- 1Floriculture, Ornamental Horticulture and Garden Design Department, Faculty of Agriculture (El-Shatby), Alexandria University, Alexandria, Egypt
- 2Department of Geography, Environmental Management and Energy Studies, University of Johannesburg, Johannesburg, South Africa
- 3Department of Biological and Geological Sciences, Faculty of Education, Ain Shams University, Cairo, Egypt
- 4Botany Department, Faculty of Science, Tanta University, Tanta, Egypt
- 5Botany and Microbiology Department, College of Science, King Saud University, Riyadh, Saudi Arabia
- 6Timber Trees Research Department, Agriculture Research Center, Sabahia Horticulture Research Station, Horticulture Research Institute, Alexandria, Egypt
The negative effects of the ongoing climate change include unusual prolonged droughts and increased salinity pressures on the agricultural lands. Consequently, crops are facing unprecedented environmental pressure, and this calls for more research toward controlling such major stresses. The current study investigates the effects of seaweed extract sprays of Ascophyllum nodosum (5 and 7 mL·L−1; 6 day intervals) on Paspalum vaginatum Salam' during prolonged irrigation intervals (2 and 6 day) and saline growing conditions (1 and 49.7 dS·m−1) for 6 weeks in containers under greenhouse conditions. Control plants showed reduced turf quality, photochemical efficiency, root length and dry weight, total non-structural carbohydrates, and K and Ca compositions. Seaweed extracts increased turf quality, leaf photochemical efficiency, root length and dry weight, total non-structural carbohydrates, K, Ca, and proline in treated plants during prolonged irrigation intervals as well as saline shock conditions. There were also increases in the antioxidant defensive mechanisms such as catalase (CAT), superoxide dismutase (SOD) and ascorbate peroxidase (APX) activities and non-enzymatic antioxidants as well as reduced lipid peroxidation. The application of SWE at 7 mL·L−1 showed higher performance in treated plants during prolonged irrigation intervals as well as saline conditions. Our findings imply that several mechanisms including drought tolerance, osmotic adjustment and antioxidant defense system may interact to enhance the performance of plants in the face of environmental stress following SWE treatments.
Introduction
Drought and salinity driven stress are two major agricultural challenges (Ondrasek, 2014) particularly for turfgrasses (Huang et al., 2014). In the face of the ongoing global warming and the multiplicity of environmental stresses, several investigations have been conducted to understand how turfgrasses would perform physiologically in response to drought and salinity stresses (Bian et al., 2009; Elansary and Yessoufou, 2015; Krishnan and Merewitz, 2015). In general, recent investigations on few horticultural crops indicated that seaweed extracts might be useful in enhancing the performance of few crops in drought and salinity conditions (Xu and Leskovar, 2015; Elansary et al., 2016b). Seaweed extracts (SWE) are marine algal species routinely accessible at any coastal regions around the world, and can be used for several economic purposes including soil fertilization as well as human consumption. Although, the composition of SWE is dependent on the algal species used, environmental conditions and method of preparation, the overall composition reported in many studies include polysaccharides, minerals, amino acids, and plant hormones that influence the performance of treated plants (Khan et al., 2009; Sharma et al., 2013).
Ascophyllum nodosum (Fucaceae) is an important species used for SWE production, and is produced commercially under several names. The SWE from A. nodosum and other algae have been proven to enhance horticultural crops production and performance while increasing antioxidants potential (Kumar et al., 2013; Lola-Luz et al., 2014; Elansary et al., 2016a), product quality (Kumari et al., 2011; Spann and Little, 2011), freezing tolerance (Nair et al., 2012), salinity and drought tolerance (Neily et al., 2010; Spann and Little, 2011; Guinan et al., 2013; Elansary et al., 2016b; Martynenko et al., 2016). However, the test of SWE on turfgrass performance has been rarely investigated (e.g., Zhang and Ervin, 2004; Zhang et al., 2010), although turfgrasses comprise dozens of grass species and hundreds of cultivars or accessions that are key in our daily life, e.g., home lawns, parks, golf courses, and many others (Turgeon, 2008; Hanna et al., 2013). Further, the mechanisms behind positive effects of SWE during water and saline stress conditions had not been studied before for turfgrasses. Recent investigations on SWE indicated that the method and dose of application have significant impacts on plant performance (Xu and Leskovar, 2015; Elansary et al., 2016a) also plant responses to SWE are species dependent (Spann and Little, 2011; Elansary et al., 2016b).
Plants known as seashore paspalum (Paspalum vaginatum) are perennial warm-season turfgrasses commonly grown worldwide in temperate, tropical and subtropical regions; they show a great diversity in texture and relatively tolerate harsh growing conditions such as salinity, drought, and water logging (Hanna et al., 2013). Newly developed cultivars of seashore paspalum tolerate low mowing heights and infrequent potable water supply, and are therefore widely grown in golf courses worldwide such as Salam, Aloha, Sea Isle 1, and Seaspray. Nonetheless, how these cultivars perform in stress conditions is poorly documented (but see Shahba et al., 2012, 2014; Elansary and Yessoufou, 2015), and particularly the potential effect of SWE on their performance is yet to be explored.
In general, turfgrasses developed three major mechanisms to resist drought. Firstly, they develop drought tolerance ability in water deficit condition, and this is achieved through osmotic adjustment driven by accumulation of solutes such as K. Secondly, they develop drought avoidance by increasing root depth to pump water and by reducing transpiration rates. The last mechanism is drought escape achieved by shortening life cycle or initiating dormancy period while in drought conditions (Nilsen and Orcutt, 1996). Due to the lack of available potable water for irrigation, there is a global trend toward the use of non-potable water, and this usually result in the accumulation of salts and the rise of salinity-driven stress for plants. Turfgrasses resist salinity conditions using physiological and biochemical mechanisms (e.g., ion homeostasis of K, fluctuation of sugar composition and production of antioxidants that detoxifying reactive oxygen species or ROS), transcriptional regulation (e.g., upregulate or downregulate specific genes such as SOS) and proteomic and metabolic regulation (e.g., production of ROS scavenging proteins including SOD and others; Mittler, 2002; Zhu, 2003; Gupta and Huang, 2014; Hussain et al., 2016). However, it is still unclear how (i.e., the mechanisms) turfgrasses respond to SWE treatments aimed to improve its physiological performance in stress conditions.
To elucidate this stress-performance relationship, the present study explores the morphological, physiological, and biochemical mechanisms involved in prolonged irrigation intervals and salinity resistance of Paspalum vaginatum “Salam” under A. nodosum SWE treatment. Studied parameters included turf quality, maximum root length and root dry weight, photochemical efficiency, total non-structural carbohydrates, K and Ca contents, and proline accumulation. The antioxidant activities by several assays were also investigated. Our rationale is that the response of turfgrasses under SWE treatment in stress conditions is driven by a complex mechanism that requires interactions among morphological, physiological, and biochemical (e.g., antioxidant) parameters.
Materials and Methods
Plant Materials
Paspalum vaginatum Salam sod pieces were obtained from local commercial companies in January 2016 and 2017. The plant was identified by Dr. Hosam Elansary and deposited at the Department of Floriculture, Faculty of Agriculture, Alexandria University, Egypt. Sod pieces were grown in sterilized sandy soil mixed with Crystalon® (19% N: 19% P: 19% K, Chema Industries, Egypt) at 24 kg N·ha−1 in PVC containers (40 cm length × 20 cm diameter) located in a greenhouse along Al-Nubariah, Alexandria-Cairo desert road. For drought stress/prolonged irrigation intervals experiment, plants were maintained for 10 h natural light conditions (23–28°C/daytime and 15–20°C/night), photosynthetically active radiation of 1000 μmol·m−2.s−1 and weekly fertilization. Plants were mowed to a 45-mm height and watered for 100% evapotranspiration rate (ET). The height of 45-mm was maintained during experiments by regular mowing. The ET was then measured on the basis of the daily change of ET in five plants through watering them and then leaving them to dry for 1 h; next, they are weighed repeatedly every 24 h and the daily weight changes, expressing the daily ET, was recorded. Volumetric water content was measured for five containers before and during the experiment through measuring the weight before and after the irrigation and by allowing free draining for 1 h. The difference between oven-dry weight (at 105°C until constant weight) and the fresh weight was recorded as the volumetric water content.
Treatments
Drought Experiment
Plants were subjected to two watering intervals of 2 and 6 days (2DWI and 6DWI) for 6 weeks. Watering intervals were accompanied by a 6 days treatment of A. nodosum SWE as a foliar spray at 5 or 7 mL·L−1 using a hand sprayer until drop off (leaves only). Seaweed extracts Stella Maris™ were obtained from Acadian Seaplants, Canada. Untreated plants with SWE were considered as controls. SWE treatments followed watering and started 1 week ahead of water regimes application, and this took place from the beginning to the end of the experiment (Seven SWE applications). Plants were grouped into three plots and each plot contained (at least) five replicates per treatment, totaling 90 plants (per season) distributed on three plots. Each DWI contained 15 plant per plot in each season and treatments started on January 2016 and repeated in 2017.
Salinity Shock Experiment
Plants were subjected to two salinity levels of 1 dS·m−1 (tap water) and 49.7 dS/m for 6 weeks. The salinity levels were obtained by gradually adding NaCl to the tank to achieve desired electrical conductivity (EC) which was measured using Metrohm™, Model 914, ON, Canada EC meter. The pH and EC levels were monitored routinely at 25°C to assure salinity levels. The salinity levels were accompanied by 6 days treatment of seaweed extracts as foliar spray at 5 or 7 mL·L−1 using a hand sprayer until drop off (leaves only); untreated plants were considered as controls. Nutrient solutions were supplied weekly to prevent sudden changes in growing conditions. Plants were grouped into three plots and each plot contained (at least) five replicates per treatment, totaling 90 plants (per season) distributed on three plots. Each DWI contained 15 plant per plot in each season and treatments started on January 2016 and repeated in 2017.
Measurements
The visual quality (TQ) were measured at the end of the experiment and included plant density, uniformity and color. TQ-values were determined on a scale of 1–9, with the value 1 indicating desiccated brown turf and increasing quality, 6 acceptable quality and the value 9 is an indication of the optimum color and uniformity. The photochemical efficiency in leaves (Fv/Fm), the maximum root length (MRL), root dry weight (RDW), and the length of the deepest root were measured as described by Elansary and Yessoufou (2015). The vegetative parts were used to measure the total non-structural carbohydrates content (TNC), K+, Ca2+, and proline contents at the end of the experiment (Elansary and Salem, 2015).
The samples were freeze-dried, ground, sieved and kept in −20°C. TNC was measured for freeze-dried samples (25 mg) spectro-photometrically at a wavelength of 515 nm (Chatterton et al., 1987). K+ and Ca2+ concentrations were determined in cell sap by pressing frozen leaves (1 g); then a dilution (1:100, v/v) was used in the inductively coupled plasma spectrophotometer (Jiang and Huang, 2001). Proline composition was measured spectro-photometrically at 520 nm in 0.5 g following the methodology of Torello and Rice (1986) and Bates et al. (1973). The DPPH and β-carotene-linoleic acid assays are widely used methods in plant biochemistry for studying antioxidant activities (Pyrzynska and Pękal, 2013; Ueno et al., 2014). The DPPH assay measures the ability of compounds to scavenge free radicals or as hydrogen donor and is mixed with reducing compounds or plant materials that cause changes in the color from purple to yellow, which could be measured spectrophotometrically. β-carotene-linoleic acid is a well-known methodology for measuring antioxidant activities (Chaillou and Nazareno, 2006) and is based on the bleaching of β-carotene by the interaction with peroxyl radicals obtained during oxidation of linoleic acid. Methanolic leaf extracts were prepared to determine the antioxidant activities by 2,2′-diphenypicrylhydrazyl (DPPH), β-carotene-linoleic acid and Thiobarbituric acid (TBARS; Elansary et al., 2016b). This is done as follows: leaf methanolic extract (50 μL, 1 mg·mL−1) was added to 0.004% DPPH methanol solution (5 mL), and incubated in darkness for 30 min and the absorbance was then determined at 517 nm. The total antioxidant activity (TAA) represented the DPPH radical inhibition percentage. In the β-carotene-linoleic acid assay, 0.5 mg β-carotene was added to a mixture of 1 mL of chloroform, 200 mg Tween 40 and 25 μL linoleic acid then mixing and removing the chloroform by vacuum evaporation. Oxygen-saturated distilled water (100 mL) was then added to the previous mixture, followed by the addition of leaf extracts (2.5 mL mixture with 50 μL of leaf methanolic extract), and then incubated at room temperature for 48 h. The absorbance was then estimated at 470 nm and expressed in percentage (%).
A blank was prepared in the same way and antioxidant capacities of sample were compared to the blank. Thiobarbituric acid (TBARS) assay was performed using 250 μL egg yolk homogenate mixed with 50 μL of leaf methanolic extract (1 mg·mL−1), then completed to 500 μL using distilled water. Fe2SO4 (0.07 M, 25 μL) was added to the previous mixture and incubated at room temperature for 30 min. A mixture of 750 μL acetate buffer (20% v/v, pH 3.3), 750 μL 0.8% TBA (in SDS), and 25 μL of 20% TCA was added to the mixture and incubated at 95°C for 1 h. In addition, 1-butanol (3 mL) was added to the mixture to extract the reddish pigment, followed by centrifugation for 10 min and the upper layer was utilized to measure the absorbance at 532 nm. Blank samples were used and experiments were performed in triplicates.
Protein was extracted according to the protocol of Bradford (1976). This is briefly explained as follows: ground leaf samples were homogenized with 0.2 M phosphate buffer 1:1 (w/v) then centrifuged and the supernatant was utilized for the assay of enzyme activity. Superoxide dismutase (SOD) was measured in leaves following the manufacturer instructions using commercial kit of SOD (19160) (Sigma-Aldrich, Egypt) and the absorbance was determined at 440 nm. SOD activity represented the percentage of the inhibition of water soluble tetrazolium salt (WST-1). Catalase (CAT) and ascorbate peroxidase (APX) enzymes activities were determined in frozen leaf tissues following (Zhang and Kirkham, 1996). In brief, for CAT frozen leaf tissues (0.25 g) were homogenized on ice in 3 mL mixture of 50 mM PBS (pH 7.0), 0.2 mM EDTA and PVP (1%, w/v). The mixture was then centrifuged and the absorbance was determined at 240 nm. For APX, frozen leaf tissues (0.25 g) were homogenized in 3 mL of a mixture of PBS, M EDTA, and PVP on ice, then centrifuged and the absorbance was determined at 290 nm. To determine ROS accumulation in plants, H2O2 contents were determined in leaves using the Beyotime Colorimetric Assay Kit following manufacturer protocol (China). Free and total ascorbate were quantified by the method described by Ma et al. (2008). The mineral composition of SWE extracts was determined using Inductively Coupled Plasma Spectroscopic Analysis (ICPSA) (Optima 4300DV, Perkin-Elmer, USA). The nitrogen (N), phosphorous pentoxide (P2O5), potassium oxide (K2O) were determined in SWE following the AOAC methods No. 990.03, 960.08, and 960.08, respectively. Heavy metals were also quantified in SWE using AOAC method No. 6020A and experiments were repeated twice in triplicates. The sugar composition was determined by hydrolyzing dried SWE in 1 M methanolic-HCI at 80°C for 16 h. Obtained sugars were derivatized using Tri-Sil then subjected to Agilent 6890N GC-FID equipped with capillary column and hydrogen as the carrier. A temperature of 250°C was maintained for the split/splitless injection port and the detector and the injection volume was 1 μL. Different carbohydrates were expressed as percentage. To measure the expression levels of APX, CAT, DHAR, and 3 SOD isoforms genes (Cu/ZnSOD, FeSOD, MnSOD) of SWE-treated and non-treated plants grown under the saline condition of 49.7 dS.m−1, we used quantitative real-time PCR assay in the current study. Total RNA was isolated from these plant tissues using RNeasy Plant kit from Qiagen, followed by using RNase-Free DNase Set to remove contaminating DNA, then first strand cDNA was synthesized using Reverse Transcription kit and qRT-PCR was then performed using QuantiTect SYBR Green PCR kit (Qiagen). Gene-specific primers and PCR amplification procedures of Hu et al. (2012) and Ara et al. (2013) were used. Actin was used as a housekeeping gene, and 2−ΔΔCt method was used for calculating the relative expression levels.
Statistical Analyses
Experimental design was used with two irrigation intervals (2 and 6DWI)/two salinity levels and three SWE treatments (5, 7 mL·L−1, and control), with three replications. The mean of five replicates represented the average of each treatment. The data of different irrigation intervals or salinity levels and SWE treatments of the two periods of 2016 and 2017 were analyzed using SPSS V.18 and differences among treatments were assessed using LSD at p = 0.05 to separate entry means in drought and salinity experiments.
Results
Prolonged Irrigation Intervals and SWE Effects on Turf Quality, Photochemical Efficiency, and Root Parameters
Under 2 day watering intervals (2DWI), SWE treatments at 5 and 7 mL·L−1 had significant effects on TQ compared to control plants in both seasons (Table 1). SWE-treated plants subjected to 6 day watering intervals (6DWI) showed higher TQ-values compared to control; also TQ-values were reduced when compared to 2DWI plants. However, TQ-values were acceptable (6) in 6DWI plants treated with SWE compared to (5) in control plants. Both seasons of 2016 and 2017 showed similar pattern. In the first season and under 6DWI, leaf photochemical efficiency Fv/Fm significantly increased from 0.46 in control plants to 0.53 and 0.58 in SWE-treated plants with 5 and 7 mL·L−1, respectively (Table 1).
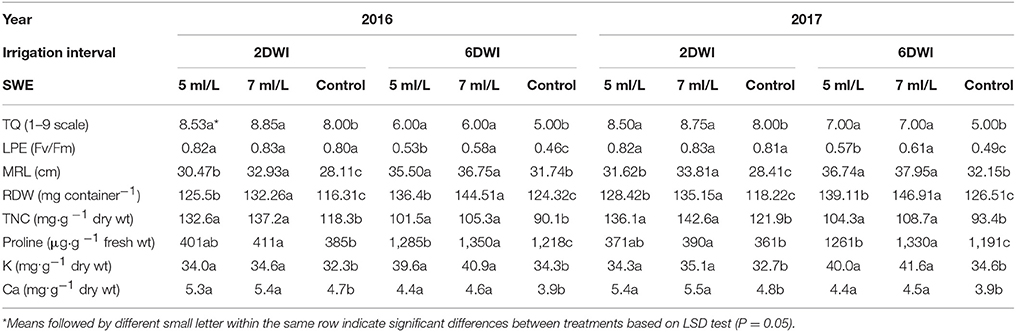
Table 1. Effect of irrigation intervals (2DWI and 6DWI) and SWE treatments (5, 7 ml/L, and control) on mean values of turf quality (TQ), leaf photochemical efficiency (LPE), maximum root length (MRL), root dry weight (RDW), total nonstructural carbohydrates (TNC), proline, K, and Ca of Salam turfgrass.
Root parameters including maximum root length and root dry weight showed significant increases following SWE treatments compared to control plants under 2 and 6DWI in both seasons. For example, under 6DWI, the maximum root length significantly increased from 31.74 cm in control to 35.5 and 36.75 cm in 5 and 7 mL·L−1 SWE treated plants, respectively, in the first season. Also, maximum root length slightly increased in control plants subjected to 6DWI compared to 2DWI in both season.
Prolonged Irrigation Intervals and SWE Effects on TNC, Proline, and K and Ca Compositions
The composition of TNC showed significant increases in SWE-treated plants compared to control under 2 and 6DWI in both seasons (Table 1). The proline composition showed significant increase under 7 mL·L−1 SWE treatment compared to control plants under 2DWI. Also, under 6DWI both of 5 and 7 mL·L−1 SWE treated plants showed significantly higher proline compositions compared to control plants in both seasons.
Leaves K and Ca compositions were significantly influenced by SWE treatments in both seasons (Table 1). There were significant increases in K composition in SWE-treated plants compared to control in the first and second seasons under 2 and 6DWI. Leaves composition of Ca showed significant increases following SWE treatments in both seasons compared to control plants.
Prolonged Irrigation Intervals and SWE Effects on Antioxidant Capacity and Lipid Peroxidation Activity
SWE treatments showed significant effects on the antioxidant capacity using linoleic acid and DPPH assays as well as lipid peroxidation activity under 2 and 6DWI (Figure 1). SWE treatment significantly promoted the antioxidant activities of treated plants compared to control plants in both seasons using the linoleic acid and DPPH assays. It was noticed the higher doses of SWE treatments of 7 mL·L−1 showed slightly higher antioxidant activities on plants compared to 5 mL·L−1 in both seasons using linoleic acid assay. Activities of lipid peroxidation were lower in plants treated with SWE under 2 and 6DWI in both seasons. Also, the higher dose of SWE under 6DWI showed lower values of lipid peroxidation activities compared to the lower SWE dose. It was noted that increasing irrigation intervals from 2 to 6DWI increased the antioxidant activities in the linoleic acid and DPPH assays and reduced lipid peroxidation activity in control plants.
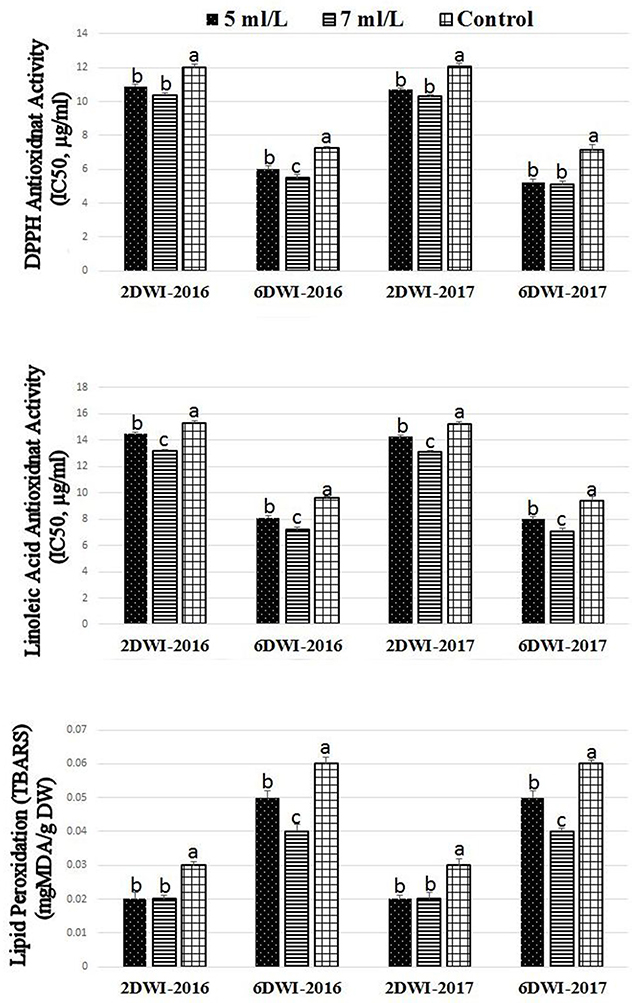
Figure 1. Effect of irrigation intervals (2DWI and 6DWI) and SWE treatments (5, 7 ml·L−1, and control) on the antioxidants activities by DPPH, β-carotene-linoleic acid, and TBARS assays in Salam turfgrass. Means followed by different small letter indicate significant differences between treatments based on LSD-test (P = 0.05).
Effects of Salinity Levels and SWE on Turf Quality, Photochemical Efficiency, and Root Parameters
Saline shock and SWE treatments showed significant effects on mean values of leaf photochemical efficiency, turf quality, root dry weight, and maximum root length (Table 2). Plant treated with SWE showed significantly higher turf quality than control under 1 dS·m−1 irrigation water in both seasons. In plants subjected to saline shock (49.7 dS·m−1 salinity), there were significantly higher turf quality in SWE-treated plants compared to control in both seasons. For example, in the first season, SWE treatment with 7 mL·L−1 had higher quality of 5.50 compared to 4.5 in control. The photochemical efficiency was higher in plants treated with SWE under saline shock (49.7 dS·m−1) only conditions. Under normal irrigation water (1 dS·m−1), SWE treatments were not effective in enhancing the photochemical efficiency in both season. The root dry weight and maximum root length were significantly higher in SWE-treated plants compared to control under both salinity levels (low and high) and in the two season.
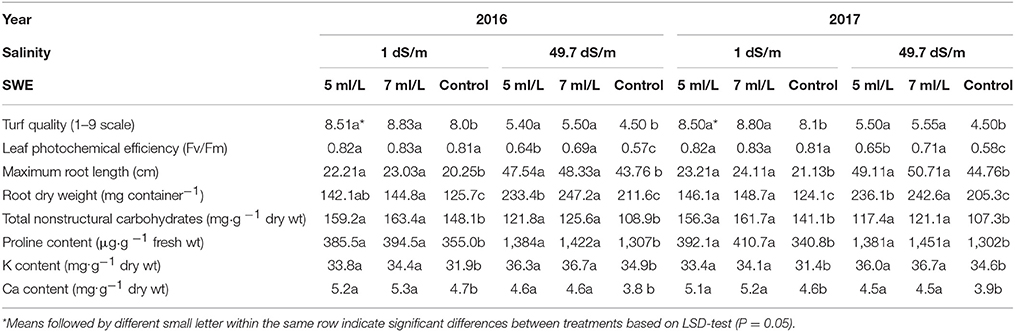
Table 2. Effect of saline levels of 1 and 49.7 dS/m and SWE treatments (5, 7 ml/L, and control) on mean values turf quality (TQ), leaf photochemical efficiency (LPE), maximum root length (MRL), root dry weight (RDW), total nonstructural carbohydrates (TNC), proline, K, and Ca of Salam turfgrass.
Effects of Saline Levels and SWE on TNC, Proline, and K and Ca Compositions
The application of SWE during low saline as well as salinity shock significantly affected plant performance by means of TNC, proline, K and Ca compositions. The TNC mean values were significantly higher in plants treated with SWE under normal and saline shock conditions in both season. For example, TNC-values were 159.2 and 163.4 mg·g −1 dry wt for SWE treated plants with 5 and 7 mL·L−1 compared to 148.1 mg·g −1 dry wt in control plants under low salinity conditions in the first season. Proline composition was significantly increased in SWE-treated plants compared to control under low and high salinity conditions. It was also noted that proline composition increased several times in control plants subjected to saline shock compared to control in low salinity conditions. K and Ca compositions significantly increased in plants treated with SWE under low and higher salinity conditions in both season.
Effects of Saline Levels and SWE on Antioxidant Capacity and Lipid Peroxidation Activity
The antioxidant activities by means of DPPH and linoleic acid assay as well as lipid peroxidation activity was significantly affected by SWE treatment under low (1 dS·m−1) and high (49.7 dS·m−1) salinity conditions (Figure 2). Higher values of antioxidant activities of DPPH were found in plants treated with SWE under high and low salinity conditions in both seasons. It was also noted that SWE treatment at 7 mL·L−1 showed higher antioxidant activities than 5 mL·L−1 treatments. The linoleic acid assay showed similar pattern to that found in the DPPH; higher values of antioxidant activities were found in SWE-treated plants compared to control under low and high salinity conditions in both seasons. General increases were detected in the antioxidant activities values in the linoleic acid and DPPH assays in plants subjected to high saline compared to low saline growing conditions. Lipid peroxidation activities were significantly reduced SWE-treated plants compared to control under low and high salinity conditions.
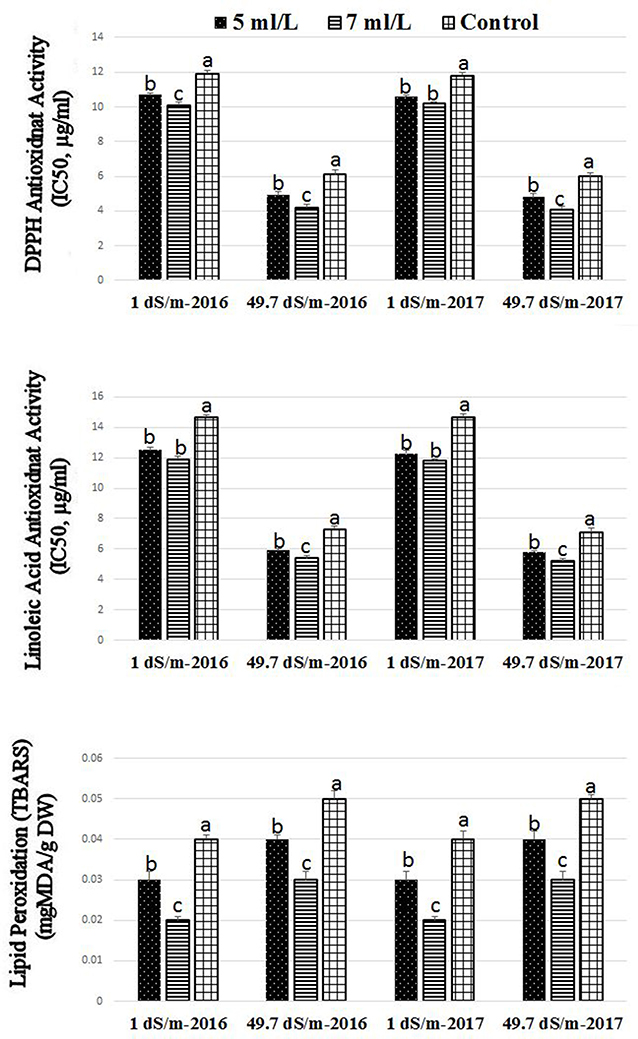
Figure 2. Effect of saline shock levels of 1 and 49.7 dS/m and SWE treatments (5, 7 ml·L−1, and control) on the antioxidants activities by DPPH, β-carotene-linoleic acid, and TBARS assays in Salam turfgrass. Means followed by different small letter indicate significant differences between treatments based on LSD-test (P = 0.05).
Effects of Prolonged Irrigation, Saline Levels and SWE on H2O2 Contents, SOD, CAT, APX Activities, and SWE Composition
The production of ROS (H2O2) significantly increased following prolonged irrigation intervals as well as saline conditions and interestingly, SWE treatments significantly reduced ROS production in both stresses compared to untreated plants (Figure 3). There were significant increases in the inhibition of WST-1 in SWE-treated plants at 5 and 7 mL·L−1 compared to control under prolonged irrigation intervals as well as saline shock conditions (Figure 4). The increases in WST-1 inhibition is an indicator of the increases in SOD activity following SWE treatment. Similarly, the activities of CAT and APX enzymes significantly increased in SWE-treated plants under prolonged irrigation intervals as well as saline shock conditions (Figures 5, 6). The free and total ascorbate composition of leaves of SWE-treated plants was significantly higher than control plants (Figures 7, 8). The SWE extract used in this study contained nitrogen (N, 0.53%), phosphorus (P2O5, 0.20%), potassium (K2O, 0.75%), magnesium (0.1%), calcium (0.1%), trace ratios (1–5 × 10−4) of some important minerals such as manganese, zinc, boron, iron, and copper as well as carbohydrates 18.8% (Table 3). Major sugars found in the carbohydrates were mannitol, fucose, xylose, and glucose. Furthermore, the results of gene expression studies showed that APX, CAT, DHAR, and 3 SOD isoforms genes exhibited higher expression levels in SWE-treated plants under saline condition (49.7 dS.m−1), as compared to non-treated plants (Figure 9), reflecting the potential effect of seaweed extract on plants and the active role of their antioxidant enzymes under environmental and abiotic stresses.
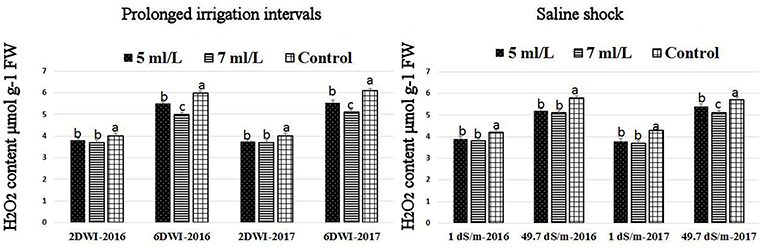
Figure 3. Effect of prolonged irrigation intervals (left) and saline shock (right) levels and SWE treatments (5, 7 mL·L−1, and control) on ROS species (H2O2) contents in Salam turfgrass leaves. Means followed by different small letter indicate significant differences between treatments based on LSD test (P = 0.05).
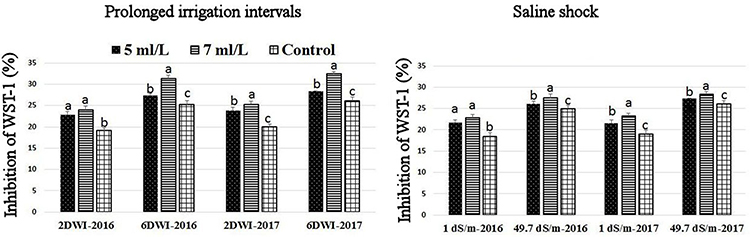
Figure 4. Effect of prolonged irrigation intervals (left) and saline shock (right) levels and SWE treatments (5, 7 mL·L−1, and control) on the inhibition of WST-1 (%) assay as an indicator of SOD enzyme activity in Salam turfgrass. Means followed by different small letter indicate significant differences between treatments based on LSD-test (P = 0.05).
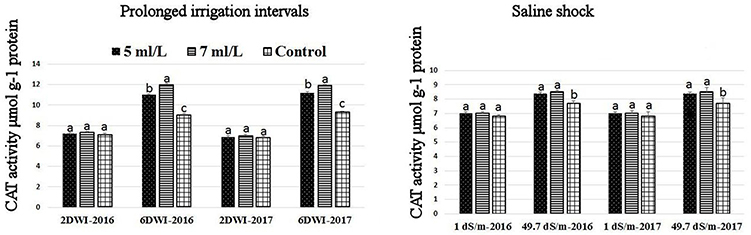
Figure 5. Effect of prolonged irrigation intervals (left) and saline shock (right) levels and SWE treatments (5, 7 ml·L−1, and control) on CAT enzyme activity in Salam turfgrass. Means followed by different small letter indicate significant differences between treatments based on LSD-test (P = 0.05).
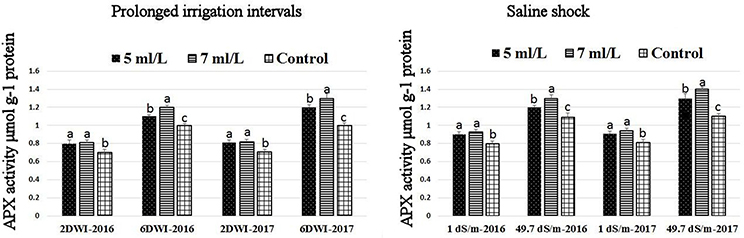
Figure 6. Effect of prolonged irrigation intervals (left) and saline shock (right) levels and SWE treatments (5, 7 mL·L−1, and control) on APX enzyme activity in Salam turfgrass. Means followed by different small letter indicate significant differences between treatments based on LSD-test (P = 0.05).
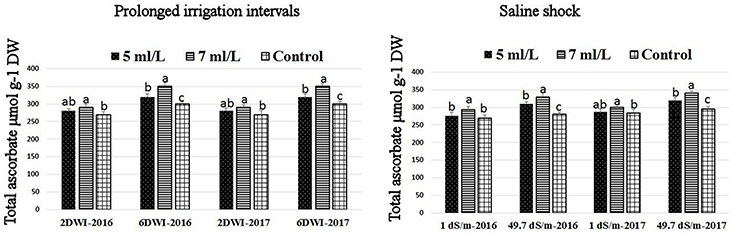
Figure 7. Effect of prolonged irrigation intervals (left) and saline shock (right) levels and SWE treatments (5, 7 mL·L−1, and control) on total ascorbate in Salam turfgrass. Means followed by different small letter indicate significant differences between treatments based on LSD-test (P = 0.05).
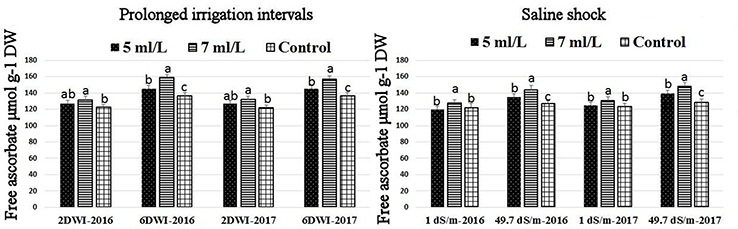
Figure 8. Effect of prolonged irrigation intervals (left) and saline shock (right) levels and SWE treatments (5, 7 mL·L−1, and control) on free ascorbate in Salam turfgrass. Means followed by different small letter indicate significant differences between treatments based on LSD-test (P = 0.05).
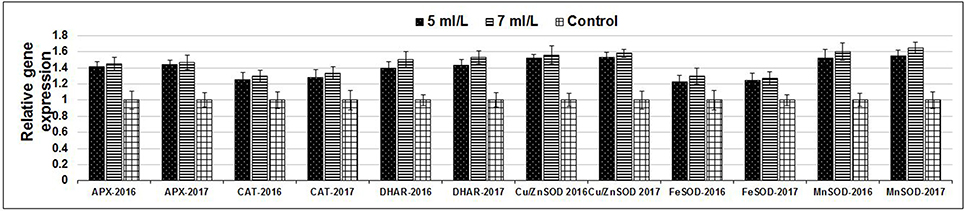
Figure 9. Expression levels of genes of APX, CAT, DHAR, and 3 SOD isoforms in SWE-treated and non-treated Salam turfgrass plants grown under saline condition (49.7 dS.m−1).
Discussion
Water shortage as well as salinity are major challenges to the establishment of lawns and golf courses (Turgeon, 2008; Huang et al., 2014). Their effects include reduction in turf quality, photochemical efficiency and increased root depth under water stress. For example, extreme saline conditions may lead to a reduction of the photochemical efficiency in different cultivars of Paspalum Seashore (Lee et al., 2004). We found an enhancement effect of SWE on turf quality under normal (2DWI) and prolonged irrigation intervals (6DWI) as well as in plants subjected to saline shock. Such effects of SWE on turf quality had been reported in other turfgrasses subjected to heat stress such as in Agrostis stolonifera L. (Zhang et al., 2010) and on photochemical efficiency in Agrostis palustris Huds. A. subjected to cytokinin-rich SWE (e.g., Zhang and Ervin, 2004). However, these studies did not investigate different mechanisms that may interact to improve turfgrass growth during SWE treatments and prolonged irrigation or saline conditions. Such enhancement effects of SWE could be attributed to the macro- and micro-elements that made up the chemical composition of SWE as found in this study and in agreement with previous investigations (e.g., Elansary et al., 2016b). Other reports indicated the presence of cytokinins (Zhang and Ervin, 2004) and abscisic acid (Khan et al., 2009; Stirk et al., 2014) in SWE and attributed the morphological and physiological effects to such growth regulators. For example, Kumari et al. (2011) indicated that SWE may promote the photosynthetic rates by improving the chlorophyll composition of leaves, and a more recent study indicated that SWE could enhance the photosynthetic rates by reducing stomatal closure and increasing gas exchange, leading to improved growth during drought conditions (Elansary et al., 2016b).
Turfgrasses may compete water shortage by increasing root extension as found in this study in plants subjected to 6DWI, matching the well-known drought avoidance mechanism (Huang et al., 2014). The increased root depth as well as root weight in SWE treated plants subjected to prolonged irrigation intervals and saline shock conditions is in agreement with Elansary et al. (2016b) who also reported a similar pattern in ornamental shrubs following SWE application in water stress condition. Such findings prove that SWE enhances root extension as well as the photochemical efficiency in turfgrasses as drought tolerance mechanism and such conclusion had not been achieved before in turfgrasses. The optimal and effective doses of SWE for enhancing Salam turfgrass had not been investigated before, and in the current study we found that SWE application at 7 mL·L−1 was more effective in enhancing the photochemical efficiency and root dry weight of the plants compared to 5 mL·L−1. However, such effects related to dosage had been studied in other crops (Spann and Little, 2011; Mattner et al., 2013).
The accumulation of carbohydrates is a common turfgrass stress tolerance mechanism associated with osmotic adjustment during drought stress conditions (McCann and Huang, 2008; Bian et al., 2009; Elansary and Yessoufou, 2015). There were significant increases in the accumulation of TNC in SWE-treated plants under normal irrigation conditions as well as prolonged irrigation compared to control plants which might be an indication that SWE enhance the composition of TNC Salam turfgrass under normal and prolonged irrigation conditions. Such effect may lead to improved energy storage and metabolism, water and ion uptake as well as osmotic adjustment and drought resistance. SWE-TNC accumulative effect might be related to enhanced photochemical efficiency in SWE-treated plants as found in this study. Few investigations reported carbohydrates in SWE-treated plants. Goyal and Thind (2015) reported an enhanced sugar content in SWE treated rice, also Ciepiela et al. (2016) found significant increases in TNC composition in fodder grass treated with Ecklonia maxima SWE. Salinity leads to the significant reductions of TNC as well as increases in reducing sugars in different turfgrasses (Qian and Fu, 2005; Shahba, 2010; Shahba et al., 2012). For example, Qian and Fu (2005) reported a reduction in TNC from 123.8 to 89.4 mg·g−1 dry wt when salinity increased from 0.2 dS·m−1 (tap water) to 15 dS·m−1, respectively and they suggested that such reduction because TNC serves as a resource for the reduced sugars which normally increase under saline conditions. In the current study, we found parallel reductions in TNC. However, SWE had significant effects in improving the performance of treated plants in saline stress conditions.
The proline as non-protein amino acid is a known marker indicating stresses in turfgrasses such as cold, drought, and salinity and plays a pivotal role in osmoregulation of turfgrasses (Sarkar et al., 2009; Arghavani et al., 2012; Uddin et al., 2012). There were significant increases in proline values in SWE-treated plants grown under well watering conditions as well as prolonged irrigation intervals. Also, similar results were achieved in plants subjected to saline shock conditions and such results might be explained by the stimulating effects of SWE on proline accumulation. Proline response to SWE application had been reported before in few plants including Agrostis stolonifera var. “Penn A4” (Butler and Hunter, 2007) and Spiraea nipponica “Snowmound” and Pittosporum eugenioides “Variegatum” (Elansary et al., 2016b). They described suppressive effects of SWE on proline accumulation. However, Munshaw et al. (2006) reported that SWE have no consistent effect on proline composition in Cynodon spp. cultivars. Shahba et al. (2012) suggested an important role of proline in salinity tolerance in seashore paspalum cultivars. Proline accumulation in plants was described as osmotic strength enhancer and stimulate plant free radical scavenging (Gill and Tuteja, 2010; Rai et al., 2012) and we found positive effects of proline accumulation in this study in response to SWE sprays on Salam turfgrass. Further, Lee et al. (2008) reported that increased proline accumulation in other paspalum seashore cultivars is directly related to salinity tolerance in specific genotypes.
The accumulation of specific ions such as K and Ca in the vegetative parts of plants had been associated with salinity and drought conditions as a mechanism for osmotic adjustment. In turfgrasses, the accumulation of ions such as K and Ca was associated with TNC build up in stressed plants (Lu et al., 2007; Gurmani et al., 2011). This accumulation of these ions plays an important role in keeping cell turgor during stress conditions and modulates Na homeostasis (Gupta and Huang, 2014), especially K, which plays a critical role in stress response in plants Wang et al. (2013) There were significant increases in K and Ca composition in the leaves of SWE-treated plants compared to control under prolonged irrigation intervals as well as saline conditions. Such effect might be attributed not only to the chemical composition of SWE, which include important minerals such as K, but also to the enhanced photosynthetic rates driven by SWE treatement, leading to TNC accumulation and improved ion transport. The positive effects of SWE on mineral composition of treated plants had been reported in several plants, e.g., Lepidium sativum (Godlewska et al., 2016) and others (Arioli et al., 2015) which is in agreement with the current study.
Under stress conditions plants tend to produce reactive oxygen species (ROS) that include superoxide, hydroxyl, perhydroxy, and alkoxy radicals and these molecules may attack and damage cellular compartments such as DNA, thus producing proteins and membranes unsaturated lipids (Gill and Tuteja, 2010; AbdElgawad et al., 2016). Plants developed mechanisms that control ROS production during stress conditions by increased production of antioxidants as well as the activation of antioxidant enzymes as defense system. Some turfgrasses tend to increase their composition in unsaturated lipids to survive stresses (Huang et al., 2014) and this production has been associated with increased lipid peroxidation in stress conditions (Bian and Jian, 2009; Liu et al., 2012). So far, only few studies investigated the effects of SWE on the antioxidant activity on turfgrasses, e.g., Zhang and Schmidt (2000) reported that SWE might enhance the antioxidant activities of Agrostis palustris Huds. A. by means of increased composition of α-tocopherol and ascorbic acid. However, our study is the first to investigate the effects of SWE application on drought and salinity tolerance of Paspalum Seashore cultivar. In addition, few studies indicated that SWE application might enhance the antioxidant activities by means of increased flavonoid and phenolic composition (Fan et al., 2011; Lola-Luz et al., 2014; Elansary et al., 2016a). In this study, significant increases in the overall antioxidant activities in plants treated with SWE during drought and saline conditions were recorded. Furthermore, we found significant increases in SOD, CAT, and APX enzymes activities following SWE applications compared to control plants under prolonged irrigation intervals as well as saline shock conditions. The expression levels of APX, CAT, DHAR, and 3 SOD isoforms genes revealed higher increase in SWE-treated plants under saline condition (49.7 dS.m−1), as compared to non-treated plants, confirming the potential effect of seaweed extract on plants and the active role of their antioxidant enzymes under environmental and abiotic stresses. Enzymes such as CAT, SOD, and APX reduce ROS (H2O2) to water; we also found that ascorbate as non-enzymatic antioxidant plays an important role in the overall reduction of ROS accumulation. Such increases in the enzymatic and non-enzymatic reducing compounds are an indication that SWE treatment in Salam turfgrass mitigates stress conditions by stimulating the antioxidant mechanism pathway. SOD enzyme catalyzes superoxide radicals to form oxygen and hydrogen peroxide. The increases in SOD activities in leaves is associated with drought (Fu and Huang, 2001) and salinity (Hussain et al., 2016). Recent investigation by Zong et al. (2015) reported increased ROS scavenging enzymes activities such as SOD and peroxidase (POD) in Paspalum vaginatum following stress conditions (e.g., water logging). Also Xu et al. (2015) found increased production of ROS (O2 and H2O2) in the root of Festuca arundinacea Schreb. in plants subjected to water stress compared to control and this increase accompanies increases in the activities of CAT and APX enzymes in the root maturation zone. However, root elongation zone did not show similar pattern. Zang et al. (2017) found that heat stress in Triticum aestivum L. increased ROS production (H2O2). In agreement with our results, Chen et al. (2016) found that salinity and tolerance genes in Paspalum vaginatum are associated with the antioxidant system and the photosynthetic metabolism. Also, a high dose of SWE (7 mL·L−1) was more effective in increasing the antioxidant activities as well as the activities of specific enzymes and reducing the lipid peroxidation values and such dose dependent variation had been reported elsewhere in few crops (Spann and Little, 2011; Mattner et al., 2013; Elansary et al., 2016a). Sugars as found in the SWE used in this study had been associated with signaling pathways of plant hormones, the accumulation of auxins and works as elicitors that accumulate secondary metabolites in plants (Rayorath et al., 2008a,b; Vera et al., 2012) which adds more complexity to the mechanisms driving SWE stress tolerance stimulated effect.
In the current study several mechanisms (e.g., drought tolerance, osmotic adjustment, and antioxidant defense system) were found to influence SWE-treated turfgrass performance during stress conditions. Indeed, other mechanisms may influence such performance including polysaccharide-driven natural defense responses (Khan et al., 2009), hormonal upregulation (Zhang and Ervin, 2004), and betaines (MacKinnon et al., 2010) which require several studies to understand such complex activity. However, from the current study, we have a strong ground to believe that SWE may mitigate side effects of prolonged irrigation intervals due to competitiveness on water resources as well as saline shock conditions when using high saline waters to overcome lack of potable water during drought periods known to occur in most agricultural areas around the world.
Conclusion
This is the first study investigating different mechanisms driving positive effects of SWE during water and saline stress conditions in turfgrasses. Environmental stresses such as prolonged irrigation intervals or deficit irrigation and salinity may reduce turf quality and photosynthetic rate; increase root length and dry weight; diminish TNC, K and Ca compositions; inhibit antioxidant defense systems and increase lipid peroxidation. Our findings provide evidence that the application of A. nodosum SWE may mitigate drought and salinity effects and enhance the overall performance of Salam turf grass by increasing turf quality, photosynthetic rate, root length and dry weight (drought tolerance), TNC, K and Ca leaf composition (osmotic adjustment), overall antioxidant activity, SOD, CAT and APX activities, and inhibiting lipid peroxidation (antioxidant defense system).
Author Contributions
HE and KY designed the experiment, HE, AA, and ME performed experiments, all authors contributed in validating, writing, and approving the final version of the manuscript.
Conflict of Interest Statement
The authors declare that the research was conducted in the absence of any commercial or financial relationships that could be construed as a potential conflict of interest.
Acknowledgments
The authors extend their appreciation to the Deanship of Scientific Research at King Saud University for funding this work through research group No. (RG1435-011). Also, the work was supported by the Faculty of Agriculture-Elshatby, Alexandria University (2016–2017), Egypt.
References
AbdElgawad, H., Zinta, G., Hegab, M. M., Pandey, R., Asard, H., and Abuelsoud, W. (2016). High salinity induces different oxidative stress and antioxidant responses in maize seedlings organs. Front. Plant Sci. 7:276. doi: 10.3389/fpls.2016.00276
Ara, N., Nakkanong, K., Lv, W., Yang, J., Hu, Z., and Zhang, M. (2013). Antioxidant enzymatic activities and gene expression associated with heat tolerance in the stems and roots of two Cucurbit species (“Cucurbita maxima” and “Cucurbita moschata”) and their interspecific inbred line “Maxchata.” Int. J. Mol. Sci. 14, 24008–24028. doi: 10.3390/ijms141224008
Arghavani, M., Kafi, M., Babalar, M., Naderi, R., Hoque, M. A., and Murata, Y. (2012). Improvement of salt tolerance in Kentucky bluegrass by Trinexapac-ethyl. HortScience 47, 1163–1170. Available online at: http://hortsci.ashspublications.org/content/47/8/1163.full
Arioli, T., Mattner, S. W., and Winberg, P. C. (2015). Applications of seaweed extracts in Australian agriculture: past, present and future. J. Appl. Phycol. 27, 2007–2015. doi: 10.1007/s10811-015-0574-9
Bates, L. S., Waldren, R. P., and Teare, I. D. (1973). Rapid determination of free proline for water stress studies. Plant Soil 39, 205–207. doi: 10.1007/BF00018060
Bian, S., and Jian, Y. (2009). Reactive oxygen species, antioxidant enzyme activities and gene expression patterns in leaves and roots of Kentucky bluegrass in response to drought stress and recovery. Sci. Hortic. 120, 264–270. doi: 10.1016/j.scienta.2008.10.014
Bian, X., Merewitz, E., and Bingru, H. (2009). Effect of Trinexapac-ethyl on drought responses in creeping bentgrass associated with water use and osmotic adjustment. J. Amer. Soc. Hortic. Sci. 134, 505–510. Available online at: http://journal.ashspublications.org/content/134/5/505.full
Bradford, M. M. (1976). A rapid and sensitive method for the quantitation of microgram quantities of protein utilizing the principle of protein-dye binding. Anal. Biochem. 72, 248–254. doi: 10.1016/0003-2697(76)90527-3
Butler, T., and Hunter, A. (2007). Impact of seaweed extract on turfgrass growth and nutrition on a Golf green to USGA specification. Acta Hort. 762, 81–90. doi: 10.17660/actahortic.2007.762.7
Chaillou, L. L., and Nazareno, M. A. (2006). New method to determine antioxidant activity of polyphenols. J. Agric. Food Chem. 54, 8397–8402. doi: 10.1021/jf061729f
Chatterton, N. J., Bennett, J. H., and Thornley, W. R. (1987). Fructan, starch, and sucrose concentrations in crested wheatgrass and redtop as affected by temperature. Plant Physiol. Biochem. 25, 617–623.
Chen, Y., Chen, C., Tan, Z., Liu, J., Zhuang, L., Yang, Z., et al. (2016). Functional identification and characterization of genes cloned from halophyte seashore paspalum conferring salinity and cadmium tolerance. Front. Pant Sci. 7:102. doi: 10.3389/fpls.2016.00102
Ciepiela, G. A., Godlewska, A., and Jankowska, J. (2016). The effect of seaweed Ecklonia maxima extract and mineral nitrogen on fodder grass chemical composition. Environ. Sci. Pollut. Res. 23, 2301–2307. doi: 10.1007/s11356-015-5417-3
Elansary, H. O., and Salem, M. Z. M. (2015). Morphological and physiological responses and drought resistance enhancement of ornamental shrubs by trinexapac-ethyl application. Sci. Hortic. 189, 1–11. doi: 10.1016/j.scienta.2015.03.033
Elansary, H. O., and Yessoufou, K. (2015). Growth regulators and mowing heights enhance the morphological and physiological performance of Seaspray turfgrass during drought conditions. Acta Physiol. Plant 37, 232–243. doi: 10.1007/s11738-015-1986-5
Elansary, H. O., Norrie, J., Ali, H. M., Salem, M. Z. M., Mahmoud, E. A., and Yessoufou, K. (2016a). Enhancement of Calibrachoa growth, secondary metabolites and bioactivity using seaweed extracts. BMC Complement. Altern. Med. 16:341. doi: 10.1186/s12906-016-1332-5
Elansary, H. O., Skalicka-Woźniakc, K., and King, I. W. (2016b). Enhancing stress growth traits as well as phytochemical and antioxidant contents of Spiraea and Pittosporum under seaweed extract treatments. Plant Physiol. Biochem. 105, 310–320. doi: 10.1016/j.plaphy.2016.05.024
Fan, D., Hodges, M., Zhang, J., Kirby, C. W., Ji, X., Locke, S. J., et al. (2011). Commercial extract of the brown seaweed Ascophyllum nodosum enhances phenolic antioxidant content of spinach (Spinacia oleracea L.) which protects Caenorhabditis elegans against oxidative and thermal stress. Food Chem. 124, 195–202.
Fu, J., and Huang, B. (2001). Involvement of antioxidants and lipid peroxidation in the adaptation of two cool-season grasses to localized drought stress. Environ. E. Bot. 45, 105–114. doi: 10.1016/S0098-8472(00)00084-8
Gill, S. S., and Tuteja, N. (2010). Reactive oxygen species and antioxidant machinery in abiotic stress tolerance in crop plants. Plant Physiol. Biochem. 48, 909–930. doi: 10.1016/j.plaphy.2010.08.016
Godlewska, K., Michalak, I., Tuhy, L., and Chojnacka, K. (2016). Plant growth biostimulants based on different methods of seaweed extraction with water. BioMed. Res. Int. 2016:5973760. doi: 10.1155/2016/5973760
Goyal, P., and Thind, S. K. (2015). Morpho-physiological indices and carbohydrate accumulation of two rice cultivars as influenced by sea weed extract application. Int. J. Emer. Res. Manage. Technol. 4, 92–95. Available online at: http://ermt.net/docs/papers/Volume_4/10_October2015/V4N10-109.pdf
Guinan, K. J., Sujeeth, N., Copeland, R. B., Jones, P. W., O'Brien, N. M., Sharma, H. S. S., et al. (2013). Discrete roles for extracts of Ascophyllum nodosum in enhancing plant growth and tolerance to abiotic and biotic stress. Acta Hortic. 1009, 127–135. doi: 10.17660/ActaHortic.2013.1009.15
Gupta, B., and Huang, B. (2014). Mechanism of salinity tolerance in plants: physiological, biochemical, and molecular characterization. Int. J. Genomics 2014:701596. doi: 10.1155/2014/701596
Gurmani, A. R., Bano, A., Khan, S. U., Din, J., and Zhang, J. L. (2011). Alleviation of salt stress by seed treatment with abscisic acid (ABA), 6-benzylaminopurine (BA) and chlormequat chloride (CCC) optimizes ion and organic matter accumulation and increases yield of rice (Oryza sativa L.). Australian J. Crop Sci. 5, 1278–1285. Available online at: http://www.cropj.com/zhang_5_10_2011_1278_1285.pdf
Hanna, W., Raymer, P., and Schwartz, B. (2013). “Warm-season grasses: biology and breeding,” in Turfgrass Biology, Use and Management, eds J. C. Stier, B. P. Horgan, and S. A. Bonos (Madison, WI: American Society of Agronomy: Soil Science Society of America: Crop Science Society of America), 563–570.
Hu, L., Huang, Z., Liu, S., and Fu, J. (2012). Growth Response and Gene Expre ession in antioxidant-related enzymes in two bermudagrass genotypes differing in salt tolerance. J. Am. Soc. Horticult. Sci. 137, 134–143.
Huang, B., DaCosta, M., and Jiang, Y. (2014). Research advances in mechanisms of turfgrass tolerance to abiotic stresses: from physiology to molecular biology. Crit. Rev. Plant Sci. 33, 141–189. doi: 10.1080/07352689.2014.870411
Hussain, S., Khan, F., Cao, W., Wu, L., and Geng, M. (2016). Seed priming alters the production and detoxification of reactive oxygen intermediates in rice seedlings grown under sub-optimal temperature and nutrient supply. Front. Plant Sci. 7:439. doi: 10.3389/fpls.2016.00439
Jiang, Y., and Huang, B. (2001). Osmotic adjustment and root growth associated with drought preconditioning-enhanced heat tolerance in Kentucky Bluegrass. Crop Sci. 41, 1168–1173. doi: 10.2135/cropsci2001.4141168x
Khan, W., Rayirath, U. P., Subramanian, S., Jithesh, M. N., Rayorath, P., Hodges, D. M., et al. (2009). Seaweed extracts as biostimulants of plant growth and development. Plant Growth Regul. 28, 386–399. doi: 10.1007/s00344-009-9103-x
Krishnan, S., and Merewitz, E. B. (2015). Drought stress and trinexapac-ethyl modify phytohormone content within Kentucky bluegrass leaves. J. Plant Growth Regul. 34, 1–12. doi: 10.1007/s00344-014-9434-0
Kumar, M., Reddy, C. R. K., and Jha, B. (2013). The ameliorating effect of Acadian marine plant extract against liquids-induce oxidative stress and DNA damage in marine macroalga Ulva lactuca. Appl. Phycol. 25, 369–378. doi: 10.1007/s10811-012-9871-8
Kumari, R., Kaur, I., and Bhatnagar, A. K. (2011). Effect of aqueous extract of Sargassum johnstonii Setchell & Gardner on growth, yield and quality of Lycopersicon esculentum Mill. Appl. Phycol. 23, 623–633. doi: 10.1007/s10811-011-9651-x
Lee, G. J., Carrow, R. N., and Duncan, R. R. (2004). Photosynthetic responses to salinity stress in halophytic seashore paspalum genotypes. Plant Sci. 166, 1417–1425. doi: 10.1016/j.plantsci.2003.12.029
Lee, G. J., Carrow, R. N., Duncan, R. R., Eiteman, M. A., and Rieger, M. W. (2008). Synthesis of organic osmolytes and salt tolerance mechanisms in Paspalum vaginatum. Environ. Exp. Bot. 63, 19–27. doi: 10.1016/j.envexpbot.2007.10.009
Liu, Y., Du, H., He, X., Huang, B., and Wang, Z. (2012). Identification of differentially expressed salt-responsive proteins in roots of two perennial grass species contrasting in salinity tolerance. Plant Physiol. 169, 117–126. doi: 10.1016/j.jplph.2011.08.019
Lola-Luz, T., Hennequart, F., and Gaffney, M. (2014). Effect on health promoting phytochemicals following seaweed application, in potato and onion crops grown under a low input agricultural system. Sci. Hortic. 170, 224–227. doi: 10.1016/j.scienta.2014.03.022
Lu, S., Peng, X., Guo, Z., Zhang, G., Fan, Z., Pang, C., et al. (2007). In vitro selection of salinity tolerant variants from triploid bermudagrass (Cynodon transvaalensis × C. dactylon) and their physiological responses to salt and drought stress. Plant Cell Rep. 26, 1413–1420. doi: 10.1007/s00299-007-0339-2
Ma, Y.-H., Ma, F.-W., Zhang, J.-K., Li, M.-J., Wang, Y.-H., and Liang, D. (2008). Effects of high temperature on activities and gene expression of enzymes involved in ascorbate–glutathione cycle in apple leaves. Plant Sci. 175, 761–766. doi: 10.1016/j.plantsci.2008.07.010
MacKinnon, S. L., Hiltz, D., Ugarte, R., and Craft, C. A. (2010). Improved methods of analysis for betaines in Ascophyllum nodosum and its commercial seaweed extracts. Appl. Phycol. 22, 489–494. doi: 10.1007/s10811-009-9483-0
Martynenko, A., Shotton, K., Astatkie, T., Petrash, G., Fowler, C., Neily, W., et al. (2016). Thermal imaging of soybean response to drought stress: the effect of Ascophyllum nodosum seaweed extract. Springerplus 5, 1393. doi: 10.1186/s40064-016-3019-2
Mattner, S. W., Wite, D., Riches, D. A., Porter, I. J., and Arioli, T. (2013). The effect of kelp extract on seedling establishment of broccoli on contrasting soil types in southern Victoria. Australia Biol. Agri. Hortic. 29, 258–270. doi: 10.1080/01448765.2013.830276
McCann, S. E., and Huang, B. (2008). “Turfgrass drought physiology and irrigation management,” in Handbook of Turfgrass Management and Physiology, ed M. Pessarakli (Boca Raton, FL: CRC Press, Taylor and Francis Group), 431–437.
Mittler, R. (2002). Oxidative stress, antioxidants and stress tolerance. Trends Plant Sci. 7, 405–410. doi: 10.1016/S1360-1385(02)02312-9
Munshaw, G. C., Ervin, E. H., Shang, C., Askew, S. D., Zhang, X., and Lemus, R. W. (2006). Influence of late-season iron, nitrogen, and seaweed extract on fall color retention and cold tolerance of four bermudagrass cultivars. Crop Sci. 46, 273–283. doi: 10.2135/cropsci2005.0078
Nair, P., Kandasamy, S., Zhang, J., Ji, X., Kirby, C., Benkel, B., et al. (2012). Transcriptional and metabolomic analysis of Ascophyllum nodosum mediated freezing tolerance in Arabidopsis thaliana. BMC Genomics 13:643. doi: 10.1186/1471-2164-13-643
Neily, W., Shishkov, L., Nickerson, S., Titus, D., and Norrie, J. (2010). Commercial extracts from the brown seaweed Ascophyllum nodosum (Acadian®) improves early establishment and helps resist water stress in vegetable and flower seedlings. HortScience 45:S234.
Nilsen, E. T., and Orcutt, D. M. (1996). Physiology of Plants under Stress: Abiotic Factors. New York, NY: Wiley.
Ondrasek, G. (2014). “Water scarcity and water stress in agriculture,” in Physiological Mechanisms and Adaptation Strategies in Plants under Changing Environment, eds P. Ahmad and M. R. Wani (New York, NY: Springer), 74–75.
Pyrzynska, K., and Pękal, A. (2013). Application of free radical diphenylpicrylhydrazyl (DPPH) to estimate the antioxidant capacity of food samples. Anal. Methods 5, 4288–4295. doi: 10.1039/c3ay40367j
Qian, Y. L., and Fu, J. M. (2005). Response of creeping bentgrass to salinity and mowing management: carbohydrate availability and ion accumulation. HortScience 40, 2170–2174. Available online at: http://hortsci.ashspublications.org/content/40/7/2170.abstract
Rai, A. C., Singh, M., and Shah, K. (2012). Effect of water withdrawal on formation of free radical, proline accumulation and activities of antioxidant enzymes in ZAT12-transformed transgenic tomato plants. Plant Physiol. Biochem. 61, 108–114. doi: 10.1016/j.plaphy.2012.09.010
Rayorath, P., Jithes, M. N., Farid, A., Khan, W., Palanisamy, R., Hankins, S. D., et al. (2008a). Rapid bioassays to evaluate the plant growth promoting activity of Ascophyllum nodosum (L.) Le Jol. using a model plant, Arabidopsis thaliana (L) Heynh. Appl. Phycol. 20, 423–429. doi: 10.1007/s10811-007-9280-6
Rayorath, P., Khan, W., Palanisamy, R., MacKinnon, S. L., Stefanova, R., Hankins, S. D., et al. (2008b). Extracts of the brown seaweed Ascophyllum nodosum induce gibberellic acid (GA3)-independent amylase activity in barley. Plant. Growth. Regul. 27, 370–379. doi: 10.1007/s00344-008-9063-6
Sarkar, D., Bhowmik, P. C., Kwon, Y.-I., and Shetty, K. (2009). Cold acclimation responses of three cool-season turfgrasses and the role of proline-associated pentose phosphate pathway. J. Amer. Soc. Hort. Sci. 134, 210–220. Available online at: http://journal.ashspublications.org/content/134/2/210.abstract
Shahba, M. A. (2010). Comparative responses of bermudagrass and seashore paspalum cultivars commonly used in Egypt to combat salinity stress. J. Hort. Environ. Biotech. 51, 383–390. doi: 10.2135/cropsci2010.04.0192
Shahba, M. A., Abbas, M. S., and Alshammary, S. F. (2014). Drought resistance strategies of seashore Paspalum cultivars at different mowing heights. HortScience 49, 221–229. Available online at: http://hortsci.ashspublications.org/content/49/2/221.full
Shahba, M. A., Alshammary, S. F., and Abbas, M. S. (2012). Effects of salinity on seashore paspalum cultivars at different mowing heights. Crop Sci. 52, 1358–1370. doi: 10.2135/cropsci2011.06.0337
Sharma, H. S. S., Fleming, C. C., Selby, C., Rao, J. R., and Martin, T. J. G. (2013). Plant biostimulants: a review on the processing of macroalgae and use of extracts for crop management to reduce abiotic and biotic stresses. Appl. Phycol. 26, 465–490. doi: 10.1007/s10811-013-0101-9
Spann, T. M., and Little, H. A. (2011). Applications of a commercial extract of the brown seaweed Ascophyllum nodosum increases drought tolerance in container-grown ‘Hamlin’ sweet orange nursery trees. Hortic. Sci. 46, 577–582. Available online at: http://hortsci.ashspublications.org/content/46/4/577.full
Stirk, W. A., Tarkowská, D., Turečová, V., Strnad, M., and van Staden, J. (2014). Abscisic acid, gibberellins and brassinosteroids in Kelpak®, commercial seaweed extract made from Ecklonia maxima. J. Appl. Phycol. 26, 561–567. doi: 10.1007/s10811-013-0062-z
Torello, W. A., and Rice, L. A. (1986). Effects of NaC1 stress on proline and cation accumulation in salt sensitive and tolerant turfgrasses. Plant Soil 93, 241–247. doi: 10.1007/BF02374226
Uddin, M. K., Juraimi, A. S., Ismail, M. R., Hossain, M. A., Othman, R., and Rahim, A. A. (2012). Physiological and growth responses of six turfgrass species relative to salinity tolerance. 2012:905468. doi: 10.1100/2012/905468
Ueno, H., Yamakura, S., Arastoo, R. S., Oshima, T., and Kokubo, K. (2014). Systematic evaluation and mechanistic investigation of antioxidant activity of fullerenols using β-carotene bleaching assay. J. Nanomaterials 2014, 7. doi: 10.1155/2014/802596
Vera, J., Castro, J., Contreras, R. A., González, A., and Moenne, A. (2012). Oligocarrageenans induce a long-term and broad-range protection against pathogens in tobacco plants (var. Xanthi). Physiol. Mol. Plant Path. 79, 31–39. doi: 10.1016/j.pmpp.2012.03.005
Wang, M., Zheng, Q., Shen, Q., and Guo, S. (2013). The critical role of potassium in plant stress response. Int. J. Mol. Sci. 14, 7370–7390. doi: 10.3390/ijms14047370
Xu, C., and Leskovar, D. I. (2015). Effects of Ascophyllum nodosum seaweed extracts on spinach growth, physiology and nutrition value under drought stress. Sci. Hortic. 183, 39–47. doi: 10.1016/j.scienta.2014.12.004
Xu, Y., Xu, Q., and Huang, B. (2015). Stress-inhibition of root growth in association with oxidative defense in tall fescue (Festuca arundinacea Schreb.). Front. Plant Sci. 6:807. doi: 10.3389/fpls.2015.00807
Zang, X., Geng, X., Wang, F., Liu, Z., Zhang, L., Zhao, Y., et al. (2017). Overexpression of wheat ferritin gene TaFER-5B enhances tolerance to heat stress and other abiotic stresses associated with the ROS scavenging. BMC Plant Biol. 17:14. doi: 10.1186/s12870-016-0958-2
Zhang, J., and Kirkham, M. B. (1996). Antioxidant responses to drought in sunflower and sorghum seedlings. NewPhytol. 132, 361–373. doi: 10.1111/j.1469-8137.1996.tb01856.x
Zhang, X. Z., and Schmidt, R. E. (2000). Hormone-containing products' impact on antioxidant status of tall fescue and creeping bentgrass subjected to drought. Crop Sci. 40, 1344–1349. doi: 10.2135/cropsci2000.4051344x
Zhang, X., and Ervin, E. H. (2004). Cytokinin-containing seaweed and humic acid extracts associated with creeping bentgrass leaf cytokinins and drought resistance. Crop Sci. 33, 1737–1745. doi: 10.2135/cropsci2004.1737
Zhang, X., Wang, K., and Ervin, E. H. (2010). Optimizing dosages of seaweed extract-based cytokininsand zeatin riboside for improving creeping bentgrass heat tolerance. Crop Sci. 50, 316–320. doi: 10.2135/cropsci2009.02.0090
Zhu, J. K. (2003). Regulation of ion homeostasis under salt stress. Curr. Opin. Plant Biol. 6, 441–445. doi: 10.1016/S1369-5266(03)00085-2
Zong, J., Gao, Y., Chen, J., Guo, H., Wang, Y., Meng, F., et al. (2015). Growth and enzymatic activity of four warm-season turfgrass species exposed to waterlogging. J. Amer. Soc. Hort. Sci. 140, 151–162. Available online at: http://journal.ashspublications.org/content/140/2/151.abstract
Keywords: Paspalum vaginatum, drought, salinity, seaweed extract, Salam, antioxidants
Citation: Elansary HO, Yessoufou K, Abdel-Hamid AME, El-Esawi MA, Ali HM and Elshikh MS (2017) Seaweed Extracts Enhance Salam Turfgrass Performance during Prolonged Irrigation Intervals and Saline Shock. Front. Plant Sci. 8:830. doi: 10.3389/fpls.2017.00830
Received: 02 March 2017; Accepted: 03 May 2017;
Published: 12 June 2017.
Edited by:
Zhulong Chan, Huazhong Agricultural University, ChinaReviewed by:
Sanalkumar Krishnan, Michigan State University, United StatesManoj Kumar, University of Technology, Sydney, Australia
Copyright © 2017 Elansary, Yessoufou, Abdel-Hamid, El-Esawi, Ali and Elshikh. This is an open-access article distributed under the terms of the Creative Commons Attribution License (CC BY). The use, distribution or reproduction in other forums is permitted, provided the original author(s) or licensor are credited and that the original publication in this journal is cited, in accordance with accepted academic practice. No use, distribution or reproduction is permitted which does not comply with these terms.
*Correspondence: Hosam O. Elansary, aG9zYW1tYWlsMjAwM0B5YWhvby5jb20=
Hayssam M. Ali, aGF5aGFzc2FuQGtzdS5lZHUuc2E=