- 1The State Key Laboratory for Conservation and Utilization of Subtropical Agro-bioresources, South China Agricultural University, Guangzhou, China
- 2The Guangdong Province Key Laboratory of Plant Molecular Breeding, College of Agriculture, South China Agricultural University, Guangzhou, China
- 3The Guangdong Subcenter of the National Center for Soybean Improvement, College of Agriculture, South China Agricultural University, Guangzhou, China
- 4The Guangdong Provincial Laboratory of Lingnan Modern Agricultural Science and Technology, South China Agricultural University, Guangzhou, China
- 5Zengcheng Teaching and Research Bases, South China Agricultural University, Guangzhou, Guangdong, China
- 6Agro-biological Gene Research Center, Guangdong Academy of Agricultural Sciences, Guangzhou, Guangdong, China
The ethylene response factor (ERF) transcription factors, which is one of the largest transcription factor families in plants, are involved in biological and abiotic stress response and play an important role in plant growth and development. In this study, the GmABR1 gene from the soybean inbred line Zhonghuang24 (ZH24)×Huaxia 3 (HX3) was investigated its aluminum (Al) tolerance. GmABR1 protein has a conserved domain AP2, which is located in the nucleus and has transcriptional activation ability. The results of real-time quantitative PCR (qRT-PCR) showed that the GmABR1 gene presented a constitutive expression pattern rich in the root tip, stem and leaf tissues of HX3. After Al stress, the GmABR1 transcript was significantly increased in the roots. The transcripts of GmABR1 in the roots of HX3 treated with 50 µM AlCl3 was 51 times than that of the control. The GmABR1 was spatiotemporally specific with the highest expression levels when Al concentration was 50 µM, which was about 36 times than that of the control. The results of hematoxylin staining showed that the root tips of GmABR1-overexpression lines were stained the lightest, followed by the control, and the root tips of GmABR1 RNAi lines were stained the darkest. The concentrations of Al3+ in root tips were 207.40 µg/g, 147.74 µg/g and 330.65 µg/g in wild type (WT), overexpressed lines and RNAi lines, respectively. When AlCl3 (pH4.5) concentration was 100 µM, all the roots of Arabidopsis were significantly inhibited. The taproot elongation of WT, GmABR1 transgenic lines was 69.6%, 85.6%, respectively. When treated with Al, the content of malondialdehyde (MDA) in leaves of WT increased to 3.03 µg/g, while that of transgenic Arabidopsis increased from 1.66-2.21 µg/g, which was lower than that of WT. Under the Al stress, the Al stress responsive genes such as AtALMT1 and AtMATE, and the genes related to ABA pathway such as AtABI1, AtRD22 and AtRD29A were up-regulated. The results indicated that GmABR1 may jointly regulate plant resistance to Al stress through genes related to Al stress response and ABA response pathways.
1 Introduction
Aluminum (Al) third only to oxygen and silicon is one of the most abundant metallic elements in the earth’s crust. When the soil pH is below 5.5, Al is mainly present in the form of Al3+ which is highly toxic to plants (Ranjan et al., 2021). Approximately 50% of the world’s arable soils are acidic, and Al toxicity is a major factor limiting crop yields under abiotic stress in acidic soils (Kochian et al., 2015). The Al absorbed by plants is first concentrated in the root tips. Previous studies have found that the Al3+ content of root tip meristem in Al-tolerant plants is generally lower than that of Al-sensitive plants (Rangel et al., 2009). After some heavy metal ions enter root cells of plants, enzyme activity is inhibited and plant metabolism is blocked (Chen, 2006). The elongation and division of root tip cells were severely inhibited under the action of Al3+. The root tip enlargement, main root coarsening and shortening, lateral root number decreased correspondingly. The photosynthesis of leaves was inhibited with yellowing and curling shape, and smaller leaf area (Chauhan et al., 2021). The root changes of plants may be due to the inhibition of cellulose synthesis in root tip cell wall by Al3+ (Matsumoto and Motoda, 2012). Plants exposed to Al for a long time will be inhibited the absorption of water and other nutrients in their roots resulting in plant production and even death (Zhang et al., 2019a).
AP2/ERF transcription factors play an important role in plant abiotic stress. They can specifically bind cis-elements in the promoter region of downstream defense genes including activating or inhibiting the expression of downstream stress-responsive genes through positive and negative regulation, and thus participate in the response to plant stress (Kumar and Sharma, 2021). The AP2/ERF transcription factor contains one or two conserved AP2/ERF domains consisting of approximately 58-70 amino acids which are involved in DNA binding (Li et al., 2022). The AP2/ERF domain consists of three β folds and one amphiphilic α helix. There are differences between the two amino acid residues at position 14 and 19 in the second β fold, which makes the AP2/ERF transcription factor specific to bind different cis-acting elements (Feng et al., 2020).
The AP2/EREBP transcription factor OPBP1 can regulate the expression of stress-related genes. The overexpression of OPBP1 in tobacco plants showed strong resistance to Pseudomonas syringaepv tabaci and Phytophthora parasiticavar nicotianae pathogens, and improved the resistance to salt stress (Guo et al., 2004). Soybean gene GmERF5 was involved in the response to Phytophthora sojae infection. The tolerance of transgenic lines to Phytophthora sojae infection was significantly improved compared with the control. GmERF5 was significantly induced. While its promoter activity was activated under the treatments of ethylene (ET), abscisic acid (ABA), Phytophthora sojae, salt and drought. The results suggested that GmERF5 was not only involved in the induced defense response but also involved in ABA-mediated pathways of salt and drought tolerance (Dong et al., 2015). In rice, OsERF922 was rapidly and strongly up-regulated by ABA, salt and blast fungus. OsERF922 knockout by RNAi enhanced rice resistance to magnaporthe oryzae and increased the expression of several genes regulating plant stress resistance synthase. In contrast, plants that overexpressed OsERF922 reduced the expression of these defense-related genes and increased susceptibility to magnaporthe oryzae. The ABA content of OsERF922 overexpressed rice was significantly higher than that of control and RNAi lines. NCED3 and NCED4 were up-regulated in OsERF922 overexpressed plants, while NCED4 was down-regulated in RNAi lines. OsERF922 may enhance plant resistance to abiotic stress by participating in the ABA pathway (Liu et al., 2012). Abscisic acid repressor ABR1, a member of ethylene responsive element binding factor superfamily of AP2/ERF transcription factors, significantly improves drought resistance of rice, crop quality and yield (Pandey et al., 2005). Fagopyrum esculentum can cope with aluminum toxicity by increasing ABA level and antioxidant system in early growth and development. The levels of reactive oxygen species (ROS), endogenous ABA and antioxidant enzyme activity in root of buckwheat seedlings increased after aluminum treatment, which may be mediated by increased ABA levels. After ABA treatment, ROS levels increased, catalase (CAT) and ascorbate peroxidase (APX) activities increased (Salazar-Chavarria et al., 2020). OsERF71 plays a positive role in drought tolerance of rice by increasing the expression of genes related to ABA signaling pathway and proline synthesis. Overexpression of OsERF71 decreased the water loss rate of rice, while the RNAi lines of OsERF71 were sensitive to drought stress and increased the water loss rate. Under drought stress, OsERF71 regulated the expression of several ABA responsive genes and proline synthesis genes, enhanced the sensitivity and proline accumulation of ABA treatment. GsERF71 overexpressing Arabidopsis has significantly higher tolerance to carbonate stress induced by NaHCO3 or KHCO3 than that of wild-type plants with greener leaves, longer roots, higher chlorophyll content and lower MDA content. GsERF71 increased the tolerance to alkaline stress by up-regulating H+, ATPase expression and regulating auxin accumulation in transgenic plants (Yu et al., 2017). SHINE (SHN) transcription factor is a branch of AP2/ERF and one of the first reported transcription factors to regulate plant cuticle formation. Cuticle plays an important role in improving plant abiotic stress, such as drought resistance, high temperature resistance, salt resistance, disease resistance (Khoudi, 2023). Recent study showed that the GsERF1 gene from BW69 wild soybean was induced by aluminum stress and ethylene (Li et al., 2022).
The quality and yield of soybean are greatly affected and limited by acidic soil in a large area of the world. In the past, there were a few reports on the involvement of ERF family genes in Al tolerance in plants. In this study, the ethylene reactive transcription factor GmABR1 of AP2/ERF family gene was identified using the population of recombinant inbred line derived from Zhonghuang 24 (ZH24) × Huaxia 3 (HX3), and its function tolerant to Al stress was investigated by heterotransformation of Arabidopsis.
2 Materials and methods
2.1 Plant materials and growth conditions
In this experiment, the HX3 soybean cultivar was bred and provided by the Guangdong Subcenter of National Soybean Improvement Center. The tissue expression pattern of GmABR1 and its response to Al stress were studied using qRT-PCR. The seeds of HX3 were germinated on vermiculite after high temperature sterilization. The culture temperature was 26 °C and the light/darkness was 16 h/8 h (Wang et al., 2019a). The cultures were incubated in 0.5 mM CaCl2 solution (containing 50 µM AlCl3) for 0, 3, 6, 9, 12, 24, 36, 48, 60 and 72 h. With the incubation in 0.5 mM CaCl2 with 0, 25, 50, 75, or 100 µM AlCl3 (pH4.5) for 24 h, all the roots of soybean are taken as samples in Al dose-effect experiments (Han et al., 2022). For tissue expression pattern, the samples of roots, stems, and leaves of soybean seedlings incubated in 0.5 mM CaCl2 (50 µM AlCl3 (pH4.5) for the experimental group, without AlCl3 for the control group) for 60 h were taken and rapidly frozen in liquid nitrogen, and stored at -80°C (Wang et al., 2022).
2.2 Bioinformatics analysis and cloning of the GmABR1 gene
The GmABR1 gene in soybean cultivar HX3 was up-regulated in the expression profile of acid-resistant aluminum genes, and the login number on the website of the National Center for Biotechnology Information (NCBI) was XP_003523249.1 by using the NCBI database for searching for the sequence information of the candidate gene. The software DNAMAN 9.0 was used for sequence alignment, phylogenetic tree was constructed by proximity method (NJ) using MAGE 7.0 software (Shu et al., 2022). The total RNA of soybean cultivar HX3 extracted by Trizol solution (Vazyme Biotech Co., Ltd., Nanjing, China) was reverse-transcribed into cDNA (HiScript® III RT SuperMix for qPCR, Vazyme Biotech Co., Ltd.; Nanjing, China) (Wang et al., 2022). The full-length of GmABR1 gene was cloned by using specific primers and high-fidelity DNA polymerase (Phanta Max, Vazyme Biotech Co., Ltd.; Nanjing, China) (Table S2). PCR products were detected by 1% agarose gel electrophoresis (GenStar Kit, GenStar Development Company, Calgary, AB, Canada), and the purified PCR products were inserted into the polyclonal site of the zero-background clone pLB vector (Tiangen Lethal Based Fast Cloning Kit, Beijing, China). The fusion vector GmABR1-pLB was transformed into Escherichia coli DH5α (Li et al., 2022). The positive clones of GmABR1 gene were identified by PCR and enzyme digestion, and the CDS sequence of GmABR1 gene was obtained by sequencing [Sangon Biotech (Shanghai) Co., Ltd., China] (Wen et al., 2021).
2.3 Construction of GmABR1 overexpression vector and genetic transformation of Arabidopsis
Using the constructed pLB plasmid as DNA template, the CDS sequence of GmABR1 amplified by PCR was inserted between the restriction sites of XbaI and SacI from the vector pTF101 by using the ClonExpressR II One Step Cloning Kit (C112, Vazyme, Nanjing, China) to construct the vector pTF101-GmABR1. The positive clones of pTF101-GmABR1 were identified by PCR using specific primers. The pTF101-GmABR1 plasmid was transformed into agrobacterium tumefaciens GV3101 cells by heat shock method (Li et al., 2022). Genetic transformation of Arabidopsis was subsequently performed using agrobacterium-mediated flower impregnation (Han et al., 2022). After the obtained transgenic plants were identified, the T3 transgenic positive lines with high expression of GmABR1 in root tip were selected for subsequent experiments including the analysis of its expression patterns response to Al stress and the phenotypes resistant to Al stress (Liu et al., 2020). The primer information used in this experiment was shown in Table S2.
2.4 Subcellular localization of GmABR1 protein
The subcellular localization of GmABR1 protein was analyzed using the method described by Collings et al. and Dong et al. (Collings, 2013; Dong et al., 2015). The subcellular localization of GmABR1 protein was predicted using a web server Cell-PLoc 2.0 (http://www.csbio.sjtu.edu.cn/bioinf/Cell-PLoc-2/). The specific operation is to cut the NcoI and SpeI sites on the expression vector pCAMBIA1302-eGFP with restriction endonuclease, and to link the recombinant amplified fragments of CDS sequences containing corresponding restriction sites with T4 ligase. After the construction of the fusion expression vector, the plasmid pCAMBIA1302-eGFP-GmABR1 and the empty plasmid pCAMBIA1302-eGFP were transformed into the cells of Agrobacterium tumefaciens GV3101 (contain the virus protein P19) and then injected into the lower epidermal cells of 4-week-old leaf in Nicotiana tabacum. The transformed leaves for 48 hours were observed with a laser confocal microscope (Carl Zeiss, Jena, Germany).
2.5 Transactivation assay of GmABR1 protein
The activation activity of GmABR1 protein was verified by using the method described by Han et al. (Han et al., 2022). The full-length sequence of GmABR1 was first cloned and inserted it into the BamHI and EcoRI restriction endonuclease sites of pGBKT7 vector to form the fusion expression vector pGBKT7-GmABR1. The vectors of pGBKT7 and pGBKT7-GmABR1 were then transformed into yeast Y2H strain, respectively. The transformed yeast cells were cultured on SD medium which was lacking Trp (SD/-Trp) at 30 °C for 3 days. Then the positive colonies were transferred from SD/-Trp medium to SD/-Trp medium containing 125 μg/L X-α-gal. After cultured for 3 days, whether the colonies appear blue or not can be observed on the selected medium.
2.6 Gene expression analysis by qRT-PCR
Total RNA of samples was extracted from soybean or Arabidopsis tissues using the Trizol solution (Vazyme Biotech Co., Ltd., Nanjing, China). qRT-PCR and first strand cDNA synthesis templates were performed with 1 μg of RNA using the HiScriptII first strand cDNA synthesis kit (Vazyme Biotech Co., Ltd., Nanjing, China) (Cai et al., 2018). qRT-PCR was performed using SYBR PremixEx-Taq™ II (Takara Bio Inc., Kusatsu, Japan) and real-time CFX96TM systems (Bio-Rad, Hercules, CA, USA) to detect RNA transcripts of related genes (Wang et al., 2022). The inner reference gene Actin3 in soybean was used to normalize the data. The expression data were calculated using the comparative cycle threshold method 2-△△ct. The experiments were repeated at three independent organisms (Xian et al., 2020). The primers used in this assay were listed in Table S2.
2.7 Phenotypic analysis of root growth in Arabidopsis treated with Al
Three homozygous lines from T3 generation of GmABR1 transgenic plants were used to measure taproot length as described previously (Yokosho et al., 2016; Li et al., 2022). The seeds of GmABR1 transgenic Arabidopsis and WT were disinfected with 10% sodium hypochlorite for 10 min respectively, during which they were vortically oscillated, and then cleaned with sterilized water for 5 times. The sterilized seeds were sown on a square plate of 1/2 MS solid medium (pH5.8), placed in a refrigerator at 4°C and vernalized at low temperature for 3-4 d. Then the seeds were germinated and grown in the growth chamber with following conditions: 23°C, 60% relative humidity, 100 mol photons m-2·s-1, 16 h light and 8 h dark cycles. When root lengths of the seedlings grew to 1-2 cm, the seedlings were selected and transferred to the medium containing different concentrations of AlCl3 (0, 25, 50 and 100 μM; pH 4.5). Phenotypic differences were observed and photographed after 3 days of Al treatment. The data were analyzed by using the software Image J (Zhou et al., 2008).
2.8 Soybean hairy root transformation and hematoxylin staining
The construction of pMU103-GmABR1 interference vector (RNAi vector) is similar to the previous construction method (Kereszt et al., 2007; Ren et al., 2019). The 159 bp coding region sequence of GmABR1 gene was selected to construct the interference vector. The vector plasmids of pTF101, pTF101-GmABR1, pMU103-GmABR1 were transformed into Agrobacterium rhizogenes K599 competent cells by heat shock, respectively. Soybean hair roots from the 5-day’s seedlings were used as experimental materials, and then transformed by the method of hypocotyl injection.
In order to analyze the expression of GmABR1 gene in soybean hairy roots, the hairy root lines were treated with 50 µM AlCl3 solution (CaCl2, pH 4.5) for 6 h, then the roots were cut and dipped into hematoxylin solution for 30 min. The stained roots were taken out and rinsed for 30 min before being observed under stereomicroscope (Li et al., 2022).
2.9 Physiological indices assay
Transgenic GmABR1 Arabidopsis and WT were treated with 0.5 mM CaCl2 solution containing 50 µM AlCl3 and 0.5 mM CaCl2 solution without AlCl3 (pH 4.5), respectively. After 10 days, the whole plant samples were taken to determine the content of MDA (Choudhury and Sharma, 2014).
The hairy root lines transferred into pTF101 plasmid were used as control. The roots of WT, GmABR1-overexpressed soybean and GmABR1-RNAi soybean were transferred to 0.5 mM CaCl2 solution (pH 4.5) containing 50 µM AlCl3. After 1 day of treatment, the root tips of 2 cm washed with ddH2O were taken as samples, weighed and recorded. Put 2 M hydrochloric acid (HCl) into a 2 ml EP tube and soak it in a shaking table for 3-4 days. After extraction and filtration, the content of Al3+ in soybean hairy roots was determined by plasma inductances coupled (Umemura et al., 2003).
2.10 Statistical analyses
Each experiment was designed at least three biological replicates. The SPSS Statistics (v.22) and GraphPad Prism 8 softwares were used to analyze and process the data. The statistical significance of the difference for the data was indicated by an asterisk (*P < 0.05; **P < 0.01) (Ma et al., 2018; Wang et al., 2022).
3 Results
3.1 Isolation and bioinformatics analysis of GmABR1
The GmABR1 gene was cloned from soybean cultivar HX3 using specific primers (Table S1). The GmABR1 gene sequence was obtained from the National Center for Biotechnology Information (NCBI) database with the accession number LOC_100793215. The length of GmABR1 gene was 1135 bp, and the length of open reading frame was 846 bp. It contains two exons encoding a 282 amino acid protein with an estimated molecular weight of 30.8504 KDa and isoelectric point of 10.0135. Sequence alignment revealed that GmABR1 protein had a conserved DNA-binding domain (AP2 domain) consisting of 60 amino acids between 135-194 amino acid residues, which was inferred to be a typical structure of ERF transcription factors. In plants, many ERF family genes have been reported to be closely associated with biotic and abiotic stresses. Amino acid sequence alignment and phylogenetic tree analysis showed that GmABR1 protein had the highest homology up to 93% with AtERF111 (At5g64750) protein in Arabidopsis (Figure 1).
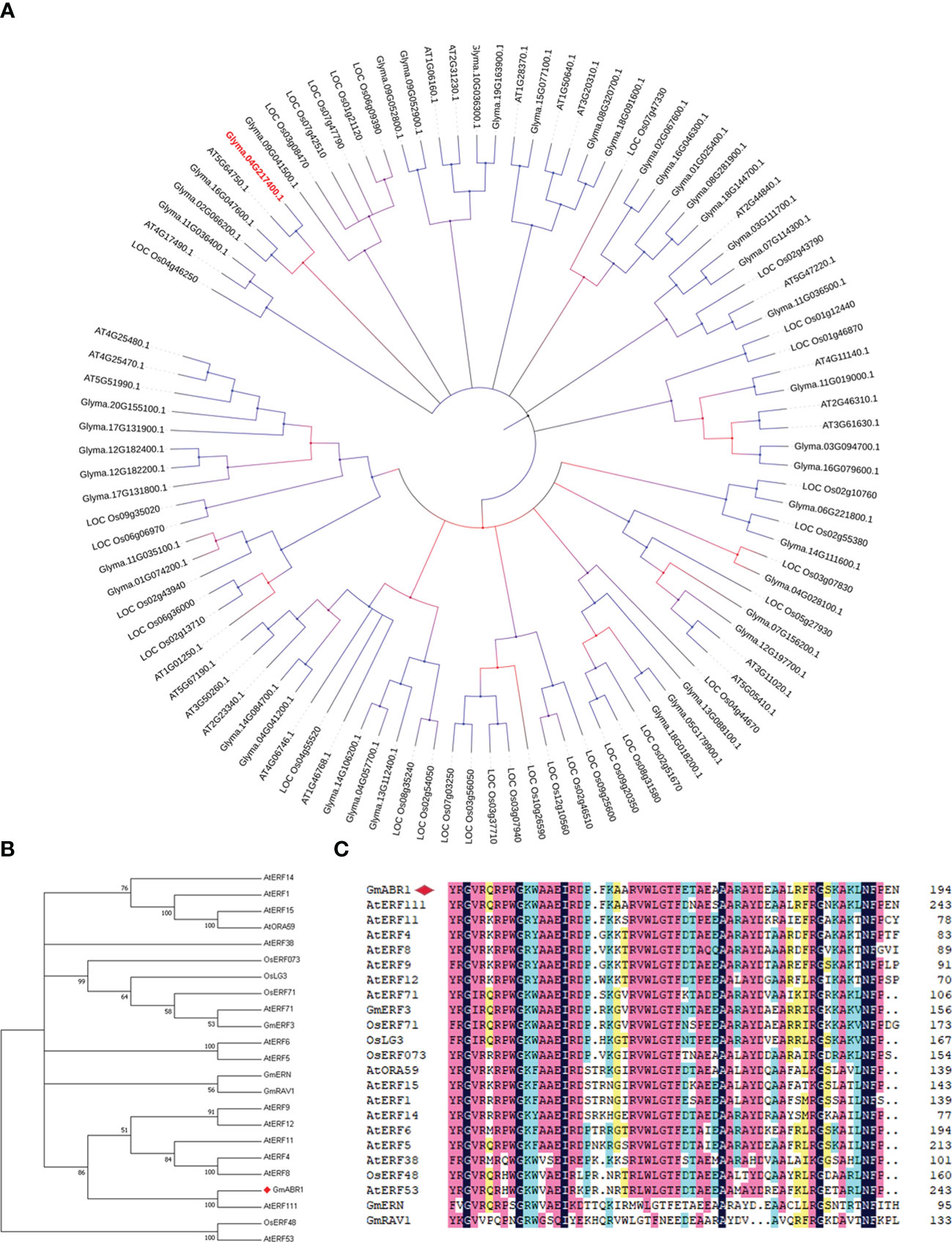
Figure 1 Amino acid sequence and phylogenetic analysis of ERF transcription factors. (A) Phylogenetic tree analysis of GmABR1 and ERF proteins in representative species of major lineage of plants. (B) Phylogenetic analysis of ERF proteins in higher plants. (C) Sequence alignment of the AP2 domain in ERF proteins of higher plants. The comparison of amino acid sequences was conducted by the software of DNAMAN9.0. The phylogenetic tree was constructed by the neighbor-joining method using the software of MEGA 7.0. The above involves the amino acid sequences of ERF family from the NCBI database (https://www.ncbi.nlm.nih.gov/). The detailed information of ERF proteins was available from the Table S3.
3.2 Subcellular localization and transcriptional activation ability of GmABR1 protein
We detected the localization of GmABR1 protein in tobacco leaf cells. The results of confocal laser microscopy showed that GFP protein stimulated green fluorescence in the whole cell, while GmABR1-GFP fusion protein only stimulated green fluorescence in the nucleus, which overlapped with the chromophore of nuclear dye DAPI (Figure 2B). The results showed that the GmABR1 protein is localized in the nucleus.
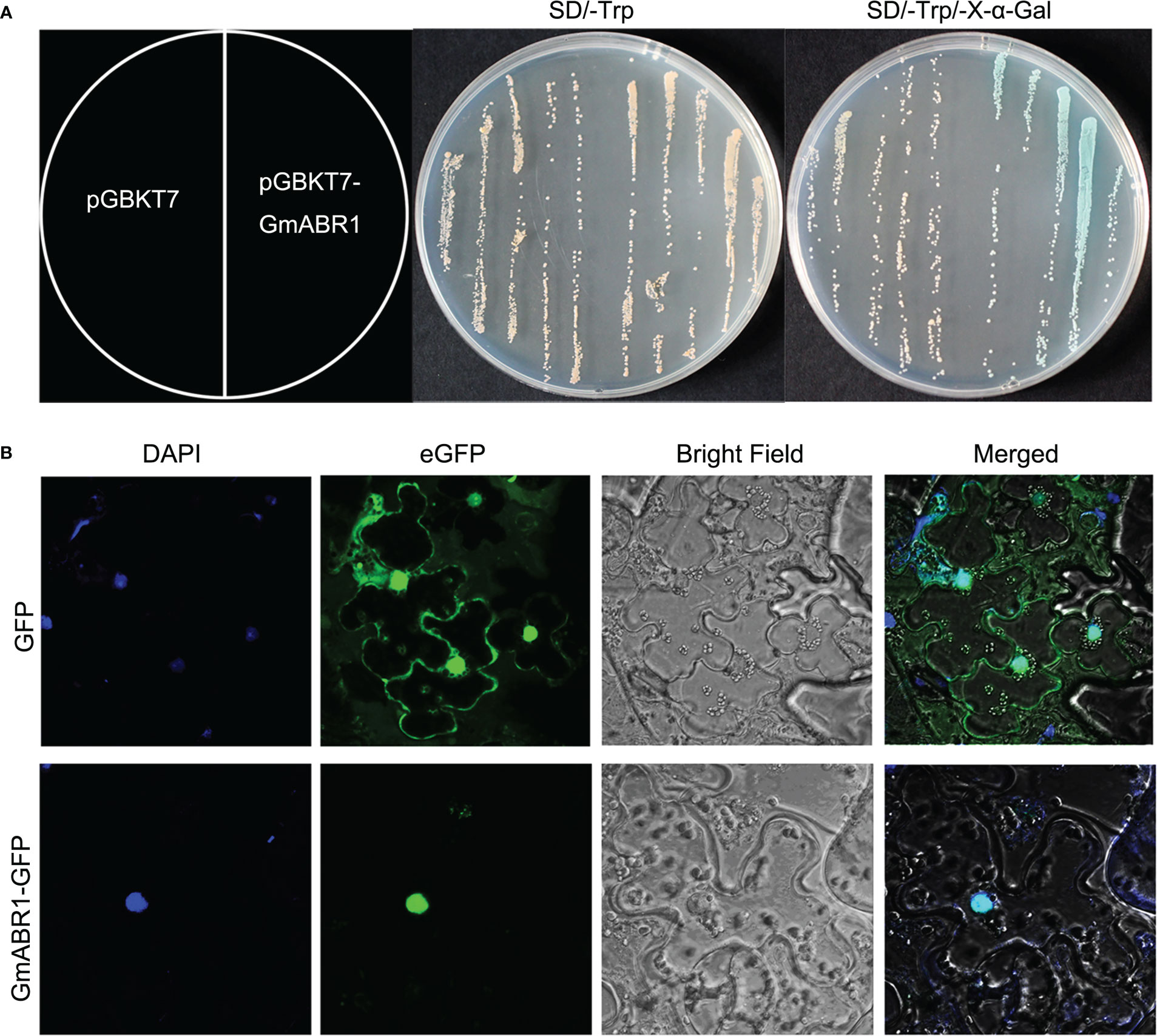
Figure 2 Transcriptional activity detection and subcellular localization of GmABR1 protein. (A) Transcriptional activation of GmABR1 protein. (B) Subcellular localization of GmABR1 protein. The CDS of GmABR1 sequence was fused with the GAL4 DNA binding region of pGBKT7 vector and expressed in yeast strain Y2H Gold. After the transformation, the two positive clones were transferred to SD/-TRP solid medium, and the other group was added with substrate X-a-Gal. The pCAMBIA1302-GFP-GmABR1 and pCAMBIA1302-GFP vectors were transformed into Agrobacterium tumefaciens GV3101 (contain the virus protein P19) respectively, and then injected into 4-week old Nicotiana tabacum L epidermal cells. After cultured for 2 days later, the leaves were observed by laser confocal microscopy (Carl Zeiss, Jena, Germany).
To determine the transcriptional activity of GmABR1 protein, we combined the ORF of GmABR1 with the pGBKT7 plasmid, and simultaneously transferred the constructed fusion plasmid pGBKT7-GmABR1 and pGBKT7 vector into the receptive cells of yeast strain Y2H. The results showed that the transformed yeast positive clones of pGBKT7-GmABR1 and pGBKT7 plasmids could grow on SD/-Trp medium. In addition, the yeast monoclonal transformed with pGBKT7-GmABR1 plasmid was blue on SD/-Trp-X-α-Gal solid medium, while the yeast monoclonal transformed with pGBKT7 vector showed no color change (Figure 2A). The results showed that GmABR1 protein had characteristic transcriptional activation.
3.3 Expression patterns of GmABR1
The expression pattern of GmABR1 was studied by using the samples of roots, stems and leaves of soybean HX3. The qRT-PCR results showed that GmABR1 was mainly expressed in stems, followed by roots and leaves without Al stress. After Al stress, the transcripts of GmABR1 gene was significantly increased in roots, but no significant change in stems and leaves (Figure 3A). In addition, the transcripts of GmABR1 increased first, then decreased and finally increased with the increase of Al3+ concentration. When treated with 50 µM AlCl3, the transcripts of GmABR1 were 51 times that of the control, while high concentration of AlCl3 inhibited the expression of GmABR1 (Figure 3B). When treated with 50 µM AlCl3, the GmABR1 transcripts increased gradually and then decreased along with time, and reached the highest level at 60 h after Al treatment, which was 36 times that of the control (Figure 3C).
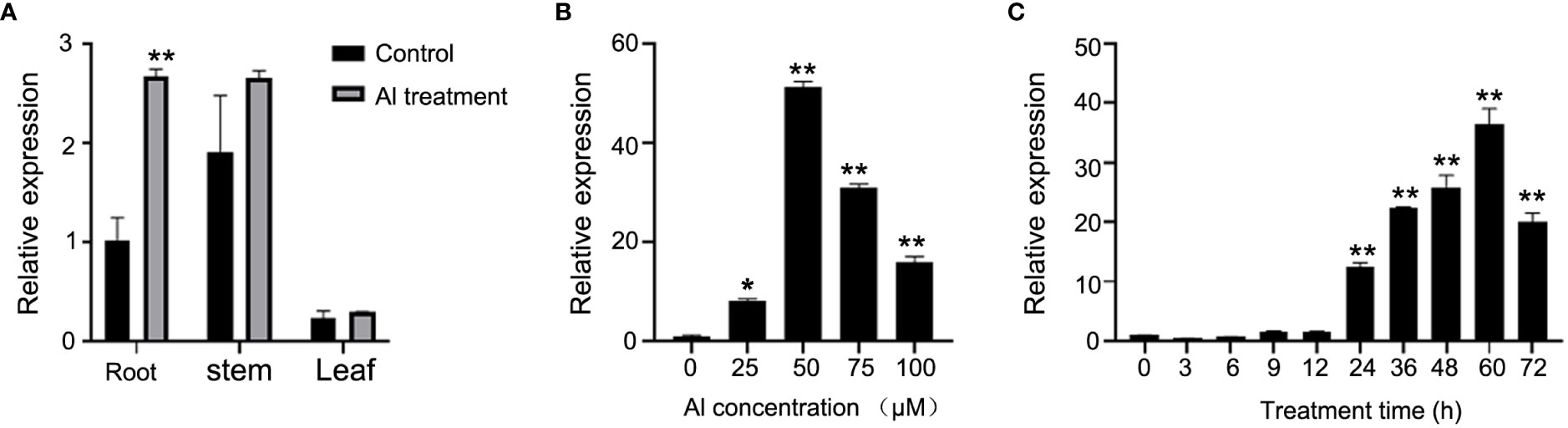
Figure 3 Expression pattern analysis of GmABR1. (A) Tissue expression pattern of GmABR1. Samples were obtained from root, stem, and leaf tissues of HX3 seedlings grown in the nutrient solutions containing 0 μM AlCl3 and 50 μM AlCl3, respectively. (B) Gene expression of GmABR1 in the root of soybean cultivar HX3. The soybean cultivar HX3 was cultured for 24 h in the nutrient solutions containing 0, 25, 50, 75 and 100 μM AlCl3, respectively. (C) Time course expression pattern of GmABR1 in the root of soybean cultivar HX3. Using soybean Actin3 as internal reference gene, three independent replicates were detected by 2^-△△CT method. The asterisks in figure A indicate significant differences between roots and other soybean tissues (*P < 0.05; **P < 0.01). The asterisk in figure B indicates that there is a significant difference between 0 μM AlCl3 and other Al concentration (25, 50, 75 and 100 μM AlCl3) treatment (*P < 0.05; **P < 0.01). The asterisk in figure C indicates that there is a significant difference between the Al treatment time of 0 h and the other Al treatments of 3, 6, 9, 12, 24, 36, 48, 60, 72 h (*P < 0.05; **P < 0.01).
3.4 Overexpression of GmABR1 enhances Al resistance in transgenic Arabidopsis
To investigate the effect of GmABR1 response to Al stress, GmABR1 was overexpressed in Arabidopsis and more than 10 homozygous lines were obtained. Three lines with high GmABR1 transcripts (L1, L4 and L9) were selected for subsequent phenotypic identification (Table S5). The results showed that there was no significant difference in taproot elongation between WT and transgenic lines without Al treatments. After AlCl3 treatments, all lines were significantly inhibited. When AlCl3 concentration was 100 µM, the taproot elongation of WT was 69.6%, while that of transgenic lines was 85.6%. The taproot elongation of GmABR1 transgenic lines was significantly higher than that of WT (Figures 4A, B). With the increase of AlCl3 concentration, the effect of inhibiting taproot elongation increased. Under the treatment of AlCl3, the concentration of MDA in WT was 1.41 µg/g, and that in GmABR1 transgenic Arabidopsis was 1.32 µg/g. After 50 µM AlCl3 (pH 4.5) treatment, the MDA content in WT increased to 3.03 µg/g, and the MDA content in transgenic Arabidopsis leaves increased slightly up to 1.66-2.21 µg/g, which was still significantly lower than that in WT (Figure 4C).
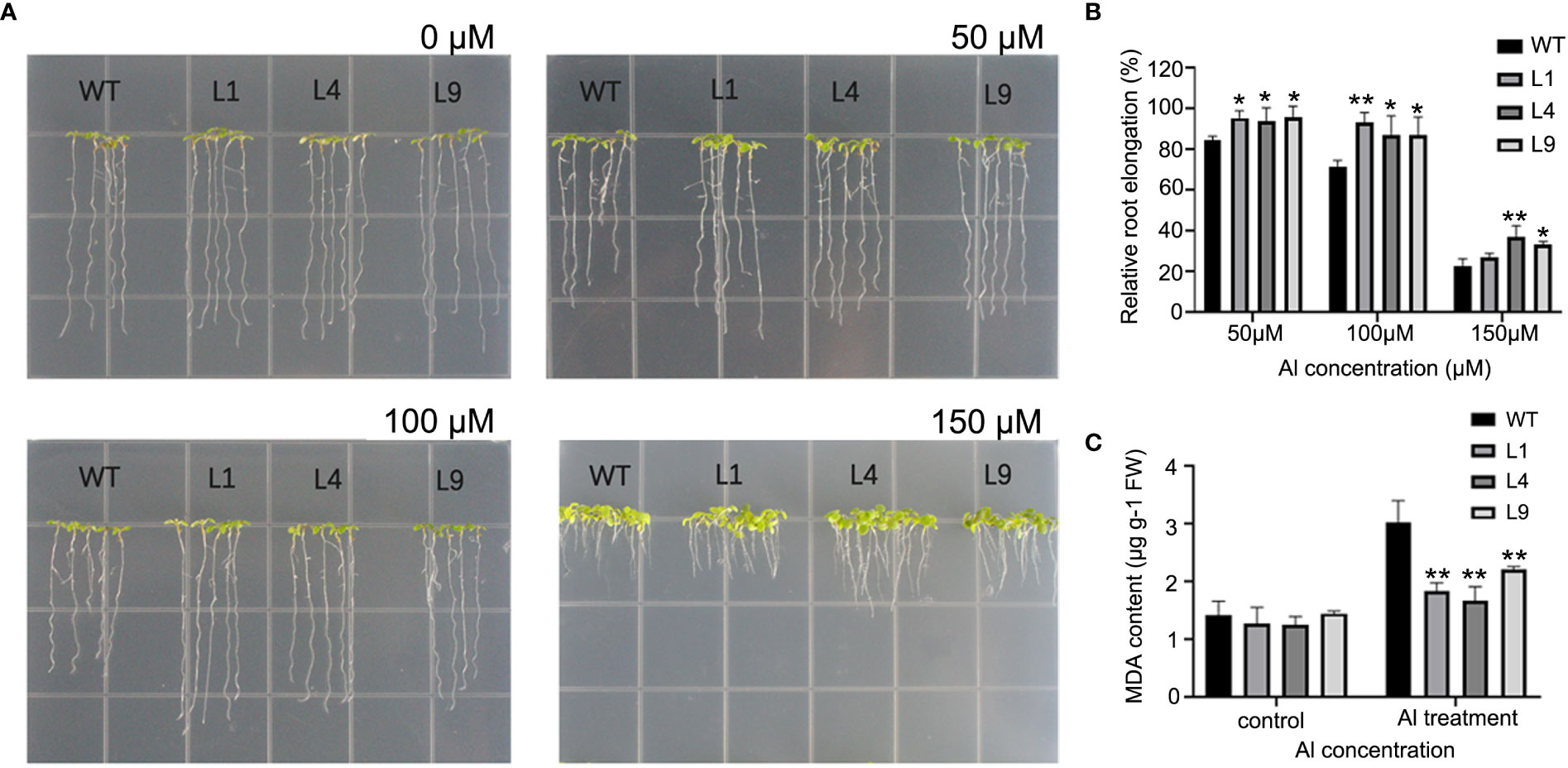
Figure 4 Overexpression of GmABR1 enhances Al resistance in transgenic Arabidopsis. (A) Root growth of WT and GmABR1 transgenic Arabidopsis under 0 µM, 50 µM, 100 µM, 150 µM AlCl3 (pH4.5). (B) Relative root elongation of WT and GmABR1 transgenic Arabidopsis. (C) MDA content in leaves of WT and GmABR1 transgenic Arabidopsis. The asterisk indicated that there was significant difference between WT and GmABR1 transgenic lines (*P < 0.05; **P < 0.01). WT: Columbia-0; L1, L4, L9: GmABR1 transgenic lines of T3 generation.
3.5 Soybean hairy roots experiment and hematoxylin staining
The effect of GmABR1 gene on Al stress was studied by soybean hairy roots experiment. Soybean gene Actin3 was used as internal reference gene, and the hair root lines transformed by pTF101 vector were used as control. The transcription level of GmABR1 in overexpressed root lines was 6.35 times that of the control, while the transcription level of GmABR1 in RNAi lines was only 0.60 time that of the control (Figure 5A). After 50 µM AlCl3 treatment, the results of hematoxylin staining showed that the overexpressed lines of GmABR1 had the lightest root tip coloring, while the RNAi lines of GmABR1 had the deepest root tip coloring (Figure 5B). The concentration of Al3+ in root tips of control, RNAi lines and overexpressed lines was 207.40 µg/g, 147.74 µg/g and 330.65 µg/g, respectively (Figure 5C). The accumulation of Al3+ in root tip of RNAi lines was significantly higher than that of GmABR1-overexpressed lines.
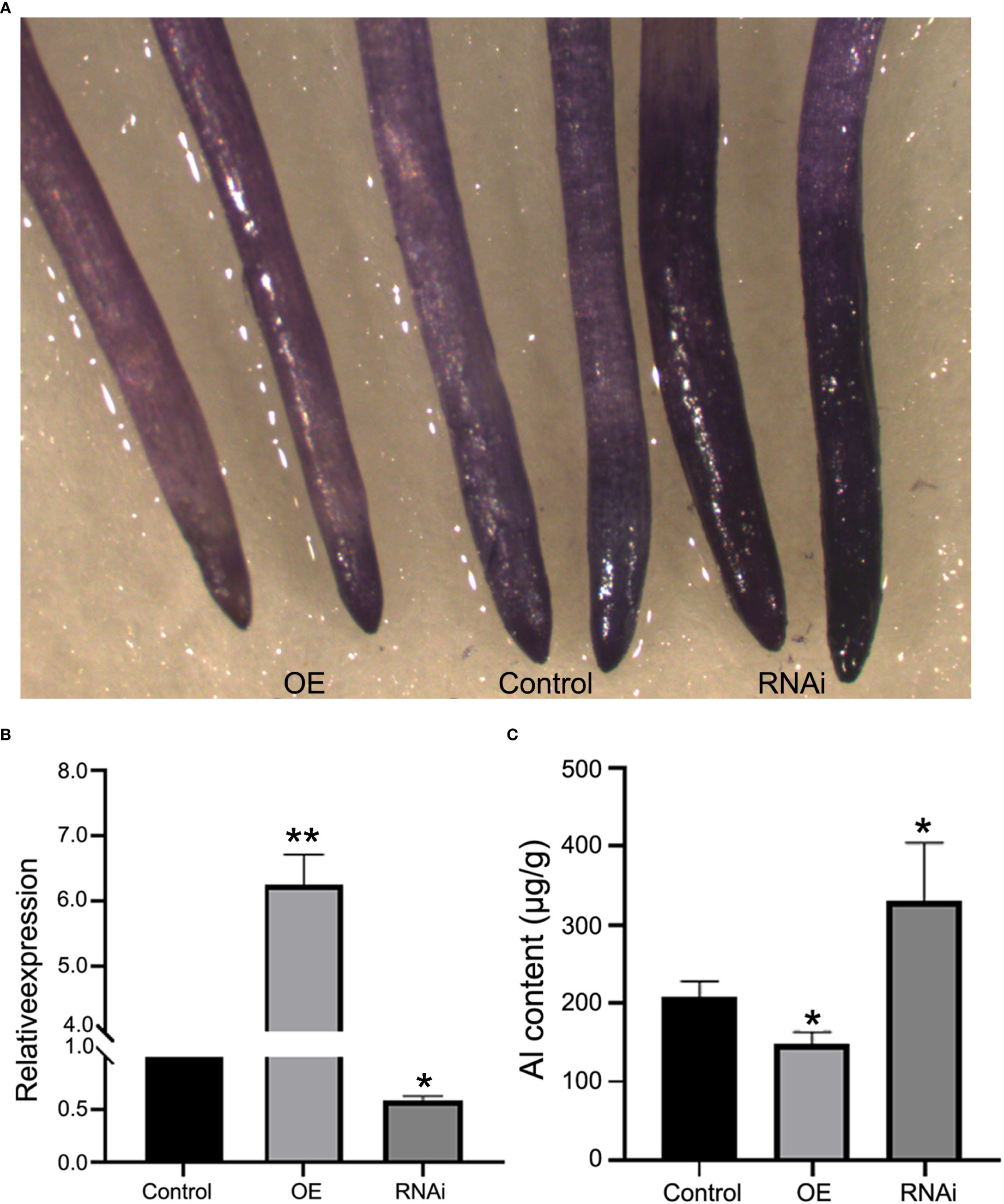
Figure 5 Soybean hairy roots experiment and hematoxylin staining. (A) Hematoxylin staining of soybean hairy roots. (B) Identification of RNA levels in hairy roots of soybean. (C) Determination of Al3+ content in root tips of soybean hairy root lines. The asterisks in figure B and C indicate significant differences between WT and OE or RNAi lines (*P < 0.05; **P < 0.01). Control: the lines of transformed pTF101 vector; OE: the lines of transformed pTF101-GmABR1 vector; RNAi: the lines of transformed pMU103-GmABR1 vector.
3.6 GmABR1 improved the transcription level of Al stress responsive genes in Arabidopsis
To further study the response mechanism of GmABR1 to Al stress, the relative genes including AtALMT1, AtMATE, AtALS3, AtSTOP1, AtPGIP1 and AtPGIP2 in Arabidopsis were identified based on previous studies. The qRT-PCR analysis showed that the expression levels of some Al stress-related genes such as AtALMT1, AtMATE, AtALS3, AtSTOP1, AtPGIP1 and AtPGIP2 in WT and transgenic Arabidopsis were up-regulated after 100 μM AlCl3 (pH4.5) treatment. In particular, the expression levels of AtALMT1 and AtMATE genes were significantly higher than those before aluminum treatment, which were 2.9 times and 3.0 times of those before aluminum treatment, respectively (Figure 6).
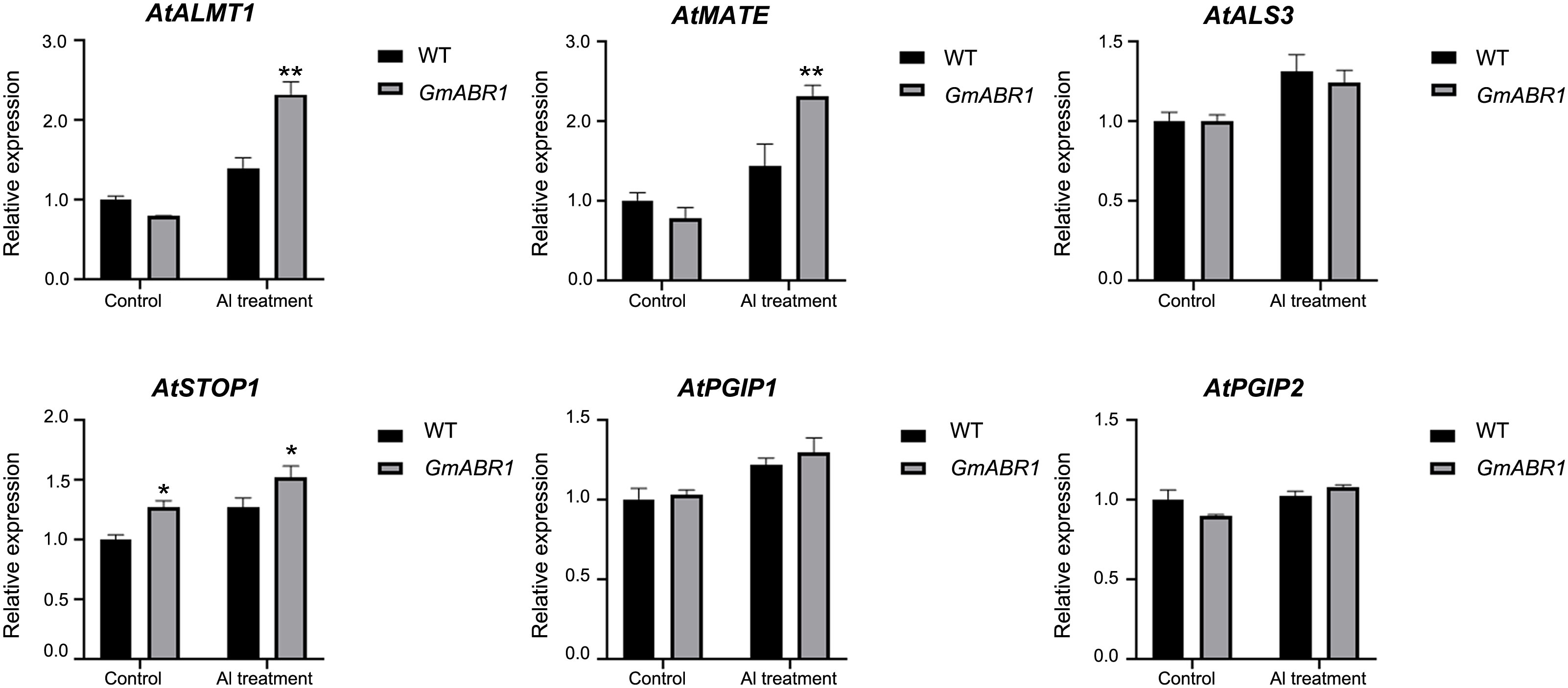
Figure 6 Expression patterns of Al stress responsive genes regulated by GmABR1 in Arabidopsis. Arabidopsis were cultured in solid medium supplemented with 0 and 100 μM AlCl3 (pH4.5) for 7 days, respectively. The expression patterns of Al stress responsive genes by GmABR1 were detected. Using Arabidopsis Tubulin as the internal reference gene, qRT-PCR was used to detect the gene expression level before and after Al treatment. The asterisks indicated that the transcripts of investigated genes in GmABR1 transgenic lines were significantly different from those of WT treated with and without AlCl3 (*P < 0.05; **P < 0.01). The information of gene sequences was from the NCBI database (https://www.ncbi.nlm.nih.gov/). qRT-PCR primer information used in this experiment was shown in Table S2.
3.7 Expression changes of ABA responsive genes in Arabidopsis
To further investigate the mechanism of GmABR1 response to Al stress, the internal reference gene Tubulin in Arabidopsis was used as a control. The qRT-PCR analysis showed that after 100 µM AlCl3 (pH 4.5) treatment, the expression levels of AtABI1, AtABI2, AtABI4, AtABI5, AtRD22 and AtRD29A were all up-regulated in WT. The up-regulations of AtABI4, AtABI5 and AtRD22 were 2.9, 3.0 and 2.1 times higher than those before Al stress, respectively. AtABI1, AtABI2 and AtRD29A were down-regulated to 0.7, 0.7 and 0.4 times of the pre-treatment levels, respectively (Figure 7).
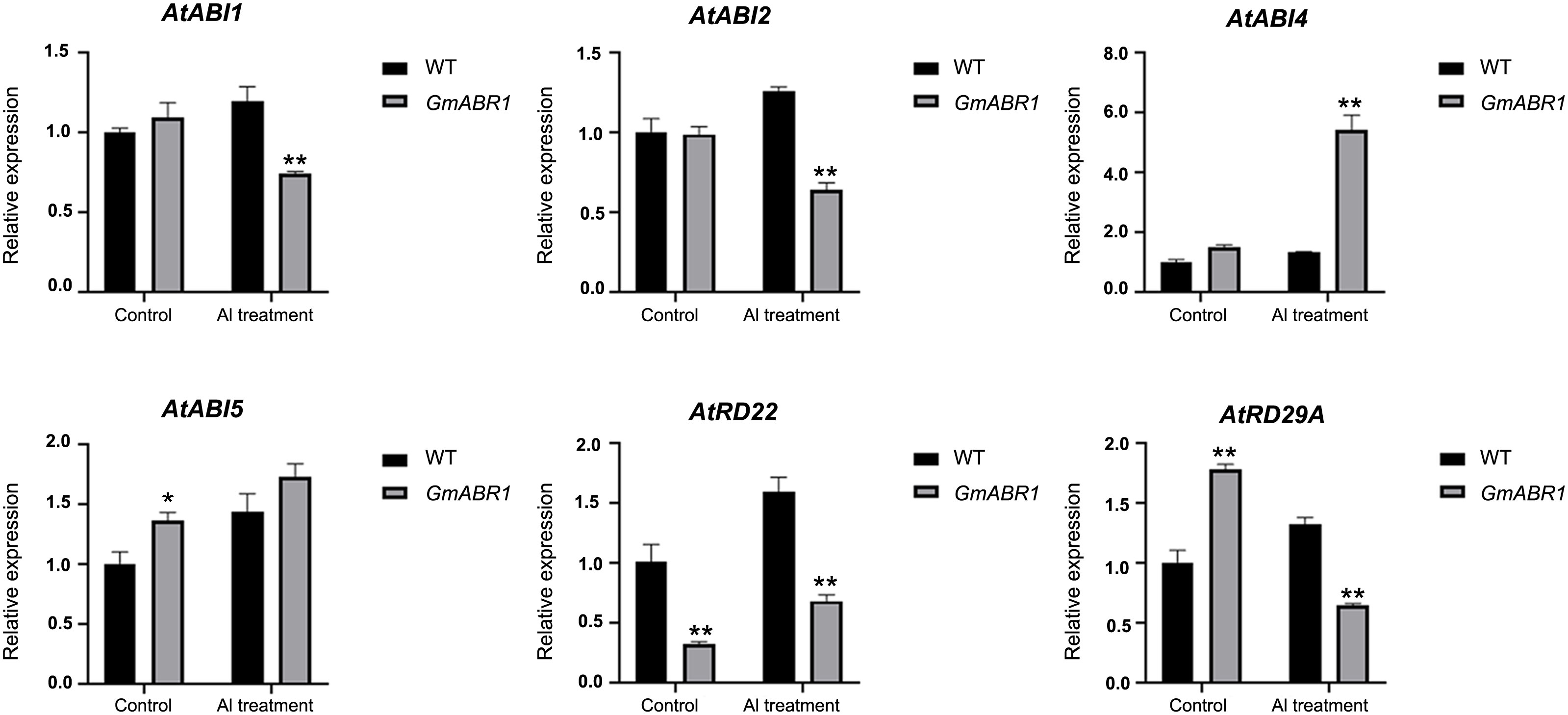
Figure 7 Expression patterns of ABA-responsive genes regulated by GmABR1 in Arabidopsis. Arabidopsis were cultured in solid medium supplemented with 0 and 100 μM AlCl3 (pH4.5) for 7 days, respectively. The expression patterns of Al stress responsive genes by GmABR1 were detected. Using Arabidopsis Tubulin as the internal reference gene, qRT-PCR was used to detect the gene expression level before and after Al treatment. The asterisks indicated that the transcripts of investigated genes in GmABR1 transgenic lines were significantly different from those of WT treated with and without AlCl3 (*P < 0.05; **P < 0.01). The information of gene sequences was from the NCBI database (https://www.ncbi.nlm.nih.gov/). qRT-PCR primer information used in this experiment was shown in Table S2.
4 Discussion
Previous studies have shown that AP2/ERF family genes play important biological functions in plants including regulating plant growth and development, and coping with various abiotic stresses. Transcription factor ERF96 is a transcriptional activator that binds GCC and positively regulates Arabidopsis resistance to necrotize pathogens by responding to jasmonic acid and ethylene (Wang et al., 2015). WRI4 which is mainly expressed in Arabidopsis stem epidermis and encodes an AP2/ERF transcription factor is up-regulated under salt stress and involved in activating epidermal wax biosynthesis to protect plants from environmental stress (Park et al., 2016). PpeERF2 regulates peach fruit ripening by inhibiting the expression of two ABA biosynthesis genes (PpeNCED2, PpeNCED3) and cell wall degradation gene (PpePG1) (Wang et al., 2019b). The expression of SlERF5 in tomato is induced not only by low temperature, injury, high salt and drought, but also by plant hormones ethylene and ABA. Transgenic plants overexpressing SlERF5 showed increased tolerance to drought and high salinity (Wang et al., 2019b).
GmABR1 protein with a highly conserved AP2 domain had the highest homology with ERF111 (AT5g64750) protein in Arabidopsis. Previous studies indicated that the AtERF111 gene was specifically induced by flooding and hypoxia. The root hair length and number of overexpressed AtERF111 plants increased, which strengthened the hypothesis that AtERF111 was involved in injury response (Baumler et al., 2019). AtERF6 plays an active role in antioxidant regulation of Arabidopsis in response to biotic and abiotic stresses (Sewelam et al., 2013). In addition, GmABR1 protein is localized in the nucleus and is self-activating like other ERF transcription factors. We speculate that GmABR1 has potential functions as a member of the ERF family as a transcription factor (Baumler et al., 2019).
In this study, Al stress can induce high expression of GmABR1 in cultivated soybean HX3, and it is expressed in soybean roots, stems and leaves (Figure 3). Previous studies have shown that Fe3+ and Al3+ can form stable complexes or chelates with the components in the dye solution of hematoxylin (Hajiboland et al., 2013). In the dyeing process, oxidation film will form on the surface of the dye solution, and blue precipitation will appear in the dye solution to color the plant nucleus. The content of Al3+ in plant can be determined by observing the color depth. Rincon et al. exposed the roots of whole wheat seedlings to Al for 24 hours with the method of hemoxylin staining, and found that the main difference of Al accumulation between the Al tolerant (Atlas 66) and sensitive (Tam 105) varieties appeared in the growth area 0~2 and 2~5 mm from the root tip (Rincon and Gonzales, 1992). Our study showed that after hemoxylin staining of Al treated hairy roots of soybean, the root tip color of RNAi lines had the deepest color, followed by control lines, and the root tip color of overexpressed lines was stained the lightest (Figure 5A). Furthermore, plasma inductively coupled technique (ICP-AES) was used to determine the root tip Al3+ data, and the root tip Al3+ content of overexpressed lines was the lowest, while the root tip Al3+ content of RNAi lines was the highest. The results indicated that GmABR1 can improve the ability of soybean Al detoxification (Figure 5C). GmABR1 was heterologous transformed into Arabidopsis, and the relative root elongation of transgenic lines after Al treatment was significantly higher than that of WT (Figure 4). MDA is one of the important products of membrane lipid oxidation, and its content reflects the degree of stress damage to plant cell membrane (Luan et al., 2018). Under Al stress, MDA concentration increased significantly in WT leaves compared with transgenic plants (Figure 4C). These results suggest that GmABR1 enhances the tolerance of plants to Al stress.
Previous studies have found that Al-activated secretion of malate and citric acid has important effects on plant Al tolerance. After MATE knockdown in Arabidopsis, the transcript level of MATE was reduced, and Al-activated root citrate secretion was eliminated. Moreover, ALMT1 and MATE double mutants lacked Al-activated root malate and citrate. Malate secretion mediated by ALMT1 and citrate secretion mediated by MATE evolved independently in Arabidopsis and together contribute to Al tolerance in Arabidopsis (Liu et al., 2009). STOP1 encodes a zinc finger protein, which is essential for Arabidopsis to resist low pH conditions, and the involvement of STOP1 is also required for ALMT1 expression (Iuchi et al., 2007). Liu et al. further demonstrated that the Al tolerance function of STOP1 also requires MATE expression and root citric acid secretion, Arabidopsis STOP1 gene plays a crucial role in the Al induced expression of MATE and ALMT1 (Liu et al., 2009). Recent studies have reported that STOP1 transcription is not affected by Al stress, but the expression of Al resistance genes ALMT1, MATE and ALS3 downstream of STOP1 is induced by Al stress. These results suggest that STOP1 may be regulated by Al at the post-transcriptional or post-translational level (Zhang et al., 2019b). ALS3 is predicted to encode a factor required for root growth in an Al-toxic environment, and mutational loss of this factor leads to severe Al-dependent consequences. This factor is specific for Al tolerance, as ALS3 loss-of-function mutants do not show increased sensitivity to other metals, including La and Cu, nor do they exhibit any abnormal growth phenotype in the absence of Al (Larsen et al., 1997). Yuriko et al. found that PGIP1 and PGIP2 were strongly inhibited in STOP1 mutants under Al stress, PGIP1 and PGIP2 may play a regulatory role in Al tolerance (Kobayashi et al., 2014). The Al-sensitive gene ALS3 encodes an ABC transporter-like protein that is required for resistance tolerance to Al to redistribute accumulated Al from sensitive tissues, thereby protecting growing roots from Al toxicity (Larsen et al., 2005).
In present study, the expression levels of AtALMT1, AtMATE, AtALS3, AtSTOP1, AtPGIP1 and AtPGIP2 genes were up-regulated in WT and transgenic Arabidopsis. The transcription levels of AtALMT1 and AtMATE in transgenic Arabidopsis were significantly increased compared with those before AlCl3 treatment (Figure 6). Therefore, the results indicated that GmABR1 may increase the secretion of malate and citrate in root tips of transgenic Arabidopsis by inducing the expression of Al stress response genes AtALMT1 and AtMATE, and then increase the resistance of transgenic Arabidopsis to Al stress through external Al exclusion mechanism.
ABA is an important endogenous messenger for plants to cope with stress. Yang et al. found that AtERF4 is a negative regulator of ethylene and abscisic acid response (Yang et al., 2005). Zhu et al. found that overexpression of RAP2.6, a member of the AP2/ERF family, could induce hypersensitivity responses to exogenous ABA and abiotic stress during seed germination and early seedling growth of Arabidopsis. ABA content in RAP2.6 overexpressing lines decreased after salt treatment (Zhu et al., 2010). The AtRAP2.6L homologous apple gene MdERF113 positively regulates abiotic stress and ABA stress (Rui et al., 2023). In recent years, Arabidopsis TCTP transcription factors have been gradually proved to be relative to abiotic stress signals. Overexpression of AtTCTP can enhance drought tolerance of plants by inducing ABA-mediated stomatal closure through interaction with microtubules (Kim et al., 2012). Merlot et al. found that double mutant plants of ABI1 and ABI2 loss function alleles were more responsive to ABA than their parental single mutants, while ABI1 and ABI2 were jointly involved in the negative feedback regulation of ABA signaling pathway (Merlot et al., 2001). ABI4 is also an important transcription factor in ABA signaling pathway, which can positively regulate NCED6, a key gene in ABA biosynthesis (Shu et al., 2016). Some studies have shown that Arabidopsis ABI5 mutants have enhanced sensitivity to Al, however, it is not related to the regulation of ALMT1 and MATE expression, indicating that ABA signal transduction pathway provides an additional regulatory mechanism for plant Al tolerance. The positive feedback regulatory transcription factor ABI5 can mediate cell wall modification and osmoregulation to enhance plant Al tolerance (Fan et al., 2019). The RD29A and RD22 genes are very sensitive to various abiotic stressors, and their expression is induced by endogenous ABA, which can enhance the ability of plants to resist abiotic stress by participating in ABA pathway (Yamaguchi-Shinozaki and Shinozaki, 1993; Lee et al., 2016). AtALMT12 is involved in ABA-induced stomatal closure and is a hypothesized target of this pathway with high nitrate and chloride transport capacity, stomatal closure may confer resistance to aluminum (Sasaki et al., 2010). Regulation of stomatal conductance by ABA signals may be an effective strategy to enhance abiotic stress in plants (Hsu et al., 2021).
In this study, the expression levels of AtABI1, AtABI2, AtABI4, AtABI5, AtRD22 and AtRD29A genes in WT were all up-regulated after Al treatment. Among them, the transcripts of AtABI4, AtABI5 and AtRD22 in transgenic lines were significantly up-regulated under Al stress. While the expressions of AtABI1, AtABI2 and AtRD29A were down-regulated with lower transcripts of the control treatment (Figure 7). The RD29A gene was up-regulated by Al stress in WT plants, but decreased in the transgenic lines. We hypothesized that this pathway has a compensating effect for the acid-resistant Al phenotype of the transgenic lines. Msanne et al. also found contrary to expectations that RD29A knockout mutant lines maintained greater root growth, photosynthesis and water use efficiency under salt stress than those of the controls. All transgenes effectively complemented resistance to salt stress-induced root growth inhibition (Msanne et al., 2011). Therefore, the results indicated that GmABR1 maybe also enhance plant resistance to abiotic stress by participating in the ABA pathway (Xu et al., 2017; Huang et al., 2022).
In summary, the GmABR1 gene encoding a AP2/ERF transcription factor in soybean was identified in present study, which was induced by Al stress and enriched in the roots, leaves, stems of soybean seedlings. GmABR1 overexpression increased the tolerance of GmABR1 transgenic plants to Al stress. The analysis of molecular mechanism showed that the enhanced tolerance to Al stress might due to the comprehensive roles in upregulating and/or downregulating transcripts of the Al stress- and/or ABA-responsive genes with MDA decrease. Therefore, these results suggested that GmABR1 may jointly regulate plant resistance to Al stress through the genes related to Al response and/or ABA response pathways.
Data availability statement
The data presented in the study are deposited in the NCBI database (https://www.ncbi.nlm.nih.gov/). The entry numbers of all genes involved in this paper (including the genetic information for making evolutionary trees) can be found in Supplementary Material Table S6. The original contributions presented in the study are included in the article/Supplementary Material, further inquiries can be directed to the corresponding author/s.
Author contributions
QM, AZ and HW conceived of and designed the study. HW, CL, LW, HZ, XX, YC, WL and HN conducted the experiments. HW, CL, LW, PC and QM performed data as well as statistical analysis. HW prepared the manuscript which was edited by QM and AZ. All authors contributed to the article and approved the submitted version.
Funding
This work was supported by the grants from the Project of China-Uruguay Joint Laboratory Cooperation (SQ2018YFE010044), the Guangdong Provincial Science and Technology Innovation Strategy Project (2020B1212060062), the Projects of the National Natural Science Foundation of China (31771816, 31971965 and 31371642), the New Varieties Cultivation of Genetically Modified Organisms (2016ZX08004002-007), the China Agricultural Research System (CARS-04-PS11), the Project of Agricultural Science and Technology Development and Resource and Environmental Protection Management from the Special Rural Revitalization Funds of Guangdong Province (2022KJ153-03), Ministry of Agriculture and Rural Affairs agricultural products quality and safety supervision special (4100-C17106, 21301091702101), the Projects of Key Area Research and Development Program of Guangdong Province (2020B020220008), the project of the Guangdong Provincial Laboratory of Lingnan Modern Agricultural Science and Technology (NZ2021012).
Conflict of interest
The authors declare that the research was conducted in the absence of any commercial or financial relationships that could be construed as a potential conflict of interest.
Publisher’s note
All claims expressed in this article are solely those of the authors and do not necessarily represent those of their affiliated organizations, or those of the publisher, the editors and the reviewers. Any product that may be evaluated in this article, or claim that may be made by its manufacturer, is not guaranteed or endorsed by the publisher.
Supplementary material
The Supplementary Material for this article can be found online at: https://www.frontiersin.org/articles/10.3389/fpls.2023.1125245/full#supplementary-material
References
Baumler, J., Riber, W., Klecker, M., Muller, L., Dissmeyer, N., Weig, A., et al. (2019). AtERF#111/ABR1 is a transcriptional activator involved in the wounding response. Plant J. 100, 969–990. doi: 10.1111/tpj.14490
Cai, Z., Cheng, Y., Xian, P., Ma, Q., Wen, K., Xia, Q, et al. (2018). Acid phosphatase gene GmHAD1 linked to low phosphorus tolerance in soybean, through fine mapping. Theor. Appl. Genet. 131, 1715–1728. doi: 10.1007/s00122-018-3109-3
Chauhan, D. K., Yadav, V., Vaculik, M., Gassmann, W., Pike, S., Arif, N., et al. (2021). Aluminum toxicity and aluminum stress-induced physiological tolerance responses in higher plants. Crit. Rev. Biotechnol. 41, 715–730. doi: 10.1080/07388551.2021.1874282
Chen, L. S. (2006). Physiological responses and tolerance of plant shoot to aluminum toxicity. Zhi. Wu. Sheng. Li. Yu. Fen. Zi. Sheng. Wu. Xue. Xue. Bao. 32, 143–155.
Choudhury, S., Sharma, P. (2014). Aluminum stress inhibits root growth and alters physiological and metabolic responses in chickpea (Cicer arietinum l.). Plant Physiol. Biochem. 85, 63–70. doi: 10.1016/j.plaphy.2014.10.012
Collings, D. A. (2013). Subcellular localization of transiently expressed fluorescent fusion proteins. Methods Mol. Biol. 1069, 227–258. doi: 10.1007/978-1-62703-613-9_16
Dong, L., Cheng, Y., Wu, J., Cheng, Q., Li, W., Fan, S., et al. (2015). Overexpression of GmERF5, a new member of the soybean EAR motif-containing ERFtranscription factor, enhances resistance to Phytophthora sojae in soybean. J. Exp. Bot. 66, 2635–2647. doi: 10.1093/jxb/erv078
Fan, W., Xu, J., Wu, P., Yang, Z., Lou, H., Chen, W., et al. (2019). Alleviation by abscisic acid of Al toxicity in rice bean is not associated with citrate efflux but depends on ABI5-mediated signal transduction pathways. J. Integr. Plant Biol. 61, 140–154. doi: 10.1111/jipb.12695
Feng, K., Hou, X., Xing, G., Liu, J., Duan, A., Xu, Z. S., et al. (2020). Advances in AP2/ERF super-family transcription factors in plant. Crit. Rev. Biotechnol. 40, 750–776. doi: 10.1080/07388551.2020.1768509
Guo, Z. J., Chen, X. J., Wu, X. L., Ling, J. Q., Xu, P. (2004). Overexpression of the AP2/EREBP transcription factor OPBP1 enhances disease resistance and salt tolerance in tobacco. Plant Mol. Biol. 55, 607–618. doi: 10.1007/s11103-004-1521-3
Hajiboland, R., Barcelo, J., Poschenrieder, C., Tolra, R. (2013). Amelioration of iron toxicity: A mechanism for aluminum-induced growth stimulation in tea plants. J. Inorg. Biochem. 128, 183–187. doi: 10.1016/j.jinorgbio.2013.07.007
Han, Z., Wang, J., Wang, X., Zhang, X., Cheng, Y., Cai, Z., et al. (2022). GmWRKY21, a soybean WRKY transcription factor gene, enhances the tolerance to aluminum stress in arabidopsis thaliana. Front. Plant Sci. 13. doi: 10.3389/fpls.2022.833326
Hsu, P. K., Dubeaux, G., Takahashi, Y., Schroeder, J. I. (2021). Signaling mechanisms in abscisic acid-mediated stomatal closure. Plant J. 105, 307–321. doi: 10.1111/tpj.15067
Huang, J., Han, R., Ji, F., Yu, Y., Wang, R., Hai, Z., et al. (2022). Glucose-6-phosphate dehydrogenase and abscisic acid mediate programmed cell death induced by aluminum toxicity in soybean root tips. J. Hazard. Mater. 425, 127964. doi: 10.1016/j.jhazmat.2021.127964
Iuchi, S., Koyama, H., Iuchi, A., Kobayashi, Y., Kitabayashi, S., Kobayashi, Y., et al. (2007). Zinc finger protein STOP1 is critical for proton tolerance in arabidopsis and coregulates a key gene in aluminum tolerance. Proc. Natl. Acad. Sci. U. S. 104, 9900–9905. doi: 10.1073/pnas.0700117104
Kereszt, A., Li, D., Indrasumunar, A., Nguyen, C., Nontachaiyapoom, S., Gresshoff, P., et al. (2007). Agrobacterium rhizogenes-mediated transformation of soybean to study root biology. Nat. Protoc. 2, 948–952. doi: 10.1038/nprot.2007.141
Khoudi, H. (2023). SHINE clade of ERF transcription factors: A significant player in abiotic and biotic stress tolerance in plants. Plant Physiol. Biochem. 195, 77–88. doi: 10.1016/j.plaphy.2022.12.030
Kim, Y., Han, Y., Hwang, O., Lee, S., Shin, A., Kim, S., et al. (2012). Overexpression of arabidopsis translationally controlled tumor protein gene AtTCTP enhances drought tolerance with rapid ABA-induced stomatal closure. Mol. Cells 33, 617–626. doi: 10.1007/s10059-012-0080-8
Kobayashi, Y., Ohyama, Y., Kobayashi, Y., Ito, H., Iuchi, S., Fujita, M., et al. (2014). STOP2 activates transcription of several genes for Al- and low pH-tolerance that are regulated by STOP1 in arabidopsis. Mol. Plant 7, 311–322. doi: 10.1093/mp/sst116
Kochian, L. V., Pineros, M. A., Liu, J., Magalhaes, J. V. (2015). Plant adaptation to acid soils: The molecular basis for crop aluminum resistance. Annu. Rev. Plant Biol. 66, 571–598. doi: 10.1146/annurev-arplant-043014-114822
Kumar, R., Sharma, A. K. (2021). Transcription factor stoichiometry in cell fate determination. J. Genet. 100, 27. doi: 10.1007/s12041-021-01278-2
Larsen, P. B., Geisler, M. J., Jones, C. A., Williams, K. M., Cancel, J. D. (2005). ALS3 encodes a phloem-localized ABC transporter-like protein that is required for aluminum tolerance in arabidopsis. Plant J. 41, 353–363. doi: 10.1111/j.1365-313X.2004.02306.x
Larsen, P. B., Kochian, L. V., Howell, S. H. (1997). Al Inhibits both shoot development and root growth in als3, an Al-sensitive arabidopsis mutant. Plant Physiol. 114, 1207–1214. doi: 10.1104/pp.114.4.1207
Lee, S. Y., Boon, N. J., Webb, A. A., Tanaka, R. J. (2016). Synergistic activation of RD29A via integration of salinity stress and abscisic acid in Arabidopsis thaliana. Plant Cell Physiol. 57, 2147–2160. doi: 10.1093/pcp/pcw132
Li, L., Li, X., Yang, C., Cheng, Y., Cai, Z., Nian, H., et al. (2022). GsERF1 enhances Arabidopsis thaliana aluminum tolerance through an ethylene-mediated pathway. BMC Plant Biol. 22, 258. doi: 10.1186/s12870-022-03625-6
Liu, D., Chen, X., Liu, J., Ye, J., Guo, Z. (2012). The rice ERF transcription factor OsERF922 negatively regulates resistance to Magnaporthe oryzae and salt tolerance. J. Exp. Bot. 63, 3899–3911. doi: 10.1093/jxb/ers079
Liu, J., Magalhaes, J. V., Shaff, J., Kochian, L. V. (2009). Aluminum-activated citrate and malate transporters from the MATE and ALMT families function independently to confer arabidopsis aluminum tolerance. Plant J. 57, 389–399. doi: 10.1111/j.1365-313X.2008.03696.x
Liu, Y. T., Shi, Q. H., Cao, H. J., Ma, Q. B., Nian, H., Zhang, X., et al. (2020). Heterologous expression of a Glycine soja C2H2 zinc finger gene improves aluminum tolerance in arabidopsis. Int. J. Mol. Sci. 21, 2754. doi: 10.3390/ijms21082754
Luan, Y., Cui, J., Li, J., Jiang, N., Liu, P., Meng, J., et al. (2018). Effective enhancement of resistance to Phytophthora infestans by overexpression of miR172a and b in Solanum lycopersicum. Planta 247, 127–138. doi: 10.1007/s00425-017-2773-x
Ma, Q., Xia, Z., Cai, Z., Li, L., Cheng, Y., Liu, J., et al. (2018). GmWRKY16 enhances drought and salt tolerance through an ABA-mediated pathway in Arabidopsis thaliana. Front. Plant Sci. 9. doi: 10.3389/fpls.2018.01979
Matsumoto, H., Motoda, H. (2012). Aluminum toxicity recovery processes in root apices. possible association with oxidative stress. Plant Sci. 185-186, 1–8. doi: 10.1016/j.plantsci.2011.07.019
Merlot, S., Gosti, F., Guerrier, D., Vavasseur, A., Giraudat, J. (2001). The ABI1 and ABI2 protein phosphatases 2C act in a negative feedback regulatory loop of the abscisic acid signalling pathway. Plant J. 25, 295–303. doi: 10.1046/j.1365-313x.2001.00965.x
Msanne, J., Lin, J., Stone, J. M., Awada, T. (2011). Characterization of abiotic stress-responsive Arabidopsis thaliana RD29A and RD29B genes and evaluation of transgenes. Planta 234, 97–107. doi: 10.1007/s00425-011-1387-y
Pandey, G. K., Grant, J. J., Cheong, Y. H., Kim, B. G., Li, L., Luan, S., et al. (2005). ABR1, an APETALA2-domain transcription factor that functions as a repressor of ABA response in arabidopsis. Plant Physiol. 139, 1185–1193. doi: 10.1104/pp.105.066324
Park, C. S., Go, Y. S., Suh, M. C. (2016). Cuticular wax biosynthesis is positively regulated by WRINKLED4, an AP2/ERF-type transcription factor, in arabidopsis stems. Plant J. 88, 257–270. doi: 10.1111/tpj.13248
Rangel, A. F., Rao, I. M., Horst, W. J. (2009). Intracellular distribution and binding state of aluminum in root apices of two common bean (Phaseolus vulgaris) genotypes in relation to Al toxicity. Physiol. Plant 135, 162–173. doi: 10.1111/j.1399-3054.2008.01183.x
Ranjan, A., Sinha, R., Sharma, T. R., Pattanayak, A., Singh, A. K. (2021). Alleviating aluminum toxicity in plants: Implications of reactive oxygen species signaling and crosstalk with other signaling pathways. Physiol. Plant 173, 1765–1784. doi: 10.1111/ppl.13382
Ren, B., Wang, X., Duan, J., Ma, J. (2019). Rhizobial tRNA-derived small RNAs are signal molecules regulating plant nodulation. Science 365, 919–922. doi: 10.1126/science.aav8907
Rincon, M., Gonzales, R. A. (1992). Aluminum partitioning in intact roots of aluminum-tolerant and aluminum-sensitive wheat (Triticum aestivum l.) cultivars. Plant Physiol. 99, 1021–1028. doi: 10.1104/pp.99.3.1021
Rui, L., Zhu, Z., Yang, Y., Wang, D., Liu, H., Zheng, P., et al. (2023). Functional characterization of MdERF113 in apple. Physiol. Plant, 175, e13853. doi: 10.1111/ppl.13853
Salazar-Chavarria, V., Sanchez-Nieto, S., Cruz-Ortega, R. (2020). Fagopyrum esculentum at early stages copes with aluminum toxicity by increasing ABA levels and antioxidant system. Plant Physiol. Biochem. 152, 170–176. doi: 10.1016/j.plaphy.2020.04.024
Sasaki, T., Mori, I. C., Furuichi, T., Munemasa, S., Toyooka, K., Matsuoka, K., et al. (2010). Closing plant stomata requires a homolog of an aluminum-activated malate transporter. Plant Cell Physiol. 51, 354–365. doi: 10.1093/pcp/pcq016
Sewelam, N., Kazan, K., Thomas-Hall, S., Kidd, B., Manners, J., Schenk, P., et al. (2013). Ethylene response factor 6 is a regulator of reactive oxygen species signaling in arabidopsis. PloS One 8, e70289. doi: 10.1371/journal.pone.0070289
Shu, K., Chen, Q., Wu, Y., Liu, R., Zhang, H., Wang, P., et al. (2016). ABI4 mediates antagonistic effects of abscisic acid and gibberellins at transcript and protein levels. Plant J. 85, 348–361. doi: 10.1111/tpj.13109
Shu, W., Zhou, Q., Xian, P., Cheng, Y., Lian, T., Ma, Q, et al. (2022). GmWRKY81 encoding a WRKY transcription factor enhances aluminum tolerance in soybean. Int. J. Mol. Sci. 23, 6518. doi: 10.3390/ijms23126518
Umemura, T., Usami, Y., Aizawa, S., Tsunoda, K., Satake, K. (2003). Seasonal change in the level and the chemical forms of aluminum in soil solution under a Japanese cedar forest. Sci. Total. Environ. 317, 149–157. doi: 10.1016/S0048-9697(03)00364-4
Wang, X., Cheng, Y., Yang, C., Yang, C., Mu, Y., Xia, Q., et al. (2019a). QTL mapping for aluminum tolerance in RIL population of soybean (Glycine max l.) by RAD sequencing. PloS One 14, e223674. doi: 10.1371/journal.pone.0223674
Wang, X., Liu, S., Tian, H., Wang, S., Chen, J. G. (2015). The small ethylene response factor ERF96 is involved in the regulation of the abscisic acid response in arabidopsis. Front. Plant Sci. 6. doi: 10.3389/fpls.2015.01064
Wang, H., Yin, X., Du, D., Liang, Z., Han, Z., Nian, H., et al. (2022). GsMYB7 encoding a R2R3-type MYB transcription factor enhances the tolerance to aluminum stress in soybean (Glycine max l.). BMC Genomics 23, 529. doi: 10.1186/s12864-022-08744-w
Wang, X., Zeng, W., Ding, Y., Wang, Y., Niu, L., Yao, J., et al. (2019b). Peach ethylene response factor PpeERF2 represses the expression of ABA biosynthesis and cell wall degradation genes during fruit ripening. Plant Sci. 283, 116–126. doi: 10.1016/j.plantsci.2019.02.009
Wen, K., Pan, H., Li, X., Huang, R., Ma, Q., Nian, H., et al. (2021). Identification of an ATP-binding cassette transporter implicated in aluminum tolerance in wild soybean (Glycine soja). Int. J. Mol. Sci. 22, 13264. doi: 10.3390/ijms222413264
Xian, P., Cai, Z., Cheng, Y., Lin, R., Lian, T., Ma, Q., et al. (2020). Wild soybean oxalyl-CoA synthetase degrades oxalate and affects the tolerance to cadmium and aluminum stresses. Int. J. Mol. Sci. 21, 8869. doi: 10.3390/ijms21228869
Xu, D., Cao, H., Fang, W., Pan, J., Chen, J., Zhang, J., et al. (2017). Linking hydrogen-enhanced rice aluminum tolerance with the reestablishment of GA/ABA balance and miRNA-modulated gene expression: A case study on germination. Ecotoxicol. Environ. Saf. 145, 303–312. doi: 10.1016/j.ecoenv.2017.07.055
Yamaguchi-Shinozaki, K., Shinozaki, K. (1993). The plant hormone abscisic acid mediates the drought-induced expression but not the seed-specific expression of rd22, a gene responsive to dehydration stress in Arabidopsis thaliana. Mol. Gen. Genet. 238, 17–25. doi: 10.1007/BF00279525
Yang, Z., Tian, L., Latoszek-Green, M., Brown, D., Wu, K. (2005). Arabidopsis ERF4 is a transcriptional repressor capable of modulating ethylene and abscisic acid responses. Plant Mol. Biol. 58, 585–596. doi: 10.1007/s11103-005-7294-5
Yokosho, K., Yamaji, N., Mitani-Ueno, N., Shen, R. F., Ma, J. F. (2016). An aluminum-inducible IREG gene is required for internal detoxification of aluminum in buckwheat. Plant Cell Physiol. 57, 1169–1178. doi: 10.1093/pcp/pcw065
Yu, Y., Duan, X., Ding, X., Chen, C., Zhu, D., Yin, K., et al. (2017). A novel AP2/ERF family transcription factor from Glycine soja, GsERF71, is a DNA binding protein that positively regulates alkaline stress tolerance in arabidopsis. Plant Mol. Biol. 94, 509–530. doi: 10.1007/s11103-017-0623-7
Zhang, X., Long, Y., Huang, J., Xia, J. (2019a). Molecular mechanisms for coping with al toxicity in plants. Int. J. Mol. Sci. 20, 1551. doi: 10.3390/ijms20071551
Zhang, Y., Zhang, J., Guo, J., Zhou, F., Singh, S., Xu, X., et al. (2019b). F-box protein RAE1 regulates the stability of the aluminum-resistance transcription factor STOP1 in arabidopsis. Proc. Natl. Acad. Sci. U. S. 116, 319–327. doi: 10.1073/pnas.1814426116
Zhou, Q., Tian, A., Zou, H., Xie, Z., Lei, G., Huang, J., et al. (2008). Soybean WRKY-type transcription factor genes, GmWRKY13, GmWRKY21, and GmWRKY54, confer differential tolerance to abiotic stresses in transgenic arabidopsis plants. Plant Biotechnol. J. 6, 486–503. doi: 10.1111/j.1467-7652.2008.00336.x
Keywords: GmABR1, soybean, Al stress, transgenic Arabidopsis thaliana, transcription factor
Citation: Wang H, Li C, Wang L, Zhong H, Xu X, Cheng Y, Nian H, Liu W, Chen P, Zhang A and Ma Q (2023) GmABR1 encoding an ERF transcription factor enhances the tolerance to aluminum stress in Arabidopsis thaliana. Front. Plant Sci. 14:1125245. doi: 10.3389/fpls.2023.1125245
Received: 16 December 2022; Accepted: 28 February 2023;
Published: 23 March 2023.
Edited by:
Girdhar Kumar Pandey, University of Delhi, IndiaReviewed by:
Haihui Fu, Jiangxi Agricultural University, ChinaYuhua Wang, Nanjing Agricultural University, China
Copyright © 2023 Wang, Li, Wang, Zhong, Xu, Cheng, Nian, Liu, Chen, Zhang and Ma. This is an open-access article distributed under the terms of the Creative Commons Attribution License (CC BY). The use, distribution or reproduction in other forums is permitted, provided the original author(s) and the copyright owner(s) are credited and that the original publication in this journal is cited, in accordance with accepted academic practice. No use, distribution or reproduction is permitted which does not comply with these terms.
*Correspondence: Qibin Ma, bWFxaWJpbkBzY2F1LmVkdS5jbg==; Aixia Zhang, OTA3MjY1ODg3QHFxLmNvbQ==
†These authors have contributed equally to this work