- 1Department Agroecology & Organic Farming, Institute of Crop Science and Resource Conservation, Rheinische Friedrich-Wilhelms-Universität Bonn, Bonn, Germany
- 2Department of Nutritional Crop Physiology (340h), Institute of Crop Science, University of Hohenheim, Stuttgart, Germany
In the European Union and worldwide there are a burgeoning markets for plant growth promoting microorganisms (PGPM) and other biological agents as soil improvers, bio-fertilizers, plant bio-stimulants, and biological control agents or bio-pesticides. Microbial agents have a major share in this development. The use of such products is often advertised with the promise of contributing to sustainable agricultural practices by increasing crop growth and yield and offering an alternative or substitute to decrease the dependency of agriculture on hazardeous agrochemicals. In contrast to registered microbial plant protection products, PGPM that are marketed in the EU as soil improvers or plant biostimulants, are not strictly required to have proven minimum efficacy levels under field conditions. Manufacturers only have to ensure that these products do not pose unacceptable risks to human, animal or plant health, safety or the environment. Uniform guidelines comparable to the EPPO - standards (European and Mediterranean Plant Protection Organisation) to test the efficacy in field trials are not available. This paper attempts to fill the gap. It proposes guidelines for PGPM field trial design and implementation, as well as recommendations for the type and scope of data collection and evaluation. Selected research papers from literature were evaluated to analyze, whether and to what extent the requirements are already met. The majority of the papers had a clear experimental design followed by proper data evaluation. Frequent deficiencies were the low number of tested environments and crop species, insufficient site and agronomic management description and missing data on soil humidity and temperature. Using the suggested standards is assumed to increase the expressive power of tested microbial products.
1 Introduction
Plant protection products (PPP) are subject to a demanding approval process including a testing of efficacy using EPPO standards (European and Mediterranean Plant Protection Organisation, Paris, France). Guidelines are available for a large range of specific indications, e.g. PP1/46(3) on efficacy evaluation of insecticides against wireworms (EPPO, 2023), or PP 1/002 (5) of fungicides against Phytophthora infestans on potato, foliar diseases on maize (PP 1/272 (1)), fungicides against Gaeumannomyces graminis causing take-all in cereal (PP 1/262 (1)), or criteria, as well as the experimental procedures, for determining the minimum effective dose of a plant protection product (PP 1/225(2)) (EPPO Bulletin, 2021b; EPPO, 2023).
Likewise, standards also exist for microbial plant protection products (PP1/276(1) published in EPPO Bullettin (2012), but without specific indications. Nowadays, various insecticides of microbial origin are well established on the market including entomopathogenic fungi such as Beauveria bassiana against locusts (Ranjan et al., 2021) or Metarhizium brunneum against wireworms (Brandl et al., 2017).
In addition to the market of strictly regulated microbial PPP, there is a burgeoning market for plant growth- promoting microorganisms (PGPM’s) and other plant biostimulants based on bioactive natural compounds. They are applied to plants with the aim to enhance nutrition efficiency, abiotic stress tolerance and/or crop quality traits without direct effects as fertilizers (Du Jardin, 2015; Weinmann and Neumann, 2020). As summarized by O’Callaghan et al. (2022) the use of these products is often advertised with the promise of contributing to sustainable agricultural practices by increasing crop growth and yield or reducing the demand for fertilizers and agrochemicals (e.g. Lantmännen BioAgri (2021), Agriges (2023); Corteva Agriscience (2023), Syngenta Biologicals (2023).
In Europe, the Regulation (EU) 2019/1009 (EU, 2023a), laying down rules on placing fertilizing products on the EU market, defines “plant biostimulants” as product with the function to stimulate plant nutrition processes independently of its nutrient content with the sole aim of improving one or more of the following characteristics of the plant or the plant rhizosphere: (i) nutrient use efficiency, (ii) tolerance to abiotic stress, (iii) quality traits, or (iv) availability of confined nutrients in the soil or rhizosphere.
The range of potential beneficial effects of living rhizosphere microorganisms, which implement their direct or indirect influence on plant performance by biological modes of actions, in particular those interfering with soil-plant-microbe interactions, is, however, scientifically well known to be much more multifaceted (Avis et al., 2008; Borriss, 2015; Weinmann, 2019).
Biostimulants have no effect against biotic stresses (e.g. pathogens and pests) and hence do not fall under the regulatory framework of pesticides. The list of biostimulants also includes PGPM such as N2-fixing bacteria genera (e.g. Azotobacter, Azospirillum, Rhizobium) or mycorrhiza fungi. Any PGPM marketed for crop production purposes must be registered as either PPP, biofertilizer or biostimulant and has to fulfil the corresponding specific requirements, as compiled for different categories of EU fertilizing products including microbial and non-microbial plant biostimulants in (Table 1).

Table 1 Categories of EU fertilizing products according to the REGULATION (EU) 2019/1009 and plant protection products according to the REGULATION (EC) No 1107/2009 in which plant growth promoting microorganisms (PGPM) and other biological agents for agriculture can be made available on the market.
Similar to registered microbial and other PPP according to Regulation (EC) No 1107/2009 (EU, 2023b), also PGPM marketed as EU fertilizing products should be sufficiently effective and not pose a risk to human, animal or plant health, to safety or to the environment. While the obligatory and visible indicator that a EU fertilizing product including microbial plant biostimulant fulfills the safety requirements is the CE (European conformity) marking, the REGULATION (EU) 2019/1009 does not further specify the requirements for a sufficient efficacy assessment. General principles governing the CE marking and its relationship to other markings are set out in Regulation (EC) No 765/2008. Furthermore, information regarding the intended application method(s), effects claimed for each target plant, and relevant instructions related to the efficacy of the product should be given. This includes soil management practices, chemical fertilisation, incompatibility with plant protection products, recommended spraying nozzles size, sprayer pressure and other anti-drift measures, if applicable. For microbial plant biostimulant products in addition, all intentionally added micro-organisms shall be indicated (REGULATION (EU) 2019/1009).
However, elaborated guidelines for efficacy testing of PGPM used as plant biostimulants are so far not available in a comprehensive collection of standards for agronomic field experiments. Some general principles have already been suggested, but they rather focus on methods how to justify the claims of biostimulants for later submission to the admission authorities (Ricci et al., 2019). To prove such a claim the principles also allow the exclusive testing under controlled conditions. Other than the proposal here, they are not targeted on testing the practical agronomic benefit for farmers, although they include various important aspects, also considered here.
At the same time the market for PGPM is continually growing offering a wide range of products of variable performance and often unspecified composition (Figure 1).
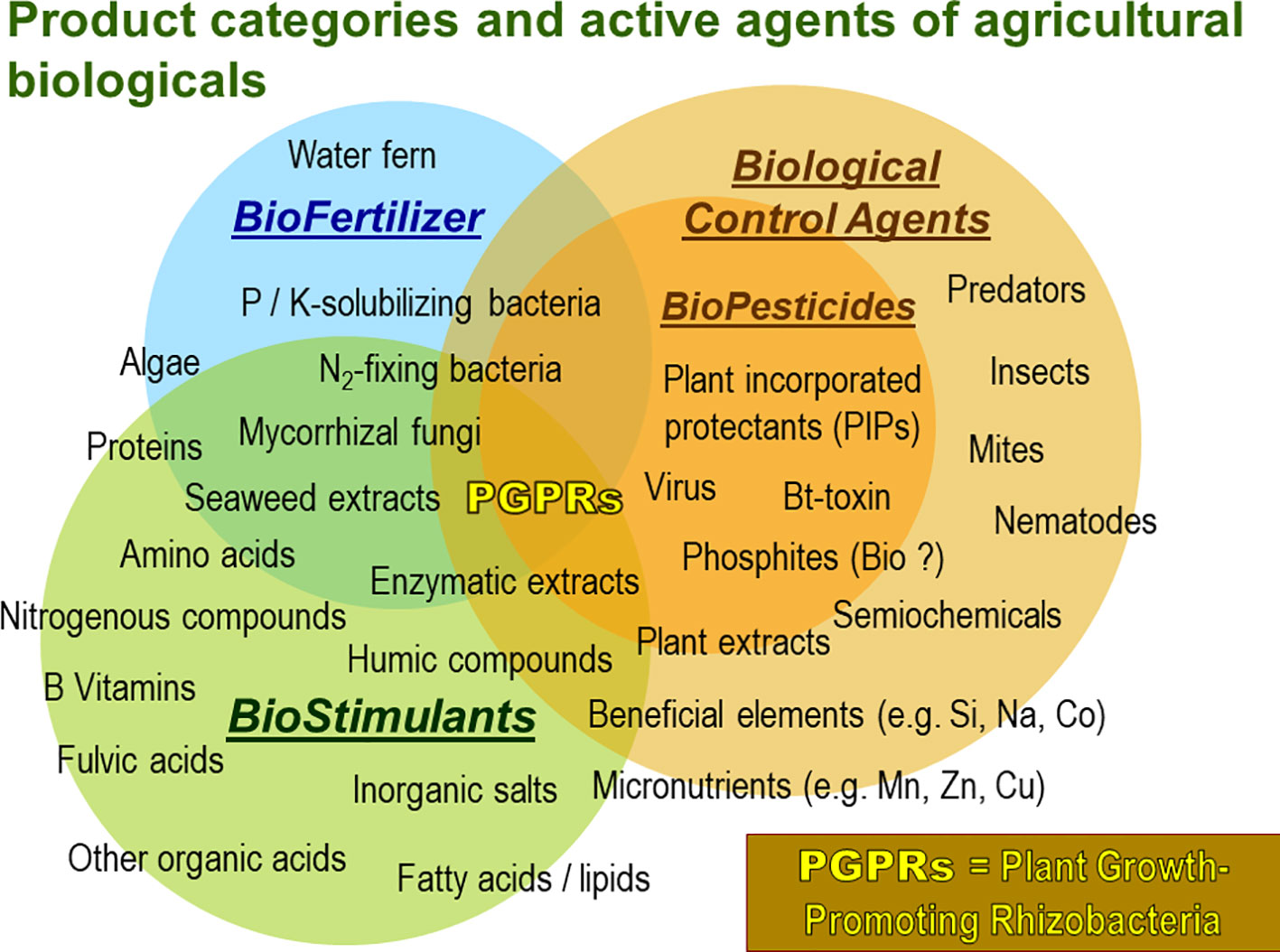
Figure 1 Product categories and respective types of active agents of agricultural biologicals for crop production. Especially microbial agents like plant growth promoting microorganisms (PGPMs) are characterized by multifaceted modes of actions. (Adapted from: Agricen Sciences’ analysis of market analysts, survey papers on Biostimulants, www.bpia.org).
The best-known example for the successful use of PGPM in crop production are rhizobia bacteria, which live in endophytic symbiosis with leguminous plants (Herridge et al., 2008; Lindström and Mousavi, 2019) and have been first patented as plant inoculants already in 1896 (Nobbe and Hiltner, 1896). Plant growth-promoting rhizobacteria (PGPR) may also live in less specific associations with plant roots, potentially resulting in growth-promoting effects on crops. In tropical and subtropical soils, for example, species of the genus Azospirillum have been shown to effectively replace N fertilizer inputs by 25-50% (Fukami et al., 2018, Santos et al., 2019). In these cases, the mode of action has been mainly linked to an improved nitrogen supply to the legume crop resulting from rhizobial atmospheric nitrogen (N2) fixation. However, a wide range of other physiological mechanisms may affect crop growth as well. According to Hett et al. (2022), the potential functions of PGPM include (i) the facilitated acquisition of water and nutrients (primarily N, P, and Fe); (ii) the modulation of phytohormonal balances by changing the levels of auxins, cytokinins, gibberellins, abscisic, jasmonic and salicylic acids, mediating, inter alia, stimulation of root growth and modifications of plant development; (iii) the release of volatile organic compounds and siderophores with functions in stress priming and nutrient mobilization and (iv) the reinforcement of resistance against abiotic stress factors (Vessey, 2003; Martínez-Viveros et al., 2010; Glick, 2012; Glick, 2014; Vejan et al., 2016 and Table 2).
Furthermore, in non-axenic soil systems, introduced PGPM interact directly (e.g. antagonistic or synergistic modes of action) or indirectly via their influence on plant physiology (e.g, alterations in phytohormonal balances) or morphology e.g. by more intensive fine root and root hair formation (Avis et al., 2008; Calvo et al., 2017; Weinmann and Neumann, 2020). A schematic illustration of the numerous facets of PGPR interferences with soil-plant-microbial interactions is illustrated in Figure 2.
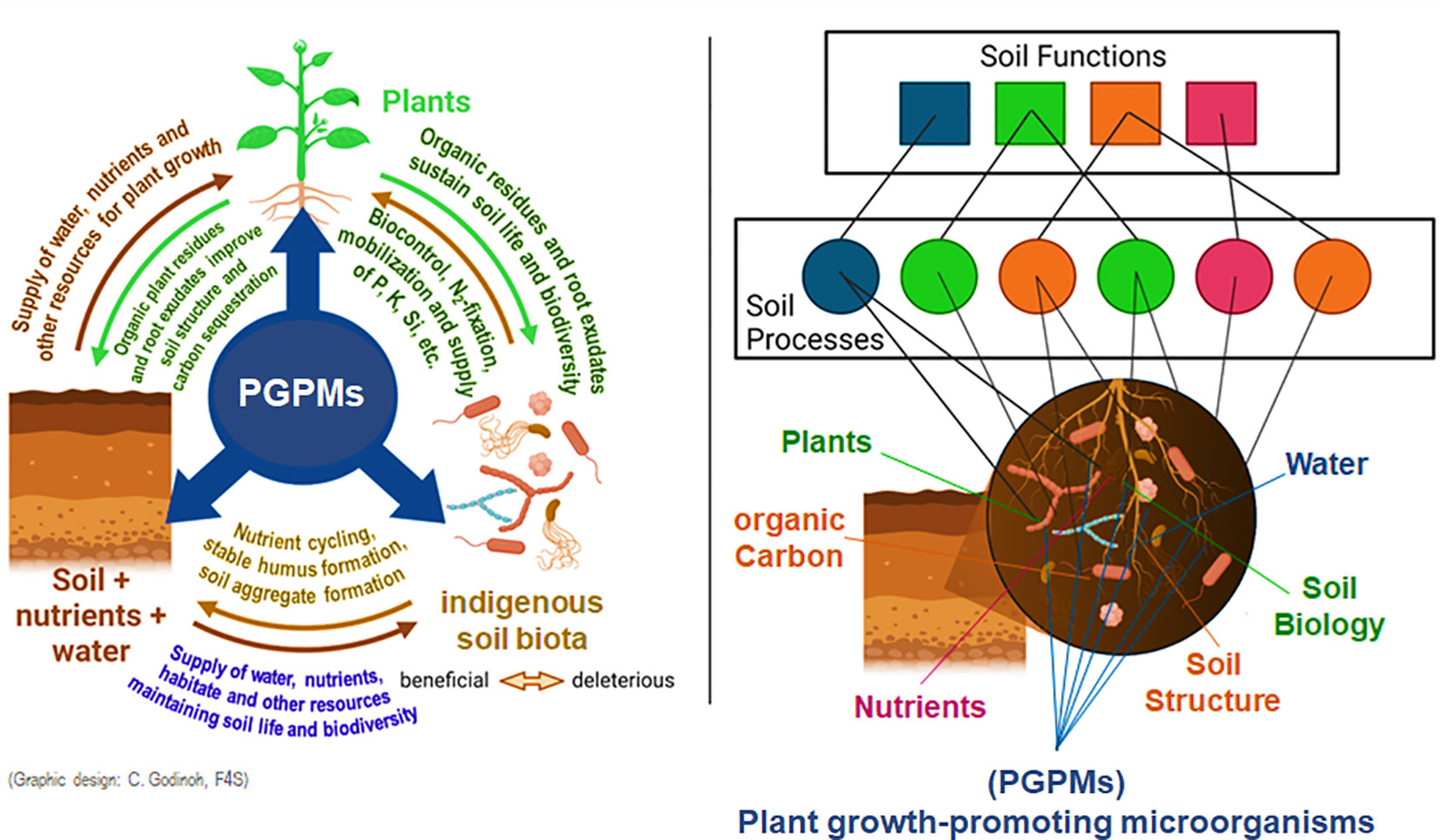
Figure 2 A better understanding of the synergies between soil, plants and soil life (Khati et al., 2020) and their impact on soil processes and ecosystem functions (Creamer et al., 2022) is of key importance for sustainable agriculture that is less dependent on the input of hazardeous agrochemicals by well-integrated PGPM applications (Weinmann and Neumann, 2020).
PGPM applications may affect various soil processes or plant physiology both expected to result in improved crop growth. However, the way from proven physiological effects of PGPM applications on plants to crop yield increases in the field is far.
Doubts on the general validity of plant growth promoting effects of microbial applications under field conditions have been raised repeatedly (Mayer et al., 2010, Meyer et al., 2019, Antoszewski et al., 2022; O’Callaghan et al., 2022). Following own experiments and published data, Hett et al. (2023) stated that there is often still no unequivocal evidence for the utility of PGPM in arable farming (Gouda et al., 2018; Santos et al., 2019; dos Santos Lopes et al., 2021).
Moreover, a so-called publication bias may reflect a certain disproportion between published results with positive effects and unpublished results with no effects. In addition, most studies have been carried out under controlled conditions in pot experiments. This type of trial is characterized by constant climatic conditions (temperature and soil humidity) and a limited soil volume. These factors significantly differ from field conditions and allow a more targeted control of environmental factors relevant for the expression of PGPM effects than field experiments. Interestingly, field experiments with missing microbial effects are rarely reported in the literature (Bashan et al., 2020), although there is increasing awareness that these results are of relevance for achieving a better understanding of the factors determining the field effectiveness of PGPM applications (Hett et al., 2023). Likewise, the number of positive reports from experiments under controlled environmental conditions is likely to provide an overly optimistic impression of the intrinsic potential effectiveness of tested agents (O’Callaghan et al., 2022). At the same time the importance of product formulations, integrated applications strategies and adapted soil and crop management strategies and other external influences for the expression of beneficial traits under practice conditions remains poorly understood (Römheld and Neumann, 2006).
Other authors, in contrast, take an optimistic view claiming that PGPM will increasingly help to make crop production more sustainable and see a great future of microbial biostimulants, despite the variable efficacy under field conditions (Santos et al., 2019; Sammauria et al., 2020; Shah et al., 2022; Singh et al., 2022). This is also reflected in the results of various forecasts on market share of biostimulants with a current global market size of approx. 3.3 Bn USD and predicted annual growth rates between 11 and 12% until 2030 (Fortune Business Insights, 2023).
In any case, a proper assessment of PGPM products under field conditions according to reproducible and comparable standards is an indispensable requirement prior to any recommendation for use in commercial farming. The validity of a study mainly depends on appropriate scientific standards including a robust experimental design (O’Callaghan et al., 2022). Here we outline a set of requirements that should be considered when testing PGPM efficacy in field trials.
2 Objectives and frame setting
Based on the challenges described above, the main objective of this paper is to propose uniform criteria for agronomic field trials suitable for scientifically testing the efficacy of PGPM based biostimulants offered for use in arable crops under temperate climate conditions. These trials are an important step prior to large-scale testing on fields with farmer equipment. Lab and pot experiments will not be considered here. These methodical tools with their controllable settings may significantly contribute to uncover distinct modes of action of a PGPM or to describe and quantify physiological processes induced by PGPM. They may also allow experimental screenings for preselection of promising microbial candidates for field testing, but they are far from being a proxy for field trials. Laboratory, pot and field experiments should carefully follow the standard rules of good experimental practice (EPPO Bulletin, 2021a). Key aspects to consider include the selection of a representative dosage suitable also for later field trials and the elimination of nutritional effects resulting from PGPM application by inclusion of appropriate controls.
A further objective of this paper is to sharpen the view of relevant stakeholders for possible sources of error, which may result in experimental artefacts and false conclusions. In contrast to EPPO standards for PPP the proposed standards are not binding for approval, but they may support producers and users to ensure a specific product quality for the benefit of all stakeholders.
In a first step we propose standards considered suitable for testing the efficacy of PGPM applications in field trials. In the subsequent discussion, we first justify the standards by underpinning them with evidence from literature. Finally, we compare the standards to the methodology described in published papers on PGPM field trials.
3 Testing the efficacy of PGPM
3.1 Field trials on efficacy
Trials need to be implemented by a skilled person with scientific aptitude. Experimenters should strictly adhere to the specifications given in the instructions with respect to crop specific mode and frequency of application, dosage and timing. They should not know treatment allocations in the field by doing a blind study. General principles of good experimental practice as outlined in EPPO guideline PP 1/181 [5] ,Conduct and reporting of efficacy evaluation trials including good experimental practice’ (EPPO Bulletin, 2021a) should be considered. These guidelines relate to various aspects including staff qualifications, use of suitable equipment and facilities, protocols, modes of operation and recording of results. In general, the following specific requirements for PGPM testing are less demanding than EPPO guidelines for PPP, since in contrast to them, results are not used for official registration purposes.
3.2 Crop and site selection
Selection of crop species as test plants should stick to the indications listed in the instructions. To rule out genotype specific treatment effects several cultivars may be tested. In general, a standard cultivar recommended for the region and already tested in published trials with other PGPM should be used. For specific indications, e.g. strengthening of plant vigor and stress tolerance, claim related choices including cultivars with known genotypic differences in stress resilience may be considered. The seed should be certified and not chemically treated unless otherwise specified. Crops should be grown on experimental plots with fairly homogenous distribution of soil properties and a known history for crop rotation and management to be performed as far as possible under ceteris paribus conditions. Field history refers to at least the last two preceding crops of the crop rotation including their management such as soil tillage, fertilization and crop protection. In situations where known gradients in soil properties and topographic factors cannot be avoided, adequate experimental designs like randomized block or Latin square can be used in order to statistically compensate for such limitation in site homogeneity. Experimental designs and statistical approaches for data evaluation that are intended to compensate for restrictions in randomization by mixed models containing both fixed and random effects need to be applied with care not to cover experimental artifacts. Optimally the persons performing the statistical data evaluations should be already involved in the planning and practical implementation of the field experiments to recognize early possible experimental biases that may lead to wrong statistical interpretations of the results.
Furthermore, a representative number of sites with well-described properties should be selected including soils with different levels of soil fertility, texture, pH, and levels of organic carbon (TOC). Selecting sites with different climatic conditions will also allow a quantitative assessment of effect stability. Annual replications may partly replace spatial variation with respect to variable weather (moisture, temperature). In case of limited test site availability, a priority might be set on testing products on less favorable soils, selected to avoid a masking of potential effects like improved root growth for better acquisition of sparingly available nutrient and water, which may not be discovered on very fertile and irrigated soils. If a specific function like improved phosphorus acquisition or enhanced tolerance to salinity stress is expected from the PGPM, site selection should consider this aspect: e.g. by conducting trials on low P or saline soils.
3.3 Treatment selection and mode of application
In the simplest case only one PGPM product is tested. In that case a minimum of two treatments would be needed (treated versus untreated). In addition to a negative control (= untreated), it is advisable to further include a positive control. Most suitable is another commercial product recommended for the same purpose. To avoid interference effects, in some cases, e.g. microbial products with additives, the inclusion of an autoclaved treatment or better the blank formulation without microbes should be taken into account. If specific effects, e.g. improved nitrogen supply or nutrient efficiency, are attributed to a PGPM - product, a second experimental factor, in that case mineral nitrogen application, should be included as a positive control, ideally in staggered treatments with lower and recommended rates. This approach allows the quantification of potential nitrogen effects resulting from PGPM application and hence the calculation of the nitrogen fertilizer equivalence (Delin et al., 2012).
Experimenters should strictly stick to the instructions on the application mode (e.g. seed coating) including dosage (in kg ha-1), timing and frequency of application. In some cases, however, it may be useful to include a treatment with higher dosage than prescribed to tickle out potential effects or to identify potential phytotoxicity. A quality check of PGPM products under use should be carried out shortly before trial implementation checking the viability of the inoculum.
3.4 Experimental design and trial implementation
The design of field trials with PGPM should be as simple as possible, i.e. a fully randomized or randomized complete block design. However, lateral contamination of adjacent plots must be avoided to obtain valid results. For that purpose, an untreated buffer stripe of at least 1 m between plots is needed. The adequate distance may depend on the type of test. For example, when spore forming pathogens (e.g. powdery mildews) or PGPM (e.g. Trichoderma fungi as mycoparasite of powdery mildews) are tested, adequate distances to obtain representative results may be found in respective EPPO guidelines.
Alternatively, this can be achieved by limiting the sampling area within a plot requiring larger plot sizes. A minimum of four field replications should be implemented, if possible, even six. Important variables to be assessed are crop yield and quality. Any other effect, except crop quality, is subordinated to yield and only becomes relevant if linked to a yield increasing effect or specific application target of the tested product (e.g. crop cultivation with less or without input of agrochemicals, while maintaining plant health, crop quality, yield level). For PGPM products which are labeled as phosphorus solubilizing bacteria for instance, empirical evidence has to be provided in field trials. Likewise, higher yields may either be additive to a given amount of fertilizer input, or substitutive, by reducing the input needed for a given yield level. Otherwise, PGPM effects such as early growth promotion or increased root growth may be advantageous, e.g. for crop competition against weeds or higher water and nutrient uptake. However, it remains to be shown, whether the observed effects sustain throughout the cropping season to produce a net benefit, or will later be compensated.
Root growth promotion may be a relevant criterion, even if aboveground biomass is not affected by PGPM application. However, complete excavation of root systems in fields, is highly demanding and labor intensive and associated with high risks of losing fine root structures. For a more standardized sampling it is possible to collect a representative number of cylinder-shaped soil cores of undisturbed soil at a defined distance and depth around the plants (Helmisaari and Brunner, 2006). Soil core samples collected under field conditions are frequently used for estimating rooting densities, specific root length and biomass or fine root distribution after root washing and digital image analysis as root growth and these variables may be influenced also by PGPM inoculants.
For proper assessment of yield data, a minimum of harvested plants and hence a sufficient plot size is needed (Stockem et al., 2022). According to EPPO Standards, representative plot sizes may vary between crop species and the tested effects (e.g. testing the effectiveness in plant protection against wind carried spore forming pathogens requires larger plot sizes than testing products for improved use efficiency of phosphorus fertilizers, since phosphorus has a low mobility in soils). Specific guidelines for the conduction of efficacy trials in wheat or other cereals are given in EPPO Standard PP1/026(4) and for maize in EPPO Standard PP1/272(2). According to these, net plot sizes for wheat and other cereals should be at least 10 m2. For maize, net plot size should be at least 2 rows x 10 m in length. The gross plot should have at least one additional treated border row on each side of the plot. With respect to accurate yield assessments it is recommended to take into account national standards for official testing of new varieties. In Germany, for instance recommended minimum plot size (harvesting area) for cereals including maize, oil seed rape and pulses such as faba bean is 10 m². Crops with lower planting density require higher minimum plots sizes, e.g. 12.5 m² for potatoes and 12 m² for sugar beet (Bundessortenamt, 2000).Whenever possible the plot size should be 15 m².
Trial establishment, in general by sowing or planting of the test plant and application of the treatments to be assessed, is of crucial importance and a relevant source of error. Good agricultural practices such as weather dependent activities, thorough seed bed preparation and optimal sowing machine settings must be considered and accurately recorded in the experimental protocol. Cross contamination of seed lots need to be avoided in any case, for instance when PGPMs are applied by seed treatment. Therefore, negative controls should be sown first. To avoid contamination of non-target plots, the sowing device should be cleaned or even be sterilized, e.g. by ethanol before applying next treatments. Seeds treated with pesticides may interfere with PGPM application and should only be used if explicitly mentioned in the instructions. In many cases it may be useful to cover the trials with bird protection nets to ensure uniform crop emergence.
3.5 Crop management
Experimental conditions and management practices can have a strong influence on the expression of beneficial PGPM traits and henceforth on the results of any trial to test their effectiveness. They need to be as close as possible to practical farming conditions. In some special cases, however, also treatments not covered by the standard practice need to be included. This applies i.e. for evaluation of stress-protective effects. For evaluation of drought stress experiments it might be necessary to use rain shelters or compare plant performance under rainfed vs. irrigated condition, [even in cases, where irrigation is not commonly used] using a drought sensitive variety. In general, the ceteris paribus approach must be followed. Some management options may interfere with PGPM application. When using mechanical weed control tools, for example, carry over effects of inoculum have to be avoided. If considered relevant precautionary measures need to be taken, such as disinfection of the working tools.
Crop management may interfere with potential mechanisms of PGPM either induced directly or indirectly. Most prominent is the direct use of pesticides that may harm the applied PGPM. Unless not specified in the product instructions at least the use of fungicides should be omitted in field trials. In a two factorial design pesticide application may be included as second factor, provided that the factor level ,no application’ is included. Likewise, tillage or fertilization intensity can affect the long-term performance particularly of fungal PGPM strains applied as soil inoculants.
The experimental protocol must include all information and dates of management practices from soil tillage, sowing, fertilization, weed control, pesticide application, irrigation and harvesting.
3.6 Data collection
It is essential to generate valid and consistent data, which help to explain the overall results. Therefore, assessments and measurements need to be carried out up from the beginning preferably from the same skilled persons. Blockwise assessments may help to avoid unsystematic errors, potentially resulting from a forced interruption of measurements, e.g. by rainfall. Treatments should not be known to the data collector during assessments. Whenever possible, published and approved methods should be used. Visual ratings, e.g. on plant vigor are important, but also a possible source of bias. An internal quality check of the rating quality might be considered. An easy way to do this is a repetition of the rating in random order followed by a statistical comparison of both data sets. Name of the data collector and date of collection need to be recorded in the experimental protocol. Any management measure, e.g. irrigation, and unusual events, e.g. abiotic stress, need to be recorded as well.
3.6.1 Site (soil and climate)
Prior to the experiment soil texture should be assessed if unknown. Important soil chemical parameters include pH, soil organic matter and nutrient concentration in the topsoil (in general 0 – 30 cm). During the field trials soil temperature and moisture should be recorded in rooted soil horizon (e.g. 5 to 10 cm depth) at least during the two weeks following PGPM application. If no weather station is available on site, public data from a nearby weather station should be collected. In that case a rain gauge should additionally be installed at the trial.
3.6.2 Crop growth
The phenological stages of the crop should be recorded. Non-destructive measurements such as plant height, stem diameter and NDVI (Pettorelli, 2013) are easy to collect and may give first hints of a growth promoting effect. For destructive measurements additional area is needed. Higher plant biomass (dry matter = dm) after oven drying at 60° C and shoot nutrient accumulation in kg ha-1 (= above ground biomass in kg dm ha-1 x % NPK in shoot dm) at a given phenological stage, often the beginning of flowering, can be an indicator for the effect of a PGPM. Depending on the crop density, i.e. plants per m², representative samples sizes may vary from 0.5 to 3 m². In general, the rule should be followed that the less plants per m² are sown (e.g. maize with 10 seeds per m² vs. wheat with 350 seeds per m²) the larger the sample area should be to compensate for genotypic effects not being related to the treatments.
The occurrence of pest and diseases needs to be regularly assessed in all plots. If available, EPPO guidelines, should be used, at least for the assessment of dominant pests and diseases.
3.6.3 Crop yield and quality
Fresh and dry matter yield need to be collected from a representative (e.g. plot) area, in general a minimum of 15 m² is compulsory for a solid assessment. It is important to analyze the yield structure as well, since it may help to better explain the obtained results. In winter wheat trials, for example, the ear density, number of grains per ear and the thousand grain weight should be reported.
Price effective quality parameters should be assessed as well. For e.g. winter wheat basic quality parameters include crude protein and gluten content of the grains. The assessment of mycotoxin contamination such as DON can be considered in justified cases. Targeted quality assessments should only be carried out, when part of the product claims.
3.6.4 Rhizosphere samples
Whenever possible rhizosphere samples should be taken in regular intervals. Metagenomic tools may help to quantify PGPM induced effects on the rhizobiome and ideally trace the fate of the inoculum after application including long-term effects on the soil microbiome and functional biodiversity. This, however, is a methodical challenge requiring considerable know-how and resources, which cannot be binding for standards.
3.7 Data evaluation and presentation of results
Prior to any statistical evaluation it is essential to check data for consistency and plausibility. The standard for field trial evaluation is Analysis of Variance (ANOVA) according to Fisher after testing the normal distribution of the residues and the homogeneity of the variances with standard tests. In general, a randomized complete block (RCB) design should be sufficient to compensate for potential field heterogeneity starting with a cross site evaluation. More sophisticated experimental designs should only be selected, if justified by an experimental factor. Testing the effect of PGPM applications on drought stress, for example, might require a split plot design with rain shelter plots. Given a sufficient number of data sets an effect size may be calculated (Hedges, 1982). Tests for mean comparisons depend on the research questions. Comparing several products among each other requires multiple comparison test such as the Tukey HSD-test. This robust post-hoc test can handle unequal sample sizes and variances, and controls the probability of making a type I error. If in specific cases individual treatments shall only be compared with an untreated control, the use of the Dunnett test may be considered.
Tables and figures need to be comprehensive allowing expert readers to quickly check statistical conditions. As a standard, both absolute and relative values for e.g. yield should be indicated. When showing efficacies against diseases, the absolute incidence level at least of the negative control should be indicated. Standard errors should be routinely reported as well.
4 Discussion
The guidelines for field trial testing of PGPM’s presented here are targeted on gaining valid results for arable crops in temperate climate. Following the guidelines can contribute to gaining realistic assessments of the practical relevance of a given PGPM product. They try to consider the cause effect relationships from both perspectives, i.e. the manufacturer and the user. A zero efficacy of a given PGPM application can be due to a fake product or to mistakes during production, storage or application. Avoiding the former protects the user, while the latter is relevant for both stakeholders.
4.1 Important factors ensuring the validity of field trial results
To exclude the use of products with insufficient performance it is important to have a quality check prior to application in field trials. Rapid screening tests under controlled conditions working with seedlings and young plants have been described in the literature (Akter et al., 2013) for pre-evaluation of the basic effectiveness of a given product. Moreover, according to the harmonized EU legislation (EU) 2019/1009, future registration of biostimulants will comprise CE certification and a documented experimental proof of efficiency.
A second important aspect is the fate of the inoculum after application. However, inoculant tracing under field conditions is not a task which can be easily integrated into routine field testing of PGPM products. It requires strain-specific DNA primers or PGPM strains carrying resistance factors against certain antibiotics which are not widely available for many products.
With respect to the experimental design key challenges are the selection of appropriate controls and the setting of minimum plot sizes for yield determination. Using an autoclaved control, or better a blank formulation may help to avoid side effects. Mayer et al. (2010) concluded on their four years experiments using also autoclaved EM (effective microorganisms) that the small effects observed were not caused by the EM microorganisms but rather by the nutrient inputs derived from Bokashi. However, even the use of autoclaved PGPM controls can induce plant responses independent of nutrient effects via modifications of rhizosphere microbial communities (Nassal et al., 2018). Likewise, autoclaving does not simply affect the active microbial agent from the product, but may alter other physical and chemical product features including the release of more or degraded cell components from the microbial agents that can still have bioactive effectiveness. Marmann et al. (2014) for instance reported that the antibiotic activity of living or autoclaved bacteria on other bacteria was similar.
Therefore, appropriate controls should rather consist of blank formulations without PGPMs either provided by the manufacturers or using the filtrates of liquid or suspended product formulations after removal of microbial cells via sterile filtration.
From an agronomic point of view, it is essential to ensure an accurate quantification of the crop yield and quality effect resulting from PGPM application. Yield may be the result of PGPM effects such as e.g. improved nitrogen supply, but improved nitrogen supply does not necessarily mean higher yield. The practical use of PGPM in arable crops is only justified if a proven benefit at least compensates for the product and application costs. This can be achieved with increases in crop yield or quality that allow for respective financial return or by improved use efficiency of fertilizers or other inputs that allow for respective cost savings. Relevance here means compensation of the product and application costs by additional revenues resulting from quality or yield increases or cost savings.
4.2 Further error sources
Soil factors can promote or restrict biological activity and effectiveness of PGPM inoculants (Figure 2). Soil pH and TOC (total soil organic carbon), but also available P and N pools have been identified as major drivers determining root traits and microbial community structures in soils (Lauber et al., 2009; Francioli et al., 2016). Accordingly, a recent meta-analysis reported that responsiveness to microbial phosphorous solubilizing PGPM and AMF inoculants decreases with increasing soil organic carbon content, whereas the response to microbial N2-fixers shows an opposite trend (Schütz et al., 2018). An increment of soil organic carbon status is reported to increase as well autochthonous populations of agronomically beneficial microorganisms, and may suppress deleterious or pathogenic microorganisms, which may be positively correlated with a higher microbial diversity (Francioli et al., 2016). This might in turn hamper the establishment or functional relevance of additionally introduced PGPM inoculants due to increased competition from the native microbial community (Paul, 2016) or decrease the need to improve soil health with additional PGPM products, because the soil indigenous PGPM already fulfil this task. In soils with high TOC content, also increased concentrations of humic substances may induce stimulating effects, which is well documented for this class of compounds (Jindo et al., 2020).
Soil pH can exert a direct selective effect on certain microbial taxa (Rousk et al., 2010) or indirectly affect plant-PGPM interactions via effects on nutrient availability in soils (Kemmitt et al., 2006; Neumann and Ludewig, 2023). All these factors need to be considered in both, experimental design and site selection, ensuring ceteris paribus conditions.
Using a field with an unknown history with respect to crop rotation, fertilization and crop protection may produce inconclusive results. For example, certain agronomic practices such as applications of fungicides or glyphosate-based herbicides, intensive tillage and fertilization or crop rotations with non-mycotrophic pre-crops, can significantly inhibit the establishment and growth of mycorrhizal associations and induce harmful alterations in the soil microbiome (e.g. predomincance of phytopathogens). These processes can also interfere adversely with arbuscular mycorrhizal fungi (AMF) applied as biostimulants (Oehl et al., 2004; Oehl et al., 2005; Helander et al., 2018; Sommermann et al., 2018). However, compatibility of PGPM products with various pesticides should be usually indicated by the application instructions provided by the manufacturers.
Missing effects of PGPM application may also be due to a masking effect resulting from high nutrient availability either in pots (Hett et al., 2022) or in the field (Hett et al. 2023) and limited impact of stress factors. On the other hand, also extreme nutrient limitations or stress factors affecting root growth and activity particularly during the sensitive establishment phase of PGPM inoculants in the rhizosphere can counteract or limit the expression of beneficial effects on the host plants. Accordingly, on soils with limited nutrient availability a starter fertilization with P and N can exert a beneficial impact on the establishment of arbuscular mycorrhizal associations or the symbiosis with N2-fixing bacteria (Bittman et al., 2006; Chekanai et al., 2018). In line with these findings also a recent meta-analysis revealed the highest efficiency of bacterial inoculants supporting plant P acquisition on soils with moderate P availability, while the benefits declined at higher and lower P levels (Schütz et al., 2018). Moreover, recent literature surveys suggest that P-solubilizing microorganisms used as inoculants contribute to the P nutrition of the host plant primarily through their long-term impact on nutrient cycling via release of sequestered P from decaying microbial biomass, rather than providing P by direct nutrient solubilization in the rhizosphere (Raymond et al., 2021).
Apart from the fertilizer dosage, also the form of fertilizer supply can affect PGPM performance. Numerous studies have reported positive PGPM effects in combination with N-rich fertilizers based on animal waste products (manures, guano, meat-, hair and feather meals) inoculants such as Bacillus, Pseudomonas, and Trichoderma (Thonar et al., 2017; Mpanga et al., 2018; Bradáčová et al., 2020; Behr et al., 2023). Particularly on soils with low TOC content, the use of these fertilisers might improve the carbon supply to the fast-growing copiotrophic inoculants, alongside with a starter fertilization effect promoting rhizosphere establishment. Also, the form of nitrogen supply (nitrate vs. ammonium) can affect plant-PGPM interactions. Particularly on soils with limited P-availability, ammonium-dominated fertilization promoted the acquisition of sparingly-soluble P sources and other nutrients in combination with various fungal and bacterial PGPM inoculants based on strains of Bacillus, Paenibacillus, Pseudomonas, Trichoderma and Penicillium (Mpanga et al., 2018; Mpanga et al., 2019a; Mpanga et al., 2019b).
PGPM products should be screened for potential non-microbial compounds that may have plant growth promoting effects as well, e.g. micronutrients or other biostimulants such as seaweed extracts or humic acids frequently applied together with PGPM in combination products. However, even pure microbial products can contain formulation additives possessing a certain biostimulatory potential. This applies e.g. for protein-based additives, such as milk powder or soybean protein which may liberate bioactive peptides (Colla et al., 2015) during degradation in the rhizosphere.
4.3 Comparison of the proposed standards with methods in published research
In total, 18 research papers were selected and checked for their conformity to the suggested standards. Paper selection criteria included the testing of an arable crop in field trials preferably under temperate climatic conditions excluding experiments with Rhizobia and on salt stress. Some 22 methodical criteria were checked. The majority of the papers fulfilled important parts of the criteria including experimental design, PGPM application technique and statistical evaluation (Table 3). However, important methodological details such as information on field history and crop management, but also data on soil humidity and temperature were rarely reported. Half of the studies did not check the quality of the inoculum prior to sowing. In most cases, the number of tested environments was low (n =2) and only one crop was tested. At least some recent studies (n = 5) traced the fate of the inoculum after application (Tab 3).
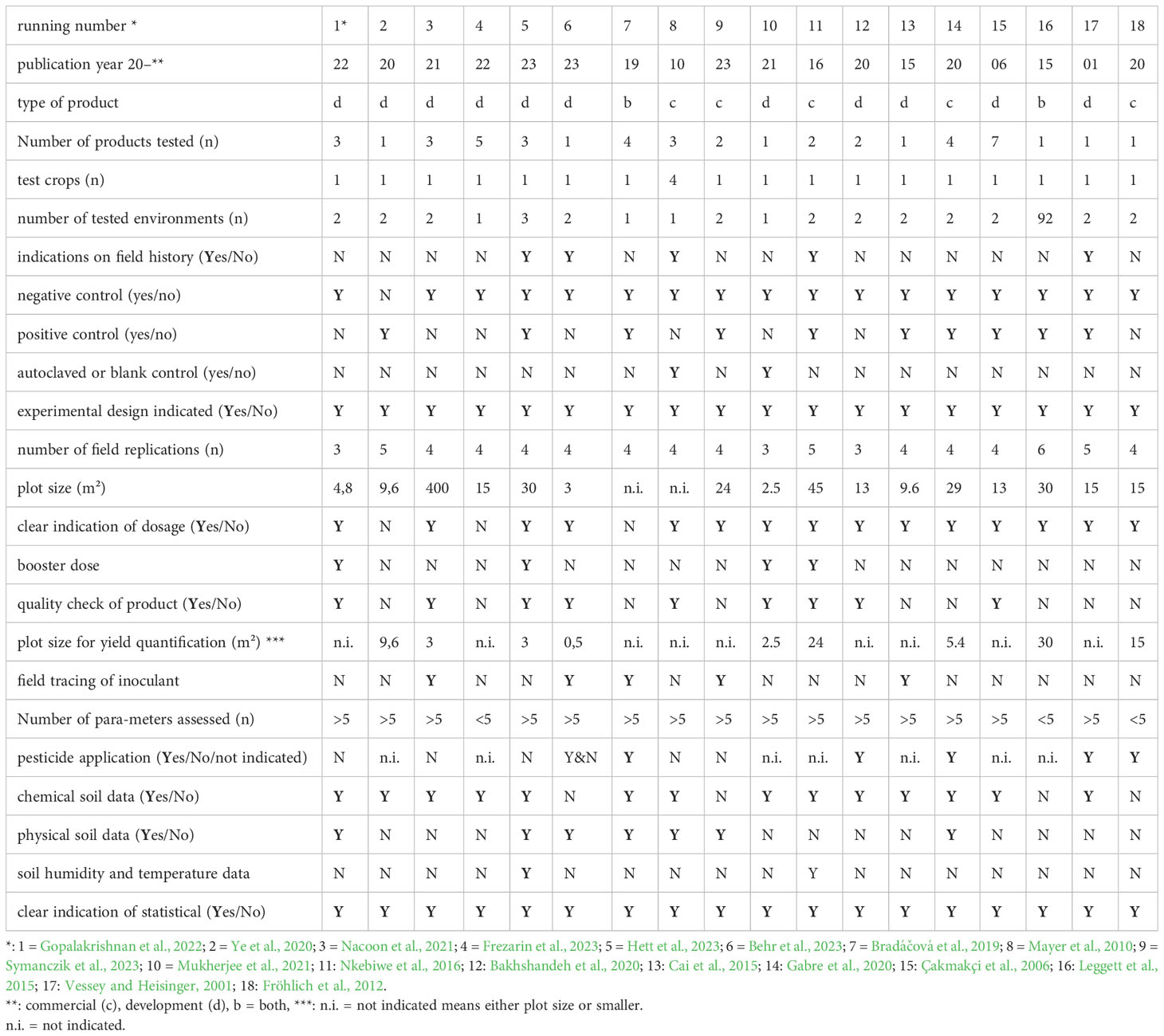
Table 3 Consistency of PGPM field trial methodology with a range of criteria based on ten published research papers.
Due to missing clarity of some of the criteria some arbitrary decisions may limit the specific validity of the evaluation. However, the rough evaluation suggests that a fulfilment of the listed criteria remains critical, even if the scientific quality of the selected papers tended to be high. Even though there are no prizes to be won for just publishing detailed data on crop yields and explaining management factors, they should be integrative part of future field research on PGPM.
Author contributions
DN: Conceptualization, Writing – original draft. GN: Methodology, Writing – review & editing. MW: Visualization, Writing – review & editing.
Funding
The author(s) declare that no financial support was received for the research, authorship, and/or publication of this article.
Acknowledgments
Valuable preparatory work for this manuscript has been carried out within the SIMBA project (Sustainable Innovation of Microbiome Applications in Food System) funded by the European Commission from 2019 to 2024 (https://simbaproject.eu/). The graphical implementation of Figure 2 was gratefully done by Cláudia P. Godinho, PhD, Food4Sustainability CoLAB, Zona Industrial, 6060-182 Idanha-a-Nova, Portugal.
Conflict of interest
The authors declare that the research was conducted in the absence of any commercial or financial relationships that could be construed as a potential conflict of interest.
The author(s) declared that they were an editorial board member of Frontiers, at the time of submission. This had no impact on the peer review process and the final decision.
Publisher’s note
All claims expressed in this article are solely those of the authors and do not necessarily represent those of their affiliated organizations, or those of the publisher, the editors and the reviewers. Any product that may be evaluated in this article, or claim that may be made by its manufacturer, is not guaranteed or endorsed by the publisher.
References
Agriges. (2023). Product catalogue Agriges Company (82035 San Salvatore Telesino (Benevento) – Italy). Available at: https://www.agriges.com/obj/catalogo_prodotti_ing-min-min.pdf (Accessed 24/04/2023).
Akter, Z., Weinmann, M., Neumann, G., Römheld, V. (2013). An in-vitro.screening method to study the activity potential of biofertilizers based on Trichoderma and Bacillus sp. J. Plant Nutr. 36, 1439–1452. doi: 10.1080/01904167.2013.793713
Antoszewski, M., Mierek-Adamska, A., Dąbrowska, G. B. (2022). The importance of microorganisms for sustainable agriculture—A review. Metabolites 12 (11), 1100. doi: 10.3390/metabo12111100
(2016). EPPO Standard PP1/272(2). Foliar diseases on maize. Bulletin OEPP/EPPO Bulletin, Vol. 46. 388–391.
Avis, T. J., Gravel, V., Antoun, H., Tweddell, R. J. (2008). Multifaceted beneficial effects of rhizosphere microorganisms on plant health and productivity. Soil Biol. Biochem. 40, 1733–1740. doi: 10.1016/j.soilbio.2008.02.013
Azeem, M., Haider, M. Z., Javed, S., Saleem, M. H., Alatawi, A. (2022). Drought stress amelioration in maize (Zea mays L.) by Inoculation of Bacillus spp. strains under sterile soil conditions. Agriculture 12, 50, 1–50,21. doi: 10.3390/agriculture12010050
Bakhshandeh, E., Pirdashti, H., Shahsavarpour Lendeh, K., Gilani, Z., Yaghoubi Khanghahi, M., Crecchio, C. (2020). Effects of plant growth promoting microorganisms inoculums on mineral nutrition, growth and productivity of rice (Oryza sativa L.). J. Plant Nutr. 43 (11), 1643–1660. doi: 10.1080/01904167.2020.1739297
Balíková, K., Vojtková, H., Duborská, E., Kim, H., Matúš, P., Urík, M. (2022). Role of exopolysaccharides of Pseudomonas in heavy metal removal and other remediation strategies. Polymers 14, 4253, 1–13. doi: 10.3390/polym14204253
Barin, M., Asadzadeh, F., Hosseini, M., Hammer, E. C., Vetukuri, R. R., Vahedi, R. (2022). Optimization of biofertilizer formulation for phosphorus solubilizing by Pseudomonas fluorescens Ur21 via Response Surface Methodology. Processes 10 650, 1–12. doi: 10.3390/pr10040650
Bashan, Y., Prabhu, S. R., De-Bashan, L. E., Kloepper, J. W. (2020). Disclosure of exact protocols of fermentation, identity of microorganisms with consortia, formation of advanced consortia with microbe-based products. Biol. Fertility Soils 56, 443–445. doi: 10.1007/s00374-020-01464-x
Basu, S., Clark, R. E., Blundell, R., Casteel, C. L., Charlton, A. M., Crowder, D. W. (2021). Reciprocal plant-mediated antagonism between a legume plant virus and soil rhizobia. Funct. Ecol. 35, 2045–2055. doi: 10.1111/1365-2435.13828
Basyal, B., Emery, S. M. (2021). An arbuscular mycorrhizal fungus alters switchgrass growth, root architecture, and cell wall chemistry across a soil moisture gradient. Mycorrhiza 31, 251–258. doi: 10.1007/s00572-020-00992-6
Behr, J. H., Kampouris, I. D., Babin, D., Sommermann, L., Francioli, D., Kuhl-Nagel, T., et al. (2023). Beneficial microbial consortium improves winter rye performance by modulating bacterial communities in the rhizosphere and enhancing plant nutrient acquisition. Front. Plant Sci. 14. doi: 10.3389/fpls.2023.1232288
Bittman, S., Kowalenko, C. G., Hunt, D. E., Forge, T. A., Wu, X. (2006). Starter phosphorus and broadcast nutrients on corn with contrasting colonization by mycorrhizae. Agron. J. 98, 394–401. doi: 10.2134/agronj2005.0093
Borriss, R. (2015). “Towards a new generation of commercial microbial disease control and plant growth promotion products,” in Principles of plant microbe interactions. Ed. Lugtenberg, B. (Switzerland: Springer International Publishing), 329–337. doi: 10.1007/978-3-319-08575-3_34
Borriss, R., Wu, H., Gao, X. (2019). “Secondary metabolites of the plant growth promoting model rhizobacterium Bacillus velezensis FZB42 are involved in direct suppression of plant pathogens and in stimulation of plant-induced systemic resistance,” in Secondary metabolites of plant growth promoting rhizomicroorganisms. Eds. Singh, H., Keswani, C., Reddy, M., Sansinenea, E., García-Estrada, C. (Singapore: Springer), 147–168. doi: 10.1007/978-981-13-5862-3_8
Bradáčová, K., Florea, A. S., Bar-Tal, A., Minz, D., Yermiyahu, U., Shawahna, R., et al. (2019). Microbial consortia versus single-strain inoculants: an advantage in PGPM-assisted tomato production? Agronomy 9 (2), 105. doi: 10.3390/agronomy9020105
Bradáčová, K., Kandeler, E., Berger, N., Ludewig, U., Neumann, G. (2020). Microbial consortia stimulate early growth of maize depending on nitrogen and phosphorus supply. Plant Soil Environ. 66 (3), 105–112. doi: 10.17221/382/2019-PSE
Brandl, M. A., Schumann, M., Przyklenk, M., Patel, A., Vidal, S. (2017). Wireworm damage reduction in potatoes with an attract-and-kill strategy using Metarhizium brunneum. J. Pest Sci. 90 (2), 479–493. doi: 10.1007/s10340-016-0824-x
Bundessortenamt. (2000). Richtlinien für die Durchführung von landwirtschaftlichen Wertprüfungen und Sortenversuchen (Hannover, Germany: Landbuch-Verlag). Available at: https://www.bundessortenamt.de/bsa/media/Files/Richtlinie_LW2000.pdf (Accessed 06 September 2023). Bundessortenamt.
Cai, F., Chen, W., Wei, Z., Pang, G., Li, R., Ran, W., et al. (2015). Colonization of Trichoderma harzianum strain SQR-T037 on tomato roots and its relationship to plant growth, nutrient availability and soil microflora. Plant Soil 388, 337–350. doi: 10.1007/s11104-014-2326-z
Çakmakçi, R., Dönmez, F., Aydın, A., Şahin, F. (2006). Growth promotion of plants by plant growth-promoting rhizobacteria under greenhouse and two different field soil conditions. Soil Biol. Biochem. 38, 1482–1487. doi: 10.1016/j.soilbio.2005.09.019
Calvo, P., Watts, D. B., Kloepper, J. W., Torbert, A. H. (2017). Effect of microbial-based inoculants on nutrient concentrations and early root morphology of corn (Zea mays). J. Plant Nutr. Soil Sci. 180, 56–70. doi: 10.1002/jpln.201500616
Chekanai, V., Chikowo, R., Vanlauwe, B. (2018). Response of common bean (Phaseolus vulgaris L.) to nitrogen, phosphorus and rhizobia inoculation across variable soils in Zimbabwe. Agr. Ecosyst. Environ. 266, 167–173. doi: 10.1016/j.agee.2018.08.010
Chen, L., Heng, J., Qin, S., Bian, K. (2018). A comprehensive understanding of the biocontrol potential of Bacillus velezensis LM2303 against Fusarium head blight. PloS One 13 (6), e0198560. doi: 10.1371/journal.pone.0198560
Chowdhury, S. P., Hartmann, A., Gao, X., Borriss, R. (2015). Biocontrol mechanism by root-associated Bacillus amyloliquefaciens FZB42–a review. Front. Microbiol. 6 (780). doi: 10.3389/fmicb.2015.00780
Colla, G., Nardi, S., Cardarelli, M., Ertani, A., Lucini, L., Canaguier, R., et al. (2015). Protein hydrolysates as biostimulants in horticulture. Scientia Hortic. 196, 2828–3838. doi: 10.1016/j.scienta.2015.08.037
Corteva Agriscience. (2023). Product information Utrisha-N. Available at: https://www.corteva.co.uk/products-and-solutions/biologicals/utrisha-n.html (Accessed 06/09/23).
Creamer, R. E., Barel, J. M., Bongiorno, G., Zwetsloot, M. J. (2022). The life of soils: Integrating the who and how of multifunctionality. Soil Biol. Biochem. 166, 108561, 1–15. doi: 10.1016/j.soilbio.2022.108561
Deka, P., Goswami, G., Das, P., Gautom, T., Chowdhury, N., Boro, R. C., et al. (2019). Bacterial exopolysaccharide promotes acid tolerance in Bacillus amyloliquefaciens and improves soil aggregation. Mol. Biol. Rep. 46, 1079–1091. doi: 10.1007/s11033-018-4566-0
Delin, S., Stenberg, B., Nyberg, A., Brohede, L. (2012). Potential methods for estimating nitrogen fertilizer value of organic residues. Soil Use Manage. 28 (3), 283–291. doi: 10.1111/j.1475-2743.2012.00417.x
Diabankana, R. G. C., Shulga, E. U., Validov, S. Z., Afordoanyi, D. M. (2022). Genetic characteristics and enzymatic activities of Bacillus velezensis KS04AU as a stable biocontrol agent against phytopathogens. Int. J. Plant Biol. 13, 201–222. doi: 10.3390/ijpb13030018
Díaz-Valle, A., López-Calleja, A. C., Alvarez-Venegas, R. (2019). Enhancement of pathogen resistance in common bean plants by inoculation with Rhizobium etli. Front. Plant Sci. 10. doi: 10.3389/fpls.2019.01317
dos Santos Lopes, M. J., Dias-Filho, M. B., Gurgel, E. S. C. (2021). Successful plant growth-promoting microbes: inoculation methods and abiotic factors. Front. Sustain. FoodSyst. 5. doi: 10.3389/fsufs.2021.606454
Du Jardin, P. (2015). Plant biostimulants: Definition, concept, main categories and regulation. Scientia Hortic. 196, 3–14. doi: 10.1016/j.scienta.2015.09.021
El-Maraghy, S. S., Tohamy, A. T., Hussein, K. A. (2020). Role of plant-growth promoting fungi (PGPF) in defensive genes expression of Triticum aestivum against wilt disease. Rhizosphere 15, 100223. doi: 10.1016/j.rhisph.2020.100223
EPPO. (2023). EOOP standards. Available at: https://www.eppo.int/RESOURCES/eppo_standards (Accessed 04 September 2023).
EPPO Bulletin. (2012). Principles of efficacy evaluation for microbial plant protection products. EPPO Bulletin, 2012) (Accessed 08 May 2023).
Eppo Bulletin. (2012). EPPO standard PP1/026(4). foliar and ear diseases on cereals. Bull. OEPP/EPPO Bull. 42, 419–425.
EPPO Bulletin. (2021a). Conduct and reporting of efficacy evaluation trials, including good experimental practice. PP 1/181 (5). EPPO Bulletin 2021. (Paris, France: EPPO), 1–13. doi: 10.1111/epp.12788
EPPO Bulletin. (2021b). Efficacy evaluation of fungicides.PP 1/002 (5) Phytophthora infestans on potato, Vol. 51. 79–82.
EU. (2023a). Regulation (EU) 2019/1009 of the European Parliament and of the Council of 5 June 2019 laying down rules on the making available on the market of EU fertilising products and amending Regulations (EC) No 1069/2009 and (EC) No 1107/2009 and repealing Regulation (EC) No 2003/2003 (Text with EEA relevance). Available at: https://eur-lex.europa.eu/legal-content/EN/TXT/?uri=celex%3A32019R1009 (Accessed 08/10/23).
EU. (2023b). Regulation (EC) No 1107/2009 of the European Parliament and of the Council of 21 October 2009 concerning the placing of plant protection products on the market and repealing Council Directives 79/117/EEC and 91/414/EEC. Available at: https://eur-lex.europa.eu/LexUriServ/LexUriServ.do?uri=OJ:L:2009:309:0001:0050:en:PDF (Accessed 08/10/23).
Fahde, S., Boughribil, S., Sijilmassi, B., Amri, A. (2023). Rhizobia: a promising source of plant growth-promoting molecules and their non-legume interactions: examining applications and mechanisms. Agriculture 13, 1279, 1–21. doi: 10.3390/agriculture13071279
Ferreira, N. S., Matos, G. F., Meneses, C. H. S. G., Reis, V. M., Rouws, J. R. C., Schwab, S., et al. (2020). Interaction of phytohormone-producing rhizobia with sugarcane mini-setts and their effect on plant development. Plant Soil 451, 221–238. doi: 10.1007/s11104-019-04388-0
Fortune Business Insights. (2023). Agricultural biologicals/biostimulants market, report ID: FBI100414. Available at: https://www.fortunebusinessinsights.com/industry-reports/biostimulants-market-100414 (Accessed 08/08/23).
Francioli, D., Schulz, E., Lentendu, G., Wubet, T., Buscot, F., Reitz, T. (2016). Mineral vs. Organic amendments: microbial community structure, activity and abundance of agriculturally relevant microbes are driven by long-term fertilization strategies. Front. Microbiol. 7. doi: 10.3389/fmicb.2016.01446
Frezarin, E. T., Santos, C. H. B., Sales, L. R., dos Santos, R. M., de Carvalho, L. A. L., Rigobelo, E. C. (2023). Promotion of peanut (Arachis hypogaea L.) growth by plant growth-promoting microorganisms. Microbiol. Res. 14 (1), 316–332. doi: 10.3390/microbiolres14010025
Fröhlich, A., Buddrus-Schiemann, K., Durner, J., Hartmann, A., von Rad, U. (2012). Response of barley to root colonization by Pseudomonas sp. DSMZ 13134 under laboratory, greenhouse, and field conditions. J. Plant Interact. 7 (1), 1–9. doi: 10.1080/17429145.2011.597002
Fukami, J., Cerezini, P., Hungria, M. (2018). Azospirillum: benefits that go far beyond biological nitrogen fixation. AMB Express 8, 73. doi: 10.1186/s13568-018-0608-1
Gabre, V. V., Venancio, W. S., Moraes, B. A., Furmam, F. D. G., Galvão, C. W., Gonçalves, D. R. P., et al. (2020). Multiple effect of different plant growth promoting microorganisms on beans (Phaseolus vulgaris L.) crop. Braz. Arch. Biol. Technol. 63, 1–9. doi: 10.1590/1678-4324-solo-2020190493
Glick, B. R. (2012). Plant growth-promoting bacteria: Mechanisms and applications. Scientifica 2012, 963401. doi: 10.6064/2012/963401
Glick, B. R. (2014). Bacteria with ACC deaminase can promote plant growth and help to feed the world. Microbiol. Res. 169, 30–39. doi: 10.1016/j.micres.2013.09.009
Gopalakrishnan, S., Srinivas, V., Chand, U., Pratyusha, S., Samineni, S. (2022). Streptomyces consortia-mediated plant growth-promotion and yield performance in chickpea. 3 Biotech. 12 (11), 318. doi: 10.1007/s13205-022-03389-8
Gouda, S., Kerry, R. G., Das, G., Paramithiotis, S., Shin, H.-S., Patra, J. K. (2018). Revitalization of plant growth promoting rhizobacteria for sustainable development in agriculture. Microbiol. Res. 206, 131–140. doi: 10.1016/j.micres.2017.08.016
Hedges, L. V. (1982). Estimation of effect size from a series of independent experiments. psychol. Bull. 92 (2), 490–499. doi: 10.1037/0033-2909.92.2.490
Helander, M., Saloniemi, I., Omacini, M., Druille, M., Salminen, J.-P., Kari Saikkonen, K. (2018). Glyphosate decreases mycorrhizal colonization and affects plant-soil feedback. Sci. Total Environ. 642, 285–291. doi: 10.1016/j.scitotenv.2018.05.377
Helmisaari, H. S., Brunner, I. (2006). Root growth and morphology. In Luster, J., Finlay, R. eds. Handbook of methods used in rhizosphere research. (Birmensdorf, Switzerland: Swiss Federal Institute for Forest, Snow, and Landscape Research), pp. 16–24.
Herridge, D. F., Peoples, M. B., Boddey, R. M. (2008). Global inputs of biological nitrogen fixation in agricultural systems. Plant Soil 311, 1–18. doi: 10.1007/s11104-008-9668-3
Hett, J., Neuhoff, D., Döring, T. F., Masoero, G., Ercole, E., Bevivino, A. (2022). Effects of multi-species microbial inoculants on early wheat growth and litterbag microbial activity. Agronomy 12 (4), 899. doi: 10.3390/agronomy12040899
Hett, J., Döring, T. F., Bevivino, A., Neuhoff, D. (2023). Impact of microbial consortia on organic maize in a temperate climate, varies with environment but not with fertilization. Eur. J. Agron. 144, 126743. doi: 10.1016/j.eja.2023.126743
Igiehon, O. N., Babalola, O. O. (2021). Rhizobium and mycorrhizal fungal species improved soybean yield under drought stress conditions. Curr. Microbiol. 78, 1615–1627. doi: 10.1007/s00284-021-02432-w
Jindo, K., Olivares, F. L., Malcher, D. J. P., Sánchez-Monedero, M. A., Kempenaar, C., Canellas, L. P. (2020). From lab to field: role of humi substances under open-field and greenhouse conditions as biostimulant and biocontrol agent. Front. Plant Sci. 11. doi: 10.3389/fpls.2020.00426
Joo, J. H., Hussein, K. A. (2022). Biological control and plant growth promotion properties of volatile organic compound-producing antagonistic Trichoderma spp. Front. Plant Sci. 13, 897668. doi: 10.3389/fpls.2022.897668
Kemmitt, S. J., Wright, D., Goulding, K. W. T., Jones, D. L. (2006). pH regulation of carbon and nitrogen dynamics in two agricultural soils. Soil Biol. Biochem. 38, 898–911. doi: 10.1016/j.soilbio.2005.08.006
Khan, R. A. A., Najeeb, S., Hussain, S., Xie, B., Li, Y. (2020). Bioactive secondary metabolites from Trichoderma spp. Against Phytopathogenic Fungi. Microorganisms 8, 817. doi: 10.3390/microorganisms8060817
Khati, P., Mishra, P. K., Parihar, M., Kumari, A., Joshi, S., Bisht, J. K., et al. (2020). “Potassium solubilization and mobilization: functional impact on plant growth for sustainable agriculture,” in Advances in plant microbiome and sustainable agriculture. Functional annotation and future challenges. Eds. Yadav, A. N., Rastegari, A. A., Yadav, N., Kourpp, D. (Cham, Switzerland: Springer Nature), 21–39.
Lantmännen BioAgri. (2021). Organic seed treatment. product information for cedoman®, cerall® and cedress® biological seed treatments (Uppsala, Sweden: Lantmännen BioAgri). Available at: https://www.lantmannenbioagri.com/biological-seed-treatment/ (Accessed 24 April 2023).
Latef, A. A. H. A., Hashem, A., Rasool, S., Abd_Allah, E. F., Alqawiri, A. A., Egamberdieva, D., et al. (2016). Arbuscular mycorrhizal symbiosis and abiotic stress in plants: A review. J. Plant Biol. 59, 407–426. doi: 10.1007/s12374-016-0237-7
Lauber, C. L., Hamady, M., Knight, R., Fierer, N. (2009). Pyrosequencing- based assessment of soil pH as a predictor of soil bacterial community structure at the Continental scale. Appl.Environ.Microbiol. 75, 5111–5120. doi: 10.1128/AEM.00335-09
Leggett, M., Newlands, N., Greenshields, D., West, L., Inman, S., Koivunen, M. (2015). Maize yield response to a phosphorus-solubilizing microbial inoculant in field trials. J. Agric. Sci. 153 (8), 1464–1478. doi: 10.1017/S0021859614001166
Lindström, K., Mousavi, S. A. (2019). Effectiveness of nitrogen fixation in rhizobia. Microbial. Biotechnol. 13, 1314–1335. doi: 10.1111/1751-7915.13517
Luo, L., Zhao, C., Wang, E., Raza, A., Yin, C. (2022). Bacillus amyloliquefaciens as an excellent agent for biofertilizer and biocontrol in agriculture: An overview for its mechanisms. Microbiological Res. 259, 127016, 1–10. doi: 10.1016/j.micres.2022.127016
Marmann, A., Aly, A. H., Lin, W., Wang, B., Proksch, P. (2014). Co-cultivation - A powerful emerging tool for enhancing the chemical diversity of microorganisms. Mar. Drugs 12, 1043–1065. doi: 10.3390/md12021043
Martínez-Viveros, O., Jorquera, M., Crowley, D., Gajardo, G., Mora, M. (2010). Mechanisms and practical considerations involved in plant growth promotion by rhizobacteria. J. Soil Sci. Plant Nutr. 2010, 10. doi: 10.4067/S0718-95162010000100006
Mayer, J., Scheid, S., Widmer, F., Fließbach, A., Oberholzer, H.-R. (2010). How effective are ‘Effective Microorganisms® (EM)’? Results from a field study in temperate climate. Appl. Soil Ecol. 46, 230–239. doi: 10.1016/j.apsoil.2010.08.007
Meyer, G., Maurhofer, M., Frossard, E., Gamper, H. A., Mäder, P., Mészáros, É., et al. (2019). Pseudomonas protegens CHA0 does not increase phosphorus uptake from 33P labeled synthetic hydroxyapatite by wheat grown on calcareous soil. Soil Biol. Biochem. 131, 217–228. doi: 10.1016/j.soilbio.2019.01.015
Miftakhov, A. K., Diabankana, R. G. C., Frolov, M., Yusupov, M. M., Validov, S. Z., Afordoanyi, D. M. (2023). Persistence as a constituent of a biocontrol mechanism (competition for nutrients and niches) in Pseudomonas putida PCL1760. Microorganisms 11, 19, 1–19,15. doi: 10.3390/microorganisms11010019
Miljaković, D., Marinković, J., Balešević-Tubić, S. (2020). The Significance of Bacillus spp. in disease suppression and growth promotion of field and vegetable crops. Microorganisms 8 (7), 1037, 1–19. doi: 10.3390/microorganisms8071037
Mpanga, I. K., Dapaah, H. K., Geistlinger, J., Ludewig, U., Neumann, G. (2018). : Soil type-dependent interactions of P-solubilizing microorganisms with organic and inorganic fertilizers mediate plant growth promotion in tomato. Agronomy 8, 213. doi: 10.3390/agronomy8100213
Mpanga, I. K., Gomez-Genao, N. J., Moradtalab, N., Wanke, D., Chrobaczek, V., Ahmed, A., et al. (2019b). The role of N form supply for PGPM-host plant interactions in maize. J. Plant Nutr. Soil Sci. 182 (6), 908–920. doi: 10.1002/jpln.201900133
Mpanga, I. A., Nkebiwe, P. M., Kuhlmann, K., Cozzolino, V., Piccolo, A., Geistlinger, G., et al. (2019a). The form of N supply determines plant growth promotion by P-solubilizing microorganisms in maize. Microorganisms 7 (2), 38. doi: 10.3390/microorganisms7020038
Mukherjee, A., Gaurav, A. K., Patel, A. K., Singh, S., Chouhan, G. K., Lepcha, A., et al. (2021). Unlocking the potential plant growth-promoting properties of chickpea (Cicer arietinum L.) seed endophytes bio-inoculants for improving soil health and crop production. Land Degradation Dev. 32 (15), 4362–4374. doi: 10.1002/ldr.4042
Nacoon, S., Jogloy, S., Riddech, N., Mongkolthanaruk, W., Ekprasert, J., Cooper, J., et al. (2021). Combination of arbuscular mycorrhizal fungi and phosphate solubilizing bacteria on growth and production of Helianthus tuberosus under field condition. Sci. Rep. 11 (1), 6501. doi: 10.1038/s41598-021-86042-3
Nadeem, S. M., Khan, M. Y., Waqas, M. R., Binyamin, R., Akhtar, S., Zahir, Z. A. (2017). “Arbuscular mycorrhizas: An overview,” in Arbuscular mycorrhizas and stress tolerance of plants. Ed. WU, Q.-S. (Singapore: Springer), 1–24.
Nanjundappa, A., Bagyaraj, D. J., Saxena, A. K., Kumar, M., Chakdar, H. (2019). Interaction between arbuscular mycorrhizal fungi and Bacillus spp. in soil enhancing growth of crop plants. Fungal Biol. Biotechnol. 6, 23, 1–23,10. doi: 10.1186/s40694-019-0086-5
Nassal, D., Spohn, M., Eltlbany, N., Jacquiod, S., Smalla, K., Marhan, S., et al. (2018). Effects of phosphorus-mobilizing bacteria on tomato growth and soil microbial activity. Plant Soil 427, 17–37. doi: 10.1007/s11104-017-3528-y
Nasslahsen, B., Prin, Y., Ferhout, H., Smouni, A., Duponnois, R. (2022). Mycorrhizae helper bacteria for managing the mycorrhizal soil infectivity. Front. Soil Sci. 2. doi: 10.3389/fsoil.2022.979246
Nazli, F., Jamil, M., Hussain, A., Hussain, T. (2020). Exopolysaccharides and indole-3-acetic acid producing Bacillus safensis strain FN13 potential candidate for phytostabilization of heavy metals. Environ. Monit Assess. 192, 738, 1–738,16. doi: 10.1007/s10661-020-08715-2
Neumann, G., Ludewig, U. (2023). “Rhizosphere chemistry in relation to plant nutrition,” in Marschner’s mineral nutrition of plants, 4th ed.. Eds. Rengel, Z., Cakmak, I., White, P. (London, United Kingdom: Academic Press, Elsevier Ltd.), 545–585.
Nkebiwe, P. M., Weinmann, M., Müller, T. (2016). Improving fertilizer-depot exploitation and maize growth by inoculation with plant growth-promoting bacteria: from lab to field. Chem. Biol. Technol. Agric. 3, 15. doi: 10.1186/s40538-016-0065-5
Nobbe, F., Hiltner, L. (1896). Inoculation of the soil for cultivating Leguminous plants, US Patent 570813 (United States Patent Office), Vol. 1896.
O’Callaghan, M., Ballard, R. A., Wright, D. (2022). Soil microbial inoculants for sustainable agriculture: Limitations and opportunities. Soil Use Manage. 38, 1340–1369. doi: 10.1111/sum.12811
Oehl, F., Sieverding, E., Ineichen, K., Ris, E. A., Boller, T., Wiemken, A. (2005). Community structure of arbuscular mycorrhizal fungi at different soil depths in extensively and intensively managed agro ecosystems. New Phytol. 165, 273–283. doi: 10.1111/j.1469-8137.2004.01235.x
Oehl, F., Sieverding, E., Mader, P., Sieverding, E., Maeder, P., Dubois, D., et al. (2004). Impact of long-term conventional and organic farming on the diversity of arbuscularmycorrhizal fungi. Oecologia 138 (4), 574–583. doi: 10.1007/s00442-003-1458-2
Paul, E. A. (2016). The nature and dynamics of soil organic matter: Plant inputs, microbial transformations, and organic matter stabilization. Soil Biol. Biochem. 98, 109–126. doi: 10.1016/j.soilbio.2016.04.001
Pettorelli, N. (2013). The normalized difference vegetation index (New York, USA: Oxford University Press), ISBN: ISBN 978-0-19-969316-0.
Poveda, J., González-Andrés, F. (2021). Bacillus as a source of phytohormones for use in agriculture. Appl. Microbiol. Biotechnol. 105 (23), 8629–8645. doi: 10.1007/s00253-021-11492-8
Ranjan, V., Sharma, M., Sur, A. (2021). Beauveria bassiana as a potent biopesticide for control of locust: A review. Res. Rep. 5, e1–e9. doi: 10.9777/rr.2020.10006
Raymond, N. S., Gómez-Muñoz, B., van der Bom, F. J., Nybroe, O., Jensen, L. S., Müller-Stöver, D. S., et al. (2021). Phosphate-solubilising microorganisms for improved crop productivity: a critical assessment. New Phytol. 229, 1268–1277. doi: 10.1111/nph.16924
Reshma, P., Naik, M. K., Aiyaz, M., Niranjana, S. R., Chennappa, G., Shaikh, S. S., et al. (2018). Induced systemic resistance by 2,4-diacetylphloroglucinol positive fluorescent Pseudomonas strains against rice sheath blight. Indian J. Exp. Biol. 56, 207–212. Available at: http://nopr.niscpr.res.in/handle/123456789/43660.
Ricci, M., Tilbury, L., Daridon, B., Sukalac, K. (2019). General principles to justify plant biostimulant claims. Front. Plant Sci. 10, 494. doi: 10.3389/fpls.2019.00494
Römheld, V., Neumann, G. (2006). “The rhizosphere: contributions of the soil-root interface to sustainable soil systems,” in Biological approaches to sustainable soil systems. Books in soils, plants, and the environment, vol. 113 . Eds. Uphoff, N., Ball, A. S., Fernandes, E., Herren, H., Husson, O., Laing, M., Palm, C., Pretty, J., Sanchez, P. (Boca Raton, USA: CRC Press, Taylor and Francis Group), 91–107.
Rousk, J., Baath, E., Brookes, P. C., Lauber, C. L., Lozupone, C., Caporaso, J. G., et al. (2010). Soil bacterial and fungal communities across a pH gradient in an arable soil. ISMEJ 4, 1340–1351. doi: 10.1038/ismej.2010.58
Saeid, A., Prochownik, E., Dobrowolska-Iwanek, J. (2018). Phosphorus solubilization by Bacillus species. Molecules 23 (11), 2897, 1–18. doi: 10.3390/molecules23112897
Sammauria, R., Kumawat, S., Kumawat, P., Singh, J., Jatwa, T. K. (2020). Microbial inoculants: potential tool for sustainability of agricultural production systems. Arch. Microbiol. 202 (4), 677–693. doi: 10.1007/s00203-019-01795-w
Sandhya, V., Ali, S. (2015). The production of exopolysaccharide by Pseudomonas putida GAP-P45 under various abiotic stress conditions and its role in soil aggregation. Microbiology 84, 512–519. doi: 10.1134/S0026261715040153
Santos, M. S., Nogueira, M. A., Hungria, M. (2019). Microbial inoculants: reviewing the past, discussing the present and previewing an outstanding future for the use of beneficial bacteria in agriculture. AMB Express 9, 205. doi: 10.1186/s13568-019-0932-0
Saxena, B., Shukla, K., Giri, B. (2017). “Arbuscular mycorrhizal fungi and tolerance of salt stress in plants,” in Arbuscular mycorrhizas and stress tolerance of plants. Ed. WU, Q.-S. (Singapore: Springer), 67–97.
Schmitz, A. M., Harrison, M. J. (2014). Signaling events during initiation of arbuscular mycorrhizal symbiosis. J. Integr. Plant Biol. 56, 250–261. doi: 10.1111/jipb.12155
Schütz, L., Gattinger, A., Meier, M., Müller, A., Boller, T., Mäder, P., et al. (2018). Improving crop yield and nutrient use efficiency via biofertilization— a global meta- analysis. Front. Plant Sci. 8. doi: 10.3389/fpls.2017.02204
Shah, A. M., Khan, I. M., Shah, T. I., Bangroo, S. A., Kirmani, N. A., Nazir, S., et al. (2022). Soil microbiome: A treasure trove for soil health sustainability under changing climate. Land 2022, 111887. doi: 10.3390/land11111887
Sibponkrung, S., Kondo, T., Tanaka, K., Tittabutr, P., Boonkerd, N., Yoshida, K.-i., et al. (2020). Co-Inoculation of Bacillus velezensis strain S141 and Bradyrhizobium strains promotes nodule growth and nitrogen fixation. Microorganisms 8 (5), 678, 1–678,24. doi: 10.3390/microorganisms8050678
Singh, P., Singh, R. K., Li, H.-B., Guo, D.-J., Sharma, A., Verma, K. K., et al. (2023). Nitrogen fixation and phytohormone stimulation of sugarcane plant through plant growth promoting diazotrophic Pseudomonas. Biotechnol. Genet. Eng. Rev. 22, 1–21. doi: 10.1080/02648725.2023.2177814
Singh, S. K., Wu, X., Shao, C., Zhang, H. (2022). Microbial enhancement of plant nutrient acquisition. Stress Biol. 2, 1–14. doi: 10.1007/s44154-021-00027-w
Sommermann, L., Geistlinger, J., Wibberg, D., Deubel, A., Zwanzig, J., Babin, D., et al. (2018). Fungal community profiles in agricultural soils of a long-term field trial under different tillage, fertilization and crop rotation conditions analysed by high-throughput ITS-amplicon sequencing. PloS One 13 (4), e0195345. doi: 10.1371/journal.pone.0195345
Soussou, S., Brunel, B., Pervent, M., van Tuinen, D., Cleyet-Marel, J.-C., Baudoin, E. (2017). Rhizobacterial Pseudomonas spp. strains harbouring acdS gene could enhance metallicolous legume lodulation in Zn/Pb/Cd mine tailings. Water Air Soil pollut. 228, 142, 1–142,15. doi: 10.1007/s11270-017-3309-5
Srivastava, P., Sahgal, M., Sharma, K., Enshasy, H. A. E., Gafur, A., Alfarraj, S., et al. (2022). Optimization and identification of siderophores produced by Pseudomonas monteilii strain MN759447 and its antagonism toward fungi associated with mortality in Dalbergia sissoo plantation forests. Front. Plant Sci. 13. doi: 10.3389/fpls.2022.984522
Stockem, J. E., Korontzis, G., Wilson, S. E., de Vries, M. E., van Eeuwijk, F. A., Struik, P. C. (2022). Optimal plot dimensions for performance testing of hybrid potato in the field. Potato Res. 65, 417–434. doi: 10.1007/s11540-021-09526-9
Symanczik, S., Lipp, C., Mäder, P., Thonar, C., Kundel, D. (2023). Limited effectiveness of selected bioeffectors combined with recycling phosphorus fertilizers for maize cultivation under Swiss farming conditions. Front. Plant Sci. 14. doi: 10.3389/fpls.2023.1239393
Syngenta Biologicals. (2023). Für die einen ist es luft. für uns nutzbarer stickstoff. product description NutribioN® (Frankfurt am Main, Germany: Syngenta Agro GmbH). Available at: https://www.syngenta.de/media/49726/download (Accessed 06 September 2023).
Tahir, H. A. S., Gu, Q., Wu, H., Raza, W., Hanif, A., Wu, L., et al. (2017). Plant growth promotion by volatile organic compounds produced by Bacillus subtilis SYST2. Front. Microbiol. 8. doi: 10.3389/fmicb.2017.00171
Tamiru, G., Muleta, D. (2018). The effect of rhizobia isolates against black root rot disease of faba bean (Vicia faba L) Caused by Fusarium solani. Open Agric. J. 12, 131–147. doi: 10.2174/1874331501812010131
Thonar, C., Lekfeldt, J. D. S., Cozzolino, V., Kundel, D., Kulhánek, M., Mosimann, C., et al. (2017). Potential of three microbial bio-effectors to promote maize growth and nutrient acquisition from alternative phosphorous fertilizers in contrasting soils. Chem. Biol. Technol. Agric. 4, 7. doi: 10.1186/s40538-017-0088-6
Vejan, P., Abdullah, R., Khadiran, T., Ismail, S., Nasrulhaq Boyce, A. (2016). Role of plant growth promoting rhizobacteria in agricultural sustainability-A review. Molecules 21, 573. doi: 10.3390/molecules21050573
Verma, M., Singh, A., Dwivedi, D. H., Kumar Arora, N. (2020). Zinc and phosphate solubilizing rhizobium radiobacter (LB2) for enhancing quality and yield of loose leaf lettuce in saline soil. Environ. Sustainability 3, 209–218. doi: 10.1007/s42398-020-00110-4
Vessey, J. K. (2003). Plant growth promoting rhizobacteria as biofertilizers. Plant Soil 255, 571–586. doi: 10.1023/A:1026037216893
Vessey, J. K., Heisinger, K. G. (2001). Effect of Penicillium bilaii inoculation and phosphorus fertilisation on root and shoot parameters of field-grown pea. Can. J. Plant Sci. 81 (3), 361–366. doi: 10.4141/P00-083
Viollet, A., Pivato, B., Mougel, C., Cleyet-Marel, J.-C., Gubry-Rangin, C., Lemanceau, P., et al. (2017). Pseudomonas fluorescens C7R12 type III secretion system impacts mycorrhization of Medicago truncatula and associated microbial communities. Mycorrhiza 27, 23–33. doi: 10.1007/s00572-016-0730-3
Weinmann, M. (2019). Bio-effectors for improved growth, nutrient acquisition and disease resistance of crops. Case studies to the development of sustainable cropping systems emphasizing soil-plant-microbial interactions. 2nd (Lörrach, Germany: Madora GmbH). Available at: http://opus.uni-hohenheim.de/volltexte/2019/1604/.
Weinmann, M., Neumann, G. (2020). “Bio-effectors to optimize the mineral nutrition of crop plants,” in Achieving sustainable crop nutrition. Ed. Rengel, Z. (Cambridge, UK: Burleigh Dodds Science Publishing Limited), 589–690.
Wu, Q.-S., Zou, Y.-N. (2017). “Arbuscular mycorrhizal fungi and tolerance of drought stress in plants,” in Arbuscular mycorrhizas and stress tolerance of plants. Ed. WU, Q.-S. (Singapore: Springer), 25–41.
Ye, L., Zhao, X., Bao, E., Li, J., Zou, Z., Cao, K. (2020). Bio-organic fertilizer with reduced rates of chemical fertilization improves soil fertility and enhances tomato yield and quality. Sci. Rep. 10 (1), 177. doi: 10.1038/s41598-019-56954-2
Zhang, Q. X., Kong, X. W., Li, S. Y., Chen, X. J., Chen Xiao, J. (2020). Antibiotics of Pseudomonas protegens FD6 are essential for biocontrol activity. Australas. Plant Pathol. 49, 307–317. doi: 10.1007/s13313-020-00696-7
Keywords: sustainable agriculture, crop yield and quality, experimental design, biostimulants, mode of action
Citation: Neuhoff D, Neumann G and Weinmann M (2024) Testing plant growth promoting microorganisms in the field - a proposal for standards. Front. Plant Sci. 14:1324665. doi: 10.3389/fpls.2023.1324665
Received: 19 October 2023; Accepted: 11 December 2023;
Published: 16 January 2024.
Edited by:
Antonio Ferrante, University of Milan, ItalyReviewed by:
Sumera Yasmin, National Institute for Biotechnology and Genetic Engineering, PakistanLuigi Lucini, Catholic University of the Sacred Heart, Italy
Copyright © 2024 Neuhoff, Neumann and Weinmann. This is an open-access article distributed under the terms of the Creative Commons Attribution License (CC BY). The use, distribution or reproduction in other forums is permitted, provided the original author(s) and the copyright owner(s) are credited and that the original publication in this journal is cited, in accordance with accepted academic practice. No use, distribution or reproduction is permitted which does not comply with these terms.
*Correspondence: Daniel Neuhoff, ZC5uZXVob2ZmQHVuaS1ib25uLmRl