- Plant Science Laboratory, Cranfield University, Cranfield, United Kingdom
Blueberries (Vaccinium corymbosum L.) are highly valued for their health-promoting potential, yet they are extremely perishable. Controlled atmosphere (CA) strategies reduce blueberry respiratory metabolism, slowing down senescence. However, the sudden change of atmosphere could elicit a physical abiotic stress in the fruit, negatively affecting quality. We propose an innovative approach based on controlled graduation to slowly reach optimum gas storage conditions as an alternative to standard CA. For two consecutive seasons, “Duke” blueberries were subjected to four different storage conditions: control (air); standard CA (sudden exposure to 5 kPa O2 and 10 kPa CO2 across the experiment); GCA3 and GCA7 (gradually reaching 5 kPa O2 and 10 kPa CO2 in 3 and 7 days, respectively). Fruit were stored for 28 days at 0 ± 0.5°C. Real-time respirometry provided an in-depth insight to the respiratory response of blueberries to their gas environment. Blueberries subjected to the graduated application of CA (GCA) treatments had a lower steady-state respiration rate compared to control and standard CA fruit. This indicated a reduction in metabolic activity that positively impacted quality and storage life extension. For example, GCA3 and GCA7 blueberries had a 25% longer storage life when compared to control, based on reduced decay incidence. In addition, GCA fruit were 27% firmer than control and CA fruit after 28 days of cold storage. GCA3 had a positive effect on maintaining individual sugars concentrations throughout the experiment, and both GCA treatments maintained ascorbic acid content close to initial values compared to a decrease of 44% in the control fruit at the end of the experiment. This work provides a paradigm shift in how CA could be applied and a better understanding of blueberry physiology and postharvest behavior.
Introduction
Blueberries (Vaccinium spp.) have become a popular soft fruit because of their organoleptic characteristics and health-promoting compound content (Manganaris et al., 2014). Yet, blueberries are highly perishable. Their storage life at 0°C varies between 14 and 20 days depending on preharvest factors (i.e., cultivar, ripeness stage, harvest method) and storage conditions (Matiacevich et al., 2013). Slowing down respiration, the main process in fruit metabolism (Gomes et al., 2010), is key to delaying senescence. Respiration is mostly affected by temperature and the respiratory gaseous environment (Wang et al., 2019). Hence, the combination of low temperature storage and controlled atmosphere (CA) technology has been used for many years to maintain physical and functional quality (Terry et al., 2009). For blueberries, a high concentration of carbon dioxide (CO2) has a positive impact on decay suppression (Retamales and Hancock, 2018). Recommendations range between 10–12 kPa CO2; higher levels elicit a negative effect on firmness, flavor, and titratable acidity (TA) content (Kader, 2003; Harb and Streif, 2004). Oxygen (O2) concentration has less impact on blueberry quality, although lowering it to 2–5 kPa is advised (Kader, 2003). O2 concentrations below 2 kPa lead to hypoxia and fermentation (Retamales and Hancock, 2018). However, applying standard CA can lead to abiotic stress derived from a sudden change in the surrounding atmosphere, negatively affecting quality (Falagán and Terry, 2018). The introduction of real-time respirometry has allowed the effects of CA to be monitored, reactivating research in this field. Previous studies have shown how targeted CA applications had a similar effect to continuous CA in extending shelf-life of onion, strawberry and avocado (Chope et al., 2007; Alamar et al., 2017). Based on these results, we hypothesized in this work that the graduated application of CA (GCA) could avoid this metabolic shock as it allows fresh produce to more slowly adapt to an optimal gaseous environment. This approach is based on the way modified atmosphere packaging (MAP) works, gradually reaching the respiratory equilibrium, instead of exposing the fresh produce to a sudden change in the atmosphere. Work on adaptive control of the gas diffusion in MAP has been successfully implemented before, e.g., preserving quality in blueberries and spinach (Lee et al., 2016). In this study, the MAP approach is translated to CA systems. The aim of this work was to study the benefits of GCA on the physiological and functional quality of “Duke” blueberries.
Materials and Methods
Plant Material
Blueberries (Vaccinium corymbosum L.) “Duke” were hand harvested at Cobrey Farms (Herefordshire, United Kingdom) on the 12 of July 2017 (season 1) and on the 27 of June 2018 (season 2) at their optimal maturity stage according to commercial standards defined by the company, considering color and maturity index. They were immediately transported in 600 g punnets to the Plant Science Laboratory at Cranfield University by refrigerated truck (2.5 h). Fruit were then directly transferred to the treatment boxes upon arrival at the laboratory and allowed to cool down for 24 h at 0°C prior treatment application.
Experimental Plan
Forty-eight 4 L air-tight polypropylene boxes (L&L Nordic OÜ, Estonia) were used to store 1 kg of sound fruit each. They were kept in a cold room at 0°C and 90–95% relative humidity (RH). Four treatments were considered: (i) Control, flushed with regular atmosphere gas concentrations (20.9 kPa O2 and 0.03 kPa CO2); (ii) standard CA (5 kPa O2 and 10 kPa CO2); (iii) graduated CA 3 (GCA3) flushed to reach the desired CA partial pressures in 3 days; and (iv) GCA7 gradually flushed to reach the standard CA conditions in 7 days (Figure 1). Each condition was performed in triplicate. The combination of 5 kPa O2 and 10 kPa CO2 was selected as optimal according to previous work (Kader, 2003; Harb and Streif, 2004; Retamales and Hancock, 2018). For each CA condition, boxes were connected to an ICA6000 (International Controlled Atmosphere Ltd., Paddock Wood, Kent, United Kingdom) via PVC tubes; gases were bubbled through water to maintain a high RH%. For each treatment, an extra empty box was used as a baseline to avoid cross-contamination and allow respirometry calculations. The ICA6000 included an automated sample sequencing system to measure and control the gas concentration introduced in CA environments. The system was regularly checked for calibration with both fresh air and bottled calibration gas. Both temperature and RH were monitored in real-time with RD Sens RFS-TH (Prodisei, Valencia, Spain). Sampling was carried out on days 0, 7, 14, 21, and 28. Day 0 (one day after harvest) analysis were considered as baseline and samples were taken before placing the fruit in the treatment boxes.
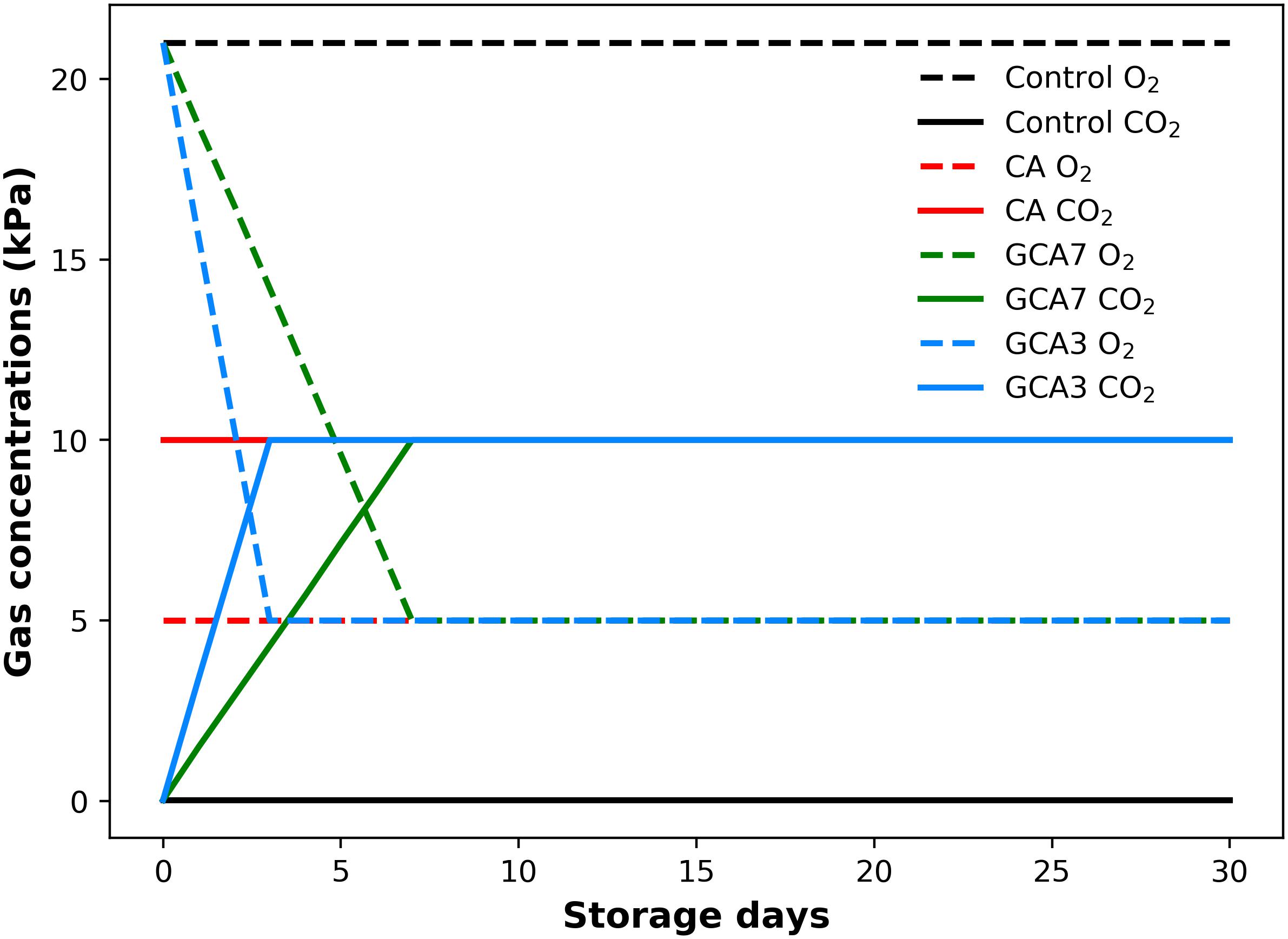
Figure 1. Gas concentration (CO2 and O2; kPa) supply throughout 28 days of storage at 0°C and 90–95 RH%; air [control]; standard CA (10 kPa CO2 + 5 kPa O2); graduated CA 7 (GCA7; 7 days to achieve standard CA values); and GCA3 (3 days to achieve standard CA values).
Fruit Quality Assessment
Respiration Rate
Real-time respiration rate was continuously recorded using a Sable Respirometry System (model 1.3.8 Pro, Sable Systems International, Nevada, United States) as described in Alamar et al. (2017) with slight modifications. Air from each box passed through a CA-10 (Firmware version 1.05) and a FC-10 sensor (Firmware version 3.0), which recorded CO2 and O2 partial pressures (kPa), respectively. For each treatment, an empty box was used as a baseline. These baseline boxes were sampled for 2 min between each replicate and for 6 min between each treatment to avoid cross-contamination. Baseline values were subtracted from sample values to obtain an accurate respiration rate expressed in mg kg–1 h–1.
Decay and Weight Loss
Fruit presenting fungal development symptoms, leakage, or collapse were considered as decayed. Decay was assessed on 100 blueberries per replicate and results were expressed as percentages. Decay severity was evaluated using a scale, where 1 = 0%, 2 = 1 to 25%, 3 = 26 to 50%, 4 = 51 to 75%, and 5 = 76 to 100% of the decayed fruit. Fruit were counted and a percentage was attributed to each level of the scale. Weight was recorded weekly using a digital balance (Precisa Ltd., Buckinghamshire, United Kingdom) with 0.1 g resolution. The same boxes were measured throughout the experiment. Weight loss was expressed as the percentage of loss compared to the initial 1 kg weight (Paniagua et al., 2014).
Firmness
A non-destructive compression test was performed on ten fruit per replicate using a uniaxial texture analyzer (model 5542, Instron, Norwood, MA, United States) equipped with a 38 mm diameter flat steel disc and a calibrated 500 N load cell. Berries were compressed by 2 mm, equatorially, at a rate of 1.2 mm s–1 according to Schotsmans et al. (2007) with slight modifications. The peak force necessary to achieve the target distance was recorded. Firmness was expressed as Newton (N).
pH, Total Soluble Solids, and Titratable Acidity
The juice of 25 blueberries per replicate (Schotsmans et al., 2007) was extracted using a commercial blender (Moulinex, Berkshire, United Kingdom). pH was recorded using a pH-meter (model 3540, Jenway, Staffordshire, United Kingdom). Total soluble solids (TSS) were determined using a digital refractometer (model PR-32α, Atago Ltd., Tokyo, Japan). TA was determined by titrating a solution of 5 mL of juice diluted in 45 mL of distilled water to pH 8.4 using 0.1 N NaOH and an automatic titrator (Mettler Toledo Ltd., Leicestershire, United Kingdom), according to Zheng et al. (2008) with slight modifications. Results were expressed as g citric acid L–1 (Schotsmans et al., 2007).
Determination of Biochemistry Attributes
Sugar Content
For each replicate (n = 3), following Downes and Terry (2010) with modifications, freeze-dried blueberry powder (150 mg) was extracted and then analyzed in an HPLC (Agilent Technologies 1200 series, Berkshire, United Kingdom) with an evaporative light scattering detector (ELSD, Agilent Technologies 1200 Series, G1362A). Sugars were quantified using the external standards glucose, fructose and sucrose purchased from Sigma-Aldrich.
Organic Acids Content
For each replicate (n = 3), following Crespo et al. (2010) with modifications, freeze-dried blueberry powder (50 mg) was extracted and analyzed in an HPLC (Agilent Technologies 1200 series, Berkshire, United Kingdom) equipped with a diode array detector (DAD Agilent Technologies 1200 Series, G1315B) set at 210 nm was used to quantify ascorbic and citric acids (Sigma Aldrich, Kent, United Kingdom). Separation was performed on a prevail organic acid column of 250 mm × 4.6 mm, 5 μm particle size (Hichrom, United Kingdom; Part no. 88645). Mobile phase was 25 mM KH2PO4 in water, adjusted to pH 2.5 using meta-phosphoric acid. Organics acids were quantified using the external standards ascorbic and citric acids purchased from Sigma-Aldrich.
Statistical Analysis
Data were subjected to analysis of variance (ANOVA) using GenStat for Windows (8.1, VSN International Ltd., Hertfordshire, United Kingdom). ANOVA assumptions were tested and found to be valid for this dataset. The differences between treatments and storage times were studied. Also, the differences between the two seasons were analyzed. Least significant difference values (LSD; p < 0.05) were calculated from each analysis.
Results
Respiratory Response to GCA
A peak in CO2 production was observed for all treatments in both seasons; its intensity and timing varied depending on treatment. Blueberries subjected to standard CA conditions showed the earliest and highest CO2 burst, while GCA7 was the most delayed and had the lowest peak. CO2 production reached a plateau after ca. 60 h in both years; control samples presented the highest CO2 production compared to any of the CA treatments during the plateau period (Figure 2).
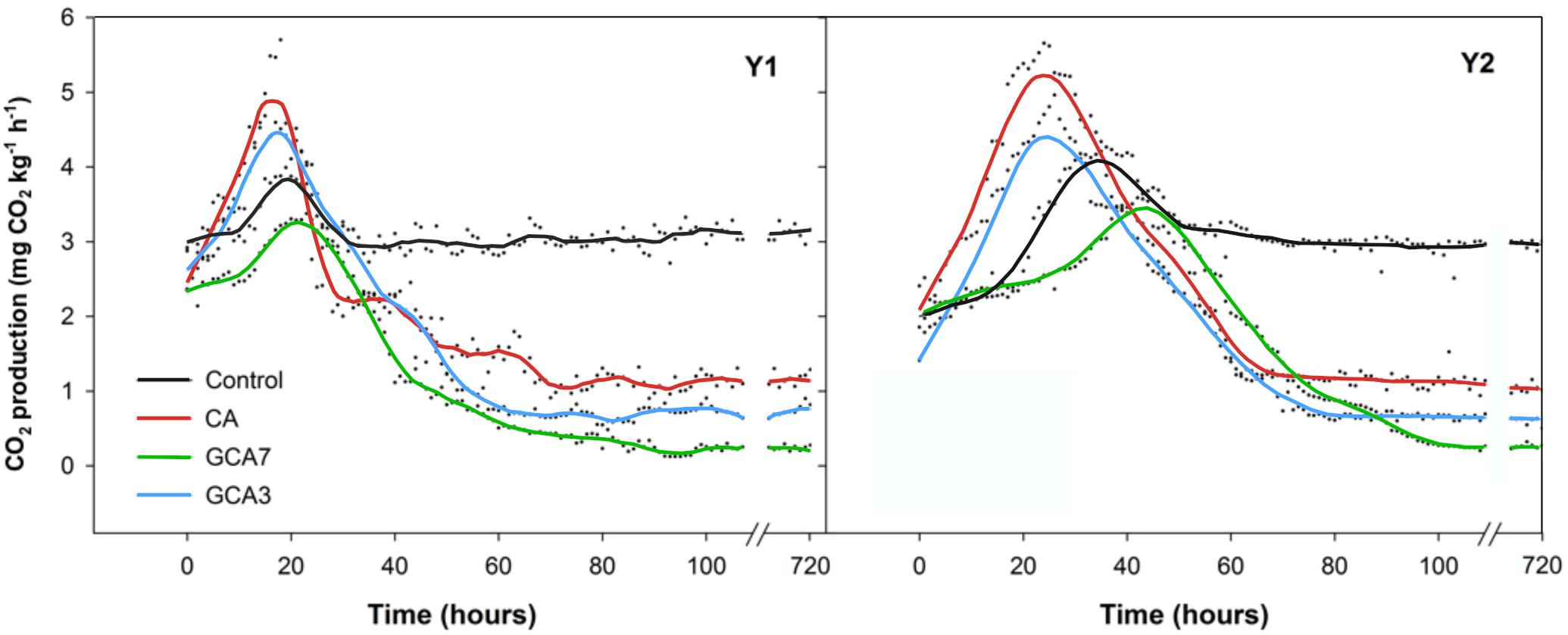
Figure 2. Real-time respiration rate (CO2 production expressed as mg kg–1 h–1) of “Duke” blueberry fruit subjected to controlled atmosphere (CA) applications throughout 28 days of storage at 0°C and 90–95 RH%; air [control]; standard CA (10 kPa CO2 + 5 kPa O2); graduated CA 7 (GCA7; 7 days to achieve standard CA values); and GCA3 (3 days to achieve standard CA values). Two consecutive seasons are considered 2017 (year 1; Y1) and 2018 (Y2). Lines represent smooth fit curves.
Physiological Response to GCA
GCA3, GCA7 and CA blueberries showed a significantly lower decay incidence than the control (92, 89, and 75% less than control, respectively; mean values between Y1 and Y2 values) after 28 storage days (Figure 3). In the case of decay severity, both GCA treatments prevented the development of fungal growth (<1%), while CA reached an average incidence value of 3.09% after 28 days (data not shown). Fruit subjected to CA and GCA showed less weight loss compared to the control. Both GCA delayed decay incidence by 14 days, compared to control and CA treatments (Figure 4). No significant differences were found between seasons (p < 0.05).
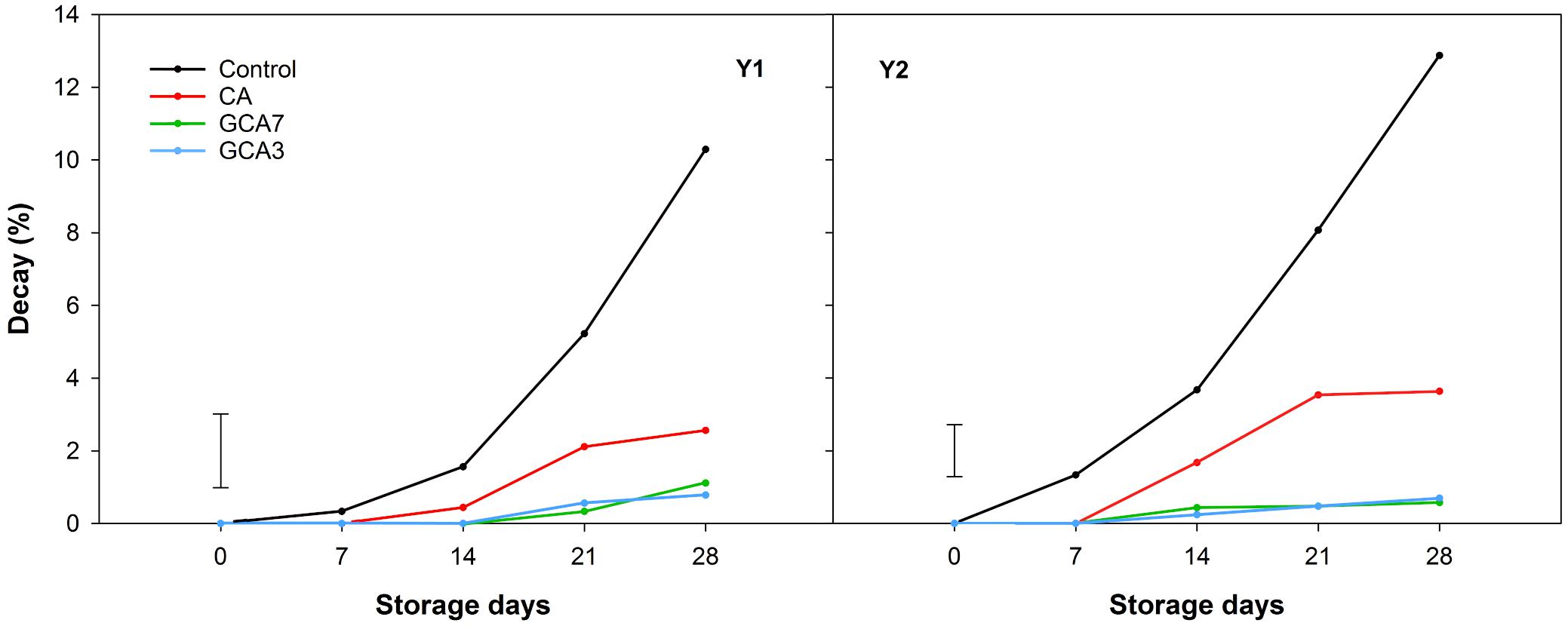
Figure 3. Decay expressed as percentage (%) of “Duke” blueberry fruit subjected to controlled atmosphere (CA) applications throughout 28 days of storage at 0°C and 90–95 RH%; air [control]; standard CA (10 kPa CO2 + 5 kPa O2); graduated CA 7 (GCA7; 7 days to achieve standard CA values); and GCA3 (3 days to achieve standard CA values). Two consecutive seasons are considered 2017 (year 1; Y1) and 2018 (Y2). Data represents means (n = 300). Least Significant Differences (LSD) are included as LSDtreatment*time = 1.0145 for Y1 and LSDtreatment*time = 0.713 for Y2.
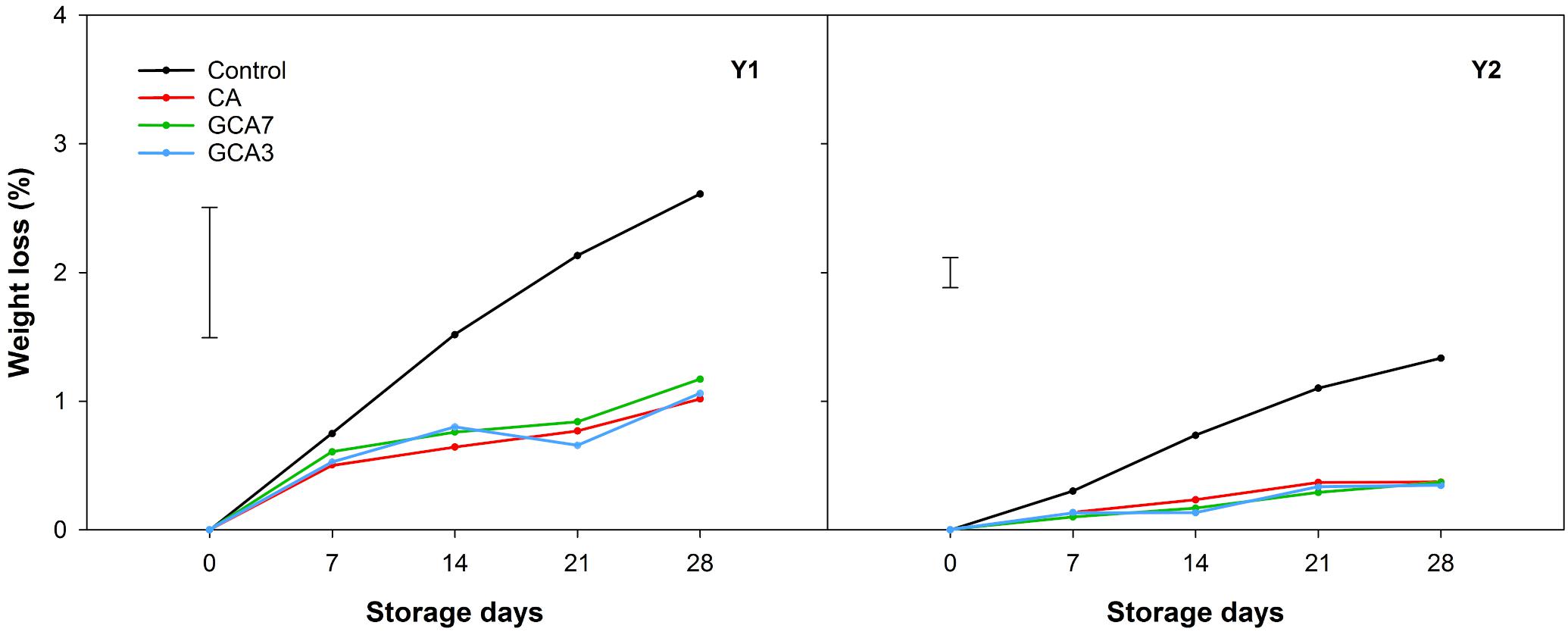
Figure 4. Weight loss expressed as percentage (%) of “Duke” blueberry fruit subjected to controlled atmosphere (CA) applications throughout 28 days of storage at 0°C and 90–95 RH%; air [control]; standard CA (10 kPa CO2 + 5 kPa O2); graduated CA 7 (GCA7; 7 days to achieve standard CA values); and GCA3 (3 days to achieve standard CA values). Two consecutive seasons are considered 2017 (year 1; Y1) and 2018 (Y2). Data represents means (n = 3). Least Significant Differences (LSD) are included as: LSDtreatment*time = 1.0145 for Y1 and LSDtreatment = LSDtime = 0.2338 for Y2.
At the end of the storage period, control and CA blueberries lost an average of 40 and 29% of their initial firmness in Y1 and Y2, respectively. Firmness of berries held under GCA3 and GCA7 showed a loss of 16% in the first season and no significant changes were observed during the second season (Figure 5). pH showed a decreasing trend in Y1 and Y2, triggered by the interaction of treatment and time in Y1 and time in Y2. No significant differences were found in TA across both years (Supplementary Table 1).
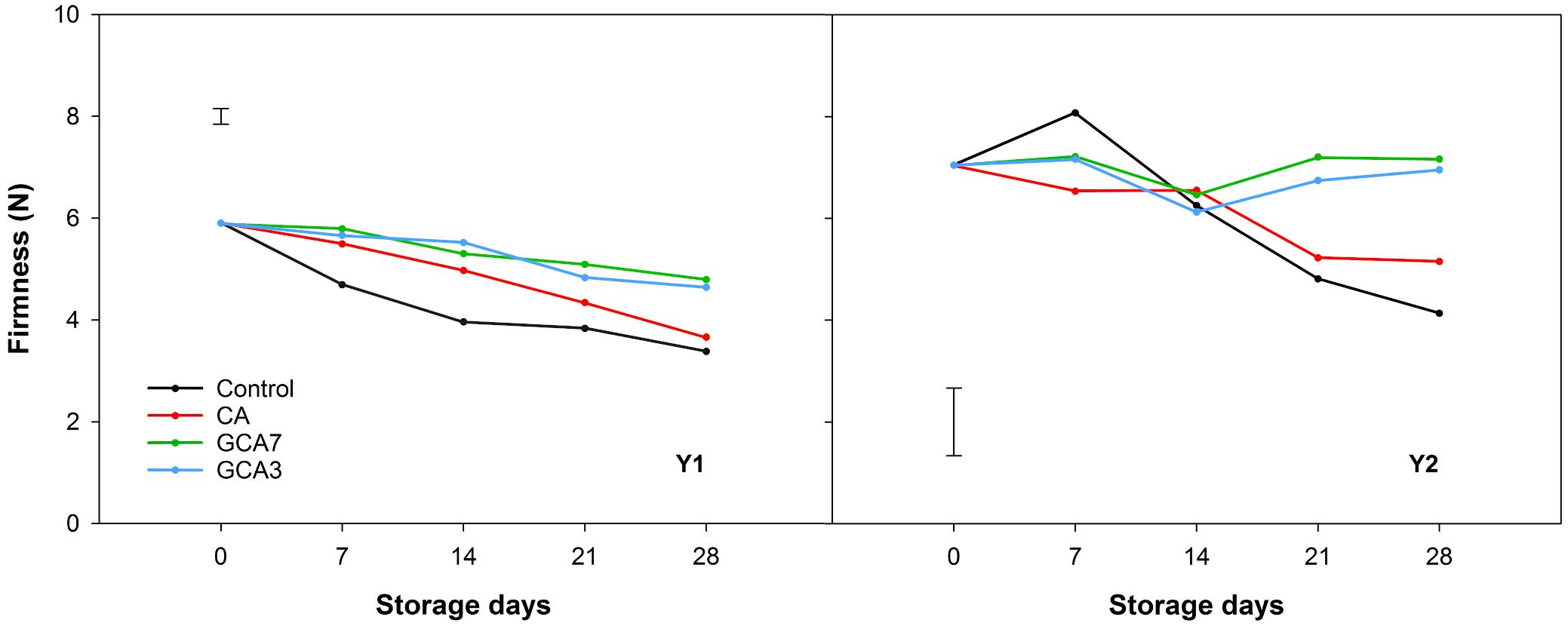
Figure 5. Firmness expressed as Newton (N) of “Duke” blueberry fruit subjected to controlled atmosphere (CA) applications throughout 28 days of storage at 0°C and 90–95 RH%; air [control]; standard CA (10 kPa CO2 + 5 kPa O2); graduated CA 7 (GCA7; 7 days to achieve standard CA values); and GCA3 (3 days to achieve standard CA values). Two consecutive seasons are considered 2017 (year 1; Y1) and 2018 (Y2). Data represents means (n = 30). Least Significant Differences (LSD) are included as: LSDtreatment = LSDtime = 0.310 for Y1 and LSDtreatment*time = 1.335 for Y2.
Biochemical Response to GCA
Total sugar content was 40% higher in Y2 compared to Y1, but showed a similar pattern during storage. CA, GCA3 and GCA7 maintained the concentration of sucrose and fructose across the experiment, while control blueberries presented a sharp decrease in sucrose (49% mean for Y1 and Y2) and fructose (12.5% mean for Y1 and Y2). Glucose concentrations were maintained in CA and GCA treated fruit, whilst they slightly increased in control fruit (Figure 6). Ascorbic acid content degraded by 34, 29, and 41% during storage for CA, GCA, and control blueberries, respectively, for Y1; and 21, 29, and 44% during storage for CA, GCA, and control blueberries, respectively, for Y2 (Figure 7). In the case of citric acid, no significant differences were found among CA treatments and time during the experiment. CA treatments maintained the initial citric acid level, while control samples suffered a decrease of 45 and 31% in Y1 and Y2, respectively (Figure 7). Significant differences were observed between Y1 and Y2 for both ascorbic and citric acids contents (Ascorbic acid: LSDY1 × Y2 = 1.56; citric acid: LSDY1 × Y2 = 0.23; p < 0.05). Despite these differences, the response of blueberries to CA and GCA was the same in both seasons (Figure 7).
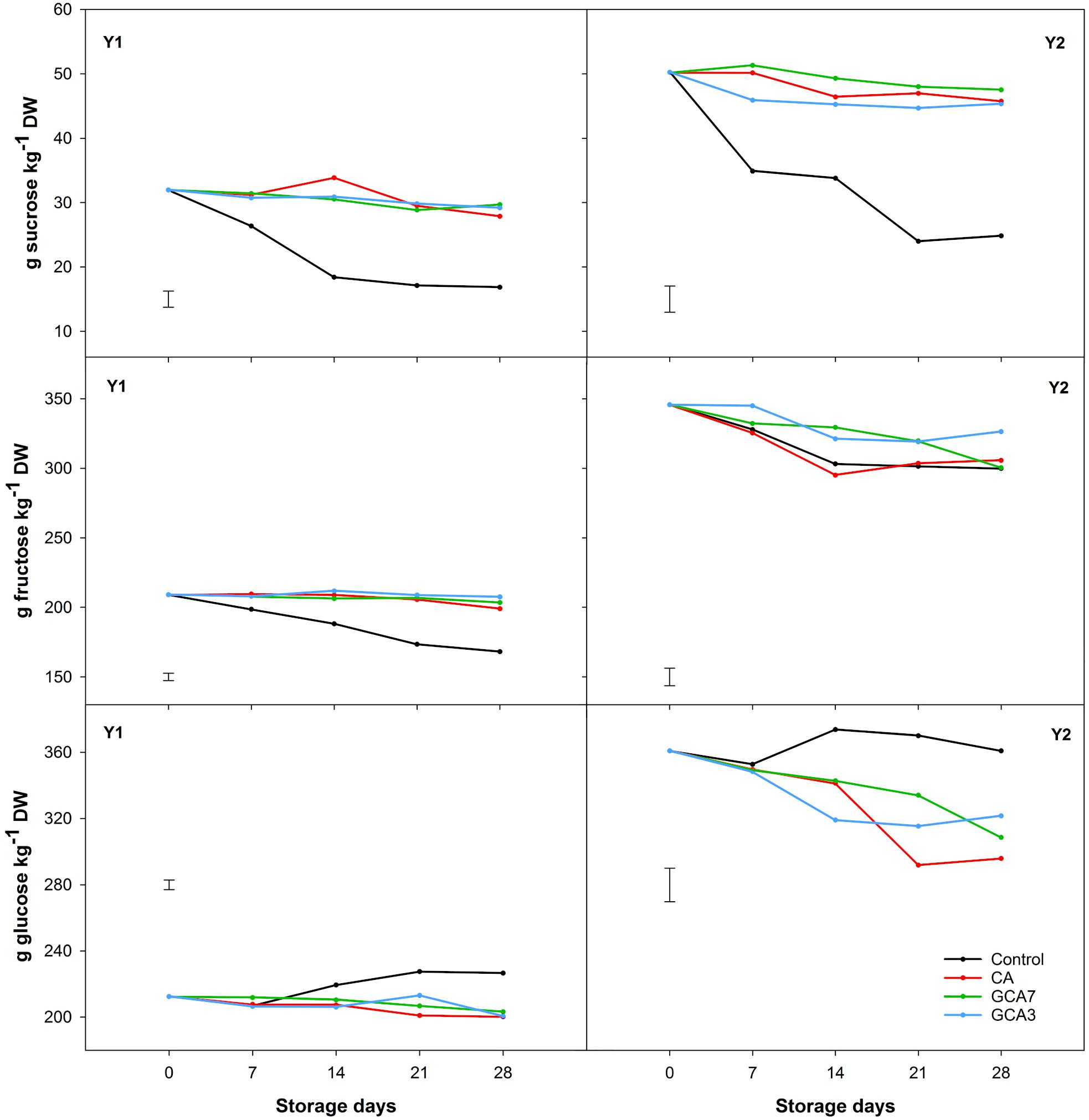
Figure 6. Individual sugar concentration (sucrose, fructose, and glucose) expressed as g kg–1 dry weight (DW) of “Duke” blueberry fruit subjected to controlled atmosphere (CA) applications throughout 28 days of storage at 0°C and 90–95 RH%; air [control]; standard CA (10 kPa CO2 + 5 kPa O2); graduated CA 7 (GCA7; 7 days to achieve standard CA values); and GCA3 (3 days to achieve standard CA values). Two consecutive seasons are considered 2017 (year 1; Y1) and 2018 (Y2). Data represents means (n = 3). Least Significant Differences (LSD) are included as: (i) Sucrose – LSDtreatment = LSDtime = 2.484 for Y1 and LSDtreatment = 4.082 for Y2; Fructose – LSDtreatment*day = 5.251 for Y1 and LSDtreatment = LSDtime = 12.63 for Y2; and Glucose – LSDtreatment*day = 5.848 for Y1 and LSDtreatment*time = 20.26 for Y2.
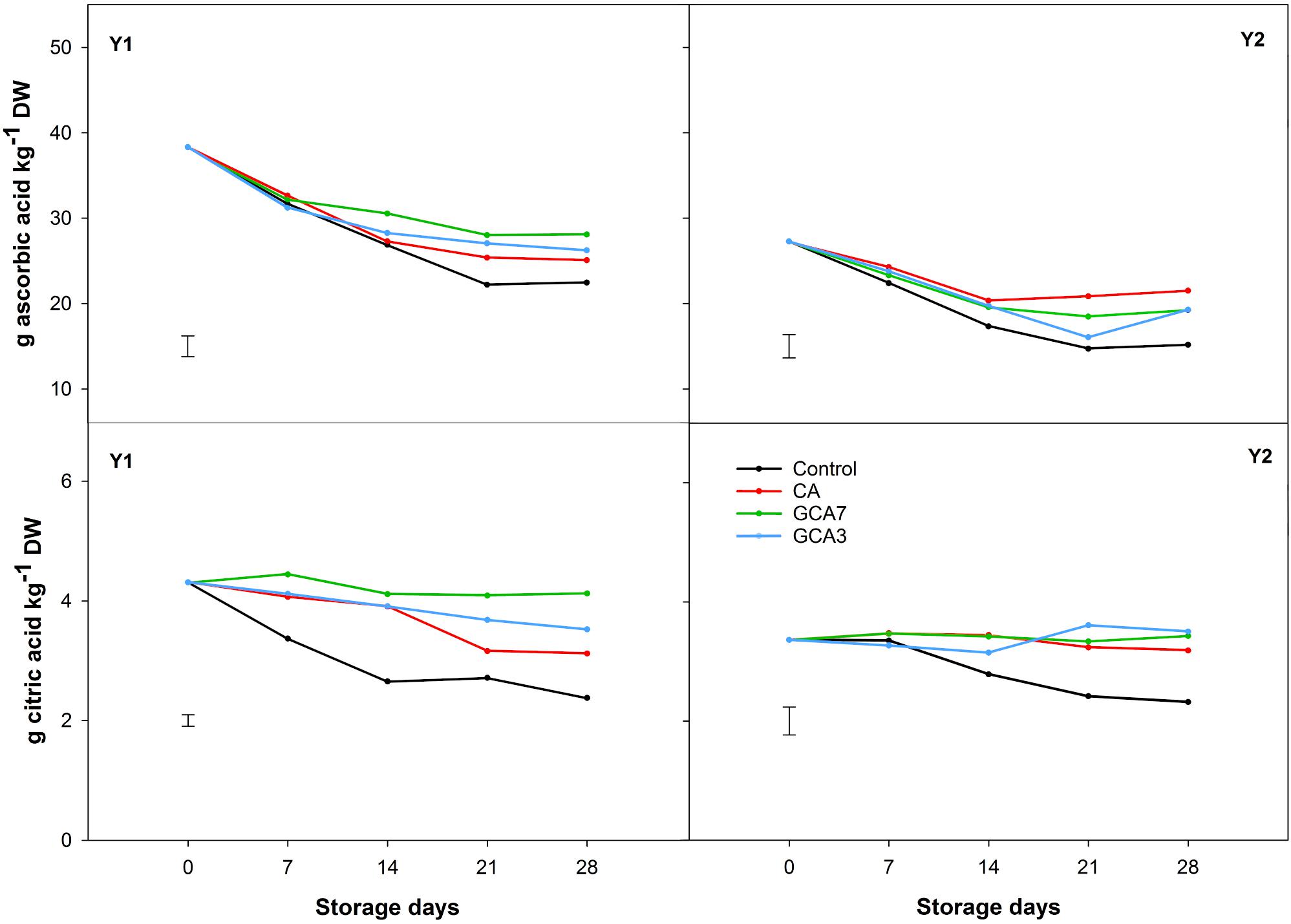
Figure 7. Organic acid concentration (ascorbic and citric acids) expressed as g kg–1 dry weight (DW) of “Duke” blueberry fruit subjected to controlled atmosphere (CA) applications throughout 28 days of storage at 0°C and 90–95 RH%; air [control]; standard CA (10 kPa CO2 + 5 kPa O2); graduated CA 7 (GCA7; 7 days to achieve standard CA values); and GCA3 (3 days to achieve standard CA values). Two consecutive seasons are considered 2017 (year 1; Y1) and 2018 (Y2). Data represents means (n = 3). Least Significant Differences (LSD) are included as: (i) ascorbic acid – LSDtreatment = LSDtime = 2.417 for Y1 and LSDtreatment = 2.731 for Y2; and citric acid – LSDtreatment = LSDtime = 0.1907 for Y1 and LSDtreatment = 0.4680 for Y2.
Discussion
Fruit and vegetables undergo physiological and functional changes during senescence, which affect their quality and storage life (Terry, 2011). Most of these changes are linked to fruit respiration, which supports the many metabolic reactions occurring in a cell (Saltveit, 2019). According to Van Hoorn (2004), the higher the respiration rate, the faster the fruit/vegetable metabolism, leading to early senescence. Until now, the main way to achieve lower blueberry respiration rate has been through cold storage and the modification of gas concentrations surrounding the fresh produce (lowering O2 availability and increasing CO2 concentration). This approach works because respiratory metabolism is affected by low O2 concentrations due to limited gas transport (Armstrong and Beckett, 2011), triggering the active regulation of respiration (Gupta et al., 2009). In this study we proposed a novel application of CA, based on reaching the optimal CA gas concentrations in a gradual way, preventing abiotic stress that results from the sudden change in the gaseous environment. The observed CO2 production bursts occurred at different times and were linked to the decrease of available O2 in the storage environment. Blueberries subjected to CA were exposed to a sudden drop in the available O2 from 21 to 5 kPa. In response to this change, CA samples presented a well-defined peak in CO2 production. Following CA response, GCA3 blueberries increased their CO2 production when the O2 availability dropped from 21 to 15.6 kPa. In the case of GCA7, its peak was delayed, coinciding with an O2 availability of ca. 15 kPa. Therefore, O2 concentrations below 15 kPa were seen to induce an abiotic stress, and consequently an increase in CO2 production. This finding agreed with Gupta et al. (2009) who highlighted the importance of the timescale in O2 availability changes for respiratory metabolism. These peaks in CO2 production represented an increase in respiration, interpreted as possible abiotic stress to the changing gaseous environment (Mathooko et al., 2001). Interestingly, control blueberries also showed a peak, although smaller than the treatments had provoked, before reaching a steady-state level. This phenomenon might be because of the handling procedure, which involved a change in temperature, RH and storage environment. More research is needed in this area to identify the mechanisms behind this response. Nevertheless, it is observed that a slower imposed GCA, such as GCA7, produced the most delayed and lowest peak in CO2 production compared to standard CA, GCA3 and control (Figure 2). We herein hypothesized that GCA allowed the adaptive response of respiration to low O2 availability, preventing the metabolic shock that could reduce the physical quality of the fruit (Armstrong and Beckett, 2011). Apart from preventing this abiotic stress, GCA achieved a lower respiration rate compared to standard CA and control treatments when it reached stability after ca. 7 days of storage.
In fresh produce, senescence is generally marked by changes in firmness, weight and decay incidence (Defraeye et al., 2015). The reduction in respiratory metabolism had a positive impact on firmness, which is a critical quality index for blueberry, as consumers associate it with freshness (Chiabrando and Giacalone, 2017). Changes in firmness are a consequence of primary cell wall component degradation, such as pectin, cellulose and hemicellulose (Liu et al., 2019). We speculated that low O2 and high CO2 storage environments inhibited the action of polygalacturonase and pectin methylesterase (Beaudry, 1999), which are the enzymes responsible for the catabolism of cell wall metabolism (Yang et al., 2018). This event was also observed in blueberries stored under standard CA conditions (Concha-Meyer et al., 2015). GCA regulated response and delayed firmness loss when compared to control and CA treatments. Previous studies showed that targeted CA application on strawberry maintained their color (flesh and calyx) and firmness when compared to control and standard CA (Alamar et al., 2017). Firmness loss is directly linked to weight loss and decay incidence, as observed in this study (Paniagua et al., 2014; Chen et al., 2017). In the case of weight loss, blueberries subjected to GCA showed the same response as CA fruit, both significantly lower than control samples. Weight is generally lost through transpiration, the gradient of water vapor pressure in the cell tissues (Díaz-Pérez et al., 2007). In blueberries, transpiration generally happens through the stem scar rather than through the cuticle and it increases with temperature (Moggia et al., 2017). The blueberries herein were stored at 0°C, so that overall low weight loss percentages were observed. Although control samples showed a significantly higher weight loss than CA and GCA blueberries, it is considered that losses up to 4–5% do not affect fruit freshness (Mannozzi et al., 2017). In this study, higher weight and firmness loss contributed to greater decay in control samples when compared to CA and GCA. In general, CA with CO2 concentrations over 6 kPa retard decay symptoms in blueberry fruit (Schotsmans et al., 2007; Rodríguez and Zoffoli, 2016). However, a long exposure to high CO2 levels can cause internal damage to fruit leading to firmness and weight loss. We assumed that a graduated CA approach prevented this damage, providing better quality fruit than CA. GCA is an innovative approach to standard CA storage, and is analogous to a MAP strategy. Alternatives to standard CA include targeted CA application, which showed that 2.5 days of CA were sufficient to extend the shelf-life of strawberry by 3 days based on delayed decay incidence (Alamar et al., 2017).
Sugars and organic acids are considered the main substrates in the respiratory metabolism (Saltveit, 2019). Recent studies have considered that glucose and sucrose can play a role as signaling molecules in the stress responses (Huang et al., 2016), rather than just as a carbon source. It has also been reported that in non-climacteric fruit such as strawberry both sucrose and glucose promote fruit ripening and senescence (Jia et al., 2013; Cherian et al., 2014). In this study, control samples showed sucrose hydrolysis (Figure 6), likely through the action of invertase and sucrose synthase (Thomas et al., 2016). This process was not observed in CA nor GCA, indicating an inhibition of sugar degradation and delayed senescence. The different sugar profiles between the control and CA/GCA blueberries was linked to observed respiratory behavior, confirming the role of sugars as substrates in the respiratory metabolism. However, there was no evidence to support the hypothesis of sugars acting as signaling molecules in the stress responses of blueberries, this being in agreement with Karppinen et al. (2018). In this study, there was a poor correlation between sugar content and TSS, as described in Terry (2012). With respect to TA, neither time or treatments had an effect on its content. In contrast, Duarte et al. (2009) found that blueberries “Brigitta” treated with CA had a higher TA than control samples. Looking at ascorbic and citric acid concentrations, both were more stable in fruit subjected to GCA and CA berries than the control. This study showed the positive impact of GCA on preserving bioactive life of blueberry and potentially for other fresh produce by suppressing senescence decline. Both pH and TA remained stable across the experiment, while changes were observed in organic acid content. We acknowledged the lack of correlation among these parameters; this could be related to the lower sensitivity of pH and TA measurement methods, compared to HPLC.
The difference in the results between seasons 1 and 2 (p < 0.05) may be potentially attributable to variable environmental preharvest factors, particularly temperature as several heat waves were registered in June and July 2018 (Met Office, 2018).
Conclusion
Blueberries require advanced postharvest techniques to maintain their physiological and functional quality. The development of an innovative CA approach, based on gradually reaching the optimal storage concentrations (GCA) allowed the reduction of blueberry respiratory metabolism when compared to standard CA and control treatments. This had a positive impact on quality parameters such as firmness and decay incidence. The reduction in respiration was also evident as sugars and organic acids were better maintained, these being the main respiratory substrates. GCA could potentially be successfully applied to other blueberry cultivars. Further research is needed to better understand the mechanisms underlying this technique and the efficacy of its use on other fresh produce.
Data Availability Statement
All datasets generated for this study can be accessed at https://doi.org/10.17862/cranfield.rd.11876544.v1.
Author Contributions
NF and LT conceived and designed the experiments. NF and TM performed the experiments. NF wrote the manuscript with the help of LT. All authors contributed to the discussion, revised, and approved the final manuscript.
Funding
This work was supported by the Biotechnology and Biological Sciences Research Council (BBSRC; BB/P00492X/1) and Johnson Matthey Plc.
Conflict of Interest
The authors declare that the research was conducted in the absence of any commercial or financial relationships that could be construed as a potential conflict of interest.
Acknowledgments
The authors thank BBSRC and Johnson Matthey Plc. for financial support.
Supplementary Material
The Supplementary Material for this article can be found online at: https://www.frontiersin.org/articles/10.3389/fpls.2020.00221/full#supplementary-material
References
Alamar, M. C., Collings, E., Cools, K., and Terry, L. A. (2017). Impact of controlled atmosphere scheduling on strawberry and imported avocado fruit. Postharvest Biol. Technol. 134, 76–86. doi: 10.1016/j.postharvbio.2017.08.003
Armstrong, W., and Beckett, P. M. (2011). Experimental and modelling data contradict the idea of respiratory down-regulation in plant tissues at an internal [O2] substantially above the critical oxygen pressure for cytochrome oxidase. New Phytol. 190, 431–441. doi: 10.1111/j.1469-8137.2010.03537.x
Beaudry, R. M. (1999). Effect of O2 and CO2 partial pressure on selected phenomena affecting fruit and vegetable quality. Postharvest Biol. Technol. 15, 293–303. doi: 10.1016/S0925-5214(98)00092-1
Chen, Y. H., Hung, Y. C., Chen, M. Y., and Lin, H. T. (2017). Effects of acidic electrolyzed oxidizing water on retarding cell wall degradation and delaying softening of blueberries during postharvest storage. LWT Food Sci. Technol. 84, 650–657. doi: 10.1016/j.lwt.2017.06.0110023-6438
Cherian, S., Figueroa, C. R., and Nair, H. (2014). ‘Movers and shakers’ in the regulation of fruit ripening: a cross-dissection of climacteric versus non-climacteric fruit. J. Exp. Bot. 65, 4705–4722. doi: 10.1093/jxb/eru280
Chiabrando, V., and Giacalone, G. (2017). Quality evaluation of blueberries coated with chitosan and sodium alginate during postharvest storage. Int. Food Res. J. 24, 1553–1561.
Chope, G., Terry, L. A., and White, P. J. (2007). The effect of the transition between controlled atmosphere and regular atmosphere storage on bulbs of onion cultivars SS1, Carlos and Renate. Postharvest Biol. Technol. 49, 61–68. doi: 10.1016/j.postharvbio.2006.12.018
Concha-Meyer, A., Eifert, J. D., Williams, R. C., Marcy, J. E., and Welbaum, G. E. (2015). Shelf life determination of fresh blueberries (Vaccinium corymbosum) stored under controlled atmosphere and ozone. Int. J. Food Sci. 164143, 1–9. doi: 10.1155/2015/164143
Crespo, P., Bordonaba, J. G., Terry, L. A., and Carlen, C. (2010). Characterisation of major taste and health-related compounds of four strawberry genotypes grown at different Swiss production sites. Food Chem. 122, 16–24. doi: 10.1016/j.foodchem.2010.02.010
Defraeye, T., Cronjé, P., Berry, T., Opara, U. L., East, A., Hertog, M., et al. (2015). Towards integrated performance evaluation of future packaging for fresh produce in the cold chain. Trends Food Sci. Technol. 44, 201–225. doi: 10.1016/j.tifs.2015.04.008
Díaz-Pérez, J. C., Muy-Rangel, M. D., and Mascorro, A. G. (2007). Fruit size and stage of ripeness affect postharvest water loss in bell pepper fruit (Capsicum annuum L.). J. Sci. Food Agric. 87, 68–73. doi: 10.1002/jsfa.2672
Downes, K., and Terry, L. A. (2010). A new acetonitrile-free mobile phase method for LC–ELSD quantification of fructooligosaccharides in onion (Allium cepa L.). Talanta 82, 118–124. doi: 10.1016/j.talanta.2010.04.003
Duarte, C., Guerra, M., Daniel, P., Camelo, A. L., and Yommi, A. (2009). Quality changes of highbush blueberries fruit stored in CA with different CO2 levels. J. Food Sci. 74, 154–159. doi: 10.1111/j.1750-3841.2009.01118.x
Falagán, N., and Terry, L. A. (2018). Recent advances in controlled and modified atmosphere of fresh produce. Johnson Matthey Tech. 62, 107–117. doi: 10.1595/205651318X696684
Gomes, M. H., Beaudry, R. M., Almeida, D. P. F., and Malcata, F. X. (2010). Modelling respiration of packaged fresh-cut ‘Rocha’ pear as affected by oxygen concentration and temperature. J. Food Eng. 96, 74–79. doi: 10.1016/j.jfoodeng.2009.06.043
Gupta, K. J., Zabalza, A., and van Dongen, J. T. (2009). Regulation of respiration when the oxygen availability changes. Physiol. Plant. 137, 383–391. doi: 10.1111/j.1399-3054.2009.01253.x
Harb, J., and Streif, J. (2004). Controlled atmosphere storage of highbush blueberries cv. ‘Duke’. Eur. J. Hort. Sci. 69, 66–72.
Huang, H., Xie, S., Xiao, Q., Wei, B., Zheng, L., Wang, Y., et al. (2016). Sucrose and ABA regulate starch biosynthesis in maize through a novel transcription factor, ZmEREB156. Sci. Rep. 6:27590. doi: 10.1038/srep27590
Jia, H., Wang, Y., Sun, M., Li, B., Han, Y., Zhao, Y., et al. (2013). Sucrose functions as a signal involved in the regulation of strawberry fruit development and ripening. New Phytol. 198, 453–465. doi: 10.1111/nph.12176
Kader, A. A. (2003). A summary of CA requirements and recommendations for fruits other than apples and pears. Acta Hortic. 600, 737–740. doi: 10.17660/ActaHortic.2003.600.112
Karppinen, K., Tegelberg, P., Häggman, H., and Jaakola, L. (2018). Abscisic acid regulates anthocyanin biosynthesis and gene expression associated with cell wall modification in ripening bilberry (Vaccinium myrtillus L.) fruits. Front. Plant Sci. 29:1259. doi: 10.3389/fpls.2018.01259
Lee, J. H., An, D. S., Song, J. M., Jung, Y. B., and Lee, D. S. (2016). An adaptively controlled modified atmosphere container system for fresh produce. Biosyst. Eng. 148, 11–17. doi: 10.1016/j.biosystemseng.2016.05.003
Liu, B., Wang, K., Shu, X., Liang, J., and Sun, L. (2019). Changes in fruit firmness, quality traits and cell wall constituents of two highbush blueberries (Vaccinium corymbosum L.) during postharvest cold storage. Sci. Hortic. 246, 557–562. doi: 10.1016/j.scienta.2018.11.042
Manganaris, G. A., Goulas, V., Vicente, A. R., and Terry, L. A. (2014). Berry antioxidants: small fruits providing large benefits. J. Sci. Food Agric. 94, 825–833. doi: 10.1002/jsfa.6432
Mannozzi, C., Cecchini, J. P., Tylewicza, U., Siroli, L., Patrignani, F., Lanciotti, R., et al. (2017). Study on the efficacy of edible coatings on quality of blueberry fruits during shelf-life. LWT Food Sci. Technol. 85, 440–444. doi: 10.1016/j.lwt.2016.12.056
Mathooko, F. M., Tsunashima, Y., Owino, W. Z. O., Kubo, Y., and Inaba, K. (2001). Regulation of genes encoding ethylene biosynthetic enzymes in peach (Prunus persica L.) fruit by carbon dioxide and 1-methylcyclopropene. Postharvest Biol. Technol. 21, 265–281. doi: 10.1016/S0925-5214(00)00158-7
Matiacevich, S., Cofré, D. C., Silva, P., Enrione, J., and Osorio, F. (2013). Quality parameters of six cultivars of blueberry using computer vision. Int. J. Food Sci. 419535, doi: 10.1155/2013/419535
Met Office (2018). Climate Summaries. Available online at: https://www.metoffice.gov.uk/climate/uk/summaries (accessed: September 2, 2018).
Moggia, C., Beaudry, R. M., Retamales, J. B., and Lobos, G. A. (2017). Variation in the impact of stem scar and cuticle on water loss in highbush blueberry fruit argue for the use of water permeance as a selection criterion in breeding. Postharvest Biol. Technol. 132:8896. doi: 10.1016/j.postharvbio.2017.05.019
Paniagua, A. C., East, A. R., and Heyes, J. A. (2014). Interaction of temperature control deficiencies and atmosphere conditions during blueberry storage on quality outcomes. Postharvest Biol. Technol. 95, 50–59. doi: 10.1016/j.postharvbio.2014.04.006
Rodríguez, J., and Zoffoli, J. P. (2016). Effect of sulfur dioxide and modified atmosphere packaging on blueberry postharvest quality. Postharvest Biol.Technol. 117, 230–238. doi: 10.1016/j.postharvbio.2016.03.008
Saltveit, M. E. (2019). “Respiratory metabolism,” in Postharvest Physiology and Biochemistry of Fruits and Vegetables, eds E. M. Yahia and A. Carrillo-López (Cambridge: Woodhead Publishing), 73–91.
Schotsmans, W., Molan, A., and MacKay, B. (2007). Controlled atmosphere storage of rabbiteye blueberries enhances postharvest quality aspects. Postharvest Biol. Technol. 44, 277–285. doi: 10.1016/j.postharvbio.2006.12.009
Terry, L. A. (2012). “Soft Fruit,” in Crop Post-harvest: Science and Technology: Perishables, eds D. Rees, G. Farrell, and J. Orchard (Hoboken, NJ: Wiley-Blackwell Publishing Ltd), 226–246.
Terry, L. A., Crisosto, C. H., and Forney, C. F. (2009). “Small fruit and berries,” in Modified and Controlled Atmospheres for the Storage, Transportation, and Packaging of Horticultural Commodities, ed. E. M. Yahia (Boca Raton, CA: CRC Press), 364–388.
Thomas, B., Murphy, D. J., and Murray, B. G. (2016). Encyclopaedia of Applied Plant Sciences. Cambridge: Academic Press.
Van Hoorn, A. T. (2004). Optimisation of Blueberry Postharvest Handling and Storage. Master’s thesis, University of Stellenbosch, Stellenbosch.
Wang, Y., Ji, S., Dai, H., Kong, X., Hao, J., Wang, S., et al. (2019). Changes in membrane lipid metabolism accompany pitting in blueberry during refrigeration and subsequent storage at room temperature. Front. Plant Sci. 10:829. doi: 10.3389/fpls.2019.00829
Yang, Y., Yu, Y., Liang, Y., Anderson, C. T., and Cao, J. (2018). A profusion of molecular scissors for pectins: classification, expression, and functions of plant polygalacturonases. Front. Plant Sci. 9:1208. doi: 10.3389/fpls.2018.01208
Keywords: Vaccinium corymbosum L, postharvest, quality, cold storage, controlled atmosphere
Citation: Falagán N, Miclo T and Terry LA (2020) Graduated Controlled Atmosphere: A Novel Approach to Increase “Duke” Blueberry Storage Life. Front. Plant Sci. 11:221. doi: 10.3389/fpls.2020.00221
Received: 25 November 2019; Accepted: 12 February 2020;
Published: 17 March 2020.
Edited by:
Peter Toivonen, Agriculture and Agri-Food Canada, CanadaReviewed by:
María Serrano, Universidad Miguel Hernández de Elche, SpainAnna Spinardi, University of Milan, Italy
Claudia Moggia, University of Talca, Chile
Copyright © 2020 Falagán, Miclo and Terry. This is an open-access article distributed under the terms of the Creative Commons Attribution License (CC BY). The use, distribution or reproduction in other forums is permitted, provided the original author(s) and the copyright owner(s) are credited and that the original publication in this journal is cited, in accordance with accepted academic practice. No use, distribution or reproduction is permitted which does not comply with these terms.
*Correspondence: Leon A. Terry, l.a.terry@cranfield.ac.uk