- 1Tropical Silviculture and Forest Ecology, Georg-August-Universität Göttingen, Göttingen, Germany
- 2Department of Forest Management, Institut Pertanian Bogor, Bogor, Indonesia
Bamboos are grasses (Poaceae) that are widespread in tropical and subtropical regions. We aimed at exploring water use patterns of four tropical bamboo species (Bambusa vulgaris, Dendrocalamus asper, Gigantochloa atroviolacea, and G. apus) with sap flux measurement techniques. Our approach included three experimental steps: (1) a pot experiment with a comparison of thermal dissipation probes (TDPs), the stem heat balance (SHB) method and gravimetric readings using potted B. vulgaris culms, (2) an in situ calibration of TDPs with the SHB method for the four bamboo species, and (3) field monitoring of sap flux of the four bamboo species along with three tropical tree species (Gmelina arborea, Shorea leprosula, and Hevea brasiliensis) during a dry and a wet period. In the pot experiment, it was confirmed that the SHB method is well suited for bamboos but that TDPs need to be calibrated. In situ, species-specific parameters for such calibration formulas were derived. During field monitoring we found that some bamboo species reached high maximum sap flux densities. Across bamboo species, maximal sap flux density increased with decreasing culm diameter. In the diurnal course, sap flux densities in bamboos peaked much earlier than radiation and vapor pressure deficit (VPD), and also much earlier than sap flux densities in trees. There was a pronounced hysteresis between sap flux density and VPD in bamboos, which was less pronounced in trees. Three of the four bamboo species showed reduced sap flux densities at high VPD values during the dry period, which was associated with a decrease in soil moisture content. Possible roles of internal water storage, root pressure and stomatal sensitivity are discussed.
Introduction
Bamboos (Poaceae, Bambuseae) are abundant in the natural vegetation of tropical and subtropical regions. They have been used by people for millennia and are still used as food and construction materials. In addition, a large variety of bamboo usages have been developed in recent decades, for example for pulp, paper, or clothing production (International Network for Bamboo and Rattan [INBAR], 2014). The increasing economic exploitation of bamboos goes along with a considerable expansion of bamboo plantations in some regions (Chen et al., 2009; Food Agriculture Organization [FAO], 2010), which may lead to changes in ecological processes such as water use patterns (Uchimura, 1994; Komatsu et al., 2010). Some bamboo stands were reported to evaporate more water than tree-dominated forests (Komatsu et al., 2010; Ichihashi et al., 2015), but studies focusing on water use patterns of bamboos are still rare thus far (Pereira and Hosegood, 1962; Dierick et al., 2010; Komatsu et al., 2010; Kume et al., 2010; Ichihashi et al., 2015).
Water use patterns of bamboos and trees differ in several aspects. In contrast to trees, bamboos are monocotyledonous species and lack secondary growth (Zimmermann and Tomlinson, 1972). Therefore, vascular conduits of bamboo xylem have to remain functional throughout the ontogeny of a bamboo culm. Bamboos consequently have great ability to avoid cavitation (Cochard et al., 1994; Cao et al., 2012; Petit et al., 2014); root pressure mechanisms may contribute to repairing embolized conduits at night (Cao et al., 2012). Such features and structural traits of bamboos may also lead to particular water use patterns.
In general, plant water use is driven by micrometeorological factors and can be limited by soil water availability (O’Brien et al., 2004; Bovard et al., 2005; Kume et al., 2007); it is regulated by stomata opening and closing (Jarvis, 1989) and can be influenced by internal water storage mechanisms (Waring and Running, 1978; Goldstein et al., 1998; Carrasco et al., 2014). Xylem sap flux reflects these multiple factors. For some tree species, for example, hysteresis in the diurnal sap flux response to radiation and vapor pressure deficit (VPD) of the air have been reported (Goldstein et al., 1998; O’Brien et al., 2004). Sap flux measurements thus appear suitable to study the water use patterns of bamboos as well as their controlling environmental factors.
Thermal dissipation probes (TDP) are widely used to measure sap flux density (Js) in trees (Granier, 1985). Several studies suggest calibrating the method before studying new species (Lu et al., 2004; Wullschleger et al., 2011; Vandegehuchte and Steppe, 2013). To our knowledge, only two studies have applied the TDP method on bamboos so far. Both reported an underestimation of bamboo sap flux compared to stem heat balance (SHB) and reference gravimetric measurements (GM) when the TDP method was not calibrated (Dierick et al., 2010; Kume et al., 2010). In contrast, the SHB method (Sakuratani, 1981) was suggested to be well suited for sap flux measurements on bamboos (Dierick et al., 2010). Bamboo culms are hollow; hence heat loss in the form of heat storage inside culms is marginal, so that steady thermal conditions as a main assumption of the method are met (Baker and Van Bavel, 1987).
The aim of this study was to analyze water use patterns of tropical bamboo species and particularly the response of Js to the principal environmental drivers. First, we calibrated the SHB and the TDP method with reference GM in an experiment on potted culms of Bambusa vulgaris. We then measured Js in the field in four bamboo species including B. vulgaris with both the TDP and SHB method, and calibrated the TDP method with the SHB method. Herein, three factors which may influence the quality of the calibration were tested: time step of the data, formula specificity and calibration formula type. After calibration of the TDP method, we applied it to monitor Js in four bamboo and three tree species in a common garden in Bogor, Indonesia. Differences in the response of Js to fluctuations in environmental conditions were assessed. The study intends to contribute to expanding the yet limited knowledge on the eco-hydrological functioning of bamboos.
Materials and Methods
Study Sites and Species Selection
The pot calibration experiment was conducted in Guangzhou, China (23°26′13″ N, 113°12′33″E, 13 m asl). The field calibration experiment and monitoring campaign were carried out in a common garden in Bogor, Indonesia (6°33′40″ S, 106°43′27″ E, 182 m asl). Average annual temperature in Bogor is 25.6°C and annual precipitation is 3978 mm. Relatively dry conditions with consecutive rainless days can occur between June and September. During this dry period, monthly precipitation is on average 40% lower than during the wet period (230 vs. 383 mm), and the number of consecutive dry days (rainfall < 1 mm) is twice that of the wet period (8 vs. 4 days, 1989–2008, Van Den Besselaar et al., 2014). During our study period (July 2012–January 2013), differences between dry and wet period were more pronounced, i.e., 155 vs. 489 mm monthly precipitation, 14 vs. 2 consecutive dry days, and 0.29 vs. 0.39 m-3 m-3 daily soil water content. In Bogor, four bamboo species (B. vulgaris, Dendrocalamus asper, Gigantochloa atroviolacea, G. apus) with five culms per species and three tree species (Gmelina arborea, Shorea leprosula and Hevea brasiliensis, Table 1) with five stems per species were selected and their Js were monitored with the TDP method for 7 months.
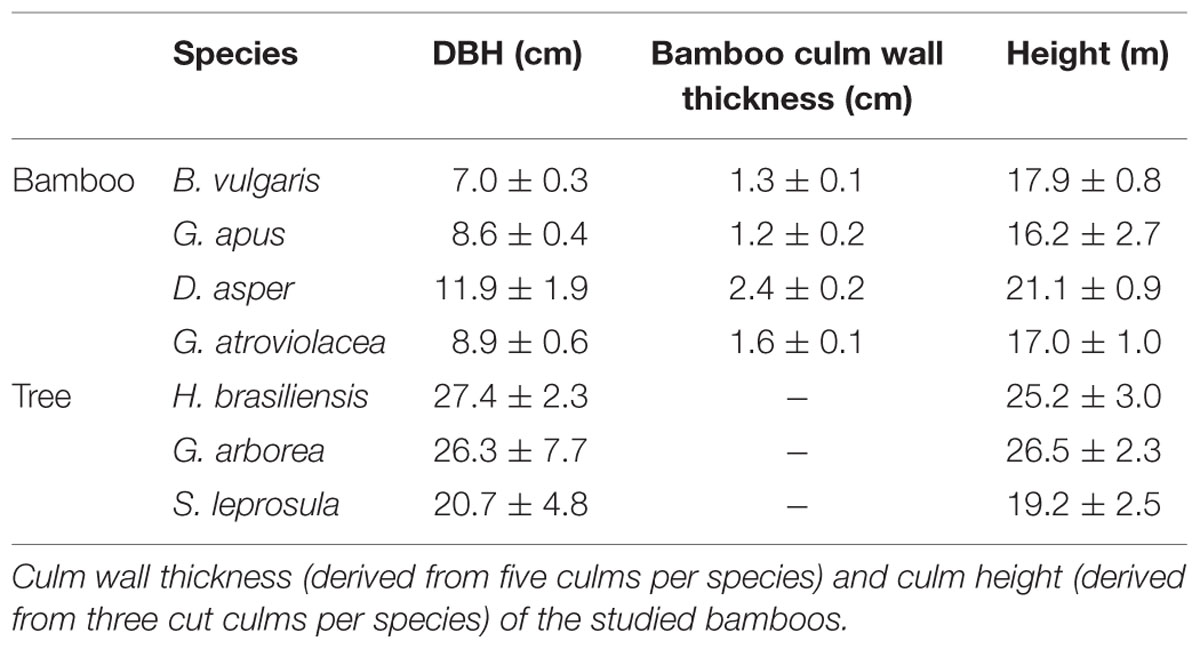
TABLE 1. Structural characteristics of the studied bamboo and tree species (n = 5 per species; mean ± SD).
TDP Construction and Installation
To measure Js in trees and bamboos, we used self-made TDP (1 and 2 cm length, respectively). In sensor design and construction, we followed Wang et al. (2012). Each TDP sensor was comprised of a heating (downstream) and a reference (upstream) probe made of steel hypodermic needles. The probes were placed 10 cm apart (vertically). For bamboos and trees, TDP installation depths in culms and stems were 1 and 2 cm, respectively. After installation, each TDP was supplied with a constant current of 120 mA; the respective power outputs of 1 and 2 cm length TDP were ∼0.1 and ∼0.2 W. TDP signals were sampled every 30 s and stored as 10-min averages for the pot calibration experiment and as 1-min averages for all other experiments by data loggers and multiplexers (CR1000, AM16/32, Campbell Scientific Inc., USA).
Calibration of the TDP Method
Pot Calibration Experiment: TDP, SHB, and GM
Five culms of B. vulgaris (diameter 5.3–7.3 cm, height 2.2–3.2 m) with trimmed canopies were transplanted into plastic bags (diameter 30 cm, height 25 cm) 6 months before the calibration experiment. One day before the experiment, the five bamboos were transplanted into bigger plastic pots (diameter 50 cm, height 65 cm). The pots were filled with cobblestones and water and were then fully sealed with plastic cover and aluminum foil to prevent evaporation of water from the pots (Figure 1A). A scaled syringe tube was attached to each pot and connected into the pot through a U-type tube. At the beginning of the experiment, the water was added into the pot through the syringe tube to a fixed level (5 cm below the pot cover). Subsequently, water was added manually every 30 min to reach the pre-defined level. The weight of the added water was determined gravimetrically (GM). To measure Js, each bamboo culm was equipped with three pairs of 1 cm length TDP which were evenly installed circumferentially, about 15 cm above the plastic cover. To minimize potential measurement errors induced by circumferential variations of Js, the thermocouple wires of the three TDP were connected in parallel to get an average voltage signal for each bamboo culm (Lu et al., 2004). For a second Js estimate, a SHB gage (SGB50 or SGA70, Dynagage Inc., USA) was installed about 1.5 m above the TDP. Both sensor types were protected by foil and the sensor signals were subsequently recorded as described in Section “TDP Construction and Installation.” For the comparison to reference GM, 10-min TDP and SHB derived values were aggregated to half-hourly values.
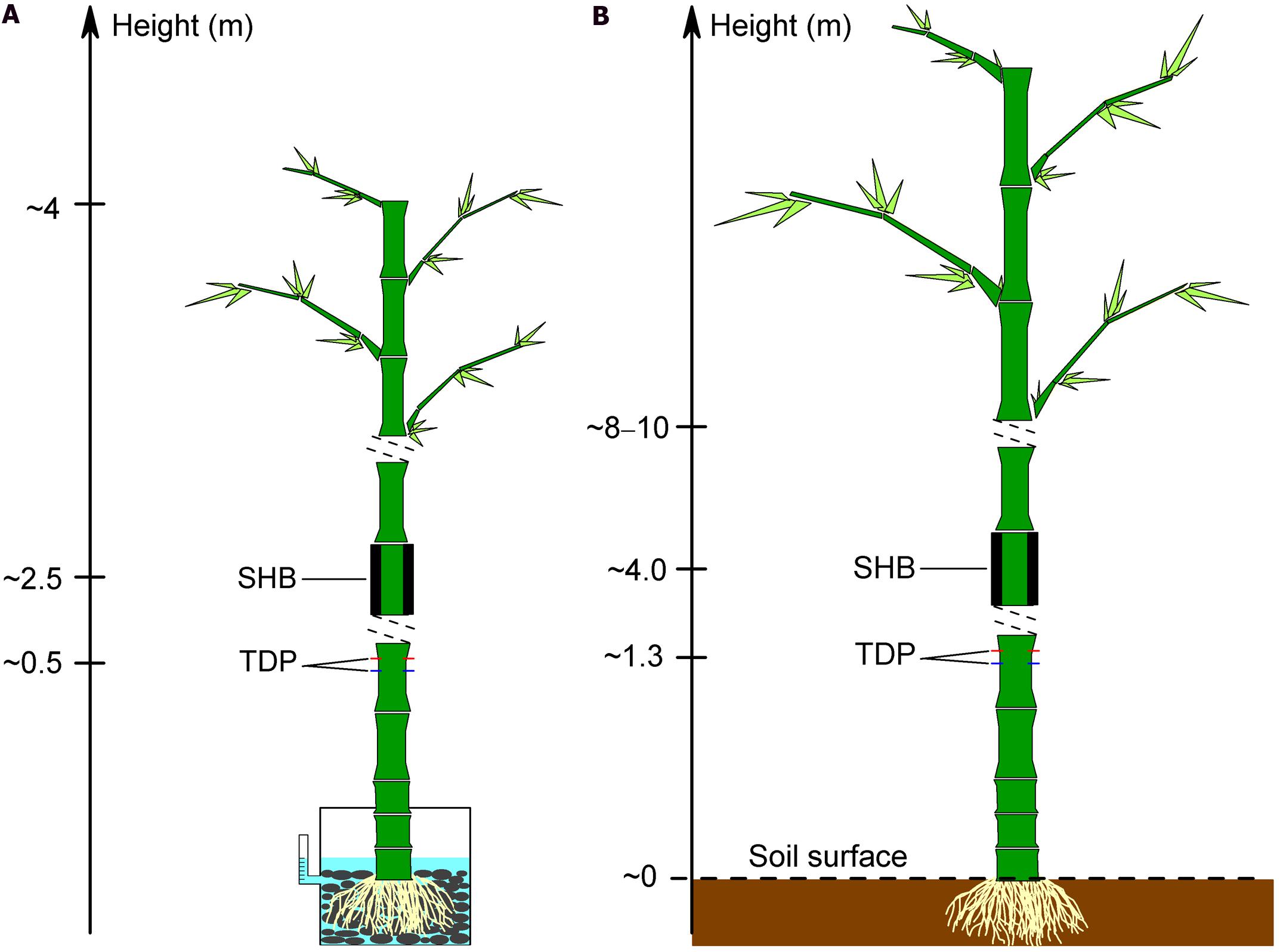
FIGURE 1. Installation of thermal dissipation probe (TDP) and stem heat balance (SHB) sensors on bamboo culms for the calibration experiments on potted plants (A) and for field calibration (B).
To assess the performance of TDP and SHB in the pot experiments, Js derived from TDP and SHB (Js_TDP and Js_SHB, respectively) on daily and 30-min scales were compared to GM derived Js (Js_GM) with paired t-tests. Additionally, the slopes of the respective linear fits between Js_TDP, Js_SHB, and Js_GM were tested for significant differences from one with the test of homogeneity of slopes. The same statistical analyses were applied again later when testing for significant differences between Js_TDP and Js_SHB in the field calibration experiments.
Field Calibration Experiment: TDP and SHB
Five culms per bamboo species (B. vulgaris, D. asper, G. atroviolacea, G. apus) were selected for TDP measurements (Table 1), three to four of which were additionally measured with SHB for a field calibration of the TDP method. TDP sensors were installed at 1.3 m height, and SHB gages (SGB50, SGA70, Dynagage Inc., USA) were installed about 2.5 m above the TDP. Simultaneous TDP-SHB measurements were conducted for a minimum of 5 days per culm (Figure 1B). Heat storage inside bamboo culms is assumed to be negligible, which was confirmed by installing thermocouple wires inside the measured segments of the respective bamboo culms to detect fluctuations in culm temperature (Dierick et al., 2010). The observed fluctuations were marginal, which meant stable thermal conditions as a requirement of the SHB method were met.
Parametrization for TDP Calibration
We derived cross-sectional water conductive areas (ATDP) from the culm wall thickness at the location of TDP sensor installation. In the pot calibration experiment, reference Js were calculated by dividing water flow rates (g h-1, GM-derived) by ATDP. In the field calibration experiment, reference Js were taken from the SHB measurements. The reference Js could subsequently be used to calibrate Js_TDP. Nighttime sap flux values were excluded in both calibration experiments.
In the field calibration, three factors were considered for obtaining a TDP calibration formula from reference (SHB) measurements: time step of the data, formula specificity and calibration formula type. To examine effects of varying time steps, the formulas were built and tested on data at varying intervals (1-, 10-, 30-, and 60-min averages, respectively). The effects of formula specificity were examined by using common (i.e., all bamboo species pooled), species-specific and culm-specific formulas, respectively. Regarding the calibration formula type, two formulas were compared: one was non-linear (Js = aKb) and generated by deriving new a and b parameters for the original Granier (1985) formula. The second was a linear formula (Js_SHB = c × Js_TDP) which was calculated from the linear relationship between Js_TDP and Js_SHB.
To obtain stable calibration formulas, pooled data sets were randomly split in half for calibration and independent validation, respectively (Niu et al., 2015). First, for each time step (1-, 10-, 30-, and 60-min, respectively), a data pool was built. Three culms of each bamboo species were randomly chosen, and for each, 3 days of data were randomly chosen from an initial common dataset. With these data pools, formula specificity was examined. For the common calibration, culms of all four species were selected for calibration. For species-specific and culm-specific calibration, only the data of the respective species or culms was selected. Next, the selected data was randomly split in half, for building the calibration formula and testing it, respectively. When testing the formula, the differences between Js_SHB and calibrated Js_TDP (Js_TDP_cali, abnormal distribution, P > 0.05) were examined with the Wilcoxon Signed-Rank Test (no significant differences at P > 0.05). The process of randomly building and testing the formula was iterated 10,000 times. Final calibration formula parameters were derived by averaging the parameters of those iterations which passed the Wilcoxon Signed-Rank Test (P > 0.05).
For an evaluation of the performance of the different formulas and the influence of the three factors (time scale, formula specificity and calibration formula type), differences in normalized Root-Mean-Square Errors (nRMSE) were assessed for each culm, species and formula factor, respectively. First, the RMSE for each day was derived with the Js_SHB and Js_TDP_cali values, and the nRMSE was calculated by normalizing the RMSE with the observed daily range of Js-SHB (difference between maximum and minimum Js_SHB). Then, the nRMSE were analyzed regarding the three formula factors (data time scale, formula specificity and calibration formula type) by ANOVA (Analysis of variance). Additionally, for each day, Js_TDP_cali with each formula type was tested for significant differences from Js_SHB with the Wilcoxon Signed-Rank Test. The rates of passing the Wilcoxon Signed-Rank Test (P > 0.05 when no significant difference between TDP and SHB derived values) were assessed for each formula.
Field Study
Monitoring Bamboo and Tree Sap Flux
Four calibrated bamboo species as well as three tree species (G. arborea, S. leprosula, and H. brasiliensis) were monitored with the TDP method for 7 months (July, 2012–January, 2013). Five bamboo culms and five tree trunks per species were selected for the measurements. On bamboos, three pairs of TDP (10 mm in length) were installed evenly around each culm at 1.3 m height and connected in parallel (see TDP Construction and Installation). On trees, two pairs of 20 mm TDP were installed in the trunk 1.3 m above the ground, in the North and South, respectively. Js for the two sensors were separately derived with the original calibration formula (Granier, 1985) and subsequently averaged to obtain values for each tree. For bamboos, Js derived with the original formula were calibrated with species-specific calibration parameters (from reference SHB field measurements) to obtain final Js values.
Environmental Measurements and Analyses
A micrometeorological station was set up in an open area. It was about 100 and 600 m away from the closer measurement sites (D. asper, G. arborea, G. atroviolacea, G. apus, S. leprosula) and farthest sites (B. vulgaris, H. brasiliensis), respectively. Air temperature (Ta, °C) and air relative humidity (RH, %) were measured with a temperature and relative humidity probe (CS215, Campbell) installed in a radiation shield. VPD (kPa) was calculated from Ta and RH. Radiation (J m-2 s-1) was measured with a pyranometer (CS300, Campbell). Data were recorded with the previously described data loggers every minute.
In addition to the mentioned micrometeorological variables, soil moisture (SM, m-3 m-3) was measured with time domain reflectometry sensors (TDR, CS616, Campbell) at 0–20 cm depth. As the clump of D. asper and the stand of G. arborea were next to each other, one TDR was positioned between them to measure soil moisture. Likewise, one sensor was used for measurements of G. atroviolacea and G. apus. One TDR each were used for the remaining species (S. leprosula, B. vulgaris, H. brasiliensis). TDR measurements ran in parallel to the sap flux field campaign and data were recorded with the described data loggers every minute.
For the day-to-day analysis of influences of fluctuations in environmental conditions (VPD, radiation, SM) on Js in the studied bamboo and tree species, daily accumulated Js (kg cm-2 d-1) were normalized by setting the highest daily observation of each species to one and the lowest to zero. For a more isolated analysis of potentially limiting influences of soil moisture on Js, we focused on ‘dry period conditions’ with consecutive rainless days, which occurred between June and September in the study area. During this period, monthly precipitation was only 32% of monthly wet period precipitation (155 vs. 489 mm), and the number of consecutive dry days (rainfall < 1 mm) was seven times higher than during the wet period (14 vs. 2 days). Dry period conditions are also characterized by higher VPD (average daily VPD > 0.74 kPa on 92% of the days). 0.74 kPa was chosen as the threshold to distinguish between dry and wet period because it constituted the mean maximum (‘turning point’) in the fitted Js response functions to VPD in three of the four studied bamboo species (except D. asper, see Figure 4B).
For the diurnal analysis of influences of fluctuations in environmental conditions on Js, time lags between Js and micrometeorological drivers (radiation and VPD) were calculated as the time difference between the respective occurrences of maximal Js (Js_max) and maximal radiation and VPD. T-tests were used to test time lags for significant differences from 0 min. 30-min Js values (average values of three selected sunny days) of each species were plotted against radiation and VPD to examine occurrences of hysteresis. The respective areas of hysteresis were compared between bamboos and trees with t-tests.
All data analyses were performed with SAS 9.3 (SAS Institute Inc., Cary, NC, USA, 2013).
Results
Calibration of the TDP Method for Bamboos
Pot Calibration Experiment: TDP, SHB, and GM
In the pot calibration experiment with B. vulgaris, SHB yielded similar absolute values of Js as GM on daily and 30-min scales (P > 0.05). The slope of the linear fit between SHB and GM on the 30-min scale was 0.98 (R2 = 0.93, P < 0.01). It did not significantly differ from 1 (P > 0.05, Figure 2A). In contrast to this, TDP estimates, with the original parameters of the calibration formula (Granier, 1985), differed substantially from GM values at both the daily (60% underestimation of accumulated Js, P < 0.01) and 30-min scale (56% underestimation, P < 0.01). The slope of the linear fit between TDP and GM on the 30-min scale was 0.44 (R2 = 0.84, P < 0.01). It was significantly different from 1 (P < 0.01, Figure 2A).
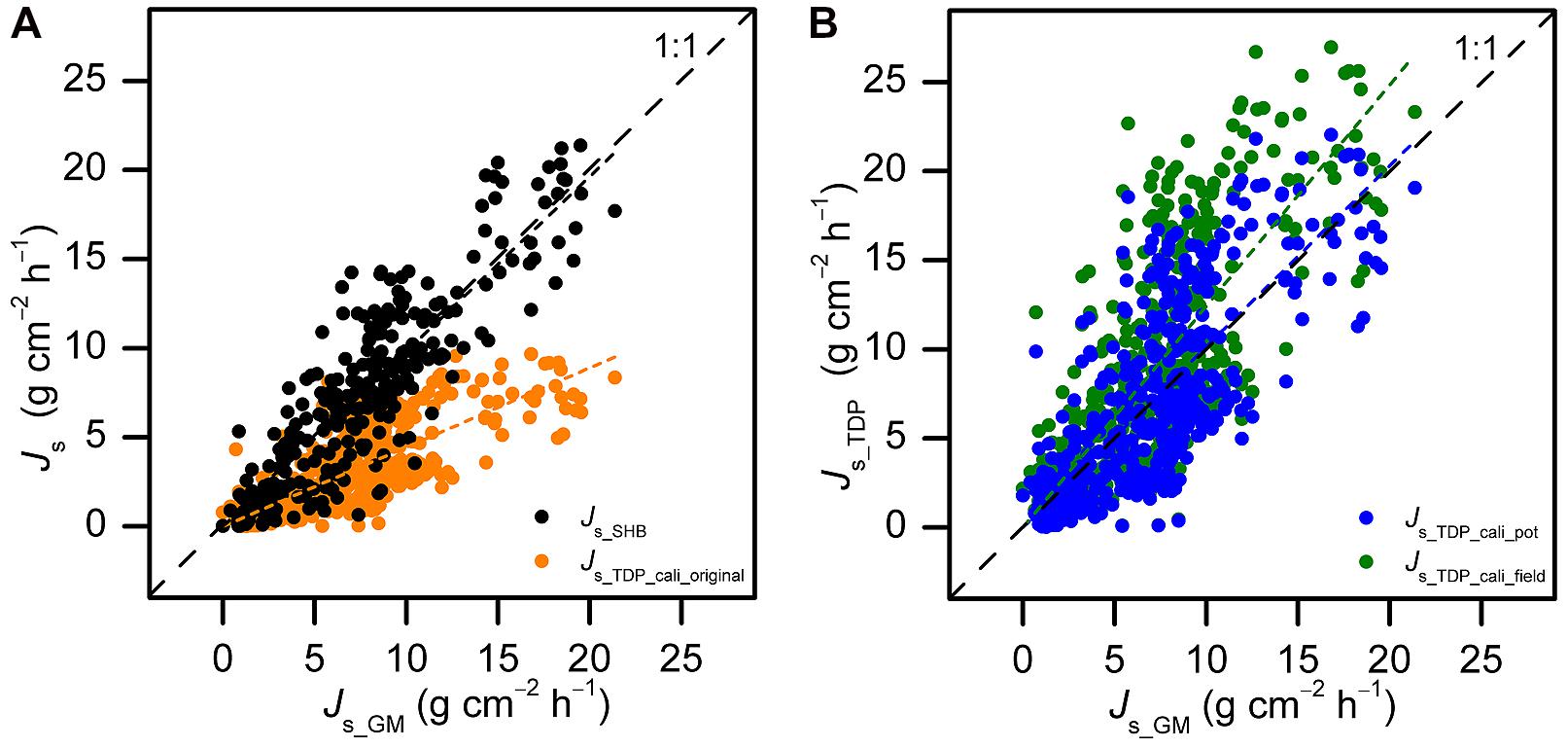
FIGURE 2. Half-hourly sap flux density (Js) measured with thermal dissipation probes (TDP) and stem heat balance (SHB) sensors on five potted Bambusa vulgaris culms plotted against GM-derived reference sap flux densities (Js_GM) before (A; Js_TDP_cali_original: Y = 0.44X, R2 = 0.84, P < 0.01; Js_SHB: Y = 0.98X, R2 = 0.93, P < 0.01) and after (B; Js_TDP_cali_field: Y = 1.24X, R2 = 0.84, P < 0.01; Js_TDP_cali_pot: Y = 1.01X, R2 = 0.84, P < 0.01) species-specific calibration and field calibrations of the TDP method. Pooled data from 2 to 5 days of simultaneous TDP, SHB, and gravimetric measurements (GM).
After applying the TDP calibration parameter for B. vulgaris derived from the pot experiment (c = 2.28), the 30-min Js_TDP were in line with those from GM. The slope was not significantly different from 1 (P > 0.05, Figure 2B). When applying the calibration parameters derived for B. vulgaris from the SHB field calibration experiment (c = 2.79), Js_TDP was 19% higher than Js-GM (P < 0.01, Figure 2B).
Field Calibration Experiment: TDP and SHB
Formula type and data time step had no significant influence on the performance of the calibration formula, but it mattered whether culm- or species-specific or a common calibration formula was used (Appendix Tables A1 and A2 Supplementary Material). Based on the nRMSE and the passing rate of the Wilcoxon test (P > 0.05) between calibrated Js_TDP and Js_SHB, culm-specific formulas performed better than species-specific and common formulas. In our study, there was no statistically significant difference between the species-specific and the common calibration parameters (Table 2, P > 0.05). For two of the four studied bamboo species (G. apus and B. vulgaris), however, using species-specific formulas slightly improved the quality of predictions as compared to applying the common formula (P = 0.06 and 0.07, respectively, Table 2). These two bamboo species had lower nRMSE and higher passing rates than D. asper and G. atroviolacea (Appendix Table A2 in Supplementary Material). The linear calibration parameters of the four bamboo species were significantly different from each other (P < 0.01). The linear calibration parameters, the slopes of Js_TDP vs. Js_SHB, were examined with the test of homogeneity of slopes and were found to differ significantly from each other (t > 0.01).
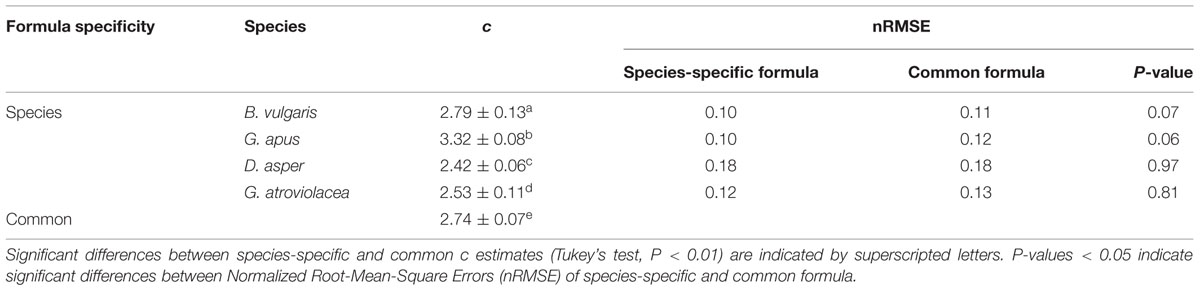
TABLE 2. Values of the parameter c of different bamboo calibrations (species-specific/common) for TDP sap flux estimates.
Before calibration, Js_TDP was on average 66 and 63% lower than SHB-derived reference values on the daily and 30-min scales, respectively (P < 0.01). This deviation was reduced to 10 and 8% underestimations (P < 0.01) when using species-specific calibration parameters (Table 2). On average, for 77 ± 6% of the days that were included in the analysis, the species-specific post-calibration 30-min Js_TDP values were not significantly different from the respective reference Js_SHB (Wilcoxon Signed-Rank test, P > 0.05).
Field Study
Monitoring Bamboo and Tree Sap Flux
Js_max in the studied bamboo species (averages from five individuals per species) were 70.5, 21.6, 49.7, and 56.2 g cm-2 h-1 for B. vulgaris, D. asper, G. apus, and G. atroviolacea, respectively. In trees, corresponding values were 17.7, 10.5, and 23.3 g cm-2 h-1 for H. brasiliensis, G. arborea, and S. leprosula, respectively. Across bamboo species, Js_max decreased with increasing culm diameter (R2 = 0.97, P = 0.02, Figure 3).
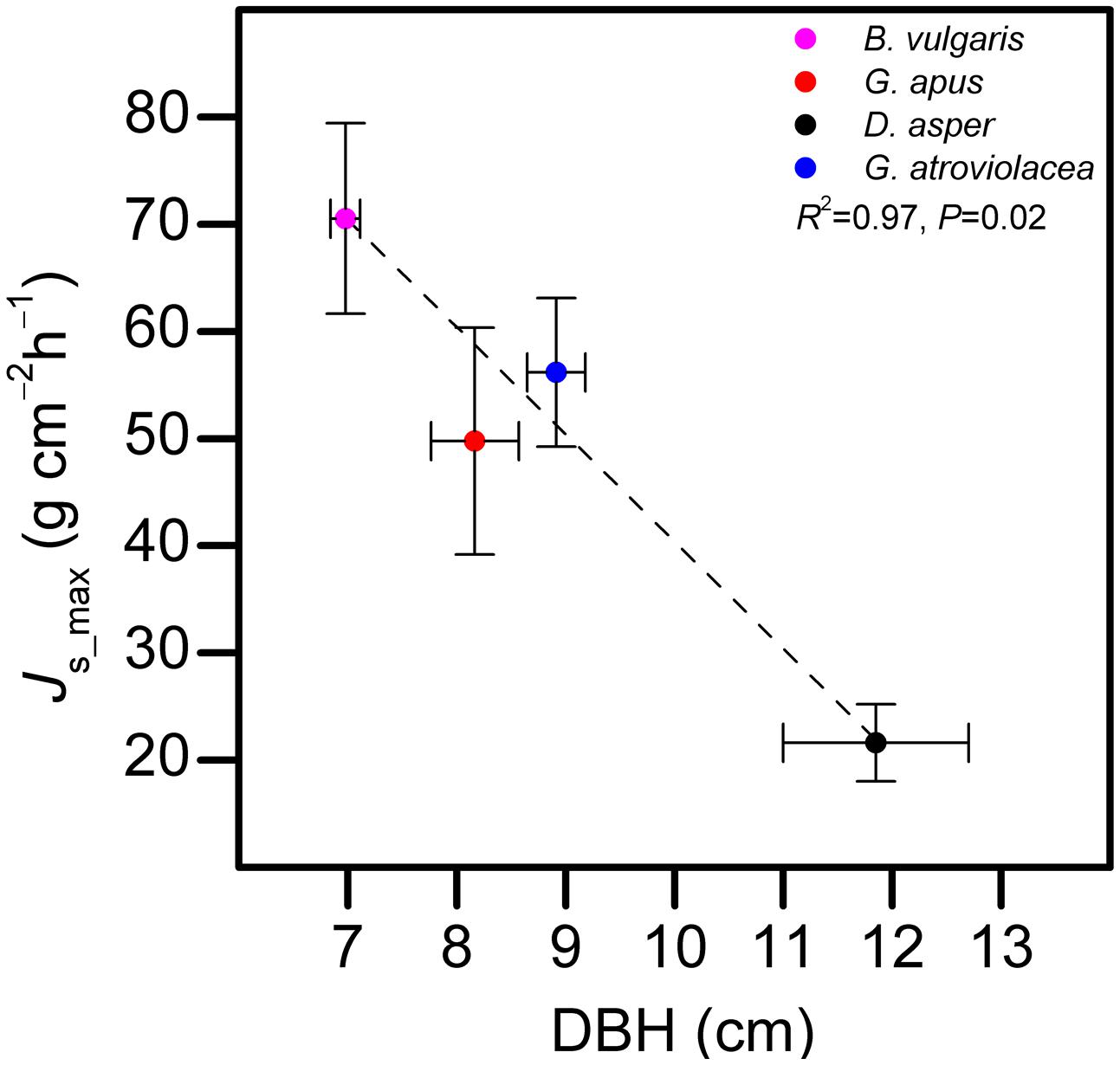
FIGURE 3. Relationship between diameter at breast height (DBH) of bamboo culms and maximum observed sap flux density (Js_max) in four bamboo species. Horizontal error bars indicate DBH standard errors, vertical bars standard errors of Js_max. Data of five culms pooled per species, average of the highest 10% of daily Js_max values of each culm used for the analysis.
Environmental Measurements and Analyses
The normalized daily accumulated Js of all studied species increased with increasing daily integrated radiation. This relationship did not fully hold up for accumulated Js and average daily VPD. In several species, daily Js increased with increasing VPD only to a certain VPD threshold (approximately 0.74 kPa, Figure 4); after this threshold, accumulated Js decreased with further increasing VPD. Such conditions of high VPD were characteristic of the dry period. For days with VPD > 0.74 kPa, daily accumulated Js of most studied species (except in D. asper and G. arborea) declined with decreasing soil moisture content (R2 = 0.39, 0.44, 0.4,0.52, and 0.55 for B. vulgaris, G. apus, G. atroviolacea, S. leprosula, and H. brasiliensis, respectively; P < 0.05, Figures 5A,B).
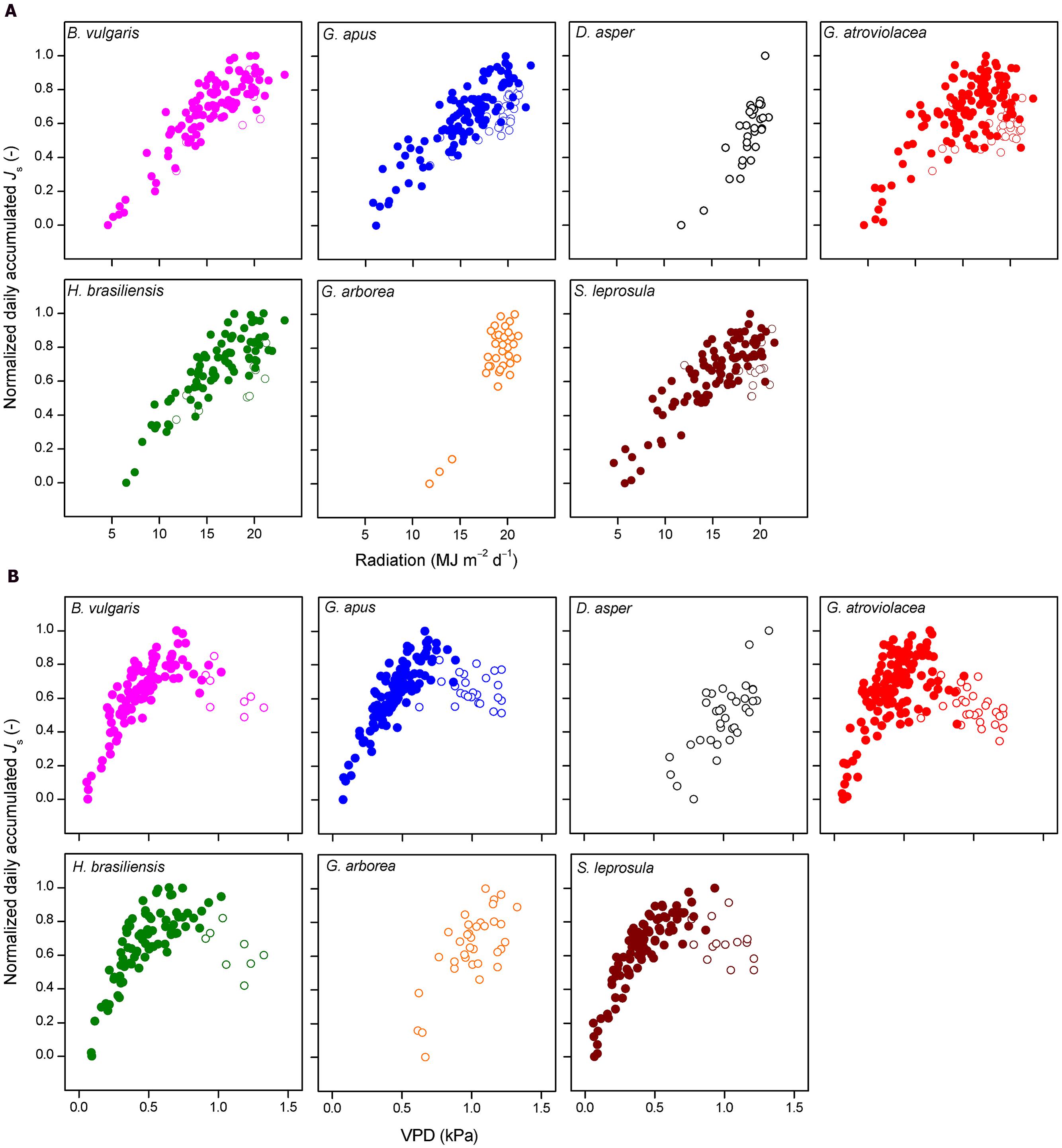
FIGURE 4. Normalized daily accumulated sap flux density (Js) plotted against absolute values of (A) integrated daily radiation and (B) average daily vapor pressure deficit (VPD). Daily values of four bamboo (upper row) and three tree species (lower row); data from 7 months of measurements (July 2012–January 2013) encompassing both wet (filled circles) and dry (open circles) periods (except for Dendrocalamus asper and Gmelina arborea, mainly dry period). Daily averages derived from measurements of five culms per species.
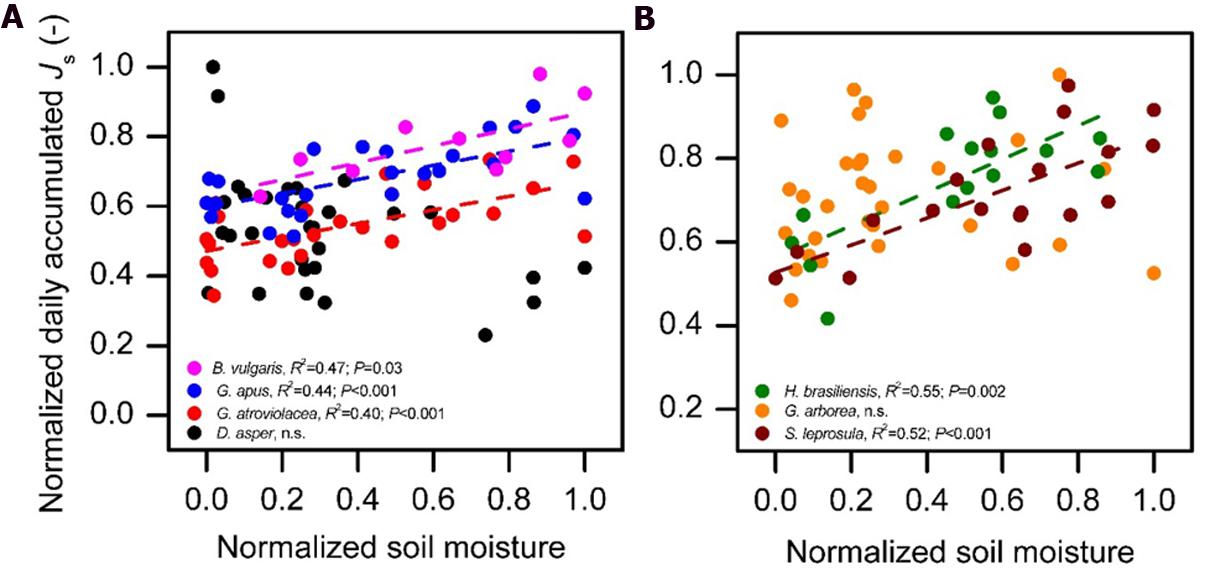
FIGURE 5. Normalized daily accumulated sap flux density (Js) of four bamboo species (A) and three tree species (B) in the ‘dry period’ (characterized with mean daily VPD > 0.74 kPa) plotted against normalized mean daily soil moisture content (SM). There was a significant linear relationship between Js and SM (P < 0.05) for all species except D. asper and G. arborea. Normalized values do not reach 1.0 for all species in the figure as the normalization was performed by setting the maximum value of the full measurement period of each species (including wet period) to one, while the figure displays only values in dry period. Daily averages derived from measurements on five culms per species, data of at least 10 dry period days per species.
Diurnal peaks in Js in the studied bamboo species occurred relatively early (on average at about 11 am), which was significantly earlier than the peaks of radiation and VPD (20–82 and 131–206 min, respectively). In the studied tree species, maximal hourly Js values were observed after the peak of radiation (3–97 min), but still before (51–108 min) VPD peaked. All time lags were significantly different from 0 min (P < 0.01; Table 3), except for the time lag to radiation for the tree species S. leprosula (P > 0.05).
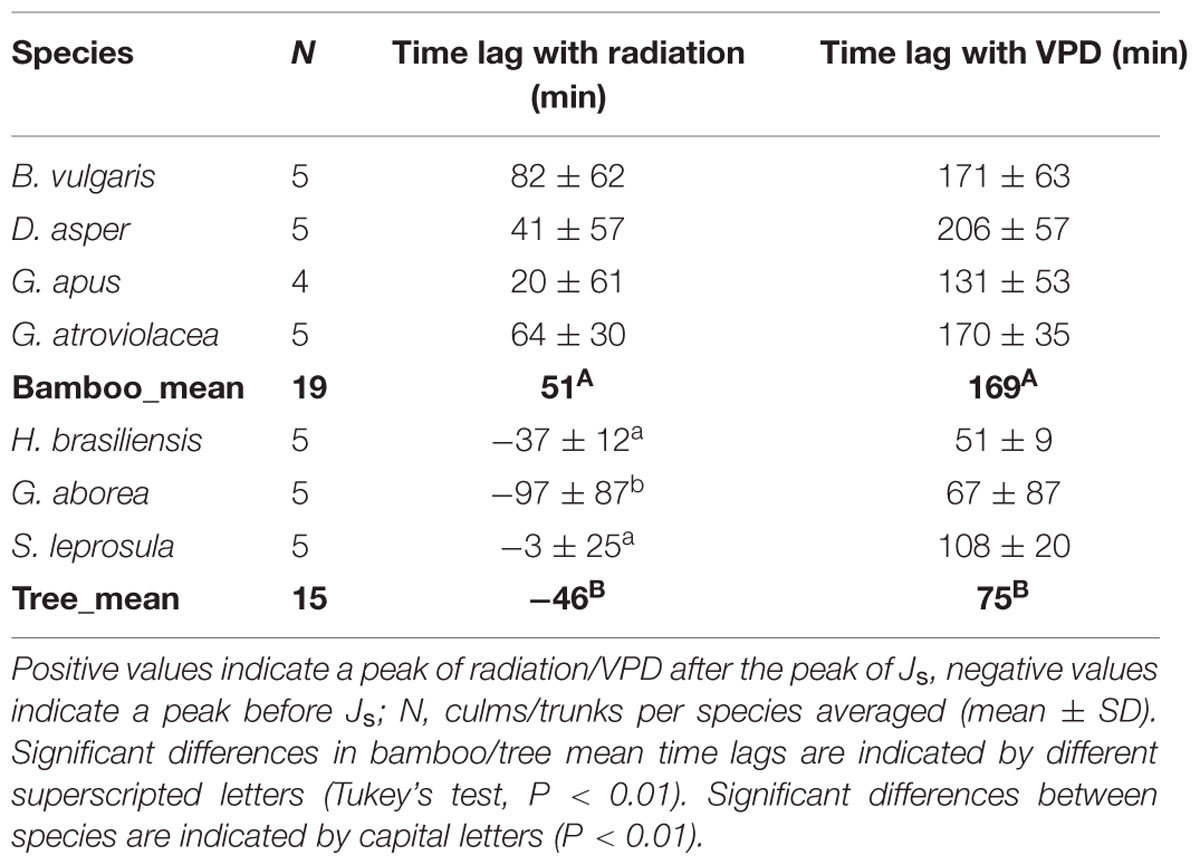
TABLE 3. Time lags between diurnal peaks of radiation and VPD and peaks of Js in studied bamboos and trees.
Diurnally, some of the studied species showed pronounced hysteresis of hourly Js to radiation and VPD. Direction of rotation (i.e., order of observations) was counter-clockwise for radiation (Figure 6A) and clockwise for VPD (Figure 6B). The area of the hysteresis to VPD was on average 32% larger in bamboos than in trees, while the area of hysteresis to radiation was on average 50% smaller in bamboos (P < 0.01).
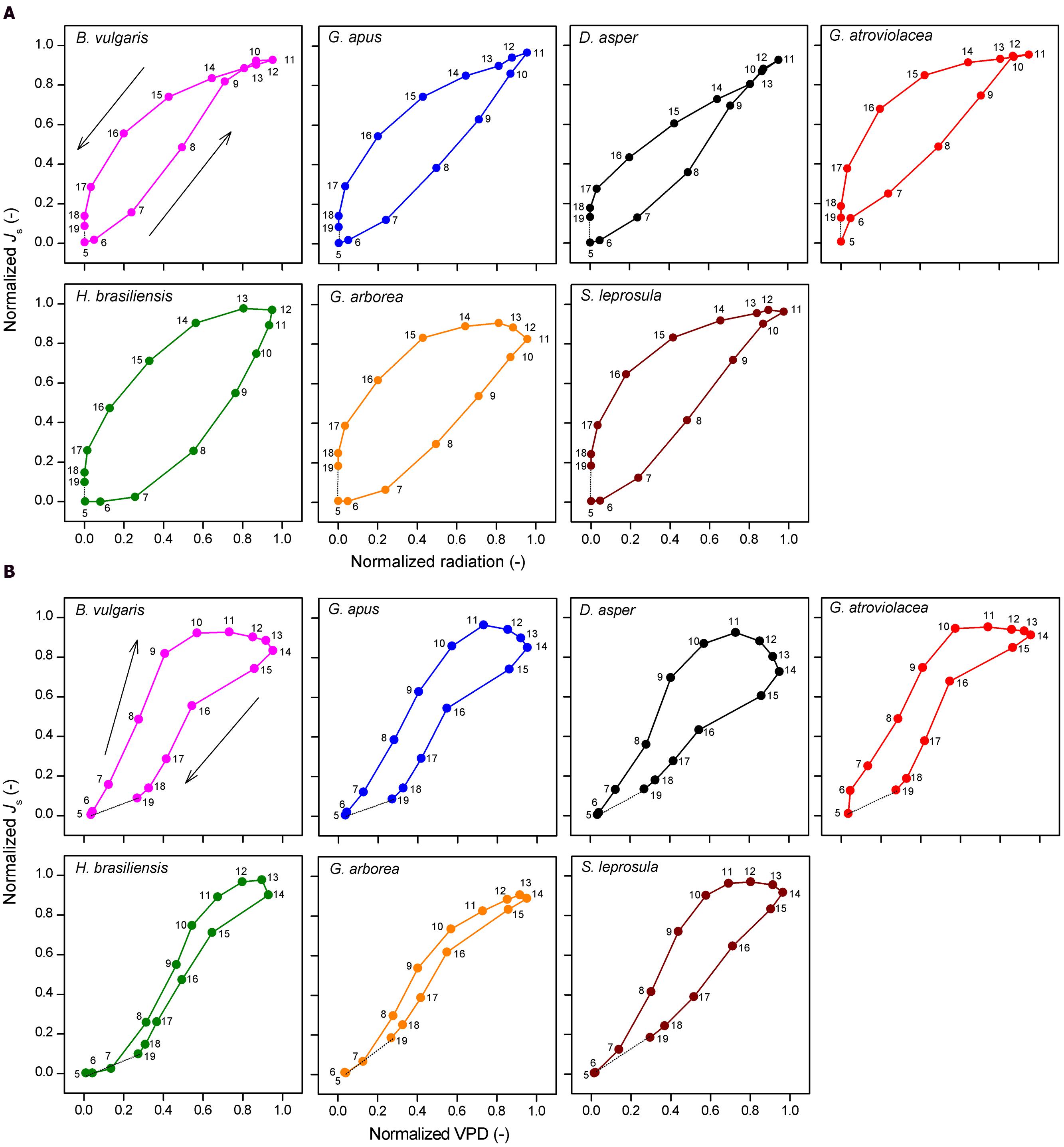
FIGURE 6. Normalized hourly sap flux density (Js) plotted against (A) normalized hourly radiation and (B) VPD. Data of four bamboo (upper row) and three tree species (lower row). Hourly averages derived from simultaneous measurements on five culms per species and by averaging the values of three sunny days to minimize influences of weather. The numbers in the sub-figures indicate the respective time of the day.
Discussion
Calibration Experiments
In the pot calibration experiment, SHB yielded similar results as reference GM measurements. Bamboos seem well suited for the SHB method (Dierick et al., 2010) due to their round shape and smooth and barkless surface, which allows for tight contact with the gages. Additionally, the hollow center and thin culm walls result in relatively low energy losses to heat storage so that the heat balance conditions required for the SHB method are met. ‘Zero sap flux’ conditions to obtain the heat conductivity of the sheath (Ksh, Sakuratani, 1981) as a further requirement of the SHB method are difficult to determine in situ due to potential root pressure induced night time sap flux in bamboos (Cao et al., 2012); however, using Ksh derived from field conditions of very low night-time sap flux likely introduced only negligible errors into the calculation of daytime sap flux (Grime and Sinclair, 1999). As we observed very low sap flux over several hours during our experiments (e.g., about 1 g cm-2 h-1 during the pot experiment), our obtained Ksh were likely reliable.
In contrast to SHB, the TDP method was found to substantially underestimate Js of bamboos in the pot and field calibration experiments. Underestimations by TDP were also reported in two other bamboo species: respective average underestimations of 13% for B. blumeana (Dierick et al., 2010) and 31% for Phyllostachys pubescens (Moso bamboo, Kume et al., 2010) were reported. Reasons for the observed underestimations could lie in the distinct hydraulic and physiological features of bamboos. Diurnal variations of stem water storage, for example, could affect the accuracy of TDP measurements (Vergeynst et al., 2014). Bamboos have approximately 50% parenchyma in culm walls (Dransfield and Widjaja, 1995), which potentially provides large water reservoirs. The depletion and refilling of the stem during the day and night, respectively, could cause diurnal fluctuations in culm thermal diffusivity. Higher water content during the night could lead to a lower maximum temperature difference (ΔTmax) between heated and reference probe under “zero sap flux” conditions. Likewise, lower water content during the day could lead to higher observed ΔT values. As ΔTmax/ΔT constitutes the basis for calculations of daytime Js, substantial underestimations of Js could be introduced when using the original calibration parameters (Granier, 1985; Vergeynst et al., 2014). This hypothesis was assessed further by comparing the linear calibration parameters of B. vulgaris from the pot and the field calibration experiment (c = 2.28 and 2.79, respectively). In the pot experiment, the bamboos were always supplied with plenty of water, so that the variability of the culm water content was likely smaller than under field conditions. Effects of varying stem water content on ΔTmax/ΔT are thus likely much smaller in the pot experiment, which may explain why pot and field calibration experiment yield different parameters for the linear calibration of the same species (B. vulgaris). Another potential factor for the divergence could be that the maximum observed Js in the field (about 70 g cm-2 h-1) was much larger than in the pot experiment (about 20 g cm-2 h-1). Higher daytime sap flux (and thus transpiration) may cause a quicker depletion of the potential culm water storage, which consequently leads to a higher variability of culm water content between night and day.
We expected the calibration formula type (linear vs. non-linear) and data time step to have an impact on the performance of TDP predictions. However, both were not as important as the factor formula specificity. Even though species-specific calibration formulas generally did not perform significantly better than the common formula, species-specific formulas tended to show slightly better performance (Table 1) for two of the studied species (G. apus and B. vulgaris). Also, the calibration parameters were significantly different among the four studied bamboo species (Table 2). Confronting this insight with results from sap flux studies on other bamboo species (Dierick et al., 2010; Kume et al., 2010), differences among species become even more apparent. We thus used the derived species-specific formulas for further analysis. The observed differences among species may be indicative of highly heterogeneous wood anatomical properties among bamboo species. For example, size and shape of vascular bundles and parenchyma of 15 bamboo species were reported to be highly variable (Rúgolo de Agrasar and Rodríguez, 2003). For two further bamboo species (Chusquea ramosissima and Merostachys claussenii), it was suggested that differences in number of vascular bundles per unit area (1000 vs. 225 per cm2) and vessel length (∼1 m vs. 20 cm) could lead to differences in xylem hydraulic conductivity (Saha et al., 2009). Differences in wood anatomical properties may also lead to heterogeneous heat conductive properties, which potentially affects applicability and accuracy of sap flux measurements and particularly of the TDP method (Wullschleger et al., 2011).
In our study, culm-specific formulas performed better at predicting Js than species-specific and common calibration formulas (Appendix Tables A1 and A2 in Supplementary Material). This result indicates heterogeneity in conductive properties among culms of the same species. Potential reasons could lie in the age and the ontogeny of individual culms. Even though we carefully selected culms of similar age (approximately 2 years old), the exact age of individual bamboo culms within a given clump is difficult to assess. As all monocot species, bamboos lack secondary growth (Zimmermann and Tomlinson, 1972), so culm diameters are not related to culm age. Additionally, over the ontogeny of a certain culm, events and processes such as conductive circuit failure (drought- or metabolism-related; Cochard et al., 1994; Liese and Weiner, 1996), lignification (Lin et al., 2002) or increasing hydraulic limitations with height (Renninger and Phillips, 2010; Cao et al., 2012) could result in overall reduced hydraulic conductivity and thus lower sap flux densities with increasing culm age. However, these processes remain difficult to assess from the outside of the culm; further studies linking the age and ontogeny of bamboos to (TDP-derived) sap flux and water use patterns are suggested.
Water Use Patterns of Bamboos and Trees
Half-hourly Js_max in the four studied bamboo species ranged from 21.6 to 70.5 g cm-2h-1 and were (on average) almost two times greater than in the studied tree species. The observed range for both bamboos and trees falls into the range of Js_max values reported for tropical tree species in a variety of sap flux studies (Meinzer et al., 2001; O’Brien et al., 2004). For D. asper, the Js_max (21.6 g cm-2 h-1) was similar to values reported for B. blumeana culms (25.7 g cm-2 h-1, Dierick et al., 2010) and Moso bamboos (approximately 20 g cm-2 h-1, Kume et al., 2010) of similar size. Our four studied bamboo species showed significant differences in Js_max, which were negatively correlated with species-specific differences in DBH (Figure 3). Consistent with this, in a study on 27 tropical tree species, the negative correlation between Js_max and DBH was also observed (Meinzer et al., 2001). It was assumed to be related to a decline of the leaf area to sapwood area ratio with increasing DBH. This was also observed in a study on Eucalyptus grandis trees (Dye and Olbrich, 1993). In our study, we harvested leaves of three bamboo species (B. vulgaris, D. asper, and G. apus) and found that the leaf weight to sapwood area ratio was positively correlated with Js_max (R2 = 0.45, P < 0.05). However, studies connecting such anatomical and eco-hydrological properties of bamboos are yet scarce (Saha et al., 2009).
On the day-to-day level, accumulated Js of both the studied bamboo and tree species were significantly correlated with radiation and VPD (Figure 4). During the long wet period, accumulated Js linearly increased with higher integrated radiation and average daily VPD. Likewise, linear relationships in the day-to-day behavior of Js to micrometeorological drivers have been reported for some tropical bamboo and several dicot tree species (Dierick and Hölscher, 2009; Köhler et al., 2009). During the dry period characterized by higher radiation and VPD (13 and 100% higher, respectively) than during the wet period, however, the observed linear relationship to VPD did not hold. Higher average daily VPD (‘dry period conditions’) led to decreases in accumulated Js of several studied species (Figure 4B). Similar decreases after a certain peak value have been reported for some previously studied tree species (Kubota et al., 2005; Jung et al., 2011), but in most species studied so far, higher average daily VPD leads to increases in accumulated Js or water use (Wullschleger and Norby, 2001; Tang et al., 2006; Kume et al., 2007; Hernández-Santana et al., 2008; Peters et al., 2010; Horna et al., 2011). This was also reported for Moso bamboo (Komatsu et al., 2010). The observed decreasing accumulated Js in bamboos under high VPD in our study were related to a reduction of soil moisture in the dry period (for three of the four bamboo and two of the three studied tree species). During the dry period, VPD was generally much higher than during the wet period. Soil moisture may become a limiting factor after several days without rainfall in the dry period. Accumulated Js decreased strongly and linearly with decreasing soil moisture under ‘dry period conditions’ (i.e., VPD > 0.74 kPa) for all studied bamboo (except D. asper) and tree species (except G. arborea, Figure 5). Similarly, in a throughfall reduction experiment in Indonesia, declines of monthly Js of Cacao and Gliricidia sepium were found to linearly correlate with reduced soil moisture (Köhler et al., 2010). Such sensitivity of daily Js to fluctuating soil moisture may be related to a relatively shallow rooting depth (Kume et al., 2007).
Regarding the diurnal course of Js, the studied bamboo species showed earlier peaks than radiation and VPD, and also earlier than the respective peaks of the studied tree species. In contrast to this, previous studies on tropical trees reported rather small time-lags between peaks of Js and radiation and VPD, respectively (Dierick and Hölscher, 2009; Köhler et al., 2009; Horna et al., 2011). Pre-noon peaks of Js have only been described for few species thus far, for example, Acer rubrum (Johnson et al., 2011) and oil palms (Niu et al., 2015). The early diurnal peaks of Js result in substantial hysteresis of Js particularly to VPD. For another monocot species, oil palm, it has been suggested that such pre-noon peaks of Js and the resulting large hysteresis to VPD could be indicative of internal trunk water storage and/or root pressure mechanisms (Niu et al., 2015; Röll et al., 2015). Early peaks of Js could be due to a pre-noon contribution of internal water storage to bamboo transpiration. Likewise, the decoupling of hourly Js particularly from VPD in the afternoon, i.e., the drop in bamboo Js (after an early peak) despite further rising VPD, could be connected to the reduced water availability for leaves after the depletion of internal water storage at a certain time of the day. The depletion of stored stem water may be compensated for during the night by root pressure mechanisms (Cao et al., 2012; Yang et al., 2012). Other potential reasons for the diurnally relatively early decline of bamboo Js and the consequent decoupling of the sap flux response from micrometeorological drivers could be a decline in leaf hydraulic conductance in the afternoon hours, which could contribute to prevent stem water potential loss and subsequent xylem cavitation (Saha et al., 2009; Yang et al., 2012).
Conclusion
Adjusting and applying the TDP method for sap flux measurements on four bamboo species pointed to substantial differences in water use patterns between the studied bamboos and three tree species studied. Bamboos had higher Js, and respective hourly maxima were reached earlier in the day than in tree species. This resulted in strong diurnal hysteresis, particularly to VPD, and in significant time lags between the peaks of Js in bamboos and the respective peaks of radiation and VPD. Both may point to a strong contribution of internal water storage mechanisms to bamboo transpiration. We found substantial differences in the day-to-day Js response of most studied bamboo and tree species to fluctuations in environmental conditions between the dry and the wet period. Reduced Js under conditions of high VPD in the dry period could largely be explained by limiting soil moisture content. The regulation of bamboo water use thus seems to involve mechanisms at the leaf-, culm-, and root- level. However, these mechanisms yet remain to be inter-connected convincingly.
Conflict of Interest Statement
The authors declare that the research was conducted in the absence of any commercial or financial relationships that could be construed as a potential conflict of interest.
Acknowledgments
The authors acknowledge the funding from the German Research Foundation (DFG, code number: HO 2119 and CRC 990). DF and FN received scholarships from the China Scholarship Council (CSC). We thank the Departments of Facility and Property, the Ecology Lab and the Agriculture Faculty of Bogor Agriculture University (IPB) for supporting our field work. We especially thank the IPB Faculty of Forestry for close cooperation in our project and our assistants Wahyu Iskandar and Popi Puspita for their work with great enthusiasm in our field experiments. We are very grateful to Diego Dierick for suggestions on experimental methods and to Michael Köhler for suggestions on data processing.
Supplementary Material
The Supplementary Material for this article can be found online at: http://journal.frontiersin.org/article/10.3389/fpls.2015.01202
References
Baker, J. M., and Van Bavel, C. H. M. (1987). Measurement of mass flow of water in the stems of herbaceous plants. Plant Cell Environ. 10, 777–782. doi: 10.1111/1365-3040.ep11604765
Bovard, B. D., Curtis, P. S., Vogel, C. S., Su, H.-B., and Schmid, H. P. (2005). Environmental controls on sap flow in a northern hardwood forest. Tree Physiol. 25, 31–38. doi: 10.1093/treephys/25.1.31
Cao, K.-F., Yang, S.-J., Zhang, Y.-J., and Brodribb, T. J. (2012). The maximum height of grasses is determined by roots. Ecol. Lett. 15, 666–672. doi: 10.1111/j.1461-0248.2012.01783.x
Carrasco, L. O., Bucci, S. J., Francescantonio, D. D., Lezcano, O. A., Campanello, P. I., Scholz, F. G., et al. (2014). Water storage dynamics in the main stem of subtropical tree species differing in wood density, growth rate and life history traits. Tree Physiol. 35, 354–365. doi: 10.1093/treephys/tpu087
Chen, X., Zhang, X., Zhang, Y., Booth, T., and He, X. (2009). Changes of carbon stocks in bamboo stands in China during 100 years. For. Ecol. Manag. 258, 1489–1496. doi: 10.1016/j.foreco.2009.06.051
Cochard, H., Ewers, F. W., and Tyree, M. T. (1994). Water relations of a tropical vine-like bamboo (Rhipidocladum racemiflorum): root pressures, vulnerability to cavitation and seasonal changes in embolism. J. Exp. Bot. 45, 1085–1089. doi: 10.1093/jxb/45.8.1085
Dierick, D., and Hölscher, D. (2009). Species-specific tree water use characteristics in reforestation stands in the Philippines. Agric. For. Meteorol. 149, 1317–1326. doi: 10.1016/j.agrformet.2009.03.003
Dierick, D., Hölscher, D., and Schwendenmann, L. (2010). Water use characteristics of a bamboo species (Bambusa blumeana) in the Philippines. Agric. For. Meteorol. 150, 1568–1578. doi: 10.1016/j.agrformet.2010.08.006
Dransfield, S., and Widjaja, E. (1995). Plant Resources of South-East Asia No. 7: Bamboos. Leiden: Backhuys Publishers.
Dye, P. J., and Olbrich, B. W. (1993). Estimating transpiration from 6-year-old Eucalyptus grandis trees: development of a canopy conductance model and comparison with independent sap flux measurements. Plant Cell Environ. 16, 45–53. doi: 10.1111/j.1365-3040.1993.tb00843.x
Food Agriculture Organization [FAO] (2010). Global Forest Resources Assessment 2010?: Main Report, FAO Forestry Paper, 0258-6150; 163. Rome: Food and Agriculture Organization of the United Nations.
Goldstein, G., Andrade, J. L., Meinzer, F. C., Holbrook, N. M., Cavelier, J., Jackson, P., et al. (1998). Stem water storage and diurnal patterns of water use in tropical forest canopy trees. Plant Cell Environ. 21, 397–406. doi: 10.1046/j.1365-3040.1998.00273.x
Granier, A. (1985). Une nouvelle méthode pour la mesure du flux de sève brute dans le tronc des arbres. Ann. For. Sci. 42:8. doi: 10.1051/forest:19850204
Grime, V. L., and Sinclair, F. L. (1999). Sources of error in stem heat balance sap flow measurements. Agric. For. Meteorol. 94, 103–121. doi: 10.1016/S0168-1923(99)00011-8
Hernández-Santana, V., David, T. S., and Martínez-Fernández, J. (2008). Environmental and plant-based controls of water use in a Mediterranean oak stand. For. Ecol. Manag. 255, 3707–3715. doi: 10.1016/j.foreco.2008.03.004
Horna, V., Schuldt, B., Brix, S., and Leuschner, C. (2011). Environment and tree size controlling stem sap flux in a perhumid tropical forest of Central Sulawesi. Indonesia. Ann. For. Sci. 68, 1027–1038. doi: 10.1007/s13595-011-0110-2
Ichihashi, R., Komatsu, H., Kume, T., Onozawa, Y., Shinohara, Y., Tsuruta, K., et al. (2015). Stand-scale transpiration of two Moso bamboo stands with different culm densities. Ecohydrology 8, 450–459. doi: 10.1002/eco.1515
International Network for Bamboo and Rattan [INBAR] (2014). International Trade in Bamboo and Rattan 2012. Beijing: International Network for Bamboo and Rattan. Available at: http://www.inbar.int/publications/?did=292 [accessed June 19, 2015].
Jarvis, N. J. (1989). A simple empirical model of root water uptake. J. Hydrol. 107, 57–72. doi: 10.1016/0022-1694(89)90050-4
Johnson, D. M., McCulloh, K. A., Meinzer, F. C., Woodruff, D. R., and Eissenstat, D. M. (2011). Hydraulic patterns and safety margins, from stem to stomata, in three eastern US tree species. Tree Physiol. 31, 659–668. doi: 10.1093/treephys/tpr050
Jung, E. Y., Otieno, D., Lee, B., Lim, J. H., Kang, S. K., Schmidt, M. W. T., et al. (2011). Up-scaling to stand transpiration of an Asian temperate mixed-deciduous forest from single tree sapflow measurements. Plant Ecol. 212, 383–395. doi: 10.1007/s11258-010-9829-3
Köhler, M., Dierick, D., Schwendenmann, L., and Hölscher, D. (2009). Water use characteristics of cacao and Gliricidia trees in an agroforest in Central Sulawesi. Indonesia. Ecohydrol. 2, 520–529. doi: 10.1002/eco.67
Köhler, M., Schwendenmann, L., and Hölscher, D. (2010). Throughfall reduction in a cacao agroforest: tree water use and soil water budgeting. Agric. For. Meteorol. 150, 1079–1089. doi: 10.1016/j.agrformet.2010.04.005
Komatsu, H., Onozawa, Y., Kume, T., Tsuruta, K., Kumagai, T., Shinohara, Y., et al. (2010). Stand-scale transpiration estimates in a Moso bamboo forest: II. Comparison with coniferous forests. For. Ecol. Manag. 260, 1295–1302. doi: 10.1016/j.foreco.2010.06.040
Kubota, M., Tenhunen, J., Zimmermann, R., Schmidt, M., Adiku, S., and Kakubari, Y. (2005). Influences of environmental factors on the radial profile of sap flux density in Fagus crenata growing at different elevations in the Naeba Mountains, Japan. Tree Physiol. 25, 545–556. doi: 10.1093/treephys/25.5.545
Kume, T., Onozawa, Y., Komatsu, H., Tsuruta, K., Shinohara, Y., Umebayashi, T., et al. (2010). Stand-scale transpiration estimates in a Moso bamboo forest: (I) Applicability of sap flux measurements. For. Ecol. Manag. 260, 1287–1294. doi: 10.1016/j.foreco.2010.07.012
Kume, T., Takizawa, H., Yoshifuji, N., Tanaka, K., Tantasirin, C., Tanaka, N., et al. (2007). Impact of soil drought on sap flow and water status of evergreen trees in a tropical monsoon forest in northern Thailand. For. Ecol. Manag. 238, 220–230. doi: 10.1016/j.foreco.2006.10.019
Liese, W., and Weiner, G. (1996). Ageing of bamboo culms. A review. Wood Sci. Technol. 30, 77–89. doi: 10.1007/BF00224958
Lin, J., He, X., Hu, Y., Kuang, T., and Ceulemans, R. (2002). Lignification and lignin heterogeneity for various age classes of bamboo (Phyllostachys pubescens) stems. Physiol. Plant. 114, 296–302. doi: 10.1034/j.1399-3054.2002.1140216.x
Lu, P., Urban, L., and Zhao, P. (2004). Granier’s thermal dissipation probe (TDP) method for measuring sap flow in trees: theory and practice. ACTA Bot. Sin. Engl. Ed. 46, 631–646.
Meinzer, F. C., Goldstein, G., and Andrade, J. L. (2001). Regulation of water flux through tropical forest canopy trees: do universal rules apply? Tree Physiol. 21, 19–26. doi: 10.1093/treephys/21.1.19
Niu, F., Röll, A., Hardanto, A., Meijide, A., Köhler, M., Hendrayanto, et al. (2015). Oil palm water use: calibration of a sap flux method and a field measurement scheme. Tree Physiol. 35, 563–573. doi: 10.1093/treephys/tpv013
O’Brien, J. J., Oberbauer, S. F., and Clark, D. B. (2004). Whole tree xylem sap flow responses to multiple environmental variables in a wet tropical forest. Plant Cell Environ. 27, 551–567. doi: 10.1111/j.1365-3040.2003.01160.x
Pereira, H. C., and Hosegood, P. H. (1962). Comparative water-use of softwood plantations and bamboo forest. J. Soil Sci. 13, 299–313. doi: 10.1111/j.1365-2389.1962.tb00709.x
Peters, E. B., McFadden, J. P., and Montgomery, R. A. (2010). Biological and environmental controls on tree transpiration in a suburban landscape. J. Geophys. Res. Biogeosci. 115:G04006. doi: 10.1029/2009JG001266
Petit, G., DeClerck, F. A. J., Carrer, M., and Anfodillo, T. (2014). Axial vessel widening in arborescent monocots. Tree Physiol. 34, 137–145. doi: 10.1093/treephys/tpt118
Renninger, H. J., and Phillips, N. (2010). Intrinsic and extrinsic hydraulic factors in varying sizes of two Amazonian palm species (Iriartea deltoidea and Mauritia flexuosa) differing in development and growing environment. Am. J. Bot. 97, 1926–1936. doi: 10.3732/ajb.1000015
Röll, A., Niu, F., Meijide, A., Hardanto, A., Hendrayanto, Knohl, A., et al. (2015). Transpiration in an oil palm landscape: effects of palm age. Biogeosciences 12, 5619–5633. doi: 10.5194/bg-12-5619-2015
Rúgolo de Agrasar, Z., and Rodríguez, M. F. (2003). Culm anatomy of native woody bamboos in argentina and neighboring areas: cross section. Bamboo Sci. Cult. J. Am. Bamboo Soc. 17, 28–43.
Saha, S., Holbrook, N. M., Montti, L., Goldstein, G., and Cardinot, G. K. (2009). Water Relations of Chusquea ramosissima and Merostachys claussenii in Iguazu National Park, Argentina. Plant Physiol. 149, 1992–1999. doi: 10.1104/pp.108.129015
Sakuratani, T. (1981). A heat balance method for measuring water flux in the stem of intact plants. J. Agric. Meteorol. 37, 9–17. doi: 10.2480/agrmet.37.9
Tang, J., Bolstad, P. V., Ewers, B. E., Desai, A. R., Davis, K. J., and Carey, E. V. (2006). Sap flux–upscaled canopy transpiration, stomatal conductance, and water use efficiency in an old growth forest in the Great Lakes region of the United States. J. Geophys. Res. Biogeosci. 111:G02009. doi: 10.1029/2005JG000083
Van Den Besselaar, E. J. M., Klein Tank, A. M. G., Van Der Schrier, G., Abass, M. S., Baddour, O., Van Engelen, A. F. V., et al. (2014). International climate assessment & dataset: climate services across borders. Bull. Am. Meteorol. Soc. 96, 16–21. doi: 10.1175/BAMS-D-13-00249.1
Vandegehuchte, M. W., and Steppe, K. (2013). Sap-flux density measurement methods: working principles and applicability. Funct. Plant Biol. 40, 213–223. doi: 10.1071/FP12233_CO
Vergeynst, L. L., Vandegehuchte, M. W., McGuire, M. A., Teskey, R. O., and Steppe, K. (2014). Changes in stem water content influence sap flux density measurements with thermal dissipation probes. Trees 28, 949–955. doi: 10.1007/s00468-014-0989-y
Wang, H., Zhao, P., Hölscher, D., Wang, Q., Lu, P., Cai, X. A., et al. (2012). Nighttime sap flow of Acacia mangium and its implications for nighttime transpiration and stem water storage. J. Plant Ecol. 5, 294–304. doi: 10.1093/jpe/rtr025
Waring, R. H., and Running, S. W. (1978). Sapwood water storage: its contribution to transpiration and effect upon water conductance through the stems of old-growth Douglas-fir. Plant Cell Environ. 1, 131–140. doi: 10.1111/j.1365-3040.1978.tb00754.x
Wullschleger, S. D., Childs, K. W., King, A. W., and Hanson, P. J. (2011). A model of heat transfer in sapwood and implications for sap flux density measurements using thermal dissipation probes. Tree Physiol. 31, 669–679. doi: 10.1093/treephys/tpr051
Wullschleger, S. D., and Norby, R. J. (2001). Sap velocity and canopy transpiration in a sweetgum stand exposed to free-air CO2 enrichment (FACE). New Phytol. 150, 489–498. doi: 10.1046/j.1469-8137.2001.00094.x
Yang, S.-J., Zhang, Y.-J., Sun, M., Goldstein, G., and Cao, K.-F. (2012). Recovery of diurnal depression of leaf hydraulic conductance in a subtropical woody bamboo species: embolism refilling by nocturnal root pressure. Tree Physiol. 32, 414–422. doi: 10.1093/treephys/tps028
Keywords: calibration, environmental drivers, hysteresis, stem heat balance, thermal dissipation probes, trees, bamboos
Citation: Mei T, Fang D, Röll A, Niu F, Hendrayanto and Hölscher D (2016) Water Use Patterns of Four Tropical Bamboo Species Assessed with Sap Flux Measurements. Front. Plant Sci. 6:1202. doi: 10.3389/fpls.2015.01202
Received: 19 August 2015; Accepted: 14 December 2015;
Published: 07 January 2016.
Edited by:
Julia Cooke, The Open University, UKReviewed by:
Teresa E. Gimeno, Institut National de la Recherche Agronomique, FranceTomonori Kume, National Taiwan University, Taiwan
Copyright © 2016 Mei, Fang, Röll, Niu, Hendrayanto and Hölscher. This is an open-access article distributed under the terms of the Creative Commons Attribution License (CC BY). The use, distribution or reproduction in other forums is permitted, provided the original author(s) or licensor are credited and that the original publication in this journal is cited, in accordance with accepted academic practice. No use, distribution or reproduction is permitted which does not comply with these terms.
*Correspondence: Tingting Mei, tmei@gwdg.de
†These authors have contributed equally to this work.