- 1Department of Agricultural and Environmental Sciences, University of Bari Aldo Moro, Bari, Italy
- 2Centro de Investigaciones Biológicas del Noroeste, Instituto Politecnico Nacional, La Paz, Mexico
Barbados aloe (Aloe vera (L.) Burm. F.) has traditionally been used for healing in natural medicine. However, aloe is now attracting great interest in the global market due to its bioactive chemicals which are extracted from the leaves and used in industrial preparations for pharmaceutical, cosmetic, and food products. Aloe originated from tropical and sub-tropical Africa, but it is also now cultivated in warm climatic areas of Asia, Europe, and America. In this review, the most important factors affecting aloe production are described. We focus on propagation techniques, sustainable agronomic practices and efficient post harvesting and processing systems.
Introduction
“Four vegetables are indispensable for the well being of man: wheat, grapes, olives and aloe. The first nourishes him, the second he refreshes the spirit, the third brings him harmony and the fourth cures him”
Christopher Columbus (1451-1506).
Aloe (Aloe vera (L.) Burm. F., Barbados aloe, Xanthorrhoeaceae, sub-family Asphodeloideae) is one of the most economically important medicinal crops worldwide with a significant bio-cultural value (Grace et al., 2009; Grace, 2011) and has a long history. Traditionally used for healing in natural medicine, for the last 20 years aloe has been at the center of a renewed interest in the global market due to its therapeutic and nutritive substances extracted from leaves, used in commercial preparations for pharmaceutical, cosmetic or alimentary uses and as a fresh food (Gutterman and Chauser-Volfson, 2007). The leaf mesophyll, commonly known as aloe gel, is subject to industrial processing to obtain derivatives.
Among its most representative organic biomolecules, aloe gel contains soluble sugars, anthraquinones, β-polysaccharides, amino acids, vitamins, glycoproteins, and enzymes (Chun-hui et al., 2007; Ahlawat and Khatkar, 2011; Chang et al., 2011; Lucini et al., 2015), with various biological properties such as antiviral, antibacterial, antifungal, anticancer, anti-inflammatory, wound healing, and many other characteristics that have prompted an increase in industrial and commercial production globally (Boudreau and Beland, 2006).
The succulent leaf tissue has an estimated annual market of $13 billion (Grace et al., 2015) which is likely to increase by 35–40% within the next 5 years. The USA dominates the market (65%), while India and China have a share of 10% each one (Biswas, 2010).
In addition to the demand from the pharmaceutical, cosmetics and processing industry, aloe has also been marketed in Europe and the USA through farmers' markets and e-commerce.
This global demand cannot be met solely by the wild harvesting of leaves aloe, which are indigenous to subtropical Africa (Van Jaarsveld, 1989; Van Wyk and Smith, 1996). Consequently, small and average-sized farms could exploit the increasing market demand worldwide, and invest in non-traditional crops, such as aloe (Liontakis and Tzouramani, 2016). Kenyan farmers see aloe cultivation as a strategy for reducing the risks linked to the fragile dependency on spontaneous aloe. This appears to have a greater potential to achieve the sustainable management of natural resources and to reduce poverty (Belmin et al., 2013). Since 2006, the Ethiopian government has recognized that the aloe plant is a source of income that integrates the diversification strategies for pastoral and agro-pastoral communities (Dirbaba, 2014).
Currently aloe is grown openly in the field in Kenya, Tanzania, Ethiopia, and Nigeria, with products exported to Europe and Asia (Oldfield, 2004); in south western Australia, China, India, Pakistan and Israel, Caribbean islands, southern USA, Mexico, and Paraguay. In Mediterranean climates (Spain, Greece, and southern Italy), it is cultivated both openly in the field and in cold greenhouses (De Lucia and Lucini, 2013).
Aloe can also be cultivated in some arid and semi-arid areas of the globe due to its eco-physiological characteristics: drought resistance, succulence, and a CAM trait.
Over the last decade, several scientific papers have dealt with the medicinal, pharmaceutical and nutritional properties of A. vera. This review brings together multiplication protocols, sustainable agronomic practices, efficient post harvesting and processing systems. Plant tissue culture techniques offer mass-production of consistently healthy planting material of a standardized quality. The most suitable agronomic management strategies can increase productivity, even surpassing that of species traditionally grown under the same conditions. Finally, suitable harvesting and post-harvesting conditions and appropriate processing methods can prevent the loss of bioactive chemical substances of the product.
Propagation Techniques
Conventional In vivo Propagation
The conventional propagation is not able to fulfill the increasing market demand for aloe, which calls for healthy, homogeneous, true-to-type, and available year-round plant material. Following, we provide an overview about the advantages and disadvantages of these propagation methods.
Propagation by Seed
The flower stalk starts from the center of the basal leaves with yellow flowers (Figure 1A); the fruit has a triangular shape and contains several seeds (Silva et al., 2010). Different drawbacks must be faced with the propagation by seed.
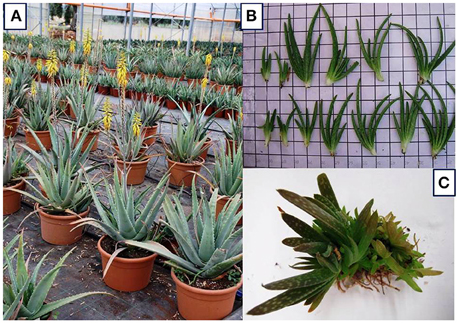
Figure 1. (A) A. vera (L.) Burm. F. plants with basal rosettes of leaves and inflorescence. Photo taken by De Lucia. (B) Suckers as in vivo planting materials. Photo taken by Cristiano. (C) In vitro culture: micropropagated shoots. Photo taken by Cardarelli.
A. vera is generally self-incompatible, seeds are only fertile if they derive from cross pollination (Botes et al., 2009); this involves a high heterogeneity of the seed population (Ghate et al., 2002). Maintaining the species or varieties of aloe using sexual reproduction is difficult because the natural hybridization of this species is very frequent (Granados-Sánchez and Castañeda-Pérez, 1998). Nayanakantha et al. (2010a) and Alagukannan and Ganesh (2016) have revealed the existence of substantial genetic diversity among the Indian accessions for yield and quality parameters.
Seedlessness is another critical aspect (Gupta et al., 2015): a study by Sharma et al. (2011) suggests that both environmental and genetic factors are responsible for the anomalous chromosomal behavior that results in seedlessness.
Low percentage (0–25%) of seed germination is scored under in vivo condition. When germination is carried out in vitro, the seed germination percentage can reach 60–70% (Das et al., 2016).
In any case, plants raised by seed propagation take a long gestation period before attain the maturity: from 3 (Pushpan, 2012) to 4 years (Eshun and He, 2004) to attain harvestable size and it has a life span of about 12 years.
Therefore, commercial nurseries prefer to propagate A. vera agamically.
Suckers and Rhizome Cuttings
Traditionally, A. vera is propagated using suckers (lateral shoots, Figure 1B) and rhizome cuttings as planting materials. In conventional production systems, each mother plant produces three to four suckers throughout the growing season (Pedroza-Sandoval and Gómez-Lorence, 2006; Smith and Van Wyk, 2009; Saggoo and Kaur, 2010; Gantait et al., 2014).
To prevent diseases, before transplantation in the field, selected suckers should be subjected to a slight moisture stress, under shade conditions (about 5–10 days). This then promotes root suberisation, which speeds up their adaptation and stimulates root growth when planting (Murillo-Amador et al., 2007). Crop is ready to harvest after 18 months of “sowing” (Das and Chattopadhay, 2004; Rajeswari et al., 2012).
Using rhizome to reproduce aloe on large scale (Rajeswari et al., 2012) is impractical due to the time required to have a completely developed plant (average 2 years), and to exposure to phytopathogens as a result of the cuts (Pedroza-Sandoval and Gómez-Lorence, 2006; Gantait et al., 2014).
Therefore, the high probability of damaging the mother plant and producing infected seedlings, the seasonality and the limited number of suckers along with the limited availability of raw material with high quality and slow vegetative growth are therefore limiting factors for a large-scale commercial production of young plants.
In vitro Propagation
To overcome the various difficulties of in vivo propagation, micropropagation can be exploited to obtain a large-scale production of uniform and healthy plantlets, which can be produced throughout the year (Gantait et al., 2014). In vitro propagation can be pursued through axillary bud stimulation and through de novo in vitro organogenesis (Rathore et al., 2011a,b; Gupta et al., 2014; Kanwar et al., 2015). In order to achieve a satisfactory production of healthy and standardized plantlets, the various stages of the in vitro cloning of A. vera need to be optimized.
Adventitious Shoot Regeneration
Adventitious aloe shoots can be obtained through direct and indirect organogenesis (Figure 2).
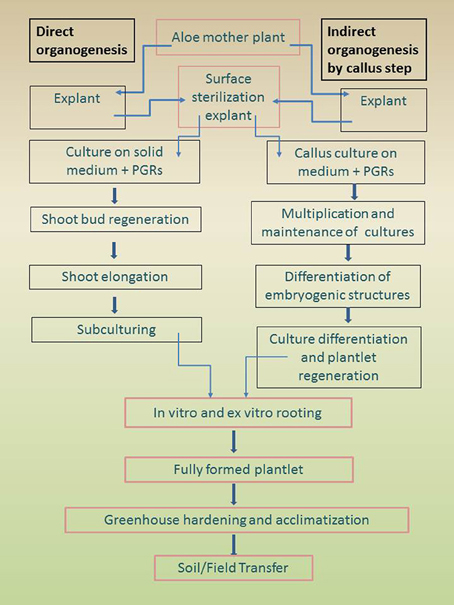
Figure 2. Outline showing summary of tissue culture processes: direct and indirect organogenesis for aloe plantlet regeneration.
During the direct pathway, the formation of a meristem proceeds without intermediate proliferation of undifferentiated callus tissue (Magyar-Tábori et al., 2010).
Direct shoot regeneration in A. vera, was achieved from leaf explants after 4–6 weeks of culture on MS medium (Murashige and Skoog, 1962) supplemented with 2.0 mg L−1 BA, 0.5 mg L−1 NAA, and 40 mg L−1 ADS (Sahoo and Rout, 2014). Rapid and large-scale indirect adventitious shoot regeneration was obtained from calli derived from the leaf base (Castorena-Sanchez et al., 1988; Lee et al., 2011), the leaf segments (Gupta et al., 2014; Kumari and Naseem, 2015), the leaf without sheaths (Abdi et al., 2013), the inflorescence axis (Rathore et al., 2011b), the stem segments (Gui et al., 1990), the stem discs (Saggoo and Kaur, 2010), and the rhizomatous stems (Roy and Sarkar, 1991). Seeds (Garro-Monge et al., 2008) and meristems (Abrie and Van Staden, 2001) have also been used as explants for callus induction.
Sterilizing the plant material is the most important step in the tissue culture protocol. Sterilization involves eliminating microbial contaminants from the surface and interior of plant material, thus giving a greater chance of survival in vitro.
Saggoo and Kaur (2010) and Rathore et al. (2011b) showed that a pre-treatment with a combination with Bavistin and streptomycin and subsequent surface sterilization, prevented fungal and bacterial growth in cultures.
The plant material can be disinfected with mercuric chloride (Liao et al., 2004; Ahmed et al., 2007; Kumar et al., 2011) which is now seldom used as it is dangerous (Kaviani, 2015), and sodium hypochlorite (Natali et al., 1990; Nayanakantha et al., 2010b; Adelberg and Naylor-Adelberg, 2012). Browning decreases the explant survival and leads to failures in shoot regeneration (Roy and Sarkar, 1991). A suitable carbon source and antioxidants (ascorbic and citric acids) need to be added to avoid excessive phenolic secretion in the culture medium (Das and Srivastav, 2015). To eliminate blacking and browning, PVP can be used (Roy and Sarkar, 1991; Liao et al., 2004; Rathore et al., 2011b). Nayanakantha et al. (2010b) reported that a medium containing 1.0 g L−1 PVP + 10 mg L−1 citric acid + 500 mg L−1 AC resulted in blocking the browning of the medium due to tissue phenols release.
The initiation of regenerative callus culture is dependent on the nature and concentration of PGRs: auxins (IAA, IBA, NAA, or 2,4-D) are also often added to the culture medium to regulate morphogenesis, alone or in combination with cytokinins.
Gui et al. (1990), in stem segments, initiated callus cultures of A. vera on MS medium with NAA alone or with 2.0 mg L−1 Zeatin; Roy and Sarkar (1991) with a combination of 1.0 mg L−1 2,4-D and 0.2 mg L−1 Kin.
The soft base of young inflorescence axis as explants, after 30–35 days, produced callus on 0.8% agar gelled MS medium supplemented with 6.0 mg L−1 2,4-D and 100 mg L−1AC (Rathore et al., 2011b). The Authors suggested that to get regenerative globular structures a decreased PGRs concentration is necessary (MS medium with 1.0 mg L−1 2,4-D). NAA only has been proved to be essential for optimum callus induction from leaf segment explants (Abdi et al., 2013).
In other studies, callus developed in the MS medium supplemented with BAP, obtaining the maximum percentage (100%) of culture within the minimum time required: 9.6 days (Gupta et al., 2014).
Rathore et al. (2011b) found that more than three to four passages lead to hyperhydricity of cultures probably caused by high concentrations of cytokinins: for this reason, once meristematic activity is achieved, the concentration of PGR needs to be decreased. The regeneration from callus culture in adventitious shoot occurred with BAP alone (Rathore et al., 2011b) and BAP in combination with NAA: Gupta et al. (2014) observed the best and most rapid microshoot formation on MS supplemented with 2.0 mg L−1 BAP + 0.5 mg L−1 NAA, which yielded the highest number (75) of regenerated shoots, with an average length of 4.0 cm.
Regenerated shoots were cultured on MS basal medium fortified with 0.5 mg L−1 NAA for adventitious rooting from the 10th day of culture, and 90% of root formation took place within a period of 3–4 weeks for maximum callus induction. The highest number of roots per shoot was 4.8, with an average length of 3.5 cm (Gupta et al., 2014).
For large or mass-scale production, the efficiency of the propagation methods is important, but perhaps even more important is the genetic stability. Rathore et al. (2011a) revealed a somaclonal variation of more than 70% in plants propagated by indirect organogenesis from the base of the inflorescence axis. This genetic instability, mainly in callus-derived plants, is a negative factor for the nursery industry, which uses axillary branching technique and to produce true-to-type clones of the aloe mother plants.
Various types of plant organs are used to promote adventitious shoot regeneration: when shoots are developed directly from leaf explants, i.e., without intervening callus phase, this is referred to as direct organogenesis.
Propagation through Axillary Branching
Axillary branching miniaturizes the natural process of branching. The cytokinins, supplemented to the culture medium, overcome apical dominance releasing lateral buds from dormancy (George et al., 2008) and BA and BAP (same compound named differently according to Teixeira da Silva, 2012) are the synthetic cytokinins commonly used. By this way, elite plant material can be efficiently produced with low or no somaclonal variation (Nayanakantha et al., 2010a). The following different steps should be faced to reach a satisfactory protocol to obtain virus-free plants.
Culture establishment
Various types of explants are used to get axillary branching: meristem in order to obtain virus-free aloe (Campestrini et al., 2006; Das et al., 2010; Rizwan et al., 2014), shoot tips (Meyer and Van Staden, 1991; Aggarwal and Barna, 2004; Ahmed et al., 2007; Supe, 2007; Hashemabadi and Kaviani, 2008; Zakia et al., 2013; Amoo et al., 2014; Molsaghi et al., 2014), axillary shoot segments (Singh et al., 2009; Shekhawat et al., 2013), shoot bud (Kanwar et al., 2015), apical buds (de Oliveira et al., 2009; Gupta et al., 2014), buds from underground stems (Liao et al., 2004), stem nodes (Singh and Sood, 2009), rhizomatous stems (Gantait et al., 2010, 2011), and suckers (Nayanakantha et al., 2010b).
In vitro culture establishment is the first stage of the protocol. It is influenced by the physiological state of the source plant and its age as well as the season of explant collection. The treatments for the explants are critical as these determine their growth and behavior in culture (Singh et al., 2009).
Aggarwal and Barna (2004) developed a cost effective and efficient method of micropropagation through axillary branching: shoot tip explants (2–3 cm), inoculated on MS medium containing 0.2 mg L−1 BA and 0.2 mg L−1 IBA for shoot initiation, showed signs of proliferation after 2 weeks. New buds appeared from the axils of leaves and developed into shoots by the 4th week of culture.
Table 1 shows that the percentage of success for the initiation phase can reach 93% (Gantait et al., 2011) or 100 % (Shekhawat et al., 2013).
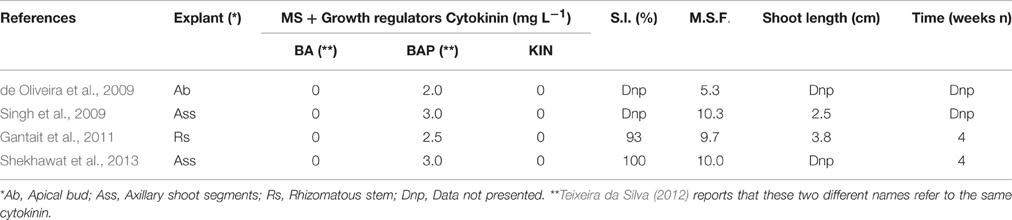
Table 1. Effect of different cytokinin concentrations on in vitro success percentage of initiation phase (S.I.) and multiple shoot formation (M.S.F.) in A. vera.
In vitro multiplication
Various basal media, such as B5 (Gamborg et al., 1968) and SH (Schenk and Hildebrandt, 1972) have been employed for micropropagation, but the most widely used culture medium is MS (Murashige and Skoog, 1962). The most used growth regulator to induce shoot initiation and multiplication was the cytokinin BAP or BA. Although Teixeira da Silva (2012) clearly explains that these two different names refer to the same cytokinin, in our review we prefer to maintain the original nomenclature used by the authors.
Shekhawat et al. (2013), Singh et al. (2009), de Oliveira et al. (2009), and Gantait et al. (2011), used BAP alone, at concentrations respectively of 3.0, 2.5, 2.0, and 0.7 mg L−1, with a shoot multiplication rate between 10 and 5.3.
Some researchers reported that a combination of PGRs improved micropropagation: Natali et al. (1990) reported multiplied shoots on MS supplemented with 0.5 mg L−1 Kin and 0.2 mg L−1 of 2,4 D; Kanwar et al. (2015) obtained maximum average number of shoots (5.5) per explant and maximum average shoot length (3.3 cm) on MS medium supplemented with 2.2 mg L−1 BA and 1.6 mg L−1 Kin.
Campestrini et al. (2006), Zakia et al. (2013), and Gupta et al. (2014) demonstrated that the best combination for the growth of aloe microshoots was MS medium supplemented with BAP and NAA. Supe (2007), Molsaghi et al. (2014), and Rizwan et al. (2014) reported that the best multiplication of shoots was obtained on medium containing BAP + IAA. Das et al. (2010) cultured aloe plantlets on medium containing BAP and IBA which produced the highest number of shoot buds (22.0) and enhanced bud proliferation within 1 to 2 weeks after the first subculture. Ahmed et al. (2007) reported 15.4 shoots per explant in MS medium containing a combination of 2.0 mg L−1 BA, 0.5 mg L−1 Kin and 0.2 mg L−1 NAA. Hashemabadi and Kaviani (2008) reported the maximum number of shoots (around 9.7 per explant) in MS medium supplemented with 0.5 mg L−1 BA and 0.5 mg L−1 NAA.
Regarding the shoot multiplication rate, Singh et al. (2009), Gantait et al. (2011) and Abdi et al. (2013) achieved a rate of more than 9, which was obtained 4 weeks after establishment, respectively with BAP 3.0, BAP 2.5, and BA 4.0 mg L−1. Variations in cytokinin level (BAP 2.0 and BA 10 mg L−1) reduced shoot proliferation (de Oliveira et al., 2009; Kanwar et al., 2015). Adelberg and Naylor-Adelberg (2012) reported that 1.3 mg L−1 BA in liquid medium is optimal for multiplication rate. Several studies (Liao et al., 2004; Ahmed et al., 2007; Supe, 2007; Gupta et al., 2014; Molsaghi et al., 2014; Rizwan et al., 2014) reported that improved in vitro multiplication can be scored when the cytokinins are associated with the auxins (multiplication rate from 1:9.5 to 1: 58 respectively on MS medium with BAP 1.5 mg L−1, NAA 0.5 mg L−1 and on the same medium with BAP 4.0 mg L−1 and IAA 1.0 mg L−1 (Table 2, Figure 1C).
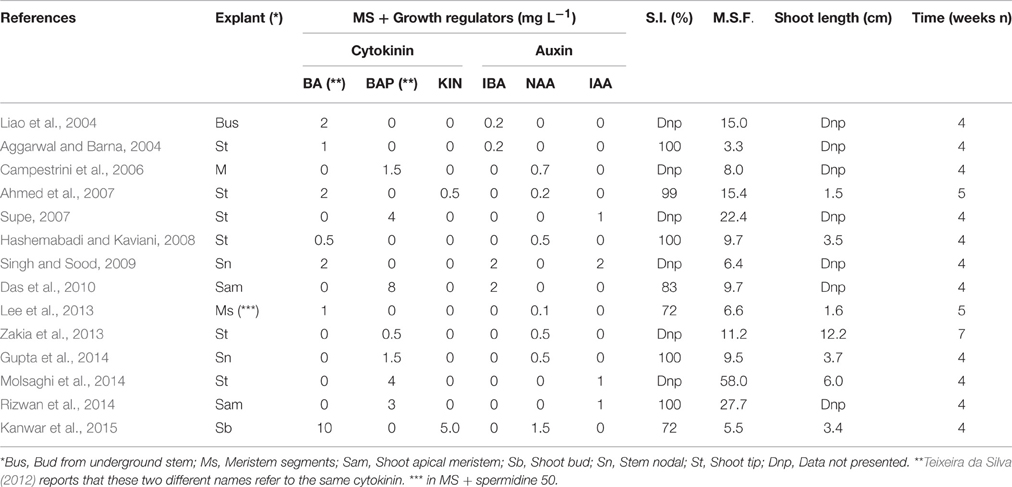
Table 2. Effect of different concentrations and combinations of BA, BAP, KIN, and IBA, NAA, IAA on success percentage of initiation phase (S.I.) and multiple shoot formation (M.S.F.) in A. vera.
Table 2 shows that the longest microshoot (12.2 cm) was obtained after 7 weeks on MS medium supplemented with 0.5 mg L−1 BAP + 0.5 mg L−1 NAA (Zakia et al., 2013).
Repeated subculturing of the micro-shoots has been suggested as a method to rejuvenate adult tissues (Kanwar et al., 2010). Gantait et al. (2010), noticed no difference in the number of shoots between the first and fifth subcultures, whereas Kanwar et al. (2015) obtained 22.7 shoots/explants after the sixth subculturing, with an average length of 9.0 cm, compared to the first with only 5.5 shoots/explant with an average length of 3.5 cm. Hashemabadi and Kaviani (2008) obtained 12 shoots/explants in the fourth subculturing. Kanwar et al. (2015) observed that the in vitro rooting response increased as a function of the in vitro culture cycle applied. At the sixth subculturing, the length root was 3.7 cm. The same authors, in another experiment, transferred explant as juvenile leaf, after every 3 days, into fresh shoot induction full strength MS medium supplemented with 0.04% AC and incubated it at 25 ± 2°C under 16 h photoperiod. They obtained a phenolic-free microshoot; AC provided the dark conditions required for the root initiation and growth and also adsorbed the substances presumed to be detrimental.
The automation of micropropagation in bioreactors on a liquid medium is one way of reducing propagation costs, as reported by Adelberg and Fári (2010). This is because the explant multiplies more rapidly, a better nutrient availability is established and once the microshoots are acclimatized under in vivo conditions, an improved growth could be observed compared to the traditional ex vitro plant material (Cardarelli et al., 2014). To override tissue hyperhydricity, Ziv (2005) and Tascan et al. (2010) proposed the addition of polyester fiber matter without mechanical ventilation.
In vitro rooting
Rooting is the last in vitro step before the plantlets are transferred to ex vitro conditions. Rooting success (Table 3) ranges from 80 (Ahmed et al., 2007) to 100% (Singh et al., 2009; Abdi et al., 2013; Molsaghi et al., 2014).
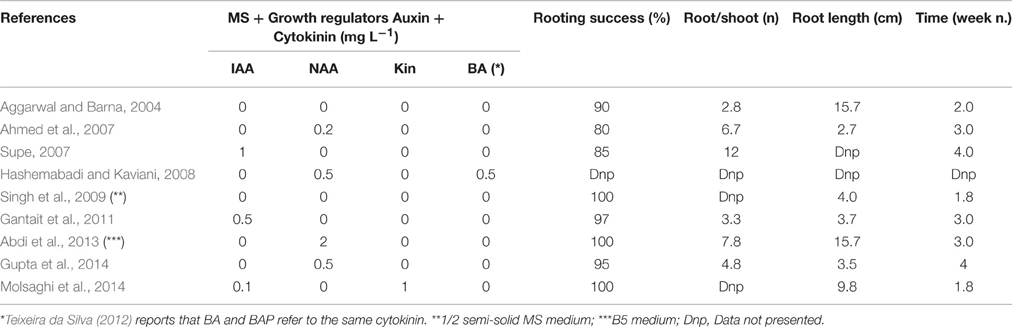
Table 3. Effect of different concentrations and combinations of IAA, NAA, KIN, and BA on in vitro rooting in A. vera.
Aggarwal and Barna (2004) observed a number of roots per shoot (2.8) in MS medium without any PGR supplementation; Supe (2007) reported 12 roots/shoot. Hashemabadi and Kaviani (2008) reported that a dose of BA during multiplication phase of more than 0.5 mg L−1 reduces the rooting percentage of the shoot as various proteolytic enzymes are suppressed.
Subsequent protocols have exploited the use of auxins (Table 3): IAA alone (Supe, 2007; Gantait et al., 2011), and NAA alone (Ahmed et al., 2007; Abdi et al., 2013; Gupta et al., 2014).
The addition of AC in the culture medium improves the root number of micropropagated shoots (Abadi and Kaviani, 2010), and also ensures a better plant growth during acclimatization (Borgognone et al., 2013). Singh et al. (2009) proposed a protocol on ex vitro rooting. They found that when the ex vitro rooting of shoots and the hardening of plantlets are achieved in the greenhouse in a single step, the protocol takes a shorter time for plantlet production and is considerably cheaper.
Acclimatization
The success of a micro-propagation protocol depends on the ex vitro acclimatization phase of the micro-propagated plantlets, which is measured by the percentage survival. A 70% survival rate was achieved by Gupta et al. (2014).
This success has been positively correlated to the dimensions of the ex vivo microplants: medium (6–8 cm long bearing 4–5 leaves) and large (13–16 cm long bearing 5–7 leaves) sizes have been found to be significantly different from small (3–4 cm long bearing 2–3 leaves) microplants (de Oliveira et al., 2009).
Supe (2006) demonstrated the need for direct sunlight throughout the acclimatization of micropropagated aloe. Hosseini and Parsa (2007) used compost, loam, and clay with 1:2:2 ratios as a substrate for acclimatization, where the plants were covered with transparent plastic pots to retain humidity throughout the process.
More than 2000 well-acclimatized plants were produced over a period of 6 months from five explants (Meyer and Van Staden, 1991).
Notes on the agronomical performance of ex vitro plantlets
The composition of aloe gel depends upon the method of propagation. Pandhair et al. (2011) showed that total sugars, fructose, sucrose, starch and phenol content were higher in micropropagated leaf gel as compared to sucker-propagated plants. Addition of 20 mg L−1 tryptophan induced a 2.43-fold increase in aloin content in multiple shoots cultures (Sharma V. et al., 2013).
Lee et al. (2011) reported that aloe-emodin content was dramatically increased in the adventitious roots grown in B5 media to 20~40 fold higher than that in MS medium.
The Figure 3 shows the average number of total weeks from in vitro shooting to rooting, in different researches.
A more extended time reference, such as a very fast protocol, was proposed by Gantait et al. (2011), which enabled them to obtain 192 true to type plants from a single explant, with a rhizomatous stem, in a total period of 85 days from bud induction to the end of acclimation (Figure 4).
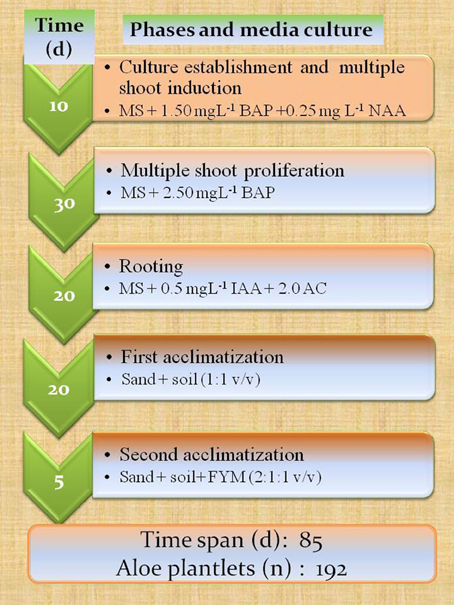
Figure 4. Fast mass propagation calendar: from multiple shoot formation to acclimatization phases. Results shown are adapted from Gantait et al. (2011). Teixeira da Silva (2012) reports that BA and BAP refer to the same cytokinin.
Singh et al. (2009) described a very cheap protocol on liquid substrate that starts from a single bud and produces 5000 plantlet in 180 days. Rathore et al. (2011b) transferred in fields under the environmental conditions 1900 tissue-cultured propagated plantlets.
The cost of a micropropagated plantlet ranges between 1.0 and 1.50 $ and is still too high for farmers in developing countries to purchase it on a large scale whereas the planting density is between 12,000 and 20,000 plants/ha.
Climatic and Soil Requirements
Although McKeown (1987) describes aloe as a subtropical species that is intolerant of low temperatures, an experiment in the Golan Heights (Israel) in 1995 showed that it can reach commercial size with an absolute minimum temperature of −3°C and up to 2 months with a temperature of ≤ 4°C (Saks and Ish-Shalom-Gordon, 1995).
Irradiance significantly affects plant development (Pedroza-Sandoval and Gómez-Lorence, 2006). In the cultivation of young plants, Paez et al. (2000) in Chile showed a direct correlation between partial shade (30% full sunlight) and yield: with 27% more leaves than plants under full sunlight.
Aloe is grown in all kinds of soil with a high organic matter content (Kent, 1980). Good drainage of the soil profile must be guaranteed to prevent puddling, which could cause root asphyxia and the spread of root apparatus diseases such as tracheo-micosis by both Fusarium oxysporum and Verticillum sp. (Ayodele and Ilondu, 2008). The channeling of drainage water must also prevent the formation of surface puddles during the rainy season.
Soil and Soilless Cultivation
Soil Cultivation
Transplant
In open fields under irrigated conditions transplant is carried out throughout the year, except in winter (Rajeswari et al., 2012). Land preparation is limited to the surface to prevent erosion, which is common in recent plantations. Rajeswari et al. (2012), in West Bengal area, reports that suckers are planted in roughly 15 cm deep pits, 60 × 60 cm apart, which are dug at the time of planting. After planting, the soil around the root zone must be firmly pressed and proper drainage must be ensured to prevent water stagnation. Saha et al. (2005) have shown that the optimal layout is with 100 cm apart rows and plants every 50 cm in the rows, with 20,000 plants per hectare. Murillo-Amador et al. (2007) reported, in Mexico, higher leaves and gel yield using 25,000 plants ha−1, with 90 cm between rows and 45 cm among plants, with a double quincunx row arrangement (Figure 5A).
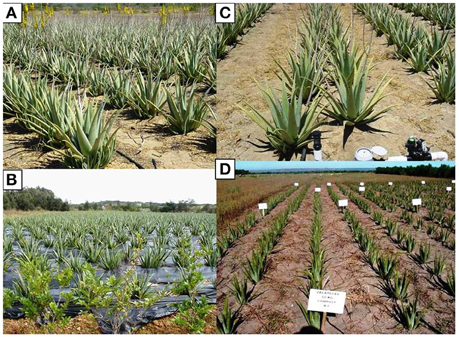
Figure 5. (A) Plantation densities as 20,000 plants ha−1 in the open field. (B) Mulching with black polyethylene film in the open field. (C) Drip irrigation system in open field cultivation. (D) Organic fertilization as manure compost. Photos taken by B. Murillo-Amador.
Hernández-Cruz et al. (2002) report that mulching the soil with a 100 μm thick black polyethylene film (Figure 5B) positively influences the gel content in the leaves.
Weed Management
According to CONAZA (1994) in areas that are less than one hectare, the manual method is the most common way to eliminate weeds in aloe plantations, which is preferred by farmers because the use of machinery decreases soil porosity, aeration and drainage. Generally, two weed eliminations per year after the rainy season are enough but in some regions farmers maintain weeds before the winter season in order to conserve microclimate conditions around the plants and thus protect them from low temperatures (Santos, 1995). Also, some farmers manually remove some parts of the plants such as suckers (5–10 cm) and the inflorescences to enable the plants to acquire energy for growth and to increase yield.
For aloe plants under warmer climate conditions and a rainfed cultivation system, four weed controls are necessary, three during the rainy season and the fourth during the dry season. Chemical control of weeds is not permitted by aloe industrial regulations. However, if the weeds are not controlled, the yield decreases and some climbing weeds can bind the leaves and damage them (Rodríguez-Canto, 1992). Most aloe growers avoid using chemical pesticides and herbicides and some let geese into the fields to eat the insects that are uncovered by plowing (Gage, 1996). According to Pedroza-Sandoval and Gómez-Lorence (2006), the weeds are most common in Aloe cultivated under irrigation systems and the type of weeds depends on the agricultural region of each country. When the aloe plants are small it is possible to use machinery to eliminate weeds, however this is not recommended when plants are growing, because the machinery can damage the plants.
The best method is to eliminate weeds manually. In fact, Gage (1996) affirms that a plants must be planted, cultivated and harvested by hand. In cultivated areas greater than one hectare, there are generally various alternative methods to eliminate weeds. For example, in some regions of Mexico, the farmers introduce goats and lambs to consume the weeds, since these animals do not feed on aloe. Another way to eliminate weeds it is to use padded plastic, which preserves the soil humidity, and controls weeds, pests and pathogens in the soil. In addition, the use of industrial aloe leaf waste, known as bagasse, is commonly used as an organic cover to prevent weeds. Bagasse also preserves the soil humidity and organic matter is incorporated to the soil. Although the use of chemical products to control weeds is not permitted in aloe, Pedroza-Sandoval and Gómez-Lorence (2006) report the use of glyphosate (Round-up®), while PLM (2015) report the use of atrazine (Atranex® 50% SC) as active ingredients to control weeds in aloe.
Water Management
CAM plants are characterized by the maintenance of a favorable water status through their ability to minimize transpiration by closing their stomata during the day and opening them at night when the vapor pressure deficit is low (Caird et al., 2007). Water loss is reduced by the nocturnal CO2 uptake with an efficient synthesis of sugars and osmolytes which allow water retention (Borland et al., 2009). Another common feature of CAM plants is succulence, characterized by cells with large vacuoles, called hydrenchyma; the internal water reserve in fleshy leaves enable aloe to survive dry seasons or cyclical droughts (Newton, 2004). The plant requires periodic rainfall or irrigation to replenish depleted reserves.
In open field cultivation, in arid and semi-arid climates, it is necessary to irrigate to ensure both continuous growth and gel production. An excessive volume of water is thus avoided, which could damage the collar and cause rotting.
A water deficit negatively influences the plant growth through a substantial reduction in the number of leaves and suckers (Genet and van Schooten, 1992). Winter et al. (2005) found that efficiency of water use, in potted aloe, can reach 54g H2O g−1 dry matter.
Rodríguez-García et al. (2007) found that low soil water content reduced the fresh weight, number of leaves and growth rate of the plants.
Silva et al. (2010) found that the water requirement of 15% of the reference evapotranspiration (ET0) led to the maximum biomass productivity.
Murillo-Amador et al. (2007) evaluated the effect of one irrigation volume (20 mm per hour), different times (1.5 and 3 h) and two intervals (once and twice per week) in the production of leaf biomass and gel in an arid zone of Mexico (Figure 5C). Their results showed that the production of leaves and gel increased when 20 mm per hour were applied for 3 h, once per week.
Nutrient Management
Fertility in agronomic management is one strategy to boost the aloe yield, however to reduce production costs aloe fields are often not fertilized. Today, considering the importance of environmental issues, increasing attention is being paid to different fertilizers and the methods of application of these organic and inorganic fertilizers. In Bangladesh, Hasanuzzaman et al. (2008) observed that aloe increases its number of leaves (+38%) and total leaf weight (+153%), with the application of 50% cowdung +50% soil, compared to the control (100% soil). García (2000) used one dose (4.6 t ha−1) of bio compost but with no clear effect on aloe growth. In Mexico, Murillo-Amador et al. (2007) showed that compost-based manure is effective and found that as the compost doses increased from 30 to 60 t ha−1, the leaves and gel yield increased (Figure 5D).
Nitrogen fertilizer may enhance both growth and yields because the nitrogen supply helps in the full expansion of the leaf, chlorophyll content, photosynthetic rates and subsequently increases the supply of carbohydrates to the plants (Khandelwal et al., 2009). The application of 150 kg N ha−1 resulted in an increased both number and size of leaves, and total yield (Bharadwaj, 2011).
Pedroza-Sandoval and Gómez-Lorence (2006) also reported the use of urea as an N source with one dose of 500 kg ha−1, and two applications per year.
The simultaneous application of nitrogen fertilizer and BA (1500 ppm), sprayed in the 17th week after planting, increases the number of leaves (Hazrati et al., 2012). The foliar application of amino acid had positive effects on the content of secondary metabolites, antioxidants, and antioxidant activity (Ardebili et al., 2012).
In order to make cultivation ever more competitive, the crop cycle should be reduced in order to obtain more rapid growth and improve quality. These results can be achieved with mycorrhizal inoculations. In many species, mycorrhizal symbiosis improves the transfer of nutritive elements to the roots, ensures greater plant growth, and stimulates the formation of a more dynamic root apparatus, making it more extensive and branched. In the literature, there are contrasting results regarding mycorrhizal applications in aloe (Tawaraya et al., 2007; Cardarelli et al., 2013; Sharma G. P. et al., 2013). This could be due to different cultivation environments, the species of fungi and the nitrogen fertilization regime. Colonization with Glomus intraradices and G. mosseae has a positive effect on the concentration of aloin and β polysaccharides (Cardarelli et al., 2013).
Soilless Cultivation
Container Cultivation
The cultivation of aloe in containers has also become a standard technique in central and southern Europe. The rooted sucker is transplanted into a plastic pot, 2.5–3.5 L in volume and re-potted after 6–9 months into a 24 L container. The “container system” is derived from the association of plant, substrate, fertilizer and irrigation. It leads to a higher quality leaf production with reduced production time. The conventional substrate is made from a mixture of peat and other mineral components (sand or pumice or perlite) in v:v 1:1. Despite the expensive cost of peat, its ever-decreasing availability due to environmental restrictions and the possibility of applying local by-products has led to the use of alternative organic materials such as compost.
Rea et al. (2008) evaluated the effect of the replacement (partially or totally) of peat, with increasing doses of green compost, derived from ornamental tree prunings. In the first half of cultivation, the replacement equaled the performances of both the plant height and diameter, and improved both leaf numbers and plant fresh weight (Table 4). In the second half, there were no significant differences between the substrates; plants did not show any phytotoxicity, and appeared disease free and with no weeds (Figure 6, Table 4).
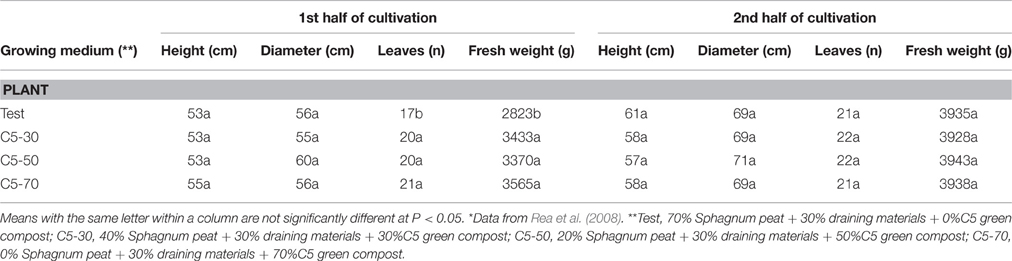
Table 4. Effect of growing media containing rates of peat (Test) and green wastes compost (C5) on some morphological traits in first and second half-year cultivation (*).
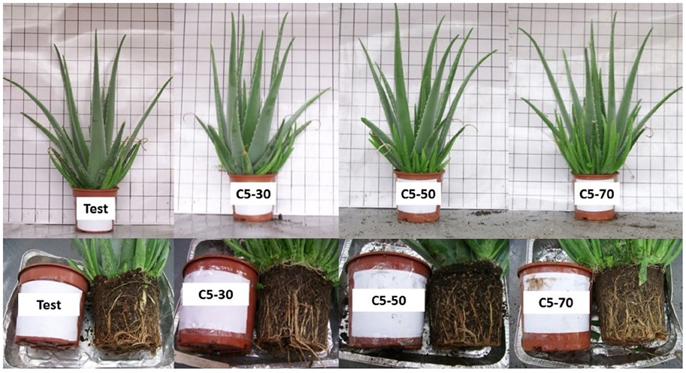
Figure 6. Potted aloes: comparison between peat cultivation (test) and increasing rates of green compost in the second half-year of cultivation. Photo taken by De Lucia.
Hydroponic Cultivation
One of the main constraints in hydroponic cultivation is to obtain a suitable nutrient solution with the highest yield and quality. However, the identification of optimal soilless practices such as nutrient solution preparation is critical in order to increase yield and quality. In A. vera, it may be possible to obtain higher yields of specific secondary metabolites or higher yields of target organs. Saliqehdar et al. (2013, 2014) developed a nutrient solution, using 9.7 meqL−1 NO3 and 5.8 meq·L−1 K, which produced the highest vegetative growth without negative effects on qualitative indices including aloin, total phenol, total antioxidative activity and element contents. Olfati et al. (2015) indicated that the NO3 supply combined with low quantities of favor aloe growth.
Abiotic Stresses and Bioactive Molecules
In arid and semi-arid areas, sustainable agriculture, should aim to store water. Aloe is naturally adapted to dry conditions and high temperatures. In the Atacama Desert of Chile, which is one of the most arid regions of the world, the plants irrigated with 75% field capacity presented the best water use efficiency in terms of dry mass and amount of gel produced by a liter of supplied water. The osmotic adjustment is highlighted by an increase in the synthesis of proline, total soluble sugars as well as polymers of β- (2-6) kestotriose (Delatorre-Herrera et al., 2010).
Saline stress is a limiting factor for production and growth, and is becoming a serious problem in many parts of the world. Fortunately, A. vera grows well on saline soils of beach areas in tropical and subtropical xerophytic conditions (Rahi et al., 2013).
Several NaCl concentrations or sea water have been used to evaluate aloe salt tolerance. Jin et al. (2007) studied the physiological response to saline stress (EC: 23 dS m−1) which causes a decrease in the water content in the plant tissues as well as in total soluble sugars and glucose. Rahimi-Dehgolan et al. (2012) irrigated plants with fresh (EC control: 0.4 dS m−1) or saline lake water (EC: 3, 6, 9, 12, 15, 18, 21 dS m−1): irrigation with saline lake water up to 9 dS m−1, increased the concentration of glucose, xylose and mannose in the leaf gel.
Murillo-Amador et al. (2014) reported that Na and Cl ions accumulated in the cells of roots, stems, leaves and sprouts, thus showing higher Na and Cl contents in sprouts followed by leaves, stems and roots.
Murillo-Amador et al. (2015) found that using moderate salt stress (0, 30, 60, 90, and 120 mM NaCl) instead of typical high salt stress (>200 mM) offers a new perspective for directly studying the salinity-resistant mechanisms of desert plants and may lead to new salt-resistant cultivars. This prevents damage under NaCl treatments, thus increasing the agronomic value of aloe plants, making this species attractive for industrial production in arid or semiarid areas with moderate salinity. Murillo-Amador et al. (2015) also found that the relative water content of aloe plants shows values close to 98%, which demonstrates that the water content is not significantly affected in the leaves of plants growing under this regime of moderate salt stress. The accumulation of soluble carbohydrates as osmolytes in plants has been widely reported as a response to salinity or drought. Soluble sugars prevent protein dehydration because of turgor pressure (Crowe et al., 1990). Aloin concentration was found to increase with salt stress up to 15 dS m−1 whichdecreased at higher salinity concentrations, perhaps due to the low water content in leaves. Proline and PEP-case increased as salinity increased in both parenchyma and chlorenchyma of aloe under NaCl stress of 0, 30, 60, 90, and 120 mM, while total protein increased in parenchyma and decreased in chlorenchyma. The increase in protein, proline and PEP-case activity, as well as the absorption and accumulation of cations under moderate NaCl stress caused osmotic adjustment, which kept the plant healthy (Murillo-Amador et al., 2014).
Under NaCl stress, Xu et al. (2006) demonstrated that the addition of silicate (Si) to a nutrient solution significantly decreased the Na and Cl contents in aloe roots, stems and leaves, while K content and K/Na ratio increased significantly. The addition of Si enhanced the selectivity of roots to K absorption and the selectivity of stems and leaves to K translocation under NaCl stress.
Harvest and Post-Harvesting
Harvest
The older outer leaves are generally harvested, leaving the fresh and young leaves at the top. The plants can be removed manually or with the help of a tractor-drawn disc harrow or cultivator. New leaves grow from the center upward. Economic yields are obtained for 5 years, after which the Aloe needs replanting. Harvesting is a labor-intensive process. Only mature well developed leaves are collected, which are 60–80 cm in length and with a width at the base of around 8–10 cm. Typically, the outermost 3–4 leaves are harvested by pulling each leaf away from the plant stem. Leaves that show signs of tip necrosis should not be harvested, as these provide entry points for microbial contamination. If harvesting is done once a year, October-November is the best period. The second year gives the maximum yield, and for about 4–5 years a good yield can be harvested. Harvested leaves are carefully stacked and then transferred to a refrigeration or processing facility.
Employing good agronomic practices and with 20,000 plants ha−1 can provide two harvests per year (May and October). Each harvest involves the removal of, on average, four leaves and therefore approximately eight leaves per year−1 per plant−1. The mean weight of a mature fresh leaf reaches 500g, thus obtaining 4 kg plant−1, corresponding to approximately 80 ton ha−1 (Bassetti and Sala, 2003). With a commercial density of 10,000 plants ha−1 and similar edaphoclimatic conditions, the maximum productivity should reach 127 ton ha−1year−1, which is comparable to the value of 108 ton ha−1year−1 estimated for the production of green biomass in Opuntia ficus indica (Flores-Hernández et al., 2004). In a greenhouse experiment, Rodríguez-García et al. (2007) found a significant reduction in leaf biomass yield due to lower water availability. They estimated yields of 44.5–58.5 ton ha−1 year−1 for a density of 10,000 plants ha−1. In India, the average yield for organically grown aloe is about 12 ton ha−1 year−1 (Rajeswari et al., 2012).
Post-harvesting
Post-harvest, aloe leaves behave differently depending on the region or country and according to the level of technology used in each aloe plantation. The gel or juice of Aloe leaves can be extracted from the complete leaf, i.e., including the yellow sap commonly called the “acibar” (Pedroza-Sandoval and Gómez-Lorence, 2006) or extracted in a way that is similar to how a fish is fileted, and the outside ring is removed, exposing the gel inside (Gage, 1996). The most precious commodity of the aloe plant is the juice, made from the clear gel stored inside the leaf. All reports of post-harvesting activities (Rodríguez-Canto, 1992; CONAZA, 1994; Gage, 1996; Granados-Sánchez and Castañeda-Pérez, 1998; Pedroza-Sandoval and Gómez-Lorence, 2006) refer to washing of the leaves using tap water alone or mixed with detergent, chloride, quaternary ammonium salts, sodium hypochlorite etc. Some processing plants also use manual or mechanized brushes to clean the leaves.
The main stages of the process of post-harvesting include: reception at processing plant, unloading, weighing, storage, washing 1, washing 2, removal of tips and bottoms, fileting, and removing the outside ring. Many aloe manufacturers use machines to filet the leaves, others believe that hand fileting produces the cleanest gel. After the leaves have been fileted, the inner jelly-like pulp is ground by a blender. The aloe is then reduced to a near-liquid state. Fresh aloe degrades when exposed to air, thus the gel must be treated to prevent oxidation and loss of the plant's vitamins, minerals, amino acids, and other ingredients.
Gel Processing
Most companies stabilize the gel by pasteurization and the addition of preservatives (sodium benzoate). However, processing techniques vary from company to company, region-to-region, and country to country, i.e., some companies use a fugitive sterilizing agent that sterilizes the product without any chemical reaction with aloe, and the agent is then completely removed, all without the need for any heat (Gage, 1996). Other preservation methods include keeping it under refrigeration or vacuum sealed in a can. After fileting, some companies dehydrate the leaves using hot air, after which they are ground by a blender and the dehydrated or granulated gel is packed to sell to other industries (Rodríguez-Canto, 1992).
One key issue with aloe juice is that it loses its nutritional quality after only 2 or 3 h. It thus needs to be stabilized by techniques such as hydrogen peroxide oxidation, ultraviolet rays in the presence of chemical catalysts, heat treatment (1.5–2 h), high temperatures (71–77°C) for 3 min, and quenching-pasteurization (Álvarez, 1987). After stabilization, the processed aloe gel may be filtered to remove the pulp, depending on the type of product the company makes. Some companies use activated carbon to discolor aloe juice so that the red color and initial bitterness of the juice are reduced. For cosmetics and some juice drinks, filtered aloe is preferred, but some people like unfiltered drinks because the pulp is a good source of fiber. After being filtered, some companies lyophilize the gel with high vacuum treatment at low temperatures to remove the moisture, thus obtaining a white-gray powder (freeze-dried gel). This aloe product has a high concentration since when 1 kg is rehydrated, 200 kg of filtered juice are obtained (Rodríguez-Canto, 1992; Pedroza-Sandoval and Gómez-Lorence, 2006).
Cosmetic quality gel is obtained after pasteurization, by centrifuging the juice. This gel is the main ingredient for shampoos, creams and body or face soaps (Pedroza-Sandoval and Gómez-Lorence, 2006). The other aloe gel category is food grade (liquid concentrate), which is the basis for certain dietetic foods (Gage, 1996) with preservatives to prevent deterioration by pollution.
Quality control is typically high in all aloe companies, and the product is tested for various properties such as color, viscosity, odor, Brix degrees, total solids, precipitable solids in methanol, sediment percentage, foreign matter, microbiological analysis such as plate full account, fungi and bacteria account, pathogens and coliforms, and pH balance (Pedroza-Sandoval and Gómez-Lorence, 2006).
Conclusions
The pharmaceutical, cosmetics, and food industries are showing ever-increasing interest in, the expansion of aloe cultivation, as an alternative to traditional crops, even in arid and semi-arid environments.
Achieving high yield in leaves and gel quality requires an integrated approach from pre cultivation, to cultivation and post cultivation.
Further research is needed with regard to:
✓ Increasing the efficiency of the protocols in vitro cultures via the callus;
✓ Assessing the eco-physiological response on marginal lands with low quality water and low supply of organic matter to compost;
✓ Improving, in soilless cultivation, the content of bioactive molecules in leaves;
✓ Preserving and maintaining the bioactive chemical entities naturally present in the leaf in processing systems.
Author Contributions
Review conception: BD, GC. Wrote, edited and revised the paper: BD, GC, BM. Critical revision: BD, GC, BM. Approved the final version of the manuscript to be published: BD, GC, BM.
Conflict of Interest Statement
The authors declare that the research was conducted in the absence of any commercial or financial relationships that could be construed as a potential conflict of interest.
Abbreviations
2,4-D, 2,4 dichlorophenoxy acetic acid; AC, activated charcoal; ADS, Adenine sulfate; B5, Gamborg medium culture; BA, N6-benzyladenine; BAP, benzyl aminopurine; CAM, crassulacean acid metabolism; FYM, farm yard manure; IAA, Indole-3-acetic acid; IBA, Indole-3-butyric acid; Kin, (Kinetin) 6-furfurylaminopurine; MS, Murashige and Skoog medium culture; NAA, α-napthalene acetic acid; PGRs, plant growth regulators; PVP, Polyvinyl pyrolidine; SH, Sckenk and Hildebrant medium culture.
References
Abadi, D. H., and Kaviani, B. (2010). In vitro proliferation of an important medicinal plant Aloe -A method for rapid production. Aust. J. Crop Sci. 4, 216–222.
Abdi, G., Hedayat, M., and Modarresi, M. (2013). In vitro micropropagation of Aloe vera - Impacts of plant growth regulators, media and type of explants. J. Biol. Environ. Sci. 7, 19–24.
Abrie, A. L., and Van Staden, J. (2001). Micropropagation of the endangered Aloe polyphylla. Plant Growth Regul. 33, 19–23. doi: 10.1023/A:1010725901900
Adelberg, J., and Fári, M. G. (2010). Applied physiology and practical bioreactors for plant propagation. Propag. Ornam. Plants. 10, 205–219.
Adelberg, J., and Naylor-Adelberg, J. (2012). Effects of cytokinin on multiplication and rooting of Aloe barbadensis during micropropagation on agar and liquid medium. J. Med. Active Plants. 1, 1–5. doi: 10.7275/R5HH6H0V
Aggarwal, D., and Barna, K. S. (2004). Tissue culture propagation of elite plant of Aloe vera Linn. J. Plant Biochem. Biot. 13, 77–79. doi: 10.1007/BF03263197
Ahlawat, K. S., and Khatkar, B. S. (2011). Processing, food applications and safety of aloe vera products: a review. J. Food Sci. Technol. 48, 525–533. doi: 10.1007/s13197-011-0229-z
Ahmed, S., Kabir, A. H., Ahmed, M. B., Razvy, M. A., and Ganesan, S. (2007). Development of rapid micropropagation method of Aloe vera L. Sjemenarstvo. 24, 121–128.
Alagukannan, G., and Ganesh, S. (2016). Genetic diversity in Aloe and its breeding strategies. Int. J. Farm Sci. 6, 312–326.
Álvarez, M. G. (1987). Estudio de Viabilidad Técnica y Financiera Del Cultivo de Sábila (Aloe vera L.) en la Zona Centro de Tamaulipas. Tesis de Maestría en Ciencias. Universidad Autónoma de Tamaulipas, Cd. Victoria.
Amoo, S. O., Aremu, A. O., and Van Staden, J. (2014). Unraveling the medicinal potential of South African Aloe species. J. Ethnopharmacol. 153, 19–41. doi: 10.1016/j.jep.2014.01.036
Ardebili, Z. O., Moghadam, A. R. L., Ardebili, N. O., and Pashaie, A. R. (2012). The induced physiological changes by foliar application of amino acids in Aloe vera L. plants. Plant Omics 5, 279–284.
Ayodele, S. M., and Ilondu, E. M. (2008). Fungi associated with base rot disease of Aloe vera (Aloe barbadensis). Afr. J. Biotechnol. 7, 4471–4474.
Belmin, R., Mulindo, C., Welimo, M., Kamau, G., and Triomphe, B. (2013). “Building on illegal value chains to achieve sustainable management of natural resources? The case of indigenous aloe exploitation and trade in Baringo, Kenya,” in Rural Resilience And Vulnerability: the Rural as Locus of Solidarity and Conflict in Times of Crisis (Florence), 382.
Bharadwaj, R. L. (2011). Effect of water regimes, nitrogen and phosphorus on crop growth, yield and economics of aloe. Indian J. Agr. Sci. 81, 44–49.
Biswas, B. C. (2010). Cultivation of medicinal plant. Indian Fertilizer Marketing News, Vol. 41, 1–4.
Borgognone, D., Marcucci, A., Colla, G., Renzaglia, M., Rea, E., and Cardarelli, M. (2013). In vitro growth of Aloe barbadensis Mill: The effect of activated charcoal on medium pH, nitrogen uptake and elements content of shoots. Propag. Ornam. Plants 13, 138–145.
Borland, A. M., Griffith, H., Hartwell, H., and Smith, A. C. (2009). Exploiting the potential plants with crassulacean acid metabolism for bioenergy production on marginal land. J. Exp. Bot. 10, 2879–2896. doi: 10.1093/jxb/erp118
Botes, C., Johnson, S. D., and Cowling, R. M. (2009). The birds and the bees: using selective exclusion to identify effective pollinators of African tree aloes. Int. J. Plant Sci. 170, 151–156. doi: 10.1086/595291
Boudreau, M. D., and Beland, F. A. (2006). An evaluation of the biological and toxicological properties of Aloe barbadensis (Miller), Aloe vera. J. Environ. Sci. Heal. C. Environ. Carcinog. Ecotoxicol. Rev. 24, 103–154. doi: 10.1080/10590500600614303
Caird, M. A., Richards, J. H., and Donovan, L. A. (2007). Nighttime stomatal conductance and transpiration in C3 and C4 plants. Plant Physiol. 143, 4–10. doi: 10.1104/pp.106.092940
Campestrini, L. H., Kuhnen, S., Lemos, P. M. M., Bach, D. B., Dias, P. F., and Maraschin, M. (2006). Cloning protocol of Aloe vera as a study-case for “Tailor-Made” biotechnology to small farmers. J. Technol. Manage. Innov. 1, 76–79.
Cardarelli, M., Cardona Suárez Carolina, M., and Colla, G. (2014). Influence of ozone treatments on in vitro propagation of Aloe barbadensis in continuous immersion bioreactor. Ind. Crop Prod. 55, 194–201. doi: 10.1016/j.indcrop.2014.02.020
Cardarelli, M., Rouphael, Y., Rea, E., Lucini, L., Pellizzoni, M., and Colla, G. (2013). Effects of fertilization, arbuscular mycorrhiza, and salinity on growth, yield, and bioactive compounds of two Aloe species. Hortscience 48, 568–575.
Castorena-Sanchez, I., Natali, L., and Cavallin, A. (1988). In vitro culture of Aloe barbadensis Mill: morphogenetic ability and nuclear DNA content. Plant Sci. 55, 53–59. doi: 10.1016/0168-9452(88)90041-6
Chang, X. L., Chen, B. Y., and Feng, Y. M. (2011). Water-soluble polysaccharides isolated from skin juice, gel juice and flower of Aloe vera Miller. J. Taiwan Inst. Chem. E. 42, 197–203. doi: 10.1016/j.jtice.2010.07.007
Chun-hui, L., Chang-hai, W., Zhi-liang, X., and Yi, W. (2007). Isolation, chemical characterization and antioxidant activities of two polysaccharides from the gel and the skin of Aloe barbadensis Miller irrigated with sea water. Process Biochem. 42, 961–970. doi: 10.1016/j.procbio.2007.03.004
CONAZA (Comisión Nacional de Zonas Áridas) (1994). La Sábila, Cultivo Alternativo Para Zonas Áridas y Semiáridas. Saltillo.
Crowe, J. H., Carpenter, J. F., Crowe, L. M., and Anchrology, T. J. (1990). Are freezing and dehydration similar stress vectors? A comparison of modes of interaction of stability solutes within biomolecules. Cryobiology 27, 219–231. doi: 10.1016/0011-2240(90)90023-W
Das, A., Haque, S. M., Ghosh, B., Nandagopal, K., and Jha, T. B. (2016). Morphological and genetic characterization of micropropagated field grown plants of Aloe vera L. Plant Tiss. Cult. Biotechnol. 25, 231–246. doi: 10.3329/ptcb.v25i2.26257
Das, A., Mukherjee, P., and Jha, T. B. (2010). High frequency micropropagation of Aloe vera L. Burm. f. as a low cost option towards commercialization. Plant Tiss. Cult. Biotechnol. 20, 29–35. doi: 10.3329/ptcb.v20i1.5962
Das, N., and Chattopadhay, R. N. (2004). Commercial cultivation of Aloe. Nat. Prod. Radiance 3, 85–87.
Das, P., and Srivastav, A. K. (2015). Phytochemical Extraction and Characterization of the Leaves of Aloe vera barbadensis for its Anti-Bacterial and Anti-Oxidant Activity. Int. J. Sci. Res. 4, 658–661.
de Oliveira, E. T., Crocomo, O. J., Farinha, T. B., and Gallo, L. A. (2009). Large-scale micropropagation of Aloe vera. HortScience 44, 1675–1678.
Delatorre-Herrera, J., Delfino, I., Salinas, C., Silva, H., and Cardemil, L. (2010). Irrigation restriction effects on water use efficiency and osmotic adjustment in Aloe vera plants (Aloe barbadensis Miller). Agric. Water Manage. 97, 1564–1570. doi: 10.1016/j.agwat.2010.05.008
De Lucia, B., and Lucini, L. (2013). Production, quality and health benefits of Aloe. Italus Hortus 20, 20–26.
Dirbaba, T. D. (2014). Aloe Soap Value Chain Initiative and Its Effect On Livelihood Diversification Strategy: The Case of Pastoralists and Agro-Pastoralists of Borana, Southern Ethiopia. Opendoc: 1–52.
Eshun, K., and He, Q. (2004). Aloe vera: a valuable ingredient for the food, pharmaceutical and cosmetic industries—a review. Crit. Rev. Food Sci. 44, 91–96. doi: 10.1080/10408690490424694
Flores-Hernández, A., Orona-Castillo, I., Murillo-Amador, B., García-Hernández, J. L., and Troyo-Diéguez, E. (2004). Yield and physiological traits of prickly pear cactus ‘nopal’ (Opuntia spp.) cultivars under drip irrigation. Agr. Water Manage. 70, 97–107. doi: 10.1016/j.agwat.2004.06.002
Gamborg, O. L., Miller, R. A., and Ojima, K. (1968). Nutrient requirements of suspension cultures of soybean root cells. Exp. Cell Res. 50, 151–158. doi: 10.1016/0014-4827(68)90403-5
Gantait, S., Mandal, N., Bhattacharyya, S., and Das, P. K. (2010). A novel strategy for in vitro conservation of Aloe vera L. through long term shoot culture. Biotechnology 9, 326–331. doi: 10.3923/biotech.2010.326.331
Gantait, S., Mandal, N., and Das, P. K. (2011). In vitro accelerated mass propagation and ex vitro evaluation of Aloe vera L. with aloin content and superoxide dismutase activity. Nat. Product Res. 25, 1370–1378. doi: 10.1080/14786419.2010.541885
Gantait, S., Sinniah, U. R., and Das, P. K. (2014). Aloe vera: a review update on advancement of in vitro culture. Agr. Scand. B Soil Plant Sci. 64, 1–12. doi: 10.1080/09064710.2013.868924
García, R. A. (2000). Informació Sobre El Cultivo de la Sábila. SAGARPA-Distrito de Desarrollo Rural No. 8. 2.
Garro-Monge, G., Gatica, A. M., and Melara, M. V. (2008). Somatic embryogenesis, plant regeneration and acemannan detection in aloe (Aloe barbadensis Mill.). Agron Costarric. 32, 41–52.
Genet, W. B., and van Schooten, C. A. (1992). Water requirement of Aloe vera in a dry Caribbean climate. Irrigation Sci. 13, 81–85. doi: 10.1007/BF00193984
George, E. F., Hall, M. A., and De Klerk, G.-J. (eds) (2008). Plant Propagation by Tissue Culture, Vol. 1, The Background, 3rd Edn. Dordrecht: Springer.
Ghate, V. S., Nagarka, R. S., and Sane, H. (2002). Floral polymorphism and fruiting in Kumari (Aloe vera). J. Med. Aromat. Plant Sci. 24, 698–702.
Grace, O. M. (2011). Current perspectives on the economic botany of the genus Aloe L. (Xanthorrhoeaceae). S. Afr. J. Bot. 77, 980–987. doi: 10.1016/j.sajb.2011.07.002
Grace, O. M., Buerki, S., Symonds, M. R., Forest, F., van Wyk, A. E., Smith, G. F., et al. (2015). Evolutionary history and leaf succulence as explanations for medicinal use in aloes and the global popularity of Aloe vera. BMC Evol. Biol. 15, 29. doi: 10.1186/s12862-015-0291-7
Grace, O. M., Simmonds, M. S., Smith, G. F., and van Wyk, A. E. (2009). Documented utility and biocultural value of Aloe L. (Asphodelaceae): a review. Econ. Bot. 63, 167–178. doi: 10.1007/s12231-009-9082-7
Granados-Sánchez, D., and Castañeda-Pérez, A. D. (1998). Sábila (Aloe barbadensis Mill.): Planta Agroindustrial (Medicinal) del Desierto. Universidad Autónoma Chapingo. Apoyos Académicos No. 2. Texcoco, 50.
Gui, Y. L., Xu, T. Y., Gu, S. R., Liu, S. Q., Zhang, Z. H. A. O., Sun, G. D., et al. (1990). Studies on stem tissue culture and organogenesis of Aloe vera. Acta Bot. Sin. 32, 606–610.
Gupta, S., Bharti, U., and Sharma, N. (2015). Seedlessness in Aloe vera L. - Role of environmental factors in failure to harness benefits of sexual reproduction. Int. J. Pharm. Bio Sci. 6, 294–305.
Gupta, S., Sahu, P. K., Sen, D. L., and Pandey, P. (2014). In-vitro Propagation of Aloe vera (L.) Burm. f. Br. Biotechnol. J. 4, 806–816. doi: 10.9734/BBJ/2014/9747
Gutterman, Y., and Chauser-Volfson, E. (2007). Secondary phenol metabolites (SPhMs), distribution and content of some Aloe species, originated from arid zones of South Africa: a review. Am. J. Food Technol. 2, 555–569. doi: 10.3923/ajft.2007.555.569
Hasanuzzaman, M., Ahamed, K. U., Khalequzzaman, K. M., Shamsuzzaman, A. M. M., and Nahar, K. (2008). Plant characteristics, growth and leaf yield of Aloe vera as affected by organic manure in pot culture. Aust. J. Crop Sci. 2, 158–163.
Hashemabadi, D., and Kaviani, B. (2008). Rapid micro-propagation of Aloe vera L. via shoot multiplication. Afr. J. Biotechnol. 7, 1899–1902. doi: 10.5897/AJB2008.000-5038
Hazrati, S., Sarvestani, Z. T., and Salehi, A. (2012). The effect of differential nitrogen fertilization on morphological and physiological traits of Aloe vera plants. Int. Res. J. Appl. Bas. Sci. 3, 682–687.
Hernández-Cruz, L. R., Rodríguez-García, R., Jasso, R. D., and Angulo-Sánchez, J. L. (2002). “Aloe vera response to plastic mulch and nitrogen,” in Trends in New Crops and New Uses, eds J. Janick and A. Whipkey (Alexandria, VA: ASHS Press), 570–574.
Hosseini, R., and Parsa, M. (2007). Micropropagation of Aloe vera L. grown in south Iran. Pak. J. Biol. Sci. 10, 1134–1137. doi: 10.3923/pjbs.2007.1134.1137
Jin, Z. M., Wang, C. H., Liu, Z. P., and Gong, W. J. (2007). Physiological and ecological characters studies on Aloe vera under soil salinity and seawater irrigation. Process Biochem. 42, 710–714. doi: 10.1016/j.procbio.2006.11.002
Kanwar, K., Joseph, J., and Deepika, R. (2010). Comparison of in vitro regeneration pathways in Punica granatum L. Plant Cell Tiss. Org. Cult. 100, 199–207. doi: 10.1007/s11240-009-9637-4
Kanwar, K., Devi, V., Sharma, S., Soni, M., and Sharma, D. (2015). Effect of physiological age and growth regulators on micropropagation of Aloe vera followed by genetic stability assessment. Natl. Acad. Sci. Lett. 38, 29–35. doi: 10.1007/s40009-014-0293-9
Khandelwal, S. K., Jain, M., Choudhary, M. R., and Gupta, K. N. (2009). Effect of nitrogen and spacing on growth and yield of Indian aloe (Aloe barbadensis L.). J. Med. Aromatic Plant Sci. 31, 203–205.
Kumar, M., Singh, S., and Singh, S. (2011). In vitro morphogenesis of a medicinal plant-Aloe vera L. Asian J. Plant Sci. Res. 1, 31–40.
Kumari, A., and Naseem, M. (2015). An efficient protocol for micropropagation of a medicinal plant Aloe Vera L. through organ culture. J. Indian bot. Soc. 94, 118–125.
Lee, Y. S., Park, H. M., Park, S. U., Baek, J. H., and Yang, T. J. (2013). Optimal protocol for mass propagation of Aloe vera. J. Crop Sci. Biotechnol. 16, 285–290. doi: 10.1007/s12892-013-0099-1
Lee, Y. S., Yang, T. J., Park, S. U., Baek, J. H., Wu, S., and Lim, K. B. (2011). Induction and proliferation of adventitious roots from Aloe vera leaf tissues for in vitro production of aloe-emodin. Plant Omics J. 4, 190.
Liao, Z., Chen, M., Tan, F., Sun, X., and Tang, K. (2004). Microprogagation of endangered Chinese aloe. Plant Cell. Tiss. Org. 76, 83–86. doi: 10.1023/A:1025868515705
Liontakis, A., and Tzouramani, I. (2016). Economic sustainability of organic Aloe Vera farming in greece under risk and uncertainty. Sustainability 8, 338. doi: 10.3390/su8040338
Lucini, L., Pellizzoni, M., Pellegrino, R., Molinari, G. P., and Colla, G. (2015). Phytochemical constituents and in vitro radical scavenging activity of different Aloe species. Food Chem. 170, 501–507. doi: 10.1016/j.foodchem.2014.08.034
Magyar-Tábori, K., Dobránszki, J., da Silva, J. A. T., Bulley, S. M., and Hudák, I. (2010). The role of cytokinins in shoot organogenesis in apple. Plant Cell Tiss. Org. Cult. 101, 251–267. doi: 10.1007/s11240-010-9696-6
Meyer, H. J., and Van Staden, J. (1991). Rapid in vitro propagation of Aloe barbadensis Mill. Plant Cell. Tiss. Org. 26, 167–171. doi: 10.1007/BF00039939
Molsaghi, M., Moieni, A., and Kahrizi, D. (2014). Efficient protocol for rapid Aloe vera micropropagation. Pharm. Biol. 52, 735–739. doi: 10.3109/13880209.2013.868494
Murashige, T., and Skoog, F. (1962). A revised medium for rapid growth and bioassay with tobacco tissues cultures. Physiol. Plant.15, 473–497. doi: 10.1111/j.1399-3054.1962.tb08052.x
Murillo-Amador, B., Córdoba-Matson, M. V., Villegas-Espinoza, J. A., Hernández-Montiel, L. G., Troyo-Diéguez, E., and García-Hernández, J. L. (2014). Mineral content and biochemical variables of Aloe vera L. under salt stress. PLoS ONE 9:e94870. doi: 10.1371/journal.pone.0094870
Murillo-Amador, B., Luna-Cisneros, M. J., Nieto-Garibay, A., Aguilar-García, M., Troyo-Diéguez, E., García-Hernández, J. L., et al. (2007). Manual Para la Producción de Sábila en el Valle del Carrizal. La Paz: Centro de Investigaciones Biológicas del Noroeste, 52.
Murillo-Amador, B., Nieto-Garibay, A., Troyo-Diéguez, E., García-Hernández, J. L., Hernández-Montiel, L., and Valdez-Cepeda, R. D. (2015). Moderate salt stress on the physiological and morphological traits of Aloe vera L. Bot. Sci. 93, 639–648. doi: 10.17129/botsci.73
Natali, L., Sanchez, I. C., and Cavallini, A. (1990). In vitro culture of Aloe barbadensis Mill.: Micropropagation from vegetative meristems. Plant Cell. Tiss. Org. 20, 71–74.
Nayanakantha, N. M. C., Singh, B. R., and Gupta, A. K. (2010a). Assessment of genetic diversity in Aloe germplasm accessions from India using RAPD and morphological markers. Ceylon J. Sci. (Bio. Sci.) 39, 1–9. doi: 10.4038/cjsbs.v39i1.2315
Nayanakantha, N. M. C., Singh, B. R., and Kumar, A. (2010b). Improved culture medium for micropropagation of Aloe vera L. Trop. Agric. Res. Ext. 13, 87–93.
Newton, L. E. (2004). “Aloes in habitat,” in Aloes The Genus Aloe, ed T. Reynolds (Boca Raton, FL: CRC Press), 3–36.
Oldfield, S. A. (2004). “Review of significant trade: East African Aloes,” in 14th Meeting of the CITES Plants Committee, Windhoek.
Olfati, J. A., Saadatian, M., and Moqbeli, E. (2015). Optimization of nitrogen and potassium for Aloe vera (L.) Burm. f. in a soilless culture system. S. Afr. J. Plant Soil. 32, 249–252. doi: 10.1080/02571862.2015.1019377
Paez, A., Gebre, G. M., Gonzalez, M. E., and Tschaplinski, T. J. (2000). Growth, soluble carbohydrates, and aloin concentration of Aloe vera plants exposed to three irradiance levels. Environ. Exp. Bot. 44, 133–139. doi: 10.1016/S0098-8472(00)00062-9
Pandhair, V., Diviya, J., Joginder, S., and Gosal, S. S. (2011). Biochemical studies of Aloe vera (Aloe barbadensis Miller) gel of the field grown and micropropagated plants at different developmental stages. J. Plant Biochem. Biotechnol. 20, 283–287. doi: 10.1007/s13562-011-0040-y
Pedroza-Sandoval, A., and Gómez-Lorence, F. (2006). La Sabila (Aloe spp.): Propiedades, Manejo Agronómico, Proceso Agroindustrial y de Mercado. Chapingo: Universidad Autonoma Chapingo.
Pushpan, R. (2012). Influence of harvesting and processing methods on the active principles of medicinal plants. Int. J. Pharm. Biol. Arch. 3, 1283–1286.
Rahi, T. S., Singh, K., and Singh, B. (2013). Screening of sodicity tolerance in Aloe vera: an industrial crop for utilization of sodic lands. Ind. Crop Prod. 44, 528–533.
Rahimi-Dehgolan, R., Tahmasebi Sarvestani, Z., Rezazadeh, S. A., and Dolatabadian, A. (2012). Morphological and physiological characters of Aloe vera subjected to saline water irrigation. J. Herbs Spices Med. Plants 18, 222–230. doi: 10.1080/10496475.2012.671802
Rajeswari, R., Umadevi, M., Rahale, C. S., Pushpa, R., Selvavenkadesh, S., Kumar, K. S., et al. (2012). Aloe vera: the miracle plant its medicinal and traditional uses in India. J. Pharmacogn. Phytochem. 1, 118–124.
Rathore, M. S., Chikara, J., Mastan, S. G., Rahman, H., Anand, K. G. V., and Shekhawat, N. S. (2011a). Assessment of genetic stability and instability of tissue culture-propagated plantlets of Aloe vera L. by RAPD and ISSR markers. Appl. Biochem. Biotechnol. 165, 1356–1365. doi: 10.1007/s12010-011-9352-6
Rathore, M. S., Chikara, J., and Shekhawat, N. S. (2011b). Plantlet regeneration from callus cultures of selected genotype of Aloe vera L. - an ancient plant for modern herbal industries. Appl. Biochem. Biotechnol. 163, 860–868. doi: 10.1007/s12010-010-9090-1
Rea, E., Pierandrei, F., Rinaldi, S., De Lucia, B., Vecchietti, L., and Ventrelli, A. (2008). Effect of compost-based alternative substrata in potted Aloe vera (L.) Burm. F. Acta Hort. 807, 541–546. doi: 10.17660/ActaHortic.2008.779.69
Rizwan, M., Soni, P., Kumar, R., Gupta, N. K., Shahzad, M., Singh, G., et al. (2014). High frequency regeneration protocol of Aloe vera L. Vegetos -an int. J. Plant Res. 27, 127–130. doi: 10.5958/2229-4473.2014.00022.6
Rodríguez-Canto, A. (1992). El Cultivo de la Sábila En Yucatán. Mérida: Universidad Autónoma Chapingo.
Rodríguez-García, R., Rodríguez, D., Gil-Marín, J. A., Angulo-Sánchez, J. L., and Lira-Saldivar, R. H. (2007). Growth, stomatal resistance, and transpiration of Aloe vera under different soil water potentials. Ind. Crop Prod. 25, 123–128. doi: 10.1016/j.indcrop.2006.08.005
Roy, S. C., and Sarkar, A. (1991). In vitro regeneration and micropropagation of Aloe vera L. Sci. Hortic. 47, 107–113.
Saggoo, M. I. S., and Kaur, R. (2010). Somaclonal variation in plants regenerated from cultures of two morphologically distinct accessions of Aloe vera Linn. Ann. Biol. Res. 1, 172–177.
Saha, R., Palit, S., Ghosh, B. C., and Mittra, B. N. (2005). Performance of Aloe vera as influenced by organic and inorganic sources of fertilizer supplied through fertigation. Acta Hortic. 676, 171–175. doi: 10.17660/ActaHortic.2005.676.22
Sahoo, S., and Rout, G. R. (2014). Plant regeneration from leaf explants of Aloe barbadensis Mill. and genetic fidelity assessment through DNA markers. Physiol. Mol. Biol. Plants 20, 235–240. doi: 10.1007/s12298-014-0226-6
Saks, Y., and Ish-Shalom-Gordon, N. (1995). Aloe vera L., a potential crop for cultivation under conditions of low-temperature winter and basalt soils. Ind. Crop Prod. 4, 85–90. doi: 10.1016/0926-6690(95)00019-9
Saliqehdar, F., Sedaghathoor, S., and Olfati, J. A. (2014). Nutrient solution on aloin content and other quality characteristics of Aloe vera. J. Med. Plants By Prod. 3, 59–62.
Saliqehdar, F., Sedaghathoor, S. S., and Olfati, J. (2013). Effects of four nutrient solutions on vegetative traits of Aloe vera L. cv. Austin at six harvest periods. J. Sci. Tech. Greenhouse Cult. 4, 15–27.
Santos, L. E. (1995). Aprovechamiento Actual de la Sábila (Aloe barbadensis Miller) y Aplicación del Análisis Dimensional en la Evaluación de Algunas Características Morfológicas. Tesis profesional. Unidad Regional Universitaria de Zonas Áridas. Universidad Autónoma Chapingo. Durango, México.
Schenk, R. U., and Hildebrandt, A. C. (1972). Medium and techniques for induction and growth of monocotyledonous and dicotyledonous plant cell cultures. Can. J. Bot. 50, 199–204. doi: 10.1139/b72-026
Sharma, D. K., Rai, S., Arora, S. S., Gupta, P. M., Sharma, R., and Chopra, A. K. (2011). Study of the trace elements in Aloe vera L. (Aloe barbandensis Miller) viz. Liliaceae and its biological and environmental importance. J. Chem. Pharm. Sci. 3, 64–68.
Sharma, G. P., Rani, A., Zala, D. A., Sain, M., Singh, A., and Rathore, S. (2013). Aloe barbadensis Miller a valuable ingredient for traditional uses and toxicological properties-A Review. Int. J. Rec. Biotechnol. 1, 48–54.
Sharma, V., Guleria, M., and Chaudhary, P. (2013). Enhanced in vitro caulogenesis and quantitative estimation of aloin through HPLC in Aloe vera. Israel J. Plant Sci. 61, 57–63. doi: 10.1080/07929978.2014.961388
Shekhawat, N. S., Rathore, M. S., Shekhawat, S., Choudhary, S. K., Phulwaria, M., Rai, M. K., et al. (2013). “Micropropagation of Aloe vera for improvement and enhanced productivity,” in Climate Change and Plant Abiotic Stress Tolerance, eds N. Tuteja and S. S. Gill (Weinheim: Wiley-VCH Verlag GmbH & Co. KGaA), 857–880. doi: 10.1002/9783527675265.ch32
Silva, H., Sagardia, S., Seguel, O., Torres, C., Tapia, C., Franck, N., et al. (2010). Effect of water availability on growth and water use efficiency for biomass and gel production in Aloe Vera (Aloe barbadensis M.). Ind. Crop Prod. 31, 20–27. doi: 10.1016/j.indcrop.2009.08.001
Singh, M., Rathore, M. S., Panwar, D., Rathore, J. S., Dagla, H. R., and Shekhawat, N. S. (2009). Micropropagation of selected genotype of Aloe vera L. - an ancient plant for modern industry. J. Sustain. Forest 28, 935–950. doi: 10.1080/10549810903344660
Singh, B., and Sood, N. (2009). Significance of explant preparation and sizing in Aloe vera L.—A highly efficient method for in vitro multiple shoot induction. Sci Hortic. 122, 146–151. doi: 10.1016/j.scienta.2009.03.023
Supe, U. (2006). In vitro propagation of Aloe barbadensis - a medicinal plant. Indian J. Agric. Biochem. 19, 19–21.
Supe, U. J. (2007). In vitro regeneration of Aloe barbadensis. Biotechnology 6, 601–603. doi: 10.3923/biotech.2007.601.603
Tascan, A., Adelberg, J., Tascan, M., Rimando, A., Joshee, N., and Yadav, A. K. (2010). Hyperhydricity and flavonoid content of Scutellaria species in vitro on polyester-supported liquid culture systems. Hortscience 45, 1723–1728.
Tawaraya, K., Turjaman, M., and Ekamawanti, H. A. (2007). Effect of arbuscular mycorrhizal colonization on nitrogen and phosphorus uptake and growth of Aloe vera L. Hortscience 42, 1737–1739.
Teixeira da Silva, J. A. (2012). Is BA (6-benzyladenine) BAP (6-benzylaminopurine)? Asian Australas. J. Plant Sci. Biotech. 6, 121–124.
Van Jaarsveld, E. (1989). The genus Aloe in South Africa with special reference to Aloe hereroensis. Veld Flora 75, 73–76.
Van Wyk, B. E., and Smith, G. (1996). Guide to the Aloes of South Africa. Pretoria: Briza Publications.
Winter, K., Aranda, J., and Holtum, J. A. (2005). Carbon isotope composition and water-use efficiency in plants with crassulacean acid metabolism. Funct. Plant Biol. 32, 381–388. doi: 10.1071/FP04123
Xu, C. X., Liu, Y. L., Zheng, Q. S., and Liu, Z. P. (2006). Silicate improves growth and ion absorption and distribution in Aloe vera under salt stress. J. Plant Physiol. Mol. Biol. 32, 73–78.
Keywords: abiotic stress tolerance, burn plant, in vitro propagation protocols, crop husbandry, leaf processing, leaf yield
Citation: Cristiano G, Murillo-Amador B and De Lucia B (2016) Propagation Techniques and Agronomic Requirements for the Cultivation of Barbados Aloe (Aloe vera (L.) Burm. F.)—A Review. Front. Plant Sci. 7:1410. doi: 10.3389/fpls.2016.01410
Received: 04 March 2016; Accepted: 05 September 2016;
Published: 23 September 2016.
Edited by:
Paul Christiaan Struik, Wageningen University and Research Centre, NetherlandsReviewed by:
Grama Nanjappa Dhanapal, University of Agricultural Sciences, Bengaluru, IndiaMargherita Irene Beruto, Istituto Regionale per la Floricoltura, Italy
Copyright © 2016 Cristiano, Murillo-Amador and De Lucia. This is an open-access article distributed under the terms of the Creative Commons Attribution License (CC BY). The use, distribution or reproduction in other forums is permitted, provided the original author(s) or licensor are credited and that the original publication in this journal is cited, in accordance with accepted academic practice. No use, distribution or reproduction is permitted which does not comply with these terms.
*Correspondence: Barbara De Lucia, YmFyYmFyYS5kZWx1Y2lhQHVuaWJhLml0