- 1Laboratory of Functional Genomics and Proteomics, National Centre for Biomolecular Research, Faculty of Science, Masaryk University, Brno, Czechia
- 2Mendel Centre for Plant Genomics and Proteomics, Central European Institute of Technology, Masaryk University, Brno, Czechia
Plants, unlike animals, possess a unique developmental plasticity, that allows them to adapt to changing environmental conditions. A fundamental aspect of this plasticity is their ability to undergo postembryonic de novo organogenesis. This requires the presence of regulators that trigger and mediate specific spatiotemporal changes in developmental programs. The phytohormone cytokinin has been known as a principal regulator of plant development for more than six decades. In de novo shoot organogenesis and in vitro shoot regeneration, cytokinins are the prime candidates for the signal that determines shoot identity. Both processes of de novo shoot apical meristem development are accompanied by changes in gene expression, cell fate reprogramming, and the switching-on of the shoot-specific homeodomain regulator, WUSCHEL. Current understanding about the role of cytokinins in the shoot regeneration will be discussed.
1 Introduction
In contrast to animals, plants possess a unique developmental plasticity that enables them to adapt to changing environmental conditions. This plasticity is possible because they can undergo postembryonic de novo organogenesis. This strategy requires that specific spatiotemporal changes to developmental programs be made, and that is accomplished by the presence of regulators – like the phytohormones cytokinins and auxin that have been known as principal regulators of plant development for a long time (Skoog and Miller, 1957). Mutual interactions between auxin and cytokinin were shown to be involved in many developmental processes in plants (reviewed in Pernisová et al., 2011; Chandler and Werr, 2015; Schaller et al., 2015). In de novo organogenesis, auxin is the principal morphogen required to induce new organ onset, while cytokinins modulate the type of organogenic response. The presence of auxin alone or high auxin-to-cytokinin concentration ratio in media induces root regeneration from various plant tissues in vitro. On the other hand, if the auxin-to-cytokinin ratio is low, shoots are formed (Skoog and Miller, 1957; Atta et al., 2009; Pernisová et al., 2009; Sugimoto et al., 2010). Over the past few years, substantial progress has been made in understanding the molecular mechanisms underlying this ability of plants to regenerate shoot meristems. In this review, we will give a brief overview of the structure and regulations of the shoot apical meristem, before shifting our focus onto shoot regeneration and the phytohormonal regulations of the process, mainly by cytokinins.
1.1 Cytokinin metabolism
The source and accessibility of cytokinins to regulate plant development is largely determined by their metabolic machinery. The initial step of cytokinin biosynthesis is catalyzed by the enzyme isopentenyltransferase (IPT) and involves the transfer of a prenyl moiety from dimethylallyl diphosphate to ATP or ADP to form N6-isopentenyladenine (iP) ribotides (Kakimoto, 2001; Takei et al., 2001). The major initial products can be hydroxylated to trans-zeatin (tZ)-type cytokinins by the action of cytochrome P450 mono-oxygenases CYP735A1 and CYP735A2 (Takei et al., 2004). The tZ-types are most probably converted by zeatin reductase to DHZ-type (Martin et al., 1989; Gaudinova et al., 2005) but the corresponding gene has not yet been identified. Cytokinins of the cis-zeatin (cZ)-type arise from tRNA degradation (Kakimoto, 2001; Miyawaki et al., 2006). Direct conversion of cytokinin ribotides to active free bases is catalysed by a nucleoside 5’-monophosphate phosphoribohydrolase named LONELY GUY (LOG) which plays a pivotal role in regulating cytokinin activity during plant growth (Kurakawa et al., 2007; Kuroha et al., 2009). Free cytokinin bases and cytokinin ribosides can be converted back to ribotides by the action of adenine phosphoribosyl transferases (APT) (Lee and Moffatt, 1993; Allen et al., 2002) and adenosine kinases (ADK) (Moffatt et al., 2000), respectively. Cytokinin deactivation readily occurs via O- and N-glycosylation catalysed by uridine diphosphate glycosyltransferases (UGT) (Hou et al., 2004). Cytokinin O-glucosides can be converted to the active free bases by β-glucosidase (Brzobohaty et al., 1993). Irreversible cytokinin degradation is catalysed by the cytokinin oxidase (CKX) (Bilyeu et al., 2001; Galuszka et al., 2001), which cleaves unsaturated N6-side chains from tZ and iP-type cytokinins (Jones and Schreiber, 1997). The combined regulation of biosynthesis, modifications and degradation maintains cytokinin homeostasis, keeping phytohormone levels optimal at each stage as well as in each tissue throughout plant growth and development.
1.2 Cytokinin signaling
Cytokinins trigger multistep phosphorelay signaling by binding to their cognate receptors. In Arabidopsis, three receptors ARABIDOPSIS HISTIDINE KINASE AHK2, AHK3 or AHK4/WOL/CRE1 (Inoue et al., 2001; Higuchi et al., 2004; Nishimura et al., 2004) sense cytokinins via their CHASE domains (Anantharaman and Aravind, 2001). This signal is sequentially transferred to ARABIDOPSIS HISTIDINE PHOSPHOTRANSFER PROTEINS (AHPs; AHP1-5) (Hutchison et al., 2006) and then to ARABIDOPSIS RESPONSE REGULATORS (ARRs) (Suzuki et al., 1998; Tanaka et al., 2004). The one exception is AHP6, which lacks a histidine and attenuates cytokinin signaling (Mahonen et al., 2006). Type-A ARRs (ARR3-9, 15-17) represent cytokinin primary response genes and are promptly upregulated by cytokinins, while simultaneously inhibiting the cytokinin signaling pathway, thus creating a negative feedback loop (Hwang and Sheen, 2001; To et al., 2004). Type-B ARRs (ARR1, 2, 10-14, 18-21) contain a DNA binding domain and control the expression of cytokinin-regulated genes, including type-A ARRs (Sakai et al., 2000; Mason et al., 2005). A third class, type-C ARRs, consists of only two members (ARR22, 24) which are structurally related to type-A ARRs, but their transcription is not induced by cytokinins (Kiba et al., 2004). Moreover, AHPs also interact with a set of cytokinin-regulated transcription factors, the CYTOKININ RESPONSE FACTORS (CRFs) (Rashotte et al., 2006; Cutcliffe et al., 2011), thus providing additional fine-tuning of cytokinin signaling output.
2 Shoot apical meristem in Arabidopsis thaliana
2.1 Definition of meristems
Plants show a remarkable plasticity in their growth over their entire lifetime, which sets them apart from mammals. In plants, two apical meristematic systems localized at opposite ends of the plant body axis – the shoot apical meristem (SAM) and the root apical meristem (RAM) – are established during embryogenesis. These meristems are responsible for giving rise to the entire plant body – both the root and the areal parts. The proper functioning of meristems is dependent on the presence of a small number of undifferentiated pluripotent stem cells, which are located in specific environments called stem cell niches (reviewed in Greb and Lohmann, 2016).
2.2 Composition of shoot apical meristem
Stem cells of SAM are located in the central zone (CZ) at the shoot apex (Fletcher et al., 1999). Stem cells undergo very slow cell division keeping the stem cell pool constant (Laux, 2003). Daughter cells on the periphery are pushed out of the CZ to neighboring zones, where they undergo faster cell divisions and subsequently differentiate giving rise to specific plant tissues and organs (Schoof et al., 2000; Reddy et al., 2004). In Arabidopsis, CZ comprises three layers called L1, L2, and L3, where cells from each layer have a different identity. Cells in the L1 and L2 layers divide anticlinally giving rise to leaf and flower primordia. L3 cells divide anticlinally and periclinally, leading to internal tissue production. A distinct group of cells that controls CZ activity, called the organizing center (OC), is located below the CZ, and furnishes signals to block differentiation, thereby keeping stem cells undifferentiated (reviewed in Greb and Lohmann, 2016).
2.3 Maintenance of shoot apical meristem
For proper plant body development, SAM must perpetuate itself. This demands general regulation factors. Homeodomain transcription factor WUSCHEL (WUS) plays a central role in SAM maintenance as a necessary and sufficient regulator of stem cells (reviewed in Lopes et al., 2021). The wus mutation causes defects in the shoot meristem at all developmental stages in Arabidopsis (Laux et al., 1996; Mayer et al., 1998). In cooperation with the CLAVATA3 (CLV3) peptide, WUS preserves the stem cell niche (Figure 1A). Originating in the OC, WUS migrates through plasmodesmata to the CZ, where it directly activates CLV3 expression (Yadav et al., 2011; Daum et al., 2014). The CLV3 peptide is perceived by leucine rich repeat (LRR)-based receptors, either by homomers of LRR-receptor-like kinases, such as CLAVATA1 (CLV1) (Ogawa et al., 2008), or by complexes of LRRs with membrane-bound kinases or pseudokinases, such as CLAVATA2 (CLV2) and CORYNE (CRN) (Bleckmann et al., 2009; Guo et al., 2010). CLV3 binding to dedicated receptors results in the activation of a signaling cascade that regulates WUS activity. Thus, the WUS-CLV interaction establishes a self-organizing feedback loop maintaining equilibrium between cell division and cell differentiation in the SAM (Fletcher et al., 1999; Schoof et al., 2000). Additionally, two CLV3-related peptides CLE16 and CLE17 contribute to SAM stem cell maintenance and organ production independently from CLV3. Their signal is precepted by BARELY ANY MERISTEM (BAM) and act upstream of WUS (Dao et al., 2022).
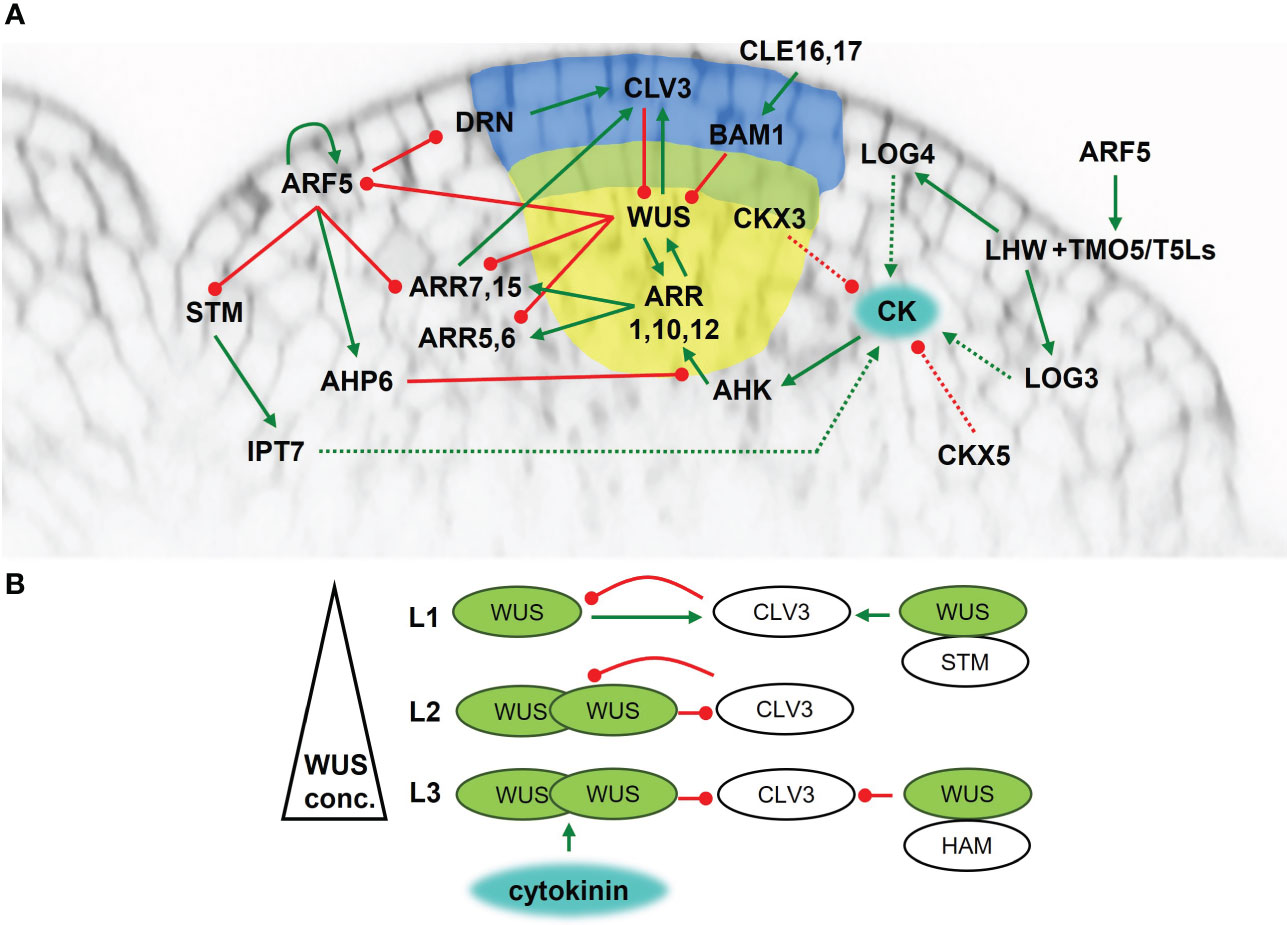
Figure 1 Maintenance of the shoot apical meristem of Arabidopsis thaliana. (A) Cytokinin signaling and metabolism network overview. Blue area – central zone; yellow area – organizing center; green line - positive regulation; red line – negative regulation; dashed line - metabolic pathway. (B) The presence of cytokinins activates WUS in the L3 layer. WUS is transported into L2 and L1, thus creating a concentration gradient. At high concentrations it forms homodimers which inhibit CLV3 activity. In the L3, WUS also forms heterodimers with HAM which strengthen the inhibitory effect on CLV3 activity. At low concentrations WUS activates CLV3 transcription. In the L1, WUS forms a heterodimer with STM, strengthening the positive regulation of CLV3.
Recently, the subcellular – particularly the nucleolar versus cytoplasmic – distribution of WUS was revealed to be an important factor in stem cell niche maintenance. In L1 and L2 layers, CLV3 inhibits WUS export from the nucleus thus blocking WUS diffusion into surrounding cells. Simultaneously, CLV3 represses WUS expression resulting in lower WUS concentrations in the nucleus than is needed for the activation of CLV3 expression. Transport of WUS from the nucleus to the cytoplasm can be enabled by direct interaction of WUS with EXPORTIN proteins via the EAR-like domain (Plong et al., 2021). The EAR-like domain could serve as a nuclear export signal and may also be required for destabilizing WUS in the cytoplasm. Mutations in the WUS EAR-like domain leads to an enlarged SAM, which was attributed to the higher pool of cytoplasmically-stable WUS available for diffusion into adjacent cells (Rodriguez et al., 2016).
Another level of WUS action control arises from the ability of WUS to form heterodimers with other proteins (Figure 1B). In the L1 and L2 layers, WUS interacts with SHOOTMERISTEMLESS (STM), a transcription factor (Long et al., 1996) required for maintaining the proliferative state of the meristematic cells (Vollbrecht et al., 2000). The WUS-STM interaction strengthens its binding to the CLV3 promoter and enhances CLV3 expression (Su et al., 2020). On the other hand, WUS can create heterodimers with proteins from HAIRY MERISTEM (HAM) family leading to the repression of CLV3 expression in the L3 layer (Zhou et al., 2015; Zhou et al., 2018b).
2.4 Role of cytokinins in the regulation of shoot apical meristem
The activity of meristematic tissues is regulated by many factors, with a crucial role played by phytohormones. Cytokinins are important regulators of SAM function and maintenance, and their abundance depends on the biosynthetic machinery. One of the rate-limiting enzymes of cytokinin biosynthesis IPT7 is triggered by STM localized in SAM (Yanai et al., 2005) (Figure 1A). The L1 layer is thought to be the source of active cytokinins as the expression of another cytokinin biosynthetic gene LOG4 was detected there (Chickarmane et al., 2012). It is hypothesized that active cytokinins move from the L1 layer into inner cell layers, where they affect SAM function. Moreover, LOG3 was showed to localize to developing primordia. Further, basic helix-loop-helix (bHLH) transcription factor heterodimers of TARGET OF MONOPTEROS5 (TMO5) and LONESOME HIGHWAY (LHW) subclades were suggested to act as a general regulator of cell proliferation in all meristems of Arabidopsis. Heterodimer complexes could regulate meristems due to heterodimer variations between subclade members, leading to diversification of target gene expression (Mor et al., 2022). The TMO5-LHW heterodimer directly regulates several enzymes of cytokinin metabolism (e.g., LOG3, LOG4, CKX3) (De Rybel et al., 2014; Yang et al., 2021) thus impacting endogenous cytokinin levels. In SAM, the phenotype and gene expression pattern of TMO5 and LHW subclade members suggest additional heterodimer combinations can affect LOG expression. LOG3 and LOG4 reporter signals are completely missing in the lhw single mutant suggesting that the LHW transcription factor is essential for LOG3 and LOG4 expression (Mor et al., 2022). Additionally, the cytokinin degrading CKX3 is expressed in a similar pattern as WUS, while CKX5 is also present in several other areas. The ckx3 ckx5 double mutant forms larger meristems with an expanded WUS domain (Bartrina et al., 2011), probably because of higher endogenous cytokinin levels.
To be functional, cytokinins need to be “visible”. The cytokinin receptors AHK2, AHK3 and AHK4 are expressed in the inner tissues of SAM including OC, but not in the L1 and L2 layers. Therefore, it seems that it is mainly the L3 layer that is the active site for cytokinin perception (Gordon et al., 2009; Chickarmane et al., 2012). The presence of cytokinins triggers cytokinin signaling leading to the activation of type-B ARRs (Figure 1A). The transcription factors ARR1, ARR10 and ARR12 bind directly to the WUS promoter and induce WUS expression (Meng et al., 2017; Zubo et al., 2017). The arr1 arr10 arr12 triple mutant forms a much smaller SAM compared to WT recalling that of the ahk2 ahk3 ahk4 triple mutant (Ishida et al., 2008), which points to a fundamental role for ARR1, ARR10 and ARR12 in SAM maintenance. Moreover, ARR1 stabilizes WUS (Snipes et al., 2018), thus setting up positive feedback between cytokinins and WUS.
Apart from activating WUS, type-B ARRs also activate type-A ARRs (Sakai et al., 2000), thus providing negative feedback regulation of cytokinin signaling (Hwang and Sheen, 2001; To et al., 2004). ARR1, ARR2 and ARR10 are responsible for activating ARR6, with ARR2 being the most effective (Hwang and Sheen, 2001). On the other hand, several type-A ARRs, particularly ARR5, ARR6, ARR7 and ARR15, are repressed by WUS, thereby potentiating cytokinin signaling. A constitutively active form of ARR7 causes significant defects in SAM function with arrested meristem in severe cases (Leibfried et al., 2005). Consistent with this, miRNA mediated attenuation of ARR7 and ARR15 expression results in SAM expansion and a severe reduction of CLV3 mRNA, pointing to ARR7 and ARR15 control of SAM maintenance by regulating CLV3 expression (Zhao et al., 2010).
It seems that local auxin accumulation is another important factor for proper functioning of ARR7 and ARR15, as revealed by their elevated transcripts in the auxin biosynthesis yucca (yuc) mutants, the auxin transport pinformed 1 (pin1) mutant, or following treatment by the auxin transport inhibitor NPA. The negative regulation of ARR7 and ARR15 by auxin is partially mediated by signaling through AUXIN RESPONSE FACTOR5/MONOPTEROS (ARF5/MP) (Zhao et al., 2010). Other targets of ARF5 involved in SAM activity and maintenance have been identified in Arabidopsis, e.g., AHP6 (Besnard et al., 2014), TMOs (Schlereth et al., 2010), DORNRÖSCHEN/ENHANCER OF SHOOT REGENERATION1 (DRN/ESR1) (Cole et al., 2009), and interestingly ARF5 itself (Lau et al., 2011). The role of auxin signaling in SAM regulation and maintenance has been reviewed recently (Pernisová and Vernoux, 2021) and we will not cover this topic in more detail here.
Taken together, the overlap between cells with threshold cytokinin levels and cells competent for cytokinin perception presumably occurs at a fixed distance from the cytokinin source (L1 layer), leading to precise WUS activation, and defining the OC domain.
3 Two-step in vitro shoot regeneration via callus formation in Arabidopsis thaliana
3.1 Acquisition of meristematic identity
Shoots can be regenerated from explants in in vitro conditions using two approaches. During two-step regeneration, explants are first placed on a callus-inducing medium (CIM), which contains a high concentration of auxin. In the second step, the callus formed on the CIM is transferred to a shoot-inducing medium (SIM), with a high concentration of cytokinins, where shoots develop (Valvekens et al., 1988). The callus maintains some level of cell organization resembling lateral root primordium pointing to the fact that a callus is not a population of undifferentiated cells, but rather a partially differentiated tissue with features resembling the lateral root developmental program (Atta et al., 2009; Sugimoto et al., 2010). Originally, it was thought that during de novo organogenesis, any somatic cell can dedifferentiate and reenter the cell cycle. However, further research uncovered that organogenesis originates from populations of partially differentiated stem cells. One such population is formed of pericycle cells located adjacent to the xylem poles in roots (Dubrovsky et al., 2000; Beeckman et al., 2001). In aerial parts of the plant body, de novo organogenesis originates from pericycle-like cells, which are present around the vasculature of multiple organs throughout the plant body (Sugimoto et al., 2010). Initiation of founder cells is the first step in lateral root development. Acquisition of founder cell status depends on auxin accumulation in pericycle cells. The specification of founder cells is followed by nuclear polarization of two adjacent pericycle cells that divide anticlinally and asymmetrically, forming two large cells and two smaller daughter cells representing stage I of the lateral root primordium (Malamy and Benfey, 1997; Casimiro et al., 2001; Dubrovsky et al., 2001). Recent observations during the early stages of regeneration have shown that the whole process likely does not originate from a single cell, but rather from a group of cells (Subban et al., 2020).
A local auxin maximum is established and auxin signaling is activated in the early stages of founder cell specification (Figure 2A). Auxin accumulation in founder cells leads to the degradation of the AUXIN/INDOLE-3-ACETIC ACID INDUCIBLE28 (AUX/IAA28) repressor, which controls the founder cell-specifying GATA23 transcription factor (De Rybel et al., 2010). Auxin also controls the division of founder cells by degrading SOLITARY ROOT/INDOLE 3 ACETIC ACID INDUCIBLE14 (SLR/IAA14), which acts as a repressor of ARF7 and ARF19. The repression of ARF7 and ARF19 is also dependent on PICKLE/SUPRESSOR OF SLR2 (PKL/SSL2). Following SLR/IAA14 degradation, ARF7 and ARF19 positively regulate the expression of LATERAL ORGAN BOUNDARIES-DOMAIN29/ASYMMETRIC LEAVES2-LIKE16 (LBD29/ASL16), LBD16/ASL18 and LBD18/ASL20 (Fukaki et al., 2002; Okushima et al., 2005; Fukaki et al., 2006), that are necessary for the initiation of lateral roots (Okushima et al., 2007; Lee et al., 2009). LBD induction promotes cell cycle progression through the G1 – S checkpoint (Feng et al., 2012). The process of callus formation seems also to be positively regulated by MORE AUXILARY GROWTH2 (MAX2) (Li et al., 2019). In the max2 mutant, LBD33 is less upregulated on CIM medium (Temmerman et al., 2022). LBD33 forms a heterodimer with a LBD18, and this is responsible for the induction of pericycle cell cycle activation via E2Fa transcription factor (Okushima et al., 2007; Berckmans et al., 2011).
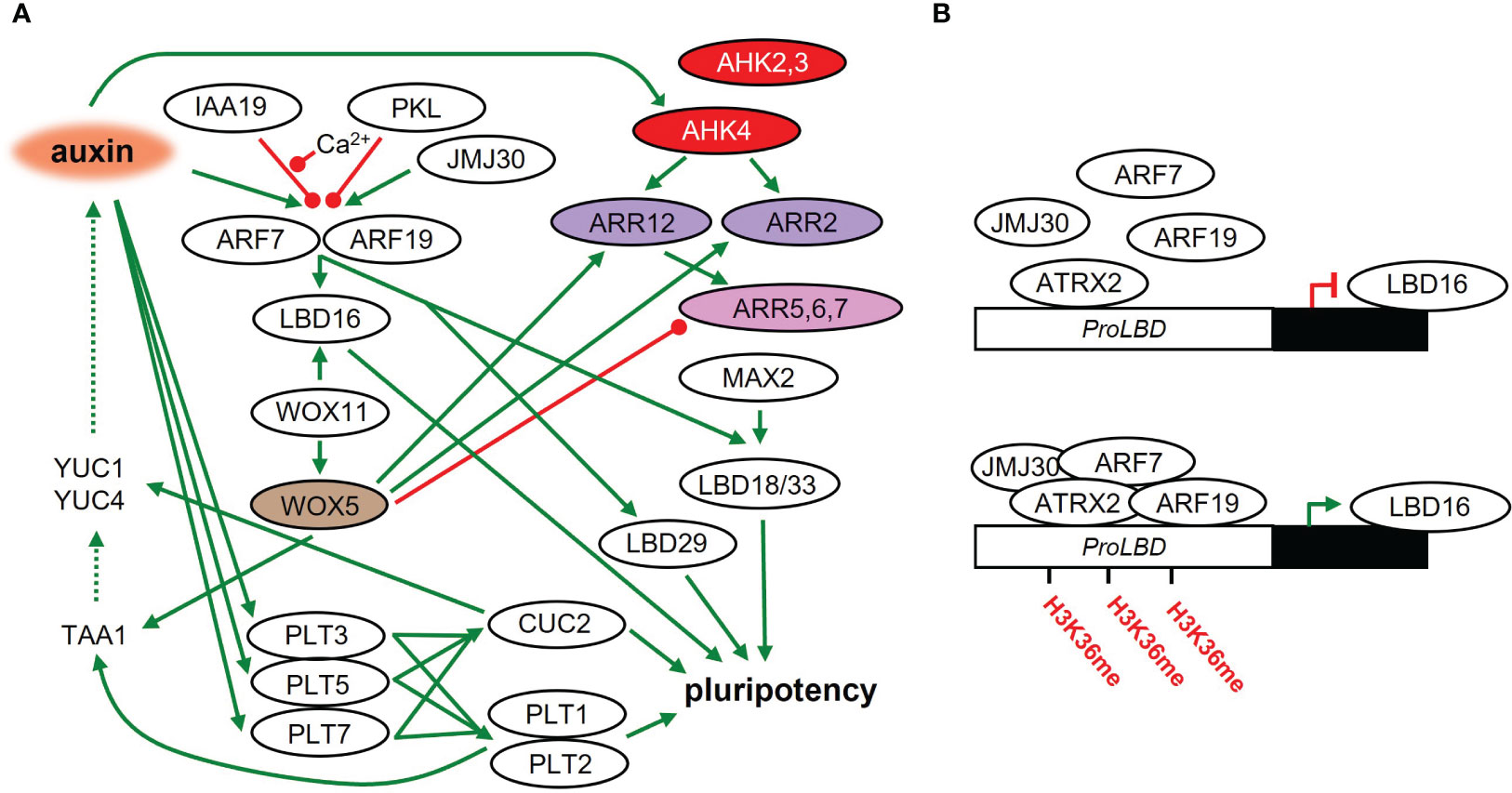
Figure 2 The acquisition of pluripotency in plant tissues on CIM. (A) Molecular regulatory network during callus formation and acquisition of pluripotency leading to shoot regeneration later on. Green line – positive regulation; red line – negative regulation; dashed line – metabolic pathway. (B) LBD16 activity leads to the acquisition of pluripotency on CIM. LBD16 is epigenetically activated (methylation of its promoter) by a complex composed of JMJ30, ARF7, ARF19 and ATRX2.
Ca2+-mediated signaling plays an important role in auxin-controlled initiation of callus formation. CALMODULIN IQ-MOTIF CONTAINING PROTEIN (CaM-IQM) physically interacts with IAA19 in a Ca2+-dependent manner, which then destabilizes the repressive interaction of IAA19 with ARF7, promoting callus formation via LBD activity (Zhang et al., 2022). The impact of Cam-IQM on other IAAs or ARFs is unclear so far.
LBD16 is controlled by WUCHEL-RELATED HOMEOBOX 11 (WOX11), which is activated shortly after the acquisition of root primordium identity by founder cells (Liu et al., 2014). WOX11 also activates another member of the WOX family, WOX5 (Hu and Xu, 2016), broadly expressed in the subepidermal layer of each callus type (Sugimoto et al., 2010). WOX5 was originally identified as a WUS homolog in SAM and is analogically responsible for the maintenance of the root quiescent center in the stem cell niche (Sarkar et al., 2007; Pi et al., 2015). Moreover, WOX5 directly affects auxin levels in cooperation with the AP2-family transcription factors PLETHORA1 (PLT1) and PLT2 by activating the auxin biosynthetic gene TRYPTOPHAN AMINOTRANSFERASE OF ARABIDOPSIS 1 (TAA1) (Zhai and Xu, 2021).
During CIM cultivation, the expression of PLT3, PLT5, and PLT7 is rapidly induced. They control PLT1 and PLT2, leading to the establishment of a pluripotent callus (Kareem et al., 2015). Analysis of the gene regulatory network uncovered PLT3 as a critical node in shoot regeneration (Ikeuchi et al., 2018). Meristem development is also dependent on the PLT1 gradient (Galinha et al., 2007), which indicates the existence of a fine-tuned transcriptional regulatory mechanism. In addition, the activity of PLT3, PLT5, and PLT7 regulates the shoot-promoting factor CUPSHAPED COTYLEDON2 (CUC2) (Kareem et al., 2015), which was shown to promote adventitious shoot formation (Daimon et al., 2003). CUC genes play an important role in the regulation of auxin abundance by controlling the auxin biosynthetic genes YUC1 and YUC4 (Yamada et al., 2022). CUC2 promotes meristem formation by activating the cell wall loosening enzyme (XTH9), whose activity is triggered in cells surrounding the meristem progenitor. This results in a mechanical conflict, triggering cell polarity in the progenitors and the subsequent promotion of meristem formation (Varapparambath et al., 2022).
Callus formation is dependent on cytokinin perception by the receptors AHK2, AHK3 and AHK4. The corresponding mutants exhibit reduced callus size and greening (Li et al., 2019). Moreover, the presence of auxin in CIM leads to strong local up-regulation of AHK4, which predestines future cytokinin-induced WUS expression (Gordon et al., 2009). Auxin signaling is therefore partially responsible for the sensitivity of the whole system to cytokinins. Further cytokinin signal transduction also plays an important role in the acquisition of pluripotency in the callus. The type-B ARR2 and ARR12 interact with the root-specific WOX5 that promotes cytokinin sensitivity of the system (Zhai and Xu, 2021). It seems that type-B ARRs also affect the recalcitrancy of plant tissues. ARR18 expression was much lower in recalcitrant Arabidopsis lines than in highly regenerative ones (Lall et al., 2004). Furthermore, WOX5 also represses type-A ARRs, negative regulators of cytokinin signaling. Overexpression of WOX5 inhibits the expression of ARR5, ARR6 and ARR7. Complementarily, the transcript level of ARR5 was higher in the wox5 mutant on both CIM and SIM (Zhai and Xu, 2021; Lee et al., 2022). The loss of ARR7 function resulted in strong induction of callus formation, which points to an inhibitory role for ARR7 in the acquisition of pluripotency (Buechel et al., 2010). Preincubation on CIM is necessary for the expression and proper function of ARR15, a direct target of ARR2 (Che et al., 2008). Thus, a proper balance between various up- and down-regulators of cytokinin signaling is crucial for the acquisition of pluripotency.
Information about changes in endogenous cytokinin levels in Arabidopsis during processes leading to de novo shoot regeneration in vitro is rather scarce. Arabidopsis hypocotyls cultivated on medium supplemented with kinetin at concentrations of 0, 300 and 1000 ng/ml had comparable dynamics of endogenous tZ-type cytokinins levels, whereas the levels of iP-type cytokinins strongly increased on media containing a high kinetin concentration (1000 ng/ml) (Pernisova et al., 2018). This seems to suggest a possible role for iP-type cytokinins in the development of de novo shoot apical meristems in vitro.
3.1.1 Epigenetic regulation of pluripotency acquisition
The importance of epigenetic regulation of de novo organogenesis has become increasingly clear over the past few years. Research has shown that histone acetyl-transferase/GENERAL CONTROL NONDEREPRESSIBLE (HAG1/CGN5) is essential for de novo shoot regeneration already during callus development. It catalyzes histone acetylation at meristem gene loci of many de novo organogenesis regulators such as WOX5, WOX14, PLT1 or PLT2, thus forming an epigenetic platform for their transcriptional activation (Kim et al., 2018).
Methylation also works as a frequent mechanism for epigenetic regulation of regeneration. One of the pathways is mediated by the JUMONJI C DOMAIN-CONTAINING PROTEIN 30 (JMJ 30), which stimulates the callus formation by binding to ARF7 and ARF15 activating the LBD genes, by removing methyl groups from H3K9me3, especially at the LBD16 and LBD29 promoters (Figure 2B). JMJ30-ARF complex further recruits ARABIDOPSIS TRITHORAX-RELATED 2 (ATXR2), a histone lysine methyltransferase promoting the accumulation of H3 histones trimethylated on lysine 36 (H3K36me3), which interacts with ARF7 and ARF19 and increases H3K36me3 accumulation on LBD promoters (Lee et al., 2017; Lee et al., 2018), and is thus responsible for stimulation of callus formation.
HISTONE THREE RELATED (H3.15) is active in processes connected to plant regeneration. It is distinguished from other Arabidopsis histones by the absence of lysine residue 27 that is trimethylated by POLYCOMB REPRESSIVE COMPLEX 2 (PRC2). Its presence results in transcriptional derepression of downstream genes such as WOX11. H3.15 expression increases after wounding of plant tissue (Yan et al., 2020), but any possible role in de novo organogenesis remains unclear.
3.1.2 Control of pluripotency acquisition regulated by miRNA
The effect of miRNA molecules on the acquisition of pluripotency and callus formation from explants is also significant. The Arabidopsis miR160 inhibits callus formation via the cleavage of ARF10 and ARF16 mRNAs, which defines the spatial expression patterns of regulators such as WOX5 and PLTs (Wang et al., 2005; Ding and Friml, 2010). Cytokinin signaling is also affected by miR160 via repression of ARF10, a negative regulator of ARR15. Activity of miR160 results in the inhibition of callus initiation by enhancing ARR15 expression (Liu et al., 2016). Thus, miR160 may play an important role in the regulation of both auxin as well as cytokinin signaling. Cytokinin responses are modulated also by the activity of miR156, which represses SQUAMOSA PROMOTER BINDING PROTEIN-LIKE (SPL) genes, defining an age-dependent pathway controlling de novo shoot organogenesis. The repression of SPL leads to the abolition of de novo shoot regenerative capacity by attenuating cytokinin responses via the modulation of ARR1 (Barrera-Rojas et al., 2020).
3.2 Meristem identity respecification
To regenerate a shoot, cells first acquire competence for organogenesis on CIM with a high concentration of auxin. The calli are then exposed to a high concentration of cytokinins on SIM, which promotes the acquisition of shoot meristem properties and subsequent shoot organogenesis (Christianson and Warnick, 1983). A rapid downregulation of root-specific markers occurs shortly after transferring the callus to SIM. Root specific WOX5 expression in the middle cell layer of callus is gradually reduced within 48 hours after transfer to SIM (Zhai and Xu, 2021). Accordingly, the ProWOX5:GFP signal is downregulated in a cytokinin concentration-dependent manner (Pernisova et al., 2018). Expression of LBD16, activated by WOX11, is also reduced after transfer to SIM (Liu et al., 2018b). Shoot-specific genes are activated at the same time as root-specific genes become attenuated. Of the transcription factors involved in shoot regeneration, WUS and STM play a key role in the initiation of shoot organogenesis (Hibara et al., 2003; Gordon et al., 2009; Chatfield et al., 2013). The shoot-specific ProWUS:tdTomato signal was detectable as soon as three days on cytokinin-rich media (Pernisova et al., 2018). Accordingly, wus mutants fail to regenerate shoots in vitro (Zhang et al., 2017b). Another member of the WOX family, WOX14 can be an important factor in shoot regeneration. A WOX14 overexpressor was able to regenerate shoots on cytokinin free medium. The ProWOX14:GUS reporter line exhibited WOX14 activity on SIM, but not on CIM (Wang et al., 2023a). However, the molecular mechanisms behind these results have yet to be uncovered.
The activity of general regulators requires the interplay and achievement of a fine balance of auxin and cytokinin function at several levels. For successful shoot regeneration, auxin must first trigger a program leading to the establishment of pluripotent status, while the presence of cytokinins modulates the organogenic response leading to shoot formation. Cytokinins quickly downregulate auxin signaling in a concentration-dependent manner, leading to a loss of root identity (Pernisova et al., 2018). The perturbation of auxin signaling was revealed to be crucial for the future induction of shoot regeneration on SIM (Ohbayashi et al., 2022). Auxin signaling can be affected by reducing the source, e.g., by decreased biosynthesis or disrupted transport. Cytokinin signaling suppresses the expression of auxin biosynthetic genes in the central region of the forming meristem, which can lead to decreased auxin levels (Figure 3). The transcription factors ARR1, ARR10, and ARR12 interact with YUC promoters leading to the inhibition of YUC1 and YUC4 expression (Meng et al., 2017). Application of auxin biosynthesis inhibitors or polar auxin transport inhibitors on CIM enhanced subsequent shoot formation on SIM (Ohbayashi et al., 2022). Also, cytokinins affect auxin distribution by regulating the expression of auxin transporters from the PIN FORMED (PIN) family (Pernisová et al., 2009). CRFs acting downstream of cytokinin perception, participate in shoot regeneration as well. Shoot formation is induced in crf2 mutants and diminished in crf5 mutants during in vitro regeneration (Rashotte et al., 2006). The expression of CRF2 is dependent on a crucial auxin signaling component ARF5 (Ckurshumova et al., 2014), thus connecting cytokinin and auxin signaling pathways. The activity of ARF5 is negatively regulated by IAA12, and in the presence of auxin, ARF5 repression is compromised by the presence of ARF4, which competes with ARF5 in binding to IAA12. ARF4 compromises the level of free IAA12, thus maintaining the activity of ARF5 (Zhang et al., 2021). Another member of auxin signaling, ARF3 acts as a negative regulator of de novo organ regeneration. It binds directly to the promoter of IPT5, which disrupts the cytokinin biosynthesis pathway (Cheng et al., 2012). ARF3 binding to the IPT5 promoter requires high auxin concentration and is thus attenuated on SIM, leading to the activation of cytokinin biosynthesis. Taken together, fine-tuning between the effects of auxins and cytokinins is fundamental for proper plant organ regeneration.
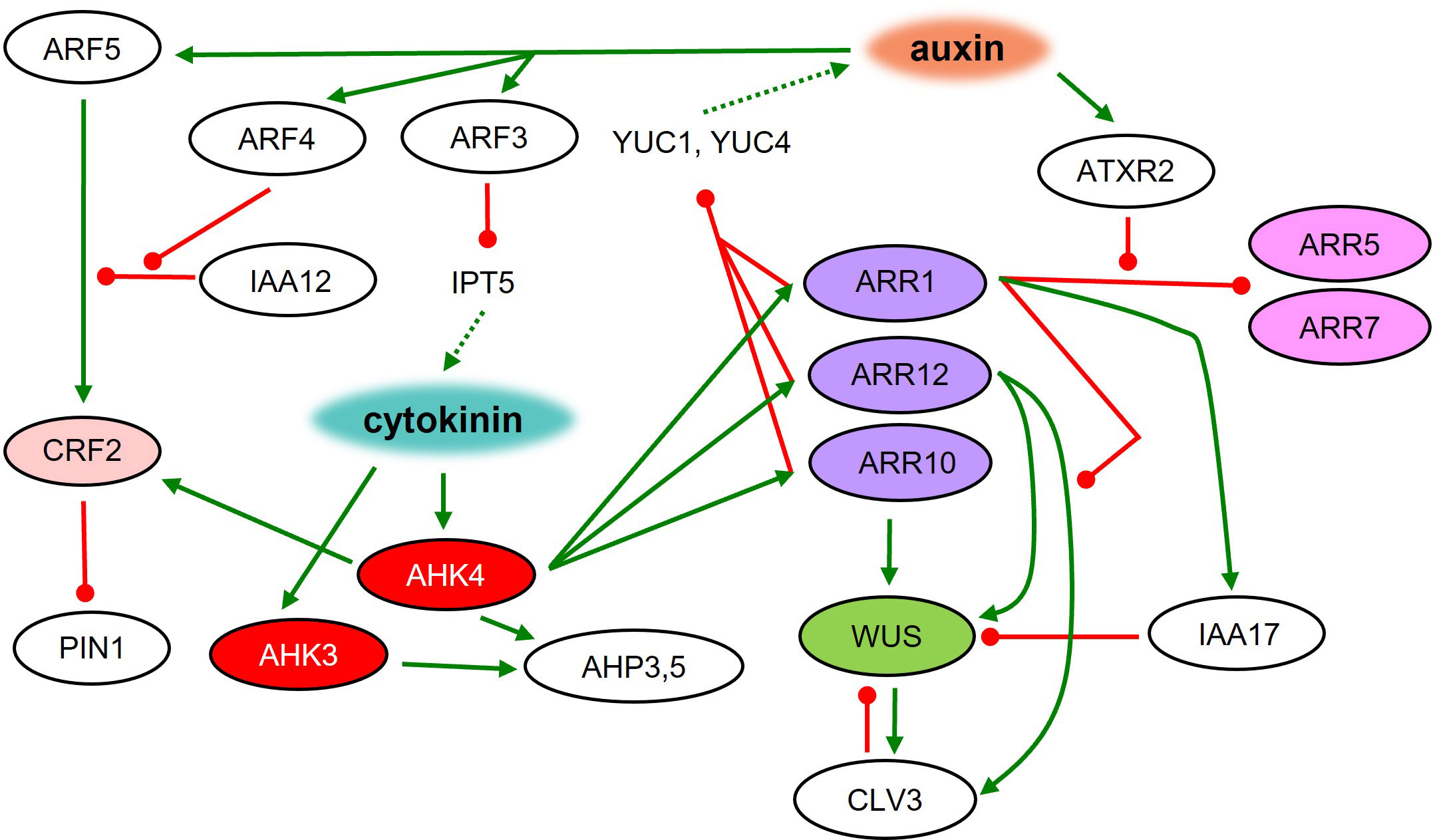
Figure 3 Root to shoot meristem identity respecification on SIM. Molecular regulatory network during shoot regeneration on cytokinin-rich medium. Green line – positive regulation; red line – negative regulation; dashed line – metabolic pathway.
The regulation of SAM formation during shoot regeneration requires WUS as a central player. WUS represents one of the first shoot-specific markers active in SAM-forming cells (Pernisova et al., 2018). Loss of WUS function leads to a severe disruption of shoot regeneration (Gordon et al., 2007; Chatfield et al., 2013). In the later stages of shoot regeneration, WUS activity can be observed only in the regions where the AHK4 cytokinin receptor was previously expressed during incubation on CIM (Gordon et al., 2009). Areas with an active AHK4 during CIM incubation also define the future positions of auxin transport protein PIN1 upregulated during SIM incubation (Gordon et al., 2007; Atta et al., 2009; Gordon et al., 2009). Application of the histidine kinase inhibitor TSCA during the first four days of SIM incubation leads to the inhibition of shoot regeneration in Arabidopsis. This inhibition seems to be at least partially connected to the disruption of cytokinin signaling via AHK4, AHK3, AHP3 and AHP5 (Lardon et al., 2022). Overall, AHK4 seems to be pivotal in the cytokinin-mediated regulation of organ identity affecting shoot-specific WUS as well as root-specific WOX5 activity (Pernisova et al., 2018).
Other members of the cytokinin signaling pathway – response regulators – are also involved in shoot regeneration. arr1 mutants generate more shoots than the wild type. It was shown that ARR1 binds to the IAA17 promoter, inducing its expression during SIM cultivation, leading to the indirect WUS inhibition and disruption of shoot regeneration (Liu et al., 2020). Another cytokinin-related transcription factor ARR12 promotes the CLV3 expression. Additionally, ARR1 causes repression of CLV3 in an ARR12 dependent manner. It was revealed that ARR1 and ARR12 compete in binding to the CLV3 promoter, which regulates its activity (Liu et al., 2020). Moreover, ARR12 positively regulates WUS by binding to its promoter and activating expression (Dai et al., 2017). Thus, ARR12 seems to be a critical player in shoot stem cell niche maintenance and shoot regeneration. Additionally, the ectopic expression of ARR2 and ARR11 gives rise to in vitro shoot regeneration in the absence of exogenous cytokinins (Hwang and Sheen, 2001; Imamura et al., 2003) as seen also with cytokinin-independent1 (CKI1) (Kakimoto, 1996).
On the other hand, the activity of type-A ARRs (negative regulators of cytokinin signaling) usually results in a reduction of shoot regeneration (Buechel et al., 2010). ARR1 binds directly to the promoters of type-A ARR5 and ARR7, which causes their repression. On SIM, ATXR2 is responsible for H3K336me3 deposition at ARR5 and ARR7 promoters thus disrupting ARR1 binding and activating type-A ARRs (Lee et al., 2021). ARR8 seems to also have an impact on shoot regeneration capacity. ARR8 overexpression abolishes SAM formation (Osakabe et al., 2002).
WUS and CLV3 activity and subsequent shoot regeneration are dependent also on abiotic factors, such as light or temperature. It was shown that ELONGATED HYPOCOTYL (HY5) a transcription factor of light signaling is responsible for inhibition of CLV3 and WUS by direct interaction with their promoter. Indirect inhibition of WUS and CLV3 is achieved through the interaction between HY5 and ARR12 (Dai et al., 2022). Temperature affects shoot regeneration competency by depleting the histone variant H2A.Z which acts as a repressor of de novo shoot organogenesis and is present at 17°C but removed at 27°C (Lambolez et al., 2022).
ALTERED MERISTEM PROGRAM 1 (AMP1) is a putative Glu carboxypeptidase present in Arabidopsis. Defective AMP1 results in the formation of enlarged shoot meristems, a larger stem cell pool and a higher rate of leaf formation (Huang et al., 2015). AMP1 affects stem cell niche patterning by controlling the downstream HD-ZIPIII/RAP2.6 transcription factor module. RAP2.6L is under direct transcriptional control of HD-ZIP III transcription factors via their binding to the RAP2.6L promoter. The presence of HD-ZIP III is elevated in the amp1 mutant. AMP1, therefore, seems to limit SAM activity via miRNA-dependent control of HD-ZIP III transcription factors and subsequent RAP2.6L activity (Yang et al., 2018). The regulatory pathways of HD-ZIP III activity also comprise auxin activity. A novel chemical inhibitor of polar auxin transport ZIC2 (LITTLE ZIPPER3 inducing compound) promotes shoot regeneration and subsequent RAP2.6L elevation in an HD-ZIP III-dependent manner (Yang et al., 2022). Moreover, previous research suggests that oxygen also works as a signal, as its spatial distribution affects the proteolysis of LITTLE ZIPPER 2 (ZPR2), controlling the activity of HD-ZIP III transcription factors (Weits et al., 2019). These findings together lift the lid on the exquisite complexity of the HD-ZIP III transcription factor regulatory network in the process of shoot regeneration.
3.2.1 Epigenetic regulation of shoot regeneration
Acquisition of shoot identity in the callus is an important step during de novo shoot organogenesis. Recent research indicates that epigenetic regulations are necessary for this process, especially in WUS activity induction. LYSINE-SPECIFIC DEMETHYLASE 1-LIKE 3 (LDL3) demethylates lysine 4 of histone H3 (H3K4me2), which leads to the reduction of the H3K4me2 tag and subsequent acquisition of shoot regeneration competency. H3K4me2 may be responsible for the control of WUS activity together with other tags such as H3K9ac, H3K4me3, and H3K9me2 (Li et al., 2011; Ishihara et al., 2019). Moreover, cytokinins launch division-dependent removal of the repressive H3K27me3 tag from WUS promoter (Zhang et al., 2017b). In this inducible state, WUS can be activated by type-B ARRs, for instance ARR10, directly targeting WUS (Zubo et al., 2017). The deposition of H3K27me3 by PRC2 (Zhou et al., 2018a) may be coordinated also by Telomeric repeat binding (TRB) proteins (Schrumpfová et al., 2016; Kusová et al., 2023). TRB proteins also recruit H3K4 demethylase JMJ14 implicated in the regulation of WUS expression (Li et al., 2011; Wang et al., 2023b).
On the other hand, shoot regeneration can be repressed by the function of DNA METHYLTRANSFERASE (MET1) by inhibiting WUS expression. MET1 activity can be promoted by a cell cycle regulator – transcription factor E2FA (Liu et al., 2018a). The ability of cells to undergo cell division is a prerequisite for shoot regeneration (Tamaki et al., 2009). Whole-genome bisulfite sequencing of the met1-3 mutant uncovered the dependence of plant regeneration on CG methylation of target genes including CRYPTOCHROME1 (CRY1) and CRY2. CRY1 is responsible for the regulation of cytokinin signaling connected to the induction of shoot regeneration mainly via type-B ARRs (Shim et al., 2021).
A recent study revealed the role of HISTONE DEACETYLASE 19 (HDA19) in de novo shoot regeneration. HDA19 is a member of the histone deacetylase family, which deacetylates histones at CUC2 and DRN/ESR1 loci. In the hda19 mutant line, histones at the CUC2 and DRN/ESR1 loci are in a hyperacetylated state, leading to their excessive expression and consequent reduced shoot regeneration capacity. In WT, CUC2 and DRN/ESR1 are active only in distinct cell groups, which will gain shoot forming identity. However, in hda19 CUC2 and DRN/ESR1 are continually expressed throughout the explant and no localized expression patterns are produced. It seems that the expression patterns of CUC2 and DRN/ESR1 are crucial for future de novo shoot regeneration (Temman et al., 2023).
3.2.2 miRNA impact on shoot regeneration
During in vitro shoot regeneration, miRNA molecules play an important role in various pathways regulating successful shoot formation. miRNA molecules are also responsible for age-regulated developmental timing, which affects the ability of plant tissue to regenerate shoots. A high concentration of miR156 is responsible for the prolongation of juvenile phases by targeting the SPL group of proteins (Xu et al., 2016). Plant regenerative capacity decreases with increasing age. It is a consequence of the gradual increase in miR156 levels targeting SPLs, which attenuate the cytokinin response by binding to type-B ARRs (Zhang et al., 2015). miRNA molecules also modulate the cytokinin response. The small RNA methyltransferase HUA ENHANCER1 (HEN1) is responsible for miR319 production. Mutations in HEN1 lead to a strong reduction of miR319 level and subsequent increase in its transcription factor targets – TEOSINTE BRANCHED 1 CYCLOIDEA AND PCF TRANSCRIPTION FACTOR 3 (TCP3) and TCP4. They are responsible for the activation of ARR16 expression, resulting in the inhibition of shoot regeneration (Yang et al., 2020). Regulation via miRNA also impacts auxin signaling. On SIM, overexpression of miR393a causes the attenuation of auxin signaling through its interaction with the auxin receptor TRANSPORT INHIBITOR RESPONSE1 (TIR1) resulting in TIR1 inhibition, disruption of auxin signaling, and subsequent defects in shoot regeneration (Wang et al., 2018). Some miRNAs can intervene in both cytokinin and auxin signaling. Based on its presence in calli incapable of shoot regeneration, miR160 was discovered as a negative regulator of SAM formation. miR160 targets and inhibits ARF10, a positive factor in SAM formation (Qiao et al., 2012; Qiao and Xiang, 2013).
Stem cell niche organization, among others, is regulated by the HD-ZIP III group of transcription factors. Their activity is partially regulated by cytokinin signaling. Type-B ARRs (ARR1, ARR2, ARR10, and ARR12) physically interact with HD ZIP III and these complexes activate WUS expression, which leads to shoot regeneration (Zhang et al., 2017a). Other than WUS-mediated stem cell niche maintenance, the activity of HD-ZIP III seems to also control a WUS-independent pathway. HD-ZIP III TFs act as repressors of this pathway, and their inactivity in the wus-1 mutant background, leads to a normal functional SAM with normal cell layering and meristem morphology (Lee and Clark, 2015). The activity of HD-ZIP III TFs is regulated at the posttranscriptional level through miR165/166 because HD-ZIP III TFs have nearly perfect complementarity with the sequence located near the 3’end of the fourth exon and the 5’end of the fifth exon with miR165/166 (Mallory et al., 2004; Zhang and Zhang, 2012). The accumulation of miR165/166 is negatively affected by the activity of an EIF2C protein ARGONAUT 10 (AGO10). ago10 mutants produce higher number of SAMs, and the stem-cell markers WUS, CLV3, and STM are strongly expressed (Xue et al., 2017).
4 Direct conversion of root primordia to shoot meristems in Arabidopsis thaliana
Apart from indirect shoot regeneration via cultivation on CIM and SIM, through the intermediate step of callus formation, direct conversion of lateral root primordia (LRP) to shoot apical meristems is also possible (Chatfield et al., 2013; Kareem et al., 2016; Rosspopoff et al., 2017). During this process, roots are cultivated on a medium with a high concentration of auxin to activate LRP development (Chatfield et al., 2013) (Figure 4) that comprises eight developmental stages (Malamy and Benfey, 1997). Subsequently, roots with LRPs are transferred to a medium containing a high concentration of cytokinins, usually of the iP-type. The whole process occurs without any signs of dedifferentiation or callus formation and is called transdifferentiation. The transition from LRP to SAM is possible only during a narrow developmental window, in which the LRPs are in developmental stages VI – VII. The organogenic programs in these tissues are remarkably plastic and their identity can be redirected multiple times by the addition of cytokinins and auxins (Rosspopoff et al., 2017). Moreover, the highest responsiveness of LRPs to conversion into shoots were seen in stages II and III (Kareem et al., 2016), whereas stages V and VI were considered the least sensitive to cytokinins (Bielach et al., 2012). Despite these discrepancies, the developmental stage of the LRP is a very important factor, influencing the success of the transition.
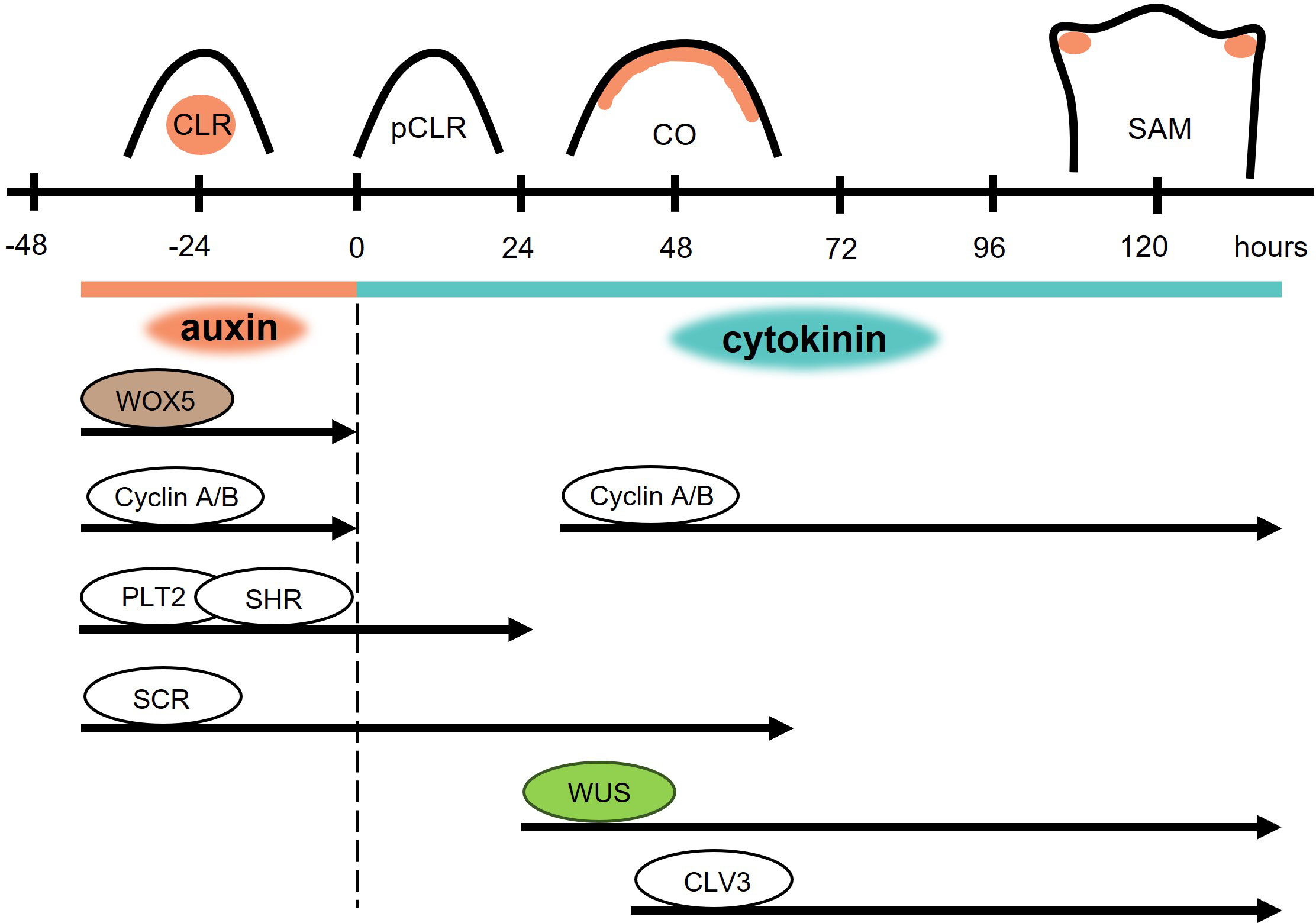
Figure 4 Direct conversion of root primordia to shoot meristems. High auxin concentration triggers the formation of a competent lateral root (CLR). After the transfer to cytokinin rich medium (dashed black line), cell division is attenuated in the paused competent lateral root (pCLR). The cell cycle is paused for 24 hours and expression of root-specific WOX5 is terminated. The activity of other root identity markers lasts for 24 hours after transfer for PLT2 and SHR, and 63 hours for SCR. The shoot identity markers WUS and CLV3 are detected after 19 and 32 hours, respectively. 48 hours after transfer to cytokinin rich medium, conversion of meristematic identity takes place in the converting organ (CO). 120 hours after transfer, a shoot apical meristem (SAM) is formed.
Few morphological changes can be observed during the first 24 hours following the transfer of explants to the cytokinin-rich medium. This is a sign of rapidly decreased mitotic activity (Rosspopoff et al., 2017); transcriptome analysis revealed a reduced expression of A- and B-type cyclins and cyclin-dependent kinases (Figure 4) - pointing to a reduction in cell division (Chatfield et al., 2013). During the second and third day, mitotic activity is restored in the provasculature and in the upper half of the primordium, creating a transitioning organ. After three days, an early shoot promeristem is formed on top of the transitioning organ and leaf primordia appear after four to five days (Rosspopoff et al., 2017).
The early changes in this direct switch of primordium identity are connected to changes in auxin signaling caused by the presence of cytokinins. Shortly after the exposure to cytokinins, auxin levels are rapidly reduced and the auxin transporter PIN1 is cleared out of the plasma membrane. Auxin levels are restored on the top of the transitioning organ with PIN1 active at the plasma membranes of L2 and L3 layers. In the fully structured shoot meristem, auxin is concentrated in the leaf primordia (Rosspopoff et al., 2017).
24 hours after cytokinin exposure, root specific PLT2 and SHR expression is undetectable (Rosspopoff et al., 2017) (Figure 4). On the other hand, SCR was detectable even after 63 hours of cytokinin treatment, but its activity was attenuated afterwards (Kareem et al., 2016). Shortly after cytokinin exposure, activity of the root-specific WOX5 rapidly decreases, and after a brief mitotic inactivity the same cells possessing root identity switch to the expression of shoot identity markers such as WUS, CLV3, and STM. The WUS reporter was first visible after 19 hours on cytokinin-rich medium and was followed by CLV3 after 32 – 48 hours (Chatfield et al., 2013). After the restoration of mitotic activity, WUS, CLV3, and STM activity is restricted to the apex of the transitioning organ and the domains of their expression overlap. When the promeristem is formed, CLV3 and WUS domains resemble their typical organization in SAM, in which CLV3 marks the central zone comprising stem cells and WUS the organizing centre. However, it seems that only WUS expression is not sufficient for direct in vitro shoot regeneration, because WUS expression was detected also in stage V of LRP development, in which the ability to form shoots was not assessed (Rosspopoff et al., 2017). Altogether, facts support the theory that neither dedifferentiation nor the formation of a new stem cell niche is necessary for de novo shoot organogenesis, and the same cells can be transdifferentiated.
5 Perspectives
In vitro shoot regeneration has been used as a reliable tool in plant propagation where seedling-based propagation is either not feasible or not appropriate. Although it has been known for more than six decades that cytokinins play a critical and irreplaceable role in shoot regeneration, we are only now beginning to uncover the detailed molecular and physiological mechanisms that are behind this role. For instance, although plant propagation protocols have most often relied on callus-based regeneration to successfully propagate viable plants, recent discoveries have proved that this two-step process is not actually necessary. One-step shoot regeneration can enable faster and more efficient production of plant material and has the potential to speed up the whole process. To achieve this goal, a better understanding of the underlying molecular mechanisms is necessary, especially the role of cytokinin signaling and metabolism, as also the interactions with other factors. Such interactions – especially with auxin – are exquisitely complex and many aspects of the process remain to be clarified. Possible differences in the molecular mechanisms during one-step and two-step in vitro shoot regeneration may also be uncovered, which would lead to greater comprehension of the whole process leading to shoot regeneration.
Cytokinins possess very complex signaling and especially metabolic pathways. Distinguishing the role of individual cytokinin metabolic enzymes taking part in shoot regeneration is complicated by redundant biosynthetic pathways leading to the production of active cytokinins. Moreover, the impact of exogenous cytokinins presented in cultivation media is not clear yet. A broad spectrum of gene editing techniques is necessary to uncover particular branches of the complex network that are behind shoot regeneration. Omics, single cell and subcellular approaches can help elucidate the fine balance present in such a complex network in even more detail.
Comprehending the central role of cytokinins in shoot apical meristem maintenance and in vitro shoot regeneration can provide a highly valuable tool enabling better control over the process. This better control can then be applied to come up with more efficient protocols for plant production. Moreover, such knowledge could be used for fine-tuning plant micropropagation and regeneration protocols. This could then mean higher efficiency in vitro regeneration of recalcitrant species or genetic lines with reduced in vitro viability. The combination of these two limitations is one of the most significant obstacles for crop improvement, because the successful transformation of crops in desirable gene targets is being hampered by the inability to establish viable tissue culture. Overcoming these obstacles may be highly beneficial for both research and commercial goals.
Author contributions
All authors listed have made a substantial, direct, and intellectual contribution to the work and approved it for publication.
Funding
Funding sources are acknowledged, particularly the European Structural and Investment Funds, Operational Programme Research, Development and Education - Project MSCAfellow@MUNI (EF17_050/0008496) to MP; Brno Ph.D. Talent Scholarship – funded by the Brno Municipality to JŠ; and Czech Science Foundation project GA21-15841S to PPS.
Conflict of interest
The authors declare that the research was conducted in the absence of any commercial or financial relationships that could be construed as a potential conflict of interest.
The reviewer KD declared a past collaboration/shared affiliation with the authors MP to the handling editor at the time of review.
Publisher’s note
All claims expressed in this article are solely those of the authors and do not necessarily represent those of their affiliated organizations, or those of the publisher, the editors and the reviewers. Any product that may be evaluated in this article, or claim that may be made by its manufacturer, is not guaranteed or endorsed by the publisher.
References
Allen, M., Qin, W., Moreau, F., Moffatt, B. (2002). Adenine phosphoribosyltransferase isoforms of Arabidopsis and their potential contributions to adenine and cytokinin metabolism. Physiol. Plantarum 115, 56–68. doi: 10.1034/j.1399-3054.2002.1150106.x
Anantharaman, V., Aravind, L. (2001). The CHASE domain: a predicted ligand-binding module in plant cytokinin receptors and other eukaryotic and bacterial receptors. Trends Biochem. Sci. 26, 579–582. doi: 10.1016/S0968-0004(01)01968-5
Atta, R., Laurens, L., Boucheron-Dubuisson, E., Guivarc’h, A., Carnero, E., Giraudat-Pautot, V., et al. (2009). Pluripotency of Arabidopsis xylem pericycle underlies shoot regeneration from root and hypocotyl explants grown in vitro. Plant J. 57, 626–644. doi: 10.1111/j.1365-313X.2008.03715.x
Barrera-Rojas, C. H., Rocha, G. H. B., Polverari, L., Pinheiro Brito, D. A., Batista, D. S., Notini, M. M., et al. (2020). miR156-targeted SPL10 controls Arabidopsis root meristem activity and root-derived de novo shoot regeneration via cytokinin responses. J. Exp. Bot. 71, 934–950. doi: 10.1093/jxb/erz475
Bartrina, I., Otto, E., Strnad, M., Werner, T., Schmülling, T. (2011). Cytokinin regulates the activity of reproductive meristems, flower organ size, ovule formation, and thus seed yield in arabidopsis thaliana. Plant Cell 23, 69–80. doi: 10.1105/tpc.110.079079
Beeckman, T., Burssens, S., Inze, D. (2001). The peri-cell-cycle in arabidopsis. J. Exp. Bot. 52, 403–411. doi: 10.1093/jexbot/52.suppl_1.403
Berckmans, B., Vassileva, V., Schmid, S. P. C., Maes, S., Parizot, B., Naramoto, S., et al. (2011). Auxin-dependent cell cycle reactivation through transcriptional regulation of arabidopsis E2Fa by lateral organ boundary proteins. Plant Cell 23, 3671–3683. doi: 10.1105/tpc.111.088377
Besnard, F., Refahi, Y., Morin, V., Marteaux, B., Brunoud, G., Chambrier, P., et al. (2014). Cytokinin signalling inhibitory fields provide robustness to phyllotaxis. Nature 505, 417–421. doi: 10.1038/nature12791
Bielach, A., Podlešáková, K., Marhavý, P., Duclercq, J., Cuesta, C., Müller, B., et al. (2012). Spatiotemporal regulation of lateral root organogenesis in arabidopsis by cytokinin. Plant Cell 24, 3967–3981. doi: 10.1105/tpc.112.103044
Bilyeu, K. D., Cole, J. L., Laskey, J. G., Riekhof, W. R., Esparza, T. J., Kramer, M. D., et al. (2001). Molecular and biochemical characterization of a cytokinin oxidase from maize. Plant Physiol. 125, 378–386. doi: 10.1104/pp.125.1.378
Bleckmann, A., Weidtkamp-Peters, S., Seidel, C. A. M., Simon, R. (2009). Stem cell signaling in arabidopsis requires CRN to localize CLV2 to the plasma membrane. Plant Physiol. 152, 166–176. doi: 10.1104/pp.109.149930
Brzobohaty, B., Moore, I., Kristoffersen, P., Bako, L., Campos, N., Schell, J., et al. (1993). Release of active cytokinin by a beta-glucosidase localized to the maize root-meristem. Science 262, 1051–1054. doi: 10.1126/science.8235622
Buechel, S., Leibfried, A., To, J. P. C., Zhao, Z., Andersen, S. U., Kieber, J. J., et al. (2010). Role of A-type ARABIDOPSIS RESPONSE REGULATORS in meristem maintenance and regeneration. Eur. J. Cell Biol. 89, 279–284. doi: 10.1016/j.ejcb.2009.11.016
Casimiro, I., Marchant, A., Bhalerao, R. P., Beeckman, T., Dhooge, S., Swarup, R., et al. (2001). Auxin transport promotes arabidopsis lateral root initiation. Plant Cell 13, 843–852. doi: 10.1105/tpc.13.4.843
Chandler, J. W., Werr, W. (2015). Cytokinin-auxin crosstalk in cell type specification. Trends Plant Sci. 20, 291–300. doi: 10.1016/j.tplants.2015.02.003
Chatfield, S. P., Capron, R., Severino, A., Penttila, P. A., Alfred, S., Nahal, H., et al. (2013). Incipient stem cell niche conversion in tissue culture: using a systems approach to probe early events in WUSCHEL-dependent conversion of lateral root primordia into shoot meristems. Plant J. 73, 798–813. doi: 10.1111/Tpj.12085
Che, P., Lall, S., Howell, S. H. (2008). Acquiring competence for shoot development in Arabidopsis: ARR2 directly targets A-type ARR genes that are differentially activated by CIM preinCubation. Plant Signaling Behav. 3, 99–101. doi: 10.4161/psb.3.2.4958
Cheng, Z. J., Wang, L., Sun, W., Zhang, Y., Zhou, C., Su, Y. H., et al. (2012). Pattern of auxin and cytokinin responses for shoot meristem induction results from the regulation of cytokinin biosynthesis by AUXIN RESPONSE FACTOR3. Plant Physiol. 161, 240–251. doi: 10.1104/pp.112.203166
Chickarmane, V. S., Gordon, S. P., Tarr, P. T., Heisler, M. G., Meyerowitz, E. M. (2012). Cytokinin signaling as a positional cue for patterning the apical–basal axis of the growing Arabidopsis shoot meristem. Proc. Natl. Acad. Sci. U.S.A. 109, 4002–4007. doi: 10.1073/pnas.1200636109
Christianson, M. L., Warnick, D. A. (1983). Competence and determination in the process of in vitro shoot organogenesis. Dev. Biol. 95, 288–293. doi: 10.1016/0012-1606(83)90029-5
Ckurshumova, W., Smirnova, T., Marcos, D., Zayed, Y., Berleth, T. (2014). Irrepressible MONOPTEROS/ARF 5 promotes de novo shoot formation. New Phytol. 204, 556–566. doi: 10.1111/nph.13014
Cole, M., Chandler, J., Weijers, D., Jacobs, B., Comelli, P., Werr, W. (2009). DORNROSCHEN is a direct target of the auxin response factor MONOPTEROS in the Arabidopsis embryo. Development 136, 1643–1651. doi: 10.1242/dev.032177
Cutcliffe, J. W., Hellmann, E., Heyl, A., Rashotte, A. M. (2011). CRFs form protein-protein interactions with each other and with members of the cytokinin signalling pathway in Arabidopsis via the CRF domain. J. Exp. Bot. 62, 4995–5002. doi: 10.1093/jxb/err199
Dai, X., Liu, Z., Qiao, M., Li, J., Li, S., Xiang, F. (2017). ARR12 promotes de novo shoot regeneration in Arabidopsis thaliana via activation of WUSCHEL expression: ARR12 promotes shoot regeneration. J. Integr. Plant Biol. 59, 747–758. doi: 10.1111/jipb.12567
Dai, X., Wang, J., Wang, L., Liu, Z., Li, Q., Cai, Y., et al. (2022). HY5 inhibits in vitro shoot stem cell niches initiation via directly repressing pluripotency and cytokinin pathways. Plant J. 110, 781–801. doi: 10.1111/tpj.15703
Daimon, Y., Takabe, K., Tasaka, M. (2003). The CUP-SHAPED COTYLEDON genes promote adventitious shoot formation on calli. Plant Cell Physiol. 44, 113–121. doi: 10.1093/pcp/pcg038
Dao, T. Q., Weksler, N., Liu, H. M.-H., Leiboff, S., Fletcher, J. C. (2022). Interactive CLV3, CLE16, and CLE17 signaling mediates stem cell homeostasis in the Arabidopsis shoot apical meristem. Development 134, 4131–4130. doi: 10.1242/dev.200787
Daum, G., Medzihradszky, A., Suzaki, T., Lohmann, J. U. (2014). A mechanistic framework for noncell autonomous stem cell induction in Arabidopsis. Proc. Natl. Acad. Sci. U.S.A. 111, 14619–14624. doi: 10.1073/pnas.1406446111
De Rybel, B., Adibi, M., Breda, A. S., Wendrich, J. R., Smit, M. E., Novák, O., et al. (2014). Integration of growth and patterning during vascular tissue formation in Arabidopsis. Science 345. doi: 10.1126/science.1255215
De Rybel, B., Vassileva, V., Parizot, B., Demeulenaere, M., Grunewald, W., Audenaert, D., et al. (2010). A novel aux/IAA28 signaling cascade activates GATA23-dependent specification of lateral root founder cell identity. Curr. Biol. 20, 1697–1706. doi: 10.1016/j.cub.2010.09.007
Ding, Z., Friml, J. (2010). Auxin regulates distal stem cell differentiation in Arabidopsis roots. Proc. Natl. Acad. Sci. U.S.A. 107, 12046–12051. doi: 10.1073/pnas.1000672107
Dubrovsky, J. G., Doerner, P. W., Colón-Carmona, A., Rost, T. L. (2000). Pericycle cell proliferation and lateral root initiation in arabidopsis. Plant Physiol. 124, 1648–1657. doi: 10.1104/pp.124.4.1648
Dubrovsky, J. G., Rost, T. L., Colón-Carmona, A., Doerner, P. (2001). Early primordium morphogenesis during lateral root initiation in Arabidopsis thaliana. Planta 214, 30–36. doi: 10.1007/s004250100598
Feng, Z., Zhu, J., Du, X., Cui, X. (2012). Effects of three auxin-inducible LBD members on lateral root formation in Arabidopsis thaliana. Planta 236, 1227–1237. doi: 10.1007/s00425-012-1673-3
Fletcher, J. C., Brand, U., Running, M. P., Simon, R., Meyerowitz, E. M. (1999). Signaling of cell fate decisions by CLAVATA3 in arabidopsis shoot meristems. Science 283, 1911–1914. doi: 10.1126/science.283.5409.1911
Fukaki, H., Tameda, S., Masuda, H., Tasaka, M. (2002). Lateral root formation is blocked by a gain-of-function mutation in the SOLITARY-ROOT/IAA14 gene of Arabidopsis: Role of SLR/IAA14 in lateral root formation. Plant J. 29, 153–168. doi: 10.1046/j.0960-7412.2001.01201.x
Fukaki, H., Taniguchi, N., Tasaka, M. (2006). PICKLE is required for SOLITARY-ROOT/IAA14-mediated repression of ARF7 and ARF19 activity during Arabidopsis lateral root initiation. Plant J. 48, 380–389. doi: 10.1111/j.1365-313X.2006.02882.x
Galinha, C., Hofhuis, H., Luijten, M., Willemsen, V., Blilou, I., Heidstra, R., et al. (2007). PLETHORA proteins as dose-dependent master regulators of Arabidopsis root development. Nature 449, 1053–1057. doi: 10.1038/nature06206
Galuszka, P., Frébort, I., Šebela, M., Sauer, P., Jacobsen, S., Peč, P. (2001). Cytokinin oxidase or dehydrogenase?: Mechanism of cytokinin degradation in cereals. Eur. J. Biochem. 268, 450–461. doi: 10.1046/j.1432-1033.2001.01910.x
Gaudinova, A., Dobrev, P. I., Solcova, B., Novak, O., Strnad, M., Friedecky, D., et al. (2005). The involvement of cytokinin oxidase/dehydrogenase and zeatin reductase in regulation of cytokinin levels in pea (Pisum sativum L.) leaves. J. Plant Growth Regul. 24, 188–200. doi: 10.1007/s00344-005-0043-9
Gordon, S. P., Chickarmane, V. S., Ohno, C., Meyerowitz, E. M. (2009). Multiple feedback loops through cytokinin signaling control stem cell number within the Arabidopsis shoot meristem. Proc. Natl. Acad. Sci. U.S.A. 106, 16529–16534. doi: 10.1073/pnas.0908122106
Gordon, S. P., Heisler, M. G., Reddy, G. V., Ohno, C., Das, P., Meyerowitz, E. M. (2007). Pattern formation during de novo assembly of the Arabidopsis shoot meristem. Development 134, 3539–3548. doi: 10.1242/dev.010298
Greb, T., Lohmann, J. U. (2016). Plant stem cells. Curr. Biol. 26, R816–R821. doi: 10.1016/j.cub.2016.07.070
Guo, Y., Han, L., Hymes, M., Denver, R., Clark, S. E. (2010). CLAVATA2 forms a distinct CLE-binding receptor complex regulating Arabidopsis stem cell specification: CLV2 forms a CLE-binding receptor complex. Plant J. 63, 889–900. doi: 10.1111/j.1365-313X.2010.04295.x
Hibara, K., Takada, S., Tasaka, M. (2003). CUC1 gene activates the expression of SAM-related genes to induce adventitious shoot formation: Role of CUC1 in adventitious SAM formation. Plant J. 36, 687–696. doi: 10.1046/j.1365-313X.2003.01911.x
Higuchi, M., Pischke, M. S., Mahonen, A. P., Miyawaki, K., Hashimoto, Y., Seki, M., et al. (2004). In planta functions of the Arabidopsis cytokinin receptor family. Proc. Natl. Acad. Sci. U.S.A. 101, 8821–8826. doi: 10.1073/pnas.04028871010402887101
Hou, B., Lim, E.-K., Higgins, G. S., Bowles, D. J. (2004). N-glucosylation of cytokinins by glycosyltransferases of arabidopsis thaliana. J. Biol. Chem. 279, 47822–47832. doi: 10.1074/jbc.M409569200
Hu, X., Xu, L. (2016). Transcription factors WOX11/12 directly activate WOX5/7 to promote root primordia initiation and organogenesis. Plant Physiol. 172, 2363–2373. doi: 10.1104/pp.16.01067
Huang, W., Pitorre, D., Poretska, O., Marizzi, C., Winter, N., Poppenberger, B., et al. (2015). ALTERED MERISTEM PROGRAM1 suppresses ectopic stem cell niche formation in the shoot apical meristem in a largely cytokinin-independent manner. Plant Physiol. 167, 1471–1486. doi: 10.1104/pp.114.254623
Hutchison, C. E., Li, J., Argueso, C., Gonzalez, M., Lee, E., Lewis, M. W., et al. (2006). The arabidopsis histidine phosphotransfer proteins are redundant positive regulators of cytokinin signaling. Plant Cell 18, 3073–3087. doi: 10.1105/tpc.106.045674
Hwang, I., Sheen, J. (2001). Two-component circuitry in Arabidopsis cytokinin signal transduction. Nature 413, 383–389. doi: 10.1038/35096500
Ikeuchi, M., Shibata, M., Rymen, B., Iwase, A., Bågman, A.-M., Watt, L., et al. (2018). A gene regulatory network for cellular reprogramming in plant regeneration. Plant Cell Physiol. 59, 770–782. doi: 10.1093/pcp/pcy013
Imamura, A., Kiba, T., Tajima, Y., Yamashino, T., Mizuno, T. (2003). In vivo and in vitro Characterization of the ARR11 Response Regulator Implicated in the His-to-Asp Phosphorelay Signal Transduction in Arabidopsis thaliana. Plant Cell Physiol. 44, 122–131. doi: 10.1093/pcp/pcg014
Inoue, T., Higuchi, M., Hashimoto, Y., Seki, M., Kobayashi, M., Kato, T., et al. (2001). Identification of CRE1 as a cytokinin receptor from Arabidopsis. Nature 409, 1060–1063. doi: 10.1038/35059117
Ishida, K., Yamashino, T., Yokoyama, A., Mizuno, T. (2008). Three Type-B Response Regulators, ARR1, ARR10 and ARR12, Play Essential but Redundant Roles in Cytokinin Signal Transduction Throughout the Life Cycle of Arabidopsis thaliana. Plant Cell Physiol. 49, 47–57. doi: 10.1093/pcp/pcm165
Ishihara, H., Sugimoto, K., Tarr, P. T., Temman, H., Kadokura, S., Inui, Y., et al. (2019). Primed histone demethylation regulates shoot regenerative competency. Nat. Commun. 10, 1786. doi: 10.1038/s41467-019-09386-5
Jones, R. J., Schreiber, B. M. N. (1997). Role and function of cytokinin oxidase in plants. Plant Growth Regul. 23, 123–134. doi: 10.1023/A:1005913311266
Kakimoto, T. (1996). CKI1, a histidine kinase homolog implicated in cytokinin signal transduction. Science 274, 982–985. doi: 10.1126/science.274.5289.982
Kakimoto, T. (2001). Identification of plant cytokinin biosynthetic enzymes as dimethylallyl diphosphate : ATP/ADP isopentenyltransferases. Plant Cell Physiol. 42, 677–685. doi: 10.1093/pcp/pce112
Kareem, A., Durgaprasad, K., Sugimoto, K., Du, Y., Pulianmackal, A. J., Trivedi, Z. B., et al. (2015). PLETHORA genes control regeneration by a two-step mechanism. Curr. Biol. 25, 1017–1030. doi: 10.1016/j.cub.2015.02.022
Kareem, A., Radhakrishnan, D., Wang, X., Bagavathiappan, S., Trivedi, Z. B., Sugimoto, K., et al. (2016). Protocol: a method to study the direct reprogramming of lateral root primordia to fertile shoots. Plant Methods 12, 27. doi: 10.1186/s13007-016-0127-5
Kiba, T., Aoki, K., Sakakibara, H., Mizuno, T. (2004). Arabidopsis response regulator, ARR22, ectopic expression of which results in phenotypes similar to the wol cytokinin-receptor mutant. Plant Cell Physiol. 45, 1063–1077. doi: 10.1093/pcp/pch12845/8/1063
Kim, J., Yang, W., Forner, J., Lohmann, J. U., Noh, B., Noh, Y. (2018). Epigenetic reprogramming by histone acetyltransferase HAG1/AtGCN5 is required for pluripotency acquisition in Arabidopsis. EMBO J. 37, e98726. doi: 10.15252/embj.201798726
Kurakawa, T., Ueda, N., Maekawa, M., Kobayashi, K., Kojima, M., Nagato, Y., et al. (2007). Direct control of shoot meristem activity by a cytokinin-activating enzyme. Nature 445, 652–655. doi: 10.1038/nature05504
Kuroha, T., Tokunaga, H., Kojima, M., Ueda, N., Ishida, T., Nagawa, S., et al. (2009). Functional analyses of LONELY GUY cytokinin-activating enzymes reveal the importance of the direct activation pathway in arabidopsis. Plant Cell 21, 3152–3169. doi: 10.1105/tpc.109.068676
Kusová, A., Steinbachová, L., Přerovská, T., Drábková, L. Z., Paleček, J., Khan, A., et al. (2023). Completing the TRB family: newly characterized members show ancient evolutionary origins and distinct localization, yet similar interactions. Plant Mol. Biol. 112, 61–83. doi: 10.1007/s11103-023-01348-2
Lall, S., Nettleton, D., DeCook, R., Che, P., Howell, S. H. (2004). Quantitative trait loci associated with adventitious shoot formation in tissue culture and the program of shoot development in arabidopsis. Genetics 167, 1883–1892. doi: 10.1534/genetics.103.025213
Lambolez, A., Kawamura, A., Takahashi, T., Rymen, B., Iwase, A., Favero, D. S., et al. (2022). Warm temperature promotes shoot regeneration in arabidopsis thaliana. Plant Cell Physiol. 63, 618–634. doi: 10.1093/pcp/pcac017
Lardon, R., Trinh, H. K., Xu, X., Vu, L. D., Van De Cotte, B., Pernisová, M., et al. (2022). Histidine kinase inhibitors impair shoot regeneration in Arabidopsis thaliana via cytokinin signaling and SAM patterning determinants. Front. Plant Sci. 13. doi: 10.3389/fpls.2022.894208
Lau, S., Smet, I. D., Kolb, M., Meinhardt, H., Jürgens, G. (2011). Auxin triggers a genetic switch. Nat. Cell Biol. 13, 611–615. doi: 10.1038/ncb2212
Laux, T. (2003). The stem cell concept in plants. Cell 113, 281–283. doi: 10.1016/S0092-8674(03)00312-X
Laux, T., Mayer, K. F. X., Berger, J., Jürgens, G. (1996). The WUSCHEL gene is required for shoot and floral meristem integrity in Arabidopsis. Development 122, 87–96. doi: 10.1242/dev.122.1.87
Lee, C., Clark, S. E. (2015). A WUSCHEL-independent stem cell specification pathway is repressed by PHB, PHV and CNA in arabidopsis. PloS One 10, e0126006. doi: 10.1371/journal.pone.0126006
Lee, H. W., Kim, N. Y., Lee, D. J., Kim, J. (2009). LBD18/ASL20 regulates lateral root formation in combination with LBD16/ASL18 downstream of ARF7 and ARF19 in arabidopsis. Plant Physiol. 151, 1377–1389. doi: 10.1104/pp.109.143685
Lee, K., Kim, J. H., Park, O.-S., Jung, Y. J., Seo, P. J. (2022). Ectopic expression of WOX5 promotes cytokinin signaling and de novo shoot regeneration. Plant Cell Rep. 41, 2415–2422. doi: 10.1007/s00299-022-02932-4
Lee, D., Moffatt, B. A. (1993). Purification and characterization of adenine phosphoribosyltransferase from Arabidopsis thaliana. Physiol. Plantarum 87, 483–492. doi: 10.1111/j.1399-3054.1993.tb02497.x
Lee, K., Park, O.-S., Go, J. Y., Yu, J., Han, J. H., Kim, J., et al. (2021). Arabidopsis ATXR2 represses de novo shoot organogenesis in the transition from callus to shoot formation. Cell Rep. 37, 109980. doi: 10.1016/j.celrep.2021.109980
Lee, K., Park, O.-S., Seo, P. J. (2017). Arabidopsis ATXR2 deposits H3K36me3 at the promoters of LBD genes to facilitate cellular dedifferentiation. Sci. Signal. 10, eaan0316. doi: 10.1126/scisignal.aan0316
Lee, K., Park, O., Seo, P. J. (2018). JMJ30-mediated demethylation of H3K9me3 drives tissue identity changes to promote callus formation in Arabidopsis. Plant J. 95, 961–975. doi: 10.1111/tpj.14002
Leibfried, A., To, J. P. C., Busch, W., Stehling, S., Kehle, A., Demar, M., et al. (2005). WUSCHEL controls meristem function by direct regulation of cytokinin-inducible response regulators. Nature 438, 1172–1175. doi: 10.1038/nature04270
Li, W., Liu, H., Cheng, Z. J., Su, Y. H., Han, H. N., Zhang, Y., et al. (2011). DNA methylation and histone modifications regulate de novo shoot regeneration in arabidopsis by modulating WUSCHEL expression and auxin signaling. PloS Genet. 7, e1002243. doi: 10.1371/journal.pgen.1002243
Li, W., Nguyen, K. H., Ha, C. V., Watanabe, Y., Tran, L.-S. P. (2019). Crosstalk between the cytokinin and MAX2 signaling pathways in growth and callus formation of Arabidopsis thaliana. Biochem. Biophys. Res. Commun. 511, 300–306. doi: 10.1016/j.bbrc.2019.02.038
Liu, Z., Dai, X., Li, J., Liu, N., Liu, X., Li, S., et al. (2020). The type-B cytokinin response regulator ARR1 inhibits shoot regeneration in an ARR12-dependent manner in arabidopsis. Plant Cell 32, 2271–2291. doi: 10.1105/tpc.19.00022
Liu, J., Hu, X., Qin, P., Prasad, K., Hu, Y., Xu, L. (2018b). The WOX11–LBD16 pathway promotes pluripotency acquisition in callus cells during de novo shoot regeneration in tissue culture. Plant Cell Physiol. 59, 739–748. doi: 10.1093/pcp/pcy010
Liu, Z., Li, J., Wang, L., Li, Q., Lu, Q., Yu, Y., et al. (2016). Repression of callus initiation by the miRNA-directed interaction of auxin-cytokinin in Arabidopsis thaliana. Plant J. 87, 391–402. doi: 10.1111/tpj.13211
Liu, J., Sheng, L., Xu, Y., Li, J., Yang, Z., Huang, H., et al. (2014). WOX11 and 12 Are Involved in the First-Step Cell Fate Transition during de Novo Root Organogenesis in Arabidopsis. Plant Cell 26, 1081–1093. doi: 10.1105/tpc.114.122887
Liu, H., Zhang, H., Dong, Y. X., Hao, Y. J., Zhang, X. S. (2018a). DNA METHYLTRANSFERASE1 -mediated shoot regeneration is regulated by cytokinin-induced cell cycle in Arabidopsis. New Phytol. 217, 219–232. doi: 10.1111/nph.14814
Long, J. A., Moan, E. I., Medford, J. I., Barton, M. K. (1996). A member of the KNOTTED class of homeodomain proteins encoded by the STM gene of Arabidopsis. Nature 379, 66–69. doi: 10.1038/379066a0
Lopes, F. L., Galvan-Ampudia, C., Landrein, B. (2021). WUSCHEL in the shoot apical meristem: old player, new tricks. J. Exp. Bot. 72, 1527–1535. doi: 10.1093/jxb/eraa572
Mahonen, A. P., Bishopp, A., Higuchi, M., Nieminen, K. M., Kinoshita, K., Tormakangas, K., et al. (2006). Cytokinin signaling and its inhibitor AHP6 regulate cell fate during vascular development. Science 311, 94–98. doi: 10.1126/science.1118875
Malamy, J. E., Benfey, P. N. (1997). Organization and cell differentiation in lateral roots of Arabidopsis thaliana. Development 124, 33–44. doi: 10.1242/dev.124.1.33
Mallory, A. C., Reinhart, B. J., Jones-Rhoades, M. W., Tang, G., Zamore, P. D., Barton, M. K., et al. (2004). MicroRNA control of PHABULOSA in leaf development: importance of pairing to the microRNA 5′ region. EMBO J. 23, 3356–3364. doi: 10.1038/sj.emboj.7600340
Martin, R. C., Mok, M. C., Shaw, G., Mok, D. W. S. (1989). An enzyme mediating the conversion of zeatin to dihydrozeatin in phaseolus embryos. Plant Physiol. 90, 1630–1635. doi: 10.1104/pp.90.4.1630
Mason, M. G., Mathews, D. E., Argyros, D. A., Maxwell, B. B., Kieber, J. J., Alonso, J. M., et al. (2005). Multiple type-B response regulators mediate cytokinin signal transduction in Arabidopsis. Plant Cell 17, 3007–3018. doi: 10.1105/tpc.105.035451
Mayer, K. F. X., Schoof, H., Haecker, A., Lenhard, M., Jürgens, G., Laux, T. (1998). Role of WUSCHEL in regulating stem cell fate in the arabidopsis shoot meristem. Cell 95, 805–815. doi: 10.1016/S0092-8674(00)81703-1
Meng, W. J., Cheng, Z. J., Sang, Y. L., Zhang, M. M., Rong, X. F., Wang, Z. W., et al. (2017). Type-B ARABIDOPSIS RESPONSE REGULATORs specify the shoot stem cell niche by dual regulation of WUSCHEL. Plant Cell 29, 1357–1372. doi: 10.1105/tpc.16.00640
Miyawaki, K., Tarkowski, P., Matsumoto-Kitano, M., Kato, T., Sato, S., Tarkowska, D., et al. (2006). Roles of Arabidopsis ATP/ADP isopentenyltransferases and tRNA isopentenyltransferases in cytokinin biosynthesis. Proc. Natl. Acad. Sci. U.S.A. 103, 16598–16603. doi: 10.1073/pnas.0603522103
Moffatt, B. A., Wang, L., Allen, M. S., Stevens, Y. Y., Qin, W., Snider, J., et al. (2000). Adenosine kinase of Arabidopsis. Kinetic properties and gene expression. Plant Physiol. 124, 1775–1785. doi: 10.1104/pp.124.4.1775
Mor, E., Pernisová, M., Minne, M., Cerutti, G., Ripper, D., Nolf, J., et al. (2022). bHLH heterodimer complex variations regulate cell proliferation activity in the meristems of Arabidopsis thaliana. iScience 25, 105364. doi: 10.1016/j.isci.2022.105364
Nishimura, C., Ohashi, Y., Sato, S., Kato, T., Tabata, S., Ueguchi, C. (2004). Histidine kinase homologs that act as cytokinin receptors possess overlapping functions in the regulation of shoot and root growth in arabidopsis. Plant Cell 16, 1365–1377. doi: 10.1105/tpc.021477
Ogawa, M., Shinohara, H., Sakagami, Y., Matsubayashi, Y. (2008). Arabidopsis CLV3 peptide directly binds CLV1 ectodomain. Science 319, 294–294. doi: 10.1126/science.1150083
Ohbayashi, I., Sakamoto, Y., Kuwae, H., Kasahara, H., Sugiyama, M. (2022). Enhancement of shoot regeneration by treatment with inhibitors of auxin biosynthesis and transport during callus induction in tissue culture of Arabidopsis thaliana. Plant Biotechnol. 39, 43–50. doi: 10.5511/plantbiotechnology.21.1225a
Okushima, Y., Fukaki, H., Onoda, M., Theologis, A., Tasaka, M. (2007). ARF7 and ARF19 regulate lateral root formation via direct activation of LBD/ASL genes in arabidopsis. Plant Cell 19, 118–130. doi: 10.1105/tpc.106.047761
Okushima, Y., Overvoorde, P. J., Arima, K., Alonso, J. M., Chan, A., Chang, C., et al. (2005). Functional genomic analysis of the AUXIN RESPONSE FACTOR gene family members in arabidopsis thaliana : unique and overlapping functions of ARF7 and ARF19. Plant Cell 17, 444–463. doi: 10.1105/tpc.104.028316
Osakabe, Y., Miyata, S., Urao, T., Seki, M., Shinozaki, K., Yamaguchi-Shinozaki, K. (2002). Overexpression of Arabidopsis response regulators, ARR4/ATRR1/IBC7 and ARR8/ATRR3, alters cytokinin responses differentially in the shoot and in callus formation. Biochem. Biophys. Res. Commun. 293, 806–815. doi: 10.1016/S0006-291X(02)00286-3
Pernisova, M., Grochova, M., Konecny, T., Plackova, L., Harustiakova, D., Kakimoto, T., et al. (2018). Cytokinin signalling regulates organ identity via the AHK4 receptor in Arabidopsis. Development 145, dev163907. doi: 10.1242/dev.163907
Pernisová, M., Klíma, P., Horák, J., Válková, M., Malbeck, J., Souček, P., et al. (2009). Cytokinins modulate auxin-induced organogenesis in plants via regulation of the auxin efflux. Proc. Natl. Acad. Sci. U.S.A. 106, 3609–3614. doi: 10.1073/pnas.0811539106
Pernisová, M., Kuderová, A., Hejátko, J. (2011). Cytokinin and auxin interactions in plant development: metabolism, signalling, transport and gene expression. Curr. Protein Pept. Sci. 12, 137–147. doi: 10.2174/138920311795684887
Pernisová, M., Vernoux, T. (2021). Auxin does the SAMba: auxin signaling in the shoot apical meristem. Cold Spring Harb. Perspect. Biol. 13, a039925. doi: 10.1101/cshperspect.a039925
Pi, L., Aichinger, E., van der Graaff, E., Llavata-Peris, C. I., Weijers, D., Hennig, L., et al. (2015). Organizer-derived WOX5 signal maintains root columella stem cells through chromatin-mediated repression of CDF4 expression. Dev. Cell 33, 576–588. doi: 10.1016/j.devcel.2015.04.024
Plong, A., Rodriguez, K., Alber, M., Chen, W., Reddy, G. V. (2021). CLAVATA3 mediated simultaneous control of transcriptional and post-translational processes provides robustness to the WUSCHEL gradient. Nat. Commun. 12, 6361. doi: 10.1038/s41467-021-26586-0
Qiao, M., Xiang, F. (2013). A set of Arabidopsis thaliana miRNAs involve shoot regeneration in vitro. Plant Signaling Behav. 8, e23479. doi: 10.4161/psb.23479
Qiao, M., Zhao, Z., Song, Y., Liu, Z., Cao, L., Yu, Y., et al. (2012). Proper regeneration from in vitro cultured Arabidopsis thaliana requires the microRNA-directed action of an auxin response factor: ARF10 in shoot regeneration in vitro. Plant J. 71, 14–22. doi: 10.1111/j.1365-313X.2012.04944.x
Rashotte, A. M., Mason, M. G., Hutchison, C. E., Ferreira, F. J., Schaller, G. E., Kieber, J. J. (2006). A subset of Arabidopsis AP2 transcription factors mediates cytokinin responses in concert with a two-component pathway. Proc. Natl. Acad. Sci. U.S.A. 103, 11081–11085. doi: 10.1073/pnas.0602038103
Reddy, G. V., Heisler, M. G., Ehrhardt, D. W., Meyerowitz, E. M. (2004). Real-time lineage analysis reveals oriented cell divisions associated with morphogenesis at the shoot apex of Arabidopsis thaliana. Development 131, 4225–4237. doi: 10.1242/dev.01261
Rodriguez, K., Perales, M., Snipes, S., Yadav, R. K., Diaz-Mendoza, M., Reddy, G. V. (2016). DNA-dependent homodimerization, sub-cellular partitioning, and protein destabilization control WUSCHEL levels and spatial patterning. Proc. Natl. Acad. Sci. U.S.A. 113, E6307–E6315. doi: 10.1073/pnas.1607673113
Rosspopoff, O., Chelysheva, L., Saffar, J., Lecorgne, L., Gey, D., Caillieux, E., et al. (2017). Direct conversion of root primordium into shoot meristem relies on timing of stem cell niche development. Development 144, 1187–1200. doi: 10.1242/dev.142570
Sakai, H., Aoyama, T., Oka, A. (2000). Arabidopsis ARR1 and ARR2 response regulators operate as transcriptional activators. Plant J. 24, 703–711. doi: 10.1046/j.1365-313x.2000.00909.x
Sarkar, A. K., Luijten, M., Miyashima, S., Lenhard, M., Hashimoto, T., Nakajima, K., et al. (2007). Conserved factors regulate signalling in Arabidopsis thaliana shoot and root stem cell organizers. Nature 446, 811–814. doi: 10.1038/nature05703
Schaller, G. E., Bishopp, A., Kieber, J. J. (2015). The yin-yang of hormones: cytokinin and auxin interactions in plant development. Plant Cell 27, 44–63. doi: 10.1105/tpc.114.133595
Schlereth, A., Möller, B., Liu, W., Kientz, M., Flipse, J., Rademacher, E. H., et al. (2010). MONOPTEROS controls embryonic root initiation by regulating a mobile transcription factor. Nature 464, 913–916. doi: 10.1038/nature08836
Schoof, H., Lenhard, M., Haecker, A., Mayer, K. F. X., Jürgens, G., Laux, T. (2000). The stem cell population of arabidopsis shoot meristems is maintained by a regulatory loop between the CLAVATA and WUSCHEL genes. Cell 100, 635–644. doi: 10.1016/S0092-8674(00)80700-X
Schrumpfová, P. P., Vychodilová, I., Hapala, J., Schořová, Š., Dvořáček, V., Fajkus, J. (2016). Telomere binding protein TRB1 is associated with promoters of translation machinery genes in vivo. Plant Mol. Biol. 90, 189–206. doi: 10.1007/s11103-015-0409-8
Shim, S., Lee, H. G., Seo, P. J. (2021). MET1-dependent DNA methylation represses light signaling and influences plant regeneration in arabidopsis. Mol. Cells 44, 746–757. doi: 10.14348/molcells.2021.0160
Skoog, F., Miller, C. O. (1957). Chemical regulation of growth and organ formation in plant tissues cultured in vitro. Symp Soc. Exp. Biol. 54, 118–130.
Snipes, S. A., Rodriguez, K., DeVries, A. E., Miyawaki, K. N., Perales, M., Xie, M., et al. (2018). Cytokinin stabilizes WUSCHEL by acting on the protein domains required for nuclear enrichment and transcription. PloS Genet. 14, e1007351. doi: 10.1371/journal.pgen.1007351
Su, Y. H., Zhou, C., Li, Y. J., Yu, Y., Tang, L. P., Zhang, W. J., et al. (2020). Integration of pluripotency pathways regulates stem cell maintenance in the Arabidopsis shoot meristem. Proc. Natl. Acad. Sci. U.S.A. 117, 22561–22571. doi: 10.1073/pnas.2015248117
Subban, P., Kutsher, Y., Evenor, D., Belausov, E., Zemach, H., Faigenboim, A., et al. (2020). Shoot regeneration is not a single cell event. Plants 10, 58. doi: 10.3390/plants10010058
Sugimoto, K., Jiao, Y., Meyerowitz, E. M. (2010). Arabidopsis regeneration from multiple tissues occurs via a root development pathway. Dev. Cell 18, 463–471. doi: 10.1016/j.devcel.2010.02.004
Suzuki, T., Imamura, A., Ueguchi, C., Mizuno, T. (1998). Histidine-containing phosphotransfer (HPt) signal transducers implicated in His-to-Asp phosphorelay in Arabidopsis. Plant Cell Physiol. 39, 1258–1268. doi: 10.1093/oxfordjournals.pcp.a029329
Takei, K., Sakakibara, H., Sugiyama, T. (2001). Identification of genes encoding adenylate isopentenyltransferase, a cytokinin biosynthesis enzyme, in Arabidopsis thaliana. J. Biol. Chem. 276, 26405–26410. doi: 10.1074/jbc.M102130200M102130200
Takei, K., Yamaya, T., Sakakibara, H. (2004). Arabidopsis CYP735A1 and CYP735A2 encode cytokinin hydroxylases that catalyze the biosynthesis of trans-Zeatin. J. Biol. Chem. 279, 41866–41872. doi: 10.1074/jbc.M406337200
Tamaki, H., Konishi, M., Daimon, Y., Aida, M., Tasaka, M., Sugiyama, M. (2009). Identification of novel meristem factors involved in shoot regeneration through the analysis of temperature-sensitive mutants of Arabidopsis. Plant J. 57, 1027–1039. doi: 10.1111/j.1365-313X.2008.03750.x
Tanaka, Y., Suzuki, T., Yamashino, T., Mizuno, T. (2004). Comparative studies of the AHP histidine-containing phosphotransmitters implicated in His-to-Asp phosphorelay in Arabidopsis thaliana. Biosci. Biotechnol. Biochem. 68, 462–465. doi: 10.1271/bbb.68.462
Temman, H., Sakamoto, T., Ueda, M., Sugimoto, K., Migihashi, M., Yamamoto, K., et al. (2023). Histone deacetylation regulates de novo shoot regeneration. PNAS Nexus 2, pgad002. doi: 10.1093/pnasnexus/pgad002
Temmerman, A., Marquez-Garcia, B., Depuydt, S., Bruznican, S., De Cuyper, C., De Keyser, A., et al. (2022). MAX2 -dependent competence for callus formation and shoot regeneration from Arabidopsis thaliana root explants. J. Exp. Bot. 73, 6272–6291. doi: 10.1093/jxb/erac281
To, J. P. C., Haberer, G., Ferreira, F. J., Deruère, J., Mason, M. G., Schaller, G. E., et al. (2004). Type-A arabidopsis response regulators are partially redundant negative regulators of cytokinin signaling[W]. Plant Cell 16, 658–671. doi: 10.1105/tpc.018978
Valvekens, D., Montagu, M. V., Lijsebettens, M. V. (1988). Agrobacterium tumefaciens -mediated transformation of Arabidopsis thaliana root explants by using kanamycin selection. Proc. Natl. Acad. Sci. U.S.A. 85, 5536–5540. doi: 10.1073/pnas.85.15.5536
Varapparambath, V., Mathew, M. M., Shanmukhan, A. P., Radhakrishnan, D., Kareem, A., Verma, S., et al. (2022). Mechanical conflict caused by a cell-wall-loosening enzyme activates de novo shoot regeneration. Dev. Cell 57, 2063–2080.e10. doi: 10.1016/j.devcel.2022.07.017
Vollbrecht, E., Reiser, L., Hake, S. (2000). Shoot meristem size is dependent on inbred background and presence of the maize homeobox gene, knotted1. Development 127, 3161–3172. doi: 10.1242/dev.127.14.3161
Wang, L., Liu, Z., Qiao, M., Xiang, F. (2018). miR393 inhibits in vitro shoot regeneration in Arabidopsis thaliana via repressing TIR1. Plant Sci. 266, 1–8. doi: 10.1016/j.plantsci.2017.10.009
Wang, J., Tan, M., Wang, X., Jia, L., Wang, M., Huang, A., et al. (2023a). WUS-RELATED HOMEOBOX 14 boosts de novo plant shoot regeneration. Plant Physiol. 192, 748–752. doi: 10.1093/plphys/kiad125
Wang, J.-W., Wang, L.-J., Mao, Y.-B., Cai, W.-J., Xue, H.-W., Chen, X.-Y. (2005). Control of root cap formation by microRNA-targeted auxin response factors in arabidopsis. Plant Cell 17, 2204–2216. doi: 10.1105/tpc.105.033076
Wang, M., Zhong, Z., Gallego-Bartolomé, J., Feng, S., Shih, Y.-H., Liu, M., et al. (2023b). Arabidopsis TRB proteins function in H3K4me3 demethylation by recruiting JMJ14. Nat. Commun. 14, 1736. doi: 10.1038/s41467-023-37263-9
Weits, D. A., Kunkowska, A. B., Kamps, N. C. W., Portz, K. M. S., Packbier, N. K., Nemec Venza, Z., et al. (2019). An apical hypoxic niche sets the pace of shoot meristem activity. Nature 569, 714–717. doi: 10.1038/s41586-019-1203-6
Xu, M., Hu, T., Zhao, J., Park, M.-Y., Earley, K. W., Wu, G., et al. (2016). Developmental Functions of miR156-Regulated SQUAMOSA PROMOTER BINDING PROTEIN-LIKE (SPL) Genes in Arabidopsis thaliana. PloS Genet. 12, e1006263. doi: 10.1371/journal.pgen.1006263
Xue, T., Dai, X., Wang, R., Wang, J., Liu, Z., Xiang, F. (2017). ARGONAUTE10 Inhibits in vitro shoot regeneration via repression of miR165/166 in Arabidopsis thaliana. Plant Cell Physiol. 58, 1789–1800. doi: 10.1093/pcp/pcx117
Yadav, R. K., Perales, M., Gruel, J., Girke, T., Jönsson, H., Reddy, G. V. (2011). WUSCHEL protein movement mediates stem cell homeostasis in the Arabidopsis shoot apex. Genes Dev. 25, 2025–2030. doi: 10.1101/gad.17258511
Yamada, M., Tanaka, S., Miyazaki, T., Aida, M. (2022). Expression of the auxin biosynthetic genes YUCCA1 and YUCCA4 is dependent on the boundary regulators CUP-SHAPED COTYLEDON genes in the Arabidopsis thaliana embryo. Plant Biotechnol. 39, 37–42. doi: 10.5511/plantbiotechnology.21.0924a
Yan, A., Borg, M., Berger, F., Chen, Z. (2020). The atypical histone variant H3.15 promotes callus formation in Arabidopsis thaliana. Development 147, dev.184895. doi: 10.1242/dev.184895
Yanai, O., Shani, E., Dolezal, K., Tarkowski, P., Sablowski, R., Sandberg, G., et al. (2005). Arabidopsis KNOXI proteins activate cytokinin biosynthesis. Curr. Biol. 15, 1566–1571. doi: 10.1016/j.cub.2005.07.060
Yang, W., Choi, M.-H., Noh, B., Noh, Y.-S. (2020). De novo shoot regeneration controlled by HEN1 and TCP3/4 in arabidopsis. Plant Cell Physiol. 61, 1600–1613. doi: 10.1093/pcp/pcaa083
Yang, S., De Haan, M., Mayer, J., Janacek, D. P., Hammes, U. Z., Poppenberger, B., et al. (2022). A novel chemical inhibitor of polar auxin transport promotes shoot regeneration by local enhancement of HD-ZIP III transcription. New Phytol. 235, 1111–1128. doi: 10.1111/nph.18196
Yang, B., Minne, M., Brunoni, F., Plačková, L., Petřík, I., Sun, Y., et al. (2021). Non-cell autonomous and spatiotemporal signalling from a tissue organizer orchestrates root vascular development. Nat. Plants 7, 1485–1494. doi: 10.1038/s41477-021-01017-6
Yang, S., Poretska, O., Sieberer, T. (2018). ALTERED MERISTEM PROGRAM1 restricts shoot meristem proliferation and regeneration by limiting HD-ZIP III-mediated expression of RAP2.6L. Plant Physiol. 177, 1580–1594. doi: 10.1104/pp.18.00252
Zhai, N., Xu, L. (2021). Pluripotency acquisition in the middle cell layer of callus is required for organ regeneration. Nat. Plants 7, 1453–1460. doi: 10.1038/s41477-021-01015-8
Zhang, T.-Q., Lian, H., Tang, H., Dolezal, K., Zhou, C.-M., Yu, S., et al. (2015). An intrinsic microRNA timer regulates progressive decline in shoot regenerative capacity in plants. Plant Cell 27, 349–360. doi: 10.1105/tpc.114.135186
Zhang, T.-Q., Lian, H., Zhou, C.-M., Xu, L., Jiao, Y., Wang, J.-W. (2017b). A Two-Step Model for de Novo Activation of WUSCHEL during Plant Shoot Regeneration. Plant Cell 29, 1073–1087. doi: 10.1105/tpc.16.00863
Zhang, F., May, A., Irish, V. F. (2017a). Type-B ARABIDOPSIS RESPONSE REGULATORs directly activate WUSCHEL. Trends Plant Sci. 22, 815–817. doi: 10.1016/j.tplants.2017.08.007
Zhang, S., Yu, R., Yu, D., Chang, P., Guo, S., Yang, X., et al. (2022). The calcium signaling module CaM–IQM destabilizes IAA–ARF interaction to regulate callus and lateral root formation. Proc. Natl. Acad. Sci. U.S.A. 119, e2202669119. doi: 10.1073/pnas.2202669119
Zhang, Z., Zhang, X. (2012). Argonautes compete for miR165/166 to regulate shoot apical meristem development. Curr. Opin. Plant Biol. 15, 652–658. doi: 10.1016/j.pbi.2012.05.007
Zhang, M. M., Zhang, H. K., Zhai, J. F., Zhang, X. S., Sang, Y. L., Cheng, Z. J. (2021). ARF4 regulates shoot regeneration through coordination with ARF5 and IAA12. Plant Cell Rep. 40, 315–325. doi: 10.1007/s00299-020-02633-w
Zhao, Z., Andersen, S. U., Ljung, K., Dolezal, K., Miotk, A., Schultheiss, S. J., et al. (2010). Hormonal control of the shoot stem-cell niche. Nature 465, 1089–1092. doi: 10.1038/nature09126
Zhou, Y., Liu, X., Engstrom, E. M., Nimchuk, Z. L., Pruneda-Paz, J. L., Tarr, P. T., et al. (2015). Control of plant stem cell function by conserved interacting transcriptional regulators. Nature 517, 377–380. doi: 10.1038/nature13853
Zhou, Y., Wang, Y., Krause, K., Yang, T., Dongus, J. A., Zhang, Y., et al. (2018a). Telobox motifs recruit CLF/SWN–PRC2 for H3K27me3 deposition via TRB factors in Arabidopsis. Nat. Genet. 50, 638–644. doi: 10.1038/s41588-018-0109-9
Zhou, Y., Yan, A., Han, H., Li, T., Geng, Y., Liu, X., et al. (2018b). HAIRY MERISTEM with WUSCHEL confines CLAVATA3 expression to the outer apical meristem layers. Science 361, 502–506. doi: 10.1126/science.aar8638
Zubo, Y. O., Blakley, I. C., Yamburenko, M. V., Worthen, J. M., Street, I. H., Franco-Zorrilla, J. M., et al. (2017). Cytokinin induces genome-wide binding of the type-B response regulator ARR10 to regulate growth and development in Arabidopsis. Proc. Natl. Acad. Sci. U.S.A. 114, E5995–E6004. doi: 10.1073/pnas.1620749114
Keywords: cytokinin, de novo organogenesis, plant stem cells, shoot apical meristem, shoot regeneration
Citation: Šmeringai J, Schrumpfová PP and Pernisová M (2023) Cytokinins – regulators of de novo shoot organogenesis. Front. Plant Sci. 14:1239133. doi: 10.3389/fpls.2023.1239133
Received: 12 June 2023; Accepted: 31 July 2023;
Published: 18 August 2023.
Edited by:
Marcus Heisler, The University of Sydney, AustraliaReviewed by:
Xian Sheng Zhang, Shandong Agricultural University, ChinaKarel Dolezal, Academy of Sciences of the Czech Republic, Czechia
Copyright © 2023 Šmeringai, Schrumpfová and Pernisová. This is an open-access article distributed under the terms of the Creative Commons Attribution License (CC BY). The use, distribution or reproduction in other forums is permitted, provided the original author(s) and the copyright owner(s) are credited and that the original publication in this journal is cited, in accordance with accepted academic practice. No use, distribution or reproduction is permitted which does not comply with these terms.
*Correspondence: Markéta Pernisová, cGVybmlzb3ZhQHNjaS5tdW5pLmN6