- 1Industial Crop Research Institute, Sichuan Academy of Agricultural Sciences, Chengdu, Sichuan, China
- 2Crop Ecophysiology and Cultivation Key Laboratory of Sichuan Province, Sichuan Agricultural University, Chengdu, Sichuan, China
- 3State Key Laboratory of Dao-di Herbs, China Academy of Chinese Medical Sciences, Beijing, China
We investigated the effects of the complex cultivation of Salvia miltiorrhiza on microbial communities, secretions, yield, and active ingredients, and the mechanism of action between microbial communities, secretions, and S. miltiorrhiza growth and development. Neither maize nor soybean was suitable to grow with S. miltiorrhiza, but sesame significantly increased salvinone content, the active ingredient of S. miltiorrhiza, and Tanshinone IIA, Tanshinone I, and Cryptotanshinone increased by 27.06%, 22.76%, and 26.41%, respectively, which increased the abundance and number of microbial communities in S. miltiorrhiza roots. 16S rRNA results showed that the most abundant bacterial phyla were Proteobacteria and Acidobacteriota, and their number increased with compound planting of sesame and S. miltiorrhiza. Salvia inter-root secretions affected the microbial community and Salvia growth and development, and lipids and lipid-like molecules significantly reduced Salvia yield and active ingredients. Overall, different plant secretions can lead to differences in the natural environment and Salvia root growth and development, and the composite planting of sesame with Salvia can improve inter-root microbial communities, enhance Salvia quality, and make fuller use of land resources.
1 Introduction
Salvia miltiorrhiza Bge. is a perennial herb in the family Labiatae, whose roots and rhizomes contain active ingredients (tanshinones and tannins) with therapeutic effects on diseases such as cardiovascular diseases, diabetes, and liver diseases (Liu et al., 2020c; Jiang et al., 2022a). S. miltiorrhiza is a medicinal herb widely grown in bulk in Henan, Sichuan, Shandong, and Shaanxi (Wang et al., 2019). However, as adoption of highly efficient monocultures can lead to a decrease in quality and yield, measures such as composite cultivation are needed to promote sustainable development (Liu et al., 2020a).
High-efficiency monocultures usually lead to disturbances in soil physicochemical properties, reduced microbial community diversity, and toxin accumulation (Xin-hui et al., 2015). Recently, compound cropping has become an important research direction to solve continuous crop challenges. Compound cropping can increase plant species diversity, improve soil physicochemical properties, and enhance microbial community abundance by growing two or more crops simultaneously (Tang et al., 2014; Maitra, 2019; Domeignoz-Horta et al., 2020; Tang et al., 2021). Compound cropping can, therefore, increase agroecosystem diversity and promote sustainable agriculture (Stomph et al., 2020). Studies have shown that complex planting can modify plant root natural environments (Wang et al., 2019), and stimulate the production of unique inter-root secretions (Casper and Castelli, 2007), which regulate plant growth and promote nutrient uptake, attract specific probiotic bacteria to form plant-soil feedbacks (PSF) (Neumann and Romheld, 2003; Bais et al., 2006), and influence microbial community formation (Sasse et al., 2018).
The beneficial effects of intercropping in complex cropping systems, such as those involving cereals and legumes, have been extensively researched (Hsiao et al., 2019). Scholars have found that maize inter-root secretions contribute to enhanced reciprocity with legumes and promote nitrogen fixation by legumes (Jiang et al., 2022b). Hu demonstrated that maize inter-root secretions promote flavonoids synthesis in faba beans (Hu et al., 2021). However, not all plant species are conducive to a good inter-root microecological environment. For example, the combination of maize and S. miltiorrhiza was detrimental to growth and yield of the latter (Deng et al., 2017). Therefore, it is crucial to investigate suitable plants for successful Salvia complex cultivation.
Lei et al. (2018) found that different plants have a significant effect on S. miltiorrhiza growth and development, with pepper showing the greatest improvement in yield and quality. S. miltiorrhiza dry weight increased by 12.52%, salvianolic acid B increased by 10.25%, and tanshinone compound content significantly increased by 58.91%. Similarly, Liu et al. (2018) investigated the intercropping effects of mint, perilla, and alfalfa with S. miltiorrhiza. They found that they produced the highest weight gain and increased content of S. miltiorrhiza active ingredients. Moreover, the content of lipid-soluble components cryptotanshinone, tanshinone I, and tanshinone IIA in S. miltiorrhiza significantly increased after intercropping treatment, with the highest tanshinone content of 1.08% in S. miltiorrhiza roots after S. miltiorrhiza–mint intercropping, which was 163.41% higher than after monocropping. However, there has been no suitable grain and oil crop identified for intercropping with S. miltiorrhiza, and little research has been conducted on the effects of different plants on S. miltiorrhiza inter-root microbial community structure and abundance. Further research is needed to explore suitable crops and the underlying mechanisms of action.
To address these shortcomings, we investigated the effects of three crop plants on S. miltiorrhiza inter-root microecology and growth and development. The study designed pot experiments using macrogenomics and metabolomics to analyze S. miltiorrhiza inter-root microecology and investigate its responses to different plant secretions, including changes in soil physicochemical properties, bacterial abundance, inter-root soil community composition, and metabolite production. To confirm our results, we conducted field plot experiments. We hypothesized that various plants would lead to different Salvia inter-root microbial populations and community structures, affect its root morphology, and alter its inter-root secretions.
2 Materials and methods
2.1 Experimental site
The experiments were conducted at the Industrial Crop Research Institute, Sichuan Academy of Agricultural Sciences, Qingbaijiang District, Chengdu City, Sichuan Province, China (30°42′N, 104°19′E), where average annual temperature was 18°C, and average annual precipitation was 558.1 mm (local weather bureau data). Salvia seedlings and maize, sesame, and soybean varieties were provided by the Institute of Economic Crop Breeding and Cultivation, Sichuan Academy of Agricultural Sciences. Basic Soil Properties: Potted Plants, pondus hydrogenii = 6.7, organic matter = 21.90 g/kg, available P = 43.76 mg/kg, available K = 115.50 mg/kg, total nitrogen = 1.48 g/kg; Field, potential of hydrogen = 6.0, organic matter = 19.78 g/kg, available P = 26.75 mg/kg, available K = 188.27 mg/kg, total nitrogen = 1.48 g/kg.
2.2 Pot experiments and field experiments
Pot experiments were set up in six planting combinations: maize + Salvia completely isolated (Salvia|Maize), maize + Salvia without isolation (SalviaMaize), soybean + Salvia completely isolated (Salvia|Soybean), soybean + Salvia without isolation (SalviaSoybean), sesame + Salvia completely isolated (Salvia|Sesame), and sesame + Salvia without isolation (SalviaSesame). S. miltiorrhiza was propagated from fresh root segments (1.3–1.8 cm in diameter) selected from seedlings dug in January 2022, and transplanted to pots and the field after seedlings were raised in the greenhouse. The pot experiment used 0.4-m-high, 0.6-m-diameter breeding bags, including two isolation treatments and was conducted in a randomized design (Figure 1): ① plastic film isolation where the two crops were completely separated, with no inter-root intercropping; and ② without any isolation, where the two crops can communicate openly between the roots, with inter-root secretions interacting with each other, and where inter-root intercropping is obvious. Five replicates of each treatment were made.
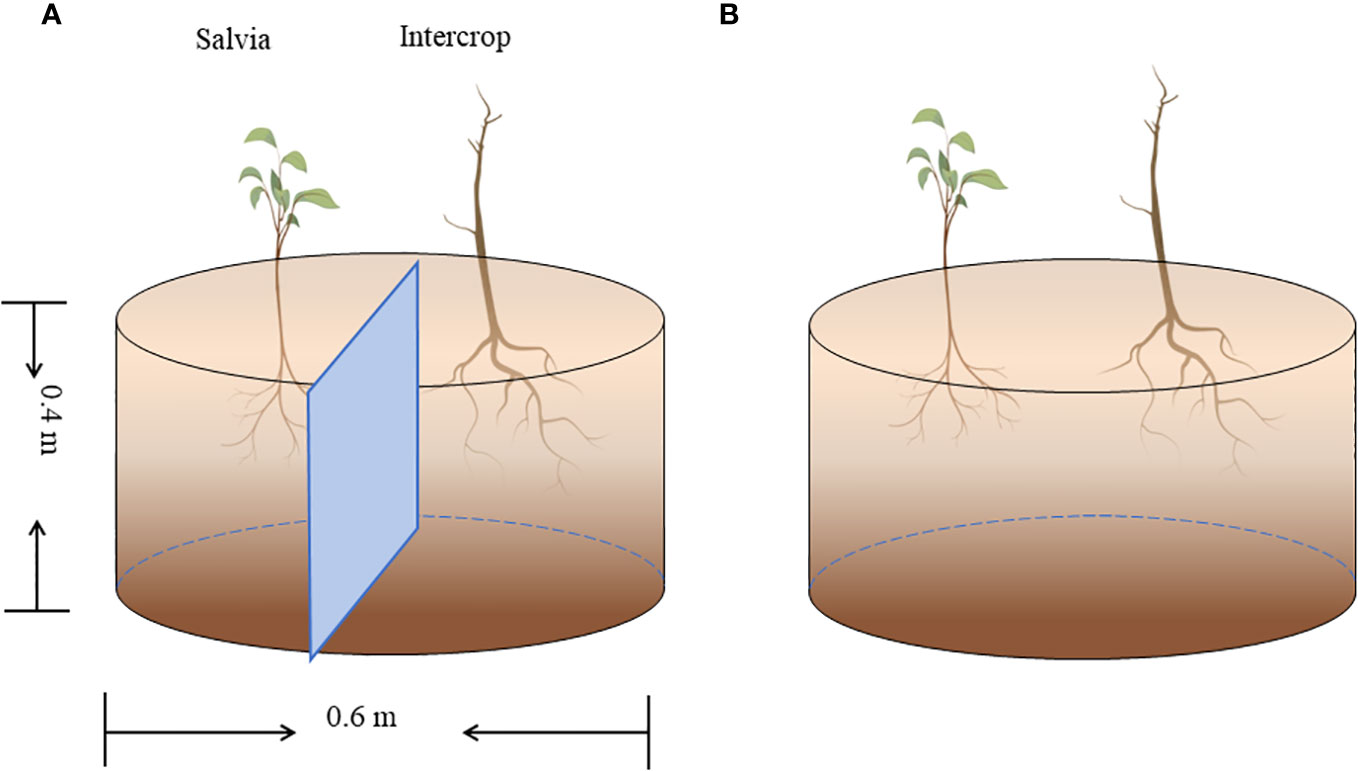
Figure 1 Pot experiment (n = 5). (A) Complete isolation of Salvia and other crops with plastic film, and (B) no isolation of Salvia and other crops.
The field experiments were conducted in a randomized design (Figure 2), including soybean monoculture (soybeanm, 0.25 m spacing), maize monoculture (maizem, 0.4 m spacing), sesame monoculture (sesamem, 0.25 m spacing), Salvia monoculture (salviam, 0.25 m spacing), Salvia-maize intercrop (Salvia + maize), Salvia-soybean intercrop (Salvia + soybean), and Salvia-sesame intercrop (Salvia + sesame). The size of each experimental area was 3.6 × 5 m, and each treatment was replicated three times.
2.3 Inter-root soil and general soil sampling
Soil samples were randomly taken from the field and pots at the time of sowing in March, and later mixed into composite samples that were naturally air-dried and stored in an oven for testing. Sampling was carried out in December 2022 on normal soil and inter-rhizosphere soil (soil close to plant roots and usually containing higher microbial populations than the surrounding normal soil) (Fierer, 2017). Inter-root soil (still attached to the roots) was shaken off gently and stored at −80°C for microbial community and inter-root metabolite analysis (Zhao et al., 2021). Simultaneously, soil around S. miltiorrhiza plants was collected, plant roots and stones were removed, and the soil was put into a self-sealing bag, and then it was dried and ground naturally, passed through a 60-mesh sieve and set aside for soil physical and chemical property testing.
2.4 Determination of soil physical and chemical properties
Soil potential of hydrogen (pH) was determined by the point method (HJ 962-2018), where soil/water is mixed at 1/2.5 (w/v), stirred vigorously for 2 min, and left for 30 min, and then pH was measured with a pH meter (PHS-SE) (Pang et al., 2021). Soil organic matter (SOM) was determined using the Walkley–Black method, which oxidizes SOM by H2SO4 and K2Cr2O7, followed by titration using FeSO4 (Fu et al., 2015). Soil active phosphorus (available P) was measured by a spectrophotometer at 700 nm (NY/T 1121.7-2014) (Liu et al., 2018). Soil available potassium (available K) was determined by a flame photometer after extraction by 1 mol/L ammonium acetate (Saber et al., 1973). Soil total nitrogen was determined by the Kelvin digestion-constant distillation–titration method, followed by digestion using an automatic Kjeldahl nitrogen tester (Hennen K1100) (Wang et al., 2018).
2.5 Microbial community structure and diversity analysis
A Soil DNA Kit was used to isolate soil microbial DNA from 5-g soil samples. The V4 hypervariable region of the bacterial gene was amplified with PCR using primers F (ACTCCTACGGGAGGCAGCA) and R (GGACTACHVGGGTWTCTAAT). After PCR processing, sequencing was conducted using an Illumina NovaSeq 6000. The raw image data files were converted to raw Sequenced Reads by Base Calling analysis. Sequenced data were filtered for connectors and low-quality using FASTP and Trimmomatic for Raw Reads to obtain high-quality valid sequences (Berg et al., 2015). Finally, the sequences were clustered with USEARCH (Edgar, 2013) (version 10.0) at the 97% similarity level, and OTUs were filtered using 0.005% of the total number of sequences sequenced as a threshold (Bokulich et al., 2013).
2.6 Salvia inter-root metabolites
To analyze metabolites in inter-rhizosphere soil, a 50-mg sample was weighed and mixed with 1,000 μL of extraction solution containing internal standard. The mixture was then ground with steel beads and sonicated for 10 min in an ice water bath, followed by centrifugation, and the supernatant was carefully collected for testing. Metabolomics analysis was conducted using a liquid mass spectrometry system composed of a Waters Acquity I-Class PLUS UPLC tandem and a Waters Xevo G2-XS QTof high-resolution mass spectrometer (Acquity UPLC HSS T3, 1.8 μm 2.1*100 mm). Positive ionization mode: mobile phase A: 0.1% formic acid aqueous solution; mobile phase B: 0.1% formic acid acetonitrile; negative ionization mode: mobile phase A: 0.1% formic acid aqueous solution; mobile phase B: 0.1% formic acid acetonitrile. The Waters Xevo G2-XS QTof high-resolution mass spectrometer is capable of primary and secondary mass spectrometry data acquisition in MSe mode under acquisition software (MassLynx V4.2, Waters) control. In each data acquisition cycle, dual-channel data acquisition is possible for both low and high collision energies. Low collision energy was 2 V, the high collision energy range was 10–40 V, and scan frequency was 0.2 s for one mass spectral map. ESI ion source parameters were as follows: capillary voltage: 2,000 V (positive ion mode) or −1,500 V (negative ion mode); cone hole voltage: 30 V; ion source temperature: 150°C; desolvent gas temperature: 500°C; backblast gas flow rate: 50 L/h; desolvent gas flow rate: 800 L/h. Raw data collected by MassLynx V4.2 were processed by Progenesis QI software for peak extraction, alignment, and other data processing operations.
2.7 Salvia root morphology and active ingredients (tanshinones and tannins)
S. miltiorrhiza was harvested in December, its inter-root morphology was investigated, and its yield was measured. The number of branches, fresh weight, and dry weight of individual roots were also measured at harvest, along with root length (measured from the base of the stem) and diameter (the largest S. miltiorrhiza diameter) (Lei et al., 2018). After harvesting, the rhizome was dried, powdered, sieved (60 mesh), and stored to determine active ingredients. Intercrops were harvested in June and July.
S. miltiorrhiza active ingredients (tanshinones and tannins) were determined using high-performance liquid chromatography. Total tanshinones and tannic acid B were determined according to the Chinese Pharmacopoeia 2015 edition, wherein tanshinones (Tanshinone IIA, Cryptotanshinone, and Tanshinone I) shall not be<0.25% in total and tanshinolic acid B shall not be<3.0%. Chromatographic analysis was performed with high-performance liquid chromatography equipment (Agilent 1260 series, USA) on a ZORBAXSB-C18 (4:6 mm × 250 mm, 5 μm) column at a temperature of 20°C. For salvianolic acid B determination, the mobile phase consisted of acetonitrile (A) and 0.1% phosphoric acid aqueous solution (B) in gradient elution mode for 20 min at 90% A with a flow rate of 1.0 mL min−1 and an injection volume of 10 μL at a detection wavelength of 286 nm. The mobile phase was composed of acetonitrile (A) and 0.02% phosphoric acid aqueous solution (B): 0–6 min, 0%–61% A; 6–20 min, 61%–90% A; 20–20.5 min, 90%–61% A; 20.5–25 min, 61% A, with a flow rate of 0.8 mL min−1, a sample volume of 10 μL, and a detection wavelength of 270 nm (Liu et al., 2020a).
2.8 Determination of field yield and land equivalent ratio
To measure land area efficiency when intercropping, Willey and Osiru (1972) introduced the land equivalent ratio (LER) concept, which reflects the yield of two crops as intercrops and as a monocrop, which can be used to evaluate the suitability of two crops for intercrop cultivation. It is:
where LER is a partial land equivalent ratio, Y1 and are the yields of seed 1 and seed 2 in interplanting, M1 and are the yields of seed 1 and seed 2 in single crop, respectively.
2.9 Data analysis
All statistical analyses were performed using various packages in R version 3.3.0 (Vermeulen et al., 2013), including ANOVA tests for multiple comparisons of soil microbial diversity, soil properties, active ingredient content, and plant biomass. Root secretion aroma diversity and evenness indices were compared among the groups by unpaired t-tests. When p< 0.05, the means of the groups were considered significantly different using the least significant difference (LSD). Principal component analysis (PCA), redundancy analysis (RDA), and heatmaps were generated using the “ggplot2” package in the R platform. PCA regroups all the metabolites originally identified linearly to form a new set of variables to determine differences between the groups of samples. To determine the proportion of variation in community structure explained by environmental factors, microbial community compositional components were determined by RDA, followed by variance decomposition analysis (VPA). Heatmaps were used to compare correlations between species abundance (OTUs) and various indicators, including environmental factors, plant growth parameters, and root base secretions.
3 Results
3.1 Effect of different planting methods on S. miltiorrhiza growth and development
In commercial trade, S. miltiorrhiza yield, quality, and appearance are very important for Salvia growers to achieve profitability. The S. miltiorrhiza fresh weight index can represent its yield, while hydrophilic and lipophilic components as well as root length and diameter indicate its quality and appearance (Liu et al., 2020a). Inter-root secretions between different plants had a significant effect on Salvia morphology (Table 1), and Salvia dry weight in the SalviaSesame treatment increased by 3.21% compared to that in Salvia|Sesame when the plants were compared with and without the isolated treatment. S. miltiorrhiza fresh weight, dry weight, and root diameter were significantly decreased by inter-root secretions from maize, and all Salvia growth indices were decreased in SalviaSoybean and SalviaMaize compared to SalviaSesame. Overall, inter-root secretions from sesame had the least effect on S. miltiorrhiza root development. The combinations SalviaMaize and SalviaMaize significantly inhibited growth, as Salvia fresh weight, dry weight, and root diameter decreased by 46.29%, 46.77%, and 27.88%, respectively.
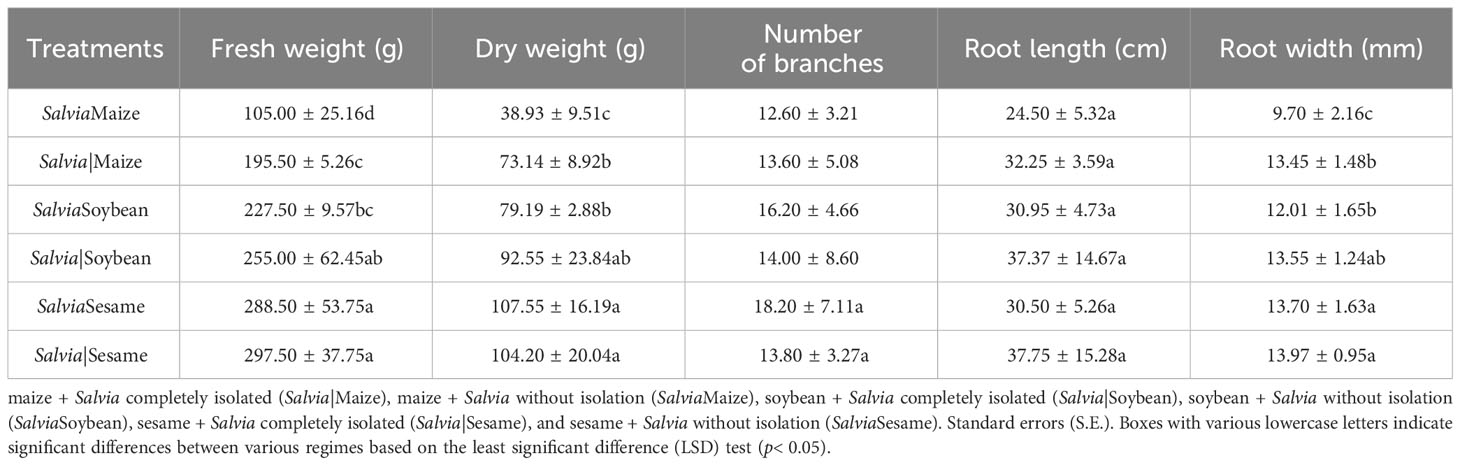
Table 1 Single plant yield and agronomic traits of Salvia Tanshinone under different cropping patterns (mean ± S.E., n = 5).
Active ingredient contents were compared under the six cultivation combinations (Figure 3). There were no significant differences in tanshinolic acid B. After SalviaSesame treatment, S. miltiorrhiza tanshinones significantly increased, with the highest levels of Tanshinone IIA, Tanshinone I, and Cryptotanshinone, showing increases of 27.06%, 22.76%, and 26.41%, respectively, compared to Salvia|Sesame. Sesame and Salvia cultivation effectively increases accumulation of Tanshinone active ingredients.
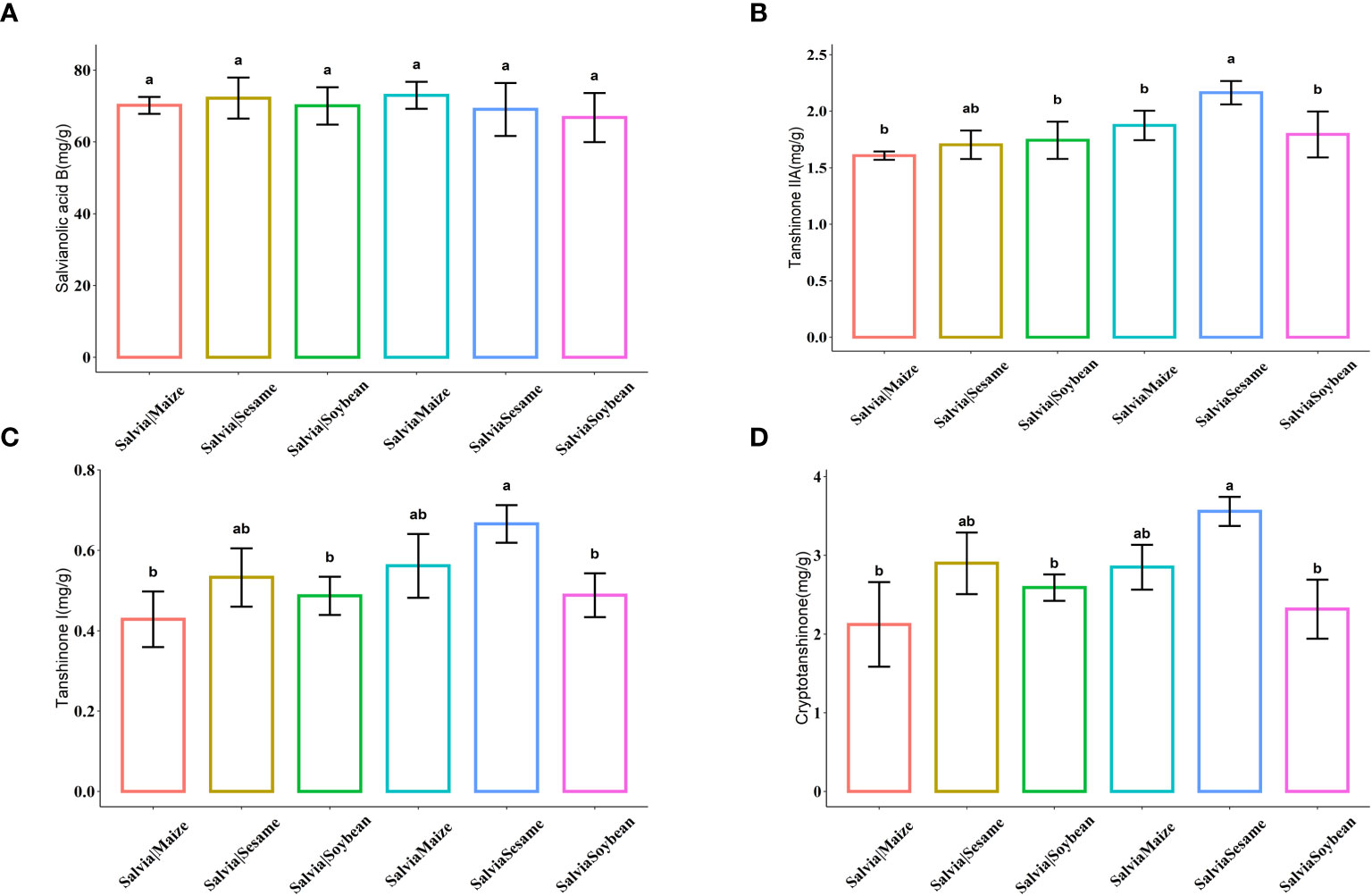
Figure 3 Histogram of active ingredient content of Salvia miltiorrhiza (n = 5). (A) Salvianolic acid B, (B) Tanshinone IIA, (C) Tanshinone I, and (D) Cryptotanshinone. Boxes with different lowercase letters indicate significant differences between various regimes based on the least significant difference (LSD) test (p< 0.05).
3.2 Effect of different planting methods on soil properties
In the potted soil after each treatment group (Salvia|Soybean, SalviaSoybean, Salvia|Maize, SalviaMaize, Salvia|Sesame, and SalviaSesame), there were no significant differences in acidity (Figure 4A), available P (Figure 4C), organic matter (Figure 4D), and total nitrogen (Figure 4E). The highest available K (Figure 4B) was found in SalviaSoybean (152.13 ± 13.69), which was significantly higher than available K in SalviaSesame (122.94 ± 15.96), while there were no significant differences between plants with and without compartment treatments.
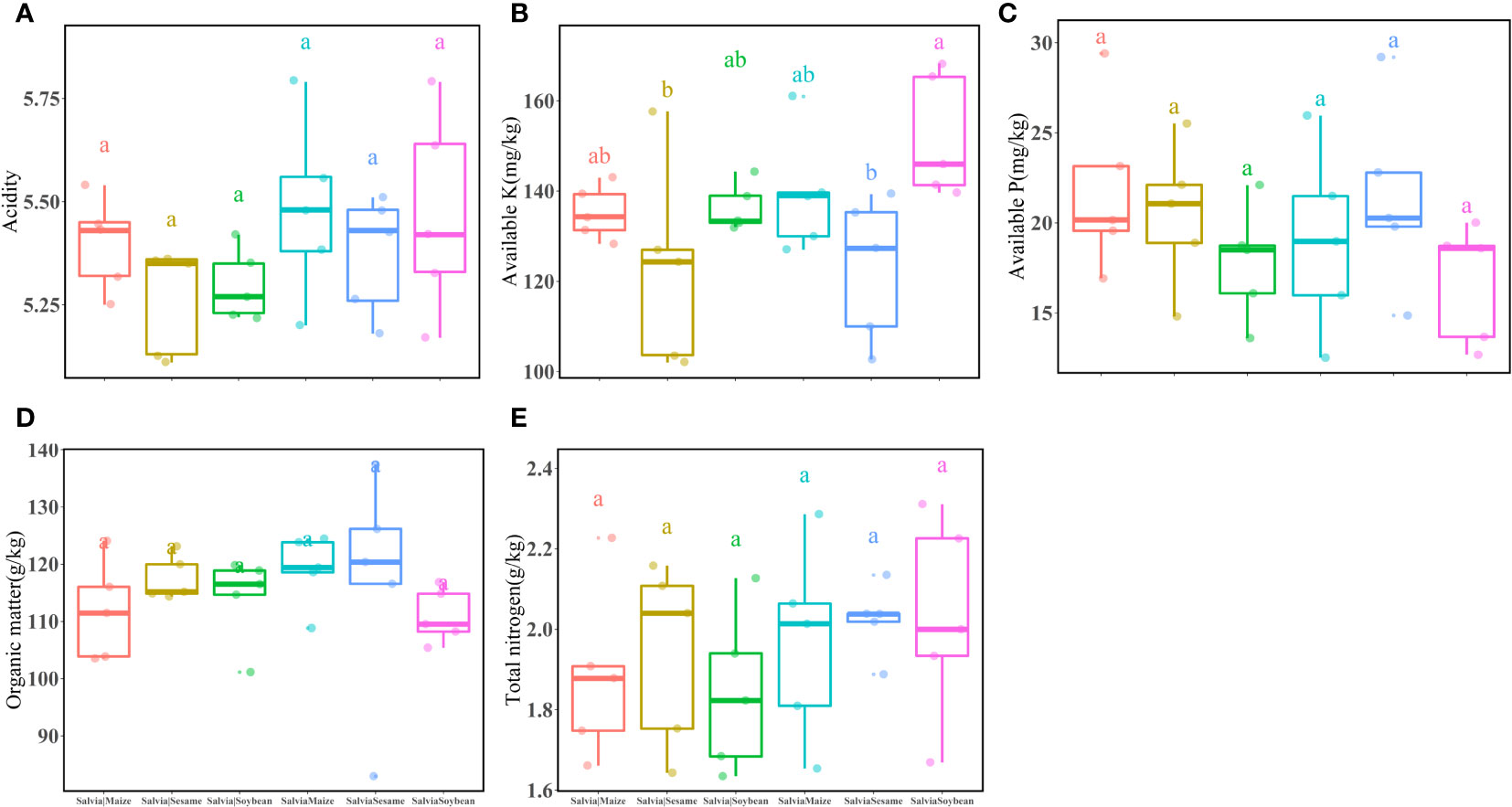
Figure 4 Inter-root soil factors under different cropping practices of Salvia and other crops (n = 3). (A) acidity, (B) available K, (C) available P, (D) organic matter, (E) total nitrogen. Boxes with different lowercase letters indicate significant differences between various regimes based on the LSD test (p< 0.05).
3.3 Effect of different planting methods on the S. miltiorrhiza inter-root bacterial community
Illumina detected a total of 1,121,526 clean reads generated from the 16S RNA gene in 18 samples, and a total of 8,654 OTUs were identified in all samples. The Chao1 index (a measure of α-diversity) was significantly lower for SalviaSoybean and SalviaMaize than for SalviaSesame, with SalviaSoybean having the lowest (275.67 ± 83.28c) number of species (Table 2). The Shannon and Simpson indices indicated that bacterial diversity and homogeneity were typically better for all treatments, with greater variation for SalviaSoybean.
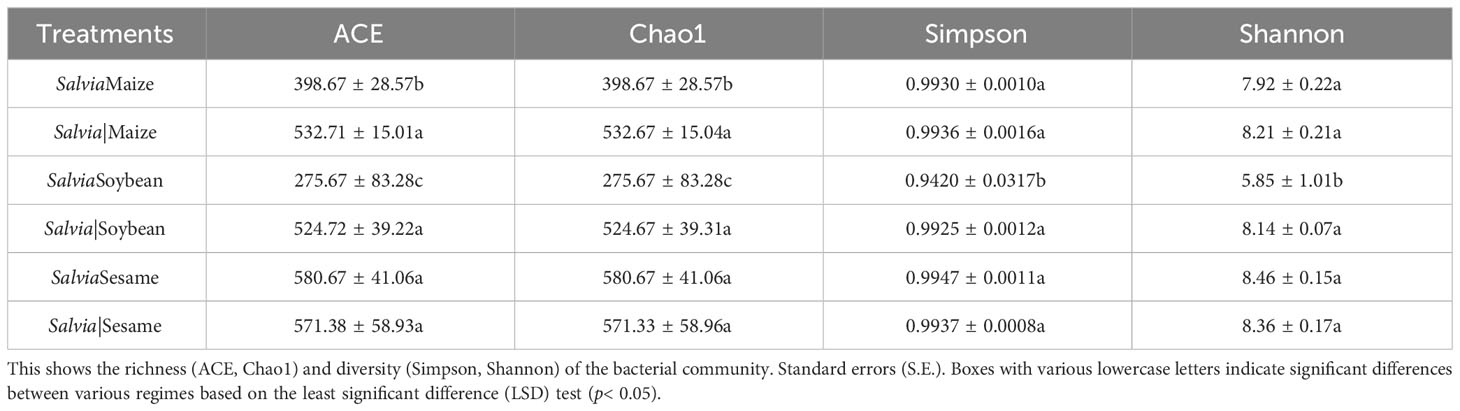
Table 2 Diversity indices of the inter-root microbial communities of Salvia miltiorrhiza under different cropping patterns (mean ± S.E., n = 3).
Additionally, there were differences in bacterial community structure after the treatment in each group. The highest abundance at bacterial phylum level was found in Proteobacteria (26.41%–44.04%), Acidobacteriota (8.37%–26.88%), Gemmatimonadota (4.62%–17.40%), Actinobacteriota (3.78%–9.13%), and unclassified_Bacteria (2.28%–11.44%) (Figure 5A). Cyanobacteria and Fusobacteriota significantly increased in the SalviaSoybean treatment, accounting for 29.11% and 4.34% of total community abundance, respectively, while both did not exceed 0.1% in any other treatment. SalviaMaize and SalviaSoybean bacterial community abundance and number showed a significant decrease compared to Salvia|Maize and Salvia|Soybean, while SalviaSesame and Salvia|Sesame bacterial community structure did not. There was no significant difference in the structure of SalviaSesame and Salvia|Sesame bacterial communities. An RDA was performed to accurately assess the relative effects of soil physicochemical properties (potential of hydrogen, organic matter, total nitrogen, available P, and available K) on the abundance and diversity of S. miltiorrhiza inter-rhizosphere bacterial communities. The first two RDA components explained 51.48% and 19.94% of the overall variation, respectively. Furthermore, potential of hydrogen, available P, available nitrogen, total nitrogen, and organic matter appeared to play a crucial role in building bacterial communities (Figure 5B). There was a negative correlation between organic matter and RDA1 (70.64%), a direct correlation between available P and RDA1 (59.13%), and a maximum contribution of 50.81% from total nitrogen to RDA2. This indicates that organic matter, available P, and total nitrogen remain the key environmental factors affecting Salvia inter-root microorganism abundance. The soil environmental factor and microbial communities correlation analysis revealed that the potential of hydrogen and Gemmatimonadota were significantly negatively correlated (r2 = 0.74; p< 0.001), and available K and Patescibacteria were significantly negatively correlated (r2 = 0.74; p< 0.001) (Figure 5C).
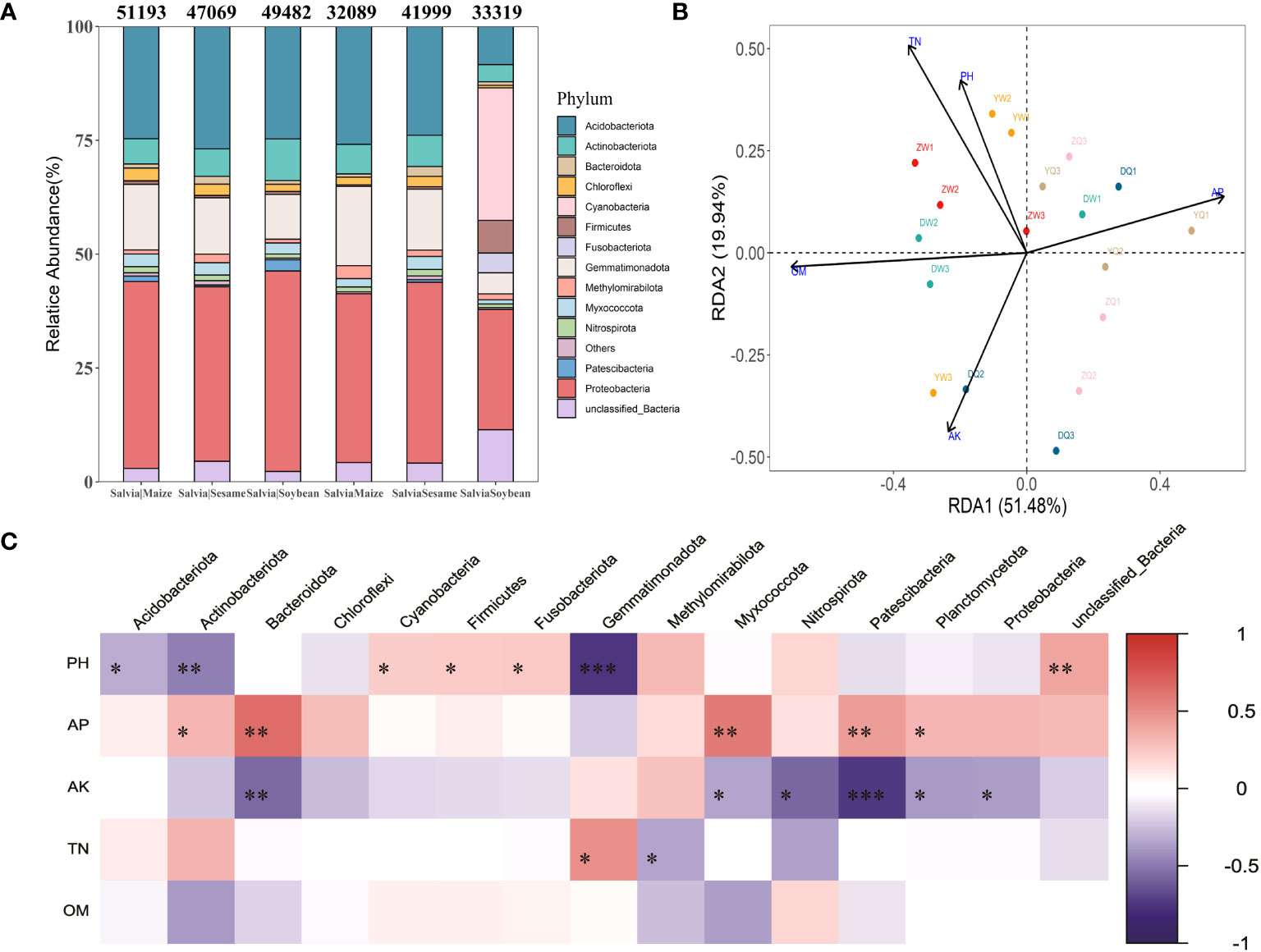
Figure 5 Distribution and composition of bacterial communities at the gate level under different cropping patterns (n = 3). (A) Relative abundance of staple microbial taxa on phylum level across all samples detected by 16S rRNA gene sequencing. The numbers above the bars indicate the number of microorganisms classified at the phylum level according to the sequence composition of feature. (B) Redundancy analysis of soil microbial communities and environmental parameters (RDA); arrows present the magnitude and direction of environmental factors associated with bacterial community structure. (C) Heatmap of soil environmental factors and phylum level bacterial correlations. Negative correlations and positive correlations are represented in blue and red. * indicates 0.01< p ≤ 0.05, ** indicates 0.001< p ≤ 0.01, *** indicates p ≤ 0.001.
3.4 Effect of different planting methods on S. miltiorrhiza inter-root secretions
After analyzing the inter-root microbial community and agronomic trait indicators of Salvia divinorum, we found that sesame was the most promising plant to grow with it. The relationship between sesame and S. miltiorrhiza inter-root secretions was further analyzed by PCA, and there were similar metabolites between SalviaSesame and Salvia|Sesame (Figure 6A). The Salvia divinorum inter-root microbial community was analyzed when the three crops were not isolated, and the lowest microbial community α-diversity was under SalviaSoybean treatment, while the highest microorganism abundance and number were found under SalviaSesame treatment. This may be due to different crops resulting in different inter-root S. miltiorrhiza feedbacks. Analysis of SalviaSoybean and SalviaSesame inter-root secretions revealed that 30 were significantly reduced in the SalviaSoybean treatment (p< 0.05 and VIP > 1.5) (Figure 6B), of which 18 belonged to the Human Metabolome Database (HMDB), with lipids and lipid-like compounds, nucleosides and analogs, organic acids and derivatives, organic oxides, and heterocyclic compounds accounting for 4.05%, 26.56%, 2.84%, 15.20%, and 8.27%, respectively. The two lowest p-value secretions screened, D-Sedoheptulose 7-phosphate and Nicotinamide-beta-riboside, both belong to the organic oxide class, and comparing the amounts of these two metabolites in SalviaSoybean and SalviaSesame, the difference multiplicity (fold change) was −1.63746 and -2.02996 (Figure 6C), respectively. The correlation heatmap between S. miltiorrhiza yield, quality, and active ingredients showed that lipids were significantly and negatively correlated with yield (fresh weight and dry weight) and quality (Tanshinone IIA, Tanshinone I, and Cryptotanshinone) (Figure 7).
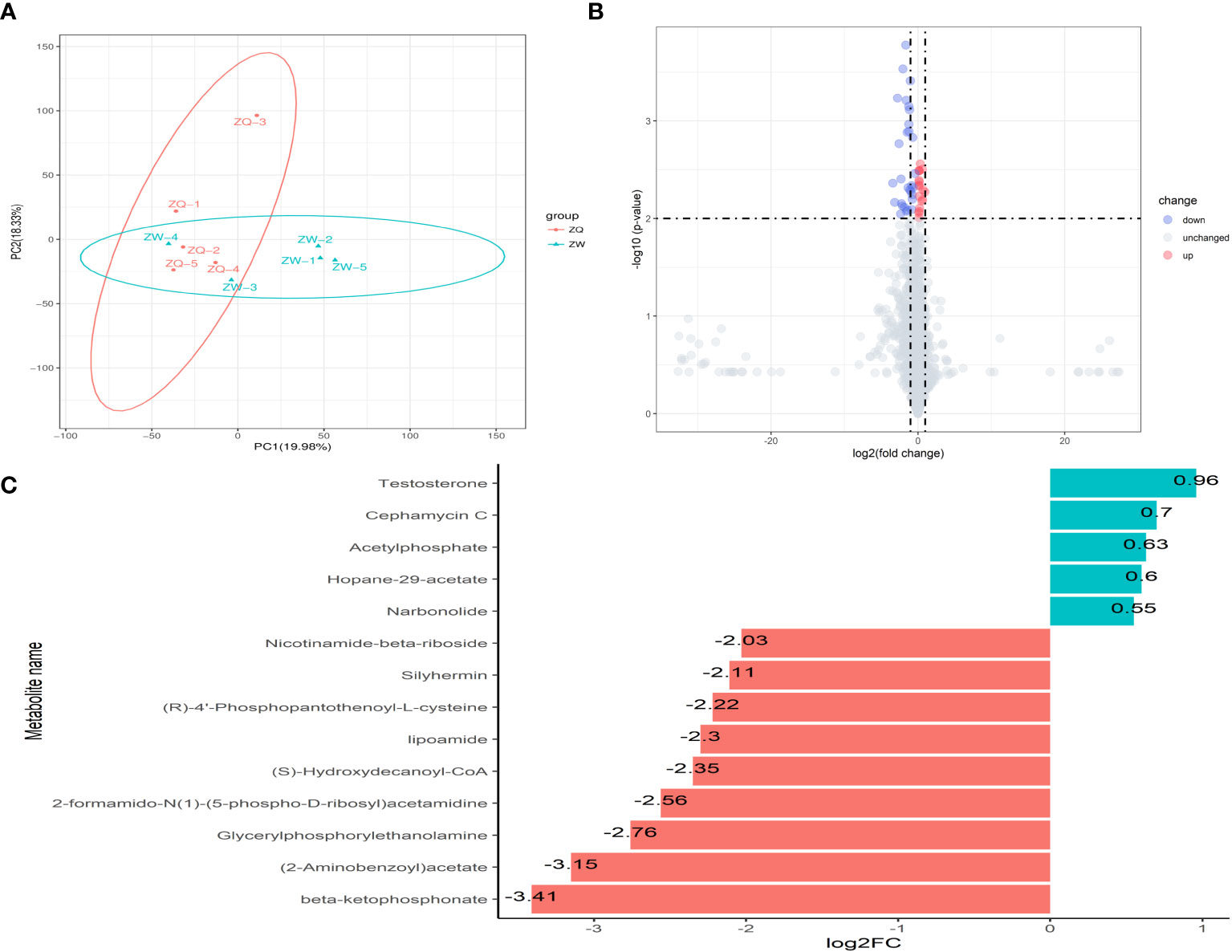
Figure 6 Analysis of inter-root secretion differences in Salvia (n = 3). (A) Principal component analysis (PCA) of inter-root secretion between Salvia|Sesame, and SalviaSesame. (B) Volcano plot depicting inter-root secretions between SalviaSesame and SalviaSoybean. (C) Fold change in the expression of inter-root metabolites of Salvia miltiorrhiza between SalviaSesame and SalviaSoybean. (B) Each point in the graph represents a metabolite; the horizontal coordinate represents the log2 value of the fold difference of a metabolite between the two samples; the vertical coordinate represents the log10 value of the p-value. Red points represent upregulated differentially expressed metabolites, blue points represent downregulated differentially expressed metabolites, and gray points represent metabolites that were detected but did not meet the filtering parameters. (C) FC (fold change).
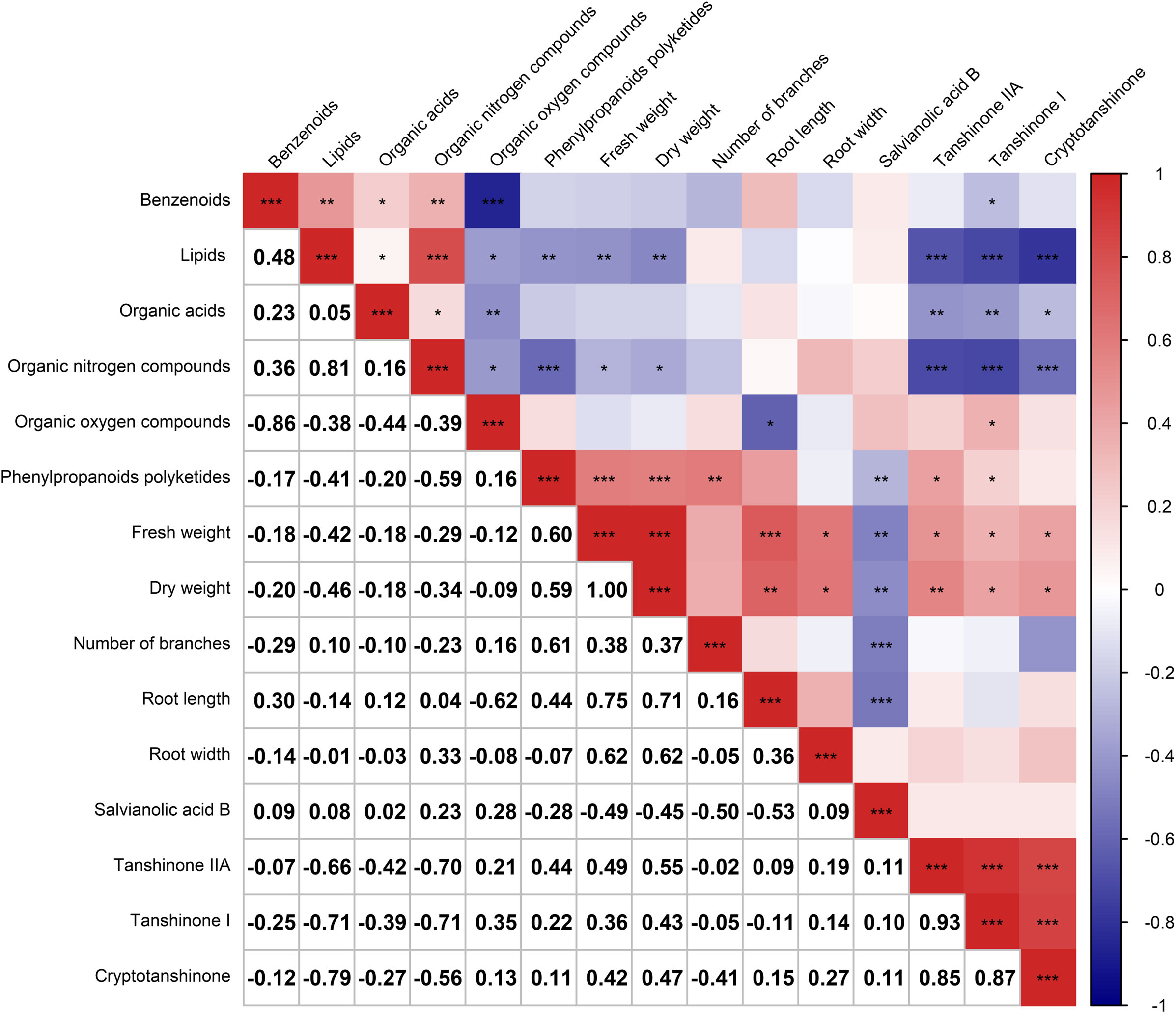
Figure 7 Correlation heatmap depicting the relationship between the six major root secretions and Salvia miltiorrhiza growth and development (n = 3). Negative correlations and positive correlations are represented in blue and red, respectively. * indicates 0.01< p ≤ 0.05, ** indicates 0.001< p ≤ 0.01, *** indicates p ≤ 0.001.
3.5 Relationships between microbial communities and Salvia root secretions, growth, and development
To accurately assess the effects of microbial communities on root secretions and S. miltiorrhiza growth and development, we investigated the relationships between the main secretions (lipids, nucleosides and analogs, organic acids and derivatives, organic oxygen compounds, organoheterocyclic compounds, phenylpropanoids, and polyketides), Salvia basic growth and developmental indicators (fresh weight, dry weight, and number of nucleosides), Salvia active constituents (Salvianolic acid B, Tanshinone IIA, Tanshinone I, and Cryptotanshinone), and the main microbial communities. Lipids and lipid-like molecules and Bacteroidota, Chloroflexi, Nitrospirota, Planctomycetota, and Proteobacteria were significantly negatively correlated (p< 0.05) (Figure 8). Organic nitrogen compounds, root width, dry weight, and Patescibacteria all showed significant positive correlations (p< 0.05). Fresh weight, dry weight and Bacteroidota, Planctomycetota were significantly positively correlated with each other (p< 0.05). Interestingly, Bacteroidota, Planctomycetota, and Patescibacteria were all significantly positively correlated with each other (p< 0.05).
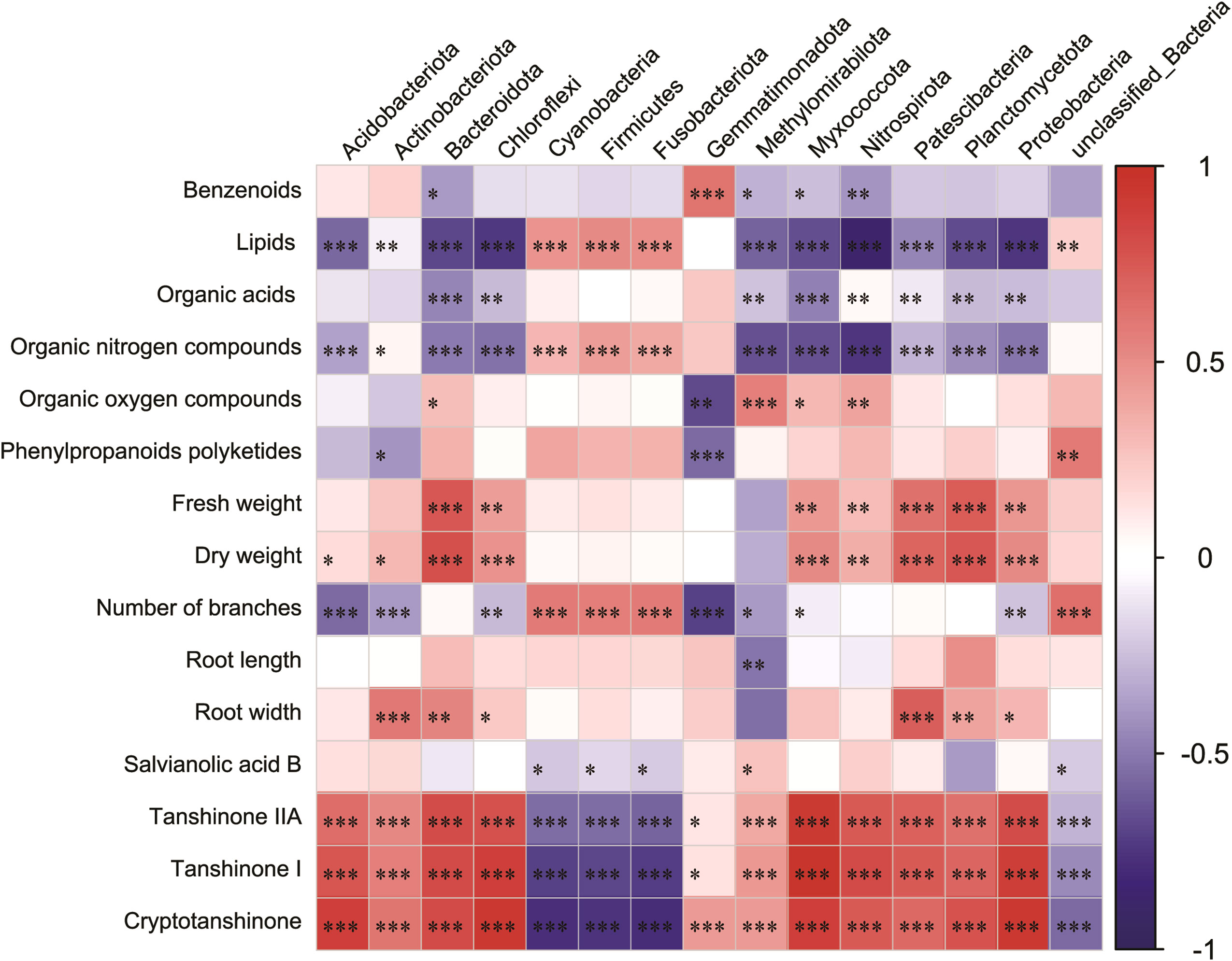
Figure 8 Correlation heatmap analysis of the association between the relative abundance of bacterial phyla and major inter-root secretions, and Salvia miltiorrhiza growth and development, including the relative richness (n = 3) of the top 15 microbial phyla. Negative correlations and positive correlations are represented by blue color and red color, respectively. Horizontal ordinate represents bacterial community abundance information, and vertical ordinate represents inter-root secretion and Salvia miltiorrhiza growth and development indicators. * indicates 0.01< p ≤ 0.05, ** indicates 0.001< p ≤ 0.01, *** indicates p ≤ 0.001.
3.6 Field experiment yield and land equivalent ratio
Compound planting of different plants and Salvia led to differences in harvest yield and LERs. Averaging the data from three replications, S. miltiorrhiza yield after intercropping maize, soybean, and sesame was 9,817.20 322.20, 14,817.451,950.15, and 15,739.65 3,061.20 kg/hm2, respectively, while monocropping maize, soybean, sesame, and Salvia yields were 17,385.001,967.7, 3,432.45 488.85, 1,800.15361.20, and 16,470.00 3,616.80 kg/hm2, respectively. The lowest Salvia yield was after intercropping with maize, where it was reduced by 40.44%. Salvia yield was reduced by 10.11% and 4.52% when intercropped with soybean and sesame, respectively. The highest LER (1.82) was achieved with sesame and Salvia intercropping, with pLER1 and of 0.96 and 0.86, respectively, and the lowest LER of 1.36 for soybean and Salvia intercropping. Field experiments verified that sesame and Salvia are a good intercropping combination.
4 Discussion
Compound planting patterns can effectively improve yield and quality of many medicinal plants, such as Salvia and Angelica, which are affected by successive crop barriers (Zhang et al., 2013; Liu et al., 2020a; Maitra et al., 2021). Compared to monocropping, Liu et al. (2018) showed that intercropping cultivation significantly enhanced Salvia root fresh weight, dry weight, and lipid-soluble component content. We showed that the combined planting of maize and Salvia reduced Salvia yield, a result consistent with previous studies (Lei et al., 2018); however, sesame and Salvia intercropping significantly increased the tanninone content of Salvia roots, while individual plant fresh and dry weight, root diameter, and branch number all increased, as did the LER (1.82) in field experiments. Many studies have demonstrated that soil factors are influenced by intercropping systems (Tang et al., 2020), e.g., hydrogen potential and available P significantly increased in both inter- and non-inter-rooted soils in the sugarcane–peanut intercropping system study group (Pang et al., 2021). In this study, there were no significant changes in potential of hydrogen, organic matter, available P, and total nitrogen, which may be due to the soil buffering effect, and/or the effects of plants on altering soil structure, which often takes several years to complete (Fierer, 2017).
Intercropping systems similarly cause changes in inter-root microbial community composition, which are mainly due to fertilization (Guo et al., 2020), crop type (Prommer et al., 2020), and changes associated with crop diversity, such as root secretions (Mommer et al., 2016) and soil environmental factors (Stefan et al., 2021). High-throughput sequencing of 16S rRNA is now an established approach (Bhakta et al., 2017) for deep exploration of soil microbial community composition and abundance. Li et al. (2022) found that Acidobacteriota and Proteobacteria were the dominant phyla in the maize intercropping system, and Proteobacteria relative abundance in the intercropped maize root soil increased significantly, primarily due to interspecific root interactions resulting in differences in bacterial community structure during intercropping. Meanwhile, Wang et al. (2019) showed that crop rotation and crop set could improve soil quality and inter-root bacterial diversity to some extent, and Symbiodinium relative abundance was higher in crop rotation and crop set than in continuous crop. In our experiment, Proteobacteria (26.41%–44.04%) and Acidobacteriota (8.37%–26.88%) were the dominant phyla, and interestingly, they (along with Salvia ketone content) were significantly and positively correlated with the fresh and dry weights of Salvia roots that were likewise positively correlated. Meanwhile, alpha diversity of the S. miltiorrhiza inter-root microbial community was highest in bacterial number and abundance when sesame and S. miltiorrhiza were grown in combination, while it was lowest when soybean and sesame were grown in combination. Thus, microbial community structure remains an important driver of S. miltiorrhiza root yield and quality.
The soil environment is the primary influencing factor in determining microbial community structure (Fierer, 2017). We found through RDA that soil environmental factors explained 51.48% and 19.94%, respectively, of the first two RDA components, which again confirmed previous studies. Available P, organic matter, and total nitrogen made the highest contribution, available P was the key factor affecting S. miltiorrhiza quality, and root Tanshinone IIA concentration was significantly and negatively correlated with soil effective phosphorus (Liang et al., 2021). Soil phosphorus content is also an important factor in soil bacterial community formation (Liu et al., 2020b). Additionally, total nitrogen is also considered the dominant factor in building microbial communities because it has key functions in cellular metabolic processes, such as energy metabolism, protein synthesis, and cell division (Rajan et al., 1991). Simultaneous analysis of soil environmental factors and microbial communities found that potential of hydrogen was negatively correlated with Gemmatimonadota, which is consistent with the findings of Guo et al. (2017).
In addition to inter-root secretions being a key factor affecting inter-root microorganisms (Wang et al., 2022), root secretion-induced autotoxin accumulation is also widely recognized as an important contributor to crop succession disorders (Xu et al., 2015). The study revealed correlations between inter-root secretion and Salvia microbial communities as well as the Salvia growth and development index. It was observed that lipids and lipid-like molecules had a significant and negative correlation with Salvia yield (fresh weight and dry weight) and quality (Tanshinone IIA, Tanshinone I, and Cryptotanshinone). Additionally, lipids and lipid-like molecules and Bacteroidota, Chloroflexi, Nitrospirota, Planctomycetota, and Proteobacteria were also significantly negatively correlated. In previous studies, different inter-root secretions had different effects on shaping microbial community structure (Li and Wu, 2018; Zhao et al., 2021). Therefore, controlling lipids and lipid-like molecule contents in soil may be an important way to improve Salvia root yield and quality and play an active role in shaping Salvia inter-root microbial structure.
5 Conclusions
The present study demonstrates that the complex cultivation of sesame and Salvia is not only a viable agricultural practice but also an effective method for optimizing land resource utilization. When sesame and Salvia are planted together, they can significantly increase Salvia tanshinone content. Furthermore, the results of our 16S rRNA sequencing analysis indicate that the number and abundance of inter-root microbial communities in Salvia are significantly higher when sesame and Salvia are planted together, compared to when Salvia is planted with either soybean or maize. Soil factors and inter-root secretions play important roles in shaping the Salvia inter-root microbial community. In particular, inter-root secretions had a significant influence on Salvia miltiorrhiza yield and quality.
Data availability statement
The original contributions presented in the study are publicly available. This data can be found here: https://www.ncbi.nlm.nih.gov/bioproject under the accession number PRJNA992203.
Author contributions
LZ and CZ designed the experiment. LZ wrote the paper and CZ revised the manuscript. ST, YZ, YY, FP, HL, CM, XW, YW, and ZX contributed to experiments and the acquisition of data. All authors contributed to the article and approved the submitted version.
Funding
The author(s) declare financial support was received for the research, authorship, and/or publication of this article. This study was supported by the Chinese Materia Medica of China Agriculture Research System (CARS-21), the Ability establishment of sustainable use for valuable Chinese medicine resources (2060302), Discipline Construction Project of SAAS (2021XKJS105).
Conflict of interest
The authors declare that the research was conducted in the absence of any commercial or financial relationships that could be construed as a potential conflict of interest.
Publisher’s note
All claims expressed in this article are solely those of the authors and do not necessarily represent those of their affiliated organizations, or those of the publisher, the editors and the reviewers. Any product that may be evaluated in this article, or claim that may be made by its manufacturer, is not guaranteed or endorsed by the publisher.
References
Bais, H. P., Weir, T. L., Perry, L. G., Gilroy, S., Vivanco, J. M. (2006). The role of root exudates in rhizosphere interactions with plants and other organisms. Annu. Rev. Plant Biol. 57, 233–266. doi: 10.1146/annurev.arplant.57.032905.105159
Berg, D., Yoon, K.-J., Will, B., Xiao, A., Kim, N.-S., Christian, K., et al. (2015). Tbr2-expressing intermediate progenitor cells in the adult mouse hippocampus are unipotent neuronal precursors with limited amplification capacity under homeostasis. Front. Biol. 10. doi: 10.1007/s11515-015-1364-0
Bhakta, J., Lahiri, S., Bhuiyna, F., Rokunuzzaaman, M., Ohonishi, K., Iwasaki, K., et al. (2017). Profiling of heavy metal(loid)-resistant bacterial community structure by metagenomic-DNA fingerprinting using PCR–DGGE for monitoring and bioremediation of contaminated environment. Energy Ecol. Environ. 3, 102–109. doi: 10.1007/s40974-017-0079-2
Bokulich, N. A., Subramanian, S., Faith, J. J., Gevers, D., Gordon, J. I., Knight, R., et al. (2013). Quality-filtering vastly improves diversity estimates from Illumina amplicon sequencing. Nat. Methods 10, 57–59. doi: 10.1038/nmeth.2276
Casper, B. B., Castelli, J. P. (2007). Evaluating plant-soil feedback together with competition in a serpentine grassland. Ecol. Lett. 10, 394–400. doi: 10.1111/j.1461-0248.2007.01030.x
Deng, G., Li, X., Zhang, H., Ren, W., Zhang, J., Wang, X., et al. (2017). Effects of intercropping maize on growth of salvia miltiorrhiza. J. Anhui Agric. Sci. 45, 122–123. doi: 10.13989/j.cnki.0517-6611.2017.05.043
Domeignoz-Horta, L. A., Pold, G., Liu, X.-J. A., Frey, S. D., Melillo, J. M., DeAngelis, K. M. (2020). Microbial diversity drives carbon use efficiency in a model soil. Nat. Commun. 11, 3684. doi: 10.1038/s41467-020-17502-z
Edgar, R. C. (2013). UPARSE: highly accurate OTU sequences from microbial amplicon reads. Nat. Methods 10, 996–998. doi: 10.1038/nmeth.2604
Fierer, N. (2017). Embracing the unknown: disentangling the complexities of the soil microbiome. Nat. Rev. Microbiol. 15, 579–590. doi: 10.1038/nrmicro.2017.87
Fu, M.-M., Huang, B., Jia, M.-M., Hu, W.-Y., Sun, W.-X., Weindorf, D. C., et al. (2015). Effect of intensive greenhouse vegetable cultivation on selenium availability in soil. Pedosphere 25, 343–350. doi: 10.1016/S1002-0160(15)30002-3
Guo, H., Nasir, M., Lv, J., Dai, Y., Gao, J. (2017). Understanding the variation of microbial community in heavy metals contaminated soil using high throughput sequencing. Ecotoxicol. Environ. Saf. 144, 300–306. doi: 10.1016/j.ecoenv.2017.06.048
Guo, Z., Wan, S., Hua, K., Yin, Y., Chu, H., Wang, D., et al. (2020). Fertilization regime has a greater effect on soil microbial community structure than crop rotation and growth stage in an agroecosystem. Appl. Soil Ecol. 149, 103510. doi: 10.1016/j.apsoil.2020.103510
Hsiao, C.-J., Sassenrath, G., Zeglin, L., Hettiarachchi, G., Rice, C. (2019). Temporal variation of soil microbial properties in a corn–wheat–soybean system. Soil Sci. Soc. Am. J. 83, 1696–1711. doi: 10.2136/sssaj2019.05.0160
Hu, H., Li, H., Hao, M., Ren, Y., Zhang, M., Liu, R., et al. (2021). Nitrogen fixation and crop productivity enhancements codriven by intercrop root exudates and key rhizosphere bacteria. J. Appl. Ecol. 58, 2243–2255. doi. 10.1111/1365-2664.13964
Jiang, J.-S., Gu, Q.-C., Feng, Z.-M., Yuan, X., Zhang, X., Zhang, P.-C., et al. (2022a). The phenolic acids from the plant of Salvia miltiorrhiza. Fitoterapia 159, 105180. doi: 10.1016/j.fitote.2022.105180
Jiang, Y., Khan, M. U., Lin, X., Lin, Z., Lin, S., Lin, W. (2022b). Evaluation of maize/peanut intercropping effects on microbial assembly, root exudates and peanut nitrogen uptake. Plant Physiol. Biochem. 171, 75–83. doi: 10.1016/j.plaphy.2021.12.024
Lei, L., Guo, Q., Wang, C., Ma, Z., Cao, W., An, J. (2018). Effects of compound planting on growth and quality of Salvia miltiorrhiza. China J. Chin. Materia Med. 43, 1818–1824. doi: 10.19540/j.cnki.cjcmm.20180307.011
Li, H., Luo, L., Tang, B., Guo, H., Cao, Z., Zeng, Q., et al. (2022). Dynamic changes of rhizosphere soil bacterial community and nutrients in cadmium polluted soils with soybean-corn intercropping. BMC Microbiol. 22, 57. doi: 10.1186/s12866-022-02468-3
Li, S., Wu, F. (2018). Diversity and co-occurrence patterns of soil bacterial and fungal communities in seven intercropping systems. Front. Microbiol. 9, 1521. doi: 10.3389/fmicb.2018.01521
Liang, H., Kong, Y., Chen, W., Wang, X., Jia, Z., Dai, Y., et al. (2021). The quality of wild Salvia miltiorrhiza from Dao Di area in China and its correlation with soil parameters and climate factors. Phytochem. Anal. 32, 318–325. doi: 10.1002/pca.2978
Liu, H., Niu, M., Zhu, S., Zhang, F., Liu, Q., Liu, Y., et al. (2020a). Effect study of continuous monoculture on the quality of salvia miltiorrhiza bge roots. BioMed. Res. Int. 2020, 4284385. doi: 10.1155/2020/4284385
Liu, H., Wang, C., Xie, Y., Luo, Y., Sheng, M., Xu, F., et al. (2020b). Ecological responses of soil microbial abundance and diversity to cadmium and soil properties in farmland around an enterprise-intensive region. J. Hazard Mater 392, 122478. doi: 10.1016/j.jhazmat.2020.122478
Liu, H., Xu, F., Xie, Y., Wang, C., Zhang, A., Li, L., et al. (2018). Effect of modified coconut shell biochar on availability of heavy metals and biochemical characteristics of soil in multiple heavy metals contaminated soil. Sci. Total Environ. 645, 702–709. doi: 10.1016/j.scitotenv.2018.07.115
Liu, Q., Zhao, Y., Qi, Y., Zhang, Z., Hao, P., Pu, G., et al. (2020c). A comprehensive analytical platform for unraveling the effect of the cultivation area on the composition of Salvia miltiorrhiza Bunge. Ind. Crops Prod. 145, 111952. doi: 10.1016/j.indcrop.2019.111952
Liu, W., Zhou, B., Wan, X., Lu, H., Guo, L., Li, F., et al. (2018). Effect of efficient ecological intercropping patterns on the growth and active ingredient content of Salvia miltiorrhiza. J. Chin. Medicinal Materials 41, 1027–1030. doi: 10.13863/j.issn1001-4454.2018.05.003
Maitra, S. (2019). Potential of intercropping system in sustaining crop productivity. Int. J. Agric. Environ. Biotechnol. 12 (1), 39–45. doi: 10.30954/0974-1712.03.2019.7
Maitra, S., Hossain, A., Brestic, M., Skalicky, M., Ondrišík, P., Gitari, H., et al. (2021). Intercropping system-A low input agricultural strategy for food and environmental security. Agronomy 11, 343. doi: 10.3390/agronomy11020343
Mommer, L., Kirkegaard, J., van Ruijven, J. (2016). Root-root interactions: towards A rhizosphere framework. Trends Plant Sci. 21, 209–217. doi: 10.1016/j.tplants.2016.01.009
Neumann, G., Romheld, V. (2003). Root-induced changes in the availability of nutrients in the rhizosphere. Cheminform 34, 617–619. doi: 10.1002/chin.200304265
Pang, Z., Fallah, N., Weng, P., Zhou, Y., Tang, X., Tayyab, M., et al. (2021). Sugarcane-peanut intercropping system enhances bacteria abundance, diversity, and sugarcane parameters in rhizospheric and bulk soils. Front. Microbiol. 12, 815129. doi: 10.3389/fmicb.2021.815129
Prommer, J., Walker, T. W. N., Wanek, W., Braun, J., Zezula, D., Hu, Y., et al. (2020). Increased microbial growth, biomass, and turnover drive soil organic carbon accumulation at higher plant diversity. Glob Chang Biol. 26, 669–681. doi: 10.1111/gcb.14777
Rajan, S. S. S., Fox, R. L., Saunders, W. M. H., Upsdell, M. (1991). Influence of pH, time and rate of application on phosphate rock dissolution and availability to pastures - I. Agronomic benefits. Nutrient Cycling Agroecosystems 28, 85–93. doi: 10.1007/bf01048860
Saber, M. S. M., Guirguis, M. A., Zanati, M. R. (1973). Biological and chemical determination of available phosphorus in soil. Zentralblatt für Bakteriologie, Parasitenkunde, Infektionskrankheiten und Hygiene. Zweite Naturwissenschaftliche Abteilung: Allgemeine Landwirtschaftliche und Technische Mikrobiologie 128, 566–571. doi: 10.1016/S0044-4057(73)80078-4
Sasse, J., Martinoia, E., Northen, T. (2018). Feed your friends: do plant exudates shape the root microbiome? Trends Plant Sci. 23, 25–41.
Stefan, L., Hartmann, M., Engbersen, N., Six, J., Schöb, C. (2021). Positive effects of crop diversity on productivity driven by changes in soil microbial composition. Front. Microbiol. 12, 660749. doi: 10.3389/fmicb.2021.660749
Stomph, T., Dordas, C., Baranger, A., de Rijk, J., Dong, B., Evers, J., et al. (2020). “Chapter One - Designing intercrops for high yield, yield stability and efficient use of resources: Are there principles?” in Advances in Agronomy. Ed. Sparks, D. L. (Academic Press), 1–50. doi: 10.1016/bs.agron.2019.10.002
Tang, X., Bernard, L., Brauman, A., Daufresne, T., Deleporte, P., Desclaux, D., et al. (2014). Increase in microbial biomass and phosphorus availability in the rhizosphere of intercropped cereal and legumes under field conditions. Soil Biol. Biochem. 75, 86–93. doi: 10.1016/j.soilbio.2014.04.001
Tang, X., Jiang, J., Huang, Z., Wu, H., Wang, J., He, L., et al. (2021). Sugarcane/peanut intercropping system improves the soil quality and increases the abundance of beneficial microbes. J. Basic Microbiol. 61, 165–176. doi: 10.1002/jobm.202000750
Tang, X., Zhong, R., Jiang, J., He, L., Huang, Z., Shi, G., et al. (2020). Cassava/peanut intercropping improves soil quality via rhizospheric microbes increased available nitrogen contents. BMC Biotechnol. 20, 13. doi: 10.1186/s12896-020-00606-1
Vermeulen, S. J., Heymans, M. W., Anema, J. R., Schellart, A. J. M., van Mechelen, W., van der Beek, A. J. (2013). Economic evaluation of a participatory return-to-work intervention for temporary agency and unemployed workers sick-listed due to musculoskeletal disorders. Scand. J. Work Environ. Health 39, 46–56. doi: 10.5271/sjweh.3314
Wang, L., Rengel, Z., Zhang, K., Jin, K., Lyu, Y., Zhang, L., et al. (2022). Ensuring future food security and resource sustainability: insights into the rhizosphere. iScience 25, 104168. doi: 10.1016/j.isci.2022.104168
Wang, Q., Wang, C., Yu, W., Turak, A., Chen, D., Huang, Y., et al. (20181543). Effects of nitrogen and phosphorus inputs on soil bacterial abundance, diversity, and community composition in chinese fir plantations. Front. Microbiol. 9. doi: 10.3389/fmicb.2018.01543
Wang, Y., Yang, B., Wang, H., Yang, C., Zhang, J., Zhu, M., et al. (2019). Variation in microbial community structure in the rhizosphere soil of Salvia miltiorrhiza Bunge under three cropping modes. Acta Ecologica Sin. 39, 4832–4843. doi: 10.5846/stxb201805151071
Willey, R. W., Osiru, D. S. O. (1972). Studies on mixtures of maize and beans (Phaseolus vulgaris) with particular reference to plant population. J. Agric. Sci. 79, 517–529. doi: 10.1017/S0021859600025909
Xin-hui, Z., Lang, D., Zhang, E., Wang, Z. (2015). Effect of autotoxicity and soil microbes in continuous cropping soil on angelica sinensis seedling growth and rhizosphere soil microbial population. Chin. Herbal Medicines 7, 88–93. doi: 10.1016/S1674-6384(15)60025-9
Xu, Y., Wu, Y.-G., Chen, Y., Zhang, J.-F., Song, X.-Q., Zhu, G.-P., et al. (2015). Autotoxicity in Pogostemon cablin and their allelochemicals. Rev. Bras. Farmacognosia 25, 117–123. doi: 10.1016/j.bjp.2015.02.003
Zhang, X., Lang, D., Zhang, E., Bai, C., Wang, H. (2013). Diurnal changes in photosynthesis and antioxidants of Angelica sinensis as influenced by cropping systems. Photosynthetica 51, 252–258. doi: 10.1007/s11099-013-0013-6
Keywords: Salvia miltiorrhiza, 16S rRNA, complex cultivation, inter-root secretions, microbial community
Citation: Zhang L, Tao S, Zhang Y, Yang Y, Peng F, Liao H, Mao C, Wan X, Wu Y, Xu Z and Zhang C (2024) Study on the effect of compound cultivation on the growth feature and active ingredients content of Salvia miltiorrhiza. Front. Plant Sci. 14:1238896. doi: 10.3389/fpls.2023.1238896
Received: 12 June 2023; Accepted: 27 December 2023;
Published: 18 January 2024.
Edited by:
Andrea Genre, University of Turin, ItalyCopyright © 2024 Zhang, Tao, Zhang, Yang, Peng, Liao, Mao, Wan, Wu, Xu and Zhang. This is an open-access article distributed under the terms of the Creative Commons Attribution License (CC BY). The use, distribution or reproduction in other forums is permitted, provided the original author(s) and the copyright owner(s) are credited and that the original publication in this journal is cited, in accordance with accepted academic practice. No use, distribution or reproduction is permitted which does not comply with these terms.
*Correspondence: Zhengjun Xu, bXl3aWxkcmljZUBhbGl5dW4uY29t; Chao Zhang, anljaGFvemhhbmdAMTYzLmNvbQ==
†These authors have contributed equally to this work and share first authorship