- 1State Key Laboratory of Eco-Hydraulic in Northwest Arid Region of China, Xi'an University of Technology, Xi'an, China
- 2School of Water Resource and Hydropower, Xi'an University of Technology, Xi'an, China
Freshwater resources in arid areas are scarce, while there are abundant brackish water reserves that have great application potential for the irrigation of desert plants. However, brackish water irrigation will lead to soil salinization, which will inhibit plant growth. Magnetized water is a new technology that makes the use of brackish water feasible. The present study assessed the effects of irrigation using three water types (fresh, brackish, and magnetized brackish water) and five irrigation amounts (W1, 81 mm; W2, 108 mm; W3, 135 mm; W4, 162mm; and W5, 189 mm) on soil salinity and Haloxylon ammodendron seedling growth. Compared with fresh water, brackish water irrigation inhibited the growth of H. ammodendron and reduced water consumption. Irrigation with magnetized brackish water effectively improved the effect of soil salt leaching, promoted the growth and water absorption of H. ammodendron roots, and stimulated the growth of plant height, basal diameter, shoot length, and crown width. Based on the principal component analysis, the first three treatments of H. ammodendron comprehensive growth state were FW4, FW3, and MBW4, respectively. This showed that magnetized brackish water combined with an appropriate irrigation amount was helpful to optimize the growth of H. ammodendron seedlings on the basis of fresh water saving. Therefore, magnetized brackish water irrigation is an effective strategy for ensuring the establishment and growth of H. ammodendron seedlings in arid and water-deficient areas.
Introduction
Xinjiang is located in the arid inland area of Northwest China, where evaporation is strong and rainfall is scarce (Danierhan et al., 2013; Wang Z. et al., 2019; Zhang et al., 2022). Its unique climate characteristics have created a serious shortage in regional water resources, and the discrepancy between water supply and demand has become large. With population growth and economic development, crop planting areas continue to expand and agricultural water used for oasis planting has increased annually. Agricultural water accounted for 86.99% of all water used in Xinjiang in 2020 (China Water Resources Bulletin, 2020). Fresh water resources are mainly used to irrigate crops such as cotton, wheat, and corn. However, it is difficult to meet the normal water supply demands during the peak water demand period, and the ecological water required by shelter forests and desert plants is not always guaranteed (Chen et al., 2015; Zhang et al., 2016, 2017, 2019; Wang et al., 2018; He et al., 2022; Hou et al., 2022). Shallow brackish water, widely distributed in Xinjiang, is a water resource, which has huge agricultural irrigation potential that could make up for the shortage of available fresh water (Wang et al., 2011; Chen et al., 2018; Ma et al., 2022). Thus, brackish water resources may be used to alleviate the discrepancy between agricultural and ecological water demands and supply.
To date, research on the use of brackish water for irrigation has mostly focused on agricultural crops such as wheat, cotton, corn, and rice, and has generally focused on variables such as crop growth, yield, water use efficiency, and physical and chemical properties of the soil (Deb et al., 2016; Tan et al., 2017; Huang et al., 2019; Liu et al., 2019; Chen et al., 2020; Zhu et al., 2021). Few studies have examined the use of brackish water to irrigate desert plants. Some studies have observed that, despite the low water quality requirements for desert plants, salt accumulation caused by brackish water irrigation still suppresses plant growth and can even lead to plant death (Wang et al., 2010; Chen et al., 2011; Zhang et al., 2020). Therefore, the key to successfully using brackish water is alleviating the damage to plants caused by salt accumulation in soil.
In recent years, the development of magnetized water technology has provided a new solution to a series of problems such as secondary soil salinization and soil structural changes caused by brackish water irrigation. Magnetized water technology involves flowing of water vertically or horizontally at a certain speed through a magnetic induction line generated by a magnetic field of a specific strength (Zhao et al., 2021). Under the action of the magnetic field, the average distance between water molecules increases, and the hydrogen bonds are weakened or even broken, causing the large clusters of associated water molecules to become smaller as free monomer molecules and dimer molecules (Amiri and Dadkhah, 2006; Toledo et al., 2008). Relevant studies have shown that, compared with the physical and chemical properties of non-magnetized water, the chemical bond angle and water-ion colloidal radius of magnetized water decrease, the osmotic pressure and solubility increase, the viscosity coefficient and surface tension of water decrease, the pH value of water increases, and the amount of dissolved oxygen in the water increases (Shimokawa, 2004; Wang Q. J. et al., 2019). To date, magnetization treatment has been thought to enhance salt leaching from the soil, which thereby reduces the damage caused by brackish water or saline water irrigation to the plants. For example, in a study by Bu et al. (2010), the desalination rate of non-magnetized water to soil was 10–20%, while that of magnetized water was 20–30%. Zhou et al. (2021) demonstrated that magnetized water irrigation could increase salt leaching from the soil and reduce the soil salt contents in the salinized soil. Mostafazadeh-Fard et al. (2011) observed that magnetized water can effectively reduce ion content in soil and improve plant survival rate. Liu X. M. et al. (2020) also observed that magnetization treatment alleviated the inhibitory effect of soil salinity on grape growth. In summary, the above applications of magnetized water have focused on crop irrigation.
Haloxylon ammodendron is a perennial shrub that is widely distributed in the desert regions of Central Asia and the arid and semi-desert regions of Northwest China (Liu et al., 2011; Buras et al., 2012; Cui et al., 2017; Lü et al., 2019, 2022; Zhou et al., 2022). H. ammodendron is planted over an area of 114,000 km2 in China, mainly distributed in the Xinjiang Junggar Basin, Tarim Basin, the Alxa Desert in Inner Mongolia, Qinghai Qaidam Basin, and Gansu Hexi Corridor (Hong et al., 2019). The Xinjiang H. ammodendron area accounts for 68.1% of the national H. ammodendron desert area (Song et al., 2021). Since H. ammodendron has high ecological adaptability, with drought resistance, cold resistance, high temperature resistance, salt-alkali resistance, and wind erosion resistance, it is the species with the highest biomass in the desert ecological zone (Tobe et al., 2000; Ma et al., 2007; Zhuang and Zhao, 2017; Yang et al., 2020). H. ammodendron is a strong pioneer plant species that has windbreak and sand fixation functions and plays a key role in the stability of the northwest desert and semi-desert ecological zone (Huang et al., 2003; Sheng et al., 2005; Dai et al., 2022; Li et al., 2022; Shi et al., 2022). In addition, H. ammodendron is utilized as a fuel species, pasture resource, and substrate for the precious parasitic medicinal plant Cistanche deserticola (Thevs et al., 2013; Yang et al., 2014; Shao et al., 2022). However, over the past few decades, under the influence of human activities and climate change, the population of H. ammodendron has decreased significantly (Zhang and Chen, 2002). Moreover, during the seed germination season, inconsistent rainfall has damaged the seedling supplementation of the H. ammodendron population, and as a large number of seedlings have died, the age structure of the H. ammodendron population has generally shifted, with the community exhibiting characteristics of retrograde succession (Huang et al., 2008). The settlement and growth of seedlings in a community is an important process that determines whether the population can be regenerated naturally (Liu et al., 2012). Given the important role of H. ammodendron in desert ecosystems, it is necessary to study the growth and development of H. ammodendron seedlings.
In recent years, studies have examined the growth of H. ammodendron seedlings under different irrigation amounts with different irrigation water qualities (Shan et al., 2008, 2009; Liu et al., 2012, 2022; Zhang et al., 2020). These studies have mainly focused on the physiological and ecological response characteristics of H. ammodendron, and a limited number of studies have analyzed the soil salt distribution, water consumption, and biomass water use efficiency. Furthermore, the effect of magnetized brackish water irrigation on the growth of H. ammodendron seedlings has not been clarified. Thus, the objectives of the study were to (1) evaluate the effects of different irrigation water types and amounts on soil salt distribution, (2) quantitatively analyze the effects of different irrigation types and amounts on the growth of H. ammodendron seedlings, and (3) determine the effects of different irrigation water types and amounts on water consumption and biomass water use efficiency.
Materials and methods
Site description
This experiment was conducted at the Bazhou Irrigation Experimental station (41°35′N, 86°10′E, 901 m above sea level), located near the edge of the oasis in Korla City on the northern margin of the Taklimakan Desert in Xinjiang Autonomous Region. The region has a temperate continental arid desert climate, with hot summers and cold winters, and is generally dry with little rain. The annual temperature is 8°C. The total precipitation at the experimental site during the H. ammodendron growth season was 55.9 mm. In spring, sandstorm activities are intense, with an average wind speed of 2.4 m/s and a maximum wind speed of 22 m/s. The soil at the site is sandy (sand 87.3%, silt 11.6%, and 1.1% cay) (USDA texture class) with little organic matter and limited mineral nutrients. The local plant community is dominated by H. ammodendron, Tamarix chinensis, and Calligonum mongolicum.
The fresh water and brackish water used for irrigation were taken from the Kongqi River and local groundwater, respectively. The electrical conductivity (EC) and main elemental contents of the brackish and freshwater are shown in Table 1.
Experimental design
The field experiment consisted of a completely randomized factorial combination of three kinds of irrigation water (fresh water, magnetized brackish water, and brackish water; F, MB, and B, respectively) and five water supply levels (81, 108, 135, 162, and 189 mm; W1, W2, W3, W4, and W5, respectively). The water supply was designed to be according to the relevant literature (Liu et al., 2018) and the actual production experience of local farmers. In total, there were 15 treatments (FW1, FW2, FW3, FW4, FW5, MBW1, MBW2, MBW3, MBW4, MBW5, BW1, BW2, BW3, BW4, and BW5) with three replicates per treatment, making a total of 45 plots. Each plot was 1.8 m × 1.8 m and the adjacent plots were separated by partitions buried to a depth of 1.8 m to eliminate the lateral movement of soil water. The planted H. ammodendron were spaced 0.6 m apart. The H. ammodendron seedlings were planted on April 24, 2021.
Brackish water was magnetized by passing through a magnetic field at a specified speed. The magnetized water device consisted of a water pipeline, water box, peristaltic pump, and permanent magnet. The permanent magnet, with a magnetic field intensity of 3000G, was mounted on the outer wall of the water pipeline. When the brackish water passed through the pipe, it was magnetized.
Measurements and calculations
Growth indicators
The growth indicators were measured throughout the growing season. From May to September, the plant height, basal diameter, new shoot length, and crown width were measured at the end of each month. The roots of H. ammodendron were excavated in late September to measure the vertical root length, and then the aboveground portion of H. ammodendron and the washed roots were weighed after drying in the oven (at 80°C for 48 h) until a constant weight was obtained. The root shoot ratio was calculated by the following formula:
Soil moisture content and soil salt content
Soil samples were collected during the middle days of each month. Soil samples were collected at 10 cm intervals from 0 to 40 cm and at a 20 cm interval from 40 to 100 cm. Soil moisture content was determined using the drying method. After the soil was dried and crushed, soil salinity was determined using a DDS-307A conductivity meter (Shanghai Precision & Scientific Instrument Inc., Shanghai, China) with a soil-to-water ratio of 1:5.
Description of the logistic function
The logistic function was originally proposed by ecologists to describe the laws of biological population growth and has subsequently been used in modeling plant height growth, leaf area expansion, and grain filling (Zahedi and Jenner, 2003; Bagheri et al., 2014; Liu Y. H. et al., 2020). The logistic growth function was used here to analyze the change in plant height, basal diameter, new shoot length, and crown width:
where, H, D, S, and C represent plant height (cm), basal diameter (mm), new shoot length (cm), and crown width (cm2) respectively; Hm, Dm, Sm, and Cm represent maximum plant height (cm), maximum basal diameter (mm), maximum new shoot length (cm), and maximum crown width (cm2), respectively; a1, a2, b1, b2, c1, c2, d1, and d2 are empirical parameters, and t is the number of days after planting (day).
Salt accumulation calculation
The salt balance formula was as follows (Zhou et al., 2021):
where S1 is the initial soil salinity, g; and S2 is the final soil salinity, g.
Soil salt accumulation or desalination rate was calculated as follows:
where G is accumulation or desalination rate, %. When G is positive, it indicates that the soil is in a salt accumulation state; and if G is negative, it indicates that the soil is in a desalination state.
Evapotranspiration and biomass water use efficiency calculation
Evapotranspiration (water consumption) was calculated based on the water-balance equation:
where P is rainfall (mm); U is groundwater recharge (mm); ΔW is the change in water storage in the 1-m soil profile (mm); I is irrigation amount (mm); D is the deep percolation (mm); R is the surface runoff (mm). The effect of groundwater was negligible due to the depth of the groundwater table (>7 m). Since there was no surface runoff during the experiment, this value was also ignored.
D was calculated using the following equation (Tan et al., 2017):
where SWS is soil water storage in the 1-m soil profile one day before irrigation (mm); and θFC is the field water holding capacity (cm3/cm3).
Biomass water use efficiency was calculated following (Liao et al., 2022):
where B is biomass of H. ammodendron, kg/hm2.
Data analysis
The Excel software was used for calculations and the SPSS 22.0 (IBM Crop., Armonk, New York, NY, USA) software was used for statistical analysis and principal component analysis (PCA). The growth indexes, soil salinity, water consumption, and biomass water use efficiency were analyzed for average and standard deviation for each treatment (n = 3). Differences were determined using Duncan's multiple range test at the 5% level of significance. OriginPro 2019 (Origin Lab Corporation, Northampton, MA, USA) was used to create the graphs.
The determination coefficient (R2) and the normalized root mean square error (nRMSE) were used to evaluate the accuracy of the growth functions. These statistical indexes were calculated as follows:
where Mi is the measured value; Si is the simulated value; is the average value of the measured data; and n is the number of measurements.
Results
Soil salt distribution
Based on the salt balance theory, the change in soil salt reserves of the 0–100 cm root layer with different irrigation treatments in 1 m2 unit during the growing season was calculated. The changes in soil salt were significantly different (p < 0.05) among all treatments during the growing season (Table 2). Compared with the initial soil salt content in April, the soil salt reserves significantly decreased in May in all treatments, and all the soils were in desalination states. Looking at the soil desalination rates in May, within each respective irrigation type, the soil desalination rate increased with increasing irrigation amount, peaking with the W5 irrigation amount at 34.15, 27.68, and 22.05% for the F, MB, and B treatments, respectively. Within the same irrigation amount, the soil desalination effects of the three water types were ordered F > MB > B. Relative to the desalination effect of freshwater irrigation, with irrigation amounts of W1, W2, W3, W4, and W5, the desalination rates of magnetized brackish water irrigation were lower by 22.27, 23.23, 11.83, 22.67, and 18.95%, while those of brackish water irrigation was lower by 51.22, 34.48, 35.08, 34.38, and 35.43%, respectively. Further comparisons of the soil salt reserves of treatments showed that the soil was in the salt accumulation state in September. Within each respective irrigation type, soil salt accumulation rate increased with increasing irrigation amount, and peaked in the W5 irrigation treatment at 19.68, 25.21, and 21.72% in the F, MB, and B treatments, respectively. Within the same irrigation amounts, the soil salt accumulation rate of different treatments was ordered MB > B > F. Compared with fresh water irrigation, under the W1, W2, W3, W4, and W5 irrigation amounts, the salt accumulation rates of magnetized brackish water irrigation were higher by 143.52, 69.98, 46.55, 39.85, and 28.10%, while those of brackish water irrigation was higher by 7.43, 31.81, 23.69, 14.10, and 10.37%, respectively.
Aboveground biomass, belowground biomass, root to shoot ratio, and root length
Figure 1 shows the effects of irrigation water type and irrigation amount on the aboveground biomass of H. ammodendron. Irrigation water type and irrigation amount both significantly affected aboveground biomass (p < 0.05). The aboveground biomass increased with an increase in irrigation amount from W1 to W4 and decreased with increasing irrigation amount over the W4 level. The aboveground biomass of the fresh, magnetized brackish, and brackish water treatments all peaked under the W4 irrigation level, reaching 110.75, 103. 73, and 98.08 g/plant, respectively. The overall mean values of aboveground dry matter of H. ammodendron under fresh, magnetized brackish, and brackish water irrigation under different irrigation amounts were 97.54, 93.04, and 84.91 g/plant, respectively. The mean values of aboveground dry matter under magnetized brackish and brackish water irrigation were 4.61 and 12.96% lower than that under fresh water irrigation, respectively.
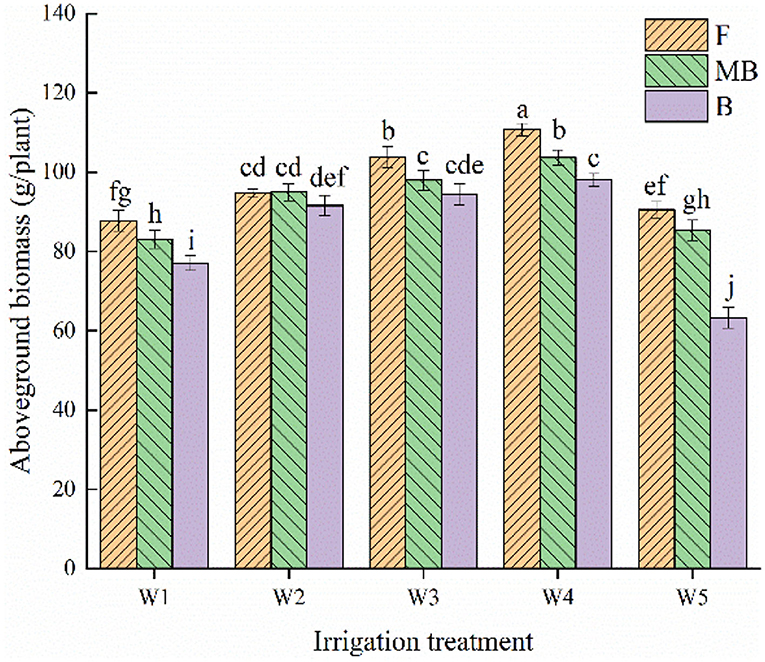
Figure 1. Effects of different irrigation treatments on aboveground biomass of H. ammodendron. F is freshwater treatment, MB is magnetized brackish water, B is brackish water. FW1, FW2, FW3, FW4, and FW5 represent irrigation amount of 81, 108, 135, 162, and 189 mm under freshwater treatment, respectively. MBW1, MBW2, MBW3, MBW4, and MBW5 represent irrigation amount of 81, 108, 135, 162, and 189 mm under magnetized brackish water treatment, respectively. BW1, BW2, BW3, BW4, and BW5 represent irrigation amount of 81, 108, 135, 162, and 189 mm under brackish water treatment, respectively. Data are mean value of the three replicates. Errors bars mean standard errors. Differences were determined using Duncan's multiple range test. Different letters above the bars indicate significant differences among treatments at p < 0.05.
Figure 2 shows the effect of different irrigation water types and irrigation amounts on the belowground biomass of H. ammodendron. Irrigation water type and irrigation amount both significantly affected the belowground biomass of H. ammodendron (p < 0.05). Within each respective irrigation type, the belowground biomass decreased with increasing irrigation amount, and the maximum value occurred under the W1 irrigation amount, reaching 36.72, 33.7, and 29.5 g/plant in the F, MB, and B treatments, respectively. Under the same irrigation amounts, the belowground dry matter of H. ammodendron was ordered F > MB > B. The mean values of the belowground dry matter of H. ammodendron under F, MB, and B irrigation under different irrigation amounts were 29.15, 23.66, and 20.70 g/plant, respectively. The mean values of belowground dry matter under MB and B irrigation were 18.82% and 28.97% lower than that under F irrigation, respectively.
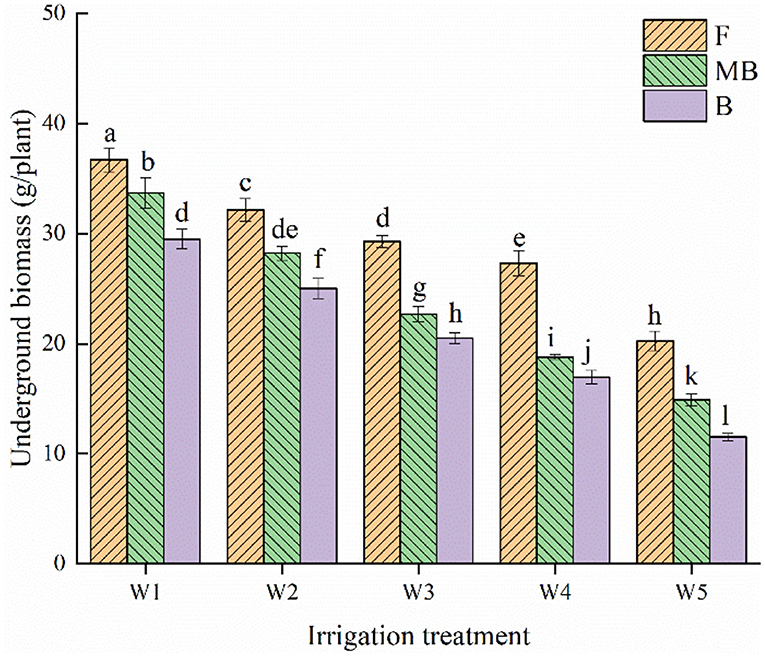
Figure 2. Effects of different irrigation treatments on underground biomass of H. ammodendron. F is freshwater treatment, MB is magnetized brackish water, B is brackish water. FW1, FW2, FW3, FW4, and FW5 represent irrigation amount of 81, 108, 135, 162, and 189 mm under freshwater treatment, respectively. MBW1, MBW2, MBW3, MBW4, and MBW5 represent irrigation amount of 81, 108, 135, 162, and 189 mm under magnetized brackish water treatment, respectively. BW1, BW2, BW3, BW4, and BW5 represent irrigation amount of 81, 108, 135, 162, and 189 mm under brackish water treatment, respectively. Data are mean value of the three replicates. Errors bars mean standard errors. Differences were determined using Duncan's multiple range test. Different letters above the bars indicate significant differences among treatments at p < 0.05.
There were similar changes in the root shoot ratios of H. ammodendron for all treatments, as shown in Figure 3. Both irrigation water type and amount had significant effects on root shoot ratio (p < 0.05). Within each irrigation water type, the root shoot ratio decreased with increasing irrigation amount. The root shoot ratio was largest under the W1 treatment, reaching 0.42, 0.41, and 0.38 g/plant in the F, MB, and B treatments, respectively. Under the same irrigation amounts, the root shoot ratios were ordered F > MB > B. The mean values of the root shoot ratios for F, MB, and B irrigation treatments under different irrigation amounts were 0.30, 0.26, and 0.24 g/plant, respectively. The mean values of the root shoot ratio under MB and B irrigation were 14.57 and 19.21% lower than that under F irrigation.
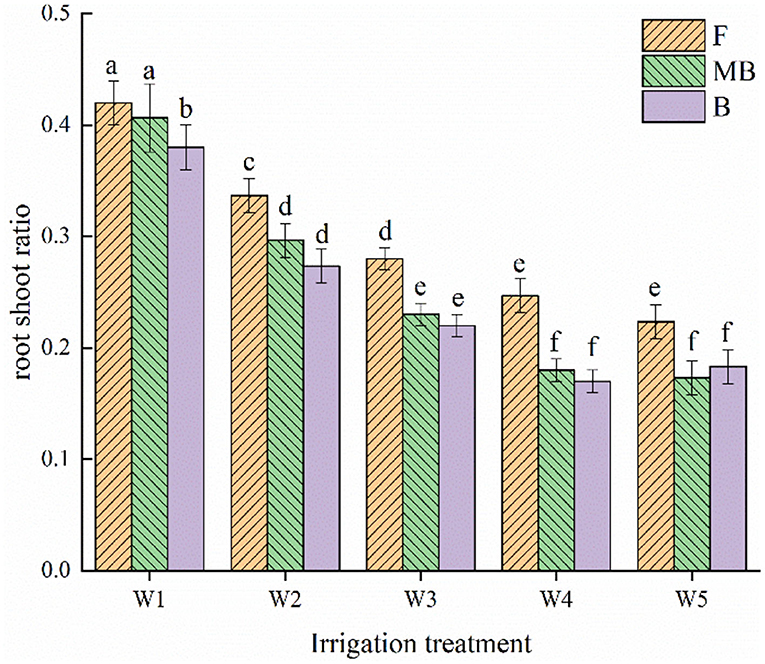
Figure 3. Effects of different irrigation treatments on root to shoot ratio of H. ammodendron. F is freshwater treatment, MB is magnetized brackish water, B is brackish water. FW1, FW2, FW3, FW4, and FW5 represent irrigation amount of 81, 108, 135, 162, and 189 mm under freshwater treatment, respectively. MBW1, MBW2, MBW3, MBW4, and MBW5 represent irrigation amount of 81, 108, 135, 162, and 189 mm under magnetized brackish water treatment, respectively. BW1, BW2, BW3, BW4, and BW5 represent irrigation amount of 81, 108, 135, 162, and 189 mm under brackish water treatment, respectively. Data are mean value of the three replicates. Errors bars mean standard errors. Differences were determined using Duncan's multiple range test. Different letters above the bars indicate significant differences among treatments at p < 0.05.
As for root length of H. ammodendron, the root length decreased with increasing irrigation amount (Figure 4). Irrigation water type and irrigation amount both significantly affected root length (p < 0.05). The root lengths under different water types were largest under the W1 treatment, reaching 72.29, 71.09, and 65.56 cm in the F, MB, and B treatments, respectively. Under the same irrigation amount, the overall mean values of root lengths under F, MB, and B irrigation under all irrigation amounts were 66.92, 64.28, and 54.15 cm, respectively. The mean values of root length under MB and B irrigation were 3.94 and 16.10% lower than that under F irrigation.
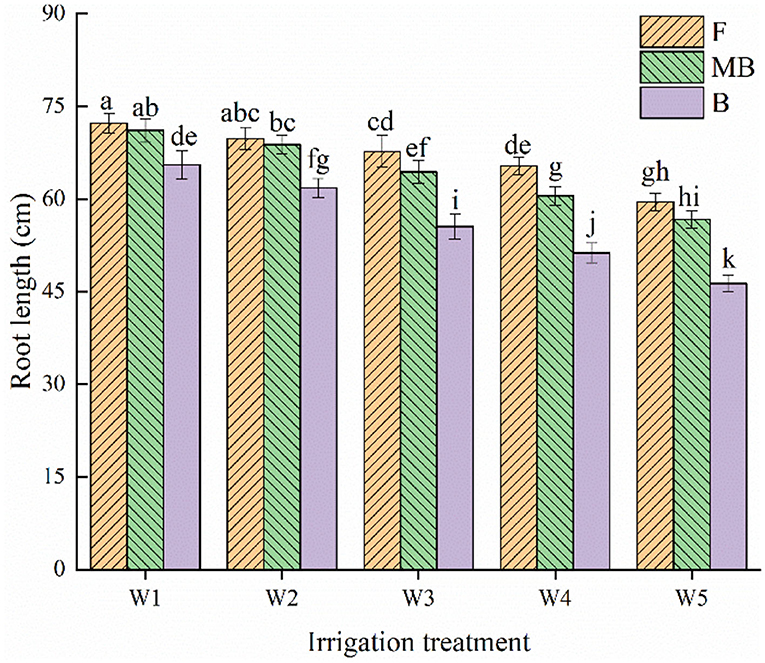
Figure 4. Effects of different irrigation treatments on root length of H. ammodendron. F is freshwater treatment, MB is magnetized brackish water, B is brackish water. FW1, FW2, FW3, FW4, and FW5 represent irrigation amount of 81, 108, 135, 162, and 189 mm under freshwater treatment, respectively. MBW1, MBW2, MBW3, MBW4, and MBW5 represent irrigation amount of 81, 108, 135, 162, and 189 mm under magnetized brackish water treatment, respectively. BW1, BW2, BW3, BW4, and BW5 represent irrigation amount of 81, 108, 135, 162, and 189 mm under brackish water treatment, respectively. Data are mean value of the three replicates. Errors bars mean standard errors. Differences were determined using Duncan's multiple range test. Different letters above the bars indicate significant differences among treatments at p < 0.05.
Plant height, basal diameter, new shoot length, and crown width
Figures 5–8 show the plant height, basal diameter, new shoot length, and crown width of H. ammodendron under different irrigation treatments. These growth indexes showed little change within 35 days of planting, but with the passage of time, the growth indexes gradually developed significant differences among different treatments (p < 0.05). Within each respective irrigation type, with increasing irrigation amount, the plant height, basal diameter, new shoot length, and crown width increased continuously, and these indicators all peaked with the W4 irrigation amount. These growth indexes were decreased when irrigation exceeded the W4 irrigation amount, suggesting that the W5 irrigation amount was not optimal for the growth of H. ammodendron. In addition, we found that under W1 irrigation, the new shoot length of H. ammodendron decreased 142 days after planting. This may have been because the W1 irrigation amount does not meet the growing conditions of H. ammodendron in the later growth stages, which will lead to the abscission of new branch growth in H. ammodendron. Under the same irrigation amounts, the H. ammodendron growth indexes of the treatments were ordered F > MB > B. Compared with fresh water irrigation, the plant height, basal diameter, new shoot length, and crown width of H. ammodendron under B irrigation decreased by 14.79–20.38%, 10.85–15.63%, 14.81–21.42%, and 15.68–27.12%, respectively. Compared with fresh water irrigation, plant height, basal diameter, shoot length, and crown width under MB water irrigation decreased by 5.64–7.49%, 3.95–7.14%, 7.21–12.99%, and 7.73–11.31%, respectively. This indicated that brackish water irrigation inhibited the growth of H. ammodendron seedlings and that the magnetization treatment alleviated that inhibition.
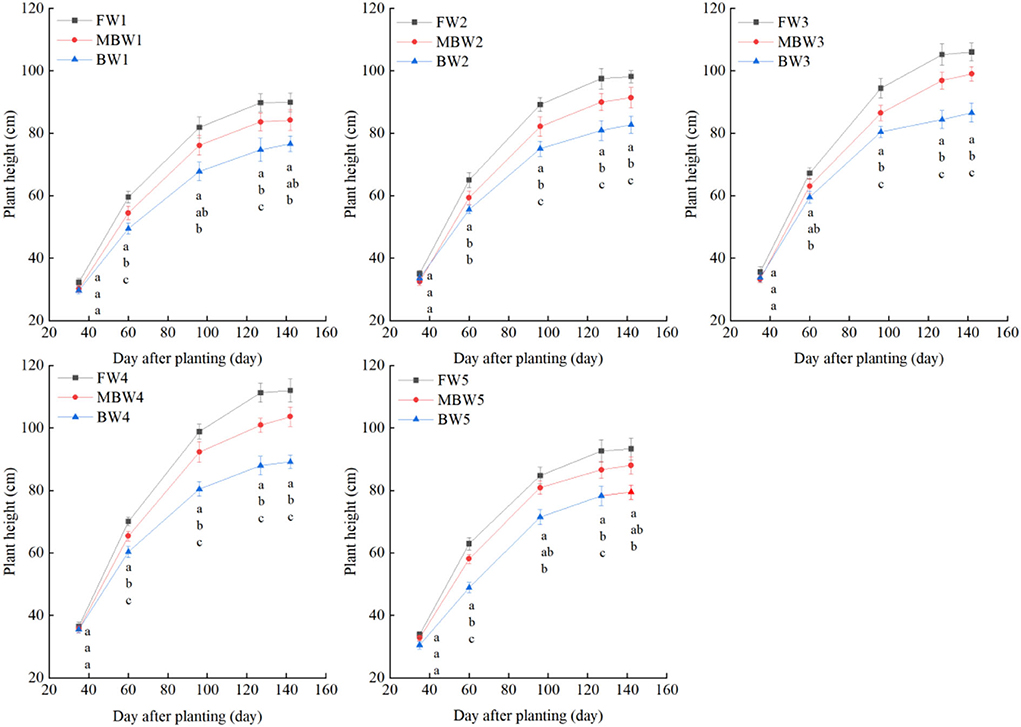
Figure 5. Effects of different irrigation treatments on plant height of H. ammodendron. FW1, FW2, FW3, FW4, and FW5 represent irrigation amount of 81, 108, 135, 162, and 189 mm under freshwater treatment, respectively. MBW1, MBW2, MBW3, MBW4, and MBW5 represent irrigation amount of 81, 108, 135, 162, and 189 mm under magnetized brackish water treatment, respectively. BW1, BW2, BW3, BW4, and BW5 represent irrigation amount of 81, 108, 135, 162, and 189 mm under brackish water treatment, respectively. Data are mean value of the three replicates. Errors bars mean standard errors. Differences were determined using Duncan's multiple range test. Different letters above the bars indicate significant differences among treatments at p < 0.05.
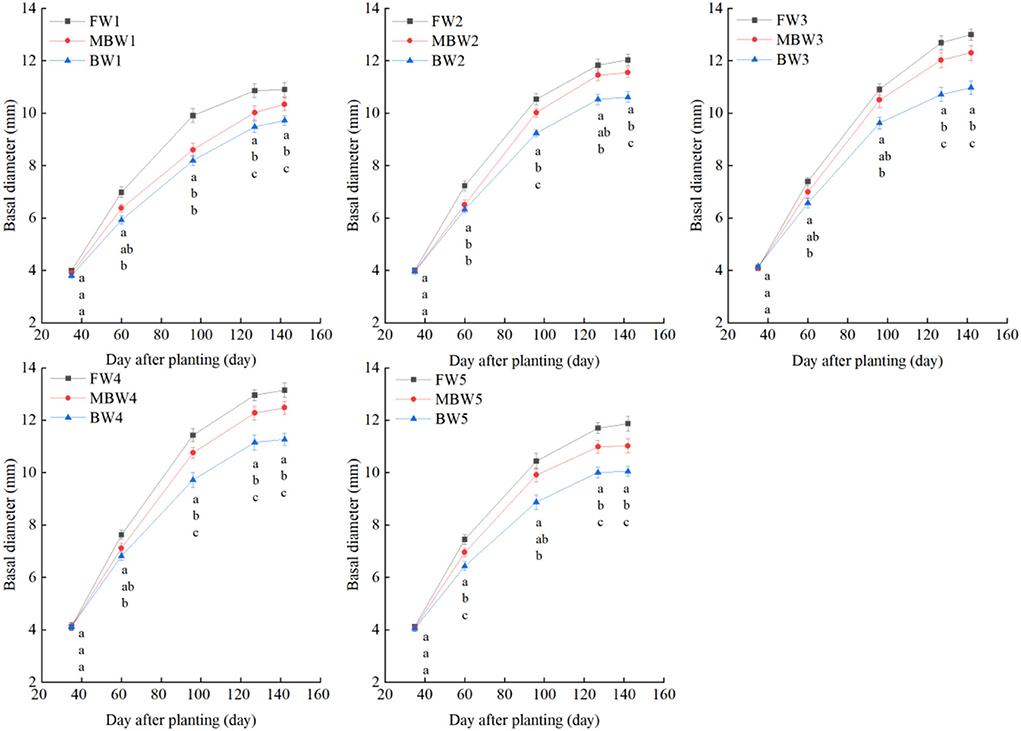
Figure 6. Effects of different irrigation treatments on basal diameter of H. ammodendron. FW1, FW2, FW3, FW4, and FW5 represent irrigation amount of 81, 108, 135, 162, and 189 mm under freshwater treatment, respectively. MBW1, MBW2, MBW3, MBW4, and MBW5 represent irrigation amount of 81, 108, 135, 162, and 189 mm under magnetized brackish water treatment, respectively. BW1, BW2, BW3, BW4, and BW5 represent irrigation amount of 81, 108, 135, 162, and 189 mm under brackish water treatment, respectively. Data are mean value of the three replicates. Errors bars mean standard errors. Differences were determined using Duncan's multiple range test. Different letters above the bars indicate significant differences among treatments at p < 0.05.
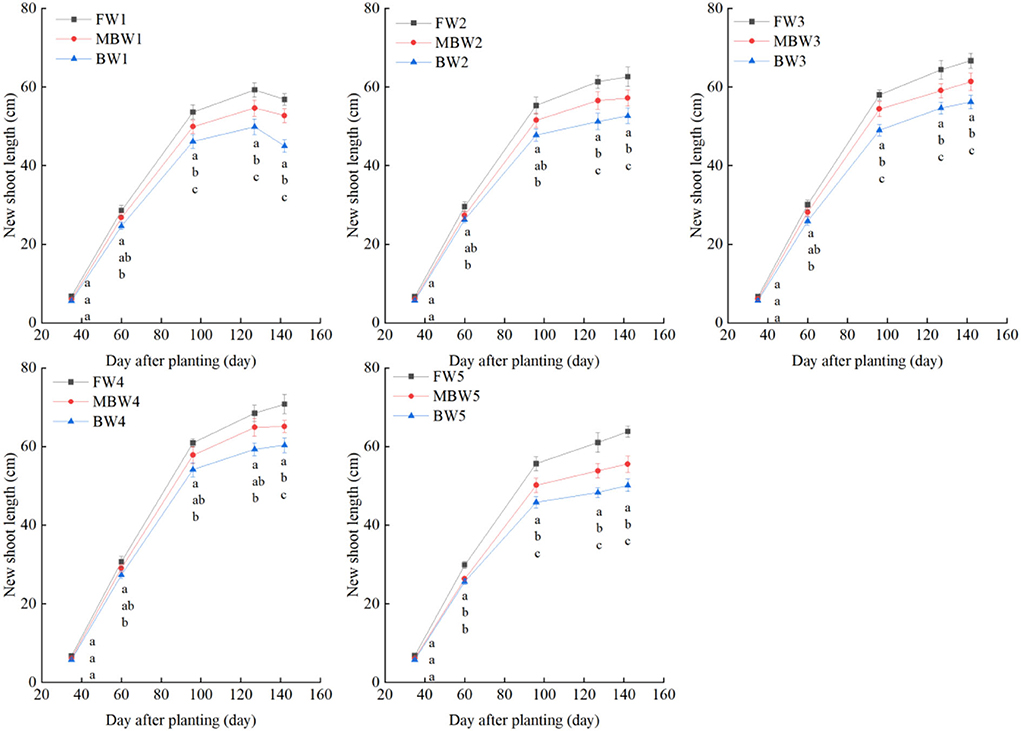
Figure 7. Effects of different irrigation treatments on new shoot length of H. ammodendron. FW1, FW2, FW3, FW4, and FW5 represent irrigation amount of 81, 108, 135, 162, and 189 mm under freshwater treatment, respectively. MBW1, MBW2, MBW3, MBW4, and MBW5 represent irrigation amount of 81, 108, 135, 162, and 189 mm under magnetized brackish water treatment, respectively. BW1, BW2, BW3, BW4, and BW5 represent irrigation amount of 81, 108, 135, 162, and 189 mm under brackish water treatment, respectively. Data are mean value of the three replicates. Errors bars mean standard errors. Differences were determined using Duncan's multiple range test. Different letters above the bars indicate significant differences among treatments at p < 0.05.
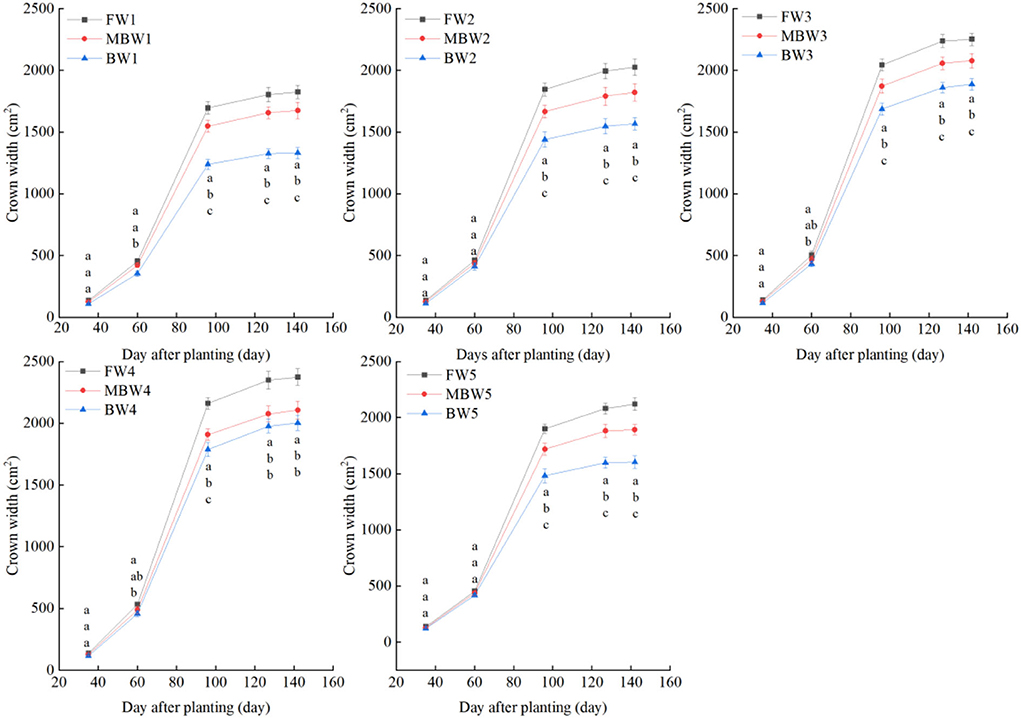
Figure 8. Effects of different irrigation treatments on crown width of H. ammodendron. FW1, FW2, FW3, FW4, and FW5 represent irrigation amount of 81, 108, 135, 162, and 189 mm under freshwater treatment, respectively. MBW1, MBW2, MBW3, MBW4, and MBW5 represent irrigation amount of 81, 108, 135, 162, and 189 mm under magnetized brackish water treatment, respectively. BW1, BW2, BW3, BW4, and BW5 represent irrigation amount of 81, 108, 135, 162, and 189 mm under brackish water treatment, respectively. Data are mean value of the three replicates. Errors bars mean standard errors. Differences were determined using Duncan's multiple range test. Different letters above the bars indicate significant differences among treatments at p < 0.05.
Simulation of the H. ammodendron growth indexes
Plant height, basal diameter, new shoot length, and crown width are important indicators to reflect the growth state of H. ammodendron. The data of plant height, basal diameter, new shoot length, and crown width of H. ammodendron under different irrigation treatments were fitted by formulas (4), (5), (6), and (7). The fitting results are shown in Table 3. Within each respective irrigation type, irrigation amount had little effect on parameters a1, a2, b1, b2, c1, c2, d1, and d2. Therefore, to simplify the functions, it was assumed that the influence of irrigation amount on parameters a1, a2, b1, b2, c1, c2, d1, and d2 can be ignored, and these parameters were averaged. However, the maximum values of plant height, basal diameter, new shoot length, and crown width first increased and then decreased with increasing irrigation water amount (Figure 9). The maximum values of the growth indexes were all significantly affected by irrigation amount. The relationship between maximum plant height, maximum basal diameter, maximum shoot length, maximum crown width, and irrigation amount was expressed by the series of formulas in Table 4.
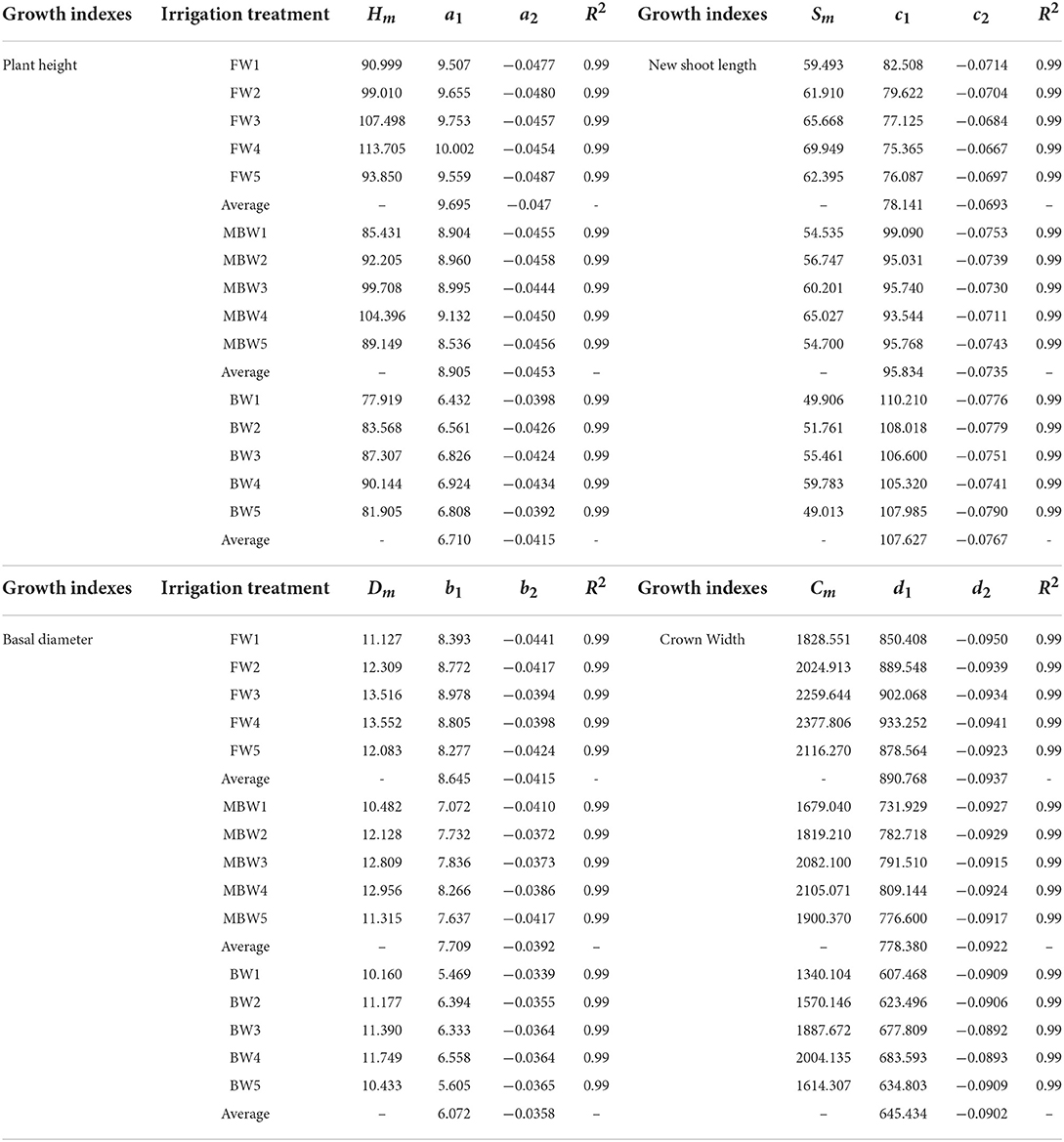
Table 3. Parameter values in logistic function of plant height, basal diameter, new shoot length, and crown width of H. ammodendron.
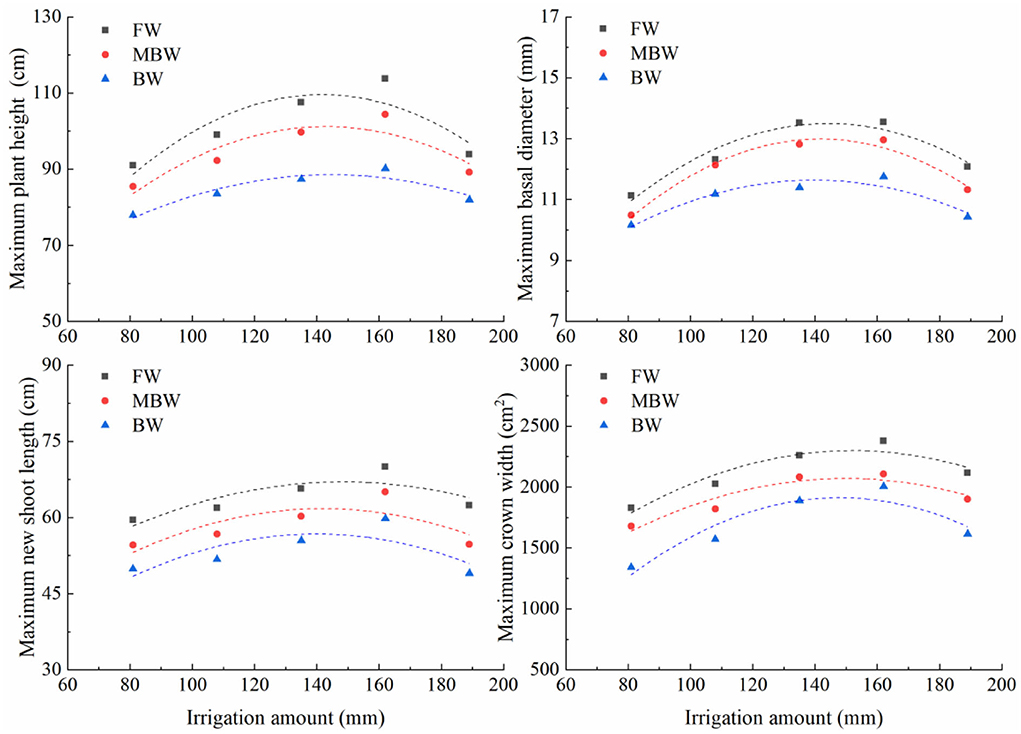
Figure 9. Relationships between maximum plant height, maximum basal diameter, maximum new shoot length, and maximum crown width and irrigation amount. FW is freshwater treatment, MBW is magnetized brackish water, BW is brackish water.
Therefore, the equations for change in H. ammodendron plant height, basal diameter, new shoot, and crown width under fresh water, magnetized brackish water, and brackish water irrigation were, respectively:
In order to test the accuracy of the modified logistic functions, they were validated against the measured data, and the simulation results are shown in Figures 10–13. R2 and nRMSE were used to calculate the error between the simulated and measured values. The results showed that under different irrigation treatments, the ranges of nRMSE and R2, respectively, were 0.94–5.28% and 0.98–0.99 for plant height; 1.74–16.39% and 0.74–0.99 for basal diameter; 3.43–6.69% and 0.98–0.99 for shoot length; and 2.53–8.78% and 0.98–0.99 for crown width. This suggested that the established series of functions performed well in simulating plant height, basal diameter, new shoot length, and crown width.
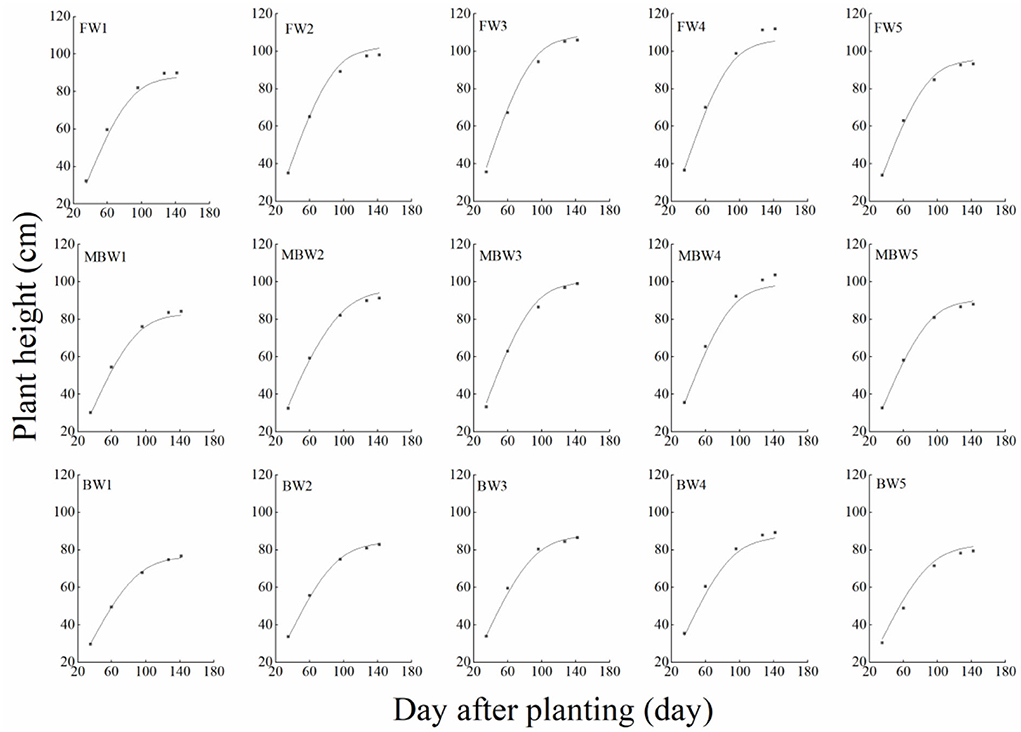
Figure 10. Comparison of simulated and measured plant heights of H. ammodendron. FW1, FW2, FW3, FW4, and FW5 represent irrigation amount of 81, 108, 135, 162, and 189 mm under freshwater treatment, respectively. MBW1, MBW2, MBW3, MBW4, and MBW5 represent irrigation amount of 81, 108, 135, 162, and 189 mm under magnetized brackish water treatment, respectively. BW1, BW2, BW3, BW4, and BW5 represent irrigation amount of 81, 108, 135, 162, and 189 mm under brackish water treatment, respectively.
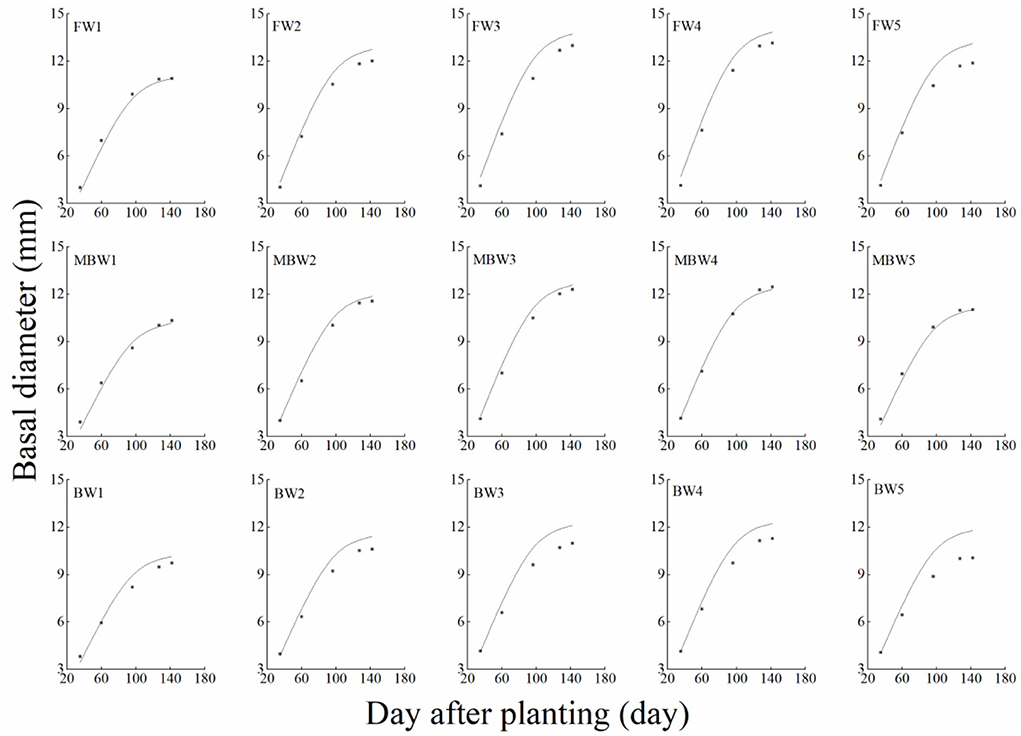
Figure 11. Comparison of simulated and measured basal diameters of H. ammodendron. FW1, FW2, FW3, FW4, and FW5 represent irrigation amount of 81, 108, 135, 162, and 189 mm under freshwater treatment, respectively. MBW1, MBW2, MBW3, MBW4, and MBW5 represent irrigation amount of 81, 108, 135, 162, and 189 mm under magnetized brackish water treatment, respectively. BW1, BW2, BW3, BW4, and BW5 represent irrigation amount of 81, 108, 135, 162, and 189 mm under brackish water treatment, respectively.
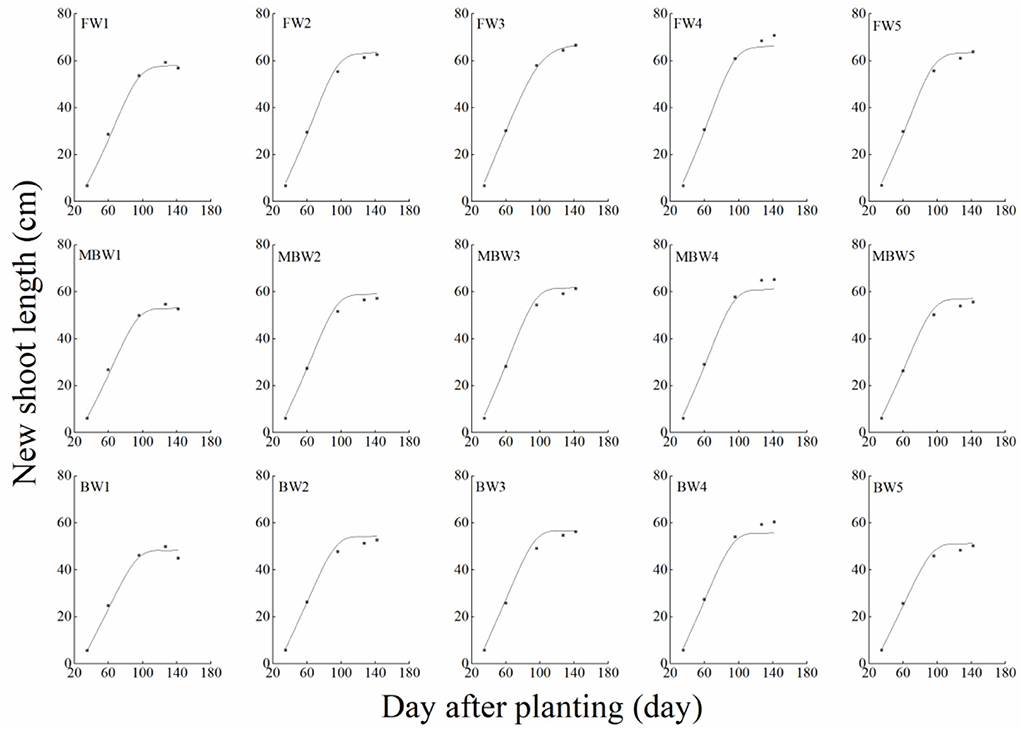
Figure 12. Comparison of simulated and measured new shoot lengths of H. ammodendron. FW1, FW2, FW3, FW4, and FW5 represent irrigation amount of 81, 108, 135, 162, and 189 mm under freshwater treatment, respectively. MBW1, MBW2, MBW3, MBW4, and MBW5 represent irrigation amount of 81, 108, 135, 162, and 189 mm under magnetized brackish water treatment, respectively. BW1, BW2, BW3, BW4, and BW5 represent irrigation amount of 81, 108, 135, 162, and 189 mm under brackish water treatment, respectively.
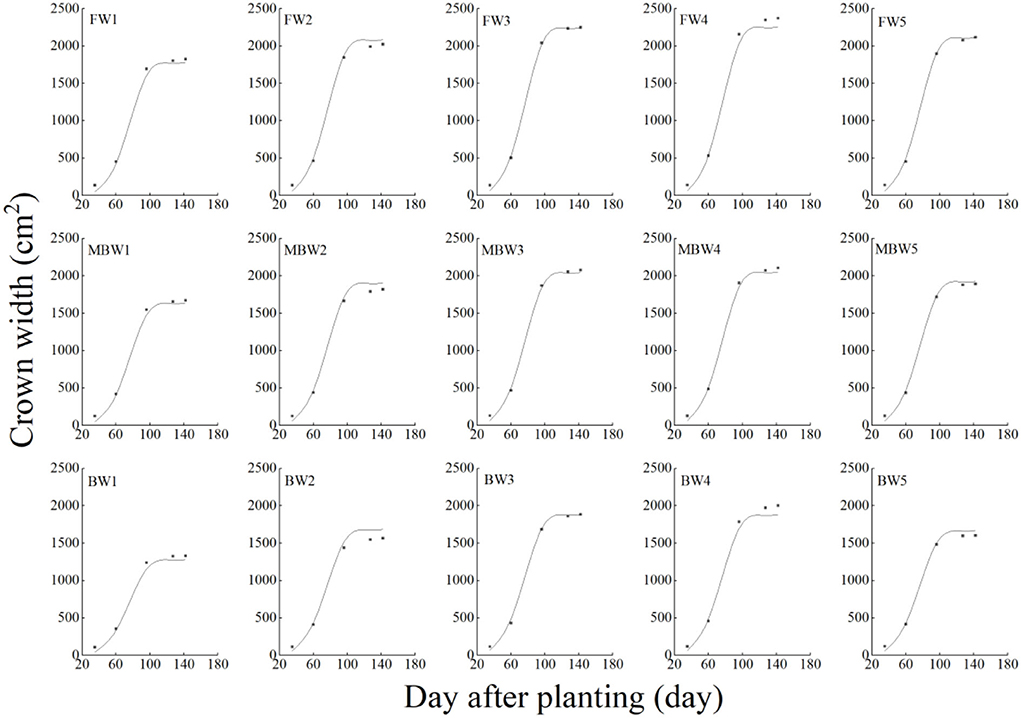
Figure 13. Comparison of simulated and measured crown widths of H. ammodendron. FW1, FW2, FW3, FW4, and FW5 represent irrigation amount of 81, 108, 135, 162, and 189 mm under freshwater treatment, respectively. MBW1, MBW2, MBW3, MBW4, and MBW5 represent irrigation amount of 81, 108, 135, 162, and 189 mm under magnetized brackish water treatment, respectively. BW1, BW2, BW3, BW4, and BW5 represent irrigation amount of 81, 108, 135, 162, and 189 mm under brackish water treatment, respectively.
Water consumption and biomass water use efficiency
The effects of different irrigation treatments on water consumption and water use efficiency of H. ammodendron are shown in Table 5. When the irrigation amounts were the same, the water consumption under fresh water irrigation is significantly higher than that under brackish water irrigation (p < 0.05). Under W1–W4 irrigation amount, the water consumption of fresh water irrigation is greater than that of magnetized brackish water irrigation, but it is not significant (p > 0.05); Under W5 irrigation, the water consumption in fresh water irrigation is significantly greater than that of magnetized brackish water irrigation (p < 0.05). The water consumption under magnetized brackish water irrigation is higher than that under brackish water irrigation, but it is not significant (p > 0.05). Compared with brackish water irrigation, the water consumption of H. ammodendron under F and MB irrigation increased by 11.57–16.65% and 4.45–6.62%, respectively. Within each respective irrigation type, the water consumption of H. ammodendron increased with increasing irrigation amount. And there were no significant differences between treatments W3, W4, and W5 (p > 0.05).
As for biomass water use efficiency, there was no significant difference (p > 0.05) in water use coefficient under the irrigation amount of W1, W2, and W3. Under W4 and W5 irrigation amount, there was no significant difference (p > 0.05) between fresh water and magnetized brackish water, and there was a significant difference (p < 0.05) between fresh water and brackish water. Within each respective irrigation type, with increasing irrigation amount, the biomass water use efficiency decreased with the increase of irrigation amount.
Principal component analysis of the H. ammodendron growth indexes
A single growth index of H. ammodendron cannot fully reflect its growth status, so it is necessary to comprehensively analyze and evaluate various indexes of H. ammodendron. Principal component analysis (PCA) is a statistical method that transforms multi-index into a few comprehensive indexes by using the idea of dimension reduction (Xin et al., 2019; Wang X. K. et al., 2020; Bi et al., 2022). These comprehensive indicators retain most of the information of the original indicators (Shi et al., 2021; Tang et al., 2021). The Kaiser–Meyer–Olkin (KMO) value was 0.582 and Bartlett's sphericity test was p < 0.001, indicating that PCA could be performed (Hao et al., 2022). PCA was performed on 10 growth indexes (aboveground biomass, belowground biomass, root shoot ratio, root length, plant height, basal diameter, new shoot length, crown width, water consumption, and biomass water use efficiency) of H. ammodendron. The variance contribution analysis table, principal component load matrix, and eigenvector of each index were shown in Tables 6, 7. The percentage of the variance of the first principal component 1 (PC1) was 54.21%, and the percentage of the variance of the second principal component 2 (PC2) was 39.43%. The cumulative percentage of the variance of PC1 and PC2 was 93.64%. The PC1 and PC2 contained most of the information of the 10 indicators. The first principal component mainly included the crown width, new shoot length, basal diameter, plant height, water consumption, and aboveground biomass. The second principal component included the belowground biomass, root length, biomass water use efficiency, and root shoot ratio.
Combining the standardized vector of the growth index and the eigenvector, the expression of the principal component was determined, and the comprehensive score was calculated:
The first principal component score was:
The second principal component score was:
The comprehensive evaluation formula was established as:
where Z1 and Z2 are the first and second principal component scores, respectively, Z is the comprehensive score, and x1, x2, x3, x4, x5, x6, x7, x8, x9, and x10 are the standardized vector of aboveground biomass, belowground biomass, root shoot ratio, root length, plant height, basal diameter, new shoot length, crown width, water consumption, and biomass water use efficiency, respectively.
The comprehensive scores of the growth characteristics are shown in Table 8.
The treatments, ordered by their comprehensive scores from high to low, were FW4, FW3, MBW4, FW2, MBW3, FW5, MBW2, FW1, BW4, BW3, MBW1, MBW5, BW2, BW1, BW5. Thus, the three treatments with the highest scores were FW4, FW3, and MBW4, respectively. And the three treatments with the lowest scores were BW2, BW1, and BW5, respectively. Compared with brackish water irrigation, the comprehensive scores under magnetized brackish water irrigation were improved.
Discussion
Effect of different irrigation treatments on soil salt distribution
In this study in May, fresh water (F), magnetized brackish water (MB), and brackish water (B) all effectively leached salt from the soil. Within each respective irrigation type, the desalination rate increased with increasing irrigation amount. This indicated that salt leaching from the soil was directly dependent on irrigation amount, which was consistent with the study by Yuan et al. (2019) and Che et al. (2021). Within each respective irrigation amount, the salt washing effects of the three irrigation water types were ordered F > MB > B. Freshwater had the best washing effect because it had the lowest salinity, and therefore introduced the least salt into the soil during irrigation, but this was inconsistent with the previous findings (Zhou et al., 2016; Huang et al., 2021; Li et al., 2021). Notably, the salt washing effect of brackish water was enhanced after magnetization. This may have been due to the fact that, under the action of the magnetic field, the average distance between water molecules was increased and some hydrogen bonds became weak or even broke, and the large associated water molecule clusters were decomposed into free single and dimer molecules. Smaller water molecules are more likely to invade the small pores of the soil and carry more soil salt away with the water, increasing the convection and diffusion of soil salt and thus improving the soil salt washing efficiency. A similar study by Zhou et al. (2021) indicated that, compared with brackish water irrigation, magnetized brackish water irrigation increased the soil desalination rate by 29.2–50.4% in a cotton field. Wang Q. J. et al. (2020) also demonstrated that magnetized water had a better ability to dissolve and leach salt. Bu et al. (2010) and Zhang et al. (2013) reported that magnetized water irrigation improved the leaching of , Cl−, and Na+ in soil.
Soil salt accumulation is mainly affected by environmental factors such as irrigation, evaporation, temperature, rainfall, and plant transpiration (Ding et al., 2016). In this study, the soil in the 0–100 cm depth range in September was in a salt accumulation state. This was due to the high temperatures and strong evaporation, resulting in the accumulation of salt in the shallower soil layers. Within each respective irrigation water type, soil salt accumulation increased with increasing irrigation water amount. This was because, as the irrigation amount increases, the salt carried by the water also increases accordingly. As the soil water evaporated, the salt in the deeper soil appeared to be carried to shallower soil layers with the water, which was consistent with previous research (Wei et al., 2021). Within the same irrigation amounts, the soil salt accumulation rate under the three irrigation water types was ordered MB > B > F. This was because the root growth indexes of H. ammodendron under magnetized brackish water irrigation were better than that under brackish water irrigation, which meant that H. ammodendron could enrich more salt when it absorbs more water under magnetized brackish water irrigation. In fact, soil salt content was affected by plant growth in the desert ecosystem. Some studies have shown that the salt content of the soil under shrubs is higher than that of the surrounding soil, which becomes the “salt island” effect. For example, Yin et al. (2012) studied the halophyte shrubs in the Tarim Basin, which showed that Tamarix ramosissima, Halostachys caspica, and Halocnemum strobilaceum all had “salt island” effects in varying degrees, and the salt island effect produced by the three enhanced the salt accumulation rate on the soil surface. Ge et al. (2007) found that after planting reed on saline-alkali soil, Na+ in soil increased significantly.
Effects of irrigation treatments on growth indexes of H. ammodendron
Roots make up the majority of the biomass in desert plants. When water is scarce, plants prioritize allocating new biomass to building roots to enhance the uptake of soil water (Lu et al., 2021). Therefore, root length is very important for the establishment and growth of desert plants, especially seedlings. In this study, the root length of H. ammodendron increased with decreasing irrigation amount, indicating that H. ammodendron adapted to the decreased water supply through root elongation and growth, which was consistent with the results of Shan et al. (2008) and Lu et al. (2021). The root lengths of H. ammodendron when irrigated with magnetized brackish water and brackish water were shorter than when irrigated with fresh water, which may have been due to the salt accumulated in the soil from the high salinity brackish and magnetized brackish waters. Li et al. (2015) reported that in soil layers with high concentrations of salt, almost no desert plant roots could be found, and roots were mainly distributed in areas where the soil EC was less than 1 mS cm−1. In addition, the experimental results showed that magnetization treatment effectively reduced the inhibition effect of water salinity on root growth. This was similar to the experimental results of Zhao et al. (2022) who reported that magnetized water promoted root vigor and growth in winter wheat. The reasons underlying this phenomenon may have been twofold, on the one hand, magnetized water may promote plant metabolism, cell division, and differentiation, all of which would promote the growth of roots; on the other hand, magnetized water irrigation may enhance the leaching effect of soil salt and improve the growth environment for the H. ammodendron root system. Zhao et al. (2016) also reported that the numbers and lengths of rice seedlings roots irrigated with magnetized water increased by 21.74 and 20.62%, respectively, compared with unmagnetized water.
Changes in the root–shoot ratio reflect the distribution strategy of assimilated nutrients in different periods, which means the study of the plant root-to-shoot ratio is central to analyzing the distribution of plant assimilates (Schenk and Jackson, 2002). In this study, the root shoot ratio of H. ammodendron seedlings decreased gradually with increasing irrigation, which showed how the biomass allocation strategy of H. ammodendron seedlings changed according to water conditions. When the water was sufficient, the seedlings allocated more biomass to aboveground growth to maximize the capture of light energy and sustain plant consumption and growth. However, when there was a water shortage, seedlings allocated more resources to root growth to increase the water absorption potential so that they could sustain competitive growth rates. This was consistent with previous research (Xu et al., 2007a,b; Tian et al., 2014). In our study, compared with brackish water irrigation, the root shoot ratio under magnetized brackish water irrigation was larger, which benefits the survival of seedlings in arid environments. Similarly, Li et al. (2020) showed that under severe drought conditions, the root shoot ratio under magnetized water irrigation was 84.6% higher than that under tap water irrigation. Wei et al. (2020) showed that the root shoot ratio under magnetized water irrigation was 26.43% higher than that under non-magnetized water irrigation. Zhao et al. (2016) also reported that the root shoot ratio of rice seedlings was 20.18% higher after magnetization treatment.
Water is the most important factor determining the growth and development of desert plants, and changes in soil water greatly influence the ecological environment in desert areas (Wang et al., 2003). In this study, irrigation amount and type had no significant effect on plant height, stem diameter, new shoot length, and crown width in the first month after planting. This could be because the growth indexes of H. ammodendron seedlings were weakly affected by the external environment at this stage. This was consistent with the research of Liu et al. (2012) and Liu et al. (2018). Then H. ammodendron entered the fastest growing period of the whole year and was affected by the irrigation treatments. During this period, the shallow soil was supplemented by irrigation, so soil water content was plentiful, especially considering that the water demand of H. ammodendron seedlings is relatively small in this stage. At the end of the growing season, air temperature gradually increased, surface evaporation was strong, the water content in the shallow soil decreased, and environmental conditions for the growth of H. ammodendron seedlings deteriorated, which led to slowed growth. Within each respective irrigation type, the plant height, basal diameter, and new shoots of H. ammodendron increased continuously and then decreased with increasing irrigation amount. This was because, as the irrigation amount increased, the water absorbed by the H. ammodendron seedlings increased, which led to better growth. However, excessive irrigation led to high soil water content, which reduced soil permeability and inhibited the growth of H. ammodendron seedlings. In addition, we found that, under W1 irrigation, new shoots fell off at the end of the growing season. This was because the assimilating branches of H. ammodendron seedlings reduce water consumption by withering. Schulze et al. (1996) also showed that desert shrubs will use abscission to reduce water consumption during drought. Here, within the same irrigation amount, the plant height, basic diameter, and new shoots of H. ammodendron under the three irrigation water types were ordered F > MB > B. This indicated that brackish water inhibited the growth of H. ammodendron. Wang et al. (2010) and Chen et al. (2011) also showed that the physiological activity and growth rate of H. ammodendron decreased with increasing salinity of irrigation water. Zhang et al. (2020) showed that, compared with fresh water irrigation, the plant height, basal diameter, and new shoots of H. ammodendron under high salinity water irrigation decreased by 13.93–34.72, 4.76–66.15, and 27.54–63.71%, respectively. The effects of magnetized brackish water and brackish water on the growth of H. ammodendron were further compared, revealing that the magnetization treatment reduced the inhibiting effect of brackish water on the growth of the plant. This was because magnetized water irrigation promoted the growth of H. ammodendron roots, improved the absorption and utilization of water and nutrients, and indirectly affected the aboveground growth indexes. A similar study reported by Wang L. et al. (2019) also showed that magnetization treatment effectively alleviated the inhibitory effect of salt water irrigation on the growth of grape stems and leaves. Zhao et al. (2021) reported that magnetized water irrigation increased plant height, leaf area index, and dry matter accumulation of winter wheat.
In this study, we found that, within the same irrigation type, the irrigation amount had little effect on the empirical parameters a1, a2, b1, b2, c1, c2, d1, and d2 in the logistic functions, but had a significant effect on the maximum growth index values. Therefore, we averaged the empirical parameters, established the regression equations between irrigation amounts and the maximum values of each growth index, and then constructed a series of mathematical equations describing the plant height, basal diameter, new shoot length, and crown width of H. ammodendron under different irrigation amounts. Ma et al. (2013) and Wang et al. (2014) also indicated that irrigation amount had little impact on the empirical parameters in the wheat logistic function. The simulation results showed that it is reasonable to predict the growth in plant height, basal diameter, new shoot length, and crown width using the established functions.
Effects of irrigation treatments on water consumption and biomass water use efficiency
In this study, water consumption of H. ammodendron increased with an increase in irrigation amount from W1 to W3. And when the irrigation amount exceeds the W3 level, the change in water consumption was not significant. When the irrigation amounts were the same, the water consumption under fresh water irrigation is significantly greater than that under brackish water irrigation and slightly greater than that under magnetized brackish water irrigation. This was because B and MB carried a large amount of salt, which resulted in the serious accumulation of salt in the soil and hindered the root system by absorbing water. Chen et al. (2011) and Hu et al. (2014) showed that brackish water and saline water irrigation significantly inhibited the physiological activity of H. ammodendron, and the water consumption decreased with increasing degree of mineralization of irrigation water. Wang et al. (2010) reported that water consumption of H. ammodendron during the growth period decreased by 22.1% when the salinity of irrigation water increased from 1 to 6 g L−1. Furthermore, the water consumption when irrigated with magnetized brackish water was slightly higher than that when using brackish water. This may be because magnetized brackish water enhanced the salt leaching effect, promoted root growth, and improved water absorption of H. ammodendron. These results were consistent with the findings of Zhou et al. (2021) and Zhao et al. (2022).
Water use efficiency reflects the ability of plants to effectively use water (Tennakoon and Milroy, 2003). In our study, over-irrigation reduced the WUE of H. ammodendron, while decreasing the irrigation amount increased WUE. There were two mechanisms driving this trend, one was because increasing irrigation amount can increase deep percolation, and the second was that H. ammodendron can resist drought by improving high water utilization efficiency during water deficits. This result agreed with many previous studies (Gong et al., 2006; Rouhi et al., 2007; Xu et al., 2007a,b; Yan et al., 2010; Yang et al., 2018). In addition, compared with fresh water irrigation, brackish water irrigation and magnetized brackish water irrigation slightly decreased the WUE. This is because brackish water and magnetized brackish water irrigation led to soil salt accumulation and inhibited root water absorption (Hu et al., 2014). Furthermore, compared with brackish water irrigation, magnetized brackish water irrigation had higher WUE because magnetized brackish water promoted root growth and enhanced water absorption. This result agreed with the earlier study by Zhao et al. (2022).
Conclusion
In the current study, we proved the potential of magnetized brackish water as an eco-friendly technology for improving soil salt leaching and then for the growth of H. ammodendron seedlings in arid areas. Compared to unmagnetized brackish water, the magnetized brackish water significantly improved the effect of soil salt leaching, promoted root growth, and stimulated the growth of plant height, basal diameter, new shoot length, and crown width. In general, magnetized brackish water treatment slightly increased water consumption and water use efficiency. Furthermore, principal component analysis indicated that the comprehensive score of magnetized brackish water irrigation was higher than that of brackish water irrigation. The first three treatments with the highest scores are FW4, FW3, and MBW4, respectively. This showed that magnetized brackish water combined with an appropriate irrigation amount was helpful to optimize the growth of H. ammodendron seedlings based on freshwater saving. Therefore, we concluded that irrigation with magnetized brackish water was an effective method to ensure the establishment and growth of H. ammodendron seedlings in arid and water-deficient areas.
Data availability statement
The raw data supporting the conclusions of this article will be made available by the authors, without undue reservation.
Author contributions
YG: investigation, data curation, formal analysis, and writing—original draft. QW: conceptualization, writing—review and editing, and supervision. XZ, ZL, ML, JZ, and KW: investigation. All authors contributed to the article and approved the submitted version.
Funding
This research is jointly supported by the National Natural Science Foundation of China (41830754, 52179042), Major science and technology projects of the XPCC (2021AA003-2) and Major Science and Technology Projects of Autonomous Region (2020A01003-3).
Conflict of interest
The authors declare that the research was conducted in the absence of any commercial or financial relationships that could be construed as a potential conflict of interest.
Publisher's note
All claims expressed in this article are solely those of the authors and do not necessarily represent those of their affiliated organizations, or those of the publisher, the editors and the reviewers. Any product that may be evaluated in this article, or claim that may be made by its manufacturer, is not guaranteed or endorsed by the publisher.
References
Amiri, M. C., and Dadkhah, A. A. (2006). On reduction in the surface tension of water due to magnetic treatment. Colloids Surfaces A Physicochem. Eng. Aspects 278, 252–255. doi: 10.1016/j.colsurfa.2005.12.046
Bagheri, S., Sepaskhah, A. R., Razzaghi, F., and Zand-Parsa, S. (2014). Developing a dynamic yield and growth model for maize under various water and nitrogen regimes. Archives Agron. Soil Sci. 60, 1173–1191. doi: 10.1080/03650340.2013.873121
Bi, H. B., Ni, Z. S., Tian, J. J., Jiang, C. L., Sun, H., Lin, Q. Z., et al. (2022). Influence of lignin on coal gangue pyrolysis and gas emission based on multi-lump parallel reaction model and principal component analysis. Sci. Total Environ. 820, 153083. doi: 10.1016/j.scitotenv.2022.153083
Bu, D. S., Feng, W. G., and Cai, L. H. (2010). Effects of magnetization water on desalinization in cotton farmland of under-film dripping irrigation in Xinjiang Province. Trans. Chin. Soc. Agri. Eng. 26, 163–166. doi: 10.3969/j.issn.1002-6819.2010.z2.031
Buras, A., Wucherer, W., Zerbe, S., Noviskiy, Z., Muchitdinov, N., Shimshikov, B., et al. (2012). Allometric variability of Haloxylon species in Central Asia. Forest Ecol. Manage. 274, 1–9. doi: 10.1016/j.foreco.2012.02.023
Che, Z., Wang, J., and Li, J. S. (2021). Effects of water quality, irrigation amount and nitrogen applied on soil salinity and cotton production under mulched drip irrigation in arid Northwest China. Agri. Water Manage. 247, 106738. doi: 10.1016/j.agwat.2021.106738
Chen, R., Cheng, W. H., Cui, J., Liao, J., Fan, H., Zheng, Z., et al. (2015). Lateral spacing in drip-irrigated wheat: the effects on soil moisture, yield, and water use efficiency. Field Crops Res. 179, 52–62. doi: 10.1016/j.fcr.2015.03.021
Chen, S. F., He, X. L., Wang, Z. F., Yang, G. S., and Li, N. (2011). Study on the relation of farm water consumption and growth of Haloxylon Ammodendron under saline water irrigation. J. Irrigation Drainage. 30, 132–135. doi: 10.13522/j.cnki.ggps.2011.02.034
Chen, W. L., Jin, M. G., Ferré, T. P., Liu, Y. F., Huang, J. O., Xian, Y., et al. (2020). Soil conditions affect cotton root distribution and cotton yield under mulched drip irrigation. Field Crops Res. 249, 107743. doi: 10.1016/j.fcr.2020.107743
Chen, W. L., Jin, M. G., Ferré, T. P., Liu, Y. F., Xian, Y., Shan, T. R., et al. (2018). Spatial distribution of soil moisture, soil salinity, and root density beneath a cotton field under mulched drip irrigation with brackish and fresh water. Field Crops Res. 215, 207–221. doi: 10.1016/j.fcr.2017.10.019
China Water Resources Bulletin (2020). Ministry of Water Resources of the People's Republic of China. Available online at: http://www.mwr.gov.cn/sj/tjgb/szygb/202107/t20210709_1528208.html (accessed July 9, 2021).
Cui, X. Q., Yue, P., Gong, Y. M., Li, K. H., Tan, D. Y., Goulding, K., et al. (2017). Impacts of water and nitrogen addition on nitrogen recovery in Haloxylon ammodendron dominated desert ecosystems. Sci. Total Environ. 601, 1280–1288. doi: 10.1016/j.scitotenv.2017.05.202
Dai, Y., Wang, H. W., and Shi, Q. D. (2022). Contrasting plant water-use responses to groundwater depth from seedlings to mature trees in the Gurbantunggut Desert. J. Hydrol. 610, 127986. doi: 10.1016/j.jhydrol.2022.127986
Danierhan, S., Shalamu, A., Tumaerbai, H., and Guan, D. (2013). Effects of emitter discharge rates on soil salinity distribution and cotton (Gossypium hirsutum L.) yield under drip irrigation with plastic mulch in an arid region of Northwest China. J. Arid Land 5, 51–59. doi: 10.1007/s40333-013-0141-7
Deb, P., Tran, D. A., and Udmale, P. D. (2016). Assessment of the impacts of climate change and brackish irrigation water on rice productivity and evaluation of adaptation measures in Ca Mau province, Vietnam. Theor. App. Climatol. 125, 641–656. doi: 10.1007/s00704-015-1525-8
Ding, X. Y., Zhou, Z. B., Xu, X. W., Wang, Y. D., and Jiang, Y. (2016). Dynamics of soil water and salt in soil under artificial plantation shelterbelt drip-irrigated with saline water in the center of the Taklimakan Desert. Acta. Pedol. Sinica. 53, 103–116. doi: 10.11766/trxb201505130096
Ge, L. P., Ma, J., and Li, Y. (2007). Soil salt and nutrient concentration in the rhizosphere of desert halophytes. Acta. Ecol. Sinica. 27, 3565–3571. doi: 10.1016/S1872-2032(07)60074-2
Gong, J. R., Zhao, A. F., Huang, Y. M., Zhang, X. S., and Zhang, C. L. (2006). Water relations, gas exchange, photochemical efficiency, and peroxidative stress of four plant species in the Heihe drainage basin of northern China. Photosynthetica. 44, 355–364. doi: 10.1007/s11099-006-0036-3
Hao, K., Liu, X. G., Wang, X. K., Fei, L. J., Liu, L. H., Jie, F. L., et al. (2022). Optimizing shade cultivation method and irrigation amount to improve photosynthetic characteristics, bean yield, and quality of coffee in a subtropical monsoon climate. Front. Plant Sci. 13, 848524. doi: 10.3389/fpls.2022.848524
He, P. R., Yu, S. E., Zhang, F. C., Ma, T., Ding, J. H., Chen, K. W., et al. (2022). Effects of soil water regulation on the cotton yield, fiber quality and soil salt accumulation under mulched drip irrigation in southern Xinjiang, China. Agronomy 12, 1246. doi: 10.3390/agronomy12051246
Hong, C., Tong, L., Wang, D. W., and Ji, X. R. (2019). Haloxylon ammodendron's potential distribution under climate change in arid areas of northwest China. J. Desert Res. 39, 110–118. doi: 10.7522/j.issn.1000-694X.2018.00048
Hou, X. H., Fan, J. L., Zhang, F. C., Hu, W. H., Yan, F. L., Xiao, C., et al. (2022). Determining water use and crop coefficients of drip-irrigated cotton in south Xinjiang of China under various irrigation amounts. Ind. Crops Products. 176, 114376. doi: 10.1016/j.indcrop.2021.114376
Hu, M., Ding, R. S., Sun, W. H., and Li, S. E. (2014). Effects of saline water irrigation on physiological and water consumption characteristics of two young shelterbelt plants. J. Irrigation Drainage 33, 145–148. doi: 10.13522/j.cnki.ggps.2014.06.033
Huang, M. Y., Zhang, Z. Y., Sheng, Z. P., Zhu, C. L., Zhai, Y. M., Lu, P. R., et al. (2019). Soil salinity and maize growth under cycle irrigation in coastal soils. Agron. J. 111, 2276–2286. doi: 10.2134/agronj2018.10.0684
Huang, M. Y., Zhang, Z. Y., Xu, H., Zhai, Y. M., Wang, C., Zhu, C. L., et al. (2021). Effects of cycle irrigation with brackish and fresh water and biochar on water salt transports of coastal saline soil. Trans. Chin. Soc. Agri. Eng. 52, 238–247. doi: 10.6041/j.issn.1000-1298.2021.01.027
Huang, P. Y., Li, Q. J., and Yuan, Q. F. (2008). Effects of climate change on Haloxylon ammodendron community in southern edge of Zhunger Basin. Acta. Ecol. Sinica. 28, 6051–6059. doi: 10.3321/j.issn:1000-0933.2008.12.033
Huang, Z. Y., Zhang, X. S., Zheng, G. H., and Gutterman, Y. (2003). Influence of light, temperature, salinity and storage on seed germination of Haloxylon ammodendron. J. Arid Environ. 55, 453–464. doi: 10.1016/S0140-1963(02)00294-X
Li, C. J., Han, H., Ablimiti, M., Liu, R., Zhang, H., Fan, J. L., et al. (2022). Morphological and physiological responses of desert plants to drought stress in a man-made landscape of the Taklimakan desert shelter belt. Ecol. Indic. 140, 109037. doi: 10.1016/j.ecolind.2022.109037
Li, C. J., Tang, J. Y., Gao, P., Sun, Y. Q., and Zhai, Z. Z. (2015). Effect of irrigation with saline water on plant root distribution and evolution of aeolian sandy soil in shelterbelts along the Taklimakan Desert highway. Acta. Pedol. Sinica. 52, 1180–1181. doi: 10.11766/trxb201410300550
Li, J., Fan, J., and Zhu, Z. M. (2020). Effects of activated water irrigation on growth characteristics of soybean under drought stress. Yingyong Shengtai Xuebao 31, 3711–3718. doi: 10.13287/j.1001-9332.202011.028
Li, Z. Z., Qu, Z. Y., Yang, W. (2021). Effects of freezing irrigation with different saline water on soil water infiltration and salt transport in severely saline-alkali soil. J. Soil Water Conserv. 35, 304–311. doi: 10.13870/j.cnki.stbcxb.2021.03.042
Liao, Q., Gu, S. J., Kang, S. Z., Du, T. S., Tong, L., Wood, J. D., et al. (2022). Mild water and salt stress improve water use efficiency by decreasing stomatal conductance via osmotic adjustment in field maize. Sci. Total Environ. 805, 150364. doi: 10.1016/j.scitotenv.2021.150364
Liu, B. X., Wang, S. Q., Kong, X. L., Liu, X. J., and Sun, H. Y. (2019). Modeling and assessing feasibility of long-term brackish water irrigation in vertically homogeneous and heterogeneous cultivated lowland in the North China Plain. Agri. Water Manage. 211, 98–110. doi: 10.1016/j.agwat.2018.09.030
Liu, G. J., Zhang, X. M., Lü, C. Y., Sun, H. Y., Wu, J. X., Deng, C. Z., et al. (2012). Seedling growth dynamic of Haloxylon ammodendron under different water supply. J. Desert Res. 32, 388–394.
Liu, J., Zhao, Y., Zhang, J. G., Hu, Q. L., and Xue, J. (2022). Effects of Irrigation Regimes on soil water dynamics of two typical woody halophyte species in taklimakan desert highway shelterbelt. Water 14, 1908. doi: 10.3390/w14121908
Liu, J. L., Wang, Y. G., Yang, X. H., and Wang, B. F. (2011). Genetic variation in seed and seedling traits of six Haloxylon ammodendron shrub provenances in desert areas of China. Agroforestry Syst. 81, 135–146. doi: 10.1007/s10457-010-9361-4
Liu, L., Jiang, J., and Song, C. W. (2018). Effect of different protection treatment on Haloxylon ammodendron growth and soil moisture. Arid Land Geograph. 41, 564–571. doi: 10.13826/j.cnki.cn65-1103/x.2018.03.015
Liu, X. M., Wang, L., Wei, Y., Zhang, Z. H., Zhu, H., Kong, L. G., et al. (2020). Irrigation with magnetically treated saline water influences the growth and photosynthetic capability of Vitis vinifera L. seedlings. Sci. Hortic. 262, 109056. doi: 10.1016/j.scienta.2019.109056
Liu, Y. H., Su, L. J., Wang, Q. J., Zhang, J. H., Shan, Y. Y., Deng, M. J., et al. (2020). Comprehensive and quantitative analysis of growth characteristics of winter wheat in China based on growing degree days. Adv. Agron. 159, 237–273. doi: 10.1016/bs.agron.2019.07.007
Lu, F. H., Shayiban, W., Liu, S. S., and Xu, G. Q. (2021). Rooting depth determined physiological response of Haloxylon ammodendron to summer drought. Acta. Ecol. Sinica. 41, 1–12. doi: 10.5846/stxb201909282038
Lü, X. P., Gao, H. J., Zhang, L., Wang, Y. P., Shao, K. Z., Zhao, Q., et al. (2019). Dynamic responses of Haloxylon ammodendron to various degrees of simulated drought stress. Plant Physiol. Biochem. 139, 121–131. doi: 10.1016/j.plaphy.2019.03.019
Lü, X. P., Shao, K. Z., Xu, J. Y., Li, J. L., Ren, W., Chen, J., et al. (2022). A heat shock transcription factor gene (HaHSFA1) from a desert shrub, Haloxylon ammodendron, elevates salt tolerance in Arabidopsis thaliana. Environ. Exp. Bot. 201, 104954. doi: 10.1016/j.envexpbot.2022.104954
Ma, K., Wang, Z. H., Li, H. Q., Wang, T. Y., and Chen, R. (2022). Effects of nitrogen application and brackish water irrigation on yield and quality of cotton. Agri. Water Manage. 264, 107512. doi: 10.1016/j.agwat.2022.107512
Ma, L., Wang, Q. J., and Fan, J. (2013). Characteristics and models of spring wheat growth by a modified logistic model in the desert oasis conditions. J. Food Agri. Environ.11, 614–624.
Ma, Q. L., Wang, J. H., and Zhu, S. J. (2007). Effects of precipitation, soil water content and soil crust on artificial Haloxylon ammodendron forest. Acta. Ecol. Sinica. 27, 5057–5067. doi: 10.1016/S1872-2032(08)60014-1
Mostafazadeh-Fard, B., and Khoshravesh, M. Mousa vi, S. F., Kiani, A. R. (2011). Effects of magnetized water and irrigation water salinity on soil moisture distribution in trickle irrigation. J. Irrigation Drainage Eng. 137, 398–402. doi: 10.1061/(ASCE)IR.1943-4774.0000304
Rouhi, V., Samson, R., Lemeur, R., and Van Damme, P. (2007). Photosynthetic gas exchange characteristics in three different almond species during drought stress and subsequent recovery. Environ. Exp. Bot. 59, 117–129. doi: 10.1016/j.envexpbot.2005.10.001
Schenk, H. J., and Jackson, R. B. (2002). Rooting depths, lateral root spreads and below-ground/above-ground allometries of plants in water-limited ecosystems. J. Ecol. 90, 480–494. doi: 10.1046/j.1365-2745.2002.00682.x
Schulze, E. D., Mooney, H. A., Sala, O. E., Jobbagy, E., Buchmann, N., Bauer, G., et al. (1996). Rooting depth, water availability, and vegetation cover along an aridity gradient in Patagonia. Oecologia 108, 503–511. doi: 10.1007/BF00333727
Shan, L. S., Zhang, X. M., Hua, Y. H., Xie, T. T., Yan, H. L., Fan, H., et al. (2009). Response of root distribution of Haloxylon ammodendron seedlings to irrigation amounts in the hinterlands of the Taklimakan Desert, China. Front. Forestry China 4, 60–67. doi: 10.1007/s11461-009-0002-x
Shan, L. S., Zhang, X. M., Wang, Y. K., Wang, H., Yan, H. N., Wei, J., et al. (2008). Influence of moisture on the growth and biomass allocation in Haloxylon ammodendron and Tamarix ramosissima seedlings in the shelterbelt along the Tarim Desert Highway, Xinjiang, China. Chin. Sci. Bullet. 53, 93–101. doi: 10.1007/s11434-008-6010-7
Shao, M. H., Wang, L., Li, B. W., Li, S. Y., Fan, J. L., Li, C. J., et al. (2022). Maxent modeling for identifying the nature reserve of cistanche deserticola ma under effects of the host (Haloxylon Bunge) forest and climate changes in Xinjiang, China. Forests 13, 189. doi: 10.3390/f13020189
Sheng, Y., Zheng, W. H., Pei, K., and Ma, K. (2005). Genetic variation within and among populations of a dominant desert tree Haloxylon ammodendron (Amaranthaceae) in China. Annal. Bot. 96, 245–252. doi: 10.1093/aob/mci171
Shi, S. B., Zhou, D. W., Wang, F. L., Shi, R., Sun, T., Li, T. C., et al. (2022). Sandstorms cause shrinkage of Haloxylon ammodendron shrubs and limit their self-renewal. Theor. Exp. Plant Physiol. 34, 197–214. doi: 10.1007/s40626-022-00242-4
Shi, S. J., Wang, E. T., Li, C. X., Zhou, H., Cai, M. L., Cao, C. G., et al. (2021). Comprehensive evaluation of 17 qualities of 84 types of rice based on principal component analysis. Foods. 10, 2883. doi: 10.3390/foods10112883
Shimokawa, I. (2004). Oxidative stress and calorie restriction. Geriatric. Gerontol. Int. 4, S149–S151. doi: 10.1111/j.1447-0594.2004.00183.x
Song, C. W., Li, C. J., Halik, Ü., Xu, X. W., Lei, J. Q., Zhou, Z. B., et al. (2021). Spatial distribution and structural characteristics for Haloxylon ammodendron plantation on the southwestern edge of the Gurbantünggüt desert. Forests 12, 633. doi: 10.3390/f12050633
Tan, S., Wang, Q. J., Xu, D., Zhang, J. H., and Shan, Y. Y. (2017). Evaluating effects of four controlling methods in bare strips on soil temperature, water, and salt accumulation under film-mulched drip irrigation. Field Crops Res. 214, 350–358. doi: 10.1016/j.fcr.2017.09.004
Tang, H., Xu, C. S., Jiang, Y. M., Wang, J. W., Wang, Z. H., Tian, L. Q., et al. (2021). Evaluation of physical characteristics of typical maize seeds in a cold area of north china based on principal component analysis. Processes 9, 1167. doi: 10.3390/pr9071167
Tennakoon, S. B., and Milroy, S. P. (2003). Crop water use and water use efficiency on irrigated cotton farms in Australia. Agri. Water Manage. 61, 179–194. doi: 10.1016/S0378-3774(03)00023-4
Thevs, N., Wucherer, W., and Buras, A. (2013). Spatial distribution and carbon stock of the Saxaul vegetation of the winter-cold deserts of Middle Asia. J. Arid Environ. 90, 29–35. doi: 10.1016/j.jaridenv.2012.10.013
Tian, Y., Tashpolat, T., Li, Y., Tang, L. S., and Fan, L. L. (2014). The survival and above/below ground growth of Haloxylon ammodendron seedling. Acta Ecol. Sinica. 34, 2012–2019. doi: 10.5846/stxb201309092241
Tobe, K., Li, X. M., and Omasa, K. (2000). Effects of sodium chloride on seed germination and growth of two Chinese desert shrubs, Haloxylon ammodendron and H. persicum (Chenopodiaceae). Aust. J. Bot. 48, 455–460. doi: 10.1071/BT99013
Toledo, E. J., Ramalho, T. C., and Magriotis, Z. M. (2008). Influence of magnetic field on physical–chemical properties of the liquid water: insights from experimental and theoretical models. J. Mol. Struct. 888, 409–415. doi: 10.1016/j.molstruc.2008.01.010
Wang, H. D., Wu, L. F., Cheng, M. H., Fan, J. L., Zhang, F. C., Zou, Y. F., et al. (2018). Coupling effects of water and fertilizer on yield, water and fertilizer use efficiency of drip-fertigated cotton in northern Xinjiang, China. Field Crops Res. 219, 169–179. doi: 10.1016/j.fcr.2018.02.002
Wang, L., Guo, J., Bi, S., Zhang, Z., Wang, H., Liu, X., et al. (2019). Effects of irrigation with magnetized saline water on Vitis vinifera growth and soil mineral nutrients. J. Fruit Sci. 36, 1683–1692. doi: 10.13925/j.cnki.gsxb.20190048
Wang, Q. J., Sun, Y., Ning, S. R., Zhang, J. H., Zhou, B. B., Su, L. J., et al. (2019). Effects of activated irrigation water on soil physicochemical properties and crop growth and analysis of the probable pathway. Adv. Earth Sci. 34, 660. doi: 10.11867/j.issn.1001-8166.2019.06.0660
Wang, Q. J., Xie, J. B., and Zhang, J. H. (2020). Effects of magnetic field strength on magnetized water infiltration and soil water and salt movement. Trans. Chin. Soc. Agri. Eng. 51, 292–298. doi: 10.6041/j.issn.1000-1298.2020.02.032
Wang, R. S., Kang, Y. H., Wan, S. Q., Hu, W., Liu, S. P., Liu, S. H., et al. (2011). Salt distribution and the growth of cotton under different drip irrigation regimes in a saline area. Agri. Water Manage. 100, 58–69. doi: 10.1016/j.agwat.2011.08.005
Wang, X. K., Guo, T., Wang, Y., Xing, Y. Y., Wang, Y. F., He, X. L., et al. (2020). Exploring the optimization of water and fertilizer management practices for potato production in the sandy loam soils of Northwest China based on PCA. Agri. Water Manage. 237, 106180. doi: 10.1016/j.agwat.2020.106180
Wang, X. Q., Jiang, J., Lei, J. Q., Zhang, W. M., and Qian, Y. B. (2003). The distribution of ephemeral vegetation on the longitudinal dune surface and its stabilization significance in the Gurbantunggut Desert. Acta. Geograph. Sinica. 58, 598–605. doi: 10.3321/j.issn:0375-5444.2003.04.015
Wang, X. X., Wang, Q. J., Fan, J., and Su, L. J. (2014). Logistic model analysis of winter wheat growth on China's Loess Plateau. Can. J. Plant Sci. 94, 1471–1479. doi: 10.4141/cjps2013-293
Wang, Z., Fan, B., and Guo, L. (2019). Soil salinization after long-term mulched drip irrigation poses a potential risk to agricultural sustainability. Eur. J. Soil Sci. 70, 20–24. 10 5 doi: 10.1111/ejss.12742
Wang, Z. F., Hei, X. L., Chen, S. F., and Lei, C. X. (2010). Haloxylon ammodendron in its infancy with different salinity under brackish water irrigation. J. Shihezi Univ. 28, 366–370. doi: 10.13880/j.cnki.65-1174/n.2010.03.019
Wei, C. C., Ren, S. M., Xu, Z. A., Zhang, M. T., Wei, R., Yang, P. L., et al. (2021). Effects of irrigation water salinity and irrigation water amount on greenhouse gas emissions and spring maize growth. Trans. Chin. Soc. Agric. Machinery 52, 251–260. doi: 10.6041/j.issn.1000-1298.2021.07.027
Wei, Y., Wang, L., Zhu, H., Meng, S. Y., Zhang, Z. H., Wang, Q., et al. (2020). Effects of magnetized water irrigation on growth and photosynthetic characteristics of grape under nitrogen application. J. Nuclear Agri. Sci. 34, 849–859. doi: 10.11869/j.issn.100-8551.2020.04.0849
Xin, X., Pang, S. S., Miguel, Mercader, D. E., and Torr, F. K. M. (2019). The effect of biomass pretreatment on catalytic pyrolysis products of pine wood by Py-GC/MS and principal component analysis. J. Anal. App. Pyrol. 138, 145–153. doi: 10.1016/j.jaap.2018.12.018
Xu, H., Li, Y., Xu, G. Q., and Zou, T. (2007a). Ecophysiological response and morphological adjustment of two Central Asian desert shrubs towards variation in summer precipitation. Plant Cell Environ. 30, 399–409. doi: 10.1111/j.1365-3040.2006.001626.x
Xu, H., Li, Y., Zou, T., Xie, J. X., and Jiang, L. X. (2007b). Ecophysiological response and morphological adjustment of Haloxylon ammodendron towards variation in summer precipitation. Acta. Ecol. Sinica. 27, 5019–5028. doi: 10.3321/j.issn:1000-0933.2007.12.010
Yan, H. L., Zhang, X. M., and Xu, H. (2010). Photosynthetic characteristics responses of three plants to drought stress in Tarim Desert Highway shelterbelt. Acta Ecol. Sinica. 30, 2519–2528. doi: 10.1016/S1872-5813(11)60001-7
Yang, G., Liu, S. H., Yan, K., Tian, L. J., Li, P. F., Li, X. L., et al. (2020). Effect of drip irrigation with brackish water on the soil chemical properties for a typical desert plant (Haloxylon Ammodendron) in the Manas River Basin. Irrigation Drainage 69, 460–471. doi: 10.1002/ird.2419
Yang, S. R., Fan, J. W., Sun, Y. Q., Li, C. J., Xu, X. W., Fan, J. L., et al. (2018). Photosynthetic characteristics and response of Haloxylon ammodendron to drought stress in hinterland of the lop nur. Arid Zone Res. 35, 379–386. doi: 10.13866/j.azr.2018.02.16
Yang, W. B., Feng, W., Jia, Z. Q., Zhu, Y. J., and Guo, J. Y. (2014). Soil water threshold for the growth of Haloxylon ammodendron in the Ulan Buh desert in arid northwest China. South Afr. J. Bot. 92, 53–58. doi: 10.1016/j.sajb.2014.02.001
Yin, C. H., Dong, J. Z., Shi, Q. M., Zhang, K., Zhao, Z. Y., Tian, C. Y., et al. (2012). Salt island effect of Halophytic shrubs in different habitats and its ecological implication. Acta. Pedol. Sinca. 49, 289–295. doi: 10.11766/10.11766/trxb201011080463
Yuan, C. F., Feng, S. Y., Huo, Z. L., and Ji, Q. Y. (2019). Effects of deficit irrigation with saline water on soil water-salt distribution and water use efficiency of maize for seed production in arid Northwest China. Agri. Water Manage. 212, 424–432. doi: 10.1016/j.agwat.2018.09.019
Zahedi, M., and Jenner, C. F. (2003). Analysis of effects in wheat of high temperature on grain filling attributes estimated from mathematical models of grain filling. J. Agri. Sci. 141, 203–212. doi: 10.1017/S0021859603003411
Zhang, D. M., Luo, Z., Liu, S. H., Li, W. J., Tang, W., Dong, H. Z., et al. (2016). Effects of deficit irrigation and plant density on the growth, yield and fiber quality of irrigated cotton. Field Crops Res. 197, 1–9. doi: 10.1016/j.fcr.2016.06.003
Zhang, G. Q., Liu, C. W., Xiao, C. H., Xie, R. Z., Ming, B., Hou, P., et al. (2017). Optimizing water use efficiency and economic return of super high yield spring maize under drip irrigation and plastic mulching in arid areas of China. Field Crops Res. 211, 137–146. doi: 10.1016/j.fcr.2017.05.026
Zhang, G. Q., Shen, D. P., Ming, B., Xie, R. Z., Jin, X. L., Liu, C. W., et al. (2019). Using irrigation intervals to optimize water-use efficiency and maize yield in Xinjiang, northwest China. Crop J. 7, 322–334. doi: 10.1016/j.cj.2018.10.008
Zhang, L. Y., and Chen, C. D. (2002). On the general characteristics of plant diversity of Gurbantunggut Sandy Desert. Acta. Ecol. Sinica. 22, 1923–1932. doi: 10.3321/j.issn:1000-0933.2002.11.018
Zhang, R. X., Chu, G. X., and Wang, W. B. (2013). Effects of magnetized water on salt leaching under drip irrigation condition. Xinjiang Agri. Sci. 50, 1656–1661. doi: 10.6048/j.issn.1001-4330.2013.09.013
Zhang, T. T., Fan, J. L., Wang, S. M., Xu, X. W., and Chai, C. H. (2020). Photosynthetic characteristics of Haloxylon ammodendron under high salinity water irrigation. J. Desert Res. 40, 112–119. doi: 10.7522/j.issn.1000-694X.2020.00023
Zhang, Y. H., Xie, P., Pu, Y. R., and Wang, P. (2022). Coupling relationship between meteorological factors and precipitation: an empirical study from Xinjiang Province, China. Theor. App. Climatol. 149, 761–771. doi: 10.1007/s00704-022-04080-x
Zhao, G. Q., Mu, Y., Wang, Y. H., and Wang, L. (2021). Response of winter-wheat grain yield and water-use efficiency to irrigation with activated water on Guan zhong Plain in China. Irrigation Sci. 39, 263–276. doi: 10.1007/s00271-020-00706-y
Zhao, G. Q., Mu, Y., Wang, Y. H., and Wang, L. (2022). Magnetization and oxidation of irrigation water to improve winter wheat (Triticum aestivum L.) production and water-use efficiency. Agri. Water Manage. 259, 1–16. doi: 10.1016/j.agwat.2021.107254
Zhao, L. M., Gu, C. M., Wang, S. Q., Wang, L. P., Wang, H., Na, Y. G., et al. (2016). Effects of magnetized water on growth of rice seedlings in solar greenhouse. J. Irrigation Rainage 35, 34–38. doi: 10.13522/j.cnki.ggps.2016.12.007
Zhou, B. B., Yang, L., Chen, X. P., Ye, S., Peng, Y., Liang, C., et al. (2021). Effect of magnetic water irrigation on the improvement of salinized soil and cotton growth in Xinjiang. Agri. Water Manage. 248, 106784. doi: 10.1016/j.agwat.2021.106784
Zhou, D. M., Si, J. H., He, X. H., Jia, B., Zhao, C. Y., Wang, C. L., et al. (2022). The process of soil desiccation under Haloxylon ammodendron Plantations: a case study of the Alxa Legue Desert, China. Plants 11, 235. doi: 10.3390/plants11030235
Zhou, G. W., Zhang, W., Ma, L. J., Guo, H. J., Min, W., Liao, N., et al. (2016). Effects of saline water irrigation and N application rate on NH3 volatilization and N use efficiency in a drip-irrigated cotton field. Water Air Soil Pollut. 227, 1–17. doi: 10.1007/s11270-016-2806-2
Zhu, M. J., Wang, Q. J., Sun, Y., and Zhang, J. H. (2021). Effects of oxygenated brackish water on germination and growth characteristics of wheat. Agri. Water Manage. 245, 106520. doi: 10.1016/j.agwat.2020.106520
Keywords: magnetized brackish water, Haloxylon ammodendron, soil salt distribution, water consumption, logistic growth function
Citation: Guo Y, Wang Q, Zhao X, Li Z, Li M, Zhang J and Wei K (2022) Field irrigation using magnetized brackish water affects the growth and water consumption of Haloxylon ammodendron seedlings in an arid area. Front. Plant Sci. 13:929021. doi: 10.3389/fpls.2022.929021
Received: 26 April 2022; Accepted: 03 August 2022;
Published: 25 August 2022.
Edited by:
Victoria Fernandez, Polytechnic University of Madrid, SpainReviewed by:
Walid Zorrig, Center of Biotechnology of Borj Cedria (CBBC), TunisiaYan Li, Zhejiang Agriculture and Forestry University, China
Copyright © 2022 Guo, Wang, Zhao, Li, Li, Zhang and Wei. This is an open-access article distributed under the terms of the Creative Commons Attribution License (CC BY). The use, distribution or reproduction in other forums is permitted, provided the original author(s) and the copyright owner(s) are credited and that the original publication in this journal is cited, in accordance with accepted academic practice. No use, distribution or reproduction is permitted which does not comply with these terms.
*Correspondence: Quanjiu Wang, d3F1YW5qaXVAMTYzLmNvbQ==