- School of Agricultural, Jilin Agricultural Science and Technology University, Jilin, China
Global warming in this century increases incidences of various abiotic stresses, restricting plant growth and productivity and posing a severe threat to global food production and security. Different phytohormones are produced by plants to mitigate the adverse effects of these stresses. One such phytohormone is melatonin (MEL), which, being a potential bio-stimulator, helps to govern a wide array of functions in horticultural crops. Recent advancements have determined the role of MEL in plants’ responses to abiotic stresses. MEL enhances physiological functions such as seed germination, growth and development, seedling growth, root system architecture, and photosynthetic efficiency. The potential function of MEL in stressful environments is to regulate the enzymatic and non-enzymatic antioxidant activity, thus playing a role in the substantial scavenging of reactive oxygen species (ROS). Additionally, MEL, as a plant growth regulator and bio-stimulator, aids in promoting plant tolerance to abiotic stress, mainly through improvements in nutrient uptake, osmolyte production, and cellular membrane stability. This review, therefore, focuses on the possible functions of MEL in the induction of different abiotic stresses in horticultural crops. Therefore, this review would help readers learn more about MEL in altered environments and provide new suggestions on how this knowledge could be used to develop stress tolerance.
Introduction
Plants, being sessile organisms, face a variety of environmental stresses (low and high temperature, metal stress, salinity, and drought stress) (Hassan et al., 2022), which have detrimental impacts on their performance in terms of growth and development (Rasheed et al., 2021; Altaf et al., 2023). It has been projected that about 90% of arable land is susceptible to one of the above-mentioned stresses (Dos Reis et al., 2012). Due to the devastating impact on the growth and productivity of agricultural crops, global attention has been diverted to these abiotic stresses. Various developmental functions and processes of plants, including morphological, physiological, and biochemical, are disrupted by these abiotic stresses (Marino, 2021; Arnao et al., 2023). Further, environmental stresses cause significant yield losses through excessive production of reactive oxygen species (ROS), nutrient deficiencies, decrease in photosynthetic efficiency, reduction in root growth, and osmolyte over-accumulation (Figure 1) (Ayyaz et al., 2022; Imran et al., 2022). With the ongoing changes in climate, these abiotic stresses are getting intensified, thus calling for the need for appropriate controlling measures (Gao et al., 2007; Andreotti, 2020; Shahid et al., 2021). In horticultural crops such as tomato, potato, pepper, and cucumber, around 70% of total yield losses are due to effects caused by environmental stresses at different growth phases (Martinez et al., 2018; Zörb et al., 2018). To promote sustainable agriculture, different management strategies have been introduced for achieving the targets (Ahammed and Li, 2023). Such techniques include plant growth regulators, different osmolyte syntheses, and accumulation to protect against stress-induced damages for maintaining cellular homoeostasis and optimum plant growth (Nawaz et al., 2017; Koza et al., 2022; Peng et al., 2023).
One of the essential plant growth regulators in stressed environments is melatonin (MEL), which is a small molecule acting as a powerful antioxidant, thus enhancing the stress resistance of plants against many environmental stressors (Hoque et al., 2021) (Table 1). This pleotropic molecule is found in various plant parts of several plant species, such as broccoli, coriander, mango, cabbage, tobacco, cucumber, and orange (Badria, 2002; Posmyk et al., 2009; Johns et al., 2013; Aguilera et al., 2015). MEL is also involved in the regulation of seed germination, seedling growth, photosynthetic efficiency, root system architecture, leaf senescence, fruit ripening, stomatal opening, and redox homeostasis (Figure 2) (Jan et al., 2022). Further, MEL has been well defined as an anti-stress promoter and growth bio-stimulator for horticultural plants, particularly in adverse environmental situations, such as cold, heat, heavy metals, salinity, drought, acidic rain, and UV stress (Sharif et al., 2018; Aghdam et al., 2021; Wu et al., 2021; Zhao et al., 2022). Plants are protected against stressful environments by the regulation of gene expressions mediated by MEL, such as the plants’ “antioxidant defense system” activation (Jahan et al., 2020) which places MEL among vital bio-stimulants to improve crop productivity in stress conditions. In stressful environments, MEL helps to trigger the antioxidant defense system, which favors the scavenging of ROS and thus acts as a stress protector (Moustafa-Farag et al., 2020). Due to this function, MEL is a promising molecule that can be applied exogenously to alleviate stress. The current review aims to explore the biochemical and physiological functioning of MEL in abiotic stress environments, along with its possible mechanism of action. Further, the future aspect of MEL-regulated stress tolerance of horticultural crops is also discussed for a detailed overview of the research.
Function of melatonin in horticultural crops
MEL promotes plant growth and development via different functions, most of which are related to different abiotic stressors such as drought, temperature fluctuation, heavy metals, and salinity (Arnao and Hernández-Ruiz, 2006; Altaf et al., 2022a). MEL functions as a growth regulator, bio-stimulator, and potential antioxidant compound (Arnao and Hernández-Ruiz, 2014) (Figure 3). A primary function attributed to MEL in plants is to act as an antioxidant, providing protection against environmental agents (Nawaz et al., 2016; Tiwari et al., 2022). However, one important function of MEL may be the scavenging of free radicals, thereby protecting plants against oxidative damage (Paredes et al., 2009). MEL is significantly involved in the process of leaf senescence (Arnao and Hernández-Ruiz, 2009). A range of different functions of MEL have been investigated in horticultural plants, some more thoroughly than others, but in all cases the data are scarce (Table 2).
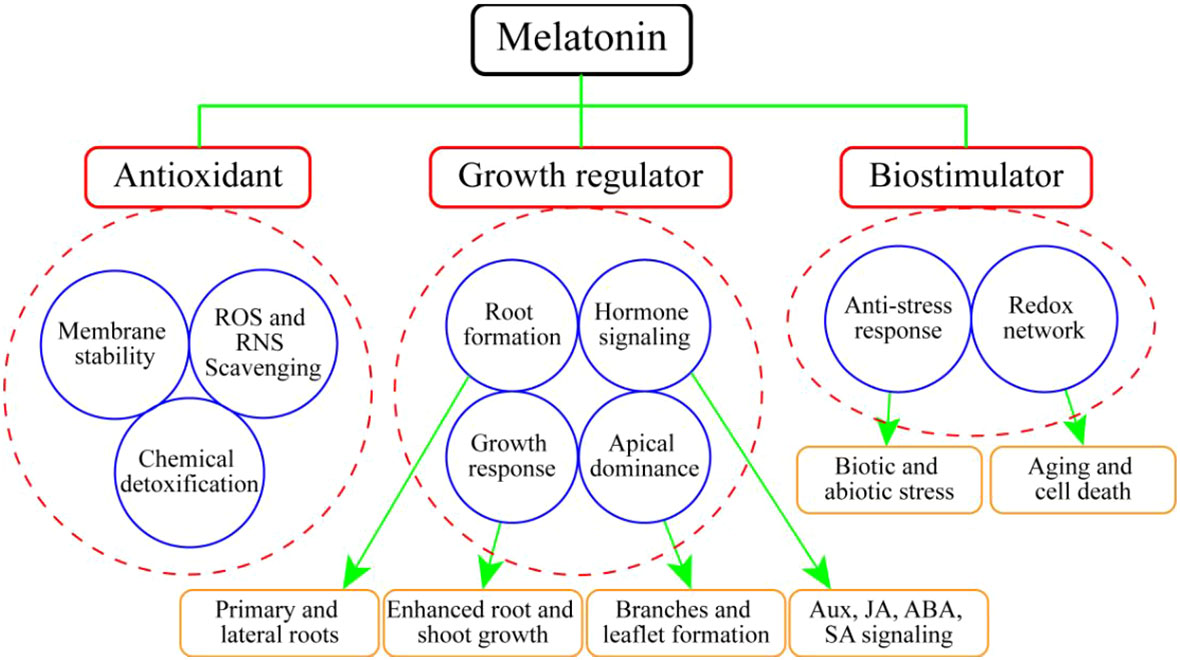
Figure 3 Action of melatonin inhorticultural plants as an antioxidant, growth regulator and bio-stimulator.
Melatonin as abiotic stress regulator in horticultural crops
Salinity
Salinity has been declared a significant hazard in modern horticulture, as it impairs and inhibits the growth and development of plants, mainly through disruption of the soil’s osmotic and ionic balances (Abdelaal et al., 2020; Zulfiqar et al., 2022). With increments in soil salt levels, an osmotic stress condition develops, which leads to declining water levels in the soil, thus less water is available for plant uptake, causing a conditional physiological drought in plants (Chang et al., 2014). Recently, MEL has emerged as an effective plant growth regulator, playing a significant role in the development of abiotic stress resistance in horticultural crops. Resultantly, several studies highlighted the stress-mitigating functions of MEL in horticultural crops under salt stress (Bose and Howlader, 2020). Plants’ antioxidant system has been reported to improve, and photosynthetic capacity is seen to get protected with exogenously applied MEL under NaCl stress in peanut (ElSayed et al., 2020), orange (Hu et al., 2022), watermelon (Li et al., 2017a), pistachio (Kamiab, 2020), and tomato (Liu et al., 2019). According to Li et al. (2012), the photosynthetic capacity of plants is maintained by the exogenous application of MEL (0.1 µM) under salinity stress, which leads to significant alleviation of growth inhibition. Further, the oxidative damage caused by the scavenging of ROS was also decreased by MEL, and antioxidant enzymes’ activity was improved, including catalase, peroxidase, and ascorbate. Salinity exerts its negative impact irrespective of the growth stage of the plants, and its effects range from seed germination to plant senescence and occur throughout the life cycle. Seed germination and plant growth are severely affected by saline stress (Nawaz et al., 2016; Zhan et al., 2019). In tomato, under salinity stress, the MEL applied exogenously reduced the uptake of sodium (Na+), hydrogen peroxide (H2O2) content, and malonaldehyde (MDA) content, while enhancing enzyme activity, relative water content (RWC), membrane stability index, gas exchange parameters, and growth attributes (Ali et al., 2021). Furthermore, MEL pre-treatment of cucumber seeds showed an increase in seed germination rate and seedling growth, along with a 5-fold increase in antioxidant enzyme activity under salinity stress (Zhang et al., 2014).
MEL supplementation improved growth traits and reduced the levels of MDA, ROS, and EL (electrolyte leakage), mainly through upregulating the enzymatic and non-enzymatic antioxidant enzyme activity. Moreover, in strawberries, MEL improved the phenolic and photosynthetic content (Zahedi et al., 2020). Importantly, Hu et al. (2021) revealed efficient reductions in the levels of MDA and ROS, increases in antioxidant activities, endogenous levels of MEL, proline, and pigment content, stomatal conductance, and the upregulation of genes related to redox, salt tolerance, and MEL biosynthesis. In addition, MEL was seen to escalate the ion homeostasis under high-NaCl stress in Malus hupehensis (Li et al., 2012). MEL further reduced ion toxicity by suppressing the accumulation of Na+ and Cl− ions (Liu et al., 2019). The tomato seedling growth showed significant improvements with the supplementation of MEL under NaCl toxicity. Additionally, MEL effectively reduced the activity of glycolate oxidase, chlorophyll degradation, and ROS levels and caused increases in antioxidant enzyme activity, proline content, and glycine betaine levels (Siddiqui et al., 2020a). According to Zhang et al. (2017a), the uniformity of seeds and germination rate of cucumber seeds increased with the regulation of energy production with the application of MEL under salinity stress. Additionally, MEL protects the photosynthetic apparatus from oxidative damage induced by NaCl stress (Zhang et al., 2020). MEL increases the antioxidant enzyme, thus leading to a decline in the accumulation of ROS in the leaves of salt-sensitive cucumber plants. In cucumber, MT was also reported to suppress the alleviation in maximum quantum efficiency of photosystem II (PSII) and net photosynthetic rate and to protect the total chlorophyll content under salinity stress (Wang et al., 2016).
Drought
Global climate change has intensified drought stress episodes, which are emerging as a serious threat to crop growth and productivity worldwide. Horticultural crops are very vulnerable to drought stress (Gao et al., 2022; Muhammad et al., 2022; Toscano et al., 2023). Drought stress is reported to cause abnormalities in the physiological and morphological states of plants (Hanaka et al., 2021). Reduced root system architecture, cellular membrane integrity, damaged photosynthetic apparatus, and imbalanced mineral and nutrient accumulation are highlighted as some of the most important abnormalities that lead to the complete devastation of a plant facing drought stress (Tabassum et al., 2021). Melatonin protects horticultural crops by preventing damage to the root architecture system, photosynthetic machinery, inducing the antioxidative defense system, regulating oxidative stress, and some other defense mechanisms (Tiwari et al., 2020). MEL pretreatment of tomato seedlings grown in field conditions under drought stress showed effective results, and the detrimental effects of drought stress were significantly reduced (Liu et al., 2015a). MEL-supplementation effectively improved seedling growth, photosynthetic efficiency, activity of antioxidant enzymes, and decreased oxidative damage (Liu et al., 2015a). Under drought stress, exogenous MEL application improved the growth, photosynthetic apparatus, and antioxidant enzyme systems of Chinese hickory plants (Sharma et al., 2020). In addition, Karaca and Cekic (2019) observed that MEL supplementation efficiently improved chlorophyll content, antioxidant enzyme systems, and reduced MDA content in Solanum lycopersicum L. under drought stress.
The photosynthetic machinery of tomato seedlings under drought stress showed significant improvements with the application of MEL (Ding et al., 2018; Ibrahim et al., 2020). Similar kinds of studies that indicate the impact of melatonin treatments on minimizing drought-induced photosynthetic damage have been performed on fenugreek (Zamani et al., 2020), kiwifruit (Xia et al., 2020), and grapes (Meng et al., 2014). Exogenous melatonin treatment showed several positive phenomena, such as preventing chloroplast photosynthetic damage (Wang et al., 2012). Further, MEL supplementation led to improved turgor pressure and water content of leaves, along with enacting the spongy tissue (Meng et al., 2014). The antioxidant defense system of plants gets triggered, and the scavenging of ROS is efficiently enhanced by the application of MEL. In horticultural plants, the mechanisms related to these phenomena are well examined, such as the scavenging of H2O2 by the regulation of the ascorbate-glutathione cycle (Li et al., 2019; Ibrahim et al., 2020). In cucumber, the seed germination rate was improved by the exogenous application of MEL (Zhang et al., 2013). In Moringa oleifera, the foliar application of MEL under drought stress showed a beneficial impact on the dry and fresh weight of shoots and leaves, number of leaves/plants, plant height, and foliage yield (Sadak et al., 2020). (Sadak et al., 2020). In Coffea arabica seedlings, the leaf area was reduced by drought stress, which was alleviated by the supplementation of MEL (Campos et al., 2019). Plant growth is maintained by the application of MT under drought stress conditions, mainly by the maintenance of homeostatic balance and vegetative tissues’ growth (Sharma and Zheng, 2019). The protective roles of MEL in plants under salinity and drought stress are summarized in Table 3.
Cold
Plant growth is significantly influenced by temperature. Low temperature stress lies amid the most detrimental environmental conditions for plants, resulting in yield and productivity losses (Malhotra, 2017). Cold stress can negatively affect seedling growth, root morphology, photosynthetic efficiency, seed germination, and pigment content (Marino, 2021). Low-temperature stress also has a negative impact on the metabolic capacity of plants. Plants’ metabolic capacity is also affected by low-temperature stress. The levels and activity of enzymes involved in important metabolic pathways are usually altered in cold-stressed plants, and as a result, the plant metabolome is completely changed (Khan et al., 2015; Liang et al., 2020). The development of commercial crop cultivars that are cold-resistant has been recently focused on by plant scientists. The use of MEL significantly reduced the adverse effects of cold stress on a diverse range of plant genera. Wang et al. (2020) reported that MEL application remarkably improved photosynthesis, metabolites, and tomato seedling growth under cold stress. MEL application efficiently improved photosynthetic performance in pepper under chilling stress (Korkmaz et al., 2021). In tomato seedlings, the application of MEL under cold stress caused significant increases in chlorophyll fluorescence parameters, pigment content, gas exchange elements, and growth characteristics (Zhou et al., 2020). Furthermore, melatonin significantly imparted cold tolerance in Citrullus lanatus stemically by regulating antioxidant capacity and the expression of defense genes (Li et al., 2017b). In a recent study, Li et al. (2022a) reported that MT supplementation efficiently enhanced root growth, antioxidant enzymes, the photosynthetic system, and reduced oxidative damage in pepper under cold stress.
MEL supplementation increased seedling growth and cold stress tolerance by balancing redox homeostasis, stomatal opening, leaf photosynthetic activity, mineral nutrient accumulation, osmolytes production, and primary and secondary metabolites, as well as improvements in antioxidant activities and ROS scavenging (Qari et al., 2022). MEL alleviates cold-induced adverse effects on tea plants. MEL pretreatment in tea plants significantly improved growth traits, photosynthesis, antioxidant profile, and balanced redox homeostasis under chilling stress (Li et al., 2018). Root pretreatment with MEL reduced aerial cold-induced suppression of photosystem II and oxidative damage in C. lanatus (Chang et al., 2020). Cao et al. (2016) revealed that in peach fruit, chilling injury was very effectively reduced by the application of MEL at a dose of 100 μM. In tomato plants under cold stress, the pretreatment of MEL showed higher levels of non-enzymatic antioxidants, greater activities of antioxidant enzymes, and reduced MDA content and EL (Ding et al., 2017). Furthermore, pretreatment of MEL declined the harmful impact of cold stress and accelerated the plants’ recovery, primarily by improving photosynthesis and antioxidant enzyme capacity in the leaves of melon (Zhang et al., 2017b). Several reports revealed that MEL positively modulates the growth of cucumber (Marta et al., 2016), tomato (Yang et al., 2018), and watermelon (Li et al., 2017a). MEL application considerably reduced the adverse effect of cold stress on eggplant seedlings. MEL supplementation enhanced leaf area, biomass production, photosynthetic mechanism, activity of antioxidant enzymes, proline content, and reduced MDA and H2O2 levels of eggplant seedlings under chilling conditions (Yakuboğlu et al., 2022).
Heat
The production of horticultural crops is severely and significantly hindered by heat stress driven by climate change. High temperature stress is a major environmental stress that limits plant growth, metabolism, and productivity worldwide. Temperature affects many of the biochemical reactions that are important for the steady growth and development of plants (Hasanuzzaman et al., 2013). High temperatures are becoming an important concern for sustainable crop production (Wahid et al., 2012). A few researchers have investigated the possible role and defensive mechanism of MEL under heat stress in plants. Recently, Ahammed et al. (2018) revealed that MEL has been declared a universal regulator of abiotic stresses, which can possibly increase the heat resistance of plants. MEL-pretreated tomato plants under heat stress showed improved root growth, chlorophyll content, activities of enzymatic and non-enzymatic antioxidants, and decreased oxidative damage. Further, Jahan et al. (2019) described that MEL efficiently increased polyamine content and considerably declined levels of MDA and EL in tomatoes. MEL supplementation effectively reduced the accumulation of ROS and increased the antioxidant profile in tomato seedlings in a high-temperature environment (Martinez et al., 2018). Ahammed et al. (2019) revealed that MEL supplementation significantly reduced MDA and EL levels and increased the antioxidant enzyme system in tomatoes under heat stress. Table 4 shows how MEL protects plants from heat and cold stress. Research to date has demonstrated the vital functions of MEL for plant survival and higher productivity of horticultural crops in high-temperature stress conditions. Yet, extensive investigations are needed to confirm the possible mechanisms of heat stress amelioration by MEL in plants.
Heavy metals
Globally, the pollution caused by heavy metals is getting worse with time, causing a wide range of toxic impacts on horticultural crop production (Shakoor et al., 2017; Behera et al., 2022). Plants are probably universally tolerant of heavy metal stress. Regardless of other stresses, the production of horticultural crops is significantly and negatively affected by heavy metal stressors, which is becoming a major concern (Noor et al., 2022). Hitherto work shows that heavy metal (lead, boron, cadmium, nickel, arsenic, and vanadium) stress remarkably reduces horticultural crop production (Dodangeh et al., 2018; Bhat et al., 2019).
One of the recently emerging potential stress-alleviating hormones is MEL, which may aid in coping mechanisms against metal-induced toxicity in plants. MEL application remarkably increased growth characteristics, root morphology, pigment content, and net photosynthetic rate. Additionally, under vanadium stress, MEL application in watermelon caused significant reductions in oxidative damage, increases in antioxidant enzymes, and levels of relevant gene expression (Nawaz et al., 2018). Under nickel toxicity, MEL application in tomato reduced the MDA and EL levels and increased the secondary metabolite content, proline level, leaf photosynthesis, and antioxidant defense mechanism (Jahan et al., 2020). MEL application considerably increased the root architecture of cucumber seedlings under copper toxicity (Cao et al., 2019), the growth status of red cabbage plants under copper toxicity (Posmyk et al., 2008), the photosynthetic efficiency of radish seedlings under aluminum toxicity (Tang et al., 2016), the antioxidant enzyme system in roses under cadmium toxicity (Nabaei and Amooaghaie, 2019), the mineral nutrient content of strawberry seedlings under cadmium toxicity (Wu et al., 2021), and lowered the MDA and EL levels in tomato seedlings under cadmium toxicity (Hasan et al., 2015). Moreover, previous findings suggested that MEL significantly reduced iron accumulation from root to shoot in cucumber, watermelon, and tomato (Ahammed et al., 2020).
Hasan et al. (2018) revealed that under low sulfur conditions, MEL efficiently enhanced different growth traits, chlorophyll fluorescence parameters, gas exchange elements, and pigment molecules, as well as declining the MDA and H2O2 levels in tomato seedlings. Siddiqui et al. (2019) observed that MEL application significantly improved the photosynthesis and growth of tomato seedlings under lanthanum toxicity. Further, MEL supplementation significantly improved aerial biomass production, carotenoid content, chlorophyll content, and carbohydrate levels in spinach under boron toxicity. Selenium (Se) toxicity impaired rapeseed growth and biomass production, decreased photosynthesis, and lowered photosynthetic pigment content. All these parameters were remarkably alleviated by MEL application. MEL significantly reduced cellular membrane damage and ROS formation. MEL effectively improved proline level, metabolite content, antioxidant enzymes, and their gene expression levels in Brassica napus under Se toxicity (Ulhassan et al., 2019). In another study, MEL treatment considerably enhanced net photosynthetic rate, growth traits, leaf gas exchange elements, and maintained macro- and micro-nutrient content in pepper leaves under B toxicity (Sarafi et al., 2017). MEL application improved antioxidant enzyme activities and root growth and reduced the MDA and proline levels in the roots of melon seedlings under Cu toxicity (Hu et al., 2020). In addition, MEL further enhanced the antioxidant enzyme system, along with a decline in the ROS level in spinach seedlings (Moussa and Algamal, 2017). MEL positively regulates the growth of tobacco under lead stress (Kobylińska et al., 2017), radish under Cd toxicity (Xu et al., 2020), and pepper under boron stress (Sarafi et al., 2017). Table 5 provides an overview of the protective functions of MEL in horticultural crops under heavy metal toxicity.
Acid rain, sodic alkaline toxic, and chemical stress
The protective role of MEL against acid rain, toxic chemicals, and sodic alkaline stresses has also been proven. Under acid rain conditions, the application of MEL on tomato plants leads to significant reductions in levels of MDA and H2O2, repairing the chloroplast’s grana lamella, along with escalations in growth parameters, antioxidant enzymes, pigment molecules, phenolic, flavonoids, and proline content (Debnath et al., 2018a). Debnath et al. (2018b) revealed that under stimulated acid-rain stress, the application of MEL to tomato plants exhibited significant improvements in yield attributes and quality traits of fruit. Moreover, pretreatment with MEL led to an increase in antioxidant enzymes’ activities, total soluble solids, soluble proteins, flavonoids, phenolics, and carotenoid content, along with remarkable reductions in MDA levels. MEL application remarkably improved the activity of antioxidant enzymes, chlorophyll content, and growth, and decreased the production of H2O2 and MDA in fenugreek under lead and acid rain stress (Xalxo and Keshavkant, 2019). Furthermore, Liu et al. (2015b) revealed that application of MEL under alkaline stress caused reductions in Na+ levels and enhanced the levels of K+ in tomato leaves, exhibiting the role of MEL in the maintenance of ion homeostasis and thus increasing the stress resistance of tomato plants to alkaline stress. The positive role of MEL in the stress resistance of plants to alkaline stress is due to the regulation of enzyme activity, and polyamine biosynthesis (Gong et al., 2017). MEL enhanced the photosynthetic pigment content of pea plants under paraquat stress (Szafrańska et al., 2017). In cucumber seedlings, the application of MEL under cinnamic acid stress increases plant stress resistance, mainly by integrating the morphology, mineral nutrient contents, and signaling crosstalk of plant hormones (Li et al., 2017c). The growth of cucumber plants is boosted by the application of MEL, which modulates mineral nutrient composition and nitrogen metabolism under nitrate stress (Zhang et al., 2017b).
Future perspectives
The review will also help and encourage plant researchers to deeply examine the mechanism of stress tolerance mediated by MEL. Exogenously applied MEL is declared a potential growth regulator for plants, which aids under abiotic stress conditions by increasing plants’ growth, yield, and quality. Various functions of plants are found to be associated with MEL, such as the regulation of physiological functions, including seed germination and seedling growth, along with the functions of MEL in stress resistance under environmental stressors. The harmful effects of abiotic stresses are alleviated by the application of MEL, either directly by scavenging ROS or indirectly by improving the photosynthetic machinery, enhancing the activities of antioxidant enzymes, regulating metal transport and growth regulators in plants, as well as increasing osmotic metabolites. Although MEL has attracted the interest of plant researchers recently and progress can be seen on the topic, there are still unexplored MEL signaling pathways that, though complicated, need to be investigated under abiotic stresses. There exists a major gap in the literature about understanding the regulation of pathways by MEL and associated genes. Moreover, there is a need to address several major problems. For instance, there is a lack of understanding regarding the mechanisms of HM uptake, sequestration, and transportation as regulated by MEL. Future research should thus aim to deeply investigate the functions of MEL and its underlying mechanisms to sustain crop production under abiotic stress environments.
Author contributions
JZ: Conceptualization, literature survey, figure designing, writing major original draft, review structure. JH: Literature survey, writing—review and editing. All authors listed have made a substantial, direct, and intellectual contribution to the work and approved it for publication.
Conflict of interest
The authors declare that the research was conducted in the absence of any commercial or financial relationships that could be construed as a potential conflict of interest.
Publisher’s note
All claims expressed in this article are solely those of the authors and do not necessarily represent those of their affiliated organizations, or those of the publisher, the editors and the reviewers. Any product that may be evaluated in this article, or claim that may be made by its manufacturer, is not guaranteed or endorsed by the publisher.
References
Abdelaal, K. A., EL-Maghraby, L. M., Elansary, H., Hafez, Y. M., Ibrahim, E. I., El Banna, M., et al. (2020). Treatment of sweet pepper with stress tolerance-inducing compounds alleviates salinity stress oxidative damage by mediating the physio-biochemical activities and antioxidant systems. Agronomy 10, 26. doi: 10.3390/agronomy10010026
Aghdam, M. S., Mukherjee, S., Flores, F. B., Arnao, M. B., Luo, Z., Corpas, F. J. (2021). Functions of melatonin during postharvest of horticultural crops. Plant Cell Physiol. 0, 1–23. doi: 10.1093/pcp/pcab175
Aguilera, Y., Herrera, T., Benítez, V., Arribas, S. M., Lopez De Pablo, A. L., Esteban, R. M. (2015). Estimation of scavenging capacity of melatonin and other antioxidants: contribution and evaluationing germinated seeds. Food Chem. 170, 203–211. doi: 10.1016/j.foodchem.2014.08.071
Ahammed, G. J., Li, X. (2023). Dopamine-induced abiotic stress tolerance in horticultural plants. Sci. Hortic. 307, 111506. doi: 10.1016/j.scienta.2022.111506
Ahammed, G. J., Wu, M., Wang, Y., Yan, Y., Mao, Q., Ren, J., et al. (2020). Melatonin alleviates iron stress by improving iron homeostasis, antioxidant defense and secondary metabolism in cucumber. Sci. Hortic. 265, 109205. doi: 10.1016/j.scienta.2020.109205
Ahammed, G. J., Xu, W., Liu, A., Chen, S. (2018). COMT1 silencing aggravates heat stress-induced reduction in photosynthesis by decreasing chlorophyll content, photosystem II activity, and electron transport efficiency in tomato. Front. Plant Sci. 9, 998. doi: 10.3389/fpls.2018.00998
Ahammed, G. J., Xu, W., Liu, A., Chen, S. (2019). Endogenous melatonin deficiency aggravates high temperature-induced oxidative stress in Solanum lycopersicum l. Environ. Exp. Bot. 161, 303–311. doi: 10.1016/j.envexpbot.2018.06.006
Ali, M., Kamran, M., Abbasi, G. H., Saleem, M. H., Ahmad, S., Parveen, A., et al. (2021). Melatonin-induced salinity tolerance by ameliorating osmotic and oxidative stress in the seedlings of two tomato (Solanum lycopersicum l.) cultivars. J. Plant Growth Regul. 40, 2236–2248. doi: 10.1007/s00344-020-10273-3
Altaf, M. A., Hao, Y., He, C., Mumtaz, M. A., Shu, H., Fu, H., et al. (2022a). Physiological and biochemical responses of pepper (Capsicum annuum l.) seedlings to nickel toxicity. Front. Plant Sci. 13, 950392. doi: 10.3389/fpls.2022.950392
Altaf, M. A., Sharma, N., Singh, J., Samota, M. K., Sankhyan, P., Singh, B., et al. (2023). Mechanistic insights on melatonin-mediated plant growth regulation and hormonal cross-talk process in solanaceous vegetables. Sci. Hortic. 308, 111570. doi: 10.1016/j.scienta.2022.111570
Altaf, M. A., Shu, H., Hao, Y., Mumtaz, M. A., Lu, X., Wang, Z. (2022b). Melatonin affects the photosynthetic performance of pepper (Capsicum annuum l.) seedlings under cold stress. Antioxidants 11, 2414. doi: 10.3390/antiox11122414
Andreotti, C. (2020). Management of abiotic stress in horticultural crops: Spotlight on biostimulants. Agronomy 10, 1514. doi: 10.3390/agronomy10101514
Arnao, M. B., Giraldo-Acosta, M., Castejón-Castillejo, A., Losada-Lorán, M., Sánchez-Herrerías, P., El Mihyaoui, A., et al. (2023). Melatonin from microorganisms, algae, and plants as possible alternatives to synthetic melatonin. Metabolites 13, 72. doi: 10.3390/metabo13010072
Arnao, M. B., Hernández-Ruiz, J. (2006). The physiological function of melatonin in plants. Plant Signal. Behav. 1, 89–95. doi: 10.4161/psb.1.3.2640
Arnao, M. B., Hernández-Ruiz, J. (2009). Protective effect of melatonin against chlorophyll degradation during the senescence of barley leaves. J. Pineal Res. 46, 58–63. doi: 10.1111/j.1600-079X.2008.00625.x
Arnao, M. B., Hernández-Ruiz, J. (2014). Melatonin: plant growth regulator and/or biostimulator during stress? Trends Plant Sci. 19, 789–797. doi: 10.1016/j.tplants.2014.07.006
Arnao, M. B., Hernández-Ruiz, J. (2020). Melatonin in flowering, fruit set and fruit ripening. Plant Reprod. 11, 77–87. doi: 10.1007/s00497-020-00388-8
Asif, M., Pervez, A., Irshad, U., Mehmood, Q., Ahmad, R. (2020). Melatonin and plant growth-promoting rhizobacteria alleviate the cadmium and arsenic stresses and increase the growth of Spinacia oleracea l. Plant Soil Environ. 66, 234–241. doi: 10.17221/135/2020-PSE
Ayyaz, A., Shahzadi, A. K., Fatima, S., Yasin, G., Zafar, Z. U., Athar, H. U. R., et al. (2022). Uncovering the role of melatonin in plant stress tolerance. Theor. Exp. Plant Physiol. 34, 335–346. doi: 10.1007/s40626-022-00255-z
Badria, F. A. (2002). Melatonin, serotonin, and tryptamine in some Egyptian food and medicinal plants. J. Medicinal Food. 5, 153–157. doi: 10.1089/10966200260398189
Behera, B., Kancheti, M., Raza, M. B., Shiv, A., Mangal, V., Rathod, G., et al. (2022). Mechanistic insight on boron-mediated toxicity in plant vis-a-vis its mitigation strategies: a review. Int. J. Phyto., 1–18. doi: 10.1080/15226514.2022.2049694
Bhat, J. A., Shivaraj, S. M., Singh, P., Navadagi, D. B., Tripathi, D. K., Dash, P. K., et al. (2019). Role of silicon in mitigation of heavy metal stresses in crop plants. Plants 8, 71. doi: 10.3390/plants8030071
Bose, S. K., Howlader, P. (2020). Melatonin plays multifunctional role in horticultural crops against environmental stresses: A review. Environ. Exp. Bot. 176, 104063. doi: 10.1016/j.envexpbot.2020.104063
Campos, C. N., Ávila, R. G., de Souza, K. R. D., Azevedo, L. M., Alves, J. D. (2019). Melatonin reduces oxidative stress and promotes drought tolerance in young Coffea arabica l. plants. Agric. Water Manage. 211, 37–47. doi: 10.1016/j.agwat.2018.09.025
Cao, Y. Y., Qi, C. D., Li, S., Wang, Z., Wang, X., Wang, J., et al. (2019). Melatonin alleviates copper toxicity via improving copper sequestration and ROS scavenging in cucumber. Plant Cell Physiol. 60, 562–574. doi: 10.1093/pcp/pcy226
Cao, S., Shao, J., Shi, L., Xu, L., Shen, Z., Chen, W., et al. (2018). Melatonin increases chilling tolerance in postharvest peach fruit by alleviating oxidative damage. Sci. Rep. 8, 1–11. doi: 10.1038/s41598-018-19363-5
Cao, S., Song, C., Shao, J., Bian, K., Chen, W., Yang, Z. (2016). Exogenous melatonin treatment increases chilling tolerance and induces defense response in harvested peach fruit during cold storage. J. Agricul Food Chem. 64, 5215–5222. doi: 10.1021/acs.jafc.6b01118
Chang, J., Guo, Y., Zhang, Z., Wei, C., Zhang, Y., Ma, J., et al. (2020). CBF-responsive pathway and phytohormones are involved in melatonin-improved photosynthesis and redox homeostasis under aerial cold stress in watermelon. Acta Physiol. Plant 42, 159. doi: 10.1007/s11738-020-03147-4
Chang, B., Yang, L., Cong, W., Zu, Y., Tang, Z. (2014). The improved resistance to high salinity induced by trehalose is associated with ionic regulation and osmotic adjustment in Catharanthus roseus. Plant Physiol. Biochem. 77, 140–148. doi: 10.1016/j.plaphy.2014.02.001
Debnath, B., Hussain, M., Irshad, M., Mitra, S., Li, M., Liu, S., et al. (2018a). Exogenous melatonin mitigates acid rain stress to tomato plants through modulation of leaf ultrastructure, photosynthesis and antioxidant potential. Molecules 23, 388. doi: 10.3390/molecules23020388
Debnath, B., Hussain, M., Li, M., Lu, X., Sun, Y., Qiu, D. (2018b). Exogenous melatonin improves fruit quality features, health promoting antioxidant compounds and yield traits in tomato fruits under acid rain stress. Molecules 23, 1868. doi: 10.3390/molecules23081868
Ding, F., Liu, B., Zhang, S. (2017). Exogenous melatonin ameliorates cold-induced damage in tomato plants. Sci. Hortic. 219, 264–271. doi: 10.1016/j.scienta.2017.03.029
Ding, F., Wang, G., Wang, M., Zhang, S. (2018). Exogenous melatonin improves tolerance to water deficit by promoting cuticle formation in tomato plants. Molecules 23, 1605. doi: 10.3390/molecules23071605
Dodangeh, H., Rahimi, G., Fallah, M., Ebrahimi, E. (2018). Investigation of heavy metal uptake by three types of ornamental plants as affected by application of organic and chemical fertilizers in contaminated soils. Environ. Earth Sci. 77, 1–15. doi: 10.1007/s12665-018-7620-2
Dos Reis, S. P., Lima, A. M., De Souza, C. R. B. (2012). Recent molecular advances on downstream plant responses to abiotic stress. Int. J. Mol. Sci. 13, 8628–8647. doi: 10.3390/ijms13078628
ElSayed, A. I., Boulila, M., Rafudeen, M. S., Mohamed, A. H., Sengupta, S., Rady, M., et al. (2020). Melatonin regulatory mechanisms and phylogenetic analyses of melatonin biosynthesis related genes extracted from peanut under salinity stress. Plants 9, 854. doi: 10.3390/plants9070854
Gao, J. P., Chao, D. Y., Lin, H. X. (2007). Understanding abiotic stress tolerance mechanisms: recent studies on stress response in rice. J. Integr. Plant Biol. 49, 742–750. doi: 10.1111/j.1744-7909.2007.00495.x
Gao, H., Lu, Z., Yang, Y., Wang, D., Yang, T., Cao, M., et al. (2018). Melatonin treatment reduces chilling injury in peach fruit through its regulation of membrane fatty acid contents and phenolic metabolism. Food Chem. 245, 659–666. doi: 10.1016/j.foodchem.2017.10.008
Gao, J., Shahid, R., Ji, X., Li, S. (2022). Climate change resilience and sustainable tropical agriculture: Farmers’ perceptions, reactive adaptations and determinants of reactive adaptations in hainan, China. Atmosphere 13, 955. doi: 10.3390/atmos13060955
Gong, X., Shi, S., Dou, F., Song, Y., Ma, F. (2017). Exogenous melatonin alleviates alkaline stress in malus hupehensis rehd. by regulating the biosynthesis of polyamines. Molecules 22, 1542. doi: 10.3390/molecules22091542
Hanaka, A., Ozimek, E., Reszczyńska, E., Jaroszuk-Ściseł, J., Stolarz, M. (2021). Plant tolerance to drought stress in the presence of supporting bacteria and fungi: An efficient strategy in horticulture. Horticulturae 7, 390. doi: 10.3390/horticulturae7100390
Hasan, M., Ahammed, G. J., Yin, L., Shi, K., Xia, X., Zhou, Y., et al. (2015). Melatonin mitigates cadmium phytotoxicity through modulation of phytochelatins biosynthesis, vacuolar sequestration, and antioxidant potential in Solanum lycopersicum l. Front. Plant Sci. 6, 601. doi: 10.3389/fpls.2015.00601
Hasan, M. K., Liu, C. X., Pan, Y. T., Ahammed, G. J., Qi, Z. Y., Zhou, J. (2018). Melatonin alleviates low-sulfur stress by promoting sulfur homeostasis in tomato plants. Sci. Rep. 8, 10182. doi: 10.1038/s41598-018-28561-0
Hasanuzzaman, M., Nahar, K., Alam, M. M., Roychowdhury, R., Fujita, M. (2013). Physiological, biochemical, and molecular mechanisms of heat stress tolerance in plants. Int. J. Mol. Sci. 14, 9643–9684. doi: 10.3390/ijms14059643
Hassan, M. U., Mahmood, A., Awan, M. I., Maqbool, R., Aamer, M., Alhaithloul, H. A., et al. (2022). Melatonin-induced protection against plant abiotic stress: mechanisms and prospects. Front. Plant Sci. 13, 902694. doi: 10.3389/fpls.2022.902694
Hoque, M. N., Tahjib-Ul-Arif, M., Hannan, A., Sultana, N., Akhter, S., Hasanuzzaman, M., et al. (2021). Melatonin modulates plant tolerance to heavy metal stress: morphological responses to molecular mechanisms. Int. J. Mol. Sci. 22, 11445. doi: 10.3390/ijms222111445
Hu, Z., Fu, Q., Zheng, J., Zhang, A., Wang, H. (2020). Transcriptomic and metabolomic analyses reveal that melatonin promotes melon root development under copper stress by inhibiting jasmonic acid biosynthesis. Hortic. Res. 7, 79. doi: 10.1038/s41438-020-0293-5
Hu, E., Liu, M., Zhou, R., Jiang, F., Sun, M., Wen, J., et al. (2021). Relationship between melatonin and abscisic acid in response to salt stress of tomato. Sci. Hortic. 285, 110176. doi: 10.1016/j.scienta.2021.110176
Hu, C. H., Zheng, Y., Tong, C. L., Zhang, D. J. (2022). Effects of exogenous melatonin on plant growth, root hormones and photosynthetic characteristics of trifoliate orange subjected to salt stress. Plant Growth Regul. 1-8. doi: 10.1007/s10725-022-00814-z
Ibrahim, M. F., Elbar, O. H. A., Farag, R., Hikal, M., El-Kelish, A., El-Yazied, A. A., et al. (2020). Melatonin counteracts drought induced oxidative damage and stimulates growth, productivity and fruit quality properties of tomato plants. Plants 9, 1276. doi: 10.3390/plants9101276
Imran, K., Zafar, H., Chattha, M. U., Mahmood, A., Maqbool, R., Athar, F. (2022). Seed priming with different agents mitigate alkalinity induced oxidative damage and improves maize growth. Not. Bot. Horti Agrobot. Cluj Napoca 50, 12615–12615. doi: 10.15835/nbha50112615
Jahan, M. S., Guo, S., Baloch, A. R., Sun, J., Shu, S., Wang, Y., et al. (2020). Melatonin alleviates nickel phytotoxicity by improving photosynthesis, secondary metabolism and oxidative stress tolerance in tomato seedlings. Ecotoxicol. Environ. Saf. 197, 110593. doi: 10.1016/j.ecoenv.2020.110593
Jahan, M. S., Shu, S., Wang, Y., Chen, Z., He, M., Tao, M., et al. (2019). Melatonin alleviates heat-induced damage of tomato seedlings by balancing redox homeostasis and modulating polyamine and nitric oxide biosynthesis. BMC Plant Biol. 19, 1–16. doi: 10.1186/s12870-019-1992-7
Jahan, M. S., Guo, S., Sun, J., Shu, S., Wang, Y., Abou El-Yazied, A., et al. (2021). Melatonin-mediated photosynthetic performance of tomato seedlings under high-temperature stress. Plant Physiology and Biochemistry 167, 309–320.
Jan, S., Singh, B., Bhardwaj, R., Singh, R., Mansoor, S., Ahmad, P. (2022). Recent advances on the pragmatic roles of phytomelatonin and its exogenous application for abiotic stress management in plants. J. Plant Growth Regul., 1–16. doi: 10.1007/s00344-022-10766-3
Jia, C., Yu, X., Zhang, M., Liu, Z., Zou, P., Ma, J., et al. (2020). Application of melatonin-enhanced tolerance to high-temperature stress in cherry radish (Raphanus sativus l. var. radculus pers). J. Plant Growth Regul. 39, 631–640. doi: 10.1007/s00344-019-10006-1
Johns, N. P., Johns, J., Porasuphatana, S., Plaimee, P., Sae-Teaw, M. (2013). Dietary intake of melatonin from tropical fruit altered urinary excretion of 6- sulfatoxymelatonin in healthy volunteers. J. Agricul. Food Chem. 61, 913–919. doi: 10.1021/jf300359a
Kamiab, F. (2020). Exogenous melatonin mitigates the salinity damages and improves the growth of pistachio under salinity stress. J. Plant Nutr. 43, 1468–1484. doi: 10.1080/01904167.2020.1730898
Karaca, P., Cekic, F. O. (2019). Exogenous melatonin-stimulated defense responses in tomato plants treated with polyethylene glycol. Int. J. Veg. Sci. 25, 601–609. doi: 10.1080/19315260.2019.1575317
Kaya, C., Sarıoglu, A., Ashraf, M., Alyemeni, M. N., Ahmad, P. (2022). The combined supplementation of melatonin and salicylic acid effectively detoxifies arsenic toxicity by modulating phytochelatins and nitrogen metabolism in pepper plants. Environ. Poll. 297, 118727. doi: 10.1016/j.envpol.2021.118727
Khan, T. A., Fariduddin, Q., Yusuf, M. (2015). Lycopersicon esculentum under low temperature stress: an approach toward enhanced antioxidants and yield. Environ. Sci. Poll. Res. 22, 14178–14188. doi: 10.1007/s11356-015-4658-5
Kobylińska, A., Reiter, R. J., Posmyk, M. M. (2017). Melatonin protects cultured tobacco cells against lead-induced cell death via inhibition of cytochrome c translocation. Front. Plant Sci. 8, 1560. doi: 10.3389/fpls.2017.01560
Kolář, J., Macháčková, I. (2005). Melatonin in higher plants: occurrence and possible functions. J. Pineal Res. 39, 333–341. doi: 10.1111/j.1600-079X.2005.00276.x
Korkmaz, A., DEĞER, Ö., SZAFRAŃSKA, K., KÖKLÜ, Ş., KARACA, A., YAKUPOĞLU, G., et al. (2021). Melatonin effects in enhancing chilling stress tolerance of pepper. Sci. Hortic. 289, 110434. doi: 10.1016/j.scienta.2021.110434
Koza, N. A., Adedayo, A. A., Babalola, O. O., Kappo, A. P. (2022). Microorganisms in plant growth and development: Roles in abiotic stress tolerance and secondary metabolites secretion. Microorganisms 10, 1528. doi: 10.3390/microorganisms10081528
Li, H., Chang, J., Chen, H., Wang, Z., Gu, X., Wei, C., et al. (2017a). Exogenous melatonin confers salt stress tolerance to watermelon by improving photosynthesis and redox homeostasis. Front. Plant Sci. 8, 295. doi: 10.3389/fpls.2017.00295
Li, H., Chang, J., Zheng, J., Dong, Y., Liu, Q., Yang, X., et al. (2017b). Local melatonin application induces cold tolerance in distant organs of Citrullus lanatus l. Via long distance transport. Sci. Rep. 7, 40858. doi: 10.1038/srep40858
Li, J., Li, Y., Tian, Y., Qu, M., Zhang, W., Gao, L. (2017c). Melatonin has the potential to alleviate cinnamic acid stress in cucumber seedlings. Front. Plant Sci. 8, 1193.
Li, C., Wang, P., Wei, Z., Liang, D., Liu, C., Yin, L., et al. (2012). The mitigation effects of exogenous melatonin on salinity-induced stress in malus hupehensis. J. Pineal Res. 53, 298–306. doi: 10.1111/j.1600-079X.2012.00999.x
Li, X., Wei, J. P., Scott, E. R., Liu, J. W., Guo, S., Li, Y., et al. (2018). Exogenous melatonin alleviates cold stress by promoting antioxidant defense and redox homeostasis in Camellia sinensis l. Molecules 23, 165. doi: 10.3390/molecules23010165
Li, J., Xie, J., Yu, J., Lyv, J., Zhang, J., Ding, D., et al. (2022a). Melatonin enhanced low-temperature combined with low-light tolerance of pepper (Capsicum annuum l.) seedlings by regulating root growth, antioxidant defense system, and osmotic adjustment. Front. Plant Sci. 13, 998293. doi: 10.3389/fpls.2022.998293
Li, J., Yang, Y., Sun, K., Chen, Y., Chen, X., Li, X. (2019). Exogenous melatonin enhances cold, salt and drought stress tolerance by improving antioxidant defense in tea plant (Camellia sinensis (L.) o. kuntze). Molecules 24, 1826. doi: 10.3390/molecules24091826
Li, M., Zhou, J., Du, J., Li, X., Sun, Y., Wang, Z., et al. (2022b). Comparative physiological and transcriptomic analyses of improved heat stress tolerance in celery (Apium graveolens l.) caused by exogenous melatonin. Int. J. Mol. Sci. 23, 11382. doi: 10.3390/ijms231911382
Liang, D., Gao, F., Ni, Z., Lin, L., Deng, Q., Tang, Y., et al. (2018). Melatonin improves heat tolerance in kiwifruit seedlings through promoting antioxidant enzymatic activity and glutathione s-transferase transcription. Molecules 23 (3), 584. doi: 10.3390/molecules23030584
Liang, S. M., Kuang, J. F., Ji, S. J., Chen, Q. F., Deng, W., Min, T., et al. (2020). The membrane lipid metabolism in horticultural products suffering chilling injury. Food Qual. Safety. 4, 9–14. doi: 10.1093/fqsafe/fyaa001
Liang, D., Ni, Z., Xia, H., Xie, Y., Lv, X., Wang, J., et al. (2019). Exogenous melatonin promotes biomass accumulation and photosynthesis of kiwifruit seedlings under drought stress. Sci. Hortic. 246, 34–43. doi: 10.1016/j.scienta.2018.10.058
Liu, N., Jin, Z., Wang, S., Gong, B., Wen, D., Wang, X., et al. (2015b). Sodic alkaline stress mitigation with exogenous melatonin involves reactive oxygen metabolism and ion homeostasis in tomato. Sci. Hortic. 181, 18–25. doi: 10.1016/j.scienta.2014.10.049
Liu, L., Li, D., Ma, Y., Shen, H., Zhao, S., Wang, Y. (2021). Combined application of arbuscular mycorrhizal fungi and exogenous melatonin alleviates drought stress and improves plant growth in tobacco seedlings. J. Plant Growth Regul. 40, 1074–1087. doi: 10.1007/s00344-020-10165-6
Liu, D. D., Sun, X. S., Liu, L., Shi, H. D., Chen, S. Y., Zhao, D. K. (2019). Overexpression of the melatonin synthesis-related gene SlCOMT1 improves the resistance of tomato to salt stress. Molecules 24, 1514. doi: 10.3390/molecules24081514
Liu, J., Wang, W., Wang, L., Sun, Y. (2015a). Exogenous melatonin improves seedling health index and drought tolerance in tomato. Plant Growth Regul. 77, 317–326. doi: 10.1007/s10725-015-0066-6
Liu, J., Wu, H., Wang, B., Zhang, Y., Wang, J., Cheng, C., et al. (2022). Exogenous melatonin enhances cold resistance by improving antioxidant defense and cold-responsive genes’ expression in banana. Horticulturae 8, 260. doi: 10.3390/horticulturae8030260
Malhotra, S. K. (2017). Horticultural crops and climate change: A review. Indian J. Agricul Sci. 87, 12–22.
Manafi, H., Baninasab, B., Gholami, M., Talebi, M., Khanizadeh, S. (2022). Exogenous melatonin alleviates heat-induced oxidative damage in strawberry (Fragaria× ananassa duch. cv. ventana) plant. J. Plant Growth Regul. 41, 52–64. doi: 10.1007/s00344-020-10279-x
Marino, S. (2021). Horticultural crop response to different environmental and nutritional stress. Horticulturae 7, 240. doi: 10.3390/horticulturae7080240
Marta, B., Szafra ´nska, K., Posmyk, M. M. (2016). Exogenous melatonin improves antioxidant defense in cucumber seeds (Cucumis sativus l.) germinated under chilling stress. Front. Plant Sci. 7, 575. doi: 10.3389/fpls.2016.00575
Martinez, V., Nieves-Cordones, M., Lopez-Delacalle, M., Rodenas, R., Mestre, T. C., Garcia-Sanchez, F., et al. (2018). Tolerance to stress combination in tomato plants: New insights in the protective role of melatonin. Molecules 23, 535. doi: 10.3390/molecules23030535
Meng, J. F., Xu, T. F., Wang, Z. Z., Fang, Y. L., Xi, Z. M., Zhang, Z. W. (2014). The ameliorative effects of exogenous melatonin on grape cuttings under water-deficient stress: antioxidant metabolites, leaf anatomy, and chloroplast morphology. J. Pineal Res. 57, 200–212. doi: 10.1111/jpi.12159
Moussa, H. R., Algamal, S. M. A. (2017). Does exogenous application of melatonin ameliorate boron toxicity in spinach plants? Int. J. Vegetable Sci. 23, 233–245. doi: 10.1080/19315260.2016.1243184
Moustafa-Farag, M., Mahmoud, A., Arnao, M. B., Sheteiwy, M. S., Dafea, M., Soltan, M., et al. (2020). Melatonin-induced water stress tolerance in plants: Recent advances. Antioxidants 9, 809. doi: 10.3390/antiox9090809
Muhammad, F., Raza, M. A. S., Iqbal, R., Zulfiqar, F., Aslam, M. U., Yong, J. W. H., et al. (2022). Ameliorating drought effects in wheat using an exclusive or Co-applied rhizobacteria and ZnO nanoparticles. Biology 11, 1564. doi: 10.3390/biology11111564
Murch, S. J., Saxena, P. K. (2002). Melatonin: a potential regulator of plant growth and development? In Vitro Cell. Dev. Biology-Plant 38, 531–536. doi: 10.1079/IVP2002333
Nabaei, M., Amooaghaie, R. (2019). Nitric oxide is involved in the regulation of melatonin-induced antioxidant responses in Catharanthus roseus roots under cadmium stress. Botany 97, 681–690. doi: 10.1139/cjb-2019-0107
Nawaz, M. A., Jiao, Y., Chen, C., Shireen, F., Zheng, Z., Imtiaz, M., et al. (2018). Melatonin pretreatment improves vanadium stress tolerance of watermelon seedlings by reducing vanadium concentration in the leaves and regulating melatonin biosynthesis and antioxidant-related gene expression. J. Plant Physiol. 220, 115–127. doi: 10.1016/j.jplph.2017.11.003
Nawaz, F., Shabbir, R. N., Shahbaz, M., Majeed, S., Raheel, M., Hassan, W., et al. (2017). Cross talk between nitric oxide and phytohormones regulate plant development during abiotic stresses. phytohormones: signaling mechanisms and crosstalk in plant development and stress responses. InTech Rijeka, 117–141. doi: 10.5772/intechopen.69812
Nawaz, M. A., Huang, Y., Bie, Z., Ahmed, W., Reiter, R. J., Niu, M., et al. (2016). Melatonin: current status and future perspectives in plant science. Front. Plant Sci. 6, 1230.
Noor, I., Sohail, H., Sun, J., Nawaz, M. A., Li, G., Hasanuzzaman, M., et al. (2022). Heavy metal and metalloid toxicity in horticultural plants: Tolerance mechanism and remediation strategies. Chemosphere 303, 135196. doi: 10.1016/j.chemosphere.2022.135196
Paredes, S. D., Korkmaz, A., Manchester, L. C., Tan, D. X., Reiter, R. J. (2009). Phytomelatonin: a review. J. Exp. Botany. 60, 57–69. doi: 10.1093/jxb/ern284
Park, S., Back, K. (2012). Melatonin promotes seminal root elongation and root growth in transgenic rice after germination. J. Pineal Res. 53, 385–389. doi: 10.1111/j.1600-079X.2012.01008.x
Peng, X., Wang, N., Sun, S., Geng, L., Guo, N., Liu, A., et al. (2023). Reactive oxygen species signaling is involved in melatonin-induced reduction of chlorothalonil residue in tomato leaves. J. Hazardous Mater. 443, 130212. doi: 10.1016/j.jhazmat.2022.130212
Posmyk, M. M., Balabusta, M., Wieczorek, M. (2009). Melatonin applied to cucumber (Cucumis sativus l.) seeds improves germination during chilling stress. J. Pineal Res. 46, 214–223. doi: 10.1111/j.1600-079X.2008.00652.x
Posmyk, M. M., Kuran, H., Marciniak, K., Janas, K. M. (2008). Pre sowing seed treatment with melatonin protects red cabbage seedlings against toxic copper ion concentrations. J. Pineal Res. 45, 24–31. doi: 10.1111/j.1600-079X.2007.00552.x
Qari, S. H., Hassan, M. U., Chattha, M. U., Mahmood, A., Naqve, M., Nawaz, M., et al. (2022). Melatonin induced cold tolerance in plants: Physiological and molecular responses. Front. Plant Sci. 13, 843071. doi: 10.3389/fpls.2022.843071
Rasheed, A., Gill, R. A., Hassan, M. U., Mahmood, A., Qari, S., Zaman, Q. U. (2021). A critical review: recent advancements in the use of CRISPR/Cas9 technology to enhance crops and alleviate global food crises. Curr. Issues Mol. Biol. 43, 1950–1976. doi: 10.3390/cimb43030135
Sadak, M. S., Abdalla, A. M., Abd Elhamid, E. M., Ezzo, M. I. (2020). Role of melatonin in improving growth, yield quantity and quality of Moringa oleifera l. plant under drought stress. Bull. Natl. Res. Cent. 44, 1–13. doi: 10.1186/s42269-019-0259-7
Sarafi, E., Tsouvaltzis, P., Chatzissavvidis, C., Siomos, A., Therios, I. (2017). Melatonin and resveratrol reverse the toxic effect of high boron (B) and modulate biochemical parameters in pepper plants (Capsicum annuum l.). Plant Physiol. Biochem. 112, 173–182. doi: 10.1016/j.plaphy.2016.12.018
Shahid, R., Shijie, L., Shahid, S., Altaf, M. A., Shahid, H. (2021). Determinants of reactive adaptations to climate change in semi-arid region of Pakistan. J. Arid Environ. 193, 104580. doi: 10.1016/j.jaridenv.2021.104580
Shakoor, A., Abdullah, M., Sarfraz, R., Altaf, M. A., Batool, S. (2017). A comprehensive review on phytoremediation of cadmium (Cd) by mustard (Brassica juncea l.) and sunflower (Helianthus annuus l.). J. Biodiversity Environ. Sci. 10, 88–98.
Sharif, R., Xie, C., Zhang, H., Arnao, M. B., Ali, M., Ali, Q., et al. (2018). Melatonin and its effects on plant systems. Molecules 23, 2352. doi: 10.3390/molecules23092352
Sharma, A., Wang, J., Xu, D., Tao, S., Chong, S., Yan, D. (2020). Melatonin regulates the functional components of photosynthesis, antioxidant system, gene expression, and metabolic pathways to induce drought resistance in grafted Carya cathayensis plants. Sci. Total Environ. 713, 136675. doi: 10.1016/j.scitotenv.2020.136675
Sharma, A., Zheng, B. (2019). Melatonin mediated regulation of drought stress: Physiological and molecular aspects. Plants 8, 190. doi: 10.3390/plants8070190
Shi, H., Reiter, R. J., Tan, D. X., Chan, Z. (2015). INDOLE-3-ACETIC ACID INDUCIBLE 17 positively modulates natural leaf senescence through melatonin-mediated pathway in Arabidopsis. J. Pineal Res. 58, 26–33. doi: 10.1111/jpi.12188
Siddiqui, M. H., Alamri, S., Alsubaie, Q. D., Ali, H. M. (2020a). Melatonin and gibberellic acid promote growth and chlorophyll biosynthesis by regulating antioxidant and methylglyoxal detoxification system in tomato seedlings under salinity. J. Plant Growth Regul. 39, 1488–1502. doi: 10.1007/s00344-020-10122-3
Siddiqui, M. H., Alamri, S., Alsubaie, Q. D., Ali, H. M., Ibrahim, A. A., Alsadon, A. (2019). Potential roles of melatonin and sulfur in alleviation of lanthanum toxicity in tomato seedlings. Ecotoxicol. Environ. Saf. 180, 656–667. doi: 10.1016/j.ecoenv.2019.05.043
Siddiqui, M. H., Alamri, S., Khan, M. N., Corpas, F. J., Al-Amri, A. A., Alsubaie, Q. D., et al. (2020b). Melatonin and calcium function synergistically to promote the resilience through ROS metabolism under arsenic-induced stress. J. Hazardous Mat. 398, 122882. doi: 10.1016/j.jhazmat.2020.122882
Szafrańska, K., Reiter, R. J., Posmyk, M. M. (2017). Melatonin improves the photosynthetic apparatus in pea leaves stressed by paraquat via chlorophyll breakdown regulation and its accelerated de novo synthesis. Front. Plant Sci. 8, 878. doi: 10.3389/fpls.2017.00878
Tabassum, S., Ossola, A., Marchin, R. M., Ellsworth, D. S., Leishman, M. R. (2021). Assessing the relationship between trait-based and horticultural classifications of plant responses to drought. Urban Forestry Urban Greening 61, 127109. doi: 10.1016/j.ufug.2021.127109
Tan, D. X., Manchester, L. C., Liu, X., Rosales-Corral, S. A., Acuna-Castroviejo, D., Reiter, R. J. (2013). Mitochondria and chloroplasts as the original sites of melatonin synthesis: a hypothesis related to melatonin's primary function and evolution in eukaryotes. J. Pineal Res. 54, 127–138. doi: 10.1111/jpi.12026
Tang, Y., Lei, F., Zhang, E., Li, H. (2016). “Soaking seeds with exogenous melatonin improve photosynthetic characteristics of radish under aluminum stress,” in 2016 2nd International Conference on Advances in Energy, Environment and Chemical Engineering (AEECE 2016). 64–67 (Atlantis Press).
Tang, Y., Li, J., Li, H. (2015). “Effects of exogenous melatonin on photosynthetic characteristics of eggplant (Solanum melongena l.) under cadmium stress,” in 2015 6th International Conference on Manufacturing Science and Engineering. 656–659 (Atlantis Press).
Tang, M., Xu, L., Wang, Y., Dong, J., Zhang, X., Wang, K., et al. (2021). Melatonin-induced DNA demethylation of metal transporters and antioxidant genes alleviates lead stress in radish plants. Hortic. Res. 8, 124. doi: 10.1038/s41438-021-00561-8
Tiwari, R. K., Kumar, R., Lal, M. K., Kumar, A., Altaf, M. A., Devi, R., et al. (2022). Melatonin-polyamine interplay in the regulation of stress responses in plants. J. Plant Growth Regul., 1–17. doi: 10.1007/s00344-022-10717-y
Tiwari, R. K., Lal, M. K., Naga, K. C., Kumar, R., Chourasia, K. N., Subhash, S., et al. (2020). Emerging roles of melatonin in mitigating abiotic and biotic stresses of horticultural crops. Sci. Hortic. 272, 109592. doi: 10.1016/j.scienta.2020.109592
Toscano, S., Franzoni, G., Álvarez, S. (2023). Drought stress in horticultural plants. Horticulturae 9, 7. doi: 10.3390/horticulturae9010007
Ulhassan, Z., Huang, Q., Gill, R. A., Ali, S., Mwamba, T. M., Ali, B., et al. (2019). Protective mechanisms of melatonin against selenium toxicity in Brassica napus: insights into physiological traits, thiol biosynthesis and antioxidant machinery. BMC Plant Biol. 19, 1–16.
Wahid, A., Farooq, M., Hussain, I., Rasheed, R., Galani, S. (2012). “Responses and management of heat stress in plants,” in Environmental adaptations and stress tolerance of plants in the era of climate change. 135–157 (New York, NY: Springer).
Wang, M., Duan, S., Zhou, Z., Chen, S., Wang, D. (2019). Foliar spraying of melatonin confers cadmium tolerance in nicotiana tabacum l. Ecotoxicol. Environ. Safety. 170, 68–76. doi: 10.1016/j.ecoenv.2018.11.127
Wang, P., Yin, L., Liang, D., Li, C., Ma, F., Yue, Z. (2012). Delayed senescence of apple leaves by exogenous melatonin treatment: toward regulating the ascorbate-glutathione cycle. J. Pineal Res. 53, 11–20. doi: 10.1111/j.1600-079X.2011.00966.x
Wang, M., Zhang, S., Ding, F. (2020). Melatonin mitigates chilling-induced oxidative stress and photosynthesis inhibition in tomato plants. Antioxidants 9, 218. doi: 10.3390/antiox9030218
Wang, L., Liu, J., Wang, W., Sun, Y. (2016). Exogenous melatonin improves growth and photosynthetic capacity of cucumber under salinity-induced stress. Photosynthetica 54, 19–27.
Wu, X., Ren, J., Huang, X., Zheng, X., Tian, Y., Shi, L., et al. (2021). Melatonin: Biosynthesis, content, and function in horticultural plants and potential application. Sci. Hortic. 288, 110392. doi: 10.1016/j.scienta.2021.110392
Wu, S., Wang, Y., Zhang, J., Gong, X., Zhang, Z., Sun, J., et al. (2021). Exogenous melatonin improves physiological characteristics and promotes growth of strawberry seedlings under cadmium stress. Hortic. Plant J. 7, 13–22. doi: 10.1016/j.hpj.2020.06.002
Xalxo, R., Keshavkant, S. (2019). Melatonin, glutathione and thiourea attenuates lead and acid rain-induced deleterious responses by regulating gene expression of antioxidants in Trigonella foenum graecum l. Chemosphere 221, 1–10. doi: 10.1016/j.chemosphere.2019.01.029
Xia, H., Ni, Z., Hu, R., Lin, L., Deng, H., Wang, J., et al. (2020). Melatonin alleviates drought stress by a non-enzymatic and enzymatic antioxidative system in kiwifruit seedlings. Int. J. Mol Sci. 21, 852. doi: 10.3390/ijms21030852
Xu, L., Xiang, G., Sun, Q., Ni, Y., Jin, Z., Gao, S., et al. (2019). Melatonin enhances salt tolerance by promoting MYB108A-mediated ethylene biosynthesis in grapevines. Hortic. Res. 6, 114. doi: 10.1038/s41438-019-0197-4
Xu, L., Zhang, F., Tang, M. J., Wang, Y., Dong, J. H. (2020). Melatonin confers cadmium tolerance by modulating critical heavy metal chelators and transporters in radish plants. J. Pineal Res. 69, e12659. doi: 10.1111/jpi.12659
Yakuboğlu, G., Ardıç, Ş.K., Cuci, Y., Korkmaz, A. (2022). Mitigation of chilling stress effects on eggplant seedlings by exogenous application of melatonin. Turkish J. Agriculture-Food Sci. Technol. 10, 562–568. doi: 10.24925/turjaf.v10i4.562-568.4601
Yang, X. L., Xu, H., Li, D., Gao, X., Li, T. L., Wang, R. (2018). Effect of melatonin priming on photosynthetic capacity of tomato leaves under low temperature stress. Photosynthetica 56, 884–892. doi: 10.1007/s11099-017-0748-6
Zahedi, S. M., Hosseini, M. S., Abadía, J., Marjani, M. (2020). Melatonin foliar sprays elicit salinity stress tolerance and enhance fruit yield and quality in strawberry (Fragaria× ananassa duch.). Plant Physiol. Biochem. 149, 313–323. doi: 10.1016/j.plaphy.2020.02.021
Zamani, Z., Amiri, H., Ismaili, A. (2020). Improving drought stress tolerance in fenugreek (Trigonella foenum-graecum) by exogenous melatonin. Plant Biosystems-An Int. J. Dealing Aspects Plant Biol. 154 (5), pp.643–pp.655. doi: 10.1080/11263504.2019.1674398
Zhan, H., Nie, X., Zhang, T., Li, S., Wang, X., Du, X., et al. (2019). Melatonin: a small molecule but important for salt stress tolerance in plants. Int. J. Mol. Sci. 20, 709. doi: 10.3390/ijms20030709
Zhang, T., Shi, Z., Zhang, X., Zheng, S., Wang, J., Mo, J. (2020). Alleviating effects of exogenous melatonin on salt stress in cucumber. Sci. Hortic. 262, 109070. doi: 10.1016/j.scienta.2019.109070
Zhang, Y. P., Xu, S., Yang, S. J., Chen, Y. Y. (2017b). Melatonin alleviates cold-induced oxidative damage by regulation of ascorbate–glutathione and proline metabolism in melon seedlings (Cucumis melo l.). J. Horticul. Sci. Biotechnol. 92, 313–324. doi: 10.1080/14620316.2016.1266915
Zhang, Y. P., Yang, S. J., Chen, Y. Y. (2017c). Effects of melatonin on photosynthetic performance and antioxidants in melon during cold and recovery. Biol. Plant 61, 571–578. doi: 10.1007/s10535-017-0717-8
Zhang, N., Zhang, H. J., Sun, Q. Q., Cao, Y. Y., Li, X., Zhao, B., et al. (2017a). Proteomic analysis reveals a role of melatonin in promoting cucumber seed germination under high salinity by regulating energy production. Sci. Rep. 7, 1–15. doi: 10.1038/s41598-017-00566-1
Zhang, H. J., Zhang, N., Yang, R. C., Wang, L., Sun, Q. Q., Li, D. B. (2014). Melatonin promotes seed germination under high salinity by regulating antioxidant systems, ABA and GA4 interaction in cucumber (Cucumis sativus l.). J. Pineal Res. 57, 269–279. doi: 10.1111/jpi.12167
Zhang, N., Zhao, B., Zhang, H. J., Weeda, S., Yang, C., Yang, Z. C., et al. (2013). Melatonin promotes water-stress tolerance, lateral root formation, and seed germination in cucumber (Cucumis sativus l.). J. Pineal Res. 54, 15–23. doi: 10.1111/j.1600-079X.2012.01015.x
Zhao, C., Nawaz, G., Cao, Q., Xu, T. (2022). Melatonin is a potential target for improving horticultural crop resistance to abiotic stress. Sci. Hortic. 291, 110560. doi: 10.1016/j.scienta.2021.110560
Zhao, H., Ye, L., Wang, Y., Zhou, X., Yang, J., Wang, J., et al. (2016). Melatonin increases the chilling tolerance of chloroplast in cucumber seedlings by regulating photosynthetic electron flux and the ascorbate-glutathione cycle. Front. Plant Sci. 7, 1814. doi: 10.3389/fpls.2016.01814
Zhao, H., Zhang, K., Zhou, X., Xi, L., Wang, Y., Xu, H., et al. (2017). Melatonin alleviates chilling stress in cucumber seedlings by up-regulation of CsZat12 and modulation of polyamine and abscisic acid metabolism. Sci. Rep. 7, 1–12. doi: 10.1038/s41598-017-05267-3
Zhou, R., Wan, H., Jiang, F., Li, X., Yu, X., Rosenqvist, E., et al. (2020). The alleviation of photosynthetic damage in tomato under drought and cold stress by high CO2 and melatonin. Int. J. Mol. Sci. 21, 5587. doi: 10.3390/ijms21155587
Zörb, C., Geilfus, C., Dietz, K. (2018). Salinity and crop yield. Plant Biol. 21, 31–38. doi: 10.1111/plb.12884
Keywords: root architecture, ion homeostasis, melatonin, redox balance, horticultural crops
Citation: Zhao J and Hu J (2023) Melatonin: Current status and future perspectives in horticultural plants. Front. Plant Sci. 14:1140803. doi: 10.3389/fpls.2023.1140803
Received: 09 January 2023; Accepted: 01 February 2023;
Published: 23 March 2023.
Edited by:
Safina Naz, Bahauddin Zakariya University, PakistanReviewed by:
Iqra Noor, Huazhong Agricultural University, ChinaRana Roy, Sylhet Agricultural University, Bangladesh
Sami Abou Fayssal, University of Forestry, Sofia, Bulgaria
Copyright © 2023 Zhao and Hu. This is an open-access article distributed under the terms of the Creative Commons Attribution License (CC BY). The use, distribution or reproduction in other forums is permitted, provided the original author(s) and the copyright owner(s) are credited and that the original publication in this journal is cited, in accordance with accepted academic practice. No use, distribution or reproduction is permitted which does not comply with these terms.
*Correspondence: Jing Zhao, zhaojing9951@sina.com