- 1Shandong Facility Horticulture Bioengineering Research Center, Weifang University of Science and Technology, Shouguang, Shandong, China
- 2Key Laboratory of Crop Water Requirement and Regulation of the Ministry of Agriculture and Rural Afairs/Farmland Irrigation Research Institute, Chinese Academy of Agricultural Sciences, Xinxiang, Henan, China
Abiotic stresses are various environmental factors that inhibit a normal plant growth and limit the crop productivity. Plant scientists have been attempting for a long time to understand how plants respond to these stresses and find an effective and feasible solution in mitigating their adverse impacts. Exogenous calcium ion as an essential element for the plant growth, development and reproduction has proven to be effective in alleviating plant stresses through enhancing its resistance or tolerance against them. With a comprehensive review of most recent advances and the analysis by VOSviewer in the researches on this focus of “exogenous calcium” and “stress” for last decade, this paper summarizes the mechanisms of exogenous calcium that are involved in plant defensive responses to abiotic stresses and classifies them accordingly into six categories: I) stabilization of cell walls and membranes; II) regulation of Na+ and K+ ratios; III) regulation of hormone levels in plants; IV) maintenance of photosynthesis; V) regulation of plant respiratory metabolism and improvement of root activities; and VI) induction of gene expressions and protein transcriptions for the stress resistance. Also, the progress and advances from the updated researches on exogenous calcium to alleviate seven abiotic stresses such as drought, flooding, salinity, high temperature, low temperature, heavy metals, and acid rain are outlined. Finally, the future research perspectives in agricultural production are discussed.
Introduction
Plants are subject to a variety of biotic or abiotic adverse attacks and stresses throughout their entire growth, development and reproduction. These adversities can force plants to suffer from a mild osmotic, oxidative and/or ionic imbalance to serious biochemical and physiological disorders such as disruption of cell membranes, reduced enzyme activity, weakened photosynthesis and respiration, and decreased uptake of mineral elements (AbdElgawad et al., 2016; Zhang et al., 2022). To some degree, plants possess limited capabilities of improving their resilience, resistance or tolerance against some mild stresses through a series of morphological, physiological and molecular adjustments, but they are not strong or sufficient to survive from the severe ones. Therefore, Seeking for exogenous substances to induce or enhance such plant positive responses against irreversible adversities have become an ultimate goal for many researchers working on this field, and a promising, realistic and feasible approach in the current research attempts. So far, many exogenous substances have proven to be beneficial and effective in alleviation of plant abiotic stresses (Feng et al., 2023).
Among exogenous substances discovered to alleviate plant stresses, calcium ion (Ca2+) has proven to be more effective and the cost/benefit efficient, not only as an essential nutrient for the plant growth actively involving in a various metabolic activities and as an intracellular messenger for many signal transductions, such as abscisic acid (ABA), reactive oxygen species (ROS), nitric oxide (NO), et al. (Besson-Bard et al., 2008; Marcec et al., 2019; Shabbir et al., 2022). Different parts of a plant differ in their absorption, translocation and utilization of exogenous Ca2+. The exogenous Ca2+ absorbed by roots is mainly through a mass flow of soil water to the root surface and the apoplastids and coplastids in root vascular bundles for its upward movement due to plant transpiration (Zhou and Wang, 2007). Once being applied on foliage, exogenous Ca2+ enters mesophyll cells mainly through stomata, hydrophilic pores in the stratum corneum, and ectoplasmic filaments distributed on the leaf surface. Once inside plants, free Ca2+ moves through Ca2+ transport systems such as the sphinoplasmic Ca2+ outflow system (Ca2+-ATPase pump), Ca2+/H+ reverse transporter, and cytosolic Ca2+ influx system so that the Ca2+ concentration increases rapidly in tissues where Ca2+ is needed for normal metabolic functions or in response to environmental changes/stresses for an internal homeostasis in plants (Zhou and Wang, 2007). Ca2+ also serves as a cellular messenger when the Ca2+ concentration in cytoplasm is different from that in intercellular space available as a Ca2+ source. A high concentration of Ca2+ in a plant seemly acts as a transmitting signal for the activation of various metabolic and molecular activities. Calcium is inert, difficult to move around in plants, hardly to be reused by cells, and prone to bind to organic acids for a further transportation to and utilization in tissues and cells that really needs it. Moreover, any stresses such as drought, high temperature, low temperature and excessive rain affecting plant transpiration can seriously further reduce the calcium absorption and movement even if soil Ca2+ is available, in which case, a foliar application of exogenous calcium can be critically important for the plant stress tolerance. It has been reported that Ca2+ can help resist adverse irritations or damages to some extent in abiotically stressed plants through five mechanisms, including the regulation of sodium/potassium ion (Na+/K+) ratio and ABA concentration, stabilization of cell walls/plasma membranes, recognition of Ca2+/Ca2+-dependent protein kinases (CDPKs) system, and initiation of specific gene expression (Gong and Wang, 2011). However, the plants under stress are less capable of absorbing and translocating Ca2+, which could seriously impair plant tolerance and resistance against abiotic stresses. Therefore, an external application of different Ca2+ supplements has become a main stream of hot research frontlines to provide a sufficient quantity of usable Ca2+ for crops to fight against abiotic stresses (Heidari et al., 2019).
VOSviewer has been used to analyze all English literature published on the Web of science database for the last decade in the scientific research community. In this review, we also used this application to connect all important subjects (dots) under the keyword of “exogenous calcium” and “stress” to generate a hotspot map of international research reports (Figure 1). In the chart generated through VOSviewer, five research hotspots, such as “plant species”, “growth status”, “accumulation of substances”, “tolerance and response mode” are closely related to “exogenous calcium” and “stress”. As far as the application of exogenous calcium for alleviating plant stresses is concerned, most published papers are focused on the salt and drought stress while fewer studies have been conducted on the stress due to the high temperature, low temperature, flooding, heavy metal and acid rain. In terms of the mechanism pertaining to plant stress alleviation by utilization of exogenous calcium, “oxidative stress”, “photosynthesis”, “osmoregulation”, “gene and protein expression” and “membrane lipid peroxidation” have surfaced as research hotspots in recent years, among which “oxidative stress” and “photosynthesis” are the most studied areas in the past two years.
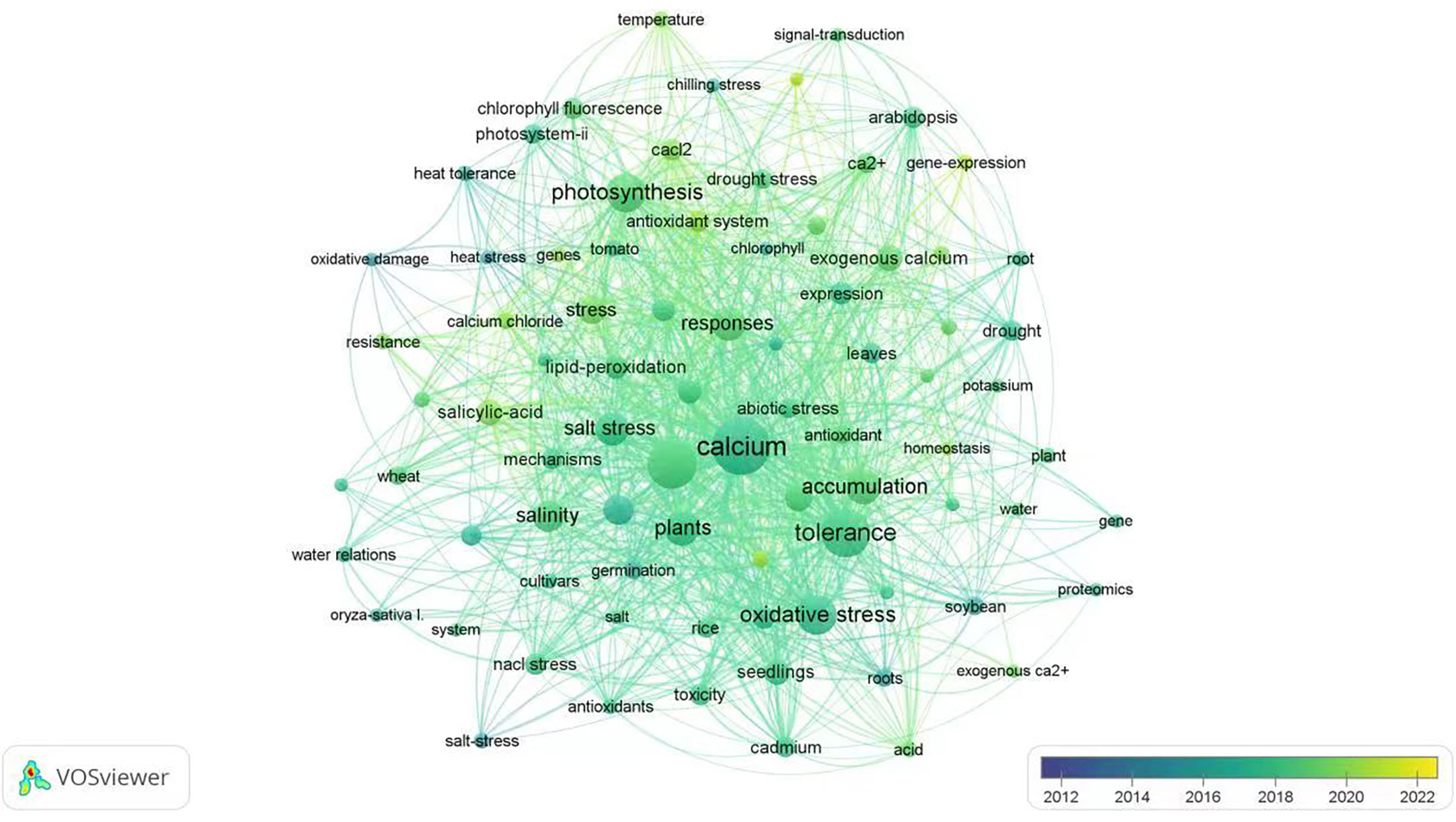
Figure 1 Hotspot analysis of English literature on “exogenous calcium” and “stress” in the last 10 years. The size of each dot represents the focal weight of each key word in the literature and the lines between two dots indicate their coupling relationships.
A number of advances have been made and many sophisticated instruments used in researches on application of exogenous calcium for plants to alleviate various stresses. Guo et al. (2021) used an inductively coupled plasma optical emission spectrometer to detect whether the exogenous calcium would increase K+ and Ca2+ abundance, decrease Na+ content in plants, and maintain the ion homeostasis in Gleditsia sinensis Lam. that was under salt stress through regulating the Na+/K+ ratio. He et al. (2015) applied the fluorescent and ultrastructural cytochemical method to determine if exogenous calcium had mitigated hazard effects of flooding stress on plant respiration by regulating the activity of respiratory metabolic enzymes in cucumber cells. Shi et al. (2014a) found that exogenous Ca2+ enhanced plant cold tolerance by promoting the differential expression of redox-related and cellular metabolism-pertaining proteins through a comparative proteomic and metabolomics analysis. Hu et al. (2018) indicated that an exogenous Ca2+ application not only had a positive effect on the integrity and function of plasma membrane but also alleviated peroxidative damages caused by draught stress on chloroplast and mitochondrial membranes. Exogenous Ca2+ have also proven to regulate the ABA content in plants under low temperature along with the hormone levels of gibberellic acid (GA), cytokinin (CTK), and indole-3-acetic acid (IAA) to maintain a balance (Liu et al., 2017). In addition, the degradation of plant chlorophylls, the damage to photosynthetic organs, and the stable performance of plant photosynthesis could be alleviated or prevented by exogenous Ca2+, which was demonstrated through using a portable chlorophyll fluorescence pulsometer (Li et al., 2022a). According to Gong and Wang (2011) the mode of actions with each exogenous calcium applied to alleviate plant stresses has much in common with the mechanism involving in plant responses to abiotic adversities, which is mainly through regulating ion balance, inducing expression of resistance genes and/or proteins, and increasing the enzyme activity and osmoregulatory substance content. However, the mechanisms involved in the plant stress alleviation are more complex and they are not easily or simply classified by one or two features based on regulating, modulating or participating in biological and physiological metabolisms or gene expression. For example, the mechanism associated with the recognition of Ca2+/CDPKs (Gong and Wang, 2011) mainly is through activating key enzymes and further inducing a series of complex physiological and biochemical responses such as production of more resistant proteins and enhancement of gene expressions for plant stress tolerance. So, the CDPKs recognition mechanism should be included into the category of the gene expression. Moreover, under flooding stress, exogenous calcium can also regulate the plant respiratory pathway to increase root activities for plants to withstand flooding stress (Yang et al., 2016; Han et al., 2019). Based on the relevant mechanisms of exogenous calcium on alleviating adversary stresses and the accumulative information shown in the hotspot analysis maps that is generated from the VOSviewer report for last 10 years, this paper intends to summarize the underlying mechanisms of exogenous Ca2+ involved in alleviation of plant abiotic stresses and tentatively place them into six major categories: I) stabilization of cell wall and membranes; II) regulation of Na+ and K+ ratios; III) regulation of hormone levels in plants; IV) maintenance of photosynthesis: V) regulation of plant respiratory metabolism and improvement of root activities; and VI) induction of gene expressions and protein transcriptions for the stress resistance. Since several outstanding papers have outlined other functional aspects of Ca2+ in plants such as its role as a signal messenger, its regulations as a molecular modulator in gene expressions, and its movement through transpiration, etc. (Edel and Kudla, 2016; Marcec et al., 2019; Wang X. et al., 2019; Liu H. et al., 2022), this review has ultimately summarized in detail the research progresses made on studies of exogenous Ca2+ and its effect on alleviation of seven abiotic stresses, drought, flooding, salt, high temperature, low temperature, heavy metals, and acid rain. At the end, a discussion of perspectives for developing experimental approaches that would take those mechanisms into account for use of different exogenous Ca2+ in increasing plant tolerance against abiotic stresses is provided.
Advances in application of exogenous Ca2+ under abiotic stresses
Drought stress
Recent studies on the drought alleviation by exogenous Ca2+ were conducted on 15 plant species and outlined in detail (Table 1), concluding that it actively involved in keeping water content and balance under check through the mechanism I, III, IV and VI when water deficit is encountered in plants under drought stress. Drought stress is one of the most important adversities causing crop yield reduction worldwide (Iqbal et al., 2020), causing imbalance in water metabolism in plants, affecting photosynthesis and bringing adverse effects on plant growth and development. Qin et al. (2019) found that exogenous Ca2+ help promote plants under drought stress to accumulate endogenous Ca2+, especially free Ca2+ content in datura plants that bind the calmodulin and thus directly or indirectly regulate intracellular related enzyme activities and cellular functions to improve seed germination. Hu et al. (2018) found that exogenous Ca2+ treatment under drought stress can stabilize the structure and function of chloroplast, mitochondrial and endosomal systems in chloroplasts, maintain net photosynthetic rate and gas exchange, alleviate the degradation of photosynthate, and ensure the normal operation of PSII. Li et al. (2012) found that exogenous Ca2+ could reduce the stomatal aperture of honeysuckle for adaptation to drought conditions. In addition, Naeem et al. (2017) indicated that the exogenous Ca2+ treatment could increase the relative water content in maize and compensate a water deficit caused by drought to some extent.
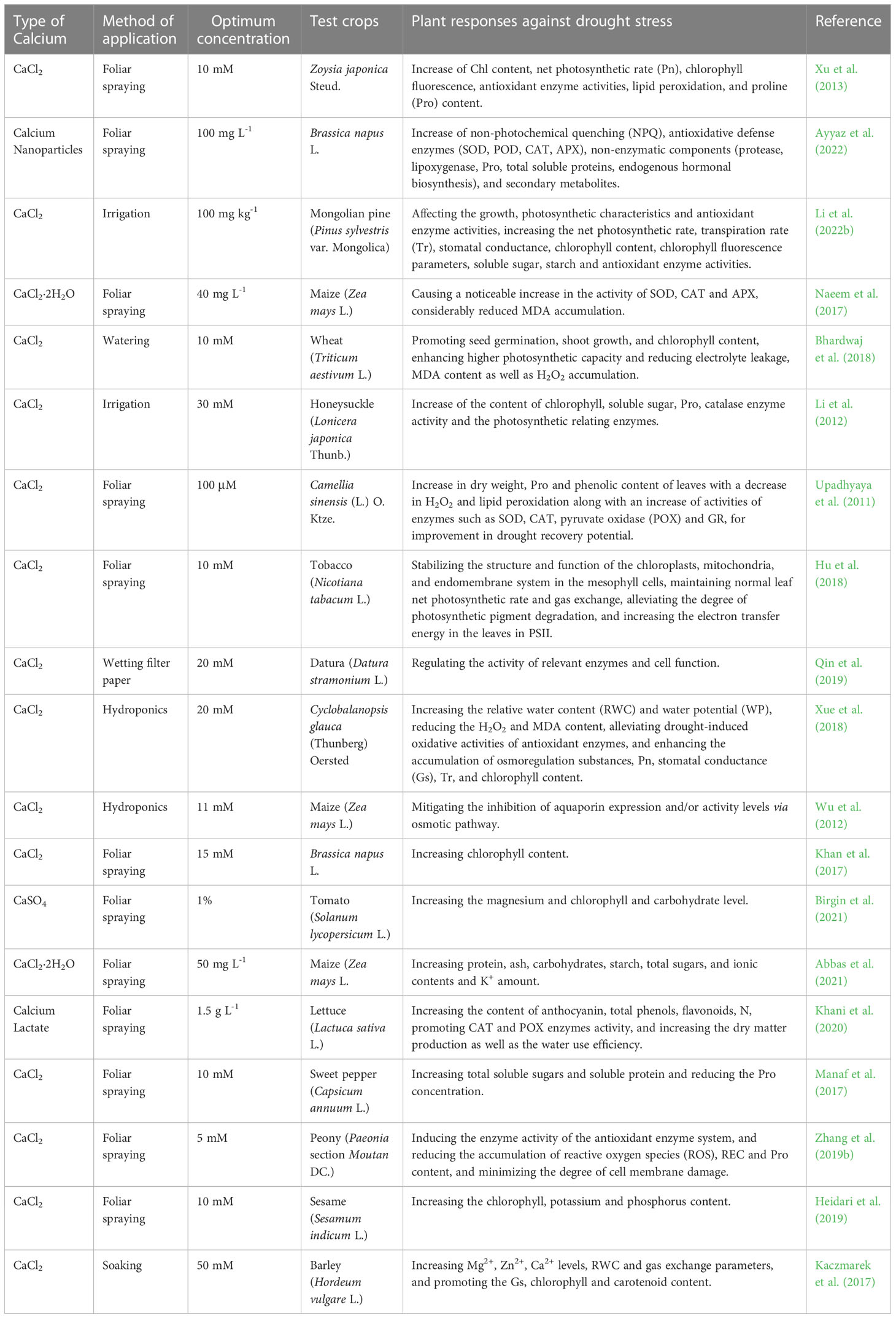
Table 1 List of optimal concentrations of exogenous calcium used to alleviate drought stress on different plants.
Flooding stress
Recent research findings have demonstrated that exogenous Ca2+ (CaCl2 only) is partially or completely participated in all structural, physiological, biochemical and genetic adjustments against flooding stress that causes temporary or prolonged hypoxia in crop roots due to lack of oxygen. The impairments or damages of hypoxia mainly include a blockage of the mitochondrial electron transport chain, a reduced aerobic respiration and an enhanced anaerobic respiration. Thus, a large amount of toxic ethanol, acetic acid, pyruvic acid and other substances are subsequently produced and accumulated in plants. Recent studies have proven that the exogenous Ca2+ treatment could have: 1) effectively minimized the ethanol, acetic acid and pyruvate content to mitigate their toxic effects in peony plants under flooding stress through reducing activities of the lactate dehydrogenase (LDH) and pyruvate decarboxylase (PDC), and increasing the alcohol dehydrogenase (ADH), malate dehydrogenase (MDH), and glucose-6-phosphate dehydrogenase (G-6-PDH) (Fan, 2019); 2) improved the catalytic capacity of pepper pentose phosphate so to produce more adenosine-triphosphate (ATP) and nicotinamide adenine dinucleotide (NADPH) and to improve plant respiratory metabolism (Yang et al., 2016); 3) promoted glycolysis and the accumulation of enzymes in the tricarboxylic acid cycle by reducing the peroxidation level of cucumber seedlings and enhanced the activity of mitochondrial antioxidant enzymes to promote the metabolism of cucumber roots and the transport of Ca2+ and K+ plasma, thus improving the hypoxia tolerance of cucumber (He et al., 2015); and 4) reduced polyamine degradation in muskmelon seedlings under anoxic conditions by promoting nitrate uptake and accelerating its conversion to amino acids, heat-stable proteins, or polyamines (Gao et al., 2011). Relevant studies of exogenous Ca2+ in mitigation of plant flooding stress are conducted on five crop species (Table 2).
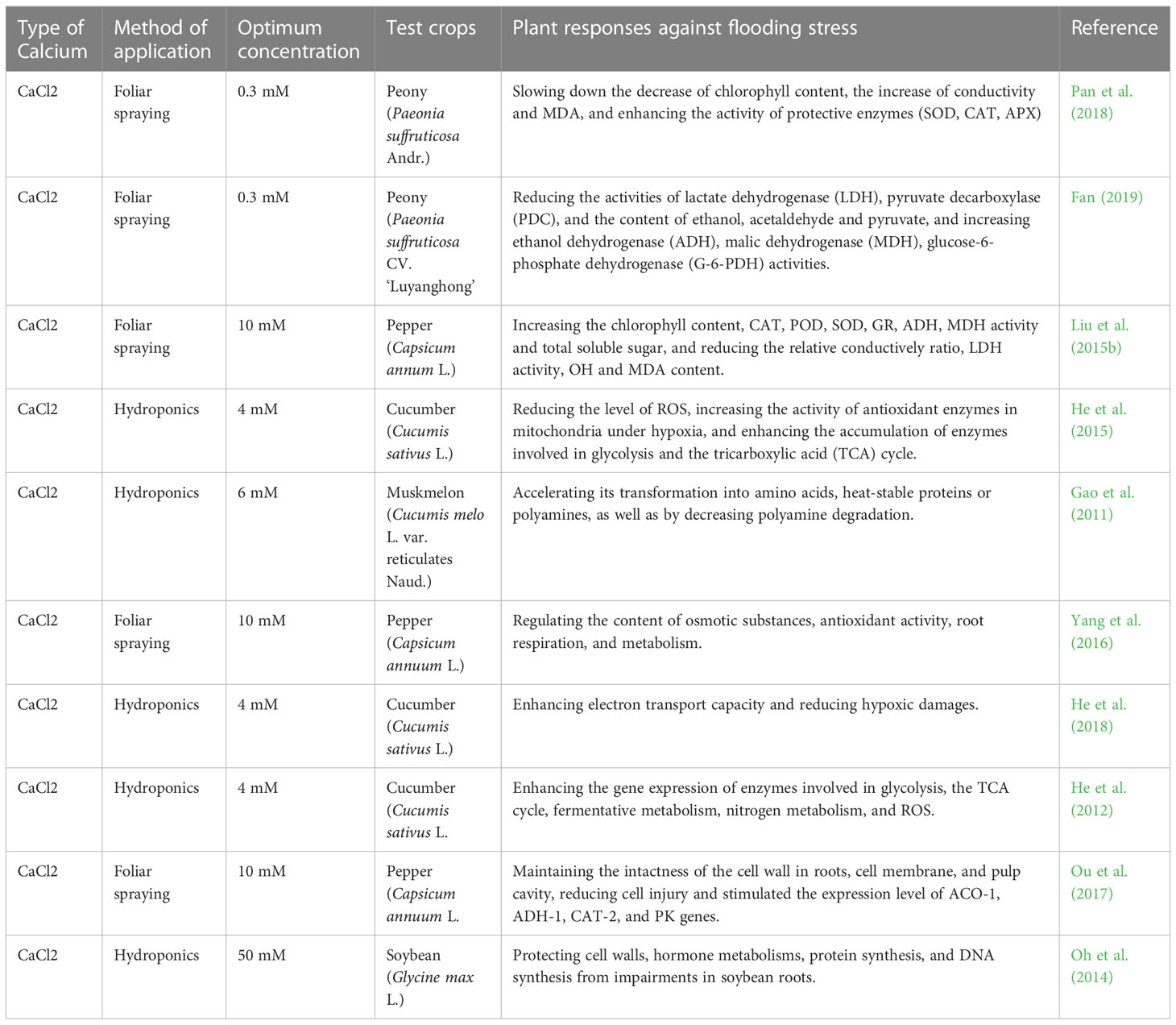
Table 2 List of optimal concentrations of exogenous calcium used to alleviate flooding stress on different plants.
Salt stress
Up to date, 19 plant species have been evaluated for their tolerance against different salt stresses through using various forms of exogenous Ca2+ in different application methods such as watering, hydroponic, soaking and foliar spray. The outcome from those studies showed that their overall mechanisms are diversified and complex mainly to maintain the ion balance and avoid the plant osmosis from impairment. Salt stress causes an ionic imbalance in plants due to the accumulation of Na+ and a great loss of Ca2+ and K+. It also causes osmotic impairments resulting in an oxidative disturbance by an excessive accumulation of ROS that affected the photosynthesis-related activities of electron transportation, phosphorylation, and dark reaction-involved enzymes (Shu et al., 2012; Manaa et al., 2014). Guo et al. (2021) found that exogenous Ca2+ promoted the function of K+ channels and its uptake through the root plasma membrane, reduced the permeability of the plasma membrane for Na+ pumping so to decrease the accumulation of passive Na+ inward flow. Li et al. (2022a) indicated that the exogenous Ca2+ treatment could increase the maximum photochemical efficiency (Fv/Fm) under salt stress in Mongolian pines and 10 mM exogenous Ca2+ could promote the growth of Salix matsudana Koidz seedlings and increase their stomatal conductance, transpiration rate (Tr) and net photosynthetic rate. Li et al. (2022c) also showed that exogenous Ca2+ significantly up-regulated genes encoding phospholipase C, inositol-3-phosphate synthase, and phosphatidylserine decarboxylase to stabilize cell membranes, up-regulated the expression of PsbQ, PsbP, and Psb28 subunits on encoded PSII, and protected PSII to increase photosynthetic rate of Pennisetum Giganteum, revealing the connections between the gene regulation and biochemical metablisms. Zehra et al. (2012) found that the concentration of exogenous Ca2+ required for alleviation of salt stress in Phragmites karka seeds varied. Relevant studies on exogenous Ca2+ mitigation of salt stress in plants involved 19 plant species, as detailed in Table 3.
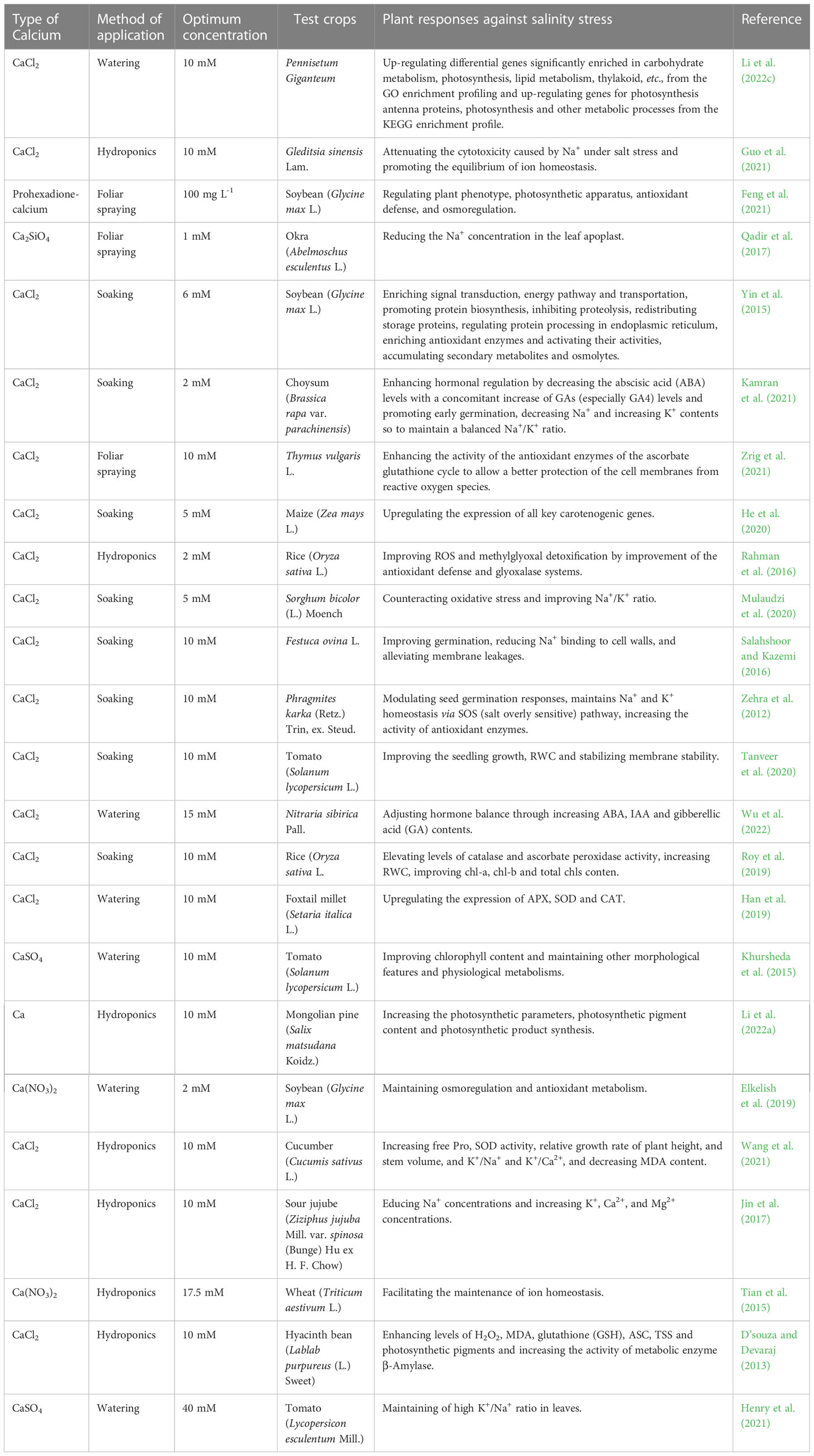
Table 3 List of optimal concentrations of exogenous calcium used to alleviate salinity stress in different plants.
High temperature stress
While being applied to plants, exogenous Ca2+ can prevent or mitigate a possible light damage caused by high temperature that assumingly disrupts the photosynthetic function of plants and severely affects their photosynthesis efficiency. Wang et al. (2022) found that the exogenous Ca2+ treatment significantly increased the chlorophyll (Chl) content, net photosynthetic rate (An), Tr, stomatal conductance (Gs), and antioxidant enzyme activities such as SOD, POD, ascorbate peroxidase (APX), and proline (Pro), as well as the content of osmoregulatory substances such as soluble sugars and soluble proteins to improve the heat tolerance of rosebay. Sun et al. (2015) indicated that exogenous Ca2+ could increase the ribulose-1,5-bisphosphate carboxylase (Rubisco) activity and leaf Fv/Fm of Capsicum fructescens L. to alleviate the photoinhibition to enhance the stomatal conductance and carbon assimilation efficiency. Tiwari et al. (2016) demonstrated that the exogenous Ca2+ treatment upregulated the levels of heat shock genes groEL and groES to maintain cell viability under high temperature stress. Bhatia and Asthir (2014) concluded that the exogenous Ca2+ treatment maintained the growth of wheat seedlings under high temperature stress by altering the carbohydrate metabolism in wheat seeds and increasing the total sugars through reducing sugar-metabolism-related a-amylase and β-amylase activities. In addition, Naeem et al. (2020) showed that exogenous Ca2+ was also involved in the process of adjusting leaf surface structure and configuration to dissipate heat by regulating the conductance of plant stomata for the purpose of alleviating heat stress in plants. Relevant studies on exogenous Ca2+ mitigation of heat stress in plants involved 11 plant species, as detailed in Table 4.
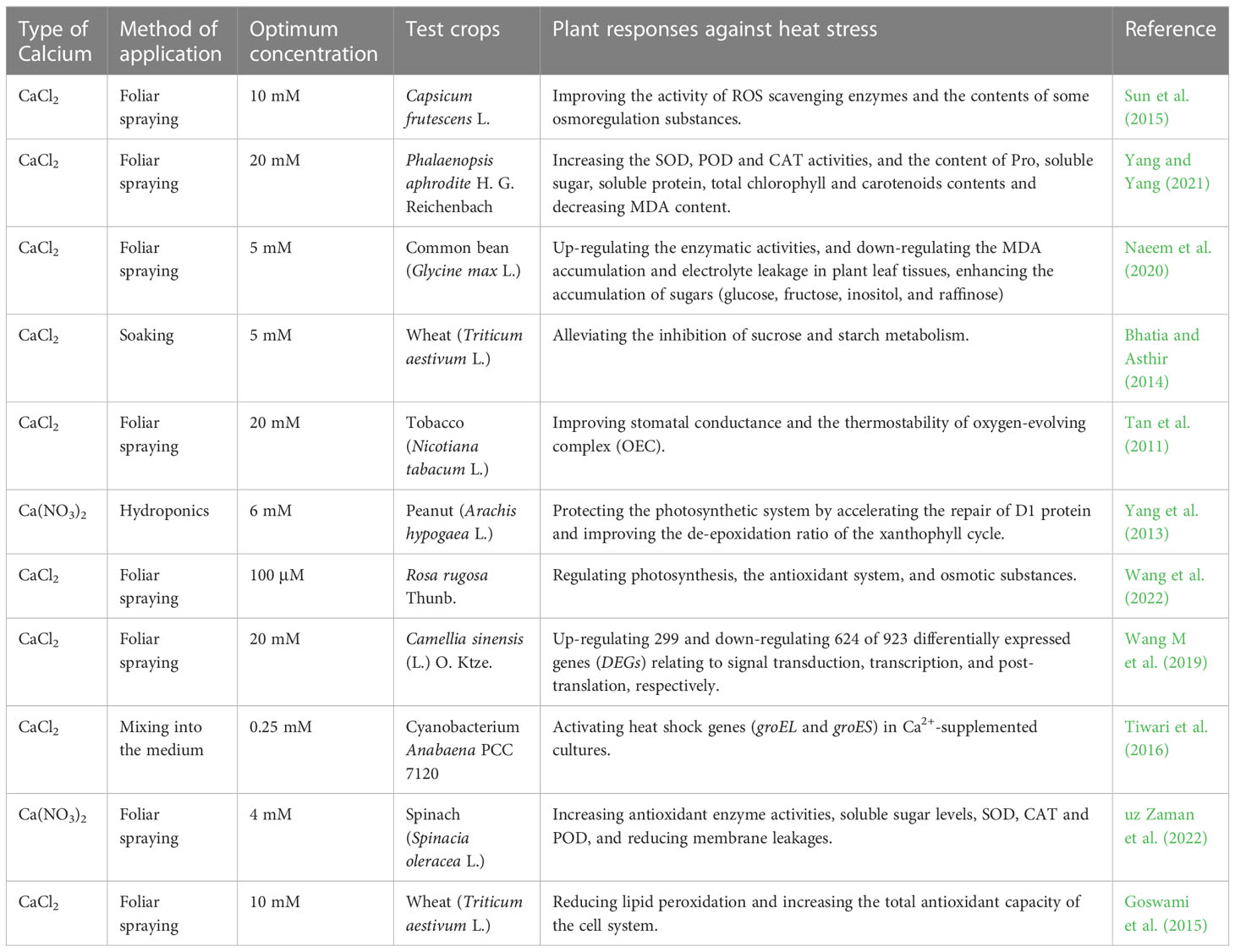
Table 4 List of optimal concentrations of exogenous calcium used to alleviate heat stress in different plants.
Low temperature stress
Low temperature as one of the main abiotic stresses reduces the cell membrane fluidity and enzyme activities before the temperature reaches a freezing point, inhibits plant physiological metabolic activities, and affects seed germination and seedling growth through the mechanism I, III, IV, VI as described in Table 5. The exogenous Ca2+ treatment could help leaves adjust their structure and configuration, promote the operation of cyclic electron transport and enhance the lutein cyclic de-cyclic oxidation in cells to alleviate photoinhibition in tomato plants (Zhang et al., 2014). It also mitigated the damage to chloroplasts due to the low temperature and promote the export of nonstructural carbohydrates to maintain normal plant photosynthesis in peanut seedlings (Wu et al., 2020b). Zhang et al. (2020) indicated that an exogenous application of CaCl2 significantly increased chlorophyll fluorescence indicators (Fv/Fo, Fv/Fm) and the photosynthetic rate in maize, while Liu J et al. (2022) demonstrated that the same treatment onto onion plants reduced cell wall porosity and lowered intracellular ice nucleus temperature. Shi et al. (2014a) concluded that adding exogenous Ca2+ enhanced ROS scavenging through increasing the activity of antioxidant enzymes and non-enzymatic GSH to maintain intracellular ROS at a low level. Relevant studies on exogenous Ca2+ in mitigation of low temperature stress in plants have been reported on nine plant species (Table 5).
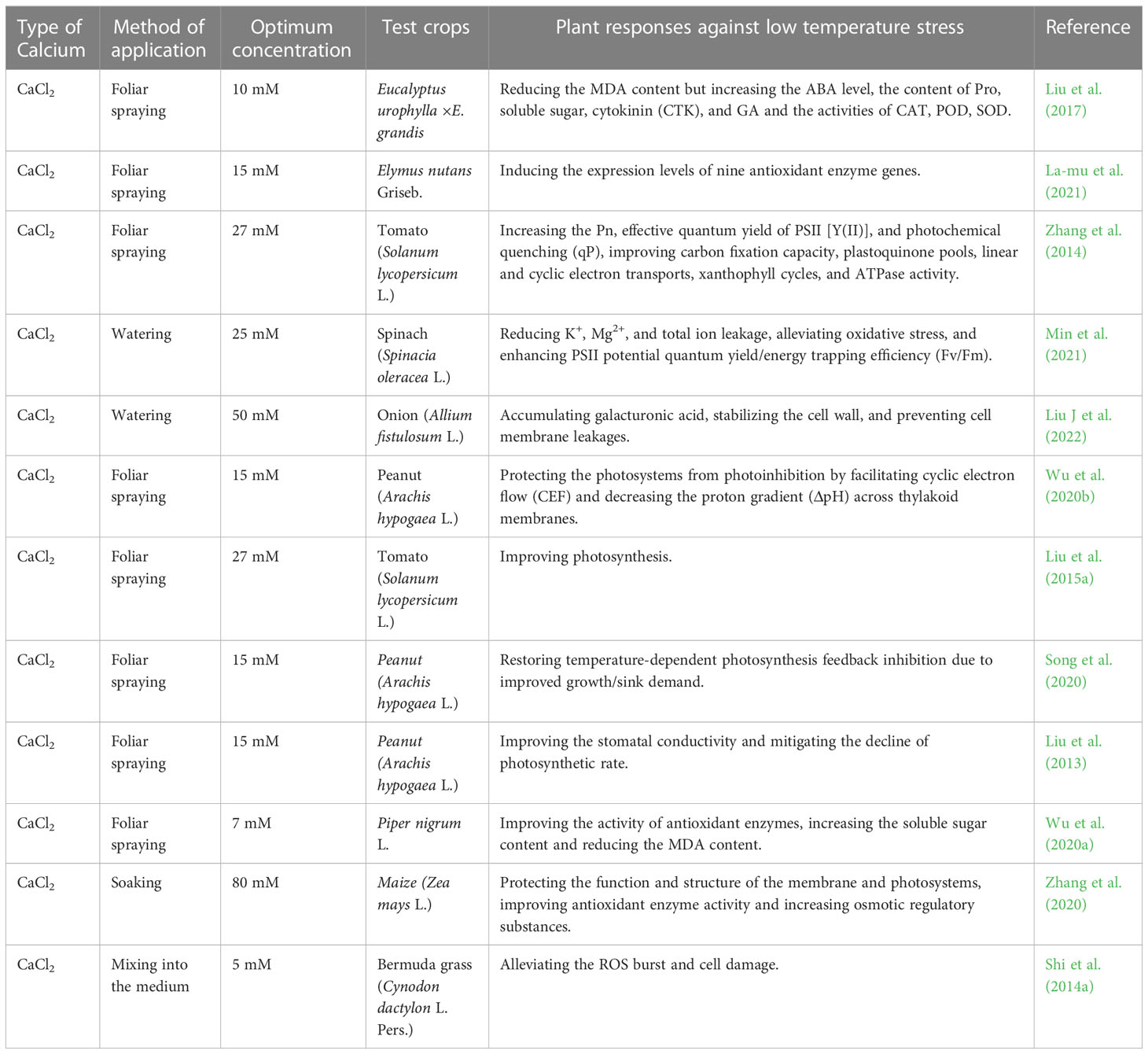
Table 5 List of optimal concentrations of exogenous calcium used to alleviate low temperature stress in different plants.
Heavy metal stress
All researches on exogenous Ca2+ in alleviation of heavy metal stress in plants have indicated that additional Ca2+ limits the uptake, movement and distribution of excessive heavy metals that might accumulate to a toxic level through five mechanisms except the mechanism V summarized in Table 6. Most studies have been focused on alleviation of Cd toxicity by an exogenous Ca2+ application. López-Climent et al. (2014) determined that exogenous Ca2+ had attenuated the Cd uptake in citrus through enhancing the metabolism to detoxicate harmful ions, which promoted the GSH synthesis, and thus increased endogenous GSH levels of the phytochelatin (PC) biosynthesis for the Cd detoxication. According to Shi et al. (2014b), exogenous Ca2+ increased the mitotic index and decreased the chromosomal aberration rate of Wedelia trilobata L. to transport Cd out of stressed cells. Li et al. (2021) suggested that exogenous Ca(OH)2 was more effective than CaCl2 in increasing the quantity of the Ca2+ channel protein (CC), ATPase, cationic/H+ antiporter (CAXs) and membrane transporter protein in Panax notoginseng plants under Cd stress. A proper application of Ca(OH)2 was also reported to increase soil pH, decrease the toxicity of heavy metals, and reduced the uptake of Cd by plants (Zu et al., 2020). Issam et al. (2012) pretreated the Faba bean (Vicia faba L.) foliage with exogenous Ca2+ and proved that the membrane integrity and lipid/fatty acid distribution were protectively stabilized to tolerate heavy metal stress. Jiang et al. (2022) hypothesized that exogenous Ca2+ might have reduced the toxicity of Pb through depositing Pb2+ in the cell wall, which might had nothing to do with soil properties. Relevant studies on exogenous Ca2+ mitigation of heavy metal stress in plants involved 11 plant species, as detailed in Table 6.
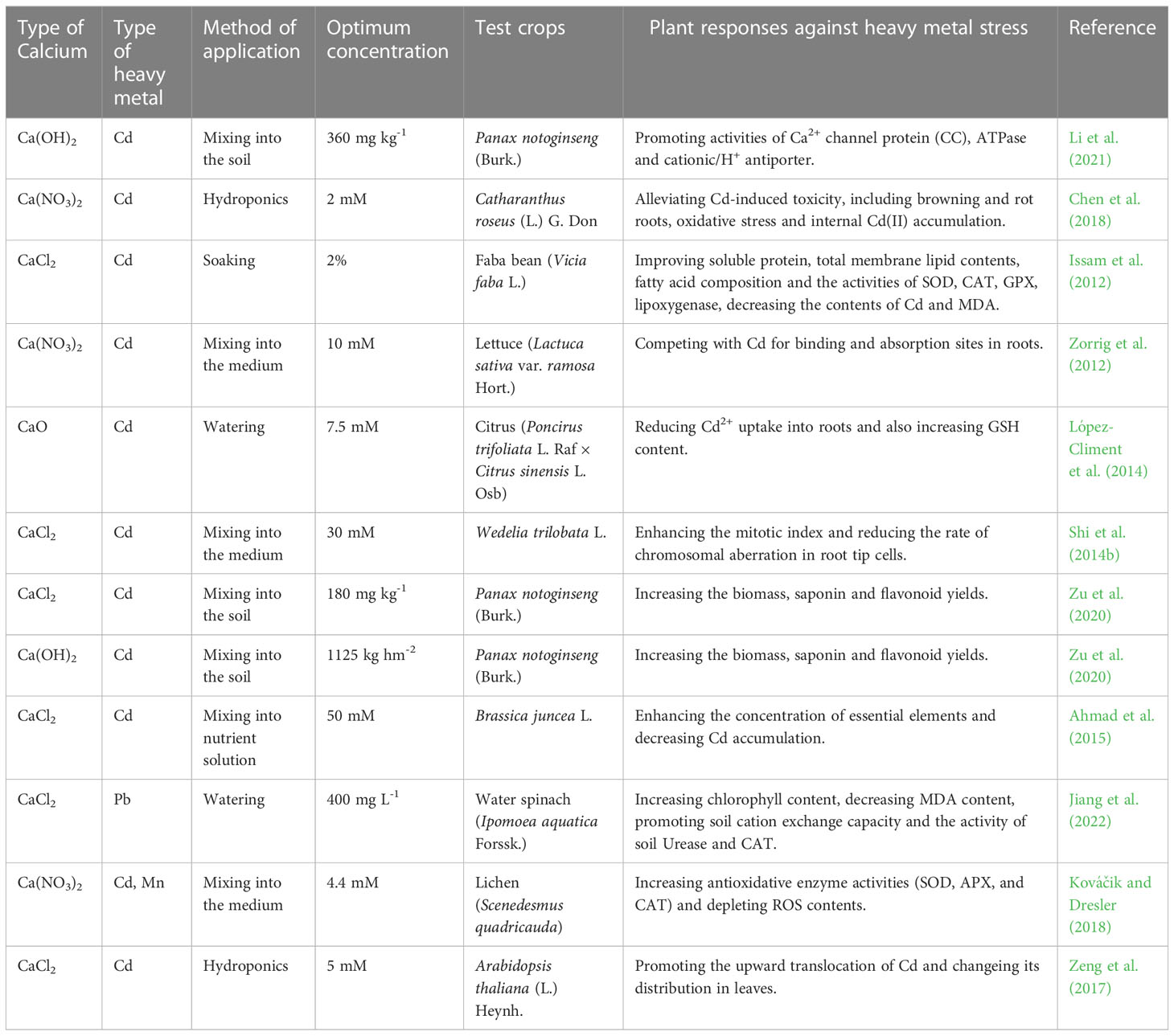
Table 6 List of optimal concentrations of exogenous calcium used to alleviate heavy metal stress in different plants.
Acid rain stress
Exogenous Ca2+ has been tried and studied on for its possible application when plants are under an acid rain situation. So far, Ca2+ has proven to be effective through the mechanism I, III, IV and VI. Acid rain is defined as any precipitation with a pH less than 5.6 due to a large amount of acid substances accumulated in the atmosphere mainly through human activities. Damages caused by acid rain on plant leaves directly breakdown the protective surface of leaves, destroy the integrity of inner plant cell membranes, and cause the organelle dysfunction. A persisted and long duration of acid rain can lead to a serious and catastrophic impairments on plant structural compositions (Zhang et al., 2021), but there have been limited studies working on use of the exogenous Ca2+ to improve plant cell membranes. One of them indicated that exogenous Ca2+ increased the H - ATPase activity with the soybean plasma membrane, kept the membranes unharmed, and initiated the GmPHA1 gene expression to generate more nutrient uptakes such as N, P, K, and Mg to keep chlorophylls from degradation (Liang and Zhang, 2018). Exogenous Ca2+ was also found to change the quantity of different forms of calcium such as water-soluble organic calcium, calcium pectinate, and calcium phosphate in Brassica napus against the acid rain stress (Cong, 2018). Also, while being evaluated on six forest tree species, exogenous Ca2+ was able to reduce the negative effects of acid rain stress imposed on the seed germination, seedling growth, leaf chlorophyll content, and plant photosynthesis (Liu et al., 2011).
Some perspectives on future research
As we have discussed above, draught, flooding, salt, high and low temperature, heavy metals and acid rain are seven commonly encountered abiotic stresses and the plant responses to those stresses are somehow related to one or several structural, physiological, biochemical and/or molecular mechanisms pertaining to the plant tolerance. With this review, we have gained sufficient understanding of basic and various underlying mechanisms that are involved in plant stress tolerance through using exogenous Ca2+, however, more and thorough researches should focus on its mitigating effect and its associated tolerant genes and use them in crop breeding for more resilient varieties and cultivars against extreme abiotic conditions. Also, all abiotic stresses are variables and their impact on plant growth are different and difficult to predict, so plants would adjust themselves constantly to adapt those fluctuations, indicating the quantity, method and timing of using different type of exogenous Ca2+ can be critically important in maximizing the Ca2+ efficacy.
Mechanisms of the role of exogenous Ca2+ in plant resistance against abiotic stresses
Stabilization of cell wall and membranes
Stresses imposed on plants such as salinity, high temperature, low temperature, and drought tend to induce more reactive oxygen species (ROS) that cause a peroxidation of cell walls and membranes and change the membrane permeability, resulting in an osmotic disturbance. While being applied, exogenous Ca2+ mainly reduced the ion leakage (Min et al., 2021), replenished the lost Ca2+, induced the synthesis of osmoregulatory substances (Hu et al., 2012; Naeem et al., 2020), increased antioxidant enzyme activities such as superoxide dismutase (SOD), peroxidase (POD), and catalase (CAT) et al., (Upadhyaya et al., 2011), and promoted biosynthesis of glutathione (GSH), ascorbate, tocopherols, and other non-enzymatic antioxidants (Ashraf, 2009; Elkelish et al., 2019). All these biological and physiological responses, secondary metabolites and pertaining enzymes have proven to maintain the stability and integrity of plant cell walls and membranes. For example, exogenous Ca2+ would facilitate the accumulation of gamma-aminobutyric acid (GABA) and free polyamines (PAs) to alleviate cell membrane damage (Yin et al., 2015), mitigate the decline of unsaturated lipids incorporated in cell membranes through producing and supplying more unsaturated fatty acids as for retaining membrane fluidity (Liang et al., 2021), and combine with phosphate, organic phosphorus, and carboxyl groups of proteins on the cell surface to stabilize the cell membrane structure and maintain cell integrity (Hu et al., 2018).
Regulation of the Na+/K+ ratio
Both Ca2+ and K+ are ions necessary in plants for the protein synthesis, antioxidant enzyme activity, and maintenance of plasma membranes and cell walls (Assaha et al., 2017) and it has been consented that the Na+/K+ ratio is mainly regulated in response to salt stress and the key factor for plants to tolerate salt stress is to keep the Na+/K+ ratio low (Shabala and Pottosin, 2014). Ca2+ incorporated in the plasma membrane in plants under a salt stress is replaced by a large amount of Na+ that are usually available due to an excessive NaCl influx, leading to an increase in cell membrane permeability and causing an intracellular K+ extravasation, a high Na+/K+ ratio, and an insalubrious ionic balance (Cabot et al., 2014). An administration of exogenous Ca2+ cannot only control the Na+ entry into plants through non-selective cation channel (NSCC), reduce K+ efflux or leakage through both NSCC and the guard cell outward rectifying potassium channels (GORK), but also increase antioxidant enzyme activities and quantity of osmoregulatory substances to reduce the ROS accumulation that opens NSCC for ion leakage and keeps the ion homeostasis balanced in stressed plants (Rahman et al., 2016). In addition, a recent research has indicated that the salt overly sensitive (SOS) pathway was initiated by the enhanced Ca2+ signals that were stimulated by exogenous Ca2+, which promoted a Na+ efflux and more K+ uptakes through the SOS pathway in wheat (Gao et al., 2021).
Regulation of hormone levels in plants
Plant growth, development and reproduction are basically regulated by endogenous hormones and their fluctuations in plant can be influenced by and responded to changes of environment conditions (Mesejo et al., 2013). Ca2+ censors include calmodulins (CaMs), CaM-like proteins (CMLs), calcineurin B-like proteins (CBLs), and CDPKs. The Ca2+ sensor is an initial stress signal detector as well as a regulator of major plant hormone signals (Ku et al., 2018). Ca2+ is reportedly involved in the ABA-induced stomatal closure process (Liu H et al., 2022), with which ABA is participated in the initiation and release of Ca2+. In addition, both Ca2+ and ABA regulating kinases target the same metabolic pathway (Edel and Kudla, 2016) through regulating the biosynthesis and signal transmission of jasmonates (JAs) that subsequently adjust the Ca2+ level, inducing an influx of extracellular Ca2+, and temporarily increase its concentration in the nucleo plasma. So, Ca2+ signal is regarded as the most important messenger in the signal cascade (Wang X et al., 2019). Ca2+ can control the transport rate of Indole-3-acetic acid (IAA) and switch the direction of IAA flow to effectively amply Ca2+ signaling for activation of cation pumps in the plasma membrane, promotion of Ca2+ influx and K+ efflux, and induction of root gravity by interacting with IAA (Vanneste and Friml, 2013). However, the Ca2+ molecular basis of mechanisms involved in the signaling, Jas pathway, IAA biosynthesis are still poorly understood. Under adverse conditions, exogenous Ca2+ proved to alleviate potentially damaging effects caused by the stress on plant growth and development through minimizing the ABA amount and increasing the production of other hormones (e.g., IAA, GA, CTK, etc.) to enhance plant resilience under stress (Liu et al., 2017; Kamran et al., 2021; Wu et al., 2022). At present, fewer studies of exogenous calcium on its effect on changes of a variety of plant hormones have been reported but mainly focused on ABA fluctuations and their associated impacts.
Maintenance of photosynthesis
Plants needs the chlorophyll as an life-supporting pigment for photosynthesis, and its quantity in plant leaves directly affects the photosynthetic capability to produce carbohydrates (Wu et al., 2019). Adversary stresses tend to impair the chloroplasts and cause a decrease in the chlorophyll quantity. Exogenous Ca2+ could prevent or minimize chlorophyll breakdowns, keep chloroplasts intact under stresses, and maintains a sufficient number of photosynthesis pertaining pigments and organelles in in leaves (Min et al., 2021; Wang et al., 2022). Ca2+ plays an important role in plant stomatal regulation as the second messenger in coupled with external signals in plant cells. Appropriate amount of Ca2+ can make plants adapt to abiotic stresses such as drought and salinity quickly by adjusting their stomatal opening/closing, optimizing their gas exchange, and improving their photosynthetic efficiency (Li et al., 2012; Li et al., 2022a). These plant adjustments and adaptations by using exogenous Ca2+ could be achieved through reshape, rearrangement and configuration of stomata during their differentiation and development for more efficient of gas exchange and water utilization (Zhang et al., 2019a). Moreover, adding exogenous Ca2+ to increase Ca2+ level improved the lutein cycle (Yang et al., 2013), mitigated the adversary effects on the photosystem II (PSII) inhibition, and preserved enzyme activities, reduced accumulations of carbohydrate, and uphold a normal plant photosynthesis (Tan et al., 2011).
Regulation of plant respiratory metabolism and improvement of root activities
Flooding causes anoxia of plant roots and weakens the respiratory metabolism. The stress due to flooding-initiated lack of oxygen can be lessened by the application of exogenous Ca2+ to improve the catalytic capacity of pentose phosphate and produce more ATP and NADPH to provide more energy for the plant respiratory metabolism (Yang et al., 2016), to promote the activity and accumulation of mitochondrial antioxidant enzymes relating to the glycolysis and tricarboxylic acid cycle (He et al., 2015), and to reduce the content of acetic acid, acetaldehyde and the activity of LDH for less lactic acid metabolism (Fan, 2019). Exogenous Ca2+ also proved to facilitate absorption of nitrate and accelerate its conversion into amino acids, heat stable proteins or polyamines to survive from hypoxia (Gao et al., 2011). In addition, some studies on plant salt stress also pointed out that exogenous Ca2+ can improve the root vitality by reducing the relative electrolyte leakage of the root, thus to improve the flooding tolerance of foxtail millets (Han et al., 2019). According to the summary of current literature, this mechanism mainly plays an active role under flooding stress, and whether it can also be activated under other stresses is unclear.
Induction of gene expressions and protein transcriptions for the stress resistance
While under the abiotic stress, the molecular mechanisms involved in plant stress tolerance are more complex and multi-layered, including stress sensing, responsive signaling, gene transcription, protein translation, and post-translational protein modification. Under various abiotic stresses, exogenous Ca2+ induces or activates a series of gene expressions and tolerant protein transcriptions to adjust and adapt to adversities accordingly. These research advances in that regards include but are not limited on: 1) upregulating the expression of antioxidant enzyme-related genes such as EnAPX, EnCAT2, EnGPX and stress-related genes to improve cold resistance of Elymus nutans (La-mu et al. 2021); 2) promoting the synthesis of plant proteins and preventing proteins from degradation through boosted activities of nucleoside diphosphate kinase (NDPK) and antioxidant enzymes and reduced expressions of heavy metal-related structural domain proteins such as PCR1, HMA2 and HMA4l (Zeng et al., 2017); 3) cutting down the Cd uptake of plants and promoting the Ca2+ internal mobility (Zeng et al., 2017); 4) stimulating ACO-1, ADH-1, CAT-2, and PK gene expression to alleviate the damage of pepper plants under flooding stress (Ou et al., 2017), and 5) inducing the expression of photosynthetic genes and stabilizing photosynthetic membrane proteins in leaves (Zhang et al., 2014). Due to the differences of gene pools in different plant species and the difficulties in monitoring those genes, the information we currently have on plant genomes is still limited, focusing only on detecting gene expressions and reflecting Ca2+ associated genetic changes after application of exogenous Ca2+, but how exactly these adjustments are induced, operated and regulated remain to be explored. In addition, how exogenous Ca2+ transduces Ca2+ signaling pathway in plants has not been determined yet.
Summary and outlook
With an extensive review of over one hundred research papers, we have sorted them according to their major mechanisms into six categories associated with the plant membranes, Na+ vs. K+ ratios, hormone regulation, gene expression and protein transcription, and photosynthesis. However, we tend to believe that this type of grouping is arbitrary, simplified and nonscientific only for the purpose of easy access and preliminary understanding of a particular aspect of main functions of exogenous Ca2+ that may have alleviated a certain type of plant stresses. The mechanisms involved in mitigating plant abiotic stresses through application of exogenous Ca2+ and their reported interrelationships are proposed and demonstrated (Figure 2) and we strongly suggest that they be perfected and completed with more fundamental information and advanced findings are available.
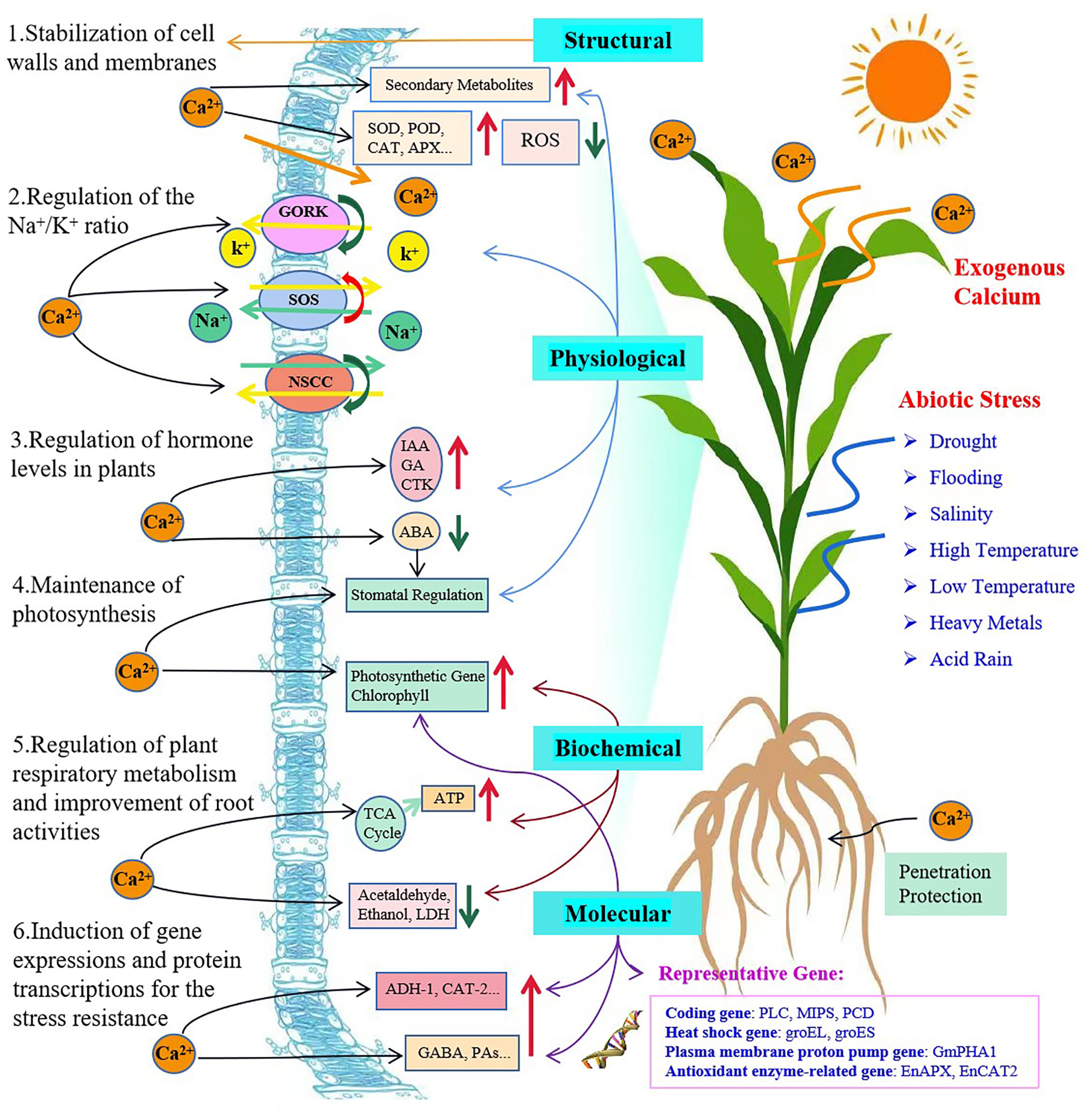
Figure 2 Plant self-responses to abiotic stresses and mechanisms of exogenous calcium involved in enhancement of plant stress tolerance. The red and green arrows indicate a promotion/increase or an inhibition/decrease, respectively.
In the process of reviewing all relevant literature, we have found that most of the studies only explored one or a few aspects of stressed plants in response to exogenous Ca2+ added to alleviate a stress, but the application of exogenous Ca2+ may have a potential to affect multiple structural, biological and physiological functions or metabolic pathways at the plant cellular level to defend plants from various stresses. Likewise, exogenous Ca2+ could be used with other exogenous substances to enhance plant defensiveness against one or multiple stresses that are related or associated with each other to intensify the adversary impact, such as the stress from drought, high temperature and high salinity since they are somehow correlated. Other studies have shown that exogenous Ca2+ is more effective when combined with other exogenous substances, which should be our research directions and objectives for our endeavor in using exogenous Ca2+ in the near future (Vafadar et al., 2020; Valivand and Amooaghaie, 2021). Therefore, more and more well-designed experiments to unearth the true underline mechanisms of exogenous Ca2+ in mitigating multiply correlated plant abiotic stresses are expected and the results derived from them should significantly help us understand how to effectively use of exogenous Ca2+.
It has come to a consensus that exogenous Ca2+ can be used to alleviate various abiotic stresses on plants through an application of leaf spray, hydroponics, seed dipping, drenching, and soil application. To avoid possible interference of NO3- and other nutrient anions to the experimental results, most of the existing studies on exogenous Ca2+ used CaCl2 as the source calcium for a foliage spraying or through hydroponics. However, with the studies of the heavy metal stress, exogenous Ca2+ such as Ca(OH)2 was mostly used in the form of mixing it into the medium or soil (Zu et al., 2020) to increase soil pH, reduced the toxicity of heavy metals, and boost the Ca2+ quantity both in soil and plants. In addition, different calcium anions also affect plant growth. For example, the application of exogenous CaCl2 on chloride-phobic cowpea plants under stress could cause the accumulation of Cl- in roots and affect the normal growth (Guimarães et al., 2011), which in turn interferes with a positive mitigating effect of Ca2+. Therefore, the research in the future should be needed as well to investigate the type of exogenous Ca2+ that is suitable for the growth and development of a specific crop with an attention to determine the amount of the usage based on the type and extent of adversary stresses.
At present, majority of the experimental studies have been carried out indoors and mainly on the plant seed germination or seedlings, however, we believe that the best way to evaluate the effect of exogenous Ca2+ for a practical and feasible application should be carried out in an actual crop production site to determine its mode of action, actual concentration and optimal time for application, etc., or if priming of plant seedlings for their fortified and prolonged tolerance against abiotic stresses really words in field trials.
In summary of most recent advances on exogenous Ca2+ applications to alleviate various plant stresses, some questions still remain unanswered in terms of: 1) how Ca2+ is further transported and translocated after it enters a plant; 2) how efficiently Ca2+ is actually utilized to function as a mitigating factor; 3) in which way the Ca2+mobility can be improved; 4) how to use modern molecular assays to reveal detailed and Ca2+-induced mechanisms pertaining to the plant tolerance against abiotic stresses; 5) what are the interactions between different biological and physiological mechanisms that are all modulated by gene expressions and protein transcriptions at the molecular level; and 6) to what degree each of the abiotic stresses causes an irreversible and permanent damage. To address those challenging questions, the modern molecular techniques and more sophisticated analytic instruments such as an fluorescence tracing technique and a laser scanning confocal microscopy analysis technique (He et al., 2015) should be used for a quantitatively and qualitatively detection of a series of changes in signaling and gene expression induced by exogenous Ca2+. With this review, we have sorted a series of complex physiological and biochemical responses and their underline mechanisms that were reported recently, but much more deserve further exploration by researchers to develop a low-cost and effective way to combat all kinds of stresses though using exogenous Ca2+.
Author contributions
Conceptualization: DF. References analysis: XW. Funding acquisition: DF. Methodology: DF, XW. Validation: DF, XS. Writing–original draft: XW, DF, JG. Writing–review & editing: XS, CZ, HL, PL. All authors contributed to the article and approved the submitted version.
Funding
This study was supported by the Natural Science Foundation of Shandong Province (No. ZR2021ME154).
Acknowledgments
We thank Nankai University and Tianjin Tianlong Technology Co., Ltd. for their joint effort in training of DF with a postdoctoral position. Our appreciation is also extended to PL with the College of Art, Weifang University of Science and Technology for her art work in completion and perfection of all figures in the manuscript.
Conflict of interest
The authors declare that the research was conducted in the absence of any commercial or financial relationships that could be construed as a potential conflict of interest.
Publisher’s note
All claims expressed in this article are solely those of the authors and do not necessarily represent those of their affiliated organizations, or those of the publisher, the editors and the reviewers. Any product that may be evaluated in this article, or claim that may be made by its manufacturer, is not guaranteed or endorsed by the publisher.
References
Abbas, M., Abdel-Lattif, H., Shahba, M. (2021). Ameliorative effects of calcium sprays on yield and grain nutritional composition of maize (Zea mays l.) cultivars under drought stress. Agriculture 11 (4), 285. doi: 10.3390/agriculture11040285
AbdElgawad, H., Zinta, G., Hegab, M. M., Pandey, R., Asard, H., Abuelsoud, W. (2016). High salinity induces different oxidative stress and antioxidant responses in maize seedlings organs. Front. Plant Sci. 7. doi: 10.3389/fpls.2016.00276
Ahmad, P., Sarwat, M., Bhat, N. A., Wani, M. R., Kazi, A. G., Tran, L.-S. P. (2015). Alleviation of cadmium toxicity in Brassica juncea L.(Czern. & coss.) by calcium application involves various physiological and biochemical strategies. PloS One 10 (1), e0114571. doi: 10.1371/journal.pone.0114571
Ashraf, M. (2009). Biotechnological approach of improving plant salt tolerance using antioxidants as markers. Biotechnol. Adv. 27 (1), 84–93. doi: 10.1016/j.biotechadv.2008.09.003
Assaha, D. V. M., Ueda, A., Saneoka, H., Al-Yahyai, R., Yaish, M. W. (2017). The role of na+ and k+ transporters in salt stress adaptation in glycophytes. Front. Physiol. 8. doi: 10.3389/fphys.2017.00509
Ayyaz, A., Fang, R., Ma, J., Hannan, F., Huang, Q., Sun, Y., et al. (2022). Calcium nanoparticles (Ca-NPs) improve drought stress tolerance in Brassica napus by modulating the photosystem II, nutrient acquisition and antioxidant performance. NanoImpact 28, 100423. doi: 10.1016/j.impact.2022.100423
Besson-Bard, A., Courtois, C., Gauthier, A., Dahan, J., Dobrowolska, G., Jeandroz, S., et al. (2008). Nitric oxide in plants: production and cross-talk with Ca2+ signaling. Mol. Plant 1, 218–228. doi: 10.1093/mp/ssm016
Bhardwaj, K., Singh, P., Raina, M., Sharma, V., Kumar, D. (2018). Exogenous application of calcium chloride in wheat genotypes alleviates negative effect of drought stress by modulating antioxidant machinery and enhanced osmolyte accumulation. In Vitro Cell. Dev. Biology-Plant 54 (5), 495–507. doi: 10.1007/s11627-018-9912-3
Bhatia, S., Asthir, B. (2014). Calcium mitigates heat stress effect in wheat seeding growth by altering carbohydrate metabolism. Indian J. Plant Physiol. 19 (2), 138–143. doi: 10.1007/s40502-014-0087-6
Birgin, Ö., Akhoundnejad, Y., Dasgan, H. Y. (2021). The effect of foliar calcium application in tomato (Solanum lycopersicum l.) under drought stress in greenhouse conditions. Appl. Ecol. Environ. Res. 19 (4), 2971–2982. doi: 10.15666/aeer/1904_29712982
Cabot, C., Sibole, J. V., Barceló, J., Poschenrieder, C. (2014). Lessons from crop plants struggling with salinity. Plant Sci. 226, 2–13. doi: 10.1016/j.plantsci.2014.04.013
Chen, Q., Lu, X., Guo, X., Pan, Y., Yu, B., Tang, Z., et al. (2018). Differential responses to cd stress induced by exogenous application of Cu, zn or Ca in the medicinal plant Catharanthus roseus. Ecotoxicology Environ. Saf. 157, 266–275. doi: 10.1016/j.ecoenv.2018.03.055
Cong, J. (2018). The impacts of different forms of calcium on physiological characters of brassica chinensis l. under the acid rain stress (Jilin: Jilin University) (Master's thesis).
D’souza, M. R., Devaraj, V. R. (2013). Role of calcium in increasing tolerance of hyacinth bean to salinity. J. Appl. Biol. Biotechnol. 1 (3), 11–20. doi: 10.7324/JABB.2013.1302
Edel, K., Kudla, J. (2016). Integration of calcium and ABA signaling. Curr. Opin. Plant Biol. 33, 83–91. doi: 10.1016/j.pbi.2016.06.010
Elkelish, A. A., Alnusaire, T. S., Soliman, M. H., Gowayed, S., Senousy, H. H., Fahad, S. (2019). Calcium availability regulates antioxidant system, physio-biochemical activities and alleviates salinity stress mediated oxidative damage in soybean seedlings. J. Appl. Bot. Food Qual 92, 258–266. doi: 10.5073/JABFQ.2019.092.036
Fan, G. (2019). Effects of exogenous calcium on respiration and metabolism between two different resistant paeonia suffruticosa varieties under waterlogging stress. Northern Horticulture 10), 80–85. doi: 10.11937/bfyy.20183200
Feng, D., Gao, Q., Liu, J., Tang, J., Hua, Z., Sun, X. (2023). Categories of exogenous substances and their effect on alleviation of plant salt stress. Eur. J. Agron. 142, 126656. doi: 10.1016/j.eja.2022.126656
Feng, N., Yu, M., Li, Y., Jin, D., Zheng, D. (2021). Prohexadione-calcium alleviates saline-alkali stress in soybean seedlings by improving the photosynthesis and up-regulating antioxidant defense. Ecotoxicology Environ. Saf. 220, 112369. doi: 10.1016/j.ecoenv.2021.112369
Gao, Q., Feng, D., Liu, J., Zhang, J. M., Han, Q. S. (2021). Main mechanisms and classification of exogenous substances alleviating plant salt stress. J. Plant Nutr. Fertilizers 27 (11), 2030–2044. doi: 10.11674/zwyf.2021155
Gao, H., Jia, Y., Guo, S., Lv, G., Wang, T., Juan, L. (2011). Exogenous calcium affects nitrogen metabolism in root-zone hypoxia-stressed muskmelon roots and enhances short-term hypoxia tolerance. J. Plant Physiol. 168 (11), 1217–1225. doi: 10.1016/j.jplph.2011.01.022
Gong, W., Wang, B. C. (2011). The role of Ca2+ in plant response to abiotic stress. Chem. Life 31, 107–111. doi: 10.13488/j.smhx.2011.01.005
Goswami, S., Kumar, R. R., Sharma, S. K., Kala, Y. K., Singh, K., Gupta, R., et al. (2015). Calcium triggers protein kinases-induced signal transduction for augmenting the thermotolerance of developing wheat (Triticum aestivum) grain under the heat stress. J. Plant Biochem. Biotechnol. 24 (4), 441–452. doi: 10.1007/s13562-014-0295-1
Guimarães, F. V. A., de Lacerda, C. F., Marques, E. C., de Miranda, M. R. A., de Abreu, C. E. B., Prisco, J. T., et al. (2011). Calcium can moderate changes on membrane structure and lipid composition in cowpea plants under salt stress. Plant Growth Regul. 65 (1), 55–63. doi: 10.1007/s10725-011-9574-1
Guo, Y., Liu, Y., Zhang, Y., Liu, J., Gul, Z., Guo, X. R., et al. (2021). Effects of exogenous calcium on adaptive growth, photosynthesis, ion homeostasis and phenolics of gleditsia sinensis lam. plants under salt stress. Agriculture 11 (10), 978. doi: 10.3390/agriculture11100978
Han, F., Sun, M., He, W., Cui, X., Pan, H., Wang, H., et al. (2019). Ameliorating effects of exogenous Ca2+ on foxtail millet seedlings under salt stress. Funct. Plant Biol. 46 (5), 407–416. doi: 10.1071/FP18314
He, L., Li, B., Lu, X., Yuan, L., Yang, Y., Yuan, Y., et al. (2015). The effect of exogenous calcium on mitochondria, respiratory metabolism enzymes and ion transport in cucumber roots under hypoxia. Sci. Rep. 5 (1), 1–14. doi: 10.1038/srep11391
He, L., Lu, X., Tian, J., Yang, Y., Li, B., Li, J., et al. (2012). Proteomic analysis of the effects of exogenous calcium on hypoxic-responsive proteins in cucumber roots. Proteome Sci. 10 (1), 1–15. doi: 10.1186/1477-5956-10-42
He, W., Wang, Y., Luo, H., Li, D., Liu, C., Song, J., et al. (2020). Effect of NaCl stress and supplemental CaCl2 on carotenoid accumulation in germinated yellow maize kernels. Food Chem. 309, 125779. doi: 10.1016/j.foodchem.2019.125779
He, L., Yu, L., Li, B., Du, N., Guo, S. (2018). The effect of exogenous calcium on cucumber fruit quality, photosynthesis, chlorophyll fluorescence, and fast chlorophyll fluorescence during the fruiting period under hypoxic stress. BMC Plant Biol. 18 (1), 1–10. doi: 10.1186/s12870-018-1393-3
Heidari, M., Amirfazli, N., Ghorbani, H., Zafarian, F. (2019). Calcium chloride and drought stress changed grain yield and physiological traits in sesame. Scientia agriculturae bohemica 50 (4), 211–218. doi: 10.2478/sab-2019-0029
Henry, E. E. Y., Sossa, E., Noumavo, A. P., Amadji, G., Baba-Moussa, L., Gandonou, C. B. (2021). Ions and organic solutes as implicated in the ameliorative effect of exogenous application of calcium on salt stressed tomato (Lycopersicon esculentum mill.) plants. Int. J. Plant Soil Sci. 33 (18), 200–212. doi: 10.9734/IJPSS/2021/v33i1830590
Hu, M., Shi, Z., Zhang, Z., Zhang, Y., Li, H. (2012). Effects of exogenous glucose on seed germination and antioxidant capacity in wheat seedlings under salt stress. Plant Growth Regul. 68 (2), 177–188. doi: 10.1007/s10725-012-9705-3
Hu, W., Tian, S. B., Di, Q., Duan, S. H., Dai, K. (2018). Effects of exogenous calcium on mesophyll cell ultrastructure, gas exchange, and photosystem II in tobacco (Nicotiana tabacum linn.) under drought stress. Photosynthetica 56 (4), 1204–1211. doi: 10.1007/s11099-018-0822-8
Iqbal, M. S., Singh, A. K., Ansari, M. I. (2020). “Effect of drought stress on crop production,” in New frontiers in stress management for durable agriculture. Eds. Rakshit, A., Singh, H., Singh, A., Singh, U., Fraceto, L. (Singapore: Springer), 35–47.
Issam, N., Kawther, M., Haythem, M., Moez, J. (2012). Effects of CaCl2 pretreatment on antioxidant enzyme and leaf lipid content of faba bean (Vicia faba l.) seedlings under cadmium stress. Plant Growth Regul. 68 (1), 37–47. doi: 10.1007/s10725-012-9691-5
Jiang, S., Liu, Y., Shu, Y. (2022). Biochar and exogenous calcium assisted alleviation of Pb phytotoxicity in water spinach (Ipomoea aquatica forsk) cultivated in Pb-spiked soil. Environ. Geochemistry Health 44 (1), 207–219. doi: 10.1007/s10653-021-00977-0
Jin, J., Cui, H., Lv, X., Yang, Y., Wang, Y., Lu, X. (2017). Exogenous CaCl2 reduces salt stress in sour jujube by reducing na+ and increasing k+, Ca2+, and Mg2+ in different plant organs. J. Hortic. Sci. Biotechnol. 92 (1), 98–106. doi: 10.1080/14620316.2016.1228435
Kaczmarek, M., Fedorowicz-Strońska, O., Głowacka, K., Waśkiewicz, A., Sadowski, J. (2017). CaCl2 treatment improves drought stress tolerance in barley (Hordeum vulgare l.). Acta Physiologiae Plantarum 39 (1), 1–11. doi: 10.1007/s11738-016-2336-y
Kamran, M., Wang, D., Xie, K., Lu, Y., Shi, C., Sabagh, A. E. L., et al. (2021). Pre-sowing seed treatment with kinetin and calcium mitigates salt induced inhibition of seed germination and seedling growth of choysum (Brassica rapa var. parachinensis). Ecotoxicology Environ. Saf. 227, 112921. doi: 10.1016/j.ecoenv.2021.112921
Khan, A., Anwar, Y., Hasan, M. M., Iqbal, A., Ali, M., Alharby, H. F., et al. (2017). Attenuation of drought stress in Brassica seedlings with exogenous application of Ca2+ and H2O2. Plants 6 (2), 20. doi: 10.3390/plants6020020
Khani, A., Barzegar, T., Nikbakht, J., Ghahremani, Z. (2020). Effect of foliar spray of calcium lactate on the growth, yield and biochemical attribute of lettuce (Lactuca sativa l.) under water deficit stress. Adv. Hortic. Sci. 34 (1), 11–24. doi: 10.13128/ahsc8252
Khursheda, P., Ahamed, K. U., Islam, M. M., Haque, M. N. (2015). Response of tomato plant under salt stress: role of exogenous calcium. J. Plant Sci. 10 (6), 222–233. doi: 10.3923/jps.2015.222.233
Kováčik, J., Dresler, S. (2018). Calcium availability but not its content modulates metal toxicity in Scenedesmus quadricauda. Ecotoxicology Environ. Saf. 147, 664–669. doi: 10.1016/j.ecoenv.2017.09.022
Ku, Y., Sintaha, M., Cheung, M., Lam, H. (2018). Plant hormone signaling crosstalks between biotic and abiotic stress responses. Int. J. Mol. Sci. 19, 3206. doi: 10.3390/ijms19103206
La-mu, Q., Zhuo-ma, P., Cui, T., Zhang, X., Jiang, H., Fu, J., et al. (2021). Effects of exogenous calcium on physiology and related gene expression of elymus nutans under cold stress. Acta Agrestia Sin. 29, 919–928. doi: 10.11733/j.issn.1007-0435.2021.05.008
Li, Q., Cao, J., Yu, L., Li, M., Liao, J., Gan, L. (2012). Effects on physiological characteristics of honeysuckle (Lonicera japonica thunb) and the role of exogenous calcium under drought stress. Plant Omics 5 (1), 1–5.
Li, H., Huang, S., Ren, C., Weng, X., Zhang, S., Liu, L., et al. (2022a). Optimal exogenous calcium alleviates the damage of snow-melting agent to Salix matsudana seedlings. Front. Plant Sci. 13. doi: 10.3389/fpls.2022.928092
Li, H., Huo, Y., Weng, X., Zhou, Y., Sun, Y., Zhang, G., et al. (2022b). Regulation of the growth of Mongolian pine (Pinus sylvestris var. mongolica) by calcium-water coupling in a semiarid region. Ecol. Indic. 137, 108736. doi: 10.1016/j.ecolind.2022.108736
Li, Z., Mei, X., Li, T., Yang, S., Qin, L., Li, B., et al. (2021). Effects of calcium application on activities of membrane transporters in Panax notoginseng under cadmium stress. Chemosphere 262, 127905. doi: 10.1016/j.chemosphere.2020.127905
Li, M., Wu, X., Xia, J., Xing, Q., Lin, H., Lin, Z., et al. (2022c). Transcriptome analysis of calcium chloride-treated leaves of Pennisetum giganteum under salt stress. Mol. Plant Breed 1-22[2023-03-14]. Available at: http://kns.cnki.net/kcms/detail/46.1068.s.20220521.1737.002.html.
Liang, C., Zhang, B. (2018). Effect of exogenous calcium on growth, nutrients uptake and plasma membrane h+-ATPase and Ca2+-ATPase activities in soybean (Glycine max) seedlings under simulated acid rain stress. Ecotoxicology Environ. Saf. 165, 261–269. doi: 10.1016/j.ecoenv.2018.09.019
Liang, C., Zhang, Y., Ren, X. (2021). Calcium regulates antioxidative isozyme activity for enhancing rice adaption to acid rain stress. Plant Sci. 306, 110876. doi: 10.1016/j.plantsci.2021.110876
Liu, Y. F., Han, X. R., Zhan, X. M., Yang, J. F., Wang, Y. Z., Song, Q. B., et al. (2013). Regulation of calcium on peanut photosynthesis under low night temperature stress. J. Integr. Agric. 12 (12), 2172–2178. doi: 10.1016/S2095-3119(13)60411-6
Liu, G. H., Lan, J. B., Liu, Y. Q., Zhu, J., Zheng, C. H. (2017). Effects of exogenous calcium on seedling physiological characteristics and endogenous hormone of Eucalyptus urophylla ×E. grandis under low temperature stress. J. Northwest Forestry Univ. 32 (6), 101–106. doi: 10.3969/j.issn.1001-7461.2017.06.15
Liu, H., Song, S., Zhang, H., Li, Y., Niu, L., Zhang, J., et al. (2022). Signaling transduction of ABA, ROS, and Ca2+ in plant stomatal closure in response to drought. Int. J. Mol. Sci. 23, 14824. doi: 10.3390/ijms232314824
Liu, J., Willick, I. R., Hiraki, H., Forand, A. D., Lawrence, J. R., Swerhone, G. D. W., et al. (2022). Cold and exogenous calcium alter Allium fistulosum cell wall pectin to depress intracellular freezing temperatures. J. Exp. Bot. 73 (11), 3807–3822. doi: 10.1093/jxb/erac108
Liu, T. W., Wu, F. H., Wang, W. H., Chen, J., Li, Z. J., Dong, X. J., et al. (2011). Effects of calcium on seed germination, seedling growth and photosynthesis of six forest tree species under simulated acid rain. Tree Physiol. 31 (4), 402–413. doi: 10.1093/treephys/tpr019
Liu, Z. B., Yang, B. Z., Ou, L. J., Zou, X. X. (2015b). The impact of different Ca2+ spraying period on alleviating pepper injury under the waterlogging stress. Acta Hortic. Sin. 42 (8), 1487–1494. doi: 10.16420/j.issn.0513-353x.2015-0068
Liu, Y. F., Zhang, G. X., Qi, M. F., Li, T. L. (2015a). Effects of calcium on photosynthesis, antioxidant system, and chloroplast ultrastructure in tomato leaves under low night temperature stress. J. Plant Growth Regul. 34 (2), 263–273. doi: 10.1007/s00344-014-9462-9
López-Climent, M. F., Arbona, V., Pérez-Clemente, R. M., Zandalinas, S. I., Gómez-Cadenas, A. (2014). Effect of cadmium and calcium treatments on phytochelatin and glutathione levels in citrus plants. Plant Biol. 16 (1), 79–87. doi: 10.1111/plb.12006
Manaa, A., Gharbi, E., Mimouni, H., Wasti, S., Aschi-Smiti, S., Lutts, S., et al. (2014). Simultaneous application of salicylic acid and calcium improves salt tolerance in two contrasting tomato (Solanum lycopersicum l.) cultivars. South Afr. J. Bot. 95, 32–39. doi: 10.1016/j.sajb.2014.07.015
Manaf, H. H., Ashour, H. M., El-Hamady, M. M. (2017). Impact of calcium chloride on resistance drought and blossom-end rot in sweet pepper plants (Capsicum annuum l.). Middle East J. Appl. Sci. 7 (2), 335–348.
Marcec, M. J., Gilroy, S., Poovaiah, B. W., Tanaka, K. (2019). Mutual interplay of Ca2+ and ROS signaling in plant immune response. Plant Sci. 283, 343–354. doi: 10.1016/j.plantsci.2019.03.004
Mesejo, C., Yuste, R., Martínez-Fuentes, A., Reig, C., Iglesias, D. J., Primo-Millo, E., et al. (2013). Self-pollination and parthenocarpic ability in developing ovaries of self-incompatible Clementine mandarins (Citrus clementina). Physiologia plantarum 148 (1), 87–96. doi: 10.1111/j.1399-3054.2012.01697.x
Min, K., Liu, B., Lee, S.-R., Arora, R. (2021). Supplemental calcium improves freezing tolerance of spinach (Spinacia oleracea l.) by mitigating membrane and photosynthetic damage, and bolstering anti-oxidant and cell-wall status. Scientia Hortic. 288, 110212. doi: 10.1016/j.scienta.2021.110212
Mulaudzi, T., Hendricks, K., Mabiya, T., Muthevhuli, M., Ajayi, R. F., Mayedwa, N., et al. (2020). Calcium improves germination and growth of Sorghum bicolor seedlings under salt stress. Plants 9 (6), 730. doi: 10.3390/plants9060730
Naeem, M., Naeem, M. S., Ahmad, R., Ahmad, R. (2017). Foliar-applied calcium induces drought stress tolerance in maize by manipulating osmolyte accumulation and antioxidative responses. Pak. J. Bot. 49 (2), 427–434.
Naeem, M., Traub, J. R., Athar, H.-u., Loescher, W. (2020). Exogenous calcium mitigates heat stress effects in common bean: a coordinated impact of photoprotection of PSII, up-regulating antioxidants, and carbohydrate metabolism. Acta Physiologiae Plantarum 42 (12), 1–13. doi: 10.1007/s11738-020-03171-4
Oh, M., Nanjo, Y., Komatsu, S. (2014). Gel-free proteomic analysis of soybean root proteins affected by calcium under flooding stress. Front. Plant Sci. 5. doi: 10.3389/fpls.2014.00559
Ou, L. J., Liu, Z. B., Zhang, Y. P., Zou, X. X. (2017). Effects of exogenous Ca2+ on photosynthetic characteristics and fruit quality of pepper under waterlogging stress. Chilean J. Agric. Res. 77 (2), 126–133. doi: 10.4067/S0718-58392017000200126
Pan, B. Q., Zhu, X. T., Pan, X. H., Dai, X. S., Fu, J., Lin, C. Y. (2018). Effects of exogenous calcium on physiological and biochemical characteristics of peony under waterlogging stress. J. Northwest Forestry Univ. 33 (1), 84–89. doi: 10.3969/j.issn.1001-7461.2018.01.14
Qadir, A., Khan, S. A., Ahmad, R., Masood, S., Irshad, M., Kaleem, F., et al. (2017). Exogenous Ca2SiO4 enrichment reduces the leaf apoplastic na+ and increases the growth of okra (Abelmoschus esculentus l.) under salt stress. Scientia Hortic. 214, 1–8. doi: 10.1016/j.scienta.2016.11.008
Qin, K., Fan, S., Zhang, F., Wang, Y. (2019). Effects of exogenous calcium on datura seed germination under drought stress. J. Botanical Res. 1 (2), 8–14. doi: 10.30564/jrb.v1i2.862
Rahman, A., Nahar, K., Hasanuzzaman, M., Fujita, M. (2016). Calcium supplementation improves Na+/K+ ratio, antioxidant defense and glyoxalase systems in salt-stressed rice seedlings. Front. Plant Sci. 7. doi: 10.3389/fpls.2016.00609
Roy, P. R., Tahjib-Ul-Arif, M., Polash, M. A. S., Hossen, M., Hossain, M. A. (2019). Physiological mechanisms of exogenous calcium on alleviating salinity-induced stress in rice (Oryza sativa l.). Physiol. Mol. Biol. Plants 25 (3), 611–624. doi: 10.1007/s12298-019-00654-8
Salahshoor, F., Kazemi, F. (2016). Effect of calcium on reducing salt stress in seed germination and early growth stage of Festuca ovina l. Plant Soil Environ. 62 (10), 460–466. doi: 10.17221/319/2016-PSE
Shabala, S., Pottosin, I. (2014). Regulation of potassium transport in plants under hostile conditions: implications for abiotic and biotic stress tolerance. Physiologia plantarum 151 (3), 257–279. doi: 10.1111/ppl.12165
Shabbir, R., Javed, T., Hussain, S., Ahmar, S., Naz, M., Zafar, H., et al. (2022). Calcium homeostasis and potential roles to combat environmental stresses in plants. South Afr. J. Bot. 148, 683–693. doi: 10.1016/j.sajb.2022.05.038
Shi, H., Ye, T., Zhong, B., Liu, X., Chan, Z. (2014a). Comparative proteomic and metabolomic analyses reveal mechanisms of improved cold stress tolerance in bermudagrass (Cynodon dactylon (L.) pers.) by exogenous calcium. J. Integr. Plant Biol. 56 (11), 1064–1079. doi: 10.1111/jipb.12167
Shi, H. P., Zhu, Y. F., Wang, Y. L., Tsang, P. K. E. (2014b). Effect of cadmium on cytogenetic toxicity in hairy roots of Wedelia trilobata l. and their alleviation by exogenous CaCl2. Environ. Sci. pollut. Res. 21 (2), 1436–1443. doi: 10.1007/s11356-013-2015-0
Shu, S., Guo, S. R., Sun, J., Yuan, L. Y. (2012). Effects of salt stress on the structure and function of the photosynthetic apparatus in Cucumis sativus and its protection by exogenous putrescine. Physiologia plantarum 146 (3), 285–296. doi: 10.1111/j.1399-3054.2012.01623.x
Song, Q., Liu, Y., Pang, J., Yong, J. W. H., Chen, Y., Bai, C., et al. (2020). Supplementary calcium restores peanut (Arachis hypogaea) growth and photosynthetic capacity under low nocturnal temperature. Front. Plant Sci. 10. doi: 10.3389/fpls.2019.01637
Sun, K. X., Yang, S., Guo, F., Liu, C. M., Meng, J. J., Hu, C. M., et al. (2015). Effects of exogenous calcium on photosynthetic chracteristics of sweet pepper (Capsicum fructescens l.) seedlings. Plant Physiol. J. 51 (3), 280–286. doi: 10.13592/j.cnki.ppj.2014.0448
Tan, W., Meng, Qw., Brestic, M., Olsovska, K., Yang, X. (2011). Photosynthesis is improved by exogenous calcium in heat-stressed tobacco plants. J. Plant Physiol. 168 (17), 2063–2071. doi: 10.1016/j.jplph.2011.06.009
Tanveer, K., Gilani, S., Hussain, Z., Ishaq, R., Adeel, M., Ilyas, N. (2020). Effect of salt stress on tomato plant and the role of calcium. J. Plant Nutr. 43 (1), 28–35. doi: 10.1080/01904167.2019.1659324
Tian, X., He, M., Wang, Z., Zhang, J., Song, Y., He, Z., et al. (2015). Application of nitric oxide and calcium nitrate enhances tolerance of wheat seedlings to salt stress. Plant Growth Regul. 77 (3), 343–356. doi: 10.1007/s10725-015-0069-3
Tiwari, A., Singh, P., Asthana, R. K. (2016). Role of calcium in the mitigation of heat stress in the cyanobacterium Anabaena PCC 7120. J. Plant Physiol. 199, 67–75. doi: 10.1016/j.jplph.2016.05.012
Upadhyaya, H., Panda, S. K., Dutta, B. K. (2011). CaCl2 improves post-drought recovery potential in Camellia sinensis (L) o. kuntze. Plant Cell Rep. 30 (4), 495–503. doi: 10.1007/s00299-010-0958-x
uz Zaman, Q., Abbasi, A., Tabassum, S., Ashraf, K., Ahmad, Z., Siddiqui, M. H., et al. (2022). Calcium induced growth, physio-biochemical, antioxidants, osmolytes adjustments and phytoconstituents status in spinach under heat stress. South Afr. J. Bot. 149, 701–711. doi: 10.1016/j.sajb.2022.06.065
Vafadar, F., Amooaghaie, R., Ehsanzadeh, P., Ghanadian, M., Talebi, M., Ghanati, F. (2020). Melatonin and calcium modulate the production of rosmarinic acid, luteolin, and apigenin in dracocephalum kotschyi under salinity stress. Phytochemistry 177, 112422. doi: 10.1016/j.phytochem.2020.112422
Valivand, M., Amooaghaie, R. (2021). Foliar spray with sodium hydrosulfide and calcium chloride advances dynamic of critical elements and efficiency of nitrogen metabolism in Cucurbita pepo l. under nickel stress. Scientia Hortic. 283, 110052. doi: 10.1016/j.scienta.2021.110052
Vanneste, S., Friml, J. (2013). Calcium: The missing link in auxin action. Plants 2, 650–675. doi: 10.3390/plants2040650
Wang, X., Lan, Z., Tian, L., Li, J., Yang, G., Gao, Y., et al. (2021). Change of physiological properties and ion distribution by synergistic effect of Ca2+ and grafting under salt stress on cucumber seedlings. Agronomy 11 (5), 848. doi: 10.3390/agronomy11050848
Wang, H., Shen, Y., Wang, K., He, S., Kim, W.-S., Shang, W., et al. (2022). Effect of exogenous calcium on the heat tolerance in Rosa hybrida ‘Carolla’. Horticulturae 8 (10), 980. doi: 10.3390/horticulturae8100980
Wang, M., Zhang, X., Li, Q., Chen, X., Li, X. (2019). Comparative transcriptome analysis to elucidate the enhanced thermotolerance of tea plants (Camellia sinensis) treated with exogenous calcium. Planta 249 (3), 775–786. doi: 10.1007/s00425-018-3039-y
Wang, X., Zhu, B., Jiang, Z., Wang, S. (2019). Review: Calcium-mediation of jasmonate biosynthesis and signaling in plants. Plant Sci. 287, 110192. doi: 10.1016/j.plantsci.2019.110192
Wu, J., Lian, W., Zeng, X., Liu, Z., Mao, L., Liu, Y., et al. (2019). Physiological response to high temperature and heat tolerance evaluation of different grape cultivars. Acta Botanica Boreali-Occidentalia Sin. 39 (6), 1075–1084. doi: 10.7606/j.issn.1000-4025.2019.06.1075
Wu, D., Liu, Y., Pang, J., Yong, J. W. H., Chen, Y., Bai, C., et al. (2020b). Exogenous calcium alleviates nocturnal chilling-induced feedback inhibition of photosynthesis by improving sink demand in peanut (Arachis hypogaea). Front. Plant Sci. 11. doi: 10.3389/fpls.2020.607029
Wu, Y., Liu, X., Wang, W., Zhang, S., Xu, B. (2012). Calcium regulates the cell-to-cell water flow pathway in maize roots during variable water conditions. Plant Physiol. Biochem. 58, 212–219. doi: 10.1016/j.plaphy.2012.07.005
Wu, B., Tang, H., Hu, L., Fan, R., Yang, J., Zhou, Y., et al. (2020a). Effects of exogenous Ca2+ on leaf physiological indexes in black pepper under low temperature stress. Chin. J. Trop. Crops 41 (2), 267–274. doi: 10.3969/j.issn.1000-2561.2020.02.009
Wu, H., Zhao, Y., Wang, H., Zhang, F., Zou, G., Yan, Y. (2022). Relieving effect of exogenous Ca2+ on salt stress injury to nitraria sibirica. Shandong Agric. Sci. 54 (8), 73–78. doi: 10.14083/j.issn.1001-4942.2022.08.011
Xu, C., Li, X., Zhang, L. (2013). The effect of calcium chloride on growth, photosynthesis, and antioxidant responses of Zoysia japonica under drought conditions. PloS One 8 (7), e68214. doi: 10.1371/journal.pone.0068214
Xue, L., Ren, H., Long, W., Leng, X., Wang, J., Yao, X., et al. (2018). Ecophysiological responses of calcicole Cyclobalanopsis glauca (Thunb.) oerst. to drought stress and calcium supply. Forests 9 (11), 667. doi: 10.3390/f9110667
Yang, B. Z., Liu, Z. B., Zhou, S. D., Ou, L. J., Dai, X. Z., Ma, Y. Q., et al. (2016). Exogenous Ca2+ alleviates waterlogging-caused damages to pepper. Photosynthetica 54 (4), 620–629. doi: 10.1007/s11099-016-0200-3
Yang, S., Wang, F., Guo, F., Meng, J.-J., Li, X.-G., Dong, S.-T., et al. (2013). Exogenous calcium alleviates photoinhibition of PSII by improving the xanthophyll cycle in peanut (Arachis hypogaea) leaves during heat stress under high irradiance. PloS One 8 (8), e71214. doi: 10.1371/journal.pone.0071214
Yang, H., Yang, Y. (2021). Effect of exogenous calcium and calcium inhibitors on the heat tolerance in phalaenopsis seedlings. Chin. J. Trop. Crops 42 (2), 436–441. doi: 10.3969/j.issn.1000-2561.2021.02.019
Yin, Y., Yang, R., Han, Y., Gu, Z. (2015). Comparative proteomic and physiological analyses reveal the protective effect of exogenous calcium on the germinating soybean response to salt stress. J. Proteomics 113, 110–126. doi: 10.1016/j.jprot.2014.09.023
Zehra, A., Gul, B., Ansari, R., Khan, M. A. (2012). Role of calcium in alleviating effect of salinity on germination of Phragmites karka seeds. South Afr. J. Bot. 78, 122–128. doi: 10.1016/j.sajb.2011.05.016
Zeng, L., Zhu, T., Gao, Y., Wang, Y., Ning, C., Björn, L. O., et al. (2017). Effects of Ca addition on the uptake, translocation, and distribution of cd in arabidopsis thaliana. Ecotoxicology Environ. Saf. 139, 228–237. doi: 10.1016/j.ecoenv.2017.01.023
Zhang, X. Y., Fang, Z. W., Liu, H. N., Zhao, D. Q., Tao, J. (2019b). Exogenous calcium-induced physiological and biochemical changes in tree peony (Paeonia section Moutan DC.) under drought stress. Photosynthetica 57, 904–911. doi: 10.32615/ps.2019.108
Zhang, G., Liu, Y., Ni, Y., Meng, Z., Lu, T., Li, T. (2014). Exogenous calcium alleviates low night temperature stress on the photosynthetic apparatus of tomato leaves. PloS One 9 (5), e97322. doi: 10.1371/journal.pone.0097322
Zhang, Q., Liu, Y., Yu, Q., Ma, Y., Gu, W., Yang, D. (2020). Physiological changes associated with enhanced cold resistance during maize (Zea mays) germination and seedling growth in response to exogenous calcium. Crop Pasture Sci. 71 (6), 529–538. doi: 10.1071/CP19510
Zhang, Y., Tian, C., Yu, T., Dayananda, B., Fu, B., Senaratne, S. L., et al. (2021). Differential effects of acid rain on photosynthetic performance and pigment composition of the critically endangered Acer amplum subsp. catalpifolium. Global Ecol. Conserv. 30, e01773. doi: 10.1016/j.gecco.2021.e01773
Zhang, H., Zheng, Y. P., Ye, J., Gao, W., Qiao, Y. J., Dai, J. C., et al. (2019a). Effects of exogenous Ca2+ on stomatal traits, photosynthesis and biomass of maize seedings under salt stress. Chin. J. Appl. Ecol. 30 (3), 923–930. doi: 10.13287/j.1001-9332.201903.020
Zhang, H., Zhu, J., Gong, Z., Zhu, J. K. (2022). Abiotic stress responses in plants. Nat. Rev. Genet. 23, 104–119. doi: 10.1038/s41576-021-00413-0
Zhou, W., Wang, H. (2007). The physiological and molecular mechanisms of calcium uptake, transport and metabolism in plants. Chin. Bull. Bot. 24 (6), 762–778.
Zorrig, W., Shahzad, Z., Abdelly, C., Berthomieu, P. (2012). Calcium enhances cadmium tolerance and decreases cadmium accumulation in lettuce (Lactuca sativa). Afr. J. Biotechnol. 11 (34), 8441–8448. doi: 10.5897/ajb11.2343
Zrig, A., AbdElgawad, H., Touneckti, T., Mohamed, H. B., Hamouda, F., Khemira, H. (2021). Potassium and calcium improve salt tolerance of thymus vulgaris by activating the antioxidant systems. Scientia Hortic. 277, 109812. doi: 10.1016/j.scienta.2020.109812
Keywords: abiotic stresses, exogenous calcium, Ca 2+, plant tolerance, plant respiratory metabolism
Citation: Feng D, Wang X, Gao J, Zhang C, Liu H, Liu P and Sun X (2023) Exogenous calcium: Its mechanisms and research advances involved in plant stress tolerance. Front. Plant Sci. 14:1143963. doi: 10.3389/fpls.2023.1143963
Received: 13 January 2023; Accepted: 09 March 2023;
Published: 21 March 2023.
Edited by:
Milan Kumar Lal, Central Potato Research Institute (ICAR), IndiaReviewed by:
Song Baiquan, Heilongjiang University, ChinaMohammad Saidur Rhaman, Bangladesh Agricultural University, Bangladesh
Copyright © 2023 Feng, Wang, Gao, Zhang, Liu, Liu and Sun. This is an open-access article distributed under the terms of the Creative Commons Attribution License (CC BY). The use, distribution or reproduction in other forums is permitted, provided the original author(s) and the copyright owner(s) are credited and that the original publication in this journal is cited, in accordance with accepted academic practice. No use, distribution or reproduction is permitted which does not comply with these terms.
*Correspondence: Xiaoan Sun, seannyx@outlook.com
†These authors have contributed equally to this work