- Department of General Biology, Federal University of Viçosa, Viçosa, Brazil
For popcorn, obtaining and identifying haploids are still challenging steps. We aimed to induce and screen haploids in popcorn using the Navajo phenotype, seedling vigor, and ploidy level. We used the Krasnodar Haploid Inducer (KHI) in crosses with 20 popcorn source germplasms and five maize controls. The field trial design was completely randomized, with three replications. We assessed the efficacy of induction and identification of haploids based on the haploidy induction rate (HIR) and false positive and negative rates (FPR and FNR). Additionally, we also measured the penetrance of the Navajo marker gene (R1-nj). All putative haploids classified by the R1-nj were germinated together with a diploid sample and evaluated for false positives and negatives based on vigor. Seedlings from 14 females were submitted to flow cytometry to determine the ploidy level. The HIR and penetrance were analyzed by fitting a generalized linear model with a logit link function. The HIR of the KHI, adjusted by cytometry, ranged from 0.0 to 1.2%, with a mean of 0.34%. The average FPR from screening based on the Navajo phenotype was 26.2% and 76.4% for vigor and ploidy, respectively. The FNR was zero. The penetrance of R1-nj ranged from 30.8 to 98.6%. The average number of seeds per ear in temperate germplasm (76) was lower than that obtained in tropical germplasm (98). There is an induction of haploids in germplasm of tropical and temperate origin. We recommend the selection of haploids associated with the Navajo phenotype with a direct method of confirming the ploidy level, such as flow cytometry. We also show that haploid screening based on Navajo phenotype and seedling vigor reduces misclassification. The origin and genetic background of the source germplasm influence the R1-nj penetrance. Because the known inducers are maize, developing doubled haploid technology for popcorn hybrid breeding requires overcoming unilateral cross-incompatibility.
Introduction
Doubled-haploid (DH) technology allows for obtaining homozygous genotypes in a shorter period, increasing genetic gains and efficiency in hybrid development, reducing costs (Melchinger et al., 2005; Chaikam et al., 2019; Molenaar et al., 2019). The development of DH lines consists of the induction of seeds with haploid embryos, identification of these seeds, duplication of the chromosome set, and multiplication of DH0 seeds (Prigge and Melchinger, 2012). Haploid induction is based on the in vivo induction method, which consists of using haploid inducers in crosses with a germplasm source. These inducers can be used as pollen donors, generating gymnogenetic haploids, or as pollen receptors, generating androgenetic haploids (Sarkar and Coe, 1966). Inducers are usually homozygotes for the R-Navajo gene (R1-nj), a dominantly acting marker that causes purple coloration of the scutellum and aleurone layer (Navajo phenotype). The staining of the scutellum and aleurone layer is used as embryo and endosperm markers, respectively, to identify haploid seeds (Kleiber et al., 2012).
The haploid induction rate (HIR) is a critical characteristic of a haploid inducer, calculated as the ratio of the number of haploid seeds to the total number of seeds produced in an induction cross. HIR is the common parameter used to compare the haploid induction efficiency of different inducers (Almeida et al., 2020). HIR is influenced by the genetic background inducer and by the source germplasm (Chase, 1952; Coe, 1959; Lashermes and Beckert, 1988; Eder and Chalyk, 2002; Prigge et al., 2011) and varies on average from 8% to 15% (Röber, 1999; Rotarenco et al., 2010; Chaikam et al., 2019). In vivo haploid induction is a quantitative trait controlled by many unknown loci (Lashermes and Beckert, 1988; Röber et al., 2005), and it has been found that the qhir11 subregion has a significant effect on the induction rate (Nair et al., 2017). Within this region, the gene encoding phospholipase A, called MATRILINEAL (MTL), has been identified as responsible for haploid induction (Kelliher et al., 2017; Liu et al., 2017; Almeida et al., 2020).
The C2, Whp1, A1, and A2 genes are required for anthocyanin biosynthesis. Homozygous recessive genotypes for any of these genes do not synthesize anthocyanin, and the absence of a dominant allele interrupts the pathway in the aleurone layer (Ford, 2000). Variation in R1-nj expression, both for the labeled area and for the intensity of anthocyanin in the endosperm and embryo, affects the accuracy of haploid identification. The low expression of the marked area and the low intensity of anthocyanin staining in the endosperm or embryo are generally associated with high rates of misclassification (Prigge et al., 2011). In a haploidy-inducing cross, depending on the homozygosity and heterozygosity of the inhibitory alleles in the population, the R1-nj marker may be completely inhibited or color expression may segregate (Chaikam et al., 2015). The advantage of R1-nj is the possibility of identifying haploid individuals even before germination.
Fertilization in maize occurs through the interaction between the male gametophyte (pollen) and the female sporophyte (silk). The in vivo induction of haploids strictly depends on this process. However, the existence of an incompatibility system that affects fertilization between some maize and popcorn genotypes can prevent the occurrence of fertilization and, consequently, the induction of haploidy. The main incompatibility system known in maize is the gametophyte factor 1 (ga1) (Kermicle and Evans, 2010; Zhang et al., 2018). For this system, three alleles are known: ga1, Ga1-s, and Ga1-m. The ga1 allele is recessive and receptive to any pollen genotype but cannot pollinate Ga1-s plants (Kermicle and Evans, 2005). The Ga1-s allele is codominant and receptive to Ga1-s and Ga1-m pollen but not to ga1 pollen (Demerec, 1929; Schwartz, 1950; Kermicle, 2006). The Ga1-m allele is dominant and capable of pollinating and being pollinated by any of these alleles (Mangelsdorf and Jones, 1926; Nelson, 1952). The Ga1-s allele is common in popcorn (Kermicle, 2006), while most other types of corn, including the known inducers, carry the ga1 allele and therefore are susceptible to the fertilization barrier imposed by Ga1-s (Moran Lauter et al., 2017).
Although DH technology could potentially accelerate maize reproduction, it has been little explored in the development of popcorn lines. This is because, despite belonging to the same species, popcorn has particularities that make obtaining DH lines from this special type of maize a challenge. For example, the smaller and rounder grains with flint endosperm make haploid screening more difficult, as it is harder to visualize the expression of the R1-nj gene in the endosperm and embryo compared to maize. Additionally, the temperate germplasm of American origin carries the Ga1-s allele, which causes unilateral cross-incompatibility, preventing popcorn pollination by the pollen inducer. Therefore, our objective was to induce maternal haploids in vivo and identify, through the Navajo phenotype, seedling vigor, and ploidy level, one of the initial steps towards developing DH technology for popcorn.
Materials and methods
Genetic material
The Krasnodar haploid inducer (KHI), a temperate population, was used as the gymnogenetic inducer (Milani et al., 2016). The females included eight popcorn populations, 10 popcorn inbred lines, seven popcorn interpopulation hybrids, three maize single crosses, a maize two-way cross, and a maize population (Table 1). The tropical populations Viçosa C4 and Beija-Flor C4 were obtained from the Viçosa and Beija-Flor populations, respectively, after four half-sib selection cycles. The tropical population Synthetic UFV was derived by crossing 40 elite inbred lines from Viçosa and Beija-Flor. The five temperate populations (UFV-MP1, UFV-MP2, UFV-MP3, UFV-MP4, and UFV-MP5) were derived from the North American hybrids P622, P625, P802, AP2501, and AP4502, respectively, developed by the Agricultural Alumni Seed Improvement Association, Romney, IN, USA. The inbreed progenies 18-303-2 and 18-390-1 were derived from UFV-MP1, 19-206-6 from UFV-MP2, 18-176-5 from UFV-MP3, 19-504-4 from UFV-MP5, 20-2039 and 20-2064 from Viçosa C4, and 20-2009, 20-2010, and 20-2024 were derived from Beija-Flor C4.
Induction crosses
The induction crosses occurred naturally in the 2021–2022 growing season in an isolated field located in an experimental area at the Federal University of Viçosa (UFV) in the municipality of Viçosa (20°50’30”S, 42°48’30”W, at an altitude of 720 m). The experiment design was completely randomized, with 25 treatments and three replications. Each pollination plot consisted of two 5-m rows of females to be induced. They were detasseled before anther dehiscence. Four rows of the inducer were planted in each plot at four different times. The first planting was performed one week after sowing the females. The other plantings were performed at five-day intervals after the first inducer planting. An outer border with two rows of inducer was also sown. The females and the inducers were planted with a spacing of 20 cm between plants and 70 cm between rows. Thinning was performed at stage v3 to remove the less developed plants so that there were five plants per m.
Identification of haploids
For each replication, the seeds resulting from the crosses were visually separated according to the appearance of purple on the endosperm and embryo (Navajo phenotype), based on the methodology described by Nanda and Chase (1966), to identify haploids; thus, seeds with purple endosperms and white embryos were classified as haploids, those with purple endosperms and purple embryos were classified as diploids, and the seeds without anthocyanin expression in the embryo and endosperm were classified as yellow (Figure 1). In addition to the classes proposed by Nanda and Chase (1966), the purple seed class was also considered a measure of R1-nj penetrance, which was defined by the sum of the haploid and diploid classes. Based on this classification, the estimates of the haploid induction rate (HIR) based on the R1-nj and gene penetrance were obtained by HIR = haploids/total and Penetrance = purples/total.
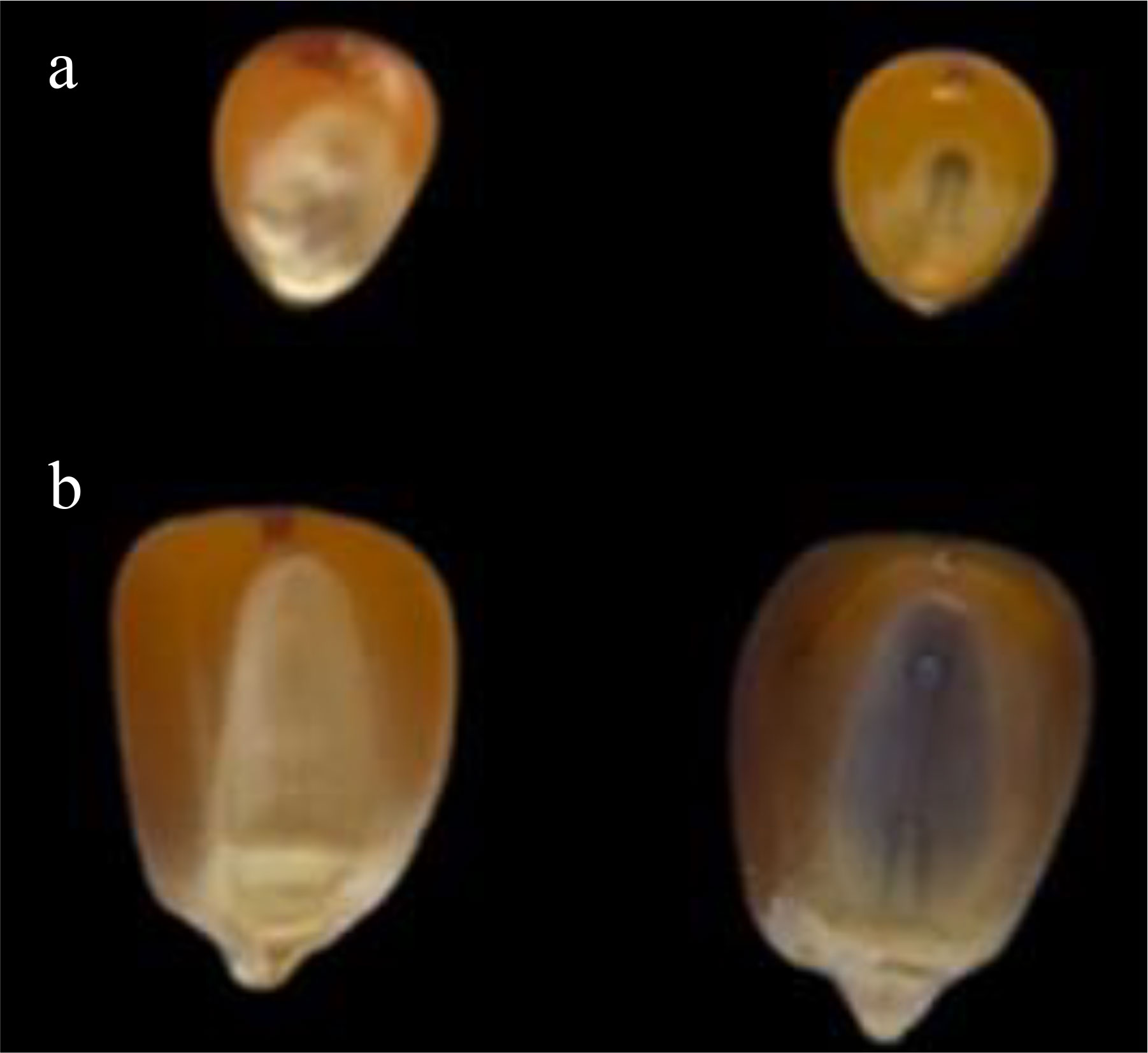
Figure 1 Classification based on the R1-nj marker. (A) Induced popcorn seeds. (B) Induced maize seeds. On the left, seeds showing purple coloration only in the endosperm were classified as putative haploids. On the right, seeds showing purple coloration in both the embryo and the endosperm were classified as diploids.
Seedling vigor
To evaluate vigor, the putative haploid seeds obtained in each pollination plot were germinated in trays containing two sheets of germitest paper and filled with sand. The number of haploid seeds obtained in each plot was variable, so the number of seedlings evaluated in each replicate and treatment was different. In addition to the haploid seeds, 10 putative diploid seeds and 10 seeds of the germplasm from which they originated were also germinated. Before planting, all seeds were treated with a fungicide. The relative vigor of the seedlings was evaluated 10 days after sowing. The evaluation was performed using the putative diploids as a reference. The taller seedlings with greater diameter leaves and stems and purple coloration were classified as diploids (Figure 2).
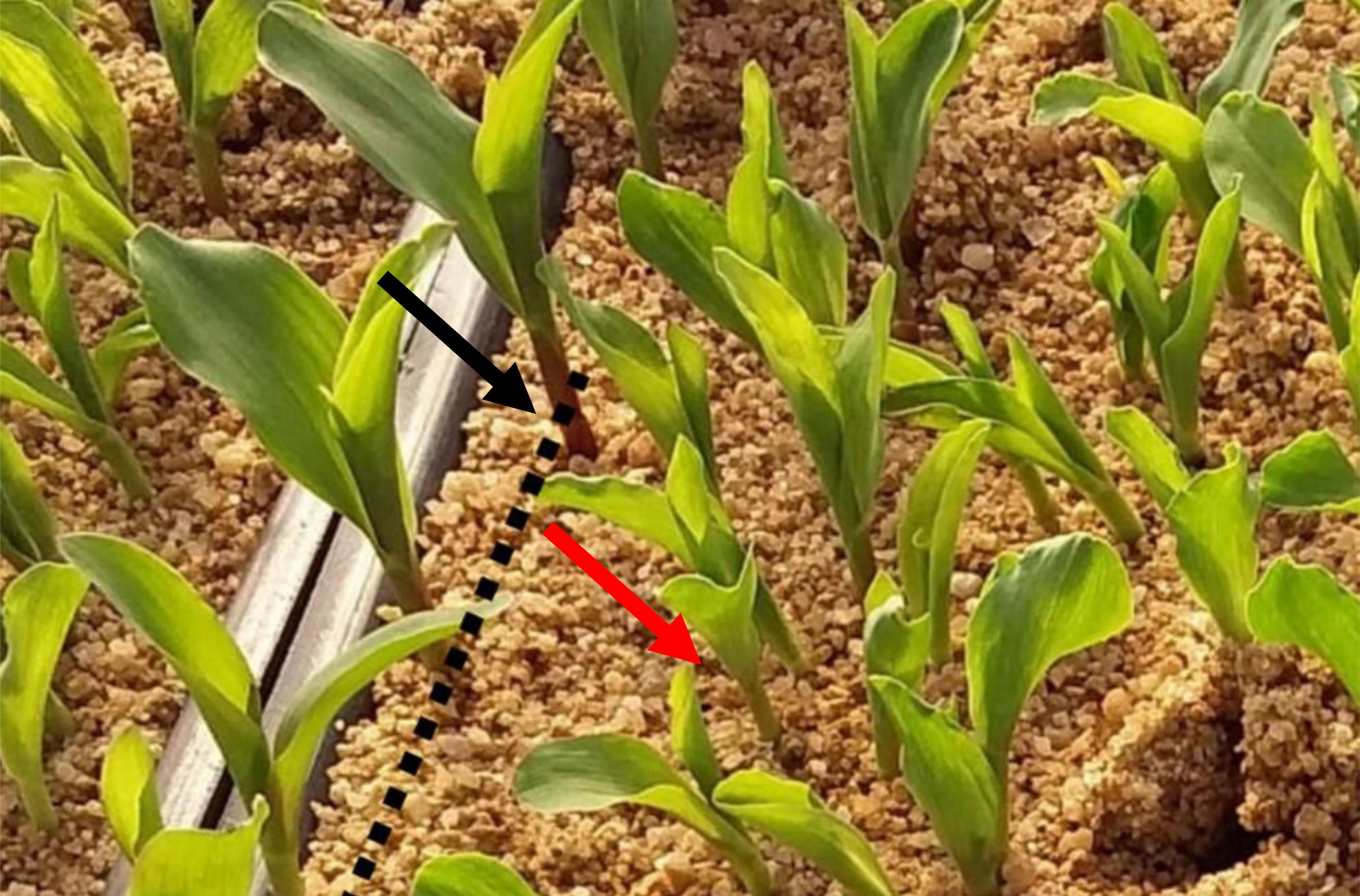
Figure 2 Seedling vigor evaluation. The dotted line separates the diploid reference line from the putative haploid group. The black arrow indicates a diploid. The red arrow indicates a putative haploid.
Ploidy level determination by flow cytometry
After evaluating vigor, the haploid seedlings from 14 of the 25 induced females, including those classified as false positives (FP) based on vigor, were subjected to flow cytometry to confirm the ploidy level. For each treatment, a sample from a diploid individual and a sample from the germplasm source were used as external standards. For nuclear extraction, fragments of young leaves of the evaluated individuals and the external standards were chopped in 500 µl of OTTO-I Otto (1990) buffer supplemented with 2.0 mM dithiothreitol (Sigma®). After 3 min, 500 µl of the same buffer was added, and the homogenate was filtered through a 30 µm nylon mesh (Partec®) and centrifuged at 100×g for 5 min. The supernatant was discarded, and the pellet was resuspended and incubated for 10 min in 100 μl of the OTTO-I buffer. The nuclear suspensions were stained in 500 µl of OTTO-I:OTTO-II buffer (1:2), supplemented with 2.0 mM dithiothreitol (Sigma®) and 75 µM propidium iodide (Sigma®) at room temperature for 30 min in the dark, filtered through 20 µm nylon mesh (Partec®) Praça-Fontes et al. (2011), and analyzed in a BD Accuri C6 flow cytometer. The histograms obtained from the nuclear suspensions of the possible haploid seedlings were compared to those provided by the external standards to determine the relative ploidy level of each sample (Supplementary Figure 1).
Statistical analysis
The putative haploids and putative diploids that were confirmed by the vigor test and flow cytometry were categorized as true positives (TP) and true negatives (TN), respectively. True diploids in the putative haploid fraction and true haploids in the putative diploid fraction that were also confirmed by the vigor test and cytometry were categorized as false positives (FP) and false negatives (FN), respectively. The numbers of TP, TN, FP, and FN were adjusted in each population based on the total number of putative haploids and diploids identified in the population based on the R1-nj. The data resulting from the level of ploidy classification were used to calculate the false positive rate (FPR), false negative rate (FNR), and Matthews correlation coefficient (MCC), according to the following equations: , , and , where FPR is the proportion of diploids in the seed fraction classified as putative haploids, FNR is the proportion of true haploids misclassified as diploid seeds, and MCC indicates the correlation between the actual and predicted ploidy level based on the marker R1-nj. The MCC has been suggested as a balanced measure of the quality of binary classifications, especially when the binary classes are of substantially different sizes (Chaikam et al., 2016). The MCC was developed by Brian W. Matthews and has become essential for measuring the performance of binary classification models. This coefficient fluctuates within the interval (−1, 1), and the higher its value is, the better the prediction ability of the classifier. On the other hand, when the assumed value is 0, there is no correlation between the two variables, i.e., the model is predicting randomly (Matthews, 1975).
For all characteristics, we assumed , where is the total number of seeds of the th genotype in replication , is the number of putative haploid seeds, and is the probability of success (probability of putative haploid seeds). We fit a generalized linear model (GLM) with a logit link function described by the following linear predictor:
To evaluate the goodness of fit of the model, we used visual inspection using seminormal plots, using the hnp package (Moral et al., 2017; Lenth, 2020). To compare the evaluated genotypes, we used asymptotic confidence intervals obtained by the emmeans package (Russell, 2020). All statistical analyses were performed in the R environment (Team, R. C 2021).
Results
Seed produced by crossing KHI × popcorn
Of the ten inbred lines pollinated by KHI, five did not produce seeds and were not considered in the analysis. The inbreed lines 19-1206-6, 19-1504-4, 18-390-1, and 18-303-2, for sure because they are derived from temperate germplasm, present unilateral cross-incompatibility alleles. Although tropical, the inbred line 20-2039 also did not produce seeds, probably because it contained these same alleles. Of the crosses that were fertile, a total of 63,232 seeds were obtained from 10 temperate (9,506), six tropical (44,019), and four tropical × temperate (19,707) germplasm sources. The average number of seeds/ear in temperate germplasm was 76, lower than that found in tropical germplasm (98).
Haploid/diploid misclassification using the Navajo phenotype
The FPR using the evaluation of seedling vigor as the “gold standard” ranged from 0 to 65.4%, with a mean of 26.2% (Table 2). Among the popcorn females, 65% presented an FPR >20%. The MCC value ranged from 0.32 to 1, with a mean of 0.77. The average FPR found in the inbred lines (28.5%) and interpopulation hybrids (26.3%) was slightly higher than the average value found for the populations (25.0%). The average FPR value found in the temperate germplasm (18.6%) was approximately 47% lower than that found in the tropical germplasm (35.0%). In the tropical × temperate germplasm, the average FPR value was 20.4%. Among the putative haploids generated from 20-2009, UFV-MP1, UFV-MP4, UFV-MP2 × UFV-MP5, and Synthetic UFV × UFV-MP1, no false positive was identified, and the MCC was 1. Among the inbred lines, the genotype 18-176-5 had the highest FPR (65.7%), but high estimates were also found for the females Beija-Flor C4 and Beija-Flor C4 × Synthetic UFV (FPR >50%). The average FPR found in the controls and popcorn was similar: 26.3 and 25.6%, respectively. There were no false negatives. However, the sample of putative diploids evaluated may have been.
The FPR, considering the level of ploidy determined by flow cytometry as the “gold standard”, ranged from 0% to 100% among the 14 germplasm sources evaluated. The average FPR obtained by the Navajo phenotype and by the Navajo phenotype associated with subsequent elimination of identified false positive by vigor was 76.4% and 69.2%, respectively (Table 3). The inbred lines had the lowest mean FPR (60.6%) compared to the populations (88.5%) and interpopulation hybrids (82.6%). Most of the studied females showed high FPR values (FPR >50%). The elimination of false positives by the vigor test reduced the FPR for most of the evaluated females, except for females 18-176-5, 20-2009, UFV-MP1, Beija-Flor C4, and Synthetic UFV × UFV-MP1. The average MCC values were low (0.38). Again, no false negatives were identified.
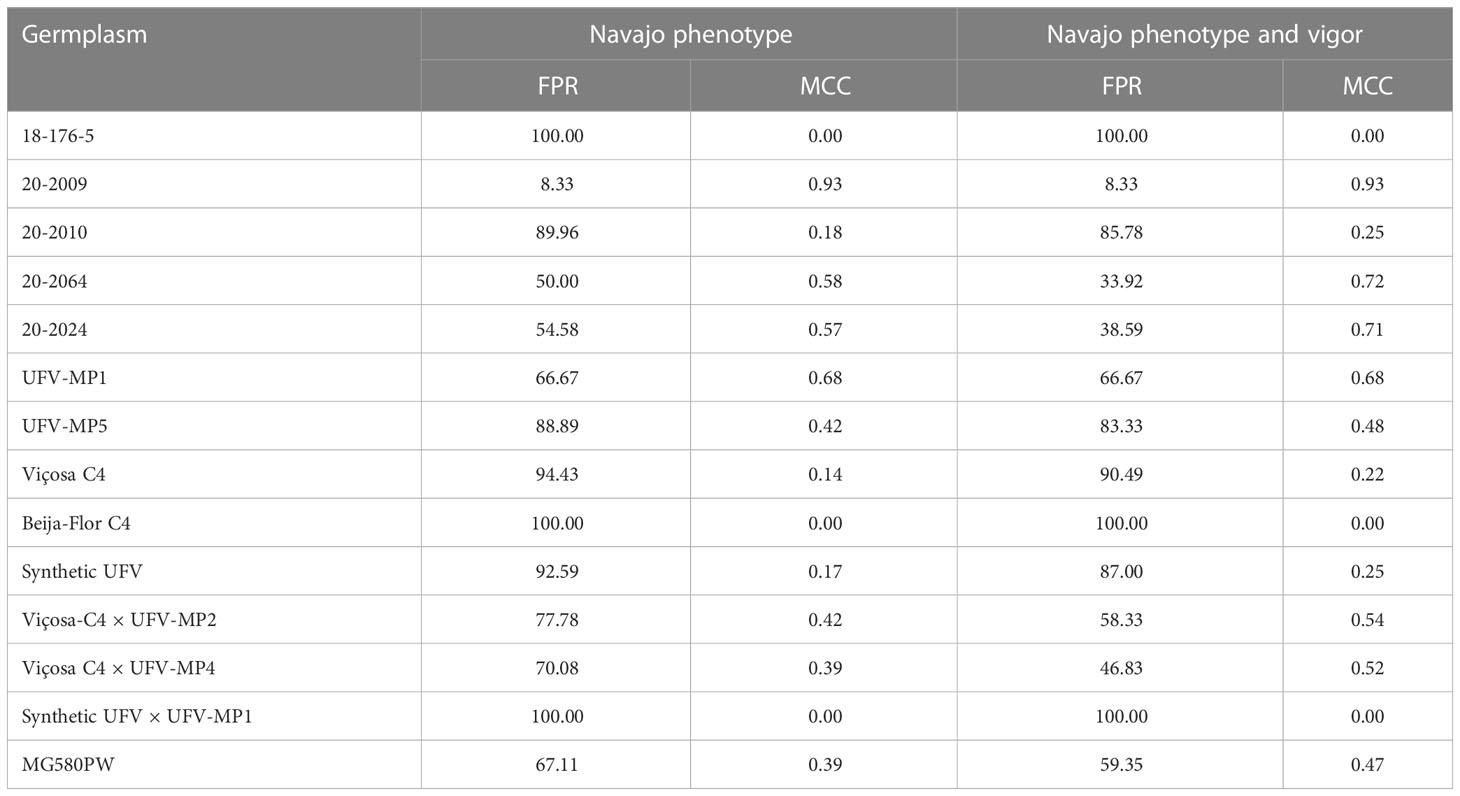
Table 3 False positive rate and Matthews correlation coefficient of Navajo phenotype and Navajo phenotype after elimination of false positives by vigor, using the ploidy level as the “gold standard.”.
Induction rate
Based on the binomial analysis of the HIR, which was adjusted after the elimination of false positives identified through the vigor test, a significant effect was found for all germplasm sources (p<0.01). The mean HIR for the KHI inducer in the evaluated germplasm sources was 0.76% (Table 4). Of the 677 putative haploid seedlings, 459 were confirmed as true haploid, including 126 of maize and 333 of popcorn (Supplementary Table 3). The overall average HIR of the KHI inducer in popcorn was 0.93%, while in maize the overall average value was much lower (0.07%). The HIR in the tropical popcorn germplasm was 1.5 times lower than in the temperate germplasm, with means of 0.89% and 1.32%, respectively. The average induction rate among the inbred lines was 1.57%, while the average in the populations was 0.85%. The lowest average induction was found in interpopulation hybrids (0.57%). Of the populations, UFV-MP4 had the highest mean induction. However, the confidence interval was large, with lower and upper limits between 0.76% and 7.07%.
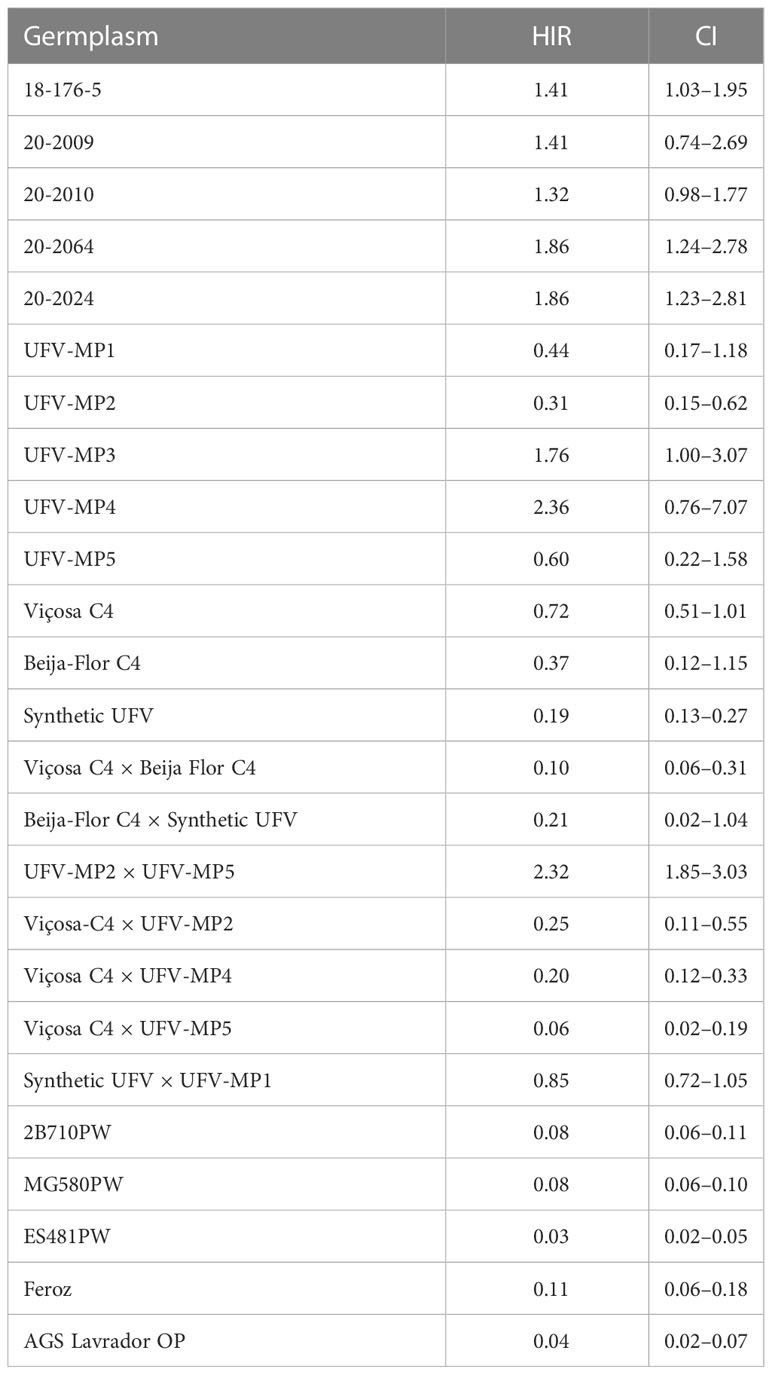
Table 4 Haploid induction rate adjusted by vigor and the confidence interval (CI) at 95% probability.
Based on the binomial analysis of the HIR, adjusted after the elimination of the false positives identified by the ploidy level, a significant effect was found for the germplasm sources (p<0.01). The overall mean HIR for the KHI inducer in the 14 germplasm sources evaluated was 0.32% (Table 5). Of the 677 putative haploid seedlings evaluated, only 78 were confirmed as true haploid by ploidy level (Supplementary Figure 1), being 18 of maize and 60 of popcorn (Supplementary Table 4). After adjusting the means by the FPR estimated through the FP identified by the ploidy level, the mean HIR of the KHI inducer in popcorn was 0.34%. The HIRs found for the inbred lines, populations, and interpopulation hybrids were 0.75%, 0.09%, and 0.07%, respectively. Only the genotypes 20-2009, 20-2064, and 20-2024 presented HIR >1%; in the other evaluated germplasm sources, the HIR values were lower than 0.25%.
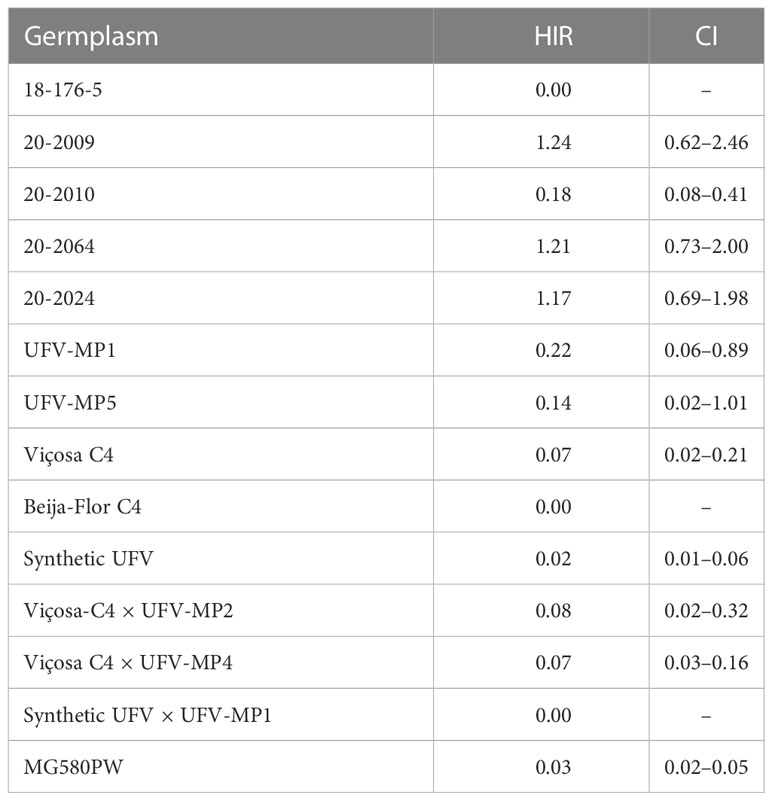
Table 5 Haploid induction rate adjusted by the ploidy level and the confidence interval (CI) at 95% probability.
Penetrance of the R1-nj
Binomial analysis of R1-nj penetrance showed significant effects on germplasm sources (p<0.01). The mean penetrance between the evaluated germplasm sources was 71.9% (Table 6). The mean penetrance of popcorn (69.9%) was lower than the mean of the control (79.9%). Seventy percent of the evaluated popcorn materials had a penetrance<65%, 40% had values between 65% and 80%, and 30% had a penetrance of >80%. The mean value of R1-nj penetrance in germplasms of tropical origin (67.2%) was lower than that of germplasms of origin (tropical × temperate) (67.8%) and temperate (74.6%). The inbred lines had the highest mean penetrance (80.2%), followed by populations (69.0%) and interpopulation hybrids (63.2%). The population Beija-Flor C4 presented the lowest penetrance value (30.8%), while genotype 20-2009 presented the highest value (98.6%).
Discussion
For the first time, we induced haploids in vivo in popcorn. We observed induction in tropical, temperate, and tropical × temperate germplasm. However, the number of seeds obtained per ear from crossing the KHI inducer with germplasm sources of temperate origin was much lower than that obtained from tropical germplasm. This can be clearly observed in ears harvested from some individuals in the UFV-MP4 temperate population, which showed a high rate of abortion (Figure 3). The temperate germplasm used for the induction of haploids is of North American origin, which would lead us to assume that they would be fixed for the incompatibility allele Ga1-s (Kermicle and Evans, 2010). However, as there was no seed production, it is more likely that they are Ga1-s/ga1-s, as this allele is codominant in relation to the ga1 allele; that is, the Ga1-s/ga1-s genotypes have partial fertility for ga1 pollen (Figure 2). Another hypothesis to explain the partial fertility observed in temperate germplasm is that the inducer is segregating. However, four inbred lines of temperate origin did not produce seeds, reducing the evidence for this hypothesis. Furthermore, these four genotypes are likely to be Ga1-s/Ga1-s, 100% incompatible with ga1 pollen. As exposed, there is evidence that the KHI inducer is ga1/ga1. In addition, it is likely that most inducers used for induction of haploids in maize have this genotypic constitution, which would prevent in vivo induction by gymnogenetic inducers in sources of Ga1-s/Ga1-s germplasm, the most common genotype in popcorn (Kermicle and Evans, 2010). In contrast, temperate germplasm is the most targeted by popcorn breeders. We advise two viable ways to overcome the inducer/popcorn incompatibility barrier: employing androgenetic inducers or modifying the genotypic constitution of gymnogenetic inducers. However, androgenetic inducers are not as effective for HIR as gymnogenetic inducers. Additionally, it is not possible to use isolated fields; that is, pollination must be done manually. Therefore, we recommend the introgression of alleles that overcome the fertility barrier imposed by Ga1-s, such as the Ga1-s allele itself or the Ga1-m allele.
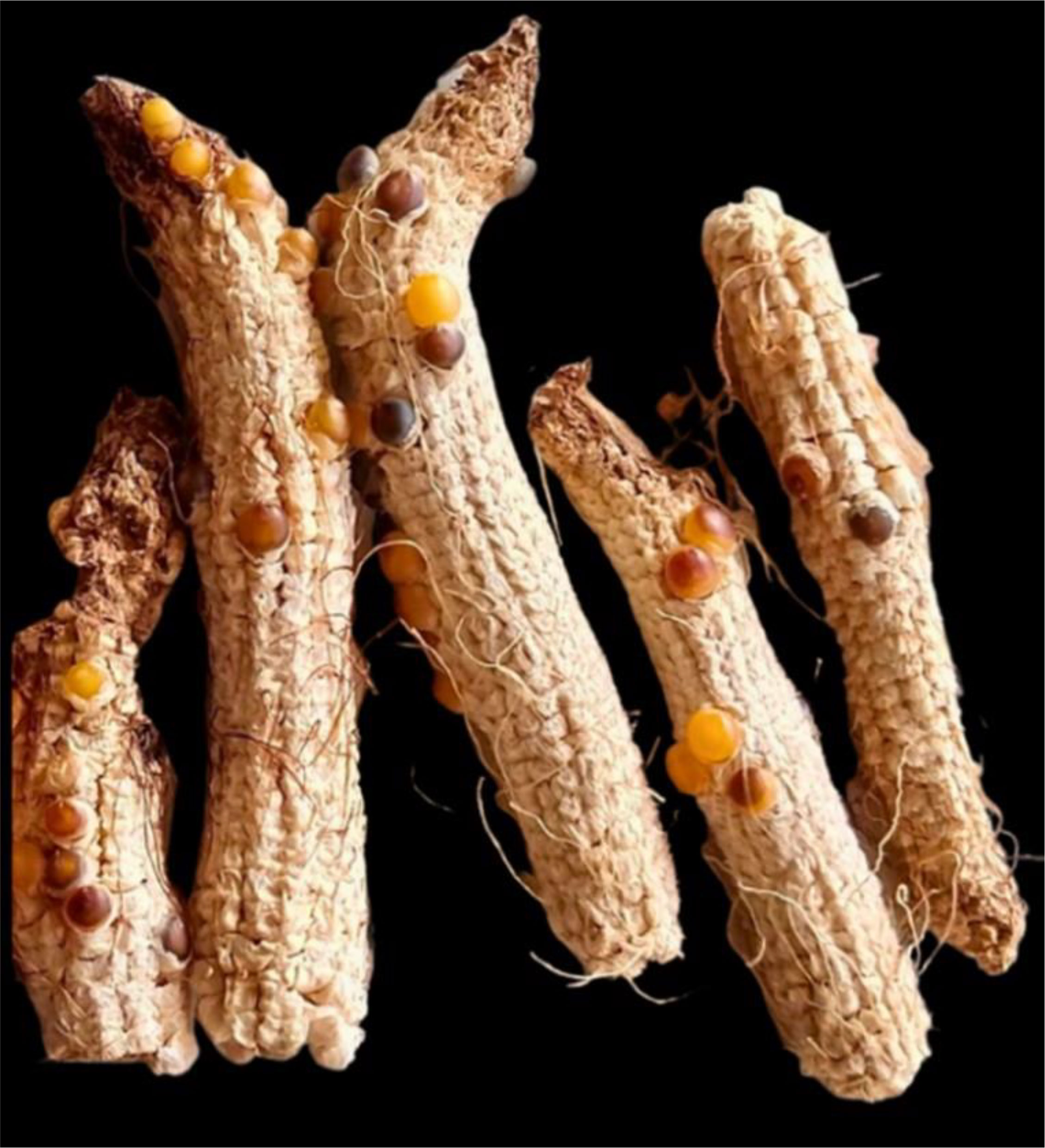
Figure 3 Ears of the UFV-MP4 population with grain abortion, indicating possible segregation for the GA1-s allele.
Concerning the efficacy of the Navajo phenotype, we expected to find higher FPR in popcorn due to the smaller size and rounded shape of the seeds, which make it difficult to visualize the embryo. However, the average FPR found in popcorn was lower than that found in the checks. Nonetheless, it is important to note that these differences may not reflect what would happen on a commercial scale. This is because the classification based on the Navajo phenotype is not automated, meaning it is done visually. Therefore, it is a laborious and tedious task that is prone to human error. Figure 4 illustrates well the difficulty in visualizing the purple coloration in the embryos of popcorn grains. The FPR values found in tropical and tropical × temperate germplasms were higher than those found in temperate germplasm. The high FPR associated with classification by the Navajo phenotype can be attributed to the presence of inhibitory genes, varied expressivity, and incomplete penetrance of this marker (Belicuas et al., 2007). In addition to environmental factors, such as the moisture content of the seeds and air pockets under the pericarp, these genes can also affect color intensity and consequently lead to an erroneous classification of the ploidy level of the evaluated classes (Rotarenco et al., 2009; Prigge et al., 2011). Although classification based on the Navajo phenotype led to high-magnitude FPR, FNR values were zero. This is especially important for inducers with low HIR, as is the case with the KHI inducer, because even with the increase in the size of plant populations in the induction fields, as recommended by Melchinger et al. (2013), there is a limited number of available haploids.
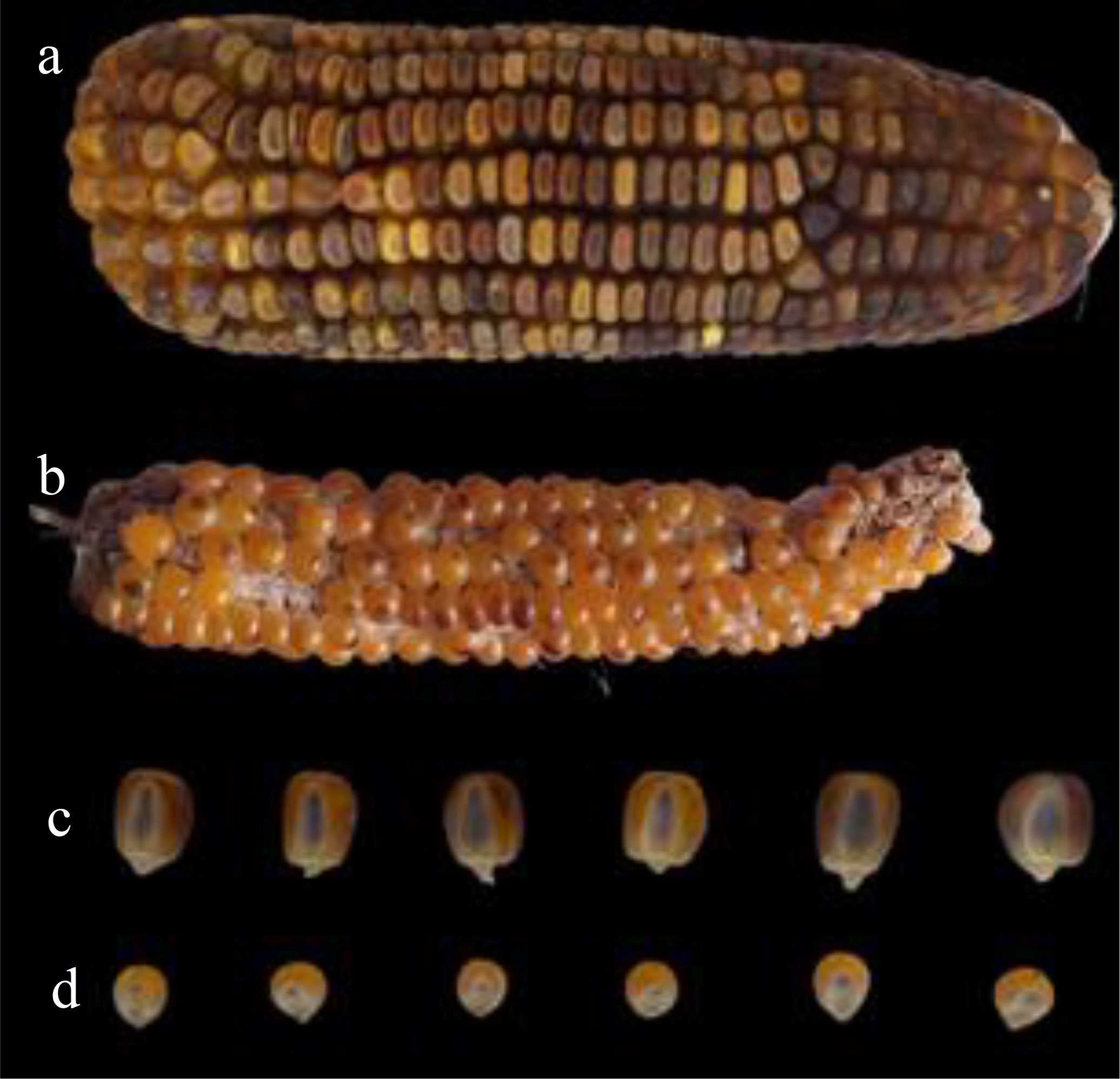
Figure 4 Morphological differences between popcorn and maize ears and grains. (A) Ear of the hybrid MG580-PW. (B) Ear of the population UFV-MP2. In (C) and (D), it is possible to observe the differences between maize and popcorn grains, respectively.
Elimination of false positives by vigor assessment reduced the FPR for most females evaluated. However, the mean values of MCC remained of low magnitude, indicating a low correlation between the ploidy determined by R1-nj and the true level of ploidy of the evaluated seedlings. Melchinger et al. (2013) found high FPR values, ranging from 7.8% to 67.6%, using the R1-nj marker. The elimination of false positives through the evaluation of seedling vigor can be useful, as it is an easy and low-tech method that allows reliably eliminating a fraction of the false positives since none of the 218 false positives identified was classified as true haploid by the ploidy level. Eliminating false positives before chromosome duplication is an important strategy to reduce costs with reagents, waste of space, inputs in greenhouses and/or DH0 seed multiplication fields, and labor resources (Choe et al., 2012; Chaikam et al., 2015).
The KHI haploidy inducer showed an average HIR of 0.93% in popcorn after adjusting the FPR by vigor and 0.34% if adjusted by ploidy level. These rates are very low, considering that inducers with HIR >10% are currently available, as in RWS and UH400 (Prigge et al., 2012; Chaikam et al., 2019). Although the HIR depends on both the inducer and the source of germplasm, the inducer has a greater contribution to make in determining this characteristic (Kebede et al., 2011). Thus, it is not possible to attribute the low HIR values found to popcorn.
To evaluate the expression of the R1-nj gene, we defined a variable obtained by the proportion between seeds that present the Navajo phenotype and the total number of induced seeds, and we called it penetrance. We found incomplete penetrance and varied expressivity of the R1-nj in the popcorn germplasm as well as in the controls (Figure 5). This was expected, as one of the main limitations of R1-nj in haploid/diploid selection is its incomplete penetrance and varied expressivity. The low values of the penetrance estimates in tropical and tropical × temperate germplasms indicate a higher frequency of inhibitors in these germplasms, such as C1-I, C2-Idf, and in-1D (Chaikam et al., 2015; Gain et al., 2022). Chaikam et al. (2015) propose a careful selection of parents that will be used in the synthesis of the source germplasm in the absence of anthocyanin inhibitors. The lower penetrance estimate of R1-nj in tropical popcorn germplasm indicates the need to use inducers equipped with other haploid/diploid classification systems that can complement R1-nj to identify haploids more efficiently. The average penetrance shown by the inbred lines was high. This result is important since the most used strategy to produce DH lines is the induction of haploids from bi-parental crosses between elite lines (Chaikam et al., 2019).
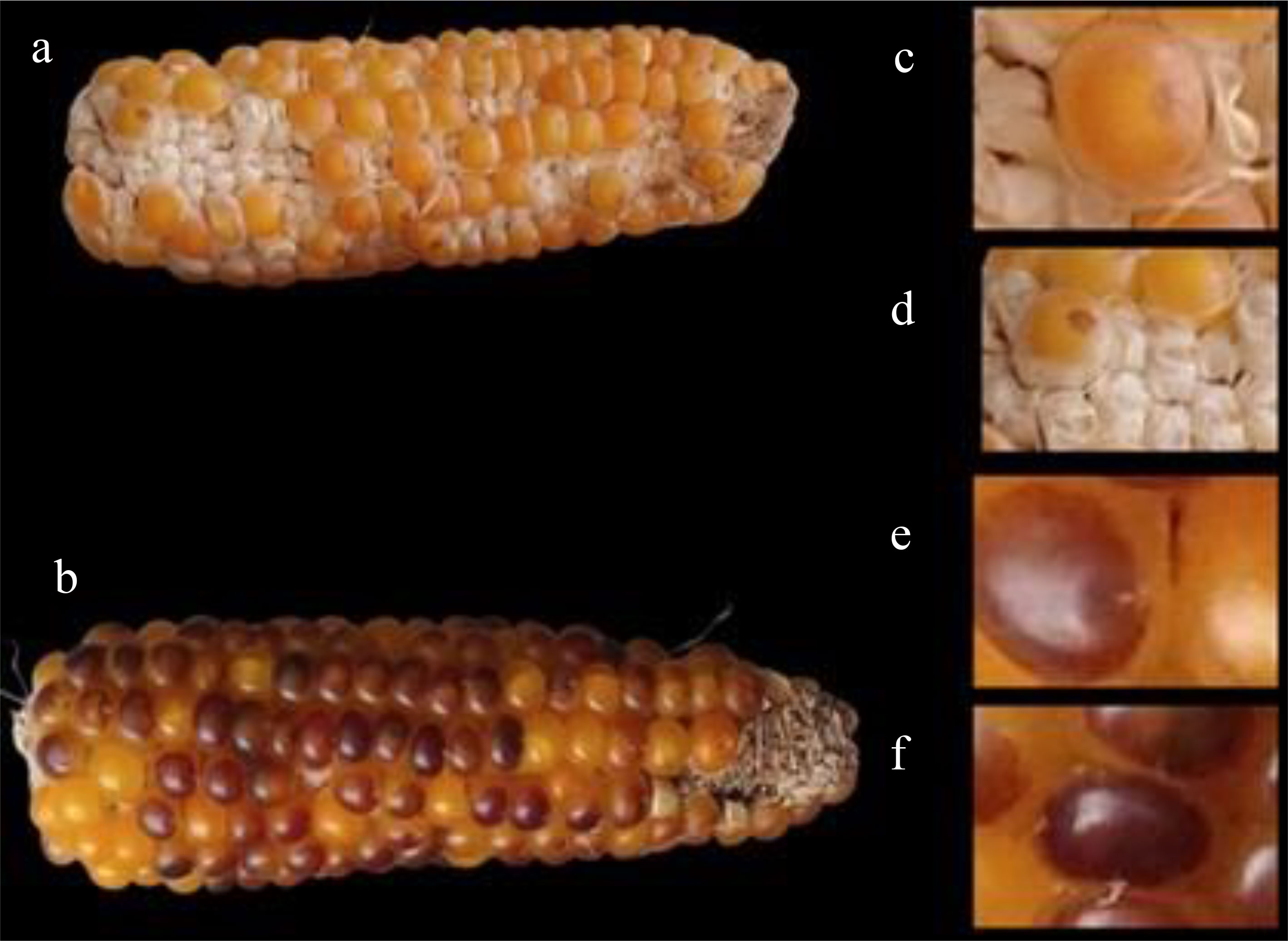
Figure 5 (A) Ear of corn with low expression of the R1-nj marker for anthocyanin intensity (B) and marked area (C). (D) Ear of corn showing high expression of the R1-nj marker for anthocyanin intensity (E) and marked area (F).
Conclusions
There is an induction of haploids in germplasm of tropical and temperate origin. We recommend the selection of haploids associating the Navajo phenotype with a direct method of confirming the ploidy level, such as flow cytometry. We also show that haploid screening based on Navajo phenotype and seedling vigor reduces misclassification. The origin and genetic background of the source germplasm influence the R1-nj penetrance. Because the known inducers are maize, developing doubled-haploid technology for popcorn hybrid breeding requires overcoming unilateral cross-incompatibility.
Data availability statement
The raw data supporting the conclusions of this article will be made available by the authors, without undue reservation.
Author contributions
JV and JPS contributed to the project planning and overall coordination. JPS performed and coordinated the field experiments. JCS, WC, JPS and VT were responsible for the flow cytometry. KD, JV and JPS carried out the analysis. JPS wrote the manuscript. All authors contributed to the article and approved the submitted version.
Acknowledgments
The authors thank the National Council for Scientific and Technological Development (CNPq), the Brazilian Federal Agency for Support and Evaluation of Graduate Education (Capes; Finance Code 001), and the Foundation for Research Support of Minas Gerais State (Fapemig) for financial support.
Conflict of interest
The authors declare that the research was conducted in the absence of any commercial or financial relationships that could be construed as a potential conflict of interest.
Publisher’s note
All claims expressed in this article are solely those of the authors and do not necessarily represent those of their affiliated organizations, or those of the publisher, the editors and the reviewers. Any product that may be evaluated in this article, or claim that may be made by its manufacturer, is not guaranteed or endorsed by the publisher.
Supplementary material
The Supplementary Material for this article can be found online at: https://www.frontiersin.org/articles/10.3389/fpls.2023.1176504/full#supplementary-material
Abbreviations
DH, doubled-haploid; FPR, false positive rate; FN; false negative; FNR, Fflse negative rate; FP, false positive; HIR, haploid induction rate; R1-nj, R1-navajo gene marker; MCC, Matthews correlation coefficient; TN, true negative; TP, true positive.
References
Almeida, V. C., Trentin, H. U., Frei, U. K., Lübberstedt, T. (2020). Genomic prediction of maternal haploid induction rate in maize. Plant Genome 13, 1–12. doi: 10.1002/tpg2.20014
Belicuas, P. R., Guimarães, C. T., Paiva, L. V., Duarte, J. M., Maluf, W. R., Paiva, E. (2007). Androgenetic haploids and SSR markers as tools for the development of tropical maize hybrids. Euphytica 156, 95–102. doi: 10.1007/s10681-007-9356-z
Chaikam, V., Martinez, L., Melchinger, A. E., Schipprack, W., Boddupalli, P. M. (2016). Development and validation of red root marker-based haploid inducers in maize. Crop Sci. 56, 1678–1688. doi: 10.2135/cropsci2015.10.0653
Chaikam, V., Molenaar, W., Melchinger, A. E., Boddupalli, P. M. (2019). Doubled haploid technology for line development in maize: technical advances and prospects. Theor. Appl. Genet. 132, 3227–3243. doi: 10.1007/s00122-019-03433-x
Chaikam, V., Nair, S. K., Babu, R., Martinez, L., Tejomurtula, J., Boddupalli, P. M. (2015). Analysis of effectiveness of R1-nj anthocyanin marker for in vivo haploid identification in maize and molecular markers for predicting the inhibition of R1-nj expression. TAG. Theor. Appl. Genet. Theoretische und angewandte Genetik 128, 159–171. doi: 10.1007/s00122-014-2419-3
Chase, S. S. (1952). Production of homozygous diploids of maize from monoploids 1. Agron. J. 44, 263–267. doi: 10.2134/agronj1952.00021962004400050010x
Choe, E., Carbonero, C. H., Mulvaney, K., Rayburn, A. L., Mumm, R. H. (2012). Improving in vivo maize doubled haploid production efficiency through early detection of false positives. Plant Breed. 131, 399–401. doi: 10.1111/j.1439-0523.2012.01962.x
Coe, E. H. (1959). A line of maize with high haploid frequency. Am. Nat. 93, 381–382. doi: 10.1086/282098
Demerec, M. (1929). Cross sterility in maize. Z. für Induktive Abstammungs- und Vererbungslehre 50, 281–291. doi: 10.1007/BF01742059
Eder, J., Chalyk, S. (2002). In vivo haploid induction in maize. Theor. Appl. Genet. 104, 703–708. doi: 10.1007/s00122-001-0773-4
Ford, R. H. (2000). Inheritance of kernel color in corn: explanations & investigations. Am. Biol. Teacher 62, 181–188. doi: 10.2307/4450870
Gain, N., Chhabra, R., Chandra, S., Zunjare, R. U., Dutta, S., Chand, G., et al. (2023). Variation in anthocyanin pigmentation by R1-navajo gene, development and validation of breeder-friendly markers specific to C1-inhibitor locus for in-vivo haploid production in maize. Mol. Biol. Rep. 50 (3), 2221–2229. doi: 10.1007/s11033-022-08214-2
Kebede, A. Z., Dhillon, B. S., Schipprack, W., Araus, J. L., Bänziger, M., Semagn, K., et al. (2011). Effect of source germplasm and season on the in vivo haploid induction rate in tropical maize. Euphytica 180, 219–226. doi: 10.1007/s10681-011-0376-3
Kelliher, T., Starr, D., Richbourg, L., Chintamanani, S., Delzer, B., Nuccio, M. L., et al. (2017). MATRILINEAL, a sperm-specific phospholipase, triggers maize haploid induction. Nature 542, 105–109. doi: 10.1038/nature20827
Kermicle, J. L. (2006). A selfish gene governing pollen-pistil compatibility confers reproductive isolation between maize relatives. Genetics 172, 499–506. doi: 10.1534/genetics.105.048645
Kermicle, J. L., Evans, M. M. S. (2005). Pollen-pistil barriers to crossing in maize and teosinte result from incongruity rather than active rejection. Sexual Plant Reprod. 18, 187–194. doi: 10.1007/s00497-005-0012-2
Kermicle, J. L., Evans, M. M. S. (2010). The zea mays sexual compatibility gene ga2: naturally occurring alleles, their distribution, and role in reproductive isolation. J. Heredity 101, 737–749. doi: 10.1093/jhered/esq090
Kleiber, D., Prigge, V., Melchinger, A. E., Burkard, F., Vicente, F. S., Palomino, G., et al. (2012). Haploid fertility in temperate and tropical maize germplasm. Crop Sci. 52, 623–630. doi: 10.2135/cropsci2011.07.0395
Lashermes, P., Beckert, M. (1988). Genetic control of maternal haploidy in maize (Zea mays l.) and selection of haploid inducing lines. Theor. Appl. Genet. 76, 405–410. doi: 10.1007/BF00265341
Liu, C., Li, X., Meng, D., Zhong, Y., Chen, C., Dong, X., et al. (2017). A 4-bp insertion at ZmPLA1 encoding a putative phospholipase a generates haploid induction in maize. Mol. Plant 10, 520–522. doi: 10.1016/j.molp.2017.01.011
Mangelsdorf, P. C., Jones, D. F. (1926). The expression of mendelian factors in the gametophyte of maize. Genetics 11, 606–606. doi: 10.1093/genetics/11.6.606b
Matthews, B. W. (1975). Comparison of the predicted and observed secondary structure of T4 phage lysozyme. Biochim. Biophys. Acta (BBA) - Protein Structure 405, 442–451. doi: 10.1016/0005-2795(75)90109-9
Melchinger, A., Longin, C., Utz, H., Reif, J. (2005) Hybrid maize breeding with doubled haploid lines: quantitative genetic and selection theory for optimum allocation of resources. Available at: http://scholar.google.com/scholar?hl=en&btnG=Search&q=intitle:Hybrid+maize+breeding+with+doubled+haploid+lines+:+quantitative+genetic+and+selection+theory+for+optimum+allocation+of+resources#0.
Melchinger, A. E., Schipprack, W., Würschum, T., Chen, S., Technow, F. (2013). Rapid and accurate identification of in vivo-induced haploid seeds based on oil content in maize. Sci. Rep. 3, 1–5. doi: 10.1038/srep02129
Milani, K. F., Baleroni, A. G., Silva, H. A., Mendes-Bonato, A. B., Pinto, R. J. B., Scapim, C. A. (2016). Effectiveness of the R1-navajo embryo marker on sorting haploids in tropical maize germplasm. Maydica 61.
Molenaar, W. S., Schipprack, W., Brauner, P. C., Melchinger, A. E. (2019). Haploid male fertility and spontaneous chromosome doubling evaluated in a diallel and recurrent selection experiment in maize. Theor. Appl. Genet. 132, 2273–2284. doi: 10.1007/s00122-019-03353-w
Moral, R. A., Hinde, J., Demétrio, C. G. B. (2017). Half-normal plots and overdispersed models in R: the hnp package. J. Stat. Software 81. doi: 10.18637/jss.v081.i10
Moran Lauter, A. N., Muszynski, M. G., Huffman, R. D., Scott, M. P. (2017). A pectin methylesterase zmpme3 is expressed in gametophyte factor1-s (Ga1-s) silks and maps to that locus in maize (zea mays l.). Front. Plant Sci. 8. doi: 10.3389/fpls.2017.01926
Nair, S. K., Molenaar, W., Melchinger, A. E., Boddupalli, P. M., Martinez, L., Lopez, L. A., et al. (2017). Dissection of a major QTL qhir1 conferring maternal haploid induction ability in maize. Theor. Appl. Genet. 130, 1113–1122. doi: 10.1007/s00122-017-2873-9
Nanda, D. K., Chase, S. S. (1966). An embryo marker for detecting monoploids of maize ( zea mays l.) 1. Crop Sci. 6, 213–215. doi: 10.2135/cropsci1966.0011183X000600020036x
Nelson, O. E. (1952). Non-reciprocal cross-sterility in maize. Genetics 37, 101–124. doi: 10.1093/genetics/37.2.101
Praça-Fontes, M. M., Carvalho, C. R., Clarindo, W. R. (2011). C-value reassessment of plant standards: an image cytometry approach. Plant Cell Rep. 30, 2303–2312. doi: 10.1007/s00299-011-1135-6
Prigge, V., Melchinger, A. E. (2012). Production of haploids and doubled haploids in maize. Methods Mol. Biol. 877, 161–172. doi: 10.1007/978-1-61779-818-4_13
Prigge, V., Sánchez, C., Dhillon, B. S., Schipprack, W., Araus, J. L., Bänziger, M., et al. (2011). Doubled haploids in tropical maize: i. effects of inducers and source germplasm on in vivo haploid induction rates. Crop Sci. 51, 1498–1506. doi: 10.2135/cropsci2010.10.0568
Prigge, V., Schipprack, W., Mahuku, G., Atlin, G. N., Melchinger, A. E. (2012). Development of in vivo haploid inducers for tropical maize breeding programs. Euphytica 185, 481–490. doi: 10.1007/s10681-012-0657-5
Röber, F. (1999). “Reproductive Biology and Genetic Studies with RFLP Markers in vivo Haploid Induction in Maize (in German).” Dissertation, University of Hohenheim.
Röber, F. K., Gordillo, G. A., Geiger, H. H. (2005). In vivo haploid induction in maize. performance of new inducers and significance of doubled haploid lines in hybrid breeding [Zea mays l.]. Maydica (Italy). 50(3-4), 275–283. Available at: https://agris.fao.org/agris-search/search.do?recordID=IT2006602235
Rotarenco, V., Dicu, G., Fuia, S. (2010). New inducers of maternal haploids in maize. Maize Genet. cooperation Newslett. 21–22.
Rotarenco, V. A., Dicu, G., Sarmaniuc, M. (2009). Induction of maternal haploids in maize. Maize Genet. Cooperation Newslett. 83, 21.
Lenth, R. V. (2020). emmeans: Estimated Marginal Means, aka Least-Squares Means. R package version 1.5.5. 5-1. Available at: https://CRAN.R-project.org/package=emmeans
Sarkar, K. R., Coe, E. H. (1966). A genetic analysis of the origin of maternal haploids in maize. Genetics 54, 453–464. doi: 10.1093/genetics/54.2.453
Schwartz, D. (1950). The analysis of a case of cross-sterility in maize. Proc. Natl. Acad. Sci. 36, 719–724. doi: 10.1073/pnas.36.12.719
Team, R. C (2021) A language and environment for statistical computing. Available at: https://www.r-project.org.
Keywords: Zea mays L. var. Everta, haploid induction, doubled haploid, unilateral cross-incompatibility, genetic improvement
Citation: Silva JPAd, Viana JMS, Dias KOdG, Silva JC, Tupper VTB and Clarindo WR (2023) Popcorn (Zea mays L. var. Everta) haploids identified by Navajo phenotype and ploidy level. Front. Plant Sci. 14:1176504. doi: 10.3389/fpls.2023.1176504
Received: 28 February 2023; Accepted: 02 May 2023;
Published: 30 May 2023.
Edited by:
Xiwen Cai, United States Department of Agriculture, United StatesReviewed by:
Pankaj Kumar Bhowmik, National Research Council Canada (NRC), CanadaPrathima Perumal Thirugnanasambandam, Indian Council of Agricultural Research, Coimbatore, India
Copyright © 2023 Silva, Viana, Dias, Silva, Tupper and Clarindo. This is an open-access article distributed under the terms of the Creative Commons Attribution License (CC BY). The use, distribution or reproduction in other forums is permitted, provided the original author(s) and the copyright owner(s) are credited and that the original publication in this journal is cited, in accordance with accepted academic practice. No use, distribution or reproduction is permitted which does not comply with these terms.
*Correspondence: Jean Paulo Aparecido da Silva, jean.p.silva@ufv.br