- 1Department of Horticulture, Faculty of Agriculture, University of Maragheh, Maragheh, Iran
- 2Department of Food Analysis and Chemistry, Faculty of Technology, Tomas Bata University in Zlin, Zlin, Czechia
- 3Department of Plant Production and Genetics, Faculty of Agriculture, University of Maragheh, Maragheh, Iran
- 4Department of Horticulture, Faculty of Agriculture, Ataturk University, Erzurum, Türkiye
- 5HGF Agro, Ata Teknokent, Erzurum, Türkiye
Introduction: Drought stress unfavorably influences the growth and physiological traits of plants in the arid and semi-arid regions of the world. This study aimed to determine the effects of arbuscular mycorrhiza fungi (AMF; Funneliformis mosseae) inoculation on the physiological and biochemical responses of summer savory (Satureja hortensis L.) under different irrigation regimes.
Methods: The first factor was different irrigation regimes, including no drought stress (100% field capacity; FC), moderate drought stress (60% FC), and severe drought stress (30% FC); the second factor included the plants without AMF (AMF0) and with AMF inoculation (AMF1).
Results: The results showed that better values, higher plant height, shoot mass (fresh and dry weight), relative water content (RWC), membrane stability index (MSI), photosynthesis pigments, Fv, Fm, Fv/Fm, and total soluble proteins were obtained in the plants inoculated with AMF. The highest values were obtained for plants with no drought stress, then the plants subjected to AMF1 under 60% FC, and the lowest ones for plants under 30% FC without AMF inoculation. Thus, these properties are reduced under moderate and severe drought stress. At the same time, the utmost activity of superoxide dismutase (SOD), ascorbate peroxidase (APX), guaiacol peroxidase (GPX), and the highest malondialdehyde (MDA), H2O2, proline, and antioxidant activity (TAA) were achieved for 30% FC + AMF0. It was also found that AMF inoculation improved essential oil (EO) composition, also as EO obtained from plants under drought stress. Carvacrol (50.84-60.03%) was the dominant component in EO; γ-terpinene (19.03-27.33%), p-cymene, α-terpinene, and myrcene, were recognized as other important components in EO. The higher carvacrol and γ-terpinene contents were obtained from summer savory plants with AMF inoculation and the lowest for plants without AMF and under 30% FC.
Conclusion: According to the present findings, using AMF inoculation could be a sustainable and eco-friendly approach to improve physiological and biochemical characteristics and the essential oil quality of summer savory plants under water shortage conditions.
1 Introduction
Summer savory (Satureja hortensis L.) is an annual Lamiaceae family plant with aromatic qualities and several medicinal properties related to its antioxidant, antifungal, antibacterial, and antiseptic characteristics (Fierascu et al., 2018). The herb is tolerant to different weather conditions and based on previous findings, the summer savory plant can be introduced into agricultural systems as a valuable drought-tolerant medicinal plant, especially in areas with low rainfall and scarce water resources (Karimi et al., 2022). Therefore, farmers focused on expanding summer savory plant cultivation in water-scarce conditions, while protecting the natural biodiversity and adequate productivity (Jaleel et al., 2007).
Drought stress is one of the most important abiotic stresses that adversely affect the physiological and biochemical characteristics and the growth and development of plants in the arid and semi-arid regions of the world (Kafi et al., 2010). The impact of drought stress on the production of secondary metabolites is related to the plant’s physiological state during stress, leading to the accumulation of reactive oxygen species (ROS) in the plants (Dossa et al., 2017). Various stresses, including drought, have been shown to affect metabolic pathways in the Lamiaceae family that are highly effective in producing terpenes and phenolics (Shariat et al., 2016). Alhaithloul et al. (Alhaithloul et al., 2019) reported that drought stress caused an increase in the level of tannins, terpenoids, and alkaloids in mint plants. In sesame, an increase in the concentration of carotenoids under drought conditions has been reported (Yousefzadeh Najafabadi and Ehsanzadeh, 2017). To prevent or reduce ROS damage, plants promote enzymatic antioxidants such as ascorbate peroxidase (APX), superoxide dismutase (SOD), catalase (CAT), and peroxidase (POD), and non-enzymatic antioxidants as carotenoids, flavonoids, phenols, anthocyanins, and some free amino acids (Agarwal and Pandey, 2004; Hanin et al., 2016).
There are several strategies for bearing the drought stress for plants, such as the use of native microorganisms. The strategy could include beneficial microbes that directly or indirectly enhance tolerance mechanisms or appropriate mineral fertilization. In sustainable agricultural systems, using arbuscular mycorrhizae fungi (AMF), especially in poor soils, can help to increase crop productivity and improve soil quality (Kyriazopoulos et al., 2014). AMF is a valuable essential microorganism that grows in the soil and can establish a symbiotic relationship with nearly 90% of plant species, including bryophytes, ferns, and flowering plants (Ahanger et al., 2014; Begum et al., 2019). AMF symbiosis can cause better plant growth, more absorption of nutrients, more tolerance against abiotic and biotic stresses, and improvement of soil structure in the host plant (Wu et al., 2013). Studies (Wu et al., 2013; Balliu et al., 2015; Hazzoumi et al., 2015) have shown that plants colonized with AMF showed higher enzyme activity, mineral nutrition content, and photosynthesis potential.
AMF inoculation is a relevant method to stimulate plant growth and enhance essential oils biosynthesis in aromatic and medicinal plants (Rydlová et al., 2016). Hazzoumi et al. (Hazzoumi et al., 2015) reported that basil plants inoculated by mycorrhiza showed higher total chlorophyll content than non-inoculated plants. In lettuce, the application of AMF caused a significant increase in the growth and development of the plant (Asadi et al., 2022). It has also been reported that indigenous microorganisms of arid and semi-arid regions have the advantage of drought tolerance that can live and help host plants to withstand drought stress (Shirinbayan et al., 2019). Under drought stress, AMF inoculation improves plant growth by increasing root length, leaf area, plant biomass, nutrient uptake, and soil structure (Rabab et al., 2016). AMF hyphae penetrate the plant roots, grow extensively between the living cells of the root cortex, and form a substantial and dynamic relationship between the symbionts (plant and fungus). As a result, this colonization increases growth and yield and reduces the effects of stress (Mathur et al., 2018). Thus, AMF increases the absorption of phosphorus, zinc, copper, and potassium. Therefore, a symbiosis occurs between the plant and the fungus, which significantly improves the plant’s physiology and nutritional conditions and mitigates the adverse effects of drought stress (Augé, 2001; Wężowicz et al., 2017). Some other phenomena that are amplified by AMF include changes in plant hormone balance, water absorption through hydraulic conduction of external hyphae, osmotic regulation [25], increased antioxidant activity, increased nutrient absorption, and increased photosynthesis [26]. Previous studies have shown that the AMF-inoculated plants achieve increased essential oil (EO) quantity and quality in plants such as thyme (Thymus vulgaris L.) (Amani Machiani et al., 2021), peppermint (Mentha x piperita L.) (Ostadi et al., 2020), and holy basil (Ocimum tenuiflorum L.) (Thokchom et al., 2020).
The interaction of drought stress and AMF inoculation in terms of essential oil profile has not been previously investigated for summer savory, while these factors were studied separately on the summer savory. The current research is presented about the hypothesis that the morphological and physiological properties of summer savory plants and the content and composition of essential oil are affected by drought stress. We then hypothesize that AMF inoculation reduces the effects of drought stress. This study, therefore, aims to investigate the inoculation of AMF on ameliorating the effect of drought stress on the summer savory plant and also to evaluate the impact of AMF on some morphological and biochemical responses of the plant.
2 Materials and methods
The current research was conducted as a factorial experiment based on a completely randomized design (CRD) with four replications, so that each plant was considered as a replication. The experiment was performed in the research greenhouse of the Department of Horticultural Sciences of Maragheh University in East Azarbaijan province with the geographical coordinates of 37° 23′ north latitude and 46°16′ east longitude and an altitude of 1485 meters above sea level. The night and day temperatures were 17-23°C, respectively, and the relative humidity was about 65-75% in the greenhouse. The study was conducted as a factorial experiment based on the completely randomized design with five replications. The experimental treatments contained two factors; the first factor included three irrigation regimes, regular irrigation (100% filed capacity (FC)), moderate (60% FC), and severe (30% FC) drought stress; the second factor was the treatment without AMF (Funneliformis mosseae) and inoculation with AMF (5 g kg-1 soil).
The savory seeds used in this experiment were purchased from the Pakan Bazr company, in Isfahan, Iran. Seeds were sown in a plastic pot (5 L volume), and the soil mix was 1:1:2; sand: animal manure: topsoil, respectively which was sandy clay loam containing 0.96% organic carbon, 0.08% total N, 10.13 and 493.25 mg kg-1 soil of available P and K, respectively, and the pH was 7.96. After seeds germination, pots were watered by graduated cylinder daily to 100% FC for two weeks. The FC was calculated by the gravimetric method. Maintenance of the drought treatments was attained by daily weighing, and the water lost in pots was replaced using a precision scale (× 0.1 accuracies). These water deficit treatments were employed for two months until harvest.
2.1 Mycorrhizal root colonization
Colonization of the savory plant roots was investigated using the crossover method (Giovannetti and Mosse, 1980). To color the roots, after cleaning and separating from the soil particles, the roots were divided into small pieces (1 cm) and placed inside the KOH solution (10%) for 10 min. After washing with distilled water, the parts were acidified with HCl (2%; v/v) for 20 min. Later, staining was done with trypan blue (0.05%) and lactic acid (80%; v/v) for 12 hours. The stained roots were washed with distilled water and kept in a solution containing water, glycerol, and lactic acid (1:1:1; v/v/v). The Olympus microscope (BH-2) discriminated and assessed the stained root pieces. The blue AMF organs and hyphae were recorded as well-sharped photos.
2.2 Measurement of morphological characteristics
The shoot mass of fresh weight (FW) was measured immediately after plant harvest. Then, the shoots were dried in an oven at 75°C for 48 hours, and their dry weight (DW) was recorded using a digital scale (A&D weighing, Japan, accuracy 0.01 mg). The height of the plants was also measured using a digital caliper.
2.3 Estimation of membrane stability index
To assay, the MSI, 200 mg of leaf sample was placed in 10 cubic centimeters of deionized water in a water bath at 40°C for 30 min, and then the EC1 (Electrical conductivity) was measured. Also, the second set of samples was boiled at 100°C in a water bath for 10 min, and its EC2 was measured. Finally, the percentage of MSI was obtained from the following formula of Sairam (Sairam et al., 2002):
2.4 Determination of relative water content
To estimate RWC, leaf pieces (1 cm2) were weighed (FW) and immediately floated in Petri dishes containing deionized water. After being placed in the darkness for 24 hours, the samples were saturated with water and weighed (TW). The dry weight of the parts was recorded after drying at 70°C for 48 hours (DW). The amount of RWC was calculated as the following formula (Hayat et al., 2007):
2.5 Determination of hydrogen peroxide content
The content of H2O2 was measured based on the method of Alexieva et al. (Alexieva et al., 2001). Briefly, 50 mg of fresh young leaves were digested with 5 ml of 0.1% TCA. After centrifugation of the homogeneous mixture at 4°C and at 12000 rpm for 15 min, 500 μl of the supernatant was mixed with 500 μl of potassium phosphate buffer (100 mM) and 2 ml of potassium iodide (KI) solution. Then, the reaction mixture was incubated for 60 minutes in the darkness at room temperature, and the absorbance was read at 390 nm (UV-1800, Shimadzu, Tokyo, Japan).
2.6 Measurement of malondialdehyde content
The MDA content as a measure of lipid peroxidation was obtained according to the method of Dhindsa et al. (Dhindsa et al., 1981). The leaf (0.5 g) sample was homogenized by adding 5 ml of 0.1% trichloroacetic acid (TCA). After centrifugation (10000 rpm at 4°C for 15 min), the supernatant (300 μL) was mixed with 1 ml of 0.5% thiobarbituric acid (TBA), and the mixture was heated at 95°C for 30 min, and then the reaction solution was cooled on ice to stop the reaction. After centrifugation at 10000 rpm for 10 min, the absorbance was recorded at 440, 532, and 600 nm wavelengths.
2.7 Determination of proline content
To measure proline content (Bates et al., 1973), 0.5 g of leaf fresh samples were extracted with 10 ml of 3% sulfosalicylic acid on an ice bath to prevent proline degradation. Then, the extracts were centrifuged at 10000 rpm for 20 min at 4°C. Afterward, 2 ml of the supernatant was separated and mixed into glass tubes along with 2 ml of ninhydrin acid and 2 ml of glacial acetic acid and heated in a hot water bath, and immediately transferred to an ice bath for one hour. Later, 4 ml of toluene was added to the mixture and vortexed for 20 s. The absorbance was read using a spectrophotometer at 520 nm (nanometer).
2.8 Total soluble proteins content
Total protein content was determined following Bradford (Bradford, 1976), with bovine serum albumin as a standard curve with the solutions containing 0, 0.2, 0.4, 0.6, 0.8, or 1 mg mL-1 to which 100 μL of the Bradford solution was added. To prepare, the Bradford reagent 50 mg of coomassie brilliant blue G-250 dissolved in methanol (50 mL) and phosphoric acid (100 mL, 85%; w/v) and then was mixed with 850 mL distilled water and filtered. Finally, 1000 μL of the Bradford reagent was added to 50 μL the extract and incubated for 5 min at room temperature. Absorbance was recorded at 595 nm with a spectrophotometer.
2.9 Measurement of total antioxidant activity
Total antioxidant activity by the ferric-reducing ability of plasma (FRAP) method was done based on the protocol of Benzie et al. (Benzie and Strain, 1996). 0.1 M acetate buffer solution (pH 3.6), 10 mM TPTZ [2,4,6-tris (2-pyridyl) - 1,3,5-triazine] were mixed (10:1:1; v/v/v) as a FRAP reagent. 200 μl of summer savory plant extract was combined with 2950 µl of FRAP reagent and then kept at 30 min. Finally, the reaction mixture was read at 593 nm using a spectrophotometer.
2.10 Assay of guaiacol peroxidase activity
To prepare a plant extract for the activity of enzymes and protein content, 3 ml of 50 mM potassium phosphate buffer was added to 0.5 g of leaf fresh weight (pH 7) and vortexed. The extract also included 1 mM EDTA and 1% polyvinylpyrrolidone (PVP; w/v). The resulting extract was centrifuged at 13000 rpm for 15 min. The supernatant was used to measure protein content and enzyme activities.
The activity of the GPX enzyme was measured by the Cakmak et al. (Cakmak et al., 2001) method. The reaction mixture consisted of 750 μL of 100 mM potassium phosphate buffer (pH 7), 100 μL of H2O2 (70 mM), 750 μL of guaiacol (10 mM), and the extract. GPX enzyme activity was measured at 470 nm. The extinction coefficient equivalent to 26.6 mM-1 cm-1 was considered in the calculation of enzyme activity.
2.11 Assay of ascorbate peroxidase activity
APX activity was measured by the method of Chen and Asada (Chen and Asada, 1992). The reaction mixture consisted of 200 μl of ascorbate (2 mM), 750 μl of potassium phosphate buffer (100 mM), 200 μl of hydrogen peroxide (2 mM), and the leaf extract. The reaction was started by adding H2O2 to the desired mixture, and the APX enzyme activity was measured by reducing the absorbance at 290 nm with a spectrophotometer due to the consumption of H2O2. The extinction coefficient of 2.8 mM-1 cm-1 was also included in the calculation of APX enzyme activity.
2.12 Assay of superoxide dismutase activity
The SOD activity was measured according to the modified method of Fu et al. (Fu and Huang, 2001). 20 μl of the enzyme extract was added to 63 μM nitrotetrazolium blue (NBT), 1.3 μM riboflavin, 13 mM methionine, and 0.1 mM EDTA in 50 mM phosphate buffer (pH 7.8). Test tubes containing each reaction mixture were placed under incandescent light for 10 minutes and absorbance was recorded at 560 nm with a spectrophotometer.
2.13 Chlorophyll fluorescence parameters
Chlorophyll fluorescence parameters were including F0 (minimum amount of chlorophyll fluorescence), (Fm) the maximum amount of chlorophyll fluorescence, (Fv) difference between F0 and Fm, (Fv/Fm) maximum PSII quantum efficiency and also, and Y (NO) non-photochemical PSII quantum efficiency. Four developed and relatively young leaves were randomly selected to measure the chlorophyll fluorescence parameters. The measurement of the mentioned parameter was performed using a pulse amplitude modulation fluorometer (PAM-2500, Heinz Walls, and Effeltrich, Germany). After the plants were adapted to the dark, the data were analyzed by PamWin software.
2.14 Photosynthetic pigments content
The content of photosynthetic pigments; chlorophyll a (Chl a), chlorophyll b (Chl b), and carotenoids (CARs) in the young developed leaves of the savory plant was determined by the method of Arnon (Arnon, 1949). Doing this, 0.5 g of fresh leaves was extracted by 10 ml of acetone (80%) and then centrifuged at 12000 rpm for 10 min. Lastly, the absorbance was read at 663, 645, and 470 nm, respectively, using a spectrophotometer (UV-1800, Shimadzu, Tokyo, Japan). The following formulas were used to calculate the content of Chl a, Chl b, and CARs:
2.15 Essential oil extraction and GC-MS analysis
Essential oil of the dried summer savory parts was isolated by distillation with water using a Clevenger-type apparatus. The amount of essential oil was measured using a calibrated burette, and the oils were stored at 5°C until analysis by gas chromatography. The GC instrument used was an Agilent 7890A (Santa Clara, CA, USA) equipped with a mass selective detector and an HP5 MS column (column length 30 m, inner diameter 0.25 lm, film thickness 0.25 mm) (voltage 70 eV). Helium was used as carrier gas with a flow rate of 0.8 ml/min. The column temperature was 280°C for the initial and the final injector temperature was 300°C. The column temperature is programmed at a rate of 4°C/min. The separation ratio was set to 40:1. 1 μL of each sample was injected into the GC with the injector in split mode (split ratio 40:1). A mixture of aliphatic hydrocarbons (C8-C40) was injected into the GC system under the same analytical conditions to calculate and identify the peaks. The compounds of the extracted essential oils were identified by comparing their gas chromatography retention times with the retention times of standard compounds and comparing mass spectral fragmentation patterns.
2.16 Statistical analysis
The two-way ANOVA analysis was conducted using MSTAT-C ver. 2.1. (Michigan State University, Michigan, USA). Mean comparisons were done via the least significant difference test (LSD) at 1% and 5% probability levels. Excel software was applied to draw graphs. Heat map cluster and Pearson correlation analysis were drawn using Rstudio ver. 14.2.1 software (github.com/talgalili/gplots).
3 Results
3.1 Arbuscular mycorrhizal fungi colonization
Microscopic images of summer savory root fragments were traced to detect arbuscular mycorrhizal colonization, which is shown in Figure 1. High-quality photographs documented the AMF organs and hyphae that appear in blue.
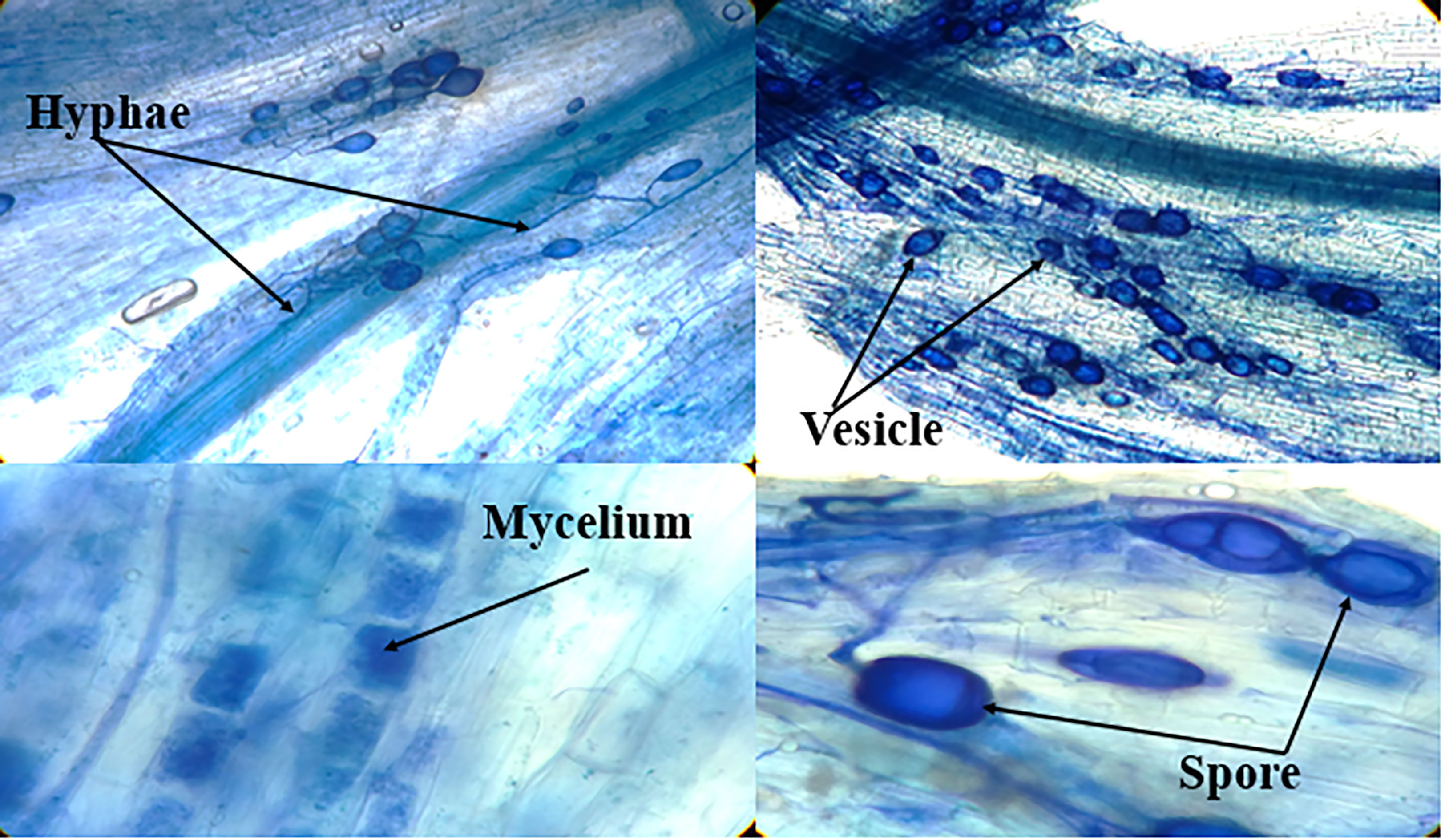
Figure 1 Microscopic images of the stained summer savory root fragments for detection of arbuscular mycorrhizae (Funneliformis mosseae) colonization.
3.2 Growth parameters
Drought stress and AMF inoculation significantly influenced some morphological traits of summer savory plants. Plant height and shoot mass (FW and DW) were significantly decreased up to 60, 78, and 80% respectively, under severe drought stress (30% filed capacity (FC)), as compared to the control plants (Table 1). The plant growth markers, plant height and shoot mass, were considerably enhanced in the plants treated with AMF inoculation (AMF1) relative to the control plants, up to 22, 15 and 21%. The highest assessment for all growth markers was documented when the plants were treated with AMF inoculation without drought stress.
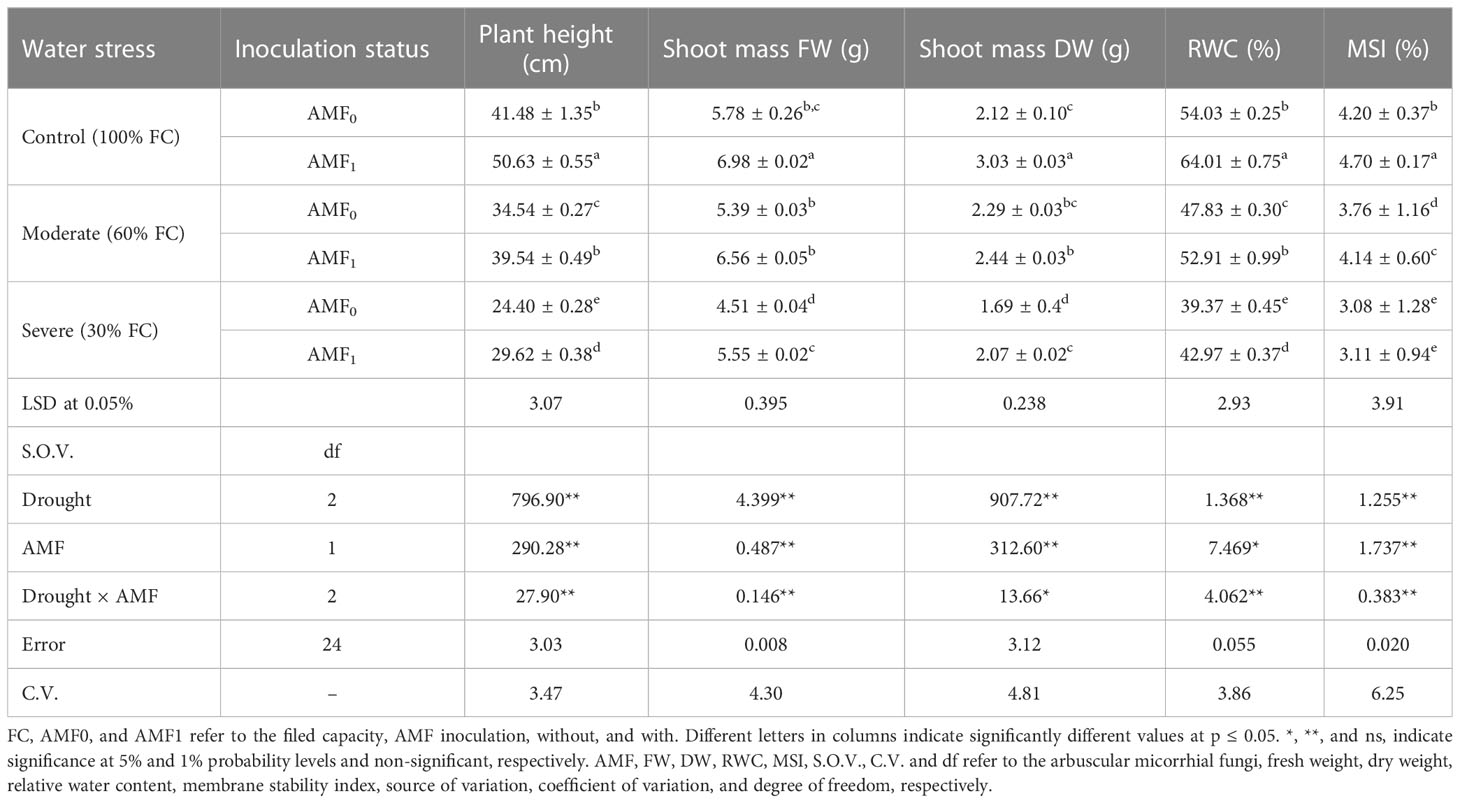
Table 1 The effect of arbuscular mycorrhiza fungi (AMF) inoculation on growth parameters, RWC and MSI in summer savory plants under drought stress conditions.
3.3 Leaf relative water content and membrane stability index
The leaf RWC and MSI have been decreased with increasing drought stress. Extreme drought stress (30% FC) significantly reduced RWC and MSI up to 64.8 and 52.6% compared to the control plants. AMF application again, similarly to growth parameters results, at both drought stress levels, improved the values of these two parameters compared to the non-inoculated ones (Table 1).
3.4 H2O2 and MDA content
Drought stress resulted in enhanced accumulation of H2O2 and MDA in summer savory plants, so the highest values for the traits were observed under the severe water deficit (30% FC), increased by 52.6% and 362%, compared to control plants, respectively. In contrast, the lowest H2O2 and MDA were documented in the plants under without drought stress. At severe drought stress; AMF inoculated plants had a decreased accumulation of H2O2 and MDA compared to the non-inoculated counterparts (Table 2).
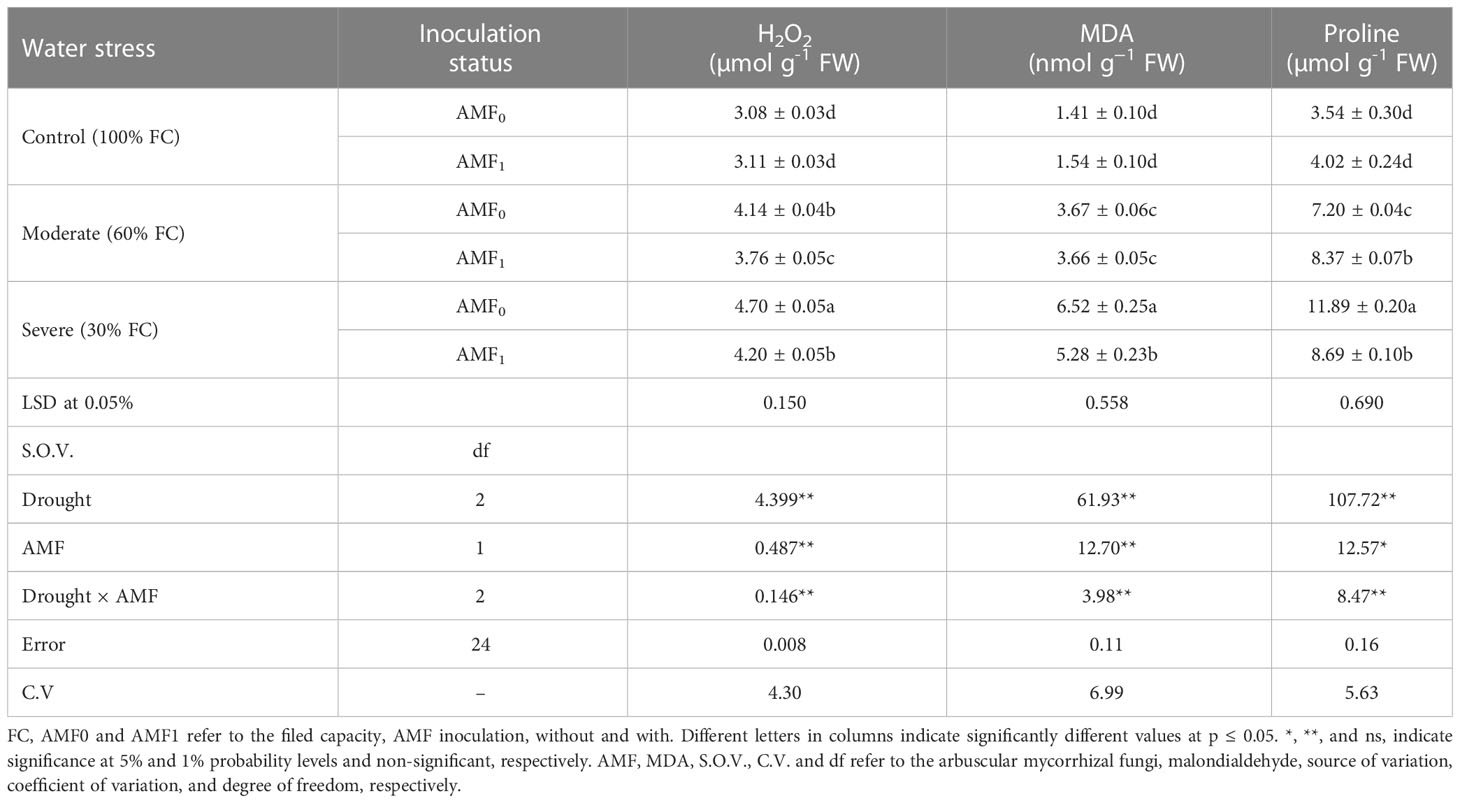
Table 2 The effect of arbuscular mycorrhiza fungi (AMF) inoculation on H2O2, MDA, and proline content in summer savory plants under drought stress conditions.
3.5 Proline content
Increased proline content was observed in response to moderate (60% FC) and severe (30% FC) drought stress (Table 2). The moderate drought-stressed plants with and without AMF had increased proline content compared to plants without AMF inoculation. Higher proline content in plants was recorded under severe drought stress, while it was reduced using AMF application (Table 2).
3.6 Total soluble proteins content
Drought stress significantly reduced the total soluble proteins (TSP) content of plants, while the trait was enhanced using AMF inoculation. AMF treatment improved the total soluble protein content by 343% compared to the severe (30%) drought-stress plants (Table 3). Moreover, the extreme drought stress caused a marked reduction in protein content over the control. In contrast, the moderate drought stress-induced reduction in protein content was nearly conquered by the AMF treatment. AMF treatment thus contributed to recovering the decline in the total protein content of summer savory plants under moderate and severe drought stress conditions.
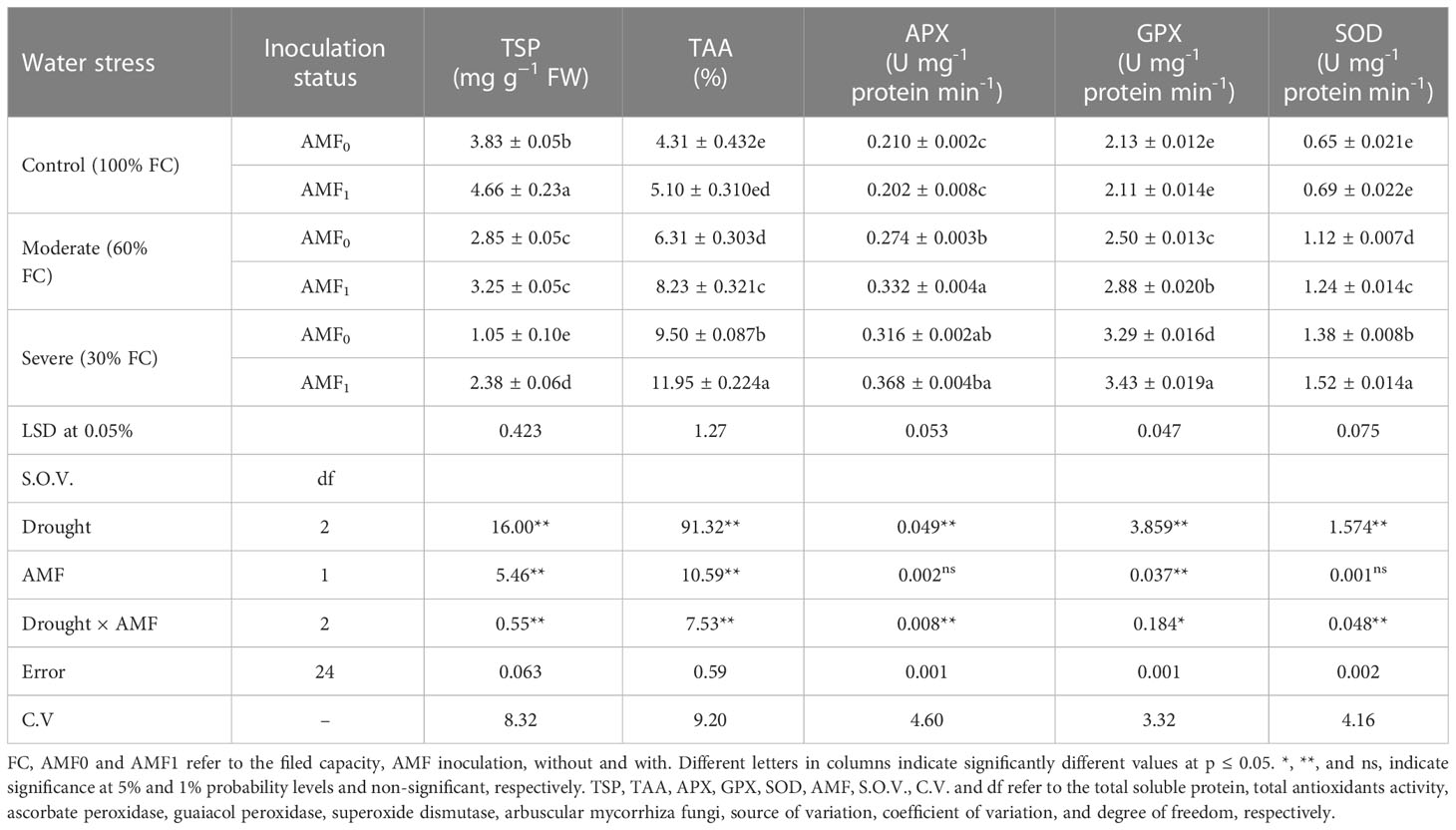
Table 3 The effect of arbuscular mycorrhiza fungi (AMF) inoculation on the total protein content, antioxidant activity, and antioxidant enzyme activities in summer savory plants under drought stress conditions.
3.7 Total antioxidant activity
TAA of summer savory plants, measured by the FRAP method, was significantly influenced by drought stress and the application of AMF. The plants grown under severe drought stress with AMF inoculation showed the maximum TAA, which increased by 177% compared to the control. The AMF inoculation increased TAA values under ideal water conditions and drought stress. The summer savory plants grown under the conditions without water stress and AMF showed a minimum of TAA (Table 3).
3.8 Activity of antioxidant enzymes
The activity of antioxidant enzymes; APX, GPX, and SOD had the same trend in response to drought stress. As the stress intensified, the activity of all three enzymes significantly increased compared to the control. So, their enhancement was 71.4%, 61.0%, and 133.8%, respectively. All of the above-mentioned activities reached the maximum activity when the plants were subjected to severe stress (30% FC). The results showed that AMF inoculation together with severe and moderated drought conditions increased the activity of these enzymes. These enhancements by AMF application and severe water deficit were 16.5%, 4.3%, and 10.1% for APX, GPX, and SOD, respectively those compared to without AMF (Table 3).
3.9 Chlorophyll fluorescence parameters
With increasing drought stress, the F0 parameter (minimum chlorophyll fluorescence yield in the light-adapted state) was enhanced, but other parameters including Fm, Fv, and Fv/Fm were reduced (Table 4). Drought stress raised the F0 parameter by 45.8% and lessened Fm, Fv, and Fv/Fm by 38.6, 63.6, and 22.9%, compared to the control, respectively. However, AMF treatment alleviated the chlorophyll fluorescence parameters under moderate and severe drought stress conditions.
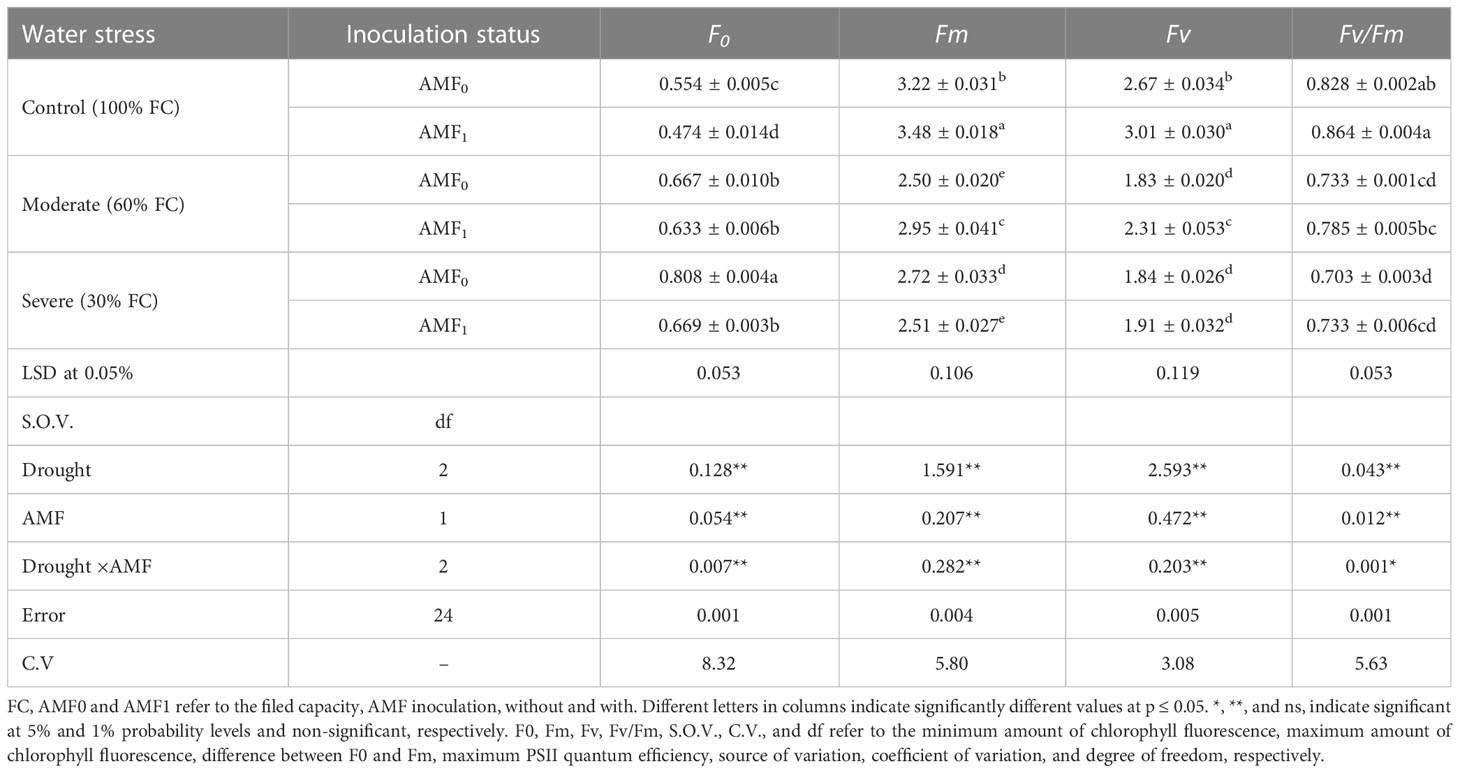
Table 4 The effect of arbuscular mycorrhiza fungi (AMF) inoculation on the chlorophyll fluorescence parameters in summer savory plants under drought stress conditions.
3.10 Photosynthetic pigments
The photosynthetic pigments, chlorophylls Chl a, b, a+b, and carotenoids (CARs) content were significantly influenced by drought stress and AMF inoculation in the summer savory plants (Table 5). Specifically, under severe drought stress, the content of Chl a, b, a+b, and CARs decreased significantly by 40.0, 19.3, 26.9, and 213%, compared to the control plants, respectively (Figures 2A–D). AMF inoculation increased the content of the photosynthetic pigments. Moreover, AMF treatment retrieved Chl a, b, a+b, and CARs contents in the plants subjected to moderate and severe stress (Figure 2).
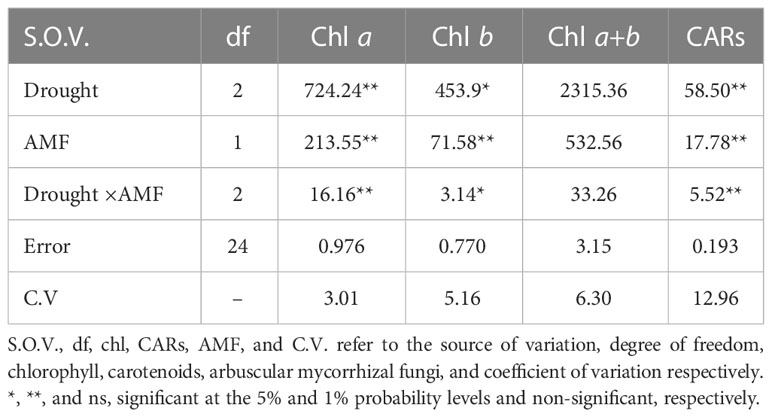
Table 5 Analysis of variance (ANOVA) for the effect of arbuscular mycorrhizal fungi (AMF) inoculation on the content of the photosynthetic pigments in summer savory plants under drought stress conditions.
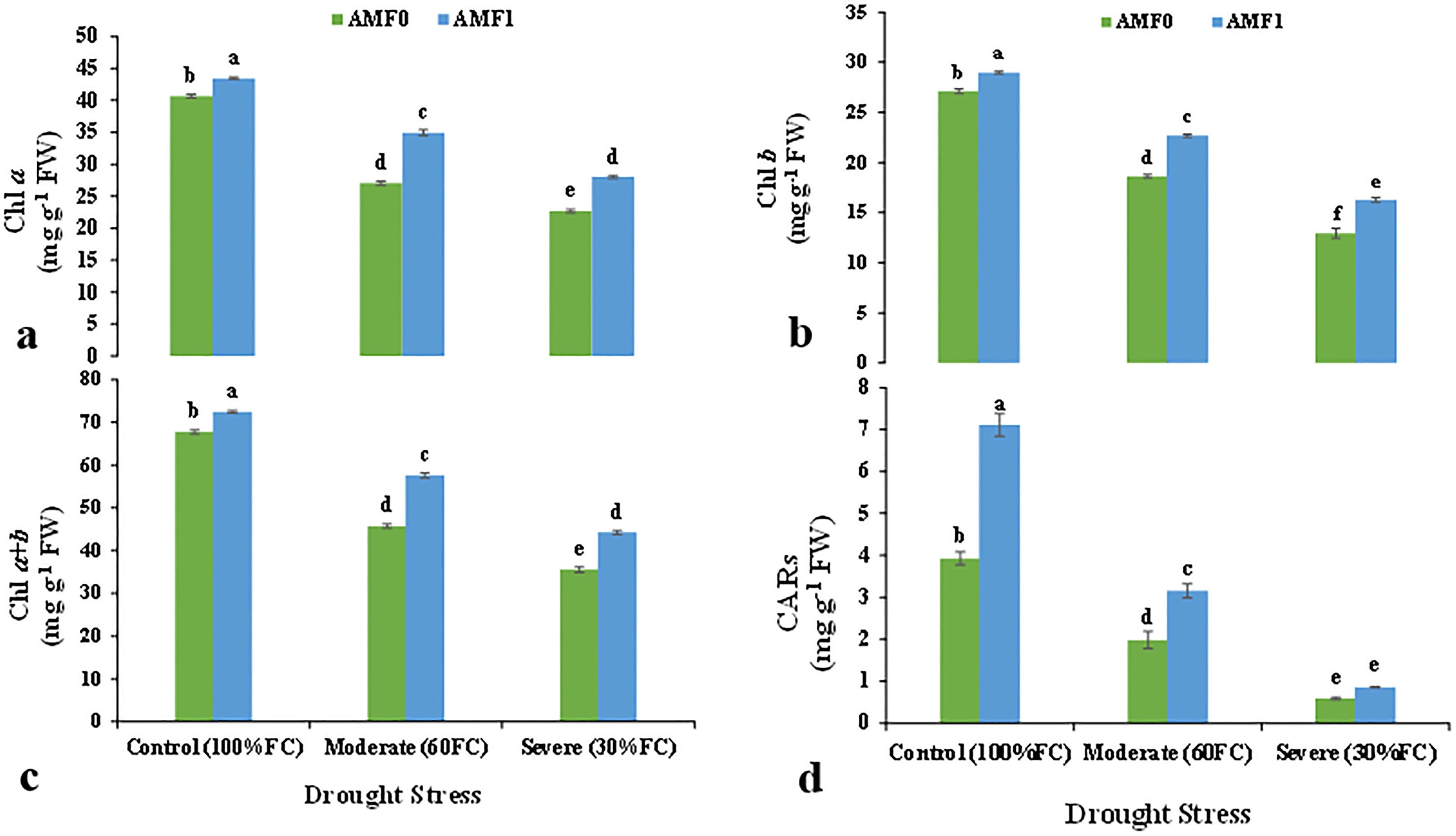
Figure 2 Effect of AMF inoculation on chlorophyll a (Chl a) (A), chlorophyll a (Chl b) (B), total chlorophyll (Chl a+b) (C), and carotenoids (CARs) (D) the content in summer savory plants under drought stress conditions. Different letters indicate significant differences according to the LSD test at p<0.05. AMF0 refer to without AMF inoculation and AMF1 with AMF inoculation.
3.11 Essential oil composition
In the essential oil of Satureja hortensis L. plants, there were identified 27 constituents, accounting for 89.2-99.8% of the total EO (Table 6). The results revealed that carvacrol (50.84-60.03%) was the main ingredient of summer savory EO. In addition, γ-terpinene (19.03-27.33%), p-cymene (1.73-3.01%), α-terpinene (2.03-2.62%), myrcene (1.28-1.58%), and aromadendrene (1.10-1.52%) were identified as other predominant constituents of summer savory essential oil. The highest carvacrol and γ-terpinene contents, up to 57.03 and 24.33%, were obtained with AMF inoculation (AMF1). The lowest content of 50.84 and 19.03% was identified under severe drought stress (30% FC) without AMF inoculation. The highest p-cymene content (3.03%) was achieved under severe drought (30% FC) with AMF inoculation, and the lowest one (1.73%) was recorded for control plants. The top of the content of α-terpinene (2.63%) was observed under severe drought (30% FC) with AMF inoculation, and the lowest (2.03%) under moderate drought (60% FC) without AMF inoculation.
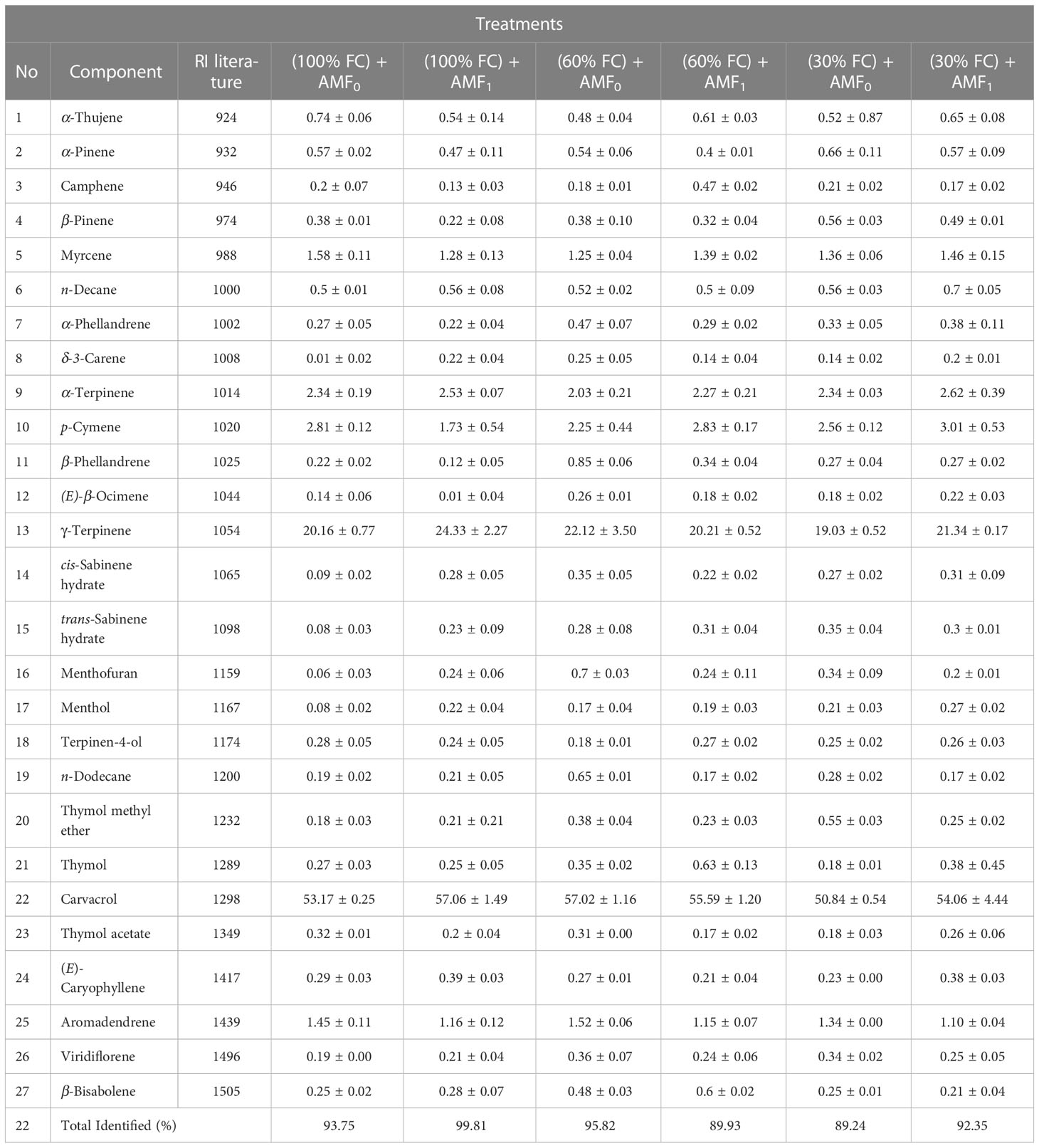
Table 6 The effect of the arbuscular mycorrhiza fungi (AMF) inoculation on essential oil (EO) composition of Satureja hortensis L. plants influenced by drought stress.
3.12 Pearson correlations, dendrogram clustering and principal component analysis
The correlation heat map exhibited a positive relationship among F0, antioxidant enzyme activities (APX, GPX, and SOD), total antioxidant activity (TAA, FRAP), the content of proline, and H2O2. Also, it was observed a strong positive correlation among growth parameters (shoot mass (FW and DW), and plant height), photosynthetic pigments (Chl a, Chl b, Chl a+b, and CARs contents), fluorescence parameters (Fm, Fv, Fv/Fm), RWC and MSI, and total soluble proteins content (TSP, protein). Finally, the results determined a significant negative correlation between the two mentioned groups above (Figure 3A). The dendrogram clustering heatmap analysis showed that the evaluated traits were classified into two clusters; cluster 1 contained F0, APX, GPX, SOD, total antioxidant activity, proline, and H2O2, and group 2 consisted of shoot mass (FW and DW), plant height, Chl a, Chl b, Chl a+b, CARs, Fm, Fv, Fv/Fm, RWC, MSI, and TSP, which confirmed the Pearson correlation. On the other hand, the plants subjected to these experimental treatments revealed two groups, as group 1 included the plants grown under regular irrigation along with AMF0 and AMF1. Group 2 had the plants grown under 60% FC without AMF inoculation and with AMF, and 30% FC without AMF inoculation and with AMF (Figure 3B). The PCA biplot showed that F0, APX, GPX, SOD, total antioxidant activity, proline, and H2O2 were associated with the severe drought stress condition without AMF inoculation and with AMF, while the traits such as shoot mass (FW, DW), plant height, Chl a, Chl b, Chl a+b, CARs, Fm, Fv, Fv/Fm, RWC, MSI, and TSP were linked to AMF inoculation under 100% FC and 60% FC (Figure 3C).
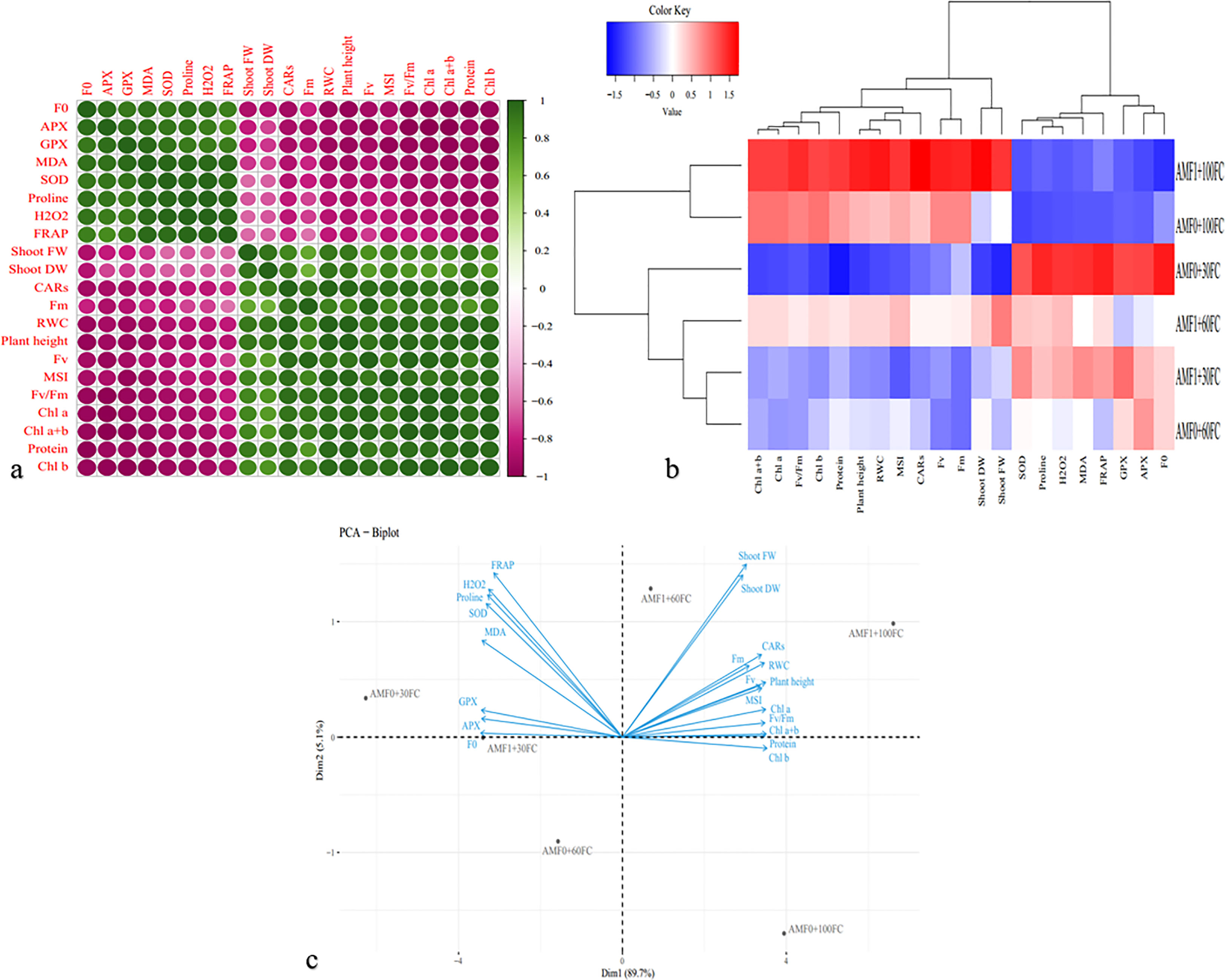
Figure 3 Heat map of Pearson correlations (A), dendrogram clustering (B), and principal component analysis PCA – Biplot (C) for the growth and biochemical responses of AMF inoculated summer savory plants exposed to drought stress conditions.
4 Discussion
Water deficit is an annoying stress factor at the global level, which excessively reduces agricultural crop production. Then, the effective methodologies for reducing the adverse water deficit effect should be considered. Over the past decades, researchers have focused on implementing and validating many tools and techniques to overcome drought stress (Begum et al., 2019). In the present study, drought stress decreased the growth characteristics, including the fresh and dry biomass and the height of the savory plant. However, AMF inoculation significantly compensated for the growth and yield reduction of the savory plant under drought stress. growth promotion, enhanced minerals, and water absorption in Sophora davidii seedlings under water stress is facilitated by the mycorrhizal hyphae expansion (Gong et al., 2013) which is in agreement with our results in summer savory plants. in the other study on coriander, AMF inoculation enhances drought tolerance by increasing water, nitrogen, phosphorus, and potassium absorption through its external hyphae, as a result, AMF-treated plants produce high biomass (Oliveira et al., 2016). In other words, through mycorrhizal hyphae, this fungus can absorb the nutrients that generally have little mobility and hardly enter the plant’s roots and increase the absorption of these nutrients by increasing the root surface and reducing the diffusion distance (Jaleel et al., 2007). In the same case, the growth and tolerance of tomato plants under drought stress were improved by inoculation with AMF (Chitarra et al., 2016) and the current findings are attuned with the mentioned reports above.
Our results showed that colonization behavior was improved by AMF inoculation in savory plants, which agrees with the results of Huang et al. (Huang et al., 2017). AMF inoculum develops from an infection point to the soil, and subsequently, secondary mycelium is formed, which can connect plants to the mycorrhizal network and finally establish colonization (Simard et al., 2012). Although there is a two-way flow of water in the hyphae, the possibility of water returning from the hyphae to the soil is much less, and the maximum water is directed from the AMF hyphae to the transpiration pathway in plants (Wu and Zou, 2009; Wu et al., 2013) that enhance the ability of water absorption by plants roots in water deficit. Besides, AMF colonization has an inverse relationship with the amount of ROS accumulation which decreases in the AMF-treated plants (Wu and Zou, 2009); most AMF species have the Sod1 gene, which increases drought resistance and growth in such conditions. These genes are regulated with high molecular diversity inside the root and improve drought tolerance to some extent (Corradi et al., 2009).
The light energy absorbed by chlorophyll molecules is employed to drive photosynthesis in chloroplast apparatus and photochemical fluorescence centers, or it is dissipated as heat (Qiu et al., 2013), which depends on different biochemical and environmental conditions (Sheng et al., 2008). Chlorophyll fluorescence reflects the photosynthetic activity of leaves in a complex manner and is often used to analyze photosynthesis and related mechanisms in plants subjected to biotic or abiotic stressors (Qiu et al., 2013). The Fv/Fm ratio can be used to measure the initial photochemical capacity of photosystem II (PSII) (Sheng et al., 2008). Under AMF colonization, the improvement of water status and stomatal opening in AMF-inoculated plants can increase the efficiency of PSII (Fv/Fm) under drought-stress conditions. In the present study, AMF treatment mitigated the Fv/Fm in savory plants under drought stress. This indicates a symbiotic relationship between plants and AMF, which can increase chloroplast’s absorption efficiency and excitation energy and improve the photochemical capacity of PSII in leaves (Gong et al., 2013). Our data support the findings of previous studies showing that AMF symbiosis increased Fv/Fm in maize plants (Sheng et al., 2008; Zhu et al., 2012). Similarly, in another experiment under drought stress conditions, AMF inoculation improved the maximum quantum yield in Ceratonia siliqua L. (Boutasknit et al., 2021). The results show that AMF treatment under drought-stress conditions can enhance the natural use of light energy in the photochemical processes of plants (Boutasknit et al., 2020). In agreement with our findings, many studies have shown that AMF inoculation significantly increased the chlorophyll content of plants (Sannazzaro et al., 2006; Sheng et al., 2008).
AMF inoculation under stress conditions improves the chloroplast cycle and protects pigments from damage caused by photosystem reaction centers and stress by increasing energy absorption efficiency (Bagheri et al., 2011; Bashir et al., 2020). On the other hand, the accumulation of osmolytes and minerals required for photosynthesis, as a result of AMF inoculation, partially increases the overall content of chlorophyll and improves the absorption of water and nutrients under drought stress (Abbaspour et al., 2012). Further, this action increases photosynthesis and biosynthesis and accumulation of protein and carbohydrate (Zaferanchi et al., 2019) which is in agreement with our findings on savory plants. Also, in the present experiment, the role of AMF in reducing the negative effect of drought stress was shown by improving the content of chlorophyll and photosynthetic pigments, which was consistent with the results of Boutasknit et al. (Boutasknit et al., 2021). Our result showed that the higher content of CARs in savory plants inoculated with AMF compared to those without AMF can also reflect the defensive reaction of plants related to higher TAA in normal and water stress conditions.
In addition to the limited cell division and reduced growth, loss of turgor is another consequence of drought stress, causing a decrease in maintaining high RWC in the plants, which is an essential factor in plant tolerance to drought stress. RWC and MSI have been introduced as the tolerance indicators of drought stress, and increasing their amount will improve the adverse conditions caused by stress (Bangar et al., 2019) which the reduction of these traits revealed mitigation of drought stress adverse effects. In the present study, despite the decrease in RWC and MSI due to drought stress, the RWC in AMF-inoculated plants improved compared to the control and is alleviated under moderate and severe drought stress. Maintaining cell mass and water relations in plants in response to water deficit largely depends on managing plant cells’ osmotically active molecules and ions (Farooq et al., 2009). While drought stress disrupts the coordination between transpiration and water uptake by plants and extensively reduces RWC; AMF mycelia help to absorb more water and nutrients (Ye et al., 2019) thus it helps to reduce RWC and MSI under water stress.
Our experiment showed that the AMF-treated plants possessed higher SOD, APX, and GPX activities under moderate stress conditions than plants without AMF inoculation, indicating that drought stress is often associated with high levels of reactive oxygen species generation (Fouad et al., 2014). In other words, in all plants, the most common technique to protect plants against oxidative stress damage is stimulating the biosynthesis of antioxidant enzymes such as SOD, APX, and GPX to reduce or eliminate ROS molecules (Mirzai et al., 2013). APX by using ascorbate as an electron donor converts H2O2 into H2O, which plays an essential role in protecting cells from the harmful effects of H2O2 and indicates the impact of AMF on the activity of the enzymes, as mentioned above. The results of this experiment were consistent with the findings of Begum et al. (Begum et al., 2022), on tobacco plants. Also, the higher activity of SOD in AMF-inoculated plants indicates that these plants have more efficient superoxide transformation, so more effective detoxification of superoxide radicals to H2O2 is done (Zou et al., 2015). Furthermore, AMF may initiate gene activation by utilizing ion and signal transfer, which can increase the expression of enzymes such as SOD under drought stress by absorbing some micronutrients such as Zn and Cu (Huang et al., 2017).
The present findings showed that plants exposed to drought stress especially severe water deficit treated with AMF showed a decrease in the accumulation of MDA and H2O2, which indicated less oxidative stress damage in plant cells compared to plants without AMF inoculation under water deficit stress. Also, the accumulation of H2O2 and MDA in plant tissues are among those that indicate the levels of oxidative stress damage to the cell membrane (Hossain et al., 2015). The decrease in MDA and H2O2 accumulation due to AMF application indicates the reduced lipid peroxidation rate in plants inoculated with AMF compared to without AMF, which was also clearly evident in our results. Aalipour et al. (Aalipour et al., 2020) in Arizona cypress plants reported a relatively lower accumulation of H2O2 and MDA in plants subjected to AMF. In addition, the lower rate of lipid peroxidation in plants accompanied by AMF indicates the increased tolerance to oxidative damage due to the enhanced activity of antioxidant enzymes, which is the most common mechanism to reduce the effect of ROS under drought stress (Talaat and Shawky, 2014). APX and GPX are also considered potential non-enzymatic antioxidants in the glutathione ascorbate cycle and play an important role in protecting plants against oxidative stress (Wu et al., 2006). The increase in the activity of these enzymes can also be a reflection of AMF inoculation in this study, which agrees with the experiment of Mirshad and Puthur (Mirshad and Puthur, 2016).
With any increase in the drought stress duration and intensity, and along with the enhancement in the activity of antioxidant enzymes, the amount of proline and other osmolytes also increases. In our research, those compound amounts were enhanced with the use of AMF in water deficit condition compared to control especially in moderate drought stress that has exhibited the importance of AMF role in enhancement of drought stress tolerance. Under certain conditions, some plants produce large quantities of proline to promote osmosis and prevent dehydration (Lu et al., 2007). These organic solutes, which act as osmotic buffers, facilitate water absorption to stabilize intracellular structures and protect them against the harmful effects of ROS (Wu et al., 2013). The increment in proline accumulation in the plants treated with AMF leads to a higher water potential gradient overcoming the osmotic imbalance. In addition to osmotic regulation, proline plays a vital role in protecting the enzymes involved in chlorophyll synthesis. Proline also reduces photodamage in the thylakoid membrane (Kishor et al., 2005). On the other hand, proline acts as a carbon and nitrogen reservoir, and its higher concentration in AMF-inoculated plants may provide energy for plant growth under drought stress (Hare and Cress, 1997). In other words, the accumulation of proline in the root can provide an osmotic mechanism for the plant to maintain a favorable potential gradient for water to enter the root, which leads to less stress damage in the plants (Porcel and Ruiz-Lozano, 2004). Abbaspour et al. (Abbaspour et al., 2012) reported similar results to the current findings in Pistacia vera L. In the present study, the AMF-inoculated savory plants had a higher content of soluble proteins under drought stress, which indicates an increase in the accumulation of proteins and their maintenance by the presence of AMF under drought stress. This action happens through the synthesis of osmolytes, whose catabolism is reduced by AMF inoculation, and thus their accumulation increases. In other words, AMF-mediated accumulation of proline, sugars, and glycine betaine may protect plants against ROS-induced oxidative damage to proteins and membranes, thereby improving protein levels (Hashem et al., 2016). The results of the present experiment were consistent with Begum et al. (Begum et al., 2019) on tobacco plants.
The results also demonstrated that the savory EO components’ percentages were enhanced under moderate drought stress. The increase in EO content under moderate drought stress conditions is one of the primary defense mechanisms in medicinal and aromatic plants, in this condition, the photosynthetic rate decreases due to the reduction in CO2 uptake (as a result of closing stomata), leading to the generation of NADPH+ (Sheteiwy et al., 2021). The increase in the compound is an inhibitory factor for the photosynthetic rate and plant productivity. Therefore, the productivity of secondary metabolites such as EO compounds, alkaloids, etc., as a function of the increase in NADPH+ consumption (accumulated in plant cells) can effectively decrease the negative impacts of drought stress in medicinal and aromatic plants (García-Caparrós et al., 2019).
Furthermore, the AMF treatment significantly increased the main constituents’ percentages under without, moderate, and severe drought stress. It seems that the application of AMF under normal and water stress circumstance improves nutrient accessibility and stimulates higher metabolic efficiency (e.g., nutrient transport), which have an essential role in the production of carbohydrates and the development of the glandular trichomes, EO channels, and secretory ducts. Additionally, AMF helps to convert inorganic N into organic N in the form of protein and chlorophyll, which leads to an increase in the photosynthetic rate. Zhao et al. (Zhao et al., 2022) noted the increase in primary photosynthesis compounds, including erythrose‐4‐phosphate, phosphoenolpyruvate, pyruvate, and glyceraldehyde‐3‐phosphate which play the leading role in increasing terpene constituents and EO productivity in medicinal and aromatic plants which it could be the reason of the EO constituents of summer savory plants improvement using AMF under normal and water stress and as a result, it led to an amelioration of water stress. In addition, Hazzoumi et al. (Hazzoumi et al., 2017) concluded that AMF inoculation stimulates the productivity of EO glands probably by increasing endogenous hormone levels, particularly cytokinin, indole‐3‐acetic acid, and gibberellin. Similarly, Hazzoumi et al. (Hazzoumi et al., 2015) noted that the EO components of Ocimum basilicum were enhanced under moderate and severe drought stress conditions.
5 Conclusions
Drought stress declined the values of morphological traits, RWC, TSP, Fm, Fv, Fv/Fm, and photosynthesis pigments in the summer savory plants, while AMF inoculation alleviated those traits in control plants, and also in plants under moderate and severe drought stress. The results showed that water stress increased H2O2, MDA, and proline contents, and APX, SOD, and GPX activities, otherwise, AMF inoculation reduced the values of these attributes. TAA values were enhanced in the savory plants exposed to water stress and without/with AMF inoculation. Overall, the findings suggest that AMF inoculation enhanced the drought tolerance of the summer savory plants by improving the antioxidant defense system and could be a hopeful approach for ameliorating the damages caused by drought stress. However, there may be a need for more in-depth studies with other AMF species or a combination of them, especially in the field conditions to decide on their actual efficiency under drought conditions.
Data availability statement
The raw data supporting the conclusions of this article will be made available by the authors, without undue reservation.
Author contributions
Conceptualization: FR, MA and TA. Formal analysis: MA. Investigation and methodology: MA and FR. Visualization: TA and MA. Writing-original draft: TA, MH, and MA. Review and editing: SE, SS, MB, MM, and JM. All authors have read and agreed to the published version of the manuscript.
Funding
This work was supported by the internal grant of Tomas Bata University in Zlin (No. IGA/FT/2023/003).
Conflict of interest
Author SE was employed by company HGF Agro.
The remaining authors declare that the research was conducted in the absence of any commercial or financial relationships that could be construed as a potential conflict of interest.
Publisher’s note
All claims expressed in this article are solely those of the authors and do not necessarily represent those of their affiliated organizations, or those of the publisher, the editors and the reviewers. Any product that may be evaluated in this article, or claim that may be made by its manufacturer, is not guaranteed or endorsed by the publisher.
References
Aalipour, H., Nikbakht, A., Etemadi, N., Rejali, F., Soleimani, M. (2020). Biochemical response and interactions between arbuscular mycorrhizal fungi and plant growth promoting rhizobacteria during establishment and stimulating growth of Arizona cypress (Cupressus arizonica g.) under drought stress. Sci. Hortic. 261, 108923. doi: 10.1016/j.scienta.2019.108923
Abbaspour, H., Saeidi-Sar, S., Afshari, H., Abdel-Wahhab, M. (2012). Tolerance of mycorrhiza infected pistachio (Pistacia vera l.) seedling to drought stress under glasshouse conditions. J. Plant Physiol. 169, 704–709. doi: 10.1016/j.jplph.2012.01.014
Agarwal, S., Pandey, V. (2004). Antioxidant enzyme responses to NaCl stress in Cassia angustifolia. Biol. Plant 48, 555–560. doi: 10.1023/B:BIOP.0000047152.07878.e7
Ahanger, M. A., Hashem, A., Abd-Allah, E. F., Ahmad, P. (2014). “Arbuscular mycorrhiza in crop improvement under environmental stress,” in Emerging technologies and management of crop stress tolerance (Elsevier), 69–95.
Alexieva, V., Sergiev, I., Mapelli, S., Karanov, E. (2001). The effect of drought and ultraviolet radiation on growth and stress markers in pea and wheat. Plant Cell Environ. 24, 1337–1344. doi: 10.1046/j.1365-3040.2001.00778.x
Alhaithloul, H. A., Soliman, M. H., Ameta, K. L., El-Esawi, M. A., Elkelish, A. (2019). Changes in ecophysiology, osmolytes, and secondary metabolites of the medicinal plants of Mentha piperita and Catharanthus roseus subjected to drought and heat stress. Biomolecules 10, 43. doi: 10.3390/biom10010043
Amani Machiani, M., Javanmard, A., Morshedloo, M. R., Aghaee, A., Maggi, F. (2021). Funneliformis mosseae inoculation under water deficit stress improves the yield and phytochemical characteristics of thyme in intercropping with soybean. Sci. Rep. 11, 1–13. doi: 10.1038/s41598-021-94681-9
Arnon, D. I. (1949). Copper enzymes in isolated chloroplasts. polyphenoloxidase in Beta vulgaris. Plant Physiol. 24, 1. doi: 10.1104/pp.24.1.1
Asadi, M., Rasouli, F., Amini, T., Hassanpouraghdam, M. B., Souri, S., Skrovankova, S., et al. (2022). Improvement of photosynthetic pigment characteristics, mineral content, and antioxidant activity of lettuce (Lactuca sativa l.) by arbuscular mycorrhizal fungus and seaweed extract foliar application. Agronomy 12, 1943. doi: 10.3390/agronomy12081943
Augé, R. M. (2001). Water relations, drought and vesicular-arbuscular mycorrhizal symbiosis. Mycorrhiza 11, 3–42. doi: 10.1007/s005720100097
Bagheri, V., Shamshiri, M., Shirani, H., Roosta, H. (2011). Effect of mycorrhizal inoculation on ecophysiological responses of pistachio plants grown under different water regimes. Photosynthetica 49, 531–538. doi: 10.1007/s11099-011-0064-5
Balliu, A., Sallaku, G., Rewald, B. (2015). AMF inoculation enhances growth and improves the nutrient uptake rates of transplanted, salt-stressed tomato seedlings. Sustainability 7, 15967–15981. doi: 10.3390/su71215799
Bangar, P., Chaudhury, A., Tiwari, B., Kumar, S., Kumari, R., Bhat, K. V. (2019). Morphophysiological and biochemical response of mungbean [Vigna radiata (L.) wilczek] varieties at different developmental stages under drought stress. Turk. J. Biol. 43, 58–69. doi: 10.3906/biy-1801-64
Bashir, A., Rizwan, M., Ur Rehman, M. Z., Zubair, M., Riaz, M., Qayyum, M. F., et al. (2020). Application of co-composted farm manure and biochar increased the wheat growth and decreased cadmium accumulation in plants under different water regimes. Chemosphere 246, 125809. doi: 10.1016/j.chemosphere.2019.125809
Bates, L. S., Waldren, R. P., Teare, I. (1973). Rapid determination of free proline for water-stress studies. Plant Soil 39, 205–207. doi: 10.1007/BF00018060
Begum, N., Ahanger, M. A., Su, Y., Lei, Y., Mustafa, N. S. A., Ahmad, P., et al. (2019). Improved drought tolerance by AMF inoculation in maize (Zea mays) involves physiological and biochemical implications. Plants 8, 579. doi: 10.3390/plants8120579
Begum, N., Wang, L., Ahmad, H., Akhtar, K., Roy, R., Khan, M. I., et al. (2022). Co-Inoculation of arbuscular mycorrhizal fungi and the plant growth-promoting rhizobacteria improve growth and photosynthesis in tobacco under drought stress by up-regulating antioxidant and mineral nutrition metabolism. Microb. Ecol. 83, 971–988. doi: 10.1007/s00248-021-01815-7
Benzie, I. F., Strain, J. J. (1996). The ferric reducing ability of plasma (FRAP) as a measure of “antioxidant power”: the FRAP assay. Anal. Biochem. 239, 70–76. doi: 10.1006/abio.1996.0292
Boutasknit, A., Baslam, M., Ait-El-Mokhtar, M., Anli, M., Ben-Laouane, R., Ait-Rahou, Y., et al. (2021). Assemblage of indigenous arbuscular mycorrhizal fungi and green waste compost enhance drought stress tolerance in carob (Ceratonia siliqua l.) trees. Sci. Rep. 11, 1–23. doi: 10.1038/s41598-021-02018-3
Boutasknit, A., Baslam, M., Ait-El-Mokhtar, M., Anli, M., Ben-Laouane, R., Douira, A., et al. (2020). Arbuscular mycorrhizal fungi mediate drought tolerance and recovery in two contrasting carob (Ceratonia siliqua l.) ecotypes by regulating stomatal, water relations, and (in) organic adjustments. Plants 9, 80. doi: 10.3390/plants9010080
Bradford, M. M. (1976). A rapid and sensitive method for the quantitation of microgram quantities of protein utilizing the principle of protein-dye binding. Anal. Biochem. 72, 248–254. doi: 10.1016/0003-2697(76)90527-3
Cakmak, O., Ozturk, L., Karanlik, S., Ozkan, H., Kaya, Z., Cakmak, I. (2001). Tolerance of 65 durum wheat genotypes to zinc deficiency in a calcareous soil. J. Plant Nutr. 24, 1831–1847. doi: 10.1081/PLN-100107315
Chen, G.-X., Asada, K. (1992). Inactivation of ascorbate peroxidase by thiols requires hydrogen peroxide. Plant Cell Physiol. 33, 117–123. doi: 10.1093/oxfordjournals.pcp.a078229
Chitarra, W., Pagliarani, C., Maserti, B., Lumini, E., Siciliano, I., Cascone, P., et al. (2016). Insights on the impact of arbuscular mycorrhizal symbiosis on tomato tolerance to water stress. Plant Physiol. 171, 1009–1023. doi: 10.1104/pp.16.00307
Corradi, N., Ruffner, B., Croll, D., Colard, A., Horák, A., Sanders, I. R. (2009). High-level molecular diversity of copper-zinc superoxide dismutase genes among and within species of arbuscular mycorrhizal fungi. Appl. Environ. Microbiol. 75, 1970–1978. doi: 10.1128/AEM.01974-08
Dhindsa, R. S., Plumb-Dhindsa, P., Thorpe, T. A. (1981). Leaf senescence: correlated with increased levels of membrane permeability and lipid peroxidation, and decreased levels of superoxide dismutase and catalase. J. Exp. Bot. 32, 93–101. doi: 10.1093/jxb/32.1.93
Dossa, K., Yehouessi, L. W., Likeng-Li-Ngue, B. C., Diouf, D., Liao, B., Zhang, X., et al. (2017). Comprehensive screening of some west and central African sesame genotypes for drought resistance probing by agromorphological, physiological, biochemical and seed quality traits. Agronomy 7, 83. doi: 10.3390/agronomy7040083
Farooq, M., Wahid, A., Kobayashi, N., Fujita, D., Basra, S. (2009). “Plant drought stress: effects, mechanisms and management,” in Sustainable agriculture (Springer), 153–188.
Fierascu, I., Dinu-Pirvu, C. E., Fierascu, R. C., Velescu, B. S., Anuta, V., Ortan, A., et al. (2018). Phytochemical profile and biological activities of Satureja hortensis l.: a review of the last decade. Molecules 23, 2458. doi: 10.3390/molecules23102458
Fouad, M. O., Essahibi, A., Benhiba, L., Qaddoury, A. (2014). Effectiveness of arbuscular mycorrhizal fungi in the protection of olive plants against oxidative stress induced by drought. Span. J. Agric. Res. 12, 763–771. doi: 10.5424/sjar/2014123-4815
Fu, J., Huang, B. (2001). Involvement of antioxidants and lipid peroxidation in the adaptation of two cool-season grasses to localized drought stress. Environ. Exp. Bot. 45, 105–114. doi: 10.1016/S0098-8472(00)00084-8
García-Caparrós, P., Romero, M. J., Llanderal, A., Cermeño, P., Lao, M. T., Segura, M. L. (2019). Effects of drought stress on biomass, essential oil content, nutritional parameters, and costs of production in six lamiaceae species. Water 11, 573. doi: 10.3390/w11030573
Giovannetti, M., Mosse, B. (1980). An evaluation of techniques for measuring vesicular arbuscular mycorrhizal infection in roots. New Phytol. 83(2), 489–500. doi: 10.1111/j.1469-8137.1980.tb04556.x
Gong, M., Tang, M., Chen, H., Zhang, Q., Feng, X. (2013). Effects of two glomus species on the growth and physiological performance of Sophora davidii seedlings under water stress. New For. 44, 399–408. doi: 10.1007/s11056-012-9349-1
Hanin, M., Ebel, C., Ngom, M., Laplaze, L., Masmoudi, K. (2016). New insights on plant salt tolerance mechanisms and their potential use for breeding. Front. Plant Sci. 7. doi: 10.3389/fpls.2016.01787
Hare, P., Cress, W. (1997). Metabolic implications of stress-induced proline accumulation in plants. Plant Growth Regul. 21, 79–102. doi: 10.1023/A:1005703923347
Hashem, A., Abd_Allah, E., Alqarawi, A., Egamberdieva, D. (2016). Bioremediation of adverse impact of cadmium toxicity on Cassia italica mill by arbuscular mycorrhizal fungi. Saudi J. Biol. Sci. 23, 39–47. doi: 10.1016/j.sjbs.2015.11.007
Hayat, S., Ali, B., Hasan, S. A., Ahmad, A. (2007). Brassinosteroid enhanced the level of antioxidants under cadmium stress in Brassica juncea. Environ. Exp. Bot. 60, 33–41. doi: 10.1016/j.envexpbot.2006.06.002
Hazzoumi, Z., Moustakime, Y., Joutei, K. A. (2015). Effect of arbuscular mycorrhizal fungi (AMF) and water stress on growth, phenolic compounds, glandular hairs, and yield of essential oil in basil (Ocimum gratissimum l). Chem. Biol. Technol. Agric. 2, 1–11. doi: 10.1186/s40538-015-0035-3
Hazzoumi, Z., Moustakime, Y., Joutei, K. A. (2017). Effect of arbuscular mycorrhizal fungi and water stress on ultrastructural change of glandular hairs and essential oil compositions in Ocimum gratissimum. Chem. Biol. Technol. Agric. 4, 1–13. doi: 10.1186/s40538-017-0102-z
Hossain, M. A., Bhattacharjee, S., Armin, S.-M., Qian, P., Xin, W., Li, H.-Y., et al. (2015). Hydrogen peroxide priming modulates abiotic oxidative stress tolerance: insights from ROS detoxification and scavenging. Front. Plant Sci. 6. doi: 10.3389/fpls.2015.00420
Huang, Y.-M., Zou, Y.-N., Wu, Q.-S. (2017). Alleviation of drought stress by mycorrhizas is related to increased root H2O2 efflux in trifoliate orange. Sci. Rep. 7, 1–9. doi: 10.1038/srep42335
Jaleel, C. A., Manivannan, P., Sankar, B., Kishorekumar, A., Gopi, R., Somasundaram, R., et al. (2007). Induction of drought stress tolerance by ketoconazole in Catharanthus roseus is mediated by enhanced antioxidant potentials and secondary metabolite accumulation. Colloids Surf. B: Biointerfaces 60, 201–206. doi: 10.1016/j.colsurfb.2007.06.010
Kafi, M., Zand, E., Kamkar, B., Mahdavi-Damghani, A., Abbasi, F. (2010). Plant physiology 2 (translate) (Jihad-e-Daneshgahi of Mashhad press).
Karimi, E., Ghasemnezhad, A., Ghorbanpour, M. (2022). Selenium-and silicon-mediated recovery of satureja (Satureja mutica fisch. & CA mey.) chemotypes subjected to drought stress followed by rewatering. Gesunde Pflanzen, 1–21. doi: 10.1007/s10343-022-00654-x
Kishor, P. K., Sangam, S., Amrutha, R., Laxmi, P. S., Naidu, K., Rao, K. S., et al. (2005). Regulation of proline biosynthesis, degradation, uptake and transport in higher plants: its implications in plant growth and abiotic stress tolerance. Curr. Sci. 84, 424–438.
Kyriazopoulos, A. P., Orfanoudakis, M., Abraham, E. M., Parissi, Z. M., Serafidou, N. (2014). Effects of arbuscular mycorrhiza fungi on growth characteristics of Dactylis glomerata l. under drought stress conditions. Not. Bot. Horti Agrobot. 42, 132–137. doi: 10.15835/nbha4219411
Lu, J., Liu, M., Mao, Y., Shen, L. (2007). Effects of vesicular-arbuscular mycorrhizae on the drought resistance of wild jujube (Zizyphs spinosus hu) seedlings. Front. Agric. China. 1, 468–471. doi: 10.1007/s11703-007-0077-9
Mathur, S., Sharma, M. P., Jajoo, A. (2018). Improved photosynthetic efficacy of maize (Zea mays) plants with arbuscular mycorrhizal fungi (AMF) under high temperature stress. J. J. Photochem. Photobiol. 180, 149–154. doi: 10.1016/j.jphotobiol.2018.02.002
Mirshad, P., Puthur, J. T. (2016). Arbuscular mycorrhizal association enhances drought tolerance potential of promising bioenergy grass (Saccharum arundinaceum retz.). Environ. Monit. Assess. 188, 1–20. doi: 10.1007/s10661-016-5428-7
Mirzai, M., Moeini, A., Ghanati, F. (2013). Effects of drought stress on the lipid peroxidation and antioxidant enzyme activities in two canola (Brassica napus l.) cultivars. JAST 15 (3), 593–602. Available at: http://jast.modares.ac.ir/article-23-9016-en.html.
Oliveira, R. S., Ma, Y., Rocha, I., Carvalho, M. F., Vosátka, M., Freitas, H. (2016). Arbuscular mycorrhizal fungi are an alternative to the application of chemical fertilizer in the production of the medicinal and aromatic plant Coriandrum sativum l. J. Toxicol. Environ. Health Part A 79, 320–328. doi: 10.1080/15287394.2016.1153447
Ostadi, A., Javanmard, A., Machiani, M. A., Morshedloo, M. R., Nouraein, M., Rasouli, F., et al. (2020). Effect of different fertilizer sources and harvesting time on the growth characteristics, nutrient uptakes, essential oil productivity and composition of Mentha x piperita l. Ind. Crops Prod. 148, 112290. doi: 10.1016/j.indcrop.2020.112290
Porcel, R., Ruiz-Lozano, J. M. (2004). Arbuscular mycorrhizal influence on leaf water potential, solute accumulation, and oxidative stress in soybean plants subjected to drought stress. J. Exp. Bot. 55, 1743–1750. doi: 10.1093/jxb/erh188
Qiu, Z., Wang, L., Zhou, Q. (2013). Effects of bisphenol a on growth, photosynthesis and chlorophyll fluorescence in above-ground organs of soybean seedlings. Chemosphere 90, 1274–1280. doi: 10.1016/j.chemosphere.2012.09.085
Rabab, A. M., Lamis, D. S., Rabie, G. H., Abdel-Fattah, G. M. (2016). The impact of the arbuscular mycorrhizal fungi on growth and physiological parameters of cowpea plants grown under salt stress conditions. IJASBT 4, 372–379. doi: 10.3126/ijasbt.v4i3.15775
Rydlová, J., Jelínková, M., Dušek, K., Dušková, E., Vosátka, M., Püschel, D. (2016). Arbuscular mycorrhiza differentially affects synthesis of essential oils in coriander and dill. Mycorrhiza 26, 123–131. doi: 10.1007/s00572-015-0652-5
Sairam, R. K., Rao, K. V., Srivastava, G. (2002). Differential response of wheat genotypes to long term salinity stress in relation to oxidative stress, antioxidant activity and osmolyte concentration. Plant Sci. 163, 1037–1046. doi: 10.1016/S0168-9452(02)00278-9
Sannazzaro, A. I., Ruiz, O. A., Alberto, E. O., Menéndez, A. B. (2006). Alleviation of salt stress in lotus glaber by Glomus intraradices. Plant Soil 285, 279–287. doi: 10.1007/s11104-006-9015-5
Shariat, A., Karimzadeh, G., Assareh, M. H., Zandi_Esfahan, E. (2016). Drought stress in Iranian endemic savory (Satureja rechingeri): in vivo and in vitro studies. JPPB 6, 1–12.
Sheng, M., Tang, M., Chen, H., Yang, B., Zhang, F., Huang, Y. (2008). Influence of arbuscular mycorrhizae on photosynthesis and water status of maize plants under salt stress. Mycorrhiza 18, 287–296. doi: 10.1007/s00572-008-0180-7
Sheteiwy, M. S., Ali, D. F. I., Xiong, Y.-C., Brestic, M., Skalicky, M., Hamoud, Y. A., et al. (2021). Physiological and biochemical responses of soybean plants inoculated with arbuscular mycorrhizal fungi and bradyrhizobium under drought stress. BMC Plant Biol. 21, 1–21. doi: 10.1186/s12870-021-02949-z
Shirinbayan, S., Khosravi, H., Malakouti, M. J. (2019). Alleviation of drought stress in maize (Zea mays) by inoculation with azotobacter strains isolated from semi-arid regions. Appl. Soil Ecol. 133, 138–145. doi: 10.1016/j.apsoil.2018.09.015
Simard, S. W., Beiler, K. J., Bingham, M. A., Deslippe, J. R., Philip, L. J., Teste, F. P. (2012). Mycorrhizal networks: mechanisms, ecology and modelling. Fungal Biol. Rev. 26, 39–60. doi: 10.1016/j.fbr.2012.01.001
Talaat, N. B., Shawky, B. T. (2014). Modulation of the ROS-scavenging system in salt-stressed wheat plants inoculated with arbuscular mycorrhizal fungi. J. Plant Nutr. Soil Sci. 177, 199–207. doi: 10.1002/jpln.201200618
Thokchom, S. D., Gupta, S., Kapoor, R. (2020). Arbuscular mycorrhiza augments essential oil composition and antioxidant properties of Ocimum tenuiflorum l.–a popular green tea additive. Ind. Crops Prod. 153, 112418. doi: 10.1016/j.indcrop.2020.112418
Wężowicz, K., Rozpądek, P., Turnau, K. (2017). Interactions of arbuscular mycorrhizal and endophytic fungi improve seedling survival and growth in post-mining waste. Mycorrhiza 27, 499–511. doi: 10.1007/s00572-017-0768-x
Wu, Q.-S., Srivastava, A. K., Zou, Y.-N. (2013). AMF-induced tolerance to drought stress in citrus: a review. Sci. Horti. 164, 77–87. doi: 10.1016/j.scienta.2013.09.010
Wu, Q., Zou, Y. (2009). Mycorrhiza has a direct effect on reactive oxygen metabolism of drought-stressed citrus. Plant Soil Environ. 55, 436–442. doi: 10.17221/61/2009-PSE
Wu, Q. S., Zou, Y. N., Xia, R. X. (2006). Effects of water stress and arbuscular mycorrhizal fungi on reactive oxygen metabolism and antioxidant production by citrus (Citrus tangerine) roots. Eur. J. Soil Biol. 42, 166–172. doi: 10.1016/j.ejsobi.2005.12.006
Ye, L., Zhao, X., Bao, E., Cao, K., Zou, Z. (2019). Effects of arbuscular mycorrhizal fungi on watermelon growth, elemental uptake, antioxidant, and photosystem II activities and stress-response gene expressions under salinity-alkalinity stresses. Front. Plant Sci. 10. doi: 10.3389/fpls.2019.00863
Yousefzadeh Najafabadi, M., Ehsanzadeh, P. (2017). Photosynthetic and antioxidative upregulation in drought-stressed sesame (Sesamum indicum l.) subjected to foliar-applied salicylic acid. Photosynthetica 55, 611–622. doi: 10.1007/s11099-017-0673-8
Zaferanchi, S., Salmasi, S. Z., Salehi Lisar, S. Y., Sarikhani, M. R. (2019). Influence of organics and bio fertilizers on biochemical properties of Calendula officinalis l. Int. J. Hortic. Sci. Technol. 6, 125–136. doi: 10.22059/ijhst.2019.266831.258
Zhao, Y., Cartabia, A., Lalaymia, I., Declerck, S. (2022). Arbuscular mycorrhizal fungi and production of secondary metabolites in medicinal plants. Mycorrhiza 1-36. doi: 10.1007/s00572-022-01079-0
Zhu, X., Song, F., Liu, S., Liu, T., Zhou, X. (2012). Arbuscular mycorrhizae improves photosynthesis and water status of Zea mays l. under drought stress. Plant Soil Environ. 58, 186–191. doi: 10.17221/23/2011-PSE
Keywords: summer savory (Satureja hortensis L.), drought stress, arbuscular mycorrhiza fungi (AMF), growth parameters, chlorophyll fluorescence, antioxidant activity, essential oil
Citation: Rasouli F, Amini T, Skrovankova S, Asadi M, Hassanpouraghdam MB, Ercisli S, Buckova M, Mrazkova M and Mlcek J (2023) Influence of drought stress and mycorrhizal (Funneliformis mosseae) symbiosis on growth parameters, chlorophyll fluorescence, antioxidant activity, and essential oil composition of summer savory (Satureja hortensis L.) plants. Front. Plant Sci. 14:1151467. doi: 10.3389/fpls.2023.1151467
Received: 26 January 2023; Accepted: 12 May 2023;
Published: 05 June 2023.
Edited by:
Alejandro Rojas, University of Arkansas, United StatesReviewed by:
Agostino Sorgona’, Mediterranea University of Reggio Calabria, ItalyAbdenour Kheloufi, University of Batna 2, Algeria
Copyright © 2023 Rasouli, Amini, Skrovankova, Asadi, Hassanpouraghdam, Ercisli, Buckova, Mrazkova and Mlcek. This is an open-access article distributed under the terms of the Creative Commons Attribution License (CC BY). The use, distribution or reproduction in other forums is permitted, provided the original author(s) and the copyright owner(s) are credited and that the original publication in this journal is cited, in accordance with accepted academic practice. No use, distribution or reproduction is permitted which does not comply with these terms.
*Correspondence: Farzad Rasouli, farzad.rasouli@maragheh.ac.ir; Jiri Mlcek, mlcek@utb.cz