- 1Key Laboratory of Vegetable Biology of Yunnan Province, College of Landscape and Horticulture, Yunnan Agricultural University, Kunming, Yunnan, China
- 2College of Agronomy and Biotechnology, Yunnan Agricultural University, Kunming, China
In the post-genomic era, virus-induced gene silencing (VIGS) has played an important role in research on reverse genetics in plants. Commonly used Agrobacterium-mediated VIGS inoculation methods include stem scratching, leaf infiltration, use of agrodrench, and air-brush spraying. In this study, we developed a root wounding–immersion method in which 1/3 of the plant root (length) was cut and immersed in a tobacco rattle virus (TRV)1:TRV2 mixed solution for 30 min. We optimized the procedure in Nicotiana benthamiana and successfully silenced N. benthamiana, tomato (Solanum lycopersicum), pepper (Capsicum annuum L.), eggplant (Solanum melongena), and Arabidopsis thaliana phytoene desaturase (PDS), and we observed the movement of green fluorescent protein (GFP) from the roots to the stem and leaves. The silencing rate of PDS in N. benthamiana and tomato was 95–100%. In addition, we successfully silenced two disease-resistance genes, SITL5 and SITL6, to decrease disease resistance in tomatoes (CLN2037E). The root wounding–immersion method can be used to inoculate large batches of plants in a short time and with high efficiency, and fresh bacterial infusions can be reused several times. The most important aspect of the root wounding–immersion method is its application to plant species susceptible to root inoculation, as well as its ability to inoculate seedlings from early growth stages. This method offers a means to conduct large-scale functional genome screening in plants.
1 Introduction
Virus-induced gene silencing (VIGS) is a reverse genetics tool for effectively silencing target gene expression by using plant antiviral defense mechanisms that inhibit invading viruses (Dommes et al., 2019). Currently, most functional gene research methods, including chemical mutagenesis, T-DNA insertion, and CRISPR/Cas, are dependent on stably transformed lines and generally require labor-intensive processes such as phenotyping and molecular screening, and several of the methods are inefficient. In some cases, the loss of function of some essential genes causes plant death during the early stages of plant growth, which may limit the application of these methods (Baulcombe, 2004; Benedito et al., 2004). In comparison, VIGS is a low-cost and rapid tool, and a large number of plant viruses have been successfully developed as VIGS vectors, including tobacco rattle virus (TRV), tobacco mosaic virus (TMV), potato virus X (PVX), tomato golden mosaic virus (TGMV), and cabbage leaf curl virus (CbLCV) (Burch-Smith et al., 2004).
Modified VIGS systems have been used in Brassicaceae (Fridborg et al., 2013; Wang et al., 2013; Yu et al., 2019), Solanaceae (Fu et al., 2005), Gramineae (Bennypaul et al., 2012; Kang et al., 2013; Martin et al., 2013), Cucurbitaceae (Bu et al., 2019; Liao et al., 2019), Asteraceae (Deng et al., 2012), Leguminosae (Ganiger et al., 2013; Atwood et al., 2014), Orchidaceae (Hsieh et al., 2013a, Hsieh et al., 2013b), and Malvaceae (Gao et al., 2011; Pang et al., 2013). The silencing efficiency of VIGS is related to the inoculation method, bacterial suspension concentration, vector selection, and environmental conditions. Virus vector-induced silencing efficiency is associated with host selection. Currently, Agrobacterium-mediated inoculation is mainly used for VIGS, and the primary methods for this type of inoculation are injection (Huang et al., 2014), agrodrench (Ryu et al., 2004), high-pressure spray (Liu et al., 2002a), and vacuum infiltration (Yan et al., 2012). TRV is widely used in VIGS vector construction owing to its high silencing efficiency, long silencing duration, and mild infection symptoms (Dinesh-Kumar et al., 2003). Studies have confirmed that low temperature and low humidity can increase VIGS silencing efficiency (Fu et al., 2006; Singh et al., 2018; Yang et al., 2018), and TRV-VIGS inoculated through agrodrench application or leaf infiltration can persist for 2 years or more (Senthil-Kumar and Mysore, 2011a). In agricultural inoculation, the concentration of the infiltration solution also considerably affects gene silencing in VIGS experiments; for example, an OD600 > 1 causes Nicotiana benthamiana leaf necrosis. In comparison, the OD600 = 1.5 of Agrobacterium results in good infection effects in tomatoes (Senthil-Kumar et al., 2007).
Several Agrobacterium-mediated inoculation methods have been developed to introduce VIGS and other related applications, such as virus-mediated genome editing (Ali et al., 2015), into host plants. Among these methods, root inoculation is a promising approach that can be applied to research on root systems and on plant hosts that are resistant to above-ground infection, as well as in other similar cases. TRV vectors can infect plant roots and efficiently express green fluorescent protein (GFP) (MacFarlane and Popovich, 2000). In addition, one study found that a modified TRV vector showed stronger infectivity and invasiveness in meristems in the rhizosphere than elsewhere (Valentine et al., 2004). The present study employed a root wounding-immersion method to trigger VIGS with TRV vectors. This method is suitable for N. benthamiana, tomato (Solanum lycopersicum), eggplant (Solanum melongena), pepper (Capsicum annuum L.), and Arabidopsis thaliana. This study provides a new VIGS inoculation approach for functional research on plant genes.
2 Materials and methods
2.1 Plant materials and growth conditions
Tomato (Micro Tom and CLN2037E), Arabidopsis thaliana, Nicotiana benthamiana, eggplant (S-34), and pepper (Capsicum annuum) were all stored by our group. The seeds were placed in cell culture dishes with moist tissue paper and covered, after which they were incubated at 25°C under high humidity for 3 days to stimulate germination. After breaking through the testa, the plants were cultured indoors with 16 h (28°C) of light and 8 h of darkness (20°C).
2.2 Plasmid construction
pTRV2-GFP was used as a backbone (Figure 1A) and GFP and phytoene desaturase (PDS) were used as reporter genes. Ryu et al. (2004) found that TRV2-NbPDS could silence endogenous PDS homologs in tomato, tobacco, and Petunia, showing that the PDS gene sequences of these species were conserved. However, TRV2-NbPDS did not inhibit the PDS homologs in pepper, potato, or eggplant (Ryu et al., 2004). To ensure accurate targeting in different hosts, we designed specific primers for different species to clone 300 bp of PDS, SITLP5, and SITLP6 silencing fragments, which were ligated to the binary vector TRV2-GFP to generate TRV2-GFP-NbPDS, TRV2-GFP-SlPDS, TRV2-GFP-CaPDS, TRV2-GFP-SmPDS, TRV2-GFP-AtPDS, TRV2-GFP-SITLP5, and TRV2-GFP-SITLP6. The designed primers are shown in Supplementary Table S1. Electroporation was used to transform Agrobacterium GV1301 using the binary vectors pTRV2-GFP-PDS and pTRV1.
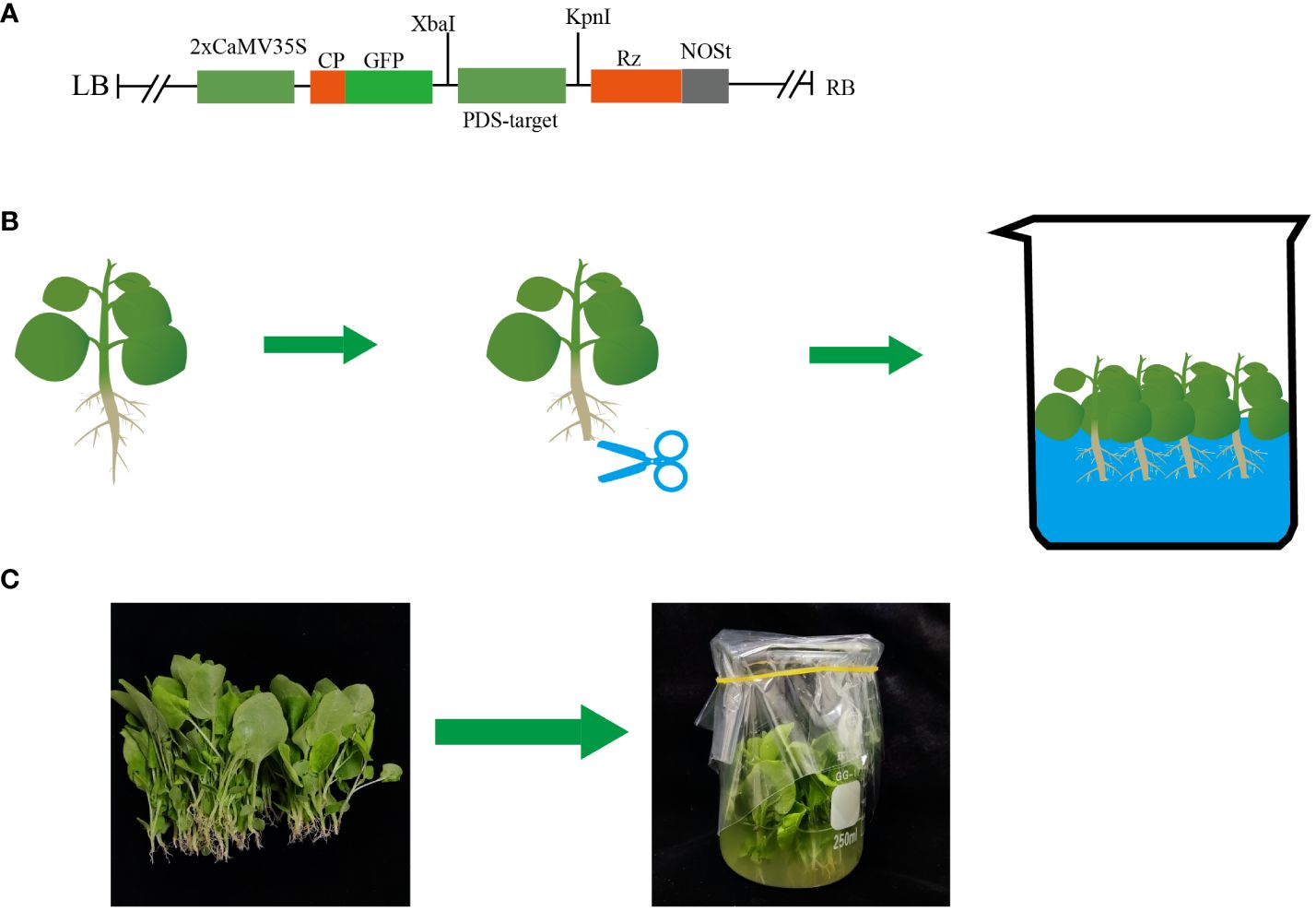
Figure 1 Schematic diagram of the root wounding–immersion method. (A) Construction of the pTRV2-GFP backbone; (B, C) Schematic of the root wounding–immersion process.
2.3 Development of root wounding–immersion method and GFP imaging
Agrobacterium GV1301 containing pTRV2-GFP-PDS and pTRV1 were cultured on LB plates containing kanamycin (50 μg/mL) and rifampin (25 μg/mL) at 28°C for 2 days. Positive colonies were selected and cultured overnight in LB broth containing the corresponding antibiotics and shaken at 200 rpm. A NanoDrop 1000 spectrophotometer (Thermo Fisher Scientific, New York, NY, USA) was used to determine that the OD600 of the inoculum was >1.
One day before VIGS, 50 μL of the above culture was added to 20 mL of LB medium containing 50 μg/mL kanamycin, 25 μg/mL rifampin, 20 μM acetosyringone, and 10 mM MES and cultured overnight at 28°C and 200 rpm. The following day, an infiltration solution (10 mM MgCl2, 10 mM MES at pH 5.6, 150 μM acetosyringone) was used to resuspend the culture until OD = 0.8. TRV1 and TRV2-carrying Agrobacterium suspensions were cultured in the dark at 28°C for 3 h (Zhang and Thomma, 2014; Meziadi et al., 2016). For the root wounding–immersion procedure, seedlings were first cultured in a tray. To increase the resistance of the plants, seedlings with 3–4 real leaves that were 3 weeks old were removed from the soil (including the roots). After pure water was used to remove soil and other impurities from the roots, a disinfected leaf knife was used to remove 1/3 of the root lengthwise. We designed two different immersion protocols: 1) “ concurrent inoculation,” where TRV1 and TRV2 were mixed and the plant was immersed for 30 min; and 2) “successive inoculation,” where the plant was immersed in TRV1 infiltration solution for 15 min and then transferred to a TRV2 infiltration solution for 15 min of immersion. To determine the effect of temperature on infection efficiency, the cut seedlings were placed in infiltration solutions with different temperature gradients in the two immersion protocols so that the roots were completely immersed. Shaking was carried out every 5 min. After 30 min, the seedlings were transferred to a 50-pore plastic tray containing sterilized soil (peat and vermiculite). After 48 h of dark treatment, the plants were cultured indoors with a 16-h (temperature was the same as immersion) light and 8-h dark (18°C) cycle with 35% humidity. Furthermore, we designed experiments with different concentrations and multiple uses of the inoculum. A fresh inoculum of 10 mL was prepared to inoculate 20 tomato plants simultaneously, using the same infection methods as described. A portable light source (LUYOR-3410RB, Luyor, USA) was used for GFP imaging.
2.4 RNA isolation and RT-qPCR analysis
To measure the silencing efficiency in TRV-infected plant leaves, a Huayueyang rapid universal plant RNA extraction kit (Huayueyang Biotechnology Co., Ltd., Beijing, China) was used to extract the total RNA from the leaves 30 days after infection. The quality and quantity of the extracted RNA were confirmed by 1.5% (w/v) agarose gel electrophoresis and a NanoDrop 1000 spectrophotometer (Thermo Fisher Scientific, New York, NY, USA). Then, 2 µg of total RNA was used to synthesize cDNA based on the manufacturer’s instructions for the TransScript one-step gDNA removal and cDNA synthesis SuperMix (TransGen, Beijing, China) kits. Primer Premier 6 (Premier, San Francisco, CA, USA) software was used to design specific primers for the segments outside the silencing regions. The species-specific internal reference genes were used as control and the primers were synthesized by Tsingke Biotechnology (Qingke Biotechnology Co., Ltd., Beijing, China). SYBR Green PCR Master Mix (TransGen) was used for RT-qPCR on a CFX96 (Bio-Rad, Hercules, CA, USA). Relative mRNA transcript abundance was calculated for TRV-GFP- and TRV-GFP-PDS-infected plants using the 2−ΔΔCt method (Zhao et al., 2013) with uninfected plants as controls. All of the primers used are shown in Supplementary Table S1.
2.5 Pathogen inoculation and disease resistance identification
The late blight pathogen (Phytophthora infestans) was obtained from the Institute of Vegetables and Flowers at the Chinese Academy of Agricultural Sciences (Kunming, Yunnan Province, China). Then, 5 × 104 sporangia/mL was used to spray six or seven leaves of VIGS-successful and control tomato seedlings. After inoculation, the seedlings were placed in a plant growth room, and 100% relative humidity (RH) and 20 ± 1°C were maintained. The seedlings were grown in the dark for 24 h. Then, the seedlings were grown under a 14 h/10 h light/dark cycle with a relative humidity of 60–80% (Jiang et al., 2018). Seven days after inoculation, the method described by Wang (2003) was used to calculate the disease severity rating (DSR), which was used as a reference for the resistance level.
2.6 Chlorophyll content measurement
The chlorophyll measurement was immediately performed after the extract was prepared based on the method of Hajirezaei et al. (2002). Then, 50 days after infection, the wild type was used as the control, and 50 mg of plant leaves were harvested and frozen in liquid nitrogen. Thereafter, 0.5 mL of 80% (v/v) acetone/water was used for extraction before 0.5 mL of 100% acetone was used for extraction to ensure that chlorophyll a and b were completely extracted. The samples were incubated at 80°C for 60 min, and spectrophotometry was used to measure the total chlorophyll, chlorophyll a, and chlorophyll b at 652, 647, and 664 nm, respectively.
2.7 Phytoene analysis
The samples were rinsed with ice-cold PBS (0.05 mol/L Tris-HCI, pH 7.4 phosphate buffered saline), and filter paper was used to remove the PBS. Then, 0.1 g of each sample was placed in 5 mL of homogenization tubes. Nine volumes of homogenization medium were added to the homogenization tube based on weight (g):volume (mL) = 1:9. The pestle was moved up and down 10 times in an ice bath to prepare 10% homogenate. Afterward, phytoene was measured using the plant phytoene ELISA Kit (Xingtai Sinobest Biotech Co., Ltd.) according to the manufacturer’s instructions.
2.8 Statistical method
Each experiment contained at least three biological replicates. IBM® SPSS® Statistics 24 statistical software (IBM, Chicago, IL, USA) was used for data processing. One-way ANOVA was used for data analysis, and Duncan’s test was used for post-hoc analysis. A p ≤ 0.05 was considered significant, and the results are presented as the means ± SD.
3 Results and discussion
3.1 Optimization of the root wounding–immersion procedure
Current inoculation methods mainly include Agrobacterium-mediated injection infiltration and vacuum infiltration. However, injecting Agrobacterium cultures into the seedling leaves of plants with tough tissues, such as soya beans and maize, can be challenging. Additionally, the leaves of these plants need to be fully unfolded to ensure successful injection (Ratcliff et al., 2001). For roots that are susceptible to inoculation and early-growth seedlings, we developed an inoculation method known as root wounding–immersion (Figure 1B). To test the feasibility of the root wounding–immersion method, we chose PDS as a reporter gene because the leaves of plants in which PDS is silenced tend to show symptoms of photobleaching (Kumagai et al., 1995; Liu et al., 2002a). To visualize TRV viral transport from the roots to the above-ground parts of plants, we cloned tobacco NbPDS fragments and inserted them into the vector pTRV2-GFP. Uninjured tobacco was infected according to the method described in Section 2.3 and the silencing rate was found to be less than 1%, so we used uninoculated plants as controls. The frequency of VIGS was defined as the number of plants that show silencing phenotype (photobleaching) after inoculation with TRV2-GFP-NbPDS. In N. benthamiana, the ratio of positive silenced plants was 95.8%, and GFP insertion did not modify the gene silencing capacity of the TRV vector (Tian et al., 2014). Under illumination with the portable excitation light source (LUYOR-3410RB), pTRV2-GFP-NbPDS was transferred from the roots to the stem and leaves (Figure 2F). We designed two different infection methods for different temperatures (Supplementary Table S1): “concurrent inoculation” and “successive inoculation.” Photobleaching was observed in N. benthamiana plants as early as day 6 (Figure 2A), which was faster than the 7–10 days reported in the study of Ryu et al. (2004).
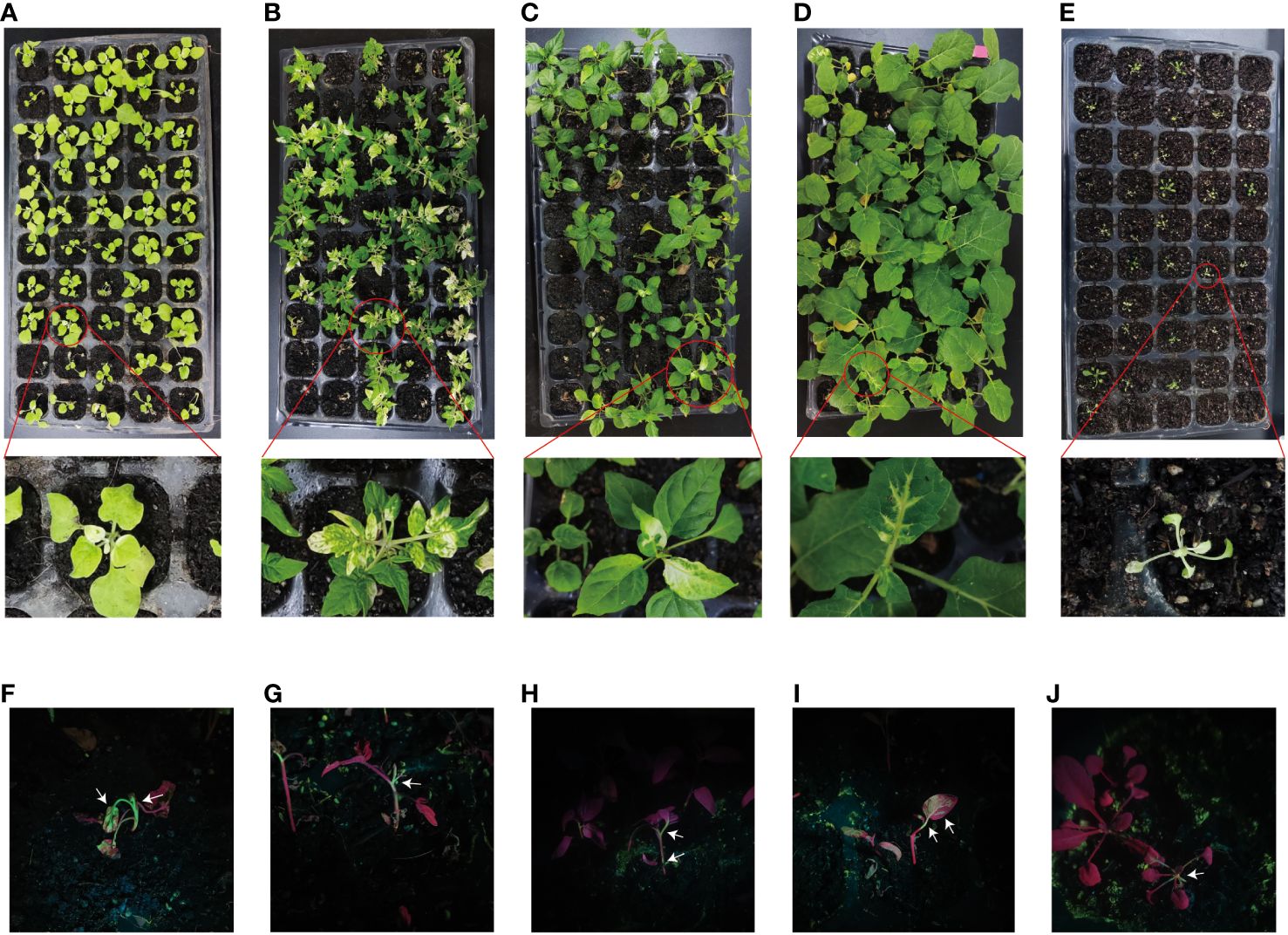
Figure 2 The VIGS system established through the root-soaking method resulted in plant bleaching. (A–E) Bleaching symptoms photographed in Nicotiana benthamiana, tomato, pepper, eggplant, and Arabidopsis thaliana, respectively, on day 30; (F–J) Green fluorescence of N. benthamiana, tomato, pepper, eggplant, and A. thaliana on days 7, 7, 10, 12, and 4, respectively.
The data show that the survival rate and silencing rate of concurrent inoculation were slightly higher than those for successive inoculation. For example, at 22°C, the survival and silencing rates of concurrent inoculation were both 100%, whereas the survival and silencing rates of successive inoculation were 96% and 92%, respectively. We believe the separate immersion in the TRV1 and TRV2 bacterial suspensions decreased opportunities for both to contact each other, which may decrease endogenous RNA-dependent RNA polymerase (RDRP) activation and the replication and production of virus dsRNA (Donaire et al., 2008). In addition, decreased TRV1 and TRV2 bacterial suspension immersion duration also decreased opportunities for entry into the plant through the wounded root.
Temperature is a key factor affecting the development of the gene-silencing phenotype of VIGS plants (Szittya et al., 2003; Fu et al., 2006; Tuttle et al., 2008), as most viruses lose their potency, decreasing virus concentration. However, low temperatures (16–21°C for tomatoes) can promote virus silencing (Szittya et al., 2003; Singh et al., 2018). Our data show that an appropriate temperature is beneficial for maximizing VIGS silencing effects. In concurrent inoculation, photobleaching occurred after 6 days at 22°C and after 12 days at 26°C, and VIGS effects were delayed. The survival and silencing rates are also affected by temperature. The survival and silencing rates of concurrent inoculation at 18°C were 72% and 79%, respectively, and the survival and silencing rates at 26°C were 60% and 56%, respectively. Because the effect of concurrent inoculation was better than that of successive inoculation, concurrent inoculation was adopted in the following studies.
A suitable concentration of permeable solution is beneficial to gene silencing efficiency in VIGS experiments. The most suitable infiltration solution concentration for N. benthamiana is OD600 = 1.0 because an inoculum with OD600 > 1.0 may cause leaf necrosis in N. benthamiana (Caplan and Dinesh-Kumar, 2006), and Dinesh-Kumar et al. (2003) found that OD600 = 1.5 shows better results in tomatoes. Burch-Smith et al. (2006a) found that the efficiency is almost 100% when the A. thaliana Col-0 ecological TRV-VIGS experiment concentration is OD = 1.5. It is necessary to determine the most favorable TRV-VIGS inoculation concentration, and we used different concentrations for concurrent inoculation at 22°C for N. benthamiana inoculation (Table 1). We found that the survival rate and PDS silencing effectiveness are dependent on the concentration of the Agrobacterium suspension. When OD = 0.4–1.2, the rate of PDS silencing was more than 95%. However, the PDS silencing rate decreased when the concentration was below 0.4, and necrosis occurred when the concentration was above 1.2. Therefore, we resuspended the bacteria to OD600 = 0.8 in the following studies to ensure a higher survival rate and silencing efficiency while considering the efficient use of the Agrobacterium resuspension.
Even though root inoculation was performed in the root wounding–immersion, the movement of virus to aboveground organs was not delayed in N. benthamiana. In contrast, we found that the root wounding–immersion method does not require leaf infiltration for every N. benthamiana plant, and batch processing can be carried out. This method is highly efficient. In addition, we used our previous bacterial suspension (guaranteed fresh) many times. Results showed that the silencing rate was more than 90% (Supplementary Table S2) even after the TRV1:TRV2 mixed bacterial suspension was used five consecutive times. This avoids the preparation of a large volume of infiltration solution for the treatment of a large quantity of materials and saves time and costs. Our method confirms the hypothesis of Ryu et al. (2004) that Agrobacterium-mediated transformation occurs when there is simulated wounding to roots in growing plants.
3.2 Application of the root wounding–immersion method in A. thaliana, tomatoes, eggplants, and pepper
Infection was carried out using concurrent inoculation at a concentration of OD = 0.8 and 22°C (Supplementary Table S3). We found that the root wounding–immersion method is similarly effective in these five plant species, but the infection efficiency differed between different plant species (Figures 2B–E). TRV typically causes mild symptoms within 10 days of inoculation in N. benthamiana and tomatoes (Liu et al., 2002a). The virus spreads from the inoculation site to the developing regions of the plant and triggers PTGS. At 2–3 weeks, the virus spreads to the completely open upper leaves (Burch-Smith et al., 2004). Using the root wounding–immersion method, we found that photobleaching occurred 1 week after inoculation. The silencing rate of the tomatoes was 95% (Supplementary Table S3). TRV-mediated VIGS silencing has similarly been used in peppers. Generally, the pale yellow (rbcS) and photobleached leaf (PDS)-silenced phenotype will become significant 2 weeks after TRV vector transformation (Chung et al., 2004). We employed the root wounding–immersion method, and these phenotypes occurred 24 days after inoculation in peppers. The silencing rate of the tomatoes was 58% (Supplementary Table S3). Liu et al. (2012) used high-pressure spraying and injection on eggplant plants, and uniform bleaching occurred in the newly formed leaves after 30 days (Liu et al., 2012). We employed root wounding–immersion, and bleaching occurred in eggplants after 29 days and spread from leaf veins to the surrounding tissue (Figure 2D). The silencing rate was 23% (Supplementary Table S3). A. thaliana is a model plant in the Brassicaceae family, and VIGS has been used for many gene functional studies (Senthil-Kumar and Mysore, 2011b). Bleaching occurred on day 5 after inoculation, and the silencing rate was 75%. Even though the root wounding–immersion method is suitable for A. thaliana, the survival rate was 48%, which is lower than that in the tomatoes, peppers, and eggplants. This may be because A. thaliana itself is weak, small, and non-viable when it is transplanted after root wounding–immersion treatment.
Under portable light excitation via LUYOR-3410RB illumination, pTRV2-GFP moved from the roots to the stems and leaves of the tomatoes, peppers, eggplants, and A. thaliana (Figures 2G–J). Moreover, we used root wounding–immersion on cabbage and cucumbers, but photobleaching was not observed (Appendix 1).
3.3 Successful application of root wounding–immersion in multiple plants
We used RT-qPCR to confirm PDS silencing at the molecular level, and showed that the average relative expression level of PDS was significantly lower in photobleached N. benthamiana, tomato, pepper, eggplant, and A. thaliana than in the control group (Figures 3A–E). The leaves became white owing to carotenoid deficiency and the photooxidative destruction of chlorophyll after PDS silencing (Kumagai et al., 1995). We measured chlorophyll a, chlorophyll b, and total chlorophyll contents upon the silencing of N. benthamiana, tomato, pepper, eggplant, and A. thaliana. The chlorophyll a, chlorophyll b, and total chlorophyll contents of N. benthamiana, tomato, eggplant, pepper, and A. thaliana TRV2-GFP-PDS-silenced plants decreased by more than 40% compared with those of the control group; specifically, the chlorophyll a, chlorophyll b, and total chlorophyll contents in N. benthamiana leaves decreased by more than 90% (Figures 4A–E).
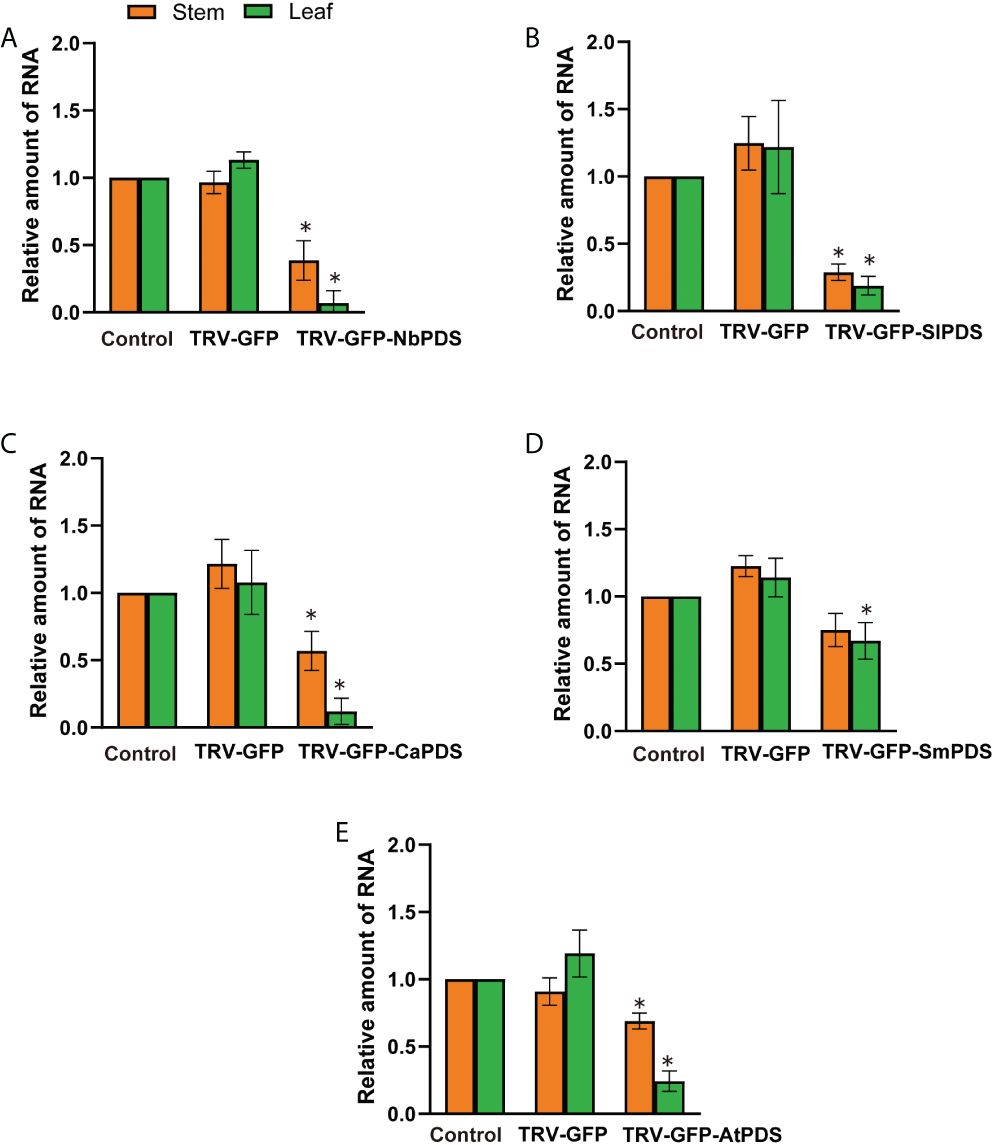
Figure 3 Virus-induced gene silencing in different plants through the root-soaking method. (A–E) Real-time fluorescence quantitative PCR measurement of PDS expression pattern in Nicotiana benthamiana, tomato, pepper, eggplant, and A. thaliana. The control expression level was assigned a value of 1. The error bars are the standard deviations of biological triplicates. The asterisk (*) means P < 0.05, based on Student’s t-test.
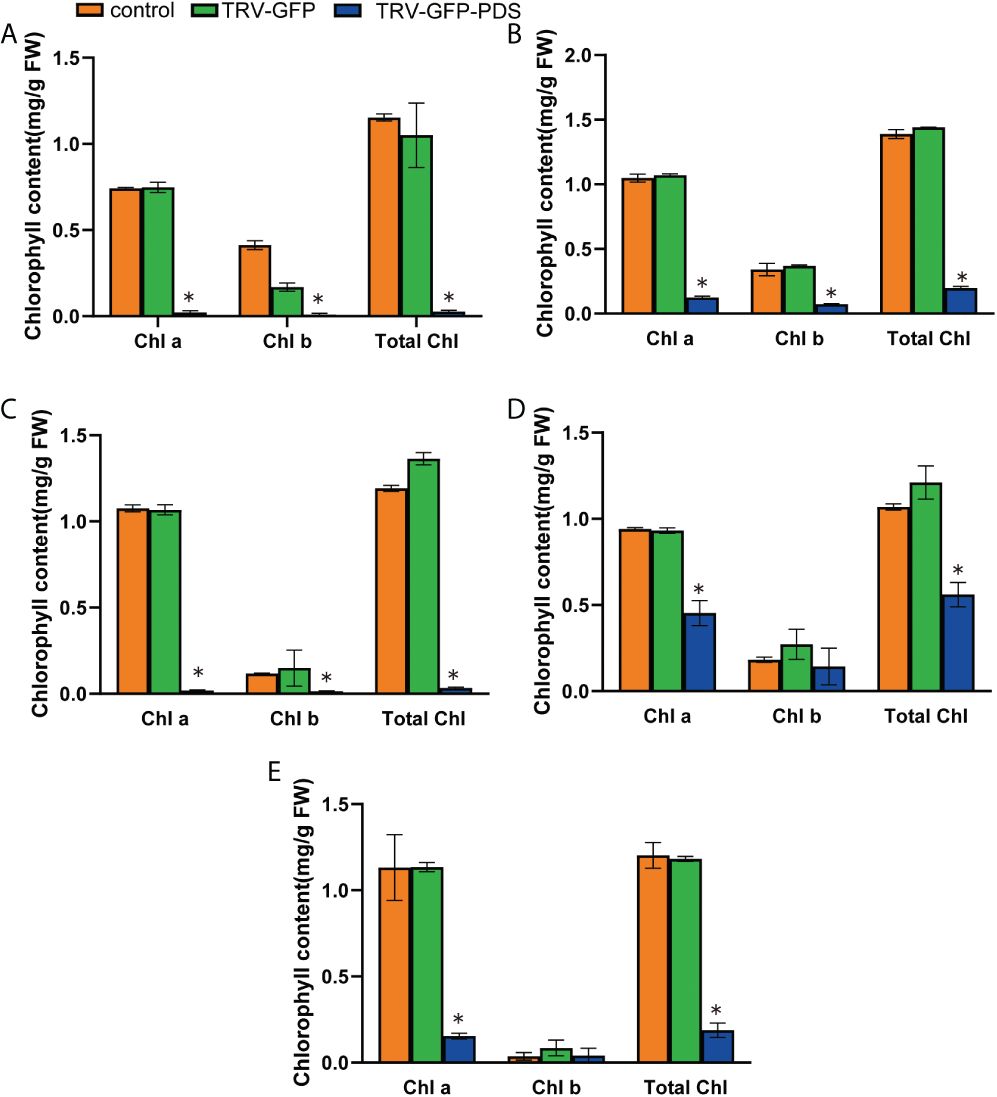
Figure 4 The VIGS system established through the root-soaking method resulted in a decrease in the chlorophyll content of plant leaves. (A–E) The decreases in chlorophyll a, chlorophyll b, and total chlorophyll contents in Nicotiana benthamiana, tomato, pepper, eggplant, and A. thaliana TRV2-PDS-infected plant leaves were measured. The error bars are the standard deviations of biological triplicates. The asterisk (*) means P < 0.05, based on Student’s t-test.
In transfected plants, PDS silencing inhibited the synthesis of carotenoids downstream of phytoene and caused high levels of phytoene (Kumagai et al., 1995; Fu et al., 2006). We measured the phytoene levels of five plants and found that phytoene content increased in the silenced plants (Figures 5A–E). These results confirm that root wounding–immersion can cause the PDS gene to be successfully silenced.
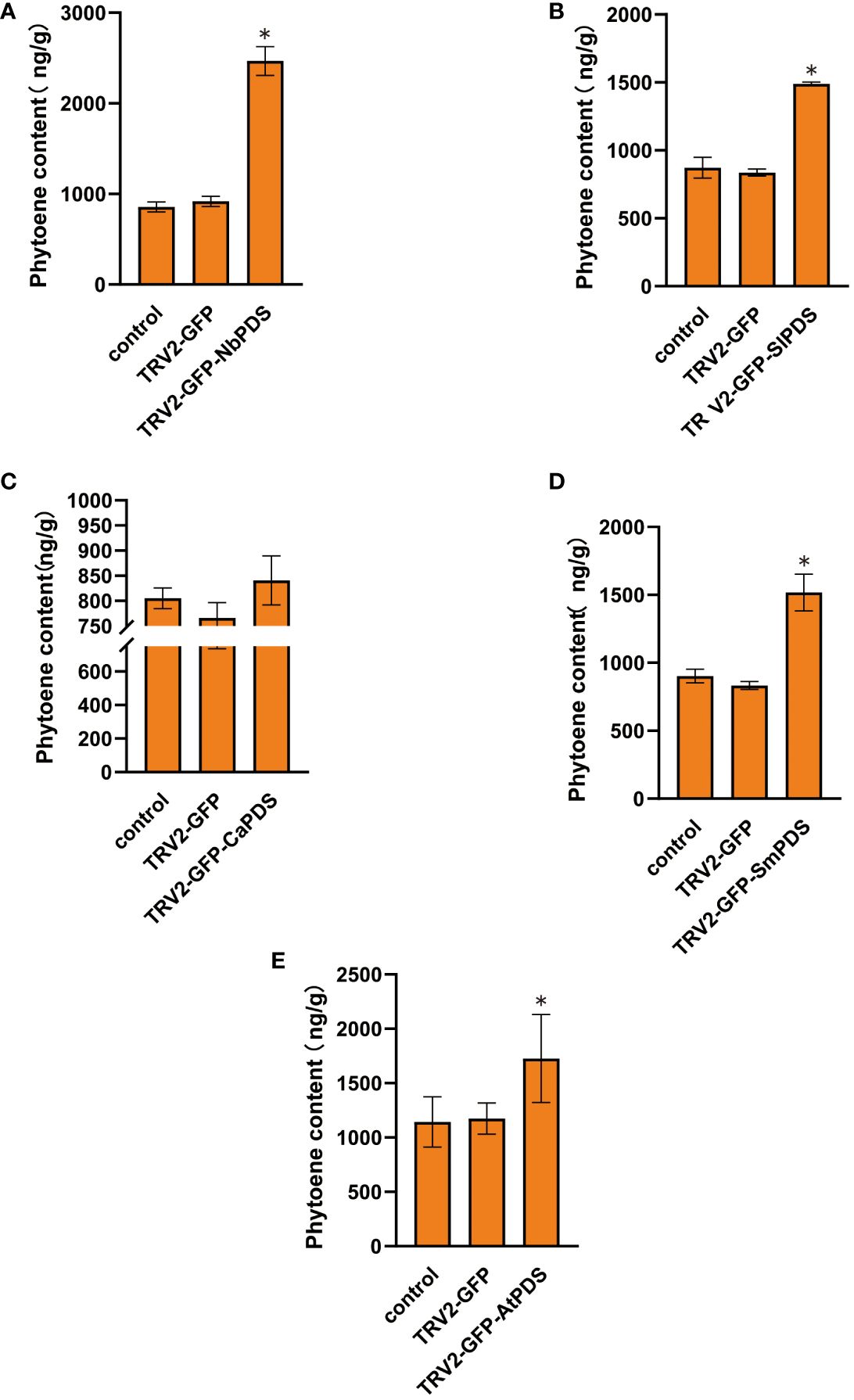
Figure 5 The VIGS system established through the root-soaking method resulted in an increase in the phytoene content of plant leaves. (A–E) The phytoene accumulation levels in Nicotiana benthamiana, tomato, pepper, eggplant, and A. thaliana TRV2-PDS infected plant leaves were measured. The error bars are the standard deviations of biological triplicates. The asterisk (*) means P < 0.05, based on Student’s t-test.
3.4 Application of root wounding–immersion in disease resistance
Inoculation of a leaf with a VIGS vector containing a gene fragment leads to the transmission of the virus throughout the entire plant (Burch-Smith et al., 2006b). To test the reliability of the root wounding–immersion method, we silenced two late blight (P. Infestans) resistance genes (SITLP5 and SITLP6) in tomatoes in addition to the commonly reported genes chalcone synthase (CHS) (Spitzer et al., 2007), H subunit of magnesium protoporphyrin chelatase (ChlH) (Hiriart et al., 2002), and anthocyanidin synthase (ANS) (Senthil-Kumar et al., 2008). SITLP5 and SITLP6 overexpression and knockout increased and decreased late blight resistance, respectively, in tomatoes (Zhu et al., 2021). The CLN2037 tomato inbred line was generated by selective breeding from Solanum pimpinellifolium and contains late blight resistance genes. Thus, it is widely used in disease-resistance selective breeding and mining of disease resistance genes (Nowicki et al., 2012; Zhang et al., 2013). We used the root wounding–immersion method to inoculate TRV1:TRV2-GFP-SITLP5 and TRV1:TRV2-GFP- SITLP6 in tomato (CLN2037E), and disease symptoms were observed 7 days after P. infestans inoculation in SITLP5- and SITLP6-silenced plants using the method of Zhu et al. (2021). RT-qPCR was used to measure SITLP5 transcript abundance in silenced plants, and the average relative expression level of SITLP5 decreased by 43% and 84% in the stems and leaves, respectively, in CLN2037E. Moreover, SITLP6 decreased by 80% and 79% in the stems and leaves, respectively (Figures 6A, B). The DSR of the control plant was 1.6, which was classified as high resistance. After SITLP5 and SITLP6 silencing, the plants showed decreased P. infestans resistance (Figures 6C, D), and the calculated DSRs were 3.0 and 2.6, respectively, which was considered moderate resistance. These results showed that the root wounding–immersion method is similarly suitable for TRV-VIGS in other biological studies.
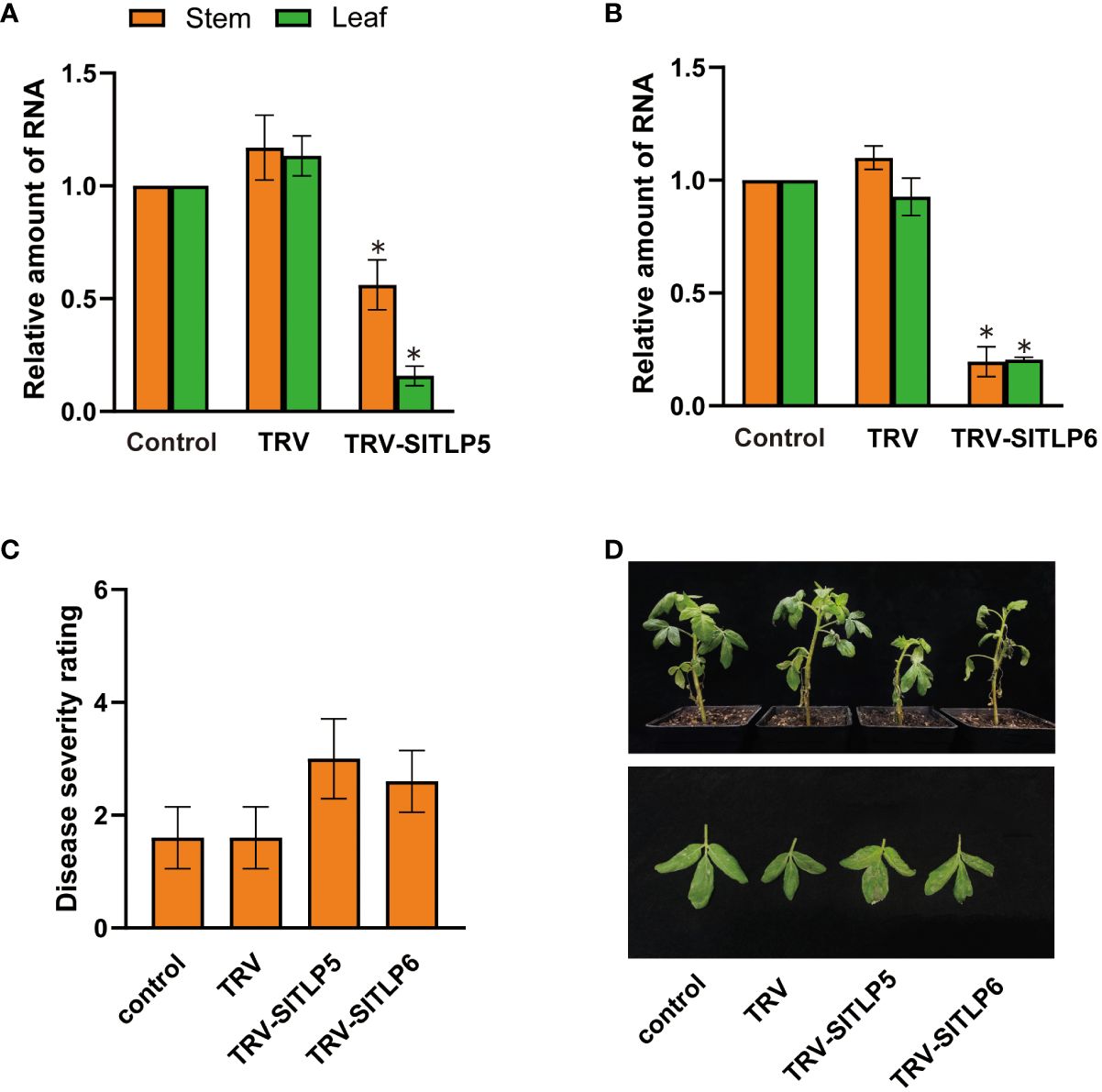
Figure 6 The VIGS system established using the root-soaking method reduced the disease resistance of tomato plants. (A, B) Real-time fluorescence quantitative PCR measurement of SITLP5 and SITLP6 expression patterns in the stems and leaves of SITLP5- and SITLP6-silenced plants. The control expression level was assigned a value of 1. (C, D) Disease severity and resistance of SITLP5- and SITLP6-silenced plants 7 days after inoculation with pathogens. The error bars are the standard deviations of biological triplicates. The asterisk (*) means P < 0.05, based on Student’s t-test.
3.5 The root wounding–immersion method can be used for large-scale, high-efficiency VIGS experiments in genetic studies
Initially, Ruiz et al. (1998) used mechanical friction to deliver potato virus X (PVX) RNA obtained from in vitro transcription to plants and successfully obtained an NbPDS gene silencing phenotype. However, this method is difficult and cumbersome, and the silencing efficiency is low. Later, stem scratch and agroinfiltration methods were successfully applied to PVX VIGS (Bhaskar et al., 2009; Du et al., 2014). Antiviral silencing in some susceptible Solanaceae roots strongly inhibited PVX replication levels (Andika et al., 2015). Ratcliff et al. (2001) silenced target genes by injection infiltration and confirmed that TRV has many advantages over PVX, TMV, and TGMV. The TRV vector is able to invade roots and express GFP efficiently, whereas the widely used PVX vector is not (MacFarlane and Popovich, 2000). Bond and Baulcombe (2015) used TRV as a VIGS-RdDM silencing vector tool to augment the production of a 24-nt sRNA. The silencing rate of tomatoes when injection is used is 50% (Liu et al., 2002b). Spraying is also widely used for tomatoes, with a silencing rate of 90% (Liu et al., 2002a; Dinesh-Kumar et al., 2003). However, it has many equipment requirements, preventing many laboratories from employing this method. We developed the root wounding–immersion method and optimized it. We selected 3-week-old seedlings, removed 1/3 of the root lengthwise, and then immersed it in TRV1 and TRV2 Agrobacterium mixtures for 30 min. The N. benthamiana silencing rate was 95–100%. The root wounding–immersion method has similar strengths as using agrodrench. Agrodrench (Ryu et al., 2004) is a 1:1 mixture of Agrobacterium sp. TRV1 and TRV2 that is used to soak the roots, and a 10-mL pipette is then used to aspirate 3–5 mL into the crown of the plant. Although this method is simple and fast, it consumes a substantial amount of materials. After the TRV1 and TRV2 mixtures are prepared for the root wounding–immersion method, they can be used to immerse hundreds of plants each time. After immersion, a fresh bacterial suspension can be used repeatedly, which can save considerable time and costs. Moreover, 60–70% of Solanaceae plants other than N. benthamiana have shown the PDS silencing phenotype when agrodrench was used. The silencing rate of tomatoes when root wounding–immersion was used was 95%. In addition to Solanaceae plants, we found that the root wounding–immersion method is similarly suitable for A. thaliana.
4 Conclusion
In the post-genomic era, the large-scale functional genomics method is essential for converting sequence information to functional information. No recent breakthroughs have been achieved regarding TRV-VIGS. Thus, we developed the root wounding–immersion method and optimized the procedure. This is an extremely simple and efficient VIGS method that can be used to process large batches of plants (Figures 1B, C). We selected PDS and GFP as reporter genes and successfully silenced N. benthamiana, tomato, pepper, eggplant, and A. thaliana. In particular, the efficiency in N. benthamiana and tomato was 95–100%. Then, two resistance genes (SITL5 and SITL6) were used to test the reliability of the root wounding–immersion method. In contrast to leaf infiltration, spray inoculation, and the use of agrodrench, the root wounding–immersion method does not require infiltration of individual leaves or preparation of large amounts of inoculation solution, and it has low laboratory requirements. VIGS experiments can be carried out in large batches and with high efficiency within 30 min. In addition, our method is suitable for plant species that are susceptible to root inoculation and seedling inoculation in early growth stages.
Data availability statement
The original contributions presented in the study are included in the article/Supplementary Material, further inquiries can be directed to the corresponding author/s.
Author contributions
XL: Writing – original draft, Writing – review & editing. NT: Writing – original draft. BX: Investigation, Writing – original draft. JX: Funding acquisition, Writing – review & editing. ZY: Funding acquisition, Writing – review & editing. CJ: Methodology, Writing – review & editing. YZ: Data curation, Writing – original draft. MD: Funding acquisition, Project administration, Writing – original draft. JL: Conceptualization, Funding acquisition, Investigation, Writing – review & editing. KZ: Formal analysis, Investigation, Methodology, Resources, Writing – review & editing.
Funding
The author(s) declare financial support was received for the research, authorship, and/or publication of this article. This research was funded by the National Natural Science Foundation of China (32160715), the Yunnan Province Basic Research Special Key Project (202301AS070078), the Research and Integrated Applications of Key Technologies in the Standardized Production of Facility Vegetables (202102AE090005), and the Innovation Guidance and Technology-Based Enterprise Cultivation Program (202204BI090006).
Conflict of interest
The authors declare that the research was conducted in the absence of any commercial or financial relationships that could be construed as a potential conflict of interest.
Publisher’s note
All claims expressed in this article are solely those of the authors and do not necessarily represent those of their affiliated organizations, or those of the publisher, the editors and the reviewers. Any product that may be evaluated in this article, or claim that may be made by its manufacturer, is not guaranteed or endorsed by the publisher.
Supplementary material
The Supplementary Material for this article can be found online at: https://www.frontiersin.org/articles/10.3389/fpls.2024.1336726/full#supplementary-material
References
Ali, Z., Abul-faraj, A., Li, L., Ghosh, N., Piatek, M., Mahjoub, A., et al. (2015). Efficient virus-mediated genome editing in plants using the CRISPR/Cas9 system. Mol. Plant 8, 1288–1291. doi: 10.1016/j.molp.2015.02.011
Andika, I. B., Maruyama, K., Sun, L., Kondo, H., Tamada, T., Suzuki, N. (2015). Differential contributions of plant Dicer-like proteins to antiviral defenses against potato virus X in leaves and roots. Plant journal: Cell Mol. Biol. 81, 781–793. doi: 10.1111/tpj.12770
Atwood, S. E., O’Rourke, J. A., Peiffer, G. A., Yin, T., Majumder, M., Zhang, C., et al. (2014). Replication protein A subunit 3 and the iron efficiency response in soybean. Plant Cell Environ. 37, 213–234. doi: 10.1111/pce.12147
Benedito, V. A., Visser, P. B., Angenent, G. C., Krens, F. A. (2004). The potential of virus-induced gene silencing for speeding up functional characterization of plant genes. Genet. Mol. Res: GMR 3, 323–341.
Bennypaul, H. S., Mutti, J. S., Rustgi, S., Kumar, N., Okubara, P. A., Gill, K. S. (2012). Virus-induced gene silencing (VIGS) of genes expressed in root, leaf, and meiotic tissues of wheat. Funct. Integr. Genomics 12, 143–156. doi: 10.1007/s10142-011-0245-0
Bhaskar, P. B., Venkateshwaran, M., Wu, L., Ané, J. M., Jiang, J. (2009). Agrobacterium-mediated transient gene expression and silencing: a rapid tool for functional gene assay in potato. PloS One 4, e5812. doi: 10.1371/journal.pone.0005812
Bond, D. M., Baulcombe, D. C. (2015). Epigenetic transitions leading to heritable, RNA-mediated de novo silencing in Arabidopsis thaliana. Proc. Natl. Acad. Sci. United States America 112, 917–922.
Bu, R., Wang, R., Wei, Q., Hu, H., Sun, H., Song, P., et al. (2019). Silencing of glycerol-3-phosphate acyltransferase 6 (GPAT6) gene using a newly established virus induced gene silencing (VIGS) system in cucumber alleviates autotoxicity mimicked by cinnamic acid (CA). Plant Soil 438, 329–346. doi: 10.1007/s11104-019-03996-0
Burch-Smith, T. M., Anderson, J. C., Martin, G. B., Dinesh-Kumar, S. P. (2004). Applications and advantages of virus-induced gene silencing for gene function studies in plants. Plant J. 39, 734–746. doi: 10.1111/j.1365-313X.2004.02158.x
Burch-Smith, T. M., Miller, J. L., Dinesh-Kumar, S. P. (2006b). Delivery of dsRNA into plants by VIGS methodology. Cold Spring Harbor Protoc. 2006, pdb–prot4327. doi: 10.1101/pdb.prot4327
Burch-Smith, T. M., Schiff, M., Liu, Y., Dinesh-Kumar, S. P. (2006a). Efficient virus-induced gene silencing in Arabidopsis. Plant Physiol. 142, 21–27. doi: 10.1104/pp.106.084624
Caplan, J., Dinesh-Kumar, S. P. (2006). Using viral vectors to silence endogenous genes. Curr. Protoc. Microbiol. 1, 16I–166. doi: 10.1002/9780471729259.mc16i06s01
Chung, E., Seong, E., Kim, Y. C., Chung, E. J., Oh, S. K., Lee, S., et al. (2004). A method of high frequency virus-induced gene silencing in chili pepper (Capsicum annuum L. cv. Bukang). Mol. Cells 17, 377–380. doi: 10.1016/S1016-8478(23)13054-8
Deng, X., Elomaa, P., Nguyen, C. X., Hytönen, T., Valkonen, J. P., Teeri, T. H. (2012). Virus-induced gene silencing for Asteraceae–a reverse genetics approach for functional genomics in Gerbera hybrida. Plant Biotechnol. J. 10, 970–978. doi: 10.1111/j.1467-7652.2012.00726.x
Dinesh-Kumar, S. P., Anandalakshmi, R., Marathe, R., Schiff, M., Liu, Y. (2003). Virus-induced gene silencing. Methods Mol. Biol. 236, 287–294. doi: 10.1385/1-59259-413-1:287
Dommes, A. B., Gross, T., Herbert, D. B., Kivivirta, K. I., Becker, A. (2019). Virus-induced gene silencing: empowering genetics in non-model organisms. J. Exp. Bot. 70, 757–770. doi: 10.1093/jxb/ery411
Donaire, L., Barajas, D., Martínez-García, B., Martínez-Priego, L., Pagán, I., Llave, C. (2008). Structural and genetic requirements for the biogenesis of tobacco rattle virus-derived small interfering RNAs. J. Virol. 82, 5167–5177. doi: 10.1128/JVI.00272-08
Du, J., Rietman, H., Vleeshouwers, V. G. (2014). Agroinfiltration and PVX agroinfection in potato and Nicotiana benthamiana. J. Vis. Exp.: JoVE (83), e50971. doi: 10.3791/50971
Fridborg, I., Johansson, A., Lagensjö, J., Leelarasamee, N., Floková, K., Tarkowská, D., et al. (2013). ML3:a novel regulator of herbivory-induced responses in Arabidopsis thaliana. J. Exp. Bot. 64, 935–948. doi: 10.1093/jxb/ers372
Fu, D. Q., Zhu, B. Z., Zhu, H. L., Jiang, W. B., Luo, Y. B. (2005). Virus-induced gene silencing in tomato fruit. Plant J. 43, 299–308. doi: 10.1111/j.1365-313X.2005.02441.x
Fu, D. Q., Zhu, B. Z., Zhu, H. L., Zhang, H. X., Xie, Y. H., Jiang, W. B., et al. (2006). Enhancement of virus-induced gene silencing in tomato by low temperature and low humidity. Mol. Cells 21, 153–160. doi: 10.1016/s1016-8478(23)12914-1
Ganiger, M., Raruang, Y., Walker, D. R., Chen, Z. (2013). VIGS study of proteins identified in soybean and Phakopsora pachyrhizi interaction. Meeting Am.-Phytopatholog.-Soc. (APS). 103 (5), 4–4.
Gao, X., Wheeler, T., Li, Z., Kenerley, C. M., He, P., Shan, L. (2011). Silencing GhNDR1 and GhMKK2 compromises cotton resistance to Verticillium wilt. Plant J. 66, 293–305. doi: 10.1111/j.1365-313X.2011.04491.x
Hajirezaei, M. R., Peisker, M., Tschiersch, H., Palatnik, J. F., Valle, E. M., Carrillo, N., et al. (2002). Small changes in the activity of chloroplastic NADP+-dependent ferredoxin oxidoreductase lead to impaired plant growth and restrict photosynthetic activity of transgenic tobacco plants. Plant J. 29, 281–293. doi: 10.1046/j.0960-7412.2001.01209.x
Hiriart, J. B., Lehto, K., Tyystjärvi, E., Junttila, T., Aro, E. M. (2002). Suppression of a key gene involved in chlorophyll biosynthesis by means of virus-inducing gene silencing. Plant Mol. Biol. 50, 213–224. doi: 10.1023/A:1016000627231
Hsieh, M. H., Lu, H. C., Pan, Z. J., Yeh, H. H., Wang, S. S., Chen, W. H., et al. (2013a). Optimizing virus-induced gene silencing efficiency with Cymbidium mosaic virus in Phalaenopsis flower. Plant Sci. 201-202, 25–41. doi: 10.1016/j.plantsci.2012.11.003
Hsieh, M. H., Pan, Z. J., Lai, P. H., Lu, H. C., Yeh, H. H., Hsu, C. C., et al. (2013b). Virus-induced gene silencing unravels multiple transcription factors involved in floral growth and development in Phalaenopsis orchids. J. Exp. Bot. 64, 3869–3884. doi: 10.1093/jxb/ert218
Huang, Y., Mei, M., Mao, Z., Lv, S., Xie, B. (2014). Molecular cloning and virus-induced gene silencing of miasb in the southern root-knot nematode, meloidogyne incognita. Eur. J. Plant Pathol. 138, 181–193. doi: 10.1007/s10658-013-0321-5
Jiang, N., Cui, J., Meng, J., Luan, Y. (2018). A tomato nucleotide binding sites-leucine-rich repeat gene is positively involved in plant resistance to phytophthora infestans. Phytopathology 108, 980–987. doi: 10.1094/PHYTO-12-17-0389-R
Kang, G., Li, G., Ma, H., Wang, C., Guo, T. (2013). Proteomic analysis on the leaves of TaBTF3 gene virus-induced silenced wheat plants may reveal its regulatory mechanism. J. Proteomics 83, 130–143. doi: 10.1016/j.jprot.2013.03.020
Kumagai, M. H., Donson, J., della-Cioppa, G., Harvey, D., Hanley, K., Grill, L. K. (1995). Cytoplasmic inhibition of carotenoid biosynthesis with virus-derived RNA. Proc. Natl. Acad. Sci. U S A. 92, 1679–1683. doi: 10.1073/pnas.92.5.1679
Liao, J. J., Wang, C. H., Xing, Q. J., Li, Y. P., Liu, X. F., Qi, H. Y. (2019). Overexpression and VIGS system for functional gene validation in oriental melon (Cucumis melo var. makuwa Makino). Plant Cell Tissue Organ Culture (PCTOC) 137, 275–284. doi: 10.1007/s11240-019-01568-9
Liu, H., Fu, D., Zhu, B., Yan, H., Shen, X., Zuo, J., et al. (2012). Virus-induced gene silencing in eggplant (Solanum melongena). J. Integr. Plant Biol. 54, 422–429. doi: 10.1111/j.1744-7909.2012.01102.x
Liu, Y., Schiff, M., Dinesh-Kumar, S. P. (2002a). irus-induced gene silencing in tomato. Plant J. 31, 777–786. doi: 10.1046/j.1365-313X.2002.01394.x
Liu, Y., Schiff, M., Marathe, R., Dinesh-Kumar, S. P. (2002b). Tobacco Rar1, EDS1 and NPR1/NIM1 like genes are required for N-mediated resistance to tobacco mosaic virus. Plant J. 30, 415–429. doi: 10.1046/j.1365-313X.2002.01297.x
MacFarlane, S. A., Popovich, A. H. (2000). Efficient expression of foreign proteins in roots from tobravirus vectors. Virology 267, 29–35. doi: 10.1006/viro.1999.0098
Martin, R. C., Glover-Cutter, K., Martin, R. R., Dombrowski, J. E. (2013). Virus induced gene silencing in Lolium temulentum. Plant Cell. 113, 163–171. doi: 10.1007/s11240-012-0257-z
Meziadi, C., Blanchet, S., Richard, M. M., Pilet-Nayel, M. L., Geffroy, V., Pflieger, S. (2016). Bean pod mottle virus: a new powerful tool for functional genomics studies in Pisum sativum. Plant Biotechnol. J. 14, 1777–1787. doi: 10.1111/pbi.12537
Nowicki, M., Foolad, M. R., Nowakowska, M., Kozik, E. U. (2012). Potato and tomato late blight caused by phytophthora infestans: an overview of pathology and resistance breeding. Plant Dis. 96, 4–17. doi: 10.1094/PDIS-05-11-0458
Pang, J., Zhu, Y., Li, Q., Liu, J., Tian, Y., Liu, Y., et al. (2013). Development of Agrobacterium-mediated virus-induced gene silencing and performance evaluation of four marker genes in Gossypium barbadense. PloS One 8, e73211. doi: 10.1371/journal.pone.0073211
Ratcliff, F., Martin-Hernandez, A. M., Baulcombe, D. C. (2001). Technical Advance. Tobacco rattle virus as a vector for analysis of gene function by silencing. Plant journal: Cell Mol. Biol. 25, 237–245. doi: 10.1046/j.0960-7412.2000.00942.x
Ruiz, M. T., Voinnet, O., Baulcombe, D. C. (1998). Initiation and maintenance of virus-induced gene silencing. Plant Cell. 10, 937–946. doi: 10.1105/tpc.10.6.937
Ryu, C. M., Anand, A., Kang, L., Mysore, K. S. (2004). Agrodrench: a novel and effective agroinoculation method for virus-induced gene silencing in roots and diverse Solanaceous species. Plant J. 40, 322–331. doi: 10.1111/j.1365-313X.2004.02211.x
Senthil-Kumar, M., Anand, A., Uppalapati, S. R., Mysore, K. S. (2008). Virus-induced gene silencing and its applications. CABI Rev. 11. doi: 10.1079/PAVSNNR20083011
Senthil-Kumar, M., Hema, R., Anand, A., Kang, L., Udayakumar, M., Mysore, K. S. (2007). A systematic study to determine the extent of gene silencing in Nicotiana benthamiana and other Solanaceae species when heterologous gene sequences are used for virus-induced gene silencing. New Phytol. 176 (4), 782–791. doi: 10.1111/j.1469-8137.2007.02225.x
Senthil-Kumar, M., Mysore, K. S. (2011a). Virus-induced gene silencing can persist for more than 2 years and also be transmitted to progeny seedlings in Nicotiana benthamiana and tomato. Plant Biotechnol. J. 9 (7), 797–806. doi: 10.1111/j.1467-7652.2011.00589.x
Senthil-Kumar, M., Mysore, K. S. (2011b). New dimensions for VIGS in plant functional genomics. Trends Plant Sci. 16, 656–665. doi: 10.1016/j.tplants.2011.08.006
Singh, D. K., Lee, H. K., Dweikat, I., Mysore, K. S. (2018). An efficient and improved method for virus-induced gene silencing in sorghum. BMC Plant Biol. 18, 123. doi: 10.1186/s12870-018-1344-z
Spitzer, B., Zvi, M. M., Ovadis, M., Marhevka, E., Barkai, O., Edelbaum, O., et al. (2007). Reverse genetics of floral scent: application of tobacco rattle virus-based gene silencing in Petunia. Plant Physiol. 145, 1241–1250. doi: 10.1104/pp.107.105916
Szittya, G., Silhavy, D., Molnár, A., Havelda, Z., Lovas, A., Lakatos, L., et al. (2003). Low temperature inhibits RNA silencing-mediated defense by the control of siRNA generation. EMBO J. 22, 633–640. doi: 10.1093/emboj/cdg74
Tian, J., Pei, H., Zhang, S., Chen, J., Chen, W., Yang, R., et al. (2014). TRV–GFP: a modified Tobacco rattle virus vector for efficient and visualizable analysis of gene function. J. Exp. Bot. 65, 311–322. doi: 10.1093/jxb/ert381
Tuttle, J. R., Idris, A. M., Brown, J. K., Haigler, C. H., Robertson, D. (2008). Geminivirus-mediated gene silencing from Cotton leaf crumple virus is enhanced by low temperature in cotton. Plant Physiol. 148, 41–50. doi: 10.1104/pp.108.123869
Valentine, T., Shaw, J., Blok, V. C., Phillips, M. S., Oparka, K. J., Lacomme, C. (2004). Efficient virus-induced gene silencing in roots using a modified tobacco rattle virus vector. Plant Physiol. 136, 3999–4009. doi: 10.1104/pp.104.051466
Wang, P., Zhang, J., Su, J., Wang, P., Liu, J., Liu, B., et al. (2013). The chloroplast min system functions differentially in two specific nongreen plastids in Arabidopsis thaliana. PloS One 8, e71190. doi: 10.1371/journal.pone.0071190
Yan, H. X., Fu, D. Q., Zhu, B. Z., Liu, H. P., Shen, X. Y., Luo, Y. B. (2012). Sprout vacuum-infiltration: a simple and efficient agroinoculation method for virus-induced gene silencing in diverse solanaceous species. Plant Cell Rep. 31, 1713–1722. doi: 10.1007/s00299-012-1285-1
Yang, J., Zhang, T. Y., Liao, Q. S., He, L., Li, J., Zhang, H. M., et al. (2018). Chinese wheat mosaic virus-induced gene silencing in monocots and dicots at low temperature. Front. Plant Sci. 9, 1627. doi: 10.3389/fpls.2018.01627
Yu, J., Gao, L., Liu, W., Song, L., Xiao, D., Liu, T., et al. (2019). Transcription coactivator ANGUSTIFOLIA3 (AN3) regulates leafy head formation in Chinese cabbage. Front. Plant Sci. 10, 520. doi: 10.3389/fpls.2019.00520
Zhang, C., Liu, L., Zheng, Z., Sun, Y., Zhou, L., Yang, Y., et al. (2013). Fine mapping of the Ph-3 gene conferring resistance to late blight (Phytophthora infestans) in tomato. TAG. Theor. Appl. Genet. 126, 2643–2653. doi: 10.1007/s00122-013-2162-1
Zhang, Z., Thomma, B. P. H. J. (2014). Virus-induced gene silencing and agrobacterium tumefaciens-mediated transient expression in nicotiana tabacum. Methods Mol. Biol. 1127, 173–181. doi: 10.1007/978-1-62703-986-4_14
Zhao, K., Shen, X., Yuan, H., Liu, Y., Liao, X., Wang, Q., et al. (2013). Isolation and characterization of dehydration-responsive element-binding factor 2C (MsDREB2C) from Malus sieversii Roem. Plant Cell Physiol. 54, 1415–1430. doi: 10.1093/pcp/pct087
Keywords: root wounding–immersion method, virus-induced gene silencing, agroinoculation, tobacco rattle virus, gene function
Citation: Li X, Tao N, Xu B, Xu J, Yang Z, Jiang C, Zhou Y, Deng M, Lv J and Zhao K (2024) Establishment and application of a root wounding–immersion method for efficient virus-induced gene silencing in plants. Front. Plant Sci. 15:1336726. doi: 10.3389/fpls.2024.1336726
Received: 11 November 2023; Accepted: 15 March 2024;
Published: 19 April 2024.
Edited by:
Xingguo Ye, Chinese Academy of Agricultural Sciences, ChinaReviewed by:
Yang Jian, Ningbo University, ChinaJinping Zhao, Texas A and M University, United States
Copyright © 2024 Li, Tao, Xu, Xu, Yang, Jiang, Zhou, Deng, Lv and Zhao. This is an open-access article distributed under the terms of the Creative Commons Attribution License (CC BY). The use, distribution or reproduction in other forums is permitted, provided the original author(s) and the copyright owner(s) are credited and that the original publication in this journal is cited, in accordance with accepted academic practice. No use, distribution or reproduction is permitted which does not comply with these terms.
*Correspondence: Junheng Lv, anVuaGVuZ2x2QHluYXUuZWR1LmNu; Kai Zhao, a2FpbGl4aWFuMTAyM0BhbGl5dW4uY29t
†These authors have contributed equally to this work