- 1College of Plant Science, Jilin University, Changchun, China
- 2Soybean Research Institute, Jilin Academy of Agricultural Sciences, Changchun, China
Introduction: Intercropping practices play a crucial role in enhancing and maintaining the biodiversity and resiliency of agroecosystems, as well as promoting stable and high crop yields. Yet the relationships between soil nitrogen, microbes, and yield in maize cultivated under maize/soybean intercropping systems remain unclear.
Methods: To fill that knowledge gap, here we collected maize rhizosphere soil at the staminate stage after 6 consecutive years of maize/soybean intercropping, to investigate how intercropping and nitrogen application rates affected nitrogen utilization by crops and soil microbial community composition and function. We also examined correlations of those responses with yields, to clarify the main ways that yield is enhanced via intercropping and by nitrogenous fertilizer gradient changes generated by different nitrogen application rates.
Results: The amount of applied fertilizer was 240 kg N ha-1 was best for obtaining a high maize yield and also led to the greatest nitrogen-use efficiency and bacterial diversity. Under the same N application rate, intercropping increased the maize yield by 31.17% and soil nitrogen (total, ammonium and nitrate nitrogen) by 14.53%, on average, in comparison to monocropping. The enrichment of Gemmatimonas and Bradyrhizobium significantly increased the soil nitrogen content, and a greater relative abundance of Sphingomonas and Gemmatimonas increased the maize yield, whereas enrichment of Candidatus_Udaeobacter and Bradyrhizobium decreased it. The benefits of intercropping mainly arise from augmenting the abundance of beneficial microorganisms and enhancing the efficiency of N use by crop plants.
Discussion: This study’s findings are of key importance to bolster the stability of agro-ecosystems, to guide the scientific rational use of nitrogen fertilizers, and to provide a sound theoretical basis for achieving the optimal management of intensive crop-planting patterns and green sustainable development.
1 Introduction
As one of the world’s staple food crops, maize figures prominently in global agricultural production (Zhou and Butterbach-Bahl, 2014). However, the continuous cultivation of a single crop—i.e., monocropping or a monoculture system—can lead to declining soil quality and its greater susceptibility to pests and diseases (Du et al., 2018). Hence, it is imperative that farmers begin to scientifically and rationally apply maize/soybean intercropping planting techniques to improve their crop yields and economic benefits. Intercropping is a type of ecological agriculture that improves the accumulation of nutrients in soil for uptake by plants, to promote their growth via complementary trait advantages among different crop species (Fan et al., 2023). Proper intercropping patterns can bolster soil structure, alter soil physicochemical properties, and increase soil’s nutrient contents and enzymatic activity (Secco et al., 2023). Improved soil conditions then enhance plants’ growth, physiology, and nutrient uptake, leading to higher biodiversity overall, which also entails a greater overall yield of intercropping systems (Yang et al., 2020). Intercropping also enriches the diversity of soil microbial communities, improves soil health, and helps to balance beneficial microorganisms vis-à-vis harmful ones, all of which is advantageous for plant growth and development (Guo et al., 2024).
Nitrogen (N) is the most basic element of life, being a key component of proteins, nucleic acids. and other essential substances of living organisms, and thus directly involved in myriad biological processes (Bai et al., 2023). Regarding N uptake and N use in grass-bean intercropping systems, the proven benefits of maize/soybean intercropping are known to include an increased effective N content of soil and fixing of N and its transfer to grasses, thus reducing their demand for chemical N fertilizers and improving the efficiency of water use and other nutrient uses (Lu et al., 2023). In recent years, whether the N fixed by legumes can be transferred to adjacent grass crops in intercropping systems has emerged as a hot research topic (Chen et al., 2023; Liu et al., 2023). Further, intercropping with cereals can reduce the “N-deterrent” effect of chemical N fertilizers on legumes, thereby promoting legume nodulation and increasing the rate of N fixation (Xu et al., 2020). Overall, the two paramount reasons for efficient N use in grass/legume intercropping systems are “N transfer” and “N repression”, with both phenomena essentially the outcome of N competition dynamics and complementarity between grass crops and legumes (Zhang et al., 2017).
A healthy soil environment underpins high and stable crop yields and is shaped by land use and land nutrition (Qi et al., 2023). One study showed that rhizosphere soil pH fell markedly in a soybean monoculture, but intercropping was able to mitigate that decline (Zaeem et al., 2019). However, intercropping has a dual effect on the organic matter content of soil. On one hand, intercropping can increase it by enhancing ecological interactions in the soil microenvironment that hasten the decomposition of plant and animal residues (Zhu et al., 2024); on the other hand, plants compete for soil nutrients, which accelerates mineralization, the decomposition of soil organic matter, and nutrient cycling, collectively reducing soil’s organic matter content (Roohi et al., 2022).
In intercropping systems, competition and facilitation effects that arise between plant species often enrich the diversity of soil microbial communities and enlarge microbial populations, culminating in mutual benefits and co-promotion achieved under appropriate intercropping modes (Zhang et al., 2023). Previous research has found a substantially increased number of soil bacteria and actinomycetes, but a considerable decrease in the number of fungi under maize/soybean intercropping vis-à-vis maize monocropping (Fu et al., 2019). According to work by Tian et al. (2019), a greater relative abundance of soil beneficial bacteria belonging to dominant taxa, such as Proteobacteria and Acidobacteria, and likewise dominant fungi, namely Ascomycota and Basidiomycota, can help to augment the overall number of beneficial microbes in soil. Recently, Li et al. (2021) showed that the structure of soil microbial populations was altered in intercropped peanut and cereal crops, resulting in less bacteria that are harmful (such as Spiroplasma) and more bacteria that are beneficial (such as Streptomycetaceae and Bacillus). The above studies uncovered impacts of intercropping for improving soil microbial population types and community structure.
While those studies do provide a good summary of recent progress towards elucidating the effects of intercropping systems upon specific soil environments, the experimental methods employed tend to differ with intercropping patterns varying widely. In recent years, research on maize/soybean intercropping has focused chiefly on the relationship between light energy use and crop yield (Yao et al., 2017; Zheng et al., 2022), leaving far less known about how maize soil N use in combination with changes in the rhizosphere soil microbial community could affect their maize yield. Here we sampled maize soils at the male withdrawal stage in a continuous 6-year maize/soybean intercrop system, then quantified the use of soil nitrogen in its transformation and determined the bacterial community composition via high-throughput sequencing. This study had three objectives: (1) to quantify the effect of maize/soybean intercropping on maize soil nitrogen-use efficiency; (2) to distinguish the influential factors and mechanisms linking the maize-soybean intercropping system to soil microbial changes in the maize rhizosphere; and (3) to clarify the interactions between the soil microenvironment and maize yield under a maize/soybean intercropping system. This study provides a theoretical basis for the implementation of optimal management and environmentally sustainable development of intensive cropping systems.
2 Materials and methods
2.1 Study area
The experiment was run at the Agricultural Experimental Base of Jilin University, Changchun City, Jilin Province (125°14.23’ E, 43°56.60’ N; elevation: 245 m a.s.l). The site has a black soil type, a temperate continental semi-moist monsoon climate, with an average annual precipitation of 600–700 mm and average annual temperature of 4.6°C (max: 40°C and min: –36.5°C). The annual frost-free period lasts 140–150 days and the annual freezing period spans 150–160 days. The soil type in the field is Phaeozems (FAO-WRB classification system, 2014).Prior to the experiment, the pH value in the soil was 5.33, and it had these characteristics: organic carbon (1.37%), total nitrogen (1.41 g·kg-1); total phosphorus (0.48 g·kg-1) and total potassium (21.42 g·kg-1).
2.2 Experimental design
This trial was established as a long-term locational trial, begun 2017. Its fertilizer application rates, and tillage practices remained consistent across all treatments from 2017 through 2023. Likewise, the same field management practices were maintained across the treatments during the fertility period in every year. While the experiment was continued from 2017 to 2023, the samples for analyses were taken in 2023 growing period. This experiment used a two-factor split-plot design, with the nitrogen supply level assigned to the main plots (blocks) and the planting mode to the subplots (strips). The maize variety used for testing was ‘Xianyu 335’ and the soybean variety was ‘Changnong 16’, both supplied by the Jilin Academy of Agricultural Sciences. Their seeds were sown on 26 April 2023, with the maize monoculture (MM) serving as the control group and intercropping of maize and soybean (IM) (maize/soybean, 2:2) set as the experimental group. Each plot was 62.4 m2 in size and contained 12 rows of maize in the monoculture plot. The maize/soybean intercropping plot consisted of three strips: each strip was planted with two rows of maize and two rows of soybean. The row spacing was 65 cm and the planting density was 90 000 plants ha-1 for maize and 180 000 plants ha-1 for soybean (Figure 1). Fertilizer application levels were the same for the intercropping and monocropping plots, with maize receiving 0 (N0), 180 (N1), 240 (N2), or 300 (N3) kg N ha-1. Basal fertilizer rates were 0, 40, 80, and 120 kg N ha-1, with the remainder of the nitrogen fertilizer applied as a top-up fertilizer at the nodulation stage of maize. The phosphorus fertilizer consisted of heavy calcium superphosphate (P2O5 46%) applied at 120 kg P2O5 ha-1; the potassium fertilizer was potassium sulfate (K2O 50%), it applied at 100 kg K2O ha-1. Both phosphorus and potassium fertilizers were applied as basal applications.
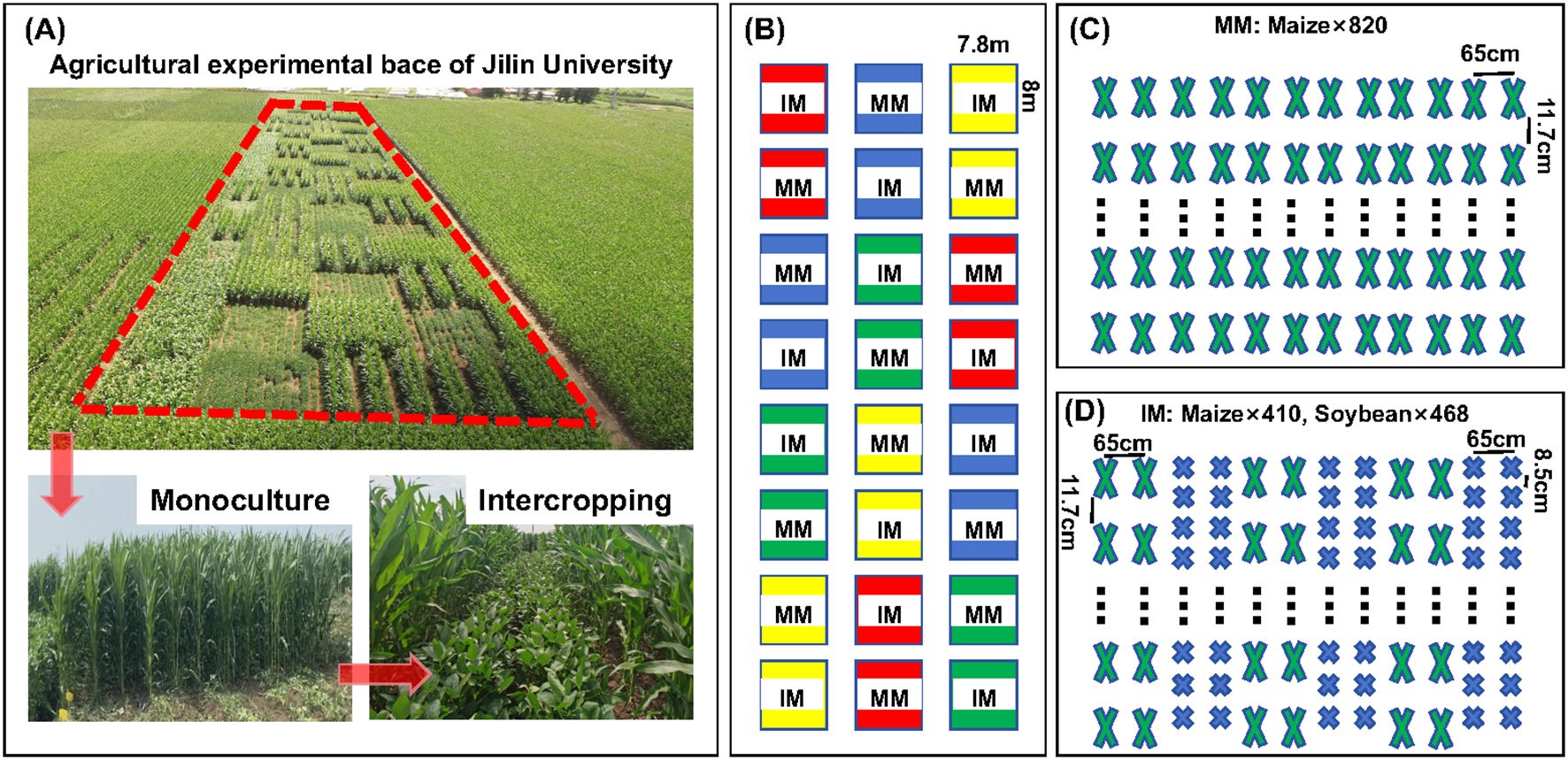
Figure 1. Aerial view (A) and schematic (B) layout of the maize/soybean intercropping experiment under different nitrogen application rates. In (B), red is the N0 level, blue is the N1 level, green is the N2 level, and yellow is the N3 level. (C) distribution of maize monoculture (MM) trial plots; (D) distribution of maize–soybean intercropping (IM) trial plots. where 820, 468, and 410 are the number of crop plants in the plot; 65 cm refers to the spacing between rows, while 8.5 cm or 11.7 cm is the spacing between plants within a given row.
2.3 Soil and plant sampling
Surface soil (0–20 cm depth, between adjacent maize on the ridge) and rhizosphere soil samples were collected on 21 July 2023 (at the maize staminate stage). Surface soil was sampled using a soil auger, at five points selected from each plot (in an X formation), with root samples were taken by drilling in situ on the ridge using an Eikelkamp root auger (auger diameter 8.0 cm, length 20.0 cm, 75% ethanol disinfection). After air-drying the soil samples indoors and passing them through a 75-mesh sieve, the ensuing fine-grained soil was used for the determination of soil chemical properties. To collect rhizosphere soil, three consecutive plants were excavated from each plot in the field, with their roots and attached soil intact. The top layer of soil was then removed; then, soil loosely bound to the root system was gently shaken and collected, and this mixed it together as a composite sample of rhizosphere soil for later testing (n = 6). All these rhizosphere soil samples were stored in a freezer at –80°C until their microbial diversity analysis. When the maize plants were mature, two rows from each plot were harvested; after threshing all their kernels, the grain weight was measured and converted to hectare yield based on the area harvested. The kernels’ moisture content was recorded with a PM8188 moisture meter, for three replicates, and the final yield data then expressed as the seed (grain) weight at 14% moisture. Panicle length, ear diameter, number of grains, 100-seed weight, and barren tip size were recorded as well.
2.4 Soil and plant sampling
2.4.1 Soil nutrients
To determine soil pH and EC (electrical conductivity), a water-to-soil ratio of 5:1 was used, this shaken at 180 r/min for 5 min and allowed to stand for 30 min before taking the respective measurements with a pH meter (pH-100A,100-2000 rpm, LICHEN, Shanghai, China) and a conductivity meter (DDSJ-11A-307, YUEPING, Shanghai, China). Soil ammonium nitrogen (NH4+-N) and nitrate nitrogen (NO3–N) fractions were extracted with sodium bicarbonate, shaken at 180 r/min for 2 h, allowed to stand for 30 min, and then filtered through a 0.45-μm PES membrane. For total soil nitrogen (TN), samples were treated with Kjeldahl digestion and filtered through a 0.45-μm PES membrane, and then measured by a continuous flow analyzer (AA3, AutoAnalyzer 3, Technician, Windows/NT) (Wang et al., 2023a). Soil total organic carbon (TOC) fractions were determined by wrapping 10-mg soil samples in aluminum foil and using an elemental analyzer (vario TOC cube, NDIR, 60 000 ppm, 2 ppb, GER) (Zhu et al., 2021).
2.4.2 Nitrogen utilization
Just before the field was harvested, five intact maize plants with uniform and typical growth were selected from each plot, and both parts oven-dried at 105°C for 30 min and then again, at 75°C, to a constant weight. After weighing the dry matter, the samples were crushed, digested with H2SO4-H2O2, and the nitrogen content of the maize plants and kernels determined using a Seal AA3 flow analyzer. Nitrogen-use efficiency (NUE) (i), nitrogen agronomic efficiency (NAE) (ii), nitrogen partial productivity (NPP) (iii) and nitrogen harvest index (NHI) (iv) were calculated as follows (Bai et al., 2024):
i. NUE (%) = (N accumulation of aboveground plants in the N-applied area – N accumulation of above-ground plants in non-N applied area)/N application × 100.
ii. NAE (kg·kg-1) = (seed yield in the N-applied area – seed yield in non-N applied area)/nitrogen application rate × 100.
iii. NPP (kg·kg-1) = seed yield/applied nitrogen.
iv. NHI (%) = total seed N accumulation/total plant N accumulation.
2.4.3 Diversity of soil bacterial communities
Total DNA was extracted from each soil sample using a DNA kit (MN NudeoSpin 96 Soi) and the DNA concentration was then determined on a NanoDrop 2000 spectrophotometer. The PCR reaction conditions were as follows: pre-denaturation at 94°C for 5 min and then 30 cycles at 94°C for 30 s, 50°C for 30 s and 72°C for 60 s, followed by a stable extension at 72°C for 7 min, and finally stored at 4°C. The extracted genomic DNA was detected by 1% agarose gel electrophoresis. For the bacterial 16S gene, the primer pair of 338F (5’-ACTCCTACGGGAGGCAGCAG-3’) and 806R (5’-GGACTACHVGGGTWTCTAAT-3’) was used to amplify the V3-V4 region, and the ensuing products were purified, quantified, and standardized. Information about the hardware and software used for sequencing is presented in Table 1.
Library construction went as follows: (1) ligation of the ‘Y’ junction; (2) removal of junction self-associated fragments by magnetic bead screening; (3) enrichment of the library template via PCR amplification; (4) denaturation by sodium hydroxide to produce single-stranded DNA fragments.
Next, these eight sequencing steps were applied: (1) one end of the DNA fragment that is complementary to the primer base is fixed on the chip; (2) its other end randomly complementary to another primer in the vicinity is also fixed, to form a ‘bridge’; (3) PCR amplification is carried out to produce DNA clusters; (4) the DNA amplicon is linearized into a single strand; (5) add the modified DNA polymerase and dNTP with four fluorescent labels, synthesizing only one base per cycle; then (6) scan the surface of the reaction plate with a laser to read the nucleotide species polymerized in the first reaction of each template sequence; (7) chemically cleave the “fluorescent group” and the “termination group” to restore the 3’-end attachment, and continue to polymerize the second nucleotide; and end by (8) counting the fluorescence signals collected in each round to obtain the sequence of the template DNA fragment (Wang et al., 2022b). All obtained sequences were deposited, and expression data uploaded to the NCBI (National Center for Biotechnology Information) (https://www.ncbi.nlm.nih.gov/) (accessed on 13 June 2024), under BioProject ID: PRJNA1132549.
2.5 Soil and plant sampling
Statistical analyses were implemented using SPSS 22.0 software to compare the effects of intercropping upon the soil response variables, using univariate two-way ANOVAs. Pearson correlations were used to evaluate the relationships between relative abundance of dominant genera, soil chemical properties, and maize yield. Beta diversity analysis was done based on the coefficient of variation of the Aitchison distance, and principal component analysis (PCA) used to compare the degree of similarity present between different samples in terms of species community diversity. The visual analysis of microbial ecological networks and derivation of topological indices were carried out in Gephi software (v0.9.6). The following topological indices were used to describe the nodes and connecting lines in the constructed microbial network: (1) the number of connecting lines of a node, which is the sum of all lines linked to each node; (2) the median centrality of a node, which is the node located on the shortest path between two nodes, this calculated according to the Formula 1 below; (3) the topological coefficient of a node, which conveys the proximity of nodes and is expressed by Formula 2 below; (4) the connecting line weight, which reflects the number of connections between a particular OTU (operation taxonomic unit) node and the sample node; (5) the connecting line centrality, a parameter that gauges the importance of each connecting line in the information transfer process of the whole network (Faust, 2021). Structural equation modeling (SEM) of the direct or indirect effects of intercropping and nitrogen application rates on yield pathways was implemented using R v4.3.1 (https://www.r-project.org/).
In the above equation, n is the destination node; s and t are nodes in the network other than n; denotes the number of shortest paths from node s to node t, and the term denotes the number of shortest paths from node s to node t that must pass through node n.
Here, is the number of all nodes adjacent to both nodes n and m, where the value of is increased by 1 if n is directly adjacent to m; is the number of all neighbours of that node.
3 Results
3.1 Maize yield
Table 2 presents the effects from the two contrasting modes of cropping and from different nitrogen application rates on the maize yield factors. When their comparing maize yields under the same nitrogen application rates, in all cases IM (intercropping maize) > MM (monoculture maize), with maize yields at N0, N1, N2, and N3 fertilizer levels increased by 45.39%, 25.57%, 25.15% and 28.58%, respectively. From a nitrogen application perspective, the strongest yield effect occurred in going from the N0 to N1 level with an increase of 53.77% and 32.49% in MM and IM, respectively. That increase fell correspondingly to 12.31% and 12.21% at the N2 level; however, when a greater nitrogen application was used, i.e., N3 level, the maize yield decreased by 8.27% and 5.76% relative to that obtained for N2.
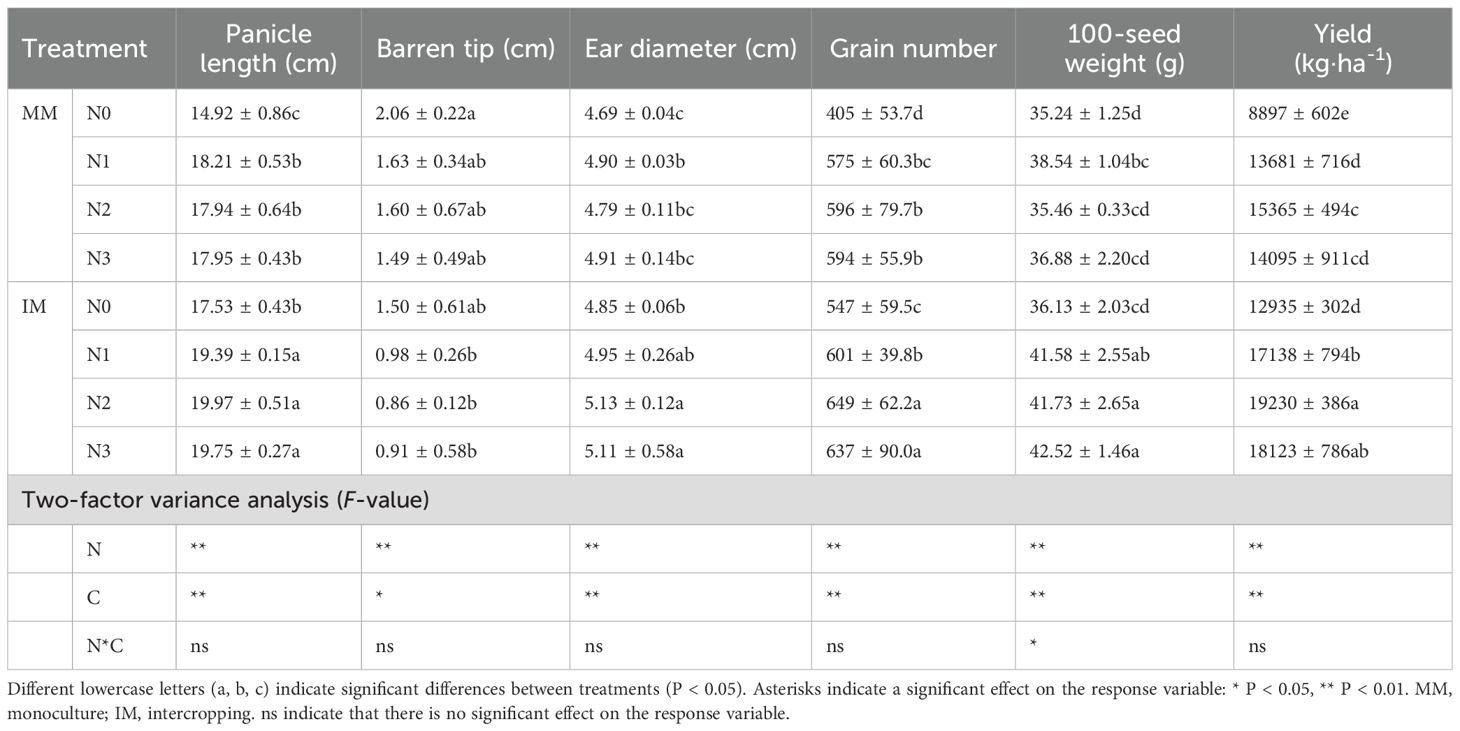
Table 2. Yields and yield factors of maize in response to two contrasting cropping systems (C) and four nitrogen application rates (N1–4).
The effects on panicle length, ear diameter, grain number, and 100-seed weight were consistent with the maize yield changes, in that all four were higher under IM than MM, but vice versa for barren tip (MM > IM). Most of the yield factors in the MM treatments showed this response, of N1 > N2, with the exception of only a lower grain number and longer barren tip for the N2; hence, the N1 yield under MM was lower than that under N2 mainly due to the effects on grain number and barren tip, while all yield factors in the IM treatment peaked at the N2 level.
The two-factor ANOVAs showed that the nitrogen application rate (N) as well as cropping mode (C) significantly affected all the indicators (P < 0.01), whereas their interaction effect (C*N) was only significant for the 100-seed weight (P < 0.05). Evidently, the N2 treatment was the better level of N application irrespective of planting mode (MM and IM), and IM coupled with N2 resulted in the highest maize yield.
3.2 Soil chemistry and nitrogen content
Soil pH under either monoculture (MM) or intercropping (IM) tended to decrease with an increasing nitrogen application level, being lowest in the N3 treatment for both, under which it was significantly (P < 0.05) reduced by 0.36 and 0.27 vis-à-vis N0 (Figure 2A). However, soil pH was similar under MM and IM at the same level of nitrogen applied. Soil EC values of treatments under MM increased with greater levels of nitrogen application (Figure 2B), significantly rising by 63.48% in N3 over the N0 treatment (P < 0.05). However, soil EC was affected differently under IM, being highest instead at the N1 level, such that with more nitrogen applied, the soil EC values decreased, even falling below that of the N0 treatment. For example, the soil EC value of N3 was reduced by 9.17% in comparison with N0. Under MM and IM, the soil TOC content reached its maximum when the nitrogen application level was increased to N2, but adding more nitrogen led to reductions in the soil TOC content (Figure 2C). In this case, relative to N0, the N2 treatment significantly increased the soil TOC content by 28.24% and 24.09% (P < 0.05) but the soil TOC content did not differ significantly between MM and IM at the same nitrogen level. Overall, different nitrogen application levels emerged as the main factor driving changes in soil pH, EC and TOC, while IM could have led to weakened soil EC.
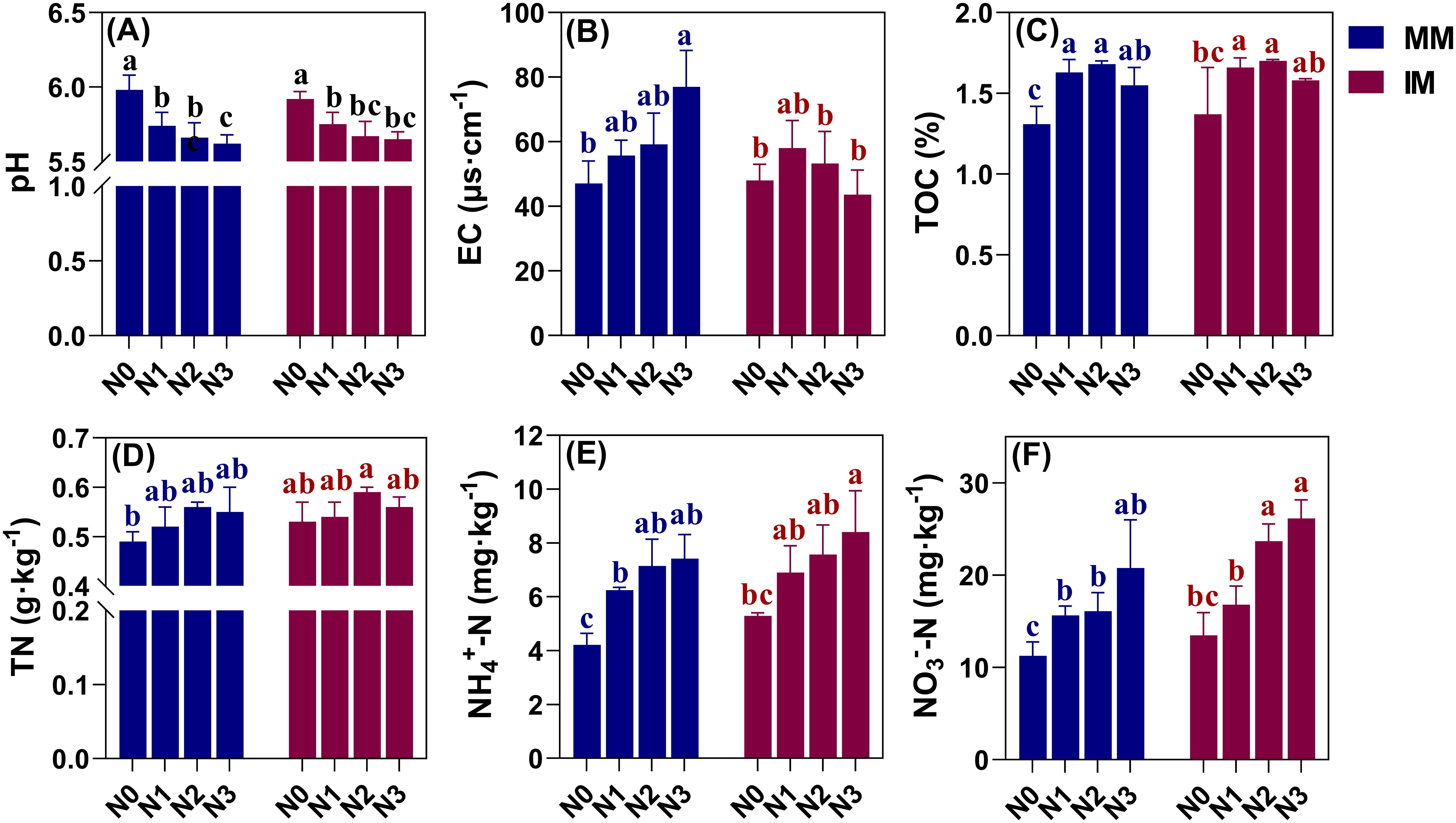
Figure 2. Effects of two contrasting cropping systems and nitrogen application rates on soil pH (A) and EC (B), and the total organic carbon (TOC) (C), total nitrogen (TN) (D), ammonium nitrogen (NH4+-N) (E), and nitrate nitrogen (NO3--N) (F) in soil. Different lowercase letters (a, b, c) above the bars (mean ± SD, n = 9) indicate significant differences between treatments (P < 0.05). MM, monoculture; IM, intercropping.
The effects of different modes of cropping and nitrogen application levels on soil nitrogen content are presented in Figures 2D–F. Evidently, the soil NH4+-N and NO3–N contents under MM and IM varied consistently, with both increasing in response to more nitrogen applied and peaking in the N3 treatment, significantly surpassing the N0 treatment by 58.98%–75.83% and 84.38%–93.93%, respectively (P < 0.05). For soil TN content, however, it was maximal at the N2 level and increasing the nitrogen application further reduced it. Notably, there was significant change in the soil TN content with increasing N application between treatments under MM or IM (P > 0.05), and only the N0 treatment under MM differed significantly from the N2 treatment under IM (P < 0.05). By comparing the different cropping systems, the outcome of a higher soil NH4+-N and NO3–N content was more pronounced under IM, increasing by 20.23% and 16.46% in response to N0 under IM vis-à-vis MM, respectively, while soil TN content increased by only 7.55%.
3.3 Nitrogen-use efficiency
To clarify the effects of different fertilizer application rates and cropping systems on nitrogen fertilizer use, we calculated the nitrogen-use efficiency (NUE), nitrogen agronomic efficiency (NAE), nitrogen partial productivity (NPP), and the nitrogen harvest index (Table 3). This analysis revealed that all indicators except NAE had the response pattern of IM > MM for a given application level, whereas NAE was always similar between the cropping systems. All indicators except NPP reached their maximum values at the N2 level, where NUE and NHI respectively increased significantly (P < 0.05) by 44.56% and 11.20% respectively, and NAE decreased by 2.67% under IM in comparison to MM. The NPP peaked at the N1 level under both IM and MM, after which it decreased significantly (P < 0.05) with more nitrogen applied. Two-way ANOVAs revealed that the application rate was the main factor influencing the nitrogen fertilizer use. Overall, the N2 level under IM had the greatest nitrogen fertilizer use.
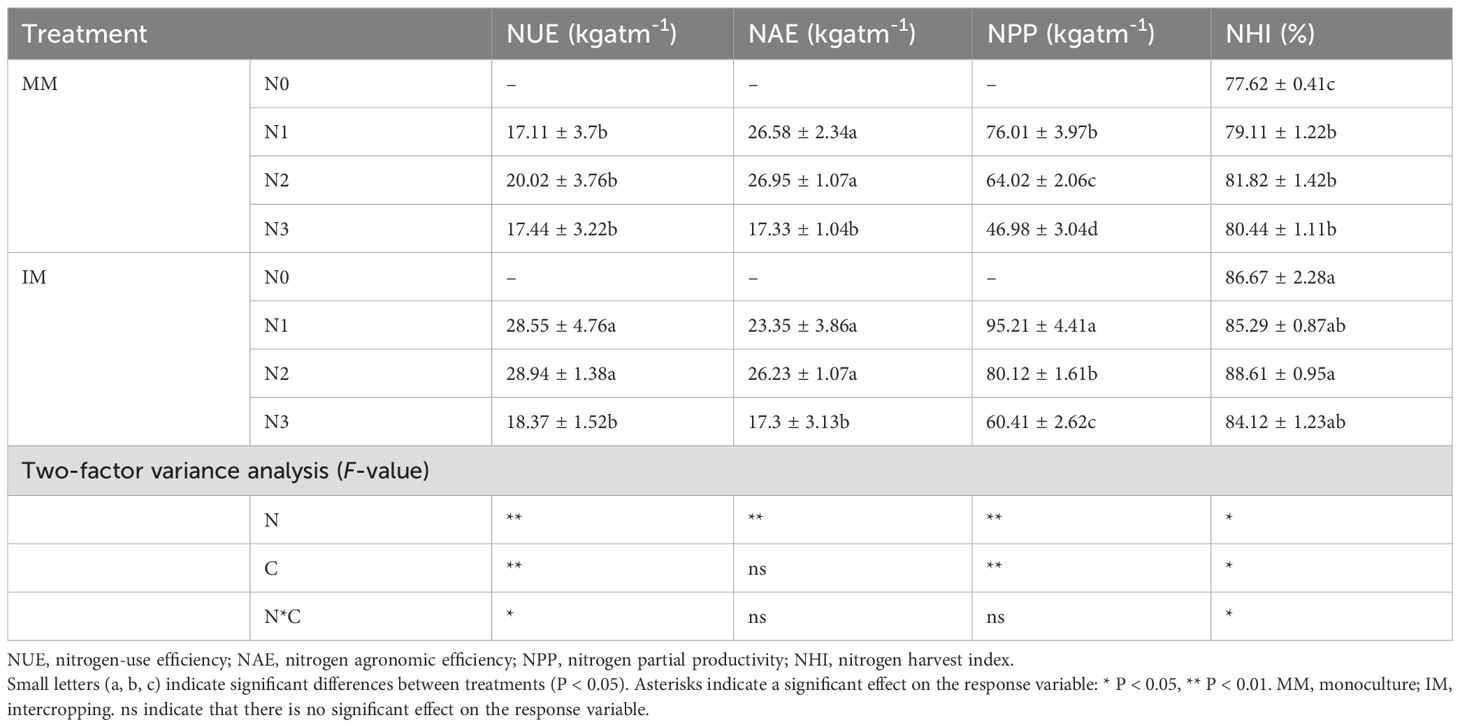
Table 3. Statistics for soil indicators of nitrogen fertilizer use under two contrasting cropping systems (C) and N application rates (N).
3.4 Changes in soil microbial communities
3.4.1 Alpha diversity of bacterial communities
Upon completing the sequencing in this study, the OTUs were screened for de-low content, after which the non-repetitive sequences (excluding single sequences) were clustered into OTUs based on 97% similarity, with any chimeras removed in the clustering process, to obtain representative sequences of OTUs. The final number of OTUs totaled 3601, resulting in a minimum of 30 566 optimized sequences per sample. To investigate the alpha diversity of individual soil samples, the richness (chao1 index), diversity (Shannon index and PD_whole_tree), and sequence depth (Goods_coverage) of the soil bacterial community were calculated for each cropping system and nitrogen fertilizer treatment. As seen in Table 4, the Goods_coverage for bacteria in all treatments was above 95%. For bacterial community diversity, the Shannon index and PD_whole_tree varied consistently among treatments, being highest at N0 level under both MM and IM modes of cropping; however, increasing the nitrogen application rate led to lower bacterial community diversity, this significantly reduced by 14.05%–17.66% and 11.73%–14.16% in N3 relative to N0 (P < 0.05). For the same level of nitrogen application, bacterial community diversity was higher under IM than MM; for example, it significantly increased by 11.86% and 10.94% (P < 0.05) under IMN0 than MMN0, but not as much (7.16%–7.88%) when more nitrogen was added up to the N3 level. In terms of bacterial community richness, the Chao1 index had an identical pattern to the diversity index, in that it was higher in IM than MM, rising by 9.13% and IMN0 compared to MMN0. Interestingly, the Chao1 index was likewise disrupted by adding more nitrogen, peaking at the N2 level in both MM and IM, with further increases in the nitrogen application significantly decreasing its value (P < 0.05). A two-way ANOVA showed that both the N application level (N) and cropping pattern (C) were highly significantly correlated (P < 0.01) with alpha diversity, and overall, intercropping increased the bacterial community’s alpha diversity regardless of N application level, with an increasing N application decreasing that diversity.
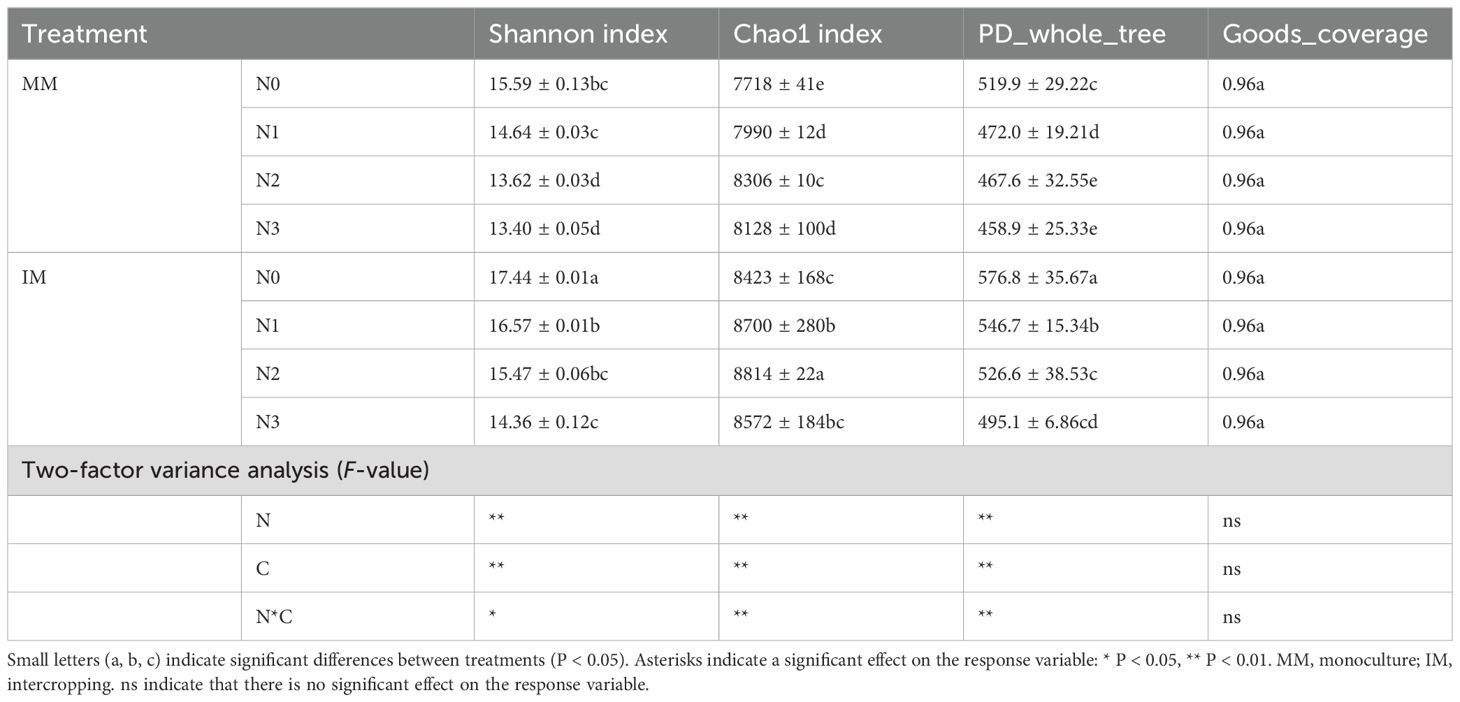
Table 4. Alpha diversity statistics of bacterial communities under two contrasting cropping systems (C) and four nitrogen application rates (N).
3.4.2 Community composition for dominant bacterial genera
Altogether, the soil samples could be annotated to 645 bacterial genera. Of these, dominant bacterial genera accounted for 17.48%–20.66% of the total, after selecting the top-50 genera by relative abundance and excluding the seven genera whose abundance was < 1% (Figure 3). At N0 level, the abundance of dominant bacterial genera was 11.49% higher in IM than MM. Across the fertilizer treatment gradient, the total abundance of dominant bacterial genera was lower in IM than in MM at each N application level. This trend was driven by the enrichment of Bryobacte in MM at each N application level, whose relative abundance was 32.80%–70.88% higher under N0–N3 than in their counterpart IM treatments. Further comparison of the relative abundance of individual genera showed that the trends of Sphingomonas and Gemmatimonas under different modes of cropping and nitrogen application levels were consistent: IM > MM. The relative abundance of these two genera responded positively to more nitrogen applied under MM, increasing most at N1, reaching 7.50% and 27.88%, respectively. Under IM, however, their relative abundance peaked at the N2 level.
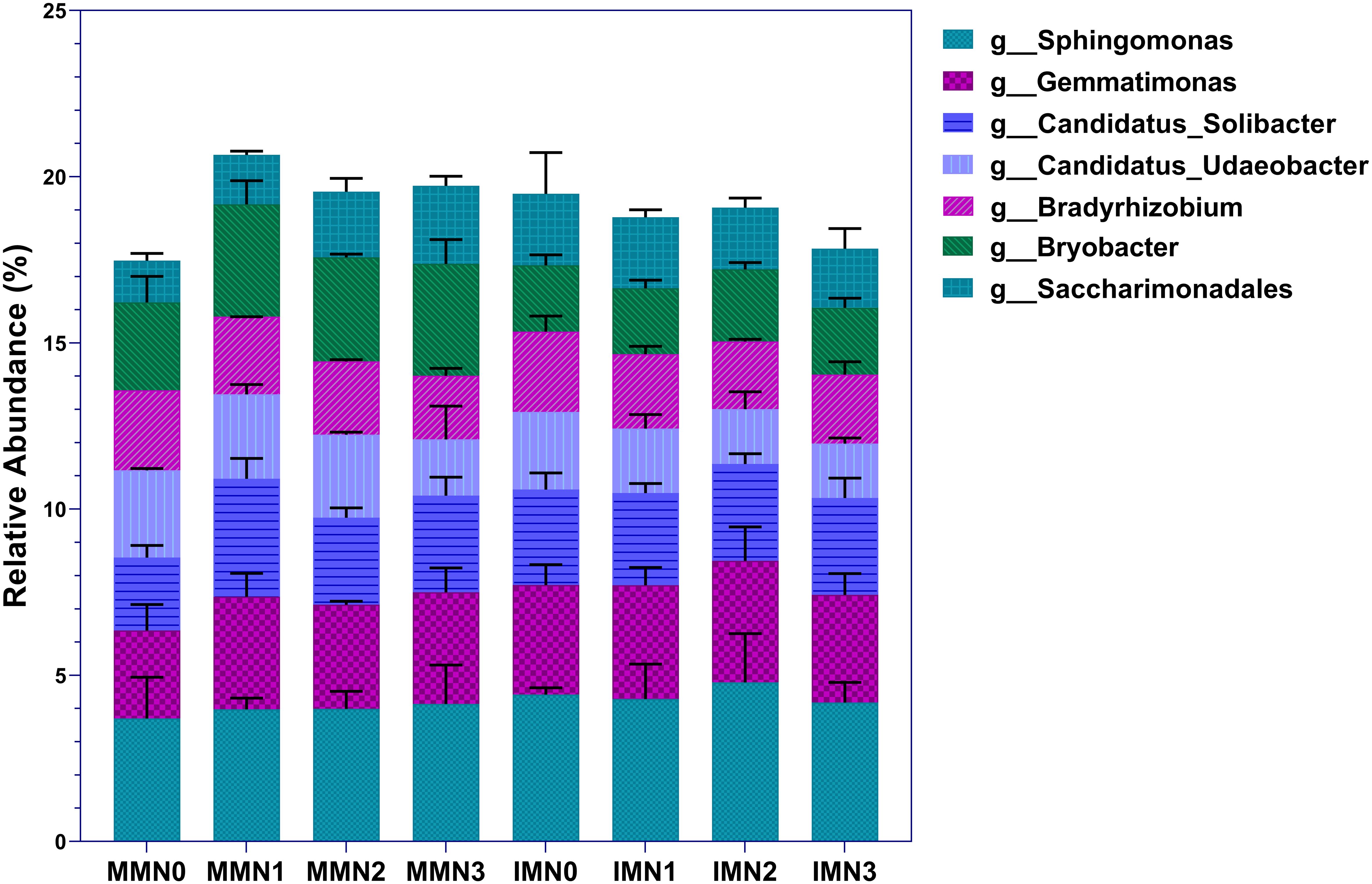
Figure 3. Horizontal community composition of bacterial genera in the eight treatment combinations of cropping system (IM, intercropping vs. MM, monoculture) and four levels of nitrogen fertilizer application (N1–4).
For Candidatus_Solibacter, the relative abundance of this genus changed the most at N0 and N1 levels, increasing by 30.84% IMN0 vis-à-vis MMN0. But in response to N1, its relative abundance increased by 61.53% compared to N0 under MM, now exceeding that of IMN1. The relative abundances of Candidatus_Udaeobacter and Bryobacter showed the same trend, namely that of MM > IM, regardless of the nitrogen application rate. However, the relative abundance of Candidatus_Udaeobacter decreased as the level of nitrogen application increased, with that at N3 being 35.52% and 29.67% lower under MM and IM, respectively, than in the N0 treatment. In stark contrast, Bryobacter abundance was maximal in the MMN1 and IMN2 treatment combinations. For Bradyrhizobium, its relative abundance was similar under MM and IM, as was its trend across nitrogen application rates: compared with the baseline N0 level, with more nitrogen, Bradyrhizobium decreased in abundance by 20.56% and 16.62% in N3 under MM and IM, respectively. The entirely opposite pattern characterized changes in Saccharimonadales under MM and IM. With more nitrogen added, its relative abundance under MM tended to increase, being 86.91% in the N3 than N0 treatment; however, under IM showed a decreasing trend in response to fertilization, being 17.47% lower at the N3 than N0 level. To sum up, IM increased the relative abundances of Sphingomonas, Gemmatimonas, and Saccharimonadales yet decreased those of Candidatus_Udaeobacter and Bryobacter, and different levels of nitrogen application had a greater impact on the enrichment of dominant bacterial genera under the MM cropping system than IM. This pointed to a superior stability of the microbial community under IM than MM.
3.4.3 PCA of the bacterial community
We employed the Aitchison distance for the beta diversity analysis of soil bacterial communities. PCA was used to compare the degree of similarity existing in the diversity of the bacterial community among different samples from the eight treatment combinations (Figure 4). The first (PC1) and second principal component (PC2) of bacterial community structure (97% similarity) explained 36.24% and 25.45% of the total variance, respectively. There was no significant effect of differing nitrogen application rates on bacterial community structure under IM or MM (P > 0.05), and most of treatment combinations were clustered in the third quadrant (lower-left) without segregation. Unlike those, the MMN0 and MMN1 treatment combinations separated well along PC1 and PC2, respectively, indicating that their community structure differed substantially from the others (P < 0.05). Overall, the IM approach had no significant effect (P > 0.05) upon soil bacterial community structure across the nitrogen fertilizer gradient, though the differences under MM for the zero and low nitrogen application rates (N0 and N1, respectively) were significant (P < 0.05). Nonetheless, with more nitrogen applied, the soil bacterial community structure of both cropping systems closely resembled each other.
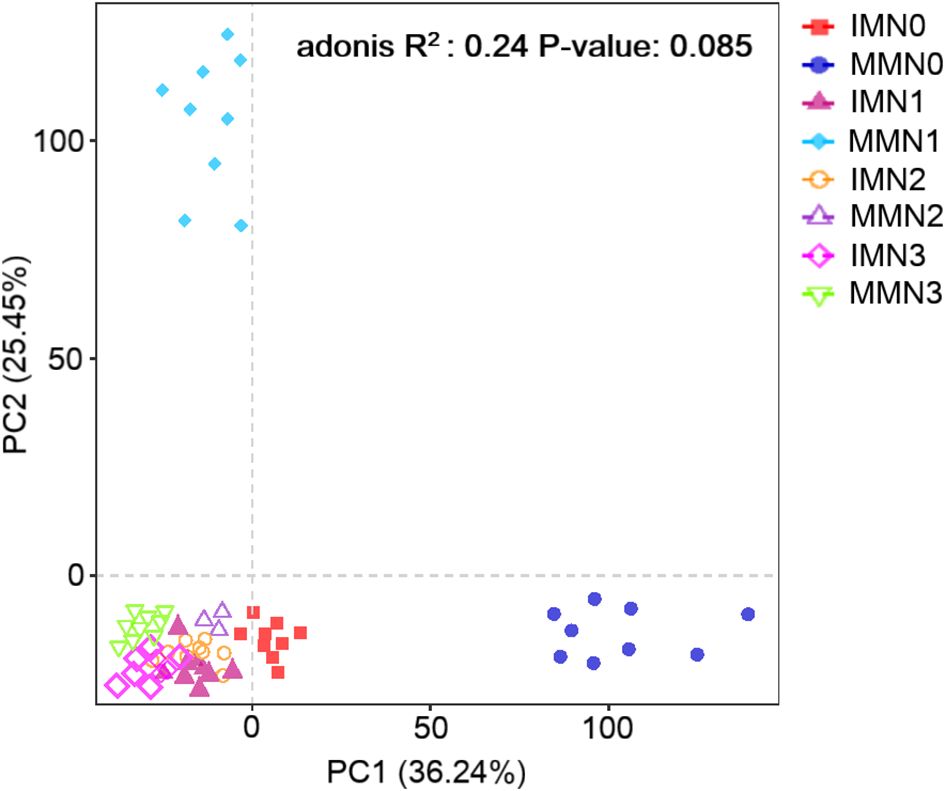
Figure 4. Principal component analysis (PCA) of bacterial community structure in the eight treatment combinations of cropping system (IM, intercropping vs. MM, monoculture) and four levels of nitrogen fertilizer application (N1–4).
3.4.4 Discrimination of differential bacterial taxa
It is evident that differential species mainly emerged in the IMN2, IMN0, and MMN0 treatment combinations (Figure 5); but not so in either MMN2 or MMN3 (thus not shown). Further analysis indicated that IMN2 harbored the most differential species, with Microscillaceae having the highest LDA values followed by 12 others, including Metagenome, Sericytochromatia, and Dojkabacteria. In the IMN0 treatment combination, the differential species consisted mainly of Bacillus, Fiemicutes, and Entomoplasmatales with more similar LDA values. While for MMN0, its differential species were mainly Bauldia and Knoellia. For IMN1, IMN3 and MMN1 their differential species respectively were Vampirovibrionaceae, Luedemannella, and Massilia_armeniaca. Altogether, the number of differential species decreased as the nitrogen application rate increased under MM, while the IM mode had the greatest number of differential species at the N2 level, indicating that the soil environment responded most to this treatment.
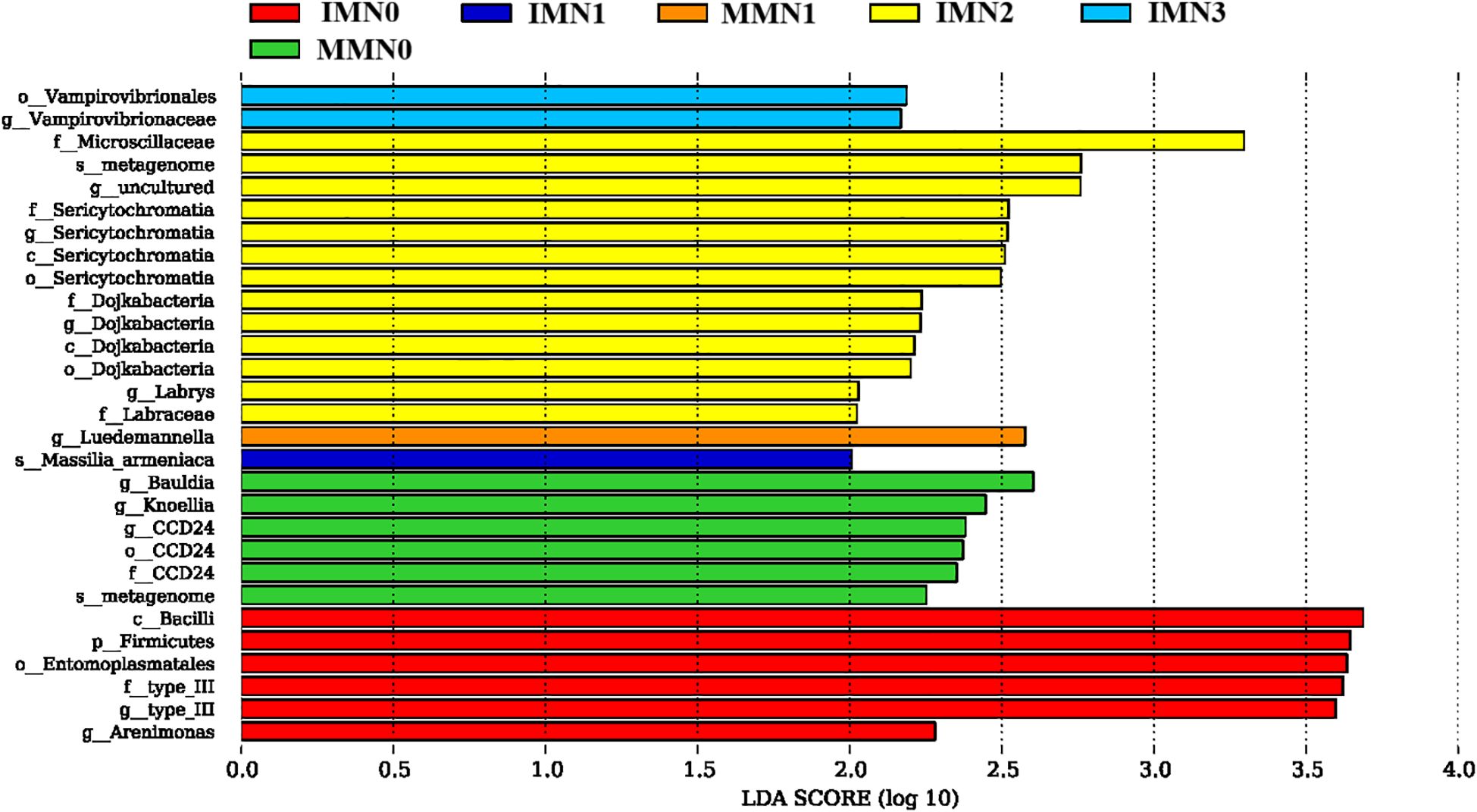
Figure 5. Determination of the most significantly different species in soil bacterial communities under contrasting cropping systems (IM, intercropping vs. MM, monoculture) and nitrogen application rates, using linear discriminant analysis (LDA) effect sizes (LEfSe). An LDA score ≥ 2 indicated a differential species, i.e. statistically different biomarkers. The bar length is proportional to the effect size.
3.4.5 Correlation analysis of dominant bacterial genera with the soil environment and yield
We summarized the dominant bacterial genera in the previous section and tallied their respective relative abundance for correlation tests with the soil environment factors and maize yield factors (Figure 6A). This analysis showed that Sphingomonas had a highly significant negative correlation with soil pH (P < 0.01) yet a positive correlation with soil TOC and EC (P < 0.05). The enrichment of Gemmatimonas and Bradyrhizobium significantly increased the soil nitrogen content; the positive correlation of Candidatus_Udaeobacter with soil EC was highly significant (P < 0.01), whereas Candidatus_Solibacter had a negative correlation (P < 0.01) with both soil TOC and EC, but a significant positive correlation with NO3–N (P < 0.01). Bryobacter was significantly positively correlated with the soil TOC content (P < 0.05), while Saccharimonadales had a highly significant negative correlation with NO3—N (P < 0.01).
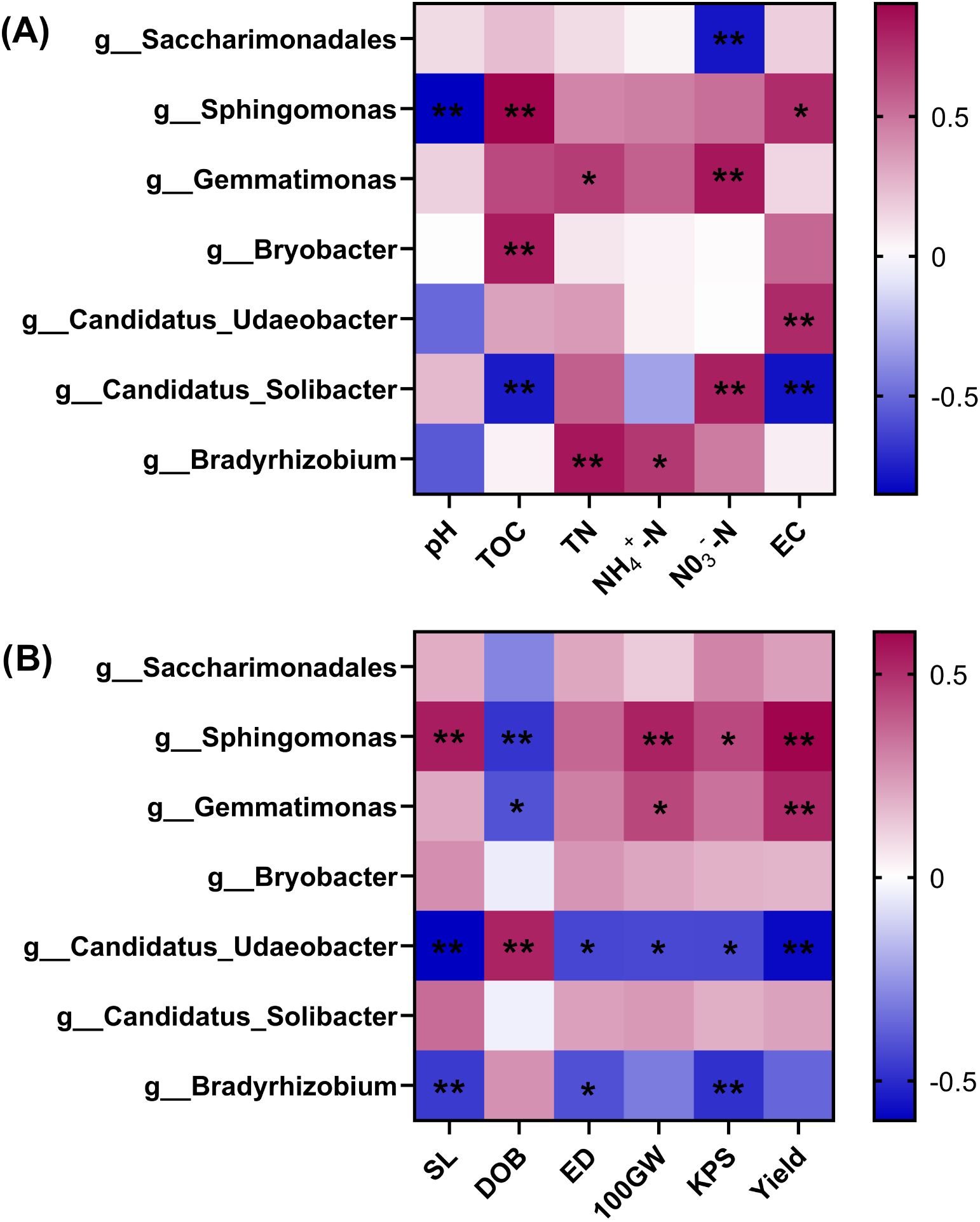
Figure 6. Heatmap of Pearson correlations of soil dominant bacterial genera with soil factors (A) and yield factors (B). Red coloring indicates a positive correlation, blue coloring indicates a negative correlation; asterisks indicate a significant correlation: *P < 0.05, **P < 0.01.TOC, TN, NH4+-N and NO3--N denote soil organic carbon, total nitrogen, ammonium nitrogen and nitrate nitrogen, respectively; SL, DOB, ED, 100GW and KPS denote maize panicle length, degree of baldness, ear diameter, 100-seed weight, and kernels in the spike, respectively; UE and CAT denote soil urease and catalase, respectively.
In terms of yield (Figure 6B), enrichment of both Sphingomonas and Gemmatimonas significantly reduced the degree of baldness (DOB) (P < 0.05) and increased the spike length (SL), number of kernels in the spike (KPS), and 100-seed [grain] weight (100GW) of maize, which in turn significantly increased maize yield (P < 0.05). However, Candidatus_Udaeobacter and Bradyrhizobium had the opposite influence on yield, with enrichment of either increasing the DOB. Still, Candidatus_Udaeobacter displayed a greater negative influence on yield, as indicated by its relative abundance being highly significantly negatively correlated with both SL and yield (P < 0. 01) and to a lesser extent with ear thickness (ED), 100GW, and KPS (P < 0.05). Finally, a higher relative abundance of Bradyrhizobium significantly reduced the maize SL, ED, and KPS (P < 0.05).
3.4.6 Co-occurrence network modeling of soil bacterial communities
To clarify the mechanism of synergistic interaction among genera, we built co-occurrence network models of soil bacterial communities at the genus level for the top 200 abundances for different nitrogen application levels (MM and IM fitted by 97% similarity) (Figures 7A–D), and likewise for different cropping modes (N0–N3 fitted by 97% similarity) (Figures 7E, F). We also examined the topology parameters of these network models (Table 5) to compare the interconnections among soil bacterial communities. When compared to the N0 level, the number of soil bacterial community edges decreased by 2.30%–8.74% at the N1–N3 levels, but the proportion of positive correlations increased by 0.66%–5.76%, being highest at the N2 level, with no significant difference detected between the N3 and N0 levels; however, the number of edges and proportion of positive correlations under IM increased by 1.91% and 4.62%, respectively, vis-à-vis MM. In comparing the average degree, average weight, average clustering coefficient, and modularity, we found that all these network topology parameters were lower for the N1–N3 levels than for N0, and always higher under IM than MM. Hence, the degree of connectivity between network nodes in the IM system was likely stronger and the connections between nodes more numerous and complex.
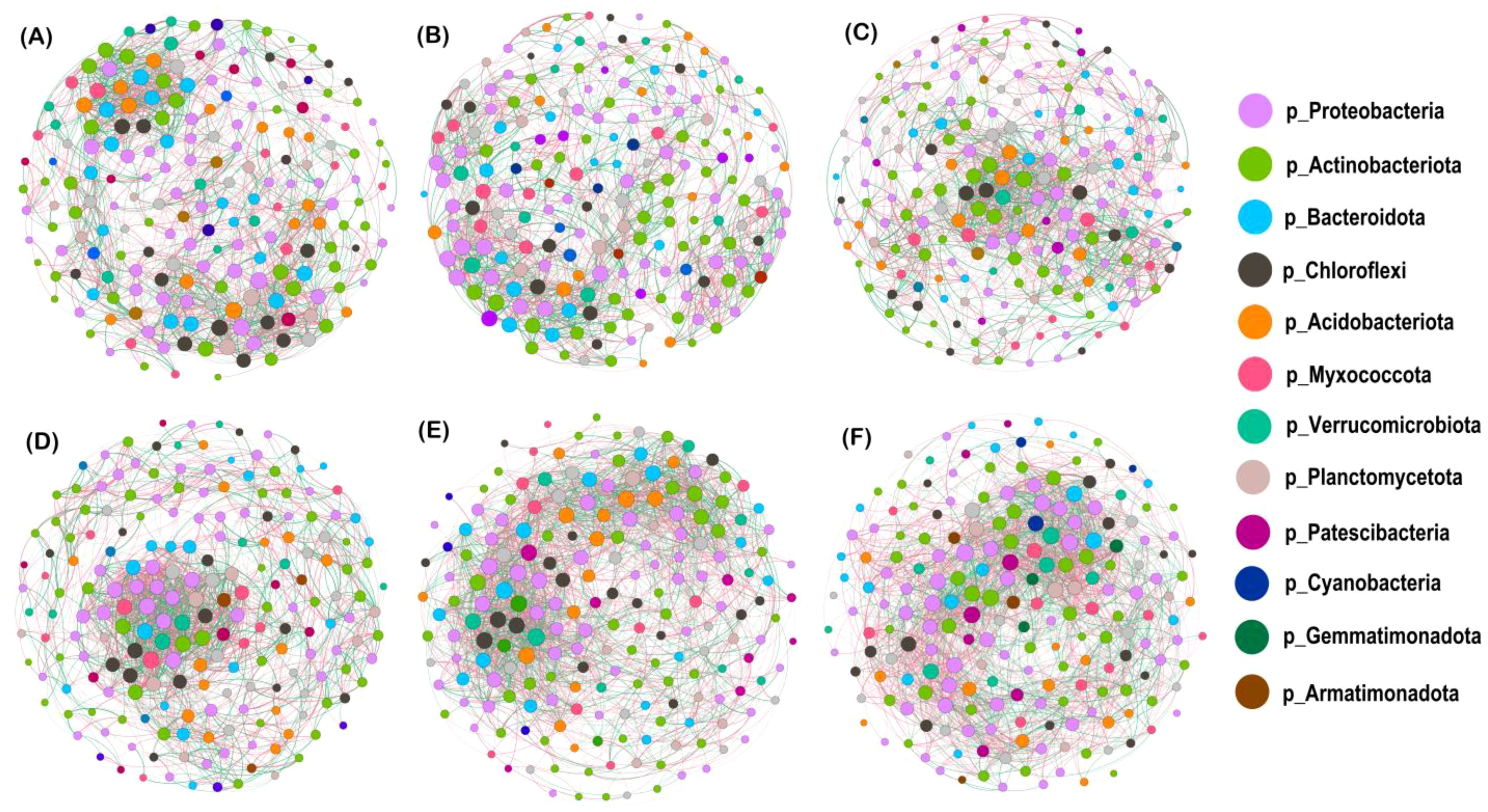
Figure 7. Co-occurrence network model of soil bacterial communities under different nitrogen application rates and cropping systems. (A–D) Different nitrogen application levels (N0, N1, N2, and N3, respectively) (MS and IS fitted by 97% similarity). (E) Maize monocropping and (F) intercropping (N0, N1, N2, and N3 fitted by 97% similarity) as contrasting cropping modes. The colored circles are different genus; the size of a circle is used to convey the average abundance of that species; line segments between circles indicate a correlation between two species, whose thickness is proportional to the correlation strengthen between them. Red lines indicate positive correlations, while green lines indicate negative correlations.
3.4.7 Structural equation modeling analysis of the effects of intercropping and nitrogen application rates on maize yield
The SEM results (Figure 8) showed that both intercropping and nitrogen application could significantly increase the soil nitrogen content and also alter bacterial community composition. Soil nitrogen content and bacterial community composition were in a mutually reinforcing relationship; accordingly, the former’s increase and the latter’s enrichment could significantly reduce the soil pH, EC, and TOC content generated a synergistic effect in soil, which in turn increased the maize yield. Therefore, intercropping not only bolstered the yield but it also was critical for maintaining soil health and providing a favorable soil environment for crop growth.
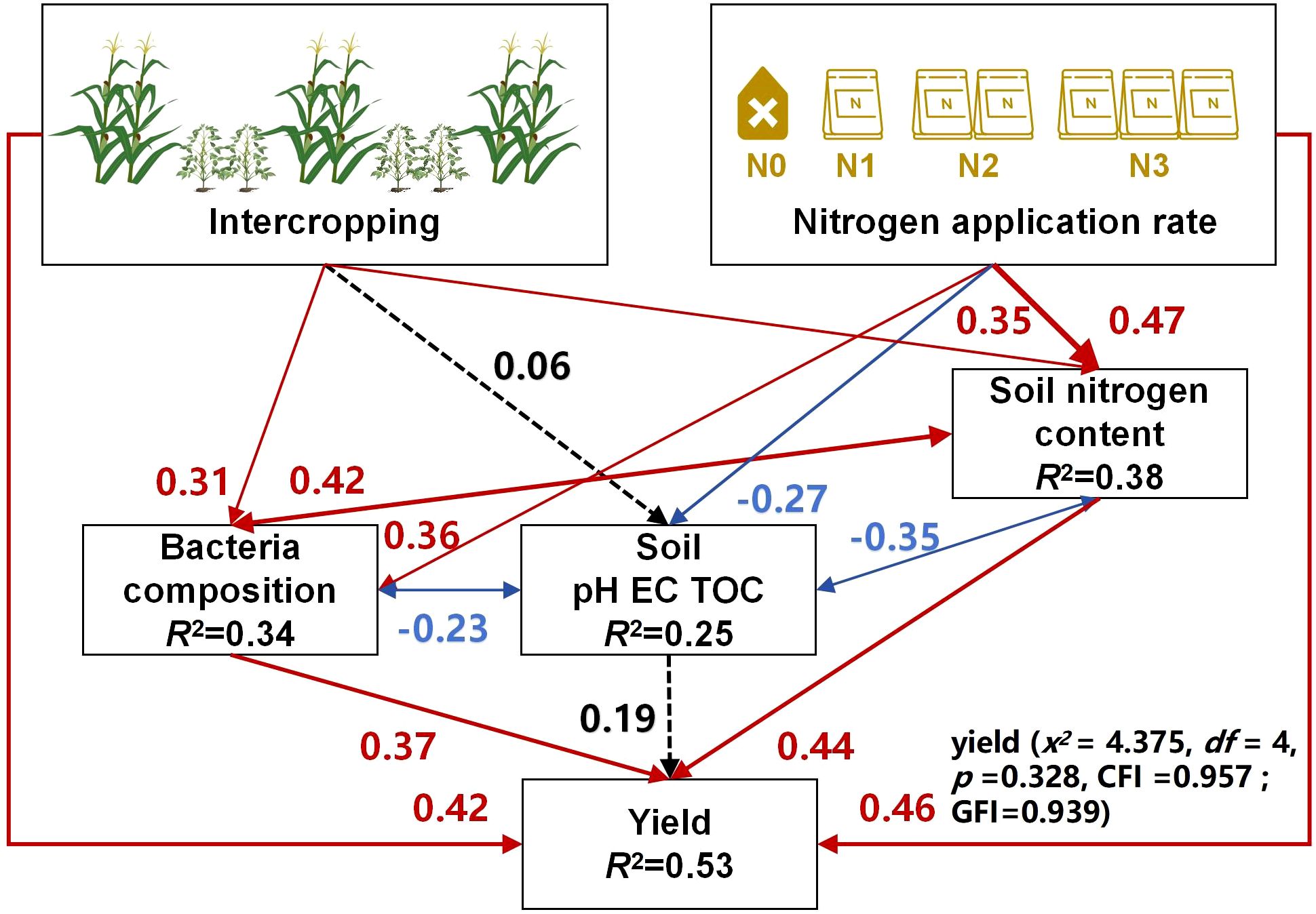
Figure 8. Structural equation model (SEM) describing the effects of intercropping and nitrogen application rates on soil chemical properties, bacterial colony composition, and maize yield. Blue arrows indicate negative relationships (correlations); red arrows indicate positive ones.
4 Discussion
The mechanism underpinning efficient nitrogen uptake in the intercropping system between maize and legume crops has three key aspects. First is interspecific plant competition, where maize outcompetes legume crops for light and nitrogen (and other resources), which promotes the superiority of maize’s uptake of nitrogen. Second, the root system’s interaction with soil microorganisms promotes the cycling of nutrients and the transfer of nitrogen between plants, such that the nitrogen fixed by legume crops can be absorbed and utilized by maize. Third, legume crops increase tuberculation and their nitrogen-fixing gene expression is enhanced, thereby increasing their own nitrogen-fixing capacity.
4.1 Soil nitrogen and chemical properties
A marked increase in soil pH and salt ion concentrations in soil will lead to the degradation of crop survival/growing environment and soil nutrient deficiencies, which heavily hinders crop growth, development, and yield. In this study, however, our results show that soil pH is negligibly affected in general, being similar at each level of nitrogen application under IM or MM. This suggests intercropping does alter soil pH, whose magnitude is instead mainly influenced by the level of nitrogen application (Wang et al., 2015). Further, we find a lower soil EC in the intercropping system than monoculture (MM) but a higher soil TOC content, which can be largely attributed to the fact that IM could balance the soil nutrient use rate, effectively alleviating the inhibitory effect of soil mono-elements and enhancing soil microbial metabolism. This would have led to a greater TOC content because of the greater decomposition capacity of organic matter (Silva et al., 2022). We also found that, under intercropping, its soil TOC content was consistent with changes in its soil EC. This could be explained by the strong adsorption capacity of soil TOC, which contributes about 20%–70% to soil EC and has a certain buffering effect, delaying the movement of surface salt ions into the deeper soil, thereby augmenting EC (Wang et al., 2023b).
The nitrogen in soil is the main source of this element for plant uptake and use, and is also the primary limiting factor for high-yielding crops, so nitrogen input as fertilizer is a key practice to maintain or improve the soil nitrogen content (Knops et al., 2002). Here, the soil nitrogen supply in IM surpassed that in MM, and the improvement was especially pronounced for the NH4+-N and NO3–N contents. We attribute this benefit to the maize/soybean intercropping system, whereby the legumes are able to efficiently fix N, which is then utilized by the same crop through various transfer pathways or available in soil to subsequent non-legume crops as residues (Raza et al., 2020).
4.2 Rhizosphere bacterial community of maize
Soil bacteria can influence the metabolic activities of crop roots, as well as soil structure and soil fertility, to varying degrees. When crops are grown in an intercropping system, plant residues and root secretions may come into close contact with each other, directly or indirectly altering the soil bacterial community structure (Duchene et al., 2017). Compared with monocropped maize, we found a more diverse soil bacterial community in the rhizosphere soil of intercropped maize, as well as a greater relative abundance of dominant genera. This result suggests that the composition of bacterial communities in intercropped soils is heavily influenced by changes in agricultural management practices (Lai et al., 2022). However, using too much nitrogen fertilizer disrupts this trend and reduces the number of endemic differential species. This can be explained by the fact that after an excessive nitrogen application, the general plant utilization rate of the fertilizer is only 30%–50%, leaving the rest to get converted to nitrate via nitrification in the soil. That would cause soil acidification and a neutralization reaction with the inorganic carbon (carbonate) in soil, leading to the loss of inorganic carbon and eventually the full deterioration of soil, which inevitably would somehow adversely impact bacterial community diversity. Recent research has shown that increasing plant diversity to ensure the diversity of the soil microbial community can significantly improve soil fertility and reduce the number of pathogenic microorganisms (Jensen et al., 2020). Earlier, Weidner et al. (2015) found that bolstering soil bacterial diversity can improve the nitrogen nutrient supply capacity of soil, thus promoting not only plant growth but also nitrogen use; Trivedi et al. (2012) reported considerably more copies of genes involved in the ecological processes of nitrogen metabolism in healthy soils than diseased soils. This indicates that maize/soybean intercropping promotes the diversity of bacterial communities in surface soil, and thus the availability of nitrogen as well as other nutrients in arable soil. Apart from being useful in maintaining the stability of soil microbial community dynamics, it could probably help to suppress pests and diseases too, collectively promoting the growth and yield increase of intercropped crops. Among dominant bacterial genera compared between modes of cropping, our results reveal that the relative abundances of Sphingomonas and Gemmatimonas are higher under IM than MM, but this is reversed for Bryobacter. Other research found that, under the action of maize root secretion, Ascomycetes came to dominate, for which Sphingomonas and Gemmatimonas are the main genus members; so it was suggested this phenomenon enables the maize root system to fully utilize inorganic nutrients in soil (Zhang et al., 2018b). Arguably, subsoil intercropping conditions most likely arise through significant changes in the composition of inter-root secretions, leading to dramatic changes in bacterial colonization outcomes and population sizes of dominant bacteria. By constructing a co-occurrence network model, we learned that IM does foster an increase in the number of soil bacterial edges, an increase in the proportion of positive correlations, and an increase in species richness, in tandem with the weakening of competitive relationships between species. This is because intercropping created ecological conditions that improved the survivorship of dominant bacterial genera in surface soil, which in turn led to the enhancement of mutually beneficial relationships and a weakening of their competitive interactions (Wang et al., 2023c). This showed that IM could promote links between the genera and, when used with a reasonable nitrogen application rate (N1 or N2 level), was capable of simplifying the interrelationship between them, presumably weakening the competitive relationship between the genera and enhancing their synergistic ability.
4.3 Soil nutrient–microbe–yield relationships
In recent years, there has been an exponential rise in studies investigating the various effects of agricultural management practices on soil microbial communities (Zhao et al., 2022). Despite that staggering research effort, it is still difficult to elucidate general soil nutrient–microbe–yield relationships and the pathways influencing their dynamics under intercropping systems. Soil microbial community structure and functional diversity are closely tied to restored soil fertility and better soil quality (Yang et al., 2023). It is reasonable to think that plant–microbe interactions between roots are closely related to the growth and yield formation of intercrops.
Here, through a comprehensive soil nutrient–microbe–yield correlation analysis, we find that enrichment of Gemmatimonas and Bradyrhizobium could drastically increase the soil nitrogen content. Firstly, Gemmatimonas is a self-photosynthesizing genus rich in bacterial chlorophyll members; in addition, this genus can reduce N2O to nitrate in soil (Zou et al., 2023). Secondly, Bradyrhizobium bacteria is able to form a nitrogen-fixing symbiosis with Rhizobium in soybean’s root system, which in turn can increase the soil nitrogen content (Cheng et al., 2023). However, maize is a plant with high fertilizer absorption capacity and high fertilizer requirement. So, in the intercropping system, due to the difference in morphological structure between maize and soybean plants, the reduced absorption of light energy due to their asymmetric competition inhibited the growth and root development of soybean to a certain extent, which indirectly constrained its symbiotic nitrogen-fixing ability (Liu et al., 2018). If so, this would explain rather well why the genus Bradyrhizobium was negatively correlated with the maize yield factors in our study. On the contrary, Saccharimonadales has a strong negative correlation with soil NO3–N, likely because this genus has the function of denitrifying and removing phosphorus, and is mainly involved in the denitrification process leading to soil nitrogen loss (Wang et al., 2022a). We also uncovered a pronounced negative correlation of Sphingomonas with soil pH but its positive correlation with soil TOC and yield. The first may be explained by the role of this genus in remediating heavy metal contamination, being vital to restoring soil fertility and promoting plant growth (Liu et al., 2022). The second result can be explained by the ability of Sphingomonas to decompose soil carbohydrates (pentoses, hexoses, disaccharides), which produces large amounts of acid during oxidation, which then lowers the soil pH (Cuartero et al., 2022). A greater relative abundance of Candidatus_Solibacte was associated with declines in TOC and EC, and an increasing content of soil NO3–N. Candidatus_Solibacte is known to effectively decompose soil organic matter and reduce soil nitrate and nitrite by consuming the soil carbon sources, which in turn slows or prevents soil acidification (Zhang et al., 2018a). Candidatus_Udaeobacter can release antibiotics that cause other microbial cells to lyse and release their nutrients (Guo et al., 2024). We did find a highly significant negative correlation between this genus and maize yield, but although nutrient contents were augmented, the lysis-induced death of other microorganisms may have disrupted the structural composition of soil bacterial communities, which in turn could have affected their functioning.
4.4 Breakthrough areas for future research
This study’s results suggest that exploring diversified intercropping patterns that provide reciprocal benefits to different plants, by harnessing inherent differences in their ecological niches, should continue to be the core focus of future research in the intercropping industry. In this study, nitrogen uptake in the intercropping system reached its maximum when 240 kg ha-1 of nitrogen fertilizer was applied. Hence, an appropriate application rate of nitrogen fertilizer could effectively improve nitrogen’s uptake and accumulation in the general corn–soybean intercropping system.
Currently, most research on intercropping mechanisms tends to focus on plant growth and nutrient uptake and utilization conditions, though some studies have analyzed changes that occur in soil enzymes and their activity. The more intertwined factors, such as the soil–soil enzyme–soil microorganism interactions, under this intercropping pattern have not been studied yet, and the vast majority of published studies are restricted to the soil or soil–soil enzyme level. Therefore, research on intercropping systems should focus on the holistic analysis of each mechanistic link involved, and future studies should also try to investigate the whole intercropping system, from its environmental factors to cultivated plants, down to its soil microorganisms. The more comprehensive the research done, the more conducive its knowledge gained is to the discovery of intercropping mechanisms, and for promoting the development of the intercropping industry. Finally, the use of high-throughput sequencing technology offers a fast and convenient way to analyze intercropping soils and to explore how the soil microbial community influences plant growth/yield changes, leading to a richer, more accurate understanding of intercropping systems and their functioning.
5 Conclusion
This study’s results show that under a maize/soybean intercropping pattern, the N2 level (240 kg ha-1) was best suited to achieve a high maize yield, for which the nitrogen-use efficiency was also maximized. We find that the maize crop yield is also correlated with the dominant soil microbial genera and soil nitrogen content. The main ways by which an intercropping mode increases yield, as well as the gradient changes under different N application levels, are also clarified. In the context of research on promoting crop yields and the efficient use of nitrogen fertilizer in intensive agriculture, we believe that intercropping’s advantages are more pronounced when an appropriate amount of nitrogen is applied, since using too much can render moot these benefits.
Data availability statement
The original contributions presented in the study are included in the article/supplementary material. Further inquiries can be directed to the corresponding authors.
Author contributions
LZ: Visualization, Writing – original draft, Writing – review & editing. YF: Writing – original draft. ZZ: Investigation, Writing – original draft. ZC: Investigation, Writing – original draft. BB: Investigation, Writing – original draft. HW: Visualization, Writing – review & editing. QL: Supervision, Writing – review & editing. JC: Supervision, Writing – review & editing.
Funding
The author(s) declare financial support was received for the research, authorship, and/or publication of this article. The work was supported by the science and technology development plan of Jilin Province, China (project nos. 20220508095RC and 20230302003NC). National Key Research and Development Project (project nos. 2023YFD1501104).
Acknowledgments
We wish to thank Dr. Wuliang Shi, Dr. Bin Li, Dr. Yubin Zhang, for valuable and encouraging discussions. We appreciate the efforts of the reviewers and the editors’ valuable suggestions in earlier versions of this manuscript.
Conflict of interest
The authors declare that the research was conducted in the absence of any commercial or financial relationships that could be construed as a potential conflict of interest.
Publisher’s note
All claims expressed in this article are solely those of the authors and do not necessarily represent those of their affiliated organizations, or those of the publisher, the editors and the reviewers. Any product that may be evaluated in this article, or claim that may be made by its manufacturer, is not guaranteed or endorsed by the publisher.
References
Bai, F. F., Qi, X. B., Li, P., Du, Z. J., Guo, W. (2023). Groundwater depth and nitrogen application amount jointly regulate the water and residual soil nitrate accumulation in agricultural soil profile. Agronomy-Basel 13, 19. doi: 10.3390/agronomy13041163
Bai, Z. T., Xie, C., Yu, J., Bai, W. Q., Pei, S. Z., Li, Y. X., et al. (2024). Effects of irrigation and nitrogen levels on yield and water-nitrogen-radiation use efficiency of drip-fertigated cotton in south Xinjiang of China. Field Crops Res. 308, 13. doi: 10.1016/j.fcr.2024.109280
Chen, G. H., Jiang, F. H., Zhang, S., Zhang, Q., Jiang, G. J., Gao, B. K., et al. (2023). Potential crop yield gains under intensive soybean/maize intercropping in China. Plant Soil 16. doi: 10.1007/s11104-023-06423-7
Cheng, Z. Y., Meng, L. B., Yin, T. J., Li, Y., Zhang, Y. H., Li, S. M. (2023). Changes in soil rhizobia diversity and their effects on the symbiotic efficiency of soybean intercropped with maize. Agronomy-Basel 13, 17. doi: 10.3390/agronomy13040997
Cuartero, J., Pascual, J. A., Vivo, J. M., Özbolat, O., Sánchez-Navarro, V., Egea-Cortines, M., et al. (2022). A first-year melon/cowpea intercropping system improves soil nutrients and changes the soil microbial community. Agric. Ecosyst. Environ. 328, 8. doi: 10.1016/j.agee.2022.107856
Du, J. B., Han, T. F., Gai, J. Y., Yong, T. W., Sun, X., Wang, X. C., et al. (2018). Maize-soybean strip intercropping: Achieved a balance between high productivity and sustainability. J. Integr. Agric. 17, 747–754. doi: 10.1016/S2095-3119(17)61789-1
Duchene, O., Vian, J. F., Celette, F. (2017). Intercropping with legume for agroecological cropping systems: Complementarity and facilitation processes and the importance of soil microorganisms. A review. Agric. Ecosyst. Environ. 240, 148–161. doi: 10.1016/j.agee.2017.02.019
Fan, Z., Deng, M. Z., Lin, Y. R., Liu, P. Z., Wang, X. L., Yang, S. F., et al. (2023). Effects of the border on yield and water use in wheat/maize intercropping in rain-fed areas with different nitrogen levels. Field Crops Res. 302, 12. doi: 10.1016/j.fcr.2023.109105
Faust, K. (2021). Open challenges for microbial network construction and analysis. Isme J. 15, 3111–3118. doi: 10.1038/s41396-021-01027-4
Fu, Z. D., Zhou, L., Chen, P., Du, Q., Pang, T., Song, C., et al. (2019). Effects of maize-soybean relay intercropping on crop nutrient uptake and soil bacterial community. J. Integr. Agric. 18, 2006–2018. doi: 10.1016/S2095-3119(18)62114-8
Guo, T. X., Yao, X. H., Wu, K. L., Guo, A. M., Yao, Y. H. (2024). Response of the rhizosphere soil microbial diversity to different nitrogen and phosphorus application rates in a hulless barley and pea mixed-cropping system. Appl. Soil Ecol. 195, 15. doi: 10.1016/j.apsoil.2023.105262
Jensen, E. S., Carlsson, G., Hauggaard-Nielsen, H. (2020). Intercropping of grain legumes and cereals improves the use of soil N resources and reduces the requirement for synthetic fertilizer N: A global-scale analysis. Agron. Sustain. Dev. 40, 9. doi: 10.1007/s13593-020-0607-x
Knops, J. M. H., Bradley, K. L., Wedin, D. A. (2002). Mechanisms of plant species impacts on ecosystem nitrogen cycling. Ecol. Lett. 5, 454–466. doi: 10.1046/j.1461-0248.2002.00332.x
Lai, H. L., Gao, F. Y., Su, H., Zheng, P., Li, Y. Y., Yao, H. Y. (2022). Nitrogen distribution and soil microbial community characteristics in a legume-cereal intercropping system: A review. Agronomy-Basel 12, 15. doi: 10.3390/agronomy12081900
Li, Q. S., Jia, X. L., Ye, J. H., Li, J. J., Luo, X. M., Lin, W. X. (2021). Effects of Maize/soybean intercropping on soil microbial community and biochemical properties. Allelopathy J. 54, 141–156. doi: 10.26651/AJ
Liu, X., Rahman, T., Song, C., Yang, F., Su, B. Y., Cui, L., et al. (2018). Relationships among light distribution, radiation use efficiency and land equivalent ratio in maize-soybean strip intercropping. Field Crops Res. 224, 91–101. doi: 10.1016/j.fcr.2018.05.010
Liu, J., Li, Y., Han, C. Q., Yang, D. L., Yang, J. J., Cade-Menun, B. J., et al. (2022). Maize-soybean intercropping facilitates chemical and microbial transformations of phosphorus fractions in a calcareous soil. Front. Microbiol. 13, 12. doi: 10.3389/fmicb.2022.1028969
Liu, X. D., Meng, L. B., Yin, T. J., Wang, X. R., Zhang, S., Cheng, Z. Y., et al. (2023). Maize/soybean intercrop over time has higher yield stability relative to matched monoculture under different nitrogen-application rates. Field Crops Res. 301, 10. doi: 10.1016/j.fcr.2023.109015
Lu, M., Zhao, J. X., Lu, Z. R., Li, M. J., Yang, J. F., Fullen, M., et al. (2023). Maize-soybean intercropping increases soil nutrient availability and aggregate stability. Plant Soil 16. doi: 10.1007/s11104-023-06282-2
Qi, W. J., Wang, Q., Mak-Mensah, E., Zhu, J. H., Zhang, D. K., Zhou, X. J., et al. (2023). Effects of soil physicochemical properties on maize, wheat, and soybean yields in maize-wheat and maize-soybean intercropping systems in China: a meta-analysis. J. Soil Sci. Plant Nutr. 24, 21–29. doi: 10.1007/s42729-023-01556-1
Raza, A., Asghar, M. A., Ahmad, B., Bin, C., Hussain, M. I., Li, W., et al. (2020). Agro-techniques for lodging stress management in maize-soybean intercropping system-A review. Plants-Basel 9, 22. doi: 10.3390/plants9111592
Roohi, M., Arif, M. S., Guillaume, T., Yasmeen, T., Riaz, M., Shakoor, A., et al. (2022). Role of fertilization regime on soil carbon sequestration and crop yield in a maize-cowpea intercropping system on low fertility soils. Geoderma 428, 11. doi: 10.1016/j.geoderma.2022.116152
Secco, D., Bassegio, D., De Marins, A. C., Chang, P. B., Savioli, M. R., Castro, M. B. S., et al. (2023). Short-term impacts of different intercropping times of maize and ruzigrass on soil physical properties in subtropical Brazil. Soil Tillage Res. 234, 9. doi: 10.1016/j.still.2023.105838
Silva, L. S., Laroca, J. V. D., Coelho, A. P., Gonsalves, E. C., Gomes, R. P., Pacheco, L. P., et al. (2022). Does grass-legume intercropping change soil quality and grain yield in integrated crop-livestock systems? Appl. Soil Ecol. 170, 10. doi: 10.1016/j.apsoil.2021.104257
Tian, X. L., Wang, C. B., Bao, X. G., Wang, P., Li, X. F., Yang, S. C., et al. (2019). Crop diversity facilitates soil aggregation in relation to soil microbial community composition driven by intercropping. Plant Soil 436, 173–192. doi: 10.1007/s11104-018-03924-8
Trivedi, P., He, Z. L., Van Nostrand, J. D., Albrigo, G., Zhou, J. Z., Wang, N. (2012). Huanglongbing alters the structure and functional diversity of microbial communities associated with citrus rhizosphere. Isme J. 6, 363–383. doi: 10.1038/ismej.2011.100
Wang, Z. G., Bao, X. G., Li, X. F., Jin, X., Zhao, J. H., Sun, J. H., et al. (2015). Intercropping maintains soil fertility in terms of chemical properties and enzyme activities on a timescale of one decade. Plant Soil 391, 265–282. doi: 10.1007/s11104-015-2428-2
Wang, W. M., Chen, Y., Zhang, F. H., Zhang, W., Liu, J. G., Wang, J. P., et al. (2023a). Cotton-maize intercropping increases rhizosphere soil phosphorus bioavailability by regulating key phosphorus cycling genes in northwest China. Appl. Soil Ecol. 182, 10. doi: 10.1016/j.apsoil.2022.104734
Wang, G. W., Jin, Z. X., Wang, X. X., George, T. S., Feng, G., Zhang, L. (2022a). Simulated root exudates stimulate the abundance of Saccharimonadales to improve the alkaline phosphatase activity in maize rhizosphere. Appl. Soil Ecol. 170, 10. doi: 10.1016/j.apsoil.2021.104274
Wang, R. T., Liu, J. X., Jiang, W. Y., Ji, P. S., Li, Y. G. (2022b). Metabolomics and microbiomics reveal impacts of rhizosphere metabolites on alfalfa continuous cropping. Front. Microbiol. 13, 20. doi: 10.3389/fmicb.2022.833968
Wang, W. M., Yin, F. T., Gu, J. C., Wang, Z., Zhang, F. H., Li, L. H., et al. (2023b). Effects of maize/soybean intercropping on rhizosphere soil phosphorus availability and functional genes involved in phosphorus cycling in Northwest China. Plant Soil 14. doi: 10.1007/s11104-023-06363-2
Wang, Y. L., Zhang, L. Q., Meng, F. A., Lou, Z. X., An, X. Y., Jiang, X. B., et al. (2023c). Responses of soil microbial communities in soybean-maize rotation to different fertilization treatments. Agronomy-Basel 13, 22. doi: 10.3390/agronomy13061590
Weidner, S., Koller, R., Latz, E., Kowalchuk, G., Bonkowski, M., Scheu, S., et al. (2015). Bacterial diversity amplifies nutrient-based plant-soil feedbacks. Funct. Ecol. 29, 1341–1349. doi: 10.1111/1365-2435.12445
Xu, Z., Li, C. J., Zhang, C. C., Yu, Y., van der Werf, W., Zhang, F. S. (2020). Intercropping maize and soybean increases efficiency of land and fertilizer nitrogen use; A meta-analysis. Field Crops Res. 246, 10. doi: 10.1016/j.fcr.2019.107661
Yang, X. Q., Wang, Y., Sun, L. Y., Qi, X. N., Song, F. B., Zhu, X. C. (2020). Impact of maize-mushroom intercropping on the soil bacterial community composition in northeast China. Agronomy-Basel 10, 15. doi: 10.3390/agronomy10101526
Yang, Z. Y., Zhang, Y. P., Luo, G. W. (2023). Regulation of soil C-N-P stoichiometry by intercropping mitigates microbial resource limitations and contributes to maize productivity. Plant Soil 498(1-2), 21–38. doi: 10.1007/s11104-023-06251-9
Yao, X. D., Zhou, H. L., Zhu, Q., Li, C. H., Zhang, H. J., Wu, J. J., et al. (2017). Photosynthetic response of soybean leaf to wide light-fluctuation in maize-soybean intercropping system. Front. Plant Sci. 8, 10. doi: 10.3389/fpls.2017.01695
Zaeem, M., Nadeem, M., Pham, T. H., Ashiq, W., Ali, W., Gilani, S. S. M., et al. (2019). The potential of corn-soybean intercropping to improve the soil health status and biomass production in cool climate boreal ecosystems. Sci. Rep. 9, 17. doi: 10.1038/s41598-019-49558-3
Zhang, L. L., Li, L. J., Pan, X. G., Shi, Z., Feng, X. H., Gong, B., et al. (2018a). Enhanced growth and activities of the dominant functional microbiota of chicken manure composts in the presence of maize straw. Front. Microbiol. 9, 11. doi: 10.3389/fmicb.2018.01131
Zhang, M. M., Wang, N., Hu, Y. B., Sun, G. Y. (2018b). Changes in soil physicochemical properties and soil bacterial community in mulberry (Morus alba L.)/alfalfa (Medicago sativa L.) intercropping system. Microbiologyopen 7, 11. doi: 10.1002/mbo3.555
Zhang, G. Z., Yang, H., Zhang, W. P., Bezemer, T. M., Liang, W. J., Li, Q., et al. (2023). Interspecific interactions between crops influence soil functional groups and networks in a maize/soybean intercropping system. Agric. Ecosyst. Environ. 355, 13. doi: 10.1016/j.agee.2023.108595
Zhang, H., Zeng, F. P., Zou, Z. G., Zhang, Z. Q., Li, Y. Z. (2017). Nitrogen uptake and transfer in a soybean/maize intercropping system in the karst region of southwest China. Ecol. Evol. 7, 8419–8426. doi: 10.1002/ece3.3295
Zhao, X. H., Dong, Q. Q., Han, Y., Zhang, K. Z., Shi, X. L., Yang, X., et al. (2022). Maize/peanut intercropping improves nutrient uptake of side-row maize and system microbial community diversity. BMC Microbiol. 22, 16. doi: 10.1186/s12866-021-02425-6
Zheng, H. Y., Wang, J. Y., Cui, Y., Guan, Z. Y., Yang, L., Tang, Q. Q., et al. (2022). Effects of row spacing and planting pattern on photosynthesis, chlorophyll fluorescence, and related enzyme activities of maize ear leaf in maize-soybean intercropping. Agronomy-Basel 12, 13. doi: 10.3390/agronomy12102503
Zhou, M. H., Butterbach-Bahl, K. (2014). Assessment of nitrate leaching loss on a yield-scaled basis from maize and wheat cropping systems. Plant Soil 374, 977–991. doi: 10.1007/s11104-013-1876-9
Zhu, Q., Liao, K. H., Lai, X. M., Lv, L. G. (2021). Scale-dependent effects of environmental factors on soil organic carbon, soil nutrients and stoichiometry under two contrasting land-use types. Soil Use Manage. 37, 243–256. doi: 10.1111/sum.12695
Zhu, Q. R., Yang, Z. Y., Zhang, Y. P., Wang, Y. Z., Fei, J. C., Rong, X. M., et al. (2024). Intercropping regulates plant- and microbe-derived carbon accumulation by influencing soil physicochemical and microbial physiological properties. Agric. Ecosyst. Environ. 364, 12. doi: 10.1016/j.agee.2023.108880
Zou, X. X., Liu, Y., Huang, M. M., Li, F., Si, T., Wang, Y. F., et al. (2023). Rotational strip intercropping of maize and peanut enhances productivity by improving crop photosynthetic production and optimizing soil nutrients and bacterial communities. Field Crops Res. 291, 13. doi: 10.1016/j.fcr.2022.108770
Keywords: maize-soybean intercropping, microbial community, nitrogen fertilizer use, soil nitrogen, yield
Citation: Zhang L, Feng Y, Zhao Z, Cui Z, Baoyin B, Wang H, Li Q and Cui J (2024) Maize/soybean intercropping with nitrogen supply levels increases maize yield and nitrogen uptake by influencing the rhizosphere bacterial diversity of soil. Front. Plant Sci. 15:1437631. doi: 10.3389/fpls.2024.1437631
Received: 24 May 2024; Accepted: 19 August 2024;
Published: 03 September 2024.
Edited by:
Khurram Shahzad, Water and Marine Sciences, PakistanReviewed by:
Xiaohui Chen, Anhui Agricultural University, ChinaShahbaz Khan, Huazhong Agricultural University, China
Vesna Dragicevic, Maize Research Institute Zemun Polje, Serbia
Copyright © 2024 Zhang, Feng, Zhao, Cui, Baoyin, Wang, Li and Cui. This is an open-access article distributed under the terms of the Creative Commons Attribution License (CC BY). The use, distribution or reproduction in other forums is permitted, provided the original author(s) and the copyright owner(s) are credited and that the original publication in this journal is cited, in accordance with accepted academic practice. No use, distribution or reproduction is permitted which does not comply with these terms.
*Correspondence: Qiuzhu Li, bGlxekBqbHUuZWR1LmNu; Jinhu Cui, Y3VpamluaHVAMTYzLmNvbQ==
†These authors share first authorship