- 1Key Laboratory of National Forestry and Grassland Administration for Orchid Conservation and Utilization at College of Landscape Architecture, Fujian Agriculture and Forestry University, Fuzhou, China
- 2College of Life Sciences, Fujian Normal University, Fuzhou, China
Pollinators are attracted to vibrant flower colors. That is why flower color is the key agent to allow successful fruit set in food or ornamental crops. However, black flower color is the least attractive to pollinators, although a number of plant species produce black flowers. Cyanidin-based anthocyanins are thought to be the key agents to induce black color in the ornamental and fruit crops. R2R3-MYB transcription factors (TFs) play key roles for the tissue-specific accumulation of anthocyanin. MYB1 and MYB11 are the key TFs regulating the expression of anthocyanin biosynthesis genes for black color accumulation. Post-transcriptional silencing of flavone synthase II (FNS) gene is the technological method to stimulate the accumulation of cyanidin-based anthocyanins in black cultivars. Type 1 promoter of DvIVS takes the advantage of FNS silencing to produce large amounts of black anthocyanins. Exogenous ethylene application triggers anthocyanin accumulation in the fruit skin at ripening. Environment cues have been the pivotal regulators to allow differential accumulation of anthocyanins to regulate black color. Heat stress is one of the most important environmental stimulus that regulates concentration gradient of anthocyanins in various plant parts, thereby affecting the color pattern of flowers. Stability of black anthocyanins in the extreme environments can save the damage, especially in fruits, caused by abiotic stress. White flowers without anthocyanin face more damages from abiotic stress than dark color flowers. The intensity and pattern of flower color accumulation determine the overall fruit set, thereby controlling crop yield and human food needs. This review paper presents comprehensive knowledge of black flower regulation as affected by high temperature stress, and the molecular regulators of anthocyanin for black color in ornamental and food crops. It also discusses the black color-pollination interaction pattern affected by heat stress for food and ornamental crops.
Introduction
Flower color is one of the most conspicuous attributes of angiosperms. Since antiquity, flower petal color has been the key to pollinator attraction. Although most of the angiosperms produce vibrant color flowers that are more attractive to pollinators, a few species generate black color in the flowers. Apparently, the black color is not much attractive to the pollinators, but it is not out of need. Both plants and pollinators are benefitted by black color. Heat stress and high temperature are closely associated with black color impacts on plants, pollinators, and pollination strategies. The most prevalent pigments to paint flowers black are the anthocyanins.
Anthocyanins are widely distributed in nature (Wu and Prior, 2005) and give attractive colors to flowers, grains, and fruits (Kong et al., 2003; Escribano-Bailón et al., 2004). Anthocyanins are important chemotaxonomic and quality indicators in plants and their antioxidant ability gains much interest for health (Kong et al., 2003; Março and Scarminio, 2007; Salehi et al., 2020; Paun et al., 2022). They are helpful to cure age-induced oxidative stress, cardiovascular disorders, and inflammatory responses (Hassellund et al., 2013). It is believed that anthocyanin is synthesized at cytosolic surface of endoplasmic reticulum (ER), and it accumulates in the vacuole (Han et al., 2022). MRP (multidrug resistance-associated protein), MATE (multidrug and toxic compound extrusion), and GST (glutathione S-transferase) are mainly responsible for the transport of anthocyanin from cytoplasm to vacuole (Hu et al., 2016; Han et al., 2022).
Anthocyanins are sugar-containing equivalents (3-glucosides) of anthocyanidins (Mekapogu et al., 2020). They are water-soluble glycosides and acylglycosides derived from anthocyanidins (Wu et al., 2004). Anthocyanidins possess two aromatic benzene rings which are separated by an oxygenated heterocycle (Noda et al., 2000; Mekapogu et al., 2020). Petal color is mainly determined by the number of hydroxyl groups in the B-ring. An increase in hydroxyl groups causes color shift to blue (Noda et al., 2000). Six anthocyanidins are widely distributed in vegetables and fruits, including malvidin, petunidin, peonidin, cyanidin, delphinidin, and pelargonidin (de Pascual-Teresa et al., 2002; Di Paola-Naranjo et al., 2004; Lohachoompol et al., 2008). The most abundant anthocyanidins in flowers include pelargonidin, delphinidin, and cyanidin (Mekapogu et al., 2020). Cyanidins usually impart magenta (reddish-purple) color and delphinidins appear purple or blue-red (Yang et al., 2022). Cyanidin causes purple-red color in chrysanthemum flowers (Kawase and Tsukamoto, 1976; Noda et al., 2000). Violet transgenic flowers of chrysanthemum are due to delphinidins, such as delphinidin 3-(3″,6″-dimalonyl) glucoside and delphinidin 3-(6″-malonyl) glucoside (Noda et al., 2013).
High accumulation of cyanidin-based anthocyanins is responsible for black color in ornamental crops (Deguchi et al., 2013, 2015). Three TF families (MYBs, bHLHs, and MBW) regulate the genes involving anthocyanin biosynthesis (Zhao et al., 2013). The PeMYB11 is the major R2R3-MYB TF that regulates the black color production (Hsu et al., 2019). The FNS and IVS are the key genes involving the biosynthesis and regulation of black anthocyanins. Non-pigmented flowers face more damages from abiotic stress than pigmented flowers. Dark color flowers get more favor in the dry conditions than light color flowers (Sullivan and Koski, 2021). High temperature upregulates the expression of most of the anthocyanin biosynthesis genes (Zhang et al., 2019). Moreover, dark petal color increases the internal flower temperature (Tikhomirov et al., 1960), attracting more pollinators during winter. Therefore, this review curtails the black color regulation by anthocyanins, the impact of black color on plant-pollinator interactions and the association of temperature fluctuations with color intensity.
Impact of High Temperature on Anthocyanin Gradient and Color Formation
Biosynthesis of anthocyanins is affected by biotic and abiotic factors, such as nutrients, light, water stress, and temperature (Ubi, 2004). High temperature affects the biosynthesis of anthocyanins. The biosynthesis pathway of anthocyanin can be divided in two phases. The early biosynthesis is regulated by genes such as CHS (chalcone synthase), CHI (chalcone isomerase), F3H (flavanone 3-hydroxylase), F3′H (flavanone-3′-hydroxylase) and F3′5′H (flavanone-3′5′-hydroxylase). The late biosynthesis is regulated by genes such as ANS (anthocyanin synthase), DFR, and UGFT (UDP-glycose: flavonoid 3-O-glycosyltransferase; Figure 1A). High temperature (35°C) significantly upregulates the expression of PAL1, ANS, 3GT, CHS2, UA5, DF4R, CHI, UA3GT2, and UA3GHT5, causing increase in anthocyanin contents in strawberries (Zhang et al., 2019; Figure 1B). High storage temperature improves UA3GT2, a UDP-glucose: anthocyanidin 3-O-glucosyltransferase (UGAT) gene correlated with high-temperature induced anthocyanin accumulation (Zhang et al., 2019). Recently, MATE TT12 is thought to involve cross-membrane anthocyanin transportation in strawberry, cotton and radish (Gao et al., 2016; Chen et al., 2018; M’mbone et al., 2018). High temperature upregulated the expression of six MATE TT12 genes in strawberry, thereby increasing anthocyanidin levels from endoplasmic reticulum to vacuole (Figure 1B). This further deepens the fruit color. High temperature upregulates MATE genes, MATE TT2, MATE DTX1, in strawberry (Zhang et al., 2019).
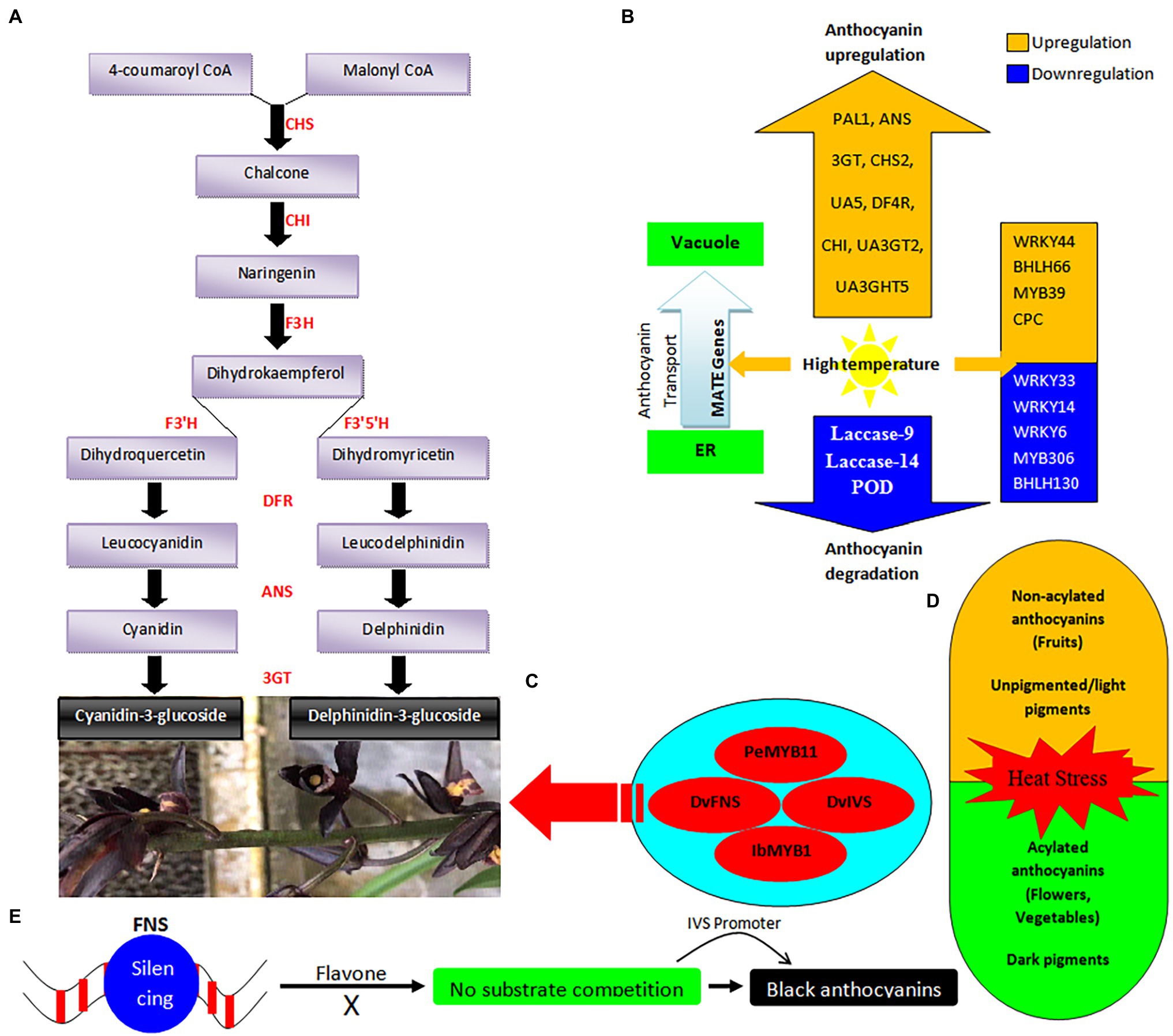
Figure 1. Summary of black color anthocyanin accumulation pathways (A); effect of high temperature on molecular regulation of deep color anthocyanins (B); the key genetic regulators of black color anthocyanin accumulation in the flower petals (C); the common effects of heat stress on plant anthocyanins (orange color shows more susceptibility towards heat stress and the green color shows stability towards heat stress; D); and the proposed pathway of black anthocyanin generation through induced gene silencing (E).
However, high temperature stress also causes low accumulation of pigments in apple (Palmer et al., 2010; Lin-Wang et al., 2011), by repressing the expression of anthocyanin biosynthesis genes and enzymes (Iglesias et al., 1999; Ban et al., 2007; Palmer et al., 2010; Lin-Wang et al., 2011). Decrease in orchard temperature improves the apple skin color, suggesting that anthocyanin biosynthesis is influenced by change in temperature (Iglesias et al., 2002, 2005).
High temperature activates the expression of anthocyanin degradation genes, such as laccase-9 and laccase-14, and it also stimulates the degradation of anthocyanins by enhancing the POD (Peng et al., 2017). Thus, high ambient temperature causes both accumulation and degradation of anthocyanin at the same time (Niu et al., 2017).
Heating magnitude and duration has a strong influence on the stability of anthocyanins (Patras et al., 2010). High ambient temperature may instigate loss of fresh color after harvesting, causing dark red appearance of strawberries leading to serious economic losses (Peng et al., 2017). Exposure of elderberry to 95°C caused 50% loss of anthocyanin contents (Sadilova et al., 2006). Researchers have documented that an arithmetic increase in temperature causes logarithmic anthocyanin destruction (Havlíková and Míková, 1985; Drdak and Daucik, 1990; Rhim, 2002). Exposure of blueberries to 95°C for 3 min causes 43% loss of anthocyanins (Brownmiller et al., 2008). However, anthocyanin from black carrot were comparatively stable up to 90°C (Rhim, 2002; Kırca et al., 2006), probably due to di-acylation of anthocyanin structure. Acylation protects anthocyanin from hydration, thereby making it more stable (Goto et al., 1979; Brouillard, 1981). High temperature of 95°C causes 53% degradation of cyanidin-3-glucoside in blackcurrant extract (Rubinskiene et al., 2005). Cyanidin-3-rutinoside is the most stable anthocyanin at 95°C in blackcurrant (Rubinskiene et al., 2005).
Temperature affects the expression of flower color (Dela et al., 2003). High temperature causes poor flower color in flowers, such as chrysanthemum (Stickland, 1974; Nozaki et al., 2005, 2006), carnation (Maekawa and Nakamura, 1977), petunia (Shvarts et al., 1997), rose (Shisa and Takano, 1964; Dela et al., 2003), Kangaroo Paw (Ben-Tal and King, 1997), and lily (Lai et al., 2011). High temperature (32°C) causes decrease in anthocyanin contents in petunia flowers (Shvarts et al., 1997), as anthocyanin synthesis is inhibited by elevated temperature conditions (Yamagishi et al., 2010; Lai et al., 2011).
Heat Stress Affects Anthocyanin Biosynthesis and Accumulation
Anthocyanins significantly affect the performance of plants during abiotic stress (Rausher, 2008; Sullivan and Koski, 2021). Anthocyanin helps plants in their tolerance against abiotic stress and presents an important biological event during ripening of fruits, such as strawberry, plum, cherry, red orange, and grape (Shin et al., 2007; Flores et al., 2015; Carmona et al., 2017; Martínez-Romero et al., 2017). Anthocyanins act as photoreceptors, anti-oxidants, and osmoregulators, making plants tolerant against abiotic stress (Gould, 2004).
White flowers without anthocyanin face more damages from abiotic stress than pigmented flowers (Figure 1D). Dark color flowers get more favor in the dry conditions than light color flowers (Sullivan and Koski, 2021). Drier Clarkia xantiana flowers accumulate more anthocyanin contents as compared to unpigmented morphs (Warren and Mackenzie, 2001; Vaidya et al., 2018). High reproductive success of pigmented Ipomoea purpurea was observed under heat stress as compared to unpigmented morphs (Coberly and Rausher, 2003). An increase in global temperature may cause decline in floral pigmentation in the case if decreased pigmentation allow proper functioning of heat-sensitive reproductive parts (van der Kooi et al., 2019). However, increased drought conditions may support pigmented morphs (Warren and Mackenzie, 2001).
The most important issue of natural colorants is their low stability under high temperature (Oancea, 2021). However, anthocyanins are comparatively more stable to heat stress (Albuquerque et al., 2021). The main anthocyanin structure contains 2-phenylbenzopyrylium heterocycle C-15 skeleton called anthocyanidin or aglycon. This skeleton contains ▬OCH3 or ▬OH groups (Oancea, 2021). Presence of ▬OH group reduces stability and increases blue color, while the presence of ▬OCH3 groups elevates stability and redness (Albuquerque et al., 2021). Changes in the structure of anthocyanins is caused by fluctuation in the number of ▬OH groups, intensity of methylation of ▬OH groups, the number, and nature of attached sugar moiety to the phenolic molecules (McGhie and Walton, 2007; Patras et al., 2010). Degradation is mainly caused by breakage of covalent bonds, oxidation or heat-triggered increase of oxidation reactions. Opening of pyrylium ring and the formation of chalcone is the first step of anthocyanin degradation stimulated by heat stress (Palamidis and Markakis, 1975; Patras et al., 2010). Upon heating, the anthocyanin decomposes into chalcone structure (Adams, 1973).
Increasing temperature negatively affects the stability of cyanidin-3-O-rutinoside and cyanidin-3-O-glucoside in black rice (Sui et al., 2014). Some anthocyanins, such as pelargonidin-3-O-glucoside and cyanidin-3-O-glucoside from strawberries and blackberries, are more susceptible to heat (Shahidi, 2012). However, the methoxylation and acylation increase anthocyanin stability against heat stress (Shahidi, 2012). Acylated anthocyanins are generated after the acylation of glycosyl groups of anthocyanins with organic acids, thereby increasing heat stability. Diacylated anthocyanins provide significantly high blue color stability to red cabbage at 50°C as compared to non-acylated anthocyanins (Fenger et al., 2020). Thus, acylation of anthocyanins is essential in technological applications to produce colorants with prolonged half-life. However, extreme heat stress (95°C) causes decomposition of acylated anthocyanins in black carrot (Sadilova et al., 2007). Acylated anthocyanins are present in flowers and vegetables, while non-acylated anthocyanins are mostly distributed in fruits (Vidana Gamage et al., 2021; Figure 1D). In black carrot, acylated anthocyanins remain stable to temperature increase of 20–50°C than non-acylated anthocyanins from blackberry (Zozio et al., 2011).
Heat stress increases the anthocyanin contents of purple wheat (De Leonardis et al., 2015; Li et al., 2018). Increased accumulation of anthocyanins was stimulated by upregulation of drought stress-related genes (Castellarin et al., 2007; Cui et al., 2017; Massonnet et al., 2017). Under water drought conditions, higher contents of anthocyanins were observed in apple (Kilili et al., 1996; Mills et al., 1996), strawberry (Ikeda et al., 2011; Rugienius et al., 2015), pomegranate (Laribi et al., 2013) and apricot (Torrecillas et al., 2000; Pérez-Pastor et al., 2007). Increase in temperature increases anthocyanin contents of dark red jujube (Jiang et al., 2020). However, draught also negatively affects anthocyanin accumulation due to reduced photosynthesis, causing poor color development (Bahar et al., 2011).
Anthocyanin degradation due to high temperature causes gradual color loss of Malus profusion fruits in summer (Rehman et al., 2017). High temperature treatment of more than 33°C significantly reduced the concentration of cyanidin 3-galactoside. This reduction is caused by the downregulation of anthocyanin biosynthesis genes (MpUFGT, MpDFR, MpLDOX, MpCHS, and MpMYB10; Steyn et al., 2004; Ubi et al., 2006; Rehman et al., 2017). High temperature also stimulated the generation of H2O2 by enhancing the activities of MDA, SOD, and cell sap pH (Rehman et al., 2017). Moreover, the expression of anthocyanin transport genes (MpVHA-B1 and MpVHA-B2) was also reduced.
Transcription Factors for Anthocyanin Formation and Color Schemes
Anthocyanin biosynthesis is regulated by a number of transcription factor families, including MYB (v-myb avian myeloblastosis viral oncogene homolog), bHLH (basic helix–loop–helix), WRKY, CPC, and WD40 (WD40-repeats proteins; Herrera Valderrama et al., 2014; Bai et al., 2017). R2R3-MYB TFs play key roles in providing the specificity for the downstream genes, causing tissue-specific accumulation of anthocyanin (Koes et al., 2005; Feller et al., 2011; Hichri et al., 2011; Petroni and Tonelli, 2011). The bHLH TFs essentially regulate the activity of R2R3-MYB partner by promoting its transcription or stabilizing the protein complexes (Hernandez et al., 2004). The WDR proteins physically interact with bHLH and MYB TFs to regulate the biosynthesis of anthocyanins (Zhang et al., 2003). Thus, MBW (MYB, bHLH, and WD40) complex primarily regulates anthocyanin biosynthesis genes (Gonzalez et al., 2008; Petroni and Tonelli, 2011). Most of the MYBs are positive regulators of anthocyanin biosynthesis (Jaakola, 2013). However, some MYBs repress it too, such as grapevine VvMYB4 and strawberry FaMYB1 and FaMYB9 (Schaart et al., 2013).
In the Phalaenopsis cultivar ‘Panda’, MYB TFs PeMYB7, PeMYB11, and miRNA156g and miR858 are responsible for purple spot formation in sepals (Zhao et al., 2019). The PeMYB11 is the major R2R3-MYB TF that regulates the black color production (Hsu et al., 2019; Figure 1C). A retrotransposon HORT1 (Harlequin Orchid RetroTransposon 1) causes very strong expression of PeMYB11, leading to extremely high anthocyanin accumulation in the harlequin flowers of Phalaenopsis (Hsu et al., 2019; Zhao et al., 2019). The miR156 and miR858 are the key interference RNAs for PeMYB7 and PeMYB11 (Zhao et al., 2019). High expression of anthocyanin biosynthesis pathway genes (PeCHI, PeANS, PeC4H, PeF3H, PeF3’H, Pe3HI, and Pe4CL2) was observed in spot tissues as compared to non-spot tissues (Figure 1B). Moreover, the ectopic MYB or bHLH expression causes dark purple color in transgenic plants, such as Leaf Color (bHLH) and Deep Purple (MYB) from petunia (Albert et al., 2009, 2011).
The black color formation has been studied in a few fruits and vegetables, such as purple cauliflower (Brassica oleracea L. var. botrytis; Chiu et al., 2010), purple sweet potato (Ipomoea batatas; Mano et al., 2007), and blood oranges (Citrus sinensis; Butelli et al., 2012). In blood oranges, insertion of a Copia-like retrotransposon in the upstream region of a R3R3-MYB TF gene, Ruby, causes extreme accumulation of anthocyanin in the fruit (Butelli et al., 2012). In the purple cauliflower, insertion of a Harbinger DNA transposon in the regulatory region of a R2R3-MYB TF encoding gene, Purple (Pr), causes upregulation of Pr, resulting in dark color accumulation (Chiu et al., 2010). Sweet potato purple color is caused by predominant expression of IbMYB1 (Mano et al., 2007). Storage of strawberries at high temperature upregulated WRKY44, bHLH128, bHLH66, MYB39, and CPC, and downregulated WRKY33, WRKY14, WRKY6, MYB306, and bHLH130 (Zhang et al., 2019; Figure 1B).
Therefore, high expression of the regulatory TFs in the biosynthesis pathway of anthocyanin may cause black flowers and fruits in plants. However, the detailed molecular mechanisms are yet to be elucidated.
Role of Hormones in Deep Coloration
Phytohormones, such as cytokinin, abscisic acid, jasmonate, and ethylene, play significant roles in color development through increasing anthocyanin accumulation (Jia et al., 2011; Colquhoun et al., 2012; Merchante et al., 2013). However, gibberellins and auxins reduce the biosynthesis of anthocyanins during fruit color development (Jaakola, 2013). Ethylene is the key hormone involving apple fruit ripening (Saure, 1990) and also a key regulator of anthocyanin development, because in the apple cultivar ‘Pink Lady’, anthocyanin biosynthesis is significantly correlated with ethylene production (Whale and Singh, 2007). Application of ethylene exogenously promotes the synthesis of anthocyanin in the fruit skin at ripening (Larrigaudiere et al., 1996; Li et al., 2002). Suppression of MdACO1, an ethylene biosynthesis gene, caused poor red pigmentation in apple (Johnston et al., 2009). Low temperature suppresses ethylene production, thereby affecting anthocyanin accumulation (Tromp, 1997; Tatsuki et al., 2011).
Dark Color Relationship With Pollination
In the drought- and heat-stressed conditions, plants with pigmented flowers can survive much better than anthocyanin-free flowers (Grace and Logan, 2000; Warren and Mackenzie, 2001; Steyn et al., 2002). Arctic flowers with dark colors can reach higher temperatures as compared to light color flowers (Büdel, 1959; Tikhomirov et al., 1960). Petals with dark and deep colors can absorb longer wavelengths of light more efficiently than light color petals, resulting in increased corolla temperature (Tikhomirov et al., 1960; Figure 2).
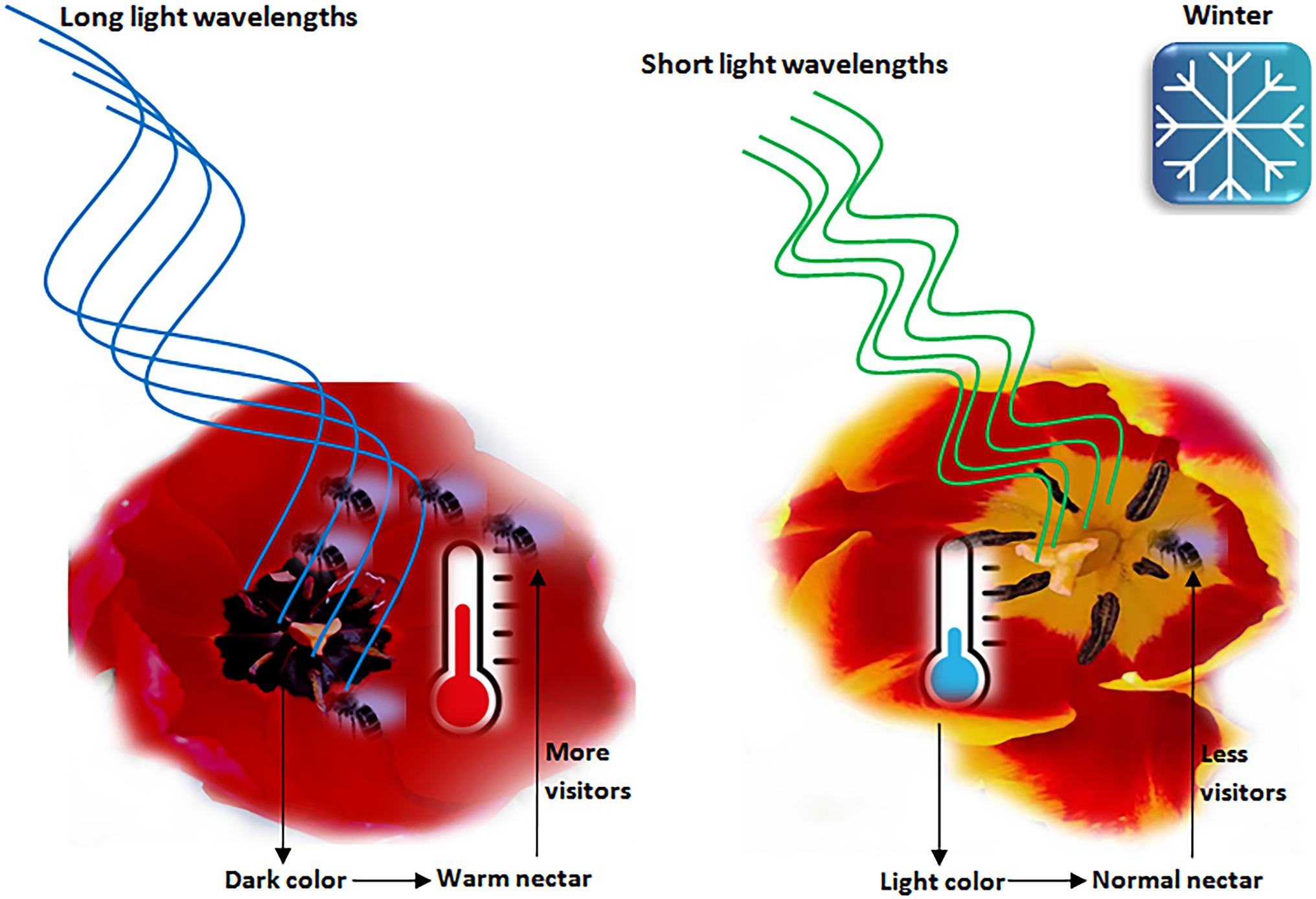
Figure 2. Flower color relationship with heat absorption and pollinators. Dark color flowers absorb sun light with longer wavelengths, which causes increase in the internal temperature of flowers that attract more pollinators in the winter season and allows better pollination. The light color flowers, however, absorb shorter wavelengths of light and do not provide much warm flowers as compared to dark color flowers.
Pollinators select flowers based on their color attributes such as hue and brightness (Caruso et al., 2010; Koski and Galloway, 2020). It is evident that pollinators contribute to disparities in flower color among populations of the same species (Streisfeld and Kohn, 2007; Sobral et al., 2015; Streinzer et al., 2019). However, differences in pollinator communities are not sufficient to explain variation of color in a number of others (Schemske and Bierzychudek, 2007; Thairu and Brunet, 2015). Therefore, non-pollinator selection agents are also involved in flower color variations (Strauss and Whittall, 2006). Temperature can be a selective factor affecting flower color (Coberly and Rausher, 2003; Lacey and Herr, 2005; Koski and Galloway, 2018). Thus, large scale flower color patterns are associated with climatic gradients (Arista et al., 2013; Koski and Ashman, 2015; Koski and Galloway, 2018). For example, dark color flowers are able to warm reproductive structures more efficiently than light color flowers. They can absorb a lot more solar radiation, thereby increasing their reproductive success in low temperature climates (Lacey et al., 2010). Warm flowers get more pollinator visitation in cold environments (Norgate et al., 2010; Figure 2). Dark colors also favor plant in drought conditions (Warren and Mackenzie, 2001). For example, heavily pigmented Boechera stricta are produced in low populations due to increased drought tolerance of pigmented morphs (Vaidya et al., 2018).
Heat absorption provides benefits to both reproductive organs of flowers and pollinators. Basking in the flowers can help insects to elevate their body temperature (Hocking and Sharplin, 1965; Kevan, 1975; Heinrich, 1979; Herrera, 1995). Bees prefer flowers with warm nectar (Dyer et al., 2006) and this choice becomes stronger with decreasing ambient temperature (Norgate et al., 2010). Warm flowers provide high quality reward to pollinators compared with low temperature flowers (Figure 2). For example, sugar production and nectar volume are increased with increasing temperature up to 38°C (Petanidou and Smets, 1996). Dark colored flowers absorb more light and emit it as heat (van der Kooi et al., 2019). Very dark flowers show high intra-floral temperature (Jewell et al., 1994; MCKEE and Richards, 1998; Figure 2). Plants developing at low temperature produce dark color spikes that are up to 2.6°C warmer in full sun (van der Kooi et al., 2019). Anthocyanin pigments regulate flower color plasticity (Lacey and Herr, 2005; Stiles et al., 2007; Anderson et al., 2013). This plasticity in response to temperature exists in most of Plantago species (Anderson et al., 2013). The Lotus corniculatus flowers with dark keel are 6°C warmer than light-keeled flowers (Jewell et al., 1994). Purple Ranunculus glacialis flowers are warmer and make more seeds than white flowers (Ida and Totland, 2014). However, no temperature difference was found in several color polymorphic species (MCKEE and Richards, 1998; Sapir et al., 2006; Mu et al., 2010; Shrestha et al., 2018; Kellenberger et al., 2019).
Black Anthocyanins
A few flowering and fruit plants with dark-purple to black colors certainly catch consumers’ eyes. They contain very high contents of anthocyanins with high antioxidant activity (Jayaprakasha and Patil, 2007; Kelebek et al., 2008). Blood orange contains activities to reduce cardiovascular risk factors (Paredes-López et al., 2010; Pascual-Teresa et al., 2010), oxidative stress (Bonina et al., 2002) and protect DNA against oxidative damage (Guarnieri et al., 2007). Eye catching purple cauliflowers are potent source of nutrition with health-promoting effects (Chiu et al., 2010). Only a few black anthocyanins have been isolated so far.
Five anthocyanin pigments are identified in blackberries, including cyanidin 3-rutinoside, cyanidin-3-(malonyl) glucoside, cyanidin-3-xyloside, cyanidin 3-glucoside, and cyanidin-3-dioxalyglucoside (Cho et al., 2004; Jordheim et al., 2011). Dark purple color of eggplant is due to anthocyanin nasunin (dalphinidin-3-(p-coumaroylrutinoside)-5-glucoside; Noda et al., 2000; Table 1). Nagai (1921) reported that the accumulation of black pigment in soybean seeds is caused by anthocyanin. Yoshikura and Hamaguchi (1969) identified anthocyanins, cyanidin-3-monoglucoside and delphinidin-3-monoglucoside, responsible for black seeds. The Cyanidin-3-(p-coumaroyl)-diglucosdie-5-glucoside is the most abundant anthocyanin in black carrot, responsible for deep purple color (Nath et al., 2022).
Higher amount of melanin pigment was found in the black seed coat of rapeseed (Zhang et al., 2008). Black rice is an important health-promoting food due to abundance of anthocyanins and thermal degradation is a major issue to food industry (Hou et al., 2013). Four anthocyanins are identified in black rice, including cyanidin-3-rutinoside, peonidin-3-glucoside, cyanidin-3-glucoside, and cyanidin-3,5-diglucoside. Cyanidin-3-glucoside is a major anthocyanin found in black rice and blackberry (Hou et al., 2013; Figure 1A). In purple barley, the most abundant anthocyanin is cyanidin 3-glucoside (Glagoleva et al., 2022) The black Phalaenopsis flowers are important breeding sources to induce color variation in floriculture crops. The harlequin/black Phalaenopsis flowers contain black spots on petals, appearing as a new color in 1996 (Chen, 2004; Hsu et al., 2019).
The Tulipa julia contains black portion on the lower side of petals and a hybrid tulip ‘Queen of the Night’ contains highly saturated violet color which appears black under specific light conditions (Markham et al., 2004; Figure 2). A study on 107 tulip cultivars identified five selections with black flowers (Shibata and Ishikura, 1960). Delphinidins were the predominant anthocyanins, including delphinidin (50%), cyaniding (29%) and pelargonidin (21%). For black ‘Queen of the Night’ tulip, tulipanin (delphinidin-3-glucorhamnoside) was the most prominent delphinidin glycoside. A p-coumroyltriglycoside of delphinidin is responsible for black color of Viola cultivar ‘Jet Black’ (Takeda and Hayashi, 1965). Violanin, delphinidin-5-O-glucoside-3-O-[4-p-coumaroylrhamnosyl(1-6)glucosie] is the black anthocyanin in the black pansy, Viola tricolor (Goto et al., 1978; Table 1). Lisanthius nigrescense is unique for its black color corolla (Markham et al., 2004). HPLC analysis showed the presence of one major and one minor anthocyanin. The anthocyanins [delphinidin-3-O-rhamnol(1-6)galactoside and its 5-O-glucoside] comprised 24% of dry weight of petals. The high anthocyanin level is thought to be responsible for complete absorption of both visible and UV wavebands (Markham et al., 2004).
Two major anthocyanins (cyanidin 3-O-glucoside and 3-O-rutinoside) were found in the back flowers of Cosmos atrosanguineus cultivar ‘Choco Mocha’ (Amamiya and Iwashina, 2016; Table 1). Total anthocyanin contents of black flower cultivars ‘Brown Rouge’ and ‘Choco Mocha’ were 3–4 folds higher than that of red flower cultivar ‘Noel Rouge’ (Amamiya and Iwashina, 2016).
In most black cultivars, high accumulation of cyanidin-based anthocyanins was induced by post-transcriptional silencing of DvFNS (flavone synthase II) gene (Deguchi et al., 2013, 2015). Cyanidin-based anthocyanins impart more black color to dahlia flowers than pelargonidin-based anthocyanins (Deguchi et al., 2016). The 3-(6″-malonylglucoside)-5-glucoside was the key cyanidin anthocyanin causing black flowers by lowering petal lightness and chroma (Deguchi et al., 2016). Abolishment of competition for substrate between flavone biosynthesis and anthocyanin biosynthesis may be related to increased accumulation of anthocyanin (Deguchi et al., 2016).
The black flower color of dahlia (Dahlia variabilis) is caused by high accumulation of cyanidin-based anthocyanins (Deguchi et al., 2013; Table 1). The black dahlia cultivars have strong Type 1 promoter of DvIVS with high expression levels (Figure 1C). However, the expression of DvFNS was significantly low in all black cultivars. Surprisingly, DvFNS suppression occurs in a post-transcriptional manner in black cultivars. Artificial silencing of FLS or FNS causes increased accumulation of anthocyanins in petunia (Davies et al., 2003). Therefore, silencing of DvFNS causing flavone absence abolishes the competition for substrates (Figure 1E). The substrate destined for the synthesis of flavone becomes available for anthocyanidin synthesis. Then using Type 1 promoter of DvIVS helps black cultivars to synthesize high amounts of anthocyanidin from large substrate including the new portion as well, leading to black color appearance (Deguchi et al., 2016; Figure 1E).
Conclusion and Future Perspectives
Black flower color is very rare in the nature and only a few species produce black flowers. A number of studies tried to justify the causes and benefits of black color in the plants. So far, the studies have found that anthocyanins are the key components of black color accumulation, especially the cyanidin-type of anthocyanins are the most important to drive black color. R2R3-MYB TFs, especially MYB11, are the key regulators of black anthocyanin accumulation in plants. Artificial induction of black color can be achieved through FNS silencing, allowing increased synthesis of black anthocyanins using IVS promoter. The major benefit of black color is the greenhouse effect it generates by absorbing long wavelengths of light, thereby providing warmth inside petals and warm nectar to attract more pollinators during the winter. Therefore, during extreme winter conditions, deep color of flowers helps plant attract more visitors than light color flowers, that increases the chances of pollination and helps plant survive during harsh conditions.
The best future aspect of black anthocyanins is their stability against temperature extremes and this can be used at industrial level to induce color stability in food products. Moreover, the ornamental flowers with deep color can withstand a long time without color deterioration. Besides, breeding plans can be adjusted for crops growing in extreme winter conditions with difficult pollination breeding. New varieties with greater ability of absorbing long wavelengths of light and deep color flowers would get maximum chances of survival.
Author Contributions
SA: conceptualization and writing—original draft. JC and GC: data curation and software. JH: investigation. YZ: visualization, investigation, and editing. KZ: data curation and conceptualization. SL: software and editing. ZL: supervision, conceptualization, and funding acquisition. DP: supervision, conceptualization, funding acquisition, and writing—reviewing and editing. All authors contributed to the article and approved the submitted version.
Funding
This work was supported by National Key Research and Development Program of China (2019YFD1001003).
Conflict of Interest
The authors declare that the research was conducted in the absence of any commercial or financial relationships that could be construed as a potential conflict of interest.
Publisher’s Note
All claims expressed in this article are solely those of the authors and do not necessarily represent those of their affiliated organizations, or those of the publisher, the editors and the reviewers. Any product that may be evaluated in this article, or claim that may be made by its manufacturer, is not guaranteed or endorsed by the publisher.
Acknowledgments
The authors are thankful to funding agencies for funding and Plant Editors for providing professional services for language editing and final polishing of our manuscript.
References
Adams, J. (1973). Thermal degradation of anthocyanins with particular reference to the 3-glycosides of cyanidin. I. In acidified aqueous solution at 100°C. J. Sci. Food Agric. 24, 747–762. doi: 10.1002/jsfa.2740240702
Albert, N. W., Lewis, D. H., Zhang, H., Irving, L. J., Jameson, P. E., and Davies, K. M. (2009). Light-induced vegetative anthocyanin pigmentation in petunia. J. Exp. Bot. 60, 2191–2202. doi: 10.1093/jxb/erp097
Albert, N. W., Lewis, D. H., Zhang, H., Schwinn, K. E., Jameson, P. E., and Davies, K. M. (2011). Members of an R2R3-MYB transcription factor family in petunia are developmentally and environmentally regulated to control complex floral and vegetative pigmentation patterning. Plant J. 65, 771–784. doi: 10.1111/j.1365-313X.2010.04465.x
Albuquerque, B. R., Oliveira, M. B. P., Barros, L., and Ferreira, I. C. (2021). Could fruits be a reliable source of food colorants? Pros and cons of these natural additives. Crit. Rev. Food Sci. Nutr. 61, 805–835. doi: 10.1080/10408398.2020.1746904
Amamiya, K., and Iwashina, T. (2016). Qualitative and quantitative analysis of flower pigments in chocolate cosmos, Cosmos atrosanguineus, and its hybrids. Nat. Prod. Commun. 11:1934578X1601100. doi: 10.1177/1934578X1601100122
Anderson, E. R., Lovin, M. E., Richter, S. J., and Lacey, E. P. (2013). Multiple Plantago species (Plantaginaceae) modify floral reflectance and color in response to thermal change. Am. J. Bot. 100, 2485–2493. doi: 10.3732/ajb.1300180
Arista, M., Talavera, M., Berjano, R., and Ortiz, P. L. (2013). Abiotic factors may explain the geographical distribution of flower colour morphs and the maintenance of colour polymorphism in the scarlet pimpernel. J. Ecol. 101, 1613–1622. doi: 10.1111/1365-2745.12151
Bahar, E., Carbonneau, A., and Korkutal, I. (2011). The effect of extreme water stress on leaf drying limits and possibilities of recovering in three grapevine (Vitis vinifera L.) cultivars. Afr. J. Agric. Res. 6, 1151–1160.
Bai, S., Sun, Y., Qian, M., Yang, F., Ni, J., Tao, R., et al. (2017). Transcriptome analysis of bagging-treated red Chinese sand pear peels reveals light-responsive pathway functions in anthocyanin accumulation. Sci. Rep. 7, 1–15. doi: 10.1038/s41598-017-00069-z
Ban, Y., Honda, C., Hatsuyama, Y., Igarashi, M., Bessho, H., and Moriguchi, T. (2007). Isolation and functional analysis of a MYB transcription factor gene that is a key regulator for the development of red coloration in apple skin. Plant Cell Physiol. 48, 958–970. doi: 10.1093/pcp/pcm066
Ben-Tal, Y., and King, R. (1997). Environmental factors involved in colouration of flowers of kangaroo paw. Sci. Hortic. 72, 35–48. doi: 10.1016/S0304-4238(97)00071-X
Bonina, F., Leotta, C., Scalia, G., Puglia, C., Trombetta, D., Tringali, G., et al. (2002). Evaluation of oxidative stress in diabetic patients after supplementation with a standardised red orange extract. Diabetes Nutr. Metab. 15, 14–19.
Brouillard, R. (1981). Origin of the exceptional colour stability of the Zebrina anthocyanin. Phytochemistry 20, 143–145. doi: 10.1016/0031-9422(81)85234-X
Brownmiller, C., Howard, L. R., and Prior, R. L. (2008). Processing and storage effects on monomeric anthocyanins, percent polymeric color, and antioxidant capacity of processed blueberry products. J. Food Sci. 73, H72–H79. doi: 10.1111/j.1750-3841.2008.00761.x
Büdel, A. (1959). The microclimate of flowers blooming near the ground. Z. Bienenforsch. 4, 131–140.
Butelli, E., Licciardello, C., Zhang, Y., Liu, J., Mackay, S., Bailey, P., et al. (2012). Retrotransposons control fruit-specific, cold-dependent accumulation of anthocyanins in blood oranges. Plant Cell 24, 1242–1255. doi: 10.1105/tpc.111.095232
Carmona, L., Alquézar, B., Marques, V. V., and Peña, L. (2017). Anthocyanin biosynthesis and accumulation in blood oranges during postharvest storage at different low temperatures. Food Chem. 237, 7–14. doi: 10.1016/j.foodchem.2017.05.076
Caruso, C. M., Scott, S. L., Wray, J. C., and Walsh, C. A. (2010). Pollinators, herbivores, and the maintenance of flower color variation: a case study with Lobelia siphilitica. Int. J. Plant Sci. 171, 1020–1028. doi: 10.1086/656511
Castellarin, S. D., Pfeiffer, A., Sivilotti, P., Degan, M., Peterlunger, E., and Di Gaspero, G. (2007). Transcriptional regulation of anthocyanin biosynthesis in ripening fruits of grapevine under seasonal water deficit. Plant Cell Environ. 30, 1381–1399. doi: 10.1111/j.1365-3040.2007.01716.x
Chen, T. (2004). “Development of harlequin flower derived from somaclone mutants of Phalaenopsis Golden Peoker “Brother,” in Proceedings of 8th Asia Pacific Orchid Conference (APOC8); Tainan, 128–137.
Chen, S.-Y., Tang, Y.-M., Hu, Y.-Y., Wang, Y., Sun, B., Wang, X.-R., et al. (2018). FaTT12-1, a multidrug and toxin extrusion (MATE) member involved in proanthocyanidin transport in strawberry fruits. Sci. Hortic. 231, 158–165. doi: 10.1016/j.scienta.2017.12.032
Chiu, L.-W., Zhou, X., Burke, S., Wu, X., Prior, R. L., and Li, L. (2010). The purple cauliflower arises from activation of a MYB transcription factor. Plant Physiol. 154, 1470–1480. doi: 10.1104/pp.110.164160
Cho, M. J., Howard, L. R., Prior, R. L., and Clark, J. R. (2004). Flavonoid glycosides and antioxidant capacity of various blackberry, blueberry and red grape genotypes determined by high-performance liquid chromatography/mass spectrometry. J. Sci. Food Agric. 84, 1771–1782. doi: 10.1002/jsfa.1885
Coberly, L., and Rausher, M. (2003). Analysis of a chalcone synthase mutant in Ipomoea purpurea reveals a novel function for flavonoids: amelioration of heat stress. Mol. Ecol. 12, 1113–1124. doi: 10.1046/j.1365-294X.2003.01786.x
Colquhoun, T. A., Levin, L. A., Moskowitz, H. R., Whitaker, V. M., Clark, D. G., and Folta, K. M. (2012). Framing the perfect strawberry: an exercise in consumer-assisted selection of fruit crops. J. Berry Res. 2, 45–61. doi: 10.3233/JBR-2011-027
Cui, Z.-H., Bi, W.-L., Hao, X.-Y., Li, P.-M., Duan, Y., Walker, M. A., et al. (2017). Drought stress enhances up-regulation of anthocyanin biosynthesis in grapevine leafroll-associated virus 3-infected in vitro grapevine (Vitis vinifera) leaves. Plant Dis. 101, 1606–1615. doi: 10.1094/PDIS-01-17-0104-RE
Davies, K. M., Schwinn, K. E., Deroles, S. C., Manson, D. G., Lewis, D. H., Bloor, S. J., et al. (2003). Enhancing anthocyanin production by altering competition for substrate between flavonol synthase and dihydroflavonol 4-reductase. Euphytica 131, 259–268. doi: 10.1023/A:1024018729349
De Leonardis, A. M., Fragasso, M., Beleggia, R., Ficco, D. B. M., De Vita, P., and Mastrangelo, A. M. (2015). Effects of heat stress on metabolite accumulation and composition, and nutritional properties of durum wheat grain. Int. J. Mol. Sci. 16, 30382–30404. doi: 10.3390/ijms161226241
De Pascual-Teresa, S., Santos-Buelga, C., and Rivas-Gonzalo, J. C. (2002). LC–MS analysis of anthocyanins from purple corn cob. J. Sci. Food Agric. 82, 1003–1006. doi: 10.1002/jsfa.1143
Deguchi, A., Ohno, S., Hosokawa, M., and Tatsuzawa, F. (2013). Endogenous post-transcriptional gene silencing of flavone synthase resulting in high accumulation of anthocyanins in black dahlia cultivars. Planta 237, 1325–1335. doi: 10.1007/s00425-013-1848-6
Deguchi, A., Tatsuzawa, F., Hosokawa, M., Doi, M., and Ohno, S. (2016). Quantitative evaluation of the contribution of four major anthocyanins to black flower coloring of dahlia petals. Hort. J. 85, 340–350. doi: 10.2503/hortj.MI-121
Deguchi, A., Tatsuzawa, F., Hosokawa, M., and Ohno, S. (2015). Tobacco streak virus (strain dahlia) suppresses post-transcriptional gene silencing of flavone synthase II in black dahlia cultivars and causes a drastic flower color change. Planta 242, 663–675. doi: 10.1007/s00425-015-2365-6
Dela, G., Or, E., Ovadia, R., Nissim-Levi, A., Weiss, D., and Oren-Shamir, M. (2003). Changes in anthocyanin concentration and composition in ‘Jaguar’rose flowers due to transient high-temperature conditions. Plant Sci. 164, 333–340. doi: 10.1016/S0168-9452(02)00417-X
Di Paola-Naranjo, R. D., Sánchez-Sánchez, J., González-Paramás, A. M. A., and Rivas-Gonzalo, J. C. (2004). Liquid chromatographic–mass spectrometric analysis of anthocyanin composition of dark blue bee pollen from Echium plantagineum. J. Chromatogr. A 1054, 205–210. doi: 10.1016/j.chroma.2004.05.023
Drdak, M., and Daucik, P. (1990). Changes of elderbery (Sambucus nigra) pigments during the production of pigment concentrates. Acta Aliment. 19, 3–7.
Dyer, A. G., Whitney, H. M., Arnold, S. E., Glover, B. J., and Chittka, L. (2006). Bees associate warmth with floral colour. Nature 442:525. doi: 10.1038/442525a
Escribano-Bailón, M. T., Santos-Buelga, C., and Rivas-Gonzalo, J. C. (2004). Anthocyanins in cereals. J. Chromatogr. A 1054, 129–141. doi: 10.1016/j.chroma.2004.08.152
Feller, A., Machemer, K., Braun, E. L., and Grotewold, E. (2011). Evolutionary and comparative analysis of MYB and bHLH plant transcription factors. Plant J. 66, 94–116. doi: 10.1111/j.1365-313X.2010.04459.x
Fenger, J.-A., Robbins, R. J., Collins, T. M., and Dangles, O. (2020). The fate of acylated anthocyanins in mildly heated neutral solution. Dyes Pigm. 178:108326. doi: 10.1016/j.dyepig.2020.108326
Flores, G., Blanch, G. P., and Del Castillo, M. L. R. (2015). Postharvest treatment with (−) and (+)-methyl jasmonate stimulates anthocyanin accumulation in grapes. LWT 62, 807–812. doi: 10.1016/j.lwt.2014.12.033
Gao, J.-S., Wu, N., Shen, Z.-L., Lv, K., Qian, S.-H., Guo, N., et al. (2016). Molecular cloning, expression analysis and subcellular localization of a transparent Testa 12 ortholog in brown cotton (Gossypium hirsutum L.). Gene 576, 763–769. doi: 10.1016/j.gene.2015.11.002
Glagoleva, A., Kukoeva, T., Mursalimov, S., Khlestkina, E., and Shoeva, O. (2022). Effects of combining the genes controlling anthocyanin and melanin synthesis in the barley grain on pigment accumulation and plant development. Agronomy. 12:112. doi: 10.3390/agronomy12010112
Gonzalez, A., Zhao, M., Leavitt, J. M., and Lloyd, A. M. (2008). Regulation of the anthocyanin biosynthetic pathway by the TTG1/bHLH/Myb transcriptional complex in Arabidopsis seedlings. Plant J. 53, 814–827. doi: 10.1111/j.1365-313X.2007.03373.x
Goto, T., Hoshino, T., and Takase, S. (1979). A proposed structure of commelinin, a sky-blue anthocyanin complex obtained from the flower petals of Commelina. Tetrahedron Lett. 20, 2905–2908. doi: 10.1016/S0040-4039(01)86447-9
Goto, T., Takase, S., and Kondo, T. (1978). PMR spectra of natural acylated anthocyanins determination of stereostructure of awobanin, shisonin and violanin. Tetrahedron Lett. 19, 2413–2416. doi: 10.1016/S0040-4039(01)94788-4
Gould, K. S. (2004). Nature’s Swiss army knife: the diverse protective roles of anthocyanins in leaves. J. Biomed. Biotechnol. 2004, 314–320. doi: 10.1155/S1110724304406147
Grace, S. C., and Logan, B. A. (2000). Energy dissipation and radical scavenging by the plant phenylpropanoid pathway. Philos. Trans. R. Soc. B Biol. Sci. 355, 1499–1510. doi: 10.1098/rstb.2000.0710
Guarnieri, S., Riso, P., and Porrini, M. (2007). Orange juice vs vitamin C: effect on hydrogen peroxide-induced DNA damage in mononuclear blood cells. Br. J. Nutr. 97, 639–643. doi: 10.1017/S0007114507657948
Han, L., Zhou, L., Zou, H., Yuan, M., and Wang, Y. (2022). PsGSTF3, an anthocyanin-related glutathione S-transferase gene, is essential for petal coloration in tree peony. Int. J. Mol. Sci. 23, 1423. doi: 10.3390/ijms23031423
Hassellund, S., Flaa, A., Kjeldsen, S., Seljeflot, I., Karlsen, A., Erlund, I., et al. (2013). Effects of anthocyanins on cardiovascular risk factors and inflammation in pre-hypertensive men: a double-blind randomized placebo-controlled crossover study. J. Hum. Hypertens. 27, 100–106. doi: 10.1038/jhh.2012.4
Havlíková, L., and Míková, K. (1985). Heat stability of anthocyanins. Z. Lebensm. Unters. Forsch. 181, 427–432. doi: 10.1007/BF01027412
Hernandez, J. M., Heine, G. F., Irani, N. G., Feller, A., Kim, M.-G., Matulnik, T., et al. (2004). Different mechanisms participate in the R-dependent activity of the R2R3 MYB transcription factor C1. J. Biol. Chem. 279, 48205–48213. doi: 10.1074/jbc.M407845200
Herrera, C. M. (1995). Floral biology, microclimate, and pollination by ectothermic bees in an early-blooming herb. Ecology 76, 218–228. doi: 10.2307/1940644
Herrera Valderrama, A., Feller, A., Martens, S., and Allan, A. (2014). “bHLH Transcription Factors involved on the regulation of anthocyanin genes during fruit development in wild strawberry (Fragaria vesca) and red raspberry (Rubus ideaus)”. in Postgraduate workshop of the DGB section “Pflanzliche Naturstoffe.” 16.
Hichri, I., Barrieu, F., Bogs, J., Kappel, C., Delrot, S., and Lauvergeat, V. (2011). Recent advances in the transcriptional regulation of the flavonoid biosynthetic pathway. J. Exp. Bot. 62, 2465–2483. doi: 10.1093/jxb/erq442
Hocking, B., and Sharplin, C. (1965). Flower basking by arctic insects. Nature 206:215. doi: 10.1038/206215b0
Hou, Z., Qin, P., Zhang, Y., Cui, S., and Ren, G. (2013). Identification of anthocyanins isolated from black rice (Oryza sativa L.) and their degradation kinetics. Food Res. Int. 50, 691–697. doi: 10.1016/j.foodres.2011.07.037
Hsu, C.-C., Su, C.-J., Jeng, M.-F., Chen, W.-H., and Chen, H.-H. (2019). A HORT1 retrotransposon insertion in the PeMYB11 promoter causes harlequin/black flowers in Phalaenopsis orchids. Plant Physiol. 180, 1535–1548. doi: 10.1104/pp.19.00205
Hu, B., Zhao, J., Lai, B., Qin, Y., Wang, H., and Hu, G. (2016). LcGST4 is an anthocyanin-related glutathioneS-transferase gene in Litchi chinensis Sonn. Plant Cell Rep. 35, 831–843. doi: 10.1007/s00299-015-1924-4
Ida, T. Y., and Totland, Ø. (2014). Heating effect by perianth retention on developing achenes and implications for seed production in the alpine herb Ranunculus glacialis. Alp. Bot. 124, 37–47. doi: 10.1007/s00035-014-0129-8
Iglesias, I., Graell, J., Faro, D., Larrigaudiere, C., Recasens, I., Echeverría, G., et al. (1999). Efecto del sistema de riego en la coloración de los frutos, contenido de antocianos y actividad de la fenilalanina amonioliasa (PAL), en manzanas cv.'Starking Delicious’. Investig. Agrar. Prod. Prot. Veg. 14, 157–172.
Iglesias, I., Salvia, J., Torguet, L., and Cabús, C. (2002). Orchard cooling with overtree microsprinkler irrigation to improve fruit colour and quality of ‘Topred delicious’ apples. Sci. Hortic. 93, 39–51. doi: 10.1016/S0304-4238(01)00308-9
Iglesias, I., Salvia, J., Torguet, L., and Montserrat, R. (2005). The evaporative cooling effects of overtree microsprinkler irrigation on ‘Mondial Gala’apples. Sci. Hortic. 103, 267–287. doi: 10.1016/j.scienta.2004.06.010
Ikeda, T., Suzuki, N., Nakayama, M., and Kawakami, Y. (2011). The effects of high temperature and water stress on fruit growth and anthocyanin content of pot-grown strawberry (Fragaria× ananassa Duch. cv.‘Sachinoka’) plants. Environ. Control. Biol. 49, 209–215. doi: 10.2525/ecb.49.209
Jaakola, L. (2013). New insights into the regulation of anthocyanin biosynthesis in fruits. Trends Plant Sci. 18, 477–483. doi: 10.1016/j.tplants.2013.06.003
Jayaprakasha, G., and Patil, B. S. (2007). In vitro evaluation of the antioxidant activities in fruit extracts from citron and blood orange. Food Chem. 101, 410–418. doi: 10.1016/j.foodchem.2005.12.038
Jewell, J., Mckee, J., and Richards, A. (1994). The keel colour polymorphism in Lotus corniculatus L.: differences in internal flower temperatures. New Phytol. 128, 363–368. doi: 10.1111/j.1469-8137.1994.tb04020.x
Jia, H.-F., Chai, Y.-M., Li, C.-L., Lu, D., Luo, J.-J., Qin, L., et al. (2011). Abscisic acid plays an important role in the regulation of strawberry fruit ripening. Plant Physiol. 157, 188–199. doi: 10.1104/pp.111.177311
Jiang, W., Li, N., Zhang, D., Meinhardt, L., Cao, B., Li, Y., et al. (2020). Elevated temperature and drought stress significantly affect fruit quality and activity of anthocyanin-related enzymes in jujube (Ziziphus jujuba mill. cv.‘Lingwuchangzao’). PLoS One 15:e0241491. doi: 10.1371/journal.pone.0241491
Johnston, J. W., Gunaseelan, K., Pidakala, P., Wang, M., and Schaffer, R. J. (2009). Co-ordination of early and late ripening events in apples is regulated through differential sensitivities to ethylene. J. Exp. Bot. 60, 2689–2699. doi: 10.1093/jxb/erp122
Jordheim, M., Enerstvedt, K. H., and Andersen, Ø. M. (2011). Identification of cyanidin 3-O-β-(6 ″-(3-Hydroxy-3-methylglutaroyl) glucoside) and other anthocyanins from wild and cultivated blackberries. J. Agric. Food Chem. 59, 7436–7440. doi: 10.1021/jf201522b
Kawase, K., and Tsukamoto, Y. (1976). Studies on flower color in Chrysanthemum morifolium Ramat III. Quantitative effects of major pigments on flower color variation, and measurement of color qualities of petals with a color difference meter. J. Jpn. Soc. Hortic. Sci. 45, 65–75. doi: 10.2503/jjshs.45.65
Kelebek, H., Canbas, A., and Selli, S. (2008). Determination of phenolic composition and antioxidant capacity of blood orange juices obtained from cvs. Moro and Sanguinello (Citrus sinensis (L.) Osbeck) grown in Turkey. Food Chem. 107, 1710–1716. doi: 10.1016/j.foodchem.2007.10.004
Kellenberger, R. T., Byers, K. J., De Brito Francisco, R. M., Staedler, Y. M., Lafountain, A. M., Schönenberger, J., et al. (2019). Emergence of a floral colour polymorphism by pollinator-mediated overdominance. Nat. Commun. 10, 1–11. doi: 10.1038/s41467-018-07936-x
Kevan, P. G. (1975). Sun-tracking solar furnaces in high arctic flowers: significance for pollination and insects. Science 189, 723–726. doi: 10.1126/science.189.4204.723
Kilili, A. W., Behboudian, M. H., and Mills, T. (1996). Composition and quality of ‘Braeburn’apples under reduced irrigation. Sci. Hortic. 67, 1–11. doi: 10.1016/S0304-4238(96)00939-9
Kırca, A., Özkan, M., and Cemeroglu, B. (2006). Stability of black carrot anthocyanins in various fruit juices and nectars. Food Chem. 97, 598–605. doi: 10.1016/j.foodchem.2005.05.036
Koes, R., Verweij, W., and Quattrocchio, F. (2005). Flavonoids: a colorful model for the regulation and evolution of biochemical pathways. Trends Plant Sci. 10, 236–242. doi: 10.1016/j.tplants.2005.03.002
Kong, J.-M., Chia, L.-S., Goh, N.-K., Chia, T.-F., and Brouillard, R. (2003). Analysis and biological activities of anthocyanins. Phytochemistry 64, 923–933. doi: 10.1016/S0031-9422(03)00438-2
Koski, M. H., and Ashman, T.-L. (2015). Floral pigmentation patterns provide an example of Gloger's rule in plants. Nat. Plants 1, 1–5. doi: 10.1038/nplants.2014.7
Koski, M. H., and Galloway, L. F. (2018). Geographic variation in pollen color is associated with temperature stress. New Phytol. 218, 370–379. doi: 10.1111/nph.14961
Koski, M. H., and Galloway, L. F. (2020). Geographic variation in floral color and reflectance correlates with temperature and colonization history. Front. Plant Sci. 11:991. doi: 10.3389/fpls.2020.00991
Lacey, E. P., and Herr, D. (2005). Phenotypic plasticity, parental effects, and parental care in plants? I. An examination of spike reflectance in Plantago lanceolata (Plantaginaceae). Am. J. Bot. 92, 920–930. doi: 10.3732/ajb.92.6.920
Lacey, E. P., Lovin, M. E., Richter, S. J., and Herington, D. A. (2010). Floral reflectance, color, and thermoregulation: what really explains geographic variation in thermal acclimation ability of ectotherms? Am. Nat. 175, 335–349. doi: 10.1086/650442
Lai, Y.-S., Yamagishi, M., and Suzuki, T. (2011). Elevated temperature inhibits anthocyanin biosynthesis in the tepals of an oriental hybrid lily via the suppression of LhMYB12 transcription. Sci. Hortic. 132, 59–65. doi: 10.1016/j.scienta.2011.09.030
Laribi, A., Palou, L., Intrigliolo, D. S., Nortes, P., Rojas-Argudo, C., Taberner, V., et al. (2013). Effect of sustained and regulated deficit irrigation on fruit quality of pomegranate cv.‘Mollar de Elche’at harvest and during cold storage. Agric Water Manag 125, 61–70. doi: 10.1016/j.agwat.2013.04.009
Larrigaudiere, C., Pinto, E., and Vendrell, M. (1996). Differential effects of Ethephon and Seniphos on color development of Starking Delicious’ apple. J. Am. Soc. Hort. Sci. 121, 746–750. doi: 10.21273/JASHS.121.4.746
Li, Z., Gemma, H., and Iwahori, S. (2002). Stimulation of ‘Fuji’apple skin color by ethephon and phosphorus–calcium mixed compounds in relation to flavonoid synthesis. Sci. Hortic. 94, 193–199. doi: 10.1016/S0304-4238(01)00363-6
Li, X., Lv, X., Wang, X., Wang, L., Zhang, M., and Ren, M. (2018). Effects of abiotic stress on anthocyanin accumulation and grain weight in purple wheat. Crop Pasture Sci. 69, 1208–1214. doi: 10.1071/CP18341
Lin-Wang, K., Micheletti, D., Palmer, J., Volz, R., Lozano, L., Espley, R., et al. (2011). High temperature reduces apple fruit colour via modulation of the anthocyanin regulatory complex. Plant Cell Environ. 34, 1176–1190. doi: 10.1111/j.1365-3040.2011.02316.x
Lohachoompol, V., Mulholland, M., Srzednicki, G., and Craske, J. (2008). Determination of anthocyanins in various cultivars of highbush and rabbiteye blueberries. Food Chem. 111, 249–254. doi: 10.1016/j.foodchem.2008.03.067
M’mbone, M. E., Cheng, W., Xu, L., Wang, Y., Karanja, B. K., Zhu, X., et al. (2018). Identification and transcript analysis of MATE genes involved in anthocyanin transport in radish (Raphanus sativus L.). Sci. Hortic. 238, 195–203. doi: 10.1016/j.scienta.2018.04.029
Maekawa, S., and Nakamura, N. (1977). Studies on the coloration of carnation flowers VII. The effects of temperature on the coloration and pigmentation for the intact flower and plant growth. J. Jpn. Soc. Hortic. Sci. 45, 375–382. doi: 10.2503/jjshs.45.375
Mano, H., Ogasawara, F., Sato, K., Higo, H., and Minobe, Y. (2007). Isolation of a regulatory gene of anthocyanin biosynthesis in tuberous roots of purple-fleshed sweet potato. Plant Physiol. 143, 1252–1268. doi: 10.1104/pp.106.094425
Março, P. H., and Scarminio, I. S. (2007). Q-mode curve resolution of UV–vis spectra for structural transformation studies of anthocyanins in acidic solutions. Anal. Chim. Acta 583, 138–146. doi: 10.1016/j.aca.2006.09.057
Markham, K. R., Bloor, S. J., Nicholson, R., Rivera, R., Shemluck, M., Kevan, P. G., et al. (2004). Black flower coloration in wild Lisianthius nigrescens: its chemistry and ecological consequences. Z. Naturforsch. C 59, 625–630. doi: 10.1515/znc-2004-9-1003
Martínez-Romero, D., Zapata, P. J., Guillén, F., Paladines, D., Castillo, S., Valero, D., et al. (2017). The addition of rosehip oil to aloe gels improves their properties as postharvest coatings for maintaining quality in plum. Food Chem. 217, 585–592. doi: 10.1016/j.foodchem.2016.09.035
Massonnet, M., Fasoli, M., Tornielli, G. B., Altieri, M., Sandri, M., Zuccolotto, P., et al. (2017). Ripening transcriptomic program in red and white grapevine varieties correlates with berry skin anthocyanin accumulation. Plant Physiol. 174, 2376–2396. doi: 10.1104/pp.17.00311
Mcghie, T. K., and Walton, M. C. (2007). The bioavailability and absorption of anthocyanins: towards a better understanding. Mol. Nutr. Food Res. 51, 702–713. doi: 10.1002/mnfr.200700092
Mckee, J., and Richards, A. (1998). Effect of flower structure and flower colour on intrafloral warming and pollen germination and pollen-tube growth in winter flowering crocus L.(Iridaceae). Bot. J. Linn. Soc. 128, 369–384. doi: 10.1111/j.1095-8339.1998.tb02127.x
Mekapogu, M., Vasamsetti, B. M. K., Kwon, O.-K., Ahn, M.-S., Lim, S.-H., and Jung, J.-A. (2020). Anthocyanins in floral colors: biosynthesis and regulation in chrysanthemum flowers. Int. J. Mol. Sci. 21:6537. doi: 10.3390/ijms21186537
Merchante, C., Vallarino, J. G., Osorio, S., Aragüez, I., Villarreal, N., Ariza, M. T., et al. (2013). Ethylene is involved in strawberry fruit ripening in an organ-specific manner. J. Exp. Bot. 64, 4421–4439. doi: 10.1093/jxb/ert257
Mills, T., Behboudian, M., and Clothier, B. (1996). Water relations, growth, and the composition of Braeburn’apple fruit under deficit irrigation. J. Am. Soc. Hort. Sci. 121, 286–291. doi: 10.21273/JASHS.121.2.286
Mu, J., Li, G., and Sun, S. (2010). Petal color, flower temperature, and behavior in an alpine annual herb, Gentiana leucomelaena (Gentianaceae). Arct. Antarct. Alp. Res. 42, 219–226. doi: 10.1657/1938-4246-42.2.219
Nagai, I. (1921). A genetico-physiological study on the formation of anthocyanin and brown pigments in plants. Tokyo Univ. Coll. Agr. J. 8, 1–92.
Nath, P., Dukare, A., Kumar, S., Kale, S., and Kannaujia, P. (2022). Black carrot (Daucus carota subsp. sativus) anthocyanin‐infused potato chips: Effect on bioactive composition, color attributes, cooking quality, and microbial stability. J. Food Process. Preserv. 46:958. doi: 10.1111/jfpp.16180
Niu, J., Zhang, G., Zhang, W., Goltsev, V., Sun, S., Wang, J., et al. (2017). Anthocyanin concentration depends on the counterbalance between its synthesis and degradation in plum fruit at high temperature. Sci. Rep. 7, 1–16. doi: 10.1038/s41598-017-07896-0
Noda, N., Aida, R., Kishimoto, S., Ishiguro, K., Fukuchi-Mizutani, M., Tanaka, Y., et al. (2013). Genetic engineering of novel bluer-colored chrysanthemums produced by accumulation of delphinidin-based anthocyanins. Plant Cell Physiol. 54, 1684–1695. doi: 10.1093/pcp/pct111
Noda, Y., Kneyuki, T., Igarashi, K., Mori, A., and Packer, L. (2000). Antioxidant activity of nasunin, an anthocyanin in eggplant peels. Toxicology 148, 119–123. doi: 10.1016/S0300-483X(00)00202-X
Norgate, M., Boyd-Gerny, S., Simonov, V., Rosa, M. G., Heard, T. A., and Dyer, A. G. (2010). Ambient temperature influences Australian native stingless bee (Trigona carbonaria) preference for warm nectar. PLoS One 5:e12000. doi: 10.1371/journal.pone.0012000
Nozaki, K., Fukai, S., and Takamura, T. (2005). Effects of growing season on flower color and flowering in pink flower genotypes of spray chrysanthemum [Dendranthema morifolium]. Hortic. Res. 4, 197–201.
Nozaki, K., Takamura, T., and Fukai, S. (2006). Effects of high temperature on flower colour and anthocyanin content in pink flower genotypes of greenhouse chrysanthemum (Chrysanthemum morifolium Ramat.). J. Hortic Sci. Biotech. 81, 728–734. doi: 10.1080/14620316.2006.11512130
Oancea, S. (2021). A review of the current knowledge of thermal stability of anthocyanins and approaches to their stabilization to heat. Antioxidants 10, 1337. doi: 10.3390/antiox10091337
Palamidis, N., and Markakis, P. (1975). Stability of grape anthocyanin in a carbonated beverage. J. Food Sci. 40, 1047–1049. doi: 10.1111/j.1365-2621.1975.tb02264.x
Palmer, J., Lozano, L., Chagné, D., Volz, R., Lin-Wang, K., Bonany, J., Micheletti, D., Troggio, M., White, A., and Kumar, S. (2010). “Physiological, molecular and genetic control of apple skin colouration under hot temperature environments.” in XXVIII International Horticultural Congress on Science and Horticulture for People (IHC2010): International Symposium on 929; August 22, 2010; 81–87.
Paredes-López, O., Cervantes-Ceja, M. L., Vigna-Pérez, M., and Hernández-Pérez, T. (2010). Berries: improving human health and healthy aging, and promoting quality life—a review. Plant Foods Hum. Nutr. 65, 299–308. doi: 10.1007/s11130-010-0177-1
Pascual-Teresa, D., Moreno, D. A., and García-Viguera, C. (2010). Flavanols and anthocyanins in cardiovascular health: a review of current evidence. Int. J. Mol. Sci. 11, 1679–1703. doi: 10.3390/ijms11041679
Patras, A., Brunton, N. P., O’donnell, C., and Tiwari, B. K. (2010). Effect of thermal processing on anthocyanin stability in foods; mechanisms and kinetics of degradation. Trends Food Sci. Technol. 21, 3–11. doi: 10.1016/j.tifs.2009.07.004
Paun, N., Botoran, O. R., and Niculescu, V.-C. (2022). Total phenolic, anthocyanins HPLC-DAD-MS determination and antioxidant capacity in black grape skins and blackberries: a comparative study. Appl. Sci. 12, 936. doi: 10.3390/app12020936
Peng, L., Yang, S., Chen, R., Johnb, S., Ye, J., Fan, G., et al. (2017). Physiological and quality changes of postharvest strawberries at different storage temperature and their relationships to fruit discoloration. Int. J. Food Nutr. Sci. 4, 81–88. doi: 10.15436/2377-0619.17.1517
Pérez-Pastor, A., Ruiz-Sánchez, M. C., Martínez, J. A., Nortes, P. A., Artés, F., and Domingo, R. (2007). Effect of deficit irrigation on apricot fruit quality at harvest and during storage. J. Sci. Food Agric. 87, 2409–2415. doi: 10.1002/jsfa.2905
Petanidou, T., and Smets, E. (1996). Does temperature stress induce nectar secretion in Mediterranean plants? New Phytol. 133, 513–518. doi: 10.1111/j.1469-8137.1996.tb01919.x
Petroni, K., and Tonelli, C. (2011). Recent advances on the regulation of anthocyanin synthesis in reproductive organs. Plant Sci. 181, 219–229. doi: 10.1016/j.plantsci.2011.05.009
Rausher, M. D. (2008). Evolutionary transitions in floral color. Int. J. Plant Sci. 169, 7–21. doi: 10.1086/523358
Rehman, R. N. U., You, Y., Zhang, L., Goudia, B. D., Khan, A. R., Li, P., et al. (2017). High temperature induced anthocyanin inhibition and active degradation in malus profusion. Front. Plant Sci. 8:1401. doi: 10.3389/fpls.2017.01401
Rhim, J.-W. (2002). Kinetics of thermal degradation of anthocyanin pigment solutions driven from red flower cabbage. Food Sci. Biotechnol. 11, 361–364.
Rubinskiene, M., Viskelis, P., Jasutiene, I., Viskeliene, R., and Bobinas, C. (2005). Impact of various factors on the composition and stability of black currant anthocyanins. Food Res. Int. 38, 867–871. doi: 10.1016/j.foodres.2005.02.027
Rugienius, R., Šikšnianas, T., Bendokas, V., and Stanys, V. (2015). Cropping and anthocyanin content in berries of wild strawberry under water deficit stress. Sodinink. Daržinink. 34, 3–14.
Sadilova, E., Carle, R., and Stintzing, F. C. (2007). Thermal degradation of anthocyanins and its impact on color and in vitro antioxidant capacity. Mol. Nutr. Food Res. 51, 1461–1471. doi: 10.1002/mnfr.200700179
Sadilova, E., Stintzing, F., and Carle, R. (2006). Thermal degradation of acylated and nonacylated anthocyanins. J. Food Sci. 71, C504–C512. doi: 10.1111/j.1750-3841.2006.00148.x
Salehi, B., Sharifi-Rad, J., Cappellini, F., Reiner, Ž., Zorzan, D., Imran, M., et al. (2020). The therapeutic potential of anthocyanins: current approaches based on their molecular mechanism of action. Front. Pharmacol. 11:1300. doi: 10.3389/fphar.2020.01300
Sapir, Y., Shmida, A., and Ne’eman, G. (2006). Morning floral heat as a reward to the pollinators of the Oncocyclus irises. Oecologia 147, 53–59. doi: 10.1007/s00442-005-0246-6
Saure, M. C. (1990). External control of anthocyanin formation in apple. Sci. Hortic. 42, 181–218. doi: 10.1016/0304-4238(90)90082-P
Schaart, J. G., Dubos, C., Romero De La Fuente, I., Van Houwelingen, A. M., De Vos, R. C., Jonker, H. H., et al. (2013). Identification and characterization of MYB-b HLH-WD 40 regulatory complexes controlling proanthocyanidin biosynthesis in strawberry (F ragaria× ananassa) fruits. New Phytol. 197, 454–467. doi: 10.1111/nph.12017
Schemske, D. W., and Bierzychudek, P. (2007). Spatial differentiation for flower color in the desert annual linanthus parryae: was wright right? Evolution 61, 2528–2543. doi: 10.1111/j.1558-5646.2007.00219.x
Shahidi, F. (2012). Dried Fruits: Phytochemicals and Health Effects. State Avenue, USA: John Wiley & Sons.
Shibata, M., and Ishikura, N. (1960). Paper chromatographic survey of anthocyanin in tulip-flowers. I. Jpn. J. Bot. 17, 230–238.
Shin, Y., Liu, R. H., Nock, J. F., Holliday, D., and Watkins, C. B. (2007). Temperature and relative humidity effects on quality, total ascorbic acid, phenolics and flavonoid concentrations, and antioxidant activity of strawberry. Postharvest Biol. Technol. 45, 349–357. doi: 10.1016/j.postharvbio.2007.03.007
Shisa, M., and Takano, T. (1964). Effect of temperature and light on the coloration of rose flowers. J. Jpn. Soc. Hortic. Sci. 33, 140–146. doi: 10.2503/jjshs.33.140
Shrestha, M., Garcia, J. E., Bukovac, Z., Dorin, A., and Dyer, A. G. (2018). Pollination in a new climate: assessing the potential influence of flower temperature variation on insect pollinator behaviour. PLoS One 13:e0200549. doi: 10.1371/journal.pone.0200549
Shvarts, M., Weiss, D., and Borochov, A. (1997). Temperature effects on growth, pigmentation and post-harvest longevity of petunia flowers. Sci. Hortic. 69, 217–227. doi: 10.1016/S0304-4238(97)00015-0
Sobral, M., Veiga, T., Domínguez, P., Guitián, J. A., Guitián, P., and Guitián, J. M. (2015). Selective pressures explain differences in flower color among Gentiana lutea populations. PLoS One 10:e0132522. doi: 10.1371/journal.pone.0132522
Steyn, W., Holcroft, D., Wand, S., and Jacobs, G. (2004). Anthocyanin degradation in detached pome fruit with reference to preharvest red color loss and pigmentation patterns of blushed and fully red pears. J. Am. Soc. Hort. Sci. 129, 13–19. doi: 10.21273/JASHS.129.1.0013
Steyn, W. J., Wand, S., Holcroft, D., and Jacobs, G. (2002). Anthocyanins in vegetative tissues: a proposed unified function in photoprotection. New Phytol. 155, 349–361. doi: 10.1046/j.1469-8137.2002.00482.x
Stickland, R. (1974). Pigment production by cultured florets of Chrysanthemum morifolium. Ann. Bot. 38, 1–6. doi: 10.1093/oxfordjournals.aob.a084779
Stiles, E. A., Cech, N. B., Dee, S. M., and Lacey, E. P. (2007). Temperature-sensitive anthocyanin production in flowers of Plantago lanceolata. Physiol. Plant. 129, 756–765. doi: 10.1111/j.1399-3054.2007.00855.x
Strauss, S. Y., and Whittall, J. B. (2006). “Non-pollinator agents of selection on floral traits,” in Ecology and Evolution of Flowers. eds. L. D. Harder and S. C. H. Barrett (Oxford: Oxford University Press), 120–138.
Streinzer, M., Roth, N., Paulus, H. F., and Spaethe, J. (2019). Color preference and spatial distribution of glaphyrid beetles suggest a key role in the maintenance of the color polymorphism in the peacock anemone (Anemone pavonina, Ranunculaceae) in northern Greece. J. Comp. Physiol. A 205, 735–743. doi: 10.1007/s00359-019-01360-2
Streisfeld, M. A., and Kohn, J. R. (2007). Environment and pollinator-mediated selection on parapatric floral races of Mimulus aurantiacus. J. Evol. Biol. 20, 122–132. doi: 10.1111/j.1420-9101.2006.01216.x
Sui, X., Dong, X., and Zhou, W. (2014). Combined effect of pH and high temperature on the stability and antioxidant capacity of two anthocyanins in aqueous solution. Food Chem. 163, 163–170. doi: 10.1016/j.foodchem.2014.04.075
Sullivan, C. N., and Koski, M. H. (2021). The effects of climate change on floral anthocyanin polymorphisms. Proc. R. Soc. B 288, 20202693. doi: 10.1098/rspb.2020.2693
Takeda, K., and Hayashi, K. (1965). Crystallization and some properties of the genuine anthocyanin inherent to the deep violet color of pansy studies on anthocyanins. XLVIII. Proc. Jpn. Acad. 41, 449–454. doi: 10.2183/pjab1945.41.449
Tatsuki, M., Hayama, H., Yoshioka, H., and Nakamura, Y. (2011). Cold pre-treatment is effective for 1-MCP efficacy in ‘Tsugaru’apple fruit. Postharvest Biol. Technol. 62, 282–287. doi: 10.1016/j.postharvbio.2011.07.005
Thairu, M. W., and Brunet, J. (2015). The role of pollinators in maintaining variation in flower colour in the Rocky Mountain columbine, Aquilegia coerulea. Ann. Bot. 115, 971–979. doi: 10.1093/aob/mcv028
Tikhomirov, B., Shamurin, V., and Shtepa, V. (1960). The temperature of arctic plants. Russ. Acad. Sci. 3, 429–442.
Torrecillas, A., Domingo, R., Galego, R., and Ruiz-Sánchez, M. (2000). Apricot tree response to withholding irrigation at different phenological periods. Sci. Hortic. 85, 201–215. doi: 10.1016/S0304-4238(99)00146-6
Tromp, J. (1997). Maturity of apple cv. Elstar as affected by temperature during a six-week period following bloom. J. Hortic. Sci. 72, 811–819.
Ubi, B. E. (2004). External stimulation of anthocyanin biosynthesis in apple fruit. J. Food Agric. Environ. 2, 65–70.
Ubi, B. E., Honda, C., Bessho, H., Kondo, S., Wada, M., Kobayashi, S., et al. (2006). Expression analysis of anthocyanin biosynthetic genes in apple skin: effect of UV-B and temperature. Plant Sci. 170, 571–578. doi: 10.1016/j.plantsci.2005.10.009
Vaidya, P., Mcdurmon, A., Mattoon, E., Keefe, M., Carley, L., Lee, C. R., et al. (2018). Ecological causes and consequences of flower color polymorphism in a self-pollinating plant (Boechera stricta). New Phytol. 218, 380–392. doi: 10.1111/nph.14998
Van Der Kooi, C. J., Kevan, P. G., and Koski, M. H. (2019). The thermal ecology of flowers. Ann. Bot. 124, 343–353. doi: 10.1093/aob/mcz073
Vidana Gamage, G. C., Lim, Y. Y., and Choo, W. S. (2021). Sources and relative stabilities of acylated and nonacylated anthocyanins in beverage systems. J. Food Sci. Technol. 59, 1–15. doi: 10.1007/s13197-021-05054-z
Warren, J., and Mackenzie, S. (2001). Why are all colour combinations not equally represented as flower-colour polymorphisms? New Phytol. 151, 237–241. doi: 10.1046/j.1469-8137.2001.00159.x
Whale, S. K., and Singh, Z. (2007). Endogenous ethylene and color development in the skin of ‘pink Lady’apple. J. Am. Soc. Hort. Sci. 132, 20–28. doi: 10.21273/JASHS.132.1.20
Wu, X., Gu, L., Prior, R. L., and Mckay, S. (2004). Characterization of anthocyanins and proanthocyanidins in some cultivars of Ribes, Aronia, and Sambucus and their antioxidant capacity. J. Agric. Food Chem. 52, 7846–7856. doi: 10.1021/jf0486850
Wu, X., and Prior, R. L. (2005). Identification and characterization of anthocyanins by high-performance liquid chromatography−electrospray ionization−tandem mass spectrometry in common foods in the United States: vegetables, nuts, and grains. J. Agric. Food Chem. 53, 3101–3113. doi: 10.1021/jf0478861
Yamagishi, M., Shimoyamada, Y., Nakatsuka, T., and Masuda, K. (2010). Two R2R3-MYB genes, homologs of petunia AN2, regulate anthocyanin biosyntheses in flower tepals, tepal spots and leaves of Asiatic hybrid lily. Plant Cell Physiol. 51, 463–474. doi: 10.1093/pcp/pcq011
Yang, G., Li, L., Wei, M., Li, J., and Yang, F. (2022). Smmyb113 is a key transcription factor responsible for compositional variation of anthocyanin and color diversity among eggplant peels. Front. Plant Sci. 13:843996. doi: 10.3389/fpls.2022.843996
Zhang, X., Chen, J., Chen, L., Wang, H., and Li, J. (2008). Imbibition behavior and flooding tolerance of rapeseed seed (Brassica napus L.) with different testa color. Genet. Resour. Crop. Evol. 55, 1175–1184. doi: 10.1007/s10722-008-9318-x
Zhang, F., Gonzalez, A., Zhao, M., Payne, C. T., and Lloyd, A. (2003). A network of redundant bHLH proteins functions in all TTG1-dependent pathways of Arabidopsis. Development 130, 4859–4869. doi: 10.1242/dev.00681
Zhang, L., Wang, L., Zeng, X., Chen, R., Yang, S., and Pan, S. (2019). Comparative transcriptome analysis reveals fruit discoloration mechanisms in postharvest strawberries in response to high ambient temperature. Food Chem. 2:100025. doi: 10.1016/j.fochx.2019.100025
Zhao, A., Cui, Z., Li, T., Pei, H., Sheng, Y., Li, X., et al. (2019). mRNA and miRNA expression analysis reveal the regulation for flower spot patterning in Phalaenopsis ‘panda’. Int. J. Mol. Sci. 20:4250. doi: 10.3390/ijms20174250
Zhao, L., Gao, L., Wang, H., Chen, X., Wang, Y., Yang, H., et al. (2013). The R2R3-MYB, bHLH, WD40, and related transcription factors in flavonoid biosynthesis. Funct. Integr. Genomics 13, 75–98. doi: 10.1007/s10142-012-0301-4
Zozio, S., Pallet, D., and Dornier, M. (2011). Evaluation of anthocyanin stability during storage of a coloured drink made from extracts of the Andean blackberry (Rubus glaucus Benth.), açai (Euterpe oleracea Mart.) and black carrot (Daucus carota L.). Fruits 66, 203–215. doi: 10.1051/fruits/2011030
Keywords: heat stress, black flower color, anthocyanins, ornamental crops, pollination
Citation: Ahmad S, Chen J, Chen G, Huang J, Zhou Y, Zhao K, Lan S, Liu Z and Peng D (2022) Why Black Flowers? An Extreme Environment and Molecular Perspective of Black Color Accumulation in the Ornamental and Food Crops. Front. Plant Sci. 13:885176. doi: 10.3389/fpls.2022.885176
Edited by:
Nasim Ahmad Yasin, University of the Punjab, PakistanReviewed by:
Zahoor Ahmad Sajid, University of the Punjab, PakistanMuhammad Akbar, University of Gujrat, Pakistan
Muhammad Imran, South China Agricultural University, China
Izhar Hussain, The University of Haripur, Pakistan
Copyright © 2022 Ahmad, Chen, Chen, Huang, Zhou, Zhao, Lan, Liu and Peng. This is an open-access article distributed under the terms of the Creative Commons Attribution License (CC BY). The use, distribution or reproduction in other forums is permitted, provided the original author(s) and the copyright owner(s) are credited and that the original publication in this journal is cited, in accordance with accepted academic practice. No use, distribution or reproduction is permitted which does not comply with these terms.
*Correspondence: Zhongjian Liu, empsaXVAZnNmdS5lZHUuY24=; Donghui Peng, ZmpwZGhAMTI2LmNvbQ==