1 Introduction
The forthcoming climatic scenario, where elevated atmospheric carbon dioxide (CO2) concentrations are expected, will challenge crop performance with a higher demand for nitrogen (N), which will further aggravate N losses from agrosystems that are already polluting air and water systems (Anas et al., 2020; IPCC, 2022), making it imperative to anticipate and develop novel and climate-smart agriculture. Research related to plants showing the ability to produce biological nitrification inhibitors (BNI) as a mitigation strategy is currently in vogue (Saud et al., 2022). Indeed, great progress has been made recently in the characterization of species with this ability, in the production of BNI molecules, and even in the development of new crop lines aimed at incorporating this trait. However, the implications of future environmental conditions on the BNI strategy remain overlooked and need to be addressed. In this study, we aimed to establish the connections between the predicted elevated eCO2 conditions and the production and activity of BNI compounds in plants and soil. We hypothesize that enhanced carbon assimilation by plants could improve their BNI capacity, promoting ammonium occurrence in the soil, which would particularly benefit ammonium-adapted crop varieties.
2 Nitrogen as centrepiece of plant adaptation to elevated CO2
Nitrogen (N) fertilization is required to improve crop yield. However, the inefficiency of agricultural systems, where only 30%–50% of applied N is used by crops, provokes significant losses to the environment in the form of N gas emissions or nitrate (NO3−) leachates, particularly because of soil microbial nitrification and denitrification (Lassaletta et al., 2014). Nitrification is an aerobic process driven by ammonium-oxidizing bacteria or archaea, where ammonium (NH4+) is oxidized to NO3−, which can be further reduced by denitrifying bacteria under anaerobic soil conditions. Both microbial pathways can yield nitrous oxide (N2O) as an end-product, which is a powerful GHG (Coskun et al., 2017a). Because N-fertilization is the main source of global anthropogenic N2O emissions (IPCC, 2022), great effort has been put into controlling N-cycling processes in agrosystems in recent decades, with the dual aim of maintaining N available for crops for longer periods while reducing its loss to the environment. Therefore, high-production agriculture needs to reconcile the double challenge of mitigating N losses and adapting to progressively changing environmental conditions, such as an elevated atmospheric CO2 (eCO2) atmosphere, rising temperatures, and water scarcity (FAO, 2018). To this end, climate-resilient crops are required, in a context where more food production will be necessary to maintain the future world population.
The predicted state atmospheric concentration of CO2 will reach 600 ppm–1,300 ppm by the end of the century (IPCC, 2022). Elevated atmospheric CO2 (eCO2) remodels plant physiology, with enhanced photosynthesis and reduced stomatal conductance as the primary effects, leading to improved water use efficiency and potentially boosting plant productivity (Gamage et al., 2018). However, long-term exposure to eCO2 often entails photosynthetic acclimation in C3 crops, limiting their growth. Although the physiological basis for acclimation to eCO2 is still unclear, one of the most accepted explanations is that increased carbohydrate biosynthesis causes C:N imbalance, leading to N depletion in tissues (Ainsworth and Rogers, 2007). Therefore, acclimation can be overcome by sufficient N supply to ensure proper sink development for excessively formed photoassimilates, thus avoiding RuBisCO inhibition (Ainsworth and Rogers, 2007). In general, using cultivars with enhanced nitrogen use efficiency (NUE) and implementing agricultural practices that ensure soil N availability are advisable to avoid N dilution in plants and maximize crop yields under eCO2. Another open debate about the plant response to eCO2 is related to the available N source. Several studies have shown similar yield stimulation in response to eCO2 regardless of the N form (NH4+ or NO3−) assimilated (Vega-Mas et al., 2015; Dier et al., 2018; Andrews et al., 2019). However, some studies have proposed that eCO2 inhibits NO3− assimilation in shoots by diminishing the reducing power of photorespiration (Bloom et al., 2020), while others argue that N limitation at eCO2 is a consequence of accelerated growth rather than impaired NO3− reduction (Andrews et al., 2020; Igarashi et al., 2021). Nonetheless, in view the possible advantage of NH4+-N sources over NO3−-N, environmental conditions favoring soil NH4+ availability to plants would certainly be desirable.
3 Biological nitrification inhibition: a promising N-management strategy in a climate change scenario
Increasing N fertilization to address crop N demand in a climate change scenario seems undesirable, as excess soil N could further aggravate the aforementioned water and air pollution (Lassaletta et al., 2014). Therefore, strategies should be developed to promote better utilization of already available N. At present, one of the extensively proven technologies to prolong N retention in soils, while reducing N losses, is the application of synthetic nitrification inhibitors (SNIs) in combination with NH4+-based fertilizers. The most widely used SNIs are nitrapyrin, dicyandiamide (DCD), and dimethylpyrazol (DMP)-based NIs (Norton and Ouyang, 2019; Huérfano et al., 2022). However, SNIs are not exempt from some disadvantages, including production or management costs that restrict their use, notably in low-income countries, their limited action over time, variable effects on yield, or potential environmental toxicity (Coskun et al., 2017b; Sadhukhan et al., 2022). As a recent alternative, exploitation of the natural capacity of different plants to exudate compounds that suppress microbial nitrification, the so-called biological nitrification inhibitors or BNIs, is a promising strategy (Subbarao and Searchinger, 2021; Lata et al., 2022; Saud et al., 2022). Since the discovery of BNIs in the tropical grass Brachiaria humidicola and Sorghum bicolor (Subbarao et al., 2007a), the search for plant species displaying this trait has led to the identification of species, including cereals of high agronomical interest such as rice and maize (Tanaka et al., 2010; Sun et al., 2016; Otaka et al., 2022). Wheat cultivars show weak BNI activity but, importantly, the recent development of elite wheat cultivars that harbor a chromosomal region introgressed from Leymus racemosus, a wild wheat relative with high BNI activity (Subbarao et al., 2021; Bozal-Leorri et al., 2022), has raised further expectations regarding the potential of crops to directly control nitrification in soils.
How N cycling, and nitrification in particular, will be affected in agrosystems by future climatic conditions, as eCO2 is still far from being understood, with variable results shown in the literature (Coskun et al., 2016). In a meta-analysis that included N-fertilized fields, Dijkstra et al. (2012) showed that eCO2 led to increased N2O emissions due to enhanced nitrification and/or denitrification. High rates of soil nitrification are predicted in the future because nitrifiers use CO2 as carbon source for growth and NH4+ as energy source (Wendeborn, 2020). Indeed, a more abundant nitrifying population was found in response to eCO2, alone or in combination with increased temperature (Diao et al., 2020; Waqas et al., 2021). Although the utility of SNIs is unquestionable, their efficiency depends on soil conditions such as water content and temperature (Menéndez et al., 2012; Nair et al., 2021). Bozal-Leorri et al. (2021) recently showed DMP-based SNIs efficiently decreased N2O losses regardless CO2 level, although further studies are needed to confirm their inhibition efficiency under eCO2 in the field and considering different soil types and environmental conditions. Additionally, anticipating how eCO2 will affect the plant’s capacity to synthesize and release BNIs, as well as their efficiency in suppressing nitrification, is of great relevance to propose effective strategies to increase NUE by crop plants under future conditions.
4 How will eCO2 influence plants biological nitrification inhibitory capacity?
From an evolutionary point of view, the BNI capacity is considered a plant response to adapt to N-scarce environments (Subbarao et al., 2006; Lata et al., 2022). Conversely, the BNI strategy has also proven to be effective in controlling soil N losses in well N-fertilized systems such as sorghum, rice, and wheat cereal cultures (Subbarao et al., 2021; Wang et al., 2021). Slowing NH4+oxidation by inhibiting soil nitrification reduces N leakage while promoting NH4+ stability, thus presumably favoring a more NH4+-based nutrition. This will surely promote greater yield potential through a more efficient assimilation of co-existent N forms (Subbarao and Searchinger, 2021), which is also crucial to match the enhanced N demands by eCO2. Nonetheless, high NH4+ content in soil may entail a stressful situation for crop performance (Britto and Kronzucker, 2002; González-Moro et al., 2021); hence, crops better adapted to NH4+ as N source are required. Because plant NH4+ assimilation is dependent on proper C-skeleton supply, conditions favoring photoassimilate production, such as eCO2 or direct carbon provision, have been shown to alleviate the symptoms associated with ammonium stress (Roosta and Schjoerring, 2008; Setién et al., 2013; Vega-Mas et al., 2015). Therefore, the predicted eCO2 may be advantageous for improving the performance of BNI-producing plants grown in the presence of enhanced NH4+ (Figure 1).
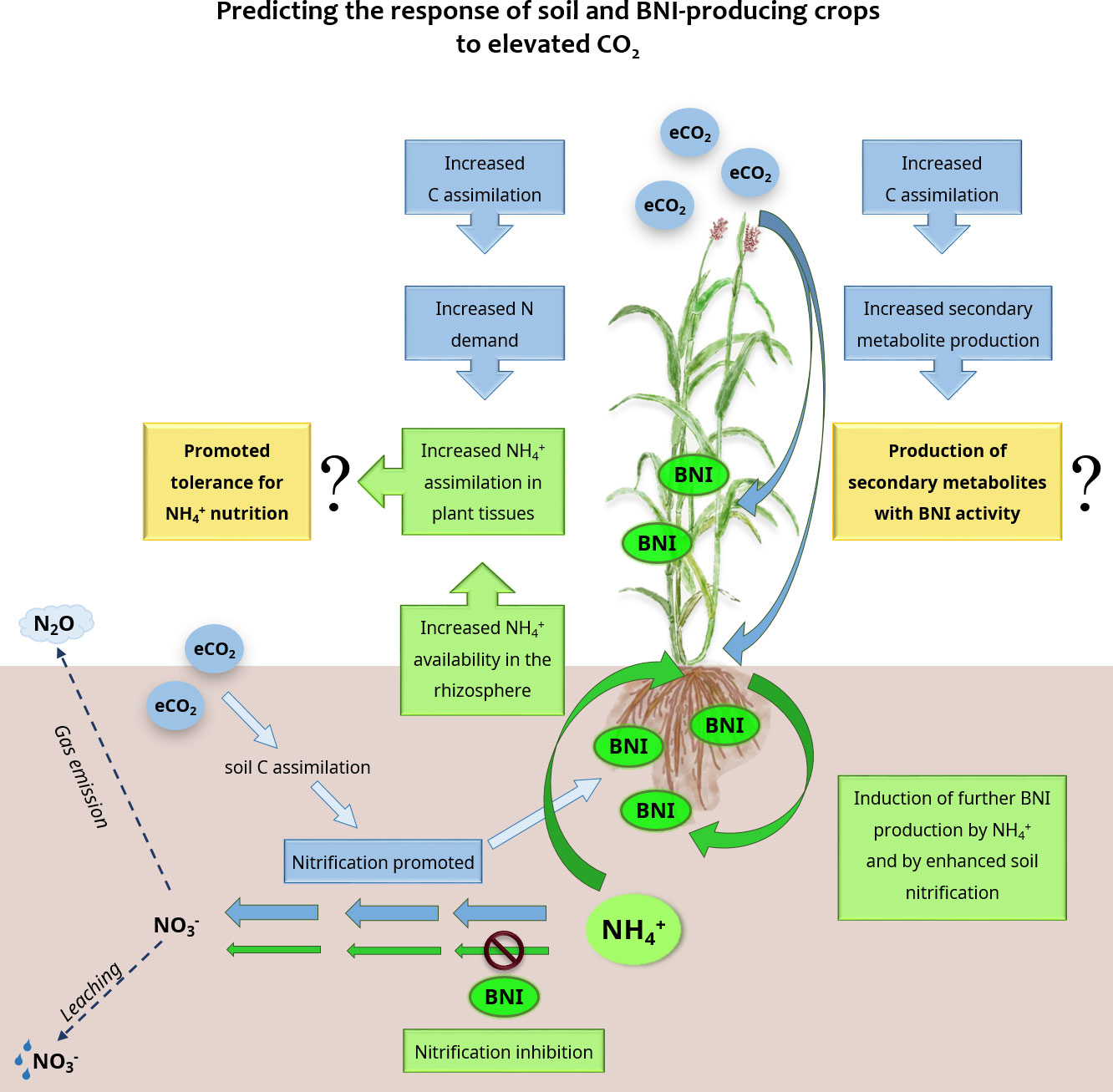
Figure 1 Main view of the predicted effects of elevated CO2 levels on soil nitrification and BNI-producing plants. Changes caused by elevated CO2 are highlighted in blue, changes due to plant BNI activity are highlighted in green and newly proposed hypotheses are highlighted in yellow.
Plant BNI capacity is dependent on soil conditions, of which rhizospheric pH, aeration, quantity, and form of available N are the main drivers of BNI synthesis and exudation (Wang et al., 2021; Zhang et al., 2022). The present working model indicates that rhizosphere acidification, associated with NH4+ assimilation and plasma membrane H+-ATPase activity, stimulates BNI release (Zhu et al., 2012; Coskun et al., 2017a; Afzal et al., 2020). Hence, more NH4+-based nutrition would act as a positive feedback regulatory strategy for BNI production and/or release (Subbarao et al., 2007b). Whether plants with higher BNI potential display specific NH4+-tolerance mechanisms needs to be explored, and results of great interest for the future. Remarkably, the presence of nitrifying bacteria, but not denitrifiers, promotes the secretion of BNI compounds in wheat (O’Sullivan et al., 2016) and rice (Zhang et al., 2019). Thus, although the specific mechanisms responsible for such BNI induction are still unknown, the existence of signaling between BNI-producing roots and nitrifying bacteria has been suggested (Wang et al., 2021). In turn, the predicted promotion of soil nitrification under eCO2 conditions (Diao et al., 2020; Waqas et al., 2021) could potentially benefit BNI production.
Elevated CO2 promotes not only whole plant and root biomass (Roy and Mathur, 2021), but also root exudate production, which accounts for up to 21% of photosynthetically fixed C (Kollah et al., 2019; Xiong et al., 2019). The BNI compounds identified to date are C-enriched secondary metabolites that belong to a wide range of different metabolic groups, such as quinones, terpenes, and phenolic compounds (Nardi et al., 2020; Chai and Schachtman, 2022). This is the case for BNIs identified as sorgoleone and methyl 3-(4-hydroxyphenyl) propionate (MHPP) from Sorghum, or brachialactone from Brachiaria (Zakir et al., 2008; Subbarao et al., 2009; Subbarao et al., 2013). Therefore, it would be expected that enhanced root exudation under eCO2 to include compounds with BNI activity (Figure 1). Overall, secondary metabolites are involved in plant–environment interactions and are produced by plants to ease their adaptation to a changing environment (Zandalinas et al., 2022). Moreover, enhanced net photosynthesis rates under eCO2 lead to the rescheduling of secondary metabolism, with enhanced C-enriched metabolite production (Matros et al., 2006; Xu et al., 2019; Roy and Mathur, 2021). Therefore, this reinforces the hypothesis of a possible positive effect of eCO2 on the production of BNI-active metabolites. However, root exudation in plants is affected by many factors; water availability is a determinant of exudation response to eCO2 (Calvo et al., 2017; Xiong et al., 2019; Chai and Schachtman, 2022). In agreement with the promotion of secondary metabolism under stress conditions, Ghatak et al. (2022) observed that drought stress in pearl millet enhanced the release of root exudates and increased total BNI activity. Deciphering how BNI production is affected by the interaction of factors such as eCO2, water availability, or temperature is the next step to further promote this trait for sustainable agriculture.
5 Concluding remarks
Many uncertainties still exist in optimizing N management under future climatic conditions. However, to make agriculture more sustainable, it is mandatory to meet crop N demand, while reducing N losses derived from N fertilization. Improving soil N availability through the exploitation of plant BNIs is an outstanding opportunity. In this study, we hypothesize that BNI production would be promoted in a climate change scenario, since eCO2 would boost both N assimilation and production of C-rich secondary metabolites. Although there are still many unresolved issues regarding factors that affect plant BNI capacity, BNI crops are promising candidates for future sustainable agrosystem production. In this context, selection of climate-resilient crop varieties adapted to the use of NH4+ as an N source is essential.
Author contributions
IV-M and MG-M conceived the manuscript and supervised the whole writing process. All authors listed have made a substantial, direct, and intellectual contribution to the work and approved it for publication.
Funding
This research was financially supported by the Department of Economic Development, Sustainability, and Environment (00048-IDA2021-45) and the Consolidated Groups program (IT1560-22) of the Basque Government, the project ERA-Net Cofund SuSCrop PCI2020-120685-2 funded by CDTI (EXP 00139688/IDI-20210754), and the project TED2021-132279B-I00 funded by MCIN/AEI/10.13039/501100011033 and by EU “NextGenerationEU”/PRTR. IV-M thanks to the Basque Government for her postdoctoral fellowship (Ref.: POS-2018-1-005) and AB-L thanks to Margarita Salas postdoctoral fellowship funded by the Ministry of Universities (Government of Spain) and by EU “NextGenerationEU” program.
Conflict of interest
The authors declare that the research was conducted in the absence of any commercial or financial relationships that could be construed as a potential conflict of interest.
Publisher’s note
All claims expressed in this article are solely those of the authors and do not necessarily represent those of their affiliated organizations, or those of the publisher, the editors and the reviewers. Any product that may be evaluated in this article, or claim that may be made by its manufacturer, is not guaranteed or endorsed by the publisher.
References
Afzal, M. R., Zhang, M., Jin, H., Wang, G., Zhang, M., Ding, M., et al. (2020). Post-translational regulation of plasma membrane H+-ATPase is involved in the release of biological nitrification inhibitors from sorghum roots. Plant Soil 450, 357–372. doi: 10.1007/s11104-020-04511-6
Ainsworth, E. A., Rogers, A. (2007). The response of photosynthesis and stomatal conductance to rising [CO2]: mechanisms and environmental interactions. Plant Cell Environ. 30, 258–270. doi: 10.1111/j.1365-3040.2007.01641.x
Anas, M., Liao, F., Verma, K. K., Sarwar, M. A., Mahmood, A., Chen, Z. L., et al. (2020). Fate of nitrogen in agriculture and environment: agronomic, eco-physiological and molecular approaches to improve nitrogen use efficiency. Biol. Res. 53, 47. doi: 10.1186/s40659-020-00312-4
Andrews, M., Condron, L. M., Kemp, P. D., Topping, J. F., Lindsey, K., Hodge, S., et al. (2019). Elevated CO2 effects on nitrogen assimilation and growth of C3 vascular plants are similar regardless of N-form assimilated. J. Exp. Bot. 70, 683–690. doi: 10.1093/jxb/ery371
Andrews, M., Condron, L. M., Kemp, P. D., Topping, J. F., Lindsey, K., Hodge, S., et al. (2020). Will rising atmospheric CO2 concentration inhibit nitrate assimilation in shoots but enhance it in roots of C3 plants? Physiol. Plant 170, 40–45. doi: 10.1111/ppl.13096
Bloom, A. J., Kasemsap, P., Rubio-Asensio, J. S. (2020). Rising atmospheric CO2 concentration inhibits nitrate assimilation in shoots but enhances it in roots of C3 plants. Physiol. Plant 168, 963–972. doi: 10.1111/ppl.13040
Bozal-Leorri, A., González-Muru, C., Marino, D., Aparicio-Tejo, P. M., Corrochano-Monsalve, M. (2021). Assessing the efficiency of dimethylpyrazole-based nitrification inhibitors under elevated CO2 conditions. Geoderma 400, 115160. doi: 10.1016/j.geoderma.2021.115160
Bozal-Leorri, A., Subbarao, G. V., Kishii, M., Urmeneta, L., Kommerell, V., Karwat, H., et al. (2022). Biological nitrification inhibitor-trait enhances nitrogen uptake by suppressing nitrifier activity and improves ammonium assimilation in two elite wheat varieties. Front. Plant Sci. 13, 1034219. doi: 10.3389/fpls.2022.1034219
Britto, D. T., Kronzucker, H. J. (2002). Review NH4+ toxicity in higher plants: a critical review I. J. Plant Physiol. 159, 567–584. doi: 10.1078/0176-1617-0774
Calvo, O. C., Franzaring, J., Schmid, I., Müller, M., Brohon, N., Fangmeier, A. (2017). Atmospheric CO2 enrichment and drought stress modify root exudation of barley. Glob. Change Biol. 23, 1292–1304. doi: 10.1111/gcb.13503
Chai, Y. N., Schachtman, D. P. (2022). Root exudates impact plant performance under abiotic stress. Trends Plant Sci. 27, 80–91. doi: 10.1016/j.tplants.2021.08.003
Coskun, D., Britto, D. T., Kronzucker, H. J. (2016). Nutrient constraints on terrestrial carbon fixation: The role of nitrogen. J. Plant Physiol. 203, 95–109. doi: 10.1016/j.jplph.2016.05.016
Coskun, D., Britto, D. T., Shi, W., Kronzucker, H. J. (2017a). Nitrogen transformations in modern agriculture and the role of biological nitrification inhibition. Nat. Plants 3, 17074. doi: 10.1038/nplants.2017.74
Coskun, D., Britto, D. T., Shi, W., Kronzucker, H. J. (2017b). How Plant Root Exudates Shape the Nitrogen Cycle. Trends Plant Sci. 22, 661–673. doi: 10.1016/j.tplants.2017.05.004
Diao, T., Peng, Z., Niu, X., Yang, R., Ma, F., Guo, L. (2020). Changes of soil microbes related with carbon and nitrogen cycling after long-term CO2 enrichment in a typical Chinese maize field. Sustainability 12, 1250. doi: 10.3390/su12031250
Dier, M., Meinen, R., Erbs, M., Kollhorst, L., Baillie, C. K., Kaufholdt, D., et al. (2018). Effects of free air carbon dioxide enrichment (FACE) on nitrogen assimilation and growth of winter wheat under nitrate and ammonium fertilization. Global Change Biol. 24, 40–54. doi: 10.1111/gcb.13819
Dijkstra, F. A., Prior, S. A., Runion, G. B., Torbert, H. A., Tian, H., Lu, C., et al. (2012). Effects of elevated carbon dioxide and increased temperature on methane and nitrous oxide fluxes: Evidence from field experiments. Front. Ecol. Environ. 10, 520–527. doi: 10.1890/120059
FAO (2018). Climate smart agriculture: building resilience to climate change. Eds. Lipper, L., McCarthy, N., Zilberman, D., Asfaw, S., Branca, G. Springer Cham (Switzerland), 477–495. doi: 10.1007/978-3-319-61194-5
Gamage, D., Thompson, M., Sutherland, M., Hirotsu, N., Makino, A., Seneweera, S. (2018). New insights into the cellular mechanisms of plant growth at elevated atmospheric carbon dioxide concentrations. Plant Cell. Environ. 41, 1233–1246. doi: 10.1111/pce.13206
Ghatak, A., Schindler, F., Bachmann, G., Engelmeier, D., Bajaj, P., Brenner, M., et al. (2022). Root exudation of contrasting drought-stressed pearl millet genotypes conveys varying biological nitrification inhibition (BNI) activity. Biol. Fert. Soils 58, 91–306. doi: 10.1007/s00374-021-01578-w
González-Moro, M. B., González-Moro, I., de la Peña, M., Estavillo, J. M., Aparicio-Tejo, P. M., Marino, D., et al. (2021). A Multi-Species analysis defines anaplerotic enzymes and amides as metabolic markers for ammonium nutrition. Front. Plant Sci. 11, 632285. doi: 10.3389/fpls.2020.632285
Huérfano, X., Estavillo, J. M., Torralbo, F., Vega-Mas, I., González-Murua, C., Fuertes-Mendizábal, T. (2022). Dimethylpyrazole-based nitrification inhibitors have a dual role in N2O emissions mitigation in forage systems under Atlantic climate conditions. Sci. Total Environ. 807, 150670. doi: 10.1016/j.scitotenv.2021.150670
Igarashi, M., Yi, Y., Yano, K. (2021). Revisiting why plants become n deficient under elevated CO2: importance to meet n demand regardless of the fed-form. Front. Plant Sci. 12, 2377. doi: 10.3389/fpls.2021.726186
IPCC, Climate Change (2022). Impacts, Adaptation, and Vulnerability. Contribution of Working Group II to the Sixth Assessment Report of the Intergovernmental Panel on Climate Change. Eds. Pörtner, H. O., Roberts, D. C., Tignor, M., Poloczanska, E. S., Mintenbeck, K., Alegría, A., Craig, M., Langsdorf, S., Löschke, S., Möller, V., Okem, A., Rama, B. (Cambridge, United Kingdom and New York: Cambridge University Press), 3056.
Kollah, B., Singh, B., Parihar, M., Ahirwar, U., Atoliya, N., Dubey, G., et al. (2019). Elevated CO2, chlorpyrifos and biochar influence nitrification and microbial abundance in the rhizosphere of wheat cultivated in a tropical vertisol. Rhizosphere 10, 100151. doi: 10.1016/j.rhisph.2019.100151
Lassaletta, L., Billen, G., Grizzetti, B., Anglade, J., Garnier, J. (2014). 50 year trends in nitrogen use efficiency of world cropping systems: The relationship between yield and nitrogen input to cropland. Environ. Res. Lett. 9, 105011. doi: 10.1088/1748-9326/9/10/105011
Lata, J. C., Le Roux, X., Koffi, K. F., Yé, L., Srikanthasamy, T., Konaré, S., et al. (2022). The causes of the selection of biological nitrification inhibition (BNI) in relation to ecosystem functioning and a research agenda to explore them. Biol. Fert. Soils 58, 207–224. doi: 10.1007/s00374-022-01630-3
Matros, A., Amme, S., Kettig, B., Buck-Sorlin, G. H., Sonnewald, U., Mock, H. P. (2006). Growth at elevated CO2 concentrations leads to modified profiles of secondary metabolites in tobacco cv. SamsunNN and to increased resistance against infection with potato virus Y. Plant Cell Environ. 29, 126–137. doi: 10.1111/j.1365-3040.2005.01406.x
Menéndez, S., Barrena, I., Setién, I., González-Murua, C., Estavillo, J. M. (2012). Efficiency of nitrification inhibitor DMPP to reduce nitrous oxide emissions under different temperature and moisture conditions. Soil Biol. Biochem. 53, 82–89. doi: 10.1016/j.soilbio.2012.04.026
Nair, D., Abalos, D., Philippot, L., Bru, D., Mateo-Marín, N., Petersen, S. O. (2021). Soil and temperature effects on nitrification and denitrification modified N2O mitigation by 3,4-dimethylpyrazole phosphate. Soil Biol. Biochem. 157, 108224. doi: 10.1016/j.soilbio.2021.108224
Nardi, P., Laanbroek, H. J., Nicol, G. W., Renella, G., Cardinale, M., Pietramellara, G., et al. (2020). Biological nitrification inhibition in the rhizosphere: determining interactions and impact on microbially mediated processes and potential applications. FEMS Microbiol. Rev. 44, 874–908. doi: 10.1093/femsre/fuaa037
Norton, J. M., Ouyang, Y. (2019). Controls and adaptive management of nitrification in agricultural soils. Front. Microbiol. 10, 931. doi: 10.3389/fmicb.2019.01931
O’Sullivan, C. A., Fillery, I. R. P., Roper, M. M., Richards, R. A. (2016). Identification of several wheat landraces with biological nitrification inhibition capacity. Plant Soil 404, 61–74. doi: 10.1007/s11104-016-2822-4
Otaka, J., Subbarao, G. V., Ono, H., Yoshihashi, T. (2022). Biological nitrification inhibition in maize-isolation and identification of hydrophobic inhibitors from root exudates. Biol. Fertil. Soils 58, 251–264. doi: 10.1007/s00374-021-01577-x
Roosta, H. R., Schjoerring, J. K. (2008). Root carbon enrichment alleviates ammonium toxicity in cucumber plants. J. Plant Nutr. 31, 941–958. doi: 10.1080/01904160802043270
Roy, S., Mathur, P. (2021). Delineating the mechanisms of elevated CO2 mediated growth, stress tolerance and phytohormonal regulation in plants. Plant Cell Rep. 40, 1345–1365. doi: 10.1007/s00299-021-02738-w
Sadhukhan, R., Jatav, H. S., Sen, S., Sharma, L. D., Rajput, V. D., Thangjam, R., et al. (2022). “Biological nitrification inhibition for sustainable crop production,” in Plant perspectives to global climate changes: Developping climate-resilient plant. Eds. Aftab, T., Roychoudhury, A. (London. United Kingdom: Academic Press, Elsevier), 135–150.
Saud, S., Wang, D., Fahad, S. (2022). Improved nitrogen use efficiency and greenhouse gas emissions in agricultural soils as producers of biological nitrification inhibitors. Front. Plant Sci. 13, 534. doi: 10.3389/fpls.2022.854195
Setién, I., Fuertes-Mendizabal, T., González, A., Aparicio-Tejo, P. M., González-Murua, C., González-Moro, M. B., et al. (2013). High irradiance improves ammonium tolerance in wheat plants by increasing N assimilation. J. Plant Physiol. 170, 758–771. doi: 10.1016/j.jplph.2012.12.015
Subbarao, G. V., Ito, O., Sahrawat, K. L., Berry, W. L., Nakahara, K., Ishikawa, T., et al. (2006). Scope and strategies for regulation of nitrification in agricultural systems-challenges and ppportunities. CRC. Crit. Rev. Plant Sci. 25, 303–335. doi: 10.1080/07352680600794232
Subbarao, G. V., Kishii, M., Bozal-Leorri, A., Ortiz-Monasterio, I., Gao, X., Ibba, M. I., et al. (2021). Enlisting wild grass genes to combat nitrification in wheat farming: A nature-based solution, Proc. Nat. Acad. Sci. (USA) 118 (35), e2106595118. doi: 10.1073/pnas.2106595118
Subbarao, G. V., Nakahara, K., Hurtado, M. P., Ono, H., Moreta, D. E., Salcedo, A. F., et al. (2009). Evidence for biological nitrification inhibition in Brachiaria pastures. Proc. Nat. Acad. Sci. (USA) 106, 17302–17307. doi: 10.1073/pnas.0903694106
Subbarao, G. V., Nakahara, K., Ishikawa, T., Ono, H., Yoshida, M., Yoshihashi, T., et al. (2013). Biological nitrification inhibition (BNI) activity in sorghum and its characterization. Plant Soil 366, 243–259. doi: 10.1007/s11104-012-1419-9
Subbarao, G. V., Rondon, M., Ito, O., Ishikawa, T., Rao, I. M., Nakahara, K., et al. (2007a). Biological nitrification inhibition (BNI)-is it a widespread phenomenon? Plant Soil 294, 5–18. doi: 10.1007/s11104-006-9159-3
Subbarao, G. V., Searchinger, T. D. (2021). A “more ammonium solution” to mitigate nitrogen pollution and boost crop yields. Proc. Nat. Acad. Sci. (USA) 118, e2107576118.
Subbarao, G. V., Wang, H. Y., Ito, O., Nakahara, K., Berry, W. L. (2007b). NH4+ triggers the synthesis and release of biological nitrification inhibition compounds in Brachiaria humidicola roots. Plant Soil 290, 245–257. doi: 10.1007/s11104-006-9156-6
Sun, L., Lu, Y., Yu, F., Kronzucker, H. J., Shi, W. (2016). Biological nitrification inhibition by rice root exudates and its relationship with nitrogen-use efficiency. New Phytol. 212, 646e656. doi: 10.1111/nph.14057
Tanaka, J. P., Nardi, P., Wissuwa, M. (2010). Nitrification inhibition activity, a novel trait in root exudates of rice. AoB PLANTS plq014. doi: 10.1093/aobpla/plq014
Vega-Mas, I., Marino, D., Sánchez-Zabala, J., González-Murua, C., Estavillo, J. M., González-Moro, M. B. (2015). CO2 enrichment modulates ammonium nutrition in tomato adjusting carbon and nitrogen metabolism to stomatal conductance. Plant Sci. 241, 32–44. doi: 10.1016/j.plantsci.2015.09.021
Wang, X., Bai, J., Xie, T., Wang, W., Zhang, G., Yin, S., et al. (2021). Effects of biological nitrification inhibitors on nitrogen use efficiency and greenhouse gas emissions in agricultural soils: A review. Ecotoxol. Environ. Saf. 220, 112338. doi: 10.1016/j.ecoenv.2021.112338
Waqas, M. A., Li, Y., Ashraf, M. N., Ahmed, W., Wang, B., Sardar, M. F., et al. (2021). Long-term warming and elevated CO2 increase ammonia-oxidizing microbial communities and accelerate nitrification in paddy soil. Appl. Soil Ecol. 166, 104063. doi: 10.1016/j.apsoil.2021.104063
Wendeborn, S. (2020). The chemistry, biology, and modulation of ammonium nitrification in soil. Angew. Chem. Int. Ed. 59, 2182–2202. doi: 10.1002/anie.201903014
Xiong, L., Liu, X., Vinci, G., Spaccini, R., Drosos, M., Li, L., et al. (2019). Molecular changes of soil organic matter induced by root exudates in a rice paddy under CO2 enrichment and warming of canopy air. Soil Biol. Biochem. 137, 107544. doi: 10.1016/j.soilbio.2019.107544
Xu, H., Xie, H., Wu, S., Wang, Z., He, K. (2019). Effects of elevated CO2 and increased N fertilization on plant secondary metabolites and chewing insect fitness. Front. Plant Sci. 10, 739. doi: 10.3389/fpls.2019.00739
Zakir, H. A. K. M., Subbarao, G. V., Pearse, S. J., Gopalakrishnan, S., Ito, O., Ishikawa, T., et al. (2008). Detection, isolation and characterization of a root-exuded compound, methyl 3-(4-hydroxyphenyl) propionate, responsible for biological nitrification inhibition by sorghum (Sorghum bicolor). New Phytol. 180, 442–451. doi: 10.1111/j.1469-8137.2008.02576.x
Zandalinas, S. I., Balfagón, D., Gómez-Cadenas, A., Mittler, R. (2022). Plant responses to climate change: metabolic changes under combined abiotic stresses. J. Exp. Bot. 73, 3339–3354. doi: 10.1093/jxb/erac073
Zhang, X., Lu, Y., Yang, T., Kronzucker, H. J., Shi, W. (2019). Factors influencing the release of the biological nitrification inhibitor 1,9-decanediol from rice (Oryza sativa L.) roots. Plant Soil 436, 253–265. doi: 10.1007/s11104-019-03933-1
Zhang, M., Zeng, H., Afzal, M. R., Gao, X., Li, Y., Subbarao, G. V., et al. (2022). BNI-release mechanisms in plant root systems: current status of understanding. Biol. Fert. Soils 58, 225–233. doi: 10.1007/s00374-021-01568-y
Keywords: ammonium, biological nitrification inhibitor (BNI), climate change, elevated CO2, nitrification, nitrogen fertilization, nitrous oxide (N2O), sustainable agriculture
Citation: Vega-Mas I, Ascencio-Medina E, Bozal-Leorri A, González-Murua C, Marino D and González-Moro MB (2023) Will crops with biological nitrification inhibition capacity be favored under future atmospheric CO2? Front. Plant Sci. 14:1245427. doi: 10.3389/fpls.2023.1245427
Received: 23 June 2023; Accepted: 03 August 2023;
Published: 24 August 2023.
Edited by:
Xue Qiang Zhao, Chinese Academy of Sciences (CAS), ChinaReviewed by:
Jacobo Arango, International Center for Tropical Agriculture (CIAT), ColombiaYufang Lu, Chinese Academy of Sciences (CAS), China
Copyright © 2023 Vega-Mas, Ascencio-Medina, Bozal-Leorri, González-Murua, Marino and González-Moro. This is an open-access article distributed under the terms of the Creative Commons Attribution License (CC BY). The use, distribution or reproduction in other forums is permitted, provided the original author(s) and the copyright owner(s) are credited and that the original publication in this journal is cited, in accordance with accepted academic practice. No use, distribution or reproduction is permitted which does not comply with these terms.
*Correspondence: Izargi Vega-Mas, izargiaida.vega@ehu.eus; María Begoña González-Moro, mariabegona.gonzalez@ehu.eus
†ORCID: Izargi Vega-Mas, orcid.org/0000-0002-9794-7078
Estefanía Ascencio-Medina, orcid.org/0000-0003-1435-0434
Adrián Bozal-Leorri, orcid.org/0000-0001-6617-1222
Carmen González-Murua, orcid.org/0000-0003-0310-5804
Daniel Marino, orcid.org/0000-0002-8788-6646
María Begoña González-Moro, orcid.org/0000-0002-9885-8296