- 1Department of Plant Protection, Faculty of Agriculture and Natural Resources, University of Mohaghegh Ardabili, Ardabil, Iran
- 2Department of Chemistry, Faculty of Science, University of Mohaghegh Ardabili, Ardabil, Iran
- 3Department of Plant Sciences, Moghan College of Agriculture and Natural Resources, University of Mohaghegh Ardabili, Ardabil, Iran
The utilization of plant essential oils (EOs) and nanomaterials due to their safety compared with synthetic chemicals has been considered in the management of plant diseases. In this study, the inhibitory effects of Zataria multiflora, Nepeta haussknechtii, Artemisia sieberi, and Citrus aurantifolia EOs in pure and Zinc Oxide (ZnO) nanocapsulated formulations were evaluated on the mycelial growth of Alternaria solani to find a suitable alternative for synthetic chemicals. The crystal structure and morphological properties of the fabricated nanomaterials were assessed via X-ray diffraction (XRD) and scanning electron microscope (SEM) analyses. The textural features of the prepared nanoparticles were investigated with Brunauer–Emmett–Teller (BET) analysis, and the presence of elements in the samples was studied with energy-dispersive X-ray (EDX) technique. The mycelial growth inhibitory (MGI) was performed in the laboratory by mixing with potato dextrose agar (PDA) medium at concentrations of 100, 300, 600, 1,000, 1,500, and 2,000 ppm. Based on the results, major differences were monitored between different concentrations. At the highest studied concentration, the inhibition of Z. multiflora EO was 100%, which was 43.20, 42.37, and 21.19% for N. haussknechtii, A. sieberi, and C. aurantifolia, respectively, and the inhibition of their nanocapsules was 100, 51.32, 55.23, and 26.58%, respectively. In the greenhouse study, Z. multiflora EO and its nanocapsule (ZnO-ZmEO) were compared with the ZnO and chlorothalonil fungicide based on the highest inhibitory of Z. multiflora in vitro. The highest antifungal effect was related to the ZnO-ZmEO by 53.33%. Therefore, the ZnO-ZmEO formulation can be recommended as a biofungicide for managing and controlling tomato early blight disease after further research.
Introduction
Tomatoes are one of the most vital vegetable crops in the human diet worldwide due to the presence of carotene (a precursor to vitamin A), a variety of vitamins, including vitamin C, ascorbic acid, and lycopene, which is one of the strongest types of natural antioxidants. Their importance to human health has been proved (Rao and Agarwal, 2000; Jacob et al., 2008). Early blight is a typical tomato fungal disease caused by Alternaria solani Sorauer, which is usually identified by the occurrence of brown to dark brown necrotic lesions, having concentric rings and drying and falling leaves prematurely, and causes quantitative and qualitative damage to the product, if not adequately managed (Chohan et al., 2015). The development of elliptical to dark brown to black cankers on the stem is another obvious symptom of this disease (El-Nagar et al., 2020).
The most common method of plant diseases management is the utilization of synthetic chemicals that are often degraded slowly in nature, and since tomatoes are commonly eaten fresh, the residual risks of these chemicals on this product are of particular importance to human health (Raza et al., 2016). Also, the application of fungicides for controlling fungal plant diseases has led to the emergence of resistant races of fungal pathogens and has resulted in more health hazards such as increasing the risk of cancer and environmental pollution (Narayanasamy, 2002; Gupta and Dikshit, 2010). Nowadays, the application of essential oils (EOs) in plant disease management has been recommended as a useful and safe approach and has been considered to minimize the side effects of synthetic chemicals, and the antifungal and antimicrobial effects of several plant compounds on some plant pathogens have been proved (Beyki and Alizadeh, 2006; Davari and Ezazi, 2017; Raveau et al., 2020). In addition to being able to control plant diseases, these compounds prevent the destruction of ecological balance (Hasanzadeh, 2005; Kizil and Uyar, 2006). In spite of having many advantages, the disadvantages of EOs are high cost, physical and chemical instability, low water solubility, decomposition with oxygen, and high vapor pressure. These compounds also have organoleptic effects and cause taste and odor in products, which are not to the satisfaction of the consumers (Donsì et al., 2011). One of the best approaches to resolve the difficulties related to the EOs’ instability and volatility is nanoencapsulation (Pavoni et al., 2020). Nanomaterials protect EOs against interactions with other compounds and increase the stability of volatile compounds and antimicrobial properties through cell adsorption (Donsì et al., 2011). Also, nanoparticles can lead to effective delivery and valid release in soil-borne disease control and management (Kutawa et al., 2021).
Nanomaterials and EOs have been widely investigated for their antifungal potential in numerous reports. In particular, EOs of various medicinal plants [Tagetes erecta L., Zataria multiflora Boiss, Melaleuca alternifolia (Maiden & Betche) Cheel] have shown beneficial antifungal effects against A. solani (Hendges et al., 2021; Karimi and Meiners, 2021; Mugao et al., 2021). The antifungal properties of Fe3O4/ZnO/AgBr nanocomposites against Fusarium oxysporum Schltdl. and F. graminearum Schwabe were proved (Hoseinzadeh et al., 2016). The antifungal characteristics of TiO2/AgBr were assessed through the inhibition of Sclerotinia sclerotiorum (Lib.) de Bary, Botrytis cinerea Pers., and F. graminearum, and the findings revealed that the TiO2/AgBr materials have better antifungal activities on F. graminearum spores than the pure TiO2 (Habibi-Yangjeh et al., 2021). Moreover, zinc oxide nanoparticles (ZnO NPs) have been employed in many antifungal assessments, and they presented the important potential to diminish the growth of phytopathogenic fungi (Kalia et al., 2020). The mechanism of the inhibitory effect of ZnO NPs on microorganisms has not been understood. Various studies have reported that the integration of ZnO NPs increased carbohydrate and nucleic acid contents of fungi. The increase in nucleic acid may be due to stress response of fungal hyphae. The increase in carbohydrates may be due to the self-protecting mechanism against the ZnO NPs (He et al., 2011). Also, the antifungal mechanisms provided by metal NPs can be related to a disturbance of fungal cell membrane integrity, and with the generation of reactive oxygen species (ROS), which enhances the membrane disintegration process (Kumari et al., 2019). The antifungal properties of encapsulated Thymus daenensis L. and Anethum graveolens L. EOs in copper nanomaterials against the phytopathogenic agent Colletotrichum nymphaeae (Pass.) Aa showed that thyme and dill EOs’ encapsulation with copper nanomaterials led to an effective 90% reduction in the mycelium growth (Weisany et al., 2019). Therefore, in this study, the antifungal effects of the EOs extracted from some Iranian medicinal plants, including Z. multiflora, N. haussknechtii Bornm., Artemisia sieberi L., Citrus aurantifolia Swingle, and their nanocapsulated formulations by ZnO were evaluated to find out the fungus causal agent of tomato early blight disease.
Materials and Methods
Preparation of Pathogenic Fungus
After visiting the tomato fields in Bilehsavar, Parsabad, and Ardabil (Samian region) cities of Ardabil province, the samples from leaves showing signs of early blight disease were collected during the summer of 2020. After surface sterilization by dipping in 0.5% sodium hypochlorite, rinsing three times in distilled water, and drying in a sterile filter paper, the samples were plated on potato dextrose agar (PDA) medium. A. solani was detected by colony color, mycelial growth appearance, and microscopic morphological characteristics based on the identification key (Yadav, 2015).
Preparation and Analysis of Essential Oils
Aerial parts of N. haussknechtii (Pune-sa) were obtained from the Heiran region of Ardabil, and A. sieberi (White wormwood) were collected from around Shurabil lake of Ardabil during the spring and summer of 2020. The plants were identified using Iranian flora sources, including flora Iranica (Rechinger, 1963–2015) and Iranian flora (Assadi, 1987–2018). Then, the dried plant samples were ground and 50 g of each sample was placed in a Clevenger-type instrument and the related EO was achieved by steam distillation method within 3 h. The as-obtained EOs were placed in dark vials at 4°C for further experiments and bioassays. C. aurantifolia (lime) and Z. multiflora (Shirazi thyme) EOs were purchased from Tabib Daroo Company.
To determine the chemical constituents of EOs, a gas chromatograph (HP-7890B) linked to a mass spectrometer (Agilent-MSD5975C) was used. Helium (99.99%) was applied as a carrier gas at a flow rate of 1 ml/min. The temperature of the injector was maintained at 250°C and a determined amount of EO was introduced. The EOs’ components were determined by employing various factors like retention time, comparing the mass spectra with those of standards such as Wiley 7n 0.1 (Wiley, NY, United States) and NIST (Standard Reference Data, Gaithersburg) (Adams, 2007; Enayati et al., 2021).
Preparation of ZnO and Nanoencapsulation of Essential Oils With ZnO
For the nanoencapsulation of EOs with ZnO, 1.8 g Zn (NO3)2.6H2O (Loba Chemie, India) was dissolved in 90 ml of water. Then, the EO (1 ml) mixed with ethanol (9 ml) was appended to the obtained solution and stirred for 60 min. Next, the pH of the solution was regulated to 10 by NaOH (1 M). The obtained precipitate was ultrasonicated for 120 min (Bandelin model HD 3100). The obtained material was rinsed with H2O and ethanol, and the sample was dried (Kedia and Kishore Dobey, 2018).
For fabrication of pure ZnO, 1.8 g Zn (NO3)2.6H2O was introduced into deionized H2O (100 ml) and stirred for 30 min. Thereafter, the pH of solution was regulated to 10 by NaOH (1 M). The solution was ultrasonicated for 2 h. The precipitate was rinsed and dried (Pirhashemi and Habibi-Yangjeh, 2017).
Characterization
The N2 adsorption–desorption isotherms were conducted using BELSORP mini II instrument. To evaluate the morphology of materials, scanning electron microscope (SEM) (LEO 1430VP) instrument was utilized. The thermal properties of samples were characterized by thermogravimetric analysis (TGA) (HORIBA model, Japan). The Fourier transform infrared (FT-IR) spectra were collected via a Perkin Elmer Spectrum RX I. The phase information of the samples was determined using a Philips Xpert diffractometer. X-ray energy-dispersive analysis (Rontec GmbH, Germany) was conducted to determine the chemical structure of the materials. A density meter (Anton paar DMA 4500M) was applied to measure the density of compounds. To explore the size of particles, a dynamic light scattering, HORIBA instrument was employed (Enayati et al., 2021).
In vitro Antifungal Study
Antifungal effect was performed in vitro on A. solani by adding various concentrations of EOs to PDA culture media. The EOs’ emulsion was obtained via Tween 80 (0.05% v/v) and mixed with PDA at 40–45°C to achieve concentrations of 100, 300, 600, 1,000, 1,500, and 2,000 μl/L for each EO. Thereafter, the mixture was introduced into 9 cm Petri dishes and coagulated. A mycelial disc (5 mm) of 7-day-old cultures of A. solani was located on the Petri dishes, and in control ones, only Tween 80 was added. The experiment was performed in three replications. Inoculated plates were sealed to hinder evaporation of each EOs and incubated at 25°C. After 48 h, the measurement of the mycelial colonies growth rate was started and continued daily until the fungus grown in the control plates filled them. The inhibitory percentage of various concentrations of EOs and nanocapsules of EOs was calculated via Abbott’s formula: IP = (C − T/C) × 100, where IP is the percentage of inhibition, C and T are the mean diameter of fungal colony in control and treated plates, respectively. For investigating the fungicide or fungistatic activity of EOs and EO nanocapsules, mycelial disks with no growth were added to PDA media without treatment and tested after 7 days (Davari and Ezazi, 2017; Enayati et al., 2021).
In vivo Antifungal Study
Tomato seeds (Solanum lycopericum L.) cultivar Super Strain B were used. The inoculum was prepared by culturing A. solani on PDA culture medium (27°C) for 15 days. Then, after adding sterile distilled H2O (10 ml) to different plates, the obtained colonies were precisely scraped with a sterile needle. The obtained conidial fungal suspension was adjusted to 5 × 106 spores/ml and employed for the inoculation. The plants were covered with polyethylenic bags for 2 days to provide high humidity conditions after inoculation. Thereafter, the bags were removed and plants were maintained under greenhouse conditions (Nashwa and Abo-Elyousr, 2013). After the onset of early symptoms (about 10 days), the most effective concentrations of Z. multiflora, ZnO-ZmEO (ZnO-Z. multiflora EO), and ZnO in five repetitions and three concentrations were evaluated under greenhouse conditions. To evaluate the effect of these materials, a healthy control treatment (without infection) and an infected control treatment (by phytopathogenic fungus only) were considered. The study was performed in a completely randomized design with five replications. The intensity of the disease was recorded 2 weeks after inoculation following the score chart from 0 to 9 scales according to Latha et al. (2009): 0 = healthy; 1 = 1–5%; 3 = 6–10%; 5 = 11–25%; 7 = 26–50%; and 9 = >51% of the leaf area infected. Percent disease index (PDI) was computed based on McKinney (1923): PDI = (Σ of ratings of infected leaves observed/no. of leaves observed × maximum disease grade) × 100.
Statistical Analysis
The analyses were done using SPSS v. 24 (IBM, Chicago, IL, United States). Data were subjected to one-way analysis of variance (ANOVA), and the comparison of means for growth inhibition percentages of A. solani was done using Tukey’s post hoc test (p < 0.05). The software was also used to calculate IP50 (concentration required to inhibit 50% mycelial growth) along with concentration-dependent regression line details and χ2 test to evaluate data heterogeneity.
Results
Chemical Composition of Essential Oils
The analysis of Z. multiflora EO identified thymol (47.08%), γ-terpinene (17.27%), p-cymene (11.95%), and carvacrol (11.63%) as the main compounds. Nepetalactone (17.78%), 1,8-cineole (13.6%), and 2-methylbicyclo[3.3.1]nonane (10.05%) were the major components of the N. haussknechtii EO. β-Thujone (20.01%), 1,8-cineole (16.25%), and camphor (12.52%) were recorded as the most abundant components in A. sieberi EO. Furthermore, limonene (32.26%), α-terpineol (12.49%), and γ-terpinene (9.31%) were the main compounds in C. aurantifolia EO (Table 1).
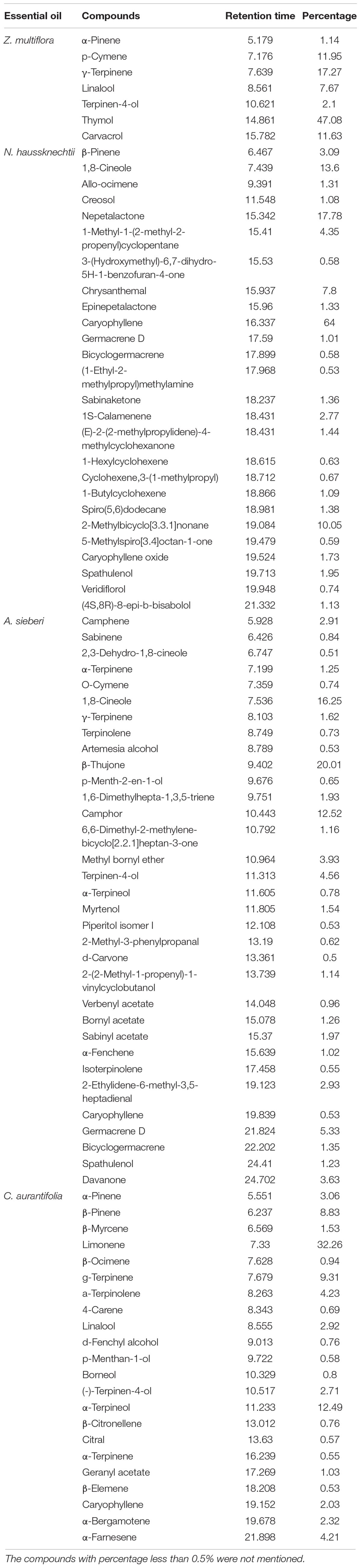
Table 1. Type and percentages of main components identified in the essential oil of Z. multiflora, N. haussknechtii, A. sieberi, and C. aurantifolia.
Characterization of the Materials
The density of Z. multiflora, N. haussknechtii, A. sieberi, and C. aurantifolia EOs was calculated as 0.92, 1.01, 0.92, and 0.86 g/ml, respectively.
The X-ray diffraction (XRD) patterns were recorded to identify the crystalline nature of the nanomaterials, and the obtained patterns are demonstrated in Figure 1A. As shown, the diffraction peaks correspond to the hexagonal crystalline structure of ZnO and are attributed to the JCPDS reference number 080-0075, confirming that encapsulations with the EOs have not changed the phase structure of ZnO particles (Beshkar et al., 2022). The absence of other diffraction peaks reveals the high purity of the fabricated materials.
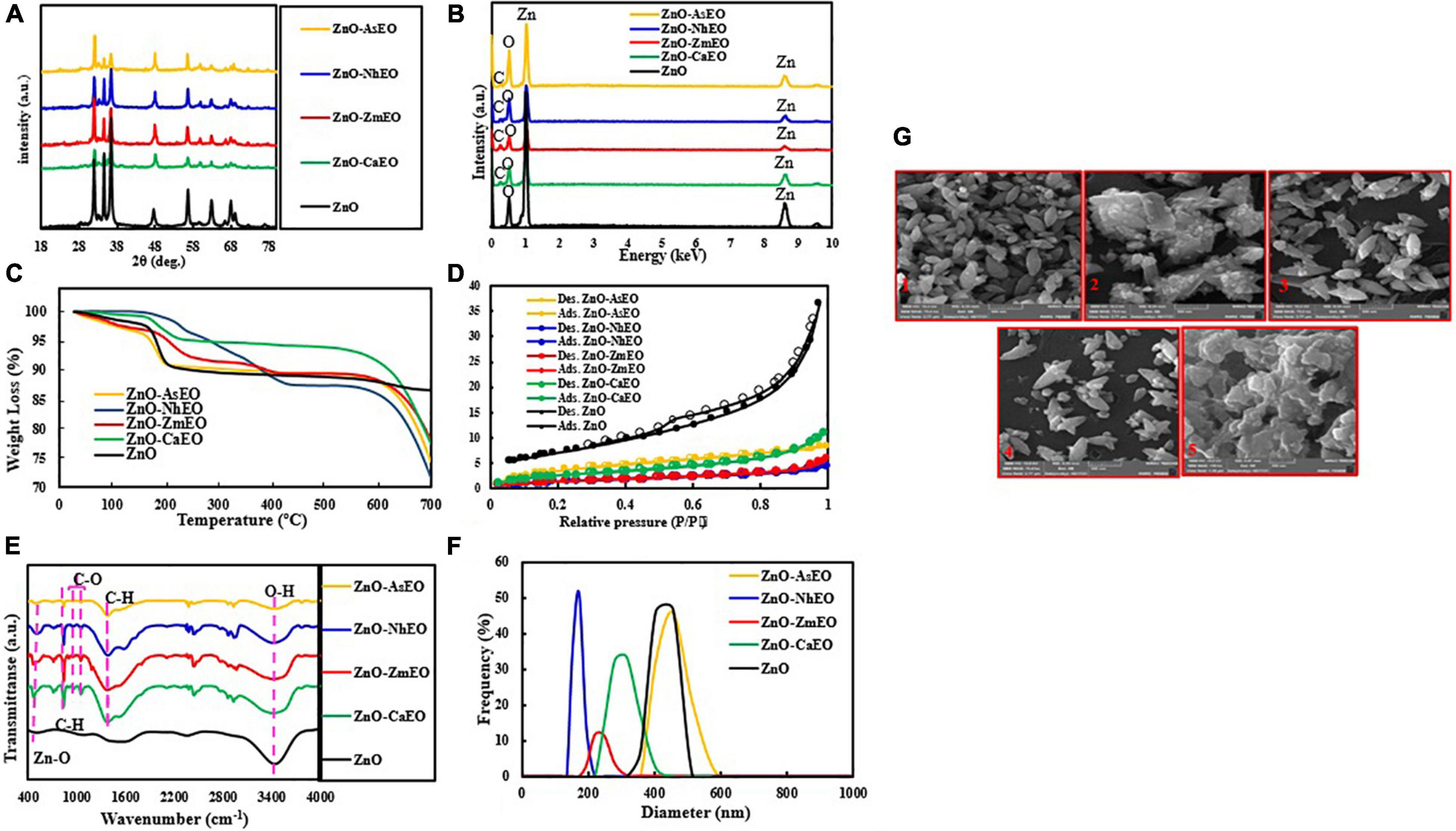
Figure 1. (A) XRD patterns, (B) EDS spectra, (C) TGA diagrams, (D) BET plots, (E) FT-IR spectra, and (F) DLS analyses of the ZnO and ZnO-EO samples, and (G) SEM images of the prepared samples: 1, ZnO; 2, ZnO-CaEO; 3, ZnO-ZmEO; 4, ZnO-NhEO; and 5, ZnO-AsEO.
The elemental characterization of the ZnO and ZnO-EO was studied by energy-dispersive X-ray spectroscopy (EDS) analysis, and the related spectra are seen in Figure 1B. The Zn and O peaks were displayed in the EDS of the ZnO NPs. The EDS spectra of the ZnO-ZmEO, ZnO-NhEO (ZnO-N. haussknechtii EO), ZnO-AsEO (ZnO-A. sieberi EO), and ZnO-CaEO (ZnO-C. aurantifolia EO) samples (Z. multiflora, N. haussknechtii, A. sieberi, and C. aurantifolia nanocapsules, respectively) demonstrate the presence of Zn, C, and O elements. The obtained spectra manifested that the EO molecules are successfully merged with ZnO particles.
Thermogravimetric analysis is an efficient method to evaluate the thermal stability of the prepared materials. As manifested in Figure 1C, the ZnO-EO samples lose more weight than the pure ZnO sample. For the pure ZnO, the weight loss is about 13.4%, which is because of the loss of adsorbed water molecules. Weight loss in biologically synthesized ZnO samples is owing to the loss of EOs as well as loss of adsorbed water molecules. From these analyses, the contents of Z. multiflora, C. aurantifolia, A. sieberi, and N. haussknechtii in the ZnO-EO samples are approximately 21.8, 22.5, 25.6, and 28.3%, respectively.
The Brunauer–Emmett–Teller (BET) test has been examined to get information on the surface area of the materials. The BET curves of ZnO, ZnO-ZmEO, ZnO-NhEO, ZnO-AsEO, and ZnO-CaEO samples are illustrated in Figure 1D. The N2 adsorption–desorption curves of the materials are consistent with the type II isotherm and mesoporous structure. Specific surface areas for ZnO, ZnO-ZmEO, ZnO-CaEO, ZnO-NhEO, and ZnO-AsEO samples were 25.5, 5.23, 9.83, 5.77, and 14.6 m2/g, respectively. The textural characteristics of the materials were estimated by BET and Barrett–Joyner–Halenda models and the outcomes tabulated (see Table 2).
To clarify the functional groups of the materials, FT-IR analysis was performed and the resulting spectra are shown in Figure 1E. In the spectra of ZnO and ZnO-EO materials, the peak at 3,400 cm–1 is assigned to the adsorbed H2O on the material’s surface. Moreover, the absorption band centered at 560 cm–1 belongs to the stretching vibration of Zn–O groups (Xue et al., 2022). For the ZnO-EO materials, the absorption bands at 840 and 1,380 cm–1 are ascribed to the C–H bending; besides, the bands at 960 and 1,052 cm–1 are related to the stretching vibration of C–O groups (Ertani et al., 2018; Luque et al., 2018; Wan Mat Khalir et al., 2020).
The particle size distribution of the ZnO, ZnO-ZmEO, ZnO-CaEO, ZnO-NhEO, and ZnO-AsEO materials was obtained by dynamic light scattering technique, and the results are given in Figure 1F and Table 3.
To evaluate the morphology of the materials, SEM images were provided. Figure 1G manifested the SEM images of the ZnO, ZnO-ZmEO, ZnO-CaEO, ZnO-NhEO, and ZnO-AsEO samples. As observed in these images, the ZnO (Figure 1G1), ZnO-ZmEO (Figure 1G3), and ZnO-NhEO (Figure 1G4) samples have spindle-shaped structures.
In vitro Antifungal Study
Macroscopic images of the mycelial growth inhibitory (MGI) effect of EOs tested on A. solani are shown in Figure 2. It can be found that as the concentration of Z. multiflora EO and ZnO-ZmEO increased, the rate of A. solani growth inhibition was augmented (Figures 2A,B). Growth inhibition percentages caused by concentrations of 1,500 and 2,000 ppm from the pure Z. multiflora EO were placed in different statistical groups in accordance with Tukey’s test (p < 0.05), but there was no significant difference in nanocapsulated formulation. Both Z. multiflora EO and its nanocapsule caused 100% growth inhibition percentage and had a fungistatic effect on the seventh day (Figures 3, 4). The results of Probit analysis showed that the calculated IP50 values for Z. multiflora EO and ZnO-ZmEO were 471.6 and 323.03 ppm, respectively. In other words, nanocapsulated EO had high antifungal activity due to lower IP50 (Table 4).
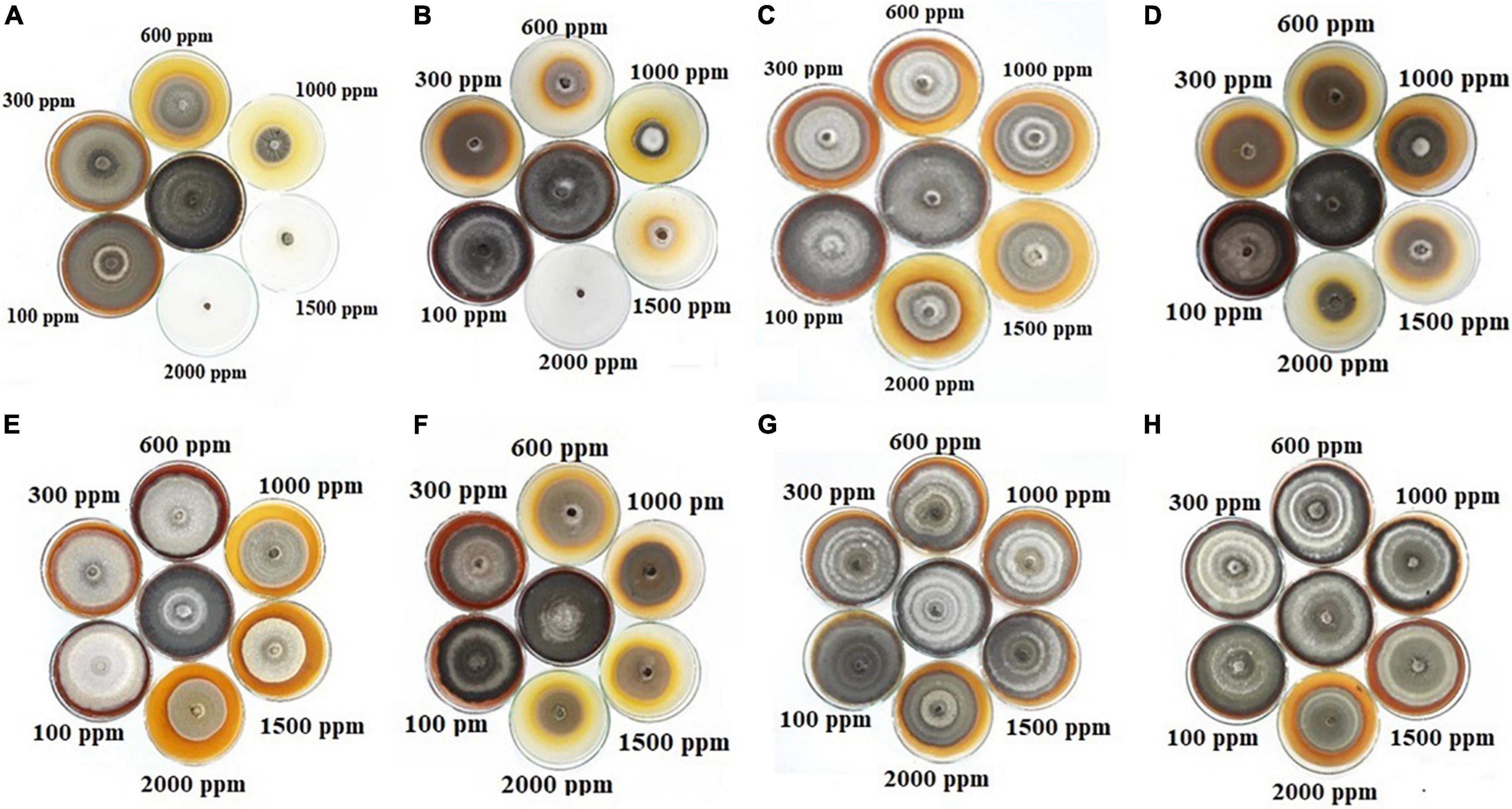
Figure 2. Antifungal activity of EOs and nanocapsules on the growth rate of A. solani at various concentrations of 0–2,000 ppm: (A) Z. multiflora, (B) ZnO-ZmEO, (C) N. haussknechtii, (D) ZnO-NhEO, (E) A. sieberi, (F) ZnO-AsEO, (G) C. aurantifolia, and (H) ZnO-CaEO.
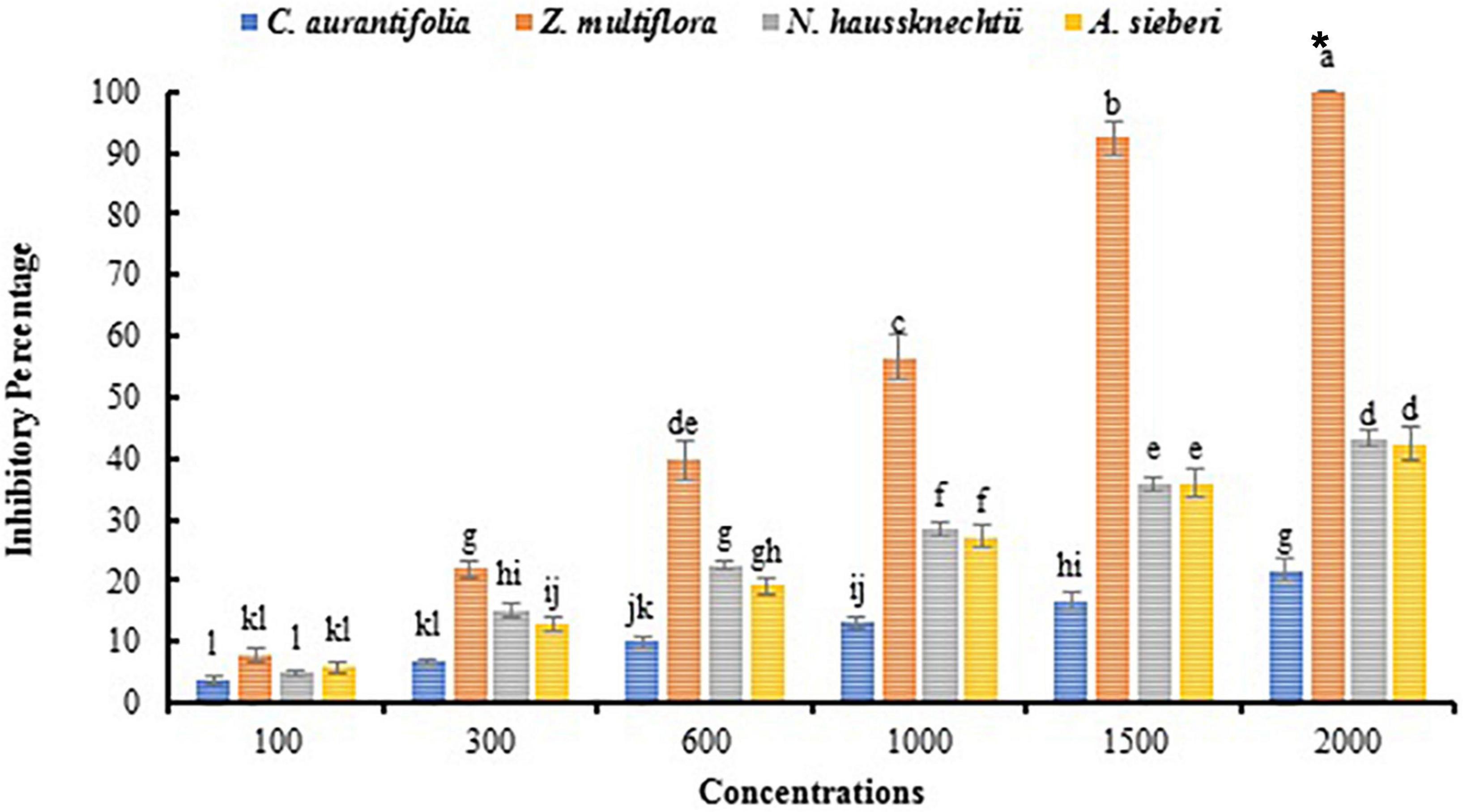
Figure 3. Comparison of mean inhibitory percentage of A. solani mycelial growth with EOs in vitro. The symbol “*” indicates the fungistatic property.
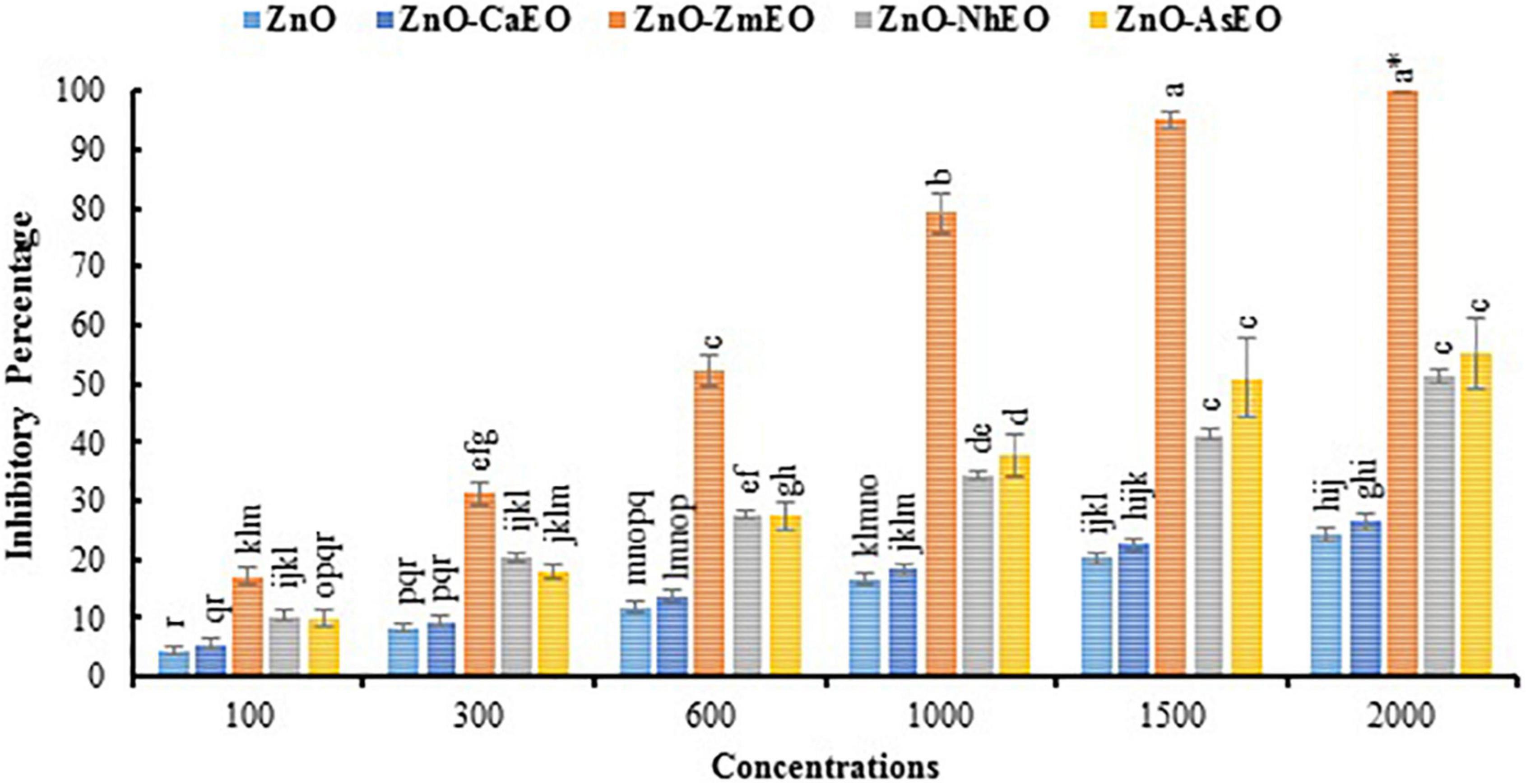
Figure 4. Comparison of mean inhibitory percentage of A. solani mycelial growth with ZnO and nanocapsules of essential oils in vitro. The symbol “*” indicates the fungistatic property.
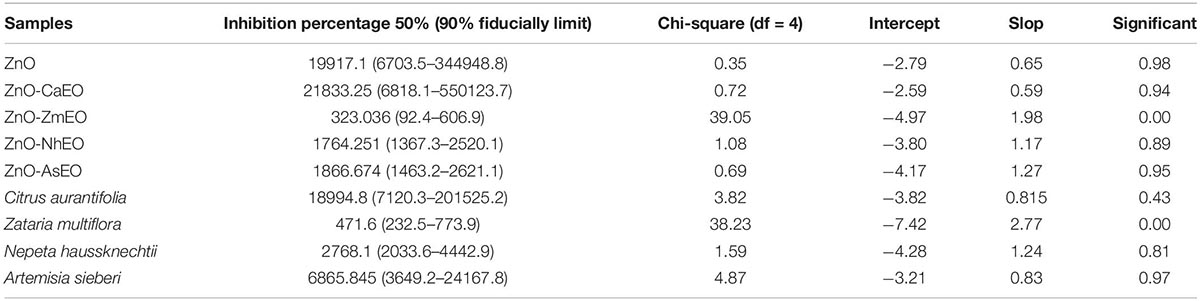
Table 4. Probit analysis of data obtained from the inhibitory effect of samples on A. solani in vitro.
Macroscopic images of the mycelial growth inhibition of A. solani affected by N. haussknechtii EO and ZnO-NhEO are illustrated in Figures 2C,D. The time of exposure and the tested concentrations of N. haussknechtii EO and ZnO-NhEO had significant inhibitory effects on the mycelial growth effect of A. solani (Figures 3, 4). The concentration of 2,000 ppm of ZnO-NhEO caused a 51.25% inhibitory effect on A. solani mycelial growth rate. Based on the results of Probit analysis, the IP50 values of N. haussknechtii EO and ZnO-NhEO were calculated as 2,768 and 1,764 ppm, respectively.
Macroscopic images of the mycelial growth inhibition of A. sieberi affected by A. sieberi EO and ZnO-AsEO are shown in Figures 2E,F. The exposure times and concentrations of A. sieberi EO and ZnO-AsEO had also significant inhibitory effects on the mycelial growth effect of A. solani (Figures 3, 4). Calculated IP50 values for the pure EO and nanocapsulated formulations were 6,865 and 1,866 ppm after 7 days, respectively.
Macroscopic images of the mycelial growth inhibition of A. sieberi treated by C. aurantifolia EO and ZnO-CaEO are presented in Figures 2G,H. The exposure times and concentrations of C. aurantifolia EO and ZnO-CaEO had also significant inhibitory effects on A. solani (Figures 3, 4). IP50 values for C. aurantifolia EO and ZnO-CaEO were 18,994 and 21,833 ppm after 7 days, respectively. Hence, it is noticed that the antifungal effects of C. aurantifolia EO and ZnO-CaEO on A. solani were lower than the other tested materials (Table 4).
As seen, the ZnO-ZmEO material has the highest effect on fungal growth. The pure Z. multiflora EO at the lowest concentration (100 μl/L) was comparable with the ZnO and ZnO-ZmEO materials on the fungal growth. Therefore, by employing a small concentration of EO, it is possible to remarkably improve the effect of ZnO NP. In the ZnO-ZmEO, the inhibition of mycelial growth was enhanced to 74.22% compared to the ZnO. In comparison with the pure Z. multiflora EO, inhibition by ZnO-ZmEO has also been improved to 53.81%. The fungal growth inhibition rate in Z. multiflora EO was 44.20% higher than in pure ZnO (Figures 3, 4).
In vivo Antifungal Study
Different concentrations of Z. multiflora EO, ZnO, and ZnO-ZmEO had diverse effects on the early blight disease on tomato plants. The disease symptoms were diminished with increasing tested agents’ concentrations so that 13.33, 31.11, and 53.33% of symptoms were reduced by the highest concentration (1,000 ppm) of ZnO, Z. multiflora EO, and ZnO-ZmEO, respectively. Plant samples treated with chlorothalonil at the same concentration showed a 48.89% reduction in disease symptoms (Figure 5). The ZnO-ZmEO had more than 75 and 41.66% inhibition than ZnO and pure EO, respectively (Figure 6). Based on the results of ANOVA, the antifungal effect of ZnO-ZmEO was significant at the tested concentrations (F = 5.17, df = 2, p = 0.036), but Z. multiflora EO and ZnO had no significant effect by concentrations used. The IP50 value for ZnO-ZmEO was 771.8 ppm, while it was 4,337.3 and 9,615.9 ppm for Z. multiflora EO and pure ZnO, respectively.
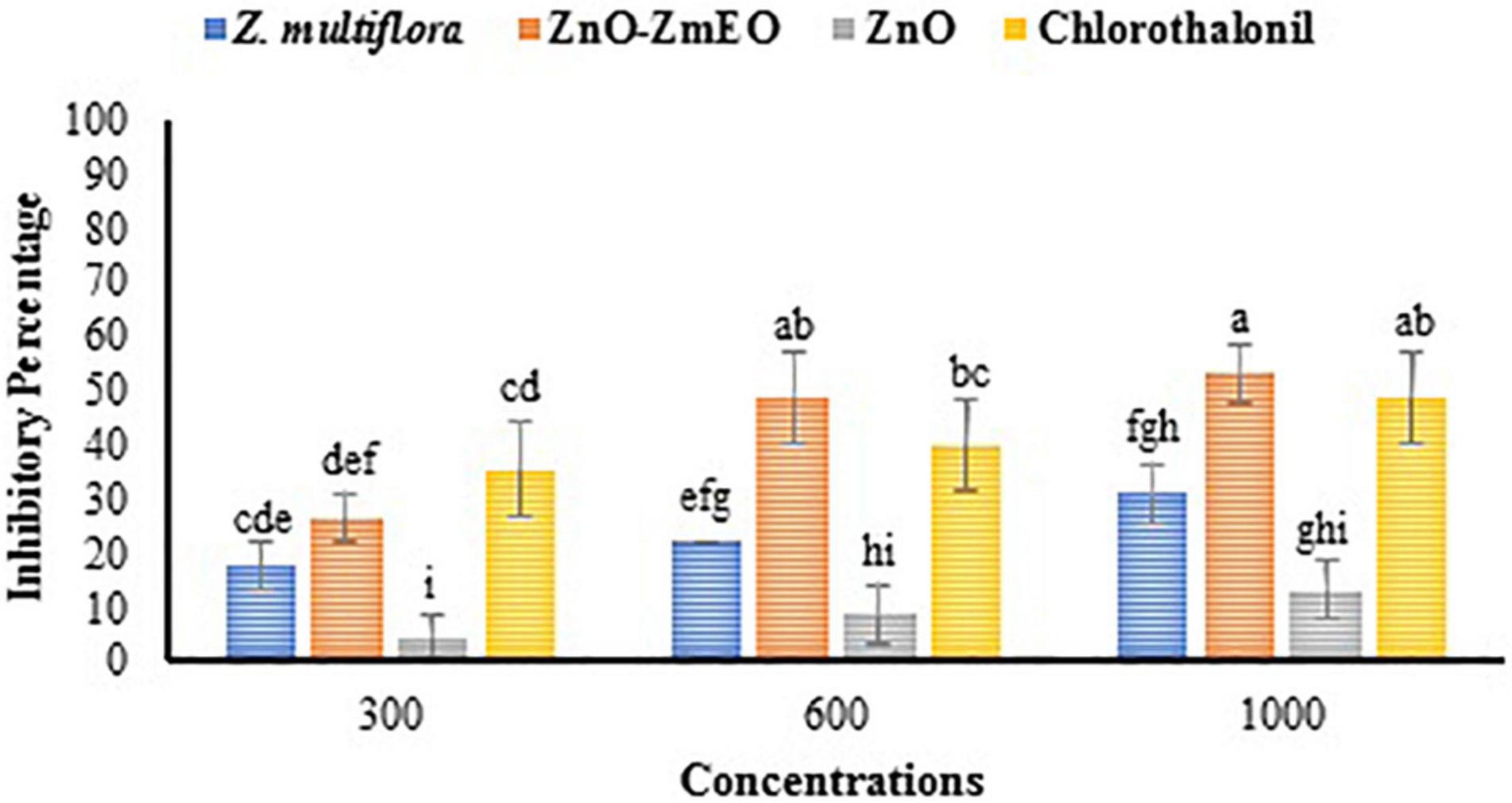
Figure 5. Comparison of mean inhibitory percentage of ZnO, ZnO-ZmEO, pure Z. multiflora EO, and chlorothalonil on early blight disease.
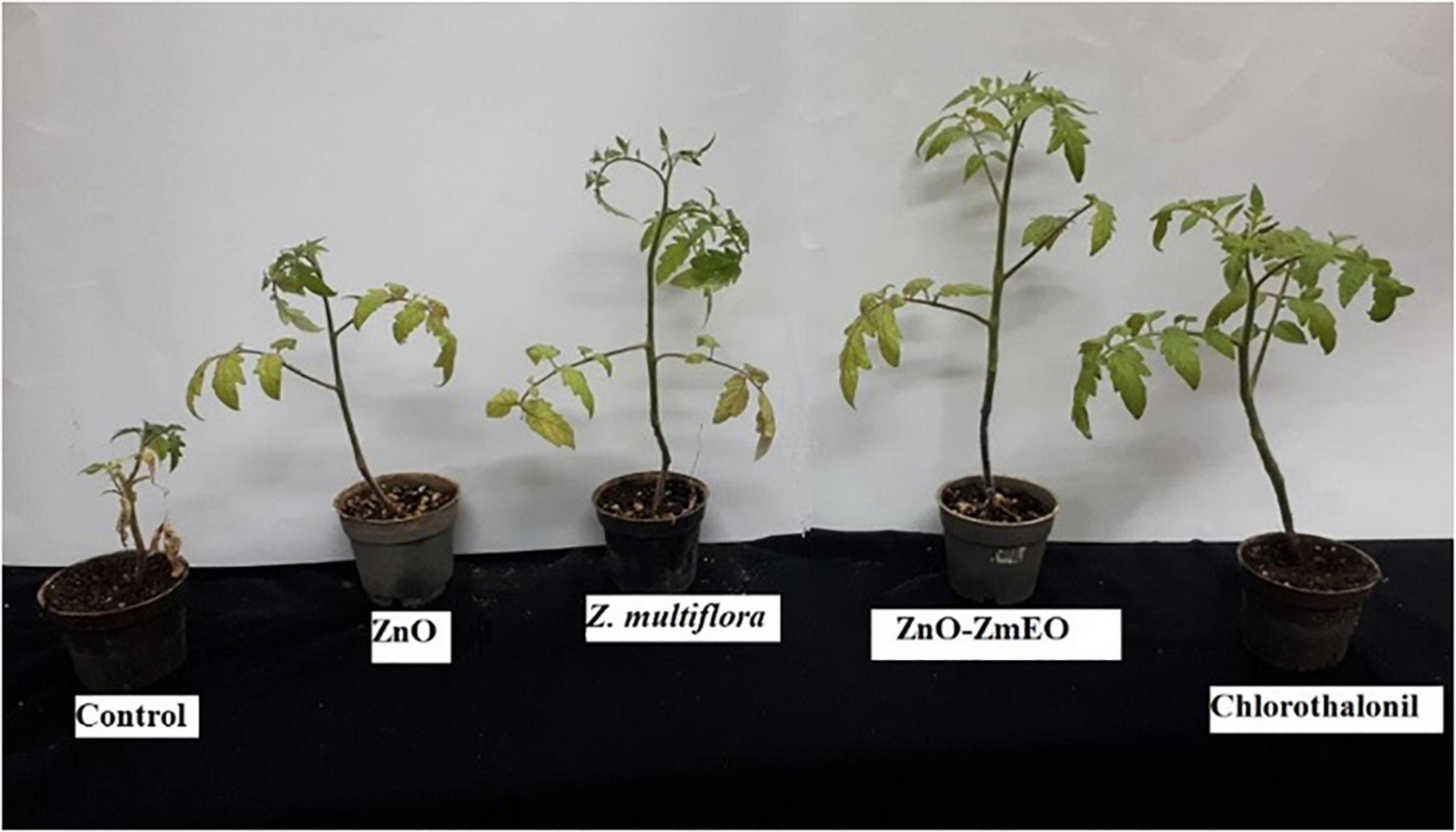
Figure 6. In vivo antifungal activity of the ZnO, pure Z. multiflora EO, ZnO-ZmEO, and chlorothalonil against Alternaria solani on tomato plants.
As phytotoxicity is a probable side effect of the application of botanical agents (Kaveh et al., 2014), high concentrations of Z. multiflora EO and ZnO-ZmEO were not tested in this study. The highest concentration used in this study was 1,000 ppm, which had more than 50% inhibition on phytopathogenic fungus and had not any phytotoxicity. The highest wet weight belonged to ZnO-ZmEO-treated plants (5.95 g), which was not statistically different from chlorothalonyl treatments (5.87 g) based on the comparison of the mean (p < 0.05). Also, the lowest wet weight of tomato plant was observed in ZnO (2.62 g) (Figure 7). Also, the highest dry weight was related to ZnO-ZmEO-treated plants (1.45 g), which was not statistically different from chlorothalonyl-treated plants (1.21 g) based on the comparison of the mean (p < 0.05). Also, the lowest dry weight of tomato plant was observed in ZnO (0.43 g) (Figure 8).
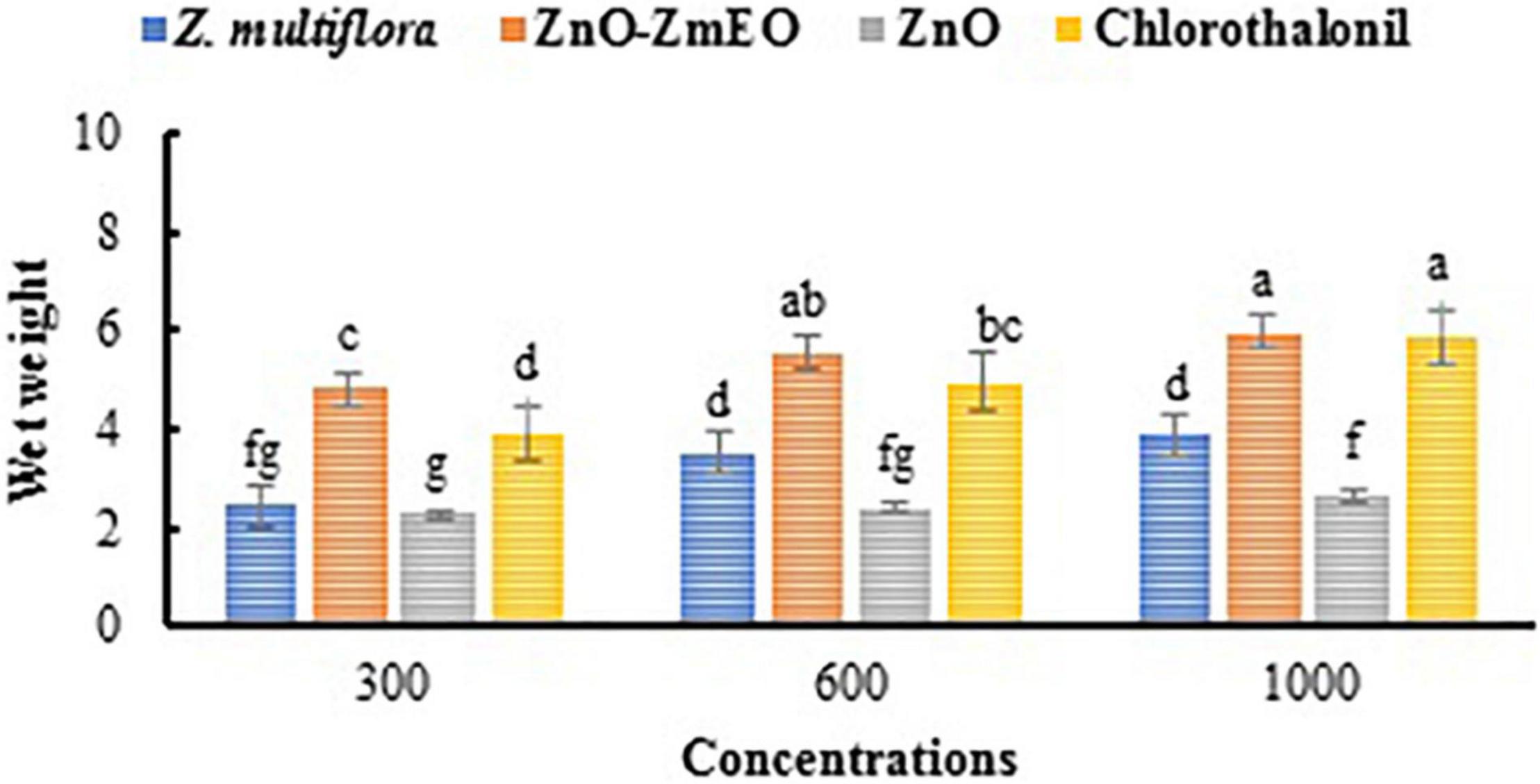
Figure 7. Comparison of mean wet weight of tomato plants treated by different concentrations of the ZnO, pure Z. multiflora EO, ZnO-ZmEO, and chlorothalonil.
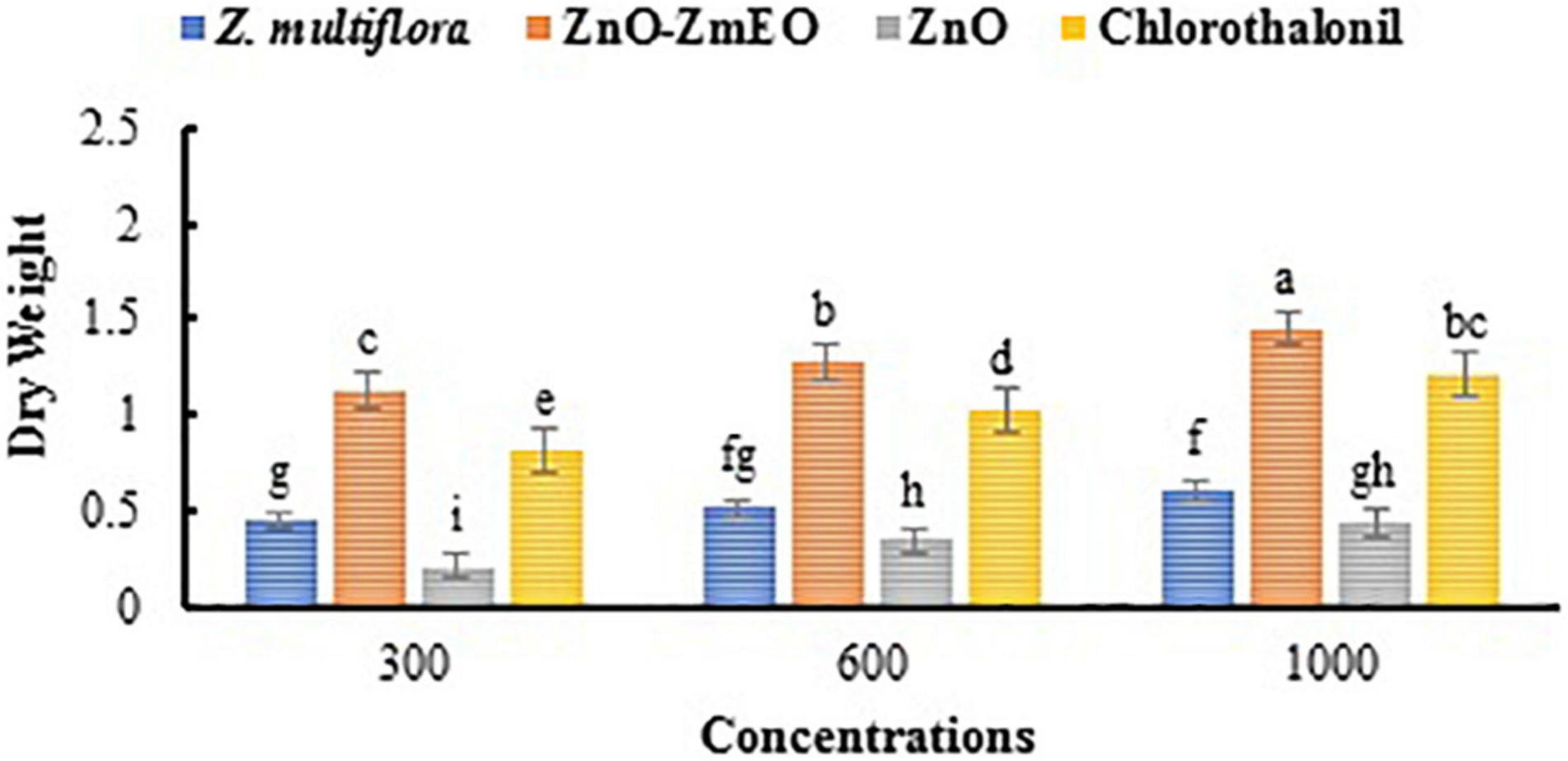
Figure 8. Comparison of mean dry weight of tomato plants treated by different concentrations of the ZnO, pure Z. multiflora EO, ZnO-ZmEO, and chlorothalonil.
Discussion
Thymol, γ-terpinene, p-cymene, and carvacrol were recognized as the main components of Z. multiflora EO, in agreement with the findings of Mahboubi et al. (2017) and Farahpour et al. (2021). However, there are quantitative differences in identified compounds. For example, the percentage of thymol in this study was 47.08%, while its corresponding amount in the Mahboubi et al. (2017) and Farahpour et al. (2021) studies was 25.80 and 52.9%, respectively. The ability of the thymol is to change the hyphal morphology and cause hyphal aggregates, resulting in reduced diameters and lyses of hyphal wall. Also, thymol is lipophilic, enabling it to interact with the cell membrane of fungus cells, altering cell membrane permeability by permitting the loss of macromolecules (Moghtader, 2012). The major constituents of A. sieberi EO are β-thujone, 1,8-cineole, camphor, germacrene D, and terpinen-4-ol. In the study by Aati et al. (2020), although camphor (24.1%) was the main compound, similar to our findings, other compounds such as β-thujone, 1,8-cineole, camphor, germacrene D, and terpinen-4-ol were not recognized. Limonene, α-terpineol, and γ-terpinene are the major compounds in C. aurantifolia EO, which were detected in the study by Song et al. (2022) with different percentage. Limonene has a variety of functions such as defense against predators and pathogens, as well as a signal to harmless organisms as pollinators for plants. Additionally, it has been reported that limonene affects the signaling pathway and cell membrane. Limonene also inhibits the growth of yeast fungi by damaging cell walls and plasma membranes. However, many ingredients in EO such as γ-terpinene and α-terpineol in addition to limonene have antifungal activity and can affect the antifungal activity of lime (Ehsanfar et al., 2020). Jamzad et al. (2008) indicated that 1,8-cineole, elemol, thymol, germacrene D, and γ-terpinene were the main compounds in N. haussknechtii EO. Although 1,8-cineole and germacrene D were also recognized in this study, there was no trace from elemol and γ-terpinene in N. haussknechtii EO. Accordingly, there are notable differences in EO compositions investigated in present and previous studies. In fact, several factors, including environmental conditions, geographical origin, genetic make-up, and even phonological stages of plants and agricultural practices, can affect the chemical composition of EOs, resulting in the abovementioned differences (Moghaddam and Mehdizadeh, 2017).
It was found that the biological effects of plant EOs are associated with their chemical compounds, and the antifungal efficiency of some identified compounds in this study was approved (Kazemi et al., 2011; Dehghani Bidgoli, 2021; Karimi and Meiners, 2021). For example, 1,8-cineole, as one of the major constituents of EOs of N. haussknechtii and A. sieberi EOs, has been shown to inhibit the growth of pathogenic fungi (Garg and Siddiqui, 1992), which may be attributed to its capability to damage cell membrane (Varshney et al., 2012). According to the study by Dehghani Bidgoli (2021), antifungal activity of Artemisia genus is due to the presence of the biological secondary metabolites such as bornyl acetate, camphor, and 1,8-cineol. Karimi and Meiners (2021) revealed that Z. multiflora EO comprises the effective phenolic compounds such as carvacrol and thymol, causing high antifungal activities on a wide range of major food and agricultural pathogens. Furthermore, it was found that thymol causes substantial morphological damage to the microbial cell membrane, changing the permeability and release of cellular contents (Di Pasqua et al., 2006; Moosavy et al., 2008). Therefore, the stronger antifungal properties of Z. multiflora EO than other ones can be due to the presence of active metabolites such as thymol, which was not found in others.
In this research, the inhibitory effect of ZnO on the mycelial growth of A. solani was investigated. The outcomes manifested that raising the concentration was useful in inhibiting the fungus mycelial growth. He et al. (2011) showed that a high concentration of ZnO possessed a good inhibitory effect on B. cinerea and Penicillium expansum Link. The antimicrobial (Kim et al., 2012) effects of nanoparticles on bacteria, fungi, and viruses have been well studied. The antibacterial performances of ZnO and silver nanomaterials have recently been demonstrated (Kumar et al., 2008). Due to the remarkable combination of superior physicochemical, chemical, optical, and considerable environmental stability, cost-effective, and non-toxic properties, ZnO has attracted much interest as one of the multifunctional metal oxide nanoparticles (Salahuddin et al., 2015). In addition to the booster effect related to zinc act as a precursor in cytokinins, auxins, and gibberellins biosynthesis, and the induction of activity of antioxidant enzymes against the pathogenic attacks, ZnO NPs have promising antifungal effects and can be applied for controlling F. oxysporum in tomatoes (González-Merino et al., 2021).
Based on previous research, metal nanoparticles are absorbed by the leaves in the atmosphere around the plant, and it has been proven that the structure of stoma and hairs is affected by this nanoparticle (Silva et al., 2006). Various studies have shown that ZnO is non-toxic to human cells and toxic to bacterial cells, and as a result, these nanoparticles are compatible with human cells (Sirelkhatim et al., 2015). The results of Mosquera-Sánchez et al. (2020) represented that the synthesized ZnO-NPs are suitable, cost-effective, and valuable antifungal alternatives to be employed in agricultural production systems, particularly in the protection of crops. Two mechanisms can be used to protect plants using nanoparticles; in the first, nanoparticles protect the plant directly, and in the second, nanoparticles act as carriers with different advantages such as high durability, improving the solubility of water-soluble poisons and reduce toxicity (Hayles et al., 2017).
Over the last decade or so, nanoencapsulation and microencapsulation technologies have been explored for their efficiency to improve the handling, dispersibility, and stability of hydrophobic substances, as well as to control their release profiles (Weisany et al., 2022). In this study, ZnO-ZmEO had the highest inhibition of growth in vitro compared to other nanocapsules of EOs and pure Z. multiflora EO, so it was compared with the chemical fungicide in the greenhouse conditions and showed a significant reduction in symptoms. Previous research has shown that nano-EO formulation has the power of gradual release and increases the durability of EOs and nanocapsulated EOs have an antifungal activity higher than the pure EO and ZnO (Emamjomeh et al., 2018). The results of Upadhyay et al. (2021) showed that nanocapsules of Cananga odorata (Lam.) Hook.f. & Thomson EO completely inhibited the growth of Aspergillus flavus and demonstrated an enhanced antioxidant activity. In Enayati et al.’s (2021) study, to obtain an effective alternative for highly toxic synthetic chemicals, the antifungal properties of Z. multiflora EO loaded on ZnO material were assessed against six isolates of Fusarium. The findings demonstrated that ZnO-EO nanocomposite possessed an antifungal effect on all investigated fungi except F. oxysporum f.sp. lentis and the fungicidal activity toward F. graminearum at a concentration of 1,000 ppm.
Conclusion
Essential oils have numerous potential applications in the agriculture, food, and pharmaceutical industries. However, their hydrophobicity, chemical instability, and volatility pose a challenge for many of their applications. One of the best approaches to solve these challenges is to encapsulate the EOs in nanosize or encapsulation EOs in colloidal delivery systems (Enayati et al., 2021; Weisany et al., 2022). In this study, nanocapsules of some EOs were successfully prepared and ZnO-Z. multiflora EO possessed the best performance compared to other EOs, nanocapsules of other EOs and ZnO in vitro because of its smaller particle size and higher distribution surface. Then, this study highlighted the advantage of nanocapsules over the common form of EOs like Z. multiflora EOs. Also, ZmEO was used with its nanocapsules and ZnO in vivo with concentrations that showed better inhibition in the laboratory, and the results illustrated that ZnO-ZmEO has the highest percentage of inhibition (53% reduction) in the control of tomato early blight disease in greenhouses and after consideration of additional research can be used as an alternative for high-risk chemical fungicides that are harmful to health and the environment.
Data Availability Statement
The original contributions presented in this study are included in the article/supplementary material, further inquiries can be directed to the corresponding author.
Author Contributions
AA performed the experiments. MD and AH-Y supervised the experiments from the beginning to the end and read and corrected the manuscript. AE advised the research, made statistical analyses, analyzed the EOs, and participated in proof-writing and editing the final version of manuscript. SF advised the research and performed the nanoparticle synthesis. All authors contributed to the article and approved the submitted version.
Funding
This research was supported by the University of Mohaghegh Ardabili, Iran.
Conflict of Interest
The authors declare that the research was conducted in the absence of any commercial or financial relationships that could be construed as a potential conflict of interest.
Publisher’s Note
All claims expressed in this article are solely those of the authors and do not necessarily represent those of their affiliated organizations, or those of the publisher, the editors and the reviewers. Any product that may be evaluated in this article, or claim that may be made by its manufacturer, is not guaranteed or endorsed by the publisher.
Acknowledgments
We are grateful to the University of Mohaghegh Ardabili for supporting this research.
References
Aati, H. Y., Perveen, S., Orfali, R., Al-Taweel, A. M., Aati, S., Wanner, J., et al. (2020). Chemical composition and antimicrobial activity of the essential oils of Artemisia absinthium, Artemisia scoparia, and Artemisia sieberi grown in Saudi Arabia. Arab. J. Chem. 13, 8209–8217. doi: 10.1016/j.arabjc.2020.09.055
Adams, R. P. (2007). Identification of Essential Oils Components by gas Chromatography/Quadrupole Mass Spectroscopy. 4th Edition. Carol Stream, IL: Allured publishing Co.
Assadi, M. (1987-2018). Flora of Iran Vols 1-149. Tehran: Research Institute of Forests and Rangelands of Iran.
Beshkar, F., Al-Nayili, A., Amiri, O., Salavati-Niasari, M., and Mousavi-Kamazani, M. (2022). Fabrication of S-scheme ZnO/Zn3 (PO4) 2 heterojunction photocatalyst toward photodegradation of tetracycline antibiotic and photocatalytic mechanism insight. Int. J. Hydrog. Energy 47, 928–939. doi: 10.1016/j.ijhydene.2021.10.060
Beyki, F., and Alizadeh, A. (2006). Antibacterial effects of some herbal essential oils and plant extracts on the causal agent of bacterial leaf streak in wheat and barley. J. Agric. Sci. Nat. Resour. 13, 70–82.
Chohan, S., Perveen, R., Abid, M., Naz, M. S., and Akram, N. (2015). Morpho-physiological studies management and screening of tomato germplasm against Alternaria solani the causal agent of tomato early blight. Int. J. Agric. Biol. 17, 111–118.
Davari, M., and Ezazi, R. (2017). Chemical composition and antifungal activity of the essential oil of Zhumeria majdae, Heracleum persicum and Eucalyptus sp. against some important phytopathogenic fungi. J. Mycol. Med. 27, 463–468. doi: 10.1016/j.mycmed.2017.06.001
Dehghani Bidgoli, R. (2021). Chemical composition of essential oil and antifungal activity of Artemisia persica Boiss. from Iran. J. Food Sci. Technol. 58, 1313–1318. doi: 10.1007/s13197-020-04640-x
Di Pasqua, R., Hoskins, N., Betts, G., and Mauriello, G. (2006). Changes in membrane fatty acids composition of microbial cells induced by addiction of thymol, carvacrol, limonene, cinnamaldehyde, and eugenol in the growing media. J. Agric. Food Chem. 54, 2745–2749. doi: 10.1021/jf052722l
Donsì, F., Annunziata, M., Sessa, M., and Ferrari, G. (2011). Nanoencapsulation of essential oils to enhance their antimicrobial activity in foods. LWT-Food Sci. Technol. 44, 1908–1914. doi: 10.1016/j.lwt.2011.03.003
Ehsanfar, P., Teimouri, M., and Pooladi, M. (2020). Investigating characterizations and antifungal effects of solid lipid nanoparticles (SLNs) loaded with essential oil of Citrus Aurantifolia on isolated Malassezia strains. Arch. Adv. Biosci. 11, 43–55.
El-Nagar, A., Elzaawely, A. A., Taha, N. A., and Nehela, Y. (2020). The antifungal activity of gallic acid and its derivatives against Alternaria solani, the causal agent of tomato early blight. Agronomy 10:1402. doi: 10.3390/agronomy10091402
Emamjomeh, L., Imani, S., Talebi, K. H., Moharramipour, S., and Larijani, K. (2018). Preparation of nanoemulsion formulation of essential oil of Zataria multiflora and comparison of contact toxicity with pure essential oil on Ephestia kuehniella. Appl. Entomol. Phytopathol. 85, 181–190.
Enayati, S., Davari, M., Habibi-Yangjeh, A., Ebadollahi, A., and Feizpoor, S. (2021). Enhancement of the antifungal properties of Zataria multiflora essential oil through nanoencapsulation with ZnO nanomaterial. Toxin Rev. 1–11. doi: 10.1080/15569543.2021.2007405
Ertani, A., Francioso, O., Tinti, A., Schiavon, M., Pizzeghello, D., and Nardi, S. (2018). Evaluation of seaweed extracts from Laminaria and Ascophyllum nodosum spp. as biostimulants in Zea mays L. using a combination of chemical, biochemical and morphological approaches. Front. Plant Sci. 9:428. doi: 10.3389/fpls.2018.00428
Farahpour, M. R., Sheikh, S., Kafshdooz, E., and Sonboli, A. (2021). Accelerative effect of topical Zataria multiflora essential oil against infected wound model by modulating inflammation, angiogenesis, and collagen biosynthesis. Pharm. Biol. 59, 1–10. doi: 10.1080/13880209.2020.1861029
Garg, S. C., and Siddiqui, N. (1992). Antifungal activity of some essential oil isolates. Pharmazie 47, 467–468.
González-Merino, A. M., Hernández-Juárez, A., Betancourt-Galindo, R., Ochoa-Fuentes, Y. M., Valdez-Aguilar, L. A., and Limón-Corona, M. L. (2021). Antifungal activity of zinc oxide nanoparticles in Fusarium oxysporum-Solanum lycopersicum pathosystem under controlled conditions. J. Phytopathol. 169, 533–544. doi: 10.1111/jph.13023
Gupta, S., and Dikshit, A. K. (2010). Biopesticides: an ecofriendly approach for pest control. J. Biopestic. 3, 186–188.
Habibi-Yangjeh, A., Davari, M., Manafi-Yeldagermani, R., Asl, A. S., Enaiati, S., Ebadollahi, A., et al. (2021). Antifungal activity of TiO2/AgBr nanocomposites on some phytopathogenic fungi. Food Sci. Nutr. 9, 3815–3823. doi: 10.1002/fsn3.2357
Hasanzadeh, N. (2005). Technological implication of natural products in plant diseases management with special emphasis on fireblight. J. Agric. Sci. 11, 53–68.
Hayles, J., Johnson, L., Worthley, C., and Losic, D. (2017). “Nanopesticides: a review of current research and perspectives,” in New Pesticides and Soil Sensors, ed. A. M. Grumezescu (Cambridge: Academic Press), 193–225. doi: 10.1016/B978-0-12-804299-1.00006-0
He, L., Liu, Y., Mustapha, A., and Lin, M. (2011). Antifungal activity of zinc oxide nanoparticles against Botrytis cinerea and Penicillium expansum. Microbiol. Res. 166, 207–215. doi: 10.1016/j.micres.2010.03.003
Hendges, C., Stangarlin, J. R., Zamban, V. C., Mascaro, M., de, H. N., and Carmelo, D. B. (2021). Antifungal activity and control of the early blight in tomato through tea tree essential oil. Crop Prot. 148:105728. doi: 10.1016/j.cropro.2021.105728
Hoseinzadeh, A., Habibi-Yangjeh, A., and Davari, M. (2016). Antifungal activity of magnetically separable Fe3O4/ZnO/AgBr nanocomposites prepared by a facile microwave-assisted method. Prog. Nat. Sci. Mater. Int. 26, 334–340. doi: 10.1016/j.pnsc.2016.06.006
Jacob, K., Periago, M. J., Böhm, V., and Berruezo, G. R. (2008). Influence of lycopene and vitamin C from tomato juice on biomarkers of oxidative stress and inflammation. Br. J. Nutr. 99, 137–146. doi: 10.1017/S0007114507791894
Jamzad, M., Rustaiyan, A., Masoudi, S., and Jamzad, Z. (2008). Composition of the essential oils of Nepeta sessilifolia Bunge and Nepeta haussknechtii Bornm. from Iran. J. Essent. Oil Res. 20, 533–535. doi: 10.1080/10412905.2008.9700081
Kalia, A., Abd-Elsalam, K. A., and Kuca, K. (2020). Zinc-based nanomaterials for diagnosis and management of plant diseases: ecological safety and future prospects. J. Fungi 6:222. doi: 10.3390/jof6040222
Karimi, A., and Meiners, T. (2021). Antifungal activity of Zataria multiflora Boiss. essential oils and changes in volatile compound composition under abiotic stress conditions. Ind. Crops Prod. 171:113888. doi: 10.1016/j.indcrop.2021.113888
Kaveh, M., Poorjavad, N., and Khajehali, J. (2014). Evaluation of contact toxicity of ten essential oils from Lamiaceae plants against Tetranychus urticae Koch (Acari: tetranychidae). Plant Pests Res. 4, 39–49.
Kazemi, M., Dakhili, M., Dadkhah, A., Yasrebifar, Z., and Larijani, K. (2011). Composition, antimicrobial and antioxidant activities of the essential oil of Artemisia kermanensis Podl., an endemic species from Iran. J. Med. Plants Res. 5, 4481–4486.
Kedia, A., and Kishore Dobey, N. (2018). Nanoencapsulation of essential oils: a possible way for an eco-friendly strategy to control postharvest spoilage of food commodities from pests. Nanomat. Plants Algae Microorganisms 1, 501–522. doi: 10.1016/B978-0-12-811487-2.00022-0
Kim, S. W., Jung, J. H., Lamsal, K., Kim, Y. S., Min, J. S., and Lee, Y. S. (2012). Antifungal effects of silver nanoparticles (AgNPs) against various plant pathogenic fungi. Mycobiology 40, 53–58. doi: 10.5941/MYCO.2012.40.1.053
Kizil, S., and Uyar, F. (2006). Antimicrobial activities of some thyme (Thymus, Staureja, Origanum and Thymbra) species against important plant pathogens. Asian J. Chem. 18:1455.
Kumar, A., Shukla, R., Singh, P., Prasad, C. S., and Dubey, N. K. (2008). Assessment of Thymus vulgaris L. essential oil as a safe botanical preservative against post harvest fungal infestation of food commodities. Innov. Food Sci. Emerg. Technol. 9, 575–580. doi: 10.1016/j.ifset.2007.12.005
Kumari, M., Giri, V. P., Pandey, S., Kumar, M., Katiyar, R., Nautiyal, C. S., et al. (2019). An insight into the mechanism of antifungal activity of biogenic nanoparticles than their chemical counterparts. Pestic. Biochem. Physiol. 157, 45–52. doi: 10.1016/j.pestbp.2019.03.005
Kutawa, A. B., Ahmad, K., Ali, A., Hussein, M. Z., Abdul Wahab, M. A., Adamu, A., et al. (2021). Trends in nanotechnology and its potentialities to control plant pathogenic fungi: a review. Biology 10:881. doi: 10.3390/biology10090881
Latha, P., Anand, T., Ragupathi, N., Prakasam, V., and Samiyappan, R. (2009). Antimicrobial activity of plant extracts and induction of systemic resistance in tomato plants by mixtures of PGPR strains and Zimmu leaf extract against Alternaria solani. Biol. Control 50, 85–93. doi: 10.1016/j.biocontrol.2009.03.002
Luque, P. A., Nava, O., Soto-Robles, C. A., Vilchis-Nestor, A. R., Garrafa-Galvez, H. E., and Castro-Beltran, A. (2018). Effects of Daucus carota extract used in green synthesis of zinc oxide nanoparticles. J. Mater. Sci. Mater. Electron. 29, 17638–17643. doi: 10.1007/s10854-018-9867-5
Mahboubi, M., Heidarytabar, R., Mahdizadeh, E., and Hosseini, H. (2017). Antimicrobial activity and chemical composition of Thymus species and Zataria multiflora essential oils. Agric. Nat. Resour. 51, 395–401. doi: 10.1016/j.anres.2018.02.001
McKinney, H. H. (1923). Influence of soil temperature and moisture on infection of wheat seedlings by Helmin. J. Agric. Res. 26:195.
Moghaddam, M., and Mehdizadeh, L. (2017). “Chemistry of essential oils and factors influencing their constituents,” in Soft Chemistry and Food Fermentation, eds A. Grumezescu and A. M. Holban (Amsterdam: Elsevier), 379–419. doi: 10.1016/B978-0-12-811412-4.00013-8
Moghtader, M. (2012). Antifungal effects of the essential oil from Thymus vulgaris L. and comparison with synthetic thymol on Aspergillus niger. J. Yeast Fungal Res. 3, 83–88.
Moosavy, M.-H., Basti, A. A., Misaghi, A., Salehi, T. Z., Abbasifar, R., Mousavi, H. A. E., et al. (2008). Effect of Zataria multiflora Boiss. essential oil and nisin on Salmonella typhimurium and Staphylococcus aureus in a food model system and on the bacterial cell membranes. Food Res. Int. 41, 1050–1057. doi: 10.1016/j.foodres.2008.07.018
Mosquera-Sánchez, L. P., Arciniegas-Grijalba, P. A., Patiño-Portela, M. C., Guerra–Sierra, B. E., Muñoz-Florez, J. E., and Rodríguez-Páez, J. E. (2020). Antifungal effect of zinc oxide nanoparticles (ZnO-NPs) on Colletotrichum sp., causal agent of anthracnose in coffee crops. Biocatal. Agric. Biotechnol. 25:101579. doi: 10.1016/j.bcab.2020.101579
Mugao, L. G., Gichimu, B. M., Muturi, P. W., and Njoroge, E. K. (2021). Essential oils as biocontrol agents of early and late blight diseases of tomato under greenhouse conditions. Int. J. Agron. 2021:5719091. doi: 10.1155/2021/5719091
Narayanasamy, P. (2002). Microbial Plant Pathogens And Crop Disease Management. Boca Raton: CRC Press. doi: 10.1201/9781482279948
Nashwa, S. M. A., and Abo-Elyousr, K. A. M. (2013). Evaluation of various plant extracts against the early blight disease of tomato plants under greenhouse and field conditions. Plant Prot. Sci. 48, 74–79. doi: 10.17221/14/2011-PPS
Pavoni, L., Perinelli, D. R., Bonacucina, G., Cespi, M., and Palmieri, G. F. (2020). An overview of micro-and nanoemulsions as vehicles for essential oils: formulation, preparation and stability. Nanomaterials 10:135. doi: 10.3390/nano10010135
Pirhashemi, M., and Habibi-Yangjeh, A. (2017). Ultrasonic-assisted preparation of plasmonic ZnO/Ag/Ag2WO4 nanocomposites with high visible-light photocatalytic performance for degradation of organic pollutants. J. Colloid Interface Sci. 491, 216–229. doi: 10.1016/j.jcis.2016.12.044
Rao, A. V., and Agarwal, S. (2000). Role of antioxidant lycopene in cancer and heart disease. J. Am. Coll. Nutr. 19, 563–569. doi: 10.1080/07315724.2000.10718953
Raveau, R., Fontaine, J., and Lounès-Hadj Sahraoui, A. (2020). Essential oils as potential alternative biocontrol products against plant pathogens and weeds: a review. Foods 9:365. doi: 10.3390/foods9030365
Raza, W., Ghazanfar, M. U., Iftikhar, Y., Ahmed, K. S., Haider, N., and Rasheed, M. H. (2016). Management of early blight of tomato through the use of plant extracts. Management 1, 1123–1133.
Rechinger, K. H. (ed.) (1963-2015). Flora Iranica, vols. 1-181. Graz: Akad. Ddruck-u Verlagsanstalt.
Salahuddin, N. A., El-Kemary, M., and Ibrahim, E. M. (2015). Synthesis and characterization of ZnO nanotubes by hydrothermal method. Int. J. Sci. Res. Publ. 5, 3–6.
Silva, L. C., Oliva, M. A., Azevedo, A. A., and De Araújo, J. M. (2006). Responses of restinga plant species to pollution from an iron pelletization factory. Water Air Soil Pollut. 175, 241–256. doi: 10.1007/s11270-006-9135-9
Sirelkhatim, A., Mahmud, S., Seeni, A., Kaus, N. H. M., Ann, L. C., Bakhori, S. K. M., et al. (2015). Review on zinc oxide nanoparticles: antibacterial activity and toxicity mechanism. Nano-Micro. Lett. 7, 219–242. doi: 10.1007/s40820-015-0040-x
Song, B. W., Lee, C. Y., Park, J.-H., Kim, B., Lee, S., Lim, S., et al. (2022). Cold-pressed oil from Citrus aurantifolia inhibits the proliferation of vascular smooth muscle cells via regulation of PI3K/MAPK signaling pathways. Exp. Ther. Med. 23:21. doi: 10.3892/etm.2021.10943
Upadhyay, N., Singh, V. K., Dwivedy, A. K., Chaudhari, A. K., and Dubey, N. K. (2021). Assessment of nanoencapsulated Cananga odorata essential oil in chitosan nanopolymer as a green approach to boost the antifungal, antioxidant and in situ efficacy. Int. J. Biol. Macromol. 171, 480–490. doi: 10.1016/j.ijbiomac.2021.01.024
Varshney, V. K., Pandey, A., Onial, P. K., and Dayal, R. (2012). Antifungal activity of phytochemicals from Eucalyptus hybrid leaves against some plant pathogenic and wood decay fungi. Arch. Phytopathol. Plant Prot. 45, 2347–2354. doi: 10.1080/03235408.2012.727073
Wan Mat Khalir, W. K. A., Shameli, K., Jazayeri, S. D., Othman, N. A., Che Jusoh, N. W., and Hassan, N. M. (2020). Biosynthesized silver nanoparticles by aqueous stem extract of Entada spiralis and screening of their biomedical activity. Front. Chem. 8:620. doi: 10.3389/fchem.2020.00620
Weisany, W., Samadi, S., Amini, J., Hossaini, S., Yousefi, S., and Maggi, F. (2019). Enhancement of the antifungal activity of thyme and dill essential oils against Colletotrichum nymphaeae by nano-encapsulation with copper NPs. Ind. Crops Prod. 132, 213–225. doi: 10.1016/j.indcrop.2019.02.031
Weisany, W., Yousefi, S., Abdul-razzak Tahir, N., Golestanehzadeh, N., McClements, D. J., Adhikari, B., et al. (2022). Targeted delivery and controlled released of essential oils using nanoencapsulation: a review. Adv. Colloid Interface Sci. 303:102655. doi: 10.1016/j.cis.2022.102655
Xue, L., Liang, E., and Wang, J. (2022). Fabrication of magnetic ZnO/ZnFe2O4/diatomite composites: improved photocatalytic efficiency under visible light irradiation. J. Mater. Sci. Mater. Electron. 33, 1405–1424. doi: 10.1007/s10854-021-07568-w
Keywords: Alternaria solani, Zataria multiflora, ZnO, essential oil nanocapsules, plant disease management
Citation: Akhtari A, Davari M, Habibi-Yangjeh A, Ebadollahi A and Feizpour S (2022) Antifungal Activities of Pure and ZnO-Encapsulated Essential Oil of Zataria multiflora on Alternaria solani as the Pathogenic Agent of Tomato Early Blight Disease. Front. Plant Sci. 13:932475. doi: 10.3389/fpls.2022.932475
Received: 03 May 2022; Accepted: 10 June 2022;
Published: 05 July 2022.
Edited by:
Filippo De Curtis, Università degli Studi del Molise, ItalyReviewed by:
Lucia Landi, Marche Polytechnic University, ItalyMuhammad Sheeraz Ahmad, Pir Mehr Ali Shah Arid Agriculture University, Pakistan
Copyright © 2022 Akhtari, Davari, Habibi-Yangjeh, Ebadollahi and Feizpour. This is an open-access article distributed under the terms of the Creative Commons Attribution License (CC BY). The use, distribution or reproduction in other forums is permitted, provided the original author(s) and the copyright owner(s) are credited and that the original publication in this journal is cited, in accordance with accepted academic practice. No use, distribution or reproduction is permitted which does not comply with these terms.
*Correspondence: Mahdi Davari, bWRhdmFyaUB1bWEuYWMuaXI=