- 1Department of Agronomy, Food, Natural Resources, Animals and the Environment, University of Padua, Padua, Italy
- 2Department of Land, Environment, Agriculture and Forestry, University of Padua, Padua, Italy
Most crops are routinely protected against seed-born and soil-borne fungal pathogens through seed-applied fungicides. The recently released succinate dehydrogenase inhibitor (SDHI), sedaxane®, is a broad-spectrum fungicide, used particularly to control Rhizoctonia spp., but also has documented growth-enhancement effects on wheat. This study investigates the potential biostimulant effects of sedaxane and related physiological changes in disease-free maize seedlings (3-leaf stage) at increasing application doses (25, 75 and 150 μg a.i. seed-1) under controlled sterilized conditions. We show sedaxane to have significant auxin-like and gibberellin-like effects, which effect marked morphological and physiological changes according to an approximate saturation dose-response model. Maximum benefits were attained at the intermediate dose, which significantly increased root length (+60%), area (+45%) and forks (+51%), and reduced root diameter as compared to untreated controls. Sedaxane enhanced leaf and root glutamine synthetase (GS) activity resulting in greater protein accumulation, particularly in the above-ground compartment, while glutamate synthase (GOGAT) activity remained almost unchanged. Sedaxane also improved leaf phenylalanine ammonia-lyase (PAL) activity, which may be responsible for the increase in shoot antioxidant activity (phenolic acids), mainly represented by p-coumaric and caffeic acids. We conclude that, in addition to its protective effect, sedaxane can facilitate root establishment and intensify nitrogen and phenylpropanoid metabolism in young maize plants, and may be beneficial in overcoming biotic and abiotic stresses in early growth stages.
Introduction
In intensive agriculture, seed coating is a technique of applying several compounds, such as pesticides, fertilizers and biostimulant substances, to the seed surface so they can start to act on the seedlings during germination and/or at the seed-soil interface immediately after sowing (Ehsanfat and Modarres-Sanavy, 2005).
Protecting field crop plants from soil- and seed-borne pathogens during germination and in early growth stages is crucial to ensure safe and fast establishment (Mathre et al., 2001). Fungicides are chemical and biological compounds that kill pathogenic fungi or inhibit fungal spore germination (McGrath, 2004), and, together with insecticides, are the molecules most frequently used in the seed coatings of many crops.
A fungicidal seed treatment is commonly composed of a trace quantity of fungicide evenly distributed among the seeds along with the adhesive substances needed to bind them to the seed surface (Sharma et al., 2015). Modern seed dressing fungicide formulations are often a mixture of several active ingredients with different modes of action (systemic and contact), which broadens the spectrum of control to include a wide range of pathogens and reduces the likelihood of resistance onset (Kitchen et al., 2016). Common fungicide combinations for cereals are triticonazole + prochloraz (Krzyzinska et al., 2005; Vermeulen et al., 2017), both sterol-inhibiting fungicides, and fludioxonil + metalaxyl-M (Mondal, 2004), the former a non-systemic phenylpyrrole, which inhibits transport-associated phosphorylation of glucose, the latter an acilalanine RNA synthesis inhibitor.
Substances on the seed surface can affect germination, as they may vary considerably in the degree to which they attract or repel moisture (Scott, 1989). When applied in high concentrations, fungicides have been reported to have potential direct negative effects on seed germination, rootlet growth, and emergence (Minamor, 2013). In many cases, the effects of seed-applied fungicides on plants vary according to growing conditions: under low pathogen pressure, they do not improve crop emergence and grain yield of wheat, but under high pressure from Fusarium graminearum they do (May et al., 2010). Environmental factors may also play a role (Cox and Cherney, 2014). Seed coating is expected to suppress arbuscular mycorrhizal fungi, hindering their colonization of roots and consequently reducing their beneficial effects on plant growth (Chiocchio et al., 2000; Channabasava et al., 2015).
In the search for highly effective active ingredients, attention is currently focused on useful secondary effects of fungicides on seedling development, regardless of genotype and growing conditions. Several fungicides have been found to have positive side-effects on plant physiology (Berdugo et al., 2012): The ubiquinol oxidase inhibitor (Qol) Strobilurin family is known to increase several morphological traits of maize, such as leaf number and area, and shoot and root biomasses (Lazo and Ascencio, 2014). Strobilurins have also been found to increase tolerance to abiotic stresses, as they can delay senescence of the photosynthetic leaf area, change the balance of the phytohormones, and increase CO2 assimilation in wheat (Wu and von Tiedemann, 2001; Köhle et al., 2002). The azole fungicide class also influences the physiology of treated plants by increasing the chlorophyll content in winter wheat plants, delaying leaf senescence, and protecting plants from several abiotic stresses (Fletcher et al., 2010).
Recent studies have demonstrated the influence of pyrazole-carboxamide succinate dehydrogenase inhibitors (SDHIs) on plant physiology (Ajigboye et al., 2014, 2016). These comprise a relatively new class of fungicide (since 2000), and now include various active ingredients, such as boscalid, bixafen, isopyrazam and sedaxane, which can disrupt fungal respiration causing a breakdown in energy/ATP production (Avenot and Michailides, 2010). The SDHI sedaxane (Syngenta Crop Protection, Basel, Switzerland) has recently been released for use as a treatment for local and systemic protection of cereal seeds, seedlings and roots against pathogenic fungi, both seed-borne (Ustilago nuda, Tilletia caries, Monographella nivalis, Pyrenophora graminea) and soil-born (Rhizoctonia solani, R. cerealis, Gaeumannomyces graminis, Typhula incarnata) (Zeun et al., 2013; Ajigboye et al., 2016). When sedaxane moves from the seed to the soil and into the plant tissues, it has been found to improve the development of the roots and lower stems of cereals (Swart, 2011). Previous research has described wheat responding positively to sedaxane in terms of greater biomass, better growth and drought resistance (Ajigboye et al., 2016). These morpho-physiological reactions are also known to be induced by biostimulants (Calvo et al., 2014), defined as substances that at low doses are able to enhance hormone biosynthesis, nutrient uptake from the soil, resistance to biotic/abiotic stresses, crop quality, and root growth (Kauffman et al., 2007).
Given all this, the present study aimed to investigate the potential biostimulant activity of seed-applied sedaxane on maize plants, and the possible physiological mechanisms underlying the morphological changes. To this end, we: (i) carried out a bioassay (Audus test) to determine the biostimulant activity of sedaxane, (ii) measured the morphological variations in pot-cultivated, disease-free maize plants at increasing fungicide doses, and (iii) studied the response of the enzymes involved in nitrogen and phenylpropanoid metabolism, and the protein, sugar and total phenol contents in the leaves and roots of the same plants.
Materials and Methods
Characteristics of Sedaxane
In this study, we used the fungicide formulation Vibrance® 500 FS, a commercial flowable concentrate for seed treatment containing 500 g sedaxane® L-1, i.e., 43.7% w/w of AI (density 1.17 g mL-1; pH 6.39). Sedaxane is the ISO common name for a mixture of two cis-isomers, 2′-[(1RS,2RS)-1,1′-bicycloprop-2-yl]-3-(difluoromethyl)-1-methylpyrazole-4-carboxanilideand two trans-isomers 2′-[(1RS,2SR)-1,1′-bicycloprop-2-yl]-3-(difluoromethyl)-1-methylpyrazole-4-carboxanilide (IUPAC). Its minimum purity is 960 g kg-1, with ranges of 820–890 g kg-1 for the 2 trans-isomers (SYN508210 – 50:50 mixture of enantiomers), and 100–150 g kg-1 for the 2 cis-isomers (SYN508211 – 50:50 mixture of enantiomers) (EFSA, 2012).
Pot Trial Set-Up and Plant Analysis
Plants of the maize hybrid Hydro (Syngenta, Basel, Switzerland) were grown in cylindrical PVC pots (50 cm high, 9 cm diameter, 3.1 L volume) in a greenhouse in the L. Toniolo experimental farm of the University of Padua (Legnaro, NE Italy). The pots were filled with a sterilized mixture (36 h in an oven at 120°C) of silty-loam soil collected from a field on the experimental farm (pH 8.4), and fine sand (1:1 w/w) to facilitate water drainage and root collection, to which was added a standard dose of pre-sowing fertilizer (about 100 kg N ha-1, 150 kg P2O5 ha-1 and 300 kg K2O ha-1). Maize seeds were treated with three increasing doses of sedaxane: 25, 75, and 150 μg AI seed-1, corresponding to label doses of 2.5, 7.5, and 15 mL of the commercial product Vibrance® 500 FS (500 g AI L-1) in 50,000 seeds. Plants grown from treated seeds were compared with untreated controls. The experimental design was completely randomized with 6 replicates.
Three seeds per pot were sown at the end of June, and immediately after emergence plants were thinned to one per pot. At harvest, growth measurements were taken from three pots/plants, and enzymatic activity assays were carried out with a further three.
Water stress was avoided throughout the experiment by regularly watering the plants. Before plant harvest, which took place 20 days after sowing (DAS) at the 3-leaf stage, SPAD (Soil Plant Analysis Development) was measured in the last fully developed leaf with a 502 chlorophyll meter (Konica-Minolta, Hong Kong). Fresh and dry (oven-dried for 24 h at 105°C) weights were measured on three replicate samples of shoots, and roots were collected, gently washed of soil, and stored in a 15% v/v ethanol solution until morphological characterization. Root length, surface area, diameter, and number of tips and forks were measured by analysis of 1-bit 400-DPI images of the roots acquired with a flatbed scanner (Epson Expression 11000XL, Epson, Suwa, Japan) using the WinRhizo software (Regent Instruments, Ville de Québec, QC, Canada).
Three replicates were stored at -80°C until analysis, then shoot and root tissue samples were taken from them for enzymatic activity assays. Each enzymatic assay (n = 9) was carried out in triplicate on each plant.
A further trial was performed following the same procedure and timing of the main experiment, and using the same sand-soil mixture (1:1 w/w), but this time it was not sterilized. We took SPAD readings, and measured fresh and dry weights, and root morphological parameters of plants grown in unsterilized soil, as reported above (Supplementary Table S1).
Bioassay to Test the Biological Activity of Sedaxane
In order to investigate the biological activity of sedaxane, we measured the reduction in root growth in the model plant watercress (Lepidium sativum L.) to assess auxin-like activity, and the increase in shoot length in lettuce (Lactuca sativa L.) to assess gibberellin-like activity (Audus, 1972).
Watercress and lettuce seeds were surface-sterilized by immersion in 8% hydrogen peroxide for 15 min. After rinsing 5 times with sterile distilled water, 20 seeds were aseptically placed on filter paper in a Petri dish. In the case of watercress, the filter paper was moistened with 1.2 mL of H2O (controls), or with 1.2 mL of 0.1, 1, 10 and 20 mg L-1 indoleacetic acid (IAA, natural auxin) (Sigma–Aldrich, St. Louis, MO, United States) to obtain the calibration curve, or with 1.2 mL of a serial dilution of the tested product Vibrance containing 500 g L-1 of AI sedaxane. The experimental design for lettuce was the same as for watercress, except that the sterile filter paper was moistened with 1.4 mL of the above solutions, while the calibration curve was a progression of 0.0001, 0.001, 0.01, and 0.1 mg L-1 gibberellic acid (GA) (Sigma–Aldrich, St. Louis, MO, United States).
Seeds were germinated in the dark at 25°C. After 48 h, the watercress seedlings were removed from the dishes and the roots measured with a digital gauge; after 72 h, the lettuce seedlings were removed and the shoots measured with a digital gauge.
A linear regression model (y = a + bx) was used to describe the dose-response relationship after logarithmic transformation of IAA, GA and the sedaxane doses, where x was the sedaxane concentration (g L-1) and y the root or shoot length (mm) (Conselvan et al., 2017).
Protein Extraction and Determination
Fresh leaf and root samples, previously stored at -80°C, were ground to a homogenous powder with liquid N2. Proteins were extracted by homogenizing 0.5 g of root or shoot materials with 5 mL of 38 mM KH2PO4 and 62 mM K2HPO4, pH 7, at 4°C. After 2 min, the extract was filtered through three layers of muslin and centrifuged at 15,000 g for 20 min at 4°C. A 50-μL supernatant sample was incubated with 50 μL of Milli-Q water and 2.5 mL of 0.00117 M Bradford reagent. After 15 min, the protein concentration in the extract was determined according to Bradford (1976), using a Jasco V-530 UV/Vis spectrophotometer (Jasco Corporation, Tokyo, Japan) at 595 nm wavelength. The protein concentration was expressed as mg of protein per g of fresh root or shoot.
Enzyme Extraction and Assay Conditions
To extract the enzymes involved in N reduction and assimilation pathways, fresh shoot and root samples were ground to a homogeneous powder with liquid N2. Each activity assay was carried out in triplicate and with 3 biological repetitions using specific buffers for enzyme extraction.
Glutamine synthetase (GS; EC 6.3.1.2) was extracted by homogenizing 0.6 g of root or shoot material at 4°C with 2.4 mL of a solution of 1 mM Tris(hydroxymethyl)aminomethane HCl (Tris-HCl), 25 mM KH2PO4, 10 mM L-cysteine hydrochloride monohydrate and 3% (w/v) bovine serum albumin at pH 7.8 (Baglieri et al., 2014). After 10 min, the extract was filtered through two layers of gauze and centrifuged at 15,000 g for 25 min at 4°C. A 200-μL sample of supernatant was incubated with 200 μL of reaction buffer (50 mM Tris-HCl, 20 mM MgSO4, 80 mM L-glutamate, 30 mM NH2OH, 24 mM ATP, pH 7.8) at 37°C for 25 min. Reaction was blocked with a stopping solution (0.5 mL of 370 mM FeCl2⋅6H2O and 670 mM HCl). Samples were centrifuged at 15,000 g for 15 min. The amount of γ-glutamyl hydroxamate in the supernatant was determined photometrically (wavelength 540 nm) against an immediately stopped parallel sample (Jezek et al., 2015). A standard curve was made using authentic γ-glutamyl hydroxamate (GHA) proportional to absorbance intensity. Enzyme activity was expressed as μmol of GHA produced per g of fresh root or leaf tissue per minute (Conselvan et al., 2017).
Glutamate synthase (GOGAT; EC 1.4.7.1) was extracted by homogenizing 0.5 g of root or shoot material with 2 mL of a solution of 100 mM Tris-HCl, pH 8.2, 10 mM MgCl2⋅6H2O, 2 mM β-mercaptoethanol, 10% (v/v) glycerol and 1 mM Na2EDTA. After 15 min, the extract was filtered through two layers of gauze and centrifuged at 15,000 g for 30 min at 4°C. The supernatant was centrifuged a second time at 15,000 g for 15 min at 4°C. For the enzyme assay, 100 μL of extract was added to 900 μL of reaction buffer (41.6 mM HEPES, pH 7.5, 1 mM NADH, 10 mM EDTA, 20 mM glutamine) and 300 μL (for leaf extract) or 900 μL (for root extract) of 10 mM α-ketoglutaric acid. The reaction time was 2 min for the shoot extract, and 1.5 min for the root extract at 30°C. GOGAT was assayed spectrophotometrically by monitoring NADH oxidation at wavelength 340 nm according to Avila et al. (1987). GOGAT activity was expressed as nmol NADH reduced per g of fresh root or shoot per minute.
For the phenylalanine ammonia-lyase (PAL; EC 4.3.1.5) assay, 1 g of shoot material was homogenized with 0.1 g of poly(vinylpolypyrrolidone) (PVPP) and 5 mL of 100 mM potassium phosphate buffer (pH 8.0) containing 1.4 mM β-mercaptoethanol. After 10 min, the extract was filtered through two layers of gauze and centrifuged at 15,000 g for 20 min at 4 °C. A 60 μL sample of supernatant was incubated with 400 μL of 100 mM Tris-HCl buffer (pH 8.8), 140 μL of 100 mM phosphate buffer and 200 μL of 40 mM phenylalanine, at 37°C for 30 min. Reaction was stopped with 200 μL 6N HCl (El-Shora, 2002). After centrifuging at 10,000 g for 15 min, the absorbance of the supernatant was measured at 280 nm against an immediately stopped parallel sample. A standard curve was made using authentic cinnamic acid at increasing dilutions. PAL activity was expressed as nmol cinnamic acid produced per mg of protein in the sample per minute.
Soluble Phenol Extraction and Determination
Soluble phenolic acids were extracted by homogenizing 200 mg of leaf material with 600 mL of pure methanol. The extract was kept on ice for 30 min then centrifuged at 15,000 g for 30 min at 4°C. Total phenols were measured according to the procedure described by Arnaldos et al. (2001). In brief, 1 mL of 2% Na2CO3 and 75 μL of Folin-Ciocalteau reagent (Sigma–Aldrich, St. Louis, MO, United States) were added to 50 μL of the phenolic extract. After incubation in the dark for 15 min at 25°C, absorbance was measured at 725 nm. A standard curve was made using authentic gallic acid. The soluble phenol content was expressed as mg of gallic acid equivalent (GAE) per g of fresh shoot material.
Free phenolic acid concentrations were revealed on 0.1 g shoot samples treated with 5 mL 80% (v/v) acetonitrile (ACN) in 10-mL tubes for 5 min at room temperature with agitation (70 rpm). After centrifugation (5 min, 10,000 RCF), clear supernatant was filtered at 0.2 μm (Acrodisc syringe filters with GHP membranes) and kept in clean tubes at -20°C until processing. HPLC analysis was carried out according to the method described by Adom et al. (2003) with modifications. Samples were manually shaken, then 200 μL was extracted and placed in vials for HPLC autosampling. The mobile phase was 0.25% (v/v) trifluoroacetic acid (TFA, solvent A) and pure ACN (solvent B). The HPLC gradient was linear: after 2 μL sample injection, solvent B was kept at 4% for 1.16 min, then increased gradually to 12% over 1.16 min, to 23% over 4.63 min, to 95% over 1.85 min, and to 100% over 1.16 min, with final rate maintained for a further 2.78 min. The duration of the analysis was 11.58 min at a solvent flow rate of 1.1 mL min-1. The HPLC equipment (Shimadzu, Kyoto, Japan) had a UV diode array detector (SPD-M20A) at wavelength 282 nm, and an Ultra Tech sphere C18 analytical column (33 mm × 4.6 mm i.d., 1.5 μm particle size; Cil Cluzeau, Sainte-Foy-La-Grande, France) kept at 36°C. Control sample solutions of shoots containing known phenolic acid concentrations were analyzed at the beginning of each new batch analysis, and measurement accuracy was verified by checking expected concentrations.
Each peak was identified by analyzing the retention time and absorbance spectrum of each pure compound (i.e., p-Coumaric, caffeic, syringic, vanillic and t-ferulic acids; Supplementary Figure S1). The coefficients of determination of all calibration curves were >99%.
Quantitative Determination of Sugars
Shoots (5 g) were homogenized in methanol (20 mL) with an Ultra Turrax T25 at 13,500 rpm for 30 s until they attained uniform consistency. Samples were filtered once through filter paper (589 Schleicher), and a second time through cellulose acetate syringe filters (0.45 μm). The extract was then ready for HPLC analysis, for which we used a Jasco X-LC liquid chromatography system (Jasco Inc., Easton, MD, United States) consisting of a PU-2080 pump, an MD-2015 multiwavelength detector, an AS-2055 autosampler, and a CO-2060 column oven interfaced to a PC using the ChromNAV chromatography data system software (Jasco Inc., Easton, MD, United States).
Sugars were separated in a HyperRez XP Carbohydrate Pbþþ analytical column (7.7 mm × 300 mm; ThermoFisher Scientific, Waltham, MA, United States), operating at 80°C. Isocratic elution was carried out with water at a flow rate of 0.6 mL min-1. D-(β)-glucose and D-(β)- fructose were quantified by a calibration method. Standards were dissolved in water and the calibration curves were generated with concentrations ranging from 100 mg L-1 to 1,000 mg L-1 (Nicoletto et al., 2013).
Statistical Analysis
The data are the means of measurements from three different pots per treatment. The analysis of variance (ANOVA) was performed in the SPSS 23 (IBM Corp) software, and was followed by pairwise post hoc analyses (Student–Newman–Keuls test) to determine significant differences among means at P ≤ 0.05.
Results
Audus Test and Effects of Sedaxane on Shoot and Root Growth
Ahead of the analysis, the Audus test was used to determine the biostimulant properties of the active ingredient sedaxane. As with the natural auxin IAA, which reduces root elongation in the model plant watercress and is dose-proportional, increasing concentrations of sedaxane led to a progressive reduction in root length, suggesting an auxin-like effect (Figure 1). We also found sedaxane to exhibit gibberellin-like activity, as it enhanced the shoot growth of lettuce and had a similar dose-proportionality to exogenous gibberellic acid (Figure 2). Both regression curves were significant (P < 0.02 for root responses, P ≤ 0.05 for shoot responses), revealing the hormone-like activity of this fungicide.
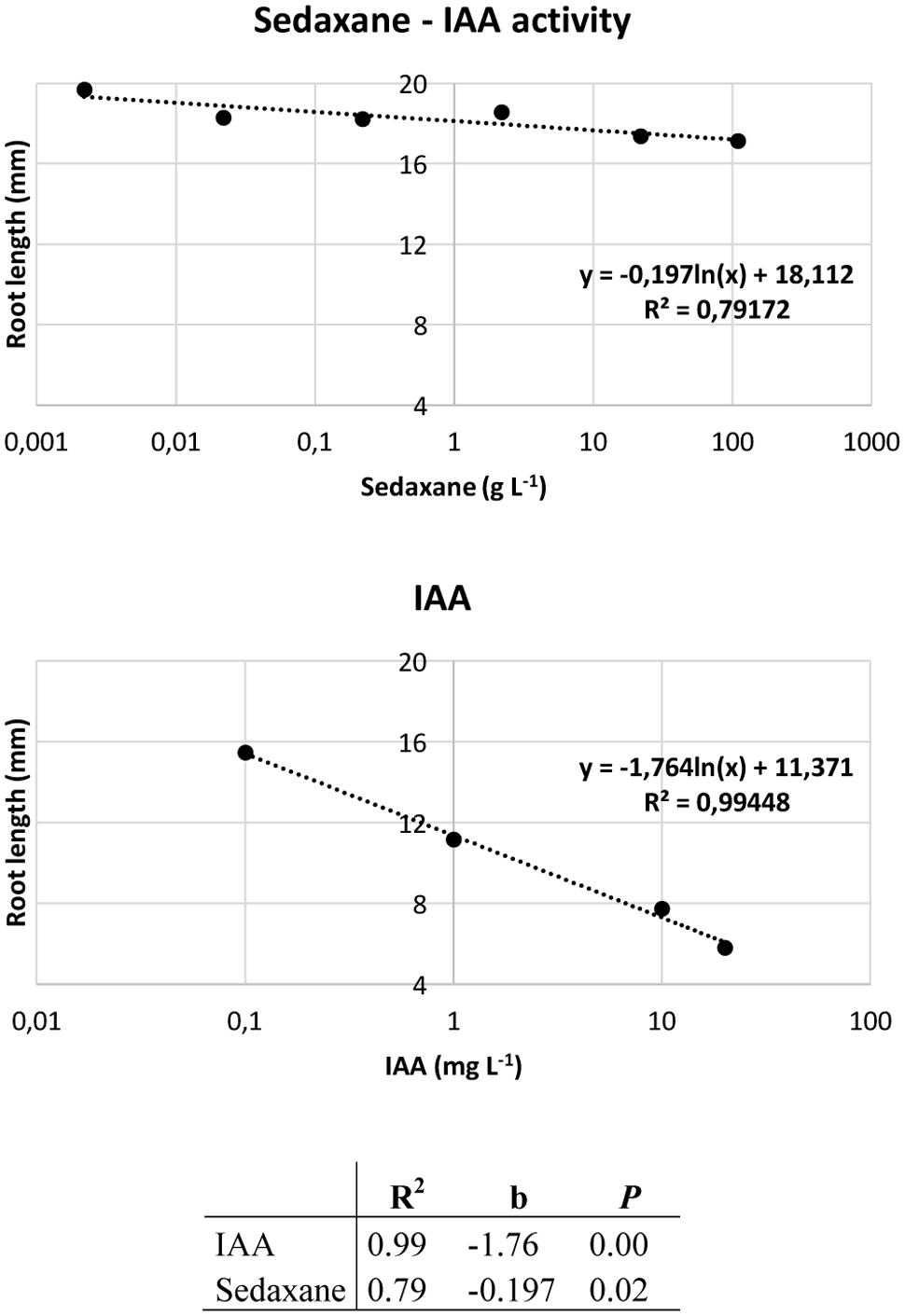
FIGURE 1. Audus test: auxin-like activity of sedaxane measured as variations in root length in watercress. Linear regression analysis (below) performed on 20 samples and averaged over 5 replicates. Note that the x axis has a logarithmic scale.
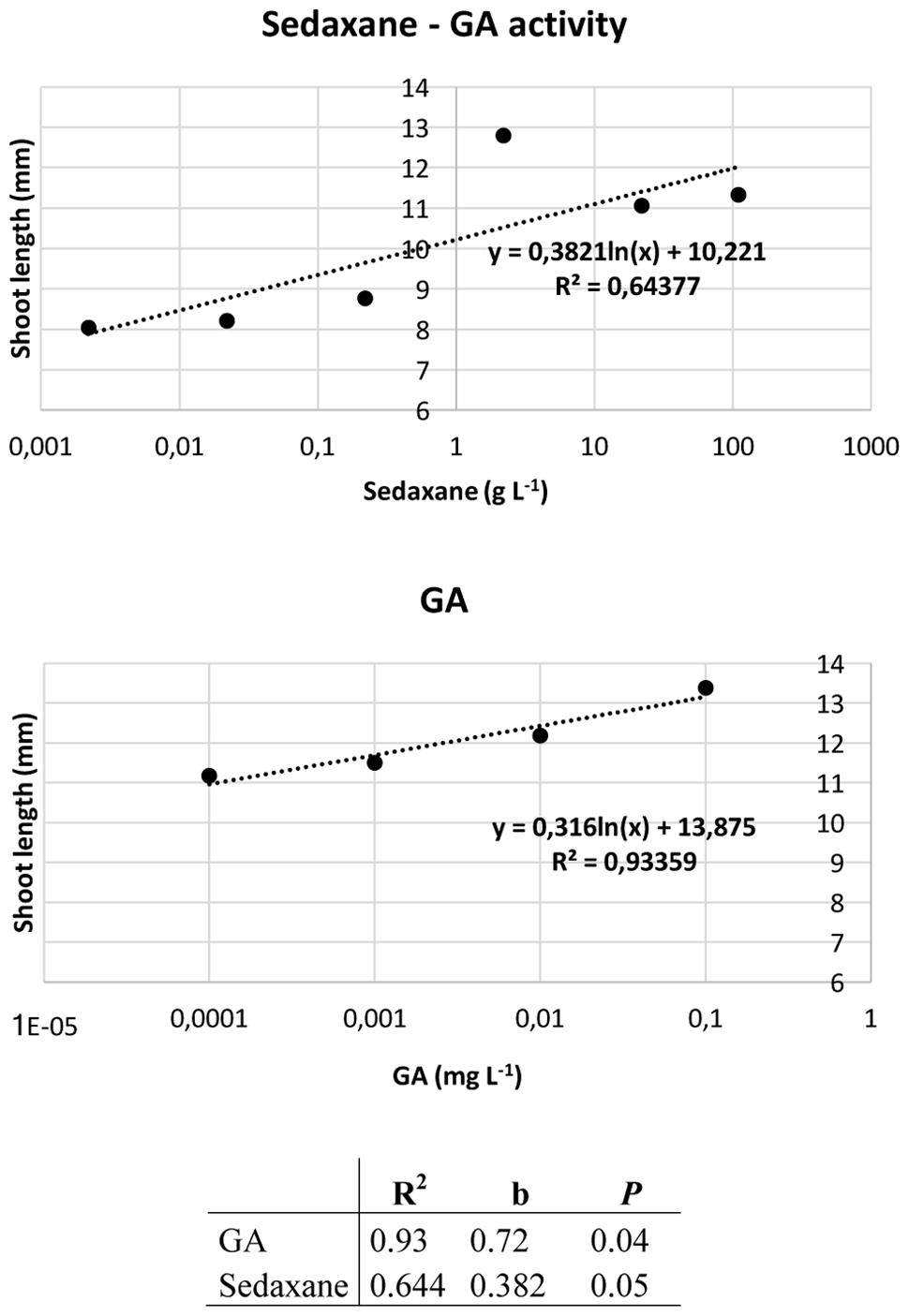
FIGURE 2. Audus test: gibberellin-like activity of sedaxane measured as variations in shoot length in lettuce. Linear regression analysis (below) was performed on 20 samples and averaged over five replicates. Note that the x-axis has a logarithmic scale.
Under sterile conditions, fungicide treatment did not significantly enhance plant growth, although the medium dose of sedaxane (75 μg seed-1) appreciably increased shoot (+21%) and root (+10%) biomasses as compared to untreated controls (Table 1). The effects of the seed treatments were more evident on other root features: Root length increased by 60% and root area by 45% at the intermediate fungicide dose. While root diameter was slightly smaller (P > 0.05), the number of root tips and forks increased, most noticeably with the intermediate (tips +27%, forks +51%) and maximum doses (tips +17%, forks +48%), although only the root branching increase was significant. These results show that root stimulation by sedaxane may be dose-dependent up to saturation.

TABLE 1. Main shoot and root parameters (mean ± SE; n = 3) in Zea mays at 20 days after sowing (DAS) in sterilized pot soil under increasing seed-applied doses of sedaxane.
Effects of Sedaxane on SPAD, Protein and Sugar Contents
Leaf greenness, measured in terms of SPAD values, was very stable across treatments at the end of the trial (Table 2), while protein content was significantly influenced by sedaxane (P < 0.001), with an increase of 14% at the intermediate and highest AI doses (Table 2). A similar effect was found in the roots, with protein content increasing significantly at the highest AI dose (+20% vs. untreated controls).

TABLE 2. Leaf SPAD values, shoot and root protein, glucose, and fructose contents (mean ± SE; n = 9) in Zea mays at 20 days after sowing (DAS) in sterilized pot soil under increasing seed-applied doses of sedaxane.
Fungicide treatment did not affect the shoot and root glucose content, the former having an average concentration of 3374 μg g-1 FW, the latter 3766 μg g-1 FW. The only variation found with regard to fructose was that it was significantly reduced in the shoot at the lowest and highest sedaxane doses (-21% and -15%, respectively, vs. untreated controls) (Table 2).
Variations in GS and GOGAT Activities with Sedaxane
Glutamine synthetase (GS) activity and glutamate synthase (GOGAT) activity were, respectively, 3.8 and 2.1 times higher, on average, in the shoots than in the roots. Seed treatment with sedaxane significantly increased GS activity in the shoots (P < 0.01) at the lowest (+145% vs. controls) and intermediate AI doses (+45%), and in the roots (P < 0.001), particularly at the intermediate and highest AI doses (both +66%, P ≤ 0.05) (Figure 3).
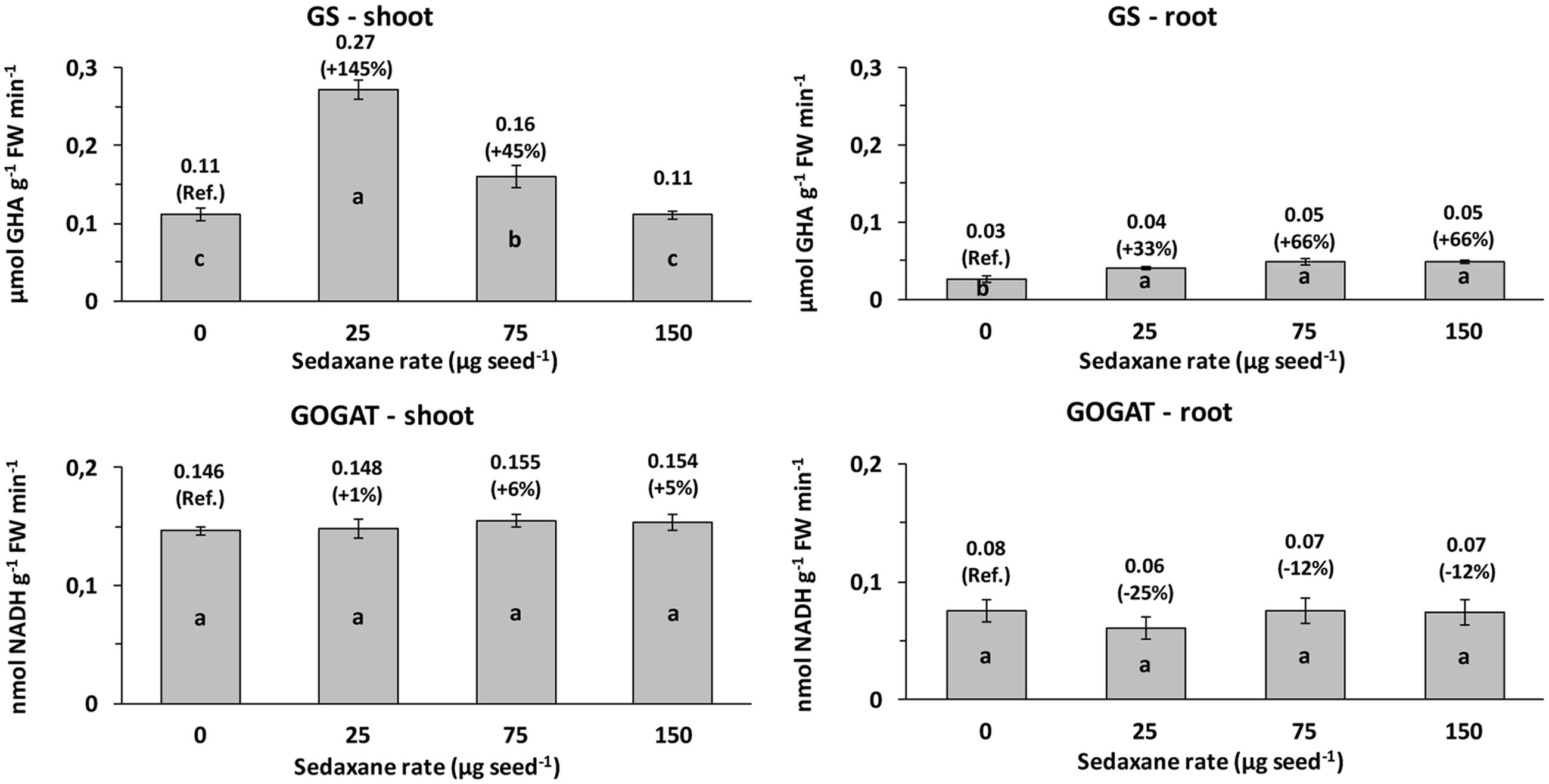
FIGURE 3. Shoot and root glutamine synthetase (GS) and glutamate synthase (GOGAT) activities (mean ± SE; n = 9) in Zea mays at 20 days after sowing (DAS) in sterilized pot soil under increasing seed-applied doses of sedaxane. Letters indicate significant differences among treatments within the same parameter (Student–Newman–Keuls test, P ≤ 0.05). In brackets: % variation vs. untreated controls.
Sedaxane treatments did not affect GOGAT activity in the shoots, while slight, but insignificant, reductions were observed in the roots (Figure 3).
Effect of Sedaxane on Leaf Phenylpropanoid Metabolism
A significant increase in soluble phenolic acids in the shoots was observed at the lowest sedaxane dose (+14% vs. untreated controls), while values similar to controls were detected at greater AI doses (P ≤ 0.05) (Table 3). However, when individual compounds were analyzed, large differences among treatments were detected for caffeic acid, and, to a lesser extent, for syringic and p-coumaric acids (P ≤ 0.05). Significantly higher concentrations of caffeic acid were found in all treated plants compared with untreated controls (P ≤ 0.05). Sedaxane increased caffeic acid by 41–58%, depending on the dose, and p-coumaric acid, the most abundant phenolic compound, by 23% at the lowest and 19% at the intermediate dose. There were only slight differences in the vanillic and t-ferulic acid contents in treated plants as compared with controls (P > 0.05).

TABLE 3. Shoot phenylalanine ammonia-lyase activity (PAL), soluble phenol content and phenolic acid profiles (mean ± SE; n = 9) of Zea mays at 20 days after sowing (DAS) in sterilized pot soil under increasing seed-applied doses of sedaxane.
The ANOVA revealed a significant increase (P ≤ 0.05) in PAL enzyme activity in the shoots with the lowest and highest fungicide doses (+29% and +43%, respectively) as compared with untreated controls (Table 3).
Discussion
Sedaxane belongs to the new class of succinate dehydrogenase inhibitors, and is currently used as a seed-coating fungicide on various crops in several countries, with registration approval being increasingly granted worldwide. It is a broad-spectrum antifungal agent, and is of particular interest in combatting Rhizoctonia solani and Mycosphaerella reliana in maize.
In light of previous results on root stimulation in wheat (Barchietto et al., 2012), we investigated the side-effects of sedaxane in maize over and above its protective capacity, and found that seed treatment significantly modified morphological traits and physiological activities in disease-free plants grown in sterile soil.
The Audus test is considered to be the most reliable bioassay in terms of reproducibility and repeatability for verifying and quantifying the biostimulant activity of molecules in plants, and can be used to ascertain whether an exogenous compound has auxin- and/or gibberellin-like activity (Conselvan et al., 2017). Auxin (IAA) is the most important hormone in plants, and is involved in several plant growth and development phases, such as embryogenesis, organogenesis, tissue patterning and tropisms (Davies, 2010). Molecular genetic studies have brought to light the central role of auxin in primary root elongation, lateral root initiation, and root hair development (De Smet et al., 2006; Overvoorde et al., 2010). The phytohormone gibberellin (GA) also modulates plant development by lengthening roots and stems, and expanding leaves (Fleet and Sun, 2005). We used an Audus bioassay to demonstrate that sedaxane has both auxin- and gibberellin-like activity, as the confirmation of its biostimulant properties.
Although the improvements in aerial and root biomasses detected in this trial were not significant, we found that root length and area, and the number of root tips and branches increased almost in proportion to the dose of sedaxane, consistent with results reported by Colla et al. (2014) on maize coleoptile elongation with protein hydrolysates. All these root morphology modifications are known responses to biostimulant compounds (Calvo et al., 2014). Root development is essential for plant survival as it plays a crucial role in water and nutrient acquisition for growth, the synthesis and accumulation of secondary metabolites, and interaction with soil organisms (Saini et al., 2013).
The data collected from this trial are consistent with Barchietto et al. (2012) regarding stimulation of wheat shoots and roots by seed-applied sedaxane. At 30 days after sowing (DAS), they observed significant increases in root length in treated plants as compared with controls, and no differences in root biomass, as in our case study at 20 DAS. Interestingly, they also found that at 60 DAS root length was unaffected by sedaxane seed treatment, whereas root biomass increased significantly (+39–87%, according to variety).
In the sterile soil conditions of our trial, the SPAD value was very stable across treatments, but this was not the case in the supplementary trial we carried out in unsterile soil conditions to investigate the potential effect of sedaxane in field-like conditions, where we found a slight but significant increase in SPAD (up to 7%) (Supplementary Table S1). This result is in line with practical expectations in the field given the correlation between SPAD and photosynthetic activity, the N status of the plant and protein contents (Prost and Jeuffroy, 2007; Sim et al., 2015).
It should be noted that sedaxane may affect not only fungal mitochondria but also the SDH complex II of plants, partially inhibiting its activity (Avenot and Michailides, 2010). Fuentes et al. (2011) reported better photosynthetic performance in Arabidopsis plants with compromised expression of the flavoprotein subunit of SDH than in wild-type plants. Inhibition of the SDH subunit also resulted in an increase in the number and aperture of leaf stomata, which significantly increased CO2 assimilation, in turn enhancing growth and protein production. Araújo et al. (2011) obtained similar results with tomato plants with antisense inhibition of the iron-sulfur subunit of SDH. However, the higher SPAD values of sedaxane-treated maize observed in our supplementary study with unsterilized soil may also be related to a slowing down of chlorophyll molecule degradation, as reported for fungicides of the Strobilurin class (Grossmann and Retzlaff, 1997; Xu and Huang, 2009). However, this hypothesis needs to be confirmed by studying SDH activity and chlorophyll content in sedaxane-treated plants.
The higher protein content in sedaxane-treated seedlings may be ascribed to better nitrogen metabolism through the activity of the enzymes involved. In fact, the GS/GOGAT metabolic pathway is the main route of N assimilation in higher plants (Mokhele et al., 2012), allowing ammonium taken up directly or originating from nitrate to be assimilated into amino acids (Xu et al., 2012). The GS enzyme is also critical for re-assimilation of the NH4+ constantly released in large amounts via photorespiration, phenylalanine consumption for lignin biosynthesis, and protein catabolism (Lea and Miflin, 2011). GS activity, which increased significantly following sedaxane application, therefore plays a pivotal role in many aspects of plant development (Seabra and Carvalho, 2014), as it is a key component in nitrogen use efficiency (NUE) and plant yield (Thomsen et al., 2014).
GS and GOGAT enzyme activities have been previously reported to be affected by biostimulants (Baglieri et al., 2014). Our data are consistent with those of Ajigboye et al. (2016), who found that improvements in the photosynthetic efficiency, growth, and biomass of sedaxane-treated wheat plants were associated with up- or down-regulation changes in gene expression, and consequent modifications of physiological processes, particularly under drought stress conditions. In particular, sedaxane is reported to induce transcriptional regulation of genes and transcriptional factors, altering the flavonoid and phenolic metabolism (Ajigboye et al., 2016). Our study confirmed that sedaxane stimulates phenylpropanoid metabolism in maize as we found an increase in PAL enzyme activity, although, unexpectedly, the effect was not observed at the intermediate dose. The PAL enzyme catalyzes the first metabolic step from primary to secondary metabolism (Douglas, 1996), deaminating phenylalanine to produce cinnamic acid. As a consequence, there was an increase in the total content of phenolic compounds in shoot tissues from seedlings treated with the lowest concentration of sedaxane, but not at the highest dose. However, there were substantial changes in the concentrations of individual phenolic acids in relation to fungicide application: In particular, there was a considerable increase in caffeic acid in treated plants, which may be of interest in view of its weak auxin-like effect (Lavee et al., 1986; Ishikura et al., 2001; Nagasawa et al., 2016). The main precursor of lignin in the cell wall of gramineous plants is p-coumaric acid, and a greater abundance of it in sedaxane-treated plants could contribute to more intense cell activity and division. Vanillic and p-coumaric acids are also reported to be antifungal phenols, meaning that sedaxane may also contribute indirectly to plant defense (Lattanzio et al., 2006; Zabka and Pavela, 2013; Pusztahelyi et al., 2015). Stimulation of the secondary metabolism may also be explained by enhanced primary metabolism activity, as evidenced by the protein and sugar contents (Table 2).
As with other SDHIs studied in wheat, all the physiological changes brought about by sedaxane may also delay senescence, and improve the yield and protein content of maize plants (Bayles, 1999; Dimmock and Gooding, 2002; Zhang et al., 2010; Abdelrahman et al., 2017), but this requires further investigation in current field conditions.
Conclusion
Sedaxane has a considerable effect on rooting power of maize, particularly on the length, surface area and number of lateral roots. This study found that sedaxane exhibits biostimulant activity in maize seedlings due to its hormone-like activities, corroborated by the fact that most of the observed effects are saturated at moderate doses, as with phytohormones. We have high expectations that seed treatment with this fungicide will facilitate plant establishment, and may provide particular benefits under adverse soil and climatic conditions. Stimulation of the enzyme activities involved in N assimilation and phenylpropanoid metabolism is in agreement with previous findings on this active ingredient and other SDHI fungicides, and is consistent with improved N status and antioxidant activity.
As the fungicide doses tested here are within the recommended label range, the biostimulant activity of sedaxane is an additional benefit, over and above its protective role against seed- and soil-borne diseases, which could be exploited in the cultivation of maize. Although further studies are needed to see whether these improvements also influence final growth and yield, our preliminary results suggest that, as things currently stand, roots may be enhanced in the early growth stages, even in non-sterile soil.
Author Contributions
CDC oversaw the greenhouse trial, assisted with the laboratory analyses, collected and analyzed the data, and wrote the first draft of the manuscript. GBC performed all the enzymatic and biological assays, and also collected and analyzed the data, carried out the literature research and improved the manuscript content. GB performed the HPLC analysis and assisted with analysis of the statistical data. PC helped design the experiment, analyzed the data and improved the manuscript content. LS helped revise the text. TV conceived the research idea, and corrected and arranged the final version of this work. All authors contributed to the interpretation and discussion of the results.
Funding
The authors gratefully thank Syngenta Crop Protection (Basel, Switzerland) for funding this research. GBC was funded by a Ph.D. grant from MIUR under “Law no. 170”, and PC received DOR1692027/16 funding.
Conflict of Interest Statement
The research was funded by Syngenta Crop Protection (Basel, Switzerland), which also provided the seeds and fungicide. Collection of data, analysis and interpretation, as well as article writing was carried out by the authors independently. The authors have the full data set available.
The authors declare that the research was conducted in the absence of any commercial or financial relationships that could be construed as a potential conflict of interest.
Acknowledgments
The authors thank Adriano Massignan for technical assistance in the greenhouse trial, Dr. Michael Feitknecht for valuable revision of the text, and Tessa Say for revising the English text.
Supplementary Material
The Supplementary Material for this article can be found online at: https://www.frontiersin.org/articles/10.3389/fpls.2017.02072/full#supplementary-material
References
Abdelrahman, M., El-Sayed, M., Jogaiah, S., Burritt, D. J., and Tran, L.-S. P. (2017). The “STAY-GREEN” trait and phytohormone signaling networks in plants under heat stress. Plant Cell Rep. 36, 1009–1025. doi: 10.1007/s00299-017-2119-y
Adom, K. K., Sorrells, M. E., and Liu, R. H. (2003). Phytochemical profiles and antioxidant activity of wheat varieties. J. Agric. Food Chem. 51, 7825–7834. doi: 10.1021/jf030404l
Ajigboye, O. O., Lu, C., Murchie, E. H., Schlatter, C., Swart, G., and Ray, R. V. (2016). Altered gene expression by sedaxane increases PSII efficiency, photosynthesis and growth and improves tolerance to drought in wheat seedlings. Pestic. Biochem. Physiol. 137, 49–61. doi: 10.1016/j.pestbp.2016.09.008
Ajigboye, O. O., Murchie, E., and Ray, R. V. (2014). Foliar application of isopyrazam and epoxiconazole improves photosystem II efficiency, biomass and yield in winter wheat. Pestic. Biochem. Physiol. 114, 52–60. doi: 10.1016/j.pestbp.2014.07.003
Araújo, W. L., Nunes-Nesi, A., Osorio, S., Usadel, B., Fuentes, D., Nagy, R., et al. (2011). Antisense inhibition of the iron-sulphur subunit of succinate dehydrogenase enhances photosynthesis and growth in tomato via an organic acid–mediated effect on stomatal aperture. Plant Cell 23, 600–627. doi: 10.1105/tpc.110.081224
Arnaldos, T. L., Munoz, R., Ferrer, M. A., and Calderon, A. A. (2001). Changes in phenol content during strawberry (Fragaria x ananassa, cv. Chandler) callus culture. Physiol. Plant. 113, 315–322. doi: 10.1034/j.1399-3054.2001.1130303.x
Avenot, H. F., and Michailides, T. J. (2010). Progress in understanding molecular mechanisms and evolution of resistance to succinate dehydrogenase inhibiting (SDHI) fungicides in phytopathogenic fungi. Crop Prot. 29, 643–651. doi: 10.1016/j.cropro.2010.02.019
Avila, C., Botella, J. R., Cánovas, F. M., de Castro, I. N., and Valpuesta, V. (1987). Different characteristics of the two glutamate synthases in the green leaves of lycopersicon esculentum. Plant Physiol. 85, 1036–1039. doi: 10.1104/pp.85.4.1036
Baglieri, A., Cadili, V., Mozzetti Monterumici, C., Gennari, M., Tabasso, S., Montoneri, E., et al. (2014). Fertilization of bean plants with tomato plants hydrolysates. Effect on biomass production, chlorophyll content and N assimilation. Sci. Hortic. 176, 194–199. doi: 10.1016/j.scienta.2014.07.002
Barchietto, T., Prévot, C., Rambach, O., Petit, M., Seng, J. M., and Schlatter, C. (2012). Sedaxane: towards a new concept in plant protection? Phytoma 653, 7–10.
Bayles, R. (1999). The interaction of strobilurin fungicides with cereal varieties. Plant Var. Seeds 12, 129–140.
Berdugo, C. A., Steiner, U., Dehne, H. W., and Oerke, E. C. (2012). Effect of bixafen on senescence and yield formation of wheat. Pestic. Biochem. Physiol. 104, 171–177. doi: 10.1016/j.pestbp.2012.07.010
Bradford, M. (1976). A rapid and sensitive method for the quantitation of microgram quantities of protein utilizing the principle of protein-dye binding. Anal. Biochem. 72, 248–254. doi: 10.1016/0003-2697(76)90527-3
Calvo, P., Nelson, L., and Kloepper, J. W. (2014). Agricultural uses of plant biostimulants. Plant Soil 383, 3–41. doi: 10.1007/s11104-014-2131-8
Channabasava, A., Lakshman, H. C., and Jorquera, M. A. (2015). Effect of fungicides on association of arbuscular mycorrhiza fungus Rhizophagus fasciculatus and growth of Proso millet (Panicum miliaceum L.). J. Soil Sci. Plant Nutr. 15, 35–45. doi: 10.4067/S0718-95162015005000004
Chiocchio, V., Venedikian, N., Martinez, A., Menendez, A., Ocampo, J., and Godeas, A. (2000). Effect of the fungicide benomyl on spore germination and hyphal length of the arbuscular mycorrhizal fungus Glomus mosseae. Int. Microbiol. 3, 173–175.
Colla, G., Rouphael, Y., Canaguier, R., Svecova, E., and Cardarelli, M. (2014). Biostimulant action of a plant-derived protein hydrolysate produced through enzymatic hydrolysis. Front. Plant Sci. 5:448. doi: 10.3389/fpls.2014.00448
Conselvan, G. B., Pizzeghello, D., Francioso, O., Di Foggia, M., Nardi, S., and Carletti, P. (2017). Biostimulant activity of humic substances extracted from leonardites. Plant Soil 420, 119–134. doi: 10.1007/s11104-017-3373-z
Cox, W. J., and Cherney, J. H. (2014). Soybean seed treatments interact with locations for populations, yield, and partial returns. Agron. J. 106, 2157–2162. doi: 10.2134/agronj14.0074
Davies, P. J. (2010). “The plant hormones: their nature, occurrence, and functions,” in Plant Hormones: Biosynthesis, Signal Transduction, Action!, ed. P. J. Davies (Dordrecht: Springer), 1–15. doi: 10.1007/978-1-4020-2686-7
De Smet, I., Vanneste, S., Inzé, D., and Beeckman, T. (2006). Lateral root initiation or the birth of a new meristem. Plant Mol. Biol. 60, 871–887. doi: 10.1007/s11103-005-4547-2
Dimmock, J., and Gooding, M. J. (2002). The effects of fungicides on rate and duration of grain filling in winter wheat in relation to maintenance of flag leaf green area. J. Agric. Sci. 138, 1–16. doi: 10.1017/S0021859601001666
Douglas, C. J. (1996). Phenylpropanoid metabolism and lignin biosynthesis: from weeds to trees. Trends Plant Sci. 1, 171–178. doi: 10.1016/1360-1385(96)10019-4
EFSA (2012). Conclusion on the peer review of the pesticide risk assessment of the active substance sedaxane. EFSA J. 10:2823.
Ehsanfat, S., and Modarres-Sanavy, S. A. M. (2005). Crop protection by seed coating. Commun. Agric. Appl. Biol. Sci. 70, 225–229.
El-Shora, H. M. (2002). Properties of phenylalanine ammonia-lyase from marrow cotyledons. Plant Sci. 162, 1–7. doi: 10.1016/s0168-9452(01)00471-x
Fleet, C. M., and Sun, T.-P. (2005). A DELLAcate balance: the role of gibberellin in plant morphogenesis. Curr. Opin. Plant Biol. 8, 77–85. doi: 10.1016/j.pbi.2004.11.015
Fletcher, R. A., Gilley, A., Sankhla, N., and Davis, T. D. (2010). “Triazoles as plant growth regulators and stress protectants,” in Horticultural Reviews, ed. J. Janick (Oxford: John Wiley & Sons), 55–138.
Fuentes, D., Meneses, M., Nunes-Nesi, A., Araújo, W. L., Tapia, R., Gómez, I., et al. (2011). A deficiency in the flavoprotein of arabidopsis mitochondrial complex II results in elevated photosynthesis and better growth in nitrogen-limiting conditions. Plant Physiol. 157, 1114–1127. doi: 10.1104/pp.111.183939
Grossmann, K., and Retzlaff, G. (1997). Bioregulatory effects of the fungicidal strobilurin kresoxim-methyl in wheat (Triticum aestivum). Pest Manag. Sci. 50, 11–20. doi: 10.1002/(SICI)1096-9063(199705)50:1<11::AID-PS556>3.0.CO;2-8
Ishikura, Y., Kojima, Y., and Terazawa, M. (2001). Effects of phenolic compounds on seed germination of shirakamba birch, Betula platyphylla var. japonica. Eur. J. For. Res. 2, 17–25.
Jezek, M., Geilfus, C. M., and Muhling, K. H. (2015). Glutamine synthetase activity in leaves of Zea mays L. as influenced by magnesium status. Planta 242, 1309–1319. doi: 10.1007/s00425-015-2371-8
Kauffman, G. L., Kneivel, D. P., and Watschke, T. L. (2007). Effects of a biostimulant on the heat tolerance associated with photosynthetic capacity, membrane thermostability, and polyphenol production of perennial ryegrass. Crop Sci. 47, 261–267. doi: 10.2135/cropsci2006.03.0171
Kitchen, J. L., van den Bosch, F., Paveley, N. D., Helps, J., and van den Berg, F. (2016). The evolution of fungicide resistance resulting from combinations of foliar-acting systemic seed treatments and foliar-applied fungicides: a modeling analysis. PLOS ONE 11:e0161887. doi: 10.1371/journal.pone.0161887
Köhle, H., Grossmann, K., Jabs, T., Gerhard, M., Kaiser, W., Glaab, J., et al. (2002). “Physiological effects of the strobilurin fungicide F 500 on plants,” in Modern Fungicides and Antifungal Compounds III, eds W. H. Dehne, H. B. Deising, U. Gisi, K. H. Kuck, P. E. Russell, and H. Lyr (Bonn: Agroconcept), 61–74.
Krzyzinska, B., Glazek, M., and Maczynska, A. (2005). Seed treatment for control leaf spot diseases of spring wheat. Acta Agrobot. 58, 37–43. doi: 10.5586/aa.2005.006
Lattanzio, V., Lattanzio, V. M., and Cardinali, A. (2006). “Role of phenolics in the resistance mechanisms of plants against fungal pathogens and insects,” in Phytochemistry: Advances in Research, ed. F. Imperato (Trivandrum: Research Signpost), 23–67.
Lavee, S., Harshemesh, H., and Avidan, N. (1986). Phenolic acids - possible involvement in regulating growth and alternate fruiting in olive trees. Acta Hortic. 179, 317–328. doi: 10.17660/ActaHortic.1986.179.46
Lazo, J. V., and Ascencio, J. (2014). Some morphometric and physiological responses induced by fungicide Opera (R) (Pyraclostrobin plus Epoxiconazole) in corn (Zea mays L.). Rev. Fac. Agron. 31, 39–59.
Lea, P. J., and Miflin, B. J. (2011). Nitrogen assimilation and its relevance to crop improvement. Annu. Plant Rev. 42, 1–40.
Mathre, D. E., Johnston, R. H., and Grey, W. E. (2001). Small Grain Cereal Seed Treatment. Saint Paul, MN: American Phytopathological Society.
May, W. E., Fernandez, M. R., and Lafond, G. P. (2010). Effect of fungicidal seed treatments on the emergence, development, and grain yield of Fusarium graminearum-infected wheat and barley seed under field conditions. Can. J. Plant Sci. 90, 893–904. doi: 10.4141/cjps09173
McGrath, M. T. (2004). What are Fungicides. Available at: http://www.apsnet.org/edcenter/intropp/topics/Pages/fungicides.aspx
Minamor, A. A. (2013). Effect of two fungicides (Caocobre and Ridomil) on germination and radicle elongation of cocoa (Theobroma cacao L) seedlings. Int. J. Pure Appl. Sci. Technol. 15, 79–86.
Mokhele, B., Zhan, X., Yang, G., and Zhang, X. (2012). Review: nitrogen assimilation in crop plants and its affecting factors. Can. J. Plant Sci. 92, 399–405. doi: 10.4141/cjps2011-135
Mondal, K. K. (2004). Evaluation of seed-dressing fungicides against sclerotinia root rot of buckwheat. Fagopyrum 21, 105–107.
Nagasawa, K., Wang, B., Nishiya, K., Ushijima, K., Zhu, Q., Fukushima, M., et al. (2016). Effects of humic acids derived from lignite and cattle manure on antioxidant enzymatic activities of barley root. J. Environ. Sci. Health B 51, 81–89. doi: 10.1080/03601234.2015.1080516
Nicoletto, C., Santagata, S., Tosini, F., and Sambo, P. (2013). Qualitative and healthy traits of different Italian typical artichoke genotypes. CYTA J. Food 11, 108–113. doi: 10.1080/19476337.2012.700951
Overvoorde, P., Fukaki, H., and Beeckman, T. (2010). Auxin control of root development. Cold Spring Harb. Perspect. Biol. 2:a001537. doi: 10.1101/cshperspect.a001537
Prost, L., and Jeuffroy, M.-H. (2007). Replacing the nitrogen nutrition index by the chlorophyll meter to assess wheat N status. Agron. Sustain. Dev. 27, 321–330. doi: 10.1051/agro:2007032
Pusztahelyi, T., Holb, I. J., and Pócsi, I. (2015). Secondary metabolites in fungus-plant interactions. Front. Plant Sci. 6:573. doi: 10.3389/fpls.2015.00573
Saini, S., Sharma, I., Kaur, N., and Pati, P. K. (2013). Auxin: a master regulator in plant root development. Plant Cell Rep. 32, 741–757. doi: 10.1007/s00299-013-1430-5
Scott, J. M. (1989). Seed coating and treatments and their effects on plant establishment. Adv. Agron. 42, 43–83. doi: 10.1016/s0065-2113(08)60523-4
Seabra, A. R., and Carvalho, H. G. (2014). Glutamine synthetase in Medicago truncatula, unveiling new secrets of a very old enzyme. Front. Plant Sci. 6:578. doi: 10.3389/fpls.2015.00578
Sharma, K. K., Singh, U. S., Sharma, P., Kumar, A., and Sharma, L. (2015). Seed treatments for sustainable agriculture - A review. J. Nat. Appl. Sci. 7, 521–539.
Sim, C., Zaharah, A., Tan, M., and Goh, K. (2015). Rapid determination of leaf chlorophyll concentration, photosynthetic activity and NK concentration of Elaeis guineensis via correlated SPAD-502 chlorophyll index. Asian J. Agric. Res. 9, 132–138. doi: 10.3923/ajar.2015.132.138
Thomsen, H. C., Eriksson, D., Møller, I. S., and Schjoerring, J. K. (2014). Cytosolic glutamine synthetase: a target for improvement of crop nitrogen use efficiency? Trends Plant Sci. 19, 656–663. doi: 10.1016/j.tplants.2014.06.002
Vermeulen, P., Flémal, P., Pigeon, O., Dardenne, P., Fernández Pierna, J., and Baeten, V. (2017). Assessment of pesticide coating on cereal seeds by near infrared hyperspectral imaging. J. Spectral Imaging 6, 1–7. doi: 10.1255/jsi.2017.a1
Wu, Y. X., and von Tiedemann, A. (2001). Physiological effects of azoxystrobin and epoxiconazole on senescence and the oxidative status of wheat. Pestic. Biochem. Physiol. 71, 1–10. doi: 10.1006/pest.2001.2561
Xu, G., Fan, X., and Miller, A. J. (2012). Plant nitrogen assimilation and use efficiency. Annu. Rev. Plant Biol. 63, 153–182. doi: 10.1146/annurev-arplant-042811-105532
Xu, Y., and Huang, B. (2009). Effects of foliar-applied ethylene inhibitor and synthetic cytokinin on creeping bentgrass to enhance heat tolerance. Crop Sci. 49, 1876–1884. doi: 10.2135/cropsci2008.07.0441
Zabka, M., and Pavela, R. (2013). Antifungal efficacy of some natural phenolic compounds against significant pathogenic and toxinogenic filamentous fungi. Chemosphere 93, 1051–1056. doi: 10.1016/j.chemosphere.2013.05.076
Zeun, R., Scalliet, G., and Oostendorp, M. (2013). Biological activity of sedaxane–a novel broad-spectrum fungicide for seed treatment. Pest Manag. Sci. 69, 527–534. doi: 10.1002/ps.3405
Zhang, Y.-J., Zhang, X., Chen, C.-J., Zhou, M.-G., and Wang, H.-C. (2010). Effects of fungicides JS399-19, azoxystrobin, tebuconazloe, and carbendazim on the physiological and biochemical indices and grain yield of winter wheat. Pestic. Biochem. Physiol. 98, 151–157. doi: 10.1016/j.pestbp.2010.04.007
Keywords: biostimulant, hormone-like activity, nitrogen metabolism, phenolic acids, root branching, succinate dehydrogenase inhibitor (SDHI)
Citation: Dal Cortivo C, Conselvan GB, Carletti P, Barion G, Sella L and Vamerali T (2017) Biostimulant Effects of Seed-Applied Sedaxane Fungicide: Morphological and Physiological Changes in Maize Seedlings. Front. Plant Sci. 8:2072. doi: 10.3389/fpls.2017.02072
Received: 19 September 2017; Accepted: 20 November 2017;
Published: 06 December 2017.
Edited by:
Youssef Rouphael, University of Naples Federico II, ItalyReviewed by:
Mostafa Abdelwahed Abdelrahman, Tohoku University, JapanTihana Teklić, Josip Juraj Strossmayer University of Osijek, Croatia
Copyright © 2017 Dal Cortivo, Conselvan, Carletti, Barion, Sella and Vamerali. This is an open-access article distributed under the terms of the Creative Commons Attribution License (CC BY). The use, distribution or reproduction in other forums is permitted, provided the original author(s) or licensor are credited and that the original publication in this journal is cited, in accordance with accepted academic practice. No use, distribution or reproduction is permitted which does not comply with these terms.
*Correspondence: Paolo Carletti, paolo.carletti@unipd.it
†These authors have contributed equally to this work.