- 1Beijing Research and Development Center for Grass and Environment, Beijing Academy of Agriculture and Forestry Sciences, Beijing, China
- 2Turfgrass Research Institute, Beijing Forestry University, Beijing, China
- 3Department of Plant Biology, University of California, Davis, Davis, CA, United States
Growing evidence indicates that some grass species are more tolerant to various abiotic and biotic stresses than many crops. Zinc finger proteins play important roles in plant abiotic and biotic stresses. Although genes coding for these proteins have been cloned and identified in various plants, their function and underlying transcriptional mechanisms in the halophyte Zoysia japonica are barely known. In the present study, ZjZFN1 was isolated from Z. japonica using RACE method. Quantitative real time PCR results revealed that the expression of ZjZFN1 was much higher in leaf than in root and stem tissues, and induced by salt, cold or ABA treatment. The subcellular localization assay demonstrated that ZjZFN1 was localized to the nucleus. Expression of the ZjZFN1 in Arabidopsis thaliana improved seed germination and enhanced plant adaption to salinity stress with improved percentage of green cotyledons and growth status under salinity stress. Physiological and transcriptional analyses suggested that ZjZFN1 might, at least in part, influence reactive oxygen species accumulation and regulate the transcription of salinity responsive genes. Furthermore, RNA-sequencing analysis of ZjZFN1-overexpressing plants revealed that ZjZFN1 may serve as a transcriptional activator in the regulation of stress responsive pathways, including phenylalanine metabolism, α-linolenic acid metabolism and phenylpropanoid biosynthesis pathways. Taken together, these results provide evidence that ZjZFN1 is a potential key player in plants’ tolerance to salt stress, and it could be a valuable gene in Z. japonica breeding projects.
Introduction
Salinity stress is becoming an increasing abiotic stress that limits crop yield and plant distribution worldwide (Zhu, 2001; Deinlein et al., 2014). Growing evidence indicates that some forage grass and turfgrass species are more tolerant to various abiotic and biotic stresses than many cultivated crop varieties (Shi et al., 2013a). Recently, several salt stress responsive genes isolated from grass species were reported as active participants in environmental responses (Huang et al., 2014). By binding to distinct cis-regulatory elements, TFs including NAC, WRKY, MYB, bZIP, and zinc finger TFs play a key role in regulating plant responses to salinity stress (Deinlein et al., 2014).
Zinc finger proteins represent a large family of TFs in plants (Kielbowicz-Matuk, 2012). According to the number and location of the histidine and cysteine residues, which surround the zinc atom to form a “zinc-finger structure,” zinc finger proteins are classified into several subfamilies, such as C2H2, CCCH, C2HC, C2HC5, and C4 (Laity et al., 2001). Specifically, 176 and 189 C2H2 zinc finger proteins were identified in Arabidopsis and rice, respectively, supporting that C2H2 is one of the most abundant families of zinc finger proteins in plants (Huang et al., 2007; Yin et al., 2017). These C2H2 zinc finger proteins have been functionally well-characterized in model plants and crops, and reported to play key roles in plant development and responses to environmental stresses. For instance, constitutive expression of ZAT7 or Zat10 enhanced the tolerance of Arabidopsis to salinity (Mittler et al., 2006; Ciftci-Yilmaz et al., 2007). Overexpression of ZFP179 improved salt tolerance but caused hypersensitivity to exogenous ABA in rice (Sun et al., 2010). Overexpression of ZAT18 (Yin et al., 2017) and GsZFP1 (Luo et al., 2012) enhanced drought tolerance of Arabidopsis, while GmZFP3 negatively regulated its drought tolerance (Zhang D.Y. et al., 2016). While AtZAT6 can modulate biotic and abiotic stress tolerance by activating the SA pathway (Shi et al., 2014), ZFP36 plays an important role in ABA induced antioxidant response in rice (Zhang et al., 2014). However, the functions of C2H2 zinc finger proteins in stress responses and the mechanism underlying the regulation of transcription in stress related genes is still unclear, especially in grasses.
Zoysiagrass (Zoysia spp. Willd.), one of the most salt-tolerant turfgrass species, can resist injury under 1% salt solution (Li et al., 2004). Zoysiagrass is now widely used in football pitches, home lawn, and ecological management (Teng et al., 2017). Studies conducted on this species so far have focused on the evaluation of abiotic stress tolerance among different cultivars, on reporting physiological mechanisms, and on the development of molecular markers (Guo et al., 2014; Xu et al., 2015). Thus, the molecular mechanism of salt tolerance in zoysiagrass remains unclear, mostly due to limited genetic resources. Functional studies of individual C2H2 zinc finger proteins will not only provide a better understanding of its detailed function in plant adaption to stress, but also provide insight into potential signaling processes occurring in plants under stress conditions. Therefore, the present study aimed to identify a C2H2 zinc finger protein in zoysiagrass, explore its role in salt stress tolerance and reveal its transcriptional regulation mechanism.
Materials and Methods
Plant Materials and Growth Conditions
Zoysia japonica cultivar “Companion” seeds were purchased from Hancock seed company (Dade City, FL, United States) and grown in a greenhouse at 28/25°C (day/night) under 400 μmol.m-2.s-1 photosynthetically active radiation (PAR). Nicotiana benthamiana seedlings were grown in a growth chamber set at 22°C with a photoperiod of 16 h. Arabidopsis thaliana ecotype “Columbia” (wild type, WT) plants were kept at 24/22°C (day/night) with 65% relative humidity and a scotophase of 8 h to yield transgenic lines. Transgenic lines were cultivated under the same growth conditions as the WT plants and T3-generation seeds were harvested for phenotype observation. The plants were manually irrigated with 1/2 strength Hoagland’s nutrient solution weekly (Hoagland and Arnon, 1950).
Isolation of ZjZFN1
Total RNA was extracted from “Companion” leaves using Trizol (Invitrogen, Carlsbad, CA, United States) according to the manufacturer’s instructions. Using the rapid amplification of cDNA ends (RACE) method, 5′/3′ full-length sequences of ZjZFN1 were isolated with a SMARTer RACE Kit (TaKaRa, Dalian, China) following the manufacturer’s instructions. Primers used for 5′/3′-RACE (Table 1) were designed based on a known cDNA sequence fragment (comp219112_c0) screened from our previous RNA-seq database (Xie et al., 2015). The amplified products were purified before the transfer into pMD18-T vectors (TaKaRa, Dalian, China). Positive clones were then sequenced at Rui-Biotech Company (Beijing, China). Based on the resulting sequences, the gene specific primers, ZjZFN1-F and ZjZFN1-R, for amplification of full-length ZjZFN1 cDNA and genomic DNA sequences.
Isolation of the ZjZFN1 Promoter
Genomic DNA was extracted from “Companion” leaves using the cetyl trimethylammonium bromide (CTAB) method and used as template for genome walking (Genome Walking Kit, TaKaRa, Dalian, China) according to the instruction. The gene specific primers ZFN-R1, ZFN-R2, and ZFN-R3 were used in the genome walking. The resulting PCR products were sequenced using the ZFN-R3 primer. Finally, promoter specific primers, namely Promoter-F and Promoter-R, were used to amplify the upstream sequence of ZjZFN1.
Bioinformatics Analysis
The ZjZFN1 amino acid sequence was deduced from the corresponding cDNA sequence using DNAMAN software (v. 7.0). Its theoretical isoelectric points (pI) and molecular weight (MW) were calculated using the Compute pI/MW tool1. Subcellular localization character was predicted using ProtComp 9.02. Promoter cis-regulatory elements analysis was carried out via PLACE database3. Potential signal peptide cleavage sites were examined with SignalP 4.1 Server4. The basic local alignment search tool (BLAST) at the National Center for Biotechnology Information (NCBI) database was used to identify homologs. Clustal W5 was employed to perform protein alignment. A phylogenetic tree was built in MEGA version 5.0 based on the neighbor-joining method.
Quantitative Real-Time PCR
The expression patterns of ZjZFN1 in the roots, stems, and leaves at different developmental stages (young, fast-growing, and mature) of Z. japonica were explored using qRT-PCR. Furthermore, ZjZFN1 expression profiles were investigated in 3-month-old Z. japonica after 24 h’ under 8°C, 150 mM NaCl, 25% PEG6000, 10 μM ABA, 5 μM SA, or 10 μM MeJA. The ZjZFN1 specific primers qZFN-F and qZFN-R were used for qRT-PCR (Table 1). Reactions were performed in 96-well blocks with a CFX Connect RT-PCR system (BIO-RAD, Hercules, CA, United States) using SYBR Premix (TaKaRa, Dalian, China) in a total volume of 25 μL. A two-step qRT-PCR program was adopted and set as follows: initial denaturation at 95°C for 30 s, followed by 40 cycles of 95°C for 5 s and 60°C for 30 s. The Z. japonica actin gene (GenBank accession GU290546) was selected as the internal reference (Table 1) and the relative gene expression levels were calculated using the comparative ΔΔCt method (Livak and Schmittgen, 2001). All data are presented as means [with corresponding standard deviations (SDs)] of at least three independent biological replicates, each including three technical replicates. To obtain sufficient samples for RNA extraction, three independent plants were pooled per biological replicate.
Binary Vector Construction
The yellow fusion protein (YFP) construct 35S::ZjZFN1:YFP and pGBKT7-ZjZFN1 were produced by transferring the complete ZjZFN1 coding sequence (CDS) into 3302Y (Jia et al., 2016) and pGBKT7 vectors, respectively. Firstly, 3302Y and pGBKT7 vector were digested by BglII or BamHI (TaKaRa, Dalian, China), respectively, and purified using a E.Z.N.A Cycle-pure Kit (Omega Bio-Tek, Norcross, GA, United States). Then primers 3302Y-ZFN1-F/R and BD-ZFN1-F/R were used to amplify the ZjZFN1 CDS (Table 1). Amplicons were then purified and infused into the linearized 3302Y and pGBKT7 plasmids using an In-fusion HD Cloning Kit (TaKaRa, Dalian, China).
The GUS fusion construct ZjZFN1pro::GUS contained a 1406-bp ZjZFN1 promoter region that was amplified from the plasmid containing the target sequence using primers 1391-ZFN1-F and 1391-ZFN1-R (Table 1). After digesting the pCAMBIA1391Z vector with NcoI (TaKaRa, Dalian, China), purified PCR product of ZjZFN1 promoter was infused into the digested vector using the In-fusion HD Cloning Kit (TaKaRa, Dalian, China) to produce ZjZFN1pro::GUS.
Subcellular Localization and Transcriptional Activity Assay of ZjZFN1
Agrobacterium tumefaciens EHA105 transformed with the 35S::ZjZFN1:YFP fusion construct were used to transform N. benthamiana to reveal the subcellular localization of ZjZFN1 using the transient overexpression method (Sparkes et al., 2006). After darkness induction for 48 h, the cells from the lower epidermis of N. benthamiana leaf cells were monitored and photographed under a SP-5 laser confocal scanning microscope (Leica, Mannheim, Germany). 1 μg/mL DAPI (Sigma-Aldrich, Munich, Germany) was used to show the nuclear.
The pGBKT7-ZjZFN1 construct was transformed into Saccharomyces cerevisiae Y2HGold competent cells using the LiAc method (Liu et al., 2016) to investigate its transcriptional activation ability. Firstly, transformed Y2HGold cells (pGBKT7 was use as control) were grown on synthetic defined (SD) medium without tryptophan (SD/-Trp). After colony-PCR verification, the positive clones were grown on SD medium without tryptophan, histidine and adenine (SD/-Trp-His-Ade) at 30°C for about 48 h. Yeast growth phenotypes were then photographed with an EOS 60D digital camera (Canon, Tokyo, Japan).
Generation of Transgenic Plants
Using floral dip method (Clough and Bent, 1998), A. tumefaciens GV3101 transformed with construct plasmids was used to infect Arabidopsis plants to generate transgenic plants expressing ZjZFN1 or ZjZFN1pro::GUS. Transgenic Arabidopsis seeds were screened using 60 mg L-1 glufosinate, or 20 μg/mL hygromycin. Positive transgenic plants were verified by reverse transcription PCR and genomic PCR. Representative T3 transgenic lines exhibiting 100% resistance to glufosinate were harvested for further phenotype observation or GUS staining assays.
GUS Staining
Using a GUS Kit (O’BioLab, Beijing, China), and according to the instructions provided by the manufacture, Arabidopsis seedlings were GUS stained. After removing chlorophyll with 70% ethanol, seedlings were then photographed under the M205FA stereomicroscope (Leica, Mannheim, Germany).
Salt Tolerance in Arabidopsis Transgenic Lines
Because lines ZFN-2 and ZFN-17 presented the highest ZjZFN1 transcript levels among the 36 T3 transgenic lines, they were selected as the representative lines for phenotype observation. For germination assessment, seeds of ZFN-2, ZNF-17, and WT were sterilized with 70% ethanol and 1% sodium hypochlorite and then sowed on Murashige and Skoog (MS) medium with or without 100 mM NaCl, respectively. After 4 and 8 days, phenotype were photographed with an EOS 60D digital camera (Canon, Tokyo, Japan). For determination salt tolerance, 3-week-old seedlings were transplanted to nutrition medium containing peat, vermiculite and pearlite (1:1:1 in volume) and moved to growth chambers. Here, seedlings were subject to incremental increases of 50 mM NaCl from the first day to the third day and then kept under 150 mM NaCl for 18 days. Leaf samples were then harvested for malondialdehyde (MDA), proline and gene expression examination at the 21st day.
For MDA and proline quantification, the leaf samples were treated as described in our previous report (Teng et al., 2017). Genes coding for stress response proteins, namely superoxide dismutase (AtMn-SOD, AT3G56350), peroxidase (AtPOD, AT3G49120), ascorbate peroxidase1 (AtAPX1, AT1G07890), sodium/hydrogen exchanger 1 (AtNHX1, AT5G27150), pyrroline-5-carboxylate synthase (AtP5CS, AT3G55610), and late embryogenesis abundant (AtLEA, AT1G02820) were selected to monitor salt stress-response transcriptional mechanisms using qRT-PCR (Table 1). The Arabidopsis AtUBQ10 gene (NM_116771) gene was adopted as internal reference.
Transcriptomic Analysis
Total RNA was isolated from 4-week-old WT (control) and ZjZFN1-overexpressing Arabidopsis seedlings (ZFN-17) cultivated under normal conditions using Trizol (Invitrogen, Carlsbad, CA, United States). Six independent RNA samples (three replicates from control and three replicates from ZNF-17), each comprising three Arabidopsis plants were obtained. Nanodrop ND-1000 spectrophotometer (NanoDrop Technologies, Rockland, DE, United States) was used for RNA quality determination. Agilent 2100 spectrophotometer (Agilent Technologies, Santa Clara, CA, United States) was used to evaluate RNA purity, concentration and integrity. Sequencing libraries and sequencing analysis were performed by Biomarker Technologies (Beijing, China) on the Illumina 4000 platform (San Diego, CA, United States) to generate 125/150 bp paired-end reads. Raw sequence reads were deposited into the NCBI Short Read Archive (SRA) repository under the accession number SRP140821. The reads containing adaptor and poly-N, as well as the low quality reads, were excluded and the Q20, Q30, and GC content of clean data reads were evaluated. High-quality clean reads were then mapped to the Arabidopsis reference genome (TAIR 106) using TopHat2 (Kim et al., 2013). Gene expression levels were calculated base on the fragments per kilobase of transcript per million fragments mapped (FPKM) method (Mortazavi et al., 2008).
Differentially expressed genes between the WT and the ZFN-17 line (each with three independent libraries) were screened using DESeq R package (1.10.1) based on adjusted false discovery rate (FDR). P-value ≤0.05 and fold change (FC) ≥2. For GO and KEGG enrichment analyze, all DEGs were mapped to GO terms in the GO database and KEGG database using the Goseq R package (Young et al., 2010) and the KOBAS software (Mao et al., 2005), respectively.
Statistical Analysis
Data were analyzed by two-tailed Student’s t test or one-way ANOVA using SPSS version 18.0 (IBM, Chicago, IL, United States). ∗P < 0.05 and ∗∗P < 0.01 were believed statistically significant. All data were presented as the means with ± SD (n = 3).
Results
Isolation and Bioinformatics Analysis of ZjZFN1 and Its Promoter
The ZjZFN1 cDNA sequence was deposited in the NCBI database with the accession number of KT596064.1. The open reading frame (ORF) of ZjZFN1 was 789 bp in length, which corresponded to 262 amino acids (Figure 1). The ZjZFN1 protein contained two typical zinc finger structures and belonged to the C2H2 superfamily (Figure 1A). Its theoretical pI was 8.76. Its MW was 27.52 KD and no potential signal peptide was found. Phylogenetic analysis showed that ZjZFN1 was most closely related to the ZFN proteins from Zea mays or Sorghum bicolor (Figure 1B).
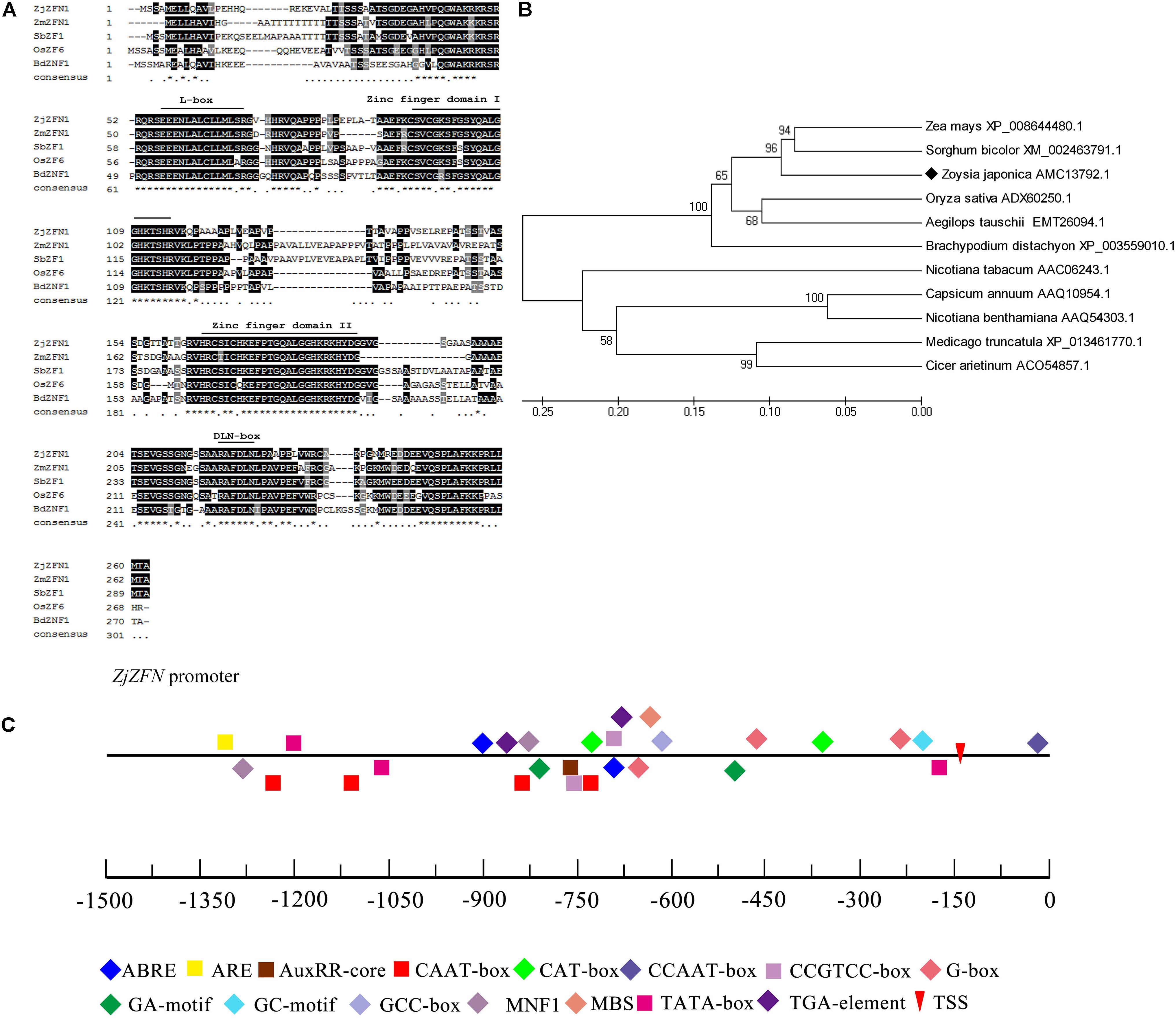
FIGURE 1. Amino acid sequence and phylogenetic analysis of ZjZFN1. (A) The alignment of C2H2-zinc finger proteins. The position of the domain of the first and second zinc fingers, L-box and DLN-box were labeled. (B) Phylogenetic analysis of ZjZFN1 protein orthologs. (C) Cis-elements analysis of ZjZFN1 promoter sequence.
The 1406-bp fragment upstream the ATG start codon, containing CAAT-box and TATA-box elements, was investigated as a potential promoter region (Figure 1C). Two ABA-responsive motifs (ABRE) and one auxin-responsive motif (AuxRR-core) were also identified. In addition, there were one cis-acting regulatory element related to meristem expression (CAT-box) and two involved in anaerobic induction (ARE).
Expression Patterns of ZjZFN1
The qRT-PCR employed to interpret the expression patterns of ZjZFN1 showed that, although ZjZFN1 expressed in all investigated tissues including leaf, stem, and root, most transcripts were observed in leaves (Figure 2A). The expression level of ZjZFN1 was higher in mature leaves than in fast-growing and young leaves (Figure 2B). The transcription of ZjZFN1 could be induced by cold and salt treatments, as the highest expression levels were observed at 6 h after NaCl or cold treatments, respectively (Figures 2C,D). However, we found that ZjZFN1 was not suspected to drought treatment in this study (Figure 2E). In addition, ZjZFN1 expression was considerably elevated by exogenous ABA, with highest transcript abundance at 24 h after 10 μM application (15.68-fold higher than that at 0 h) (Figure 2F). On the contrary, ZjZFN1 expression was prone to suppression by 10 μM MeJA during 24 h after its application (Figure 2G). Although 0.5 mM SA suppressed the expression of ZjZFN1 1 h after application, it then stimulated the expression of ZjZFN1, which was highest 3 h after MeJA application (Figure 2H).
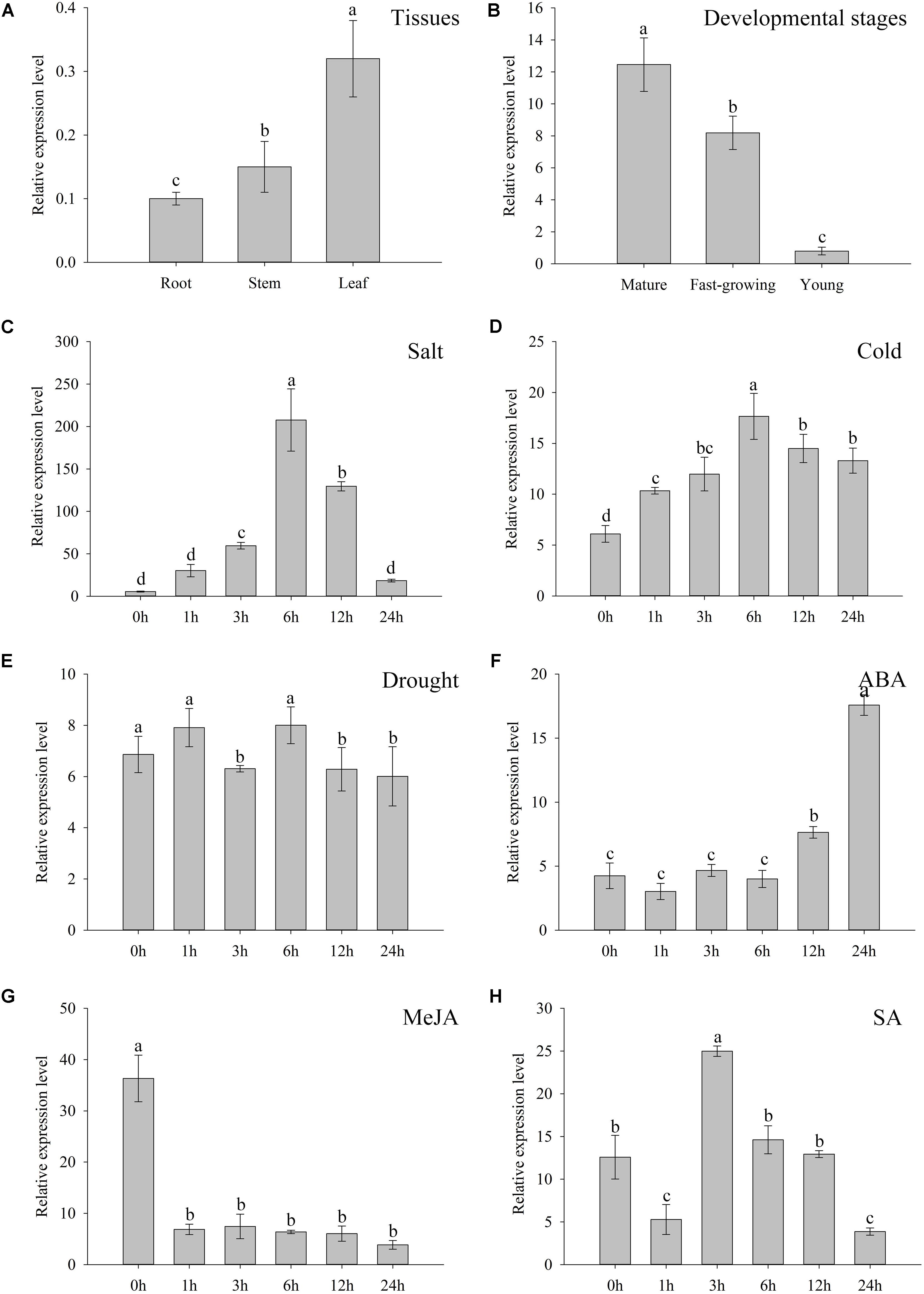
FIGURE 2. Expression characters of ZjZFN1. (A) ZjZFN1 expression levels in root, stem, and leaf of Z. japonica. (B) Expression levels of ZjZFN1 in Z. japonica leaves at different developmental stages. (C–F) ZjZFN1 expression levels in Z. japonica exposed to salt (C), cold (D), drought (E), 10 μM ABA (F), 10 μM MeJA (G), and 0.5 mM SA (H). Mean ± SDs (n = 3). Different letters indicate significant differences at 5% level of probability.
ZjZFN1 Was Localized to Nucleus and Had Transcriptional Activity
Bioinformatics analysis showed that ZjZFN1 contained a nuclear localization signal. To confirm the exact subcellular localization of ZjZFN1, we performed transient overexpression of 35S::ZjZFN1:YFP in N. benthamiana leaf cells. Laser confocal microscopic observations revealed strong YFP signal in the whole cells of the control while the YFP signal was only found in nucleus in 35S::ZjZFN1:YFP overexpressing cells (Figure 3A). It proved that ZjZFN1 was localized to the nucleus.
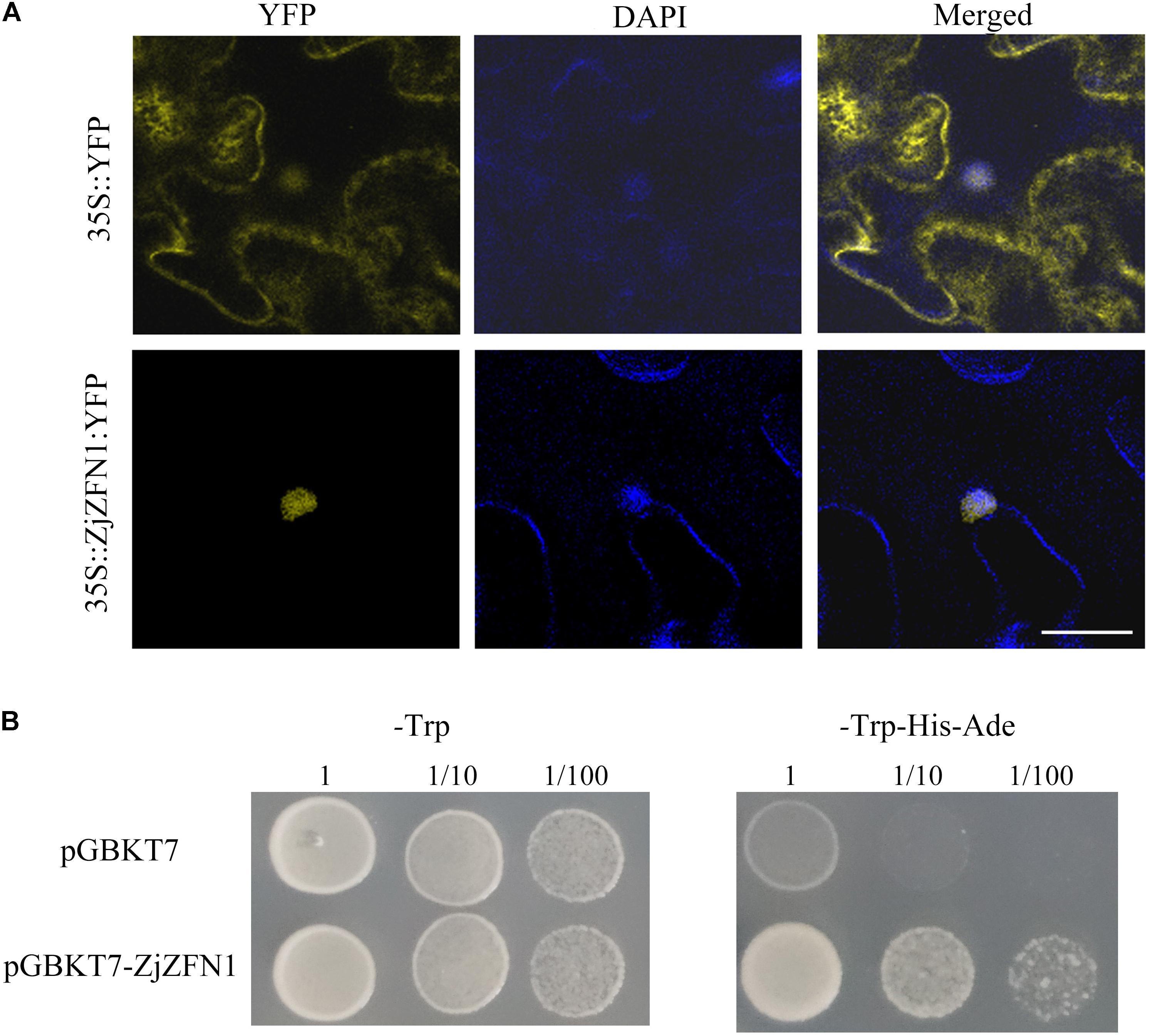
FIGURE 3. Subcellular localization and transcriptional activation ability assay of ZjZFN1. (A) Subcellular localization of ZjZFN1 under UV radiation in N. benthamiana leaf epidermis. Fluorescence of 35S::ZjZFN1:YFP was dominantly distributed in the nucleus. (B) Transcriptional activation ability assay using Y2HGold yeast system.
The Y2HGold yeast cells transformed with pGBKT7-ZjZFN1 and pGBKT7 empty vectors could grow normally on SD/-Trp medium (Figure 3B) suggesting that the target plasmids were successfully transformed in the host cells. Further analysis showed that the yeast cells transformed with the pGBKT7-ZjZFN1 vector could grow on SD/-Trp-His-Ade medium whereas control yeast cells (Figure 3B). Thus ZjZFN1 had transcriptional activation ability.
Abundant ZjZFN1 Expression in Leaf
The native promoter of ZjZFN1 was infused with GUS to generate the ZjZFN1pro::GUS construct, and transgenic Arabidopsis lines were generated to examine promoter activity. Twenty-two independent transgenic lines were examined for GUS staining. Histochemical analysis showed obvious GUS signal in the seedlings transformed with the 35S::GUS construct (control) (Figure 4A). However, strong GUS activity was only detected in the leaves and petioles of the ZjZFN1pro::GUS transgenic seedlings (Figures 4B–D). These results proposed that ZjZFN1 was mostly expressed in leaf and petioles.
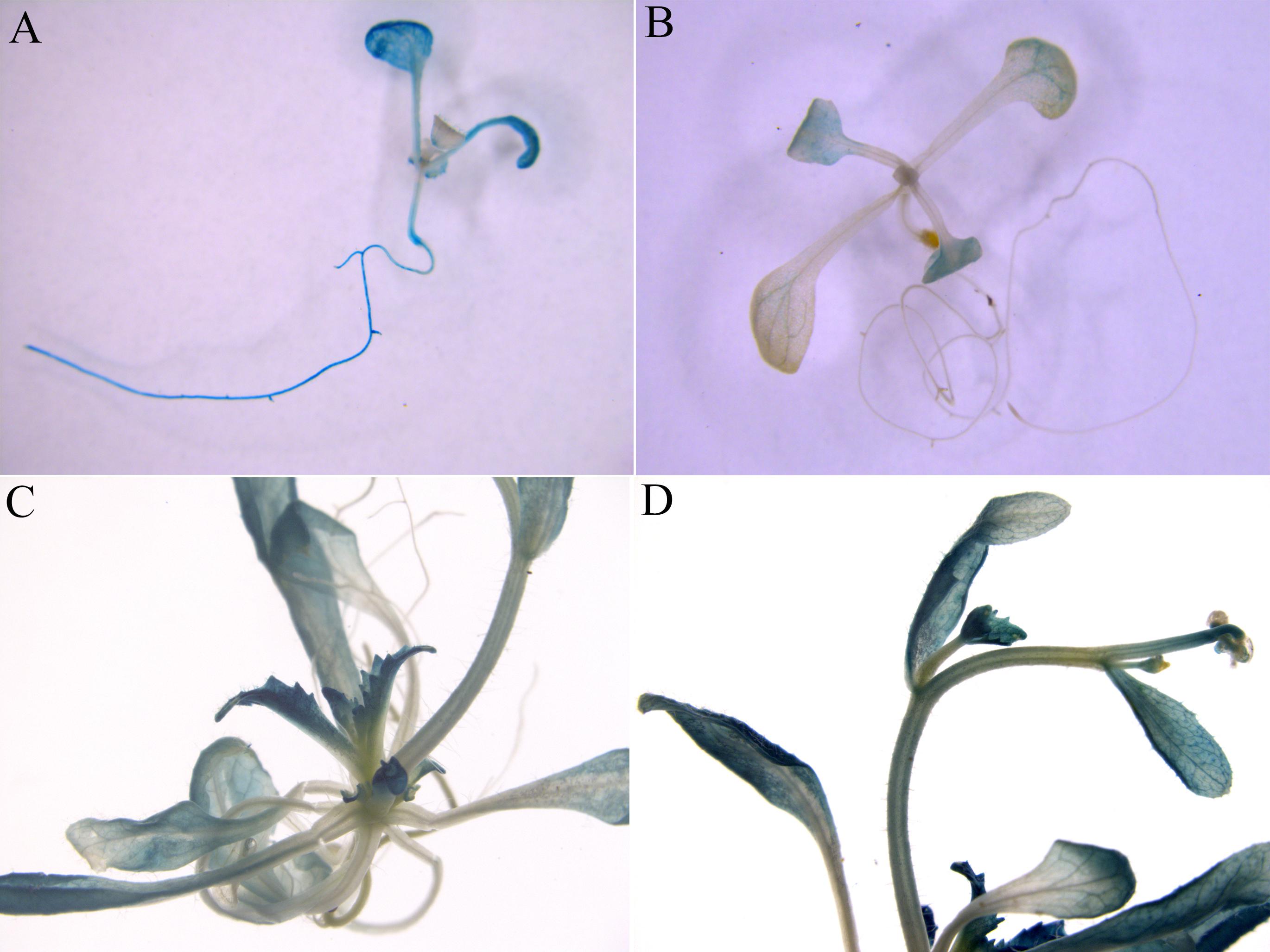
FIGURE 4. β-Glucuronidase (GUS) staining in transgenic Arabidopsis seedlings. (A) 35S::GUS (control), (B) 2-week-old ZjZFN1Pro::GUS-overexpressing Arabidopsis seedlings, (C,D) Mature ZjZFN1Pro::GUS-overexpressing Arabidopsis seedlings.
ZjZFN1 Positively Regulated Seed Germination Under Salt Stress
Arabidopsis plants overexpressing ZjZFN1 were generated to characterize the function of ZjZFN1 in response to salt stress. No significant difference in germination determination was observed between WT and transgenic lines in the absence of NaCl (Figures 5A–C). However, the ZjZFN1-overexpressing lines showed enhanced salt tolerance in relation to that of WT in MS medium containing 150 mM NaCl before day 6. In detail, on the fourth day after being sowed in the presence of 150 mM NaCl, approximately 60% of WT seeds germinated with emerged radicles, while nearly 75% transgenic seeds germinated with emerged radicles (Figure 5D). Obvious differences were also observed in terms of the percentage of green cotyledons at the eighth day after sowing (Figure 5B).
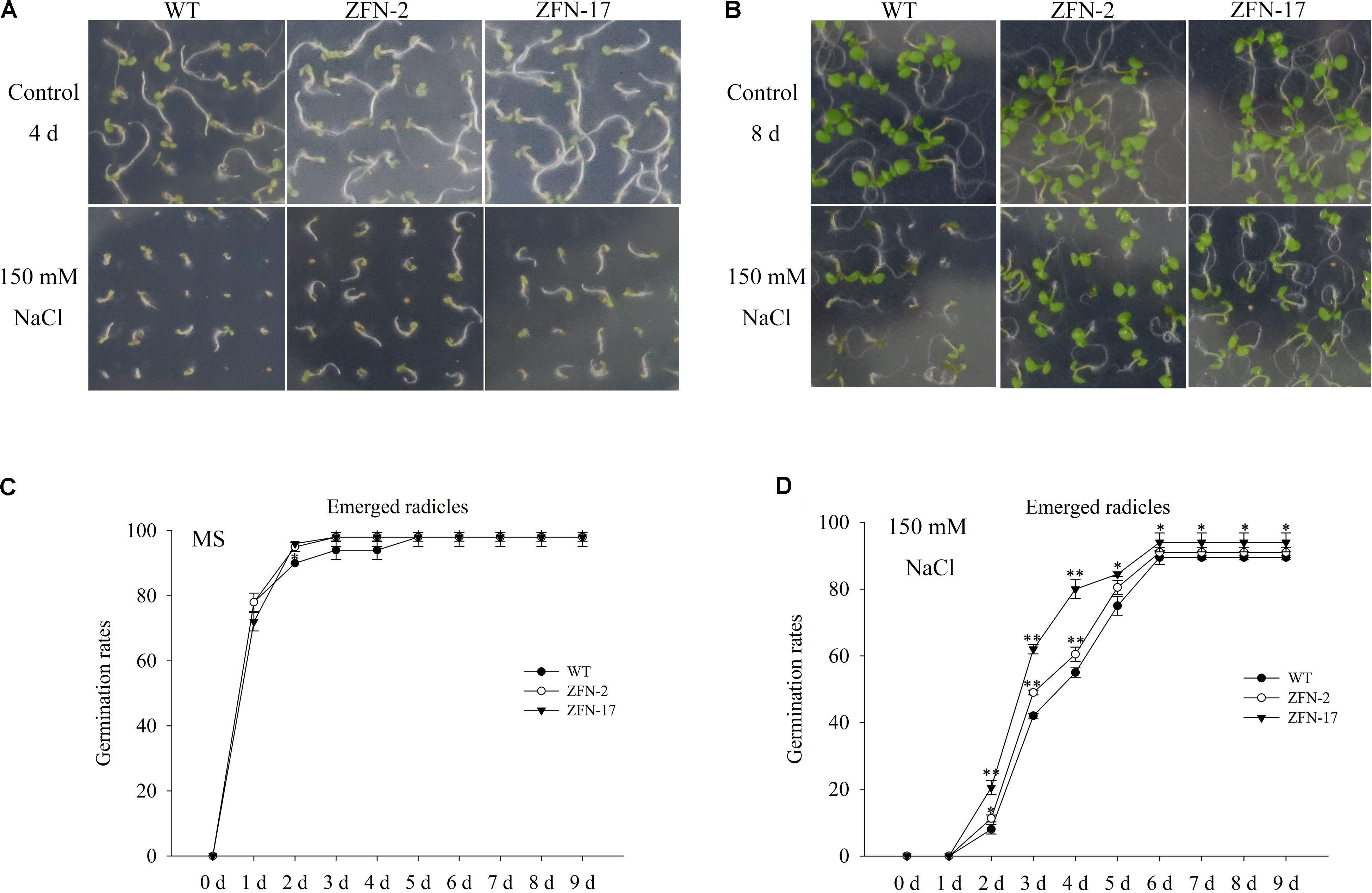
FIGURE 5. Germination assay of ZjZFN1-overexpressing lines in 150 mM NaCl. Growth of the transgenic and WT plants in the presence of 150 mM NaCl for 4 days (A) and 8 days (B), respectively. Rates of emerged radicles of control and the transgenic plants in MS medium (C) and MS medium containing 150 mM NaCl (D).
Overexpression of ZjZFN1 Enhanced Plant Tolerance to Salt Stress
To further examine the function of ZjZFN1, 3-week-old ZjZFN1-overexpressing plants were subjected to 150 mM NaCl for 21 days. All surveyed plants showed delayed growth and leaves were dehydrated, although ZFN-2 and ZFN-17 plants showed less damage compared to that observed in WT plants (Figure 6A). Accordingly, no significant difference in MDA or proline content was detected between ZjZFN1-overexpressing and WT plants under normal conditions. Nevertheless, MDA contents in WT increased to 1.78 nmol g-1, which was 7.5 and 16.9% higher than that in ZFN-2 and ZFN-17, respectively, at the end of the experiment (Figure 6B). In addition, under salt stress, ZFN-2 and ZFN-17 showed 15.6 and 27.7% increased proline content in relation to that of WT, respectively (Figure 6C).
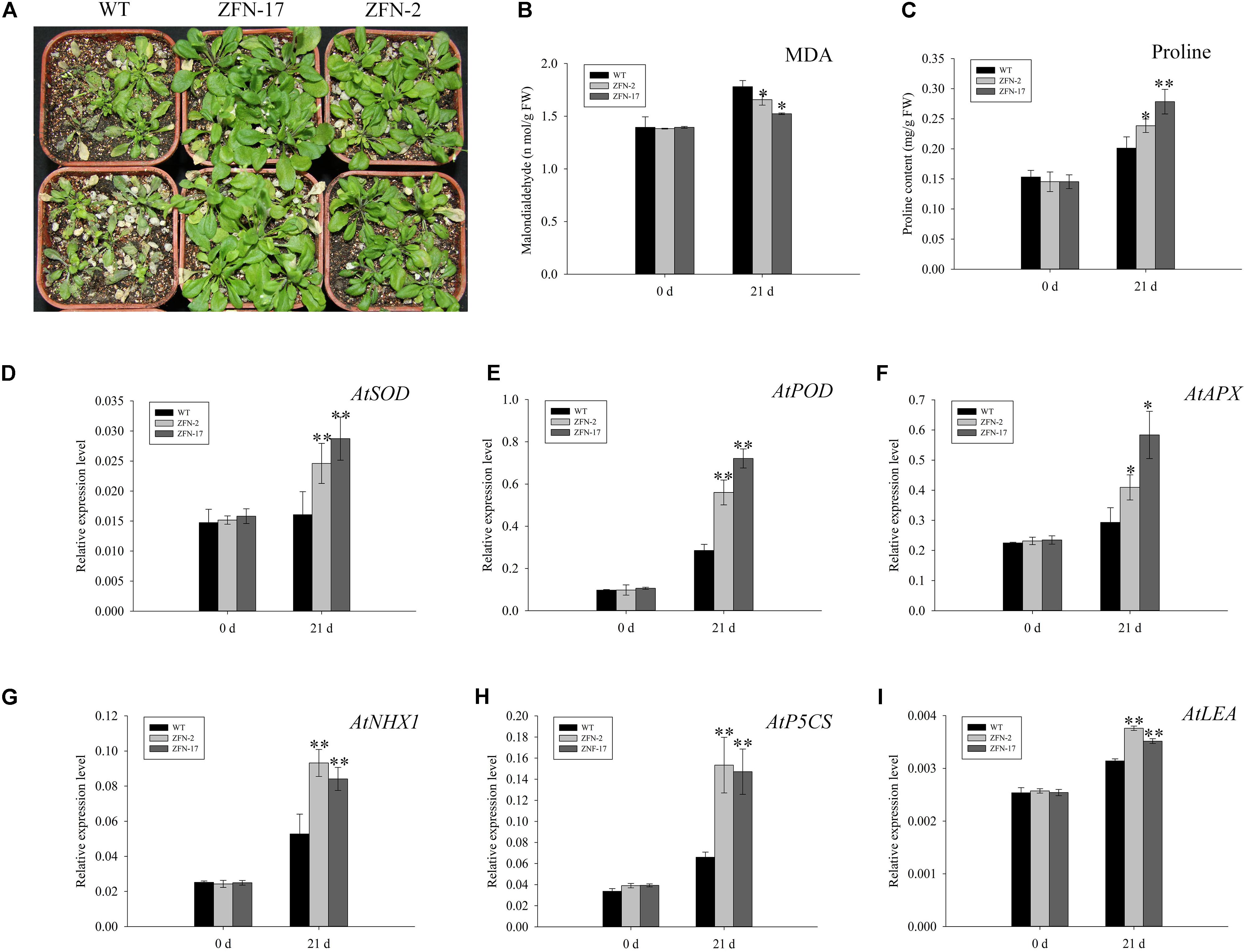
FIGURE 6. Phenotype observation and indexes determination. (A) Phenotype of transgenic lines and control under 150 mM NaCl treatment for 21 days. (B) Comparison of MDA. (C) Proline contents. (D) AtSOD expression. (E) AtPOD expression. (F) AtAPX expression. (G) AtNHX1 expression. (H) AtP5CS expression. (I) AtLEA expression. ∗ and ∗∗, respectively, represent significant differences from the control at values of P < 0.05 and P < 0.01 as determined by Student’s t-test.
To interpret the underlying transcriptional mechanism, a qRT-PCR was performed to evaluate gene expression differences between ZjZFN1-overexpressing and WT plants. Six salinity responsive genes involved in antioxidation (AtSOD, AtPOD, and AtAPX), ion transport (AtNHX1), and osmotic regulation (AtP5CS and AtLEA) were selected to monitor transcriptional variation. No obvious difference in gene expression was found between WT and transgenic plants under normal growth conditions. However, under salinity stress, the transcription of all genes was up-regulated, particularly in ZjZFN1-overexpressing plants (Figures 6D–I).
Global Expression Analysis of Abiotic Stress-Related Genes in ZjZFN1-Overexpressing Plants
To provide a comprehensive landscape of the transcriptional regulation network of ZjZFN1 and gain insight into the performance of ZjZFN1-overexpressing plants, we carried out an RNA-seq assessment to screen DEGs between WT and transgenic plants (ZFN-17). Among the 289 DEGs identified, 274 were up-regulated and 15 were down-regulated in ZFN-17 (Figure 7A and Supplementary Table S1). The DEGs were classified into 51 groups based on their allocated GO terms (Supplementary Figure S1). Further classification of the DEGs within the “biological process” group revealed a large number of abiotic stress responsive DEGs including “response to stress,” “response to abiotic stimulus,” and “response to salt stress” (Figure 7B). All the 21 DEGs classified within the “response to salt stress” term were up-regulated, and these included genes SOS2, WRK33, MYB15, and peroxidase (isoforms 22 and 23), among other genes (Table 2).
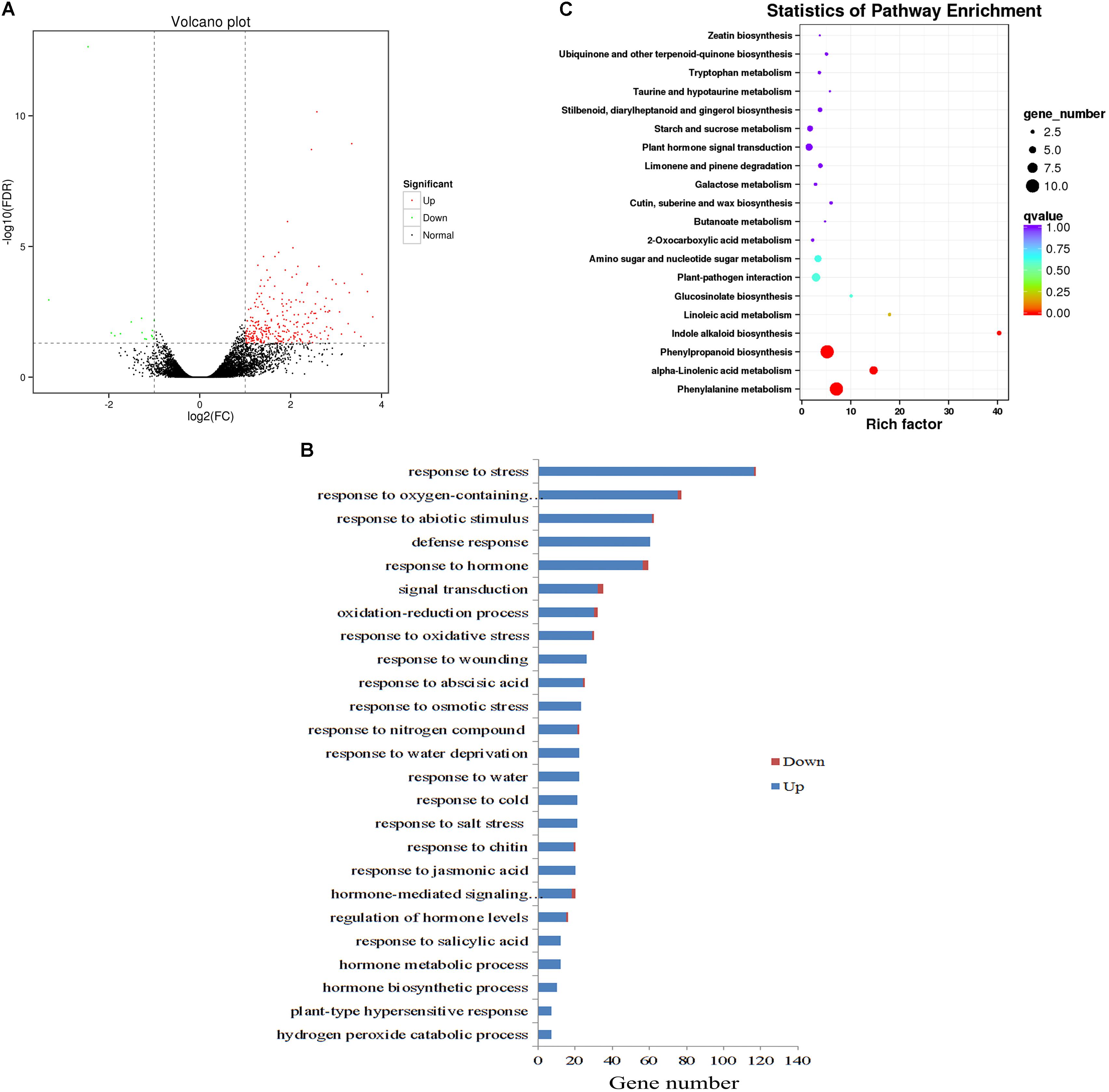
FIGURE 7. Global transcriptome analysis of WT and ZjZFN1-overexpressing Arabidopsis plants. (A) Volcano plot of the DEGs. (B) Gene ontology (GO) classification for abiotic stress-related DEGs. Only the biological processes were collected for GO term analysis. (C) Statistic of KEGG pathway enrichment.
All DEGs were analyzed regarding the pathways underlying the regulation of ZjZFN1. The 36 enriched KEGG pathways included 33 metabolic pathways (Supplementary Figure S1). Among these, the “phenylalanine metabolism” and “phenylpropanoid biosynthesis” pathways, both containing 10 annotated DEGs, were the most abundant pathways. Statistics of pathway enrichment revealed that “phenylalanine metabolism,” “alpha-linolenic acid metabolism,” “phenylpropanoid biosynthesis,” and “indole alkaloid biosynthesis” pathways were significantly enriched (Corrected P < 0.05) (Figure 7C).
Discussion
Genetic studies on halophytic species lag far behind those in other species and their potential remain unexplored (Orsini et al., 2010). Zinc finger proteins, a large gene family in plants, plays a key role in environmental adaption. Here, we isolated a novel zinc finger protein, ZjZFN1, from Z. japonica aiming to better understand its function and underlying transcriptional regulation mechanism. Homology analysis showed that ZjZFN1 belonged to the C2H2 zinc finger protein family and contained two typical zinc finger structures, suggesting its potential for high DNA binding affinity (Kielbowicz-Matuk, 2012). The localization of ZjZFN1 to the nucleus was consistent with that of ZAT6 (Shi et al., 2014) and ZAT18 (Yin et al., 2017) in Arabidopsis, OsMSR15 in Oryza sativa (Zhang X. et al., 2016), and VvZFP11 in Vitis vinifera (Yu et al., 2016). The yeast assay provided evidence that ZjZFN1 has transcriptional activation ability, which was consistent with the results obtained for VvZFP11 and OsMSR15. As extensively reported, the nuclear localization attribute together with transcriptional activation ability suggest that ZjZFN1 might function as a typical plant TF.
Although ZjZFN1 was widely expressed in different tissues of Z. japonica, the highest expression levels were found in leaves. Additionally, ZjZFN1 transcript abundance was highest in mature leaves. In agreement, GUS staining results corroborated those of the qRT-PCR, suggesting that ZjZFN1 might be related with leaf development. The motifs exhibited in the ZjZFN1 promoter suggested that its expression might be regulated by different abiotic and hormone factors. In the present study, the transcription of ZjZFN1 was induced by cold and salt treatments but not by drought stress, which is consistent with that found for ZAT6, ZAT10, and ZAT18 (Yin et al., 2017), except for drought stress. Hormone induction experiments revealed that ZjZFN1 expression changed in response to SA, which is consistent with the results obtained for AtZAT6 (Shi et al., 2014) and VvZFP11 (Yu et al., 2016). The expression level of ZjZFN1 was also up-regulated by exogenous ABA, in agreement with the attributes of GmZFP3 (Zhang D.Y. et al., 2016), StZFP1 (Tian et al., 2010), and TaDi19A (Li et al., 2010). These results indicate that ZjZFN1 might play several roles in plants’ response to environmental stresses and might be an important factor for signal transduction.
C2H2 zinc finger proteins have key roles in the response to salinity stress in different plant species. In the present study, the germination and growth of ZjZFN1-overexpressing lines was obviously improved under salinity stress compared with that of WT plants. MDA is regarded as a typical indicator of salinity stress (Shi et al., 2013b). Additionally, increase in proline content is a primary metabolic defense for protecting plants from abiotic stresses (Zhang et al., 2011). Consistent with phenotype observations, transgenic lines showed lower MDA but higher proline content than WT plants under salt stress, supporting the hypothesis that ZjZFN1 overexpression of enhances plant tolerance to salinity stress.
In plant responses to environmental stresses, transcriptional regulation plays a dominant role (Teng et al., 2017). Under salt stress, plants employ reactive oxygen species (ROS) scavenging enzymes, such as SOD, POD, and APX, to eliminate ROS accumulation. The improved transcript abundance of SOD, POD, and APX reflected an enhanced ROS-scavenging ability in ZjZFN1-overexpressing lines, which was consistent with that reported for ZFP179 (Sun et al., 2010). As an important ion transporter, the overexpression of NHX1 improves plant resistance to salinity stress (Shi et al., 2003). P5CS activates proline biosynthesis to protect protein integrity and enhance the activities of different enzymes under osmotic stress (Szabados and Savoure, 2010). LEA contributes to plant responses to abiotic stresses (Olvera-Carrillo et al., 2010). In the present study, the overexpression of ZjZFN1 induced the transcription of all selected genes under salinity stress in relation to shown by control (WT) plants, suggesting that ZjZFN1 may regulate salt tolerance via multiple pathways, in agreement with phenotype observations.
Genes encoding stress-activated C2H2 TFs also fine-tune the transcription of stress-responsive genes, leading to parallel changes in plant adaptation to various stresses (Kielbowicz-Matuk, 2012; Yin et al., 2017). Transcriptome analysis is a powerful tool for the identification of novel C2H2-manipulating genes. Among the 289 screened DEGs, 274 (94.81%) were up-regulated and 15 (5.19%) were down-regulated by ZjZFN1, corroborating that ZjZFN1 may function as a transcriptional activator. Specifically, some of the 21 DEGs enriched in the “response to salt stress” term, corresponded to genes SOS2, peroxidase (isoforms 22 and 23), WRKY33, and MYB15, are directly involved in plant salt tolerance. Increasing evidence has shown that phenylalanine ammonia-lyase (PAL) metabolism pathway actively participates in plant environmental stresses (Chen et al., 2008). Phenylpropanoid is an important enzyme involved in plant cell defense and response to salinity stress (Gao et al., 2008). Alpha-linolenic acid metabolism is one of the major fatty acids in leaf membrane lipids, and it has been reported to contribute to the osmotic tolerance of rice (Lenka et al., 2011). Pathway enrichment analysis demonstrated that the three pathways above were over-represented in ZjZFN1-overexpressing plants, indicating that ZjZFN1 could modulate stress responsive pathways, which in turn contribute to the improved adaptive capacity of transgenic plants.
Conclusion
In summary, we isolated ZjZFN1 and interpreted its functions in salinity stress responses. The overexpression of ZjZFN1 improved stress responses in seed germination and enhanced salt tolerance of transgenic lines. The ZjZFN1 transgene decreased leaf MDA content and improved proline content, and improved transcriptional activities of several salt-stress-related genes under salinity stress conditions. The RNA-seq results indicated that ZjZFN1 could function as a transcriptional activator, actively participating in the improvement of stress responsive genes’ expression and regulating stress-responsive pathways, including phenylalanine metabolism, α-linolenic acid metabolism, and phenylpropanoid biosynthesis pathways. Overall, our results suggest that ZjZFN1 might be a valuable gene in Z. japonica breeding projects.
Author Contributions
KT and JW conceived the study and designed the experiments. KT and PT performed the experiments. KT and WG analyzed the data with suggestions by YY, XF, and JW. KT wrote the manuscript.
Funding
This research was supported by the China Postdoctoral Science Foundation (2017M620677), Beijing Postdoctoral Research Foundation (No. 2017ZZ-087), Postdoctoral Fund of the Beijing Academy of Agriculture and Forestry Sciences, Scientific Funds of Beijing Academy of Agriculture and Forestry Sciences (KJCX20161502-1 and KJCX20180101), and Beijing Technology Plan Project (No. 171100007217001).
Conflict of Interest Statement
The authors declare that the research was conducted in the absence of any commercial or financial relationships that could be construed as a potential conflict of interest.
Acknowledgments
We are very grateful to Dr. Ning Jia (Institute of Food Science and Technology, Chinese Academy of Agricultural Science) for providing the pGBKT7 vector and Dr. Chuanjing An (School of Pharmaceutical Sciences, Peking University) for technical assistance with the microscopy.
Supplementary Material
The Supplementary Material for this article can be found online at: https://www.frontiersin.org/articles/10.3389/fpls.2018.01159/full#supplementary-material
FIGURE S1. Gene ontology and KEGG annotation of the identified DEGs. (A) GO classification. (B) KEGG annotation.
TABLE S1. List of differently expressed genes between ZjZFN1-overexpressing line (ZFN-17) and WT screened by RNA-sequencing.
Abbreviations
ABA, abscisic acid; DAPI, 4′,6-diamidino-2-phenylindole; DEGs, differently expressed genes; GO, Gene ontology; GUS, β-glucuronidase; KEGG, Kyoto Encyclopedia of Genes and Genomes; MeJA, methyl jasmonate; qRT-PCR, quantitative real-time PCR; RNA-seq, RNA sequencing; SA, salicylic acid; TFs, transcript factors.
Footnotes
- ^ http://web.expasy.org/compute_pi/
- ^ http://www.softberry.com
- ^ http://sogo.dna.affrc.go.jp/cgi-bin/sogo.cgi?lang=en&pj=640&action=page&page=newplace
- ^ http://www.cbs.dtu.dk/services/SignalP/
- ^ https://www.genome.jp/tools-bin/clustalw
- ^ ftp://ftp.ensemblgenomes.org/pub/plants/release-25/fasta/arabidopsis_thaliana/
References
Chen, J. Y., He, L. H., Jiang, Y. M., Wang, Y., Joyce, D. C., Ji, Z. L., et al. (2008). Role of phenylalanine ammonia-lyase in heat pretreatment-induced chilling tolerance in banana fruit. Physiol. Plant. 132, 318–328. doi: 10.1111/j.1399-3054.2007.01013.x
Ciftci-Yilmaz, S., Morsy, M. R., Song, L., Coutu, A., Krizek, B. A., Lewis, M. W., et al. (2007). The EAR-motif of the Cys2/His2-type zinc finger protein Zat7 plays a key role in the defense response of Arabidopsis to salinity stress. J. Biol. Chem. 282, 9260–9268. doi: 10.1074/jbc.M611093200
Clough, S. J., and Bent, A. F. (1998). Floral dip: a simplified method for Agrobacterium-mediated transformation of Arabidopsis thaliana. Plant J. 16, 735–743. doi: 10.1046/j.1365-313x.1998.00343.x
Deinlein, U., Stephan, A. B., Horie, T., Luo, W., Xu, G., and Schroeder, J. I. (2014). Plant salt-tolerance mechanisms. Trends Plant Sci. 19, 371–379. doi: 10.1016/j.tplants.2014.02.001
Gao, S., Ouyang, C., Wang, S., Xu, Y., Tang, L., and Chen, F. (2008). Effects of salt stress on growth, antioxidant enzyme and phenylalanine ammonia-lyase activities in Jatropha curcas L. seedlings. Plant Soil and Environ. 54, 374–381. doi: 10.17221/410-PSE
Guo, H. L., Ding, W. W., Chen, J. B., Chen, X., Zheng, Y. Q., Wang, Z. Y., et al. (2014). Genetic linkage map construction and QTL mapping of salt tolerance traits in Zoysiagrass (Zoysia japonica). PLoS One 9:e107249. doi: 10.1371/journal.pone.0107249
Hoagland, D. R., and Arnon, D. I. (1950). The water-culture method for growing plants without soil. Circ. Calif. Agric. Exp. Station 347:32.
Huang, B. R., Dacosta, M., and Jiang, Y. W. (2014). Research advances in mechanisms of turfgrass tolerance to abiotic stresses: from physiology to molecular biology. Crit. Rev. Plant Sci. 33, 141–189. doi: 10.1080/07352689.2014.870411
Huang, J., Yang, X., Wang, M. M., Tang, H. J., Ding, L. Y., Shen, Y., et al. (2007). A novel rice C2H2-type zinc finger protein lacking DLN-box/EAR-motif plays a role in salt tolerance. Biochim. Biophys. Acta 1769, 220–227. doi: 10.1016/j.bbaexp.2007.02.006
Jia, N., Liu, X. M., and Gao, H. B. (2016). A DNA2 homolog is required for dna damage repair, cell cycle regulation, and meristem maintenance in plants. Plant Physiol. 171, 318–333. doi: 10.1104/pp.16.00312
Kielbowicz-Matuk, A. (2012). Involvement of plant C2H2-type zinc finger transcription factors in stress responses. Plant Sci. 185, 78–85. doi: 10.1016/j.plantsci.2011.11.015
Kim, D., Pertea, G., Trapnell, C., Pimentel, H., Kelley, R., and Salzberg, S. L. (2013). TopHat2: accurate alignment of transcriptomes in the presence of insertions, deletions and gene fusions. Genome Biol. 14:R36. doi: 10.1186/gb-2013-14-4-r36
Laity, J. H., Lee, B. M., and Wright, P. E. (2001). Zinc finger proteins: new insights into structural and functional diversity. Curr. Opin. Struct. Biol. 11, 39–46. doi: 10.1016/S0959-440X(00)00167-6
Lenka, S. K., Katiyar, A., Chinnusamy, V., and Bansal, K. C. (2011). Comparative analysis of drought-responsive transcriptome in Indica rice genotypes with contrasting drought tolerance. Plant Biotechnol. J. 9, 315–327. doi: 10.1111/j.1467-7652.2010.00560.x
Li, S. O., Xu, C. H., Yang, Y. A., and Xia, G. M. (2010). Functional analysis of TaDi19A, a salt-responsive gene in wheat. Plant Cell Environ. 33, 117–129. doi: 10.1111/j.1365-3040.2009.02063.x
Li, Y., Geng, L., and Liu, J. (2004). Assessment on salt–tolerance of Zoysia spp. in China. Acta Agrestia Sin. 12, 8–11.
Liu, J. Q., Chen, X. J., Liang, X. X., Zhou, X. G., Yang, F., Liu, J., et al. (2016). Alternative splicing of rice wrky62 and wrky76 transcription factor genes in pathogen defense. Plant Physiol. 171, 1427–1442. doi: 10.1104/pp.15.01921
Livak, K. J., and Schmittgen, T. D. (2001). Analysis of relative gene expression data using real-time quantitative PCR and the 2- ΔΔCT method. Methods 25, 402–408. doi: 10.1006/meth.2001.1262
Luo, X., Bai, X., Zhu, D., Li, Y., Ji, W., Cai, H., et al. (2012). GsZFP1, a new Cys2/His2-type zinc-finger protein, is a positive regulator of plant tolerance to cold and drought stress. Planta 235, 1141–1155. doi: 10.1007/s00425-011-1563-0
Mao, X. Z., Cai, T., Olyarchuk, J. G., and Wei, L. P. (2005). Automated genome annotation and pathway identification using the KEGG orthology (KO) as a controlled vocabulary. Bioinformatics 21, 3787–3793. doi: 10.1093/bioinformatics/bti430
Mittler, R., Kim, Y., Song, L., Coutu, J., Coutu, A., Ciftci-Yilmaz, S., et al. (2006). Gain- and loss-of-function mutations in Zat10 enhance the tolerance of plants to abiotic stress. FEBS Lett. 580, 6537–6542. doi: 10.1016/j.febslet.2006.11.002
Mortazavi, A., Williams, B. A., Mccue, K., Schaeffer, L., and Wold, B. (2008). Mapping and quantifying mammalian transcriptomes by RNA-Seq. Nat. Methods 5, 621–628. doi: 10.1038/nmeth.1226
Olvera-Carrillo, Y., Campos, F., Reyes, J. L., Garciarrubio, A., and Covarrubias, A. A. (2010). Functional analysis of the group 4 late embryogenesis abundant proteins reveals their relevance in the adaptive response during water deficit in arabidopsis. Plant Physiol. 154, 373–390. doi: 10.1104/pp.110.158964
Orsini, F., D’urzo, M. P., Inan, G., Serra, S., Oh, D. H., Mickelbart, M. V., et al. (2010). A comparative study of salt tolerance parameters in 11 wild relatives of Arabidopsis thaliana. J. Exp. Bot. 61, 3787–3798. doi: 10.1093/jxb/erq188
Shi, H. Z., Lee, B. H., Wu, S. J., and Zhu, J. K. (2003). Overexpression of a plasma membrane Na+/H+ antiporter gene improves salt tolerance in Arabidopsis thaliana. Nat. Biotechnol. 21, 81–85. doi: 10.1038/nbt766
Shi, H. T., Wang, X., Ye, T. T., Chen, F. F., Deng, J., Yang, P. F., et al. (2014). The Cysteine2/Histidine2-Type Transcription Factor ZINC FINGER OF Arabidopsis thaliana6 modulates biotic and abiotic stress responses by activating salicylic acid-related genes and c-repeat-binding factor genes in Arabidopsis. Plant Physiol. 165, 1367–1379. doi: 10.1104/pp.114.242404
Shi, H. T., Ye, T. T., and Chan, Z. L. (2013a). Comparative proteomic and physiological analyses reveal the protective effect of exogenous polyamines in the Bermudagrass (Cynodon dactylon) response to salt and drought stresses. J. Proteome Res. 12, 4951–4964. doi: 10.1021/pr400479k
Shi, H. T., Ye, T. T., and Chan, Z. L. (2013b). Exogenous application of hydrogen sulfide donor sodium hydrosulfide enhanced multiple abiotic stress tolerance in bermudagrass (Cynodon dactylon (L). pers.). Plant Physiol. Biochem. 71, 226–234. doi: 10.1016/j.plaphy.2013.07.021
Sparkes, I. A., Runions, J., Kearns, A., and Hawes, C. (2006). Rapid, transient expression of fluorescent fusion proteins in tobacco plants and generation of stably transformed plants. Nat. Protoc. 1, 2019–2025. doi: 10.1038/nprot.2006.286
Sun, S. J., Guo, S. Q., Yang, X., Bao, Y. M., Tang, H. J., Sun, H., et al. (2010). Functional analysis of a novel Cys2/His2-type zinc finger protein involved in salt tolerance in rice. J. Exp. Bot. 61, 2807–2818. doi: 10.1093/jxb/erq120
Szabados, L., and Savoure, A. (2010). Proline: a multifunctional amino acid. Trends Plant Sci. 15, 89–97. doi: 10.1016/j.tplants.2009.11.009
Teng, K., Tan, P. H., Xiao, G. Z., Han, L. B., Chang, Z. H., and Chao, Y. H. (2017). Heterologous expression of a novel Zoysia japonica salt-induced glycine-rich RNA-binding protein gene, ZjGRP, caused salt sensitivity in Arabidopsis. Plant Cell Rep. 36, 179–191. doi: 10.1007/s00299-016-2068-x
Tian, Z. D., Zhang, Y., Liu, J., and Xie, C. H. (2010). Novel potato C2H2-type zinc finger protein gene, StZFP1, which responds to biotic and abiotic stress, plays a role in salt tolerance. Plant Biology 12, 689–697. doi: 10.1111/j.1438-8677.2009.00276.x
Xie, Q., Niu, J., Xu, X. L., Xu, L. X., Zhang, Y. B., Fan, B., et al. (2015). De novo assembly of the Japanese lawngrass (Zoysia japonica Steud.) root transcriptome and identification of candidate unigenes related to early responses under salt stress. Front. Plant Sci. 6:610. doi: 10.3389/fpls.2015.00610
Xu, L. X., Zhang, M. L., Zhang, X. Z., and Han, L. B. (2015). Cold acclimation treatment-induced changes in abscisic acid, cytokinin, and antioxidant metabolism in Zoysiagrass (Zoysia japonica). HortScience 50, 1075–1080. doi: 10.1007/s10142-011-0213-8
Yin, M. Z., Wang, Y. P., Zhang, L. H., Li, J. Z., Quan, W. L., Yang, L., et al. (2017). The Arabidopsis Cys2/His2 zinc finger transcription factor ZAT18 is a positive regulator of plant tolerance to drought stress. J. Exp. Bot. 68, 2991–3005. doi: 10.1093/jxb/erx157
Young, M. D., Wakefield, M. J., Smyth, G. K., and Oshlack, A. (2010). Gene ontology analysis for RNA-seq: accounting for selection bias. Genome Biol. 11:R14. doi: 10.1186/gb-2010-11-2-r14
Yu, Y. H., Li, X. Z., Wu, Z. J., Chen, D. X., Li, G. R., Li, X. Q., et al. (2016). VvZFP11, a Cys2His2-type zinc finger transcription factor, is involved in defense responses in Vitis vinifera. Biol. Plant. 60, 292–298. doi: 10.1007/s10535-016-0598-2
Zhang, D. Y., Tong, J. F., Xu, Z. L., Wei, P. P., Xu, L., Wan, Q., et al. (2016). Soybean C2H2-type Zinc finger protein GmZFP3 with conserved QALGGH motif negatively regulates drought responses in transgenic Arabidopsis. Front. Plant Sci. 7:325. doi: 10.3389/fpls.2016.00325
Zhang, H., Liu, Y. P., Wen, F., Yao, D. M., Wang, L., Guo, J., et al. (2014). A novel rice C2H2-type zinc finger protein, ZFP36, is a key player involved in abscisic acid-induced antioxidant defence and oxidative stress tolerance in rice. J. Exp. Bot. 65, 5795–5809. doi: 10.1093/jxb/eru313
Zhang, X., Zhang, B., Li, M. J., Yin, X. M., Huang, L. F., Cui, Y. C., et al. (2016). OsMSR15 encoding a rice C2H2-type zinc finger protein confers enhanced drought tolerance in transgenic Arabidopsis. J. Plant Biol. 59, 271–281. doi: 10.1007/s12374-016-0539-9
Zhang, X. Z., Wang, K. H., Ervin, E. H., Waltz, C., and Murphy, T. (2011). Metabolic changes during cold acclimation and deacclimation in five bermudagrass varieties. I. proline, total amino acid, protein, and dehydrin expression. Crop Sci. 51, 838–846. doi: 10.2135/cropsci2010.06.0345
Keywords: Zoysia japonica, C2H2 zinc finger protein, transgenic Arabidopsis, salinity tolerance, RNA-sequencing
Citation: Teng K, Tan P, Guo W, Yue Y, Fan X and Wu J (2018) Heterologous Expression of a Novel Zoysia japonica C2H2 Zinc Finger Gene, ZjZFN1, Improved Salt Tolerance in Arabidopsis. Front. Plant Sci. 9:1159. doi: 10.3389/fpls.2018.01159
Received: 22 April 2018; Accepted: 23 July 2018;
Published: 14 August 2018.
Edited by:
Kyung-Nam Kim, Sejong University, South KoreaReviewed by:
Kazuo Nakashima, Japan International Research Center for Agricultural Sciences, JapanDong-Ha Oh, Louisiana State University, United States
Copyright © 2018 Teng, Tan, Guo, Yue, Fan and Wu. This is an open-access article distributed under the terms of the Creative Commons Attribution License (CC BY). The use, distribution or reproduction in other forums is permitted, provided the original author(s) and the copyright owner(s) are credited and that the original publication in this journal is cited, in accordance with accepted academic practice. No use, distribution or reproduction is permitted which does not comply with these terms.
*Correspondence: Juying Wu, d3VqdXlpbmdAZ3Jhc3MtZW52LmNvbQ==