- 1College of Resources and Environment, Hunan Agricultural University, Changsha, China
- 2Hunan Provincial Key Laboratory of Rural Ecosystem Health in Dongting Lake Area, Hunan Agricultural University, Changsha, China
- 3Hunan Academy of Forestry, Changsha, China
- 4Hunan Dongting Lake Wetland Ecosystem National Positioning Observation and Research Station, Yueyang, China
Plant non-harvest changes element circulation and has a marked effect on element sinks in the ecosystem. In this study, a field investigation was conducted on the fixation of nitrogen and phosphorus in Miscanthus lutarioriparius, the most dominant plant species in the Dongting Lake wetlands. Further, to quantitatively compare the difference in nitrogen and phosphorus sinks between harvest and non-harvest, an in situ experiment on the release of the two elements from two types of litters (leaves and stems) was studied. The nitrogen concentrations in the plant had no significant relationship with the environmental parameters. The phosphorus concentrations were positively related to the plot elevation, soil organic matter, and soil total potassium and were negatively related to the soil moisture. The leaves demonstrated a higher decomposition coefficient than that of the stems in the in situ experiment. The half decomposition time was 0.61 years for leaves and 1.12 years for stems, and the complete decomposition time was 2.83 years for leaves and 4.95 years for stems. Except for the nitrogen concentration in the leaves, all the concentrations increased during the flood period. All concentrations unsteadily changed in the backwater period. Similarly, except for the relative release index of nitrogen in the leaves, all the relative release indices decreased in the flood period. At the end of the in situ decomposition experiment, the relative release indices of both the nitrogen and phosphors were greater than zero, indicating that there was a net release of nitrogen and phosphorus. Under the harvest scenario, the aboveground parts of the plant were harvested and moved from the wetlands, thus increasing the nitrogen and phosphorus sinks linearly over time. The fixed nitrogen and phosphorus in the aboveground parts were released under the non-harvest scenario, gradually accumulating the nitrogen and phosphorus sinks from the first year to the fifth year after non-harvest, reaching a maximum value after the fifth year. This study showed that the nitrogen and phosphorus sinks greatly decreased after the non-harvest of M. lutarioriparius compared to that after harvest. It is recommended to continue harvesting the plant for enhancing the capacity of element sinks.
Introduction
A large amount of nitrogen and phosphorus has been released into the environment in the past several decades, causing great threats to the ecosystem (Grizzetti et al., 2020). Approximately 605 million moles of nitrogen and 36 million moles of phosphorus are reportedly inputted into the Narragansett Bay each year, eventually getting deposited in the sediment (Nixon et al., 2008). Recently, the nitrogen and phosphorus concentrations in the sediments of the major rivers of China (such as the Yangtze River, Yellow River, and Huai River) were found to be much higher than the soil background values (Yang et al., 2017). This nitrogen and phosphorus will be released from the sediments again and decrease the water quality and accelerate eutrophication (Jin et al., 2006). Due to their important roles in element sinks, plant species such as Pistia stratiotes, Eichhornia crassipes, and Phragmites australis are commonly used to remove the nitrogen and phosphorus from aquatic ecosystems (Lu et al., 2010; Nash et al., 2019; Rezania et al., 2019).
For plants, the capacity of nitrogen and phosphorus sinks depends on the amount of elements fixed in the plant. Nitrogen and phosphorus fixation is a biological process and is associated with certain environmental factors. Studies have shown soil pH to be one of the main factors affecting the fixation of phosphorus (Barrow, 2017). In high soil moisture conditions, low oxygen availability limits nitrogen fixation (Sajedi et al., 2012). Under the comprehensive influence of environmental factors, plant organs exhibit different nitrogen and phosphorus allocation patterns. For example, compared with stems and roots, the leaves in 30 species of desert plants demonstrated higher nitrogen and phosphorus concentrations (Luo et al., 2021). Unlike terrestrial plants, aquatic plants can obtain nitrogen and phosphorus from both soil and water, thus demonstrating higher element concentrations in aboveground parts than in underground parts (Granéli and Solander, 1988). Detailed studies on nitrogen and phosphorus fixation are therefore necessary for quantitatively evaluating the capacity of element sinks.
The fixed nitrogen and phosphorus in plants is released into the environment with the decomposition of litter. The release of nitrogen and phosphorus from litter is also a biological process, and the amount of the released elements can be reflected by the weight of residual litter and elemental concentrations (van der Valk et al., 1991; Kuehn and Suberkropp, 1998). The negative exponential decay model is usually adopted for estimating the mass of residual litters in the decomposition process (Olson, 1963). The nitrogen and phosphorus concentrations in litters show a dynamic balance between element release from litters into the environment and element fixation by litters from the environment (Zhang et al., 2020). A study conducted on the decomposition of forest litter showed that nitrogen concentration in litters increased in the first and second years of the decomposition and decreased in the third year (McClaugherty et al., 1985). Studies on the release process of nitrogen and phosphorus in litter decomposition are therefore also necessary for quantitatively evaluating the capacity of element sinks.
Dongting Lake is the second largest freshwater lake in China and is connected to the Yangtze River and the “four rivers” of Hunan (Xiang, Zi, Yuan, and Li rivers). In the catchment, a large amount of nitrogen, phosphorus, and heavy metals are imported into the lake and deposited in the sediments. Miscanthus lutarioriparius, a perennial, emergent macrophyte, is distributed on the beach of the lake and is the most dominant species in this area (Xu et al., 2021). In the past several decades, the aboveground parts of this plant have been harvested as a raw material for papermaking, causing the removal of a great number of elements from the lake (Yao et al., 2018). However, it is now forbidden to harvest M. lutarioriparius owing to the important role of the lake in biodiversity protection and the disappearance of papermaking industries in the region. Typically, the aboveground parts of M. lutarioriparius will decompose, and the fixed elements will be released into aquatic ecosystems. The non-harvest of the plant, therefore, evokes high concerns regarding the potential ecological risk caused by the possible decline in element sinks. Few studies have quantitatively estimated the difference in element sink between plant harvest and non-harvest to date (Morton et al., 2010). In this context, harvest refers to harvesting the aboveground parts of plants once a year, while non-harvest means removing human disturbance and letting plants fall and decompose in the wild.
To this end, a field investigation and an in situ experiment were conducted to explore the fixation of nitrogen and phosphorus in the aboveground parts of the plants and the release of the two elements to reveal the changes in nitrogen and phosphorus sinks after the policy change from harvest to non-harvest.
Materials and methods
Study area
Dongting Lake has an area of 2,625 km2 (28(382gt98452gting15402gti30102gting Lake has an area of 2n a by floods from the Yangtze, Xiang, Zi, Yuan, and Li rivers. The annual fluctuation in the water level is approximately 12–14 m, with the maxima in July–August and minima in January–February (Xie et al., 2015). Since the 1960s, M. lutarioriparius was planted in Dongting Lake for paper manufacture. This plant generally germinates in March–April and is harvested during November–December. The total distribution area of M. lutarioriparius in the lake is approximately 585 km2 and accounts for 22.3% of the total area of Dongting Lake (Xu et al., 2021). Currently, the lake is a global hotspot for biodiversity conservation, with three international important wetlands and two national wetland nature reserves.
Field investigation on nitrogen and phosphorus fixation
In November 2016, 24 vegetated plots were selected on the beach of Dongting Lake (Figure 1). The geographical information of each plot was recorded using a hand-held GPS (UniStrong, China). A quadrat (1 m × 1 m) was chosen for investigation in each vegetated plot. The stems in each sampled quadrat were cut from the soil surface and cut into pieces, and the leaves were collected from the stems. The roots were dug from a soil depth of 0–30 cm and carefully washed such that they were free of soil. The mixed soil samples of 0–30 cm were collected for determining the environmental parameters in the soil. These vegetation and soil samples were sealed in plastic bags and brought back to the laboratory for further analysis.
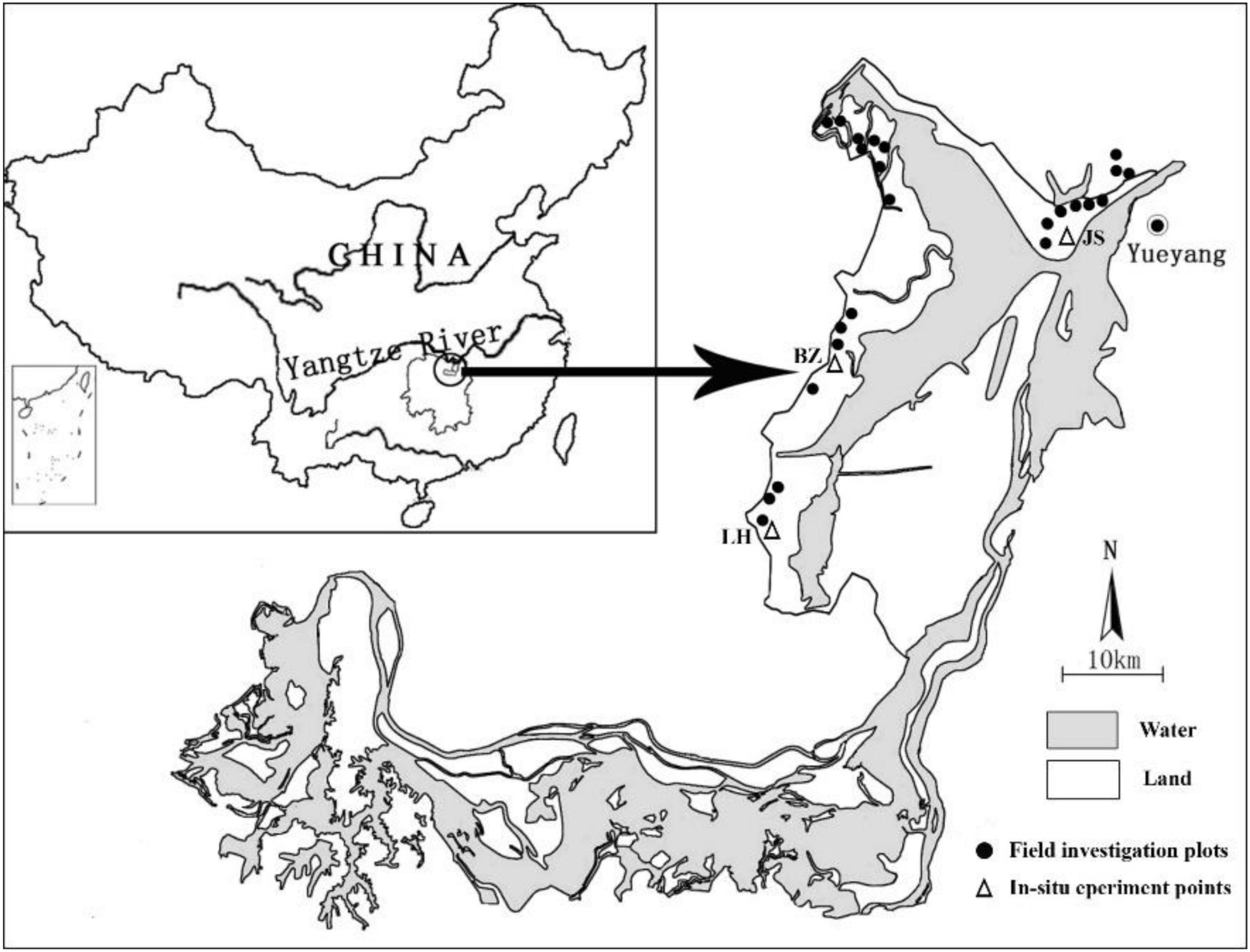
Figure 1. The field investigation plots (circles) and in situ experiment points (triangles; JS: Junshan; BZ: Beizhouzi; LH: Luhu) in the Dongting Lake wetlands.
In situ experiment on nitrogen and phosphorus release
In November 2019, the leaves and stems of M. lutarioriparius were collected from the beach of Dongting Lake and air-dried. In April 2020, 5 g each of leaves and stems were put into separate nylon mesh bags (15 cm × 25 cm) with 1 mm apertures. These bags were taken into M. lutarioriparius communities on three beaches (Junshan, Beizhouzi, and Luhu) of Dongting Lake. In each plant community, 72 bags, including 36 leaf bags and 36 stem bags, were placed on the soil surface. These bags were placed in the center of the plant communities with an area of 30 width and 50 m length; the distance between bags was 1 m. After the plant bags were put into the plant communities for 30, 210, 240, 270, 300, and 330 days, the samples were taken with six replicates and brought back to the laboratory for further analysis (Figure 2).
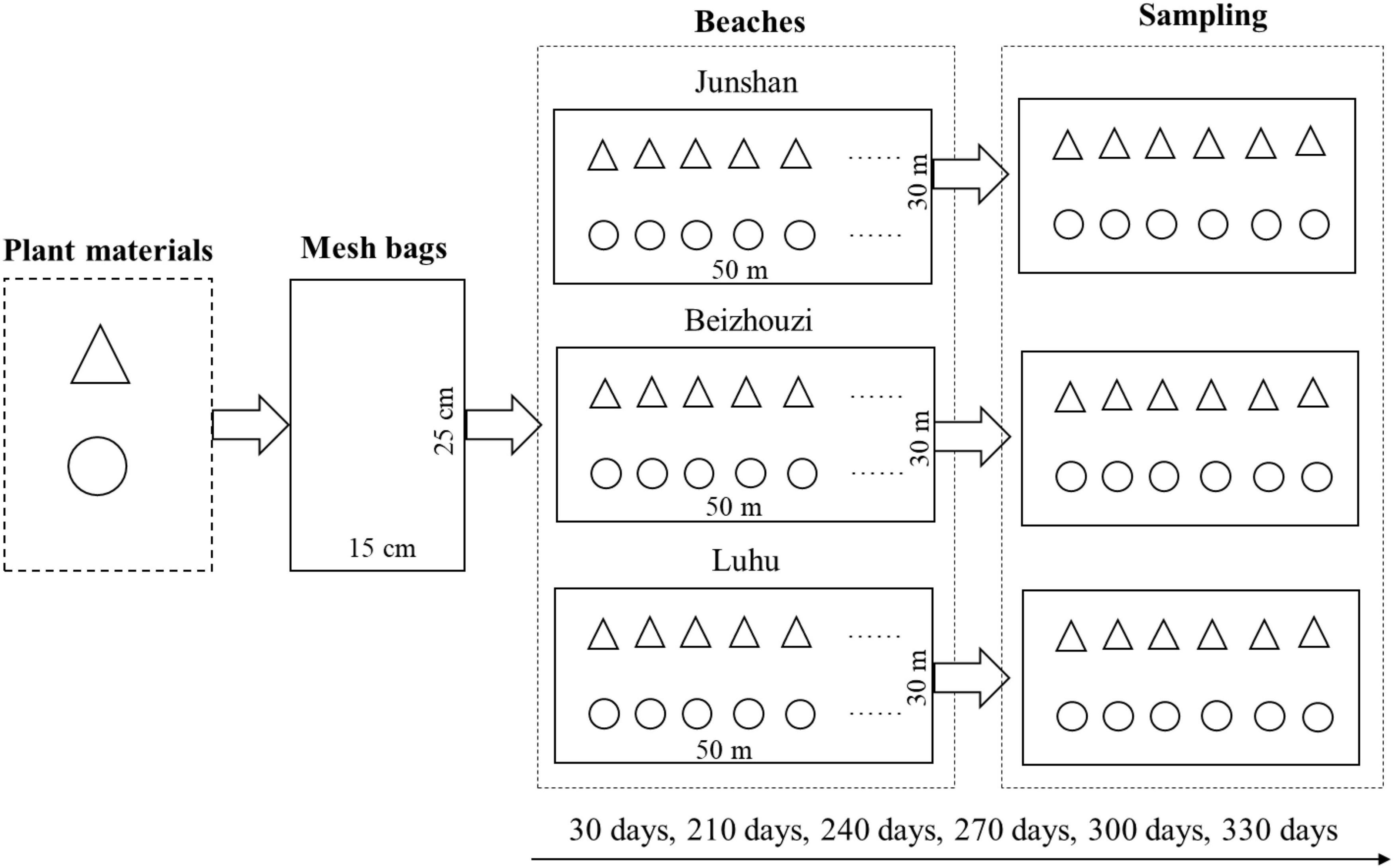
Figure 2. Flow picture of the implementation of the in situ experiment (triangles, leaves; circles, stems).
Measurements of biomass, and nitrogen and phosphorus concentrations in plants
In the field investigation and in situ experiment, samples of roots, stems, and leaves were dried separately in an oven at 80°C for 48 h before measuring their dry weight. The biomass of plant organs was defined as dry mass per square meter, and plant total biomass was calculated as the total weight of roots, stems, and leaves. Plant organs were ground to a fine powder and passed through a 0.15 mm mesh. Material less than 0.15 mm was then used to test the concentrations of nitrogen and phosphorus. The nitrogen concentrations in plants were analyzed using a continuous flow analyzer (Auto Analyzer 3 HR, Seal Analytical, Germany), and phosphorus concentrations were measured by the molybdenum-antimony anti-colorimetric method (NY/T 2017-2011).
Measurement of soil properties
In the field investigation, soil properties were measured according to the Chinese national standards (Liu, 1996). Soil moisture was calculated as [(FW - DW)/FW] × 100%, where FW is the soil fresh weight and DW is the soil dry weight after drying in an oven at 105°C for 48 h. The remaining fresh soil was air-dried in the shade for the analysis of soil variables. The pH, electrolyte leakage, organic matter concentration, total nitrogen concentration, alkali-hydrolyzable nitrogen concentration, total phosphorus concentration, available phosphorus concentration, and total potassium concentration were then measured (Yao et al., 2018).
Data calculation
The amount of nitrogen or phosphorus fixed by a plant organ was calculated using the following equation:
where Ef is the amount of an element (nitrogen or phosphorus) fixed in the plant organ (leaf, stem, or root), M is the dry mass of the plant organ, and C is the concentration of an element in the organ. The amount of elements fixed by the aboveground parts of plants was the sum of the amount of the elements fixed by leaves and stems.
The mass residual ratio of litter (leaf or stem) was calculated using the following equation:
where R is the mass residual ratio of litter, Mt is the litter mass after decomposing time t, and M0 is the initial litter mass.
A negative exponential decay model was used to analyze the mass residual ratio of litter in litter decomposition (Olson, 1963):
where y is the mass residual ratio of litter, a is the fitting parameter, k is the annual decomposition coefficient, and t is the decomposing time.
The amount of an element released in litter decomposition (Er) and the relative release index (RRI) were calculated using the following equations:
where M0 is the initial litter mass, C0 is the initial concentration of an element in the litter, Mt is litter mass after decomposing time t, and Ct is the concentration of an element in the litter at time t. The litter on the 360th day could not be collected due to uncontrollable factors in the field, and thus, the RRI in the time was calculated according to the regression model of RRI in the leaves and stems (Figure 3).
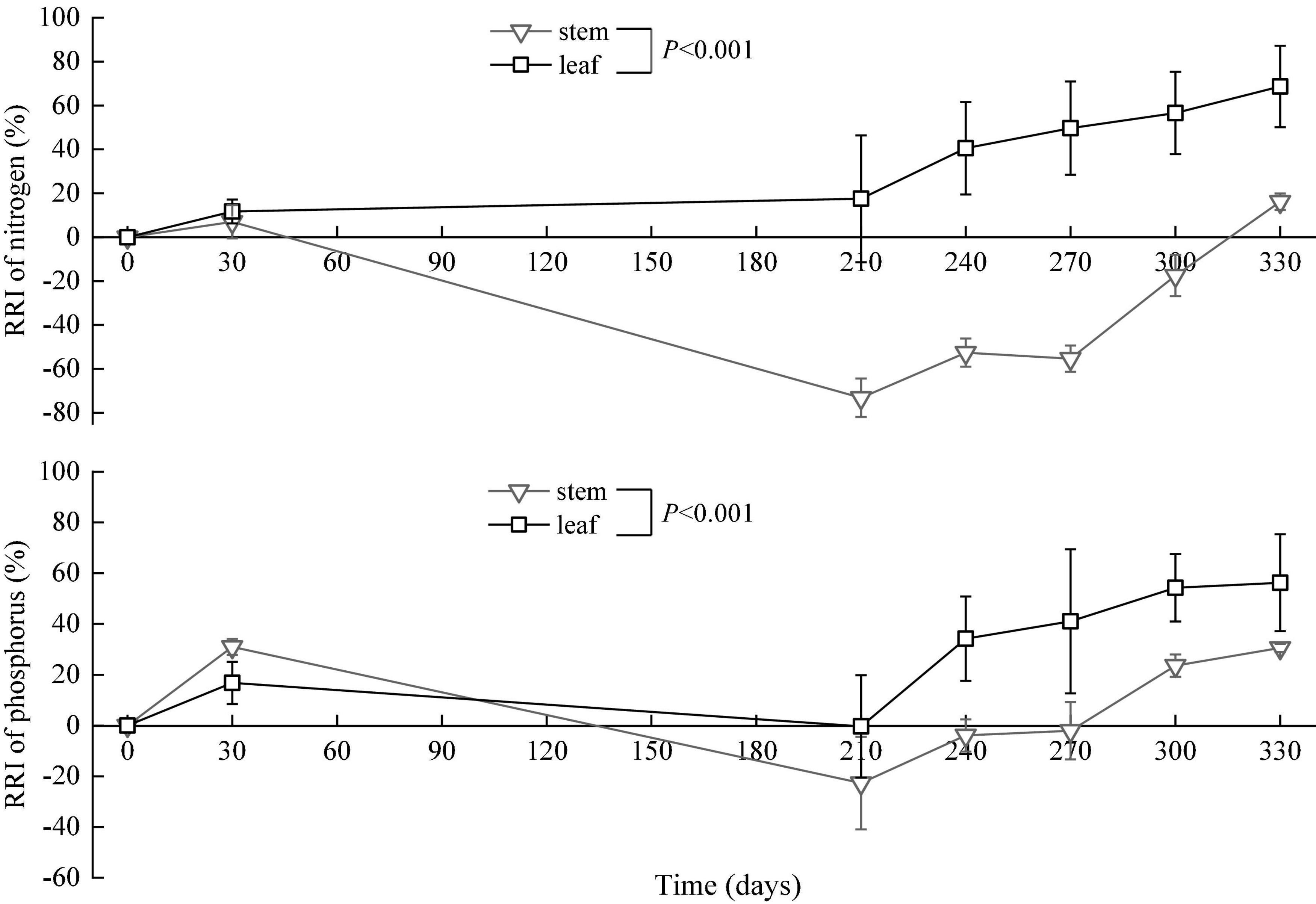
Figure 3. Dynamics of relative release indices (RRI, means ± SD) of nitrogen and phosphorus in the in situ experiment.
Nitrogen and phosphorus sinks were evaluated for two scenarios. If the plant is harvested, the annual element sinks were considered equal to Ef. If the plant is not harvested, the element sinks were calculated as follows:
where Sn is the element sink in the non-harvest scenario; Erl and Ers are the element contents in residual leaves and stems after a year’s litter decomposition, respectively; and n and m are the complete decomposition times of leaves and stems, respectively. Considering that the in situ experiment was conducted in 1 year, the amounts of both elements in the residual litters in the following years (the second year, third year, etc.) were estimated as the mean element concentrations in the in situ experiment of 1 year multiplied by the weight of residual litters calculated with the exponential decay model of the residual litters (y = ae–kt).
Statistical analysis
Multiple comparisons were conducted to test the difference in the biomass, concentrations of nitrogen and phosphorus in plants, and amount of nitrogen and phosphorus fixed in plants using Tukey’s test at the 0.05 significance level. Linear regression analysis between mean concentrations of nitrogen and phosphorus in plants and environmental parameters was performed at the 0.05 significance level. Before analysis, the homogeneity of variances was tested using Levene’s test, and data were log10-transformed when necessary to reduce the heterogeneity of variances. The statistical analyses were performed using SPSS Statistics 23.0 (IBM Corp., United States) software, and figures were produced using OriginPro 2021 (OriginLab Corp., United States) software.
Results
Concentrations and amount of nitrogen and phosphorus in plants in the field investigation
The concentrations and amounts of nitrogen and phosphorus were significantly different in the three plant organs (Table 1). For concentrations, both nitrogen and phosphorus were the highest in the leaves (16.27 and 1.34 g kg–1 for nitrogen and phosphorus, respectively), intermediate in the roots (7.13 and 1.03 g kg–1, respectively), and lowest in the stems (3.83 and 0.44 g kg–1, respectively). However, the amounts of fixed nitrogen and phosphorus were the highest in the roots (9.42 and 1.55 g m2 for nitrogen and phosphorus, respectively), intermediate in the stems (4.46 and 0.49 g m2, respectively), and lowest in the leaves (2.66 and 0.21 g m2, respectively).

Table 1. Plant biomass, concentrations, and amounts of nitrogen and phosphorus (means ± SD) fixed in M. lutarioriparius in the field investigation.
The total amounts of elements fixed by plants were 16.544 g m2 for nitrogen and 2.239 g m2 for phosphorus. Combined with the area of M. lutarioriparius in the Dongting Lake wetlands, approximately 9,680 t nitrogen and 1,310 t phosphorus were fixed by this plant per year, and 4,168 t nitrogen and 404 t phosphorus were fixed by the aboveground parts.
The relationship between nitrogen and phosphorus concentrations in plants and environmental parameters in the field investigation
The mean nitrogen concentrations in the plant did not exhibit a significant relationship with the studied parameters (Table 2). The mean phosphorus concentrations in the plant were positive related to the plot elevation, soil organic matter, and soil total potassium, and were negatively correlated to the soil moisture.
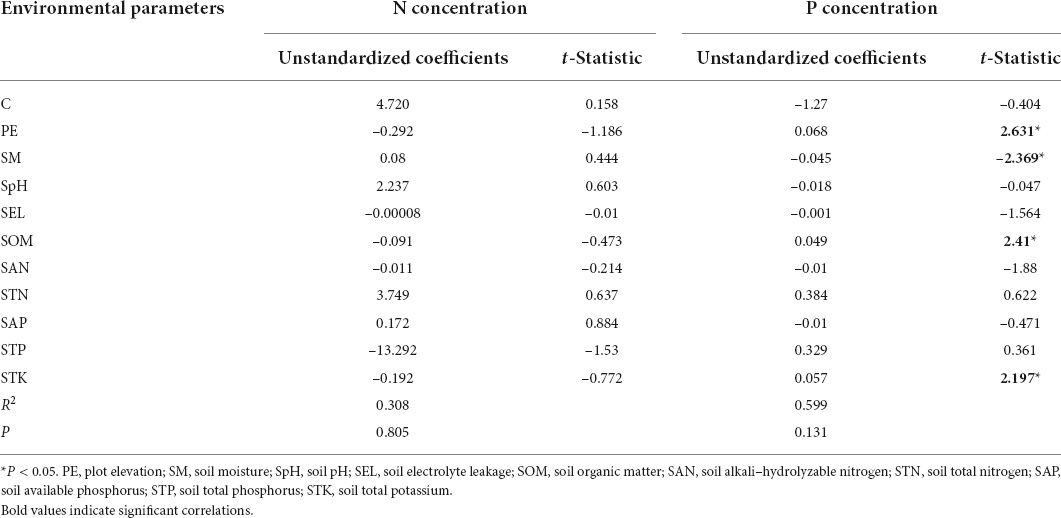
Table 2. Linear regression analysis of nitrogen (N) and phosphorus (P) concentrations in M. lutarioriparius and environmental parameters in the field investigation.
Mass residual ratio of leaves and stems in the in situ experiment
There was an obvious difference in the mass residual ratio between leaves and stems (Figure 4). Over time, the mass residual ratio of the leaves and stems demonstrated negative exponential decay models. The decomposition coefficient was 1.037 for stems and 0.6018 for leaves. Based on the negative exponential decay model, it may be deduced that the half decomposition (50% decomposition) time was 0.61 years for leaves and 1.12 years for stems, and the complete decomposition (95% decomposition) time was 2.83 years for leaves and 4.95 years for stems.
Release of nitrogen and phosphorus in leaves and stems in the in situ experiment
The concentrations of nitrogen and phosphorus in the leaves and stems changed with time gradually. The nitrogen concentration in the leaves increased in the period from the 0th to the 30th day, decreased from the 30th to the 210th day, and unsteadily changed from the 210th to the 330th day. However, the phosphorus concentration in the leaves increased from the 0th to the 300th day and decreased from the 300th to the 330th day. Overall, the concentrations of nitrogen and phosphorus in the stems increased from the 0th to the 270th day and decreased from the 270th to the 330th day (Figure 5).
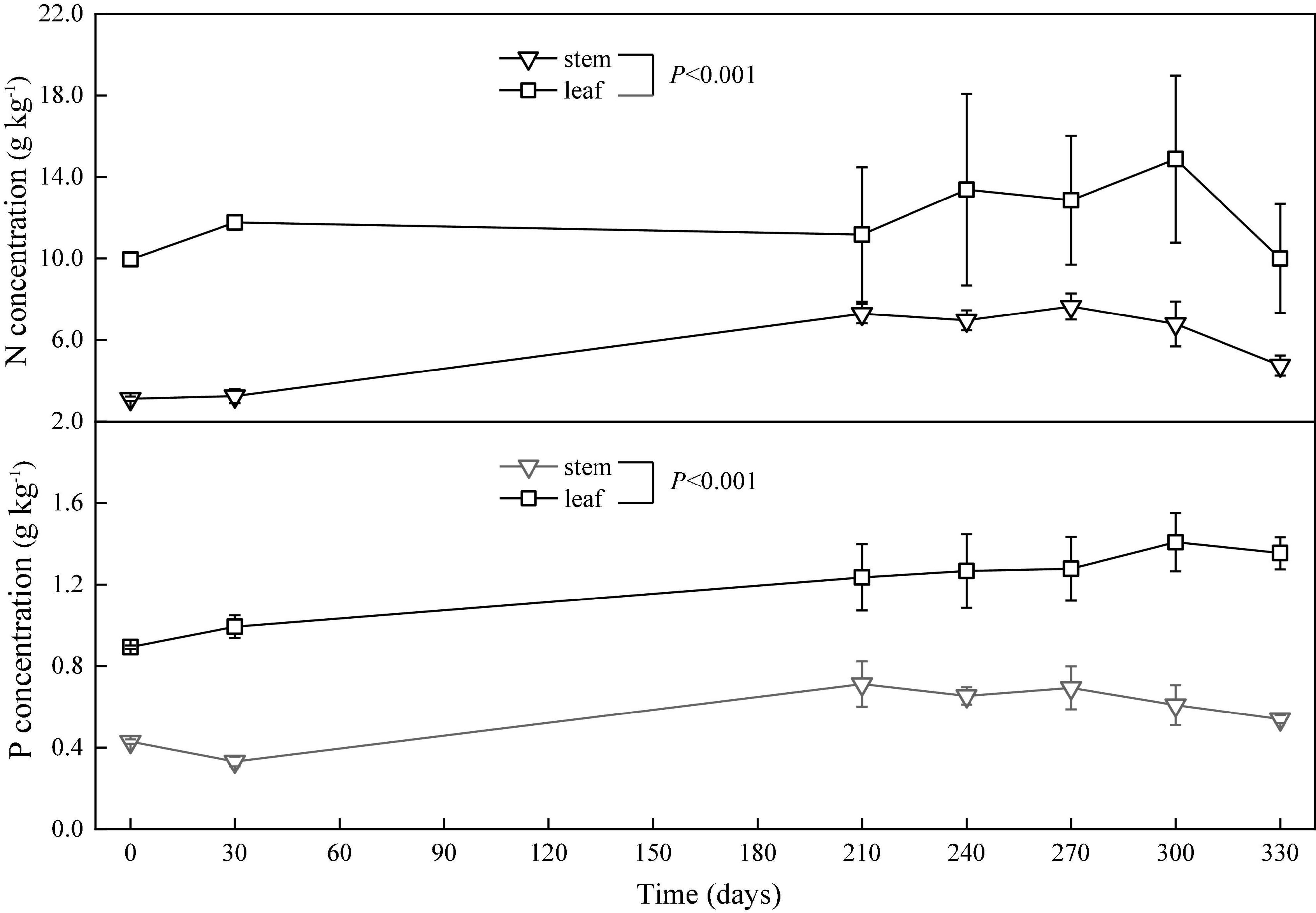
Figure 5. Concentrations (means ± SD) of nitrogen (N) and phosphorus (P) in the leaves and stems in the in situ experiment.
The relative release index of nitrogen in the leaves gradually increased in the period from the 0th to the 330th day. However, the relative release index of nitrogen in the stems increased in the period from the 0th to the 30th day, decreased from the 30th to the 210th day (flood period), and then increased from the 210th to the 330th day (backwater period). The relative release index of phosphorus in the leaves and stems increased in the period from the 0th to the 30th day, decreased from the 30th to the 210th day, and then increased from the 210th to the 330th day. At the end of the in situ decomposition experiment, the relative release indices of nitrogen and phosphors were greater than zero (Figure 3).
Nitrogen and phosphorus sinks in the scenario of harvest and non-harvest of the plant
In the scenario of the harvest of the plant, the nitrogen and phosphorus sinks increased linearly over time (Figure 6). In the scenario of non-harvest, the nitrogen and phosphorus sinks gradually increased during the period from the first year to the fifth year after non-harvest. However, after the fifth year of non-harvest, the element sinks reached a constant value (4,300 t for nitrogen sinks and 411 t for phosphorus sinks). The nitrogen and phosphorus sinks therefore greatly decreased after the non-harvest of M. lutarioriparius for 5 years compared to the harvest scenario.
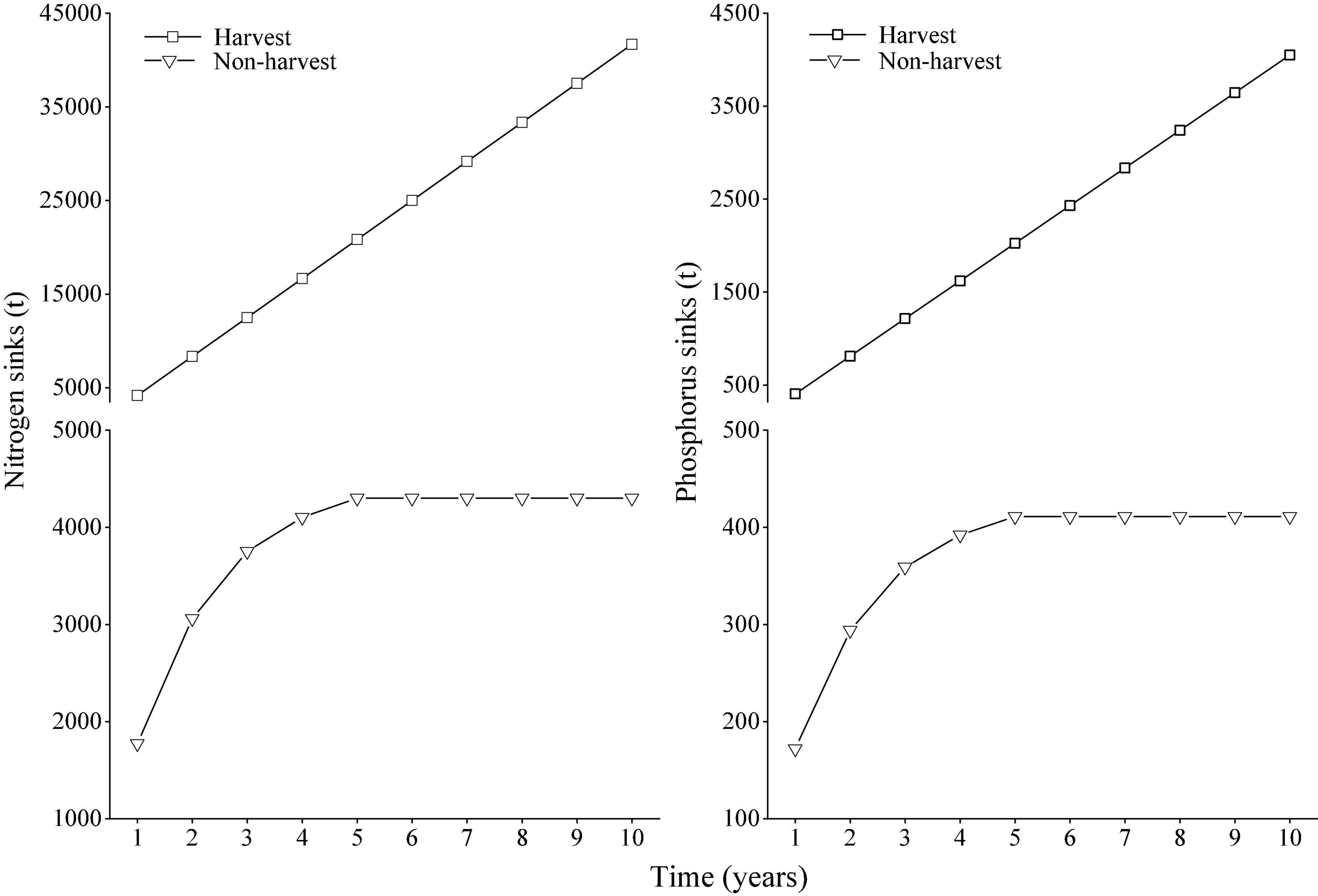
Figure 6. Nitrogen and phosphorus sinks in the scenarios of harvest and non-harvest of M. lutarioriparius in the Dongting Lake wetlands over time.
Discussion
Amount of nitrogen and phosphorus fixed in plants in the field investigation
This study showed that element concentrations differed across plant organs. The concentrations of both nitrogen and phosphorus were the highest in the leaves, intermediate in the roots, and lowest in the stems, which was consistent with observations from previous studies on the wetland plant P. australis (Song and Shan, 2012). One possible reason for this may be that leaves are the main photosynthetic organ, with vigorous metabolism and high demand for nitrogen and phosphorus. Another possible reason could be that leaves can absorb some elements from water during prolonged flood periods (Bonanno and Giudice, 2010). Combined with the biomass of plant organs, the amount of nitrogen and phosphorus fixed in the three plant organs was the highest in the roots, intermediate in the stems, and lowest in the leaves. In Dongting Lake, the annual amount of nitrogen and phosphorus fixed by the aboveground parts of M. lutarioriparius reached 4,168 and 404 t, respectively. M. lutarioriparius demonstrated great potential for removing nitrogen and phosphorus from the lake as industrial raw materials.
Since elements in plants come from their environment, there is an obvious relationship between the contents of elements in the plants and their environment (Fernández-Aláez et al., 1999; Baldantoni et al., 2004; Chen et al., 2015). However, in this study, we found that the mean nitrogen and phosphorus concentrations in plants were not significantly correlated with their concentrations in soil. Kern-Hansen and Dawson (1978) also found that nutrient concentrations in plants had no obvious correlation with the concentrations in environments. The possible reasons for this may be as follows. Large amounts of nitrogen and phosphorus are deposited in the lake during the flood period from April to October in Dongting Lake, which changes the concentrations of nitrogen and phosphorus in soils and thus weakens the relationship between the element concentrations in plants and those in soils. On the other hand, during the flood period, plants can absorb nitrogen and phosphorus from the water, reducing the links between the element concentrations in plants and soils. Additionally, significant regression coefficients were observed between the mean phosphorus concentrations in plants with plot elevation, soil moisture, soil organic matter concentration, and soil total potassium in this study.
Amounts of nitrogen and phosphorus released in the in situ experiment
In this study, the decomposition rate of stems was significantly lower than that of leaves. Similar results were obtained from a study on Salix triandroides, a native plant species in the Dongting Lake wetlands (Bian, 2020). Compared with leaves, stems and branches contain higher concentrations of lignin and cellulose, which contribute to the lower decomposition rate in these plant organs (dos Santos Fonseca et al., 2013). The stems, therefore, require a longer time to complete decomposition compared to the leaves (Xie et al., 2017). It was found that the half decomposition time was 1.12 years for stems and 0.61 years for leaves, and the complete decomposition time was 4.95 years for stems and 2.83 years for leaves. Unlike the stems on the soil surface designed in the in situ experiment, some stems in the field remained standing in the non-harvest scenario. These stems that remain standing are often colonized slowly by decomposing organisms (Mäkinen et al., 2006); hence, standing stems have a lower decomposition rate than that of fallen stems. Therefore, the half and complete decomposition time for the stems of M. lutarioriparius would be slightly longer than the estimated time.
The relative release indices changed obviously over time. For leaves, the relative release indices of nitrogen and phosphorus were above zero, indicating that there was a net release of nitrogen and phosphorus. This result is consistent with previous research conducted on the wetland plant Carex cinerascens (Zhang et al., 2020). Litters were buried during flooding, and many decomposition products were either absorbed on soil exchange surfaces or remained available for direct root uptake (Shure et al., 1986). For stems, the relative release indices of nitrogen and phosphorus were lower than zero in the middle stage of the experiment and higher than zero in the early and later stages, indicating a net fixation of nitrogen and phosphorus in the middle stage. Unlike other experiment conditions, the litter in situ experiment in this study was in a special environment condition with alternate flooding and non-flooding. During the long flood period, large amounts of nutrients, such as phosphorus, were carried by water into the lake and deposited into the sediment (Huang et al., 2022). Meanwhile, some nutrients were partially absorbed by the litter (Wang et al., 2018). Exogenous nitrogen and phosphorus supplementation, therefore, leads to increased concentrations of nitrogen and phosphorus in litters in the flood period (Figure 5) and contributes to negative relative release indices of the two elements in the period (Figure 3).
After litter decomposition of 1 year, the relative release indices of nitrogen and phosphorus in the leaves reached 87.2 and 78.4%, and those in the stems reached 39.7 and 48.6%, respectively. Combined with the distribution area of M. lutarioriparius in the Dongting Lake wetlands and the plant biomass in the unit area, the release amounts of nitrogen and phosphorus from the aboveground parts in the first year after the non-harvest of the plant were 2,394, and 232 t, respectively.
Nitrogen and phosphorus sinks between harvest and non-harvest
In the harvest scenario, the aboveground parts of M. lutarioriparius were harvested and removed from the Dongting Lake wetlands, and annual element sinks were 4,168 t for nitrogen and 404 t for phosphorus. In the non-harvest scenario, considering that the aboveground parts were not harvested and would decompose in the wetland ecosystems, the element sinks in the first year after the non-harvest were 1,774 t for nitrogen and 172 t for phosphorus. Based on the model of mass residual ratio in litters in the in situ experiment, it was found that the nitrogen and phosphorus sinks increased greatly over time in the harvest scenario, while they increased gradually from the first year to the fifth year in the non-harvest scenario and reached a maximum value after the fifth year. Nitrogen and phosphorus sinks therefore greatly decreased after the non-harvest of M. lutarioriparius compared to that after harvest.
Besides nitrogen and phosphorus, plant harvest also removes several other pollutants, such as heavy metals. A study conducted by Yao et al. (2018) demonstrated that 0.7 t cadmium, 22.9 t copper, 77.5 t manganese, 3.1 t lead, and 95.9 t zinc were removed per year from the Dongting Lake wetlands through the annual harvest of the aboveground parts of M. lutarioriparius. Additionally, a large amount of the fixed elements (nitrogen, phosphorus, heavy metals, etc.) would be released into the environment, leading to new ecological and environmental problems in the non-harvest scenario. A new study demonstrated that the decomposition of M. lutarioriparius consumed the dissolved oxygen and increased the carbon, nitrogen, and phosphorus concentrations in the water (Zhao et al., 2021). The non-harvest of M. lutarioriparius may therefore have far-reaching impacts on the Dongting Lake wetlands. On the other hand, M. lutarioriparius is used to make diverse products, such as acetylated lignin, 2,5-furandicarboxylic acid, and particleboard (Chen et al., 2018; Chai et al., 2021; Liao et al., 2021). It is therefore recommended to continue to harvest M. lutarioriparius for ecosystem health and stability.
Data availability statement
The raw data supporting the conclusions of this article will be made available by the authors, without undue reservation.
Author contributions
YL designed the study. ZP and YD conducted the field investigation and the in situ experiment. SN, LX, and YN participated the two experiments. ZP and YL wrote the manuscript and other authors revised it. All authors contributed to the article and approved the submitted version.
Funding
This study was supported by the Joint Fund for Regional Innovation and Development of NSFC (U21A2009), the Leading Plan for Scientific and Technological Innovation of High-tech Industries in Hunan Province (2020SK2019), the Key Program of Research and Development of Hunan Province (2022SK2088 and 2019SK2336), and the Natural Science Foundation of Hunan Province (2021JJ30330).
Conflict of interest
The authors declare that the research was conducted in the absence of any commercial or financial relationships that could be construed as a potential conflict of interest.
Publisher’s note
All claims expressed in this article are solely those of the authors and do not necessarily represent those of their affiliated organizations, or those of the publisher, the editors and the reviewers. Any product that may be evaluated in this article, or claim that may be made by its manufacturer, is not guaranteed or endorsed by the publisher.
Supplementary material
The Supplementary Material for this article can be found online at: https://www.frontiersin.org/articles/10.3389/fpls.2022.989931/full#supplementary-material
References
Baldantoni, D., Alfani, A., Di Tommasi, P., Bartoli, G., and De Santo, A. V. (2004). Assessment of macro and microelement accumulation capability of two aquatic plants. Environ. Pollut. 130, 149–156. doi: 10.1016/j.envpol.2003.12.015
Barrow, N. (2017). The effects of pH on phosphate uptake from the soil. Plant Soil 410, 401–410. doi: 10.1007/s11104-016-3008-9
Bian, H. L. (2020). Carbon sink in Communities of Salix Triandroides and Carex Brevicuspis in Dongting Lake Wetlands, Ph.D Thesis. Changsha: Hunan Agricultural University.
Bonanno, G., and Giudice, R. L. (2010). Heavy metal bioaccumulation by the organs of Phragmites australis (common reed) and their potential use as contamination indicators. Ecol. Indic. 10, 639–645. doi: 10.1016/j.ecolind.2009.11.002
Chai, Y. Z., Yang, H. C., Bai, M., Chen, A. W., Peng, L., Yan, B. H., et al. (2021). Direct production of 2, 5-Furandicarboxylicacid from raw biomass by manganese dioxide catalysis cooperated with ultrasonic-assisted diluted acid pretreatment. Bioresou. Technol. 337:125421. doi: 10.1016/j.biortech.2021.125421
Chen, T. Y., Li, Z. W., Zhang, X. M., Min, D. Y., Wu, Y. Y., Wen, J. L., et al. (2018). Effects of hydrothermal pretreatment on the structural characteristics of organosolv lignin from Triarrhena lutarioriparia. Polymers 10:1157. doi: 10.3390/polym10101157
Chen, X. S., Li, X., Xie, Y. H., Li, F., Hou, Z. Y., and Zeng, J. (2015). Combined influence of hydrological gradient and edaphic factors on the distribution of macrophyte communities in Dongting Lake wetlands. China. Wetl. Ecol. Manag. 23, 481–490.
dos Santos Fonseca, A. L., Bianchini, I., Pimenta, C. M. M., Soares, C. B. P., and Mangiavacchi, N. (2013). The flow velocity as driving force for decomposition of leaves and twigs. Hydrobiologia 703, 59–67. doi: 10.1007/s10750-012-1342-3
Fernández-Aláez, M., Fernández-Aláez, C., and Bécares, E. (1999). “Nutrient content in macrophytes in Spanish shallow lakes,” in Shallow Lakes’ 98, eds N. Walz and B. Nixdorf (Dordrecht: Springer), 317–326.
Granéli, W., and Solander, D. (1988). Influence of aquatic macrophytes on phosphorus cycling in lakes. Hydrobiologia 170, 245–266. doi: 10.1007/978-94-017-2986-4_35
Grizzetti, B., Billen, G., Davidson, E. A., Winiwarter, W., Vries, W. D., Fowler, D., et al. (2020). “Global nitrogen and phosphorus pollution,” in Just Enough Nitrogen, eds M. A. Sutton, K. E. Mason, and A. Bleeker (Cham: Springer), 421–431. doi: 10.1007/978-3-030-58065-0_28
Huang, Y., Chen, X. S., Li, F., Hou, Z. Y., Li, X., Zeng, J., et al. (2022). Concurrent effects of flooding regimes and floodwater quality on sediment properties in a Yangtze River-connected floodplain wetland: Insights from field investigations during 2011-2020. Sci. Total Environ. 827:154225. doi: 10.1016/j.scitotenv.2022.154225
Jin, X., Wang, S., Pang, Y., and Wu, F. C. (2006). Phosphorus fractions and the effect of pH on the phosphorus release of the sediments from different trophic areas in Taihu Lake. China. Environ. Pollut. 139, 288–295. doi: 10.1016/j.envpol.2005.05.010
Kern-Hansen, V., and Dawson, F. H. (1978). “The standing crop of aquatic plants of lowland streams in Denmark and the inter-relationships of nutrients in plant, sediment and water,” in Proceedings 5th EWRS International Symposium on Aquatic Weeds, (Amsterdam), 143–150.
Kuehn, K. A., and Suberkropp, K. (1998). Decomposition of standing litter of the freshwater emergent macrophyte Juncus effusus. Freshwater Biol. 40, 717–727. doi: 10.1046/j.1365-2427.1998.00374.x
Liao, Q. Y., Zhang, J., Yi, Z. L., and Li, Y. Z. (2021). Do Miscanthus lutarioriparius-Based Oriented Strand Boards Provide Environmentally Benign Alternatives? An LCA Case Study of Lake Dongting District in China. Sustainability 13:12976. doi: 10.3390/su132312976
Liu, G. S. (1996). Soil Physical and Chemical Analysis and Description of Profile. Beijing: China Standards Press.
Lu, Q., He, Z. L., Graetz, D. A., Stoffella, P. J., and Yang, X. (2010). Phytoremediation to remove nutrients and improve eutrophic stormwaters using water lettuce (Pistia stratiotes L.). Environ. Sci. Pollut. R. 17, 84–96. doi: 10.1007/s11356-008-0094-0
Luo, Y., Peng, Q. W., Li, K. H., Gong, Y. M., Liu, Y. Y., and Han, W. X. (2021). Patterns of nitrogen and phosphorus stoichiometry among leaf, stem and root of desert plants and responses to climate and soil factors in Xinjiang. China. Catena 199:105100. doi: 10.1016/j.catena.2020.105100
Mäkinen, H., Hynynen, J., Siitonen, J., and Sievänen, R. (2006). Predicting the decomposition of Scots pine, Norway spruce, and birch stems in Finland. Ecol. Appl. 16, 1865–1879. doi: 10.1890/1051-07612006016
McClaugherty, C. A., Pastor, J., Aber, J. D., and Melillo, J. M. (1985). Forest litter decomposition in relation to soil nitrogen dynamics and litter quality. Ecology 66, 266–275. doi: 10.2307/1941327
Morton, C., Cameron, R., and Duinker, P. (2010). Modeling carbon budgets for four protected wilderness areas in Nova Scotia, eds S. Bondrup-Nielsen, K. Beazley, G. Bissix, D. Colville, and S. Flemming Ecosystem Based Management: Beyond Boundaries. (Wolfville, NS: Science and Management of Protected Areas Association), 429–440.
Nash, D. A. H., Abdullah, S. R. S., Hasan, H. A., Mushrifah, I., Muhammad, N. F., Al-Baldawi, I. A., et al. (2019). Phytoremediation of Nutrients and Organic Carbon from Sago Mill Effluent using Water Hyacinth (Eichhornia crassipes). J. Eng. Technol. Sci 51, 573–584.12. doi: 10.5614/j.eng.technol.sci.2019.51.4.8
Nixon, S. W., Buckley, B. A., Granger, S. L., Harris, L. A., Oczkowski, A. J., Fulweiler, R. W., et al. (2008). Nitrogen and Phosphorus Inputs to Narragansett Bay: Past, Present, and Future. Science for Ecosystem-Based Management. New York, NY: Springer, 101–175. doi: 10.1007/978-0-387-35299-2_5
Olson, J. S. (1963). Energy storage and the balance of producers and decomposers in ecological systems. Ecology 44, 322–331. doi: 10.2307/1932179
Rezania, S., Park, J., Rupani, P. F., Darajeh, N., Xu, X., and Shahrokhishahraki, R. (2019). Phytoremediation potential and control of Phragmites australis as a green phytomass: An overview. Environ. Sci. Pollut. R. 26, 7428–7441. doi: 10.1007/s11356-019-04300-4
Sajedi, T., Prescott, C. E., Seely, B., and Lavkulich, L. M. (2012). Relationships among soil moisture, aeration and plant communities in natural and harvested coniferous forests in coastal British Columbia. Canada. J. Ecol. 100, 605–618. doi: 10.1111/j.1365-2745.2011.01942.x
Shure, D. J., Gottschalk, M. R., and Parsons, K. A. (1986). Litter decomposition processes in a floodplain forest. Am. Midl. Nat 115, 314–327. doi: 10.2307/2425868
Song, J. Y., and Shan, B. Q. (2012). Nitrogen and phosphorus absorption capacity of Phragmites australis in constructed pond-wetland system for reclaimed water treatment. Environ. Sci. Technol. 35, 16–19.
van der Valk, A. G., Rhymer, J. M., and Murkin, H. R. (1991). Flooding and the decomposition of litter of four emergent plant species in a prairie wetland. Wetlands 11, 1–16. doi: 10.1007/bf03160837
Wang, L. Z., Liu, Q. J., Hu, C. W., Liang, R. J., Qiu, J. C., and Wang, Y. (2018). Phosphorus release during decomposition of the submerged macrophyte Potamogeton crispus. Limnology 19, 355–366. doi: 10.1007/s10201-018-0538-2
Xie, Y. H., Tang, Y., Chen, X. S., Li, F., and Deng, Z. M. (2015). The impact of Three Gorges Dam on the downstream eco-hydrological environment and vegetation distribution of East Dongting Lake. Ecohydrology 8, 738–746. doi: 10.1002/eco.1543
Xie, Y. J., Xie, Y. H., Xiao, H. Y., Chen, X. S., and Li, F. (2017). Controls on litter decomposition of emergent macrophyte in Dongting Lake wetlands. Ecosystems 20, 1383–1389. doi: 10.1007/s10021-017-0119-y
Xu, Y. Z., Fu, G. Y., Tang, N., He, Z. H., Jian, L. C., and Zhao, Y. Y. (2021). Distribution of Triarrhena lutarioriparia and its reserve characteristics of nitrogen and phosphorus in Dongting Lake. In E3S Web of Conferences. EDP Sci. 237:01004. doi: 10.1051/e3sconf/202123701004
Yang, Y., Gao, B., Hao, H., Zhou, H. D., and Lu, J. (2017). Nitrogen and phosphorus in sediments in China: A national-scale assessment and review. Sci. Total Environ. 576, 840–849. doi: 10.1016/j.scitotenv.2016.10.136
Yao, X., Niu, Y. D., Li, Y. L., Zou, D. S., Ding, X. H., and Bian, H. L. (2018). Heavy metal bioaccumulation by Miscanthus sacchariflorus and its potential for removing metals from the Dongting Lake wetlands. China. Environ. Sci. Pollut. R. 25, 20003–20011. doi: 10.1007/s11356-018-2174-0
Zhang, Q. J., Zhang, G. S., Yu, X. B., Liu, Y., Xia, S. X., Meng, Z. J., et al. (2020). Dynamic characteristics of the decomposition rate and carbon, nitrogen and phosphorus release of the dominant plants in Poyang Lake Wetland. Acta Ecol. Sinaca 40, 8905–8916. doi: 10.5846/stxb201909161925
Keywords: nitrogen sinks, phosphorus sinks, harvest, non-harvest, litter decomposition, relative release indices
Citation: Peng Z, Du Y, Niu S, Xi L, Niu Y and Li Y (2022) Differences in nitrogen and phosphorus sinks between the harvest and non-harvest of Miscanthus lutarioriparius in the Dongting Lake wetlands. Front. Plant Sci. 13:989931. doi: 10.3389/fpls.2022.989931
Received: 09 July 2022; Accepted: 22 August 2022;
Published: 08 September 2022.
Edited by:
Yong Li, Chinese Academy of Forestry, ChinaReviewed by:
Peng Li, Hainan Normal University, ChinaXinsheng Chen, Anhui University, China
Yonghong Xie, Key Laboratory of Agro-Ecological Processes in Subtropical Region, Institute of Subtropical Agriculture (CAS), China
Copyright © 2022 Peng, Du, Niu, Xi, Niu and Li. This is an open-access article distributed under the terms of the Creative Commons Attribution License (CC BY). The use, distribution or reproduction in other forums is permitted, provided the original author(s) and the copyright owner(s) are credited and that the original publication in this journal is cited, in accordance with accepted academic practice. No use, distribution or reproduction is permitted which does not comply with these terms.
*Correspondence: Youzhi Li, bGl5b3V6aGkyMDA0QDE2My5jb20=