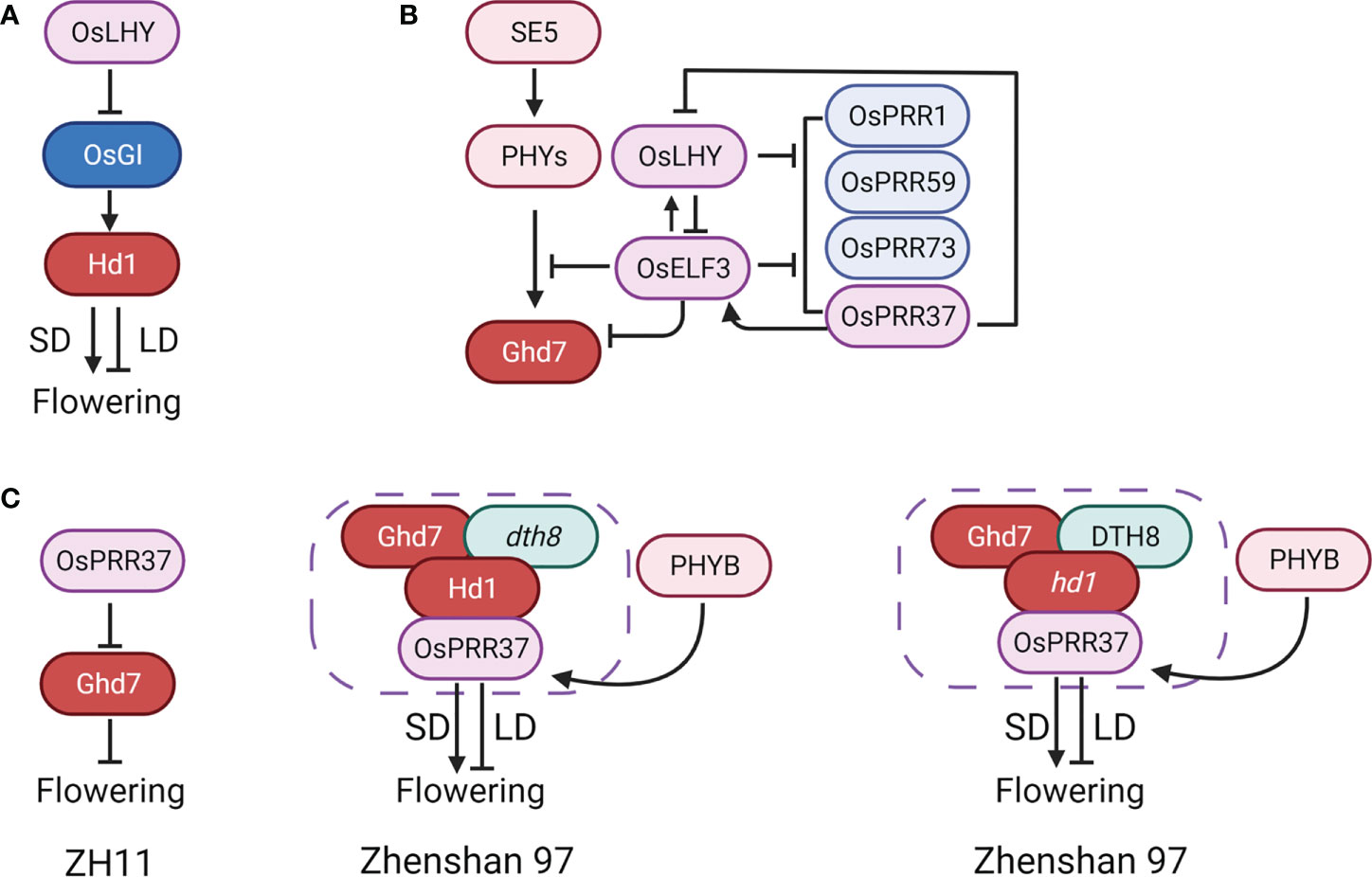
Figure 5 Circadian clock pathway is critical to the reversal mechanism. (A) The OsLHY-OsGI-Hd1 pathway could fine-tune the CDL by adjusting the degree of coincidence between Hd1 expression and day length. (B) The possible flowering mechanism of OsELF3. OsELF3 suppresses the phytochrome signal, prevents light from entering Ghd7 and impresses Ghd7 expression. (C) OsPRR37 acts as an activator or a suppressor depending on the background, and three genes, Hd1, Ghd7, and DTH8. Arrows represent positive regulation, inhibitors represent negative regulation, and graphical overlap represents protein physical interaction.
Furthermore, OsGI and phytochromes play antagonists in regulating Ghd7 protein stability and flowering time. PhyA, PhyB and OsGI could directly interact with Ghd7, and PhyA and PhyB could inhibit the interaction between OsGI and Ghd7, thus helping to stabilize the Ghd7 protein (Zheng et al., 2019). OsGI is also a main distinct element between PhyB and PhyA downstream pathways, primarily activated by PhyA but unaffected in the phyB mutant (Lee et al., 2010). As mentioned above, PhyA induces flowering under SD but represses it under LD without other phytochromes (Osugi et al., 2011). One possible reason is that PhyA primarily affects the expression of OsGI under SD and Ghd7 under LD in PhyB deficiency background (Lee et al., 2016) (Figure 4F).
OsLHY
Recently, we demonstrated that OsLHY is a critical circadian rhythm gene in rice. The oslhy mutant delays flowering under LD but induces flowering under SD partly through the OsGI-Hd1 pathway by binding to the CBS element in OsGI promoter (Figure 5A). Moreover, the CDL for OsLHY in oslhy (11-12 h) was prolonged in oslhy osgi double mutant (about 13.5 h), indicating the CDL set by OsLHY was OsGI dependent. Additionally, as the oslhy hd1 double mutant headed simultaneously with hd1 under both SD and LD, the reversible function of OsLHY entirely relied on Hd1. Therefore, the OsLHY-OsGI-Hd1 pathway could fine-tune the CDL through the biological clock (Sun et al., 2021; Sun et al., 2022).
OsELF3
The rice genome carries two ELF3 homologs, OsELF3 (OsELF3-1) and OsELF3-2. OsELF3 can negatively regulate the expression of OsPRR1, OsPRR37, OsPRR73, and OsPRR95, while positively regulating the expression of OsLHY (Zhao et al., 2012; Itoh et al., 2019) (Figure 5B). Though oself3-1 mutant flowered later under both SD and LD, the OsELF3 overexpression transgenic plant showed 6 days earlier and 14 days later heading under LD and SD, respectively (Yang et al., 2013). It is consistent with earlier research about Hd3, which contains the OsELF3 locus. Hd3-NIL plants with Kasalath allele locus shorten flowering time under SD and delay heading under LD (Lin et al., 2000). Then, Hd3 was identified as two tightly linked loci, Hd3a and Hd3b (Monna et al., 2002). Further genetic evidence supports the assumption that the genes for Hd17 (OsELF3-1) and Hd3b are located at the same locus (Matsubara et al., 2012).
The mechanism of flowering time regulation in OsELF3 overexpression plants is still unclear. However, subsequent studies give some hints. Defective OsELF3 did not appear to alter Hd1 expression; however, its effect on flowering time vanished in hd1 background, indicating that OsELF3 may modulate the Hd1 protein activate through a post-transcriptional mechanism (Matsubara et al., 2012; Saito et al., 2012; Zhao et al., 2012). OsELF3 also suppresses the phytochrome signal, prevents light from entering Ghd7 and represses Ghd7 expression (Saito et al., 2012). Recent research further proves this speculation. Compared with se5, the double mutant se5 oself3-1 recovered Ghd7 transcription and the photoperiodic flowering response. However, as Ghd7 expression is not restored by oself3-1 in the phyAphyBphyC triple mutant background, the triple mutant appears to be different from se5 (Itoh et al., 2019) (Figure 5B).
OsPRR37
OsPRR37 encodes a pseudo-response regulator (PRR) protein, functions as a transcriptional repressor of clock genes, and delays flowering time through Ehd1 in an expression level-dependent manner (Koo et al., 2013; Liu et al., 2013; Yan et al., 2013; Gao et al., 2014; Liu et al., 2018). However, OsPRR37 could also function as a flowering promoter in cv. Zhonghua 11 (ZH11) background, mainly by inhibited Ghd7 expression (Hu et al., 2021) (Figure 5C). Furthermore, OsPRR37 could act as an activator or a suppressor depending on the status of three genes, Ghd7, DTH8, and Hd1 (Zhang et al., 2019). For example, in the background of Ghd7DTH8 hd1 and Ghd7 dth8 Hd1 in Zhenshan97, OsPRR37 promoted the heading under nature short day (nSD) but delayed the heading date under nLD (Hu et al., 2021). It has been reported that the OsPRR37 (DTH7) transcript level was decreased in the phyB mutant, suggesting that OsPRR37 might act downstream of PhyB (Gao et al., 2014). Further genetic analysis indicated that the function of OsPRR37 required an intact Ghd7-related regulatory pathway, its upstream regulators OsGI and PhyB, and its interacting partner Hd1 (Hu et al., 2021) (Figure 5C).
Other bifunctional regulators
Hd6 and Hd16
Casein kinases (CKs) function in various eukaryotic signal transduction systems (Knippschild et al., 2005; Mulekar and Huq, 2014). In plants, CKI and CK2 are involved in phosphorylating circadian clock components and regulating flowering time (Sugano et al., 1998; Sugano et al., 1999). Heading date 6 (Hd6) and Heading date 16 (Hd16) encode CK2 alpha and CKI, respectively, are two flowering regulators in rice (Yamamoto et al., 2000; Takahashi et al., 2001; Ogiso et al., 2010). However, Hd6 and Hd16 were not likely to be involved in controlling the circadian clock. Interestingly, one non-synonymous alteration in Nipponbare-NIL (Hd16) shortens flowering time by 20 days under LDs and prolongs heading date by 3 days under SD, which is proved mostly because of the decreased phosphorylation of Ghd7 (Hori et al., 2013; Kwon et al., 2014) (Figure 6A). Additionally, Hd6 and Hd16 also act upstream of OsPRR37 and phosphorylate different regions of OsPRR37 (Kwon et al., 2015). More important, Hd6 and Hd16 could also function as an enhancer of the bifunctional action of Hd1, and fine-tune the CDL of flowering (Figure 6A). Therefore, plants with functioning Hd6 and Hd16 show earlier flowering under 10 h day length, while delayed flowering with 14.5 h photoperiod (Nemoto et al., 2018).
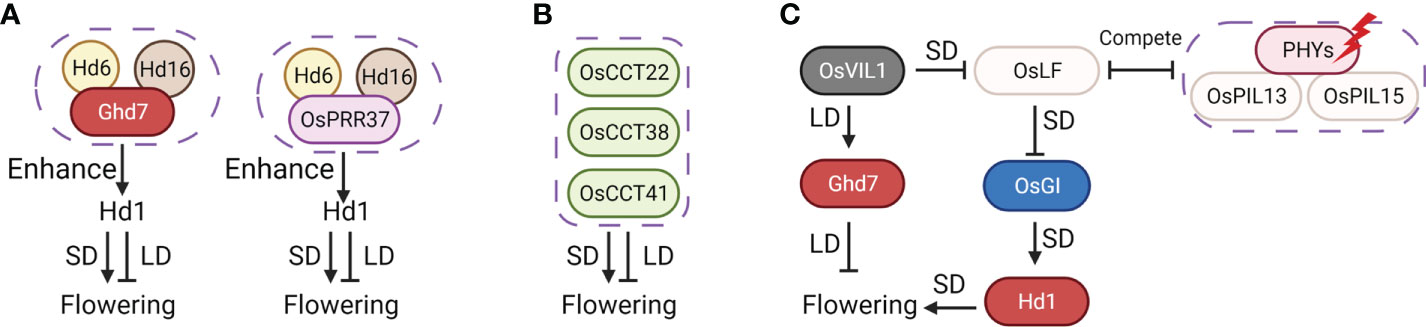
Figure 6 Other bifunctional regulators in rice. (A) Hd6 and Hd16 function as enhancers of the bifunctional action of Hd1, and fine-tune the CDL of flowering. (B) Three new dual-function flowering regulators in CCT domain-containing protein family. (C) OsVIL1 could activate flowering by suppressing OsLF under SD and delay flowering by inducing Ghd7 under LD. Arrows represent positive regulation, inhibitors represent negative regulation, and graphical overlap represents protein physical interaction.
CCT family genes
The CONSTANS (CO), CO-LIKE, and TIMING OF CAB EXPRESSION1 (TOC1) domain-containing protein family (CCT), which was initially discovered in Arabidopsis thaliana, is involved in controlling flowering time (Putterill et al., 1995; Strayer et al., 2000). There are 41 CCT domain-containing genes in rice, and at least 18 have been linked to flowering control, including 6 dual-function flowering regulators: Hd1, Ghd7, OsPRR37, OsCCT22, OsCCT38, and OsCCT41. OsCCT22, OsCCT38, and OsCCT41 suppress heading under LD and promote it under SD, indicating that they could enhance the photoperiod sensitivity of flowering in rice (Zhang et al., 2021) (Figure 6B).
OsVIL1
A specific group of basic helix-loop-helix (bHLH) factors, which are known as phytochrome-interacting factor-like (PIL or PIF) family proteins, are important factors connecting light signal, phytochrome and downstream regulators (Yamashino et al., 2003). OsLF encodes an atypical HLH protein. By competing with OsPRR1 for interaction with Oryza sativa PHYTOCHROME-INTERACTING FACTOR-LIKE 13 (OsPIL13) and Oryza sativa PHYTOCHROME-INTERACTING FACTOR-LIKE 15 (OsPIL15) and repressing the expression of OsGI and Hd1, OsLF inhibits heading in rice (Zhao et al., 2011). Interestingly, Oryza sativa VERNALIZATION INSENSITIVE 3-LIKE 1 (OsVIL1), a component of Polycomb Repressive Complex 2 (PRC2) complex, could activate flowering by suppressing OsLF under SD and delay flowering by inducing Ghd7 under LD, suggesting a relation between histone modification and flowering reversal pathways (Jeong et al., 2016) (Figure 6C).
Conclusions and perspectives
The most consistent environmental characteristic on the earth is day length. Plants employ photoperiods to detect seasonal variations for making proper flowering time. In Arabidopsis, CO functions as a network hub to integrate numerous external and internal signals into the photoperiodic flowering pathway. Identifying and characterizing regulators that physically interact with CO and affect its activity are two important research advances (Shim et al., 2017). In rice, more than 80 flowering regulators have been identified, most of which are involved in the photoperiodic pathway. Thus, the photoperiod might be the most important flowering pathway in rice. As SDPs, rice has a CDL that fixes the schedule for flowering time, limiting the range of cultivation areas (Nemoto et al., 2018). Investigating the CDL control mechanism in rice requires thoroughly studying the functionally reversible gene.
Hd1 is a homolog of CO and was the first bifunctional flowering regulator identified in rice. Numerous studies have shown that the direct effect of light on Hd1 protein complex activity but not the protein stability or transcription level is the primary determinant of photoperiodic flowering. Subsequently, other reversal regulators, such as Ghd7, DTH8, and OsPRR37, have proved to help rice judge photoperiod for flowering (Yano et al., 2000; Ishikawa et al., 2011). Interestingly, reversible factors are not found in more than 300 flowering regulators in Arabidopsis, suggesting a completely different mechanism for sensing day length between rice and Arabidopsis (Bouche et al., 2016). Revealing the molecular mechanism of CDL recognition in rice could unravel the nature of the difference in day length recognition in SDPs and LDPs. These molecular mechanisms could help us transform SD/LD crops into LD/SD crops at the genetic level to expand planting areas as needed to ensure food security.
Several issues need attention in future research to explore the reversal mechanism in the rice flowering pathway. First, there is still a key issue regarding the nature of Hd1 protein reversal activity. Is it a change in Hd1 protein activity due to phosphorylation or a functional inversion due to different protein complexes? What do light, dark, and photoperiod do to Hd1? We still lack the means to detect, for example, Hd1 site-specific phosphorylation antibodies and protein complexes with different functions where Hd1 is located in spatial and temporal features. Second, loss of Hd1 function did not completely abolish rice’s critical day length setting in some background. As mutation of Hd1 could not cause OsPRR37 and Hd16 to lose their flowering inversion function, other reversal mechanisms independent of Hd1 in rice should be explored in the future. Third, many flowering genes have more than one interacting factor. Polygenic mutants construction is much more difficult and time-consuming than Arabidopsis, which makes genetic analysis more difficult. Fourth, according to our previous study, different bi-functional genes have different reverse CDLs (Sun et al., 2021). Although many bi-functional regulators have been reported, most studies did not examine their CDLs. Identifying these CDL regulation pathways will unravel the molecular mechanisms of CDL regulation in the future. Finally, though an atypical HLH protein OsLF was identified as a flowering regulator in rice, there is still less identification of physical or direct interacting regulators with phytochrome. Which genes play the role of PIL/PIF and to which downstream genes the day length signal transmitted, leading to the mechanism for CDL recognition, are far from fully understood.
Author contributions
CS, CH, CZ, and XD wrote the paper. All authors contributed to the article and approved the submitted version.
Funding
This study was supported by grants from the National Natural Science Foundation of China (32172022, 31371602, and 31401358) and Sichuan Science and Technology Program (2020YJ0408).
Acknowledgments
All the figures were created with BioRender.com.
Conflict of interest
The authors declare that the research was conducted in the absence of any commercial or financial relationships that could be construed as a potential conflict of interest.
Publisher’s Note
All claims expressed in this article are solely those of the authors and do not necessarily represent those of their affiliated organizations, or those of the publisher, the editors and the reviewers. Any product that may be evaluated in this article, or claim that may be made by its manufacturer, is not guaranteed or endorsed by the publisher.
References
Amasino, R. (2010). Seasonal and developmental timing of flowering. Plant J. 61 (6), 1001–1013. doi: 10.1111/j.1365-313X.2010.04148.x
Andres, F., Coupland, G. (2012). The genetic basis of flowering responses to seasonal cues. Nat. Rev. Genet. 13 (9), 627–639. doi: 10.1038/nrg3291
Andres, F., Galbraith, D. W., Talon, M., Domingo, C. (2009). Analysis of PHOTOPERIOD SENSITIVITY5 sheds light on the role of phytochromes in photoperiodic flowering in rice. Plant Physiol. 151 (2), 681–690. doi: 10.1104/pp.109.139097
Anwer, M. U., Davis, S. J. (2013). An overview of natural variation studies in the Arabidopsis thaliana circadian clock. Semin. Cell Dev. Biol. 24 (5), 422–429. doi: 10.1016/j.semcdb.2013.03.006
Bae, G., Choi, G. (2008). Decoding of light signals by plant phytochromes and their interacting proteins. Annu. Rev. Plant Biol. 59, 281–311. doi: 10.1146/annurev.arplant.59.032607.092859
Bouche, F., Lobet, G., Tocquin, P., Perilleux, C. (2016). FLOR-ID: an interactive database of flowering-time gene networks in arabidopsis thaliana. Nucleic Acids Res. 44 (D1), D1167–D1171. doi: 10.1093/nar/gkv1054
Bunning, E. (1936). Die endogene tagesrhythmik als grundlage der photoperiodischen reaktion. Berichte der deutschen botanischen Gesellschaft 54, 590–607. doi: 10.1111/j.1438-8677.1937.tb01941.x
Cao, S., Luo, X., Xu, D., Tian, X., Song, J., Xia, X., et al. (2021). Genetic architecture underlying light and temperature mediated flowering in arabidopsis, rice, and temperate cereals. New Phytol. 230 (5), 1731–1745. doi: 10.1111/nph.17276
Chen, R., Deng, Y., Ding, Y., Guo, J., Qiu, J., Wang, B., et al. (2022). Rice functional genomics: decades' efforts and roads ahead. Sci. China Life Sci. 65 (1), 33–92. doi: 10.1007/s11427-021-2024-0
Cheng, M. C., Kathare, P. K., Paik, I., Huq, E. (2021). Phytochrome signaling networks. Annu. Rev. Plant Biol. 72, 217–244. doi: 10.1146/annurev-arplant-080620-024221
Cho, L. H., Yoon, J., Pasriga, R., An, G. (2016). Homodimerization of Ehd1 is required to induce flowering in rice. Plant Physiol. 170 (4), 2159–2171. doi: 10.1104/pp.15.01723
Dai, X., Ding, Y., Tan, L., Fu, Y., Liu, F., Zhu, Z., et al. (2012). LHD1, an allele of DTH8/Ghd8, controls late heading date in common wild rice (Oryza rufipogon). J. Integr. Plant Biol. 54 (10), 790–799. doi: 10.1111/j.1744-7909.2012.01166.x
Doi, K., Izawa, T., Fuse, T., Yamanouchi, U., Kubo, T., Shimatani, Z., et al. (2004). Ehd1, a b-type response regulator in rice, confers short-day promotion of flowering and controls FT-like gene expression independently of Hd1. Genes Dev. 18 (8), 926–936. doi: 10.1101/gad.1189604
Du, A., Tian, W., Wei, M., Yan, W., He, H., Zhou, D., et al. (2017). The DTH8-Hd1 module mediates day-Length-Dependent regulation of rice flowering. Mol. Plant 10 (7), 948–961. doi: 10.1016/j.molp.2017.05.006
Eichenberg, K., Bäurle, I., Paulo, N., Sharrock, R. A., Rüdiger, W., Schäfer, E. (2000). Arabidopsis phytochromes c and e have different spectral characteristics from those of phytochromes a and b. FEBS Lett. 470 (2), 107–112. doi: 10.1016/S0014-5793(00)01301-6
Elich, T. D., Chory, J. (1997). Biochemical characterization of arabidopsis wild-type and mutant phytochrome b holoproteins. Plant Cell 9 (12), 2271–2280. doi: 10.1105/tpc.9.12.2271
Fankhauser, C. (2001). The phytochromes, a family of red/far-red absorbing photoreceptors. J. Biol. Chem. 276 (15), 11453–11456. doi: 10.1074/jbc.R100006200
Fujino, K., Yamanouchi, U., Yano, M. (2013). Roles of the Hd5 gene controlling heading date for adaptation to the northern limits of rice cultivation. Theor. Appl. Genet. 126 (3), 611–618. doi: 10.1007/s00122-012-2005-5
Gao, H., Jin, M., Zheng, X. M., Chen, J., Yuan, D., Xin, Y., et al. (2014). Days to heading 7, a major quantitative locus determining photoperiod sensitivity and regional adaptation in rice. Proc. Natl. Acad. Sci. U.S.A. 111 (46), 16337–16342. doi: 10.1073/pnas.1418204111
Garner, W. W., Allard, H. A. (1920). Effect of the relative length of day and night and other factors of the environment on growth and reproduction in plants. Monthly Weather Rev. 48 (7), 415–415. doi: 10.1175/1520-0493(1920)48<415b:EOTRLO>2.0.CO;2
Garner, W. W., Allard, H. A. (1923). Further studies in photoperiodism: the response of the plant to relative length of day and night (US Government Printing Office).
Hamner, K. C., Bonner, J. (1938). Photoperiodism in relation to hormones as factors in floral initiation and development. Botanical Gazette 100 (2), 388–431. doi: 10.1086/334793
Hayama, R., Coupland, G. (2004). The molecular basis of diversity in the photoperiodic flowering responses of arabidopsis and rice. Plant Physiol. 135 (2), 677–684. doi: 10.1104/pp.104.042614
Hayama, R., Yokoi, S., Tamaki, S., Yano, M., Shimamoto, K. (2003). Adaptation of photoperiodic control pathways produces short-day flowering in rice. Nature 422 (6933), 719–722. doi: 10.1038/nature01549
Hori, K., Ogiso-Tanaka, E., Matsubara, K., Yamanouchi, U., Ebana, K., Yano, M. (2013). Hd16, a gene for casein kinase I, is involved in the control of rice flowering time by modulating the day-length response. Plant J. 76 (1), 36–46. doi: 10.1111/tpj.12268
Hu, W., Franklin, K. A., Sharrock, R. A., Jones, M. A., Harmer, S. L., Lagarias, J. C. (2013). Unanticipated regulatory roles for arabidopsis phytochromes revealed by null mutant analysis. Proc. Natl. Acad. Sci. U.S.A. 110 (4), 1542–1547. doi: 10.1073/pnas.1221738110
Hu, Y., Zhou, X., Zhang, B., Li, S., Fan, X., Zhao, H., et al. (2021). OsPRR37 alternatively promotes heading date through suppressing the expression of Ghd7 in the japonica variety zhonghua 11 under natural long-day conditions. Rice (N Y) 14 (1), 20. doi: 10.1186/s12284-021-00464-1
Imaizumi, T., Kay, S. A. (2006). Photoperiodic control of flowering: not only by coincidence. Trends Plant Sci. 11 (11), 550–558. doi: 10.1016/j.tplants.2006.09.004
Ishikawa, R., Aoki, M., Kurotani, K., Yokoi, S., Shinomura, T., Takano, M., et al. (2011). Phytochrome b regulates Heading date 1 (Hd1)-mediated expression of rice florigen Hd3a and critical day length in rice. Mol. Genet. Genomics 285 (6), 461–470. doi: 10.1007/s00438-011-0621-4
Ishikawa, R., Shinomura, T., Takano, M., Shimamoto, K. (2009). Phytochrome dependent quantitative control of Hd3a transcription is the basis of the night break effect in rice flowering. Genes Genet. Syst. 84 (2), 179–184. doi: 10.1266/ggs.84.179
Itoh, H., Nonoue, Y., Yano, M., Izawa, T. (2010). A pair of floral regulators sets critical day length for Hd3a florigen expression in rice. Nat. Genet. 42 (7), 635–638. doi: 10.1038/ng.606
Itoh, H., Tanaka, Y., Izawa, T. (2019). Genetic relationship between phytochromes and OsELF3-1 reveals the mode of regulation for the suppression of phytochrome signaling in rice. Plant Cell Physiol. 60 (3), 549–561. doi: 10.1093/pcp/pcy225
Izawa, T. (2007). Daylength measurements by rice plants in photoperiodic short-day flowering. Int. Rev. Cytology - Survey Cell Biol. 256, 191–19+. doi: 10.1016/S0074-7696(07)56006-7
Izawa, T., Mihara, M., Suzuki, Y., Gupta, M., Itoh, H., Nagano, A. J., et al. (2011). Os-GIGANTEA confers robust diurnal rhythms on the global transcriptome of rice in the field. Plant Cell 23 (5), 1741–1755. doi: 10.1105/tpc.111.083238
Izawa, T., Oikawa, T., Sugiyama, N., Tanisaka, T., Yano, M., Shimamoto, K. (2002). Phytochrome mediates the external light signal to repress FT orthologs in photoperiodic flowering of rice. Genes Dev. 16 (15), 2006–2020. doi: 10.1101/gad.999202
Izawa, T., Oikawa, T., Tokutomi, S., Okuno, K., Shimamoto, K. (2000). Phytochromes confer the photoperiodic control of flowering in rice (a short-day plant). Plant J. 22 (5), 391–399. doi: 10.1046/j.1365-313x.2000.00753.x
Jeong, H. J., Yang, J., Cho, L. H., An, G. (2016). OsVIL1 controls flowering time in rice by suppressing OsLF under short days and by inducing Ghd7 under long days. Plant Cell Rep. 35 (4), 905–920. doi: 10.1007/s00299-015-1931-5
Klose, C., Viczian, A., Kircher, S., Schafer, E., Nagy, F. (2015). Molecular mechanisms for mediating light-dependent nucleo/cytoplasmic partitioning of phytochrome photoreceptors. New Phytol. 206 (3), 965–971. doi: 10.1111/nph.13207
Knippschild, U., Gocht, A., Wolff, S., Huber, N., Lohler, J., Stoter, M. (2005). The casein kinase 1 family: participation in multiple cellular processes in eukaryotes. Cell Signal 17 (6), 675–689. doi: 10.1016/j.cellsig.2004.12.011
Komiya, R., Ikegami, A., Tamaki, S., Yokoi, S., Shimamoto, K. (2008). Hd3a and RFT1 are essential for flowering in rice. Development 135 (4), 767–774. doi: 10.1242/dev.008631
Koo, B. H., Yoo, S. C., Park, J. W., Kwon, C. T., Lee, B. D., An, G., et al. (2013). Natural variation in OsPRR37 regulates heading date and contributes to rice cultivation at a wide range of latitudes. Mol. Plant 6 (6), 1877–1888. doi: 10.1093/mp/sst088
Kwon, C. T., Koo, B. H., Kim, D., Yoo, S. C., Paek, N. C. (2015). Casein kinases I and 2alpha phosphorylate oryza sativa pseudo-response regulator 37 (OsPRR37) in photoperiodic flowering in rice. Mol. Cells 38 (1), 81–88. doi: 10.14348/molcells.2015.2254
Kwon, C. T., Yoo, S. C., Koo, B. H., Cho, S. H., Park, J. W., Zhang, Z., et al. (2014). Natural variation in Early flowering1 contributes to early flowering in japonica rice under long days. Plant Cell Environ. 37 (1), 101–112. doi: 10.1111/pce.12134
Lee, Y. S., An, G. (2015). OsGI controls flowering time by modulating rhythmic flowering time regulators preferentially under short day in rice. J. Plant Biol. 58 (2), 137–145. doi: 10.1007/s12374-015-0007-y
Lee, Y. S., Jeong, D. H., Lee, D. Y., Yi, J., Ryu, C. H., Kim, S. L., et al. (2010). OsCOL4 is a constitutive flowering repressor upstream of Ehd1 and downstream of OsphyB. Plant J. 63 (1), 18–30. doi: 10.1111/j.1365-313X.2010.04226.x
Lee, Y. S., Yi, J., An, G. (2016). OsPhyA modulates rice flowering time mainly through OsGI under short days and Ghd7 under long days in the absence of phytochrome b. Plant Mol. Biol. 91 (4-5), 413–427. doi: 10.1007/s11103-016-0474-7
Li, X., Liu, H., Wang, M., Liu, H., Tian, X., Zhou, W., et al. (2015b). Combinations of Hd2 and Hd4 genes determine rice adaptability to heilongjiang province, northern limit of China. J. Integr. Plant Biol. 57 (8), 698–707. doi: 10.1111/jipb.12326
Li, J., Li, G., Wang, H., Wang Deng, X. (2011). Phytochrome signaling mechanisms. Arabidopsis Book 9, e0148. doi: 10.1199/tab.0148
Li, F. W., Melkonian, M., Rothfels, C. J., Villarreal, J. C., Stevenson, D. W., Graham, S. W., et al. (2015a). Phytochrome diversity in green plants and the origin of canonical plant phytochromes. Nat. Commun. 6 (1), 1–12. doi: 10.1038/ncomms8852
Lin, H. X., Yamamoto, T., Sasaki, T., Yano, M. (2000). Characterization and detection of epistatic interactions of 3 QTLs, Hd1, Hd2, and Hd3,controlling heading date in rice using nearly isogenic lines. Theor. Appl. Genet. 101 (7), 1021–1028. doi: 10.1007/s001220051576
Liu, T., Liu, H., Zhang, H., Xing, Y. (2013). Validation and characterization of Ghd7.1, a major quantitative trait locus with pleiotropic effects on spikelets per panicle, plant height, and heading date in rice (Oryza sativa l.). J. Integr. Plant Biol. 55 (10), 917–927. doi: 10.1111/jipb.12070
Liu, C., Qu, X., Zhou, Y., Song, G., Abiri, N., Xiao, Y., et al. (2018). OsPRR37 confers an expanded regulation of the diurnal rhythms of the transcriptome and photoperiodic flowering pathways in rice. Plant Cell Environ. 41 (3), 630–645. doi: 10.1111/pce.13135
Mancinelli, A. (1994). “The physiology of phytochrome action,” in Photomorphogenesis in plants. Eds. Kendrick, R. E., Kronenberg, G. H. M. (The Netherlands: Kluwer Academic Publishers).
Mathews, S., Sharrock, R. (1997). Phytochrome gene diversity. Plant Cell Environ. 20 (6), 666–671. doi: 10.1046/j.1365-3040.1997.d01-117.x
Matsubara, K., Ogiso-Tanaka, E., Hori, K., Ebana, K., Ando, T., Yano, M. (2012). Natural variation in Hd17, a homolog of Arabidopsis ELF3 that is involved in rice photoperiodic flowering. Plant Cell Physiol. 53 (4), 709–716. doi: 10.1093/pcp/pcs028
Monna, L., Lin, X., Kojima, S., Sasaki, T., Yano, M. (2002). Genetic dissection of a genomic region for a quantitative trait locus, Hd3, into two loci, Hd3a and Hd3b, controlling heading date in rice. Theor. Appl. Genet. 104 (5), 772–778. doi: 10.1007/s00122-001-0813-0
Mulekar, J. J., Huq, E. (2014). Expanding roles of protein kinase CK2 in regulating plant growth and development. J. Exp. Bot. 65 (11), 2883–2893. doi: 10.1093/jxb/ert401
Nemoto, Y., Hori, K., Izawa, T. (2018). Fine-tuning of the setting of critical day length by two casein kinases in rice photoperiodic flowering. J. Exp. Bot. 69 (3), 553–565. doi: 10.1093/jxb/erx412
Nemoto, Y., Nonoue, Y., Yano, M., Izawa, T. (2016). Hd1,a CONSTANS ortholog in rice, functions as an Ehd1 repressor through interaction with monocot-specific CCT-domain protein Ghd7. Plant J. 86 (3), 221–233. doi: 10.1111/tpj.13168
Nishida, H., Inoue, H., Okumoto, Y., Tanisaka, T. (2002). A novel gene ef1-h conferring an extremely long basic vegetative growth period in rice. Crop Sci. 42 (2), 348–354. doi: 10.2135/cropsci2002.3480
Ogiso, E., Takahashi, Y., Sasaki, T., Yano, M., Izawa, T. (2010). The role of casein kinase II in flowering time regulation has diversified during evolution. Plant Physiol. 152 (2), 808–820. doi: 10.1104/pp.109.148908
Osugi, A., Itoh, H., Ikeda-Kawakatsu, K., Takano, M., Izawa, T. (2011). Molecular dissection of the roles of phytochrome in photoperiodic flowering in rice. Plant Physiol. 157 (3), 1128–1137. doi: 10.1104/pp.111.181792
Pham, V. N., Kathare, P. K., Huq, E. (2018). Phytochromes and phytochrome interacting factors. Plant Physiol. 176 (2), 1025–1038. doi: 10.1104/pp.17.01384
Putterill, J., Robson, F., Lee, K., Simon, R., Coupland, G. (1995). The CONSTANS gene of arabidopsis promotes flowering and encodes a protein showing similarities to zinc finger transcription factors. Cell 80 (6), 847–857. doi: 10.1016/0092-8674(95)90288-0
Quail, P. (1997). An emerging molecular map of the phytochromes. Plant Cell Environ. 20 (6), 657–665. doi: 10.1046/j.1365-3040.1997.d01-108.x
Saito, H., Ogiso-Tanaka, E., Okumoto, Y., Yoshitake, Y., Izumi, H., Yokoo, T., et al. (2012). Ef7 encodes an ELF3-like protein and promotes rice flowering by negatively regulating the floral repressor gene Ghd7 under both short- and long-day conditions. Plant Cell Physiol. 53 (4), 717–728. doi: 10.1093/pcp/pcs029
Sharrock, R. A., Quail, P. H. (1989). Novel phytochrome sequences in arabidopsis thaliana: structure, evolution, and differential expression of a plant regulatory photoreceptor family. Genes Dev. 3 (11), 1745–1757. doi: 10.1101/gad.3.11.1745
Shim, J. S., Kubota, A., Imaizumi, T. (2017). Circadian clock and photoperiodic flowering in arabidopsis: CONSTANS is a hub for signal integration. Plant Physiol. 173 (1), 5–15. doi: 10.1104/pp.16.01327
Song, Y. H., Shim, J. S., Kinmonth-Schultz, H. A., Imaizumi, T. (2015). Photoperiodic flowering: time measurement mechanisms in leaves. Annu. Rev. Plant Biol. 66, 441–464. doi: 10.1146/annurev-arplant-043014-115555
Strasser, B., Sanchez-Lamas, M., Yanovsky, M. J., Casal, J. J., Cerdan, P. D. (2010). Arabidopsis thaliana life without phytochromes. Proc. Natl. Acad. Sci. U.S.A. 107 (10), 4776–4781. doi: 10.1073/pnas.0910446107
Strayer, C., Oyama, T., Schultz, T. F., Raman, R., Somers, D. E., Mas, P., et al. (2000). Cloning of the arabidopsis clock gene TOC1, an autoregulatory response regulator homolog. Science 289 (5480), 768–771. doi: 10.1126/science.289.5480.768
Sugano, S., Andronis, C., Green, R. M., Wang, Z. Y., Tobin, E. M. (1998). Protein kinase CK2 interacts with and phosphorylates the arabidopsis circadian clock-associated 1 protein. Proc. Natl. Acad. Sci. U.S.A. 95 (18), 11020–11025. doi: 10.1073/pnas.95.18.11020
Sugano, S., Andronis, C., Ong, M. S., Green, R. M., Tobin, E. M. (1999). The protein kinase CK2 is involved in regulation of circadian rhythms in arabidopsis. Proc. Natl. Acad. Sci. U.S.A. 96 (22), 12362–12366. doi: 10.1073/pnas.96.22.12362
Sun, C., Chen, D., Fang, J., Wang, P., Deng, X., Chu, C. (2014). Understanding the genetic and epigenetic architecture in complex network of rice flowering pathways. Protein Cell 5 (12), 889–898. doi: 10.1007/s13238-014-0068-6
Sun, C., Liu, S., He, C., Zhong, C., Liu, H., Luo, X., et al. (2022). Crosstalk between the circadian clock and histone methylation. Int. J. Mol. Sci. 23 (12), 6465. doi: 10.3390/ijms23126465
Sun, C., Zhang, K., Zhou, Y., Xiang, L., He, C., Zhong, C., et al. (2021). Dual function of clock component OsLHY sets critical day length for photoperiodic flowering in rice. Plant Biotechnol. J. 19 (8), 1644–1657. doi: 10.1111/pbi.13580
Takahashi, Y., Shomura, A., Sasaki, T., Yano, M. (2001). Hd6, a rice quantitative trait locus involved in photoperiod sensitivity, encodes the alpha subunit of protein kinase CK2. Proc. Natl. Acad. Sci. U.S.A. 98 (14), 7922–7927. doi: 10.1073/pnas.111136798
Takano, M., Inagaki, N., Xie, X., Yuzurihara, N., Hihara, F., Ishizuka, T., et al. (2005). Distinct and cooperative functions of phytochromes a, b, and c in the control of deetiolation and flowering in rice. Plant Cell 17 (12), 3311–3325. doi: 10.1105/tpc.105.035899
Thomas, B. (1998). Photoperiodism: an overview. In Lumsden, P. J., Millar, A. J.. Biological rhythms and photoperiodism in plants, 151–165. (Oxford: BIOS Scientific Publishers Ltd).
Van Buskirk, E. K., Decker, P. V., Chen, M. (2012). Photobodies in light signaling. Plant Physiol. 158 (1), 52–60. doi: 10.1104/pp.111.186411
Wang, X., He, Y., Wei, H., Wang, L. (2021a). A clock regulatory module is required for salt tolerance and control of heading date in rice. Plant Cell Environ. 44 (10), 3283–3301. doi: 10.1111/pce.14167
Wang, X., Zhou, P., Huang, R., Zhang, J., Ouyang, X. (2021b). A daylength recognition model of photoperiodic flowering. Front. Plant Sci. 12. doi: 10.3389/fpls.2021.778515
Wei, X., Xu, J., Guo, H., Jiang, L., Chen, S., Yu, C., et al. (2010). DTH8 suppresses flowering in rice, influencing plant height and yield potential simultaneously. Plant Physiol. 153 (4), 1747–1758. doi: 10.1104/pp.110.156943
Xue, W., Xing, Y., Weng, X., Zhao, Y., Tang, W., Wang, L., et al. (2008). Natural variation in Ghd7 is an important regulator of heading date and yield potential in rice. Nat. Genet. 40 (6), 761–767. doi: 10.1038/ng.143
Xu, X., Paik, I., Zhu, L., Huq, E. (2015). Illuminating progress in phytochrome-mediated light signaling pathways. Trends Plant Sci. 20 (10), 641–650. doi: 10.1016/j.tplants.2015.06.010
Yamamoto, T., Lin, H., Sasaki, T., Yano, M. (2000). Identification of heading date quantitative trait locus Hd6 and characterization of its epistatic interactions with Hd2 in rice using advanced backcross progeny. Genetics 154 (2), 885–891. doi: 10.1093/genetics/154.2.885
Yamashino, T., Matsushika, A., Fujimori, T., Sato, S., Kato, T., Tabata, S., et al. (2003). A link between circadian-controlled bHLH factors and the APRR1/TOC1 quintet in arabidopsis thaliana. Plant Cell Physiol. 44 (6), 619–629. doi: 10.1093/pcp/pcg078
Yang, Y., Peng, Q., Chen, G. X., Li, X. H., Wu, C. Y. (2013). OsELF3 is involved in circadian clock regulation for promoting flowering under long-day conditions in rice. Mol. Plant 6 (1), 202–215. doi: 10.1093/mp/sss062
Yan, W., Liu, H., Zhou, X., Li, Q., Zhang, J., Lu, L., et al. (2013). Natural variation in Ghd7.1 plays an important role in grain yield and adaptation in rice. Cell Res. 23 (7), 969–971. doi: 10.1038/cr.2013.43
Yano, M., Katayose, Y., Ashikari, M., Yamanouchi, U., Monna, L., Fuse, T., et al. (2000). Hd1, a major photoperiod sensitivity quantitative trait locus in rice, is closely related to the arabidopsis flowering time gene CONSTANS. Plant Cell 12 (12), 2473–2484. doi: 10.1105/tpc.12.12.2473
Yano, M., Kojima, S., Takahashi, Y., Lin, H., Sasaki, T. (2001). Genetic control of flowering time in rice, a short-day plant. Plant Physiol. 127 (4), 1425–1429. doi: 10.1104/pp.010710
Yanovsky, M. J., Kay, S. A. (2002). Molecular basis of seasonal time measurement in Arabidopsis. Nature 419 (6904), 308–312. doi: 10.1038/nature00996
Yanovsky, M. J., Kay, S. A. (2003). Living by the calendar: how plants know when to flower. Nat. Rev. Mol. Cell Biol. 4 (4), 265–275. doi: 10.1038/nrm1077
Yan, W. H., Wang, P., Chen, H. X., Zhou, H. J., Li, Q. P., Wang, C. R., et al. (2011). A major QTL, Ghd8, plays pleiotropic roles in regulating grain productivity, plant height, and heading date in rice. Mol. Plant 4 (2), 319–330. doi: 10.1093/mp/ssq070
Wang, P., Gong, R., Yang, Y., Yu, S (2019). Ghd8 regulates rice photosensitivity by forming a complex that interacts with Ghd7. BMC plant biology 19 (1), 1–14 doi: 10.1186/s12870-019-2053-y
Zhang, J., Fan, X., Hu, Y., Zhou, X., He, Q., Liang, L., et al. (2021). Global analysis of CCT family knockout mutants identifies four genes involved in regulating heading date in rice. J. Integr. Plant Biol. 63 (5), 913–923. doi: 10.1111/jipb.13013
Zhang, Z., Hu, W., Shen, G., Liu, H., Hu, Y., Zhou, X., et al. (2017). Alternative functions of Hd1 in repressing or promoting heading are determined by Ghd7 status under long-day conditions. Sci. Rep. 7 (1), 5388. doi: 10.1038/s41598-017-05873-1
Zhang, B., Liu, H., Qi, F., Zhang, Z., Li, Q., Han, Z., et al. (2019). Genetic interactions among Ghd7, Ghd8, OsPRR37 and Hd1 contribute to Large variation in heading date in rice. Rice (N Y) 12 (1), 48. doi: 10.1186/s12284-019-0314-x
Zhao, J., Huang, X., Ouyang, X., Chen, W., Du, A., Zhu, L., et al. (2012). OsELF3-1, an ortholog of Arabidopsis early flowering 3, regulates rice circadian rhythm and photoperiodic flowering. PloS One 7 (8), e43705. doi: 10.1371/journal.pone.0043705
Zhao, X. L., Shi, Z. Y., Peng, L. T., Shen, G. Z., Zhang, J. L. (2011). An atypical HLH protein OsLF in rice regulates flowering time and interacts with OsPIL13 and OsPIL15. N Biotechnol. 28 (6), 788–797. doi: 10.1016/j.nbt.2011.04.006
Zheng, T., Sun, J., Zhou, S., Chen, S., Lu, J., Cui, S., et al. (2019). Post-transcriptional regulation of Ghd7 protein stability by phytochrome and OsGI in photoperiodic control of flowering in rice. New Phytol. 224 (1), 306–320. doi: 10.1111/nph.16010
Keywords: flowering, photoperiod, bifunctional regulators, critical day length, phytochrome, circadian clock
Citation: Sun C, He C, Zhong C, Liu S, Liu H, Luo X, Li J, Zhang Y, Guo Y, Yang B, Wang P and Deng X (2022) Bifunctional regulators of photoperiodic flowering in short day plant rice. Front. Plant Sci. 13:1044790. doi: 10.3389/fpls.2022.1044790
Received: 15 September 2022; Accepted: 07 October 2022;
Published: 20 October 2022.
Edited by:
Liang Wu, Zhejiang University, ChinaReviewed by:
Liangyu Liu, Capital Normal University, ChinaQingyun Bu, Chinese Academy of Sciences (CAS), China
Copyright © 2022 Sun, He, Zhong, Liu, Liu, Luo, Li, Zhang, Guo, Yang, Wang and Deng. This is an open-access article distributed under the terms of the Creative Commons Attribution License (CC BY). The use, distribution or reproduction in other forums is permitted, provided the original author(s) and the copyright owner(s) are credited and that the original publication in this journal is cited, in accordance with accepted academic practice. No use, distribution or reproduction is permitted which does not comply with these terms.
*Correspondence: Changhui Sun, c3VuaHVpMDMwN0AxNjMuY29t; Xiaojian Deng, eGpkZW5nQHNpY2F1LmVkdS5jbg==
†These authors have contributed equally to this work