- 1Department of Plant Physiology, University of Silesia in Katowice, Katowice, Poland
- 2Institute for Ecology of Industrial Areas, Katowice, Poland
- 3Laboratoire de Physiologie et de Génétique Moléculaire des Plantes, Université Libre de Bruxelles, Brussels, Belgium
Hyperaccumulation and hypertolerance of Trace Metal Elements (TME) like Cd and Zn are highly variable in pseudo-metallophytes species. In this study we compared the impact of high Cd or Zn concentration on the photosynthetic apparatus of the Arabidopsis arenosa and Arabidopsis halleri pseudo-metallophytes growing on the same contaminated site in Piekary Slaskie in southern Poland. Plants were grown in hydroponic culture for 6 weeks, and then treated with 1.0 mM Cd or 5.0 mM Zn for 5 days. Chlorophyll a fluorescence and pigment content were measured after 0, 1, 2, 3, 4, and 5 days in plants grown in control and exposed to Cd or Zn treatments. Moreover, the effect of TME excess on the level of oxidative stress and gas-exchange parameters were investigated. In both plant species, exposure to high Cd or Zn induced a decrease in chlorophyll and an increase in anthocyanin contents in leaves compared to the control condition. After 5 days Cd treatment, energy absorbance, trapped energy flux and the percentage of active reaction centers decreased in both species. However, the dissipated energy flux in the leaves of A. arenosa was smaller than in A. halleri. Zn treatment had more toxic effect than Cd on electron transport in A. halleri compared with A. arenosa. A. arenosa plants treated with Zn excess did not react as strongly as in the Cd treatment and a decrease only in electron transport flux and percentage of active reaction centers compared with control was observed. The two species showed contrasting Cd and Zn accumulation. Cd concentration was almost 3-fold higher in A. arenosa leaves than in A. halleri. On the opposite, A. halleri leaves contained 3-fold higher Zn concentration than A. arenosa. In short, our results showed that the two Arabidopsis metallicolous populations are resistant to high Cd or Zn concentration, however, the photosynthetic apparatus responded differently to the toxic effects.
Introduction
Due to industrial and agricultural activities, such as mining, smelting, traffic, using of fertilizers, and sewage sludges, as well as natural processes including atmospheric deposition and weathering of minerals metal contamination has become serious environmental problem worldwide (Alloway, 2013; Su et al., 2014).
Cadmium (Cd) is considered as one of the most toxic non-essential elements for plants (Clemens and Ma, 2016). Despite zinc (Zn) being an essential microelement (Kabata-Pendias, 2011), its excess in plants can also induce phytotoxic effects (Chaney, 1993). It was found that excess of both trace metal elements (TME) can have similar negative influence on different elements of photosynthetic apparatus, for example: pigment biosynthesis, light capture, electron transport, stomatal conductance, CO2 assimilation, and activity of enzymes in Calvin cycle (Clijsters and Van Assche, 1985; Van Assche and Clijsters, 1986; Myśliwska-Kurdziel et al., 2002; Sagardoy et al., 2010; Vassilev et al., 2011; Verbruggen et al., 2013). However, the toxic effects of high Cd and excess Zn concentration on the complex process of photosynthesis are still poorly understood (Paunov et al., 2018).
Arabidopsis halleri and A. arenosa are pseudo-metallophytes closely related to A. thaliana (Clauss and Koch, 2006), which are used to study the adaptation to environments highly contaminated with TME. Both species can be commonly found on metalliferous and non-metalliferous sites in southern Poland (Fiałkiewicz and Rostanski, 2006; Szarek-Łukaszewska and Grodzinska, 2007, 2011; Preite et al., 2018). It was documented that metalliferous populations of A. halleri and A. arenosa are hypertolerant to Cd and Zn (Przedpełska and Wierzbicka, 2007; Nadgórska-Socha et al., 2013; Sitko et al., 2017; Stein et al., 2017). While Zn hyperaccumulation is a constitutive trait, Cd accumulation is highly variable within A. halleri populations (Pauwels et al., 2012; Meyer et al., 2015; Sitko et al., 2017; Stein et al., 2017; Corso et al., 2018; Frérot et al., 2018; Schvartzman et al., 2018), whereas these traits are poorly studied in A. arenosa.
Measurements of chlorophyll a fluorescence is a noninvasive and sensitive method for monitoring physiological status of plants (Baker, 2008; Kalaji et al., 2012, 2014; Sitko et al., 2017). In recent years such measurements have become more and more commonly used under various conditions such as TME stress (Kalaji et al., 2014; Daszkowska-Golec et al., 2017; Sitko et al., 2017; Paunov et al., 2018). Analysis of chlorophyll a fluorescence kinetics, known as OJIP transient, can provide information on electron transport reactions mainly inside PSII and parts of PSI (Baker, 2008; Kalaji et al., 2014; Goltsev et al., 2016; Paunov et al., 2018). In order to obtain more complete picture of effect of TME excess on photosynthesis, measurements of leaf gas-exchange parameters such as photosynthetic rate based on CO2 assimilation and parameters based on chlorophyll a fluorescence are necessary (Arshad et al., 2015; Rusinowski et al., 2019).
Measurements of pigment contents such as chlorophyll, flavonols and anthocyanins have also become increasingly popular due to the development of portable devices, which enable measurements of these pigment contents in laboratory as well as in situ (Cerovic et al., 2015; Lefebvre et al., 2016; Gonzalez-Mendoza et al., 2017; Hosseini et al., 2017; Sitko et al., 2017). Flavonols and anthocyanins belong to flavonoid secondary metabolites, which are the largest class of polyphenols (around 8,000 metabolites) in plants (Mattivi et al., 2006; Tohge et al., 2017). Flavonoids are characterized by two benzene rings linked by a heterocyclic pyran ring and primarily occurring in plants as O-glycosides. They play a major role in plant protection against negative effects of abiotic and biotic stress factors in model and crop plants (Jaakola et al., 2004; Corso et al., 2015; Tohge et al., 2017). Flavonols and anthocyanins can serve as protection against damage caused by TME, free oxygen radicals and excessive light radiation (Gould, 2004; Emiliania et al., 2013; Landi et al., 2015; Peng et al., 2017; Tohge et al., 2017; Moustaka et al., 2018).
High concentrations of TME such as Cd and Zn can interfere with numerous physiological processes in plants. As a result the overproduction of reactive oxygen species (ROS) such as H2O2 occurs and oxidative stress in plants is observed (Sandalio et al., 2009; Moura et al., 2012). One of the enzymes, which is responsible for the decomposition of H2O2 is catalase. For this reason, the changes in activity of this enzyme are used as a marker of plant resistance to oxidative stress (Moura et al., 2012; Rusinowski et al., 2019). When excess of ROS and/or H2O2 appeared in plants and oxidative stress develops, lipid peroxidation takes place. One of the products of lipid degradation is malondialdehyde (MDA). Thus, the increase in the content of this chemical compound in plant tissues is frequently taken into account as a measure of oxidative stress level (Bouazizi et al., 2010; Rusinowski et al., 2019).
The aim of this study was to compare the response of the photosynthetic apparatus of two hypertolerant plant species to high concentration of Cd or Zn living on the same contaminated site. In this work we compared physiological responses of two metallicolous A. arenosa and A. halleri populations from the contaminated site of Piekary Slaskie in South Poland. Presented in the current paper results for chlorophyll a fluorescence indicate that A. arenosa shows similar tolerance to Cd and Zn as A. halleri.
Materials and Methods
Plant Material and Culture Conditions
Plants of Arabidopsis halleri and Arabidopsis arenosa were grown hydroponically in controlled greenhouse conditions. Seeds of both populations were collected from the same metalliferous site in Piekary Slaskie (50°22′00.6“N, 18°58′18.4”E). Vernalized seeds were sown onto vermiculite watered with deionized water for the first 2 weeks and for the next 2 weeks with 1/10 strength Hoagland solution. Four-week-old seedlings were transferred into hydroponic containers (three seedlings of each species per container) filled with 2 L of ½ strength Hoagland solution (Bloom, 2006) with initial pH adjusted to 5.8 ± 0.05. Seedlings were grown in a greenhouse under artificial light with high pressure sodium lamps (HPS), photoperiod 12 h light (150 μE m−2 s−1)/12 h darkness. The temperature in the greenhouse was 20 ± 1°C and air humidity was 60 ± 5%. The medium was changed twice a week. Plants were grown for 6 weeks in the control solution. After 6 weeks of growth containers were divided into 3 experimental groups: Control (2 containers, 6 plants per species), Cd treatment (3 containers, 9 plants per species), and Zn treatment (3 containers, 9 plants per species). Cd was added at the concentration of 1,000 μM and Zn at the concentration of 5,000 μM, two concentrations that are lethal for non-tolerant plant species, as Arabidopsis thaliana. These concentrations were chosen in order to induce toxic effect on photosynthetic apparatus. The concentrations were chosen on the basis of preliminary studies, where half of mentioned concentrations did not show toxic effect on photosynthetic apparatus performance in short-term experiment (data not shown). The experiment was finished after 5 days and all measurements were made after 0, 1, 2, 3, 4, and 5 days, except growth parameters (shoot and root fresh weight, and root length) and accumulation of TME, which was performed on plant material harvested at the end of experiment. The experiment was repeated three times.
Measurements of Plant Growth
At the end of the experiment, plants were harvested and root length was measured. Afterwards, shoot and root fresh biomasses were measured separately.
Analysis of Cd and Zn Accumulation in Leaves
At the end of the experiment leaves of plants were harvested and dried at 80°C for 72 h. Dry plant material was ground in the mortar and subsequently digested in a microwave-assisted wet digestion system (ETHOS 1, Milestone, Italy) according to the procedure provided by the manufacturer (concentrated HNO3 and 30% H2O2, 4:1 v/v). The Cd and Zn concentrations in leaves were analyzed in the digests using flame atomic absorption spectrophotometer (iCE 3,500 FAAS, Thermo Scientific, USA). Reference plant material (Oriental Basma Tobacco Leaves (INCT-OBTL-5), Institute of Nuclear Chemistry and Technology, Poland) was used for the quality assurance of the analytical data.
Measurements of Chlorophyll a Fluorescence and Pigment Index
Measurements were done on fully developed Arabidopsis halleri and A. arenosa leaves which entirely filled the area of the sensor. Chlorophyll a fluorescence was measured at 0, 1, 2, 3, 4, and 5 days using the Plant Efficiency Analyzer (PocketPEA fluorimeter, Hansatech Instruments Ltd., England). Before measurement, each selected leaf was adapted in the dark for 30 min using dedicated leaf clips. After adaptation, a saturating light pulse of 3,500 μE m−2 s−1 was applied for 1 s, which closed all the reaction centers, and the fluorescence parameters were measured. Measurements were done without damaging the plant material.
Chlorophyll, flavonol, and anthocyanin index were measured with the use of Dualex Scientific+ sensor (Force-A, France). Measurements of pigment index were performed at 0, 1, 2, 3, 4, and 5 days on the same leaves as for chlorophyll a fluorescence measurements. Measurements were done without damaging the plant material.
Gas-Exchange Parameters
Plant gas exchange parameters, such as intracellular CO2 concentration (Ci), photosynthetic rate (A), stomatal conductance (gs), and transpiration rate (E) were conducted on fully developed leaves. Measurements were carried out at the end of experiment, after 5 days using an infrared gas analyzer with special chamber for Arabidopsis (LCpro+, ADC Bioscientific, UK) under controlled climate conditions (T = 24°C, Ambient light PAR = 150 μmol m−2 s−1). Measurements were performed at noon.
Oxidative Stress Parameters
Hydrogen peroxide concentration was determined as described by Bouazizi et al. (2010) with minor modifications. Fresh leaf tissues (150 mg) were homogenized in 1.5 ml of 0.1% trichloroacetic acid (TCA). The homogenate was centrifuged at 12,000 g for 15 min and 0.5 ml of the supernatant was added to 0.5 ml potassium phosphate buffer (10 mM, pH 7.0) and 1 ml potassium iodide (KI) (1 M). The absorbance of the supernatant was measured at 390 nm, and the content of H2O2 was obtained using a standard curve.
The level of lipid peroxidation estimated by malondialdehyde (MDA) concentration was determined as described by Bouazizi et al. (2010) with minor modifications. Fresh leaf tissues (150 mg) were homogenized in 4 ml 0.25% thiobarbituric acid (TBA) prepared in 10% TCA. The homogenate was incubated in a water bath at 95°C for 30 min and then cooled in an ice bath. After centrifugation at 10,000 g for 10 min, the absorbance of the supernatant was measured at 532 nm and corrected by subtracting the non-specific absorbance at 600 nm. Concentration of malondialdehyde (MDA) was calculated using an extinction coefficient of 155 mM−1 cm−1.
The activity of catalase was determined as described by Bouazizi et al. (2010) with minor modifications. Fresh leaf tissues (150 mg) were homogenized in 1.5 ml potassium phosphate buffer (10 mM, pH 7.0). The homogenate was centrifuged at 12,000 g for 20 min. The supernatant (0.02 ml) was added to 2 ml 10 mM peroxide prepared in potassium phosphate buffer. Catalase activity was determined spectrophotometrically by monitoring the changes of absorbance caused by H2O2 reduction at 240 nm.
Statistical Analysis
The statistically significant differences among mean values were determined using one-way ANOVA and post hoc Tukey HSD test (P < 0.05). Additionally two-way ANOVA analysis was performed to determine effect of treatment (Ctr, 1.0 mM Cd and 5.0 mM Zn), species (A. arenosa and A. halleri) as well as interaction between these two factors on measured physiological parameters (Table S1). The statistical analysis was performed using the computer software Statistica v.13.1 (Dell Inc., USA). The pipeline models of energy fluxes through leaf's cross section were done using CorelDRAW X6 (Corel Corp., Canada).
Results
Cd and Zn Content in A. halleri and A. arenosa Leaves
Cadmium and zinc concentrations were measured in leaves of both plant species (Figure 1). Remarkably, A. arenosa accumulated 3-fold more Cd in the leaves with respect to A. halleri (6 and 2 g Cd kg−1 DW in A. arenosa and A. halleri, respectively) (Figure 1A). In contrast, A. halleri leaves accumulated 13 g Zn kg−1 DW, which was 3-fold more compared to Zn accumulated by A. arenosa (Figure 1B).
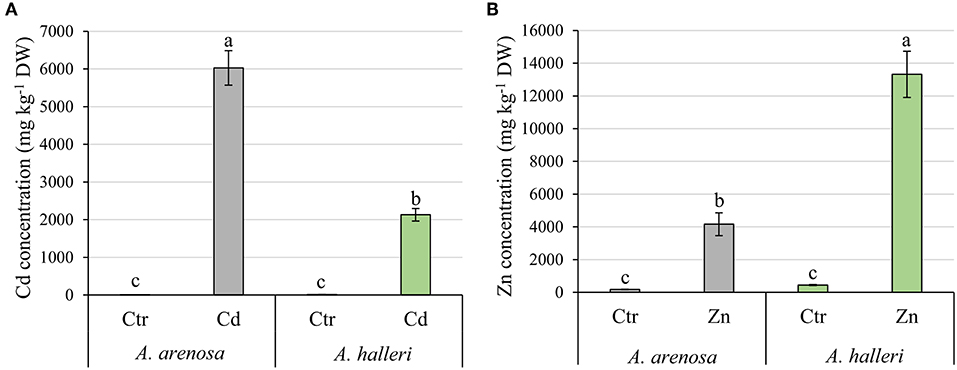
Figure 1. Accumulation of Cd (A) and Zn (B) in A. arenosa (gray) and A. halleri (green) leaves after 120 h of experiment. Ctr—control; Cd−1.0 mM Cd treatment; Zn−5.0 mM Zn treatment. Values are means ± SE (n = 6). Means followed by the same letter for each metal treatment are not significantly different from each other using the HSD test (P < 0.05).
Plant Growth
In our control conditions A. arenosa plants grew better than A. halleri, with a biomass over 2-fold higher than A. halleri (Figures 2A,B). The biomass of A. arenosa shoots was more affected by Cd treatment (60% of control) than by Zn (78% of the control), whereas the biomass of A. halleri shoots was similarly affected, irrespective of the metal (53% of the control) (Figure 2A). The root biomass in Cd treatment was significantly lower only in A. arenosa (23% of the control), whereas Zn treatment considerably diminished root growth (33% of the control) of both species (Figure 2B). Only plants of A. arenosa had their root length significantly lowered (73% of the control) under Zn treatment (Figure S1). Pictures of plants at the end of experiment are presented in Figure S2. Two-way ANOVA analysis showed that both treatment type and species significantly influenced shoot and root biomass, however, interaction between treatment type and species was significant only for root biomass (Table S1).
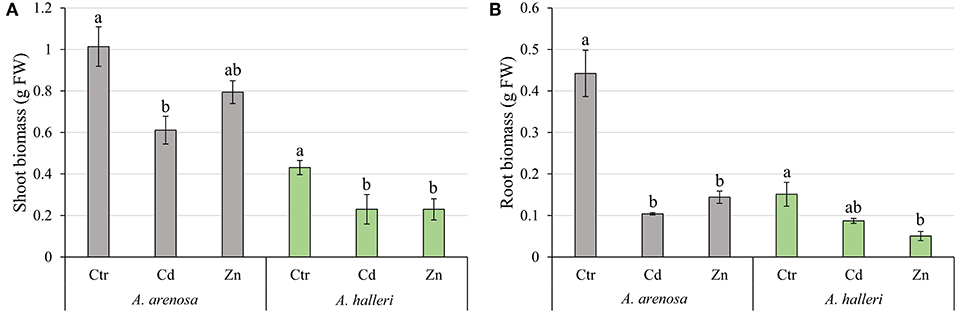
Figure 2. Growth of the shoots (A) and roots (B) of A. arenosa (gray) and A. halleri (green) after 120 h of experiment. Ctr—control; Cd−1.0 mM of Cd treatment; Zn−5.0 mM of Zn treatment. Values are means ± SE (n = 6). Means followed by the same letter for each species are not significantly different from each other using the HSD test (P < 0.05).
Oxidative Stress
Concentrations of H2O2 and MDA were significantly increased by Cd treatment in leaves of both species, but not Zn (Figures 3A,B). The treatment type had significant impact on both H2O2 and MDA concentration, whereas species had significant impact only on MDA concentration. There was no significant interaction between species and treatment for both parameters (Table S1).
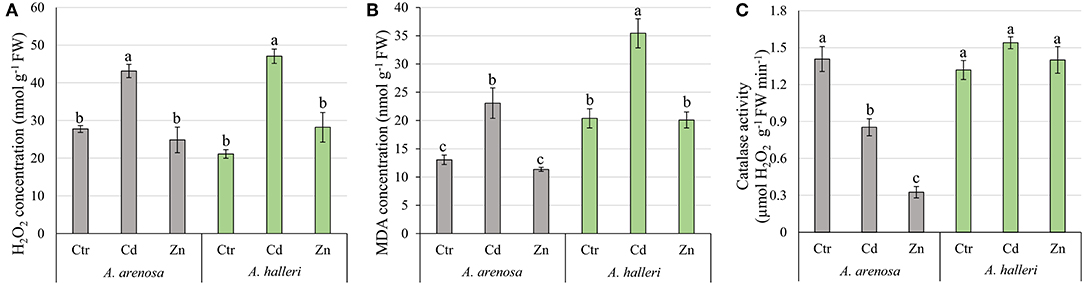
Figure 3. Concentration of H2O2 (A), MDA (B), and catalase activity (C) in shoots of A. arenosa (gray) and A. halleri (green) after 120 h of experiment. Ctr—control; Cd−1.0 mM Cd treatment; Zn−5.0 mM Zn treatment. Values are means ± SE (n = 6). Means followed by the same letter are not significantly different from each other using the HSD test (P < 0.05).
Catalase activity was lowered by Cd and Zn treatments in leaves of A. arenosa compared with control, but not in in A. halleri (Figure 3C). Both factors (species and treatment) had significant influence on catalase activity, moreover effect of treatment was dependent on the species (Table S1).
Pigment Indices
The chlorophyll index in A. arenosa and A. halleri was similar after 5 days Cd or Zn treatments compared to their respective controls (Figure 4A).
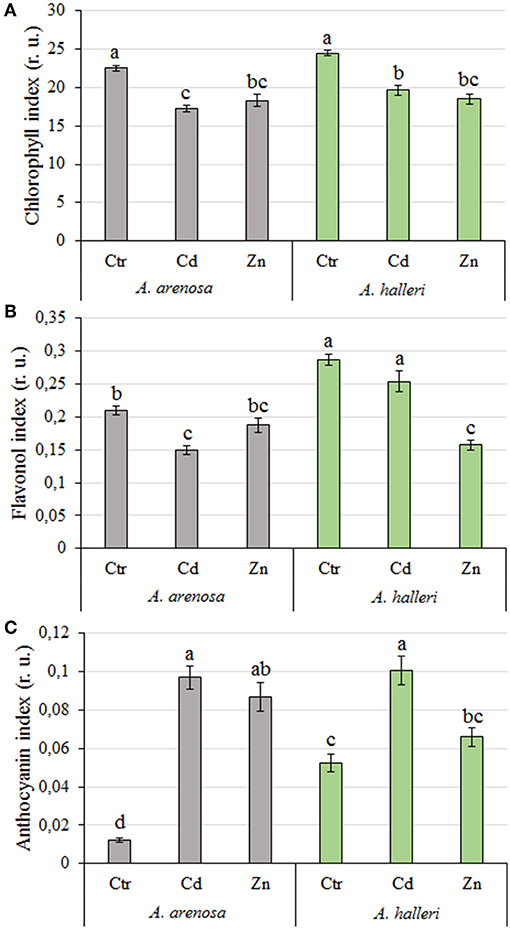
Figure 4. Chlorophyll (A), flavonol (B), and anthocyanin (C) index (relative units) in A. arenosa (gray) and A. halleri (green) leaves after 120 h of experiment. Ctr—control; Cd−1.0 mM Cd treatment; Zn−5.0 mM Zn treatment. Values are means (n = 30). Means followed by the same letter are not significantly different from each other, using Tukey HSD test (P < 0.05).
The flavonol and anthocyanin index was significantly higher in leaves of A. halleri compared to A. arenosa in control conditions (Figures 4B,C). After 5 days Cd treatment the flavonol index substantially decreased in leaves of A. arenosa compared to the control, which was not observed in A. halleri. In marked contrast, Zn treatment did not affect the flavonol index of A. arenosa leaves, whereas it decreased by 50% the one of A. halleri in comparison to the control (Figure 4B). Five days Cd treatment caused similar significant increase in the anthocyanin index of A. arenosa and A. halleri leaves compared with the control, whereas anthocyanin index in Zn treated plants was increased only in A. arenosa (Figure 4C).
Both factors (species and treatment) had significant influence on chlorophyll and flavonol indices, whereas anthocyanin index was differentiated only by treatment (Table S1).
Changes over time in the chlorophyll, flavonol and anthocyanin contents during 5 days treatments are presented in supplementary material (Figure S3).
Photosynthetic Apparatus Performance
The parameters describing photosynthesis performance are listed in Table S2. Photosynthetic apparatus performance was differently affected by Cd in A. arenosa and A. halleri (Figures 5A,B). Minimal fluorescence (F0) gradually increased in A. halleri treated with Cd, whereas it was not affected in A. arenosa in the same conditions (Table 1). The presence of the positive ΔK-band in ΔVt curves in A. arenosa may be correlated with damage or uncoupling of Oxygen Evolving Complex (OEC) caused by Cd treatment (Figure 5A). Toxic effect of Cd in point ΔJ was observed in A. arenosa after 48 h of treatment and it remained unchanged till the end of experiment, whereas no significant changes were observed for ΔI compared with the control. Changes observed between ΔJ-ΔI can be attributed to the reduced rate of electron transfer between quinone acceptors (from QA to QB). Slight increase in values compared with the control in points ΔH and ΔG observed in A. arenosa treated with Cd can be connected with damage to plastoquinone pool and PSI end of electron acceptors such as Ferredoxin-NADP+ Reductase (FNR). In contrast to A. arenosa, Cd seemed to stimulate performance of photosynthetic apparatus of A. halleri compared with control, with slight negative effect observed only after 5 days in points ΔK and ΔH (Figure 5B). Maximal fluorescence (Fm) in A. arenosa gradually decreased during Cd exposure and was significantly lower after 4 days, whereas Fm in A. halleri significantly decreased only after 5 days (Table 1). Zn treatment affected differently A. arenosa and A. halleri performance of photosynthetic apparatus. Minimal fluorescence (F0) was not affected by Zn treatment in A. arenosa (Table 1), whereas in A. halleri F0 increased considerably with the exposure to high concentration of Zn (Table 1). A. arenosa was more affected by Zn treatment compared with A. halleri (Figures 5C,D). Toxic effect of Zn excess in A. arenosa compared with control was observed earlier than in A. halleri. Moreover, in A. halleri at the beginning of Zn treatment slight stimulation of photosynthetic apparatus was observed and at the end of the experiment the toxic effect was not as high as in A. arenosa (Figures 5C,D). Fm was significantly lowered in A. arenosa after 5 days of Zn treatment, whereas in A. halleri this parameter was not affected by the treatment (Table 1).
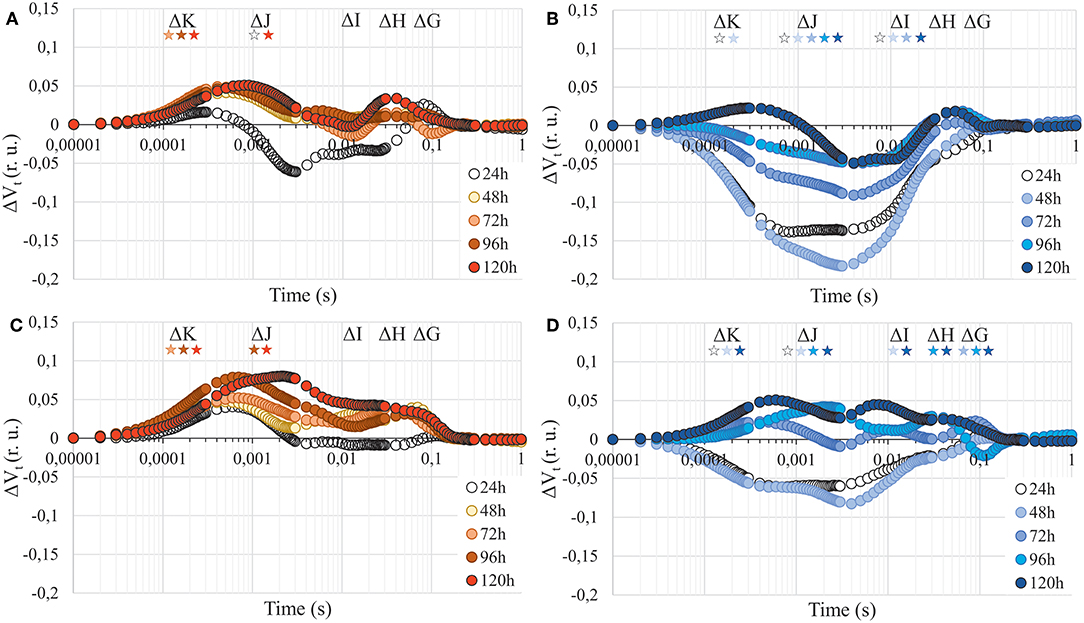
Figure 5. Chlorophyll a fluorescence induction curves presented as relative variable fluorescence (ΔVt) after 24, 48, 72, 96, and 120 h of experiment. (A)—A. arenosa treated with 1.0 mM Cd, (B)—A. halleri treated with 1.0 mM Cd, (C)—A. arenosa treated with 5.0 mM Zn, (D)—A. halleri treated with 5.0 mM Zn. For ΔVt analysis, the fluorescence of control was the reference and equaled 0. Values are means (n = 8). Asterisks indicate statistically significant differences (Fisher's LSD test, P < 0.05) between fluorescence of plants treated with HM and the control for each band (ΔK, ΔJ, ΔI, ΔH, and ΔG). Asterisk are arranged chronologically from 24 to 120 h and asterisks color corresponds to point color, which represent the time of metal treatment. (r. u.), relative units.
Parameters describing characteristics of photosynthetic apparatus in general did not significantly change for both species in the control (Table 1). Changes observed in chlorophyll a fluorescence kinetics for Cd treated plants were confirmed by the value of maximum quantum efficiency of the PSII (ϕP0) which decreased with the trace metal elements (TME) exposure time (Table 1). Parameters such as quantum yield (ϕE0) and probability for electron transport from reduced plastoquinone (Q) to plastoquinone (ψE0) significantly decreased toward the end of the experiment only in A. halleri. Moreover, the quantum yield (ϕR0) and probability for the reduction of the end electron acceptors at the PSI acceptor side (δR0) were decreased in both species over the duration of Cd treatment. Quantum yield of energy dissipation (ϕD0) increased in both species under Cd treatment (Table 1). ϕP0 of A. arenosa was not affected by the Zn treatment, whereas in A. halleri it was significantly lowered at the end of the experiment (Table 1). ϕE0 and ψE0 in A. arenosa were considerably decreased after 1 days, whereas in A. halleri the significant decrease in these parameters was observed at the end of experiment (5 days). δR0 was not substantially changed over time in both species treated with Zn, whereas ϕR0 was significantly lowered after 1 and 3 days of Zn treatment in A. arenosa and A. halleri, respectively. ϕD0 did not change during the Zn treatment, however, in A. halleri it significantly increased only after 5 days (Table 1).
Overall efficiency of PSII, showed as models of phenomenological energy fluxes per cross section (CS) of A. arenosa and A. halleri, was differently affected by Cd or Zn treatment after 5 days compared with the control (Figure 6). After 5 days of Cd treatment absorption flux (ABS/CS) was considerably lower in both species compared with the control, whereas Zn treatment did not cause a significant change in both species (Figure 6). Trapped energy flux was significantly diminished by Cd and Zn treatment in both species, except in A. arenosa treated with Zn. Electron transport flux (ET/CS) was not lowered compared with the control only in A. halleri treated with Cd. Both Cd and Zn treatment caused significant increase in percentage of inactive reaction centers (RC) in A. arenosa, whereas in A. halleri significant increase in this parameter was observed only in Cd treatment (Figure 6).
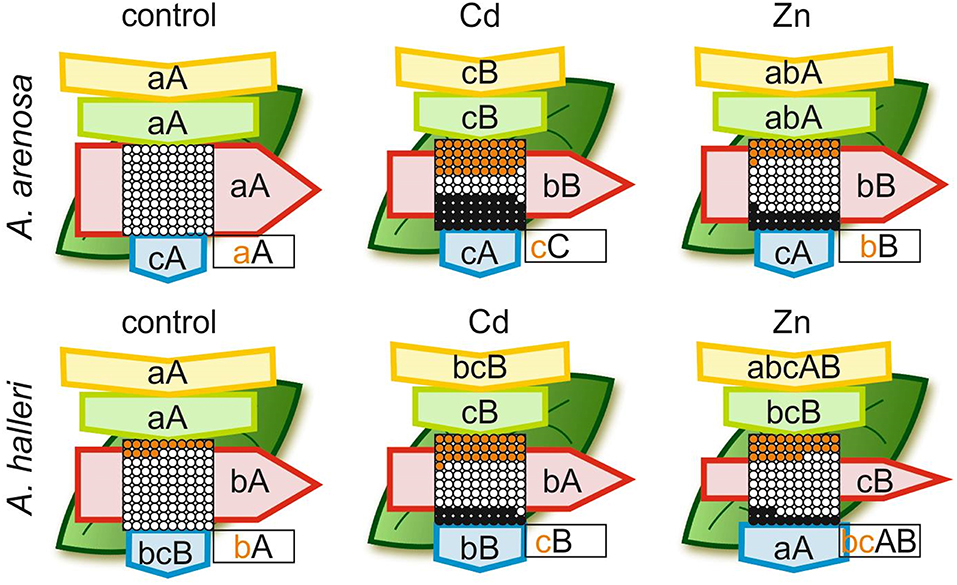
Figure 6. Leaf model showing the phenomenological energy fluxes per the excited cross sections (CS) of the leaves of the A. arenosa and A. halleri at the end of experiment (120 h). Cd−1.0 mM Cd treatment; Zn−5.0 mM Zn treatment. Each relative value of the measured parameters is the mean (n = 20) and the width of each arrow corresponds to the intensity of the flux. Yellow arrow—ABS/CS, absorption flux per CS approximated; green arrow—TR/CS, trapped energy flux per CS; red arrow—ET/CS, electron transport flux per CS; blue arrow—DI/CS, dissipated energy flux per CS; circles inscribed in squares—RC/CS, % of active/inactive reaction centers. White circles inscribed in squares represent reduced QA reaction centers (active), black (or orange) circles represent non-reduced QA reaction centers (inactive), 100% of the active reaction centers responded with the highest mean value observed in the control conditions. Orange circles present the difference in % of inactive RC between A. arenosa and A. halleri. Means followed by the same letter for each parameter in a row (upper case letters) or in a column (lower case letters) are not significantly different from each other using the Tukey HSD test (P < 0.05). Letters are inscribed into arrows, except for RC/CS, where they are placed in a box in the bottom right corner of the square with circles. Lower case letters describe statistical differences between species in all treatments, upper case letters describe changes for each species during experiment. Orange lower case letters describe statistical differences in RC/CS between species under each HM treatment.
Gas-Exchange Parameters
The concentration of intercellular CO2 (Ci) was considerably decreased by 5 days Cd treatment in A. arenosa and A. halleri (Figure 7A). In control conditions the photosynthetic rate (A) of A. arenosa was more than twice that of A. halleri (Figure 7B). Both Cd ad Zn treatments caused a considerable decrease of the photosynthetic rate only in A. arenosa (38 and 49% of control for Cd and Zn, respectively) after 5 days (Figure 7B). In control conditions the transpiration rate (E) of A. arenosa was twice that of A. halleri (Figure 7C). Cd treatment caused a significant decrease in E for both species (19 and 30% of the control for A. arenosa and A. halleri, respectively). On the contrary, E significantly decreased during Zn treatment only in A. arenosa (35% of the control) (Figure 7C). In the control conditions, the stomatal conductance (gs) of A. arenosa was more than twice that of A. halleri (Figure 7D). A significant decrease in gs was observed in Cd treated A. arenosa (12% of the control) and A. halleri (26% of the control). In Zn treated plants, significant decrease in gs was observed only in A. arenosa (22% of the control) (Figure 7D). All gas-exchange parameters were considerably affected by both treatments and species. Moreover, interaction between treatment type and species was not substantial only for Ci (Table S1).
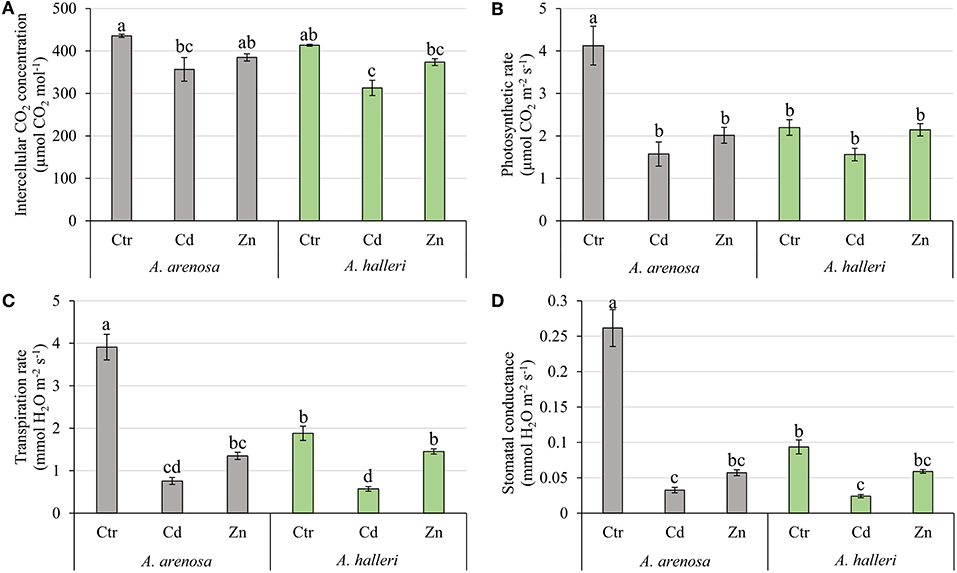
Figure 7. Intercellular CO2 concentration (A), Photosynthetic rate (B), Transpiration rate (C), and Stomatal conductance (D) in A. arenosa (gray) and A. halleri (green) after 120 h of treatment. Ctr—control; Cd−1.0 mM Cd treatment; Zn−5.0 mM Zn treatment. Values are means ± SE (n = 20). Means followed by the same letter are not significantly different from each other using the HSD test (P < 0.05).
Discussion
In order to gain a better understanding of mechanisms underlying metal tolerance of A. halleri and A. arenosa, metal content, photosynthetic activity, levels of oxidative stress, gas-exchange parameters, chlorophyll, flavonol, and anthocyanin indices were analyzed in metallicolous populations from the same contaminated site in southern Poland grown in control, and exposed to high Cd and Zn concentrations.
Cd and Zn contents in leaves (Figures 1A,B) suggest that the two species differ in the mechanisms involved in the uptake and transport of these metals, with a clear preference for Zn accumulation in A. halleri and for Cd accumulation in A. arenosa. Since there are no reports, which compare both plant species in control conditions it is impossible to explain these differences. However, it is tempting to suggest that the higher accumulation of Zn by A. halleri and higher accumulation of Cd by A. arenosa is connected with different transport activity of transporters responsible for the uptake and translocation of both metals in plants. In order to elucidate this deference between the species further research on gene expression levels are needed. Nevertheless, our results showed that the studied metallicolous populations of A. arenosa and A. halleri are capable of accumulating high concentrations of Cd and Zn. Moreover, the concentrations of Cd and Zn in A. arenosa observed in the current study are comparable to the concentrations reported in hyperaccumulator plant species by other authors (Meyer et al., 2010; Farinati et al., 2011; Corso et al., 2018; Schvartzman et al., 2018).
We observed that high Cd treatment (1.0 mM) decreased the growth of shoots in both A. arenosa and A. halleri (Figure 2A). Despite the higher accumulation of Cd in A. arenosa (Figure 1A), the decrease in shoot biomass was slightly different (60% of control) than that observed in A. halleri (53% of control). This finding shows high tolerance to Cd of A. arenosa and A. halleri. In plants treated with high Zn concentration (5.0 mM), the shoot biomass was considerably lowered only in A. halleri, but it is important to note that accumulation of Zn in A. halleri was almost three-times higher than in A. arenosa (Figure 1B).
Our results showed that Cd caused similar and significant increase in the concentrations of hydrogen peroxide and MDA, while Zn treatment did not cause any change compared to the control conditions in A. arenosa and A. halleri (Figures 3A,B). However, considering contrasting accumulation of Cd and Zn in both species, our data suggests that anti-oxidative mechanisms in A. arenosa can better cope with Cd-induced oxidative stress compared to A. halleri, while the opposite trend was observed for Zn. Furthermore, large difference in catalase activity in the response to Cd or Zn treatment was found between A. arenosa and A. halleri, which suggests that various anti-oxidative mechanisms may function in both species. Cd induced oxidative stress in A. halleri has been already reported by other authors (Baliardini et al., 2015; Ahmadi et al., 2018).
Measurements of chlorophyll content can be useful for assessing plant tolerance to stress (Rong-hua et al., 2006; Ramirez et al., 2014; Xue et al., 2018). Relatively low alterations in chlorophyll content measured after exposure to high concentrations of the metals (1 mM Cd and 5 mM Zn) suggest that A. arenosa is as tolerant to Cd and Zn excess as A. halleri, which was not reported so far (Figure 4A). In contrast, Paunov et al. (2018) reported a substantial decrease in chlorophyll content in leaves of durum wheat after 7 days treatment with only 50 μM Cd and 600 μM Zn. Küpper et al. (2007) also showed a significantly higher decrease in chlorophyll content in non-hyperaccumulator Thlaspi fendleri after exposure to Cd when compared with Noccaea caerulescens.
It was documented in the current study that flavonol and anthocyanin content was constitutively higher in A. halleri than in A. arenosa (Figures 4B,C). Generally, the content of flavonols lowered in both species after exposure to Cd or Zn, whereas the content of anthocyanins increased. The role of flavonols and anthocyanins during metallic stress has been poorly investigated in plants so far. Nonetheless, Corso et al. (2018) showed recently that flavonols and anthocyanins play an important role in plant response to TME stress in hyperaccumulating and non-hyperaccumulating plant species, most likely through the enhancement of antioxidant capacity and/or metal chelation in plants (Corso et al., 2018). Our results may suggest that A. arenosa and A. halleri favor biosynthesis of anthocyanins over flavonols in response to the toxic effect of Cd or Zn (Figures 4B,C). However, it is possible that flavonols made the chelates with Cd and Zn and were not detected by the method used in the current study. Such lower detectability of flavonols by fluorometric methods, as a result of chelation with TME, was documented by Kasprzak et al. (2015). Hence, the role of flavonols in response to Cd and Zn toxicity in both plant species should be further investigated.
There is a dearth of data focusing on toxic effects of Cd and Zn on photosynthesis presented as chlorophyll a induction transients in metal hyperaccumulator and hypertolerant species such as A. halleri, moreover, there are no such reports for A. arenosa. Our data showed the presence of ΔK, ΔJ, ΔI, ΔH, and ΔG peaks on ΔVt curves. Positive peaks on ΔVt curves can be linked to the toxic effect of TME on different components of photosynthetic apparatus, whereas negative values might suggest stimulatory effect compared with the control. Under 1.0 mM Cd treatment in A. arenosa, which may suggest damage to the OEC (positive ΔK peak), electron transport between QA and QB (ΔJ) and connectivity between PSII and PSI, as well as the end electron acceptors of PSI like FNR (ΔI, ΔH, and ΔG) (Figure 5A) (Kalaji and Loboda, 2007; Paunov et al., 2018). On the other hand, in A. halleri that accumulated significantly less Cd (Figure 1A), we observed a stimulation of most of components of electron transport chain compared with the control (Figure 5B). Our research for the first time shows such stimulatory effect of Cd on photosynthetic apparatus of A. halleri. However, Tang et al. (2016) reported increase in plant growth as well as induction of some photosynthetic parameters (e.g., ϕP0) and up-regulation of genes involved in photosynthesis in hyperaccumulator Sedum alfredii exposed to low Cd concentration (5 μM). Moreover, similar stimulation of growth of another hyperaccumulator Noccea caerulescens was reported by Lombi et al. (2000) that was exposed to Cd (100 μM). By contrast, Paunov et al. (2018) observed a presence of positive ΔK and ΔJ, and negative ΔI and ΔH peaks in ΔVt curve for durum wheat plants treated with 50 μM of Cd for 7 days, whereas Kalaji and Loboda (2007) and Kalaji et al. (2018) reported the complete flattening of chlorophyll a fluorescence induction curve for barley treated with only 25 μM Cd for 24 h, which suggested lethal effect of investigated treatment.
Little information is available on the toxic effect of Zn on chlorophyll a fluorescence induction curves in plants and PSII functionality under Zn stress (Paunov et al., 2018; Moustakas et al., 2019). Paunov et al. (2018) showed the toxic effect of high Zn (600 μM) treatment, visible as a presence of positive ΔK and ΔJ peaks on chlorophyll a fluorescence induction transient in durum wheat. In current research we used 8-fold higher Zn concentration than that used by Paunov et al. (2018) and we showed that OEC (positive ΔK peak), QA pool (positive ΔJ peak) and PSI components (positive ΔI, ΔH, and ΔG peak) were more affected in A. arenosa (Figure 5C) compared with A. halleri (Figure 5D) despite higher Zn accumulation in leaves of A. halleri (Figure 1B). It should be stressed, however, that the Zn concertation used in the present study was 8-fold higher compared to the concentration used by Paunov et al. (2018).
Our results show that both Cd and Zn have contrasting effects on photosynthetic apparatus of A. arenosa and A. halleri. Based on presented chlorophyll a fluorescence induction curves and available literature we can conclude that photosynthetic apparatus of metallicolous A. arenosa population is hypertolerant to Cd and Zn stresses. Although Przedpełska and Wierzbicka (2007) reported that metallicolous population of A. arenosa are more tolerant to Cd and Zn than non-metallicolous populations, they did not compare them with any well-known hyperaccumulator plant of both metals.
Maximum quantum efficiency of the PSII (ϕP0), otherwise known as Fv/Fm, is the most commonly used parameter which describes the state of photosynthetic apparatus, and many reports show its decrease in various plant species under Cd (Huang et al., 2017 Paunov et al., 2018; Xue et al., 2018) or Zn stress treatment (Vassilev et al., 2011; Santos et al., 2014; Paunov et al., 2018). Our results showed that Cd treatment caused ϕP0 of A. arenosa and A. halleri to decrease near the end of experiment compared with the control plants, however, considering extremely high Cd concentration the overall physiological status of both species was not significantly reduced. Moreover, ψE0 and ϕE0 that are associated with O-J phase were not considerably affected by Cd treatment in both species (Table 1). In this report, exposure for 5 days to 5 mM Zn did not affect ϕP0 of A. arenosa, whereas in A. halleri significant decrease was observed only at the end of experiment (Table 1). Other reports showing decreasing values of ϕP0 in A. arenosa, A. halleri and Noccea caerulescens under Zn treatment (from 10 μM to 3.0 mM) are in good agreement with hypertolerance of photosynthetic apparatus of A. arenosa and A. halleri presented in the current study (Table 1) (Cho et al., 2003; Küpper et al., 2007; Przedpelska-Wasowicz and Wasowicz, 2013). Kalaji and Loboda (2007) reported that energy fluxes per excited cross section in two cultivars of Hordeum vulgare were significantly disrupted after 24 h treatment with 25 μM Cd. In their research in both cultivars, almost all RC were deactivated by Cd and DI/CS was extremely higher compared to the control, causing ET/CS to be almost completely inhibited. The current study shows that 5 days treatment with 1 mM Cd or 5 mM Zn caused in both species that most energy fluxes per excited cross section, although significantly lowered compared with the control, had relatively high values and most of RC were still active (Figure 6). These results suggest that PSII of both species is hypertolerant to high concentration of Cd and Zn. For the first time, we show complete analysis of photosynthetic apparatus (Table 1) and phenomenological energy fluxes per excited cross section (Figure 6) for A. arenosa and A. halleri treated with high concentration of Cd and excess Zn in controlled conditions.
Our data showed that the studied metallicolous population of A. arenosa was extremely tolerant to Cd and Zn, similarly to the Zn, Cd hyperaccumulating A. halleri metallicolous population. This study also highlighted contrasting responses of plants growing at the same contaminated site to Cd and Zn treatments, in particular in metal accumulation, photosynthetic parameters [e.g., quantum yield (ϕP0)], gas-exchange parameters (e.g., photosynthetic rate) as well as in the content of chlorophyll, flavonols, and anthocyanins.
Author Contributions
MS, KS, NV, and EM conceived and designed the research. MS, KS, and ŻG conducted the experiments. MS, KS, SR, MC, and CH analyzed the data. MS wrote the first draft of the manuscript, which was edited by all the authors.
Funding
This work was financed by the National Science Center (Poland), granted on the basis of decision 2018/29/N/NZ8/01336.
Conflict of Interest Statement
The authors declare that the research was conducted in the absence of any commercial or financial relationships that could be construed as a potential conflict of interest.
Acknowledgments
CH is researcher of F.R.S.-FNRS. MC was post-doctoral researcher of FNRS until 30 September 2018.
Supplementary Material
The Supplementary Material for this article can be found online at: https://www.frontiersin.org/articles/10.3389/fpls.2019.00748/full#supplementary-material
References
Ahmadi, H., Corso, M., Weber, M., Verbruggen, N., and Clemens, S. (2018). CAX1 suppresses Cd-induced generation of reactive oxygen species in Arabidopsis halleri. Plant Cell Environ. 41, 2435–2448. doi: 10.1111/pce.13362
Alloway, B. J. (2013). “Sources of heavy metals and metalloids in soils,” in Heavy Metals in Soils, ed B. J. Alloway (Dordrecht: Springer), 11–50.
Arshad, M., Ali, S., Noman, A., Ali, Q., Rizwan, M., Farid, M., et al. (2015). Phosphorus amendment decreased cadmium (Cd)uptake and ameliorates chlorophyll contents, gas exchange attributes, antioxidants, and mineral nutrients in wheat (Triticum aestivum L.) under Cd stress. Arch. Agron. Soil Sci. 62, 533–546. doi: 10.1080/03650340.2015.1064903
Baker, N. R. (2008). Chlorophyll fluorescence: a probe of photosynthesis in vivo. Annu. Rev. Plant Biol. 59, 89–113. doi: 10.1146/annurev.arplant.59.032607.092759
Baliardini, C., Meyer, C.-L., Salis, P., Samitou-Laprade, P., and Verbruggen, N. (2015). CAX1 co-segregates with Cd tolerance in the metal hyperaccumulator Arabidopsis halleri and plays a role in limiting oxidative stress in Arabidopsis. Plant Physiol. 169, 549–559. doi: 10.1104/pp.15.01037
Bloom, A. J. (2006). “Mineral nutrition,” in Plant Physiology, eds L. Taiz and E. Zeiger (Sunderland: Sinauer Associates, Inc., Publishers).
Bouazizi, H., Jouili, H., Geitmann, A., and El Ferjani, E. (2010). Copper toxicity in expanding leaves of Phaseolus vulgaris L.: antioxidant enzyme response and nutrient element uptake. Ecotox. Environ. Saf. 73, 1304–1308. doi: 10.1016/j.ecoenv.2010.05.014
Cerovic, Z. G., Ben Ghozlen, N., Milhade, C., Obert, M., Debuisson, S., and Le Moigne, M. (2015). Nondestructive diagnostic test for nitrogen nutrition of grapevine (Vitis vinifera L.) based on dualex leaf-clip measurements in the field. J. Agric. Food Chem. 63, 3669–3680. doi: 10.1021/acs.jafc.5b00304
Chaney, R. (1993). “Zinc phytotoxicity,” in Zinc in Soils and Plants, ed A. D. Robson (Dordrecht: Kluwer Academic Publisher), 135–150. doi: 10.1007/978-94-011-0878-2_10
Cho, M., Chardonnens, A. N., and Dietz, K. J. (2003). Differential heavy metal tolerance of Arabidopsis halleri and Arabidopsis thaliana: a leaf slice test. New Phytol. 158, 287–293. doi: 10.1046/j.1469-8137.2003.00746.x
Clauss, M. J., and Koch, M. A. (2006). Poorly known relatives of Arabidopsis thaliana. Trends Plant Sci. 11, 449–459. doi: 10.1016/j.tplants.2006.07.005
Clemens, S., and Ma, J. F. (2016). Toxic heavy metal and metalloid accumulation in crop plants and foods. Ann. Rev. Plant Biol. 67, 489–512. doi: 10.1146/annurev-arplant-043015-112301
Clijsters, H., and Van Assche, F. (1985). Inhibition of photosynthesis by heavy metals. Photosynth. Res. 7, 31–40. doi: 10.1007/BF00032920
Corso, M., Schvartzman, M. S., Guzzo, F., Souard, F., Małkowski, E., Hanikenne, M., et al. (2018). Contrasting cadmium resistance strategies in two metallicolous populations of Arabidopsis halleri. N. Phytol. 218, 283–297. doi: 10.1111/nph.14948
Corso, M., Vannozzi, A., Maza, E., Vitulo, N., Meggio, F., Pitacco, A., et al. (2015). Comprehensive transcript profiling of two grapevine rootstock genotypes contrasting in drought susceptibility links the phenylpropanoid pathway to enhanced tolerance. J. Exp. Bot. 66, 5739–5752. doi: 10.1093/jxb/erv274
Daszkowska-Golec, A., Skubacz, A., Marzec, M., Slota, M., Kurowska, M., Gajecka, M., et al. (2017). Mutation in HvCBP20 (Cap Binding Protein 20) adapts barley to drought stress at phenotypic and transcriptomic levels. Front. Plant Sci. 8:00942. doi: 10.3389/fpls.2017.00942
Emiliania, J., Grotewold, E., Ferreyra, M. L., and Casti, P. (2013). Flavonols protect Arabidopsis plants against UV-B deleterious effects. Mol. Plant. 6, 1376–1379. doi: 10.1093/mp/sst021
Farinati, S., Dal Corso, G., Panigati, M., and Furini, A. (2011). Interaction between selected bacterial strains and Arabidopsis halleri modulates shoot proteome and cadmium and zinc accumulation. J. Exp. Bot. 62, 3433–3447. doi: 10.1093/jxb/err015
Fiałkiewicz, B., and Rostanski, A. (2006). Morphological variability of Cardaminopsis halleri (L.) Hayek from selected habitats in the Silesian Upland (Southern Poland). Biodiv. Res. Conserv. 1, 37–44. Available online at: https://www.researchgate.net/publication/285723505_Morphological_variability_of_Cardaminopsis_halleri_L_Hayek_from_selected_habitats_in_the_Silesian_Upland_Southern_Polan
Frérot, H., Hautekèete, N.-C., Decombeix, I., Bouchet, M.-H., Créach, A., Saumitou-Laprade, P., et al. (2018). Habitat heterogeneity in the pseudometallophyte Arabidopsis halleri and its structuring effect on natural variation of zinc and cadmium hyperaccumulation. Plant Soil. 423, 157–174. doi: 10.1007/s11104-017-3509-1
Goltsev, V. N., Kalaji, H. M., Paunov, M., Baba, W., Horaczek, T., Mojski, J., et al. (2016). Variable chlorophyll fluorescence and its use for assessing physiological condition of plant photosynthetic apparatus. Rus. J. Plant Physiol. 63, 869–893. doi: 10.1134/S1021443716050058
Gonzalez-Mendoza, D., Mendez-Trujillo, V., Grimaldo-Juarez, O., Ceceña-Duran, C., Tzintzun –Camacho, O., Gutierrez-Miceli, F., et al. (2017). Changes of photochemical efficiency and epidermal polyphenols content of Prosopis glandulosa and Prosopis juliflora leaves exposed to cadmium and copper. Open Life Sci. 12, 373–378. doi: 10.1515/biol-2017-0043
Gould, K. S. (2004). Nature's Swiss army knife: the diverse protective roles of anthocyanins in leaves. J. Biomed. Biotech. 5, 314–320. doi: 10.1155/S1110724304406147
Hosseini, S. A., Maillard, A., Hajirezaei, M. R., Ali, N., Schwarzenberg, A., Jamois, F., et al. (2017). Induction of barley silicon transporter HvLsi1 and HvLsi2, increased silicon concentration in the shoot and regulated starch and ABA homeostasis under osmotic stress and concomitant potassium deficiency. Front. Plant Sci. 8:1359. doi: 10.3389/fpls.2017.01359
Huang, M., Zhu, H., Zhang, J., Tang, D., Han, X., Chen, L., et al. (2017). Toxic effects of cadmium on tall fescue and different responses of the photosynthetic activities in the photosystem electron donor and acceptor sides. Sci. Rep. 7:14387. doi: 10.1038/s41598-017-14718-w
Jaakola, L., Määttä-Riihinen, K., Kärenlampi, S., and Hohtola, A. (2004). Activation of flavonoid biosynthesis by solar radiation in bilberry (Vaccinium myrtillus L.) leaves. Planta 218, 721–728. doi: 10.1007/s00425-003-1161-x
Kabata-Pendias, A. (2011). Trace Elements in Soils and Plants. Boca-Raton, FL: CRC press. doi: 10.1201/b10158
Kalaji, H. M., Goltsev, V., Bosa, K., Allakhverdiev, S. I., Strasser, R. J., and Govindjee (2012). Experimental in vivo measurements of light emission in plants: a perspective dedicated to David Walker. Photosynth. Res. 114, 69–96. doi: 10.1007/s11120-012-9780-3
Kalaji, H. M., and Loboda, T. (2007). Photosystem II of barley seedlings under cadmium and lead stress. Plant Soil Environ. 53, 511–516. doi: 10.17221/2191-PSE
Kalaji, H. M., Oukarroum, A., Alexandrov, V., Kouzmanova, M., Brestic, M., Zivcak, M., et al. (2014). Identification of nutrient deficiency in maize and tomato plants by in vivo chlorophyll a fluorescence measurements. Plant Physiol. Biochem. 81, 16–25. doi: 10.1016/j.plaphy.2014.03.029
Kalaji, H. M., Rastogi, A., Živčák, M., Brestic, M., Daszkowska-Golec, A., Sitko, K., et al. (2018). Prompt chlorophyll fluorescence as a tool for crop phenotyping: an example of barley landraces exposed to various abiotic stress factors. Photosynthetica 56, 953–961. doi: 10.1007/s11099-018-0766-z
Kasprzak, M. M., Erxleben, A., and Ochocki, J (2015): Properties applications of flavonoid metal complexes. RSC Adv. 5, 45853–45877. doi: 10.1039/C5RA05069C
Küpper, H., Parameswaran, A., Leitenmaier, B., Trtílek, M., and Šetlík, I. (2007). Cadmium-induced inhibition of photosynthesis and long-term acclimation to cadmium stress in the hyperaccumulator Thlaspi caerulescens. N. Phytol. 175, 655–674. doi: 10.1111/j.1469-8137.2007.02139.x
Landi, M., Tattini, M., and Gould, K. S. (2015). Multiple functional roles of anthocyanins in plant- environment interactions. Environ. Exp. Bot. 119, 4–17. doi: 10.1016/j.envexpbot.2015.05.012
Lefebvre, T., Millery-Vigues, A., and Gallet, C. (2016). Does leaf optical absorbance reflect the polyphenol content of alpine plants along an elevational gradient? Alp. Bot. 126, 177–185. doi: 10.1007/s00035-016-0167-5
Lombi, E., Zhao, F. J., Dunham, S. J., and McGrath, S. P. (2000). Cadmium accumulation in populations of Thlaspi caerulescens and Thlaspi goesingense. N. Phytol. 145, 11–20. doi: 10.1046/j.1469-8137.2000.00560.x
Mattivi, F., Guzzon, R., Vrhovsek, U., Stefanini, M., and Velasco, R. (2006). Metabolite profiling of grape: flavonols and anthocyanins. J. Agric. Food Chem. 54, 7692–7702. doi: 10.1021/jf061538c
Meyer, C. L., Juraniec, M., Huguet, S., Chaves-Rodriguez, E., Salis, P., Isaure, M. P., et al. (2015). Intraspecific variability of cadmium tolerance and accumulation, and cadmium-induced cell wall modifications in the metal hyperaccumulator Arabidopsis halleri. J. Exp. Bot. 66, 3215–3227. doi: 10.1093/jxb/erv144
Meyer, C. L., Kostecka, A. A., Saumitou-Laprade, P., Créach, A., Castric, V., Pauwels, M., et al. (2010). Variability of zinc tolerance among and within populations of the pseudometallophyte species Arabidopsis halleri and possible role of directional selection. N. Phytol. 185, 130–142. doi: 10.1111/j.1469-8137.2009.03062.x
Moura, D. J., Péres, V. F., Jacques, R. A., and Saffi, J. (2012). “Heavy metal toxicity: oxidative stress and DNA repair,” in Metal Toxicity in Plants: Perception, Signaling and Remediation, eds D. K. Gupta, and L. M. Sandalio (Granada: Springer-Verlag), 187–205. doi: 10.1007/978-3-642-22081-4_9
Moustaka, J., Panteris, E., Adamakis, I. D. S., Tanou, G., Giannakoula, A., Eleftheriou, E. P., et al. (2018). High anthocyanin accumulation in poinsettia leaves is accompanied by thylakoid membrane unstacking, acting as a photoprotective mechanism, to prevent ROS formation. Environ. Exp. Bot. 154, 44–55. doi: 10.1016/j.envexpbot.2018.01.006
Moustakas, M., Bayçu, G., Gevrek, N., Moustaka, J., Csatári, I., and Rogens, S. E. (2019). Spatiotemporal heterogeneity of photosystem II function during acclimation to zinc exposure and mineral nutrition changes in the hyperaccumulator Noccaea caerulescens. Environ. Sci. Pollut. Res. 26, 6613–6624. doi: 10.1007/s11356-019-04126-0
Myśliwska-Kurdziel, B., Prasad, M. N. V., and Strzałka, K. (2002). “Heavy metal influence on the light phase of photosynthesis,” in Physiology and Biochemistry of Metal Toxicity and Tolerance in Plants, eds M. N. V. Prasad and K. Strzałka (Berlin; Heidelberg: Luwer Academic Publishers), 229–255. doi: 10.1007/978-94-017-2660-3_9
Nadgórska-Socha, A., Ptasinski, B., and Kita, A. (2013). Heavy metal bioaccumulation and antioxidative responses in Cardaminopsis arenosa and Plantago lanceolata leaves from metalliferous and non-metalliferous sites: a field study. Ecotoxicology 22, 1422–1434. doi: 10.1007/s10646-013-1129-y
Paunov, M., Koleva, L., Vassilev, A., Vangronsveld, J., and Goltsev, V. (2018). Effects of different metals on photosynthesis: cadmium and zinc affect chlorophyll fluorescence in durum wheat. Int. J. Mol. Sci. 19:787. doi: 10.3390/ijms19030787
Pauwels, M., Vekemans, X., Godé, C., Frérot, H., Castic, V., and Saumitou-Laprade, P. (2012). Nuclear and chloroplast DNA phylogeography reveals vicariance among European populations of the model species for the study of metal tolerance, Arabidopsis halleri (Brassicaceae). N. Phytol. 193, 916–928. doi: 10.1111/j.1469-8137.2011.04003.x
Peng, M., Shazad, R., Gul, A., Subthain, H., Shen, S., Lei, L., et al. (2017). Differentially evolved glucosyltransferases determine natural variation of rice flavone accumulation and UV-tolerance. Nat. Commun. 8:1975. doi: 10.1038/s41467-017-02168-x
Preite, V., Sailer, C., Syllwasschy, L., Bray, S., Kraemer, U., and Yant, L. (2018). Convergent evolution in Arabidopsis halleri and Arabidopsis arenosa on calamine metalliferous soils. bioRxiv, 459362. doi: 10.1101/459362
Przedpełska, E., and Wierzbicka, M. (2007). Arabidopsis arenosa (Brassicaceae) from a lead–zinc waste heap in southern Poland – a plant with high tolerance to heavy metals. Plant Soil 299, 43–53. doi: 10.1007/s11104-007-9359-5
Przedpelska-Wasowicz, E., and Wasowicz, P. (2013). Does zinc concentration in the substrate influence the onset of flowering in Arabidopsis arenosa (Brassicaceae)? Plant Growth Regul. 69, 87–97. doi: 10.1007/s10725-012-9750-y
Ramirez, D. A., Yactayo, W., Guterrez, R., Mares, V., De Mendiburu, F., Posadas, A., et al. (2014). Chlorophyll concentration in leaves is an indicator of potato tuber yield in water-shortage conditions. Sci. Hortic. 168, 202–209. doi: 10.1016/j.scienta.2014.01.036
Rong-hua, L., Pei-guo, G., Baum, M., Grando, S., and Ceccarelli, S. (2006). Evaluation of chlorophyll content and fluorescence parameters as indicators of drought tolerance in barley. Agri. Sci. China 5, 751–757. doi: 10.1016/S1671-2927(06)60120-X
Rusinowski, S., Szada-Borzyszkowska, A., Zieleznik-Rusinowska, P., Małkowski, E., Krzyzak, J., Wozniak, G., et al. (2019). How autochthonous microorganisms influence physiological status of Zea mays L. cultivated on heavy metal contaminated soils? Environ. Sci. Pollut. Res. 26, 4746–4763. doi: 10.1007/s11356-018-3923-9
Sagardoy, R., Vázquez, S., Florez-Sarasa, I. D., Albacete, A., Ribas-Carb,ó, M., Flexas, J., et al. (2010). Stomatal and mesophyll conductances to CO2 are the main limitations to photosynthesis in sugar beet (Beta vulgaris) plants grown with excess zinc. N. Phytol. 187, 145–158. doi: 10.1111/j.1469-8137.2010.03241.x
Sandalio, L. M., Rodriguez-Serrano, M., del Rio, L. A., and Romero-Puertas, M. C. (2009). “Reactive oxygen species and signaling in cadmium toxicity,” in Reactive Oxygen Species in Plant Signaling, eds L. A. del Rio, A. Puppo (Berlin; Heidelberg: Springer-Verlag), 175–189. doi: 10.1007/978-3-642-00390-5_11
Santos, D., Duarte, B., and Caçador, I. (2014). Unveiling Zn hyperaccumulation in Juncus acutus: implications on the electronic energy fluxes and on oxidative stress with emphasis on non-functional Zn-chlorophylls. J. Photochem. Photobiol. Biol. 140, 228–239. doi: 10.1016/j.jphotobiol.2014.07.019
Schvartzman, M. S., Corso, M., Fataftah, N., Scheepers, M., Nouet, C., Bosman, B., et al. (2018). Adaptation to high zinc depends on distinct mechanisms in metallicolous populations of Arabidopsis halleri. N. Phytol. 218, 269–282. doi: 10.1111/nph.14949
Sitko, K., Rusinowski, S., Kalaji, H. M., Szopinski, M., and Małkowski, E. (2017). Photosynthetic efficiency as bioindicator of environmental pressure in A. halleri. Plant Physiol. 175, 290–302. doi: 10.1104/pp.17.00212
Stein, R. J., Höreth, S., de Melo, J. R. F., Syllwasschy, L., Lee, G., Garbin, M. L., et al. (2017). Relationships between soil and leaf mineral composition are element-specific, environment-dependent and geographically structured in the emerging model Arabidopsis halleri. N. Phytol. 213, 1274–1286. doi: 10.1111/nph.14219
Su, C., Jiang, L. Q., and Zhang, W. J. (2014). A review on heavy metal contamination in the soil worldwide: Situation, impact and remediation techniques. Environ. Skept. Crit. 3, 24–38.
Szarek-Łukaszewska, G., and Grodzinska, K. (2007). Vegetation of a post-mining open pit (Zn/Pb ores): three-year study of colonization. Pol. J. Ecol. 55, 261–282. Available online at: https://www.miiz.waw.pl/pliki/article/ar55_2_05.pdf
Szarek-Łukaszewska, G., and Grodzinska, K. (2011). Grasslands of Zn-Pb post-mining area (Olkusz ore-bearing region, S Poland). Pol. Bot. J. 56, 245–260. Available online at: http://bomax.botany.pl/pubs-new/#article-2901
Tang, L., Yao, A., Yuan, M., Tang, Y., Liu, J., Liu, X., et al. (2016). Transcriptional up-regulation of genes involved in photosynthesis of the Zn/Cd hyperaccumulator Sedum alfredii in response to zinc and cadmium. Chemosphere 164, 190–200. doi: 10.1016/j.chemosphere.2016.08.026
Tohge, T., de Souza, L. P., and Fernie, A. R. (2017). Current understanding of the pathways of flavonoid biosynthesis in model and crop plants. J. Exp. Bot. 68, 4013–4028. doi: 10.1093/jxb/erx177
Van Assche, F. V., and Clijsters, H. (1986). Inhibition of photosynthesis by treatment of Phaseolus vulgaris with toxic concentration of zinc: effects on electron transport and photophosphorylation. Physiol. Plant. 66, 717–721. doi: 10.1111/j.1399-3054.1986.tb05605.x
Vassilev, A., Nikolova, A., Koleva, L., and Lidon, F. (2011). Effects of Excess Zn on growth and photosynthetic performance of young bean plants. J. Phytol. 3, 58–62. Available online at: https://updatepublishing.com/journal/index.php/jp/article/view/2279
Verbruggen, N., Juraniec, M., Balliardini, C., and Meyer, C. L. (2013). Tolerance to cadmium in plants: the special case of hyperaccumulators. Biometals 26, 633–638. doi: 10.1007/s10534-013-9659-6
Keywords: photosystem II, Cadmium, Zinc, Arabidopsis, chlorophyll a fluorescence
Citation: Szopiński M, Sitko K, Gieroń Ż, Rusinowski S, Corso M, Hermans C, Verbruggen N and Małkowski E (2019) Toxic Effects of Cd and Zn on the Photosynthetic Apparatus of the Arabidopsis halleri and Arabidopsis arenosa Pseudo-Metallophytes. Front. Plant Sci. 10:748. doi: 10.3389/fpls.2019.00748
Received: 07 December 2018; Accepted: 21 May 2019;
Published: 06 June 2019.
Edited by:
Marcin Rapacz, University of Agriculture of Krakow, PolandReviewed by:
Nadia Bazihizina, University of Florence, ItalyRosario Vera-Estrella, National Autonomous University of Mexico, Mexico
Copyright © 2019 Szopiński, Sitko, Gieroń, Rusinowski, Corso, Hermans, Verbruggen and Małkowski. This is an open-access article distributed under the terms of the Creative Commons Attribution License (CC BY). The use, distribution or reproduction in other forums is permitted, provided the original author(s) and the copyright owner(s) are credited and that the original publication in this journal is cited, in accordance with accepted academic practice. No use, distribution or reproduction is permitted which does not comply with these terms.
*Correspondence: Michał Szopiński, bXN6b3BpbnNraUB1cy5lZHUucGw=; Eugeniusz Małkowski, ZXVnZW5pdXN6Lm1hbGtvd3NraUB1cy5lZHUucGw=
†Present Address: Massimiliano Corso, Institute for Integrative Biology of the Cell (I2BC), CEA, CNRS, Université Paris-Sud, Université Paris-Saclay, Gif-sur-Yvette, France