- 1College of Horticulture, Shenyang Agricultural University, Shenyang, Liaoning, China
- 2Key Lab of Fruit Quality Development and Regulation of Liaoning Province, Shenyang Agricultural University, Shenyang, Liaoning, China
Cadmium (Cd) is a nonessential element and highly toxic to apple tree. However, Cd accumulation, translocation and tolerance in apple trees planted in different soils remain unknown. To investigate soil Cd bioavailability, plant Cd accumulation, physiological changes as well as gene expression patterns in apple trees grown in five different soils, ‘Hanfu’ apple seedlings were planted in orchard soils collected from Maliangou village (ML), Desheng village (DS), Xishan village (XS), Kaoshantun village (KS) and Qianertaizi village (QT), and subjected to 500 μM CdCl2 for 70 d. Results showed that soils of ML and XS had higher content of organic matter (OM), clay and silt, and cation exchange capacity (CEC) but lower sand content than the other soils, thereby reduced Cd bioavailability, which could be reflected by lower concentrations and proportions of acid-soluble Cd but higher concentrations and proportions of reducible and oxidizable Cd. The plants grown in soils of ML and XS had relatively lower Cd accumulation levels and bio-concentration factors than those grown in the other soils. Excess Cd reduced plant biomass, root architecture, and chlorophyll content in all plants but to relatively lesser degree in those grown in soils of ML and XS. The plants grown in soils of ML, XS and QT had comparatively lower reactive oxygen species (ROS) content, less membrane lipid peroxidation, and higher antioxidant content and enzyme activity than those grown in soils of DS and KS. Transcript levels of genes regulating Cd uptake, transport and detoxification such as HA11, VHA4, ZIP6, IRT1, NAS1, MT2, MHX, MTP1, ABCC1, HMA4 and PCR2 displayed significant differences in roots of plants grown in different soils. These results indicate that soil types affect Cd accumulation and tolerance in apple plants, and plants grown in soils with higher OM content, CEC, clay and silt content and lower sand content suffer less Cd toxicity.
1 Introduction
Extensive sewage irrigation and metal-based pesticide and chemical fertilizer application in recent years have contaminated fruit orchards with cadmium (Cd) to varying degrees (Li et al., 2006; Karimi Nezhad et al., 2011; Argüello et al., 2019). Cd is water-soluble and readily transported from the soil to roots (Luo et al., 2016; He et al., 2020). In plants, Cd induces reactive oxygen species (ROS) production, causes oxidative stress, destroys subcellular structure, reduces photosynthesis, inhibits growth, and may cause death (Liu and Kottke, 2004; Mobin and Khan, 2007; Podazza et al., 2012; Podazza et al., 2016). Moreover, Cd in agricultural products may be ingested by humans and cause various diseases such as hypertension, kidney dysfunction and lung cancers (Chen et al., 2015).
The Cd content in plant tissues depends largely upon the Cd bioavailability in the soil and the ability of the plants to absorb soil Cd. Therefore, it is important to understand the factors affecting Cd migration from the soil to roots. After Cd enters the soil, it undergoes physicochemical transformations such as dissolution, precipitation, complexation, and adsorption and assumes different forms (Qasim and Motelica-Heino, 2014). Cd bioavailability in plants is controlled by various soil physicochemical factors, such as pH value, organic matter (OM) content, soil texture, and cation exchange capacity (CEC) (Golia et al., 2008; Karimi Nezhad et al., 2011). This relationship has been established in some crops where soil Cd mobility and plant Cd uptake are decreased at high soil pH, OM content and CEC (Vega et al., 2010; Chen et al., 2015; Chen et al., 2016; Argüello et al., 2019). In addition, soil texture is also an important factor affecting heavy metal bioavailability. Finer soil particles have relatively larger surface areas, higher secondary mineral and OM content (Hardy and Cornu, 2006; Yu et al., 2017), and greater heavy metal adsorption capacity (Luo et al., 2011). Soils of fruit orchards differ greatly in terms of these key properties in different planting regions. However, little is known about Cd accumulation and toxicity in apple trees planted in different soils.
Excess Cd induces the production of ROS including superoxide anion () and hydrogen peroxide (H2O2) which cause cell membrane lipid peroxidation, nucleic acid and protein denaturation, abnormal cell metabolism, and even cell death (PÉRez-Chaca et al., 2014; Ahammed et al., 2021). Plants activate their antioxidant defense systems including antioxidant enzymes and non-enzymatic antioxidant metabolites to mitigate oxidative damage induced by Cd (Anjum et al., 2015; Zhou et al., 2017). The antioxidant capacity of soybean under heavy metal stress displayed significant differences when planted in different soils (Melo et al., 2011). In addition, application of organic amendments affected the antioxidant defense system and alleviated Cd toxicity in mung bean (Ramzani et al., 2017), possibly because they altered the pH, CEC, OM, and other physicochemical properties of the soil (Fellet et al., 2014; Cui et al., 2016). To our knowledge, however, little is known about how Cd stress affects the antioxidant defense system of apple trees grown in different soils.
Plant Cd absorption, transport, and detoxification are key processes controlled by several genes (He et al., 2020). Plasma membrane (PM) H+-ATPase pumps protons extracellularly at the expense of ATP and creates an electrochemical gradient for transmembrane ion transport (Zhang et al., 2017). Upregulation of the gene encoding PM H+-ATPase enhanced Cd2+ absorption in plants (He et al., 2015). ZRT-IRT-like protein 6.2 (ZIP6.2) and iron transporter 1 (IRT1) are plant cells membrane localized protein and regulate extracellular Cd2+ uptake (Migeon et al., 2010; Zhu et al., 2012). Upon entry into root cells, Cd may complex with Cd effective chelators including nicotianamine (NA; encoded by nicotianamine synthase 1 (NAS1)) and metallothionein (He et al., 2020). Metal tolerance protein 1 (MTP1), magnesium proton exchangers (MHX), and ATP-binding cassette transporter C1 (ABCC1) are localized to the tonoplasts. MTP1 and MHX transport Cd2+ and ABCC1 transports PC-Cd complexes into the vacuoles (Berezin et al., 2008; Gaillard et al., 2008; Migeon et al., 2010). Plant Cd resistance protein 2 (PCR2) and HM ATPase4 (HMA4) are localized to the PMs, pump Cd into the apoplast, and play critical roles in Cd translocation from the roots to the aerial parts (Hanikenne et al., 2008; Song et al., 2010). Up to now, little is known about the gene expression patterns related to Cd absorption, transport and tolerance in apple plants grown in different soils.
‘Hanfu’ apple (Malus domestica Borkh.) is a high quality variety with cold resistance and high yield, and has become the main variety grown in Liaoning Province. In this study, according to the different characteristics of the apple orchard soil in the Liaoning Province, orchards soils in five regions were collected. To explore the differences of Cd absorption, accumulation and tolerance of apple seedlings grown in different soils, ‘Hanfu’ apple grafted onto M. baccata Borkh. with relative higher Cd tolerance (Zhou et al., 2017) were planted in five soils with different physicochemical properties and subjected to 500 μM Cd for 70 d. We hypothesized that (i) there would be variations in Cd accumulation and tolerance in apple plants grown in different soils, and (ii) these variations are associated with physiological acclimation and transcriptional regulation. To examine these hypotheses, we compared and analyzed various soil Cd forms, growth characteristics of plants, Cd concentrations, ROS, antioxidants, and the transcription level of key genes related to Cd uptake, transport, and detoxification. The obtained results are of great significance for mitigating the risk of Cd phytotoxicity in apple as well as other fruit trees through the application of amendments to modify soil properties.
2 Materials and methods
2.1 Soil sources and physicochemical properties
Soils were sampled at 0–20 cm depth from ‘Hanfu’ apple (Malus domestica Borkh.) orchards in Maliangou village in Liaoyang city (ML), Desheng village in Panjin city (DS), Xishan village in Chaoyang city (XS), Kaoshantun village in Xinmin city (KS), and Qianertaizi village in Xinmin city (QT) in Liaoning Province, China. Visible residues were removed from the samples and they were then passed through a 2-mm mesh sieve and air-dried for the subsequent experiment.
The pH value was determined for each 1:2.5 (soil/water (w/v)) suspension by pH meter (PHS-3C; Shanghai Leici Equipment Factory, China). OM was analyzed according to Sparks et al. (1996). CEC was measured as described previously (Gaskin et al., 2008). The content of clay, silt, and sand were determined using a laser particle size analyzer (MS3000; Malvern PANanalytical, Malvern, UK). The initial soil Cd concentration was measured by the procedure described by Wu et al. (2014).
2.2 Plant material and Cd exposure
Full buds on branches of ‘Hanfu’ apple were grafted on annual seedlings of M. baccata Borkh., and planted in a plastic pots (one plant pot-1) containing 2.5 kg soil in a greenhouse with natural photoperiod (day/night temperature, 25/17°C; relative air humidity, 50%–60%), and watered every dusk. When the plants grew to about 20 cm, 120 plants with similar heights were divided equally to ten groups, and transplanted them in different soils collected from five regions. After two weeks, 100 mL of 500 μM CdCl2 or distilled water was irrigated to the plants of each group in every day, and the final soil Cd content was 156.8 mg kg-1. A plastic tray was placed under each pot to collect the soil leachate which was poured back into the pot to prevent Cd leaching. The soil was regularly irrigated with distilled water, and the soil moisture of each pot was maintained at approximately 70% of field water capacity. The concentration of Cd treatment was used on the basis of previous study (Abdel Latef, 2013). Three replicates for each treatment were conducted, and each replicate contained 4 plants. Plants were harvested after Cd treatment for 70 d.
2.3 Soil collection and plant harvest
The rhizosphere soil of each treatment was collected by shaking off the roots in the air, and then air-dried and then passed through a 2-mm mesh sieve. The dust and soil on the surface of plants were washed with deionized water, and then the roots of each plant were rinsed in 50 mM CaCl2 solution for 5 minutes to remove Cd from the root surface (He et al., 2013b). Subsequently, the roots were carefully washed with deionized water (He et al., 2013b). After separating into the roots, stems and leaves and recording their fresh weight, different tissues were immediately frozen in liquid nitrogen. The frozen samples were then ground to fine powder using a ball mill (MM400; Retsch, Haan, Germany) and subsequently stored at -80°C. The fresh material of each tissue per plant was dried at 60°C for 72 h to analyze the fresh-to-dry mass ratio.
2.4 Determination of Cd forms in soil
The content of Cd chemical forms including acid-soluble Cd, reducible Cd, oxidizable Cd and residual Cd in soil samples was measure by European Community Bureau of Reference (BCR) sequential extraction method (Sungur et al., 2014b). After digestion of residues with a mixture of acids [HNO3/H2O2/HF (3/2/1 (v/v/v)] using a microwave digestion system (MARS 6 CLASSIC; CEM Corporation, Matthews, NC, USA) as described by Wu et al. (2014), the Cd levels in the extract and digest were measured using a flame atomic absorbance spectrometry (FAAS; Hitachi 180-80; Hitachi Ltd., Tokyo, Japan).
2.5 Analysis of root characteristics and photosynthetic pigments
The WinRHIZO Root Analyzer System (WinRHIZO 2012b; Regent Instruments Canada Inc., Montreal, Canada) was used to measure the total root length, total root surface area and total root volume based on the method of Ma et al. (2014). Chlorophyll and carotenoids contents in the leaves of plants were determined using a spectrophotometer (UV-3802; Unico Instruments Co. Ltd, Shanghai, China) according to the method of He et al. (2011).
2.6 Analysis of Cd concentration, total Cd and bio-concentration factor (BCF)
Frozen samples (ca. 100 mg) of each tissue were digested at 170°C in a mixture containing HNO3/HClO4 (7/1 (v/v)) as previously described (Zhou et al., 2016). The content of Cd in each tissue was determined by flame atomic absorption spectrometry (Hitachi 180-80; Hitachi Ltd, Tokyo, Japan). The total Cd amounts of each tissue were calculated based on the concentration of Cd in each tissue multiplied by the dry mass of the corresponding tissue. BCF was calculated using Cd concentration (μg g-1 DW) in root or aerial parts tissues divided by the Cd concentration (μg g-1 DW) in the soil (He et al., 2015).
2.7 Measurement of O2 ·−, H2O2 and MDA
The and H2O2 concentrations of each tissue were determined spectrophotometrically at 530 and 410 nm, respectively, as recommended by He et al. (2011). According to Lei et al. (2007), the MDA concentrations in each tissue of plants were analyzed at 450, 532 and 600 nm with a spectrophotometer.
2.8 Analysis of non-enzymatic and enzymatic antioxidants
The contents of non-enzymatic metabolites including free proline, ascorbate (ASC), T-SH and soluble phenolics in each tissue of plants were measured by the procedure described by He et al. (2013a).
The soluble protein contents in each sample were measured by the procedure described by Luo et al. (2008). The activities of SOD, CAT, POD and ascorbate peroxidase (APX) were measured as previously described by He et al. (2011), and the activities of glutathione reductase (GR) were determined as described by Wang et al. (2013).
2.9 Analysis of gene transcription levels
According to Zhou et al. (2016), the transcript level of genes was analyzed. Root total RNA was extracted and purified based on the manufacturer’s instructions by a RNA extraction kit (R6827, Omega Bio-Tek, Norcross, GA, USA). Then a spectrophotometer (NanoDrop 2000; Thermo Fisher Scientific, Waltham, MA, USA) and agarose gel electrophoresis was used to determine the concentration and quality of total RNA, respectively. Subsequently, 1 μg of total RNA was used to synthesize first-strand cDNA using a PrimeScript RT Reagent Kit with gDNA Eraser (DRR037A, Takara, Dalian, China) following the manufacturer’s protocol. Quantitative PCR for each gene was run with 10 μL 2× SYBR Green Premix Ex Taq II (DRR820A, Takara), 0.5 μL cDNA and 0.2 μM each gene-specific primer (Table S1). β-Actin was used as a reference gene (Table S1). 2-ΔΔCT method was used to calculate the relative mRNA expression. For each gene in the roots, expression level was set to 1 in ‘Hanfu’ apple plants grown in soil of ML subjected to 0 μM CdCl2. Corresponding fold changes in other treatments were then calculated accordingly. Gene expression heatmap was generated based on log2 average expression fold values.
2.10 Statistical analysis
All statistical analyses were processed using Statgraphics (STN, St Louis, MO, USA) after confirming their normality. For all physiological parameters, two-way ANOVA was employed using Cd treatment (Cd) and soil (S) as factors. To reduce the error of type I, Tukey-HSD method was used to correct the P-value obtained from multiple comparisons. When the P-value was < 0.05, differences between treatment means were considered significant. Pearson’s correlation analysis was used to determine the relationships between soil Cd forms and soil properties as well as Cd content in plant tissues. For the principal component analysis (PCA), the data of plant growth related parameters, photosynthetic pigments, Cd concentrations, ROS and antioxidant levels in the roots, stems, and leaves were standardized and subsequently computed by the command prcomp () in R (http://www.r-project.org/), as suggested by He et al. (2015).
3 Results
3.1 Soil physicochemical properties
There were significant differences among the five soil types in terms of their physicochemical properties and initial Cd content (Table 1). The pH value of soil of KS was the lowest while those of soils of DS and QT were considerably higher than those of all others. Soils of DS, KS, and QT had relatively lower OM content, CEC, clay and silt content and relatively higher sand content than soils of ML and XS. Initial Cd content was higher in soil of XS than that in all others (Table 1).
3.2 Cd content and proportion in various soils
The acid-soluble Cd content was lower in soils of ML and XS than soils of DS and KS (Figure 1A). However, the reducible and oxidizable Cd contents in soils of ML and XS were dramatically higher than those in the other soils (Figures 1B, C). The residual Cd content was remarkably higher in soils of DS and QT than the other soils (Figure 1D).
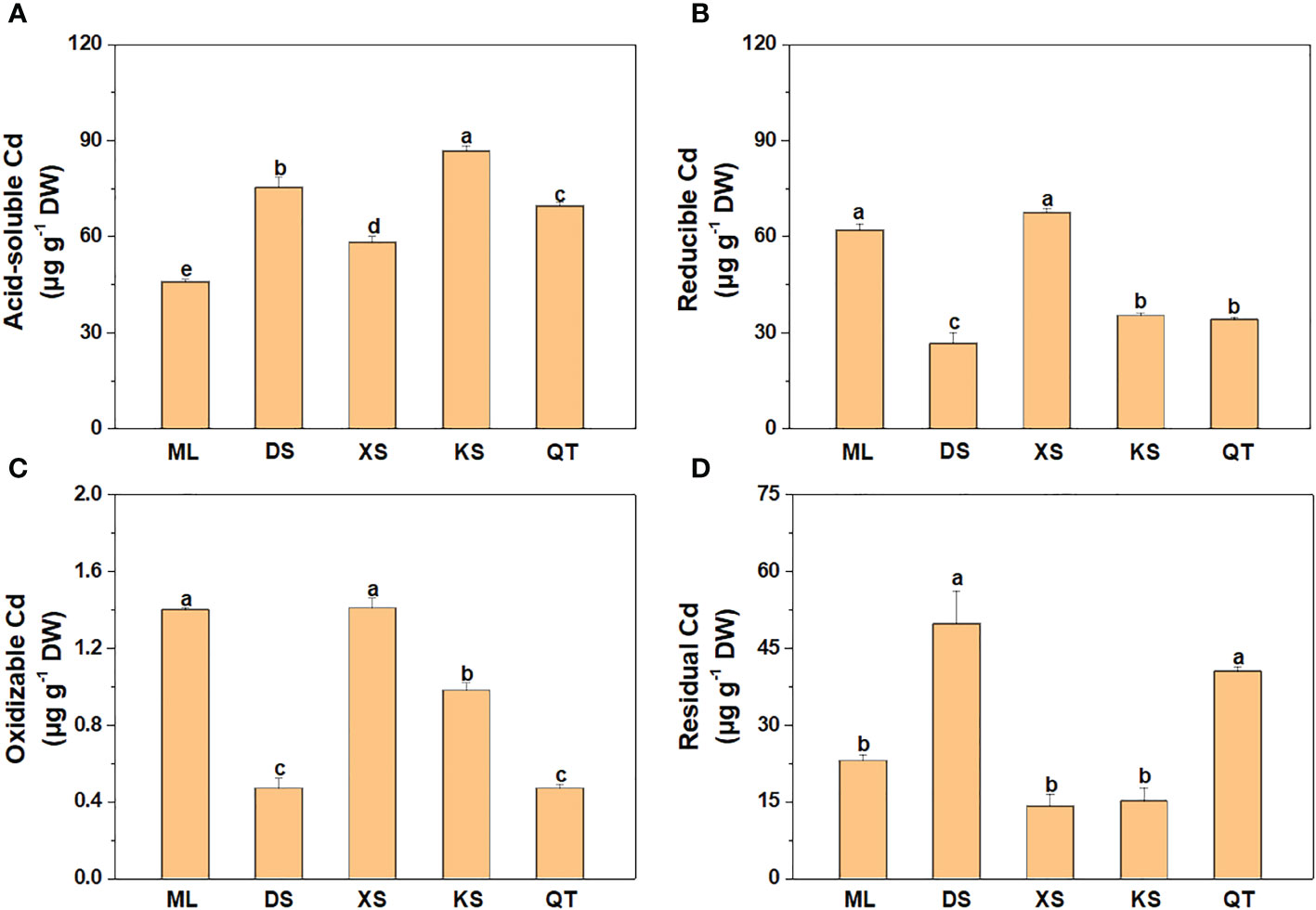
Figure 1 Cd concentrations of acid-soluble (A), reducible (B), oxidizable (C), and residual (D) forms in soils sampled from five apple orchards subjected to 500 μM CdCl2 for 70 d. Data are means ± SE (n = 3). Different letters on bars indicate significant differences between soils. ML, Maliangou village in Liaoyang city; DS, Desheng village in Panjin city; XS, Xishan village in Chaoyang city; KS, Kaoshantun village in Xinmin city; QT, Qianertaizi village in Xinmin city.
The proportions of acid-soluble and reducible Cd were highest in all five soils followed by residual and oxidizable Cd (Figure 2). The proportions of acid-soluble Cd were significantly lower in soils of ML and XS than soils of DS and KS. By contrast, the proportions of reducible and oxidizable Cd were markedly higher in soils of ML and XS than the other soils. The percentages of residual Cd were highest in soils of DS and QT (Figure 2).
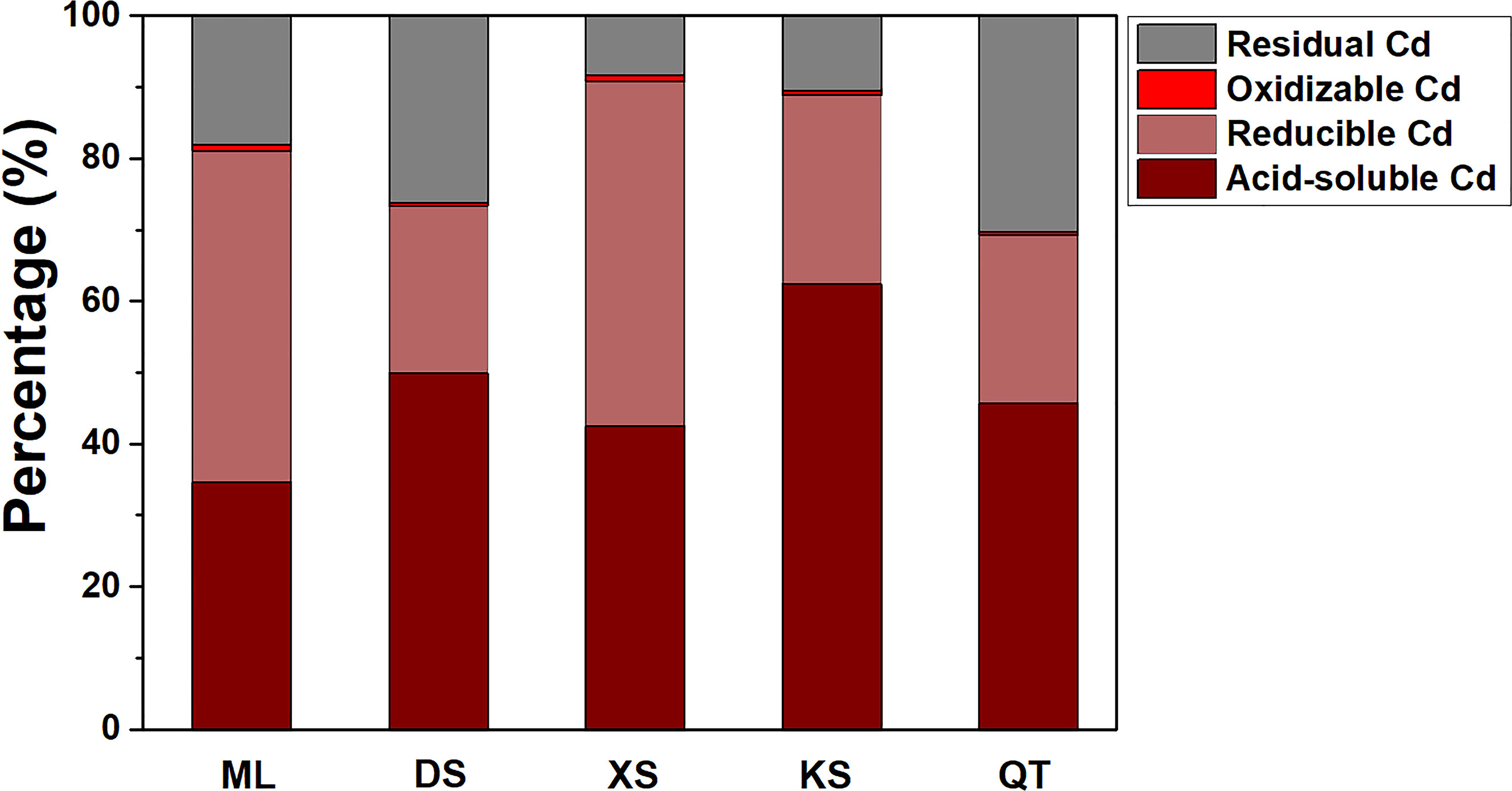
Figure 2 Proportions of various Cd fractions in soils sampled from five apple orchards subjected to 500 μM CdCl2 for 70 d. Data are means ± SE (n = 3). ML, Maliangou village in Liaoyang city; DS, Desheng village in Panjin city; XS, Xishan village in Chaoyang city; KS, Kaoshantun village in Xinmin city; QT, Qianertaizi village in Xinmin city.
3.3 Plant Cd concentration, total Cd and BCF
Under Cd stress, the roots of the plants grown in soils of DS and KS accumulated more Cd than those of the plants grown in soils of ML, XS, and QT (Figure 3A). The Cd concentrations in the stems of the plants grown in soils of ML and XS were significantly lower than those of the plants grown in the other three soils (Figure 3B). The Cd concentrations were 135.5–212.5% higher in the leaves of the plants grown in soil of DS than they were in those of the plants grown in the other four soils (Figure 3C).
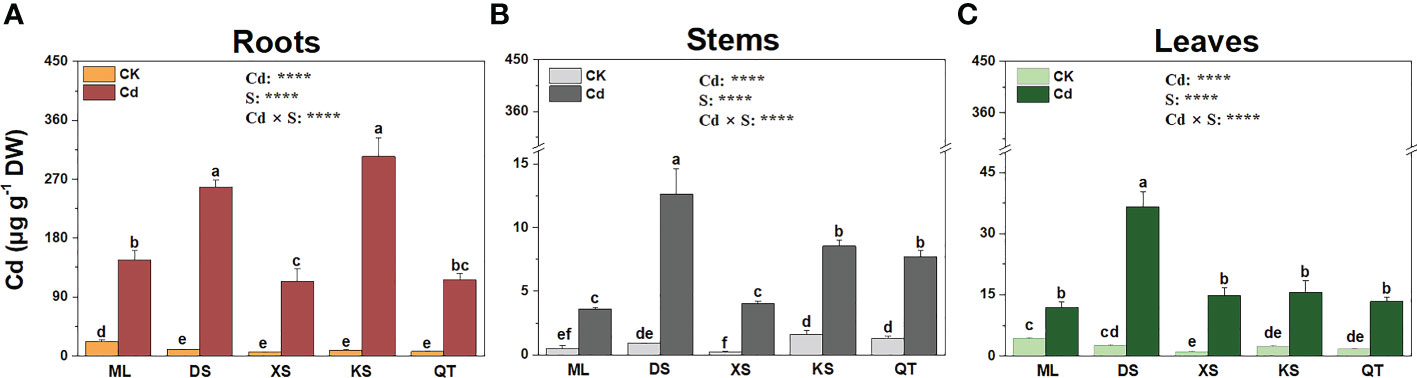
Figure 3 Cd concentrations in roots (A), stems (B), and leaves (C) of ‘Hanfu’ apple plants grown in soils sampled from five apple orchards subjected to 0 (CK) or 500 μM CdCl2 (Cd) for 70 d. Data are means ± SE (n = 3). Different letters on bars indicate significant differences between treatments. P-values indicated for ANOVA of Cd treatment (Cd), soil (S), and their interaction (Cd × S). ****P < 0.0001. ML, Maliangou village in Liaoyang city; DS, Desheng village in Panjin city; XS, Xishan village in Chaoyang city; KS, Kaoshantun village in Xinmin city; QT, Qianertaizi village in Xinmin city.
Under Cd stress, the total Cd was highest in the roots of the plants grown in soil of KS and lowest in those of the plants grown in soil of XS. The total Cd was significantly lower in the aerial parts of the plants grown in soils of ML and XS than those of the plants grown in other soils (Figure 4A). After Cd exposure for 70 d, the BCFs were markedly higher in the roots and aerial parts of the plants grown in soils of DS and KS than they were in those of the plants grown in soils of ML, XS, and QT (Figure 4B).
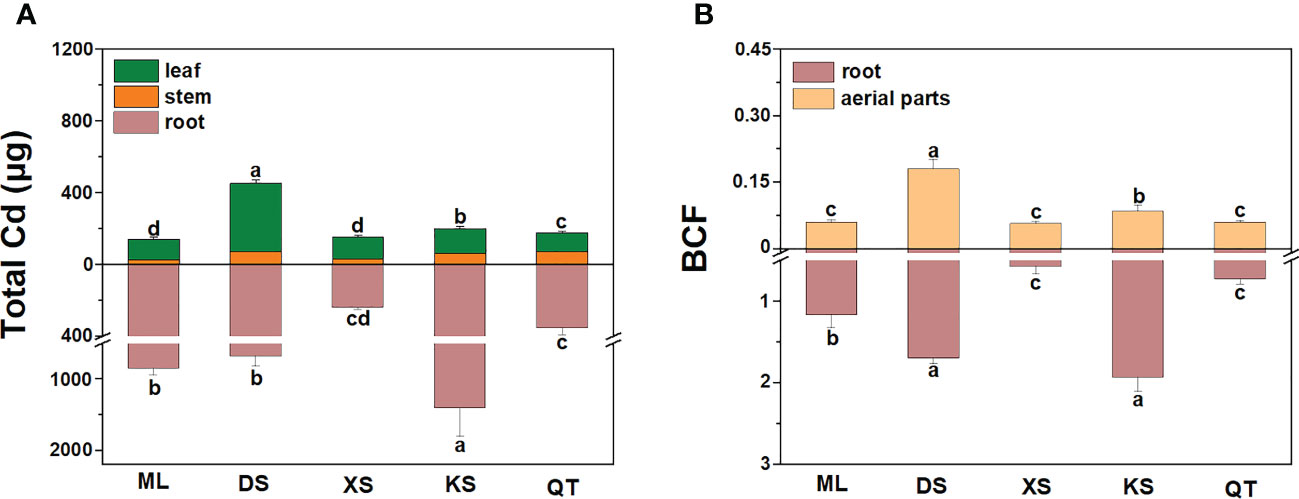
Figure 4 Total plant Cd content (A) and bio-concentration factor (BCF, B) of roots and aerial parts of ‘Hanfu’ apple plants grown in soils sampled from five apple orchards subjected to 500 μM CdCl2 for 70 d. Data are means ± SE (n = 3). Different letters on bars indicate significant differences between soils. ML, Maliangou village in Liaoyang city. DS, Desheng village in Panjin city; XS, Xishan village in Chaoyang city; KS, Kaoshantun village in Xinmin city; QT, Qianertaizi village in Xinmin city.
3.4 Coefficients of correlation between content of different forms of Cd and soil physicochemical properties, and Cd content in various ‘Hanfu’ apple plant tissues
Acid-soluble Cd was significantly negatively correlated with CEC and silt content but significantly positively correlated with sand content (Table S2). Reducible and oxidizable Cd was significantly positively correlated with OM content, CEC, and clay and silt content but significantly negatively correlated with sand content (Table S2). Oxidizable Cd was significantly positively correlated with pH value (Table S2). Residual Cd was significantly positively correlated with pH value and sand content but significantly negatively correlated with OM content, CEC, and content of clay and silt (Table S2). In general, root, stem and leaf Cd concentrations of apple plants were significantly positively correlated with acid-soluble Cd but negatively correlated with reducible and oxidizable Cd (Table S3).
3.5 Influences of Cd stress on growth characteristics of apple plants grown in different soils
Relative to the untreated control, Cd stress markedly reduced the dry mass of root, stem, and leaf of the plants grown in all five soils (Table 2). Whereas the root dry mass of the plants grown in soils of ML and XS was only slightly inhibited, the root dry mass of the plants grown in soil of DS was inhibited to the greatest extent (Table 2). There were no marked differences in the inhibition degree of Cd stress on stem dry mass of plants grown in different soil types. After Cd exposure, foliar biomass was inhibited in all plants but to relatively lesser degree in those grown in soils of ML and KS (Table 2).
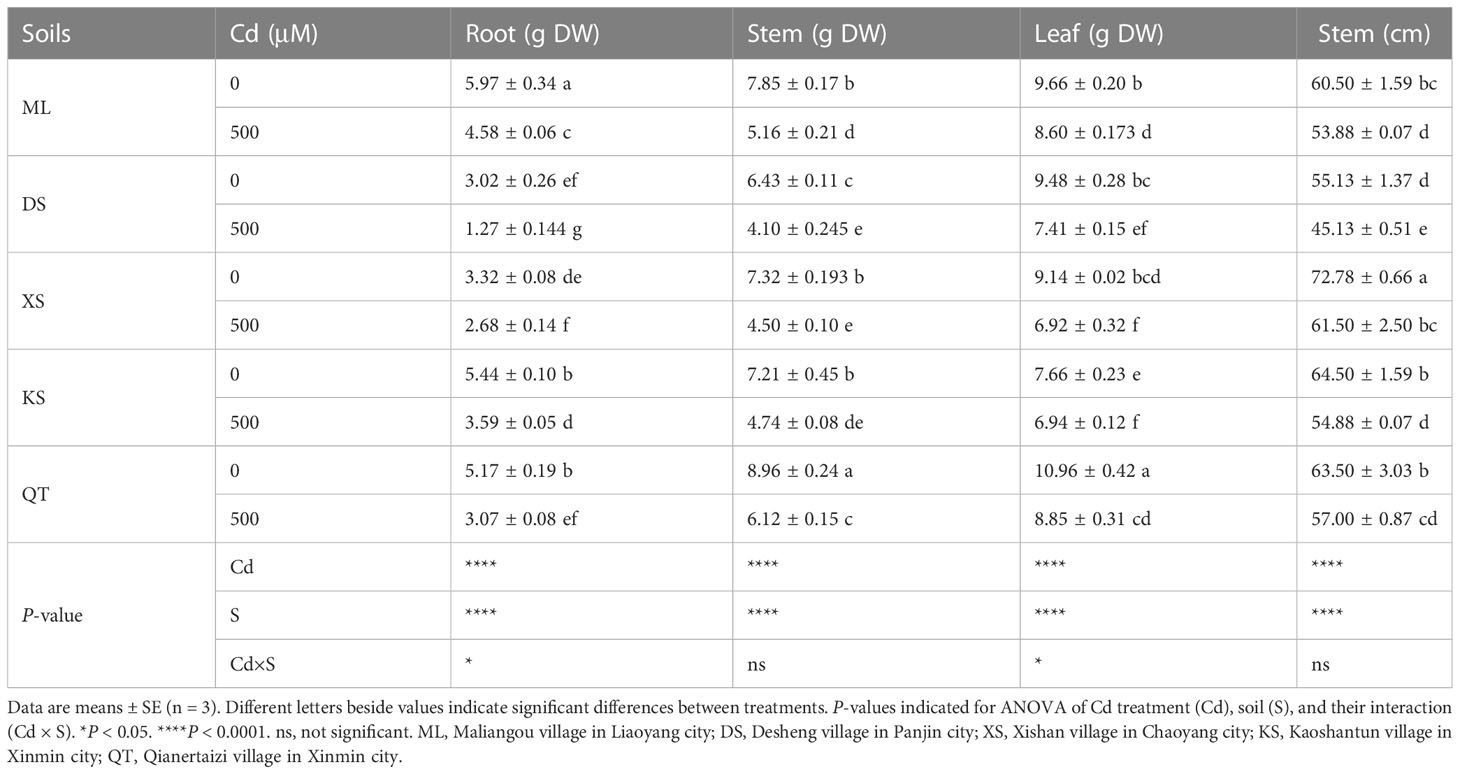
Table 2 Dry mass (g) of roots, stems, and leaves and lengths of stems of ‘Hanfu’ apple plants grown in soils sampled from five apple orchards subjected to 0 or 500 μM CdCl2 for 70 d.
The total root length, total root surface area, and total root volume of the ‘Hanfu’ plants significantly decreased after Cd stress. However, these negative effects were relatively less pronounced in the plants grown in soil of ML and relatively more pronounced in those grown in soil of DS (Table S4). The chlorophyll content considerably differed among the plants grown in different soils (Table S4). Cd stress reduced chlorophylls a, b, (a+b), and carotenoid in all plants but to a greater extent in those grown in soil of KS than the others (Table S4).
3.6 Effects of Cd stress on O2 ·−, H2O2, and MDA in plants grown under different soil conditions
Cd exposure significantly increased accumulation in the roots of the plants grown in soils of DS, XS and KS (Figure 5). Cd exposure induced a more pronounced increase in concentrations in the stems of plants grown in the soils of DS and QT (Figure 5). After Cd exposure, the concentrations were significantly increased in the leaves of the plants grown in all soils except for soil of ML (Figure 5). Cd exposure significantly increased the H2O2 content in the roots of all plants except those grown in soil of XS, in the stems of all plants except those grown in soils of ML and XS, and in the leaves of all plants except those grown in soil of DS (Figure 5). Cd induced H2O2 accumulation to the greatest extent in the roots and leaves of the plants grown in soil of KS and in the stems of the plants grown in soil of DS (Figure 5). Compared with the control, Cd exposure had no effects on the MDA concentration in the roots, stems, or leaves of the plants grown in soils of ML, XS, and QT but significantly increased it in the same organs of the plants grown in soils of DS and KS (Figure 5).
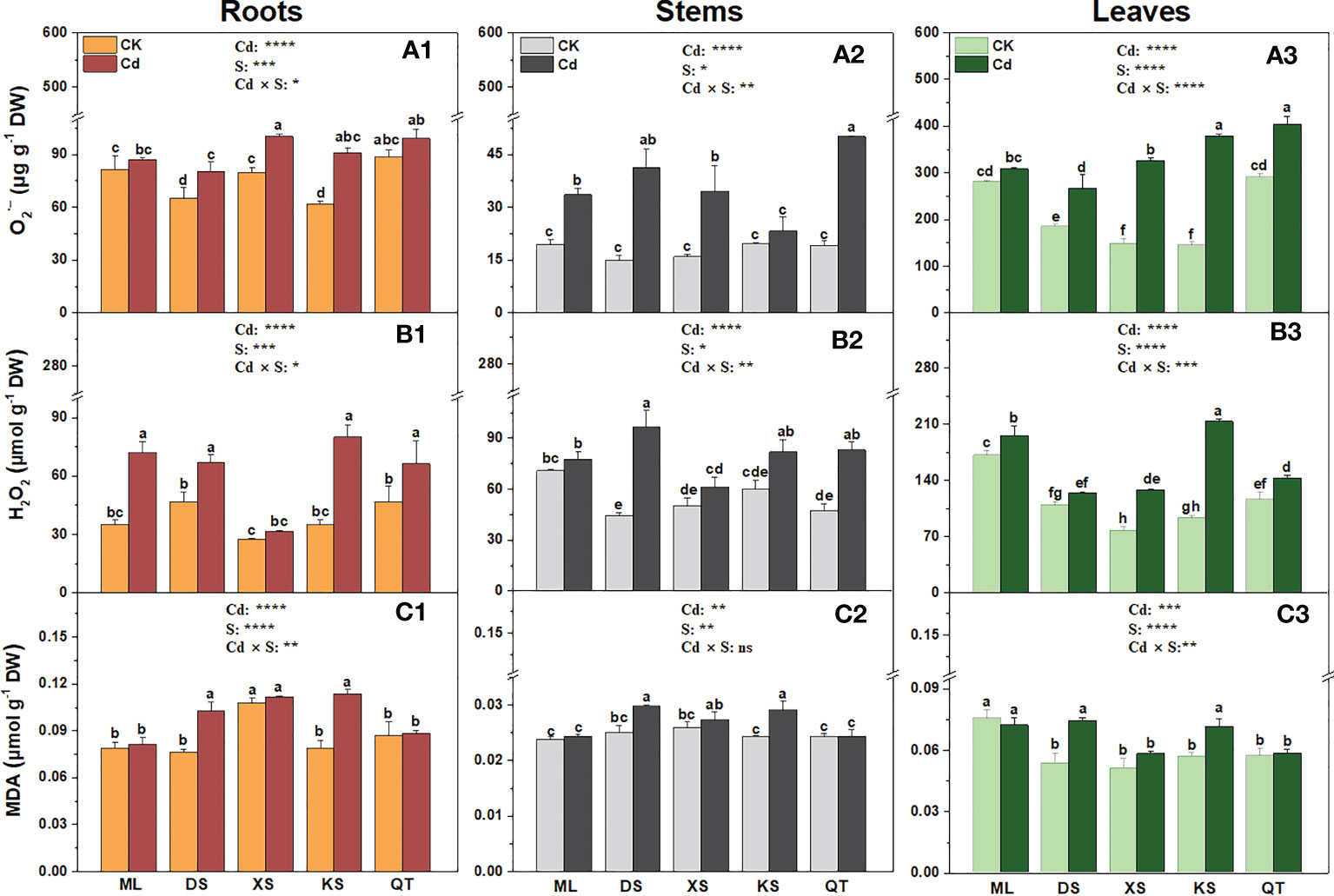
Figure 5 Superoxide anion (, A1-A3), hydrogen peroxide (H2O2, B1-B3) and malondialdehyde (MDA, C1-C3) in roots, stems and leaves of ‘Hanfu’ apple plants grown in soils sampled from five apple orchards subjected 0 (CK) or 500 μM CdCl2 (Cd) for 70 d. Data are means ± SE (n = 3). Different letters on bars indicate significant differences between treatments. P-values indicated for ANOVA of Cd treatment (Cd), soil (S), and their interaction (Cd × S). *P < 0.05. **P < 0.01. ***P < 0.001. ****P < 0.0001. ns, not significant. ML, Maliangou village in Liaoyang city; DS, Desheng village in Panjin city; XS, Xishan village in Chaoyang city; KS, Kaoshantun village in Xinmin city; QT, Qianertaizi village in Xinmin city.
3.7 Effects of Cd stress on the antioxidant systems of plants grown in different soils
Compared to the control, Cd stress significantly increased the free proline content in the roots of the plants grown in all soils except soil of ML (Figure 6A1). Cd exposure significantly lowered the free proline concentration in the stems of the plants grown in soils of DS and KS and in the leaves of the plants grown in soil of KS (Figures 6A2, A3). After Cd exposure, the ASC content increased in the roots and stems of the plants grown in soil of ML and the leaves of the plants grown in all soils except for soil of QT. However, it decreased in the roots of the plants grown in soils of DS and KS (Figures 6B1–B3). Under Cd stress, the T-SH content was highest in the roots of the plants grown in soil of ML, the stems of the plants grown in soil of XS, the leaves of the plants grown in soil of KS (Figures 6C1–C3). Cd exposure significantly decreased the soluble phenolic content in the roots of the plants grown in soils of DS and XS, but slightly increased the soluble phenolic content in the stems of the plants grown in soils of XS and KS and the leaves of the plants grown in soil of ML (Figures 6D1–D3).
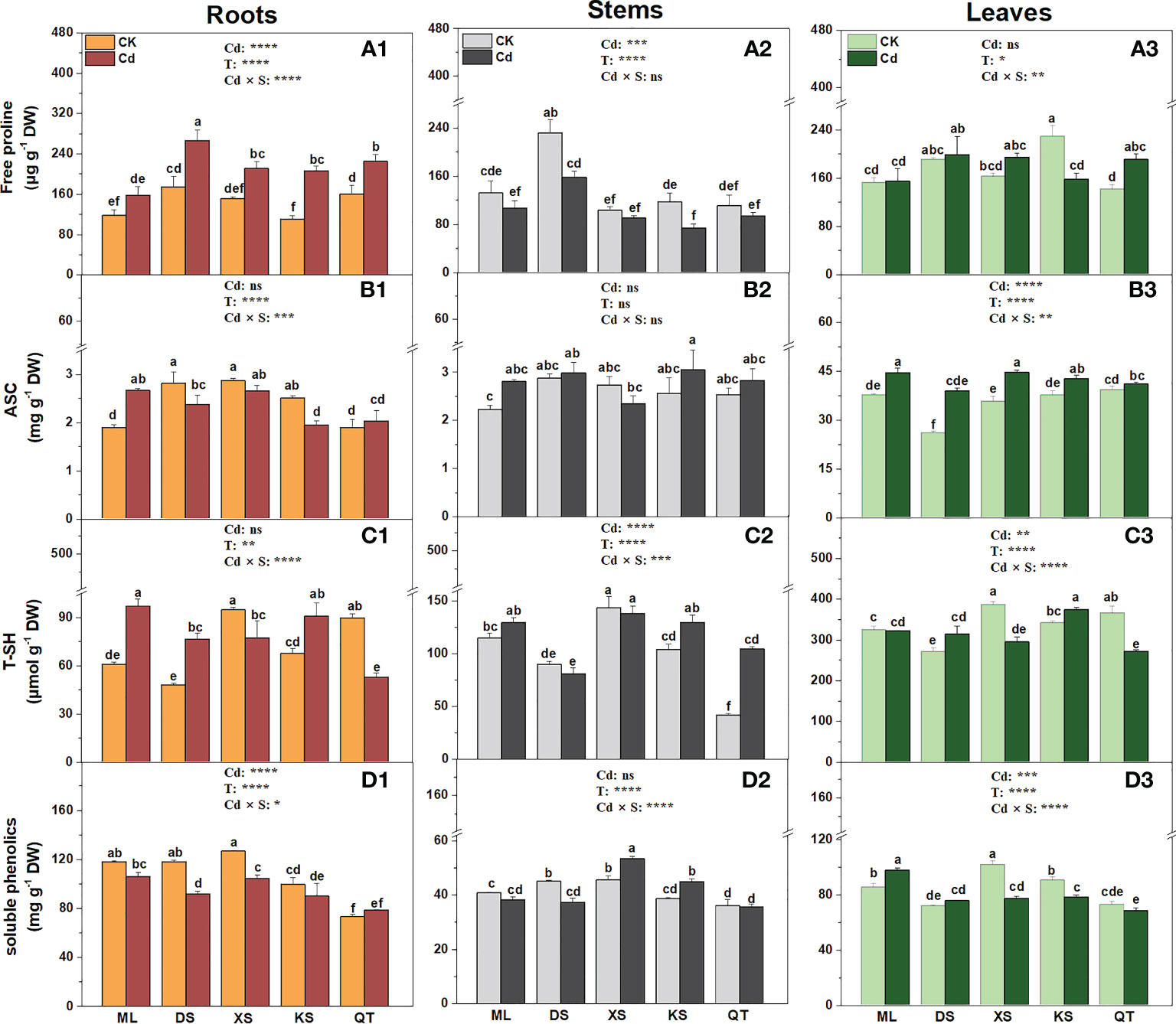
Figure 6 Free proline (A1-A3), ascorbate (ASC) (B1-B3), total thiols (T-SH) (C1-C3) and soluble phenolics (D1-D3) in the roots (A1-D1), stems (A2-D2) and leaves (A3-D3) of ‘Hanfu’ apple plants grown in the soils sampled from five apple orchards subjected to 0 (CK) or 500 μM CdCl2 (Cd) for 70 d. Data indicate means ± SE (n = 3). Different letters on the bars indicate significant differences between the treatments. P-values indicated for ANOVA of Cd treatment (Cd), soil (S) and their interaction (Cd × S). *P < 0.05. **P < 0.01. ***P <0.001. ****P < 0.0001. ns, not significant. ML, Maliangou village in Liaoyang city; DS, Desheng village in Panjin city; XS, Xishan village in Chaoyang city; KS, Kaoshantun cillage in Xinmin city; QT, Qianertaizi village in Xinmin city.
After Cd exposure, the SOD was significantly enhanced by 98.7%, 344.5%, and 105.9% in the roots of the plants grown in soils of DS, XS, and KS, respectively (Figure S1A1). In general, Cd exposure increased SOD activity in the stems and leaves of all plants except the leaves of plants grown in soil of XS and QT (Figures S1A2, A3). Irrespective of Cd treatment, SOD activity was always higher in the stems of the plants grown in soil of ML than it was in those of the plants grown in all other soils (Figure S1A2). Generally, Cd exposure increased POD activity in all tissues of the plants grown in most soils compared to those under control conditions (Figures S1B1–B3). The highest POD activity was observed in the roots of the plants grown in soil of QT and the stems of the plants grown in soil of ML (Figures S1B1, B2). Cd exposure generally increased CAT in the roots and stems of most plants especially in the stems of the plants grown in soil of XS (Figures S1C1, C2). By contrast, CAT activity increased only in the leaves of the plants grown in soil of DS (Figure S1C3). Compared with the control, APX activity was elevated in the roots of all plants except those grown in soil of KS (Figure S1D1). By contrast, APX activity was only increased in the stems of the plants grown in soils of DS and QT. The leaves of the plants grown in all five soil types did not differ in terms of APX activity following Cd exposure (Figures S1D2, D3). GR activity increased only in the roots of the plants grown in soil of QT, the stems of the plants grown in soils of ML and XS, and the leaves of the plants grown in soil of DS (Figures S1E1–E3).
3.8 PCA of physiological responses to Cd in apple plants grown under different soil conditions
To elucidate the response patterns of ‘Hanfu’ apple plants to Cd stress, a PCA was conducted using data associated with plant growth, photosynthetic pigments, Cd concentrations, and the ROS and antioxidant levels in the roots, stems, and leaves (Figure 7; Table S5). The effect of Cd treatment was separated by PC1 and its contribution rate was 31.4% (Figure 7). The dominant components in PC1 were root Cd concentration and free proline content, stem dry mass and Cd content, and leaf Cd content (Table S5). However, PC2 was affected by soil and its contribution rate was 15.2% (Figure 7). The dominant factors in PC2 were root POD and GR content, stem SOD content, and leaf H2O2 and SOD content (Table S5). The PCA showed that plants grown in various soils demonstrated different physiological responses to Cd exposure mainly because of the relative differences in their root POD and GR content, their stem SOD content, and their leaf H2O2 and SOD content. In the PCA plot, the shorter distances between CK and Cd treatment in soils of ML and XS indicated that the plants grown in these soils were relatively less affected by Cd exposure at the physiological level.
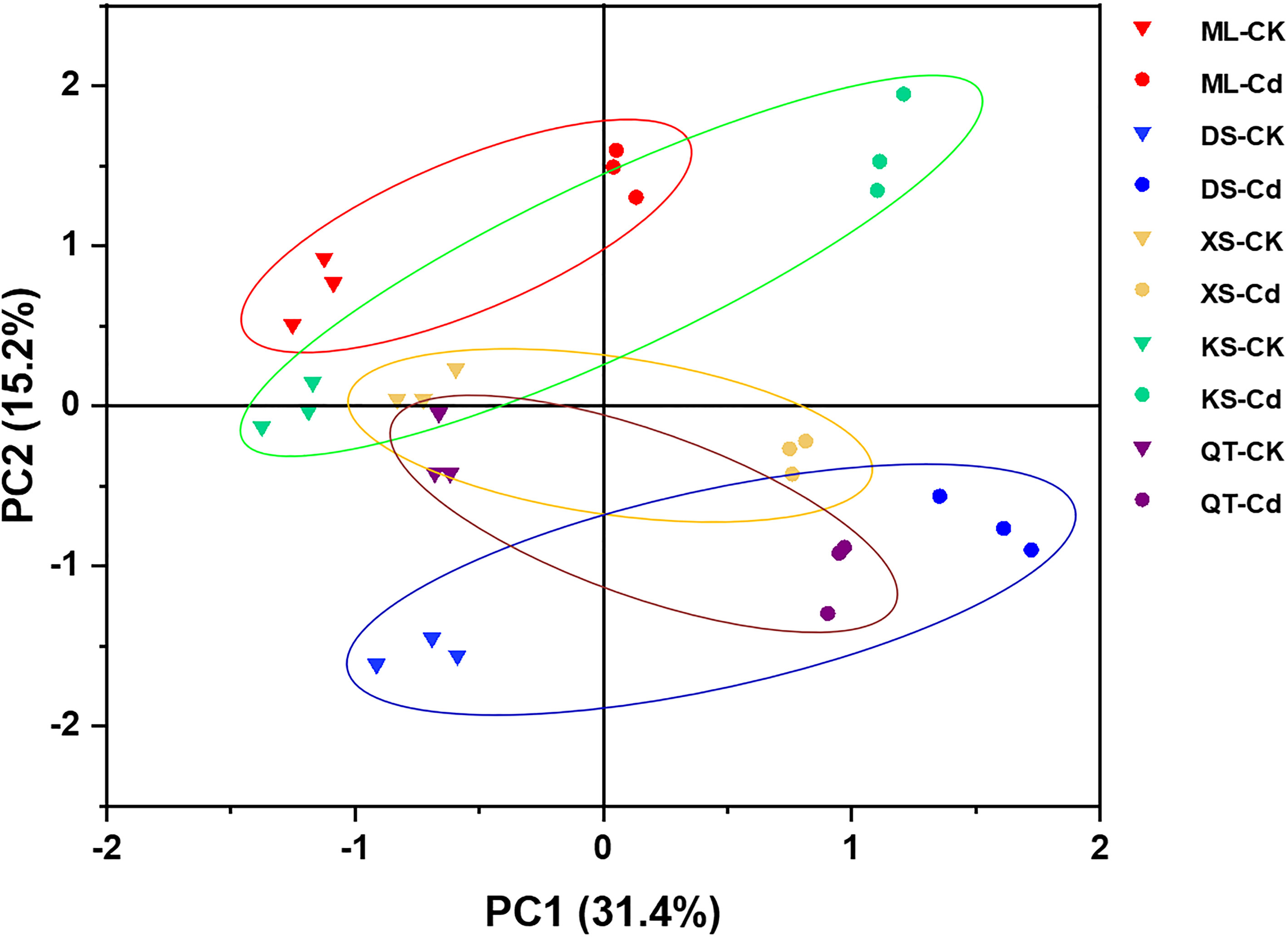
Figure 7 Principal component analysis (PCA) of plant growth related parameters, photosynthetic pigments, Cd concentrations, ROS and antioxidant levels in roots, stems, and leaves of apple plants grown in soils sampled from five apple orchards subjected to 0 (CK) or 500 μM CdCl2 (Cd) for 70 d. Triangles and circles represent 0 (CK) and 500 μM CdCl2 (Cd) treatments, respectively. Different colors represent different soils. Red, Maliangou village in Liaoyang city. Blue, Desheng village in Panjin city. Yellow, Xishan village in Chaoyang city. Green, Kaoshantun village in Xinmin city. Purple, Qianertaizi village in Xinmin city. PCA loadings presented in Table S5.
3.9 Transcriptional changes of the genes involved in Cd absorption, transport, and detoxification
PM H+-ATPase pumps protons out of cells by consuming ATP, creates an electrochemical gradient for transmembrane ion transport (Zhang et al., 2017), and is encoded by several genes including HA11 and VHA4. Cd exposure downregulated HA11 in the roots of the plants grown in soils of ML, XS, and KS by 7.14-fold, 1.43-fold, and 1.58-fold, respectively (Figure 8). By contrast, Cd exposure upregulated HA11 in the roots of the plants grown in soils of DS and QT, relative to their respective controls (Figure 8). Compared to untreated controls, the roots of the plants grown in soils of ML and QT presented with VHA4 downregulation. However, the opposite trend was observed in the roots of the plants grown in all other soils. ZIP and IRT1 transport Cd2+ intracellularly and regulate plant Cd absorption (Migeon et al., 2010; Wu et al., 2015). Compared with the control, Cd exposure upregulated ZIP6 in the roots of the plants grown in soil of DS but downregulated it in the roots of the plants grown in all other soils and especially those of the plants grown in soil of XS. Cd exposure upregulated IRT1 in the roots of the plants grown in all soils but especially in those of the plants grown in soil of DS (Figure 8).
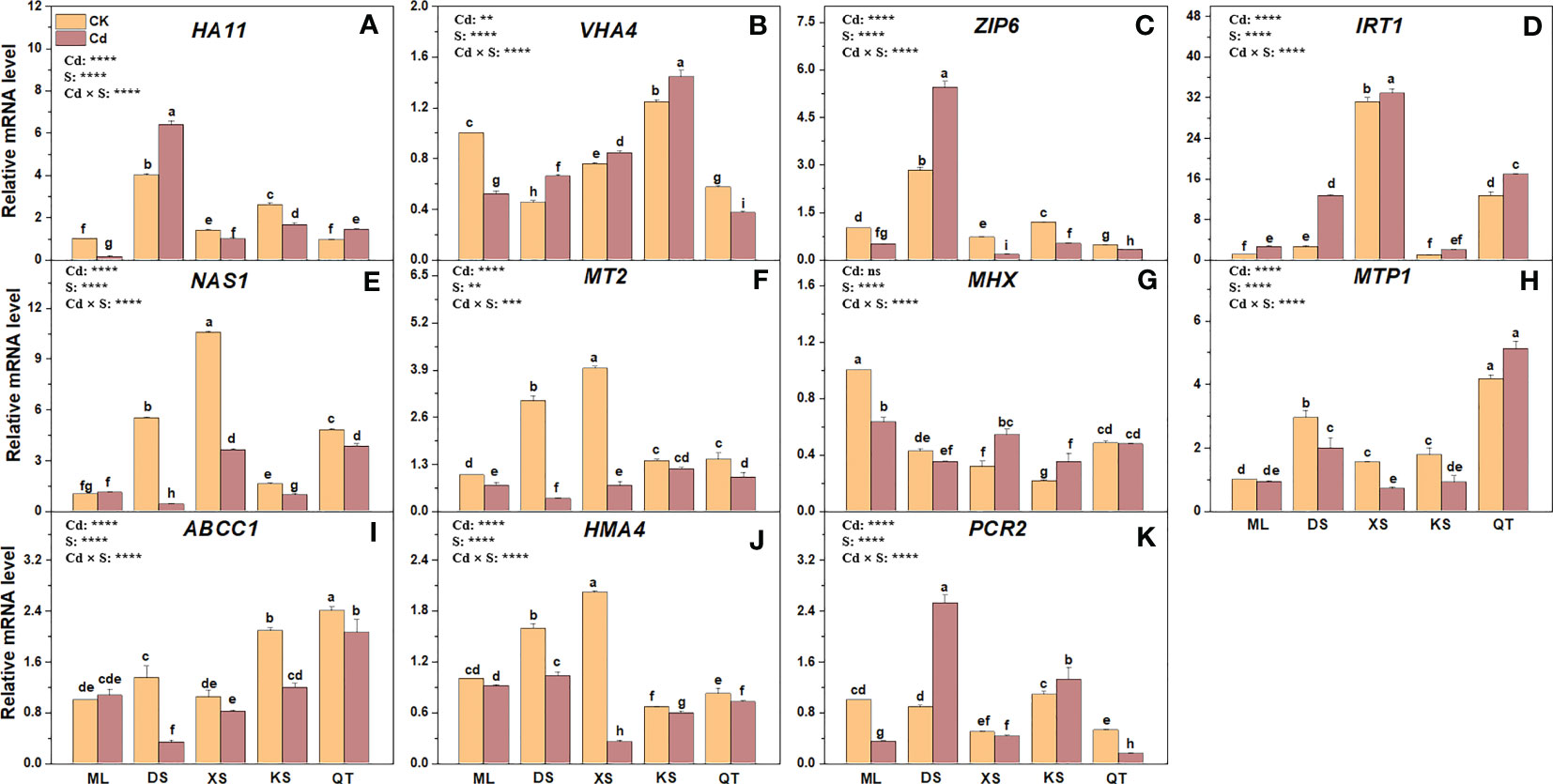
Figure 8 Relative expression levels of genes encoding proteins regulating Cd absorption (A–D) detoxification (E–I) and transport (J, K) in roots of ‘Hanfu’ apple plants grown in soils sampled from five apple orchards subjected to 0 (CK) or 500 μM CdCl2 (Cd) for 70 d. Data are means ± SE (n = 3). Different letters on the bars indicate significant differences between the treatments. For each gene, expression level was normalized to the reference gene β-Actin and the expression level was set to 1 in the roots of ‘Hanfu’ apple plants grown in soil of ML subjected to 0 μM CdCl2. Corresponding fold changes in other treatments were then calculated accordingly. P-values indicated for ANOVA of Cd treatment (Cd), soil (S) and their interaction (Cd × S). **P < 0.01. ***P < 0.001. ****P < 0.0001. ns, not significant. ML, Maliangou village in Liaoyang city; DS, Desheng village in Panjin city; XS, Xishan village in Chaoyang city; KS, Kaoshantun village in Xinmin city; QT, Qianertaizi village in Xinmin city.
NAS1 and MT2 may regulate Cd detoxification in plants (Luo et al., 2016). Cd exposure did not affect NAS1 expression in the roots of the plants grown in soil of ML but markedly downregulated NAS1 in those of the plants grown in the other soils and especially soil of DS (Figure 8). Cd exposure downregulated MT2 in the roots of the plants grown in all soils and especially in those of the plants grown in soil of DS (Figure 8). MHX, MTP1 and ABCC1 are localized to the tonoplast and transfer Cd2+ or the Cd-PC complex to the vacuole for storage (Berezin et al., 2008; Lin and Aarts, 2012; Park et al., 2012). Compared with the control, Cd exposure upregulated MHX only in the roots of the plants grown in soils of XS and KS. Relative to the control, Cd exposure markedly downregulated MTP1 in the roots of all plants except those grown in soils of ML and QT (Figure 8). Compared with the control, Cd exposure downregulated ABCC1 in the roots of the plants grown in soils of DS and KS but not in the roots of the plants grown in soils of ML and XS (Figure 8).
PM-bound transporters such as HMA4 and PCR2 export Cd from the cytosol, facilitating Cd translocation into the central cylinder for xylem transport (Hanikenne et al., 2008; Song et al., 2010). Cd treatment caused downregulation of HMA4 in the roots of the plants grown in all soils but to a greater extent in the roots of those grown in soil of XS (Figure 8). After Cd exposure, PCR2 was downregulated in the roots of the plants grown in soils of ML and QT but upregulated in the roots of the plants grown in soils of DS and KS (Figure 8).
4 Discussion
4.1 Variations in Cd accumulation and tolerance in apple plants grown in different soils
Heavy metals in soils are compartmentalized into the acid-soluble, reducible, oxidizable, and residual fractions (Meng et al., 2018). Of these, the acid-soluble fraction has the highest mobility (Matong et al., 2016; Zhang et al., 2018b). Soil Cd fractions with different mobility are readily affected by the soil physicochemical properties (Mohamed et al., 2015; Filipović et al., 2018; Xiao et al., 2019). Elevated OM content, CEC, and silty clay loam content provide abundant exchange sites for Cd adsorption, reduce the soil available Cd content, and lower plant Cd uptake (Mohamed et al., 2015; Filipović et al., 2018). In contrast, high soil sand content decreases the surface area and the total negative charge and is not, therefore, conducive to metal cation retention in the soil (Sungur et al., 2014a). Consistent with these results, higher OM, CEC, silt content and lower sand content in soils of ML and XS led to relatively lower acid-soluble Cd and higher reducible and oxidizable Cd content and proportions. He et al. (2017) found that soil Cd availability decreased with the increase of soil pH value. Soil of KS had the lowest soil pH, which may be an important reason for the higher acid-soluble Cd content in soil of KS. Interestingly, the correlations between pH value and acid-soluble and reducible Cd were not significant, indicating that pH value played less important roles in regulating soil Cd forms than other soil characteristics in the present study.
Plant Cd content is usually positively correlated with soil bioavailable Cd (Geebelen et al., 2003). In this study, Cd accumulation varied greatly in apple plants grown in different soils. Plants grown in soils of ML and XS presented with lower root and stem Cd accumulation and BCF, which is attributed to relatively lower Cd bioavailability in these soils. Biomass, root architecture, and plant pigment content reflect heavy metal phytotoxicity (Shi and Cai, 2009). Plants grown in different soils with distinct physicochemical properties varied greatly in their growth parameters (Patel et al., 2015). Apple plants grown in soils ML and XS displayed lower inhibition of tissue biomass and root architecture as well as photosynthetic pigment, while the corresponding indexes of soils of DS, KS and QT showed opposite results. Thus, the plants grown in soils of ML and XS have a greater Cd tolerance as these substrates had relatively higher OM content, CEC, and silt content, lower sand content, and, therefore, low Cd mobility. These results are consistent with those reported in cabbage and maize under Cd stress (Mohamed et al., 2015).
4.2 Physiological basis of the differences in Cd tolerance among plants grown under various soil conditions
Cd stress induces the accumulation of ROS and MDA, therefore, lead to oxidative stress in plants (Muradoglu et al., 2015). Here, the reductions in growth and photosynthetic pigment content of plant grown in five different soils under Cd stress are associated with Cd-induced oxidative stress. However, as the Cd accumulation was relatively lower in the plants grown in soils of ML and XS, there was relatively less oxidative stress in the plants grown in these two soils, indicating that these plants may adopt more effective coordinated regulatory mechanisms than the plants grown in the other soils.
Cd stress induces plants to produce non-enzymatic antioxidants, for example free proline, ASC, soluble phenolics, and T-SH to remove ROS and mitigate Cd phytotoxicity (He et al., 2020). Hussain et al. (2022) found that biochar application to wheat (Triticum aestivum L.) plants under Cd stress increased their foliar free proline, and total soluble phenolics content, which may be attributed to high pH and CEC of biochar (Cui et al., 2016; Moradi et al., 2019). Here, relative higher levels of non-enzymatic antioxidants in plants grown in soils of ML and XS are probably contributed to lower ROS and MDA accumulation. SOD, POD, CAT, APX, and GR are key antioxidant enzymes, playing pivotal roles in overcoming Cd-induced oxidative stress in plants. Biochar amendment significantly increased these enzyme activities in the leaves of pak choi (Brassica chinensis L.) under Cd stress possibly because biochar alters soil physicochemical properties (Kamran et al., 2019). Under Cd treatment, the lower ROS and MDA levels in the plants grown in soils of ML and XS were associated with increased antioxidant enzymes activities in tissues of plants grown in these two soils.
4.3 Variations in Cd accumulation and tolerance are related to different gene expression patterns in apple plants grown under various soil conditions
Our previous study showed that PM H+-ATPase gene downregulation inhibited Cd uptake by apple rootstocks (He et al., 2020) and that PM H+-ATPase activity might be affected by soil physicochemical properties (Zhang et al., 2018a; Olaetxea et al., 2019). Under Cd exposure conditions, the expression levels of HA11 in the plants grown in soils of ML and XS and the expression level of VHA4 in the plants grown in soil of ML were lower than those in the plants grown in soils of DS and KS. These observations were consistent with the fact that Cd concentrations were relatively lower in the roots of the plants grown in soils of ML and XS. ZIP and IRT1 regulated Cd absorption in Populus × canescens and Chinese cabbage, respectively (Wu et al., 2015; Ding et al., 2017). Nevertheless, there is little empirical evidence about the expression of ZIP and IRT1 regulating Cd uptake in plants grown under different soil conditions. Here, the downregulation of ZIP6 in the plants grown in soils of ML and XS, and the upregulation of ZIP and IRT1 in the plants grown in soil of DS were consistent with root Cd concentration in these plants. Therefore, variations in Cd accumulation are related to differential transcriptional regulation of genes related to Cd uptake in apple plants grown in different soils.
Arabidopsis thaliana harboring overexpressing Brassica juncea gene encoding MT2 had lower Cd sensitivity and higher Cd tolerance than its wild type counterpart (Zhigang et al., 2006). Under Cd stress, three NAS genes were strongly upregulated in the roots of durum wheat, thereby maintaining NA production and lowering Cd phytotoxicity (Aprile et al., 2018). Jaskulak et al. (2019) found that soil and metal cation type affected MT expression. In this study, the lowest mRNA levels of MT2 and NAS1 were found in the plants grown in soil of DS subjected to Cd stress. These findings were in line with the fact that Cd phytotoxicity was more severe in the plants grown in soil of DS. By contrast, the less repression of transcript levels of MT2 in plants grown in soil of ML, and NAS1 in plants grown in soils of ML and XS by Cd than in soil of DS corresponding well to its relative lower Cd toxicity than those grown in the soils of DS.
Transporters such as MHX, MTP1, and ABCC1 are located on the tonoplast, transport Cd or Cd complexes from the cytoplasm to vacuole, reduce cytoplasmic free Cd content (Berezin et al., 2008; Lin and Aarts, 2012), and mitigate Cd phytotoxicity. However, there is little empirical evidence that soil physicochemical properties affect transcription of the genes regulating Cd detoxification. Only Jaskulak et al. (2020) found that relative expression of ABCC in the shoots of Sinapis alba L. varied with soil CEC and total organic carbon content. We found that MHX was relatively upregulated in the roots of the plants grown in soil of XS subjected to Cd stress. Cd exposure downregulated MTP1 in the roots of the plants grown in all soils except in soils of ML and QT and downregulated ABCC1 in roots of the plants grown in soils of DS and KS. The foregoing results might indicate that the plants grown in soils of ML and XS had relatively lower Cd toxicity as they sequestered the Cd in vacuoles or formed relatively nontoxic Cd-containing complexes.
HMA4 and PCR2 are localized to the plasma membrane and transport Cd2+ extracellularly (Hanikenne et al., 2008; Song et al., 2010). Our previous study showed that exogenous melatonin restricted root-to-shoot Cd transport in apple rootstock by downregulating root HMA4 and PCR2 (He et al., 2020). In the present study, compared with soils of DS and KS, the HMA4 levels were relatively lower in the roots of the plants grown in soil of XS and the PCR2 levels were relatively lower in the roots of the plants grown in soils of ML, XS, and QT under Cd stress. For these reasons, these plants effectively inhibited Cd root-to-shoot transport and mitigated its shoot toxicity.
5 Conclusions
There were significant differences in Cd accumulation, translocation and tolerance in apple plants grown in soils with different physicochemical properties. Lower Cd accumulation and BCF were found in apple plants grown in soils of ML and XS, which could be ascribed to lower Cd bioavailability in these soils. The negative effects of Cd stress on the growth were less pronounced in the plants grown in soils of ML and XS. Moreover, the plants grown in these two soils had relatively lower ROS and MDA production, but higher antioxidant content and enzyme activity. The expression of genes related to Cd absorption and transport was lower in the roots of plants grown in the soils of ML and XS while the transcription levels of the key genes related to Cd detoxification were higher. Our data demonstrated that apple plants grown in soils of ML and XS with higher OM content, CEC, clay and silt content and lower sand content accumulated less Cd and had relative higher Cd tolerance, indicating that the soil types affect Cd accumulation and tolerance in apple plants. These results lay the foundation for mitigation strategies by application of amendments to modify soil properties to decrease soil Cd bioavailability.
Data availability statement
The raw data supporting the conclusions of this article will be made available by the authors, without undue reservation.
Author contributions
XZ and JH conceived and designed research. XZ, HXW and HYW performed the experiment. XZ and JH analyzed data and prepared the manuscript. JH, SQ and DL are responsible for conceptualization, funding acquisition, review and editing. All authors read and approved the final manuscript.
Funding
This work was funded by the Liaoning Revitalization Talents Program [Grant No. XLYC1907105], Scientific Research Foundation of Liaoning [LSNQN201916], the China Agriculture Research System of MOF and MARA [Grant No. CARS-27], Program for Liaoning Innovative Talents in University (LR2019063), and the Scientific Research Foundation of Talent Introduction of Shenyang Agricultural University [20153007].
Conflict of interest
The authors declare that the research was conducted in the absence of any commercial or financial relationships that could be construed as a potential conflict of interest.
Publisher’s note
All claims expressed in this article are solely those of the authors and do not necessarily represent those of their affiliated organizations, or those of the publisher, the editors and the reviewers. Any product that may be evaluated in this article, or claim that may be made by its manufacturer, is not guaranteed or endorsed by the publisher.
Supplementary material
The Supplementary Material for this article can be found online at: https://www.frontiersin.org/articles/10.3389/fpls.2023.1188241/full#supplementary-material
References
Abdel Latef, A. A. (2013). Growth and some physiological activities of pepper (Capsicum annuum l.) in response to cadmium stress and mycorrhizal symbiosis. J. Agric. Sci. Technol. 15, 1437–1448. doi: 10.1016/j.biosystemseng.2013.07.010
Ahammed, G. J., Li, C. X., Li, X., Liu, A., Chen, S., Zhou, J. (2021). Overexpression of tomato RING E3 ubiquitin ligase gene SlRING1 confers cadmium tolerance by attenuating cadmium accumulation and oxidative stress. Physiol. Plant 173, 449–459. doi: 10.1111/ppl.13294
Anjum, S. A., Tanveer, M., Hussain, S., Bao, M., Wang, L., Khan, I., et al. (2015). Cadmium toxicity in maize (Zea mays l.): consequences on antioxidative systems, reactive oxygen species and cadmium accumulation. Environ. Sci. Pollut. Res. 22, 17022–17030. doi: 10.1007/s11356-015-4882-z
Aprile, A., Sabella, E., Vergine, M., Genga, A., Siciliano, M., Nutricati, E., et al. (2018). Activation of a gene network in durum wheat roots exposed to cadmium. BMC Plant Biol. 18, 238. doi: 10.1186/s12870-018-1473-4
Argüello, D., Chavez, E., Lauryssen, F., Vanderschueren, R., Smolders, E., Montalvo, D. (2019). Soil properties and agronomic factors affecting cadmium concentrations in cacao beans: a nationwide survey in Ecuador. Sci. Total Environ. 649, 120–127. doi: 10.1016/j.scitotenv.2018.08.292
Berezin, I., Mizrachy-Dagry, T., Brook, E., Mizrahi, K., Elazar, M., Zhuo, S., et al. (2008). Overexpression of AtMHX in tobacco causes increased sensitivity to Mg2+, Zn2+, and Cd2+ ions, induction of V-ATPase expression, and a reduction in plant size. Plant Cell Rep. 27, 939–949. doi: 10.1007/s00299-007-0502-9
Chen, H., Teng, Y., Lu, S., Wang, Y., Wang, J. (2015). Contamination features and health risk of soil heavy metals in China. Sci. Total Environ. 512, 143–153. doi: 10.1016/j.scitotenv.2015.01.025
Chen, H., Yuan, X., Li, T., Hu, S., Ji, J., Wang, C. (2016). Characteristics of heavy metal transfer and their influencing factors in different soil-crop systems of the industrialization region, China. Ecotoxicol. Environ. Saf. 126, 193–201. doi: 10.1016/j.ecoenv.2015.12.042
Cui, L., Pan, G., Li, L., Bian, R., Liu, X., Yan, J., et al. (2016). Continuous immobilization of cadmium and lead in biochar amended contaminated paddy soil: a five-year field experiment. Ecol. Eng. 93, 1–8. doi: 10.1016/j.ecoleng.2016.05.007
Ding, S., Ma, C., Shi, W., Liu, W., Lu, Y., Liu, Q., et al. (2017). Exogenous glutathione enhances cadmium accumulation and alleviates its toxicity in Populus × canescens. Tree Physiol. 37, 1697–1712. doi: 10.1093/treephys/tpx132
Fellet, G., Marmiroli, M., Marchiol, L. (2014). Elements uptake by metal accumulator species grown on mine tailings amended with three types of biochar. Sci. Total Environ. 468, 598–608. doi: 10.1016/j.scitotenv.2013.08.072
Filipović, L., Romic, M., Romic, D., Filipović, V., Ondrasek, G. (2018). Organic matter and salinity modify cadmium soil (phyto)availability. Ecotoxicol. Environ. Saf. 147, 824–831. doi: 10.1016/j.ecoenv.2017.09.041
Gaillard, S., Jacquet, H., Vavasseur, A., Leonhardt, N., Forestier, C. (2008). AtMRP6/AtABCC6, an ATP-binding cassette transporter gene expressed during early steps of seedling development and up-regulated by cadmium in Arabidopsis thaliana. BMC Plant Biol. 8, 22–32. doi: 10.1186/1471-2229-8-22
Gaskin, J. W., Steiner, C., Harris, K., Das, K. C., Bibens, B. (2008). Effect of low-temperature pyrolysis conditions on biochar for agricultural use. T. Asabe 51, 2061–2069. doi: 10.13031/2013.25409
Geebelen, W., Adriano, D. C., van der Lelie, D., Mench, M., Carleer, R., Clijsters, H., et al. (2003). Selected bioavailability assays to test the efficacy of amendment-induced immobilization of lead in soils. Plant Soil 249, 217–228. doi: 10.1023/A:1022534524063
Golia, E. E., Dimirkou, A., Mitsios, I. K. (2008). Influence of some soil parameters on heavy metals accumulation by vegetables grown in agricultural soils of different soil orders. B. Environ. Contam. Tox. 81, 80–84. doi: 10.1007/s00128-008-9416-7
Hanikenne, M., Talke, I. N., Haydon, M. J., Lanz, C., Nolte, A., Motte, P., et al. (2008). Evolution of metal hyperaccumulation required cis-regulatory changes and triplication of HMA4. Nature 453, 391–395. doi: 10.1038/nature06877
Hardy, M., Cornu, S. (2006). Location of natural trace elements in silty soils using particle-size fractionation. Geoderma 133, 295–308. doi: 10.1016/j.geoderma.2005.07.015
He, Y. B., Huang, D. Y., Zhu, Q. H., Wang, S., Liu, S. L., He, H. B., et al. (2017). A three-season field study on the in-situ remediation of cd-contaminated paddy soil using lime, two industrial by-products, and a low-cd-accumulation rice cultivar. Ecotoxicol. Environ. Saf. 136, 135–141. doi: 10.1016/j.ecoenv.2016.11.005
He, J., Li, H., Luo, J., Ma, C., Li, S., Qu, L., et al. (2013a). A transcriptomic network underlies microstructural and physiological responses to cadmium in Populus × canescens. Plant Physiol. 162, 424–439. doi: 10.1104/pp.113.215681
He, J., Li, H., Ma, C., Zhang, Y., Polle, A., Rennenberg, H., et al. (2015). Overexpression of bacterial gamma-glutamylcysteine synthetase mediates changes in cadmium influx, allocation and detoxification in poplar. New Phytol. 205, 240–254. doi: 10.1111/nph.13013
He, J., Ma, C., Ma, Y., Li, H., Kang, J., Liu, T., et al. (2013b). Cadmium tolerance in six poplar species. Environ. Sci. pollut. Res. 20, 163–174. doi: 10.1007/s11356-012-1008-8
He, J., Qin, J., Long, L., Ma, Y., Li, H., Li, K., et al. (2011). Net cadmium flux and accumulation reveal tissue-specific oxidative stress and detoxification in Populus × canescens. Physiol. Plant 143, 50–63. doi: 10.1111/j.1399-3054.2011.01487.x
He, J., Zhuang, X., Zhou, J., Sun, L., Wan, H., Li, H., et al. (2020). Exogenous melatonin alleviates cadmium uptake and toxicity in apple rootstocks. Tree Physiol. 40, 746–761. doi: 10.1093/treephys/tpaa024
Hussain, S., Irfan, M., Sattar, A., Hussain, S., Ullah, S., Abbas, T., et al. (2022). Alleviation of cadmium stress in wheat through the combined application of boron and biochar via regulating morpho-physiological and antioxidant defense mechanisms. Agronomy 12, 434. doi: 10.3390/agronomy12020434
Jaskulak, M., Grobelak, A., Vandenbulcke, F. (2020). Effects of sewage sludge supplementation on heavy metal accumulation and the expression of ABC transporters in Sinapis alba l. during assisted phytoremediation of contaminated sites. Ecotoxicol. Environ. Saf. 197, 110606. doi: 10.1016/j.ecoenv.2020.110606
Jaskulak, M., Rorat, A., Grobelak, A., Chaabene, Z., Kacprzak, M., Vandenbulcke, F. (2019). Bioaccumulation, antioxidative response, and metallothionein expression in Berezin l. exposed to heavy metals and silver nanoparticles. Environ. Sci. Pollut. Res. 26, 16040–16052. doi: 10.1007/s11356-019-04972-y
Kamran, M., Malik, Z., Parveen, A., Zong, Y., Abbasi, G. H., Rafiq, M. T., et al. (2019). Biochar alleviates cd phytotoxicity by minimizing bioavailability and oxidative stress in pak choi (Brassica chinensis l.) cultivated in cd-polluted soil. J. Environ. Manage. 250, 109500. doi: 10.1016/j.jenvman.2019.109500
Karimi Nezhad, M. T., Ghahroudi Tali, M., Hashemi Mahmoudi, M., Pazira, E. (2011). Assessment of as and cd contamination in topsoils of northern ghorveh (Western iran): role of parent material, land use and soil properties. Environ. Earth Sci. 64, 1203–1213. doi: 10.1007/s12665-011-0935-x
Lei, Y., Korpelainen, H., Li, C. (2007). Physiological and biochemical responses to high Mn concentrations in two contrasting populus cathayana populations. Chemosphere 68, 686–694. doi: 10.1016/j.chemosphere.2007.01.066
Li, J. T., Qiu, J. W., Wang, X. W., Zhong, Y., Lan, C. Y., Shu, W. S. (2006). Cadmium contamination in orchard soils and fruit trees and its potential health risk in guangzhou, China. Environ. Pollut. 143, 159–165. doi: 10.1016/j.envpol.2005.10.016
Lin, Y. F., Aarts, M. G. (2012). The molecular mechanism of zinc and cadmium stress response in plants. Cell. Mol. Life Sci. 69, 3187–3206. doi: 10.1007/s00018-012-1089-z
Liu, D., Kottke, I. (2004). Subcellular localization of cadmium in the root cells of allium cepa by electron energy loss spectroscopy and cytochemistry. J. Biosci. 29, 329–335. doi: 10.1007/BF02702615
Luo, Z. B., Calfapietra, C., Scarascia-Mugnozza, G., Liberloo, M., Polle, A. (2008). Carbon-based secondary metabolites and internal nitrogen pools in populus nigra under free air CO2 enrichment (FACE) and nitrogen fertilisation. Plant Soil 304, 45–57. doi: 10.1007/s11104-007-9518-8
Luo, Z. B., He, J., Polle, A., Rennenberg, H. (2016). Heavy metal accumulation and signal transduction in herbaceous and woody plants: paving the way for enhancing phytoremediation efficiency. Biotechnol. Adv. 34, 1131–1148. doi: 10.1016/j.biotechadv.2016.07.003
Luo, X. S., Yu, S., Li, X. D. (2011). Distribution, availability, and sources of trace metals in different particle size fractions of urban soils in Hong Kong: implications for assessing the risk to human health. Environ. Pollut. 159, 1317–1326. doi: 10.1016/j.envpol.2011.01.013
Ma, Y., He, J., Ma, C., Luo, J., Li, H., Liu, T., et al. (2014). Ectomycorrhizas with paxillus involutus enhance cadmium uptake and tolerance in Populus × canescens. Plant Cell Environ. 37, 627–642. doi: 10.1111/pce.12183
Matong, J. M., Nyaba, L., Nomngongo, P. N. (2016). Fractionation of trace elements in agricultural soils using ultrasound assisted sequential extraction prior to inductively coupled plasma mass spectrometric determination. Chemosphere 154, 249–257. doi: 10.1016/j.chemosphere.2016.03.123
Melo, L. C. A., Alleoni, L. R. F., Carvalho, G., Azevedo, R. A. (2011). Cadmium- and barium-toxicity effects on growth and antioxidant capacity of soybean (Glycine max l.) plants, grown in two soil types with different physicochemical properties. J. Plant Nutr. Soil Sci. 174, 847–859. doi: 10.1002/jpln.201000250
Meng, J., Zhong, L., Wang, L., Liu, X., Tang, C., Chen, H., et al. (2018). Contrasting effects of alkaline amendments on the bioavailability and uptake of cd in rice plants in a cd-contaminated acid paddy soil. Environ. Sci. Pollut. Res. 25, 8827–8835. doi: 10.1007/s11356-017-1148-y
Migeon, A., Blaudez, D., Wilkins, O., Montanini, B., Campbell, M. M., Richaud, P., et al. (2010). Genome-wide analysis of plant metal transporters, with an emphasis on poplar. Cell. Mol. Life Sci. 67, 3763–3784. doi: 10.1007/s00018-010-0445-0
Mobin, M., Khan, N. A. (2007). Photosynthetic activity, pigment composition and antioxidative response of two mustard (Brassica juncea) cultivars differing in photosynthetic capacity subjected to cadmium stress. J. Plant Physiol. 164, 601–610. doi: 10.1016/j.jplph.2006.03.003
Mohamed, I., Zhang, G.-S., Li, Z.-G., Liu, Y., Chen, F., Dai, K. (2015). Ecological restoration of an acidic cd contaminated soil using bamboo biochar application. Ecol. Eng. 84, 67–76. doi: 10.1016/j.ecoleng.2015.07.009
Moradi, R., Pourghasemian, N., Naghizadeh, M. (2019). Effect of beeswax waste biochar on growth, physiology and cadmium uptake in saffron. J. Clean. Prod. 229, 1251–1261. doi: 10.1016/j.jclepro.2019.05.047
Muradoglu, F., Gundogdu, M., Ercisli, S., Encu, T., Balta, F., Jaafar, H. Z., et al. (2015). Cadmium toxicity affects chlorophyll a and b content, antioxidant enzyme activities and mineral nutrient accumulation in strawberry. Biol. Res. 48, 11. doi: 10.1186/s40659-015-0001-3
Olaetxea, M., Mora, V., Bacaicoa, E., Baigorri, R., Garnica, M., Fuentes, M., et al. (2019). Root ABA and h+-ATPase are key players in the root and shoot growth-promoting action of humic acids. Plant Direct. 3, 1–12. doi: 10.1002/pld3.175
Park, J., Song, W. Y., Ko, D., Eom, Y., Hansen, T. H., Schiller, M., et al. (2012). The phytochelatin transporters AtABCC1 and AtABCC2 mediate tolerance to cadmium and mercury. Plant J. 69, 278–288. doi: 10.1111/j.1365-313X.2011.04789.x
Patel, A., Pandey, V., Patra, D. D. (2015). Influence of tannery sludge on oil yield, metal uptake and antioxidant activities of Ocimum basilicum l. grown in two different soils. Ecol. Eng. 83, 422–430. doi: 10.1016/j.ecoleng.2015.06.046
PÉRez-Chaca, M. V., RodrÍGuez-Serrano, M., Molina, A. S., Pedranzani, H. E., Zirulnik, F., Sandalio, L. M., et al. (2014). Cadmium induces two waves of reactive oxygen species in Glycine max (L.) roots. Plant Cell Environ. 37, 1672–1687. doi: 10.1111/pce.12280
Podazza, G., Arias, M., Prado, F. E. (2012). Cadmium accumulation and strategies to avoid its toxicity in roots of the citrus rootstock citrumelo. J. Hazard. Mater. 215-216, 83–89. doi: 10.1016/j.jhazmat.2012.02.031
Podazza, G., Arias, M., Prado, F. E. (2016). Early interconnectivity between metabolic and defense events against oxidative stress induced by cadmium in roots of four citrus rootstocks. Funct. Plant Biol. 43, 973–985. doi: 10.1071/FP16153
Qasim, B., Motelica-Heino, M. (2014). Potentially toxic element fractionation in technosoils using two sequential extraction schemes. Environ. Sci. Pollut. Res. 21, 5054–5065. doi: 10.1007/s11356-013-2457-4
Ramzani, P. M. A., Coyne, M. S., Anjum, S., Khan, W. U., Iqbal, M. (2017). In situ immobilization of cd by organic amendments and their effect on antioxidant enzyme defense mechanism in mung bean (Vigna radiata l.) seedlings. Plant Physiol. Biochem. 118, 561–570. doi: 10.1016/j.plaphy.2017.07.022
Shi, G. R., Cai, Q. S. (2009). Cadmium tolerance and accumulation in eight potential energy crops. Biotechnol. Adv. 27, 555–561. doi: 10.1016/j.biotechadv.2009.04.006
Shi, W. G., Liu, W., Yu, W., Zhang, Y., Ding, S., Li, H., et al. (2019). Abscisic acid enhances lead translocation from the roots to the leaves and alleviates its toxicity in Populus x canescens. J. Hazard. Mater. 362, 275–285. doi: 10.1016/j.jhazmat.2018.09.024
Song, W. Y., Choi, K. S., Kim, D. Y., Geisler, M., Park, J., Vincenzetti, V., et al. (2010). Arabidopsis PCR2 is a zinc exporter involved in both zinc extrusion and long-distance zinc transport. Plant Cell 22, 2237–2252. doi: 10.1105/tpc.109.070185
Sparks, D. L., Page, A. L., Helmke, P. A., Loeppert, R. H., Soltanpour, P. N., Tabatabai, M. A., et al. (1996). Methods of soil analysis, part 3-chemical methods (Madison, America: Soil Science Society of America Inc).
Sungur, A., Soylak, M., Yilmaz, S., Özcan, H. (2014b). Determination of heavy metals in sediments of the ergene river by BCR sequential extraction method. Environ. Earth Sci. 72, 3293–3305. doi: 10.1007/s12665-014-3233-6
Sungur, A., Soylak, M., Yilmaz, E., Yilmaz, S., Ozcan, H. (2014a). Characterization of heavy metal fractions in agricultural soils by sequential extraction procedure: the relationship between soil properties and heavy metal fractions. Soil Sediment Contam. 24, 1–15. doi: 10.1080/15320383.2014.907238
Vega, F. A., Andrade, M. L., Covelo, E. F. (2010). Influence of soil properties on the sorption and retention of cadmium, copper and lead, separately and together, by 20 soil horizons: comparison of linear regression and tree regression analyses. J. Hazard. Mater. 174, 522–533. doi: 10.1016/j.jhazmat.2009.09.083
Wang, P., Sun, X., Li, C., Wei, Z., Liang, D., Ma, F. (2013). Long-term exogenous application of melatonin delays drought-induced leaf senescence in apple. J. Pineal Res. 54, 292–302. doi: 10.1111/jpi.12017
Wu, Z., McGrouther, K., Huang, J., Wu, P., Wu, W., Wang, H. (2014). Decomposition and the contribution of glomalin-related soil protein (GRSP) in heavy metal sequestration: field experiment. Soil Biol. Biochem. 68, 283–290. doi: 10.1016/j.soilbio.2013.10.010
Wu, Q., Su, N., Cai, J., Shen, Z., Cui, J. (2015). Hydrogen-rich water enhances cadmium tolerance in Chinese cabbage by reducing cadmium uptake and increasing antioxidant capacities. J. Plant Physiol. 175, 174–182. doi: 10.1016/j.jplph.2014.09.017
Xiao, R., Wang, P., Mi, S., Ali, A., Liu, X., Li, Y., et al. (2019). Effects of crop straw and its derived biochar on the mobility and bioavailability in cd and zn in two smelter-contaminated alkaline soils. Ecotox. Environ. Safe. 181, 155–163. doi: 10.1016/j.ecoenv.2019.06.005
Yu, K., Xu, J., Jiang, X., Liu, C., McCall, W., Lu, J. (2017). Stabilization of heavy metals in soil using two organo-bentonites. Chemosphere 184, 884–891. doi: 10.1016/j.chemosphere.2017.06.040
Zhang, X., Chen, L., Wu, H., Liu, L., Wan, X. (2018a). Root plasma membrane h+-ATPase is involved in low pH-inhibited nitrogen accumulation in tea plants (Camellia sinensis l.). Plant Growth Regul. 86, 423–432. doi: 10.1007/s10725-018-0440-2
Zhang, Y., Sa, G., Zhang, Y., Zhu, Z., Deng, S., Sun, J., et al. (2017). Paxillus involutus-facilitated Cd2+ influx through plasma membrane Ca2+-permeable channels is stimulated by H2O2 and h+-ATPase in ectomycorrhizal Populus × canescens under cadmium stress. Front. Plant Sci. 7. doi: 10.3389/fpls.2016.01975
Zhang, Z., Wang, J. J., Ali, A., DeLaune, R. D. (2018b). Physico-chemical forms of copper in water and sediments of lake pontchartrain basin, USA. Chemosphere 195, 448–454. doi: 10.1016/j.chemosphere.2017.12.115
Zhigang, A., Cuijie, L., Yuangang, Z., Yejie, D., Wachter, A., Gromes, R., et al. (2006). Expression of BjMT2, a metallothionein 2 from Brassica juncea, increases copper and cadmium tolerance in Escherichia coli and Arabidopsis thaliana, but inhibits root elongation in Arabidopsis thaliana seedlings. J. Exp. Bot. 57, 3575–3582. doi: 10.1093/jxb/erl102
Zhou, J., Wan, H., He, J., Lyu, D., Li, H. (2017). Integration of cadmium accumulation, subcellular distribution, and physiological responses to understand cadmium tolerance in apple rootstocks. Front. Plant Sci. 8. doi: 10.3389/fpls.2017.00966
Zhou, J., Wan, H., Qin, S., He, J., Lyu, D., Li, H. (2016). Net cadmium flux and gene expression in relation to differences in cadmium accumulation and translocation in four apple rootstocks. Environ. Exp. Bot. 130, 95–105. doi: 10.1016/j.envexpbot.2016.05.012
Keywords: apple, cadmium, soil physicochemical property, oxidative stress, gene expression
Citation: Zhuang X, Wan H, Wang H, Qin S, He J and Lyu D (2023) Characteristics of cadmium accumulation and tolerance in apple plants grown in different soils. Front. Plant Sci. 14:1188241. doi: 10.3389/fpls.2023.1188241
Received: 17 March 2023; Accepted: 18 May 2023;
Published: 02 June 2023.
Edited by:
Jiawen Wu, Yan’an University, ChinaReviewed by:
Stefan Trapp, Technical University of Denmark, DenmarkLiu Haitao, Henan Agricultural University, China
Copyright © 2023 Zhuang, Wan, Wang, Qin, He and Lyu. This is an open-access article distributed under the terms of the Creative Commons Attribution License (CC BY). The use, distribution or reproduction in other forums is permitted, provided the original author(s) and the copyright owner(s) are credited and that the original publication in this journal is cited, in accordance with accepted academic practice. No use, distribution or reproduction is permitted which does not comply with these terms.
*Correspondence: Jiali He, aGVqaWFsaTEwMTdAMTYzLmNvbQ==; aGVqaWFsaTEwMTdAc3lhdS5lZHUuY24=; Deguo Lyu, bHZkZWd1b0AxNjMuY29t