- 1Department of Biology, Al-Jumum University College, Umm Al-Qura University, Makkah, Saudi Arabia
- 2Research Center on Ecological Sciences, Jiangxi Agricultural University, Nanchang, China
- 3Department of Agronomy, University of Agriculture, Faisalabad, Pakistan
- 4Department of Botany, University of Agriculture, Faisalabad, Pakistan
- 5Department of Agricultural Engineering, Khwaja Fareed University of Engineering and Information Technology, Rahim Yar Khan, Pakistan
- 6Department of Agricultural and Food Sciences, University of Bologna, Bologna, Italy
- 7Department of Biology, Faculty of Applied Sciences, Umm Al-Qura University, Makkah, Saudi Arabia
- 8Department of Biology, Research Laboratories Centre, Faculty of Applied Science, Umm Al-Qura University, Makkah, Saudi Arabia
Cold stress is one of the most limiting factors for plant growth and development. Cold stress adversely affects plant physiology, molecular and biochemical processes by determining oxidative stress, poor nutrient and water uptake, disorganization of cellular membranes and reduced photosynthetic efficiency. Therefore, to recover impaired plant functions under cold stress, the application of bio-stimulants can be considered a suitable approach. Melatonin (MT) is a critical bio-stimulant that has often shown to enhance plant performance under cold stress. Melatonin application improved plant growth and tolerance to cold stress by maintaining membrane integrity, plant water content, stomatal opening, photosynthetic efficiency, nutrient and water uptake, redox homeostasis, accumulation of osmolytes, hormones and secondary metabolites, and the scavenging of reactive oxygen species (ROS) through improved antioxidant activities and increase in expression of stress-responsive genes. Thus, it is essential to understand the mechanisms of MT induced cold tolerance and identify the diverse research gaps necessitating to be addressed in future research programs. This review discusses MT involvement in the control of various physiological and molecular responses for inducing cold tolerance. We also shed light on engineering MT biosynthesis for improving the cold tolerance in plants. Moreover, we highlighted areas where future research is needed to make MT a vital antioxidant conferring cold tolerance to plants.
Introduction
Cold stress is a severe abiotic stress that significantly limits crop growth and productivity, particularly in temperate areas (Aazami et al., 2021; Feng et al., 2021). Cold stress induces severe alterations in plant physiological, biochemical, metabolic and molecular processes, resulting in a significant reduction in crop productivity (Hu et al., 2016; Repkina et al., 2021). The plasma membrane is considered the first place affected by cold stress (Barrero et al., 2017). Exposure to cold stress substantially alters lipid composition and increases fatty acid saturation (Lado et al., 2016). Low temperature reduces water uptake, and inadequate moisture in aboveground organs leads to drought stress (Aroca et al., 2012; Hussain et al., 2018). In turn, the onset of drought owing to cold stress causes a significant reduction in root growth, nutrient, and water uptake (Nezhadahmadi et al., 2013; Hassan et al., 2017). Low temperature also induces the production of reactive oxygen species (ROS) (Hu et al., 2016; Dey et al., 2021) that damage the proteins, lipids and resultantly inhibit plant growth and, eventually, its productivity (Hassan et al., 2019, 2020, 2021). However, plants have an excellent antioxidant system to cope with ROS under stress conditions (Chattha et al., 2021; Dustgeer et al., 2021; Imran et al., 2021; Seleiman et al., 2021; Sultan et al., 2021). Additionally, plants can neutralize the impact of cold stress by accumulating various osmolytes such as proline, glycine betaine, soluble sugars, and proteins (Erdal, 2012; Ghosh et al., 2021). However, the accumulation of these osmolytes varies depending on crop species and stress conditions (Erdal, 2012). The accumulation of these osmolytes protects the membranes and ensures better growth and production in cold stress (Sun et al., 2020; Ghosh et al., 2021).
Melatonin (N-acetyl-5-methoxytryptamine) (MT) is an imperious endogenous molecule that possesses excellent antioxidant properties (Arnao and Hernández-Ruiz, 2015a; Kołodziejczyk et al., 2021). MT is involved in different processes ranging from root growth (Zhang et al., 2014), flower development, fruit ripening, photosynthesis (Tan et al., 2012), leaf senescence (Byeon et al., 2012; Wang et al., 2013), and alleviation of stress-induced oxidative damage (Wang et al., 2014; Arnao and Hernández-Ruiz, 2015b; Shi et al., 2015a; Kołodziejczyk et al., 2021). The application of MT improves antioxidant activities performing ROS scavenging and conferring cold tolerance to plants (Zhang H. et al., 2021). Melatonin improves gene expression, which regulates the antioxidant activities and redox status under cold stress (Wang et al., 2017; Li et al., 2018a). MT reduces cold-induced inhibition in photosynthesis and photosystem-II (PS-II) activities by increasing antioxidant activities (Han et al., 2017). Moreover, MT also improves the cold tolerance by degradation of starch and increasing the electron transport and antioxidant activities (Li et al., 2018b). In recent years, many functions of MT have been identified, among which contributing to stress tolerance. Therefore, in this review we systematically discussed the potential regulatory mechanism of MT to induce cold tolerance. Further, we also focused on the future directions to make MT an essential antioxidant for cold tolerance.
Melatonin Biosynthesis in Plants
Tryptophan (TP) is considered a precursor of MT. The conversion of TP into MT involves four enzymatic reactions (Figure 1). The first step consists of converting TP into tryptamine by tryptophan decarboxylase (TDC). Tryptamine is converted into serotonin (ST) by the action of an enzyme named tryptamine 5-hydroxylase (T5H) (Posmyk and Janas, 2009). Later on, ST is converted into N-acetyl-serotonin by means of N-acetyltransferase (SNAT) or arylalkylamine N-acetyltransferase (AANAT). Afterward, N-acetyl-serotonin is converted into MT by N-acetyl-serotonin methyltransferase (ASMT) or hydroxyindole-O-methyltransferase (HIOMT) (Zuo et al., 2014). In parallel to this, ST is also converted by HIOMT into the 5-methoxytryptamine, which in turn is converted by SNAT into the final product, MT (Tan et al., 2016). A recent study also identified the reverse pathway for MT biosynthesis, in which N-acetyl-serotonin deacetylase catalyzes N-acetyl-serotonin into serotonin (Lee et al., 2017). As a precursor of MT, tryptophan is also a precursor of indole-3-acetic-acid (IAA). Tryptamine pathway is one of the pathways of IAA synthesis, in which TP is converted into tryptamine, and then tryptamine is converted into IAA by indole-3-acetaldehyde (Wang J. et al., 2012; Wang Y. Y. et al., 2012). This similarity explains why MT has effects similar to those of IAA, as it has been reported that MT improves vegetative growth to an extent comparable with IAA (Hernandez-Ruiz et al., 2004).
Endogenous Melatonin Biosynthesis in Plants
Plant chloroplast and mitochondria are considered important sites of MT biosynthesis (Tan et al., 2013). MT biosynthesis has been reported in many plants, including fruit trees, herbs, and crops (Byeon et al., 2012). The levels of MT synthesis in plants are subjected to seasons and circadian rhythms (Beilby et al., 2015). Additionally, MT concentrations vary among plant species, organs, and growth stages (Hernández-Ruiz and Arnao, 2008). For instance, in morning glory, the MT concentration was significantly increased during the maturation period (Van-Tassel et al., 2001). Lastly, environmental conditions significantly affect MT synthesis in plants; for instance, MT concentration was significantly higher in field grown rice compared to the growth chamber (Byeon et al., 2012). Similarly, MT levels were also significantly higher in grapevine plants grown under illumination than under darkness, indicating that light signals induce MT synthesis (Boccalandro et al., 2011). In contrast to this, another source reports that MT synthesis in grapevine was significantly higher during the night compared to the day, which indicates that light inhibits the MT biosynthesis in these species (Arnao and Hernández-Ruiz, 2013b).
Abiotic Stress Induced Melatonin Biosynthesis in Plants
Melatonin, an excellent antioxidant, interacts with ROS and reduces ROS production and its damaging effects under stress conditions (Arnao and Hernández-Ruiz, 2013a). Therefore, in stress conditions, the increases in MT synthesis is linked with an increase in ROS (Arnao and Hernández-Ruiz, 2013b). The concentration of MT in grapevine and barley was significantly increased in stress conditions, and the level of MT was further enhanced by increasing the stress intensity (Arnao and Hernández-Ruiz, 2009). Moreover, MT synthesis in rice seedlings was also significantly increased under heat stress (Byeon and Back, 2013). MT biosynthesis considerately increased on exposure to stress, proving that MT plays an imperative role in plants’ response to different stresses (Hardeland, 2016).
Melatonin biosynthesis in plants is related to gene expression and enzymatic activities responsible for MT biosynthesis. For instance, an increase in genes expression (TDC: tryptophan decarboxylase: TDC, T5H: tyrosine gene) significantly increased MT synthesis in rice seedlings grown under cadmium stress (Byeon et al., 2015). Moreover, an increase in MT in rice was also linked with SNAT and ASMT under high temperatures (Byeon and Back, 2013). Generally, the concentration of MT in plants is strongly correlated with the availability of its precursors (Byeon et al., 2015), and ST plays a crucial role to improve cold tolerance (Kang et al., 2010). Moreover, a higher level of 2-hydroxymelatonin under cold and drought stress in rice indicates its role in plant resistance to these stresses (Lee and Back, 2016). Additionally, in the tomato crop, the concentration of MT was significantly increased by direct binding of a transcription factor (HsfA1a) to the caffeic acid O-methyltransferase 1 (COMT1) gene promoter under Cd stress (Cai et al., 2017).
Effect of Cold Stress on Plants
Cold stress induces several morphological alterations in plants and causes a reduction in growth and productivity (Equiza et al., 2001). Cold stress determines leaf chlorosis and wilting, leading to necrosis and stunted growth (Janowiak et al., 2002). Cold stress delayed and reduced wheat germination, reducing stand establishment and final productivity (Jame and Cutforth, 2004). Cold stress limits root proliferation, growth and surface areas (Figure 2), leading to a substantial reduction in nutrient and water uptake (Hussain et al., 2018; Kul et al., 2020). The reproductive stage of plant life is also susceptible to cold stress (Thakur et al., 2010). For instance, cold stress causes shedding of flowers, deforms pollen tubes (Chakrabarti et al., 2011), induces pollen sterility (Ji et al., 2017), and disrupts grain development (Barton et al., 2014), consequently causing a reduction in final productivity (Hussain et al., 2018).
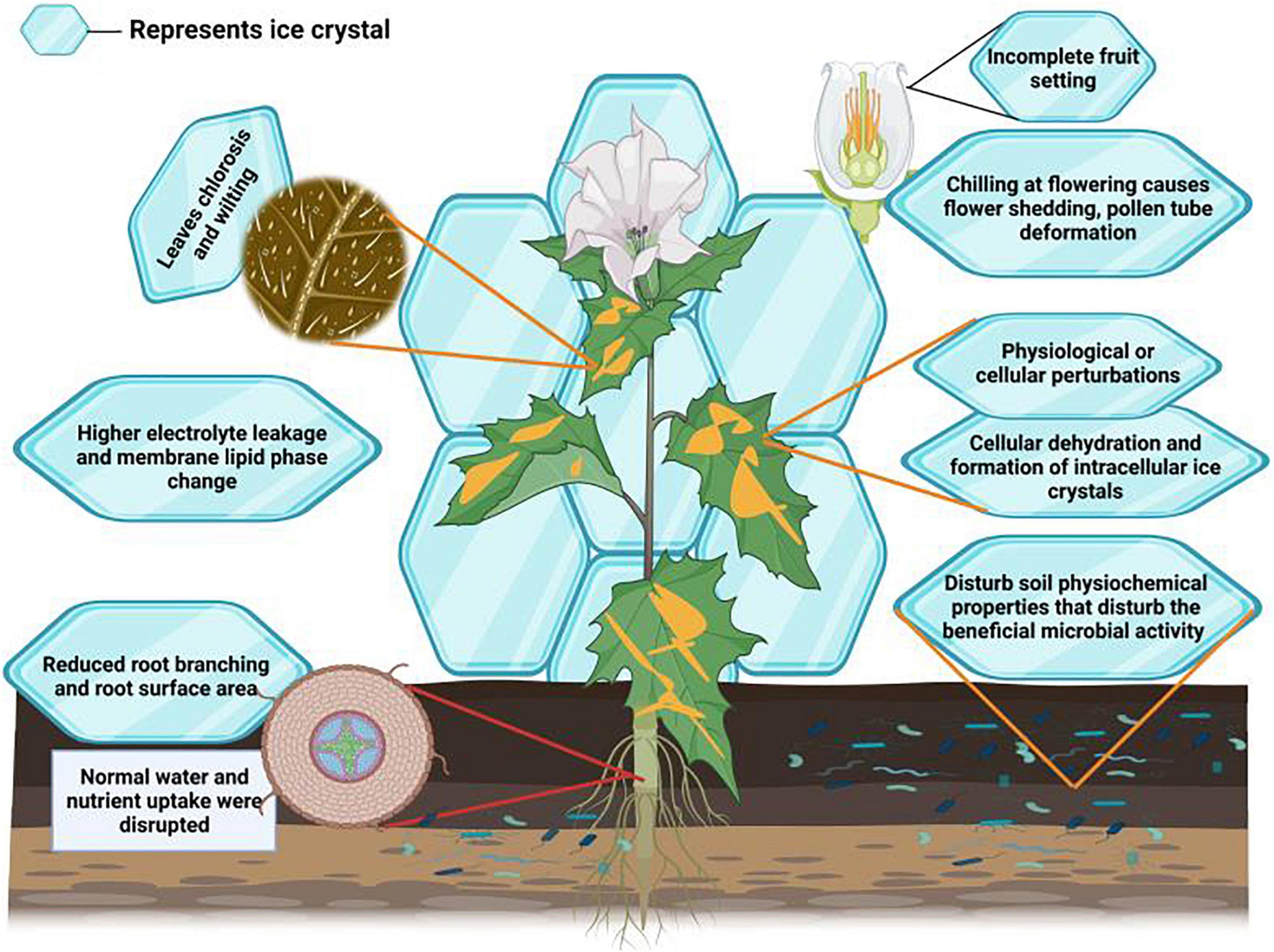
Figure 2. Effect of cold stress on plants. Cold stress induces the formation of crystal which reduces membrane integrity, causes electrolyte leakage and lipid saturation, reduces root growth which in turn decreases the water and nutrient uptake. Moreover, cold stress also causes leaf wilting and chlorosis and disturbs photosynthetic performance and microbial activities, and induces flowering shedding, deformation of pollen tube, incomplete fruit setting and results in significant growth and yield losses.
Cold stress severely alters plant physiological processes. Plants need to maintain membrane stability in stress conditions; however, cold stress reduces membrane stability (Table 1) and protein structures (Chen et al., 2018). Cold stress induces the formation of ice crystals in plant tissues (Puhakainen, 2004), which reduces apoplastic water potential and results in the flow of water from cells. Thus, cold stress at the cellular level, often followed by drought stress, seriously reduces growth and productivity (Hassan et al., 2021). This onset of drought stress reduces root growth (Table 1), root hydraulic conductivity and turgidity of plant leaves (Siddique et al., 2000). Resultantly, plant water and nutrient uptake and carbohydrate metabolism are seriously disrupted, involving significant yield losses (Hassan et al., 2021). Besides this, lower temperature also disturbs soil microbial activities, affecting plant nutrient relationships (Massenssini et al., 2015). Ice crystals’ formation also increases electrolyte leakage and causes lipid peroxidation (Hassan et al., 2021). Ice crystals can also puncture the cells, resulting in cytosol outflow and causing plant death (Zhang F. et al., 2011; Demidchik et al., 2014; Sun et al., 2019). Thus, preventing the formation of ice crystals is considered an essential cold tolerance mechanism in plants. Plants accumulate various cryoprotective polypeptides (e.g., COR15a) and osmolytes to cope with the damaging effects of cold stress (Ritonga and Chen, 2020).
Photosynthesis is a major source of grain production, and this process is seriously affected by cold stress (Rinalducci et al., 2011; Khan et al., 2017). Cold stress causes the over-excitation of PS-II, which increases the energy loss by non-radiative reactions (Venzhik et al., 2011; Cvetkovic et al., 2017). Further, cold stress reduces chlorophyll synthesis, photosynthetic efficiency, Rubisco activity, electron transport, stomatal conductivity, which reduce the assimilates production and cause severe yield losses (Bota et al., 2004; Yamori et al., 2009; Hussain et al., 2018). Moreover, cold stress also damages mitochondria’s structure, disturbs enzymatic activities, and slows down the flow of kinetic energy, consequently diminishing the respiration rate (Ikkonen et al., 2020).
Reactive oxygen species increase under cold stress improved the oxygenation response in plant chloroplast and increased glycolate accumulation. This glycolate is converted to glyoxylate in plant peroxisomes, accompanied by accumulation of hydrogen peroxide (H2O2) (Hassan et al., 2021). However, plants have an excellent antioxidant defense system to scavenge these ROS (Ritonga and Chen, 2020). The response of plants to cold stress consists of different steps, including dictation of stress followed by signal perception, transduction, and increased expression of stress-responsive genes (Ganeshan et al., 2008). Many genes have been identified in plants that initiate a cascade of transcriptional, biochemical, and physiological processes crucial to chilling tolerance (Kosová et al., 2008). Moreover, plants accumulate various osmolytes, reduce water content, scavenge ROS and maintain carbon metabolism to counter the effects of cold stress (Ruelland and Zachowski, 2010; Thakur and Nayyar, 2013; Hassan et al., 2021). Plants also accumulate various soluble sugars that stabilize the cellular membrane on exposure to cold stress (Yokota et al., 2015). Moreover, the accumulation of osmolytes and sugars also decrease the ROS and malondialdehyde (MDA) contents under cold stress by improving catalase (CAT), peroxidase (POD), and superoxide dismutase (SOD) activities (Sun et al., 2019).
Melatonin Improves Growth and Yield Under Cold Stress
Cold stress is a severe abiotic stress that substantially limits crop productivity by imposing serious alterations in plant physiological and metabolic processes, and hormonal imbalance, nutritional disorders, poor photosynthetic efficiency, and production of ROS (Turk and Genisel, 2020). MT is an major signaling molecule that promotes plant growth (Table 2) and development, and protects against abiotic stresses (Posmyk and Janas, 2009). Low temperature inhibits plant growth and development, in response to which, MT possesses excellent potential to counter cold influence (Table 2; Bajwa et al., 2014). Cold stress induces reduction in photosynthetic pigments; however, MT application (100 μM) significantly increases the synthesis of photosynthetic pigments, and therefore maintains plant growth under cold stress (Han et al., 2017). Cold stress induces a marked increase in MDA accumulation, lipid peroxidation and electrolyte leakage (Hulya et al., 2014; Hu et al., 2016). However, MT supplementation was shown to markedly reduce MDA accumulation and ROS deleterious impact on cellular membranes of rice seedlings, which in turn resulted in appreciably improved plant growth under cold stress (Han et al., 2017).
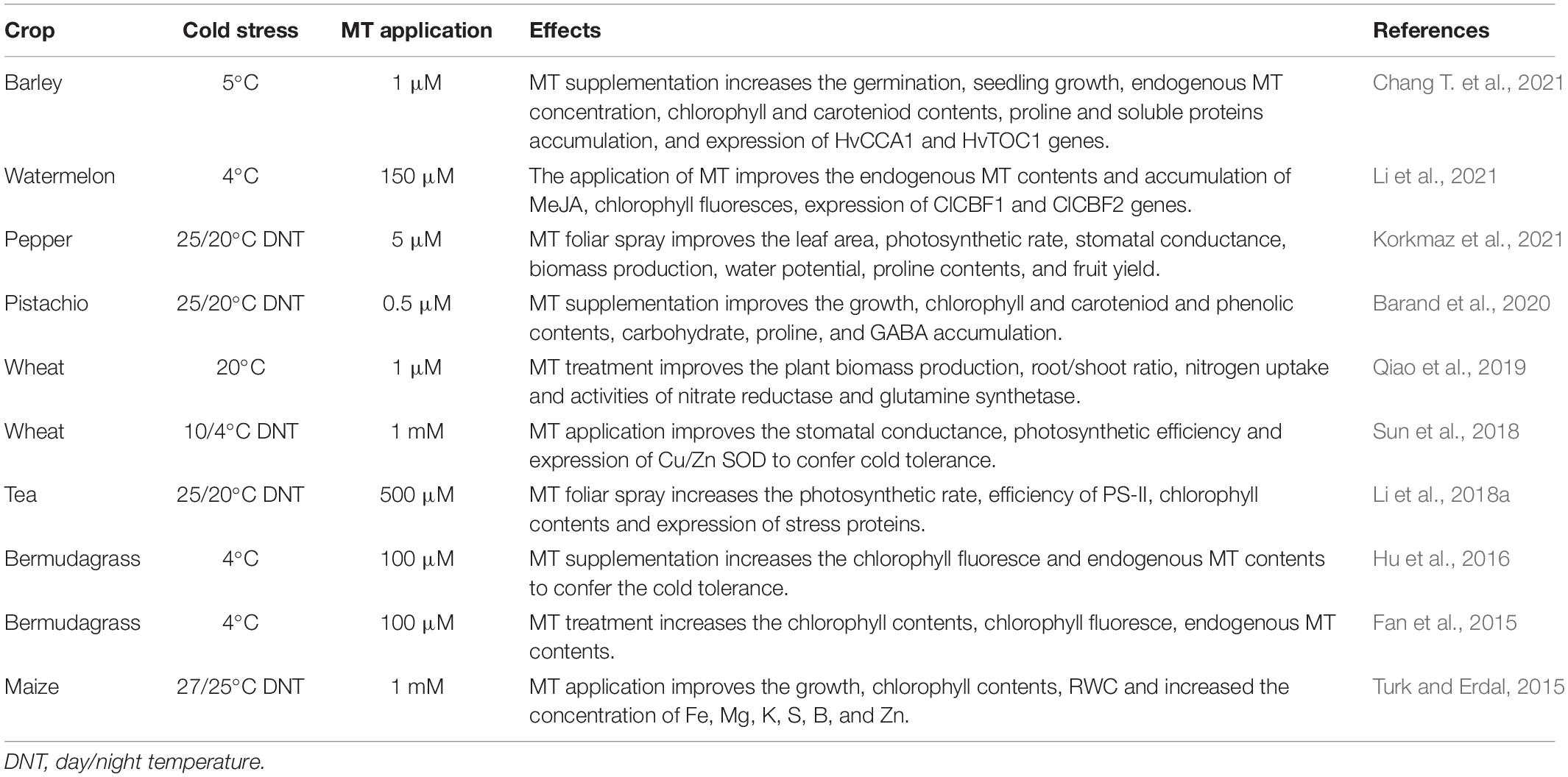
Table 2. Effect of melatonin application on growth and physiological and molecular attributes under cold stress.
In the same experiment (Han et al., 2017), MT reduced the cold-induced inhibition in plant photosynthetic activities, and protected the photosynthetic apparatus by improving the antioxidant activities; all this determined better plant performance under cold stress. However, MT mediated improvement in plant growth largely depends on methods and rate of MT application under cold stress (Han et al., 2017). MT supplementation maintains higher Fv/Fm and plant water relationships, while it reduces MDA and H2O2 by improving antioxidant activities (Table 2: ascorbate peroxidase: APX, CAT, POD, and SOD), enhancing plants tolerance to cold stress (Li et al., 2018a). MT supplementation also protects the photosynthetic machinery, maintains the redox homeostasis, and enhances gene expression so as to mitigate the deleterious impacts of cold stress and improve plant growth (Li et al., 2018a). Exogenous application of MT improved plant defense to counter the harmful effects of cold stress in Bermudagrass (Fan et al., 2015). MT application also improved osmolyte accumulation, nutrient and water uptake, hormonal accumulation and enzymatic activities, which countered the effects of cold stress by strengthening the anti-oxidant defense system and improving plant growth (Irshad et al., 2021). In addition, MT supplementation also improved carbon assimilation, osmotic potential, enhanced plant water content and photosynthetic efficiency, resulting in substantial growth improvement and unconstrained development under freezing temperature (Irshad et al., 2021).
Melatonin Maintains Membrane Stability and Improves Plant Water Relations Under Cold Stress
Membrane stability is a major damage in plants caused due to cold stress. Cold stress decreases membrane fluidity and changes the balance between transpiration and water uptake, and cause water dehydration in plant shoots (Turk et al., 2014). Eventually, it also affects the stomata movements and substantially decreases the photosynthetic rate (Hassan et al., 2021). However, MT application protects membranes and improves membrane stability by scavenging ROS through enhanced antioxidant activities (Turk et al., 2014). The foliar and seed priming with MT appreciably improved the membrane stability linked with reduced MDA and H2O2 (Table 3) accumulation (Sun et al., 2018). The increase in membrane stability reduced the EL and loss of osmolytes and conferred the cold tolerance in plants (Sun et al., 2018). Additionally, exogenous MT also improved the enzymatic and non-enzymatic antioxidant activities, maintaining the membrane integrity and conferring cold tolerance with corresponding lower electrolyte leakage (EL), MDA, and H2O2 accumulation (Table 3; Fan et al., 2015). The regulation of plant water relationships is plants are linked with plants adaptation to cold stress (Turk et al., 2014). Cold stress significantly decreased the plant relative water contents (RWC); however, MT application reduces the negative impacts of cold stress and maintains higher RWC (Pu et al., 2021). The larger leaf surface area with MT treatment may be associated with improved water contents under cold stress (Turk et al., 2014). Moreover, MT also protects the plant membranes, reducing water loss and maintaining the higher RWC under cold stress (Turk et al., 2014).
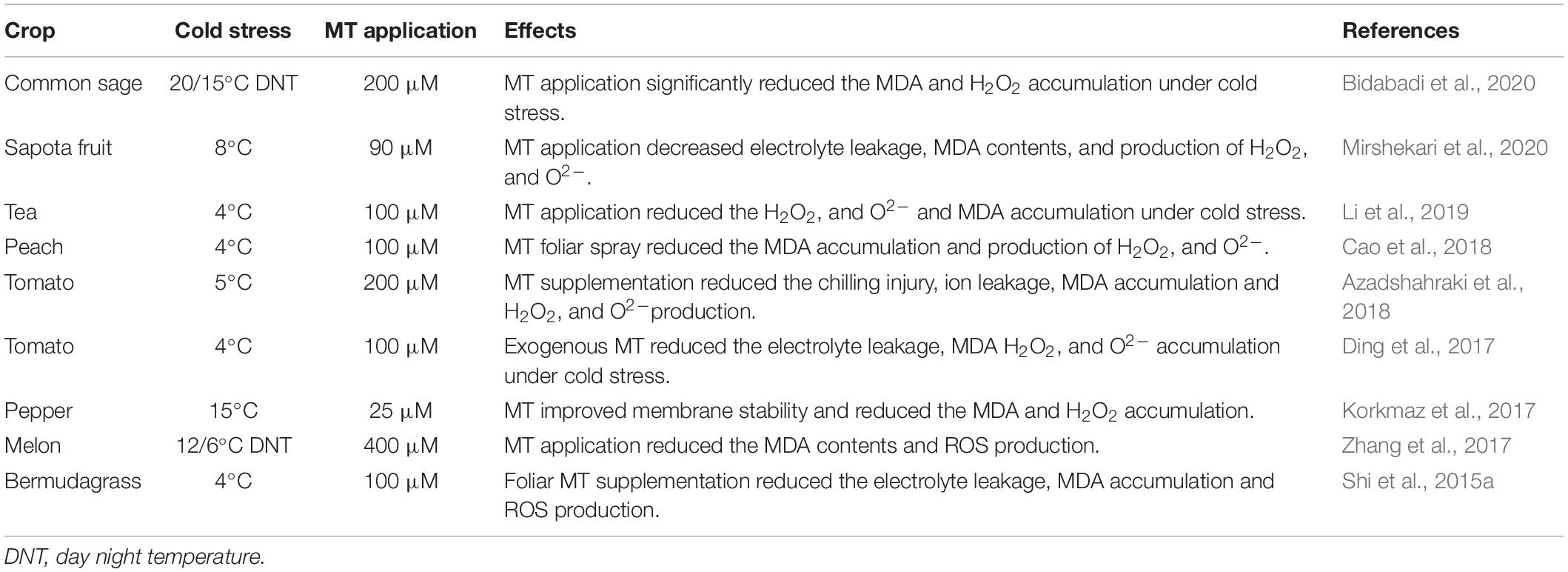
Table 3. Effect of melatonin supplementation on different oxidative stress markers under cold stress.
Melatonin Improves Water and Nutrient Uptake Under Cold Stress
The potential water reduction is considered the fastest effect of chilling stress. Cold stress diminishes the water influx through plants roots due to increased water viscosity and a decrease in membrane fluidity, which reduces the cell turgor pressure (Turk et al., 2014). However, MT application improves the plant water uptake under cold stress, which indicates that MT can reduce the negative impacts of cold stress (Turk et al., 2014; Hussain et al., 2018). Exogenous MT application increases the vapor pressure deficit between the plant leaf surface and atmosphere, enabling the plant roots to improve the water uptake (Pu et al., 2021).
Cold stress alters membrane structure by disturbing various physiological and biochemical properties, disturbing multiple processes, including nutrient and water uptake (Nayyar et al., 2005). Optimum nutrient uptake and transportation is necessary for plants to maintain their physiological processes and structural integrity under cold stress (Dumlupinar et al., 2011). MT application significantly improved the nutrient uptake under cold stress. Likewise, MT application causes a significant increase in calcium (Ca) uptake under cold stress, which shows that MT achieved its protective role on membranes under cold by increasing the Ca uptake. Moreover, increased Ca uptake following MT application protects the membranes and reduces electrolyte leakage and MDA accumulation under cold stress (Turk and Erdal, 2015). MT application also maintained higher uptake of potassium (K), phosphorus (P), sulfar (S), boron (B), copper (Cu), iron (Fe), magnesium (Mg), manganese (Mn), and zinc (Zn), which improved the plant performance and confer the cold tolerance (Turk and Erdal, 2015).
Cold stress also decreased the Mg uptake which in turn decreased the chlorophyll synthesis owing to fact Mg is important constituent of chlorophyll. However, MT treatment improves the Mg uptake and ensures the better chlorophyll synthesis and subsequent photosynthetic performance under cold stress (Turk and Erdal, 2015). MT application also improved the N uptake and maintained higher N contents in plant shoot under cold stress. The increase in N uptake following MT application is attributed to higher activities of nitrate reductase (NR) and glutamine synthetase (GS) and resultantly improve the plant growth and productivity (Qiao et al., 2019). Cold stress substantially reduced the NPK however, MT application improved the NPK under cold stress (Irshad et al., 2021). Cold stress reduced the N uptake by reducing the root activities (Feng et al., 2011) nonetheless, exogenous MT application upregulates nutrient uptake by increasing root activity and enzymatic activities under cold stress (Turk and Erdal, 2015; Irshad et al., 2021).
Melatonin Improves Hormones and Osmo-Lytes Accumulation to Confer Cold Tolerance
Osmo-lytes accumulation is one of the most important mechanisms used by plants to improve the stress tolerance (Hassan et al., 2021). The formation of viscous among cells is imperious to improve the cold tolerance; however, this formation largely depends on carbohydrate contents. The application of MT improved the carbohydrate contents which in turn improve the cold tolerance in plants (Sarropoulou et al., 2012; Turk et al., 2014). Amino acids and proteins also play an imperative role in plants tolerance to cold stress. The application MT substantially increased the MT accumulation in plants which in turns improve the plant anti-oxidant performance and confer the cold tolerance (Turk et al., 2014). Melatonin application also maintained higher proline contents under cold stress that keeps cell water contents, maintain membrane stability and increases the anti-oxidant activities to confer cold stress in plants (Turk et al., 2014). The application of MT appreciably improved the synthesis of proline enzymes including the 1-pyrroline-5-carboxylate syntheses (P5CS) and ornithine aminotransferase (OAT) which in turn improve the proline synthesis under cold stress and confer the cold tolerance were (Madebo et al., 2021). Melatonin application also improved the endogenous MT, glycine betaine and soluble sugars accumulation and resulting in substantial increase in anti-oxidant activities and subsequently in cold stress (Irshad et al., 2021).
Different hormones including, auxins (IAA), abscisic acid (ABA), gibberellins (GA3), and cytokinins (CK) play a significant role in chilling tolerance (Khan et al., 2017). The response of plants to various stresses are depends on the cross talk among the hormonal signaling pathways (Verma et al., 2016). The exogenous MT supplementation improved the IAA and GA3 concentration while MT application reduced the ABA accumulation under cold stress (Pu et al., 2021). ABA induces stomata closing and reduced the photosynthetic rate under cold stress (Lata and Prasad, 2011). Cold stress significantly increases the ABA contents (Zhang et al., 2014), however, MT application markedly reduced the ABA accumulation in cold stress (Zhao et al., 2016). The reduction in ABA accumulation under cold stress is attributed to re-opening of stomata following MT application (Pu et al., 2021). Melatonin application also induced significant increase in methyl jasmonate (MeJA) that leads to an increase in H2O2 accumulation and cold tolerance (Li et al., 2021). Nitric oxide (NO) maintains cellular homeostasis under stress conditions by repairing the stress induced oxidative damages (Zhao et al., 2007; Kaya et al., 2020). The increase in NO following MT initiate the signaling processes involved in maintenance of cellular redox homeostasis that neutralize the adverse impacts of ROS and provide NO induced defense against oxidative by improving anti-oxidant activities, carotenoid contents and electron transport under cold stress (Irshad et al., 2021).
Melatonin Improves Photosynthetic Performance Under Cold Stress
Photosynthesis is an imperative physiological process that occurs in plants and it is considered as a basis of biological world, however, this process is considered to be very sensitive to cold stress (Dalal and Tripathy, 2012). Cold stress decreases the plant photosynthetic pigments, destroy chloroplasts structure, close stomata, and decreases photosynthetic rate and stomata conductance (Fan et al., 2015; Cai et al., 2016; Han et al., 2017). Melatonin supply alleviate the cold induce inhibition in plant photosynthetic efficiency, maintain lower non-photochemical quenching (NPQ) and protect the photosynthetic apparatus from cold stress (Han et al., 2017). MT application also improves the chlorophyll synthesis (Figure 3) by improving the anti-oxidant activities and protecting the photosynthetic apparatus (Irshad et al., 2021). MT supplementation improved the endogenous MT contents that decreased the expression of oxygenase (PAO) gene that is involved in chlorophyll degradation and senescence-related hexokinase-1 (HXK1) gene (Wang et al., 2013; Weeda et al., 2014). MT supply also improved the stomata conductance and improve the plant photosynthetic efficiency by increasing the carbon dioxide (CO2) absorption (Zhong et al., 2020).
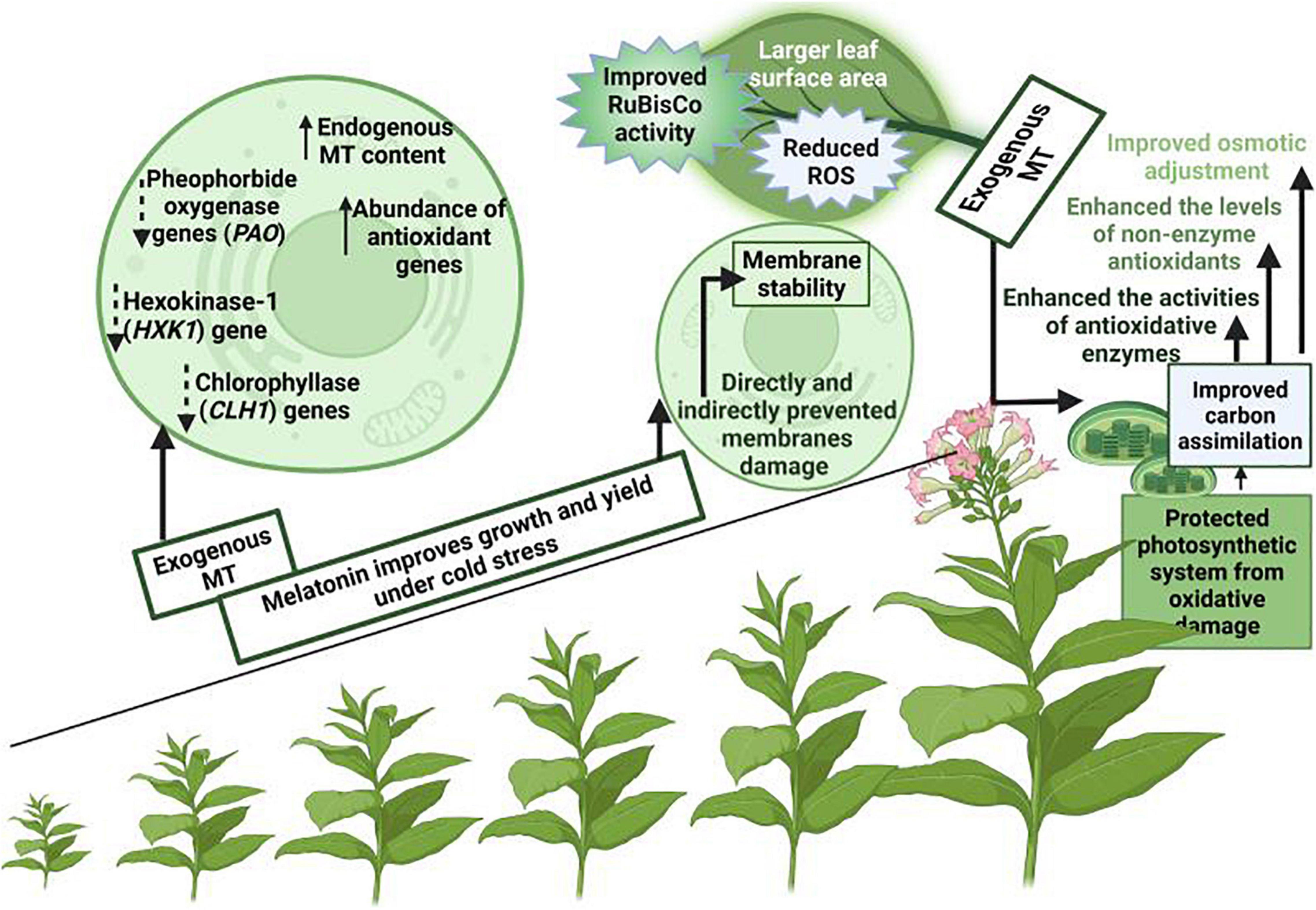
Figure 3. A proposed model for MT induced increase in photosynthetic under cold stress. MT supplementation protects photosynthetic apparatus, and maintains genes expression linked with chlorophyll synthesis and improves the osmotic adjustment, carbon assimilation and anti-oxidant activities and resulting in significant improvement in photosynthesis under cold stress.
Cold stress decreases the activities of enzymes involved in photosynthesis and RuBisCo is considered as a most important enzyme of photosynthetic process (Turk et al., 2014). Cold stress inhibited RuBisCo activity, however; MT maintained the higher RuBisCo activity and improves the photosynthetic efficiency under cold stress owing to reduced ROS production (Turk et al., 2014). The improvement in photosynthetic efficiency with MT application under cold stress is attributed to decreased ROS production, increase in light perception and RuBisCo activity (Turk et al., 2014; Erland et al., 2018; Yang et al., 2018). Additionally, MT also protects the chlorophyll degradation addition, and delays the leaf senesces which also leads to marked improvement in photosynthetic efficiency under cold stress (Han et al., 2017; Ye et al., 2020).
The exogenous application of MT also maintains the higher Fv/Fm and reduced the MDA and H2O2 accumulation which favors an increase in photosynthetic efficiency (Tan et al., 2012; Bajwa et al., 2014; Li et al., 2018a). The effect of MT on photosynthesis is concentration dependent. Since the endogenous MT varies among the species, therefore, different concentrations of exogenous MT may exert different effects on plant photosynthetic efficiency (Lazar et al., 2013). The photo-inhibition of photosystem-I (PS-I) is considered to be more dangerous as compared to PS-II, however, MT application protect the thylakoid membranes and recover the photo-inhibition of PS-1 and PS-II and maintain the higher photosynthetic efficiency under cold stress (Yang et al., 2018).
Melatonin Improves Accumulation of Secondary Metabolites in Cold Stress
Phenolic compounds possess excellent anti-oxidant properties and they accumulate in plants in response to different stress conditions (Agati et al., 2007). Cold stress increased the levels of phenolic compounds while exogenous application of MT further enhanced the phenolic contents to confer the cold tolerance (Szafranska et al., 2012; Turk et al., 2014). Polyamines maintain enzymatic activities, membrane integrity and protein structures by scavenging ROS and phospholipid binding capacity (Aghdam et al., 2019). MT application improves the cold stress defense mechanism by increasing the concentration of polyamines (Figure 4) (Put, Spd, and Spm) (Cao et al., 2016). Moreover, increased expression of LeARG1 and LeARG2 encoding arginase genes, arginine decarboxylase (LeADC) and ornithine decarboxylase (LeODC) improved the chilling tolerance in plants (Zhang X. et al., 2011). Additionally, MT pre-treatments increased the accumulation of spermine, spermidine, and putrescine by regulating the S-adenosylmethionine decarboxylase (SAMDC) and tranglutaminase (TGase) activities and resulting in increase in cold tolerance (Du et al., 2021).
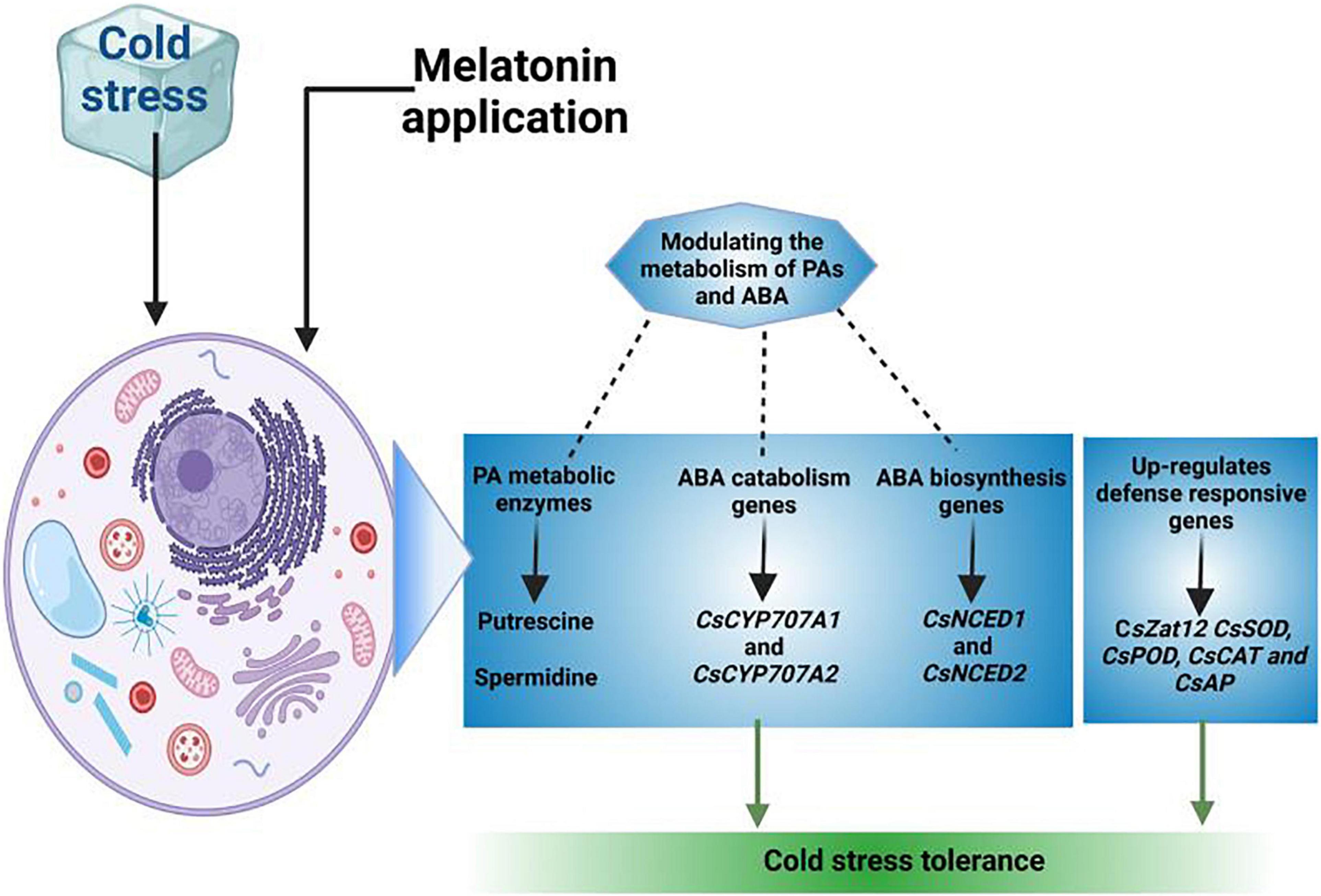
Figure 4. A proposed model of MT medicated polyamines accumulation for improving cold tolerance in plants. MT application upregulates genes expression linked with polyamines accumulation and genes linked with ABA synthesis and ABA catabolism. The application of MT improves polyamines accumulation and increase the genes expression to for catabolism of ABA and resulting in significant improvement in photosynthetic efficiency and cold tolerance in plants.
The increase in zinc finger protein (Zat12) gene expression involved in putrescine accumulation is also upregulated by expression of ADC1 and ADC2 genes following MT application that improved the cold tolerance in plants (Zhao et al., 2017). Moreover, MT supplementation also increased the enzymatic activities and encoding genes (CsADC and CsODC) expression level which in turn improved the polyamines accumulation and improved the cold tolerance by increasing anti-oxidant activities (Madebo et al., 2021). Gamma-aminobutyric acid (GABA) is a non-protein amino acid which is found in most of organisms (Madebo et al., 2021). The MT treatment improved the upregulation of PpGAD expression and increases ascertain of GABA in chilling stress (Cao et al., 2016). The increase in GABA accumulation following MT application serves as H2O2 scavenger which protects the membranes and improved the plant performance under cold stress (Cao et al., 2016; Wang et al., 2016).
Melatonin Strengthens Antioxidant Defense Activities to Confer Cold Tolerance
Cold stress induces certain changes in plant anti-oxidant activities and these alterations are considered as mechanisms to alleviate the adverse impacts of ROS (Barand et al., 2020). MT application scavenges the ROS directly or indirectly by raising the activities of anti-oxidant (Figure 5) enzymes (Li et al., 2012; Arnao and Hernández-Ruiz, 2015a). APX, CAT and glutathione peroxidase (GPX) are considered as (Table 4) essential enzymes responsible for breaking the H2O2 into H2O in plant cells (Mittler et al., 2004; Aamer et al., 2018). MT application enhances the activities of aforementioned enzymes and counters the deleterious impacts of various abiotic stresses (Fan et al., 2015). MT application appreciably increased the APX, CAT, POD, and SOD (Table 4) activities which is attributed to drop in leaf temperature and increase in ROS accumulation in plant leaves (Li et al., 2018c). The reduction in ROS production by MT improved the plant performance under cold stress (Li et al., 2018c). MT seed priming and foliar application upregulated the APX and SOD activities which reduced the ROS production and conferred the cold tolerance in barley (Li et al., 2016). The exogenous MT application increased the expression of anti-oxidant genes including Cu/Zn-SOD and Fe-SOD that increased the SOD activities and improved the cold tolerance in MT treated plants (Sun et al., 2018).
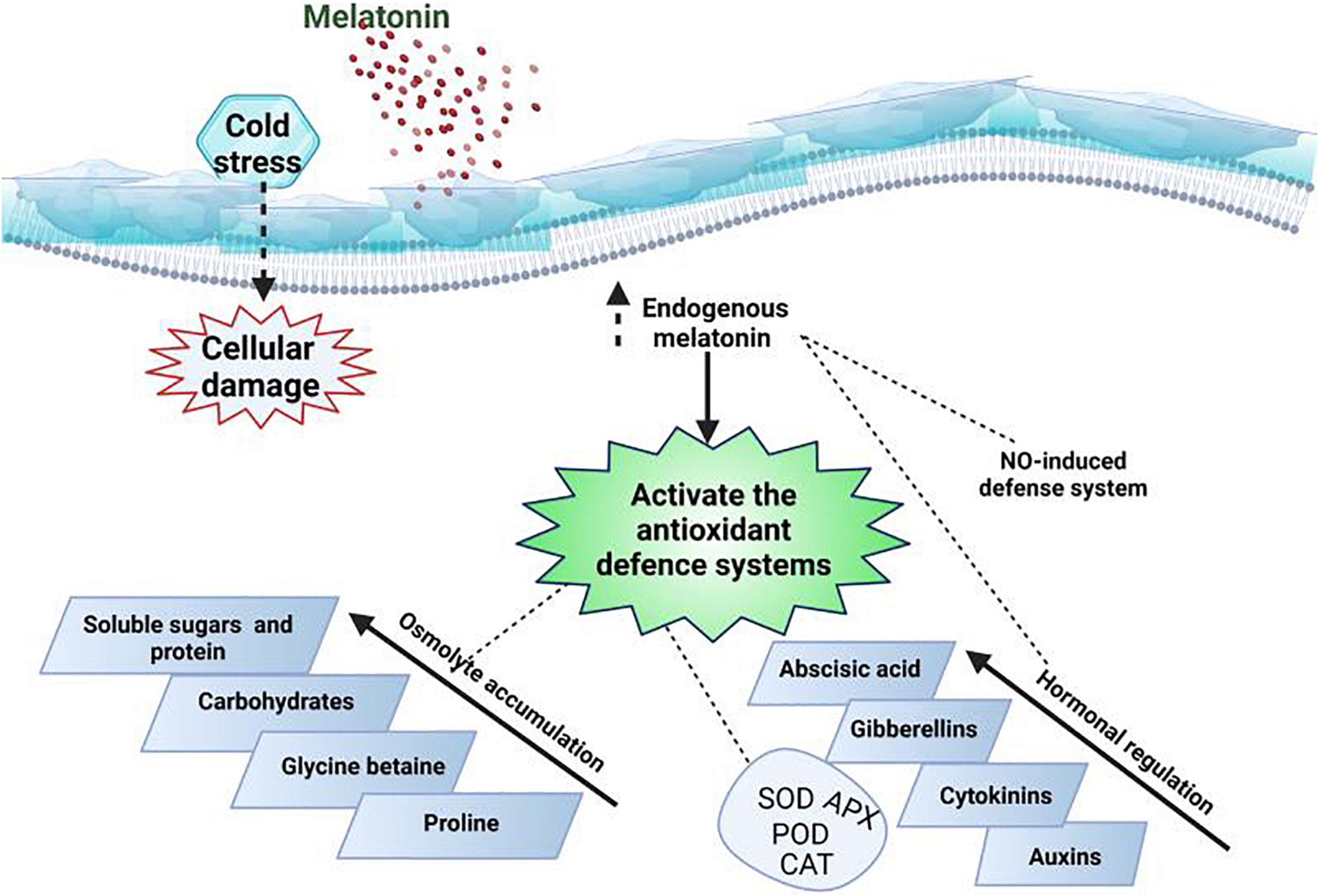
Figure 5. A proposed model of MT mediated increase in anti-oxidant activities and osmolytes accumulation for conferring cold stress in plants. NO: nitric acid.
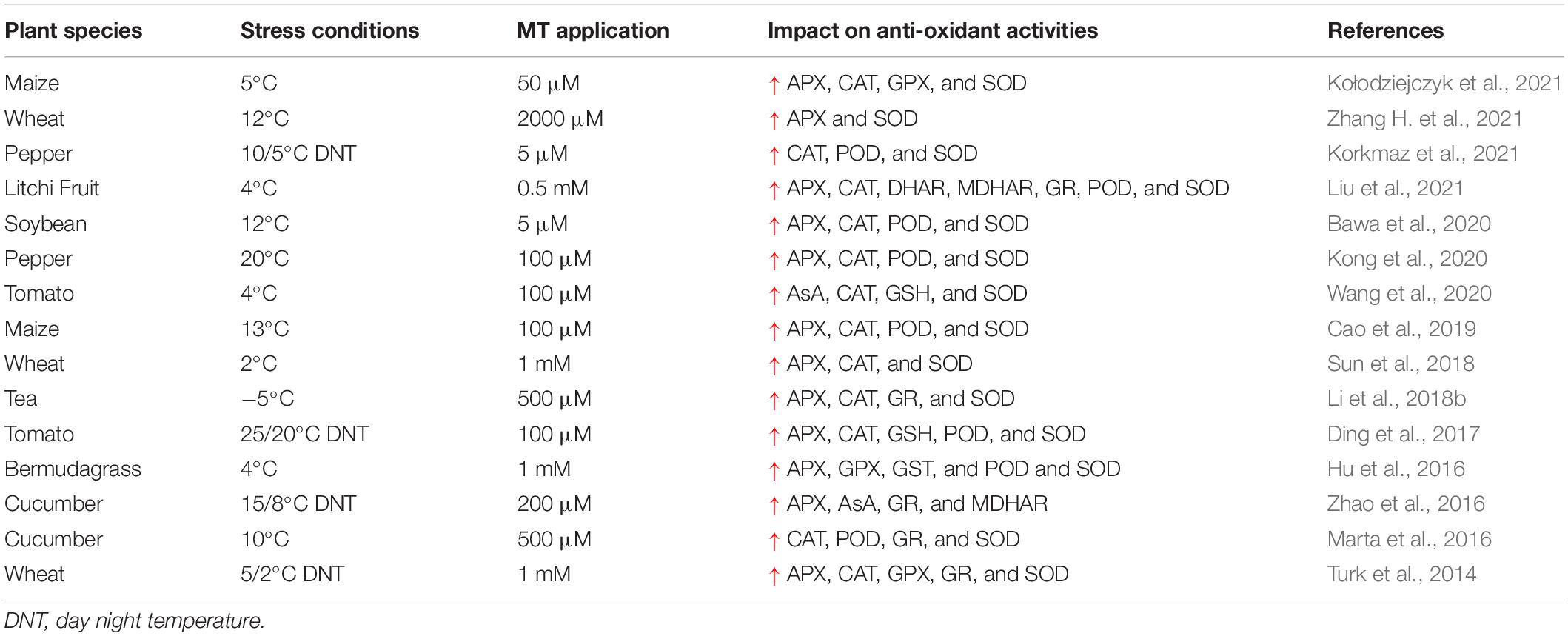
Table 4. Effect of melatonin application on enzymatic and non-enzymatic activities under cold stress.
Melatonin also induced the accumulation of anti-oxidant metabolism-related proteins and increases the potential of anti-oxidant system to scavenge the ROS under cold stress (Tan et al., 2012; Turk et al., 2014; Shi et al., 2015b). MT application also increases the stress tolerance in different plant species by inducing H2O2 as defense signaling (Shi et al., 2015b; Li et al., 2016). Plants also have to maintain optimum cellular redox homeostasis to continue normal functioning under stress conditions (Kocsy et al., 2001). Glutathione being a redox active compound maintains cellular homeostasis by affecting the different biological pathways and maintain plant performance under cold stress (Suzuki et al., 2012). MT pretreatment maintains higher GSH:GSSG ratio and reduce the ROS production under cold stress (Li et al., 2018a). Moreover, MT application also improved the AsA activity in cold stress, additionally, MT also improved GSH content by improving the activity of c-glutamylcysteine enzymes involved in glutathione (GSH) synthesis (Xu et al., 2010; Li et al., 2018a). All these findings finding indicated that MT application upregulates the activities both enzymatic and non-enzymatic anti-oxidant to confer cold tolerance in plants.
Melatonin Increases the Expression of Stress Responsive Genes to Confer Cold Tolerance
The increase in genes expression plays an imperious role to mitigate the adverse impacts of cold stress. MT treatment appreciably improved the genes expression and improved the plant tolerance to cold stress. Likewise, MT seed treatment and foliar spray considerably increased the expression of Cu/Zn SOD, Fe/SOD gene and CAT genes which in turn improves overall plant performance and anti-oxidant activities under cold stress (Sun et al., 2018). MT markedly upregulate the expression of C-repeat-binding factors (CBFs)/drought response element binding factors (DREBs) and different cold responsive genes (COR15a and CAMTA1) and anti-oxidant genes (ZAT10 and ZAT12) that contributes to improved growth and cold tolerance in plants (Bajwa et al., 2014). MT application increased the IAA and jasmonic acid levels, however, it decreased the ABA concentration in cold stress. This indicates that MT works synergistically with IAA and jasmonic acid (JA) and anta-agonistically with ABA to regulate the plant responses to cold stress (Chang et al., 2020).
Cold stress also increased the expression of fatty acid desaturase (FAD2), conversely MT treatment lower the FAD2 expression and consequently reduced the lipid per-oxidation under cold stress (García et al., 2014; Barand et al., 2020). MT treatment upregulates stress responsive gene (CsZat12) and increases the accumulation of polyamines (Put, Spm, Spd) by altering the activity of polyamine metabolic enzymes. Moreover, MT also modulates the expression of ABA synthesis genes (CsNCED1 and CsNCED2) and ABA catabolism genes (CsCYP707A1 and CsCYP707A2) to confer cold tolerance in plants (Zhao et al., 2017). The application of MT also induces the RBOHD-dependent H2O2 generation in cold stress and increase in H2O2 promotes Ca2+ accumulation that sends signals for anti-oxidant activities and improve the cold tolerance (Chang T. et al., 2021). MT application also upregulate the expression of anti-oxidant genes (CsSOD, CsPOD, CsCAT, and CsAPX) that increases the anti-oxidant activities of and resultantly increased the ROS scavenging (Li et al., 2019).
Engineering Melatonin Biosynthesis Improves Cold Tolerance
The efforts are being made to develop the transgenic plants with improved MT bio-synthesis for ensuring the cold tolerance in plants. For instance higher SNA (Serotonin N-acetyltransferase) specific enzyme activities were noticed in transgenic plants, and higher expression of SNA induces a significant increase in MT biosynthesis and subsequent cold tolerance (Kang et al., 2010). Likewise, over-expression of SNAT2 in rice lines increased the MT biosynthesis, which improved plant tolerance to cold stress (Hwang and Back, 2019). The oAANAT gene’s over-expression plays a significant role in MT biosynthesis under stress conditions. The increase in expression of the oAANAT gene enhanced the MT contents and promoted the plant growth and spike length of switchgrass under cold stress (Yuan et al., 2016). In cotton crops, over-expression of GhM2H gene improved the tolerance against heat and cold stress by increasing endogenous MT contents and antioxidant activities and reducing ABA accumulation (Zhang Y. et al., 2021).
The insertion of ClCOMT1 in transgenic watermelon plants significantly increased the MT bio-synthesis. ClCOMT1 expression in watermelon was also substantially increased under cold, drought, and salt stress following increased MT accumulation. Therefore, ClCOMT1 over-expression is considered a positive plant growth regulator in response to heat, cold and drought stresses (Chang J. et al., 2021). Another group of researchers identified that inserted the ASMT genes apple plant. They noted that ASMT genes were significantly upregulated under cold, drought and heat stress. The expression of these genes appreciably increased MT biosynthesis, which increased the plant tolerance to cold, drought, and heat stresses (Wang et al., 2022).
Conclusion and Future Perspectives
Melatonin application effectively modulates plant growth and confers cold tolerance in plants. The exogenous MT application improved the synthesis of photosynthetic pigments and maintains membrane stability, plant water status, increasing the nutrient and water uptake, which improved plant growth under cold stress. Melatonin supplementation also alleviates the cold-induced osmotic imbalance by increasing the accumulation of different osmolytes, endogenous MT, hormones, and secondary metabolites. Moreover, exogenous MT supply also helps the cold-induced deleterious impacts by increasing the expression of different defensive genes responsible for the higher antioxidant activities under cold stress. The genes manipulation associated with enhanced MT biosynthesis also appreciably improved the cold tolerance in plants by favoring the antioxidant activities, photosynthetic performance and accumulation of different osmolytes.
Still, the role of MT in cold tolerance is not fully explored, and more research is direly needed to uncover its potential benefits under cold tolerance. The exact position of MT biosynthesis in plants requires further investigation. MT is also an unstable molecule; therefore, its transportation in plants organs under cold stress must also be studied in future research programs. The role of MT in improving root growth under cold stress is well studied; however, its role in nutrient uptake is poorly studied. Therefore, the role of MT in nutrient uptake and transportation must be explored in future research studies.
Moreover, increased endogenous MT level in plants under cold stress occurs by upregulation of MT bio-synthesis genes or MT absorption from the exogenous MT application; both mechanisms need more investigation to ensure better MT biosynthesis in plants. The role of MT on pollen viability, abscission and crop quality under cold stress must be explored at the field level. Further studies are also direly needed to identify the interaction of MT with other osmolytes and hormones under cold stress. Recent improvements in genetic engineering have provided clues to diverse complex gene-protein interactions and interconnected networks. Therefore, genetic engineering will enable us to understand better the interaction of MT with other hormones under cold stress. The role of MT in stomatal signaling under cold stress is also unknown; therefore, future research direction on this aspect would also fascinate. Plant chloroplast and mitochondria are e significant sites of ROS production. MT works as signaling molecules; therefore, it would be fascinating to explore the inter-organelle MT signaling under cold stress. Additionally, molecular mechanisms of MT in increasing the expression of antioxidant as stress-responsive genes must also be examined under cold pressure in future research studies. All these efforts will increase our understanding of the roles of MT as a potential antioxidant to be used in cold stress conditions.
Data Availability Statement
The original contributions presented in the study are included in the article/supplementary material, further inquiries can be directed to the corresponding author.
Author Contributions
SHQ and MUH conceived the idea and wrote the original draft. MUC, AM, MS, MaN, MuN, LB, MAA, and MA helped with organization and editing. All authors contributed to the article and approved the submitted version.
Conflict of Interest
The authors declare that the research was conducted in the absence of any commercial or financial relationships that could be construed as a potential conflict of interest.
Publisher’s Note
All claims expressed in this article are solely those of the authors and do not necessarily represent those of their affiliated organizations, or those of the publisher, the editors and the reviewers. Any product that may be evaluated in this article, or claim that may be made by its manufacturer, is not guaranteed or endorsed by the publisher.
References
Aamer, M., Muhammad, U. H., Li, Z., Abid, A., Su, Q., Liu, Y., et al. (2018). Foliar application of glycinebetaine (GB) alleviates the cadmium (Cd) toxicity in spinach through reducing Cd uptake and improving the activity of anti-oxidant system. Appl. Ecol. Environ. Res. 16, 7575–7583.
Aazami, M. A., Asghari-Aruq, M., Hassanpouraghdam, M. B., Ercisli, S., Baron, M., and Sochor, J. (2021). Low Temperature stress mediates the antioxidants pool and chlorophyll fluorescence in Vitis vinifera L. cultivars. Plants 10:1877. doi: 10.3390/plants10091877
Agati, G., Matteini, P., Goti, A., and Tattini, M. (2007). Chloroplast-located flavonoids can scavenge singlet oxygen. New Phytol. 174, 77–89. doi: 10.1111/j.1469-8137.2007.01986.x
Aghdam, M. S., Luo, Z., Jannatizadeh, A., Sheikh-Assadi, M., Sharafi, Y., Farmani, B., et al. (2019). Employing exogenous melatonin applying confers chilling tolerance in tomato fruits by upregulating ZAT2/6/12 giving rise to promoting endogenous polyamines, proline, and nitric oxide accumulation by triggering arginine pathway activity. Food Chem. 275, 549–556. doi: 10.1016/j.foodchem.2018.09.157
Arnao, M. B., and Hernández-Ruiz, R. J. (2015a). Functions of melatonin in plants: a review. J. Pineal Res. 59, 133–150. doi: 10.1111/jpi.12253
Arnao, M. B., and Hernández-Ruiz, R. J. (2015b). “Chapter 11 – Phytomelatonin: searching for plants with high levels for use as a natural nutraceutical,” in Studies in Natural Products Chemistry, ed. F. R. S. Atta-ur-Rahman (Netherlands: Elsevier Science Publishers), 519–545.
Arnao, M. B., and Hernández-Ruiz, J. (2009). Chemical stress by different agents affects the melatonin content of barley roots. J. Pineal Res. 46, 295–299. doi: 10.1111/j.1600-079X.2008.00660.x
Arnao, M. B., and Hernández-Ruiz, J. (2013b). Growth conditions determine different melatonin levels in Lupinus albus L. J. Pineal Res. 55, 149–155. doi: 10.1111/jpi.12055
Arnao, M. B., and Hernández-Ruiz, J. (2013a). Growth conditions influence the melatonin content of tomato plants. Food Chem. 138, 1212–1214. doi: 10.1016/j.foodchem.2012.10.077
Aroca, R., Porcel, R., and Ruiz-Lozano, J. M. (2012). Regulation of root water uptake under abiotic stress conditions. J. Exp. Bot. 63, 43–57. doi: 10.1093/jxb/err266
Azadshahraki, F., Jamshidi, B., and Mohebbi, S. (2018). Postharvest melatonin treatment reduces chilling injury and enhances antioxidant capacity of tomato fruit during cold storage. Adv. Hortic. Sci. 32, 299–309.
Bajwa, V. S., Shukla, R., Sherif, S. M., Murch, S. J., and Saxena, P. K. (2014). Role of melatonin in alleviating cold stress in Arabidopsis thaliana. J. Pineal Res. 56, 238–245. doi: 10.1111/jpi.12115
Barand, A., Nasibi, F., Manouchehri Kalantari, K., and Moradi, M. (2020). The effects of foliar application of melatonin on some physiological and biochemical characteristics and expression of fatty acid desaturase gene in pistachio seedlings (Pistacia vera L.) under freezing stress. J. Plant Interact. 15, 257–265. doi: 10.1080/17429145.2020.1781271
Barrero, S. C., Silvestre, S., Haslam, R. P., and Michaelson, L. V. (2017). Lipid remodelling: unravelling the response to cold stress in Arabidopsis and its extremophile relative Eutrema salsugineum. Plant Sci. 263, 194–200. doi: 10.1016/j.plantsci.2017.07.017
Barton, D. A., Cantrill, L. C., Law, A. M. K., Phillips, C. G., Sutton, B. G., and Overall, R. L. (2014). Chilling to zero degrees disrupts pollen formation but not meiotic microtubule arrays in Triticum aestivum L. Plant Cell Environ. 37, 2781–2794. doi: 10.1111/pce.12358
Bawa, G., Feng, L., Shi, J., Chen, G., Cheng, Y., Luo, J., et al. (2020). Evidence that melatonin promotes soybean seedlings growth from low-temperature stress by mediating plant mineral elements and genes involved in the antioxidant pathway. Funct. Plant Biol. 47, 815–824. doi: 10.1071/FP19358
Beilby, M. J., Turi, C. E., Baker, T. C., Tymm, F. J., and Murch, S. J. (2015). Circadian changes in endogenous concentrations of indole-3-acetic acid, melatonin, serotonin, abscisic acid and jasmonic acid in Characeae (Chara australis Brown). Plant Signal. Behav. 10:e1082697. doi: 10.1080/15592324.2015.1082697
Bibi, A., Majid, S. A., Ulfat, A., Khatoon, S., Munir, A., and Javed, G. (2017). Effect of nitric oxide seed priming on chilling induced water related physiological attributes in germinating wheat. J. Anim. Plant Sci. 1, 186–191.
Bidabadi, S. S., Vander-Weide, J., and Sabbatini, P. (2020). Exogenous melatonin improves glutathione content, redox state and increases essential oil production in two Salvia species under drought stress. Sci. Rep. 10:6883. doi: 10.1038/s41598-020-63986-6
Boccalandro, H. E., González, C. V., Wunderlin, D. A., and Silva, M. F. (2011). Melatonin levels, determined by LC-ESI-MS/MS, fluctuate during the day/night cycle in Vitis vinifera cv Malbec: evidence of its antioxidant role in fruits. J. Pineal Res. 51, 226–232. doi: 10.1111/j.1600-079X.2011.00884.x
Bota, J., Medrano, H., and Flexas, J. (2004). Is photosynthesis limited by decreased Rubisco activity and RuBP content under progressive water stress? New Phytol. 162, 671–681. doi: 10.1111/j.1469-8137.2004.01056.x
Byeon, Y., and Back, K. (2013). Melatonin synthesis in rice seedlings in vivo is enhanced at high temperatures and under dark conditions due to increased serotonin N-acetyltransferase and N-acetylserotonin methyltransferase activities. J. Pineal Res. 56, 189–195. doi: 10.1111/jpi.12111
Byeon, Y., Lee, H. Y., Hwang, O. J., Lee, H. J., Lee, K., and Back, K. (2015). Coordinated regulation of melatonin synthesis and degradation genes in rice leaves in response to cadmium treatment. J. Pineal Res. 58, 470–478. doi: 10.1111/jpi.12232
Byeon, Y., Park, S., Kim, Y. S., Park, D. H., Lee, S., and Back, K. (2012). Light regulated melatonin biosynthesis in rice during the senescence process in detached leaves. J. Pineal Res. 53, 107–111. doi: 10.1111/j.1600-079X.2012.00976.x
Cai, H. M., Dong, Y. Y., Li, Y. Y., Li, D. X., Peng, C. Y., Zhang, Z. Z., et al. (2016). Physiological and cellular responses to fluoride stress in tea (Camellia sinensis) leaves. Acta Physiol. Plant. 38, 144–155.
Cai, S. Y., Zhang, Y., Xu, Y. P., Qi, Z. Y., Li, M. Q., Ahammed, G. J., et al. (2017). Hsfa1a upregulates melatonin biosynthesis to confer cadmium tolerance in tomato plants. J. Pineal Res. 62:e12387. doi: 10.1111/jpi.12387
Cao, Q., Li, G., Cui, Z., Yang, F., Jiang, X., Diallo, L., et al. (2019). Seed priming with melatonin improves the seed germination of waxy maize under chilling stress via promoting the antioxidant system and starch metabolism. Sci. Rep. 9:15044. doi: 10.1038/s41598-019-51122-y
Cao, S., Shao, J., Shi, L., Xu, L., Shen, Z., Chen, W., et al. (2018). Melatonin increases chilling tolerance in postharvest peach fruit by alleviating oxidative damage. Sci. Rep. 8:806. doi: 10.1038/s41598-018-19363-5
Cao, S., Song, C., Shao, J., Bian, K., Chen, W., and Yang, Z. (2016). Exogenous melatonin treatment increases chilling tolerance and induces defense response in harvested peach fruit during cold storage. J. Agric. Food Chem. 64, 5215–5222. doi: 10.1021/acs.jafc.6b01118
Chakrabarti, B., Singh, S., Nagarajan, S., and Aggarwal, P. (2011). Impact of Temperature on Phenology and Pollen Sterility of Wheat Varieties. Brisbane: Southern Cross Publishing.
Chang, J., Guo, Y., Yan, J., Zhang, Z., Yuan, L., Wei, C., et al. (2021). The role of watermelon caffeic acid O-methyltransferase (ClCOMT1) in melatonin biosynthesis and abiotic stress tolerance. Hortic. Res. 8:210. doi: 10.1038/s41438-021-00645-5
Chang, J., Guo, Y., Zhang, Z., Wei, C., Zhang, Y., Ma, J., et al. (2020). CBF-responsive pathway and phytohormones are involved in melatonin-improved photosynthesis and redox homeostasis under aerial cold stress in watermelon. Acta Physiol. Plant. 42:159.
Chang, T., Zhao, Y., He, H., Xi, Q., Fu, J., and Zhao, Y. (2021). Exogenous melatonin improves growth in hulless barley seedlings under cold stress by influencing the expression rhythms of circadian clock genes. PeerJ 9:e10740. doi: 10.7717/peerj.10740
Chattha, M. U., Arif, W., Khan, I., Soufan, W., Bilal Chattha, M., Hassan, M. U., et al. (2021). Mitigation of cadmium induced oxidative stress by using organic amendments to improve the growth and yield of mash beans [Vigna mungo (L.)]. Agronomy 11:2152.
Chen, L., Zhao, Y., Xu, S., Zhang, Z., Xu, Y., Zhang, J., et al. (2018). Os MADS 57 together with Os TB 1 coordinates transcription of its target Os WRKY 94 and D14 to switch its organogenesis to defense for cold adaptation in rice. New Phytol. 218, 219–231. doi: 10.1111/nph.14977
Cvetkovic, J., Müller, K., and Baier, M. (2017). The effect of cold priming on the fitness of Arabidopsis thaliana accessions under natural and controlled conditions. Sci. Rep. 7:44055. doi: 10.1038/srep44055
Dalal, V. K., and Tripathy, B. C. (2012). Modulation of chlorophyll biosynthesis by water stress in rice seedlings during chloroplast biogenesis. Plant Cell Environ. 35, 1685–1703. doi: 10.1111/j.1365-3040.2012.02520.x
Demidchik, V., Straltsova, D., Medvedev, S. S., Pozhvanov, G. A., Sokolik, A., and Yurin, V. (2014). Stress-induced electrolyte leakage: the role of K+-permeable channels and involvement in programmed cell death and metabolic adjustment. J. Exp. Bot. 65, 1259–1270. doi: 10.1093/jxb/eru004
Dey, S., Biswas, A., Huang, S., Li, D., Liu, L., Deng, Y., et al. (2021). Low temperature effect on different varieties of Corchorus capsularis and Corchorus olitorius at seedling stage. Agronomy 11:2547.
Ding, F., Liu, B., and Zhang, S. (2017). Exogenous melatonin ameliorates cold-induced damage in tomato plants. Sci. Hortic. 219, 264–271.
Du, H., Liu, G., Hua, C., Liu, D., He, Y., Liu, H., et al. (2021). Exogenous melatonin alleviated chilling injury in harvested plum fruit via affecting the levels of polyamines conjugated to plasma membrane. Postharvest Biol. Technol. 179:111585.
Dumlupinar, R., Genisel, M., Erdal, S., Korkut, T., Taspinar, M. S., and Taskin, M. (2011). Effects of progesterone, beta-estradiol, and androsterone on the changes of inorganic element content in barley leaves. Biol. Trace Elem. Res. 143, 1740–1745. doi: 10.1007/s12011-011-8980-6
Dustgeer, Z., Seleiman, M. F., Imran, K. H. A. N., Chattha, M. U., Alhammad, B. A., Jalal, R. S., et al. (2021). Glycine-betaine induced salinity tolerance in maize by regulating the physiological attributes, antioxidant defense system and ionic homeostasis. Not. Bot. Hort. Agrobot. Cluj Napoca 49:12248.
Equiza, M. A., Miravé, J. P., and Tognetti, J. A. (2001). Morphological, anatomical and physiological responses related to differential shoot vs. root growth inhibition at low temperature in spring and winter wheat. Ann. Bot. 87, 67–76.
Erdal, S. (2012). Androsterone-induced molecular and physiological changes in maize seedlings in response to chilling stress. Plant Physiol. Biochem. 57, 1–7. doi: 10.1016/j.plaphy.2012.04.016
Erland, L. A. E., Saxena, P. K., and Murch, S. J. (2018). Melatonin in plant signalling and behaviour. Funct. Plant Biol. 45, 58–69. doi: 10.1071/FP16384
Fan, J., Hu, Z., Xie, Y., Chan, Z., Chen, K., Amombo, E., et al. (2015). Alleviation of cold damage to photosystem II and metabolisms by melatonin in Bermudagrass. Front. Plant Sci. 6:925. doi: 10.3389/fpls.2015.00925
Feng, H., Yan, M., Fan, X., Li, B., Shen, Q., Miller, A. J., et al. (2011). Spatial expression and regulation of rice high-affinity nitrate transporters by nitrogen and carbon status. J. Exp. Bot. 62, 2319–2332. doi: 10.1093/jxb/erq403
Feng, Q., Yang, S., Wang, Y., Lu, L., Sun, M., He, C., et al. (2021). Physiological and molecular mechanisms of aba and cacl2 regulating chilling tolerance of cucumber seedlings. Plants 10:2746. doi: 10.3390/plants10122746
Ganeshan, S., Vitamvas, P., Fowler, D. B., and Chibbar, R. N. (2008). Quantitative expression analysis of selected COR genes reveals their differential expression in leaf and crown tissues of wheat (Triticum aestivum L.) during an extended low temperature acclimation regimen. J. Exp. Bot. 59, 2393–2402. doi: 10.1093/jxb/ern112
García, J. J., López-Pingarrón, L., Almeida-Souza, P., Tres, A., Escudero, P., García-Gil, F. A., et al. (2014). Protective effects of melatonin in reducing oxidative stress and in preserving the fluidity of biological membranes: a review. J. Pineal Res. 56, 225–237. doi: 10.1111/jpi.12128
Ghosh, U. K., Islam, M. N., Siddiqui, M. N., and Khan, M. A. R. (2021). Understanding the roles of osmolytes for acclimatizing plants to changing environment: a review of potential mechanism. Plant Signal. Behav 16:1913306. doi: 10.1080/15592324.2021.1913306
Golizadeh, F., and Kumleh, H. H. (2019). Physiological Responses and Expression Changes of Fatty Acid Metabolism–Related Genes in Wheat (Triticum aestivum) Under Cold Stress. Plant Mol. Biol. Rep. 37, 224–236. doi: 10.1007/s11105-019-01150-9
Hajihashemi, S., Noedoost, F., Geuns, J., Djalovic, I., and Siddique, K. H. (2018). Effect of cold stress on photosynthetic traits, carbohydrates, morphology, and anatomy in nine cultivars of Stevia rebaudiana. Front. Plant Sci. 9:1430. doi: 10.3389/fpls.2018.01430
Han, Q. H., Huang, B., Ding, C. B., Zhang, Z. W., Chen, Y. E., Hu, C., et al. (2017). Effects of melatonin on anti-oxidative systems and photosystem II in cold-stressed rice seedlings. Front. Plant Sci. 8:785. doi: 10.3389/fpls.2017.00785
Hardeland, R. (2016). Melatonin in plants—Diversity of levels and multiplicity of functions. Front. Plant Sci. 7:198. doi: 10.3389/fpls.2016.00198
Hassan, M. U., Aamer, M., Chattha, M. U., Ullah, M. A., Sulaman, S., Nawaz, M., et al. (2017). The role of potassium in plants under drought stress: mini Review. J. Basic Appl. Sci. 13, 268–271. doi: 10.6000/1927-5129.2017.13.44
Hassan, M. U., Aamer, M., Umer Chattha, M., Haiying, T., Shahzad, B., Barbanti, L., et al. (2020). The critical role of zinc in plants facing the drought stress. Agriculture 10:396.
Hassan, M. U., Chattha, M. U., Khan, I., Chattha, M. B., Aamer, M., Nawaz, M., et al. (2019). Nickel toxicity in plants: reasons, toxic effects, tolerance mechanisms, and remediation possibilities:a review. Environ. Sci. Pollut. Res. 26, 12673–12688. doi: 10.1007/s11356-019-04892-x
Hassan, M. U., Chattha, M. U., Khan, I., Chattha, M. B., Barbanti, L., Aamer, M., et al. (2021). Heat stress in cultivated plants: nature, impact, mechanisms, and mitigation strategies:a review. Plant Biosyst. 155, 211–234.
Helena, H., Hejnák, V., Němcová, L., Martinková, J., Skalický, M., Hnilička, F., et al. (2017). The effect of freezing temperature on physiological traits in sunflower. Plant Soil Environ. 63, 375–380.
Hernández-Ruiz, J., and Arnao, M. B. (2008). Distribution of melatonin in different zones of lupin and barley plants at different ages in the presence and absence of light. J. Agric. Food Chem. 56, 10567–10573. doi: 10.1021/jf8022063
Hernandez-Ruiz, J., Cano, A., and Arnao, M. B. (2004). Melatonin: a growth-stimulating compound present in lupin tissues. Planta 220, 140–144. doi: 10.1007/s00425-004-1317-3
Hu, Z. R., Fan, J. B., Xie, Y., Amombo, E., Liu, A., Gitau, M. M., et al. (2016). Comparative photosynthetic and metabolic analyses reveal mechanism of improved cold stress tolerance in bermudagrass by exogenous melatonin. Plant Physiol. Biochem. 100, 94–104. doi: 10.1016/j.plaphy.2016.01.008
Hulya, T., Serkan, E., Mucip, G., Okkes, A., Yavuz, D., and Derya, Y. (2014). The regulatory effect of melatonin on physiological, biochemical and molecular parameters in cold-stressed wheat seedlings. Plant Growth Regul. 74, 139–152.
Hussain, H. A., Hussain, S., Khaliq, A., Ashraf, U., Anjum, S. A., Men, S., et al. (2018). Chilling and drought stresses in crop plants: implications, cross talk, and potential management opportunities. Front. Plant Sci. 9:393. doi: 10.3389/fpls.2018.00393
Hwang, O. J., and Back, K. (2019). Melatonin deficiency confers tolerance to multiple abiotic stresses in rice via decreased brassinosteroid levels. Int. J. Mol. Sci. 20:5173. doi: 10.3390/ijms20205173
Ikkonen, E. N., Shibaeva, T. G., Sherudilo, E. G., and Titov, A. F. (2020). Response of winter wheat seedlings respiration to long-term cold exposure and short-term daily temperature drops. Russ. J. Plant Physiol. 67, 538–544.
Imran, K., Seleiman, M. F., Chattha, M. U., Jalal, R. S., Mahmood, F., Hassan, F. A., et al. (2021). Enhancing antioxidant defense system of mung bean with a salicylic acid exogenous application to mitigate cadmium toxicity. Not. Bot. Hort. Agrobot. Cluj Napoca 49, 12303–12303.
Irshad, A., Rehman, R. N. U., Kareem, H. A., Yang, P., and Hu, T. (2021). Addressing the challenge of cold stress resilience with the synergistic effect of Rhizobium inoculation and exogenous melatonin application in Medicago truncatula. Ecotoxicol. Environ. Saf. 226:112816. doi: 10.1016/j.ecoenv.2021.112816
Jame, Y. W., and Cutforth, H. W. (2004). Simulating the effects of temperature and seeding depth on germination and emergence of spring wheat. Agric. For. Meteorol. 124, 207–218. doi: 10.1016/j.agrformet.2004.01.012
Janowiak, F., Maas, B., and Dörffling, K. (2002). Importance of abscisic acid for chilling tolerance of maize seedlings. J. Plant Physiol. 159, 635–643.
Ji, H., Xiao, L., Xia, Y., Song, H., Liu, B., Tang, L., et al. (2017). Effects of jointing and booting low temperature stresses on grain yield and yield components in wheat. Agric. For. Meteorol. 243, 33–42. doi: 10.1016/j.agrformet.2017.04.016
Kang, K., Lee, K., Park, S., Kim, Y. S., and Back, K. (2010). Enhanced production of melatonin by ectopic overexpression of human serotonin N-acetyltransferase plays a role in cold resistance in transgenic rice seedlings. J. Pineal Res. 49, 176–182. doi: 10.1111/j.1600-079X.2010.00783.x
Karami, M. S., Maali-Amiri, R., and Kazemi-Shahandashti, S. S. (2018). Effect of cold stress on oxidative damage and mitochondrial respiratory properties in chickpea. Plant Physiol. Biochem. 122, 31–39. doi: 10.1016/j.plaphy.2017.11.011
Kaya, C., Ashraf, M., Alyemeni, M. N., and Ahmad, P. (2020). The role of nitrate reductase in brassinosteroid-induced endogenous nitric oxide generation to improve cadmium stress tolerance of pepper plants by upregulating the ascorbate-glutathione cycle. Ecotoxicol. Environ. Saf. 196:110483. doi: 10.1016/j.ecoenv.2020.110483
Khan, T. A., Fariduddin, Q., and Yusuf, M. (2017). Low-temperature stress: is phytohormones application a remedy? Environ. Sci. Pollut. Res. 24, 21574–21590. doi: 10.1007/s11356-017-9948-7
Kocsy, G., Galiba, G., and Brunold, C. (2001). Role of glutathione in adaptation and signalling during chilling and cold acclimation in plants. Physiol. Plant. 113, 158–164.
Kołodziejczyk, I., Kaźmierczak, A., and Posmyk, M. M. (2021). Melatonin Application Modifies Antioxidant Defense and Induces Endoreplication in Maize Seeds Exposed to Chilling Stress. Int. J. Mol. Sci. 22:8628. doi: 10.3390/ijms22168628
Kong, X. M., Ge, W. Y., Wei, B. D., Zhou, Q., Zhou, X., Zhao, Y. B., et al. (2020). Melatonin ameliorates chilling injury in green bell peppers during storage by regulating membrane lipid metabolism and antioxidant capacity. Postharvest Biol. Technol. 170:111315.
Korkmaz, A., Deger, O., Szafranska, K., Koklu, S., Karaca, A., Yakupoglu, G., et al. (2021). Melatonin effects in enhancing chilling stress tolerance of pepper. Sci. Hortic. 289:110434.
Korkmaz, A., Karaca, A., Kocacinar, F., and Cuci, Y. (2017). The effects of seed treatment with melatonin on germination and emergence performance of pepper seeds under chilling stress. J. Agric. Sci. 23, 167–176.
Kosová, K., Prášil, I. T., and Vítámvás, P. (2008). The relationship between vernalization- and photoperiodically-regulated genes and the development of frost tolerance in wheat and barley. Biol. Plant. 52, 601–615. doi: 10.1007/s10535-008-0120-6
Kul, R., Ekinci, M., Turan, M., Ors, S., and Yildirim, E. (2020). “How abiotic stress conditions affects plant roots,” in Plant Roots [Working Title], ed. E. Yildirim (London: IntechOpen), 6–10.
Lado, J., Rodrigo, M. J., López-Climent, M., Gómez-Cadenas, A., and Zacarías, L. (2016). Implication of the antioxidant system in chilling injury tolerance in the red peel of grapefruit. Postharvest Biol. Tecchnol. 111, 214–223.
Lata, C., and Prasad, M. (2011). Role of DREBs in regulation of abiotic stress responses in plants. J. Exp. Bot. 62, 4731–4748. doi: 10.1093/jxb/err210
Lazar, D., Murch, S. J., Beilby, M. J., and Al Khazaaly, S. (2013). Exogenous melatonin affects photosynthesis in characeae Chara australis. Plant Signal. Behav. 8:e23279. doi: 10.4161/psb.23279
Lee, H. Y., and Back, K. (2016). 2-hydroxymelatonin promotes the resistance of rice plant to multiple simultaneous abiotic stresses (combined cold and drought). J. Pineal Res. 61, 303–316. doi: 10.1111/jpi.12347
Lee, K., Choi, G. H., and Back, K. (2017). Cadmium-induced melatonin synthesis in rice requires light, hydrogen peroxide, and nitric oxide: key regulatory roles for tryptophan decarboxylase and caffeic acid O-methyltransferase. J Pineal Res. 63:e12441. doi: 10.1111/jpi.12441
Li, C., Wang, P., Wei, Z. W., Liang, D., Liu, C. H., Yin, L. J. D. F., et al. (2012). The mitigation effects of exogenous melatonin on salinityinduced stress in Malus hupehensis. J. Pineal Res. 53, 298–306. doi: 10.1111/j.1600-079X.2012.00999.x
Li, H., Guo, Y., Lan, Z., Xu, K., Chang, J., Ahammed, G. J., et al. (2021). Methyl jasmonate mediates melatonin-induced cold tolerance of grafted watermelon plants. Hortic. Res. 8:57. doi: 10.1038/s41438-021-00496-0
Li, J., Yang, Y., Sun, K., Chen, Y., Chen, X., and Li, X. (2019). Exogenous melatonin enhances cold, salt and drought stress tolerance by improving antioxidant defense in tea plant (Camellia sinensis (L.) O. Kuntze). Molecules. 24:1826. doi: 10.3390/molecules24091826
Li, X., Tan, D. X., Jiang, D., and Liu, F. (2016). Melatonin enhances cold tolerance in drought-primed wild-type and abscisic acid-deficient mutant barley. J. Pineal Res. 61, 328–339. doi: 10.1111/jpi.12350
Li, X., Wei, J. P., Scott, E., Liu, J. W., Guo, S., Li, Y., et al. (2018a). Exogenous melatonin alleviates cold stress by promoting antioxidant defense and redox homeostasis in Camellia sinensis L. Molecules 23:165. doi: 10.3390/molecules23010165
Li, X., Brestic, M., Tan, D. X., Zivcak, M., Zhu, X., Liu, S., et al. (2018b). Melatonin alleviates low PS I limited carbon assimilation under elevated CO2 and enhances the cold tolerance of offspring in chlorophyll b-deficient mutant wheat. J. Pineal Res. 64:e12453. doi: 10.1111/jpi.12453
Li, J., Arkorful, E., Cheng, S., Zhou, Q., Li, H., Chen, X., et al. (2018c). Alleviation of cold damage by exogenous application of melatonin in vegetatively propagated tea plant (Camellia sinensis (L.) O. Kuntze). Sci. Hortic. 238, 356–362.
Liu, J., Sun, J., Pan, Y., Yun, Z., Zhang, Z., Jiang, G., et al. (2021). Endogenous melatonin generation plays a positive role in chilling tolerance in relation to redox homeostasis in litchi fruit during refrigeration. Postharvest Biol. Technol. 178:111554. doi: 10.1016/j.postharvbio.2021.111554
Madebo, M. P., Lou, S. M., Li, W., Zheng, Y. H., and Peng, J. (2021). Melatonin treatment induces chilling tolerance by regulating the contents of polyamine, γ-aminobutyric acid, and proline in cucumber fruit. J. Integr. Agric. 20, 3060–3074.
Marta, B., Szafrańska, K., and Posmyk, M. M. (2016). Exogenous melatonin improves antioxidant defense in cucumber seeds (Cucumis sativus L.) germinated under chilling stress. Front. Plant Sci. 7:575. doi: 10.3389/fpls.2016.00575
Massenssini, A. M., Bonduki, V. H. A., Melo, C. A. D., Tótola, M. R., Ferreira, F. A., and Costa, M. D. (2015). Relative importance of soil physico-chemical characteristics and plant species identity to the determination of soil microbial community structure. Appl. Soil Ecol. 91, 8–15. doi: 10.1016/j.apsoil.2015.02.009
Mirshekari, A., Madani, B., Yahia, E. M., Golding, J. B., and Vand, S. H. (2020). Postharvest melatonin treatment reduces chilling injury in sapota fruit. J. Sci. Food Agric. 100, 1897–1903. doi: 10.1002/jsfa.10198
Mittler, R., Vanderauwera, S., Gollery, M., and Breusegem, F. V. (2004). Reactive oxygen gene network of plants. Trends Plant Sci. 9:490. doi: 10.1016/j.tplants.2004.08.009
Nayyar, H., Bains, T. S., and Kumar, S. (2005). Chilling stressed chickpea seedlings: effect of cold acclimation, calcium and abscisic acid on cryoprotective solutes and oxidative damage. Environ. Exp. Bot. 54, 275–285. doi: 10.1016/j.envexpbot.2004.09.007
Nezhadahmadi, A., Prodhan, Z. H., and Faruq, G. (2013). Drought tolerance in wheat. Sci. World J. 2013:610721.
Pu, Y. J., Zhang, L. J., Miao, L. F., Nawaz, M., and Yang, F. (2021). Coupling exogenous melatonin with Ca2+ alleviated chilling stress in Dalbergia odorifera T. Chen. Trees 35, 1–14.
Puhakainen, T. (2004). Short-Day Potentiation of Low Temperature-Induced Gene Expression of a C-Repeat-Binding Factor-Controlled Gene during Cold Acclimation in Silver Birch. Plant Physiol. 136, 4299–4307. doi: 10.1104/pp.104.047258
Qiao, Y., Yin, L., Wang, B., Ke, Q., Deng, X., and Wang, S. (2019). Melatonin promotes plant growth by increasing nitrogen uptake and assimilation under nitrogen deficient condition in winter wheat. Plant Physiol. Biochem. 139, 342–349. doi: 10.1016/j.plaphy.2019.03.037
Repkina, N., Ignatenko, A., Holoptseva, E., MiszalskI, Z., Kaszycki, P., and Talanova, V. (2021). Exogenous methyl jasmonate improves cold tolerance with parallel induction of two cold-regulated (COR) genes expression in Triticum aestivum L. Plants 10:1421. doi: 10.3390/plants10071421
Rinalducci, S., Egidi, M. G., Karimzadeh, G., Jazii, F. R., and Zolla, L. (2011). Proteomic analysis of a spring wheat cultivar in response to prolonged cold stress. Electrophoresis 32, 1807–1818. doi: 10.1002/elps.201000663
Ritonga, F. N., and Chen, S. (2020). Physiological and molecular mechanism involved in cold stress tolerance in plants. Plant 9:560. doi: 10.3390/plants9050560
Ruelland, E., and Zachowski, A. (2010). How plants sense temperature. Environ. Exp. Bot. 69, 225–232. doi: 10.1016/j.envexpbot.2010.05.011
Sarropoulou, V., Dimassi-Theriou, K., Therios, I., and Koukourikou-Petridou, M. (2012). Melatonin enhances root regeneration, photosynthetic pigments, biomass, total carbohydrates and proline content in the cherry rootstock PHL-C (Prunus avium×Prunuscerasus). Plant Physiol. Biochem. 61, 162–168. doi: 10.1016/j.plaphy.2012.10.001
Seleiman, M. F., Aslam, M. T., Alhammad, B. A., Hassan, M. U., Maqbool, R., Chattha, M. U., et al. (2021). Salinity stress in wheat: effects, mechanisms and management strategies. Phyton Int. J. Exp. Bot. 91, 667–694. doi: 10.3389/fpls.2016.00957
Shi, H., Jiang, C., Ye, T. T., Tan, D. X., Reiter, R. J., Zhang, H., et al. (2015a). Comparative physiological, metabolomic, and transcriptomic analyses reveal mechanisms of improved abiotic stress tolerance resistance in bermudagrass [Cynodon dactylon (L). Pers.] by exogenous melatonin. J. Exp. Bot. 66, 681–694. doi: 10.1093/jxb/eru373
Shi, H., Chen, Y., Tan, D. X., Reiter, R. J., Chan, Z., and He, C. (2015b). Melatonin induces nitric oxide and the potential mechanisms relate to innate immunity against bacterial pathogen infection in Arabidopsis. J. Pineal Res. 59, 102–108. doi: 10.1111/jpi.12244
Siddique, M. R. B., Hamid, A., and Islam, M. S. (2000). Drought stress effects on water relations of wheat. Bot. Bull. Acad. Sin. 41, 35–39.
Staniak, M., Czopek, K., Stȩpień-Warda, A., Kocira, A., and Przybyś, M. (2021). Cold Stress during Flowering Alters Plant Structure, Yield and Seed Quality of Different Soybean Genotypes. Agronomy 11:2059.
Sultan, I., Khan, I., Chattha, M. U., Hassan, M. U., Barbanti, L., Calone, R., et al. (2021). Improved salinity tolerance in early growth stage of maize through salicylic acid foliar application. Ital. J. Agron. 16:1810.
Sun, B., Guang-Ling, L., Thi, T. P., Li-Tao, Y., Yang-Rui, L., and Yong-Xiu, X. (2017). Effects of cold stress on root growth and physiological metabolisms in seedlings of different sugarcane varieties. Sugar Tech. 19, 165–175.
Sun, L., Li, X., Wang, Z., Sun, Z., Zhu, X., Liu, S., et al. (2018). Cold priming induced tolerance to subsequent low temperature stress is enhanced by melatonin application during recovery in wheat. Molecules 23:1091. doi: 10.3390/molecules23051091
Sun, X., Zhu, Z., Zhang, L., Fang, L., Zhang, J., Wang, Q., et al. (2019). Overexpression of ethylene response factors VaERF080 and VaERF087 from Vitis amurensis enhances cold tolerance in Arabidopsis. Sci. Hortic. 243, 320–326. doi: 10.1111/tpj.14378
Sun, Y., He, Y., Irfan, A. R., Liu, X., Yu, Q., Zhang, Q., et al. (2020). Exogenous brassinolide enhances the growth and cold resistance of maize (Zea mays L.) seedlings under chilling stress. Agronomy 10:488.
Suzuki, N., Koussevitzky, S., Mittler, R. O. N., and Miller, G. A. D. (2012). ROS and redox signalling in the response of plants to abiotic stress. Plant Cell Environ. 35, 259–270. doi: 10.1111/j.1365-3040.2011.02336.x
Szafranska, K., Glinska, S., and Janas, K. M. (2012). Changes in the nature of phenolic deposits after re-warming as a result of melatonin presowing treatment of Vigna radiata seeds. J. Plant Physiol. 169, 34–40. doi: 10.1016/j.jplph.2011.08.011
Tan, D. X., Hardeland, R., Back, K., Manchester, L. C., Alatorre-Jimenez, M. A., and Reiter, R. J. (2016). On the significance of an alternate pathway of melatonin synthesis via 5-methoxytryptamine: comparisons across species. J. Pineal Res. 61, 27–40. doi: 10.1111/jpi.12336
Tan, D. X., Hardeland, R., Manchester, L. C., Korkmaz, A., Ma, S., Rosales-Corral, S., et al. (2012). Functional roles of melatonin in plants, and perspectives in nutritional and agricultural science. J. Exp. Bot. 63, 577–597. doi: 10.1093/jxb/err256
Tan, D. X., Manchester, L. C., Liu, X., Rosales-Corral, S. A., Acuna-Castroviejo, D., and Reiter, R. J. (2013). Mitochondria and chloroplasts as the original sites of melatonin synthesis: a hypothesis related to melatonin’s primary function and evolution in eukaryotes. J. Pineal Res. 54, 127–138. doi: 10.1111/jpi.12026
Thakur, P., and Nayyar, H. (2013). “Facing the cold stress by plants in the changing environment: sensing, signaling, and defending mechanisms,” in Plant Acclimation to Environmental Stress, eds N. Tuteja and Gill, S. S. (New York, NY: Springer Science+Business Media), 29–69.
Thakur, P., Kumar, S., Malik, J. A., Berger, J. D., and Nayyar, H. (2010). Cold stress effects on reproductive development in grain crops: an overview. Environ. Exp. Bot. 67, 429–443. doi: 10.1016/j.envexpbot.2009.09.004
Turk, H., and Erdal, S. (2015). Melatonin alleviates cold-induced oxidative damage in maize seedlings by up-regulating mineral elements and enhancing antioxidant activity. J. Plant Nutr. Soil Sci. 178, 433–439.
Turk, H., Erdal, S., Genisel, M., Atici, O., Demir, Y., and Yanmis, D. (2014). The regulatory effect of melatonin on physiological, biochemical and molecular parameters in cold-stressed wheat seedlings. Plant Growth Regul. 74, 139–152. doi: 10.1007/s10725-014-9905-0
Turk, H., and Genisel, M. (2020). Melatonin-related mitochondrial respiration responses are associated with growth promotion and cold tolerance in plants. Cryobiology 92, 76–85. doi: 10.1016/j.cryobiol.2019.11.006
Valizadeh, K. R., Toorchi, M., Mogadam, M., Mohammadi, H., and Pessarakli, M. (2018). Effects of freeze and cold stress on certain physiological and biochemical traits in sensitive and tolerant barley (Hordeum vulgare) genotypes. J. Plant Nutr. 41, 102–111.
Van-Tassel, D. L., Roberts, N., Lewy, A., and O’Neill, S. D. (2001). Melatonin in plant organs. J. Pineal Res. 31, 8–15. doi: 10.1034/j.1600-079x.2001.310102.x
Venzhik, Y. V., Titov, A. F., Talanova, V. V., Frolova, S. A., Talanov, A. V., and Nazarkina, Y. A. (2011). Influence of lowered temperature on the resistance and functional activity of the photosynthetic apparatus of wheat plants. Biol. Bull. 38, 132–137.
Verma, V., Ravindran, P., and Kumar, P. P. (2016). Plant hormone-mediated regulation of stress responses. BMC Plant Biol. 16:86. doi: 10.1186/s12870-016-0771-y
Wang, H., Song, C., Fang, S., Wang, Z., Song, S., Jiao, J., et al. (2022). Genome-wide identification and expression analysis of the ASMT gene family reveals their role in abiotic stress tolerance in apple. Sci. Hortic. 293:110683.
Wang, J., Liu, D., Guo, X., and Zhang, A. (2012). Research Progress in the Pathway of Auxin Synthesis. Acta Bot. Sin. 47, 292–301.
Wang, Y. Y., Hsu, P. K., and Tsay, Y. F. (2012). Uptake, allocation and signaling of nitrate. Trend Plant Sci. 17, 458–467.
Wang, L., Feng, C., Zheng, X., Guo, Y., Zhou, F., Shan, D., et al. (2017). Plant mitochondria synthesize melatonin and enhance the tolerance of plants to drought stress. J. Pineal Res. 63:e1242. doi: 10.1111/jpi.12429
Wang, L., Zhao, Y., Reiter, R. J., He, C., Liu, G., Lei, Q., et al. (2014). Changes in melatonin levels in transgenic ‘Micro-Tom’ tomato overexpressing ovine AANAT and ovine HIOMT genes. J. Pineal Res. 56, 134–142. doi: 10.1111/jpi.12105
Wang, M., Zhang, S., and Ding, F. (2020). Melatonin mitigates chilling-induced oxidative stress and photosynthesis inhibition in tomato plants. Antioxidants 9:218. doi: 10.3390/antiox9030218
Wang, P., Sun, X., Li, C., Wei, Z., Liang, D., and Ma, F. (2013). Long-term exogenous application of melatonin delays drought-induced leaf senescence in apple. J. Pineal Res. 54, 292–302. doi: 10.1111/jpi.12017
Wang, Y., Luo, Z., Mao, L., and Ying, T. (2016). Contribution of polyamines metabolism and GABA shunt to chilling tolerance induced by nitric oxide in cold-stored banana fruit. Food Chem. 197, 333–339. doi: 10.1016/j.foodchem.2015.10.118
Weeda, S., Zhang, N., Zhao, X., Ndip, G., Guo, Y., Buck, G. A., et al. (2014). Arabidopsis transcriptome analysis reveals key roles of melatonin in plant defense systems. PLoS One 9:93462. doi: 10.1371/journal.pone.0093462
Xu, X. D., Sun, Y., Guo, X. Q., Sun, B., and Zhang, J. (2010). Effects of exogenous melatonin on ascorbate metabolism system in cucumber seedlings under high temperature stress. Ying Yong Sheng Tai Xue Bao 21, 2580–2586.
Yamori, W., Noguchi, K., Hikosaka, K., and Terashima, I. (2009). Cold-tolerant crop species have greater temperature homeostasis of leaf respiration and photosynthesis than cold-sensitive species. Plant Cell Physiol. 50, 203–215. doi: 10.1093/pcp/pcn189
Yang, X. L., Xu, H., Li, D., Gao, X., Li, T. L., and Wang, R. (2018). Effect of melatonin priming on photosynthetic capacity of tomato leaves under low-temperature stress. Photosynthetica 56, 884–892.
Ye, J., Yang, W., Li, Y., Wang, S., Yin, L., and Deng, X. (2020). Seed pre-soaking with melatonin improves wheat yield by delaying leaf senescence and promoting root development. Agronomy 10:84.
Yokota, H., Iehisa, J. C. M., Shimosaka, E., and Takumi, S. (2015). Line differences in Cor/Lea and fructan biosynthesis-related gene transcript accumulation are related to distinct freezing tolerance levels in synthetic wheat hexaploids. J. Plant Physiol. 176, 78–88. doi: 10.1016/j.jplph.2014.12.007
Yuan, S., Huang, Y., Liu, S., Guan, C., Cui, X., Tian, D., et al. (2016). RNA-seq analysis of overexpressing ovine AANAT gene of melatonin biosynthesis in switchgrass. Front. Plant Sci. 7:1289. doi: 10.3389/fpls.2016.01289
Zhang, X., Shen, L., Li, F., Meng, D., and Sheng, J. (2011). Methyl salicylate-induced arginine catabolism is associated with up-regulation of polyamine and nitric oxide levels and improves chilling tolerance in cherry tomato fruit. J. Agric. Food Chem. 59, 9351–9357. doi: 10.1021/jf201812r
Zhang, F., Jiang, Y., Bai, L. P., Zhang, L., Chen, L. J., Li, H. G., et al. (2011). The ICE-CBF-COR Pathway in Cold Acclimation and AFPs in Plants. Middle East J. Sci. Res. 8, 493–498.
Zhang, H., Liu, L., Wang, Z., Feng, G., Gao, Q., and Li, X. (2021). Induction of Low Temperature Tolerance in Wheat by Pre-Soaking and Parental Treatment with Melatonin. Molecules 26:1192. doi: 10.3390/molecules26041192
Zhang, Y., Wang, J., Chen, X., Lu, X., Wang, D., Wang, J., et al. (2021). Genome-wide identification and characteristic analysis of the downstream melatonin metabolism gene GhM2H in Gossypium hirsutum L. Biol. Res. 54:36. doi: 10.1186/s40659-021-00358-y
Zhang, H. J., Zhang, N., Yang, R. C., Wang, L., Sun, Q., Cao, Y. Y., et al. (2014). Melatonin promotes seed germination under high salinity by regulating antioxidant systems, ABA and GA4 interaction in cucumber (Cucumis sativus L.). J Pineal Res. 57, 269–279. doi: 10.1111/jpi.12167
Zhang, Q., Li, D., Wang, Q., Song, X., Wang, Y., Yang, X., et al. (2021). Exogenous Salicylic Acid Improves Chilling Tolerance in Maize Seedlings by Improving Plant Growth and Physiological Characteristics. Agronomy 11:1341. doi: 10.3390/agronomy11071341
Zhang, Y. P., Yang, S. J., and Chen, Y. Y. (2017). Effects of melatonin on photosynthetic performance and antioxidants in melon during cold and recovery. Biol. Plant. 61, 571–578. doi: 10.1007/s10535-017-0717-8
Zhao, H., Ye, L., Wang, Y., Zhou, X., Yang, J., Wang, J., et al. (2016). Melatonin increases the chilling tolerance of chloroplast in cucumber seedlings by regulating photosynthetic electron flux and the ascorbate-glutathione cycle. Front. Plant Sci. 7:1814. doi: 10.3389/fpls.2016.01814
Zhao, H., Zhang, K., Zhou, X., Xi, L., Wang, Y., Xu, H., et al. (2017). Melatonin alleviates chilling stress in cucumber seedlings by up-regulation of CsZat12 and modulation of polyamine and abscisic acid metabolism. Sci. Rep. 7:4998. doi: 10.1038/s41598-017-05267-3
Zhao, M. G., Tian, Q. Y., and Zhang, W. H. (2007). Nitric oxide synthase-dependent nitric oxide production is associated with salt tolerance in Arabidopsis. Plant Physiol. 144, 206–217. doi: 10.1104/pp.107.096842
Zhong, L., Lin, L., Yang, L., Liao, M. A., Wang, X., Wang, J., et al. (2020). Exogenous melatonin promotes growth and sucrose metabolism of grape seedlings. PLoS One 15:0232033. doi: 10.1371/journal.pone.0232033
Keywords: antioxidants, cold stress, gene expression, melatonin, oxidative stress, photosynthesis
Citation: Qari SH, Hassan MU, Chattha MU, Mahmood A, Naqve M, Nawaz M, Barbanti L, Alahdal MA and Aljabri M (2022) Melatonin Induced Cold Tolerance in Plants: Physiological and Molecular Responses. Front. Plant Sci. 13:843071. doi: 10.3389/fpls.2022.843071
Received: 24 December 2021; Accepted: 31 January 2022;
Published: 14 March 2022.
Edited by:
Yingfang Zhu, Henan University, ChinaReviewed by:
Jing Zhang, Nanjing Agricultural University, ChinaJen-Tsung Chen, National University of Kaohsiung, Taiwan
Copyright © 2022 Qari, Hassan, Chattha, Mahmood, Naqve, Nawaz, Barbanti, Alahdal and Aljabri. This is an open-access article distributed under the terms of the Creative Commons Attribution License (CC BY). The use, distribution or reproduction in other forums is permitted, provided the original author(s) and the copyright owner(s) are credited and that the original publication in this journal is cited, in accordance with accepted academic practice. No use, distribution or reproduction is permitted which does not comply with these terms.
*Correspondence: Sameer H. Qari, c2hxYXJpQHVxdS5lZHUuc2E=