- 1College of Agriculture and Biotechnology, Hunan University of Humanities, Science and Technology, Loudi, China
- 2Research Center on Ecological Sciences, Jiangxi Agricultural University, Nanchang, China
- 3College of Agronomy, Sichuan Agricultural University, Chengdu, China
- 4Sichuan Engineering Research Center for Crop Strip Intercropping System, Key Laboratory of Crop Eco-physiology and Farming System in Southwest, Ministry of Agriculture and Rural Affairs, Chengdu, China
- 5Department of Agricultural Engineering, Khwaja Fareed University of Engineering and Information Technology, Rahim Yar Khan, Pakistan
- 6Department of Biology, Al-Jumum University College, Umm Al-Qura University, Makkah, Saudi Arabia
- 7School of Computer Information and Engineering, Jiangxi Agricultural University, Nanchang, China
Drought stress (DS) is a serious abiotic stress and a major concern across the globe as its intensity is continuously climbing. Therefore, it is direly needed to develop new management strategies to mitigate the adverse effects of DS to ensure better crop productivity and food security. The use of arbuscular mycorrhizal fungi (AMF) has emerged as an important approach in recent years to improve crop productivity under DS conditions. AMF establishes a relationship with 80% of land plants and it induces pronounced impacts on plant growth and provides protection to plants from abiotic stress. Drought stress significantly reduces plant growth and development by inducing oxidative stress, disturbing membrane integrity, plant water relations, nutrient uptake, photosynthetic activity, photosynthetic apparatus, and anti-oxidant activities. However, AMF can significantly improve the plant tolerance against DS. AMF maintains membrane integrity, improves plant water contents, nutrient and water uptake, and water use efficiency (WUE) therefore, improve the plant growth under DS. Moreover, AMF also protects the photosynthetic apparatus from drought-induced oxidative stress and improves photosynthetic efficiency, osmolytes, phenols and hormone accumulation, and reduces the accumulation of reactive oxygen species (ROS) by increasing anti-oxidant activities and gene expression which provide the tolerance to plants against DS. Therefore, it is imperative to understand the role of AMF in plants grown under DS. This review presented the different functions of AMF in different responses of plants under DS. We have provided a detailed picture of the different mechanisms mediated by AMF to induce drought tolerance in plants. Moreover, we also identified the potential research gaps that must be fulfilled for a promising future for AMF. Lastly, nitrogen (N) is an important nutrient needed for plant growth and development, however, the efficiency of applied N fertilizers is quite low. Therefore, we also present the information on how AMF improves N uptake and nitrogen use efficiency (NUE) in plants.
Introduction
Drought stress (DS) is a serious abiotic stress, negatively affecting plant growth and development across the globe (Amiri et al., 2015; Hassan et al., 2020). The recent increase in climate change has increased the intensity of DS which is posing a serious challenge to global food security (Behrooz et al., 2019; Huang et al., 2020). Drought stress is the greatest threat to field crops and it has a direct impact on crop yields and the global economy (Dolan et al., 2021; He et al., 2022). Drought stress negatively affects the plant process ranging from seed germination, growth, and final productivity. Seed germination is an essential process in the growth of plants (El-Badri et al., 2021). Successful crop productivity mainly depends on seed germination and early plant growth has direct linking with seeds’ ability to sprout under DS (Khan et al., 2019). Drought stress severely affects the activities of hormones, mobilization of stored materials, and protein structure that negatively affect the seed germination and subsequent growth of plants (Abdel-Ghani et al., 2015; Basal et al., 2020). DS also affects enzymatic activities and reduces nutrient assimilation and nutrient uptake resulting in huge yield losses (Ahanger and Agarwal, 2017). Moreover, DS also impairs the photosynthetic process, and plant water contents and reduces the synthesis of photosynthetic pigments which negatively affects plant growth (Hellal et al., 2018; Mamnabi et al., 2020). Besides this, DS also impairs the structural integrity of photosynthetic apparatus which is the main reason for reduced growth under DS (Zhang et al., 2015).
Drought stress also reduces photosynthesis by reducing cell turgor and plants’ access to CO2 owing to the closure of stomata (Jaleel et al., 2007). One of the major detriments of DS is the production of reactive oxygen species (ROS) that damage the structural integrity of membranes, proteins, and DNA (Ahanger and Agarwal, 2017; Sultan et al., 2021; Qari et al., 2022; Rehman et al., 2022). However, plants have developed different mechanisms to reduce the harmful effects of ROS to protect the stability of cellular structures and improve the yield under DS (Ahanger and Ahmad, 2019). The key mechanisms developed by plants to mitigate the adverse impacts of DS are better accretion of osmolytes and secondary metabolites and activation of the anti-oxidant defense system (Ahmad, 2010; Amiri et al., 2015).
Arbuscular mycorrhizal fungi (AMF) is one of the most distributed fungi across the globe that forms symbiosis association with more than 80% of terrestrial plant species (Behrooz et al., 2019). The symbiosis relationship formed between plants and AMF is beneficial for plant growth, nutrient uptake, soil quality, and stress resistance (Bi et al., 2019; Ahmed et al., 2020; Gupta, 2020; Hu et al., 2022). The symbiosis relationship between the host plant and AMF substantially improved the resistance to drought stress (Hashem et al., 2019; Zhang et al., 2019). The regulation of DS in plants by AMF is a complex process that involves diverse metabolic pathways and metabolites (Aalipour et al., 2020; Huang et al., 2020). AMF improves the survival of seedlings (Wu and Zou, 2017), and promote water uptake and transportation in the host plant (Quiroga et al., 2019a,b; Ren et al., 2019; Ortas et al., 2021), improve plant water use efficiency and gas change abilities (Quiroga et al., 2019a; Huang et al., 2020), change the morphology of roots (Quiroga et al., 2019b; Zhang et al., 2019), regulate hormone levels (Begum et al., 2020; Rydlová and Püschel, 2020), and reduce the production of ROS (Amiri et al., 2015) and thereby reduce the adverse impacts of DS. Additionally, AMF also produces glomalin, which is also known as glomalin-related soil protein (GRSP), which works as a glue that promotes the formation of water-stable aggregates by physical entanglement of extraradical hyphae, thus improving the soil water holding capacity and stabilization of soil structure (Santander et al., 2017; Gupta, 2020). Additionally, AMF also up-regulates anti-oxidant activities, osmolytes accumulation, gene expression and maintains the plant water status and photosynthetic performance under DS and resulting in a significant increase in DS tolerance (Al-Arjani et al., 2020; Seutra et al., 2021; Wang et al., 2022).
Nitrogen (N) is an essential nutrient needed for plant growth (Nishida and Suzaki, 2018). However, the efficiency of applied nitrogenous fertilizers to fulfill the plant needs is quite low (40–45%) which needs to be improved for reducing the impact on the environment (Chien et al., 2016). The excessive use of N fertilizers increases the emissions of greenhouse gases (GHGs) particular nitrous oxide (N2O) which is serious concern across the globe (Abeydeera et al., 2019). Globally, different efforts are being made to improve the nitrogen use efficiency (NUE) in plants. Among these efforts the use of microbes has emerged as an excellent strategy to improve NUE. Among different microbes AMF can significant improve the efficiency of N and other nutrients in field crops by increasing the surface area of roots to ensure the better absorption of nutrients (Tajini et al., 2011). AMF induce NO3– and NH4+ transporters in plants, therefore, effect N uptake in plants (Koegel et al., 2013). AMF also bring changes in compositions of soil microbial communities by changing the development of denitrifying, nitrifying and diazotrophic symbiotic or free-living bacteria which in turn affect the N uptake and subsequent N availability to plants (Veresoglou et al., 2011). In this review, we have presented detailed information about the role of AMF in inducing the DS tolerance in plants. We have systematically presented different mechanisms of AMF mediated DS tolerance in plants. We have also discussed the research gaps that need to be filled in future studies for improving the crop production under DS with AMF. Additionally, we have also discussed the role of AMF in improving NUE in plants for ensuring better growth of plants.
Plant Responses to Drought Stress
Drought stress affects each stage of plant growth, however, germination is a very crucial stage of plant life. Drought reduces seed germination and leads to poor seedling growth and development (Khan et al., 2019). Water deficiency reduces seed germination owing (Figure 1) to a reduction in water uptake and availability of stored food, and inactivation of enzymes involved in the germination process (Jabbari et al., 2013). Drought also causes a significant decrease in plant growth by decreasing the cell expansion, increasing leaf shedding, and impairing the processes of mitosis (Liu et al., 2013; Yang et al., 2021; Batool et al., 2022a,b). DS also brings many morphological changes in plants, likewise, it reduces leaf area, leaf size, and root and shoot growth owing to activation of abscisic acid (ABA) precursor (ACC) which prevents root growth (Hewedy et al., 2021; Sayer et al., 2021). Despite this the activation of the ABA precursor also induces early maturity, leaf rolling and folding, and stomata closing which negatively affect the photosynthetic process and subsequent growth and development (Hewedy et al., 2021; Sayer et al., 2021). Moreover, DS also reduces the nodule growth and their functioning which in turn reduces the N fixation and leads to a significant decrease in the growth and production of legume crops (Furlan et al., 2012; Wilmowicz et al., 2020).
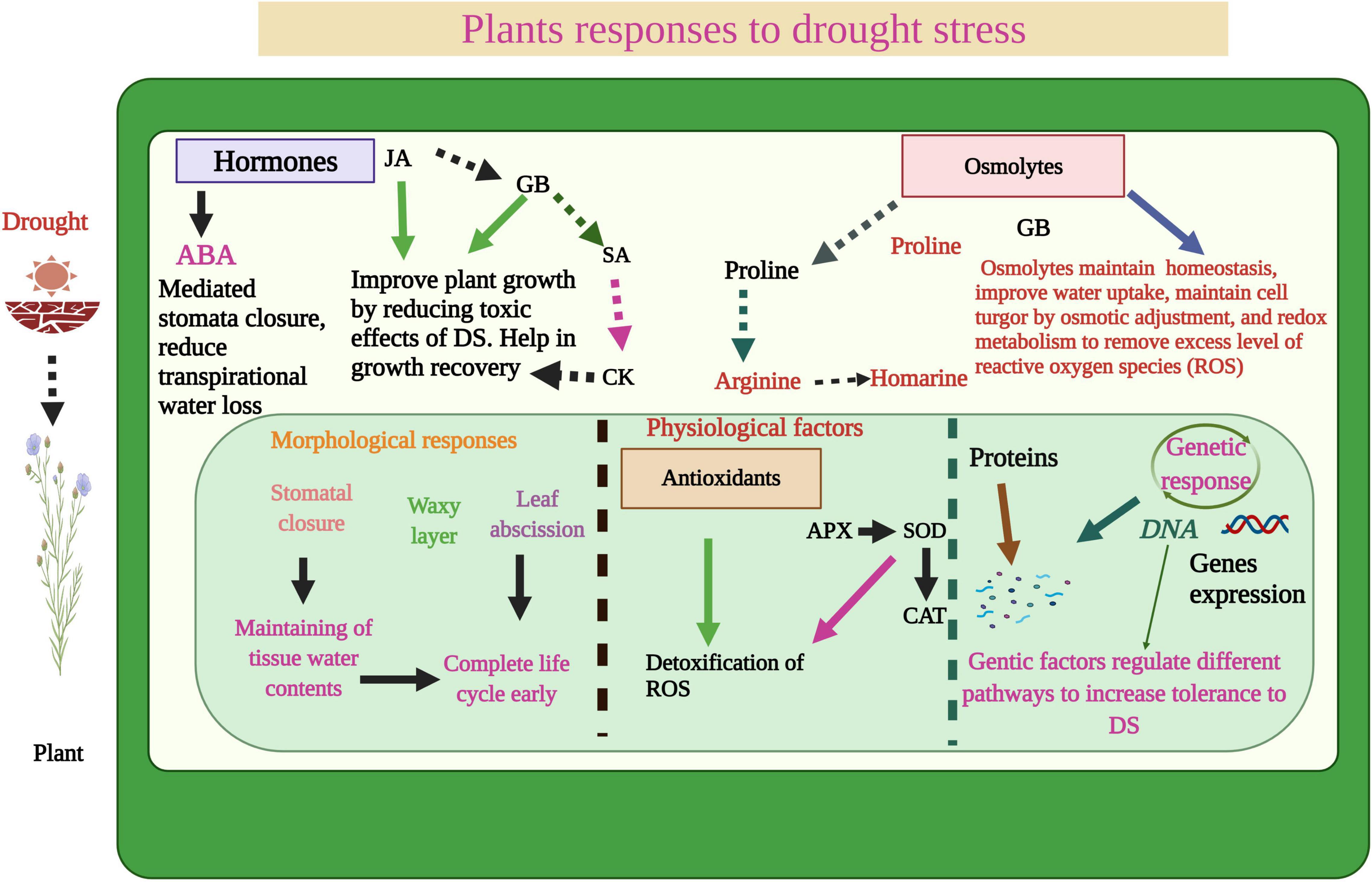
Figure 1. Plant responses to DS. The water deficiency disturbs plant physiological processes, plant photosynthetic efficiency, induces stomata closure, and ROS production which significant reduction in plant growth. However, plants activate an antioxidant defense system, accumulate different osmolytes, increase gene expression, produce a waxy layer and complete the early life cycle to mitigate the adverse impacts of DS.
Photosynthesis is the main process negatively affected by DS. The decrease in the photosynthetic process under DS occurs due to stomatal and non-stomatal limitations (Bogati and Walczak, 2022). The stomata limitations are considered to be the main reason for decrease in the photosynthetic rate under mild DS, whereas non-stomata limitations are the main reason for reduction in photosynthetic rate under severe DS (Bogati and Walczak, 2022). Stomata closing limits the carbon dioxide (CO2) absorption and prevents transpiration losses owing to reduced water potential (Yang et al., 2021). In the case of non-stomata factors, the reduced activity of RuBisCO and reduction in efficiency of PS-II substantially reduce the photosynthetic rate under DS (Ma et al., 2015).
Drought also induces various biochemical changes in plants (Table 1). Likewise, DS reduces the synthesis of chlorophyll contents and increases the proline contents, and causes oxidative damage by inducing the production of ROS (Hassan et al., 2017). Reactive oxygen species (ROS) produced by DS synthesis of chlorophyll and photosynthetic apparatus and lead to a serious reduction in photosynthesis and subsequent assimilate production (Bogati and Walczak, 2022). Drought stress also disturbs electron transport and decreases the pool size of electron acceptors (Feng and Cao, 2005; Azzeme et al., 2016), also leading to marked reduction in photosynthesis. Drought-induced ROS also damage membrane integrity and cause oxidation of proteins, DNA, nucleic acid, lipids, and carbohydrates (Sabra et al., 2012; Dossa et al., 2017; Hassan et al., 2019, 2021; Bao et al., 2020; Jinhu et al., 2022; Khan et al., 2022a,b). However, plants accumulate different osmolytes to counter the effects of DS. Among different osmolytes, proline (Pro) is an important osmolyte that reduces the ROS by stimulating the activity of catalase (CAT), peroxidase (POD), superoxide dismutase (SOD), and other different antioxidant enzymes (Bogati and Walczak, 2022). Proline has an appreciable ability to bind and hydrate enzymes thereby it stabilizes and protects the macro-molecules and maintains their structural integrity and their functioning under DS (Yang et al., 2021).
Glycine betaine (GB) is important osmolyte that plays a crucial role in plant responses against DS (Bogati and Walczak, 2022). It improves growth, photosynthetic apparatus, and anti-oxidant activities and it also protects key enzymes of the dicarboxylic acid cycle and photosystem, which play an imperious role in proper photosynthesis and respiration in plants (Ma et al., 2007). Plants under DS also synthesized different proteins including late embryogenesis abundant proteins, protein kinases, phospholipase C, phospholipase D, and G proteins, which play a significant role against DS (Bogati and Walczak, 2022). These proteins protect the functioning of ion channels, scavenge ROS, improve anti-oxidant activities, gene expression, membrane integrity, and water transportation at the cellular and sub-cellular levels and therefore improve plant tolerance against DS (Yang et al., 2021). Plants also accumulate different hormones and amino acids that play an important role in stomata regulation, osmolytes accumulation, and scavenging of ROS (Bowne et al., 2012; You et al., 2019).
Effect of Drought Stress on Growth and Diversity of Arbuscular Mycorrhizal Fungi
The diversity of AMF species largely depends on the application and mode of the new methodology (Bahadur et al., 2019a). Around 250 AMF species have been recognized in different ecosystems (Opik et al., 2013). AMF improves plant responses against the DS which in turn improves growth and final productivity (Basu et al., 2018; Ouledali et al., 2018). Conversely, DS also has a direct impact on AMF, and water shortage significantly reduces the germination of AMF spores, AMF growth, colonization, and elongation of AMF extra-radical hyphae (Zhang et al., 2018). Over the years the major focus of researchers is to understand how DS affects the diversity as well as the composition of AMF. Generally, the behavior of AMF is opportunistic and they use their energy to produce more descendants. In addition, AMF also develop many promising characteristics to perform better in water deficit conditions (Sykorova et al., 2007). Many authors noted that Glomus AMF species are considered typical species of semi-arid conditions and they have an appreciable ability to grow well under DS conditions (Verma et al., 2008; Tian et al., 2009).
The Association of Host Plant and Arbuscular Mycorrhizal Fungi Under Drought Stress
Plants use different strategies to avoid the deleterious impacts of DS. AMF modifies plant root traits which in turn increase the water uptake and reduce water loss (Bahadur et al., 2019b). Interestingly, upon exposure to DS most plants quickly ask the AMF to help them by secreting a rhizosphere molecule that is known as strigolactone (Oldroyd and Speak, 2013). In recent years AMF have gained attention globally to reduce the adverse impacts of DS (Kumar and Verma, 2018). AMF association improves plant water status at the whole plant level as indicated by high leaf relative water contents (LRWC; Barros et al., 2018). AMF association with plants under DS significantly improves plant growth by improving water and nutrient water (Kapoor et al., 2013; Pavithra and Yapa, 2018). AMF forms an extensive hyphal network which ensures better nutrient as well as water uptake by plant roots (Gong et al., 2012). Despite this AMF also affect diverse plant mechanisms including root architecture, root hydraulic conductivity, and plant photosynthetic rate (Lee et al., 2012).
Arbuscular mycorrhizal fungi-mediated response against DS involves different mechanisms including the activation of genes and metabolic pathways (Fiorilli et al., 2022). AMF improves water uptake by the host plant by triggering hormonal signaling and increasing the accumulation of osmolytes (Diagne et al., 2020). Plants tolerate drought-induced osmotic stress by increasing the accumulation of sugars, proline, and GB (Latef et al., 2016). These metabolites reduce the osmotic potential and leaf water potential (Wu et al., 2013) which allows the AMF inoculated plants to maintain higher turgor pressure which in turn improves the physiological activities particularly linked with photosynthetic apparatus (Smith et al., 2010). AMF plants also counter the drought-induced oxidative stress by scavenging ROS through enhanced anti-oxidant activities (Begum et al., 2019a). AMF also increases root growth and root hydraulic characteristics and consequently increases root’s ability to uptake more nutrients and water (Ouledali et al., 2018). Moreover, AMF hyphae also establish some beneficial pathways in soil for better nutrient acquisition and transportation and leading to a significant increase in plant growth under DS. AMF also activates plant molecular responses including activation of genes (aquaporins membrane transporters), ions, and sugar transporters (Bahadur et al., 2019a). Moreover, AMF substantially improves nutrient and water uptake which improves drought tolerance by affecting different physiological and biochemical processes (Diagne et al., 2020). Lastly, AMF also improves the plant DS tolerance through secondary responses such as improving soil stability and water holding capacity (Bahadur et al., 2019b; Hamedani et al., 2022).
The Role of Arbuscular Mycorrhizal Fungi in Plants Under Drought Stress
Drought stress imposes serious threats to crop productivity and global food security. In recent years the role of AMF under DS is well explored. AMF protects the plants against DS by mediating biochemical, morphological, and physiological mechanisms.
Arbuscular Mycorrhizal Fungi Maintains Membrane Stability and Plant Water Relationships Against Drought Stress
Drought stress impedes plant growth by damaging the membrane integrity (Hasanuzzaman et al., 2013); nonetheless, AMF effectively improves the membrane stability and improves the plant performance under DS (Mirshad and Puthur, 2016). Malondialdehyde (MDA) is an important indicator of membrane damage (Hassan et al., 2020) and AMF can effectively reduce MDA by 30–50% by increasing the activities of anti-oxidants (CAT and SOD), and therefore maintain the membrane integrity under DS (Li et al., 2019). AMF improves Pro, GB, and soluble sugar accumulation which protects plant proteins and membranes from under DS (Hashem et al., 2016a; Begum et al., 2019a). The mycorrhizal association also maintains higher water status in host plants which in turn maintains plant functioning under DS (Barros et al., 2018). AMF association improves root hydraulic conductivity which improves water uptake and maintains higher plant water status under DS (Augé et al., 2008). The large root surface area in the AMF association increases the water exploration area in soil which has a direct impact on LRWC, water potential, photosynthetic and transpiration rates, and crop yield (Meddich et al., 2015). Living hyphae involved in water transportation have a smaller diameter of 2–5 μm which allows them to penetrate soils that are inaccessible to root hairs and thereby absorb water and maintain higher water status in AMF inoculated plants (Allen, 2009).
Arbuscular mycorrhizal fungi also showed beneficial impacts on soil aggregate stability owing to the production of a glycoprotein known as glomalin (Wu et al., 2008) which ensures better water uptake and maintains higher plant water status (Augé et al., 2007). The glomalin in AMF association maintains soil structure stability and improves the water holding capacity which in turn maintains higher plant water content under DS (Santander et al., 2017; Gupta, 2020). AMF also regulate gene (TFT1-TFT12) expression involved in the ABA signaling pathway and improve the water status of plants in DS (Xu et al., 2018). Besides this AMF also improves plant root growth which allows plants to take more nutrient and water and resultantly maintains higher RWC under DS (Hashem et al., 2018). In conclusion, AMF regulates antioxidant activities, and gene expression, therefore maintaining membrane stability under DS.
Arbuscular Mycorrhizal Fungi Maintains Water Use Efficiency and Nutrient Uptake Under Drought Stress
Stomata conductance plays an important role in the photosynthetic process. DS significantly reduces the stomata conductance (Hassan et al., 2020), however, AMF improves stomata conductance resultantly improves the plant WUE (Ruíz-Sánchez et al., 2011). AMF improve gene expression coding for aquaporins and AMF mediated increase in gene expression linked with aquaporins improve water absorption (Figure 2) by plants and ensures a higher WUE under DS (Porcel et al., 2005). In some other plants like Poncirus trifoliata and Rosmarinus officinalis AMF has substantially improved the stomata conductance and plant WUE under DS (Ruíz-Sánchez et al., 2011). AMF also modify the accumulation of different hormones including ABA, jasmonic acid (JA), and strigolactones which maintain the higher LRWC and plant WUE under DS (Fernández-Lizarazo and Moreno-Fonseca, 2016). The better water uptake and higher WUE in AMF association are also linked with better root activity and higher hydraulic conductance of roots (Avio et al., 2006). In addition, increasing the ABA level works as an anti-transpirant, which reduces water loss by stomata closing thus maintaining higher WUE under DS (Mohanta et al., 2017; Egamberdieva et al., 2018). AMF also produce glomalin secretions which assist in nutrient and water uptake and lead to a significant increase in WUE under DS (Gong et al., 2012). Likewise, AMF colonization also improves root growth and root hydraulic properties and consequently maintains higher water uptake and WUE in plants facing DS (Ouledali et al., 2018). AMF hyphae also establish beneficial pathways in soils for better nutrient and water uptake which leads to a substantial increase in WUE under DS (Hamedani et al., 2022).
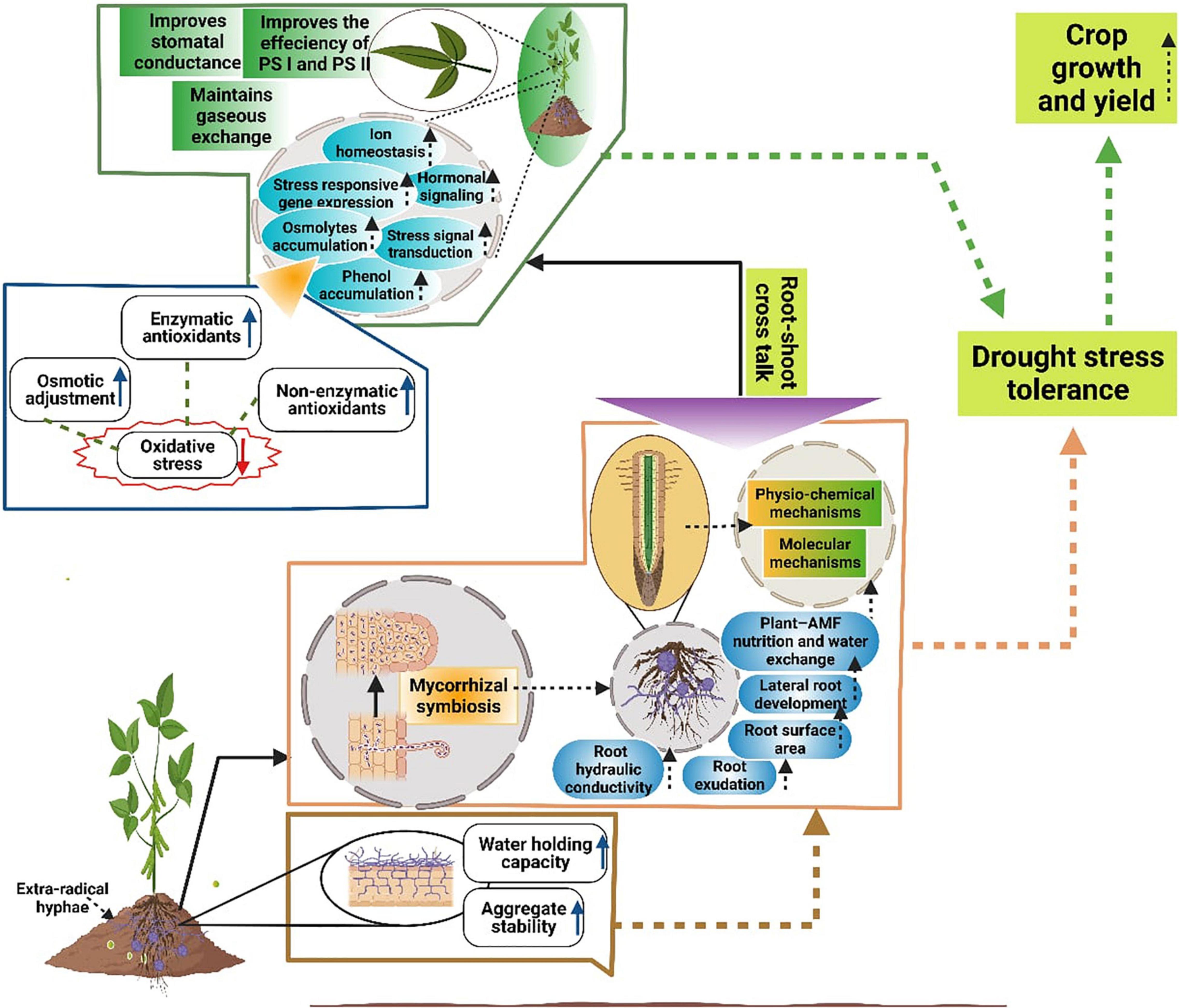
Figure 2. Schematic representation of different mechanisms mediated by AMF to improve growth and yield under DS. AMF improves soil aggregate stability, soil water holding capacity, root hydraulic conductance, water uptake, plant water status, root exudation, root surface area, root development, uptake of nutrients and water and leads to a significant increase in DS tolerance. Moreover, AMF improves hormonal crosstalk, osmolytes accumulations and induces signaling transduction, phenol accumulation, maintains ionic homeostasis, the efficiency of PS-I, PS-II, intercellular CO2 concentration resultantly improves the DS and plant growth and yield under DS.
Arbuscular mycorrhizal fungi also maintains better nutrient uptake under DS and ensures better plant performance under DS. AMF improves calcium (Ca2+) uptake and Ca2+ is considered to play an imperative role in plant stress signaling system to attain drought tolerance (Abd_Allah et al., 2017). Potassium (K+) also plays an important role in the activation of various enzymes and it also up-regulates the anti-oxidant activities and osmolytes accumulation (Hashem et al., 2016b). AMF inoculation improves the plant growth under DS by increasing nitrogen (N), phosphorus (P), potassium (K), and magnesium (Mg) uptake (Egamberdieva et al., 2017). AMF inoculated plants have better root growth which maintains the higher nutrient uptake and ensures better growth under DS (Egamberdieva et al., 2017). DS impedes nutrient uptake by making nutrients inaccessible to plants owing to a reduction in roots exploration capacity in dried soils (Hameed et al., 2014). AMF improves the growth of plant roots and their exploration ability, therefore, maintains better nutrient uptake in AMF inoculated plants under DS (Bowles et al., 2018). AMF hyphae can also explore the soil pores which plant roots cannot explore, thereby, AMF maintains better nutrient uptake in plants under DS (Zhao et al., 2015). In another study, it was noted that AMF application appreciably improved the N, P, and Mg uptake and ensured better nutrient uptake for plants growing under DS (Miransari et al., 2007).
Arbuscular mycorrhizal fungi makes better assimilation of N in plants by increasing the activity of nitrate reductase (NR) enzymes (Garg, 2013). An increase in N status in mycorrhizal is due to increase transportation of N through AMF hyphae which also increases the concentration of P which is needed for the phosphorylation of NR where there is a low concentration of N (Caravaca et al., 2003). Phosphatases enzyme plays an important role in the absorption, assimilation, and metabolism of P (Abd_Allah et al., 2015). AMF improves phosphatase activity, which increases the organically bound P and therefore makes it available for uptake and transport (Egamberdieva et al., 2017). In conclusion, AMF increases gene expression is linked with aquaporins that improve water absorption and ensures a higher WUE under DS. Moreover, an increase in nutrients owing to AMF substantially improves the plant growth and development under DS.
Arbuscular Mycorrhizal Fungi Maintains Plant Photosynthetic Performance Under Drought Stress
Drought stress negatively affects photosynthesis and causes a significant reduction in assimilate production for plants. DS reduces photosynthesis by decreasing the chlorophyll contents and increasing the production of ROS (Sochacki et al., 2013). AMF effectively improve chlorophyll synthesis and maintains better photosynthesis and subsequent assimilate production by reducing the ROS formation (Hashem et al., 2016a). DS also reduces photosynthesis by reducing adenosine triphosphate (ATP) production, stomata, and non-stomata limitations (Hassan et al., 2020). However, AMF significantly improved the gas exchange characteristics and fluorescence parameters, nutrient and water uptake, and RuBisCO activities, therefore, ensuring better photosynthesis under DS (Begum et al., 2019b).
Drought stress triggers the reduction in photosynthesis by reducing RuBisCO synthesis and up-regulating the activity of chlorophyll degrading enzyme (chlorophyllase) (Dalal and Tripathy, 2012; Fatima et al., 2014). However, AMF substantially increased the chlorophyll contents and maintain better synthesis of RuBisCO and leading to a significant increase in photosynthetic rate under DS (Abdel-Salam et al., 2018). AMF also increased photosynthesis by affecting both stomatal and non-stomatal parameters. Likewise, AMF improved stomata conductance which increases the entry of CO2 into plant leaf tissues which in turn increases the efficiency of PS-II resultantly improving photosynthetic efficiency under DS (Zhou et al., 2015; Mo et al., 2016). Drought stress down-regulated the enzymes responsible for the synthesis of chlorophyll, however, at the same time DS increases the activity of chlorophyll degrading enzyme (chlorophyllase) (Zhu et al., 2017). However, AMF substantially decreases the activity of chlorophyllase and maintains the activity of genes and enzymes involved in chlorophyll synthesis thereby maintaining higher chlorophyll synthesis under DS (Hashem et al., 2016b; Alwhibi et al., 2017; Zhu et al., 2017). In another study, it was noted that AMF inoculation improved the chlorophyll contents, efficiency of PS-II, and root hydraulic conductivity (Calvo-Polanco et al., 2016). AMF inoculation also improved the carotenoid contents which protect photosynthetic apparatus from drought-induced oxidative stress and ensured optimum photosynthesis under DS (Abd Allah et al., 2015).
It is a well-known fact that plants facing the DS show a significant reduction in photosynthesis owing to ROS that damage the photosynthetic apparatus which can reduce/limit the supply of ATP and reduce nicotinamide adenine dinucleotide phosphate (NADPH) to the Calvin cycle (Abbaspour et al., 2012). However, AMF symbiosis improves plant water status which increases stomata conductance and therefore increases CO2 fixing and improves the ATP and NADPH supply to Calvin supply and resulting in better photosynthesis and assimilate production (Boldt et al., 2011; Estrada et al., 2013). DS also decreases the efficiency of PS-II and Fv/Fm ratio, and it has been reported that AMF shows a positive effect on PS-II efficiency which in turn improves the plant photosynthesis under DS (Sánchez-Blanco et al., 2004; Manoharan et al., 2010). To summarize, AMF improves antioxidant activities which protect the photosynthetic apparatus, therefore maintaining higher photosynthetic efficiency under DS.
Arbuscular Mycorrhizal Fungi Maintains Osmolyte Accumulation and Confers Drought Tolerance
Osmolyte accumulation is an important strategy used by plants to cope with the deleterious impacts of DS. AMF inoculation significantly increased the accumulation of sugars, proline, and GB, which confer DS tolerance in plants. DS also triggers the synthesis of glucose and proline, however, AMF further increased the synthesis of these osmolytes and improves the drought tolerance (Mona et al., 2017; Wu et al., 2017). AMF is considered to upregulate the synthesis of enzymes involved in proline synthesis and AMF mediated increase in proline accumulation protects proteins (Table 2) and membranes from ROS (Abd Allah et al., 2015; Hashem et al., 2015). The accumulation of proline in plants also affects the metabolism of N which is needed for stress recovery. AMF significantly improves the activity of N metabolizing enzymes resulting in a marked increase in the accumulation of energy-rich amino acids (Hameed et al., 2014) which enhanced the drought tolerance (Doubková et al., 2013; Ouledali et al., 2018) and biomass production in plants grown under DS (Zou et al., 2013).
Arbuscular mycorrhizal fungi inoculation also increases the accumulation of nitrogenous compounds and free polyamines in water-deficient plants (Rapparini and Peñuelas, 2014). The increase in the accumulation of polyamines adjusts the plant osmotic potential in DS which is considered to be an important protective mechanism of AMF against DS (Bakr et al., 2018). Some authors noted that AMF decreased the accumulation of soluble sugars and improve the plant tolerance against DS (Zhang et al., 2010). Contrarily, some authors also noted a positive correlation between AMF and accumulation of soluble sugars which might be due to the sink effect on the AMF that demands sugar from plant shoot tissues (Yooyongwech et al., 2013). AMF also caused a substantial increase in the accretion of free amino acids (FAA) to increase NR activity which contributes toward to a great accumulation of FAA which in turn improve tolerance against DS (Zarea et al., 2011; Ahanger et al., 2017). Improved GB accumulation protects the photosynthesis and RuBisCO activity and plants from the damaging effects of DS (Khan and Hakeem, 2014). Drought tolerance in plants is upregulated by anti-oxidant activities which are further enhanced by the accumulation of GB and proline (Hashem et al., 2016a). Additionally, AMF-mediated increase in osmolytes accumulation improved the functioning of RuBisCO, and protects the photosynthetic apparatus from ROS which in turn improved the plant performance under DS (Mo et al., 2016).
Arbuscular Mycorrhizal Fungi Maintains Hormonal Crosstalk to Confer Drought Tolerance
Drought stress causes a significant increase in ABA bio-synthesis which increases the ABA level in plants and induces the stomata closure which minimizes the water loss by transpiration (Pozo et al., 2015; Xie et al., 2018). ABA is an abiotic stress hormone and a reduction in ABA level may explain why an AMF-associated plant has more tolerance against DS. AMF are considered to be indispensable for sustaining the AMF colonization, especially in unfavorable conditions such as drought conditions (Ludwig-Müller, 2010). ABA improves AMF colonization, functionality as well as development (Herrera-Medina et al., 2007; Aroca et al., 2013). Strigolactones are newly discovered plant hormones that regulate plant architecture and reproductive development (Foo and Reid, 2013). However, they were initially identified for their intermediation capacity in the AMF symbiosis, where they work as a signaling molecule for plants under unfavorable conditions (López et al., 2010).
Arbuscular mycorrhizal fungi colonization in the host plant also activates the jasmonic acid (JA) signaling pathway (Tejeda et al., 2008; Asensio et al., 2012). Jasmonic acid together with ABA plays an important role development and functionality of AMF. Auxins (IAA) and gibberellic acid (GA) play an imperious role in the growth of plants under stress conditions (Vishwakarma et al., 2017). AMF colonization significantly increases the accumulation of IAA, GA, and JA which improved the plant performance under stress and drought tolerance (Egamberdieva et al., 2018; Hashem et al., 2018; Sánchez et al., 2018). Drought tolerance in plants is up-regulated by anti-oxidant activities which are further enhanced by the accumulation of GB and proline (Hashem et al., 2016a). To summarize, AMF inoculation improves the synthesis and accumulation of osmolytes and hormones which maintains better plant performance under DS.
Arbuscular Mycorrhizal Fungi Improves the Accumulation of Phenols and Enzyme Activity to Confer Drought Stress
Phenolic compounds have excellent anti-oxidant activity and they play a significant role in stress conditions. AMF significantly increases the accumulation of phenolic substances, which strengthens the anti-oxidant defense system and improves the tolerance against DS (Begum et al., 2019a). Polyphenolic substances are active scavengers of free radicals and protect cell structures and their functioning (Hazzoumi et al., 2015). AMF substantially increase the accumulation of phenolic compounds by 50–60% which substantially improves the DS tolerance (Amiri et al., 2015; Kasote et al., 2015). AMF inoculation increases the accumulation of phenols and flavonoids which play an important in mycorrhization, adaptation, and growth of plants by inducing cellular signaling (Steinkellner et al., 2007; Mandal et al., 2010).
Phenylalanine ammonia-lyase (PAL) is found abundantly in higher plants that play a crucial role in plant metabolism and this enzyme also improves plant protection against biotic and abiotic stresses (Osakabe et al., 2007). AMF inoculation substantially improved the up-regulation of PAL which in turn improved the plant growth and provide protection against DS (Osakabe et al., 2007; Hao et al., 2016; Datrindade et al., 2019). Further AMF also increases the concentration of total GRSP (T-GRSP), easily extractable (EE-GRSP), and difficultly extractable (DE-GRSP) under DS. Glomalin-related soil proteins (GRSP) contain different cations, carbohydrates, proteins, and aliphatic components and work as glue to bind soil particles (Rillig et al., 2001). AMF substantially increases T-GRSP, EE-GRSP, and DE-GRSP under DS and ensures better water uptake and plant tolerance against DS (Begum et al., 2021).
Arbuscular Mycorrhizal Fungi Strengthens the Anti-Oxidant Defense System to Confer Drought Tolerance
The major effect of DS is the production of ROS that damage plant cellular membranes, proteins, and lipids. Nonetheless, AMF improve anti-oxidant activities and mitigate the drought-induced ROS effects on plants (Egamberdieva et al., 2017). Stress conditions up-surge the ROS which causes lipid-peroxidation and affects the functioning and fluidity of cellular membranes. However, AMF significantly increases the activity of antioxidants [superoxide dismutase (SOD), catalase (CAT), ascorbate peroxidase (APX), glutathione reductase (GR), ascorbic acid (AsA), and glutathione synthetase (GSH)] which in turn increases the cellular stability (Hashem et al., 2016a,b). Superoxide dismutase is the first line of defense against oxidative stress and APX, GR, AsA, and GSH (Table 3) are key components of ROS scavenging pathways. AMF increases the activity of the aforementioned antioxidant and neutralizes H2O2 by preventing the formation of toxic OH and protecting plant mitochondria and electron transport (Abd_Allah et al., 2015; Mona et al., 2017). The better maintenance of AsA-GSH components ensures NADP availability in order to keep electron transport at a normal rate. AMF substantially improves the activities of AsA and GSH which in turn maintain NADP availability and electron transport thus improving photosynthesis under DS (Scheibe and Dietz, 2012; Abd Allah et al., 2015). Many other authors also reported that AMF significantly increases the APX, CAT, SOD, and GR activities which demonstrated greater protection of the photosynthetic apparatus and subsequently improve plant growth (Mirzai et al., 2013; Weng et al., 2015; Yang et al., 2015).
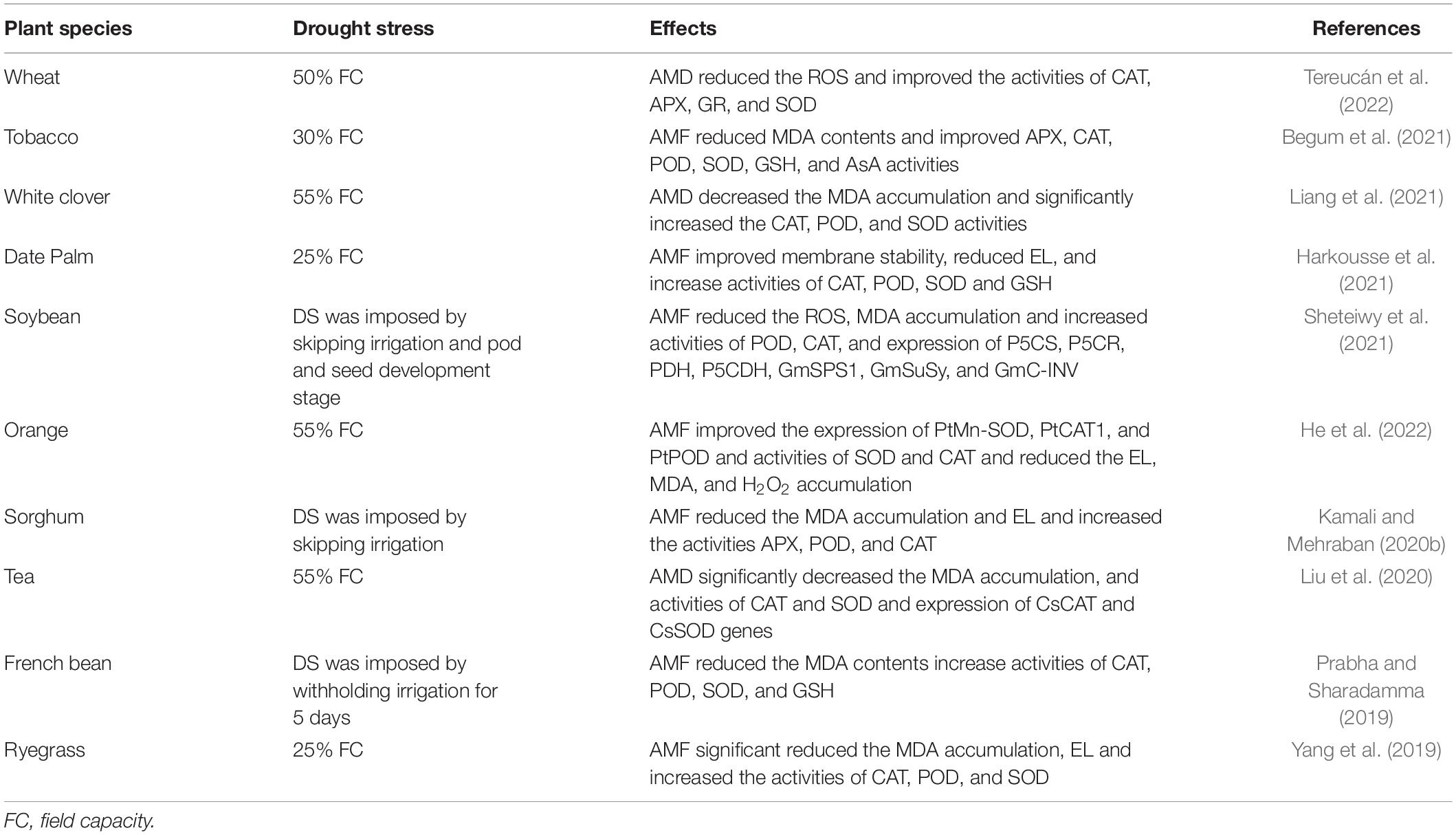
Table 3. Effect of AMF on oxidative stress markers, antioxidant activities and genes expression under DS.
Likewise, an increase in AsA and glutathione (GT) has been reported in Brassica napus and Sesbania sesban plants inoculated with AMF resulting in greater stress tolerance (Shafiq et al., 2014; Abd Allah et al., 2015). The Brassica napus are considered to fall among the plants that do not form arbuscules within the roots, however, beneficial impacts of AMF association can be attained without a complete mycorrhizal association (Fernandez et al., 2020). SOD induced dis-mutation of superoxide radicals produced in plant chloroplast prevent damage to photosynthetic apparatus (Per et al., 2016). AMF-mediated increase in SOD activity prevents the production of hydroxyl radicals through the Haber–Weiss reaction, which can otherwise pose serious damage to plant membranes and organelle functioning (Ahanger et al., 2017). Likewise, AMF-mediated increases in CAT and POD neutralize excessive H2O2 in cytosol whereas APX, GR, AsA, and GSH work as an intriguing pathway for neutralizing the H2O2 in plant chloroplast and mitochondria (Ahanger et al., 2017). Many other authors also demonstrated that AMF inoculation upregulates anti-oxidant activities, increases photosynthesis, and maintains the redox homeostasis in plants grown under DS (Yang et al., 2015; Mo et al., 2016). AsA and GSH are key components of redox buffer and they can also scavenge ROS directly and AMF up-regulates these anti-oxidants to counter the stress conditions (Weng et al., 2015; Begum et al., 2019b). In conclusion, the AMF-mediated increase in antioxidant activities alleviates drought-induced toxic effects and maintains better plant performance.
Arbuscular Mycorrhizal Fungi Regulates Gene Expression Aquaporins Activity to Confer Drought Stress Tolerance
Aquaporins are involved in the transportation of water in plant roots and shoots. They also play an important role in plant growth, fixation of CO2, nutrient allocation, and plant interactions with different abiotic stresses (Maurel et al., 2015). Recently, AQP functions in plant-water relations received considerable attention as an important way to improve crop performance (Moshelion et al., 2015; Tharanya et al., 2018). AQP maintains the root water balance with transpiration (Sadok and Sinclair, 2010) by increasing root hydraulic conductivity upon high transpiration demand (Sakurai et al., 2011). In some crops, AQP functioning is also improved under lower transpiration which is also considered to be beneficial for crop yields (Kholová et al., 2012). AQP is considered to be involved in diverse physiological mechanisms that determine the pattern as well as the rate of plant water usage (Vadez et al., 2013). Further AMF also enhanced the root hydraulic conductance and maintains hydraulic continuity between plant roots thereby reducing the drop in matric potential at the root-soil furnace and enhancing the water uptake by AMA inoculated plants (Quiroga et al., 2019a; Pauwels et al., 2020; Abdalla and Ahmed, 2021).
Arbuscular mycorrhizal fungi symbiosis activates different molecular mechanisms including activation of gene expression, aquaporins (AQP) membrane transporters, sugar, and ion transporters to cope with the impacts of DS (Bahadur et al., 2019a). The AMF-mediated increase in expression of AQP improves nutrient and water uptake and mitigates the drought-induced toxic effects (Bahadur et al., 2019a). Likewise, in tomato (Solanum lycopersicum) plants AMF increased the DS by regulating the expression of genes (TFT1-TFT12) involved in the ABA signaling pathway (Xu et al., 2018). The AMF-mediated increase in D-myo-inositol-3-phosphate synthase (IPS) and 14-3-3-like protein GF14 (14-3GF) expression improved the plant tolerance against DS (Li et al., 2016). In another study, it was noted that expression of two AQP genes (GintAQPF1 and GintAQPF2) was markedly increased in mycorrhizal roots in response to DS which supports the evidence of direct AMF involvement in improving the plant tolerance against DS (Li et al., 2013). Consistently, another group of authors also noted a significant increase in AQPs gene expression in AMF-inoculated roots cells under DS which in turn increased the tolerance against DS by increasing nutrient and water uptake and better architecture of the root system (Neumann et al., 2009; Zou et al., 2015). AMF symbiosis clearly regulates AQP expression and improves root hydraulic conductivity, root water status, and tolerance against DS (Aroca et al., 2012; Bárzana et al., 2014).
The increase in expression of AQPs in AMF inoculated plants substantially increased the root hydraulic conductivity which in turn increased plant performance under DS (Sanchez-Romera et al., 2016). In another study, it was noted that AMF increases the expression of PtAHA2 genes in plant leaves and roots under DS and leads to a significant increase in photosynthetic and transpiration rates, intercellular CO2 concentration, and stomata conductance (Cheng et al., 2021). TdSHN1 is considered to be involved in plant tolerance against different abiotic Djemal and Khoudi (2015) AMF considerably increased the expression of SHN1 which in turn improved the plant WUE by modifying the leaf diffusive characteristics owing to the accumulation of high levels of wax (Aharoni et al., 2004; Fiorilli et al., 2022). Another gene named dehydration responsive element binding protein (DREB) has been reported to play an important role against DS (Latini et al., 2013). In a study, it was noted AMF improve the expression of TdDRF1 and induce drought tolerance in wheat plants (Fiorilli et al., 2022). AMF also up-regulates the expression of MdGH3-2 and MdGH3-12 which improve the plant RWC, photosynthetic capacity, chlorophyll contents, and scavenging of ROS in apple plants (Huang et al., 2021). Conversely, silencing of MdGH3-2/12 genes from apple plants negatively affected AMF colonization, plant growth, and subsequent development under DS (Huang et al., 2021). AMF increase the genes’ expression which in turn increased the tolerance against DS by increasing nutrient and water uptake and better architecture of the root system.
Arbuscular Mycorrhizal Fungi Improves Growth, Yield, and Quality Under Drought Stress
Arbuscular mycorrhizal fungi colonization significantly improves plant growth and development under DS (Table 2). AMF-mediated increases in growth under stress can be attributed to an increase in the accumulation of growth-promoting hormones, better nutrient and water uptake, and scavenging of ROS (Egamberdieva et al., 2017; Vishwakarma et al., 2017). AMF also maintain optimum nutrient availability and plant tissue water status which in turn improves the overall performance of plants under DS (Abdel-Salam et al., 2018). AMF also modifies root characteristics including root diameter, root morphology, and promotes a dense root system which allows better nutrient (N, P, and K) and water uptake and resultantly ensures better plant growth under DS (Chitarra et al., 2016; Begum et al., 2019b). Moreover, AMF inoculation also increases the plant height, leaves per plant, and biomass production by improving P uptake, water acquisition, and cellular signaling in plants under DS (Li et al., 2013; Jayne and Quigley, 2014; Begum et al., 2021). In another study, Sheteiwy et al. (2021) noted that DS significantly reduces the grain yield of plants. However, AMF (Bradyrhizobium) inoculation considerably increased the growth and yield by decreasing lipid peroxidation through enhanced antioxidant activities (CAT and POD) and accumulation of proline under DS (Sheteiwy et al., 2021).
Arbuscular mycorrhizal fungi also improve the plant metabolism, which affects both the quality and quantity of secondary metabolites quality (Karagiannidis et al., 2011; Fokom et al., 2019). Likewise, in tobacco plants, AMF improved the accumulation of essential oil (EO) which was linked with a change in the secondary metabolism of the plant (Begum et al., 2021). In another study, it was reported that AMF in combination with vermicompost significantly improved plant height, root and shoot length, biomass, and grain yield of quinoa plants under well water and DS (Cozzolino et al., 2016; Lahbouki et al., 2022). Likely, the beneficial impacts of AMF in improving the plants growth are also linked with improved nutrient cycling, better nutrient and water uptake, bio-degradation of organic matter, hormone production, and improvement in soil properties (Cavagnaro, 2015; Zhang et al., 2020; Benaffari et al., 2022).
Implications of Arbuscular Mycorrhizal Fungi for Improving Nitrogen Use Efficiency
Nitrogen (N) is an essential nutrient needed for plant growth and development. However, its deficiency in agricultural soils is a major problem. Therefore, to fulfill plant nutrient needs farmers use a large quantity of N fertilizers, which is also posing a serious threat to the environment (Varinderpal-Singh et al., 2020). The poor recovery efficiency of N fertilizers poses serious economical, environmental, and ecological losses (Varinderpal-Singh et al., 2020). Inappropriate timing and excessive use of N fertilizers are major reasons for the lower recovery efficiency of N fertilizers (Varinderpal et al., 2021). Globally, different strategies are being used to improve the efficiency of applied nutrients. Among these, the use of microbes is considered an important and effective approach to improve N use efficiency. Many authors have reported the role of AMF in the uptake of nutrients (Figure 3) which can appreciably improve the growth of the host plant (Smith et al., 2011). The formation of a hyphal network in AMF inoculated plants limits the inefficient use of applied N fertilizers. AMF play an imperative part in N cycling by altering microbial compositions and modifying the development of denitrifying, nitrifying, and free-living bacteria (Veresoglou et al., 2011).
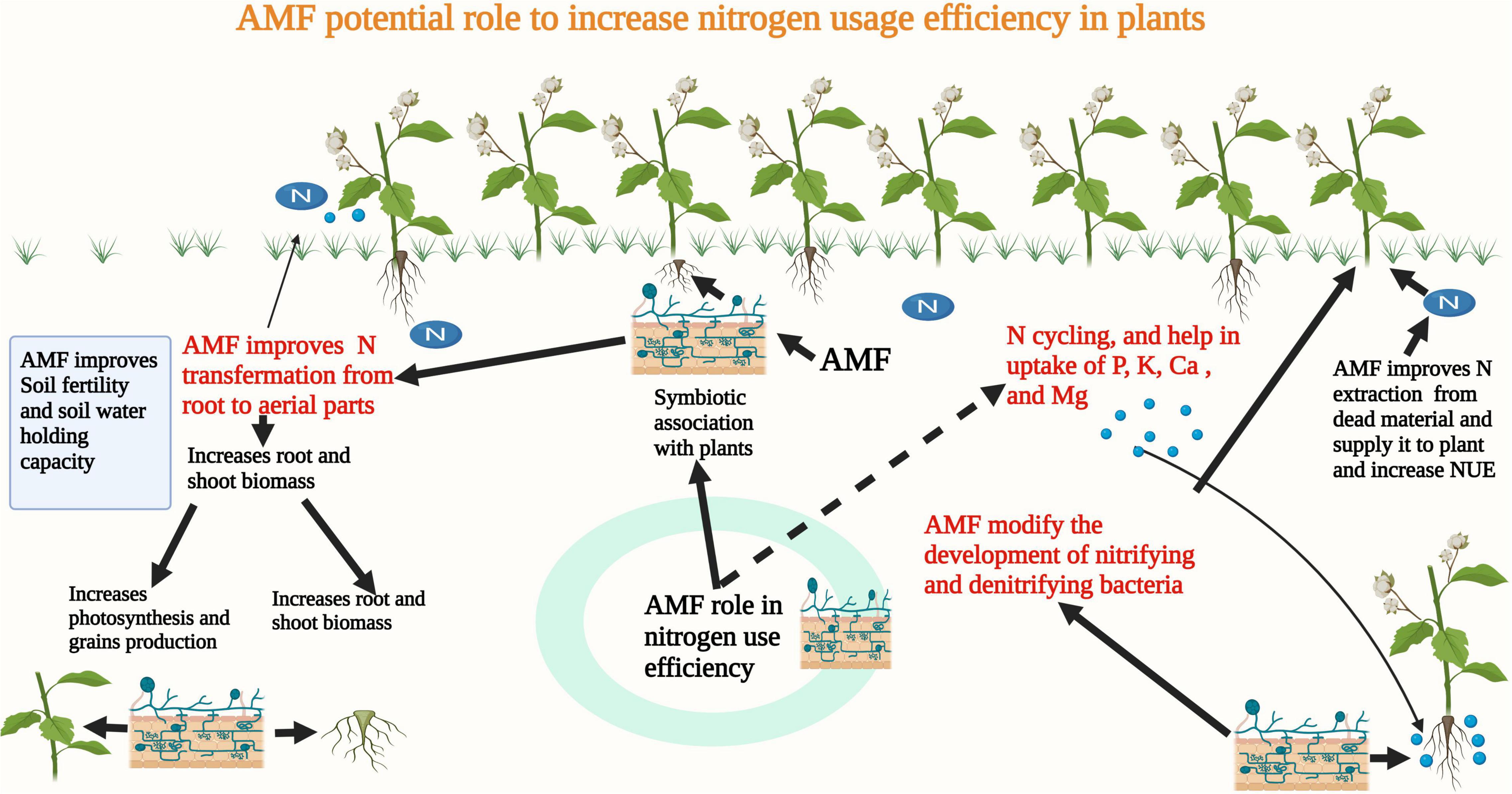
Figure 3. AMF forms a symbiotic association with plants and it improves N extraction from dead materials and it also improves microbial activities and N uptake, thereby improving NUE in plants.
In many crops, N is considered to be a limiting factor and many authors have noted that AMF absorbs and transfers N to nearby plants and improves their growth and development (Battini et al., 2017; Turrini et al., 2018). In another study, Zhang et al. (2018b) noted that AMF increases the shoot biomass and panicles and grains production by increasing the N uptake and its redistribution to plants. AMF form a symbiotic relationship that improves nutrient uptake particular N which in turn improves the plant growth and development (Battini et al., 2017). The interaction between the AMF and salinity stress significantly affects the N and P concentration in plant parts. AMF significantly improve N contents and NUE in crop plants (Turrini et al., 2018). It has been reported that AMF can take a significant amount of N from dead and decomposed materials then provide N to host plants and improve N uptake and NUE of the host plant (Turrini et al., 2018).
The extra-radical hyphae of AMF absorb and assimilate inorganic N (Jin et al., 2005) and it has been reported that 20–75% N uptake by AMF is transferred to the host plant which in turn improves NUE in host plants (Hashem et al., 2018). The increase in the N uptake in AMF-inoculated plants increases chlorophyll synthesis which improves the plant’s photosynthetic efficiency and subsequent assimilate production (Deandrade et al., 2015). AMF symbiosis also improves C and N accumulation under elevated CO2 concentration and ambient conditions (Zhu et al., 2016). Moreover, AMF also improve the N, P, K, Ca, and Mg uptake under DS (Asrar et al., 2012; Bati et al., 2015). Additionally, AMF also proved beneficial in accumulating the Fe, Mn, Mg, and Na and protecting the plants from the toxic effects of these metals (Bati et al., 2015). A significant positive relationship was noted between N accumulation and biomass production which suggested that an increase in dry matter production was associated with an increase in N uptake in AMF inoculated plants (Zhu et al., 2016). In another study, Fellbaum et al. (2012) noted that C supply increases the N uptake and transportation in AMF symbiosis. Moreover, NUE of AMF inoculated plants is considered to be higher at elevated CO2 as compared to non-AMF-inoculated plants which suggested that AMF colonization may change the balance of N and C under elevated CO2 by changing the C demands of AMF and supply of N from AMF to host plants (Cavagnaro et al., 2011).
Arbuscular mycorrhizal fungi plays an imperative role in N cycling by absorbing N and then supplying it to host plants (Bonfante and Genre, 2010). The extraradical mycelium of AMF can directly absorb ammonium, nitrate, and amino acids and improve the N uptake and subsequently N use efficiency in plants (Basu et al., 2018). Additionally, AMF are also vital for the translocation of N and which in turn increases the NUE and neutralizes the excessive N fertilization (Leifheit-Eva et al., 2014; Rosolem et al., 2017). AMF assist plant roots to supply soil nutrients to host plants which are largely depend on symbiosis reaction between strigolactones, AMF “Myc factors” and root exudates of host plant roots (Basu, 2018) thereby this association improve the N uptake resultantly NUE in plants (Xing et al., 2019). Additionally, strigolactones also adjust root development and shoot branching (Seto et al., 2012) and significantly influence the N distribution as well as N translocation to different shoot tissues (Luo et al., 2018). Moreover, AMF forms an interconnected hyphal network which improves the N uptake and NUE in host plant (Verzeaux et al., 2017). The beneficial impact of AMF in improving N uptake and NUE has been highlighted in a meta-analysis of more than 300 field studies. The results of the meta-analysis indicated that the use of AMF is a profitable practice and has many agronomic uses and improves N uptake and subsequently NUE in plants (Mensah et al., 2015).
Conclusion and Future Prospective
Drought stress causes a serious reduction in plant growth and development by disturbing plant physiological, biochemical, and molecular responses. However, AMF protects plants under DS and substantially improves their growth and development. AMF inoculation maintains membrane integrity and plant water status, protects photosynthetic apparatus from drought-induced oxidative stress, and improves the synthesis of photosynthetic pigments thereby improving plant growth and development under DS. AMF also improves the accumulation of osmolytes and, hormones and gene expression and anti-oxidant activities which leads to an appreciable improvement in plant performance in water deficit conditions. Besides this, AMF also improves the expression of aquaporins and improves the water uptake and water use efficiency which are major reasons for AMF-mediated improvement in plant growth under DS. Additionally, AMF also improves soil health and plays an important role in soil nutrient cycling and improves nutrient uptake and nutrient use efficiency and alleviates negative impacts of DS, and improves plant growth and yield.
Despite recent progress about the role of AMF in mitigating the adverse effects of DS, there are many unanswered questions. The role of AMF in seed germination mechanism is poorly studied, therefore it is important to determine how AMF affect the germination mechanism and improve seed germination under DS. The role of AMF in nutrient uptake is well studied by authors, however, its role in nutrient signaling, ion transport, and nutrient channels is not explored. Therefore, it would be fascinating to perform research on these aspects to increase our understanding of the role of AMF against DS. The role of AMF in improving photosynthesis is clearly explored nonetheless, its role in stomata movements is still unknown therefore, it is suggested to explore the role of AMF in stomata signaling and its impacts on anion channels in leaf guard cells under DS.
The role of AMF on hormones (ABA, GA, and IAA) and osmolytes (proline and GB) accumulation under DS is will be studied by the authors. However, it would be interesting to explore the relationship between AMF cytokinin, ethylene, salicylic acid, proline, and GB at the transcriptomic level. Also, it would also be interesting to understand the effect of AMF on genes and enzymes involved in the synthesis of these compounds under DS. The role of AMF on pollen viability and quality of crops is not explored well, therefore, it is needed to understand the role of AMF in improving pollen viability and final crop quality under DS. Likewise, the identification of host plant and AMF-specific protein factors regulating the symbiotic relationship and major cellular as well as metabolic pathways under DS can also be an important area of research. The role of AMF on nitrogen use efficiency (NUE) is well explored in plants. However, more studies are needed at the transcriptomic, proteomics, and metabolomics level to elucidate the role of AMF in N uptake and its effect on NUE. Lastly, more studies are direly needed to determine the effect of AMF on aquaporins and their role in plant water uptake under DS.
Author Contributions
HT and MH: conceptualization and writing original draft. LF, JM, YL, MN, AS, and SQ: reviewing and editing. All authors contributed to the article and approved the submitted version.
Funding
This work was supported and funded by the National Natural Science Foundation of China (32060318), and Project of the Youth Fund of Hunan University of Humanities, Science and Technology (2010QN23).
Conflict of Interest
The authors declare that the research was conducted in the absence of any commercial or financial relationships that could be construed as a potential conflict of interest.
Publisher’s Note
All claims expressed in this article are solely those of the authors and do not necessarily represent those of their affiliated organizations, or those of the publisher, the editors and the reviewers. Any product that may be evaluated in this article, or claim that may be made by its manufacturer, is not guaranteed or endorsed by the publisher.
References
Aalipour, H., Nikbakht, A., Etemadi, N., Rejali, F., and Soleimani, M. (2020). Biochemical response and interactions between arbuscular mycorrhizal fungi and plant growth promoting rhizobacteria during establishment and stimulating growth of Arizona cypress (Cupressus arizonica G.) under drought stress. Sci. Hortic. 261:108923.
Abbaspour, H., Saeidi-Sar, S., Afshari, H., and Abdel-Wahhab, M. A. (2012). Tolerance of mycorrhiza infected pistachio (Pistacia vera L.) seedling to drought stress under glasshouse conditions. J. Plant Physiol. 169, 704–709. doi: 10.1016/j.jplph.2012.01.014
Abdalla, M., and Ahmed, M. A. (2021). Arbuscular mycorrhiza symbiosis enhances water status and soil-plant hydraulic conductance under drought. Front. Plant Sci. 12:722954. doi: 10.3389/fpls.2021.722954
Abd_Allah, E. F., Abeer, H., Alqarawi, A. A., and Hend, A. A. (2015). Alleviation of adverse impact of cadmium stress in sunflower (Helianthus annuus L.) by arbuscular mycorrhizal fungi. Pak. J. Bot 47, 785–795.
Abd_Allah, E. F., Alqarawi, A. A., Hashem, A., Radhakrishnan, R., Al-Huqail, A. A., Al-Otibi, F. O. N., et al. (2017). Endophytic bacterium Bacillus subtilis (BERA 71) improves salt tolerance in chickpea plants by regulating the plant defense mechanisms. J. Plant Interact. 13, 37–44.
Abd Allah, E. F., Hashem, A., Alqarawi, A. A., Bahkali, A. H., and Alwhibi, M. S. (2015). Enhancing growth performance and systemic acquired resistance of medicinal plant Sesbania sesban (L.) Merr using arbuscular mycorrhizal fungi under salt stress. Saudi J. Biol. Sci. 22, 274–283. doi: 10.1016/j.sjbs.2015.03.004
Abdel-Ghani, A. H., Neumann, K., Wabila, C., Sharma, R., Dhanagond, S., Owais, S. J., et al. (2015). Diversity of germination and seedling traits in a spring barley (Hordeum vulgare L.) collection under drought simulated conditions. Genet. Resour. Crop Evol. 62, 275–292.
Abdel-Salam, E., Alatar, A., and El-Sheikh, M. A. (2018). Inoculation with arbuscular mycorrhizal fungi alleviates harmful effects of drought stress on damask rose. Saudi J. Biol. Sci. 25, 1772–1780. doi: 10.1016/j.sjbs.2017.10.015
Abeydeera, L. H. U. W., Mesthrige, J. W., and Samarasinghalage, T. I. (2019). Global research on carbon emissions: a scientometric review. Sustainability 11:3972.
Ahanger, M. A., and Agarwal, R. M. (2017). Salinity stress induced alterations in antioxidant metabolism and nitrogen assimilation in wheat (Triticum aestivum L) as influenced by potassium supplementation. Plant Physiol. Biochem. 115, 449–460. doi: 10.1016/j.plaphy.2017.04.017
Ahanger, M. A., and Ahmad, P. (2019). Role of Mineral Nutrients in Abiotic Stress Tolerance. Amsterdam: Elsevier Inc, 2019.
Ahanger, M. A., Hashem, A., Abd-Allah, E. F., and Ahmad, P. (2017). “Arbuscular mycorrhiza in crop improvement under environmental stress,” in Emerging Technologies and Management of Crop Stress Tolerance, eds P. Ahmad and S. Rasool (Cambridge, MA: Academic Press), 69–95.
Aharoni, A., Dixit, S., Jetter, R., Thoenes, E., Van Arkel, G., and Pereira, A. (2004). The SHINE clade of AP2 domain transcription factors activates wax biosynthesis, alters cuticle properties, and confers drought tolerance when overexpressed in Arabidopsis. Plant Cell 16, 2463–2480. doi: 10.1105/tpc.104.022897
Ahmad, P. (2010). Growth and antioxidant responses in mustard (Brassica juncea L.) plants subjected to combined effect of gibberellic acid and salinity. Arch. Agron. Soil Sci. 56, 575–588.
Ahmed, A., Abdelmalik, A., Alsharani, T., Al-Qarawi, B. A., and Aref, I. (2020). Response of growth and drought tolerance of Acacia seyal Del. seedlings to arbuscular mycorrhizal fungi. Plant Soil Environ. 66, 264–271.
Al-Arjani, A. B. F., Hashem, A., and Abd-Allah, E. F. (2020). Arbuscular mycorrhizal fungi modulates dynamics tolerance expression to mitigate drought stress in Ephedra foliata Boiss. Saudi J. Biol. Sci. 27, 380–394. doi: 10.1016/j.sjbs.2019.10.008
Allen, M. F. (2009). Bidirectional water flows through the soil-fungal-plant mycorrhizal continuum. New Phytol. 182, 290–293. doi: 10.1111/j.1469-8137.2009.02815.x
Alwhibi, M. S., Khalil, M. I., Ibrahim, M. M., El-Gaaly, G. A., and Sultan, A. S. (2017). Potential antitumor activity and apoptosis induction of Glossostemon bruguieri root extract against hepatocellular carcinoma cells. Evid. Based Complement. Alternat. Med. 2017:7218562. doi: 10.1155/2017/7218562
Amiri, R., Nikbakht, A., and Etemadi, N. (2015). Alleviation of drought stress on rose geranium [Pelargonium graveolens (L.) Herit.] in terms of antioxidant activity and secondary metabolites by mycorrhizal inoculation. Sci. Hortic. 197, 373–380.
Aroca, R., Porcel, R., and Ruiz-Lozano, J. M. (2012). Regulation of root water uptake under abiotic stress conditions. J. Exp. Bot. 63, 43–57. doi: 10.1093/jxb/err266
Aroca, R., Ruiz-Lozano, J. M., Zamarreño, ÁM., Paz, J. A., García-Mina, J. M., Pozo, M. J., et al. (2013). Arbuscular mycorrhizal symbiosis influences strigolactone production under salinity and alleviates salt stress in lettuce plants. J. Plant Physiol. 170, 47–55. doi: 10.1016/j.jplph.2012.08.020
Asensio, D., Rapparini, F., and Peñuelas, J. (2012). AM fungi root colonization increases the production of essential isoprenoids vs. nonessential isoprenoids especially under drought stress conditions or after jasmonic acid application. Phytochemistry 77, 149–161. doi: 10.1016/j.phytochem.2011.12.012
Asrar, A. A., Abdel-Fattah, G. M., and Elhindi, K. M. (2012). Improving growth, flower yield, and water relations of snapdragon (Antirhinum majus L.) plants grown under well-watered and water-stress conditions using arbuscular mycorrhizal fungi. Photosynthetica 50, 305–316.
Augé, R. M., Toler, H. D., Moore, J. L., Cho, K., and Saxton, A. M. (2007). Comparing contributions of soil versus root colonization to variations in stomatal behavior and soil drying in mycorrhizal Sorghum bicolor and Cucurbita pepo. J. Plant Physiol. 164, 1289–1299. doi: 10.1016/j.jplph.2006.08.005
Augé, R. M., Toler, H. D., Sams, C. E., and Nasim, G. (2008). Hydraulic conductance and water potential gradients in squash leaves showing mycorrhiza-induced increases in stomatal conductance. Mycorrhiza 18, 115–121. doi: 10.1007/s00572-008-0162-9
Avio, L., Pellegrino, E., Bonari, E., and Giovannetti, M. (2006). Functional diversity of arbuscular mycorrhizal fungal isolates in relation to extraradical mycelial networks. New Phytol. 172, 347–357. doi: 10.1111/j.1469-8137.2006.01839.x
Azzeme, A. M., Abdullah, S. N. A., Aziz, M. A., and Wahab, P. E. M. (2016). Oil palm leaves and roots differ in physiological response, antioxidant enzyme activities and expression of stress-responsive genes upon exposure to drought stress. Acta Physiol. Plant. 38, 1–12.
Bahadur, A., Jin, Z., Jiang, S., Chai, Y., Zhang, Q., Pan, J., et al. (2019a). Arbuscular mycorrhizal spores distribution across different ecosystems of Qinghai Tibetan Plateau. Pak. J. Bot. 51, 1481–1492.
Bahadur, A., Batool, A., Nasir, F., Jiang, S., Mingsen, Q., Zhang, Q., et al. (2019b). Mechanistic insights into arbuscular mycorrhizal fungi-mediated drought stress tolerance in plants. Int. J. Mol. Sci. 20:4199. doi: 10.3390/ijms20174199
Bakr, J., Pék, Z., Helyes, L., and Posta, K. (2018). Mycorrhizal inoculation alleviates water deficit impact on field-grown processing tomato. Pol. J. Environ. Stud. 27, 1949–1958.
Bao, G., Tang, W., An, Q., Liu, Y., Tian, J., Zhao, N., et al. (2020). Physiological effects of the combined stresses of freezing-thawing, acid precipitation and deicing salt on alfalfa seedlings. BMC Plant Biol. 20:204. doi: 10.1186/s12870-020-02413-4
Barros, V., Frosi, G., Santos, M., Ramos, D. G., Falcão, H. M., and Santos, M. G. (2018). Arbuscular mycorrhizal fungi improve photosynthetic energy use efficiency and decrease foliar construction cost under recurrent water deficit in woody evergreen species. Plant Physiol. Biochem. 127, 469–477. doi: 10.1016/j.plaphy.2018.04.016
Bárzana, G., Aroca, R., Bienert, G. P., Chaumont, F., and Ruiz-Lozano, J. M. (2014). New insights into the regulation of aquaporins by the arbuscular mycorrhizal symbiosis in maize plants under drought stress and possible implications for plant performance. Mol. Plant Microbe Interact. 27, 349–363. doi: 10.1094/MPMI-09-13-0268-R
Basal, O., Szabó, A., and Veres, S. (2020). PEG-induced drought stress effects on soybean germination parameters. J. Plant Nutr. 43, 1768–1779.
Basu, S., Rabara, R. C., and Negi, S. (2018). AMF: the future prospect for sustainable agriculture. Physiol. Mol. Plant Pathol. 102, 36–45.
Bati, B. C., Santilli, E., and Lombardo, L. (2015). Effect of arbuscular mycorrhizal fungi on growth and on micronutrient and macronutrient uptake and allocation in olive plantlets growing under high total Mn levels. Mycorrhiza 25, 97–108. doi: 10.1007/s00572-014-0589-0
Batool, M., El-Badri, A. M., Hassan, M. U., Haiyun, Y., Chunyun, W., Zhenkun, Y., et al. (2022a). Drought stress in Brassica napus: effects, tolerance mechanisms, and management strategies. J. Plant Growth Reg. [Preprint].
Batool, M., El-Badri, A. M., Wang, Z., Mohamed, I. A., Yang, H., Ai, X., et al. (2022b). Rapeseed morpho-physio-biochemical responses to drought stress induced by PEG-6000. Agronomy 12:579.
Battini, F., Grønlund, M., Agnolucci, M., Giovannetti, M., and Jakobsen, I. (2017). Facilitation of phosphorus uptake in maize plants by mycorrhizosphere bacteria. Sci. Rep. 7:4686. doi: 10.1038/s41598-017-04959-0
Begum, N., Qin, C., Ahanger, M. A., Raza, S., Khan, M. I., Ashraf, M., et al. (2019b). Role of arbuscular mycorrhizal fungi in plant growth regulation: implications in abiotic stress tolerance. Front. Plant Sci. 10:1068. doi: 10.3389/fpls.2019.01068
Begum, N., Ahanger, M. A., Su, Y., Lei, Y., Mustafa, N. S. A., Ahmad, P., et al. (2019a). Improved drought tolerance by AMF inoculation in maize (Zea mays) involves physiological and biochemical implications. Plant 8:579. doi: 10.3390/plants8120579
Begum, N., Ahanger, M. A., and Zhang, L. (2020). AMF inoculation and phosphorus supplementation alleviates drought induced growth and photosynthetic decline in Nicotiana tabacum by up-regulating antioxidant metabolism and osmolyte accumulation. Environ. Exp. Bot. 176:104088.
Begum, N., Akhtar, K., Ahanger, M. A., Iqbal, M., Wang, P., Mustafa, N. S., et al. (2021). Arbuscular mycorrhizal fungi improve growth, essential oil, secondary metabolism, and yield of tobacco (Nicotiana tabacum L.) under drought stress conditions. Environ. Sci. Pollut. Res. 28, 45276–45295. doi: 10.1007/s11356-021-13755-3
Behrooz, A., Vahdati, K., Rejali, F., Lotfi, M., Sarikhani, S., and Leslie, C. (2019). Arbuscular mycorrhiza and plant growth-promoting bacteria alleviate drought stress in walnut. Hortscience 54, 1087–1092.
Benaffari, W., Boutasknit, A., Anli, M., Ait-El-Mokhtar, M., Ait-Rahou, Y., Ben-Laouane, R., et al. (2022). The native arbuscular mycorrhizal fungi and vermicompost-based organic amendments enhance soil fertility, growth performance, and the drought stress tolerance of quinoa. Plant 11:393. doi: 10.3390/plants11030393
Bi, Y., Xiao, L., and Sun, J. (2019). An arbuscular mycorrhizal fungus ameliorates plant growth and hormones after moderate root damage due to simulated coal mining subsidence: a microcosm study. Environ. Sci. Pollut. Res. 26, 11053–11061. doi: 10.1007/s11356-019-04559-7
Bian, Z., Wang, D., Liu, Y., Xi, Y., Wang, X., and Meng, S. (2021). Analysis of Populus glycosyl hydrolase family I members and their potential role in the ABA treatment and drought stress response. Plant Physiol. Biochem. 163, 178–188.
Bogati, K., and Walczak, M. (2022). The impact of drought stress on soil microbial community, enzyme activities and plants. Agronomy 12:189.
Boldt, K., Pörs, Y., Haupt, B., Bitterlich, M., Kühn, C., Grimm, B., et al. (2011). Photochemical processes, carbon assimilation and RNA accumulation of sucrose transporter genes in tomato arbuscular mycorrhiza. J. Plant Physiol. 168, 1256–1263. doi: 10.1016/j.jplph.2011.01.026
Bonfante, P., and Genre, A. (2010). Mechanisms underlying beneficial plant–fungus interactions in mycorrhizal symbiosis. Nat. Commun. 1:48. doi: 10.1038/ncomms1046
Bowles, T. M., Jackson, L. E., and Cavagnaro, T. R. (2018). Mycorrhizal fungi enhance plant nutrient acquisition and modulate nitrogen loss with variable water regimes. Glob. Chang. Biol. 24, e171–e182. doi: 10.1111/gcb.13884
Bowne, J. B., Erwin, T. A., Juttner, J., Schnurbusch, T., Langridge, P., Bacic, A., et al. (2012). Drought responses of leaf tissues from wheat cultivars of differing drought tolerance at the metabolite level. Mol. Plant 5, 418–429. doi: 10.1093/mp/ssr114
Calvo-Polanco, M., Sánchez-Castro, I., Cantos, M., García, J. L., Azcón, R., Ruiz-Lozano, J. M., et al. (2016). Effects of different arbuscular mycorrhizal fungal backgrounds and soils on olive plants growth and water relation properties under well-watered and drought conditions. Plant Cell Environ. 39, 2498–2514. doi: 10.1111/pce.12807
Caravaca, F., Diaz, E., Barea, J. M., Azcón-Aguilar, C., and Roldan, A. (2003). Photosynthetic and transpiration rates of Olea europaea subsp. sylvestris and Rhamnus lycioides as affected by water deficit and mycorrhiza. Biol. Plant 46, 637–639.
Cavagnaro, T. R. (2015). Biologically regulated nutrient supply systems: compost and arbuscular mycorrhizas—a review. Adv. Agron. 129, 293–321.
Cavagnaro, T. R., Gleadow, R. M., and Miller, R. E. (2011). Plant nutrient acquisition and utilisation in a high carbon dioxide world. Funct. Plant Biol. 38, 87–96. doi: 10.1071/FP10124
Cheng, H. Q., Zou, Y. N., Wu, Q. S., and Kuča, K. (2021). Arbuscular mycorrhizal fungi alleviate drought stress in trifoliate orange by regulating H+-ATPase activity and gene expression. Front. Plant Sci. 12:659694. doi: 10.3389/fpls.2021.659694
Chien, S. H., Teixeira, L. A., Cantarella, H., Rehm, G. W., Grant, C. A., and Gearhart, M. M. (2016). Agronomic effectiveness of granular nitrogen/phosphorus fertilizers containing elemental sulfur with and without ammonium sulfate: a review. Agron. J. 108, 1203–1213.
Chitarra, W., Pagliarani, C., Maserti, B., Lumini, E., Siciliano, I., Cascone, P., et al. (2016). Insights on the impact of arbuscular mycorrhizal symbiosis on tomato tolerance to water stress. Plant Physiol. 171, 1009–1023. doi: 10.1104/pp.16.00307
Chowdhury, M. K., Hasan, M. A., Bahadur, M. M., Islam, M., Hakim, M., Iqbal, M. A., et al. (2021). Evaluation of drought tolerance of some wheat (Triticum aestivum L.) genotypes through phenology, growth, and physiological indices. Agronomy 11:1792.
Cozzolino, V., Di Meo, V., Monda, H., Spaccini, R., and Piccolo, A. (2016). The molecular characteristics of compost affect plant growth, arbuscular mycorrhizal fungi, and soil microbial community composition. Biol. Fertil. Soil 52, 15–29.
Dalal, V. K., and Tripathy, B. C. (2012). Modulation of chlorophyll biosynthesis by water stress in rice seedlings during chloroplast biogenesis. Plant Cell Environ. 35, 1685–1703. doi: 10.1111/j.1365-3040.2012.02520.x
Datrindade, R., Almeida, L., Xavier, L., Lins, A. L., Andrade, E. H., Maia, J. G., et al. (2019). Arbuscular mycorrhizal fungi colonization promotes changes in the volatile compounds and enzymatic activity of lipoxygenase and phenylalanine ammonia lyase in Piper nigrum L.‘Bragantina’. Plant 8:442. doi: 10.3390/plants8110442
Das, D., Basar, N. U., Ullah, H., Salin, K. R., and Datta, A. (2021). Interactive effect of silicon and mycorrhizal inoculation on growth, yield and water productivity of rice under water-deficit stress. J. Plant Nutr. 44, 2756–2769.
Deandrade, S. A. L., Domingues, A. P., and Mazzafera, P. (2015). Photosynthesis is induced in rice plants that associate with arbuscular mycorrhizal fungi and are grown under arsenate and arsenite stress. Chemosphere 134, 141–149. doi: 10.1016/j.chemosphere.2015.04.023
Diagne, N., Ngom, M., Djighaly, P. I., Fall, D., Hocher, V., and Svistoonoff, S. (2020). Roles of arbuscular mycorrhizal fungi on plant growth and performance: importance in biotic and abiotic stressed regulation. Diversity 12:370.
Djemal, R., and Khoudi, H. (2015). Isolation and molecular characterization of a novel WIN1/SHN1 ethylene-responsive transcription factor TdSHN1 from durum wheat (Triticum turgidum, L. subsp. durum). Protoplasma 252, 1461–1473. doi: 10.1007/s00709-015-0775-8
Dolan, F., Lamontagne, J., Link, R., Hejazi, M., Reed, P., and Edmonds, J. (2021). Evaluating the economic impact of water scarcity in a changing world. Nat. Commun. 12:1915. doi: 10.1038/s41467-021-22194-0
Dossa, K., Li, D., Wang, L., Zheng, X., Liu, A., Yu, J., et al. (2017). Transcriptomic, biochemical and physio-anatomical investigations shed more light on responses to drought stress in two contrasting sesame genotypes. Sci. Rep. 7:8755. doi: 10.1038/s41598-017-09397-6
Doubková, P., Vlasáková, E., and Sudová, R. (2013). Arbuscular mycorrhizal symbiosis alleviates drought stress imposed on Knautia arvensis plants in serpentine soil. Plant Soil 370, 149–161.
Egamberdieva, D., Wirth, S., and Abd-Allah, E. F. (2018). “Plant hormones as key regulators in plant-microbe interactions under salt stress,” in Plant Microbiome: Stress Response, D. Egamberdieva and P. Ahmad (Singapore: Springer), 165–182.
Egamberdieva, D., Wirth, S. J., Alqarawi, A. A., Abd-Allah, E. F., and Hashem, A. (2017). Phytohormones and beneficial microbes: essential components for plants to balance stress and fitness. Front. Microbiol 8:2104. doi: 10.3389/fmicb.2017.02104
El-Badri, A. M., Batool, M., Aa Mohamed, I., Wang, Z., Khatab, A., Sherif, A., et al. (2021). Antioxidative and metabolic contribution to salinity stress responses in two rapeseed cultivars during the early seedling stage. Antioxidants 10:1227. doi: 10.3390/antiox10081227
Eid, M. A., El-hady, M. A. A., Abdelkader, M. A., Abd-Elkrem, Y. M., El-Gabry, Y. A., El-temsah, M. E., et al. (2022). Response in physiological traits and antioxidant capacity of two cotton cultivars under water limitations. Agronomy 12:803.
Estrada, B., Aroca, R., Barea, J. M., and Ruiz-Lozano, J. M. (2013). Native arbuscular mycorrhizal fungi isolated from a saline habitat improved maize antioxidant systems and plant tolerance to salinity. Plant Sci. 201, 42–51. doi: 10.1016/j.plantsci.2012.11.009
Fatima, M., Asgher, M., Masood, A., and Khan, N. A. (2014). Excess sulfur supplementation improves photosynthesis and growth in mustard under salt stress through increased production of glutathione. Environ. Exp. Bot. 107, 55–63.
Fellbaum, C. R., Gachomo, E. W., Beesetty, Y., Choudhari, S., Strahan, G. D., Pfeffer, P. E., et al. (2012). Carbon availability triggers fungal nitrogen uptake and transport in arbuscular mycorrhizal symbiosis. Proc. Nat. Acad. Sci. U.S.A. 109, 2666–2671. doi: 10.1073/pnas.1118650109
Feng, Y. L., and Cao, K. F. (2005). Photosynthesis and photoinhibition after night chilling in seedlings of two tropical tree species grown under three irradiances. Photosynthetica 43, 567–574.
Fernandez, C. W., See, C. R., and Kennedy, P. G. (2020). Decelerated carbon cycling by ectomycorrhizal fungi is controlled by substrate quality and community composition. New Phytol. 226, 569–582. doi: 10.1111/nph.16269
Fernández-Lizarazo, J. C., and Moreno-Fonseca, L. P. (2016). Mechanisms for tolerance to water-deficit stress in plants inoculated with arbuscular mycorrhizal fungi. A review. Agron. Colombon. 34, 179–189. doi: 10.3390/ijms20071769
Fiorilli, V., Maghrebi, M., Novero, M., Votta, C., Mazzarella, T., Buffoni, B., et al. (2022). Arbuscular Mycorrhizal Symbiosis differentially affects the nutritional status of two durum wheat genotypes under drought conditions. Plant 11:804. doi: 10.3390/plants11060804
Fokom, R., Adamou, S., Essono, D., Ngwasiri, D. P., Eke, P., Mofor, C. T., et al. (2019). Growth, essential oil content, chemical composition and antioxidant properties of lemongrass as affected by harvest period and arbuscular mycorrhizal fungi in field conditions. Ind. Crop. Prod. 138:111477.
Foo, E., and Reid, J. B. (2013). Strigolactones: new physiological roles for an ancient signal. J. Plant Growth Reg. 32, 429–442.
Furlan, A., Llanes, A., Luna, V., and Castro, S. (2012). Physiological and biochemical responses to drought stress and subsequent rehydration in the symbiotic association peanut-Bradyrhizobium sp. Int. Sch. Res. Notices 2012, 890–895.
Garg, S. K. (2013). Role and hormonal regulation of nitrate reductase activity in higher plants: a review. Plant Sci. Feed. 3, 13–20. doi: 10.1007/s11356-021-18232-5
Gong, M., Tang, M., Chen, H., Zhang, Q., and Feng, X. (2012). Effects of two Glomus species on the growth and physiological performance of Sophora davidii seedlings under water stress. New For. 4, 399–408.
Gupta, M. M. (2020). “Arbuscular mycorrhizal fungi: the potential soil health indicators,” in Soil Health, eds B. Giri and A. Varma (Cham: Springer), 183–195.
Hamedani, N. G., Gholamhoseini, M., Bazrafshan, F., Habibzadeh, F., and Amiri, B. (2022). Yield, irrigation water productivity and nutrient uptake of arbuscular mycorrhiza inoculated sesame under drought stress conditions. Agric. Water Manag. 266:107569.
Hameed, A., Wu, Q. S., Abd-Allah, E. F., Hashem, A., Kumar, A., Lone, H. A., et al. (2014). “Role of AM fungi in alleviating drought stress in plants,” in Use of microbes for the alleviation of soil stresses, M. Miransari (New York, NY: Springer), 55–75.
Hao, C., Xia, Z., Fan, R., Tan, L., Hu, L., Wu, B., et al. (2016). De novo transcriptome sequencing of black pepper (Piper nigrum L.) and an analysis of genes involved in phenylpropanoid metabolism in response to Phytophthora capsici. BMC Genet. 17:822. doi: 10.1186/s12864-016-3155-7
Harkousse, O., Slimani, A., Jadrane, I., Aitboulahsen, M., Mazri, M. A., Zouahri, A., et al. (2021). Role of local biofertilizer in enhancing the oxidative stress defence systems of date palm seedling (Phoenix dactylifera) against abiotic stress. Appl. Environ. Soil Sci. 2021:6628544. doi: 10.1155/2021/6628544
Hasanuzzaman, M., Nahar, K., Gill, S. S., and Fujita, M. (2013). “Drought stress responses in plants, oxidative stress, and antioxidant defense,” in Climate Change and Plant Abiotic Stress Tolerance, eds N. Tuteja and S. S. Gill (Hoboken, NJ: Wiley-VCH Verlag GmbH & Co. KGaA).
Hashem, A., Abd-Allah, E. F., Alqarawi, A. A., Al Huqail, A. A., Egamberdieva, D., and Wirth, S. (2016a). Alleviation of cadmium stress in Solanum lycopersicum L. by arbuscular mycorrhizal fungi via induction of acquired systemic tolerance. Saudi J. Biol. Sci. 23, 272–281. doi: 10.1016/j.sjbs.2015.11.002
Hashem, A., Abd-Allah, E. F., Alqarawi, A. A., and Egamberdieva, D. (2016b). Bioremediation of adverse impact of cadmium toxicity on Cassia italica Mill by arbuscular mycorrhizal fungi. Saudi J. Biol. Sci. 23, 39–47. doi: 10.1016/j.sjbs.2015.11.007
Hashem, A., Abd_Allah, E. F., Alqarawi, A. A., Aldubise, A., and Egamberdieva, D. (2015). Arbuscular mycorrhizal fungi enhances salinity tolerance of Panicum turgidum Forssk by altering photosynthetic and antioxidant pathways. J. Plant Interact. 10, 230–242.
Hashem, A., Abd-Allah, E. F., Alqarawi, A. A., and Egamberdieva, D. (2018). “Arbuscular mycorrhizal fungi and plant stress tolerance,” in Plant Microbiome: Stress Response. Microorganisms for Sustainability, eds D. Egamberdieva and P. Ahmad (Singapore: Springer).
Hashem, A., Kumar, A., Al-Dbass, A. M., Alqarawi, A. A., Al-Arjani, A. B. F., Singh, G., et al. (2019). Arbuscular mycorrhizal fungi and biochar improves drought tolerance in chickpea. Saudi J. Biol. Sci. 26, 614–624. doi: 10.1016/j.sjbs.2018.11.005
Hassan, M. U., Aamer, M., Chattha, M. U., Ullah, M. A., Sulaman, S., Nawaz, M., et al. (2017). The role of potassium in plants under drought stress: Mini review. J. Basic Appl. Sci. 13, 268–271.
Hassan, M. U., Aamer, M., Umer Chattha, M., Haiying, T., Shahzad, B., Barbanti, L., et al. (2020). The critical role of zinc in plants facing the drought stress. Agriculture 10:396.
Hassan, M. U., Chattha, M. U., Khan, I., Chattha, M. B., Aamer, M., Nawaz, M., et al. (2019). Nickel toxicity in plants: reasons, toxic effects, tolerance mechanisms, and remediation possibilities-a review. Environ. Sci. Poll. Res. 26, 12673–12688. doi: 10.1007/s11356-019-04892-x
Hassan, M. U., Chattha, M. U., Khan, I., Chattha, M. B., Barbanti, L., Aamer, M., et al. (2021). Heat stress in cultivated plants: Nature, impact, mechanisms, and mitigation strategies-A review. Plant Biosyst. 155, 211–234.
Hazzoumi, Z., Moustakime, Y., and Joutei, K. A. (2015). Effect of arbuscular mycorrhizal fungi (AMF) and water stress on growth, phenolic compounds, glandular hairs, and yield of essential oil in basil (Ocimum gratissimum L). Chem. Biol. Tech. Agric. 2:10.
He, Y., Fang, J., Xu, W., and Shi, P. (2022). Substantial increase of compound droughts and heatwaves in wheat growing seasons worldwide. Int. J. Climatol. doi: 10.1002/joc.7518
Hellal, F. A., El-Shabrawi, H. M., Abd El-Hady, M., Khatab, I. A., El-Sayed, S. A. A., and Abdelly, C. (2018). Influence of PEG induced drought stress on molecular and biochemical constituents and seedling growth of Egyptian barley cultivars. J. Genet. Engr. Biotechnol. 16, 203–212. doi: 10.1016/j.jgeb.2017.10.009
Herrera-Medina, M. J., Steinkellner, S., Vierheilig, H., Ocampo Bote, J. A., and Garcia Garrido, J. (2007). Abscisic acid determines arbuscule development and functionality in the tomato arbuscular mycorrhiza. New Phytol. 175, 554–564. doi: 10.1111/j.1469-8137.2007.02107.x
Hewedy, O. A., Abdel Lateif, K. S., Seleiman, M. F., Shami, A., Albarakaty, F. M., and El-Meihy, R. M. (2021). Phylogenetic diversity of Trichoderma strains and their antagonistic potential against soil-borne pathogens under stress conditions. Biology 9:189. doi: 10.3390/biology9080189
Hu, Y., Pandey, A. K., Wu, X., Fang, P., and Xu, P. (2022). The role of arbuscular mycorrhiza fungi in drought tolerance in legume crops: a review. Legume Res. Intern. J. 1:9.
Huang, D., Ma, M., Wang, Q., Zhang, M., Jing, G., Li, C., et al. (2020). Arbuscular mycorrhizal fungi enhanced drought resistance in apple by regulating genes in the MAPK pathway. Plant Physiol. Biochem. 149, 245–255. doi: 10.1016/j.plaphy.2020.02.020
Huang, D., Wang, Q., Zhang, Z., Jing, G., Ma, M., Ma, F., et al. (2021). Silencing MdGH3-2/12 in apple reduces drought resistance by regulating AM colonization. Hortic. Res. 8:84. doi: 10.1038/s41438-021-00524-z
Igiehon, N. O., Babalola, O. O., Cheseto, X., and Torto, B. (2021). Effects of rhizobia and arbuscular mycorrhizal fungi on yield, size distribution and fatty acid of soybean seeds grown under drought stress. Microbiol. Res. 242:126640.
Istanbuli, T., Baum, M., Touchan, H., and Hamwieh, A. (2020). Evaluation of morpho-physiological traits under drought stress conditions in barley (Hordeum vulgare L.). Photosynthetica 58, 1059–1067.
Jabbari, H., Akbari, G. A., Sima, N. A. K. K., Rad, A. H. S., Alahdadi, I., Hamed, A., et al. (2013). Relationships between seedling establishment and soil moisture content for winter and spring rapeseed genotypes. Ind. Crops Prod. 49, 177–187.
Jaleel, C. A., Manivannan, P., Kishorekumar, A., Sankar, B., Gopi, R., Somasundaram, R., et al. (2007). Alterations in osmoregulation, antioxidant enzymes and indole alkaloid levels in Catharanthus roseus exposed to water deficit. Coll. Surf. Biointer. 59, 150–157. doi: 10.1016/j.colsurfb.2007.05.001
Jayne, B., and Quigley, M. (2014). Influence of arbuscular mycorrhiza on growth and reproductive response of plants under water deficit: a meta-analysis. Mycorrhiza 24, 109–119. doi: 10.1007/s00572-013-0515-x
Jin, H., Pfeffer, P. E., Douds, D. D., Piotrowski, E., Lammers, P. J., and Shachar-Hill, Y. (2005). The uptake, metabolism, transport and transfer of nitrogen in an arbuscular mycorrhizal symbiosis. New Phytol. 168, 687–696. doi: 10.1111/j.1469-8137.2005.01536.x
Jinhu, S., Weixiong, W., Rasul, F., Munir, H., Huang, K., Albishi, T. S., et al. (2022). Trehalose induced drought tolerance in plants: Physiological and molecular responses. Not. Bot. Horti Agrobot. Cluj Napoca 50, 12584–12584.
Kamali, S., and Mehraban, A. (2020a). Effects of Nitroxin and arbuscular mycorrhizal fungi on the agro-physiological traits and grain yield of sorghum (Sorghum bicolor L.) under drought stress conditions. PLoS One 15:e0243824. doi: 10.1371/journal.pone.0243824
Kamali, S., and Mehraban, A. (2020b). Nitroxin and arbuscular mycorrhizal fungi alleviate negative effects of drought stress on Sorghum bicolor yield through improving physiological and biochemical characteristics. Plant Signal. Behav. 15:1813998.
Kapoor, R., Evelin, H., Mathur, P., and Giri, B. (2013). “Arbuscular mycorrhiza: approaches for abiotic stress tolerance in crop plants for sustainable agriculture,” in Plant Acclimation to Environmental Stress, eds N. Tuteja and S. S. Gill (New York, NY: Springer), 359–401.
Karagiannidis, N., Thomidis, T., Lazari, D., Panou-Filotheou, E., and Karagiannidou, C. (2011). Effect of three Greek arbuscular mycorrhizal fungi in improving the growth, nutrient concentration, and production of essential oils of oregano and mint plants. Sci. Hortic. 129, 329–334.
Kasote, D. M., Katyare, S. S., Hegde, M. V., and Bae, H. (2015). Significance of antioxidant potential of plants and its relevance to therapeutic applications. Int. J. Boil. Sci. 11:982. doi: 10.7150/ijbs.12096
Kenawy, E. R., Rashad, M., Hosny, A., Shendy, S., Gad, D., and Saad-Allah, K. M. (2022). Enhancement of growth and physiological traits under drought stress in faba bean (Vicia faba L.) using nanocomposite. J. Plant Interact. 17, 404–418.
Khan, F., and Hakeem, K. R. (2014). “Cell signaling during drought and salt stress,” in Plant Signaling: Understanding the Molecular Crosstalk, eds K. R. Hakeem, R. Rehman, and I. Tahir (New Delhi: Springer), 227–239.
Khan, I., Muhammad, A., Chattha, M. U., Skalicky, M., Bilal Chattha, M., Ahsin Ayub, M., et al. (2022a). Mitigation of salinity induced oxidative damage, growth and yield reduction in fine rice by sugarcane press-mud application. Front. Plant Sci. 13:840900. doi: 10.3389/fpls.2022.840900
Khan, I., Zafar, H., Chattha, M. U., Mahmood, A., Maqbool, R., Athar, F., et al. (2022b). Seed priming with different agents mitigate alkalinity induced oxidative damage and improves maize growth. Not. Bot. Horti Agrobot. Cluj-Napoca 50, 12615–12615.
Khan, M. N., Zhang, J., Luo, T., Liu, J., Ni, F., Rizwan, M., et al. (2019). Morpho-physiological and biochemical responses of tolerant and sensitive rapeseed cultivars to drought stress during early seedling growth stage. Acta Physiol. Plant. 41:25.
Kholová, J., Nepolean, T., Tom, H. C., Supriya, A., Rajaram, V., and Senthilvel, S. (2012). Water saving traits co-map with a major terminal drought tolerance quantitative trait locus in pearl millet [Pennisetum glaucum (L.) R. Br.]. Mol. Breed. 30, 1337–1353.
Koegel, S., Ait-Lahmidi, N., Arnould, C., Chatagnier, O., Walder, F., Ineichen, K., et al. (2013). The family of ammonium transporters (AMT) in Sorghum bicolor: two AMT members are induced locally, but not systemically in roots colonized by arbuscular mycorrhizal fungi. New Phytol. 198, 853–865. doi: 10.1111/nph.12199
Kumar, A., and Verma, J. P. (2018). Does plant-microbe interaction confer stress tolerance in plants: a review? Microbiol. Res. 207, 41–52. doi: 10.1016/j.micres.2017.11.004
Lahbouki, S., Ben-Laouane, R., Anli, M., Boutasknit, A., Ait-Rahou, Y., Ait-El-Mokhtar, M., et al. (2022). Arbuscular mycorrhizal fungi and/or organic amendment enhance the tolerance of prickly pear (Opuntia ficus-indica) under drought stress. J. Arid Environ. 199:104703.
Latef, A. A. H. A., Hashem, A., Rasool, S., Abd-Allah, E. F., Alqarawi, A. A., Egamberdieva, D., et al. (2016). Arbuscular mycorrhizal symbiosis and abiotic stress in plants: a review. J. Plant Biol. 59, 407–426.
Latini, A., Sperandei, M., Cantale, C., Arcangeli, C., Ammar, K., and Galeffi, P. (2013). Variability and expression profile of the DRF1 gene in four cultivars of durum wheat and one triticale under moderate water stress conditions. Planta 237, 967–978. doi: 10.1007/s00425-012-1816-6
Lee, B. R., Muneer, S., Avice, J. C., Jung, W. J., and Kim, T. H. (2012). Mycorrhizal colonisation and P-supplement effects on N uptake and N assimilation in perennial ryegrass under well-watered and drought-stressed conditions. Mycorrhiza 22, 525–534. doi: 10.1007/s00572-012-0430-6
Leifheit-Eva, F., Stavros, D., Veresoglou, A., Lehmann, E., Kathryn, M., and Matthias, C. R. (2014). Multiple factors influence the role of arbuscular mycorrhizal fungi in soil aggregation—a meta-analysis. Plant Soil 374, 523–537.
Li, J., Meng, B., Chai, H., Yang, X., Song, W., Li, S., et al. (2019). Arbuscular mycorrhizal fungi alleviate drought stress in C3 (Leymus chinensis) and C4 (Hemarthria altissima) grasses via altering antioxidant enzyme activities and photosynthesis. Front. Plant Sci. 10:499. doi: 10.3389/fpls.2019.00499
Li, T., Hu, Y. J., Hao, Z. P., Li, H., Wang, Y. S., and Chen, B. D. (2013). First cloning and characterization of two functional aquaporin genes from an arbuscular mycorrhizal fungus Glomus intraradices. New Phytol. 197, 617–630. doi: 10.1111/nph.12011
Li, T., Sun, Y., Ruan, Y., Xu, L., Hu, Y., Hao, Z., et al. (2016). Potential role of D-myo-inositol-3-phosphate synthase and 14-3-3 genes in the crosstalk between Zea mays and Rhizophagus intraradices under drought stress. Mycorrhiza 26, 879–893. doi: 10.1007/s00572-016-0723-2
Liang, S.-M., Jiang, D.-J., Xie, M.-M., Zou, Y.-N., Wu, Q.-S., and Kuča, K. (2021). Physiological responses of mycorrhizal symbiosis to drought stress in white clover. Not. Bot. Horti Agrobot. Cluj Napoca 49:12209.
Liu, C.-Y., Wang, Y.-J., Wu, Q.-S., Yang, T.-Y., and Kuča, K. (2020). Arbuscular mycorrhizal fungi improve the antioxidant capacity of tea (Camellia sinensis) seedlings under drought stress. Not. Bot. Horti Agrobot. Cluj Napoca 48, 1993–2005. doi: 10.15835/nbha48412066
Liu, D., Wu, L., Naeem, M. S., Liu, H., Deng, X., Xu, L., et al. (2013). 5-Aminolevulinic acid enhances photosynthetic gas exchange, chlorophyll fluorescence and antioxidant system in oilseed rape under drought stress. Acta Physiol. Plant 35, 2747–2759.
López, J., Kohlen, W., Charnikhova, T., Mulder, P., Undas, A. K., Sergeant, M. K., et al. (2010). Does abscisic acid affect strigolactone biosynthesis? New Phytol. 187, 343–354. doi: 10.1111/j.1469-8137.2010.03291.x
Ludwig-Müller, J. (2010). “Hormonal responses in host plants triggered by arbuscular mycorrhizal fungi,” in Arbuscular Mycoorrhizas: Physiology and Function, eds H. Koltai and Y. Kapulnik (Dordrecht: Springer.), 169–190. doi: 10.1016/j.pbi.2009.06.001
Luo, L., Wang, H., Liu, X., Hu, J., Zhu, X., Pan, S., et al. (2018). Strigolactones affect the translocation of nitrogen in rice. Plant Sci. 270, 190–197. doi: 10.1016/j.plantsci.2018.02.020
Ma, P., Bai, T. H., and Ma, F. W. (2015). Effects of progressive drought on photosynthesis and partitioning of absorbed light in apple trees. J. Integr. Agric. 14, 681–690.
Ma, X. L., Wang, Y. J., Xie, S. L., Wang, C., and Wang, W. (2007). Glycinebetaine application ameliorates negative effects of drought stress in tobacco. Russ. J. Plant Physiol. 54:472.
Mamnabi, S., Nasrollahzadeh, S., Ghassemi-Golezani, K., and Raei, Y. (2020). Improving yield-related physiological characteristics of spring rapeseed by integrated fertilizer management under water deficit conditions. Saudi J. Biol. Sci. 27, 797–804. doi: 10.1016/j.sjbs.2020.01.008
Mandal, S. M., Chakraborty, D., and Dey, S. (2010). Phenolic acids act as signaling molecules in plant-microbe symbioses. Plant Signal. Behav. 5, 359–368. doi: 10.4161/psb.5.4.10871
Manoharan, P. T., Shanmugaiah, V., Balasubramanian, N., Go-mathinayagam, S., Sharma, M. P., and Muthuchelian, K. (2010). Influence of AM fungi on the growth and physiological status of Erythrina variegata Linn. grown under different water stress conditions. Eur. J. Soil Biol. 46, 151–156.
Mathur, S., Tomar, R. S., and Jajoo, A. (2019). Arbuscular mycorrhizal fungi (AMF) protects photosynthetic apparatus of wheat under drought stress. Photosynth. Res. 139, 227–238.
Maurel, C., Boursiac, Y., Luu, D. T., Santoni, V., Shahzad, Z., and Verdoucq, L. (2015). Aquaporins in plants. Physiol. Rev. 95, 1321–1358.
Meddich, A., Jaiti, F., Bourzik, W., El-Asli, A., and Hafidi, M. (2015). Use of mycorrhizal fungi as a strategy for improving the drought tolerance in date palm (Phoenix dactylifera). Sci. Hortic. 192, 468–474.
Mehmood, M., Khan, I., Chattha, M. U., Hussain, S., Ahmad, N., Aslam, M. T., et al. (2021). Thiourea application protects maize from drought stress by regulating growth and physiological traits. Pak. J. Sci. 73, 355–363.
Mensah, J. A., Koch, A. M., Antunes, P. M., Kiers, E. T., Hart, M., and Bücking, H. (2015). High functional diversity within species of arbuscular mycorrhizal fungi is associated with difference in phosphate and nitrogen uptake and fungal phosphate metabolism. Mycorrhiza 25, 533–546. doi: 10.1007/s00572-015-0631-x
Miransari, M., Bahrami, H. A., Rejali, F., Malakouti, M. J., and Torabi, H. (2007). Using arbuscular mycorrhiza to reduce the stressful effects ofsoil compaction on corn (Zea mays L.) growth. Soil Biol. Biochem. 39, 2014–2026.
Mirshad, P. P., and Puthur, J. T. (2016). Arbuscular mycorrhizal association enhances drought tolerance potential of promising bioenergy grass (Saccharum arundinaceum retz). Environ. Monit. Assess. 188:425. doi: 10.1007/s10661-016-5428-7
Mirzai, M., Moeini, A., and Ghanati, F. (2013). Effects of drought stress on the lipid peroxidation and antioxidant enzyme activities in two canola (Brassica napus L.) cultivars. J. Agri. Sci. Tech. 15, 593–602.
Mo, Y., Wang, Y., Yang, R., Zheng, J., Liu, C., Li, H., et al. (2016). Regulation of plant growth, photosynthesis, antioxidation and osmosis by an arbuscular mycorrhizal fungus in watermelon seedlings under well-watered and drought conditions. Front. Plant Sci. 7:644. doi: 10.3389/fpls.2016.00644
Mohanta, T. K., Bashir, T., Hashem, A., and Abd-Allah, E. F. (2017). Systems biology approach in plant abiotic stresses. Plant Physiol. Biochem. 121, 58–73.
Mona, S. A., Hashem, A., Abd-Allah, E. F., Alqarawi, A. A., Soliman, D. W. K., Wirth, S., et al. (2017). Increased resistance of drought by Trichoderma harzianum fungal treatment correlates with increased secondary metabolites and proline content. J. Integr. Agric. 16, 1751–1757.
Moradtalab, N., Hajiboland, R., Aliasgharzad, N., Hartmann, T. E., and Neumann, G. (2019). Silicon and the association with an arbuscular-mycorrhizal fungus (Rhizophagus clarus) mitigate the adverse effects of drought stress on strawberry. Agronomy 9:41.
Moshelion, M., Halperin, O., Wallach, R., Oren, R., and Way, D. A. (2015). Role of aquaporins in determining transpiration and photosynthesis in water-stressed plants: Crop water-use efficiency, growth and yield. Plant Cell Environ. 38, 1785–1793. doi: 10.1111/pce.12410
Neumann, E., Schmid, B., Romheld, V., and George, E. (2009). Extraradical development and contribution to plant performance of an arbuscular mycorrhizal symbiosis exposed to complete or partial rootzone drying. Mycorrhiza 20, 3–23. doi: 10.1007/s00572-009-0259-9
Nishida, H., and Suzaki, T. (2018). Nitrate-mediated control of root nodule symbiosis. Curr. Opin. Plant Biol. 44, 129–136. doi: 10.1016/j.pbi.2018.04.006
Oldroyd, G. E. D., and Speak, F. (2013). Signalling systems that promote beneficial symbiotic associations in plants. Nat. Rev. Microbiol. 11, 252–263. doi: 10.1038/nrmicro2990
Opik, M., Zobel, M., Cantero, J. J., Davison, J., Facelli, J. M., Hiiesalu, I., et al. (2013). Global sampling of plant roots expands the described molecular diversity of arbuscular mycorrhizal fungi. Mycorrhiza 23, 411–430. doi: 10.1007/s00572-013-0482-2
Ortas, I., Rafique, M., and Çekiç, F. Ö (2021). “Do Mycorrhizal Fungi enable plants to cope with abiotic stresses by overcoming the detrimental effects of salinity and improving drought tolerance?,” in Symbiotic Soil Microorganisms, eds N. Shrivastava, S. Mahajan, and A. Varma (Cham: Springer), 391–428.
Osakabe, Y., Nishikubo, N., and Osakabe, K. (2007). Phenylalanine ammonia-lyase in woody plants: a key switch of carbon accumulation in biomass. Jpn. J. Plant Sci. 1, 103–108.
Ouledali, S., Ennajeh, M., Zrig, A., Gianinazzi, S., and Khemira, H. (2018). Estimating the contribution of arbuscular mycorrhizal fungi to drought tolerance of potted olive trees (Olea europaea). Acta Physiol. Plant 40:81.
Pauwels, R., Jansa, J., Püschel, D., Müller, A., Graefe, J., and Kolb, S. (2020). Root growth and presence of Rhizophagus irregularis distinctly alter substrate hydraulic properties in a model system with Medicago truncatula. Plant Soil 457, 131–151.
Pavithra, D., and Yapa, N. (2018). Arbuscular mycorrhizal fungi inoculation enhances drought stress tolerance of plants. Groundw. Sustain. Dev. 7, 490–494.
Per, T. S., Khan, N. A., Masood, A., and Fatma, M. (2016). Methyl jasmonate alleviates cadmium-induced photosynthetic damages through increased S-assimilation and glutathione production in mustard. Front. Plant Sci. 7:1933. doi: 10.3389/fpls.2016.01933
Porcel, R., Aroca, R., Azcón, R., and Ruiz-Lozano, J. M. (2005). PIP aquaporin gene expression in arbuscular mycorrhizal Glycine max and Lactuca sativa plants in relation to drought stress tolerance. Plant Mol. Biol. 60, 389–404. doi: 10.1007/s11103-005-4210-y
Pour, A. A., Mohammadi, R., Etminan, A., Shooshtari, L., Maleki-Tabrizi, N., and Poczai, P. (2020). Effects of drought stress on some agronomic and morpho-physiological traits in durum wheat genotypes. Sustainability 12:5610.
Pozo, M. J., Lopez-Raez, J. A., Azcon-Aguilar, C., and Garcia-Garrido, J. M. (2015). Phytohormones as integrators of environmental signals in the regulation of mycorrhizal symbioses. New Phytol. 205, 1431–1436. doi: 10.1111/nph.13252
Prabha, C., and Sharadamma, N. (2019). Arbuscular mycorrhizal influence on oxidative stress in French bean (Phaseolus vulgaris) under drought conditions. GSC Biol. Pharm. Sci. 7, 021–029.
Qari, S. H., Hassan, M. U., Chattha, M. U., Mahmood, A., Naqve, M., Nawaz, M., et al. (2022). Melatonin induced cold tolerance in plants: physiological and molecular responses. Front. Plant Sci. 13:843071. doi: 10.3389/fpls.2022.843071
Quiroga, G., Erice, G., Aroca, R., Chaumont, F., and Ruiz-Lozano, J. M. (2019a). Contribution of the arbuscular mycorrhizal symbiosis to the regulation of radial root water transport in maize plants under water deficit. Environ. Exp. Bot. 167:103821.
Quiroga, G., Erice, G., Ding, L., Chaumont, F., Aroca, R., and Ruiz-Lozano, J. M. (2019b). The arbuscular mycorrhizal symbiosis regulates aquaporins activity and improves root cell water permeability in maize plants subjected to water stress. Plant Cell Environ. 42, 2274–2290.
Rapparini, F., and Peñuelas, J. (2014). “Mycorrhizal fungi to alleviate drought stress on plant growth,” in Use of Microbes for the Alleviation of Soil Stresses, ed. M. Miransari (New York, NY: Springer.), 21–42.
Rehman, S., Chattha, M. U., Khan, I., Mahmood, A., Hassan, M. U., Al-Huqail, A. A., et al. (2022). Exogenously applied trehalose augments cadmium stress tolerance and yield of mung bean (Vigna radiata L.) grown in soil and hydroponic systems through reducing Cd uptake and enhancing photosynthetic efficiency and antioxidant defense systems. Plants 11:822. doi: 10.3390/plants11060822
Ren, A. T., Zhu, Y., Chen, Y. L., Ren, H. X., Li, J. Y., Abbott, L. K., et al. (2019). Arbuscular mycorrhizal fungus alters root-sourced signal (abscisic acid) for better drought acclimation in Zea mays L. seedlings. Environ. Exp. Bot. 167, 103–824.
Rillig, M. C., Wright, S. F., Nichols, K. A., Schmidt, W. F., and Torn, M. S. (2001). Large contribution of arbuscular mycorrhizal fungi to soil carbon pools in tropical forest soils. Plant Soil 233, 167–177.
Rosolem, Ciro, A., Ritz, K., Cantarella, H., Galdos, M. V., Hawkesford, M. J., et al. (2017). “Enhanced plant rooting and crop system management for improved N use efficiency,” in Advances in Agronomy, ed. D. L. Sparks (Cambridge, MA: Academic Press).
Ruíz-Sánchez, M., Armada, E., Muñoz, E., García-de-Salamone, I. E., Aroca, R., Ruíz-Lozano, J. M., et al. (2011). Azospirillum and arbuscular mycorrhizal colonization enhance rice growth and physiological traits under well-watered and drought conditions. J. Plant Physiol. 168, 1031–1037. doi: 10.1016/j.jplph.2010.12.019
Rydlová, J., and Püschel, D. (2020). Arbuscular mycorrhiza, but not hydrogel, alleviates drought stress of ornamental plants in peat-based substrate. Appl. Soil Ecol. 146, 103–394.
Sabra, A., Daayf, F., and Renault, S. (2012). Differential physiological and biochemical responses of three Echinacea species to salinity stress. Sci. Hort. 135, 23–31.
Sadok, W., and Sinclair, T. R. (2010). Transpiration response of slow-wilting and commercial soybean (Glycine max (L.) Merr.) genotypes to three aquaporin inhibitors. J. Exp. Bot. 61, 821–829. doi: 10.1093/jxb/erp350
Sakurai, I. J., Murai-Hatano, M., Hayashi, H., Ahamed, A., Fukushi, K., and Matsumoto, T. (2011). Transpiration from shoots triggers diurnal changes in root aquaporin expression. Plant Cell Environ. 34, 1150–1163. doi: 10.1111/j.1365-3040.2011.02313.x
Sánchez, R. B., Calvo-Polanco, M., Ruiz-Lozano, J. M., Zamarreño, ÁM., Arbona, V., García-Mina, J. M., et al. (2018). Involvement of the def-1 mutation in the response of tomato plants to arbuscular mycorrhizal symbiosis under well-watered and drought conditions. Plant Cell Physiol. 59, 248–261. doi: 10.1093/pcp/pcx178
Sánchez-Blanco, M. J., Ferrández, T., Morales, M. A., Morte, A., and Alarcón, J. J. (2004). Variations in water status, gas exchange, and growth in Rosmarinus officinalis plants infected with Glomus deserticola under drought conditions. J. Plant Physiol. 161, 675–182. doi: 10.1078/0176-1617-01191
Sanchez-Romera, B., Ruiz-Lozano, J. M., Zamarreno, A. M., Garcia-Mina, J. M., and Aroca, R. (2016). Arbuscular mycorrhizal symbiosis and methyl jasmonate avoid the inhibition of root hydraulic conductivity caused by drought. Mycorrhiza 26, 111–122. doi: 10.1007/s00572-015-0650-7
Santander, C., Aroca, R., Ruiz-Lozano, J. M., Olave, J., Cartes, P., Borie, F., et al. (2017). Arbuscular mycorrhiza effects on plant performance under osmotic stress. Mycorrhiza 27, 639–657. doi: 10.1007/s00572-017-0784-x
Sayer, E. J., Crawford, J. A., Edgerley, J., Askew, A. P., Hahn, C. Z., Whitlock, R., et al. (2021). Adaptation to chronic drought modifies soil microbial community responses to phytohormones. Commun. Biol. 4:516. doi: 10.1038/s42003-021-02037-w
Scheibe, R., and Dietz, K. J. (2012). Reduction–oxidation network for flexible adjustment of cellular metabolism in photoautotrophic cells Plant. Cell Environ. 35, 202–216. doi: 10.1111/j.1365-3040.2011.02319.x
Seto, Y., Kameoka, H., Yamaguchi, S., and Kyozuka, J. (2012). Recent advances in strigolactone research: Chemical and biological aspects. Plant Cell Physiol. 53:1843. doi: 10.1093/pcp/pcs142
Seutra, K. J., Abunyewa, A. A., Kugbe, J., Kwashie, G. K., Owusu, A. E., and Andoh, H. (2021). Arbuscular mycorrhizal fungi and potassium fertilizer as plant biostimulants and alternative research for enhancing plants adaptation to drought stress: Opportunities for enhancing drought tolerance in cocoa (Theobroma cacao L.). Sustain. Environ. 7:1963927.
Shafiq, S., Akram, N. A., Ashraf, M., and Arshad, A. (2014). Synergistic effects of drought and ascorbic acid on growth, mineral nutrients and oxidative defense system in canola (Brassica napus L.) plants. Acta Physiol. Plant. 36, 1539–1553.
Sheteiwy, M. S., Ali, D. F. I., Xiong, Y. C., Brestic, M., Skalicky, M., Hamoud, Y. A., et al. (2021). Physiological and biochemical responses of soybean plants inoculated with Arbuscular mycorrhizal fungi and Bradyrhizobium under drought stress. BMC Plant Biol. 21:195. doi: 10.1186/s12870-021-02949-z
Varinderpal-Singh, V., Sharma, S., Kunal, Gosal, S. K., Choudhary, R., Singh, R., et al. (2020). Synergistic use of plant growth-promoting rhizobacteria, arbuscular mycorrhizal fungi, and spectral properties for improving nutrient use efficiencies in wheat (Triticum aestivum L.). Commun. Soil Sci. Plant Anal. 51, 14–27.
Smith, S. E., Facelli, E., Pope, S., and Smith, F. A. (2010). Plant performance in stressful environments: interpreting new and established knowledge of the roles of arbuscular mycorrhizas. Plant Soil 326, 3–20.
Smith, S. E., Jakobsen, I., Grnlund, M., and Smith, F. A. (2011). Roles of arbuscular mycorrhizas in plant phosphorus nutrition: interactions between pathways of phosphorus uptake in arbuscular mycorrhizal roots have important implications for understanding and manipulating plant phosphorus acquisition. Plant Physiol. 156, 1050–1057. doi: 10.1104/pp.111.174581
Sochacki, P., Ward, J. R., and Cruzan, M. B. (2013). Consequences of mycorrhizal colonization for Piriqueta morphotypes under drought stress. Int. J. Plant Sci. 174, 65–73.
Shafighi, A., Ardakani, M. R., Rad, A. H. S., Alavifazel, M., and Rafiei, F. (2021). Grain yield and associated physiological traits of rapeseed (Brassica napus L.) cultivars under different planting dates and drought stress at the flowering stage. Ital. J. Agron. 16:1648. doi: 10.4081/ija.2020.1648
Steinkellner, S., Lendzemo, V., Langer, I., Schweiger, P., Khaosaad, T., Toussaint, J. P., et al. (2007). Flavonoids and strigolactones in root exudates as signals in symbiotic and pathogenic plant-fungus interactions. Molecules 12, 1290–1306. doi: 10.3390/12071290
Sultan, I., Khan, I., Chattha, M. U., Hassan, M. U., Barbanti, L., Calone, R., et al. (2021). Improved salinity tolerance in early growth stage of maize through salicylic acid foliar application. Ita. J. Agron. 16:1810.
Sykorova, Z., Ineichen, K., Wiemken, A., and Redecker, D. (2007). The cultivation bias: different communities of arbuscular mycorrhizal fungi detected in roots from the field, from bait plants transplanted to the field, and from a greenhouse trap experiment. Mycorrhiza 18, 1–14. doi: 10.1007/s00572-007-0147-0
Tajini, F., Trabelsi, M., and Drevon, J. J. (2011). Co-inoculation with Glomus intraradices and Rhizobium tropici CIAT899 increases P use efficiency for N2 fixation in the common bean (Phaseolus vulgaris L.) under P deficiency in hydroaeroponic culture. Symbiosis 53, 123–129.
Tejeda, M., Martínez, O., Vega, D. L., and Délano-Frier, J. P. (2008). Jasmonic acid influences mycorrhizal colonization in tomato plants by modifying the expression of genes involved in carbohydrate partitioning. Physiol. Plant 133, 339–353. doi: 10.1111/j.1399-3054.2008.01081.x
Tharanya, M., Kholova, J., Sivasakthi, K., Seghal, D., Hash, C. T., and Raj, B. (2018). Quantitative trait loci (QTLs) for water use and crop production traits co-locate with major QTL for tolerance to water deficit in a fine-mapping population of pearl millet (Pennisetum glaucum L. R.Br.). Theor. Appl. Genet. 131, 1509–1529. doi: 10.1007/s00122-018-3094-6
Tian, H., Gai, J. P., Zhang, J. L., Christie, P., and Li, X. L. (2009). Arbuscular mycorrhizal fungi associated with wild forage plants in typical steppe of eastern Inner Mongolia. Eur. J. Soil Biol. 45, 321–332.
Tereucán, G., Ruiz, A., Nahuelcura, J. Oyarzún, P., Santander, C., Winterhalter, P., et al. (2022). Shifts in biochemical and physiological responses by the inoculation of arbuscular mycorrhizal fungi in Triticum aestivum growing under drought conditions. J. Sci. Food Agric. 102, 1927–1938.
Turrini, A., Bedini, A., Loor, M. B., Santini, G., Sbrana, C., and Giovannetti, M. (2018). Local diversity of native arbuscular mycorrhizal symbionts differentially affects growth and nutrition of three crop plant species. Biol. Fertil. Soils 54, 203–217.
Vadez, V., Kholova, J., Zaman-Allah, M., and Belko, N. (2013). Water: The most important “molecular” component of water stress tolerance research. Funct. Plant Biol. 40, 1310–1322. doi: 10.1071/FP13149
Varinderpal, S., Kaur, S., Singh, J., Kaur, A., and Gupta, R. K. (2021). Rescheduling fertilizer nitrogen topdressing timings for improving productivity and mitigating N2O emissions in timely and late sown irrigated wheat (Triticum aestivum L.). Arch. Agron. Soil Sci. 67, 647–659.
Veresoglou, S., Chen, B., and Rillig, M. C. (2011). Arbuscular mycorrhiza and soil nitrogen cycling. Soil Biol. Biochem. 46, 53–62.
Verma, N., Tarafdar, J. C., Srivastava, K. K., and Panwar, J. (2008). Arbuscular mycorrhizal (AM) diversity in Prosopis cineraria (L.) Druce under arid agroecosystems. Agric. Sci. China 7, 754–761.
Verzeaux, J., Hirel, B., Dubois, F., Peter Lee, J., and Tetu, T. (2017). Agricultural practices to improve nitrogen use efficiency through the use of arbuscular mycorrhizae: Basic and agronomic aspects. Plant Sci. 264, 48–56. doi: 10.1016/j.plantsci.2017.08.004
Vishwakarma, N., Upadhyay, N., Kumar, G., Yadav, J., Singh, R. K., Mishra, V., et al. (2017). Abscisic acid signaling and abiotic stress tolerance in plants: a review on current knowledge and future prospects. Front. Plant Sci. 8:161. doi: 10.3389/fpls.2017.00161
Wang, Y., Lin, J., Yang, F., Tao, S., Yan, X., Zhou, Z., et al. (2022). Arbuscular mycorrhizal fungi improve the growth and performance in the seedlings of Leymus chinensis under alkali and drought stresses. PeerJ 10:12890. doi: 10.7717/peerj.12890
Weng, M., Cui, L., Liu, F., Zhang, M., Shan, L., Yang, S., et al. (2015). Effects of drought stress on antioxidant enzymes in seedlings of different wheat genotypes. Pak. J. Bot. 47, 49–45.
Wilmowicz, E., Kućko, A., Golińska, P., Burchardt, S., Przywieczerski, T., Świdziński, M., et al. (2020). Abscisic acid and ethylene in the control of nodule-specific response on drought in yellow lupine. Environ. Exp. Bot. 169:103900.
Wu, H. H., Zou, Y. N., Rahman, M. M., Ni, D., and Wu, Q. S. (2017). Mycorrhizas alter sucrose and proline metabolism in trifoliate orange exposed to drought stress. Sci. Rep. 7:42389. doi: 10.1038/srep42389
Wu, Q. S., Srivastava, A. K., and Zou, Y. N. (2013). AMF-induced tolerance to drought stress in citrus: A review. Sci. Hortic. 164, 77–87.
Wu, Q. S., Xia, R. X., and Zou, Y. N. (2008). Improved soil structure and citrus growth after inoculation with three arbuscular mycorrhizal fungi under drought stress. Eur. J. Soil Biol. 44, 122–128.
Wu, Q. S., and Zou, Y. N. (2017). “Arbuscular mycorrhizal fungi and tolerance of drought stress in plants,” in Arbuscular Mycorrhizas and Stress Tolerance of Plants, ed. Q. S. Wu (singapore: springer), 25–41.
Xie, W., Hao, Z., Zhou, X., Jiang, X., Xu, L., Wu, S., et al. (2018). Arbuscular mycorrhiza facilitates the accumulation of glycyrrhizin and liquiritin in Glycyrrhiza uralensis under drought stress. Mycorrhiza 28, 285–300. doi: 10.1007/s00572-018-0827-y
Xing, Y., Jiang, W., He, X., Fiaz, S., Ahmad, S., Lei, X., et al. (2019). A review of nitrogen translocation and nitrogen-use efficiency. J. Plant Nutr. 42, 2624–2641.
Xu, L., Li, T., Wu, Z., Feng, H., Yu, M., Zhang, X., et al. (2018). Arbuscular mycorrhiza enhances drought tolerance of tomato plants by regulating the 14-3-3 genes in the ABA signaling pathway. Appl. Soil Ecol. 125, 213–221.
Yang, X., Lu, M., Wang, Y., Wang, Y., Liu, Z., and Chen, S. (2021). Response mechanism of plants to drought stress. Horticulturae 7:50.
Yang, Y., Han, X., Liang, Y., Ghosh, A., Chen, J., and Tang, M. (2015). The combined effects of arbuscular mycorrhizal fungi (AMF) and lead (Pb) stress on Pb accumulation, plant growth parameters, photosynthesis, and antioxidant enzymes in Robinia pseudoacacia L. PLoS One 10:e0145726. doi: 10.1371/journal.pone.0145726
Yang, Q., Zhao, Z., Bai, Z., Hou, H., Yuan, Y., Guo, A., et al. (2019). Effects of mycorrhizae and water conditions on perennial ryegrass growth in rare earth tailings. RSC Adv. 9, 10881–10888.
Yooyongwech, S., Phaukinsang, N., Cha-um, S., and Supaibulwatana, K. (2013). Arbuscular mycorrhiza improved growth performance in Macadamia tetraphylla L. grown under water deficit stress involves soluble sugar and proline accumulation. Plant Growth Reg. 69, 285–293.
You, J., Zhang, Y., Liu, A., Li, D., Wang, X., Dossa, K., et al. (2019). Transcriptomic and metabolomic profiling of drought-tolerant and susceptible sesame genotypes in response to drought stress. BMC Plant Biol. 19:267. doi: 10.1186/s12870-019-1880-1
Zarea, M. J., Karimi, N., Goltapeh, E. M., and Ghalavand, A. (2011). Effect of cropping systems and arbuscular mycorrhizal fungi on soil microbial activity and root nodule nitrogenase. J. Saudi Soc. Agric. Sci. 10, 109–120.
Zhang, F., Zou, Y. N., and Wu, Q. S. (2018). Quantitative estimation of water uptake by mycorrhizal extraradical hyphae in citrus under drought stress. Sci. Hortic. 229, 132–136.
Zhang, F., Jia-Dong, H. E., Qiu-Dan, N. I., Qiang-Sheng, W. U., and Zou, Y. N. (2018b). Enhancement of drought tolerance in trifoliate orange by mycorrhiza: changes in root sucrose and proline metabolisms. Not. Bot. Horti. Agrobot. Cluj-Napoca 46:270.
Zhang, F., Zou, Y. N., Wu, Q. S., and Ku-ca, K. (2020). Arbuscular mycorrhizas modulate root polyamine metabolism to enhance drought tolerance of trifoliate orange. Environ. Exp. Bot. 171:103926.
Zhang, F. J., Zhang, K. K., Du, C. Z., Li, J., Xing, Y. X., Yang, L. T., et al. (2015). Effect of drought stress on anatomical structure and chloroplast ultrastructure in leaves of sugarcane. Sugar. Tech. 17, 41–48.
Zhang, H. H., Tang, M., Chen, H., Zheng, C. L., and Niu, Z. C. (2010). Effect of inoculation with AM fungi on lead uptake, translocation and stress alleviation of Zea mays L. seedlings planting in soil with increasing lead concentrations. Eur. J. Soil Biol. 46, 306–311.
Zhang, Z., Zhang, J., Xu, G., Zhou, L., and Li, Y. (2019). Arbuscular mycorrhizal fungi improve the growth and drought tolerance of Zenia insignis seedlings under drought stress. New For. 50, 593–604.
Zhao, R., Guo, W., Bi, N., Guo, J., Wang, L., Zhao, J., et al. (2015). Arbuscular mycorrhizal fungi affect the growth, nutrient uptake and water status of maize (Zea mays L.) grown in two types of coal mine spoils under drought stress. Appl. Soil Ecol. 88, 41–49.
Zhou, Q., Ravnskov, S., Jiang, D., and Wollenweber, B. (2015). Changes in carbon and nitrogen allocation, growth and grain yield induced by arbuscular mycorrhizal fungi in wheat (Triticum aestivum L.) subjected to a period of water deficit. Plant Growth Reg. 75, 751–760.
Zhu, X., Song, F., Liu, S., and Liu, F. (2016). Arbuscular mycorrhiza improve growth, nitrogen uptake, and nitrogen use efficiency in wheat grown under elevated CO2. Mycorrhiza 26, 133–140. doi: 10.1007/s00572-015-0654-3
Zhu, X. Q., Tang, M., and Zhang, H. Q. (2017). Arbuscular mycorrhizal fungi enhanced the growth, photosynthesis, and calorific value of black locust under salt stress. Photosynthetica 55, 378–385.
Zhao, B., Ma, B. L., Hu, Y., and Liu, J. (2021). Source–sink adjustment: a mechanistic understanding of the timing and severity of drought stress on photosynthesis and grain yields of two contrasting oat (Avena sativa L.) genotypes. J. Plant Growth Regul. 40, 263–276.
Zou, Y. N., Srivastava, A. K., Ni, Q. D., and Wu, Q. S. (2015). Disruption of mycorrhizal extraradical mycelium and changes in leaf water status and soil aggregate stability in rootbox-grown trifoliate orange. Front. Microbiol. 6:203. doi: 10.3389/fmicb.2015.00203
Zou, Y. N., Wu, Q. S., Huang, Y. M., Ni, Q. D., and He, X. H. (2013). Mycorrhizal-mediated lower proline accumulation in Poncirus trifoliata under water deficit derives from the integration of inhibition of proline synthesis with increase of proline degradation. PLoS One 8:e80568. doi: 10.1371/journal.pone.0080568
Keywords: AMF, antioxidant defense system, aquaporins, drought stress, genes expression, hormones, NUE
Citation: Tang H, Hassan MU, Feng L, Nawaz M, Shah AN, Qari SH, Liu Y and Miao J (2022) The Critical Role of Arbuscular Mycorrhizal Fungi to Improve Drought Tolerance and Nitrogen Use Efficiency in Crops. Front. Plant Sci. 13:919166. doi: 10.3389/fpls.2022.919166
Received: 13 April 2022; Accepted: 09 May 2022;
Published: 06 July 2022.
Edited by:
Muhammad Azhar Nadeem, Sivas University of Science and Technology, TurkeyReviewed by:
Monica Calvo-Polanco, University of Salamanca, SpainTianxu Cao, Yangtze Normal University, China
Ahmad Sher, Bahauddin Zakariya University, Pakistan
Copyright © 2022 Tang, Hassan, Feng, Nawaz, Shah, Qari, Liu and Miao. This is an open-access article distributed under the terms of the Creative Commons Attribution License (CC BY). The use, distribution or reproduction in other forums is permitted, provided the original author(s) and the copyright owner(s) are credited and that the original publication in this journal is cited, in accordance with accepted academic practice. No use, distribution or reproduction is permitted which does not comply with these terms.
*Correspondence: Jianqun Miao, anFtaWFvOTk4QHNpbmEuY29t; Ying Liu, bHkzOTY2MTAyNkAxMjYuY29t