- 1Centre for Environmental and Marine Studies (CESAM), Department of Biology, University of Aveiro, Aveiro, Portugal
- 2Department of Organisms and Systems Biology, University of Oviedo, Oviedo, Spain
- 3University Institute of Biotechnology of Asturias, University of Oviedo, Oviedo, Spain
- 4Department of Vegetal Production and Forest Resources, University of Valladolid, Palencia, Spain
- 5Sustainable Forest Management Research Institute, University of Valladolid-INIA, Palencia, Spain
In an era of climate change and global trade, forests sustainability is endangered by several biotic threats. Pine pitch canker (PPC), caused by Fusarium circinatum, is one of the most important disease affecting conifers worldwide. To date, no effective control measures have been found for this disease. Earlier studies on PPC were mainly focused on the pathogen itself or on determining the levels of susceptibility of different hosts to F. circinatum infection. However, over the last years, plenty of information on the mechanisms that may explain the susceptibility or resistance to PPC has been published. This data are useful to better understand tree response to biotic stress and, most importantly, to aid the development of innovative and scientific-based disease control measures. This review gathers and discusses the main advances on PPC knowledge, especially focusing on multi-disciplinary studies investigating the response of pines with different levels of susceptibility to PPC upon infection. After an overview of the general knowledge of the disease, the importance of integrating information from physiological and Omics studies to unveil the mechanisms behind PPC susceptibility/resistance and to develop control strategies is explored. An extensive review of the main host responses to PPC was performed, including changes in water relations, signalling (ROS and hormones), primary metabolism, and defence (resin, phenolics, and PR proteins). A general picture of pine response to PPC is suggested according to the host susceptibility level and the next steps and gaps on PPC research are pointed out.
Introduction: An overview of pine pitch canker
Forests cover nearly 31% of the total land area globally (4.06 billion ha), representing important economic, environmental, and social assets [Food and Agriculture Organization of the United Nations (FAO), 2020]. In particular, European forests are mainly composed of conifers (46%), with pine species being the greatest growing stock, key for CO2 sink and a vital source of resin and timber (Forest Europe-Ministerial Conference on the Protection of Forests in Europe, 2020). Forest sustainability is currently threatened by several biotic and abiotic factors that negatively affect its productivity and health. The vulnerability to these stresses is increased by climate change and the global trade of plants and derivates, especially regarding the importation of plant pathogens which may find further opportunities to colonise new environments (Sturrock et al., 2011; Santini et al., 2013; Wingfield et al., 2015).
Pine Pitch Canker (PPC), caused by the ascomycete fungus Fusarium circinatum Nirenberg & O’Donnell (sexual morph Gibberella circinata Nirenberg & O’Donnell), is one of the most important diseases affecting conifers worldwide (Wingfield et al., 2008). Although PPC was first reported in the United States (Hepting and Roth, 1946), phylogenetic analyses indicate that F. circinatum is originally an endemic member of the native Mexican forest (Wikler and Gordon, 2000). Introduction into Europe, Japan, and South Africa seems to have occurred through United States or Mexico (Wikler and Gordon, 2000; Berbegal et al., 2013). PPC has now been reported globally (Figure 1; reviewed by Drenkhan et al., 2020). As the natural spread of F. circinatum via rain, wind, and insects is limited by short spore dispersal and vector-insect flight distances, longer distance dissemination is mainly human-assisted (Zamora-Ballesteros et al., 2019). This occurs mainly through the movement of infected seeds worldwide, and of asymptomatic seedlings, and contaminated substrates, or containers regionally.
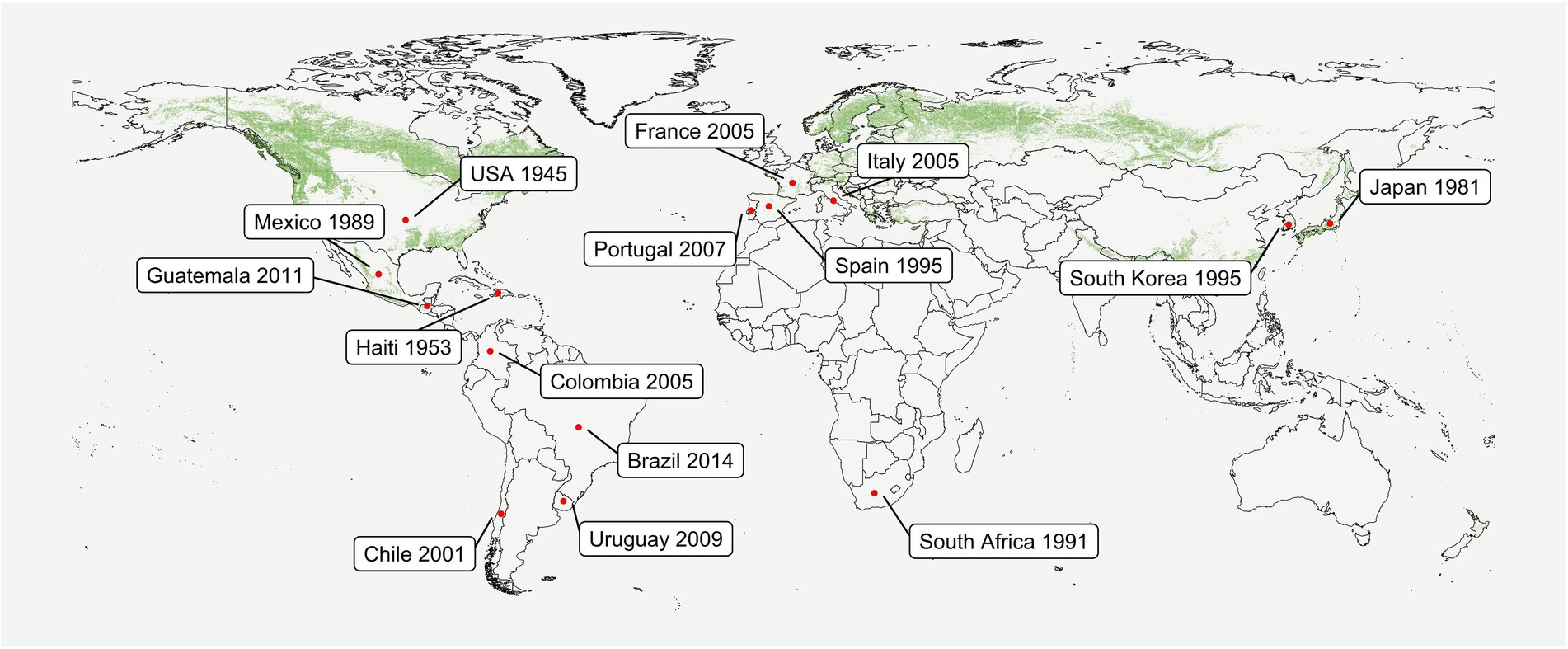
Figure 1. Conifer forest distribution and first reports of Fusarium circinatum worldwide. Green dots represent evergreen, deciduous, and mixed needle forests (min. area of 1 km2) according to the Corine Land Cover (CLC) 2012 v. 2020_20u1 provided by the European Union Copernicus Land Monitoring Service, European Environment Agency (EEA). Red points indicate areas in which PPC has been reported and the year of the first observation. This data have been recently reviewed by Drenkhan et al. (2020), where further detail regarding the sampling site (nursery or field), identification method, and host species can be found. The report of F. circinatum in Guatemala (Dvorak et al., 2011) was also included. Databases and figures were built using R v3.6.0.
After a new introduction occurs, moisture and warm temperatures are crucial for the establishment and spread of PPC (Wingfield et al., 2008; Ganley et al., 2009; Baker et al., 2010; Quesada et al., 2019; Elvira-Recuenco et al., 2021). A large area of European pine forests has been reported to be suitable or optimal for PPC development, with F. circinatum distribution potentially changing towards northern Europe due to the predicted reduction of cold and drought (Ganley et al., 2009; Baker et al., 2010; Watt et al., 2011; Möykkynen et al., 2015). This is of particular concern giving that F. circinatum showed to be able to infect conifers present in these currently disease-free areas such as Pinus sylvestris L. and Picea abies (L.) H. Karst (Martín-García et al., 2017, 2018), and that these areas are populated with a high density of conifers (Figure 1). In nurseries, optimal conditions may be easily achieved for disease development in imported infected seeds or plants independently of its location (Drenkhan et al., 2020).
Pine pitch canker affects more than 60 species of Pinus and Pseudotsuga menziesii (Mirb.) Franco (Bezos et al., 2017) at all stages of tree development infecting seeds, seedlings, and mature trees [European and Mediterranean Plant Protection Organization (EPPO), 2021]. Disease symptoms include excessive pitch flow, resinous canker formation, and branch/canopy dieback in adult trees and tip dieback and seedling damping-off in nurseries (Wingfield et al., 2008; Martín-Rodrigues et al., 2013; Santana et al., 2016). These results in significant economic losses in nurseries and pine plantations/stands (Aegerter et al., 2003; Wingfield et al., 2008; Carrasco et al., 2016). Although natural infections occur through wounds (Iturritxa et al., 2011), the pathogen is also able to colonise seedlings or roots of mature trees without damaging the adjacent tissues allowing plants to remain asymptomatic (Elvira-Recuenco et al., 2015; Swett et al., 2016, 2018; Martín-García et al., 2018; Hernandez-Escribano et al., 2018a). This hemibiotrophic nature represents an additional challenge to manage PPC (Vettraino et al., 2018); as well as its ability to naturally infect grasses surrounding pine species, where it may represent a source of inoculum (Swett and Gordon, 2012; Swett et al., 2014; Hernandez-Escribano et al., 2018b; Herron et al., 2020).
Several studies focused on F. circinatum infection mechanism and virulence. After conidia germination, the pathogen colonises the host stem radially advancing towards the pith, likely releasing cell wall degrading enzymes (e.g. endopolygalacturonase) to liberate nutrients from the host tissues (Chimwamurombe et al., 2001; Martín-Rodrigues et al., 2013). Vertical colonization of the host occurs later through the spread of conidiophores and conidia when the pathogen reaches the phloem or the traumatic resin ducts in the xylem (Martín-Rodrigues et al., 2013). Physical obstruction by F. circinatum growth, generalised cell death in the xylem and increased resin production may restrict water supply and result in plant death (Gordon, 2011; Martín-Rodrigues et al., 2013). Different phenotypical and molecular characteristics have been associated to F. circinatum pathogenicity or virulence (Mullett et al., 2017; Muñoz-Adalia et al., 2018a,b; Phasha et al., 2021a). Also, several compounds have been proposed to allow host colonization, including beauvericin, ergosterol, fusaric acid, and laccases (Fotso et al., 2002; Muñoz-Adalia et al., 2016; Visser et al., 2019; Phasha et al., 2021b).
In general, earlier research on PPC was focused on the characterization of F. circinatum populations and on testing the susceptibility of different hosts to PPC, with information on the mechanisms behind these differential responses remaining scarce. Over the last few years, a new perspective has gained relevance aiming to unveil these mechanisms based on an integrative approach (from physiology to Omics). This review highlights the main advances on PPC knowledge, with a special focus on multi-disciplinary studies exploring host response to F. circinatum and on how these contribute for tree biotic stress response knowledge and for the development of effective disease control measures.
PPC resistance: Exploiting genetic variance using an integrated approach, from physiology to omics
Exploring genetic resistance based on intra- and inter-specific variation has been proposed as a promising environmentally-friendly strategy to avoid the natural spread of PPC (Martín-García et al., 2019; Zamora-Ballesteros et al., 2019). This would allow for the selection/development of resistant trees to reforest high-risk sites. Host susceptibility to F. circinatum is known to vary at the species, population, family, and clone levels (reviewed by Drenkhan et al., 2020). While some species, such as Pinus radiata D. Don and Pinus patula Schltdl. & Cham., are highly susceptible to F. circinatum, others are moderately susceptible (P. pinaster Aiton) or resistant (P. pinea L.). Pinus radiata has been extensively studied as it is the most planted pine worldwide due to its fast growth and wood quality (Mead, 2013). The among-population variation found in some species has been attributed to geographical and environmental gradients (e.g., resistance in low elevation Pinus tecunumanii Eguiluz & J. P. Perry). Moreover, variation in PPC resistance showed to be quantitative and dependent on polygenic mechanism (i.e. results from the integration of small effects of several genes; information mainly based on SNPs associations; Gordon et al., 1998; Kayihan et al., 2005; Quesada et al., 2010), and presents variable heritability estimates (reviewed by Martín-García et al., 2019).
Although resistance to diseases in forest trees has been mostly achieved based on phenotypic selection, advances on genomic techniques and resources contributed for the identification of candidate genes related to biotic stress tolerance (Kovalchuk et al., 2013; Muranty et al., 2014; Sniezko and Koch, 2017). For example, the integration of multiple Omics level data from trees with varying levels of susceptibility to the disease may be used to identify candidate genes or metabolic pathways for manipulation (Naidoo et al., 2019). Innovative bioinformatic and statistical tools allow to build networks that model the dynamics and complexity of a given biological system linking molecular interactions and complex traits (zu Castell and Ernst, 2012; Valledor et al., 2018; Naidoo et al., 2019), such as PPC. This systems biology view may provide foundation for defining specific gene sets relevant for PPC resistance. Despite possible legislative barriers, these may be targeted by novel gene editing tools if the desired combination of genes cannot be achieved through classical breeding (Chang et al., 2018).
The use of high-throughput Omics technologies in forest trees has lagged behind that of model or crop species, especially due to trees long-life cycles, large genome sizes, and the lack of genomic tools (Neale and Kremer, 2011). The availability of Pinus taeda L. whole-sequenced genome allowed for the identification of several single nucleotide polymorphisms (SNPs) associated with PPC resistance (Quesada et al., 2010; Lu et al., 2017; De La Torre et al., 2019; Figure 2). Moreover, the increasing performance and cost reduction of next-generation sequencing technologies make whole-transcriptome sequencing (RNA-Seq) attractive for the evaluation of gene expression in organism for which genome sequencing is not available, in particular for species with megagenomes such as conifers (e.g. Santos et al., 2012). Also, dual RNA-Seq strategies may address changes in both the host and the pathogen transcriptomes at once allowing to better decipher its interaction (Naidoo et al., 2018). RNA-Seq and dual RNA-Seq studies have been performed in pine species with different levels of susceptibility to PPC to unveil host responses to F. circinatum infection and explore how the pathogen is able to overcome them; as well as target transcriptomic studies (check Figure 2 for references).
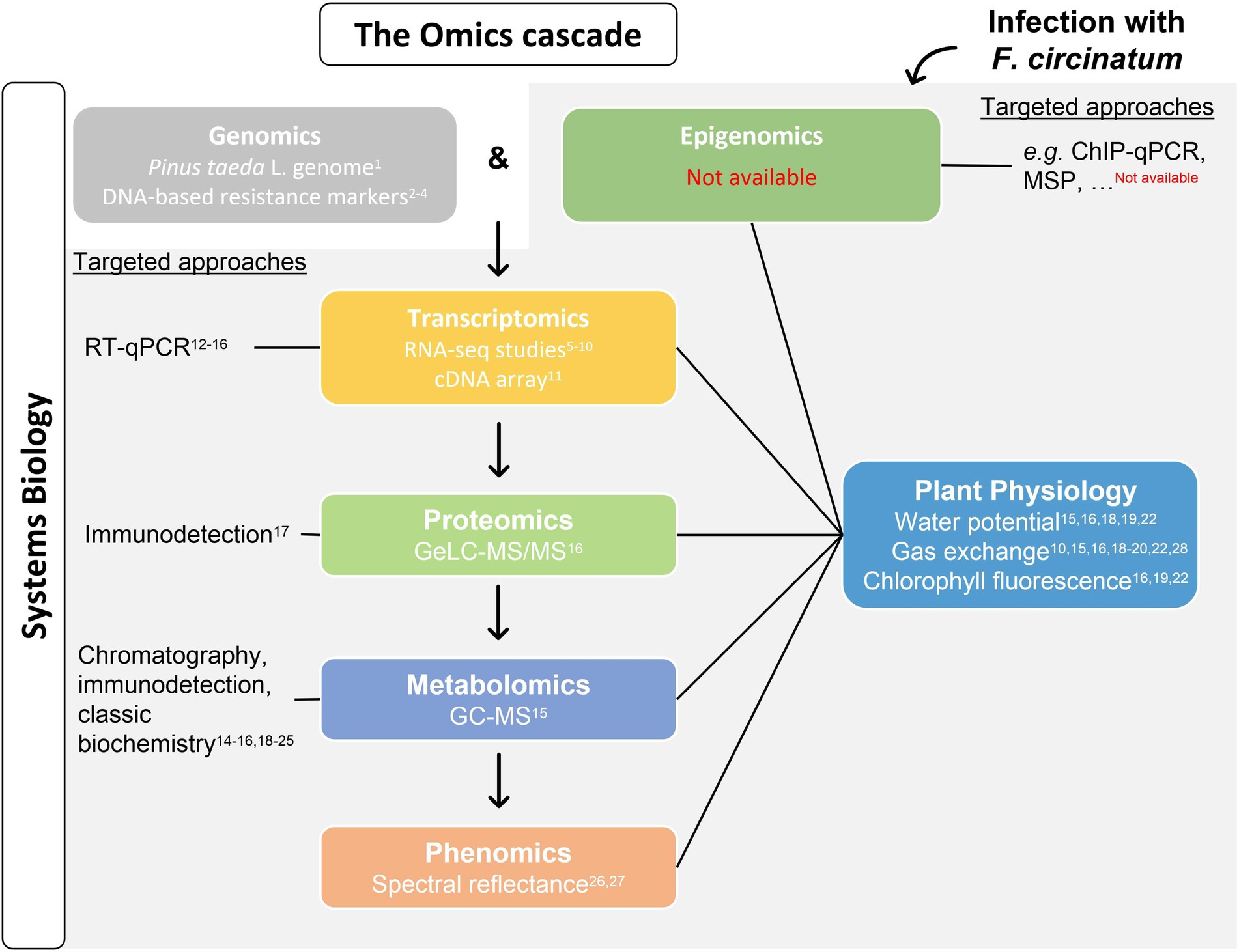
Figure 2. Advances and knowledge gaps on Pine pitch canker (PPC) research regarding the different levels of the Omics cascade. The availability of P. taeda genome is a useful tool for exploring Pinus-Fusarium circinatum interaction and allowed to identify DNA markers that determine the resistance of a certain genotype to PPC. In addition, F. circinatum infection induces changes at the DNA, RNA, and protein and metabolite levels, ultimately resulting in different phenotypes (grey background). While epigenetic studies were not performed on PPC, several transcriptomics studies included hosts with different susceptibilities and overtime analysis near the inoculation point. The single proteomic and metabolomic analyses available were performed using needles of pines with contrasting responses to F. circinatum infection. Phenomics studies are also available. Changes on the abundance of putative transcripts, proteins, and metabolites of interest have been explored and may complement/validate Omics data together with plant physiology measurements. The integration of this data provides a systems biology view of pine response to PPC. References: 17Davis et al. (2002), 11Morse et al. (2004), 24Kim et al. (2010), 2Quesada et al. (2010), 12Fitza et al. (2013), 1Neale et al. (2014), 21Vivas et al. (2014a), 13Donoso et al. (2015), 5–7Visser et al. (2015, 2018, 2019), 28López-López et al. (2016), 26Reynolds et al. (2016), 8Carrasco et al. (2017), 22Cerqueira et al. (2017), 3Lu et al. (2017), 23Silva-Castro et al. (2018a), 27Lee and Lee (2019), 15,16,18,19Amaral et al. (2019a,b, 2021a,b), 4De La Torre et al. (2019), 25Lombardero et al. (2019), 9Hernandez-Escribano et al. (2020), 14Otero et al. (2020), 20Leitão et al. (2021), and 10Zamora-Ballesteros et al. (2021). GC–MS, gas chromatography coupled to mass spectrometry; GeLC-MS/MS, gel-enhanced liquid chromatography–tandem mass spectrometry; RT-qPCR, reverse transcription quantitative PCR; ChIP-qPCR, chromatin immunoprecipitation-qPCR; and MSP, methylation-specific PCR.
However, changes at the transcriptional level are not always reflected at the downstream levels of the Omics cascade. Proteomics allow to study the proteins regulating disease development, contributing for the understanding of complex host defence mechanisms (Kaur et al., 2017; Rustagi et al., 2018). Although several of the proteomic studies in forest trees are based on two-dimensional electrophoresis (2-DE) coupled to MS analysis, resulting in low proteome coverage, forest proteomics have evolved, especially regarding LC–MS-based systems for protein identification and quantification using shotgun/bottom-up proteomics (Rodrigues et al., 2021). Early proteomics studies regarding PPC focused only on the role of a particular protein family (chitinases) in response to F. circinatum infection (Davis et al., 2002); while recent studies explored the needle proteome of pine species with contrasting PPC response phenotypes followed by integration with physiological data (Amaral et al., 2021b).
In addition, metabolites, at the end of the Omics cascade, represent a phenotypic signature of genetic variance, and of epigenetic, transcriptomic, and proteomic changes (Fiehn, 2002). As for proteomics, advances in MS approaches currently allow a large-scale profiling of plant metabolites (Rodrigues et al., 2021), allowing to identify biomarkers of forest diseases (e.g. Hantao et al., 2014; Wu et al., 2015) or screen disease resistant trees for breeding programs (e.g. Sambles et al., 2017; Wang et al., 2017). However, to distinguish whether the metabolites analysed are of plant or of pathogen origin may represent a great challenge. Unlike transcripts and proteins, metabolites identification does not depend on known genetic sequences. While some techniques have been proposed to address this issue, these are still associated to several drawbacks (see Tenenboim and Brotman, 2016). Regarding PPC, the quantification of specific metabolites, such as sugars, proline, hormones, terpenoids, phenolics, or lignin has been carried out using mainly traditional biochemical methods (check Figure 2 for references). GC–MS analysis of the needle primary metabolism of pines with different levels of susceptibility to F. circinatum infection were only recently performed together with physiological and targeted gene expression measurements (Amaral et al., 2019a).
Despite phenomics approaches are usually scarce in forest trees, some advances have been reported for PPC (Reynolds et al., 2016; Lee and Lee, 2019). Phenomics combine well-established plant physiology methodologies (e.g., gas exchange, chlorophyll fluorescence, water relations, nutrient uptake, and growth rate measurements) with recent technological advances to develop high-throughput phenotyping capture technologies, such as unmanned aerial vehicle remote sensing, to obtain a large-scale physiological overview of plants under different environmental scenarios (Flexas and Gago, 2018; Naidoo et al., 2019). The integration of different Omics levels with plant physiology theoretical knowledge and techniques is expected to maximise the understanding of plants functioning (Flexas and Gago, 2018); especially given that plant physiology has also proven useful for the understanding of plant-pathogen interaction (Berger et al., 2007). Studying the mechanism behind Pinus-F. circinatum interaction in hosts with varying levels of susceptibility to PPC based on a multidisciplinary approach unveiled defence response associated to each phenotype and is predicted to support innovative disease control measures.
Host response to pathogen infection: State of the art on PPC
Plants possess a complex innate immune system that enables them to respond to pathogen attack, including non-specific constitutive defences (e.g., lignosuberised trees outer bark, needles cuticular surface, and phytoanticipins, such as phenolics, terpenoids, and alkaloids) and induced defences (Kovalchuk et al., 2013). The latter may be activated by the recognition of Pathogen-Associated Molecular Patterns (PAMPs) by extracellular domains of membrane receptor proteins (pattern-recognition receptors—PRRs) and result in PAMP-triggered immunity (PTI; Chisholm et al., 2006; Jones and Dangl, 2006; Nishad et al., 2020). The activation of PTI in response to F. circinatum infection in P. pinaster has been proposed due to the early upregulation of genes encoding a lysine motif receptor-like kinase (LysM-RLK), which has been associated to the recognition of fungal chitin (Hernandez-Escribano et al., 2020). Several genes coding for PRR containing domains have also been upregulated in the resistant P. pinea but not in the susceptible P. radiata, which may explain the weak downstream signalling in the latter (Zamora-Ballesteros et al., 2021). Regarding effector-triggered immunity (ETI), a second line of defence activated by the recognition of fungal effector proteins by plant resistance (R) proteins (Hammond-Kosack and Parker, 2003), there is still no evidence of its role in PPC.
After pathogen recognition, plants activate several defence responses to avoid its spread. These include morphological modifications (e.g., cytoskeletal reorganization and cell wall fortification); the production of reactive oxygen species (ROS) and secondary metabolites (such as phytohormones, phytoalexins, and plant volatiles), which may interact directly with the pathogen or function as signalling molecules distant from the infection site (Bolton, 2009; Eyles et al., 2010; Rojas et al., 2014); and the induction of pathogenesis-related (PR) proteins (van Loon et al., 2006) and a programmed cell death mechanism known as hypersensitive response (HR; Coll et al., 2011). The production of PR proteins in uninfected tissues is associated to the development of systemic acquired resistance (SAR), a systemic induced resistance (SIR) phenomena mediated by salicylic acid (SA) that protects plants against future infections (Fu and Dong, 2013). SIR is known to occur in trees in response to necrotrophic pathogens, including F. circinatum, although there is no sufficient information on the signalling pathways involved to identify the specific mechanism developed (Bonello et al., 2001, 2006; Eyles et al., 2010; Gordon et al., 2011). The use of chitosan as an elicitor of pine defence against F. circinatum has showed promising results based on the systemic induction of host resistance (e.g., activation of the phenylpropanoids pathway) and/or its fungistatic effect (Reglinski et al., 2004; Fitza et al., 2013; Silva-Castro et al., 2018a,b). The potential of using biotic (pathogens or beneficial microbes) and abiotic (e.g. wounding and chemicals) elicitors to trigger the establishment of induced responses in pines and enhance resistance to F. circinatum has been reviewed by Martín-García et al. (2019). Promising promoters of PPC resistance may be part of an integrated management approach to control PPC in an environmentally friendly manner.
A general reprogramming of host’s metabolism occurs to fuel plant defence responses against pathogens instead of growth, reproduction, and yield; but also to meet the nutritional requirements of the pathogen (accumulation of C and N sources; Berger et al., 2007; Bolton, 2009; Fagard et al., 2014; Rojas et al., 2014). For example, transcriptomic studies evidenced this metabolic trade-off between growth and defence in the P. pinaster-F. circinatum interaction: genes related to growth, morphogenesis, and photosynthesis were downregulated; while genes involved in phytohormone signalling, ROS regulation, oxidative stress, regulation of cell death, signal transduction, synthesis of flavonoids, anthocyanins, and other defence-related secondary metabolites were increased (Hernandez-Escribano et al., 2020). However, the late production of secondary metabolites was insufficient to fight pathogen infection.
The main aspects of pine response to F. circinatum are integrated in the following sections, including changes in plant water relations, signalling pathways (ROS and hormones), primary metabolism, and induction of common conifer defences (resin, phenolics, and PR proteins). These advances were achieved fusing techniques from different disciplines (from physiology to Omics) to explore changes in hosts with different susceptibility to PPC.
Changes in plant water relations
The impact of canker development after F. circinatum infection in hydraulic failure and consequent tree death is well-documented. The dieback observed from the tips of pine branches to the F. circinatum infection site was shown to occur as a result of girdling cankers and pathogen growth obstructing water flow (Gordon et al., 2001; Martín-Rodrigues et al., 2013). Earlier studies demonstrated that the wilting preceding this dieback correlated in fact with the hampering of the water flow through stem segments showing similar canker sizes in P. taeda, although the possible effect of the phytotoxins produced by the pathogen has not been excluded (Solel and Bruck, 1990). These could include, e.g., beauvericin or fusaric acid which have shown to contribute to F. circinatum pathogenicity (Muñoz-Adalia et al., 2016; Phasha et al., 2021b).
At the molecular level, a SNP was located on a PPC resistance-related gene in P. taeda (dehydration-responsive protein RD22-like), suggesting that water deficit resistance was also induced as part of the defence mechanism against F. circinatum infection (Lu et al., 2017). The upregulation of pi307a (usually associated to drought responses) in Pinus elliottii Engelm. var. elliottii was suggested to reflect the shoot desiccation observed being an example of a “molecular symptom” (Morse et al., 2004). The authors propose that the obstruction/destruction of the vascular system by the pathogen results in the loss of solute transport, inducing a drought response in the upper parts of the shoot including the regulation of drought-responsive genes. A gene involved in stomatal closure was also found to be upregulated in P. patula seedlings only 1 day post-inoculation with F. circinatum (Visser et al., 2015).
Changes in plant water relations associated to PPC showed to modulate foliar gas exchange through stomata regulation. Physiological measurements revealed a decreased water potential coupled to a reduction of needle transpiration rate and stomatal conductance in symptomatic P. radiata and P. pinaster (Cerqueira et al., 2017; Amaral et al., 2019a,b, 2021a). However, the water stress-like scenario observed showed to be more intense for P. pinaster (lower water potential, decreased relative water content, overexpression of the drought-responsive SnRK2.6 and PR5, and accumulation of the osmolyte proline; Amaral et al., 2019a,b). The greater resistance to PPC observed in P. pinaster has thus been attributed to its greater tolerance to water-limiting conditions in comparison with P. radiata, which would explain the earlier development of PPC symptoms at lower thresholds of water deficit in the highly susceptible species. This observation paves the way for exploring shared mechanisms underlying pine resistance to both drought and PPC and offers an opportunity to develop trees resistant to multiple stresses, which is of utmost importance in the current climate change context.
In contrast with the susceptible P. radiata, which opens its stomata immediately after infection with F. circinatum closing them after 1 day, the resistant P. pinea maintains this response overtime (Amaral et al., 2021a). Although the involvement of the glutathione-ascorbate cycle in this process has been proposed (Amaral et al., 2019a), this hypothesis has been excluded after enzymatic activity analysis (Amaral et al., 2021a). The role of stomata opening/closure in plant immune defence is well-described regarding foliar bacterial pathogens which use them as entering points for infection, but little is known about stomatal defence in pathogens that enter the host through other vias. This represents an exciting field of research as it could represent, e.g., a resistance mechanisms that relies on the maintenance of host photosynthetic capacity to avoid carbon starvation after pathogen infection and/or a strategy to optimise water uptake by decreasing needle water potential to compensate transpiration as ‘water spender’ species do under drought.
Signalling mechanisms in the response to pathogen infection
ROS signalling, oxidative damage, and antioxidant mechanisms
In addition to its direct antimicrobial activity, many of the defences induced by pathogen invasion are associated to the rapid production of ROS (the ‘oxidative burst’; O’Brien et al., 2012). These include the induction of the expression of defence genes, HR, cell wall protein cross-linking, phytoalexin production, callose deposition, SAR, and the inactivation of key photosynthetic genes. However, at high levels, ROS may become toxic and interact with several organic molecules (e.g. proteins, nucleic acids, lipids, and carbohydrates) leading to oxidative-induced damages (Sharma et al., 2012; Soares et al., 2019). Therefore, plants have developed a complex antioxidant system composed of enzymatic and (low-molecular weight) non-enzymatic elements that help maintain cell redox homeostasis (Soares et al., 2019).
Several studies reported the involvement of these mechanisms in PPC. In P. radiata, (i) proline accumulation may explain unchanged malondialdehyde and electrolyte leakage values—indicators of cell membrane integrity—as proline protects proteins and membranes from denaturation and degradation and is linked to ROS scavenging (Cerqueira et al., 2017) and (ii) a long non-coding RNA (lncRNAPiRa.19024.1) seems to be involved in cell detoxification processes after an oxidative burst is triggered by F. circinatum infection (Zamora-Ballesteros et al., 2022). For relatively resistant species it is known that: (i) P. pinaster seedlings originated from a favourable maternal environment presented an effective antioxidant capacity which allowed to limit oxidative damage (necrosis) and F. circinatum growth (Vivas et al., 2014b); (ii) non-enzymatic components, such as L-ascorbate, glycerol, and vitamin B6 were associated to PPC resistance (Amaral et al., 2019a, 2021b; Zamora-Ballesteros et al., 2021); and (iii) the induction of phenolic compounds seems key in pine antioxidant response to F. circinatum infection (further discussed). The exogenous application of some of these natural compounds or others that induce its biosynthesis could be further explored for the development of environmentally friendly measures to control PPC.
Although the activity of important antioxidant enzymes from the ascorbate-glutathione and water–water cycles was not altered after F. circinatum infection in needles of the resistant P. pinea, the water–water cycle is compromised in symptomatic P. radiata (Amaral et al., 2021a). The failure of this photoprotective mechanism to dissipate the excess excitation energy generated after photosynthesis impairment may explain the increased susceptibility to photoinhibition observed, which suggests oxidative damages in PSII. Proteomic studies further discussed the importance of chloroplastic redox regulation in pine response to PPC (Amaral et al., 2021b). While in P. radiata it is hypothesised that the pathogen may target chloroplastic proteins for the redox regulation of key metabolic pathways, in the resistant P. pinea some chloroplastic proteins have been identified as part of its antioxidant response upon infection (chloroplastic peroxiredoxin Q and nucleoside diphosphate kinase 2). However, further studies are needed to better understand these mechanisms.
Regulation of hormone signalling pathways
Phytohormones are important signalling components of plant defence, establishing a complex crosstalk in response to infection depending on the plant-pathogen interaction (Marzec, 2016; Huang et al., 2020). However, fungal pathogens also have the ability to produce hormones contributing to plant disease (Chanclud and Morel, 2016). Interestingly, gibberellins (GAs; well-known growth-promoting phytohormones) were named after Gibberella fujikuroi (Sawada) S. Ito (= Fusarium fujikuroi Nirenberg) in which these were first identified (Stępień et al., 2020). Fusarium circinatum is part of the F. fujikuroi species complex and is thus also able to produce GAs (Stępień et al., 2020) although it possesses only one gene from the gene cluster related to its biosynthesis found in other Fusarium species (Bömke and Tudzynski, 2009). Besides fungal GAs are suggested to participate in pathogenicity (Chanclud and Morel, 2016), its role in PPC has not yet been determined.
On the other hand, the importance of hormone-mediate defence responses against F. circinatum infection has been highlighted by recent transcriptomic studies in different pine species (Carrasco et al., 2017; Visser et al., 2019; Hernandez-Escribano et al., 2020; Zamora-Ballesteros et al., 2021). While abscisic acid (ABA) signalling-related genes were part of the second most relevant category of genes differentially expressed in P. radiata upon inoculation (Carrasco et al., 2017) and ABA catabolism seems to be activated (Zamora-Ballesteros et al., 2021); a complex integration and coordination of SA, jasmonic acid (JA), and ethylene (ET), and auxins signalling is suggested to be involved in the resistance of P. tecunumanii and P. pinea to PPC (Visser et al., 2019; Zamora-Ballesteros et al., 2021), as well as the moderate resistance shown by P. pinaster at early stages of infection (Hernandez-Escribano et al., 2020). In particular, lncRNAPiRa.85000.6 has been suggested as a key regulator of ET levels after infection: while P. pinaster presents a fine-tuned ET response against F. circinatum, this does not occur in P. radiata likely due to the influence of this lncRNA located upstream of its transcription (Zamora-Ballesteros et al., 2022).
The initial response of P. pinaster has been suggested to be then manipulated by the ability of the pathogen to: stop SA biosynthesis through the chorismate pathway by the synthesis of isochorismatase family hydrolase (ICSH); block JA signalling by the suppression of a key regulatory element of this pathway, the receptor coronatine insensitive 1 (COI1), and perturb ET homeostasis by the production of fungal ET which can act as a virulence factor and interfere with ET signalling to suppress effective defence pathways (Hernandez-Escribano et al., 2020). However, the authors acknowledged that further physiological measurements (including hormone quantification) and functional studies with F. circinatum mutants are needed. The blocking of JA signalling by F. circinatum has been also proposed by Zamora-Ballesteros et al. (2021). Moreover, besides increased levels of JA were found in P. radiata and P. pinaster needles, these species were still susceptible to F. circinatum infection (Amaral et al., 2019a,b). This further supports that the pathogen can overcome JA-dependent defence responses.
Different studies report an increase of the endogenous levels of ABA in needles of P. radiata and P. pinaster upon inoculation with F. circinatum associated to stomata closure and photosynthesis impairment (Cerqueira et al., 2017; Amaral et al., 2019a,b, 2021a). Again, the authors discuss that this may result either from the host defence response or from its manipulation by the pathogen to suppress plant basal resistance. In accordance, lower levels of ABA were verified when a delay on disease symptom development was induced by the foliar application of phosphite (Cerqueira et al., 2017). The catabolism of ABA is also activated early in the infection process leading to the accumulation of the inactive dihydrophaseic acid in P. radiata (to control de pool of bio-active ABA) and of the weakly ABA-like active phaseic acid in the resistant P. pinea, which may be a key defence mechanism (Amaral et al., 2021a). Proteomic studies further suggest an ABA-mediated epigenetic reprogramming of gene expression, although this still lacks validation (Amaral et al., 2021b).
Despite the effort to untangle the intricate regulation of hormone balance during Pinus-F. circinatum interaction and understand how the pathogen may interfere with it has contributed to elucidate these signalling networks, there is still a long way towards the development of hormone-based control strategies for PPC. The constitutive activation of particular hormone signalling pathways (in mutants or transgenic lines) may enhance resistance to a certain pathogen but also impact plant fitness and increase susceptibility to other stresses (Denancé et al., 2013). A promising alternative to this would be “priming,” i.e., treat plants with a defensive compound, such as an hormone, in order to modulate its “immunological memory” so that a faster and stronger resistance response occurs upon attack (Conrath, 2011). The exogenous application of both SA and methyl jasmonate (MeJA) failed to enhance resistance to PPC (Vivas et al., 2012; Fitza et al., 2013). Given the relevance of ABA signalling in PPC outcome, the next step would be to explore the potential of ABA and its catabolites as inducers of resistance; as well as the chemical manipulation of key control points in ABA signalling, as this has been pointed out as an innovative approach to manage stress in agriculture (Hewage et al., 2020).
Rearrangement of host primary metabolism: Photosynthesis, carbohydrates, and nitrogen
RNA-Seq analysis of two contrasting P. radiata genotypes in their response against F. circinatum revealed that the most interesting differentially expressed genes included genes related to primary metabolism (Carrasco et al., 2017). In general, it is known that the high demands of energy for defence response upon biotic challenge are associated with a reprogramming of the host primary metabolism, including changes in photosynthesis, carbohydrate and N metabolism, plant respiration, and aerobic fermentation (Berger et al., 2007; Bolton, 2009; Rojas et al., 2014).
Most photosynthesis-related studies in PPC report photosynthesis limitations at late stages of disease in susceptible hosts, likely due to oxidative damages in the photosynthetic apparatus. The expression of photosynthetic genes was decreased in the stem of the resistant P. pinea but not in P. radiata, although no changes on needle photosynthetic rate were verified (Zamora-Ballesteros et al., 2021). However, RuBisCO was downregulated in needles of symptomatic P. radiata and P. pinaster and independent gas exchange measurements revealed photosynthesis impairment in these species due to stomatal and non-stomatal processes, either maintaining or increasing its total chlorophyll content (López-López et al., 2016; Cerqueira et al., 2017; Amaral et al., 2019a,b, 2021a). Decreased photochemical efficiency (Fv/Fm – maximum quantum yield of PSII and ΦPSII – effective quantum yield of PSII) was also reported in P. radiata (Cerqueira et al., 2017), as well as increased susceptibility to photoinhibition (Amaral et al., 2021a).
Photosynthesis decrease may induce a metabolic shift towards respiration (from source-to-sink) through the transformation of the stored sucrose into fructose and hexoses (Berger et al., 2007; Bolton, 2009; Rojas et al., 2014). Sugars and its phosphate derivates are also key signalling molecules in several defence-related pathways (Morkunas and Ratajczak, 2014). Besides Cerqueira et al. (2017) did not found significant changes in P. radiata total soluble sugars content after F. circinatum infection; Vivas et al. (2014a) highlighted the role of carbohydrates in P. pinaster response to PPC, with seedlings originated from mother trees growing on a favourable environment showing greater tolerance to the disease and an altered carbohydrate type proportion (increased glucose vs. decreased uronic acids content). The authors suggested the involvement of epigenetic mechanisms in transmitting carbohydrate changes to offspring and highlighted the influence that the inherited endophyte community may have, but no further studies are available. Moreover, two genes linked to sugar metabolism were shown to be upregulated after inoculation of P. patula seedlings at very early stages of infection (Visser et al., 2015), and the downregulation of RuBisCO in symptomatic P. radiata and P. pinaster has been associated to a source-to-sink metabolic shift which was reflected in the induction of several alternative energy-producing pathways (e.g. respiration, aerobic fermentation, and gluconeogenesis/glyoxylate cycle) and in the accumulation of amino acids (Amaral et al., 2019a, 2021b).
Strong changes on host N metabolism are expected after pathogen infection as a result of the activation of defence response and of fungal manipulation of host metabolism in its benefit (Fagard et al., 2014). The relevance of N recycling, transport, and storage in P. radiata defence response against F. circinatum has been suggested by the enrichment of several lncRNAs related to allantoin metabolism (Zamora-Ballesteros et al., 2022). On the other hand, the general accumulation of amino acids in P. radiata and P. pinaster after F. circinatum infection suggests the ability of the pathogen to manipulate hosts’ primary metabolism to favour its nutritional conditions by increasing the availability of N sources (Amaral et al., 2019a). In accordance, an upregulation of F. circinatum nutrient transporter genes was associated to N uptake when infecting the susceptible P. radiata (Zamora-Ballesteros et al., 2021). Several studies evidence the impact of N availability on F. circinatum infection ability. Increased amounts of N predispose pines to F. circinatum infection and increase the severity of PPC symptoms (e.g., Lopez-Zamora et al., 2007; Swett et al., 2016). In nurseries, this could be explained by the stimulation of succulent shoot growth which would allow the infection of young pine seedlings without wounding (Mitchell et al., 2011). Fertilization of P. radiata using aerated compost tea reduced F. circinatum concentration likely because it is rich in N-fixing bacteria which decrease the inorganic N available restricting fungi growth and sporulation (Otero et al., 2020); while the reduction of PPC incidence in P. taeda seedlings grown under elevated CO2 has been hypothesised to be related to decreased N levels and increased C-based defence compounds (Runion et al., 2010).
Defence response in conifers: Resin, phenolics, and PR proteins
Given that conifers are often exposed to several insects and fungal pathogens during their long lifetime, these possess a strong arsenal of defence mechanisms for protection (Franceschi et al., 2005; Kolosova and Bohlmann, 2012). These include the production of toxic secondary metabolites against the biotic agent (such as oleoresin terpenoids and phenolics), of anatomical structures to store and transport these molecules, and of PR proteins. A simple explanation of each of these mechanisms is presented in the context of Pinus response to F. circinatum.
Increase of resin production: Traumatic resin ducts and terpenoids
One of the first lines of defence against pests and pathogens in conifers is the production of resin (or oleoresin) in the resin ducts (Franceschi et al., 2005), representing both a mechanical and chemical defence mechanism (Kolosova and Bohlmann, 2012). Although resin ducts are constitutively distributed throughout the plant, new resin ducts (traumatic resin ducts) may be formed after pathogen attack or application of the defence elicitor MeJA to further increase conifer defence capacity (Franceschi et al., 2005; Kolosova and Bohlmann, 2012; Celedon and Bohlmann, 2019). Several studies explored the role of resin in PPC response as this is one of the most characteristic symptoms of the disease.
Although increased resin production is observed in infected trees, F. circinatum has shown to have the ability to grow in both constitutive and traumatic resin ducts in the susceptible P. radiata and use them in its favour to colonise regions of the host far from the infection site through the spread of conidiophores and conidia (Martín-Rodrigues et al., 2013). Slinski et al. (2015) reported that F. circinatum showed greater tolerance to P. radiata resin and to isolated monoterpenes than the non-pathogenic Fusarium temperatum Scaufl. & Munaut. Moreover, the application of MeJA as an elicitor of plant defence in P. pinaster led to an increase in resin duct density and thus failed to confer resistance against F. circinatum infection (Vivas et al., 2012), and the increased resistance of young P. patula seedlings to PPC using chitosan as elicitor does not seem to be associated to the synthesis of terpenoids (based on the downregulation of DXS1, involved in its biosynthesis) (Fitza et al., 2013). In accordance, Amaral et al. (2019b) hypothesise that the activation of the JA-dependent signalling pathway in P. radiata and P. pinaster may increase traumatic resin ducts formation and facilitate stem vertical colonization.
However, the decrease of PPC severity after wounding pre-treatment (artificial or caused by the bark-feeding PPC vector insect Tomicus piniperda L.) was associated to increased resin flow and/or terpene accumulation (Kim et al., 2010; Lombardero et al., 2019), and the enhanced resistance of P. radiata to F. circinatum infection after aerated compost tea fertilization was associated to the overexpression of a gene involved in terpenoids biosynthesis (hydroxymethylglutaryl-CoA reductase 2; Otero et al., 2020). Also, a general overexpression of genes related to terpenoids biosynthesis was observed in the resistant P. pinea upon F. circinatum infection (Zamora-Ballesteros et al., 2021). Despite this, it seems that the typical constitutive and induced resin production-associated defence responses in conifers fail to fight F. circinatum infection, which is able to resist terpenoids activity and take advantage of the formation of traumatic resin ducts to further colonise the plant. Moreover, the excessive production of resin during PPC development may lead to the hampering of plant water flow and result in plant mortality.
Phenylpropanoids pathway: The swiss knife of plant secondary metabolism
Phenolics are important constitutive and induced components of conifer defence against fungal infection (Franceschi et al., 2005), including flavonoids (such as anthocyanins), lignin, lignans, stilbenes, condensed tannins, and SA (Pascual et al., 2016). These are produced through the phenylpropanoids pathway, which is initiated by phenylalanine ammonia lyase (PAL) and represents the greatest sink of primary metabolism (Kolosova and Bohlmann, 2012; Pascual et al., 2016). ‘Non-structural’ phenolics such as flavonoids often have a direct toxic effect on pathogens (phytoalexins) and offer protection against the oxidative stress resulting from the activation of defence against pathogens through ROS signalling (Grace, 2005). The activation of this pathway and associated defences has been extensively studied in PPC.
The overexpression of genes involved in the phenylpropanoids pathway, including pal, was associated to the resistance of a P. radiata genotype (Donoso et al., 2015; Carrasco et al., 2017) and of P. pinea (Zamora-Ballesteros et al., 2021) to PPC; as well as to the onset of induced resistance to PPC in seedlings of P. radiata fertilised with aerated tea compost (Otero et al., 2020) and of P. patula treated with chitosan (Fitza et al., 2013). In accordance, the application of chitosan avoided the reduction of both total phenolic content and radical scavenging activity verified in P. sylvestris upon inoculation with F. circinatum (Silva-Castro et al., 2018a). In addition, early lignification and production of lignans at the infection site to avoid the spread of F. circinatum has been proposed to confer resistance to PPC in a P. radiata genotype showing increased expression of phenylcoumaran benzylic ether reductase (pcber; involved in the synthesis of secondary metabolites such as lignans) (Donoso et al., 2015) and in P. pinea (Zamora-Ballesteros et al., 2021). This response may protect the host against the early oxidation of phenolic compounds (e.g. lignin) by F. circinatum laccases (Muñoz-Adalia et al., 2016; Zamora-Ballesteros et al., 2021). An early overexpression of pinosylvin synthase (pst) was also observed in this resistant P. radiata genotype, which is related to the synthesis of one the most common stilbene phytoalexins in conifers (Kolosova and Bohlmann, 2012). The role of lncRNAs in the transcriptional regulation of genes involved in the phenylpropanoids pathway has been recently described in P. radiata after F. circinatum inoculation, highlighting the regulatory mechanisms behind cell wall remodelling and lignification processes upon pathogen attack (Zamora-Ballesteros et al., 2022).
Therefore, the induction of the production of several phenolics through the phenylpropanoids pathway seems a key on determining PPC resistance. Only Visser et al. (2015) reported a downregulation of PAL transcripts in P. patula after F. circinatum inoculation and Donoso et al. (2015) did not found changes in chalcone synthase expression (chs; first pathway-specific step in flavonoids biosynthesis) between a PPC resistant and susceptible P. radiata genotypes; but both authors hypothesise that the sampling times considered were not adequate to evaluate these responses. Although an upregulation of pal has been observed in needles of pine species with contrasting susceptibilities to PPC, the importance of increased phenolics contents (such as anthocyanins) to resist F. circinatum has been suggested both upon inoculation, at the constitutive level and after the application of phosphite as a resistance elicitor (Cerqueira et al., 2017; Amaral et al., 2019a,b; Leitão et al., 2021). Furthermore, proteomic studies hypothesised that P. radiata secondary metabolism may also be targeted by F. circinatum to negatively regulate immune response (Amaral et al., 2021b).
Induction of PR proteins
Antimicrobial PR proteins are only induced upon pathogen attack, being associated to HR and SAR as these offer protection from further infection at the infection site and in uninfected tissues (Kolosova and Bohlmann, 2012; Jain and Khurana, 2018). The production of PR proteins is induced by (i) molecules derived from pathogens (e.g., chitin from fungal cell wall), (ii) ROS, and (iii) by SA or JA/ET defence signalling, activating PRs involved in SAR or local acquired resistance, respectively (Ali et al., 2018; Jain and Khurana, 2018). However, the signalling behind the induction of PR gene expression is still poorly understood and it is expected not to be that straight-forward for PPC given the complexity of the phytohormone signalling proposed.
The analysis of the susceptible P. patula and the resistant P. tecunumanii (low elevation provenance) transcriptomes upon F. circinatum inoculation allowed to identify 801 and 646 putative PR genes, respectively (Visser et al., 2018). The lower number of transcripts in P. patula PR5, PR2, PR9, and PR1 orthogroups could reveal transcripts that are absent in its defence response. For example, the relative expansion of the PR9 peroxidase orthogroups in P. tecunumanii supports a more robust cell wall reinforcement or oxidative burst response. These enzymes are involved in the polymerization of hydroxycinnamyl alcohols—a H2O2-dependent reaction-, essential for lignin polymerization and suberin disposal (Koutaniemi et al., 2007), which can potentially enhance plant resistance against pathogens (van Loon et al., 2006). The overexpression of PR9 genes has been associated to the PPC resistant response of a P. radiata genotype (Donoso et al., 2015), but also to P. elliottii and P. pinaster disease development (Morse et al., 2004; Hernandez-Escribano et al., 2020). Moreover, PR9 has been suggested to be a target of F. circinatum effectors in P. pinea (Zamora-Ballesteros et al., 2021).
The upregulation of the antifungal PR1 protein transcripts has been reported in (relatively) resistant PPC hosts in association with increased expression of SA biosynthesis-related genes, which supports its involvement in SAR during F. circinatum infection (Carrasco et al., 2017; Visser et al., 2019; Hernandez-Escribano et al., 2020; Zamora-Ballesteros et al., 2021). Moreover, the downregulation of F. circinatum ergosterol biosynthesis genes could increase its susceptibility to PR1 in the resistant P. tecunumanii (Visser et al., 2019) as these are known to bind and sequester sterols directly inhibiting sterol-auxotrophic pathogens and sterol-prototrophic pathogens with compromised sterol biosynthesis (Gamir et al., 2017).
The upregulation of PR2 β-1,3-glucanase, PR3 chitinase, and PR5 thaumatin-like protein upon F. circinatum infection also showed to be related to hormone signalling and suggest an effort of the host to degrade fungal cell wall increasing its susceptibility to cell lysis and plant defence molecules (Davis et al., 2002; Morse et al., 2004; Donoso et al., 2015; Carrasco et al., 2017; Visser et al., 2019; Zamora-Ballesteros et al., 2021). PR5 was associated to PPC resistance in a P. radiata genotype mediated by ET (Carrasco et al., 2017) and to the relative resistance of P. pinaster to PPC (Amaral et al., 2019a); while PR3 upregulation occurred in susceptible and resistant hosts associated to both SA or JA/ET signalling (Davis et al., 2002; Morse et al., 2004; Carrasco et al., 2017; Visser et al., 2019; Amaral et al., 2019a), and was related to the enhanced resistance of P. radiata to PPC through fertilization with aerated compost tea (Otero et al., 2020).
Davis et al. (2002) performed proteomic studies regarding the role of chitinase in the P. elliottii-F. circinatum interaction finding that these were overexpressed at the mRNA level in both the susceptible and resistant genotypes but only in the symptomatic susceptible genotype at the protein level. This evidenced the importance of employing proteomic studies to further explore the role of PR proteins in hosts with varying susceptibilities to PPC upon F. circinatum challenge. Recent needle proteomics analysis of the PPC susceptible P. radiata and resistant P. pinea did not highlighted any key PR protein in response to F. circinatum infection (Amaral et al., 2021b).
Final considerations and future perspectives
The use of a comprehensive number of physiological and Omics techniques to evaluate the response of pine species with different levels of susceptibility to PPC allowed to unravel several aspects of Pinus-F. circinatum interaction (see Figure 3). While susceptible hosts are generally highly responsive to pathogen infection either as a result of pathogenesis or of a late and insufficient activation of plant immune defence; resistant species seem to activate more specific mechanisms that may enable them to fight F. circinatum.
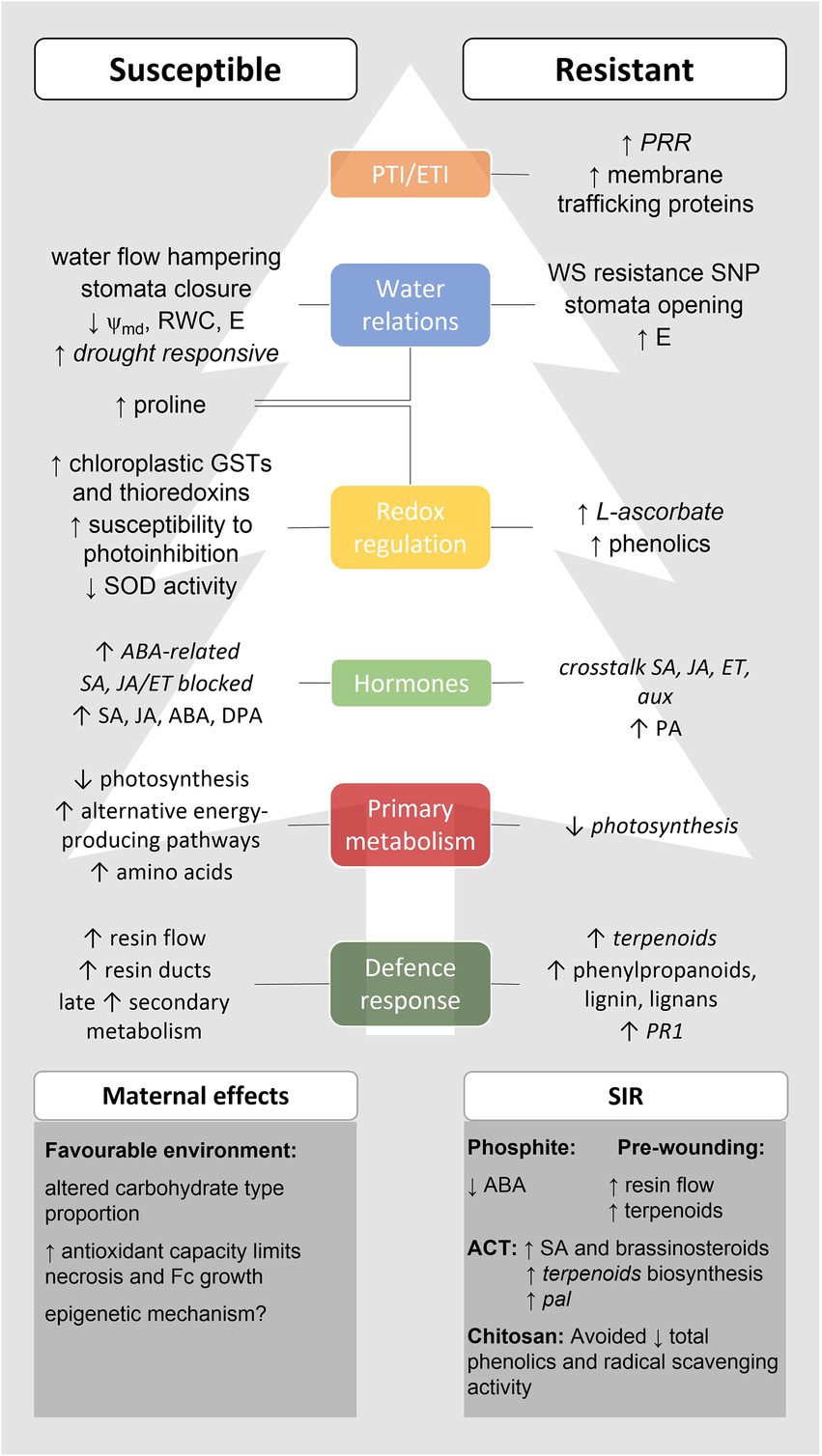
Figure 3. Main responses to Fusarium circinatum infection in susceptible and resistant hosts and effects of maternal environments and SIR in these responses. This scheme was built based on the multi-disciplinary studies focusing on pine response to PPC herein reviewed, which contributed to unveil several layers of PPC response. Italics indicate results obtained exclusively through gene expression analysis. Altogether, PPC resistant species seem to activate PTI early in the infection process and avoid F. circinatum effectors through membrane trafficking processes, resulting in a prompt induction of hormone signalling and defence-related responses, including the production of antioxidant compounds. Resistance to WS has been proposed as part of PPC resistance, and stomata opening and increased transpiration occurs in PPC resistant hosts upon infection although the significance of this response remains elusive. On the other hand, PTI/ETI fails in PPC susceptible pines allowing F. circinatum to grow inside the host and manipulate its metabolism in its benefit. The pathogen resists to the increased resin production and uses resin ducts to further colonise the host. A WS-like scenario is then established due to resin accumulation and fungal growth, leading to stomata closure (reducing water loss by transpiration), induction of drought-responsive genes, and accumulation of the osmolyte and antioxidant amino acid proline. A greater susceptibility to photoinhibition, the failure of the water–water cycle and the accumulation of chloroplastic GSTs and thioredoxins were also reported. The latter may result from fungal manipulation of host metabolism to activate alternative energy-producing pathways (e.g. respiration, aerobic fermentation, and gluconeogenesis) under photosynthesis impairment to produce amino acids as an N source. The late induction of PPC susceptible host secondary metabolism is insufficient to fight PPC. Moreover, ABA accumulation is associated to PPC susceptibility and conceivably results from fungal manipulation, which is also able to block SA and JA/ET signalling. The resistance to PPC observed in hosts originated from mother trees grown under favourable environments and after treatment with phosphite, ACT, chitosan, or pre-wounding has been attributed to changes in both primary and secondary metabolism, antioxidant capacity, and hormone signalling. ABA, abscisic acid; ACT, aerated compost tea; aux, auxins; DPA, dihydrophaseic acid; E, transpiration rate; ET, ethylene; GSTs, glutathione S-transferase; JA, jasmonic acid; PA, phaseic acid; PPC, pine pitch canker; PR, pathogenesis-related protein; PRR, pattern recognition receptors; RWC, relative water content; SA, salicylic acid; SIR, systemic induced resistance; SNP, single nucleotide polymorphism; SOD, superoxide dismutase; WS, water stress; and ψmd, midday water potential.
Besides contributing for the identification of potential pathways associated to PPC susceptibility/resistance, the studies herein reviewed pinpoint fields of research that deserve deeper investigation and may contribute for the development of innovative disease control measures. These include exploring the convergence between F. circinatum- and drought-induced responses (including stomata regulation), taking advantage of the advanced knowledge on tree response to water limiting conditions. Also, the importance of ABA-signalling and of several antioxidants on PPC response should be further examined based on the systemic induction of resistance through chemical priming, comprising either its direct application or the application of other compounds that influence its biosynthesis. Different application timings, methods, and concentrations should be tested on seedlings with different ages to assure broad-spectrum and long-lasting resistance against several isolates of F. circinatum.
The recent advent of microbiome studies in hosts with varying levels of susceptibility to PPC (Leitão et al., 2021; Romeralo et al., 2022) also represents an opportunity to identify microorganisms that impact host response with potential to be employed in biocontrol strategies, such as the development of disease suppressive soils. Other advances such as the publication of the chromosome-level assembly and methylome of the 25.4-Gb genome of Pinus tabuliformis (Niu et al., 2022) offer new venues for genomics studies on PPC, as well as for epigenetics which are still absent. In addition, the study of F. circinatum secretome and exometabolome would be key to better understand its interaction with Pinus species allowing to determine the secreted proteins and metabolites that favour tissue penetration and colonization in susceptible hosts. Given the complexity of the next steps proposed for studying PPC and developing effective control measures, it is required that scientists from different disciplines collaborate in order to speed up the understanding of the disease and thus contribute for improving the current forest management policies.
Author contributions
JA and GP designed the article. JA wrote the manuscript and built the figures. All authors contributed to the article and approved the submitted version.
Funding
This work was performed under the framework of the URGENTpine project (PTDC/AGR-FOR/2768/2014), which is supported by FEDER through COMPETE (Programa Operacional Fatores de Competitividade; POCI-01-FEDER-016785) and by national funds through the Portuguese Foundation for Science and Technology (FCT), and of the F4F—Forest For Future project (CENTRO-08-5864-FSE-000031) from Programa Operacional Regional do Centro (Centro 2020), Portugal 2020 and Fundo Social Europeu. Thanks are due to FCT/MCTES (Ministry for Science, Technology and Higher Education) for financial support to CESAM (UID/50017/2020 + UIDB/50017/2020 + LA/P/0094/2020) through national funds. FCT also supported JA (SFRH/BD/120967/2016). The Spanish Ministry of Economy and Competitiveness supported LV through the Ramón y Cajal programme (RYC-2015-17871).
Acknowledgments
The authors would like to acknowledge Eduardo Batista (University of Aveiro) for assistance on building Figure 1.
Conflict of interest
The authors declare that the research was conducted in the absence of any commercial or financial relationships that could be construed as a potential conflict of interest.
Publisher’s note
All claims expressed in this article are solely those of the authors and do not necessarily represent those of their affiliated organizations, or those of the publisher, the editors and the reviewers. Any product that may be evaluated in this article, or claim that may be made by its manufacturer, is not guaranteed or endorsed by the publisher.
References
Aegerter, B. J., Gordon, T. R., Storer, A. J., and Wood, D. L. (2003). Pitch Canker: A Technical Review. Oakland, CA, USA: University of California Agriculture and Natural Resources.
Ali, S., Ganai, B. A., Kamili, A. N., Bhat, A. A., Mir, Z. A., Bhat, J. A., et al. (2018). Pathogenesis-related proteins and peptides as promising tools for engineering plants with multiple stress tolerance. Microbiol. Res. 212, 29–37. doi: 10.1016/j.micres.2018.04.008
Amaral, J., Correia, B., António, C., Rodrigues, A. M., Gómez-Cadenas, A., Valledor, L., et al. (2019a). Pinus susceptibility to pitch canker triggers physiological responses in symptomatic plants: an integrated approach. Front. Plant Sci. 10:509. doi: 10.3389/fpls.2019.00509
Amaral, J., Correia, B., Escandón, M., Jesus, C., Serôdio, J., Valledor, L., et al. (2021a). Temporal physiological response of pine to Fusarium circinatum infection is dependent on host susceptibility level: the role of ABA catabolism. Tree Physiol. 41, 801–816. doi: 10.1093/treephys/tpaa143
Amaral, J., Lamelas, L., Valledor, L., Castillejo, M. A., Alves, A., and Pinto, G. (2021b). Comparative proteomics of Pinus-Fusarium circinatum reveals metabolic clues to biotic stress resistance. Physiol. Plant. 173, 2142–2154. doi: 10.1111/ppl.13563
Amaral, J., Pinto, G., Flores-Pacheco, J. A., Díez-Casero, J., Cerqueira, A., Monteiro, P., et al. (2019b). Effect of Trichoderma viride pre-inoculation in pine species with different levels of susceptibility to Fusarium circinatum: physiological and hormonal responses. Plant Pathol. 68, 1645–1653. doi: 10.1111/ppa.13080
Baker, R., Candresse, T., Simon, D., Gilioli, G., Grégoire, J.-C., Jeger, M. J., et al. (2010). Risk assessment of Gibberella circinata for the EU territory and identification and evaluation of risk management options. EFSA J. 8:1620. doi: 10.2903/j.efsa.2010.1620
Berbegal, M., Pérez-Sierra, A., Armengol, J., and Grünwald, N. J. (2013). Evidence for multiple introductions and clonality in Spanish populations of Fusarium circinatum. Phytopathology 103, 851–861. doi: 10.1094/PHYTO-11-12-0281-R
Berger, S., Sinha, A. K., and Roitsch, T. (2007). Plant physiology meets phytopathology: plant primary metabolism and plant pathogen interactions. J. Exp. Bot. 58, 4019–4026. doi: 10.1093/jxb/erm298
Bezos, D., Martínez-Alvarez, P., Fernández, M., and Diez, J. J. (2017). Epidemiology and management of pine pitch canker disease in Europe—a review. Balt. For. 23, 279–293.
Bolton, M. D. (2009). Primary metabolism and plant defense—fuel for the fire. Mol. Plant-Microbe Interact. 22, 487–497. doi: 10.1094/MPMI-22-5-0487
Bömke, C., and Tudzynski, B. (2009). Diversity, regulation, and evolution of the gibberellin biosynthetic pathway in fungi compared to plants and bacteria. Phytochemistry 70, 1876–1893. doi: 10.1016/j.phytochem.2009.05.020
Bonello, P., Gordon, T. R., Herms, D. A., Wood, D. L., and Erbilgin, N. (2006). Nature and ecological implications of pathogen-induced systemic resistance in conifers: a novel hypothesis. Physiol. Mol. Plant Pathol. 68, 95–104. doi: 10.1016/j.pmpp.2006.12.002
Bonello, P., Gordon,, and Storer, A. J. (2001). Systemic induced resistance in Monterey pine. For. Pathol. 31, 99–106. doi: 10.1046/j.1439-0329.2001.00230.x
Carrasco, A., Sanfuentes, E., Durán, Á., and Valenzuela, S. (2016). Cancro resinoso del pino: ¿una amenaza potencial para las plantaciones de Pinus radiata en Chile? Gayana. Botánica 73, 369–380. doi: 10.4067/S0717-66432016000200369
Carrasco, A., Wegrzyn, J. L., Durán, R., Fernández, M., Donoso, A., Rodriguez, V., et al. (2017). Expression profiling in Pinus radiata infected with Fusarium circinatum. Tree Genet. Genomes 13:46. doi: 10.1007/s11295-017-1125-0
Celedon, J. M., and Bohlmann, J. (2019). Oleoresin defenses in conifers: chemical diversity, terpene synthases and limitations of oleoresin defense under climate change. New Phytol. 224, 1444–1463. doi: 10.1111/nph.15984
Cerqueira, A., Alves, A., Berenguer, H., Correia, B., Gómez-Cadenas, A., Diez, J. J., et al. (2017). Phosphite shifts physiological and hormonal profile of Monterey pine and delays Fusarium circinatum progression. Plant Physiol. Biochem. 114, 88–99. doi: 10.1016/j.plaphy.2017.02.020
Chanclud, E., and Morel, J. (2016). Plant hormones: a fungal point of view. Mol. Plant Pathol. 17, 1289–1297. doi: 10.1111/mpp.12393
Chang, S., Mahon, E. L., MacKay, H. A., Rottmann, W. H., Strauss, S. H., Pijut, P. M., et al. (2018). Genetic engineering of trees: progress and new horizons. In vitro Cell. Dev. Biol. Plant 54, 341–376. doi: 10.1007/s11627-018-9914-1
Chimwamurombe, P. M., Wingfield, B. D., Botha, A.-M., and Wingfield, M. J. (2001). Cloning and sequence analysis of the endopolygalacturonase gene from the pitch canker fungus, Fusarium circinatum. Curr. Microbiol. 42, 350–352. doi: 10.1007/s002840010228
Chisholm, S. T., Coaker, G., Day, B., and Staskawicz, B. J. (2006). Host-microbe interactions: shaping the evolution of the plant immune response. Cell 124, 803–814. doi: 10.1016/j.cell.2006.02.008
Coll, N. S., Epple, P., and Dangl, J. L. (2011). Programmed cell death in the plant immune system. Cell Death Differ. 18, 1247–1256. doi: 10.1038/cdd.2011.37
Conrath, U. (2011). Molecular aspects of defence priming. Trends Plant Sci. 16, 524–531. doi: 10.1016/j.tplants.2011.06.004
Davis, J. M., Wu, H., Cooke, J. E. K., Reed, J. M., Luce, K. S., and Michler, C. H. (2002). Pathogen challenge, salicylic acid, and jasmonic acid regulate expression of chitinase gene homologs in pine. Mol. Plant Microb. Interact. 15, 380–387. doi: 10.1094/MPMI.2002.15.4.380
De La Torre, A. R., Puiu, D., Crepeau, M. W., Stevens, K., Salzberg, S. L., Langley, C. H., et al. (2019). Genomic architecture of complex traits in loblolly pine. New Phytol. 221, 1789–1801. doi: 10.1111/nph.15535
Denancé, N., Sánchez-Vallet, A., Goffner, D., and Molina, A. (2013). Disease resistance or growth: the role of plant hormones in balancing immune responses and fitness costs. Front. Plant Sci. 4:155. doi: 10.3389/fpls.2013.00155
Donoso, A., Rodriguez, V., Carrasco, A., Ahumada, R., Sanfuentes, E., and Valenzuela, S. (2015). Relative expression of seven candidate genes for pathogen resistance on Pinus radiata infected with Fusarium circinatum. Physiol. Mol. Plant Pathol. 92, 42–50. doi: 10.1016/j.pmpp.2015.08.009
Drenkhan, R., Ganley, B., Martín-García, J., Vahalík, P., Adamson, K., Adamčíková, K., et al. (2020). Global geographic distribution and host range of Fusarium circinatum, the causal agent of pine pitch canker. Forests 11:724. doi: 10.3390/f11070724
Dvorak, W. S., Hodge, G. R., López, J. L., Espinoza, J. A., Woodbridge, W. C., Jump, R., et al. (2011). 2011 CAMCORE Annual Report. International tree breeding and conservation. Raleigh, North Carolina, USA: North Carolina State University.
Elvira-Recuenco, M., Iturritxa, E., and Raposo, R. (2015). Impact of seed transmission on the infection and development of pitch canker disease in Pinus radiata. Forests 6, 3353–3368. doi: 10.3390/f6093353
Elvira-Recuenco, M., Pando, V., Berbegal, M., Manzano Muñoz, A., Iturritxa, E., and Raposo, R. (2021). Influence of temperature and moisture duration on pathogenic life-history traits of predominant haplotypes of Fusarium circinatum on Pinus spp. in Spain. Phytopathology 111, 2002–2009. doi: 10.1094/PHYTO-10-20-0445-R
European and Mediterranean Plant Protection Organization (EPPO) (2021). Fusarium circinatum. EPPO datasheets on pests recommended for regulation.
Eyles, A., Bonello, P., Ganley, R., and Mohammed, C. (2010). Induced resistance to pests and pathogens in trees. New Phytol. 185, 893–908. doi: 10.1111/j.1469-8137.2009.03127.x
Fagard, M., Launay, A., Clement, G., Courtial, J., Dellagi, A., Farjad, M., et al. (2014). Nitrogen metabolism meets phytopathology. J. Exp. Bot. 65, 5643–5656. doi: 10.1093/jxb/eru323
Fiehn, O. (2002). Metabolomics--the link between genotypes and phenotypes. Plant Mol. Biol. 48, 155–171. doi: 10.1023/A:1013713905833
Fitza, K. N. E., Payn, K. G., Steenkamp, E. T., Myburg, A. A., and Naidoo, S. (2013). Chitosan application improves resistance to Fusarium circinatum in Pinus patula. S. Afr. J. Bot. 85, 70–78. doi: 10.1016/j.sajb.2012.12.006
Flexas, J., and Gago, J. (2018). A role for ecophysiology in the’ omics’ era. Plant J. 96, 251–259. doi: 10.1111/tpj.14059
Food and Agriculture Organization of the United Nations (FAO) (2020). Global forest resources assessment 2020: main report.
Forest Europe-Ministerial Conference on the Protection of Forests in Europe (2020). State of Europe’s Forests 2020.
Fotso, J., Leslie, J. F., and Smith, J. S. (2002). Production of beauvericin, moniliformin, fusaproliferin, and fumonisins B1, B2, and B3 by fifteen ex-type strains of Fusarium species. Appl. Environ. Microbiol. 68, 5195–5197. doi: 10.1128/AEM.68.10.5195-5197.2002
Franceschi, V. R., Krokene, P., Christiansen, E., and Krekling, T. (2005). Anatomical and chemical defenses of conifer bark against bark beetles and other pests. New Phytol. 167, 353–375. doi: 10.1111/j.1469-8137.2005.01436.x
Fu, Z. Q., and Dong, X. (2013). Systemic acquired resistance: turning local infection into global defense. Annu. Rev. Plant Biol. 64, 839–863. doi: 10.1146/annurev-arplant-042811-105606
Gamir, J., Darwiche, R., van’t Hof, P., Choudhary, V., Stumpe, M., Schneiter, R., et al. (2017). The sterol-binding activity of PATHOGENESIS-RELATED PROTEIN 1 reveals the mode of action of an antimicrobial protein. Plant J. 89, 502–509. doi: 10.1111/tpj.13398
Ganley, R. J., Watt, M. S., Manning, L., and Iturritxa, E. (2009). A global climatic risk assessment of pitch canker disease. Can. J. For. Res. 39, 2246–2256. doi: 10.1139/X09-131
Gordon, T. R. (2011). “Biology and management of Gibberella circinata, the cause of pitch canker in pines,” in Control of Fusarium Diseases. eds. F. M. Alves-Santos and J. J. Diez (Trivandrum, Kerala, India: Research Signpost), 195–207.
Gordon, T. R., Kirkpatrick, S. C., Aegerter, B. J., Fisher, A. J., Storer, A. J., and Wood, D. L. (2011). Evidence for the occurrence of induced resistance to pitch canker, caused by Gibberella circinata (anamorph Fusarium circinatum), in populations of Pinus radiata. For. Pathol. 41, 227–232. doi: 10.1111/j.1439-0329.2010.00678.x
Gordon, T. R., Storer, A. J., and Wood, D. L. (2001). The pitch canker epidemic in California. Plant Dis. 85, 1128–1139. doi: 10.1094/PDIS.2001.85.11.1128
Gordon, T. R., Wikler, K. R., Clark, S. L., Okamoto, D., Storer, A. J., and Bonello, P. (1998). Resistance to pitch canker disease, caused by Fusarium subglutinans f.sp. pini, in Monterey pine (Pinus radiata). Plant Pathol. 47, 706–711.
Grace, S. C. (2005). “Phenolics as antioxidants” in Antioxidants and Reactive oxygen Species in Plants. ed. N. Smirnoff (Oxford, UK: Blackwell Publishing Ltd.), 141–168.
Hammond-Kosack, K. E., and Parker, J. E. (2003). Deciphering plant-pathogen communication: fresh perspectives for molecular resistance breeding. Curr. Opin. Biotechnol. 14, 177–193. doi: 10.1016/S0958-1669(03)00035-1
Hantao, L. W., de Lima Ribeiro, F. A., Passador, M. M., Furtado, E. L., Poppi, R. J., Gozzo, F. C., et al. (2014). Metabolic profiling by ultra-performance liquid chromatography-mass spectrometry and parallel factor analysis for the determination of disease biomarkers in eucalyptus. Metabolomics 10, 1318–1325. doi: 10.1007/s11306-014-0666-6
Hepting, G., and Roth, E. (1946). Pitch canker, a new disease of some southern pines. J. For. 44, 742–744.
Hernandez-Escribano, L., Iturritxa, E., Aragonés, A., Mesanza, N., Berbegal, M., Raposo, R., et al. (2018a). Root infection of canker pathogens, Fusarium circinatum and Diplodia sapinea, in asymptomatic trees in Pinus radiata and Pinus pinaster plantations. Forests 9:128. doi: 10.3390/f9030128
Hernandez-Escribano, L., Iturritxa, E., Elvira-Recuenco, M., Berbegal, M., Campos, J. A., Renobales, G., et al. (2018b). Herbaceous plants in the understory of a pitch canker-affected Pinus radiata plantation are endophytically infected with Fusarium circinatum. Fungal Ecol. 32, 65–71. doi: 10.1016/j.funeco.2017.12.001
Hernandez-Escribano, L., Visser, E. A., Iturritxa, E., Raposo, R., and Naidoo, S. (2020). The transcriptome of Pinus pinaster under Fusarium circinatum challenge. BMC Genomics 21, 1–18. doi: 10.1186/s12864-019-6444-0
Herron, D. A., Wingfield, M. J., Fru, F., Wingfield, B. D., and Steenkamp, E. T. (2020). Grasses as a refuge for Fusarium circinatum L.—evidence from South Africa. South. For. J. For. Sci. 82, 253–262. doi: 10.2989/20702620.2020.1813649
Hewage, K. A. H., Yang, J.-F., Wang, D., Hao, G.-F., and Yang, G.-G. (2020). Chemical manipulation of abscisic acid signaling: a new approach to abiotic and biotic stress management in agriculture. Advanc. Sci. 7:2001265. doi: 10.1002/advs.202001265
Huang, S., Zhang, X., and Fernando, W. G. D. (2020). Directing trophic divergence in plant-pathogen interactions: antagonistic phytohormones with NO doubt? Front. Plant Sci. 11:600063. doi: 10.3389/fpls.2020.600063
Iturritxa, E., Ganley, R. J., Wright, J., Heppe, E., Steenkamp, E. T., Gordon, T. R., et al. (2011). A genetically homogenous population of Fusarium circinatum causes pitch canker of Pinus radiata in the Basque Country, Spain. Fungal Biol. 115, 288–295. doi: 10.1016/j.funbio.2010.12.014
Jain, D., and Khurana, J. P. (2018). “Role of pathogenesis-related (PR) proteins in plant defense mechanism,” in Molecular Aspects of Plant-Pathogen Interaction. eds. A. Singh and I. K. Singh (Singapore: Springer), 265–281.
Jones, J. D. G., and Dangl, J. L. (2006). The plant immune system. Nature 444, 323–329. doi: 10.1038/nature05286
Kaur, A., Kumar, A., and Sudhakara, R. M. (2017). “Plant–pathogen interactions: a proteomic approach,” in Understanding host-Microbiome Interactions—An Omics Approach. eds. R. Singh, R. Kothari, P. Koring, and S. Singh (Singapore: Springer), 207–225.
Kayihan, G. C., Huber, D. A., Morse, A. M., White, T. L., and Davis, J. M. (2005). Genetic dissection of fusiform rust and pitch canker disease traits in loblolly pine. Theor. Appl. Genet. 110, 948–958. doi: 10.1007/s00122-004-1915-2
Kim, K.-W., Lee, I.-J., Kim, C.-S., Eom, I.-Y., Choi, J.-W., Lee, D.-K., et al. (2010). Resin flow, symptom development, and lignin biosynthesis of two pine species in response to wounding and inoculation with Fusarium circinatum. Plant Pathol. J. 26, 394–401. doi: 10.5423/PPJ.2010.26.4.394
Kolosova, N., and Bohlmann, J. (2012). “Conifer defense against insects and fungal pathogens,” in Growth and Defence in Plants. eds. R. Matyssek, H. Schnyder, W. Obwald, D. Ernst, J. C. Munch, and H. Pretzsch (Berlin: Springer), 85–109.
Koutaniemi, S., Warinowski, T., Kärkönen, A., Alatalo, E., Fossdal, C. G., Saranpää, P., et al. (2007). Expression profiling of the lignin biosynthetic pathway in Norway spruce using EST sequencing and real-time RT-PCR. Plant Mol. Biol. 65, 311–328. doi: 10.1007/s11103-007-9220-5
Kovalchuk, A., Keriö, S., Oghenekaro, A. O., Jaber, E., Raffaello, T., and Asiegbu, F. O. (2013). Antimicrobial defenses and resistance in forest trees: challenges and perspectives in a genomic era. Annu. Rev. Phytopathol. 51, 221–244. doi: 10.1146/annurev-phyto-082712-102307
Lee, H.-S., and Lee, K.-S. (2019). Multi-temporal analysis of high-resolution satellite images for detecting and monitoring canopy decline by pine pitch canker. Kor. J. Remote Sens. 35, 545–560. doi: 10.7780/kjrs.2019.35.4.5
Leitão, F., Pinto, G., Amaral, J., Monteiro, P., and Henriques, I. (2021). New insights into the role of constitutive bacterial rhizobiome and phenolic compounds in two Pinus spp. with contrasting susceptibility to pine pitch canker. Tree Physiol. 42, 600–615. doi: 10.1093/treephys/tpab119
Lombardero, M. J., Solla, A., and Ayres, M. P. (2019). Pine defenses against the pitch canker disease are modulated by a native insect newly associated with the invasive fungus. For. Ecol. Manag. 437, 253–262. doi: 10.1016/j.foreco.2019.01.041
López-López, N., Segarra, G., Vergara, O., López-Fabal, A., and Trillas, M. I. (2016). Compost from forest cleaning green waste and Trichoderma asperellum strain T34 reduced incidence of Fusarium circinatum in Pinus radiata seedlings. Biol. Control 95, 31–39. doi: 10.1016/j.biocontrol.2015.12.014
Lopez-Zamora, I., Bliss, C., Jokela, E. J., Comerford, N. B., Grunwald, S., Barnard, E., et al. (2007). Spatial relationships between nitrogen status and pitch canker disease in slash pine planted adjacent to a poultry operation. Environ. Pollut. 147, 101–111. doi: 10.1016/j.envpol.2006.08.025
Lu, M., Krutovsky, K. V., Nelson, C. D., West, J. B., Reilly, N. A., and Loopstra, C. A. (2017). Association genetics of growth and adaptive traits in loblolly pine (Pinus taeda L.) using whole-exome-discovered polymorphisms. Tree Genet. Genomes 13:57. doi: 10.1007/s11295-017-1140-1
Martín-García, J., Lukačevičová, A., Flores-Pacheco, J., Diez, J., and Dvořák, M. (2018). Evaluation of the susceptibility of several Czech conifer provenances to Fusarium circinatum. Forests 9:72. doi: 10.3390/f9020072
Martín-García, J., Paraschiv, M., Flores-Pacheco, J., Chira, D., Diez, J., and Fernández, M. (2017). Susceptibility of several northeastern conifers to Fusarium circinatum and strategies for biocontrol. Forests 8:318. doi: 10.3390/f8090318
Martín-García, J., Zas, R., Solla, A., Woodward, S., Hantula, J., Vainio, E. J., et al. (2019). Environmentally friendly methods for controlling pine pitch canker. Plant Pathol. 68, 843–860. doi: 10.1111/ppa.13009
Martín-Rodrigues, N., Espinel, S., Sanchez-Zabala, J., Ortíz, A., González-Murua, C., and Duñabeitia, M. K. (2013). Spatial and temporal dynamics of the colonization of Pinus radiata by Fusarium circinatum, of conidiophora development in the pith and of traumatic resin duct formation. New Phytol. 198, 1215–1227. doi: 10.1111/nph.12222
Marzec, M. (2016). Strigolactones as part of the plant defence system. Trends Plant Sci. 21:900–903. doi: 10.1016/j.tplants.2016.08.010
Mead, D. (2013). Sustainable Management of Pinus radiata Plantations. Vol. 170. Rome: FAO Forestry Paper.
Mitchell, R., Steenkamp, E., Coutinho, T., and Wingfield, M. (2011). The pitch canker fungus, Fusarium circinatum: implications for south African forestry. South. For. J. For. Sci. 73, 1–13. doi: 10.2989/20702620.2011.574828
Morkunas, I., and Ratajczak, L. (2014). The role of sugar signaling in plant defense responses against fungal pathogens. Acta Physiol. Plant. 36, 1607–1619. doi: 10.1007/s11738-014-1559-z
Morse, A. M., Nelson, C. D., Covert, S. F., Holliday, A. G., Smith, K. E., and Davis, J. M. (2004). Pine genes regulated by the necrotrophic pathogen Fusarium circinatum. Theor. Appl. Genet. 109, 922–932. doi: 10.1007/s00122-004-1719-4
Möykkynen, T., Capretti, P., and Pukkala, T. (2015). Modelling the potential spread of Fusarium circinatum, the causal agent of pitch canker in Europe. Ann. For. Sci. 72, 169–181. doi: 10.1007/s13595-014-0412-2
Mullett, M., Pérez-Sierra, A., Armengol, J., and Berbegal, M. (2017). Phenotypical and molecular characterisation of Fusarium circinatum: correlation with virulence and fungicide sensitivity. Forests 8:458. doi: 10.3390/f8110458
Muñoz-Adalia, E., Cañizares, M., Fernández, M., Diez, J., and García-Pedrajas, M. (2018a). The Fusarium circinatum gene Fcrho1, encoding a putative Rho1 GTPase, is involved in vegetative growth but dispensable for pathogenic development. Forests 9:684. doi: 10.3390/f9110684
Muñoz-Adalia, E. J., Fernández, M., Wingfield, B. D., and Diez, J. J. (2018b). In silico annotation of five candidate genes associated with pathogenicity in Fusarium circinatum. For. Pathol. 48:e12417. doi: 10.1111/efp.12417
Muñoz-Adalia, E. J., Flores-Pacheco, J. A., Martínez-Álvarez, P., Martín-García, J., Fernández, M., and Diez, J. J. (2016). Effect of mycoviruses on the virulence of Fusarium circinatum and laccase activity. Physiol. Mol. Plant Pathol. 94, 8–15. doi: 10.1016/j.pmpp.2016.03.002
Muranty, H., Jorge, V., Bastien, C., Lepoittevin, C., Bouffier, L., and Sanchez, L. (2014). Potential for marker-assisted selection for forest tree breeding: lessons from 20 years of MAS in crops. Tree Genet. Genomes 10, 1491–1510. doi: 10.1007/s11295-014-0790-5
Naidoo, S., Slippers, B., Plett, J. M., Coles, D., and Oates, C. N. (2019). The road to resistance in forest trees. Front. Plant Sci. 10:273. doi: 10.3389/fpls.2019.00273
Naidoo, S., Visser, E. A., Zwart, L., du Toit, Y., Bhadauria, V., and Shuey, L. S. (2018). Dual RNA-sequencing to elucidate the plant-pathogen duel. Curr. Issues Mol. Biol. 27, 127–142. doi: 10.21775/cimb.027.127
Neale, D. B., and Kremer, A. (2011). Forest tree genomics: growing resources and applications. Nat. Rev. Genet. 12, 111–122. doi: 10.1038/nrg2931
Neale, D. B., Wegrzyn, J. L., Stevens, K. A., Zimin, A. V., Puiu, D., Crepeau, M. W., et al. (2014). Decoding the massive genome of loblolly pine using haploid DNA and novel assembly strategies. Genome Biol. 15:R59. doi: 10.1186/gb-2014-15-3-r59
Nishad, R., Ahmed, T., Rahman, V. J., and Kareem, A. (2020). Modulation of plant defense systems in response to microbial interactions. Front. Microbiol. 11:1298. doi: 10.3389/fmicb.2020.01298
Niu, S., Li, J., Bo, W., Yang, W., Zuccolo, A., Giacomello, S., et al. (2022). The Chinese pine genome and methylome unveil key features of conifer evolution. Cell 185, 204–217.e14. doi: 10.1016/j.cell.2021.12.006
O’Brien, J. A., Daudi, A., Butt, V. S., and Paul, B. G. (2012). Reactive oxygen species and their role in plant defence and cell wall metabolism. Planta 236, 765–779. doi: 10.1007/s00425-012-1696-9
Otero, M., Salcedo, I., Txarterina, K., González-Murua, C., and Duñabeitia, M. K. (2020). Compost tea reduces the susceptibility of Pinus radiata to Fusarium circinatum in nursery production. Phytopathology 110, 813–821. doi: 10.1094/PHYTO-04-19-0139-R
Pascual, M. B., El-Azaz, J., de la Torre, F. N., Cañas, R. A., Avila, C., and Cánovas, F. M. (2016). Biosynthesis and metabolic fate of phenylalanine in conifers. Front. Plant Sci. 7:1030. doi: 10.3389/fpls.2016.01030
Phasha, M. M., Wingfield, M. J., Wingfield, B. D., Coetzee, M. P. A., Hallen-Adams, H., Fru, F., et al. (2021a). Ras2 is important for growth and pathogenicity in Fusarium circinatum. Fungal Genet. Biol. 150:103541. doi: 10.1016/j.fgb.2021.103541
Phasha, M. M., Wingfield, B. D., Wingfield, M. J., Coetzee, M. P. A., Hammerbacher, A., and Steenkamp, E. T. (2021b). Deciphering the effect of FUB1 disruption on fusaric acid production and pathogenicity in Fusarium circinatum. Fungal Biol. 25, 1036–1047. doi: 10.1016/j.funbio.2021.07.002
Quesada, T., Gopal, V., Cumbie, W. P., Eckert, A. J., Wegrzyn, J. L., Neale, D. B., et al. (2010). Association mapping of quantitative disease resistance in a natural population of loblolly pine (Pinus taeda L.). Genetics 186, 677–686. doi: 10.1534/genetics.110.117549
Quesada, T., Lucas, S., Smith, K., and Smith, J. (2019). Response to temperature and virulence assessment of Fusarium circinatum isolates in the context of climate change. Forests 10:40. doi: 10.3390/f10010040
Reglinski, T., Taylor, J. T., and Dick, M. A. (2004). Chitosan induces resistance to pitch canker in Pinus radiata. N.Z. J. For. Sci. 34, 49–58.
Reynolds, G., Gordon, T., and McRoberts, N. (2016). Quantifying the impacts of systemic acquired resistance to pitch canker on Monterey pine growth rate and hyperspectral reflectance. Forests 7:20. doi: 10.3390/f7010020
Rodrigues, A. M., Miguel, C., Chaves, I., and António, C. (2021). Mass spectrometry-based forest tree metabolomics. Mass Spectrom. Rev. 40, 126–157. doi: 10.1002/mas.21603
Rojas, C. M., Senthil-Kumar, M., Tzin, V., and Mysore, K. S. (2014). Regulation of primary plant metabolism during plant-pathogen interactions and its contribution to plant defense. Front. Plant Sci. 5:17. doi: 10.3389/fpls.2014.00017
Romeralo, C., Martín-García, J., Matínez-Álvarez, P., Muñoz-Adalia, E. J., Gonçalves, D. R., Torres, E., et al. (2022). Pine species determine fungal microbiome composition in a common garden experiment. Fungal Ecol. 56:101137. doi: 10.1016/j.funeco.2021.101137
Runion, G. B., Prior, S. A., Rogers, H. H., and Mitchell, R. J. (2010). Effects of elevated atmospheric CO2 on two southern forest diseases. New For. 39, 275–285. doi: 10.1007/s11056-009-9170-7
Rustagi, A., Singh, G., Agrawal, S., and Gupta, P. K. (2018). “Proteomic studies revealing enigma of plant–pathogen interaction” in Molecular Aspects of Plant-Pathogen Interaction. eds. A. Singh and I. Singh (Singapore: Springer), 239–264.
Sambles, C. M., Salmon, D. L., Florance, H., Howard, T. P., Smirnoff, N., Nielsen, L. R., et al. (2017). Ash leaf metabolomes reveal differences between trees tolerant and susceptible to ash dieback disease. Sci. Data 4:170190. doi: 10.1038/sdata.2017.190
Santana, Q. C., Coetzee, M. P. A., Wingfield, B. D., Wingfield, M. J., and Steenkamp, E. T. (2016). Nursery-linked plantation outbreaks and evidence for multiple introductions of the pitch canker pathogen Fusarium circinatum into South Africa. Plant Pathol. 65, 357–368. doi: 10.1111/ppa.12437
Santini, A., Ghelardini, L., De Pace, C., Desprez-Loustau, M. L., Capretti, P., Chandelier, A., et al. (2013). Biogeographical patterns and determinants of invasion by forest pathogens in Europe. New Phytol. 197, 238–250. doi: 10.1111/j.1469-8137.2012.04364.x
Santos, C. S., Pinheiro, M., Silva, A. I., Egas, C., and Vasconcelos, M. W. (2012). Searching for resistance genes to Bursaphelenchus xylophilus using high throughput screening. BMC Genomics 13, 1–16. doi: 10.1186/1471-2164-13-599
Sharma, P., Jha, A. B., Dubey, R. S., and Pessarakli, M. (2012). Reactive oxygen species, oxidative damage, and antioxidative defense mechanism in plants under stressful conditions. Aust. J. Bot. 2012, 1–26. doi: 10.1155/2012/217037
Silva-Castro, I., Diez, J., Martín-Ramos, P., Pinto, G., Alves, A., Martín-Gil, J., et al. (2018a). Application of bioactive coatings based on chitosan and propolis for Pinus spp. protection against Fusarium circinatum. Forests 9:685. doi: 10.3390/f9110685
Silva-Castro, I., Martín-García, J., Diez, J. J., Flores-Pacheco, J. A., Martín-Gil, J., and Martín-Ramos, P. (2018b). Potential control of forest diseases by solutions of chitosan oligomers, propolis and nanosilver. Eur. J. Plant Pathol. 150, 401–411. doi: 10.1007/s10658-017-1288-4
Slinski, S. L., Zakharov, F., and Gordon, T. R. (2015). The effect of resin and monoterpenes on spore germination and growth in Fusarium circinatum. Phytopathology 105, 119–125. doi: 10.1094/PHYTO-02-14-0027-R
Sniezko, R. A., and Koch, J. (2017). Breeding trees resistant to insects and diseases: putting theory into application. Biol. Invasions 19, 3377–3400. doi: 10.1007/s10530-017-1482-5
Soares, C., Carvalho, M. E. A., Azevedo, R. A., and Fidalgo, F. (2019). Plants facing oxidative challenges—a little help from the antioxidant networks. Environ. Exp. Bot. 161, 4–25. doi: 10.1016/j.envexpbot.2018.12.009
Solel, Z., and Bruck, R. I. (1990). Relation between wilt rate and obstruction of water flow in stems of two families of loblolly pine affected by pitch canker. For. Pathol. 20, 317–320. doi: 10.1111/j.1439-0329.1990.tb01143.x
Stępień, Ł., Lalak-Kańczugowska, J., Witaszak, N., and Urbaniak, M. (2020). “Fusarium secondary metabolism biosynthetic pathways: so close but so far away,” in Co-Evolution of Secondary Metabolites. Reference Series in Phytochemistry. eds. J. M. Merillon and K. Ramawat (Cham: Springer), 211–247.
Sturrock, R. N., Frankel, S. J., Brown, A. V., Hennon, P. E., Kliejunas, J. T., Lewis, K. J., et al. (2011). Climate change and forest diseases. Plant Pathol. 60, 133–149. doi: 10.1111/j.1365-3059.2010.02406.x
Swett, C. L., and Gordon, T. R. (2012). First report of grass species (Poaceae) as naturally occurring hosts of the pine pathogen Gibberella circinata. Plant Dis. 96:908. doi: 10.1094/PDIS-02-12-0136-PDN
Swett, C. L., Kirkpatrick, S. C., and Gordon, T. R. (2016). Evidence for a hemibiotrophic association of the pitch canker pathogen Fusarium circinatum with Pinus radiata. Plant Dis. 100, 79–84. doi: 10.1094/PDIS-03-15-0270-RE
Swett, C. L., Porter, B., Fourie, G., Steenkamp, E. T., Gordon, T. R., and Wingfield, M. J. (2014). Association of the pitch canker pathogen Fusarium circinatum with grass hosts in commercial pine production areas of South Africa. South. For. J. For. Sci. 76, 161–166. doi: 10.2989/20702620.2014.916087
Swett, C. L., Reynolds, G. J., and Gordon, T. R. (2018). Infection without wounding and symptomless shoot colonization of Pinus radiata by Fusarium circinatum, the cause of pitch canker. For. Pathol. 48:e12422. doi: 10.1111/efp.12422
Tenenboim, H., and Brotman, Y. (2016). Omic relief for the Biotically stressed: metabolomics of plant biotic interactions. Trends Plant Sci. 21, 781–791. doi: 10.1016/j.tplants.2016.04.009
Valledor, L., Carbó, M., Lamelas, L., Escandón, M., Colina, F. J., Cañal, M. J., et al. (2018). “When the tree let us see the forest: systems biology and natural variation studies in forest species,” in Progress in Botany. eds. F. Cánovas, U. Lüttge, C. Leuschner, and M. C. Risueño (Cham: Springer), 353–375.
van Loon, L. C., Rep, M., and Pieterse, C. M. J. (2006). Significance of inducible defense-related proteins in infected plants. Annu. Rev. Phytopathol. 44, 135–162. doi: 10.1146/annurev.phyto.44.070505.143425
Vettraino, A., Potting, R., and Raposo, R. (2018). EU Legislation on forest plant health: an overview with a focus on Fusarium circinatum. Forests 9:568. doi: 10.3390/f9090568
Visser, E. A., Wegrzyn, J. L., Myburg, A. A., and Naidoo, S. (2018). Defence transcriptome assembly and pathogenesis related gene family analysis in Pinus tecunumanii (low elevation). BMC Genomics 19:632. doi: 10.1186/s12864-018-5015-0
Visser, E. A., Wegrzyn, J. L., Steenkamp, E. T., Myburg, A. A., and Naidoo, S. (2019). Dual RNA-Seq analysis of the pine-Fusarium circinatum interaction in resistant (Pinus tecunumanii) and susceptible (Pinus patula) hosts. Microorganisms 7:315. doi: 10.3390/microorganisms7090315
Visser, E. A., Wegrzyn, J. L., Steenkmap, E. T., Myburg, A. A., and Naidoo, S. (2015). Combined de novo and genome guided assembly and annotation of the Pinus patula juvenile shoot transcriptome. BMC Genomics 16:1057. doi: 10.1186/s12864-015-2277-7
Vivas, M., Martín, J. A., Gil, L., and Solla, A. (2012). Evaluating methyl jasmonate for induction of resistance to Fusarium oxysporum, F. circinatum and Ophiostoma novo-ulmi. For. Syst. 21, 289–299. doi: 10.5424/fs/2012212-02172
Vivas, M., Nunes, C., Coimbra, M. A., and Solla, A. (2014a). Maternal effects and carbohydrate changes of Pinus pinaster after inoculation with Fusarium circinatum. Trees 28, 373–379. doi: 10.1007/s00468-013-0955-0
Vivas, M., Nunes, C., Coimbra, M. A., and Solla, A. (2014b). Antioxidant activity of Pinus pinaster infected with Fusarium circinatum is influenced by maternal effects. For. Pathol. 44, 337–340. doi: 10.1111/efp.12111
Wang, L., Qu, L., Hu, J., Zhang, L., Tang, F., and Lu, M. (2017). Metabolomics reveals constitutive metabolites that contribute resistance to fall webworm (Hyphantria cunea) in Populus deltoides. Environ. Exp. Bot. 136, 31–40. doi: 10.1016/j.envexpbot.2017.01.002
Watt, M. S., Ganley, R. J., Kriticos, D. J., and Manning, L. K. (2011). Dothistroma needle blight and pitch canker: the current and future potential distribution of two important diseases of Pinus species. Can. J. For. Res. 41, 412–424. doi: 10.1139/X10-204
Wikler, K., and Gordon, T. R. (2000). An initial assessment of genetic relationships among populations of Fusarium circinatum in different parts of the world. Can. J. Bot. 78, 709–717.
Wingfield, M. J., Brockerhoff, E. G., Wingfield, B. D., and Slippers, B. (2015). Planted forest health: the need for a global strategy. Science 349, 832–836. doi: 10.1126/science.aac6674
Wingfield, M. J., Hammerbacher, A., Ganley, R. J., Steenkamp, E. T., Gordon, T. R., Wingfield, B. D., et al. (2008). Pitch canker caused by Fusarium circinatum—a growing threat to pine plantations and forests worldwide. Australas. Plant Pathol. 37:319. doi: 10.1071/AP08036
Wu, Q., Chen, M., Zhou, H., Zhou, X., and Wang, Y. (2015). Metabolite profiles of Populus in response to pathogen stress. Biochem. Biophys. Res. Commun. 465, 421–426. doi: 10.1016/j.bbrc.2015.08.025
Zamora-Ballesteros, C., Diez, J. J., Martín-García, J., Witzell, J., Solla, A., Ahumada, R., et al. (2019). Pine pitch canker (PPC): pathways of pathogen spread and preventive measures. Forests 10:1158. doi: 10.3390/f10121158
Zamora-Ballesteros, C., Martin-Garcia, J., Suarez-Vega, A., and Diez, J. J. (2022). Genome-wide identification of Fusarium circinatum-responsive lncRNAs in Pinus radiata. BMC Genomics 23:194. doi: 10.1186/s12864-022-08408-9
Zamora-Ballesteros, C., Pinto, G., Amaral, J., Valledor, L., Alves, A., Diez, J. J., et al. (2021). Dual RNA-sequencing analysis of resistant (Pinus pinea) and susceptible (Pinus radiata) hosts during Fusarium circinatum challenge. Int. J. Mol. Sci. 22:5231. doi: 10.3390/ijms22105231
Keywords: physiology, omics, immune defence, plant pathogen, biotic stress, forest disease, control measures, Pinus
Citation: Amaral J, Valledor L, Alves A, Martín-García J and Pinto G (2022) Studying tree response to biotic stress using a multi-disciplinary approach: The pine pitch canker case study. Front. Plant Sci. 13:916138. doi: 10.3389/fpls.2022.916138
Edited by:
Luciano Kayser Vargas, State Secretariat of Agriculture, Livestock, and Irrigation, BrazilReviewed by:
Rodrigo Ahumada, Bioforest S.A., ChileIsabel Alvarez Munck, United States Forest Service (USDA), United States
Ricardo Alia, Instituto Nacional de Investigación y Tecnología Agroalimentaria (INIA), Spain
Copyright © 2022 Amaral, Valledor, Alves, Martín-García and Pinto. This is an open-access article distributed under the terms of the Creative Commons Attribution License (CC BY). The use, distribution or reproduction in other forums is permitted, provided the original author(s) and the copyright owner(s) are credited and that the original publication in this journal is cited, in accordance with accepted academic practice. No use, distribution or reproduction is permitted which does not comply with these terms.
*Correspondence: Joana Amaral, jsamaral@ua.pt; Glória Pinto, gpinto@ua.pt