- 1The Research Center of Soil and Water Conservation and Ecological Environment, Chinese Academy of Sciences and Ministry of Education, Yangling, Shaanxi, China
- 2Institute of Soil and Water Conservation, Chinese Academy of Sciences and Ministry of Water Resources, Yangling, Shaanxi, China
- 3University of Chinese Academy of Sciences, Beijing, China
- 4Institute of Soil and Water Conservation, Northwest A&E University, Yangling, China
- 5College of Urban, Rural Planning and Architectural Engineering, Shangluo University, Shangluo, China
Large-scale afforestation is considered a natural way to address climate challenges (e.g., the greenhouse effect). However, there is a paucity of evidence linking plant diversity to soil carbon sequestration pathways during long-term natural restoration of temperate vegetation. In particular, the carbon sequestration mechanisms and functions of woody plants require further study. Therefore, we conducted a comparative study of plant diversity and soil carbon sequestration characteristics during 150 years of natural vegetation restoration in the temperate zone to provide a comprehensive assessment of the effects of long-term natural vegetation restoration processes on soil organic carbon stocks. The results suggested positive effects of woody plant diversity on carbon sequestration. In addition, fine root biomass and deadfall accumulation were significantly positively correlated with soil organic carbon stocks, and carbon was stored in large grain size aggregates (1–5 mm). Meanwhile, the diversity of Fabaceae and Rosaceae was observed to be important for soil organic carbon accumulation, and the carbon sequestration function of shrubs should not be neglected during vegetation restoration. Finally, we identified three plants that showed high potential for carbon sequestration: Lespedeza bicolor, Sophora davidii, and Cotoneaster multiflorus, which should be considered for inclusion in the construction of local artificial vegetation. Among them, L. bicolor is probably the best choice.
1 Introduction
The loss of plant diversity is a major challenge faced by humans in maintaining the stability and functional sustainability of ecosystems (Hautier et al., 2015; Hua et al., 2022). Anthropogenic activity such as deforestation and landuse changes causes 30% reduction in C (carbon) stock (Bargali et al., 2018; Awasthi et al., 2022a Manral et al., 2020; Manral et al., 2022; Bisht et al., 2023). Population pressure, agricultural expansion/intensification and development of infrastructure have been considered as major threats to biodiversity (Davidar et al., 2010; Baboo et al., 2017; Bargali et al., 2019; Bargali et al., 2022; Bisht et al., 2022) which causes an increase in CO2 in the atmosphere. Carbon sequestration is a key ecosystem function influenced by plant diversity (Bartelt-Ryser et al., 2005; Isbell et al., 2018). Forests are an important component of the global carbon cycle as they store 70–90% of terrestrial above and belowground biomass and are a major carbon sink, which is strongly linked to the diversity of plants in different forest types (Aponte et al., 2020; Besnard et al., 2021). There is much uncertainty about whether tropical forests are carbon sinks or sources, while temperate forests are known to play a significant role as terrestrial carbon sinks (Clark, 2004; Yang et al., 2022).
In recent decades, the increasing emissions of CO2-based greenhouse gases in the atmosphere (CO2 emissions have increased from 280 ppm in the pre-industrial era to 400 ppm today) have led to a series of environmental problems, such as global warming, sea level rise, and increase in extreme weather events, which seriously threaten the sustainable development of natural ecosystems and socio-economic systems (Zhang et al., 2011; Ramachandra and Bharath, 2020). Vegetation restoration is often used to increase soil organic carbon (SOC) storage and sequestration to reduce CO2 emissions and restore ecosystem functions (Zhao et al., 2015; Pandey et al., 2023; Shahi et al., 2023). Such restoration promotes the accumulation of SOC, making SOC the largest component of the terrestrial SOC pool, which is consequently two to three times greater than the vegetation carbon pool and plays a crucial role in regulating global warming (Davidson et al., 2000; Lal, 2004). In forest ecosystems, soil is an important participant and carrier of matter and energy. It provides essential mineral nutrients and water for plant growth. It can be a carbon sink or source, and has become a hot topic in the discussion on global climate change (Deng et al., 2013). Plant-soil interactions are important intrinsic drivers of ecosystem evolution. Many studies have focused on plant dynamics during vegetation succession (Teixeira et al., 2020; Prach et al., 2021), the physical and chemical properties of soils (Gu et al., 2019; Zhang et al., 2021), microbial turnover and change (Gavazov et al., 2022; Hu et al., 2022), and the effect of vegetation type changes caused by natural restoration and afforestation on soil carbon sequestration capacity (Liu et al., 2015; Yang et al., 2019a; Wang et al., 2020; Yan et al., 2020; Augusto and Boča, 2022). However, these factors are usually considered to influence soil carbon accumulation individually or independently. Currently, experimental evidence from grasslands and subtropical and tropical studies indicates that plant diversity increases biomass production and SOC storage (Craven et al., 2018; Chai et al., 2019; Yang et al., 2019b; Osuri et al., 2020; Yu et al., 2020). It has also been recognized that increasing plant diversity may improve plant productivity through complementary effects of ecological niches (Duffy et al., 2017). This consequently promotes plant input of carbon-containing material into the soil and increases SOC accumulation (Chen et al., 2020). However, there is a paucity of evidence linking vegetation diversity to soil carbon sequestration pathways on a broad scale during long-term natural recovery of temperate vegetation. Furthermore, tree-based ecosystems are critical for climate change mitigation; however, the mechanisms of carbon sequestration by woody plants are poorly understood, and there are also few reports on the plants that participate in carbon sequestration.
To elucidate these processes, in the present study, we investigated the influence of plant diversity on soil carbon sequestration by examining the natural recovery process of vegetation in the temperate zone for up to 150 years, considering sample sites, vegetation composition at different time periods, plant diversity, fine-root biomass, litter accumulation, soil aggregates, and SOC characteristics. Representative stand types and major species assemblages were selected and combined with partial least squares path modelling (PLS-PM) to quantify the contribution of plant diversity to soil carbon storage and evaluate the direct and indirect effects of the diversity of key plant families on carbon sequestration pathways. The aim of this study was to answer the following questions: 1) What are the characteristics of changes in vegetation and SOC during long-term vegetation recovery in the temperate zone? 2) How does plant diversity drive soil carbon sequestration? 3) What are the plants associated with soil carbon sequestration? Understanding how they are connected and how they work is important for managing ecosystem carbon pools, restoring vegetation to a near-natural state, and improving ecological management, which is important for addressing global climate change.
2 Materials and methods
2.1 Study area
The Ziwuling Nature Reserve is located in China’s temperate zone on the Loess Plateau (34°50′–36°50′N, 107°30′–109°40′E) (Figure 1). This reserve represents the best-preserved natural vegetation area on the Loess Plateau, with a representative plant germplasm resource base and the most important secondary primary forest (Guo and Wang, 2005). The vegetation on the ground is mainly represented by a temperate deciduous broadleaf forest dominated by Quercus mongolica and a temperate coniferous forest dominated by Pinus oleifera (Cheng et al., 2012). Zonal soil in the mountains is either primary or secondary loess with a pH of 7.5-8.2, and the soil profile is shallow overall (Tian et al., 2022).
2.2 Experimental design
The “space-for-time” replacement method has been widely utilized by ecologists to anticipate vegetation chronosequence change since 1899 (Cowles, 1899; Blois et al., 2013). In the present study, we used a “space for time” strategy to analyze 48 plots. From 2020 to 2021, standard sample plots of typical vegetation types were established with basically equal stand conditions and similar soil texture, with six duplicates for each restoration stage (Table 1). The restoration phase was determined by the findings of the relevant forestry department survey and an in-depth review of all relevant reports (Zou et al., 2002; Fan et al., 2006; Deng et al., 2013; Zhao et al., 2015; Liu et al., 2020).
2.3 Vegetation investigation and sampling
The species and their abundance in each standard sample plot (random selection) were recorded for plots of 20 m × 20 m (trees), 10 m × 10 m (shrubs), and 1 m × 1 m (grasses) (Table 1). The species’ Latin names were verified against the Flora of China (Li, 2007) and The Plant List (http://www.plantlist.org/). Owing to the shallow soil layer in the Ziwuling area, the improvement effect of vegetation restoration on the soil is mainly concentrated in the 0–40 cm surface soil layer (Gu et al., 2019). Therefore, a root auger with an inner diameter of 7 cm was used to collect fine root (≤2 mm), and soil samples were collected from this soil layer using a root auger with four replicates for each type of soil sample. The complete harvest method was used to collect litter from 31.7 cm × 31.7 cm plots (Ravindranath and Ostwald, 2007). The bulk density of the soil was determined using the cutting ring (100 cm3) method. Soil water-stable aggregates were determined using the wet sieve method (Yoder, 1936), and SOC content was determined using the dichromate oxidation method (Nelson and Sommers, 1996).
2.4 Data processing
The total plant diversity, woody plant diversity, and herbaceous plant diversity were determined using the following equations (Simpson, 1949; Hill, 1973):
where S is the total number of plant species in the sample quadrat, pi is the relative abundance of plant species i in one quadrat, and H is the Shannon–Wiener index.
SOC (g/m2) was calculated using Equation (5):
where X. is the SOC content of the soil (g/kg), BD is the density of the bulk soil (g/cm3), and T is the soil layer thickness (cm).
2.5 Statistical analyses
Prior to analysis, all data were examined for normality and homogeneity of variance; data having non-normal distribution and/or non-homogeneous variance were log- or power function transformed to meet the assumptions for statistical analysis. Regression analysis was performed to evaluate the relationships between plant diversity and restoration stage. Carbon sequestration characteristics among the different periods were compared using one-way ANOVA. If significant effects were observed using ANOVA, the least significant difference [LSD (0.05)] test was used. Pearson’s correlations were used to determine the variables that were significantly correlated with plant diversity, restoration stage, and carbon sequestration characteristics. PLS-PM was performed to further link plant diversity with carbon sequestration characteristics. PLS-PM is a type of structural equation modelling algorithm based on correlation. The concept of causality is expressed in terms of linear conditional expectations to seek the best linear prediction relationship and allow the use of latent variables to estimate complex causality or prediction models (Sanchez, 2013). The PLS-PM method was selected because it requires a small sample size, and the algorithm can optimize the prediction of dependent variables and fitting of data to a predetermined model (Hulland, 1999; Götz et al., 2010; Ali et al., 2017). Models with different structures were evaluated using the goodness-of-fit (GOF) statistics, a measure of their overall predictive power, with GOF > 0.7 considered an acceptable value (Tian et al., 2019; Zhong et al., 2020). All statistical analyses were performed using the R software (v. 4.1.1, R Core Team, 2020).
3 Results and analysis
3.1 Vegetation composition characteristics
Figure 2 shows that the vegetation in the study area has been naturally restored for 150 years. A total of 128 species of seed plants were recorded in the area, belonging to 39 families and 99 genera, including two species of gymnosperms belonging to two families and two genera and 126 species of angiosperms belonging to 37 families and 97 genera. Asteraceae (17 genera and 28 species), Rosaceae (13 genera and 17 species), Poaceae (10 genera and 10 species), and Fabaceae (six genera and eight species) accounted for 49.22% of the total number of species and were the dominant families. Asteraceae and Poaceae are primarily herbaceous plants, whereas Rosaceae and Fabaceae are primarily woody plants. The plant species richness of all species and four major families showed a single-peaked curve, increasing and then decreasing with the progress of restoration, and reaching a maximum after 70 years of restoration.
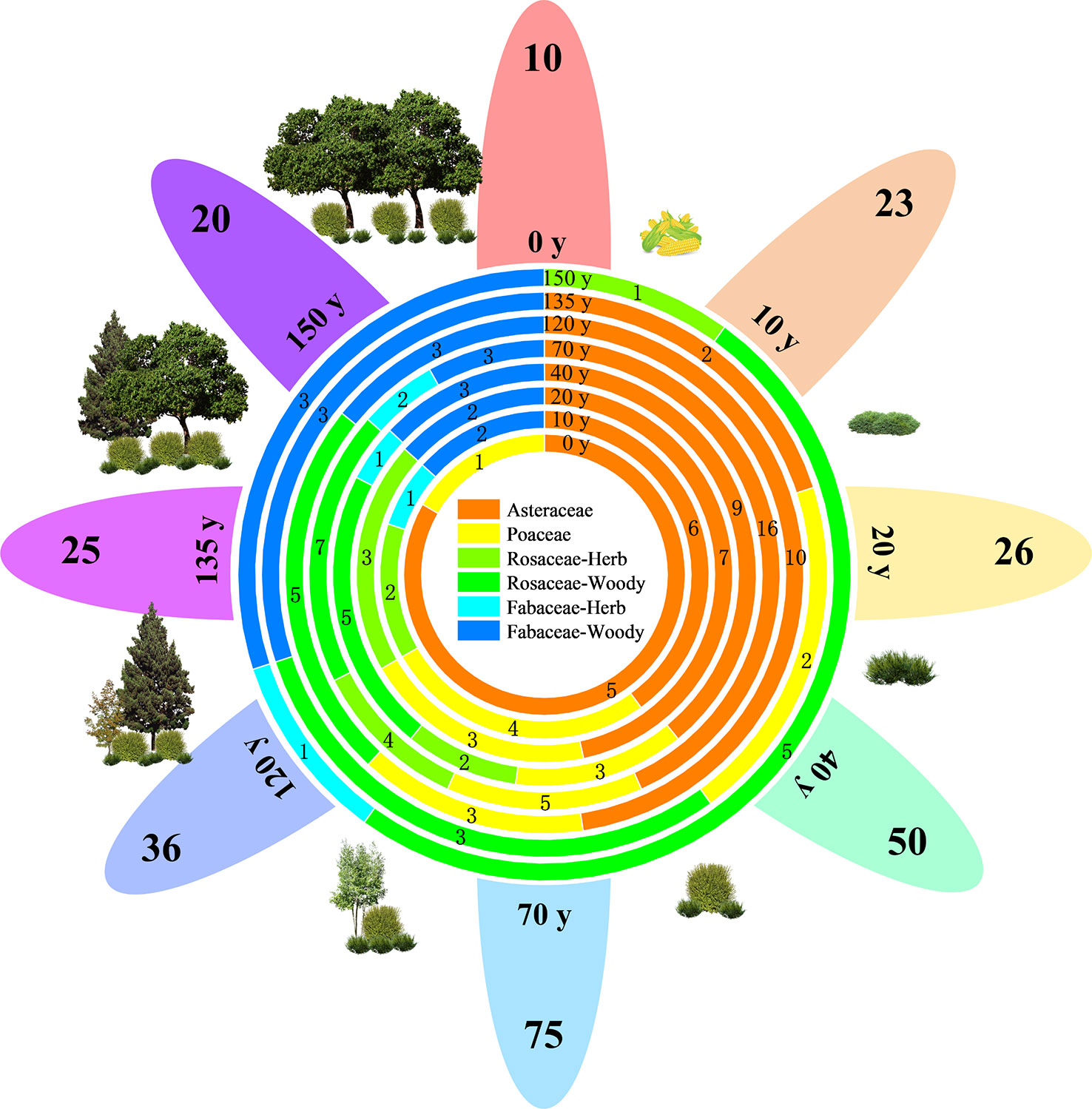
Figure 2 Vegetation composition and life forms of different vegetation restoration stages on the Chinese Loess Plateau. The numbers on the petal margins indicate the richness of plant species at each stage (e.g., 10). The combination containing y indicates the recovery time (e.g., 20 y). The circles in the center of the flowers indicate the species richness of different families at different stages.
3.2 Plant diversity characteristics
The fitted curves showed that the plant diversity indices had a single-peaked curve in relation to the restoration years during the 150 years of natural vegetation restoration succession (Figure 3). The Species richness, Shannon-Wiener, and Simpson indices showed a similar pattern, increasing and then decreasing, with the peak of herbaceous plants occurring earlier, between 40 and 70 years of vegetation restoration, the peak of total plant diversity occurring in the middle of the restoration period, between 70 and 100 years, and the peak of woody plants occurring later, between 100 and 120 years of restoration. However, the Pielou indices of herbaceous plants and total plants showed similar trends, with their peaks occurring earlier, at approximately 20 years of natural recovery, and decreasing thereafter. The Pielou index of woody plants increased at first, peaked at approximately 90 years, and then started to decrease. This indicated that the evenness of species distribution in the community was mainly influenced by herbaceous plants. In addition, we found that herbaceous plant diversity was graphically symmetric with respect to woody plant diversity at 70 years of restoration. This reflected the differentiation of ecological niches to capture maximum possible resources.
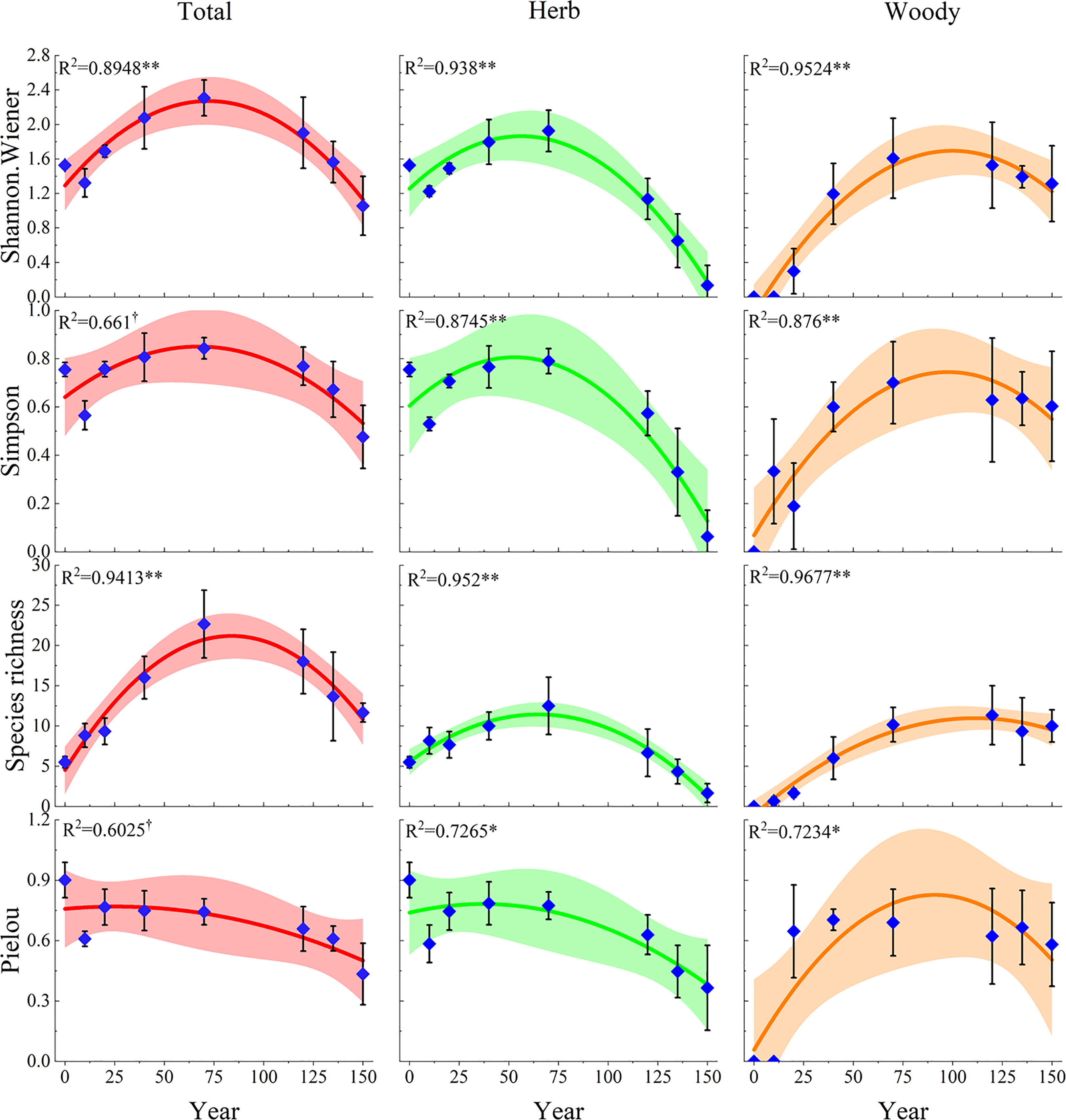
Figure 3 Multiple linear regression relationships of plant diversity with the natural restoration succession periods. The dark lines indicate the fits of the linear model to the plant diversity and succession ages, while the ribbon are the 95% confidence intervals of these linear models. †, P< 0.1; *, P< 0.05; **, P< 0.01. Total represents the overall diversity of plant communities; Herb represents the herb diversity in plant communities; Woody represents the woody plant diversity in plant communities.
3.3 Carbon sequestration characteristics
Figures 4A, C and D show that with the increase in the natural vegetation recovery time, the difference in vegetation compared with the agricultural stage became more significant (P< 0.05). Litter accumulation, fine-root biomass, and SOC storage increased with the progress of vegetation restoration. The most considerable increase in litter accumulation was 773 g/m2 after 120 years of restoration compared to that after 70 years of restoration, while the differences were smaller in the later stages (Figure 4A). A significant increase in fine-root biomass was observed during the 120- to 135-year stage of vegetation recovery (Figure 4C). As the SOC storage peaked at about 9447 g/m2, the disparity between the agricultural stage and the 120 years of restoration stage was at its greatest (Figure 4D). From the agricultural stage to the 10-year natural recovery stage, the content of large-sized water-stable agglomerates increased the fastest at 24.7%, followed by 10.9% at the 40–70-year recovery stage. The >5 mm size agglomerates increased rapidly at first, peaked at 25.1% in the 10-year natural recovery stage, and then decreased gradually; the 1–5 mm size agglomerates initially increased (but fluctuated), peaked at 70 years, and then decreased gradually, while the 0.25–1 mm size agglomerates showed relatively smaller variations (Figure 4B).
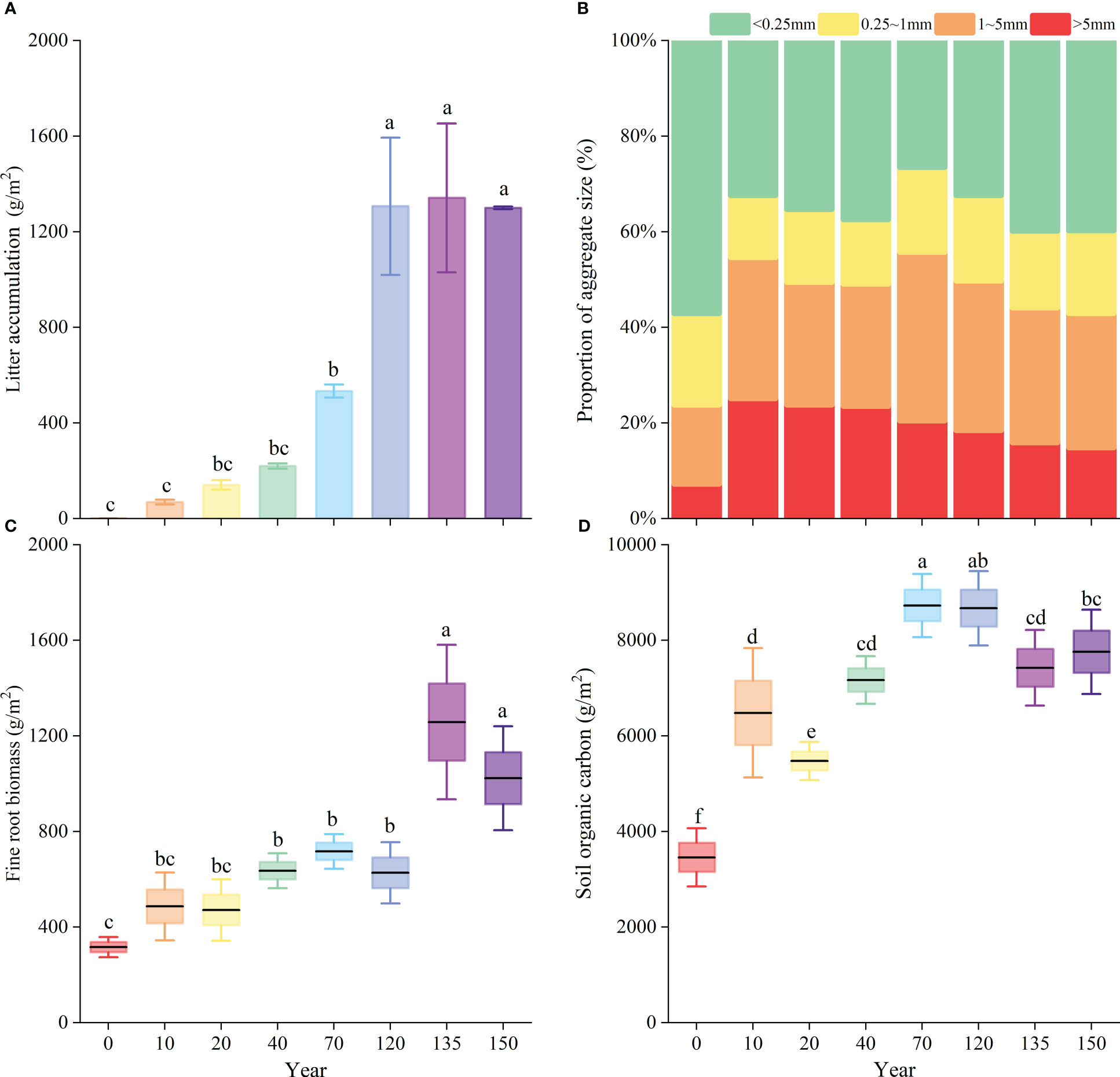
Figure 4 Comparison of litter (A), soil aggregate (B), fine-root biomass (C) and soil organic carbon (D) in each restoration succession period. Different lowercase letters (a–f) indicate significant differences (P< 0.05) within each variable among different succession periods.
3.4 Relationship between plant diversity and carbon sequestration characteristics
Pearson correlation analysis revealed that the total species richness, woody plant diversity index, litter, fine-root biomass, and SOC were significantly positively correlated with recovery time, whereas the herb diversity index and total Pielou index were significantly negatively correlated with recovery time (Figure 5). The total species richness of the plant communities was strongly positively correlated with woody plant diversity. Herb diversity was significantly positively correlated with the total Pielou, Simpson, and Shannon–Wiener indexes of the plant community. This indicated that the species variety of the entire community was controlled by woody plants, and the species number was influenced by herbaceous plants. In addition, herbaceous plant diversity was negatively correlated with fine-root biomass and litter accumulation. Woody plant diversity significantly affected the SOC storage and was positively correlated with fine-root biomass and litter accumulation, as well as with 1–5 mm grain size aggregates, which had multiple functions. Litter and fine roots were significantly positively correlated with SOC (P< 0.05). At the same time, agglomerates of 1–5 mm particle size were significantly positively correlated with SOC. This indicated that litter and fine roots are important for SOC accumulation, and 1–5 mm agglomerates are important carriers of SOC.
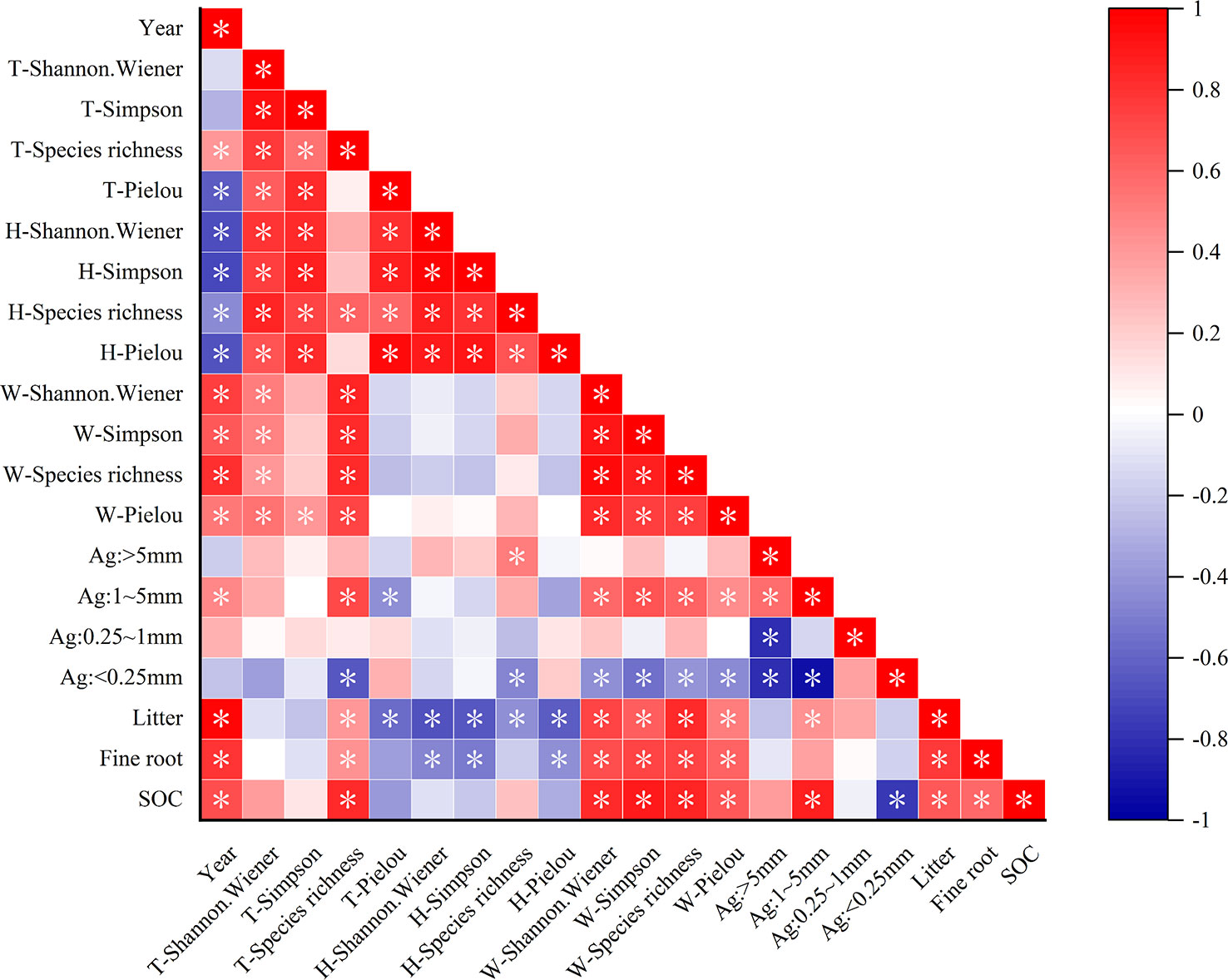
Figure 5 Relationship between plant diversity and carbon sequestration characteristics. *P < 0.05. T represents the overall diversity of plant communities; H represents the herb diversity in plant communities; W represents the woody plant diversity in plant communities; Ag represents the aggregate size; SOC represents the soil organic carbon. The darker the color, the stronger the correlation.
3.5 Plant diversity drives soil carbon sequestration
PLS-PM revealed a significant association between plant diversity and soil carbon sequestration-related pathways involving carbon inputs, carbon storage, and a direct path (Figure 6A). It explained 93.8% of the SOC sequestration variance and provided the best fit to our data (GOF = 0.805). Woody plant diversity showed the greatest positive effect on soil carbon sequestration via direct and indirect effects, whereas herb diversity was a negative determinant of the effects of carbon inputs on natural restoration succession periods. Based on our findings (Figure 6A), we performed a PLS-PM analysis using the number of woody Fabaceae and Rosaceae species at different recovery periods (Figure 6B). PLS-PM explained 92.6% of the SOC sequestration variance and provided the best fit to our data (GOF = 0.752). The results showed that Fabaceae and Rosaceae diversity, through direct and indirect effects of improving soil aggregation, significantly affected the SOC accumulation. This indicated the presence of carbon sequestration plants in these two families, which are the key species related to SOC accumulation during natural vegetation recovery.
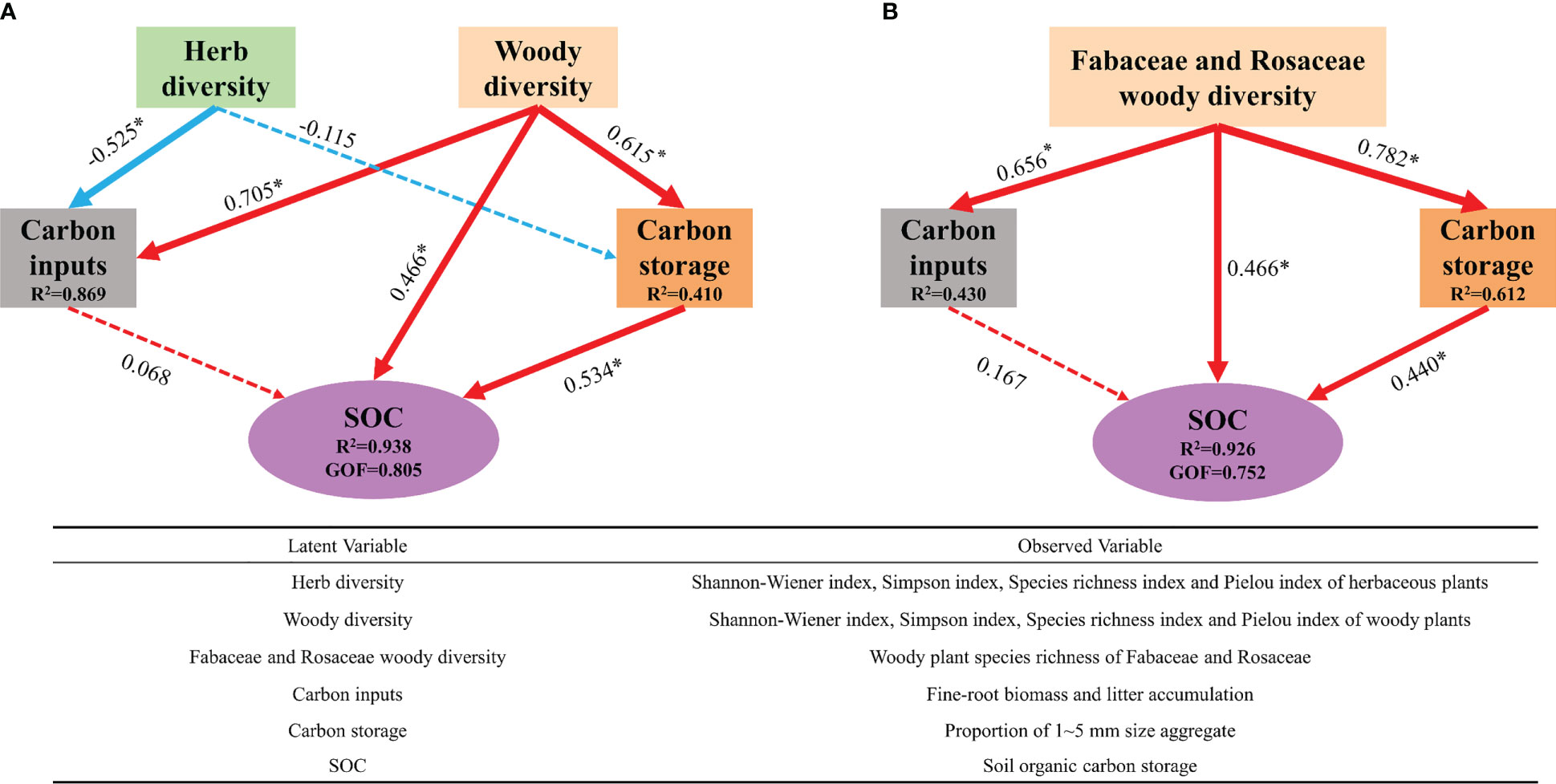
Figure 6 (A) Plant diversity drives soil carbon sequestration. (B) Carbon capturing plants drive soil carbon sequestration. *P < 0.05. Numbers on arrows are path coefficients indicating a positive (positive number) or negative effect (negative number) effect. The red lines represent positive effects, and blue lines represent negative effects. Solid lines represent significant effects, while dashed lines represent nonsignificant effects. The width of each arrow is proportional to the standardized path coefficients.
4 Discussion
4.1 Plant diversity drives soil carbon sequestration
The present study confirmed the consistency of plant diversity recovery and soil carbon accumulation, which is consistent with the findings of several previous studies (Chen et al., 2018; Madrigal-González et al., 2020; Osuri et al., 2020; Furey and Tilman, 2021; Suryaningrum et al., 2021). However, it is important to note that soil carbon sequestration was mainly driven by woody plant diversity during the 150 years of natural recovery of temperate vegetation. This indicated the significant role of forests in carbon sequestration, not only in the ground but also in carbon input to the soil, a view that is widely shared (Besnard et al., 2021; Ding et al., 2021). As shown by the direct relationship in PLS-PM (Figure 6A), SOC accumulation was mediated by woody plant diversity through increased proportions of large soil aggregates and other hitherto unidentified mechanisms. The mechanisms that have been identified so far include, first and foremost, that communities with high plant diversity have higher litter accumulation, and the input of litter increases future soil carbon (Gregorich et al., 2017). Second, the input of root secretions acts as a source of soil carbon (Rasse et al., 2005; Kramer et al., 2010; Wang et al., 2021). Simultaneously, changes in deadfall and root systems can alter the activity and composition of microbial communities and affect microbial carbon turnover and carbon sequestration rates (Bais et al., 2006; Craig et al., 2022). Third, soil microbial metabolic activity can influence anabolic changes in plant diversity, which in turn affects the carbon input from vegetation to the soil (Lange et al., 2015; Manral et al., 2022; Padalia et al., 2022; Manral et al., 2023). In addition, herbaceous plant diversity decreased with increasing time of revegetation and showed a highly significant negative correlation with carbon input, suggesting that dominant populations established by herbaceous plants through competitive exclusion had a positive effect on litter and fine roots. Herbaceous plants may also influence SOC accumulation through the three pathways described above (Yang et al., 2019b). In summary, vegetation plays a significant role in the formation of soil organic matter and influences fundamental soil forming processes such as aggregation or podzolization (Awasthi et al., 2022b; Awasthi et al., 2022c). Notably, soil aggregates are known to be the main sites of SOC fixation (Shankar and Suresha, 2006; Bai et al., 2020). It is hypothesized that approximately 90% of SOC in the surface soil of terrestrial ecosystems is fixed in soil aggregates (Six and Paustian, 2014). Soil fixation of organic carbon depends on soil agglomeration (Oades and Waters, 1991), and the physical segregation of soil aggregates, which provides them a good C sequestration capacity, can slow down the rate of organic carbon loss (Six et al., 2004; De Gryze et al., 2006). Moreover, increase in the proportion of large-size aggregates due to woody plant diversity enhances the SOC storage capacity.
In the present study, the carbon input pathway for SOC accumulation in PLS-PM was not significant, probably because the entry of litter into the soil requires microbial mediation. Fine roots are mostly living roots, and their main role is exchanging material with the soil, during which they produce carbon input as biological residues, thereby affecting future soil carbon (Gregorich et al., 2017). However, in the present study, Pearson’s correlation analysis found that both deadfall and fine roots increased significantly with the natural vegetation recovery process, and SOC accumulated gradually (Figures 4, 5). In summary, from our observations of 150 years of natural vegetation recovery succession, we hypothesized that the positive effect of plant diversity on carbon sequestration was driven by the input of carbon into the soil by litter and roots that was stored in large particle size aggregates, in which soil microorganisms play an important role. This mechanism is more evident in woody plants than in other plants. Some evidence suggests that our proposed mechanism is of general interest (Prommer et al., 2020; Jia et al., 2021; Wang et al., 2021; Feng et al., 2022). This study emphasized that a wide variety of plants is important and can have many ecological and environmental benefits, such as greater carbon sequestration.
4.2 Carbon capturing plants drive soil carbon sequestration
In the present study, we found that Fabaceae and Rosaceae plant diversity is important for SOC accumulation, and that plants belonging to these two families can directly transport organic carbon to the soil through the mechanisms described previously. It should not be overlooked that most of these species are well-rooted plants, and they can increase the content of large particle size aggregates (1–5 mm) in the soil and enhance the soil carbon sequestration capacity. This inter-rooting effect can also significantly improve the stability of soil aggregates and promote carbon sequestration by soil aggregates (Li et al., 2020). An increase in nitrogen content has been shown to be highly correlated with an increase in SOC (Li et al., 2022), and an increase in SOC content under high-nitrogen conditions corresponds to a 33% reduction in CO2 outflow (Tian et al., 2019). Fabaceae and nitrogen-fixing bacteria are known to form specific host relationships (Dassen et al., 2017). Several carbon sequestration pathways showed that both families contained species that could efficiently sequester carbon.
We screened three key species (L. bicolor, S. davidii, and C. multiflorus) taking into account the two criteria that the plants must be woody and in either Rosaceae or Leguminosae, as well as the reported plant importance values (Tian et al., 2022). All three species are shrubs; therefore, the carbon- sequestration function of shrubs should not be neglected in the process of revegetation. All three plants grow in temperate conditions, which is consistent with the climatic characteristics of the study area. Furthermore, Tian et al. (2022) showed that the ecological niche breadth of L. bicolor, S. davidii, and C. multiflorus was 9.42, 4.36, and 3.34, respectively, and they easily co-occur with other species. This indicates that they are well adapted and are important for maintaining community stability and active vegetation recovery in the area. According to Kou (2016), these three species are highly erosion-resistant and have high ecological value. Overall, we concluded that reasonable allocation of shrubs in artificial vegetation restoration can improve the vegetation carbon sequestration capacity, ecological service function, and soil health. In this study area, these three species can be considered for inclusion in artificial vegetation construction. Among them, L. bicolor is probably the best choice because it has been found to contain a variety of effective rhizobacteria (Yao et al., 2002). Moreover, the nitrogen in its senescing leaves is rarely transferred and is fed back to the soil in the form of litter and it has a significant impact on soil nitrogen and SOC accumulation because of the appropriate rate of litter decomposition (Hendricks and Boring, 1992). It has also been found that transplantation of this species to poorly eroded soils resulted in SOC enrichment and significantly improved cluster stability (Bin and Xin-Hua, 2006; Yao et al., 2009). This evidence is consistent with the results of the present study and suggests that L. bicolor has great potential for improving vegetation carbon sequestration capacity and deserves further study.
5 Conclusions
This study confirmed that the restoration of plant diversity is consistent with soil carbon accumulation. Plant diversity showed a single-peaked curve during 150 years of vegetation restoration. The peak of herbaceous plant diversity occurred between 40 and 70 years of vegetation recovery, and the peak of woody plant diversity occurred between 70 and 120 years of vegetation recovery. Litter accumulation, fine-root biomass, and SOC storage increased with the progress of vegetation restoration. The positive effect of plant diversity on carbon sequestration may be related to litter accumulation and fine-root biomass, which drive carbon input and storage in large-particle-size (1–5 mm) aggregates. Furthermore, woody plant diversity was revealed to be the primary driver of soil carbon sequestration, whereas plant diversity in Fabaceae and Rosaceae was discovered to be crucial for SOC accumulation. The vegetation carbon sequestration function of shrubs should not be neglected during vegetation restoration. In the study area, three species, namely L. bicolor, S. davidii, and C. multiflorus, should be considered for plantation. Among them, L. bicolor was observed to be the best choice. Overall, this study provides important insights into the driving mechanisms underlying plant diversity and soil carbon sequestration and its implications for addressing the effects of global climate. To quantitatively identify the contribution of different mechanisms to soil carbon sequestration, future research needs to incorporate more biotic (e.g., microbial) and abiotic factors. This will be useful for fully clarifying the connections between the above- and belowground components of temperate vegetation.
Data availability statement
The original contributions presented in this study are included in the article/supplementary material. Further inquiries can be directed to the corresponding author.
Author contributions
Conception and design of study: QT, XZ. Acquisition of data: QT, XZ, HY, XX, JH, LH. Analysis and/or interpretation of data: QT, XZ, YL. Drafting the manuscript: QT. Revising the manuscript critically for important intellectual content: XZ, YL. All authors contributed to the article and approved the submitted version.
Funding
This research was funded by the National Key R & D Program of China (2022YFE0115300) and National Natural Science Foundation of China, grant number 41877083, 41440012 and 41230852.
Acknowledgments
We would like to thanks Yadong Zou, Fan Xue and Weinan Sun for help with data processing and discussion for this article.
Conflict of interest
The authors declare that the research was conducted in the absence of any commercial or financial relationships that could be construed as a potential conflict of interest.
Publisher’s note
All claims expressed in this article are solely those of the authors and do not necessarily represent those of their affiliated organizations, or those of the publisher, the editors and the reviewers. Any product that may be evaluated in this article, or claim that may be made by its manufacturer, is not guaranteed or endorsed by the publisher.
References
Ali, H. E., Reineking, B., Münkemüller, T. (2017). Effects of plant functional traits on soil stability: intraspecific variability matters. Plant Soil 411, 359–375. doi: 10.1007/s11104-016-3036-5
Aponte, C., Kasel, S., Nitschke, C. R., Tanase, M. A., Vickers, H., Parker, L., et al. (2020). Structural diversity underpins carbon storage in Australian temperate forests. Global Ecol. Biogeog. 29 (5), 789–802. doi: 10.1111/geb.13038
Augusto, L., Boča, A. (2022). Tree functional traits, forest biomass, and tree species diversity interact with site properties to drive forest soil carbon. Nat. Commun. 13 (1), 1–12. doi: 10.1038/s41467-022-28748-0
Awasthi, P., Bargali, K., Bargali, S. S., Jhariya, M. K. (2022a). Structure and functioning of coriaria nepalensis dominated shrublands in degraded hills of kumaun himalaya. I. Dry Matter Dynam. Land Degradation Dev. 33 (9), 1474–1494. doi: 10.1002/ldr.4235
Awasthi, P., Bargali, K., Bargali, S. S., Khatri, K. (2022b). Nutrient return through decomposing coriaria nepalensis litter in degraded hills of kumaun himalaya, India. Front. Forests Global Change 5. doi: 10.3389/ffgc.2022.1008939
Awasthi, P., Bargali, K., Bargali, S. S., Khatri, K., Jhariya, M. K. (2022c). Nutrient partitioning and dynamics in coriaria nepalensis wall dominated shrublands of degraded hills of kumaun himalaya. Front. Glob Change 5. doi: 10.3389/ffgc.2022.913127
Baboo, B., Sagar, R., Bargali, S. S., Verma, H. (2017). Tree species composition, regeneration and diversity of an Indian dry tropical forest protected area. Trop. Ecol. 58 (2), 409–423.
Bai, Y. X., Zhou, Y. C., He, H. Z. (2020). Effects of rehabilitation through afforestation on soil aggregate stability and aggregate-associated carbon after forest fires in subtropical China. Geoderma 376, 114548. doi: 10.1016/j.geoderma.2020.114548
Bais, H. P., Weir, T. L., Perry, L. G., Gilroy, S., Vivanco, J. M. (2006). The role of root exudates in rhizosphere interactions with plants and other organisms. Annu. Rev. Plant Biol. 57, 233–266. doi: 10.1146/annurev.arplant.57.032905.105159
Bargali, K., Manral, V., Padalia, K., Bargali, S. S., Upadhyay, V. P. (2018). Effect of vegetation type and season on microbial biomass carbon in central Himalayan forest soils, India. Catena 171, 125–135. doi: 10.1016/j.catena.1018.07.001
Bargali, S. S., Padalia, K., Bargali, K. (2019). Effects of tree fostering on soil health and microbial biomass under different land use systems in the central Himalayas. Land Degradation Dev. 30 (16), 1984–1998. doi: 10.1002/ldr.3394
Bargali, S. S., Shahi, C., Bargali, K., Negi, B., Khatri, K. (2022). Energy and monetary efficiencies at the different altitudinal agroecosystems in central himalaya, India. Heliyon 8 (11), e11500. doi: 10.1016/j.heliyon.2022.e11500
Bartelt-Ryser, J., Joshi, J., Schmid, B., Brandl, H., Balser, T. (2005). Soil feedbacks of plant diversity on soil microbial communities and subsequent plant growth. Perspect. Plant Ecology Evol. Systematics 7 (1), 27–49. doi: 10.1016/j.ppees.2004.11.002
Besnard, S., Santoro, M., Cartus, O., Fan, N., Linscheid, N., Nair, R., et al. (2021). Global sensitivities of forest carbon changes to environmental conditions. Global Change Biol. 27 (24), 6467–6483. doi: 10.1111/gcb.15877
Bin, Z., Xin-Hua, P. E. N. G. (2006). Organic matter enrichment and aggregate stabilization in a severely degraded ultisol after reforestation. Pedosphere 16 (6), 699–706. doi: 10.1016/S1002-0160(06)60105-7
Bisht, S., Bargali, S. S., Bargali, K., Rawat, G. S., Rawat, Y. S., Fartyal, A. (2022). Influence of anthropogenic activities on forest carbon stocks–a case study from gori valley, Western himalaya. Sustainability 14 (24), 16918. doi: 10.3390/su142416918
Bisht, S., Rawat, G. S., Bargali, S. S., Rawat, Y. S., Mehta, A. (2023). Forest vegetation response to anthropogenic pressures: a case study from askot wildlife sanctuary, Western himalaya. Environment Dev. Sustain. 1–25. doi: 10.1007/s10668-023-03130-2
Blois, J. L., Williams, J. W., Fitzpatrick, M. C., Jackson, S. T., Ferrier, S. (2013). Space can substitute for time in predicting climate-change effects on biodiversity. Proc. Natl. Acad. Sci. 110 (23), 9374–9379. doi: 10.1073/pnas.1220228110
Chai, Q., Ma, Z., Chang, X., Wu, G., Zheng, J., Li, Z., et al. (2019). Optimizing management to conserve plant diversity and soil carbon stock of semi-arid grasslands on the loess plateau. Catena 172, 781–788. doi: 10.1016/j.catena.2018.09.034
Chen, X., Chen, H. Y., Chen, C., Ma, Z., Searle, E. B., Yu, Z., et al. (2020). Effects of plant diversity on soil carbon in diverse ecosystems: a global meta-analysis. Biol. Rev. 95 (1), 167–183. doi: 10.1111/brv.12554
Chen, S., Wang, W., Xu, W., Wang, Y., Wan, H., Chen, D., et al. (2018). Plant diversity enhances productivity and soil carbon storage. Proc. Natl. Acad. Sci. 115 (16), 4027–4032. doi: 10.1073/pnas.1700298114
Cheng, J., Cheng, J., Shao, H., Zhao, L., Yang, X. (2012). Soil seed banks and forest succession direction reflect soil quality in ziwuling mountain, loess plateau, China. Clean–Soil Air Water 40 (2), 140–147. doi: 10.1002/clen.201000377
Clark, D. A. (2004). Sources or sinks? the responses of tropical forests to current and future climate and atmospheric composition. philosophical transactions of the royal society of London. Ser. B: Biol. Sci. 359 (1443), 477–491. doi: 10.1098/rstb.2003.1426
Cowles, H. C. (1899). The ecological relations of the vegetation on the sand dunes of lake michigan. part i.-geographical 567 relations of the dune floras. Botanical gazette 27 (2), 95–117.
Craig, M. E., Geyer, K. M., Beidler, K. V., Brzostek, E. R., Frey, S. D., Stuart Grandy, A., et al. (2022). Fast-decaying plant litter enhances soil carbon in temperate forests but not through microbial physiological traits. Nat. Commun. 13 (1), 1–10. doi: 10.1038/s41467-022-28715-9
Craven, D., Eisenhauer, N., Pearse, W. D., Hautier, Y., Isbell, F., Roscher, C., et al. (2018). Multiple facets of biodiversity drive the diversity–stability relationship. Nat. Ecol. Evol. 2 (10), 1579–1587. doi: 10.1038/s41559-018-0647-7
Dassen, S., Cortois, R., Martens, H., de Hollander, M., Kowalchuk, G. A., van der Putten, W. H., et al. (2017). Differential responses of soil bacteria, fungi, archaea and protists to plant species richness and plant functional group identity. Mol. Ecol. 26 (15), 4085–4098. doi: 10.1111/mec.14175
Davidar, P., Sahoo, S., Mammen, P. C., Acharya, P., Puyravaud, J. P., Arjunan, M., et al. (2010). Assessing the extent and causes of forest degradation in India: where do we stand? Biol. Conserv. 143 (12), 2937–2944. doi: 10.1016/j.biocon.2010.04.032
Davidson, E. A., Trumbore, S. E., Amundson, R. (2000). Biogeochemistry: soil warming and organic carbon content. Nature 408, 789–790. doi: 10.1038/35048672
De Gryze, S., Six, J., Merckx, R. (2006). Quantifying water-stable soil aggregate turnover and its implication for soil organic matter dynamics in a model study. Eur. J. Soil Sci. 57 (5), 693–707. doi: 10.1111/j.1365-2389.2005.00760.x
Deng, L., Wang, K. B., Chen, M. L., Shangguan, Z. P., Sweeney, S. (2013). Soil organic carbon storage capacity positively related to forest succession on the loess plateau, China. Catena 110, 1–7. doi: 10.1016/j.catena.2013.06.016
Ding, J., Delgado-Baquerizo, M., Wang, J. T., Eldridge, D. J. (2021). Ecosystem functions are related to tree diversity in forests but soil biodiversity in open woodlands and shrublands. J. Ecol. 109 (12), 4158–4170. doi: 10.1111/1365-2745.13788
Duffy, J. E., Godwin, C. M., Cardinale, B. J. (2017). Biodiversity effects in the wild are common and as strong as key drivers of productivity. Nature 549 (7671), 261–264. doi: 10.1038/nature23886
Fan, W. Y., Wang, X. A., Guo, H. (2006). Analysis of plant community successional series in the ziwuling area on the loess plateau. Acta Ecologica Sin. , 26 (3), 706–714.
Feng, J., He, K., Zhang, Q., Han, M., Zhu, B. (2022). Changes in plant inputs alter soil carbon and microbial communities in forest ecosystems. Global Change Biol. 28 (10), 3426–3440. doi: 10.1111/gcb.16107
Furey, G. N., Tilman, D. (2021). Plant biodiversity and the regeneration of soil fertility. Proc. Natl. Acad. Sci. 118 (49), e2111321118. doi: 10.1073/pnas.211132111
Gavazov, K., Canarini, A., Jassey, V. E., Mills, R., Richter, A., Sundqvist, M. K., et al. (2022). Plant-microbial linkages underpin carbon sequestration in contrasting mountain tundra vegetation types. Soil Biol. Biochem. 165, 108530. doi: 10.1016/j.soilbio.2021.108530
Götz, O., Liehr-Gobbers, K., Krafft, M. (2010). “Evaluation of structural equation models using the partial least squares (PLS) approach,” in In handbook of partial least squares (Berlin, Heidelberg: Springer), 691–711). doi: 10.1007/978-3-540-32827-8_30
Gregorich, E. G., Janzen, H., Ellert, B. H., Helgason, B. L., Qian, B., Zebarth, B. J., et al. (2017). Litter decay controlled by temperature, not soil properties, affecting future soil carbon. Global Change Biol. 23 (4), 1725–1734. doi: 10.1111/gcb.13502
Gu, C., Mu, X., Gao, P., Zhao, G., Sun, W., Tatarko, J., et al. (2019). Influence of vegetation restoration on soil physical properties in the loess plateau, China. J. Soils Sediments 19 (2), 716–728. doi: 10.1007/s11368-018-2083-3
Guo, H., Wang, X. A. (2005). Canopy characteristics of the man-made pinus tabulaeformis forests in the ziwuling mountain of the loess plateau. Acta Botanica Boreal-Occidentalia Sin. 25 (7), 1335–1339. doi: 10.1360/biodiv.050022
Hautier, Y., Tilman, D., Isbell, F., Seabloom, E. W., Borer, E. T., Reich, P. B. (2015). Anthropogenic environmental changes affect ecosystem stability via biodiversity. Science 348 (6232), 336–340. doi: 10.1126/science.aaa1788
Hendricks, J. J., Boring, L. R. (1992). Litter quality of native herbaceous legumes in a burned pine forest of the Georgia piedmont. Can. J. For. Res. 22 (12), 2007–2010. doi: 10.1139/x92-263
Hill, M. (1973). Diversity and evenness: a unifying notation and its consequences. Ecology 54, 427–432. doi: 10.2307/1934352
Hu, L., Li, Q., Yan, J., Liu, C., Zhong, J. (2022). Vegetation restoration facilitates belowground microbial network complexity and recalcitrant soil organic carbon storage in southwest China karst region. Sci. Total Environ. 820, 153137. doi: 10.1016/j.scitotenv.2022.153137
Hua, F., Bruijnzeel, L. A., Meli, P., Martin, P. A., Zhang, J., Nakagawa, S., et al. (2022). The biodiversity and ecosystem service contributions and trade-offs of forest restoration approaches. Science 376 (6595), 839–844. doi: 10.1126/science.Abl4649
Hulland, J. (1999). Use of partial least squares (PLS) in strategic management research: a review of four recent studies. Strategic Manage. J. 20 (2), 195–204. doi: 10.1002/(SICI)1097-0266(199902)20:2<195:AID-SMJ13<3.0.CO
Isbell, F., Cowles, J., Dee, L. E., Loreau, M., Reich, P. B., Gonzalez, A., et al. (2018). Quantifying effects of biodiversity on ecosystem functioning across times and places. Ecol. Lett. 21 (6), 763–778. doi: 10.1111/ele.12928
Jia, Y., Zhai, G., Zhu, S., Liu, X., Schmid, B., Wang, Z., et al. (2021). Plant and microbial pathways driving plant diversity effects on soil carbon accumulation in subtropical forest. Soil Biol. Biochem. 161, 108375. doi: 10.1016/j.soilbio.2021.108375
Kou, M. (2016). The characteristics of erosion-resistant plant and its community in the hill and gully loess plateau region. Res. Center Soil Water Conserv. Ecol. Environment Chin. Acad. Sci. Ministry Educ.
Kramer, C., Trumbore, S., Fröberg, M., Dozal, L. M. C., Zhang, D., Xu, X., et al. (2010). Recent (< 4 year old) leaf litter is not a major source of microbial carbon in a temperate forest mineral soil. Soil Biol. Biochem. 42 (7), 1028–1037. doi: 10.1016/j.soilbio.2010.02.021
Lal, R. (2004). Soil carbon sequestration impacts on global climate change and food security. science 304 (5677), 1623–1627. doi: 10.1126/science.1097396
Lange, M., Eisenhauer, N., Sierra, C. A., Bessler, H., Engels, C., Griffiths, R. I., et al. (2015). Plant diversity increases soil microbial activity and soil carbon storage. Nat. Commun. 6 (1), 1–8. doi: 10.1038/ncomms7707
Li, H., Wu, Y., Liu, S., Xiao, J., Zhao, W., Chen, J., et al. (2022). Decipher soil organic carbon dynamics and driving forces across China using machine learning. Global Change Biol. 28 (10), 3394–3410. doi: 10.1111/gcb.16154
Li, J., Yuan, X., Ge, L., Li, Q., Li, Z., Wang, L., et al. (2020). Rhizosphere effects promote soil aggregate stability and associated organic carbon sequestration in rocky areas of desertification. Agriculture Ecosyst. Environ. 304, 107126. doi: 10.1016/j.agee.2020.107126
Liu, S., Zhang, W., Wang, K., Pan, F., Yang, S., Shu, S. (2015). Factors controlling accumulation of soil organic carbon along vegetation succession in a typical karst region in southwest China. Sci. Total Environ. 521, 52–58. doi: 10.1016/j.scitotenv.2015.03.074
Liu, Y., Zhu, G., Hai, X., Li, J., Shangguan, Z., Peng, C., et al. (2020). Long-term forest succession improves plant diversity and soil quality but not significantly increase soil microbial diversity: evidence from the loess plateau. Ecol. Eng. 142, 105631. doi: 10.1016/j.ecoleng.2019.105631
Madrigal-González, J., Calatayud, J., Ballesteros-Cánovas, J. A., Escudero, A., Cayuela, L., Rueda, M., et al. (2020). Climate reverses directionality in the richness–abundance relationship across the world’s main forest biomes. Nat. Commun. 11 (1), 1–7. doi: 10.1038/s41467-020-19460-y
Manral, V., Bargali, K., Bargali, S. S., Jhariya, M. K., Padalia, K. (2022). Relationships between soil and microbial biomass properties and annual flux of nutrients in central himalaya forests, India. Land Degradation Dev. 33 (12), 2014–2025. doi: 10.1002/ldr.4283
Manral, V., Bargali, K., Bargali, S. S., Karki, H., Chaturvedi, R. K. (2023). Seasonal dynamics of soil microbial biomass c, n and p along an altitudinal gradient in central himalaya, India. Sustainability 15 (2), 1651. doi: 10.3390/su15021651
Manral, V., Bargali, K., Bargali, S. S., Shahi, C. (2020). Changes in soil biochemical properties following replacement of banj oak forest with chir pine in central himalaya, India. Ecol. processes 9, 1–9. doi: 10.1186/s13717-020-00235-8
Nelson, D. W., Sommers, L. E. (1996). “Total carbon, organic carbon, and organic matter.” in Methods of soil analysis: part 3 chemical methods (Wisconsin, USA: Soil Science Society of America and American Society of Agronomy), 961–1010.
Oades, J. M., Waters, A. G. (1991). Aggregate hierarchy in soils. Soil Res. 29 (6), 815–828. doi: 10.1071/SR9910815
Osuri, A. M., Machado, S., Ratnam, J., Sankaran, M., Ayyappan, N., Muthuramkumar, S., et al. (2020). Tree diversity and carbon storage cobenefits in tropical human-dominated landscapes. Conserv. Lett. 13 (2), e12699. doi: 10.1111/conl.12699
Padalia, K., Bargali, S. S., Bargali, K., Manral, V. (2022). Soil microbial biomass phosphorus under different land use systems of central himalaya. Trop. Ecol. 63, 30–48. doi: 10.1007/s42965-021-00184-z
Pandey, R., Bargali, S. S., Bargali, K., Karki, H., Kumar, M., Sahoo, U. K. (2023). Fine root dynamics and associated nutrient flux in Sal dominated forest ecosystems of central himalaya, India. Front. Forests Global Change 5, 1064502. doi: 10.3389/ffgc.2022.1064502
Prach, K., Ujházy, K., Knopp, V., Fanta, J. (2021). Two centuries of forest succession, and 30 years of vegetation changes in permanent plots in an inland sand dune area, the Netherlands. PloS One 16 (4), e0250003. doi: 10.1371/journal.pone.0250003
Prommer, J., Walker, T. W., Wanek, W., Braun, J., Zezula, D., Hu, Y., et al. (2020). Increased microbial growth, biomass, and turnover drive soil organic carbon accumulation at higher plant diversity. Global Change Biol. 26 (2), 669–681. doi: 10.1111/gcb.14777
Ramachandra, T. V., Bharath, S. (2020). Carbon sequestration potential of the forest ecosystems in the Western ghats, a global biodiversity hotspot. Natural Resour. Res. 29 (4), 2753–2771. doi: 10.1007/s11053-019-09588-0
Rasse, D. P., Rumpel, C., Dignac, M. F. (2005). Is soil carbon mostly root carbon? mechanisms for a specific stabilisation. Plant Soil 269 (1), 341–356. doi: 10.1007/s11104-004-0907-y
Ravindranath, N. H., Ostwald, M. (2007). “Carbon inventory methods: handbook for greenhouse gas inventory, carbon mitigation and roundwood production projects,”in Advances in Global Change Research (Springer Dordrecht. Headquartered in Berlin, Germany: Springer Science & Business Media), vol. 29.
R Core Team (2020). R: a language and environment for statistical computing (Vienna, Austria: R Foundation for Statistical Computing). Available at: https://www.R-project.org.
Shahi, C., Bargali, S. S., Bargali, K. (2023). 8Dry matter dynamics and CO2 mitigation in the herb layer of central Himalayan agroecosystems along an altitudinal gradient, India. Trop. Ecol. 64 (1), 180–192. doi: 10.1007/s42965-022-00258-6
Shankar, A. U. R., Suresha, S. N. (2006). Strength behaviour of geogrid reinforced shedi soil subgrade and aggregate system. Road materials pavement design 7 (3), 313–330. doi: 10.1080/14680629.2006.9690040
Six, J., Bossuyt, H., Degryze, S., Denef, K. (2004). A history of research on the link between (micro) aggregates, soil biota, and soil organic matter dynamics. Soil tillage Res. 79 (1), 7–31. doi: 10.1016/j.still.2004.03.008
Six, J., Paustian, K. (2014). Aggregate-associated soil organic matter as an ecosystem property and a measurement tool. Soil Biol. Biochem. 68, A4–A9. doi: 10.1016/j.soilbio.2013.06.014
Suryaningrum, F., Jarvis, R. M., Buckley, H. L., Hall, D., Case, B. S. (2021). Large-Scale tree planting initiatives as an opportunity to derive carbon and biodiversity co-benefits: a case study from aotearoa New Zealand. New Forests 53, 589–602. doi: 10.1007/s11056-021-09883-w
Teixeira, H. M., Cardoso, I. M., Bianchi, F. J., da Cruz Silva, A., Jamme, D., Peña-Claros, M. (2020). Linking vegetation and soil functions during secondary forest succession in the Atlantic forest. For. Ecol. Manage. 457, 117696. doi: 10.1016/j.foreco.2019.117696
Tian, J., Dungait, J. A., Lu, X., Yang, Y., Hartley, I. P., Zhang, W., et al. (2019). Long-term nitrogen addition modifies microbial composition and functions for slow carbon cycling and increased sequestration in tropical forest soil. Global Change Biol. 25 (10), 3267–3281. doi: 10.1111/gcb.14750
Tian, Q., Zhang, X., Xu, X., Yi, H., He, J., He, L., et al. (2022). Knowledge about plant coexistence during vegetation succession for forest management on the loess plateau, China. Forests 13 (9), 1456. doi: 10.3390/f13091456
Wang, H. H., Yue, C., Mao, Q. Q., Zhao, J., Ciais, P., Li, W., et al. (2020). Vegetation and species impacts on soil organic carbon sequestration following ecological restoration over the loess plateau, China. Geoderma 371, 114389. doi: 10.1016/j.geoderma.2020.114389
Wang, Q., Zhang, Z., Guo, W., Zhu, X., Xiao, J., Liu, Q., et al. (2021). Absorptive and transport roots differ in terms of their impacts on rhizosphere soil carbon storage and stability in alpine forests. Soil Biol. Biochem. 161, 108379. doi: 10.1016/j.soilbio.2021.108379
Yoder, R. E. (1936). A direct method of aggregate analysis of soils and a study of the physical nature of erosion losses. J. Am. Soc. Agro. 28, 337–351. doi: 10.2134/agronj1936.00021962002800050001x
Yan, M., Cui, F., Liu, Y., Zhang, Z., Zhang, J., Ren, H., et al. (2020). Vegetation type and plant diversity affected soil carbon accumulation in a postmining area in shanxi province, China. Land Degradation Dev. 31 (2), 181–189. doi: 10.1002/ldr.3438
Yang, Y., Dou, Y., Cheng, H., An, S. (2019a). Plant functional diversity drives carbon storage following vegetation restoration in loess plateau, China. J. Environ. Manage. 246, 668–678. doi: 10.1016/j.jenvman.2019.06.054
Yang, Y., Shi, Y., Sun, W., Chang, J., Zhu, J., Chen, L., et al. (2022). Terrestrial carbon sinks in China and around the world and their contribution to carbon neutrality. Sci. China Life Sci. 65 (5), 861–895. doi: 10.1007/s11427-021-2045-5
Yang, Y., Tilman, D., Furey, G., Lehman, C. (2019b). Soil carbon sequestration accelerated by restoration of grassland biodiversity. Nat. Commun. 10 (1), 1–7. doi: 10.1038/s41467-019-08636-w
Yao, Z. Y., Kan, F. L., Wang, E. T., Wei, G. H., Chen, W. X. (2002). Characterization of rhizobia that nodulate legume species of the genus lespedeza and description of bradyrhizobium yuanmingense sp. nov. Int. J. Systemat. Evolution. Microbiol. 52 (6), 2219–2230. doi: 10.1086/421712
Yao, S., Qin, J., Peng, X., Zhang, B. (2009). The effects of vegetation on restoration of physical stability of a severely degraded soil in China. Ecol. Eng. 35 (5), 723–734. doi: 10.1016/j.ecoleng.2008.11.008
Yu, Q., Rao, X., Chu, C., Liu, S., Lin, Y., Sun, D., et al. (2020). Species dominance rather than species asynchrony determines the temporal stability of productivity in four subtropical forests along 30 years of restoration. For. Ecol. Manage. 457, 117687. doi: 10.1016/j.foreco.2019.117687
Zhang, Y., Duan, B., Xian, J., Korpelainen, H., Li, C. (2011). Links between plant diversity, carbon stocks and environmental factors along a successional gradient in a subalpine coniferous forest in southwest China. For. Ecol. Manage. 262 (3), 361–369. doi: 10.1016/j.foreco.2011.03.042
Zhang, Y., Xu, X., Li, Z., Xu, C., Luo, W. (2021). Improvements in soil quality with vegetation succession in subtropical China karst. Sci. Total Environ. 775, 145876. doi: 10.1016/j.scitotenv.2021.145876
Zhao, Y. G., Liu, X. F., Wang, Z. L., Zhao, S. W. (2015). Soil organic carbon fractions and sequestration across a 150-yr secondary forest chronosequence on the loess plateau, China. Catena 133, 303–308. doi: 10.1016/j.catena.2015.05.028
Zhong, Z., Zhang, X., Wang, X., Fu, S., Wu, S., Lu, X., et al. (2020). Soil bacteria and fungi respond differently to plant diversity and plant family composition during the secondary succession of abandoned farmland on the loess plateau, China. Plant Soil 448 (1), 183–200. doi: 10.1007/s11104-019-04415-0
Keywords: biodiversity, carbon sink, vegetation succession, forest plantation, soil organic carbon
Citation: Tian Q, Zhang X, Yi H, Li Y, Xu X, He J and He L (2023) Plant diversity drives soil carbon sequestration: evidence from 150 years of vegetation restoration in the temperate zone. Front. Plant Sci. 14:1191704. doi: 10.3389/fpls.2023.1191704
Received: 22 March 2023; Accepted: 15 May 2023;
Published: 06 June 2023.
Edited by:
Qing Zhang, Inner Mongolia University, ChinaReviewed by:
Guofang Liu, Chinese Academy of Sciences (CAS), ChinaSurendra Singh Bargali, Kumaun University, India
Copyright © 2023 Tian, Zhang, Yi, Li, Xu, He and He. This is an open-access article distributed under the terms of the Creative Commons Attribution License (CC BY). The use, distribution or reproduction in other forums is permitted, provided the original author(s) and the copyright owner(s) are credited and that the original publication in this journal is cited, in accordance with accepted academic practice. No use, distribution or reproduction is permitted which does not comply with these terms.
*Correspondence: Xiaoping Zhang, emhhbmd4cEBtcy5pc3djLmFjLmNu